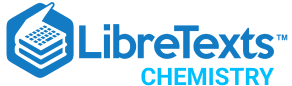
- school Campus Bookshelves
- menu_book Bookshelves
- perm_media Learning Objects
- login Login
- how_to_reg Request Instructor Account
- hub Instructor Commons
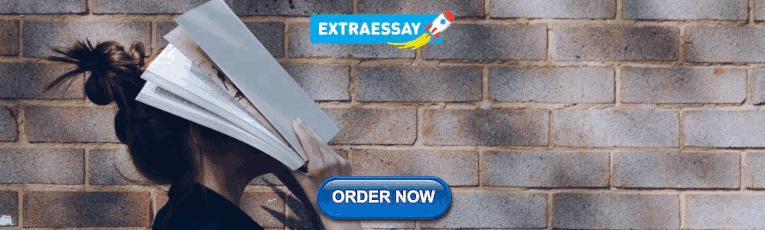
Margin Size
- Download Page (PDF)
- Download Full Book (PDF)
- Periodic Table
- Physics Constants
- Scientific Calculator
- Reference & Cite
- Tools expand_more
- Readability
selected template will load here
This action is not available.
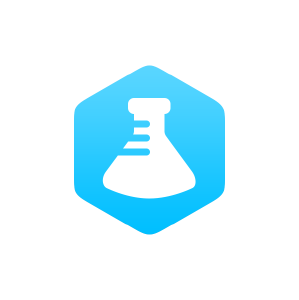
3.1 Modern Atomic Theory
- Last updated
- Save as PDF
- Page ID 218040
\( \newcommand{\vecs}[1]{\overset { \scriptstyle \rightharpoonup} {\mathbf{#1}} } \)
\( \newcommand{\vecd}[1]{\overset{-\!-\!\rightharpoonup}{\vphantom{a}\smash {#1}}} \)
\( \newcommand{\id}{\mathrm{id}}\) \( \newcommand{\Span}{\mathrm{span}}\)
( \newcommand{\kernel}{\mathrm{null}\,}\) \( \newcommand{\range}{\mathrm{range}\,}\)
\( \newcommand{\RealPart}{\mathrm{Re}}\) \( \newcommand{\ImaginaryPart}{\mathrm{Im}}\)
\( \newcommand{\Argument}{\mathrm{Arg}}\) \( \newcommand{\norm}[1]{\| #1 \|}\)
\( \newcommand{\inner}[2]{\langle #1, #2 \rangle}\)
\( \newcommand{\Span}{\mathrm{span}}\)
\( \newcommand{\id}{\mathrm{id}}\)
\( \newcommand{\kernel}{\mathrm{null}\,}\)
\( \newcommand{\range}{\mathrm{range}\,}\)
\( \newcommand{\RealPart}{\mathrm{Re}}\)
\( \newcommand{\ImaginaryPart}{\mathrm{Im}}\)
\( \newcommand{\Argument}{\mathrm{Arg}}\)
\( \newcommand{\norm}[1]{\| #1 \|}\)
\( \newcommand{\Span}{\mathrm{span}}\) \( \newcommand{\AA}{\unicode[.8,0]{x212B}}\)
\( \newcommand{\vectorA}[1]{\vec{#1}} % arrow\)
\( \newcommand{\vectorAt}[1]{\vec{\text{#1}}} % arrow\)
\( \newcommand{\vectorB}[1]{\overset { \scriptstyle \rightharpoonup} {\mathbf{#1}} } \)
\( \newcommand{\vectorC}[1]{\textbf{#1}} \)
\( \newcommand{\vectorD}[1]{\overrightarrow{#1}} \)
\( \newcommand{\vectorDt}[1]{\overrightarrow{\text{#1}}} \)
\( \newcommand{\vectE}[1]{\overset{-\!-\!\rightharpoonup}{\vphantom{a}\smash{\mathbf {#1}}}} \)
Skills to Develop
By the end of this section, you will be able to:
- State the postulates of Dalton’s atomic theory
- Use postulates of Dalton’s atomic theory to explain the laws of definite and multiple proportions
The language used in chemistry is seen and heard in many disciplines, ranging from medicine to engineering to forensics to art. The language of chemistry includes its own vocabulary as well as its own form of shorthand. Chemical symbols are used to represent atoms and elements. Chemical formulas depict molecules as well as the composition of compounds. Chemical equations provide information about the quality and quantity of the changes associated with chemical reactions.
This chapter will lay the foundation for our study of the language of chemistry. The concepts of this foundation include the atomic theory, ion formation, the composition and mass of an atom, the variability of the composition of isotopes, nuclear stability, and atomic electronic structure. We will also discuss in greater detail one of the most powerful tools for organizing chemical knowledge: the periodic table.
Atomic Theory through the Nineteenth Century
The earliest recorded discussion of the basic structure of matter comes from ancient Greek philosophers, the scientists of their day. In the fifth century BC, Leucippus and Democritus argued that all matter was composed of small, finite particles that they called atomos , a term derived from the Greek word for “indivisible.” They thought of atoms as moving particles that differed in shape and size, and which could join together. Later, Aristotle and others came to the conclusion that matter consisted of various combinations of the four “elements”—fire, earth, air, and water—and could be infinitely divided. Interestingly, these philosophers thought about atoms and “elements” as philosophical concepts, but apparently never considered performing experiments to test their ideas.
The Aristotelian view of the composition of matter held sway for over two thousand years, until English schoolteacher John Dalton helped to revolutionize chemistry with his hypothesis that the behavior of matter could be explained using an atomic theory. First published in 1807, many of Dalton’s hypotheses about the microscopic features of matter are still valid in modern atomic theory. Here are the postulates of Dalton’s atomic theory .
- Matter is composed of exceedingly small particles called atoms. An atom is the smallest unit of an element that can participate in a chemical change.
- An element consists of only one type of atom, which has a mass that is characteristic of the element and is the same for all atoms of that element (Figure \(\PageIndex{1}\)). A macroscopic sample of an element contains an incredibly large number of atoms, all of which have identical chemical properties.
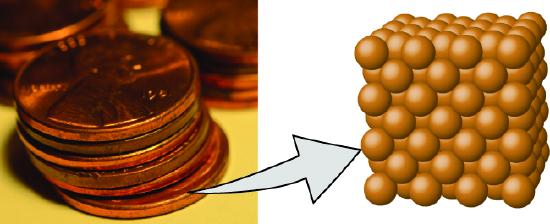
Figure \(\PageIndex{1}\): A pre-1982 copper penny (left) contains approximately 3 \(\times\) 10 22 copper atoms (several dozen are represented as brown spheres at the right), each of which has the same chemical properties. (credit: modification of work by “slgckgc”/Flickr)
- Atoms of one element differ in properties from atoms of all other elements.
- A compound consists of atoms of two or more elements combined in a small, whole-number ratio. In a given compound, the numbers of atoms of each of its elements are always present in the same ratio (Figure \(\PageIndex{2}\)).
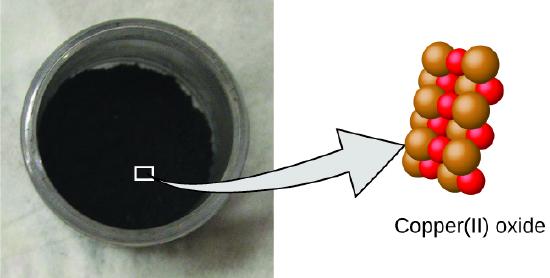
Figure \(\PageIndex{2}\): Copper(II) oxide, a powdery, black compound, results from the combination of two types of atoms—copper (brown spheres) and oxygen (red spheres)—in a 1:1 ratio. (credit: modification of work by “Chemicalinterest”/Wikimedia Commons)
- Atoms are neither created nor destroyed during a chemical change, but are instead rearranged to yield substances that are different from those present before the change (Figure \(\PageIndex{3}\)).
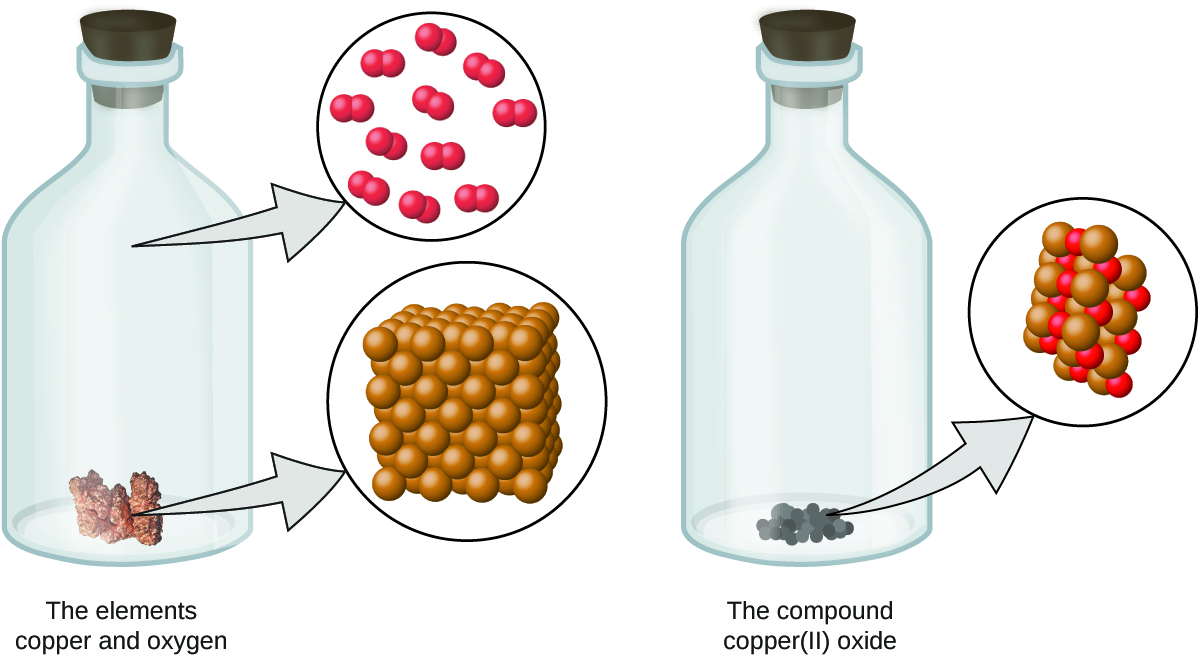
Figure \(\PageIndex{3}\) : When the elements copper (a shiny, red-brown solid, shown here as brown spheres) and oxygen (a clear and colorless gas, shown here as red spheres) react, their atoms rearrange to form a compound containing copper and oxygen (a powdery, black solid). (credit copper: modification of work by http://images-of-elements.com/copper.php ).
Dalton’s atomic theory provides a microscopic explanation of the many macroscopic properties of matter that you’ve learned about. For example, if an element such as copper consists of only one kind of atom, then it cannot be broken down into simpler substances, that is, into substances composed of fewer types of atoms. And if atoms are neither created nor destroyed during a chemical change, then the total mass of matter present when matter changes from one type to another will remain constant (the law of conservation of matter). We will see that Dalton's atomic theory was not entirely correct because Dalton was unaware of the existence of both isotopes and nuclear reactions.
Discovery of the Electron
Long before the end of the 19th century, it was well known that applying a high voltage to a gas contained at low pressure in a sealed tube (called a gas discharge tube) caused electricity to flow through the gas, which then emitted light (Figure \(\PageIndex{4}\)). Researchers trying to understand this phenomenon found that an unusual form of energy was also emitted from the cathode, or negatively charged electrode; this form of energy was called a cathode ray.

Figure 2.4. 1
Figure \(\PageIndex{4}\) : A Gas Discharge Tube Producing Cathode Rays. When a high voltage is applied to a gas contained at low pressure in a gas discharge tube, electricity flows through the gas, and energy is emitted in the form of light. Image used with Permission (CC BY-SA-NC).
In 1897, the British physicist J. J. Thomson (1856–1940) proved that atoms were not the most basic form of matter. He demonstrated that cathode rays could be deflected, or bent, by magnetic or electric fields, which indicated that cathode rays consist of charged particles (Figure \(\PageIndex{5}\)) More important, by measuring the extent of the deflection of the cathode rays in magnetic or electric fields of various strengths, Thomson was able to calculate the mass-to-charge ratio of the particles. These particles were emitted by the negatively charged cathode and repelled by the negative terminal of an electric field. Because like charges repel each other and opposite charges attract, Thomson concluded that the particles had a net negative charge; these particles are now called electrons. Most relevant to the field of chemistry, Thomson found that the mass-to-charge ratio of cathode rays is independent of the nature of the metal electrodes or the gas, which suggested that electrons were fundamental components of all atoms.

Figure \(\PageIndex{5}\) : Deflection of Cathode Rays by an Electric Field. As the cathode rays travel toward the right, they are deflected toward the positive electrode (+), demonstrating that they are negatively charged. Image used with Permission (CC BY-SA-NC).
Subsequently, the American scientist Robert Millikan (1868–1953) carried out a series of experiments using electrically charged oil droplets, which allowed him to calculate the charge on a single electron. With this information and Thomson’s mass-to-charge ratio, Millikan determined the mass of an electron:
\(\dfrac{mass}{charge} \: x \: charge = mass \)
It was at this point that two separate lines of investigation began to converge, both aimed at determining how and why matter emits energy. The video below shows how JJ Thompson used such a tube to measure the ratio of charge over mass of an electron
Measuring e/m For an Electron. Video from Davidson College demonstrating Thompson's e/m experiment.
Radioactivity
The second line of investigation began in 1896, when the French physicist Henri Becquerel (1852–1908) discovered that certain minerals, such as uranium salts, emitted a new form of energy. Becquerel’s work was greatly extended by Marie Curie (1867–1934) and her husband, Pierre (1854–1906); all three shared the Nobel Prize in Physics in 1903. Marie Curie coined the term radioactivity (from the Latin radius , meaning “ray”) to describe the emission of energy rays by matter. She found that one particular uranium ore, pitchblende, was substantially more radioactive than most, which suggested that it contained one or more highly radioactive impurities. Starting with several tons of pitchblende, the Curies isolated two new radioactive elements after months of work: polonium, which was named for Marie’s native Poland, and radium, which was named for its intense radioactivity. Pierre Curie carried a vial of radium in his coat pocket to demonstrate its greenish glow, a habit that caused him to become ill from radiation poisoning well before he was run over by a horse-drawn wagon and killed instantly in 1906. Marie Curie, in turn, died of what was almost certainly radiation poisoning.
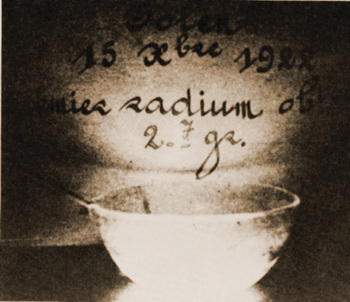
Figure \(\PageIndex{6}\) : Radium bromide illuminated by its own radioactive glow. This 1922 photo was taken in the dark in the Curie laboratory.
Building on the Curies’ work, the British physicist Ernest Rutherford (1871–1937) performed decisive experiments that led to the modern view of the structure of the atom. While working in Thomson’s laboratory shortly after Thomson discovered the electron, Rutherford showed that compounds of uranium and other elements emitted at least two distinct types of radiation. One was readily absorbed by matter and seemed to consist of particles that had a positive charge and were massive compared to electrons. Because it was the first kind of radiation to be discovered, Rutherford called these substances α particles. Rutherford also showed that the particles in the second type of radiation, β particles, had the same charge and mass-to-charge ratio as Thomson’s electrons; they are now known to be high-speed electrons. A third type of radiation, γ rays, was discovered somewhat later and found to be similar to the lower-energy form of radiation called x-rays, now used to produce images of bones and teeth.
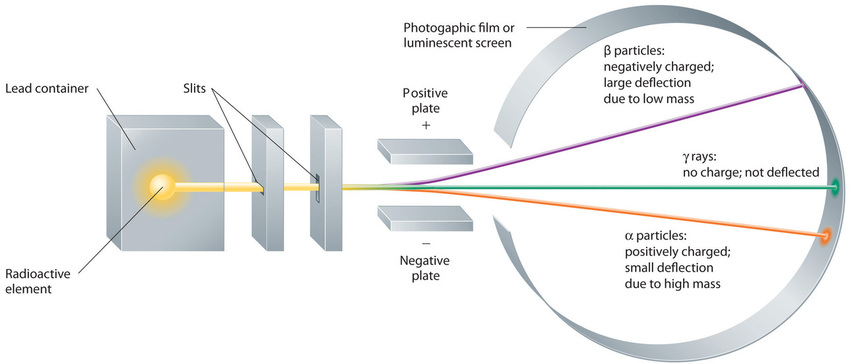
Figure \(\PageIndex{7}\): Effect of an Electric Field on α Particles, β Particles, and γ Rays. A negative electrode deflects negatively charged β particles, whereas a positive electrode deflects positively charged α particles. Uncharged γ rays are unaffected by an electric field. (Relative deflections are not shown to scale.) Image used with Permission (CC BY-SA-NC).
These three kinds of radiation—α particles, β particles, and γ rays—are readily distinguished by the way they are deflected by an electric field and by the degree to which they penetrate matter. As (Figure \(\PageIndex{7}\)) illustrates, α particles and β particles are deflected in opposite directions; α particles are deflected to a much lesser extent because of their higher mass-to-charge ratio. In contrast, γ rays have no charge, so they are not deflected by electric or magnetic fields. Figure \(\PageIndex{8}\) hows that α particles have the least penetrating power and are stopped by a sheet of paper, whereas β particles can pass through thin sheets of metal but are absorbed by lead foil or even thick glass. In contrast, γ-rays can readily penetrate matter; thick blocks of lead or concrete are needed to stop them.
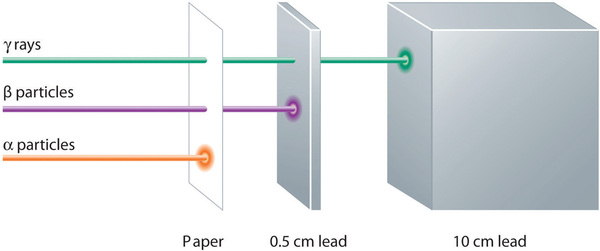
Figure \(\PageIndex{8}\): Relative Penetrating Power of the Three Types of Radiation. A sheet of paper stops comparatively massive α particles, whereas β particles easily penetrate paper but are stopped by a thin piece of lead foil. Uncharged γ rays penetrate the paper and lead foil; a much thicker piece of lead or concrete is needed to absorb them. Image used with Permission (CC BY-SA-NC).
The Atomic Model
Once scientists concluded that all matter contains negatively charged electrons, it became clear that atoms, which are electrically neutral, must also contain positive charges to balance the negative ones. Thomson proposed that the electrons were embedded in a uniform sphere that contained both the positive charge and most of the mass of the atom, much like raisins in plum pudding or chocolate chips in a cookie (Figure \(\PageIndex{9}\))

Figure \(\PageIndex{9}\): Thomson’s Plum Pudding or Chocolate Chip Cookie Model of the Atom. In this model, the electrons are embedded in a uniform sphere of positive charge. Image used with Permission (CC BY-SA-NC).
In a single famous experiment, however, Rutherford showed unambiguously that Thomson’s model of the atom was incorrect. Rutherford aimed a stream of α particles at a very thin gold foil target (Figure \(\PageIndex{10a}\)) and examined how the α particles were scattered by the foil. Gold was chosen because it could be easily hammered into extremely thin sheets, minimizing the number of atoms in the target. If Thomson’s model of the atom were correct, the positively-charged α particles should crash through the uniformly distributed mass of the gold target like cannonballs through the side of a wooden house. They might be moving a little slower when they emerged, but they should pass essentially straight through the target (Figure \(\PageIndex{10b}\))To Rutherford’s amazement, a small fraction of the α particles were deflected at large angles, and some were reflected directly back at the source (Figure \(\PageIndex{10c}\)). According to Rutherford, “It was almost as incredible as if you fired a 15-inch shell at a piece of tissue paper and it came back and hit you.”

Figure \(\PageIndex{10}\): A Summary of Rutherford’s Experiments. (a) A representation of the apparatus Rutherford used to detect deflections in a stream of α particles aimed at a thin gold foil target. The particles were produced by a sample of radium. (b) If Thomson’s model of the atom were correct, the α particles should have passed straight through the gold foil. (c) However, a small number of α particles were deflected in various directions, including right back at the source. This could be true only if the positive charge were much more massive than the α particle. It suggested that the mass of the gold atom is concentrated in a very small region of space, which he called the nucleus. Image used with Permission (CC BY-SA-NC).
Rutherford’s results were not consistent with a model in which the mass and positive charge are distributed uniformly throughout the volume of an atom. Instead, they strongly suggested that both the mass and positive charge are concentrated in a tiny fraction of the volume of an atom, which Rutherford called the nucleus. It made sense that a small fraction of the α particles collided with the dense, positively charged nuclei in either a glancing fashion, resulting in large deflections, or almost head-on, causing them to be reflected straight back at the source.
Although Rutherford could not explain why repulsions between the positive charges in nuclei that contained more than one positive charge did not cause the nucleus to disintegrate, he reasoned that repulsions between negatively charged electrons would cause the electrons to be uniformly distributed throughout the atom’s volume.Today it is known that strong nuclear forces, which are much stronger than electrostatic interactions, hold the protons and the neutrons together in the nucleus. For this and other insights, Rutherford was awarded the Nobel Prize in Chemistry in 1908. Unfortunately, Rutherford would have preferred to receive the Nobel Prize in Physics because he considered physics superior to chemistry. In his opinion, “All science is either physics or stamp collecting.”
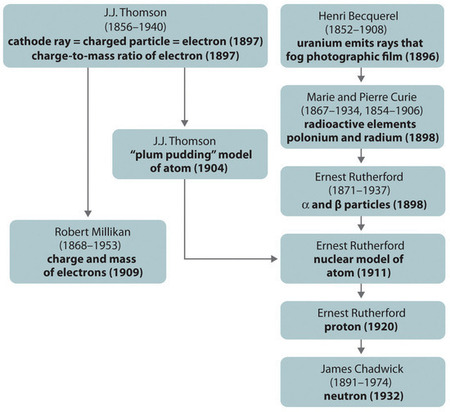
Figure \(\PageIndex{11}\) : A Summary of the Historical Development of Models of the Components and Structure of the Atom. The dates in parentheses are the years in which the key experiments were performed. Image used with Permission (CC BY-SA-NC).
The historical development of the different models of the atom’s structure is summarized in (Figure \(\PageIndex{11}\)) Rutherford established that the nucleus of the hydrogen atom was a positively charged particle, for which he coined the name proton in 1920. He also suggested that the nuclei of elements other than hydrogen must contain electrically neutral particles with approximately the same mass as the proton. The neutron, however, was not discovered until 1932, when James Chadwick (1891–1974, a student of Rutherford; Nobel Prize in Physics, 1935) discovered it. As a result of Rutherford’s work, it became clear that an α particle contains two protons and neutrons, and is therefore the nucleus of a helium atom.
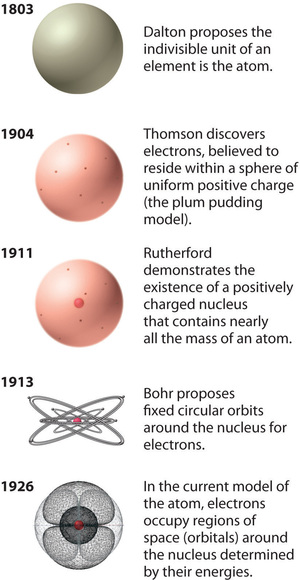
Figure \(\PageIndex{12}\) : The Evolution of Atomic Theory, as Illustrated by Models of the Oxygen Atom. Bohr’s model and the current model are described in Chapter 6, "The Structure of Atoms." Image used with Permission (CC BY-SA-NC).
Rutherford’s model of the atom is essentially the same as the modern model, except that it is now known that electrons are not uniformly distributed throughout an atom’s volume. Instead, they are distributed according to a set of principles described by Quantum Mechanics. Figure \(\PageIndex{12}\) shows how the model of the atom has evolved over time from the indivisible unit of Dalton to the modern view taught today.
The ancient Greeks proposed that matter consists of extremely small particles called atoms. Dalton postulated that each element has a characteristic type of atom that differs in properties from atoms of all other elements, and that atoms of different elements can combine in fixed, small, whole-number ratios to form compounds. During any chemical change, atoms are neither created nor destroyed. Atoms are the ultimate building blocks of all matter. The modern atomic theory establishes the concepts of atoms and how they compose matter.
Atoms consist of negatively charged electrons around a central nucleus composed of more massive positively charged protons and electrically neutral neutrons. Radioactivity is the emission of energetic particles and rays (radiation) by some substances. Three important kinds of radiation are α particles (helium nuclei), β particles (electrons traveling at high speed), and γ rays (similar to x-rays but higher in energy).
Contributors
Paul Flowers (University of North Carolina - Pembroke), Klaus Theopold (University of Delaware) and Richard Langley (Stephen F. Austin State University) with contributing authors. Textbook content produced by OpenStax College is licensed under a Creative Commons Attribution License 4.0 license. Download for free at http://cnx.org/contents/[email protected] ).
- Modified by Tom Neils (Grand Rapids Community College)
Incorporate STEM journalism in your classroom
- Exercise type: Activity
- Topic: Particle Physics
Dig into atomic models
- Download Student Worksheet
Purpose: Students will work in groups to research, create and present different models of the atom that show the evolution of physicists’ understanding of atomic structure. The class also will learn about the standard model of particle physics and brainstorm how it could be shown in two or three dimensions.
Procedural overview: This activity is designed for three class periods and includes two homework assignments. To prepare for the first class, students will read the online Science News article “ How matter’s hidden complexity unleashed the power of nuclear physics ,” and answer three questions for homework.
During the first class, students will work in groups to research a classic atomic model and begin preparing a presentation about it. For homework, students can work with their groups or individually to create a 2-D or 3-D physical model of their group’s classic model. During the second class, the groups can finalize their physical models and make their presentations to the rest of the class.
In the final session for this activity, teachers will discuss the standard model of particle physics and have the students brainstorm ways they might create a 2-D or 3-D interpretation of it. To develop their ideas, the class should draw on current scientific knowledge and use resources such as CERN: The Standard Model .
Approximate class time: 3 class periods
Want to make it a virtual lesson? This activity can be delivered remotely using meeting software that allows you to convene the whole class and also to divide students into groups.
Various building materials (examples could include beans, candies. cotton balls, pipe cleaners, packing materials, yarn, tennis balls, baseballs, baggies and other items that can be found at home or in school)
Internet access
“ Dig into atomic models ” student worksheet
A projector (optional)
Whiteboard (optional)
Directions for teachers:
Before the first class for this activity, have students read the online Science News article “ How matter’s hidden complexity unleashed the power of nuclear physics ” and answer the first set of questions on the student worksheet for homework. A version of the story, “Cracking the atom,” appears in the April 10, 2021 issue of Science News .
1. What is an atom and why do we care about its structure?
Atoms make up matter; they are the building blocks of chemistry. The basic components of atoms are protons, neutrons and electrons. Knowing how many protons, neutrons and electrons a particular atom has will tell you what chemical element it is and how it will behave with atoms of different elements. Atomic structure helps scientists understand and predict the properties of matter.
2. Why has the model of the atom changed over time?
As scientists do experiments and make discoveries, they change their models to reflect new knowledge.
3. What are some practical implications of knowing the structure of the atom?
Answers will vary. Students may note that knowing the structure of atoms can help scientists determine how chemicals will react with each other. For instance, chemists can predict melting and freezing points for chemicals they create when they know the structures of their starting molecules. Knowing the structure of atoms also aids in the design of medicines and materials such as those used in environmental monitoring equipment and solar panels.
First class
After reviewing the homework answers, divide the students into groups. Assign one of the classic models — Dalton, Thomson, Rutherford, Bohr and Schrödinger — to each group.
The groups will work in class and if needed, at home, to research and prepare 5- to 7-minute presentations about their models, to be delivered at the next class. Each presentation should include a description of the model’s appearance, information about the scientists that developed the model and what was known about atoms at the time the model was developed. Groups should build physical representations of their assigned models to incorporate in their presentations. Creativity is encouraged, but students should still aim for accuracy. Alternatively, you could assign model building to students individually. When students bring their models to class, each group can come together to learn from each member’s attempt and put forth the best model or models for their presentation.
As students develop their presentations and build their models, have groups answer the following questions. You can use the questions to evaluate the presentations.
1. What other names have been used to describe the model assigned to you, and why did the model receive the names it did?
Answers will vary depending on the model assigned . T he Dalton model is called the billiard ball model or the solid sphere model. Dalton thought of atoms as solid spheres that could not be broken down into smaller parts. The Thomson model is known as the plum pudding model. He thought that electrons were studded over a “sea of positive charge” — something like plums in an English plum pudding. The Rutherford and Bohr models are called planetary models because both have electrons orbiting around an atomic nucleus similar to how planets orbit a star. The Schrödinger model is known as the quantum mechanical model because it incorporates the uncertainty inherent in quantum mechanics. Schrödinger used mathematics to predict the likely location of electrons in atoms.
2. When was your assigned model developed?
Answers will vary depending on the model assigned. The Dalton model was proposed in 1803, the Thoms on model was proposed in 1904, the Rutherford model was advanced in 1911, the Bohr model was developed in 1913, and the Schrödinger model was developed in 1926.
3. Although each classic model is named after the scientist who proposed it, these scientists either worked with or were influenced by other scientists. Name some of the influencers.
Answers will vary based on the model assigned. Dalton based his model partly on the work of Antoine Lavoisier and Joseph Proust. The Thomson model is named for Sir J. J. Thomson, who discovered the electron. A physicist named George Johnstone Stoney proposed the existence of electrons in 1874. The Rutherford model was derived from work Ernest Rutherford performed with his colleagues Hans Geiger and Ernest Marsden. Niels Bohr, for whom the Bohr model is named, was influenced by the work of Albert Einstein, Max Planck, Wolfgang Pauli and others. Erwin Schrödinger was influenced by the work Louis de Broglie, Werner Heisenberg and other physicists.
4. What discoveries or new evidence led to the development of this model?
Answers will vary depending on the model assigned. For instance, the Thomson model was developed after the discovery of the electron, while the Rutherford model was created after an experiment yielded results that contradicted the Thomson model. Rutherford and colleagues shot alpha particles at a piece of gold foil and found that some of the particles bounced off the foil instead of passing straight through it as predicted by the Thomson model. The finding led Rutherford to realize that the atom had to have a nucleus of positively charged particles.
5. How is the model that you were assigned different from previous models?
Answers will vary depending on the model assigned. For instance, the Bohr model incorporated the knowledge that electrons tend to occupy specific, discrete energy levels, while the Rutherford model assumed all electrons have the same energy potential. This was conveyed by having the electrons appear to orbit the nucleus in rings akin to planets around the sun instead of equally sized elliptical orbits around the nucleus as it was in the Rutherford model.
6. Are there places your assigned model is still used, and where have you seen it before?
You can still see influences of the Dalton model in depictions of molecules. Atoms that make up the molecule are shown as solid circles or spheres that are bonded together. The Thomson model is not often referenced these days. The Rutherford model is commonly used in popular media. The Bohr model is often used to teach students about electron shells. Students may learn the basics of the Schrödinger model when classes talk about quantum numbers.
Second class
At the start of the second class, have the groups finalize their presentations and models. While the students are working, you can walk around to evaluate the models and offer guidance. If there are several good models made by individuals in the group, you can help the students figure out which components to use from the different models to make an improved group model.
The presentations should be given in the order that the models were developed during the 19th and 20th centuries. Doing the presentations chronologically will help the students better understand how the field advanced.
Third class
In the third class, introduce students to the standard model of particle physics and the current science that supports it. If you need more information to prepare for this lesson, go to CERN: The Standard Model and DOE Explains…the Standard Model of Particle Physics .
Have the class brainstorm a way to visualize the standard model. Students can use paper, a computer with internet access and a whiteboard for their brainstorming work.
Cover the following questions in the discussion.
1. What is the standard model of particle physics? What are some key things to know about the standard model?
The standard model is a well-established theory in particle physics that describes elementary particles (also called fundamental particles) and explains how these particles behave. The model accounts for three forces in nature: electromagnetism, the strong nuclear force and the weak nuclear force. Elementary particles called quarks make up the protons and neutrons in an atom’s nucleus. Electrons are a kind of lepton, another type of elementary particle. There are force-carrying elementary particles called bosons that allow other particles to interact. For example, a boson called gluon transmits the strong nuclear force, which keeps the quarks in protons and neutrons “glued” together. There are 17 known elementary particles.
2. How is antimatter different from matter? Give an example of each.
Many elementary particles have an antimatter counterpart. Those counterparts have the same mass, but the opposite charge. For example, the antimatter counterpart of the electron is the positron. The electron has a negative charge, and the positron has a positive charge. One of the puzzling things about matter and antimatter is they are not present in equal amounts in the universe. Physicists are trying to understand why there is so much more matter than antimatter.
3. Who developed the standard model of particle physics?
The standard model of particle physics was not the work of one scientist. Many physicists investigating the structure and interactions of subatomic particles did the experimental and theoretical work that made the standard model possible. Notable contributors include James Chadwick, Paul Dirac, Carl Anderson, Enrico Fermi, Peter Higgs and Sheldon Glashow.
4. When was the standard model developed?
From the 1930s onward, physicists began finding more subatomic particles. They wanted to understand what the particles had to do with each other and determine which ones were fundamental (or elementary) particles. Using mathematics and theoretical and experimental work, physicists were able to establish the standard model of particle physics as a theory by the mid-1970s. Since then, more elementary particles predicted by the theory have been discovered. The most recent was in 2012. It is called the Higgs boson.
5. Are there any other common names for the standard model?
Not really.
6.What discoveries or ideas contributed to the standard model?
Many scientists’ work contributed to the standard model. Here are a few examples. Chadwick discovered the neutron, which helped explain isotopes and broke physics out of the two-particle model of atoms made from only electrons and protons. Fermi advanced the idea that beta decay, the decay of a neutron into a proton within an atom, created an electron and another particle now called an antineutrino. Fermi also discovered many subatomic particles using first natural cosmic rays and then particle accelerators. Higgs and others independently developed a theory for how particles obtain mass in the 1960s; their theory was confirmed in 2012 with the discovery of the Higgs boson, the last of the elementary particles to be discovered.
7. How is the standard model different from the classic models you have studied?
The standard model of particle physics is much more complex than the atomic models that preceded it. Even the most complicated classic models included only three components: electrons, protons and neutrons. There are 17 elementary particles in the standard model — most of them probably unimagined by earlier generations of scientists. The standard model accounts for matter and antimatter, and it contains particles that transmit forces within atoms.
8. What are some weaknesses of the standard model?
The standard model does not take into account gravity, the fourth force of nature, and does not answer why there is more matter than antimatter in the universe. The model also does not explain dark matter, an unidentified, invisible type of matter in the universe. Dark matter appears to interact weakly with all regular matter except through gravity.
9. What visual depictions of the standard model have you seen, and what do you think of them?
Student answers will vary. Visual representations are rare. Most often, the standard model is represented as a “periodic table” of elementary particles with no mention of how they combine to create electrons, neutrons and protons ( CERN: The Standard Model ). The U.S. Department of Energy website uses a graphic that places the Higgs boson in the center surrounded by a ring of bosons with leptons and quarks in an outer ring ( DOE Explains…the Standard Model of Particle Physics ).
10. If you were doing a 2-D or 3-D visualization of the standard model, would you include all of the known elementary particles? Why or why not?
Student answers will vary. For instance, a student may say that it would be too complicated to use all of the elementary particles in a 3-D visualization of the standard model. It would be like trying to make a 3-D version of the periodic table. Also, many of these particles disappear quickly, so it would be particularly hard to depict them. Instead, the student may say they would focus on a few elementary particles.
11. Describe how you would depict the standard model.
Student answers will vary. An example answer is provided below: In my depiction, I want to focus on the basic atom. I think it is important to know that protons are made up of 2 up quarks and one down quark and that neutrons are made up on one up quark and two down quarks. In my 3-D visualization, I would use Starbursts (in their wrappers) to represent the quarks. The up quark would be yellow; the down quark would be red. The proton would have one yellow Starburst (up quark) on each end and the red one (down quark) would be in the middle. The neutrons would be shown with red Starbursts (down quarks) on the ends and a yellow one (up quark). I’d use glitter glue to hold my “protons” and “neutrons” together. The glue represents the gluon, the boson that holds the particles together. I will use tiny zip-lock bags to hold my “atomic nuclei.” The bag can represent the electrons around each “atomic nucleus.”
To convey the idea of antiquarks and positrons, I would again use yellow and red Starbursts and tiny baggies, but everything would be marked with an “A” so the people will know they represent antimatter. To get across the idea that there is a lot less antimatter in the universe than matter, I would put my antiatoms in a quart zip-lock bag and my atoms in a garbage bag. The two bags would be connected with tape or string so people viewing my model would know there is a relationship between the two. The difference in the scale of the two bags is a visual reminder that matter is more common than antimatter.
12. How would you convey the interactions among the various elementary particles?
Student answers will vary. For instance, a student may say that they don’t think the interactions can be shown in a physical model because those interactions happen very fast and some elementary particles don’t last very long. The student may suggest that the concepts covered by the standard model could instead be explained in an animated film .
Additional resources
CERN: The Standard Model
PBS LearningMedia: Atomic Structure
Institute of Physics: The Standard Model
Stanford ATLAS: Introduction to Particle Physics
Berkeley Lab: The Particle Adventure Five mysteries the Standard Model can’t explain
If you're seeing this message, it means we're having trouble loading external resources on our website.
If you're behind a web filter, please make sure that the domains *.kastatic.org and *.kasandbox.org are unblocked.
To log in and use all the features of Khan Academy, please enable JavaScript in your browser.
High school chemistry
Course: high school chemistry > unit 1, the bohr model.
- Understand: Bohr models
- Apply: Bohr models
- The electromagnetic spectrum
- Atomic spectra
- Absorption/emission lines
- Apply: atomic spectra
The electron cloud model
Limitations, how are electrons arranged in bohr models, energy levels, valence electrons, additional notes about bohr models.
- The rings of a Bohr model do NOT represent circular paths followed by electrons. Electrons do NOT orbit the nucleus like planets orbit the sun. The rings are simply a convenient way to represent electron energy levels .
- Sometimes, you may see a Bohr model with rings that get closer together as they get farther out. This represents how the difference between energy levels decreases with greater n . However, since the rings are not intended to perfectly represent electron positions or energies, the exact spacing of the rings is not important.
- Bohr models are not meant to represent what real atoms "look" like. In fact, real atoms are too small to look like anything! The particles inside them have no color and no definite shape. Everything is fuzzy and fluctuating.
Want to join the conversation?
- Upvote Button navigates to signup page
- Downvote Button navigates to signup page
- Flag Button navigates to signup page

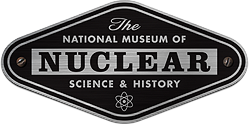
National Museum of Nuclear Science & History

Atom Lesson Plan
- High School
- Middle School
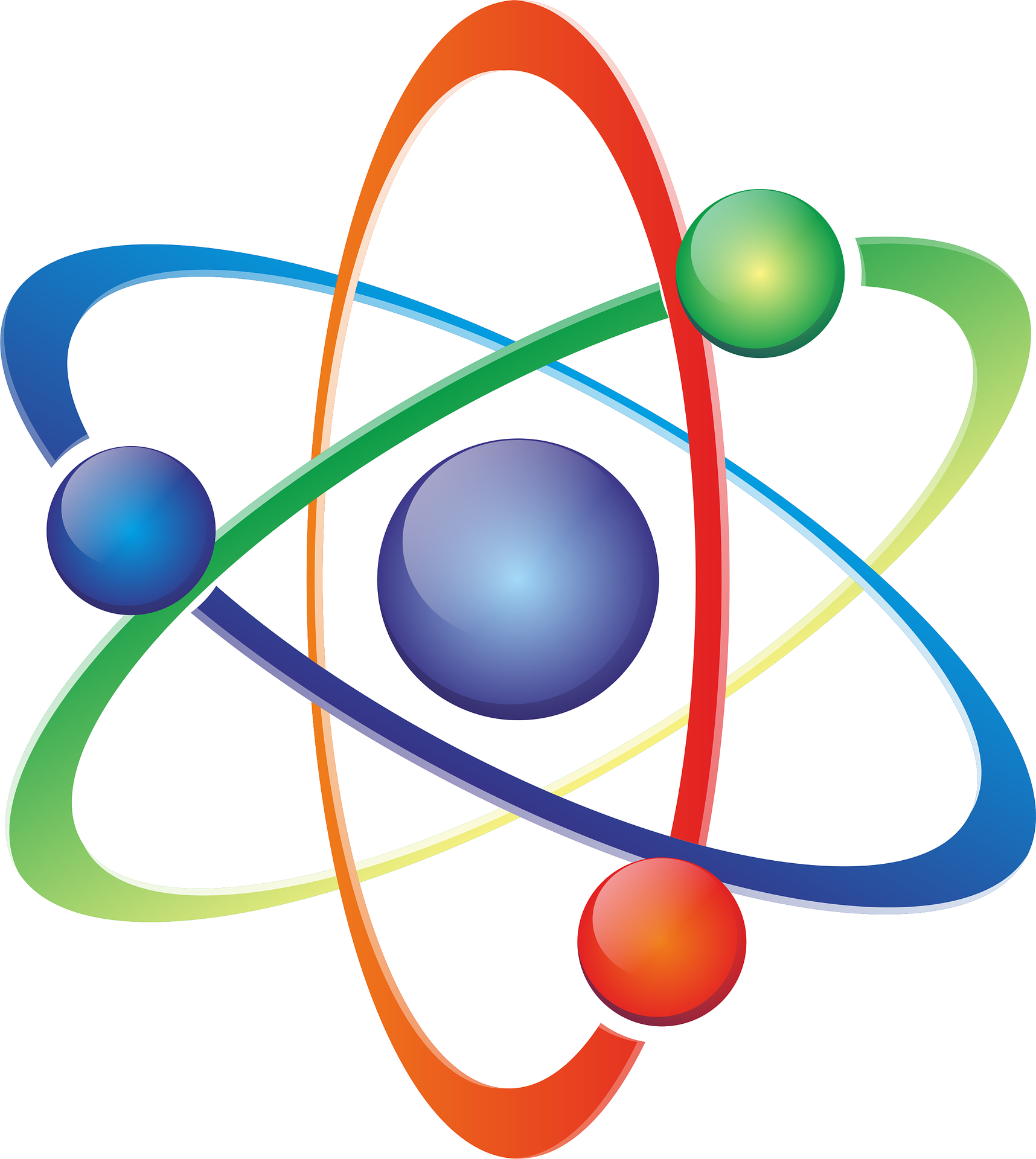
This activity compliments the teacher’s lesson on the atom. In the basic version, students learn about the atom, its structure, the particles. They will also learn how to calculate the atomic mass and find the number of protons, neutrons, and electrons. In the advanced version, students learn about isotopes and ions.
Three sets of signs: red with a plus (protons), yellow (neutron), and blue with a minus (electron). Periodic tables for students to reference later on in the activity.
Helpful visual aid: Bohr’s Model of the atom
Assignment:
Demonstration: The teacher calls on 6 volunteers to the front of the classroom and hands a “proton” to 2 students, a “neutron” to 2 students, and 2 “electrons” to the last student. The “protons” and “neutrons” stand together, while the “electrons” “orbit” around them.
***Students will be forming a helium atom
Explanation: ATOMS are the basic building blocks of matter. They make up everything around you–including air! There are three basic parts of an atom: the PROTON , which has a positive charge; the NEUTRON , which as no charge and is neutral ; and the ELECTRON , which has a negative charge.
***Helpful visual aid: Bohr’s Model of the atom
Practice: The teacher calls on students to point to which volunteers are the proton, neutron, and electron particles. The students explain why they know which volunteer is which particle.
Explanation: The protons and neutrons make up the nucleus, which is the core of the atom. The electrons orbit around the nucleus in electron shells. In general, there are the same number of protons and electrons in an atom. We can tell what element the atom is by counting the number of protons in the nucleus. How many protons are in our atom? The volunteers formed the helium atom, which normally has 2 protons, 2 neutrons, and 2 electrons.
Demonstration: The teacher shows the students how to use the periodic table.
The ATOMIC NUMBER is the number of protons there are in the atom.
The ELEMENT SYMBOL is the element’s shorthand or abbreviation
The ATOMIC WEIGHT / ATOMIC MASS is the average mass of an element. You can find it by adding the protons and neutrons together. The unit of measurement is the ATOMIC MASS UNIT , or AMU .
PUTTING IT ALL TOGETHER
Activity 1: The teacher calls on 4 volunteers to pick up 4 proton signs. What is this element? What are the atomic number, atomic mass, and element symbol? How many neutrons and electrons should there be? The teacher can call on more volunteers to pick up neutron and electron signs, and the volunteers must form the Beryllium atom.
***Element: Beryllium
***Atomic Number: 4
***Atomic Mass: ~9 amu
***Element Symbol: Be
***Hint: To find the number of neutrons, students must subtract the atomic number from the atomic mass (9amu – 4protons = 5neutrons)
***Number of Electrons: 4
The teacher can follow this activity with other elements, too. This activity can also be done without calling volunteers to the front. The teacher calls the atomic number, and students answer the same question. Eg, “This element has 92 protons. What is this element? What are the atomic number, atomic mass, and element symbol? How many neutrons and electrons should there be?”
Activity 2: The teacher names an element, and the students must answer how many protons, neutrons, and electrons there are. Volunteers go to the front of the classroom and form the element’s atom. Students can answer additional questions about the atomic number, atomic mass, and element symbol. This activity can also be done without volunteers. Eg, “What can you tell me about the element uranium?”
ADVANCED VERSION
Explanation: Different versions of the same element can exist. For example, there is U-235 and U-238. U-235 is uranium with an atomic mass of 235 amu and U-238 is uranium with an atomic mass of 238 amu.
Activity: Have the students look on the periodic table and find the atomic mass of uranium. What would change the mass of an atom?
Explanation: Atoms that have the same atomic number (same number of protons) but have a different number of neutrons are called ISOTOPES . Isotopes also have different atomic masses because of the neutrons. For example, U-235 has 143 neutrons, and U-238 has 146 neutrons.
Activity 3: The teacher calls on volunteers to form a hydrogen atom. Students answer questions about the atomic number and atomic mass. Then, the teacher adds a “neutron” to form deuterium. Students answer questions about the atomic number, number of neutrons, and atomic mass. The teacher can add one more “neutron” to form tritium. [Good segue into radiation]
***Remember: a hydrogen atom does NOT have a neutron
***Deuterium: 1 proton, 1 neutron, and ~ 2 amu
***Tritium: 1 proton, 2 neutrons, and about ~3 amu
Explanation: Atoms can also have “positive” and “negative” charges, and atoms with a positive or negative charge are called “ IONS .”
Activity 4: The teacher calls on volunteers to form a neutrally charged lithium atom (3 protons, 3 electrons, and 4 neutrons). The teacher can call on a volunteer to add an electron to form a CATION (a negatively charged atom) or take away an electron to form an ANION (a positively charged atom).

The History of the Atom – Theories and Models
All matter is made up of atoms. This is something we now take as a given and one of the things you learn right back at the beginning of high school or secondary school chemistry classes. Despite this, our ideas about what an atom is are surprisingly recent: as little as one hundred years ago, scientists were still debating what exactly an atom looked like. This graphic takes a look at the key models proposed for the atom, and how they changed over time.
Though our graphic starts in the 1800s, the idea of atoms was around long before. In fact, we have to go all the way back to Ancient Greece to find its genesis. The word ‘atom’ actually comes from Ancient Greek and roughly translates as ‘indivisible’. The Ancient Greek theory has been credited to several different scholars but is most often attributed to Democritus (460–370 BC) and his mentor Leucippus. Though their ideas about atoms were rudimentary compared to our concepts today, they outlined the idea that everything is made of atoms, invisible and indivisible spheres of matter of infinite type and number.
These scholars imagined atoms as varying in shape depending on the type of atom. They envisaged iron atoms as having hooks which locked them together, explaining why iron was a solid at room temperature. Water atoms were smooth and slippery, explaining why water was a liquid at room temperature and could be poured. Though we now know that this is not the case, their ideas laid the foundations for future atomic models.
It was a long wait, however, before these foundations were built upon. It wasn’t until 1803 that the English chemist John Dalton started to develop a more scientific definition of the atom. He drew on the ideas of the Ancient Greeks in describing atoms as small, hard spheres that are indivisible, and that atoms of a given element are identical to each other. The latter point is one that pretty much still holds true, with the notable exception being isotopes of different elements, which differ in their number of neutrons. However, since the neutron wouldn’t be discovered until 1932, we can probably forgive Dalton for this oversight. He came up with theories about how atoms combine to make compounds and also came up with the first set of chemical symbols for the known elements.
Dalton’s outlining of atomic theory was a start, but it still didn’t really tell us much about the nature of atoms themselves. What followed was another, shorter lull where our knowledge of atoms didn’t progress all that much. There were some attempts to define what atoms might look like, such as Lord Kelvin’s suggestion that they might have a vortex-like structure , but it wasn’t until just after the turn of the 20th Century that progress on elucidating atomic structure really started to pick up.
The first breakthrough came in the late 1800s when English physicist Joseph John (JJ) Thomson discovered that the atom wasn’t as indivisible as previously claimed. He carried out experiments using cathode rays produced in a discharge tube and found that the rays were attracted by positively charged metal plates but repelled by negatively charged ones. From this, he deduced the rays must be negatively charged.
By measuring the charge on the particles in the rays, he was able to deduce that they were two thousand times lighter than hydrogen, and by changing the metal the cathode was made from he could tell that these particles were present in many types of atoms. He had discovered the electron (though he referred to it as a ‘corpuscle’), and shown that atoms were not indivisible, but had smaller constituent parts. This discovery would win him a Nobel Prize in 1906.
In 1904, he put forward his model of the atom based on his findings. Dubbed ‘The Plum Pudding Model’ (though not by Thomson himself), it envisaged the atom as a sphere of positive charge, with electrons dotted throughout like plums in a pudding. Scientists had started to peer into the atom’s innards, but Thomson’s model would not hang around for long – and it was one of his students who provided the evidence to consign it to history.
Ernest Rutherford was a physicist from New Zealand who studied at Cambridge University under Thomson. It was his later work at the University of Manchester which would provide further insights into the insides of an atom. This work came after he had already received a Nobel Prize in 1908 for his investigations into the chemistry of radioactive substances.
Rutherford devised an experiment to probe atomic structure which involved firing positively charged alpha particles at a thin sheet of gold foil . The alpha particles were so small they could pass through the gold foil, and according to Thomson’s model which showed the positive charge diffused over the entire atom, they should do so with little or no deflection. By carrying out this experiment, he hoped to be able to confirm Thomson’s model, but he ended up doing exactly the opposite.
During the experiment, most of the alpha particles did pass through the foil with little or no deflection. However, a very small number of the particles were deflected from their original paths at very large angles. This was completely unexpected; as Rutherford himself observed, “It was almost as incredible as if you fired a 15-inch shell at a piece of tissue paper and it came back and hit you”. The only possible explanation was that the positive charge was not spread throughout the atom, but concentrated in a small, dense centre: the nucleus. Most of the rest of the atom was simply empty space.
Rutherford’s discovery of the nucleus meant the atomic model needed a rethink. He proposed a model where the electrons orbit the positively charged nucleus. While this was an improvement on Thomson’s model, it didn’t explain what kept the electrons orbiting instead of simply spiralling into the nucleus.
Enter Niels Bohr. Bohr was a Danish physicist who set about trying to solve the problems with Rutherford’s model. He realised that classical physics could not properly explain what was going on at the atomic level; instead, he invoked quantum theory to try and explain the arrangement of electrons. His model postulated the existence of energy levels or shells of electrons. Electrons could only be found in these specific energy levels; in other words, their energy was quantised, and couldn’t take just any value. Electrons could move between these energy levels (referred to by Bohr as ‘stationary states’), but had to do so by either absorbing or emitting energy.
Bohr’s suggestion of stable energy levels addressed the problem of electrons spiralling into the nucleus to an extent, but not entirely. The exact reasons are a little more complex than we’re going to discuss here because we’re getting into the complex world of quantum mechanics; and as Bohr himself said, “If quantum mechanics hasn’t profoundly shocked you, you haven’t understood it yet”. In other words, it gets kind of weird.
Bohr’s model didn’t solve all the atomic model problems. It worked well for hydrogen atoms, but couldn’t explain observations of heavier elements. It also violates the Heisenberg Uncertainty Principle, one of the cornerstones of quantum mechanics, which states we can’t know both the exact position and momentum of an electron. Still, this principle wasn’t postulated until several years after Bohr proposed his model. Despite all this, Bohr’s is probably still the model of the atom you’re most familiar with since it’s often the one first introduced during high school or secondary school chemistry courses. It still has its uses too; it’s quite handy for explaining chemical bonding and the reactivity of some groups of elements at a simple level.
At any rate, the model still required refining. At this point, many scientists were investigating and trying to develop the quantum model of the atom. Chief amongst these was Austrian physicist Erwin Schrödinger, who you’ve probably heard of before ( he’s the guy with the cat and the box ). In 1926 Schrödinger proposed that, rather than the electrons moving in fixed orbits or shells, the electrons behave as waves. This seems a little weird, but you probably already recall that light can behave as both a wave and a particle (what’s known as a wave-particle duality), and it turns out electrons can too.
Schrödinger solved a series of mathematical equations to come up with a model for the distributions of electrons in an atom. His model shows the nucleus surrounded by clouds of electron density. These clouds are clouds of probability; though we don’t know exactly where the electrons are, we know they’re likely to be found in given regions of space. These regions of space are referred to as electron orbitals. It’s perhaps understandable why high school chemistry lessons don’t lead in straight with this model, though it’s the accepted model today, because it takes a little more time to get your head around!
Schrödinger’s wasn’t quite the last word on the atom. In 1932, the English physicist James Chadwick (a student of Ernest Rutherford) discovered the existence of the neutron, completing our picture of the subatomic particles that make up an atom. The story doesn’t end there either; physicists have since discovered that the protons and neutrons that make up the nucleus are themselves divisible into particles called quarks – but that’s beyond the scope of this post! At any rate, the atom gives us a great example of how scientific models can change over time and shows how new evidence can lead to new models.
Enjoyed this post & graphic? Consider supporting Compound Interest on Patreon!
The graphic in this article is licensed under a Creative Commons Attribution-NonCommercial-NoDerivatives 4.0 International License . See the site’s content usage guidelines .
References & Further Reading
- The development of the atomic model – R Allain, Wired
- Models of the atom – M Fowler
- History and philosophy of science through models: some challenges in the case of the atom (£) – R Justi and J Gilbert
Antonio Balordi
Why bohr’s model couldn’t explain obsvervations of heavier elements?
Justin Mason
Because the Bohr model assumes that electrons are just added as though we were just adding more and more hydrogen. This is incorrect, because things get very complicated after hydrogen, with electron orbitals, the uncertainty principle and wave functions of electrons and many other things.
- Trackback: Atomic Theory | Mrs. Petersen's Site
- Trackback: Atomes | Pearltrees
- Trackback: Atomes et molécules | Pearltrees
- Trackback: Whewell’s Gazette: Year 3, Vol. #09 | Whewell's Ghost
- Trackback: A Brief History of Atomic Theory - Relatively Interesting
Comments are closed.
You May Also Like

Today in Chemistry History: Susan Solomon, ozone depletion, and CFCs

Today in chemistry history: J. J. Thomson and the discovery of the electron

Today in Chemistry History – Ernest Rutherford’s Birthday
Browse Course Material
Course info.
- Prof. Donald Sadoway
Departments
- Materials Science and Engineering
As Taught In
- Chemical Engineering
Learning Resource Types
Introduction to solid state chemistry, self-assessment: structure of the atom.
« Previous | Next »
This self-assessment page completes the Structure of the Atom module, and covers material from the following sessions.
- Session 1: Introduction to Solid State Chemistry
- Session 2: The Periodic Table
- Session 3: Atomic Models: Rutherford & Bohr
- Session 4: Matter/Energy Interactions: Atomic Spectra, Quantum Numbers
- Session 5: Electron Shell Model & Quantum Numbers
- Session 6: Particle-Wave Duality
- Session 7: The Aufbau Principle, Photoelectron Spectroscopy
On this page are a simple weekly quiz and solutions ; relevant exam problems and solutions from the 2009 class; help session videos that review selected solutions to the exam problems; and supplemental exam problems and solutions for further study.
Weekly Quiz and Solutions
This short quiz is given approximately once for every three lecture sessions. You should work through the quiz problems in preparation for the exam problems.
- Quiz problems (PDF)
- Quiz solutions key (PDF)
Exam Problems and Solutions
These exam problems are intended for you to demonstrate your personal mastery of the material, and should be done alone, closed-book, with just a calculator, the two permitted reference tables (periodic table, physical constants), and one 8 1/2" x 11" aid sheet of your own creation.
- Exam problems (PDF)
- Exam solutions key (PDF - 1.9MB)
After you’ve taken the exam, watch the help session videos below for insights into how to approach some of the exam problems.
Exam Help Session Videos
In these videos, 3.091 teaching assistants review some of the exam problems, demonstrating their approach to solutions, and noting some common mistakes made by students.
Clip 1: Exam 1, Problem 1
Clip 2: Exam 1, Problem 2
Clip 3: Exam 1, Problem 4
Clip 4: Exam 1, Problem 6
Clip 5: Exam 2, Problem 2
Supplemental Exam Problems and Solutions
These additional exam problems from prior years’ classes are offered for further study.
- Supplemental exam problems (PDF)
- Supplemental exam solutions key (PDF)

You are leaving MIT OpenCourseWare
- Atoms and Molecules
Atomic Model (Atomic Chemistry)
What is atomic chemistry.
An atom is the smallest unit of ordinary matter that forms a chemical element.
Atoms are made of fundamental particles called protons, neutrons, and electrons. Protons and neutrons clump together to form a central nucleus. The electrons move in a cloud-like region around the nucleus. Most atoms are stable. Their protons, neutrons, and electrons balance.
The electrons of an atom are attracted to the protons in an atomic nucleus by the electromagnetic force. The protons and neutrons in the nucleus are attracted to each other by the nuclear force.
Some atoms have too many neutrons in the nucleus, which makes them unstable. They’re radioactive, giving off particles until they become stable. Atoms with extra or missing electrons are called ions. They have a positive or negative electric charge and are responsible for many chemical reactions.
Table of Contents
Atomic theory, atomic structure.
- Atomic symbol
- Atomic number
- Atomic mass
- Different atomic species
Dalton’s Atomic Theory
According to the postulates proposed by Dalton, the atomic structure comprised atoms, the smallest particle responsible for the chemical reactions to occur.
Postulates of Dalton’s Atomic Theory
The following are the postulates of his theory:
- Every matter is made up of atoms.
- Atoms are indivisible.
- Specific elements have only one type of atom in them.
- Each atom has its own constant mass that varies from element to element..
- Atoms can neither be created nor be destroyed but can be transformed from one form to another.
Dalton’s atomic theory successfully explained the Laws of chemical reactions , namely, the Law of conservation of mass, Law of constant properties, Law of multiple proportions, and Law of reciprocal proportions.
Discovery of subatomic particles electrons, protons, and neutrons discarded the indivisible nature of the atom proposed by John Dalton.
From the several experiments scientists proved that an atom was not the smallest indivisible particle but had a complex structure of its own and is made up of smaller particles like electrons, protons, and neutrons, etc. These particles are termed as subatomic particles.
To explain the distribution of various subatomic particles inside the atom several models were proposed. These were called atomic models .
Thomson Model of Atom
It was proposed by JJ Thomson, in 1898. According to this model, an atom possesses a spherical shape in which the positive charge is uniformly distributed.
This model can be visualized as a pudding with plums or watermelon of positive charge with seeds (electrons) embedded into it. Hence, it is also called plum pudding, raisin pudding, or watermelon model.
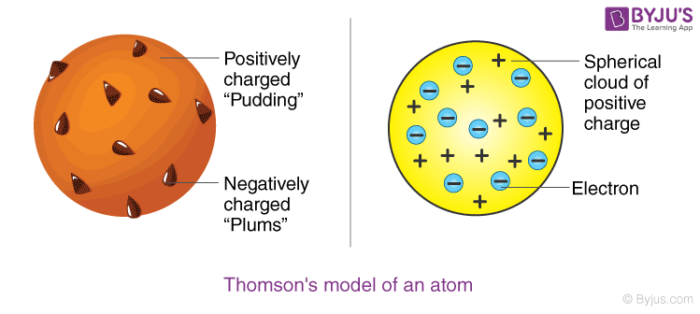
Limitations of Thomson Model
Although this model was able to explain the overall neutrality of the atom, it was not consistent with the results of later experiments, like the α-ray scattering experiment.
Rutherford Model of Atom
- The positively charged particles and most of the mass of an atom were concentrated in an extremely small volume called the nucleus. Nucleus is made up of nucleons.( neutrons + protons)
- There is an empty space around the nucleus called the extranuclear part, in this part electrons are present. Electrons revolve around the nucleus in closed orbits with high speed. The centrifugal force acting on the revolving electrons is being counterbalanced by the force of attraction between the electrons and the nucleus.
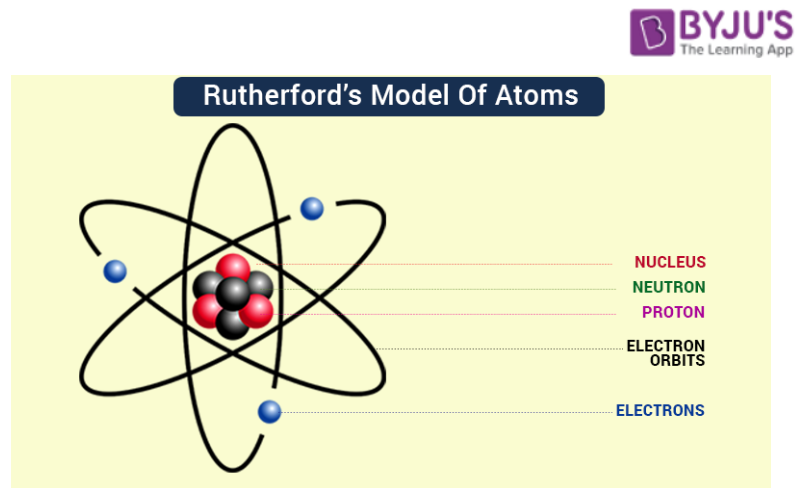
Limitations of Rutherford Model
According to classical electromagnetic theory, accelerated charged particles emit electromagnetic radiation and hence an electron revolving around the nucleus should emit electromagnetic radiation. This radiation would carry energy from the motion of the electron which would come at the cost of shrinking of orbits. Ultimately the electrons would collapse in the nucleus. So Rutherford model was not in accordance with classical electromagnetic and could not explain the stability of an atom .
Bohr’s Model of Atom
Postulates of Bohr’s model are:
- Electrons revolve around the nucleus in a specific circular path known as orbital, energy of each orbital is constant.
- Energy absorbed or emitted during the transition is given by ΔE = hν
- The angular momentum of an electron present in any orbital must be an integral multiple of h/2π
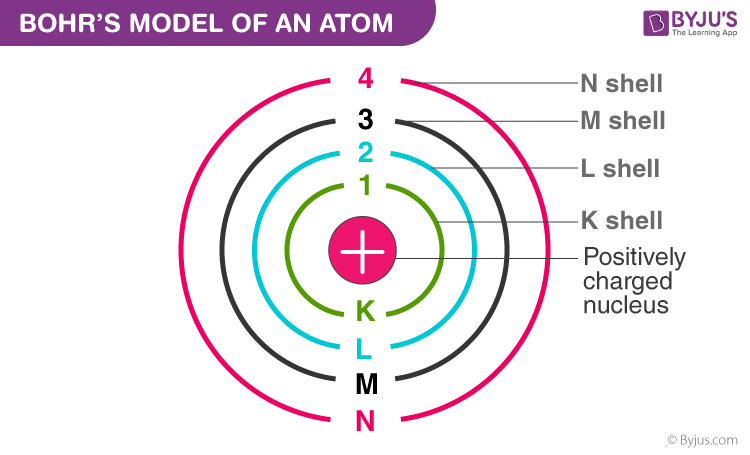
Limitations of Bohr’s Model
- Bohr’s model was not able to explain the Heisenberg uncertainty principle.
- It failed to explain the Stark effect (effect of electric field on the spectra of atoms).
- It is also failed to explain the Zeeman Effect (effect of magnetic field on the spectra of atoms)
Recommended Video
Atomic models – structure of atom.
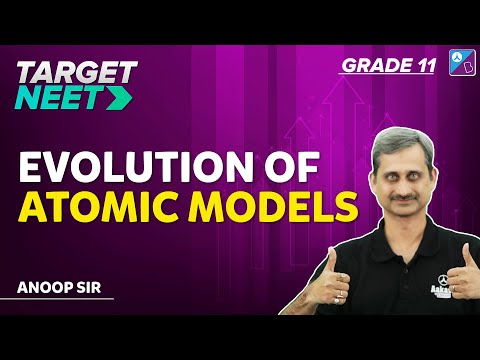
Atomic Symbol
Atom of an element is represented in the form of an atomic symbol.
To know the atomic symbol of 118 element you may visit ➡ Atomic symbol
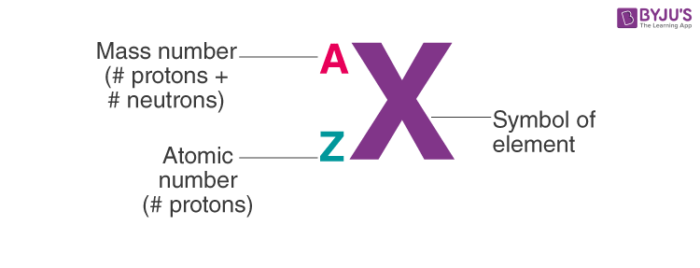
Atomic Number (z)
The number of protons present in the nucleus of an atom is equal to its atomic number (Z).
Thus Atomic number (Z) = number of protons in the nucleus of an atom
Example Atomic number of Carbon(C) = 6 = number of protons
To know the atomic number of 118 elements you may visit ➡ Atomic number
Atomic Mass (A)
Atomic mass is equal to the sum of protons and neutrons present in the nucleus of the atom.
Thus Atomic mass = number of protons + number of neutrons
For Example Atomic mass of carbon = 12
To know the atomic mass of element you may visit ➡ Atomic mass
Different Atomic Species
The types of atomic species are:
Atoms of the same element which have the same atomic number (or number of protons) but have different mass numbers (or number of neutrons) are called isotopes .
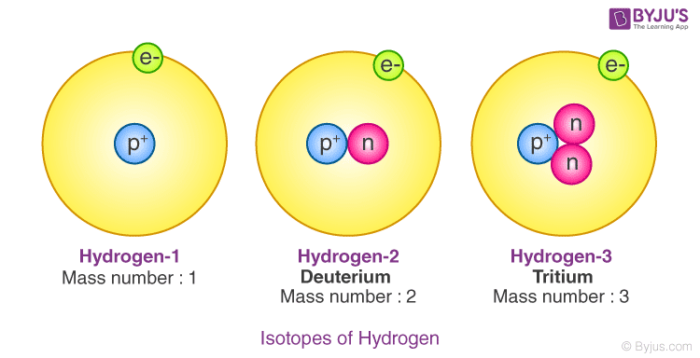
To learn more about its properties you may visit Isotopes of elements
Atoms of different elements that have the same mass number but different atomic numbers are called Isobars.
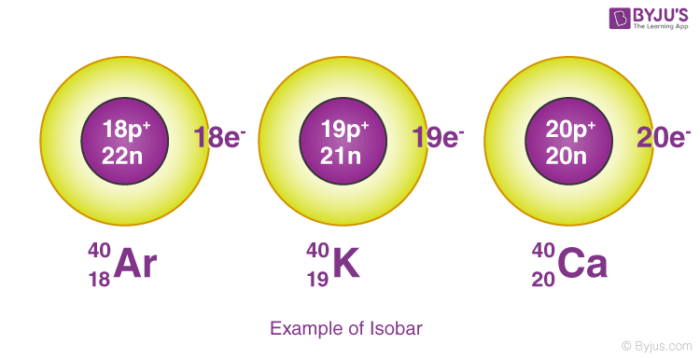
To learn more about the difference between isotopes and isobars visit Isotopes and isobar
Frequently Asked Questions on Atomic
Who discovered atoms.
The idea that everything is made of atoms was pioneered by John Dalton.
What is an atom made of?
Atoms are made of fundamental particles called protons, neutrons, and electrons. Protons and neutrons clump together to form a central nucleus. The electrons move in a cloud-like region around the nucleus.
Are atoms particles or waves?
All matter, which appears to be particulate, can also behave like waves. Atoms are both particles or waves.
Can an element be one atom?
The simplest structural unit of an element is an atom. An element is a substance that is made entirely from one type of atom.
What are atomic mass and atomic number?
Atomic mass is equal to the sum of protons and neutrons present in the nucleus of the atom. The number of protons present in the nucleus of an atom is equal to its atomic number.
Leave a Comment Cancel reply
Your Mobile number and Email id will not be published. Required fields are marked *
Request OTP on Voice Call
Post My Comment

- Share Share
Register with BYJU'S & Download Free PDFs
Register with byju's & watch live videos.


- My Storyboards
History of The Atomic Model
In this activity, activity overview, template and class instructions, more storyboard that activities.
- This Activity is Part of Many Teacher Guides
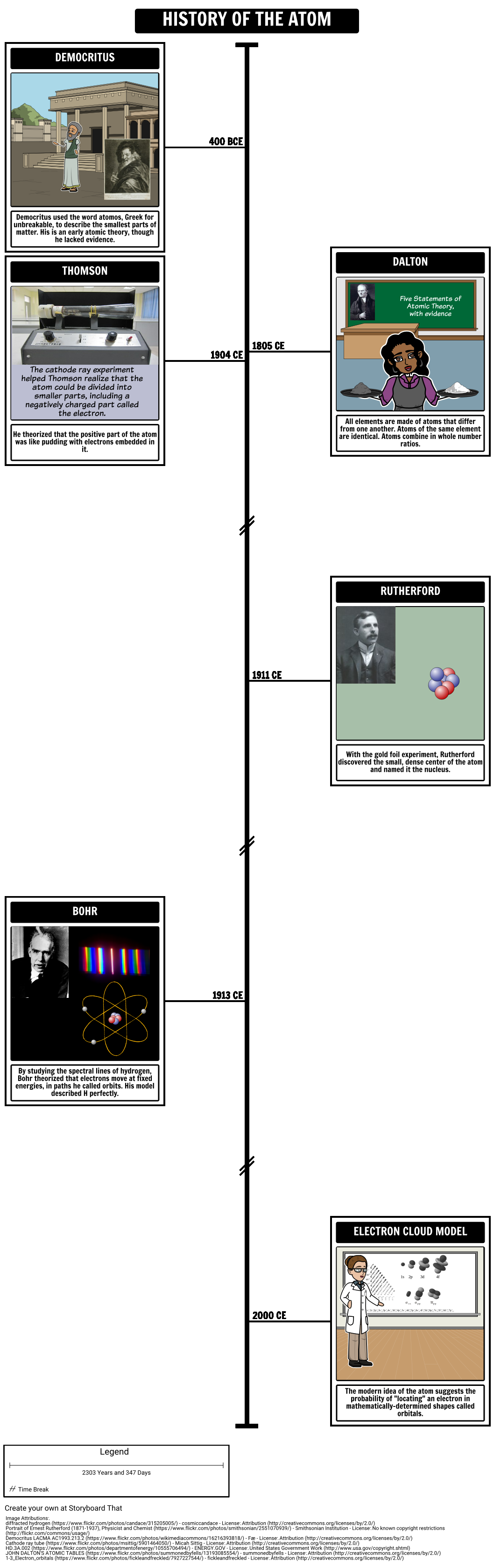
Many lessons on atomic structure begin with a recitation of the historic developments that led to the current theory. It is a valuable experience for students to understand that scientific ideas evolve over time in response to new and better data. Using a timeline layout, students will illustrate major atomic developments and outline how ideas changed over time. They should be sure to include a variety of different scientists and discoveries related to atomic theory in their timeline.
Students can upload pictures of these scientists or, in some cases, of their equipment, using Photos for Class. They can also describe the major advancements in each description box to make as detailed or general a timeline as is required by the level of chemistry they are studying.
For an alternative to the timeline layout, have students create a timeline poster to incorporate into a presentation or gallery walk. You can add more than one template to this assignment to give students lots of options!
Suggested Scientists
- Democritus is often credited with the first atomic theory, though it was a philosophical idea without evidence. He is also credited with using the word " atomos " to describe a small unit of matter that was unbreakable.
- John Dalton completed scientific experiments to develop his atomic model with evidence. His theory included the ideas that atoms make up all matter, are indivisible, and that atoms of an element are identical.
- JJ Thomson is credited with proving the first part of Dalton’s theory incorrect when he discovered the electron, as a result of his cathode ray tube experiment.
- Ernest Rutherford later showed that most of the mass and all of the positive charge of an atom is found in a small dense core of the atom that we call the nucleus .
- Niels Bohr suggested that electrons move on orbits like planets around a sun. This was later found to be too specific a model and was pushed aside for the current theory of quantum mechanics which is based on mathematics and describes a probability of “locating” an electron.
(These instructions are completely customizable. After clicking "Copy Activity", update the instructions on the Edit Tab of the assignment.)
Student Instructions
Create a timeline detailing the important events and scientists in the history of the Atomic Model.
- Click "Start Assignment".
- Add cells to your timeline as needed.
- List out each of the events and scientists in sequential order.
- In the description boxes, describe the events.
- Create an image in each cell that depicts the events, using appropriate scenes, characters, and items.
- Save the storyboard and submit it to the assignment.
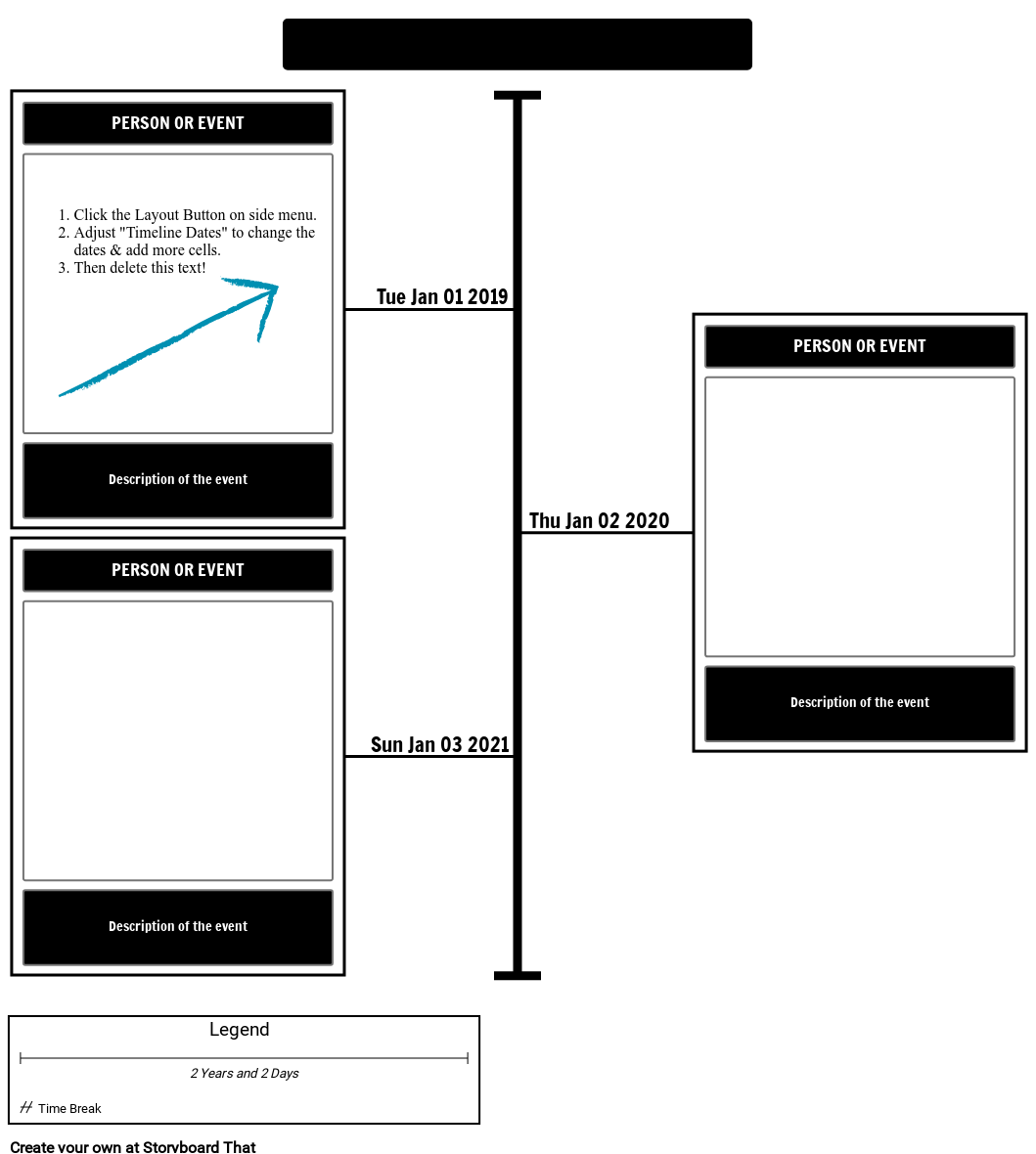
Lesson Plan Reference
Grade Level 9-12
Difficulty Level 3 (Developing to Mastery)
Type of Assignment Individual or Partner
Type of Activity: Timelines
(You can also create your own on Quick Rubric .)
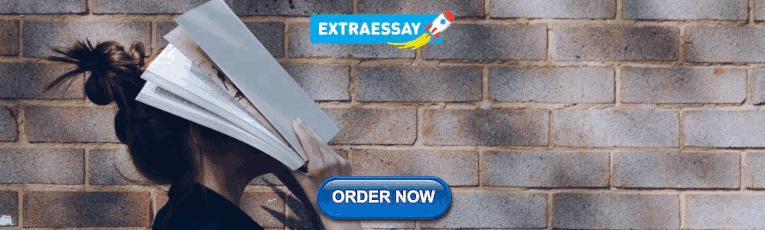
Understanding Atomic Structures
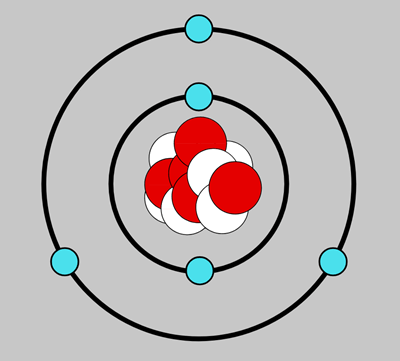
- 1-3_Electron_orbitals • fickleandfreckled • License Attribution (http://creativecommons.org/licenses/by/2.0/)
- Cathode ray tube • Micah Sittig • License Attribution (http://creativecommons.org/licenses/by/2.0/)
- Democritus LACMA AC1993.213.2 • Fæ • License Attribution (http://creativecommons.org/licenses/by/2.0/)
- diffracted hydrogen • cosmiccandace • License Attribution (http://creativecommons.org/licenses/by/2.0/)
- HD.3A.002 • ENERGY.GOV • License United States Government Work (http://www.usa.gov/copyright.shtml)
- JOHN DALTON'S ATOMIC TABLES • summonedbyfells • License Attribution (http://creativecommons.org/licenses/by/2.0/)
- Portrait of Ernest Rutherford (1871-1937), Physicist and Chemist • Smithsonian Institution • License No known copyright restrictions (http://flickr.com/commons/usage/)
Try 1 Month For
30 Day Money Back Guarantee New Customers Only Full Price After Introductory Offer
Learn more about our Department, School, and District packages
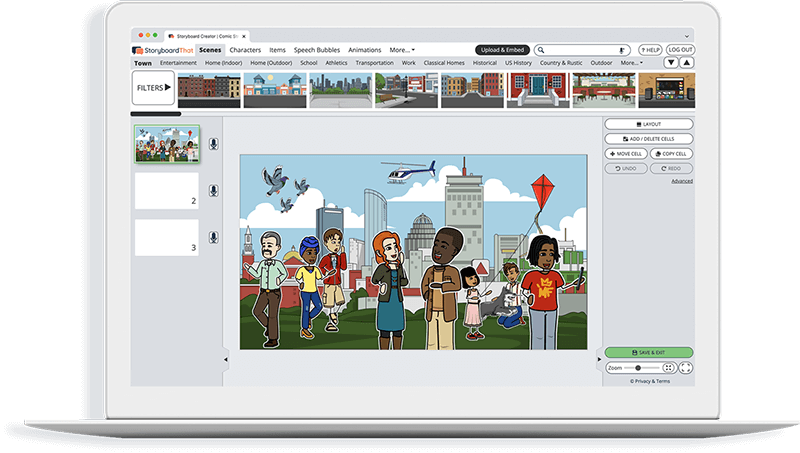
- Thousands of images
- Custom layouts, scenes, characters
- And so much more!!
Create a Storyboard
Module 2: Atoms, Molecules, and Ions
Assignment: atoms, molecules, and ions.
This assignment can be found in Google Docs: Chemistry for Majors Assignment: Atoms, Molecules, and Ions
To make your own copy to edit:
- If you want a Google Doc : in the file menu of the open document, click “Make a copy.” This will give you your own Google Doc to work from.
- If you want a PDF or Word file : in the file menu of the open document, click “Download” and select the file type you would like to have (note: depending on the file type you select, the formatting could get jumbled).
Instructions
As you work these problems, consider and explain:
- What type of question is it?
- How do you know what type of question it is?
- What information are you looking for?
- What information do they give?
- How will you go about solving this?
- Show how to solve the problem.
- Be able to answer for a different reaction, number, set of conditions, etc.
- NH 4 and NH 4 Cl
- ZnO 2 and ZnCl 2
- H 2 O and HCl
- NO and NO 2
- CH 4 and CO 2
- A sample of chemical X is found to contain 5.0 grams of oxygen, 10.0 grams of carbon, and 20.0 grams of nitrogen. The law of definite proportion would predict that a 70 gram sample of chemical X should contain how many grams of carbon?
- All atoms of the same element are identical.
- Compounds are combinations of different atoms.
- A chemical reaction changes the way atoms are grouped together.
- Atoms are indestructible.
- Who was the first scientist to show that atoms emit any negative particles?
- The Rutherford experiment proved the Thomson “plum-pudding” model of the atom to be essentially correct.
- The Rutherford experiment was useful in determining the nuclear charge on the atom.
- Millikan’s oil-drop experiment showed that the charge on any particle was a simple multiple of the charge on the electron.
- The electric discharge tube proved that electrons have a negative charge.
- All of the above experiments gave the results described.
- An atom is mostly empty space.
- Almost all of the mass of the atom is concentrated in the nucleus.
- The protons and neutrons in the nucleus are very tightly packed.
- The number of protons and neutrons is always the same in the neutral atom.
- All of the above statements (A–D) are true.
- [latex]{}_{14}^{16}\text{C}[/latex]
- [latex]{}_{17}^{37}\text{Cl}[/latex]
- [latex]{}_{15}^{32}\text{P}[/latex]
- [latex]{}_{19}^{39}\text{K}[/latex]
- [latex]{}_{14}^{8}\text{N}[/latex]
- The element rhenium (Re) exists as two stable isotopes and 18 unstable isotopes. What does Rhenium-185 have in its nucleus?
- 20 protons and 20 neutrons
- 21 protons and 19 neutrons
- 22 neutrons and 18 protons
- 20 protons and 22 neutrons
- 21 protons and 20 neutrons
- How many protons, neutrons, and electrons does [latex]{}_{20}^{40}\text{Ca}^{2+}[/latex] have?
- How many protons, neutrons, and electrons are in [latex]{}_{19}^{39}\text{K}^+[/latex]
- There is twice as much mass of hydrogen as oxygen in each molecule.
- There are two hydrogen atoms and one oxygen atom per water molecule.
- There is twice as much mass of oxygen as hydrogen in each molecule.
- There are two oxygen atoms and one hydrogen atom per water molecule.
- None of these.
- K, alkali metal
- Ba, alkaline earth metal
- Ne, noble gas
- Ni, transition metal
- Calcium, Ca
- good conductors of heat
- often lustrous
- tend to gain electrons in chemical reactions
- cobalt(II) chloride
- magnesium oxide
- aluminum(III) oxide
- diphosphorus pentoxide
- All of the above names are correct
- iodine trichloride, ICl 3
- phosophorus pentoxide, P 2 O 5
- ammonia, NH 3
- sulfur hexafluoride, SF 6
- All of the above pairs are correct.
- How many oxygen atoms are there in one formula unit of Ca 2+ ?
- What is the correct name for FeO?
- What is the correct name for Ca 2+ ?
- What is the correct name for V 3+ ?
- What is the subscript of barium in the formula of barium sulfate?
- What is the formula for calcium bisulfate?
- Pb(NO 3 ) 2 , lead(II) nitrate
- NH 4 ClO 4 , ammonium perchlorate
- PO 4 3− , phosphate ion
- Mg(OH) 2 , magnesium hydroxide
- NO 3− , nitrite ion
- Authored by : Jessica Garber. Provided by : Tidewater Community College. License : CC BY: Attribution
- Earth & Space
- Life Science
- Physical Science
- Earth and Space Science Curriculum Map
- Physical Science Curriculum Map
- Life Science Curriculum Map
- Biology Curriculum Map
- Chemistry Curriculum Map
NGSS Science Curriculum for Science Teachers

- All Courses
- Earth Science Materials
- Physical Science Materials
- Biology Materials
- Chemistry Materials
- Teacher Forums
1-3 Models of an Atom
Here is your free content for your lesson on models of an atom.
Models of an Atom Worksheet - Word Docs, PowerPoint, & PDF's
Please note this lesson is split into 2 parts because of the length and depth of the lesson.
ADDITIONAL RESOURCES 1-3 Additional Resources - Models of the Atom (Doc) 1-3 Additional Resources - Models of the Atom (PDF) GUIDED NOTES 1-3 Guided Notes SE - Models of the Atom (Doc) 1-3 Guided Notes SE - Models of the Atom (PDF) 1-3 Guided Notes TE - Models of the Atom (Doc) 1-3 Guided Notes TE - Models of the Atom (PDF) POWERPOINT PRESENTATION 1-3 Slide Show - Models of the Atom (PPT) 1-3 Slide Show - Models of the Atom (PDF) PART A BELL RINGER 1-3a Bell Ringer SE - Models of the Atom (Doc) 1-3a Bell Ringer SE - Models of the Atom (PDF) 1-3a Bell Ringer TE - Models of the Atom (Doc) 1-3a Bell Ringer TE - Models of the Atom (PDF) EXIT QUIZ 1-3a Exit Quiz SE - Models of the Atom (Doc) 1-3a Exit Quiz SE - Models of the Atom (PDF) 1-3a Exit Quiz TE - Models of the Atom (Doc) 1-3a Exit Quiz TE - Models of the Atom (PDF) HOMEWORK ASSIGNMENT 1-3a Homework Questions SE - Models of the Atom (Doc) 1-3a Homework Questions SE - Models of the Atom (PDF) 1-3a Homework Questions TE - Models of the Atom (Doc) 1-3a Homework Questions TE - Models of the Atom (PDF) LESSON PLAN 1-3a Lesson Plan - Models of the Atom (Doc) 1-3a Lesson Plan - Models of the Atom (PDF) VOCABULARY 1-3a Vocabulary Worksheet SE - Models of the Atom (Doc) 1-3a Vocabulary Worksheet SE - Models of the Atom (PDF) 1-3a Vocabulary Worksheet TE - Models of the Atom (Doc) 1-3a Vocabulary Worksheet TE - Models of the Atom (PDF) PART B BELL RINGER 1-3b Bell Ringer SE - Models of the Atom (Doc) 1-3b Bell Ringer SE - Models of the Atom (PDF) 1-3b Bell Ringer TE - Models of the Atom (Doc) 1-3b Bell Ringer TE - Models of the Atom (PDF) EXIT QUIZ 1-3b Exit Quiz SE - Models of the Atom (Doc) 1-3b Exit Quiz SE - Models of the Atom (PDF) 1-3b Exit Quiz TE - Models of the Atom (Doc) 1-3b Exit Quiz TE - Models of the Atom (PDF) HOMEWORK ASSIGNMENT 1-3b Homework Questions SE - Models of the Atom (Doc) 1-3b Homework Questions SE - Models of the Atom (PDF) 1-3b Homework Questions TE - Models of the Atom (Doc) 1-3b Homework Questions TE - Models of the Atom (PDF) LESSON PLAN 1-3b Lesson Plan - Models of the Atom (Doc) 1-3b Lesson Plan - Models of the Atom (PDF) VOCABULARY 1-3b Vocabulary Doodle Notes SE - Models of the Atom (Doc) 1-3b Vocabulary Doodle Notes SE - Models of the Atom (PDF)
Want access to our Full Chemistry Curriculum?
Simply click the image below to get access to all of our lessons.
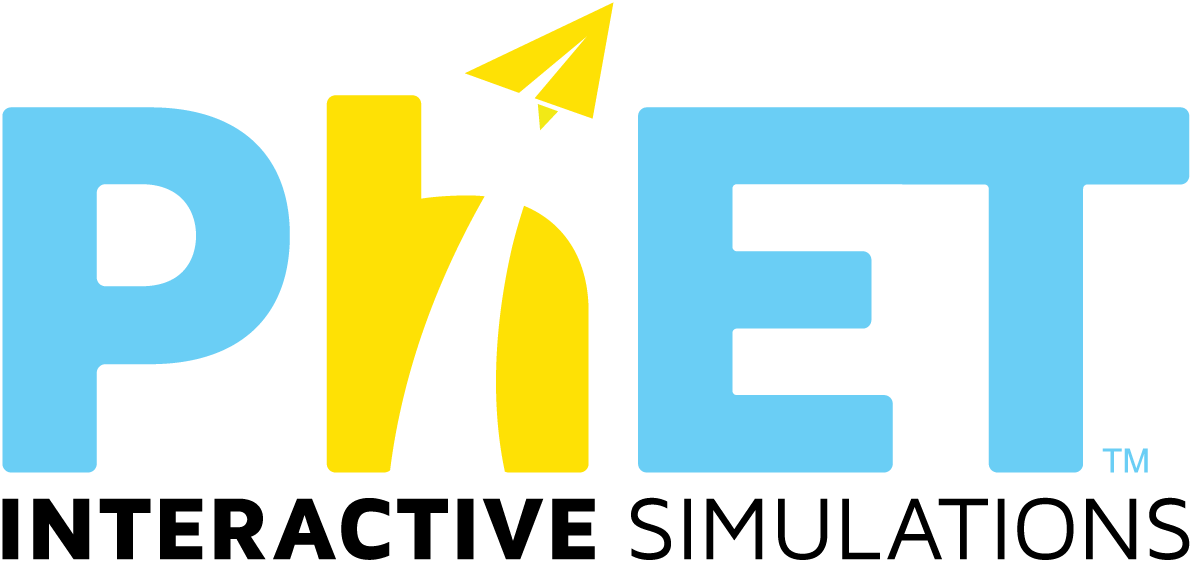
- Sign in / Register
- Administration
- Edit profile
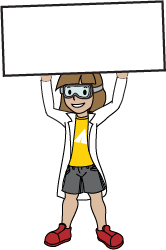
Models of the Atom
- Rutherford Alpha Scattering - Comparing Models.docx - 257 kB