Create an account
Create a free IEA account to download our reports or subcribe to a paid service.
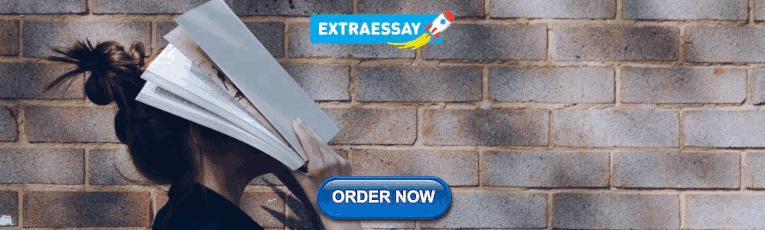
EV City Casebook
About this report, cite report.
IEA (2012), EV City Casebook , IEA, Paris https://www.iea.org/reports/ev-city-casebook, Licence: CC BY 4.0
Share this report
- Share on Twitter Twitter
- Share on Facebook Facebook
- Share on LinkedIn LinkedIn
- Share on Email Email
- Share on Print Print
Subscription successful
Thank you for subscribing. You can unsubscribe at any time by clicking the link at the bottom of any IEA newsletter.
Advertisement
The circular economy of electric vehicle batteries: a Finnish case study
- Open access
- Published: 23 May 2023
- Volume 44 , pages 100–113, ( 2024 )
Cite this article
You have full access to this open access article
- Pasi Rönkkö 1 ,
- Jukka Majava 1 ,
- Tatu Hyvärinen 1 ,
- Ilari Oksanen 1 ,
- Pekka Tervonen 1 &
- Ulla Lassi 2
4034 Accesses
3 Citations
9 Altmetric
Explore all metrics
The automotive industry faces challenges because of the electrification of vehicles and the rapidly increasing need for electric vehicle batteries (EVBs). Raw materials availability is limited; however, there will also be a significant number of end-of-life (EOL) batteries. This creates various circular economy (CE) business opportunities for EVB manufacturers, third-party providers, and other stakeholders. However, not all CE solutions are sustainable or economically feasible. In this study, through the use of case studies, expert interviews, and a survey, we determined the current state of the EVB CE in Finland, the possible options for utilizing EOL vehicle batteries, and the greatest barriers for the EVB CE. We found that some EVB-related CE applications are not supported by the government and legislation, for example environmental regulations and building standards. CE opportunities include a shorter lead time for some components, which makes them attractive for EOL applications.
Similar content being viewed by others
Managing Circular Electric Vehicle Battery Lifecycles Using Standards
Life-Cycle Analysis for the Automotive Sector
Review of recent lifecycle assessments of energy and greenhouse gas emissions for electric vehicles.
Avoid common mistakes on your manuscript.
1 Introduction
The number of electric vehicles (EVs) has rapidly increased during the past decade (Bobba et al. 2019 ; Hu et al. 2022 ) because of the environmental advantages (Fallah et al. 2021 ; Harper et al. 2019 ), aims to improve air quality (Harper et al. 2019 ; Thorne et al. 2021 ), customer needs (Fallah et al. 2021 ; Harper et al. 2019 ), and reduced taxes and grants for the EV purchasers (Fallah et al. 2021 ). At the end of 2021, there were roughly 12 million EVs worldwide, and some estimates show that the number may be as high as 54 million by 2025 (Statista 2022 ). The rapid electrification of transportation in turn increases the demand for electric vehicle batteries (EVBs) (Deng 2015 ; Harper et al. 2019 ; Jaffe 2017 ; Sanclemente Crespo et al. 2022 ). According to many studies, the suggested lifecycle guaranteed by the vehicle manufacturers for EVBs in vehicle use is roughly 8–10 years (Hu et al. 2022 ). After this, the batteries can still hold 60–80% of their original capacity, which enables other uses (Saxena et al. 2015 ; Shahjalal et al. 2022 ). In the near future a large quantity of end-of-life (EOL) batteries will be available (Sanclemente Crespo et al. 2022 ). Even though EVs bring various environmental benefits during their lifetime, they may become a burden if waste is not managed correctly (Fallah et al. 2021 ; Harper et al. 2019 ). Thus, it is important to enable efficient EOL operations (Yanamandra et al. 2022 ).
There are already numerous second-life solutions for EOL EVBs, such as in household energy storage, balancing electricity grids, or using them in less demanding EVs (Ai et al. 2019 ; Sanclemente Crespo et al. 2022 ). All the second-life options outlined above may reduce environmental impacts and bring economic benefits but also extend the life of EVBs (Ai et al. 2019 ; Bobba et al. 2019 ). EVBs can be recycled at the end of their lifetime, which may also provide local access to critical materials such as cobalt (Harper et al. 2019 ).
The European Union (EU) has recently made a proposal for a new battery regulation which includes provisions for a CE (European Commission 2020 ), and several studies have been conducted related to the CE of EVBs (Hu et al. 2022 ; Olsson et al. 2018 ; Shahjalal et al. 2022 ). For example, Hu et al. ( 2022 ) developed an EVB recycling model that minimizes the total carbon dioxide emissions and total costs. Shahjalal et al. ( 2022 ) made a comprehensive study of the current status of recycled Li-ion batteries (LIBs), and Albertsen et al. ( 2021 ) reviewed current LIB CE strategies and their adoption among European vehicle manufacturers. However, the EV industry is rapidly changing, and new CE models related to EOL EV battery management are being established. In addition, several generic challenges and barriers of CE exist, and these have been addressed, for example, by Kirchherr et al. ( 2018 ), Govindan & Hasangic ( 2018 ), Ranta et al. ( 2018 ). and Farooque et al. ( 2019 ). Barriers specifically related to EVB CE are covered in the studies of da Silva et al. ( 2023 ), Gephardt et al. ( 2022 ), Fallah & Fitzpatrick ( 2022 ), Sopha et al. 2022 , Azadnia et al. ( 2021 ), Schulz et al. ( 2021 ), and Olsson et al. ( 2018 ).
However, previous studies on EVC CE have mostly focused on countries with a large population, and sparsely populated countries with small population have received insufficient attention. To address this gap, we define through the research literature and an empirical data the best available solutions for EOL EVB utilization in Finland. The study focuses mainly on EVBs used in passenger cars. Finland is a sparsely populated country with long distances, and it is located far from its main markets, which creates challenges for a CE (Työ- ja elinkeinoministeriö 2021 ; Leppänen et al. 2022 ). Finland has also established a national battery strategy to support the development of a battery ecosystem in Finland (Työ- ja elinkeinoministeriö 2021 ). One goal in the strategy is to promote a CE and digital solutions. The EVB CE studies concerning Finland are missing, and because of rapid increase in the number of EVs, studies are needed to cover this research gap. Additionally, an in-depth understanding of challenges and barriers of EVB CE should be created to develop the CE of EVBs in Finland.
The above-described objectives can be formed into the following research questions:
RQ 1 What is the current state of the EVB CE in Finland?
RQ 2 What are the challenges and barriers of a CE of EVBs?
The structure of this paper is as follows. Section 2 presents the literature background, Sect. 3 describes the research method and process, and Sect. 4 presents the results. The findings are discussed in Sect. 5 , and the conclusions are provided in Sect. 6 .
2 Literature review
2.1 the circular economy.
CE is considered an umbrella term for different activities and methods that aim for sustainability, including economic, environmental, and social dimensions (Geissdoerfer et al. 2017 ; Kirchherr et al. 2017 ). The framework for the CE has been introduced by Ellen MacArthur Foundation (Ellen MacArthur Foundation 2013 ). According to Korhonen et al. ( 2018 ), the concept of a CE is not fully defined but it has been considered as an alternative for the currently dominating ‘take-make-dispose’ culture (Korhonen et al. 2018 ; Stahel 2016 ). One of the key issues in the CE concept is to utilize the remaining value of the product (Olsson et al. 2018 ) through reusing, refurbishing, remanufacturing, and recycling (Kirchherr et al. 2017 ; Korhonen et al. 2018 ). One additional option, which is not usually considered as circular, is to use the material for energy through combustion. This order enables the highest possible value for the product in its whole lifecycle, as well as the highest energy efficiency (Korhonen et al. 2018 ).
Reusing is typically considered as the most viable EOL option because it does not consume additional resources (Stahel 2016 ). In other options, additional resources are used to extract the value (recycling) or to return the product in functional (refurbishing) or even like-new or better condition (remanufacturing) (Korhonen et al. 2018 ; Soh et al. 2015 ). In remanufacturing and refurbishing, the product is returned to its original function, whereas in recycling the materials of the product can be used for something else. The main benefit of remanufacturing is that the same core materials can be used over and over again, which saves resources compared to the value chain of manufacturing totally new products. One dimension of the CE is the sharing economy, meaning that the products are shared between users to enable a higher use rate (Korhonen et al. 2018 ).
Even though the CE is promoted by the European Union (EU), several national governments, and businesses around the world (Korhonen et al. 2018 ), some authors such as Kirchherr et al. ( 2017 ) and Korhonen et al. ( 2018 ) have criticized the CE concept for meaning different things to different people or being a collection of separate ideas from several fields and semi-scientific concepts (Corvellec et al. 2022 ). Net sustainability of the CE concept is not always clear; for example, land originally used for mining may be used in the production of renewable energy, which cannot always be considered as clean (Korhonen et al. 2018 ). The CE may also increase logistics and transportation because of the need to recycle materials (van Buren et al. 2016 ; Korhonen et al. 2018 ). The CE also requires cultural change to current customer habits (Korhonen et al. 2018 ). Gupta et al. ( 2020 ) propose that more resources will be needed for research and capability building to support sustainable development, as well as governmental incentives and tax benefits for organizations working towards sustainable development.
2.2 The circular economy of electric vehicle batteries
The manufacturing of EV LIBs is energy intensive and polluting, and it requires scarce or non-sustainable materials such as lithium, cobalt, and nickel (Albertsen et al. 2021 ; Jaffe 2017 ). Cobalt is mostly mined in the Democratic Republic of the Congo, sometimes in socially non-sustainable ways. Virgin lithium resources are significant and the price is relatively low, but its mining has high environmental impacts (Deng 2015 ; Jaffe 2017 ). Jaffe ( 2017 ) states that without CE practices, increasing the capacity of battery manufacturing using only virgin raw materials would require the establishment of new mines and building infrastructure for different manufacturing stages.
EV batteries reach their EOL in 8–10 years after a certain number of charging cycles (Hu et al. 2022 ), when they still have 60–80% of their original capacity left (Olsson et al. 2018 ; Saxena et al. 2015 ). However, Saxena et al. ( 2015 ) highlight that 70–80% capacity still meets the needs of most vehicle users, and they propose that the EOL of batteries should be defined according to the needs of certain vehicle user groups. Additionally, the price of an EVB is relatively high, accounting for up to half of the cost of the EV (Jiao and Evans 2016 ; Shahjalal et al. 2022 ). Thus, EVBs have a high remaining value when they are reused or repurposed, but they also contain economically valuable materials that make them a significant material source when the batteries are recycled (Harper et al. 2019 ; Hu et al. 2022 .
Currently, secondary lithium is not used due to the relatively low price and abundance of virgin lithium (Helbig et al. 2018 ; Jaffe 2017 ). Still, Deng ( 2015 ) mentions that it may be difficult to meet future lithium needs, which makes it difficult to lower the price of an LIB. However, the new EU proposal for battery regulation aims to recycle up to 70% of lithium by 2030 (European Commission 2022). Cobalt can make the recycling of batteries a viable and even mandatory option (Yanamandra et al. 2022 ), and the recycling of EV batteries may ensure stable supply chains (Harper et al. 2019 ). Wrålsen et al. ( 2021 ) state that raw material prices and availability may accelerate interest in applying circular business models.
CE principles play an important role in EOL EVB management. With these principles, environmental benefits can be increased while at the same time meeting the increasing demand of materials needed for new batteries (Hu et al. 2022 ; Olsson et al. 2018 ). The primary EOL management options for EV batteries are reusing or repurposing (Ai et al. 2019 ; Hu et al. 2022 ), and recycling (Olsson et al. 2018 ; Wegener et al. 2015 ). Refurbishing or remanufacturing are challenging to implement and they are not common in the EVB CE, but they have been investigated in some studies (e.g., Ai et al. 2019 ). However, EOL management of EV batteries may require several different circular model activities (Wrålsen et al. 2021 ); for example, Olsson et al. (Olsson et al. 2018 ) propose that the largest benefits can be achieved when the batteries are first reused and then recycled. Extending battery life has been seen as an option to reduce the negative environmental impacts of EV batteries related to hazardous and rare raw materials (Olsson et al. 2018 ).
Because batteries consist of cells, suitable cells can be re-used in batteries, whereas cells in poor condition can be replaced with new ones and the removed cells can be recycled for materials (Hu et al. 2022 ). Even though battery reusing seems to be a common EOL activity, Shahjalal et al. (Shahjalal et al. 2022 ) state that used EVBs cannot be used straight after removing from the vehicles, but they should be first inspected, and any possibly damaged cells should be replaced to avoid harm, such as the risk of explosion.
The first steps of EOL EVB management include collection and logistics of the batteries (Hu et al. 2022 ; Slattery et al. 2021 ). The appearance and performance of the batteries can be tested, and good quality batteries can be used as such for similar or other purposes, whereas low quality batteries can be disassembled and recycled for useable materials (Hu et al. 2022 ). Disassembly and recycling should be performed in a controlled atmosphere with no atmospheric air (Shahjalal et al. 2022 ). Disassembly is usually performed manually because of inflexibility and the high capital costs of automation. Also, faulty (e.g., corroded) parts may require human intervention (Soh et al. 2015 ). Battery recycling methods are pyrometallurgical recovery, physical material separation, hydrometallurgical metals reclamation, direct recycling, and biological metals reclamation (Harper et al. 2019 ).
2.3 Applications for second-life batteries
Because EOL EV batteries can be in relatively good condition, they may enable straight reusing and repurposing applications, providing an environmentally and economically alternative for various functions (Jiao and Evans 2016 ; Shahjalal et al. 2022 ). A potential profit for the original battery manufacturer would be achieved when selling EOL batteries for the second-life user instead of paying for the recycling cost (Olsson et al. 2018 ). For example, used vehicle batteries can create revenue for the vehicle manufacturers and offer low-priced batteries (Volan et al. 2021 ). Some second-life applications for used EV batteries are off-the grid applications such as forklift trucks and other EVs which are used in less demanding environments (Albertsen et al. 2021 ; Olsson et al. 2018 ), energy peak smoothing in renewable energy networks (Albertsen et al. 2021 ; Saez-De-Ibarra et al. 2016 ), or home electricity storage (Olsson et al. 2018 ; Volan et al. 2021 ).
2.4 Barriers to an EVB circular economy
Despite the environmental benefits, there are a number of recognized challenges and barriers that may impact an EOL EVB CE (Govindan & Hasangic 2018 ; Kirchherr et al. 2018 ). In this study, we define CE barriers and challenges as issues that may limit the EOL options of the certain CE applications, for example, because of unprofitability in economic or environmental view. Barriers to EVB CE recognized in the literature have been listed in Table 1 .
One major challenge is the overall management of EOL batteries when the number of batteries increases (Harper et al. 2019 ). The process is also costly and it requires technology innovations (Albertsen et al. 2021 ; Deng 2015 ). The original equipment manufacturers (OEMs) may not have a willingness for EOL battery management because the return may not cover the costs, and customers may be unwilling to pay for used products (Albertsen et al. 2021 ). Other recognized challenges are reverse logistics and potential risks during transportation (Ai et al. 2019 ; Shahjalal et al. 2022 ), and the technical development of EV batteries and, thus, a rising variation in battery types and physical properties such as mass, cell number, and chemistry (Shahjalal et al. 2022 ; Wrålsen et al. 2021 ). Variations in chemistry between different battery manufacturers brings challenges for third-party EOL activities if labeling is insufficient. According to Ai et al. ( 2019 ), collection and treatment facilities are currently inadequate.
Reverse logistics include collection, handling, storage, and recycling (Shahjalal et al. 2022 ). The transportation and collection of EOL EVBs is considered to be one of the most expensive parts of EOL management (Olsson et al. 2018 ; Slattery et al. 2021 ). It is also highly regulated due to their nature as hazardous waste; some transportation companies do not transport used LIBs, and air transportation is totally prohibited (Olsson et al. 2018 ).
Technical issues also include battery design: current battery packs are not designed for easy disassembly, which may significantly affect EOL battery CE operations (Harper et al. 2019 ; Soh et al. 2015 ). Each battery type has a specific battery management system (BMS) tailored for that specific battery type (Olsson et al. 2018 ). Disassembly of batteries may require breaking the material, which lowers the value of packing material in the waste hierarchy (Harper et al. 2019 ). Second-life batteries may also have higher chemical imbalances between different cells compared to new batteries, which predisposes them to premature failure. Variances can be balanced using active balancing technologies, but this increases the number of components and, thus, the complexity of the batteries (Khalid et al. 2021 ). Second-life batteries require close inspection, assessment of their performance capability, and strict monitoring and protection. Battery disassembly also requires specialized tools and high-voltage training to prevent short-circuits, explosions, toxication, or damage to the operators (Harper et al. 2019 ; Wegener et al. 2015 ).
Vehicle batteries are mostly disassembled manually, which is labor intensive, expensive, and includes many risks (Harper et al. 2019 ; Shahjalal et al. 2022 ). There have been attempts to automate disassembly operations to reduce costs and time, improve the purity of the separated materials, and ensure the safety of the disassembly operators (Harper et al. 2019 ; Shahjalal et al. 2022 ). The purity of separated materials would be a benefit in the downstream of production. However, barriers for automation include variations in design and chemistries of batteries from different manufacturers due to a of lack of standardization, which also leads to a low volume of certain battery types (Harper et al. 2019 ; Shahjalal et al. 2022 ). Wegener et al. ( 2015 ) claim that car manufacturers only make small changes for the current vehicle models to fit the electric system to current car models. Cell designs and chemistries are also rapidly developing, which challenges recyclers in developing economical automated recycling processes (Yanamandra et al. 2022 ). However, Wegener et al. ( 2015 ) suggest a combination of robots and humans in disassembly tasks in which robots can undertake the simpler tasks.
Hu et al. ( 2022 ) claim that building a recycling network for EV batteries is expensive, impeding the sustainable development of EVs. Even though the OEMs may not have profit-related incentives for managing EOL batteries, they are always responsible for the product until it is considered as waste (Albertsen et al. 2021 ; Olsson et al. 2018 ). Producer responsibility requires the manufacturers to manage EOL batteries, but that responsibility can be shifted to other parties if the EOL batteries are reused in other purposes. If there are many actors in the second-life battery value chain, the producer responsibility may not always be clear (Olsson et al. 2018 ).
Despite the rapidly increasing number of EVs, Fallah et al. ( 2021 ) claim that estimation of the future material flows would be challenging because of the uncertain future trend. Even though a CE of battery materials has been seen as an important way to improve resource availability, economies of scale cannot be achieved with the current waste streams (Wang et al. 2014 ). Even though the average age of current EVs is quite low, they have not yet reached their EOLs. Returning EVBs come mostly from accidents, recalls, and malfunctions (Thorne et al. 2021 ).
In conclusion, EVB EOL management could be more economically viable with better sorting technologies and methods to separate electrode materials, greater process flexibility, a redesign of the recycling process, and standardization of batteries from different manufacturers (Harper et al. 2019 ; Shahjalal et al. 2022 ). Furthermore, a supportive environment created by policies, standards, and law has been mentioned by several authors (Ai et al. 2019 ; Albertsen et al. 2021 ; Fallah et al. 2021 ). For example, the EU seeks to secure the supply of some critical materials that are necessary for EVs, meaning that import of these materials should be reduced (Albertsen et al. 2021 ). Ai et al. ( 2019 ) state that with a landfilling ban, all batteries would be recycled even though the operation may not be economically viable. Yanamandra et al. ( 2022 ) compare LIB recycling to lead acid (LA) batteries, which is a mature battery technology having an almost 99% recycling rate. Deng ( 2015 ) mentions that it is challenging to manufacture low carbon footprint electrode materials, which could be considered as green batteries. While battery chemistry changes can mean less expensive batteries, for example if the quantity of cobalt decreases in the batteries, battery value recovery decreases correspondingly (Wang et al. 2014 ).
3 Research methodology
3.1 research process, method, and data.
A qualitative research approach with abductive reasoning was chosen for this study. We adopted abductive approach to claim for multiple contributions in a more convincing way, for example, related to new barriers relevant for the EVB CE context; relative importance of these barriers as perceived by different actors; insights on how these barriers are influencing the EVB CE in Finland; and recommendations for possible improvements. Thus, it is suitable for studying issues that have not yet been widely studied. Research patterns in abductive reasoning are not strictly defined, and multiple methods can be used. For a wide view, we used triangulation using Finnish cases related to EOL EVB management, statistics, survey, and expert interviews. The literature review was carried out by utilizing the most recent research papers related to EVB EOL operations. The literature was searched through Scopus database, but also Google Scholar was used as a supportive database. For the barriers of the EVB CE, the literature review was done in a systematic way using keywords “electric vehicle battery” OR “EVB” AND “circular economy” OR “CE” AND “barrier*”. This led only to seven publications that were published between 2018 and 2023 with a mention of these keywords in the abstract, title, or the keywords. Additionally, some publications cited in these publications were screened for further sources and cornerstone publications in the field (snowball sampling method). Other relevant topics in the literature review were covered rather in a narrative than systematic way. The literature was used in the section two to form a body for the literature review, but also to define questions for the survey, and to compare the literature with the empirical evidence in discussion. The main empirical data were collected through expert interviews and a Webropol survey, which was aimed at experts in the battery industry but also in the field of the CE in general. The research process is illustrated in Fig. 1 .

Research process
The common questions in the survey and the interview are presented in Table 2 .
The survey was sent through different battery-and circular-economy-related networks, and also personally to actors in Finnish battery-related industries. The survey reached 90 + experts in both academia and industry and led to 10 responses. Five respondents represented the field of research and education, two of whom had planned or ongoing research related to the CE of EVBs. The average working experience of the respondents was 15 years. In addition, three extensive semi-structured interviews were conducted with experts in the field. The participants for the interviews were chosen because of their strong expertise and unique know-how in the field. The results from the survey and interviews were analyzed qualitatively using a content analysis approach. The interview and survey data were coded and organized into emerging themes and issues. The findings are presented in Sect. 4 . The background information of the interviewed persons is presented in Table 3 .
3.2 EVB CE operators in Finland
In Finland, the EOL EVB is managed by Suomen Autokierrätys. The actual operations are done by four independent operators. In total, there are 288 dedicated collection points and 450 registered car repair shops to collect EVBs. The collection points are scrapyards or authorized repair shops that can replace the battery for still operational vehicles. The customers can return the batteries to any collection point free of charge. After collection, the batteries are transported to the operators for evaluation of the future steps (Suomen Autokierrätys 2022 ).
Fortum Waste Solutions both repurpose and recycle batteries, currently focusing on recycling. Fortum also provides services such as safe handling, transportation, and storing of EVBs for other companies through their logistics network. Fortum currently has a plant in Finland that can recycle around 10,000 EVBs annually using mechanical recycling processes, but a second facility is under construction for hydrometallurgical recycling. Together, these would allow as much as 95% of the critical metals and 80% of the entire battery to be recycled. Fortum has also piloted repurposing EVBs as stationary energy storage in hydropower plants (Fortum 2022a , 2022b ).
Eurajoen Romu has a history of recycling EOL vehicles and lead batteries but is currently also recycling EVBs. The recycling methods have not been revealed, but they have a mechanical battery plant focused on recycling lead batteries. They handle the batteries at cell level and salvage recyclable materials to enable a more than 50% recycling rate. (Eurajoen Romu 2022).
Stena Recycling has recycling centers in several European countries, and also plans to develop a recycling site in Finland. The largest recycling and sorting site is located in Halmstad, Sweden, where all the batteries collected in Finland are transported. In Halmstad, the batteries are inspected and their state of health is determined. If a battery is suitable for reusing, it can be prepared and transported to the place of use. If the batteries do not meet the required standards, they are disassembled and short-circuited, and the remaining electricity is fed to the internal grid of the facility. Stena Recycling also repurpose batteries through their subsidiary company Batteryloop, which provides stationary power storage for EV recharging stations (Stena Recycling 2022 ).
Cactos transforms used Tesla Model S batteries for energy storage applications. Tesla batteries include liquid cooling and they are originally designed to tolerate challenging conditions, whereas in energy storage use, the batteries are in a stable environment, which enables efficient transformation for energy storage. The individual battery modules are assembled as energy storage units that are leased to customers for storing self-produced energy and smoothing consumption spikes between high and low energy prices. Additionally, Cactos provides software with artificial intelligence and cloud services for efficient recharging and discharging. The model lowers the need for manufacturing new batteries and, thus, the environmental impact (HS Visio 2022 ; Cactos 2022 ).
Akkurate is a Finnish software company that focuses on LIBs and their diagnostics. Services are provided for battery manufacturers and energy storage businesses. In EVs, the focus is on optimizing performance and maximizing the useful life of batteries. The benefit is prolonging the useful life of EVBs and evaluation of their reuse potential. By inspecting batteries proactively during their use, failures can be recognized in real time well in advance and batteries can be repaired before bigger faults occur. Through the collected data, still-useful cells can be identified for reuse. (Akkurate 2022 ).
There are other companies operating in similar fields. AkkuSer has recycled batteries since 2006, and it has been responsible of recycling 100% of the portable and non-chargeable batteries in Finland. The company has also attempted to develop a process for EV battery recycling. One goal of the company is to develop a new recycling process for batteries with low cobalt content (AkkuSer 2022 ).
4.1 How the EV battery CE is seen
Generally, the CE of EV batteries was seen as a good or even a great opportunity (nine respondents), but also a ‘necessary evil’ to some extent (three respondents). For example, the EVB is an expensive item to manufacture, and straight utilization after the main use would create value further down its lifecycle before recycling. A common view was that batteries should be utilized for as long as possible. One respondent mentioned that the battery CE has been seen more as a mandatory activity based on the 2006 Battery Directive, which required the recycling of batteries, but that more recently it has become more of an opportunity because of the high price of metals. Some respondents also saw that certain minerals have become even rarer, and more attention will be paid to where and under what conditions the minerals are mined. A new battery regulation proposal of EU obligates manufacturers to intensify the battery CE and increase recovery rate of the batteries. What is new in this regulation is that EVBs and their EOL management has been included, including traceability of batteries and their materials.
Company representatives generally considered how to get benefits from CE, and they were active members in different CE networks. Additionally, because of the current world political situation, EOL operations of EVBs are seen as increasingly important. One company representative saw the CE of EVB as very beneficial due to the six months shorter lead-time in receiving used battery cells compared to new ones. Used battery cells of some high-end vehicles (e.g., Tesla) can even be more expensive compared to some new mass-produced cells, but they are highly developed and are of much higher quality. Thus, they are suitable for energy storage use, because in this use the capacity per volume unit is not as critical as in EV use.
Battery manufacturers have producer responsibility, which follows all the operations related to EOL batteries, and the companies are responsible for recycling when batteries can no longer be utilized. However, according to one interviewee, it is unclear how this really works. Currently, most EOL EV batteries are only recycled, and one interviewee saw that ‘all the other CE activities are just tinkering in Finland’ . For example, if half of the battery is damaged, it is not utilized, but the whole battery is recycled even if the other half is in good condition. They also saw that there are some recyclers and other CE actors, but they do not have yet significant activities.
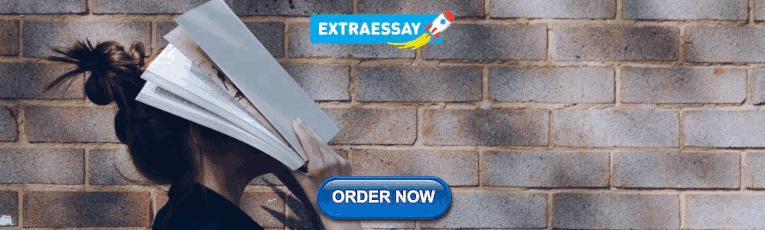
4.2 Challenges related to the CE of EVBs
The mostly recognized challenges related to CE of EVBs are presented in Fig. 2 .
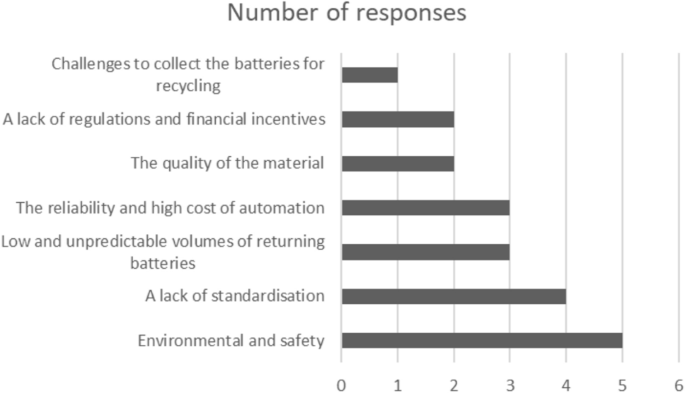
The mostly recognized challenges related to CE of EVBs
Additionally, one respondent mentioned that there are still few actors in the current (Finnish) markets to develop high-value products from EOL EVBs. Also, issues related to complexity and the fast technology evolution of batteries were recognized. One respondent questioned if reuse makes sense after 10 years, and three respondents recognized the issue that the low cost of virgin raw materials does not promote the circular use of secondary materials. They also pointed out that because of small material flows, the material availability would be challenging to predict, and thus EVBs would be challenging items to utilize.
Two respondents mentioned that EVBs are challenging for mechanical recycling, but also for logistics and safety, and some respondents recognized that currently the quality and use history of used EVBs is not sufficiently known. They also mentioned that ‘ there is not sufficient data to know what is possible in CE and where are the CE actors’ . Some challenges are related to the physical properties, such as mass and the large physical size of the battery pack. The weight of a vehicle battery can be as much as 600 kg, so they often cannot be handled by a single person.
EVBs are not basically designed for CE, which makes EOL management challenging (two respondents). According to one expert in the field, it is possible to design batteries for CE, but the price may be too high for some customer segments. In addition, smaller customers with low volumes do not always know what they really need, which makes product development challenging, whereas larger customer segments with higher volumes are usually more aware of the possibilities and they also know what they need.
According to one interviewee, ‘everything that happens before recycling is challenging’ . When hundreds of batteries are received daily, handicraft is no longer enough. There are also variances in battery cells even between different models from the same manufacturer. In some vehicles there can be thousands of battery cells in a battery, which presents its own challenges. In Finland, mechanical recycling is mostly used, and it requires disassembling modules to the cell level. Cells can be shredded to create black mass. Another option is the thermal process, where certain materials are recovered.
One interviewee mentioned that suppliers of used batteries are usually reliable and it can be trusted that the batteries are in good condition. If there are damaged cells, a discount is given by the supplier. However, there are no standards for battery recycling and there is a small risk whether the battery is in good condition. Diagnostics can be used, but everything cannot be revealed by reading the BMS. The respondent also stated that ‘generally the regulations related to EVB handling are not clear. CE should be supported, but it is not clear if the utilizer of EOL EV batteries requires environmental permissions, and whether the activities equate to waste handling’ .
4.3 Applications with the best potential in EVB CE
The repurposing of batteries or battery cells for energy storage was seen as a very relevant option (six respondents) because of the low cost and increasing amount of renewable energy and, thus, the growing need for energy storage. As supportive solutions, diagnostics and digital battery passports were seen to be a great opportunity in Finland. A common view was repurposing followed by environmentally sound recycling (six respondents), which may eventually become more economically viable as the volumes of EOL EV batteries rise. Only two respondents mentioned remanufacturing for EV purposes and one mentioned the ‘ reusing of dismantled parts in many applications ’. The issue of using less resources to produce batteries was considered, but as one respondent stated, ‘vehicle batteries should not only become raw material for other applications, but instead batteries should be developed to become more sustainable’ . A few respondents mentioned that EOL EVBs could be repaired and refurbished to be used again in EVs, but that does not seem to be always possible. The reusing of waste material from cell manufacturing as raw material for new battery cells was also suggested by one respondent.
4.4 Strengths and weaknesses of the Finnish EVB ecosystem
According to the responses, Finland has an almost complete value chain from raw materials (mining) to recycling solutions. However, one interviewee stated that mining cannot be considered as a strength because it is not related to a CE. The special strengths are related to strong metal processing beginning from the education. According to one respondent, the metal processing industry could take responsibility for recycling so that third-party actors would not be required to do so.
Additional factors considered by the respondents were knowhow and interest, and also plans related to the Finnish EVB CE. According to three respondents, one totally missing part is cell manufacturing. According to one interviewee, there is little EVB CE activity in Finland, and the existing actors are mainly scrapyards. Because of so few actors, there are many missing parts in the value chain.
Some respondents mentioned that Finland is small enough to enable the connection of key actors (two respondents), seeing Finland as a ‘test bed’ for fully circular and local value chains in the future. Additionally, Finnish industrial actors were seen to have a willingness to collaborate ‘for the sake of sustainable development’ . On the other hand, the Finnish domestic market area was seen as too small, thus lacking the local material for recycling. Finland was also seen to be too far from central Europe, where the highest material flows are. Some of the respondents recognized that big actors can more easily enter the market, and that manufacturers seek to lock waste batteries into their own value chain, preventing efficient circularity. However, it was also stated that ‘we should also be more integrated with big companies and key players in the field, because the battery value chain is now more comprehensive’ . Overall, the small volumes of EOL EVBs in Finland seemed to be a weakness because ‘the number of EVs on the road is not yet significant enough to provide a reliable source for circular batteries’ . The more pessimistic respondents saw that ‘the government seems to be against CE solutions’ because there are no public subsidies available for profitable EVB reuse. When discussing battery recycling, permissions such as environmental permissions are needed. Currently, the permit process is slow and inflexible. There is also a safety point of view because battery recycling is not possible everywhere.
Some respondents mentioned that investments in research and development are needed to ensure efficient value chains. As a solution for increasing CE activities in Finland, one interviewee saw that business opportunities for companies should be made easier. Legislation related to EVBs, for example building regulations and official regulations related to the CE of EVBs, is largely unclear . ‘Companies and their operating conditions should be supported; for example, the migration of foreign experts to Finland should be supported by lower personal taxation’ .
4.5 Future opportunities for the EVB CE
Overall, the EVB CE offers potential for new business models because more and more batteries will be received for recycling in the coming years. In particular, the Finnish national battery strategy was seen to provide new business opportunities, ‘but it is interesting to see if this is going to create possibilities for new companies, or if the large companies who know that the value can be on their own hands are going to keep the operations on their own hands’ . According to some respondents, battery value chains seem to be closed, and the actors seek to be responsible for the whole value chain. The EVB CE also seems to be a very fragmented business area so that ‘one does something and another does something else’ . There are many actors in the value chains, and the trend has been that companies take operations into their own hands. Some of the respondents stated that ‘if a company is not in the chain, it is totally out of the chain’ , meaning that nowadays it is hard for a small individual company to join the value chain. For example, Tesla has made plans for starting its own mining operations.
To get the full potential of battery utilization, it seems to be beneficial to obtain data on how and in what kinds of conditions batteries were used during their lifetime. Some respondents saw that it would be best that the materials were as close the factories as possible, which would be a more environmentally sound solution. One interviewee mentioned that there seems to be some potential in using recycled materials for manufacturing new batteries, and one mentioned lower quality products, such as fertilizers, if there are no viable options for other use. Some battery types, such as small LIBs, have already been recycled for 15 years in Finland; however, the recovered quantities of materials are small. If recycled materials are used in further production, the content is currently only 3–5%. This amount will probably increase, ‘but not a single factory would fully work with recycled material’ .
One interviewee felt that there should be sufficient virgin battery materials for the applications where they are really needed, which justifies reusing EOL batteries. Some of the respondents did not see the use of recycled materials as reasonable because ‘batteries are needed in Finland more than the raw materials’, and ‘batteries for certain applications can be replaced with used batteries’ . Some interviewees stated that in the future there will probably be energy storing systems in many buildings, and the most sustainable solution would be to utilize batteries that have already been in use. Carbon-free energy production is a significant driver of the increasing need for energy storing systems, but investments should also be made in the infrastructure. Because there is demand elasticity in the electrical network, batteries are needed. ‘The more wind and solar energy are built, the more demand elasticity is needed . ’ However, most of the interviewees saw that second-life batteries can be recycled for raw materials.
5 Discussion
The demand for EVBs is constantly growing, which increases the demand for critical raw materials such as cobalt. The CE of EOL EVBs has been seen as a solution to manage the increasing number of batteries, but also to meet the increasing need for materials. However, vehicle markets may still rapidly change due to new technologies, such as hydrogen, or changes in the energy market and prices. For example, Russia’s invasion to Ukraine has led to a Europe-wide energy crisis. The traditional thinking of owning a car might also shift towards shared cars, which would enable the more effective use of vehicles but also lower the number of new vehicles (Thorne et al. 2021 ). Because of these changes, the old estimations of EV markets may not be accurate, which may also affect the economic viability of EOL management of EV batteries.
Our study focused on the current state, challenges, and future of the EVB CE in Finland. The current state and actors of the EVB CE in Finland (RQ1 of this study) are presented in Fig. 3 . As can be seen, most of the actors are in primary material production, and there are also some actors in the recycling and production stages. However, the number of companies representing reuse/repurpose or extending the life of batteries was relatively low, and currently there are no actors in the remanufacturing/refurbishing of EV batteries. This indicates a low maturity of the Finnish EVB ecosystem, caused by the low number of EVs in Finland.
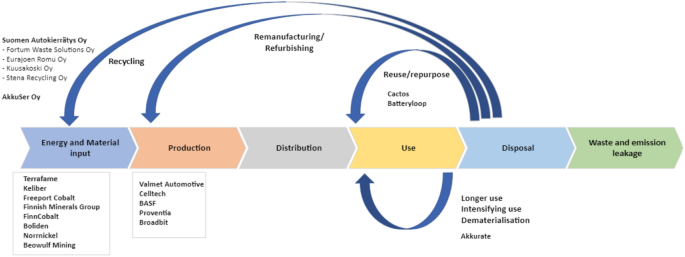
The current actors in the Finnish EVB CE ecosystem
As identified in the literature review, the EVB CE involves various challenges. To address RQ2 of this study, the challenges to an EVB CE found from Finnish empirical data are compared with the earlier studies in Table 4 . Table 4 has been derived from the analysis of empirical data and barriers listed in Table 1 in Sect. 2.4 . The individual barriers identified in earlier studies have been synthetised into common challenges in EVB CE, and they are compared with the empirical findings. The significance of the challenge in the context of Finland is indicated using + + (significant challenge), + (challenge), and – (not identified in the empirical study).
Several earlier findings were confirmed in our empirical study. These are, for example, the lack of standardization, unpredictable material flows, labor-intensive disassembly, and a lack of regulations. Even though LIBs in general are an extensively studied and mature technology (Deng 2015 ), vehicle battery technologies and physical properties such as chemistries and cell structures are still rapidly developing. Standardization of battery technologies could be promoted through legislation, but this may significantly harm the natural development of technologies, which would be necessary to improve some critical properties such as recharging time, durability, and driving range. Thus, the technology should be mature enough before legislative standardization. This means that all EOL batteries cannot be properly managed yet with costs low enough to enable viable CE solutions. An example of a mature technology is lead batteries, which are currently 99% recycled (Yanamandra et al. 2022 ). This example implies that EV battery technologies may eventually be naturally matured to enable cost-efficient CE.
Our empirical study also identified some issues that have not been extensively studied in the literature. One of these was insufficient quality and use historical data. Currently, only a few studies can be found that cover the use history of products through product passports. Other new findings in the empirical study were ‘missing data related to CE possibilities and locations of current CE actors’, and ‘few actors in the current second-life battery market’. These issues are relevant also in other sparsely populated areas besides Finland and indicate a need for developing innovative solutions to achieve sufficient economies of scale for local EVB ecosystems. Respectively, some issues found in literature, such as expensive collection and transportation, were not highlighted in the empirical study. This will probably become an issue in Finland as well when the volumes of EOL EVB grow in the future.
Because the electrification of transportation is currently a very hot topic, there will probably be a lot of research and development related to EV batteries. For example, more sustainable materials can be used in batteries, or batteries can be developed for better circularity, which will change the whole value chain. As found in the empirical study, batteries should be developed to be more sustainable, not just being raw material for new batteries. However, according to Wang et al. (Wang et al. 2014 ), changing chemistries may lower the viability of the EOL battery CE. Based on our findings, it is possible to design batteries for circularity, but some customer segments are not willing to pay extra for that.
Although reused or repurposed batteries may provide cost effective alternatives for new batteries in various applications, concerns about used batteries may slow down the development of the reuse battery market. In general, the economic benefits of CE activities are related to the price gap between old and new products, transportation costs, and economies of scale of EOL management (Ai et al. 2019 ; Leppänen et al. 2022 ; Rönkkö et al. 2021 ). However, as an original result in our empirical study we found that the CE may also provide a faster lead time of some components compared to new products.
It was also found that Finnish domestic market for CE activities may be too small, which correlates with Ai et al. (Ai et al. 2019 ) who argue that EOL batteries can be sent to other countries to be managed. This is also related to the currently low volumes of EV batteries; economies of scale have not yet been achieved. Currently, the battery industry is largely controlled by China, which has access to all the necessary raw materials. To prevent total dependency on China, self-sufficiency would be required in the European battery industry. This could be done by improving intra-European material flows through a more efficient CE. The results of this study indicate that in Finland there are knowhow and willingness to promote the CE of EV batteries, but to some extent there seems to be a lack of government support for some CE applications. Based on the results of this study, the CE related to EV batteries should be supported by policies or legislation, which is also in line with several authors in the field (Ai et al. 2019 ; Albertsen et al. 2021 ; Fallah et al. 2021 ). Based on our empirical study, we see Finland as an innovative country where it is possible to pilot different technological innovations and solutions. Finland is also a relatively large actor in the wind energy sector, and significant plans have been formulated to develop green hydrogen production. To be effective, excess renewable energy should be stored to be used during times of low production, which requires energy storage solutions. To meet the growing demand, used battery cells would provide a circular solution for these applications.
6 Conclusions
In this study, we investigated the current state of the Finnish EV battery CE by studying literature and utilizing expert interviews and a survey. We analyzed the current state, the potential challenges and barriers, and the requirements for an EV battery CE in Finland.
Through this study, we recognized that there are many actors in the field, but the main EOL management is in the hands of only one main actor and its key partners. This enables the effective take-back of batteries through nationwide collection, which is a prerequisite for economies of scale of CE operations. We also found that there are some innovative companies who are developing diagnostics solutions and repurposing batteries, for example, for energy storage. However, only EV batteries with certain properties, such as liquid cooling, are used for this purpose.
The greatest challenges for the circularity of vehicle batteries are related to varying battery technologies and chemistries, which lead to a high variety of batteries and, thus, lower the economies of scale of CE activities or possibilities for automated disassembly. Sometimes batteries have been designed for easy assembly, which may lower the effectiveness of disassembly. What can be concluded is that varying battery technologies and chemistries that are not designed for circular application may significantly limit the effective CE of EVBs. Thus, it may eventually be necessary to standardize EV battery technologies. However, this may limit the natural development of batteries, and it should not be done before a certain maturity point is reached. Our results indicate that it is possible to design products to be circular, but on the other hand, customers do not always have the willingness to pay extra for products designed to be circular.
The circular economy will play a large role in Finland in enabling efficient material flows and sufficient availability of certain materials. However, according to our findings, only a small fraction of materials can be obtained through circularity for future needs, and huge amounts of virgin materials are still needed to meet increasing requirements. Instead, repurposing batteries for energy storage systems seems to be a very attractive solution because fewer new batteries are required, which may be both an environmentally and economically sound solution. However, this does not solve the problem of the growing demand for vehicle batteries. New battery technologies with less critical materials will be needed to solve the material availability challenges. This requires significant investment in the research and development of vehicle batteries. Before this, the repurposing of batteries seems to be a viable solution to meet the growing needs of storing renewable energy. Furthermore, other innovations and innovative companies would be needed in the value chain to enable the best solutions for the various battery types on the market.
This study provides a current state analysis of Finnish EV battery EOL operations and the CE, which has both managerial and academic implications. Even though the CE of EVBs has been extensively studied during recent years, the field is rapidly changing and maturing, and thus there is a need for continuous research. For the companies in the field, this study provides new ideas how to utilize EOL batteries and information on the barriers for economic viability. Naturally, this study has limitations. The limitations are mostly related to the qualitative research method, which makes generalization of this study challenging. Additionally, the number of the respondents was low, which also makes generalization difficult. The respondents do not cover all the actors in the EVB CE value chain. However, the respondents were able to bring new knowledge because of their expertise in the field and their geographical residence, thus representing a case where the studied phenomena occur (Eisenhardt 2021 ). This study was also carried out in a regionally challenging environment where EV markets are not yet fully developed, EOL management of used EVBs is not yet matured, and there are still missing links in the value chains. Finland is also a sparsely populated but geographically large country far from international markets, which presents further challenges, especially for logistics.
For future research, the Finnish EVB CE should be studied again in a few years when there are more EVs in the road. Also, the CE of special vehicle batteries could be studied. Generally, it seems that there are not yet extensive studies related to the remanufacturing of vehicle batteries, which is a significant research gap. Additionally, new battery technologies, such as solid-state batteries or battery cells manufactured using environmentally friendly materials, may bring up unique challenges related to the CE of EV batteries, which could also provide a significant topic for future research. Another research gap was found related to historical data on the EVB CE.
Data availability
The data and materials are available upon request.
Ai N, Junjun Z, Wei QC (2019) U.S. end-of-life electric vehicle batteries: dynamic inventory modeling and spatial analysis for regional solutions. Resour Conserv Recycl. https://doi.org/10.1016/j.resconrec.2019.01.021
Article Google Scholar
Akkurate 2022. AKKURATE - Your Battery Analytics and Diagnostics Specialist. Retrieved 18 Aug 2022 ( https://www.akkurate.fi/ ).
AkkuSer (2022) Prosessi. Retrieved 19 Aug 2022 ( https://www.akkuser.fi/prosessi/ ).
Albertsen L, Richter JL, Peck P, Dalhammar C, Plepys A (2021) Circular business models for electric vehicle lithium-ion batteries: an analysis of current practices of vehicle manufacturers and policies in the EU. Resour Conserv Recycl. https://doi.org/10.1016/j.resconrec.2021.105658
Azadnia AH, Onofrei G, Pezhman G (2021) Electric vehicles lithium-ion batteries reverse logistics implementation barriers analysis: A TISM-MICMAC approach. Resour Conserv Recycl 174:105751. https://doi.org/10.1016/j.resconrec.2021.105751
Bobba S, Fabrice M, Blengini GA (2019) How will second-use of batteries affect stocks and flows in the EU? A model for traction Li-ion batteries. Resour Conserv Recycl. https://doi.org/10.1016/j.resconrec.2019.02.022
Article PubMed PubMed Central Google Scholar
Bonsu NO (2020) Towards a circular and low-carbon economy: Insights from the transitioning to electric vehicles and net zero economy. J Clean Prod 256:120659. https://doi.org/10.1016/j.jclepro.2020.120659
Cactos 2022. Älykkäät Sähkövarastot-Cactos. Retrieved 18 Aug 2022 ( https://www.cactos.fi/ )
Corvellec H, Stowell AF, Johansson N (2022) Critiques of the circular economy. J Ind Ecol. https://doi.org/10.1111/jiec.13187
da Silva ER, Lohmer J, Rohla M, Angelis J (2023) Unleashing the circular economy in the electric vehicle battery supply chain: a case study on data sharing and blockhain potential. Resour Conserv Recycl 193:106969. https://doi.org/10.1016/j.resconrec.2023.106969
Deng D (2015) Li-ion batteries: basics, progress, and challenges. Energy Sci Eng. https://doi.org/10.1002/ese3.95
Eisenhardt KM (2021) What is Eisenhardt method, really? Strateg Organ 19(1):147–160. https://doi.org/10.1177/1476127020982866
Ellen MacArthur Foundation (2013) Towards the Circular Economy - Economic and business rationale or an accelerated transition. Ellen MacArthur Foundation.
Eurajoki (2022) Monipuoliset Kierrätyspalvelut’. Retrieved 18 August 2022 ( http://eurajokigroup.com/eurajoki-group-eurajoki-yritys/ ).
European Commission (2020) Regulation of the European Parliament and of the Council concerning batteries and waste batteries, repealing Directive 2006/66/EC and Ameding Regulation (EU) No 2019/1020. European Commission: Brussels.
Fallah N, Fitzpatrick C (2022) How will retired electric vehicle batteries perform in grid-based second-life applications? A comparative techno-economic evaluation of used batteries in different scenarios. J Clean Prod 361:132281. https://doi.org/10.1016/j.jclepro.2022.132281
Fallah N, Fitzpatrick C, Killian S, Johnson M (2021) End-of-life electric vehicle battery stock estimation in Ireland through integrated energy and circular economy modelling. Resour Conserv Recycl. https://doi.org/10.1016/j.resconrec.2021.105753
Farooque M, Zhang A, Thürer M, Qu T, Huisingh D (2019) Circular supply chain management: a definition and structured literature review. J Clean Prod 228:882–900. https://doi.org/10.1016/j.jclepro.2019.04.303
Fortum (2022a) End of Life Services for Lithium-Ion Batteries. Retrieved 18 August 2022a ( https://www.fortum.com/products-and-services/fortum-battery-solutions/recycling/end-of-life ).
Fortum (2022b) New Nordic Export Permits Enhance Fortum’s Recycling of Electric Car Batteries in Finland. Retrieved 18 Aug 2022b ( https://www.fortum.com/media/2021/05/new-nordic-export-permits-enhance-fortums-recycling-electric-car-batteries-finland ).
Geissdoerfer M, Savaget P, Bocken NMP, Hultink EJ (2017) The circular economy–a new sustainability paradigm? J Clean Prod. https://doi.org/10.1016/j.jclepro.2016.12.048
Gephardt M, Beck J, Kopyto M, Spieske A (2022) Determining requirements and challenges for a sustainable and circular electric vehicle battery supply chain: a mixed-methods approach. Sustain Prod Consum 33:203–217. https://doi.org/10.1016/j.spc.2022.06.024
Govindan K, Hasangic M (2018) A systematic review on drivers, barriers, and practices towards circular economy: a supply chain perspective. Int J Prod Res. https://doi.org/10.1080/00207543.2017.1402141
Gupta H, Kusi-Sarpong S, Rezaei J (2020) Barriers and overcoming strategies to supply chain sustainability innovation. Resour Conserv Recycl. https://doi.org/10.1016/j.resconrec.2020.104819
Harper G, Sommerville R, Kendrick E, Driscoll L, Slater P, Stolkin R, Walton A, Christensen P, Heidrich O, Lambert S, Abbott A, Ryder K, Gaines L, Anderson P (2019) Recycling lithium-ion batteries from electric vehicles. Nature 575(7781):75–86
Article PubMed ADS CAS Google Scholar
Helbig C, Bradshaw AM, Wietschel L, Thorenz A, Tuma A (2018) Supply risks associated with lithium-ion battery materials. J Clean Prod. https://doi.org/10.1016/j.jclepro.2017.10.122
Hu X, Yan W, Zhang X, Feng Z, Wang Y, Ying B, Zhang H (2022) LRP-based design of sustainable recycling network for electric vehicle batteries. Processes. https://doi.org/10.3390/pr10020273
Jaffe S (2017) Vulnerable links in the lithium-ion battery supply chain. Joule. https://doi.org/10.1016/j.joule.2017.09.021
Jiao N, Evans S (2016) Business models for sustainability: the case of second-life electric vehicle batteries. Procedia CIRP. https://doi.org/10.1016/j.procir.2016.01.114
Khalid A, Stevenson A, Sarwat AI (2021) Performance analysis of commercial passive balancing battery management system operation using a hardware-in-the-loop testbed. Energies. https://doi.org/10.3390/en14238037
Kirchherr J, Reike D, Hekkert M (2017) Conceptualizing the Circular Economy: An Analysis of 114 Definitions. Resour Conserv Recycl 127 221-232. https://doi.org/10.1016/j.resconrec.2017.09.005
Kirchherr J, Piscicelli L, Bour R, Kostense-Smit E, Muller J, Huibrechtse-Truijens A, Hekkert M (2018) Barriers to the circular economy: evidence from the European Union (EU). Ecol Econ 150:264–272. https://doi.org/10.1016/j.ecolecon.2018.04.028
Korhonen J, Honkasalo A, Seppälä J (2018) Circular economy: the concept and its limitations. Ecol Econ. https://doi.org/10.1016/j.ecolecon.2017.06.041
Kuusakoski (2022) Akkujen Kierrätys. Retrieved 19 August 2022 ( https://www.kuusakoski.com/fi/finland/toimialat/sahko-ja-energia/akkujen-kierratys/ ).
Leppänen T, Rönkkö P, Haapasalo H, Tervonen P (2022) The role of logistics in industrial side stream utilisation–case: aqueous paint sludge. Int J Manag Knowl Learn 5:35
Google Scholar
Olsson L, Fallahi S, Schnurr M, Diener D, van Loon P (2018) Circular business models for extended Ev battery life. Batteries. https://doi.org/10.3390/batteries4040057
Ranta V, Aarikka-Stenroos L, Ritala P, Mäkinen SJ (2018) Exploring institutional drivers and barriers of the circular economy: a cross-regional comparison of China, the US, and Europe. Resour Conserv Recycl 135:70–82. https://doi.org/10.1016/j.resconrec.2017.08.017
Rönkkö P, Ayati SM, Majava J (2021) Remanufacturing in the heavy vehicle industry—case study of a Finnish machine manufacturer. Sustainability (switzerland). https://doi.org/10.3390/su131911120
Saez-De-Ibarra A, Martinez-Laserna E, Stroe DI, Swierczynski M, Rodriguez P (2016) Sizing study of second life Li-ion batteries for enhancing renewable energy grid integration. IEEE Trans Ind Appl. https://doi.org/10.1109/TIA.2016.2593425
Sanclemente CM, van Ginkel GM, Peiró LT (2022) Prospects on end of life electric vehicle batteries through 2050 in Catalonia. Resour Conserv Recycl. https://doi.org/10.1016/j.resconrec.2021.106133
Saxena S, le Floch C, Macdonald J, Moura S (2015) Quantifying EV battery end-of-life through analysis of travel needs with vehicle powertrain models. J Power Sources. https://doi.org/10.1016/j.jpowsour.2015.01.072
Schulz M, Monia N, Rehmann L-M, Susse G (2021) Exploration of decision-contexts for circular economy in automotive industry. Procedia CIRP 98:19–24. https://doi.org/10.1016/j.procir.2020.11.005
Shahjalal M, Roy PK, Shams T, Fly A, Chowdhury JI, Ahmed R, Liu K (2022) A Review on second-life of Li-ion batteries: prospects, challenges, and issues. Energy. https://doi.org/10.1016/j.energy.2021.122881
Slattery M, Dunn J, Kendall A (2021) Transportation of electric vehicle lithium-ion batteries at end-of-life: a literature review. Resour Conserv Recycl. https://doi.org/10.1016/j.resconrec.2021.105755
Soh SL, Ong SK, Nee AYC (2015) Application of design for disassembly from remanufacturing perspective. Procedia CIRP. https://doi.org/10.1016/j.procir.2014.07.028
Sopha BM, Purnamasari DM, Ma’mun S (2022) Barriers and enablers of circular economy implementation for electric-vehicle batteries: from systematic literature review to conceptual framework. Sustainability (switzerland) 14:6359. https://doi.org/10.3390/su14106359
Article CAS Google Scholar
Stahel WR (2016) The Circular Economy. Nature 531:435-438
Statista (2022). Number of electric passenger vehicles in use globally 2021–2025. Retrieved 18 Aug 2022 ( https://www.statista.com/statistics/970958/worldwide-number-of-electric-vehicles/ ).
Stena Recycling (2022) Stena Recycling. Retrieved 18 Aug 2022 ( https://www.stenarecycling.fi/top-menu/yritys/stena-recycling-oy/ ).
Suomen Autokierrätys (2022) Sähköauton Ajovoima-Akkujen Kierrätys - Suomen Autokierrätys. Retrieved 18 Aug 2022 ( https://autokierratys.fi/kuluttajille/kierratysjarjestelma/sahkoauton-akkujen-kierratys/ ).
Thorne R, Lopez FA, Figenbaum E, Fridstrøm L, Müller DB (2021) Estimating stocks and flows of electric passenger vehicle batteries in the norwegian fleet from 2011 to 2030. J Ind Ecol. https://doi.org/10.1111/jiec.13186
Työ- ja elinkeinoministeriö (2021) Kansallinen akkustrategia 2025 - Suomi vastuullisen akkutuotannon ja kestävän kehityksen kärkimaaksi: trategiset tavoitteet ja toimenpiteet. Työ- ja elinkeinoministeriön julkaisuja 2021:2. ISBN: 978-952-327-635-2
van Buren N, Demmers M, van der Heijden R, Witlox F (2016) Towards a circular economy: the role of dutch logistics industries and governments. Sustainability (switzerland). https://doi.org/10.3390/su8070647
HS Visio (2022). Suomalaisyritys Alkaa Vuokrata Käytetyistä Teslan Akuista Rakennettuja Sähkövarastoja. Retrieved 19 Aug 2022 ( https://www.hs.fi/visio/art-2000008536480.html ).
Volan T, Vaz CR, Uriona-Maldonado M (2021) Scenarios for end-of-life (EOL) electric vehicle batteries in China. Revista De Gestao. https://doi.org/10.1108/REGE-12-2020-0143
Walden J, Steinbrecher A, Marinkovic M (2021) Digital product passports as enabler of the circular economy. Chem-Ing-Tech. https://doi.org/10.1002/cite.202100121
Wang X, Gaustad G, Babbitt CW, Bailey C, Ganter MJ, Landi BJ (2014) Economic and environmental characterization of an evolving Li-ion battery waste stream. J Environ Manage. https://doi.org/10.1016/j.jenvman.2014.01.021
Article PubMed Google Scholar
Wegener K, Chen WH, Dietrich F, Dröder K, Sami K (2015) Robot assisted disassembly for the recycling of electric vehicle batteries. Procedia CIRP. https://doi.org/10.1016/j.procir.2015.02.051
Wrålsen B, Prieto-Sandoval V, Mejia-Villa A, O’Born R, Hellström M, Faessler B (2021) Circular business models for lithium-ion batteries-stakeholders, barriers, and drivers. J Clean Prod. https://doi.org/10.1016/j.jclepro.2021.128393
Yanamandra K, Pinisetty D, Daoud A, Gupta N (2022) Recycling of Li-ion and lead acid batteries: a review. J Indian Inst Sci. https://doi.org/10.1007/s41745-021-00269-7
Download references
Acknowledgements
The authors would like to thank all the people who took a part in this research, especially the interviewed persons and those who participated on the Webropol survey.
Open Access funding provided by University of Oulu including Oulu University Hospital. This research was funded by the Academy of Finland, InStreams profiling (Grant No. 326291).
Author information
Authors and affiliations.
Industrial Engineering and Management Research Unit, University of Oulu, P.O. Box 4610, 90014, Oulu, Finland
Pasi Rönkkö, Jukka Majava, Tatu Hyvärinen, Ilari Oksanen & Pekka Tervonen
Sustainable Chemistry Research Unit, University of Oulu, P.O. Box 4610, 90014, Oulu, Finland
You can also search for this author in PubMed Google Scholar
Contributions
PR is responsible of data collection and analysis, and writing the original manuscript including literature review. TH, IO, JM and UL were responsible of studying the background of Finnish EVB CE. The study was supervised and reviewed by JM and PT, and validated by all the authors.
Corresponding author
Correspondence to Pasi Rönkkö .
Ethics declarations
Conflict of interest.
On behalf of all authors, the corresponding author states that there is no competing interest.
Ethical approval
Not applicable.
Consent to participate
Consent for publication.
All the authors have approved the manuscript and they have given their consent for publication.
Rights and permissions
Open Access This article is licensed under a Creative Commons Attribution 4.0 International License, which permits use, sharing, adaptation, distribution and reproduction in any medium or format, as long as you give appropriate credit to the original author(s) and the source, provide a link to the Creative Commons licence, and indicate if changes were made. The images or other third party material in this article are included in the article's Creative Commons licence, unless indicated otherwise in a credit line to the material. If material is not included in the article's Creative Commons licence and your intended use is not permitted by statutory regulation or exceeds the permitted use, you will need to obtain permission directly from the copyright holder. To view a copy of this licence, visit http://creativecommons.org/licenses/by/4.0/ .
Reprints and permissions
About this article
Rönkkö, P., Majava, J., Hyvärinen, T. et al. The circular economy of electric vehicle batteries: a Finnish case study. Environ Syst Decis 44 , 100–113 (2024). https://doi.org/10.1007/s10669-023-09916-z
Download citation
Accepted : 15 May 2023
Published : 23 May 2023
Issue Date : March 2024
DOI : https://doi.org/10.1007/s10669-023-09916-z
Share this article
Anyone you share the following link with will be able to read this content:
Sorry, a shareable link is not currently available for this article.
Provided by the Springer Nature SharedIt content-sharing initiative
- Circular economy
- Vehicle battery
- Repurposing
- Electric vehicle
- Find a journal
- Publish with us
- Track your research
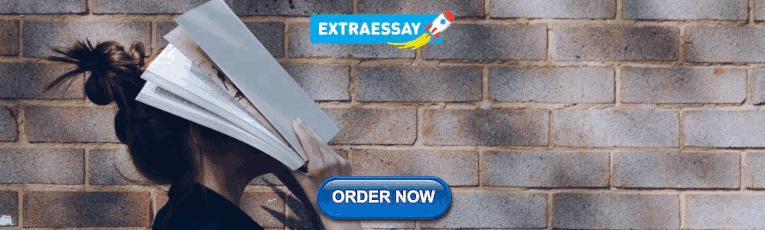
IMAGES
VIDEO
COMMENTS
Electric vehicles (EVs) have advanced significantly this decade, owing in part to decreasing battery costs. Yet EVs remain more costly than gasoline fueled vehicles over their useful life. This paper analyzes the additional advances that will be needed, if electric vehicles are to sig-nificantly penetrate the passenger vehicle fleet. Battery Prices
automobile giants that have introduced electric cars are Hyundai, Tata Motors, Mahindra, and MG. Motors [6]. The total sales figures for all EVs increased by 20% when compared to 2018-2019. In ...
Electric Vehicles Rollout—Two Case Studies. 133. Electric Vehicles Rollout—Two Case Studies. Fridrik M. Baldursson,aNils-Henrik M. von der Fehr,b* and Ewa Lazarczykc. abstract. We present and discuss evidence on electric-vehicle rollout in The Netherlands and Norway, two forerunners in this area. We demonstrate that the uptake of electric.
The purpose of the EV City Casebook is to share experiences on EV demonstration and deployment, identify challenges and opportunities, and highlight best practices for creating thriving EV ecosystems. These studies seek to enhance understanding of the most effective policy measures to foster the uptake of electric vehicles in urban areas. EV ...
An expansion of this vision is necessary to have a future of complete electric mobility by maximising the usage of electric vehicles. The aim of the study to find the possible factors that can influence the adoption of EV's and provide the Government to come out with the policies and to help the automakers to understand the needs and wants of ...
Case Study on Electric Vehicles: City of Durham and Durham County, North Carolina Introduction T h e Ci t y o f Du rh a m a n d Du rh a m Co u n t y g o ve rn me n t s o p e ra t e se p a ra t e f l e e t s wi t h a t o t a l o f a p p ro xi ma t e l y 3 4 0 l i g h t d u t y ve h i cl e s (L DV s), n o t i n cl u d i n g p u b l i c ...
By contrast, electric motors are very efficient, using 90-95% of the input energy to power the movement of the vehicle. But the challenge with electric vehicles is storing enough energy in batteries to provide adequate driving range, as well as recharging that battery without excessive inconvenience to drivers.
companies. The E vehicle is an emerging con cept and the. automobile industry is conducting extensive research to make the. option feasible and commercially viable. Th ere are already some. first ...
Electric vehicle charging and end-user motivation for exibility: a case study from Norway Ida Marie Henriksen*, William Throndsen, Marianne Ryghaug and Tomas Moe Skjølsvold Abstract Background: Norway is currently in the process of replacing internal combustion engine (ICE) vehicles with electric vehicles (EVs).
The market share of battery electric vehicles (EVs) is expected to increase in the near future, but so far little is known about the actual usage of this emergent technology. Consumer preference studies have indicated that the current limitation on driving distance is important. At the same time studies on the actual use of household vehicles ...
Higher upfront cost of most EVs compared to internal combustion engine (ICE) counterpart is due to cost of battery. Battery pack prices have fallen 89% since 2010, despite recent supply chain issues. Figure. Projected decrease in cost of batteries, 2020-2050. Figure.
View PDF; Download full issue; Search ScienceDirect. Procedia Computer Science. Volume 131, ... (ICICT-2018) Investigating Real-World Energy Consumption of Electric Vehicles: A Case Study of Shanghai Zhang Qia, Jie Yangb, Ruo Jiaa,一, Fan Wangc aJiangsu Key Laboratory of Urban ITS, Jiangsu Province Collaborative Innovation Center of Modern ...
Abstract and Figures. In the last years, most European countries have developed strategies to implement the use of electric vehicles. This paper uses a qualitative case study research in the ...
For example, as seen in Figure 6, within the San Francisco Peninsula and 1 million electric vehicles in California, the majority of feeders contribute less than 5% (with the highest around 7%) of peak load. By the time 6 million vehicles are adopted in California, charging demand is responsible for upwards of 20% of peak load (and reaching as ...
In this case study, we start by exploring the motivations behind advances in vehicle autonomy and the key areas of progress that have been made so far. We also explain how autonomous driving is linked to the development of electric vehicles. We explore the key technological, commercial, legal, and ethical challenges of driverless vehicles and ...
PEB 1: I will save on fuel expenses, as running cost should be lower in case of an electric vehicle. ←: PEB: 0.7*** PEB 2: The maintenance cost for an electric vehicle will be less. ←: PEB: 0.6*** PEB 3: Overall cost of owning an electric vehicle will be low due to government incentives (incentives = lower road tax/less insurance premium ...
Piëch in 2005. It produced 10.2 million vehicles, with 12 brands and had an employment base in excess of 600,000—45% from Germany. While Germany itself represented 12% of VW sales, 26% of its production came from Germany—a high cost production base by global standards. It was, and remains, an important source of employment (and tax revenue ...
Download book PDF. Download book EPUB. Shopan Dey 41, Aniruddha Mukherjee 41 & ... Pedrosa et al. done a case study on electric vehicle conversion. They present the conversion process of an internal combustion engine vehicle into an electric vehicle using a three-phase inverter . Karki et al. converted a Maruti 800 into an electric vehicle for ...
The automotive industry faces challenges because of the electrification of vehicles and the rapidly increasing need for electric vehicle batteries (EVBs). Raw materials availability is limited; however, there will also be a significant number of end-of-life (EOL) batteries. This creates various circular economy (CE) business opportunities for EVB manufacturers, third-party providers, and other ...
The engine of Nexon generates 245 Nm of instant torque from a standstill. This assists Nexon EV with speeding up from 0 to 100 kmph in 9.9 seconds. The EV accompanies a best-in-industry dust and water verification battery pack which satisfies IP67 guidelines. This high-density battery pack is fluid cooled to dominate in Indian conditions.
REVENGE OF THE ELECTRIC CAR IN THE 2020S: A CASE STUDY . Ahmed Maamoun, University of Minnesota . ABSTRACT . To say Elon Musk is a disrupter is quite an understatement. The self-made billionaire has transformed several industries (Electric Vehicles, financial ervices, space travel, hyperloopss , artificial intelligence, etc.).
Tel. +45 4525 1510 - Email: [email protected]. Abstract. The market share of battery electric vehicles (EVs) is expected to increase in the near future, but so. far little is known about the actual ...
The study concerns the life cycle assessment (LCA) of a prototype electric racing car, Formula Student, developed by students of the Poznan University of Technology under the name of eVarta. The main objective of this study is to identify critical environmental points and indicate key elements of the vehicle's life cycle, along with the impact of the assumptions made.
Due to the increasing number of battery electric vehicles (BEV) on the roads and the number of BEV accidents with the occurrence of a fire, full-scale fire tests of BEVs were carried out. For initiation, the BEVs were mechanically damaged, forming a gap with a size of 15 cm × 15 cm. The external heat source was a 300 kW propane burner with a maximum power of 54.0 kW and a length of 54 cm. The ...
Download Charged Logistics: The Cost of Electric Vehicle Conversion for U.S. Commercial Fleets, Ryder's data-driven analysis on the potential economic impacts of state and federal legal requirements aimed at converting commercial diesel vehicles to zero-emission vehicles.In the analysis, Ryder uses historical data and current market conditions to uncover the cost to convert diesel commercial ...
Tesla: Case Study Analysis Name: Parisha Sanjay Chande Student ID: 46316728 Word Count: 1547 Online Monday Workshop. Summary Tesla is a company that produces electric cars and energy storage solutions, aiming to disrupt the auto industry with eco-friendly and advanced vehicles. Its marketing strategy includes a range of premium electric cars ...