Deforestation, warming flip part of Amazon forest from carbon sink to source
- July 14, 2021
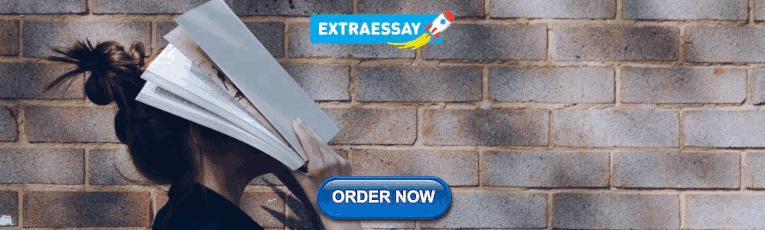
The study area, which represents about 20 percent of the Amazon basin, has lost 30 percent of its rainforest
New results from a nine-year research project in the eastern Amazon rainforest finds that significant deforestation in eastern and southeastern Brazil has been associated with a long-term decrease in rainfall and increase in temperature during the dry season, turning what was once a forest that absorbed carbon dioxide into a source of planet-warming carbon dioxide emissions.
The study, published in the journal Nature , explored whether these changes had altered how much carbon the Amazon stored in its vast forests.
“Using nearly 10 years of CO 2 (carbon dioxide ) measurements, we found that the more deforested and climate-stressed eastern Amazon, especially the southeast, was a net emitter of CO 2 to the atmosphere, especially as a result of fires,” said John Miller, a scientist with NOAA’s Global Monitoring Laboratory and a co-author. “On the other hand, the wetter, more intact western and central Amazon, was neither a carbon sink nor source of atmospheric CO 2 , with the absorption by healthy forests balancing the emissions from fires.”
In addition to storing vast amounts of carbon, Amazonia is also one of the wettest places on Earth, storing immense amounts of water in its soils and vegetation. Transpired by leaves, this moisture evaporates into the atmosphere, where it fuels prodigious rainfall, averaging more than seven feet per year across the basin. For comparison the average annual rainfall in the contiguous U.S. is two and half feet. Several studies have estimated that water cycling through evaporation is responsible for 25 to 35 percent of total rainfall in the basin.
But deforestation and global warming over the last 40 years have affected rainfall and temperature with potential impacts for the Amazon’s ability to store carbon. Conversion of rainforest to agriculture has caused a 17 percent decrease in forest extent in the Amazon, which stretches over an area almost as large as the continental U.S.. Replacing dense, humid forest canopies with drier pastures and cropland has increased local temperatures and decreased evaporation of water from the rainforest, which deprives downwind locations of rainfall. Regional deforestation and selective logging of adjacent forests further reduces forest cover, amplifying the cycle of drying and warming. This, in turn, can reduce the capacity of the forests to store carbon, and increase their vulnerability to fires.
The 2.8 million square miles of jungle in the Amazon basin represents more than half of the tropical rainforest remaining on the planet. The Amazon is estimated to contain about 123 billion tons of carbon above and below ground, and is one of Earth’s most important terrestrial carbon reserves. As global fossil-fuel burning has risen, the Amazon has absorbed CO 2 from the atmosphere, helping to moderate global climate. But there are indications from this study and previous ones that the Amazon’s capacity to act as a sink may be disappearing.
Over the past several decades, intense scientific interest has focused on the question of whether the combined effects of climate change and the ongoing conversion of jungle to pasture and cropland could cause the Amazon to release more carbon dioxide than it absorbs.
In 2010, lead author Luciana Gatti, who led the international team of scientists from Brazil, the United Kingdom, New Zealand and the Netherlands, set out to explore this question. During the next nine years, Gatti, a scientist with Brazil’s National Institute for Space Research and colleagues obtained airborne measurements of CO 2 and carbon monoxide concentrations above Brazilian Amazonia. Analysis of CO 2 measurements from over 600 aircraft vertical profiles, extending from the surface to around 2.8 miles above sea level at four sites, revealed that total carbon emissions in eastern Amazonia are greater than those in the west.
“The regions of southern Pará and northern Mato Grosso states represent a worst-case scenario,” said Gatti.
The southeast region, which represents about 20 percent of the Amazon basin, and has experienced 30 percent deforestation over the previous 40 years. Scientists recorded a 25 percent reduction in precipitation and a temperature increase of at least 2.7 degrees Fahrenheit during the dry months of August, September and October, when trees are already under seasonal stress. Airborne measurements over nine years revealed this region was a net emitter of carbon, mainly as a result of fires, while areas further west, where less than 20 percent of the forest had been removed, sources balanced sinks. The scientists said the increased emissions were likely due to conversion of forest to cropland by burning, and by reduced uptake of CO 2 by the trees that remained.
These findings help scientists better understand the long-term impacts of interactions between climate and human disturbances on the carbon balance of the world’s largest tropical forest.
“The big question this research raises is if the connection between climate, deforestation, and carbon that we see in the eastern Amazon could one day be the fate of the central and western Amazon, if they become subject to stronger human impact,” Miller said. Changes in the capacity of tropical forests to absorb carbon will require downward adjustments of the fossil fuel emissions compatible with limiting global mean temperature increases to less than 2.0 or 1.5 degrees Celsius, he added.
This research was supported by NOAA’s Global Monitoring Laboratory and by funding from the State of Sao Paulo Science Foundation, UK Environmental Research Council, NASA, and the European Research Council.
For more information, contact Theo Stein, NOAA Communications: [email protected]
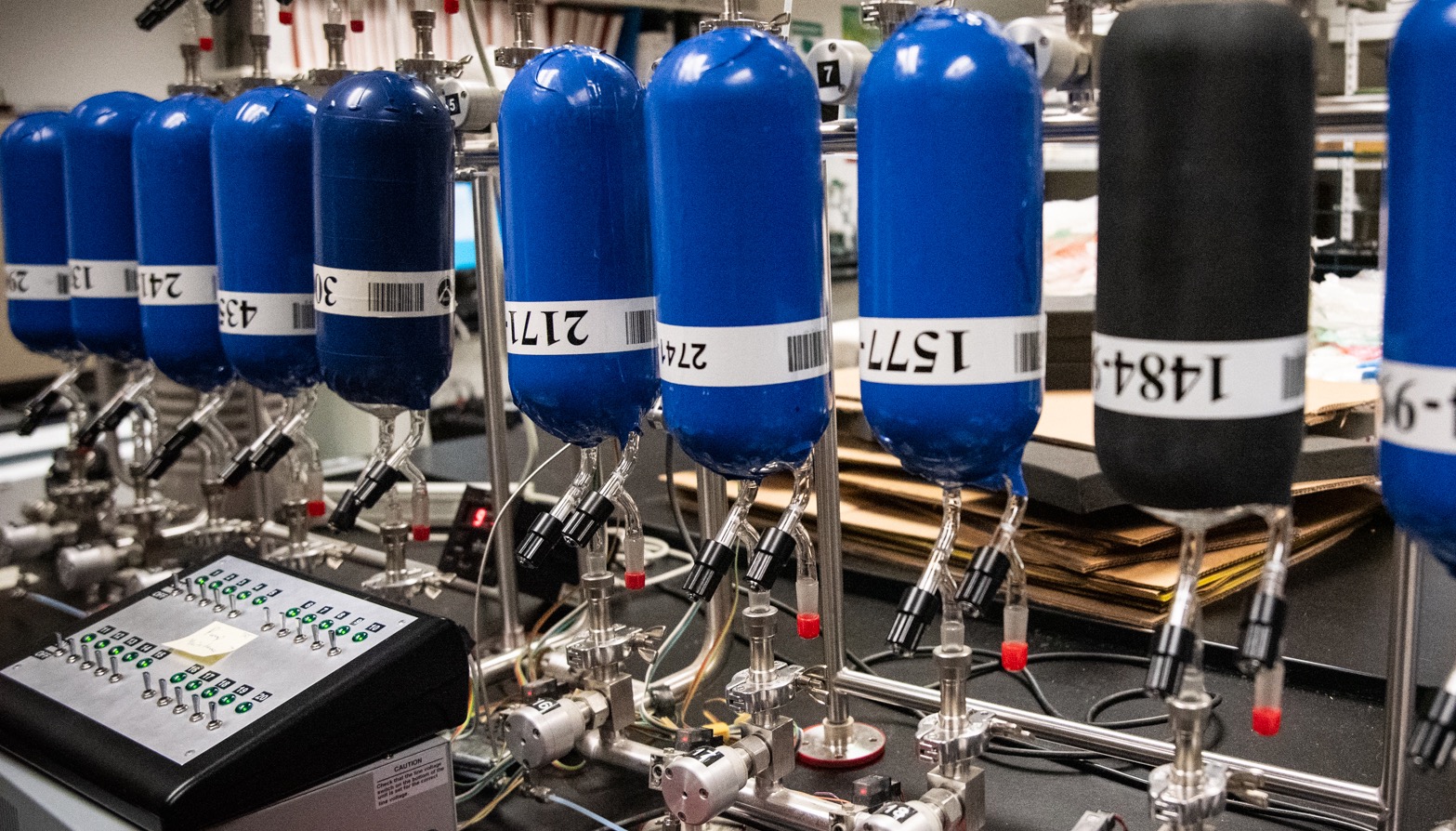
No sign of greenhouse gases increases slowing in 2023
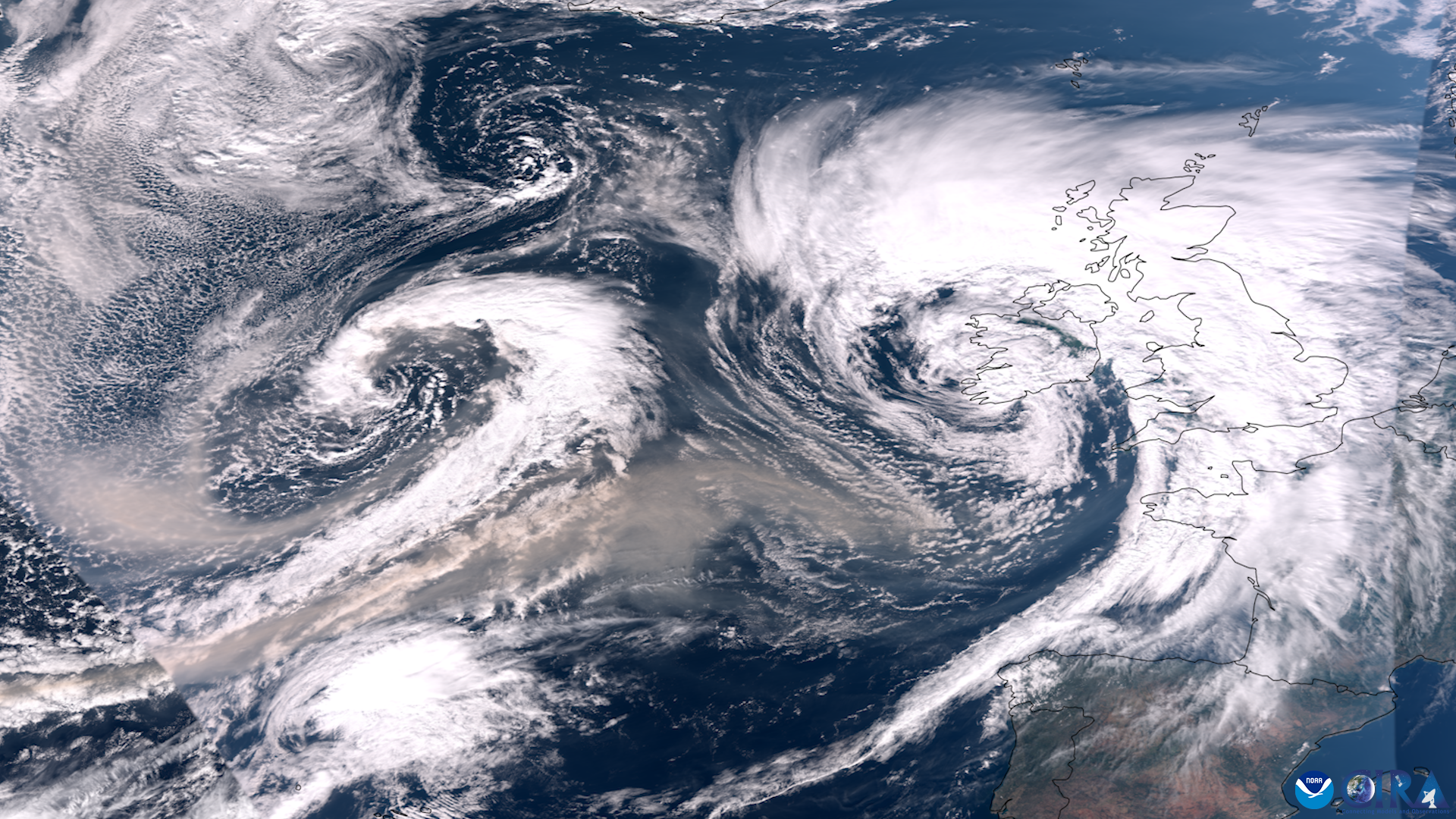
5 science wins from the 2023 NOAA Science Report
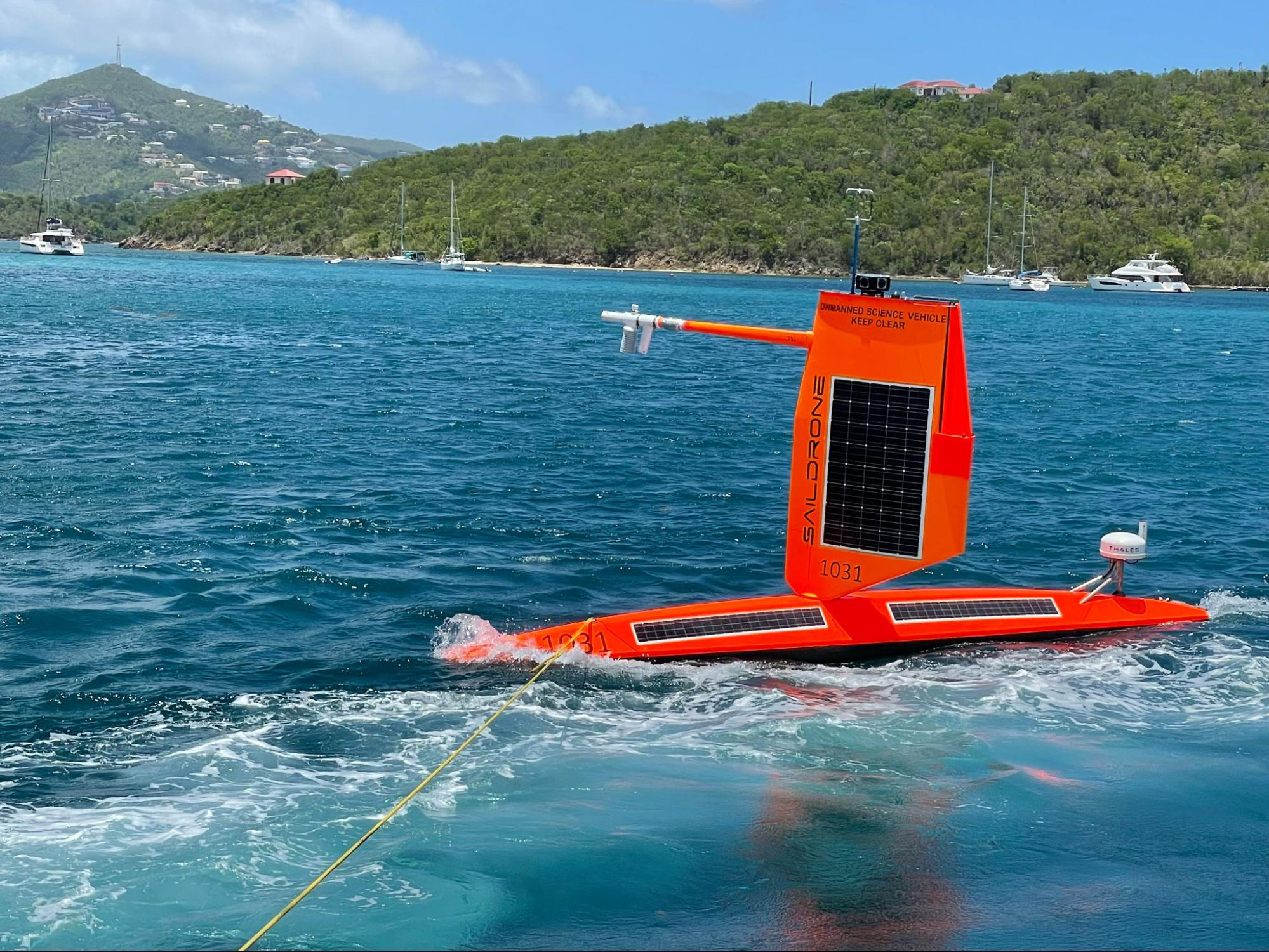
Filling A Data Gap In The Tropical Pacific To Reveal Daily Air-Sea Interactions
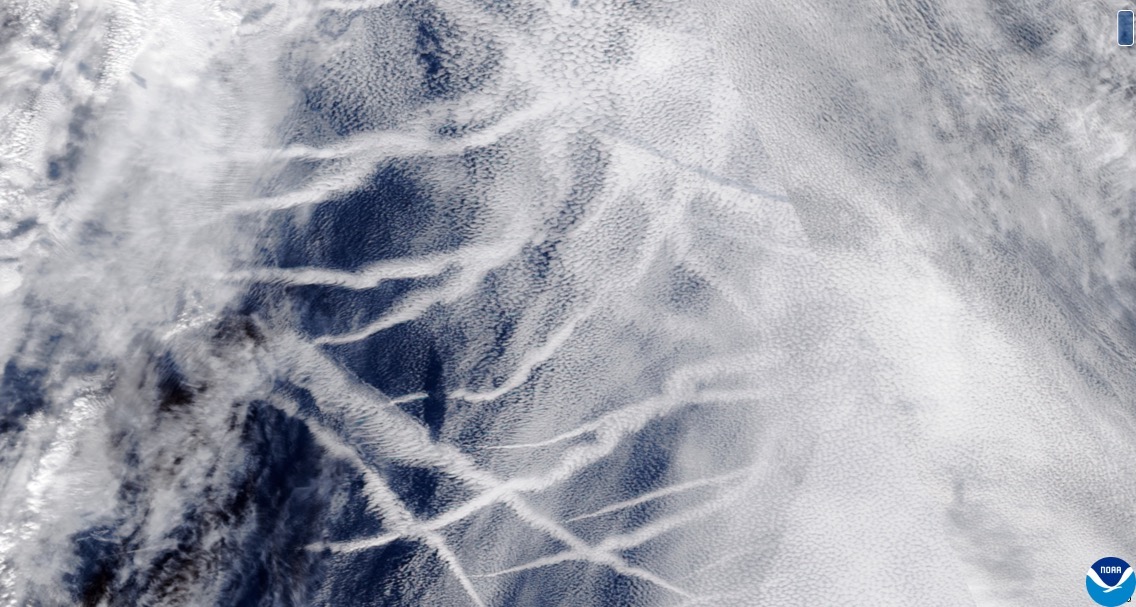
Scientists detail research to assess viability and risks of marine cloud brightening
Popup call to action.
A prompt with more information on your call to action.
Amazon Deforestation and Climate Change
Join Gisele Bundchen when she meets with one of Brazil’s top climate scientists to discuss the complexity of the Amazon rainforest and its connection to Earth’s atmosphere.
Anthropology, Geography
High on a tower overlooking the lush Amazon canopy, Gisele Bundchen and Brazilian climate scientist Antonio Nobre talk about the importance of the rain forest and the impact of cutting down its trees.
As Nobre explains, the rainforest is not only home to an incredible diversity of species, it also has a critical cooling effect on the planet because its trees channel heat high into the atmosphere. In addition, forests absorb and store carbon dioxide (CO2) from the atmosphere—CO2 that is released back into the atmosphere when trees are cut and burned.
Nobre warns that if deforestation continues at current levels, we are headed for disaster. The Amazon region could become drier and drier, unable to support healthy habitats or croplands.
Find more of this story in the “Fueling the Fire” episode of the National Geographic Channel’s Years of Living Dangerously series.
The Amazon rain forest absorbs one-fourth of the CO2 absorbed by all the land on Earth. The amount absorbed today, however, is 30% less than it was in the 1990s because of deforestation. A major motive for deforestation is cattle ranching. China, the United States, and other countries have created a consumer demand for beef, so clearing land for cattle ranching can be profitable—even if it’s illegal. The demand for pastureland, as well as cropland for food such as soybeans, makes it difficult to protect forest resources.
Many countries are making progress in the effort to stop deforestation. Countries in South America and Southeast Asia, as well as China, have taken steps that have helped reduce greenhouse gas emissions from the destruction of forests by one-fourth over the past 15 years.
Brazil continues to make impressive strides in reducing its impact on climate change. In the past two decades, its CO2 emissions have dropped more than any other country. Destruction of the rain forest in Brazil has decreased from about 19,943 square kilometers (7,700 square miles) per year in the late 1990s to about 5,180 square kilometers (2,000 square miles) per year now. Moving forward, the major challenge will be fighting illegal deforestation.
Articles & Profiles
Instructional links, media credits.
The audio, illustrations, photos, and videos are credited beneath the media asset, except for promotional images, which generally link to another page that contains the media credit. The Rights Holder for media is the person or group credited.
Last Updated
March 11, 2024
User Permissions
For information on user permissions, please read our Terms of Service. If you have questions about how to cite anything on our website in your project or classroom presentation, please contact your teacher. They will best know the preferred format. When you reach out to them, you will need the page title, URL, and the date you accessed the resource.
If a media asset is downloadable, a download button appears in the corner of the media viewer. If no button appears, you cannot download or save the media.
Text on this page is printable and can be used according to our Terms of Service .
Interactives
Any interactives on this page can only be played while you are visiting our website. You cannot download interactives.
Related Resources
Case Study: The Amazonian Road Decision
The proposed Pucallpa–Cruzeiro do Sul will connect the Amazon’s interior to urban centers and export markets in Peru and Brazil. However, critics are worried that the road will also create new opportunities for illegal logging and infringe on the territory of indigenous communities and wildlife.
Biology, Geography, Human Geography
Loading ...
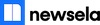
On the western edge of the Amazon River, there is a proposal to construct a road. This road would connect the remote town of Cruzeiro do Sul, Brazil, with the larger city of Pucallpa, Peru. The construction of the road has become a subject of contentious debate. Proponents of the road claimed that it would provide an efficient way for rural farmers and tradesmen to get their goods to city markets. They claimed it would also allow loggers to more easily transport timber from the depths of the Amazon rainforest to sawmills. From the sawmills in Pucallpa, goods could be transported to Peru’s Pacific coast and shipped to international buyers. Critics of the Pucallpa-Cruzeiro do Sul road, however, argue that it would cut right through traditional territories of the Ashéninka, an indigenous people of eastern Peru. Many leaders fear the road will increase access to previously undeveloped rainforest, threatening the ecosystem and the Ashéninka way of life. Large trees, such as mahogany, for example, will catch the eye of illegal loggers because of their high market value. The great mahogany trees also serve as protection to the Ashéninka from the outside world and are essential for the health of the Amazonian rainforest. The trees provide shelter, food, and nesting grounds that sustain the vast biodiversity within the ecosystem, an ecosystem the Ashéninka have come to depend on for their own food, shelter, and life sustenance. Geography The Amazon Basin is located in South America, covering an area of seven million square kilometers (2.7 million square miles). Nearly 70 percent of the basin falls within Brazil with remaining areas stretching into parts of Peru, Ecuador, Bolivia, Colombia, Venezuela, and Guyana. The Amazon’s massive drainage basin is made of dozens of smaller watersheds , including the Tamaya. Its watershed lies at the headwaters of the Purus and Juruá Rivers, near the border of Peru and Brazil. The Ashéninka people have lived in this region for centuries, surviving on game, fish, and cultivated crops, such as yucca roots, sweet potato, corn, coffee, and sugar cane. Background The rainforest surrounding the Amazon is the largest on the entire planet. In addition to 33 million human inhabitants, including 385 distinct Indigenous groups, it hosts the greatest diversity of plant and animal life in the world. More than two million species of insects are native to the region, including many tree-living species and hundreds of spiders and butterflies. Primates are abundant—including howler, spider, and capuchin monkeys—along with sloths, snakes, and iguanas. Brightly colored parrots, toucans, and parakeets are just some of the region’s native birds. Many of these species are unique to the Amazon rainforest, which means they cannot be found anywhere else in the world. At a global level, the Amazon rainforest helps to regulate climate and acts as a carbon sink for greenhouse gases . At a national level, the Amazon is considered a source of energy and income, based on production and commercialization of raw materials. Some of the most valued tree species in the world thrive in the rainforest. Mahogany is one of the most valuable resources from the Amazon forest. The tree’s rich, red grain and durability make it one of the most coveted building materials in the world. A single mahogany tree can fetch thousands of U.S. dollars on the international market. Even though logging is prohibited in much of the Amazon River, it is legal in some areas in large part because the sale of the wood is so lucrative. The high demand for mahogany has left many of Peru’s watersheds—such as the Tamaya—stripped of their most valuable trees. Without large trees, and their roots, the watershed risks heavy flooding and soil erosion. Conflict The Pucallpa-Cruzeiro do Sul road is part of a larger development plan to link South America’s remote, isolated economies through new transportation, energy, and telecommunications projects. Tension exists between communities that favor developing the rural economies of the Amazon Basin and those who favor preserving its forested areas and diversity of life. The Initiative for the Integration of the Regional Infrastructure of South America (IIRSA) is a proposal for the construction of several highways throughout the continent, five of them within the western Amazon Basin. The Pucallpa-Cruzeiro do Sul road is one such proposed highway. Supporters of the Pucallpa-Cruzeiro do Sul road say international demand for Amazonian resources could help develop the rural economies that are scattered throughout the basin. In addition to providing a route of access for rural goods to enter the global market, the road will allow members of rural communities to access better health care, education, and welfare. This could lead to improved living conditions, healthier lifestyles, and longer life spans. Conservationists are concerned that infrastructure such as the Pucallpa-Cruzeiro do Sul road will devastate an already weakened Amazonian ecosystem, as road access is highly correlated with deforestation . In Brazil, for instance, 80 percent of deforestation occurs within 48.28 kilometers (30 miles) of a road. Critics argue that the construction of a road along the Brazil-Peru corridor will provide easier access for loggers to reach mahogany and other trees. Indigenous communities like the Ashéninka will also be affected. These communities have largely chosen to maintain a traditional way of life, and conservationists are concerned that the Pucallpa-Cruzeiro do Sul road may expose them to disease and land theft. Identification of Stakeholders Indigenous Communities: Members of the Ashéninka community are trying to protect the forest and their native lands. Yet, like other Indigenous communities in the area, they are in turmoil, largely divided between those favoring conservation and those seeking greater economic opportunities. While the Ashéninka want to preserve their culture and connections to the forest, they also need access to things like clothes, soap, and medicine. The road could establish trade routes that make these goods more accessible. However, isolated peoples could be exposed to disease and land theft. Wildlife: The proposed Pucallpa-Cruzeiro do Sul road runs through Serra do Divisor National Park, Brazil, and other reserves that are home to threatened and rare species, including mammals, reptiles, and birds. For some of these species, such as the spider monkey and red howler monkey, the construction of the road could make their populations vulnerable to fragmentation and more visible to hunters. As mahogany and other canopy giants are removed, any wildlife that relies on the trees for shelter, nesting, or food will need to relocate. Amazonian Ecosystem: In addition to the detrimental effects to the flora and fauna in the area, the construction of the Pucallpa-Cruzeiro do Sul road could accelerate erosion, reduce water quality, and increase deforestation for agriculture and timber extraction. Tropical forest accounts for 40 percent of the global terrestrial carbon sink. A reduced number of trees could exacerbate global warming. Fewer forests means larger amounts of greenhouse gases entering the atmosphere. Logging Companies: If a road is constructed, loggers will have easier access to mahogany and other trees, allowing them to generate more income and provide a higher standard of living for their families and communities. A higher standard of living might include expanded educational opportunities, improved healthcare facilities, and the chance to participate in political debate. Residents of Rural Communities: The Pucallpa-Cruzeiro do Sul road would allow local farmers and business people to transfer goods from the Amazonian interior to Peru’s Pacific coast. Right now, merchants who want to travel between Cruzeiro do Sul and Pucallpa must do so by plane. A reliable road would improve basic infrastructure, transportation, and communication for greater commercial and social integration between Peru and Brazil, which meets part of the larger objective of the Initiative for the Integration of Regional Infrastructure in South America. International Consumers: The global demand for mahogany makes it a multimillion dollar business. Mahogany is used to create bedroom sets, cabinets, flooring, and patio decks throughout the world, mostly in the United States and Europe. Conflict Mitigation Groups are seeking to mitigate conflict in the Pucallpa-Cruzeiro do Sul road conflict through dialogue and alternate infrastructure plans. Environmental conservation groups have suggested that the Pucallpa-Cruzeiro do Sul road be removed from the list of approved projects until the community engages in greater communication surrounding two aspects of the project. First, conservationists are seeking more information on the environmental impact of the construction. This discussion involves local environmental groups, government representatives, and businesses. Second, conservationists are seeking full consent to the project from indigenous communities. Some critics of the Pucallpa-Cruzeiro do Sul road argue that roads are not the only option for the Pucallpa business community to extend its commerce. Traditional river systems are already in place. These critics think the fluvial network should be explored as a viable alternative to road construction. The Upper Amazon Conservancy is working with indigenous peoples to help protect their native territories. One initiative involves organizing community “vigilance committees” that consist of members of indigenous peoples who help park services by patrolling the edges of national parks and keeping illegal loggers out.
Media Credits
The audio, illustrations, photos, and videos are credited beneath the media asset, except for promotional images, which generally link to another page that contains the media credit. The Rights Holder for media is the person or group credited.
Research Manager
Educator reviewer, expert reviewer, last updated.
October 19, 2023
User Permissions
For information on user permissions, please read our Terms of Service. If you have questions about how to cite anything on our website in your project or classroom presentation, please contact your teacher. They will best know the preferred format. When you reach out to them, you will need the page title, URL, and the date you accessed the resource.
If a media asset is downloadable, a download button appears in the corner of the media viewer. If no button appears, you cannot download or save the media.
Text on this page is printable and can be used according to our Terms of Service .
Interactives
Any interactives on this page can only be played while you are visiting our website. You cannot download interactives.
Related Resources
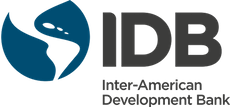
Our websites may use cookies to personalize and enhance your experience. By continuing without changing your cookie settings, you agree to this collection. For more information, please see our University Websites Privacy Notice .
UConn Today
- School and College News
- Arts & Culture
- Community Impact
- Entrepreneurship
- Health & Well-Being
- Research & Discovery
- UConn Health
- University Life
- UConn Voices
- University News
September 7, 2021 | Combined Reports - UConn Communications
Study Shows the Impacts of Deforestation and Forest Burning on Biodiversity in the Amazon
Since 2001, between 40,000 and 73,400 square miles of Amazon rainforest have been impacted by fires
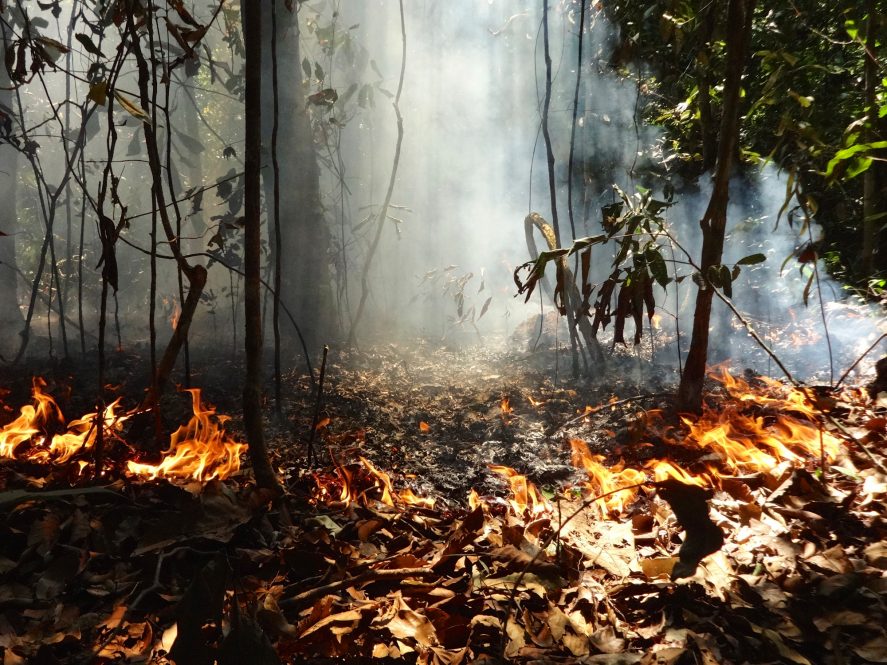
Ring of fire: Smoke rises through the understory of a forest in the Amazon region. Plants and animals in the Amazonian rainforest evolved largely without fire, so they lack the adaptations necessary to cope with it. (Credit: Paulo Brando)
A new study, co-authored by a team of researchers including UConn Ecology and Evolutionary Biology researcher Cory Merow provides the first quantitative assessment of how environmental policies on deforestation, along with forest fires and drought, have impacted the diversity of plants and animals in the Amazon. The findings were published in the Sept. 1 issue of Nature .
Researchers used records of more than 14,500 plant and vertebrate species to create biodiversity maps of the Amazon region. Overlaying the maps with historical and current observations of forest fires and deforestation over the last two decades allowed the team to quantify the cumulative impacts on the region’s species.
They found that since 2001, between 40,000 and 73,400 square miles of Amazon rainforest have been impacted by fires, affecting 95% of all Amazonian species and as many as 85% of species that are listed as threatened in this region. While forest management policies enacted in Brazil during the mid-2000s slowed the rate of habitat destruction, relaxed enforcement of these policies coinciding with a change in government in 2019 has seemingly begun to reverse this trend, the authors write. With fires impacting 1,640 to 4,000 square miles of forest, 2019 stands out as one of the most extreme years for biodiversity impacts since 2009, when regulations limiting deforestation were enforced.
“Perhaps most compelling is the role that public pressure played in curbing forest loss in 2019,” Merow says. “When the Brazilian government stopped enforced forest regulations in 2019, each month between January and August 2019 was the worse month on record (e.g. comparing January 2019 to previous January’s) for forest loss in the 20-year history of available data. However, based on international pressure, forest regulation resumed in September 2019, and forest loss declined significantly for the rest of the year, resulting in 2019 looking like an average year compared to the 20-year history. This was big: active media coverage and public support for policy changes were effective at curbing biodiversity loss on a very rapid time scale.”
The findings are especially critical in light of the fact that at no point in time did the Amazon get a break from those increasing impacts, which would have allowed for some recovery, says senior study author Brian Enquist, a professor in UArizona’s Department of Ecology and Evolutionary Biology .
“Even with policies in place, which you can think of as a brake slowing the rate of deforestation, it’s like a car that keeps moving forward, just at a slower speed,” Enquist says. “But in 2019, it’s like the foot was let off the brake, causing it to accelerate again.”
Known mostly for its dense rainforests, the Amazon basin supports around 40% of the world’s remaining tropical forests. It is of global importance as a provider of ecosystem services such as scrubbing and storing carbon from the atmosphere, and it plays a vital role in regulating Earth’s climate. The area also is an enormous reservoir of the planet’s biodiversity, providing habitats for one out of every 10 of the planet’s known species. It has been estimated that in the Amazon, 1,000 tree species can populate an area smaller than a half square mile.
“Fire is not a part of the natural cycle in the rainforest,” says study co-author Crystal N. H. McMichael at the University of Amsterdam. “Native species lack the adaptations that would allow them to cope with it, unlike the forest communities in temperate areas. Repeated burning can cause massive changes in species composition and likely devastating consequences for the entire ecosystem.”
Since the 1960s, the Amazon has lost about 20% of its forest cover to deforestation and fires. While fires and deforestation often go hand in hand, that has not always been the case, Enquist says. As climate change brings more frequent and more severe drought conditions to the region, and fire is often used to clear large areas of rainforest for the agricultural industry, deforestation has spillover effects by increasing the chances of wildfires. Forest loss is predicted reach 21 to 40% by 2050, and such habitat loss will have large impacts on the region’s biodiversity, according to the authors.
“Since the majority of fires in the Amazon are intentionally set by people, preventing them is largely within our control,” says study co-author Patrick Roehrdanz, senior manager of climate change and biodiversity at Conservation International. “One way is to recommit to strong antideforestation policies in Brazil, combined with incentives for a forest economy, and replicate them in other Amazonian countries.”
Policies to protect Amazonian biodiversity should include the formal recognition of Indigenous lands, which encompass more than one-third of the Amazon region, the authors write, pointing to previous research showing that lands owned, used or occupied by Indigenous peoples have less species decline, less pollution and better-managed natural resources.
The authors say their study underscores the dangers of continuing lax policy enforcement. As fires encroach on the heart of the Amazon basin, where biodiversity is greatest, their impacts will have more dire effects, even if the rate of forest burning remains unchanged.
The research was made possible by strategic investment funds allocated by the Arizona Institutes for Resilience at UArizona and the university’s Bridging Biodiversity and Conservation Science group. Additional support came from the National Science Foundation’s Harnessing the Data Revolution program . Data and computation were provided through the Botanical Information and Ecology Network , which is supported by CyVerse , the NSF’s data management platform led by UArizona.
Recent Articles
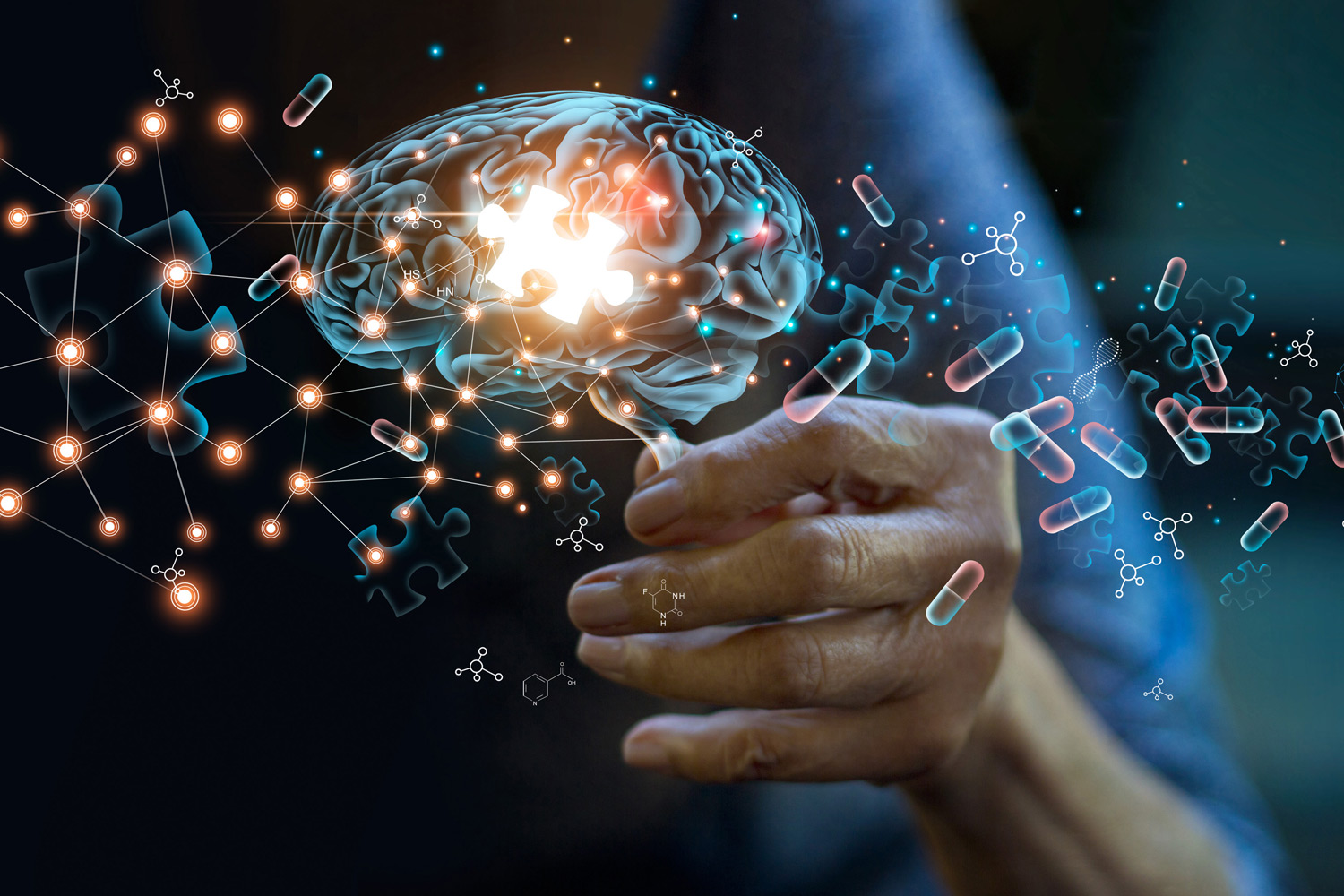
April 26, 2024
New Alzheimer’s Treatment Available at UConn Health
Read the article
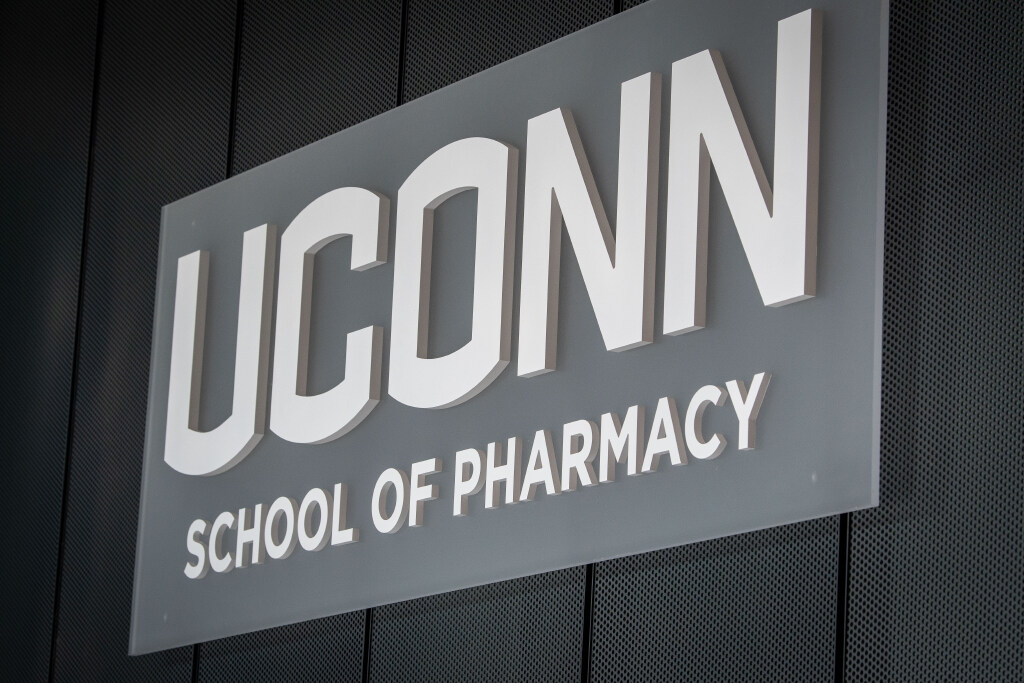
Marissa Salvo Named the 2024 School of Pharmacy Faculty Service Award Recipient
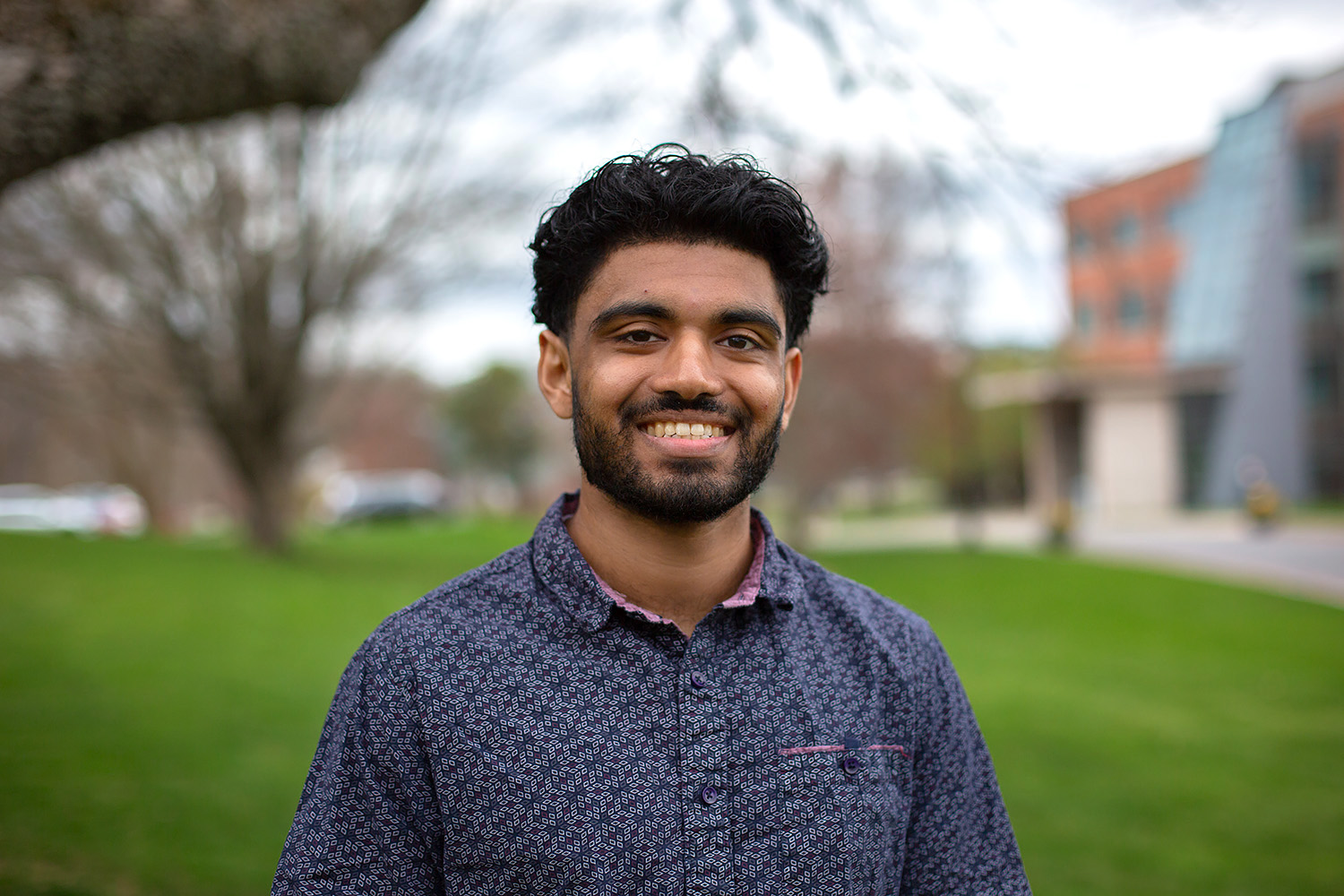
Mathew Chandy ’24, College of Liberal Arts and Sciences
- 0 Shopping Cart
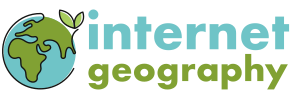
Sustainable Management of the Amazon Rainforest
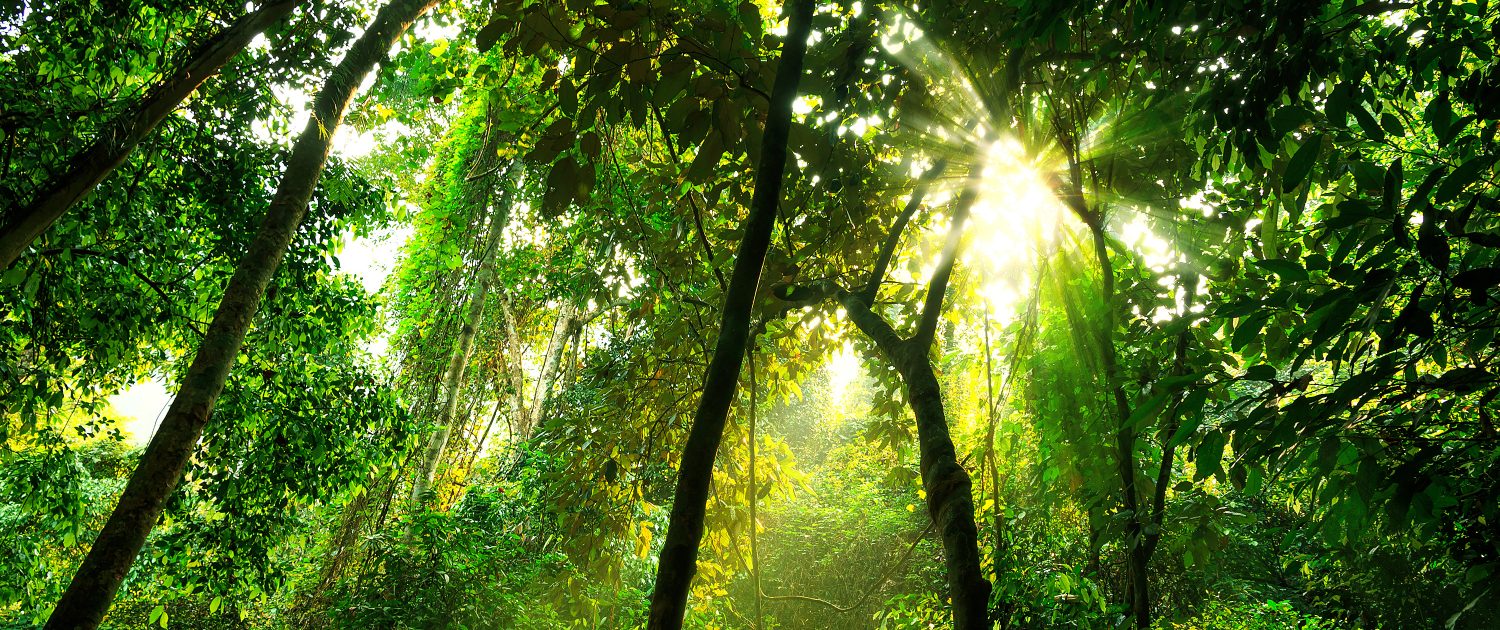
The Amazon rainforest is located in the north of South America, spanning an area of around 8 million km2 including parts of Brazil, Columbia, Peru, Venezuela, Ecuador Bolivia, Suriname, Guyana and French Guyana.
In some areas of the Amazon rainforest, sustainable management strategies are in place to ensure people today can get the resources they need in a way that ensures future generations can also benefit from the ecosystem .
Sustainable management strategies are affected by political and economic factors .
Governance
Governance relates to control of rainforests and who has a say in how rainforests are used. In some areas, rainforests are protected by national and international laws.
In Brazil, the largest protected area of rainforest is the Central Amazon Conservation Complex (CACC) . The CACC covers 60000 km2 as is classified as a World Heritage Site by the United Nations, which means it is protected by international treaties. Limits are placed on hunting , logging and fishing and access is limited.
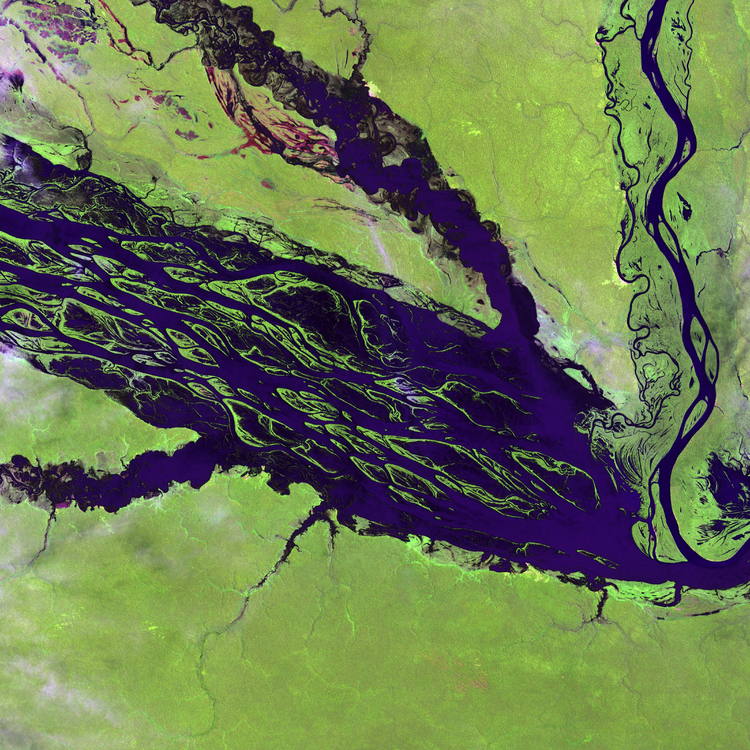
Central Amazon Conservation Complex (CACC)
In other areas local communities, with the help of NGOs, are involved in rainforest governance. In Columbia, an organisation known as Natütama is working with the local community in Puerto Nariño to protect river species such as the Amazon River dolphin. Local people are employed to teach members of the community on how to protect habitats and endangered river species. Local fishermen collect information about the number and distribution of species and report illegal hunting.
Commodity Value
Commodity value means assigning a value to different good and services in a rainforest. Sustainable management ensures rainforests are worth. more than the value of the timber and other resources that can be extracted, such as gold. An example of this is sustainable foresty, which balances the removal of trees to sell with the conservation of the forest.
Selective logging involves only removing a small number of trees, allowing the forest to regenerate naturally. This saves money in the long run as logging companies do not need to replace felled trees.
Sustainable logging companies such as Precious Woods Amazon place limits on the number of trees being cut down so the rainforest can recover. They also use a range of species so that none are over-exploited.
International agreements try to reduce illegal logging and ensure timber comes from sustainable sources. The Forestry Stewardship Council allows the use of its logo by companies that operate in a sustainable way so consumers know they are buying sustainable timber.
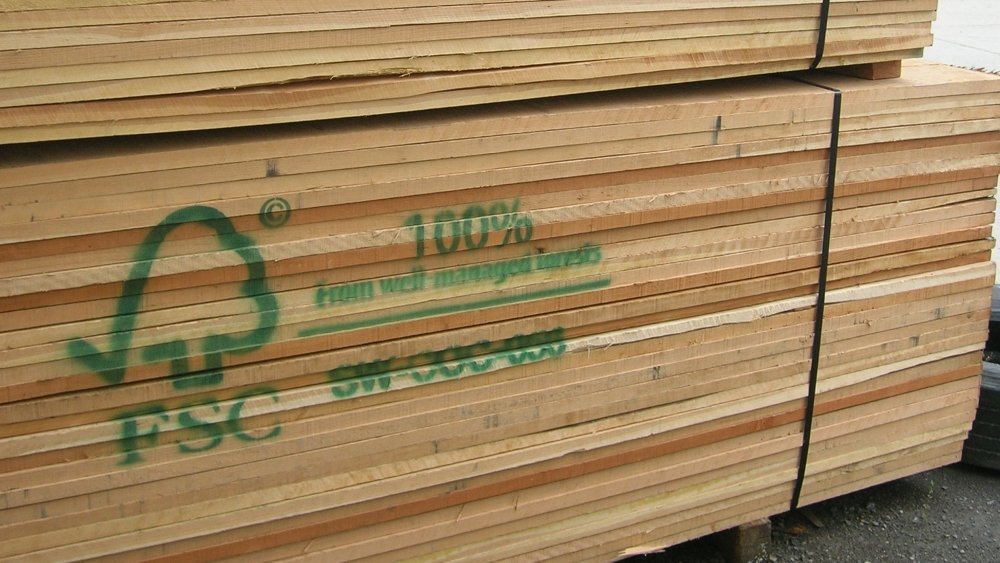
FSC certified wood
Ecotourism is a type of tourism that minimises damage to the environment and benefits local people.
An example of an ecotourism project is the Yachana Lodge in Equador. It is located in a remote area of the Amazon Rainforest where local people rely on subsistence farming.
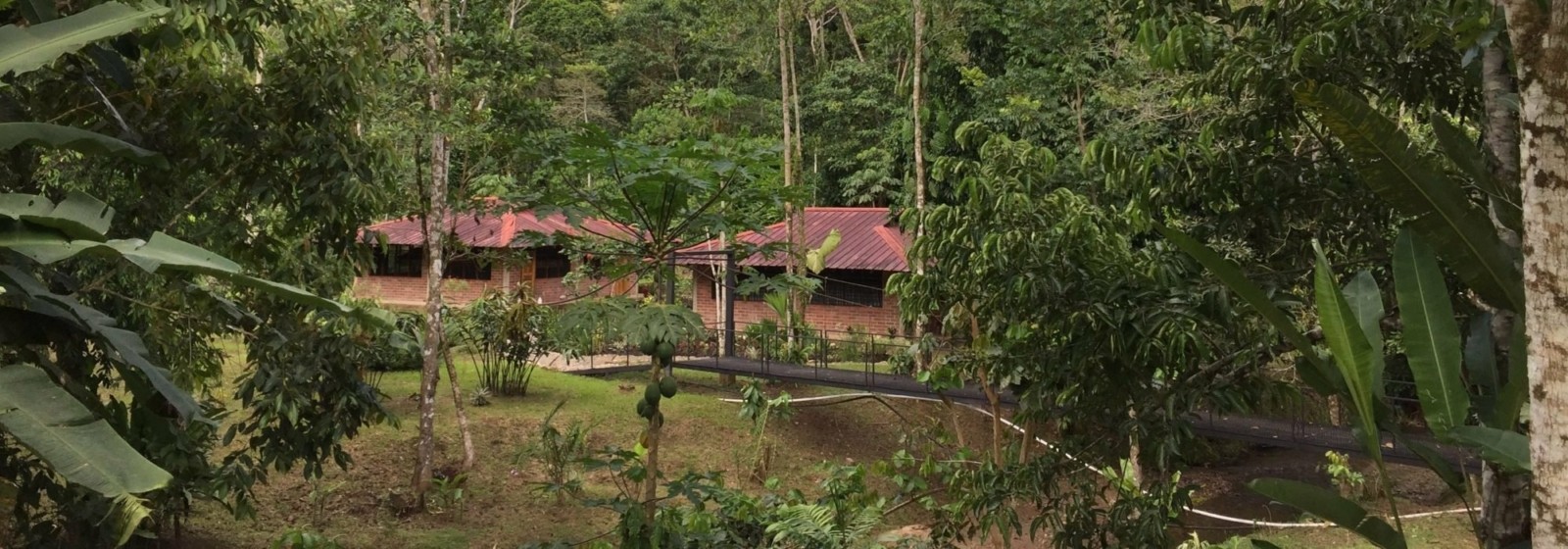
Yachana Lodge
The project employs local people. This provides a reliable source of income and a better quality of life. The project encourages local people to use the rainforest in a sustainable way so tourists continue to visit.
Volunteers work with local Amazon youth who study at the Yachana Technical High School where learning is focused on five main areas:
- Rainforest conservation
- Sustainable agriculture
- Renewable energy
- Animal husbandry
- Micro-enterprise development .
Tourists are only allowed to visit in small groups, minimising their impact on the environment. Tourists take part in activities that help raise awareness of conservation issues.
Entrance fees are paid by the tourists which are invested in conservation and education projects.
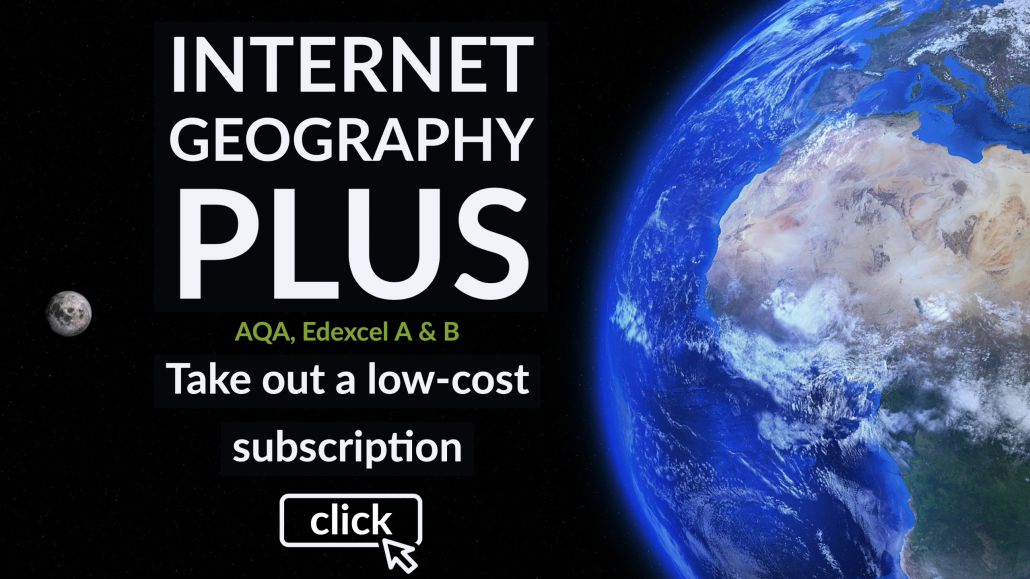
Premium Resources
Please support internet geography.
If you've found the resources on this page useful please consider making a secure donation via PayPal to support the development of the site. The site is self-funded and your support is really appreciated.
Related Topics
Use the images below to explore related GeoTopics.
Sustainable Management of the Rainforest
Topic home, share this:.
- Click to share on Twitter (Opens in new window)
- Click to share on Facebook (Opens in new window)
- Click to share on Pinterest (Opens in new window)
- Click to email a link to a friend (Opens in new window)
- Click to share on WhatsApp (Opens in new window)
- Click to print (Opens in new window)
If you've found the resources on this site useful please consider making a secure donation via PayPal to support the development of the site. The site is self-funded and your support is really appreciated.
Search Internet Geography
Top posts and pages.

Latest Blog Entries

Pin It on Pinterest
- Click to share
- Print Friendly
April 24, 2024
A Singular Climate Experiment Takes Shape in the Amazon
After years of delay, researchers are ready to inject carbon dioxide into jungle plots.
By Daniel Grossman
Patrick Vanier/Scientific American
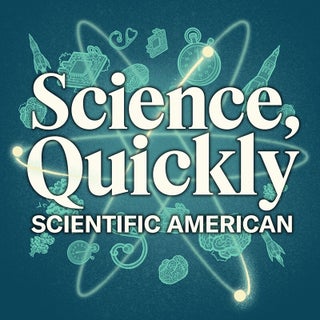
This story was produced with assistance from the Pulitzer Center and additional support from the Pendleton Mazer Family Fund.
[CLIP: Sound of milling crowd and forest noises]
Daniel Grossman: Several dozen people pile out of a charter bus into a clearing in the central Amazon jungle. They’ve driven to a research site called ZF2 from Manaus, the Amazon’s largest city. It’s October 2023.
On supporting science journalism
If you're enjoying this article, consider supporting our award-winning journalism by subscribing . By purchasing a subscription you are helping to ensure the future of impactful stories about the discoveries and ideas shaping our world today.
Beto Quesada: Please, everybody, come closer, come closer.
Grossman: They’re the scientific advisers for one of the largest—and some say most important—ecological experiments in the world, now in its final stages of construction. Beto Quesada , an ecologist and one of the three leaders of the project, herds them into a semicircle.
Quesada: It is so great to have you all here.
Grossman: I’m Daniel Grossman, and this is Science, Quickly . Today we present the second of three episodes about AmazonFACE —FACE is an acronym I’ll explain later—an experiment that could help forecast the future health of the Amazon forest—and the rest of the planet as a result.
The experiment’s elaborate setup in six circular plots is almost done. Around the perimeter of each of the two plots that are closest to completion, 16 metal spires rise up off the forest floor, pierce the tree canopy and lord over the tallest crowns. The trees inside these circles have been monitored as closely as patients in an intensive care unit. Researchers have measured trunks, photographed roots, collected dead leaves and quantified sap flow. And now these experts are getting their first look at what, thanks to the cost of construction and long-term monitoring, Beto says are the world’s most expensive square feet of jungle.
Quesada: And I hope you enjoy what we are going to see. [Applause]
[CLIP: Crowd noise fades, followed by faint forest sounds]
Grossman: Okay, so here’s the background.
[CLIP: “Working It Out,” by Jon Björk ]
Amazon trees soak up more than a billion tons of carbon dioxide from the air every year and convert it into starches and sugars. The carbon in these compounds is sequestered for decades—or even centuries—in wood and soil, slowing the advance of global warming.
But scientists worry that increasing temperatures and drought caused by climate change will weaken the forest. If that were to happen, this forest’s ingesting of carbon could slow or stop entirely . And CO 2 would build up faster in the atmosphere and worsen the climate emergency.
Climate researchers realized decades ago that an experiment like this one was essential for forecasting the Amazon’s future. But it took years to get traction. I first heard about the project in 2015, a couple of years after it got underway.
[CLIP: Faint forest noises, the sound of feet climbing the metal steps of a tower, forest sounds at the top of the tower and various bird calls]
Grossman: At dawn one day early the next year, I slogged up the 18-flight open-air staircase of a metal observation tower. Looking down I saw beams of early morning sunlight bathing the canopy in orange.
Next to me stood David Lapola , a climate scientist at Brazil’s State University of Campinas and another of the project’s leaders. He described to me one of his nightmares. It’s called the dieback hypothesis. We heard about it briefly in Episode One.
David Lapola: The forest dieback hypothesis is the transformation of this forest—a catastrophic loss of biomass—due to global climate change.
Grossman: A dieback would occur if the Amazon jungle and global climate interact in a disastrous, vicious cycle. It would work like this: Hotter, drier weather caused by climate change weakens and kills Amazon trees, reducing the amount of CO 2 from fossil fuel burning that the forest soaks up. As a result, more CO 2 accumulates in the atmosphere, which causes more warming, degrading the forest more, and so on, until this place—and the rest of the planet—is catastrophically hot.
Lapola: Certain scenarios predict that you would have a climate here—regional climate—that would not sustain a forest anymore.
Grossman: Some scientists say there is still hope—that an effect called carbon fertilization will disrupt this feedback loop and save the forest. The idea is that extra CO 2 in the air might help trees grow better, counteracting the impacts of increased heat and drought.
Lapola: If we have CO 2 fertilization, the forest will be conserved. If we don't have it, the forest wouldn’t survive anymore.
Grossman: But scientists can’t yet say if carbon fertilization will save the Amazon, in part because the forest is so diverse. This jungle has something like 10 times as many species of trees as North America—and scientists know practically nothing about a lot of them.
Lapola: When you look here, you see this number of species here, this high number.
[CLIP: “Let There Be Rain,” by Silver Maple ]
Grossman: In the first episode of this miniseries I mentioned an important carbon fertilization experiment at Duke University. It started in the mid-1990s and continued for more than a decade. It showed that when sprayed with extra CO 2 , plots of pine trees grew faster and absorbed more carbon. The benefit of the carbon dioxide was sustained—at least over the course of the experiment. It did vary from plot to plot, suggesting that other factors, like natural variation in water and nutrient availability, could affect how much of a boost trees get from additional CO 2 .
What I didn't say was that a related experiment that started a couple of years later highlighted the important role that nutrients play. Plots of sweet gum trees in Tennessee that were sprayed with extra CO 2 initially took up additional carbon. But after the first several years, the benefit dissipated. The researchers concluded that enhanced growth caused by the increase in CO 2 reduced the supply of plant-available nitrogen in the soil. That, in turn, limited the benefit that extra carbon dioxide could provide.
Will the Amazon see a benefit from extra CO 2 , like what happened at Duke? That could protect it from some of the hazards climate change is expected to continue bringing, like higher temperatures and reduced rainfall. Or will it act more like the sweet gums in Tennessee and not get the long-term CO 2 boost and the protection from climate change that could bring?
Lapola: That’s the important thing this experiment will bring about: how the forest may be resilient to this future increase in temperature and CO 2 .
Grossman: Researchers have called for this experiment for decades. But there are good reasons why nobody has conducted it before.
Amazon trees are huge—up to 110 feet tall here at ZF2—so they require extra-large plots. That means bigger, more expensive gear for spraying CO 2 . Infrastructure such as roads and technical support is relatively poor. And funding for tropical forest research is scarce, a problem that we’ll see hindered AmazonFACE.
Incidentally, the “FACE” in AmazonFACE stands for Free-Air CO 2 Enrichment. It’s called “free air” because the plots have no walls, making the experiment more realistic than any lab study.
[CLIP: Bird vocalizations and other forest sounds]
Grossman: That was 2016, when I climbed that tower with David. Several years earlier he and his collaborators had staked out the first two plots and had begun making measurements of the trees there, establishing their behavior under natural conditions. The researchers said the site could be the world’s most intensely studied patch of tropical forest.
Then in 2017 AmazonFACE ran out of money. David told me that they wanted about $20 million for construction and staff salaries, an amount that only a country or a very rich person could afford. But Brazil was in political turmoil. The president had recently been removed from office, and the government was stuck. Undaunted, David, Beto and their collaborators in the U.S. and Europe reached out to other governments.
Lapola: You never know where a yes will come from the middle of many noes.
Grossman: They even tried Jeff Bezos, then president and CEO of the other Amazon. He seemed like a good candidate. He’d just christened a mini rain forest in his Seattle headquarters. But courting the world’s then-richest person didn’t work, and for four years they got one no after another.
Lapola: I was about to give up. I confess, I wasn’t believing this would happen anymore.
Grossman: Beto also despaired at times. Fortunately Bruno Takeshi , the third core member of the team, never gave up hope.
Bruno Takeshi: I actually never thought that we would just end the idea to do the AmazonFACE, to be honest. I just imagined it’s a matter to find the proper door to be knocked because the AmazonFACE idea is a lot good that we cannot just forget about it.
Grossman: In 2021 the U.K. finally agreed to chip in funding.
Lapola: The support from the British government was decisive, so the Brazilian government came to match it.
Grossman: Brazil gave roughly $6 million, and the U.K. funding would eventually total about $9 million.
Lapola: And then this became a reality.
Grossman: According to David, Brazil is currently considering another multimillion-dollar grant, money that he says AmazonFACE needs to buy the massive amount of carbon dioxide required to run the experiment in the years ahead.
[CLIP: Sounds of trucks going by and backing up and other construction noises]
Grossman: I go back to the ZF2 site for my fourth or fifth visit in July 2023. Now construction is in full swing. The formerly tranquil forest sounds, feels and smells like a mining camp. Diesel fumes waft through the air. Bulldozers, dump trucks and backhoes lumber up and down a new road that snakes into the jungle to the six experimental plots.
[CLIP: Clinking of metal parts, ratchet sound from socket wrench and other construction noises]
Grossman: In a clearing, a group of men wearing hard hats and carrying oversized wrenches swarm like ants around a long lattice of metal tubes laid out on the ground. They’re assembling a section of a tower they’ve bolted together from precut metal parts. The 16 towers assembled here will be secured to huge concrete blocks around the perimeter of one of the plots. The purpose of the towers is to hold up the tubing that will spread CO 2 into the forest.
Takeshi: And then we’re now in this stage to make the assembly.
Grossman: Bruno is the logistics whiz who oversees all this activity. Today he brought a guest, Susan Trumbore , to see the progress.
Takeshi: So we have two teams—actually, three teams on the field.
Grossman: Susan is practically scientific royalty. She is a director of the Max Planck Institute for Biogeochemistry in Jena, Germany, and is an expert in the stability of carbon in ecosystems.
Susan Trumbore: Amazing. I never thought it would actually happen.
Takeshi: Yes, yes, thank you so much.
Grossman: Bruno says it’s essential to get every detail right. The hostile climate—with intense heat and humidity year-round—and the great distance from the city make building and running equipment here tricky at best. He’s just noticed that workers have installed some bolts upside down, making them more likely to fail.
[CLIP: Takeshi speaks with metal workers in Portuguese]
Grossman: He tells them to start over.
“Are you this fastidious about everything?” I ask Bruno. He says, “Yes.”
Takeshi: I’m a guy that, every day before I move my car from the garage, I open my hood and take a look in the oil level, the water level.
Grossman (tape): Every day?
Takeshi: Every single day [laughs].
Grossman: Looming above the worksite and reaching high over the forest horizontally is the bright yellow arm of a crane. It built skyscrapers and bridges before being shipped to the Amazon. The operator, Mabel Marques, says lifting loads here is a challenge.
Mabel Marques (speaking in Portuguese with English translation): It’s quite different from other places I’ve worked at. There are many trees near the areas where the towers will be set up. There will be moments of difficulty, but I’m sure we’ll find the best and most efficient way to do the work.
Grossman: Mabel holds the crane’s remote control, which looks like an industrial version of an XBox controller. Using two small joysticks operated with his fingertips, he lifts a 30-foot section of tower—four stout legs laced together with braces—off the ground.
[CLIP: Walkie-talkie beeps and voices]
Once it’s upright and dangling above the forest, he swings it over the plot. Guided by helpers equipped with walkie-talkies, he threads the steel latticework through the canopy. Waiting workers bolt it to the concrete base. Next he’ll swing over a second piece and then a third.
Several completed towers already stick up above the treetops like shiny, leafless stalks. The circle of towers is taking shape.
[CLIP: Construction sounds]
Grossman: A brawny man holding a handsaw has just shimmied up a tall, thin trunk and tied himself to a branch. Maria Juliana Monte , one of Bruno’s assistants, says the climber is preparing the canopy here for the next tower.
Maria Juliana Monte: He’s trying to come to the middle of the branch, so we need to clear the way of this tower. So he needs to get closer to the side.
Grossman (tape): So you have to have a complete hole right there, right? There has to be a hole in the canopy right above me?
Monte: Yes, they have to have the space clear.
Grossman: It’s tricky because a mass of vines wraps around the tree’s limbs. The man in the tree has to untangle the vines so other limbs or trunks aren’t ripped down when the branches are cut. That could complicate calculations of the carbon that the plot absorbs and put the crew in danger.
Monte: You see, it’s one of the highest trees. Also, its canopy—it’s really, really big. You know, a branch could kill all of us if it’s not cut the right way.
[CLIP: Plot sounds fade]
Quesada and others: Let’s go inside! Let’s go inside.
Grossman: I’m back at the science advisers’ tour of ZF2 last October.
Beto leads them to the two completed plots, each now ringed by a full set of towers.
One of these plots will be exposed to twice as much carbon dioxide as existed in the air before the beginning of global industrialization in the 19th century.
The other plot, for comparison, will not receive extra CO 2 . Two more matched sets are still under construction.
But the feeling this day is not about the details—it’s about the moment. It’s emotional for the project’s leaders to be showing it off to some of the world’s foremost carbon fertilization experts.
Quesada: Humans, we need crazy ideas, right? To really push boundaries. And for a very long time it sounded, like, too crazy. But here we are—we are doing it.
Grossman: These plots will test CO 2 in the next few months. The full set should be running in early 2025.
In the next and final episode of this three-part podcast, we’ll learn what the researchers running the experiment think will happen when they turn on the carbon dioxide.
[CLIP: Theme music]
Science, Quickly is produced by Jeff DelViscio, Rachel Feltman, Kelso Harper and Madison Goldberg. Our theme music was composed by Dominic Smith.
Shayna Posses and Aaron Shattuck fact-checked this miniseries.
Special thanks to Dado Galdieri and Patrick Vanier for logistical support and to Lucas Pinheiro for providing translations.
Don’t forget to subscribe to Science, Quickly . And for more in-depth science news, visit ScientificAmerican.com.
For Scientific American ’s Science, Quickly , I’m Daniel Grossman.

Tropical rainforest case study
Case study of a tropical rainforest setting to illustrate and analyse key themes in water and carbon cycles and their relationship to environmental change and human activity.
Amazon Forest The Amazon is the largest tropical rainforest on Earth. It sits within the Amazon River basin, covers some 40% of the South American continent and as you can see on the map below includes parts of eight South American countries: Brazil, Bolivia, Peru, Ecuador, Colombia, Venezuela, Guyana, and Suriname. The actual word “Amazon” comes from river. Amazing Amazon facts; • It is home to 1000 species of bird and 60,000 species of plants • 10 million species of insects live in the Amazon • It is home to 20 million people, who use the wood, cut down trees for farms and for cattle. • It covers 2.1 million square miles of land • The Amazon is home to almost 20% of species on Earth • The UK and Ireland would fit into the Amazon 17 times! The Amazon caught the public’s attention in the 1980s when a series of shocking news reports said that an area of rainforest the size of Belgium was being cut down and subsequently burnt every year. This deforestation has continued to the present day according to the Sao Paulo Space Research Centre. Current statistics suggest that we have lost 20% of Amazon rainforest. Their satellite data is also showing increased deforestation in parts of the Amazon.

Water The water cycle is very active within the Amazon rainforest and it interlinks the lithosphere, atmosphere and biosphere. The basin is drained by the Amazon River and its tributaries. The average discharge of water into the Atlantic Ocean by the Amazon is approximately 175,000 m 3 per second, or between 1/5th and 1/6th of the total discharge into the oceans of all of the world's rivers. 3 The Rio Negro, a tributary of the Amazon, is the second largest river in the world in terms of water flow, and is 100 meters deep and 14 kilometers wide near its mouth at Manaus, Brazil. Rainfall across the Amazon is very high. Average rainfall across the whole Amazon basin is approximately 2300 mm annually. In some areas of the northwest portion of the Amazon basin, yearly rainfall can exceed 6000 mm. 3 Only around 1/3 of the rain that falls in the Amazon basin is discharged into the Atlantic Ocean. It is thought that; 1. Up to half of the rainfall in some areas may never reach the ground, being intercepted by the forest and re-evaporated into the atmosphere. 2. Additional evaporation occurs from ground and river surfaces, or is released into the atmosphere by transpiration from plant leaves (in which plants release water from their leaves during photosynthesis) 3. This moisture contributes to the formation of rain clouds, which release the water back onto the rainforest. In the Amazon, 50-80 percent of moisture remains in the ecosystem’s water cycle. 4
This means that much of the rainfall re-enters the water cycling system of the Amazon, and a given molecule of water may be "re-cycled" many times between the time that it leaves the surface of the Atlantic Ocean and is carried by the prevailing westerly winds into the Amazon basin, to the time that it is carried back to the ocean by the Amazon River. 4 It is thought that the water cycle of the Amazon has global effects. The moisture created by rainforests travels around the world. Moisture created in the Amazon ends up falling as rain as far away as Texas, and forests in Southeast Asia influence rain patterns in south eastern Europe and China. 4 When forests are cut down, less moisture goes into the atmosphere and rainfall declines, sometimes leading to drought. These have been made worse by deforestation. 4 Change to the water and carbon cycles in the Amazon The main change to the Amazon rainforest is deforestation. Deforestation in the Amazon is generally the result of land clearances for; 1. Agriculture (to grow crops like Soya or Palm oil) or for pasture land for cattle grazing 2. Logging – This involves cutting down trees for sale as timber or pulp. The timber is used to build homes, furniture, etc. and the pulp is used to make paper and paper products. Logging can be either selective or clear cutting. Selective logging is selective because loggers choose only wood that is highly valued, such as mahogany. Clear-cutting is not selective. Loggers are interested in all types of wood and therefore cut all of the trees down, thus clearing the forest, hence the name- clear-cutting. 3. Road building – trees are also clear for roads. Roads are an essential way for the Brazilian government to allow development of the Amazon rainforest. However, unless they are paved many of the roads are unusable during the wettest periods of the year. The Trans Amazonian Highway has already opened up large parts of the forest and now a new road is going to be paved, the BR163 is a road that runs 1700km from Cuiaba to Santarem. The government planned to tarmac it making it a superhighway. This would make the untouched forest along the route more accessible and under threat from development. 4. Mineral extraction – forests are also cleared to make way for huge mines. The Brazilian part of the Amazon has mines that extract iron, manganese, nickel, tin, bauxite, beryllium, copper, lead, tungsten, zinc and gold! 5. Energy developmen t – This has focussed mainly on using Hydro Electric Power, and there are 150 new dams planned for the Amazon alone. The dams create electricity as water is passed through huge pipes within them, where it turns a turbine which helps to generate the electricity. The power in the Amazon is often used for mining. Dams displace many people and the reservoirs they create flood large area of land, which would previously have been forest. They also alter the hydrological cycle and trap huge quantities of sediment behind them. The huge Belo Monte dam started operating in April 2016 and will generate over 11,000 Mw of power. A new scheme the 8,000-megawatt São Luiz do Tapajós dam has been held up because of the concerns over the impacts on the local Munduruku people. 6. Settlement & population growth – populations are growing within the Amazon forest and along with them settlements. Many people are migrating to the forest looking for work associated with the natural wealth of this environment. Settlements like Parauapebas, an iron ore mining town, have grown rapidly, destroying forest and replacing it with a swath of shanty towns. The population has grown from 154,000 in 2010 to 220,000 in 2012. The Brazilian Amazon’s population grew by a massive 23% between 2000 and 2010, 11% above the national average.
The WWF estimates that 27 per cent, more than a quarter, of the Amazon biome will be without trees by 2030 if the current rate of deforestation continues. They also state that Forest losses in the Amazon biome averaged 1.4 million hectares per year between 2001 and 2012, resulting in a total loss of 17.7 million hectares, mostly in Brazil, Peru and Bolivia. 12
The impacts of deforestation Atmospheric impacts Deforestation causes important changes in the energy and water balance of the Amazon. Pasturelands and croplands (e.g. soya beans and corn) have a higher albedo and decreased water demand, evapotranspiration and canopy interception compared with the forests they replace. 9 Lathuillière et al. 10 found that forests in the state of Mato Grosso; • Contributed about 50 km 3 per year of evapotranspiration to the atmosphere in the year 2000. • Deforestation reduced that forest flux rate by approximately 1 km 3 per year throughout the decade. • As a result, by 2009, forests were contributing about 40 km 3 per year of evapotranspiration in Mato Grosso.
Differences such as these can affect atmospheric circulation and rainfall in proportion to the scale of deforestation The agriculture that replaces forest cover also decreases precipitation. In Rondônia, Brazil, one of the most heavily deforested areas of Brazil, daily rainfall data suggest that deforestation since the 1970s has caused an 18-day delay in the onset of the rainy season. 11 SSE Amazon also has many wild fires, which are closely associated with deforestation, forest fragmentation and drought intensity. According to Coe et al (2015) “ the increased atmospheric aerosol loads produced by fires have been shown to decrease droplet size, increase cloud height and cloud lifetime and inhibit rainfall, particularly in the dry season in the SSE Amazon. Thus, fires and drought may create a positive feedback in the SSE Amazon such that drought is more severe with continued deforestation and climate change .” 9

The impacts of climate change on the Amazon According to the WWF: • Some Amazon species capable of moving fast enough will attempt to find a more suitable environment. Many other species will either be unable to move or will have nowhere to go. • Higher temperatures will impact temperature-dependent species like fish, causing their distribution to change. • Reduced rainfall and increased temperatures may also reduce suitable habitat during dry, warm months and potentially lead to an increase in invasive, exotic species, which then can out-compete native species. • Less rainfall during the dry months could seriously affect many Amazon rivers and other freshwater systems. • The impact of reduced rainfall is a change in nutrient input into streams and rivers, which can greatly affect aquatic organisms. • A more variable climate and more extreme events will also likely mean that Amazon fish populations will more often experience hot temperatures and potentially lethal environmental conditions. • Flooding associated with sea-level rise will have substantial impacts on lowland areas such as the Amazon River delta. The rate of sea-level rise over the last 100 years has been 1.0-2.5 mm per year, and this rate could rise to 5 mm per year. • Sea-level rise, increased temperature, changes in rainfall and runoff will likely cause major changes in species habitats such as mangrove ecosystems. 15 Impacts of deforestation on soils Removing trees deprives the forest of portions of its canopy, which blocks the sun’s rays during the day, and holds in heat at night. This disruption leads to more extreme temperature swings that can be harmful to plants and animals. 8 Without protection from sun-blocking tree cover, moist tropical soils quickly dry out. In terms of Carbon, Tropical soils contain a lot of carbon. The top meter holds 66.9 PgC with around 52% of this carbon pool held in the top 0.3 m of the soil, the layer which is most prone to changes upon land use conversion and deforestation. 14 Deforestation releases much of this carbon through clearance and burning. For the carbon that remains in the soil, when it rains soil erosion will wash much of the carbon away into rivers after initial deforestation and some will be lost to the atmosphere via decomposition too.
Impacts of deforestation on Rivers Trees also help continue the water cycle by returning water vapor to the atmosphere. When trees are removed this cycle is severely disrupted and areas can suffer more droughts. There are many consequences of deforestation and climate change for the water cycle in forests; 1. There is increased soil erosion and weathering of rainforest soils as water acts immediately upon them rather than being intercepted. 2. Flash floods are more likely to happen as there is less interception and absorption by the forest cover. 3. Conversely, the interruption of normal water cycling has resulted in more droughts in the forest, increasing the risk of wild fires 4. More soil and silt is being washed into rivers, resulting in changes to waterways and transport 5. Disrupt water supplies to many people in Brazil
References 1 - Malhi, Y. et al. The regional variation of aboveground live biomass in old-growth Amazonian forests. Glob. Chang. Biol. 12, 1107–1138 (2006). 2 - Fernando D.B. Espírito-Santo et al. Size and frequency of natural forest disturbances and the Amazon forest carbon balance. Nature Communications volume 5, Article number: 3434 (2014) Accessed 3rd of January 2019 retrieved from https://www.nature.com/articles/ncomms4434#ref4 3 - Project Amazonas. Accessed 3rd of January 2019 retrieved from https://www.projectamazonas.org/amazon-facts 4 - Rhett Butler, 2012. IMPACT OF DEFORESTATION: LOCAL AND NATIONAL CONSEQUENCES. Accessed 3rd of January 2019 retrieved from https://rainforests.mongabay.com/0902.htm 5 – Mark Kinver. Amazon: 1% of tree species store 50% of region's carbon. 2015. BBC. Accessed 3rd of January 2019 retrieved from https://www.bbc.co.uk/news/science-environment-32497537 6 - Sophie Fauset et al. Hyperdominance in Amazonian forest carbon cycling. Nature Communications volume 6, Article number: 6857 (2015). Accessed 3rd of January 2019 retrieved from https://www.nature.com/articles/ncomms7857 7- Brienen, R.J.W et al. (2015) Long-term decline of the Amazon carbon sink, Nature, h ttps://www.nature.com/articles/nature14283 8 – National Geographic – Deforestation - Learn about the man-made and natural causes of deforestation–and how it's impacting our planet. Accessed 20th of January 2019 retrieved from https://www.nationalgeographic.com/environment/global-warming/deforestation/
9 - Michael T. Coe, Toby R. Marthews, Marcos Heil Costa, David R. Galbraith, Nora L. Greenglass, Hewlley M. A. Imbuzeiro, Naomi M. Levine, Yadvinder Malhi, Paul R. Moorcroft, Michel Nobre Muza, Thomas L. Powell, Scott R. Saleska, Luis A. Solorzano, and Jingfeng Wang. (2015) Deforestation and climate feedbacks threaten the ecological integrity of south–southeastern Amazonia. 368, Philosophical Transactions of the Royal Society B: Biological Sciences. Accessed 20th of January 2019 retrieved from http://rstb.royalsocietypublishing.org/content/368/1619/20120155
10 - Lathuillière MJ, Mark S, Johnson MS & Donner SD. (2012). Water use by terrestrial ecosystems: temporal variability in rainforest and agricultural contributions to evapotranspiration in Mato Grosso, Brazil. Environmental research Letters Volume 7 Number 2. http://iopscience.iop.org/article/10.1088/1748-9326/7/2/024024/meta
11- Nathalie Butt, Paula Afonso de Oliveira & Marcos Heil Costa (2011). Evidence that deforestation affects the onset of the rainy season in Rondonia, Brazil JGR Atmospheres, Volume 116, Issue D11. https://doi.org/10.1029/2010JD015174
12 – WWF, Amazon Deforestation. Accessed 20th of January 2019 retrieved from http://wwf.panda.org/our_work/forests/deforestation_fronts/deforestation_in_the_amazon/
13 - Berenguer, E., Ferreira, J., Gardner, T. A., Aragão, L. E. O. C., De Camargo, P. B., Cerri, C. E., Durigan, M., Oliveira, R. C. D., Vieira, I. C. G. and Barlow, J. (2014), A large-scale field assessment of carbon stocks in human-modified tropical forests. Global Change Biology, 20: 3713–3726. https://onlinelibrary.wiley.com/doi/full/10.1111/gcb.12627
14 - N.HBatjes, J.ADijkshoorn, (1999). Carbon and nitrogen stocks in the soils of the Amazon Region. Geoderma, Volume 89, Issues 3–4, Pages 273-286. Accessed 20th of January 2019 retrieved from https://www.sciencedirect.com/science/article/pii/S001670619800086X
15 – WWF, Impacts of climate change in the Amazon. Accessed 20th of January 2019 retrieved from http://wwf.panda.org/knowledge_hub/where_we_work/amazon/amazon_threats/climate_change_amazon/amazon_climate_change_impacts/
Written by Rob Gamesby
©2015 Cool Geography
- Copyright Policy
- Privacy & Cookies
- Testimonials
- Feedback & support

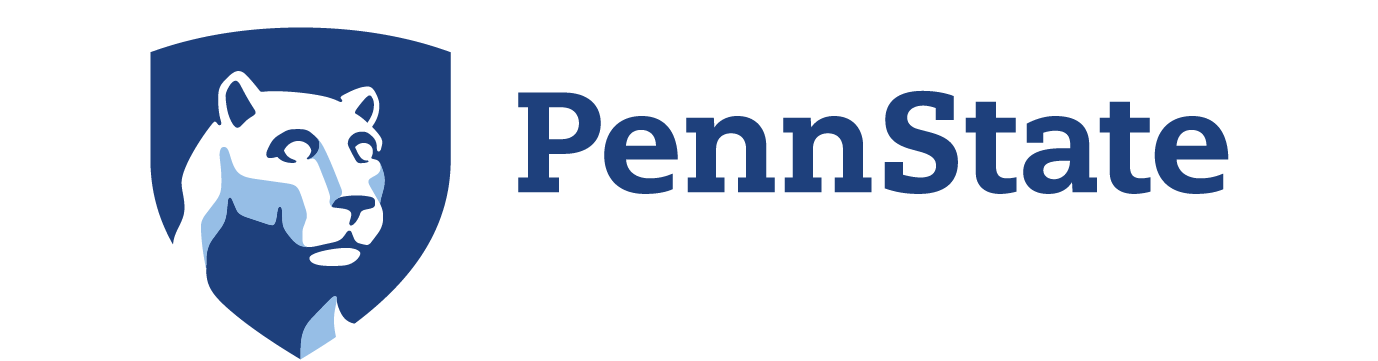
Want to create or adapt books like this? Learn more about how Pressbooks supports open publishing practices.
73 Case Study: The Amazon Rainforest
The amazon in context.
Tropical rainforests are often considered to be the “cradles of biodiversity.” Though they cover only about 6% of the Earth’s land surface, they are home to over 50% of global biodiversity. Rain forests also take in massive amounts of carbon dioxide and release oxygen through photosynthesis, which has also given them the nickname “lungs of the planet.” They also store very large amounts of carbon, and so cutting and burning their biomass contributes to global climate change. Many modern medicines are derived from rainforest plants, and several very important food crops originated in the rainforest, including bananas, mangos, chocolate, coffee, and sugar cane.
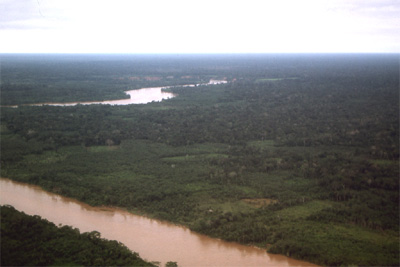
In order to qualify as a tropical rainforest, an area must receive over 250 centimeters of rainfall each year and have an average temperature above 24 degrees centigrade, as well as never experiencing frosts. The Amazon rainforest in South America is the largest in the world. The second largest is the Congo in central Africa, and other important rainforests can be found in Central America, the Caribbean, and Southeast Asia. Brazil contains about 40% of the world’s remaining tropical rainforest. Its rainforest covers an area of land about 2/3 the size of the continental United States.
There are countless reasons, both anthropocentric and ecocentric, to value rainforests. But they are one of the most threatened types of ecosystems in the world today. It’s somewhat difficult to estimate how quickly rainforests are being cut down, but estimates range from between 50,000 and 170,000 square kilometers per year. Even the most conservative estimates project that if we keep cutting rainforests as we are today, within about 100 years there will be none left.
How does a rainforest work?
Rainforests are incredibly complex ecosystems, but understanding a few basics about their ecology will help us understand why clear-cutting and fragmentation are such destructive activities for rainforest biodiversity.
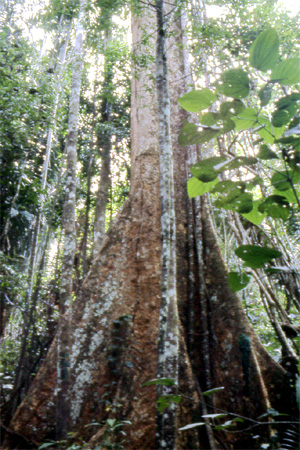
High biodiversity in tropical rainforests means that the interrelationships between organisms are very complex. A single tree may house more than 40 different ant species, each of which has a different ecological function and may alter the habitat in distinct and important ways. Ecologists debate about whether systems that have high biodiversity are stable and resilient, like a spider web composed of many strong individual strands, or fragile, like a house of cards. Both metaphors are likely appropriate in some cases. One thing we can be certain of is that it is very difficult in a rainforest system, as in most others, to affect just one type of organism. Also, clear cutting one small area may damage hundreds or thousands of established species interactions that reach beyond the cleared area.
Pollination is a challenge for rainforest trees because there are so many different species, unlike forests in the temperate regions that are often dominated by less than a dozen tree species. One solution is for individual trees to grow close together, making pollination simpler, but this can make that species vulnerable to extinction if the one area where it lives is clear cut. Another strategy is to develop a mutualistic relationship with a long-distance pollinator, like a specific bee or hummingbird species. These pollinators develop mental maps of where each tree of a particular species is located and then travel between them on a sort of “trap-line” that allows trees to pollinate each other. One problem is that if a forest is fragmented then these trap-line connections can be disrupted, and so trees can fail to be pollinated and reproduce even if they haven’t been cut.
The quality of rainforest soils is perhaps the most surprising aspect of their ecology. We might expect a lush rainforest to grow from incredibly rich, fertile soils, but actually, the opposite is true. While some rainforest soils that are derived from volcanic ash or from river deposits can be quite fertile, generally rainforest soils are very poor in nutrients and organic matter. Rainforests hold most of their nutrients in their live vegetation, not in the soil. Their soils do not maintain nutrients very well either, which means that existing nutrients quickly “leech” out, being carried away by water as it percolates through the soil. Also, soils in rainforests tend to be acidic, which means that it’s difficult for plants to access even the few existing nutrients. The section on slash and burn agriculture in the previous module describes some of the challenges that farmers face when they attempt to grow crops on tropical rainforest soils, but perhaps the most important lesson is that once a rainforest is cut down and cleared away, very little fertility is left to help a forest regrow.
What is driving deforestation in the Amazon?
Many factors contribute to tropical deforestation, but consider this typical set of circumstances and processes that result in rapid and unsustainable rates of deforestation. This story fits well with the historical experience of Brazil and other countries with territory in the Amazon Basin.
Population growth and poverty encourage poor farmers to clear new areas of rainforest, and their efforts are further exacerbated by government policies that permit landless peasants to establish legal title to land that they have cleared.
At the same time, international lending institutions like the World Bank provide money to the national government for large-scale projects like mining, construction of dams, new roads, and other infrastructure that directly reduces the forest or makes it easier for farmers to access new areas to clear.
The activities most often encouraging new road development are timber harvesting and mining. Loggers cut out the best timber for domestic use or export, and in the process knock over many other less valuable trees. Those trees are eventually cleared and used for wood pulp, or burned, and the area is converted into cattle pastures. After a few years, the vegetation is sufficiently degraded to make it not profitable to raise cattle, and the land is sold to poor farmers seeking out a subsistence living.
Regardless of how poor farmers get their land, they often are only able to gain a few years of decent crop yields before the poor quality of the soil overwhelms their efforts, and then they are forced to move on to another plot of land. Small-scale farmers also hunt for meat in the remaining fragmented forest areas, which reduces the biodiversity in those areas as well.
Another important factor not mentioned in the scenario above is the clearing of rainforest for industrial agriculture plantations of bananas, pineapples, and sugar cane. These crops are primarily grown for export, and so an additional driver to consider is consumer demand for these crops in countries like the United States.
These cycles of land use, which are driven by poverty and population growth as well as government policies, have led to the rapid loss of tropical rainforests. What is lost in many cases is not simply biodiversity, but also valuable renewable resources that could sustain many generations of humans to come. Efforts to protect rainforests and other areas of high biodiversity is the topic of the next section.
Introduction to Geography Copyright © by Petra Tschakert; Karl Zimmerer; Brian King; Seth Baum; and Chongming Wang is licensed under a Creative Commons Attribution-NonCommercial-ShareAlike 4.0 International License , except where otherwise noted.
Share This Book
Thank you for visiting nature.com. You are using a browser version with limited support for CSS. To obtain the best experience, we recommend you use a more up to date browser (or turn off compatibility mode in Internet Explorer). In the meantime, to ensure continued support, we are displaying the site without styles and JavaScript.
- View all journals
- My Account Login
- Explore content
- About the journal
- Publish with us
- Sign up for alerts
- Open access
- Published: 07 March 2022
Pronounced loss of Amazon rainforest resilience since the early 2000s
- Chris A. Boulton ORCID: orcid.org/0000-0001-7836-9391 1 ,
- Timothy M. Lenton ORCID: orcid.org/0000-0002-6725-7498 1 &
- Niklas Boers ORCID: orcid.org/0000-0002-1239-9034 1 , 2 , 3
Nature Climate Change volume 12 , pages 271–278 ( 2022 ) Cite this article
99k Accesses
177 Citations
4678 Altmetric
Metrics details
- Climate-change ecology
- Climate-change impacts
- Climate sciences
Matters Arising to this article was published on 09 November 2023
The resilience of the Amazon rainforest to climate and land-use change is crucial for biodiversity, regional climate and the global carbon cycle. Deforestation and climate change, via increasing dry-season length and drought frequency, may already have pushed the Amazon close to a critical threshold of rainforest dieback. Here, we quantify changes of Amazon resilience by applying established indicators (for example, measuring lag-1 autocorrelation) to remotely sensed vegetation data with a focus on vegetation optical depth (1991–2016). We find that more than three-quarters of the Amazon rainforest has been losing resilience since the early 2000s, consistent with the approach to a critical transition. Resilience is being lost faster in regions with less rainfall and in parts of the rainforest that are closer to human activity. We provide direct empirical evidence that the Amazon rainforest is losing resilience, risking dieback with profound implications for biodiversity, carbon storage and climate change at a global scale.
Similar content being viewed by others
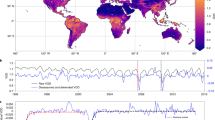
Empirical evidence for recent global shifts in vegetation resilience
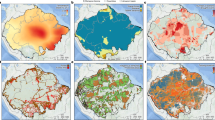
Critical transitions in the Amazon forest system
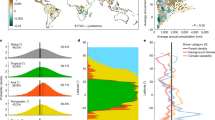
Emerging signals of declining forest resilience under climate change
There is widespread concern about the resilience of the Amazon rainforest to land-use change and climate change. The Amazon is recognized as a potential tipping element in the Earth’s climate system 1 , is a crucible of biodiversity 2 and usually acts as a large terrestrial carbon sink 3 , 4 . The net ecosystem productivity (carbon uptake flux) of the Amazon has, however, been declining over the last four decades and, during two major droughts in 2005 and 2010, the Amazon temporarily turned into a carbon source, due to increased tree mortality 5 , 6 , 7 . Several studies have suggested that deforestation 8 and anthropogenic global warming 9 , 10 , especially in combination, could push the Amazon rainforest past critical thresholds 11 , 12 where positive feedbacks propel abrupt and substantial further forest loss. Two types of positive feedback are particularly important. First, localized fire feedbacks amplify drought and associated forest loss by destroying trees 13 and the fire regime itself may ‘tip’ from localized to ‘mega-fires’ 14 . Second, deforestation and forest degradation, whether due to direct human intervention or droughts, reduce evapotranspiration and hence the moisture transported further westward, reducing rainfall and forest viability there 15 and establishing a large-scale moisture recycling feedback. Net rainfall reduction may in turn reduce latent heating over the Amazon to the extent that it weakens the low-level circulation of the South American monsoon 8 . Model projections of future changes in the Amazon rainforest differ widely 9 , 16 , 17 , 18 . Early studies showed that the Amazon rainforest may exhibit strong dieback by the end of the twenty-first century 9 , 19 . Both pronounced drying in tropical South America and a weak CO 2 fertilization effect 18 contributed to this result, with dieback also more common under stronger greenhouse gas emission scenarios 17 . Other studies based on varying general circulation and vegetation model components show a wider range of results 20 , 21 . Nevertheless, the forest may be ‘committed’ to dieback despite appearing stable at the end of model runs 16 . This highlights the importance of measuring the changing dynamic stability of the forest alongside its mean state. Given the uncertainty in model projections, we directly analyse observational data for signs of resilience loss in the Amazon.
The mean state of a system is not usually informative of changes in resilience; either one can change whilst the other remains constant 16 , 17 . Thus, higher-order statistical characteristics that respond more sensitively to destabilization than the mean need to be considered to quantify resilience. To measure the changing resilience of the Amazon rainforest, we use a stability indicator used to predict the approach of a dynamical system towards a bifurcation-induced critical transition. The predictability arises from the phenomenon of critical slowing down 22 , 23 (CSD): as the currently occupied equilibrium state of a system becomes less stable, it responds more sluggishly to short-term perturbations (for example, weather variability for the Amazon). This loss of resilience, which is itself typically defined 24 as the return rate from perturbations, reflects a weakening of negative feedbacks that maintain stability. The behaviour can be detected by an increase in lag-1 autocorrelation (AR(1)) in time series capturing the system dynamics 25 , 26 . It may also manifest as an increase in variance over time but variance can also be easily influenced by changing variability of the perturbations driving the system 27 . Increasing AR(1) has been used to detect CSD before bifurcation-induced state transitions in a number of systems, including but not limited to climate 25 , 28 and ecology 29 . In particular, CSD has recently been detected in reconstructions of western Greenland ice sheet height changes 30 as well as of the variability of the Atlantic Meridional Overturning Circulation 31 . A caveat, highlighted by analysis of model projections before Amazon dieback 27 , is that a system should be forced slower than its intrinsic response time scale for CSD to occur ( Methods ). Hence, the absence of CSD may not rule out the possibility of a forthcoming critical transition. Conversely, increasing AR(1) can sometimes occur for other physical reasons. A space-for-time substitution has previously revealed that tropical forest resilience as measured by mean AR(1) (on a grid cell basis) is lower for less annual rainfall sums 32 but changes of Amazon resilience over time have not been investigated so far.
We investigate controls on the resilience of the Amazon vegetation system and how its resilience has changed over the last three decades, in terms of a changing AR(1) coefficient as estimated from satellite-derived vegetation data. For comparison, we also investigate changes in variance over time, as a secondary indicator for CSD. The main dataset we use is from the Vegetation Optical Depth Climate Archive (VODCA) 33 but we also analyse the NOAA Advanced Very-High-Resolution Radiometer’s (AVHRR) Normalized Difference Vegetation Index (NDVI) 34 for comparison. Vegetation optical depth (VOD) has been previously used to estimate changes in vegetation biomass 35 , whereas NDVI is more commonly used to measure the greenness of vegetation (that is, photosynthetic activity 36 ), which can saturate at dense grass cover. VOD, a microwave-derived product, does not saturate and remains sensitive to changes also at high biomass density 35 . We use the longer, Ku-band product from VODCA, which has a resolution of 0.25° × 0.25°, from which we create a monthly dataset by taking the mean values and for direct comparison we rescale the NDVI data to the same resolution. For a check of robustness, we also use the C-band product from VODCA, which spans a shorter time period. We focus on two stressors of the Amazon that may cause resilience changes—precipitation decline and human influence.
We use the Amazon basin as our study region and focus on those grid cells that have a ≥80% evergreen broadleaf (BL) fraction according to the MODIS Land Cover Type product in 2001 37 ( Methods ). Figure 1 shows that mean changes in BL fraction in this region correspond well to changes in VOD. Averaged across the Amazon study region we find overall decreasing VOD over 2001–2016, corresponding to the observed decrease in the number of grid cells that have BL ≥ 80% each year (Fig. 1a ). Between 2001 and 2016, the BL fraction has changed most prominently in the south-eastern parts of the Amazon basin, along parts of the Amazon River and in some northern parts of the basin (Fig. 1b ). Changes in VOD have a similar spatial pattern to changes in BL fraction, with decreases concentrated around the south-eastern edges of the forest (Fig. 1c ). NDVI, in contrast, does not agree temporally or spatially with the changes in BL fraction (Supplementary Fig. 1 ), with NDVI increases observed in the south-eastern parts of the Amazon where deforestation rates are known to be highest. On the individual grid cell level, changes in BL are strongly correlated with changes in VOD (Pearson’s r = 0.556; Supplementary Fig. 2a ) compared to changes in NDVI ( r = −0.133; Supplementary Fig. 2b ). This echoes previous in-situ comparisons between VOD and NDVI 38 . Hence, we focus our analysis on VOD in the following, with results for NDVI in the Supplementary Figures .
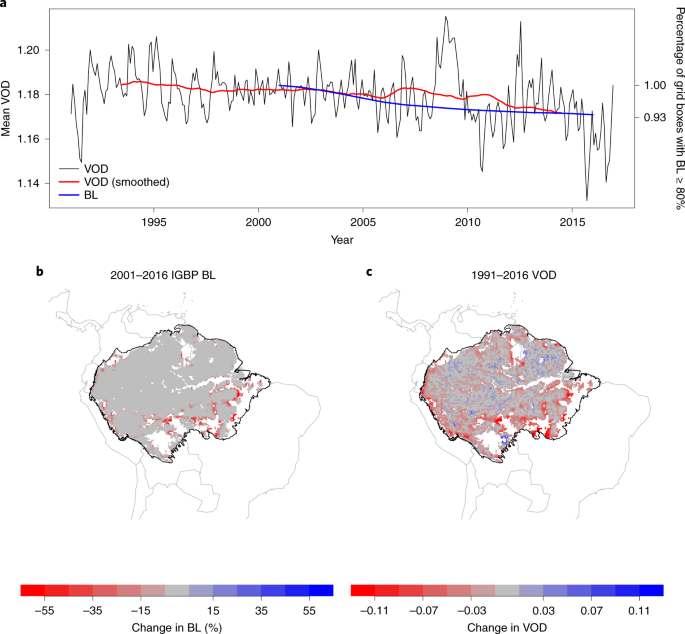
a , Time series of MODIS Land Cover evergreen BL fraction and VODCA Ku-band product. Changes in BL fraction expressed as the percentage of grid cells that have BL fraction ≥80% in each year, compared to the number of grid cells that had BL fraction ≥80% in 2001, and VOD is the monthly mean. b , Changes in the BL fraction from 2001 to 2016 for grid cells where the BL fraction is ≥80% in 2001. c , Changes in VOD from 1991 to 2016 (difference between the 2012–2016 and 1991–1995 means) for the grid cells where the BL fraction was ≥80% in 2001. Country outlines were provided by the ‘maps’ package in R and Amazon basin outline was created from http://worldmap.harvard.edu/data/geonode:amapoly_ivb ( Methods ).
We begin our resilience analysis by focusing on the temporal changes of AR(1), computed in sliding windows from the nonlinearly detrended and deseasonalized VOD time series (Fig. 2 and Methods ). We remove forested (BL ≥ 80%) grid cells that have any human land use in them ( Methods ), resulting in 6,369 grid cells being analysed. The spatial distribution of the AR(1) tendency, measured by the Kendall’s rank correlation coefficient τ ( Methods ) at each grid cell, shows that the AR(1) increases in most of the grid cells comprising the Amazon rainforest (Fig. 2a,b ). Likewise, the time series calculated from the mean AR(1) value across our study area each month shows a substantial increase over time, particularly from the early 2000s (Fig. 2c ). We observe some stable or decreasing AR(1) values around the tributaries of the Amazon River, suggesting increasing resilience. VOD values can be influenced by open water but this should be minimized by looking only at grid cells where BL ≥ 80%. Previously, floodplain forests near the river, which cover 14% of the basin, have been shown to be much less resilient than the non-flooded forests throughout the Amazon 39 . However, when we compare grid cells in the Amazon basin floodplains and those outside ( Methods ), we find no significant differences between the resilience signals (Mann–Whitney U -test P = 0.579), except for a smaller Kendall τ value for floodplains from 2003 onwards (0.863 compared to 0.915; Supplementary Fig. 3 ).
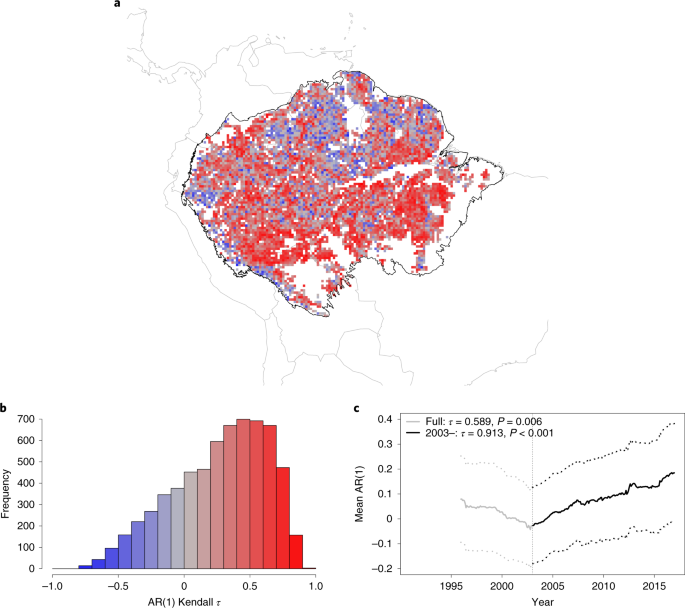
a , A map of the Kendall τ values of individual grid cells from 2003. b , Histogram of the Kendall τ values for the Amazon rainforest, considering data from 2003 onwards. Of the grid cells, 76.2% have a positive Kendall τ value from 2003 onwards and 77.8% have this for the full time series. c , Mean VOD AR(1) time series (solid line) along with ±1 s.d. (dotted lines) created from grid cells that have BL fraction ≥80% in the Amazon basin and also contain no human land use (main text and Methods ). The full AR(1) time series from 1991 (grey) has a Kendall τ value of 0.589 ( P = 0.006) and from 2003 (black), a value of 0.913 ( P < 0.001). Note that the AR(1) values are plotted at the end of each 5-yr sliding window. Country and Amazon basin outlines produced as described in Fig. 1 .
Overall, most (76.2%) of the grid cells show increasing AR(1) values from the early 2000s onwards and hence, loss of resilience (Fig. 2b ), as well as 77.8% of grid cells over the full time period. Using alternative methods of detrending the VOD time series ( Methods ) yields similar results (Supplementary Figs. 4 and 5 ), as does varying the window length used to estimate AR(1) (Supplementary Fig. 6 ). The results are also robust to the choice of the BL fraction threshold used to determine forest, finding increasing AR(1) also when either BL ≥ 90% or BL ≥ 40% is used (Supplementary Fig. 7 ). Furthermore, restricting the analysis to those grid points that have BL ≥ 80% through the period 2001–2016, rather than only checking grid cells for BL ≥ 80% in 2001, shows similar signals of resilience loss (Supplementary Fig. 8 ). A predominance of increasing AR(1) trends is also found for the NDVI time series since 2003 (Supplementary Fig. 9 ).
To try and determine the causes for the detected resilience loss across the Amazon basin, we explore the relationships between the AR(1) trends and mean annual precipitation (MAP, estimated from the CHIRPS dataset 40 ), as well as between the AR(1) trends and the distance from human land use ( Methods ). It has previously been suggested that drier forest is less resilient 32 as well as forest nearer human land use 41 . We also include distance from roads alongside land use but this restricts the domain of analysis to Brazil to avoid biases by heterogeneities in road data across different countries (Supplementary Fig. 10 ; Methods ). Figure 3 compares the spatial patterns of the AR(1) trends, MAP and human land use. Relationships with both explanatory variables are discernible, with (less common) decreases in AR(1) (Fig. 3a ) being found in regions of high MAP in the north of the region (Fig. 3b ) and further away from human land use (Fig. 3c ). We find no confounding relationship between MAP and human land use; they are only very weakly correlated with each other (Spearman’s ρ = 0.057, P < 0.001). Hence, we can consider them as separate relationships. The computed minimum distances to human land use and roads should be interpreted as upper bounds because for the full region we do not include roads, and for the Brazil distances our dataset will not include non-federal or non-state roads, which also have human activity associated with them. Furthermore, the classification of grid cells that contain human land use at the spatial resolution used in this analysis is likely to only detect large farms and settlements.
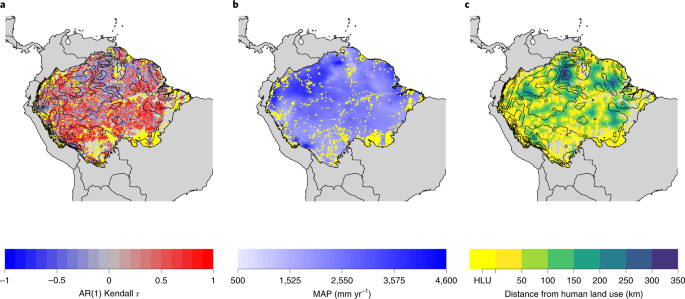
a , VOD AR(1) Kendall τ values (as in Fig. 2a ). b , MAP from the CHIRPS dataset from 1991 to 2016. c , Distance from human land use (HLU) ( Methods ). In a – c , MAP contours are shown, along with HLU grid cells (yellow). Supplementary Fig. 10 shows the distance from HLU or Brazilian roads for the grid cells in Brazil only. Country and Amazon basin outlines produced as described in Fig. 1 .
To further explore the relationship between MAP and AR(1) trends, we create mean AR(1) time series on a moving MAP band of 500 mm ( Methods ). These bands show broadly the same behaviour as the region overall (Fig. 4a ), with all bands showing a significant decrease in resilience post-2003 ( P < 0.001 for all MAP bands). The increase in AR(1) post-2003 appears least pronounced for the highest rainfall band (3,500–4,000 mm). The strength of resilience loss increases as the MAP band decreases below 3,500–4,000 mm (Fig. 4b ). For NDVI, the same relationship is also observed (Supplementary Fig. 11a,b ). However, due to a large decrease in NDVI AR(1) pre-2003 across the region, analysing the full NDVI AR(1) time series yield decreasing AR(1) Kendall τ coefficients for the higher MAP bands.
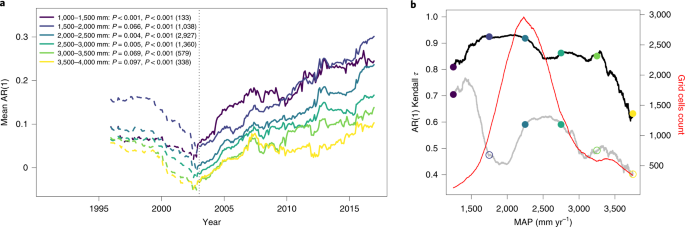
a , VOD AR(1) time series for 500 mm MAP bands from 1996 (including data going back to 1991; dashed lines) and from 2003 (including data going back to 1998; solid lines). The P values of the trend significance test ( Methods ) are given in the legend; from 2003 onwards, they are all >0.001. b , VOD AR(1) Kendall τ series for a sliding MAP band with a width of 500 mm, from 1996 (grey) and from 2003 (black). Circles are coloured according to the corresponding time series shown in a and filled if the Kendall τ value is significantly positive ( P < 0.05) and open otherwise. The tendencies of the relationships in b are τ = −0.403 (grey) and τ = −0.463 (black), confirming there is a more severe decrease in resilience with lower rainfall values. See Supplementary Fig. 15 for an uncertainty quantification of the results shown in b . The number of grid cells used to calculate the AR(1) time series and thus the Kendall τ values are shown in red in b . This never falls below 100 grid cells and so we can be confident in the mean estimation of the AR(1) time series. The number of grid cells used in the calculation of the time series in a is shown in brackets in the legend. Note that the AR(1) values are plotted at the end of each 5-yr sliding window.
To further explore the relationship between resilience and the distance to human land use, we calculate mean AR(1) time series on 50 km distance bands. Our results show that increases in AR(1) post-2003 are stronger for grid cells closer to human land use (Fig. 5a ). Above 200–250 km away from human land use the signal of loss of resilience becomes less pronounced (Fig. 5b ). Using the subset of Brazilian grid cells ( n = 3,797) to include roads in our measurement of distance to human activity (Supplementary Fig. 10 ; Methods ) generally reduces distances but we find a similar relationship, with decreases in Kendall τ coefficients observed up to 75 km (Fig. 5c,d ). We note in both cases that, at longer distances, the number of grid cells used to calculate the AR(1) time series is lower (red lines and right-hand y axis in Fig. 5b,d ). The remaining areas also tend to become more separated (the two vertical lines in Fig. 5b,d mark where <100 and <50 grid cells were left for the calculations, respectively). This helps to explain the more variable AR(1) time series at greater distances (for example, the yellow line in Fig. 5a ) and the more fluctuating results for Kendall τ coefficients at greater distances (Fig. 5b,d ). NDVI time series also show a loss of resilience from the early 2000s, in grid cells that are closer than 200 km from human land use (Supplementary Fig. 11c,d ). We reiterate that the stated minimum distances to human land use should be viewed as upper limits with, for example, selective logging and other intrusions expected to be closer to the forest than the grid cells with major human land use and major roads. Comprehensive robustness tests, using alternative datasets and indicators, are presented in the Methods . In particular, we find overall consistent results when using the variance instead of the AR(1) as measure of resilience.
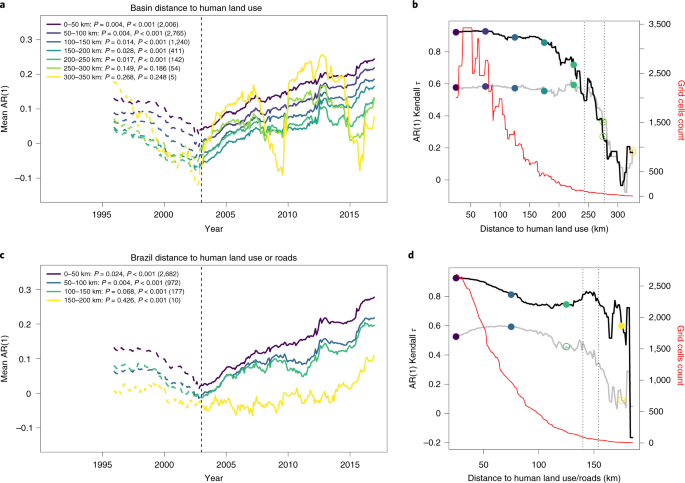
a , VOD AR(1) time series for 50 km bands measuring the minimum distance a forested grid cell is from a grid cell with human land use (defined in the Methods from the MODIS Land Cover product), from 1996 (dashed lines, these include data going back to 1991 due to the 5-yr sliding windows used to estimate the AR(1)) and from 2003 (solid lines, including data going back to 1998), with the significance of these respective tendencies shown in the legend ( Methods ). b , VOD AR(1) Kendall τ series for the sliding 50 km bands, from 1996 (grey, again including data going back to 1991) and from 2003 (black, including data going back to 1998). Circles are coloured according to the corresponding time series in a and are filled if the Kendall τ value is significantly positive ( P < 0.05) and open otherwise. The tendencies of these relationships are τ = −0.574 (grey) and τ = −0.858 (black), showing that there is a more severe decrease in resilience with increasing proximity to human land use. The number of grid cells used to calculate the AR(1) time series and thus the Kendall τ values are shown in red in b , with vertical dotted lines denoting where there are 100 and 50 grid cells available for the calculation. The number of grid cells used in the calculation of the time series in a is shown in brackets in the legend. c , d , The same as a and b , respectively, but for the subset of grid cells in Brazil, where reliable road data are available (as shown in Supplementary Fig. 10 ). For this case, where the distances from any given forested grid cell to human land use or roads are computed, the trends in the Kendall τ series are τ = −0.688 and τ = −0.679, respectively.
We reiterate that changes in the mean state of a system do not directly relate to changes in resilience. Model studies show that large parts of the Amazon rainforest can be committed to dieback 16 before showing a strong change in mean state. Indeed, from our CSD indicators we infer a marked loss of Amazon rainforest resilience since the early 2000s, in vast areas where the BL fraction has not strongly decreased (compare Figs. 1b and 2a or Supplementary Fig. 8b ).
Given that lower baseline MAP (Fig. 4 ) and greater proximity to human interference (Fig. 5 ) are both statistically associated with greater loss of resilience, we hypothesize that low MAP and increasing human interference could both be contributing to the large-scale loss of resilience (Fig. 2 ). What remains to be explained is why these two factors might play such an important role and why the large-scale resilience loss started in the early 2000s.
Previous work 32 has shown that regions with lower MAP have lower absolute forest resilience, conceivably because vegetation is more water stressed and struggles to regulate its internal water content. VOD, being dependent on this water content, would consequently adjust more slowly to perturbations. Our finding that resilience has been lost faster in lower MAP regions, additionally suggests that vegetation in regions with more pronounced aridity stress is at greater risk of losing resilience. Large parts of the study region show decreasing MAP. However, the spatial pattern of MAP change (as measured by the difference between the means for January 1998 to December 2002 and January 2012 to December 2016; Supplementary Fig. 12 ) is different to that of AR(1) increases (Fig. 2 ) and we find no spatial correlation between these MAP changes and VOD AR(1) Kendall τ (Spearman’s ρ between the spatial field of MAP change and the spatial field of VOD AR(1) Kendall τ equals 0.04). Increases in dry-season length (DSL; Supplementary Fig. 12 ) reported in several recent studies 42 , 43 , 44 , 45 , might conceivably be a better explanatory variable but again we find no spatial correlation between DSL change and VOD AR(1) Kendall τ ( ρ = −0.08). The lack of spatial correlations for both MAP and DSL could be due to the relatively short period to measure rainfall trends and for DSL due to the discrete nature of DSL values, which are given in units of full months, compared to the continuous τ values.
Despite a lack of spatial correlations, existing understanding and larger-scale aggregate measures suggest that climate variability may be among multiple factors contributing to the observed Amazon resilience loss since the early 2000s (Fig. 6 ). Notably, the Amazon shows signs of resilience loss during a period with three ‘one-in-a-century’ droughts 10 , 46 , 47 , 48 . Sea surface temperature (SST) anomalies in the northern tropical Atlantic Ocean (from the HadISST 49 datatset; Methods ) from around 2000 onwards have been generally positive compared to climatology, consistent with a shift of the Atlantic Multidecadal Oscillation (AMO) to its positive phase (Supplementary Fig. 13 ), although reductions in anthropogenic northern-hemisphere aerosol cooling may also play a role 10 . These positive northern tropical Atlantic SST anomalies led—via the associated northward shift of the Intertropical Convergence Zone—to drier conditions in the Amazon and, in particular, to two severe droughts in 2005 and 2010 46 , 48 (Fig. 6a ). These two drought events are associated with corresponding peaks in the spatial-mean AR(1) time series, superimposed on the overall positive trend (see arrows in Fig. 6b ). These peaks are also found in the separate AR(1) time series in Fig. 4a , appearing about 2.5 yr ahead due to the time series being plotted at the end of the 5-yr sliding windows used to calculate the AR(1) there. Moreover, a third, El Niño-driven drought in 2015/16 is accompanied by an increased overall rate of resilience loss at the very end of the time range for which the VOD data are available. The decrease in AR(1) before the early 2000s may also be linked to internal climate variability; the AMO was in its negative phase (Supplementary Fig. 13 ), consistent with negative SST anomalies in the northern tropical Atlantic (Fig. 6 ) and wetter conditions in the Amazon. The fact that the AR(1) increase since the early 2000s is statistically strongly significant suggests that it is not just due to natural climate variability.
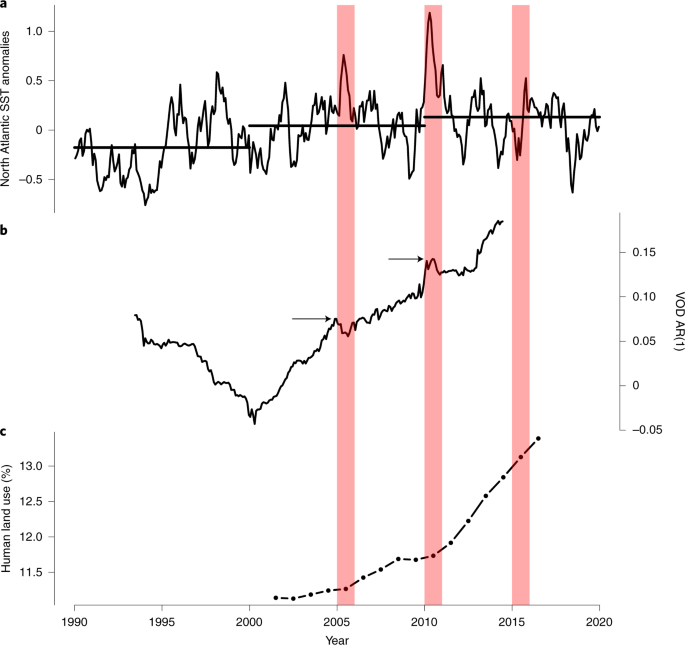
a , Northern tropical Atlantic SST anomalies averaged over 15–70° W, 5–25° N, once a mean monthly cycle has been removed. Horizontal black lines denote the decade-mean anomalies. b , Spatially averaged Amazon basin VOD AR(1) time series as in Fig. 2 , plotted at the midpoint of the window used to calculate AR(1) rather than at the end of the window. c , Annual time series of percentage of grid cells in the Amazon basin that have human land use (as described in Methods ). Red bands refer to 2005, 2010 and 2015, which were severe drought years in the Amazon basin. Arrows in b show the peak value in AR(1) in or near the drought years. These peak values may appear earlier due to the AR(1) time series being calculated on a moving window, compared to the SST anomalies being a monthly mean.
Increasing human land use also appears to be contributing to the observed Amazon resilience loss, with human land-use areas increasing in both reach and intensity (Fig. 6c and Supplementary Fig. 14 ). Notably, the expansion of human land use accelerates after 2010, in an interval that also shows accelerated resilience loss (Fig. 6b ) but less striking northern tropical Atlantic SST anomalies (Fig. 6a ). Greater proximity to human land use can increase disturbance factors such as direct removal of trees, construction of roads and fires, conceivably reducing absolute resilience (Fig. 5 ) and making the forest more prone to resilience loss.
Other factors, including rising atmospheric temperatures in response to anthropogenic greenhouse gas emissions, may additionally have negative effects on Amazon resilience (and are contributing to the warming of northern tropical Atlantic SSTs; Fig. 6a ). Furthermore, the rapid change in climate is triggering ecological changes but ecosystems are having difficulties in keeping pace. In particular, the replacement of drought-sensitive tree species by drought-resistant ones is happening slower than changes in (hydro)meteorological conditions 50 , potentially reducing forest resilience further.
In summary, we have revealed empirical evidence that the Amazon rainforest has been losing resilience since the early 2000s, risking dieback with profound implications for biodiversity, carbon storage and climate change at a global scale. We further provided empirical evidence suggesting that overall drier conditions, culminating in three severe drought events, combined with pronounced increases in human land-use activity in the Amazon, probably played a crucial role in the observed resilience loss. The amplified loss of Amazon resilience in areas closer to human land use suggests that reducing deforestation will not just protect the parts of the forest that are directly threatened but also benefit Amazon rainforest resilience over much larger spatial scales.
We use the Amazon basin ( http://worldmap.harvard.edu/data/geonode:amapoly_ivb , accessed 28 January 2021) as our region of study. To determine the grid cells that are contained within Brazil for a subset of analysis, we use the ‘maps’ package in R (v.3.3.0; https://CRAN.R-project.org/package=maps ). This is also used in the plotting of country outlines. The main dataset used to determine forest health is from VODCA 33 , of which we use the Ku-band product. These data are available at 0.25° × 0.25° at a monthly resolution from January 1988 to December 2016. We also use NOAA AVHRR NDVI 34 . For precipitation data, we use the CHIRPS dataset 40 downloaded from Google Earth Engine at a monthly resolution. Finally, to determine land cover types, we used the IGBP MODIS land cover dataset MCD12C1 (ref. 37 ). All these datasets are at a higher spatial resolution than the VODCA dataset and thus we downscale them to match the lower resolution. Our SST data comes from HadISST 49 , where we define a North Atlantic region (15–70° W, 5–25° N), for which we take the spatial mean. The mean monthly cycle is then removed to produce anomalies.
For the vegetation datasets that we measure the resilience indicators on (below), we use STL decomposition (seasonal and trend decomposition using Loess) 51 using the stl() function in R. This splits time series in each grid cell into an overall trend, a repeating annual cycle (by using the ‘periodic’ option for the seasonal window) and a residual component. We use the residual component in our resilience analysis. The first 3 yr of data had large jumps in VOD which were seen when testing other regions of the world as well as in the Amazon region. Hence, we restrict our analysis to the period January 1991 to December 2016.
To test the robustness of the detrending, we also vary the size of the trend window in the stl() function. The results from these alternatively detrended time series are shown in Supplementary Fig. 4 . The results are also robust to varying the window used to calculate the seasonal component rather than using ‘periodic’; at the strictest plausible value of 13, we still see the same increases in AR(1) (Supplementary Fig. 5 ).
For the AMO index shown in Supplementary Fig. 13 , data come from the Kaplan SST dataset and can be downloaded from https://psl.noaa.gov/data/timeseries/AMO/ .
Grid cell selection
We use the IGBP MODIS land cover dataset at the resolution described above to determine which grid cells to use in our analysis. The dataset is available at an annual resolution from 2001 to 2018 (but we only use the time series up to 2016 to match the time span of our VOD and NDVI datasets). To focus on changes in forest resilience, we use grid cells where the evergreen BL fraction is ≥80% in 2001. Grid cells are treated as human land-use area if the built-up, croplands or vegetation mosaics fraction is >0%. We remove grid cells that have human land use in them from our forest analysis, regardless of if there is ≥80% BL fraction in the grid cell.
We measure the minimum distance between forested Amazon basin grid cells and human land-use grid cells in 2016 (believing this to be the most cautious and least biased way to measure distance) using the latitude and longitude of each grid point and computing the great-circle distance. We use human land-use grid cells over a larger area than the basin, so that we can determine the closest distance to human land use, regardless of whether this human land use lies within the basin. We also measure the minimum distance from human land use or roads in Brazil, where we have reliable data on state and federal roads ( https://datacatalog.worldbank.org/dataset/brazil-road-network-federal-and-state-highways ). As in the main text, we reiterate that these minimum distances can be viewed as the maximum distance from human land use as our data will not include roads for the full Amazon basin, or non-federal or non-state roads in Brazil that will have human activity associated with them.
To ensure that the pattern of changes in resilience is not a consequence of more settlements being in the southeast of the region, combined with the gradient of rainfall from northwest to southeast typical of the rainforest, we measure the correlation between MAP and the distances from the urban grid cells, which is very weak (Spearman’s ρ = 0.109, P < 0.001) and as such we are confident that there are separate processes that causes these relationships.
Resilience indicator AR(1)
We measure our resilience indicator on the residual component of the decomposed vegetation time series. We focus on AR(1), which provides a robust indicator for CSD before bifurcation-induced transitions and has been widely used for this purpose 23 , 25 , 32 . We measure it on a sliding window length equal to 5 yr (60 months). The sliding window creates a time series of the AR(1) coefficient in each location. Our results are robust to the sliding window length used, as shown in Supplementary Fig. 6 .
From linearization and the analogy to the Ornstein–Uhlenbeck process, it holds approximately that for discrete time steps of width Δ t (1 month in the case at hand):
where κ is the linear recovery rate. A decreasing recovery rate κ implies that the system’s capability to recover from perturbations is progressively lost, corresponding to diminishing stability or resilience of the attained equilibrium state. From the above equation it is clear that the AR(1) increases with decreasing κ . The point at which stability is lost and the system will undergo a critical transition to shift to a new equilibrium state, corresponds to κ = 0 and AR(1) = 1, respectively.
Measuring AR(1) across the whole time series provides information about the characteristic time scales of the two vegetation datasets we use 26 . Inverting κ gives the characteristic time scale of the system; for the VOD, we find 1/ κ = 1.240 months, whereas for the NDVI, we find 1/ κ = 0.838 months when using the mean AR(1) value across the region. This suggests that, in accordance with our interpretation of the two satellite-derived variables, the NDVI is more sensitive to shorter-term vegetation changes such as leaf greenness, while the VOD’s Ku-band is sensitive to longer-term changes such as variability in the thickness of forest stems.
Creation and tendency of AR(1) and variance time series
For analyses where either MAP bands or distance bands are used to create an AR(1) or variance series, we calculate the mean AR(1) or variance value in each month for forested (BL ≥ 80%), non-human land-use Amazon basin grid cells, from which the tendency of this mean series can be calculated. Alternatively, the Kendall τ for each band can be calculated by taking the mean Kendall τ for each individual grid cell that is within the band. Results from the first option are shown in Figs. 4 and 5 and results from the second method in Supplementary Fig. 15 for AR(1).
The tendencies of the CSD indicators are determined in terms of Kendall τ . This is a rank correlation coefficient with one variable taken to be time. Kendall τ values of 1 imply that the time series is always increasing, −1 implies that the time series is always decreasing and 0 indicates that there is no overall trend. Following previous work 25 , 52 , 53 , we test the statistical significance of positive tendencies using a test based on phase surrogates that preserve both the variance and the serial correlations of the time series from which the surrogates are constructed. Specifically, we compute the Fourier transform of each time series for which we want to test the significance of Kendall τ , then randomly permute the phases and finally apply the inverse Fourier transform. Since this preserves the power spectral density, it also preserves the autocorrelation function due to the Wiener–Khinchin theorem. For each time series this procedure is repeated 100,000 times to obtain the surrogates. Kendall τ is computed for each surrogate to obtain the null model distribution (corresponding to the assumption of the same variance and autocorrelation but no underlying trend), from which we calculate a P value by calculating the proportion of surrogates that have a higher Kendall τ value.
Robustness tests
To account for the possibility of human deforestation interfering with the signals we observe (which may not necessarily be detected by the MODIS Land Cover dataset) we also use the Hansen forest loss dataset 54 to determine grid cells to remove in an alternate analysis. The original Hansen dataset is at a 0.00025° resolution, 1,000 times higher than the VOD dataset and as such for each VOD grid cell we measure the percentage of Hansen grid cells that show some forest loss over the time period. Note that this dataset does not specify if the observed loss is natural or caused by human deforestation. Excluding any VOD grid cells that contain more than a conservative 5% of lost forest grid cells according to this dataset and running the analysis in the main paper shows similar results. Supplementary Figs. 16–18 are recreations of Figs. 2 , 4 and 5 , respectively.
The loss of forest resilience observed as increasing AR(1) in both vegetation indices is supported by another indicator of CSD, namely increasing variance 28 —of both VOD (Supplementary Fig. 19 ) and NDVI (Supplementary Fig. 20 ). Variance is more strongly affected by changes in the frequency and amplitude of the forcing of a system and as such results could be biased towards individual events. However, we assessed the precipitation time series for changes in variance and found no relationship with the variance signals of VOD and NDVI (Supplementary Fig. 21 ). Nevertheless, AR(1) is considered the more robust indicator 55 . As another test of robustness, we partition the grid cells into those that are in floodplains and those that are not (Supplementary Fig. 3 ). Floodplain data are part of the NASA Large Scale Biosphere–Atmosphere Experiment (LBA-ECO) 56 . We also calculate the resilience signals for the C-band product of VOD for comparison (Supplementary Fig. 22 ). Despite the smaller temporal scale of this product, we still see increases in both AR(1) and variance. To account for a change in the number of satellites used to calculate VOD, for the Ku-band we also recreate the dataset by sampling a single random day per month rather than taking a monthly average, to mimic a constant satellite pass for the whole time period (Supplementary Fig. 23 ). Although this expectedly affects the absolute values of AR(1) and variance, their relative changes over time remain unaffected. To further test the robustness of our results, we looked for similar signals of resilience change in terms of trends in AR(1) in addition to variance in the precipitation time series used, as a change in the forcing could have an impact on the forest that could mistakenly be interpreted as a vegetation resilience loss. However, as for the variance, there is no clear increase in the AR(1) of precipitation, nor do the spatial patterns of both indicators reveal any relationship between changing precipitation AR(1) and variance and the observed vegetation resilience loss. Hence, we are confident that changes in precipitation forcing are not driving the vegetation AR(1) signals.
Data availability
The VOD dataset is available from https://zenodo.org/record/2575599#.YHRE6RRKj7E . The NDVI dataset is available from https://www.ncei.noaa.gov/metadata/geoportal/rest/metadata/item/gov.noaa.ncdc:C01558/html . The MODIS Land Cover dataset is available from https://lpdaac.usgs.gov/products/mcd12c1v006 . The CHIRPS precipitation dataset is available from https://data.chc.ucsb.edu/products/CHIRPS-2.0/global_2-monthly/tifs . The Amazon basin shapefile is available from http://worldmap.harvard.edu/data/geonode:amapoly_ivb . Brazilian road data are available from https://datacatalog.worldbank.org/dataset/brazil-road-network-federal-and-state-highways . SST data from HadISST are available from https://www.metoffice.gov.uk/hadobs/hadisst/ .
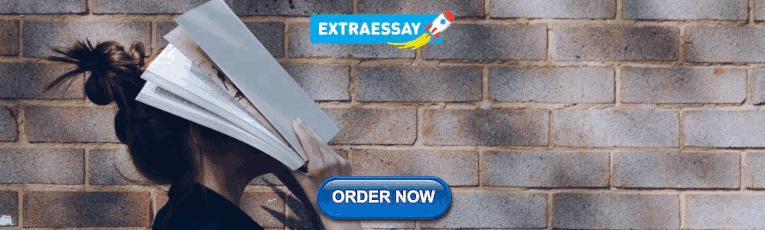
Code availability
All data and code used for the analysis are available on request from the corresponding author and are published online 57 .
Lenton, T. M. et al. Tipping elements in the Earth’s climate system. Proc. Natl Acad. Sci. USA 105 , 1786–1793 (2008).
Article CAS Google Scholar
Dirzo, R. & Raven, P. H. Global state of biodiversity and loss. Ann. Rev. Environ. Res. 28 , 137–167 (2003).
Article Google Scholar
Malhi, Y. et al. Climate change, deforestation, and the fate of the Amazon. Science 319 , 169–172 (2008).
Gatti, L. V. et al. Amazonia as a carbon source linked to deforestation and climate change. Nature 595 , 388–393 (2021).
Brienen, R. J. W. et al. Long-term decline of the Amazon carbon sink. Nature 519 , 344–348 (2015).
Feldpausch, T. R. et al. Amazon forest response to repeated droughts. Glob. Biogeochem. Cycles 30 , 964–982 (2016).
Hubau, W. et al. Asynchronous carbon sink saturation in African and Amazonian tropical forests. Nature 579 , 80–87 (2020).
Boers, N., Marwan, N., Barbosa, H. M. J. & Kurths, J. A deforestation-induced tipping point for the South American monsoon system. Sci. Rep. 7 , 41489 (2017).
Cox, P. M., Betts, R. A., Jones, C. D., Spall, S. A. & Totterdell, I. J. Acceleration of global warming due to carbon-cycle feedbacks in a coupled climate model. Nature 408 , 184–187 (2000).
Cox, P. M. et al. Increasing risk of Amazonian drought due to decreasing aerosol pollution. Nature 453 , 212–215 (2008).
Lovejoy, T. E. & Nobre, C. Amazon tipping point. Sci. Adv. 4 , eaat2340 (2018).
Lovejoy, T. E. & Nobre, C. Amazon tipping point: last chance for action. Sci. Adv. 5 , eaba2949 (2019).
Brando, P. M. et al. Abrupt increases in Amazonian tree mortality due to drought–fire interactions. Proc. Natl Acad. Sci. USA 111 , 6347–6352 (2014).
Pueyo, S. et al. Testing for criticality in ecosystem dynamics: the case of Amazonian rainforest and savanna fire. Ecol. Lett. 13 , 793–802 (2010).
Salati, E., Dall’Olio, A., Matsui, E. & Gat, J. R. Recycling of water in the Amazon basin: an isotopic study. Water Resour. Res. 15 , 1250–1258 (1979).
Jones, C., Lowe, J., Liddicoat, S. & Betts, R. Committed terrestrial ecosystem changes due to climate change. Nat. Geosci. 2 , 484–487 (2009).
Boulton, C. A., Booth, B. B. B. & Good, P. Exploring uncertainty of Amazon dieback in a perturbed parameter Earth system ensemble. Glob. Change Biol. 23 , 5032–5044 (2017).
Huntingford, C. et al. Simulated resilience of tropical rainforests to CO 2 -induced climate change. Nat. Geosci. 6 , 268–273 (2013).
Friend, A. D., Stevens, A. K., Knox, R. G. & Cannell, M. G. R. A process-based, terrestrial biosphere model of ecosystem dynamics (Hybrid v3.0). Ecol. Model. 95 , 249–287 (1997).
Poulter, B. et al. Robust dynamics of Amazon dieback to climate change with perturbed ecosystem model parameters. Glob. Change Biol. 16 , 2476–2495 (2010).
Sitch, S. et al. Evaluation of the terrestrial carbon cycle, future plant geography and climate-carbon cycle feedbacks using five Dynamic Global Vegetation Models (DGVMs). Glob. Change Biol. 14 , 2015–2039 (2008).
Wissel, C. A universal law of the characteristic return time near thresholds. Oecologia 65 , 101–107 (1984).
Scheffer, M. et al. Early-warning signals for critical transitions. Nature 461 , 53–59 (2009).
Pimm, S. L. The complexity and stability of ecosystems. Nature 307 , 321–326 (1984).
Dakos, V. et al. Slowing down as an early warning signal for abrupt climate change. Proc. Natl Acad. Sci. USA 105 , 14308–14312 (2008).
Held, H. & Kleinen, T. Detection of climate system bifurcations by degenerate fingerprinting. Geophys. Res. Lett. https://doi.org/10.1029/2004gl020972 (2004).
Boulton, C. A., Good, P. & Lenton, T. M. Early warning signals of simulated Amazon rainforest dieback. Theor. Ecol. 6 , 373–384 (2013).
Lenton, T. M. Early warning of climate tipping points. Nat. Clim. Change 1 , 201–209 (2011).
Biggs, R., Carpenter, S. R. & Brock, W. A. Turning back from the brink: detecting an impending regime shift in time to avert it. Proc. Natl Acad. Sci. USA 106 , 826–831 (2009).
Boers, N. & Rypdal, M. Critical slowing down suggests that the western Greenland Ice Sheet is close to a tipping point. Proc. Natl Acad. Sci. USA 118 , e2024192118 (2021).
Boers, N. Observation-based early-warning signals for a collapse of the Atlantic Meridional Overturning Circulation. Nat. Clim. Change 11 , 680–688 (2021).
Verbesselt, J. et al. Remotely sensed resilience of tropical forests. Nat. Clim. Change 6 , 1028–1031 (2016).
Moesinger, L. et al. The global long-term microwave Vegetation Optical Depth Climate Archive (VODCA). Earth Syst. Sci. Data 12 , 177–196 (2020).
NOAA Climate Data Record (CDR) of AVHRR Normalized Difference Vegetation Index (NDVI), Version 5 (NOAA National Centers for Environmental Information, accessed 10 February 2021); https://doi.org/10.7289/V5ZG6QH9
Liu, Y. Y. et al. Recent reversal in loss of global terrestrial biomass. Nat. Clim. Change 5 , 470–474 (2015).
Myneni, R. B., Keeling, C. D., Tucker, C. J., Asrar, G. & Nemani, R. R. Increased plant growth in the northern high latitudes from 1981 to 1991. Nature 386 , 698–702 (1997).
Friedl, M. & Sulla-Menashe, D. MCD12C1, Version 6 (NASA EOSDIS Land Processes DAAC, accessed 20 April 2020); https://doi.org/10.5067/MODIS/MCD12C1.006
Tian, F. et al. Remote sensing of vegetation dynamics in drylands: evaluating vegetation optical depth (VOD) using AVHRR NDVI and in situ green biomass data over West African Sahel. Remote Sens. Environ. 177 , 265–276 (2016).
Flores, B. M. et al. Floodplains as an Achilles’ heel of Amazonian forest resilience. Proc. Natl Acad. Sci. USA 114 , 4442–4446 (2017).
Funk, C. et al. The climate hazards infrared precipitation with stations—a new environmental record for monitoring extremes. Sci. Data 2 , 150066 (2015).
Wuyts, B., Champneys, A. R. & House, J. I. Amazonian forest–savanna bistability and human impact. Nat. Commun. 8 , 15519 (2017).
Fu, R. et al. Increased dry-season length over southern Amazonia in recent decades and its implication for future climate projection. Proc. Natl Acad. Sci. USA 110 , 18110–18115 (2013).
Marengo, J. A. et al. Changes in climate and land use over the Amazon region: current and future variability and trends. Front. Earth Sci. https://doi.org/10.3389/feart.2018.00228 (2018).
Leite-Filho, A. T., Sousa Pontes, V. Y. & Costa, M. H. Effects of deforestation on the onset of the rainy season and the duration of dry spells in southern Amazonia. J. Geophys. Res. Atmos. 124 , 5268–5281 (2019).
Leite‐Filho, A. T., Costa, M. H. & Fu, R. The southern Amazon rainy season: the role of deforestation and its interactions with large‐scale mechanisms. Int. J. Climatol. https://doi.org/10.1002/joc.6335 (2019).
Lewis, S. L., Brando, P. M., Phillips, O. L., Van Der Heijden, G. M. F. & Nepstad, D. The 2010 Amazon drought. Science 331 , 554 (2011).
Erfanian, A., Wang, G. & Fomenko, L. Unprecedented drought over tropical South America in 2016: significantly under-predicted by tropical SST. Sci. Rep. 7 , 5811 (2017).
Ciemer, C. et al. An early-warning indicator for Amazon droughts exclusively based on tropical Atlantic sea surface temperatures. Environ. Res. Lett. 15 , 094087 (2020).
Rayner, N. A. et al. Global analyses of sea surface temperature, sea ice, and night marine air temperature since the late nineteenth century. J. Geophys. Res. https://doi.org/10.1029/2002jd002670 (2003).
Esquivel‐Muelbert, A. et al. Compositional response of Amazon forests to climate change. Glob. Change Biol. 25 , 39–56 (2019).
Cleveland, R. B., Cleveland, W. S. & Terpenning, I. STL: a seasonal-trend decomposition procedure based on Loess. J. Off. Stat. 6 , 3 (1990).
Google Scholar
Rypdal, M. Early-warning signals for the onsets of Greenland interstadials and the younger dryas–preboreal transition. J. Clim. 29 , 4047–4056 (2016).
Boers, N. Early-warning signals for Dansgaard–Oeschger events in a high-resolution ice core record. Nat. Commun. 9 , 2556 (2018).
Hansen, M. C. et al. High-resolution global maps of 21st-century forest cover change. Science 342 , 850–853 (2013).
Dakos, V., Van Nes, E. H., D’Odorico, P. & Scheffer, M. Robustness of variance and autocorrelation as indicators of critical slowing down. Ecology 93 , 264–271 (2012).
Hess, L. L. et al. LBA-ECO LC-07 Wetland Extent, Vegetation, and Inundation: Lowland Amazon Basin (ORNL DAAC, 2005); https://doi.org/10.3334/ORNLDAAC/1284
Boulton, C. A. Amazon rainforest resilience data. Zenodo https://doi.org/10.5281/zenodo.5837469 (2022).
Download references
Acknowledgements
We thank B. Sakschewski for his helpful comments on the research. N.B. acknowledges funding by the Volkswagen Foundation. This is TiPES contribution no. 107. The TiPES project (‘Tipping Points in the Earth System’) has received funding from the European Union’s Horizon 2020 research and innovation programme under grant agreement no. 820970. C.A.B. and T.M.L. were supported by the Leverhulme Trust (RPG-2018-046). T.M.L. was also supported by a grant from The Alan Turing Institute under a Turing Fellowship (R-EXE-001).
Author information
Authors and affiliations.
Global Systems Institute, University of Exeter, Exeter, UK
Chris A. Boulton, Timothy M. Lenton & Niklas Boers
Earth System Modelling, School of Engineering & Design, Technical University of Munich, Munich, Germany
Niklas Boers
Potsdam Institute for Climate Impact Research, Potsdam, Germany
You can also search for this author in PubMed Google Scholar
Contributions
N.B. and C.A.B. conceived and designed the study with input from T.M.L. C.A.B. performed the numerical analysis with contributions from N.B. All authors discussed and interpreted results, drew conclusions and wrote the paper.
Corresponding author
Correspondence to Chris A. Boulton .
Ethics declarations
Competing interests.
The authors declare no competing interests.
Peer review
Peer review information.
Nature Climate Change thanks Albertus J. Dolman, Bernardo Flores and the other, anonymous, reviewer(s) for their contribution to the peer review of this work.
Additional information
Publisher’s note Springer Nature remains neutral with regard to jurisdictional claims in published maps and institutional affiliations.
Supplementary information
Supplementary information.
Supplementary Figs. 1–23.
Rights and permissions
Open Access This article is licensed under a Creative Commons Attribution 4.0 International License, which permits use, sharing, adaptation, distribution and reproduction in any medium or format, as long as you give appropriate credit to the original author(s) and the source, provide a link to the Creative Commons license, and indicate if changes were made. The images or other third party material in this article are included in the article’s Creative Commons license, unless indicated otherwise in a credit line to the material. If material is not included in the article’s Creative Commons license and your intended use is not permitted by statutory regulation or exceeds the permitted use, you will need to obtain permission directly from the copyright holder. To view a copy of this license, visit http://creativecommons.org/licenses/by/4.0/ .
Reprints and permissions
About this article
Cite this article.
Boulton, C.A., Lenton, T.M. & Boers, N. Pronounced loss of Amazon rainforest resilience since the early 2000s. Nat. Clim. Chang. 12 , 271–278 (2022). https://doi.org/10.1038/s41558-022-01287-8
Download citation
Received : 31 March 2021
Accepted : 13 January 2022
Published : 07 March 2022
Issue Date : March 2022
DOI : https://doi.org/10.1038/s41558-022-01287-8
Share this article
Anyone you share the following link with will be able to read this content:
Sorry, a shareable link is not currently available for this article.
Provided by the Springer Nature SharedIt content-sharing initiative
Quick links
- Explore articles by subject
- Guide to authors
- Editorial policies
Sign up for the Nature Briefing: Anthropocene newsletter — what matters in anthropocene research, free to your inbox weekly.

- Environment
Everything you need to know about the fires in the Amazon
Why are the fires burning and why is it such a big deal.
By Justine Calma , a senior science reporter covering climate change, clean energy, and environmental justice with more than a decade of experience. She is also the host of Hell or High Water: When Disaster Hits Home, a podcast from Vox Media and Audible Originals.
Share this story
If you buy something from a Verge link, Vox Media may earn a commission. See our ethics statement.
:format(webp)/cdn.vox-cdn.com/uploads/chorus_asset/file/19125948/1164446768.jpg.jpg)
Record-breaking fires are ripping through the Amazon — an ecosystem on which the whole world depends. The Verge will update this page with news and analysis on the fires and the effects that could linger once the ash settles.
Table of Contents:
Why is the Amazon burning?
Why is this a big deal?
Why is this a hot topic politically?
- How are the fires being fought?
An unprecedented number of fires raged throughout Brazil in 2019, intensifying in August. That month, the country’s National Institute for Space Research (INPE) reported that there were more than 80,000 fires, the most that it had ever recorded. It was a nearly 80 percent jump compared to the number of fires the country experienced over the same time period in 2018. More than half of those fires took place in the Amazon.
The number of blazes decreased in September , after president Jair Bolsonaro bowed to mounting pressure to address the flames and announced a 60-day ban on setting fires to clear land. Some exceptions were made for indigenous peoples who practice subsistence agriculture and those who’ve received clearance by environmental authorities to use controlled burning to prevent larger fires.
“There is no doubt that this rise in fire activity is associated with a sharp rise in deforestation”
“These are intentional fires to clear the forest,” Cathelijne Stoof, coordinator of the Fire Center at Wageningen University (WUR) in the Netherlands, tells The Verge . “People want to get rid of the forest to make agricultural land, for people to eat meat.” The INPE found that deforestation in Brazil’s Amazon hit an 11-year high in 2019.
“There is no doubt that this rise in fire activity is associated with a sharp rise in deforestation,” Paulo Artaxo, an atmospheric physicist at the University of São Paulo, told Science Magazine . He explained that the fires are expanding along the borders of new agricultural development, which is what’s often seen in fires related to forest clearing.
President Jair Bolsonaro’s administration, which had pledged to open up the Amazon to more development, has sought to shift attention away from deforestation. Bolsonaro initially pointed a finger at NGOs opposing his policies for allegedly intentionally setting fires in protest, without giving any evidence to back his claim. In August, he fired the director of the National Institute for Space Research over a dispute over data it released showing the sharp uptick in deforestation that’s taken place since Bolsonaro took office. On August 20th, Brazil’s Minister of the Environment Ricardo Salles tweeted that dry weather, wind, and heat caused the fires to spread so widely. But even during the dry season, large fires aren’t a natural phenomenon in the Amazon’s tropical ecosystem.
:format(webp)/cdn.vox-cdn.com/uploads/chorus_asset/file/19126415/1163385128.jpg.jpg)
Everyone on the planet benefits from the health of the Amazon. As its trees take in carbon dioxide and release oxygen, the Amazon plays a huge role in pulling planet-warming greenhouse gases out of the atmosphere. Without it, climate change speeds up. But as the world’s largest rainforest is eaten away by logging, mining, and agribusiness, it may not be able to provide the same buffer.
“The Amazon was buying you some time that it is not going to buy anymore,” Carlos Quesada, a scientist at Brazil’s National Institute for Amazonian Research, told Public Radio International in 2018. Scientists warn that the rainforest could reach a tipping point , turning into something more like a savanna when it can no longer sustain itself as a rainforest. That would mean it’s not able to soak up nearly as much carbon as it does now. And if the Amazon as we know it dies, it wouldn’t go quietly. As the trees and plants perish, they would release billions of tons of carbon that has been stored for decades — making it nearly impossible to escape a climate catastrophe.
Everyone on the planet benefits from the health of the Amazon
Of course, those nearest to the fires will bear the most immediate effects. Smoke from the fires got so bad, it seemed to turn day into night in São Paulo on August 20th. Residents say the air quality is still making it difficult to breathe. On top of that, a massive global study on air pollution found that among the two dozen countries it observed, Brazil showed one of the sharpest increases in mortality rates whenever there’s more soot in the air.
And because fire isn’t a natural phenomenon in the region, it can have outsized impacts on local plants and animals. One in ten of all animal species on Earth call the Amazon home, and experts expect that they will be dramatically affected by the fires in the short term . In the Amazon, plants and animals are “exceptionally sensitive” to fire, Jos Barlow, a professor of conservation science at Lancaster University in the UK, said to The Verge in an email. According to Barlow, even low-intensity fires with flames just 30 centimeters tall can kill up to half of the trees burned in a tropical rainforest.
When Jair Bolsonaro was campaigning for office as a far-right candidate, he called for setting aside less land in the Amazon for indigenous tribes and preservation, and instead making it easier for industry to come into the rainforest. Since his election in October 2018, Bolsonaro put the Ministry of Agriculture in charge of the demarcation of indigenous territories instead of the Justice Ministry, essentially “letting the fox take over the chicken coop,” according to one lawmaker . His policies have been politically popular among industry and agricultural interests in Brazil, even as they’ve been condemned by Brazilian environmental groups and opposition lawmakers. Hundreds of indigenous women stormed the country’s capital on August 13th to protest Bolsonaro’s environmental rollbacks and encroachment of development on indigenous lands. The hashtag #PrayforAmazonia blew up on Twitter.
:format(webp)/cdn.vox-cdn.com/uploads/chorus_asset/file/19126393/1161492194.jpg.jpg)
About 60 percent of the Amazon can be found within Brazil’s borders, which gives the nation a massive amount of influence over the region. Not surprisingly, the fires have called international attention to the plight of the Amazon and have turned up the heat on Bolsonaro’s environmental policies.
French President Emmanuel Macron took to Twitter to call for action, pushing for emergency international talks on the Amazon at the G7 summit. On August 26th, the world’s seven largest economies offered Brazil more than $22 million in aid to help it get the fires under control. Bolsonaro promptly turned down the money, accusing Macron on Twitter of treating Brazil like a colony. Some in Brazil, including Bolsonaro, see the international aid as an attack on Brazil’s sovereignty , and its right to decide how to manage the land within its borders.
“Letting the fox take over the chicken coop”
President Donald Trump, on the other hand, congratulated Bolsonaro on his handling of the fires. “He is working very hard on the Amazon fires and in all respects doing a great job for the people of Brazil,” he tweeted on the 27th.
Bolsonaro has since said that he’ll reconsider the deal, as long as Macron takes back his “ insults ” and Brazil has control over how the money is spent. On the 27th, Bolsonaro accepted $12.2 million in aid from the UK.
:format(webp)/cdn.vox-cdn.com/uploads/chorus_asset/file/19126423/1164459408.jpg.jpg)
How are the fires being fought?
After weeks of international and internal pressure, Bolsonaro deployed the military to help battle the fires on August 24, sending 44,000 troops to six states. Reuters reported the next day that warplanes were dousing flames.
“It’s a complex operation. We have a lot of challenges,” Paulo Barroso tells The Verge. Barroso is the chairman of the national forest fire management committee of the National League of Military Firefighters Corps in Brazil. He has spent three decades fighting fires in Mato Grosso, one of the regions most affected by the ongoing fires. According to Barroso, more than 10,400 firefighters are spread thin across 5.5 million square kilometers in the Amazon and “hotspots” break out in the locations they’re unable to cover.
“We don’t have an adequate structure to prevent, to control, and to fight the forest fires”
Barroso contends that they need more equipment and infrastructure to adequately battle the flames. There are 778 municipalities throughout the Amazon, but according to Barroso, only 110 of those have fire departments. “We don’t have an adequate structure to prevent, to control, and to fight the forest fires,” Barroso says. He wants to establish a forest fire protection system in the Amazon that brings together government entities, indigenous peoples, local communities, the military, large companies, NGOs, and education and research centers. “We have to integrate everybody,” Barroso says, adding, “we need money to do this, we have to receive a great investment.”
Barroso and other experts agree that it’s important to look ahead to prevent fires like we’re seeing now. After all, August is just the beginning of Brazil’s largely manmade fire season, when slashing-and-burning in the country peaks and coincides with drier weather.
:format(webp)/cdn.vox-cdn.com/uploads/chorus_asset/file/19126433/1163748175.jpg.jpg)
Controlled burns are also a popular deforestation technique in other countries where the Amazon is burning, including Bolivia . There, the government brought in a modified Boeing 747 supertanker to douse the flames.
Using planes to put out wildfires in the Amazon isn’t a typical method of firefighting in tropical forests, and is likely to get expensive, Lancaster University’s Jos Barlow tells The Verge . He says that large-scale fires in areas cleared by deforestation “are best contained with wide firebreaks created with bulldozers — not easy in remote regions.” If the fires enter the forest itself, they require different tactics. “They can normally be contained by clearing narrow fire breaks in the leaf litter and fine fuel,” Barlow says. “But this is labour intensive over large scales, and fires need to be reached soon, before they get too big.”
Fires that have been intentionally set, as we’re seeing in Brazil, can be even more difficult to control compared to a sudden wildland fire. “They’re designed to be deliberately destructive,” says Timothy Ingalsbee, co-founder and executive director of Firefighters United for Safety, Ethics, and Ecology and research associate at the University of Oregon. Slashing before burning produces a lot of very dry, very flammable fuel. And at this scale, Ingalsbee calls the fires “an act of global vandalism.”
Barlow says, “The best fire fighting technique in the Amazon is to prevent them in the first place — by controlling deforestation and managing agricultural activities.”
WUR’s Cathelijne Stoof agrees: “Fighting the fires is of course important now,” she says. “For the longer term, it is way more important to focus on deforestation.”
I traded in my MacBook and now I’m a desktop convert
Tesla’s autopilot and full self-driving linked to hundreds of crashes, dozens of deaths, this self-transforming megatron is as badass as it is expensive, blizzcon 2024 has been canceled, dji might get banned next in the us.

More from Science
:format(webp)/cdn.vox-cdn.com/uploads/chorus_asset/file/23935561/acastro_STK103__04.jpg)
Amazon — like SpaceX — claims the labor board is unconstitutional
:format(webp)/cdn.vox-cdn.com/uploads/chorus_asset/file/25288452/246992_AI_at_Work_REAL_COST_ECarter.png)
How much electricity does AI consume?
:format(webp)/cdn.vox-cdn.com/uploads/chorus_asset/file/25287681/1371856480.jpg)
A Big Tech-backed campaign to plant trees might have taken a wrong turn
:format(webp)/cdn.vox-cdn.com/uploads/chorus_asset/file/25287408/2003731596.jpg)
SpaceX successfully launches Odysseus in bid to return US to the lunar surface
Explainer: Causes and consequences of Amazon fires and deforestation
- Medium Text
WHAT CAUSES THE FIRES?
Why are brazil's fires so bad at this time of year, is climate change affecting amazon forest fires, have brazil's fires been worse than usual in recent years, how much worse is forest loss since 2019.
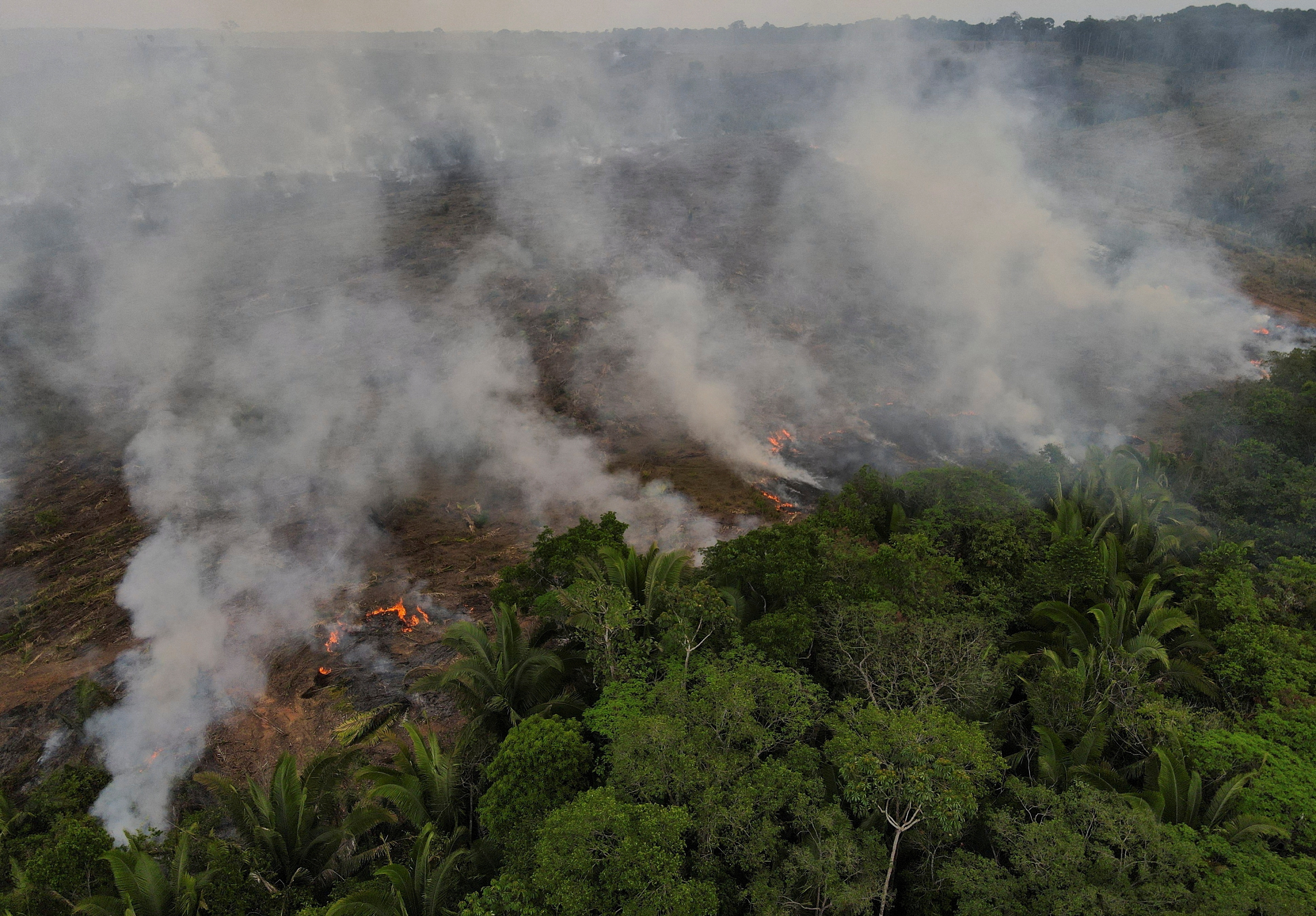
WILL THIS YEAR'S FIRE SEASON BE DIFFERENT?
Are the amazon fires contributing to climate change.
Sign up here.
Reporting by Jake Spring in Sao Paulo and Gloria Dickie in London; Editing by Katy Daigle
Our Standards: The Thomson Reuters Trust Principles. New Tab , opens new tab

Thomson Reuters
Jake Spring reports primarily on forests, climate diplomacy, carbon markets and climate science. Based in Brazil, his investigative reporting on destruction of the Amazon rainforest under ex-President Jair Bolsonaro won 2021 Best Reporting in Latin America from the Overseas Press Club of America (https://opcofamerica.org/Awardarchive/the-robert-spiers-benjamin-award-2021/). His beat reporting on Brazil’s environmental destruction won a Covering Climate Now award and was honored by the Society of Environmental Journalists. He joined Reuters in 2014 in China, where he previously worked as editor-in-chief of China Economic Review. He is fluent in Mandarin Chinese and Brazilian Portuguese.

Gloria Dickie reports on climate and environmental issues for Reuters. She is based in London. Her interests include biodiversity loss, Arctic science, the cryosphere, international climate diplomacy, climate change and public health, and human-wildlife conflict. She previously worked as a freelance environmental journalist for 7 years, writing for publications such as the New York Times, the Guardian, Scientific American, and Wired magazine. Dickie was a 2022 finalist for the Livingston Awards for Young Journalists in the international reporting category for her climate reporting from Svalbard. She is also an author at W.W. Norton.
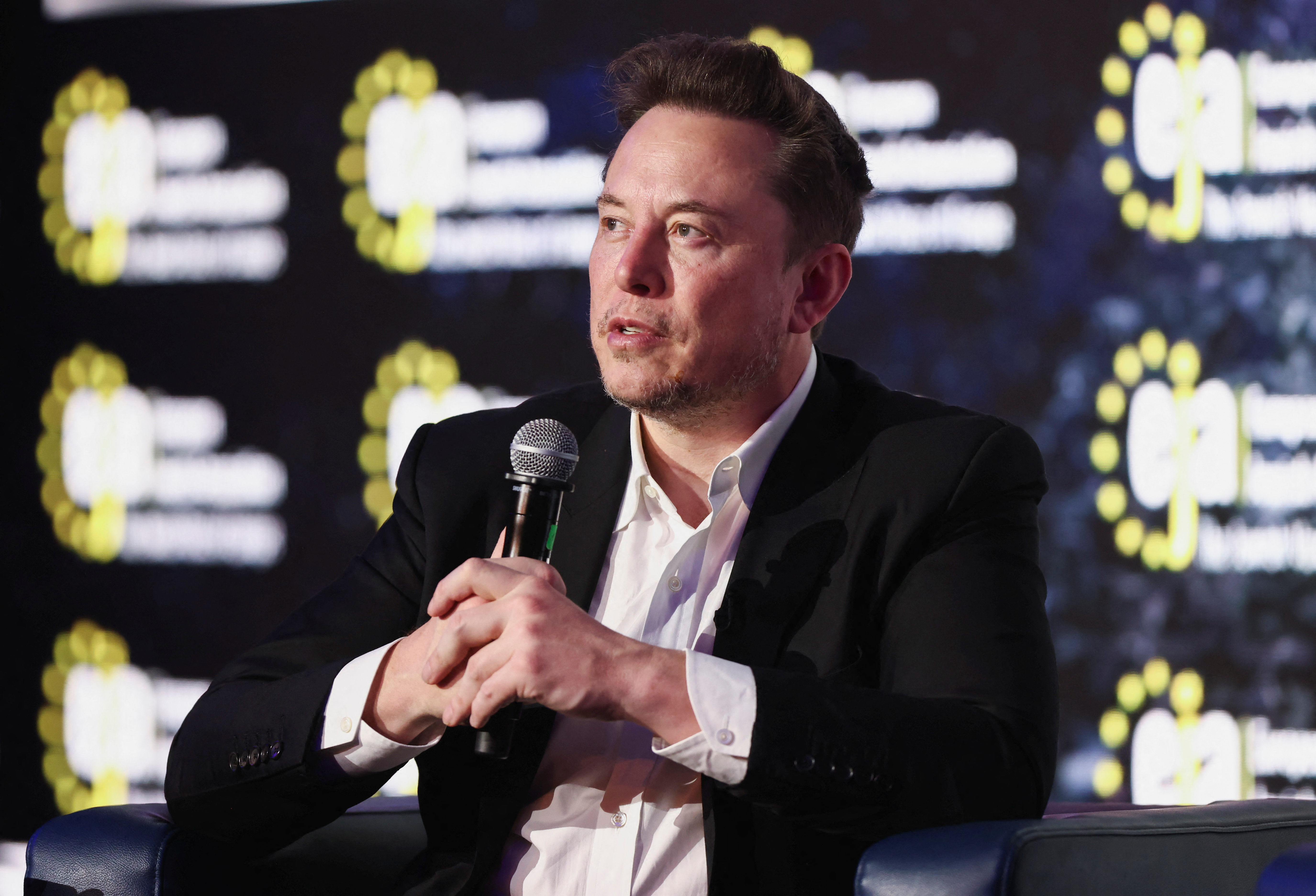
Mexico on Friday said it has prevailed in a labor dispute centered on a mine operated by one of the world's top copper producers in which the United States had sought a probe, with a labor panel under the trade agreement between the two countries and Canada saying it lacked jurisdiction.
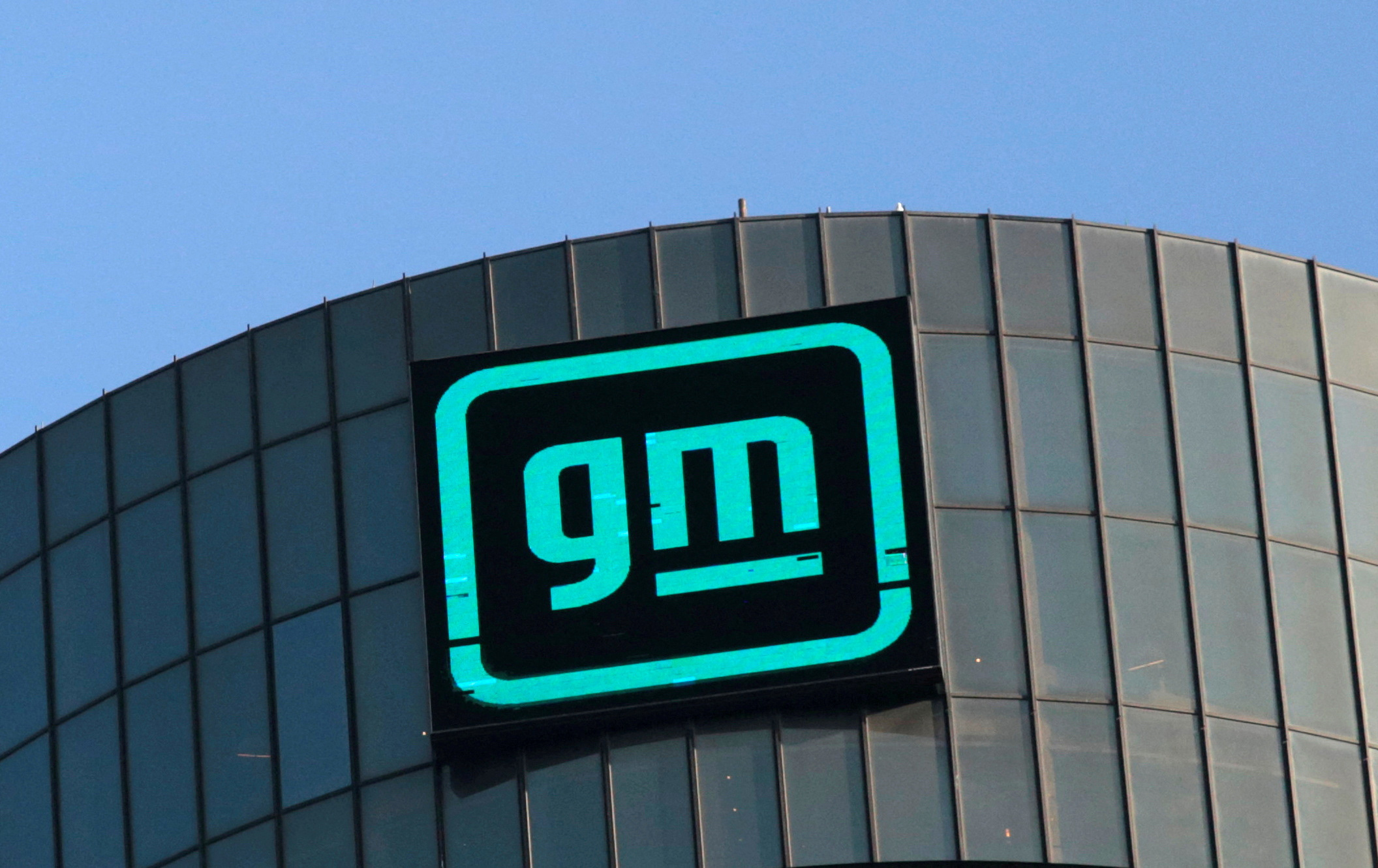
Canada's Aamjiwnaang First Nation declared a state of emergency due to the excessive release of harmful chemicals from INEOS Styrolution's plastic manufacturing plant, the Indigenous group said.

World Chevron
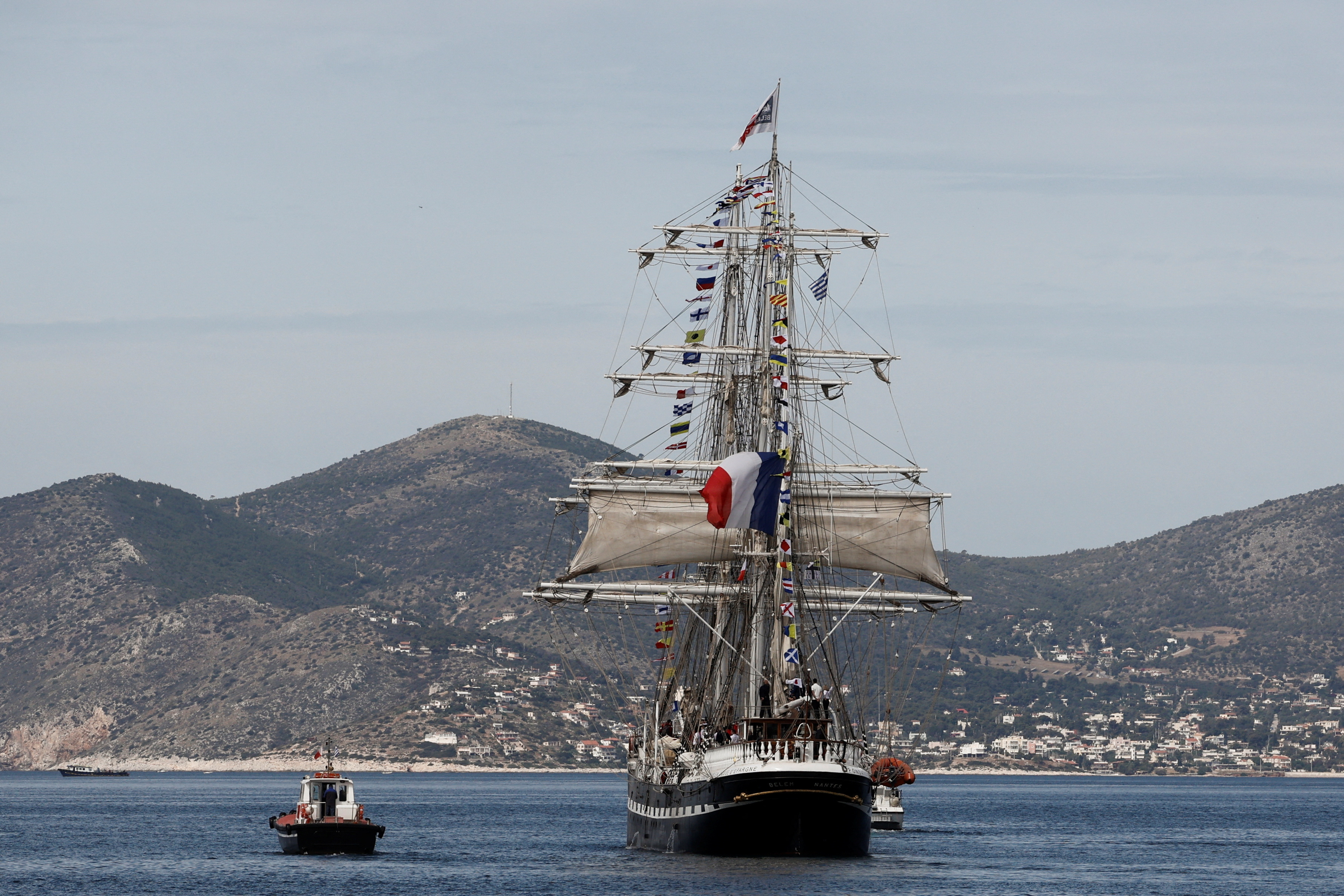
Paris 2024 Olympics flame sets sail for France in final relay leg
The Paris 2024 Olympic flame sailed for France on Saturday on board a three-masted ship to mark the final sprint of preparations ahead of the Olympic Games opening ceremony on July 26.
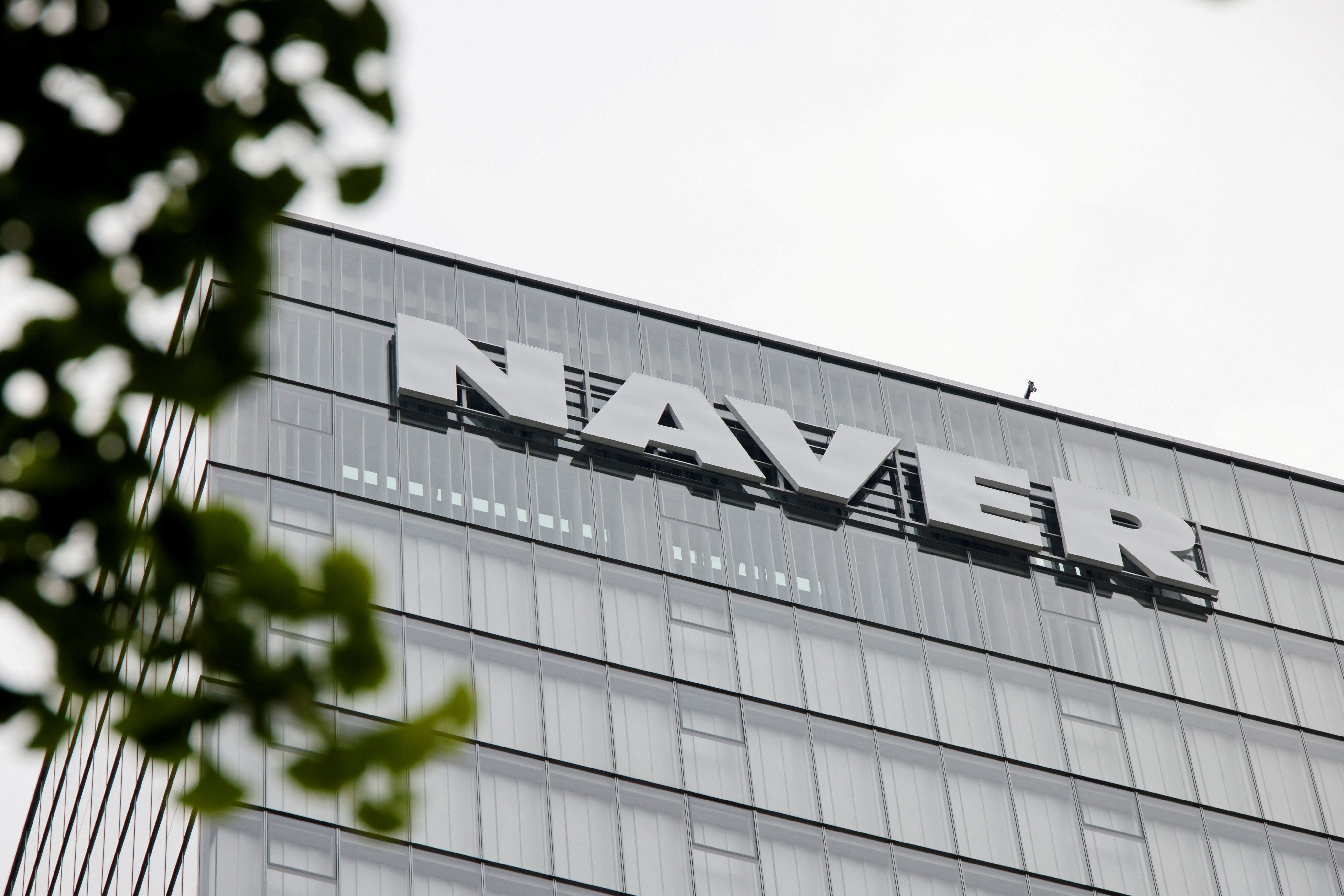
Ukraine attacked the Ilsky and Slavyansk oil refineries in Russia's Krasnodar region with drones early on Saturday, causing fires at the facilities, a Ukrainian intelligence source told Reuters.
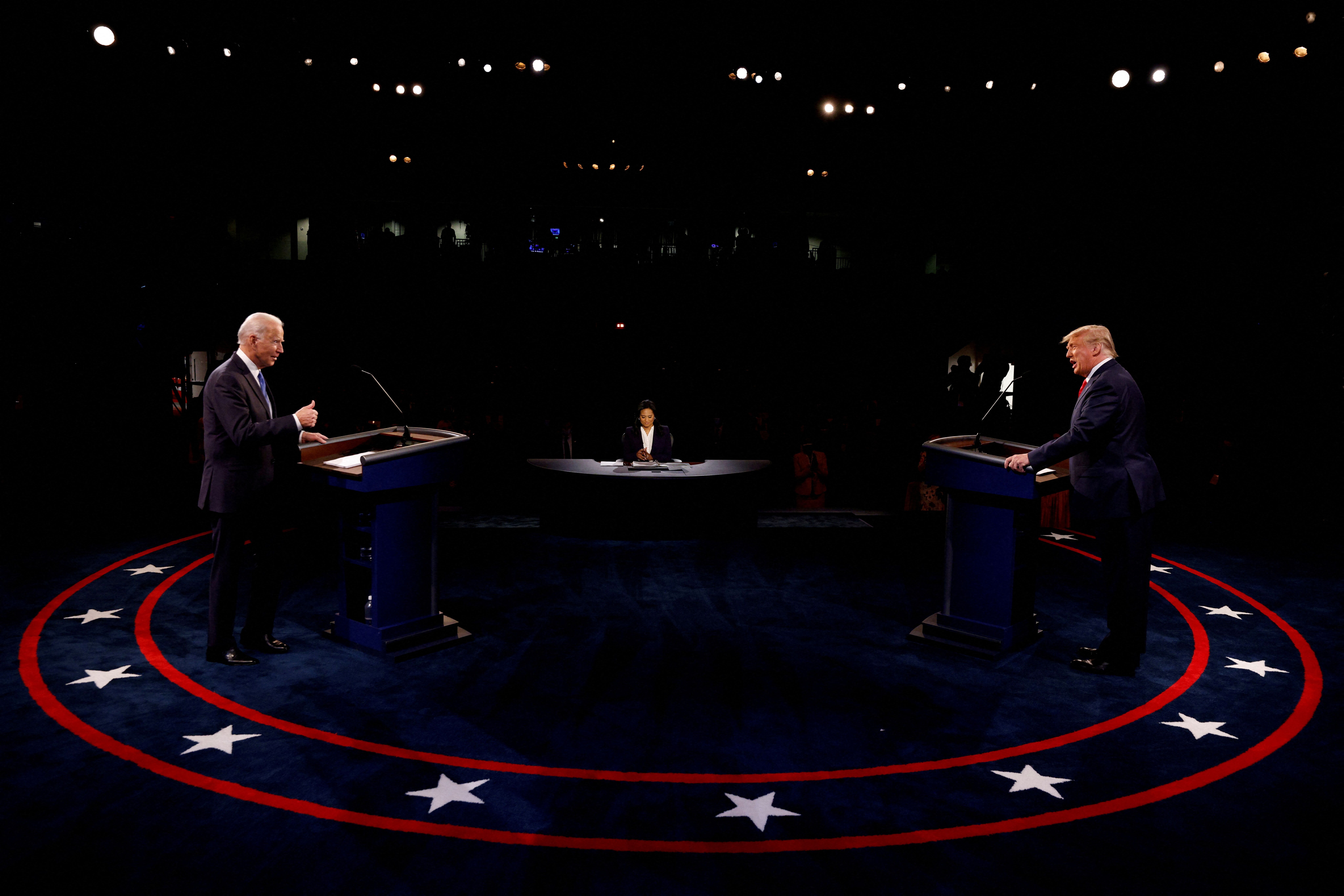
An earthquake of magnitude 6.5 struck Japan's Bonin Islands, the United States Geological Survey (USGS) said on Saturday.
Case Study: Deforestation in the Amazon Rainforest
Deforestation in the amazon rainforest.
The Amazon rainforest area spans about 8,200,000km 2 across 9 countries, making it the largest rainforest in the world. The tree coverage in 1970 was 4.1m km 2 . In 2018, it was 3.3m km 2 . Between 2001 and 2013, the causes of Amazonian deforestation were:
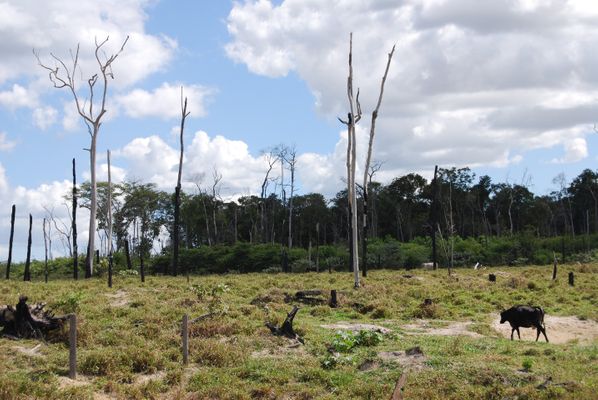
Pasture and cattle ranching = 63%
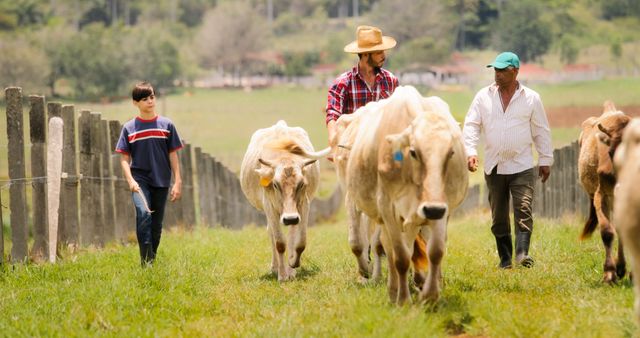
Small-scale, subsistence farmers = 12%
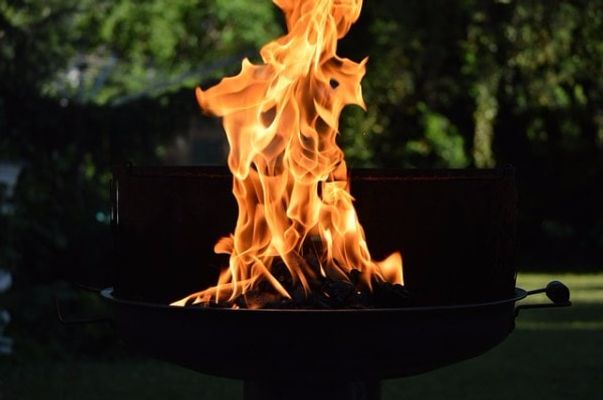
Commercial crop farming = 7%
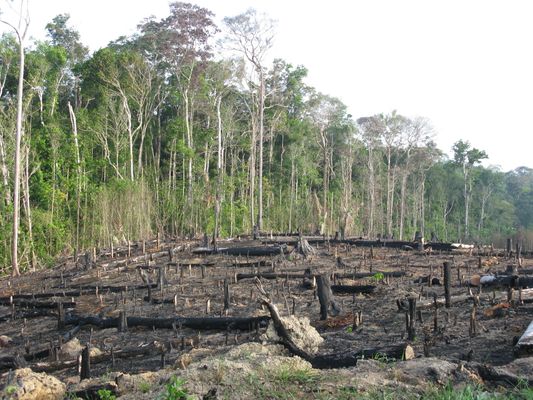
Tree felling and logging = 6%
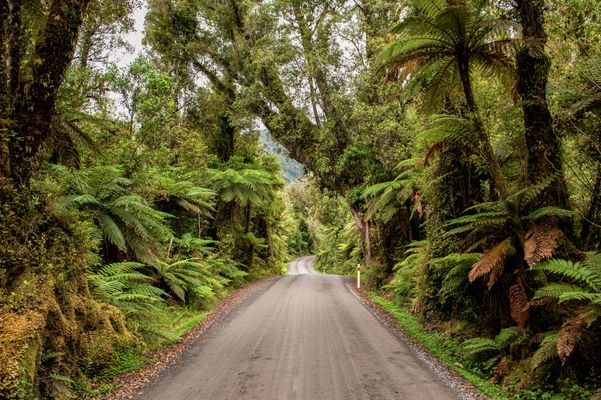
Other activities = 3%
- E.g. plantations, mining, road-building, and construction.
Impacts of Deforestation in the Amazon
Deforestation in the Amazon rainforest has the following environmental and economic impacts:
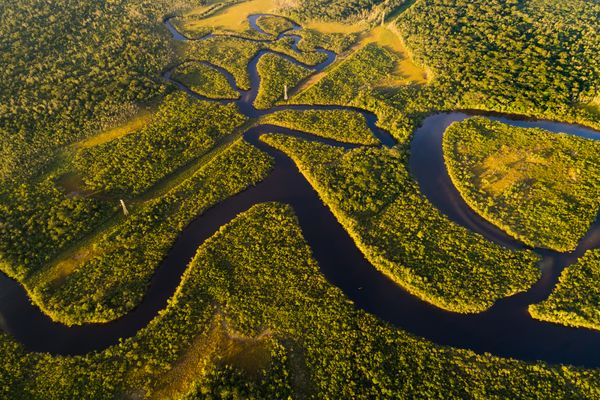
Environmental impact of Amazonian deforestation
- Photosynthesis by trees in the Amazon absorbs 5% of the world's carbon emissions each year (2bn tons of CO2).
- 100 billion tonnes of carbon are stored in the wood of the trees in the Amazon.
- If the Amazon were completely deforested, it would release the 100bn tonnes and also reduce the amount of carbon dioxide taken out of the atmosphere by 2bn tons each year.
- Trees anchor soil in the ground, bound to their roots. Deforestation damages the topsoil and once this has happened, the fertility of the ground is seriously damaged.
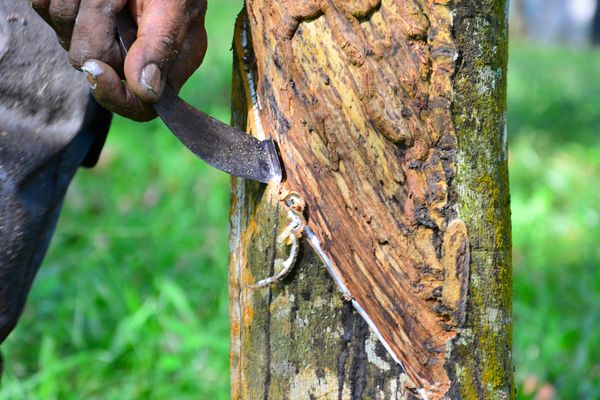
Economic impact of Amazonian deforestation
- Deforestation has fuelled the economic development of poor countries.
- In 2018, Brazil exported $28bn worth of metals. The mining industry creates jobs, exports and helps increase Brazilian people's standard of living.
- Similarly, hydroelectric power plants and cattle farms help to create jobs.
- In 2018, Brazil became the world's largest exporter of beef.
- Rio Tinto, an iron ore mining company employs 47,000 people globally and thousands of these are in Brazil.
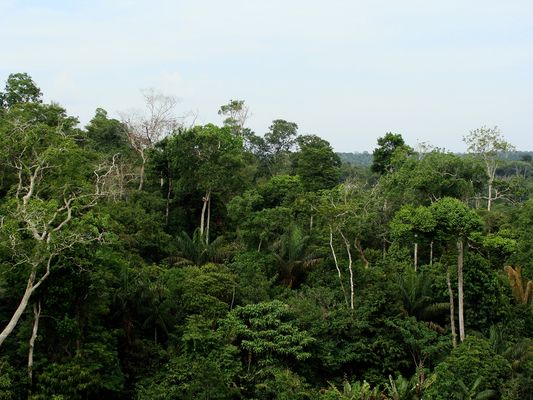
The rate of deforestation in the Amazon
- In 2015, the Brazilian President Dilma Rousseff claimed that the rate of deforestation had fallen by 83% and that actually Brazil was going to reforest the Amazon.
- However, the policies under President Temer and President Bolsonaro has reversed Rousseff's plan. In 2019, under Bolsonaro, the rate of deforestation was increasing again.
1 The Challenge of Natural Hazards
1.1 Natural Hazards
1.1.1 Types of Natural Hazards
1.1.2 Hazard Risk
1.1.3 Consequences of Natural Hazards
1.1.4 End of Topic Test - Natural Hazards
1.1.5 Exam-Style Questions - Natural Hazards
1.2 Tectonic Hazards
1.2.1 Tectonic Plates
1.2.2 Tectonic Plates & Convection Currents
1.2.3 Plate Margins
1.2.4 Volcanoes
1.2.5 Effects of Volcanoes
1.2.6 Responses to Volcanic Eruptions
1.2.7 Earthquakes
1.2.8 Earthquakes 2
1.2.9 Responses to Earthquakes
1.2.10 Case Studies: The L'Aquila & Kashmir Earthquakes
1.2.11 Earthquake Case Study: Chile 2010
1.2.12 Earthquake Case Study: Nepal 2015
1.2.13 Living with Tectonic Hazards 1
1.2.14 Living with Tectonic Hazards 2
1.2.15 End of Topic Test - Tectonic Hazards
1.2.16 Exam-Style Questions - Tectonic Hazards
1.2.17 Tectonic Hazards - Statistical Skills
1.3 Weather Hazards
1.3.1 Global Atmospheric Circulation
1.3.2 Surface Winds
1.3.3 UK Weather Hazards
1.3.4 Tropical Storms
1.3.5 Features of Tropical Storms
1.3.6 Impact of Tropical Storms 1
1.3.7 Impact of Tropical Storms 2
1.3.8 Tropical Storms Case Study: Katrina
1.3.9 Tropical Storms Case Study: Haiyan
1.3.10 UK Weather Hazards Case Study: Somerset 2014
1.3.11 End of Topic Test - Weather Hazards
1.3.12 Exam-Style Questions - Weather Hazards
1.3.13 Weather Hazards - Statistical Skills
1.4 Climate Change
1.4.1 Evidence for Climate Change
1.4.2 Causes of Climate Change
1.4.3 Effects of Climate Change
1.4.4 Managing Climate Change
1.4.5 End of Topic Test - Climate Change
1.4.6 Exam-Style Questions - Climate Change
1.4.7 Climate Change - Statistical Skills
2 The Living World
2.1 Ecosystems
2.1.1 Ecosystems
2.1.2 Ecosystem Cascades & Global Ecosystems
2.1.3 Ecosystem Case Study: Freshwater Ponds
2.2 Tropical Rainforests
2.2.1 Tropical Rainforests - Intro & Interdependence
2.2.2 Adaptations
2.2.3 Biodiversity of Tropical Rainforests
2.2.4 Deforestation
2.2.5 Case Study: Deforestation in the Amazon Rainforest
2.2.6 Sustainable Management of Rainforests
2.2.7 Case Study: Malaysian Rainforest
2.2.8 End of Topic Test - Tropical Rainforests
2.2.9 Exam-Style Questions - Tropical Rainforests
2.2.10 Deforestation - Statistical Skills
2.3 Hot Deserts
2.3.1 Overview of Hot Deserts
2.3.2 Biodiversity & Adaptation to Hot Deserts
2.3.3 Case Study: Sahara Desert
2.3.4 Desertification
2.3.5 Case Study: Thar Desert
2.3.6 End of Topic Test - Hot Deserts
2.3.7 Exam-Style Questions - Hot Deserts
2.4 Tundra & Polar Environments
2.4.1 Overview of Cold Environments
2.4.2 Adaptations in Cold Environments
2.4.3 Biodiversity in Cold Environments
2.4.4 Case Study: Alaska
2.4.5 Sustainable Management
2.4.6 Case Study: Svalbard
2.4.7 End of Topic Test - Tundra & Polar Environments
2.4.8 Exam-Style Questions - Cold Environments
3 Physical Landscapes in the UK
3.1 The UK Physical Landscape
3.1.1 The UK Physical Landscape
3.2 Coastal Landscapes in the UK
3.2.1 Types of Wave
3.2.2 Weathering & Mass Movement
3.2.3 Processes of Erosion & Wave-Cut Platforms
3.2.4 Headlands, Bays, Caves, Arches & Stacks
3.2.5 Transportation
3.2.6 Deposition
3.2.7 Spits, Bars & Sand Dunes
3.2.8 Case Study: Landforms on the Dorset Coast
3.2.9 Types of Coastal Management 1
3.2.10 Types of Coastal Management 2
3.2.11 Coastal Management Case Study - Holderness
3.2.12 Coastal Management Case Study: Swanage
3.2.13 Coastal Management Case Study - Lyme Regis
3.2.14 End of Topic Test - Coastal Landscapes in the UK
3.2.15 Exam-Style Questions - Coasts
3.3 River Landscapes in the UK
3.3.1 The River Valley
3.3.2 River Valley Case Study - River Tees
3.3.3 Erosion
3.3.4 Transportation & Deposition
3.3.5 Waterfalls, Gorges & Interlocking Spurs
3.3.6 Meanders & Oxbow Lakes
3.3.7 Floodplains & Levees
3.3.8 Estuaries
3.3.9 Case Study: The River Clyde
3.3.10 River Management
3.3.11 Hard & Soft Flood Defences
3.3.12 River Management Case Study - Boscastle
3.3.13 River Management Case Study - Banbury
3.3.14 End of Topic Test - River Landscapes in the UK
3.3.15 Exam-Style Questions - Rivers
3.4 Glacial Landscapes in the UK
3.4.1 Erosion
3.4.2 Landforms Caused by Erosion
3.4.3 Landforms Caused by Transportation & Deposition
3.4.4 Snowdonia
3.4.5 Land Use in Glaciated Areas
3.4.6 Tourism in Glacial Landscapes
3.4.7 Case Study - Lake District
3.4.8 End of Topic Test - Glacial Landscapes in the UK
3.4.9 Exam-Style Questions - Glacial Landscapes
4 Urban Issues & Challenges
4.1 Urban Issues & Challenges
4.1.1 Urbanisation
4.1.2 Urbanisation Case Study: Lagos
4.1.3 Urbanisation Case Study: Rio de Janeiro
4.1.4 UK Cities
4.1.5 Case Study: Urban Regen Projects - Manchester
4.1.6 Case Study: Urban Change in Liverpool
4.1.7 Case Study: Urban Change in Bristol
4.1.8 Sustainable Urban Life
4.1.9 End of Topic Test - Urban Issues & Challenges
4.1.10 Exam-Style Questions - Urban Issues & Challenges
4.1.11 Urban Issues -Statistical Skills
5 The Changing Economic World
5.1 The Changing Economic World
5.1.1 Measuring Development
5.1.2 Classifying Countries Based on Wealth
5.1.3 The Demographic Transition Model
5.1.4 Physical & Historical Causes of Uneven Development
5.1.5 Economic Causes of Uneven Development
5.1.6 How Can We Reduce the Global Development Gap?
5.1.7 Case Study: Tourism in Kenya
5.1.8 Case Study: Tourism in Jamaica
5.1.9 Case Study: Economic Development in India
5.1.10 Case Study: Aid & Development in India
5.1.11 Case Study: Economic Development in Nigeria
5.1.12 Case Study: Aid & Development in Nigeria
5.1.13 Economic Development in the UK
5.1.14 Economic Development UK: Industry & Rural
5.1.15 Economic Development UK: Transport & North-South
5.1.16 Economic Development UK: Regional & Global
5.1.17 End of Topic Test - The Changing Economic World
5.1.18 Exam-Style Questions - The Changing Economic World
5.1.19 Changing Economic World - Statistical Skills
6 The Challenge of Resource Management
6.1 Resource Management
6.1.1 Global Distribution of Resources
6.1.2 Food in the UK
6.1.3 Water in the UK 1
6.1.4 Water in the UK 2
6.1.5 Energy in the UK
6.1.6 Resource Management - Statistical Skills
6.2.1 Areas of Food Surplus & Food Deficit
6.2.2 Food Supply & Food Insecurity
6.2.3 Increasing Food Supply
6.2.4 Case Study: Thanet Earth
6.2.5 Creating a Sustainable Food Supply
6.2.6 Case Study: Agroforestry in Mali
6.2.7 End of Topic Test - Food
6.2.8 Exam-Style Questions - Food
6.2.9 Food - Statistical Skills
6.3.1 The Global Demand for Water
6.3.2 What Affects the Availability of Water?
6.3.3 Increasing Water Supplies
6.3.4 Case Study: Water Transfer in China
6.3.5 Sustainable Water Supply
6.3.6 Case Study: Kenya's Sand Dams
6.3.7 Case Study: Lesotho Highland Water Project
6.3.8 Case Study: Wakel River Basin Project
6.3.9 Exam-Style Questions - Water
6.3.10 Water - Statistical Skills
6.4.1 Global Demand for Energy
6.4.2 Factors Affecting Energy Supply
6.4.3 Increasing Energy Supply: Renewables
6.4.4 Increasing Energy Supply: Non-Renewables
6.4.5 Carbon Footprints & Energy Conservation
6.4.6 Case Study: Rice Husks in Bihar
6.4.7 Exam-Style Questions - Energy
6.4.8 Energy - Statistical Skills
Jump to other topics
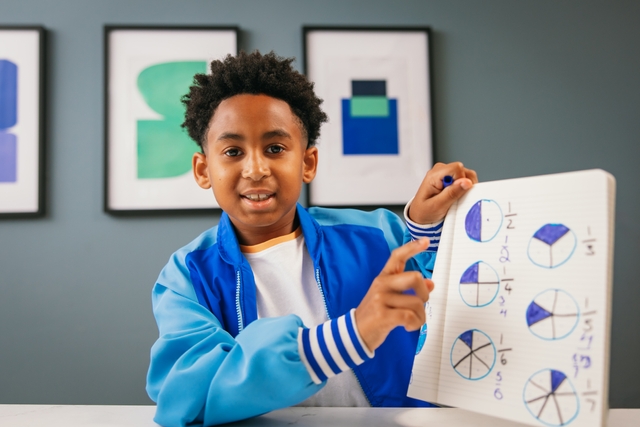
Unlock your full potential with GoStudent tutoring
Affordable 1:1 tutoring from the comfort of your home
Tutors are matched to your specific learning needs
30+ school subjects covered
Deforestation
Sustainable Management of Rainforests
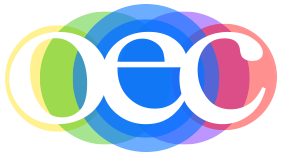
Site Search
- How to Search
- Advisory Group
- Editorial Board
- OEC Fellows
- History and Funding
- Using OEC Materials
- Collections
- Research Ethics Resources
- Ethics Projects
- Communities of Practice
- Get Involved
- Submit Content
- Open Access Membership
- Become a Partner
Case: Conservation in the Amazon
In this case, Armando, a Brazilian Federal Senator with a BS and MS in Biology, must confront ethical dilemmas concerning the Amazon forest. Topics for consideration include: 1) the biodiversity and ecosystem services associated with the forest, 2) the social and economic issues surrounding deforestation, and the 3) options available to Armando as he makes his recommendation on how to use the US funds. Finally, we will explore the role of Maria as a science advisor and address her responsibilities, as well as her mistake in addressing the press.
This biodiversity case is part of a larger collection of Life and Environmental Science ethics education resource sets on ethics of emerging biotechnologies, big data in the life sciences, human enhancement, and biodiversity. Doctoral students from Arizona State University’s Center for Biology and Society developed the resources under the direction of Karin Ellison and Joseph Herkert between 2014 and 2019.
Armando Santos grew up the son of rubber tappers in the city of Tailândia, Brazil, in the northern state of Pará. Throughout his childhood, Armando would often accompany his parents on their trips into the Amazon forest to harvest latex from the trees (a sustainable, legal process). His rubber tapper parents often directly competed with illegal loggers, slash-and-burn farmers, and cattle ranchers. Logging, farming and ranching typically destroy mature, “mother-trees” that rubber tappers harvest from. Entrenched in the Tailândia community, however, Armando did not blame his fellow citizens for the Amazon’s destruction; he knew that many people log the forest out of financial or personal desperation. Most citizens of Tailândia rely on soybean agriculture, cattle ranching, and logging for their income.
Armando became interested in how people and the forest could co-exist, and he went on to complete undergraduate and masters degrees in biology at the local university, with a special focus on coupled social and ecological systems. Throughout his education, he became more aware of the political and economic implications of forest management and realized that as a biologist he wouldn’t have the type of political power he wanted to affect the ways in which the government manages the Amazon. Thus, Armando ventured into a political career. Having worked through positions in local and regional government over the last 15 years, today Armando represents the state of Pará in the Federal Senate, the upper house of the national congress. Armando is a proud member of the Worker’s Party, a left of center party with a social and economic platform that resonates with the citizens of Pará who struggle to live in harsh working and financial conditions.
In the upcoming Federal Senate session, senators will vote on a bill to appropriate funds from an investment by the US of around $750 million (USD) to be distributed over 10 years as a part of the Amazon Fund organized by the Brazilian Government in 2008. As a well-liked senator from Pará with considerable expertise in forest ecology and management, Armando will be expected to weigh in on the bill. Armando’s many responsibilities as a senator necessitate hiring advisors of different expertise to aid him in decision-making. Maria Duarte, Ph.D., is the new science advisor to Armando. Though raised in the large capital city of Pará, Belém, Maria completed undergraduate and graduate schooling in the US, studying the ecology of tropical forests. Regarding the upcoming Senate session, Maria argues that Armando should recommend putting the US funds towards creating a new swath of protected area in Pará resembling a US National Park. This, she argues, is the best and most ethical way to ensure the ecosystem remains intact for generations to come and to demonstrate to the US that funds are being used in an effective and discernible manner. Should resistance to this idea arise in the Senate, she notes that Armando could emphasize the global and regional ecosystem services offered by the forest to justify protection, though she feels the intrinsic value of the ecosystem is justification enough.
Before presenting her policy recommendations to Armando, Maria takes the liberty of replying to an inquiry from the Pará newspaper, O Liberal . Asked about Armando’s response to the US investment, Maria states that she is recommending that he heed her suggestions: If so, “Pará will soon be home to a new, US-funded, US-inspired national park.” O Liberal , however, misquotes Maria and reports that Armando has already agreed to Maria’s plan for the US investment. Upset that Maria spoke to the press without permission, Armando reminds her that he is not obligated to follow her advice. Yet Armando understands that the press can occasionally take statements out of context to the point of miscommunication, so he allows the mistake so long as Maria promises to leave news communication to the press secretary in the future.
Though he loves the forest, Armando explains to Maria that there is more to the Amazon than the trees. The social system in Pará is deeply tied to the ecological system. Armando believes it is unethical to exclude humans from the forest and is doubtful a protected area restricting human use will be popular with his constituents or his Senate colleagues.
First, monitoring protected areas in the region is difficult. More money will buy more personnel, but Armando feels it is unlikely to halt violent encounters among police, illegal loggers, rubber tappers, and farmers. Second, Armando’s constituents elected him on the Worker Party platform, hopeful that he could improve infrastructure and broaden economic opportunities. Investment in a protected area would be unpopular with citizens of Pará who rely on developing and using the forest for income. Armando is up for re-election at the end of the year. If he disappoints his constituents, he could lose his Senate seat. Finally, both his constituents and his Senate colleagues will be sensitive to attempted replication of a US National Park in Pará. Brazilians in general are wary of foreign influence, and particularly look out for foreign powers that threaten to “annex the Amazon forest unless the country can find something useful to do with it” (Economist 2009). Efforts by the international community to conserve the Amazon in Brazil are met with suspicion and frustration: “Do you care about us, or just our forest?” (Nordhaus and Shellenberger 2009, 61).
Armando has one month to deliberate with Maria and the rest of his team on a plan for the US investment. He will present his suggestions in front of the Federal Senate, a nationally televised event.
Discussion Questions:
- Briefly summarize the competing interests involved in conservation of biodiversity in the Brazilian Amazon Rain Forest, categorized by scale (local, regional, global).
- How would you describe the ethical stance of Armando Santos? Do you agree or disagree with his views? Why or why not? Summarize the competing interests he must manage in his decision. Which do you feel he should prioritize? Why?
- How would you describe the ethical stance of Maria Duarte? Do you agree or disagree with her views? Why or why not? Provide a critique of Maria’s argument to preserve the Amazon in Pará as a national park.
- What is Maria’s job as a science advisor? Is Maria performing her job as science advisor in a responsible manner?
- Was it ethical for Maria to talk with the press without permission? To what extent does Maria bare responsibility for the reporter’s error? Should Armando discipline her? Is there a positive lesson Armando can teach Maria about dealing with the press?
- How can the US investment be appropriated to avoid tension in foreign relations?
- How could the US funds be used to serve both economic and ecological interests?
Bibliography:
Börner, J., S. Wunder, S. Wertz- Kanounnikoff, M. Rügnitz Tito, L. Pereira, and N. Nascimento. 2010. “Direct conservation payments in the Brazilian Amazon: Scope and equity implications.” Ecological Economics 69: 1272-1282.
Eltahir, E. A., and R. L. Bras. 1994. “Precipitation recycling in the Amazon basin.” Quarterly Journal of the Royal Meteorological Society 120: 861-880.
Garcia-Navarro, L. 2015. “Deep in the Amazon, an Unseen Battle Over the Most Valuable Trees.” NPR , November 4.
Gibbs, H. K, L. Rausch, J. Munger, I. Schelly, D. C. Morton, P. Noojipady, B. Soares-Filho, P. Barreto, L. Micol, and N. F. Walker. 2015. “Brazil’s Soy Moratorium.” Science 347: 377-378.
Greenpeace. 2016. “The Amazon Soya Moratorium.” Accessed July 25. http://www.greenpeace.org/international/Global/international/code/2014/amazon/index.html
IPCC. 2014. Climate Change 2014 Synthesis Report. Geneva, Switzerland.
Lewinsohn, T. M., and P. I. Prado. 2005. “How Many Species are there in Brazil?” Conservation Biology 19: 619-624.
Malhi, Y., J. T. Roberts, R. A. Betts, T. J. Killeen, W. Li, and C. A. Nobre. 2008. “Climate Change, Deforestation, and the Fate of the Amazon.” Science 319: 169-172.
Myers, N., R. A. Mittermeier, C. G. Mittermeier, G. A. B. da Fonseca, and J. Kent. 2000. “Biodiversity Hotspots for Conservation Priorities.” Nature 403: 853-858.
Nordhaus, T., and M. Shellenberger. 2009. “The Forest for the Trees.” In Break Through: Why We Can’t Leave Saving the Planet to Environmentalists, 41-65 . Boston, MA: Mariner Books.
Pielke, Jr., R. A. 2007. The Honest Broker: Making Sense of Science in Policy and Politics . Cambridge: Cambridge University Press.
Soares-Filho, B. S., D. C. Nepstad, L. M. Curran, G. C. Cerqueira, R. A. Garcia, C. A. Ramos, E. Voll, A. McDonald, P. Lefebvre, and P. Schlesinger. 2006. “Modeling conservation in the Amazon Basin.” Nature 440: 520-523.
The Economist. 2009. “The Amazon: The Future of the Forest.” The Economist , June 11. Accessed July 25, 2016. http://www.nature.com/news/stopping-deforestation-battle-for-the-amazon-1.17223
The Nature Conservancy. 2016. “The Amazon Rainforest.” Accessed July 18. http://www.nature.org/ourinitiatives/regions/southamerica/brazil/placesweprotect/amazon-rainforest-infographic.xml
Tollefson, J. 2015. “Stopping Deforestation: Battle for the Amazon.” Nature News Feature , April 1.
World Wildlife Fund (WWF). 2016. “Amazon Threats.” Accessed July 18. http://wwf.panda.org/what_we_do/where_we_work/amazon/amazon_threats/
The Amazon Fund: http://www.amazonfund.gov.br/en/amazon-fund/
Greenpeace: http://www.greenpeace.org/international/Global/international/code/2014/amazon/index.html
REDD: https://unredd.net/about/what-is-redd-plus.html
The Nature Conservancy: http://www.nature.org/ourinitiatives/regions/southamerica/brazil/placesweprotect/amazon.xml
The author wishes to acknowledge the contributions of Karin Ellison, OEC - Life and Environmental Sciences Editor, and Joseph Herkert, OEC Engineering Co-Editor. They provided valuable input in selecting topics and crafting the resources.
This case study addresses several important ethical issues around the topic of biodiversity and tropical deforestation in the Amazon. I also see it as a useful starting point for guiding OEC users to a wider range of issues around the ethics of international biodiversity research and environmental action.
By having the study’s protagonists be native to and working in Brazil, the case study misses the chance to ask what ethical issues OEC users based at American institutions might consider when they work in foreign countries in areas such as biodiversity research or conservation. I propose a few, but this is not an exhaustive set.
First, for anyone doing biodiversity research, a fundamental tenet of international law (i.e., the Convention on Biological Diversity or CBD) is that nations have sovereign control of their biological resources and that scientific needs do not override a country’s rules. This means that all researchers must secure the required research permits, collections permits and/or material transfer agreements for each area in which they work; the requirements and processes can vary within and between countries. In the past, non-compliance with such requirements has had serious negative consequences for some researchers, students, projects, U.S. universities and even international relationships between the United States and other countries. Alas, right now, the ethical path, i.e., following those rules, can be especially cumbersome and time-consuming because many countries are working to develop or revise their biodiversity-related rules to comply with the 2014 addition to the CBD treaty, known as the Nagoya Protocol (NP) on Access and Benefit Sharing of Genetic Resources. The NP website , and the CBD’s Access and benefit-Sharing Clearing-House , have a vast array of country-specific information that could assist many OEC users, especially researchers, follow the right steps; a guest blog that I wrote on this shifting landscape also provides some tips for researchers.
A second issue in foreign countries is that the ethical landscape around biodiversity, i.e., the sets of values held by different groups, can be vastly different than those familiar to Americans scientists. When I taught a course on tropical deforestation with an economist several decades ago, I discovered a rich literature on the various value constructs underlying human-nature relationships, including the Judeo-Christian stewardship perspective that underlies many conservation efforts, as well as beliefs shaped by other religions, and by secular, utilitarian/economical, interconnectivity, and deep ecology/intrinsic value views, for example. Understanding how these views play out in different countries is difficult; one cannot assume that various foreign communities will embrace the mainstream scientific mindset that U.S. researchers might carry. For example, many countries in South America have adopted the view that “Mother Earth” has her own rights and that countries need to protect the rights of wildlife and forests . (Note that this is not just an international issue – Native American peoples can also have different values that guide human-nature interactions in their sovereign nations within the United States.) More in-depth consideration of these topics can be found by OEC readers in journals such as Environmental Ethics ; Culture Matters , a report by a National Academies of Science group, also touches on the importance of many facets of culture in arranging fair and equitable international research partnerships, including in environmental fields.
In the case study the Senator and his Science Advisor bring differing perspectives on the value of the rain forest, with the former weighing heavily the economic and social benefits and his advisor arguing for the intrinsic value of the forest and its ecosystem. OEC readers can examine this potential conflict more deeply by tapping into the worldwide IPBES community that is forging consensus on the valuation and conservation of ecosystem services. IPBES, the Intergovernmental Platform on Biodiversity and Ecosystem Services , is an international agreement established in 2012 and signed by 126 countries, including the United States. (I was honored to be part of the U.S. delegation to the First IPBES Plenary in 2013). OEC readers will find that a recent paper by the IPBES Expert Group on Ecosystem Values, with 48 authors from 31 countries, brings a wide range of international, cultural and scientific perspectives to the valuation of ecosystem services and most importantly, expands the discussion from an intrinsic vs. human economic valuation to a pluralistic approach that incorporates diverse values: “ Valuing nature’s contributions to people: the IPBES approach ."
Author: Elizabeth Lyons, NSF Office of International Science and Engineering.
This material is based upon work done while serving at the National Science Foundation. Any opinion, findings, and conclusions or recommendations expressed in this material are those of the author and do not necessarily reflect the views of the National Science Foundation.
In this case, Armando must consider competing interests among his constituents, colleagues, and advisors while being mindful of the myriad ethical dilemmas in which the forest is implicated. Here, we will consider the biodiversity and ecosystem services associated with the forest, the social and economic issues surrounding deforestation, and the options available to Armando as he makes his recommendation on how to use the US funds. Finally, we will explore the role of Maria as a science advisor and address her responsibilities, as well as her mistake in addressing the press.
The Amazon Rain Forest is the largest tropical forest on earth (6.7 million km 2 … eight times the size of Texas!); it contains more than half the planet’s rainforests and record amounts of biodiversity. Brazil, the largest country in South America, encompasses the largest portion of the Amazon within its borders, particularly in the states of Amazonas and Pará in Northern Brazil. Although there is no current, official species count, Brazil likely contains around 50,000 species of plants alone, accounting for one-sixth of the earth’s plant species (Myers et al. 2000). A 2005 estimate put the total number of species (plants and animals, both vertebrates and invertebrates) in Brazil at between 170,000 and 210,000 species, or approximately 9.5% the world total (Lewinsohn and Prado 2005). Deep within the forest, it is easy to feel isolated from human issues, totally encompassed by wild nature. But the forest is very much central to social, economic, and environmental interests at local, national, and global scales.
In the last 50 years, around 20% of the forest cover has been lost to logging, development, and agriculture (WWF 2016, The Nature Conservancy 2016). Deforestation leads to loss of biodiversity, particularly through habitat loss and habitat fragmentation. Biodiversity loss in the Amazon can affect the global pharmacology sector, the regional tourism sector, and the scientists and environmentalists the world over who praise the intrinsic value of the unique and unparalleled plants and animals found there.
In addition to threats to biodiversity, the regional and global regulatory ecosystem services provided by the Brazilian Amazon are also in jeopardy. Plants in the Amazon contain between 90 and 140 billion tons of carbon (Soares-Filho et al. 2006). In perspective, human induced carbon emissions occur at a rate around 35 billion tons a year (and growing) (IPCC Report 2014). When trees are logged, the forest’s capacity to absorb and hold CO 2 is greatly reduced. In particular, land use change for agriculture contributes to human emissions by burning off CO 2 contained within slashed and burned portions of the forest. In addition to being a carbon reservoir, the Amazon plays a crucial role in the water cycle. The forest recycles 25-50% of regional rainfall (Eltahir and Bras 1994), but large-scale deforestation could break the cycle and reduce average regional rainfall. The Amazon also impacts the amount of cloudiness, thermal insolation, land surface reflectance, atmospheric aerosol loading (which could effect global rain patterns), and surface roughness (affecting regional wind speeds) (reviewed in Malhi et al. 2008).
Besides regional and global ecosystem services, the forest holds local economic value to Brazilian citizens living in the forest. Though there are sustainable ways to live off of the forest, such as rubber tapping, many more people either directly or indirectly derive their income from logging. For example, in Tailândia, Pará, 70% of the 25,000 people living in the city depend financially on deforestation in some way (Economist 2009). Loggers take the best and most valuable trees for their lumber business, while farmers and cattle ranchers depend on cleared lands for their agricultural purposes.
Social issues in the forest abound. In the 1960s through the 1980s, the Brazilian government subsidized the mass migration of citizens into the forest in efforts to begin economically utilizing the country’s vast interior (Economist 2009; Nordhaus and Shellenberger 2009). However, once citizens moved into the forest, promises of free land and prosperity were met only for the lucky few. Members of the elite class bought land ahead of the mass migration, and to demonstrate ownership they immediately began to log and burn the land. Still today, the top 1% of affluent landlords oversees 45% of the land (Nordhaus and Shellenberger 2009). And “only 14% of privately owned land is backed by secure title deed,” while the rest is backed with fake documents (Imazon study quoted, Economist 2009).
Many low-income families living in the forest are forced to work in harsh conditions governed largely by landlords, gangs, poachers, and illegal loggers (Garcia-Navarro 2015; Economist 2009; Nordhaus and Shellenberger 2009). It is said, “the law of the Amazon is made by the bullet” (Nordhaus and Shellenberger 2009, 49). For example, a recent NPR report details the ongoing “war over wood” between rubber-tappers and illegal loggers in protected portions of the forest. Illegal loggers are barely penalized, due to a lacking and understaffed police force. When rubber tappers who depend on the intact ecosystem encounter loggers, arguments are settled with bullets, knives, and murder. Rubber-tappers fight to defend their way of life. Loggers not only fight to protect their illegal jobs, but also their own lives. Many are impoverished and treated no better than slave laborers, overseen by cruel gang lords who run the illegal logging operations (Garcia-Navarro 2015).
Finally, there is a long history of fear over foreign involvement in Brazilian affairs. Nordhaus and Shellenberger (2009) summarize:
“As a country of great artists, architects, diplomats, designers, and engineers, and as people who speak Portuguese in a region where Spanish dominates, Brazilians justifiably see themselves as special and unique. At the same time, many Brazilians are ashamed of the persistence of widespread poverty, violence, and lawlessness… This stew of national pride and shame results in Brazil’s love-hate relationship with the United States. Environmentalists’ efforts to reassure Brazilians that their attempts to save the Amazon are in Brazil’s best interests not only fail to assuage Brazilian concerns, they trigger Brazil’s fear of being patronized…
Brazilians continue to see environmental proposals [such as working with US funds to establish a new national park] as suspicious. …Brazilians ask themselves … ‘Do you care about us or just our forest?’” (60-61).
Armando will consider these multi-scale social, economic, and environmental interests as he decides how to recommend using the US funds. He must also manage input from his constituents, his Senate colleagues, the US government, and Maria and his other trusted advisors. His personal compass further complicates his decision. Armando’s anthropocentric ethic and utilitarian view of the forest make him partial to social and economic interests. So although he is aware of the global and regional benefits of a protected area, his constituents have local, immediate needs that he feels more answerable to. Having grown up as a rubber tapper and having studied the forest extensively in school, Armando loves the Amazon, but he believes that people can co-exist with nature and there is no need for a national park that would restrict human use of the forest.
On the other hand, Maria’s ecocentric ethic fuels her bias toward preservation of the forest, particularly for its intrinsic value. She would like the forest protected in pure form, free from human influence. Her US-based education may contribute to her admiration of the US National Parks. And having come from the large city, Belém, Maria is detached from the direct interactions between people and nature occurring at the border of and within the Amazon in Pará, such as in Tailândia. Thus, it is understandable that she would believe the best policy is to create a national park, and as an advisor she can provide this opinion to Armando. It is his decision whether or not to act on Maria’s suggestion.
Maria should be able to perform her job as a science advisor despite her personal biases. It remains up to her how she elects to use her expertise. For example, Maria might choose to be an “Issue Advocate,” and “[focus] on the implications of [her] research for a particular political agenda” (Pielke 2007, 15). In the case study above, she did just that, aligning her policy suggestion to her ecocentric ethic and using media outreach to push her agenda. Maria could also be an “Honest Broker,” with a responsibility to provide information regarding a suite of different policy alternatives and how they will effect or be affected by the state of the forest. A politician or the political process (e.g., popular vote) takes on the responsibility of making a choice among the alternatives (Pielke 2007, 17). Armando’s negative reaction to Maria’s media statement and his disapproval of her narrow vision for a policy suggestion alludes to his preference for science advisors that act as “Honest Brokers.”
There is also a workplace authority problem in this case. It is possible that Maria could advocate her position and Armando would not mind. But, whether Armando agrees with her recommendation or not, she should not be talking to the press without permission from her boss. Typically, institutions (including Armando’s Senate office) have press secretaries that are charged with communicating to media outlets. Because she spoke to the press without a clear message, Maria and the reporter and editor at O Liberal who misunderstood her all share responsibility for the misquoted phrase. However, there is a possibility that the reporter did understand but decided to embellish the story. If that is the case Maria bears less responsibility, but speaking via a press secretary may have helped, as press secretaries are knowledgeable about how to communicate clearly and consistently with reputable news sources. If in the future Maria is allowed to speak directly with the press, she should be honest and she should be clear that her statements in no way reflect the as of yet unannounced opinions of Armando or any other members of Armando’s team. The press secretary will release statements that represent the views of the institution as a whole.
Considering that Armando does not plan to adopt Maria’s vision of a national park, below are a few alternative policy options that are more in line with Armando’s desire to both protect the forest and support his constituents with the funds (by no means, an exhaustive list). An “honest broker” analysis by a Maria might have revealed some of these policy alternatives. Note that many of them are not strictly scientific, an indicator that it may also be the job of a science advisor to collaborate with experts and advisors of different expertise to come up with a more thorough and inclusive field of potential policies.
Although law enforcement efforts in the forest have increased in recent years, “the basic factors driving deforestation — including poverty and the profitability of agricultural land — have not changed” (Tollefson 2015). To address these “basic factors,” Armando could use the funds to invest in local economic issues. For example, investing in public education and stimulating creation of service-economy jobs could help to eliminate the need for a logging-derived income. Another option, subsidization of advanced faming equipment, could preclude the need for slash and burn agriculture. Armando could also advocate using the funds to bolster efforts to put together a rural land registry in Pará (Tollefson 2015). A land registry could aid in easing violent disagreements over land ownership and allow the government to more easily enforce land-use laws. These locally minded suggestions would also be appealing to Brazilians who would like to minimize replicating or depending on the American environmental movement.
Funds could also be used for a “payment-for-ecosystem-services” (PES) scheme for the farmers within Pará. Such a scheme would reward farmers for keeping parts of the forest that fall within their property intact. For example, a farmer could be paid for the economic hit they take for not planting on a forested portion of their property. However, PES schemes are difficult to implement (e.g., how do you decide the value of the intact forest? How do you monitor the scheme?), and an Amazon forest PES scheme may only benefit large land owners because they are the ones who own the majority of land (or at least appear to own the majority of land based on documents, both legal and fake) (Börner et al. 2010). PES schemes could also be internationally scaled, such as REDD (“reducing emissions from deforestation and forest degradation”), in which foreign countries pay Brazil to keep the forest intact to prevent the carbon emissions associated with deforestation. In 2015, Norway paid the last installment of an unprecedented $1 billion investment in the program. However, Brazilian farmers and local programs complain that they’ve seen few direct benefits from the REDD program (Tollefson 2015).
Another idea, Armando could use funds to advertise and implement a boycott of products and goods that are implicated in deforestation. For example, initially backed and promoted by the international environmental organization, Greenpeace, the Soyabean Moratorium is essentially an agreement among soyabean exporters (led by McDonald’s) to cease buying from growers in the Amazon and to fund monitoring programs to ensure the success of the moratorium (Greenpeace 2016; Tollefson 2015; Economist 2009). The moratorium appears successful thus far. A 2015 study found that deforestation was higher in areas not under the moratorium, compared to those that were (Gibbs et al. 2015). Funding could potentially be used to maintain and expand the soyabean moratorium, or to replicate it in the cattle or logging industries.
What remains for Armando, is to decide which of the options (among these and among others) he thinks could be successful and desirable. Then he will advocate his selection to his colleagues and constituents in the upcoming Federal Senate session.
Related Resources
Submit Content to the OEC Donate
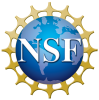
This material is based upon work supported by the National Science Foundation under Award No. 2055332. Any opinions, findings, and conclusions or recommendations expressed in this material are those of the author(s) and do not necessarily reflect the views of the National Science Foundation.
Case Study: The Amazon Rainforest
The amazon in context.
Tropical rainforests are often considered to be the “cradles of biodiversity.” Though they cover only about 6% of the Earth’s land surface, they are home to over 50% of global biodiversity. Rainforests also take in massive amounts of carbon dioxide and release oxygen through photosynthesis, which has also given them the nickname “lungs of the planet.” They also store very large amounts of carbon, and so cutting and burning their biomass contributes to global climate change. Many modern medicines are derived from rainforest plants, and several very important food crops originated in the rainforest, including bananas, mangos, chocolate, coffee, and sugar cane.
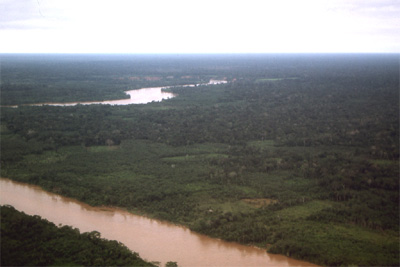
In order to qualify as a tropical rainforest, an area must receive over 250 centimeters of rainfall each year and have an average temperature above 24 degrees centigrade, as well as never experience frosts. The Amazon rainforest in South America is the largest in the world. The second largest is the Congo in central Africa, and other important rainforests can be found in Central America, the Caribbean, and Southeast Asia. Brazil contains about 40% of the world’s remaining tropical rainforest. Its rainforest covers an area of land about 2/3 the size of the continental United States.
There are countless reasons, both anthropocentric and ecocentric, to value rainforests. But they are one of the most threatened types of ecosystems in the world today. It’s somewhat difficult to estimate how quickly rainforests are being cut down, but estimates range from between 50,000 and 170,000 square kilometers per year. Even the most conservative estimates project that if we keep cutting down rainforests as we are today, within about 100 years there will be none left.
How does a rainforest work?
Rainforests are incredibly complex ecosystems, but understanding a few basics about their ecology will help us understand why clear-cutting and fragmentation are such destructive activities for rainforest biodiversity.
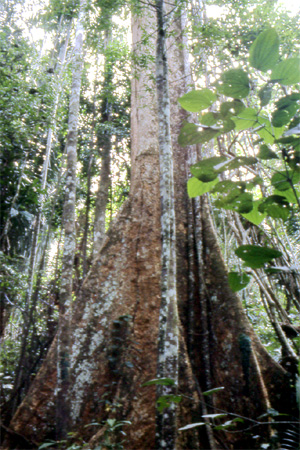
High biodiversity in tropical rainforests means that the interrelationships between organisms are very complex. A single tree may house more than 40 different ant species, each of which has a different ecological function and may alter the habitat in distinct and important ways. Ecologists debate about whether systems that have high biodiversity are stable and resilient, like a spider web composed of many strong individual strands, or fragile, like a house of cards. Both metaphors are likely appropriate in some cases. One thing we can be certain of is that it is very difficult in a rainforest system, as in most other ecosystems, to affect just one type of organism. Also, clear cutting one small area may damage hundreds or thousands of established species interactions that reach beyond the cleared area.
Pollination is a challenge for rainforest trees because there are so many different species, unlike forests in the temperate regions that are often dominated by less than a dozen tree species. One solution is for individual trees to grow close together, making pollination simpler, but this can make that species vulnerable to extinction if the one area where it lives is clear cut. Another strategy is to develop a mutualistic relationship with a long-distance pollinator, like a specific bee or hummingbird species. These pollinators develop mental maps of where each tree of a particular species is located and then travel between them on a sort of “trap-line” that allows trees to pollinate each other. One problem is that if a forest is fragmented then these trap-line connections can be disrupted, and so trees can fail to be pollinated and reproduce even if they haven’t been cut.
The quality of rainforest soils is perhaps the most surprising aspect of their ecology. We might expect a lush rainforest to grow from incredibly rich, fertile soils, but actually, the opposite is true. While some rainforest soils that are derived from volcanic ash or from river deposits can be quite fertile, generally rainforest soils are very poor in nutrients and organic matter. Rainforests hold most of their nutrients in their live vegetation, not in the soil. Their soils do not maintain nutrients very well either, which means that existing nutrients quickly “leech” out, being carried away by water as it percolates through the soil. Also, soils in rainforests tend to be acidic, which means that it’s difficult for plants to access even the few existing nutrients. The section on slash and burn agriculture in the previous module describes some of the challenges that farmers face when they attempt to grow crops on tropical rainforest soils, but perhaps the most important lesson is that once a rainforest is cut down and cleared away, very little fertility is left to help a forest regrow.
What is driving deforestation in the Amazon?
Many factors contribute to tropical deforestation, but consider this typical set of circumstances and processes that result in rapid and unsustainable rates of deforestation. This story fits well with the historical experience of Brazil and other countries with territory in the Amazon Basin.
Population growth and poverty encourage poor farmers to clear new areas of rainforest, and their efforts are further exacerbated by government policies that permit landless peasants to establish legal title to land that they have cleared.
At the same time, international lending institutions like the World Bank provide money to the national government for large-scale projects like mining, construction of dams, new roads, and other infrastructure that directly reduces the forest or makes it easier for farmers to access new areas to clear.
The activities most often encouraging new road development are timber harvesting and mining. Loggers cut out the best timber for domestic use or export, and in the process knock over many other less valuable trees. Those trees are eventually cleared and used for wood pulp, or burned, and the area is converted into cattle pastures. After a few years, the vegetation is sufficiently degraded to make it not profitable to raise cattle, and the land is sold to poor farmers seeking out a subsistence living.
Regardless of how poor farmers get their land, they often are only able to gain a few years of decent crop yields before the poor quality of the soil overwhelms their efforts, and then they are forced to move on to another plot of land. Small-scale farmers also hunt for meat in the remaining fragmented forest areas, which reduces the biodiversity in those areas as well.
Another important factor not mentioned in the scenario above is the clearing of rainforest for industrial agriculture plantations of bananas, pineapples, and sugar cane. These crops are primarily grown for export, and so an additional driver to consider is consumer demand for these crops in countries like the United States.
These cycles of land use, which are driven by poverty and population growth as well as government policies, have led to the rapid loss of tropical rainforests. What is lost in many cases is not simply biodiversity, but also valuable renewable resources that could sustain many generations of humans to come. Efforts to protect rainforests and other areas of high biodiversity is the topic of the next section.
Help | Advanced Search
Computer Science > Cryptography and Security
Title: ransomware detection and classification using random forest: a case study with the ugransome2024 dataset.
Abstract: Cybersecurity faces challenges in identifying and mitigating ransomware, which is important for protecting critical infrastructures. The absence of datasets for distinguishing normal versus abnormal network behaviour hinders the development of proactive detection strategies against ransomware. An obstacle in proactive prevention methods is the absence of comprehensive datasets for contrasting normal versus abnormal network behaviours. The dataset enabling such contrasts would significantly expedite threat anomaly mitigation. In this study, we introduce UGRansome2024, an optimised dataset for ransomware detection in network traffic. This dataset is derived from the UGRansome data using an intuitionistic feature engineering approach that considers only relevant patterns in network behaviour analysis. The study presents an analysis of ransomware detection using the UGRansome2024 dataset and the Random Forest algorithm. Through encoding and feature relevance determination, the Random Forest achieved a classification accuracy of 96% and effectively identified unusual ransomware transactions. Findings indicate that certain ransomware variants, such as those utilising Encrypt Decrypt Algorithms (EDA) and Globe ransomware, have the highest financial impact. These insights have significant implications for real-world cybersecurity practices, highlighting the importance of machine learning in ransomware detection and mitigation. Further research is recommended to expand datasets, explore alternative detection methods, and address limitations in current approaches.
Submission history
Access paper:.
- Other Formats

References & Citations
- Google Scholar
- Semantic Scholar
BibTeX formatted citation

Bibliographic and Citation Tools
Code, data and media associated with this article, recommenders and search tools.
- Institution
arXivLabs: experimental projects with community collaborators
arXivLabs is a framework that allows collaborators to develop and share new arXiv features directly on our website.
Both individuals and organizations that work with arXivLabs have embraced and accepted our values of openness, community, excellence, and user data privacy. arXiv is committed to these values and only works with partners that adhere to them.
Have an idea for a project that will add value for arXiv's community? Learn more about arXivLabs .
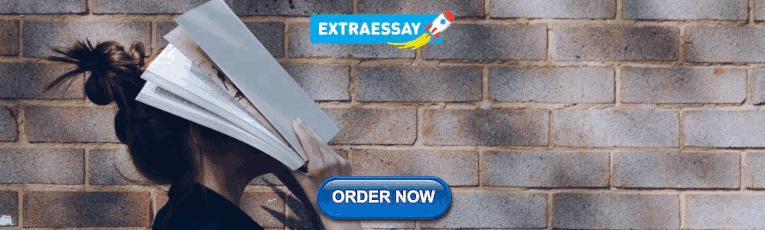
IMAGES
VIDEO
COMMENTS
Case Study: The Amazon Rainforest The Amazon in context. Tropical rainforests are often considered to be the "cradles of biodiversity." Though they cover only about 6% of the Earth's land surface, they are home to over 50% of global biodiversity. Rainforests also take in massive amounts of carbon dioxide and release oxygen through ...
The study area, which represents about 20 percent of the Amazon basin, has lost 30 percent of its rainforest. New results from a nine-year research project in the eastern Amazon rainforest finds that significant deforestation in eastern and southeastern Brazil has been associated with a long-term decrease in rainfall and increase in temperature during the dry season, turning what was once a ...
The possibility that the Amazon forest system could soon reach a tipping point, inducing large-scale collapse, has raised global concern1-3. For 65 million years, Amazonian forests remained ...
Fast Fact. The Amazon rain forest absorbs one-fourth of the CO2 absorbed by all the land on Earth. The amount absorbed today, however, is 30% less than it was in the 1990s because of deforestation. A major motive for deforestation is cattle ranching. China, the United States, and other countries have created a consumer demand for beef, so ...
Case Study: The Amazonian Road Decision. The proposed Pucallpa-Cruzeiro do Sul will connect the Amazon's interior to urban centers and export markets in Peru and Brazil. However, critics are worried that the road will also create new opportunities for illegal logging and infringe on the territory of indigenous communities and wildlife.
We restricted our study area to the Amazon forest, defined as at least 60% forest cover in MODIS Vegetation Continuous Fields (MOD44Bv006; see section 1) during 2001-2018 within the Amazon Basin ...
The Amazon forest contains about 123 ± 23 petagrams carbon ... In the case of CO, ... The Amazon study area was defined according to subregions from Eva et al. 33 and biomes from Olson et al. 34.
A new study, co-authored by a team of researchers including UConn Ecology and Evolutionary Biology researcher Cory Merow provides the first quantitative assessment of how environmental policies on deforestation, along with forest fires and drought, have impacted the diversity of plants and animals in the Amazon.
The study area is defined by the Amazon biogeographic limit (RAISG 2020). The Amazon rainforest comprises different climate zones. ... Amazon forest cover and deforestation data are currently available from several sources, such as INPE (2019) or RAISG (2020). ... (1990-2010 in the case of Gloor et al. 2015), ...
Sustainable management ensures rainforests are worth. more than the value of the timber and other resources that can be extracted, such as gold. An example of this is sustainable foresty, which balances the removal of trees to sell with the conservation of the forest. Selective logging involves only removing a small number of trees, allowing ...
Based on estimates of 1% annual tropical forest loss, the Amazon may be losing as many as 11 to 16 species per day (Wilson 1989), and the resulting ecosystems are often highly degraded (Buschbacher 1986). Te deforestation of Amazonia presents a challenging study of the interactions among people, their values, and the environment.
Living World - Amazon Case Study The Amazon is the largest tropical rainforest on Earth. It sits within the Amazon River basin, covers some 40% of the South American continent and as you can see on the map below includes parts of eight South American countries: Brazil, Bolivia, Peru, Ecuador, Colombia, Venezuela, Guyana, and Suriname.
Amazon Deforestation: A Regional Conservation Case Study. GIS analysis of select strictly protected areas supported by the Amazon Region Protected Areas Program (ARPA)
Amazon trees are huge—up to 110 feet tall here at ZF2—so they require extra-large plots. That means bigger, more expensive gear for spraying CO 2 . Infrastructure such as roads and technical ...
Case study of a tropical rainforest setting to illustrate and analyse key themes in water and carbon cycles and their relationship to environmental change and human activity. Amazon Forest The Amazon is the largest tropical rainforest on Earth. It sits within the Amazon River basin, covers some 40% of the South American continent and as you can ...
73 Case Study: The Amazon Rainforest The Amazon in context. Tropical rainforests are often considered to be the "cradles of biodiversity." Though they cover only about 6% of the Earth's land surface, they are home to over 50% of global biodiversity. Rain forests also take in massive amounts of carbon dioxide and release oxygen through ...
Early studies showed that the Amazon rainforest may exhibit strong dieback by the end of the twenty-first century 9,19. ... the case of Amazonian rainforest and savanna fire. Ecol.
That month, the country's National Institute for Space Research (INPE) reported that there were more than 80,000 fires, the most that it had ever recorded. It was a nearly 80 percent jump ...
Item 1 of 4 Smoke from a fire rises into the air as trees burn amongst vegetation in Brazil's Amazon rainforest, in Apui, Amazonas state, Brazil, September 5, 2021. ... One study last year ...
The Amazon rainforest area spans about 8,200,000km 2 across 9 countries, making it the largest rainforest in the world. The tree coverage in 1970 was 4.1m km 2 . In 2018, it was 3.3m km 2 . Between 2001 and 2013, the causes of Amazonian deforestation were:
In this case, Armando, a Brazilian Federal Senator with a BS and MS in Biology, must confront ethical dilemmas concerning the Amazon forest. Topics for consideration include: 1) the biodiversity and ecosystem services associated with the forest, 2) the social and economic issues surrounding deforestation, and the 3) options available to Armando as he makes his recommendation on how to use the ...
The Amazon Region Protected Areas (ARPA) - created in 2002 by the Brazilian government: - By 2018, 60 million hectares of new parks and reserves were created under ARPA. - protect habitats, ecosystems and biodiversity. - reduce conflicts over land ownership. - provide sustainable use options to local communities.
Forests drive tourism growth in China, offering ecologic and economic benefits over urbanization trends. Wellness tourism, aligned with UN goals, thrives in forest settings, though challenges remain, particularly in the underinvested forest regions of Western China. This study aims to identify and rank the forest wellness tourism industry development strategies for Chongqing Tea Mountain and ...
Case Study: The Amazon Rainforest The Amazon in context. Tropical rainforests are often considered to be the "cradles of biodiversity." Though they cover only about 6% of the Earth's land surface, they are home to over 50% of global biodiversity. Rainforests also take in massive amounts of carbon dioxide and release oxygen through ...
Satellite-based near-real-time forest disturbance alerting systems have been widely used to support law enforcement actions against illegal and unsustainable human activities in tropical forests. The availability of multiple optical and radar-based forest disturbance alerts, each with varying detection capabilities depending mainly on the satellite sensor used, poses a challenge for users in ...
The study presents an analysis of ransomware detection using the UGRansome2024 dataset and the Random Forest algorithm. Through encoding and feature relevance determination, the Random Forest achieved a classification accuracy of 96% and effectively identified unusual ransomware transactions.