- Search Menu
- Advance articles
- Author Guidelines
- Submission Site
- Open Access
- Why Publish?
- About Research Evaluation
- Editorial Board
- Advertising and Corporate Services
- Journals Career Network
- Self-Archiving Policy
- Dispatch Dates
- Journals on Oxford Academic
- Books on Oxford Academic

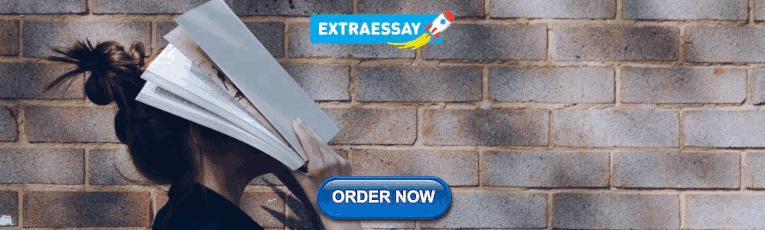
Article Contents
1. introduction, 2. analytical framework, 3. literature search, 5. discussion, 6. conclusion, acknowledgement.
- < Previous
Research impact assessment in agriculture—A review of approaches and impact areas
- Article contents
- Figures & tables
- Supplementary Data
Peter Weißhuhn, Katharina Helming, Johanna Ferretti, Research impact assessment in agriculture—A review of approaches and impact areas, Research Evaluation , Volume 27, Issue 1, January 2018, Pages 36–42, https://doi.org/10.1093/reseval/rvx034
- Permissions Icon Permissions
Research has a role to play in society’s endeavour for sustainable development. This is particularly true for agricultural research, since agriculture is at the nexus between numerous sustainable development goals. Yet, generally accepted methods for linking research outcomes to sustainability impacts are missing. We conducted a review of scientific literature to analyse how impacts of agricultural research were assessed and what types of impacts were covered. A total of 171 papers published between 2008 and 2016 were reviewed. Our analytical framework covered three categories: (1) the assessment level of research (policy, programme, organization, project, technology, or other); (2) the type of assessment method (conceptual, qualitative, or quantitative); and (3) the impact areas (economic, social, environmental, or sustainability). The analysis revealed that most papers (56%) addressed economic impacts, such as cost-effectiveness of research funding or macroeconomic effects. In total, 42% analysed social impacts, like food security or aspects of equity. Very few papers (2%) examined environmental impacts, such as climate effects or ecosystem change. Only one paper considered all three sustainability dimensions. We found a majority of papers assessing research impacts at the level of technologies, particularly for economic impacts. There was a tendency of preferring quantitative methods for economic impacts, and qualitative methods for social impacts. The most striking finding was the ‘blind eye’ towards environmental and sustainability implications in research impact assessments. Efforts have to be made to close this gap and to develop integrated research assessment approaches, such as those available for policy impact assessments.
Research has multiple impacts on society. In the light of the international discourse on grand societal challenges and sustainable development, the debate is reinforced about the role of research on economic growth, societal well-being, and environmental integrity ( 1 ). Research impact assessment (RIA) is a key instrument to exploring this role ( 2 ).
A number of countries have begun using RIA to base decisions for allocation of funding on it, and to justify the value of investments in research to taxpayers ( 3 ). The so-called scientometric assessments with a focus on bibliometric and exploitable results such as patents are the main basis for current RIA practices ( 4–6 ). However, neither academic values of science, based on the assumption of ‘knowledge as progress’, nor market values frameworks (‘profit as progress’) seem adequate for achieving and assessing broader public values ( 7 ). Those approaches do not explicitly acknowledge the contribution of research to solving societal challenges, although they are sufficient to measure scientific excellence ( 8 ) or academic impact.
RIA may however represent a vital element for designing socially responsible research processes with orientation towards responsibility for a sustainable development ( 9 , 10 ). In the past, RIAs occurred to focus on output indicators and on links between science and productivity while hardly exploring the wider societal impacts of science ( 11 ). RIA should entail the consideration of intended and non-intended, positive and negative, and long- and short-term impacts of research ( 12 ). Indeed, there has been a broadening of impact assessments to include, for example, cultural and social returns to society ( 13 ). RIA is conceptually and methodologically not yet sufficiently equipped to capture wider societal implications, though ( 14 ). This is due to the specific challenges associated with RIA, including inter alia unknown time lags between research processes and their impacts ( 15–17 ). Independent from their orientation, RIAs are likely to influence research policies for years to come ( 18 ).
Research on RIA and its potential to cover wider societal impacts has examined assessment methods and approaches in specific fields of research, and in specific research organizations. The European Science Foundation ( 19 ) and Guthrie et al. ( 20 ) provided overviews of a range of methods usable in assessment exercises. They discuss generic methods (e.g. economic analyses, surveys, and case studies) with view to their selection for RIAs. Methods need to fit the objectives of the assessment and the characteristics of the disciplines examined. Econometric methods consider the rate of return over investment ( 21 ), indicators for ‘productive interactions’ between the stakeholders try to capture the social impact of research ( 22 ), and case study-based approaches map the ‘public values’ of research programmes ( 8 , 23 ). No approach is generally favourable over another, while challenges exist in understanding which impact areas are relevant in what contexts. Penfield et al. ( 6 ) looked at the different methods and frameworks employed in assessment approaches worldwide, with a focus on the UK Research Excellence Framework. They argue that there is a need for RIA approaches based on types of impact rather than research discipline. They point to the need for tools and systems to assist in RIAs and highlight different types of information needed along the output-outcome-impact-chain to provide for a comprehensive assessment. In the field of public health research, a minority of RIAs exhibit a wider scope on impacts, and these studies highlight the relevance of case studies ( 24 ). However, case studies often rely on principal investigator interviews and/or peer review, not taking into account the views of end users. Evaluation practices in environment-related research organizations tend to focus on research uptake and management processes, but partially show a broader scope and longer-term outcomes. Establishing attribution of environmental research to different types of impacts was identified to be a key challenge ( 25 ). Other authors tested impact frameworks or impact patterns in disciplinary public research organizations. For example, Gaunand et al. ( 26 ) analysed an internal database of the French Agricultural research organization INRA with 1,048 entries to identify seven impact areas, with five going beyond traditional types of impacts (e.g. conservation of natural resources or scientific advice). Besides, for the case of agricultural research, no systematic review of RIA methods exists in the academic literature that would allow for an overview of available approaches covering different impact areas of research.
Against this background, the objective of this study was to review in how far RIAs of agricultural research capture wider societal implications. We understand agricultural research as being a prime example for the consideration of wider research impacts. This is because agriculture is a sector which has direct and severe implications for a range of the UN Sustainable Development Goals. It has a strong practice orientation and is just beginning to develop a common understanding of innovation processes ( 27 ).
The analysis of the identified literature on agricultural RIA (for details, see next section ‘Literature search’) built on a framework from a preliminary study presented at the ImpAR Conference 2015 ( 28 ). It was based on three categories to explore the impact areas that were addressed and the design of RIA. In particular, the analytical framework consisted of: ( 1 ) the assessment level of research; ( 2 ) the type of assessment method; and ( 3 ) the impact areas covered. On the side, we additionally explored the time dimension of RIA, i.e. whether the assessment was done ex ante or ex post (see Fig. 1 ).

Analytical framework for the review of non-scientometric impact assessment literature of agricultural research.
Agricultural research and the ramifications following from that refer to different levels of assessment (or levels of evaluation, ( 29 )). We defined six assessment levels that can be the subject of a RIA: policy, programme, organization, project, technology, and other. The assessment level of the RIA is a relevant category, since it shapes the approach to the RIA (e.g. the impact chain of a research project differs to that at policy level). The assessment level was clearly stated in all of the analysed papers and in no case more than one assessment level was addressed. Articles were assigned to the policy level, if a certain public technology policy ( 30 ) or science policy, implemented by governments to directly or indirectly affect the conduct of science, was considered. Exemplary topics are research funding, transfer of research results to application, or contribution to economic development. Research programmes were understood as instruments that are adopted by government departments, or other organizational entities to implement research policies and fund research activities in a specific research field (e.g. programmes to promote research on a certain crop or cultivation technique). Articles dealing with the organizational level assess the impact of research activities of a specific research organization. The term research organization comprises public or private research institutes, associations, networks, or partnerships (e.g. the Consultative Group on International Agricultural Research (CGIAR) and its research centres). A research project is the level at which research is actually carried out, e.g. as part of a research programme. The assessment of a research project would consider the impacts of the whole project, from planning through implementation to evaluation instead of focusing on a specific project output, like a certain agricultural innovation. The technology level was considered to be complementary to the other assessment levels of research and comprises studies with a strong focus on specific agricultural machinery or other agricultural innovation such as new crops or crop rotations, fertilizer applications, pest control, or tillage practices, irrespective of the agricultural system (e.g. smallholder or high-technology farming, or organic, integrated, or conventional farming). The category ‘other’ included one article addressing RIA at the level of individual researchers (see ( 31 )).
We categorized the impact areas along the three dimensions of sustainable development by drawing upon the European Commission’s impact assessment guidelines (cf. ( 32 )). The guidelines entail a list of 7 environmental impacts, such as natural resource use, climate change, or aspects of nature conservation; 12 social impacts, such as employment and working conditions, security, education, or aspects of equity; and 10 economic impacts, including business competitiveness, increased trade, and several macroeconomic aspects. The European Commission’s impact assessment guidelines were used as a classification framework because it is one of the most advanced impact assessment frameworks established until to date ( 33 ). In addition, we opened a separate category for those articles exploring joint impacts on the three sustainability dimensions. Few articles addressed impacts in two sustainability dimensions which we assigned to the dominating impact area.
To categorize the type of RIA method, we distinguished between conceptual, qualitative, and quantitative. Conceptual analyses include the development of frameworks or concepts for measuring impacts of agricultural research (e.g. tracking of innovation pathways or the identification of barriers and supporting factors for impact generation). Qualitative and quantitative methods were identified by the use of qualitative data or quantitative data, respectively (cf. ( 34–36 )). Qualitative data can be scaled nominally or ordinally. It is generated by interviews, questionnaires, surveys or choice experiments to gauge stakeholder attitudes to new technologies, their willingness to pay, and their preference for adoption measures. The generation of quantitative data involves a numeric measurement in a standardized way. Such data are on a metric scale and are often used for modelling. The used categorization is rather simple. We assigned approaches which employed mixed-method approaches according to their dominant method. We preferred this over more sophisticated typologies to achieve a high level of abstraction and because the focus of our analysis was on impact areas rather than methods. However, to show consistencies with existing typologies of impact assessment methods ( 19 , 37 ), we provide an overview of the categorization chosen and give examples of the most relevant types of methods.
To additionally explore the approach of the assessment ( 38 ), the dimensions ex ante and ex post were identified. The two approaches are complementary: whereas ex ante impact assessments are usually conducted for strategic and planning purposes to set priorities, ex post impact assessments serve as accountability validation and control against a baseline. The studies in our sample that employed an ex ante approach to RIA usually made this explicit, while in the majority of ex post impact assessments, this was indicated rather implicitly.
This study was performed as a literature review based on Thomson Reuters Web of Science TM Core Collection, indexed in the Science Citation Index Expanded (SCI-Exp) and the Social Sciences Citation Index (SSCI). The motivation for restricting the analysis to articles from ISI-listed journals was to stay within the boundaries of internationally accepted scientific quality management and worldwide access. The advantages of a search based on Elsevier’s Scopus ® (more journals and alternative publications, and more articles from social and health science covered) would not apply for this literature review, with regard to the drawbacks of an index system based on abstracts instead of citation indexes, which is not as transparent as the Core Collection regarding the database definable by the user. We selected the years of 2008 to mid-2016 for the analysis (numbers last updated on 2 June 2016) . First, because most performance-based funding systems have been introduced since 2000, allowing sufficient time for the RIA approaches to evolve and literature to be published. Secondly, in 2008 two key publications on RIA of agricultural research triggered the topic: Kelley, et al. ( 38 ) published the lessons learned from the Standing Panel on Impact Assessment of CGIAR; Watts, et al. ( 39 ) summarized several central pitfalls of impact assessment concerning agricultural research. We took these publications as a starting point for the literature search. We searched in TOPIC and therefore, the terms had to appear in the title, abstract, author keywords, or keywords plus ® . The search query 1 filtered for agricultural research in relation to research impact. To cover similar expressions, we used science, ‘R&D’, and innovation interchangeably with research, and we searched for assessment, evaluation, criteria, benefit, adoption, or adaptation of research.
We combined the TOPIC search with a less strict search query 2 in TITLE using the same groups of terms, as these searches contained approximately two-thirds non-overlapping papers. Together they consisted of 315 papers. Of these, we reviewed 282 after excluding all document types other than articles and reviews (19 papers were not peer-reviewed journal articles) and all papers not written in English language (14 papers). After going through them, 171 proved to be topic-relevant and were included in the analysis.
Analysis matrix showing the number of reviewed articles, each categorized to an assessment level and an impact area (social, economic, environmental, or all three (sustainability)). Additionally, the type of analytical method (conceptual, quantitative, and qualitative) is itemized
In the agricultural RIA, the core assessment level of the reviewed articles was technology (39%), while the other levels were almost equally represented (with the exception of ‘other’). Generally, most papers (56%) addressed economic research impacts, closely followed by social research impacts (42%); however, only three papers (2%) addressed environmental research impacts and only 1 of 171 papers addressed all three dimensions of sustainable development. Assessments at the level of research policy slightly emphasized social impacts over economic impacts (18 papers, or 58%), whereas assessments at the level of technology clearly focused primarily on economic impacts (46 papers, or 68%).
The methods used for agricultural RIA showed no preference for one method type (see Table 1 ). Approximately 31% of the papers assessed research impacts quantitatively, whereas 37% used qualitative methods. Conceptual considerations on research impact were applied by 32% of the studies. A noticeable high number of qualitative studies were conducted to assess social impacts. At the evaluation level of research policy and research programmes, we found a focus on quantitative methods, if economic impacts were assessed.
Overview on type of methods used for agricultural RIA
a Mix of conceptual and qualitative methods.
b Mix of conceptual, qualitative, and quantitative methods.
Additionally, 37 ex ante studies, compared to 134 ex post studies, revealed that the latter clearly dominated, but no robust relation to any other investigated characteristic was found. Of the three environmental impact studies, none assessed ex ante , while the one study exploring sustainability impacts did. The share of ex ante assessments regarding social impacts was very similar to those regarding economic impacts. Within the assessment levels of research (excluding ‘others’ with only one paper), no notable difference between the shares of ex ante assessments occurred as they ranged between 13 and 28%.
The most relevant outcome of the review analysis was that only 3 of the 171 papers focus on the environmental impacts of agricultural research. This seems surprising because agriculture is dependent on an intact environment. However, this finding is supported by two recent reviews: one from Bennett, et al. ( 40 ) and one from Maredia and Raitzer ( 41 ). Both note that not only international agricultural research in general but also research on natural resource management shows a lack regarding large-scale assessments of environmental impacts. The CGIAR also recognized the necessity to deepen the understanding of the environmental impacts of its work because RIAs had largely ignored environmental benefits ( 42 ).
A few papers explicitly include environmental impacts of research in addition to their main focus. Raitzer and Maredia ( 43 ) address water depletion, greenhouse gas emissions, and landscape effects; however, their overall focus is on poverty reduction. Ajayi et al. ( 44 ) report the improvement of soil physical properties and soil biodiversity from introducing fertilizer trees but predominantly measure economic and social effects. Cavallo, et al. ( 45 ) investigate users’ attitudes towards the environmental impact of agricultural tractors (considered as technological innovation) but do not measure the environmental impact. Briones, et al. ( 46 ) configure an environmental ‘modification’ of economic surplus analysis, but they do not prioritize environmental impacts.
Of course, the environmental impacts of agricultural practices were the topic of many studies in recent decades, such as Kyllmar, et al. ( 47 ), Skinner, et al. ( 48 ), Van der Werf and Petit ( 49 ), among many others. However, we found very little evidence for the impact of agricultural research on the environment. A study on environmental management systems that examined technology adoption rates though not the environmental impacts is exemplarily for this ( 50 ). One possible explanation is based on the observation made by Morris, et al. ( 51 ) and Watts, et al. ( 39 ). They see impact assessments tending to accentuate the success stories because studies are often commissioned strategically as to demonstrate a certain outcome. This would mean to avoid carving out negative environmental impacts that conflict with, when indicated, the positive economic or societal impacts of the assessed research activity. In analogy to policy impact assessments, this points to the need of incentives to equally explore intended and unintended, expected and non-expected impacts from scratch ( 52 ). From those tasked with an RIA, this again requires an open attitude in ‘doing RIA’ and towards the findings of their RIA.
Another possible explanation was given by Bennett, et al. ( 40 ): a lack of skills in ecology or environmental economics to cope with the technically complex and data-intensive integration of environmental impacts. Although such a lack of skills or data could also apply to social and economic impacts, continuous monitoring of environmental data related to agricultural practices is particularly scarce. A third possible explanation is a conceptual oversight, as environmental impacts may be thought to be covered by the plenty of environmental impact assessments of agricultural activities itself.
The impression of a ‘blind eye’ on the environment in agricultural RIA may change when publications beyond Web of Science TM Core Collection are considered ( 53 ) or sources other than peer-reviewed journal articles are analysed (e.g. reports; conference proceedings). See, for example, Kelley, et al. ( 38 ), Maredia and Pingali ( 54 ), or FAO ( 55 ). Additionally, scientific publications of the highest quality standard (indicated by reviews and articles being listed in the Web of Science TM Core Collection) seem to not yet reflect experiences and advancements from assessment applications on research and innovation policy that usually include the environmental impact ( 56 ).
Since their beginnings, RIAs have begun to move away from narrow exercises concerned with economic impacts ( 11 ) and expanded their scope to social impacts. However, we only found one sustainability approach in our review that would cover all three impact areas of agricultural research (see ( 57 )). In contrast, progressive approaches to policy impact assessment largely attempt to cover the full range of environmental, social, and economic impacts of policy ( 33 , 58 ). RIAs may learn from them.
Additionally, the focus of agricultural research on technological innovation seems evident. Although the word innovation is sometimes still used for new technology (as in ‘diffusion of innovations’), it is increasingly used for the process of technical and institutional change at the farm level and higher levels of impact. Technology production increasingly is embedded in innovation systems ( 59 ).
The review revealed a diversity of methods (see Table 2 ) applied in impact assessments of agricultural research. In the early phases of RIA, the methods drawn from agricultural economics were considered as good standard for an impact assessment of international agricultural research ( 39 ). However, quantitative methods most often address economic impacts. In addition, the reliability of assessments based on econometric models is often disputed because of strong relationships between modelling assumptions and respective results.
Regarding environmental (or sustainability) impacts of agricultural research, the portfolio of assessment methods could be extended by learning from RIAs in other impact areas. In our literature sample, only review, framework development (e.g. key barrier typologies, environmental costing, or payments for ecosystem services), life-cycle assessment, and semi-structured interviews were used for environmental impacts of agricultural research.
In total, 42 of the 171 analysed papers assessed the impact of participatory research. A co-management of public research acknowledges the influence of the surrounding ecological, social, and political system and allows different types of stakeholder knowledge to shape innovation ( 60 ). Schut, et al. ( 36 ) conceptualize an agricultural innovation support system, which considers multi-stakeholder dynamics next to multilevel interactions within the agricultural system and multiple dimensions of the agricultural problem. Another type of participation in RIAs is the involvement of stakeholders to the evaluation process. A comparatively low number of six papers considered participatory evaluation of research impact, of them three in combination with impact assessment of participatory research.
Approximately 22% of the articles in our sample on agricultural research reported that they conducted their assessments ex ante , but most studies were ex post assessments. Watts, et al. ( 39 ) considered ex ante impact assessment to be more instructive than ex post assessment because it can directly guide the design of research towards maximizing beneficial impacts. This is particularly true when an ex ante assessment is conducted as a comparative assessment comprising a set of alternative options ( 61 ).
Many authors of the studies analysed were not explicit about the time frames considered in their ex post studies. The potential latency of impacts from research points to the need for ex post (and ex ante) studies to account for and analyse longer time periods, either considering ‘decades’ ( 62 , 63 ) or a lag distribution covering up to 50 years, with a peak approximately in the middle of the impact period ( 64 ). This finding is in line with the perspective of impact assessments as an ongoing process throughout a project’s life cycle and not as a one-off process at the end ( 51 ). Nevertheless, ex post assessments are an important component of a comprehensive evaluation package, which includes ex ante impact assessment, impact pathway analysis, programme peer reviews, performance monitoring and evaluation, and process evaluations, among others ( 38 ).
RIA is conceptually and methodologically not yet sufficiently equipped to capture wider societal implications, though ( 14 ). This is due to the specific challenges associated with RIA, including inter alia unknown time lags between research processes and their impacts ( 15–17 ). Independent from their orientation, RIAs are likely to influence research policies for years to come ( 18 ).
However, in the cases in which a RIA is carried out, an increase in the positive impacts (or avoidance of negative impacts) of agricultural research does not follow automatically. Lilja and Dixon ( 65 ) state the following methodological reasons for the missing impact of impact studies: no accountability with internal learning, no developed scaling out, the overlap of monitoring and evaluation and impact assessment, the intrinsic nature of functional and empowering farmer participation, the persistent lack of widespread attention to gender, and the operational and political complexity of multi-stakeholder impact assessment. In contrast, a desired impact of research could be reached or boosted by specific measures without making an impact assessment at all. Kristjanson, et al. ( 66 ), for example, proposed seven framework conditions for agricultural research to bridge the gap between scientific knowledge and action towards sustainable development. RIA should develop into process-oriented evaluations, in contrast to outcome-oriented evaluation ( 67 ), for addressing the intended kind of impacts, the scope of assessment, and for choosing the appropriate assessment method ( 19 ).
This review aimed at providing an overview of impact assessment activities reported in academic agricultural literature with regard to their coverage of impact areas and type of assessment method used. We found a remarkable body of non-scientometric RIA at all evaluation levels of agricultural research but a major interest in economic impacts of new agricultural technologies. These are closely followed by an interest in social impacts at multiple assessments levels that usually focus on food security and poverty reduction and rely slightly more on qualitative assessment methods. In contrast, the assessment of the environmental impacts of agricultural research or comprehensive sustainability assessments was exceptionally limited. They may have been systematically overlooked in the past, for the reason of expected negative results, thought to be covered by other impact studies or methodological challenges. RIA could learn from user-oriented policy impact assessments that usually include environmental impacts. Frameworks for RIA should avoid narrowing the assessment focus and instead considering intended and unintended impacts in several impact areas equally. It seems fruitful to invest in assessment teams’ environmental analytic skills and to expand several of the already developed methods for economic or social impact to the environmental impacts. Only then, the complex and comprehensive contribution of agricultural research to sustainable development can be revealed.
The authors would like to thank Jana Rumler and Claus Dalchow for their support in the Web of Science analysis and Melanie Gutschker for her support in the quantitative literature analysis.
This work was supported by the project LIAISE (Linking Impact Assessment to Sustainability Expertise, www.liaisenoe.eu ), which was funded by Framework Programme 7 of the European Commission and co-funded by the Leibniz-Centre for Agricultural Landscape Research. The research was further inspired and supported by funding from the ‘Guidelines for Sustainability Management’ project for non-university research institutes in Germany (‘Leitfaden Nachhaltigkeitsmanagement’, BMBF grant 311 number 13NKE003A).
Seidl R. et al. ( 2013 ) ‘ Science with Society in the Anthropocene ’, Ambio , 42 / 1 : 5 – 12 .
Google Scholar
OECD . ( 2010 ) ‘Performance-Based Funding for Public Research in Tertiary Education Institutions’, Workshop Proceedings ' 2010. Paris : Organisation for Economic Co-operation and Development .
Hicks D. ( 2012 ) ‘ Performance-based University Research Funding Systems ’, Research Policy , 41 / 2 : 251 – 61 .
Martin B. R. ( 1996 ) ‘ The Use of Multiple Indicators in the Assessment of Basic Research ’, Scientometrics , 36 / 3 : 343 – 62 .
Moed H. F. , Halevi G. ( 2015 ) ‘ Multidimensional Assessment of Scholarly Research Impact ’, Journal of the Association for Information Science and Technology , 66 : 1988 – 2002 .
Penfield T. et al. ( 2014 ) ‘ Assessment, Evaluations, and Definitions of Research Impact: A Review ’, Research Evaluation , 23 / 1 : 21 – 32 .
Meyer R. ( 2011 ) ‘ The Public Values Failures of Climate Science in the US ’, Minerva , 49 / 1 : 47 – 70 .
Bozeman B. , Sarewitz D. ( 2011 ) ‘ Public Value Mapping and Science Policy Evaluation ’, Minerva , 49 / 1 : 1 – 23 .
Helming K. et al. ( 2016 ) ‘ Forschen für nachhaltige Entwicklung. Kriterien für gesellschaftlich verantwortliche Forschungsprozesse (Research for Sustainable Development. Criteria for Socially Responsible Research Processes) ’, GAIA , 25 / 3 : 161 – 5 .
Cagnin C. , Amanatidou E. , Keenan M. ( 2012 ) ‘ Orienting European Innovation Systems Towards Grand Challenges and the Roles that FTA Can Play ’, Science and Public Policy , 39 / 2 : 140 – 52 .
Godin B. , Doré C. ( 2004 ) Measuring the Impacts of Science: Beyond the Economic Dimension . Montréal (Québec) : Centre Urbanisation Culture Société (INRS) .
Ferretti J. et al. ( 2016 ) Reflexionsrahmen für Forschen in gesellschaftlicher Verantwortung. (Framework for Reflecting Research in Societal Responsibility) . Berlin : Federal Ministry of Education and Research (BMBF) .
Jacobsson S. , Vico E. P. , Hellsmark H. ( 2014 ) ‘ The Many Ways of Academic Researchers: How is Science Made Useful? ’, Science and Public Policy , 41 : 641 – 57 .
Levitt R. et al. ( 2010 ) Assessing the Impact of Arts and Humanities Research at the University of Cambridge . Cambridge : University of Cambridge .
Donovan C. ( 2011 ) ‘ State of the Art in Assessing Research Impact: Introduction to a Special Issue ’, Research Evaluation , 20 / 3 : 175 – 9 .
Ekboir J. ( 2003 ) ‘ Why Impact Analysis Should not be Used for Research Evaluation and what the Alternatives Are ’, Agricultural Systems , 78 / 2 : 166 – 84 .
Morton S. ( 2015 ) ‘ Progressing Research Impact Assessment: A ‘Contributions’ Approach ’, Research Evaluation , 24 : 405 – 19 .
Reinhardt A. ( 2013 ) ‘Different Pathways to Impact? “Impact” and Research Fund Allocation in Selected European Countries’, in Dean A. , Wykes M. , Stevens H. (eds) 7 Essays on Impact. DESCRIBE Project Report for Jisc , pp. 88 – 101 . Exeter : University of Exeter .
Google Preview
European Science Foundation . ( 2012 ) The Challenges of Impact Assessment. Working Group 2: Impact Assessment . Strasbourg : European Science Foundation .
Guthrie S. et al. ( 2013 ) Measuring Research. A Guide to Research Evaluation Frameworks and Tools . Cambridge : RAND Corporation .
Alston J. M. et al. ( 2011 ) ‘ The Economic Returns to US Public Agricultural Research ’, American Journal of Agricultural Economics , 93 / 5 : 1257 – 77 .
Spaapen J. , Drooge L. ( 2011 ) ‘ Introducing' Productive Interactions' in Social Impact Assessment ’, Research Evaluation , 20 / 3 : 211 – 18 .
Bozeman B. ( 2003 ) Public Value Mapping of Science Outcomes: Theory and Method . Washington : Center for Science, Policy and Outcomes .
Milat A. J. , Bauman A. E. , Redman S. ( 2015 ) ‘ A Narrative Review of Research Impact Assessment Models and Methods ’, Health Research Policy and Systems , 13 / 1 : 18.
Bell S. , Shaw B. , Boaz A. ( 2011 ) ‘ Real-world Approaches to Assessing the Impact of Environmental Research on Policy ’, Research Evaluation , 20 / 3 : 227 – 37 .
Gaunand A. et al. ( 2015 ) ‘ How Does Public Agricultural Research Impact Society? A Characterization of Various Patterns ’, Research Policy , 44 / 4 : 849 – 61 .
Bokelmann W. et al. ( 2012 ) Sector Study on the Analysis of the Innovation of German Agriculture (Sektorstudie zur Untersuchung des Innovationssystems der deutschen Landwirtschaft) . Berlin : Federal Office for Agriculture and Food (BLE) .
Weißhuhn P. , Helming K. ( 2015 ) ‘Methods for Assessing the Non-Scientometric Impacts of Agricultural Research: A Review’. In ImpAR Conference 2015: Impacts of Agricultural Research-Towards an Approach of Societal V alues. Paris: INRA.
European Science Foundation . ( 2009 ) Evaluation in National Research Funding Agencies: Approaches, Experiences and Case Studies . Strasbourg : European Science Foundation .
Bozeman B. ( 2000 ) ‘ Technology Transfer and Public Policy: A Review of Research and Theory ’, Research Policy , 29 / 4 : 627 – 55 .
Hummer K. E. , Hancock J. F. ( 2015 ) ‘ Vavilovian Centers of Plant Diversity: Implications and Impacts ’, Hortscience , 50 / 6 : 780 – 3 .
EC . ( 2015 ) Better Regulation “Toolbox” . Brussels : European Commission .
Helming K. et al. ( 2013 ) ‘ Mainstreaming Ecosystem Services in European Policy Impact Assessment ’, Ecosystem Services in EIA and SEA , 40 : 82 – 7 .
Thapa D. B. et al. ( 2009 ) ‘ Identifying Superior Wheat Cultivars in Participatory Research on Resource Poor Farms ’, Field Crops Research , 112 / 2–3 : 124 – 30 .
Holdsworth M. et al. ( 2015 ) ‘ African Stakeholders' Views of Research Options to Improve Nutritional Status in Sub-Saharan Africa ’, Health Policy and Planning , 30 / 7 : 863 – 74 .
Schut M. et al. ( 2015 ) ‘ RAAIS: Rapid Appraisal of Agricultural Innovation Systems (Part I). A Diagnostic Tool for Integrated Analysis of Complex Problems and Innovation Capacity ’, Agricultural Systems , 132 : 1 – 11 .
Jones M. M. , Grant J. ( 2013 ) ’Making the Grade: Methodologies for assessing and evidencing research impact’. In Dean A. , Wykes M. , Stevens H. (eds) 7 Essays on Impact. DESCRIBE Project Report for Jisc , pp. 25 – 43 . Exeter : University of Exeter .
Kelley T. , Ryan J. , Gregersen H. ( 2008 ) ‘ Enhancing Ex Post Impact Assessment of Agricultural Research: The CGIAR Experience ’, Research Evaluation , 17 / 3 : 201 – 12 .
Watts J. et al. ( 2008 ) ‘ Transforming Impact Assessment: Beginning the Quiet Revolution of Institutional Learning and Change ’, Experimental Agriculture , 44 / 1 : 21 – 35 .
Bennett J. W. , Kelley T. G. , Maredia M. K. ( 2012 ) ‘ Integration of Environmental Impacts Into Ex-post Assessments of International Agricultural Research: Conceptual Issues, Applications, and the Way Forward ’, Research Evaluation , 21 / 3 : 216 – 28 .
Maredia M. K. , Raitzer D. A. ( 2012 ) ‘ Review and Analysis of Documented Patterns of Agricultural Research Impacts in Southeast Asia ’, Agricultural Systems , 106 / 1 : 46 – 58 .
Renkow M. , Byerlee D. ( 2010 ) ‘ The Impacts of CGIAR Research: A Review of Recent Evidence ’, Food Policy , 35 / 5 : 391 – 402 .
Raitzer D. A. , Maredia M. K. ( 2012 ) ‘ Analysis of Agricultural Research Investment Priorities for Sustainable Poverty Reduction in Southeast Asia ’, Food Policy , 37 / 4 : 412 – 26 .
Ajayi O. C. et al. ( 2011 ) ‘ Agricultural Success from Africa: The Case of Fertilizer Tree Systems in Southern Africa (Malawi, Tanzania, Mozambique, Zambia and Zimbabwe) ’, International Journal of Agricultural Sustainability , 9 / 1 : 129 – 36 .
Cavallo E. et al. ( 2014 ) ‘ Strategic Management Implications for the Adoption of Technological Innovations in Agricultural Tractor: The Role of Scale Factors and Environmental Attitude ’, Technology Analysis and Strategic Management , 26 / 7 : 765 – 79 .
Briones R. M. et al. ( 2008 ) ‘ Priority Setting for Research on Aquatic Resources: An Application of Modified Economic Surplus Analysis to Natural Resource Systems ’, Agricultural Economics , 39 / 2 : 231 – 43 .
Kyllmar K. et al. ( 2014 ) ‘ Small Agricultural Monitoring Catchments in Sweden Representing Environmental Impact ’, Agriculture, Ecosystems and Environment , 198 : 25 – 35 .
Skinner J. et al. ( 1997 ) ‘ An Overview of the Environmental Impact of Agriculture in the UK ’, Journal of Environmental Management , 50 / 2 : 111 – 28 .
Van der Werf H. M. , Petit J. ( 2002 ) ‘ Evaluation of the Environmental Impact of Agriculture at the Farm Level: A Comparison and Analysis of 12 Indicator-based Methods ’, Agriculture, Ecosystems and Environment , 93 / 1 : 131 – 45 .
Carruthers G. , Vanclay F. ( 2012 ) ‘ The Intrinsic Features of Environmental Management Systems that Facilitate Adoption and Encourage Innovation in Primary Industries ’, Journal of Environmental Management , 110 : 125 – 34 .
Morris M. et al. ( 2003 ) ‘ Assessing the Impact of Agricultural Research: An Overview ’, Quarterly Journal of International Agriculture , 42 / 2 : 127 – 48 .
Podhora A. et al. ( 2013 ) ‘ The Policy-Relevancy of Impact Assessment Tools: Evaluating Nine Years of European Research Funding ’, Environmental Science and Policy , 31 : 85 – 95 .
Rodrigues G. S. , de Almeida Buschinelli C. C. , Dias Avila A. F. ( 2010 ) ‘ An Environmental Impact Assessment System for Agricultural Research and Development II: Institutional Learning Experience at Embrapa ’, Journal of Technology Management and Innovation , 5 / 4 : 38 – 56 .
Maredia M. , Pingali P. ( 2001 ) Environmental Impacts of Productivity-Enhancing Crop Research: A Critical Review . Durban : CGIAR .
FAO . ( 2011 ) ‘ Environmental Impact Assessment', Guideline for FAO field projects . Rome : Food and Agriculture Organization of the United Nations .
Miedzinski M. et al. ( 2013 ) Assessing Environmental Impacts of Research and Innovation Policy .
Ervin D. E. , Glenna L. L. , Jussaume R. A. ( 2011 ) ‘ The Theory and Practice of Genetically Engineered Crops and Agricultural Sustainability ’, Sustainability , 3 / 6 : 847 – 74 .
Jacob K. et al. ( 2012 ) ‘Sustainability in Impact Assessments - A Review of Impact Assessment Systems in selected OECD countries and the European Commission’ . Paris : Organisation for Economic Co-operation and Development .
Röling N. ( 2009 ) ‘ Pathways for Impact: Scientists' Different Perspectives on Agricultural Innovation ’, International Journal of Agricultural Sustainability , 7 / 2 : 83 – 94 .
Dentoni D. , Klerkx L. ( 2015 ) ‘ Co-managing Public Research in Australian Fisheries Through Convergence-Divergence Processes ’, Marine Policy , 60 : 259 – 71 .
Helming K. et al. ( 2011 ) ‘ Ex Ante Impact Assessment of Policies Affecting Land Use, Part A: Analytical Framework ’, Ecology and Society , 16 / 1 : 27 .
Stads G. J. , Beintema N. ( 2015 ) ‘ Agricultural R&D Expenditure in Africa: An Analysis of Growth and Volatility ’, European Journal of Development Research , 27 / 3 : 391 – 406 .
Raitzer D. A. , Kelley T. G. ( 2008 ) ‘ Benefit-cost Meta-analysis of Investment in the International Agricultural Research Centers of the CGIAR ’, Agricultural Systems , 96 / 1-3 : 108 – 23 .
Andersen M. A. ( 2015 ) ‘ Public Investment in US Agricultural R&D and the Economic Benefits ’, Food Policy , 51 : 38 – 43 .
Lilja N. , Dixon J. ( 2008 ) ‘ Responding to the Challenges of Impact Assessment of Participatory Research and Gender Analysis ’, Experimental Agriculture , 44 / 1 : 3 – 19 .
Kristjanson P. et al. ( 2009 ) ‘ Linking International Agricultural Research Knowledge with Action for Sustainable Development ’, Proceedings of the National Academy of Sciences United States of America , 106 / 13 : 5047 – 52 .
Upton S. , Vallance P. , Goddard J. ( 2014 ) ‘ From Outcomes to Process: Evidence for a New Approach to Research Impact Assessment ’, Research Evaluation , 23 : 352 – 65 .
The exact TOPIC query was: agricult* NEAR/1 (research* OR *scien* OR "R&D" OR innovati*) AND (research* OR *scien* OR "R&D" OR innovati*) NEAR/2 (impact* OR assess* OR evaluat* OR criteria* OR benefit* OR adoption* OR adaptation*)
The exact TITLE query was: agricult* AND (research* OR *scien* OR "R&D" OR innovati*) AND (impact* OR assess* OR evaluat* OR criteria* OR benefit* OR adoption* OR adaptation*)
Email alerts
Citing articles via.
- Recommend to your Library
Affiliations
- Online ISSN 1471-5449
- Print ISSN 0958-2029
- Copyright © 2024 Oxford University Press
- About Oxford Academic
- Publish journals with us
- University press partners
- What we publish
- New features
- Open access
- Institutional account management
- Rights and permissions
- Get help with access
- Accessibility
- Advertising
- Media enquiries
- Oxford University Press
- Oxford Languages
- University of Oxford
Oxford University Press is a department of the University of Oxford. It furthers the University's objective of excellence in research, scholarship, and education by publishing worldwide
- Copyright © 2024 Oxford University Press
- Cookie settings
- Cookie policy
- Privacy policy
- Legal notice
This Feature Is Available To Subscribers Only
Sign In or Create an Account
This PDF is available to Subscribers Only
For full access to this pdf, sign in to an existing account, or purchase an annual subscription.
Thank you for visiting nature.com. You are using a browser version with limited support for CSS. To obtain the best experience, we recommend you use a more up to date browser (or turn off compatibility mode in Internet Explorer). In the meantime, to ensure continued support, we are displaying the site without styles and JavaScript.
- View all journals
- Explore content
- About the journal
- Publish with us
- Sign up for alerts
- Perspective
- Published: 23 December 2021
On-Farm Experimentation to transform global agriculture
- Myrtille Lacoste ORCID: orcid.org/0000-0001-6557-1865 1 , 2 ,
- Simon Cook ORCID: orcid.org/0000-0003-0902-1476 1 , 3 ,
- Matthew McNee 4 ,
- Danielle Gale ORCID: orcid.org/0000-0003-3733-025X 1 ,
- Julie Ingram ORCID: orcid.org/0000-0003-0712-4789 5 ,
- Véronique Bellon-Maurel 6 , 7 ,
- Tom MacMillan ORCID: orcid.org/0000-0002-2893-6981 8 ,
- Roger Sylvester-Bradley 9 ,
- Daniel Kindred ORCID: orcid.org/0000-0001-7910-7676 9 ,
- Rob Bramley ORCID: orcid.org/0000-0003-0643-7409 10 ,
- Nicolas Tremblay ORCID: orcid.org/0000-0003-1409-4442 11 ,
- Louis Longchamps ORCID: orcid.org/0000-0002-4761-6094 12 ,
- Laura Thompson ORCID: orcid.org/0000-0001-5751-7869 13 ,
- Julie Ruiz ORCID: orcid.org/0000-0001-5672-2705 14 ,
- Fernando Oscar García ORCID: orcid.org/0000-0001-6681-0135 15 , 16 ,
- Bruce Maxwell 17 ,
- Terry Griffin ORCID: orcid.org/0000-0001-5664-484X 18 ,
- Thomas Oberthür ORCID: orcid.org/0000-0002-6050-9832 19 , 20 ,
- Christian Huyghe 21 ,
- Weifeng Zhang 22 ,
- John McNamara 23 &
- Andrew Hall ORCID: orcid.org/0000-0002-8580-6569 24
Nature Food volume 3 , pages 11–18 ( 2022 ) Cite this article
3832 Accesses
74 Citations
141 Altmetric
Metrics details
- Agriculture
- Science, technology and society
- Scientific community
- Sustainability
Restructuring farmer–researcher relationships and addressing complexity and uncertainty through joint exploration are at the heart of On-Farm Experimentation (OFE). OFE describes new approaches to agricultural research and innovation that are embedded in real-world farm management, and reflects new demands for decentralized and inclusive research that bridges sources of knowledge and fosters open innovation. Here we propose that OFE research could help to transform agriculture globally. We highlight the role of digitalization, which motivates and enables OFE by dramatically increasing scales and complexity when investigating agricultural challenges.
This is a preview of subscription content, access via your institution
Access options
Access Nature and 54 other Nature Portfolio journals
Get Nature+, our best-value online-access subscription
24,99 € / 30 days
cancel any time
Subscribe to this journal
Receive 12 digital issues and online access to articles
111,21 € per year
only 9,27 € per issue
Buy this article
- Purchase on Springer Link
- Instant access to full article PDF
Prices may be subject to local taxes which are calculated during checkout
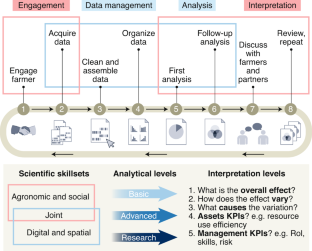
Similar content being viewed by others
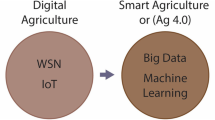
Digital Regenerative Agriculture
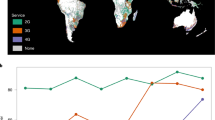
The global divide in data-driven farming
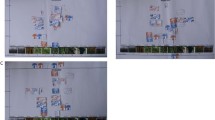
Transdisciplinary participatory-action-research from questions to actionable knowledge for sustainable viticulture development
Data availability.
The authors declare that the data supporting the findings of this study are available within the paper and its Supplementary Information (sources of Figs. 1 – 3 ).
Whitfield, S., Challinor, A. J. & Rees, R. M. Frontiers in climate smart food systems: outlining the research space. Front. Sustain. Food Syst. 2 , https://doi.org/10.3389/fsufs.2018.00002 (2018).
Scoones, I. & Thompson, J. (eds) Farmer First Revisited: Innovation for Agricultural Research and Development 1st edn (Practical Action Publishing, 2009).
Stone, G. D. Towards a general theory of agricultural knowledge production: environmental, social, and didactic learning. Cult. Agric. Food Environ. 38 , 5–17 (2016).
Hansson, S. O. Farmers’ experiments and scientific methodology. Euro. J. Phil. Sci. 9 , 32 (2019).
Maat, H. & Glover, D. in Contested Agronomy: Agricultural Research in a Changing World (eds Sumberg, J. & Thompson, J.) 131–145 (Routledge, 2012).
Šūmane, S. et al. Local and farmers’ knowledge matters! How integrating informal and formal knowledge enhances sustainable and resilient agriculture. J. Rural Stud. 59 , 232–241 (2018).
Article Google Scholar
de Janvry, A., Sadoulet, E. & Rao, M. Adjusting Extension Models to the Way Farmers Learn Policy Brief No. 159 (FERDI, 2016).
Cross, R. & Ampt, P. Exploring agroecological sustainability: unearthing innovators and documenting a community of practice in Southeast Australia. Soc. Nat. Resour. 30 , 585–600 (2016).
Rickards, L., Alexandra, J., Jolley, C., Farhey, K. & Frewer, T. Review of Agricultural Extension (ACIAR, 2019).
MacMillan, T. & Benton, T. G. Engage farmers in research. Nature 509 , 25–27 (2014).
Article ADS CAS Google Scholar
Waters-Bayer, A. et al. Exploring the impact of farmer-led research supported by civil society organisations. Agric. Food Secur. 4 , 4 (2015).
Berthet, E. T. A., Barnaud, C., Girard, N., Labatut, J. & Martin, G. How to foster agroecological innovations? A comparison of participatory design methods. J. Environ. Plan. Manage. 59 , 280–301 (2015).
Cook, S. et al. An on-farm experimental philosophy for farmer-centric digital innovation. In 14th International Conference on Precision Agriculture (ISPA, 2018).
Cook, S. E., Cock, J., Oberthür, T. & Fisher, M. On-farm experimentation. Better Crops 97 , 17–20 (2013).
Google Scholar
Richardson, M. et al. Farmer research networks in principle and practice. Int. J. Agric. Sustain. https://doi.org/10.1080/14735903.2021.1930954 (2021).
Thompson, L. J. et al. Farmers as researchers: in‐depth interviews to discern participant motivation and impact. Agron. J. 111 , 2670–2680 (2019).
Sewell, A. M. et al. Hatching new ideas about herb pastures: learning together in a community of New Zealand farmers and agricultural scientists. Agric. Syst. 125 , 63–73 (2014).
Bramley, R. G. V., Lawes, R. & Cook, S. in Precision Agriculture for Sustainability and Environmental Protection (eds Oliver, M. A., Bishop, T. F. A. & Marchant, B. M.) 205–218 (Routledge, 2013).
Marchant, B. et al. Establishing the precision and robustness of farmers’ crop experiments. Field Crops Res. 230 , 31–45 (2019).
Briggs, J. Indigenous knowledge: a false dawn for development theory and practice? Progr. Dev. Stud. 13 , 231–243 (2013).
Caron, P., Biénabe, E. & Hainzelin, E. Making transition towards ecological intensification of agriculture a reality: the gaps in and the role of scientific knowledge. Curr. Opin. Environ. Sustain. 8 , 44–52 (2014).
Kool, H., Andersson, J. A. & Giller, K. E. Reproducibility and external validity of on-farm experimental research in Africa. Exp. Agric . 56 , 587–607 (2020).
de Roo, N., Andersson, J. A. & Krupnik, T. J. On-farm trials for development impact? The organisation of research and the scaling of agricultural technologies. Exp. Agric. 55 , 163–184 (2019).
Möhring, N. et al. Pathways for advancing pesticide policies. Nat. Food 1 , 535–540 (2020).
Sylvester-Bradley, R. et al. Agronōmics: transforming crop science through digital technologies. Adv. Anim. Biosci. 8 , 728–733 (2017).
Ruiz, J., Dumont, A. & Zingraff, V. in Penser le Gouvernement des Ressources Naturelles (eds Busca, D. & Lew, N.) 293–330 (Presses de l’Université Laval, 2019).
Fabregas, R., Kremer, M. & Schilbach, F. Realizing the potential of digital development: the case of agricultural advice. Science https://doi.org/10.1126/science.aay3038 (2019).
Dowd, A.-M. et al. The role of networks in transforming Australian agriculture. Nat. Clim. Change 4 , 558–563 (2014).
Article ADS Google Scholar
Klerkx, L., van Mierlo, B. & Leeuwis, C. in Farming Systems Research into the 21st Century: The New Dynamic (eds Darnhofer, I., Gibbon, D. & Dedieu, B.) 457–483 (Springer, 2012).
Ingram, J., Gaskell, P., Mills, J. & Dwyer, J. How do we enact co-innovation with stakeholders in agricultural research projects? Managing the complex interplay between contextual and facilitation processes. J. Rural Stud. 78 , 65–77 (2020).
Jackson, L. et al. Biodiversity and agricultural sustainagility: from assessment to adaptive management. Curr. Opin. Environ. Sustain. 2 , 80–87 (2010).
Laurent, A., Kyveryga, P., Makowski, D. & Miguez, F. A framework for visualization and analysis of agronomic field trials from on‐farm research networks. Agron. J. 111 , 2712–2723 (2019).
Kyveryga, P. M. On‐farm research: experimental approaches, analytical frameworks, case studies, and impact. Agron. J. 111 , 2633–2635 (2019).
Tremblay, N. in Precision Agriculture for Sustainability (ed. Stafford, J.) 145–168 (Burleigh Dodds Science Limited, 2019); https://doi.org/10.1201/9781351114592
Bullock, D. S. et al. The data‐intensive farm management project: changing agronomic research through on‐farm precision experimentation. Agron. J. 111 , 2736–2746 (2019).
Wyatt, J., Brown, T. & Carey, S. The next chapter in design for social innovation. Stanford Soc. Innov. Rev. 19 , 40–47 (2021).
Griffin, T. W., Fitzgerald, G. J., Lowenberg‐DeBoer, J. & Barnes, E. M. Modeling local and global spatial correlation in field‐scale experiments. Agron. J . https://doi.org/10.1002/agj2.20266 (2020).
Coudel, E., Tonneau, J.-P. & Rey-Valette, H. Diverse approaches to learning in rural and development studies: review of the literature from the perspective of action learning. Knowl. Manage. Res. Pract. 9 , 120–135 (2017).
Browning, D. M. et al. Emerging technological and cultural shifts advancing drylands research and management. Front. Ecol. Environ. 13 , 52–60 (2015).
Maxwell, B. et al. Can optimization associated with on-farm experimentation using site-specific technologies improve producer management decisions? In 14th International Conference on Precision Agriculture (2018).
Kindred, D. et al. Supporting and analysing on-farm nitrogen tramline trials so farmers, industry, agronomists and scientists can learn together. In 14th International Conference on Precision Agriculture (2018).
Oberthür, T. et al. Plantation intelligence applied oil palm operations: unlocking value by analysing commercial data. Planter 93 , 339–351 (2017).
Jin, H., Shuvo Bakar, K., Henderson, B. L., Bramley, R. G. V. & Gobbett, D. L. An efficient geostatistical analysis tool for on-farm experiments targeted at localised treatment. Biosys. Eng. 205 , 121–136 (2021).
Berthet, E. T., Hickey, G. M. & Klerkx, L. Opening design and innovation processes in agriculture: insights from design and management sciences and future directions. Agric. Syst. 165 , 111–115 (2018).
Curley, M. Twelve principles for open innovation 2.0. Nature 533 , 315–316 (2016).
Ryan, S. F. et al. The role of citizen science in addressing grand challenges in food and agriculture research. Proc. Biol. Sci . 285 , 20181977 (2018).
Herrero, M. et al. Innovation can accelerate the transition towards a sustainable food system. Nat. Food 1 , 266–272 (2020).
Fielke, S. J. et al. Conceptualising the DAIS: implications of the ‘digitalisation of agricultural innovation systems’ on technology and policy at multiple levels. NJAS 90–91 , 100296 (2019).
Cook, S., Jackson, E. L., Fisher, M. J., Baker, D. & Diepeveen, D. Embedding digital agriculture into sustainable Australian food systems: pathways and pitfalls to value creation. Int. J. Agric. Sustain. https://doi.org/10.1080/14735903.2021.1937881 (2021).
van Etten, J. et al. Crop variety management for climate adaptation supported by citizen science. Proc. Natl Acad. Sci. USA 116 , 4194–4199 (2019).
Ingram, J. & Maye, D. What are the implications of digitalisation for agricultural knowledge? Front. Sustain. Food Syst. 4 , https://doi.org/10.3389/fsufs.2020.00066 (2020).
McNee, M. Government Support for Farmer-Based Research in the Falkland Islands AAC Agenda 07.11.2019, Item 10 (Agricultural Advisory Committee, Falkland Islands Government, 2019).
Zhang, W. et al. Closing yield gaps in China by empowering smallholder farmers. Nature 537 , 671–674 (2016).
Lechenet, M., Dessaint, F., Py, G., Makowski, D. & Munier-Jolain, N. Reducing pesticide use while preserving crop productivity and profitability on arable farms. Nat. Plants 3 , 17008 (2017).
García, F. et al. La Red de Nutrición de la Región Crea Sur de Santa Fe: Resultados y Conclusiones de los Primeros Diez Años 2000-2009 (AACREA, 2010).
Posner, S. M., McKenzie, E. & Ricketts, T. H. Policy impacts of ecosystem services knowledge. Proc. Natl. Acad Sci. USA 113 , 1760–1765 (2016).
Moore, M.-L., Riddell, D. & Vocisano, D. Scaling out, scaling up, scaling deep. Strategies of non-profits in advancing systemic social innovation. J. Corp. Citizenship 58 , 67–84 (2015).
Payan, J.‐C. & Pichon, L. ApeX‐Vigne, Version 2020: Une Application Mobile Gratuite pour Faciliter le Suivi de la Croissance des Vignes et Estimer la Contrainte Hydrique (Institut Français de la Vigne et du Vin and Institut Agro, Montpellier SupAgro, 2020).
Samberg, L. H. A collaboration worth its weight in grain. Nature 537 , 624–625 (2016).
Download references
Acknowledgements
This study was funded by the Premier’s Agriculture and Food Fellowship Program of Western Australia. This Fellowship is a collaboration between Curtin and Murdoch Universities and the State Government. The Fellowship is the centrepiece of the Science and Agribusiness Connect initiative, made possible by the State Government’s Royalties for Regions program. Additional support was provided by the MAK’IT-FIAS Fellowship programme (Montpellier Advanced Knowledge Institute on Transitions – French Institutes for Advanced Study) co-funded by the University of Montpellier and the European Union’s Horizon 2020 Marie Skłodowska-Curie Actions (co-fund grant agreement no. 945408), the Digital Agriculture Convergence Lab #DigitAg (grant no. ANR-16-CONV-0004) supported by ANR/PIA, and the Elizabeth Creak Charitable Trust. Contributions toward enabling workshops were made by the USDA (USDA AFRI FACT Los Angeles 2017), the International Society for Precision Agriculture (ICPA Montreal 2018 OFE-C, On-Farm Experimentation Community), the National Key Research and Development Program of China (2016YFD0201303) and ADAS (Cambridge 2018), the European Conference for Precision Agriculture (ECPA Montpellier 2019) and the OECD Co-operative Research Program for ‘Biological resource management for sustainable agricultural systems – Transformational technologies and innovation’ towards ‘#OFE2021, the first Conference on farmer-centric On-Farm Experimentation – Digital Tools for a Scalable Transformative Pathway’. L. Tresh assisted with the design and preparation of Figs. 2 and 3. Members of the #OFE2021 Working Groups also contributed their experiences and insights.
Author information
Authors and affiliations.
Centre for Digital Agriculture, Curtin University, Perth, Western Australia, Australia
Myrtille Lacoste, Simon Cook & Danielle Gale
Montpellier Advanced Knowledge Institute on Transitions (MAK’IT), University of Montpellier, Montpellier, France
Myrtille Lacoste
Centre for Digital Agriculture, Murdoch University, Perth, Western Australia, Australia
Department of Agriculture, Falkland Islands Government, Stanley, Falkland Islands
Matthew McNee
Countryside and Community Research Institute, University of Gloucestershire, Cheltenham, UK
Julie Ingram
Technologies and methods for the agricultures of tomorrow (ITAP), University of Montpellier–National Research Institute for Agriculture, Food and Environment (INRAE)–L’Institut Agro, Montpellier, France
Véronique Bellon-Maurel
Digital Agriculture Convergence Lab (#DigitAg), National Research Institute for Agriculture, Food and Environment (INRAE), Montpellier, France
Centre for Effective Innovation in Agriculture, Royal Agricultural University, Cirencester, UK
Tom MacMillan
ADAS, Cambridge, UK
Roger Sylvester-Bradley & Daniel Kindred
Commonwealth Scientific and Industrial Research Organisation (CSIRO), Adelaide, South Australia, Australia
Rob Bramley
Saint-Jean-sur-Richelieu Research and Development Centre, Agriculture and Agri-Food Canada (AAFC), St-Jean-sur-Richelieu, Quebec, Canada
Nicolas Tremblay
School of Integrative Plant Science, Cornell University, Ithaca, NY, USA
Louis Longchamps
Institute of Agriculture and Natural Resources, University of Nebraska-Lincoln, Falls City, NE, USA
Laura Thompson
Watershed and Aquatic Ecosystem Interactions Research Centre (RIVE), Université du Québec à Trois-Rivières, Trois-Rivières, Québec, Canada
Latin America Southern Cone Group, International Plant Nutrition Institute (IPNI), Buenos Aires, Argentina
Fernando Oscar García
Faculty of Agricultural Sciences, National University of Mar del Plata, Balcarce, Argentina
Montana Institute on Ecosystems, Montana State University, Bozeman, MT, USA
Bruce Maxwell
Department of Agricultural Economics, Kansas State University, Manhattan, KS, USA
Terry Griffin
Southeast Asia Group, International Plant Nutrition Institute (IPNI), Penang, Malaysia
Thomas Oberthür
Business and Partnership Development, African Plant Nutrition Institute (APNI), Benguérir, Morocco
Scientific Direction of Agriculture, National Research Institute for Agriculture, Food and Environment (INRAE), Paris, France
Christian Huyghe
College of Resources and Environmental Sciences and National Academy of Agriculture Green Development, China Agricultural University, Beijing, China
Weifeng Zhang
National Animal Nutrition Program (NANP), United States Department of Agriculture (USDA), Pullman, WA, USA
John McNamara
Commonwealth Scientific and Industrial Research Organisation (CSIRO), Canberra, Australian Capital Territory, Australia
Andrew Hall
You can also search for this author in PubMed Google Scholar
Contributions
M.L. and S.C. developed the study concept. M.M., D.G., J.I., V.B.-M., T.M., R.S.-B. and A.H. contributed additional concept development. M.L. and D.G. obtained the data and prepared the results. M.L., M.M., L.T., D.K., F.O.G., B.M., V.B.-M., J.R., C.H. and W.Z. contributed data. M.L. wrote the manuscript with input from all other authors.
Corresponding author
Correspondence to Myrtille Lacoste .
Ethics declarations
Competing interests.
The authors declare no competing interests.
Additional information
Peer review information Nature Food thanks Carol Shennan, Petro Kyveryga, Nicolas Martin and the other, anonymous, reviewer(s) for their contribution to the peer review of this work.
Publisher’s note Springer Nature remains neutral with regard to jurisdictional claims in published maps and institutional affiliations.
Supplementary information
Supplementary information.
Sources for Figs. 1–3.
Rights and permissions
Reprints and permissions
About this article
Cite this article.
Lacoste, M., Cook, S., McNee, M. et al. On-Farm Experimentation to transform global agriculture. Nat Food 3 , 11–18 (2022). https://doi.org/10.1038/s43016-021-00424-4
Download citation
Received : 13 August 2020
Accepted : 05 November 2021
Published : 23 December 2021
Issue Date : January 2022
DOI : https://doi.org/10.1038/s43016-021-00424-4
Share this article
Anyone you share the following link with will be able to read this content:
Sorry, a shareable link is not currently available for this article.
Provided by the Springer Nature SharedIt content-sharing initiative
This article is cited by
Can machine learning models provide accurate fertilizer recommendations.
- Takashi S. T. Tanaka
- Gerard B. M. Heuvelink
- David S. Bullock
Precision Agriculture (2024)
Digital strategies for nitrogen management in grain production systems: lessons from multi-method assessment using on-farm experimentation
- A. F. Colaço
- B. M. Whelan
- R. A. Lawes
Fully Bayesian economically optimal design for a spatially varying coefficient linear stochastic plateau model over multiple years
- Davood Poursina
- B. Wade Brorsen
Stochastic Environmental Research and Risk Assessment (2024)
Analytic transparency is key for reproducibility of agricultural research
- Gudeta W. Sileshi
CABI Agriculture and Bioscience (2023)
Pesticide reduction amidst food and feed security concerns in Europe
- Kevin Schneider
- Jesus Barreiro-Hurle
- Emilio Rodriguez-Cerezo
Nature Food (2023)
Quick links
- Explore articles by subject
- Guide to authors
- Editorial policies
Sign up for the Nature Briefing: Anthropocene newsletter — what matters in anthropocene research, free to your inbox weekly.


An official website of the United States government
Official websites use .gov A .gov website belongs to an official government organization in the United States.
Secure .gov websites use HTTPS A lock ( Lock A locked padlock ) or https:// means you’ve safely connected to the .gov website. Share sensitive information only on official, secure websites.
Research and Science
From fostering continued economic growth to adapting to the effects of climate change and addressing food security, the United States can continue to be a leader in global agriculture. Each day, the work of USDA scientists and researchers touches the lives of all Americans - from the farm field to the kitchen table and from the air we breathe to the energy that powers our country.
The challenges facing agriculture, natural resources, and conservation are immense and can be addressed through robust research enterprise and educational programs. USDA intramural and extramural science helps to protect, secure, and improve our food, agricultural and natural resources systems.
USDA Science and Research Strategy, 2023-2026: Cultivating Scientific Innovation
The “ USDA Science and Research Strategy, 2023-2026: Cultivating Scientific Innovation (PDF, 21.4 MB)” presents a near-term vision for transforming U.S. agriculture through science and innovation, and outlines USDA’s highest scientific priorities. The S&RS is a call to action for USDA partners, stakeholders, and customers to join the conversation and help identify innovative research strategies that lead to real-world, practical solutions that help farmers, producers, and communities thrive.
Learn more and engage below:
USDA Science and Research Strategy
AGARDA: A Vision for Disruptive Science to Confront Audacious Challenges
Agriculture Advanced Research and Development Authority (AGARDA) Implementation Strategy (PDF, 1.8 MB) is a framework outlining a new approach for delivering disruptive breakthrough discoveries for agriculture.
Strengthening Our Research System
USDA has refocused its science agencies to ensure the most effective and efficient use of its resources, while leveraging the strengths of our partners across the scientific community.
The Office of the Chief Scientist (OCS) coordinates USDA research, education and Extension with scientists and researchers across the federal government and university and private partners, to make the best use of taxpayer investments. In 2012, OCS continued focus on the Research, Education and Economics Action Plan (PDF, 486 KB) and identified seven priority research topics:
- Global Food Supply and Security
- Climate and Energy Needs
- Sustainable Use of Natural Resources
- Nutrition and Childhood Obesity
- Food Safety
- Education and Science Literacy
- Rural-urban Interdependence/Rural Prosperity
The Agricultural Research Service (ARS) conducts research to develop and transfer solutions to agricultural problems of high national priority.
The Economic Research Service (ERS) , through science-based economic research and analysis, informs public policy and other decisions about agriculture, food, rural development, and environmental challenges.
The National Agricultural Statistics Service (NASS) conducts hundreds of surveys every year and prepares reports covering virtually every aspect of U.S. agriculture.
The National Institute of Food and Agriculture (NIFA) supports research, education and Extension programs in the Land-Grant University System and other partner organizations.
Enhancing the Productivity of American Agriculture and Ensuring the Safety of our Food Supply
USDA invests in research, development, and outreach of new varieties and technologies to mitigate animal/plant diseases and increase productivity, sustainability, and product quality. USDA research has supported America's farmers and ranchers in their work to produce a safe and abundant food supply for over 100 years. This work has helped feed the nation and sustain an agricultural trade surplus since the 1960s.
An additional focus is to establish more sustainable systems that enhance crop and animal health. Our scientists and university partners have revealed the genetic blueprints of a host of plants and animals including the genomes of apples, pigs, and turkeys, and in 2012, they furthered understanding of the tomato, bean, wheat and barley genomes -- key drivers in developing the resilience of those crops to feed growing populations.
NASS has developed animated U.S. crop progress and topsoil moisture maps , along with other resources, to help experts assess farmland data. USDA researchers also created the Maize Genome Database, an important tool to help farmers improve traits in a crop vital to the world. Meeting growing global demand for food, fiber, and biofuel requires robust investment in agricultural research and development (R&D) from both public and private sectors. USDA is a leader in remote sensing and mapping to visualize data in support of agricultural policy and business decision making as well as program operation. We ranked first worldwide among research institutions publishing on priority diseases in animal health including salmonellosis, avian influenza , mycobacterial disease, coccidiosis, campylobacterosis, mastitis and others.
USDA conducts and supports science that informs decisions and policies contributing to a safe food supply and the reduction of foodborne hazards. Our scientists found the primary site where the virus that causes foot-and-mouth disease begins infection in cattle and developed an improved vaccine against the disease. They are also working on new strategies to control mites and other major honey bee problems such as colony collapse disorder .
Improving Nutrition and Confronting Obesity
USDA builds the evidence base for food-based and physical activity strategies and develops effective education activities to promote health and reduce malnutrition and obesity in children and high-risk populations. For example, ARS evaluated school characteristics associated with healthier or less healthy food preparation practices and offerings and found that the school nutrition environment could be improved by requiring food service managers to hold nutrition-related college degrees, pass a food service training program, and by participating in a school-based nutrition program such as USDA Team Nutrition .
USDA-supported science is investigating the causes of childhood obesity so that our country can address the epidemic. In these efforts, USDA supports nutrition education programs and encourages Americans to consume more nutritious foods like fruits and vegetables. Our scientists are part of an international team that has found a way to boost the nutritional value of broccoli, tomatoes and corn, and have worked to find ways to bolster the nutritional content of other staple crops like oats and rice. USDA research has supported these efforts, showing how healthy foods can often cost less than foods that are high in saturated fat, added sugar and/or sodium.
In 2013, USDA updated the national assessment of urban and rural food deserts - low-income areas with limited access to affordable and nutritious food - and provided information on the socioeconomic and demographic characteristics that distinguish food deserts from other areas, for decision-makers and stakeholders concerned about access to healthy foods.
Conserving Natural Resources and Combating Climate Change
USDA develops and delivers science-based knowledge that empowers farmers, foresters, ranchers, landowners, resource managers, policymakers, and Federal agencies to manage the risks, challenges, and opportunities of climate variability, and that informs decision-making and improves practices in environmental conservation.
Our scientists are developing rice and corn crops that are drought- and flood-resistant and helping to improve the productivity of soil, as well as production systems that require increasing smaller amounts of pesticides or none at all.
Vegetation indices contained in VegScape have proven useful for assessing crop condition and identifying the aerial extent of floods, drought, major weather anomalies, and vulnerabilities of early/late season crops. This tool allows users to monitor and track weather anomalies' effects on crops in near real time and compare this information to historical data on localized levels or across States.
Additionally, our researchers have examined the potential impacts of a suite of climate scenarios on U.S. crop production. Studies like these will help policymakers, farmers, industry leaders and others better understand and adapt to a changing climate on America's crop production.
Our researchers created i-Tree , urban forest management software to help cities understand the value of urban trees through carbon sequestration, erosion protection, energy conservation and water filtration, and since 2009 have continued building on the success of the tool and expanding its use. Our scientists are conducting research on uses of wood, helping companies meet green building design standards and creating jobs using forest products. We have also worked with Major League Baseball to reduce the occurrence of broken baseball bats.
USDA supports families managing through tough economic times by helping residents save energy at home and conserve water, with a program run by Cooperative Extension and our land-grant university partners. Cooperative Extension-affiliated volunteer monitoring programs have engaged citizens in water monitoring to better understand the effects of climate change and/or aquatic invasive species on local waters. Collectively, these programs interacted with hundreds of local, State, and Federal partners. The programs help citizens detect the presence of invasive species and harmful algal blooms.
Science Education and Extension
USDA recognizes the importance of recruiting, cultivating, and developing the next generation of scientists, leaders, and a highly skilled workforce for food, agriculture, natural resources, forestry, environmental systems, and life sciences.
The NIFA interagency agreement with the U.S. Fish and Wildlife Service leverages technology and innovation and involves youth in STEM outreach and exposure. Youth participants developed science process skills related to using GIS and research design, analyzing and interpreting data, and reporting findings to the community which has enabled them to become better consumers of science and citizens capable of making wise STEM policy choices.
USDA strives to provide effective research, education, and extension activities that inform public and private decision-making in support of rural and community development . NASS holds outreach events throughout the Census cycle with underserved and minority and disadvantaged farming groups to promote participation in the Census of Agriculture . With funding and support from NIFA, many Tribal Colleges are offering Reservation citizens training ranging from basic financial literacy to business start-up and marketing information so that families not only survive, but thrive.
In addition, the ERS Atlas of Rural and Small Town America brings together over 80 demographic, economic, and agricultural statistics for every county in all 50 states and assembles statistics in four broad categories -- people, jobs, agriculture, and geography.
Research and Science Centers and Databases
- Agricultural Network Information Center (AGNIC)
- Agricultural Online Access (AGRICOLA)
- Alternative Farming Systems Information Center (AFSIC)
- Animal Welfare Information Center (AWIC)
- Current Research Information Center (CRIS)
- Digital Desktop (DigiTop) for Employees
- Food and Nutrition Assistance Research Database
- Food and Nutrition Information Center
- Production, Supply and Distribution Online (PSD Online) Database
- Rural Information Center
- Water and Agricultural Information Center
- Understanding Poverty
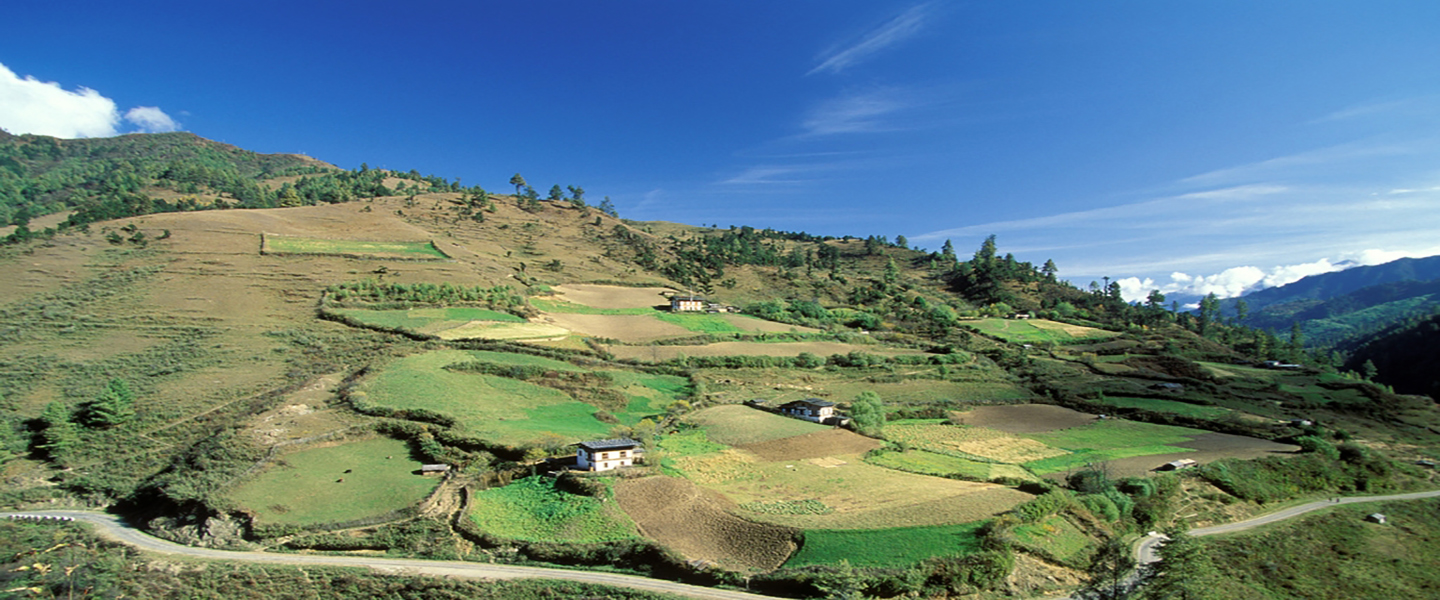
Climate-smart agriculture
Climate-smart agriculture (csa) is an integrated approach to managing landscapes—cropland, livestock, forests and fisheries--that address the interlinked challenges of food security and climate change..
Climate change and food and nutrition insecurity pose two of the greatest development challenges of our time. Yet a more sustainable food system can not only heal the planet, but ensure food security for all.
Today, the global agrifood system emits one-third of all emissions. Global food demand is estimated to increase to feed a projected global population of 9.7 billion people by 2050. Traditionally, the increase in food production has been linked to agricultural expansion, and unsustainable use of land and resources. This creates a vicious circle, leading to an increase in emissions.
Food systems are the leading source of methane emissions and biodiversity loss, and they use around 70% of fresh water. If food waste were a country, it would be the third highest emitter in the world. Meanwhile, emissions from agriculture are increasing in developing countries – a worrying trend which must be reversed.
Without significant climate mitigation action in the agri-food sector, the Paris Agreement goals cannot be reached. Agriculture is the primary cause of deforestation, threatening pristine ecosystems such as the Amazon and the Congo Basin. Without action, emissions from food systems will rise even further, with increasing food production.
Achieving the Triple Win of CSA
The global agrifood system must therefore deliver on multiple fronts. It must feed the world, adapt to climate change, and drastically reduce its greenhouse gas emissions. In response to these challenges, the concept of Climate-smart Agriculture (CSA) has emerged as a holistic approach to end food security and promote sustainable development while addressing climate change issues.
CSA is a set of agricultural practices and technologies which simultaneously boost productivity, enhance resilience and reduce GHG emissions. Although it is built on existing agricultural knowledge, technologies, and sustainability principles, CSA is distinct in several ways. First, it has an explicit focus on addressing climate change in the agrifood system. Second, CSA systematically considers the synergies and tradeoffs that exist between productivity, adaptation, and mitigation. And third, CSA encompasses a range of practices and technologies that are tailored to specific agro-ecological conditions and socio-economic contexts including the adoption of climate-resilient crop varieties, conservation agriculture techniques, agroforestry, precision farming, water management strategies, and improved livestock management. By implementing these practices, triple win results can be achieved:
1. Increased productivity: Produce more and higher quality food without putting an additional strain on natural resources, to improve nutrition security and boost incomes, especially for 75 percent of the world’s poor who live in rural areas and mainly rely on agriculture for their livelihoods.
2. Enhanced resilience: Reduce vulnerability to droughts, pests, diseases and other climate-related risks and shocks; and improve the capacity to adapt and grow in the face of longer-term stresses like increased seasonal variability and more erratic weather patterns.
3. Reduced emissions: Reduce greenhouse gas emissions of the food system, avoid deforestation due to cropland expansion, and increase the carbon sequestration of plants and soils.
Finally, funding for CSA needs to be increased to align available finance with the relevance of the sector. Despite causing one third of global greenhouse gas emissions, agrifood systems receive 4% of climate finance, with only a fifth of this going to smallholders. Current financial flows need to be realigned in order to support a sustainable agrifood system transformation.
Climate-Smart Agriculture and the World Bank Group
The World Bank has significantly scaled up its engagement and investment in climate-smart agriculture (CSA). In its Climate Change Action Plan (2021- 2025), the World Bank has identified Agriculture, Food, Water and Land as one of the five key transitions needed to tackle the Paris Agreement. Since the adoption of the Paris Agreement, the World Bank has increased financing for CSA by eight times, to almost $3 billion annually.
As of July 2023, all new World Bank operations must be aligned with the goals of the Paris Agreement , meaning that CSA is at the core of all the World Bank’s new agriculture and food operations. To this end, the World Bank has prepared a Sector Note of Paris Alignment of its Agriculture and Food operations. Furthermore, all projects are screened for climate and disaster risks. Climate change indicators are used to measure outputs and outcomes, and greenhouse gas accounting of projects is conducted prior to approval . These actions will help client countries implement their Nationally Determined Contributions (NDCs) in the agriculture sector, and will contribute to progress on the Sustainable Development Goals (SDGs) for climate action, poverty, and the eradication of hunger.
The World Bank engages strategically with countries, supporting them to enhance productivity, improve resilience and reduce greenhouse gas emissions. The World Bank uses the following tools, diagnostics and other analytics to help countries in the transition towards sustainable agriculture.
- Country Climate and Development Reports (CCDRs), new core diagnostics, help countries prioritize the most impactful actions that can reduce greenhouse gas emissions and boost adaptation, while delivering on broader development goals. CCDRs identify climate impacts on countries’ agrifood systems, such as reduced yields and increased food prices, and present a variety of country-specific technology options as well as policy reforms under the umbrella of CSA.
- Climate-Smart Agriculture (CSA) Country Profiles developed by the World Bank and partners, give an overview of the agricultural challenges in countries around the world, and how CSA can help them adapt to and mitigate climate change. They bridge knowledge gaps by providing clarity on CSA terminology, components, relevant issues, and how to contextualize them under different country conditions.
- Climate-Smart Agriculture Investment Plans (CSAIPs) developed for a subset of client countries aim to mainstream CSA into national agricultural policies and to identify investment opportunities in CSA. The World Bank provides technical assistance and financial support to help countries develop and implement CSAIPs. These plans prioritize investments in climate-resilient infrastructure, capacity building, and knowledge sharing to promote sustainable agricultural practices. CSAIPs are available, or currently under preparation, for Bangladesh , Belize, Burkina Faso, Cote D’Ivoire , Cameroon, the Republic of Congo, Ethiopia, Ghana , Iraq, Jordan, Kenya, Lesotho , Madagascar, Mali , Morocco , Nepal , Senegal, Zambia , and Zimbabwe .
- The World Bank also supports research programs such as with the CGIAR , which develops and supports climate-smart technologies and management methods, early warning systems, risk insurance, and other innovations that promote resilience and combat climate change.”
Working Toward Resilience and Food and Nutrition Security, while Curbing GHG Emissions
The Bank’s support of CSA is making a difference across the globe, for example:
- A new US$345 million loan for the China Green Agricultural and Rural Revitalization Program for Results will support China’s global public goods agenda by promoting the greening of agriculture and rural development in Hubei and Hunan provinces in central China. The program will reduce greenhouse gas (GHG) emissions from crop and livestock farming, increase carbon sequestration in farmlands, and improve biodiversity protection and restoration in agricultural ecosystems, while strengthening the institutional capacity of local governments to integrate environmental and decarbonization objectives in government rural revitalization plans and investments. World Bank financing will complement a US$4.1 billion commitment by the Government of China (GoC).
- The US$621 million Food Systems Resilience Program for Eastern and Southern Africa (Phase 3) FSRP Project in Kenya, Comoros, Malawi, Somalia aims to increase the resilience of food systems and the recipients’ preparedness for food insecurity. The project has six components, including building resilient agricultural production capacity to strengthen the productivity and resilience of domestic food production to shocks and stressors, by supporting the development and adoption of improved agricultural inputs and services and climate-smart and gender-sensitive farming technologies in the crops, livestock, and fisheries sectors.
- A US$200 million credit for the Punjab Resilient and Inclusive Agriculture Transformation Project (PRIAT) will help Pakistan enhance access to, and productivity of, agricultural water, and improve incomes of farmers supported by the project. PRIAT will notably reduce the differences in water availability among head, middle, and tail end users of watercourses, increase agricultural output per unit of water used at farm level for selected crops, increase the share of area under high-value crops cultivation, and increase agriculture incomes of households participating in project activities, yielding important climate change adaptation and mitigation co-benefits.
- The US$125 million Agriculture Resilience, Value Chain Development and Innovation (ARDI) program will play a pivotal role in strengthening the transition Jordan’s agri-food sector. It supports Jordan's National Sustainable Agriculture Plan and aims to enhance climate resilience, competitiveness, and inclusivity of the agri-food sector. Over the next five years, it will support 30,000 farming household with the adoption of climate-smart and water-efficient agricultural practices, provide needs-based training, create about 12,000 employment opportunities, and promote value chain and export promotion through advanced market diagnostics. A particular focus will be on strengthening the participation of women, youth and refugees.
Last Updated: Feb 26, 2024
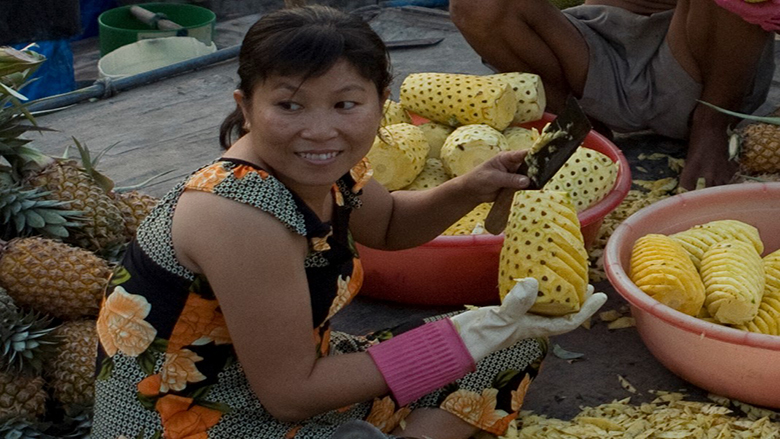
Agriculture and Food
Go back to main topic.
- 'Recipe for a Livable Planet' report launch event, May 22 - Watch live stream
- Recipe for a Livable Planet: Achieving Net Zero Emissions in the Agrifood System
- Climate-Smart Agriculture Country Profiles
- Climate-Smart Agriculture Investment Plans
- Future of Food: Shaping a Climate-Smart Global Food System
- Podcast: Can the World Feed Itself as the Climate Changes?
- Brief: Livestock and Sustainability at the World Bank
- Bringing the Concept of Climate-Smart Agriculture to Life
A Better Food System for Healthier People, Planet and Economy

An official website of the United States government
The .gov means it's official. Federal government websites often end in .gov or .mil. Before sharing sensitive information, make sure you're on a federal government site.
The site is secure. The https:// ensures that you are connecting to the official website and that any information you provide is encrypted and transmitted securely.
- Publications
- Account settings
- Browse Titles
NCBI Bookshelf. A service of the National Library of Medicine, National Institutes of Health.
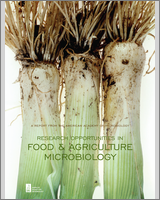
Research Opportunities in Food & Agriculture Microbiology
- Copyright and Permissions
This report presents a wealth of research opportunities in food and agriculture microbiology. The backdrop for these research opportunities is a world of microorganisms teeming with threats and benefits to abundant, healthy food and associated environments. Threats come from microbial pathogens that perpetrate a wide range of plant and animal diseases, destroying agricultural productivity. The constant spread and evolution of agricultural pathogens provides a continually renewed source of challenges to productivity and food safety. Pathogens continue to cause harm once food has left the farm, causing spoilage, and in some cases poisoning and diseases of humans and animals. New vulnerabilities are generated for agriculture by global movement of agricultural products, trading policies, industrial agricultural practices, and the potential for malicious releases of pathogens by “bioterrorists.” In addition to the threats, benefits also come from the many microorganisms associated with, or introduced into, our food supply where they serve important roles in bioprocessing, fermentation, or as probiotics.
Science and technology emerging from microbiology research can help meet these challenges to food and agriculture. Knowledge of microbial pathogens will lead to tools for surveillance and disease prevention. Beneficial microbes may find uses in protecting agriculture, preserving food, enhancing the value of food products and providing general benefits to health and well being. Complex interactions among microbes and agricultural systems must be better understood to facilitate the optimal use of beneficial microorganisms and maximal control of pathogens.
Opportunities in microbiology research are the gateway to sustaining and improving agriculture and food production, quality, and safety. Multidisciplinary research must be undertaken to capitalize on advances in different disciplines, such as genomics, nanotechnology, and computational biology. Research into the interactions of animal and plant hosts with pathogens and beneficial microbes is essential to preventing disease and encouraging mutualistic interactions. On a more holistic scale, interactions occurring among organisms within a microbial community require study so that a healthy balance between the highly managed ecosystems of industrial agriculture and the unmanaged ecosystems of the natural environment can be achieved. Finally, research is critical to determine why pathogens continue to emerge and where and how newly developed technologies should be put to use.
Barriers to seizing these research opportunities must be overcome. The lagging priority of food and agriculture research will be reversed as funding programs and research institutions recognize its importance and improve resources, infrastructure, and incentives accordingly. Endeavors, such as long-term research projects and the banking of diverse microbial specimens, require support so that a foundation of future innovation and discovery is established and sustained. A decline in the number of young scientists entering the fields of food and agriculture research will have to be reversed with funding and fellowship opportunities to provide a highly trained core that will carry out the research of the future. Regulatory hurdles impose stringent processes for research on certain organisms, but are viewed as out of step with actual hazards and must be revised consistent with scientific assessment of risk. Changes that are needed will have to be advocated by scientists, research institutions, professional societies, non-governmental institutions, and companies that are committed to food and agriculture.
This report offers recommendations for research priorities and identifies barriers to a strong food and agriculture research agenda.
- Front Matter
The American Academy of Microbiology is the honorific leadership group of the American Society for Microbiology. The mission of the American Academy of Microbiology is to recognize scientific excellence and foster knowledge and understanding in the microbiological sciences. The Academy strives to include underrepresented scientists in all its activities.
The opinions expressed in this report are those solely of the colloquium participants and may not necessarily reflect the official positions of the American Society for Microbiology.
BOARD OF GOVERNORS, AMERICAN ACADEMY OF MICROBIOLOGY
R. John Collier, Ph.D. (Chair) Harvard Medical School
Kenneth I. Berns, M.D., Ph.D. University of Florida Genetics Institute
Arnold L. Demain, Ph.D. Drew University
E. Peter Greenberg, Ph.D. University of Washington
Carol A. Gross, Ph.D. University of California, San Francisco
J. Michael Miller, Ph.D. Centers for Disease Control and Prevention
Stephen A. Morse, Ph.D. Centers for Disease Control and Prevention
Harriet L. Robinson, Ph.D. Emory University
George F. Sprague, Jr., Ph.D. University of Oregon
David A. Stahl, Ph.D. University of Washington
Judy A. Wall, University of Missouri
COLLOQUIUM STEERING COMMITTEE
Michael Doyle, Ph.D., (Co-Chair) University of Georgia
Lee-Ann Jaykus, Ph.D. (Co-Chair) North Carolina State University
Charles Rice, Ph.D. Kansas State University
Sue Tolin, Ph.D. Virginia Tech University
Anne Vidaver, Ph.D. University of Nebraska
Carol A. Colgan, Director, American Academy of Microbiology
COLLOQUIUM PARTICIPANTS
Thomas E. Besser, D.V.M., Ph.D. Washington State University
Michael Doyle, Ph.D. University of Georgia
Paul Hall, Ph.D. Kraft Food of North America
Craig Hedberg, Ph.D. University of Minnesota
Lee-Ann Jaykus, Ph.D. North Carolina State University
Vivek Kapur, Ph.D. University of Minnesota
Todd Klaenhammer, Ph.D. North Carolina State University
Jan Leach, Ph.D. Colorado State University
Harley W. Moon, Ph.D. Iowa State University
Cindy Nakatsu, Ph.D. Purdue University
Donald Nuss, Ph.D. University of Maryland Biotechnology Institute
John L. Sherwood, Ph.D. University of Georgia
William Sischo, Ph.D. University of California, Davis
Trevor V. Suslow, Ph.D. University of California, Davis
Paul White, Ph.D. Kansas State University
- EXECUTIVE SUMMARY
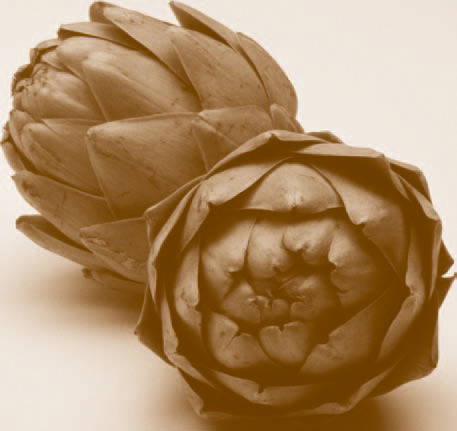
Microbes permeate the entire food and agricultural process. While the most visible role of agriculture is probably that of producing and delivering food, microbiology is critical to other agricultural sectors as well, e.g., for production of energy and for bioremediation of agricultural wastes. Some microorganisms are a constant source of trouble for agricultural endeavors, while others are an integral part of successful food production. Microbial influences on food and agriculture have produced both advancements and disasters that have punctuated human history. Some examples of microbe-driven outcomes set the stage for describing how important it is to seize research opportunities in food and agriculture microbiology.
MICROBES ON THE FARM & IN OUR FOOD
In the fall of 1844 a horde of hungry microbes, whose name Phytopthora infestans was earned from the damage they were about to cause, lurked in the soil of Western Europe. This pathogen causes a disease known as Potato Late Blight, and over the next several years they spread to the fields of Ireland where subsistence farmers were completely reliant on growing potatoes. Devastation of the Irish potato crop led to a terrible famine where almost one million people died and more than twice that many fled their country in abject poverty. A new variant of this blight emerged in the United States in the 1980s, causing serious losses and even bankruptcy for modern potato growers.
The relationship of microbes to the human food supply also includes many examples of organisms that preserve rather than destroy. Early Mediterranean societies discovered that fermentation could be used to help create yogurt and cheese from dairy products. These products were flavorful, safe, and could be stored for extended periods of time. Different types of bacteria and fungi are now known to be involved in fermentation processes. For example, fermentation of sugars in extracts from grain or grape juice produces alcohol that serves as a preservative and provides its own added value. The ancient Egyptians are frequently credited with inventing beer.
Every category of microorganism has members that impact food and agriculture. These include bacteria, single-cell organisms without special compartments for storing their genes; fungi, which can be single- or multicellular, and like plants and animals store their more complex genomes in a compartment called a nucleus; and viruses, which are little more than an infectious set of genes that must operate inside a host cell to reproduce. Among all of these different organisms there are those that benefit agriculture and food, enhancing productivity or nutrition through their interactions with plants and animals. Some microorganisms provide benefit by virtue of their ability to harm other organisms that would cause damage or spoilage if not disrupted. Agriculture and food are prey to many microorganisms that, in the course of their life cycles, destroy crops, animals, and foodstuffs. Some of these microbial pathogens create toxins or are infectious enough to cause disease in humans exposed to the products they have tainted.
MICROBIOLOGY RESEARCH IN FOOD & AGRICULTURE
The wide-ranging impact that microbes have on agriculture and food has always been, and is expected to remain, a challenge. To eat and survive, humans have answered this challenge with ingenuity. Answers sometimes begin as empirical approaches to problems, like the early development of fermentation. Such solutions are subject to improvement and refinement through scientific study and discovery. The better the understanding of the living organisms involved in the agriculture and food chain, the better equipped people are to steer the course of these interactions in our favor.
Basic research is a critical driver for innovation in agriculture and food. For example, despite centuries of using fermentation to ward off spoilage, people still found their wine and beer spoiling over time. In the mid 1800s Louis Pasteur was embroiled in a scientific conflict, disputing the favored belief at the time that microorganisms could appear through “spontaneous generation” in nutrient broth. To disprove this contemporary view, Pasteur devised a method for heat sterilizing broth and keeping it sealed off from contamination. Lengthy demonstrations that the treatment prevented any growth of microorganisms, however, did not win his theory immediate acceptance in the intellectual community. The French navy, then struggling to deal with spoilage on its ships and eruptions of mutiny due to shortages of wine, was ready to perform a large scale test of the principle. “Pasteurization” proved effective, and a basic research problem led to a fundamental technological advancement.
REAPING BENEFITS FROM RESEARCH
Technological advancements do not always find immediate or the most opportune applications. In the case of pasteurization, the technology was shown to effectively rid milk of dangerous pathogens before the end of the 19th century. Despite promotion of the benefits of pasteurization, it was adopted very slowly due to reluctant producers and suspicious consumers. Milk remained responsible for one quarter of all food borne illness throughout the first third of the 20th century in the United States. Wide scale use of pasteurization now provides the invisible benefit of a much safer food supply. In another example, the new technology of genetic modification or engineering has led indirectly to decreases in mycotoxins produced by fungi in some growing crops. These toxins are highly detrimental to animals and humans, including being implicated in several cancers.
“MILK REMAINED RESPONSIBLE FOR ONE QUARTER OF ALL FOOD BORNE ILLNESS THROUGHOUT THE FIRST THIRD OF THE 20TH CENTURY IN THE UNITED STATES.”
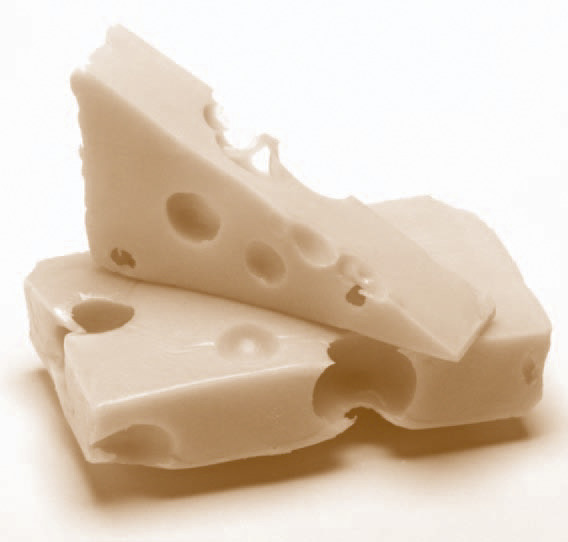
On the opposite end of the spectrum, some technologies experience rapid and extensive adoption before their impact is sufficiently understood. Extensive use of antibiotics in livestock and poultry production came into practice to protect large populations of closely quartered animals from infection, and for its poorly understood growth-promoting effect. The practice is associated with the appearance of some strains of antibiotic resistant microbial pathogens. Furthermore, this practice may provide a pool of resistance genes that can be transferred among organisms in both the gut of animals and the production environment.
The contribution of research towards providing a plentiful, healthy, and safe food supply reaches beyond the cycle of basic research and applied science. Research is also required after development of a technology to direct its prudent or appropriate use. For example, research predicted the selection of antibiotic resistant microbes in agriculture, but did not predict the potential consequences of changing the formulation and processing methods for feed used in British cattle production. Most scientists believe that the origin of bovine spongi-form encephalopathy, the so-called “Mad Cow” disease, was the supplementation of cattle feed with animal protein derived from other ruminants. This resulted in a disease caused by a replicating protein that was propagated through British cattle herds and was subsequently epidemiologically linked to a deadly neurological condition in humans. Assurances of the safety of the meat supply were provided without scientific backing as an animal epidemic gained momentum. As of 2003, there have been over 180,000 confirmed diagnoses of BSE in British cattle and the most recent 2005 statistics cite over 100 confirmed deaths from variant Creutzfeldt-Jakob disease, the human form of the disease believed to be linked to BSE.
In an endeavor such as food production and distribution, tension is always present between technological advances and avoidance of unintended consequences. This tension can be heightened when disasters, such as the Mad Cow episode in Britain, are amplified by policies made without sufficient scientific understanding. Even with scientific understanding of benefits and risks, a technology may be scuttled by lingering mistrust or poor public understanding of complex issues. This is part of what caused the slow adoption of pasteurization, and still has the adoption of food irradiation mostly ham-strung in the United States. Irradiation, proven for decades to destroy pathogens in spices and food and protect against spoilage, lacks a confidence-inspiring name and popular understanding. Similarly, the use of modern molecular biology to add genes into crops, animals, or microbes, creating so-called Genetically Modified Organisms (GMOs), has met with a cool reception in many parts of the globe. Despite their benefits in terms of production, reduced pesticide use, and now a record of safe use after one decade, there remains rancorous dispute about the potential risks of GMOs. Government-sponsored research and oversight, with reasonable transparency in some countries, has enabled commercialization of several crops. Use of rigorous science to study risks and weigh them against the benefits of any new technology has not yet been convincing enough to diffuse the tension that interrupts progress.
Nineteen scientists with expertise in areas ranging from plant pathology to food microbiology to microbial ecology were brought together for a two-and-a-half-day colloquium to examine the future of food and agriculture microbiology. Their deliberations and conclusions are captured in this report.
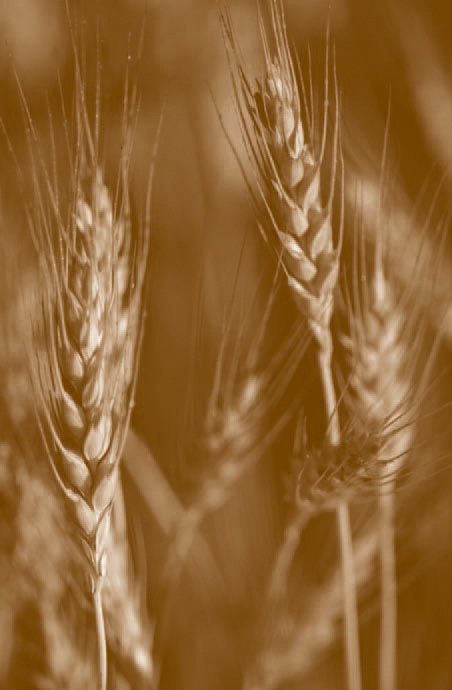
MICROBIOLOGICAL CHALLENGES TO FOOD & AGRICULTURE
An abundant and healthy food supply is expected from our agricultural systems. Fulfilling this demand is a complicated process involving plant cultivation, animal husbandry, soil and water management, harvesting, processing, storage, and transport. At each step the microbial world presents obstacles to success.
MICROBIAL DISEASE
Disease-causing microbes continually assault the animals and crops that humans raise for food. These unwelcome guests make their living off of our agriculture as well. Each animal or plant that we raise is host to an assortment of bacterial, viral, and fungal pathogens. One of the more famous examples of viral diseases in animals, Foot and Mouth Disease, infects cloven hoofed animals such as cattle and sheep. It is extremely contagious and persists in susceptible animals in the wild and in husbandry. The severe blisters and cankers caused by the virus are usually not deadly, but the lifespan and productivity of infected animals is severely reduced. The disease is dreaded globally as a problem for trade because it can be spread easily, not only by sick animals, but by meat products and even on clothing.
The most virulent diseases are crippling or deadly to agriculture, draining or even annihilating a crop harvest or animal population. Some of these diseases have consequences for humans reaching beyond their impact on the availability or expense of our food. Pathogens known as “zoonotic” are those that can be transmitted from animals to humans and include transmission via vectors (i.e., insects, rodents), food, or water that have become contaminated from these animal sources. The most notorious contemporary example is Anthrax. This soil dwelling bacterium can kill cattle and other herd animals that encounter it while grazing. It is also known to cause skin lesions, gastrointestinal infection, and serious systemic disease in people exposed to infected animals. This bacterium has some choice properties as a potential biological weapon, including deadly toxins it produces while growing in the warm tissues of an animal host. In the fall of 2001, illnesses and deaths resulted from letters containing special preparations of Anthrax spores, which infected individuals who had contact with the contaminated mail.
Plant diseases also impact humans. Most significantly, fungi leave behind toxins poisonous to people and animals that eat them, as well as to the host plant. As unlikely as it seems, there is some evidence that plant pathogens can also be infectious to people. The greatest number of documented cases so far are pathologies found in the immune compromised, but an increasing number are associated with apparently healthy humans. However, this is a neglected field of study, and it is not known how widespread or important such infections might be and with what types of syndromes these agents might be associated.
Fungal, bacterial, and viral pathogens are problems in any system where dense populations of the same kind of plants or animals are being cultivated for food. This principle extends beyond fields and pastures, to areas like ponds or net-cages, where aquaculture is performed, and caves, where mushrooms are grown. Diseases that assail agriculture can also be more complicated than an infection by a single pathogen; polymicrobial diseases result from the compound effects of multiple pathogens acting together. Diseases of this kind can be more difficult to predict, diagnose, and respond to than those caused by one organism alone.
“FOOT AND MOUTH DISEASE OF LIVESTOCK COMES IN ABOUT 80 DIFFERENT “SEROTYPES” AROUND THE WORLD; EACH ONE IS SEROLOGICALLY DIFFERENT. ”
MICROBIAL PATHOGENS MOVING & MORPHING
Pathogens have a variety of ways to invade agricultural plants, animals, and products, such as sliced meats and cheeses. Vector transmission, seed and aerial dispersion, environmental persistence, and living on alternate, often perennial hosts are some of the ways that pathogens can break into an agricultural setting. Vector transmission occurs when another organism, such as an insect, carries the pathogen from an infected host and inoculates a healthy host. For example, the glassy-winged sharpshooter can suck sap from a grape vine infected with Pierces' Disease, and be able to transmit the disease-causing bacteria to other healthy vines for several days. Infected plants rapidly show complete loss of productivity. Vector transmission, seed and aerial dispersion, environmental persistence, and living on alternate, often perennial hosts are some of the ways that pathogens can break into an agricultural setting. The spores of the Anthrax bacterium mentioned above can remain viable in the soil for decades until one finds its way into the nutrient rich, warm setting of a skin scratch or the lung of a mammal. Some pathogens are not able to survive long without a host, but are able to linger on what are called “alternate hosts.” This allows the pathogen to last over a winter or for several years, with the alternate host providing a reservoir of infectious material upon reappearance of the susceptible agricultural host and the right conditions for infection.
Agricultural pathogens not only have diverse ways of infecting plants and animals, but also have ways to overcome host defenses directed against them. The sheer enormity of microbial populations provides them with an evolutionary advantage. In vast microbial populations which replicate very quickly, variations in genetic makeup become statistically more probable when compared to slower growing plant and animal populations. Genetic variation allows for the emergence of pathogens that are no longer recognizable to the immune system of a host, or that have improved mechanisms for inflicting disease. Foot and Mouth disease of livestock comes in about 80 different “serotypes” around the world; each one is serologically different. An animal resistant to one serotype through vaccination or exposure will still have an immune system that is unprepared for most of the other serotypes.
Evolution frequently produces pathogens resistant to pesticides and antibiotics through genetic routes more complex than simple mutation. For example, the problem of antibiotic resistant bacteria is driven by the swapping of genetic material between organisms. Genes can be transferred on mobile pieces of DNA, or shuttled from one cell to another by plasmids or bacteria-infecting viruses called bacteriophages. Not recognized by opponents of GMOs, microbial pests of agriculture and public health readily take advantage of “natural” gene transfer or genetic engineering for survival and spread.
Viruses are capable of even greater wholesale shuffling of genetic material. Virus genomes reproduce inside of a host cell. Co-infection of a cell with different viruses allows the opportunity for a broad array of hybrid genomes to result. These new “hybrid” variants are usually inactive, but occasional variants gain properties, such as increased virulence, enhanced infectivity, or altered host range or vector specificity. A frightening agricultural example of this phenomenon is Avian Influenza. In 1918, a pandemic flu emerged that decimated cities and countries around the world. Today, with high density poultry production and many people in close contact with flocks, zoonotic episodes of Avian Influenza are being reported. Experts are concerned that it is only a matter of time before another highly virulent pandemic strain of human influenza evolves, perhaps this time of avian origin.
MICROBES ROTTING & POISONING THE FOOD SUPPLY
Microbial interference with an abundant and healthy food supply continues once plant and animal products leave the farm. Since pathogens can be part of the normal gastrointestinal flora of many animals, they are difficult, if not impossible, to completely eradicate and may contaminate our food supply. Bacteria such as Salmonella, Campylobacter , and certain strains of Escherichia coli , as well as enteropathogenic viruses, can persist, and some bacteria will multiply. If not killed before the food is eaten, these microbes can cause serious illness. There are an estimated 76 million incidents of foodborne illness in the United States each year. This is despite all of the advancements in food handling and processing hygiene, such as pasteurization, already in practice. Pathogens can also reach the food supply through contaminated water, transmission between infected animals, using animal manure as fertilizer, and even from contaminated or infected food handlers. Some contaminated seafood acquires viruses, for example, from contaminated harvest waters.
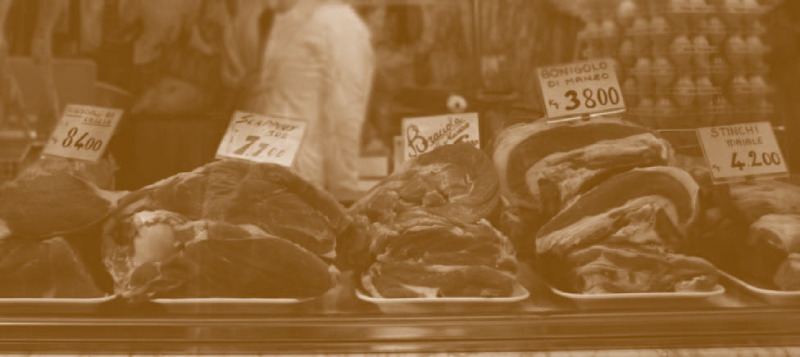
Illness due to microbes is also caused by toxins left behind in food as a consequence of microbial growth. Fungi, such as those in the genus Fusarium and Aspergillus , grow well on grains and other crops. As they grow, they produce toxic substances called mycotoxins. These toxins remain in edible tissues, or can accumulate after harvest if the fungus continues growing, and are poisonous to humans and animals that eat them. The toxins cause a variety of damaging effects on the nervous, digestive, immune and vascular systems. Some are also highly carcinogenic, including one of the most potent cancer causing chemicals known, aflatoxin. The most potent neurotoxin known causes Botulism, and is a product of a bacterium that grows in food in the absence of oxygen.
Even without directly causing human disease, microbes can have a chilling effect on the efficiency and cost of food production. Most people are familiar with vegetables left too long in the refrigerator drawer which are transformed into black puddles of mush. This spoilage is caused by bacteria and/or fungi that eat, or rot, the food. The process of spoilage erodes the quality and availability of our food supply at every step in the food production, processing, transportation, and marketing chain. Its costs are borne by producers, shippers, processors and consumers.
PRACTICES THAT INCREASE THE MICROBIAL THREAT TO FOOD & AGRICULTURE
A newly realized threat to agricultural production and food safety is the purposeful use of disease and damage-causing organisms. Whether thought of as “bioterrorism” or simply malicious sabotage, this is a real threat derived from the microbial world. The systems we have in place to detect and deal with the broad array of diseases and toxins discussed above now must take into account human-made versions of the threats as well.
Many elements of the modern world interact with the microbial world to create new problems. Regional and international shipment of agricultural products means that pathogens do not necessarily require natural dispersion. Although some pathogens, such as soybean rust, can be transported by wind, others that might not spread so easily by natural forces can arrive by plane or boat. Stiff trade restrictions are established to prevent the entry and spread of certain diseases, but these restrictions are only as good as the inspection and detection measures used. Foot and Mouth Disease is a notable example. Countries that are free of FMD prohibit import of any meat products from countries not considered free of the disease. However, because of limitations to current detection methods, countries that vaccinate against the disease are automatically treated as though they have infectious animals. Countries that are able to operate without using vaccine have privileged access to certain markets, but at the cost of having completely vulnerable herds if the disease were introduced. Many microbiological issues are thus serious international agricultural trade issues and often are a reason for blocking trade of certain commodities.
Modern, intensive agricultural practices provide advancements in efficiency and product uniformity, but also bear some elements contributing to their own demise. Overuse of antibiotics and pesticides can select for resistance in the microorganisms that they target. Many of these chemicals are also pollutants, contaminating the environment and perhaps reaching people or organisms that were never their intended targets. Heavy use of fertilizers feeds nutrients into waterways, fueling microbial growth that can kill fish and other wildlife. And animal manure, used as a fertilizer, can contaminate water sources used for animal and plant production, providing a source of foodborne pathogens if not applied using best management practices.
In the drive for uniformity, intensive agriculture, such as planting row upon row of genetically identical crops, or raising large herds or flocks with little genetic diversity in close quarters, may result in expansive populations of vulnerable hosts upon the emergence of pathogens that are newly resistant to pesticides, vaccines, or other critical barriers.
FOOT & MOUTH DISEASE & TRADE BARRIERS.
MEETING CHALLENGES WITH MICROBIOLOGICAL SCIENCE & TECHNOLOGY
The microbial world is not just a source of endless problems for food and agriculture. Some of the solutions to disease and spoilage lie in the application of microbiology. Solutions to non microbe-derived problems may also be provided through microbiology. An ever growing human population is demanding more food, and is also using more energy and creating more waste. Food and agriculture microbiology can present opportunities to address some of these problems. Furthermore, some of the solutions to agricultural problems, such as soil salinization and drought, may lie with microbes.
UNDERSTANDING MICROBIAL PATHOGENS & COMBATING THEM
Better understanding of the microorganisms that cause disease and spoilage in agriculture and food will lead to better ways of controlling them. Pesticides are needed that are more environmentally friendly and that have added barriers to the production of resistance. Improved vaccines and immunomodulators are needed to make immunization of herds and flocks against pathogens more effective. Advances in these areas are dependent on knowing more about the “enemy,” i.e., microbes that cause the diseases.
It also pays to know about the enemies of one's enemies. Pathogens that attack crops and animals frequently have competitors and pathogens of their own. Harnessing the capabilities of these antagonists is an approach called biological control. Through biological control, relatively harmless microorganisms (or their metabolic products) that inhibit or kill a harmful organism are mass produced and applied to food or crops as a protective measure. Large scale production of biological control organisms is a difficult process, and their performance in a field setting is often unpredictable as a match with each local ecosytem's conditions is needed. Nevertheless, genetic engineering of biological control organisms is a possible way to overcome these shortcomings. As biological control using GMOs is more difficult to clear through regulatory and political hurdles due to the current climate, little work is in progress.
A strategy of containment and destruction is required in instances whereby plant and animal diseases cannot be controlled by selective breeding, pesticides, vaccines, drugs, or biological control. The approach of quarantining and then depopulating possibly infected plants and animals becomes more expensive and devastating with increased disease spread. This is illustrated by the expense of destroying millions of chickens during the 2003-04 outbreaks of Avian Influenza, and by the 2001 FMD outbreak in Great Britain. The appearance of citrus canker in Florida has required extensive cutting of citrus trees, even in urban areas, at the cost of millions of dollars. Reducing these losses relies upon the ability to detect and react quickly to the appearance of a pathogen.
SURVEILLANCE FOR MICROBIAL PATHOGENS
Improved surveillance of, and response to, disease outbreaks relies on a variety of technologies. Accurate models of pathogen spread are required to prevent costly overestimates of pathogen dispersion, or underestimates that render control measures incomplete or ineffective. Better coordination and networking between the different entities handling surveillance operations and the standardization of detection technologies will facilitate more rapid, thorough response to outbreaks.
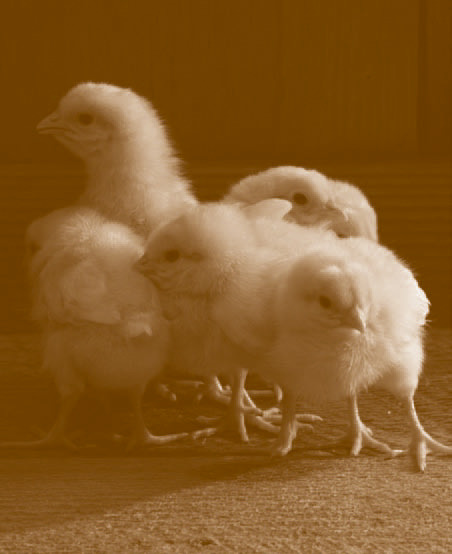
Ideally, surveillance networks should also be capable of tracing the cause of an outbreak to its point of origin. Knowledge of how incidents were initiated is critical to instituting changes that will prevent future incidents. Under ideal circumstances, surveillance networks should be capable of distinguishing among incidents caused by natural, accidental, and purposeful release of pathogenic organisms. This would require a much greater knowledge of microbial communities than we presently have, as well as forensic capabilities. Robust systems for disease surveillance advance the capability of responding to microbiological threats, thereby reducing damage. This is the case in preemptive actions against some agricultural diseases. For example, planting of certain genotypes of wheat in North America is guided each year by a forecasting system that observes what wheat rust virulence types are appearing to the South, and then recommending what available resistance genotypes will fare best in the upcoming planting season. Surveillance is also applied to respond as early as possible to outbreaks such as Avian Influenza. Another form of surveillance is routinely applied when we monitor for contaminants in the food supply. For example, grains are screened for mycotoxin contamination, and raw meats are sometimes tested for the presence of enteropathogenic bacteria.
“… SURVEILLANCE NETWORKS SHOULD BE CAPABLE OF DISTINGUISHING AMONG INCIDENTS CAUSED BY NATURE, ACCIDENTAL, AND PURPOSEFUL RELEASE OF PATHOGENIC ORGANISMS.”
Successful surveillance depends on accurate, fast, and practical detection technologies. Most immediately, there is a need for diagnostics that can test for multiple organisms in a single test, a concept termed multiplexing. In addition, diagnostic tests must be robust enough to be applied to complex sample materials, such as soil, food, and fecal material. The complexity of these materials can cause so-called matrix effects, severely hindering the sensitivity and specificity of a diagnostic test that would otherwise perform perfectly when applied to a pristine sample matrix, such as a pure culture of the target organism.
Other needed improvements to diagnostics are ones that enable them to be more widely accessible for use and more widely relied upon. Improving the accuracy and versatility, as described above, will help accomplish this. Standardization of tests nationally and internationally will be a challenge. The technologies need to be more portable and more rapid, enabling field sampling and real time analysis. Diagnostics should be made less expensive so they can be used more frequently and by programs with restricted budgets. Finally, diagnostic technologies must contribute useable information to inform risk management. Wrong information in a practical sense can consist of more than a simple false-positive result; information can also be useless if the test correctly detects the presence of the target organism, but it is dead, or not present at levels of concern. To help resolve these issues, it is necessary for diagnostic tests to be made more quantitative. Along similar lines, test specificity needs to be refined so that we are detecting the presence of pathogenic variants of microorganisms. There are many cases where diagnostic specificity to the species level is insufficient to accurately reflect risk because different strains within a species can differ significantly in their pathogenic characteristics.
The versatility and ruggedness that is needed from diagnostic technologies is also dependent on improving methodologies for handling specimens before testing. Improved methods for the pre-analytical processing of specimens are required. Means of cultivating organisms that have previously been non-culturable will improve diagnostic capabilities, although knowledge of microbial genomics will enable identification of many non-culturable micro-organisms as well as viruses.
PRESERVING FOOD & ENHANCING ITS VALUE
A major point of inefficiency in food production will be improved with reductions in post-harvest spoilage. Finding ways to slow or even prevent microbial spoilage will provide one set of solutions to this problem. Inactivation of spoilage-causing microbes is only one way to preserve food. Better understanding the spoilage process itself will open opportunities to alternatives to spoilage control, such as the biocontrol option. A time honored example of this principle is in the production of yogurt. The bacteria that grow in milk to generate yogurt convert the nutrients into byproducts that make the food environment much less amenable to the growth of spoilage organisms, thereby extending the shelf life of the food.
Beneficial microbes cultivated in food can provide added value far beyond delay or prevention of spoilage. Many of these microbes have “probiotic” properties that can help exclude disease-causing organisms and prevent infections. Probiotic properties of beneficial microbes are thought to be derived in part from competitive exclusion of pathogenic microbial species. However, the phenomenon is complex and may include other elements, such as the release of compounds antagonistic to pathogens or stimulation of the host immune system. Deepening understanding of the nature of such probiotic effects and elucidated ways that these can be strengthened will allow scientists to capitalize further on the beneficial effects of these microbes.
The presence of beneficial microbes in agriculture and food holds the possibility of generating added value to products. Some microbes have properties that convey nutritional enhancement to food. For example, yeast is a source of B-complex vitamins. There is also speculation that interactions between plants and certain microbes can stimulate enhanced production of compounds with pharmaceutical properties. This possibility may provide opportunities to generate health-promoting foods with so-called nutraceutical properties.
A HELPING HAND IN AGRICULTURE FROM MICROBES
Other interactions with beneficial microbes can be of direct benefit to agricultural plants and animals. A classic example of mutualism in action is the partnership between legumes and bacteria called Rhizobia. The bacteria take up residence in plant roots, receiving nutrients. In exchange, they fix nitrogen from the air into a form that the plants can use, replacing a need for nitrogen-containing fertilizer. There are other cases of microbes helping a host organism scavenge essential nutrients, or fend off pathogens. In the intestinal tract of ruminants, a complex mixture of bacteria enables the animal to extract sufficient nutrients from a diet of grasses.
Even the best-recognized and most-studied forms of mutualism are not understood well enough to be effectively controlled or expanded to cover hosts previously unknown to benefit from a particular interaction. Scientists have been unsuccessful in getting Rhizobia to form a mutualistic relationship with wheat roots, for instance. For the few classic examples of mutualism in agricultural systems, there are likely to be many more interactions taking place in obscurity. Study of interactions between organisms that boost agricultural success is a field rich with opportunities. More knowledge of microbial ecology and mutualistic interactions will pave the way for advances that enhance agricultural organisms' nutrient use, pathogen resistance, and hardiness.
Microbial ecology will likely be found to have impacts on agricultural systems beyond those currently recognized. Complex interactions between plants and the consortia of microbes found in soil probably extend beyond resisting pathogens and scavenging nutrients. Properly tuned interactions could help improve drought resistance and salt tolerance of plants and have other growth-promoting activities. Understanding and managing microbial ecology will have major benefits for stressed agricultural systems.
The massive scale of human agricultural and food production enterprises brings with it an array of problems that microbiology can help address. Any technological advances that increase resistance to pathogens or nutrient scavenging will also contribute to reduced use of pesticides and fertilizers. This represents a corresponding reduction in pollutants. Other pollutants are a direct consequence of agricultural production itself, rather than production practices. Waste produced by animals, particularly when produced in high densities, frequently represents a serious environmental and health hazard. Animal manure is typically accumulated in bulk and some of the material is used as fertilizer on agricultural fields. Technology to harness microbes for digestion of animal waste could alleviate some of the environmental and health hazards generated by large-scale animal rearing operations. Microbes may also be harnessed for the remediation of agricultural chemicals or for mitigating greenhouse gases.
Microbial digestion, another form of fermentation, can be harnessed to produce alternative fuels. Fermentation of animal wastes can create flammable gases, such as methane. Devising bioreactors that efficiently convert animal waste on a large scale would help eliminate an environmental and health hazard, while also satisfying growing energy needs. Fermentative processes also produce fuels, such as ethanol from plant material. The inefficiency of this type of fermentation for fuel production has kept it from being widely adopted. Improvements in fuel generating technology would allow the gradual replacement of highly polluting fossil fuels with more environmentally friendly fuel sources. However, continued removal of plant waste from fields may have unintended effects, such as altering the composition and characteristics of the soil, affecting microbial populations and subsequent plant growth. Thus, this practice needs to be examined and followed over multiple years to monitor its effects.
ETHANOL FUEL FROM CELLULOSE.
MICROBIOLOGY RESEARCH OPPORTUNITIES TO ADVANCE FOOD & AGRICULTURE
The previous lineup of microbiology-related problems confronting agriculture and food, followed by approaches to solving these problems, provides an empirical appreciation of the value of agriculture and food research. There have been attempts to measure this value. Estimates of the return on investment in agricultural research, based on a purely economic level, range from approximately 30-60%. This means that for every dollar invested in agricultural research, there is an annual net flow of return to society of 30 to 60 cents. However, many of the benefits of research in agriculture and food microbiology carry over into other important areas, such as public health and economic development. These returns have not yet been estimated.
PROGRESS THROUGH MULTIDISCIPLINARY RESEARCH
Food and agriculture microbiology research intersects with many other fields. This overlap is evident in the research opportunities associated with multidisciplinary research. For example, the need for improved detection technologies will be addressed by research that combines such diverse fields as microbiology, molecular biology, statistics, and engineering. Multidisciplinary research approaches will also lead the way in developing better tools to model the behavior of microbiological hazards, and successful application of massive amounts of biological information to the management of the living systems that comprise agriculture.
Assays and detectors used in surveillance for agricultural diseases and human pathogens in food have the potential to be improved as a result of several types of multidisciplinary research. One approach that is rapidly improving detection technology is the combination of microfluidic engineering and molecular biology. Devices arising from the marriage of these fields will be inexpensive to operate, portable, and rapid. The combination of genetically engineered microorganisms and optical devices is facilitating the creation of biosensors in which a living microbe is actually the interface that detects targeted microbes or toxins.
The ability to predict pathogen spread and persistence is being boosted by multidisciplinary research. Combining Global Information Systems (GIS) tools, mathematical modeling, and microbial physiology, it is now possible to simulate microbial behavior in the environment. This will enable integration of climate information and biology to predict or track microbial dispersion or viability upon release into the environment. Improving predictive capability is the key to predicting, recognizing, and containing outbreaks and enabling intervention efforts to be properly and efficiently applied in a timely manner.
Application of massive computing power to the handling and analysis of biological information has expanded our understanding of many biological systems and processes in ways not imagined previously. Computational analysis of genetic, protein, and metabolic data has spawned new approaches to studying complex, networked events that take place within living organisms to give rise to particular phenotypes. Genomics, proteomics, and metabolomics rely on microscopic handling of molecular samples (e.g., microarrays) to generate enormous data sets that measure the state of genes, proteins, or metabolic products in an organism. As computing advances allow processing of ever increasing amounts of data and nanotechnology improves the ability to precisely handle and detect molecular samples, genomic, proteomic, and metabolomic studies will become increasingly effective. These kinds of analyses will enable discoveries that can advance agricultural efficiency and food quality and safety. The power of these analyses is currently expanding to enable study of the interactions between diverse microbial species and the interactions between microbes and their environments.
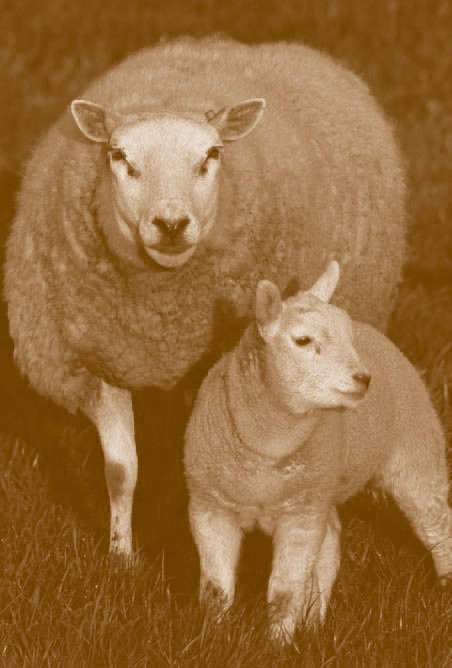
MULTI-ORGANISM BIOLOGY – INTERACTIONS BETWEEN HOSTS, PATHOGENS & MUTUALISTS
An exciting and relevant area in food and agriculture microbiology is the study of disease and infection. Agricultural productivity and food safety can be improved by disruption of pathogen inflicted disease in plants and animals. Traditional studies of pathogen virulence and host range continue to contribute to our understanding of disease processes. Breeding of disease resistance into plants and animals, for example, remains an important effort to continue. Creation of improved vaccines is another area where sustaining the pursuit of previously successful approaches can continue to reap benefits. Many vaccines could be improved by increasing their breadth of immunogenic activity, facilitating the ability to differentiate vaccinated animals from disease carriers, and increasing the duration of immunity that is conferred.
Beyond traditional methods of combating pathogens, research into the disease process itself and the role of innate host resistance will open new insights into the complex set of interactions between hosts and pathogens associated with food and agricultural systems. Budding areas of research, such as the use of immunomodulators to fortify host innate immunity against pathogens, will contribute to this end. Research is revealing that, in many cases, a critical part of the disease process occurs when pathogens disrupt immune responses in the host. As multi-organism genomic, proteomic, and metabolomic studies reveal precisely how pathogens attack a host, subsequent studies can investigate immunomodulation as a way to interfere with the infection process of specific pathogens.
Organisms that are participating in complex biological interactions represent a hugely underexploited pool of interventions to prevent disease through efforts such as immunological fortification, production of antibiotics/probiotics, and other mechanisms. Current investigation and knowledge of probiotics scratches the surface of this area of research, and even in this area, specific knowledge is lacking about what interactions occur between microbes and the host and how those interactions can be capitalized upon to prevent disease. For example, beneficial organisms may contribute to the prevention of disease by producing substances that interfere with successful colonization or infection by a pathogen in a host, and/or the beneficial organisms may exclude pathogens by competing for resources while not damaging the host. Past methodological restraints have limited our ability to understand complex host-microbe interactions. However, functional genomics, proteomics, and metabolomic approaches can all be harnessed to answer basic science questions about these interactions. In so doing, probiotic approaches can be refined, providing public health benefits and enhancing the sustainability of agriculture. This is one area where agriculture may come face-to-face with human and animal clinical medicine.
Another related phenomenon where microorganisms protect against pathogens is biological control. Many microorganisms can actively antagonize or kill the organisms that damage or cause disease in our agricultural crops and animals. A famous example is the soil bacterium Bacillus thuringiensis (Bt) that produces insect-killing toxins. Microbiology research has extracted a wide range of toxin specificities from different strains of Bt and enabled these toxins to be expressed directly in genetically modified crops, providing the plants with their own protective compounds. Continued research will undoubtedly produce more discoveries from this bio-control organism. Many other biological control options, such as fungi and viruses that are pathogenic to a wide variety of specific agricultural pests, are largely unexplored and may be exploited for protection of crops and animals. Genetic engineering of biocontrol organisms has promise for ensuring their effectiveness in targeted, large scale use against pests.
“RESEARCH IN MICROBIAL ECOLOGY WILL HELP TO DETERMINE HOW TO PRESERVE A BALANCE IN MICROBIAL COMMUNITIES THAT FAVORS AGRICULTURE.”
MICROBIAL ECOLOGY & HEALTHY AGRICULTURAL SYSTEMS
The role of beneficial organisms in promoting the health of agricultural plants and animals extends beyond combating pathogens. Research into how beneficial microorganisms can promote growth, improve stress tolerance, and aid in the uptake of nutrients are research areas ripe for discovery and innovation. Research into these complex and often delicate interactions between different organisms should ultimately pay off by revealing ways to assure that agriculture can become heartier and less environmentally taxing.
The same communities of microbes that benefit agricultural health and efficiency are likely to be disturbed by some of the practices of industrialized agriculture. One way to fortify agriculture against disease and stress is to supplement systems with probiotic and biocontrol organisms, but a complementary and sometimes alternative approach is to protect beneficial organisms that may already be present in the environment. Research in microbial ecology will help to determine how to preserve a balance in microbial communities that favors agriculture. Heavy pesticide and fertilizer use, in particular, are two practices that should be studied using a holistic or integrated approach to determine their impact on microbial ecology within the context of tradeoffs between risks and benefits. More knowledge in this area will help determine optimal tradeoffs such that the benefits of use outweigh the disruption caused by chemical inputs into our agricultural systems.
Microbial communities are both vulnerable to, and contribute to, removal of pollutants. Research into how multiple organisms work in partnership to degrade complex molecules is essential to increase options for dealing with the byproducts of industrialized agriculture. Understanding this aspect of microbial ecology may also lead to improvements in waste disposal and energy generation through fermentation, as well as bioremediation.
Organisms that cannot currently be cultivated in a laboratory setting are likely to be pivotal in advances in probiotics, biocontrol, and microbial ecology. Research efforts targeted on identification of these previously uncharacterized organisms, whether through new cultivation methods or by indirect detection approaches made possible by genomic knowledge, will strengthen our ability to use beneficial organisms effectively.
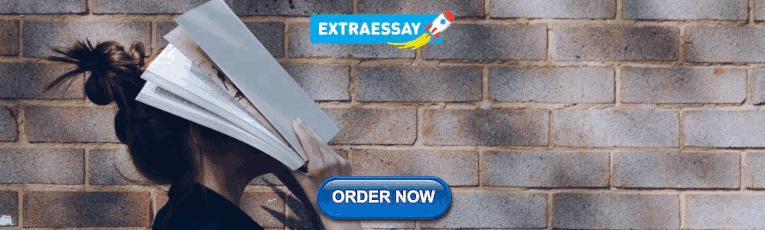
MAKING THE BEST USE OF AGRICULTURAL TECHNOLOGY
Research offers opportunities for understanding more completely the impacts of technologies that are already used in agriculture and food production. Questions remain concerning if genetically modified organisms interact differently in the environment as compared to their non-engineered counterparts. Whether or not there are negative impacts associated with the use of GMOs needs to be considered and balanced within the broader context of sustained use and potential benefits.
Increased attention to food hygiene, which has occurred in the developed world over the last century, has dramatically reduced incidence of foodborne infection. However the void of microbially-based immune stimulation has been proposed as a culprit in weakening immune systems in animals and people. Further research into this area will determine whether this is a real phenomenon and, if so, how to balance tradeoffs between a microbiologically safer food supply and maintaining a healthy immune system throughout life.
Food and agriculture microbiology research provides potential solutions to problems that cut across many fields. The scientific principles and the implications of food and agricultural research are increasingly linked to public health and the environment, topics that historically have received more public attention. For example, interventions against Avian Influenza, which has been devastating poultry production, may also prevent dissemination of another human influenza epidemic. Providing biologically-based alternatives for protection of crops and animals against pathogens will help reduce pesticide and antibiotic use, both of which have public health and environmental implications.
OVERCOMING BARRIERS TO SUCCESSFUL AGRICULTURAL & FOOD MICROBIOLOGY RESEARCH
Despite the need for continued advances in agriculture and food microbiology, and the proven track record of agricultural research, research support for these fields over the last few decades has been lean and is, in fact, decreasing. Reversing the decline in funding and recognition of the value of agricultural research requires fundamental changes, in addition to an infusion of financial support. The major barriers to advancing agriculture and food research are institutional and perception based.
PUTTING FOOD & AGRICULTURE MICROBIOLOGY IN THE SPOTLIGHT
The profile and priority of agricultural research needs to be raised. Designated research centers of excellence, similar to those in the biomedical and defense arenas, would make strides in this respect. There are numerous institutions that provide a backbone of exceptional scientific work for U.S. agriculture, but their programs run in aging facilities and with limited financial resources. Within U.S. research institutions, agriculture is too often a subordinate priority. One way to reverse this would be to raise the institutional overhead rate that is currently allowed on USDA grants from its uniquely low level to a level on par with that provided by other funding agencies, such as the National Science Foundation (NSF) or the National Institutes of Health (NIH). With limited overhead capital, administrators and investigators are restricted in their efforts to build strong programs and recruit personnel to pursue state-of-the-art agricultural research. Increases in overall research expenditures for U.S. Department of Agriculture (USDA) programs would then be needed to maintain even the current level of direct funds for research.
A healthy agricultural research community depends on an influx of young scientific talent. Trouble recruiting and maintaining graduate students is impacting programs and will ultimately affect the field. Several measures can be taken to alleviate this problem. A program of prestigious and remunerative fellowships for graduate students and postdoctoral fellows would provide some needed recruiting leverage. Internships involving industry, non-governmental organizations (NGOs), and government agencies would have mutually beneficial value. Such internships would infuse awareness and technical knowledge of agricultural science to institutions and provide the visiting scientists with networking and training opportunities. The recent security-motivated tightening of immigration procedures has limited access of the U.S. scientific community to international talent. Making the U.S. more accessible to legitimate international students and scientists again would help all scientific endeavors, including invigorating the base for revival of agricultural science.
Funding for agriculture and food research is essential to fulfilling any of the potential benefits that have been proposed. Because of the shallow profit margin in agriculture and food, it is to be expected that industry/commodity funding for research in these areas will be minimal, and when it does occur, it is usually focused on short-term payoffs. Such sources of funding traditionally have provided no indirect costs, further perpetuating the declining research facility infrastructure. Therefore, basic research on high priority agriculture and food problems is deeply dependent on government sources to provide sufficient funding. Currently the National Research Initiative of the USDA explicitly favors “translational research,” i.e., those projects with the promise of providing near-term, technological products or advances. By nature, many agriculture and food research endeavors are long-term, but competitive funding for long-term projects is more or less unavailable. An expansion of research priorities to emphasize basic and long-term research and dedicated funding to back this expansion will help revive agricultural research.
“ LONG-TERM AGRICULTURAL RESEARCH PROJECTS ARE THE ONLY WAY TO OBTAIN SCIENTIFIC ANSWERS.”
RESEARCH PRIORITIES WITH REACH
Long-term agricultural research projects are the only way to obtain scientific answers to many questions in areas such as microbial ecology and epidemiology. Unfortunately, the formula-funded land grant university system once could support such long-term research, but funds have diminished to the point that this is no longer possible, even though the land facilities are still there under university ownership. One mechanism for overcoming barriers to long-term research in agriculture would be through projects like the Long-Term Ecological Research (LTER) stations. Sustained study of particular agriculture and food science problems is also hampered by certain institutionalized incentive structures. Tenure evaluation procedures draw heavily on publication records of individuals, but long-term projects may be incomplete and unpublished by the time of tenure or promotion review. It is also the case that research grants generally demand a structure that promises completion of a project within two or three, and at most, five years. Allowing for longer project duration, including sustained funding, and evaluation of productivity based on alternative measures, would make long-term research a more viable scientific pursuit.
Collections of microbial specimens are an essential asset to agriculture and food microbiology research. Availability of specimens for initiation and verification of research can be assured only if the resources and expertise are maintained to properly curate collections of microbes. In the same way that institutions and funding agencies determine the viability of long-term research, their commitment of resources and recognition to the maintenance of microbe collections will determine whether this asset is sustained or lost.
With multidisciplinary research at the center of many needed advances in food and agriculture research, some of the disincentives to this type of work should be lifted. Institutions frequently fail to recognize the effort required to coordinate across different research groups and disciplines to make a project run successfully. The many-author publications that result from this type of work are also not as highly regarded as ones where credit is less widely distributed or—in a view that is not necessarily justified—less diluted.
The research community is also responsible for some barriers to more successful food and agriculture science. Compartmentalization between fields of research and industrial practice prevent effective cross-fertilization and sharing of lessons learned. Divisions also stand in the way of greater multidisciplinary efforts. Research opportunities will expand as scientists become more aware and more engaged across fields dealing with microbiology, veterinary and human medicine, plant diseases (pathology), epidemiology, statistics and mathematical modeling, environmental sciences, and engineering, to name a few.
PAVING THE WAY FOR ESSENTIAL RESEARCH
Various regulations present obstacles to research because they are inappropriately stringent or lacking in discrimination. For example, listing certain pathogen species as “select agents” dramatically increases the expense and legal liability of working with these microorganisms. In many cases, agents that are on the select agent lists are already prevalent in the environment, and their study in a laboratory represents no extraordinary safety or security risk. For example, soybean rust recently reached and spread throughout the southeastern United States, creating the potential to cause havoc with growers in the current growing season. But because of the pathogen's select agent rating before its arrival into the country, there was a scarcity of research at a time when studies of the pathogen were urgently needed. Fortunately, the action by USDA to remove it from the select agent list will permit a greater number of researchers to work with the organism and monitor its progress across the soybean fields of the country. Regulations that are more science-based and flexible will allow essential scientific investigation to proceed. For the cases for which the select agent regulation remains sensible, it is important that funding programs allow investigators to budget for the biosafety and security measures that are legally required to work with these organisms. These requirements are becoming necessary for many non-select agent plant pathogens as well and must be met for basic research to continue.
Basic research is stifled by a number of forces in the research funding arena. The push for applied results in a short timescale as seen in the USDA's National Research Initiative is one such force. While understandable that agencies are under pressure to demonstrate an immediate payoff from research supported by taxpayers, this ultimately drains the scientific foundation of innovation. If every grant is charged with generating specific, applied solutions to problems that are most politically compelling at the time, there will not be time or resources for the occasional unexpected but remarkable discovery. This phenomenon is currently at its worst in the area of biodefense research. In fact, biological research funding programs are currently skewed in favor of supporting proposals with a biodefense spin, and the agricultural arena is no exception.
The product-oriented leaning of research funding programs also spills over to discourage proposals that are not stoked with preliminary data. This puts innovative but perhaps “high-risk” proposals at a funding disadvantage because they are seen as exploratory or speculative rather than hypothesis-driven. It also puts younger investigators at a disadvantage.
FOOD & AGRICULTURE ADVOCACY
Successful institution of the changes necessary to revitalize food and agriculture research must be influenced by various players. Consumers, commodity groups, and legislators need to be persuaded of the importance of food and agriculture microbiology research, particularly basic research. Organizations whose members are part of this research community include the American Society for Microbiology, the American Phytopathological Society, the Institute of Food Technologists, the Council on Agricultural Science and Technology, and the National Association of State Universities and Land-Grant Colleges.
More successful communication of the benefits of food and agricultural microbiological research is critical. Interested organizations and academic institutions can contribute by training some of their scientists in speaking to and writing for the lay public. They can further contribute by training educators of non-scientists to be able to convey basic scientific literacy. As scientific information can reach and be understood by the public, facts become more likely to be used for decision making. This will be of benefit to many arenas, including those that impact food quality and safety, public health, and environmental protection. It will also encourage the public to be a more informed participant in decisions regarding food and agriculture technology, rather than being either complacent or frenzied by rumor and conjecture.
Building awareness of the critical value of a broad, basic research agenda in food and agriculture microbiology is an important step to overcoming obstacles that have in fact hindered the progress of these disciplines.
- RECOMMENDATIONS
RESEARCH AGENDA
- Study the impact of production and processing practices on microorganism evolution, persistence, and antibiotic/pesticide resistance as they affect agricultural animals, plants, and environments.
- Apply systems biology approaches to understanding communities of microorganisms within agricultural hosts, food matrices, and production/processing environments.
- Develop more sophisticated understanding of the nature, specificity and adaptation of microorganisms to food environments, hosts (human/animal/plant), and host responses to both pathogenic and beneficial microbes.
- Use a comparative pathobiology approach to understand the importance of pathogens that cross from animals or plants to humans and what characteristics enable their pathogenicity to multiple hosts.
- Develop microbial technologies that can be applied in agricultural contexts for reduction of inputs, bioremediation of pollution, conversion of biomass, and converting wastes to fuel.
- Pursue multidisciplinary strategies for developing knowledge and technologies to solve food and agriculture problems.
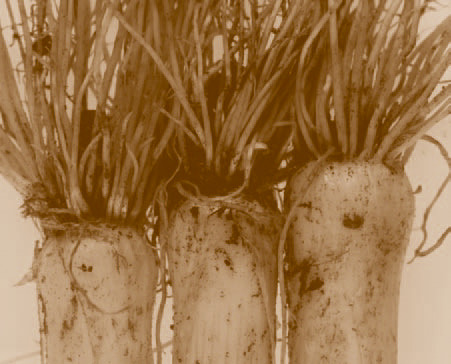
REBUILDING THE FOUNDATION FOR TECHNOLOGY USE & RESEARCH
- Coordinate development and standardize use of diagnostic tests across agricultural production, food processing, and public health systems to provide a foundation for integrated surveillance systems.
- Provide, through integrated educational initiatives, scientifically trained professionals who will serve the food and agricultural communities.
- Facilitate implementation of systems approaches, long-term projects, and multidisciplinary research in food and agricultural microbiology.
REFERENCES & BIBLIOGRAPHY
- Fiehn, O. 2001. Combining genomics, metabolome analyis, and biochemical modelling to understand metabolic networks. Comparative and Functional Genomics. 2:155–168. [ PMC free article : PMC2447208 ] [ PubMed : 18628911 ]
- Harmon, PF, MT Momol, JJ Marois, H Dankers, and CL Harmon. 2005. Asian soybean rust caused by Phakopsora pachyrhizi on soybean and kudzu in Florida. Online. Plant Health Progress doi: 10 .1094/PHP-2005-0613-01-RS .
- Institute of Food Technologists. 2002. Emerging microbiological food safety issues: implications for control in the 21 st century.
- National Research Council. 2000. National Research Initiative: A Vital Competitive Grants Program in Food, Fiber, and Natural-Resources Research. National Academy Press, Washington, DC. [ PubMed : 25032473 ]
- Schumann, GL. 1991. Plant Diseases: Their Biology and Social Impact. American Phytopathologial Society, St. Paul, MN.
- http://www .dh.gov.uk /PublicationsAndStatistics /PressReleases /PressReleasesNotices /fs/en?CONTENT_ID=4112474&chk =tbjtJp
- http://www .fsis.usda .gov/Fact_Sheets/Bovine _Spongiform_Encephalopathy_BSE/index .asp
- http://www .plantmanagementnetwork .org/infocenter /topic/soybeanrust/
This work is licensed under a Creative Commons Attribution-NonCommercial-NoDerivatives 4.0 International License .
- Cite this Page Research Opportunities in Food & Agriculture Microbiology: This report is based on a colloquium, sponsored by the American Academy of Microbiology, held March 12-14, 2005, in Washington, DC. Washington (DC): American Society for Microbiology; 2005. doi: 10.1128/AAMCol.12Mar.2005
- PDF version of this title (1004K)
In this Page
- MICROBIOLOGICAL CHALLENGES TO FOOD & AGRICULTURE
- MEETING CHALLENGES WITH MICROBIOLOGICAL SCIENCE & TECHNOLOGY
- MICROBIOLOGY RESEARCH OPPORTUNITIES TO ADVANCE FOOD & AGRICULTURE
- OVERCOMING BARRIERS TO SUCCESSFUL AGRICULTURAL & FOOD MICROBIOLOGY RESEARCH
- REFERENCES & BIBLIOGRAPHY
Other titles in this collection
- American Academy of Microbiology Colloquia Reports
Related information
- NLM Catalog Related NLM Catalog Entries
Similar articles in PubMed
- Review The Global Genome Question: Microbes as the Key to Understanding Evolution and Ecology: This report is based on a colloquium, “The Global Genome Question: Microbes as the Key to Understanding Evolution and Ecology,” sponsored by the American Academy of Microbiology and held October 11-13, 2002, in Longboat Key, Florida [ 2004] Review The Global Genome Question: Microbes as the Key to Understanding Evolution and Ecology: This report is based on a colloquium, “The Global Genome Question: Microbes as the Key to Understanding Evolution and Ecology,” sponsored by the American Academy of Microbiology and held October 11-13, 2002, in Longboat Key, Florida . 2004
- Review Microbial Forensics: A Scientific Assessment: This report is based on a colloquium sponsored by the American Academy of Microbiology held June 7-9, 2002, in Burlington, Vermont [ 2003] Review Microbial Forensics: A Scientific Assessment: This report is based on a colloquium sponsored by the American Academy of Microbiology held June 7-9, 2002, in Burlington, Vermont . 2003
- Review The Uncharted Microbial World: Microbes and Their Activities in the Environment: This report is based on a colloquium, sponsored by the American Academy of Microbiology, convened February 9-11, 2007, in Seattle, Washington [ 2008] Review The Uncharted Microbial World: Microbes and Their Activities in the Environment: This report is based on a colloquium, sponsored by the American Academy of Microbiology, convened February 9-11, 2007, in Seattle, Washington . 2008
- Review Preharvest Food Safety and Security: This report is based on a colloquium sponsored by the American Academy of Microbiology held December 5-7, 2003, in Perthshire, Scotland [ 2004] Review Preharvest Food Safety and Security: This report is based on a colloquium sponsored by the American Academy of Microbiology held December 5-7, 2003, in Perthshire, Scotland . 2004
- Review The Fungal Kingdom: diverse and essential roles in earth's ecosystem: This report is based on a colloquium, sponsored by the American Academy of Microbiology, convened November 2–4, 2007 in Tucson, Arizona [ 2008] Review The Fungal Kingdom: diverse and essential roles in earth's ecosystem: This report is based on a colloquium, sponsored by the American Academy of Microbiology, convened November 2–4, 2007 in Tucson, Arizona Buckley M. 2008
Recent Activity
- Research Opportunities in Food & Agriculture Microbiology Research Opportunities in Food & Agriculture Microbiology
Your browsing activity is empty.
Activity recording is turned off.
Turn recording back on
Connect with NLM
National Library of Medicine 8600 Rockville Pike Bethesda, MD 20894
Web Policies FOIA HHS Vulnerability Disclosure
Help Accessibility Careers
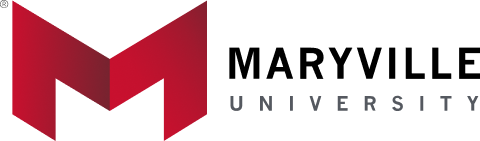
- Bachelor’s Degrees
- Master’s Degrees
- Doctorate Degrees
- Certificate Programs
- Nursing Degrees
- Cybersecurity
- Human Services
- Science & Mathematics
- Communication
- Liberal Arts
- Social Sciences
- Computer Science
- Admissions Overview
- Tuition and Financial Aid
- Incoming Freshman and Graduate Students
- Transfer Students
- Military Students
- International Students
- Early Access Program
- About Maryville
- Our Faculty
- Our Approach
- Our History
- Accreditation
- Tales of the Brave
- Student Support Overview
- Online Learning Tools
- Infographics
Home / Blog
Why Is Agriculture Important? Benefits and Its Role
July 12, 2022
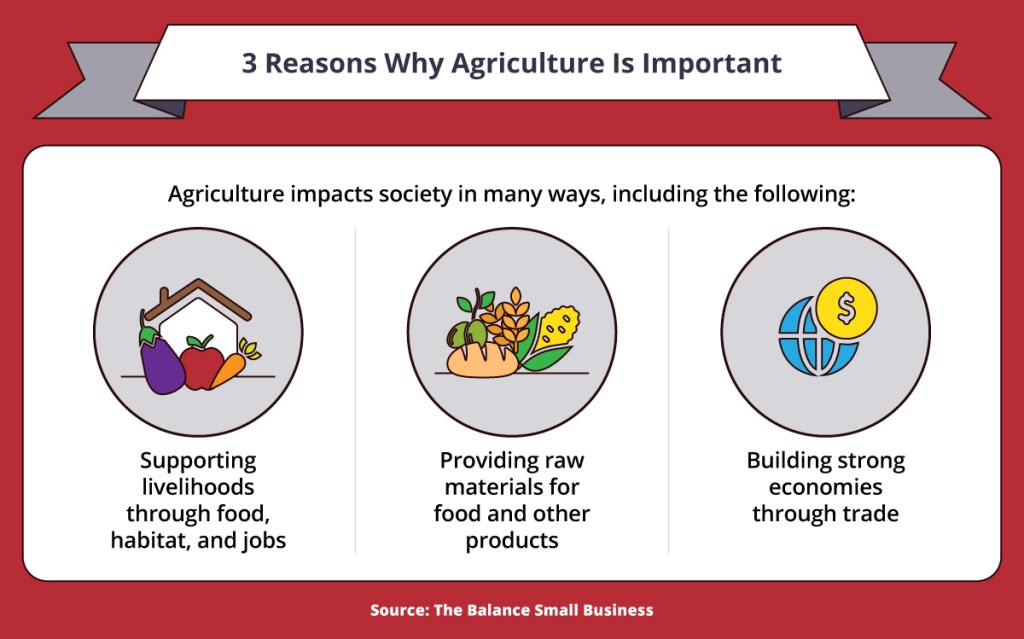
Tables of Contents
What Is Agriculture?
Why is agriculture important, how is agriculture important, importance of agriculture in everyday life, how does agriculture affect the economy, importance of agricultural biodiversity, why is agriculture important for the future.
When people think of agriculture, they often envision crop farming: soil and land preparation and sowing, fertilizing, irrigating, and harvesting different types of plants and vegetation.
However, according to the U.S. Census Bureau’s North American Industry Classification System (NAICS) , crop farming is just one element of the Agriculture, Forestry, Fishing, and Hunting sector. Agriculture also encompasses raising livestock; industrial forestry and fishing; and agricultural support services, such as agricultural equipment repair and trucking operations.
Why is agriculture important? It helps sustain life by providing the food we need to survive. It also contributes $7 trillion to the U.S. economy. Despite agriculture’s importance, the Economic Policy Institute reports that farmworkers are among the lowest-paid workers in the U.S.
However, agriculture also provides opportunities for economic equity and helps people prosper around the world. For example, since 2000, the agricultural growth rate in Sub-Saharan Africa has surpassed that of any other region in the world (approximately 4.3% annually), contributing to the region’s economic gains, according to the United States Agency for International Development (USAID). While there’s been a global decline in agricultural jobs — from 1 billion in 2000 to 883 million in 2019, according to employment indicators from the Food and Agriculture Organization of the United Nations — agriculture remains the second-highest source of employment (26.7% of total work).
Study the impact of access to natural resources, such as water, air, plants and animals
The online BS in sustainability from Maryville University will prepare you to build a better, more sustainable future. Accelerate your degree completion with our transfer credit-friendly program. No SAT or ACT scores required.
- Engage in a range of relevant course topics, from biology to business statistics.
- Learn how human health is connected to the environment through environmental science and health courses.
Agriculture is the practice of cultivating natural resources to sustain human life and provide economic gain. It combines the creativity, imagination, and skill involved in planting crops and raising animals with modern production methods and new technologies.
Agriculture is also a business that provides the global economy with commodities: basic goods used in commerce, such as grain, livestock, dairy, fiber, and raw materials for fuel. For example, fiber is a top crop in U.S. agricultural production , according to The Balance Small Business, and a necessary commodity for the clothing sector.
Back To Top
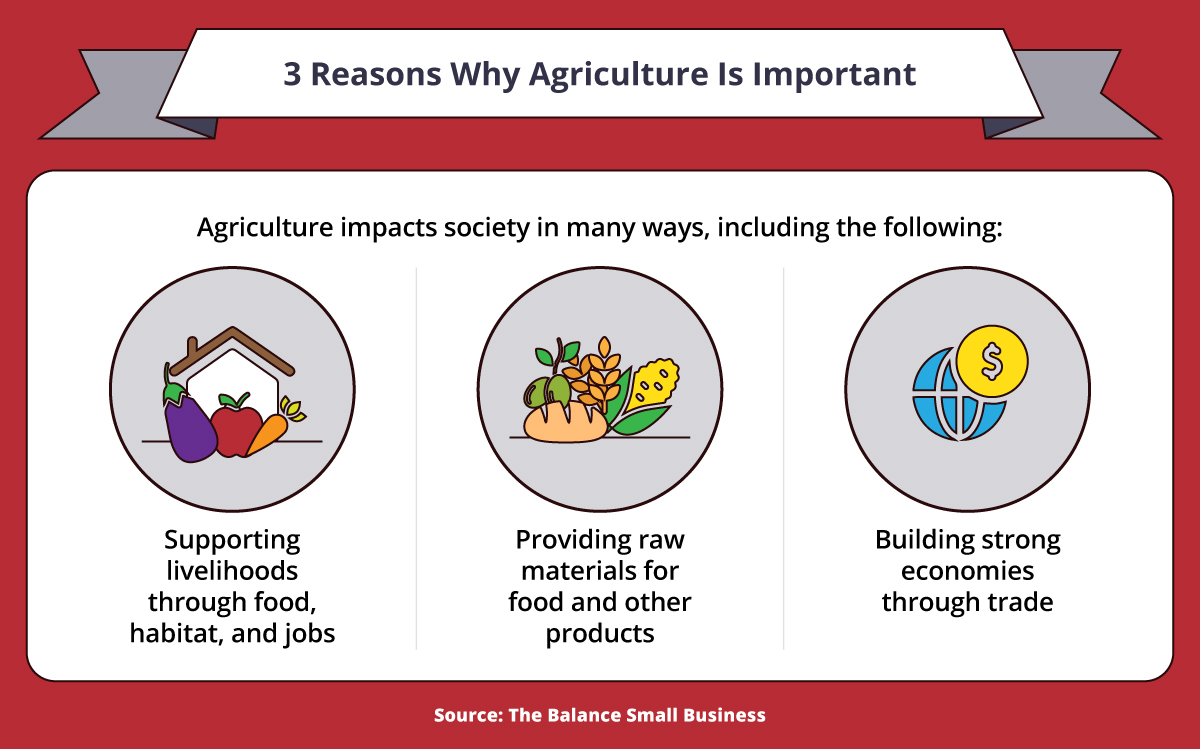
Agriculture impacts society in many ways, including: supporting livelihoods through food, habitat, and jobs; providing raw materials for food and other products; and building strong economies through trade. Source: The Balance Small Business.
A key to why agriculture is important to business and society is its output — from producing raw materials to contributing to the global supply chain and economic development.
Providing Raw Materials
Raw materials are a core building block of the global economy. Without access to raw materials, manufacturers can’t make products. Nonagricultural raw materials include steel, minerals, and coal. However, many raw materials derive from agriculture — from lumber for construction materials to herbs for adding flavor to food. Corn, for example, is used to produce foods and serves as a foundation for ethanol, a type of fuel. Another example is resins : plant products used in various industrial applications, such as adhesives, coatings, and paints used in construction.
Creating a Strong Supply Chain
Importing and exporting goods such as agricultural products requires shipping methods such as ocean freight, rail, and trucking. Delays in shipping agricultural products from a Los Angeles port can create problems in China, and vice versa, impacting the global supply chain.
For example, sales of soybean crops from Iowa skyrocketed in 2021 due to various factors including delays in South American crop shipments, according to the Iowa Soybean Association. In this example, Iowa benefited from a competitive standpoint. However, delays in shipping crops could also be detrimental to regions expecting shipment, limiting availability of products on store shelves and affecting livelihoods.
Encouraging Economic Development
Agriculture impacts global trade because it’s tied to other sectors of the economy, supporting job creation and encouraging economic development. Countries with strong agricultural sectors experience employment growth in other sectors, according to USAID. Countries with agricultural productivity growth and robust agriculture infrastructure also have higher per capita incomes, since producers in these countries innovate through technology and farm management practices to boost agricultural productivity and profitability.
Resources on the Importance of Agriculture
The following resources provide information about the importance of agriculture as a source of raw materials and its impact on transportation and contribution to economic development:
- American Farm Bureau Federation, Fast Facts About Agriculture & Food : Provides various statistics demonstrating why agriculture is important.
- The Western Producer, “Suddenly Agriculture Is Important ”: Highlights agriculture’s role as a stable commodity provider even amid disruption.
- LinkedIn, “What Is Agriculture and Its Importance? ”: Discusses the importance of agriculture in 10 areas.
When global supply chains are disrupted , considerable attention is given to the technology sector. For example, the lack of computer chips — made from silicon, a nonagricultural raw material — limits a manufacturer’s ability to make computers, cars, and other products. This impacts many areas of society and business.
Agriculture also plays a central role in meeting consumer and business market demand in a world with interconnected economies. Here are different types of products derived from agriculture.
Fruits and Vegetables
Fruits and vegetables are essential sources of fiber, proteins, and carbohydrates in human diets. Vitamins, such as A, C, and E, and minerals, such as magnesium, zinc, and phosphorus, are naturally occurring in many fruits and vegetables. In addition to health benefits, fruits and vegetables add flavors to the human palette.
Animal Feed
Some fruits and vegetables are grown to provide feed for animals, from poultry to livestock. The American Industry Feed Association reports that about 900 animal feed ingredients are approved by law in the U.S. These include ingredients that come from agricultural production, including hay, straw, oils, sprouted grains, and legumes.
Natural Rubber Production
The number of vehicles in the world is more than 1.4 billion, according to Hedges & Company market research. Every single one runs on rubber tires. According to GEP, the top rubber-producing countries are Thailand, Indonesia, and Malaysia — collectively representing approximately 70% of global natural rubber production — and about 90% of suppliers are small-scale farmers.
Cotton for Clothing
From cotton to clothes, the journey starts with agricultural production. Cotton is grown, harvested, and then processed, spun, and woven into fabric before it becomes a piece of clothing. Cotton production encompasses an expansive global supply chain, and according to Forum for the Future , it’s a leading commodity, making up approximately 31% of all textile fibers globally.
The U.S. Environmental Protection Agency (EPA) reports favorable economics of biofuels , produced from biomass sources including agricultural products such as corn, soybeans, sugarcane, and algae. The benefits include reduced greenhouse gas and pollutant emissions and the potential for increased incomes for farmers. However, biodiesel production requires the use of land and water resources that can affect food costs.
Industrial Products
Bio-based chemistry involves using raw materials derived from biomass to develop industrial products. Different industrial products derived from bio-based chemicals include bioplastics, plant oils, biolubricants, inks, dyes, detergents, and fertilizers. Bio-based chemicals and products offer an alternative to conventional products derived from petroleum products. Bio-based chemistry is considered a type of green chemistry because it promotes the reduction of environmental impacts in industrial production.
Pharmaceutical Products
For thousands of years, humans have turned to plants to help treat what ails them. For example, ginger, a plant root typically consumed in tea, can help aid digestion. Substances derived from plants and herbs can also help in healthcare. For example, extracted chemicals from the foxglove plant are used for digoxin, a drug used for heart failure. Another example is polylactic acid (PLA), a chemical produced when glucose is fermented into lactic acid in green plants. PLA has applications in tissue engineering, cardiovascular implants, orthopedic interventions, cancer therapy, and fabrication of surgical implants, according to a study published in Engineered Regeneration .
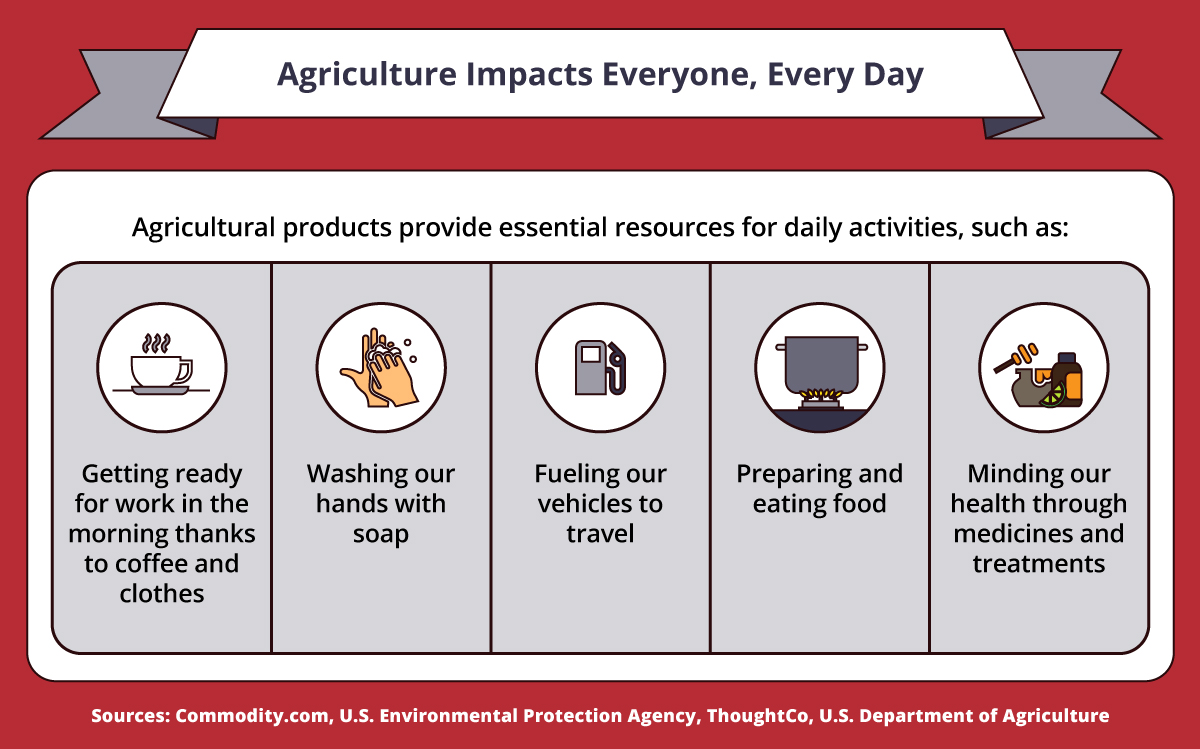
Agricultural products provide essential resources for daily activities, such as: getting ready for work in the morning, thanks to coffee and clothes; washing hands with soap; fueling vehicles to travel; preparing and eating food; and minding health through medicines and treatments. Sources: Commodity.com, the U.S. Environmental Protection Agency, ThoughtCo, and the U.S. Department of Agriculture.
For thousands of years, agriculture has played an important role in everyday life. Before agriculture, hunting and gathering enabled humans to survive. It wasn’t until the transition to the planned sowing and harvesting of crops that humans began to thrive. Humans developed tools and practices to improve agricultural output with more efficient means of sustaining themselves. From there, innovations that created industries led to the modern era.
Today, the importance of agriculture in everyday life can’t be minimized. Without the agriculture sector, activities such as getting dressed for work and cleaning the home wouldn’t be possible. Here are examples of the agricultural products we use in our everyday lives:
- Shelter . Wood and plant-based materials, such as bamboo, can be used for indoor décor and construction materials.
- Morning routine. Mint is often an ingredient in toothpaste, adding flavor while brushing your teeth, and the caffeine in coffee that keeps you awake is derived from the coffee bean.
- Dressing up. In addition to cotton, clothing can be manufactured from hemp, ramie, and flax. Bio-based materials can be used to produce grooming products such as skin creams and shampoos.
- Cleaning. Two types of chemicals used in detergents, cleaning products, and bath or hand soap — surfactants and solvents — can be produced from biomass.
- Driving to work. Plants make it possible to get to and from work. Think of rubber (sourced from rubber trees) and biodiesel fuel, which often includes ethanol (sourced from corn).
- Entertainment. Paper from trees enables you to write, and some musical instruments, such as reed instruments, require materials made from plants.
- Education. From pencils (still often made of wood) to paper textbooks, students rely on agricultural products every day.
Agriculture can have a significant effect on the economy. The U.S. Department of Agriculture (USDA) Economic Research Service reports that agricultural and food sectors provided 10% of all U.S. employment in 2020 — nearly 20 million full- and part-time jobs. Additionally, the USDA reported that cash receipts from crops totaled nearly $198 billion in 2020. Animal and animal product receipts weren’t far behind in 2020, totaling $165 billion.
The interdependence of the food and agriculture sector with other sectors, including water and wastewater systems, transportation systems, energy, and chemical, makes it a critical engine for economic activity, according to the Cybersecurity and Infrastructure Security Agency (CISA).
Agriculture also impacts economic development by contributing to the overall U.S. gross domestic product (GDP), directly and indirectly. It does so through farm production, forestry, fishing activities, textile mills and products, apparel and food and beverage sales, and service and manufacturing.
- Farm production. The latest USDA data on farming and farming income report the U.S. had a little over 2 million farms, encompassing 897 million acres, in 2020. Farm production includes producing fruits, vegetables, plants, and varieties of crops to meet demand for agricultural products throughout the country and abroad.
- Forestry and fishing activities. Agricultural activities include forestry and harvesting fish in water farms or in their natural habitat. Agroforestry is focused on “establishing, managing, using, and conserving forests, trees and associated resources in a sustainable manner to meet desired goals, needs, and values,” according to the USDA. A form of fishing activity known as aquaculture involves the production of fish and other sea animals under controlled conditions to provide food.
- Textile mills and products. The S. cotton industry produces $21 billion in products and services annually, according to the USDA. The industry has created various employment roles, such as growers, ginners, and buyers working on farms and in textile mills, cotton gins, offices, and warehouses.
- Apparel and food and beverage sales. Since agriculture is a business, selling products made from agricultural production is essential. A key aspect of the sales component in agriculture is to help growers build capacity and understand the market dynamics to meet the needs of customers, many of whom care deeply about Food services and eating and drinking places accounted for 10.5 million jobs in 2020, the largest share among all categories within the agriculture and food sectors, according to the USDA.
- Manufacturing. Agricultural products contribute to the manufacturing of a huge variety of goods, including food and beverage products, textiles, cleaning and personal products, construction materials, fuels, and more. According to the USDA, food and beverage manufacturing companies employ about 1.7 million people in the U.S.
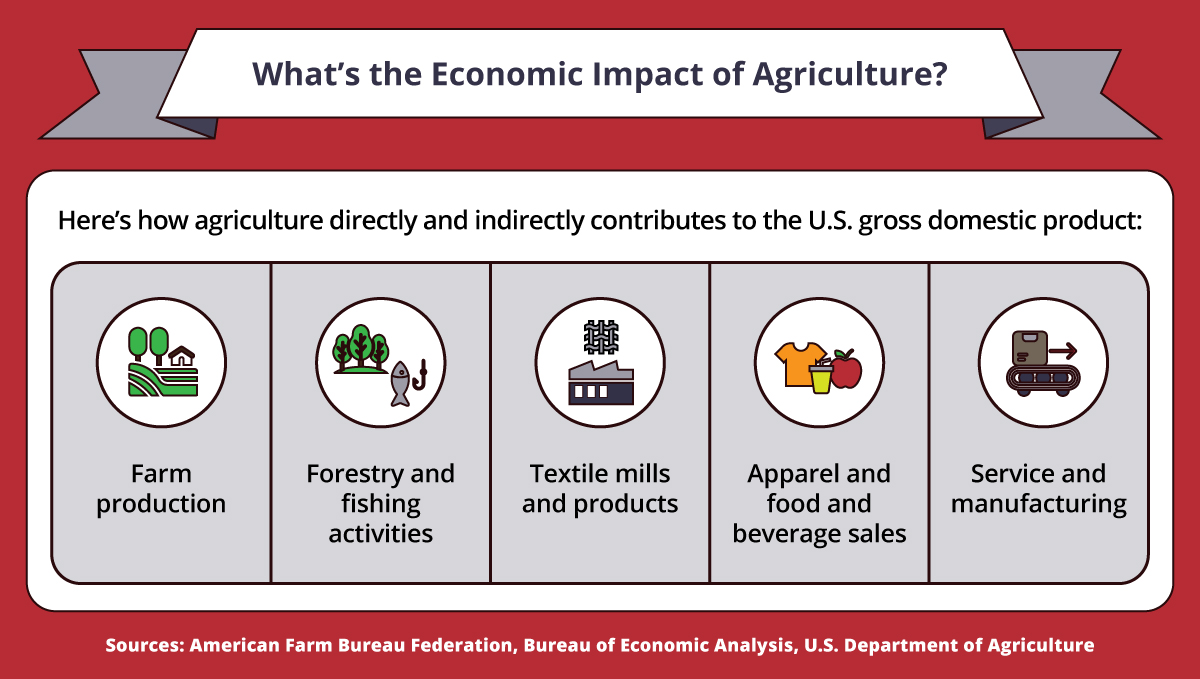
Here’s how agriculture directly and indirectly contributes to the U.S. gross domestic product: farm production, forestry and fishing activities, textile mills and products, apparel and food and beverage sales, and service and manufacturing. Sources: American Farm Bureau Federation, the Bureau of Economic Analysis, and the USDA.
Here are ways agriculture and related industries impact economic development:
Agribusiness
Agribusiness consists of the companies that perform the commercial activities involved in getting agricultural goods to market. It includes all types of businesses in the food sector, from small family farms to global agricultural conglomerates. In the U.S., farms contributed about $136 billion to GDP (about 0.6% of total GDP) in 2019, according to the USDA.
However, farms are just one component of agribusiness. Agribusiness also includes businesses involved in manufacturing agricultural equipment (such as tractors) and chemical-based products (like fertilizers) and companies involved in the production and refinement of biofuels. USDA data reports that in total, farms and related industries contributed more than $1.1 trillion to GDP, a little over 5% of the GDP, in 2019.
The economics of agribusiness also entails building production systems and supply chains that help maintain a country’s economic and social stability. Through the development of organizational and technological knowledge, agribusiness plays a vital role in protecting the environment and biodiversity near farms and using natural resources sustainably.
Food Security
Food security is central to the agricultural industry: Sustainable agriculture is a key to fulfilling the United Nations’s Sustainable Development Goals (SDGs), including SDG 2 : Zero Hunger . In addition to food security, the agricultural sector raises the incomes among the poorest communities up to four times more effectively than other sectors, according to the World Bank.
Job Creation
Throughout the world, agriculture plays an important role in job creation. For example, agriculture accounts for 25% of exports in developing countries in Latin America, about 5% of their regional GDP, according to a report about the importance of agribusiness from BBVA, a corporate and investment bank. This activity is a source of economic activity and jobs in these countries. In the U.S., agriculture and related industries provide 19.7 million full- and part-time jobs, about 10.3% of all employment.
Resources on the Economic Impact of Agriculture
The following resources highlight agriculture’s impact on the economy, from how disruption affects the business and the benefits of the sector to people’s livelihoods:
- Economic Research Service, Farming and Farm Income : Provides an overview of trends in farming and economic development statistics.
- American Journal of Agricultural Economics, “The Importance of Agriculture in the Economy: Impacts from COVID-19” : Highlights why agriculture is important based on the impact of COVID-19’s disruptions to the sector.
- Canadian Journal of Agricultural Economics, “Agriculture, Transportation, and the COVID-19 Crisis” : Discusses how transportation services that COVID-19 has disrupted can impact agricultural supply chains.
Advanced farming equipment and the increased use of fertilizers and pesticides have resulted in higher crop yields. At the same time, they’ve impacted the environment, contributing to soil and water pollution and climate change. NASA projects a 24% decline in corn crop yields by 2030, thanks to climate change. However, ensuring a healthy biodiversity can help mitigate the impact. Here are some factors to consider:
- Sustainable agriculture. Through sustainable agricultural practices , farmers and ranchers help ensure the profitability of their land while improving soil fertility, helping promote sound environmental practices, and minimizing environmental impacts through climate action .
- Climate change regulation. The agricultural sector produced about 10% of U.S. greenhouse gas emissions in 2019, according to the EPA. Regulation and policy changes can help promote sustainable practices in the sector and provide guidance on agricultural adaptation to address the challenges that climate change poses.
- Agriculture technology and innovation. From temperature- and moisture-sensing devices to GPS technologies for land surveys to robots, agriculture technology can result in higher crop yields, less chemical runoff, and lower impact on natural resources.
Agricultural Biodiversity Resources
Find information about agricultural biodiversity and its impacts in the following resources:
- Our World in Data, “Environmental Impacts of Food Production” : Discusses how sustainable agriculture offers a path to addressing food and nutrition issues.
- IBM, “The Benefits of Sustainable Agriculture and How We Get There” : Addresses how artificial intelligence (AI) and analytics technologies help farmers maximize food production and minimize their environmental impact.
- S. Environmental Protection Agency, The Sources and Solutions: Agriculture : Explains how agriculture can contribute to reducing nutrient pollution.
- FoodPrint, Biodiversity and Agriculture : Provides answers to what it will take to preserve the health of the planet to safeguard our own food supply.
- Brookings, “What Is the Future of Work in Agri-Food? ”: Discusses the future of agricultural automation and its impact on work.
Agriculture offers an opportunity to improve the lives of millions of food-insecure people and help countries develop economies that create jobs and raise incomes. Today’s agriculture also impacts future generations. To ensure the long-term success of the global agricultural sector, building a more sustainable economic system aligned with the U.N.’s Sustainable Development Goals is a crucial imperative to help create a more equitable society.
Infographic Sources
American Farm Bureau Federation, “Farm Contribution to Agricultural GDP at Record Low”
Bureau of Economic Analysis, “Gross Domestic Product (Third Estimate), Corporate Profits (Revised Estimate), and GDP by Industry, Second Quarter 2021”
Commodity.com, “Learn All About Agricultural Commodities and Market Trends”
Environmental Protection Agency, Commonly Consumed Food Commodities
The Balance Small Business, “What Is Agricultural Production?”
ThoughtCo, “List of Medicines Made From Plants”
USDA, Ag and Food Sectors and the Economy
USDA National Agricultural Library, Industrial, Energy, and Non-food Crops
Bring us your ambition and we’ll guide you along a personalized path to a quality education that’s designed to change your life.
Take Your Next Brave Step
Receive information about the benefits of our programs, the courses you'll take, and what you need to apply.
- Interesting
- Scholarships
- UGC-CARE Journals
45 Research Project Ideas in Agriculture – Innovative Approaches to Sustainable Farming
Explore 45 research project ideas in agriculture for sustainable farming.
Agriculture is a vast and dynamic field that plays a critical role in feeding the world’s population. As the global population continues to grow, the demand for food production is also increasing, making agriculture one of the most important sectors for ensuring food security and sustainable development. However, the challenges facing the agriculture industry today are numerous, ranging from climate change, soil degradation, water scarcity, and pest infestation to biodiversity loss and food waste.
To tackle these issues and promote sustainable agriculture, researchers and professionals in the field are continuously exploring new and innovative ways to improve agricultural practices, increase productivity, and reduce environmental impact. In this article, we will present 45 research project ideas in agriculture that can help address some of the most pressing issues facing the industry today.
These research projects cover a wide range of topics, from soil health and crop yields to livestock farming, aquaculture, and food systems, providing a comprehensive overview of the latest trends and innovations in agricultural research.
Whether you are a student, researcher, or professional in the field, these research project ideas can help guide your work and contribute to a more sustainable and resilient agriculture industry.
- Evaluating the effectiveness of natural pest control methods in agriculture.
- Investigating the effects of climate change on crop yields and food security.
- Studying the impact of soil quality on plant growth and crop yields.
- Analyzing the potential of precision agriculture techniques to increase yields and reduce costs.
- Assessing the feasibility of vertical farming as a sustainable solution to food production.
- Investigating the impact of sustainable agriculture practices on soil health and ecosystem services.
- Exploring the potential of agroforestry to improve soil fertility and crop yields.
- Developing strategies to mitigate the effects of drought on crop production.
- Analyzing the impact of irrigation management techniques on crop yields and water use efficiency.
- Studying the potential of biochar as a soil amendment to improve crop productivity.
- Investigating the effects of soil compaction on crop yields and soil health.
- Evaluating the impact of soil erosion on agriculture and ecosystem services.
- Developing integrated pest management strategies for organic agriculture.
- Assessing the potential of cover crops to improve soil health and reduce erosion.
- Studying the effects of biofertilizers on crop yields and soil health.
- Investigating the potential of phytoremediation to mitigate soil pollution in agriculture.
- Developing sustainable practices for livestock farming and manure management.
- Studying the effects of climate change on animal health and productivity.
- Analyzing the impact of animal feeding practices on meat quality and safety.
- Investigating the potential of aquaponics to increase food production and reduce environmental impact.
- Developing strategies to reduce food waste and loss in agriculture.
- Studying the effects of nutrient management practices on crop yields and environmental impact.
- Evaluating the potential of organic agriculture to improve soil health and reduce environmental impact.
- Investigating the effects of land use change on agriculture and biodiversity.
- Developing strategies to reduce greenhouse gas emissions from agriculture.
- Analyzing the impact of agricultural policies on food security and sustainability.
- Studying the potential of precision livestock farming to improve animal welfare and productivity.
- Investigating the impact of agrochemicals on soil health and biodiversity.
- Developing sustainable practices for fisheries and aquaculture.
- Studying the potential of bioremediation to mitigate pollution in aquaculture.
- Investigating the effects of climate change on fisheries and aquaculture.
- Developing strategies to reduce water pollution from agriculture and aquaculture.
- Studying the impact of land use change on water resources and aquatic ecosystems.
- Evaluating the potential of agroecology to promote sustainable agriculture and food systems.
- Investigating the impact of climate-smart agriculture practices on food security and resilience.
- Studying the potential of agrobiodiversity to improve crop productivity and resilience.
- Analyzing the impact of agricultural trade on food security and sustainability.
- Investigating the effects of urbanization on agriculture and food systems.
- Developing strategies to promote gender equity in agriculture and food systems.
- Studying the potential of agroforestry to promote biodiversity and ecosystem services.
- Analyzing the impact of food systems on public health and nutrition.
- Investigating the effects of climate change on pollination and crop yields.
- Developing strategies to promote agrotourism and rural development.
- Studying the potential of agroforestry to promote carbon sequestration and mitigate climate change.
- Analyzing the impact of agricultural subsidies on food security and sustainability.
I hope this article would help you to know the new project topics and research ideas in Agricultural.
- agriculture research
- crop yields
- food systems
- livestock farming
- Project Topics
- Research Ideas
- soil health
- sustainable farming
How to Write a Research Paper? A Complete Guide
14 websites to download research paper for free – 2024, 42 digital signal processing project ideas to explore, most popular, phd in india 2024 – cost, duration, and eligibility for admission, 100 connective words for research paper writing, phd supervisors – unsung heroes of doctoral students, india-canada collaborative industrial r&d grant, call for mobility plus project proposal – india and the czech republic, effective tips on how to read research paper, iitm & birmingham – joint master program, anna’s archive – download research papers for free, fulbright-kalam climate fellowship: fostering us-india collaboration, fulbright specialist program 2024-25, best for you, 24 best free plagiarism checkers in 2024, what is phd, popular posts, how to check scopus indexed journals 2024, 480 ugc-care list of journals – science – 2024, popular category.
- POSTDOC 317
- Interesting 258
- Journals 234
- Fellowship 128
- Research Methodology 102
- All Scopus Indexed Journals 92
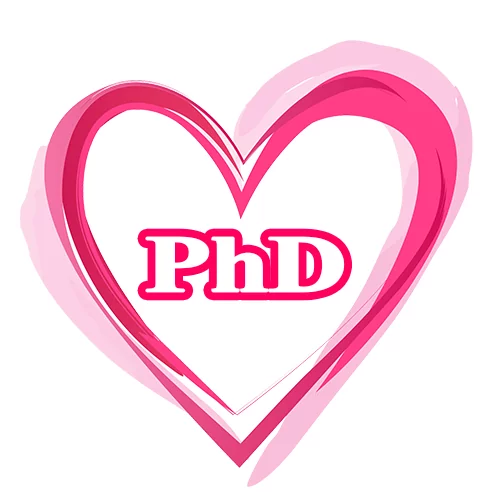
iLovePhD is a research education website to know updated research-related information. It helps researchers to find top journals for publishing research articles and get an easy manual for research tools. The main aim of this website is to help Ph.D. scholars who are working in various domains to get more valuable ideas to carry out their research. Learn the current groundbreaking research activities around the world, love the process of getting a Ph.D.
Contact us: [email protected]
Google News
Copyright © 2024 iLovePhD. All rights reserved
- Artificial intelligence

Advertisement
Cultivating Green Champions: The Role of High-Quality Farmer Training in Sustainable Agriculture
- Published: 20 May 2024
Cite this article
- Xiankai Lei 1 , 3 &
- Dongmei Yang 2
This study examines the transformative impact of cultivating high-quality farmers on the green production efficiency of agricultural practices in Henan Province, China. Utilizing a robust dataset from 2263 exemplary farmers and employing the Data Envelopment Analysis-Slack Based Measure (DEA-SBM) and Tobit models, we explore the nexus between farmer training programs and sustainable agricultural outcomes. Our findings underscore a statistically significant positive correlation between the cultivation of high-quality farmers and green production efficiency, highlighting the pivotal role of comprehensive training and skill development in enhancing sustainable farming practices. Further analysis reveals the nuanced effects of technological extension in amplifying the benefits of high-quality farmer cultivation on green production. This study contributes to the discourse on sustainable development within the agricultural sector by demonstrating how targeted educational initiatives and the promotion of advanced technologies can foster environmental stewardship and optimize resource utilization. The insights presented herein advocate for the scaling and customization of farmer training programs to meet the diverse needs of various farm types, promoting a knowledge-driven approach toward sustainable agriculture within the knowledge economy framework. Our research offers valuable implications for policymakers, agricultural stakeholders, and the broader academic community, stressing the importance of integrating knowledge, innovation, and technology in cultivating a resilient and sustainable agricultural landscape.
This is a preview of subscription content, log in via an institution to check access.
Access this article
Price includes VAT (Russian Federation)
Instant access to the full article PDF.
Rent this article via DeepDyve
Institutional subscriptions
Data Availability
The datasets used and/or analyzed during the current study are available from the corresponding author on reasonable request.
Achour, Y., Ouammi, A., & Zejli, D. (2021). Technological progresses in modern sustainable greenhouses cultivation as the path towards precision agriculture. Renewable and Sustainable Energy Reviews, 147 , 111251.
Article Google Scholar
Ali, A., Hussain, T., Tantashutikun, N., Hussain, N., & Cocetta, G. (2023). Application of smart techniques, internet of things and data mining for resource use efficient and sustainable crop production. Agriculture, 13 (2), 397.
Allioui, H., & Mourdi, Y. (2023). Exploring the full potentials of IoT for better financial growth and stability: A comprehensive survey. Sensors, 23 (19), 8015.
Barman, R. B. (2021). Sustaining high economic growth requires a different strategy: An integrated approach for broad-based knowledge economy. Strategic Management, Decision Theory, and Decision Science . https://doi.org/10.1007/978-981-16-1368-5_1
Baweja, P., Kumar, S., & Kumar, G. (2020). Fertilizers and pesticides: Their impact on soil health and environment. Soil Biology, 59 , 265–285. https://doi.org/10.1007/978-3-030-44364-1_15
Bui, T. L., Tran, H. C., Azadi, H., & Lebailly, P. (2018). Improving the technical efficiency of Sengcu rice producers through better financial management and sustainable farming practices in mountainous areas of Vietnam. Sustainability, 10 (7), 2279.
Cai, B., Shi, F., Huang, Y., & Abatechanie, M. (2021). The impact of agricultural socialized services to promote the farmland scale management behavior of smallholder farmers: Empirical evidence from the rice-growing region of southern China. Sustainability, 14 (1), 316.
Cao, Z., Meng, Q., & Gao, B. (2021). The consumption patterns and determining factors of rural household energy: A case study of Henan Province in China. Renewable and Sustainable Energy Reviews, 146 , 111142.
Castrignanò, A., Buttafuoco, G., Khosla, R., Mouazen, A., Moshou, D., & Naud, O. (Eds.). (2020). Agricultural internet of things and decision support for precision smart farming . Academic Press. Paperback ISBN: 9780128183731. eBook ISBN: 9780128183748
Chen, W. (2020). The transformation of commercialized rural service system with taking agricultural informatization as a breakthrough. China and Globalization 2.0 , 341–417. https://doi.org/10.1007/978-981-15-3536-9_6
Chen, T., & Lu, X. (2022). Factors affecting the adoption of green prevention and control techniques by family farms: Evidence from Henan province of China. Frontiers in Psychology, 13 , 1015802.
Chen, J., & Zhou, H. (2023). The role of contract farming in green smart agricultural technology. Sustainability, 15 (13), 10600.
Chiles, R. M., Broad, G., Gagnon, M., Negowetti, N., Glenna, L., Griffin, M., & A., ... & Beck, K. (2021). Democratizing ownership and participation in the 4th industrial revolution: Challenges and opportunities in cellular agriculture. Agriculture and Human Values, 38 (4), 943–961.
Cimino, C., Negri, E., & Fumagalli, L. (2019). Review of digital twin applications in manufacturing. Computers in Industry, 113 , 103130.
Clapp, J., Newell, P., & Brent, Z. W. (2018). The global political economy of climate change, agriculture and food systems. The Journal of Peasant Studies, 45 (1), 80–88.
Cramer, C., Di John, J., & Sender, J. (2022). Classification and roundabout production in high-value agriculture: A fresh approach to industrialization. Development and Change, 53 (3), 495–524.
Demestichas, K., & Daskalakis, E. (2020). Data lifecycle management in precision agriculture supported by information and communication technology. Agronomy, 10 (11), 1648.
Deng, Q., Li, E., & Zhang, P. (2020). Livelihood sustainability and dynamic mechanisms of rural households out of poverty: An empirical analysis of Hua County, Henan Province. China. Habitat International, 99 , 102160.
Dubey, A., & Lal, R. (2009). Carbon footprint and sustainability of agricultural production systems in Punjab, India, and Ohio, USA[J]. Journal of Crop Improvement, 23 (4), 332–350.
Edmondson, A. C., & Harvey, J. F. (2018). Cross-boundary teaming for innovation: Integrating research on teams and knowledge in organizations. Human Resource Management Review, 28 (4), 347–360.
Gao, Y., Zhao, D., Yu, L., & Yang, H. (2020). Influence of a new agricultural technology extension mode on farmers’ technology adoption behavior in China. Journal of Rural Studies, 76 , 173–183.
Gebresenbet, G., Bosona, T., Patterson, D., Persson, H., Fischer, B., & Mandaluniz, N., ... & Nasirahmadi, A. (2023). A concept for application of integrated digital technologies to enhance future smart agricultural systems. Smart Agricultural Technology, 5 , 100255.
Giantari, I. G. A. K., & Sukaatmadja, I. P. G. (2021). Effects of environmental orientation, green marketing mix and social capital on the competitive advantage of real estate developers in Bali. Property Management, 39 (2), 193–209.
Gladju, J., Kamalam, B. S., & Kanagaraj, A. (2022). Applications of data mining and machine learning framework in aquaculture and fisheries: A review. Smart Agricultural Technology, 2 , 100061.
Gulati, A., Zhou, Y., Huang, J., Tal, A., & Juneja, R. (2021). From food scarcity to surplus . Springer Nature. Publisher: Springer Singapore. ISBN (Electronic): 9789811594847. ISBN (Print): 9789811594830.
Guo, A., Wei, X., Zhong, F., Wang, P., & Song, X. (2022). Does cognition of resources and the environment affect farmers’ production efficiency? Study of oasis agriculture in China. Agriculture, 12 (5), 592.
Hassanein, N. (1999). Changing the way America farms: Knowledge and community in the sustainable agriculture movement (Vol. 12). U of Nebraska Press. 978-0-8032-7321-4.
He, G., Zhao, Y., Wang, L., Jiang, S., & Zhu, Y. (2019). China’s food security challenge: Effects of food habit changes on requirements for arable land and water. Journal of Cleaner Production, 229 , 739–750.
Hong, M., Yu, S., & Long, J. (2023). Agricultural land rights confirmation, clan network, and farmers’ investment in production and operation. Land, 12 (10), 1951.
Javaid, M., Haleem, A., Singh, R. P., Suman, R., & Gonzalez, E. S. (2022). Understanding the adoption of industry 4.0 technologies in improving environmental sustainability. Sustainable Operations and Computers, 3 , 203–217.
Kareem, A., Farooqi, Z. U. R., Kalsom, A., Mohy-Ud-Din, W., Hussain, M. M., Raza, M. A., & Khursheed, M. M. (2021). Organic farming for sustainable soil use, management, food production and climate change mitigation. Sustainable Agriculture . https://doi.org/10.1007/978-3-030-83066-3_3
Karlsson, M., & Hovelsrud, G. K. (2021). “Everyone comes with their own shade of green”: Negotiating the meaning of transformation in Norway’s agriculture and fisheries sectors. Journal of Rural Studies, 81 , 259–268.
Karuri, A. N. (2020). Adaptation of small-scale tea and coffee farmers in Kenya to climate change. African Handbook of Climate Change Adaptation . https://doi.org/10.1007/978-3-030-42091-8_70-1
Klerkx, L., Jakku, E., & Labarthe, P. (2019). A review of social science on digital agriculture, smart farming and agriculture 4.0: New contributions and a future research agenda. NJAS - Wageningen Journal of Life Sciences, 90–91 (1), 100315. https://doi.org/10.1016/j.njas.2019.100315
Liu, Y., Sun, D., Wang, H., Wang, X., Yu, G., & Zhao, X. (2020). An evaluation of China’s agricultural green production: 1978–2017. Journal of Cleaner Production, 243 , 118483.
Liu, S., & Zhong, C. (2024). Green growth: Intellectual property conflicts and prospects in the extraction of natural resources for sustainable development. Resources Policy, 89 , 104588.
Mogili, U. R., & Deepak, B. B. V. L. (2018). Review on application of drone systems in precision agriculture. Procedia Computer Science, 133 , 502–509.
Mohanta, B. K., Jena, D., Satapathy, U., & Patnaik, S. (2020). Survey on IoT security: Challenges and solution using machine learning, artificial intelligence and blockchain technology. Internet of Things, 11 , 100227.
Molina, N., Brunori, G., Favilli, E., Grando, S., & Proietti, P. (2021). Farmers’ participation in operational groups to foster innovation in the agricultural sector: An Italian case study. Sustainability, 13 (10), 5605.
Niu, Z., Chen, C., Gao, Y., Wang, Y., Chen, Y., & Zhao, K. (2022). Peer effects, attention allocation and farmers’ adoption of cleaner production technology: Taking green control techniques as an example. Journal of Cleaner Production, 339 , 130700.
Olorunfemi, T. O., Olorunfemi, O. D., & Oladele, O. I. (2020). Borich needs model analysis of extension agents’ competence on climate smart agricultural initiatives in South West Nigeria. The Journal of Agricultural Education and Extension, 26 (1), 59–73.
Osrof, H. Y., Tan, C. L., Angappa, G., Yeo, S. F., & Tan, K. H. (2023). Adoption of smart farming technologies in field operations: A systematic review and future research agenda. Technology in Society, 75 , 102400. https://doi.org/10.1016/j.techsoc.2023.102400
Park, C. W., MacInnis, D. J., Priester, J., et al. (2010). Brand attachment and brand attitude strength: Conceptual and empirical differentiation of two critical brand equity drivers[J]. Journal of Marketing, 74 (6), 1–17.
Patel, S. K., Sharma, A., & Singh, G. S. (2020). Traditional agricultural practices in India: An approach for environmental sustainability and food security. Energy, Ecology and Environment, 5 (4), 253–271.
Pawlak, K., & Kołodziejczak, M. (2020). The role of agriculture in ensuring food security in developing countries: Considerations in the context of the problem of sustainable food production. Sustainability, 12 (13), 5488.
Qaim, M. (2020). Role of new plant breeding technologies for food security and sustainable agricultural development. Applied Economic Perspectives and Policy, 42 (2), 129–150.
Qayyum, M., Zhang, Y., Wang, M., Yu, Y., Li, S., & Ahmad, W., ... & Gan, J. (2023). Advancements in technology and innovation for sustainable agriculture: Understanding and mitigating greenhouse gas emissions from agricultural soils. Journal of Environmental Management, 347 , 119147.
Qi, X., Liang, F., Yuan, W., Zhang, T., & Li, J. (2021). Factors influencing farmers’ adoption of eco-friendly fertilization technology in grain production: An integrated spatial–econometric analysis in China. Journal of Cleaner Production, 310 , 127536.
Rajabzadeh, M., & Fatorachian, H. (2023). Modelling factors influencing IoT adoption: With a focus on agricultural logistics operations. Smart Cities, 6 (6), 3266–3296.
Rani, S., Borodynko-Filas, N., Aminuzzaman, F. M., Das, K., & Ayim, B. Y. (2023). Harnessing the future: cutting-edge technologies for plant disease control. Journal of Plant Protection Research, 63 (4), 387–398. https://journals.pan.pl/dlibra/publication/147829/edition/129614/content
Roy, R., Gain, A. K., Samat, N., Hurlbert, M., Tan, M. L., & Chan, N. W. (2019). Resilience of coastal agricultural systems in Bangladesh: Assessment for agroecosystem stewardship strategies. Ecological Indicators, 106 , 105525.
Sarfraz, S., Ali, F., Hameed, A., Ahmad, Z., & Riaz, K. (2023). Sustainable agriculture through technological innovations. Sustainable agriculture in the era of the OMICs revolution (pp. 223–239). Springer International Publishing.
Chapter Google Scholar
Sarkar, D., Kar, S. K., Chattopadhyay, A., Rakshit, A., Tripathi, V. K., Dubey, P. K., & Abhilash, P. C. (2020). Low input sustainable agriculture: A viable climate-smart option for boosting food production in a warming world. Ecological Indicators, 115 , 106412.
Sharma, B., Tiwari, S., Kumawat, K. C., & Cardinale, M. (2023). Nano-biofertilizers as bio-emerging strategies for sustainable agriculture development: Potentiality and their limitations. Science of the Total Environment, 860 , 160476.
Shepherd, M., Turner, J. A., Small, B., & Wheeler, D. (2020). Priorities for science to overcome hurdles thwarting the full promise of the ‘digital agriculture’ revolution. Journal of the Science of Food and Agriculture, 100 (14), 5083–5092.
Srivastava, P. K., Singh, V. P., Singh, A., Tripathi, D. K., Singh, S., Prasad, S. M., & Chauhan, D. K. (Eds.). (2020). Pesticides in crop production: Physiological and biochemical action . John Wiley & Sons. ISBN: 978-1-119-43223-4.
Subeesh, A., & Mehta, C. R. (2021). Automation and digitization of agriculture using artificial intelligence and internet of things. Artificial Intelligence in Agriculture, 5 , 278–291.
Šūmane, S., Kunda, I., Knickel, K., Strauss, A., Tisenkopfs, T., & des Ios Rios, I., ... & Ashkenazy, A. (2018). Local and farmers’ knowledge matters! How integrating informal and formal knowledge enhances sustainable and resilient agriculture. Journal of Rural Studies, 59 , 232–241.
Tang, G., Chen, Y., Jiang, Y., Paillé, P., & Jia, J. (2018). Green human resource management practices: Scale development and validity. Asia Pacific Journal of Human Resources, 56 (1), 31–55.
Taylor, M., & Bhasme, S. (2018). Model farmers, extension networks and the politics of agricultural knowledge transfer. Journal of Rural Studies, 64 , 1–10.
Vasileiou, M., Kyrgiakos, L. S., Kleisiari, C., Kleftodimos, G., Vlontzos, G., Belhouchette, H., & Pardalos, P. M. (2024). Transforming weed management in sustainable agriculture with artificial intelligence: a systematic literature review towards weed identification and deep learning. Crop Protection, 176, p. 106522. https://www.iamm.ciheam.org/ress_doc/opac_css/index.php?lvl=notice_display&id=50649
Wang, X., Feng, Y., Yu, L., Shu, Y., Tan, F., & Gou, Y., ... & Wang, J. (2020). Sugarcane/soybean intercropping with reduced nitrogen input improves crop productivity and reduces carbon footprint in China. Science of the Total Environment, 719 , 137517.
Wu, H., Wang, B., Lu, M., Irfan, M., Miao, X., Luo, S., & Hao, Y. (2023). The strategy to achieve zero-carbon in agricultural sector: Does digitalization matter under the background of COP26 targets? Energy Economics, 126 , 106916.
Xie, Y., Chen, Z., Tang, H., Boadu, F., & Yang, Y. (2023). Effects of executives’ pro-environmental education and knowledge sharing activities on eco-friendly agricultural production: Evidence from China. Journal of Cleaner Production, 395 , 136469.
Xie, H., & Huang, Y. (2021). Influencing factors of farmers’ adoption of pro-environmental agricultural technologies in China: Meta-analysis. Land Use Policy, 109 , 105622.
Xu, G., Li, J., Schwarz, P. M., Yang, H., & Chang, H. (2022). Rural financial development and achieving an agricultural carbon emissions peak: An empirical analysis of Henan Province, China. Environment, Development and Sustainability , 1–27.
Yan, A., Luo, X., Tang, L., Huang, Y., & Du, S. (2024). Can socialized pest control service reduce the intensity of pesticide use? Evidence from rice farmers in China. Pest Management Science, 80 (2), 317–332.
Zhong, Y. P., Tang, L. R., & Li, Y. (2022). Role of digital empowerment in developing farmers’ green production by agro-tourism integration in Xichong. Sichuan. Agriculture, 12 (11), 1761.
Download references
This article was supported by the Henan Province Soft Science Project (Grant No. 232400411094), the General Projects of Humanities and Social Sciences Research in Henan Province Universities (2024-ZDJH-289) and Postgraduate Education Reform and Quality Improvement Project of Henan Province(YJS2022JD30).
Author information
Authors and affiliations.
Business School, Xinyang Normal University, Xinyang. 464000, China
Xiankai Lei
School of Marxism, Xinyang Normal University, Xinyang, 464000, China
Dongmei Yang
Dabie Mountain Economic and Social Development Research Center, Xinyang, 464000, China
You can also search for this author in PubMed Google Scholar
Contributions
Conceptualization: X.L. Writing—original draft preparation and writing—review and editing: X.L. Writing—review and editing: D.Y. Methodology: D.Y. All authors have read and agreed to the published version of the manuscript.
Corresponding author
Correspondence to Xiankai Lei .
Ethics declarations
Competing interest.
The authors declare no competing interests.
Additional information
Publisher's note.
Springer Nature remains neutral with regard to jurisdictional claims in published maps and institutional affiliations.
Rights and permissions
Springer Nature or its licensor (e.g. a society or other partner) holds exclusive rights to this article under a publishing agreement with the author(s) or other rightsholder(s); author self-archiving of the accepted manuscript version of this article is solely governed by the terms of such publishing agreement and applicable law.
Reprints and permissions
About this article
Lei, X., Yang, D. Cultivating Green Champions: The Role of High-Quality Farmer Training in Sustainable Agriculture. J Knowl Econ (2024). https://doi.org/10.1007/s13132-024-02014-8
Download citation
Received : 11 December 2023
Accepted : 15 April 2024
Published : 20 May 2024
DOI : https://doi.org/10.1007/s13132-024-02014-8
Share this article
Anyone you share the following link with will be able to read this content:
Sorry, a shareable link is not currently available for this article.
Provided by the Springer Nature SharedIt content-sharing initiative
- Sustainable agriculture
- Green production efficiency
- High-quality farmers
- Farmer training programs
- Environmental stewardship
- Agricultural innovation
- Rural development
- Find a journal
- Publish with us
- Track your research
- Skip to main content
- Skip to footer
AgriLife Today
Texas A&M AgriLife's digital magazine and newsroom
Texas A&M AgriLife Research gets $5.2 million grant for onion improvement
National institute of food and agriculture-funded project will address multiple aspects of successful short-day onion production.
January 6, 2024 - by Paul Schattenberg
Texas A&M AgriLife Research received more than $5.2 million in grant funding from the U.S. Department of Agriculture’s National Institute of Food and Agriculture for a project to address multiple aspects of the southern U.S. onion harvest system.
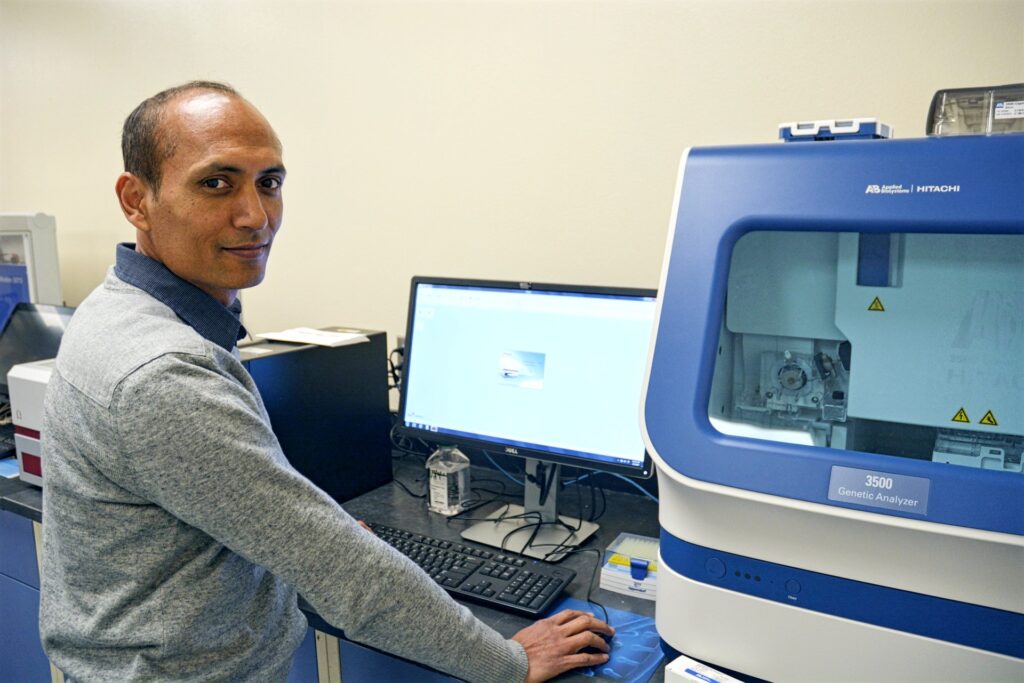
The director for the “Ensuring Future Economic Viability of U.S. Short-Day Onion Production Through Mechanical Harvesting” project will be Subas Malla, Ph.D. Malla is an AgriLife Research associate professor of vegetable breeding in the Texas A&M Department of Horticultural Sciences at the Texas A&M AgriLife Research and Extension Center in Uvalde.
The Texas A&M AgriLife team is comprised of Stephen Searcy, Ph.D., Professor Emeritus and former head of the Department of Biological and Agricultural Engineering , Bryan-College Station; Juan Anciso, Ph.D., professor and Texas A&M AgriLife Extension Service vegetable specialist, Weslaco; Francisco Abello, Ph.D., assistant professor and AgriLife Extension economist – management, Vernon; and Larry Stein, Ph.D., professor, Regents Fellow and associate head of the Department of Horticultural Sciences, Uvalde. The University of California, the University of Georgia and New Mexico State University will also partner on the project.
Searcy will serve as co-director and lead the project’s agricultural engineering research. Anciso and Abello will lead the AgriLife Extension and economic analysis components, respectively.
“The goal of this proposal is to improve profitability and ultimately market share for short-day onions by mechanizing short-day onion harvesting,” Malla said. “We intend to do this through development and selection of cultivars, optimization of production practices, improved harvest systems, and communication of the associated socioeconomic benefits to growers and packers.”
Short-day onions long on production challenges
The majority of onions grown across the southern region of the U.S. are short-day onions. Short-day onions require about 10-12 hours of daylight to produce bulbs, while long-day onions require 14-16 hours.
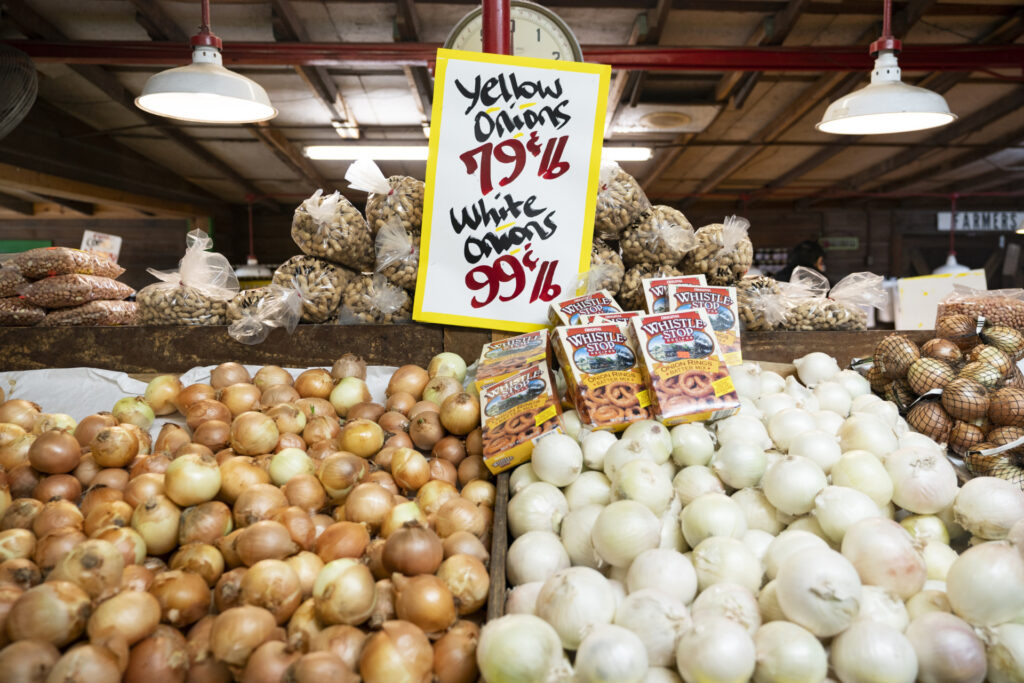
According to South Texas Growers Inc., a wholesale nursery, in 2023, the state’s fresh market onion industry — sweet, yellow, red and white onions combined — grew a total of 75,460 tons across 7,158 acres in the 35 South Texas counties. The average farm-gate value of those onions is around $39 million .
Short-day onions are a high-value vegetable crop in many southern U.S. states, including Texas, but their biology and structure present some difficulties, especially during harvest.
Onion harvesters developed for long-day onions in northern states have been tested with short-day onions, Searcy said, but growers judged the results to be less than satisfactory.
“Dry matter content in the short-day sweet onion is low but the water content is high,” Malla said. “Due to the high water content in the bulb, there is a greater likelihood of bruise damage since the bulbs can’t withstand a higher-pressure impact when harvested. That is why short-day onions have traditionally been harvested by hand.”
In efforts to use mechanized harvesting, too many bulbs were damaged to be acceptable for the fresh market, he said.
“However, these past attempts were limited to substituting the mechanical harvester for manual labor and did not involve a whole-system approach.”
The new project aimed at mechanizing harvest will involve the whole system and include short-day onion areas in Georgia, Texas, New Mexico and California to represent a full range of growing conditions.
“Limited availability and increasing cost of labor has resulted in decreased U.S. short-day onion production and a lack of competitiveness with foreign sources,” Malla said. “A viable mechanized harvest system is a high priority for growers and the industry.”
Building a better short-day onion harvest system
Malla said the project will address the many facets of developing a successful harvest system. These include:
– Identifying cultivars and production practices suitable for mechanical harvesting.
– Modifying the harvest system to minimize the potential damage to onion bulbs.
– Evaluating the influence of mechanical harvest on profitability and risk faced by onion growers.
– Communicating the benefits and drawbacks of adopting mechanical harvesting to growers.
Malla said the adoption of any advances by growers will rely on partnerships with equipment manufacturers, seed companies and technology providers. A stakeholder advisory panel and scientific advisers will participate in the project’s proposed research and outreach activities.
“We are already in the process of evaluating diverse Texas A&M onion germplasm to understand the genetic mechanism of host resistance against diseases and insects,” Malla said. “We are working with the Texas A&M Vegetable and Fruit Improvement Center to test bulb quality and storability in onions. We are also testing Texas A&M onion germplasm in a way that will help shorten the cultivar development cycle.”
To his knowledge, Malla said, this project is one of the broadest and most comprehensive ever undertaken to address the many challenges to short-day onion production.
“Our ultimate goal is identifying the best cultivars and improving the short-day onion harvest system in a way that provides better results and better profitability for the producer,” he said.
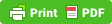
A member of Texas A&M AgriLife
Texas A&M AgriLife Extension Service | Texas A&M AgriLife Research | Texas A&M Forest Service | Texas A&M Veterinary Medical Diagnostic Lab | College of Agriculture & Life Sciences
Texas A&M AgriLife
Phone: (979) 803-1287 [email protected]
More Information
- Resources for Press and Media
- Story Suggestion
Find Us on Social Media
About texas a&m university.

Understanding Cattle Grazing Personalities May Foster Sustainable Rangelands
Matching herds to landscape can support animal growth and ecological needs.
- by Emily C. Dooley
- May 20, 2024
Original article on the College of Agriculture and Environmental Science website .
Not all cattle are the same when it comes to grazing. Some like to wander while others prefer to stay close to water and rest areas.
Recognizing those personality differences could help ranchers select herds that best meet grazing needs on rangelands, leading to better animal health and environmental conditions, according to a new paper from the University of California, Davis, published in the journal Applied Animal Behaviour Science.
“Cattle can actually be beneficial for the rangelands,” said lead author Maggie Creamer, who recently earned her Ph.D. in animal behavior at UC Davis. “Vegetation in rangelands actually need these kinds of disturbances like grazing.”
Ranchers can add elements to the rangeland such as water, mineral supplements and fencing to influence where cattle graze, but little research has been done on how those efforts affect individual cows. Considering personalities could save money.
“If you're spending all this money to add a management tool in order to change the distribution of your animals, that's a huge cost to ranchers,” said Creamer. “Thinking about other tools or selecting certain animals with these grazing traits, might be a better way to optimize the distribution on rangeland rather than spending a bunch of money for something that may ultimately not pan out for all your animals.”
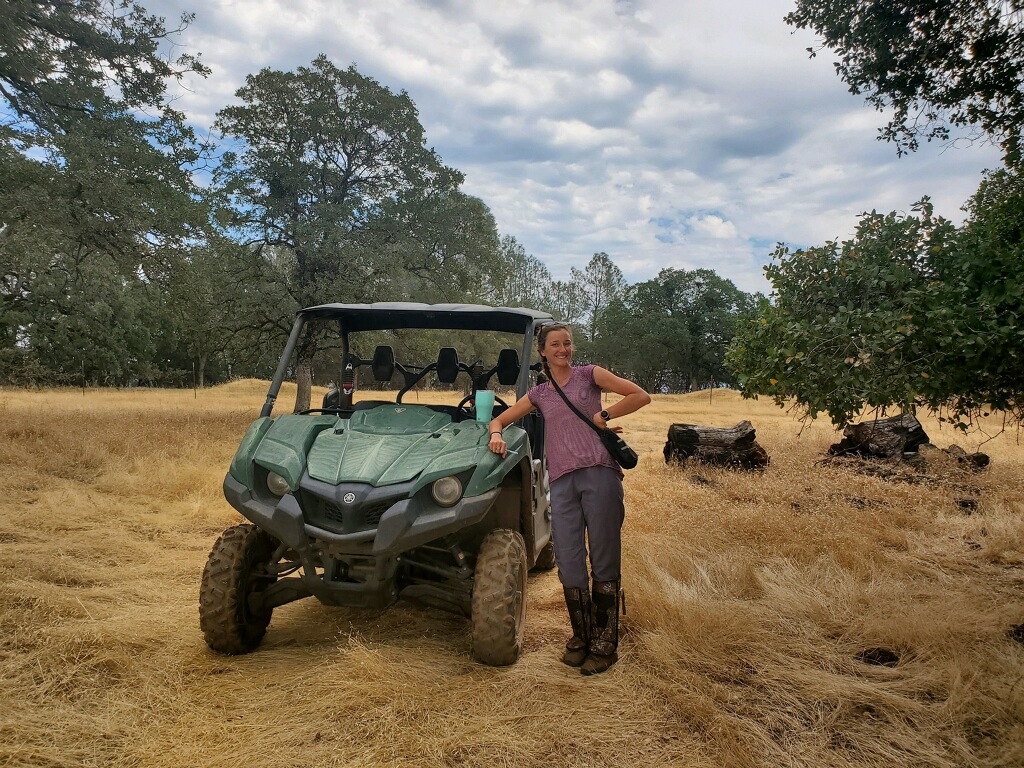
Effects of grazing
Livestock graze on an estimated 56 million acres in California and healthy rangelands host native vegetation and animals, foster nutrient cycling and support carbon sequestration.
Uneven grazing can degrade water quality, soil health and habitats. Optimizing grazing – including the even spread of cow pies – can improve the ecosystem while also reducing fuel loads for wildfires.
To better understand individual grazing patterns, researchers went to the UC Sierra Foothill Research and Extension Center in Browns Valley and tracked 50 pregnant Angus and Hereford beef cows fitted with GPS collars.
The research
The cattle, which were tracked from June to August over two years, had access to 625 acres of grasslands and treed areas ranging in elevation from 600 to 2028 feet. In the second year, a new watering site was added at a higher elevation.
Across the two years, the cows showed consistent and distinct grazing patterns even when water sources changed. Age and stage of pregnancy did not affect patterns, though cattle tended to clump near water and rest sites on hotter days.
The cows that ventured into higher elevations and farther from watering sites had more variability in their grazing patterns than those that stayed at lower elevations near water. That suggests it may be harder for non-wanderers to adjust to some landscapes.
“Thinking about the topography of your rangeland and your herd of cows can benefit both the animals and the sustainability of the land,” said Creamer, who next month begins work as a postdoctoral scholar in North Carolina.
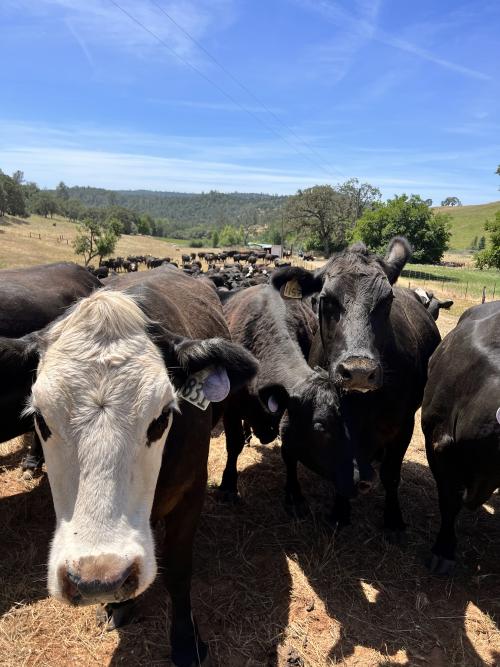
Gauging personalities
Keying in on personality type may sound difficult but the researchers also found some clues as to how to pinpoint the wanderers and homebodies. Unlike cattle at feedlots, the breeding cow population, especially on rangelands in California and other western states, live largely “wild” lives and are rarely handled, save for vaccinations and weaning.
Research due to be published later this year found that paying attention to individual cow reactions during those events can help determine personalities. The cows that appeared more passive during those handling interactions tended to be nomadic.
“We found that you can maybe predict those hill climbers if you kind of look at how they act when the veterinarian or rancher handle them,” said senior author Kristina Horback, an associate professor in the Department of Animal Science at UC Davis.
Informing practices
For ranchers, the findings could be invaluable, said Dan Macon, a livestock and natural resources Cooperative Extension advisor in Placer and Nevada counties for UC Agriculture and Natural Resources.
“Any time we can improve our understanding of cattle behavior, particularly at the individual level, it can improve how we handle livestock and manage the landscape,” he said.
Macon said that during the recent drought, it was hard to get cattle into higher country but if ranchers could have selected the nomads, it may have saved money in terms of ranch labor and other efforts.
“If you ask a rancher who has been attentive to their cattle over many years, they know the personalities,” Macon said.
For Creamer and Horback, the research opens new doors into understanding herd behavior and dynamics, one that could be a cheaper alternative to high-tech solutions.
“Animal science tends to look overlook the mind of the animal when searching for solutions to challenges,” Horback said. “It's always been a direct line to genetics for immunity or nutrition, but nothing about the mind of the animal. And that's such a loss. There's so much we can learn from behavior in the end.”
The Russell L. Rustici Rangeland and Cattle Research Endowment supported the research.
Media Resources
- Maggie Creamer, Department of Animal Science, [email protected]
- Kristina Horback, Department of Animal Science, 530-752-7018, [email protected]
- Dan Macon, UC Agriculture and Natural Resources, 530-889-7385, [email protected]
- Emily C. Dooley, College of Agricultural and Environmental Sciences, 530-650-6807, [email protected]
Primary Category
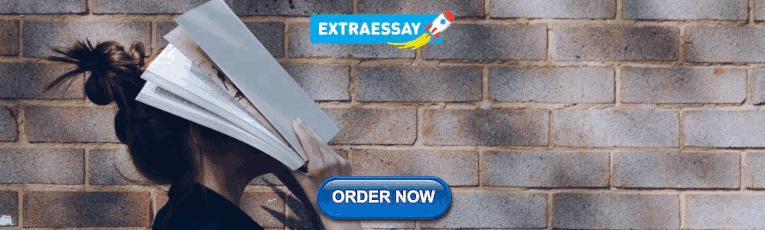
IMAGES
VIDEO
COMMENTS
A research project is the level at which research is actually carried out, e.g. as part of a research programme. The assessment of a research project would consider the impacts of the whole project, from planning through implementation to evaluation instead of focusing on a specific project output, like a certain agricultural innovation.
Transforming research into results for rural people. Agricultural research is essential for sustainable and inclusive agricultural development. Research generates new technologies and improved policies which are essential for small-scale farmers who face the interconnected challenges of climate change, land degradation, gender biases, hunger ...
Agriculture can help reduce poverty for 75% of the world's poor, who live in rural areas and work mainly in farming. It can raise incomes, improve food security and benefit the environment. The World Bank Group is a leading financier of agriculture, with $8.1 billion in new commitments in 2013.
Global volatility of public agricultural R&D expenditure. Stuti Rawat, in Advances in Food Security and Sustainability, 2020. Abstract. Public investment in agricultural research and development (R&D) is important for global food security and environmental sustainability. Although public agricultural R&D projects are associated with high economic returns, they are characterized by long time ...
(Washington, D.C., January 12, 2021) - Today, the U.S. Department of Agriculture (USDA) released its U.S. Agriculture Innovation Strategy Directional Vision for Research (PDF, 4.8 MB) summary and dashboard that will help to guide future research decisions within USDA. The strategy synthesizes the information USDA collected as part of a public announcement earlier this year engaging the ...
Agricultural economists have used supply and demand models of commodity markets to represent agricultural research impacts, beginning with Schultz (1953) and Griliches (1958), with important subsequent contributions by Petersen (1967), Duncan and Tisdell (1971), Duncan (1972), Akino and Hayami (1975), and Scobie (1976), among others.
Building productive relationships between farmers and scientists is critical to developing new innovation pathways to solve the challenges of contemporary agriculture. On-farm experimentation (OFE ...
This report contains a review of the literature on the role of agricultural research and development in fostering innovation and productivity in agriculture. The review seeks to clarify concepts and terminology used in the area, provide a critical assessment of approaches found in the literature, report main results, and draw inferences.
The " USDA Science and Research Strategy, 2023-2026: Cultivating Scientific Innovation (PDF, 21.4 MB)" presents a near-term vision for transforming U.S. agriculture through science and innovation, and outlines USDA's highest scientific priorities. The S&RS is a call to action for USDA partners, stakeholders, and customers to join the ...
FAO's role in Research and Extension. Research and extension systems play a crucial role in agricultural and rural development. Moreover, they are central to realizing the potential of agricultural innovation. In developing countries, innovation can address most of the challenges facing agriculture and natural resources management.
About 4 percent of Federal support for research at universities and colleges was for agri-culture ($408 million of $10 billion). Agriculture's future share of Federal resources for science and research may depend on how society judges the benefits of agri-cultural research compared with other public investments.
Farmer participatory research (FPR) is an important approach to help ensure relevance and define locally adapted solutions for enhanced adoption of sustainable agriculture (SA) technologies. The mother and baby trial (MBT) design has proved effective as a FPR approach to address challenges on smallholder farms.
Agricultural Research, Development and Extension BY DELY PASCUAL-GAPASIN ... Decentralized Agriculture and Forestry Extension Project DAR - Department of Agrarian Reform DAR-MO - Department of Agrarian Reform - Municipal Office ... Research investment can also provide important direct and indirect impacts on rural poverty. Byerlee and Alex ...
particularly important stakeholder in public agricultural research when commercialization is the targeted pathway for scaling that research. This section builds on the concepts outlined in the Supplemental Guidance by providing additional recommendations for involving the private sector in research strategy and project design.
Participatory modes of agricultural research have gained significant attention over the last 40 years. While many scholars and practitioners agree that engaging farmers and other stakeholders is a valuable complement to traditional scientific research, there is significant diversity in the goals and approaches used by participatory projects. Building on previous conceptual frameworks on ...
In response to these challenges, the concept of Climate-smart Agriculture (CSA) has emerged as a holistic approach to end food security and promote sustainable development while addressing climate change issues. CSA is a set of agricultural practices and technologies which simultaneously boost productivity, enhance resilience and reduce GHG ...
The U.S. agricultural system is organized at f ederal, state, university, private, and commodity group levels. The U nited States Department of Agriculture (USDA) is a federal agency that funds research projects of national or regional relevance. The USDA also plays a regulatory role at the national level.
This report presents a wealth of research opportunities in food and agriculture microbiology. The backdrop for these research opportunities is a world of microorganisms teeming with threats and benefits to abundant, healthy food and associated environments. Threats come from microbial pathogens that perpetrate a wide range of plant and animal diseases, destroying agricultural productivity. The ...
Agricultural research is a very general term f or an activity that involves finding out solutions for the value chains in productivity, production, processing, marketing, an d
Agriculture also impacts economic development by contributing to the overall U.S. gross domestic product (GDP), directly and indirectly. It does so through farm production, forestry, fishing activities, textile mills and products, apparel and food and beverage sales, and service and manufacturing. Farm production.
Complex agriculture projects directly affect the welfare of over half the world's population, are a key Sustainable Development Goal (SDG), and account for the single most extensive human activity ...
This publication, Learning Knowledge and Skills for Agriculture to Improve Rural Livelihoods, offers rich insights into young peoples' learning, livelihoods and aspirations. The publication is the result of a joint qualitative research project implemented by the International Fund for Agricultural Development and UNESCO in Cambodia, Egypt and ...
Explore 45 Research Project Ideas in Agriculture for Sustainable Farming. Evaluating the effectiveness of natural pest control methods in agriculture. Investigating the effects of climate change on crop yields and food security. Studying the impact of soil quality on plant growth and crop yields. Analyzing the potential of precision agriculture ...
This study examines the transformative impact of cultivating high-quality farmers on the green production efficiency of agricultural practices in Henan Province, China. Utilizing a robust dataset from 2263 exemplary farmers and employing the Data Envelopment Analysis-Slack Based Measure (DEA-SBM) and Tobit models, we explore the nexus between farmer training programs and sustainable ...
The Seed Bank component of this project holds paramount importance as it addresses the challenges faced by Iraq's agricultural sector due to the of challenges that the country has experienced. The main objectives are the rehabilitation of the Abu Gharib seed bank in Baghdad, which has been operational since 1977 but has suffered from infrastructural damage. Additionally, the project aims to ...
January 6, 2024 - by Paul Schattenberg. Texas A&M AgriLife Research received more than $5.2 million in grant funding from the U.S. Department of Agriculture's National Institute of Food and Agriculture for a project to address multiple aspects of the southern U.S. onion harvest system. Subas Malla, Ph.D., Texas A&M AgriLife Research associate ...
[email protected]. UMass Extension. Gloucester Marine Station. 932 Washington Street. Gloucester, MA 01930. Profile: Jynessa is a research scientist in the Department of Environmental Conservation, based full-time at the Gloucester Marine Station. She works with integrative fisheries sciences and human dimension of fisheries, engaging local ...
A cow at the Sierra Foothill Research and Extension Center in Browns Valley being tracked as part of research on cattle grazing personalities. ... a livestock and natural resources Cooperative Extension advisor in Placer and Nevada counties for UC Agriculture and Natural Resources. ... The Jawless Fish Play an Important Ecosystem Role February ...
Water is an important resource and is a free gift of nature. Potable water for drinking and water for agriculture both maintains the life on the earth. It is available in plenty but face acute ...
The INITIATE project proposes an innovative process to produce urea from residual gases of steel making and demonstrates the importance of industrial symbiosis between different industrial sectors ...