Thank you for visiting nature.com. You are using a browser version with limited support for CSS. To obtain the best experience, we recommend you use a more up to date browser (or turn off compatibility mode in Internet Explorer). In the meantime, to ensure continued support, we are displaying the site without styles and JavaScript.
- View all journals
- Explore content
- About the journal
- Publish with us
- Sign up for alerts
- 08 August 2023
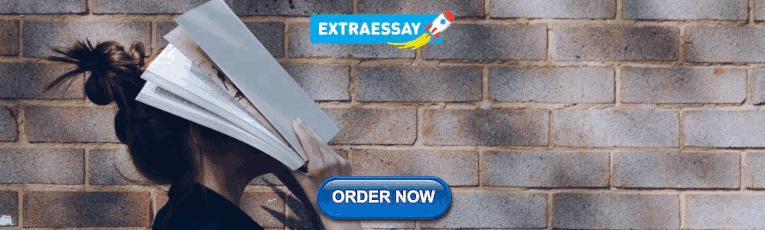
Clean energy can fuel the future — and make the world healthier
You have full access to this article via your institution.
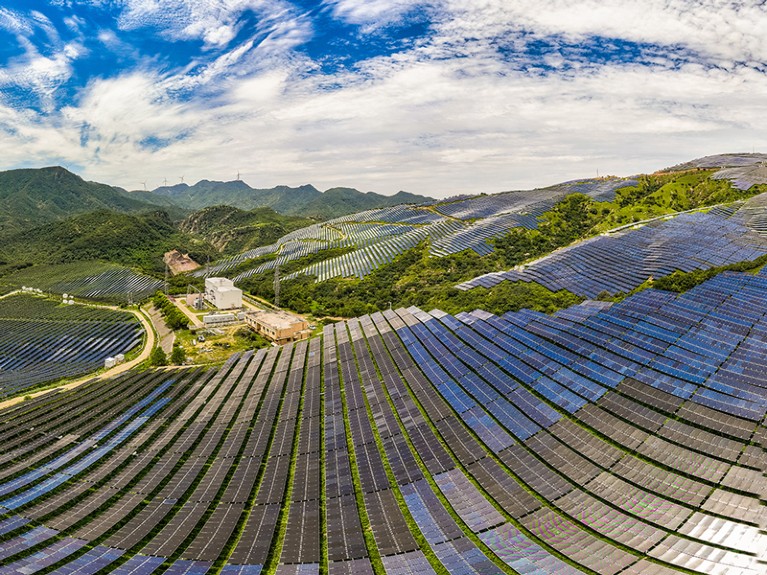
China is on track to reach its solar-power target for 2030. Credit: Zhao Yongtao/VCG/Getty
The 2030 targets laid out by the United Nations for the seventh Sustainable Development Goal (SDG 7) are clear enough: provide affordable access to energy; expand use of renewable sources; improve energy efficiency year on year; and enhance international cooperation in support of clean-energy research, development and infrastructure. Meeting those goals, however, will be anything but simple. As seen in many of the editorials in this series examining the SDGs at their halfway stage , the world is falling short.
This is due, at least in part, to the influence of the fossil-fuel industry, which drives the economics and, often, the politics of countries large and small, rich and poor. Rising human prosperity, as measured by economic growth, has long been linked to an abundance of fossil fuels. Many politicians fear that the pursuit of clean-energy sources will compromise that economic development. The latest science clearly counters this view — but the voice of the research community is not being heard in the right places. To meet the targets embodied in SDG 7, that has to change.
There is much to be done. In 2021, some 675 million people worldwide still did not have access to electricity. This is down from 1.1 billion a decade or so ago, but the pace of progress has slowed. On the basis of current trends, 660 million people, many of them in sub-Saharan Africa, will remain without electricity by 2030. And projections indicate that some 1.9 billion people will still be using polluting and inefficient cooking systems fuelled by coal and wood (see go.nature.com/3s8d887 ). This is bad news all round: for health, biodiversity and the climate.
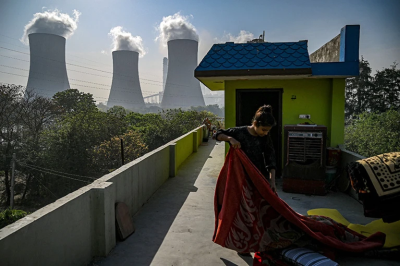
Carbon emissions hit new high: warning from COP27
Achieving the energy-access targets was always going to be a stretch, but progress has been slow elsewhere, too. Take energy efficiency. More energy efficiency means less pollution, and energy efficiency has increased by around 2% annually in the past few years. But meeting the target for 2030 — to double the rate of the 1990–2010 average — would require gains of around 3.4% every year for the rest of this decade.
The picture for renewable energy is similarly mixed. Despite considerable growth in wind and solar power to generate grid electricity, progress in the heat and transport sectors remains sluggish. Renewable energy’s share of total global energy consumption was just 19.1% in 2020, according to the latest UN tracking report, but one-third of that came from burning resources such as wood.
One reason for the slow progress is the continued idea that aggressive clean-energy goals will get in the way of economic development. It’s easier and more profitable for major fossil-fuel producers to simply maintain the status quo. Just last month, ministers from the G20 group of the world’s biggest economies, including the European Union, India, Saudi Arabia and the United States, failed to agree on a plan to phase out fossil fuels and triple the capacity of renewable energy by 2030.
But this is where science has a story to tell. In the past, researchers say, many models indicated that clean energy would be more expensive than that from fossil fuels, potentially pricing the poorest nations out of the market as well as driving up people’s food bills and exacerbating hunger. But the latest research suggests that the picture is more complex. Energy is a linchpin for most of the SDGs, and research that merges climate, energy and the SDGs underscores this 1 . For example, the agriculture and food-transport sectors still depend on fossil fuels, and that generates pollution that kills millions of people each year. Other links are indirect: lack of access to light at night and to online information — as a result of energy poverty — hampers educational attainment and contributes to both long- and short-term inequality.
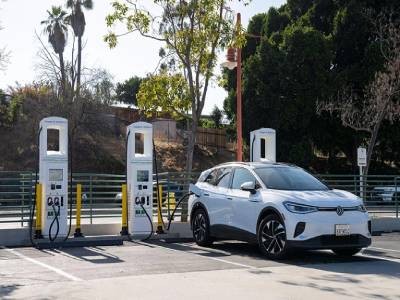
US aims for electric-car revolution — will it work?
The lesson from research is that it might be easier, not harder, to address these challenges together. In 2021, researcher Gabriela Iacobuţă at the German Institute of Development and Sustainability in Bonn and her colleagues showed that technologies centred on renewable resources and efficiency tend to come with few trade-offs and many benefits, including improved public health and wealth, thanks to a cleaner environment and better jobs 2 . And climate scientist Bjoern Soergel at the Potsdam Institute for Climate Impact Research in Germany and his colleagues found that a coordinated package of climate and development policies could achieve most of the SDGs while limiting global warming to 1.5 °C above pre-industrial levels 3 .
The study assessed 56 indicators across all 17 SDGs. One proposed intervention is an international climate finance mechanism that would levy fees on carbon emissions that would be redistributed through national programmes to reduce poverty. A second focuses on promoting healthy diets — including reducing the consumption of meat, the production of which requires a lot of water, energy and land. This would benefit people on low incomes by lowering both food and energy prices.
The biggest challenge lies in translating these models to the real world. To do so, we need leaders who are not bound by outmoded thinking, are aware of the latest science and can draw on the research to build public support for the necessary energy transition. We require more national and international public institutions that are willing to address problems at the system level. And all of this needs a science community that is willing and able to champion knowledge and evidence.
Nature 620 , 245 (2023)
doi: https://doi.org/10.1038/d41586-023-02510-y
Vohra, K. et al. Environ. Res. 195 , 110754 (2021).
Article PubMed Google Scholar
Iacobuţă, G. I., Höhne, N., van Soest, H. L. & Leemans, R. Sustainability 13 , 10774 (2021).
Article Google Scholar
Soergel, B. et al. Nature Clim. Change 11 , 656–664 (2021).
Download references
Reprints and permissions
Related Articles
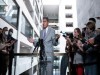
- Environmental sciences
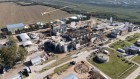
Argentina’s pioneering nuclear research threatened by huge budget cuts
News 07 MAY 24
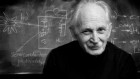
Allen J. Bard obituary: electrochemist whose techniques underpin clinical diagnostics, materials discovery and more
Obituary 01 MAY 24
It’s time to talk about the hidden human cost of the green transition
Correspondence 16 APR 24
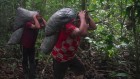
Forestry social science is failing the needs of the people who need it most
Editorial 15 MAY 24
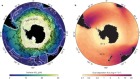
One-third of Southern Ocean productivity is supported by dust deposition
Article 15 MAY 24
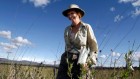
Diana Wall obituary: ecologist who foresaw the importance of soil biodiversity
Obituary 10 MAY 24
Real-world plastic-waste success stories can help to boost global treaty
Correspondence 14 MAY 24
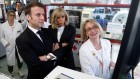
A DARPA-like agency could boost EU innovation — but cannot come at the expense of existing schemes
Editorial 14 MAY 24
Japan can embrace open science — but flexible approaches are key
Correspondence 07 MAY 24
Senior Research Assistant in Human Immunology (wet lab)
Senior Research Scientist in Human Immunology, high-dimensional (40+) cytometry, ICS and automated robotic platforms.
Boston, Massachusetts (US)
Boston University Atomic Lab
Postdoctoral Fellow in Systems Immunology (dry lab)
Postdoc in systems immunology with expertise in AI and data-driven approaches for deciphering human immune responses to vaccines and diseases.
Global Talent Recruitment of Xinjiang University in 2024
Recruitment involves disciplines that can contact the person in charge by phone.
Wulumuqi city, Ürümqi, Xinjiang Province, China
Xinjiang University
Tenure-Track Assistant Professor, Associate Professor, and Professor
Westlake Center for Genome Editing seeks exceptional scholars in the many areas.
Westlake Center for Genome Editing, Westlake University
Faculty Positions at SUSTech School of Medicine
SUSTech School of Medicine offers equal opportunities and welcome applicants from the world with all ethnic backgrounds.
Shenzhen, Guangdong, China
Southern University of Science and Technology, School of Medicine
Sign up for the Nature Briefing newsletter — what matters in science, free to your inbox daily.
Quick links
- Explore articles by subject
- Guide to authors
- Editorial policies
Sustainable Energy Research
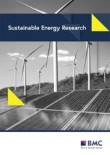
For articles submitted in 2023 or 2024, Sustainable Energy Research has waivers available to offer that can be allocated on acceptance. Once the standard peer review process has been completed and the Editor-in-Chief has made a decision to accept the article, authors without funding available won’t need to pay an APC.
Please direct all waiver-related enquiries to the journal Publisher .
Design models for small run-of-river hydropower plants: a review
This review analyzes several existing models to estimate the energy produced and expected cost of small hydropower projects for grid-connected and off-grid/micro-grid applications and aims to suggest the most appropriate model according to the context of study and proposes methods to use them more efficiently.
Can wind and solar replace coal in Texas?
An investigation into the potential for new wind and solar projects already proposed in the ERCOT interconnection queue as of June 2020 to replace the coal power that remained in 2019. The authors conclude that the wind and solar portfolio would outproduce retired coal on summer afternoons when demand peaks, leaving small gaps in evenings and shoulder seasons when demand is lower.
As of the 1st of January 2023, Renewables: Wind, Water, and Solar is published under the new title Sustainable Energy Research . The journal welcomes contributions on all sources of energy that support a sustainable approach to energy transformation, including renewable energy, energy efficient systems, and innovative and green systems that contribute to reducing energy poverty and the use of polluting and inefficient energy systems.
While focusing primarily on basic science and technological aspects, Sustainable Energy Research considers reviews and policy issue articles on themes that affect sustainable energy technologies and their implementation.
Call for Papers
Advances in Green Energy Solutions for Harnessing Wastewater Management Practices
Guest Editors:
Maulin Pramod Shah, PhD, Enviro Technology Limited, India
Sema Sevinç Şengör, PhD, Middle East Technical University, Turkey
Submission Status: Open until 30 January 2025
Integration of renewable energy sources and energy infrastructure
Do you have an idea for an article collection? Please let us know by filling in this form here .
- Most accessed
- Collections
Harnessing the power of wind: a comprehensive analysis of wind energy potential in Dhaka city
Authors: Mohammad Liton Hossain, S. M. Nasif Shams and Saeed Mahmud Ullah
Combatting energy poverty in eastern Turkey: innovative debt solutions and the power-cut index
Authors: Tamer Emre and Adnan Sözen
Assessment of the locational potential of floating offshore wind energy in South Africa
Authors: Kubiat Umoh, Abbas Hasan, Amangeldi Kenjegaliev and Ayman Al-Qattan
Investigating the role of subsistence renewables in alleviating power poverty within Nigeria’s energy-mix strategy
Authors: David Oluseun Olayungbo, Ayodele Adekunle Faiyetole and Adenike Anike Olayungbo
Modeling, design and optimization of integrated renewable energy systems for electrification in remote communities
Authors: Kuanrong Qiu and Evgueniy Entchev
Most recent articles RSS
View all articles
An experimental study on effect of dust on power loss in solar photovoltaic module
Authors: Athar Hussain, Ankit Batra and Rupendra Pachauri
Wind turbine performance analysis for energy cost minimization
Authors: Yassine Charabi and Sabah Abdul-Wahab
The Correction to this article has been published in Renewables: Wind, Water, and Solar 2021 8 :2
Estimation of solar radiation from cloud cover data of Bangladesh
Authors: Md. Nazmul Islam Sarkar
Low-cost virtual instrumentation of PV panel characteristics using Excel and Arduino in comparison with traditional instrumentation
Authors: Aboubakr El Hammoumi, Saad Motahhir, Abdelilah Chalh, Abdelaziz El Ghzizal and Aziz Derouich
A comprehensive decade review and analysis on designs and performance parameters of passive solar still
Authors: Himanshu Manchanda and Mahesh Kumar
Most accessed articles RSS
Open For Submissions
Edited by: Maulin Pramod Shah, Sema Sevinç Şengör
Edited by: Zafar Said, Haris M. Khalid
Closed For Submissions
Energy and material recovery in waste management
Edited by: Shrikaant Kulkarni
Check out the Springer Nature SDG 7 hub for content related to Affordable and Clean Energy
Trending articles
Click here to view which articles have been shared the most
Aims and scope
Sustainable Energy Research (formerly Renewables: Wind, Water, and Solar ) provides a multidisciplinary and international forum for research in the basic science, technologies, industrial R&D, products and system implementation that accelerate the transition to sustainable energy on a local and global scale. Sustainable Energy Research welcomes contributions on all sources of energy that support a sustainable approach to energy transformation, including renewable energy, energy efficient systems, and innovative and green systems that contribute to reducing energy poverty and the use of polluting and inefficient energy systems. While focusing primarily on basic science and technological aspects, Sustainable Energy Research considers reviews and policy issue articles on themes that affect sustainable energy technologies and their implementation. Furthermore, articles are welcome as they contribute to the achievement of the United Nations Sustainable Development Goal 7 ‘Affordable and Clean Energy’, particularly in increasing substantially the share of renewable energy in the global energy mix, and doubling the global rate of improvement in energy efficiency. Sustainable Energy Research serves as a forum for specialists from both academic institutions, research laboratories and industries involved in R&D projects, system implementation and policy formulations in this field. Topics in scope include:
- Solar energy
- Wind energy
- Wave energy
- Geothermal energy
- Hydroelectricity
- Energy harvesting devices
- Hydrogen energy
- Low carbon energy generation
- Batteries and energy storage
- Supercapacitors
- Electrocatalysis and photocatalysis
- Carbon capture and utilization
- Energy systems, processes, planning and policy
- Energy grids and networks
- Energy economics
- Editorial Board
- Sign up for article alerts and news from this journal
Article Types
The journal offers the following article types:
- Original Research Articles
- Policy Issue Articles
- Case Studies
- Letters to the Editor
- Design and simulation studies
Annual journal metrics
2023 Speed 8 days submission to first editorial decision for all manuscripts (Median) 106 days submission to accept (Median)
2023 Usage 132,400 downloads 12 Altmetric mentions
- More about our metrics
Institutional membership
Building a sustainable energy future calls for leaps forward in both technology and policy leadership. State governments, major corporations and nations around the world have pledged to address the worsening climate crisis by transitioning to 100% renewable energy over the next few decades. Turning those statements of intention into a reality means undertaking unprecedented efforts and collaboration between disciplines ranging from environmental science to economics.
There are highly promising opportunities for green initiatives that could deliver a better future. However, making a lasting difference will require both new technology and experts who can help governments and organizations transition to more sustainable practices. These leaders will be needed to source renewables efficiently and create environmentally friendly policies, as well as educate consumers and policymakers. To maximize their impact, they must make decisions informed by the most advanced research in clean energy technology, economics, and finance.
Current Trends in Sustainability
The imperative to adopt renewable power solutions on a worldwide scale continues to grow even more urgent as the global average surface temperature hits historic highs and amplifies the danger from extreme weather events . In many regions, the average temperature has already increased by 1.5 degrees , and experts predict that additional warming could drive further heatwaves, droughts, severe hurricanes, wildfires, sea level rises, and even mass extinctions.
In addition, physicians warn that failure to respond to this dire situation could unleash novel diseases : Dr. Rexford Ahima and Dr. Arturo Casadevall of the Johns Hopkins University School of Medicine contributed to an article in the Journal of Clinical Investigation that explained how climate change could affect the human body’s ability to regulate its own temperature while bringing about infectious microbes that adapt to the warmer conditions.
World leaders have accepted that greenhouse gas emissions are a serious problem that must be addressed. Since the Paris Agreement was first adopted in December 2015, 197 nations have signed on to its framework for combating climate change and preventing the global temperature increase from reaching 2 degrees Celsius over preindustrial levels.
Corporate giants made their own commitments to become carbon neutral by funding offsets to reduce greenhouse gases and gradually transitioning into using 100% renewable energy. Google declared its operations carbon neutral in 2017 and has promised that all data centers and campuses will be carbon-free by 2030. Facebook stated that it would eliminate its carbon footprint in 2020 and expand that commitment to all the organization’s suppliers within 10 years. Amazon ordered 100,000 electric delivery vehicles and has promised that its sprawling logistics operations will arrive at net-zero emissions by 2040.
Despite these promising developments, many experts say that nations and businesses are still not changing fast enough. While carbon neutrality pledges are a step in the right direction, they don’t mean that organizations have actually stopped using fossil fuels . And despite the intentions expressed by Paris Agreement signatories, total annual carbon dioxide emissions reached a record high of 33.5 gigatons in 2018, led by China, the U.S., and India.
“The problem is that what we need to achieve is so daunting and taxes our resources so much that we end up with a situation that’s much, much worse than if we had focused our efforts,” Ferraro said.
Recent Breakthroughs in Renewable Power
An environmentally sustainable infrastructure requires innovations in transportation, industry, and utilities. Fortunately, researchers in the private and public sectors are laying the groundwork for an energy transformation that could make the renewable energy of the future more widely accessible and efficient.
Some of the most promising areas that have seen major developments in recent years include:
Driving Electric Vehicles Forward
The technical capabilities of electric cars are taking great strides, and the popularity of these vehicles is also growing among consumers. At Tesla’s September 22, 2020 Battery Day event, Elon Musk announced the company’s plans for new batteries that can be manufactured at a lower cost while offering greater range and increased power output .
The electric car market has seen continuing expansion in Europe even during the COVID-19 pandemic, thanks in large part to generous government subsidies. Market experts once predicted that it would take until 2025 for electric car prices to reach parity with gasoline-powered vehicles. However, growing sales and new battery technology could greatly speed up that timetable .
Cost-Effective Storage For Renewable Power
One of the biggest hurdles in the way of embracing 100% renewable energy has been the need to adjust supply based on demand. Utilities providers need efficient, cost-effective ways of storing solar and wind power so that electricity is available regardless of weather conditions. Most electricity storage currently takes place in pumped-storage hydropower plants, but these facilities require multiple reservoirs at different elevations.
Pumped thermal electricity storage is an inexpensive solution to get around both the geographic limitations of hydropower and high costs of batteries. This approach, which is currently being tested , uses a pump to convert electricity into heat so it can be stored in a material like gravel, water, or molten salts and kept in an insulated tank. A heat engine converts the heat back into electricity as necessary to meet demand.
Unlocking the Potential of Microgrids
Microgrids are another area of research that could prove invaluable to the future of power. These systems can operate autonomously from a traditional electrical grid, delivering electricity to homes and business even when there’s an outage. By using this approach with power sources like solar, wind, or biomass, microgrids can make renewable energy transmission more efficient.
Researchers in public policy and engineering are exploring how microgrids could serve to bring clean electricity to remote, rural areas . One early effort in the Netherlands found that communities could become 90% energy self-sufficient , and solar-powered microgrids have now also been employed in Indian villages. This technology has enormous potential to change the way we access electricity, but lowering costs is an essential step to bring about wider adoption and encourage residents to use the power for purposes beyond basic lighting and cooling.
Advancing the Future of Sustainable Energy
There’s still monumental work to be done in developing the next generation of renewable energy solutions as well as the policy framework to eliminate greenhouse gases from our atmosphere. An analysis from the International Energy Agency found that the technologies currently on the market can only get the world halfway to the reductions needed for net-zero emissions by 2050.
To make it the rest of the way, researchers and policymakers must still explore possibilities such as:
- Devise and implement large-scale carbon capture systems that store and use carbon dioxide without polluting the atmosphere
- Establish low-carbon electricity as the primary power source for everyday applications like powering vehicles and heat in buildings
- Grow the use of bioenergy harnessed from plants and algae for electricity, heat, transportation, and manufacturing
- Implement zero-emission hydrogen fuel cells as a way to power transportation and utilities
However, even revolutionary technology will not do the job alone. Ambitious goals for renewable energy solutions and long-term cuts in emissions also demand enhanced international cooperation, especially among the biggest polluters. That’s why Jonas Nahm of the Johns Hopkins School of Advanced International Studies has focused much of his research on China’s sustainable energy efforts. He has also argued that the international community should recognize China’s pivotal role in any long-term plans for fighting climate change.
As both the leading emitter of carbon dioxide and the No. 1 producer of wind and solar energy, China is uniquely positioned to determine the future of sustainability initiatives. According to Nahm, the key to making collaboration with China work is understanding the complexities of the Chinese political and economic dynamics. Because of conflicting interests on the national and local levels, the world’s most populous nation continues to power its industries with coal even while President Xi Jinping advocates for fully embracing green alternatives.
China’s fraught position demonstrates that economics and diplomacy could prove to be just as important as technical ingenuity in creating a better future. International cooperation must guide a wide-ranging economic transformation that involves countries and organizations increasing their capacity for producing and storing renewable energy.
It will take strategic thinking and massive investment to realize a vision of a world where utilities produce 100% renewable power while rows of fully electric cars travel on smart highways. To meet the challenge of our generation, it’s more crucial than ever to develop leaders who understand how to apply the latest research to inform policy and who can take charge of globe-spanning sustainable energy initiatives .
About the MA in Sustainable Energy (online) Program at Johns Hopkins SAIS
Created by Johns Hopkins University School of Advanced International Studies faculty with input from industry experts and employers, the Master of Arts in Sustainable Energy (online) program is tailored for the demands of a rapidly evolving sector. As a top-11 global university, Johns Hopkins is uniquely positioned to equip graduates with the skills they need to confront global challenges in the transition to renewable energy.
The MA in Sustainable Energy curriculum is designed to build expertise in finance, economics, and policy. Courses from our faculty of highly experienced researchers and practitioners prepare graduates to excel in professional environments including government agencies, utility companies, energy trade organizations, global energy governance organizations, and more. Students in the Johns Hopkins SAIS benefit from industry connections, an engaged network of more than 230,000 alumni, and high-touch career services.
Request Information
To learn more about the MA in Sustainable Energy (online) and download a brochure , fill out the fields below, or call +1 410-648-2495 or toll-free at +1 888-513-5303 to talk with one of our admissions counselors.
Johns Hopkins University has engaged AllCampus to help support your educational journey. AllCampus will contact you shortly in response to your request for information. About AllCampus . Privacy Policy . You may opt out of receiving communications at any time.
* All Fields are Required. Your Privacy is Protected.
Connect with us
- Email: [email protected]
- Local Phone: +1 410-648-2495 (Local)
- Toll-Free Phone: +1 888-513-5303 (Toll-Free)
Notification: View the latest site access restrictions, updates, and resources related to the coronavirus (COVID-19) »
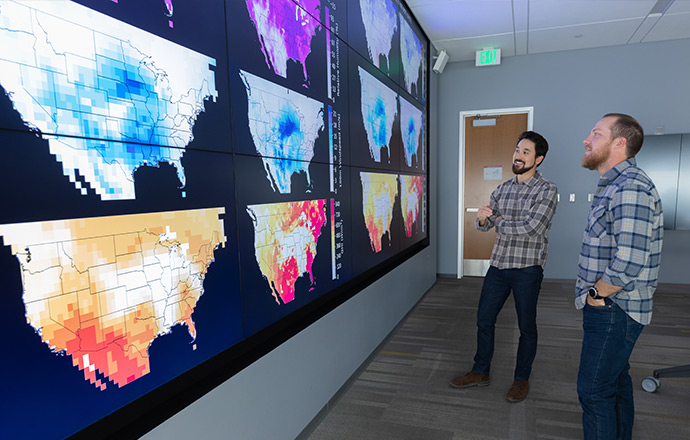
Machine Learning Model To Simulate Future Energy-Climate Impacts
NREL's new open-source generative machine learning model, Sup3rCC, rapidly produces detailed future climate data to support energy system planning.
The National Renewable Energy Laboratory (NREL) is transforming energy through research, development, commercialization, and deployment of renewable energy and energy efficiency technologies.
Partner with us to accelerate the transition of renewable energy and energy efficiency technologies to the marketplace.
Data and Tools
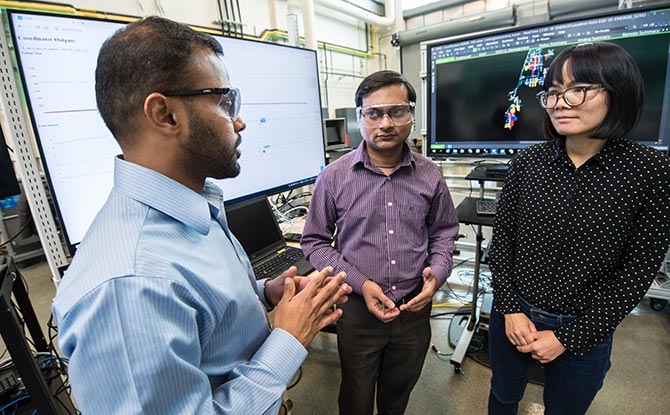
Find NREL-developed data sets, maps, models, and tools used for the analysis of energy and energy efficiency technologies.
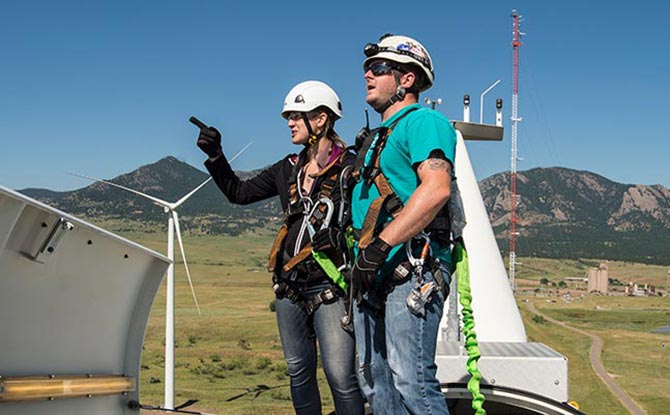
Explore our current job postings, which include research positions and other career opportunities in a variety of fields.
Energy Basics
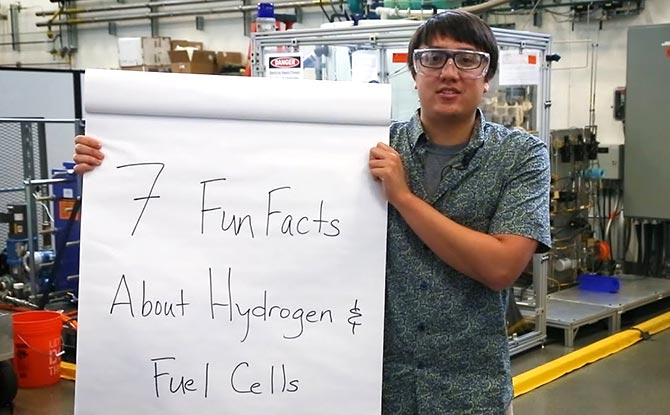
Learn about the basics of our research areas—bioenergy, geothermal, hydrogen, solar, transportation, wind, and water.
NREL Battery Supply Chain Database Maps Out the State of North America's Manufacturing Base
May 15, 2024
Resilience at the Edge: City of Eastport Considers Harnessing Tidal Power for Island Microgrid
New Device Architecture Enables Streamlined Production of Formic Acid From CO₂ Using Renewable Electricity
May 14, 2024
NREL Celebrates Fiscal Year 2023 Staff Awards and Tech Awards
May 13, 2024
Popular Publications
- Technical Potential and Meaningful Benefits of Community Solar in the United States
- Energy I-Corps Annual Report 2023
- Puerto Rico Grid Resilience and Transitions to 100% Renewable Energy Study (PR100): Summary Report
- Major Drivers of Long-Term Distribution Transformer Demand
- Winter 2024 Solar Industry Update
Research Areas
Advanced Manufacturing
Chemistry and Nanoscience
Computational Science
Energy Analysis
Energy Security and Resilience
Energy Storage
Grid Modernization
Hydrogen and Fuel Cells
Integrated Energy Solutions
International Activities
Materials Science
State, Local, and Tribal Governments
Transportation and Mobility
Text version
Future Energy Systems Center
Studies and reports, funding opportunities.
- Basic energy science
- Built environment and infrastructure
- Climate and environment
- Conventional energy
- Developing world
- Energy efficiency
- Nuclear energy
- Policy and economics
- Power distribution and energy storage
- Renewable energy
- Transportation
Undergraduate education
Graduate & postdoctoral, online education, education research.
- Current members
- Energy Futures
- In the media
- Affiliations
Linking science , innovation , and policy to transform the world's energy systems.
The MIT Energy Initiative, MIT’s hub for energy research , education , and outreach , is advancing zero- and low-carbon solutions to combat climate change and expand energy access.
Read our full mission statement →
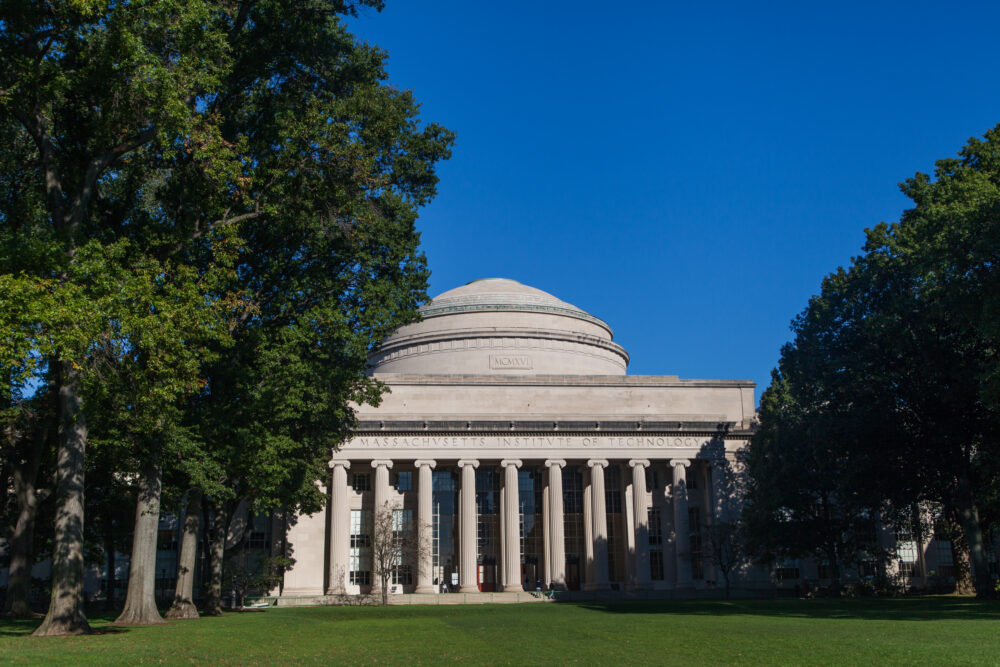
At the 2024 MIT Energy Initiative Spring Symposium, experts weighed whether hydrogen stored in the earth might be a practical energy source of the future.
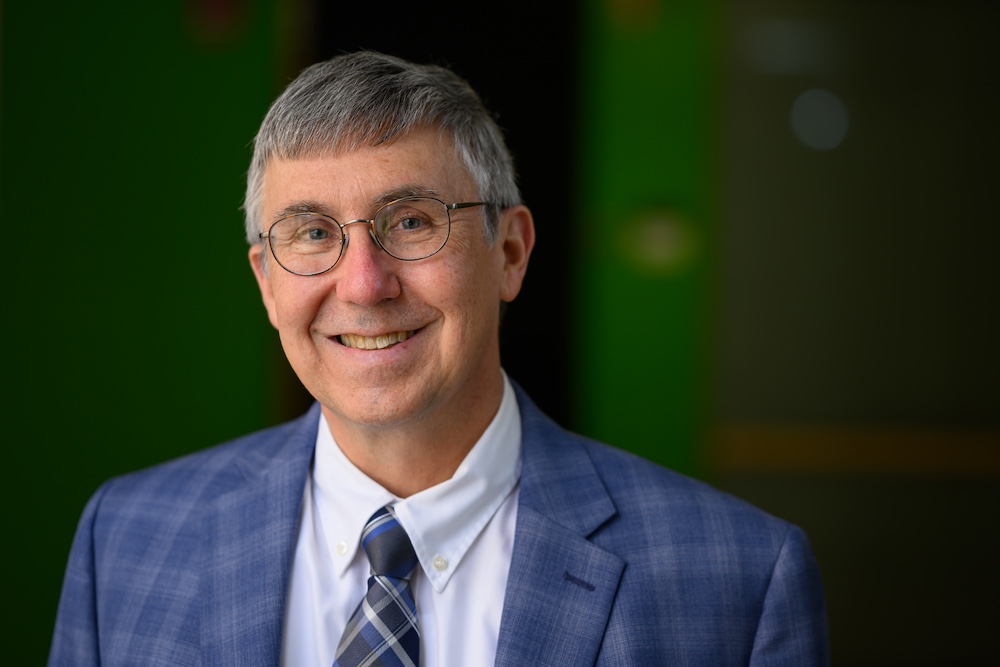
In his new role, the professor of chemical engineering plans to speed up the consensus process among academics, business leaders, and policymakers for a successful energy transition.
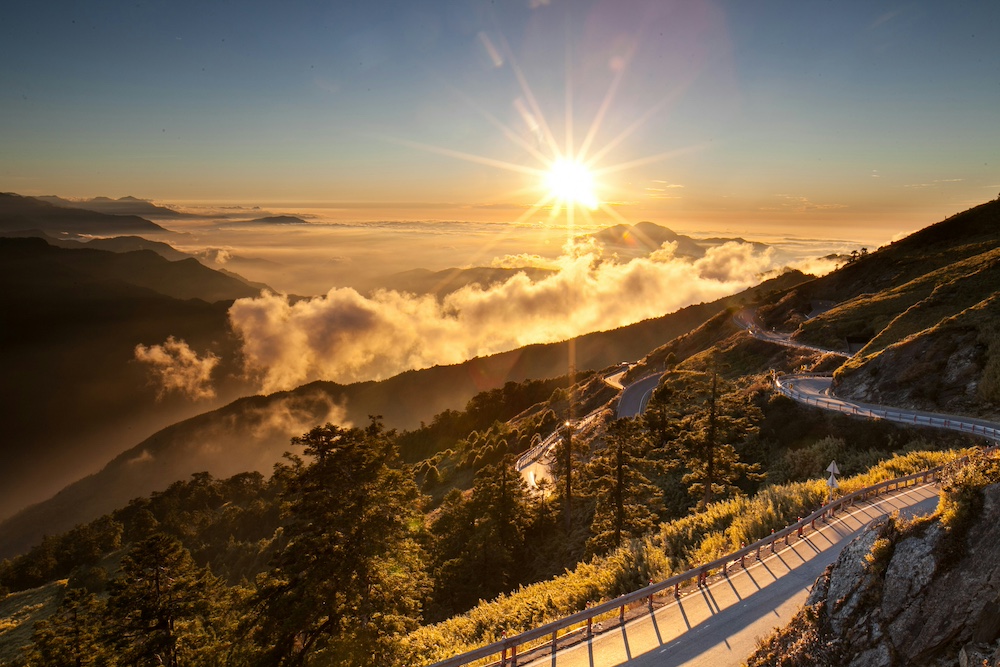
The MIT Energy Initiative and a consortium of Taiwanese companies are exploring how Taiwan can secure its energy future as the world transitions away from fossil fuels.
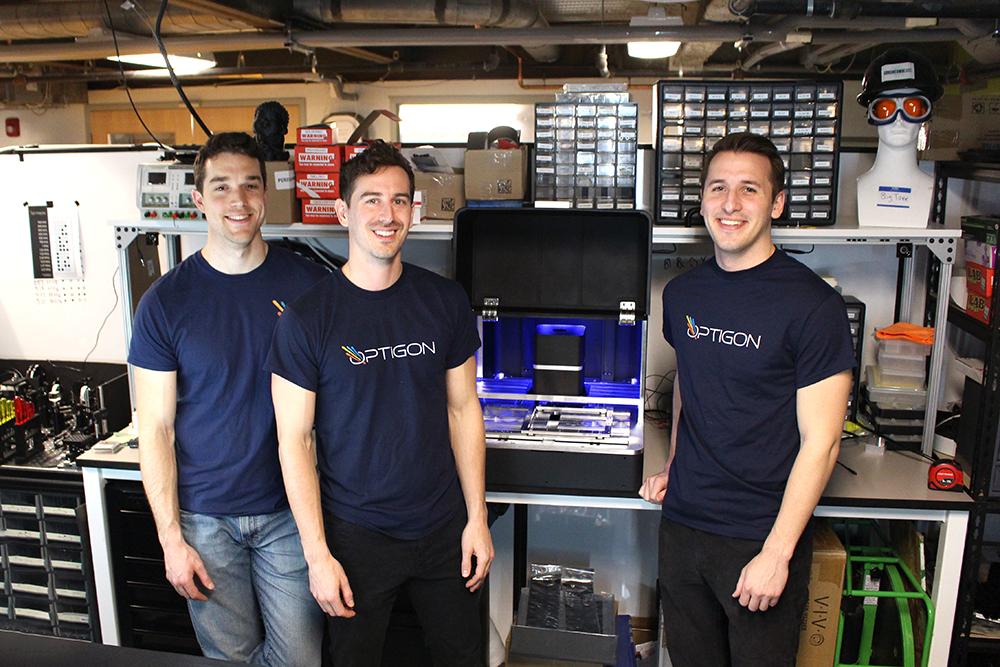
With laser-based precision tools for measuring and tuning materials, MIT-spinout Optigon aims to rev up the energy transition
Eni membership continues through 2027
05/14/2024 05/15/2024
07/11/2024 2:00 pm-3:00 pm ET
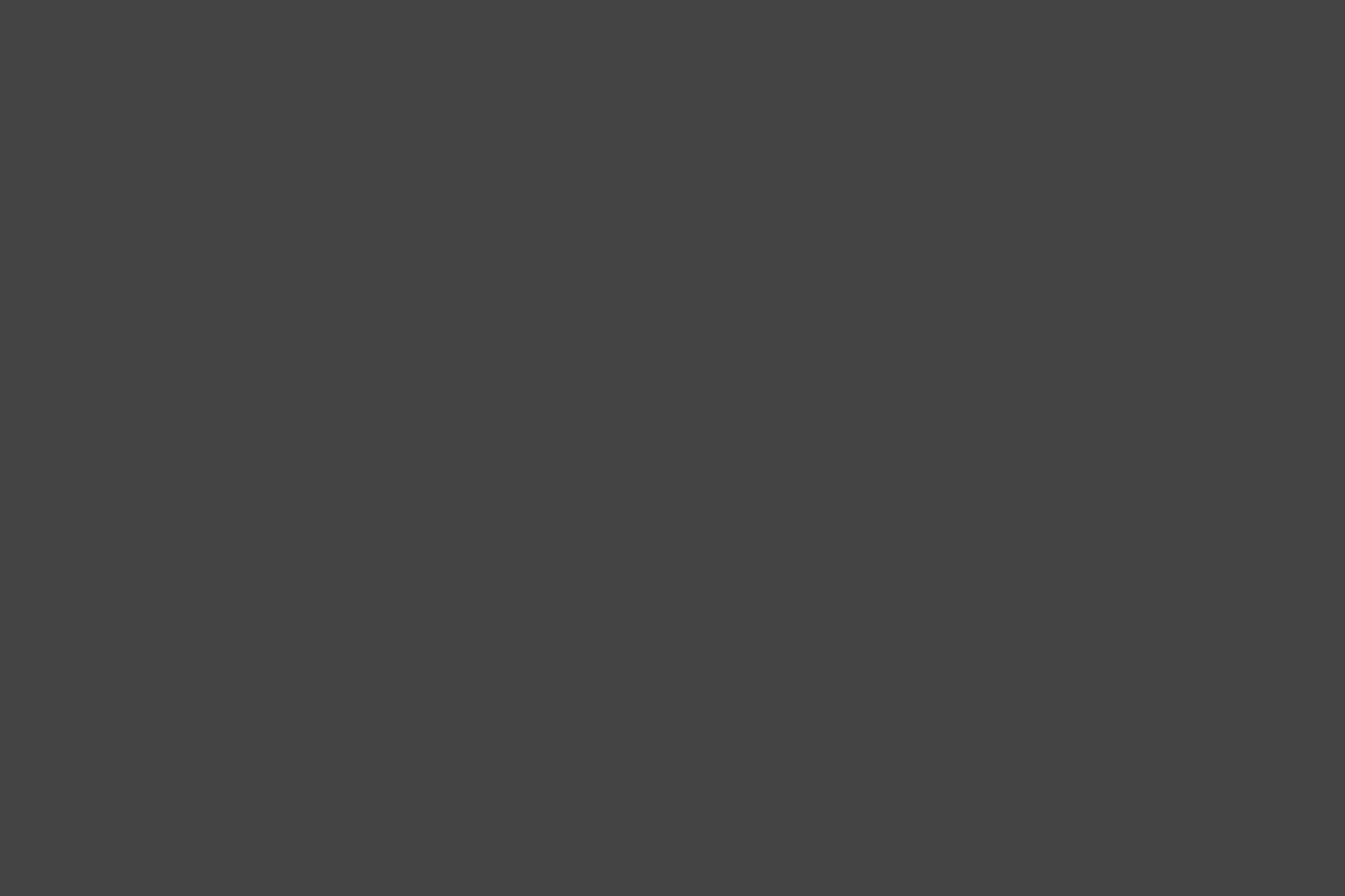
Collaborative research with industry in key technology areas to address the energy transition.
Analyses that shape and influence policy, technology development, and future research.
Learn about funding opportunities for MIT faculty.
- ENVIRONMENT
Renewable energy, explained
Solar, wind, hydroelectric, biomass, and geothermal power can provide energy without the planet-warming effects of fossil fuels.
In any discussion about climate change , renewable energy usually tops the list of changes the world can implement to stave off the worst effects of rising temperatures. That's because renewable energy sources such as solar and wind don't emit carbon dioxide and other greenhouse gases that contribute to global warming .
Clean energy has far more to recommend it than just being "green." The growing sector creates jobs , makes electric grids more resilient, expands energy access in developing countries, and helps lower energy bills. All of those factors have contributed to a renewable energy renaissance in recent years, with wind and solar setting new records for electricity generation .
For the past 150 years or so, humans have relied heavily on coal, oil, and other fossil fuels to power everything from light bulbs to cars to factories. Fossil fuels are embedded in nearly everything we do, and as a result, the greenhouse gases released from the burning of those fuels have reached historically high levels .
As greenhouse gases trap heat in the atmosphere that would otherwise escape into space, average temperatures on the surface are rising . Global warming is one symptom of climate change, the term scientists now prefer to describe the complex shifts affecting our planet’s weather and climate systems. Climate change encompasses not only rising average temperatures but also extreme weather events, shifting wildlife populations and habitats, rising seas , and a range of other impacts .
Of course, renewables—like any source of energy—have their own trade-offs and associated debates. One of them centers on the definition of renewable energy. Strictly speaking, renewable energy is just what you might think: perpetually available, or as the U.S. Energy Information Administration puts it, " virtually inexhaustible ." But "renewable" doesn't necessarily mean sustainable, as opponents of corn-based ethanol or large hydropower dams often argue. It also doesn't encompass other low- or zero-emissions resources that have their own advocates, including energy efficiency and nuclear power.
Types of renewable energy sources
Hydropower: For centuries, people have harnessed the energy of river currents, using dams to control water flow. Hydropower is the world's biggest source of renewable energy by far, with China, Brazil, Canada, the U.S., and Russia the leading hydropower producers . While hydropower is theoretically a clean energy source replenished by rain and snow, it also has several drawbacks.
For Hungry Minds
Large dams can disrupt river ecosystems and surrounding communities , harming wildlife and displacing residents. Hydropower generation is vulnerable to silt buildup, which can compromise capacity and harm equipment. Drought can also cause problems. In the western U.S., carbon dioxide emissions over a 15-year period were 100 megatons higher than they normally would have been, according to a 2018 study , as utilities turned to coal and gas to replace hydropower lost to drought. Even hydropower at full capacity bears its own emissions problems, as decaying organic material in reservoirs releases methane.
Dams aren't the only way to use water for power: Tidal and wave energy projects around the world aim to capture the ocean's natural rhythms. Marine energy projects currently generate an estimated 500 megawatts of power —less than one percent of all renewables—but the potential is far greater. Programs like Scotland’s Saltire Prize have encouraged innovation in this area.
Wind: Harnessing the wind as a source of energy started more than 7,000 years ago . Now, electricity-generating wind turbines are proliferating around the globe, and China, the U.S., and Germany are the leading wind energy producers. From 2001 to 2017 , cumulative wind capacity around the world increased to more than 539,000 megawatts from 23,900 mw—more than 22 fold.
Some people may object to how wind turbines look on the horizon and to how they sound, but wind energy, whose prices are declining , is proving too valuable a resource to deny. While most wind power comes from onshore turbines, offshore projects are appearing too, with the most in the U.K. and Germany. The first U.S. offshore wind farm opened in 2016 in Rhode Island, and other offshore projects are gaining momentum . Another problem with wind turbines is that they’re a danger for birds and bats, killing hundreds of thousands annually , not as many as from glass collisions and other threats like habitat loss and invasive species, but enough that engineers are working on solutions to make them safer for flying wildlife.
You May Also Like
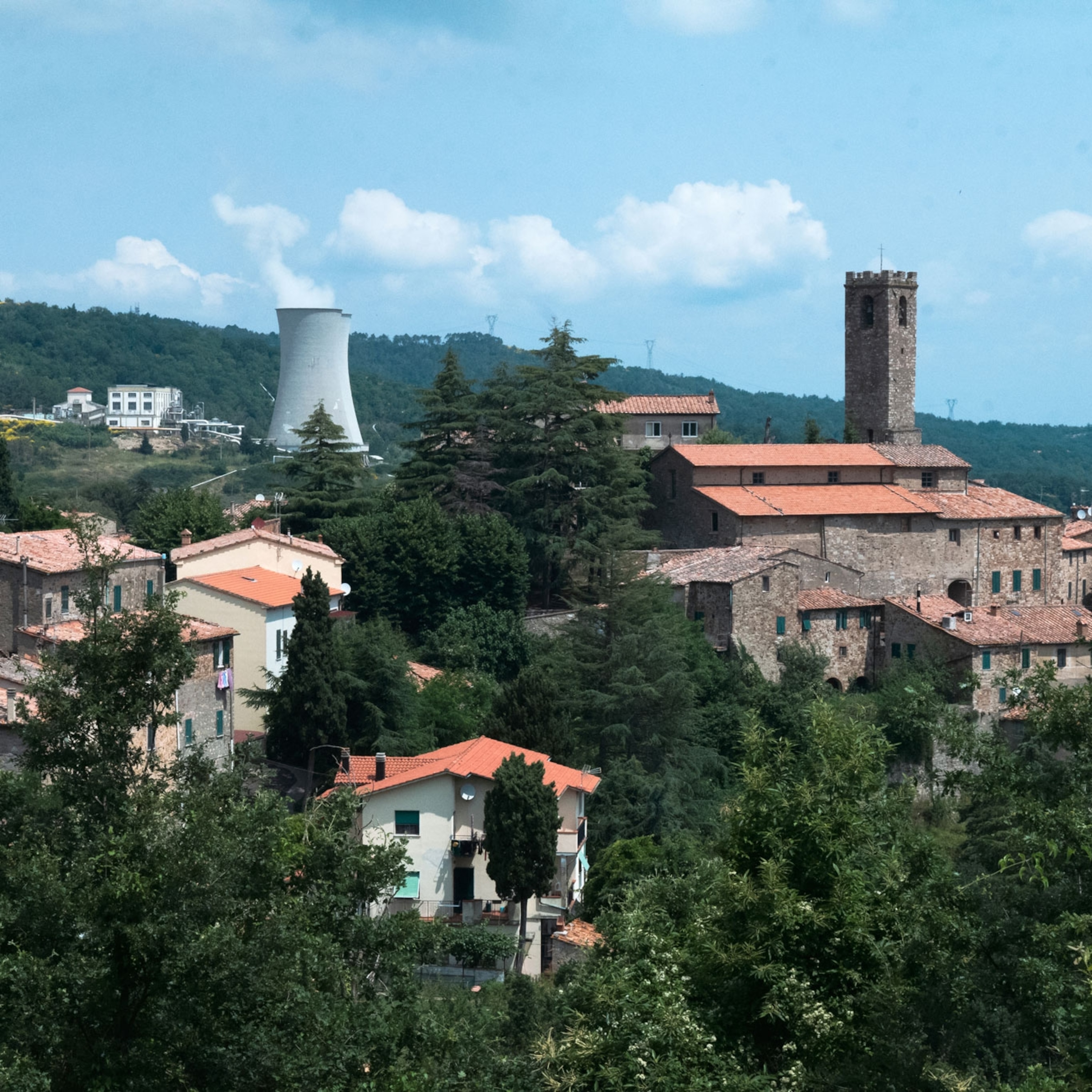
Can energy harnessed from Earth’s interior help power the world?
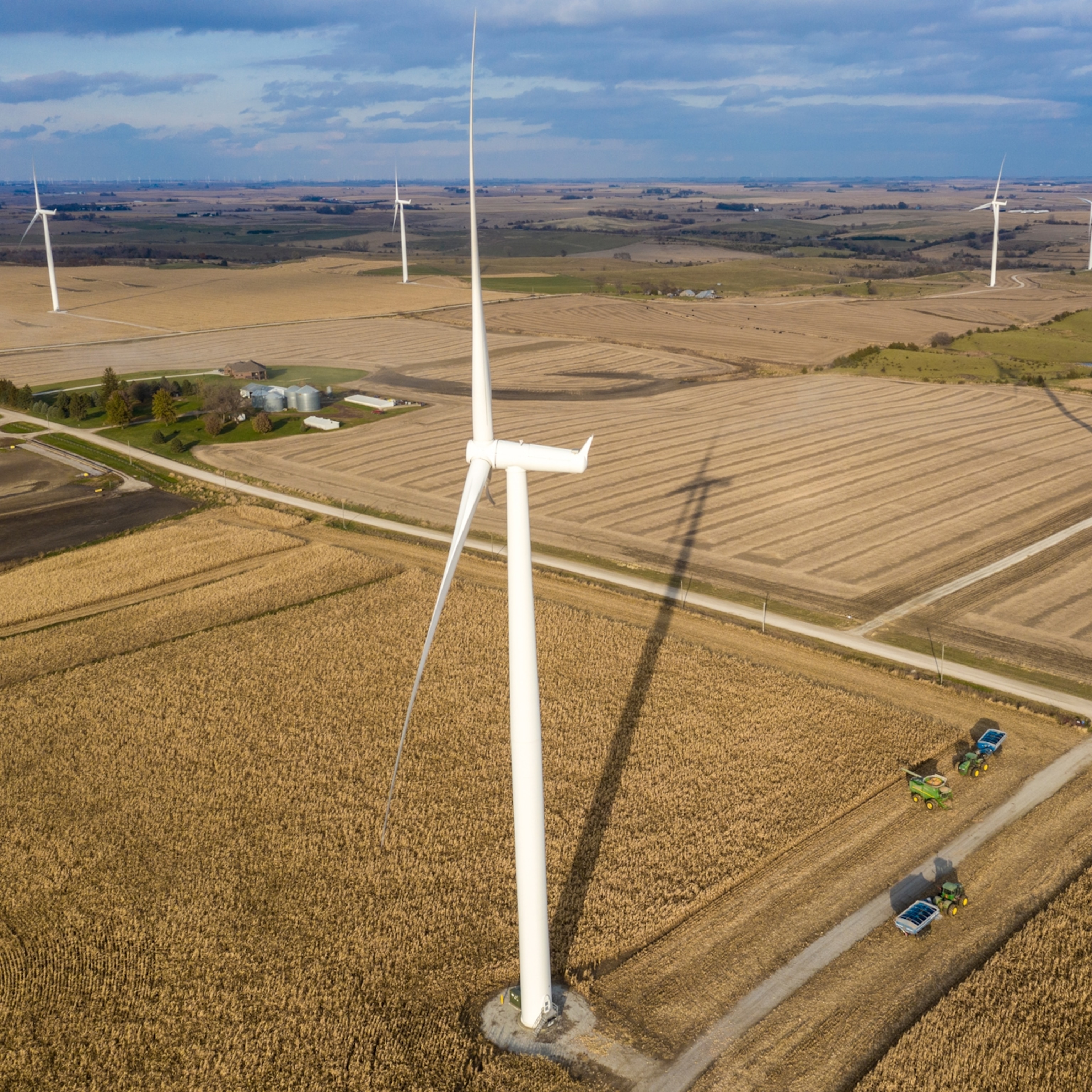
How the historic climate bill will dramatically reduce U.S. emissions
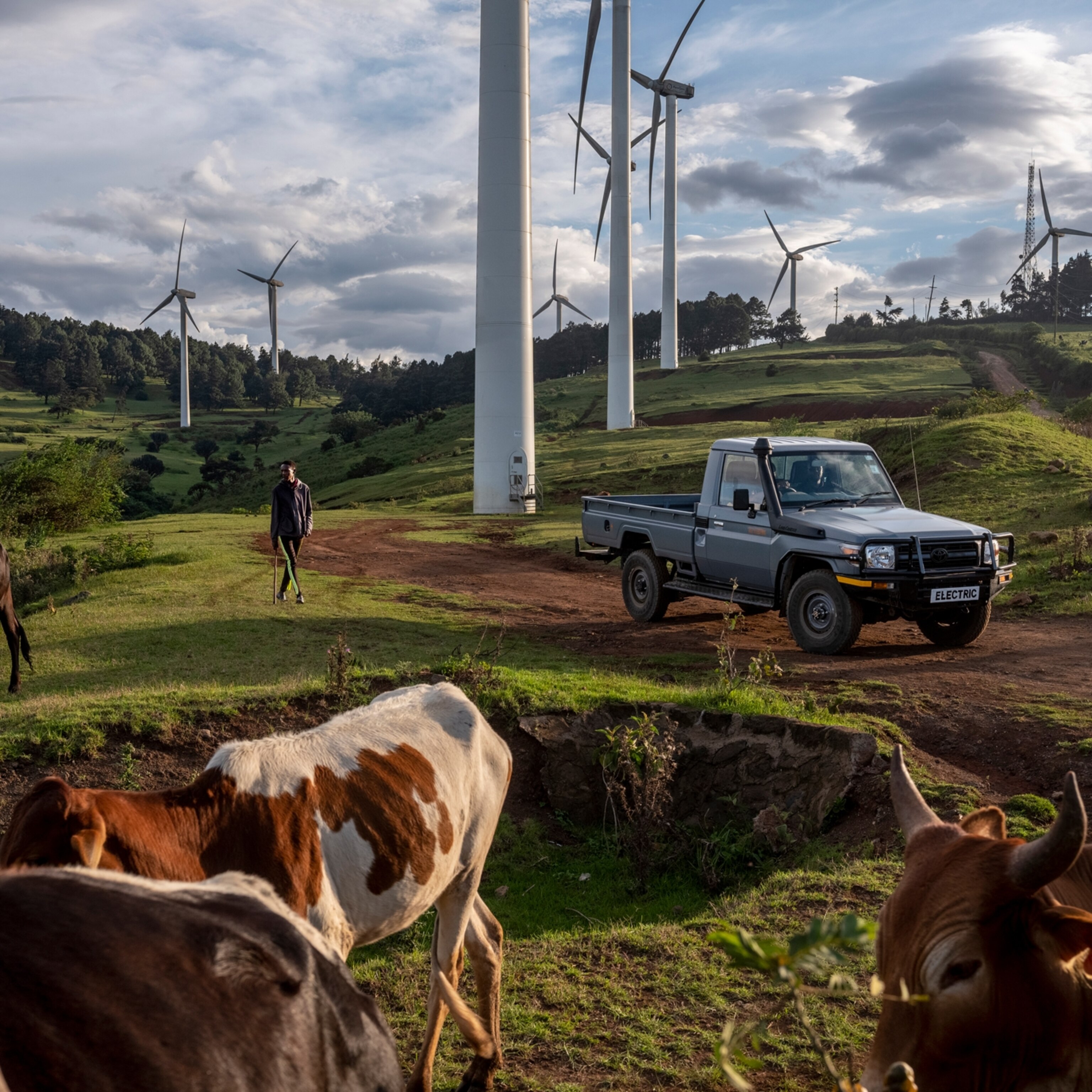
5 environmental victories from 2021 that offer hope
Solar: From home rooftops to utility-scale farms, solar power is reshaping energy markets around the world. In the decade from 2007 and 2017 the world's total installed energy capacity from photovoltaic panels increased a whopping 4,300 percent .
In addition to solar panels, which convert the sun's light to electricity, concentrating solar power (CSP) plants use mirrors to concentrate the sun's heat, deriving thermal energy instead. China, Japan, and the U.S. are leading the solar transformation, but solar still has a long way to go, accounting for around two percent of the total electricity generated in the U.S. in 2017. Solar thermal energy is also being used worldwide for hot water, heating, and cooling.
Biomass: Biomass energy includes biofuels such as ethanol and biodiesel , wood and wood waste, biogas from landfills, and municipal solid waste. Like solar power, biomass is a flexible energy source, able to fuel vehicles, heat buildings, and produce electricity. But biomass can raise thorny issues.
Critics of corn-based ethanol , for example, say it competes with the food market for corn and supports the same harmful agricultural practices that have led to toxic algae blooms and other environmental hazards. Similarly, debates have erupted over whether it's a good idea to ship wood pellets from U.S. forests over to Europe so that it can be burned for electricity. Meanwhile, scientists and companies are working on ways to more efficiently convert corn stover , wastewater sludge , and other biomass sources into energy, aiming to extract value from material that would otherwise go to waste.
Geothermal: Used for thousands of years in some countries for cooking and heating, geothermal energy is derived from the Earth’s internal heat . On a large scale, underground reservoirs of steam and hot water can be tapped through wells that can go a mile deep or more to generate electricity. On a smaller scale, some buildings have geothermal heat pumps that use temperature differences several feet below ground for heating and cooling. Unlike solar and wind energy, geothermal energy is always available, but it has side effects that need to be managed, such as the rotten egg smell that can accompany released hydrogen sulfide.
Ways to boost renewable energy
Cities, states, and federal governments around the world are instituting policies aimed at increasing renewable energy. At least 29 U.S. states have set renewable portfolio standards —policies that mandate a certain percentage of energy from renewable sources, More than 100 cities worldwide now boast at least 70 percent renewable energy, and still others are making commitments to reach 100 percent . Other policies that could encourage renewable energy growth include carbon pricing, fuel economy standards, and building efficiency standards. Corporations are making a difference too, purchasing record amounts of renewable power in 2018.
Wonder whether your state could ever be powered by 100 percent renewables? No matter where you live, scientist Mark Jacobson believes it's possible. That vision is laid out here , and while his analysis is not without critics , it punctuates a reality with which the world must now reckon. Even without climate change, fossil fuels are a finite resource, and if we want our lease on the planet to be renewed, our energy will have to be renewable.
Related Topics
- SUSTAINABILITY
- RENEWABLE ENERGY
- GEOTHERMAL ENERGY
- SOLAR POWER
- HYDROELECTRIC POWER
- CLIMATE CHANGE
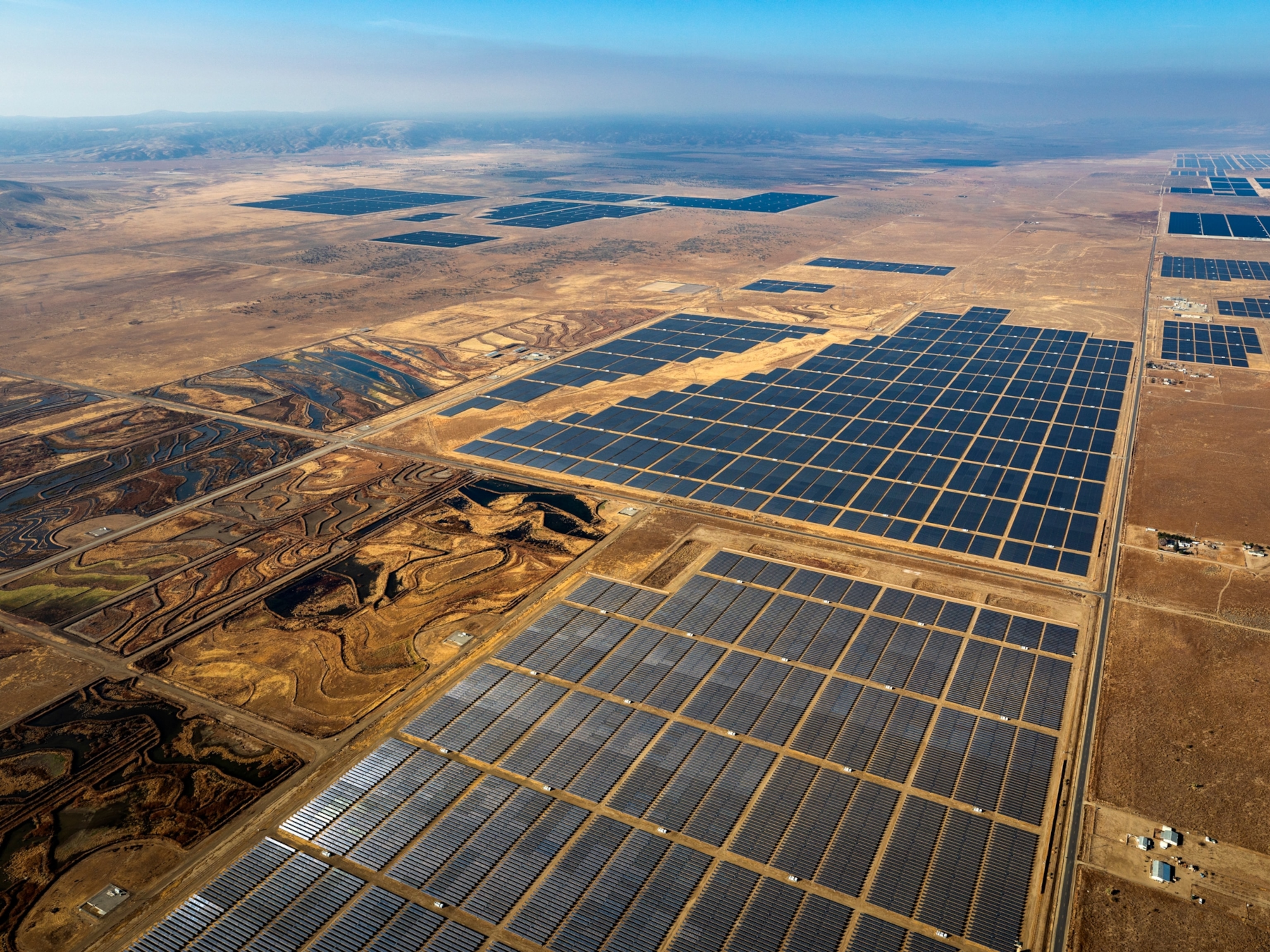
Activists fear a new threat to biodiversity—renewable energy
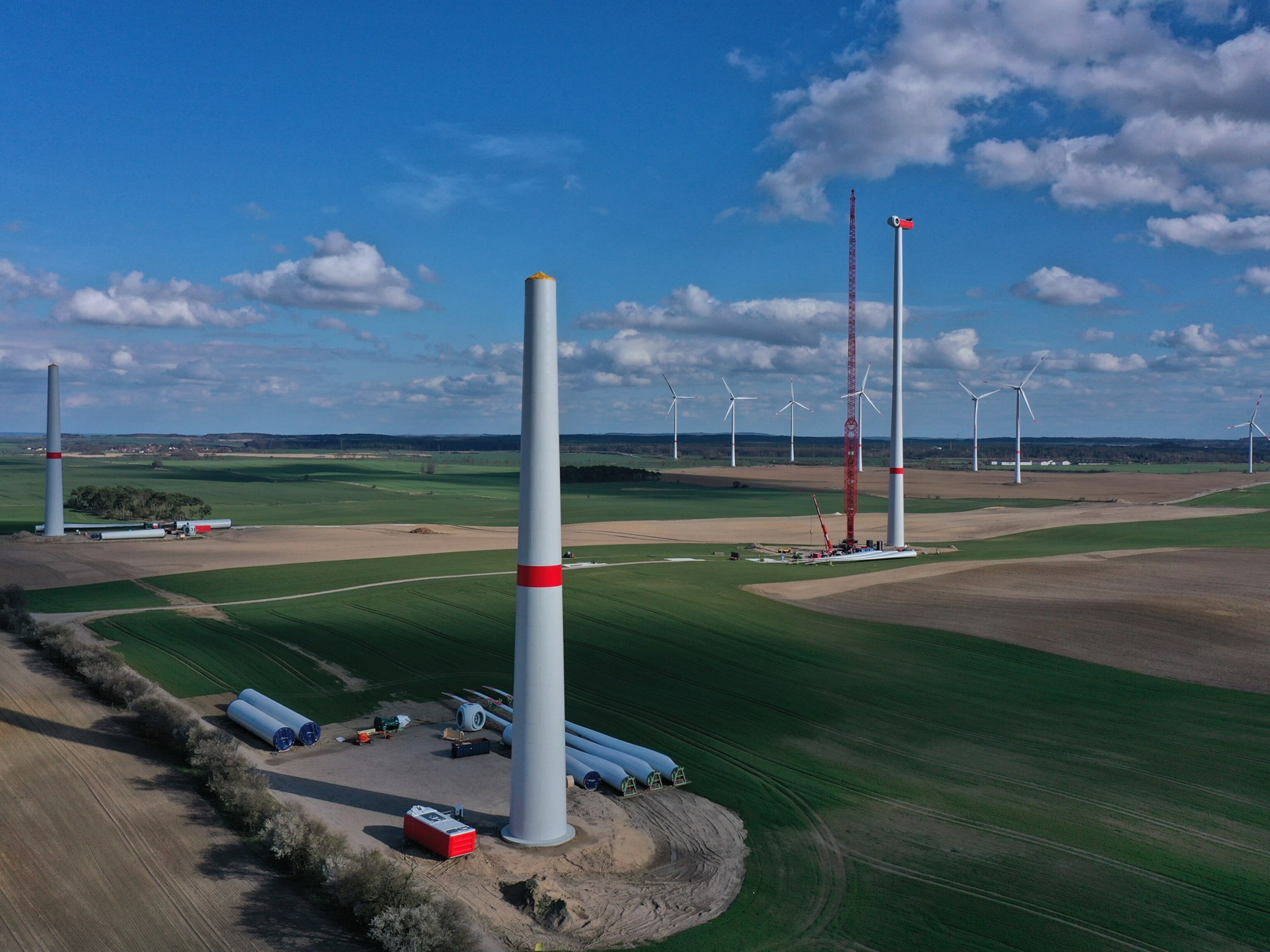
How the Ukraine war is accelerating Germany's renewable energy transition
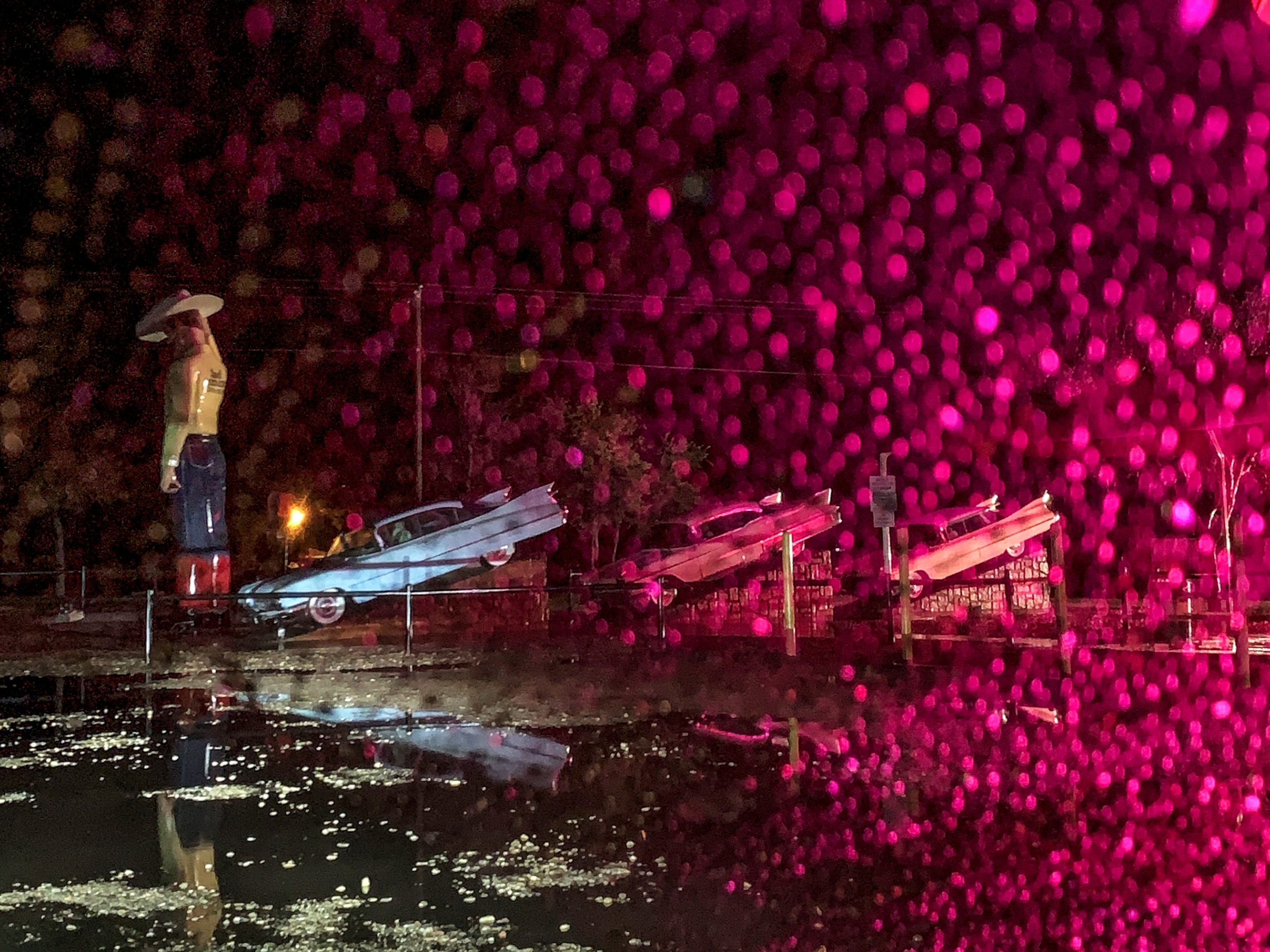
We took the Great American Road Trip—in electric cars
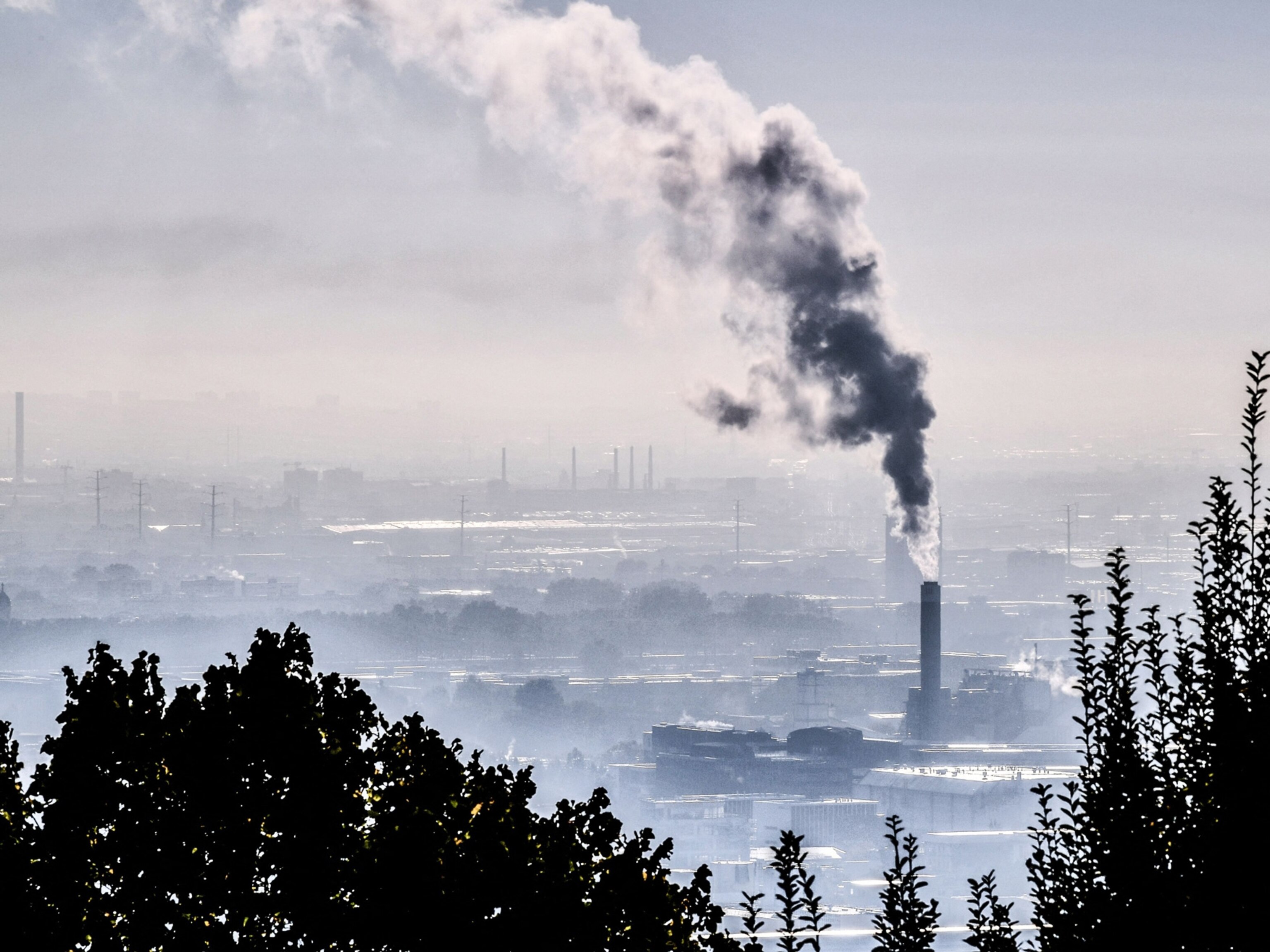
What’s at stake at COP26—the crucial global climate summit
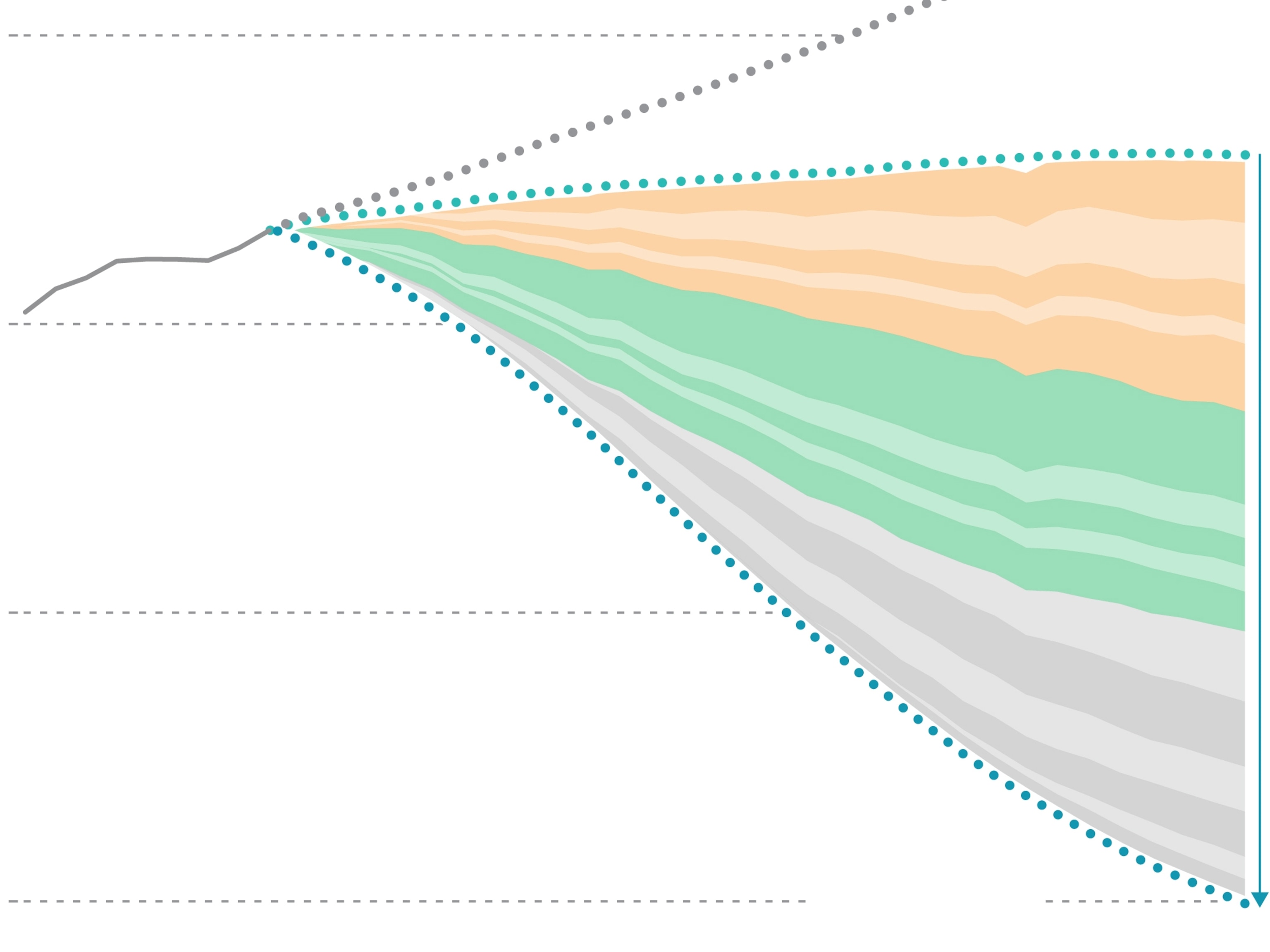
3 ways COVID-19 is making us rethink energy and emissions
- Environment
- Perpetual Planet
History & Culture
- History & Culture
- History Magazine
- Mind, Body, Wonder
- Terms of Use
- Privacy Policy
- Your US State Privacy Rights
- Children's Online Privacy Policy
- Interest-Based Ads
- About Nielsen Measurement
- Do Not Sell or Share My Personal Information
- Nat Geo Home
- Attend a Live Event
- Book a Trip
- Inspire Your Kids
- Shop Nat Geo
- Visit the D.C. Museum
- Learn About Our Impact
- Support Our Mission
- Advertise With Us
- Customer Service
- Renew Subscription
- Manage Your Subscription
- Work at Nat Geo
- Sign Up for Our Newsletters
- Contribute to Protect the Planet
Copyright © 1996-2015 National Geographic Society Copyright © 2015-2024 National Geographic Partners, LLC. All rights reserved
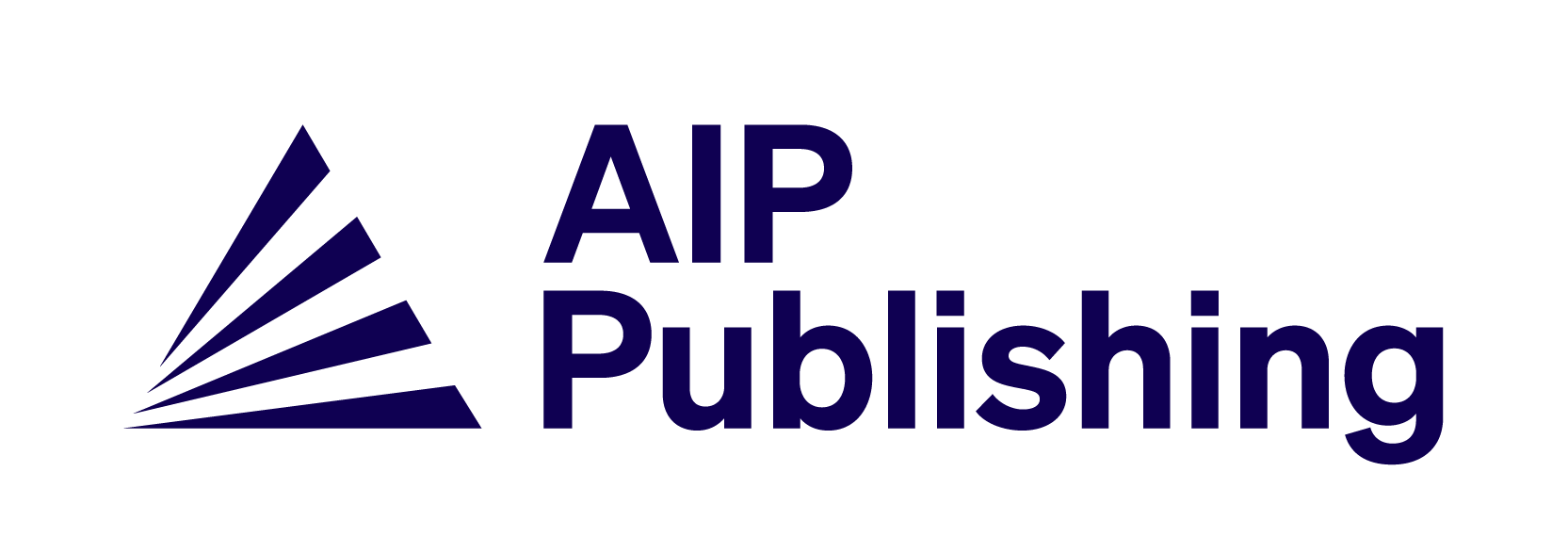
Focus and Coverage
The Journal of Renewable and Sustainable Energy is an interdisciplinary journal covering specific areas of renewable and sustainable energy relevant to the physical science and engineering communities. The journal has a strong focus on integration of disciplines for renewable power technologies at global scales that have the potential to mitigate abrupt climate change. Since volume 12, the journal has increasingly focused on discoveries related to weather-dependent renewable generation (solar and wind).
Read more about the journal
Editor-in-Chief
Professor Zhenhong Lin
Current issue
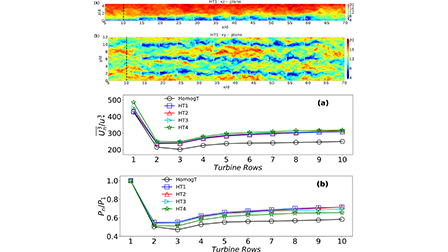
Submit your article
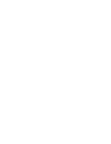
Sign up for alerts

Active Topics
- Energy technology
- Renewable energy
- Mathematical analysis
- Computer science and technology
- Energy production, transmission and distribution
- Fluid mechanics
Latest Physics Jobs
- Online ISSN 1941-7012
- For Researchers
- For Librarians
- For Advertisers
- Our Publishing Partners
- Physics Today
- Conference Proceedings
- Special Topics
pubs.aip.org
- Privacy Policy
- Terms of Use
Connect with AIP Publishing
This feature is available to subscribers only.
Sign In or Create an Account

Along with Stanford news and stories, show me:
- Student information
- Faculty/Staff information
We want to provide announcements, events, leadership messages and resources that are relevant to you. Your selection is stored in a browser cookie which you can remove at any time using “Clear all personalization” below.
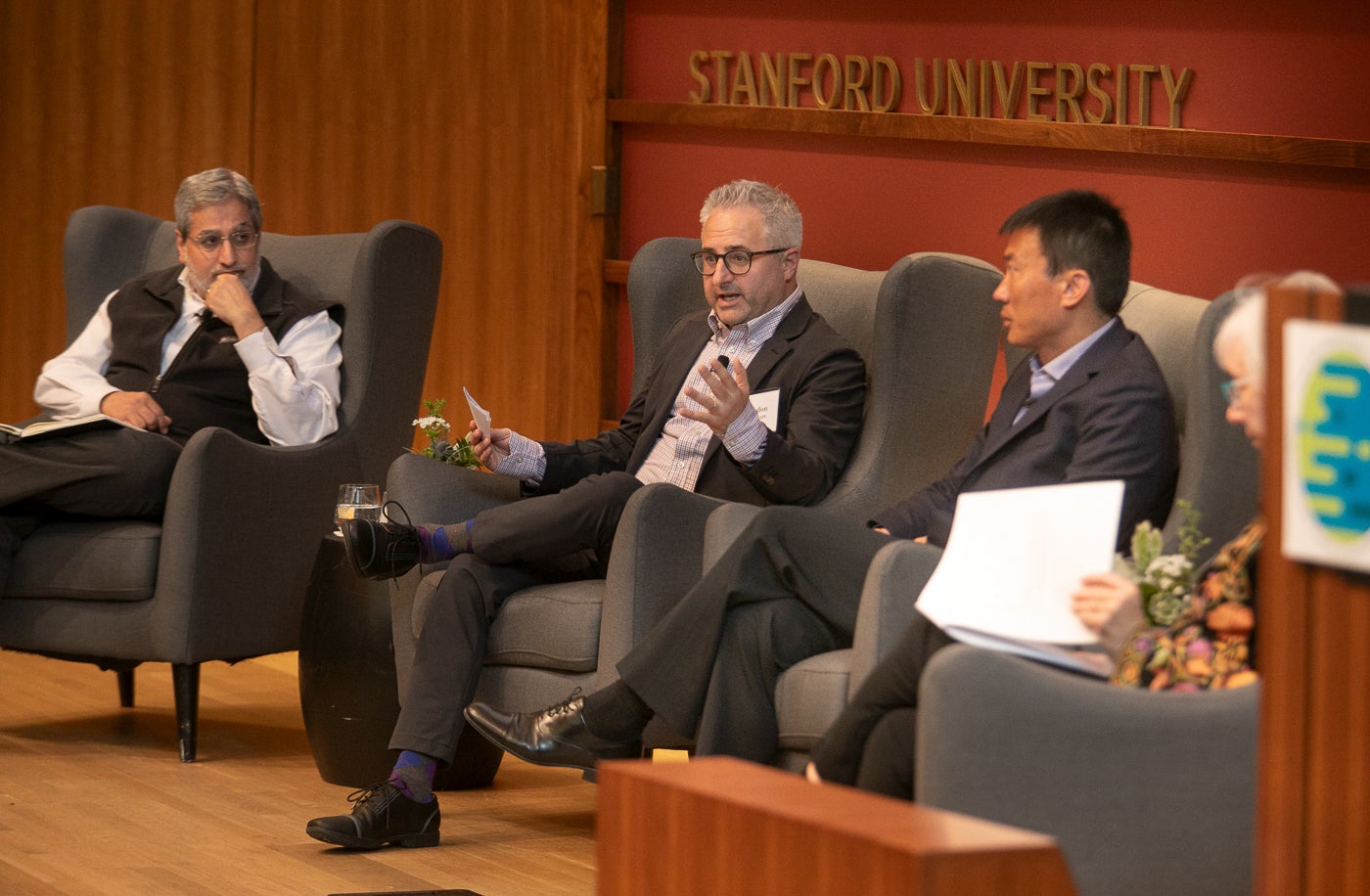
From left to right: Rajeev Ram of MIT; Brandon Hurlbut, a co-founder of Boundary Stone Partners; and Stanford’s Yi Cui and Sally Benson discuss challenges and innovations in transforming the grid. (Image credit: Saul Bromberger)
How do we transition to clean energy with enough speed and scale to prevent the most extreme impacts of climate change? This question loomed large for many of the speakers and participants at the Stanford Forum on the Science of Energy Transition , held on campus April 10 for an audience of students and invited guests.
To stabilize global temperatures, we need to find ways to reduce and remove our carbon emissions from Earth’s atmosphere by tens of gigatons every single year. By comparison, gas-powered vehicles in the U.S. together produce about a gigaton of carbon dioxide emissions each year.
Many of the speakers agreed action over the next few decades is critical, and addressing climate change will require coordinated efforts across the scientific community, climate technology innovators, government, the private sector, and others to transition the world’s $100 trillion economy to clean energy.
The forum, co-hosted by the Stanford Doerr School of Sustainability and Stanford Management Company , convened experts to explore challenges and opportunities to transform the power grid, rethink renewable fuels, remove greenhouse gases from our atmosphere, and address energy issues in tandem with other sustainability concerns.
The forum served as a powerful example of how Stanford leaders are educating changemakers in energy and facilitating connections that will help bring insights from scientific research to decisions that will affect global sustainability.
“Today, the energy transition will require us to forge new pathways, but we can’t just blindly strike out. That will lead us down too many dead ends, and time is of the essence. Instead, the paths we choose must be informed by science,” said Robert Wallace, the chief executive officer of Stanford Management Company.
Consider speed and scale from the get-go
Decarbonizing global energy production is a tall order. That’s before you consider the rising demand for energy as countries develop and look for opportunities to increase mobility, communication, security, and economic prosperity, multiple speakers said.
U.S. clean energy projects are on hold due to bottlenecks in the process for permitting new transmission lines and grid interconnections. The queue of projects waiting for approval by transmission operators would effectively triple the size of our generating resource, said Rajeev Ram , a professor of electrical engineering at MIT. Removing some of those logistical barriers through AI, modeling, and software tools can help accelerate the timeline for projects that could provide clean energy to the grid, said Ram.
Yi Cui , the director of Stanford’s Sustainability Accelerator , is one of the leading experts developing batteries for renewable energy storage. Scholars in the Stanford Doerr School of Sustainability – which celebrated its first anniversary in September – have embraced a core philosophy of thinking about scale at the beginning of the design process, which is a good sign, Cui said.
For example, the relative scarcity of some elements – such as lithium, cobalt, and nickel – in current battery designs could limit their potential for large-scale production at low cost. Cui emphasized that scientists could focus on designing battery materials based on more widely available minerals, like zinc, manganese, and iron .
“We need to figure out for each of these technologies what is already going on at the gigaton-scale – like natural cycles, like agriculture – and see what we can do to tweak it in the right way so that you can create a market and use market mechanisms to scale it,” said Arun Majumdar , the inaugural dean of the Stanford Doerr School of Sustainability.
Others highlighted the importance of driving down costs for companies and consumers. If innovators can eliminate the green premium – the cost of choosing a clean energy technology over a traditional source – for their products, they will be competitive in the market.
Meanwhile, incentives like subsidies for renewable energy will need to be supplemented with policies that actively discourage use of carbon-intensive resources. “If we’re serious about addressing climate change, we’ve got to have a price on carbon,” whether through direct pricing per ton or indirectly through regulation, said Majumdar.
Powered by electrons versus molecules
The grid is essentially a system of wires that transports electrons from power plants to consumers. However, grid electrification will only get us about halfway to reducing greenhouse gas emissions, noted Sally Benson , the Precourt Family Professor, who moderated one of the panels.
Eighty percent of global energy comes from fossil fuels. Much of this comes in the form of liquid fossil fuels for transportation – cars, planes, ships, and some trains. The challenges of developing batteries and grid storage capable of providing electricity without interruption could limit electrification of some parts of the transportation sector. Instead, renewable fuels like hydrogen, biofuels, and fuels made primarily from captured carbon dioxide may help reduce carbon emissions from heavy-duty transportation.
“Our challenge is to think that the future is electrification, but that doesn’t mean that electrons are going to do everything for us directly,” said Anthony Kovscek , the Keleen and Carlton Beal Professor in the Stanford Doerr School of Sustainability.
Hydrogen could help bridge the gap to carbon neutrality, said Eric Toone , managing director and technical lead at Breakthrough Energy Ventures.
“Hydrogen is pure, reactive chemical energy. If you have enough hydrogen, you can do anything,” he said.
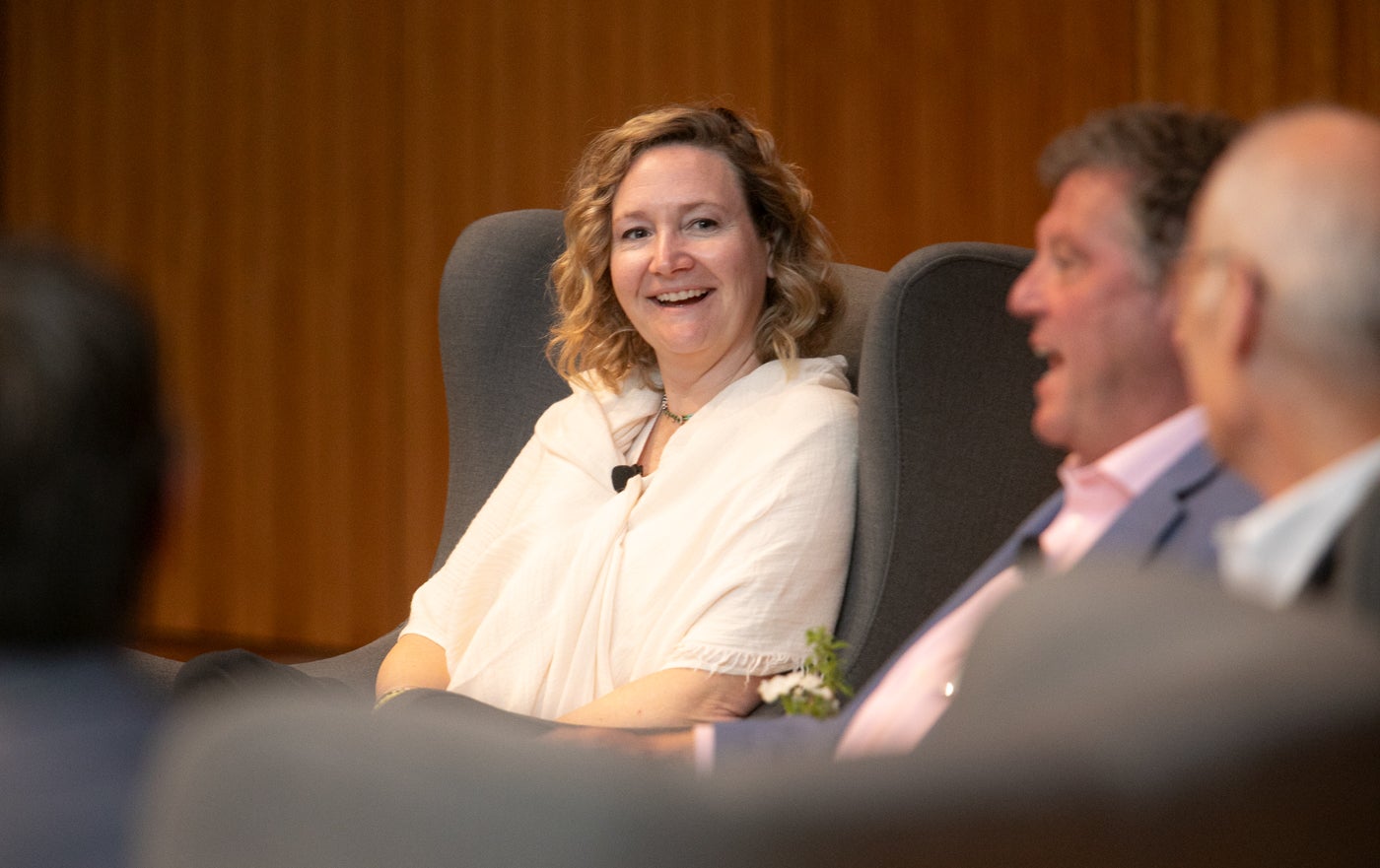
Zara Summers is the chief science officer at LanzaTech. (Image credit: Saul Bromberger)
Zara Summers is the chief science officer at LanzaTech, a company that makes chemicals and fuels from carbon dioxide captured from factories and other industrial sources.
LanzaTech has been working to develop ethanol alternatives that make use of carbon from municipal waste or industrial emissions. These alternatives could serve as effective drop-in replacements for liquid fuels like gasoline, with the added benefit of easy adoption by the public and ability to tap into existing supply chains, but many current economic incentives specifically benefit corn-based ethanol.
“If you’re going to go big, you have to be at cost parity or better. But, you also have to fight against policy that’s written with a solution in mind, not an outcome,” said Summers. She highlighted how designing policies that are flexible and adaptable to new innovations will help bring solutions to scale.
Science as the bedrock
In addition to the challenge of rapidly reducing greenhouse gas emissions, the forum also explored solutions for removing historic carbon emissions from the atmosphere. During a panel on greenhouse gas removal strategies , speakers turned the focus to more low-tech solutions: carbon cycles in nature. For example, Benson enthusiastically described enhanced weathering, which builds on a naturally occurring process where rain interacts with certain rocks to form a mild acid. This acid then reacts with carbon dioxide in the atmosphere to form solid compounds that permanently store carbon.
Chris Field , the director of the Stanford Woods Institute for the Environment , cited research published last month which found that adding crushed basalt rocks for enhanced weathering could increase productivity and soil health on croplands .
“It really highlights where you can potentially accomplish these big co-benefits that can make something that’s a real challenge logistically or financially come into the realm of possibility,” said Field.
The energy transition touches all of the major social-environmental systems. A final panel brought together experts on freshwater, oceans and aquatic foods, and agricultural technology to explore cultivating resilience amid climate pressures. “Agriculture sits at the very center of many of the pressures that we’re putting on Earth’s systems,” said Jim Leape , co-director of the Stanford Center for Ocean Solutions .
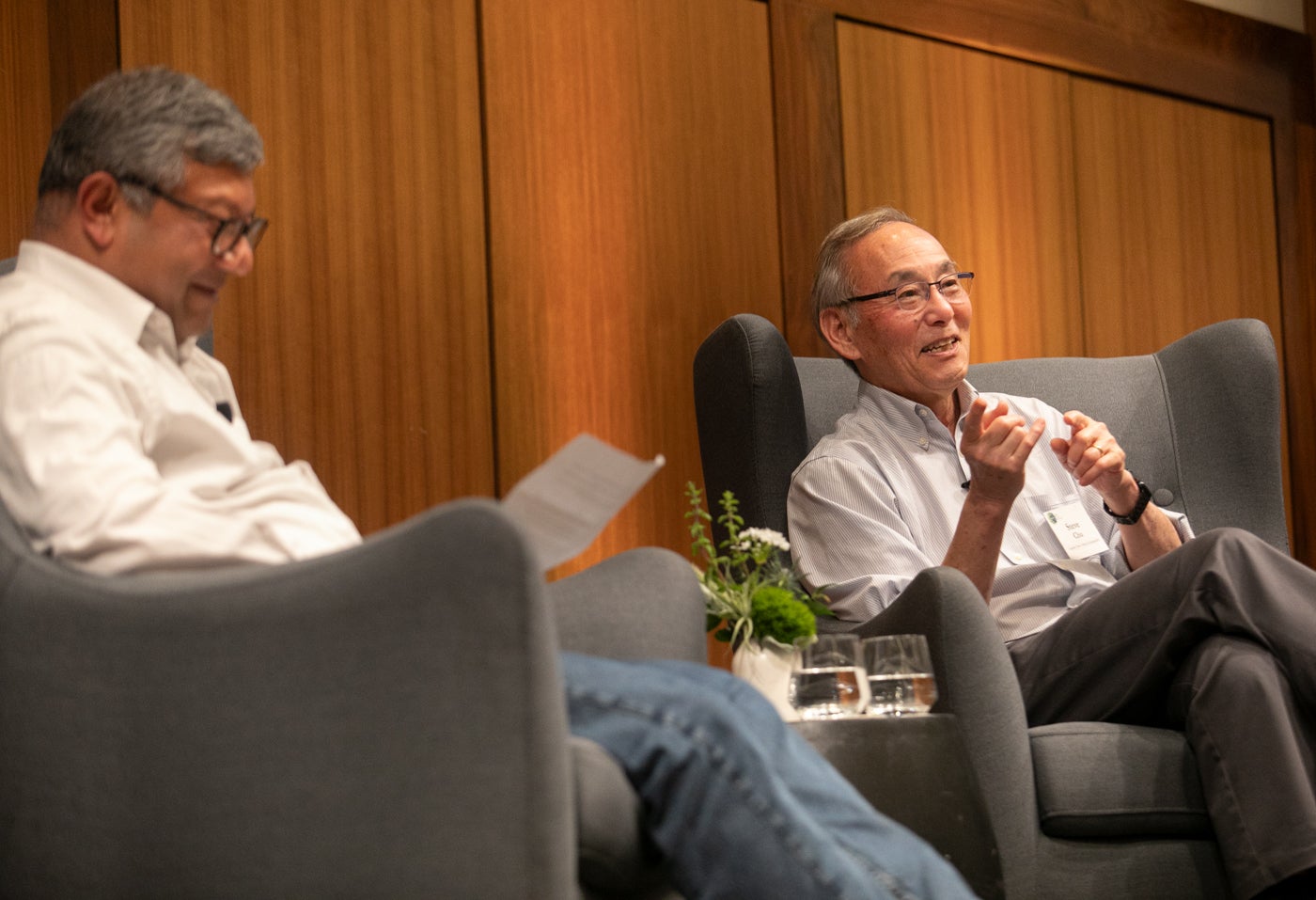
Arun Majumdar and Steven Chu discussed energy efficiency, nuclear fusion, national security, and more during a fireside chat. (Image credit: Saul Bromberger)
During a fireside chat, Majumdar discussed emerging trends in clean energy with Steven Chu , the William R. Kenan, Jr. Professor in the School of Humanities and Sciences . They mentioned the “low-hanging fruit” of energy efficiency, the future of nuclear fusion, and balancing the energy transition with national and economic security. Nevertheless, Chu brought the conversation back to the critical role of new inventions.
“As a physicist, when I stand back and look at things: What really changed the world? New materials are actually what changed the world,” said Chu, a former U.S. Secretary of Energy who embodies the impact of science-based decision-making in energy systems as the first scientist to hold a Cabinet position.
From the steam engine and the agricultural revolution to semiconductors and nuclear fission, leaps forward in technology have enabled global-scale changes and development. Highlighting the vision for the Stanford Doerr School of Sustainability, Majumdar noted that when research institutions like Stanford collaborate with public and private entities, they can serve as a place where scholars can serve as incubators for translating novel ideas into impact.
Yi Cui is also the Fortinet Founders Professor and a professor of materials science and engineering in the School of Engineering . He is a professor of energy science and engineering in the Stanford Doerr School of Sustainability, a professor, by courtesy, of chemistry in the School of Humanities and Sciences, and a professor in the Photon Science Directorate. Cui is also a senior fellow at the Precourt Institute for Energy , and the institute’s immediate past director, and a senior fellow at the Stanford Woods Institute for the Environment.
Arun Majumdar is the Chester Naramore Dean of the Stanford Doerr School of Sustainability, the Jay Precourt Provostial Chair Professor, and a senior fellow at the Precourt Institute for Energy. He is also a professor of mechanical engineering and, by courtesy, of materials science and engineering in the School of Engineering, and a professor in the Photon Science Directorate. He is a senior fellow, by courtesy, at the Hoover Institution.
Sally Benson is the Precourt Family Professor in the Stanford Doerr School of Sustainability, where she is a professor of energy science and engineering; a senior fellow at the Precourt Institute for Energy; and a senior fellow at the Woods Institute.
Anthony Kovcsek is a professor of energy science and engineering, and a senior fellow at the Precourt Institute for Energy.
Chris Field is also the Melvin and Joan Lane Professor in Interdisciplinary Environmental Studies in the School of Humanities and Sciences; the Perry L. McCarty Director of the Woods Institute; a professor of Earth system science in the Stanford Doerr School of Sustainability; and a senior fellow at the Precourt Institute for Energy.
Jim Leape is the William and Eva Price Senior Fellow at the Woods Institute and a professor, by courtesy, of oceans.
Steven Chu is a Nobel laureate and a professor of energy science and engineering in the Stanford Doerr School of Sustainability. He is also a professor of molecular and cellular physiology at Stanford Medicine and of physics in the School of Humanities and Sciences.

An official website of the United States government
The .gov means it’s official. Federal government websites often end in .gov or .mil. Before sharing sensitive information, make sure you’re on a federal government site.
The site is secure. The https:// ensures that you are connecting to the official website and that any information you provide is encrypted and transmitted securely.
- Publications
- Account settings
Preview improvements coming to the PMC website in October 2024. Learn More or Try it out now .
- Advanced Search
- Journal List

Energy Sustainability with a Focus on Environmental Perspectives
Marc a. rosen.
Faculty of Engineering and Applied Science, University of Ontario Institute of Technology, 2000 Simcoe Street North, Oshawa, ON L1G 0C5 Canada
Energy sustainability is a key consideration for anthropogenic activity and the development of societies, and more broadly, civilization. In this article, energy sustainability is described and examined, as are methods and technologies that can help enhance it. As a key component of sustainability, the significance and importance of energy sustainability becomes clear. Requirements to enhance energy sustainability are described, including low environmental and ecological impacts, sustainable energy resources and complementary energy carriers, high efficiencies, and various other factors. The latter are predominantly non-technical, and include living standards, societal acceptability and equity. The outcomes and results are anticipated to inform and educate about energy sustainability, to provide an impetus to greater energy sustainability.
Introduction
Energy is utilized pervasively to provide energy services of all types. These include the provision of electricity, transportation, lighting, heating, cooling, industrial processes (e.g., refining and manufacturing) and many more. The full life cycle of energy is complex, and includes obtaining energy sources, converting them to useful forms, transporting, distributing, storing energy, and utilizing energy (Karunathilake et al. 2019 ). The services provided by energy allow for good living standards and support societal development.
Most countries today use energy in a manner that is not sustainable (Baleta et al. 2019 ). This applies to countries of all kinds (developing, industrialized, etc.) (Kumar and Majid 2020 ). Despite this general view, it is observed that wealthy countries appear to be using energy in a manner that is more sustainable today than before 1970. This phenomenon is illustrated in Table Table1. 1 . For G7 countries, for instance, energy use per capita and real gross domestic product per capita both rose in step by about 60% between 1960 and 1973, but between 1973 and 2015, energy use per capital remained roughly constant while real gross domestic product per capita continued to rise, by roughly 100% (World Bank Group 2021 ). These data suggest that energy usage and GDP growth per capita became in part decoupled, implying countries can continue to generate wealth without necessarily using increasing amounts of energy through a higher energy intensity. Note that, as the data in Table Table1 1 are just for the G7 countries, the rest of the world may not follow this behavior. G7 countries have outsourced portions of their heavy industry, which tends to be energy intensive, to developing and recently developed countries (e.g., Mexico). Hence, the net effect globally in terms of reducing energy consumption is likely less that that observed for G7 countries.
Change in energy use and real gross domestic product per capita for G7 countries between 1960 and 2015
Data source: World Bank Group ( 2021 )
Energy sustainability involves the use of energy during all aspects of its life cycle in a manner that supports the various facets of sustainable development. Energy sustainability is, therefore, a comprehensive concept that reaches beyond the use of sustainable energy resources, and can be viewed as a component of overall sustainability.
A universally accepted definition for energy sustainability does not exist, even though some definitions have been proposed ( 2017a ; Zvolinschi et al. 2007 ; Chen et al. 2020a ; Razmjoo et al. 2020 ; Suganthi 2020 ; Kumar and Majid 2020 ). A general definition can perhaps be developed by extending definitions of sustainability or sustainable development. For instance, Kutscher et al. ( 2019 ) define sustainable energy as energy produced and used in such a way that it “meets the needs of the present without compromising the ability of future generations to meet their own needs.” Grigoroudis et al. ( 2019 ) suggest that “energy sustainability is related with the provision of adequate, reliable, and affordable energy, in conformity with social and environmental requirements.” Nonetheless, defining energy sustainability is challenging due to the multidisciplinary and complex nature of energy sustainability. The present author defines energy sustainability as the provision of energy services for all people now and in the future in a manner that is sustainable, i.e., adequate to meet basic necessities, not unduly environmentally detrimental, affordable by all, and acceptable to people and their communities. Note that the author’s definition has a temporal persistence element, and that it includes communities, which adds a collective element such as can be represented by culture. Note also that the concept ‘basic necessities’ has an element of vagueness as do other aspects of definitions of energy sustainability or overall sustainability. This can be problematic, although it also provides room for interpretation by individual countries or regions. Since overall sustainability is often viewed as the simultaneous attainment of environmental, economic and societal sustainability, it is clear that energy processes affect each these facets of sustainability. This highlights the importance of energy sustainability to sustainability overall. The relevance of these ideas is increasingly in the fore, as many countries and cities are seeking to become more sustainable, and view energy sustainability as a component of this objective.
Notable environmental, economic and societal challenges are associated with energy. These need to be addressed adequately as part of achieving energy sustainability, although the process can be complex and challenging. Some of the notable challenges relate to societal inequities, excessive resource consumption, climate change and the environmental and ecological affects of other emissions, and limited energy affordability. These are made more challenging by the fact that energy prices are skewed by taxes and incentives, and political factors affect energy issues, sometimes greatly. In addition, wealth and living standards as well as population, culture and level of urbanization often vary among countries, further affecting energy sustainability. The challenges are often greater for developing and non-industrialized countries, due to lack of wealth, education, technology and many other factors. The objective of this article is to assist in addressing these challenges, by informing about energy sustainability and enhancing efforts supporting energy sustainability.
It is noted that this extends earlier work by the author, including an effort to develop a pragmatic approach to energy sustainability with relevant illustrations (Rosen 2009 ). The first illustration considers a thermal energy storage that receives and holds heat (or cold) until it is required, while the second assesses a heat pump that uses electricity to extract heat from a low-temperature region and to deliver it to a region of higher temperature for heating. The third illustration is cogeneration of thermal and electrical energy as well as trigeneration of electricity, heat and cold, while the final illustration considers hydrogen production based on thermochemical water decomposition driven by nuclear or solar energy.
Energy resources are obtained from the environment. Some energy resources are renewable and some are finite in quantity and thus non-renewable. Energy systems in most countries today are principally driven by fossil fuels, but renewable energy utilization is increasing (Karunathilake et al. 2019 ; Hansen et al. 2019 ; Mehrjerdi et al. 2019 ; Kumar and Majid 2020 ). Renewable energy resources are listed with details on the main basis from which they are derived in Table Table2, 2 , while non-renewable energy resources grouped by resource type are given in Table Table3. 3 . Data from the IEA ( 2020 , 2021 ) on global production of the energy resources are also provided for the most significant resources in terms of quality. It is seen that many types of renewable energy are derived from solar energy, including hydraulic, biomass, wind and geothermal energy (as ground energy at ground temperature) (Rosen and Koohi-Fayegh 2017 ). Constraints on long-term energy supplies help to determine the sustainability of the energy resources and have been discussed by Weisz ( 2004 ).
Renewable energy resources
a Data source: IEA ( 2020 , 2021 )
Non-renewable energy resources
Energy carriers are the forms of energy that are utilized in processes and systems, and include fuels, electricity and heat (Rosen 2018 ). Some energy carriers exist in the environment while others do not and need to be produced artificially. Energy carriers, divided by energy carrier type, are listed in Table Table4 4 for non-chemical energy carriers and in Table Table5 5 for chemical energy carriers. Note that energy carriers do not include energy storages, which are simply temporary buffers for energy resources or carriers. Energy storages are indeed important and discussed subsequently in the article.
Non-chemical energy carriers
Chemical energy carriers
Energy is seen in Tables Tables2, 2 , ,3, 3 , ,4 4 and and5 5 to exist in various forms. Energy-conversion processes and technologies convert energy from one form to another, and can be described with thermodynamics. Of particular use are the first law of thermodynamics (the principle of conservation of energy) and the second law (the principle of non-conservation of entropy). The latter in particular helps determine energy quality and is the basis for the quantity exergy.
Sustainability and Sustainable Development
There are various understandings of sustainability and sustainable development, embodying various viewpoints (Rosen 2018 ; Baleta et al. 2019 ; Hengst et al. 2020 ; Pauliuk 2020 ; Dragicevic 2020 ; Chen et al. 2020a ; Rezaie and Rosen 2020 ). Some of the more significant of these are illustrated in Fig. 1 and examined below (Fig. 2 ):
- Multidisciplinary . Sustainability is often viewed as multidimensional with economic, social and environmental facets (see Fig. Fig.2). 2 ). Achieving sustainability is a challenge as these three facets are often opposing, e.g., economic sustainability may necessitate sacrificing environmental sustainability, and vice versa. Jose and Ramakrishna ( 2021 ) point out the multidisciplinary nature of sustainability in their assessment of the comprehensiveness of research in the field.
- Carrying capacity . Sustainability can be considered in terms of carrying capacity, i.e., the maximum population supportable, given the ability of the environment to provide resources and receive wastes. This involves an environmental perspective, but is focused more on limitations. The demand and supply of resources affects carrying capacity significantly. For example, Park et al. ( 2020 ) have evaluated the carrying capacity as a measure of sustainability, for Jeju Island, South Korea.
- Temporal . Sustainability is usually understood as temporally lasting. The temporal scale to be considered is subjective, although a period of 50–100 years is fairly often viewed as reasonable for many sustainability considerations (Graedel and Allenby 2010 ). Yet, this time frame can be disputed, especially for energy issues that can straddle centuries or more. For example, the lifetimes in terms of reserve base for fossil fuels have been estimated to be 51 years for oil, 53 years for natural gas and 114 years for coal, based on annual consumption rates (BP 2016 ). Thus coal-burning could be viewed as sustainable for the next 100 years or so based on the available resources, but then they would be practically exhausted clearly making them coal use not sustainable (and that is not considering the pollution and climate change effects from coal combustion). This contrasts with solar and wind energy, which have no date to exhaustion (until the sun ‘dies’ through running out of hydrogen, in about 5 billion years). Clearly, too short a period for evaluating sustainability is not helpful since most activities are sustainable for years, but too long a period is intractable.

United Nations Sustainable Development Goals (SDGs) (public domain material provided by United Nations at http://www.un.org/sustainabledevelopment/news/communications-material/ )

Selected understandings of sustainability and sustainable development, embodying various viewpoints

Sustainability viewed as having three principal facets: environmental, economic and societal
Sustainability and Energy
Based on the present author’s definition of energy sustainability cited earlier (the provision of energy services for all people now and in the future in a manner that is adequate to meet basic necessities, not unduly environmentally detrimental, affordable by all, and acceptable to people and their communities), it is evident that various issues impact how energy resources can be sustainable. Many of these issues are illustrated in Fig. 4 . Through these issues, key needs for energy sustainability can be developed. These are listed in Table Table6 6 along with interpretations of them.

Principal issues for achieving or shifting towards energy sustainability
Principal requirements for energy sustainability
The key needs for energy sustainability are examined in the remainder of this section.
Low Environmental and Ecological Impacts
Numerous environmental and ecological impacts are associated with energy systems over their lifetimes, ranging from local to national and international. Energy-related environmental and ecological impacts must be adequately addressed to attain energy sustainability, as their mitigation supports energy sustainability (Rosen 2012 , 2018 ; Sciubba 2019 ; Veiga and Romanelli 2020 ).
Some of the more notable environmental and ecological impacts linked to energy are as follows:
- Global climate change due to greenhouse gas emissions (Almazroui et al. 2019 ; Scott 2007 ).
- Abiotic resource depletion, due to the excessive use of non-biological and non-renewable raw materials (Graedel and Allenby 2010 ).
- Acid precipitation and acidification due to emissions of substances such as sulfur dioxide and nitrogen oxides (Rosen 2012 ).
- Stratospheric ozone depletion, which allows increased levels of ultraviolet radiation to reach the surface of the earth, causing adverse health effects (Razmjoo et al. 2020 ).
- Ecotoxicity and radiological exposures, and the health problems they can cause, such as those due to radioactivity in building materials (Pillai et al. 2017 ).
Climate change, as a consequence of global warming, is caused mainly by emissions of greenhouse gases (especially carbon dioxide), and is particularly concerning due to its potentially severe consequences (loss of land fertility in near equatorial regions, rising ocean levels and flooding of many cities, more frequent and stronger storms, etc.). These effects and others have recently been quantitatively assessed (Chen et al. 2020b ). By disrupting the earth–sun–space energy balance, these emissions lead to increases in mean global temperatures and consequential changes in climates. Low-carbon and carbon-free energy options are needed for climate change mitigation, as they can significantly lower emissions of the primary greenhouse gas, carbon dioxide, which is emitted through carbon fuel combustion.
Many effects of climate change have been studied, such as its impacts on hydro-meteorological variables and water resources (Almazroui and Şen 2020 ) and on water engineering structures (Almazroui et al. 2019 ). In addition, responses to climate change in the form of mitigation efforts have been examined, including carbon sequestration (Were et al. 2019 ) and carbon emission reduction (Khalil et al. 2019 ). Many of the effects and responses mentioned here relate to energy use, directly or indirectly.
For comprehensive and meaningful assessments of environmental and ecological impact, the overall life cycle of an energy system or activity needs to be considered, starting with the harvesting and processing of energy and other resources, and on to their utilization and ultimate disposal. Life cycle assessment (LCA) is an effective methodology for analyses (Graedel and Allenby 2010 ). LCA has been applied extensively to a broad range of activities (Ben-Alon et al. 2019 ; Lodato et al. 2020 ; Lu and Halog 2020 ), including energy processes (Sadeghi et al. 2020 ; Mendecka et al. 2020 ) and communities (Karunathilake et al. 2019 ).
Sustainable Energy Resources and Complementary Energy Carriers
Sustainable energy resources are crucial to energy sustainability, as are complementary energy carriers that allow those energy resources to be exploited or facilitate sustainable energy options. On the one hand, fossil fuels (see Table Table3), 3 ), the most common non-renewable energy resources, are finite in nature. On the other hand, renewable energy sources, including solar, hydraulic, wind, biomass, and geothermal energy (see Table Table2), 2 ), can be sustained for extremely long. Renewable energy resources also mitigate greatly or avoid greenhouse gas emissions, among other advantages. Some special cases are worth noting:
- Uranium (nuclear energy fuel) is a non-renewable energy resource but it does not contribute significantly to climate change, and the lifetimes of nuclear fuel assuming their use in advanced breeder reactors is thought to exceed 1000 years, so it is often viewed as a sustainable energy option (Al-Zareer et al. 2020a ). For example, Fetter ( 2009 ) estimated the extraction of uranium from seawater would make available 4.5 billion metric tons of uranium, representing a 60,000-year supply at present usage rates, while fuel-recycling fast-breeder reactors could match today’s nuclear output for 30,000 years, based on data of the Nuclear Energy Association (NEA). But this is contentious, as these very long nuclear fuel lifetimes remain hypothetical, while current actual nuclear power plants consume uranium at a much faster rate relative to reserves, in the process generating significant amounts of waste with half-lives that are significantly longer than 1000 years. The supply was estimated at 230 years in 2009 (Fetter 2009 ), based on identified uranium resources of total 5.5 million metric tons and an additional 10.5 million metric tons still undiscovered and the consumption rate at that time. Moreover, only very few nuclear plants are “fast breeder reactors”.
- Biomass may or may not be considered a renewable energy option, depending on its rates of utilization and replenishment. Regardless of the classification, decisions on using various types of biomass depend on both their costs (in terms of energy use quantity and rate, net quantity of carbon used, economics), and their benefits (net quantity of carbon emissions avoided, financial savings, etc.). The potential for biomass use to be sustainable often includes energy return on investment (EROI), which is the ratio of the amount of usable energy delivered from a particular energy resource to the amount of energy used to obtain that energy resource (Hall et al. 2014 ; Wang et al. 2021 ). This value has ranged from 0.64 (below the breakeven value of 1) for early biomass uses for producing ethanol to as high as 48 for some particular processes involving molasses, and typical values today are 4–5. Biomass is generally not sustainable when EROI values are near or below 1. In addition, it is noted that biomass typically has a low-energy conversion efficiency (relative to values for fossil fuels) and its production sometimes displaces food production, reducing in those cases its prospects as a sustainable energy resource.
- Wastes, which can include some forms of biomass, are sometimes viewed as a renewable energy resource and sometimes are not, given people can modify behaviors to reduce wastes greatly.
Much research on energy resources has been reported, including electricity generation from food waste through anaerobic digestion (Ali et al. 2019 ; Rezaie and Rosen 2020 ) and hydroelectric generation (Udayakumara and Gunawardena 2018 ), and solar energy applications (Hachem-Vermette et al. 2019 ; Sun et al. 2019 ). These studies collectively demonstrate the importance of energy sources in discussions of sustainability, and illustrate the feasibility of such technologies in practical applications.
Energy carriers, which include electricity, thermal energy and secondary fuels (see Tables Tables4, 4 , ,5), 5 ), play an important although less prominent role in energy sustainability. Before they can be utilized, energy resources often require conversion to other energy forms or carriers, e.g., solar photovoltaic panels to produce electricity for renewable energy resources, petroleum refineries for non-renewable energy resources, and hydrogen production from both types of energy resources. The latter example supports the idea of a hydrogen economy, in which hydrogen and electricity are the two main energy carriers (Scott 2007 ; Rosen 2017b ; Gnanapragasam and Rosen 2017 ; Moharamian et al. 2019 ; Abe et al. 2019 ; Endo et al. 2019 ; Fonseca et al. 2019 ; Chapman et al. 2020 ; Al-Zareer et al. 2020a ; Mehrjerdi et al. 2019 ) . Energy sustainability is supported well by this combination of energy carriers since most chemical energy needs can be satisfied by hydrogen (and select hydrogen-derived fuels) and non-chemical energy needs by electricity.
High Efficiencies
High efficiency in a holistic sense is broad, covering:
- high device and system efficiencies,
- energy conservation,
- energy management and matching of energy demands and supplies,
- appropriate utilization of energy quality, and
- advantageous fuel substitution.
This holistic sense is adopted here. High efficiency supports energy sustainability by expanding the benefits of energy technologies, whether renewable or not, although the benefits are more pronounced for non-renewable energy resources. High efficiency elongates the lives of finite-energy resources and lowers the capacities needed for energy devices. High efficiencies often can improve societal metrics such as standard of living, quality of life, and satisfaction. For instance, the US and Sweden have similar gross domestic products (per capita), but the latter exceeds the US in most social indicators and utilizes 40% less energy (per capita) through more efficient buildings, smaller automobiles and better public transit, and higher gasoline taxes (Rosen 2018 ). Advanced methods are available to help attain high efficiencies, e.g., exergy analysis provides insights not available via conventional energy methods (Rosen 2012 ; Dincer and Rosen 2021b , 2015 ) and has been applied widely (Morosuk and Tsatsaronis 2019 ; Sciubba 2019 ; Veiga and Romanelli 2020 ; Kumar et al. 2020 ).
Economic Sustainability and Affordability
Energy sustainability necessitates that the energy services required for basic needs be economically affordable by most if not all people and societies (Rosen 2011 ). However, the economics of energy sustainability measures usually need to be reasonably competitive with conventional approaches to find acceptance and adoption, although it is noted that some efficiency measures, like some environmental impact mitigation measures, can over time sometimes pay for themselves or save money. Government incentives also can enhance affordability.
Of course, many other factors affect economic sustainability and affordability. First, the economic “externalities” associated with fossil fuel combustion, i.e., the environmental costs that are not accounted for in the cost of production, are normally not counted. When externalities are properly accounted for, the economics improve for non-polluting energy forms such as wind and solar, and can become more favorable than the economics for fossil fuels. For example, Bielecki et al. ( 2020 ) show that the costs of externalities for fossil fuels and peat are typically 10–100 times greater than those for sustainable energy forms such as hydraulic, solar, wind, biomass and nuclear. Furthermore, economies of scale are an important factor in lowering economic costs, thereby making energy sources more sustainable. In addition, the economics of energy often fluctuates in response to energy resource scarcity or abundance, political instability for the case of finite-energy resources such as oil, natural gas, and uranium. Finally, the intermittency of some renewable energy forms such as wind and solar can raise their costs.
Other needs exist for energy sustainability and need to be addressed, and a great number of these are non-technical. Selected needs are shown in Fig. 5 and discussed below:
- Geographic and intergenerational equity. For energy sustainability, equity is needed among present and future generations and among developed and developing countries in terms of energy access. Being concerned about future generations is important to the temporal aspect of sustainability, and involves considering the responsibility of people to consider the effects of their actions today, and their motivations, on any harm that may be brought to future generations. This involves trade offs. Concerns about energy access developed and developing countries raises issues of fairness and other trade offs, e.g., is it reasonable for countries that became wealthy in large part through extensive use of fossil fuels to ask developing and poorer nations to forego the use of fossil fuels and to use more sustainable energy forms, even if the costs are higher.
- Increasing population, energy demands and living standards. Increasing global population must be accounted for in energy sustainability measures and strategies, as it places stresses on the carrying capacity of the planet and the environment. Furthermore, the rising demand and desire for energy resources with increasing wealth, especially as developing countries attain higher living standards, also makes energy sustainability more challenging. Energy sustainability can be assisted by measures involving transformations in lifestyles and reductions in energy demands, although this is usually very challenging in general and especially for policy makers. Behavioral modification requires recognition that present development trends are unsustainable over time. Many of these issues have been studied previously, such as the vulnerability of livelihoods in regions and countries (Qaisrani et al. 2018 ).
- Resource and land use. Balances are often necessary to preserve resources and land for the uses for which they are most needed. For instance, land uses for growing biomass for biofuels needs to be appropriately weighed against agriculture needs, flooding large tracts of land needs to be balanced against hydroelectric generation requirements, and ecosystem preservation needs to be balanced against long-distance electrical transmission corridors.
- Societal acceptability and involvement. For acceptance of energy sustainability measures, societies and their populations must be informed, involved in decisions, and supportive of them. This normally necessitates thorough consultation, and is particularly important when special or disadvantaged communities are involved, such as some indigenous communities.
- Aesthetics and cleanliness. Energy sustainability measures should not degrade unduly the aesthetic appeal and cleanliness of the environment, for societal and other reasons. Even renewable energy resources can be aesthetically problematic, e.g., large solar PV installations and wind farms. Of course, aesthetics are a personal matter and vary from one person to another, sometimes considerably, often making it challenging to find the appropriate trade off.
- Health and safety. In strategies and plans for energy sustainability, energy options must be healthy and safe, as evidenced by concerns associated with the COVID-19 pandemic that began in 2019. This issue has spawned much research, e.g., an investigation of the impact of daily weather on the temporal pattern of COVID-19 outbreaks (Gupta et al. 2020 ).

Selected non-technical aspects of energy sustainability
Note that these non-technical factors of energy sustainability are at times interconnected, related and overlapping. Note also that many of the non-technical factors are often addressed if the technical factors discussed previously are addressed suitably. An example: factors such as public acceptability, economics, and equity need to be accounted for when choosing among sustainable energy options. Examining these issues makes it apparent that energy sustainability is politically sensitive, due to the political nature of many of the issues raised in the above points. Even though these points may be recognized already, they are included here for completeness, especially in light of their importance.
Methods for Enhancing Energy Sustainability
A selection of energy methods that can help enhance energy sustainability directly or indirectly, shown in Fig. 6 , are now described.

Selected methods for enhancing energy sustainability
Efficiency, loss prevention and waste recovery can all help enhance energy sustainability. Appropriately high-efficiency devices and systems facilitate and contribute to energy sustainability, e.g., heaters, chillers and air conditioners, pumps and compressors, motors and fans, and lighting have higher efficiencies today than in the past, the latter due to more efficient bulbs, lower lighting intensities, task lighting, and lighting occupancy sensors. Efficiency can also be improved by preventing losses, e.g., with better insulation, and by recovering energy wastes, e.g., by waste heat recovery.
Exergy analysis and other advanced tools can support energy sustainability. Thermodynamic performance can be better assessed, improved and optimized with exergy analysis rather than energy analysis, since the former evaluates more meaningful efficiencies and better pinpoints inefficiencies. Based on exergy, a measure of energy usefulness or quality or value (Dincer and Rosen 2021b ), exergy methods have been applied increasingly in recent years (Dincer et al. 2017 ; Moharamian et al. 2019 ). In addition, quality matching of energy supply and demand can also support energy efficiency. It is usually more efficient to supply an energy quality better matched to energy demand instead of supplying an exceedingly high-quality energy form, and thus having a quality mismatch, a result well illustrated with exergy analysis. For example, supplying heating for aquaculture at 20 °C with a natural gas combustor capable of heating to 1000 °C is mismatched compared to using simple solar thermal collectors operating at 40 °C.
Governments can also apply incentives (technically and/or societally directed) and enforcement activities to support energy measures. These can be mandatory or voluntary, depending on circumstances and needs. Modifications to lifestyles and societal structures can also reduce energy use, e.g., shifting North America’s transportation preference to mass transit from automotive, in part by changing energy taxation and environmental restrictions.
Technologies for Enhancing Energy Sustainability
Sample energy technologies that can help enhance energy sustainability directly or indirectly, shown in Fig. 7 , are now described. Note that the methods discussed in the prior section are intended to include techniques and approaches for improving energy sustainability, while the technologies covered in this section focus on specific technologies that can be employed to improve energy sustainability. Of course the methods can be applied to technologies, but the focus of the prior section was on methods and techniques.

Selected technologies for enhancing energy sustainability
Utilizing renewable energy sources (e.g., hydraulic, solar, wind, geothermal, biomass, wave, tidal and ocean thermal energy) can contribute to energy sustainability, as they can be sustained for long time periods and have low environmental emissions and impacts. These sources have been extensively investigated, e.g., the amplitudes and phases of tides near power stations (Madah 2020 ) as well as the impacts of potential sea-level rise on tides (Lafta et al. 2020 ). Energy storage can also support energy sustainability, in part by offsetting the intermittency of some renewable energy resources (Krishan and Suhag 2019 ). Energy storage can also store energy until it is economic to deploy, and enhance efficiency and energy management (Al-Zareer et al. 2020b ). There are various types of energy storage (Koohi-Fayegh and Rosen 2020 ), including thermal energy storage (Dincer and Rosen 2021a ), underground storage using borehole heat exchangers (Sliwa et al. 2019 ) and batteries (Al-Zareer et al. 2020b ). Energy storage is increasingly being employed in building and HVAC systems (Dincer and Rosen 2015 ), and in renewable energy systems involving hybrid energy schemes (Rekioua 2020 ) and microgrids (Al-Ghussain et al. 2020 ).
Integrated energy systems, based on renewable and/or non-renewable energy technologies, can enhance energy sustainability and efficiency, e.g., polygeneration systems (Rosen and Koohi-Fayegh 2016 ; Calise et al. 2019 ; Rokni 2020 ; Mendecka et al. 2020 ; Kasaeian et al. 2020 ), and linking separate systems advantageously such as in cascading energy systems (Campana et al. 2019 ; Liu et al. 2020 ; Rokni 2020 ).
Building energy systems can be modified to enhance energy sustainability, e.g., using active systems such as renewable energy resources and passive technologies such as Trombe walls, multiple glazing windows and selective window coatings, daylight harvesting, insulation, weatherstripping and caulking. Note that behavior, culture and lifestyle also can affect the success of energy efficiency measures in buildings, as was illustrated for China (Zhang and Wang 2013 ). Energy sustainability can also be enhanced via district energy systems, in which thermal energy can be generated in heating or cooling facilities, using renewable energy or conventional resources, and transported to users. District energy systems are used in many cities and traverse a wide range of distances (Rosen and Koohi-Fayegh 2016 ). Buildings in many cities are connected through district energy systems that provide space and water heating and space cooling.
Illustration
In this illustration, we consider net-positive energy buildings. A net-positive energy building over an average year generates more energy from renewable energy sources than it uses, as shown in Fig. 8 , and can support energy sustainability (Rosen 2015 ; Endo et al. 2019 ; Delavar and Sahebi 2020 ; Tumminia et al. 2020 ; Singh and Das 2020 ). A net-positive energy building uses energy for a variety of tasks and generates energy from various renewable energy resources, and achieves net-positive energy status through advanced design and exploitation of technologies such as advanced automation, controls, component integration, energy storage, lighting and HVAC. One of the main upcoming “other energy uses” for electricity in Fig. 8 will likely be for vehicle energy (e.g., for electric automobiles). Such utilization of energy is likely to prove both cost effective and environmentally friendly. A net-positive energy building generates more electrical plus thermal energy from renewable energy sources than it uses over an average year, as shown in Fig. 9 . Such buildings are net energy generators, rather than net energy users, like most buildings today. Research on net-zero and net-positive energy buildings has been reported (Athienitis and O’Brien 2015 ; Mehrjerdi et al. 2019 ; Sun et al. 2019 ), while the International Energy Agency included an annex on “Towards Net-zero Energy Solar Building” and Canada launched in 2011 the Smart Net-zero Energy Buildings Strategic Research Network ( http://www.solarbuildings.ca ). The net-zero and net-positive energy building concepts can be expanded to include transportation devices that are part of the building (Garmsiri et al. 2016 ; Sun et al. 2019 ) and to net-zero and net-positive energy communities (Rad et al. 2017 ; Hachem-Vermette et al. 2019 ; Karunathilake et al. 2019 ; Nematchoua et al. 2021 ).

Net-positive energy building, in which energy generation from renewable energy resources exceeds energy use over a typical year

Imbalance of a net-positive energy building, highlighting how energy use over a typical year is less than energy generation from renewable energy resources
As a numerical example that correlates with the qualitative presentation in Fig. 8 , a performance assessment by Zomer et al. ( 2020 ) of PV systems installed at a positive energy building is considered here. The building is the Fotovoltaica/UFSC solar energy laboratory ( http://www.fotovoltaica.ufsc.br ) in Florianópolis, Brazil (27° S, 48° W). Although originally designed as a zero-energy building with PV systems on rooftops and façades, additional PV systems were installed on the same location on a carport and an electric bus (eBus) shelter and charging station, and ground-mount PV systems with single-axis solar tracking installed. The system then had a peak PV generation capacity of 111 kW. Energy generation and consumption were analyzed on monthly bases, and the key results are listed in Table Table7. 7 . The total PV generation in the period could supply 97% of the building (including eBus) energy consumption, accounting for actual performance and downtime for R&D activities. In that case, the building was almost a net-zero energy building (for which the energy supply would meet 100% of the consumption). However, when the systems operate all the time at their optimal performance, the PV system can supply 134% of the building (including eBus) energy consumption, making it a positive energy building.
Energy generation and consumption for Fotovoltaica/UFSC solar energy laboratory
Conclusions
Energy sustainability is described, with a focus on environmental perspectives, as are methods and technologies to enhance it. In essence being a key component of sustainability, the significance and importance of energy sustainability becomes clear. Requirements to increase energy sustainability are discussed, including low environmental and ecological impacts, sustainable energy resources and complementary energy carriers, high efficiencies, low environmental impacts, and various other predominantly non-technical factors. The latter include living standards, societal acceptability and equity. Interrelations among these are examined. Examples and illustrations are described that help to indicate the benefits of enhancing energy sustainability. The illustrations also indicate the complexity of energy sustainability and the factors that contribute to it, showing how challenging it can be to enhance energy sustainability. Net-positive energy buildings in particular illustrate the benefits and challenges. The outcomes and results serve to inform and educate about energy sustainability, to provide an impetus to move people in particular and civilization in general towards it.
Acknowledgements
The author is grateful for support provided by the Natural Sciences and Engineering Research Council of Canada, and the contributions of students and colleagues over the years that have helped in developing the ideas presented.
Declarations
The author has no conflicts of interest to declare that are relevant to the content of this article.
- Abe JO, Popoola API, Ajenifuja E, Popoola OM. Hydrogen energy, economy and storage: review and recommendation. Int J Hydrogen Energy. 2019; 44 (29):15072–15086. [ Google Scholar ]
- Al-Ghussain L, Samu R, Taylan O, Fahrioglu M. Sizing renewable energy systems with energy storage systems in microgrids for maximum cost-efficient utilization of renewable energy resources. Sustain Urban Areas. 2020; 55 :102059. [ Google Scholar ]
- Ali A, Mahar RB, Sheerazi STH. Renewable electricity generation from food waste through anaerobic digestion in Pakistan: a mini-review. Earth Syst Environ. 2019; 3 :95–100. [ Google Scholar ]
- Almazroui M, Şen Z. Trend analyses methodologies in hydro-meteorological records. Earth Syst Environ. 2020; 4 :713–738. [ Google Scholar ]
- Almazroui M, Şen Z, Mohorji AM, et al. Impacts of climate change on water engineering structures in arid regions: case studies in Turkey and Saudi Arabia. Earth Syst Environ. 2019; 3 :43–57. [ Google Scholar ]
- Al-Zareer M, Dincer I, Rosen MA. Assessment and analysis of the integrated generation IV gas-cooled fast nuclear reactor and copper–chlorine cycle for hydrogen and electricity production. Energy Convers Manag. 2020; 205 :112387. [ Google Scholar ]
- Al-Zareer M, Dincer I, Rosen MA. A thermal performance management system for lithium-ion battery packs. Appl Therm Eng. 2020; 165 :114378. [ Google Scholar ]
- Athienitis A, O'Brien W, editors. Modeling, design, and optimization of net-zero energy buildings. Berlin: Ernst & Sohn; 2015. [ Google Scholar ]
- Baleta J, Mikulčić H, Klemeš JJ, Urbaniec K, Duić N. Integration of energy, water and environmental systems for a sustainable development. J Clean Prod. 2019; 215 :1424–1436. [ Google Scholar ]
- Ben-Alon L, Loftness V, Harries KA, DiPietro G, Hameen EC. Cradle to site life cycle assessment (LCA) of natural vs conventional building materials: a case study on cob earthen material. Build Environ. 2019; 160 :106150. [ Google Scholar ]
- Bielecki A, Ernst S, Skrodzka W, Wojnicki I. The externalities of energy production in the context of development of clean energy generation. Environ Sci Pollut Res. 2020; 27 :11506–11530. [ PMC free article ] [ PubMed ] [ Google Scholar ]
- BP . BP statistical review of world energy 2016. 65. London: BP; 2016. [ Google Scholar ]
- Calise F, Dentice d’Accadia M, Vicidomini M. Optimization and dynamic analysis of a novel polygeneration system producing heat, cool and fresh water. Renew Energy. 2019; 143 :1331–1347. [ Google Scholar ]
- Campana PE, Wästhage L, Nookuea W, Tan Y, Yan J. Optimization and assessment of floating and floating-tracking PV systems integrated in on- and off-grid hybrid energy systems. Sol Energy. 2019; 177 :782–795. [ Google Scholar ]
- Chapman A, Itaoka K, Farabi-Asl H, Fujii Y, Nakahara M. Societal penetration of hydrogen into the future energy system: Impacts of policy, technology and carbon targets. Int J Hydrogen Energy. 2020; 45 (7):3883–3898. [ Google Scholar ]
- Chen S, Kharrazi A, Liang S, Fath BD, Lenzen M, Yan J. Advanced approaches and applications of energy footprints toward the promotion of global sustainability. Appl Energy. 2020; 261 :114415. [ Google Scholar ]
- Chen Y, Liu A, Cheng X. Quantifying economic impacts of climate change under nine future emission scenarios within CMIP6. Sci Total Environ. 2020; 703 :134950. [ PubMed ] [ Google Scholar ]
- Delavar H, Sahebi H. A sustainable mathematical model for design of net zero energy buildings. Heliyon. 2020; 6 (1):e03190. [ PMC free article ] [ PubMed ] [ Google Scholar ]
- Dincer I, Rosen MA. Exergy analysis of heating, refrigerating and air conditioning: methods and applications. Oxford: Elsevier; 2015. [ Google Scholar ]
- Dincer I, Rosen MA. Thermal energy storage: systems and applications. 3. London: Wiley; 2021. [ Google Scholar ]
- Dincer I, Rosen MA. Exergy: energy, environment and sustainable development. 3. Oxford: Elsevier; 2021. [ Google Scholar ]
- Dincer I, Rosen MA, Ahmadi P. Optimization of energy systems. London: Wiley; 2017. [ Google Scholar ]
- Dragicevic AZ. Concentric framework for sustainability assessment. J Clean Prod. 2020; 248 :119268. [ Google Scholar ]
- Endo N, Shimoda E, Goshome K, Yamane T, Nozu T, Maeda T. Construction and operation of hydrogen energy utilization system for a zero emission building. Int J Hydrogen Energy. 2019; 44 (29):14596–14604. [ Google Scholar ]
- Fetter S. How long will the world’s uranium supplies last? Sci Am. 2009; 300 :84. [ Google Scholar ]
- Fonseca JD, Camargo M, Commenge J-M, Falk L, Gil ID. Trends in design of distributed energy systems using hydrogen as energy vector: a systematic literature review. Int J Hydrogen Energy. 2019; 44 (19):9486–9504. [ Google Scholar ]
- Garmsiri S, Kouhi-Fayegh S, Rosen MA, Smith GR. Integration of transportation energy processes with a net zero energy community using captured waste hydrogen from electrochemical plants. Int J Hydrogen Energy. 2016; 41 (19):8337–8346. [ Google Scholar ]
- Gnanapragasam NV, Rosen MA. A review of hydrogen production using coal, biomass and other solid fuels. Biofuels. 2017; 8 (6):725–745. [ Google Scholar ]
- Graedel TE, Allenby BR. Industrial ecology and sustainable engineering. Upper Saddle River: Prentice Hall; 2010. [ Google Scholar ]
- Grigoroudis E, Kouikoglou VS, Phillis YA, Kanellos FD. Energy sustainability: a definition and assessment model. Oper Res Int J. 2019 doi: 10.1007/s12351-019-00492-2. [ CrossRef ] [ Google Scholar ]
- Gupta A, Pradhan B, Maulud KNA. Estimating the impact of daily weather on the temporal pattern of COVID-19 outbreak in India. Earth Syst Environ. 2020; 4 :523–534. [ PMC free article ] [ PubMed ] [ Google Scholar ]
- Hachem-Vermette C, Guarino F, La Rocca V, Cellura M. Towards achieving net-zero energy communities: investigation of design strategies and seasonal solar collection and storage net-zero. Sol Energy. 2019; 192 :169–185. [ Google Scholar ]
- Hall CAS, Lambert JG, Balogh SB. EROI of different fuels and the implications for society. Energy Policy. 2014; 64 :141–152. [ Google Scholar ]
- Hansen K, Breyer C, Lund H. Status and perspectives on 100% renewable energy systems. Energy. 2019; 175 :471–480. [ Google Scholar ]
- Hengst I-A, Jarzabkowski P, Hoegl M, Muethel M. Toward a process theory of making sustainability strategies legitimate in action. Acad Manag J. 2020; 63 :246–271. [ Google Scholar ]
- IEA . World Energy Statistics Database Documentation. 2020. Paris: International Energy Agency; 2020. [ Google Scholar ]
- IEA (2021) Explore energy data by category, indicator, country or region. International Energy Agency. https://www.iea.org/data-and-statistics/data-tables?country=WORLD&energy=Balances&year=2018 . Accessed 16 Mar 2021
- Jose R, Ramakrishna S. Comprehensiveness in the research on sustainability. Mater Circu Econ. 2021; 3 :1. doi: 10.1007/s42824-020-00015-x. [ CrossRef ] [ Google Scholar ]
- Karunathilake H, Hewage K, Mérida W, Sadiq R. Renewable energy selection for net-zero energy communities: life cycle based decision making under uncertainty. Renew Energy. 2019; 130 :558–573. [ Google Scholar ]
- Kasaeian A, Bellos E, Shamaeizadeh A, Tzivanidis C. Solar-driven polygeneration systems: recent progress and outlook. Appl Energy. 2020; 264 :114764. [ Google Scholar ]
- Khalil A, Javed A, Bashir H. Evaluation of carbon emission reduction via GCIP projects: creating a better future for Pakistan. Earth Syst Environ. 2019; 3 :19–28. [ Google Scholar ]
- Koohi-Fayegh S, Rosen MA. A review of energy storage types, applications and recent developments. J Energy storage. 2020; 27 :101047. [ Google Scholar ]
- Krishan O, Suhag S. An updated review of energy storage systems: classification and applications in distributed generation power systems incorporating renewable energy resources. Int J Energy Res. 2019; 43 :6171–6210. [ Google Scholar ]
- Kumar J, C. R., Majid, M. A. Renewable energy for sustainable development in India: current status, future prospects, challenges, employment, and investment opportunities. Energy Sustain Soc. 2020; 10 :2. [ Google Scholar ]
- Kumar A, Sharma M, Thakur P, Kumar Thakur V, Rahatekar SS, Kumar R. A review on exergy analysis of solar parabolic collectors. Sol Energy. 2020; 197 :411–432. [ Google Scholar ]
- Kutscher CF, Milford JB, Kreith F. Principles of sustainable energy systems. Mechanical and aerospace engineering series. 3. Boca Raton: CRC Press; 2019. [ Google Scholar ]
- Lafta AA, Altaei SA, Al-Hashimi NH. Impacts of potential sea-level rise on tidal dynamics in Khor Abdullah and Khor Al-Zubair, Northwest of Arabian Gulf. Earth Syst Environ. 2020; 4 :93–105. [ Google Scholar ]
- Liu Z, Yu H, Liu R, Wang M, Li C. Configuration optimization model for data-center-park-integrated energy systems under economic, reliability, and environmental considerations. Energies. 2020; 13 :448. [ Google Scholar ]
- Lodato C, Tonini D, Damgaard A, Astrup TF. A process-oriented life-cycle assessment (LCA) model for environmental and resource-related technologies (EASETECH) Int J Life Cycle Assess. 2020; 25 :73–88. [ Google Scholar ]
- Lu T, Halog A. Towards better life cycle assessment and circular economy: on recent studies on interrelationships among environmental sustainability, food systems and diet. Int J Sustain Dev World. 2020 doi: 10.1080/13504509.2020.1734984. [ CrossRef ] [ Google Scholar ]
- Madah FA. The amplitudes and phases of tidal constituents from harmonic analysis at two stations in the Gulf of Aden. Earth Syst Environ. 2020; 4 :321–328. [ Google Scholar ]
- Mehrjerdi H, Iqbal A, Rakhshani E, Torres JR. Daily-seasonal operation in net-zero energy building powered by hybrid renewable energies and hydrogen storage systems. Energy Convers Manag. 2019; 201 :112156. [ Google Scholar ]
- Mendecka B, Tribioli L, Cozzolino R. Life cycle assessment of a stand-alone solar-based polygeneration power plant for a commercial building in different climate zones. Renew Energy. 2020 doi: 10.1016/j.renene.2020.03.063. [ CrossRef ] [ Google Scholar ]
- Moharamian A, Soltani S, Rosen MA, Mahmoudi SMS, Bhattacharya T. Modified exergy and modified exergoeconomic analyses of a solar based biomass co-fired cycle with hydrogen production. Energy. 2019; 167 :715–729. [ Google Scholar ]
- Morosuk T, Tsatsaronis G. Advanced exergy-based methods used to understand and improve energy-conversion systems. Energy. 2019; 169 :238–246. [ Google Scholar ]
- Nematchoua MK, Marie-Reine Nishimwe A, Reiter S. Towards nearly zero-energy residential neighbourhoods in the European Union: a case study. Renew Sustain Energy Rev. 2021; 135 :110198. [ Google Scholar ]
- Park J, Kim S, Kim Y, Hong S, Suh K. Evaluation of carrying capacity and sustainability of Jeju Island using Onishi Model. J Korean Soc Rural Plan. 2020; 26 (02):95–106. doi: 10.7851/ksrp.2020.26.2.095. [ CrossRef ] [ Google Scholar ]
- Pauliuk S. Making sustainability science a cumulative effort. Nat Sustain. 2020; 3 :2–4. [ Google Scholar ]
- Pillai GS, Jeevarenuka K, Hameed PS. Radioactivity in building materials of Pudukkottai Geological Region, Tamil Nadu, India. Earth Syst Environ. 2017; 1 :4. [ Google Scholar ]
- Qaisrani A, Umar MA, Siyal GEA, et al. What defines livelihood vulnerability in rural semi-arid areas? Evidence from Pakistan. Earth Syst Environ. 2018; 2 :455–475. [ Google Scholar ]
- Rad FM, Fung AS, Rosen MA. An integrated model for designing a solar community heating system with borehole thermal storage. Energy Sustain Dev. 2017; 36C :6–15. [ Google Scholar ]
- Razmjoo AA, Sumper A, Davarpanah A. Energy sustainability analysis based on SDGs for developing countries. Energy Sources Part A Recovery Util Environ Eff. 2020; 42 (9):1041–1056. [ Google Scholar ]
- Rekioua D (2020) Storage in hybrid renewable energy systems. In: Hybrid renewable energy systems: optimization and power management control. Springer, Cham, pp 139–172
- Rezaie B, Rosen MA. The energy-water-food nexus: a framework for sustainable development modeling. Energy Equip Syst. 2020; 8 (2):179–201. [ Google Scholar ]
- Rokni MM. Waste to energy and polygeneration systems based on solid oxide fuel cells. ASME J Electrochem Energy Convers Storage. 2020; 17 (3):031011. [ Google Scholar ]
- Rosen MA. Energy sustainability: a pragmatic approach and illustrations. Sustainability. 2009; 1 (1):55–80. [ Google Scholar ]
- Rosen MA. Economics and exergy: an enhanced approach to energy economics. Hauppauge: Nova Science Publishers; 2011. [ Google Scholar ]
- Rosen MA. Environment, ecology and exergy: enhanced approaches to environmental and ecological management. Hauppauge: Nova Science Publishers; 2012. [ Google Scholar ]
- Rosen MA. Net-zero energy buildings and communities: potential and the role of energy storage. J Power Energy Eng. 2015; 3 (4):470–474. [ Google Scholar ]
- Rosen MA. How can we achieve the UN sustainable development goals? Eur J Sustain Dev Res. 2017; 1 (2):06. [ Google Scholar ]
- Rosen MA. Sustainable development: a vital quest. Eur J Sustain Dev Res. 2017; 1 (1):2. [ Google Scholar ]
- Rosen MA. Enhancing renewable energy prospects via hydrogen energy systems. J Fundam Renew Energy Appl. 2017; 7 (5):22. [ Google Scholar ]
- Rosen MA. Issues, concepts and applications for sustainability. Glocalism J Cult Polit Innov. 2018; 3 :1–21. [ Google Scholar ]
- Rosen MA, Koohi-Fayegh S. Cogeneration and district energy systems: modelling, analysis and optimization. London: Institution of Engineering and Technology; 2016. [ Google Scholar ]
- Rosen MA, Koohi-Fayegh S. Geothermal energy: sustainable heating and cooling using the ground. London: Wiley; 2017. [ Google Scholar ]
- Sadeghi S, Ghandehariun S, Rosen MA. Comparative economic and life cycle assessment of solar-based hydrogen production for oil and gas industries. Energy. 2020; 208 :118347. [ Google Scholar ]
- Sciubba E. Exergy-based ecological indicators: from thermo-economics to cumulative exergy consumption to thermo-ecological cost and extended exergy accounting. Energy. 2019; 168 :462–476. [ Google Scholar ]
- Scott DS. Smelling land: the hydrogen defense against climate catastrophe. Ottawa: Canadian Hydrogen Association; 2007. [ Google Scholar ]
- Singh G, Das R. Comparative assessment of different air-conditioning systems for nearly/net zero-energy buildings. Int J Energy Res. 2020; 44 :3526–3546. [ Google Scholar ]
- Sliwa T, Sojczynska A, Rosen MA, Kowalski T. Evaluation of temperature profiling quality in determining energy efficiencies of borehole heat exchangers. Geothermics. 2019; 78 :129–137. [ Google Scholar ]
- Suganthi L. Sustainability indices for energy utilization using a multi-criteria decision model. Energy Sustain Soc. 2020; 10 :16. [ Google Scholar ]
- Sun D, Athienitis A, D'Avignon K. Application of semitransparent photovoltaics in transportation infrastructure for energy savings and solar electricity production: toward novel net-zero energy tunnel design. Prog Photovolt Res Appl. 2019; 27 :1034–1044. [ Google Scholar ]
- Tumminia G, Guarino F, Longo S, Aloisio D, Cellura S, Sergi F, Brunaccini G, Antonucci V, Ferraro M. Grid interaction and environmental impact of a net zero energy building. Energy Convers Manag. 2020; 203 :112228. [ Google Scholar ]
- Udayakumara EPN, Gunawardena UADP. Cost–benefit analysis of Samanalawewa Hydroelectric Project in Sri Lanka: an ex post analysis. Earth Syst Environ. 2018; 2 :401–412. [ Google Scholar ]
- United Nations (2015) Resolution adopted by the general assembly on 25 September 2015. A/RES/70/1. Seventieth session. United Nations
- Veiga JPS, Romanelli TL. Mitigation of greenhouse gas emissions using exergy. J Clean Prod. 2020; 260 :121092. [ Google Scholar ]
- Wang C, Zhang L, Chang Y, Pang M. Energy return on investment (EROI) of biomass conversion systems in China: meta-analysis focused on system boundary unification. Renew Sustain Energy Rev. 2021; 137 :110652. [ Google Scholar ]
- Weisz PB. Basic choices and constraints on long-term energy supplies. Phys Today. 2004; 57 (7):47–52. doi: 10.1063/1.1784302. [ CrossRef ] [ Google Scholar ]
- Were D, Kansiime F, Fetahi T, et al. Carbon sequestration by wetlands: a critical review of enhancement measures for climate change mitigation. Earth Syst Environ. 2019; 3 :327–340. [ Google Scholar ]
- World Bank Group (2021) DataBank: World Development Indicators (series “GDP (Constant 2005 US$)”, “Energy Use (kt of oil equivalent)” and “Population (Total)”. http://www.databank.worldbank.org . Accessed 19 March 2021
- World Commission on Environment and Development . Our common future. New York: Oxford University Press; 1987. [ Google Scholar ]
- Zhang Y, Wang Y. Barriers’ and policies’ analysis of China’s building energy efficiency. Energy Policy. 2013; 62 :768–773. [ Google Scholar ]
- Zomer C, Custódio I, Goulart S, Mantelli S, Martins G, Campos R, Pinto G, Rüther R. Energy balance and performance assessment of PV systems installed at a positive-energy building (PEB) solar energy research centre. Sol Energy. 2020; 212 :258–274. [ Google Scholar ]
- Zvolinschi A, Kjelstrup S, Bolland O, van der Kooi HJ. Exergy sustainability indicators as a tool in industrial ecology. J Ind Ecol. 2007; 11 :85–98. [ Google Scholar ]
- Reference Manager
- Simple TEXT file
People also looked at
Review article, sustainable energy transition for renewable and low carbon grid electricity generation and supply.
- Industrial Engineering Department, Durban University of Technology, Durban, South Africa
The greatest sustainability challenge facing humanity today is the greenhouse gas emissions and the global climate change with fossil fuels led by coal, natural gas and oil contributing 61.3% of global electricity generation in the year 2020. The cumulative effect of the Stockholm, Rio, and Johannesburg conferences identified sustainable energy development (SED) as a very important factor in the sustainable global development. This study reviews energy transition strategies and proposes a roadmap for sustainable energy transition for sustainable electricity generation and supply in line with commitments of the Paris Agreement aimed at reducing greenhouse gas emissions and limiting the rise in global average temperature to 1.5°C above the preindustrial level. The sustainable transition strategies typically consist of three major technological changes namely, energy savings on the demand side, generation efficiency at production level and fossil fuel substitution by various renewable energy sources and low carbon nuclear. For the transition remain technically and economically feasible and beneficial, policy initiatives are necessary to steer the global electricity transition towards a sustainable energy and electricity system. Large-scale renewable energy adoption should include measures to improve efficiency of existing nonrenewable sources which still have an important cost reduction and stabilization role. A resilient grid with advanced energy storage for storage and absorption of variable renewables should also be part of the transition strategies. From this study, it was noted that whereas sustainable development has social, economic, and environmental pillars, energy sustainability is best analysed by five-dimensional approach consisting of environmental, economic, social, technical, and institutional/political sustainability to determine resource sustainability. The energy transition requires new technology for maximum use of the abundant but intermittent renewable sources a sustainable mix with limited nonrenewable sources optimized to minimize cost and environmental impact but maintained quality, stability, and flexibility of an electricity supply system. Technologies needed for the transition are those that use conventional mitigation, negative emissions technologies which capture and sequester carbon emissions and finally technologies which alter the global atmospheric radiative energy budget to stabilize and reduce global average temperature. A sustainable electricity system needs facilitating technology, policy, strategies and infrastructure like smart grids, and models with an appropriate mix of both renewable and low carbon energy sources.
• The Paris Agreement of 2015 set targets to be realized to limit greenhouse gas emissions and related global warming with the objective of reducing greenhouse gas emissions and global average temperature rise.
• The cumulative effect of the Stockholm, Rio, and Johannesburg conferences made sustainable energy development (SED) a very important factor in the sustainable development.
• The energy transition seeks to transform the world order with respect to development and environment and particularly the use of energy in its many forms with priority to electricity.
• Sustainable energy transition should incorporate the three dimensions of sustainable development of social, environment and economic in addition to technical and political/institutional dimension.
• A sustainable global electricity transition will entail increased use of renewable energy sources particularly wind and solar, nuclear energy as a low carbon energy source, electrification of transport and thermal processes in industry, bioenergy, and waste to energy conversion, shift from coal and petroleum to natural gas, hydrogen as a fuel with low carbon footprint, increased energy efficiency.
• This transition can only take place successfully through collaboration between players locally and international with effective facilitating policy framework, facilitating infrastructure and technology development and adaptation, use of smart grids and various modelling and optimization facilities in decision support.
1 Introduction
Decarbonization of the global energy systems is one of the greatest and most important challenges facing man in the 21st Century. The energy sector is vital in tacking the climate change since it accounts for about two thirds of global carbon dioxide ( Quitzow, 2021 ). Energy in form of electricity and primary energy resources drive the prosperity of the world economy ( Quitzow, 2021 ). From the 1970s, the global gross domestic product (GDP) has grown by about 4.5 times, while the consumption of primary energy has grown from 155.22 EJ in 1965 to 556.63 EJ in the year 2020. The total proved energy reserves of the dominant fuels, i.e., oil, natural gas, and coal at the end of 2020 could last just 53.5 years for oil, 48.8 years for natural gas, and 139 years for coal ( BP, 2021 ). These fossil fuels account for 85% of the total primary energy consumption globally. The current era is faced with the challenge of global warming as the most prominent environmental issue thus reduction of carbon emissions is at the center of global environmental policy. It is therefore important to understand the relationship between economic development and energy consumption, and effectively improve energy efficiency for a better relationship and sustainable development ( Barasa Kabeyi and Olanrewaju, 2022 ; Jin et al., 2022 ). Electricity plays a very important role in modern economies as it provides a rising share of energy generation and consumption in all countries ( Solarin et al., 2021 ). Electricity demand is poised to increase further due to increasing household incomes, and electrification of transport and thermal energy applications as well as continues growth for digital connected devices and air conditioning ( International Energy Agency, 2019 ). Energy is a critical requirement for sustainable development and therefore optimum selection of low carbon and green energy sources remains a key objective for all nations ( Bhowmik et al., 2020 ). Electric power plays an important role in human life because all vital activities and operations today need electricity directly or indirectly ( Beaudin and Zareipour, 2015 ; Bayram and Ustun, 2017 ).
Electricity as a form of energy is extremely important for socioeconomic development. Global electricity generation stood at 4,114 GW in 2005 and increased to 5,699.3 GW in 2014 and it continues to grow annually. Fossil fuel-based electricity accounted for over 60% of this generation in 2014 and 42% of CO 2 . There is need to develop evaluation index system and models for sustainable electricity generation to sustainably cope with this ever growing demand ( Li et al., 2016 ). According to the emissions gap report, the total greenhouse gas emissions in 2018 was about 55.3 GtCO 2e of which 37.5 GtCO 2 were on account of fossil fuels combustion in various operations and activities including electricity generation [ United Nations Environmental Program(UNEP), 2019 ].
Energy sustainability or energy for sustainable development is a challenge for many countries developed and developing countries. There is need for a transition roadmap to renewable energy sources that may be unique to each country based on local resources and prevailing circumstances ( Iddrisu and Bhattacharyya, 2015 ). Energy transition is a reality for all nations because of the targets set in the Paris agreement. The global community is developing decarbonization plans aimed at reducing greenhouse gas emission in a sustainable manner ( Kabeyi and Olanrewaju, 2020b ). The process is unique to different countries because the transition is affected by local social and economic conditions. The complexity and comprehensiveness of the energy transition is influenced by the diversity of actors involved in their interests which are often in conflict with one another ( Krzywda et al., 2021 ). Electricity is a very important form of end-use energy, and it is a leading factor for economic growth and development. However, electricity generation is a leading source of greenhouse gas emissions which cause global warming and climate change which threatens sustainable development. This is because most of the global electricity is generated from fossil fuel sources of energy. Electricity accounts for a significant share of the three components that make up total energy production and consumption are electricity, transport, and heating ( Ritchie and Roser, 2021 ). The main challenges facing the electricity sector are the ever growing electricity demand, growing need to reduce greenhouse gas emissions and the need realize zero-net carbon emissions in power generation in line with the Paris Agreement which seeks to limit the increase in average global temperature to 1.5°C ( Colangelo et al., 2021 ). This calls for an energy transition from the fossil fuel dominated electricity mix to one dominated by renewable sources of energy and low carbon nuclear as well as clean fuel and conversion technologies ( Kabeyi and Olanrewaju, 2020b ; Kabeyi and Olanrewaju, 2021a ).
The world has so far witnessed three typical energy transitions. The first transition involved replacement of wood with coal as the main energy source. In the second transition, oil replaced coal as the dominant energy resource. In the third transition, there is global commitment to replace fossil fuels with renewable energy. As in 2018, 80% of the global energy was derived from fossil fuel energy resources with 36% being petroleum, 13.2% for coal, and 31% was from natural gas ( Lu et al., 2020 ). Energy transition refers changes undertaken in fundamental processes in charge of evolution of human societies that drive and are driven by technical, economic, and social changes ( Smil, 2010 ). It is a new path for economic development and innovation that does not compromise the environmental integrity and sustainability motivated by challenges caused by greenhouse gas emissions, climate change and natural resource depletion ( Mostafa, 2014 ). Energy transition consists of processes of structural changes to the subsystems of society which lead to greater sustainability in the society ( Barasa Kabeyi, 2019a ). Therefore energy transitions call for changes in existing policies, technology as well as supply and demand patterns for electricity and other energy resources ( Mostafa, 2014 ). The world is said to be undergoing a fourth energy transition today having witnessed three energy transitions in the past. The main objective of this fourth transition is to fight the global climate change through decarbonization of the energy supply and consumption patterns ( Mitrova and Melnikov, 2019 ). Therefore a sustainable energy transition system must be driven by the climate change agenda, technology developments and innovation, increased energy efficiency, competitive economies, enhanced energy security, development of affordable energy solutions and measures and modernization of the energy sector from traditional energy systems ( Smil, 2010 ; Mitrova and Melnikov, 2019 ). The International Renewable Energy Agency (IRA) defines energy transition as the pathway in the transformation of the global energy sector from fossil-dominated mix to zero-carbon by the second half of the 21st century ( Inglesi-Lotz, 2021 ).
The selection criteria for development sustainable energy transition should consider the environmental, technical, social, institutional, and economic dimensions of sustainability. While choosing or selecting energy sources for electricity generation, the choice of conversion technology and cost involved play a crucial role in modern economies and societies ( Bhowmik et al., 2020 ; Kabeyi and Olanrewaju, 2020b ). With continuous increase in global population and socio-economic activities leading to increased urbanization, and industrialization around the world, the demand for natural energy resources and more so renewable energy is gradually increasing ( Ebrahimi and Rahmani, 2019 ). It is notable that the world’s population has grown by 2.5 times since 1950, while energy demand over the same period has grown by 7 times ( Şengül et al., 2015 ). These increasing energy demand is predominantly met by fossil fuel combustion and nuclear power plants ( Tunc et al., 2012 ). With ever increasing energy demand, the related challenges are depletion of fossil fuel reserves, their price volatility, and global climate change which have attracted much attention to renewable energy sources and other low carbon and cheap sources of energy for power generation. As a result, many countries have adopted policies, strategic and operational measures to support the growth of renewable energy sources and other sustainable energy measures in the energy transition ( Ebrahimi and Rahmani, 2019 ).
Sustainable energy transitions require formulation of effective policies that promote the biomass resources, increased use of renewable and low carbon sources and penalize as well as discourage the use of fossil fuels and unsustainable natural resource use. Directing agricultural resources toward food production ( Andress et al., 2011 ). Renewable energy like solar, wind power, or hydropower can be used as viable options for generating electricity. Solar power plants, for example, could be constructed in countries with vast expanses of desert land. With developing countries like China having huge coal reserves and high electricity demand, coal fired power plants will continue to dominate electricity generation in these countries and options like clean coal technologies and carbon capture and sequestration are critical options. Production of hydrogen from coal is another strategy. For countries with high electricity demand, nuclear power generation is an option for reducing GHG emissions although with a danger of proliferation for politically unstable governments with weapons agenda. Large scale penetration of renewable energy requires development of advanced batteries, high efficiency conversion technologies, and stable and resilient grids to absorb variable renewable energy sources. Electrification of transport with most electricity coming from low carbon and green sources is another strategy for the sustainable energy transition ( Andress et al., 2011 ).
There are various strategies, measures and technologies that can be used to improve sustainability. They include energy efficiency, increasing the contribution of renewable energy in electricity generation, use of Carbon Capture and Storage (CCS) in fossil and biomass power plants, use of low carbon nuclear power, use of hydrogen in the transportation sector and reductions in the demand for energy and electrification as well as use of biofuels in transport services. The main challenges facing various options and technologies include lack of acceptance and behavioral changes as well as cost limitations and availability of cheap fossil fuels ( Hildingsson and Johansson, 2016 ).
1.1 Problem Statement
Most of the electricity generated globally is comes from fossil fuel-based power plants. These energy resources are generally expensive, scarce, exhaustible, polluting, and insecure since not all nations are endowed with the primary resources hence a source of energy insecurity, while the combustion of fossil fuels produces greenhouse gases like carbon dioxide (CO 2 ), Sulphur dioxide (SO 2 ), Nitrous oxides (NOx), which are the main causes of the global warming that is threatening the very existence of humanity and mother nature. This concern is the main motivation behind sustainable energy transition by increased use of renewable and low carbon clean energy sources especially solar, wind, biomass, hydro and nuclear. These renewable and low carbon sources improve and widen power supply, enhance long term access and utility in energy production, decrease dependence on fossil fuel, and reduce greenhouse gas emissions ( Rathor and Saxena, 2020a ; Nguyen et al., 2020 ).
Natural increases in CO 2 concentrations have historically been warming the Earth during ice age cycles for millions of years. These warm episodes are said to have started with slight increase in solar radiations reaching the Earth due to a slight wobble in Earth’s axis and path of rotation around the Sun that caused some notable warming. This phenomenon caused the warming of oceans leading to an increase in carbon dioxide (CO 2 ) emissions from the oceans. However, CO 2 concentration never exceeded 300 ppm during these periods that took place about a million years ago. Before the industrial revolution that started in of mid-1700s, the global average amount of carbon dioxide was about 280 ppm ( Lindsey, 2021 ). Figure 1 shows the historical growth of CO 2 emissions and concentration between 1750 and 2020.
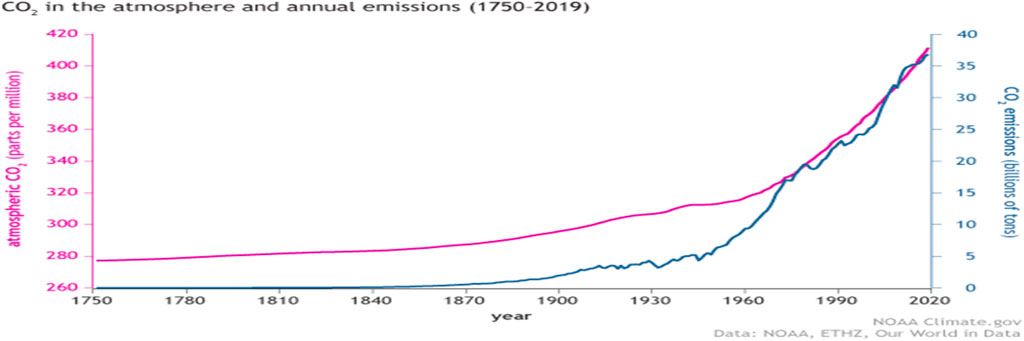
FIGURE 1 . Concentration of carbon dioxide emissions between the year 1750 and 2020 ( Lidsey, 2020 ).
Figure 1 shows that the global CO 2 emissions remained constant between 1750 and about 1840 where they started to increase rapidly. The atmospheric concentration increased slightly between 1750 and 1960 before the rate increased between 1960 and the year 2020 mainly due to higher level of industrial activities and increasing use of fossil fuels. Therefore, it is the entry of fossil fuels in the energy mix that triggered rapid increase in CO 2 emissions as the level of industrialization developed.
Fossil fuels continue to dominate the current energy systems and therefore significantly contribute to the global carbon dioxide (CO2) and other greenhouse gases emissions to the atmosphere. To realize the global climate targets and avoid destructive climate change, there is need for a global transition in electricity generation, transmission, and distribution and as well as its consumption. Humanity must strike a balance between developmental needs and the environmental conservation and protection. The main challenges facing renewable energy sources is resource availability, resource access, resource location, security of supply, sustainability, and affordability ( Samaras et al., 2019 ). The growing demand for electricity has led to increased demand and consumption of fossil fuels and growing level of economic activities have contributed to growth of the greenhouse gas emissions and consequently, global warming ( Wang, 2019 ). The transition challenges are further compounded by the fact that efforts to promote more sustainable, more resilient, and equitable energy disrupts economic, political, and institutional relationships. As a result, issues of power and politics are now central themes in sustainability transition in energy sectors ( Lenhart and Fox, 2021 ).
This study examined sustainability in energy and particularly electricity generation systems and the challenges and opportunities of sustainability. The overall objective was to lay a framework for a sustainable transition to a green and low carbon electricity grid system as a contribution to the global effort to fight the climate change and greenhouse gas emissions. Various pathways to sustainable electricity generation are examined and proposals made on a feasible roadmap to a sustainable energy transition, particularly grid electricity generation, transmission, distribution, and consumption. The transition acknowledges the significance of nonrenewable sources and their main challenges of intermittence and variability as the global community seeks to transition to green energy sources. Using a critical discourse analysis, the study attempts to develop a roadmap that can be adopted by nations based on their local conditions to sustainably transition their electricity production and supply. Of particular concern is how the available energy sources can be used to realize the Paris targets without compromising the socio-economic and environmental set up and hence achieve sustainable energy transition.
1.2 Rationale of the Study
The Paris Agreement of the 21st UNFCCC Conference of Parties (COP21) of 2015 seeks to limit average global temperature increase below 2°C above pre-industrial levels look for measures to limit the average temperature rise to 1.5°C the pre-industrial temperature. This calls for drastic measures to reduce anthropogenic emissions and removals by sinks of greenhouse gases by second half of 21st century ( Lawrence et al., 2018 ). Studies have shown that the climate is changing mostly because of the anthropogenic activities. The report by Intergovernmental Panel on Climate Change (IPCC) for 2021 indicates that several climate changes are already irreversible but adds that we still have hope for the future if action is taken. To mitigate further changes ( Inglesi-Lotz, 2021 ). The climate is very important to man and other living organisms on the planet, yet there is overwhelming evidence that the world is facing changing climatic conditions due to the greenhouse effect as demonstrated by the increase in average global temperature, high incidents of climate related issues like drought, storms and desertification ( Wallington et al., 2004 ). The global anthropogenic activities have led to about 1°C rise in average global temperature above prehistoric level and is further projected to reach 1.5°C between the year 2030 and 2052 if current greenhouse gas emission rates are maintained ( Fawzy et al., 2020 ).
Electricity generation accounts for about 26% of total greenhouse gas emissions making it an important target for emissions control in the war against climate change ( Kabeyi and Oludolapo, 2020a ). The Intergovernmental Panel on Climate Change (IPCC) sought to stabilize the atmospheric air carbon concentration with a target to limit concentration to 350 ppm for CO 2 while maintaining temperature rise of 2° C above the preindustrial level, a target that was ratified by many nations globally. This calls for limitation in the consumption of fossil fuels particularly in electricity generation and transport industries through substitution with renewable energy sources and electrification of transport among other measures. This calls for massive expansion in power generation capacity using renewable energy and low carbon energy sources ( Burger et al., 2012 ). The future of humanity as defined by the sustainable development goals in the face of climate change has made sustainability the concern for all major systems including energy or electricity generation, supply and consumption systems ( Vine, 2019 ). There is need for a shift from current dependence on fossil fuels for power generation, transport, and thermal applications ( Burger et al., 2012 ).
The global greenhouse gas emissions can be presented based on economic activities that lead to their production and emission. Greenhouse gases are mainly released by electricity and heat generation in the energy and related sectors, manufacturing activities, industrial operations, transportation, agriculture and forestry as well as the building industry ( Marcus, 1992 ). The sources of greenhouse gases can be classified into five economic sectors. These sectors are energy, industry, transport, buildings and AFOLU, i.e., agriculture, forestry, and other land uses ( Lamb et al., 2021 ). In Figure 4 below, the greenhouse gas emissions by sector are presented for the years 1990–2018 for the entire world and across 10 ten global regions, namely Asia pacific, Africa, East Asia, Eurasia, Europe, Latin America, Middle east, North America, South Asia, and Southeast Asia. Figure 2 below shows the contribution of greenhouse gases by five economic sectors.
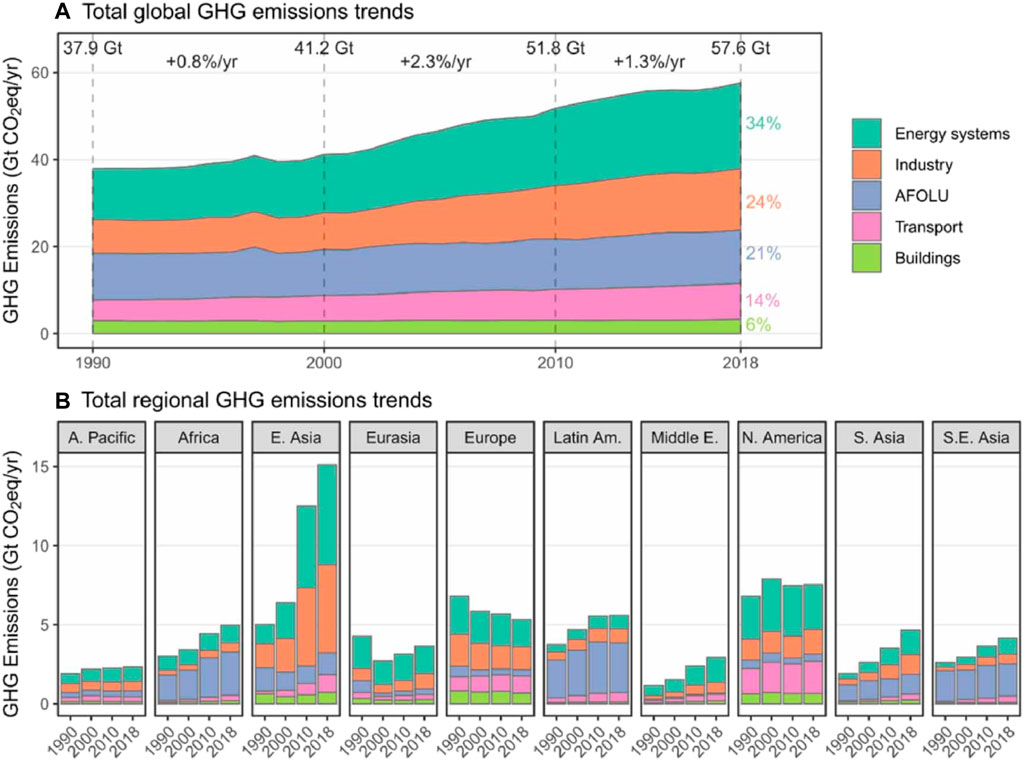
FIGURE 2 . Greenhouse gas contribution by economic sector ( Boden et al., 2017 ; Lamb et al., 2021 ).
From Figure 2 , it is noted that the energy sector inn form of electricity and heat production is the largest contributor of green house gases with about 34%, industry at 24% followed by agriculture, forestry and other land activities accounting for 21%, transportation with 14%, while buildings contributed about 6% while the building sector is least with 6% in 2018 ( Lamb et al., 2021 ). Figure 2 further demonstrates that for the African region, most emissions.
The greenhouse gas emissions (GHG) for 2018 were about 11% (5.8 GtCO 2 eq) higher than GHG emission levels in 2010 (51.8 GtCO 2 eq). The energy sector accounted for close to 1/3rd of the increase in GHG emissions between 2010 and 2018 of about 1.9 GtCO 2 eq, followed by industrial sector with 1.8 GtCO 2 eq which was about 30% of the increase, then the transport accounted for 1.2 GtCO 2 eq or 20% of the increase. Emissions that came from AFOLU increased by about 0.72 GtCO 2 eq equivalent to 12% increase while the buildings sector recorded the lowest increase in emissions with about 0.22 GtCO 2 eq, or 4% ( Lamb et al., 2021 ).
A sustainable energy transition should address the energy sources, energy conversion, transmission, and consumption especially the leading sectors in energy consumptions like heat and power production, transport related activities including fuel use and conversion. These measures include a shift from fossil fuel sources to renewable and low carbon sources, efficient conversion technologies, electrification of transport with most electricity coming from renewable resources, energy conservation measures and elimination of unnecessary energy demand and consumption.
Energy is far much more than just the technical infrastructure. It is through the energy transition that we realize emergence of innovative business models and organization that drives the establishment of new practices and procedures, and new ways of life, reassign responsibilities, reorganize governance, and redistributes power structure. It is for these reasons that the sustainable energy transition calls for consideration of the social, technical, economic, political, and institutional dimensions of sustainability in addressing challenges of the energy sector in order to sustainably shift to a low-carbon economy and electricity systems which is the main focus of this research ( Quitzow, 2021 ).
2 Methodology and Novelity of the Study
In this study, low-carbon energy transitions options and strategies are considered governed and proposed in line with broader sustainability goals and requirements as specified by the dimensions of sustainability. The study sought to identify conflicts and synergies between low-carbon strategies and the attainment of both short term and longer-term environmental, economic, technical, social, and institutional objectives. The research framework is organized across the five dimensions of sustainable energy and specifically electricity development which are environmental, economic dimensions, social aspects, technical dimensions, and institutional, political dimensions. The study adopted secondary method of data collection and analysis from recent primary and secondary data found in original research findings and reports from credible peer reviewed sources. This would facilitate the voice of the common people, professional, experts, and authorities as well as governments for a cleaner global future. For this research, the term primary data refers to the data originated and carried out and presented as peer reviewed academic and professional papers and reports through personal interviews with the expert team or analysis based on primary data and presented as original reports or peer reviewed journal article. Therefore, this study is a review of the energy sustainable transitions globally. Published literature in the form of technical reports, peer reviewed journals and conference papers were reviewed by the authors. The study is a survey using credible literature from peer reviewed journals papers and energy data from various authorities globally.
Most studies on sustainable energy transition are narrow in scope as they tend to concentrate on the environmental dimension of sustainability. Researchers that look at sustainability more wholistically also tend to concentrate on the three pillars of sustainability, namely economic, environmental, and social dimensions of sustainability ( Kabeyi, 2019a ; Barasa Kabeyi, 2019b ; Krzywda et al., 2021 ). Past reviews also concentrate on energy sustainability in general. However, in this study, the focus is electricity as a secondary form of energy derived from primary sources of energy like wind, solar, hydro, nuclear, coal, and gas through indirect conversion through a generator or direct conversion as in fuel cells and solar photovoltaics.
In most studies undertaken on energy transitions, the focus has been on energy technologies and sources with main objective being minimizing emissions. Others have gone further to address variability and intermittence and to rank sources in order of potential within the framework of the three pillars of sustainability. This study is unique as it looks at technical and institutional dimensions in addition to the economic, social, and environmental and hence the role of energy storage and electrification of transport which induces additional variability to demand side. The study also brings into focus the role of the smart grid in managing the dynamic nature of electricity demand and supply in decentralized generation and with the intermittence and variability of wind and solar. The study therefore recognizes that social foundations and human behaviors have a significant role to play in the future sustainability of the energy sector ( Inglesi-Lotz, 2021 ). Therefore, the study pays attention and extensively analyses to energy and electricity models and modelling tools that wholistically considers sustainability in the energy sector and electricity systems.
3 Greenhouse Gas Emissions and Their Environmental Impacts
Greenhouse effect refers to a natural process that has taken place over millions of years but was first discovered by Jean Baptiste-Joseph de Fourier in 1827 ( Wallington et al., 2004 ; Wang, 2019 ). Greenhouse gas effect is the long-term increase in the temperature of the planet Earth as a result of accumulation of greenhouse gases in the atmosphere ( İpek Tunç et al., 2007 ). The discovery of greenhouse effect was demonstrated experimentally by John Tyndall in 1861 and quantified in 1896 by Svante Arrhenius. In the work of Svante Arrhenius, the author observed that the release of large amounts of CO 2 emissions from the combustion of fossil and doubling of atmospheric CO 2 concentration warmed the Earth by 5–6°C, as compared to current climate models which predict a 1.5–4.5°C rise by doubling the concentration of CO 2 ( Kabeyi and Oludolapo, 2020a ; Kabeyi and Oludolapo, 2020b ). Greenhouse gas effect results from the interaction between solar energy and greenhouse gases in the atmosphere. The work of Roger Revelle and Hans Suess in 1957, remarked that the build-up of carbon dioxide in the atmosphere constituted a large-scale geophysical experiment whose consequences were unknown and so should be monitored and controlled. As a result, the year 1958 was designated as the International Geophysical Year. This marked the beginning of the ongoing program of continuous measurements of atmospheric CO 2 levels at Mauna Loa, Hawaii, in the United States by Charles Keeling. This measurement demonstrated that the levels of carbon dioxide were rising steadily from 315 ppm in 1958 to 370 ppm in 2001 ( Wallington et al., 2004 ).
The global climate change is one of the leading challenges facing humanity today ( del RíoJaneiro, 2016 ). This is because the climate has been changing over time with manifestations like the global increase in average temperature, rising sea level, heat waves, several incidents of flooding, both stronger and frequent ocean waves, and drought in many parts of the world ( Intergovernmental Panel on Climate Change(IPCC), 2007 ; Butt et al., 2012 ). The United Nations Framework Convention on climate change, attributes these directly or indirectly to human activities that change the atmospheric composition leading variation in the natural climate as observed over a long period of time. There has always been evidence that the global climate has been changing since the beginning of creation, but the current rate is alarming with several consequences already being witnessed today.
Greenhouse gases like carbon dioxide (CO 2 ) can absorb and radiate thermal energy. It is this greenhouse effect that warms the Earth and keeps its average annual temperature above the freezing point which implies that normal global warming maintains life and so is necessary ( Lindsey, 2021 ). Although carbon dioxide (CO 2 ) is less potent than methane and nitrous oxide, it is more abundant and stays longer in the atmosphere thereby making it more significant. It is for this reason that the increase in atmospheric carbon dioxide is responsible for about two-thirds of the total energy imbalance and temperature rise. Additionally, carbon dioxide has a negative impact to the sea water because when dissolved in ocean water, CO 2 reacts with water molecules to produce carbonic acid which lowers the PH of the ocean water. It is for this reason that the PH of the ocean’s water has reduced from 8.21 to 8.10 since the beginning of the industrial revolution. This is quite significant because a drop by 0.1 in PH causes about 30% increase in acidity of the ocean. The biological effect of ocean acidification is interference with marine life’s ability to extract calcium from the water to build their shells and skeletons ( Lindsey, 2021 ).
The global atmospheric of carbon dioxide (CO 2 ) has been increasing particularly on the account of fossil fuel combustion in powerplants, transport and several industrial processes. This is not sustainable because the fossil fuels took many millions of years to form, only to be returned to the atmosphere in just few years, hence their lack of renewability [ United Nations Economic and Social Commission (ESCAP), 2016 ]. It is estimated that the last time the atmospheric CO₂ amounts were relatively higher was about 3 million years ago, when temperature was 2–3°C (3.6–5.4°F) above the pre-industrial era, and the sea level was 15–25 m (50–80 feet) higher than it is today ( Lindsey, 2021 ). Industrialization is the main cause for the steady rise in the concentration of carbon dioxide between 1970 and 2020 because of the use of fossil fuels in transportation, industry, and power generation. This trend is demonstrated in Figure 3 .
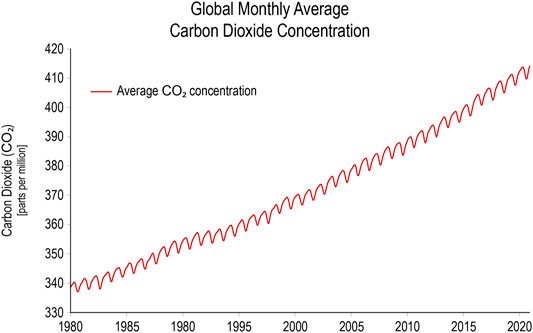
FIGURE 3 . Changes in carbon dioxide concentration between 1970 and 2020 ( Lindsey, 2021 ).
From Figure 3 , it is noted that the concentration of atmospheric carbon dioxide has increased steadily from about 328 ppm in 1970 to 412.5 ppm in 2020 up from 409.8 ppm in 2019 having increased steadily from the value of 328 in 1970. The annual rate of increase in CO 2 in the atmosphere is about 100 times faster than previous natural increases over the past 60 years, e.g., last ice age 11,000–17,000 years ago. Additionally, the ocean has absorbed enough carbon dioxide to lower its pH by 0.1 units causing a 30% increase in ocean acidity ( Lindsey, 2021 ). Although the concentration dropped in 2020, global energy-related CO 2 emissions stood 31.5 Gt, which contributed to CO 2 that made atmospheric concentration reach about 50% higher than it was at the beginning of the industrial revolution ( International Energy Agency, 2021a ).
3.1 Greenhouse Gases in Power Generation
Electricity and heat generation is a leading source of CO 2 emissions and they generated 13 billion tons of CO 2 emissions accounting for 41% of all CO 2 emissions coming from fuel combustion globally in 2017. This was due to the use of fossil fuels to generate about 16,947 TWhrs of electricity representing 63% of the total electricity generation ( Solarin et al., 2021 ) At the global scale, the key greenhouse gases emitted by human activities are carbon dioxide, methane, nitrous oxide, and F gases. Energy supply is the largest contributor to global greenhouse gas emissions. About 35% of total anthropogenic GHG emissions in 2010 originated in the energy sector. The global annual growth in greenhouse gas emissions from energy supply sector increased from 1.7% per year in 1990–2000 to 3.1% in 2000–2010 mainly due to faster economic growth which increase demand for heat and electricity consumption. Most of these heat and power demand is met by fossil fuel sources of energy ( Bruckner et al., 2014a ).
3.1.1 Carbon Dioxide (CO 2 )
The Natural increases in CO 2 concentrations have been warming the Earth over time with increase in temperature during ice age cycles over millions of years. These warm episodes or interglacial started with a small increase in sunlight when the Earth had a tiny wobble in its axis of rotation around the Sun. This event led to some warming of the Earth’s surface and oceans causing increased release of carbon dioxide. The extra CO 2 in the atmosphere only magnified the initial natural warming. Among the anthropogenic greenhouse gases, CO 2 is responsible for about 60% of the greenhouse gas effect ( İpek Tunç et al., 2007 ). With the industrial revolution, manmade sources have become significant sources of atmospheric carbon dioxide through activities like fossil fuel combustion for power generation, transportation, industrial as well as domestic activities ( Lindsey, 2021 ). Other leading sources are industrial chemical reactions and operations like cement production. Carbon dioxide is mainly removed from the atmosphere or sequestration through photosynthesis [ United States Environmental Protection Agency (EPA), 2017 ]. When carbon dioxide is heated, it absorbs and radiates heat in the form of thermal infrared energy. This radiation has the positive side because without it, the annual average temperature would be just close to 60°F. However, with the rapid increase in greenhouse gas emission, additional heat has been trapped leading to raising planet Earth’s average temperature above the pre-industrial level.
3.1.2 Methane (CH 4 )
Methane is generated from various natural and artificial processes like anerobic digestion and during the production and transport of fossil fuels like coal, natural gas, and oil [ United States Environmental Protection Agency (EPA), 2017 ]. Therefore, methane is added to the atmosphere through natural and anthropogenic sources, with 30% of the methane flux originating from natural sources while about 70% is contributed by anthropogenic sources ( Wallington et al., 2004 ).
3.1.3 Nitrous Oxide (N 2 O)
Food production particularly the use of fertilizers is the primary source of N 2 O emissions. As well as fossil fuel combustion in power generation, industry, and transportation as a product of combustion and wastewater treatment process ( Viet et al., 2020 ). Nitrous oxide (N 2 O) is the third most abundant well mixed greenhouse gas after CO 2 and CH 4 with a life span of about 130 years. The natural sources of N 2 O come from soils and the oceans. Anthropogenic emissions originate from biomass combustion, fossil fuel combustion, and industrial production of adipic and nitric acids, and nitrogen fertilizer use in agriculture ( Wallington et al., 2004 ).
3.1.4 F Gases
The F gases result from industrial processes, refrigeration systems, and the use of some consumer products associated with emissions of F-gases. These gases include hydro fluorocarbons (HFCs), perfluorocarbons (PFCs), and sulfur hexafluoride (SF 6 ). Hydro fluorocarbons, perfluorocarbons, sulfur hexafluoride, and nitrogen trifluoride are synthetic, and come from industrial processes. These Fluorinated gases are occasionally used as substitutes for ozone-depleting substances , e.g., chlorofluorocarbons, hydro chlorofluorocarbons, and halons. These gases have high global warming potential gases even though they are released in small quantities [ United States Environmental Protection Agency (EPA), 2017 ].
Figure 4 below shows the global composition of the greenhouse gases in the atmosphere ( Lamb et al., 2021 ).
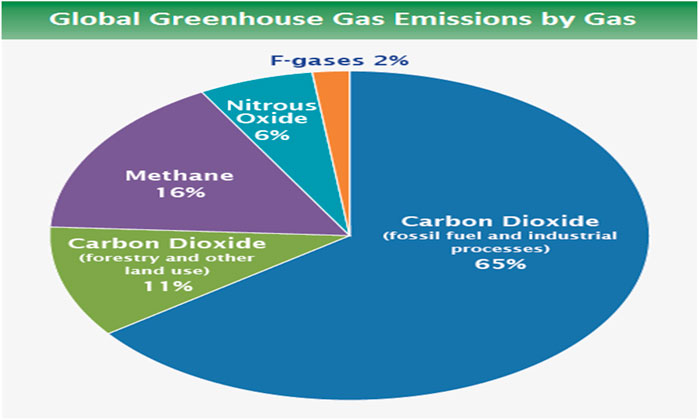
FIGURE 4 . Global greenhouse gas emissions by gas ( Boden et al., 2017 ).
From Figure 4 , it is noted that carbon dioxide remains the dominant greenhouse gas with most of it coming from fossil fuel combustion in industry, heat, and power generation which accounts for 65% of global emissions. Other sources of carbon dioxide are agriculture and forestry related activities which contribute 11% of the greenhouse gas emission in form of CO 2 . Methane is the second largest greenhouse gas accounting for 16% of greenhouse gas emissions, followed by nitrous oxide and F-gases respectively with 6 and 2% contribution respectively.
The global average CO 2 concentration in 2018 was about 407.4 ppm with uncertainty of + and −0.1 ppm. Today, carbon dioxide levels are the highest over the past 800,000 years ( Lindsey, 2021 ). Over 400 billion metric tons of carbon dioxide has been released to the atmosphere from fossil fuels combustion and cement production since the year 1751, of which about 200 billion metric tons originate from fossil fuel combustion since the late 1980s.
In 2014, the global carbon emissions to the atmosphere were about 36 billion tons, which is approximately 1.6 times the 1990s rates, an increase which has been associated with average global temperature rises ( Gamil et al., 2020 ). Close to 9,855 million metric tons of fossil-fuel based carbon dioxide emissions were emitted between 2013 and 2014, representing an increase of 0.8%. Liquid and solid fuels accounted for 75.1% of the global carbon emissions from fossil-fuel burning and cement production in 2014. Gaseous fuel accounted for 18.5% of the emissions which is about 1,823 million metric tons of carbon emissions from fossil fuels in 2014. In 2014, emissions from cement production were 568 million metric tons of carbon indicating, which shows a more than double increase in the last decade to represent 5.8% of global CO 2 emissions from fossil-fuel burning and cement production. Emissions from gas flaring, which accounted for about 2% of global emissions during the 1970s, reduced to less than 1% of global carbon emissions from fossil-fuel ( Boden et al., 2017 ). These statistics show that fossil fuel combustion which mainly comes from power generation and cement production in the manufacturing industry are leading polluters of the atmosphere in terms of carbon emissions.
3.2 Global Warming/Greenhouse Effect
Global warming is the effect of the imbalance between the heat received by the Earth and, the heat reradiated to the space. Terrestrial longwave radiative flux is emitted by the Earth’s surface beyond the 3–100 µm wavelength range while the surface incoming radiation is shortwave solar radiation also called global irradiance or solar surface irradiance. This is the radiation flux density reaching a horizontal unit of Earth surface in the 0.2–3 µm wavelength range ( Ming et al., 2014 ). Global warming refers to the process which leads to the average rise in the Earth’s temperature and that of the atmospheric layers close to Earth because of human activities. Climate change on the other hand is the phenomenon where other climatic factors change due to global warming. Any slight increase in the ocean temperature causes hydrological events which effectively change the physical and chemical characteristics of the water. Aquatic life is affected by change in water as it affects their life cycle, physiology, and behaviors ( Ninawe et al., 2018 ).
Global warming potential (GWP) is a measure of how much a given mass of a chemical substance adds to global warming over a specified time. It refers to the ratio of the warming caused by a substance to the warming caused by a similar mass of carbon dioxide. These therefore allocates 1 as the global warming potential of carbon dioxide. Water has the lowest global warming potential of 0, while Chlorofluorocarbon-12 has a high global warming potential of 8,500. Chlorofluorocarbon-11 has GWP of 5,000. Several hydrochlorofluorocarbons and hydrofluorocarbons have GWP potentials varying between 93 and 12,100 based on a 100-years period ( Demirel and Demirel, 2014 ). The three-time scales used to compute the GWP are 20, 100 or 500 years respectively. The main greenhouse gases from fossil fuel combustion are nitrous oxide (N 2 O), methane (CH 4 ) and carbon dioxide (CO 2 ). The value of the three main greenhouse gases varies in GWP for the time scales used in evaluation, i.e., 20, 100, and 500 years. The GWP of CO 2 is used as a reference in the computation of the relative GWP of other gases ( MacLeod et al., 2011 ; Demirel and Demirel, 2014 ). Table 1 below shows the relative global warming potential (GWP) for water, carbon dioxide, methane and nitrous oxide based on the 20-, 100- and 500-years’ time scale.

TABLE 1 . Global warming potential for various substances/gases ( MacLeod et al., 2011 ; Demirel and Demirel, 2014 ).
From Table 1 , it is noted that the global warming potential of the major greenhouse gases various in value over the three timescales. It shows that N 2 O has the highest individual and average GWP followed by methane and carbon dioxide while water has no global warming potential.
Carbon dioxide accounts for about 60% of the anthropogenic greenhouse gases which are the cause the greenhouse gas effect with use of fossil fuels especially in power being cited as the major cause ( İpek Tunç et al., 2007 ; Tunc et al., 2012 ). The global increase in carbon emissions was about 1.4 ppm before 1995 but thereafter increased to 2.0 ppm ( Owusu and Asumadu-Sarkodie, 2016 ). Carbon dioxide concentration has grown from 277 ppm in 1750 to 397 ppm in 2014 representing about 43% and peaked at 400 ppm between March and December 2015 ( Berga, 2016 ). According to Moriarty and Honnery (2019) and Lindsey (2021) , the concentration of CO 2 in the atmosphere passed 407 ppm in 2018 with energy sector emerging as the largest emitter of the greenhouse gases ( Intergovernmental Panel o, 2007 ; Butt et al., 2012 ; Berga, 2016 ; Owusu and Asumadu-Sarkodie, 2016 ). In 2010, the energy supply sector contributed 35% of all the anthropogenic greenhouse gas emissions realized. The emissions increased at average rate of 1.7% between 1990 and 2000 which increased to average of 3.1% between 2000 and 2010 on account of rapid economic growth experienced due to increased use of coal in the energy mix ( Sagan, 2011 ). Fossil fuel sources like coal, heavy fuel oil and natural gas used in power generation are significant sources of greenhouse gases ( Butt et al., 2012 ), yet fossil fuels inform of gas and coal account for over 60% of global electricity generation ( International Energy Agency, 2018 ).
With the increase in energy demand and continuous use of fossil fuels in power generation, a 62% increase in CO 2 emissions is expected between 2011 and 2050 with two thirds of these emissions coming from China and India ( Butt et al., 2012 ). It is for this reason that the Intergovernmental panel on climate change (IPCC) recommended that greenhouse gas emissions should be reduced by 50–80% by the year 2050 to avoid serious consequences of global warming, ( Butt et al., 2012 ). Among the potential consequences are more frequent extreme weather events like heat waves, storms, flooding and droughts, stress due to higher temperatures for plants and humans, rising sea level, and altering occurrence of pathogenic organisms ( Streimikienea et al., 2012 ).
3.3 Other Impacts of Greenhouse Gas Emissions
Besides global warming, some gaseous emissions have other significant negative impacts to the global and local environment. They include contamination of air by pollutants like Sulphur oxides, nitrogen oxides, particulate matter, and volatile organic compounds, which are released into the atmosphere by the combustion of fossil fuels in power plants, vehicles exhausts, industry, and building and equipment. Whereas some pollutants harm human health directly, others lead to atmospheric chemical reactions that yield harmful conditions like depletion of ozone layer besides global warming. This pollution can be controlled or limited through fuel substitution and conversion technologies that are less polluting ( United States Department, 2015 ). For example, power plants should be equipped with pollution monitoring and control systems like desulphurization systems, carbon capture systems and fuel substitution technologies.
The rise in sea level and its acidification is another threat to the global environment and mankind. GHG emissions in the atmosphere lead to changes include sea-level rise, and an increase in the frequency and intensity of certain extreme weather events like drought and storms. Carbon dioxide is also by the oceans, leading to ocean acidification. The solution is increased use of nonpolluting energy sources, carbon capture and energy efficiency measures ( Nag, 2008 ; United States Department, 2015 ; Kabeyi and Olanrewaju, 2020b ).
4 Global Electricity Generation
The ever-growing electricity demand is the main reason for growth in global CO 2 emissions from electricity which have now reached a record high. The commercial availability of low emissions generation technologies and energy sources has placed electricity at the center of the global effort to combat climate change and pollution. Decarbonization of electricity has a significant potential to provide a platform for CO 2 emissions reduction by means of increased use of electricity-based fuels such as hydrogen and biofuels. Renewable energy sources and efficiency in resource use and generation has a special role to play in increasing access to electricity globally ( International Energy Agency, 2019 ).
The shift and growth in electricity demand adopts two distinct global routes or paths. For most developed countries, demand growth is linked to increasing digitalization and electrification which is largely offset by energy efficiency measures and improvements in process energy efficiency. For the developing countries like China and India, the reasons for growing electricity demand are the growing incomes and better quality of life, industrialization, and growing services sector. It is also worth noting that the developed countries account for about 90% of global electricity demand growth, and the trend may remain so until the year 2040 ( International Energy Agency, 2019 ).
Today, Industry and building sectors are the main users of electricity accounting for over 90% of global electricity demand. Moving forward, the main drivers of electricity demand growth are motors in industry which may account for over 30% of the total growth to 2040. It is projected that industrial and domestic space cooling will account for 17% while large electrical appliances are projected to account for 10% growth while electric vehicles are projected to account for 10% growth in electricity demand. Further growth in electricity demand of about 2% is projected to come from provision of electricity access to 530 million first time users of electricity. The Sustainable Development Scenario, projects that electric vehicles will become a leading source of electricity demand moving to the future towards the year 2040 ( International Energy Agency, 2019 ).
The global energy demand and supply has been growing with supply increase of about 60% between 1990 and 2016 when supply hit 568 EJ. The international bunkers was 16.3 EJ in 2016 accounting for 3% of global total energy supply and was marked by a double growth since 1990, an indication of growing activity and hence energy consumption internationally [ United Nations(UN), 2019 ]. The global electricity generation more than doubled between 1990 and 2016, to reach about 25,000 TWh. Between 1990 and 2016, the largest absolute growth in terms of energy sources came from coal with about 5,300 TWh representing +116% growth. Natural gas supply reached 3,500 TWh representing a growth of +213%.
Renewable sources of energy represented by mainly solar, wind grew by +2,224% or 1,370 TWh over the same period. This was the fastest growth recorded for renewable sources of energy. However, over 75% of electricity in 2016 came from non-renewable sources, mainly from thermal energy accounting for 65% or 16,186 TWh and nuclear 10% or 2,608 TWh. On the positive note, between 2000 and 2016, 50% of new electricity generating capacity came from renewable energy sources [ United Nations(UN), 2019 ; Wanga et al., 2020 ]. Figure 5 below shows the changes in total energy supply between 1971 and 2019.
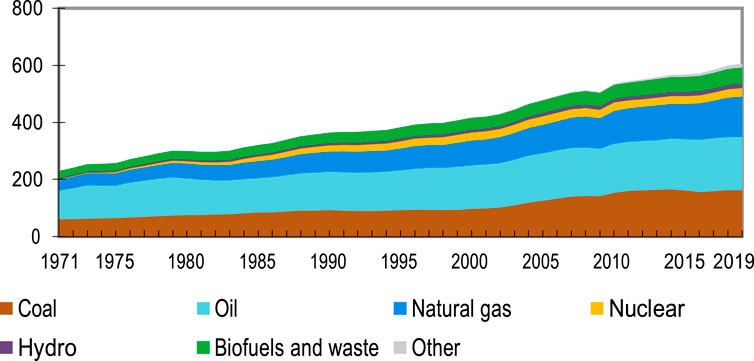
FIGURE 5 . World total energy supply between 1971 and 2019 ( United Nations, 2019a ; International Energy Agency, 2021b ).
From Figure 5 above, it is noted that between 1971 and 2019, the proportionate composition of primary energy mix has been changing. The total primary energy consumption increased from 254 EJ in 1973 to 606 EJ in 2019. Biofuels and wastes reduced from 10.5% in 1973 to 9.3% in 2019. Coal consumption increased from 24.7% of total primary energy consumption to 26.8% in 2019. Oil reduced from 46.2% in 1973 to 30.9% in 2019. Natural gas increased from 16.2% of consumption in 1973 to 23.1%. Nuclear increased from 0.9 to 5% while hydro increased from 1.8 to 2.5% of total primary energy consumption in 2019 ( International Energy Agency, 2021b ).
Fossil fuels generated 61% of global in the year 2020 while combined nuclear, wind and solar accounted for 35% of global electricity generation in the year. Solar energy also surpassed oil in global electricity generation 2020 where solar accounted for 3.2% compared to oil that contributed 2.8% of global electricity generation for the year 2020 ( World Energy Data, 2021 ). Figure 6 below shows global electricity generation from different sources for the year 2020.
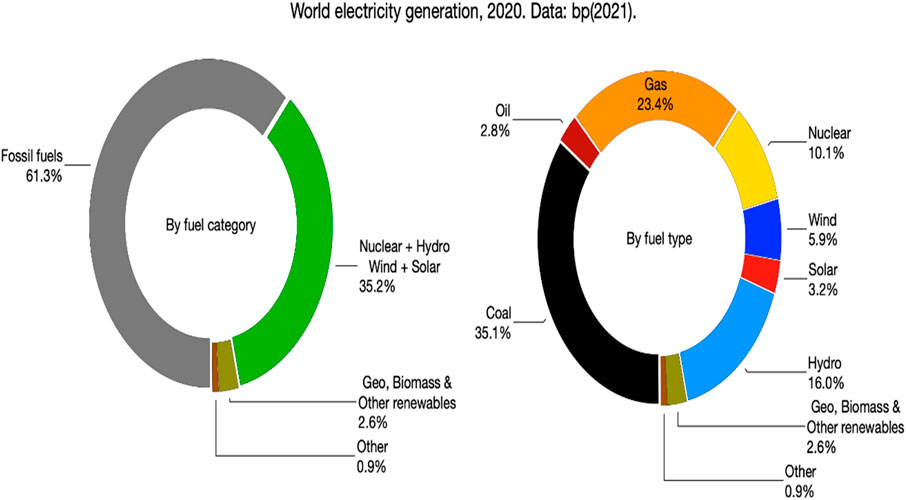
FIGURE 6 . World electricity generation by source for 2020 ( BP, 2021 ).
From Figure 6 , it is noted that for the year 2020, fossil fuels contributed 61.3% of global electricity production, 35.2% was on account of combination of nuclear, hydro, and solar, other renewable s accounted for 2.6% while other sources accounted for 0.9%. Coal contributed 35.1% of global electricity while gas accounted for 23.4% of global electricity.
5 Sustainable Energy Development
The road to a sustainable energy future has twin challenges of energy access and mitigation of global warming by control of greenhouse gas emissions ( Kaygusuz, 2012 ). Energy is at the center of several Sustainable Development Goals. They include expansion of electricity access, improving clean cooking fuels, curbing pollution, and reducing wasteful energy subsidies. Goal number 7 also referred to as SDG 7–aims to ensure access to reliable, affordable and modern energy for all by the end of the next decade ( Birol, 2021 ). The global adoption of energy specific sustainable development goals was an important milestone towards a more sustainable and equitable society. Although energy must be at the heart of efforts to lead the world to a more sustainable pathway, the current and planned policies fall well short of realizing the critical energy-related sustainable development targets. On the positive note, there is tremendous progress in delivering universal electricity access (SDG 7.1.1) for Asia and parts of sub-Saharan Africa ( Birol, 2021 ). As in the year 2012, about 1.4 billion people globally had no access to electricity of which 85% are based in rural areas. It is projected that by the year 20130, about 2.8 billion people globally will be relying on traditional forms of energy mainly biomass, which is an increase from 2.7 billion people in the year 2012 ( Kaygusuz, 2012 ), where the number of people without access to electricity declined to 1.1 billion in 2016, from about 1.7 billion in the year 2000. However, it is projected that more than 670 million people will still have no access to electricity in 2030. Therefore a lot more remains to be done in terms of electricity access ( Birol, 2021 ).
Lack of access to affordable electricity and reliance on the inefficient and unsustainable traditional energy like fuelwood, charcoal, agricultural waste, and animal dung) are a clear manifestation as well as an indicator of poverty. Modern energy sources and electricity plays an important role in socio-economic development ( Tracey and Anne, 2008 ; Kaygusuz, 2012 ). Reliable electricity and light lengthen the day activities hence provide extra hours for economic activities. Positive contribution of electricity includes saving women and children from exposure to poisonous smoke and long hours of looking for firewood. Hospitals can better sterilise instruments and store medicines in refrigerators. Electricity improves manufacturing and service enterprises by extending the quality and range of their products hence creating more jobs and higher wage ( Kaygusuz, 2012 ).
5.1 Sustainable Development
There is need to eliminate energy poverty to achieve the Millennium Development Goals but in a way that takes the world away from dependence on the fossil fuels to avoid global warming by moving rapidly towards a green economy ( Vezzoli Vezzoliet al., 2018 ) . The three interlinked objectives that must be achieved by the year 2030 to realise sustainable energy for all are ensuring universal access to modern energy services, double the share of renewable energy in the global energy mix and double the rate of energy efficiency improvement ( Kaygusuz, 2012 ; Vezzoli Vezzoliet al., 2018 ).
Philosophers, economists and scientists introduced the closely related concepts of sustainable development and sustainability in the 18th, 19th, and early 20th centuries ( Seghezzo, 2009 ). Sustainable development can further be defined socio-economic growth that delivers the traditional positive progress and targets in an ecologically acceptable manner and with due regard of the future generations’ welfare and rights to the same ( Kabeyi and Olanrewaju, 2020b ; Kabeyi and Oludolapo, 2021a ). Sustainable is defined as sustained growth, or sustained change or can also be defined simply as development that is successful ( Lélé, 1991 ). Sustainability is necessary in energy and other resources exploitation so as man exploits resources to meet his ever-growing energy demand, he does not compromise the ability of future generations to meet their own energy needs and a stable environment ( Broman and Robèrt, 2017 ; Kabeyi and Olanrewaju, 2020b ). Because of these requirement and expectations, society must strike a balance between economic growth and the social wellbeing of the society as a whole, now and in future to realize sustainability, which is a technical, political and economic challenge ( Dyllick and Hockerts, 2002 ). Therefore, the concepts of sustainable development and sustainability has the objective of achieving economic advancement and progress while at the same time conserve the value and integrity of the environment. This calls for a tradeoff between environmental sustainability goals and economic development objectives and targets ( Emas, 2015 ).
The publication of Carson’s book called “Silent Spring” in 1962 was used as the starting point of the global concern over proper use of natural resources. This can be demonstrated by what emerged 10 years later in 1972 as the “Club of Rome” that styled itself as an independent analysts and think tank who later published a book called “The Limits to Growth” ( Jacobs et al., 1987 ; Intergovernmental Panel o, 2007 ; Akella et al., 2009 ). In this book, the authors observed that if the global economy and population grew unchecked, the planet Earth’s natural resources would approach depletion at a point in future. These narrative led to the formation of the UN “World Commission on Development and Environment”, also called the Brundtland Commission, named after its chair, Gro Harlem Brundtland, who was a former Norwegian Prime Minister ( Seghezzo, 2009 ; University of Alberta, 2021 ). The “Brundtland Commission” released its final report that was entitled, “Our Common Future” 4 years later that defined sustainable development. The report defined sustainable development as a positive change that meets the needs of the present generation without compromising the ability of future generations to meet their own needs in future ( World Commission on Envir, 1987 ; University of Alberta, 2021 ). Therefore the concept of sustainable development is more concerned with whether what is acceptable today and is acceptable or not acceptable to the next generation ( Jonathan, 2001 ). Today, the strategies for sustainable development aim at promoting harmony and wellbeing among human beings and between humanity and Mother nature. Obviously, energy especially in the form of electricity has a central role in any effort to achieve sustainable development. From the use of wastes by industry to generate power and stabilize the grid, and conversion of polluting and eye soring slaughterhouse waste to clean electricity and create jobs keeping humanity clean and healthy fee of diseases, poverty, and physical harm ( Kabeyi and Olanrewaju, 2021b ; Kabeyi and Olanrewaju, 2021c ; Kabeyi and Olanrewaju, 2021d ; Kabeyi and Olanrewaju, 2021e ).
Sustainable development and the concept of sustainability calls for integration of economic benefits, social considerations and progress with environmental protection and considerations for maximum positive outcome ( Mohamad and Anuge, 2021 ). The United Nations General Assembly in 2015 adopted the 2030 Agenda for sustainable development which as a framework of 17 sustainable development goals (SDGs). This agenda calls for sustainable development which recognizes need to reduce poverty and guarantee equity and integrity of the entire global human community. These 2030 agenda calls for member countries to protect the planet Earth from further degradation by taking sustainability measures which include sustainable resource production and consumption, and sustainable management and conservation of the Earth’s natural resources and prevent climate change ( United States Department, 2015 ; Kabeyi and Oludolapo, 2020a ).
There is inherent interdependence between environmental stability and the economy which then lays a strong foundation for sustainable development ( Emas, 2015 ). There is however need for public policy that promotes investment in economic and industrial activities that seek to protect the natural environment, promote human, and social capital, and prevent the damage caused by pollution, social clashes, resource waste, and greenhouse gas emissions which are both indicators and effects of unsustainable practices ( United States Department, 2015 ). Fortunately, policies that seek to protect the environment and mother nature also promote innovation and profitability by organizations, and this should encourage enforcement, either voluntarily or by legislations. Promotion of innovation and strict environmental regulations can enhance competitiveness and hence economic performance and progress. The link between the environmental integrity and development provides a strong rationale for environmental protection ( Liu, 2014 ; Kabeyi and Olanrewaju, 2020b ).
The use of polluter pay principle in environmental protection requires authorities to impose penalties upon those who pollute the environment and hence make them bear the cost of their impact instead of leaving it with the environment or others. There is need to integrate economic, environmental, and social objects across sectors, territories, and even generations if sustainable development can be achieved. This implies that energy policy should be an integral part of the entire national and international agenda and should be therefore be integrated in other policies touching on the economy, society and the environment ( Emas, 2015 ). Sustainable energy development should also be taken as a continuous process integrating all aspects of national, local, and international development agendas ( Mohamad and Anuge, 2021 ). Therefore, sustainable energy development can only be realized through integration of energy objectives, development goals and environmental protection to avoid conflict by creating a critical synergy.
5.2 Relationship Between Sustainable Development and Energy
Energy is currently recognized as one of the most important factors that influence the rate of progress as well as sustainable development of all nations ( Kolagar et al., 2020 ). To meet the ever-growing energy demand especially electricity, increase access to electricity for the billions of people with no access to electricity and high-quality low carbon fuels, as well as to reduce greenhouse gas emissions requires a radical shift from the fossil-fuel focused energy systems. There is need for a new energy paradigm to encourage the transformation of the predominantly fossil fuel-based energy systems. Sustainability is an important paradigm in the global energy transition where all dimensions of sustainability are addressed any policy formulation and implementation, planning, operation, and dispatch of the energy resources in both generation and consumption ( Davidsdottir and Sayigh, 2012 ). For a longtime, energy did not seriously factor in sustainable development. However, sustainable development and sustainability issues now play a central role in energy and electricity by anchoring the evolution of the sustainable development paradigm ( Iddrisu and Bhattacharyya, 2015 ; Kabeyi and Olanrewaju, 2021f ).
Specific energy projects influence the economic, social, and environmental dimensions of the sustainability country or region. The triangular approach to the three dimensions of sustainable development consisting of economic, social, and environmental is used to assess the sustainability of a specific energy project ( Kolagar et al., 2020 ). Figure 7 below illustrates the triangular approach in energy project assessment.
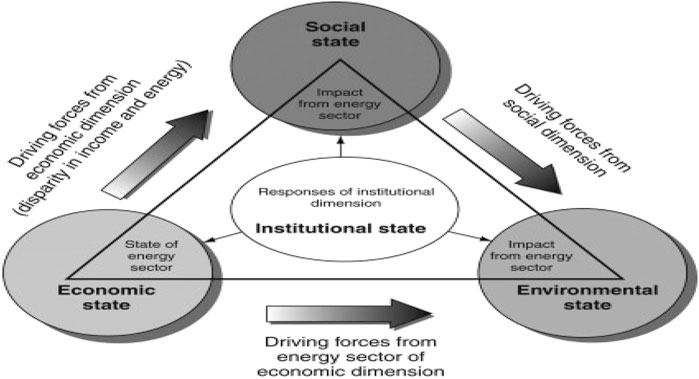
FIGURE 7 . The dimensions of sustainability and their interrelationships ( Kolagar et al., 2020 ).
From Figure 7 , it is noted that the three dimensions of sustainability are held together by the institutional state. The economic state is the main driver of social and environmental states with the institutional state playing the coordination role.
It is at the Stockholm conference, energy was identified as a source of environmental stress, thus linking energy to the environmental dimension of sustainable development. The United Nations Conference on Environment and Development (UNCED) which was also referred to as the “Earth Summit,” that was held in Rio de Janeiro, Brazil, from 3 to 14 June 1992 led to the Rio Declaration on Environment and Development, that had no specific reference to energy. Energy was however a central them theme in Chapter 9 in Agenda 21 on the protection of the atmosphere in which energy was identified as a major source of atmospheric pollution ( Quitzow, 2021 ). Agenda 21 further illustrated the need to draw a balance between economic growth, energy consumption, and its environmental impacts. Although the Commission for Sustainable Development (CSD) was established by the Rio conference of 1992, it is in 1997 when energy was placed in the agenda of the Commission for Sustainable Development ( Gibbes et al., 2020 ; Kolagar et al., 2020 ). Based on the progress of the Commission for Sustainable Development (CSD9), the 2002 Johannesburg conference made direct reference to energy as crucial for sustainable development. It is in this conference that energy was addressed within the three dimensions of sustainable development, i.e., economic, social, and environmental. The conference further clearly treated energy as a specific issue of concern rather than a subset of other issues as it was in the Rio Conference. There was strong and specific emphasis on energy use and its social attributes like access to high-quality energy as a basic human right. It is therefore from the Johannesburg conference of 2002 that the social dimension of energy was incorporated in addition to environmental and economic dimensions which had already been incorporated curtesy of the Rio and Stockholm conferences ( Gibbes et al., 2020 ; Kolagar et al., 2020 ).
Important energy issues that affect sustainability are energy research and development, training and capacity building, and technology development and transfers. Therefore, it is the cumulative effect of the Stockholm, Rio, and Johannesburg conferences that the notion of sustainable energy development (SED) as a very important factor in the sustainable development was mooted by linking energy to the environmental dimension in the Stockholm conference, economy in the Rio conference and society in the Johannesburg conference. Over time, energy consumption and energy development have become a specific issue in the three dimensions of sustainable development.
It is Article 8 from the Johannesburg declaration that we get the most comprehensive definition of sustainable energy development. Therefore, sustainable energy development involves improving access to reliable, affordable, economically viable, socially acceptable, and environmentally sound energy services and resources that consider the national specificities and circumstances. These can be achieved through means like enhanced rural electrification, decentralized electricity generation, greater use of renewable energy, use of clean gaseous and liquid fuels and improved energy use efficiency while recognizing the poor and vulnerable and their right of access to clean energy ( Davidsdottir and Sayigh, 2012 ).
5.3 Characteristics of Sustainable Energy
For energy sources and systems to contribute to sustainable development, they should possess the following characteristics.
• Energy resources and systems are sustainable if they are renewable or perpetual in nature.
• Sustainable energy system should not be wasteful but efficiently produced and used with minimum resource wastage.
• Sustainable energy and energy systems should be economically and financially viable.
• Energy is sustainable if the source is secure and diverse.
• Sustainable energy and energy systems should be equitable or readily accessible, available, and affordable.
• Sustainable energy development should bring positive social impacts.
• Sustainable energy should be associated with minimal environmental impacts ( Kolagar et al., 2020 ).
5.4 Themes/Goals of Sustainable Energy Development
By combining the characteristics or features of the Johannesburg definition with the International Atomic Energy Agency (IAEA) definition, there ae four central goals/themes of sustainable energy development. These are.
5.4.1 Improving Energy Efficiency
This involves improvement in economic and the technical efficiency of energy systems in generation and consumption. With investment in efficient energy systems, costs will reduce as well as output from available energy resources This can be achieved through technology transfer, research, and development and good energy management practices ( Kolagar et al., 2020 ).
5.4.2 Improving Energy Security
Energy security covers the security of both supply and the energy resources infrastructure. Energy security refers to the availability of energy at all times in various forms, in sufficient quantities, and at fair prices that are affordable and predictable. Therefore, for energy to be regarded as secure, it must meet all dimensions of sustainable energy and development. Strategies to improve energy security include decentralizing power generation, wide use of renewable energy resources, investment in redundancy, diversifying energy sources, enhancing supply, more use of local energy resources. Common indicators of energy insecurity include power rationing, frequent blackouts, energy related conflicts and price instability and supply instability ( Gibbes et al., 2020 ; Kolagar et al., 2020 ).
5.4.3 Reduce Environmental Impact
Sustainable energy development calls for reduction in emission of greenhouse gas emissions, which cause global warming. This can be achieved through reduction in the lifecycle environmental impact of energy systems use and production or generation. Other strategies include waste recycling and treatment and adoption of clean technologies that ensure that disposal of wastes does not exceed the Earth’s assimilative capacity ( Kolagar et al., 2020 ). Decarbonization of the energy supply is a very important function in the transition to low carbon energy grid and economy. Besides technology, the deployment of a powerplant depends on the availability of resources, socioeconomic impact, and smooth integration with the existing electricity system. Energy system planners should consider all these to determine and prioritize energy projects and programs ( Colla et al., 2020 ).
5.4.4 Expand Access, Availability, and Affordability
Sustainable energy should be reliable in supply and access at affordable price or cost and quality. There is need to expand energy resources to ensure supply reliability. Goals one and two correspond to the economic dimension of sustainable development, while the third goal deals with environmental dimension of sustainable development. On the other hand, the fourth goal deals with the social dimension of sustainable development. Progress towards sustainable energy development can be measured by various indicators which are critical in the energy transition to sustainable energy ( Davidsdottir and Sayigh, 2012 ).
6 Sustainable Energy and Electricity Generation
Sustainable energy or electricity refers to the generation and supply of electricity in a way that does not compromise the ability of future generations to meet their own energy or electricity needs ( Hollaway and Bai, 2013 ). It can also be defined as energy sources that do not get depleted in a time frame that is relevant to humanity and hence contribute to the sustainability of all species ( Lund and Lund, 2010 ). Sustainable Energy, just like sustainable development requires significant changes in the way things are done and the exact things that we do with effects on the industrial, production, social infrastructure, and value systems. The development of clean energy would unlock many challenges to sustainable development ( Kabeyi and Oludolapo, 2020c ; Kabeyi and Olanrewaju, 2021a ; Kabeyi and Olanrewaju, 2022 ). Sustainability is a major concern today as a direct result of the serious concerns over the climate change, of which electricity generation is an important contributor ( Vine, 2019 ). Electricity is a critical product needed to support life, welfare, and global sustainable development ( Berga, 2016 ). Currently, humanity is faced with a significant challenge to realize new sustainable development Goals (SDGs) by the year 2030 ( Berga, 2016 ; Kabeyi and Oludolapo, 2020b ). Sustainable development and its correlation with energy became a significant global concern and issue in the 2002 Johannesburg world summit on sustainable development ( CS-UNIDO, 2008 ) . Determination of the most appropriate energy systems in an electricity mix is considered as a strategic approach in realization of sustainable development ( Ebrahimi and Rahmani, 2019 ; Kabeyi and Olanrewaju, 2020b ). Electricity generation systems can be assessed by a five-dimensional approach consisting of environmental, economic, social, technical, and institutional sustainability as a strong measure of energy sustainability ( Ebrahimi and Rahmani, 2019 ). Therefore, sustainability in energy development seeks to achieve technical sustainability, political or institutional sustainability, social sustainability, environmental sustainability, and economic sustainability which is greatly realized by the development and use of renewable energy resources ( Kabeyi, 2020a ). Figure 8 illustrates the five main dimensions of energy sustainability.
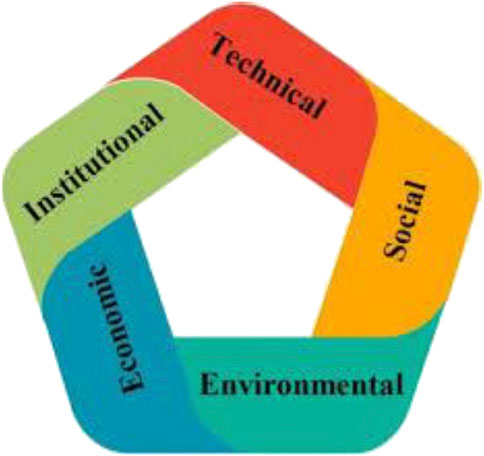
FIGURE 8 . Dimensions of energy sustainability ( Kabeyi, 2020a ).
Figure 8 above summarizes the main dimensions of energy sustainability particularly electricity. The main dimensions of sustainability in energy development and consumption are environmental, social, political, economic, and technical sustainability.
6.1 Technical Sustainability
Technical sustainability of electricity generation refers to the ability to meet the current and future demand in a safe and efficient manner with the use of clean sources of energy and technology ( Kabeyi, 2020a ). Unsustainable production and consumption of energy resources is the main cause of environmental damage in many organizations and countries globally ( Liu, 2014 ). To realise sustainable development calls for changes in industrial processes and systems, in terms of the type and quantity of energy and other resources for waste management, emission control as well as product and service range or type ( Krajnc and Glavic, 2003 ). Through the development and adoption of appropriate technology, humanity can make development of energy sustainable. Engineering and technology are closely linked to sustainability, but the engineering input so sustainability must be in partnership with other interests by application of constraints to the three pillars of sustainability which are social, environmental, and economic pillars. All engineering systems, products, operations, services, and infrastructure have designed and actual life after which they should be subjected to sustainability analysis by application of three measures in order of priority. These measures are reuse, recycle, and disposal ( The Royal Academy of Engineering, 2005 ).
For evaluation of energy or electricity sources, a number technical and operational indicators have to be analyzed ( Dobranskyte-Niskota et al., 2009 ). The typical technical evaluation criteria for energy systems and sources include efficiency, exergy efficiency, primary energy ration, system reliability, maturity, and safety ( Şengül et al., 2015 ). Other important powerplant performance indicators in power plant generation are specific fuel consumption, specific emissions, power plant availability, load factor, among others.
The transformative and disruptive potential of rapid technological changes, and the danger of using primitive technologies should be avoided for a sustainable transition. Even with advanced science, technology and innovation policies, and technologies, it is unlikely to deliver progress regarding global development unless the environment nurtures learning and innovation for effective management of innovation systems. Both national and international policies should promote international technology assessment and foresight and cooperation including collaborations and technology transfer. This cross border partnerships and cooperation will facilitate rapid sustainable energy development ( United Nations, 2019b ).
6.2 Environmental Sustainability
Environmental sustainability is concerned with managing the negative impact of energy production and use to the society and to magnify or extend the good ones. The environment should never be allowed to absorb more than it can contain, naturally or artificially ( Iddrisu and Bhattacharyya, 2015 ). Environmental sustainability is concerned with the integrity of natural environment and its ability to remain resilient and productive in support of humanity ( Kolagar et al., 2020 ). Environmental sustainability is further concerned with the integrity and carrying capacity of the natural environment to sustain humanity as a waste sink and source of raw materials ( Mensah, 2019 ). Thus, the environment or ecological dimension of sustainability is concerned with preservation of the environment and habitats, especially against the impacts of waste disposal, excessive consumption of Earth’s resources, and greenhouse gas emissions. The gases that lead global warming include, carbon dioxide, methane, and nitrous oxides. Carbon emissions in the atmosphere have increased from about 280 ppm by volume during pre-industrial times to over 400 ppm today representing more than 40% increase. In the United States, fossil fuel consumption results in average annual emission of about 5.3 billion metric tons of CO 2 into the atmosphere ( Nag, 2008 ). The origin of these carbon emissions is from energy consumption, non-energy use and industrial processes like iron and cement production.
The typical environmental evaluation criteria of energy systems include emission levels for SO 2 , NO x , CO 2 , particulate emissions, non-methane volatile organic compounds, land use and requirements ( Şengül et al., 2015 ). The main environmental dimension indicators for energy technology assessment include: GHG emissions, environmental external costs, radionuclides external costs, severe accidents perceived in future and fatal accidents from the experience. Additional environmental indicators are land use and solid waste. Life cycle emissions of GHG emissions in kg (CO 2-eq .)/kWh are selected to assess electricity generation technologies according policies like the EU environmental policy on climate change mitigation ( Streimikienea et al., 2012 ). GHC emissions in kg CO 2 eq./kWh were selected instead of external costs of GHG emissions because of the large uncertainties related to evaluation of external costs of GHG emissions. Climate change is the dominating environmental concern of the international environ-mental political discussion of today. Global warming is not only an issue for the environment, but rather for human society, since rising global temperatures might have serious consequences on the economy and social life. The indicator reflects the potential negative impacts of the global climate change caused by emissions of greenhouse gases to produce 1 kWh of electricity. This indicator was used in almost all studies on energy technologies assessment survived ( Streimikienea et al., 2012 ).
Therefore, an environmentally sustainable energy systems should maintain a stable resource base, avoiding energy resource over-exploitation, and avoid depleting non-renewable resources through development of adequate substitutes. These approaches include biodiversity maintenance, atmospheric stability, as well other ecosystem functions that are not necessarily economic resources ( Jonathan, 2001 ).
6.2.1 Atmospheric Temperature Changes
For the world to have a stable atmosphere, it is recommended to maintain temperature increase of 1.5–2°C above preindustrial level which then translates to 400–450 ppm of CO 2 equivalence ( Dyllick and Hockerts, 2002 ). The most widely accepted climate change scenarios and projections predict annual temperature increase of 1–3.5°C in coming decades based on existing scenarios ( Butt et al., 2012 ), hence the need for more global commitment. The Intergovernmental panel on climate change (IPCC) predicted that greenhouse gas emissions (GHG) will lead to global temperature increase of between 1.1 and 6.4°C by the end of the 21st Century with cities like London, Los Angeles and Phoenix having already experienced about 1°C average temperature rise within a decade. The United Kingdom (UK) is expected to experience temperature rise of about 3.5°C by 2050 which will also be accompanied by increased winter precipitation of up to 20% as well as increased incidences of storms ( Hulme et al., 2002 ; Intergovernmental Panel o, 2007 ). This predicted temperature rise is higher than the target set by the Paris Conference of 2015 (COP21) of 2°C above pre-industrial levels ( Berga, 2016 ; Lu, 2017 ). These statistics paint a picture of a world that is already missing its targets that are necessary to save mother nature and humanity.
6.2.2 Ecological Sustainability
Ecologically sustainability requires organizations to use only natural resources that are consumed at rates that are below their natural reproduction or replenishment rates or at a rate less than the development rate of substitute products or resources. They should also ensure that emissions which have potential of accumulation should not be allowed to accumulate at rates that are more than the capacity of the natural removal or absorption from the ecosystem system ( Kabeyi, 2019a ; Barasa Kabeyi, 2019b ; Kabeyi, 2020b ). Sustainable organizations should never engage in actions or activities that degrade any part of their eco-system ( Dyllick and Hockerts, 2002 ). Natural resources should be consumed by mankind at rates that allow them to replenish themselves ( University of Alberta, 2021 ). Therefore, environmentally sustainable systems should maintain a stable resource base free from over exploitation of energy resources. Ecological sustainability also advocates for maintenance of biodiversity, stability of the atmosphere, and other economic and non-economic ecosystem functions and resources ( Jonathan, 2001 ), while non-renewable resource substitution by renewable sources is a solution for the depleting ad polluting resources like fossil fuels namely petroleum, coal, and gas.
Environmental management and protection are a significant indicator of peoples’ culture, values, and ethical principles. Ethics involve making decisions based on acceptable values. This calls for social movements that constitute useful sources of cultural values and environmental movement to influence the environment is managed ( Kabeyi, 2018a ; Kabeyi, 2018b ). Therefore, sustainable environmental management is a result of multiple actors and factors. Environmental management for sustainability is concerned with control of interaction with the environment to protect and enhance human health and welfare together with environmental quality. Energy production, conversion, delivery, and end use can have serious environmental consequences to air, land, and water quality which are important for preservation of human life. These environmental issues related to energy are discussed below ( Kabeyi and Olanrewaju, 2020b ).
6.2.3 Water Pollution
Energy-related processes impact on water through discharge of water polluting solid and liquid wastes, thermal pollution from waste process heat, and excessive consumption of freshwater, and negative impact on aquatic life. Chemical contaminants like acids from mining operations, and discharge of coal ash to water bodies, radioactive wastes from nuclear power plants as well as oil spills from diesel power plants are common scenes from power plants ( Raja et al., 2006 ; Rajput, 2010 ). Although considered as gaseous and hence air contaminant, Carbon dioxide (CO 2 ) causes acidification of oceans with serious negative impacts on aquatic life. Process improvements and technology can help mitigate this pollution of water from energy related activities ( Nag, 2008 ; United States Department, 2015 ). Buried nuclear wastes can cause contamination of underground water source. Energy-related atmospheric emissions of conventional pollutants such as particulates, sulfur, and nitrogen compounds have been reduced through improved combustion strategies and exhaust scrubbing while transition to cleaner energy sources is also proving to be effective ( Raja et al., 2006 ; Nag, 2008 ; Rajput, 2010 ; Kabeyi, 2020a ). Used brine can also contaminate surface water in geothermal powerplants in powerplants with no reinjection ( Kabeyi, 2019b ; Kabeyi, 2020c ). Some technologies may not be water polluting but the process through which the plant and equipment are producing may be highly polluting.
6.2.4 Land Contamination
Energy and power generation related activities contaminate or pollute land in various ways . Land pollution takes many forms like deposition of atmospheric pollutants with precipitation, direct discharge, and accumulation of pollutants like coal ash from coal power plants. Oil spills from diesel power plants, soil extraction or excavation for fuel mining and production or associated with energy plant and infrastructure siting and development. Although regarded as clean power sources, wind, and solar power plants occupy huge tracts of land which may no longer be used for other economic activities like farming and human settlements. Cases of induced seismicity have been experienced in geothermal power development and generation which affects land use ( Barasa Kabeyi, 2019a ; Kabeyi, 2020c ; Kabeyi et al., 2020 ). Radioactive wastes from nuclear power plants may also be buried underground rendering the area useless for other economic activities ( Rajput, 2010 ). While liquid and gaseous emissions and effluents still must be handled by nuclear power plants. Buried nuclear wastes can cause contamination of underground water source. Energy-related atmospheric emissions of conventional pollutants such as particulates, sulfur, and nitrogen compounds have been reduced through improved combustion strategies and exhaust scrubbing while transition to cleaner energy sources is also proving to be effective ( Raja et al., 2006 ; Nag, 2008 ; Rajput, 2010 ; Kabeyi, 2020a ). Lack of reinjection in geothermal power plants can lead to land contamination by the used geothermal fluid ( Kabeyi, 2020c ; Kabeyi and Olanrewaju, 2021a ).
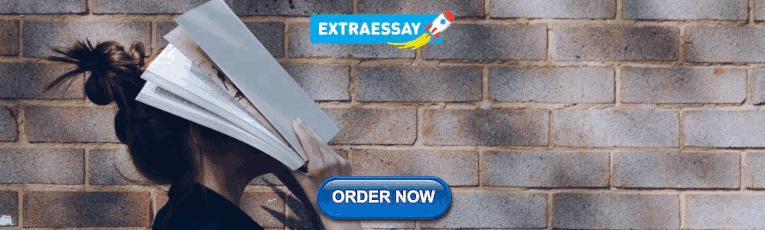
6.3 Economic Sustainability
Economic sustainability in energy and electricity production and use refers to the ability to meet demand in a cost-effective manner. It also a measure of access to requisite financing for energy resource development. The cost-effective operation will ensure that the energy system is s economically viable and feasible and hence makes the investment attractive to investors and financiers ( Kabeyi, 2020a ). All economies are made up of markets where transactions are made. The main activity in an economy is production of goods and services, distribution and consumption ( Mensah, 2019 ). Therefore, economic dimension of energy sustainability is concerned with the viability of individuals and organizations, products, and services in production and consumption of energy or electricity, distribution, and interactions.
Economic sustainability seeks to maintain the operational stability in terms of liquidity and cash flow and ensure fair or reasonable income and benefits to investors and other stakeholders in energy systems ( Dyllick and Hockerts, 2002 ). Electricity is the most multipurpose energy carrier globally, and therefore it is highly linked to human and economic development ( Bazmi and Zahedi, 2011 ). For economic sustainability to thrive, organizational policies, and operations should not retard economic progress, development, or affluence of society ( Hasna, 2007 ). It is through economic sustainability that humanity can maintain independence and have unlimited access to the required energy resources. Economic sustainability is realized from energy systems if they remain intact while activities and processes are equitably accessible to all to secure their livelihoods in a fair manner ( University of Alberta, 2021 ). Therefore, energy or electricity system must continuously produce goods and services to manage debts, pay bills, pay workers ensure sectorial balance with stable agricultural and industrial production ( United States Department, 2015 ). Energy systems and organizations should remain profitable and useful from one generation to another generation ( Kabeyi, 2018b ). Therefore, this implies that energy systems are economically sustainable if they are operated profitably by investors or organizations.
Energy costs are embedded in every commodity and service in an economy and therefore the economy requires better and efficient energy technologies to reduce energy costs leading to affordable electricity. This will enhance the level of economic activities through better supply reliability, reduced import bill, and a bigger market for energy goods and services. This leads to higher gross domestic product and balance of payments at a macroeconomic level ( United States Department, 2015 ). The various elements of economic dimension of energy sustainability of include corporate sustainability, energy costs, supply disruption loses, energy import bills, energy technology, and service market.
Renewable energy projects make use of local labor from rural areas, businesses, local material and business, local investors, and other services. Therefore revenue from renewable energy electricity revenue is invested back to local communities in form of taxes, payments for materials and labor as well as profits to investors which leave more economic benefits than imported fossil fuels or imported grid power ( Kumar and Okedu, 2019 ). Different renewable energy sources have different socioeconomic value. For example, biofuel projects create more jobs as compared to jobs created by solar and wind powerplants which gives the different projects a unique rank in socioeconomic evaluation of energy options. The cost and price of generated electricity is another important economic aspect of power generation projects and has a bearing on electricity sustainability ( Akella et al., 2009 ).
In economic evaluation of energy systems, typical evaluation criteria include total cost of investment, operation, and maintenance cost, fuel cost of generation, electricity cost, net present value (NPV), service value and equivalent cost of the energy system ( Şengül et al., 2015 ). The Economic dimension in sustainability assessment of energy technologies and projects is significant since energy or electricity supply cost influences technology adoption and penetration. Indicators that address economic dimension of energy sustainability assessment in electricity and heat generation and supply include the private or investors costs involved, the fuel price increase sensitivity, energy or plant average availability factor, costs involved in grid connection, energy or plant peak load response, and energy security of supply. Very important economic investment indicators are private costs, availability factor and costs of electricity grid connection ( Streimikienea et al., 2012 ). Goods and services should be produced in a continuous manner in an economically sustainable system to be realized. Should produce goods and services continuously, to maintain sustainable levels of debt, and ( Jonathan, 2001 ).
6.3.1 Energy Costs
Several factors influence the cost of energy and electricity and hence the price paid by consumers. These factors include the type of primary energy commodity used, availability of supplies or resources, primary sources price or cost, the capital costs of the power plant and operating costs incurred to convert or process the supplies into energy services like electricity, and prevailing energy demand. The variation in energy cost for various sources of energy leads to market competition among energy resources and services, with alternatives sources of energy. Unfortunately, the costs associated with energy security and environmental factors are often not fully included in the market price of energy sources. Reduced energy costs generally contribute to improved performance in many sectors of the economy, hence the need for low-cost energy and electricity supply. The reduction in cost of solar and wind power generation can significantly affect the competition with other, more traditional generation options like fossil fuels ( United States Department, 2015 ).
Energy systems respond to changes in input price and technology at different rates to in the energy sector and markets. The price of energy responds to the supply and prevailing demand which is dynamic. The factors influencing price include inventory level, level of economic activities, political factors, environmental factors, and market speculation which can drive market price volatility. These instability and volatility in energy price makes planning complicated and difficult, and hence negatively impact the entire economy. It is desirable to have a diversified portfolio comprising of many different sources of energy supply and enabling technologies to provide feasible options that can allow one to hedge the risk of being dependent on a single energy supply ( United States Department, 2015 ).
6.3.2 Energy Related Disruption and Losses
Energy disruptions can occur on the supply side, consumer side or transmission and distribution infrastructure any time, whether planned or not. Any electric power outage causes substantial economic costs and losses to the businesses and activities most of which depend on electricity. As an example, study by Lawrence Berkeley National Laboratory in 2006 on the cost of power outages estimated that disruptions to the U.S. electric power system cost between $22 and $135 billion per year with common causes identified as weather-related events like falling trees, and equipment failures like transformer failures. In another study, it was found outage-related costs ranging from $20 to $50 billion per year for weather-related outages alone. These losses are worse off if damages due to extreme weather events like Hurricanes are considered. The solution to this significant loss is improvements to the transmission and distribution systems ( United States Department, 2015 ). Sustainable engage should not only be affordable but reliable in supply, generation, and transmission.
6.3.3 Energy Import Liabilities
Energy business has significant impact on the balance of payment positions for importing and exporting countries for example the US spent approximately $190 billion in 2014 on petroleum imports. Oil importing countries must content with energy insecurity and fluctuating price and supply of petroleum. Electricity can also be imported and exported between countries leading and sharing of resources with resource rich countries especially with desirable renewable and low carbon sources like hydro and nuclear. Reducing dependence on energy imports reduces the impact of supply disruptions while promoting local investment in sustainable energy options ( United States Department, 2015 ).
6.3.4 Energy Technology Markets
Electricity generation and distribution is a big business in all countries while production and export of energy resources like fossil fuel sustains many countries’ economies with some almost entirely dependent on oil and gas exports. Other commercial primary resources include coal while some countries have relied on charcoal business to generate significant revenue. Export of energy production equipment like generators, turbines, boilers, and other plant equipment represents a substantial market opportunity for many countries like the United States and often generate high-value jobs. The International Energy Agency (IEA) predicts that clean energy will supply between $7 trillion and $10 trillion investment in electricity generation of which $6 trillion will be renewable sources and $1 trillion in low carbon nuclear power generation over between 2015 and 2025. It is observed further about two-thirds of this investment will be done in emerging economies. Additionally, energy efficiency investments will account for a further $8 trillion of investment ( United States Department, 2015 ).
6.4 Social Sustainability
Social sustainability is concerned with the rights of the community as measured by the level of social acceptance and access to the energy resource and systems by the people ( Iddrisu and Bhattacharyya, 2015 ). Social sustainability is the ability to preserve desirable social values, institutions, traditions, and social characteristics of the society before and after a project or an intervention. It is also concerned with social justice and therefore addresses aspects like labour practices, variance in production standards, and promotion of equity among all people ( Kabeyi, 2018c ; Kabeyi, 2019a ; Kabeyi, 2019c ). Social sustainability can be achieved by the selection and development of technology and powerplants that provide adequate power and employment to local communities and that don’t interrupt or interfere with their established way of living and value system ( Liu, 2014 ; Kabeyi, 2020a ; Kolagar et al., 2020 ). Therefore, social sustainability implies that people are important because development is basically about the people themselves. Principles that should be applied in energy development to realize social sustainability include accountability, empowerment, participation, cultural identity, and institutional stability ( Mensah, 2019 ).
At institutional level, sustainability is grounded in environmental initiatives which were sometimes referred to as green corporate initiatives. This is the ability of an organization to endure, take care of the needy in society and ensure institutional responsibility for greater good of the human society ( Samaras et al., 2019 ), by taking care of the world’s most vulnerable people in society. Any effort to achieve financial gains for the few while ignoring the needs of the majority is no longer acceptable, reasonable, productive, or justifiable ( Kabeyi, 2020b ). Therefore, socially sustainable organizations attempt and succeed in adding value to the communities within which they operate or do business. This is achieved by increasing the human capital base of individual partners and social capital of the community. Organizations should manage social capital in a way that stakeholders do understand the objectives and motivations for general agreement and cooperation ( Dyllick and Hockerts, 2002 ). Therefore key requirements for social sustainability of energy transformation is openness and democracy in the process ( Miller et al., 2013 ). Therefore, engaging in community social responsibility (CSR) activities is a strategy for increasing social sustainability of energy activities.
Social sustainability assumes two types of sustainability, i.e., social capital and human capital. Social capital is concerned with quality of public services like good education, water, infrastructure or a supporting culture and value system. Human capital is primarily concerned human skills, level of motivation, and loyalty of employees and business partners in their own capacity ( Dyllick and Hockerts, 2002 ). Therefore, human capital is an intrinsic phenomenon of an individual or individuals while social capital is concerned with communal or common physical projects, infrastructure or facilities meant to improve the quality of human beings in each social system. This implies that sustainable energy systems should have a positive impact on both human and social capital of the society.
Individual organizations have a critical role to play to ensure social sustainability of energy systems during the energy transition. Social sustainability requires organizations to act in a manner that creates welfare to society and all its people and should be ready to take responsibility for their actions ( Mohamed et al., 2020 ). A socially sustainable organization should internalize the social costs, develop the capital stock, exceed the social carrying capacity, enhance self-renewal of natural systems, nature openness, accountability, and democracy, enhance human choices and practice fair distribution of available but scarce energy and other resources among all stakeholders. It is necessary that social sustainability should preserve human rights and human dignity and guarantee equitable access to necessities which leads to a healthy and secure society. Communities that are healthy have fair leadership which ensures that personnel, labor, and cultural rights of all are respected to the later ( University of Alberta, 2021 ). Therefore for energy resources and electricity systems to be socially sustainable, they should be characterized by equity, reliable supply of social services, address gender equity, facilitate political stability, address accountability issues, and nature participation in their governance systems ( Jonathan, 2001 ). Social sustainability requirements call for energy practitioners, organizations, and countries, local and international organizations to go beyond just energy solutions and ensure holistic approach in as far as energy transition is concerned. Energy transition should address human physical and health, system and human safety, human rights, and dignity for sustainable energy development to be achieved.
Energy policies necessary to realize sustainability should be guided by a mixture of robust, objective, empirical, and theoretical principles that consider the impact not just to the current generation, but equally on future generations. Energy resources and electricity systems should consider socio-technical impacts on man and machines for them to be socially acceptable. Adoption of new electricity or energy technologies should bear in mind that systems are operated by man and should therefore be acceptable and comfortable by design and adequate capacity building should be done for man to be comfortable and therefore embrace new ways and systems. The impact of the technology change or acquisition on current and future financial systems, school system, labour market composition, organizational culture and political aspirations of the people should be considered in the energy transition if it has to be sustainable and avoid failure or negative perception by the people ( Miller et al., 2013 ). This implies that man is a very important aspect of the energy transition and should be at the center of the transition and be involved using various participatory methodologies, otherwise the transition may never succeed.
Any social evaluation of energy systems should consider social acceptability, expected job creation, and other social benefits of the energy systems ( Şengül et al., 2015 ). Significant social indicators for energy and electricity technologies are related job opportunities, health effects, food safety and security risk and work-related accidents and fatalities. Technology specific job opportunities in person-year/kWh indicator are based on the average amount of labor used to produce a unit of electricity ( Berga, 2016 ; Lu, 2017 ). The quality of electricity or energy related work is addressed by Work Quality indicator is based on knowledge and training of average worker in each technology chain, using an ordinal scale indicator ( Streimikienea et al., 2012 ). A system is said to be socially sustainable if it guarantees distributional equity, provides social services like education, health, guarantees gender equity as well as political accountability and adequate public participation of stakeholders ( Jonathan, 2001 ).
Therefore, social sustainability of the energy transition is concerned with the value to community, democratic participation, direct benefits through addition of human and social capital, job creation and other community benefits through activities like corporate social responsibility and respect for local customs, traditions, and beliefs.
6.5 Political and Institutional Sustainability
The development of new energy technologies, new business models, and new policy priorities and frameworks need new market participation and control models and rules and regulations which require new governance and new institutional design ( De, 2021 ; Lenhart and Fox, 2021 ). Transition historical studies show that whereas technological innovations and market actors are the main drivers of change, extensive studies claims that it is governance systems that influence the distribution of the benefits of new technologies to the society which is an important requirement of sustainability ( Kuzemko et al., 2016 ; Kabeyi, 2020a ). The road to the global low-carbon transformation should deal with the climate crisis is within reach, but this requires political actions from world leaders. There is need for action along multiple approaches and models globally that can be scaled up and adapted to suit specific national prevailing circumstances. Cost-effective a low-carbon technologies are available in many fields with several under research but the rate of adoption is still a serious concern because governments need to put in place the right policies, regulations and a facilitating legal framework for faster and successful adaption ( Watson, 2014 ; Krzywda et al., 2021 ). Political or institutional dimension of energy sustainability is concerned with governance of sustainable energy transformation at all levels. This is achieved by setting and implementing policies and regulations with different political institutions influencing governance choices ( Kuzemko et al., 2016 ). This implies that the political dimension of energy sustainability is concerned with the strategic planning and definition of the energy system and related systems and processes. Therefore, political sustainability concerns address the future structure and indicates some issue on political stability and foreign policies of the energy system ( Kabeyi, 2020a ). For development to be sustainable, there is need for adequate management of the tradeoff between social equity, protection and integrity of the environment, real economic development and progress and preservation and use of natural resources for equitable use by all ( Robyns et al., 2012 ). This can only be achieved effectively when we have the political will from all if not many nations and groups that have power to influence energy policy ( Kabeyi, 2019a ).
The institutional dimension of sustainability defines the role of local participation in the control and management of energy resources and energy systems ( Kabeyi, 2019a ; Kabeyi, 2019c ). The institutional dimension embodies elements like local ownership, participation, local contributions, local skill base, local policy and regulation, protection of investors and consumers and sharing of resources and benefits accruing. This dimension is the one that defines the system structure and framework of processes, systems and policy decisions which affect the project or investment ( Iddrisu and Bhattacharyya, 2015 ; Kolagar et al., 2020 ). There is evidence that highly or adequately institutionalized countries with efficient and effective energy related institutions are more successful in managing the energy transition. This is because the institutions encourage innovation, efficient resource allocation and set desirable policy, legal and financial measures and instruments which are enables of sustainable energy transition ( Inglesi-Lotz, 2021 ).
6.5.1 Politics and Sustainable Energy Transition
Energy activities, products and services constitute big business globally. In 2015, four out of eight top Fortune 500 companies, were energy related companies like Exxon Mobil, Chevron, Phillips 66, and General Electric. Therefore, energy represents a big portion of global economy and therefore it affects jobs, people’s incomes, company performance, profits, and personal or individual economics. Within these dynamics, politics become important especially as an enabler of business through various instruments at their disposal. Internationally, government subsidies have helped the development of new technology for solar and wind power. Many governments subsidize the oil and nuclear power industries which complicates the viability of renewable energy resources and technologies ( United States Department, 2015 ).
Politics play a leading role in the coal industry which continues to survive and thrive in many countries like the United States because of exemptions from federal pollution regulations making its use competitive. The same is true for hydrologic fracturing or fracking, which has survived in the US because of the 2005 Clean Water Act. The US government also subsidizes pipelines and supports military actions in the Middle East as a strategy to ensure a stable and reliable supply of fossil fuels. Energy has also been a leading cause of most political tensions between countries. These tensions or conflicts shape the decisions all countries make about their energy resources which ultimately affects their electricity mix so as to manage costs, security and environmental concerns besides shaping international relationships ( Dufour, 2018 ).
6.5.2 Energy Policies, Regulations, and Governance
Governments should strive to meet the growing energy demand but also meet environmental requirements. To realize these demand and sustainability challenges, Governments should develop regulations and policies that seek to meet the growing energy needs and concerns over emissions and global warming. It is necessary to develop sustainable energy policies to provide relevant and suitable policy recommendations for end-users ( Lu et al., 2020 ). Policymakers should develop sustainable solutions and a conducive environment for a sustainable energy transition. Good governance in the energy sector is an important tool to realise climate change mitigation by investing in sustainable measures and projects. There is need for public intervention by putting in place what is considered as a conducive energy transition’s regulatory framework. Renewable energy projects and other sustainable energy investments, just like any other investment, require political stability, proper regulatory frameworks, good and effective governance and secure property rights ( Inglesi-Lotz, 2021 ).
Energy policy issues are political in nature, and act as instruments through which governments can influence sustainability in the society. Governments should develop energy policies which can alter consumption habits and patterns, reduce fossil fuel dependency and environmental conservation and protection while stimulating investment and development of clean energy technologies. Interest groups represent interests from energy conservation proponents to nuclear power opponents ( Marcus, 1992 ). Interest groups representing various groups and stakeholders from energy conservation to nuclear power opponents need to be heard during policy formulation on sustainable electricity ( Marcus, 1992 ). Energy policy seeks to establish security of supply, energy affordability, and minimum impact on the environment.
Institutions in energy transition generally refers to the formal and informal rules and their enforcement ( Inglesi-Lotz, 2021 ). The quality of these institutions is measured in terms of ability to create a conducing environment characterised by the following indicators and dimensions.
6.5.2.1 Voice and Accountability
This refers to the extent to which the people in a country can choose and challenge the government of the day which limits executive authority.
6.5.2.2 Peace and Political Stability
The citizens of a country have no incentive to invest in their future in environments of political instability or civil strife. This therefore makes sustainability concerns secondary to the need for immediate survival.
6.5.2.3 Government Effectiveness
Government effectiveness is the quality of public services and the degree of freedom or independence from political pressures and interference. This creates an enabling environment for private sector investment in energy.
6.5.2.4 Regulatory Quality
Regulatory quality is the ability to formulate and implement appropriate policies and regulations that facilitate private sector growth and development. This requires that government lays down fair and uniform rules of economic engagement.
6.5.2.5 Rule of Law
Investment in sustainable energy requires suitable laws governing quality of contract enforcement, private and public property rights, effective police, and courts for arbitration and the enforcement of the rules of society.
6.5.2.6 Control of Corruption
There is need for a strong anticorruption prevention for more the more economic success as a Corruption inhibits investment and increases the cost of doing business and lowers competence and efficiency of performance which in itself and indicator of lack of sustainability ( Kabeyi, 2020b ).
6.5.2.7 Ease of Doing Business
This is a measure of the multitude of aspects that influence the extent to which the regulatory environment is facilitates business operations. Investment in sustainable renewable energy projects can delayed or abandoned by too complex and lengthy bureaucratic procedures and corruption ( Inglesi-Lotz, 2021 ).
A sustainable energy transition calls for the design of an appropriate market structure for proper performance of the energy sector in terms of prices, energy efficiency, supply, and technological innovation. Governance mechanisms directly influence the market structures and influence investment decisions. Therefore, bad or improper market structure designs and policies can lead to higher costs of the energy sector unnecessarily which impacts negatively on the welfare of consumers ( Inglesi-Lotz, 2021 ).
6.5.3 Energy Security, Risks, and System Resilience
Energy security generally refers to low probability of damage to acquired values. Energy security is best defined by the four As of energy security which refer to availability, affordability, acceptability, and access to energy resources and systems ( Cherp and Jewell, 2014 ). Energy security is therefore the degree of vulnerability on vital energy resources and systems which is influenced by the degree of exposure to energy related risks, its resilience and links to important energy and social systems. Energy security issues emerged in the early 20th century with respect to the supply of oil to the military more so in the frontline. Academic reflections on energy security emerged in 1960s and became real in 1970s with the oil crisis. It remerged in the 2000s with concerns over rapid demand growth for energy in Asia and gas supply disruptions in Europe and the current pressure over emissions and global warming concerns ( Cherp and Jewell, 2014 ; Austvik, 2016 ).
Energy systems are entangled with human and national security with reliability concerns shaping public opinion and policy as well as political decisions and agenda with implications on the economy and political systems ( Austvik, 2016 ). It is the desire of everybody and every nation to have uninterrupted supply of vital energy services and hence, energy security is a priority for all nations ( Jansen and Seebregts, 2010 ). The security concerns are robustness, i.e., resource sufficiency, system reliability, stability, and affordability; sovereignty which include protection from internal and external threats; and resilience which is ability to withstand disruptions of energy systems. For many countries, energy insecurity means lack of self-sufficiency and having aging infrastructure, while insecurity issues among developing countries additionally includes lack of adequate capacity, high energy intensity, and high demand growth that is more than ability to supply. For low-income countries, multiple vulnerabilities overlap, making them seriously energy insecure ( Jacobs et al., 1987 ).
Energy security is a very important policy driver with privatization of the electricity sector being used as a tool to secure cheaper energy supplies in some countries in the short term. However, this has led to contrary effects in some places because of stiff competition, resulting in delayed and deferred development of power plant and infrastructure caused by prolonged uncertainties on viability ( Bazmi and Zahedi, 2011 ). Renewable energy sources have the potential to help nations become independent from foreign energy supplies and mitigate risks from conflicts and other disruptions to vital energy resource supplies because most of them do not rely on imports unlike fossil fuels sources ( Ölz et al., 2007 ). A typical example of an energy conflict involved the expansion of the existing pipeline between Germany and Russia through the Baltic Sea. This caused international disputes with the US warning Germany with sanctions ( Dettmer, 2019 ). In this energy project, countries like Poland and Ukraine heavily criticized the pipeline, fearing that Russia would use it for political gain and escalate regional conflicts through arming Eastern European countries ( Gurzu, 2019 ). This is because of the concern that the pipelines can deliver gas to German directly and hence by-pass Ukraine and thus escalate the conflict with Ukraine.
In another case of energy insecurity, the first oil crisis in 1973 brought about a reduction of about 30% in the supply to of oil to Japan leading to Japanese economic downturn and recession in 1974. These reminded policy makers in Japan that energy supply is a serious security issue and should be managed ( Cheng, 2009 ; Mihut and Daniel, 2013 ). The case was similar in South Korea which was also seriously affected by the first and second oil shocks of 1973 and 1979 ( Miller et al., 2013 ; Azad, 2015 ). The supply shocks demonstrated that energy supply and national security are seriously intertwined. In 2011, the Tohoku earthquake in Japan brought about massive disruptions to energy supply after Japan was forced to shut down its nuclear power plants after the nuclear accident at Fukushima Daiichi power plant leading to increased use of fossil fuels. This caused significant increase in fossil fuel demand and supply ( McCurry, 2015 ; Energy Information A, 2020 ). Today, Japan and South Korea have shifted their electricity generation from oil based and to liquefied natural gas and coal which is steal a fossil fuel but with less environmental impact ( Korea Energy Economics In, 2017 ; Ministry of Economy, 2018 ).
In yet another case of energy insecurity, Taiwan, experienced a massive power outage in the northern half of the island in 201 that lasted 5 h causing an estimated damage of three million US dollars. Although this was partly blamed on human error, and structural challenges within Taiwan Power Corporation, a critical analysis showed that operating electricity reserves had significantly reduced from 6% to just 1% within 1 week to the blackout ( Yu, 2017 ). Therefore, adequate energy planning has an important role to play in energy supply security and that unreliable or insecure energy system can be quite destructive and costly to any economy.
There are several energy related risks to national energy and electricity security, and can be broadly categorized into physical, cyber, economic, and conflict-related risks although with significant overlaps among these categories. Energy technologies must be robust and resistant to these vulnerabilities if they must be sustainable and secure.
6.5.3.1 Physical Energy Risks
Energy security risks are related to the damage and disruption of energy supply, energy storage, and delivery infrastructures. Several energy infrastructure and assets are susceptible to damage and disruptions. Energy infrastructure include the electrical grid infrastructure system, pipelines for oil and gas, and rail transport network and infrastructure, as well as road and marine systems. Examples of damages to the infrastructure include Hurricane Sandy effects and the attacks on substation facilities and power plants as a result of extreme weather with climate change raises these risks ( United States Department, 2015 ).
6.5.3.2 Cyber Security Risks
Cyber security refers to vulnerabilities that compromise the computer-based systems and related operations and functions like data inputs, data analysis, and data processing, the real time operation and coordination of electricity supply systems, energy delivery, and end-use systems control in smart grids. There is need to validate and manage all data inputs, monitor the systems for intrusion, and the need to address other vulnerabilities which come with challenges of maintaining the integrity of these systems. Electricity networks encounter cyber security threats which increase with access to the internet and other computer supported operations as in the case of the smart grid ( United States Department, 2015 ).
6.5.3.3 Economic Energy Risks
Economic energy security risks are related to resource supply and price shocks like the oil crisis of 1973 and 1978. Energy resources that are traded internationally are particularly subject to price and supply fluctuations due to various reasons that include civil war, international war, economic sanctions, and deliberate supply control. The price and supply shocks create uncertainties for energy-dependent businesses, which then invest in energy security measures including research and development of renewable and sustainable energy systems. Supplier related risks include price and supply manipulations. Manufacture of complex energy infrastructure components is often dependent on global supply chains which can be adversely affected by long lead times, long-range shipping logistics, and price volatility ( United States Department, 2015 ).
6.5.3.4 Conflict-Related Energy Insecurity
These are risks that are related to unrest in foreign countries as well as energy fueled domestic or local conflicts. International security risks include those that involve unrest in locations that are critical to global energy supply ( Austvik, 2016 ). They include conflicts in the Middle East which seriously disrupt the supply of petroleum resources. These conflicts also cause deaths, economic meltdown, and environmental disaster like oil spills and destruction of oil resources by enemies in conflicts ( United States Department, 2015 ).
In many countries, conflicts over energy resources are a common denominator. In most of these conflicts, prevailing historical differences and injustices among neighbors has been cited in various areas like religion, tribe, race or even clans and political inclinations. For example, in Syria and Iran, the conflicts appear like they are religious in nature between Sunnis, Shiite Muslims, Kurds, Turkmen, and others while. In Nigeria, it may appear as a conflict is over energy between Muslims, Christians, and other traditional groups, while in the South Sudan, the conflict looks like just differences between the Dinka and Nuer tribes. In Eastern Europe, conflicts in Ukraine, are between Ukrainian loyalists and Russian speakers aligned with Moscow. A deep scrutiny of these conflicts highlighted place energy at the epicenter of the differences, hence the reality is that these conflicts are struggles for control over the principal source of national income which is energy ( Marcus, 1992 ; Dufour, 2018 ). Therefore, it is only through proper energy resource management that social, political, and economic conflicts can be avoided or resolved. Hence, for energy to be sustainable, governments and groups have a duty to maintain peace and stability and prevent or manage existing and potential energy conflicts within their political environment.
6.5.4 Corporate Sustainability
This is an extension of institutional and political dimension of energy sustainability ( Kabeyi and Olanrewaju, 2020b ). Organizations and individuals create social impacts that are both positive and negative, through their operational activities. Societies rely on organizations for individual and common good and benefits like employment and social infrastructure, while organizations or corporations need societies to provide workforce and other critical inputs like raw materials to sustain their operations. While we have the interdependence between organizations and society, it is only a healthy and positive society that will create good workers sought organizations ( Tharp, 2012 ). Individuals within the community or society play a significant role in developing sustainable situations and circumstances but organizations can influence this by acting sustainably in their operations and relationships with society ( University of Alberta, 2021 ). Since there is mutual dependence between institution or organizations and society, there is need to have the principle of shared value while making choices and decisions. Sustainability demands for responsibility and facilitates human creativity to develop innovative ways that will further protect the shared environment, respect for all, and empower stakeholders. For organizations, sustainability is important because it creates value and provides them with competitive advantage and leaves a greater positive value to society and stakeholders as well ( Nawaz and Koç, 2019 ).
Energy sustainability programs and activities should facilitate the bridging of the gap between laws and the requirements of good business practice, which include prevention of exploitation, transparence, and accountability to all stakeholders in business or a given system under consideration. This calls for proactive risk identification, assessment, mitigation, and management. This makes sustainability a necessary value and an integral business goal and objective of all organizations including energy companies. Long term success of business operations should incorporate social, environmental, and supply issues in all their undertakings with suppliers, customers, and all other stakeholders. This in return adds value to the respective organizations in terms of corporate reputation, brand visibility, value and equity, better risk management, easier access to capital, talent attraction and retention and higher profitability and return on investment ( Kanter, 2021 ).
Corporate sustainability involves the integration of the economic, ecological, and social aspects in business practice and operations ( Dyllick and Hockerts, 2002 ). The consumption of goods and services is pivotal to enhanced organization’s operational efficiency because sustainable use of goods and services leads to reduced generation of waste during productive operations. The general objective of sustainable corporate development is to realize economic, social, and low carbon sustainability by companies. By embracing sustainable innovation practices, organizations can reduce adverse social and economic impacts of their operations which leads to better corporate performance ( Kabeyi and Oludolapo, 2020a ; Mohamed et al., 2020 ).
Environmental sustainability of an organization is an important element of corporate sustainability since it is associated with social consequences of business activities and the environment ( Darkwah et al., 2018 ; Mohamed et al., 2020 ). An organization that is environmentally proactive should accommodate their stakeholder concerns which leads to better corporate performance and profitability. Environmentally friendly practices are positively related to corporate sustainable performance because of low carbon innovation attitude ( Rosen, 2009 ; Darkwah et al., 2018 ; Mohamed et al., 2020 ). Therefore, socially responsible corporate behavior often affects environmental sustainability.
Corporate sustainability strategies incorporate sustainable development principles into business activities and mediation of the relationship between environmental, social sustainability and technology in organizational operations. Nurturing creativity helps to increase environmental, social, and economic efficiency and effectiveness by organizations and in the process, it facilitates advancement of environmentally friendly measures. Therefore, creativity impacts green innovation within organizations and so should be encouraged ( Musango et al., 2011 ; Moriarty and Honnery, 2019 ; Mohamed et al., 2020 ).
Various elements have an impact on corporate sustainability which also affects energy sustainability. Energy activities constitute a very important social enterprise with 9 out of 12 most capitalized companies globally engaging in energy business ( Miller et al., 2013 ). Organizations that are economically sustainable have guarantee that they always have sufficient cashflow to ensure liquidity while at the same time they produce above average returns to the investment. Organizations that are ecologically sustainable consume natural resources at a pace lower than the resource reproduction or substitution and they do not pollute the environment with emissions that accumulate beyond the capacity of natural systems to absorb and assimilate them ( Kabeyi, 2018b ; Barasa Kabeyi, 2019b ; Kabeyi and Oludolapo, 2020b ; Kabeyi et al., 2020 ). They also do not engage in ecosystem degrading services. Socially sustainable companies usually add value to the communities where they operate through increment of the human capital of partners. They also improve the societal capital of surrounding communities and are transparent in their activities and operations. ( Dyllick and Hockerts, 2002 ). Therefore, corporate sustainability is a critical part of the wider energy and global sustainable transition.
Therefore, institutional dimension is a very important dimension in determining and influencing investment, competitiveness, prices, investment, and performance of energy sector. The political function sets energy sector institutions, policies and regulations that govern the energy sector and therefore directly influence choices and performance of the energy transition measures and investment.
7 Strategies for Sustainable Energy and Electricity Systems
The sustainable transition strategies typically consist of three major technological changes namely, energy savings on the demand side, generation efficiency at production level and fossil fuel substitution by various renewable energy sources and low carbon nuclear ( Lund, 2007 ; Kabeyi and Oludolapo, 2021b ; Kabeyi and Olanrewaju, 2022 ). For the transition remain technically and economically feasible and beneficial, policy initiatives are necessary to steer the global electricity transition towards a sustainable energy and electricity system. ( Bruckner et al., 2014b ; IRENA, 2018 ). Whereas renewable sources energy holds the key for sustainable energy transition, large-scale renewable energy adoption should include measures to improve efficiency of existing nonrenewable sources which still have an important cost reduction and stabilization role ( Lund, 2007 ). A resilient grid with advanced energy storage for storage and absorption of variable renewables should also be part of the transition strategies ( Kabeyi and Olanrewaju, 2020b ).
7.1 Policy Measures and Initiatives for the Energy Transition
A successful energy transition requires a stable political and economic framework, and support systems, financial measures, technical and as well as well as administrative policy measures to overcome barriers existing as a result of a distorted energy market ( Fouquet, 2013 ). At the center of any sustainable energy strategies is the objective of improving the production and use of energy resources so that they contribute to sustainable development. These requires policies that seek to widen access to reliable and affordable modern energy supplies and while mitigating the negative health and environmental impacts associated with the energy processes and systems. To increase energy supplies comes with economic burdens and so the policies should also foster real socio-economic development to sustain any expansion and access. Measures be taken to make markets work more effectively, and develop new markets while modernizing and expanding old once for efficiency and sustainability in general ( Jefferson, 2000 ).
The focus areas where policy and decision makers should act are as follows.
7.1.1 Develop a Strong Synergy Between Energy Efficiency and Renewable Energy
Policy makers policy design policies that combine the effect of bulk of energy-related decarbonization needs by 2050 in a cost-effective manner through efficient energy production and use ( Bruckner et al., 2014b ; IRENA, 2018 ).
7.1.2 Develop an Electric System in Which Renewables Provide a High Share of the Energy
There is need to transform the global energy system through fundamental shift in the way electric power systems are conceived and deployed. This calls for long-term system planning and a shift to policymaking that is more holistic and coordinated across all sectors and nations. There should be timely deployment of infrastructure and the redesign of rules and regulations to achieve cost-effective large-scale integration of solar and wind generation. With their massive potential and renewability with negligible emissions and environmental impact, wind and solar should be made the backbone of electric power systems by the year 2050 to meet the targets set in the Paris agreement ( Bruckner et al., 2014b ; IRENA, 2018 ).
7.1.3 Accelerate Electrification in Transport, Building, and Industry
There is need for deep and cost-effective decarbonization of transport and heat sectors by electrification with the bulk of power coming from renewable electricity. Where it is not possible to electrify transport, industry and buildings then other renewable solutions will have to be adopted ( Fouquet, 2013 ). Alternative sources of energy for direct use include modern bioenergy, solar thermal, and geothermal heat applications. The realization of this shift needs the deployment of an enabling policy framework and development of supporting technology and other related initiatives in urban planning, building, transport and industrial sectors ( Bruckner et al., 2014b ; IRENA, 2018 ).
7.1.4 Foster System-Wide Innovation
There is need to have continued technological innovation to achieve a successful global energy transition, just the same way new technologies have played a leading role in renewable energy development and deployment. Innovation effort must cover a technology’s full life cycle, that includes demonstration, technology deployment, commercialization, and final disposal. It is worth noting that innovation is broader than technology research and development (R&D) as it includes new approaches to existing energy systems and markets and development of new technical and business models. There is need for coordinated effort by regional, national governments, national and international actors, and the private sector to deliver the needed innovations to facilitate energy ( Bruckner et al., 2014b ; IRENA, 2018 ).
7.1.5 Align Socio-Economic Structures and Investment With the Transition
There is need for a globally integrated and holistic approach by alignment of socio-economic systems with the requirements of the energy transition. Implementation of the energy transition requires investments, in addition to those already incurred with respect to the adaptation to climate change. Faster realization of the energy transition would lower the climate change adaptation costs and in addition to reduced socio-economic disruption. This calls for alignment of the financial systems with broader sustainability and energy transition demands that today’s investment decisions made define the energy system of many years ahead. For smooth investment in energy transitions, there is need to allow urgent flows of capital investment to low-carbon solutions, avoid locking economies into a carbon-intensive energy systems and to minimize incidents of stranded assets ( Mullen and Dong, 2021 ).
A smooth and successful energy transition calls for establishment of regulatory and policy frameworks that give all relevant stakeholders a clear, firm, and long-term guarantee of energy systems transformations to meet the emissions and climate goals set. This will create economic incentives that are aligned to the environmental and social costs of fossil fuels and remove barriers to accelerated deployment of low carbon energy systems and solutions ( Mullen and Dong, 2021 ). Energy transition would require increased participation of both private and public institutional investors as well as community-based finance who should be facilitated and motivated with relevant incentives. The requirements and specifications of distributed investment needs including energy efficiency and distributed generation need to be addressed within the socioeconomic structures and investment transformation ( Bruckner et al., 2014b ; IRENA, 2018 ).
7.1.6 Ensure That Transition Costs and Benefits are Distributed Fairly
Policies put in place should ensure that the whole society should be involved in a collaborative manner and process to achieve the desired transition. Effective participation is only achievable if the energy transformation costs and benefits are shared and the transition itself is implemented in a fair and just manner. A key requirement and component of a fair and just transition is the universal energy access where all benefit. There is need for the transition scenarios and planning to incorporate access and convergence factors in the transition because there are huge disparities presently in availability of energy services, hence the need for energy services to cover all regions ( IRENA, 2018 ).
A successful energy transition will require the promotion and facilitation of a social accounting framework which enables and visualizes the transition contributions and obligations from the stakeholders in the transition. There is need to make advances in the definition and implementation of a fair context to share costs of transition while at the same time promoting and facilitate structures which enable fair distribution of the benefits of the transition. There is need to explicitly address a just transition considerations from the onset, at both macro, and micro levels, which will enable the creation of structures needed to provide alternatives that allow parties who have been trapped into the fossil fuel dynamics to participate effectively from the transition benefits.
The economic, social, political and technological realities and developments continuously influence the energy mix hence the need to have a rationale in energy system decision making in energy planning and generation deployment ( Streimikienea et al., 2012 ). Renewable energy is considered as a solution for mitigating climate change and environmental pollution; however, an important problem of the application of renewable energy systems (RESs) is that the evaluation of the sustainability of these systems is extremely complicated.
7.1.7 Specific Policy and Legal Measures
There is need to have appropriate policy frameworks, attractive prices for investors and consumers, and a facilitating regulatory framework to realize a sustainable energy transition. Whereas strategies to encourage sustainable energy systems are straightforward, there is need for wider acknowledgement of the challenges and stronger commitment to specific enabling policies ( Wanga et al., 2020 ). It is also necessary to ensure that electricity utilities have adequate generation capacity and that they are financially healthy for them to contribute to sustainable energy and power ( Karekezi and Kimani, 2002 ). Governments should play a proactive role in the transformation, but they cannot single handedly create desired change and the right speed without the involvement and participation of non-state actors to nature the transformation ( Pegels, 2010 ).
With electricity generation being an important contributor to global greenhouse gas emissions, a viable option in the transition is to decarbonize the grid electricity energy sources by use of low carbon and renewable sources ( Jefferson, 2000 ; Colla et al., 2020 ). Several measures should be put in place to ensure that energy systems promote sustainable socioeconomic development. The main challenges to overcome are expansion of access to affordable, reliable, and adequate energy supplies while addressing environmental impacts at all levels ( Jefferson, 2000 ). With the right policies, prices, and regulations in place, energy markets can achieve many of these objectives. But where markets do not operate or where they fail to protect important public benefits, targeted government policies, programs, and regulations are justified to achieve policy goals. Although strategies to encourage sustainable energy systems are straightforward, there is need for a wider acknowledgement of the challenges and a stronger commitment to specific policies aimed at enhancing sustainability ( Wang, 2019 ; Wanga et al., 2020 ).
7.2 Electricity/Energy Planning and Resource Allocation for the Energy Transition
It should be noted that a competitive and sustainable energy market is the most efficient allocator of energy resources and provides high levels of consumer service and satisfaction as expected. Thus, a key requirement for any sustainable energy strategy should be to maintain competitive market conditions. However, the market alone cannot meet the needs and expectations of the most vulnerable groups, protect, or preserve the natural environment, and ensure energy security in the face of a complex political environment. In general, governments and societies should put in place proper frameworks to enable competitive pricing and effective regulation of energy markets so as to achieve many of the objectives of sustainable energy ( Jefferson, 2000 ). Therefore, it is critical to have a working policy and supportive political environment.
The selection and deployment of energy and power systems is a multicriterial approach that considers all the dimensions of sustainable energy systems and sources. Figure 9 below illustrates the stages in multicriterial sustainable energy decision analysis.
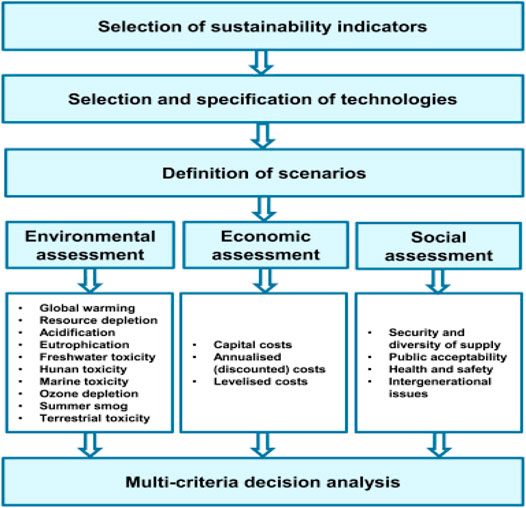
FIGURE 9 . Planning for electricity sustainability.
Figure 9 above shows the recommended steps in the planning for sustainable energy and electricity solutions and options using the multicriteria approach. The process starts with the selection of sustainability indicators for use in selection and planning. Based on the appropriate indicators, appropriate technology is selected from the desirable specifications that were specified. In addition to the social, environmental, and economic indicators which define sustainable development, further considerations are needed to capture the technical and institutional/political dimensions which will guide in the deployment of the best or most appropriate energy and electric power systems.
The multicriteria analysis and decision making often requires the application of energy and electricity models for more effective decision making. The same will also become critical in real time electricity generation and supply for efficient deployment of powerplants and electricity supply as well as consumption by all category of consumers ( Bruckner et al., 2014b ; IRENA, 2018 ).
7.3 Renewable Energy in the Energy Transition
Renewable energy sources come from naturally occurring sources which replenish themselves through natural forces. As a source of clean energy that is inexhaustible, renewable energy sources have a significant role to play in the energy transition ( del RíoJaneiro, 2016 ). Although renewable in nature, their consumption should allow for natural replenishment for them to be renewable and competitive ( Owusu and Asumadu-Sarkodie, 2016 ). Strategies to improve access and consumption of renewable energy sources include improvement in conversion efficiency, use of energy storage technologies to deal with fluctuating nature and policies that discourage more consumption of fossil fuels ( Sasmaz et al., 2020 ). Renewable energy sources have been identified as the main solution for mitigation of greenhouse gas emissions and climate change, and environmental pollution ( Liu, 2014 ). Renewable energy sources (RES) can greatly contribute to economic, social, and environmental energy sustainability. The renewable energy sources can be used to improve energy access for most of the population because they are often locally available, reduce greenhouse gas emissions and may create local socioeconomic development opportunities through job creation and improved local economy. ( Jaramillo-Nieves and Del Río, 2010 ). Before the industrial revolution, solar energy was the most readily available form of energy for direct solar application like drying. This changed with the industrial revolution as fossil fuels became dominant source of energy. Fossil fuels constitute the main class of energy sources that cause severe environmental pollution and are thus the main target of substitution with renewable and low carbon sources. The challenge of this substitution is that it may impact negatively on human development ( Sasmaz et al., 2020 ). Sustainable social and economic development goals cannot be achieved without access to clean, reliable, and affordable energy resources and supplies ( Kabeyi and Oludolapo, 2020b ; Kabeyi and Olanrewaju, 2020b ; Sasmaz et al., 2020 ).
As the world’s population and economy keeps growing, so is the energy demand, a scenario that automatically increase the demand and consumption of conventional sources of energy, particularly fossil fuels ( United States Department, 2015 ; Owusu and Asumadu-Sarkodie, 2016 ). Renewable energy resources can be used as substitutes for fossil fuels. These sources are characteristically ideal to achieve sustainability in energy use as one of the basic requirements of sustainable development ( Wanga et al., 2020 ). Therefore, replacement of fossil fuels with renewable energy sources in electricity generation is an important measure to reduce carbon emissions ( Mohamad and Anuge, 2021 ).
There are increasing opportunities for companies to increase the use of renewable energy through development in data-driven technology which can help to better understand on real-time basis, internal energy consumption and demand, which may provide information required to control cost. Such information can assist investors in negotiating energy supply contracts that are more appropriate for their unique consumption and demand patterns ( Scheneider Electric Company, 2019 ). Energy planners face challenges in energy planning because of challenges like unequal distribution of natural resource, limited financial resources and other considerations. Factors considered in energy evaluation include economic, institutional, technological development, energy security, environmental protection, and prevailing state of the energy market ( Wanga et al., 2020 ). For energy systems to be sustainable, the consumption should bear in mind the limits of resource supply and the environmental and social impact ( Jefferson, 2000 ). Maximum advantage should be taken of immense resource supply for renewables like geothermal, solar, hydro and wind ( Jonathan, 2001 ).
Although renewable energy sources have significant advantages, their consumption is also associated with challenges like low conversion efficiency, unsteady supply, low conversion efficiency, and general variability and unpredictability in supply. These weaknesses can be addressed by technological advances and application of computer hardware and software which can enhance optimization and hence create stability and reliability in renewable energy supply and use ( Bishoge et al., 2019 ). Since energy production and consumption accounts for over two thirds of the total greenhouse gas emissions and over 80% of Carbon dioxide emissions, countries that seek to meet the long-term climate objectives of the Paris Agreement must develop measures and strategies to mitigate emissions from power plants and other energy related activities. Countries should individually and collectively tackle their challenges through right and effective energy policy measures ( Dufour, 2018 ). Countries may adopt different transition routes due to relative differences in endowment and competitiveness of renewable and nonrenewable energy resources.
Strategies and measures that can be adopted by countries to exploit their renewable energy resources include on site power generation which involves generating power at the location where it is used like photovoltaic panels installed on buildings, farm-based biogas plants, use of geothermal heat pumps located next to the building, and combined heat and power or cogeneration as well as energy saving and efficiency measures. Another strategy to use renewable and low carbon energy is the use of interconnectors whose role is to physically link different grids or countries for more interconnection needed to ensure the countries can import electricity from low carbon producers like France mainly from nuclear and Sweden and Ethiopia with huge hydro potential as a strategy for de-carbonization of their grid electricity ( Dutton, 2019 ). The purchase of green power through Renewable Energy Certificates (RECS) also known as green tags, green energy certificates, also called tradable renewable certificates is another important strategy in promoting electricity generation from renewable sources ( Wanga et al., 2020 ).
7.3.1 Renewable and Non-Renewable Energy Options in the Transition
Through sustainable energy, the dependence on fossil fuel sources is reduced while increasing the use of renewable sources of energy thus reducing greenhouse gases. Renewable energy technologies may be divided into three generations. The first generation commenced in the nineteenth century and relied on hydropower biomass and geothermal energy. The second generation started in the 1980s and consisted of consists of tidal, wind power, wave power, and solar energy. The third stage or generation is still under development today and is based on gasification, bio-refinery, and ocean thermal power ( Hollaway and Bai, 2013 ). As the global fossil fuel reserves and nuclear diminish, the world has an urgent need to increase the use of renewable energy resources and diversify other available resources and efficiency options. Currently renewable energy power generation has focused on solar photovoltaic (PV), hydro, and wind energy resources with limited use of geothermal and biomass. This is despite the abundance of these energy resources, underlined, for instance, by the importance of sugar-mill power generation ( CS-UNIDO, 2008 ).
There is a global energy transition back to renewable energy, after a century of fossil fuel dominance. Solar energy, wind, bioenergy and geothermal among others will play a leading role in the current transition ( Moriarty and Honnery, 2019 ). The transition will however require creativity and enhanced innovation in form of technology and institutional reforms ( Pegels et al., 2018 ). There are various options for future use of renewable energy.
1) Electricity from Intermittent wind, solar, and wave energy.
2) Dispatchable electricity from hydropower, i.e., major, mini, micro, and others based on resource availability.
3) Energy in form of thermal dispatchable power from solar, geothermal, and biomass.
4) Direct use of thermal energy from bioenergy and low-temperature geothermal energy for heating and cooling applications.
5) Biochemical conversion like biomass to biogas and fermentation to produce gaseous and liquid forms energy ( Moriarty and Honnery, 2019 ).
7.3.1.1 Hydropower
Hydroelectric plants convert energy in moving water to electricity. Conventional hydropower plants have a reservoir developed behind a dam to supply water to the hydraulic turbine for generation of a highly flexible, dispatchable electricity supply. Hydropower can be combined with wind, solar and other sources to supply reliable steady and affordable grid electricity. Hydropower can also be exploited from, run-of-the-river resources which have less environmental impact but with overreliance on steady supply of rain water whose supply is unsteady and unpredictable. Apart from power generation, reservoirs can control floods, supply water, and power from stored water even during drought ( Wikipedia, 2021 ). Hydroelectric electric power plants are useful for grid electricity sustainability particularly during peak hours where plants that generate flexible and cheaper electricity are on high demand ( Kolagar et al., 2020 ).
In 2017, whereas fossil fuels supplied 16,947 TWh or 63% of the total global electricity generation, 4,222 TWh or 16% came from hydropower ( BP, 2021 ) while in 2020, hydropower contributed 16% of global electricity generation as fossil fuels supplied 61.3% of global electricity ( International Energy Agency, 2019 ; BP, 2021 ). Hydropower is environmental-friendly and releases much less greenhouse gases (GHG) compared to fossil fuel sources like oil, natural gas, coal, and diesel. Hydropower also provides energy security as it decreases reliance on fossil fuels, besides other benefits of developing dams like irrigation, supply of water for industrial and domestic use, flood control and employment opportunities ( Solarin et al., 2021 ).
Hydropower has very low emission which vary with the size of the reservoir. Decomposing organic matter release methane and carbon dioxide while deforestation affects the local hydrology and promotes desertification besides displacing many people from their settlements ( Wikipedia, 2021 ).
7.3.1.2 Solar Energy
Solar energy is cheap because the cost of solar energy is usually negligible, beyond the initial cost outlay. The operational costs of solar are also significantly lower than the conventional power plants. Solar is an important source of energy security since it is locally available. Energy security which is guaranteed by solar energy makes a country less susceptible to external interruptions or events which may influence supply or cost. Socially and economically, solar power generation creates employment opportunities, for example in the year 2018, the solar photovoltaic industry supported more than over 3.6 million jobs globally ( Solarin et al., 2021 ). The main challenge facing solar energy is variability and intermittence in supply and relatively low electricity conversion efficiency.
7.3.1.3 Geothermal Energy
Geothermal energy is produced by drilling deep into the Earth’s crust for harnessing to generate electricity or thermal energy. Feasible geothermal resources are available where the thermal gradient is above 30°C/km, permeable rock structure, natural or artificial water replenishment, and an impervious cap rock. Geothermal contributes less than 1% of global electricity generation even though we have significant potential such that it can meet the entire energy needs of humanity at current rates of consumption ( Barasa Kabeyi, 2019a ; Kabeyi, 2020c ).
As a renewable energy resources, geothermal energy is constantly replenished from neighboring hotter regions and the radioactive decay of naturally occurring isotopes deep in the Earth’s crust. The greenhouse gas emissions from geothermal-based electricity are less than 5% of total emissions from coal-based electricity generation ( Kabeyi and Olanrewaju, 2022 ). The risks associated with geothermal energy exploitation include the risk of inducing earthquakes, water and soil pollution from brine, and releases toxic emissions like hydrogen sulphide and greenhouse gas emissions like carbon dioxide ( Kabeyi and Olanrewaju, 2021f ). The main challenge facing geothermal electricity generation is long project development period, high upfront risks and huge project costs for conventional technologies which also have low electricity conversion efficiency. The adoption of wellhead generators as a project development option can reduce the period and risks involved in development of geothermal powerplants ( Kabeyi and Oludolapo, 2020a ; Kabeyi et al., 2020 ; Kabeyi and Olanrewaju, 2021a ).
7.3.1.4 Wind Energy and Power
Wind has been used by man for a very long period to drive windmills, pumps, sailing ships and mechanical energy for industrial processes. Wind turbine generators are used to generate electric power and provided about 6% of global electricity in 2019 ( Enerdata, 2021 ). Wind generated electricity is competitive with nuclear and natural gas and is cheaper than electricity from coal Other than installing onshore, wind turbogenerators can be installed offshore where wind is stronger but will cost more in construction and maintenance ( Dreyer, 2021 ).
Wind generators have environmental impact in form of visual impact on the landscape. collisions between turbine blades with birds and bats is common while noise and flickering lights can cause annoyance and constrain human settlement near the installations ( Wang and Wang, 2015 ). Advantages of wind power is low construction energy and the plants have low water requirements but need more land and the turbine blade materials are not fully recyclable ( Huang et al., 2017 ).
7.3.1.5 Bioenergy
Bioenergy is energy that comes from biomass which is organic material that comes from animals and plants. Biomass produce heat and electricity on combustion and can also be converted into biofuels like biodiesel, ethanol, methanol, etc. for use in combustion engines ( Ayompe et al., 2021 ). Biomass or bioenergy resources include solid and liquid waste, industrial and domestic wastewater, forest resource waste, agricultural waste, and livestock waste ( Kabeyi, 2020a ). All countries around the word have bioenergy in one form or another. This makes biomass an important energy or electricity source that guarantees energy security with limited environmental harm ( Kolagar et al., 2020 ).
The feedstocks used to include how they are grown, harvested, and processed determines the climate impact of biomass sources of energy. As an example, burning wood fuel produces carbon dioxide which can be offset by photosynthesis in fast growing energy trees and well-managed forest cover since trees absorb carbon dioxide as they grow. The negative impact of bioenergy crops is that they displace natural ecosystems, cause soil degradation, and also they consume water resources and synthetic fertilizers which have some carbon value. About a 1/3 of wood used globally is unsustainably harvested and consumed. Additionally the harvesting and processing of bioenergy feedstocks requires energy for harvest, drying, and transportation which adds to its carbon footprint as greenhouse gases are emitted, although in significantly less quantities than fossil fuels ( Correa, 2019 ).
7.3.1.6 Hydrogen Energy
Hydrogen produces electricity with zero emissions at the point of usage. However, the overall lifecycle emissions of hydrogen are determined by the production process used in its production. Currently, hydrogen is mainly produced from fossil fuel sources ( Chant, 2021 ). The main method of hydrogen production is by steam methane reforming where hydrogen is made by chemical reaction between steam and methane. About 6.6–6.9 tons of CO 2 are emitted by of this process to produce one ton of hydrogen ( Bonheure et al., 2021 ). Carbon capture process can then be used to remove a large percentage of the CO 2 produced making the process cleaner. Although the overall carbon footprint of hydrogen as a fuel is yet to be fully established, it remains a cleaner fuel than natural gas, biogas or methane ( Griffiths et al., 2021 ).
In another method of hydrogen production, electrolysis by use of electricity can be used to split water molecules to hydrogen fuel. However, the process is more expensive compared to methane reforming and sustainability requires that the electricity is from green sources which for now is still a challenge in many parts of the world. Hydrogen fuel can be produced during surplus of intermittent renewable electricity and stored for use during peak and when the variable renewable disappears ( Palys and Daoutidis, 2020 ). Hydrogen can also be processed into synthetic fuels sources of energy like ammonia and methanol ( Blank and Molly, 2020 ).
Research and development are encouraged to develop hydrogen electrolyzers for use in large-scale production of hydrogen for power generation competitively. Hydrogen produces intense heat suitable for industrial production of steel, cement, glass, and chemicals. Therefore, hydrogen can act as a clean fuel in the steal steelmaking, can act as a clean energy carrier and simultaneously as low-carbon catalyst in place of coke ( Blank and Molly, 2020 ). The main limitation of hydrogen as an energy carrier is high storage and distribution costs since it is explosive and occupies a large volume. The gas also embrittles pipes hence it needs special handling facilities which have to be developed ( Griffiths et al., 2021 ).
7.3.1.7 Natural Gas
It is important to note that several cities globally have reached or are reaching epidemically poor-quality atmospheric air quality requirements and urgently need to reduce pollution from engines emissions. There is need for an immediate remedy to pollution caused by urban diesel vehicles. Natural gas as a fuel is also cheaper than refined petroleum products. In most markets around the world [ Group of Experts on Pollution & Energy (GRPE), 2001 ].
Besides direct combustion, natural gas has more hydrogen atoms making it an excellent raw material or feedstock for hydrogen production by using high-temperature steam also called, steam methane reforming, and by partial oxidation. Steam reforming and partial oxidation both produce “synthesis gas,” that produces more hydrogen when reacted with water. These processes make natural gas a pathway to the hydrogen future. This is mainly because several aspects of hydrogen and natural gas distribution and storage, fueling, station siting, and training of technicians and drivers are similar. Hence knowledge from handling of natural gas will make the transition to a hydrogen fuel smoother. Hydrogen and natural gas can also be blended with hydrogen to make transportation fuel. These can take the form of 20% by volume hydrogen also called Hythane or 30% by volume hydrogen called HCNG ( Werpy et al., 2010 ).
7.3.1.8 Marine Energy
This energy resource has one of the smallest contributions to the global energy market. Marine energy consists of tidal power, which is a maturing technology and wave power, which is steal under early stages of research and development as well as ocean thermal energy. A typical example of these resources is the Two Tidal Barrage Systems in France and in South Korea which account for 90% of world marine energy production. The environmental impact of small and single marine energy devices is little except for larger devices are less well known ( Wikipedia, 2021 ). Tidal power is a form of green energy resource, as it emits near zero greenhouse gases and occupies less space per unit power. The largest tidal powerplant project globally is the Sihwa Lake Tidal Power Station in South Korea, which has installed capacity of 254 MW established in 2011, as a development to a 12.5 km-long seawall that was built in the year 1994 to for flood control and support of farming ( Husseini, 2021 ). Tidal power has the benefit of predictability as the gravitational forces of celestial bodies won’t be going anywhere soon. The equipment is also about four times longer lasting than wind equipment and the plant has high power density with limited surface area requirements ( Wikipedia, 2021 ; Husseini, 2021 ).
The Ocean Thermal Energy Conversion (OTEC) is a marine technology that utilizes the solar energy absorbed by sea water to generate electricity. It takes advantage of the thermal difference between cooler deep waters and warmer shallow or surface ocean waters to operate a heat engine to generate work. The main challenge is that the temperature difference is small which poses a challenge to the technical and economic sustainability ( World Energy Council, 2013 ). This calls for more research and development for ocean thermal energy.
7.3.1.9 Nuclear Power
Nuclear power plants have been in operation since 1950s as sources of low carbon base load electricity. Nuclear power plants operate in over 30 countries generating over 10% of global electric power ( Rhodes, 2021 ; World Nuclear Association, 2021 ). As of the year 2019, over 25% of the low carbon electricity was generated from nuclear making it the 2nd largest source after hydropower. The main environmental benefit of nuclear power is that the lifecycle greenhouse gas emissions including that of mining and processing of uranium are close to emissions from renewable energy sources ( Bruckner and Fulton, 2014 ). Additionally, its land requirement per unit power output is or less than that of the major renewables, and it doesn’t pollute the local environment. Although uranium ore is a non-renewable resource, its available quantities can provide power for hundreds to thousands of years to come. Therefore, increased use of nuclear power will reduce emissions and related environmental impact ( Bruckner and Fulton, 2014 ; Dunai and De Clercq, 2019 ).
The main sustainability challenges of nuclear power generation which should be addressed are challenges of nuclear waste handling and disposal, weapon proliferation, and catastrophic accidents ( Gill et al., 2014 ). There is need to manage radioactive nuclear waste over long time scales before final disposal ( Gill et al., 2014 ), while low energy fissile material created is a feasible raw material for low energy nuclear applications including military use in weapon development ( Gill et al., 2014 ). Statistically, nuclear energy has caused fewer accidents and pollution related deaths than fossil fuel ( Ritchie, 2021 ). The challenge to the investment and hence development of nuclear power is politically motivated and is mainly over fear for weapons proliferation ( Gill et al., 2014 ).
The main challenges facing nuclear power development is long developing period, and high cost of capacity and powerplant development, hence the need to reduce cost and delivery time ( Timmer, 2021 ). Technology options include Fast breeder reactors which are capable of recycling nuclear waste hence reduce disposal challenges by reducing waste but they are yet to be commercially deployed ( Joint Research Centre, 2021 ). In terms of energy security, countries with no Uranium can resort to the use of thorium rather than uranium ( Gill et al., 2014 ). Another sustainable option is use of Small modular reactors which are smaller, cheaper and faster to deploy. While their modularization allows flexibility in capacity development for countries with low electricity demand. Modular units also generate less waste and have less risks of explosion ( Bruckner and Fulton, 2014 ; Gill et al., 2014 ).
7.3.1.10 Coal and Petroleum Resources
Coal is a leading source of energy for grid electricity while countries like Poland rely on coal for significant energy applications and source of revenue to the economy. Poland for example is the largest producer of hard coal and the second largest producer of lignite in the European union followed by Germany. Therefore, coal is an important economic product for several countries, besides being a secure energy resource. Due to abundant supply of coal as a secure energy source, coal accounted for 76.8% of its electricity in Poland. For Germany, which is another leading producer of coal, it accounted for 35.6% of electricity generation, followed by the United Kingdom which produced 5.1% of its electricity from coal. The entire European union produced 18.9% of its electricity from coal. Poland produced 68.3 million tons of hard coal in 2019, which was an 85 reduction over the 2018 production. Of all coal produced, 60.1% was consumed by the energy sector, 24.6 was used by the industry and construction while households used 15.2%. The coal industry has a positive social value worth noting. For example, in 2019, coal mining employed 94% of people in coal mining or 78,500 people in Upper Silesian Basin, of Poland while the remaining 6% worked at the Bogdanka mine in the Lublin province of Poland with monthly salaries in 2020 being twice the average salary in Poland ( Krzywda et al., 2021 ).
Although coal is a fossil fuel with huge environmental impact, it will continue to play an important role in power generation with application of clean coal technologies. Gradual substitution is recommended as coal rich economies continue transition to renewable and low carbon energy resources and diverse their economies to substitute declining coal revenue.
7.3.1.11 Shift to Natural Gas From Coal and Petroleum Fuels
The switching from coal and diesel to natural gas in power generation has significant benefits in terms of sustainability. Natural gas generates about half the emissions of coal when used in power generation and about two-thirds the emissions of coal when applied in heat production. Additionally, natural gas produces less air pollution than coal, but the challenge is to limit gas leakages since methane is highly potent as a greenhouse gas ( Information Adminitstr, 2021 ).
The shift from coal to natural gas reduces emissions as a short term measure but does not provide a long term path to the desirable net-zero emissions. Therefore these transition has the danger of causing carbon lock-in and stranded assets which must be written off or they are allowed to continue operating against the emission targets ( Gürsan and de Gooyert, 2021 ; Plumer, 2021 ).
7.3.2 Benefits and Challenges of Renewable Energy
There is a relationship between total greenhouse gas emissions and consumption of renewable energy resources. For example between 1990 and 2012, greenhouse gas emissions (GHG) in European Environmental Agency (EEA) with 33 member countries reduced by 14% while GHG emissions per capita declined by 22% over the same period ( European Environment Agen, 2016 ) due to increased use of renewable energy, a scenario that was also witnessed in the United States between 2006 and 2014 ( Owusu and Asumadu-Sarkodie, 2016 ). This brings both environmental and socioeconomic benefits with less environmental impact through substituting polluting fossils with renewable and low carbon energy sources and creation of jobs and social capital in the society [ United States Environmental Protection Agency (EPA), 2017 ].
Renewable energy sources and technologies are competitive energy options particularly for remote areas but encounter barriers to their diffusion like lack of access to capital for the for low and medium-income population. There is need for financing for renewable energy technologies, such as solar PV, micro-hydro, wind power for water pumping and electric power generation, bio digesters and biogas installation costs and improved woodstoves production and installation ( Kabeyi and Oludolapo, 2021b ). Other barriers include:
1) Lack of competitiveness since most of these renewable energy power plants have higher investment and energy costs as compared to conventional or nonrenewable options particularly in terms of initial cost of the project.
2) Uncoordinated planning, policy and legal and financial instruments has ensured that renewable energy renewable energy projects need support against nonrenewable sources in form carbon tax, tax incentives and subsidies and regulations support to enhance their diffusion and interconnection to electricity grid and general adoption.
3) There is Lack of information, supportive infrastructure, and maintenance, for example in some cases, there is lack of technologies and infrastructure or capabilities to develop renewable energy projects or markets ( Kabeyi and Oludolapo, 2021b ).
7.4 Decentralized and Distributed Power Generation
Power transmission and distribution networks where initially conceived and designed to distribute electricity from central power stations to consumers kilometers away. This approach is no longer valid because of the increasing presence of distributed generation systems that are mainly based on variable renewable energy sources and a growing number of variable load users like, plug-in electric cars connected to the grid and lower voltage points ( Zarco-Soto et al., 2021 ). There has been a shift to the use of small and distributed powerplants as the world gradually adopts the use of renewable sources of energy for grid electricity generation which requires a bi-directional flow of power through transformers ( Colangelo et al., 2021 ). The centralized model of power generation and distribution has dominated the electricity sector in many countries while distributed energy resources (DER), are slowly being accommodated and remain dominant in remote and isolated areas. The decentralization of electricity generation gathered momentum when economies of scale stopped being significant factor owing to innovation and technology development. The main motivation was the use of diesel engines and gas turbines and the adoption of smart grids. Traditionally, decentralised systems consisted of dispatchable resources; but we have increasing use of non-dispatchable PV as a recent development. The development of decentralised generation is such that today, the global annual distributed generation capacity additions have surpassed the centralized electricity systems ( Mitrova and Melnikov, 2019 ).
For maximum use of DER technologies to be achieved, there is need to develop a systemic architecture and put in place policy measures in the power sector to balance the interests of new players with the existing centralized model players. However, an optimal combination of centralized generation and DER seems to be the most effective and efficient approach in the energy transition. This implementation requires principles and mechanisms for seamless for the integration of the centralized and decentralized for reliability in operations ( Mitrova and Melnikov, 2019 ). The main system benefit distributed renewable energy sources is that it leads to increase in nodal voltages. The growing use of variable loads on distribution networks like electric cars puts significant pressure on the need for nodal voltage control through a flexible and resilient electricity grid that goes beyond mere decentralization of grid power generation ( Zarco-Soto et al., 2021 ). With the new decentralized generation technologies, economies of scale have been turned upside down with improved viability of small energy systems. Increased use of information technologies has generated new opportunities for energy e infrastructure management in a less hierarchical and flexible manner. This combined with consumer demands for control over their energy systems has created energy communities (ECs) on the agenda hence higher opportunities for transition towards more sustainable energy through improvement in efficiency, less emissions, reduced costs, and hence a sustainable energy future.
7.5 Transition From Traditional to Smart Grids
About 10% of the total grid power is lost to transmission and distribution of which 40% is lost at the distribution side alone in the traditional grid ( Rathor and Saxena, 2020b ). The solutions to the energy or electricity related pollution and losses are significant reduction in use of fossil fuels, increased use of renewable energy sources like photovoltaics and wind, use of fuel cells and integration of energy and battery storage systems and use of plug-in electric cars ( Conejo et al., 2010 ; Azzouz et al., 2015 ; Rathor and Saxena, 2020b ). The world has witnessed significant advances in technology which includes development of better electricity carriers, decentralization of generation and increasing contribution of variable renewable sources energy to grid electricity and electrification of transport which introduces unpredictable load on the grid. All these developments call for development and use of smart grids. The smart grid uses computer programs and hardware to manage electricity generation and distribution resources and hence can help in optimization of the energy mix of both renewable and non-renewable energy for sustainable power generation and supply through smart grids. Smart grids can facilitate increased absorption of variable renewable sources of energy like wind and solar and thus displace fossil fuels from the grid. They enhance decentralization of generation provide the infrastructure and capacity needed to facilitate increased use of renewable energy help increase participation of all stakeholders in the operation and power delivery between sources and users in a two-way manner. This will greatly contribute to the dream of a sustainable grid electricity system.
The current global trend is to develop digital technologies for the entire economies and hence digitalization of the power systems is part of the global technological transition. Digitalization of electricity sector brings opportunities particularly the increased absorption of variable renewable like wind and solar which makes control very difficult ( Schiffer and Trüby, 2018 ). Lack of system flexibility reduces its resilience and hence capacity to absorb the variable sources of energy hence the need to adopt power system digitalization as a transition strategy ( Mitrova and Melnikov, 2019 ). Digitalization of operation and controls in power-generating and supply assets will increase efficiency, security of power systems as well as resilience more efficient, the electric grid more secure and resilient, thus reducing emissions and the threat of global climate change.
7.6 Research and Development of Sustainable Energy Technologies
It is not practical to achieve significant contribution of the intermittent renewable energy sources like solar, wind and hydro in power production without a combination of flexible dispatch able power, a reliable electricity transmission system, energy storage facilities, the smart grids, and demand side electricity management. To realize maximum renewable energy contribution, it is necessary to develop effective business models and policies, modern innovative energy technologies, system operational flexibility, and efficiency through continues research and development ( Gielen et al., 2019 ). The technology and approaches to enable sustainable electricity include the development of smart grids and replacement of the traditional electricity grid, decentralization of grid electricity generation and use which will lead to better absorption of renewable energy and adequate participation of consumers in demand management, electrification transport, development of energy storage technologies, demand side management strategies through measures like time dependent electricity tariff system and smart solutions like smart meters for consumers within a smart grid. Most of the critical technologies for the energy transition like smart grids and cheaper energy storage technologies are still under research and development and require funding and other forms of research support to mature.
7.6.1 Carbon Capture and Storage
Carbon capture and storage technology is an effective technology to absorb emissions, either by natural processes in bio crops or industrial scale plant processes. This process is known as bioenergy with carbon capture and storage (BECCS) can lead to net CO 2 removal from the atmosphere while carbon dioxide and other emissions from powerplants and other process plants can be absorbed and stored or buried. Unfortunately, BECCS may lead to net positive emissions based on how the biomass is produced, harvested, transported, and processed. Biomass material is grown, harvested, and transported ( Ayompe et al., 2021 ).
7.6.2 Energy Storage
Energy storage is an important solution to intermittent renewable energy supply and hence a critical aspect of a sustainable energy system. Various storage methods for use include pumped-storage hydroelectricity ( Hunt, 2020 ), and batteries especially lithium-ion batteries ( Blanco and Faaij, 2018 ). The challenge with batteries is that they have limited storage periods which calls for more research into storage technology for both utility-scale batteries and low energy density batteries makes them impractical for the very large energy storage needed to balance inter-seasonal variations in energy production. Other than pumped hydro storage and power-to-gas like hydrogen needs further research ( Koohi-Fayegh and Rosen, 2020 ).
7.6.3 Fuel Cells in Sustainable Electricity
Fuel cells are electrochemical systems that convert chemical energy of a fuel like hydrogen and oxidizing agent often oxygen to electricity through a pair of redox reactions. Unlike batteries they continuously require supply of fuel and oxygen to sustain the process. In batteries the chemical energy comes from metals and their ions or oxides that are already in the battery except for flow batteries ( Saikia et al., 2018 ). The fuel cell will produce power continuously on condition that there is a steady supply of the fuel and oxygen and are more efficient than combustion systems. The heat generated in the process of power generation can be put into thermal application thus further increasing the efficiency through cogeneration. They are able to reduce building facility energy service cost by 20–40% ( Fuel Cells, 2000 ).
Stationary fuel cells are often used for commercial, industrial, and residential primary and backup electricity generation and can be very useful for power supply in remote locations, like spacecraft, isolated weather stations, large parks, telecommunication communication centers, off grid stations including research stations, remote military applications, and standby power supply sources for power stations ( Saikia et al., 2018 ). The main advantage of fuel cell systems like hydrogen fuel cells is that they are compact, light, and have limited moving parts to attend to hence low maintenance and can realize 99.9999% reliability ( Fuel Cells, 2000 ).
7.6.4 Electrification Transport Industrial Processes and Rural Areas
It is possible to reduce emissions faster in electricity systems than many other systems because as in 2019, about 37% of global electricity generation came from low-carbon sources, i.e., renewables and nuclear energy with the rest coming from coal and other fossil fuel sources ( Bruckner et al., 2014b ). Phasing out coal fired power plants is among the easiest and fastest ways to controlling greenhouse gas emissions and in its place increase the share of renewable and low carbon electricity generation ( Ritchie, 2021 ). A leading limitation in provision of universal access to electric power is rural electrification where both off-grid and on-grid systems based on renewable energy can power villages who predominantly rely on wood fuel, kerosene and diesel generators for heat lighting and power ( Rosen, 2009 ). Wider access to reliable electricity would lead to less use of kerosene lighting particularly for the developing countries ( United Nations Develpment programme, 2016 ).
Electrification of transport is significant because transport sector accounts for about 14% of global greenhouse gas emissions which can be reduced by use of electric cars, buses, and electric trains that consume green electricity ( Bigazzi, 2019 ). The various climate change scenarios predict extensive electrification and substitution of direct fossil fuel combustion with clean electricity for heating building and for transport ( Miller et al., 2013 ). A deliberate climate policy should see double increase in energy share from electric power by 2015 from the 20% of the year 2020 ( Bruckner et al., 2014b ; IRENA, 2018 ; United Nations Develpment programme, 2016 ).
7.6.5 Energy Efficiency and Conservation
Energy efficiency and conservation have potential to provide a means to achieve global emissions and climate change targets set by the Paris agreement and other national and international protocols. Energy efficiency and conservation measures will lead to reduction in greenhouse gas emissions, reduce fuel consumption, reduce the load and strain on the electricity grid, and reduce cost of both generation and cost of electricity consumed ( Clark and Clark, 2019 ). The main challenge facing adoption of efficiency and conservation measures is lack of appropriate technology and high capital requirements which creates financial management risk and undetermined return-on-investment and hence undetermined payback periods which significantly limit their adoption ( Clark and Clark, 2019 ). Significant amount of energy in many forms including heat, electricity and even primary resource id lost or wasted through transmission, heat loss, and application of inefficient technology. This is a huge cost to consumers who must pay for the lost energy as more energy is consumed to carter for the losses resulting in more pollution for every extra unit consumed due to losses ( Department of Energy, 2021 ). Therefore, putting in place various energy efficiency measures is one of the easiest and cost-effective means of combatting climate change, limit emissions and related pollution, reduce energy costs and improve the competitiveness of businesses ( Department of Energy, 2021 ). In a sustainable energy scenario by the International Energy Agency, energy efficiency is expected to deliver more than 40% of targeted reduction in energy-related greenhouse gas emissions between 2020 and 2040 as a strategy to put the world on track to achieve international emissions and related climate change targets ( Clark and Clark, 2019 ; International Energy Agency, 2021c ).
7.6.6 Microgrids
Microgrids are becoming an important solution in the sustainable energy transition by improving reliability and resilience of electric power grids, necessary to manage distributed clean energy resources like wind and solar photovoltaic (PV) as well as generation to reduce emissions as well as supply power to off grid locations ( Wilson, 2021 ). A microgrid can be defined as a group of interconnected loads and distributed energy resources existing in a well-defined electrical boundary that is operated as single controllable unit with respect to the grid. It can connect and disconnect from the main grid to enable it to operate in both grid and off grid mode ( Valencia et al., 2021 ; Wilson, 2021 ).
A grid basically consists of a power source, consumers, wires to connect them, and a system to control generation and supply. On the other hand, a microgrid is a grid but a smaller version of it. A microgrid can cover one or several buildings and can be used to supply power to critical infrastructure, remote or small communities or business and industrial installation ( Valencia et al., 2021 ). Microgrids enable supply of clean and efficient energy, with more resiliency, and improves the operation and stability of the local electric power systems ( Wilson, 2021 ). Microgrids constitute a very important segment of the energy transition representing a shift from centralized power towards more localized and distributed generation solutions. The main benefit of microgrids is ability to isolate from the central or larger grid hence a feasible and attractive option for cities, rural areas, industrial parks, suburbs, and remote installations. With use of microgrids, it possible to balance generation from variable renewable power sources such as solar, wind, and hydro and conventional sources like gas-fueled combustion turbines, coal, and diesel powerplants ( Wilson, 2021 ).
7.7 Earth Radiation Management and the Solar Radiation Management
The current global warming mitigation efforts and future commitments are inadequate to achieve the Paris Agreement temperature targets. Although the various techniques show the physical potential to contribute to limiting climate change, many are still in the early stages of development. For this reason, the climate geoengineering techniques provide alternative or additional measures to contribute to meeting the Paris Agreement temperature goals ( Lawrence et al., 2018 ). The best way so far to reduce global warming is reduction in the anthropogenic emissions of greenhouse gases. However, the global economy with its ever-growing population cannot do without energy most of which is generated from fossil fuels. Replacing this energy with carbon dioxide-free renewable energies, and energy efficiency is a long term, costly, and difficult venture. By use of geoengineering schemes which use solar radiation management technologies to modify terrestrial albedo or reflect incoming shortwave solar radiation back to space provide an alternative solution to the challenge of global warming ( Lenton and Vaughan, 2009 ). We also have power-generating systems that have potential to transfer heat from the Earth surface to the upper layers of the troposphere and then to the space ( Ming et al., 2014 ). The main objective of Geoengineering is to stabilize global climate, reduce global warming and reduce anthropogenic climate changes by two main strategies namely, shortwave (0.3–3 μm) reflection where sunlight is reflected back and then secondly the use of carbon dioxide removal technologies ( Ming et al., 2014 ).
The solar radiation management geoengineering systems work by the parasol effect, i.e., reducing solar incoming radiation, but the carbon dioxide still traps the reduced heat both day and night over the entire world. The effect of solar radiation management would be only experienced during the day particularly at the equator ( Ming et al., 2014 ).
8 Results and Discussion
A good concept of sustainable development should facilitate social equity, prevent environmental degradation, and maintain a sound economic base. There is need for sustainable preservation of natural capital for sustained economic production and equity in intergeneration equity in resource exploitation. Fulfillment of basic health and participatory democracy is crucial in energy resource planning and exploitation to ensure sustainability. Sustainable transition requires governments to use policy instruments and an effective institutional mechanism to deliver working solutions to a sustainable energy future. The three main dimensions of sustainable development are economic, social, and environmental sustainability. However, sustainability in energy resource use and electricity systems has extra dimensions of technical, and political or institutional sustainability.
Humanity has increased the concentration of carbon dioxide in our atmosphere, amplifying Earth’s natural greenhouse effect. This is still ongoing and hence a continuous threat to the global environment. The global average amount of carbon dioxide hit a new record high in 2020: of 412.5 ppm. The annual rate of global increase in atmospheric CO 2 over the last 60 years is about 100 times faster than previous natural increases like those that occurred at the end of the last ice age about 11,000–17,000 years ago. As a result, the ocean has absorbed enough carbon dioxide to lower its PH by 0.1 units, from 8.21 to 8.10 since the beginning of the industrial revolution which represents about 30% increase in acidity of the ocean. This is dangerous to aquatic ecological balance due to the biological effect of ocean acidification which interferes with marine life’s ability to extract calcium from the water to build their shells and skeletons.
The energy sector is the largest contributor of global carbon dioxide emissions and second largest contributor of non-carbon dioxide greenhouse gas emissions globally. With electricity being the leading source of greenhouse gases, which are the cause of global warming, any effort to minimize greenhouse gases should address emissions from power generation. Sustainable grid electricity requires facilitating technologies and infrastructure like smart grids, decentralization of generation. A mixture of options is necessary to lower the unit cost and carbon intensity of energy systems to achieve a truly sustainable energy with low carbon world. Energy related GHG emissions are a result from conversion and delivery sectors like extraction/refining, power generation and direct transport of energy carriers in pipelines, cables, ships, tracks an end use sectors and industries like transport, buildings and construction, manufacturing, agriculture, forestry, households, and waste and hence cannot be blamed entirely on one sector or process alone.
The Intergovernmental panel on climate change (IPCC) predicted that a greenhouse gas emission (GHG) will lead to global temperature increase of between 1.1 and 6.4°C by the end of the 21st Century. The world would experience about 62% increase in CO 2 emissions between 2011 and 2050 if energy demand and use of fossil fuels to meet the demand does not change. To maintain ecologically sustainability, organizations should consume natural resources whose consumption rates are lower than the rate of natural replenishment or reproduction. Where substitutes exist, the rate of consumption should be lower than the rate of substitute development. The greenhouse gas emissions should be reduced by between 50 and 80% by the year 2050 if the world must avoid the looming consequences of global warming. The composition of atmospheric carbon dioxide (CO 2 ) has been rising as summarized in Table 2 above.
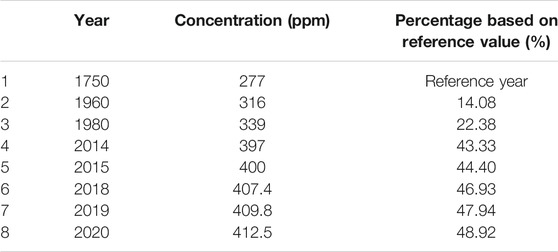
TABLE 2 . Increase in CO 2 concentration between 1750 and 2018.
Table 2 shows that between 1750 and 2020, the atmospheric concentration of carbon dioxide has increased from 277 to 412.5 ppm representing an increase of 48.92%.
For a stable atmosphere, the global average temperature increase should be maintained between 1.5 and 2°C above the preindustrial level which translates to atmospheric carbon dioxide concentration of 400–450 Energy resources are sources of various past and current political and social conflicts and therefore it is through proper energy resource management that many social, political, and economic conflicts can be avoided or resolved globally. Countries can use renewable energy sources like solar, wind and even hydro to enhance their national energy security because these resources do not need international trade to secure them and hence cushions countries against energy instigated insecurity.
Today, industry and building sectors are the main users of electricity accounting for over 90% of global electricity demand. Moving forward, the main drivers of electricity demand growth are motors in industry which may account for over 30% of the total growth to 2040, industrial and domestic space cooling will account for 17% while large electrical appliances are projected to account for 10% growth and electric vehicles are projected to account for 10% growth in electricity demand. Further growth in electricity demand of about 2% is projected to come from provision of electricity access to 530 million first time users of electricity. The sustainable development scenario, projects that electric vehicles will become a leading source of electricity demand moving to the future towards the year 2040. Therefore, a sustainable electricity transition should prepare for wider use of variable renewables, low carbon nuclear power, electrification of transportation and industrial processes, better and efficient conversion, and efficient energy use technologies and electrification of thermal application of energy.
Table 3 , it is noted that fossil fuels in the form of coal, natural gas and oil contributed 61.3% of the global electricity generation in the year 2020. Low carbon nuclear and renewable energy sources which should be the basis of the sustainable electricity transition accounted for about 38% of global electricity with undefined sources accounting for about 0.7% of the global electricity generation in the year 2020.
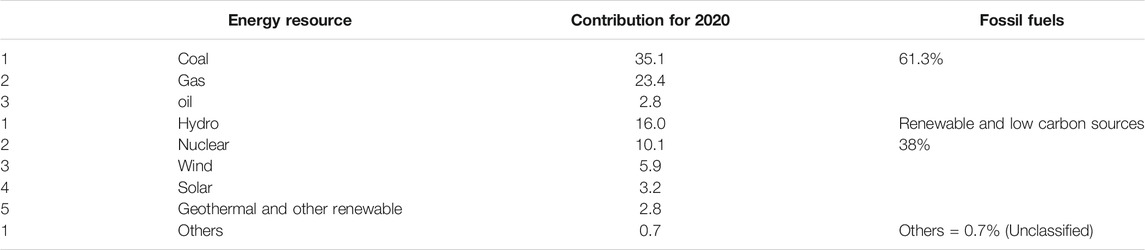
TABLE 3 . The global electricity generation can be summarized in Table 3 below.
A sustainable electricity transition calls for eventual transition of the 61.3% of the global electricity production to low carbon and renewable energy sources. In the short and to some extend middle term, natural gas can substitute oil and coal although with the risk of delaying the zero emissions transition and creating transition related carbon lock-in and stranded assets by developing natural gas infrastructure. Since countries with huge coal and oil reserves may find it unsustainable to make immediate transition, increase in the share of natural gas, and investment in efficient technologies like cogeneration and clean coal technology can reduce the carbon footprint.
Sustainable energy transition should address the five major dimensions. They are technical, social, economic, environmental, and institutional dimensions. These dimensions of energy sustainability are summarized in Table 4 below.
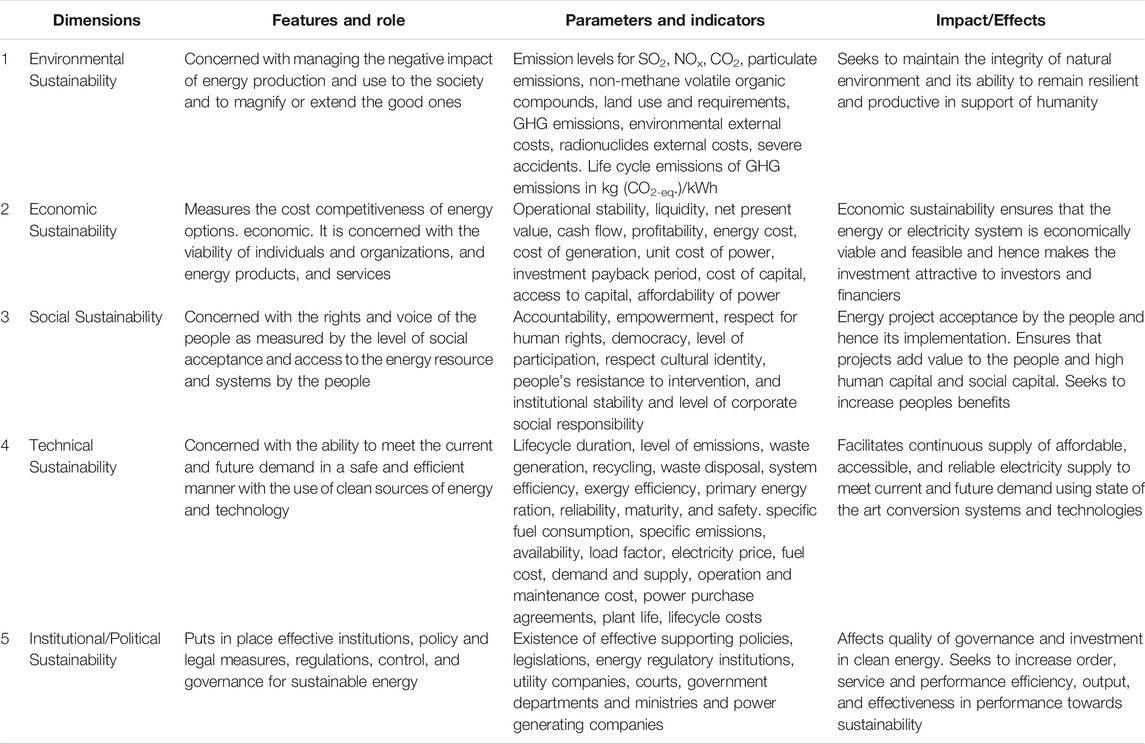
TABLE 4 . The five dimensions of energy sustainability.
From Table 4 , it is noted that there are five dimensions of energy sustainability namely environmental, economic, social, technical, and institutional/political sustainability which can be used to design and analyses energy sustainability measures.
Various energy resources have been identified as potential solution to the global transition. They are discussed in summary form in Table 5 below.
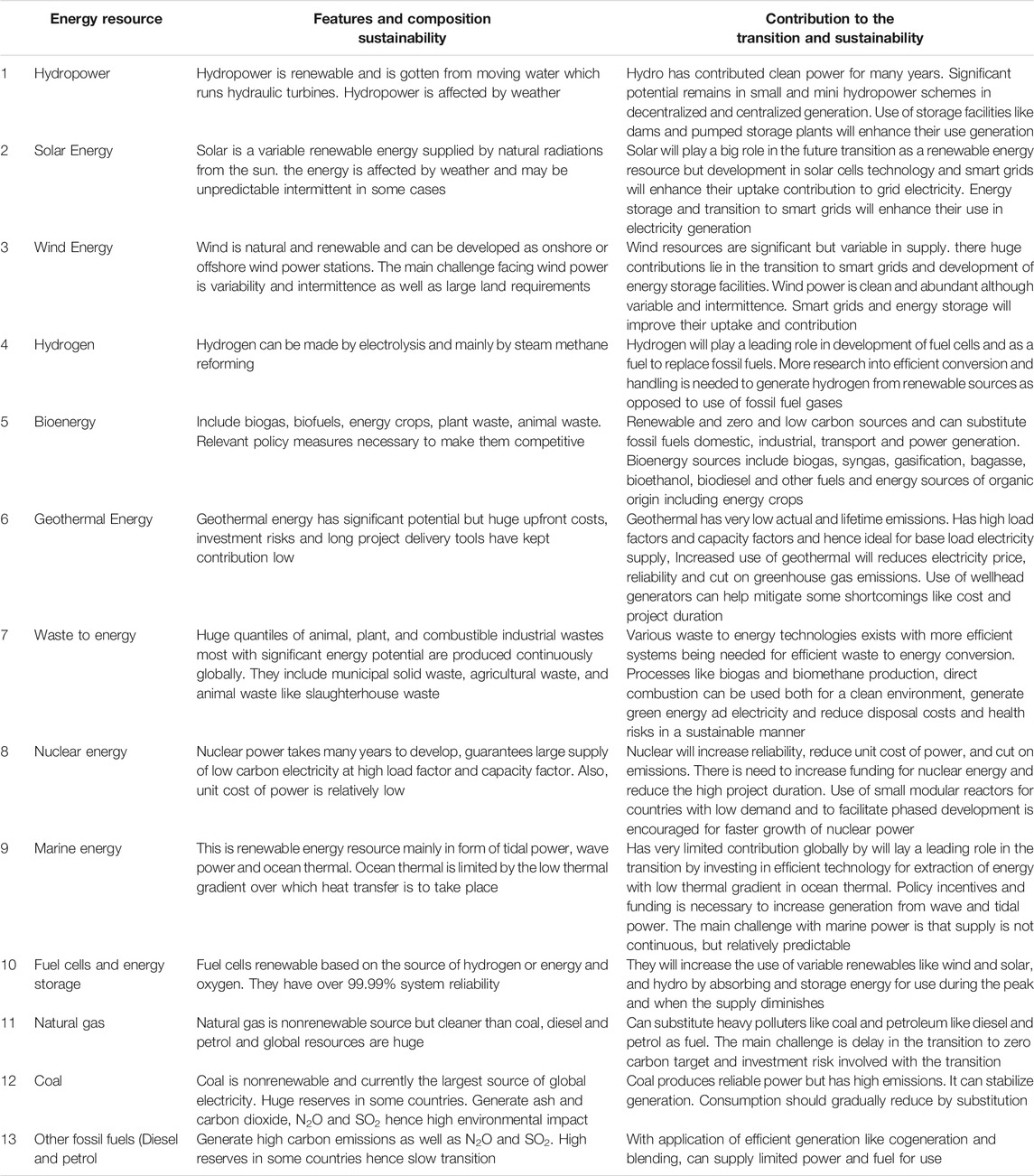
TABLE 5 . Summary of energy options for the global transition.
From Table 5 , it is noted that both renewable and nonrenewable sources of energy have a role to play in the energy transition. The nonrenewable sources like coal and oil are abundant in several countries and therefore are readily available and offer energy security. The steady release of energy by fossil fuel sources is important for reliability and stability and hence quality which are sustainable energy requirements. However, their high carbon footprint, finite supply, price, and supply interruptions and resource related conflicts make fossil fuels unreliable and unsustainable source of energy and hence the need for gradual substitution with renewable and low carbon sources of energy. The use of highly efficient conversion technology and clean coal as well as carbon capture and sequestration can greatly reduce the carbon footprint of fossil fuel sources. For natural gas, controlling leakages along the entire supply chain is paramount due to the high global warming potential of methane which is the main constituent of natural gas.
Solar and wind offer the greatest potential but suffer from unpredictable and unreliable supply challenge hence the need for advanced energy storage facilities. For grid electricity, the unreliability and unpredictable supply nature of wind and solar is a danger to energy security and the grid stability for the traditional grid. However, the use of smart grids with ability to absorb small scale producers and variable supply will greatly increase the absorption of wind and solar energy as well as small hydro sources and decentralized generation.
Various strategies or methods that can be adopted to reduce carbon emissions and hence realize the global energy/electricity transition are summarized in Table 6 below.
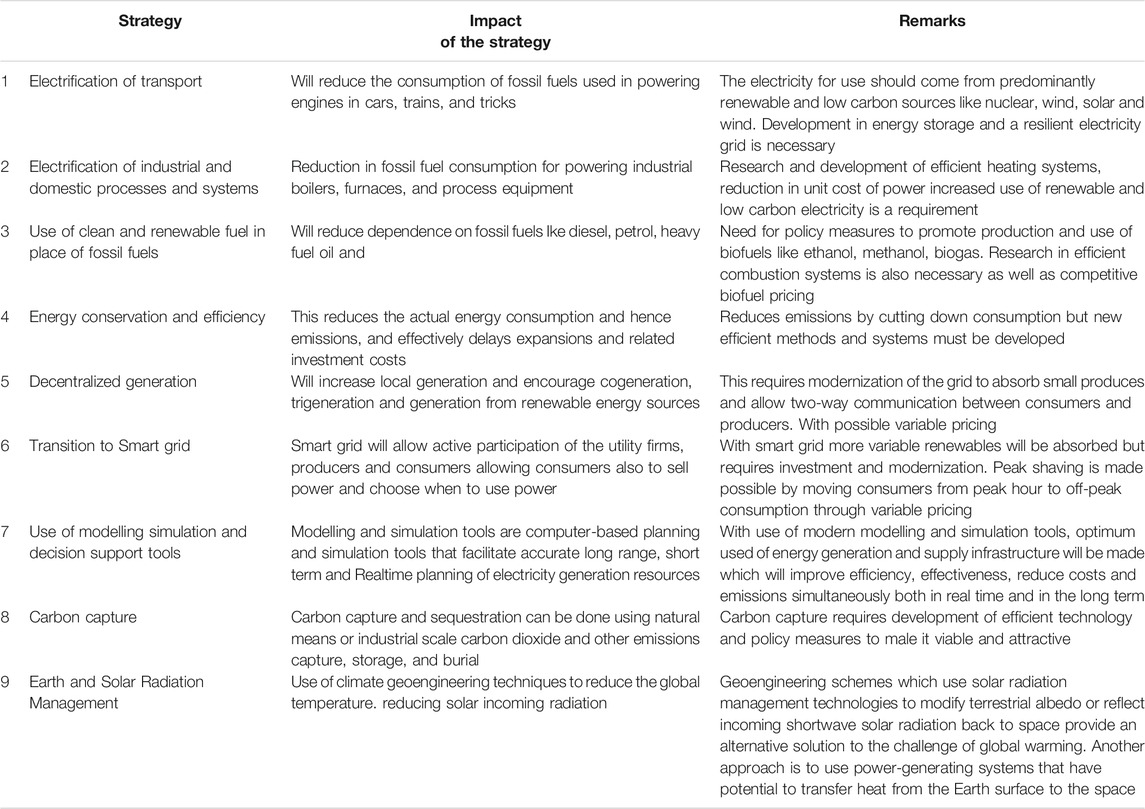
TABLE 6 . Summary of sustainable energy transition strategies.
From Table 6 above it is noted that various technological strategies can be adopted separately or in combination to reduce emissions and hence achieve a sustainable energy transition. They include electrification of transport which requires efficient and cost-effective energy storage systems and a resilient electricity grid to handle multiple variable consumer and supplier load. This creates the need to transition from the traditional grid to a more resilient smart grid. Electrification of the rural populations that are not electrified also provide an opportunity for increased use of renewable sources of electricity especially through decentralized generation systems. The use of decentralized generation will widen feasible grid connected generation which mostly comes from renewable energy sources. Energy efficiency by consumers will reduce demand and wastage hence avoid emissions while efficiency in generations leads to less fuel consumption and hence emissions and reduced environmental impact.
Although fossil fuels are major contributors of greenhouse gas emissions leading to global warming, most of them are price competitive, have steady release of energy hence the power plants operate at high load and capacity factors with high reliability of electricity supply, and thus provide grid stability. Therefore, their consumption now and soon is key to a stable and reliable electricity grid which is a key requirement of energy sustainability. The consumption of fossil fuels should be reduced but they will continue to supplement the intermittent and unpredictable but abundant wind and solar energy which have the key to the future sustainable energy supply in a highly optimized electricity generation and supply system where technology will play a key role in planning and decision support. The carbon footprint of fossil fuels used in power generation should minimized by adoption of efficient conversion technologies like cogeneration and trigeneration to minimize which reduce fuel consumption and maximize generation from limited energy resources. Other technologies include dual fuel diesel power, use f biofuel substitutes fuel blending with biofuels and use of combined cycle powerplants. Therefore, a sustainable transition for now should involve increase in energy efficiency to reduce the total demand and wastage of fossil fuels in an optimized system in which the grid absorbs all variable renewables. The smart grid and advanced storage technologies will play a significant role in the sustainable electricity transition.
From this study, the technical options to the energy transition can be grouped into three categories. They are substitution technologies, carbon capture and sequestration and climate geoengineering techniques.
9 Conclusion
Sustainable development cannot be achieved without sustainable energy which facilitates sustainable electricity generation. Whereas sustainable development can be analyzed within three dimensions representing the three pillar of sustainable development namely economic, social and environmental dimensions, sustainable energy is best analyzed with five dimensions namely social, economic, environmental, institutional or political, and technical, The sustainable transition strategies typically consist of three major technological changes namely, energy savings on the demand side, generation efficiency at production level and fossil fuel substitution by various renewable energy sources and low carbon nuclear. For the transition to remain technically and economically feasible and beneficial, policy initiatives are necessary to steer the global electricity transition towards a sustainable energy and electricity system. Whereas renewable sources energy holds the key for sustainable energy transition, large-scale renewable energy adoption should include measures to improve efficiency of existing nonrenewable sources which still have an important cost reduction and stabilization role. A resilient grid with advanced energy storage for storage and absorption of variable renewables should also be part of the transition strategies. The world has so far witnessed three typical energy transitions. The first transition involved replacement of wood with coal as the main energy source. In the second transition, oil replaced coal as the dominant energy resource. In the third transition, there is global commitment to replace fossil fuels with renewable energy. Through the cumulative effect of the Stockholm, Rio, and Johannesburg conferences, sustainable energy development (SED) was identified as a key requirement for sustainable development and so energy was linked energy to the environmental dimension in the Stockholm conference, economy in the Rio conference and society in the Johannesburg conference. Sustainable development is expected to bring economic and progress in an environmentally benign manner free from wastage, pollution, destructive emissions, and social strive in a facilitating political environment. Sustainable development has got three main dimensions of economic, social, and environmental aspects while sustainable energy has got two additional dimensions of technical and institutional or political environment.
The greatest sustainability challenge facing humanity today is the greenhouse gas emissions and the global climate change with fossil fuels led by coal, natural gas, and oil contributing 61.3% of global electricity generation in the year 2020. Through sustainable energy, the dependence on fossil fuel sources is reduced while increasing the use of renewable sources of energy thus reducing greenhouse gases. Renewable energy technologies may be divided into three generations. The first generation commenced in the nineteenth century and relied on hydropower biomass and geothermal energy. The second generation started in the 1980s and consisted of consists of tidal, wind power, wave power, and solar energy. The third stage or generation is still under development today and is based on gasification, bio-refinery, and ocean thermal power. Electricity is the most dominant form of energy deriving its supply from both renewable and nonrenewable sources. The optimum operation of the grid electricity system is influenced by many dynamic variables which must be determined and controlled, managed, or accommodated to deliver reliable, affordable, and clean electricity. Sustainable grid electricity transformation needs competitive and cost-effective financing mechanisms to accelerate the transition, needs reliable energy supplies, and application of effective business and operation modelling tools that can deliver sustainable electricity which also needs new technology and data capability to analyze, and optimize results on real-time basis and in medium- and long-term planning.
Technology has very important role to play in the transition to a low carbon electricity grid and economy. Technology options to facilitate the energy transition will include rapid digitalization of the energy sector which will enhance its flexibility and resilience to absorb variable renewable sources of energy particularly wind and solar. Important technology include transition from the traditional grid based on centralized generation to smart grids which support decentralized generation and ability to absorb the fluctuating renewable energy sources like solar and wind and fluctuating demand like electric cars while guaranteeing a stable and reliable electricity supply. Decentralization and enhanced use of variable renewables will further be enhanced by use of microgrid technology. With use of microgrids, it possible to balance generation from variable renewable power sources such as solar, wind, and hydro and conventional sources like gas-fueled combustion turbines, coal, and diesel powerplants. Since greenhouse gas emissions come from sectors like extraction/refining, power generation and direct transport, agriculture, industry, and homes, electrification of all industries and homes with power coming from renewable sources of energy will greatly succeed in cutting down global emissions. The broad strategies adopted for sustainable transition include liberalisation and restructuring of electricity and other energy markets which is made attractive by ever growing energy demand globally. Key polices adopted should aim at making electricity markets work better. More research and development into efficient, environmentally friendly, and competitive technology is needed to facilitate innovation and diffusion of sustainable energy technologies. To successful implement these policies calls for reduction unit cost of electricity and affordable cost of appropriate energy carriers and services, plus regulations to increase efficiency and reduce energy related environmental for greater public benefits.
The implementation of effective sustainable energy technologies to minimize carbon emissions will require the use of renewable and low carbon sources of energy and adoption of three main strategies namely conventional mitigation, negative emissions technologies which capture and sequester carbon emissions and finally technologies which alter the global atmospheric radiative energy budget to stabilize and reduce global average temperature. Besides low emissions, a sustainable electricity grid system should be stable and supply reliable, affordable, and socially acceptable electricity.
Although there is no consensus on quantitative factors and their magnitude there is agreement on the need for supportive policies, regulations, programmes, and international commitments. Other measures are the development and expansion of financial sector, and improvement on the performance and quality of energy sector institutions. This study concludes that both renewable and non-renewable sources of energy have a leading role to play in the short and long-term energy transition. They include energy sources like solar, wind, hydro, hydrogen, bioresources, marine energy, nuclear, natural gas substitute of other fossil fuels and application of clean and efficient technologies for existing fossil fuel and non-renewable systems. Important strategies include electrification of thermal applications and household, and technologies like smart grids and energy storage for variable renewables and carbon capture and sequestration, cogeneration, and energy efficiency measures to limit consumption and wastage of energy resources. Waste to energy and particularly in form of electricity will minimize solid waste load and reduce environmental pollution like water and soil contamination. With the grid connecting different energy sources and infrastructure, decision support systems and optimization models will play a key role in realizing cost effective and environmentally friendly and reliable electricity generation and supply. Technology measures to control global warming can be classified into three broad categories of carbon capture and sequestration, emission mitigation strategies and technologies that alter the radiative properties of incoming and outgoing solar radiations.
10 Recommendations for Future Research
This study lays the foundation for further research into technical and non-technical measures to ensure a sustainable transition to a low carbon electricity grid from different sources, both renewable and nonrenewable and will form a firm foundation for effective policy formulation and implementation necessary to drive the energy transition globally. Further research into practical details of the technologies and measures is recommended to guide the actual implementation of the transition measures and strategies like smart grids, decentralized generation, energy storage, decentralized generation, and computerization and optimization of electricity generation, transmission, and distribution resources to facilitate a sustainable energy future. The study further recommends identification and development of transition specific energy and electricity models to aid in planning and execution of sustainable energy and electricity production, supply and consumption by end users, utilities, and prosumers.
Author Contributions
MK developed the draft manuscript with OO reviewing and making further input including editorial.
Conflict of Interest
The authors declare that the research was conducted in the absence of any commercial or financial relationships that could be construed as a potential conflict of interest.
Publisher’s Note
All claims expressed in this article are solely those of the authors and do not necessarily represent those of their affiliated organizations, or those of the publisher, the editors and the reviewers. Any product that may be evaluated in this article, or claim that may be made by its manufacturer, is not guaranteed or endorsed by the publisher.
Acknowledgments
The authors sincerely acknowledge the contribution of all individuals, reviewers, and editors for their contribution towards the production of this manuscript.
Abbreviations
CHP, Combined heat and power; CO 2-eq , Carbon dioxide equivalent; CSR, Corporate social responsibility; EJ, Exajoules; GHG, Greenhouse gases; KenGen, Kenya Electricity Generating Company PLC; KWS, Kenya Wildlife Services; PPM, Parts per million; NEMA, National Environment Management Authority.
Akella, A. K., Saini, R., and Sharma, M. (2009). Social, Economical and Environmental Impacts of Renewable Energy Systems. Renew. Energ. 34 (2), 390–396. doi:10.1016/j.renene.2008.05.002
CrossRef Full Text | Google Scholar
Andress, D., Nguyen, T. D., and Das, S. (2011). Reducing GHG Emissions in the United States' Transportation Sector. Energ. Sustainable Development 15, 117–136. doi:10.1016/j.esd.2011.03.002
Austvik, O. G. (2016). The Energy Union and Security-Of-Gas Supply. Energy Policy 96, 372–382. doi:10.1016/j.enpol.2016.06.013
Ayompe, L. M., Schaafsma, M., and Egoh, B. N. (2021). Towards Sustainable palm Oil Production: The Positive and Negative Impacts on Ecosystem Services and Human Wellbeing. J. Clean. Prod. 278, 123914. doi:10.1016/j.jclepro.2020.123914
Azad, S. (2015). Koreans in the Persian Gulf: Policies and International Relations . New York: USA Routledge .
Google Scholar
Azzouz, M. A., Shaaban, M. F., and El‐Saadany, E. F. (2015). Real‐time Optimal Voltage Regulation for Distribution Networks Incorporating High Penetration of PEVs. IEEE Trans. Power Syst. 30 (6), 3234–3245. doi:10.1109/tpwrs.2014.2385834
Barasa Kabeyi, M. J., and Olanrewaju, O. A. (2022). Geothermal Wellhead Technology Power Plants in Grid Electricity Generation: A Review. Energ. Strategy Rev. 39, 100735. doi:10.1016/j.esr.2021.100735
Barasa Kabeyi, M. J. (2019). Geothermal Electricity Generation, Challenges, Opportunities and Recommendations. Ijasre 5 (8), 53–95. doi:10.31695/IJASRE.2019.33408
Barasa Kabeyi, M. J. (2019). Project and Program Evaluation Process, Consultancy and Terms of Reference with Challenges, Opportunities and Recommendations. Ijsrp 9 (12), p9622–194. doi:10.29322/IJSRP.9.12.2019.p9622
Bayram, I. S., and Ustun, T. S. (2017). A Survey on behind the Meter Energy Management Systems in Smart Grid. Renew. Sustainable Energ. Rev. 72, 1208–1232. doi:10.1016/j.rser.2016.10.034
Bazmi, A. A., and Zahedi, G. (2011). Sustainable Energy Systems: Role of Optimization Modeling Techniques in Power Generation and Supply—A Review. Renew. Sustainable Energ. Rev. 15, 3480–3500. doi:10.1016/j.rser.2011.05.003
Beaudin, M., and Zareipour, H. (2015). Home Energy Management Systems: A Review of Modelling and Complexity. Renew. Sustainable Energ. Rev. 45, 318–335. doi:10.1016/j.rser.2015.01.046
Berga, L. (2016). The Role of Hydropower in Climate Change Mitigation and Adaptation: A Review. Engineering 2, 313–318. doi:10.1016/J.ENG.2016.03.004
Bhowmik, C., Bhowmik, S., and Ray, A. (2020). Optimal green Energy Source Selection: An eclectic Decision. Energ. Environ. 31 (5), 842–859. doi:10.1177/0958305X19882392
Bigazzi, A. (2019). Comparison of Marginal and Average Emission Factors for Passenger Transportation Modes. Appl. Energ. 242, 1460–1466. doi:10.1016/j.apenergy.2019.03.172
Birol, F. (2021). Energy Is at the Heart of the Sustainable Development Agenda to 2030 . Paris, France: International Energy Agency . Available at: https://www.iea.org/commentaries/energy-is-at-the-heart-of-the-sustainable-development-agenda-to-2030 (accessed, 2021).
Bishoge, O. K., Zhang, L., and Mushi, W. G. (2019). The Potential Renewable Energy for Sustainable Development in Tanzania: A Review. Clean. Tech. 1 (1), 70–88. doi:10.3390/cleantechnol1010006
Blanco, H., and Faaij, A. (2018). A Review at the Role of Storage in Energy Systems with a Focus on Power to Gas and Long-Term Storage. Renew. Sustainable Energ. Rev. 81, 1049–1086. doi:10.1016/j.rser.2017.07.062
Blank, T. K., and Molly, P. (2020). “Hydrogen’s Decarbonization Impact for Industry,” in Near-term Challenges and Long-Term Potential (New York, NY, January: Rocky Mountain Institute ). [Online]. Available: https://rmi.org/wp-content/uploads/2020/01/hydrogen_insight_brief.pdf .
Boden, T. A., Marland, G., and Andres, R. J. (2017). Global, Regional, and National Fossil-Fuel CO2 Emissions . Oak Ridge, Tenn., U.S.A.: U.S. Department of Energy . [Online]. Available: https://cdiac.ess-dive.lbl.gov/trends/emis/overview_2014.html .
Bonheure, M., Vandewalle, L. A., Marin, G. B., and Geem, K. M. V. (2021). Dream or Reality? Electrification of the Chemical Process Industries. Chem. Eng. Prog. 117 (7), 37–42. [Online]. Available: https://www.aiche.org/resources/publications/cep/2021/march/dream-or-reality-electrification-chemical-process-industries .
Bp, (2021). Statistical Review of World Energy . [Online]. Available: https://www.bp.com/en/global/corporate/energy-economics/statistical-review-of-world-energy.html .
Broman, G. I., and Robèrt, K.-H. (2017). A Framework for Strategic Sustainable Development. J. Clean. Prod. 140, 17–31. doi:10.1016/j.jclepro.2015.10.121
Bruckner, T., Fulton, L., Hertwich, E., McKinnon, A., Perczyk, D., Roy, J., et al. (2014). Annex III: Technology-specific Cost and Performance Parameters . [Online]. Available: https://www.ipcc.ch/site/assets/uploads/2018/02/ipcc_wg3_ar5_annex-iii.pdf .
Bruckner, T. (2014). “Energy Systems,” in Climate Change 2014: Mitigation of Climate Change. Contribution of Working Group III to the Fifth Assessment Report of the Intergovernmental Panel on Climate Change . Editors K. Parikh, and J. Skea (Cambridge, United Kingdom and New York, NY, USA: Cambridge University Press ), 77. ch. 7.
Bruckner, T., et al. (2014). “Energy Systems,” in Climate Change 2014: Mitigation of Climate Change. Contribution of Working Group III to the Fifth Assessment Report of the Intergovernmental Panel on Climate Change . Editors K. Parikh, and J. Skea (United Kingdom and New York, NY, USA: Cambridge University Press ). ch. 7.
Burger, A., Lünenbürger, B., and Osiek, D. (2012). “Sustainable Electricity for the Future,” in Costs and Benefits of Transformation to 100% Renewable Energy (Dessau-Roßlau, Germany: German Federal Environment Agency ). [Online]. Available: https://www.umweltbundesamt.de/sites/default/files/medien/378/publikationen/sustainable_electricity_for_the_future_-neu.pdf .
Butt, T. E., Giddings, R. D., and Jones, K. G. (2012). Environmental Sustainability and Climate Change Mitigation-CCS Technology, Better Having it Than Not Having it at All!. Environ. Prog. Sustainable Energ. 31 (4), 642–649. doi:10.1002/ep.10590
Chant, News. (2021). Hydrogen Is One Answer to Climate Change. Getting it Is the Hard Part . Chant: News Chant . Available at: https://us.newschant.com/business/hydrogen-is-one-answer-to-climate-change-getting-it-is-the-hard-part/ (accessed, 2021).
Cheng, J. Y. S. (2009). The 1979 Oil Shock and the “Flying Geese Model” in East AsiaThe 1979 “Oil Shock:” Legacy, Lessons, and Lasting Reverberations . Washington, DC: The Middle East Institute . [Online]. Available: https://www.mei.edu/sites/default/files/publications/2009.09.The%201979%20Oil%20Shock%20-%20Legacy%20Lessons%20and%20Lasting%20Reverberations.pdf .
Cherp, A., and Jewell, J. (2014). The Concept of Energy Security: Beyond the Four as. Energy Policy 75, 415–421. doi:10.1016/j.enpol.2014.09.005
Clark, W. W. (2019). “Chapter 11 - Conclusion: The Global Green Paradigm Shift,” in Climate Preservation in Urban Communities Case Studies . Editor W. W. Clark. Ed. ( Butterworth-Heinemann ), 439–451. doi:10.1016/b978-0-12-815920-0.00011-3
Colangelo, G., Spirto, G., Milanese, M., and de Risi, A. (2021). Progresses in Analytical Design of Distribution Grids and Energy Storage. Energies 14 (14), 4270. doi:10.3390/en14144270
Colla, M., Ioannou, A., and Falcone, G. (2020). Critical Review of Competitiveness Indicators for Energy Projects. Renew. Sustainable Energ. Rev. 125, 109794. doi:10.1016/j.rser.2020.109794
Conejo, A. J., Morales, J. M., and Baringo, L. (2010). Real-time Demand Response Model. IEEE Trans. Smart Grid 1 (3), 236–242. doi:10.1109/tsg.2010.2078843
Correa, D. F. (2019). Towards the Implementation of Sustainable Biofuel Production Systems. Renew. Sustainable Energ. Rev. 107, 250–263. doi:10.1016/j.rser.2019.03.005
Cs-Unido, (2008). “Renewable Energy Technologies: Wind, mini-hydro,thermal, Photovoltaic Biomass and Waste,” in Survey of Appropriate Technologies and Perspectives for Latin America and the Caribbean (Trieste, Italy: International Centre for Science and High Technology ). [Online]. Available: https://www.academia.edu/5958430/Renewable_Energy_Technologies_wind_mini_hydro .
Darkwah, W. K., Odum, B., Addae, M., and Koomson, D. (2018). Greenhouse Gas Effect: Greenhouse Gases and Their Impact on Global Warming. J. Scientific Res. Rep. 17 (6), 1–9. doi:10.9734/JSRR/2017/39630
Davidsdottir, B. (2012). “7.10 - Sustainable Energy Development: The Role of Geothermal Power,” in Comprehensive Renewable Energy . Editor A. Sayigh (Oxford: Elsevier ), 273–297. doi:10.1016/b978-0-08-087872-0.00715-0
De, A. (2021). Institutional Structures for Making Electricity Sustainable . India , 31–38. [Online]. Available: https://www.brookings.edu/wp-content/uploads/2015/01/renewable-energy_ch4.pdf .
del Río, P., and Janeiro, L. (2016). Overcapacity as a Barrier to Renewable Energy Deployment: The Spanish Case. J. Energ. 2016, 1–10. doi:10.1155/2016/8510527
Demirel, Y. (2014). “Thermoeconomics,” in Nonequilibrium Thermodynamics . Editor Y. Demirel. Third Edition (Amsterdam: Elsevier ), 265–302. doi:10.1016/b978-0-444-59557-7.00005-9
UD Department of Energy (2021). Energy Efficiency . Washington, DC: United States Department of Energy . Available at: https://www.energy.gov/eere/energy-efficiency (accessed, 2021).
Dettmer, J. (2019). US Officials Issue Sanctions Warnings to Europe over Russian Gas . USA: VOA .
Dobranskyte-Niskota, A., Perujo, A., Jesinghaus, J., and Jensen, P. (2009). Indicators to Assess Sustainability ofTransport Activities . Ispra (VA) -ITALY: European Commission, Institute for Environment and Sustainability , 88. [Online]. Available: https://publications.jrc.ec.europa.eu/repository/handle/JRC54971 .
Dreyer, J. (2021). The Benefits and Drawbacks of Offshore Wind Farms . Available at: http://large.stanford.edu/courses/2017/ph240/dreyer2/ (accessed, 2021).
Dufour, F. (2018). The Costs and Implications of Our Demand for Energy: A Comparative and Comprehensive Analysis of the Available Energy Resources . [Online]. Available: https://www.academia.edu/36768579/The_Costs_and_Implications_of_Our_Demand_for_Energy_A_Comparative_and_Comprehensive_Analysis_of_the_Available_Energy_Resources?auto=download&email_work_card=view-paper .
Dunai, M., and De Clercq, G. (2019). Nuclear Energy Too Slow, Too Expensive to Save Climate: Report . Reuters . Available at: https://www.reuters.com/article/us-energy-nuclearpower-idUSKBN1W909J .
Dutton, J. (2019). JSTOR . [Online]. Available: https://www.jstor.org/tc/accept?origin=%2Fstable%2Fpdf%2Fresrep21758.pdf&is_image=False .UK-EU Electricity Interconnection: THE UK'S Low Carbon Future and Regional Cooperation after Brext
Dyllick, T., and Hockerts, K. (2002). Beyond the Business Case for Corporate Sustainability. Business Strategy Environ. 11 (2), 130–141. doi:10.1002/bse.323
Ebrahimi, M., and Rahmani, D. (2019). A Five-Dimensional Approach to Sustainability for Prioritizing Energy Production Systems Using a Revised GRA Method: A Case Study. Renew. Energ. 135, 345–354. doi:10.1016/j.renene.2018.12.008
Emas, J. (2015). The Concept of Sustainable Development: Definition and Defining Principles . Florida: Florida International University . [Online]. Available: https://sustainabledevelopment.un.org/content/documents/5839GSDR%202015_SD_concept_definiton_rev.pdf .
Enerdata (2021). Share of Wind and Solar in Electricity Production . Enerdata . Available at: https://yearbook.enerdata.net/renewables/wind-solar-share-electricity-production.html (accessed, 2021).
U.S. Energy Information Administration (2020). Country Analysis Executive Summary: Japan . United States Energy Information Administartion . [Online]. Available: https://www.eia.gov/beta/international/analysis_includes/countries_long/Japan/japan.pdf .
European Environment Agency (2016). Energy and Environment in the European Union . Copenhagen, Denmark . [Online]. Available: No 8/2006.
Fawzy, S., Osman, A. I., Doran, J., and Rooney, D. W. (2020). Strategies for Mitigation of Climate Change: a Review. Environ. Chem. Lett. 18 (6), 2069–2094. doi:10.1007/s10311-020-01059-w
Fouquet, D. (2013). Policy Instruments for Renewable Energy – from a European Perspective. Renew. Energ. 49, 15–18. doi:10.1016/j.renene.2012.01.075
Fuel Cells (2000). Fuel Oil Basics . Rochester, New York: Fuel Cells . Available at: https://web.archive.org/web/20070928225430/http://www.fuelcells.org/basics/benefits.html (accessed, 2021).
Gamil, M. M., Sugimura, M., Nakadomari, A., Senjyu, T., Howlader, H. O. R., Takahashi, H., et al. (2020). Optimal Sizing of a Real Remote Japanese Microgrid with Sea Water Electrolysis Plant under Time-Based Demand Response Programs. Energies 13 (14), 3666. doi:10.3390/en13143666
Gibbes, C., Hopkins, A. L., Díaz, A. I., and Jimenez-Osornio, J. (2020). Defining and Measuring Sustainability: a Systematic Review of Studies in Rural Latin America and the Caribbean. Environ. Development Sustainability 22 (1), 447–468. doi:10.1007/s10668-018-0209-9
Gielen, D., Boshell, F., Saygin, D., Bazilian, M. D., Wagner, N., and Gorini, R. (2019). The Role of Renewable Energy in the Global Energy Transformation. Energ. Strategy Rev. 24, 38–50. doi:10.1016/j.esr.2019.01.006
Gill, M., Livens, F., and Peakman, A. (2014). “Chapter 9 - Nuclear Fission,” in Future Energy . Editor T. M. Letcher. Second Edition (Boston: Elsevier ), 181–198. doi:10.1016/b978-0-08-099424-6.00009-0
Griffiths, S., Sovacool, B. K., Kim, J., Bazilian, M., and Uratani, J. M. (2021). Industrial Decarbonization via Hydrogen: A Critical and Systematic Review of Developments, Socio-Technical Systems and Policy Options. Energ. Res. Soc. Sci. 80, 102208. doi:10.1016/j.erss.2021.102208
Group of Experts on Pollution & Energy (Grpe), (2001). Dual Fuel (Natural Gas/diesel) Engines: Operation, Applications & Contribution . Geneva, Swizerland: The European Natural Gas Vehicle Association . Available at: https://unece.org/DAM/trans/doc/2001/wp29grpe/TRANS-WP29-GRPE-42-inf18.pdf (accessed.
Gürsan, C., and de Gooyert, V. (2021). The Systemic Impact of a Transition Fuel: Does Natural Gas Help or Hinder the Energy Transition? Renew. Sustainable Energ. Rev. 138, 110552. doi:10.1016/j.rser.2020.110552
Gurzu, A. (2019). Nord Stream 2: Who Fared Best. Politico . Brussels: Politico .
Hasna, A. M. (2007). “Dimensions of Sustainability,” in presented at the 3rd International SIIV Conference , Bari, Italy , September 22-24 .1.
Hildingsson, R., and Johansson, B. (2016). Governing Low-Carbon Energy Transitions in Sustainable Ways: Potential Synergies and Conflicts between Climate and Environmental Policy Objectives. Energy Policy 88, 245–252. doi:10.1016/j.enpol.2015.10.029
Hollaway, L. C. (2013). “19 - Sustainable Energy Production: Key Material Requirements,” in Advanced Fibre-Reinforced Polymer (FRP) Composites for Structural Applications . Editor J. Bai (Sawston, United Kingdom: Woodhead Publishing ), 705–736. doi:10.1533/9780857098641.4.705
Huang, Y.-F., Gan, X.-J., and Chiueh, P.-T. (2017). Life Cycle Assessment and Net Energy Analysis of Offshore Wind Power Systems. Renew. Energ. 102, 98–106. doi:10.1016/j.renene.2016.10.050
Hulme, M., Jenkins, G. J., Lu, X., Turnpenny, J. R., Mitchell, T. D., Jones, R. G., et al. (2002). “Climate Change Scenarios for the United Kingdom,” in The UKCIP 2002 Scientific Report (Norwich, UK: Tyndall Centre for Climate Change Research ). [Online]. Available: https://www.academia.edu/2986789/Climate_Change_Scenarios_for_the_United_Kingdom_The_UKCIP02_Scientific_Report .
Hunt, J. D. (2020). Global Resource Potential of Seasonal Pumped Hydropower Storage for Energy and Water Storage. Nat. Commun. 11 (1), 947. doi:10.1038/s41467-020-14555-y
PubMed Abstract | CrossRef Full Text | Google Scholar
Husseini, T. (2021). Riding the Renewable Wave: Tidal Energy Advantages and Disadvantages . New York, USA: Power Tech. . Available at: https://www.power-technology.com/features/tidal-energy-advantages-and-disadvantages/ (Accessed, 2021).
Iddrisu, I., and Bhattacharyya, S. C. (2015). Sustainable Energy Development Index: A Multi-Dimensional Indicator for Measuring Sustainable Energy Development. Renew. Sustainable Energ. Rev. 50, 513–530. doi:10.1016/j.rser.2015.05.032
UD Information Adminitstration (2021). Natural Gas and the Environment . Available at: https://www.eia.gov/energyexplained/natural-gas/natural-gas-and-the-environment.php (accessed, 2021).
Inglesi-Lotz, R. (2021). Energy Transitions: The Role of Institutions and Market Structures . Available at: https://theconversation.com/energy-transitions-the-role-of-institutions-and-market-structures-168156 (accessed, 2021).
Intergovernmental Panel on Climate Change(IPCC) (2007). “Climate Change 2007:The Physical Science Basis,” in Contribution of Working Group I to the Fourth Assessment Report of the Intergovernmental Panel on Climate Change " Intergovernmental Panel on Climate Change 2007 (Cambridge, United Kingdom and New York, NY: Cambridge University ). [Online]. Available: https://www.ipcc.ch/site/assets/uploads/2020/02/ar4-wg1-sum_vol_en.pdf .
International Energy Agency (2018). CO2 Emissions from Fuel Combustion 2018 . Soregraph, France: International Energy Agency .
International Energy Agency (2019). World Energy Outlook 2019 . Paris, France: International Energy Agency . [Online]. Available: https://www.iea.org/reports/world-energy-outlook-2019/electricity .
International Energy Agency (2021a). Global Energy Review 2021 . Paris, France: International Energy Agency . Available at: https://www.iea.org/reports/global-energy-review-2021/co2-emissions (accessed, 2021).
International Energy Agency (2021b). Key World Energy Statistics 2021 . Paris: International Energy Agency . [Online]. Available: https://www.iea.org/reports/key-world-energy-statistics-2021 .
International Energy Agency (2021c). Energy Efficiency the First Fuel of a Sustainable Global Energy System . Paris, France: International Energy Agency . Available at: https://www.iea.org/topics/energy-efficiency (accessed, 2021).
İpek Tunç, G., Türüt-Aşık, S., and Akbostancı, E. (2007). CO2 Emissions vs. CO2 Responsibility: An Input-Output Approach for the Turkish Economy. Energy Policy 35, 855–868. doi:10.1016/j.enpol.2006.02.012
IRENA (2018). “A Roadmap to 2050,” in Global Energy Transformation (Abu Dhabi: International Renewable Energy Agency ). [Online]. Available: https://www.irena.org/-/media/Files/IRENA/Agency/Publication/2018/Apr/IRENA_Report_GET_2018.pdf .
Jacobs, P., Gardner, J., and Munro, D. (1987). “Sustainable and Equitable Development: An Emerging Paradigm,” in Conservation with Equitable Development: Strategies for Sustainable Development . Editors P. Jacobs, and D. A. Munro (Cambridge: International Union for onservation of Nature and Natural Resource ).
Jansen, J. C., and Seebregts, A. J. (2010). Long-term Energy Services Security: What Is it and How Can it Be Measured and Valued? Energy Policy 38 (4), 1654–1664. doi:10.1016/j.enpol.2009.02.047
Jaramillo-Nieves, L., and Del Río, P. (2010). Contribution of Renewable Energy Sources to the Sustainable Development of Islands: An Overview of the Literature and a Research Agenda. Sustainability 2 (3), 783–811. doi:10.3390/su2030783
Jefferson, M. (2000). “Energy Policies for Sustainable Development,” in World Energy Assessment: Energy and the Challenge of Sustainability (London, UK: United Nations Development Program ), 416–452. ch. 12.
Jin, Z., Wang, J., Yang, M., and Tang, Z. (2022). The Effects of Participation in Global Value Chains on Energy Intensity: Evidence from International Industry-Level Decomposition. Energ. Strategy Rev. 39, 100780. doi:10.1016/j.esr.2021.100780
Joint Research Centre (JRC) (2021). Technical Assessment of Nuclear Energy with Respect to the ‘do No Significant Harm’ Criteria of Regulation (EU) 2020/852 (‘Taxonomy Regulation’) . Netherlands: European Atomic Energy Community . [Online]. Available: https://ec.europa.eu/info/sites/default/files/business_economy_euro/banking_and_finance/documents/210329-jrc-report-nuclear-energy-assessment_en.pdf .
Jonathan, M. H. (2001). Basic Principles of Sustainable Development " Global Developement and Environment Institute . Medford, MA, USA: Tufts University . [Online]. Available: https://notendur.hi.is/bdavids/UAU101/Readings/Harris_2000_Sustainable_development.pdf .
Kabeyi, M. J. B., and Olanrewaju, O. A. (2021a). Central versus Wellhead Power Plants in Geothermal Grid Electricity Generation. Energ Sustain. Soc. 11 (1), 7. doi:10.1186/s13705-021-00283-8
Kabeyi, M. J. B., and Olanrewaju, O. A. (2021b). “Development of a Cereal Grain Drying System Using Internal Combustion Engine Waste Heat,” in presented at the 11th Annual International Conference on Industrial Engineering and Operations Management , Singapore , March 7-11, 2021 . [Online]. Available: http://www.ieomsociety.org/singapore2021/papers/188.pdf .
Kabeyi, M. J. B., and Olanrewaju, O. A. (2021c). “Fuel from Plastic Wastes for Sustainable Energy Transition,” in presented at the 11th Annual International Conference on Industrial Engineering and Operations Management , Singapore , March 7-11, 2021 . [Online]. Available: http://www.ieomsociety.org/singapore2021/papers/199.pdf .
Kabeyi, M. J. B., and Olanrewaju, O. A. (2021d). “Dual Cycle Cogeneration Plant for an Operating Diesel Powerplant,” in presented at the 11th Annual International Conference on Industrial Engineering and Operations Management , Singapore , March 7-11, 2021 . [Online]. Available: http://www.ieomsociety.org/singapore2021/papers/200.pdf .
Kabeyi, M. J. B., and Olanrewaju, O. A. (2021e). “Performance Analysis of a Sugarcane Bagasse Cogeneration Power Plant in Grid Electricity Generation,” in presented at the 11th Annual International Conference on Industrial Engineering and Operations Management , Singapore , March 7-11, 2021 . [Online]. Available: http://www.ieomsociety.org/singapore2021/papers/201.pdf .
Kabeyi, M. J. B., and Olanrewaju, A. O. (2021f). Geothermal Wellhead Technology Power Plants in Grid Electricity Generation: a Review. Energ. Strategy Rev. 27, 1. doi:10.1016/j.esr.2021.100735 ,
Kabeyi, M. j. B., and Olanrewaju, O. A. (2022). Geothermal Wellhead Technology Power Plants in Grid Electricity Generation: A Review. Energ. Strategy Rev. 39, 100735. doi:10.1016/j.esr.2021.100735
Kabeyi, M. J. B., and Oludolapo, A. O. (2020c). “Viability of Wellhead Power Plants as Substitutes of Permanent Power Plants,” in presented at the 2nd African International Conference on Industrial Engineering and Operations Management , December 7-10 ( Harare, Zimbabwe , 77. [Online]. Available: http://www.ieomsociety.org/harare2020/papers/77.pdf .
Kabeyi, M. J. B., and Oludolapo, A. O. (2020d). “Performance Analysis of an Open Cycle Gas Turbine Power Plant in Grid Electricity Generation,” in presented at the 2020 IEEE International Conference on Industrial Engineering and Engineering Management (IEEM) , Singapore, 2020 . [Online]. Available: https://ieeexplore.ieee.org/stamp/stamp.jsp?tp=&arnumber=9309840 .
Kabeyi, M. J. B., and Oludolapo, A. O. (2020e). “Managing Sustainability in Electricity Generation,” in presented at the 2020 IEEE International Conference on Industrial Engineering and Engineering Management , Singapore , 14-17 December 2020 . doi:10.1109/ieem45057.2020.9309994
Kabeyi, M. J. B., and Oludolapo, A. O. (2021g). “Preliminary Design of a Bagasse Based Firm Power Plant for a Sugar Factory,” in presented at the South African Universities Power Engineering Conference (SAUPEC), Nortn West University , South Africa , 27-28 January 2021 , 104. [Online]. Available: https://ieeexplore.ieee.org/abstract/document/9377242 .
Kabeyi, M. J. B., and Oludolapo, A. O. (2021h). Central versus Wellhead Power Plants in Geothermal Grid Electricity Generation. Energ. Sustainability Soc. 1, 1. doi:10.1186/s13705-021-00283-8
Kabeyi, M. J. B., and Oludolapo, A. O. (2020a). “Characteristics and Applications of Geothermal Wellhead Powerplants in Electricity Generation,” in SAIIE31 Proceedings, South Africa . Editor H. Teresa (South Africa: South African Institution of Industrial Engineers ), 31222–31235. [Online]. Available: https://www.dropbox.com/s/o0sj1l08v8n9sgh/SAIIE31%20Conference%20Proceedings.pdf?dl= .
Kabeyi, M. J. B. (2018). Ethical and Unethical Leadership Issues, Cases, and Dilemmas with Case Studies. Int. J. Appl. Res. 4 (7), 373–379. doi:10.22271/allresearch.2018.v4.i7f.5153
Kabeyi, M. J. B. (2018). Transformational vs Transactional Leadership with Examples. Int. J. Business Management 6 (5), 191–193. [Online]. Available: http://www.internationaljournalcorner.com/index.php/theijbm/article/view/129786/90079 .
Kabeyi, M. J. B. (2018). Michael porter’s Five Competitive Forces and Generic Strategies, Market Segmentation Strategy and Case Study of Competition in Global Smartphone Manufacturing Industry. Int. J. Appl. Res. 10 (4), 39–45. doi:10.22271/allresearch.2018.v4.i10a.5275
Kabeyi, M. J. B. (2019). Evolution of Project Management, Monitoring and Evaluation, with Historical Events and Projects that Have Shaped the Development of Project Management as a Profession. Int. J. Sci. Res. (Ijsr) 8 (12), 1. doi:10.21275/ART20202078
Kabeyi, M. J. B. (2019). Geothermal Electricity Generation, Challenges, Opportunities and Recommendations. Int. J. Adv. Scientific Res. Eng. (Ijasre) 5 (8), 53–95. doi:10.31695/IJASRE.2019.33408
Kabeyi, M. J. B. (2019). Organizational Strategic Planning, Implementation and Evaluation with Analysis of Challenges and Benefits for Profit and Nonprofit Organizations. Int. J. Appl. Res. 5 (6), 27–32. doi:10.22271/allresearch.2019.v5.i6a.5870
Kabeyi, M. J. B. (2020). Investigating the Challenges of Bagasse Cogeneration in the Kenyan Sugar Industry. Int. J. Eng. Sci. Res. Technology 9 (5), 7–64. doi:10.5281/zenodo.3828855
Kabeyi, M. J. B. (2020). Corporate Governance in Manufacturing and Management with Analysis of Governance Failures at Enron and Volkswagen Corporations. Am. J. Operations Management Inf. Syst. 4 (4), 109–123. doi:10.11648/j.ajomis.20190404.11
Kabeyi, M. J. B. (2020). Feasibility of Wellhead Technology Power Plants for Electricity Generation. Int. J. Computer Eng. Res. Trends 7 (2), 1–16. doi:10.22362/ijcert/2020/v7/i02/v7i0201
Kabeyi, M. J.B., and Olanrewaju, O. A. (2020b). Managing Sustainability in Electricity Generation " Presented at the 2020. IEEE Int. Conf. Ind. Eng. Eng. Management (Ieem) Singapore 14. doi:10.1109/IEEM45057.2020.9309994
Kanter, R. M. (2021). How to Do Well and Do Good . MIT Sloan Management Review . [Online]. Available: http://sloanreview.mit.edu/the-magazine/2010-fall/52118/how-to-do-well .
Karekezi, S., and Kimani, J. (2002). Status of Power Sector Reform in Africa: Impact on the Poor. Energy Policy 30, 923–945. doi:10.1016/S0301-4215(02)00048-4
Kaygusuz, K. (2012). Energy for Sustainable Development: A Case of Developing Countries. Renew. Sustainable Energ. Rev. 16 (2), 1116–1126. doi:10.1016/j.rser.2011.11.013
Kolagar, M., Hosseini, S. M. H., Felegari, R., and Fattahi, P. (2020). Policy-making for Renewable Energy Sources in Search of Sustainable Development: a Hybrid DEA-FBWM Approach. Environ. Syst. Decisions 40 (4), 485–509. doi:10.1007/s10669-019-09747-x
Koohi-Fayegh, S., and Rosen, M. A. (2020). A Review of Energy Storage Types, Applications and Recent Developments. J. Energ. Storage 27, 101047. doi:10.1016/j.est.2019.101047
Korea Energy Economics Institute (KEEI) (2017). Energy Info Korea " Joo-Heon Park, South Korea . [Online]. Available: http://www.keei.re.kr/keei/download/EnergyInfo2017.pdf .
Krajnc, D., and Glavic, P. (2003). Indicators of Sustainable Production. Clean. Tech. Environ. Pol. 5, 279–288. doi:10.1007/s10098-003-0221-z
Krzywda, J., Krzywda, D., and Androniceanu, A. (2021). Managing the Energy Transition through Discourse. The Case of Poland. Energies 14 (20), 6471. doi:10.3390/en14206471
Kumar, M. (2019). “Social, Economic, and Environmental Impacts of Renewable Energy Resources,” in Wind, Solar, Renewable Energy Hybrid Systems . Editor K. E. Okedu, A. Tahour, and A. G. Aissaou (London, UK: IntertechOpen ).
Kuzemko, C., Lockwood, M., Mitchell, C., and Hoggett, R. (2016). Governing for Sustainable Energy System Change: Politics, Contexts and Contingency. Energ. Res. Soc. Sci. 12, 96–105. doi:10.1016/j.erss.2015.12.022
Lamb, W. F., Wiedmann, T., Pongratz, J., Andrew, R., Crippa, M., Olivier, J. G. J., et al. (2021). A Review of Trends and Drivers of Greenhouse Gas Emissions by Sector from 1990 to 2018. Environ. Res. Lett. 16 (7), 073005. doi:10.1088/1748-9326/abee4e
Lawrence, M. G., Schäfer, S., Muri, H., Scott, V., Oschlies, A., Vaughan, N. E., et al. (2018). Evaluating Climate Geoengineering Proposals in the Context of the Paris Agreement Temperature Goals. Nat. Commun. 9 (1), 3734. doi:10.1038/s41467-018-05938-3
Lélé, S. M. (1991). Sustainable Development: A Critical Review. World Development 19 (6), 607–621. doi:10.1016/0305-750X(91)90197-P
Lenhart, S., and Fox, D. (2021). Structural Power in Sustainability Transitions: Case Studies of Energy Storage Integration into Regional Transmission Organization Decision Processes (In English), Frontiers in Climate. Front. Clim. 3 (145), 1. doi:10.3389/fclim.2021.749021
Lenton, T. M., and Vaughan, N. E. (2009). The Radiative Forcing Potential of Different Climate Geoengineering Options. Atmos. Chem. Phys. 9 (15), 5539–5561. doi:10.5194/acp-9-5539-2009
Li, J., Geng, X., and Li, J. (2016). A Comparison of Electricity Generation System Sustainability Among G20 Countries. Sustainability 8 (12), 1276. doi:10.3390/su8121276
Lidsey, R. (2020). “Climate Change: Atmospheric Carbon Dioxide,” in Understanding Climate . Washington, DC: US Government . [Online]. Available: https://www.climate.gov/news-features/understanding-climate/climate-change-atmospheric-carbon-dioxide .
Lindsey, R. (2021). “Climate Change: Atmospheric Carbon Dioxide,” in Understanding Climate (Washington, DCM: US Government ). [Online]. Available: https://www.climate.gov/sites/default/files/BAMS_SOTC_2019_co2_paleo_1000px.jpg .
Liu, G. (2014). Development of a General Sustainability Indicator for Renewable Energy Systems: A Review. Renew. Sustainable Energ. Rev. 31, 611–621. doi:10.1016/j.rser.2013.12.038
Lu, Y., Khan, Z. A., Alvarez-Alvarado, M. S., Zhang, Y., Huang, Z., and Imran, M. (2020). A Critical Review of Sustainable Energy Policies for the Promotion of Renewable Energy Sources. Sustainability 12 (12), 5078. doi:10.3390/su12125078
Lu, W.-C. (2017). Electricity Consumption and Economic Growth: Evidence from 17 Taiwanese Industries. Sustainability 9 (1), 50. . doi:10.3390/su9010050
Lund, H. (2010). “Chapter 1 - Introduction,” in Renewable Energy Systems . Editor H. Lund (Boston: Academic Press ), 1–12. doi:10.1016/b978-0-12-375028-0.00001-7
Lund, H. (2007). Renewable Energy Strategies for Sustainable Development. Energy 32 (6), 912–919. doi:10.1016/j.energy.2006.10.017
MacLeod, M. G. (2011). “19 - Environmental Sustainability of Egg Production and Processing,” in Improving the Safety and Quality of Eggs and Egg Products . Editors Y. Nys, M. Bain, and F. Van Immerseel (United Kingdom: Woodhead Publishing ), 445–462. doi:10.1533/9780857093912.4.445
Marcus, A. A. (1992). Energy Policy . Phoenix, AZ: Sage Press . [Online]. Available: https://www.encyclopedia.com/environment/encyclopedias-almanacs-transcripts-and-maps/energy-policy .
McCurry, J. (2015). Can Japan’s Climate Policy Get Back on Track after Fukushima? London, UK: The Guardian . Available at: https://www.theguardian.com/environment/2015/apr/17/can-japans-climate-policy-get-back-on-track-after-fukushima .
Mensah, J. (2019). Sustainable Development: Meaning, History, Principles, Pillars, and Implications for Human Action: Literature Review. Cogent Soc. Sci. 1 (5), 1–21. doi:10.1080/23311886.2019.1653531
Mihut, M. I., and Daniel, D. L. (2013). First Oil Shock Impact on the Japanese Economy. Proced. Econ. Finance 3 (12), 1042–1048. doi:10.1016/S2212-5671(12)00271-7
Miller, C. A., Iles, A., and Jones, C. F. (2013). The Social Dimensions of Energy Transitions. Sci. as Cult. 22 (2), 135–148. doi:10.1080/09505431.2013.786989
Ming, T., de_Richter, R., Liu, W., and Caillol, S. (2014). Fighting Global Warming by Climate Engineering: Is the Earth Radiation Management and the Solar Radiation Management Any Option for Fighting Climate Change? Renew. Sustainable Energ. Rev. 31, 792–834. doi:10.1016/j.rser.2013.12.032
Ministry of Economy, T. A. I. M. (2018). Japan’s Energy 2017," Ministry of Economy . Japan: Trade and Industry . [Online]. Available: http://www.enecho.meti.go.jp/en/category/brochures/pdf/japan_energy_2017.pdf .
Mitrova, T., and Melnikov, Y. (2019). Energy Transition in Russia. Energy Transit 3, 73–80. doi:10.1007/s41825-019-00016-8
Mohamad, M. R. A., and Anuge, J. (2021). The challenge of Future Sustainable Development in Power Sector . [Online]. Available: https://usir.salford.ac.uk/id/eprint/37674/7/Mostafa%20Mohamad.pdf .
Mohamed, R. K. M. H., Raman, G., Mani, R., and Subramaniam, V. (2020). Green HRM Practices towards Sustainable Performance in Facility Management Industry. Int. J. Management (Ijm) 11 (9), 137–147. doi:10.34218/IJM.11.9.2020.015
Moriarty, H., and Honnery, D. (2019). Energy Accounting for a Renewable Energy Future, (in English). Energies 12 (4280), 1–16. doi:10.3390/en12224280
Mostafa, M. (2014). Challenges to Energy Transition in Egypt: A Study of Wind and Solar Sectors . Germany: Masters, Public Management University of Potsdam .
Mullen, J. D., and Dong, L. (2021). Effects of State and Federal Policy on Renewable Electricity Generation Capacity in the United States. Energ. Econ. 105, 105764. doi:10.1016/j.eneco.2021.105764
Musango, J. K., Amigun, B., and Brent, A. C. (2011). Sustainable Electricity Generation Technologies in South Africa: Initiatives, Challenges and Policy Implications. Energ. Environ. Res. 1 (1), 124–138. doi:10.5539/eer.v1n1p124
Nag, K. (2008). Power Plant Engineering . New Delhi: Tata McGraw-Hill Publishing Company Limited .
Nawaz, W., and Koç, M. (2019). Exploring Organizational Sustainability: Themes, Functional Areas, and Best Practices. Sustainability 11 (16), 4307. doi:10.3390/su11164307
Nguyen, T.-H., Nguyen, L. V., Jung, J. J., Agbehadji, I. E., Frimpong, S. O., and Millham, R. C. (2020). Bio-Inspired Approaches for Smart Energy Management: State of the Art and Challenges. Sustainability 12 (20), 8495. doi:10.3390/su12208495
Ninawe, A. S., Indulkar, S. T., and Amin, A. (2018). “Impact of Climate Change on Fisheries,” in Biotechnology for Sustainable Agriculture . Editors R. L. Singh, and S. Mondal (United Kingdom: Woodhead Publishing ), 257–280. doi:10.1016/b978-0-12-812160-3.00009-x
Ölz, S., Sims, R., and Kirchner, N. (2007). Contribution of Renewables to Energy Security . Paris, France: INTERNATIONAL ENERGY AGENCY . [Online]. Available: https://www.iea.org/reports/contribution-of-renewables-to-energy-security .
Owusu, A., and Asumadu-Sarkodie, S. (2016). A Review of Renewable Energy Sources, Sustainability Issues and Climate Change Mitigation. Cogent Eng. 3 (1167990), 1–14. doi:10.1080/23311916.2016.1167990
Palys, M. J., and Daoutidis, P. (2020). Using Hydrogen and Ammonia for Renewable Energy Storage: A Geographically Comprehensive Techno-Economic Study. Comput. Chem. Eng. 136, 106785. doi:10.1016/j.compchemeng.2020.106785
Pegels, A. V.-A., Lutkenhorst, G., and W Altenburg, T. (2018). Politics of Green Energy Policy. J. Environ. Development 27 (1), 26–45. doi:10.1177/1070496517747660
Pegels, A. (2010). Renewable Energy in South Africa: Potentials, Barriers and Options for Support. Energy Policy 38 (9), 4945–4954. doi:10.1016/j.enpol.2010.03.077
Plumer, B. (2021). As Coal Fades in the U.S., Natural Gas Becomes the Climate Battleground . Manhattan, New York City: The New York Times Company . Available at: https://www.nytimes.com/2019/06/26/climate/natural-gas-renewables-fight.html (Accessed, 2021).
Quitzow, R. (2021). Energy Transitions and Societal Change . Berliner Strasse, Germany: Institute of Advanced Sustainability Studies . Available: https://www.iass-potsdam.de/en/research-area/energy-systems-and-societal-change (Accessed, 2021).
Raja, A. K., Srivastava, A., and Dwivedi, M. (2006). Power Plant Engineering . New Delhi, India: New Age International Publishers Ltd .
Rajput, R. K. (2010). Power Plant Engineering . New Delhi, India: Laxami Publications .
Rathor, S. K., and Saxena, D. (2020). Energy Management System for Smart Grid: An Overview and Key Issues. Int. J. Energ. Res 44, 4067–4109. doi:10.1002/er.4883
Rathor, S. K., and Saxena, D. (2020). Energy Management System for Smart Grid: An Overview and Key Issues. Int. J. Energ. Res. 44, 4067–4109. doi:10.1002/er.4883
Rhodes, R. (2021). Why Nuclear Power Must Be Part of the Energy Solution . New Haven, USA: Yale school of the Environment . Available at: https://e360.yale.edu/features/why-nuclear-power-must-be-part-of-the-energy-solution-environmentalists-climate (accessed, 2021).
Ritchie, H., and Roser, M. (2021). Electricity Mix . Oxford, UK: Our World of data . Available at: https://ourworldindata.org/electricity-mix#citation (accessed, 2021).
Ritchie, H. (2021). What Are the Safest and Cleanest Sources of Energy? Oxford, UK: Our World in Data . Available at: https://ourworldindata.org/safest-sources-of-energy . (accessed, 2021).
Robyns, B., Davigny, A., Francois, B., Henneton, A., and Sprooten, J. (2012). Electricity Production from Renewable Energies . New Jersey, USA: John Wiley & Sons .
Rosen, M. A. (2009). Energy Sustainability: A Pragmatic Approach and Illustrations. Sustainability 1 (1), 55–80. doi:10.3390/su1010055
Sagan, S. D. (2011). The Causes of Nuclear Weapons Proliferation. Annu. Rev. Polit. Sci. 14, 225–244. [Online]. Available: http://www.scopus.com/inward/record.url?eid=2-s2.0-79955949040&partnerID=40&md5=a53c9fe0cb5f3e6b2a071b3afd868b06 . doi:10.1146/annurev-polisci-052209-131042
Saikia, K., Kakati, B. K., Boro, B., and Verma, A. (2018). “Current Advances and Applications of Fuel Cell Technologies,” in Recent Advancements in Biofuels and Bioenergy Utilization . Editors P. K. Sarangi, S. Nanda, and P. Mohanty (Singapore: Springer ), 303–337. doi:10.1007/978-981-13-1307-3_13
Samaras, C., Nuttall, W. J., and Bazilian, M. (2019). Energy and the Military: Convergence of Security, Economic, and Environmental Decision-Making. Energ. Strategy Rev. 26, 100409. doi:10.1016/j.esr.2019.100409
Sasmaz, M. U., Sakar, E., Yayla, Y. E., and Akkucuk, U. (2020). The Relationship between Renewable Energy and Human Development in OECD Countries: A Panel Data Analysis. Sustainability 12 (18), 7450. doi:10.3390/su12187450
Scheneider Electric Company (2019). Schneider Sustainability Report 2017 – 2018 . Nanterre, France: Schneider Electric SE, France . [Online]. Available: https://www.se.com/ww/en/assets/564/document/46127/schneider-sustainability-report-2017-2018.pdf .
Schiffer, H., and Trüby, J. (2018). A Review of the German Energy Transition: Taking Stock, Looking Ahead, and Drawing Conclusions for the Middle East and North Africa. Energy Transitions 2, 1. doi:10.1007/s41825-018-0010-2
Seghezzo, L. (2009). The Five Dimensions of Sustainability. Environ. Polit. 18 (4). doi:10.1080/09644010903063669
Şengül, Ü., Eren, M., Eslamian Shiraz, S., Gezder, V., and Şengül, A. B. (2015). Fuzzy TOPSIS Method for Ranking Renewable Energy Supply Systems in Turkey. Renew. Energ. 75, 617–625. doi:10.1016/j.renene.2014.10.045
Smil, V. (2010). Energy Transitions: History, Requirements, Prospects . Santa Barbara, CA, USA: Praeger .
Solarin, S. A., Bello, M. O., and Bekun, F. V. (2021). Sustainable Electricity Generation: the Possibility of Substituting Fossil Fuels for Hydropower and Solar Energy in Italy. Int. J. Sustainable Development World Ecol. 28 (5), 429–439. doi:10.1080/13504509.2020.1860152
Streimikienea, D., BalezentisbIrena, T., Krisciukaitienėb, K., and Balezentisa, A. (2012). Prioritizing Sustainable Electricity Production Technologies: MCDM Approach. Renew. Sustainable Energ. Rev. 16 (5), 3302–3311. doi:10.1016/j.rser.2012.02.067
Tharp, J. (2012). “Project Management and Global Sustainability,” in Global Congress 2012—EMEA, Marsailles, France. Newtown Square (PA: Project Management Institute ).
The Royal Academy of Engineering, (2005). in The Royal Academy of Engineering, Engineering for Sustainable Development: Guiding Principles . Editors R. Dodds, and R. Venables (London, UK: The Royal Academy of Engineering ), 52. [Online]. Available: https://www.raeng.org.uk/publications/reports/engineering-for-sustainable-development (Accessed on September, 2005).
Timmer, J. (2021). Why Are Nuclear Plants So Expensive? Safety’s Only Part of the story . California, USA: Technica . Available at: https://arstechnica.com/science/2020/11/why-are-nuclear-plants-so-expensive-safetys-only-part-of-the-story (accessed, 2021).
Tracey, S., and Anne, B. (2008). OECD Insights Sustainable Development Linking Economy, Society, Environment: Linking Economy, Society, Environment . Paris, France: OECD Publishing .
Tunc, M., Sisbot, S., and Camdali, U. (2012). Exergy Analysis of Electricity Generation for the Geothermal Resources Using Organic Rankine Cycle: Kızıldere-Denizli Case. Environ. Prog. Sustainable Energ. 32 (3), 830–836. doi:10.1002/ep.11662
United Nations Develpment programme, (2016). Delivering Sustainable Energy in a Changing Climate . [Online]. Available at: http://www.un-energy.org/wp-content/uploads/2017/01/UNDP-Energy-Strategy-2017-2021.pdf .
United Nations Economic and Social Commission (Escap), (2016). Regional Trends Report on Energy for Sustainable Development in Asia and the Pacific 2016 . Bangkok, Thailand: United Nations Economic Commision fo Asia and the Pacific . [Online]. Available: https://www.unescap.org/sites/default/files/publications/publication_RTRWEB%20%285%29v2.pdf .
United Nations Environmental Program(Unep), (2019). Emissions Gap Report 2019 . Nairobi, Kenya: United Nations Environmental Program . [Online]. Available: https://wedocs.unep.org/bitstream/handle/20.500.11822/30797/EGR2019.pdf?sequence=1&isAllowed=y (Accessed June 16, 2021).
United Nations(Un), (2019). Energy Statistics Pocketbook in Statistics Papers " Department of Economic and Social Affairs ( New York, USA . [Online]. Available: https://unstats.un.org/unsd/energy/pocket/2019/2019pb-web.pdf .
United Nations (2019). Energy Statistics Pocketbook . New York: Department of Economic and Social Aairs . [Online]. Available:at: https://unstats.un.org/unsd/energy/pocket/2019/2019pb-web.pdf .
United Nations (2019). The Impact of Rapid Technological Change on Sustainable Development . Geneva, Switzerland: United Nations Commission on Science and Technology for Development , 17. [Online]. Available: https://www.un.org/pga/74/event/the-impact-of-rapid-technological-change-on-the-sustainable-development-goals-and-targets/ .
United States Department of Energy (2015). “An Assessment of Energy Technologies and Research Opportunities,” in Quadrennial Technology Review . [Online]. Available: https://www.energy.gov/sites/prod/files/2017/03/f34/qtr-2015-chapter1.pdf .
United States Environmental Protection Agency (Epa), (2017). Energy Resources for State and Local Governments . January: United States Environmental Protection Agency " United States Environmental Protection Agency . [Online]. Available: https://www.epa.gov/statelocalenergy/local-renewable-energy-benefits-and-resources .
University of Alberta (2021). What Is Sustainability? Edmonton, AB, Canada: Office of Sustainability . Available at: https://www.mcgill.ca/sustainability/files/sustainability/what-is-sustainability.pdf (accessed, 2021).
Valencia, F., Billi, M., and Urquiza, A. (2021). Overcoming Energy Poverty through Micro-grids: An Integrated Framework for Resilient, Participatory Sociotechnical Transitions. Energ. Res. Soc. Sci. 75, 102030. doi:10.1016/j.erss.2021.102030
Vezzoli, C., Ceschin, F., Osanjo, L., M’Rithaa, M. K., Moalosi, R., Nakazibwe, V., et al. (2018). “Energy and Sustainable Development,” in Designing Sustainable Energy for All: Sustainable Product-Service System Design Applied to Distributed Renewable Energy . Editor C. Vezzoli, F. Ceschin, L. Osanjo, M. K. M’Rithaa, R. Moalosi, V. Nakazibweet al. (Cham: Springer International Publishing ), 3–22.
Viet, D. T., Phuong, V. V., Duong, M. Q., and Tran, Q. T. (2020). Models for Short-Term Wind Power Forecasting Based on Improved Artificial Neural Network Using Particle Swarm Optimization and Genetic Algorithms. Energies 13 (11), 2873. doi:10.3390/en13112873
Vine, E. (2019). Building a Sustainable Organizational Energy Evaluation System in the Asia Pacific. Glob. Energ. Interconnection 2 (5), 378–385. doi:10.1016/j.gloei.2019.11.012
Wallington, T. J., Srinivasan, J., Nielsen, O. J., and Highwood, E. J. (2004). “Greenhouse Gases and Global Warming,” in Environmental and Ecological Chemistry in Encyclopedia of Life Support Systems (EOLSS), Developed under the Auspices of the UNESCO . Editor A. Sabljic (Oxford, UK: Eolss Publishers ).
Wang, S., and Wang, S. (2015). Impacts of Wind Energy on Environment: A Review. Renew. Sustainable Energ. Rev. 49, 437–443. doi:10.1016/j.rser.2015.04.137
Wang, H. K. H. (2019). Climate Change and Clean Energy Management Challenges and Growth Strategies . 1st ed.. London: Routledge , 192. [Online]. Available: https://www.taylorfrancis.com/books/mono/10.4324/9781351050715/climate-change-clean-energy-management-henry-wang .
Wanga, Y., Zhanga, D., Ji, Q., and Shi, X. (2020). Regional Renewable Energy Development in China: A Multidimensional Assessment. Renew. Sustainable Energ. Rev. 124, 1–12. doi:10.1016/j.rser.2020.109797
Watson, R. (2014). Tackling the challenge of Climate Change . Alliance of Small Island States AOSIS . [Online]. Available: https://www.aosis.org/wp-content/uploads/2014/09/Tackling-Climate-Change-K.pdf .
Werpy, M. R., Santini, D., Burnham, A., and Mintz, M. (2010). Natural Gas Vehicles: Status, Barriers, and Opportunities . Argonne, Illinois: Energy Systems Division, U.S. Department of Energy .
Wikipedia (2021). Sustainable Energy . San Francisco, California: Wikimedia Foundation, Inc. .
Wilson, M. (2021). Providing Reliable, Resilient, and Sustainable Energy Solutions . Edmonton, Canada: Stantec . Available at: https://www.stantec.com/en/ideas/content/blog/2021/microgrids-a-critical-key-to-the-energy-transition (accessed, 2021).
World Commission on Environment and Development (WCED) (1987). Our Common Future . New York, USA: Oxford University Press .
World Energy Council (2013). Marine Energy . [Online]. Available: https://www.worldenergy.org/assets/images/imported/2013/10/WER_2013_11_Marine_Energy.pdf .
World Energy Data (2021). World Electricity Generation . Newcastle, Australia: World Energy Data . Available at: https://www.worldenergydata.org/world-electricity-generation/ , (accessed, 2021).
World Nuclear Association (2021). Nuclear Power in the World Today. World Nuclear Association. June 2021. Archivedfrom the Original on 16 July 2021 . London, United Kingdom: World Nuclear ssociation . Retrieved 19 July 2021. Available at: https://world-nuclear.org/information-library/current-and-future-generation/nuclear-power-in-the-world-today.aspx (accessed, 2021).
Yu, J. M. (2017). Taiwan Power Outage Affected 151 Companies, Caused $3 Million in Damages . Available at: https://www.reuters.com/article/us-taiwan-power-Outages/taiwan-power-outage-affected-151-companies-caused-3-million-in-damages-dUSKCN1AX0S3 .
Zarco-Soto, F. J., Zarco-Periñán, J., and Martínez-Ramos, J. L. (2021). Centralized Control of Distribution Networks with High Penetration of Renewable Energies. Energies 14 (14), 4283. doi:10.3390/en14144283
Keywords: renewable energy, sustainable electricity, energy and electricity sustainability, energy transition, energy security, energy transition strategies, global climate change, greenhouse gas emissions
Citation: Kabeyi MJB and Olanrewaju OA (2022) Sustainable Energy Transition for Renewable and Low Carbon Grid Electricity Generation and Supply. Front. Energy Res. 9:743114. doi: 10.3389/fenrg.2021.743114
Received: 17 July 2021; Accepted: 28 December 2021; Published: 24 March 2022.
Reviewed by:
Copyright © 2022 Kabeyi and Olanrewaju. This is an open-access article distributed under the terms of the Creative Commons Attribution License (CC BY). The use, distribution or reproduction in other forums is permitted, provided the original author(s) and the copyright owner(s) are credited and that the original publication in this journal is cited, in accordance with accepted academic practice. No use, distribution or reproduction is permitted which does not comply with these terms.
*Correspondence: Moses Jeremiah Barasa Kabeyi, [email protected] , [email protected]
This article is part of the Research Topic
Advances Towards Deep Decarbonization of Energy Systems
Towards Sustainable Energy: A Systematic Review of Renewable Energy Sources, Technologies, and Public Opinions
Ieee account.
- Change Username/Password
- Update Address
Purchase Details
- Payment Options
- Order History
- View Purchased Documents
Profile Information
- Communications Preferences
- Profession and Education
- Technical Interests
- US & Canada: +1 800 678 4333
- Worldwide: +1 732 981 0060
- Contact & Support
- About IEEE Xplore
- Accessibility
- Terms of Use
- Nondiscrimination Policy
- Privacy & Opting Out of Cookies
A not-for-profit organization, IEEE is the world's largest technical professional organization dedicated to advancing technology for the benefit of humanity. © Copyright 2024 IEEE - All rights reserved. Use of this web site signifies your agreement to the terms and conditions.
- Contact Contact icon Contact
- Training Training icon Training

- Portuguese, Brazil
- Chinese, Simplified
Check the spelling in your query or search for a new term.
Site search accepts advanced operators to help refine your query. Learn more.
Top 10 areas of green energy research
Magesh Ganesan , Scientist, ACS International India Pvt. Ltd.
February 29, 2024
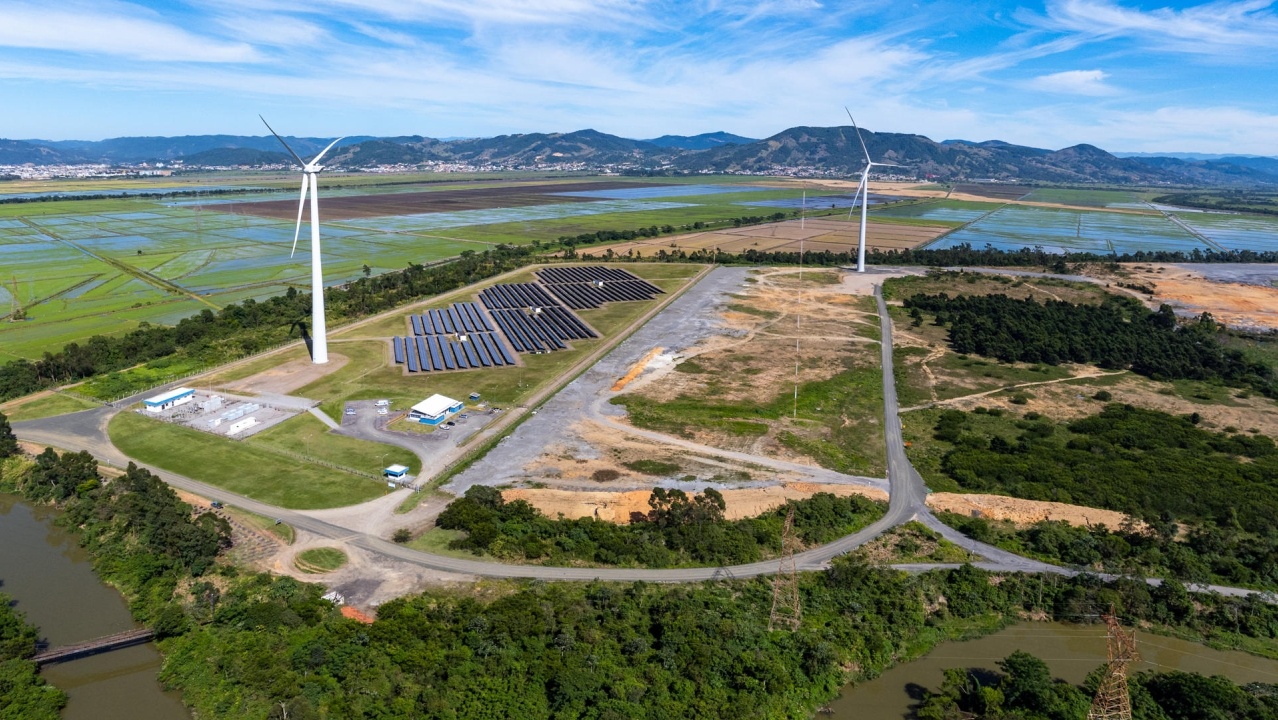
With so much being published on green energy and sustainability, how can you identify the fastest growing areas of research? Learn how CAS Insights provides a unique view of the green energy research landscape and can help you identify emerging trends sooner. Subscribe to be the first to know when we publish new scientific insights.
Fossil fuels remain our primary energy source, but their limited availability and negative environmental impact have led to events like the 2023 United Nations Climate Change Conference (COP28) to seek green energy alternatives. These are sources that can be replenished in the average human lifespan and have a net zero environmental impact.
Every year, millions of journal articles and patent applications are created, so it can be difficult to identify the signals from the noise. CAS curates, connects, and analyzes the world’s published science inside the CAS Content Collection™ to provide a unique view of the scientific landscape. This enables novel insights that show emerging trends in new research areas. Five broad areas of research were identified that contained the fastest-growing trends: batteries, hydrogen energy, solar cells, new materials, and photothermal energy.
How was the analysis done?
First, CAS identified almost one million indexed documents in the CAS Content Collection™ that were relevant to the green energy space. Then, our researchers analyzed the hidden connections between key concepts using advanced analytics, knowledge graphing, and natural language processing to identify emerging trends in this area. Finally, our expert scientists with dozens of years of experience derived unique insights from the landscape of connections created. Several exciting growth patterns emerged between 2018 and 2022 that are early indicators of opportunities ahead. While many areas of green energy are growing quickly, we identified and prioritized the top ten emerging topics (Figure 1) that will help us reach a more sustainable future.
Batteries, energy storage, and battery recycling
Batteries are the leading method of storing electricity worldwide. Lithium-ion batteries have become commonplace, being used in portable devices and electric vehicles due to their high energy density, while lead-acid batteries are conventionally used for portable and stationary power storage. However, lithium-ion batteries are a fire hazard while lead-acid batteries are notably toxic. This has led to researchers looking for alternate, safer ways to store electricity.
- Aqueous zinc-ion batteries: These batteries are being studied as alternatives to lead-acid batteries because they are naturally occurring, much more environmentally friendly, and notably cheaper and non-toxic.
- Solid-state lithium-ion battery: Standard lithium-ion batteries degrade quickly, are fire hazards, and have high toxicity. However, they are still widely used because of faster charging and easy manufacturing. Solid-state lithium-ion batteries can be charged and discharged many times more than lithium-ion batteries and hold more electricity.
The successful development of these new battery types will make the industry much safer. Not only will solid-state lithium batteries be less of a fire hazard, but the overall pollution will drop, owing to the absence of toxic liquid electrolytes and more sustainable production. Learn more about lithium-ion batteries , the landscape of recyclin g legislation and regulations , and the new breakthroughs that are driving innovation.
Hydrogen energy, green hydrogen economy, and hydrogen storage
Hydrogen has emerged as a promising alternative to fossil fuels, being more environmentally friendly, having higher energy per given weight than gasoline, and more applicable in many energy-related fields.
- Liquid hydrogen storage: Hydrogen has three times the gravimetric density of gasoline but only one-fourth of the volumetric energy density. This means liquid hydrogen is considered the most efficient method of storing hydrogen in its base form, and researchers are seeking new ways to take advantage of it. If properly harnessed, liquid hydrogen storage could enable new fuel cell-driven automobiles and decrease costs in petroleum refining, fertilizer production, and more.
- Water splitting using heterojunction photocatalysts: Photocatalysts have emerged as a sustainable energy source, producing hydrogen using only water and sunlight. The main challenge, however, is identifying or developing them due to low efficiency and unsuitable band positions. Once these hurdles are overcome, the cost of hydrogen is expected to decrease, which could make it the preferred fuel source.
The benefits of these hydrogen production and storage technologies are immense. Urea oxidation will be dual purpose, cleaning water and providing energy simultaneously. More efficient storage methods could facilitate the utilization of hydrogen fuel cells, revolutionizing commercial products like automobiles. These changes would be further bolstered by new water-splitting methods, which would make accessing the necessary hydrogen for these processes much cheaper. Learn more about photocatalysis and new breakthroughs in the landscape of green hydrogen production.
Solar cells
Solar cells have seen an increased interest both academically and commercially. As industries look for more sustainable options, there will be more studies on how to optimize this technology for higher efficiency and lower cost.
- Non-fullerene acceptors for solar cells: The performance of organic solar cells has increased, but development is already underway to replace their most used acceptor, fullerene, with an alternative. These non-fullerene acceptors have more tunable properties, higher thermal and photochemical stability, and can lead to longer device lifetimes. This could lead to more stable, longer-lasting, and cheaper solar cells.
- Stable perovskite solar cells: As researchers look to enhance the efficiency of solar cells, one area of interest is perovskite-based solar cells. These easy-to-fabricate, low-cost cells have reported some of the highest energy efficiencies. However, the current materials used are unstable in certain conditions. The advantages of stable perovskite solar cells remain substantial and could diminish manufacturing costs if this challenge is overcome.
The main hurdle that these two innovations could overcome is cost. By cutting out fullerene or developing cells with perovskite, solar energy could be more affordable to many consumers. Learn more about emerging technologies in materials .
Sustainable chemistry, new materials, and greener alternatives
Noble and toxic metals are frequently used in the energy field. While functional, risks and challenges remain, hindering the industry’s progress. This has led studies to examine more sustainable and efficient alternatives.
- Mxenes: These are two-dimensional materials that incorporate a transition metal and a functional group. Their layered nature makes them strong candidates for energy storage applications like capacitors and batteries while their optical and catalytic properties have potential in photocatalysis and electrocatalysis. Mxenes also contain earth-abundant elements, circumventing the risks associated with noble or toxic metals. Any breakthrough with this material could result in significant cost, environmental, and energy storage benefits.
- Covalent organic frameworks: These are two or three-dimensional structures formed by organic precursor reactions. Forming covalently bonded, porous structures, they are being studied for hydrogen/methane and catalytic/electrocatalytic energy storage applications. Successful covalent organic framework applications could lead to many economic and environmental benefits in energy storage, chemical synthesis, catalysis, and gas separation with special interest in the automobile industry.
These materials have many possible applications. Both being made of earth-abundant materials, they will be more available and sustainable compared to other materials. By replacing current materials with covalent organic frameworks and mxenes, there will also be significant environmental and economic benefits across many industries. Learn more about sustainable catalysts , new biomaterials, and carbon nanotubes that can help build upon new opportunities ahead.
Solar energy, photothermal conversion, and green energy sources
As researchers try to find ways of reducing energy’s environmental impact, special interest is placed on renewable sources. Solar is already gaining commercial and industrial popularity, but further breakthroughs could lead to wider adoption.
- Photothermal energy conversion: The conversion of solar energy to heat, which generates steam, can generate electricity without using other sources. Researchers are studying inorganic and polymeric materials to find a suitable photothermal candidate. Success in this area could lead to a drastic energy cost reduction, owing to the sole reliance on solar energy. It would also have a substantial positive environmental impact by ideally removing the need for fossil fuels.
Unlocking the potential of photothermal energy conversion could lead to immense energy cost savings and cleaner energy sources. Additionally, there has always been a desire to use solar energy to split water atoms for clean hydrogen production. This process of photocatalysis would be critical to using solar energy for clean hydrogen production and could reshape the future for a green hydrogen economy .
Looking ahead
Green energy will remain a large research focus as we seek a net-zero environmental impact thanks to events like COP28. Suitable alternatives are being examined, but there remain challenges and risks that must be overcome before we see real-world applications. As more green energy advances are made, it can be challenging to keep up with the new developments. Subscribe to CAS Insights™ for unique views and the latest updates on green energy alternatives.
Gain new perspectives for faster progress directly to your inbox.
Related Insights
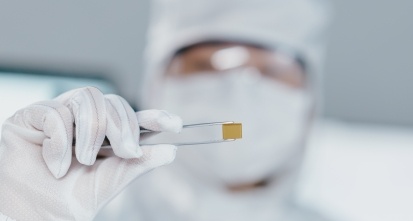
Nuclear power in your pocket? 50-year battery...
March 11, 2024
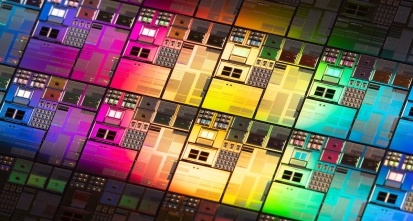
Science Fact Fiction: Can we really recycle...
March 8, 2024
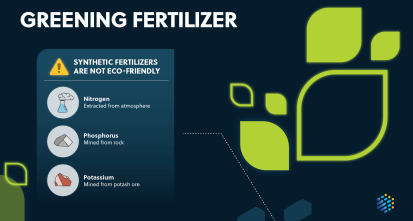
Infographic: Sustainable crops start with green(er)...
Infographic
February 16, 2024
Research Area: Energy Sustainability
Main navigation, the future of energy.
Improved efficiency of energy systems and development of sustainable, low-carbon-emission energy generation processes are essential for the long-term health of the environment.
Recent increases in energy prices provide a graphic reminder of the importance of energy to our economy and our quality of life. Most of our endeavors — economic, social and societal — are fueled by a near-transparent infrastructure of relatively inexpensive, highly reliable and easily accessible energy. However, the traditional model — one based on plentiful, inexpensive fossil fuels — will not carry us past the middle of the century.
In the Mechanical Engineering Department at Stanford University, we recognize that developing sustainable energy solutions requires efforts in multiple disciplines and by large teams of faculty and students. It will require that we identify attractive fuel sources and that we develop the technologies required to use those sources in efficient, environmentally benign ways. Many ME faculty are focused on advanced energy carrier technologies and energy conversion devices such as fuel cells, hydrogen storage systems, hybrid transportation and power systems, as well as “smart” ways of accomplishing chemical-to-work energy conversion.
Energy faculty
Faculty in the Thermofluids, Energy, and Propulsion Systems and Flow Physics & Computational Engineering Groups have a long tradition of experimental and simulation leadership for energy systems. These efforts include a world-leading set of laboratories for the study of reacting flows and combustion processes including a massive engine laboratory and shock tube facility. Our laboratories also include facilities to study clean-coal energy conversion, thermoelectric energy conversion for waste-heat recovery, and fuel cell devices and systems. Our faculty are at the forefront of computational engineering of advanced energy conversion processes, and have led the way in the use of parallel computing and the development of strategies for handling multi-physics energy transport and conversion phenomena. These activities, as a group, provide compelling simulations and data for systems such as fuel cells, thermoelectrics, clean coal and high-efficiency gas turbine engines. In the Design and Mechanics & Computation Groups, faculty are studying the basic materials physics for novel energy conversion systems including solid oxide and PEM fuel cells.
Multi-disciplinary solutions are required
These developments will take place both within the traditional boundaries of mechanical engineering and at the boundaries where ME intersects with material science (such as membranes), electrical engineering (sensors, actuators and controls), biology (biosynthesis of fuels) and other fields. Our current, highly diverse approach to research positions us well to contribute to this rapidly changing landscape.
Sustainability News

Stanford University launches research initiative on hydrogen as a climate solution
The Stanford Energy Hydrogen Initiative will fund research to evaluate hydrogen’s role in the transition to sustainable energy and the technologies, policies, and financial mechanisms to fulfill that role.

Empowering the next generation of sustainable energy researchers
Stanford students take local high schoolers behind the scenes of renewable energy and battery research.
Read all stories from ME Sustainability Research

How systems transitions can improve sustainable development
Clark, William, et al. Systems transitions research and sustainable development: Challenges, progress, and prospects ,” Proceedings of the National Academy of Sciences (Vol. 120, No. 47, Nov. 2023).
Faculty Authors

William Clark
What’s the issue.
Making development sustainable is a central challenge of our age. The field of sustainability science tries to figure out how interactions of nature and society can support sustainable development. One key goal is figuring out how to restructure complex consumption-production systems. Examples of these systems are production and consumption of food, energy, and mobility. Scientists are studying how transitions in these production-consumption systems can bend development pathways toward sustainability.
What does the research say?
Writing in a special edition of the Proceedings of the National Academy of Sciences, scholars Frank Geels of the University of Manchester, Florian Kern of the Institute for Ecological Economy Research in Berlin, and William C. Clark of Harvard Kennedy School bring together insights from experts on system transitions that shape sustainable development. This work weighs how potential solutions might fundamentally shift consumption-production systems. The authors note that radical innovations in small niches can lead to major transitions in large consumption-production systems. The three lead authors call on more than a dozen sustainable development scholars to examine specific innovations and obstacles to progress. These experts look at the drivers and barriers to sustainability in electricity, food, and mobility systems; they also consider larger cross-cutting systems issues. For example, one essay analyzes the British electricity system since World War Two, and the role that increasing concerns about climate change played in generating a more interventionist policy approach to shift electricity production and consumption. These systems changes led to an 86% reduction in emissions from 2006 to 2019. The authors note that political and economic struggles as well as technological advances drove this transition. Another paper analyzes how recent technology advances in energy storage, microgrids, and digitized systems in the United States have helped undermine long-time obstacles to small-scale solar energy development. Other essays look at the adoption of electric vehicles in Norway, the systems issues involved in car-sharing, agroecology in Nicaragua, and the shift to plant-based alternatives to meat production.
More from HKS
For more than four decades, professor bill clark has championed sustainability, walking the talk: how students can contribute to the hks sustainability agenda, pursuing sustainability: a guide to the science and practice.
Get smart & reliable public policy insights right in your inbox.
How renewable energy serves as a catalyst to broader social change
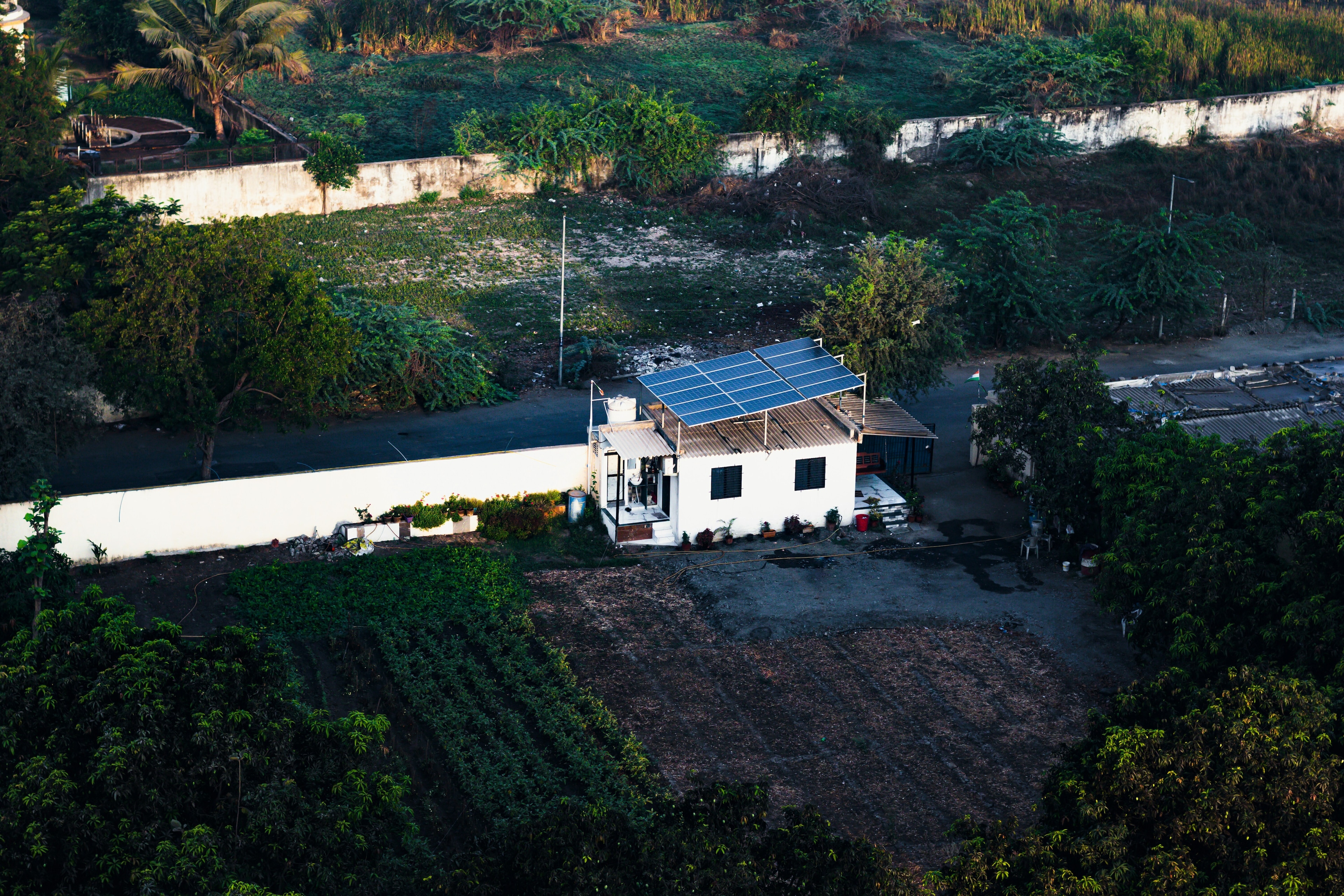
Renewable energy boosts livelihoods worldwide Image: Unsplash/VD Photography
.chakra .wef-1c7l3mo{-webkit-transition:all 0.15s ease-out;transition:all 0.15s ease-out;cursor:pointer;-webkit-text-decoration:none;text-decoration:none;outline:none;color:inherit;}.chakra .wef-1c7l3mo:hover,.chakra .wef-1c7l3mo[data-hover]{-webkit-text-decoration:underline;text-decoration:underline;}.chakra .wef-1c7l3mo:focus,.chakra .wef-1c7l3mo[data-focus]{box-shadow:0 0 0 3px rgba(168,203,251,0.5);} Jennifer Rosen
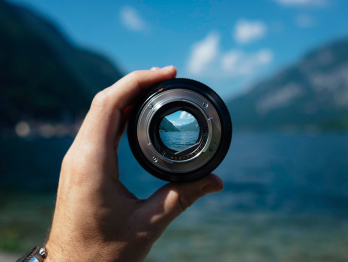
.chakra .wef-1nk5u5d{margin-top:16px;margin-bottom:16px;line-height:1.388;color:#2846F8;font-size:1.25rem;}@media screen and (min-width:56.5rem){.chakra .wef-1nk5u5d{font-size:1.125rem;}} Get involved .chakra .wef-9dduvl{margin-top:16px;margin-bottom:16px;line-height:1.388;font-size:1.25rem;}@media screen and (min-width:56.5rem){.chakra .wef-9dduvl{font-size:1.125rem;}} with our crowdsourced digital platform to deliver impact at scale
- From fostering innovation to job creation, renewable energy solutions drive progress towards a more equitable and sustainable world.
- Many renewable energy solutions create opportunities for economic development while reducing greenhouse gas emissions.
- Here are some examples of how renewable energy solutions are changing lives all over the world.
Renewable energy solutions mitigate climate change and promote a healthier environment and they often serve as catalysts for broader social change. From fostering innovation and job creation to promoting gender equality or making civic participation more accessible, renewable energy solutions drive progress towards a more equitable and sustainable world.
With support from the Skoll Foundation, the Solutions Insights Lab (SIL), a new initiative of the Solutions Journalism Network (SJN), created What’s Working . This is a searchable portal that combines published solutions journalism and interviews with a wide array of leaders whose work has been supported by the Skoll Foundation over the past 20 years to uncover insights that can help address social problems worldwide.
Have you read?
A new study reveals how renewables could power africa by 2040.
The SIL is a targeted research and analysis service focused on identifying and interrogating what’s working and what’s not in a particular sector or field. It employs interviewing techniques drawn from the solutions journalism approach but is not a work of journalism and is appropriately separated with a firewall from SJN’s core journalism work.
The interviews are not works of journalism themselves. The interviewing approach was standardized and the over 200 individuals interviewed were specifically selected as part of a project supported by the Skoll Foundation. They do not represent any form of endorsement by SJN, which is an independent, non-partisan organization that does not advocate for any particular approach to social change.
The interviews follow a solutions framework to explore how successful approaches work. They look at evidence of impact and replicable insights and their limitations. We analyzed these interviews, in combination with relevant stories within SJN’s Solutions Story Tracker , to distil the lessons learned by those doing this work on the ground and surface insights related to the role renewable energy solutions play in mitigating climate change and making progress on several other Sustainable Development Goals, from eradicating poverty to achieving gender equality.
Solutions for multiple problems
We’ve consistently seen that changemakers can and do successfully design solutions to address more than one issue. This is perhaps most evident among renewable energy solutions that seek to create opportunities for economic development while reducing greenhouse gas emissions. Bringing affordable and clean renewable energy to rural and underdeveloped communities benefits the health of their environment and their economy, creating jobs and providing resources that foster innovation and entrepreneurship.
Lifeline Energy , for example, designs, manufactures and distributes solar-powered and wind-up media players across sub-Saharan Africa to connect communities to important information. Classrooms can listen to school lessons, farmers can listen to agricultural radio broadcasts about pesticides, frontline health workers can listen to pre-recorded health content and villagers can access information that allows them to participate in their communities in more informed ways.
Moving to clean energy is key to combating climate change, yet in the past five years, the energy transition has stagnated.
Energy consumption and production contribute to two-thirds of global emissions, and 81% of the global energy system is still based on fossil fuels, the same percentage as 30 years ago. Plus, improvements in the energy intensity of the global economy (the amount of energy used per unit of economic activity) are slowing. In 2018 energy intensity improved by 1.2%, the slowest rate since 2010.
Effective policies, private-sector action and public-private cooperation are needed to create a more inclusive, sustainable, affordable and secure global energy system.
Benchmarking progress is essential to a successful transition. The World Economic Forum’s Energy Transition Index , which ranks 115 economies on how well they balance energy security and access with environmental sustainability and affordability, shows that the biggest challenge facing energy transition is the lack of readiness among the world’s largest emitters, including US, China, India and Russia. The 10 countries that score the highest in terms of readiness account for only 2.6% of global annual emissions.
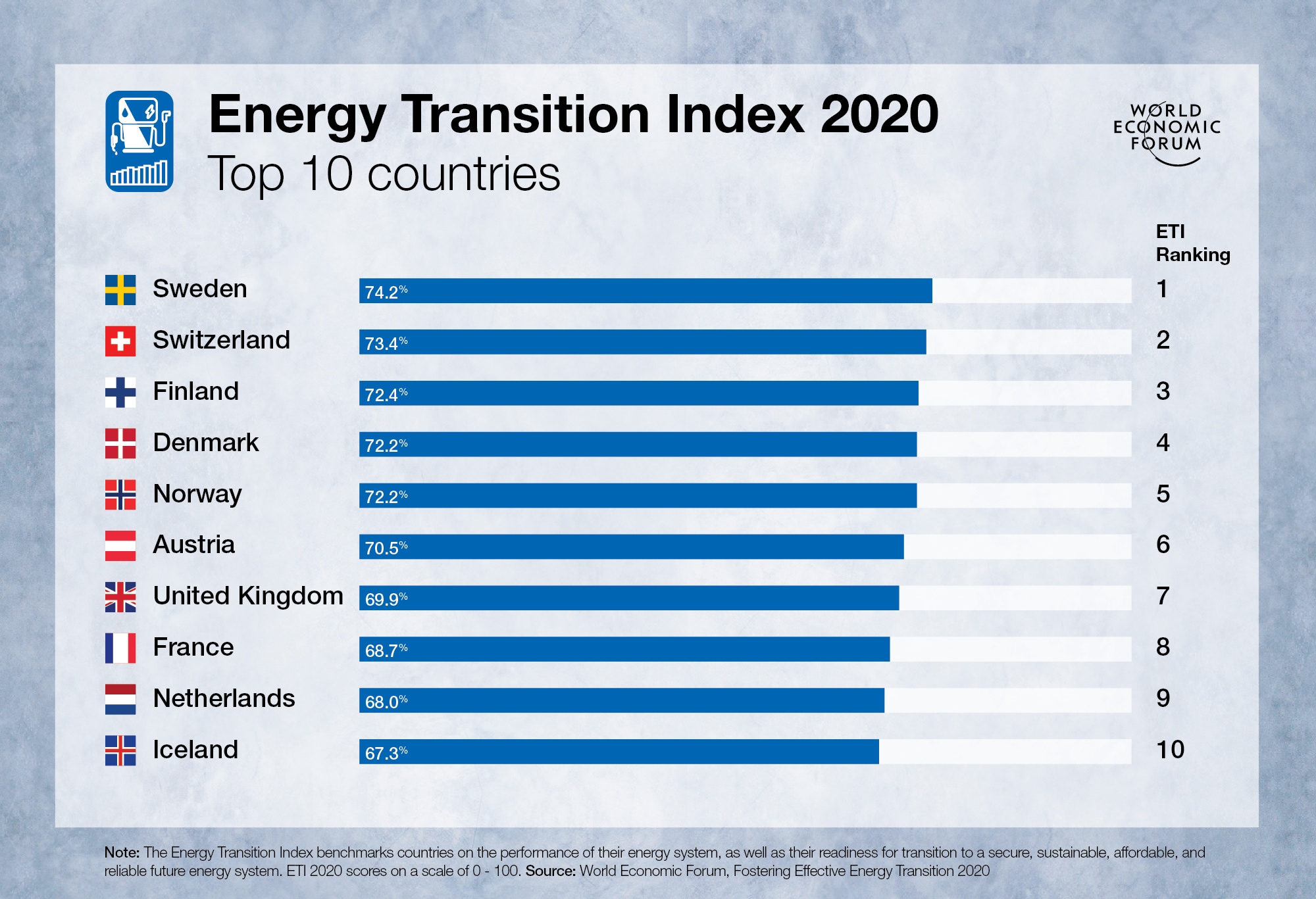
To future-proof the global energy system, the Forum’s Centre for Energy & Materials is working on initiatives including Clean Power and Electrification , Energy and Industry Transition Intelligence, Industrial Ecosystems Transformation , and Transition Enablers to encourage and enable innovative energy investments, technologies and solutions.
Additionally, the Mission Possible Partnership (MPP) is working to assemble public and private partners to further the industry transition to set heavy industry and mobility sectors on the pathway towards net-zero emissions. MPP is an initiative created by the World Economic Forum and the Energy Transitions Commission.
Is your organisation interested in working with the World Economic Forum? Find out more here .
The more of these solutions I looked at, the more I saw that their benefits often have third and fourth layers. They are improving women’s lives and reducing gender inequalities, for example, or making it easier for communities to access information and services, like healthcare. Solutions that address multiple needs are the most powerful and cost-effective. They also bridge the silos that so many solutions exist in.
How to finance the transition to climate-smart agriculture
Earth day: we are almost certainly all eating plastics, says report, and other nature and climate stories you need to read this week, powering up gender equality.
Barefoot College International , for example, uses clean energy to promote socio-economic development, protect the environment and improve women’s lives. Its theory of change places women at the centre as key changemakers. CEO Rodrigo París told us that putting resources towards women is key because “Women have roots in the communities, they have the knowledge... They have a good understanding about the past, about family and the roots, but they have a clear vision on how to solve problems.”
The organization trains mostly older women with little to no formal education in over 90 countries to install, repair and maintain solar lighting units in their villages. Women like Jullietta, a 69-year-old mother of seven and grandmother of 30 in Guatemala, receive the skills and resources they need to electrify homes and schools with solar energy. In doing so, they earn an income for themselves, better the environment and expand economic development opportunities for their entire village.
As one of the 20 trained 'solar mamas' in Guatemala (there are over 3,500 across the world), Jullietta brought power to 35 families in her village and is helping increase the status of women more broadly. The women’s new skills and financial independence puts them at the centre of important community-wide changes too and give them more agency to impact decision-making.
Global Gender Gap Report 2023
Transforming agriculture.
Almost 10,000 miles away, a group of women farmers in Harpur, India purchased and installed solar pumps that use affordable and clean energy to irrigate their crops. The pumps have increased their yields and enabled more diverse crops, which has led to greater profits. They also make a profit by selling irrigation services to others.
Despite facing discrimination, their increased financial independence and greater self-reliance has challenged gender norms in the traditionally male-dominated village. The women report deciding how to use the money they’ve earned and having more control over their economic well-being. The state government in Bihar has also used solar-operated pump projects as a means to improve livelihoods among rural women and is looking to replicate the model in other districts.
Solar lights, in particular, have expanded opportunities for women to improve their livelihoods. From women in Kenya leaving the sex trade once they had lights to fish at night to women in a rural Pakistani village being able to earn money making pottery after the sun goes down and women in Mali creating cooking solutions that don’t produce harmful indoor pollutants , renewable energy solutions have expanded choices and resources for women across the world.
Improving access to healthcare
Other renewable energy solutions seek to alleviate poverty by increasing access to information and services, like healthcare, which also impact women. To reduce high maternal mortality rates among women in rural Zimbabwe, Mobility for Africa uses Hambas , electric three-wheel tricycles that run on rechargeable batteries. Hambas transport pregnant women and new mothers to health facilities for pre- and post-natal care. The clean and renewable energy source mitigates harm to the environment.
Many solutions use renewable energy to expand health services, which is a foundational step in reducing poverty. From the Selco Foundation using solar panels to increase treatment capacities of rural health facilities to using mini solar grids for lighting homes to avoid venomous snake bites , these creative solutions address multiple issues in a single package.
An important aspect of the solutions framework is acknowledging the limitations of a solution. While renewable energy solutions have the potential to provide benefits across three or more areas of people’s lives, they are not without challenges. The biggest hurdle is the upfront costs of purchasing and installing the infrastructure, as well as ensuring there is a system in place to maintain it.
Renewable energy sources, such as solar, can also be less reliable than traditional energy sources, so the power may be more intermittent. But solutions that combine renewable energy with other important issues clearly play important roles in achieving several Sustainable Development Goals, from climate action to eradicating poverty to achieving gender equality. Clean, affordable and renewable energy stands as a pivotal solution with the potential to create a more equitable and healthy future for all.
Don't miss any update on this topic
Create a free account and access your personalized content collection with our latest publications and analyses.
License and Republishing
World Economic Forum articles may be republished in accordance with the Creative Commons Attribution-NonCommercial-NoDerivatives 4.0 International Public License, and in accordance with our Terms of Use.
The views expressed in this article are those of the author alone and not the World Economic Forum.
Related topics:
The agenda .chakra .wef-n7bacu{margin-top:16px;margin-bottom:16px;line-height:1.388;font-weight:400;} weekly.
A weekly update of the most important issues driving the global agenda
.chakra .wef-1dtnjt5{display:-webkit-box;display:-webkit-flex;display:-ms-flexbox;display:flex;-webkit-align-items:center;-webkit-box-align:center;-ms-flex-align:center;align-items:center;-webkit-flex-wrap:wrap;-ms-flex-wrap:wrap;flex-wrap:wrap;} More on Energy Transition .chakra .wef-17xejub{-webkit-flex:1;-ms-flex:1;flex:1;justify-self:stretch;-webkit-align-self:stretch;-ms-flex-item-align:stretch;align-self:stretch;} .chakra .wef-nr1rr4{display:-webkit-inline-box;display:-webkit-inline-flex;display:-ms-inline-flexbox;display:inline-flex;white-space:normal;vertical-align:middle;text-transform:uppercase;font-size:0.75rem;border-radius:0.25rem;font-weight:700;-webkit-align-items:center;-webkit-box-align:center;-ms-flex-align:center;align-items:center;line-height:1.2;-webkit-letter-spacing:1.25px;-moz-letter-spacing:1.25px;-ms-letter-spacing:1.25px;letter-spacing:1.25px;background:none;padding:0px;color:#B3B3B3;-webkit-box-decoration-break:clone;box-decoration-break:clone;-webkit-box-decoration-break:clone;}@media screen and (min-width:37.5rem){.chakra .wef-nr1rr4{font-size:0.875rem;}}@media screen and (min-width:56.5rem){.chakra .wef-nr1rr4{font-size:1rem;}} See all
How wind energy is reshaping the future of global power and politics
Rishabh Mishra
May 14, 2024

This bus in Oman is powered by dates
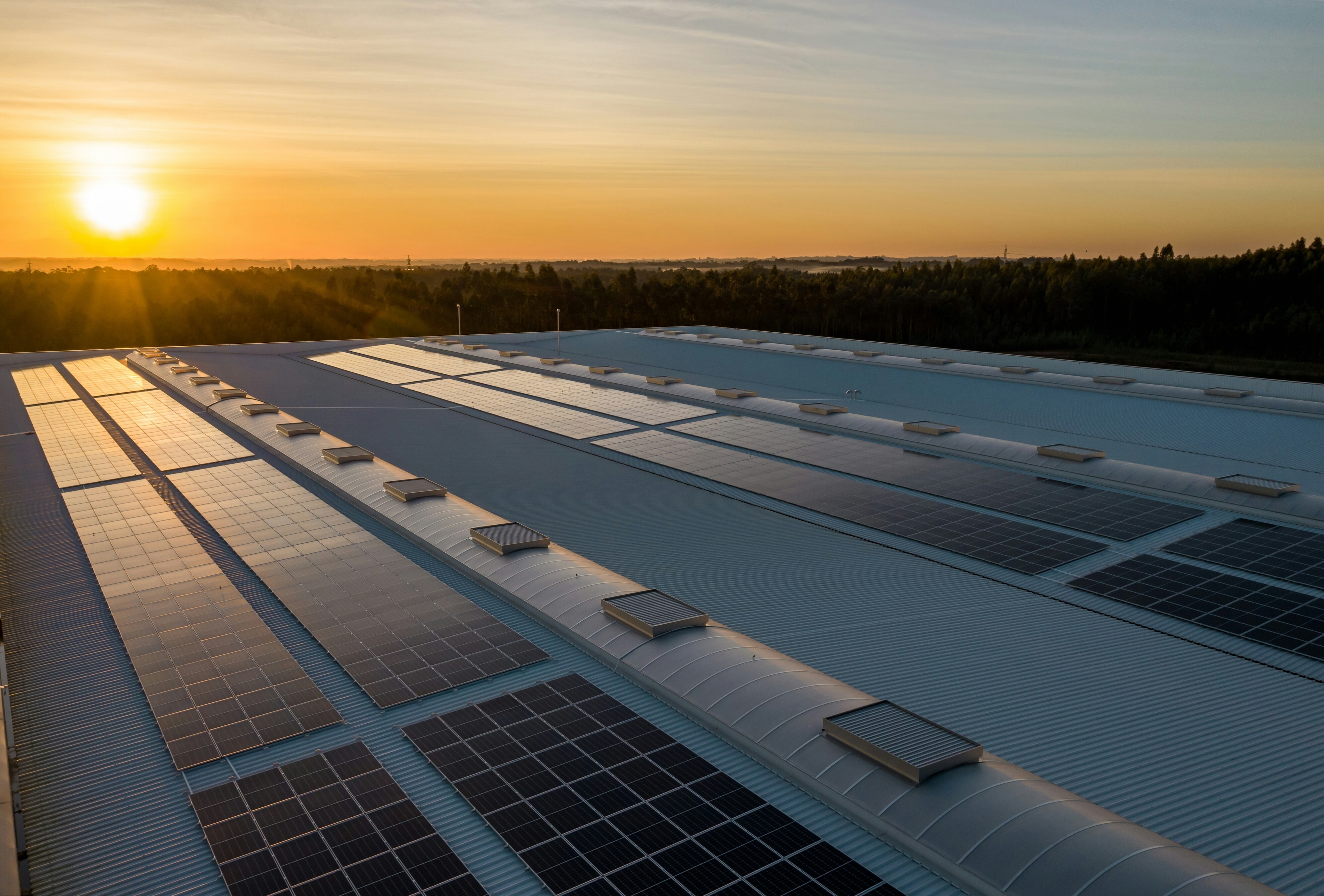
Boosting renewable energy growth through responsible value chains: A case study from China
Vee Li and Zhang Xun
May 13, 2024
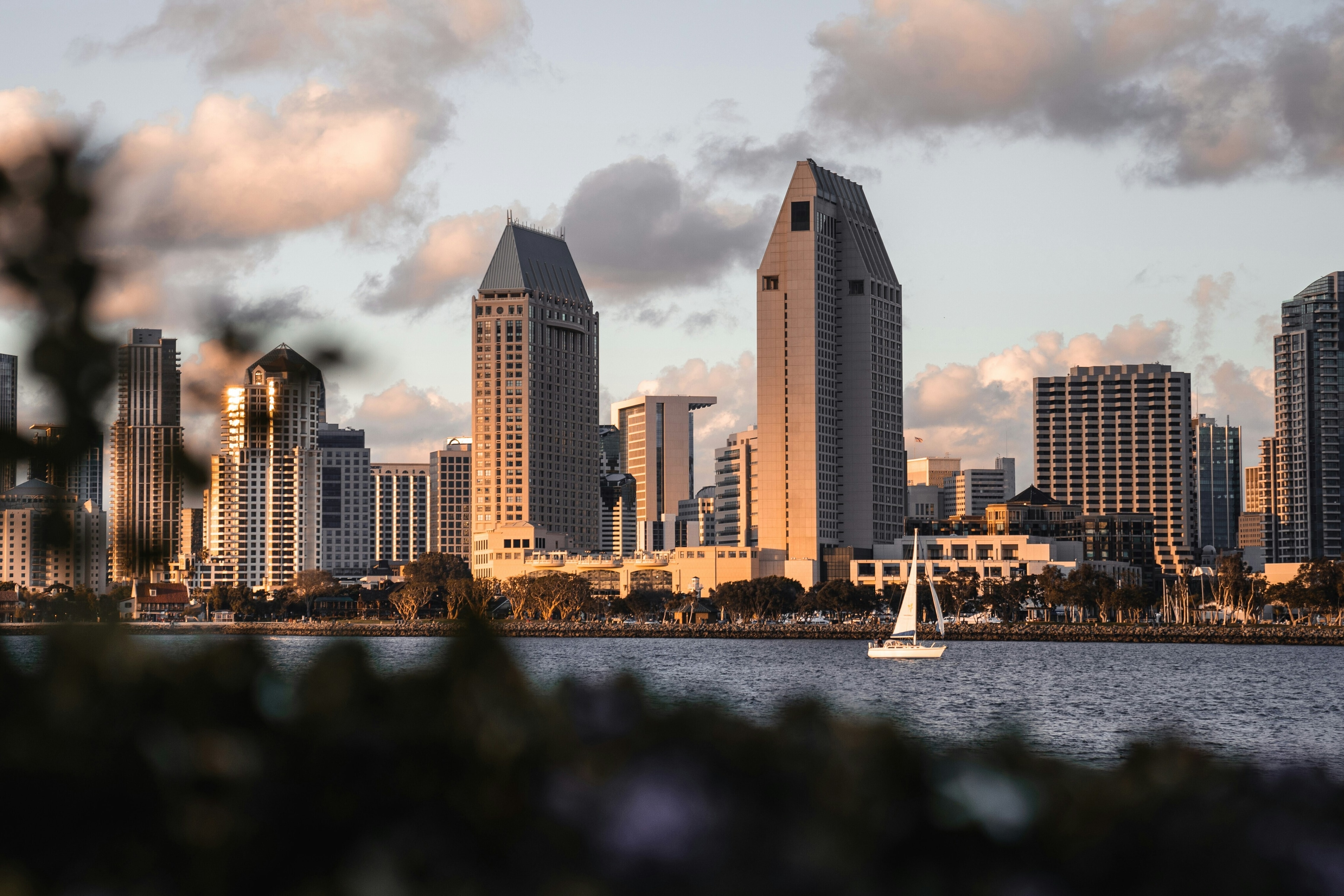
4 strategies to decarbonize existing buildings
Jesse Saldivar, Alaina Ladner, Marc Starkey and Brittany Syz
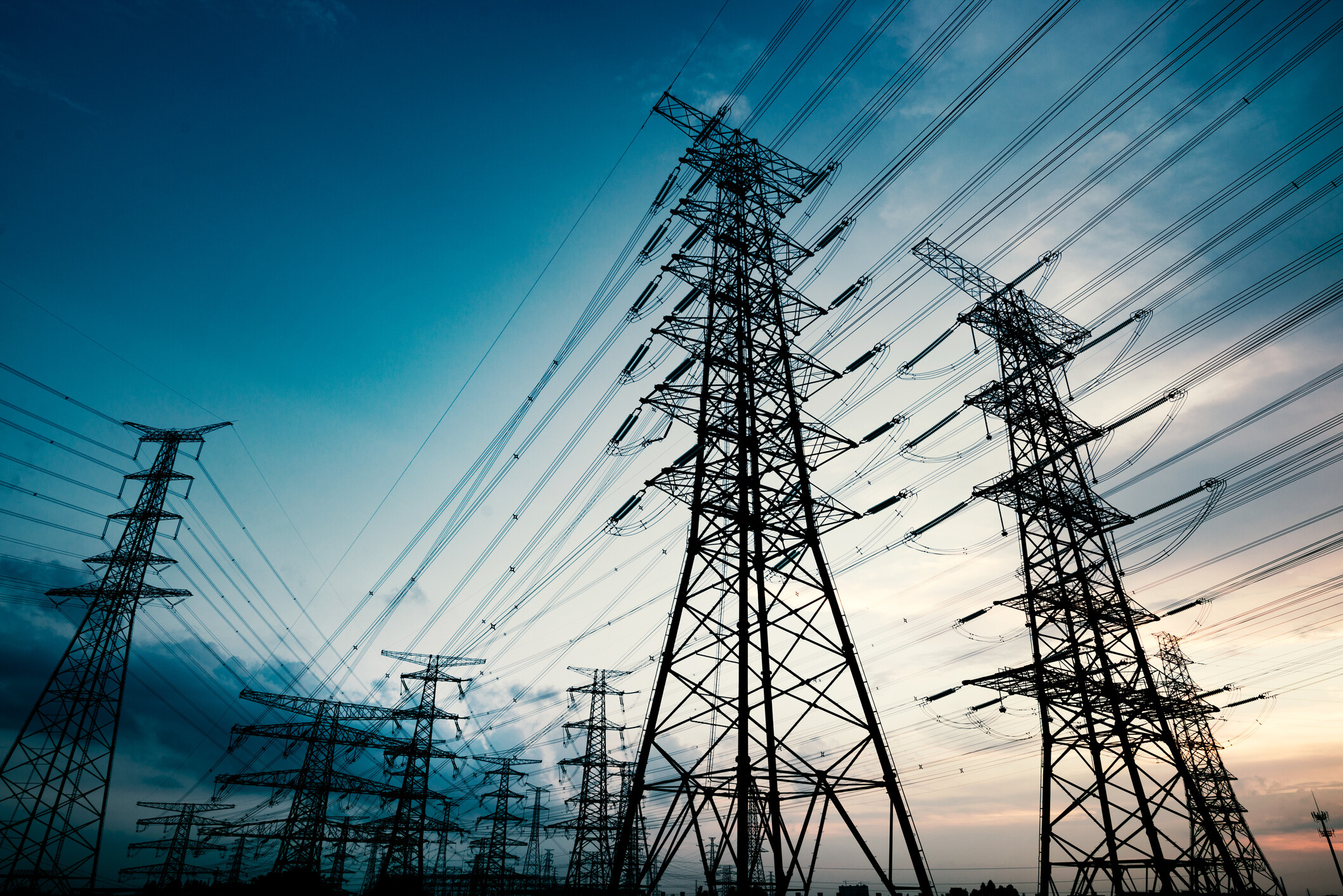
Why realism is key as we balance the energy transition with global growth
Fahad Al-Dhubaib
May 10, 2024
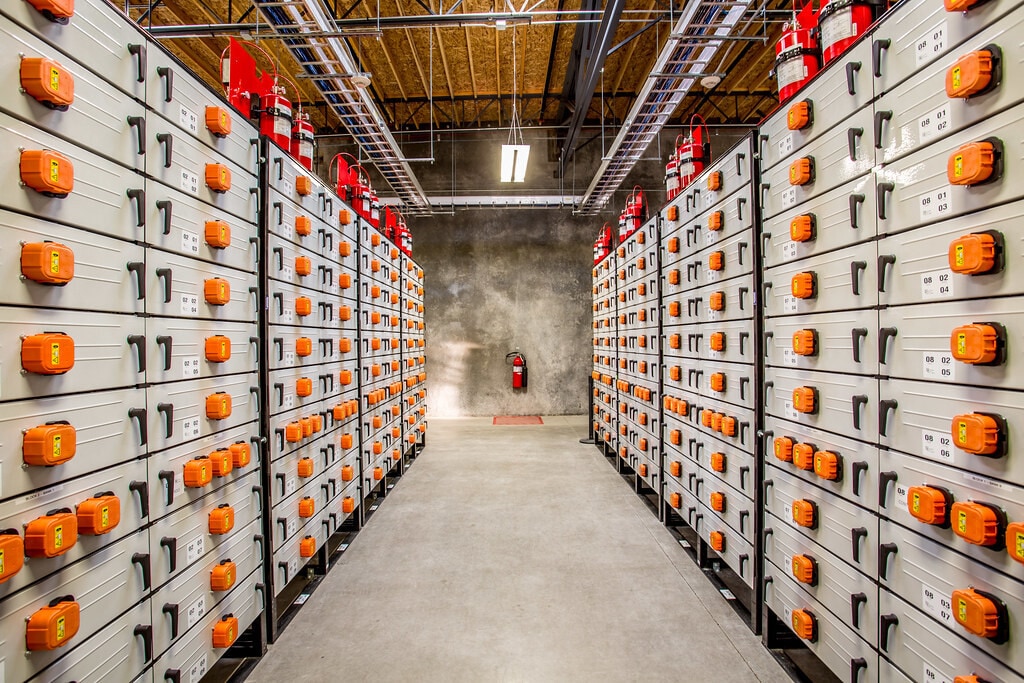
How to finance battery energy storage and ensure constant clean energy
Prasad Thakur and Labanya Prakash Jena
Sustainable Energy Research
Biofuels from biomass.

Faculty : Larry Baxter
Dr. Baxter researches sustainable energy in several forms. His current projects include biomass gasification and combustion, nuclear reactor neutronics, isotope-specific gas kinetics, natural gas processing, and carbon capture. He does both laboratory and theoretical/modeling work and produces both academic and industrial results, including commercialization of academic results into useful industrial processes.
More information :
- Carbon Capture
- Energy Storage
- Coal Combustion
Direct Carbohydrate Fuel Cells

Faculty : Dean R. Wheeler
Glucose and other carbohydrates are among the most abundant and renewable sources of energy in the world. Yet, there has been a longstanding unmet need to utilize efficiently such biologically based sources of energy in batteries and fuel cells. As part of a team of researchers (chemical engineering and chemistry) we recently uncovered a class of organic catalysts that are able to extract electrical energy from a variety of carbohydrate fuels (i.e. sugars) to a degree that has not previously been reported. This catalyst system has the potential to operate at low temperatures and relatively high efficiencies, while avoiding use of expensive noble metals (like platinum) as do competing fuel cell technologies. Our best catalyst, methyl viologen, is also used industrially as an herbicide, and so is inexpensive and widely available. The promise of these catalysts is to enable a new type of fuel cell that can directly power remote and portable electrical devices from biologically derived fuels. Current work is to improve the performance of this catalyst system and bring a new type of fuel cell product to market. This work is supported by the National Science Foundation.
- Fuel cell research
- Publications
Economic complexity, greenfield investments, and energy innovation: policy implications for sustainable development goals in newly industrialised economies
- Research Article
- Published: 15 May 2024
Cite this article
- Muhammad Farhan Bashir ORCID: orcid.org/0000-0001-5103-4639 1 ,
- Roula Inglesi-Lotz 2 ,
- Ummara Razi 3 , 4 &
- Luqman Shahzad 5
The crucial role of environmental assessment quality has been recognised by environmental and sustainable development goals in addressing climate change challenges. By focusing on the key identifier of environmental assessment, progress can be made towards overcoming climate change issues effectively. The current study considers environmental commitments under COP28 to study the role of economic complexity, greenfield investments, and energy innovation in environmental degradation in newly industrialised economies from 1995 to 2021. We employ novel panel estimations from CS-ARDL, CS-DL, AMG, and CCEMG to confirm that economic growth and greenfield investments degrade environmental quality. On the other hand, energy innovation and urbanisation improve environmental sustainability. Lastly, we confirm the EKC hypothesis for economic complexity as well. Given the reported empirical findings, the study suggests policymakers must focus on economic complexity to transform industrial sectors’ economic potential. Furthermore, foreign investment projects must be linked with environmental goals to increase renewable energy capacity.
This is a preview of subscription content, log in via an institution to check access.
Access this article
Price excludes VAT (USA) Tax calculation will be finalised during checkout.
Instant access to the full article PDF.
Rent this article via DeepDyve
Institutional subscriptions
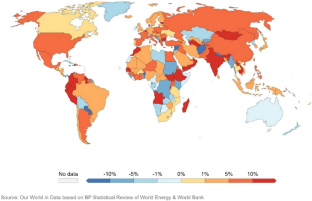
Data availability
Data and relevant materials will be available from the corresponding author.
Turkey, Thailand, South Africa, Philippines, Mexico, Malaysia, Indonesia, India, China, Brazil.
Hydrofluorocarbons sulphur, hexafluoride, and perfluorocarbons.
Abu Bakar NA (2019) Greenfield, mergers and acquisitions, energy consumption, and environmental performance in selected SAARC and ASEAN countries. Int J Energy Econ Policy 9(2):216–224
Adeel-Farooq RM, Abu Bakar NA, Olajide Raji J (2018) Green field investment and environmental performance: a case of selected nine developing countries of Asia. Environ Prog Sustain Energy 37:1085–1092
Article CAS Google Scholar
Adom PK, Opoku EEO, Yan IKM (2019) Energy demand–FDI nexus in Africa: do FDIs induce dichotomous paths? Energy Econ 81:928–941. https://doi.org/10.1016/j.eneco.2019.05.030
Article Google Scholar
Ahmed Z, Asghar MM, Malik MN, Nawaz K (2020a) Moving towards a sustainable environment: the dynamic linkage between natural resources, human capital, urbanization, economic growth, and ecological footprint in China. Resour Policy 67:101677
Ahmed Z, Zafar MW, Ali S (2020b) Linking urbanization, human capital, and the ecological footprint in G7 countries: an empirical analysis. Sustain Cities Soc 55:102064
Akadiri S saint, Adebayo TS, Asuzu OC et al (2022) Testing the role of economic complexity on the ecological footprint in China: a nonparametric causality-in-quantiles approach. Energy Environ 34(7):2290–2316
Al-Mulali U, Ozturk I (2015) The effect of energy consumption, urbanization, trade openness, industrial output, and the political stability on the environmental degradation in the MENA (Middle East and North African) region. Energy 84:382–389
Al-Mulali U, Weng-Wai C, Sheau-Ting L, Mohammed AH (2015) Investigating the environmental Kuznets curve (EKC) hypothesis by utilizing the ecological footprint as an indicator of environmental degradation. Ecol Indic 48:315–323
Aluko OA, Opoku EEO, Acheampong AO (2022) Economic complexity and environmental degradation: evidence from OECD countries. Bus Strategy Environ n/a. https://doi.org/10.1002/bse.3269
Álvarez-Herránz A, Balsalobre D, Cantos JM, Shahbaz M (2017) Energy innovations-GHG emissions nexus: fresh empirical evidence from OECD countries. Energy Policy 101:90–100. https://doi.org/10.1016/j.enpol.2016.11.030
Baek J (2016) A new look at the FDI–income–energy–environment nexus: dynamic panel data analysis of ASEAN. Energy Policy 91:22–27
Bashir MF (2022) Discovering the evolution of Pollution Haven Hypothesis: a literature review and future research agenda. Environ Sci Pollut Res 29:48210–48232. https://doi.org/10.1007/s11356-022-20782-1
Bashir MF, Ma B, Shahbaz M, Jiao Z (2020) The nexus between environmental tax and carbon emissions with the roles of environmental technology and financial development. PLoS One 15:e0242412
Bashir MF, MA B, Hussain HI et al (2022a) Evaluating environmental commitments to COP21 and the role of economic complexity, renewable energy, financial development, urbanization, and energy innovation: empirical evidence from the RCEP countries. Renew Energy 184:541–550. https://doi.org/10.1016/j.renene.2021.11.102
Bashir MF, Sadiq M, Talbi B et al (2022b) An outlook on the development of renewable energy, policy measures to reshape the current energy mix, and how to achieve sustainable economic growth in the post COVID-19 era. Environ Sci Pollut Res 29:43636–43647. https://doi.org/10.1007/s11356-022-20010-w
Bashir MF, Pan Y, Shahbaz M, Ghosh S (2023) How energy transition and environmental innovation ensure environmental sustainability? Contextual evidence from Top-10 manufacturing countries. Renew Energy 204:697–709. https://doi.org/10.1016/j.renene.2023.01.049
Bekun FV, Alola AA, Sarkodie SA (2019) Toward a sustainable environment: nexus between CO2 emissions, resource rent, renewable and nonrenewable energy in 16-EU countries. Sci Total Environ 657:1023–1029
Bilgili F, Kuşkaya S, Khan M et al (2021) The roles of economic growth and health expenditure on CO2 emissions in selected Asian countries: a quantile regression model approach. Environ Sci Pollut Res 28:44949–44972
Bukhari WAA, Pervaiz A, Zafar M et al (2023) Role of renewable and non-renewable energy consumption in environmental quality and their subsequent effects on average temperature: an assessment of sustainable development goals in South Korea. Environ Sci Pollut Res. https://doi.org/10.1007/s11356-023-30493-w
Can M, Gozgor G (2017) The impact of economic complexity on carbon emissions: evidence from France. Environ Sci Pollut Res 24:16364–16370
Charfeddine L (2017) The impact of energy consumption and economic development on ecological footprint and CO2 emissions: evidence from a Markov switching equilibrium correction model. Energy Econ 65:355–374
Charfeddine L, Mrabet Z (2017) The impact of economic development and social-political factors on ecological footprint: a panel data analysis for 15 MENA countries. Renew Sustain Energy Rev 76:138–154
Chu LK (2021) Economic structure and environmental Kuznets curve hypothesis: new evidence from economic complexity. Appl Econ Lett 28:612–616
Chu LK, Le NTM (2022) Environmental quality and the role of economic policy uncertainty, economic complexity, renewable energy, and energy intensity: the case of G7 countries. Environ Sci Pollut Res 29:2866–2882
Danish, Wang Z (2019) Investigation of the ecological footprint’s driving factors: what we learn from the experience of emerging economies. Sustain Cities Soc 49:101626
Destek MA, Manga M (2021) Technological innovation, financialization, and ecological footprint: evidence from BEM economies. Environ Sci Pollut Res 28:21991–22001
Dogan E, Ulucak R, Kocak E, Isik C (2020) The use of ecological footprint in estimating the environmental Kuznets curve hypothesis for BRICST by considering cross-section dependence and heterogeneity. Sci Total Environ 723:138063
Doğan B, Balsalobre-Lorente D, Nasir MA (2020) European commitment to COP21 and the role of energy consumption, FDI, trade and economic complexity in sustaining economic growth. J Environ Manag 273:111146
Doğan B, Driha OM, Balsalobre Lorente D, Shahzad U (2021) The mitigating effects of economic complexity and renewable energy on carbon emissions in developed countries. Sustain Dev 29:1–12
Doytch N (2020) The impact of foreign direct investment on the ecological footprints of nations. Environ Sustain Indic 8:100085
Google Scholar
Doytch N, Ashraf A (2022) The ecological footprints of greenfield FDI and cross-border M&A Sales. Environ Model Assess 27:935–951
Doytch N, Narayan S (2016) Does FDI influence renewable energy consumption? An analysis of sectoral FDI impact on renewable and non-renewable industrial energy consumption. Energy Econ 54:291–301
Dumitrescu EI, Hurlin C (2012) Testing for Granger non-causality in heterogeneous panels. Econ Model 29:1450–1460. https://doi.org/10.1016/j.econmod.2012.02.014
García-Quevedo J, Jové-Llopis E (2021) Environmental policies and energy efficiency investments. An industry-level analysis. Energy Policy 156:112461
Gormus S, Aydin M (2020) Revisiting the environmental Kuznets curve hypothesis using innovation: new evidence from the top 10 innovative economies. Environ Sci Pollut Res 27:27904–27913
Hassan ST, Wang P, Khan I, Zhu B (2023) The impact of economic complexity, technology advancements, and nuclear energy consumption on the ecological footprint of the USA: Towards circular economy initiatives. Gondwana Res 113:237–246
He K, Ramzan M, Awosusi AA et al (2021) Does globalization moderate the effect of economic complexity on CO2 emissions? Evidence from the top 10 energy transition economies. Front Environ Sci 9:778088
IEA (2020) World energy outlook 2020. International Energy Agency: Paris, France, p 2050
Ke H, Yang W, Liu X, Fan F (2020) Does innovation efficiency suppress the ecological footprint? Empirical evidence from 280 Chinese cities. Int J Environ Res Public Health 17:6826
Khezri M, Heshmati A, Khodaei M (2022) Environmental implications of economic complexity and its role in determining how renewable energies affect CO2 emissions. Appl Energy 306:117948
Kihombo S, Ahmed Z, Chen S et al (2021) Linking financial development, economic growth, and ecological footprint: what is the role of technological innovation? Environ Sci Pollut Res 28:61235–61245
Kosifakis G, Kampas A, Papadas CT (2020) Economic complexity and the environment: some estimates on their links. Int J Sustain Agric Manag Inform 6:261–271
Lee C-C, Chen M-P, Wu W (2022) The criticality of tourism development, economic complexity, and country security on ecological footprint. Environ Sci Pollut Res 29:37004–37040
Leitão NC, Balsalobre-Lorente D, Cantos-Cantos JM (2021) The impact of renewable energy and economic complexity on carbon emissions in BRICS countries under the EKC scheme. Energies (basel) 14:4908
Li S, Sun H, Sharif A et al (2024) Economic complexity, natural resource abundance and education: implications for sustainable development in BRICST economies. Resour Policy 89:104572. https://doi.org/10.1016/j.resourpol.2023.104572
Liang W, Yang M (2019) Urbanization, economic growth and environmental pollution: evidence from China. Sustai Comput: Inform Syst 21:1–9. https://doi.org/10.1016/j.suscom.2018.11.007
Long X, Ji X, Ulgiati S (2017) Is urbanization eco-friendly? An energy and land use cross-country analysis. Energy Policy 100:387–396
Luo W, Bai H, Jing Q et al (2018) Urbanization-induced ecological degradation in Midwestern China: an analysis based on an improved ecological footprint model. Resour Conserv Recycl 137:113–125
Ma B, Lin S, Bashir MF et al (2023a) Revisiting the role of firm-level carbon disclosure in sustainable development goals: research agenda and policy implications. Gondwana Res 117:230–242. https://doi.org/10.1016/j.gr.2023.02.002
Ma B, Sharif A, Bashir M, Bashir MF (2023b) The dynamic influence of energy consumption, fiscal policy and green innovation on environmental degradation in BRICST economies. Energy Policy 183:113823. https://doi.org/10.1016/j.enpol.2023.113823
Mahmood N, Zhao Y, Lou Q, Geng J (2022) Role of environmental regulations and eco-innovation in energy structure transition for green growth: evidence from OECD. Technol Forecast Soc Chang 183:121890
Majeed MT, Mazhar M, Samreen I, Tauqir A (2022) Economic complexities and environmental degradation: evidence from OECD countries. Environ Dev Sustain 24:5846–5866
Martins JM, Adebayo TS, Mata MN et al (2021) Modeling the relationship between economic complexity and environmental degradation: evidence from top seven economic complexity countries. Front Environ Sci 9(2021):744781
Mealy P, Teytelboym A (2022) Economic complexity and the green economy. Res Policy 51:103948
Mensah CN, Long X, Boamah KB et al (2018) The effect of innovation on CO2 emissions of OCED countries from 1990 to 2014. Environ Sci Pollut Res 25:29678–29698
Mrabet Z, AlSamara M, Hezam Jarallah S (2017) The impact of economic development on environmental degradation in Qatar. Environ Ecol Stat 24:7–38
Nathaniel S, Khan SAR (2020) The nexus between urbanization, renewable energy, trade, and ecological footprint in ASEAN countries. J Clean Prod 272:122709
Neagu O (2021) Economic complexity: a new challenge for the environment. Earth 2:1059–1076
Okamoto S (2013) Impacts of growth of a service economy on CO 2 emissions: Japan’s case. J Econ Struct 2:1–21
Pesaran MH (2007) A simple panel unit root test in the presence of cross-section dependence. J Appl Economet 22:265–312. https://doi.org/10.1002/jae.951
Ponce P, Álvarez-García J, Álvarez V, Irfan M (2022) Analysing the influence of foreign direct investment and urbanization on the development of private financial system and its ecological footprint. Environ Sci Pollut Res 30(4):9624–9641
Rafei M, Esmaeili P, Balsalobre-Lorente D (2022) A step towards environmental mitigation: How do economic complexity and natural resources matter? Focusing on different institutional quality level countries. Resour Policy 78:102848
Sadiq M, Wen F, Bashir MF, Amin A (2022) Does nuclear energy consumption contribute to human development? Modeling the effects of public debt and trade globalization in an OECD heterogeneous panel. J Clean Prod 375:133965. https://doi.org/10.1016/j.jclepro.2022.133965
Shahbaz M, Balsalobre D, Shahzad SJH (2019) The influencing factors of CO 2 emissions and the role of biomass energy consumption: statistical experience from G-7 countries. Environ Model Assess 24:143–161
Shahbaz M, Sinha A, Raghutla C, Vo XV (2022) Decomposing scale and technique effects of financial development and foreign direct investment on renewable energy consumption. Energy 238:121758
Swart J, Brinkmann L (2020) Economic complexity and the environment: evidence from Brazil. In: Universities and sustainable communities: meeting the goals of the agenda 2030. pp 3–45. Springer International Publishing, 2020
Wang Y, Kang L, Wu X, Xiao Y (2013) Estimating the environmental Kuznets curve for ecological footprint at the global level: a spatial econometric approach. Ecol Indic 34:15–21
World Bank (2022) World development indicators 2022. The World Bank 2022
Yahya F, Rafiq M (2020) Brownfield, greenfield, and renewable energy consumption: moderating role of effective governance. Energy Environ 31:405–423
Yilanci V, Pata UK (2020) Investigating the EKC hypothesis for China: the role of economic complexity on ecological footprint. Environ Sci Pollut Res 27:32683–32694
Yu Y, Jiang T, Li S et al (2020) Energy-related CO2 emissions and structural emissions’ reduction in China’s agriculture: an input–output perspective. J Clean Prod 276:124169
Download references
Moreover, we acknowledge funding support from Project (Grant No:2023JJ40061) supported by Natural Science Foundation of Hunan Province.
Author information
Authors and affiliations.
College of Management, Shenzhen University, Shenzhen, Guangzhou, People’s Republic of China
Muhammad Farhan Bashir
Department of Economics, University of Pretoria, Pretoria, South Africa
Roula Inglesi-Lotz
Department of Economic and Finance, Sunway Business School, Sunway University, Subang Jaya, Malaysia
Ummara Razi
Department of Business Administration, ILMA University, Karachi, Pakistan
Independent Researcher, Guangzhou, Guangdong, People’s Republic of China
Luqman Shahzad
You can also search for this author in PubMed Google Scholar
Contributions
Muhammad Farhan Bashir: conceptualization, methodology, writing draft, writing—revision; Roula Inglesi-Lotz: data curation, writing draft, methodology, data analysis; Ummara Razi: writing draft; Luqman Shahzad: data curation; methodology.
Corresponding author
Correspondence to Muhammad Farhan Bashir .
Ethics declarations
Ethics approval, consent to participate, consent for publication, competing interests.
The author declares no competing interest.
Additional information
Responsible Editor: Philippe Garrigues
Publisher's Note
Springer Nature remains neutral with regard to jurisdictional claims in published maps and institutional affiliations.
Rights and permissions
Springer Nature or its licensor (e.g. a society or other partner) holds exclusive rights to this article under a publishing agreement with the author(s) or other rightsholder(s); author self-archiving of the accepted manuscript version of this article is solely governed by the terms of such publishing agreement and applicable law.
Reprints and permissions
About this article
Bashir, M.F., Inglesi-Lotz, R., Razi, U. et al. Economic complexity, greenfield investments, and energy innovation: policy implications for sustainable development goals in newly industrialised economies. Environ Sci Pollut Res (2024). https://doi.org/10.1007/s11356-024-33433-4
Download citation
Received : 07 November 2023
Accepted : 18 April 2024
Published : 15 May 2024
DOI : https://doi.org/10.1007/s11356-024-33433-4
Share this article
Anyone you share the following link with will be able to read this content:
Sorry, a shareable link is not currently available for this article.
Provided by the Springer Nature SharedIt content-sharing initiative
- Newly industrialised countries
- Energy transition
- Environmental degradation
- Economics complexity
- Greenfield investment
- Find a journal
- Publish with us
- Track your research

UCL Institute for Sustainable Resources
- Partnerships
- Publications

New research shows the damage caused by the ‘Green grabbing’ of Brazilian public and common lands
13 May 2024
New research from UCL and Boku University shows that a lack of transparency is allowing for the privatisation of land for renewable energy infrastructure in Brazil by large international corporations
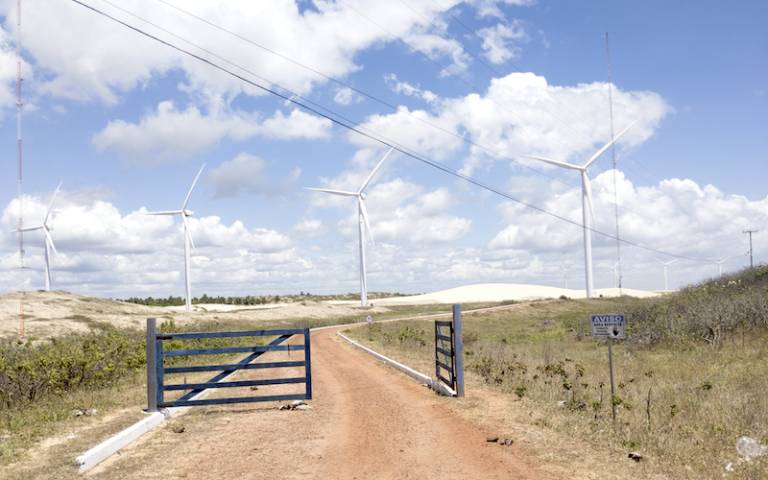
The study, published in Nature Sustainability, found that land privatisation is the dominant means for solar and wind companies to secure land for their power generation infrastructure, which includes the transfer of control of formerly public and common lands to often international companies.
In the years between 2011and 2021, wind power in Brazil grew from 1.2% of its power generation capacity to 11.4%, and solar power grew from 0.1% to 2.6%, with plans for significant growth in place over the next several decades. While the transition from fossil fuels to renewable energy reduces carbon emissions, wind parks and solar photovoltaic parks require a significant land footprint.
The researchers combined databases of geographic data with ownership and investment information, and digital land registries from 2000 through 2021. They found that wind parks take up a total of about 2,148 square kilometres and solar parks take up about 102 square kilometres in Brazil. However, they found that international investors, primarily from Europe, claimed a 78% stake in wind parks and 96% share of solar assets. While on paper Brazilian entities own 89% of wind parks, the majority (68%) are subsidiaries of internationally controlled companies, particularly from Italy and France.
Most of the land that these companies build their energy infrastructure on is private property. However, there is a growing concern that the privatisation process is stimulated by the expansion of renewable energies and acquired by dispossessing local residents. A significant portion of wind parks have been built on legally dubious ground, including 28% that only rely on environmental registries without legal property titles, and 7% that are built on public common land.
Co-author Dr Nadia Ameli (UCL Bartlett School of Environment, Energy and Resources) noted:
“ Our work highlights the importance of striking a balance between sustainable energy development and the protection of public land and commons. Privatisation with poor oversight and little transparency is being used to appropriate and transfer land from traditional communities to large international corporations, despite great land tenure insecurity.
This kind of appropriation is common in Brazil because of historical inequities in land ownership, regulatory gaps and weak governance. The phenomenon of ‘land grabbing’ is well documented across Brazil, commonly for agricultural purposes like creating large cattle ranches and soybean farms. It has led to environmental degradation and numerous conflicts over land ownership between Brazil’s government, farmers and ranchers, and the country’s traditional and indigenous population. The researchers are concerned that the trend will continue as ‘green grabbing,’ which uses the need for clean and renewable energy as a justification for the further appropriation of public and common lands.
Lead author, Dr Michael Klingler of Boku University, added:
“ The land issue is central to driving effective climate action and promoting 'just' low-carbon energy pathways. The necessity for the energy transition must not be questioned, but rather the way in which these projects are implemented. The collaboration with affected rural communities, NGOs and scientists in the Brazilian state of Bahia showed that traditional and marginalized population groups are confronted with 'new' land conflicts due to the expansion of renewable energies. However, these are increasingly being legitimized by the climate change mitigation imperative.
The research was supported by the European Research Council.
- Read the research paper in Nature Sustainability
- Dr Nadia Ameli's academic profile
- Dr Michael Klinger's academic profile
- UCL Bartlett School of Environment, Energy & Resources
- UCL Faculty of the Built Environment
- Boku University
- Image credit: Michael Klingler
- Image description: Wind turbines visible on sandy land fenced off with barbed wire in the state of Ceará, Brazil.
Related News
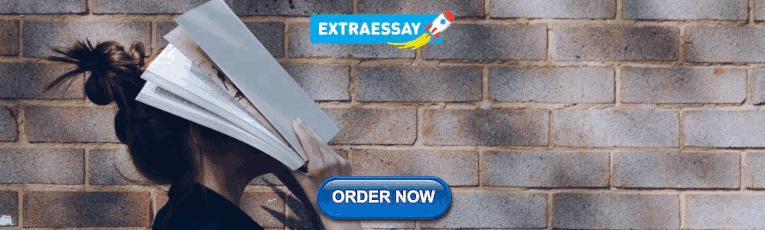
IMAGES
COMMENTS
Energy is a linchpin for most of the SDGs, and research that merges climate, energy and the SDGs underscores this 1. For example, the agriculture and food-transport sectors still depend on fossil ...
Sustainable Energy Research (formerly Renewables: Wind, Water, and Solar) provides a multidisciplinary and international forum for research in the basic science, technologies, industrial R&D, products and system implementation that accelerate the transition to sustainable energy on a local and global scale.Sustainable Energy Research welcomes contributions on all sources of energy that support ...
To learn more about the MA in Sustainable Energy (online) and download a brochure, fill out the fields below, or call +1 410-648-2495 or toll-free at +1 888-513-5303 to talk with one of our admissions counselors. By checking this box, I authorize Johns Hopkins University and its representatives to contact me via SMS. Participation is voluntary.
NREL's new open-source generative machine learning model, Sup3rCC, rapidly produces detailed future climate data to support energy system planning. The National Renewable Energy Laboratory (NREL) is transforming energy through research, development, commercialization, and deployment of renewable energy and energy efficiency technologies.
The MIT Energy Initiative, MIT's hub for energy research, education, and outreach, is advancing zero- and low-carbon solutions to combat climate change and expand energy access. Read our full mission statement →. H2 Underground. At the 2024 MIT Energy Initiative Spring Symposium, experts weighed whether hydrogen stored in the earth might be ...
Hydropower is the world's biggest source of renewable energy by far, with China, Brazil, Canada, the U.S., and Russia the leading hydropower producers. While hydropower is theoretically a clean ...
Focus and Coverage. The Journal of Renewable and Sustainable Energy is an interdisciplinary journal covering specific areas of renewable and sustainable energy relevant to the physical science and engineering communities. The journal has a strong focus on integration of disciplines for renewable power technologies at global scales that have the ...
He is a professor of energy science and engineering in the Stanford Doerr School of Sustainability, a professor, by courtesy, of chemistry in the School of Humanities and Sciences, and a professor ...
Sustainable energy resources are crucial to energy sustainability, as are complementary energy carriers that allow those energy resources to be exploited or facilitate sustainable energy options. ... Much research on energy resources has been reported, including electricity generation from food waste through anaerobic digestion (Ali et al. 2019 ...
The greatest sustainability challenge facing humanity today is the greenhouse gas emissions and the global climate change with fossil fuels led by coal, natural gas and oil contributing 61.3% of global electricity generation in the year 2020. The cumulative effect of the Stockholm, Rio, and Johannesburg conferences identified sustainable energy development (SED) as a very important factor in ...
The U.S. Department of Energy's Office of Energy Efficiency and Renewable Energy (EERE) has three core divisions: Renewable Energy, Sustainable Transportation and Fuels, and Buildings and Industry. The Renewable Energy pillar comprises four technology offices: Geothermal Technologies Office. Solar Energy Technologies Office.
Initiatives. Advanced and Sustainable Energy. A core mission of the DOE Office of Science is to lay the scientific groundwork for the next generation of energy technologies. We do so by supporting basic research across a wide range of disciplines, as well the construction and operation of major scientific facilities, to provide the foundation ...
Find a journal Publish with us Track your research Search. Cart. Sustainable Energy Research. Search within journal. Search. Volumes and issues. Volume 11 December 2024. December 2024, issue 1; Volume 10 December 2023. December 2023, issue 1; Volume 9 December 2022. December 2022, issue 1; Volume 8 December 2021 ...
The use of renewable energy resources, such as solar, wind, and biomass will not diminish their availability. Sunlight being a constant source of energy is used to meet the ever-increasing energy need. This review discusses the world's energy needs, renewable energy technologies for domestic use, and highlights public opinions on renewable energy. A systematic review of the literature was ...
While many areas of green energy are growing quickly, we identified and prioritized the top ten emerging topics (Figure 1) that will help us reach a more sustainable future. Figure 1: Normalized growth in the number of publications between 2013-2022 for the emerging research topics. Batteries, energy storage, and battery recycling
This research contributes to the overarching objectives of achieving carbon neutrality and enhancing environmental governance by examining the role of artificial intelligence-enhanced multi-energy optimization in rural energy planning within the broader context of a sustainable energy economy. By proposing an innovative planning framework that accounts for geographical and economic disparities ...
Improved efficiency of energy systems and development of sustainable, low-carbon-emission energy generation processes are essential for the long-term health of the environment. Recent increases in energy prices provide a graphic reminder of the importance of energy to our economy and our quality of life. Most of our endeavors — economic ...
The Ralph O'Connor Sustainable Energy Institute (ROSEI) is a community of researchers at Johns Hopkins University (JHU) that is committed to advancing sustainable energy, and we would love for you to join us. PhD programs are housed within the academic departments at JHU, so PhD students working in sustainable energy span many parts of the ...
The field of sustainability science tries to figure out how interactions of nature and society can support sustainable development. One key goal is figuring out how to restructure complex consumption-production systems. Examples of these systems are production and consumption of food, energy, and mobility.
From fostering innovation to job creation, renewable energy solutions drive progress towards a more equitable and sustainable world. Many renewable energy solutions create opportunities for economic development while reducing greenhouse gas emissions. Here are some examples of how renewable energy solutions are changing lives all over the world.
Sustainable energy includes any energy source that cannot be depleted and can remain viable forever. It does not need to be renewed or replenished; sustainable energy meets our demand for energy without any risk of going bad or running out. This is why sustainable energy is the answer to our energy needs. Furthermore, sustainable energy doesn ...
Dr. Baxter researches sustainable energy in several forms. His current projects include biomass gasification and combustion, nuclear reactor neutronics, isotope-specific gas kinetics, natural gas processing, and carbon capture. He does both laboratory and theoretical/modeling work and produces both academic and industrial results, including ...
Sustainable Energy. Our research program in sustainable energy has five highly interrelated research areas including fuel cells, flow batteries, photovoltaic materials, catalysis, biocatalysis, and bio-fuels. Some current research projects include inorganic and hybrid polymeric/biological photovoltaic materials; polyelectrolyte membrane ...
The crucial role of environmental assessment quality has been recognised by environmental and sustainable development goals in addressing climate change challenges. By focusing on the key identifier of environmental assessment, progress can be made towards overcoming climate change issues effectively. The current study considers environmental commitments under COP28 to study the role of ...
New research from UCL and Boku University shows that a lack of transparency is allowing for the privatisation of land for renewable energy infrastructure in Brazil by large international corporations. ... " Our work highlights the importance of striking a balance between sustainable energy development and the protection of public land and ...