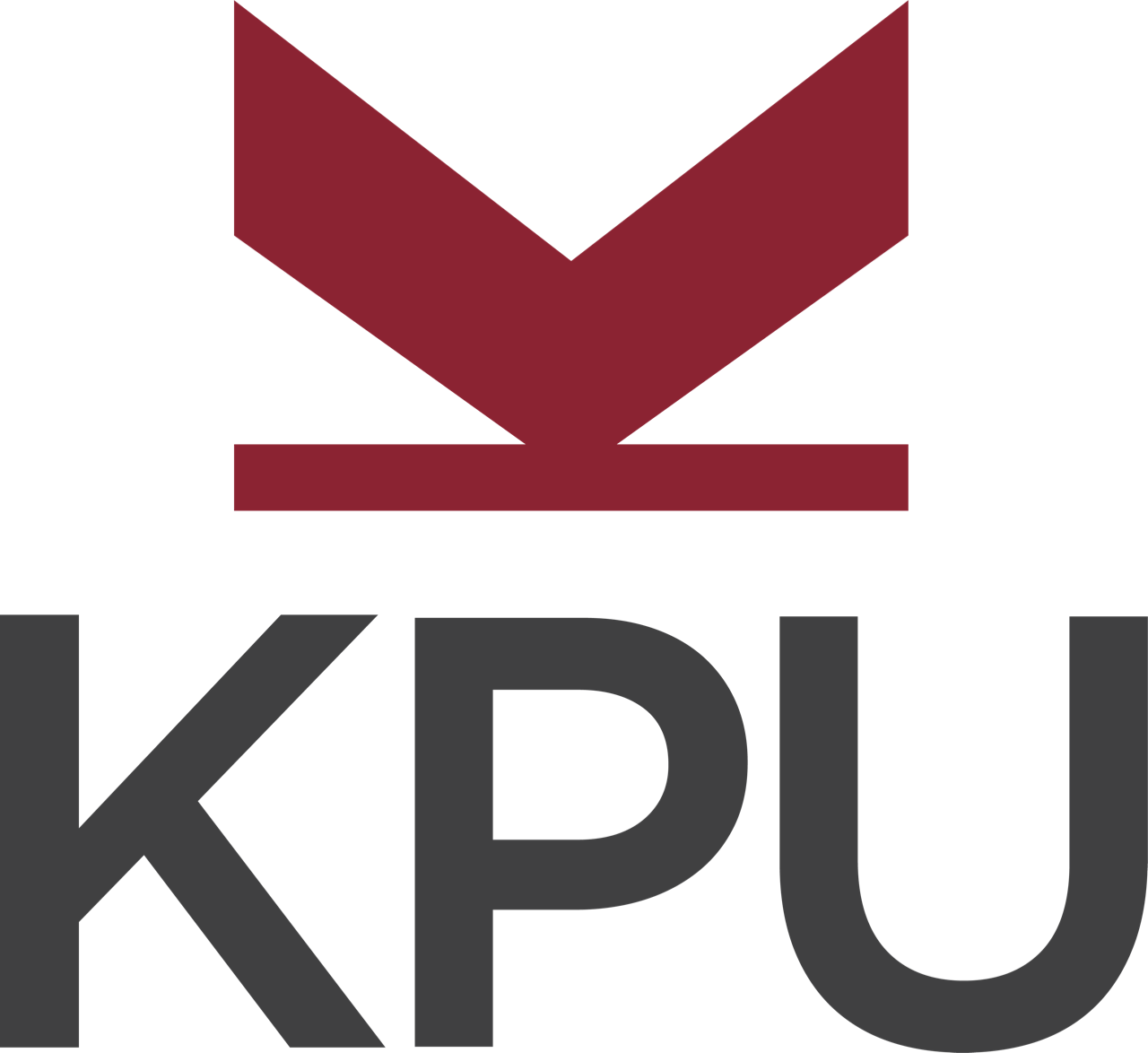
Want to create or adapt books like this? Learn more about how Pressbooks supports open publishing practices.
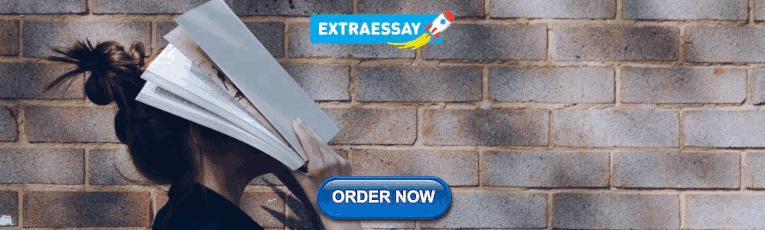
11 Hypothesis testing
The process of induction is the process of assuming the simplest law that can be made to harmonize with our experience. This process, however, has no logical foundation but only a psychological one. It is clear that there are no grounds for believing that the simplest course of events will really happen. It is an hypothesis that the sun will rise tomorrow: and this means that we do not know whether it will rise. – Ludwig Wittgenstein 157
In the last chapter, I discussed the ideas behind estimation, which is one of the two “big ideas” in inferential statistics. It’s now time to turn out attention to the other big idea, which is hypothesis testing . In its most abstract form, hypothesis testing really a very simple idea: the researcher has some theory about the world, and wants to determine whether or not the data actually support that theory. However, the details are messy, and most people find the theory of hypothesis testing to be the most frustrating part of statistics. The structure of the chapter is as follows. Firstly, I’ll describe how hypothesis testing works, in a fair amount of detail, using a simple running example to show you how a hypothesis test is “built”. I’ll try to avoid being too dogmatic while doing so, and focus instead on the underlying logic of the testing procedure. 158 Afterwards, I’ll spend a bit of time talking about the various dogmas, rules and heresies that surround the theory of hypothesis testing.
11.1 A menagerie of hypotheses
Eventually we all succumb to madness. For me, that day will arrive once I’m finally promoted to full professor. Safely ensconced in my ivory tower, happily protected by tenure, I will finally be able to take leave of my senses (so to speak), and indulge in that most thoroughly unproductive line of psychological research: the search for extrasensory perception (ESP). 159
Let’s suppose that this glorious day has come. My first study is a simple one, in which I seek to test whether clairvoyance exists. Each participant sits down at a table, and is shown a card by an experimenter. The card is black on one side and white on the other. The experimenter takes the card away, and places it on a table in an adjacent room. The card is placed black side up or white side up completely at random, with the randomisation occurring only after the experimenter has left the room with the participant. A second experimenter comes in and asks the participant which side of the card is now facing upwards. It’s purely a one-shot experiment. Each person sees only one card, and gives only one answer; and at no stage is the participant actually in contact with someone who knows the right answer. My data set, therefore, is very simple. I have asked the question of \(N\) people, and some number \(X\) of these people have given the correct response. To make things concrete, let’s suppose that I have tested \(N = 100\) people, and \(X = 62\) of these got the answer right… a surprisingly large number, sure, but is it large enough for me to feel safe in claiming I’ve found evidence for ESP? This is the situation where hypothesis testing comes in useful. However, before we talk about how to test hypotheses, we need to be clear about what we mean by hypotheses.
11.1.1 Research hypotheses versus statistical hypotheses
The first distinction that you need to keep clear in your mind is between research hypotheses and statistical hypotheses. In my ESP study, my overall scientific goal is to demonstrate that clairvoyance exists. In this situation, I have a clear research goal: I am hoping to discover evidence for ESP. In other situations I might actually be a lot more neutral than that, so I might say that my research goal is to determine whether or not clairvoyance exists. Regardless of how I want to portray myself, the basic point that I’m trying to convey here is that a research hypothesis involves making a substantive, testable scientific claim… if you are a psychologist, then your research hypotheses are fundamentally about psychological constructs. Any of the following would count as research hypotheses :
- Listening to music reduces your ability to pay attention to other things. This is a claim about the causal relationship between two psychologically meaningful concepts (listening to music and paying attention to things), so it’s a perfectly reasonable research hypothesis.
- Intelligence is related to personality . Like the last one, this is a relational claim about two psychological constructs (intelligence and personality), but the claim is weaker: correlational not causal.
- Intelligence is* speed of information processing . This hypothesis has a quite different character: it’s not actually a relational claim at all. It’s an ontological claim about the fundamental character of intelligence (and I’m pretty sure it’s wrong). It’s worth expanding on this one actually: It’s usually easier to think about how to construct experiments to test research hypotheses of the form “does X affect Y?” than it is to address claims like “what is X?” And in practice, what usually happens is that you find ways of testing relational claims that follow from your ontological ones. For instance, if I believe that intelligence is* speed of information processing in the brain, my experiments will often involve looking for relationships between measures of intelligence and measures of speed. As a consequence, most everyday research questions do tend to be relational in nature, but they’re almost always motivated by deeper ontological questions about the state of nature.
Notice that in practice, my research hypotheses could overlap a lot. My ultimate goal in the ESP experiment might be to test an ontological claim like “ESP exists”, but I might operationally restrict myself to a narrower hypothesis like “Some people can `see’ objects in a clairvoyant fashion”. That said, there are some things that really don’t count as proper research hypotheses in any meaningful sense:
- Love is a battlefield . This is too vague to be testable. While it’s okay for a research hypothesis to have a degree of vagueness to it, it has to be possible to operationalise your theoretical ideas. Maybe I’m just not creative enough to see it, but I can’t see how this can be converted into any concrete research design. If that’s true, then this isn’t a scientific research hypothesis, it’s a pop song. That doesn’t mean it’s not interesting – a lot of deep questions that humans have fall into this category. Maybe one day science will be able to construct testable theories of love, or to test to see if God exists, and so on; but right now we can’t, and I wouldn’t bet on ever seeing a satisfying scientific approach to either.
- The first rule of tautology club is the first rule of tautology club . This is not a substantive claim of any kind. It’s true by definition. No conceivable state of nature could possibly be inconsistent with this claim. As such, we say that this is an unfalsifiable hypothesis, and as such it is outside the domain of science. Whatever else you do in science, your claims must have the possibility of being wrong.
- More people in my experiment will say “yes” than “no” . This one fails as a research hypothesis because it’s a claim about the data set, not about the psychology (unless of course your actual research question is whether people have some kind of “yes” bias!). As we’ll see shortly, this hypothesis is starting to sound more like a statistical hypothesis than a research hypothesis.
As you can see, research hypotheses can be somewhat messy at times; and ultimately they are scientific claims. Statistical hypotheses are neither of these two things. Statistical hypotheses must be mathematically precise, and they must correspond to specific claims about the characteristics of the data generating mechanism (i.e., the “population”). Even so, the intent is that statistical hypotheses bear a clear relationship to the substantive research hypotheses that you care about! For instance, in my ESP study my research hypothesis is that some people are able to see through walls or whatever. What I want to do is to “map” this onto a statement about how the data were generated. So let’s think about what that statement would be. The quantity that I’m interested in within the experiment is \(P(\mbox{“correct”})\) , the true-but-unknown probability with which the participants in my experiment answer the question correctly. Let’s use the Greek letter \(\theta\) (theta) to refer to this probability. Here are four different statistical hypotheses:
- If ESP doesn’t exist and if my experiment is well designed, then my participants are just guessing. So I should expect them to get it right half of the time and so my statistical hypothesis is that the true probability of choosing correctly is \(\theta = 0.5\) .
- Alternatively, suppose ESP does exist and participants can see the card. If that’s true, people will perform better than chance. The statistical hypotheis would be that \(\theta > 0.5\) .
- A third possibility is that ESP does exist, but the colours are all reversed and people don’t realise it (okay, that’s wacky, but you never know…). If that’s how it works then you’d expect people’s performance to be below chance. This would correspond to a statistical hypothesis that \(\theta < 0.5\) .
- Finally, suppose ESP exists, but I have no idea whether people are seeing the right colour or the wrong one. In that case, the only claim I could make about the data would be that the probability of making the correct answer is not equal to 50. This corresponds to the statistical hypothesis that \(\theta \neq 0.5\) .
All of these are legitimate examples of a statistical hypothesis because they are statements about a population parameter and are meaningfully related to my experiment.
What this discussion makes clear, I hope, is that when attempting to construct a statistical hypothesis test the researcher actually has two quite distinct hypotheses to consider. First, he or she has a research hypothesis (a claim about psychology), and this corresponds to a statistical hypothesis (a claim about the data generating population). In my ESP example, these might be
And the key thing to recognise is this: a statistical hypothesis test is a test of the statistical hypothesis, not the research hypothesis . If your study is badly designed, then the link between your research hypothesis and your statistical hypothesis is broken. To give a silly example, suppose that my ESP study was conducted in a situation where the participant can actually see the card reflected in a window; if that happens, I would be able to find very strong evidence that \(\theta \neq 0.5\) , but this would tell us nothing about whether “ESP exists”.
11.1.2 Null hypotheses and alternative hypotheses
So far, so good. I have a research hypothesis that corresponds to what I want to believe about the world, and I can map it onto a statistical hypothesis that corresponds to what I want to believe about how the data were generated. It’s at this point that things get somewhat counterintuitive for a lot of people. Because what I’m about to do is invent a new statistical hypothesis (the “null” hypothesis, \(H_0\) ) that corresponds to the exact opposite of what I want to believe, and then focus exclusively on that, almost to the neglect of the thing I’m actually interested in (which is now called the “alternative” hypothesis, \(H_1\) ). In our ESP example, the null hypothesis is that \(\theta = 0.5\) , since that’s what we’d expect if ESP didn’t exist. My hope, of course, is that ESP is totally real, and so the alternative to this null hypothesis is \(\theta \neq 0.5\) . In essence, what we’re doing here is dividing up the possible values of \(\theta\) into two groups: those values that I really hope aren’t true (the null), and those values that I’d be happy with if they turn out to be right (the alternative). Having done so, the important thing to recognise is that the goal of a hypothesis test is not to show that the alternative hypothesis is (probably) true; the goal is to show that the null hypothesis is (probably) false. Most people find this pretty weird.
The best way to think about it, in my experience, is to imagine that a hypothesis test is a criminal trial 160 … the trial of the null hypothesis . The null hypothesis is the defendant, the researcher is the prosecutor, and the statistical test itself is the judge. Just like a criminal trial, there is a presumption of innocence: the null hypothesis is deemed to be true unless you, the researcher, can prove beyond a reasonable doubt that it is false. You are free to design your experiment however you like (within reason, obviously!), and your goal when doing so is to maximise the chance that the data will yield a conviction… for the crime of being false. The catch is that the statistical test sets the rules of the trial, and those rules are designed to protect the null hypothesis – specifically to ensure that if the null hypothesis is actually true, the chances of a false conviction are guaranteed to be low. This is pretty important: after all, the null hypothesis doesn’t get a lawyer. And given that the researcher is trying desperately to prove it to be false, someone has to protect it.
11.2 Two types of errors
Before going into details about how a statistical test is constructed, it’s useful to understand the philosophy behind it. I hinted at it when pointing out the similarity between a null hypothesis test and a criminal trial, but I should now be explicit. Ideally, we would like to construct our test so that we never make any errors. Unfortunately, since the world is messy, this is never possible. Sometimes you’re just really unlucky: for instance, suppose you flip a coin 10 times in a row and it comes up heads all 10 times. That feels like very strong evidence that the coin is biased (and it is!), but of course there’s a 1 in 1024 chance that this would happen even if the coin was totally fair. In other words, in real life we always have to accept that there’s a chance that we did the wrong thing. As a consequence, the goal behind statistical hypothesis testing is not to eliminate errors, but to minimise them.
At this point, we need to be a bit more precise about what we mean by “errors”. Firstly, let’s state the obvious: it is either the case that the null hypothesis is true, or it is false; and our test will either reject the null hypothesis or retain it. 161 So, as the table below illustrates, after we run the test and make our choice, one of four things might have happened:
As a consequence there are actually two different types of error here. If we reject a null hypothesis that is actually true, then we have made a type I error . On the other hand, if we retain the null hypothesis when it is in fact false, then we have made a type II error .
Remember how I said that statistical testing was kind of like a criminal trial? Well, I meant it. A criminal trial requires that you establish “beyond a reasonable doubt” that the defendant did it. All of the evidentiary rules are (in theory, at least) designed to ensure that there’s (almost) no chance of wrongfully convicting an innocent defendant. The trial is designed to protect the rights of a defendant: as the English jurist William Blackstone famously said, it is “better that ten guilty persons escape than that one innocent suffer.” In other words, a criminal trial doesn’t treat the two types of error in the same way~… punishing the innocent is deemed to be much worse than letting the guilty go free. A statistical test is pretty much the same: the single most important design principle of the test is to control the probability of a type I error, to keep it below some fixed probability. This probability, which is denoted \(\alpha\) , is called the significance level of the test (or sometimes, the size of the test). And I’ll say it again, because it is so central to the whole set-up~… a hypothesis test is said to have significance level \(\alpha\) if the type I error rate is no larger than \(\alpha\) .
So, what about the type II error rate? Well, we’d also like to keep those under control too, and we denote this probability by \(\beta\) . However, it’s much more common to refer to the power of the test, which is the probability with which we reject a null hypothesis when it really is false, which is \(1-\beta\) . To help keep this straight, here’s the same table again, but with the relevant numbers added:
A “powerful” hypothesis test is one that has a small value of \(\beta\) , while still keeping \(\alpha\) fixed at some (small) desired level. By convention, scientists make use of three different \(\alpha\) levels: \(.05\) , \(.01\) and \(.001\) . Notice the asymmetry here~… the tests are designed to ensure that the \(\alpha\) level is kept small, but there’s no corresponding guarantee regarding \(\beta\) . We’d certainly like the type II error rate to be small, and we try to design tests that keep it small, but this is very much secondary to the overwhelming need to control the type I error rate. As Blackstone might have said if he were a statistician, it is “better to retain 10 false null hypotheses than to reject a single true one”. To be honest, I don’t know that I agree with this philosophy – there are situations where I think it makes sense, and situations where I think it doesn’t – but that’s neither here nor there. It’s how the tests are built.
11.3 Test statistics and sampling distributions
At this point we need to start talking specifics about how a hypothesis test is constructed. To that end, let’s return to the ESP example. Let’s ignore the actual data that we obtained, for the moment, and think about the structure of the experiment. Regardless of what the actual numbers are, the form of the data is that \(X\) out of \(N\) people correctly identified the colour of the hidden card. Moreover, let’s suppose for the moment that the null hypothesis really is true: ESP doesn’t exist, and the true probability that anyone picks the correct colour is exactly \(\theta = 0.5\) . What would we expect the data to look like? Well, obviously, we’d expect the proportion of people who make the correct response to be pretty close to 50%. Or, to phrase this in more mathematical terms, we’d say that \(X/N\) is approximately \(0.5\) . Of course, we wouldn’t expect this fraction to be exactly 0.5: if, for example we tested \(N=100\) people, and \(X = 53\) of them got the question right, we’d probably be forced to concede that the data are quite consistent with the null hypothesis. On the other hand, if \(X = 99\) of our participants got the question right, then we’d feel pretty confident that the null hypothesis is wrong. Similarly, if only \(X=3\) people got the answer right, we’d be similarly confident that the null was wrong. Let’s be a little more technical about this: we have a quantity \(X\) that we can calculate by looking at our data; after looking at the value of \(X\) , we make a decision about whether to believe that the null hypothesis is correct, or to reject the null hypothesis in favour of the alternative. The name for this thing that we calculate to guide our choices is a test statistic .
Having chosen a test statistic, the next step is to state precisely which values of the test statistic would cause is to reject the null hypothesis, and which values would cause us to keep it. In order to do so, we need to determine what the sampling distribution of the test statistic would be if the null hypothesis were actually true (we talked about sampling distributions earlier in Section 10.3.1 ). Why do we need this? Because this distribution tells us exactly what values of \(X\) our null hypothesis would lead us to expect. And therefore, we can use this distribution as a tool for assessing how closely the null hypothesis agrees with our data.
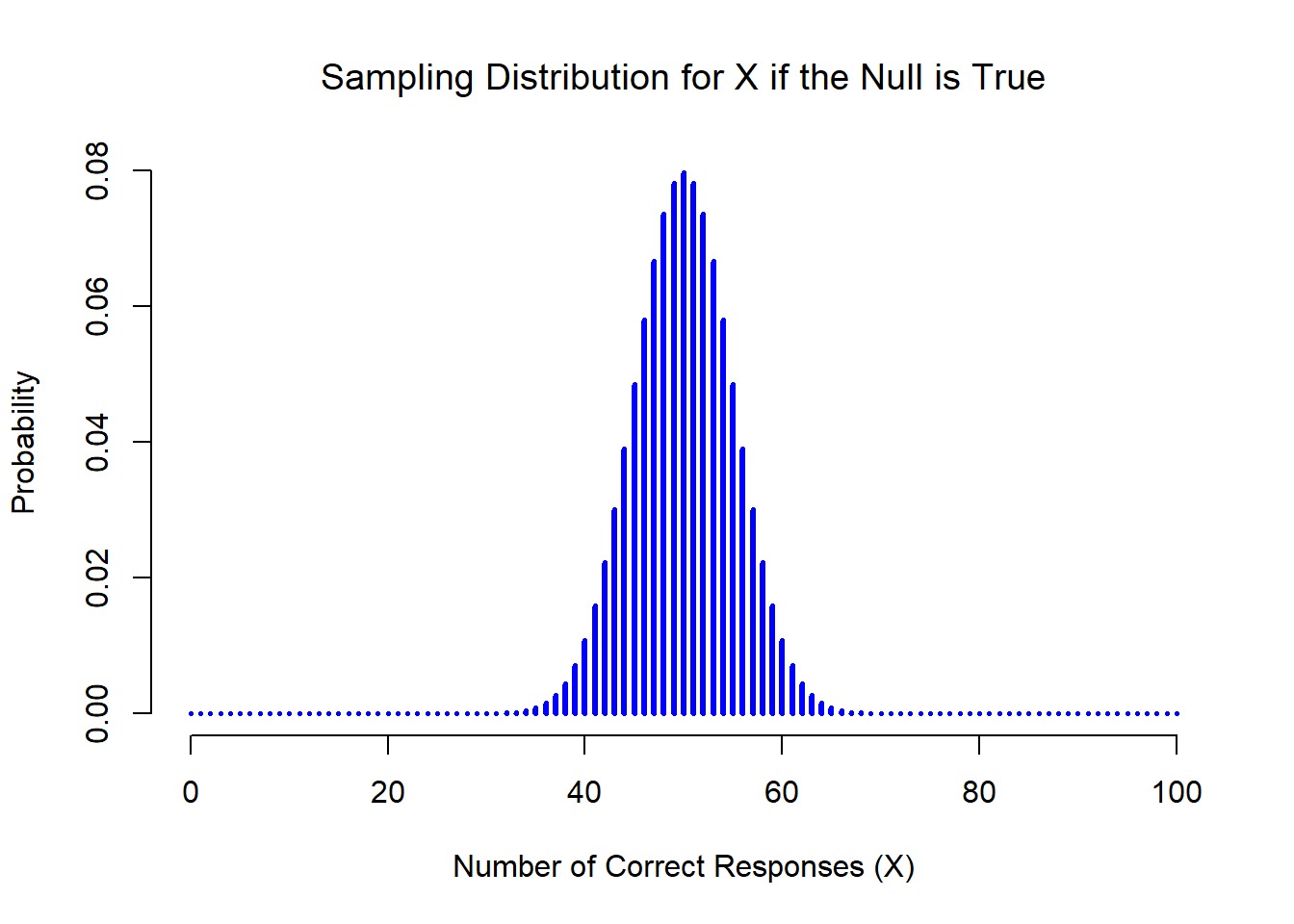
Figure 11.1: The sampling distribution for our test statistic \(X\) when the null hypothesis is true. For our ESP scenario, this is a binomial distribution. Not surprisingly, since the null hypothesis says that the probability of a correct response is \(\theta = .5\) , the sampling distribution says that the most likely value is 50 (our of 100) correct responses. Most of the probability mass lies between 40 and 60.
How do we actually determine the sampling distribution of the test statistic? For a lot of hypothesis tests this step is actually quite complicated, and later on in the book you’ll see me being slightly evasive about it for some of the tests (some of them I don’t even understand myself). However, sometimes it’s very easy. And, fortunately for us, our ESP example provides us with one of the easiest cases. Our population parameter \(\theta\) is just the overall probability that people respond correctly when asked the question, and our test statistic \(X\) is the count of the number of people who did so, out of a sample size of \(N\) . We’ve seen a distribution like this before, in Section 9.4 : that’s exactly what the binomial distribution describes! So, to use the notation and terminology that I introduced in that section, we would say that the null hypothesis predicts that \(X\) is binomially distributed, which is written \[ X \sim \mbox{Binomial}(\theta,N) \] Since the null hypothesis states that \(\theta = 0.5\) and our experiment has \(N=100\) people, we have the sampling distribution we need. This sampling distribution is plotted in Figure 11.1 . No surprises really: the null hypothesis says that \(X=50\) is the most likely outcome, and it says that we’re almost certain to see somewhere between 40 and 60 correct responses.
11.4 Making decisions
Okay, we’re very close to being finished. We’ve constructed a test statistic ( \(X\) ), and we chose this test statistic in such a way that we’re pretty confident that if \(X\) is close to \(N/2\) then we should retain the null, and if not we should reject it. The question that remains is this: exactly which values of the test statistic should we associate with the null hypothesis, and which exactly values go with the alternative hypothesis? In my ESP study, for example, I’ve observed a value of \(X=62\) . What decision should I make? Should I choose to believe the null hypothesis, or the alternative hypothesis?
11.4.1 Critical regions and critical values
To answer this question, we need to introduce the concept of a critical region for the test statistic \(X\) . The critical region of the test corresponds to those values of \(X\) that would lead us to reject null hypothesis (which is why the critical region is also sometimes called the rejection region). How do we find this critical region? Well, let’s consider what we know:
- \(X\) should be very big or very small in order to reject the null hypothesis.
- If the null hypothesis is true, the sampling distribution of \(X\) is Binomial \((0.5, N)\) .
- If \(\alpha =.05\) , the critical region must cover 5% of this sampling distribution.
It’s important to make sure you understand this last point: the critical region corresponds to those values of \(X\) for which we would reject the null hypothesis, and the sampling distribution in question describes the probability that we would obtain a particular value of \(X\) if the null hypothesis were actually true. Now, let’s suppose that we chose a critical region that covers 20% of the sampling distribution, and suppose that the null hypothesis is actually true. What would be the probability of incorrectly rejecting the null? The answer is of course 20%. And therefore, we would have built a test that had an \(\alpha\) level of \(0.2\) . If we want \(\alpha = .05\) , the critical region is only allowed to cover 5% of the sampling distribution of our test statistic.
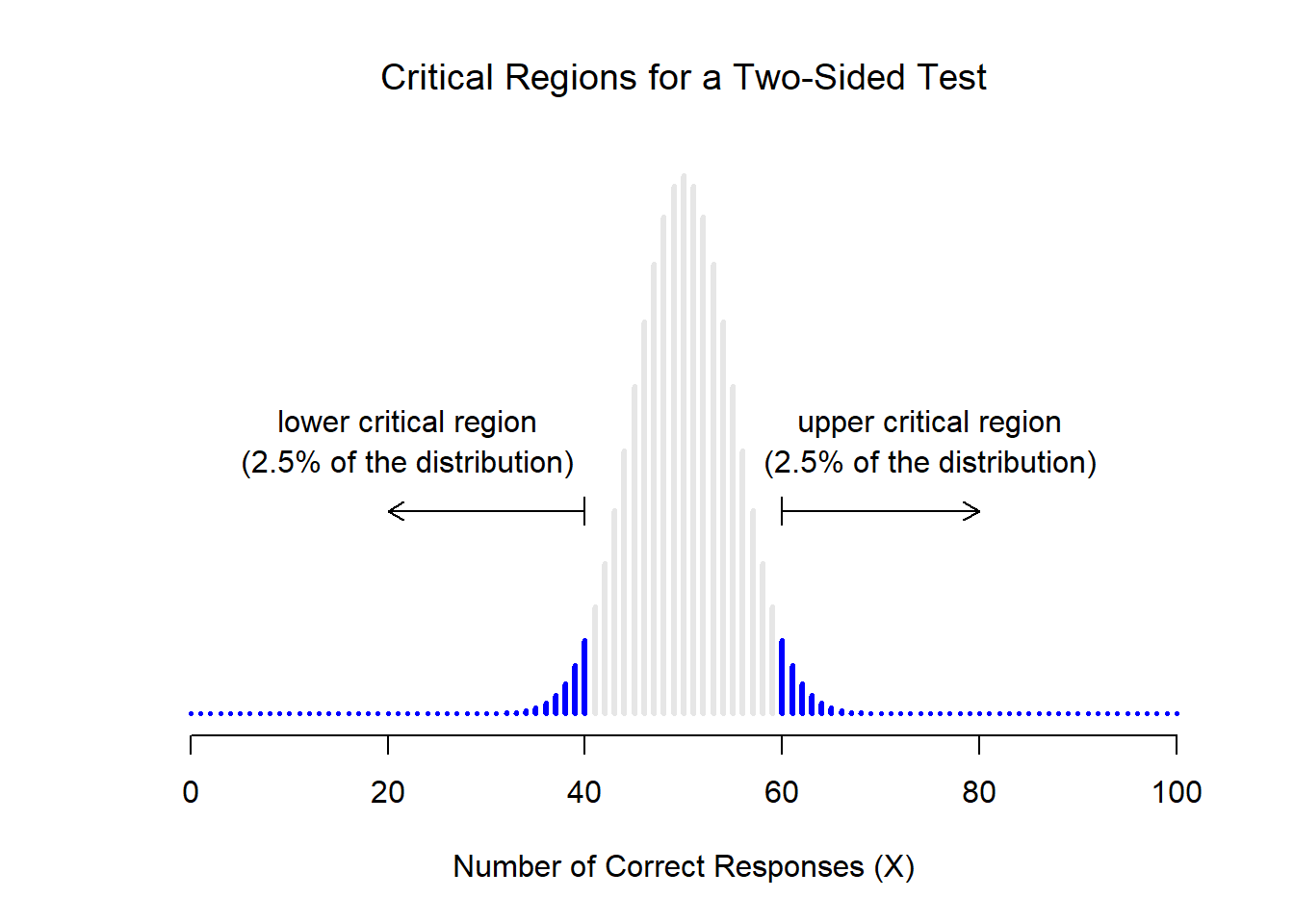
Figure 11.2: The critical region associated with the hypothesis test for the ESP study, for a hypothesis test with a significance level of \(\alpha = .05\) . The plot itself shows the sampling distribution of \(X\) under the null hypothesis: the grey bars correspond to those values of \(X\) for which we would retain the null hypothesis. The black bars show the critical region: those values of \(X\) for which we would reject the null. Because the alternative hypothesis is two sided (i.e., allows both \(\theta <.5\) and \(\theta >.5\) ), the critical region covers both tails of the distribution. To ensure an \(\alpha\) level of \(.05\) , we need to ensure that each of the two regions encompasses 2.5% of the sampling distribution.
As it turns out, those three things uniquely solve the problem: our critical region consists of the most extreme values , known as the tails of the distribution. This is illustrated in Figure 11.2 . As it turns out, if we want \(\alpha = .05\) , then our critical regions correspond to \(X \leq 40\) and \(X \geq 60\) . 162 That is, if the number of people saying “true” is between 41 and 59, then we should retain the null hypothesis. If the number is between 0 to 40 or between 60 to 100, then we should reject the null hypothesis. The numbers 40 and 60 are often referred to as the critical values , since they define the edges of the critical region.
At this point, our hypothesis test is essentially complete: (1) we choose an \(\alpha\) level (e.g., \(\alpha = .05\) , (2) come up with some test statistic (e.g., \(X\) ) that does a good job (in some meaningful sense) of comparing \(H_0\) to \(H_1\) , (3) figure out the sampling distribution of the test statistic on the assumption that the null hypothesis is true (in this case, binomial) and then (4) calculate the critical region that produces an appropriate \(\alpha\) level (0-40 and 60-100). All that we have to do now is calculate the value of the test statistic for the real data (e.g., \(X = 62\) ) and then compare it to the critical values to make our decision. Since 62 is greater than the critical value of 60, we would reject the null hypothesis. Or, to phrase it slightly differently, we say that the test has produced a significant result.
11.4.2 A note on statistical “significance”
Like other occult techniques of divination, the statistical method has a private jargon deliberately contrived to obscure its methods from non-practitioners. – Attributed to G. O. Ashley 163
A very brief digression is in order at this point, regarding the word “significant”. The concept of statistical significance is actually a very simple one, but has a very unfortunate name. If the data allow us to reject the null hypothesis, we say that “the result is statistically significant ”, which is often shortened to “the result is significant”. This terminology is rather old, and dates back to a time when “significant” just meant something like “indicated”, rather than its modern meaning, which is much closer to “important”. As a result, a lot of modern readers get very confused when they start learning statistics, because they think that a “significant result” must be an important one. It doesn’t mean that at all. All that “statistically significant” means is that the data allowed us to reject a null hypothesis. Whether or not the result is actually important in the real world is a very different question, and depends on all sorts of other things.
11.4.3 The difference between one sided and two sided tests
There’s one more thing I want to point out about the hypothesis test that I’ve just constructed. If we take a moment to think about the statistical hypotheses I’ve been using, \[ \begin{array}{cc} H_0 : & \theta = .5 \\ H_1 : & \theta \neq .5 \end{array} \] we notice that the alternative hypothesis covers both the possibility that \(\theta < .5\) and the possibility that \(\theta > .5\) . This makes sense if I really think that ESP could produce better-than-chance performance or worse-than-chance performance (and there are some people who think that). In statistical language, this is an example of a two-sided test . It’s called this because the alternative hypothesis covers the area on both “sides” of the null hypothesis, and as a consequence the critical region of the test covers both tails of the sampling distribution (2.5% on either side if \(\alpha =.05\) ), as illustrated earlier in Figure 11.2 .
However, that’s not the only possibility. It might be the case, for example, that I’m only willing to believe in ESP if it produces better than chance performance. If so, then my alternative hypothesis would only covers the possibility that \(\theta > .5\) , and as a consequence the null hypothesis now becomes \(\theta \leq .5\) : \[ \begin{array}{cc} H_0 : & \theta \leq .5 \\ H_1 : & \theta > .5 \end{array} \] When this happens, we have what’s called a one-sided test , and when this happens the critical region only covers one tail of the sampling distribution. This is illustrated in Figure 11.3 .
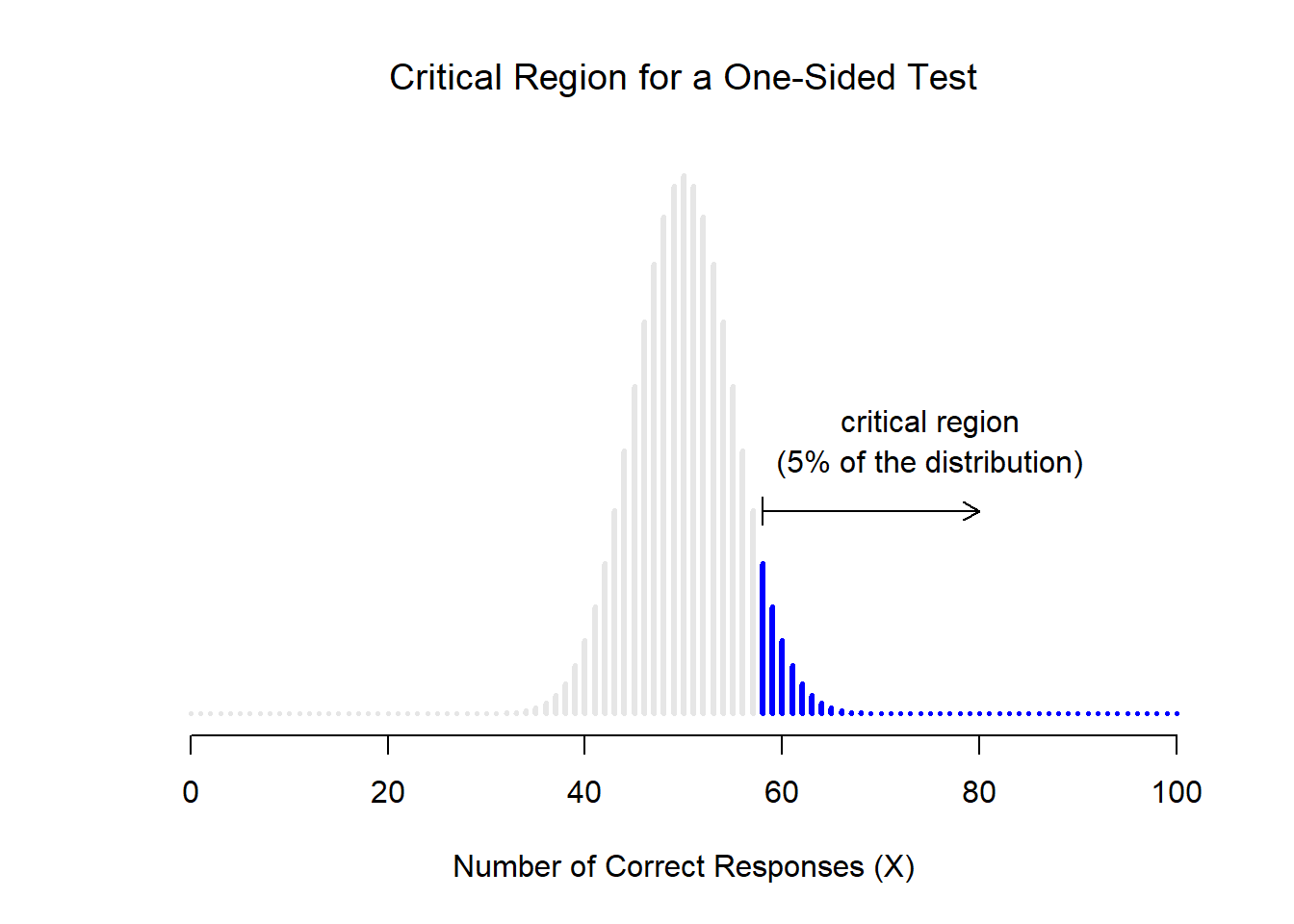
Figure 11.3: The critical region for a one sided test. In this case, the alternative hypothesis is that \(\theta > .05\) , so we would only reject the null hypothesis for large values of \(X\) . As a consequence, the critical region only covers the upper tail of the sampling distribution; specifically the upper 5% of the distribution. Contrast this to the two-sided version earlier)
11.5 The \(p\) value of a test
In one sense, our hypothesis test is complete; we’ve constructed a test statistic, figured out its sampling distribution if the null hypothesis is true, and then constructed the critical region for the test. Nevertheless, I’ve actually omitted the most important number of all: the \(p\) value . It is to this topic that we now turn. There are two somewhat different ways of interpreting a \(p\) value, one proposed by Sir Ronald Fisher and the other by Jerzy Neyman. Both versions are legitimate, though they reflect very different ways of thinking about hypothesis tests. Most introductory textbooks tend to give Fisher’s version only, but I think that’s a bit of a shame. To my mind, Neyman’s version is cleaner, and actually better reflects the logic of the null hypothesis test. You might disagree though, so I’ve included both. I’ll start with Neyman’s version…
11.5.1 A softer view of decision making
One problem with the hypothesis testing procedure that I’ve described is that it makes no distinction at all between a result this “barely significant” and those that are “highly significant”. For instance, in my ESP study the data I obtained only just fell inside the critical region – so I did get a significant effect, but was a pretty near thing. In contrast, suppose that I’d run a study in which \(X=97\) out of my \(N=100\) participants got the answer right. This would obviously be significant too, but my a much larger margin; there’s really no ambiguity about this at all. The procedure that I described makes no distinction between the two. If I adopt the standard convention of allowing \(\alpha = .05\) as my acceptable Type I error rate, then both of these are significant results.
This is where the \(p\) value comes in handy. To understand how it works, let’s suppose that we ran lots of hypothesis tests on the same data set: but with a different value of \(\alpha\) in each case. When we do that for my original ESP data, what we’d get is something like this
When we test ESP data ( \(X=62\) successes out of \(N=100\) observations) using \(\alpha\) levels of .03 and above, we’d always find ourselves rejecting the null hypothesis. For \(\alpha\) levels of .02 and below, we always end up retaining the null hypothesis. Therefore, somewhere between .02 and .03 there must be a smallest value of \(\alpha\) that would allow us to reject the null hypothesis for this data. This is the \(p\) value; as it turns out the ESP data has \(p = .021\) . In short:
\(p\) is defined to be the smallest Type I error rate ( \(\alpha\) ) that you have to be willing to tolerate if you want to reject the null hypothesis.
If it turns out that \(p\) describes an error rate that you find intolerable, then you must retain the null. If you’re comfortable with an error rate equal to \(p\) , then it’s okay to reject the null hypothesis in favour of your preferred alternative.
In effect, \(p\) is a summary of all the possible hypothesis tests that you could have run, taken across all possible \(\alpha\) values. And as a consequence it has the effect of “softening” our decision process. For those tests in which \(p \leq \alpha\) you would have rejected the null hypothesis, whereas for those tests in which \(p > \alpha\) you would have retained the null. In my ESP study I obtained \(X=62\) , and as a consequence I’ve ended up with \(p = .021\) . So the error rate I have to tolerate is 2.1%. In contrast, suppose my experiment had yielded \(X=97\) . What happens to my \(p\) value now? This time it’s shrunk to \(p = 1.36 \times 10^{-25}\) , which is a tiny, tiny 164 Type I error rate. For this second case I would be able to reject the null hypothesis with a lot more confidence, because I only have to be “willing” to tolerate a type I error rate of about 1 in 10 trillion trillion in order to justify my decision to reject.
11.5.2 The probability of extreme data
The second definition of the \(p\) -value comes from Sir Ronald Fisher, and it’s actually this one that you tend to see in most introductory statistics textbooks. Notice how, when I constructed the critical region, it corresponded to the tails (i.e., extreme values) of the sampling distribution? That’s not a coincidence: almost all “good” tests have this characteristic (good in the sense of minimising our type II error rate, \(\beta\) ). The reason for that is that a good critical region almost always corresponds to those values of the test statistic that are least likely to be observed if the null hypothesis is true. If this rule is true, then we can define the \(p\) -value as the probability that we would have observed a test statistic that is at least as extreme as the one we actually did get. In other words, if the data are extremely implausible according to the null hypothesis, then the null hypothesis is probably wrong.
11.5.3 A common mistake
Okay, so you can see that there are two rather different but legitimate ways to interpret the \(p\) value, one based on Neyman’s approach to hypothesis testing and the other based on Fisher’s. Unfortunately, there is a third explanation that people sometimes give, especially when they’re first learning statistics, and it is absolutely and completely wrong . This mistaken approach is to refer to the \(p\) value as “the probability that the null hypothesis is true”. It’s an intuitively appealing way to think, but it’s wrong in two key respects: (1) null hypothesis testing is a frequentist tool, and the frequentist approach to probability does not allow you to assign probabilities to the null hypothesis… according to this view of probability, the null hypothesis is either true or it is not; it cannot have a “5% chance” of being true. (2) even within the Bayesian approach, which does let you assign probabilities to hypotheses, the \(p\) value would not correspond to the probability that the null is true; this interpretation is entirely inconsistent with the mathematics of how the \(p\) value is calculated. Put bluntly, despite the intuitive appeal of thinking this way, there is no justification for interpreting a \(p\) value this way. Never do it.
11.6 Reporting the results of a hypothesis test
When writing up the results of a hypothesis test, there’s usually several pieces of information that you need to report, but it varies a fair bit from test to test. Throughout the rest of the book I’ll spend a little time talking about how to report the results of different tests (see Section 12.1.9 for a particularly detailed example), so that you can get a feel for how it’s usually done. However, regardless of what test you’re doing, the one thing that you always have to do is say something about the \(p\) value, and whether or not the outcome was significant.
The fact that you have to do this is unsurprising; it’s the whole point of doing the test. What might be surprising is the fact that there is some contention over exactly how you’re supposed to do it. Leaving aside those people who completely disagree with the entire framework underpinning null hypothesis testing, there’s a certain amount of tension that exists regarding whether or not to report the exact \(p\) value that you obtained, or if you should state only that \(p < \alpha\) for a significance level that you chose in advance (e.g., \(p<.05\) ).
11.6.1 The issue
To see why this is an issue, the key thing to recognise is that \(p\) values are terribly convenient. In practice, the fact that we can compute a \(p\) value means that we don’t actually have to specify any \(\alpha\) level at all in order to run the test. Instead, what you can do is calculate your \(p\) value and interpret it directly: if you get \(p = .062\) , then it means that you’d have to be willing to tolerate a Type I error rate of 6.2% to justify rejecting the null. If you personally find 6.2% intolerable, then you retain the null. Therefore, the argument goes, why don’t we just report the actual \(p\) value and let the reader make up their own minds about what an acceptable Type I error rate is? This approach has the big advantage of “softening” the decision making process – in fact, if you accept the Neyman definition of the \(p\) value, that’s the whole point of the \(p\) value. We no longer have a fixed significance level of \(\alpha = .05\) as a bright line separating “accept” from “reject” decisions; and this removes the rather pathological problem of being forced to treat \(p = .051\) in a fundamentally different way to \(p = .049\) .
This flexibility is both the advantage and the disadvantage to the \(p\) value. The reason why a lot of people don’t like the idea of reporting an exact \(p\) value is that it gives the researcher a bit too much freedom. In particular, it lets you change your mind about what error tolerance you’re willing to put up with after you look at the data. For instance, consider my ESP experiment. Suppose I ran my test, and ended up with a \(p\) value of .09. Should I accept or reject? Now, to be honest, I haven’t yet bothered to think about what level of Type I error I’m “really” willing to accept. I don’t have an opinion on that topic. But I do have an opinion about whether or not ESP exists, and I definitely have an opinion about whether my research should be published in a reputable scientific journal. And amazingly, now that I’ve looked at the data I’m starting to think that a 9% error rate isn’t so bad, especially when compared to how annoying it would be to have to admit to the world that my experiment has failed. So, to avoid looking like I just made it up after the fact, I now say that my \(\alpha\) is .1: a 10% type I error rate isn’t too bad, and at that level my test is significant! I win.
In other words, the worry here is that I might have the best of intentions, and be the most honest of people, but the temptation to just “shade” things a little bit here and there is really, really strong. As anyone who has ever run an experiment can attest, it’s a long and difficult process, and you often get very attached to your hypotheses. It’s hard to let go and admit the experiment didn’t find what you wanted it to find. And that’s the danger here. If we use the “raw” \(p\) -value, people will start interpreting the data in terms of what they want to believe, not what the data are actually saying… and if we allow that, well, why are we bothering to do science at all? Why not let everyone believe whatever they like about anything, regardless of what the facts are? Okay, that’s a bit extreme, but that’s where the worry comes from. According to this view, you really must specify your \(\alpha\) value in advance, and then only report whether the test was significant or not. It’s the only way to keep ourselves honest.
11.6.2 Two proposed solutions
In practice, it’s pretty rare for a researcher to specify a single \(\alpha\) level ahead of time. Instead, the convention is that scientists rely on three standard significance levels: .05, .01 and .001. When reporting your results, you indicate which (if any) of these significance levels allow you to reject the null hypothesis. This is summarised in Table 11.1 . This allows us to soften the decision rule a little bit, since \(p<.01\) implies that the data meet a stronger evidentiary standard than \(p<.05\) would. Nevertheless, since these levels are fixed in advance by convention, it does prevent people choosing their \(\alpha\) level after looking at the data.
Nevertheless, quite a lot of people still prefer to report exact \(p\) values. To many people, the advantage of allowing the reader to make up their own mind about how to interpret \(p = .06\) outweighs any disadvantages. In practice, however, even among those researchers who prefer exact \(p\) values it is quite common to just write \(p<.001\) instead of reporting an exact value for small \(p\) . This is in part because a lot of software doesn’t actually print out the \(p\) value when it’s that small (e.g., SPSS just writes \(p = .000\) whenever \(p<.001\) ), and in part because a very small \(p\) value can be kind of misleading. The human mind sees a number like .0000000001 and it’s hard to suppress the gut feeling that the evidence in favour of the alternative hypothesis is a near certainty. In practice however, this is usually wrong. Life is a big, messy, complicated thing: and every statistical test ever invented relies on simplifications, approximations and assumptions. As a consequence, it’s probably not reasonable to walk away from any statistical analysis with a feeling of confidence stronger than \(p<.001\) implies. In other words, \(p<.001\) is really code for “as far as this test is concerned, the evidence is overwhelming.”
In light of all this, you might be wondering exactly what you should do. There’s a fair bit of contradictory advice on the topic, with some people arguing that you should report the exact \(p\) value, and other people arguing that you should use the tiered approach illustrated in Table 11.1 . As a result, the best advice I can give is to suggest that you look at papers/reports written in your field and see what the convention seems to be. If there doesn’t seem to be any consistent pattern, then use whichever method you prefer.
11.7 Running the hypothesis test in practice
At this point some of you might be wondering if this is a “real” hypothesis test, or just a toy example that I made up. It’s real. In the previous discussion I built the test from first principles, thinking that it was the simplest possible problem that you might ever encounter in real life. However, this test already exists: it’s called the binomial test , and it’s implemented by an R function called binom.test() . To test the null hypothesis that the response probability is one-half p = .5 , 165 using data in which x = 62 of n = 100 people made the correct response, here’s how to do it in R:
Right now, this output looks pretty unfamiliar to you, but you can see that it’s telling you more or less the right things. Specifically, the \(p\) -value of 0.02 is less than the usual choice of \(\alpha = .05\) , so you can reject the null. We’ll talk a lot more about how to read this sort of output as we go along; and after a while you’ll hopefully find it quite easy to read and understand. For now, however, I just wanted to make the point that R contains a whole lot of functions corresponding to different kinds of hypothesis test. And while I’ll usually spend quite a lot of time explaining the logic behind how the tests are built, every time I discuss a hypothesis test the discussion will end with me showing you a fairly simple R command that you can use to run the test in practice.
11.8 Effect size, sample size and power
In previous sections I’ve emphasised the fact that the major design principle behind statistical hypothesis testing is that we try to control our Type I error rate. When we fix \(\alpha = .05\) we are attempting to ensure that only 5% of true null hypotheses are incorrectly rejected. However, this doesn’t mean that we don’t care about Type II errors. In fact, from the researcher’s perspective, the error of failing to reject the null when it is actually false is an extremely annoying one. With that in mind, a secondary goal of hypothesis testing is to try to minimise \(\beta\) , the Type II error rate, although we don’t usually talk in terms of minimising Type II errors. Instead, we talk about maximising the power of the test. Since power is defined as \(1-\beta\) , this is the same thing.
11.8.1 The power function
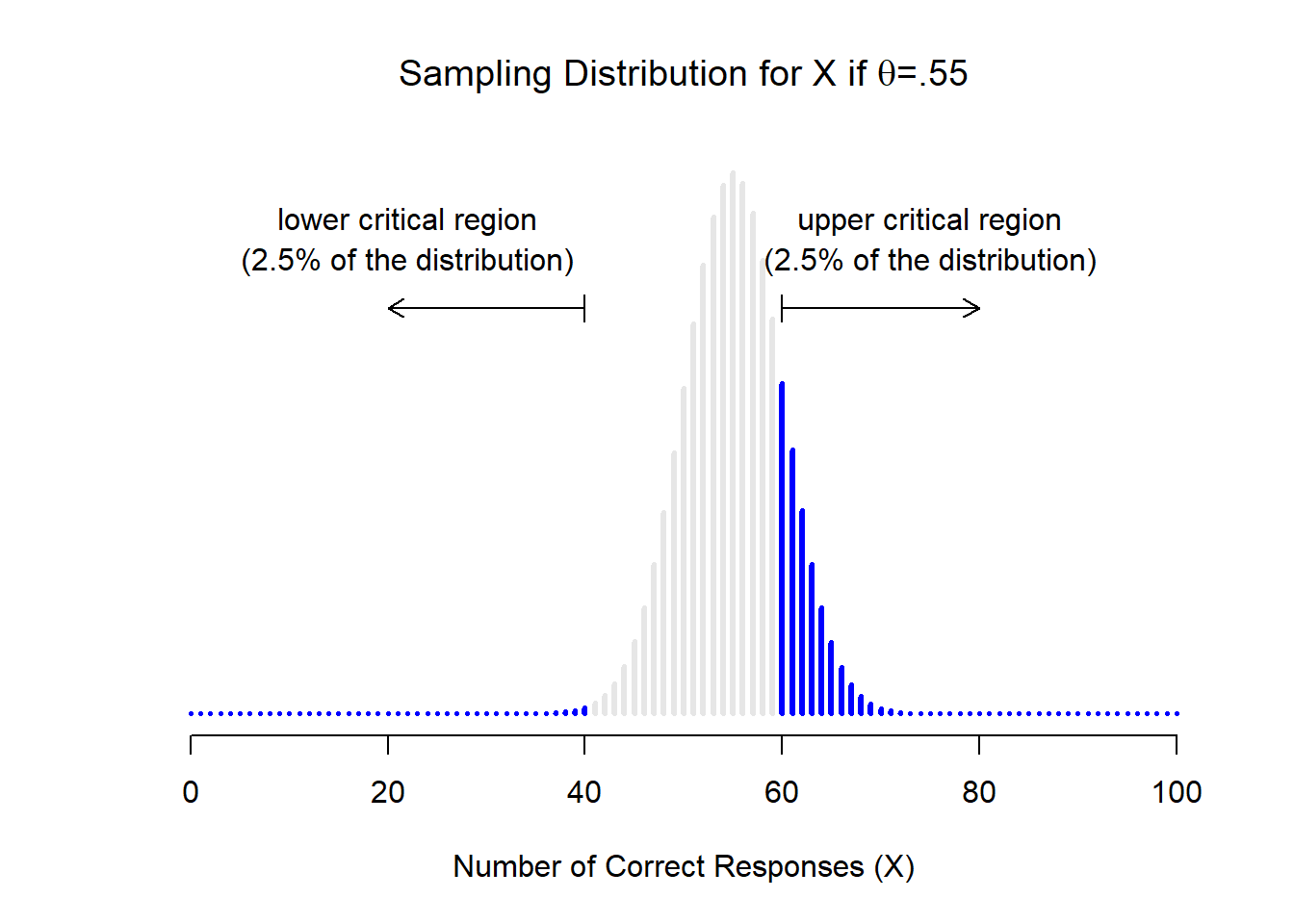
Figure 11.4: Sampling distribution under the alternative hypothesis, for a population parameter value of \(\theta = 0.55\) . A reasonable proportion of the distribution lies in the rejection region.
Let’s take a moment to think about what a Type II error actually is. A Type II error occurs when the alternative hypothesis is true, but we are nevertheless unable to reject the null hypothesis. Ideally, we’d be able to calculate a single number \(\beta\) that tells us the Type II error rate, in the same way that we can set \(\alpha = .05\) for the Type I error rate. Unfortunately, this is a lot trickier to do. To see this, notice that in my ESP study the alternative hypothesis actually corresponds to lots of possible values of \(\theta\) . In fact, the alternative hypothesis corresponds to every value of \(\theta\) except 0.5. Let’s suppose that the true probability of someone choosing the correct response is 55% (i.e., \(\theta = .55\) ). If so, then the true sampling distribution for \(X\) is not the same one that the null hypothesis predicts: the most likely value for \(X\) is now 55 out of 100. Not only that, the whole sampling distribution has now shifted, as shown in Figure 11.4 . The critical regions, of course, do not change: by definition, the critical regions are based on what the null hypothesis predicts. What we’re seeing in this figure is the fact that when the null hypothesis is wrong, a much larger proportion of the sampling distribution distribution falls in the critical region. And of course that’s what should happen: the probability of rejecting the null hypothesis is larger when the null hypothesis is actually false! However \(\theta = .55\) is not the only possibility consistent with the alternative hypothesis. Let’s instead suppose that the true value of \(\theta\) is actually 0.7. What happens to the sampling distribution when this occurs? The answer, shown in Figure 11.5 , is that almost the entirety of the sampling distribution has now moved into the critical region. Therefore, if \(\theta = 0.7\) the probability of us correctly rejecting the null hypothesis (i.e., the power of the test) is much larger than if \(\theta = 0.55\) . In short, while \(\theta = .55\) and \(\theta = .70\) are both part of the alternative hypothesis, the Type II error rate is different.
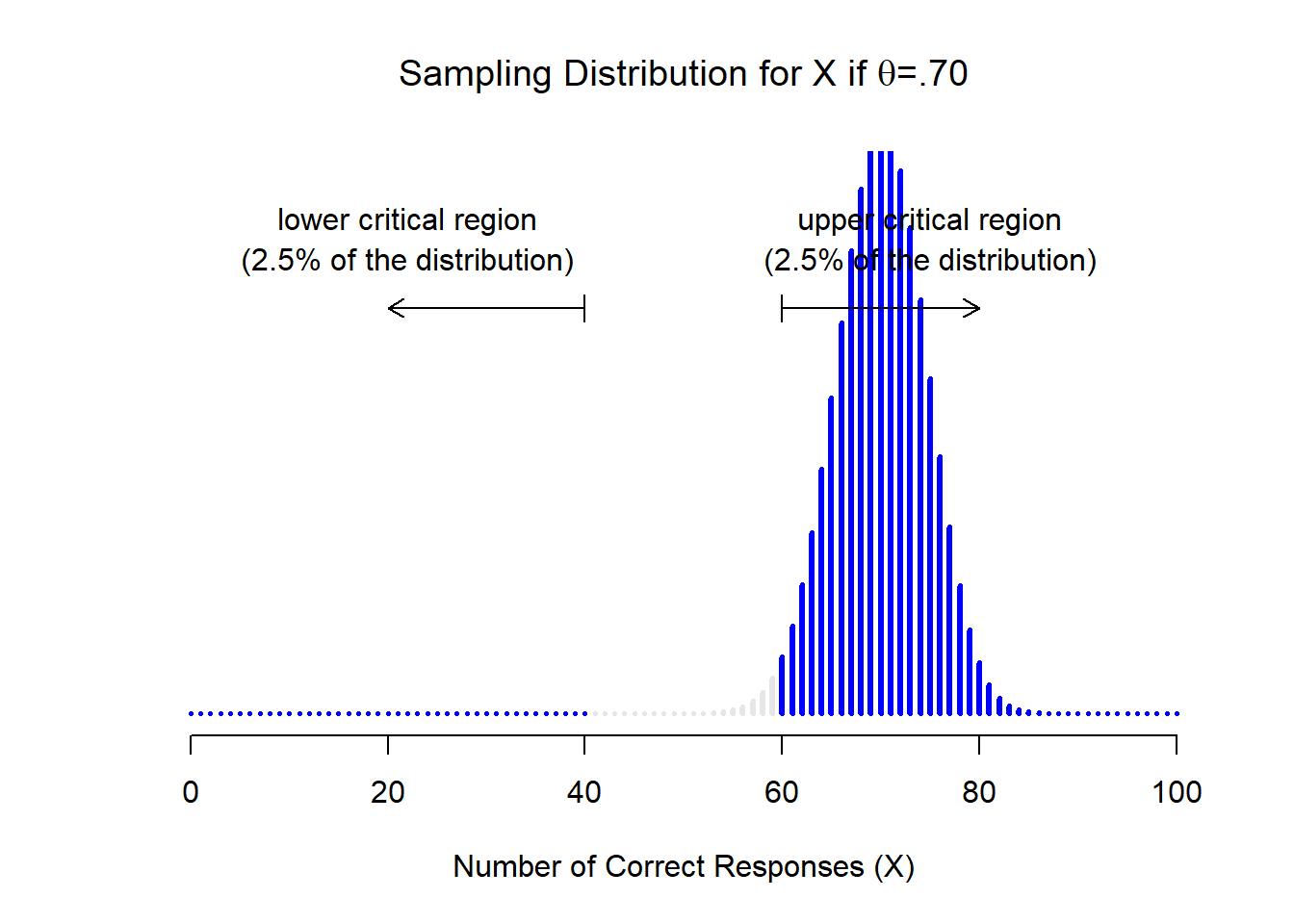
Figure 11.5: Sampling distribution under the alternative hypothesis, for a population parameter value of \(\theta = 0.70\) . Almost all of the distribution lies in the rejection region.
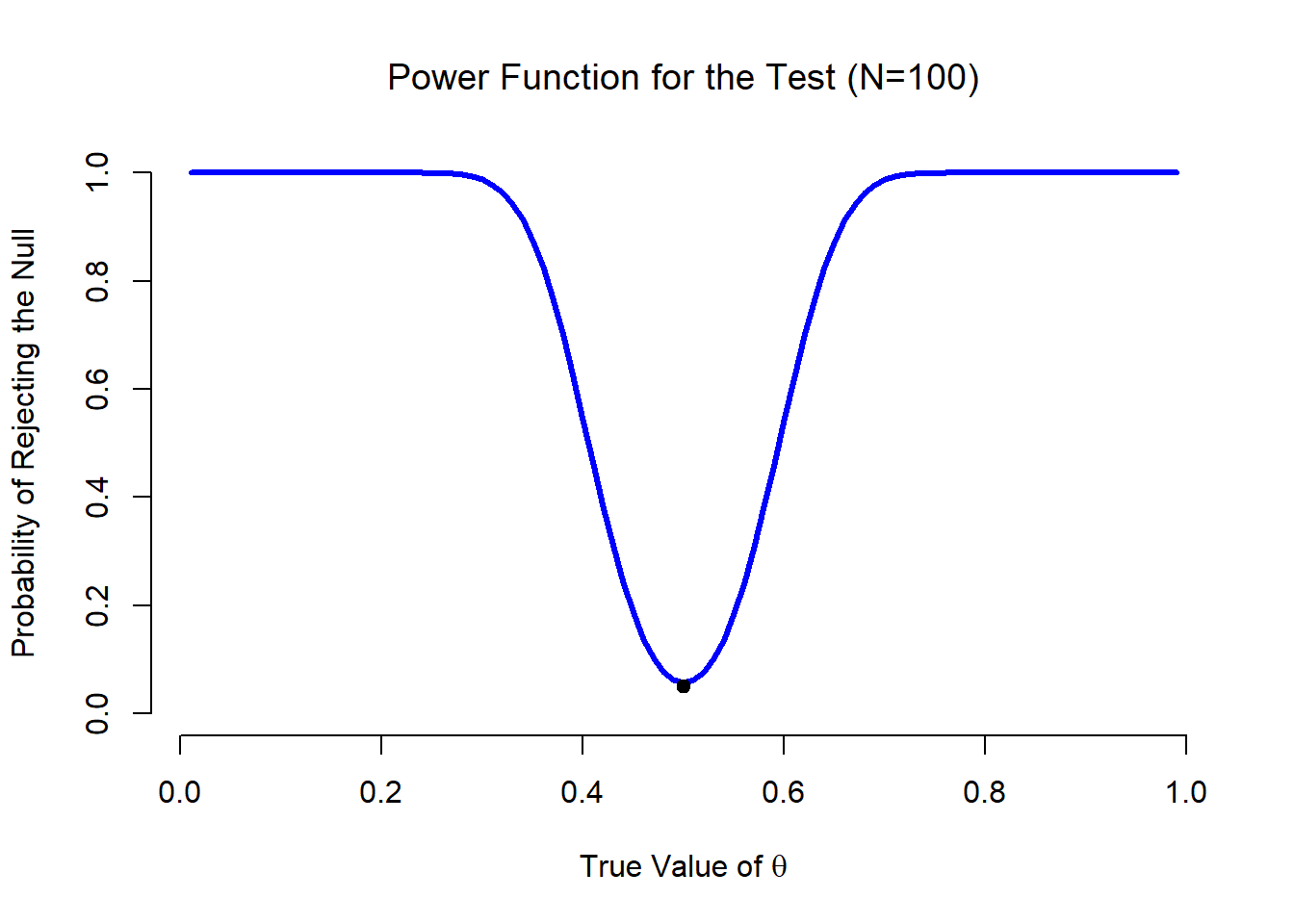
Figure 11.6: The probability that we will reject the null hypothesis, plotted as a function of the true value of \(\theta\) . Obviously, the test is more powerful (greater chance of correct rejection) if the true value of \(\theta\) is very different from the value that the null hypothesis specifies (i.e., \(\theta=.5\) ). Notice that when \(\theta\) actually is equal to .5 (plotted as a black dot), the null hypothesis is in fact true: rejecting the null hypothesis in this instance would be a Type I error.
What all this means is that the power of a test (i.e., \(1-\beta\) ) depends on the true value of \(\theta\) . To illustrate this, I’ve calculated the expected probability of rejecting the null hypothesis for all values of \(\theta\) , and plotted it in Figure 11.6 . This plot describes what is usually called the power function of the test. It’s a nice summary of how good the test is, because it actually tells you the power ( \(1-\beta\) ) for all possible values of \(\theta\) . As you can see, when the true value of \(\theta\) is very close to 0.5, the power of the test drops very sharply, but when it is further away, the power is large.
11.8.2 Effect size
Since all models are wrong the scientist must be alert to what is importantly wrong. It is inappropriate to be concerned with mice when there are tigers abroad – George Box 1976
The plot shown in Figure 11.6 captures a fairly basic point about hypothesis testing. If the true state of the world is very different from what the null hypothesis predicts, then your power will be very high; but if the true state of the world is similar to the null (but not identical) then the power of the test is going to be very low. Therefore, it’s useful to be able to have some way of quantifying how “similar” the true state of the world is to the null hypothesis. A statistic that does this is called a measure of effect size (e.g. Cohen 1988 ; Ellis 2010 ) . Effect size is defined slightly differently in different contexts, 166 (and so this section just talks in general terms) but the qualitative idea that it tries to capture is always the same: how big is the difference between the true population parameters, and the parameter values that are assumed by the null hypothesis? In our ESP example, if we let \(\theta_0 = 0.5\) denote the value assumed by the null hypothesis, and let \(\theta\) denote the true value, then a simple measure of effect size could be something like the difference between the true value and null (i.e., \(\theta – \theta_0\) ), or possibly just the magnitude of this difference, \(\mbox{abs}(\theta – \theta_0)\) .
Why calculate effect size? Let’s assume that you’ve run your experiment, collected the data, and gotten a significant effect when you ran your hypothesis test. Isn’t it enough just to say that you’ve gotten a significant effect? Surely that’s the point of hypothesis testing? Well, sort of. Yes, the point of doing a hypothesis test is to try to demonstrate that the null hypothesis is wrong, but that’s hardly the only thing we’re interested in. If the null hypothesis claimed that \(\theta = .5\) , and we show that it’s wrong, we’ve only really told half of the story. Rejecting the null hypothesis implies that we believe that \(\theta \neq .5\) , but there’s a big difference between \(\theta = .51\) and \(\theta = .8\) . If we find that \(\theta = .8\) , then not only have we found that the null hypothesis is wrong, it appears to be very wrong. On the other hand, suppose we’ve successfully rejected the null hypothesis, but it looks like the true value of \(\theta\) is only .51 (this would only be possible with a large study). Sure, the null hypothesis is wrong, but it’s not at all clear that we actually care , because the effect size is so small. In the context of my ESP study we might still care, since any demonstration of real psychic powers would actually be pretty cool 167 , but in other contexts a 1% difference isn’t very interesting, even if it is a real difference. For instance, suppose we’re looking at differences in high school exam scores between males and females, and it turns out that the female scores are 1% higher on average than the males. If I’ve got data from thousands of students, then this difference will almost certainly be statistically significant , but regardless of how small the \(p\) value is it’s just not very interesting. You’d hardly want to go around proclaiming a crisis in boys education on the basis of such a tiny difference would you? It’s for this reason that it is becoming more standard (slowly, but surely) to report some kind of standard measure of effect size along with the the results of the hypothesis test. The hypothesis test itself tells you whether you should believe that the effect you have observed is real (i.e., not just due to chance); the effect size tells you whether or not you should care.
11.8.3 Increasing the power of your study
Not surprisingly, scientists are fairly obsessed with maximising the power of their experiments. We want our experiments to work, and so we want to maximise the chance of rejecting the null hypothesis if it is false (and of course we usually want to believe that it is false!) As we’ve seen, one factor that influences power is the effect size. So the first thing you can do to increase your power is to increase the effect size. In practice, what this means is that you want to design your study in such a way that the effect size gets magnified. For instance, in my ESP study I might believe that psychic powers work best in a quiet, darkened room; with fewer distractions to cloud the mind. Therefore I would try to conduct my experiments in just such an environment: if I can strengthen people’s ESP abilities somehow, then the true value of \(\theta\) will go up 168 and therefore my effect size will be larger. In short, clever experimental design is one way to boost power; because it can alter the effect size.
Unfortunately, it’s often the case that even with the best of experimental designs you may have only a small effect. Perhaps, for example, ESP really does exist, but even under the best of conditions it’s very very weak. Under those circumstances, your best bet for increasing power is to increase the sample size. In general, the more observations that you have available, the more likely it is that you can discriminate between two hypotheses. If I ran my ESP experiment with 10 participants, and 7 of them correctly guessed the colour of the hidden card, you wouldn’t be terribly impressed. But if I ran it with 10,000 participants and 7,000 of them got the answer right, you would be much more likely to think I had discovered something. In other words, power increases with the sample size. This is illustrated in Figure 11.7 , which shows the power of the test for a true parameter of \(\theta = 0.7\) , for all sample sizes \(N\) from 1 to 100, where I’m assuming that the null hypothesis predicts that \(\theta_0 = 0.5\) .
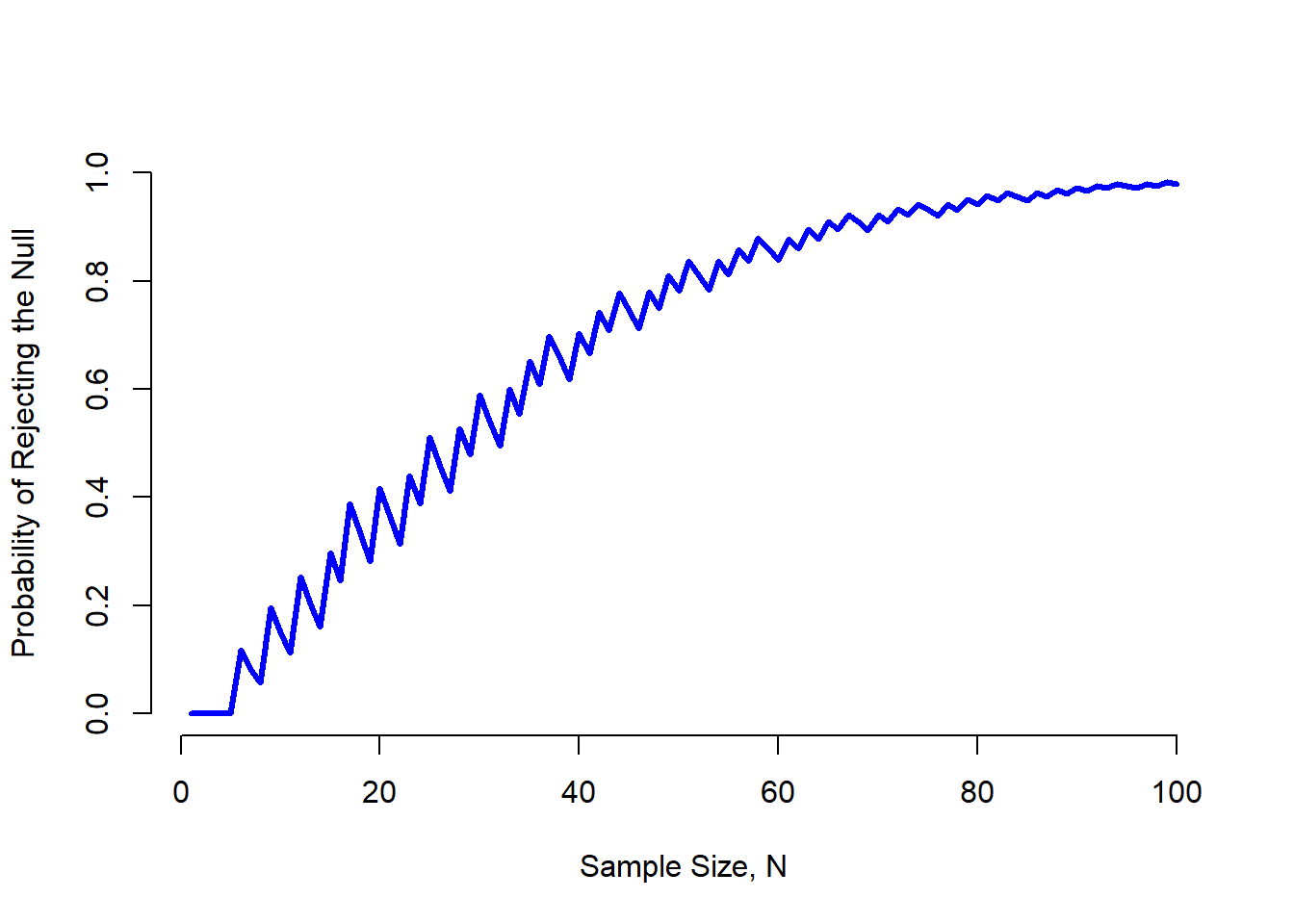
Figure 11.7: The power of our test, plotted as a function of the sample size \(N\) . In this case, the true value of \(\theta\) is 0.7, but the null hypothesis is that \(\theta = 0.5\) . Overall, larger \(N\) means greater power. (The small zig-zags in this function occur because of some odd interactions between \(\theta\) , \(\alpha\) and the fact that the binomial distribution is discrete; it doesn’t matter for any serious purpose)
Because power is important, whenever you’re contemplating running an experiment it would be pretty useful to know how much power you’re likely to have. It’s never possible to know for sure, since you can’t possibly know what your effect size is. However, it’s often (well, sometimes) possible to guess how big it should be. If so, you can guess what sample size you need! This idea is called power analysis , and if it’s feasible to do it, then it’s very helpful, since it can tell you something about whether you have enough time or money to be able to run the experiment successfully. It’s increasingly common to see people arguing that power analysis should be a required part of experimental design, so it’s worth knowing about. I don’t discuss power analysis in this book, however. This is partly for a boring reason and partly for a substantive one. The boring reason is that I haven’t had time to write about power analysis yet. The substantive one is that I’m still a little suspicious of power analysis. Speaking as a researcher, I have very rarely found myself in a position to be able to do one – it’s either the case that (a) my experiment is a bit non-standard and I don’t know how to define effect size properly, (b) I literally have so little idea about what the effect size will be that I wouldn’t know how to interpret the answers. Not only that, after extensive conversations with someone who does stats consulting for a living (my wife, as it happens), I can’t help but notice that in practice the only time anyone ever asks her for a power analysis is when she’s helping someone write a grant application. In other words, the only time any scientist ever seems to want a power analysis in real life is when they’re being forced to do it by bureaucratic process. It’s not part of anyone’s day to day work. In short, I’ve always been of the view that while power is an important concept, power analysis is not as useful as people make it sound, except in the rare cases where (a) someone has figured out how to calculate power for your actual experimental design and (b) you have a pretty good idea what the effect size is likely to be. Maybe other people have had better experiences than me, but I’ve personally never been in a situation where both (a) and (b) were true. Maybe I’ll be convinced otherwise in the future, and probably a future version of this book would include a more detailed discussion of power analysis, but for now this is about as much as I’m comfortable saying about the topic.
11.9 Some issues to consider
What I’ve described to you in this chapter is the orthodox framework for null hypothesis significance testing (NHST). Understanding how NHST works is an absolute necessity, since it has been the dominant approach to inferential statistics ever since it came to prominence in the early 20th century. It’s what the vast majority of working scientists rely on for their data analysis, so even if you hate it you need to know it. However, the approach is not without problems. There are a number of quirks in the framework, historical oddities in how it came to be, theoretical disputes over whether or not the framework is right, and a lot of practical traps for the unwary. I’m not going to go into a lot of detail on this topic, but I think it’s worth briefly discussing a few of these issues.
11.9.1 Neyman versus Fisher
The first thing you should be aware of is that orthodox NHST is actually a mash-up of two rather different approaches to hypothesis testing, one proposed by Sir Ronald Fisher and the other proposed by Jerzy Neyman (for a historical summary see Lehmann 2011 ) . The history is messy because Fisher and Neyman were real people whose opinions changed over time, and at no point did either of them offer “the definitive statement” of how we should interpret their work many decades later. That said, here’s a quick summary of what I take these two approaches to be.
First, let’s talk about Fisher’s approach. As far as I can tell, Fisher assumed that you only had the one hypothesis (the null), and what you want to do is find out if the null hypothesis is inconsistent with the data. From his perspective, what you should do is check to see if the data are “sufficiently unlikely” according to the null. In fact, if you remember back to our earlier discussion, that’s how Fisher defines the \(p\) -value. According to Fisher, if the null hypothesis provided a very poor account of the data, you could safely reject it. But, since you don’t have any other hypotheses to compare it to, there’s no way of “accepting the alternative” because you don’t necessarily have an explicitly stated alternative. That’s more or less all that there was to it.
In contrast, Neyman thought that the point of hypothesis testing was as a guide to action, and his approach was somewhat more formal than Fisher’s. His view was that there are multiple things that you could do (accept the null or accept the alternative) and the point of the test was to tell you which one the data support. From this perspective, it is critical to specify your alternative hypothesis properly. If you don’t know what the alternative hypothesis is, then you don’t know how powerful the test is, or even which action makes sense. His framework genuinely requires a competition between different hypotheses. For Neyman, the \(p\) value didn’t directly measure the probability of the data (or data more extreme) under the null, it was more of an abstract description about which “possible tests” were telling you to accept the null, and which “possible tests” were telling you to accept the alternative.
As you can see, what we have today is an odd mishmash of the two. We talk about having both a null hypothesis and an alternative (Neyman), but usually 169 define the \(p\) value in terms of exreme data (Fisher), but we still have \(\alpha\) values (Neyman). Some of the statistical tests have explicitly specified alternatives (Neyman) but others are quite vague about it (Fisher). And, according to some people at least, we’re not allowed to talk about accepting the alternative (Fisher). It’s a mess: but I hope this at least explains why it’s a mess.
11.9.2 Bayesians versus frequentists
Earlier on in this chapter I was quite emphatic about the fact that you cannot interpret the \(p\) value as the probability that the null hypothesis is true. NHST is fundamentally a frequentist tool (see Chapter 9 ) and as such it does not allow you to assign probabilities to hypotheses: the null hypothesis is either true or it is not. The Bayesian approach to statistics interprets probability as a degree of belief, so it’s totally okay to say that there is a 10% chance that the null hypothesis is true: that’s just a reflection of the degree of confidence that you have in this hypothesis. You aren’t allowed to do this within the frequentist approach. Remember, if you’re a frequentist, a probability can only be defined in terms of what happens after a large number of independent replications (i.e., a long run frequency). If this is your interpretation of probability, talking about the “probability” that the null hypothesis is true is complete gibberish: a null hypothesis is either true or it is false. There’s no way you can talk about a long run frequency for this statement. To talk about “the probability of the null hypothesis” is as meaningless as “the colour of freedom”. It doesn’t have one!
Most importantly, this isn’t a purely ideological matter. If you decide that you are a Bayesian and that you’re okay with making probability statements about hypotheses, you have to follow the Bayesian rules for calculating those probabilities. I’ll talk more about this in Chapter 17 , but for now what I want to point out to you is the \(p\) value is a terrible approximation to the probability that \(H_0\) is true. If what you want to know is the probability of the null, then the \(p\) value is not what you’re looking for!
11.9.3 Traps
As you can see, the theory behind hypothesis testing is a mess, and even now there are arguments in statistics about how it “should” work. However, disagreements among statisticians are not our real concern here. Our real concern is practical data analysis. And while the “orthodox” approach to null hypothesis significance testing has many drawbacks, even an unrepentant Bayesian like myself would agree that they can be useful if used responsibly. Most of the time they give sensible answers, and you can use them to learn interesting things. Setting aside the various ideologies and historical confusions that we’ve discussed, the fact remains that the biggest danger in all of statistics is thoughtlessness . I don’t mean stupidity, here: I literally mean thoughtlessness. The rush to interpret a result without spending time thinking through what each test actually says about the data, and checking whether that’s consistent with how you’ve interpreted it. That’s where the biggest trap lies.
To give an example of this, consider the following example (see Gelman and Stern 2006 ) . Suppose I’m running my ESP study, and I’ve decided to analyse the data separately for the male participants and the female participants. Of the male participants, 33 out of 50 guessed the colour of the card correctly. This is a significant effect ( \(p = .03\) ). Of the female participants, 29 out of 50 guessed correctly. This is not a significant effect ( \(p = .32\) ). Upon observing this, it is extremely tempting for people to start wondering why there is a difference between males and females in terms of their psychic abilities. However, this is wrong. If you think about it, we haven’t actually run a test that explicitly compares males to females. All we have done is compare males to chance (binomial test was significant) and compared females to chance (binomial test was non significant). If we want to argue that there is a real difference between the males and the females, we should probably run a test of the null hypothesis that there is no difference! We can do that using a different hypothesis test, 170 but when we do that it turns out that we have no evidence that males and females are significantly different ( \(p = .54\) ). Now do you think that there’s anything fundamentally different between the two groups? Of course not. What’s happened here is that the data from both groups (male and female) are pretty borderline: by pure chance, one of them happened to end up on the magic side of the \(p = .05\) line, and the other one didn’t. That doesn’t actually imply that males and females are different. This mistake is so common that you should always be wary of it: the difference between significant and not-significant is not evidence of a real difference – if you want to say that there’s a difference between two groups, then you have to test for that difference!
The example above is just that: an example. I’ve singled it out because it’s such a common one, but the bigger picture is that data analysis can be tricky to get right. Think about what it is you want to test, why you want to test it, and whether or not the answers that your test gives could possibly make any sense in the real world.
11.10 Summary
Null hypothesis testing is one of the most ubiquitous elements to statistical theory. The vast majority of scientific papers report the results of some hypothesis test or another. As a consequence it is almost impossible to get by in science without having at least a cursory understanding of what a \(p\) -value means, making this one of the most important chapters in the book. As usual, I’ll end the chapter with a quick recap of the key ideas that we’ve talked about:
- Research hypotheses and statistical hypotheses. Null and alternative hypotheses. (Section 11.1 ).
- Type 1 and Type 2 errors (Section 11.2 )
- Test statistics and sampling distributions (Section 11.3 )
- Hypothesis testing as a decision making process (Section 11.4 )
- \(p\) -values as “soft” decisions (Section 11.5 )
- Writing up the results of a hypothesis test (Section 11.6 )
- Effect size and power (Section 11.8 )
- A few issues to consider regarding hypothesis testing (Section 11.9 )
Later in the book, in Chapter 17 , I’ll revisit the theory of null hypothesis tests from a Bayesian perspective, and introduce a number of new tools that you can use if you aren’t particularly fond of the orthodox approach. But for now, though, we’re done with the abstract statistical theory, and we can start discussing specific data analysis tools.
Cohen, J. 1988. Statistical Power Analysis for the Behavioral Sciences . 2nd ed. Lawrence Erlbaum.
Ellis, P. D. 2010. The Essential Guide to Effect Sizes: Statistical Power, Meta-Analysis, and the Interpretation of Research Results . Cambridge, UK: Cambridge University Press.
Lehmann, Erich L. 2011. Fisher, Neyman, and the Creation of Classical Statistics . Springer.
Gelman, A., and H. Stern. 2006. “The Difference Between ‘Significant’ and ‘Not Significant’ Is Not Itself Statistically Significant.” The American Statistician 60: 328–31.
- The quote comes from Wittgenstein’s (1922) text, Tractatus Logico-Philosphicus . ↩
- A technical note. The description below differs subtly from the standard description given in a lot of introductory texts. The orthodox theory of null hypothesis testing emerged from the work of Sir Ronald Fisher and Jerzy Neyman in the early 20th century; but Fisher and Neyman actually had very different views about how it should work. The standard treatment of hypothesis testing that most texts use is a hybrid of the two approaches. The treatment here is a little more Neyman-style than the orthodox view, especially as regards the meaning of the \(p\) value. ↩
- My apologies to anyone who actually believes in this stuff, but on my reading of the literature on ESP, it’s just not reasonable to think this is real. To be fair, though, some of the studies are rigorously designed; so it’s actually an interesting area for thinking about psychological research design. And of course it’s a free country, so you can spend your own time and effort proving me wrong if you like, but I wouldn’t think that’s a terribly practical use of your intellect. ↩
- This analogy only works if you’re from an adversarial legal system like UK/US/Australia. As I understand these things, the French inquisitorial system is quite different. ↩
- An aside regarding the language you use to talk about hypothesis testing. Firstly, one thing you really want to avoid is the word “prove”: a statistical test really doesn’t prove that a hypothesis is true or false. Proof implies certainty, and as the saying goes, statistics means never having to say you’re certain. On that point almost everyone would agree. However, beyond that there’s a fair amount of confusion. Some people argue that you’re only allowed to make statements like “rejected the null”, “failed to reject the null”, or possibly “retained the null”. According to this line of thinking, you can’t say things like “accept the alternative” or “accept the null”. Personally I think this is too strong: in my opinion, this conflates null hypothesis testing with Karl Popper’s falsificationist view of the scientific process. While there are similarities between falsificationism and null hypothesis testing, they aren’t equivalent. However, while I personally think it’s fine to talk about accepting a hypothesis (on the proviso that “acceptance” doesn’t actually mean that it’s necessarily true, especially in the case of the null hypothesis), many people will disagree. And more to the point, you should be aware that this particular weirdness exists, so that you’re not caught unawares by it when writing up your own results. ↩
- Strictly speaking, the test I just constructed has \(\alpha = .057\) , which is a bit too generous. However, if I’d chosen 39 and 61 to be the boundaries for the critical region, then the critical region only covers 3.5% of the distribution. I figured that it makes more sense to use 40 and 60 as my critical values, and be willing to tolerate a 5.7% type I error rate, since that’s as close as I can get to a value of \(\alpha = .05\) . ↩
- The internet seems fairly convinced that Ashley said this, though I can’t for the life of me find anyone willing to give a source for the claim. ↩
- That’s \(p = .000000000000000000000000136\) for folks that don’t like scientific notation! ↩
- Note that the p here has nothing to do with a \(p\) value. The p argument in the binom.test() function corresponds to the probability of making a correct response, according to the null hypothesis. In other words, it’s the \(\theta\) value. ↩
- There’s an R package called compute.es that can be used for calculating a very broad range of effect size measures; but for the purposes of the current book we won’t need it: all of the effect size measures that I’ll talk about here have functions in the lsr package ↩
- Although in practice a very small effect size is worrying, because even very minor methodological flaws might be responsible for the effect; and in practice no experiment is perfect, so there are always methodological issues to worry about. ↩
- Notice that the true population parameter \(\theta\) doesn’t necessarily correspond to an immutable fact of nature. In this context \(\theta\) is just the true probability that people would correctly guess the colour of the card in the other room. As such the population parameter can be influenced by all sorts of things. Of course, this is all on the assumption that ESP actually exists! ↩
- Although this book describes both Neyman’s and Fisher’s definition of the \(p\) value, most don’t. Most introductory textbooks will only give you the Fisher version. ↩
- In this case, the Pearson chi-square test of independence (Chapter 12 ; chisq.test() in R) is what we use; see also the prop.test() function. ↩
Learning Statistics with R Copyright © by Danielle Navarro is licensed under a Creative Commons Attribution-ShareAlike 4.0 International License , except where otherwise noted.
Share This Book

Statistics Made Easy
The Complete Guide: Hypothesis Testing in R
A hypothesis test is a formal statistical test we use to reject or fail to reject some statistical hypothesis.
This tutorial explains how to perform the following hypothesis tests in R:
- One sample t-test
- Two sample t-test
- Paired samples t-test
We can use the t.test() function in R to perform each type of test:
- x, y: The two samples of data.
- alternative: The alternative hypothesis of the test.
- mu: The true value of the mean.
- paired: Whether to perform a paired t-test or not.
- var.equal: Whether to assume the variances are equal between the samples.
- conf.level: The confidence level to use.
The following examples show how to use this function in practice.
Example 1: One Sample t-test in R
A one sample t-test is used to test whether or not the mean of a population is equal to some value.
For example, suppose we want to know whether or not the mean weight of a certain species of some turtle is equal to 310 pounds. We go out and collect a simple random sample of turtles with the following weights:
Weights : 300, 315, 320, 311, 314, 309, 300, 308, 305, 303, 305, 301, 303
The following code shows how to perform this one sample t-test in R:
From the output we can see:
- t-test statistic: -1.5848
- degrees of freedom: 12
- p-value: 0.139
- 95% confidence interval for true mean: [303.4236, 311.0379]
- mean of turtle weights: 307.230
Since the p-value of the test (0.139) is not less than .05, we fail to reject the null hypothesis.
This means we do not have sufficient evidence to say that the mean weight of this species of turtle is different from 310 pounds.
Example 2: Two Sample t-test in R
A two sample t-test is used to test whether or not the means of two populations are equal.
For example, suppose we want to know whether or not the mean weight between two different species of turtles is equal. To test this, we collect a simple random sample of turtles from each species with the following weights:
Sample 1 : 300, 315, 320, 311, 314, 309, 300, 308, 305, 303, 305, 301, 303
Sample 2 : 335, 329, 322, 321, 324, 319, 304, 308, 305, 311, 307, 300, 305
The following code shows how to perform this two sample t-test in R:
- t-test statistic: -2.1009
- degrees of freedom: 19.112
- p-value: 0.04914
- 95% confidence interval for true mean difference: [-14.74, -0.03]
- mean of sample 1 weights: 307.2308
- mean of sample 2 weights: 314.6154
Since the p-value of the test (0.04914) is less than .05, we reject the null hypothesis.
This means we have sufficient evidence to say that the mean weight between the two species is not equal.
Example 3: Paired Samples t-test in R
A paired samples t-test is used to compare the means of two samples when each observation in one sample can be paired with an observation in the other sample.
For example, suppose we want to know whether or not a certain training program is able to increase the max vertical jump (in inches) of basketball players.
To test this, we may recruit a simple random sample of 12 college basketball players and measure each of their max vertical jumps. Then, we may have each player use the training program for one month and then measure their max vertical jump again at the end of the month.
The following data shows the max jump height (in inches) before and after using the training program for each player:
Before : 22, 24, 20, 19, 19, 20, 22, 25, 24, 23, 22, 21
After : 23, 25, 20, 24, 18, 22, 23, 28, 24, 25, 24, 20
The following code shows how to perform this paired samples t-test in R:
- t-test statistic: -2.5289
- degrees of freedom: 11
- p-value: 0.02803
- 95% confidence interval for true mean difference: [-2.34, -0.16]
- mean difference between before and after: -1.25
Since the p-value of the test (0.02803) is less than .05, we reject the null hypothesis.
This means we have sufficient evidence to say that the mean jump height before and after using the training program is not equal.
Additional Resources
Use the following online calculators to automatically perform various t-tests:
One Sample t-test Calculator Two Sample t-test Calculator Paired Samples t-test Calculator
Featured Posts

Hey there. My name is Zach Bobbitt. I have a Masters of Science degree in Applied Statistics and I’ve worked on machine learning algorithms for professional businesses in both healthcare and retail. I’m passionate about statistics, machine learning, and data visualization and I created Statology to be a resource for both students and teachers alike. My goal with this site is to help you learn statistics through using simple terms, plenty of real-world examples, and helpful illustrations.
Leave a Reply Cancel reply
Your email address will not be published. Required fields are marked *
User Preferences
Content preview.
Arcu felis bibendum ut tristique et egestas quis:
- Ut enim ad minim veniam, quis nostrud exercitation ullamco laboris
- Duis aute irure dolor in reprehenderit in voluptate
- Excepteur sint occaecat cupidatat non proident
Keyboard Shortcuts
10: hypothesis testing, lesson overview section .
In Lesson 2 we saw the value of random assignment in designed experiments. Random assignment alleviates the bias that might cause a systematic difference between groups unrelated to the treatment itself. Precautions like blinding that ensure that the subjects are treated the same during the experiment then leave us with just two possibilities for the cause of differences seen between groups. Either:
- the treatment was effective in producing the changes (the research hypothesis), or
- differences were just the result of the luck of the draw (the null hypothesis).
This shows the importance of addressing the concept of statistical significance. If it is very unlikely that the results of a randomized experiment are just the result of random chance, then we are left with the treatment itself as the probable cause of any relationship seen. Even in an observational study, being able to show that random chance is a poor explanation of the data is still good evidence for a true association in the population (even though it is poor evidence of causality).
This lesson focuses on Statistical hypothesis testing. In a significance test, you carry out a probability calculation assuming the null hypothesis is true to see if random chance is a plausible explanation for the data. Let's illustrate the process with an example.
Example 10.1 Section
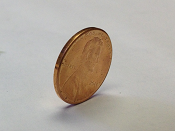
Physical theory suggests that when a coin is spun on a table (rather than flipped in the air) the probability it lands heads up is less than 0.5. We are hesitant to believe this without proof.
To test the theory we carry out an experiment and independently spin a penny 100 times getting 37 heads and 63 tails. Thus, the observed proportion of heads is 37 / 100 = 0.37
We have two possible explanations for the data:
Null Hypothesis : The data is merely a reflection of chance variation. The probability of heads when a penny is spun is really p = 0.5
Alternative Hypothesis : The probability of heads when a penny is spun is really < 0.5.
A statistical hypothesis test is designed to answer the question: "Does the Null Hypothesis provide a reasonable explanation of the data?”
To answer this question we carry out a probability calculation. First, we can calculate a
Test Statistic = a measure of the difference between the data and what is expected when the null hypothesis is true.
In our example, the null hypothesis says the number of heads in 100 spins would closely follow the normal distribution with p = 0.5. So, if the null hypothesis is true, we expect half (0.5) heads give or take a standard deviation of
\[\sqrt{\frac{0.5(1-0.5)}{100}}=0.05\]
Further, we can see how unusual our data is if the null hypothesis is true by finding the standard score z for the test statistic and using the normal curve:
\[z = (0.37-0.5)/0.05 = -2.6\]
How unusual is the value we got, assuming the null hypothesis (i.e., the real proportion is 0.5) is true? We know that standard scores of -2.6 or lower only happen about 0.5% of the time. So the null hypothesis provides a poor explanation for our data. This would seem to provide strong evidence that spinning a coin has less than a 50% chance of landing heads.
- Formulate appropriate null and alternative hypotheses.
- Identify the type 1 and the type 2 error in the context of the problem.
- Use the four basic steps to carry out a significance test in some basic situations.
- Interpret a p -value in terms of the problem.
- State an appropriate conclusion for a hypothesis test.
Language Testing and Assessment
Cite this chapter.
- April Ginther 5 &
- Kyle McIntosh 6
6970 Accesses
5 Citations
We begin by examining the history of language testing and assessment as parallel to the development of large-scale, high-stakes language proficiency tests (e.g., TOEFL) used primarily for admission into institutions of higher learning. We then discuss core concepts in the field and provide an overview of the most commonly used research methods. Lastly, we address a number of challenges and concerns arising from tensions between those who see the growing emphasis on testing as a way to ensure fairness and accountability and those who believe it results in bias and inequality. Consequential validity, assessment literacy, and world Englishes/English as a lingua franca are discussed in relation to language tests and assessments as used for decision-making purposes in various domains.
This is a preview of subscription content, log in via an institution to check access.
Access this chapter
- Available as PDF
- Read on any device
- Instant download
- Own it forever
- Available as EPUB and PDF
- Compact, lightweight edition
- Dispatched in 3 to 5 business days
- Free shipping worldwide - see info
- Durable hardcover edition
Tax calculation will be finalised at checkout
Purchases are for personal use only
Institutional subscriptions
ACTFL. (2012). ACTFL proficiency guidelines (Revised). Alexandria, VA: American Council on the Teaching of Foreign Languages.
Google Scholar
Alderson, C. (1991). Language testing in the 1990s: How far have we come? How much further have we to go? In A. Sarinee (Ed.), Current developments in language testing: Anthology Series 25 (pp. 1–27). Singapore: Regional Language Centre.
American Educational Research Association, American Psychological Association, & National Council on Measurement in Education. (1985). Standards for educational and psychological testing . Washington, DC: American Educational Research Association.
American Educational Research Association, American Psychological Association, & National Council on Measurement in Education. (1999). Standards for educational and psychological testing . Washington, DC: American Educational Research Association.
Angoff, W. H. (1988). Validity: An evolving concept. In H. Wainer & H. Braun (Eds.), Test validity (pp. 9–13). Hillsdale, NJ: Lawrence Erlbaum.
Bachman, L. (1988). Problems in examining the validity of the ACTFL oral proficiency interview. Studies in Second Language Acquisition, 10 , 149–164.
Article Google Scholar
Bachman, L., & Savignon, S. (1986). The evaluation of communicative language proficiency: A critique of the ACTFL oral interview. Modern Language Journal, 70 , 380–391.
Bachman, L. F. (1990). Fundamental considerations in language testing . Oxford: Oxford University Press.
Bachman, L. F., & Palmer, A. S. (1996). Language testing in practice . Oxford: Oxford University Press.
Banerjee, J., & Luoma, S. (1997). Qualitative approaches to test validation. In C. Clapham & D. Corson (Eds.), Encyclopedia of language and education, Volume 7: Language testing and assessment (pp. 275–287). Dordrecht: Kluwer Academic.
Chapter Google Scholar
Berns, M. (2008). World Englishes, English as a lingua franca, and intelligibility. World Englishes, 27 , 327–334.
Canale, M. (1983). From communicative competence to communicative language pedagogy. In J. C. Richards & R. W. Schmidt (Eds.), Language and communication (pp. 2–27). New York: Longman.
Canale, M., & Swain, M. (1980). Theoretical bases of communicative approaches to second language teaching and testing. Applied Linguistics, 1 , 1–47.
Carroll, J. B. (1961). Fundamental considerations in testing for English language proficiency of foreign students. In H. B. Allen & R. N. Campbell (Eds.), Teaching English as a second language: A book of readings (2nd ed., pp. 313–321). New York: McGraw Hill.
Carroll, J. B. (1986). LT + 25, and beyond. Language Testing, 3 , 123–129.
Chapelle, C., Chung, Y., Hegelheimer, V., Pendar, N., & Xu, J. (2010). Towards a computer-delivered test of productive grammatical ability. Language Testing, 27 , 443–469.
Chapelle, C. A., Enright, M. K., & Jamieson, J. (2008). Building a validity argument for the test of English as a foreign language . New York: Routledge.
Chomsky, N. (1957). Syntactic structures . The Hague: Mouton.
Chomsky, N. (1965). Aspects of the theory of syntax . Cambridge: M.I.T. Press.
Council of Europe. (2003). Relating language examinations to the Common European Framework of Reference for languages: Learning, teaching and assessment . Cambridge: Cambridge University Press.
Cronbach, L. J. (1984). Essentials of psychological testing (4th ed.). New York: Harper and Row.
Davidson, F. (2006). World Englishes and test construction. In B. B. Kachru, Y. Kachru, & C. Nelson (Eds.), The handbook of world Englishes (pp. 709–717). Hoboken, NJ: Wiley-Blackwell.
Davidson, F., & Fulcher, G. (2007). The Common European Framework of Reference (CEFR) and the design of language tests: A matter of effect. Language Teaching, 40 , 231–24I.
Davies, A. (1984). Validating three tests of language proficiency. Language Testing, 1 , 50–69.
Davis, L. (2016). The influence of training and experience on rater performance in scoring spoken language. Language Testing, 33 , 117–135.
Davis, L., Laughlin, V., Gu, L., & Ockey, G. (2016, March). Face-to-face speaking assessment in the digital age: Interactive speaking tasks on-line . Paper presented at the Georgetown University Roundtable, Washington, DC.
Dimova, S. (2017). Pronunciation assessment in the context of world Englishes. In O. Kang & A. Ginther (Eds.), Assessment in second language pronunciation (pp. 49–66). New York: Routledge.
Eckes, T. (2008). Rater types in writing performance assessments: A classification approach to rater variability. Language Testing, 25 , 155–185.
Fulcher, G. (1996). Invalidating validity claims for the ACTFL oral rating scale. System, 24 , 163–172.
Fulcher, G. (1997). An English language placement test: Issues in reliability and validity. Language Testing, 14 , 113–138.
Fulcher, G. (2004). Deluded by artifices? The Common European Framework and harmonization. Language Assessment Quarterly, 1 , 253–266.
Fulcher, G. (2012). Assessment literacy for the language classroom. Language Assessment Quarterly, 9 , 113–132.
Gardener, H. (1985). The mind’s new science . New York: Basic Books.
Ginther, A., & Elder, C. (2014). A comparative investigation into understandings and uses of the TOEFL iBT test, the International English Language Testing Service (academic) test, and the Pearson Test of English for Graduate Admissions in the United States and Australia: A case study of two university contexts . ETS research report No. TOEFLiBT-24. Retrieved from https://www.ets.org/research/policy_research_reports/publications/report/2014/jtms
Ginther, A., & Stevens, J. (1998). Language background, ethnicity, and the internal construct validity of the Advanced Placement Spanish language examination. In A. Kunnan (Ed.), Validation in language assessment (pp. 169–194). Mahwah, NJ: Lawrence Erlbaum.
Hawkins, J., & Filipović, L. (2012). Criterial features in L2 English: Specifying the reference levels of the Common European Framework . Cambridge: Cambridge University Press.
Henning, G. (1984). Advantages of latent trait measurement in language testing. Language Testing, 1 , 123–133.
Hsu, T. H.-L. (2016). Removing bias towards World Englishes: The development of a rater attitude instrument using Indian English as a stimulus. Language Testing, 33 , 367–389.
Hymes, D. H. (1972). On communicative competence. In J. B. Pride & J. Holmes (Eds.), Sociolinguistics. Selected readings (pp. 269–293). Harmondsworth: Penguin.
Inbar-Lourie, O. (2008). Constructing a language assessment knowledge base: A focus on language assessment courses. Language Testing, 25 , 385–402.
Jenkins, J. (2006). Current perspectives on teaching world Englishes and English as a lingua Franca. TESOL Quarterly, 40 , 157–181.
Kachru, B. (1985). Standards, codification and sociolinguistic realism: The English language in the Outer Circle. In R. Quirk & H. Widdowson (Eds.), English in the world, teaching and learning the language and literatures (pp. 11–30). Cambridge: Cambridge University Press.
Kane, M. T. (2013). Validating the interpretation and uses of test scores. Journal of Educational Measurement, 50 , 1–73.
Lado, R. (1961). Language testing: The construction and use of foreign language tests . London: Longman.
Linn, R. L. (1998). Partitioning responsibility for the evaluation of the consequences of assessment programs. Educational Measurement: Issues and Practice, 17 , 28–30.
Lowenberg, P. H. (1993). Issues in validity in tests of English as a world language: Whose standards? World Englishes, 12 , 95–106.
Major, R. C., Fitzmaurice, S. F., Bunta, F., & Balasubramanian, C. (2005). Testing the effects of regional, ethnic and international dialects of English on listening comprehension. Language Learning, 55 , 37–69.
McNamara, T. F. (1995). Modelling performance: Opening Pandora’s box. Applied Linguistics, 16 , 159–179.
McNamara, T. F. (1996). Measuring second language performance: A new era in language testing . New York: Longman.
Mehrens, W. A. (1997). The consequences of consequential validity. Educational Measurement: Issues and Practice, 16 , 16–18.
Messick, S. (1975). The standard program: Meaning and values in measurement and evaluation. American Psychologist, 30 , 955–966.
Messick, S. (1989). Validity. In R. L. Linn (Ed.), Educational measurement (3rd ed., pp. 13–103). New York: American Council on Education and Macmillan.
Miller, G. (2003). The cognitive revolution: A historical perspective. Trends in Cognitive Sciences, 7 , 141–144.
Morrow, K. (1981). Communicative language testing: Revolution or evolution? In J. C. Alderson & A. Hughes (Eds.), Issues in language testing, 38 (pp. 9–26). London: The British Council.
Nelson, C. (2011). Intelligibility in world Englishes . Hoboken, NJ: Blackwell.
O’Loughlin, K. (2013). Developing the assessment literacy of university proficiency test users. Language Testing, 30 , 363–380.
Papageorgiou, S., Tannenbaum, R. J., Bridgeman, B., & Cho, Y. (2015). The association between TOEFL iBT test scores and the Common European Framework of Reference (CEFR) levels . Research Memorandum-15-06. Princeton, NJ: ETS.
Phakiti, A. (2008). Construct validation of Bachman and Palmer’s (1996) strategic competence model over time in EFL reading tests. Language Testing, 25 , 237–272.
Popham, W. J. (1997). Consequential validity: Right concern – Wrong concept. Educational Measurement: Issues and Practice, 16 , 9–13.
Rea-Dickins, P. (2001). Mirror, mirror on the wall: Identifying processes of classroom assessment. Language Testing, 18 , 429–462.
Sawaki, Y., Stricker, L. J., & Oranje, A. H. (2009). Factor structure of the TOEFL Internet-based test. Language Testing, 26 , 5–30.
Seidlhofer, B. (2001). Closing a conceptual gap: The case for a description of English as a lingua franca. International Journal of Applied Linguistics, 11 , 133–158.
Shepard, L. A. (1993). Evaluating test validity. In L. Darling-Hammond (Ed.), Review of Research in Education, 19 (pp. 405–450). Washington, DC: AERA.
Shiotsu, T., & Weir, C. J. (2007). The relative significance of syntactic knowledge and vocabulary breadth in the prediction of reading comprehension test performance. Language Testing, 24 , 99–128.
Spolsky, B. (1981). Some ethical questions about language testing. In C. Klein-Braley & D. K. Stevenson (Eds.), Practice and problems in language testing (pp. 5–30). Frankfurt am Main: Peter Lang.
Spolsky, B. (1986). A multiple choice for language testers. Language Testing, 3 , 147–158.
Spolsky, B. (1993). Testing across cultures: An historical perspective. World Englishes, 12 , 87–93.
Spolsky, B. (1995). Measured words: The development of objective language testing . Oxford: Oxford University Press.
Stansfield, C. (2008). Where we have been and where we should go? Language Testing, 25 , 311–326.
Stiggins, R. J. (1991). Assessment literacy. Phi Delta Kappan, 72 , 534–539.
Torkildsen, L. G., & Erickson, G. (2016). “If they’d written more…” – On students’ perceptions of assessment and assessment practices. Education Inquiry, 7 , 137–157.
Toulmin, S. (1958). The uses of argument . Cambridge: Cambridge University Press.
Toulmin, S. (2001). Return to reason . Cambridge, MA: Harvard University Press.
Weigle, S. C. (2007). Teaching writing teachers about assessment. Journal of Second Language Writing, 16 , 194–209.
Wind, S. A., & Peterson, M. E. (2017). A systematic review of methods for evaluating rating quality in language assessment. Language Testing, 35 , 161–192.
Yan, X., Thirakunkovit, S., Kauper, N., & Ginther, A. (2016). What do test takers say: Test-taker feedback as input for quality control. In J. Read (Ed.), Post-admission language assessments of university students (pp. 157–183). Switzerland: Springer.
Zhang, Y., & Elder, C. (2011). Judgments of oral proficiency by non-native and native English speaking teacher raters: Competing or complementary constructs? Language Testing, 28 , 31–50.
Download references
Author information
Authors and affiliations.
Department of English, Purdue University, West Lafayette, IN, USA
April Ginther
Department of English and Writing, University of Tampa, Tampa, FL, USA
Kyle McIntosh
You can also search for this author in PubMed Google Scholar
Corresponding author
Correspondence to April Ginther .
Editor information
Editors and affiliations.
Sydney School of Education and Social Work, University of Sydney, Sydney, NSW, Australia
Aek Phakiti
Department of Linguistics, Germanic, Slavic, Asian and African Languages, Michigan State University, East Lansing, MI, USA
Peter De Costa
Applied Linguistics, Northern Arizona University, Flagstaff, AZ, USA
Luke Plonsky
School of Education, UNSW Sydney, Sydney, NSW, Australia
Sue Starfield
Copyright information
© 2018 The Author(s)
About this chapter
Ginther, A., McIntosh, K. (2018). Language Testing and Assessment. In: Phakiti, A., De Costa, P., Plonsky, L., Starfield, S. (eds) The Palgrave Handbook of Applied Linguistics Research Methodology. Palgrave Macmillan, London. https://doi.org/10.1057/978-1-137-59900-1_39
Download citation
DOI : https://doi.org/10.1057/978-1-137-59900-1_39
Publisher Name : Palgrave Macmillan, London
Print ISBN : 978-1-137-59899-8
Online ISBN : 978-1-137-59900-1
eBook Packages : Social Sciences Social Sciences (R0)
Share this chapter
Anyone you share the following link with will be able to read this content:
Sorry, a shareable link is not currently available for this article.
Provided by the Springer Nature SharedIt content-sharing initiative
- Publish with us
Policies and ethics
- Find a journal
- Track your research
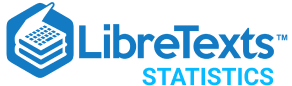
- school Campus Bookshelves
- menu_book Bookshelves
- perm_media Learning Objects
- login Login
- how_to_reg Request Instructor Account
- hub Instructor Commons
- Download Page (PDF)
- Download Full Book (PDF)
- Periodic Table
- Physics Constants
- Scientific Calculator
- Reference & Cite
- Tools expand_more
- Readability
selected template will load here
This action is not available.
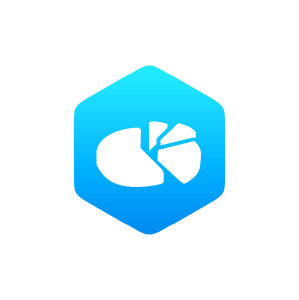
7.1: Basics of Hypothesis Testing
- Last updated
- Save as PDF
- Page ID 16360
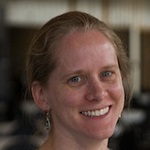
- Kathryn Kozak
- Coconino Community College
To understand the process of a hypothesis tests, you need to first have an understanding of what a hypothesis is, which is an educated guess about a parameter. Once you have the hypothesis, you collect data and use the data to make a determination to see if there is enough evidence to show that the hypothesis is true. However, in hypothesis testing you actually assume something else is true, and then you look at your data to see how likely it is to get an event that your data demonstrates with that assumption. If the event is very unusual, then you might think that your assumption is actually false. If you are able to say this assumption is false, then your hypothesis must be true. This is known as a proof by contradiction. You assume the opposite of your hypothesis is true and show that it can’t be true. If this happens, then your hypothesis must be true. All hypothesis tests go through the same process. Once you have the process down, then the concept is much easier. It is easier to see the process by looking at an example. Concepts that are needed will be detailed in this example.
Example \(\PageIndex{1}\) basics of hypothesis testing
Suppose a manufacturer of the XJ35 battery claims the mean life of the battery is 500 days with a standard deviation of 25 days. You are the buyer of this battery and you think this claim is inflated. You would like to test your belief because without a good reason you can’t get out of your contract.
What do you do?
Well first, you should know what you are trying to measure. Define the random variable.
Let x = life of a XJ35 battery
Now you are not just trying to find different x values. You are trying to find what the true mean is. Since you are trying to find it, it must be unknown. You don’t think it is 500 days. If you did, you wouldn’t be doing any testing. The true mean, \(\mu\), is unknown. That means you should define that too.
Let \(\mu\)= mean life of a XJ35 battery
You may want to collect a sample. What kind of sample?
You could ask the manufacturers to give you batteries, but there is a chance that there could be some bias in the batteries they pick. To reduce the chance of bias, it is best to take a random sample.
How big should the sample be?
A sample of size 30 or more means that you can use the central limit theorem. Pick a sample of size 30.
Example \(\PageIndex{1}\) contains the data for the sample you collected:
Now what should you do? Looking at the data set, you see some of the times are above 500 and some are below. But looking at all of the numbers is too difficult. It might be helpful to calculate the mean for this sample.
The sample mean is \(\overline{x} = 490\) days. Looking at the sample mean, one might think that you are right. However, the standard deviation and the sample size also plays a role, so maybe you are wrong.
Before going any farther, it is time to formalize a few definitions.
You have a guess that the mean life of a battery is less than 500 days. This is opposed to what the manufacturer claims. There really are two hypotheses, which are just guesses here – the one that the manufacturer claims and the one that you believe. It is helpful to have names for them.
Definition \(\PageIndex{1}\)
Null Hypothesis : historical value, claim, or product specification. The symbol used is \(H_{o}\).
Definition \(\PageIndex{2}\)
Alternate Hypothesis : what you want to prove. This is what you want to accept as true when you reject the null hypothesis. There are two symbols that are commonly used for the alternative hypothesis: \(H_{A}\) or \(H_{I}\). The symbol \(H_{A}\) will be used in this book.
In general, the hypotheses look something like this:
\(H_{o} : \mu=\mu_{o}\)
\(H_{A} : \mu<\mu_{o}\)
where \(\mu_{o}\) just represents the value that the claim says the population mean is actually equal to.
Also, \(H_{A}\) can be less than, greater than, or not equal to.
For this problem:
\(H_{o} : \mu=500\) days, since the manufacturer says the mean life of a battery is 500 days.
\(H_{A} : \mu<500\) days, since you believe that the mean life of the battery is less than 500 days.
Now back to the mean. You have a sample mean of 490 days. Is this small enough to believe that you are right and the manufacturer is wrong? How small does it have to be?
If you calculated a sample mean of 235, you would definitely believe the population mean is less than 500. But even if you had a sample mean of 435 you would probably believe that the true mean was less than 500. What about 475? Or 483? There is some point where you would stop being so sure that the population mean is less than 500. That point separates the values of where you are sure or pretty sure that the mean is less than 500 from the area where you are not so sure. How do you find that point?
Well it depends on how much error you want to make. Of course you don’t want to make any errors, but unfortunately that is unavoidable in statistics. You need to figure out how much error you made with your sample. Take the sample mean, and find the probability of getting another sample mean less than it, assuming for the moment that the manufacturer is right. The idea behind this is that you want to know what is the chance that you could have come up with your sample mean even if the population mean really is 500 days.
You want to find \(P\left(\overline{x}<490 | H_{o} \text { is true }\right)=P(\overline{x}<490 | \mu=500)\)
To compute this probability, you need to know how the sample mean is distributed. Since the sample size is at least 30, then you know the sample mean is approximately normally distributed. Remember \(\mu_{\overline{x}}=\mu\) and \(\sigma_{\overline{x}}=\dfrac{\sigma}{\sqrt{n}}\)
A picture is always useful.
.png?revision=1)
Before calculating the probability, it is useful to see how many standard deviations away from the mean the sample mean is. Using the formula for the z-score from chapter 6, you find
\(z=\dfrac{\overline{x}-\mu_{o}}{\sigma / \sqrt{n}}=\dfrac{490-500}{25 / \sqrt{30}}=-2.19\)
This sample mean is more than two standard deviations away from the mean. That seems pretty far, but you should look at the probability too.
On TI-83/84:
\(P(\overline{x}<490 | \mu=500)=\text { normalcdf }(-1 E 99,490,500,25 \div \sqrt{30}) \approx 0.0142\)
\(P(\overline{x}<490 \mu=500)=\text { pnorm }(490,500,25 / \operatorname{sqrt}(30)) \approx 0.0142\)
There is a 1.42% chance that you could find a sample mean less than 490 when the population mean is 500 days. This is really small, so the chances are that the assumption that the population mean is 500 days is wrong, and you can reject the manufacturer’s claim. But how do you quantify really small? Is 5% or 10% or 15% really small? How do you decide?
Before you answer that question, a couple more definitions are needed.
Definition \(\PageIndex{3}\)
Test Statistic : \(z=\dfrac{\overline{x}-\mu_{o}}{\sigma / \sqrt{n}}\) since it is calculated as part of the testing of the hypothesis.
Definition \(\PageIndex{4}\)
p – value : probability that the test statistic will take on more extreme values than the observed test statistic, given that the null hypothesis is true. It is the probability that was calculated above.
Now, how small is small enough? To answer that, you really want to know the types of errors you can make.
There are actually only two errors that can be made. The first error is if you say that \(H_{o}\) is false, when in fact it is true. This means you reject \(H_{o}\) when \(H_{o}\) was true. The second error is if you say that \(H_{o}\) is true, when in fact it is false. This means you fail to reject \(H_{o}\) when \(H_{o}\) is false. The following table organizes this for you:
Type of errors:
Definition \(\PageIndex{5}\)
Type I Error is rejecting \(H_{o}\) when \(H_{o}\) is true, and
Definition \(\PageIndex{6}\)
Type II Error is failing to reject \(H_{o}\) when \(H_{o}\) is false.
Since these are the errors, then one can define the probabilities attached to each error.
Definition \(\PageIndex{7}\)
\(\alpha\) = P(type I error) = P(rejecting \(H_{o} / H_{o}\) is true)
Definition \(\PageIndex{8}\)
\(\beta\) = P(type II error) = P(failing to reject \(H_{o} / H_{o}\) is false)
\(\alpha\) is also called the level of significance .
Another common concept that is used is Power = \(1-\beta \).
Now there is a relationship between \(\alpha\) and \(\beta\). They are not complements of each other. How are they related?
If \(\alpha\) increases that means the chances of making a type I error will increase. It is more likely that a type I error will occur. It makes sense that you are less likely to make type II errors, only because you will be rejecting \(H_{o}\) more often. You will be failing to reject \(H_{o}\) less, and therefore, the chance of making a type II error will decrease. Thus, as \(\alpha\) increases, \(\beta\) will decrease, and vice versa. That makes them seem like complements, but they aren’t complements. What gives? Consider one more factor – sample size.
Consider if you have a larger sample that is representative of the population, then it makes sense that you have more accuracy then with a smaller sample. Think of it this way, which would you trust more, a sample mean of 490 if you had a sample size of 35 or sample size of 350 (assuming a representative sample)? Of course the 350 because there are more data points and so more accuracy. If you are more accurate, then there is less chance that you will make any error. By increasing the sample size of a representative sample, you decrease both \(\alpha\) and \(\beta\).
Summary of all of this:
- For a certain sample size, n , if \(\alpha\) increases, \(\beta\) decreases.
- For a certain level of significance, \(\alpha\), if n increases, \(\beta\) decreases.
Now how do you find \(\alpha\) and \(\beta\)? Well \(\alpha\) is actually chosen. There are only three values that are usually picked for \(\alpha\): 0.01, 0.05, and 0.10. \(\beta\) is very difficult to find, so usually it isn’t found. If you want to make sure it is small you take as large of a sample as you can afford provided it is a representative sample. This is one use of the Power. You want \(\beta\) to be small and the Power of the test is large. The Power word sounds good.
Which pick of \(\alpha\) do you pick? Well that depends on what you are working on. Remember in this example you are the buyer who is trying to get out of a contract to buy these batteries. If you create a type I error, you said that the batteries are bad when they aren’t, most likely the manufacturer will sue you. You want to avoid this. You might pick \(\alpha\) to be 0.01. This way you have a small chance of making a type I error. Of course this means you have more of a chance of making a type II error. No big deal right? What if the batteries are used in pacemakers and you tell the person that their pacemaker’s batteries are good for 500 days when they actually last less, that might be bad. If you make a type II error, you say that the batteries do last 500 days when they last less, then you have the possibility of killing someone. You certainly do not want to do this. In this case you might want to pick \(\alpha\) as 0.10. If both errors are equally bad, then pick \(\alpha\) as 0.05.
The above discussion is why the choice of \(\alpha\) depends on what you are researching. As the researcher, you are the one that needs to decide what \(\alpha\) level to use based on your analysis of the consequences of making each error is.
If a type I error is really bad, then pick \(\alpha\) = 0.01.
If a type II error is really bad, then pick \(\alpha\) = 0.10
If neither error is bad, or both are equally bad, then pick \(\alpha\) = 0.05
The main thing is to always pick the \(\alpha\) before you collect the data and start the test.
The above discussion was long, but it is really important information. If you don’t know what the errors of the test are about, then there really is no point in making conclusions with the tests. Make sure you understand what the two errors are and what the probabilities are for them.
Now it is time to go back to the example and put this all together. This is the basic structure of testing a hypothesis, usually called a hypothesis test. Since this one has a test statistic involving z, it is also called a z-test. And since there is only one sample, it is usually called a one-sample z-test.
Example \(\PageIndex{2}\) battery example revisited
- State the random variable and the parameter in words.
- State the null and alternative hypothesis and the level of significance.
- A random sample of size n is taken.
- The population standard derivation is known.
- The sample size is at least 30 or the population of the random variable is normally distributed.
- Find the sample statistic, test statistic, and p-value.
- Interpretation
1. x = life of battery
\(\mu\) = mean life of a XJ35 battery
2. \(H_{o} : \mu=500\) days
\(H_{A} : \mu<500\) days
\(\alpha = 0.10\) (from above discussion about consequences)
3. Every hypothesis has some assumptions that be met to make sure that the results of the hypothesis are valid. The assumptions are different for each test. This test has the following assumptions.
- This occurred in this example, since it was stated that a random sample of 30 battery lives were taken.
- This is true, since it was given in the problem.
- The sample size was 30, so this condition is met.
4. The test statistic depends on how many samples there are, what parameter you are testing, and assumptions that need to be checked. In this case, there is one sample and you are testing the mean. The assumptions were checked above.
Sample statistic:
\(\overline{x} = 490\)
Test statistic:
.png?revision=1)
Using TI-83/84:
\(P(\overline{x}<490 | \mu=500)=\text { normalcdf }(-1 \mathrm{E} 99,490,500,25 / \sqrt{30}) \approx 0.0142\)
\(P(\overline{x}<490 | \mu=500)=\operatorname{pnorm}(490,500,25 / \operatorname{sqrt}(30)) \approx 0.0142\)
5. Now what? Well, this p-value is 0.0142. This is a lot smaller than the amount of error you would accept in the problem -\(\alpha\) = 0.10. That means that finding a sample mean less than 490 days is unusual to happen if \(H_{o}\) is true. This should make you think that \(H_{o}\) is not true. You should reject \(H_{o}\).
In fact, in general:
Reject \(H_{o}\) if the p-value < \(\alpha\) and
Fail to reject \(H_{o}\) if the p-value \(\geq \alpha\).
6. Since you rejected \(H_{o}\), what does this mean in the real world? That is what goes in the interpretation. Since you rejected the claim by the manufacturer that the mean life of the batteries is 500 days, then you now can believe that your hypothesis was correct. In other words, there is enough evidence to show that the mean life of the battery is less than 500 days.
Now that you know that the batteries last less than 500 days, should you cancel the contract? Statistically, there is evidence that the batteries do not last as long as the manufacturer says they should. However, based on this sample there are only ten days less on average that the batteries last. There may not be practical significance in this case. Ten days do not seem like a large difference. In reality, if the batteries are used in pacemakers, then you would probably tell the patient to have the batteries replaced every year. You have a large buffer whether the batteries last 490 days or 500 days. It seems that it might not be worth it to break the contract over ten days. What if the 10 days was practically significant? Are there any other things you should consider? You might look at the business relationship with the manufacturer. You might also look at how much it would cost to find a new manufacturer. These are also questions to consider before making any changes. What this discussion should show you is that just because a hypothesis has statistical significance does not mean it has practical significance. The hypothesis test is just one part of a research process. There are other pieces that you need to consider.
That’s it. That is what a hypothesis test looks like. All hypothesis tests are done with the same six steps. Those general six steps are outlined below.
- State the random variable and the parameter in words. This is where you are defining what the unknowns are in this problem. x = random variable \(\mu\) = mean of random variable, if the parameter of interest is the mean. There are other parameters you can test, and you would use the appropriate symbol for that parameter.
- State the null and alternative hypotheses and the level of significance \(H_{o} : \mu=\mu_{o}\), where \(\mu_{o}\) is the known mean \(H_{A} : \mu<\mu_{o}\) \(H_{A} : \mu>\mu_{o}\), use the appropriate one for your problem \(H_{A} : \mu \neq \mu_{o}\) Also, state your \(\alpha\) level here.
- State and check the assumptions for a hypothesis test. Each hypothesis test has its own assumptions. They will be stated when the different hypothesis tests are discussed.
- Find the sample statistic, test statistic, and p-value. This depends on what parameter you are working with, how many samples, and the assumptions of the test. The p-value depends on your \(H_{A}\). If you are doing the \(H_{A}\) with the less than, then it is a left-tailed test, and you find the probability of being in that left tail. If you are doing the \(H_{A}\) with the greater than, then it is a right-tailed test, and you find the probability of being in the right tail. If you are doing the \(H_{A}\) with the not equal to, then you are doing a two-tail test, and you find the probability of being in both tails. Because of symmetry, you could find the probability in one tail and double this value to find the probability in both tails.
- Conclusion This is where you write reject \(H_{o}\) or fail to reject \(H_{o}\). The rule is: if the p-value < \(\alpha\), then reject \(H_{o}\). If the p-value \(\geq \alpha\), then fail to reject \(H_{o}\).
- Interpretation This is where you interpret in real world terms the conclusion to the test. The conclusion for a hypothesis test is that you either have enough evidence to show \(H_{A}\) is true, or you do not have enough evidence to show \(H_{A}\) is true.
Sorry, one more concept about the conclusion and interpretation. First, the conclusion is that you reject \(H_{o}\) or you fail to reject \(H_{o}\). Why was it said like this? It is because you never accept the null hypothesis. If you wanted to accept the null hypothesis, then why do the test in the first place? In the interpretation, you either have enough evidence to show \(H_{A}\) is true, or you do not have enough evidence to show \(H_{A}\) is true. You wouldn’t want to go to all this work and then find out you wanted to accept the claim. Why go through the trouble? You always want to show that the alternative hypothesis is true. Sometimes you can do that and sometimes you can’t. It doesn’t mean you proved the null hypothesis; it just means you can’t prove the alternative hypothesis. Here is an example to demonstrate this.
Example \(\PageIndex{3}\) conclusion in hypothesis tests
In the U.S. court system a jury trial could be set up as a hypothesis test. To really help you see how this works, let’s use OJ Simpson as an example. In the court system, a person is presumed innocent until he/she is proven guilty, and this is your null hypothesis. OJ Simpson was a football player in the 1970s. In 1994 his ex-wife and her friend were killed. OJ Simpson was accused of the crime, and in 1995 the case was tried. The prosecutors wanted to prove OJ was guilty of killing his wife and her friend, and that is the alternative hypothesis
\(H_{0}\): OJ is innocent of killing his wife and her friend
\(H_{A}\): OJ is guilty of killing his wife and her friend
In this case, a verdict of not guilty was given. That does not mean that he is innocent of this crime. It means there was not enough evidence to prove he was guilty. Many people believe that OJ was guilty of this crime, but the jury did not feel that the evidence presented was enough to show there was guilt. The verdict in a jury trial is always guilty or not guilty!
The same is true in a hypothesis test. There is either enough or not enough evidence to show that alternative hypothesis. It is not that you proved the null hypothesis true.
When identifying hypothesis, it is important to state your random variable and the appropriate parameter you want to make a decision about. If count something, then the random variable is the number of whatever you counted. The parameter is the proportion of what you counted. If the random variable is something you measured, then the parameter is the mean of what you measured. (Note: there are other parameters you can calculate, and some analysis of those will be presented in later chapters.)
Example \(\PageIndex{4}\) stating hypotheses
Identify the hypotheses necessary to test the following statements:
- The average salary of a teacher is more than $30,000.
- The proportion of students who like math is less than 10%.
- The average age of students in this class differs from 21.
a. x = salary of teacher
\(\mu\) = mean salary of teacher
The guess is that \(\mu>\$ 30,000\) and that is the alternative hypothesis.
The null hypothesis has the same parameter and number with an equal sign.
\(\begin{array}{l}{H_{0} : \mu=\$ 30,000} \\ {H_{A} : \mu>\$ 30,000}\end{array}\)
b. x = number od students who like math
p = proportion of students who like math
The guess is that p < 0.10 and that is the alternative hypothesis.
\(\begin{array}{l}{H_{0} : p=0.10} \\ {H_{A} : p<0.10}\end{array}\)
c. x = age of students in this class
\(\mu\) = mean age of students in this class
The guess is that \(\mu \neq 21\) and that is the alternative hypothesis.
\(\begin{array}{c}{H_{0} : \mu=21} \\ {H_{A} : \mu \neq 21}\end{array}\)
Example \(\PageIndex{5}\) Stating Type I and II Errors and Picking Level of Significance
- The plant-breeding department at a major university developed a new hybrid raspberry plant called YumYum Berry. Based on research data, the claim is made that from the time shoots are planted 90 days on average are required to obtain the first berry with a standard deviation of 9.2 days. A corporation that is interested in marketing the product tests 60 shoots by planting them and recording the number of days before each plant produces its first berry. The sample mean is 92.3 days. The corporation wants to know if the mean number of days is more than the 90 days claimed. State the type I and type II errors in terms of this problem, consequences of each error, and state which level of significance to use.
- A concern was raised in Australia that the percentage of deaths of Aboriginal prisoners was higher than the percent of deaths of non-indigenous prisoners, which is 0.27%. State the type I and type II errors in terms of this problem, consequences of each error, and state which level of significance to use.
a. x = time to first berry for YumYum Berry plant
\(\mu\) = mean time to first berry for YumYum Berry plant
\(\begin{array}{l}{H_{0} : \mu=90} \\ {H_{A} : \mu>90}\end{array}\)
Type I Error: If the corporation does a type I error, then they will say that the plants take longer to produce than 90 days when they don’t. They probably will not want to market the plants if they think they will take longer. They will not market them even though in reality the plants do produce in 90 days. They may have loss of future earnings, but that is all.
Type II error: The corporation do not say that the plants take longer then 90 days to produce when they do take longer. Most likely they will market the plants. The plants will take longer, and so customers might get upset and then the company would get a bad reputation. This would be really bad for the company.
Level of significance: It appears that the corporation would not want to make a type II error. Pick a 10% level of significance, \(\alpha = 0.10\).
b. x = number of Aboriginal prisoners who have died
p = proportion of Aboriginal prisoners who have died
\(\begin{array}{l}{H_{o} : p=0.27 \%} \\ {H_{A} : p>0.27 \%}\end{array}\)
Type I error: Rejecting that the proportion of Aboriginal prisoners who died was 0.27%, when in fact it was 0.27%. This would mean you would say there is a problem when there isn’t one. You could anger the Aboriginal community, and spend time and energy researching something that isn’t a problem.
Type II error: Failing to reject that the proportion of Aboriginal prisoners who died was 0.27%, when in fact it is higher than 0.27%. This would mean that you wouldn’t think there was a problem with Aboriginal prisoners dying when there really is a problem. You risk causing deaths when there could be a way to avoid them.
Level of significance: It appears that both errors may be issues in this case. You wouldn’t want to anger the Aboriginal community when there isn’t an issue, and you wouldn’t want people to die when there may be a way to stop it. It may be best to pick a 5% level of significance, \(\alpha = 0.05\).
Hypothesis testing is really easy if you follow the same recipe every time. The only differences in the various problems are the assumptions of the test and the test statistic you calculate so you can find the p-value. Do the same steps, in the same order, with the same words, every time and these problems become very easy.
Exercise \(\PageIndex{1}\)
For the problems in this section, a question is being asked. This is to help you understand what the hypotheses are. You are not to run any hypothesis tests and come up with any conclusions in this section.
- Eyeglassomatic manufactures eyeglasses for different retailers. They test to see how many defective lenses they made in a given time period and found that 11% of all lenses had defects of some type. Looking at the type of defects, they found in a three-month time period that out of 34,641 defective lenses, 5865 were due to scratches. Are there more defects from scratches than from all other causes? State the random variable, population parameter, and hypotheses.
- According to the February 2008 Federal Trade Commission report on consumer fraud and identity theft, 23% of all complaints in 2007 were for identity theft. In that year, Alaska had 321 complaints of identity theft out of 1,432 consumer complaints ("Consumer fraud and," 2008). Does this data provide enough evidence to show that Alaska had a lower proportion of identity theft than 23%? State the random variable, population parameter, and hypotheses.
- The Kyoto Protocol was signed in 1997, and required countries to start reducing their carbon emissions. The protocol became enforceable in February 2005. In 2004, the mean CO2 emission was 4.87 metric tons per capita. Is there enough evidence to show that the mean CO2 emission is lower in 2010 than in 2004? State the random variable, population parameter, and hypotheses.
- The FDA regulates that fish that is consumed is allowed to contain 1.0 mg/kg of mercury. In Florida, bass fish were collected in 53 different lakes to measure the amount of mercury in the fish. The data for the average amount of mercury in each lake is in Example \(\PageIndex{5}\) ("Multi-disciplinary niser activity," 2013). Do the data provide enough evidence to show that the fish in Florida lakes has more mercury than the allowable amount? State the random variable, population parameter, and hypotheses.
- Eyeglassomatic manufactures eyeglasses for different retailers. They test to see how many defective lenses they made in a given time period and found that 11% of all lenses had defects of some type. Looking at the type of defects, they found in a three-month time period that out of 34,641 defective lenses, 5865 were due to scratches. Are there more defects from scratches than from all other causes? State the type I and type II errors in this case, consequences of each error type for this situation from the perspective of the manufacturer, and the appropriate alpha level to use. State why you picked this alpha level.
- According to the February 2008 Federal Trade Commission report on consumer fraud and identity theft, 23% of all complaints in 2007 were for identity theft. In that year, Alaska had 321 complaints of identity theft out of 1,432 consumer complaints ("Consumer fraud and," 2008). Does this data provide enough evidence to show that Alaska had a lower proportion of identity theft than 23%? State the type I and type II errors in this case, consequences of each error type for this situation from the perspective of the state of Arizona, and the appropriate alpha level to use. State why you picked this alpha level.
- The Kyoto Protocol was signed in 1997, and required countries to start reducing their carbon emissions. The protocol became enforceable in February 2005. In 2004, the mean CO2 emission was 4.87 metric tons per capita. Is there enough evidence to show that the mean CO2 emission is lower in 2010 than in 2004? State the type I and type II errors in this case, consequences of each error type for this situation from the perspective of the agency overseeing the protocol, and the appropriate alpha level to use. State why you picked this alpha level.
- The FDA regulates that fish that is consumed is allowed to contain 1.0 mg/kg of mercury. In Florida, bass fish were collected in 53 different lakes to measure the amount of mercury in the fish. The data for the average amount of mercury in each lake is in Example \(\PageIndex{5}\) ("Multi-disciplinary niser activity," 2013). Do the data provide enough evidence to show that the fish in Florida lakes has more mercury than the allowable amount? State the type I and type II errors in this case, consequences of each error type for this situation from the perspective of the FDA, and the appropriate alpha level to use. State why you picked this alpha level.
1. \(H_{o} : p=0.11, H_{A} : p>0.11\)
3. \(H_{o} : \mu=4.87 \text { metric tons per capita, } H_{A} : \mu<4.87 \text { metric tons per capita }\)
5. See solutions
7. See solutions
Tutorial Playlist
Statistics tutorial, everything you need to know about the probability density function in statistics, the best guide to understand central limit theorem, an in-depth guide to measures of central tendency : mean, median and mode, the ultimate guide to understand conditional probability.
A Comprehensive Look at Percentile in Statistics
The Best Guide to Understand Bayes Theorem
Everything you need to know about the normal distribution, an in-depth explanation of cumulative distribution function, a complete guide to chi-square test, a complete guide on hypothesis testing in statistics, understanding the fundamentals of arithmetic and geometric progression, the definitive guide to understand spearman’s rank correlation, a comprehensive guide to understand mean squared error, all you need to know about the empirical rule in statistics, the complete guide to skewness and kurtosis, a holistic look at bernoulli distribution.
All You Need to Know About Bias in Statistics
A Complete Guide to Get a Grasp of Time Series Analysis
The Key Differences Between Z-Test Vs. T-Test
The Complete Guide to Understand Pearson's Correlation
A complete guide on the types of statistical studies, everything you need to know about poisson distribution, your best guide to understand correlation vs. regression, the most comprehensive guide for beginners on what is correlation, what is hypothesis testing in statistics types and examples.
Lesson 10 of 24 By Avijeet Biswal
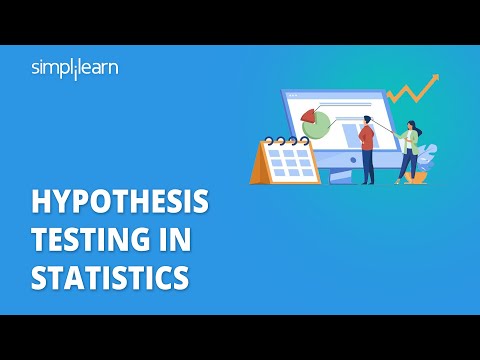
Table of Contents
In today’s data-driven world , decisions are based on data all the time. Hypothesis plays a crucial role in that process, whether it may be making business decisions, in the health sector, academia, or in quality improvement. Without hypothesis & hypothesis tests, you risk drawing the wrong conclusions and making bad decisions. In this tutorial, you will look at Hypothesis Testing in Statistics.
What Is Hypothesis Testing in Statistics?
Hypothesis Testing is a type of statistical analysis in which you put your assumptions about a population parameter to the test. It is used to estimate the relationship between 2 statistical variables.
Let's discuss few examples of statistical hypothesis from real-life -
- A teacher assumes that 60% of his college's students come from lower-middle-class families.
- A doctor believes that 3D (Diet, Dose, and Discipline) is 90% effective for diabetic patients.
Now that you know about hypothesis testing, look at the two types of hypothesis testing in statistics.
Hypothesis Testing Formula
Z = ( x̅ – μ0 ) / (σ /√n)
- Here, x̅ is the sample mean,
- μ0 is the population mean,
- σ is the standard deviation,
- n is the sample size.
How Hypothesis Testing Works?
An analyst performs hypothesis testing on a statistical sample to present evidence of the plausibility of the null hypothesis. Measurements and analyses are conducted on a random sample of the population to test a theory. Analysts use a random population sample to test two hypotheses: the null and alternative hypotheses.
The null hypothesis is typically an equality hypothesis between population parameters; for example, a null hypothesis may claim that the population means return equals zero. The alternate hypothesis is essentially the inverse of the null hypothesis (e.g., the population means the return is not equal to zero). As a result, they are mutually exclusive, and only one can be correct. One of the two possibilities, however, will always be correct.
Your Dream Career is Just Around The Corner!
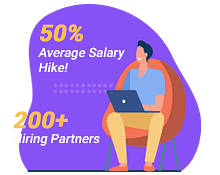
Null Hypothesis and Alternate Hypothesis
The Null Hypothesis is the assumption that the event will not occur. A null hypothesis has no bearing on the study's outcome unless it is rejected.
H0 is the symbol for it, and it is pronounced H-naught.
The Alternate Hypothesis is the logical opposite of the null hypothesis. The acceptance of the alternative hypothesis follows the rejection of the null hypothesis. H1 is the symbol for it.
Let's understand this with an example.
A sanitizer manufacturer claims that its product kills 95 percent of germs on average.
To put this company's claim to the test, create a null and alternate hypothesis.
H0 (Null Hypothesis): Average = 95%.
Alternative Hypothesis (H1): The average is less than 95%.
Another straightforward example to understand this concept is determining whether or not a coin is fair and balanced. The null hypothesis states that the probability of a show of heads is equal to the likelihood of a show of tails. In contrast, the alternate theory states that the probability of a show of heads and tails would be very different.
Become a Data Scientist with Hands-on Training!
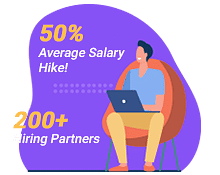
Hypothesis Testing Calculation With Examples
Let's consider a hypothesis test for the average height of women in the United States. Suppose our null hypothesis is that the average height is 5'4". We gather a sample of 100 women and determine that their average height is 5'5". The standard deviation of population is 2.
To calculate the z-score, we would use the following formula:
z = ( x̅ – μ0 ) / (σ /√n)
z = (5'5" - 5'4") / (2" / √100)
z = 0.5 / (0.045)
We will reject the null hypothesis as the z-score of 11.11 is very large and conclude that there is evidence to suggest that the average height of women in the US is greater than 5'4".
Steps of Hypothesis Testing
Step 1: specify your null and alternate hypotheses.
It is critical to rephrase your original research hypothesis (the prediction that you wish to study) as a null (Ho) and alternative (Ha) hypothesis so that you can test it quantitatively. Your first hypothesis, which predicts a link between variables, is generally your alternate hypothesis. The null hypothesis predicts no link between the variables of interest.
Step 2: Gather Data
For a statistical test to be legitimate, sampling and data collection must be done in a way that is meant to test your hypothesis. You cannot draw statistical conclusions about the population you are interested in if your data is not representative.
Step 3: Conduct a Statistical Test
Other statistical tests are available, but they all compare within-group variance (how to spread out the data inside a category) against between-group variance (how different the categories are from one another). If the between-group variation is big enough that there is little or no overlap between groups, your statistical test will display a low p-value to represent this. This suggests that the disparities between these groups are unlikely to have occurred by accident. Alternatively, if there is a large within-group variance and a low between-group variance, your statistical test will show a high p-value. Any difference you find across groups is most likely attributable to chance. The variety of variables and the level of measurement of your obtained data will influence your statistical test selection.
Step 4: Determine Rejection Of Your Null Hypothesis
Your statistical test results must determine whether your null hypothesis should be rejected or not. In most circumstances, you will base your judgment on the p-value provided by the statistical test. In most circumstances, your preset level of significance for rejecting the null hypothesis will be 0.05 - that is, when there is less than a 5% likelihood that these data would be seen if the null hypothesis were true. In other circumstances, researchers use a lower level of significance, such as 0.01 (1%). This reduces the possibility of wrongly rejecting the null hypothesis.
Step 5: Present Your Results
The findings of hypothesis testing will be discussed in the results and discussion portions of your research paper, dissertation, or thesis. You should include a concise overview of the data and a summary of the findings of your statistical test in the results section. You can talk about whether your results confirmed your initial hypothesis or not in the conversation. Rejecting or failing to reject the null hypothesis is a formal term used in hypothesis testing. This is likely a must for your statistics assignments.
Types of Hypothesis Testing
To determine whether a discovery or relationship is statistically significant, hypothesis testing uses a z-test. It usually checks to see if two means are the same (the null hypothesis). Only when the population standard deviation is known and the sample size is 30 data points or more, can a z-test be applied.
A statistical test called a t-test is employed to compare the means of two groups. To determine whether two groups differ or if a procedure or treatment affects the population of interest, it is frequently used in hypothesis testing.
Chi-Square
You utilize a Chi-square test for hypothesis testing concerning whether your data is as predicted. To determine if the expected and observed results are well-fitted, the Chi-square test analyzes the differences between categorical variables from a random sample. The test's fundamental premise is that the observed values in your data should be compared to the predicted values that would be present if the null hypothesis were true.
Hypothesis Testing and Confidence Intervals
Both confidence intervals and hypothesis tests are inferential techniques that depend on approximating the sample distribution. Data from a sample is used to estimate a population parameter using confidence intervals. Data from a sample is used in hypothesis testing to examine a given hypothesis. We must have a postulated parameter to conduct hypothesis testing.
Bootstrap distributions and randomization distributions are created using comparable simulation techniques. The observed sample statistic is the focal point of a bootstrap distribution, whereas the null hypothesis value is the focal point of a randomization distribution.
A variety of feasible population parameter estimates are included in confidence ranges. In this lesson, we created just two-tailed confidence intervals. There is a direct connection between these two-tail confidence intervals and these two-tail hypothesis tests. The results of a two-tailed hypothesis test and two-tailed confidence intervals typically provide the same results. In other words, a hypothesis test at the 0.05 level will virtually always fail to reject the null hypothesis if the 95% confidence interval contains the predicted value. A hypothesis test at the 0.05 level will nearly certainly reject the null hypothesis if the 95% confidence interval does not include the hypothesized parameter.
Simple and Composite Hypothesis Testing
Depending on the population distribution, you can classify the statistical hypothesis into two types.
Simple Hypothesis: A simple hypothesis specifies an exact value for the parameter.
Composite Hypothesis: A composite hypothesis specifies a range of values.
A company is claiming that their average sales for this quarter are 1000 units. This is an example of a simple hypothesis.
Suppose the company claims that the sales are in the range of 900 to 1000 units. Then this is a case of a composite hypothesis.
One-Tailed and Two-Tailed Hypothesis Testing
The One-Tailed test, also called a directional test, considers a critical region of data that would result in the null hypothesis being rejected if the test sample falls into it, inevitably meaning the acceptance of the alternate hypothesis.
In a one-tailed test, the critical distribution area is one-sided, meaning the test sample is either greater or lesser than a specific value.
In two tails, the test sample is checked to be greater or less than a range of values in a Two-Tailed test, implying that the critical distribution area is two-sided.
If the sample falls within this range, the alternate hypothesis will be accepted, and the null hypothesis will be rejected.
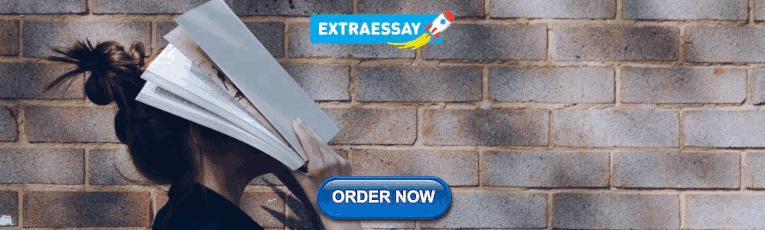
Become a Data Scientist With Real-World Experience
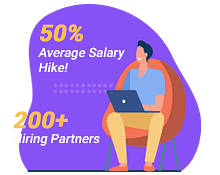
Right Tailed Hypothesis Testing
If the larger than (>) sign appears in your hypothesis statement, you are using a right-tailed test, also known as an upper test. Or, to put it another way, the disparity is to the right. For instance, you can contrast the battery life before and after a change in production. Your hypothesis statements can be the following if you want to know if the battery life is longer than the original (let's say 90 hours):
- The null hypothesis is (H0 <= 90) or less change.
- A possibility is that battery life has risen (H1) > 90.
The crucial point in this situation is that the alternate hypothesis (H1), not the null hypothesis, decides whether you get a right-tailed test.
Left Tailed Hypothesis Testing
Alternative hypotheses that assert the true value of a parameter is lower than the null hypothesis are tested with a left-tailed test; they are indicated by the asterisk "<".
Suppose H0: mean = 50 and H1: mean not equal to 50
According to the H1, the mean can be greater than or less than 50. This is an example of a Two-tailed test.
In a similar manner, if H0: mean >=50, then H1: mean <50
Here the mean is less than 50. It is called a One-tailed test.
Type 1 and Type 2 Error
A hypothesis test can result in two types of errors.
Type 1 Error: A Type-I error occurs when sample results reject the null hypothesis despite being true.
Type 2 Error: A Type-II error occurs when the null hypothesis is not rejected when it is false, unlike a Type-I error.
Suppose a teacher evaluates the examination paper to decide whether a student passes or fails.
H0: Student has passed
H1: Student has failed
Type I error will be the teacher failing the student [rejects H0] although the student scored the passing marks [H0 was true].
Type II error will be the case where the teacher passes the student [do not reject H0] although the student did not score the passing marks [H1 is true].
Level of Significance
The alpha value is a criterion for determining whether a test statistic is statistically significant. In a statistical test, Alpha represents an acceptable probability of a Type I error. Because alpha is a probability, it can be anywhere between 0 and 1. In practice, the most commonly used alpha values are 0.01, 0.05, and 0.1, which represent a 1%, 5%, and 10% chance of a Type I error, respectively (i.e. rejecting the null hypothesis when it is in fact correct).
Future-Proof Your AI/ML Career: Top Dos and Don'ts
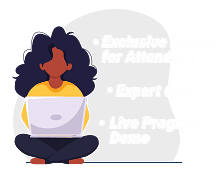
A p-value is a metric that expresses the likelihood that an observed difference could have occurred by chance. As the p-value decreases the statistical significance of the observed difference increases. If the p-value is too low, you reject the null hypothesis.
Here you have taken an example in which you are trying to test whether the new advertising campaign has increased the product's sales. The p-value is the likelihood that the null hypothesis, which states that there is no change in the sales due to the new advertising campaign, is true. If the p-value is .30, then there is a 30% chance that there is no increase or decrease in the product's sales. If the p-value is 0.03, then there is a 3% probability that there is no increase or decrease in the sales value due to the new advertising campaign. As you can see, the lower the p-value, the chances of the alternate hypothesis being true increases, which means that the new advertising campaign causes an increase or decrease in sales.
Why is Hypothesis Testing Important in Research Methodology?
Hypothesis testing is crucial in research methodology for several reasons:
- Provides evidence-based conclusions: It allows researchers to make objective conclusions based on empirical data, providing evidence to support or refute their research hypotheses.
- Supports decision-making: It helps make informed decisions, such as accepting or rejecting a new treatment, implementing policy changes, or adopting new practices.
- Adds rigor and validity: It adds scientific rigor to research using statistical methods to analyze data, ensuring that conclusions are based on sound statistical evidence.
- Contributes to the advancement of knowledge: By testing hypotheses, researchers contribute to the growth of knowledge in their respective fields by confirming existing theories or discovering new patterns and relationships.
Limitations of Hypothesis Testing
Hypothesis testing has some limitations that researchers should be aware of:
- It cannot prove or establish the truth: Hypothesis testing provides evidence to support or reject a hypothesis, but it cannot confirm the absolute truth of the research question.
- Results are sample-specific: Hypothesis testing is based on analyzing a sample from a population, and the conclusions drawn are specific to that particular sample.
- Possible errors: During hypothesis testing, there is a chance of committing type I error (rejecting a true null hypothesis) or type II error (failing to reject a false null hypothesis).
- Assumptions and requirements: Different tests have specific assumptions and requirements that must be met to accurately interpret results.
After reading this tutorial, you would have a much better understanding of hypothesis testing, one of the most important concepts in the field of Data Science . The majority of hypotheses are based on speculation about observed behavior, natural phenomena, or established theories.
If you are interested in statistics of data science and skills needed for such a career, you ought to explore Simplilearn’s Post Graduate Program in Data Science.
If you have any questions regarding this ‘Hypothesis Testing In Statistics’ tutorial, do share them in the comment section. Our subject matter expert will respond to your queries. Happy learning!
1. What is hypothesis testing in statistics with example?
Hypothesis testing is a statistical method used to determine if there is enough evidence in a sample data to draw conclusions about a population. It involves formulating two competing hypotheses, the null hypothesis (H0) and the alternative hypothesis (Ha), and then collecting data to assess the evidence. An example: testing if a new drug improves patient recovery (Ha) compared to the standard treatment (H0) based on collected patient data.
2. What is hypothesis testing and its types?
Hypothesis testing is a statistical method used to make inferences about a population based on sample data. It involves formulating two hypotheses: the null hypothesis (H0), which represents the default assumption, and the alternative hypothesis (Ha), which contradicts H0. The goal is to assess the evidence and determine whether there is enough statistical significance to reject the null hypothesis in favor of the alternative hypothesis.
Types of hypothesis testing:
- One-sample test: Used to compare a sample to a known value or a hypothesized value.
- Two-sample test: Compares two independent samples to assess if there is a significant difference between their means or distributions.
- Paired-sample test: Compares two related samples, such as pre-test and post-test data, to evaluate changes within the same subjects over time or under different conditions.
- Chi-square test: Used to analyze categorical data and determine if there is a significant association between variables.
- ANOVA (Analysis of Variance): Compares means across multiple groups to check if there is a significant difference between them.
3. What are the steps of hypothesis testing?
The steps of hypothesis testing are as follows:
- Formulate the hypotheses: State the null hypothesis (H0) and the alternative hypothesis (Ha) based on the research question.
- Set the significance level: Determine the acceptable level of error (alpha) for making a decision.
- Collect and analyze data: Gather and process the sample data.
- Compute test statistic: Calculate the appropriate statistical test to assess the evidence.
- Make a decision: Compare the test statistic with critical values or p-values and determine whether to reject H0 in favor of Ha or not.
- Draw conclusions: Interpret the results and communicate the findings in the context of the research question.
4. What are the 2 types of hypothesis testing?
- One-tailed (or one-sided) test: Tests for the significance of an effect in only one direction, either positive or negative.
- Two-tailed (or two-sided) test: Tests for the significance of an effect in both directions, allowing for the possibility of a positive or negative effect.
The choice between one-tailed and two-tailed tests depends on the specific research question and the directionality of the expected effect.
5. What are the 3 major types of hypothesis?
The three major types of hypotheses are:
- Null Hypothesis (H0): Represents the default assumption, stating that there is no significant effect or relationship in the data.
- Alternative Hypothesis (Ha): Contradicts the null hypothesis and proposes a specific effect or relationship that researchers want to investigate.
- Nondirectional Hypothesis: An alternative hypothesis that doesn't specify the direction of the effect, leaving it open for both positive and negative possibilities.
Find our Data Analyst Online Bootcamp in top cities:
About the author.
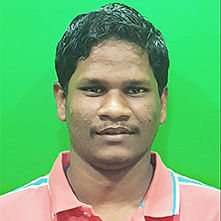
Avijeet is a Senior Research Analyst at Simplilearn. Passionate about Data Analytics, Machine Learning, and Deep Learning, Avijeet is also interested in politics, cricket, and football.
Recommended Resources

Free eBook: Top Programming Languages For A Data Scientist
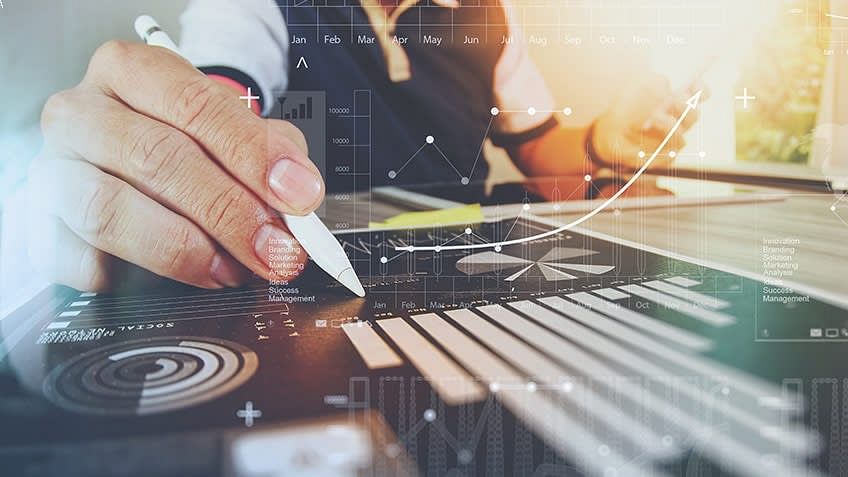
Normality Test in Minitab: Minitab with Statistics

Machine Learning Career Guide: A Playbook to Becoming a Machine Learning Engineer
- PMP, PMI, PMBOK, CAPM, PgMP, PfMP, ACP, PBA, RMP, SP, and OPM3 are registered marks of the Project Management Institute, Inc.
Get science-backed answers as you write with Paperpal's Research feature
How to Write a Hypothesis? Types and Examples
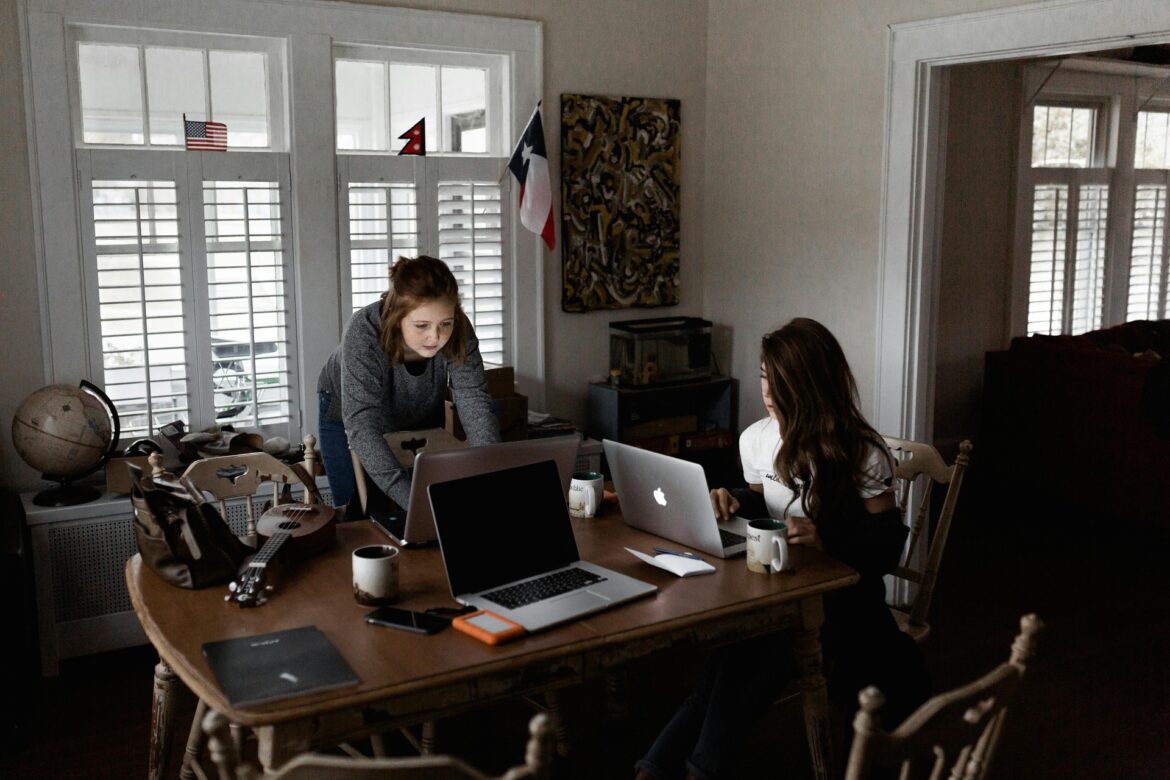
All research studies involve the use of the scientific method, which is a mathematical and experimental technique used to conduct experiments by developing and testing a hypothesis or a prediction about an outcome. Simply put, a hypothesis is a suggested solution to a problem. It includes elements that are expressed in terms of relationships with each other to explain a condition or an assumption that hasn’t been verified using facts. 1 The typical steps in a scientific method include developing such a hypothesis, testing it through various methods, and then modifying it based on the outcomes of the experiments.
A research hypothesis can be defined as a specific, testable prediction about the anticipated results of a study. 2 Hypotheses help guide the research process and supplement the aim of the study. After several rounds of testing, hypotheses can help develop scientific theories. 3 Hypotheses are often written as if-then statements.
Here are two hypothesis examples:
Dandelions growing in nitrogen-rich soils for two weeks develop larger leaves than those in nitrogen-poor soils because nitrogen stimulates vegetative growth. 4
If a company offers flexible work hours, then their employees will be happier at work. 5
Table of Contents
- What is a hypothesis?
- Types of hypotheses
- Characteristics of a hypothesis
- Functions of a hypothesis
- How to write a hypothesis
- Hypothesis examples
- Frequently asked questions
What is a hypothesis?
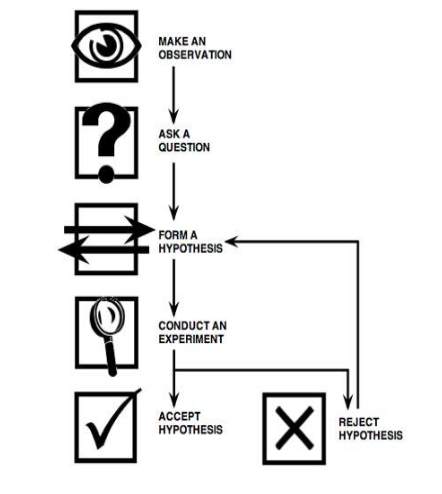
A hypothesis expresses an expected relationship between variables in a study and is developed before conducting any research. Hypotheses are not opinions but rather are expected relationships based on facts and observations. They help support scientific research and expand existing knowledge. An incorrectly formulated hypothesis can affect the entire experiment leading to errors in the results so it’s important to know how to formulate a hypothesis and develop it carefully.
A few sources of a hypothesis include observations from prior studies, current research and experiences, competitors, scientific theories, and general conditions that can influence people. Figure 1 depicts the different steps in a research design and shows where exactly in the process a hypothesis is developed. 4
There are seven different types of hypotheses—simple, complex, directional, nondirectional, associative and causal, null, and alternative.
Types of hypotheses
The seven types of hypotheses are listed below: 5 , 6,7
- Simple : Predicts the relationship between a single dependent variable and a single independent variable.
Example: Exercising in the morning every day will increase your productivity.
- Complex : Predicts the relationship between two or more variables.
Example: Spending three hours or more on social media daily will negatively affect children’s mental health and productivity, more than that of adults.
- Directional : Specifies the expected direction to be followed and uses terms like increase, decrease, positive, negative, more, or less.
Example: The inclusion of intervention X decreases infant mortality compared to the original treatment.
- Non-directional : Does not predict the exact direction, nature, or magnitude of the relationship between two variables but rather states the existence of a relationship. This hypothesis may be used when there is no underlying theory or if findings contradict prior research.
Example: Cats and dogs differ in the amount of affection they express.
- Associative and causal : An associative hypothesis suggests an interdependency between variables, that is, how a change in one variable changes the other.
Example: There is a positive association between physical activity levels and overall health.
A causal hypothesis, on the other hand, expresses a cause-and-effect association between variables.
Example: Long-term alcohol use causes liver damage.
- Null : Claims that the original hypothesis is false by showing that there is no relationship between the variables.
Example: Sleep duration does not have any effect on productivity.
- Alternative : States the opposite of the null hypothesis, that is, a relationship exists between two variables.
Example: Sleep duration affects productivity.
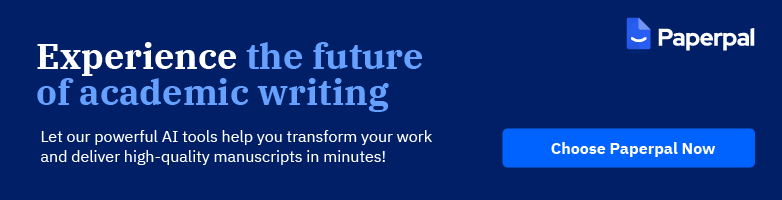
Characteristics of a hypothesis
So, what makes a good hypothesis? Here are some important characteristics of a hypothesis. 8,9
- Testable : You must be able to test the hypothesis using scientific methods to either accept or reject the prediction.
- Falsifiable : It should be possible to collect data that reject rather than support the hypothesis.
- Logical : Hypotheses shouldn’t be a random guess but rather should be based on previous theories, observations, prior research, and logical reasoning.
- Positive : The hypothesis statement about the existence of an association should be positive, that is, it should not suggest that an association does not exist. Therefore, the language used and knowing how to phrase a hypothesis is very important.
- Clear and accurate : The language used should be easily comprehensible and use correct terminology.
- Relevant : The hypothesis should be relevant and specific to the research question.
- Structure : Should include all the elements that make a good hypothesis: variables, relationship, and outcome.
Functions of a hypothesis
The following list mentions some important functions of a hypothesis: 1
- Maintains the direction and progress of the research.
- Expresses the important assumptions underlying the proposition in a single statement.
- Establishes a suitable context for researchers to begin their investigation and for readers who are referring to the final report.
- Provides an explanation for the occurrence of a specific phenomenon.
- Ensures selection of appropriate and accurate facts necessary and relevant to the research subject.
To summarize, a hypothesis provides the conceptual elements that complete the known data, conceptual relationships that systematize unordered elements, and conceptual meanings and interpretations that explain the unknown phenomena. 1
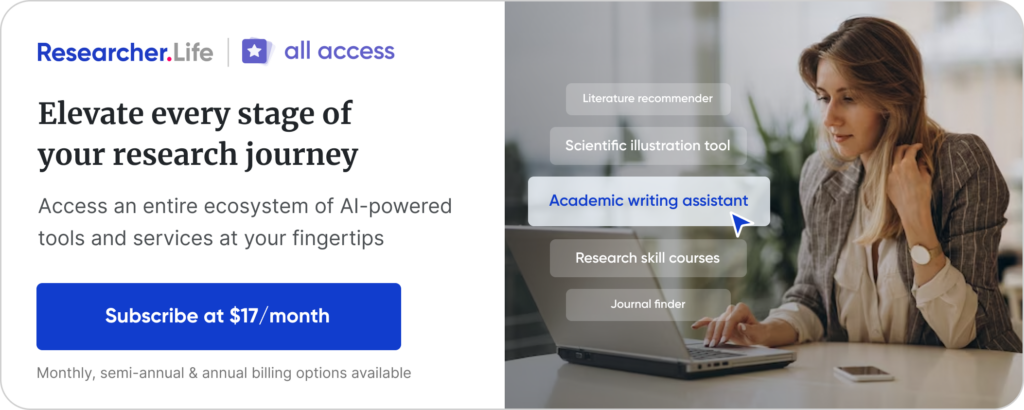
How to write a hypothesis
Listed below are the main steps explaining how to write a hypothesis. 2,4,5
- Make an observation and identify variables : Observe the subject in question and try to recognize a pattern or a relationship between the variables involved. This step provides essential background information to begin your research.
For example, if you notice that an office’s vending machine frequently runs out of a specific snack, you may predict that more people in the office choose that snack over another.
- Identify the main research question : After identifying a subject and recognizing a pattern, the next step is to ask a question that your hypothesis will answer.
For example, after observing employees’ break times at work, you could ask “why do more employees take breaks in the morning rather than in the afternoon?”
- Conduct some preliminary research to ensure originality and novelty : Your initial answer, which is your hypothesis, to the question is based on some pre-existing information about the subject. However, to ensure that your hypothesis has not been asked before or that it has been asked but rejected by other researchers you would need to gather additional information.
For example, based on your observations you might state a hypothesis that employees work more efficiently when the air conditioning in the office is set at a lower temperature. However, during your preliminary research you find that this hypothesis was proven incorrect by a prior study.
- Develop a general statement : After your preliminary research has confirmed the originality of your proposed answer, draft a general statement that includes all variables, subjects, and predicted outcome. The statement could be if/then or declarative.
- Finalize the hypothesis statement : Use the PICOT model, which clarifies how to word a hypothesis effectively, when finalizing the statement. This model lists the important components required to write a hypothesis.
P opulation: The specific group or individual who is the main subject of the research
I nterest: The main concern of the study/research question
C omparison: The main alternative group
O utcome: The expected results
T ime: Duration of the experiment
Once you’ve finalized your hypothesis statement you would need to conduct experiments to test whether the hypothesis is true or false.
Hypothesis examples
The following table provides examples of different types of hypotheses. 10 ,11
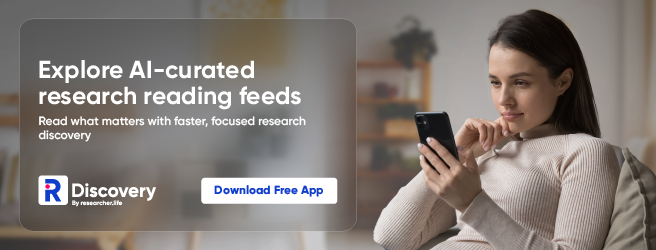
Key takeaways
Here’s a summary of all the key points discussed in this article about how to write a hypothesis.
- A hypothesis is an assumption about an association between variables made based on limited evidence, which should be tested.
- A hypothesis has four parts—the research question, independent variable, dependent variable, and the proposed relationship between the variables.
- The statement should be clear, concise, testable, logical, and falsifiable.
- There are seven types of hypotheses—simple, complex, directional, non-directional, associative and causal, null, and alternative.
- A hypothesis provides a focus and direction for the research to progress.
- A hypothesis plays an important role in the scientific method by helping to create an appropriate experimental design.
Frequently asked questions
Hypotheses and research questions have different objectives and structure. The following table lists some major differences between the two. 9
Here are a few examples to differentiate between a research question and hypothesis.
Yes, here’s a simple checklist to help you gauge the effectiveness of your hypothesis. 9 1. When writing a hypothesis statement, check if it: 2. Predicts the relationship between the stated variables and the expected outcome. 3. Uses simple and concise language and is not wordy. 4. Does not assume readers’ knowledge about the subject. 5. Has observable, falsifiable, and testable results.
As mentioned earlier in this article, a hypothesis is an assumption or prediction about an association between variables based on observations and simple evidence. These statements are usually generic. Research objectives, on the other hand, are more specific and dictated by hypotheses. The same hypothesis can be tested using different methods and the research objectives could be different in each case. For example, Louis Pasteur observed that food lasts longer at higher altitudes, reasoned that it could be because the air at higher altitudes is cleaner (with fewer or no germs), and tested the hypothesis by exposing food to air cleaned in the laboratory. 12 Thus, a hypothesis is predictive—if the reasoning is correct, X will lead to Y—and research objectives are developed to test these predictions.
Null hypothesis testing is a method to decide between two assumptions or predictions between variables (null and alternative hypotheses) in a statistical relationship in a sample. The null hypothesis, denoted as H 0 , claims that no relationship exists between variables in a population and any relationship in the sample reflects a sampling error or occurrence by chance. The alternative hypothesis, denoted as H 1 , claims that there is a relationship in the population. In every study, researchers need to decide whether the relationship in a sample occurred by chance or reflects a relationship in the population. This is done by hypothesis testing using the following steps: 13 1. Assume that the null hypothesis is true. 2. Determine how likely the sample relationship would be if the null hypothesis were true. This probability is called the p value. 3. If the sample relationship would be extremely unlikely, reject the null hypothesis and accept the alternative hypothesis. If the relationship would not be unlikely, accept the null hypothesis.
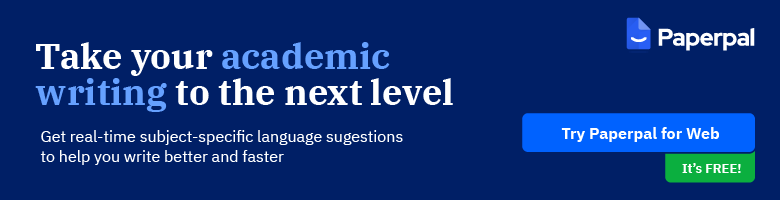
To summarize, researchers should know how to write a good hypothesis to ensure that their research progresses in the required direction. A hypothesis is a testable prediction about any behavior or relationship between variables, usually based on facts and observation, and states an expected outcome.
We hope this article has provided you with essential insight into the different types of hypotheses and their functions so that you can use them appropriately in your next research project.
References
- Dalen, DVV. The function of hypotheses in research. Proquest website. Accessed April 8, 2024. https://www.proquest.com/docview/1437933010?pq-origsite=gscholar&fromopenview=true&sourcetype=Scholarly%20Journals&imgSeq=1
- McLeod S. Research hypothesis in psychology: Types & examples. SimplyPsychology website. Updated December 13, 2023. Accessed April 9, 2024. https://www.simplypsychology.org/what-is-a-hypotheses.html
- Scientific method. Britannica website. Updated March 14, 2024. Accessed April 9, 2024. https://www.britannica.com/science/scientific-method
- The hypothesis in science writing. Accessed April 10, 2024. https://berks.psu.edu/sites/berks/files/campus/HypothesisHandout_Final.pdf
- How to develop a hypothesis (with elements, types, and examples). Indeed.com website. Updated February 3, 2023. Accessed April 10, 2024. https://www.indeed.com/career-advice/career-development/how-to-write-a-hypothesis
- Types of research hypotheses. Excelsior online writing lab. Accessed April 11, 2024. https://owl.excelsior.edu/research/research-hypotheses/types-of-research-hypotheses/
- What is a research hypothesis: how to write it, types, and examples. Researcher.life website. Published February 8, 2023. Accessed April 11, 2024. https://researcher.life/blog/article/how-to-write-a-research-hypothesis-definition-types-examples/
- Developing a hypothesis. Pressbooks website. Accessed April 12, 2024. https://opentext.wsu.edu/carriecuttler/chapter/developing-a-hypothesis/
- What is and how to write a good hypothesis in research. Elsevier author services website. Accessed April 12, 2024. https://scientific-publishing.webshop.elsevier.com/manuscript-preparation/what-how-write-good-hypothesis-research/
- How to write a great hypothesis. Verywellmind website. Updated March 12, 2023. Accessed April 13, 2024. https://www.verywellmind.com/what-is-a-hypothesis-2795239
- 15 Hypothesis examples. Helpfulprofessor.com Published September 8, 2023. Accessed March 14, 2024. https://helpfulprofessor.com/hypothesis-examples/
- Editage insights. What is the interconnectivity between research objectives and hypothesis? Published February 24, 2021. Accessed April 13, 2024. https://www.editage.com/insights/what-is-the-interconnectivity-between-research-objectives-and-hypothesis
- Understanding null hypothesis testing. BCCampus open publishing. Accessed April 16, 2024. https://opentextbc.ca/researchmethods/chapter/understanding-null-hypothesis-testing/#:~:text=In%20null%20hypothesis%20testing%2C%20this,said%20to%20be%20statistically%20significant
Paperpal is a comprehensive AI writing toolkit that helps students and researchers achieve 2x the writing in half the time. It leverages 21+ years of STM experience and insights from millions of research articles to provide in-depth academic writing, language editing, and submission readiness support to help you write better, faster.
Get accurate academic translations, rewriting support, grammar checks, vocabulary suggestions, and generative AI assistance that delivers human precision at machine speed. Try for free or upgrade to Paperpal Prime starting at US$19 a month to access premium features, including consistency, plagiarism, and 30+ submission readiness checks to help you succeed.
Experience the future of academic writing – Sign up to Paperpal and start writing for free!
Related Reads:
- Empirical Research: A Comprehensive Guide for Academics
- How to Write a Scientific Paper in 10 Steps
- What is a Literature Review? How to Write It (with Examples)
- What are Journal Guidelines on Using Generative AI Tools
Measuring Academic Success: Definition & Strategies for Excellence
What are scholarly sources and where can you find them , you may also like, what are scholarly sources and where can you..., what is academic writing: tips for students, why traditional editorial process needs an upgrade, paperpal’s new ai research finder empowers authors to..., what is hedging in academic writing , how to use ai to enhance your college..., ai + human expertise – a paradigm shift..., how to use paperpal to generate emails &..., ai in education: it’s time to change the....
- Machine Learning Tutorial
- Data Analysis Tutorial
- Python - Data visualization tutorial
- Machine Learning Projects
- Machine Learning Interview Questions
- Machine Learning Mathematics
- Deep Learning Tutorial
- Deep Learning Project
- Deep Learning Interview Questions
- Computer Vision Tutorial
- Computer Vision Projects
- NLP Project
- NLP Interview Questions
- Statistics with Python
- 100 Days of Machine Learning
- Data Analysis with Python
Introduction to Data Analysis
- What is Data Analysis?
- Data Analytics and its type
- How to Install Numpy on Windows?
- How to Install Pandas in Python?
- How to Install Matplotlib on python?
- How to Install Python Tensorflow in Windows?
Data Analysis Libraries
- Pandas Tutorial
- NumPy Tutorial - Python Library
- Data Analysis with SciPy
- Introduction to TensorFlow
Data Visulization Libraries
- Matplotlib Tutorial
- Python Seaborn Tutorial
- Plotly tutorial
- Introduction to Bokeh in Python
Exploratory Data Analysis (EDA)
- Univariate, Bivariate and Multivariate data and its analysis
- Measures of Central Tendency in Statistics
- Measures of spread - Range, Variance, and Standard Deviation
- Interquartile Range and Quartile Deviation using NumPy and SciPy
- Anova Formula
- Skewness of Statistical Data
- How to Calculate Skewness and Kurtosis in Python?
- Difference Between Skewness and Kurtosis
- Histogram | Meaning, Example, Types and Steps to Draw
- Interpretations of Histogram
- Quantile Quantile plots
- What is Univariate, Bivariate & Multivariate Analysis in Data Visualisation?
- Using pandas crosstab to create a bar plot
- Exploring Correlation in Python
- Mathematics | Covariance and Correlation
- Factor Analysis | Data Analysis
- Data Mining - Cluster Analysis
- MANOVA Test in R Programming
- Python - Central Limit Theorem
- Probability Distribution Function
- Probability Density Estimation & Maximum Likelihood Estimation
- Exponential Distribution in R Programming - dexp(), pexp(), qexp(), and rexp() Functions
- Mathematics | Probability Distributions Set 4 (Binomial Distribution)
- Poisson Distribution - Definition, Formula, Table and Examples
- P-Value: Comprehensive Guide to Understand, Apply, and Interpret
- Z-Score in Statistics
- How to Calculate Point Estimates in R?
- Confidence Interval
- Chi-square test in Machine Learning
Understanding Hypothesis Testing
Data preprocessing.
- ML | Data Preprocessing in Python
- ML | Overview of Data Cleaning
- ML | Handling Missing Values
- Detect and Remove the Outliers using Python
Data Transformation
- Data Normalization Machine Learning
- Sampling distribution Using Python
Time Series Data Analysis
- Data Mining - Time-Series, Symbolic and Biological Sequences Data
- Basic DateTime Operations in Python
- Time Series Analysis & Visualization in Python
- How to deal with missing values in a Timeseries in Python?
- How to calculate MOVING AVERAGE in a Pandas DataFrame?
- What is a trend in time series?
- How to Perform an Augmented Dickey-Fuller Test in R
- AutoCorrelation
Case Studies and Projects
- Top 8 Free Dataset Sources to Use for Data Science Projects
- Step by Step Predictive Analysis - Machine Learning
- 6 Tips for Creating Effective Data Visualizations
Hypothesis testing involves formulating assumptions about population parameters based on sample statistics and rigorously evaluating these assumptions against empirical evidence. This article sheds light on the significance of hypothesis testing and the critical steps involved in the process.
What is Hypothesis Testing?
Hypothesis testing is a statistical method that is used to make a statistical decision using experimental data. Hypothesis testing is basically an assumption that we make about a population parameter. It evaluates two mutually exclusive statements about a population to determine which statement is best supported by the sample data.
Example: You say an average height in the class is 30 or a boy is taller than a girl. All of these is an assumption that we are assuming, and we need some statistical way to prove these. We need some mathematical conclusion whatever we are assuming is true.
Defining Hypotheses

Key Terms of Hypothesis Testing

- P-value: The P value , or calculated probability, is the probability of finding the observed/extreme results when the null hypothesis(H0) of a study-given problem is true. If your P-value is less than the chosen significance level then you reject the null hypothesis i.e. accept that your sample claims to support the alternative hypothesis.
- Test Statistic: The test statistic is a numerical value calculated from sample data during a hypothesis test, used to determine whether to reject the null hypothesis. It is compared to a critical value or p-value to make decisions about the statistical significance of the observed results.
- Critical value : The critical value in statistics is a threshold or cutoff point used to determine whether to reject the null hypothesis in a hypothesis test.
- Degrees of freedom: Degrees of freedom are associated with the variability or freedom one has in estimating a parameter. The degrees of freedom are related to the sample size and determine the shape.
Why do we use Hypothesis Testing?
Hypothesis testing is an important procedure in statistics. Hypothesis testing evaluates two mutually exclusive population statements to determine which statement is most supported by sample data. When we say that the findings are statistically significant, thanks to hypothesis testing.
One-Tailed and Two-Tailed Test
One tailed test focuses on one direction, either greater than or less than a specified value. We use a one-tailed test when there is a clear directional expectation based on prior knowledge or theory. The critical region is located on only one side of the distribution curve. If the sample falls into this critical region, the null hypothesis is rejected in favor of the alternative hypothesis.
One-Tailed Test
There are two types of one-tailed test:

Two-Tailed Test
A two-tailed test considers both directions, greater than and less than a specified value.We use a two-tailed test when there is no specific directional expectation, and want to detect any significant difference.

What are Type 1 and Type 2 errors in Hypothesis Testing?
In hypothesis testing, Type I and Type II errors are two possible errors that researchers can make when drawing conclusions about a population based on a sample of data. These errors are associated with the decisions made regarding the null hypothesis and the alternative hypothesis.

How does Hypothesis Testing work?
Step 1: define null and alternative hypothesis.

We first identify the problem about which we want to make an assumption keeping in mind that our assumption should be contradictory to one another, assuming Normally distributed data.
Step 2 – Choose significance level

Step 3 – Collect and Analyze data.
Gather relevant data through observation or experimentation. Analyze the data using appropriate statistical methods to obtain a test statistic.
Step 4-Calculate Test Statistic
The data for the tests are evaluated in this step we look for various scores based on the characteristics of data. The choice of the test statistic depends on the type of hypothesis test being conducted.
There are various hypothesis tests, each appropriate for various goal to calculate our test. This could be a Z-test , Chi-square , T-test , and so on.
- Z-test : If population means and standard deviations are known. Z-statistic is commonly used.
- t-test : If population standard deviations are unknown. and sample size is small than t-test statistic is more appropriate.
- Chi-square test : Chi-square test is used for categorical data or for testing independence in contingency tables
- F-test : F-test is often used in analysis of variance (ANOVA) to compare variances or test the equality of means across multiple groups.
We have a smaller dataset, So, T-test is more appropriate to test our hypothesis.
T-statistic is a measure of the difference between the means of two groups relative to the variability within each group. It is calculated as the difference between the sample means divided by the standard error of the difference. It is also known as the t-value or t-score.
Step 5 – Comparing Test Statistic:
In this stage, we decide where we should accept the null hypothesis or reject the null hypothesis. There are two ways to decide where we should accept or reject the null hypothesis.
Method A: Using Crtical values
Comparing the test statistic and tabulated critical value we have,
- If Test Statistic>Critical Value: Reject the null hypothesis.
- If Test Statistic≤Critical Value: Fail to reject the null hypothesis.
Note: Critical values are predetermined threshold values that are used to make a decision in hypothesis testing. To determine critical values for hypothesis testing, we typically refer to a statistical distribution table , such as the normal distribution or t-distribution tables based on.
Method B: Using P-values
We can also come to an conclusion using the p-value,

Note : The p-value is the probability of obtaining a test statistic as extreme as, or more extreme than, the one observed in the sample, assuming the null hypothesis is true. To determine p-value for hypothesis testing, we typically refer to a statistical distribution table , such as the normal distribution or t-distribution tables based on.
Step 7- Interpret the Results
At last, we can conclude our experiment using method A or B.
Calculating test statistic
To validate our hypothesis about a population parameter we use statistical functions . We use the z-score, p-value, and level of significance(alpha) to make evidence for our hypothesis for normally distributed data .
1. Z-statistics:
When population means and standard deviations are known.

- μ represents the population mean,
- σ is the standard deviation
- and n is the size of the sample.
2. T-Statistics
T test is used when n<30,
t-statistic calculation is given by:

- t = t-score,
- x̄ = sample mean
- μ = population mean,
- s = standard deviation of the sample,
- n = sample size
3. Chi-Square Test
Chi-Square Test for Independence categorical Data (Non-normally distributed) using:

- i,j are the rows and columns index respectively.

Real life Hypothesis Testing example
Let’s examine hypothesis testing using two real life situations,
Case A: D oes a New Drug Affect Blood Pressure?
Imagine a pharmaceutical company has developed a new drug that they believe can effectively lower blood pressure in patients with hypertension. Before bringing the drug to market, they need to conduct a study to assess its impact on blood pressure.
- Before Treatment: 120, 122, 118, 130, 125, 128, 115, 121, 123, 119
- After Treatment: 115, 120, 112, 128, 122, 125, 110, 117, 119, 114
Step 1 : Define the Hypothesis
- Null Hypothesis : (H 0 )The new drug has no effect on blood pressure.
- Alternate Hypothesis : (H 1 )The new drug has an effect on blood pressure.
Step 2: Define the Significance level
Let’s consider the Significance level at 0.05, indicating rejection of the null hypothesis.
If the evidence suggests less than a 5% chance of observing the results due to random variation.
Step 3 : Compute the test statistic
Using paired T-test analyze the data to obtain a test statistic and a p-value.
The test statistic (e.g., T-statistic) is calculated based on the differences between blood pressure measurements before and after treatment.
t = m/(s/√n)
- m = mean of the difference i.e X after, X before
- s = standard deviation of the difference (d) i.e d i = X after, i − X before,
- n = sample size,
then, m= -3.9, s= 1.8 and n= 10
we, calculate the , T-statistic = -9 based on the formula for paired t test
Step 4: Find the p-value
The calculated t-statistic is -9 and degrees of freedom df = 9, you can find the p-value using statistical software or a t-distribution table.
thus, p-value = 8.538051223166285e-06
Step 5: Result
- If the p-value is less than or equal to 0.05, the researchers reject the null hypothesis.
- If the p-value is greater than 0.05, they fail to reject the null hypothesis.
Conclusion: Since the p-value (8.538051223166285e-06) is less than the significance level (0.05), the researchers reject the null hypothesis. There is statistically significant evidence that the average blood pressure before and after treatment with the new drug is different.
Python Implementation of Hypothesis Testing
Let’s create hypothesis testing with python, where we are testing whether a new drug affects blood pressure. For this example, we will use a paired T-test. We’ll use the scipy.stats library for the T-test.
Scipy is a mathematical library in Python that is mostly used for mathematical equations and computations.
We will implement our first real life problem via python,
In the above example, given the T-statistic of approximately -9 and an extremely small p-value, the results indicate a strong case to reject the null hypothesis at a significance level of 0.05.
- The results suggest that the new drug, treatment, or intervention has a significant effect on lowering blood pressure.
- The negative T-statistic indicates that the mean blood pressure after treatment is significantly lower than the assumed population mean before treatment.
Case B : Cholesterol level in a population
Data: A sample of 25 individuals is taken, and their cholesterol levels are measured.
Cholesterol Levels (mg/dL): 205, 198, 210, 190, 215, 205, 200, 192, 198, 205, 198, 202, 208, 200, 205, 198, 205, 210, 192, 205, 198, 205, 210, 192, 205.
Populations Mean = 200
Population Standard Deviation (σ): 5 mg/dL(given for this problem)
Step 1: Define the Hypothesis
- Null Hypothesis (H 0 ): The average cholesterol level in a population is 200 mg/dL.
- Alternate Hypothesis (H 1 ): The average cholesterol level in a population is different from 200 mg/dL.
As the direction of deviation is not given , we assume a two-tailed test, and based on a normal distribution table, the critical values for a significance level of 0.05 (two-tailed) can be calculated through the z-table and are approximately -1.96 and 1.96.

Step 4: Result
Since the absolute value of the test statistic (2.04) is greater than the critical value (1.96), we reject the null hypothesis. And conclude that, there is statistically significant evidence that the average cholesterol level in the population is different from 200 mg/dL
Limitations of Hypothesis Testing
- Although a useful technique, hypothesis testing does not offer a comprehensive grasp of the topic being studied. Without fully reflecting the intricacy or whole context of the phenomena, it concentrates on certain hypotheses and statistical significance.
- The accuracy of hypothesis testing results is contingent on the quality of available data and the appropriateness of statistical methods used. Inaccurate data or poorly formulated hypotheses can lead to incorrect conclusions.
- Relying solely on hypothesis testing may cause analysts to overlook significant patterns or relationships in the data that are not captured by the specific hypotheses being tested. This limitation underscores the importance of complimenting hypothesis testing with other analytical approaches.
Hypothesis testing stands as a cornerstone in statistical analysis, enabling data scientists to navigate uncertainties and draw credible inferences from sample data. By systematically defining null and alternative hypotheses, choosing significance levels, and leveraging statistical tests, researchers can assess the validity of their assumptions. The article also elucidates the critical distinction between Type I and Type II errors, providing a comprehensive understanding of the nuanced decision-making process inherent in hypothesis testing. The real-life example of testing a new drug’s effect on blood pressure using a paired T-test showcases the practical application of these principles, underscoring the importance of statistical rigor in data-driven decision-making.
Frequently Asked Questions (FAQs)
1. what are the 3 types of hypothesis test.
There are three types of hypothesis tests: right-tailed, left-tailed, and two-tailed. Right-tailed tests assess if a parameter is greater, left-tailed if lesser. Two-tailed tests check for non-directional differences, greater or lesser.
2.What are the 4 components of hypothesis testing?
Null Hypothesis ( ): No effect or difference exists. Alternative Hypothesis ( ): An effect or difference exists. Significance Level ( ): Risk of rejecting null hypothesis when it’s true (Type I error). Test Statistic: Numerical value representing observed evidence against null hypothesis.
3.What is hypothesis testing in ML?
Statistical method to evaluate the performance and validity of machine learning models. Tests specific hypotheses about model behavior, like whether features influence predictions or if a model generalizes well to unseen data.
4.What is the difference between Pytest and hypothesis in Python?
Pytest purposes general testing framework for Python code while Hypothesis is a Property-based testing framework for Python, focusing on generating test cases based on specified properties of the code.
Please Login to comment...
Similar reads.
- data-science
- Data Science
- Machine Learning
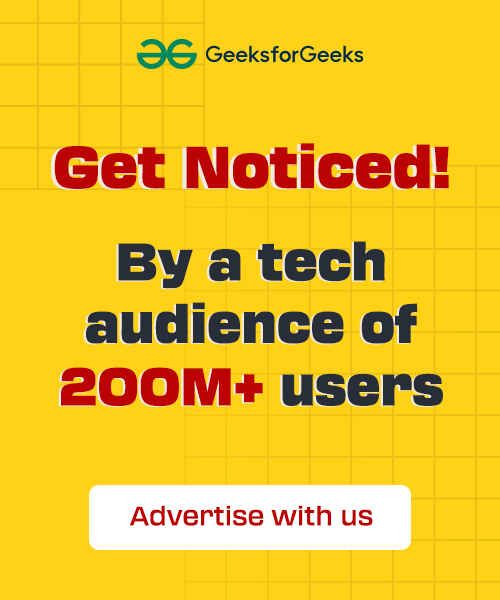
Improve your Coding Skills with Practice
What kind of Experience do you want to share?
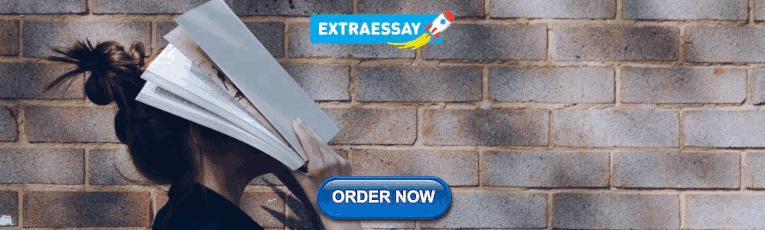
IMAGES
VIDEO
COMMENTS
Hypothesis testing is a formal procedure for investigating our ideas about the world using statistics. It is most often used by scientists to test specific predictions, called hypotheses, that arise from theories. ... In the formal language of hypothesis testing, we talk about rejecting or failing to reject the null hypothesis. You will ...
8-2 Basics of hypothesis testing In this section, 1st we introduce the language of hypothesis testing, then we discuss the formal process of testing a hypothesis. A hypothesis is a statement or claim regarding a characteristic of one or more population Hypothesis testing (or test of significance) is a procedure, based on a sample
The Four Steps in Hypothesis Testing. STEP 1: State the appropriate null and alternative hypotheses, Ho and Ha. STEP 2: Obtain a random sample, collect relevant data, and check whether the data meet the conditions under which the test can be used. If the conditions are met, summarize the data using a test statistic.
This page titled 9.1: Introduction to Hypothesis Testing is shared under a CC BY 2.0 license and was authored, remixed, and/or curated by Kyle Siegrist ( Random Services) via source content that was edited to the style and standards of the LibreTexts platform; a detailed edit history is available upon request. In hypothesis testing, the goal is ...
We will try to find evidence to support the alternative hypothesis. There are three general ways in this chapter that we'll set up the null and alternative hypothesis. two tailed. H 0: parameter = some value. H 1: parameter ≠ the value. left-tailed. H 0: parameter = some value. H 1: parameter < the value. right-tailed.
Online lesson: https://faculty.elgin.edu/dkernler/statistics/ch10/10-1.htmlIn this video, we introduce the idea of hypothesis testing, define the null and al...
Testing Hypotheses using Confidence Intervals. We can start the evaluation of the hypothesis setup by comparing 2006 and 2012 run times using a point estimate from the 2012 sample: x¯12 = 95.61 x ¯ 12 = 95.61 minutes. This estimate suggests the average time is actually longer than the 2006 time, 93.29 minutes.
Likelihood ratio. In the likelihood ratio test, we reject the null hypothesis if the ratio is above a certain value i.e, reject the null hypothesis if L(X) > 𝜉, else accept it. 𝜉 is called the critical ratio.. So this is how we can draw a decision boundary: we separate the observations for which the likelihood ratio is greater than the critical ratio from the observations for which it ...
11. Hypothesis testing. The process of induction is the process of assuming the simplest law that can be made to harmonize with our experience. This process, however, has no logical foundation but only a psychological one. It is clear that there are no grounds for believing that the simplest course of events will really happen.
Let's translate into hypothesis testing language: Null Hypothesis: Probability of landing on Heads = 0.5. Alt Hypothesis: Probability of landing on Heads != 0.5. Each coin flip is a Bernoulli trial, which is an experiment with two outcomes — outcome 1, "success", (probability p) and outcome 0, "fail" (probability 1-p). The reason it ...
The assessment model, characterized as a hypothesis-testing process, is covered in three sections. First is an overview of several types of oral and written language impairment variations found in the school-aged population. Awareness of these variations informs the hypothesis-testing process.
The students came from different language backgrounds: native English, Spanish-speaking language minority, and Filipino-speaking language minority. The data were collected from the students over the course of two testing periods during their sixth-grade year. The authors tested their proposed model on all four language-background groups.
The above image shows a table with some of the most common test statistics and their corresponding tests or models.. A statistical hypothesis test is a method of statistical inference used to decide whether the data sufficiently support a particular hypothesis. A statistical hypothesis test typically involves a calculation of a test statistic.Then a decision is made, either by comparing the ...
A hypothesis test is a formal statistical test we use to reject or fail to reject some statistical hypothesis. This tutorial explains how to perform the following hypothesis tests in R: One sample t-test. Two sample t-test. Paired samples t-test. We can use the t.test () function in R to perform each type of test:
Test Statistic = a measure of the difference between the data and what is expected when the null hypothesis is true. In our example, the null hypothesis says the number of heads in 100 spins would closely follow the normal distribution with p = 0.5. So, if the null hypothesis is true, we expect half (0.5) heads give or take a standard deviation of.
The Unitary Trait Hypothesis. Language testing research in the 1970s was so preoccupied with John Oller's Unitary Trait Hypothesis and cloze procedure that Stansfield remarked, "one could call this the decade of John Oller" (p. 312, italics original). Oller was influenced by Carroll's research on intelligence, as well as his use of ...
Test Statistic: z = ¯ x − μo σ / √n since it is calculated as part of the testing of the hypothesis. Definition 7.1.4. p - value: probability that the test statistic will take on more extreme values than the observed test statistic, given that the null hypothesis is true.
A hypothesis is an assumption made by the researchers that are not mandatory true. In simple words, a hypothesis is a decision taken by the researchers based on the data of the population collected. Hypothesis Testing in R Programming is a process of testing the hypothesis made by the researcher or to validate the hypothesis. To perform ...
Hypothesis testing is a statistical method used to determine if there is enough evidence in a sample data to draw conclusions about a population. It involves formulating two competing hypotheses, the null hypothesis (H0) and the alternative hypothesis (Ha), and then collecting data to assess the evidence.
HYPOTHESIS TESTING IN SECOND-LANGUAGE ACQUISITION THEORY. Robert Bley-Vroman, Robert Bley-Vroman. University of Texas. Search for more papers by this author ... Learner hypotheses may be classified logically into two kinds depending on the relationship of the hypothesis to the data needed to test it. Type-N hypotheses require "negative ...
Characteristics of a hypothesis. So, what makes a good hypothesis? Here are some important characteristics of a hypothesis. 8,9 . Testable: You must be able to test the hypothesis using scientific methods to either accept or reject the prediction. Falsifiable: It should be possible to collect data that reject rather than support the hypothesis.
Hypothesis testing is a statistical method that is used to make a statistical decision using experimental data. Hypothesis testing is basically an assumption that we make about a population parameter. It evaluates two mutually exclusive statements about a population to determine which statement is best supported by the sample data.
Type-P hypotheses can be tested on the basis of "positive data" (comprehensible input, for example) alone. The difference between hypotheses is related to the intuitive concepts of ...