If you're seeing this message, it means we're having trouble loading external resources on our website.
If you're behind a web filter, please make sure that the domains *.kastatic.org and *.kasandbox.org are unblocked.
To log in and use all the features of Khan Academy, please enable JavaScript in your browser.
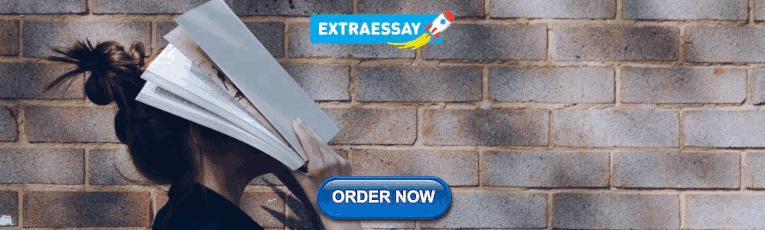
Unit 2: Structure of atom
Discovery of sub-atomic particles.
- The history of atomic chemistry (Opens a modal)
- Discovery of the electron and nucleus (Opens a modal)
Atomic models
- Rutherford’s gold foil experiment (Opens a modal)
- Atomic number, mass number, and isotopes (Opens a modal)
- Isotopes (Opens a modal)
- Worked example: Identifying isotopes and ions (Opens a modal)
- Isotope composition: Counting protons, electrons, and neutrons Get 5 of 7 questions to level up!
Wave nature of electromagnetic radiation
- Light: Electromagnetic waves, the electromagnetic spectrum and photons (Opens a modal)
- Electromagnetic waves and the electromagnetic spectrum (Opens a modal)
- Introduction to light (Opens a modal)
- Spectroscopy: Interaction of light and matter (Opens a modal)
- Properties of periodic waves (Opens a modal)
Particle Nature of electromagnetic radiation: Planck's quantum theory
- Photon Energy (Opens a modal)
- Photoelectric effect (Opens a modal)
Bohr's model of hydrogen atom
- Bohr's model of hydrogen (Opens a modal)
- Absorption/emission lines (Opens a modal)
- Absorption and emission (Opens a modal)
- Emission spectrum of hydrogen (Opens a modal)
- Bohr model radii (derivation using physics) (Opens a modal)
- Bohr model radii (Opens a modal)
- Bohr model energy levels (derivation using physics) (Opens a modal)
- Bohr model energy levels (Opens a modal)
- Atomic Energy Levels (Opens a modal)
Towards Quantum mechanical model of the atom
- De Broglie wavelength (Opens a modal)
- Heisenberg uncertainty principle (Opens a modal)
Quantum mechanical model of hydrogen atom
- Quantum Wavefunction (Opens a modal)
- The quantum mechanical model of the atom (Opens a modal)
- Quantum numbers (Opens a modal)
- Quantum numbers for the first four shells (Opens a modal)
- Shells, subshells, and orbitals (Opens a modal)
- The periodic table, electron shells, and orbitals (Opens a modal)
Filling of electrons in the orbitals
- The Aufbau principle (Opens a modal)
Electronic configuration of atom
- Introduction to electron configurations (Opens a modal)
- Electron configurations article (Opens a modal)
- Noble gas configuration (Opens a modal)
- Electron configurations for the first period (Opens a modal)
- Electron configurations for the second period (Opens a modal)
- Electron configurations for the third and fourth periods (Opens a modal)
- Electron configurations of the 3d transition metals (Opens a modal)
- Electron configurations of ions (Opens a modal)
- Electron configurations Get 5 of 7 questions to level up!
- Atomic structure and electron configuration Get 3 of 4 questions to level up!
Incorporate STEM journalism in your classroom
- Exercise type: Activity
- Topic: Particle Physics
Dig into atomic models
- Download Student Worksheet
Purpose: Students will work in groups to research, create and present different models of the atom that show the evolution of physicists’ understanding of atomic structure. The class also will learn about the standard model of particle physics and brainstorm how it could be shown in two or three dimensions.
Procedural overview: This activity is designed for three class periods and includes two homework assignments. To prepare for the first class, students will read the online Science News article “ How matter’s hidden complexity unleashed the power of nuclear physics ,” and answer three questions for homework.
During the first class, students will work in groups to research a classic atomic model and begin preparing a presentation about it. For homework, students can work with their groups or individually to create a 2-D or 3-D physical model of their group’s classic model. During the second class, the groups can finalize their physical models and make their presentations to the rest of the class.
In the final session for this activity, teachers will discuss the standard model of particle physics and have the students brainstorm ways they might create a 2-D or 3-D interpretation of it. To develop their ideas, the class should draw on current scientific knowledge and use resources such as CERN: The Standard Model .
Approximate class time: 3 class periods
Want to make it a virtual lesson? This activity can be delivered remotely using meeting software that allows you to convene the whole class and also to divide students into groups.
Various building materials (examples could include beans, candies. cotton balls, pipe cleaners, packing materials, yarn, tennis balls, baseballs, baggies and other items that can be found at home or in school)
Internet access
“ Dig into atomic models ” student worksheet
A projector (optional)
Whiteboard (optional)
Directions for teachers:
Before the first class for this activity, have students read the online Science News article “ How matter’s hidden complexity unleashed the power of nuclear physics ” and answer the first set of questions on the student worksheet for homework. A version of the story, “Cracking the atom,” appears in the April 10, 2021 issue of Science News .
1. What is an atom and why do we care about its structure?
Atoms make up matter; they are the building blocks of chemistry. The basic components of atoms are protons, neutrons and electrons. Knowing how many protons, neutrons and electrons a particular atom has will tell you what chemical element it is and how it will behave with atoms of different elements. Atomic structure helps scientists understand and predict the properties of matter.
2. Why has the model of the atom changed over time?
As scientists do experiments and make discoveries, they change their models to reflect new knowledge.
3. What are some practical implications of knowing the structure of the atom?
Answers will vary. Students may note that knowing the structure of atoms can help scientists determine how chemicals will react with each other. For instance, chemists can predict melting and freezing points for chemicals they create when they know the structures of their starting molecules. Knowing the structure of atoms also aids in the design of medicines and materials such as those used in environmental monitoring equipment and solar panels.
First class
After reviewing the homework answers, divide the students into groups. Assign one of the classic models — Dalton, Thomson, Rutherford, Bohr and Schrödinger — to each group.
The groups will work in class and if needed, at home, to research and prepare 5- to 7-minute presentations about their models, to be delivered at the next class. Each presentation should include a description of the model’s appearance, information about the scientists that developed the model and what was known about atoms at the time the model was developed. Groups should build physical representations of their assigned models to incorporate in their presentations. Creativity is encouraged, but students should still aim for accuracy. Alternatively, you could assign model building to students individually. When students bring their models to class, each group can come together to learn from each member’s attempt and put forth the best model or models for their presentation.
As students develop their presentations and build their models, have groups answer the following questions. You can use the questions to evaluate the presentations.
1. What other names have been used to describe the model assigned to you, and why did the model receive the names it did?
Answers will vary depending on the model assigned . T he Dalton model is called the billiard ball model or the solid sphere model. Dalton thought of atoms as solid spheres that could not be broken down into smaller parts. The Thomson model is known as the plum pudding model. He thought that electrons were studded over a “sea of positive charge” — something like plums in an English plum pudding. The Rutherford and Bohr models are called planetary models because both have electrons orbiting around an atomic nucleus similar to how planets orbit a star. The Schrödinger model is known as the quantum mechanical model because it incorporates the uncertainty inherent in quantum mechanics. Schrödinger used mathematics to predict the likely location of electrons in atoms.
2. When was your assigned model developed?
Answers will vary depending on the model assigned. The Dalton model was proposed in 1803, the Thoms on model was proposed in 1904, the Rutherford model was advanced in 1911, the Bohr model was developed in 1913, and the Schrödinger model was developed in 1926.
3. Although each classic model is named after the scientist who proposed it, these scientists either worked with or were influenced by other scientists. Name some of the influencers.
Answers will vary based on the model assigned. Dalton based his model partly on the work of Antoine Lavoisier and Joseph Proust. The Thomson model is named for Sir J. J. Thomson, who discovered the electron. A physicist named George Johnstone Stoney proposed the existence of electrons in 1874. The Rutherford model was derived from work Ernest Rutherford performed with his colleagues Hans Geiger and Ernest Marsden. Niels Bohr, for whom the Bohr model is named, was influenced by the work of Albert Einstein, Max Planck, Wolfgang Pauli and others. Erwin Schrödinger was influenced by the work Louis de Broglie, Werner Heisenberg and other physicists.
4. What discoveries or new evidence led to the development of this model?
Answers will vary depending on the model assigned. For instance, the Thomson model was developed after the discovery of the electron, while the Rutherford model was created after an experiment yielded results that contradicted the Thomson model. Rutherford and colleagues shot alpha particles at a piece of gold foil and found that some of the particles bounced off the foil instead of passing straight through it as predicted by the Thomson model. The finding led Rutherford to realize that the atom had to have a nucleus of positively charged particles.
5. How is the model that you were assigned different from previous models?
Answers will vary depending on the model assigned. For instance, the Bohr model incorporated the knowledge that electrons tend to occupy specific, discrete energy levels, while the Rutherford model assumed all electrons have the same energy potential. This was conveyed by having the electrons appear to orbit the nucleus in rings akin to planets around the sun instead of equally sized elliptical orbits around the nucleus as it was in the Rutherford model.
6. Are there places your assigned model is still used, and where have you seen it before?
You can still see influences of the Dalton model in depictions of molecules. Atoms that make up the molecule are shown as solid circles or spheres that are bonded together. The Thomson model is not often referenced these days. The Rutherford model is commonly used in popular media. The Bohr model is often used to teach students about electron shells. Students may learn the basics of the Schrödinger model when classes talk about quantum numbers.
Second class
At the start of the second class, have the groups finalize their presentations and models. While the students are working, you can walk around to evaluate the models and offer guidance. If there are several good models made by individuals in the group, you can help the students figure out which components to use from the different models to make an improved group model.
The presentations should be given in the order that the models were developed during the 19th and 20th centuries. Doing the presentations chronologically will help the students better understand how the field advanced.
Third class
In the third class, introduce students to the standard model of particle physics and the current science that supports it. If you need more information to prepare for this lesson, go to CERN: The Standard Model and DOE Explains…the Standard Model of Particle Physics .
Have the class brainstorm a way to visualize the standard model. Students can use paper, a computer with internet access and a whiteboard for their brainstorming work.
Cover the following questions in the discussion.
1. What is the standard model of particle physics? What are some key things to know about the standard model?
The standard model is a well-established theory in particle physics that describes elementary particles (also called fundamental particles) and explains how these particles behave. The model accounts for three forces in nature: electromagnetism, the strong nuclear force and the weak nuclear force. Elementary particles called quarks make up the protons and neutrons in an atom’s nucleus. Electrons are a kind of lepton, another type of elementary particle. There are force-carrying elementary particles called bosons that allow other particles to interact. For example, a boson called gluon transmits the strong nuclear force, which keeps the quarks in protons and neutrons “glued” together. There are 17 known elementary particles.
2. How is antimatter different from matter? Give an example of each.
Many elementary particles have an antimatter counterpart. Those counterparts have the same mass, but the opposite charge. For example, the antimatter counterpart of the electron is the positron. The electron has a negative charge, and the positron has a positive charge. One of the puzzling things about matter and antimatter is they are not present in equal amounts in the universe. Physicists are trying to understand why there is so much more matter than antimatter.
3. Who developed the standard model of particle physics?
The standard model of particle physics was not the work of one scientist. Many physicists investigating the structure and interactions of subatomic particles did the experimental and theoretical work that made the standard model possible. Notable contributors include James Chadwick, Paul Dirac, Carl Anderson, Enrico Fermi, Peter Higgs and Sheldon Glashow.
4. When was the standard model developed?
From the 1930s onward, physicists began finding more subatomic particles. They wanted to understand what the particles had to do with each other and determine which ones were fundamental (or elementary) particles. Using mathematics and theoretical and experimental work, physicists were able to establish the standard model of particle physics as a theory by the mid-1970s. Since then, more elementary particles predicted by the theory have been discovered. The most recent was in 2012. It is called the Higgs boson.
5. Are there any other common names for the standard model?
Not really.
6.What discoveries or ideas contributed to the standard model?
Many scientists’ work contributed to the standard model. Here are a few examples. Chadwick discovered the neutron, which helped explain isotopes and broke physics out of the two-particle model of atoms made from only electrons and protons. Fermi advanced the idea that beta decay, the decay of a neutron into a proton within an atom, created an electron and another particle now called an antineutrino. Fermi also discovered many subatomic particles using first natural cosmic rays and then particle accelerators. Higgs and others independently developed a theory for how particles obtain mass in the 1960s; their theory was confirmed in 2012 with the discovery of the Higgs boson, the last of the elementary particles to be discovered.
7. How is the standard model different from the classic models you have studied?
The standard model of particle physics is much more complex than the atomic models that preceded it. Even the most complicated classic models included only three components: electrons, protons and neutrons. There are 17 elementary particles in the standard model — most of them probably unimagined by earlier generations of scientists. The standard model accounts for matter and antimatter, and it contains particles that transmit forces within atoms.
8. What are some weaknesses of the standard model?
The standard model does not take into account gravity, the fourth force of nature, and does not answer why there is more matter than antimatter in the universe. The model also does not explain dark matter, an unidentified, invisible type of matter in the universe. Dark matter appears to interact weakly with all regular matter except through gravity.
9. What visual depictions of the standard model have you seen, and what do you think of them?
Student answers will vary. Visual representations are rare. Most often, the standard model is represented as a “periodic table” of elementary particles with no mention of how they combine to create electrons, neutrons and protons ( CERN: The Standard Model ). The U.S. Department of Energy website uses a graphic that places the Higgs boson in the center surrounded by a ring of bosons with leptons and quarks in an outer ring ( DOE Explains…the Standard Model of Particle Physics ).
10. If you were doing a 2-D or 3-D visualization of the standard model, would you include all of the known elementary particles? Why or why not?
Student answers will vary. For instance, a student may say that it would be too complicated to use all of the elementary particles in a 3-D visualization of the standard model. It would be like trying to make a 3-D version of the periodic table. Also, many of these particles disappear quickly, so it would be particularly hard to depict them. Instead, the student may say they would focus on a few elementary particles.
11. Describe how you would depict the standard model.
Student answers will vary. An example answer is provided below: In my depiction, I want to focus on the basic atom. I think it is important to know that protons are made up of 2 up quarks and one down quark and that neutrons are made up on one up quark and two down quarks. In my 3-D visualization, I would use Starbursts (in their wrappers) to represent the quarks. The up quark would be yellow; the down quark would be red. The proton would have one yellow Starburst (up quark) on each end and the red one (down quark) would be in the middle. The neutrons would be shown with red Starbursts (down quarks) on the ends and a yellow one (up quark). I’d use glitter glue to hold my “protons” and “neutrons” together. The glue represents the gluon, the boson that holds the particles together. I will use tiny zip-lock bags to hold my “atomic nuclei.” The bag can represent the electrons around each “atomic nucleus.”
To convey the idea of antiquarks and positrons, I would again use yellow and red Starbursts and tiny baggies, but everything would be marked with an “A” so the people will know they represent antimatter. To get across the idea that there is a lot less antimatter in the universe than matter, I would put my antiatoms in a quart zip-lock bag and my atoms in a garbage bag. The two bags would be connected with tape or string so people viewing my model would know there is a relationship between the two. The difference in the scale of the two bags is a visual reminder that matter is more common than antimatter.
12. How would you convey the interactions among the various elementary particles?
Student answers will vary. For instance, a student may say that they don’t think the interactions can be shown in a physical model because those interactions happen very fast and some elementary particles don’t last very long. The student may suggest that the concepts covered by the standard model could instead be explained in an animated film .
Additional resources
CERN: The Standard Model
PBS LearningMedia: Atomic Structure
Institute of Physics: The Standard Model
Stanford ATLAS: Introduction to Particle Physics
Berkeley Lab: The Particle Adventure Five mysteries the Standard Model can’t explain
Browse Course Material
Course info.
- Prof. Donald Sadoway
Departments
- Materials Science and Engineering
As Taught In
- Chemical Engineering
Learning Resource Types
Introduction to solid state chemistry, 3. atomic models: rutherford & bohr.
« Previous | Next »
Session Overview
Prerequisites.
Before starting this session, you should be familiar with:
- Session 2: The Periodic Table
Looking Ahead
Prof. Sadoway discusses the atomic spectra of hydrogen ( Session 4 ).
Learning Objectives
After completing this session, you should be able to:
- Understand Thomson’s “plum pudding” model .
- Understand Rutherford’s “nuclear” model .
- Explain the Bohr model of hydrogen .
- Understand Bohr’s quantization condition.
Archived Lecture Notes #1 (PDF) , Sections 1-3
Lecture Video
- Download video
- Download transcript
Lecture Slides (PDF - 9.3MB)
Periodic Table and Table of Constants
Lecture Summary
Prof. Sadoway talks about the principles of modern chemistry and how that led to the understanding of the structure of the atom . He details Bohr’s postulates for the hydrogen atom and discusses how the Planck-Einstein relationship applies to electron transitions. He defines the different isotopes of hydrogen.
This lecture includes the following:
- Electrons are distributed uniformly throughout the atom
- Conclusions from the gold foil experiment
- Majority of the mass is found in the nucleus
- Electrons orbit around the nucleus
- Explanation of blackbody radiation and atomic spectra
- Electrons follow circular orbits around a nucleus
- Orbital angular momentum is quantized hence only certain orbits are possible
- Electrons in stable orbits do not radiate
- Electrons change orbits by radiating or absorbing photons
Problems (PDF)
Solutions (PDF)
Textbook Problems
For further study, supplemental readings.
Ottaviani, J. Suspended in Language: Niels Bohr’s Life, Discoveries, and the Century He Shaped . GT Labs: Ann Arbor, MI, 2004. ISBN: 9780978803728.
Rozental, S. Niels Bohr: His Life and Work as Seen by His Friends and Colleagues . New York, NY: Wiley, 1967.
Bohr, Niels H. D. On the Constitution of Atoms and Molecules . New York, NY: W.A. Benjamin, 1963.
Bohr, Niels H. D. Atomic Physics and Human Knowledge . New York, NY: Wiley, 1958.
Bohr, Niels. “ On the Constitution of Atoms and Molecules. ” Philosophical Magazine Series 6 26 (July 1913): 1-15.
Cathcart, B. The Fly in the Cathedral: How a Small Group of Cambridge Scientists Won the Race to Split the Atom . New York, NY: Penguin, 2005. ISBN: 9780670883219.
Andrade, E. N. Rutherford and the Nature of the Atom . Garden City, NY: Doubleday, 1964.
Frayn, M. Copenhagen: A Play in Two Acts . New York, NY: S. French, 2000.
Miller, D. P. Discovering Water: James Watt, Henry Cavendish and the Nineteenth Century Water Controversy . Burlington, VT: Ashgate, 2004. ISBN: 9780754631774.
Cavendish Laboratory
How Atoms Work
Joseph Thompson - 1906 Nobel Prize in Physics
Ernest Rutherford - 1908 Nobel Prize in Chemistry
Johannes Geiger
Ernest Marsden
Max Planck - 1918 Nobel Prize in Physics
Albert Einstein - 1921 Nobel Prize in Physics
Niels Bohr - 1922 Nobel Prize in Physics
Robert Millikan - 1923 Nobel Prize in Physics
Henry Cavendish
Werner Heisenberg - 1932 Nobel Prize in Physics
Harold Urey - 1934 Nobel Prize in Chemistry
Charles-Augustin de Coulomb
James Prescott Joule
Other OCW and OER Content

You are leaving MIT OpenCourseWare
Log In | Join AACT | Renew Membership
Save Your Favorite AACT Resources! ×
Log in or join now to start building your personalized "My Favorites" page. Easily save all the resources you love by logging in and clicking on the star icon next to any resource title.
- AACT member benefits »
- Forgot User Name or Password?
Atomic Structure Unit Plan Mark as Favorite (148 Favorites)
LESSON PLAN in Atomic Spectra , Model of the Atom , Isotopes , Atomic Theory , Subatomic Particles , Emission Spectrum , Electrons , Orbitals , Ions , Unit Plans . Last updated September 18, 2023.
The AACT high school classroom resource library has everything you need to put together a unit plan for your classroom: lessons, activities, labs, projects, videos, simulations, and animations. We constructed a unit plan using AACT resources that is designed to teach Atomic Structure to your students.
Grade Level
High School
By the end of this unit, students should be able to
- Identify that all matter is made of one or more of the atoms of the 118 elements on the periodic table.
- Begin to understand how small atoms are and how many of them are in small objects.
- Explain the specific contributions of Democritus, Aristotle, John Dalton, J.J. Thomson, Ernest Rutherford, Niels Bohr, Edwin Schrodinger, Albert Einstein, and Werner Heisenberg.
- Understand past and current theories regarding the structure of the atom.
- Use the number of protons, neutrons, and electrons in an element to predict an atom’s identity.
- Calculate atomic mass.
- Calculate atomic number.
- Understand the meaning of ion .
- Convert mass data for an element or a compound into values of moles, atoms or molecules.
- Explain how the atomic mass shown on the periodic table for an element was determined.
- Define an isotope .
- Accurately calculate the average atomic mass of an element given the atomic mass of each isotope and its abundancy.
- Explain the meaning of a weighted average and calculate it.
- Identify which subatomic particle(s) affect the atomic mass of an atom.
- Define an ion.
- Predict the charge an ion will have.
- Determine the atomic mass from a mixture of isotopes.
- Discuss the mass number in relation to an atom’s protons and neutrons.
- Understand the law of conservation of matter.
- Visualize how orbitals are superimposed upon one another within an atom.
- Explain the meaning of conservation of energy.
- Describe that when energy is added to an atom its electron(s) are able to temporarily move to an excited state. Energy is emitted when the atom returns to its ground state.
- Understand that energy is quantized.
- Use flame tests to identify a metal or metallic salt by the color that it produces when it is put into a flame.
- Calculate the frequency of light given its wavelength.
- Calculate the wavelength of light given its frequency.
- Identify an unknown metal by the color it emits when passed through a flame.
Chemistry Topics
This unit supports students’ understanding of
- Atomic Structure
- Atomic Theory
- Model of the Atom
- Subatomic Particles
- Atomic Mass
- Atomic Number
- Atomic Spectra
- Electromagnetic Spectrum
- Quantitative Chemistry
- Measurement
- History of Chemistry
- Chemical Reactions
- Balancing Chemical Equations
- Law of Conservation of Matter
- Law of Conservation of Energy
- Activity series
Teacher Preparation : See individual resources.
Lesson : 7-10 class periods, depending on class level.
- Refer to the materials list given with each individual activity.
- Refer to the safety instructions given with each individual activity.
Teacher Notes
- The activities shown below are listed in the order that they should be completed.
- The teacher notes, student handouts, and additional materials can be accessed on the page for each individual activity.
- Please note that most of these resources are AACT member benefits.
Atomic Theory:
- The Atoms Animation uses analogies to help students visualize the size of an atom. It’s a great introductory video to start off the unit. The accompanying lesson plan contains general and extension questions to check for understanding.
- Founders of Chemistry Series : Introduce the development of atomic theory by using one or more of our Founders of Chemistry videos. The Ancient Chemistry Video traces the history of chemistry from the discovery of fire, through the various metal ages, and finally to the great philosophers. Students can learn about Rutherford's initial research on alpha particles in the Ernest Rutherford Video and hear about how he hypothesized that they were helium nuclei. The Niels Bohr Video tells the story of Niels Bohr, a great scientist who redefined how we think about atoms and the electron. Each of these includes an activity sheet that include questions for students to answer as they watch the videos.
- The Scientists Behind the Atom Project : After viewing the videos, use this project to have your students create a digital or paper book about the scientists who contributed to our understanding of the atom. This project will help students explain the specific contributions of several scientists and understand past and current theories regarding the structure of the atom.
- Indirectly Measuring the Atom : To help students better connect with Rutherford’s Gold Foil Experiment, consider having students complete this activity. Students will drop a marble 150 times on a sheet of carbon paper and use the resulting marks where the marble fell to make estimates about the measurement of the diameter of a small circle on the paper. Each marble drop is similar to an alpha particle fired at the gold foil.
- Modeling Atomic Theories with Food . One of the benefits of this activity is that is has students model what an atom looks like based on their prior knowledge. Students then amend the model over time as they are introduced to each new model of the atom, ending with a model that shows the closest representation to what we know the atom to be like today. The focus on the science and engineering practice of Developing and Using Models makes this a welcome NGSS addition.
- Building an Atom Simulation : Additionally, use this simulation to have your students manipulate the number of protons, neutrons, and electrons in an element and determine how these effect the mass number, atomic number, and other properties of an atom. This lesson, which is guided by a PhET simulation , allows students to see how they can use the number of protons, neutrons, and electrons in an element to predict its identity.
Ions, Isotopes, and Average Atomic Mass:
- Introduce the concept of isotopes with the What are Isotopes? Video Questions . Students watch a video that is part of the American Chemical Society video series Chemistry Basics and then answer questions about isotopes. This activity will help them learn about the discovery of isotopes, the difference between chemical and nuclear reactions, different kinds of radioactive decay, and some uses of radioactive isotopes.
- Isotopes & Calculating Average Atomic Mass : Students can learn how the average atomic mass is determined through a tutorial based on the isotope abundance for Carbon with this simulation. They then select the number of isotopes, the mass of each isotope, as well as the abundance of each to successfully build a mystery element and calculate its average atomic mass.
- Beanium Isotopes : If you prefer a hands-on activity, this lab will lead your students through the steps that they will take to calculate the average atomic mass of an imaginary element called Beanium.
- Candy Isotopes & Atomic Mass : This is an alternate activity you can use to introduce the concept of average atomic mass. This simple and inexpensive lesson uses M&M candies to model ratios that approximate real world atomic mass values on the periodic table. The resource can be used with middle or high school students and includes alignment with NGSS performance expectations.
- The Electrons and Ions Explained with Balloons activity is a great analogy for how gaining or losing electrons affects the properties of an atom. Clothespins that represent electrons are added or removed from a helium balloon, causing the height of the balloon in the air to change. Consider using this for visual learners.
Conservation of Mass:
- Antoine Lavoisier : Introduce the concepts of conservation of mass and the law of definite proportion with this Founders of Chemistry video, which tells the story of how, who many consider to be the father of modern chemistry, discovered oxygen and hydrogen and first proposed the Law of Conservation of Mass. It also includes an activity sheet that includes questions for students to answer as they watch the videos.
- Balancing Legos : Following the video, have your students use this hands on activity to model the reactants and products in a chemical reaction. They then use these “atoms” and “molecules” to balance the chemical reaction to demonstrate the law of conservation of matter.
- Chemistry in a Bag : Use this lesson as an activity or a demonstration to have your students make observations about the Law of Conservation of Mass.
Electrons, Electron Configurations, and Electromagnetic Radiation:
- Emissions of Light : This makes a great phenomena-based lab. Students can explore different emissions of light. You can ask probing questions about where the energy came from to give off light and then return to the questions once you have taught students about electron transitions and light.
- Orbitals Animation : With the use of the Bohr model to introduce atomic structure, students visualize electrons “orbiting” around the nucleus. Help them expand their knowledge with this animation, which allows students to visualize how orbitals are superimposed upon one another within an atom in three dimensions. The orbitals depicted in this animation are 1s, 2s, 2p, 3s, 3p, 4s, and 3d.
- Follow up with the lesson, Electrons and Orbitals to help students differentiate between energy levels, sublevels, orbitals, and electrons. Students often confuse these terms related to electrons and this activity helps them develop a stronger understanding of how to distinguish between them.
- Expand on the topic of electrons and orbitals with the Electromagnetic Spectrum animation and lesson plan . The animation helps students learn about the electromagnetic spectrum, with a focus on the visible spectrum. It addresses the relationship between color, wavelength, frequency, and energy of light waves, as well as how an object absorbs and reflects certain wavelengths of light to contribute to the color we perceive.
- Exciting Electrons : Then use this simulation from the March 2015 issue of Chemistry Solutions to let your students explore what happens when electrons within a generic atom are excited from their ground state. They will see that when an electron returns to its ground state from an excited state, energy is released in the form of electromagnetic radiation.
- Flame Test (Rainbow Demo) : Finish up your study of electrons with this safer version of the traditional demonstration that is commonly used in high school chemistry classrooms. It can be used to show students the variety of colors that are produced when different metallic salts are heated in a flame. You can view a video of this demonstration on The Flame Test page of the ACS website. Read more about this in the ACS Safety Alert about the Rainbow Demonstration .
- Flame Test: Going Further : In additions to the demonstration, have your students investigate the colors produced when several mixtures of metallic ions are placed in a flame. Note that this procedure also uses wooden splints and aqueous solutions in place of the traditional flammable solvent.
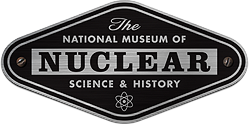
National Museum of Nuclear Science & History

Atom Lesson Plan
- High School
- Middle School
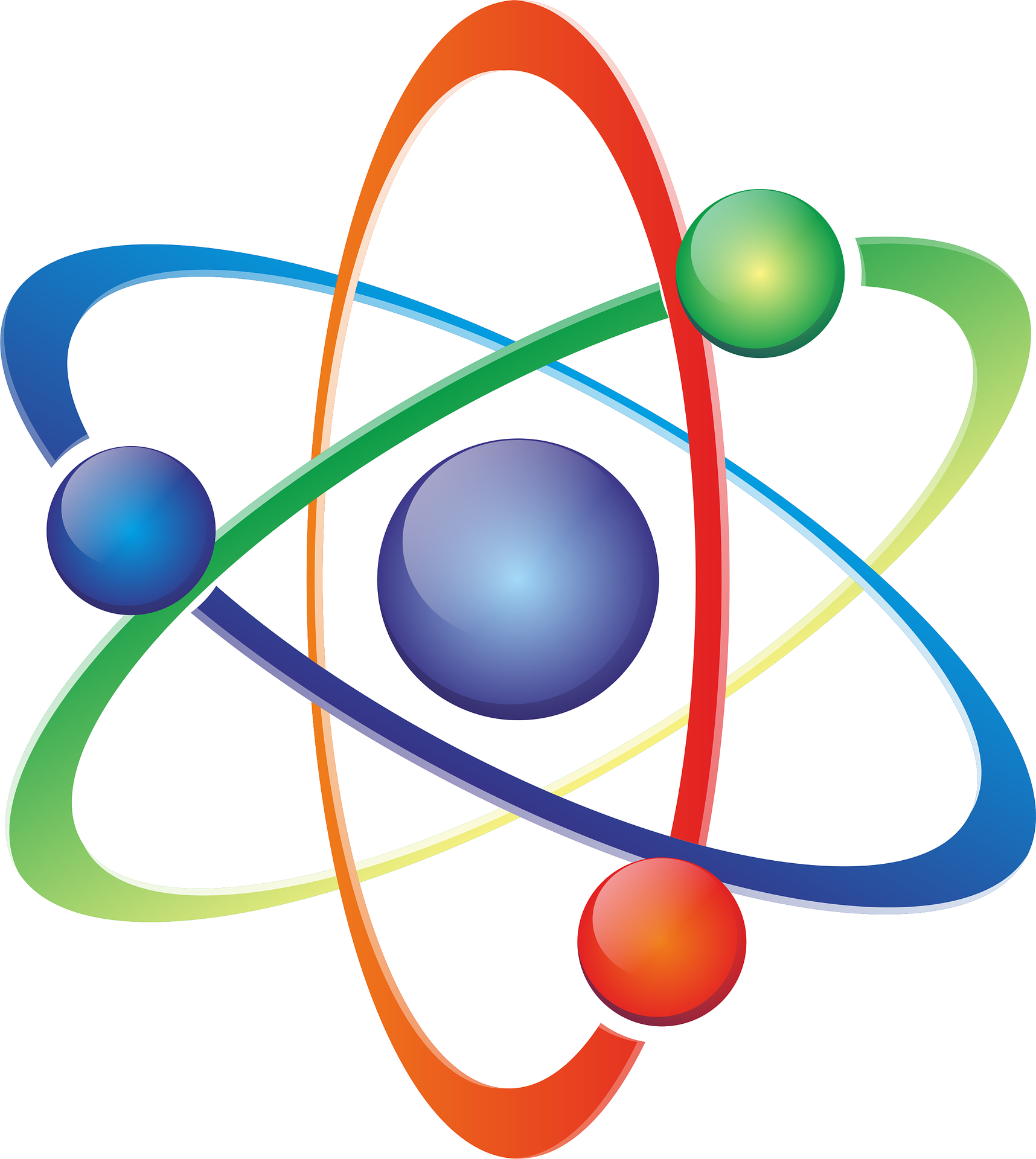
This activity compliments the teacher’s lesson on the atom. In the basic version, students learn about the atom, its structure, the particles. They will also learn how to calculate the atomic mass and find the number of protons, neutrons, and electrons. In the advanced version, students learn about isotopes and ions.
Three sets of signs: red with a plus (protons), yellow (neutron), and blue with a minus (electron). Periodic tables for students to reference later on in the activity.
Helpful visual aid: Bohr’s Model of the atom
Assignment:
Demonstration: The teacher calls on 6 volunteers to the front of the classroom and hands a “proton” to 2 students, a “neutron” to 2 students, and 2 “electrons” to the last student. The “protons” and “neutrons” stand together, while the “electrons” “orbit” around them.
***Students will be forming a helium atom
Explanation: ATOMS are the basic building blocks of matter. They make up everything around you–including air! There are three basic parts of an atom: the PROTON , which has a positive charge; the NEUTRON , which as no charge and is neutral ; and the ELECTRON , which has a negative charge.
***Helpful visual aid: Bohr’s Model of the atom
Practice: The teacher calls on students to point to which volunteers are the proton, neutron, and electron particles. The students explain why they know which volunteer is which particle.
Explanation: The protons and neutrons make up the nucleus, which is the core of the atom. The electrons orbit around the nucleus in electron shells. In general, there are the same number of protons and electrons in an atom. We can tell what element the atom is by counting the number of protons in the nucleus. How many protons are in our atom? The volunteers formed the helium atom, which normally has 2 protons, 2 neutrons, and 2 electrons.
Demonstration: The teacher shows the students how to use the periodic table.
The ATOMIC NUMBER is the number of protons there are in the atom.
The ELEMENT SYMBOL is the element’s shorthand or abbreviation
The ATOMIC WEIGHT / ATOMIC MASS is the average mass of an element. You can find it by adding the protons and neutrons together. The unit of measurement is the ATOMIC MASS UNIT , or AMU .
PUTTING IT ALL TOGETHER
Activity 1: The teacher calls on 4 volunteers to pick up 4 proton signs. What is this element? What are the atomic number, atomic mass, and element symbol? How many neutrons and electrons should there be? The teacher can call on more volunteers to pick up neutron and electron signs, and the volunteers must form the Beryllium atom.
***Element: Beryllium
***Atomic Number: 4
***Atomic Mass: ~9 amu
***Element Symbol: Be
***Hint: To find the number of neutrons, students must subtract the atomic number from the atomic mass (9amu – 4protons = 5neutrons)
***Number of Electrons: 4
The teacher can follow this activity with other elements, too. This activity can also be done without calling volunteers to the front. The teacher calls the atomic number, and students answer the same question. Eg, “This element has 92 protons. What is this element? What are the atomic number, atomic mass, and element symbol? How many neutrons and electrons should there be?”
Activity 2: The teacher names an element, and the students must answer how many protons, neutrons, and electrons there are. Volunteers go to the front of the classroom and form the element’s atom. Students can answer additional questions about the atomic number, atomic mass, and element symbol. This activity can also be done without volunteers. Eg, “What can you tell me about the element uranium?”
ADVANCED VERSION
Explanation: Different versions of the same element can exist. For example, there is U-235 and U-238. U-235 is uranium with an atomic mass of 235 amu and U-238 is uranium with an atomic mass of 238 amu.
Activity: Have the students look on the periodic table and find the atomic mass of uranium. What would change the mass of an atom?
Explanation: Atoms that have the same atomic number (same number of protons) but have a different number of neutrons are called ISOTOPES . Isotopes also have different atomic masses because of the neutrons. For example, U-235 has 143 neutrons, and U-238 has 146 neutrons.
Activity 3: The teacher calls on volunteers to form a hydrogen atom. Students answer questions about the atomic number and atomic mass. Then, the teacher adds a “neutron” to form deuterium. Students answer questions about the atomic number, number of neutrons, and atomic mass. The teacher can add one more “neutron” to form tritium. [Good segue into radiation]
***Remember: a hydrogen atom does NOT have a neutron
***Deuterium: 1 proton, 1 neutron, and ~ 2 amu
***Tritium: 1 proton, 2 neutrons, and about ~3 amu
Explanation: Atoms can also have “positive” and “negative” charges, and atoms with a positive or negative charge are called “ IONS .”
Activity 4: The teacher calls on volunteers to form a neutrally charged lithium atom (3 protons, 3 electrons, and 4 neutrons). The teacher can call on a volunteer to add an electron to form a CATION (a negatively charged atom) or take away an electron to form an ANION (a positively charged atom).
Module 2: Atoms, Molecules, and Ions
Assignment: atomic structure and the periodic table.
This assignment can be found in Google Docs: Chemistry for Majors Assignment: Atomic Structure and the Periodic Table
To make your own copy to edit:
- If you want a Google Doc : in the file menu of the open document, click “Make a copy.” This will give you your own Google Doc to work from.
- If you want a PDF or Word file : in the file menu of the open document, click “Download” and select the file type you would like to have (note: depending on the file type you select, the formatting could get jumbled).
Instructions
As you work these problems, consider and explain:
- What type of question is it?
- How do you know what type of question it is?
- What information are you looking for?
- What information do they give?
- How will you go about solving this?
- Show how to solve the problem.
- Be able to answer for a different reaction, number, set of conditions, etc.
- Which form of electromagnetic radiation has the longest wavelengths?
- A line in the spectrum of atomic mercury has a wavelength of 254 nm. When mercury emits a photon of light at this wavelength, what is the frequency of the light?
- Consider an atom traveling at 1% of the speed of light. The de Broglie wavelength is found to be 1.46 × 10 –3 pm. Which element is this?
- What is the energy of a photon of blue light that has a wavelength of 453 nm?
- The hydrogen molecules they came from have the formula H 4 .
- We could observe more lines if we had a stronger prism.
- There are four electrons in an excited hydrogen atom.
- Only certain energies are allowed for the electron in a hydrogen atom.
- The spectrum is continuous.
For questions 6–8, consider the following portion of the energy-level diagram for hydrogen:
- n = 4 to n = 3
- n = 4 to n = 2
- n = 4 to n = 1
- n = 3 to n = 2
- n = 2 to n = 1
- In the hydrogen spectrum, what is the wavelength of light associated with the n = 3 to n = 1 electron transition?
- Energy is emitted.
- Energy is absorbed.
- The electron loses energy.
- The electron gains energy.
- The electron cannot make this transition.
- It makes no attempt to explain why the negative electron does not eventually fall into the positive nucleus.
- It does not adequately predict the line spectrum of hydrogen.
- It does not adequately predict the ionization energy of the valence electron(s) for elements other than hydrogen.
- It does not adequately predict the ionization energy of the first energy level electrons for one-electron species for elements other than hydrogen.
- It shows the electrons to exist outside of the nucleus.
- The energy of the light emitted when a hydrogen electron goes from n = 2 to n = 1 is what fraction of its ground-state ionization energy?
- The emission spectrum of hydrogen contains a continuum of colors.
- Diffraction produces both constructive and destructive interference.
- All matter displays both particle and wavelike characteristics.
- Niels Bohr developed a quantum model for the hydrogen atom.
- The lowest possible energy state of a molecule or atom is called its ground state.
- A gamma ray of wavelength 1.00 × 10 –8 cm has enough energy to remove an electron from a hydrogen atom.
- space where electrons are unlikely to be found in an atom
- space which may contain electrons, protons, and/or neutrons
- the space in an atom where an electron is most likely to be found
- small, walled spheres that contain electrons
- a single space within an atom that contains all electrons of that atom
- How many f orbitals have the value n = 3?
- If n = 2, how many orbitals are possible?

- The electrons move along the outer surface of the p -orbital, similar to a “figure 8” type of movement.
- The electrons move within the two lobes of the p -orbital, but never beyond the outside surface of the orbital.
- The electrons are concentrated at the center (node) of the two lobes.
- The electrons are only moving in one lobe at any given time.
- The electron movement cannot be exactly determined.
- How many electrons in an atom can have the quantum numbers n = 3, l = 2?
- How many electrons can be contained in all of the orbitals with n = 4?
- n = 1, l = 1, m l = 0, m s = 1/2
- n = 3, l = 0, m l = 0, m s = -1/2
- n = 2, l = 1, m l = -1, m s = 1/2
- n = 4, l = 3, m l = -2, m s = -1/2
- n = 4, l = 2, m l = 0, m s = 1/2
- What is the electron configuration for the barium atom?
- What is the complete electron configuration of tin?
- The exact location of an electron can be determined if we know its energy.
- An electron in a 2 s orbital can have the same n , l , and ml quantum numbers as an electron in a 3 s orbital.
- Ni has two unpaired electrons in its 3 d orbitals.
- In the buildup of atoms, electrons occupy the 4 f orbitals before the 6 s orbitals.
- Only three quantum numbers are needed to uniquely describe an electron.
- What is the statement that “the lowest energy configuration for an atom is the one having the maximum number of unpaired electrons allowed by the Pauli principle in a particular set of degenerate orbitals” known as?
- An element with the electron configuration [Xe] 6 s 2 4 f 14 5 d 7 would belong to which class on the periodic table?
- Ti has __________ in its d orbitals.
- Authored by : Jessica Garber. Provided by : Tidewater Community College. License : CC BY: Attribution

The History of the Atom – Theories and Models
All matter is made up of atoms. This is something we now take as a given and one of the things you learn right back at the beginning of high school or secondary school chemistry classes. Despite this, our ideas about what an atom is are surprisingly recent: as little as one hundred years ago, scientists were still debating what exactly an atom looked like. This graphic takes a look at the key models proposed for the atom, and how they changed over time.
Though our graphic starts in the 1800s, the idea of atoms was around long before. In fact, we have to go all the way back to Ancient Greece to find its genesis. The word ‘atom’ actually comes from Ancient Greek and roughly translates as ‘indivisible’. The Ancient Greek theory has been credited to several different scholars but is most often attributed to Democritus (460–370 BC) and his mentor Leucippus. Though their ideas about atoms were rudimentary compared to our concepts today, they outlined the idea that everything is made of atoms, invisible and indivisible spheres of matter of infinite type and number.
These scholars imagined atoms as varying in shape depending on the type of atom. They envisaged iron atoms as having hooks which locked them together, explaining why iron was a solid at room temperature. Water atoms were smooth and slippery, explaining why water was a liquid at room temperature and could be poured. Though we now know that this is not the case, their ideas laid the foundations for future atomic models.
It was a long wait, however, before these foundations were built upon. It wasn’t until 1803 that the English chemist John Dalton started to develop a more scientific definition of the atom. He drew on the ideas of the Ancient Greeks in describing atoms as small, hard spheres that are indivisible, and that atoms of a given element are identical to each other. The latter point is one that pretty much still holds true, with the notable exception being isotopes of different elements, which differ in their number of neutrons. However, since the neutron wouldn’t be discovered until 1932, we can probably forgive Dalton for this oversight. He came up with theories about how atoms combine to make compounds and also came up with the first set of chemical symbols for the known elements.
Dalton’s outlining of atomic theory was a start, but it still didn’t really tell us much about the nature of atoms themselves. What followed was another, shorter lull where our knowledge of atoms didn’t progress all that much. There were some attempts to define what atoms might look like, such as Lord Kelvin’s suggestion that they might have a vortex-like structure , but it wasn’t until just after the turn of the 20th Century that progress on elucidating atomic structure really started to pick up.
The first breakthrough came in the late 1800s when English physicist Joseph John (JJ) Thomson discovered that the atom wasn’t as indivisible as previously claimed. He carried out experiments using cathode rays produced in a discharge tube and found that the rays were attracted by positively charged metal plates but repelled by negatively charged ones. From this, he deduced the rays must be negatively charged.
By measuring the charge on the particles in the rays, he was able to deduce that they were two thousand times lighter than hydrogen, and by changing the metal the cathode was made from he could tell that these particles were present in many types of atoms. He had discovered the electron (though he referred to it as a ‘corpuscle’), and shown that atoms were not indivisible, but had smaller constituent parts. This discovery would win him a Nobel Prize in 1906.
In 1904, he put forward his model of the atom based on his findings. Dubbed ‘The Plum Pudding Model’ (though not by Thomson himself), it envisaged the atom as a sphere of positive charge, with electrons dotted throughout like plums in a pudding. Scientists had started to peer into the atom’s innards, but Thomson’s model would not hang around for long – and it was one of his students who provided the evidence to consign it to history.
Ernest Rutherford was a physicist from New Zealand who studied at Cambridge University under Thomson. It was his later work at the University of Manchester which would provide further insights into the insides of an atom. This work came after he had already received a Nobel Prize in 1908 for his investigations into the chemistry of radioactive substances.
Rutherford devised an experiment to probe atomic structure which involved firing positively charged alpha particles at a thin sheet of gold foil . The alpha particles were so small they could pass through the gold foil, and according to Thomson’s model which showed the positive charge diffused over the entire atom, they should do so with little or no deflection. By carrying out this experiment, he hoped to be able to confirm Thomson’s model, but he ended up doing exactly the opposite.
During the experiment, most of the alpha particles did pass through the foil with little or no deflection. However, a very small number of the particles were deflected from their original paths at very large angles. This was completely unexpected; as Rutherford himself observed, “It was almost as incredible as if you fired a 15-inch shell at a piece of tissue paper and it came back and hit you”. The only possible explanation was that the positive charge was not spread throughout the atom, but concentrated in a small, dense centre: the nucleus. Most of the rest of the atom was simply empty space.
Rutherford’s discovery of the nucleus meant the atomic model needed a rethink. He proposed a model where the electrons orbit the positively charged nucleus. While this was an improvement on Thomson’s model, it didn’t explain what kept the electrons orbiting instead of simply spiralling into the nucleus.
Enter Niels Bohr. Bohr was a Danish physicist who set about trying to solve the problems with Rutherford’s model. He realised that classical physics could not properly explain what was going on at the atomic level; instead, he invoked quantum theory to try and explain the arrangement of electrons. His model postulated the existence of energy levels or shells of electrons. Electrons could only be found in these specific energy levels; in other words, their energy was quantised, and couldn’t take just any value. Electrons could move between these energy levels (referred to by Bohr as ‘stationary states’), but had to do so by either absorbing or emitting energy.
Bohr’s suggestion of stable energy levels addressed the problem of electrons spiralling into the nucleus to an extent, but not entirely. The exact reasons are a little more complex than we’re going to discuss here because we’re getting into the complex world of quantum mechanics; and as Bohr himself said, “If quantum mechanics hasn’t profoundly shocked you, you haven’t understood it yet”. In other words, it gets kind of weird.
Bohr’s model didn’t solve all the atomic model problems. It worked well for hydrogen atoms, but couldn’t explain observations of heavier elements. It also violates the Heisenberg Uncertainty Principle, one of the cornerstones of quantum mechanics, which states we can’t know both the exact position and momentum of an electron. Still, this principle wasn’t postulated until several years after Bohr proposed his model. Despite all this, Bohr’s is probably still the model of the atom you’re most familiar with since it’s often the one first introduced during high school or secondary school chemistry courses. It still has its uses too; it’s quite handy for explaining chemical bonding and the reactivity of some groups of elements at a simple level.
At any rate, the model still required refining. At this point, many scientists were investigating and trying to develop the quantum model of the atom. Chief amongst these was Austrian physicist Erwin Schrödinger, who you’ve probably heard of before ( he’s the guy with the cat and the box ). In 1926 Schrödinger proposed that, rather than the electrons moving in fixed orbits or shells, the electrons behave as waves. This seems a little weird, but you probably already recall that light can behave as both a wave and a particle (what’s known as a wave-particle duality), and it turns out electrons can too.
Schrödinger solved a series of mathematical equations to come up with a model for the distributions of electrons in an atom. His model shows the nucleus surrounded by clouds of electron density. These clouds are clouds of probability; though we don’t know exactly where the electrons are, we know they’re likely to be found in given regions of space. These regions of space are referred to as electron orbitals. It’s perhaps understandable why high school chemistry lessons don’t lead in straight with this model, though it’s the accepted model today, because it takes a little more time to get your head around!
Schrödinger’s wasn’t quite the last word on the atom. In 1932, the English physicist James Chadwick (a student of Ernest Rutherford) discovered the existence of the neutron, completing our picture of the subatomic particles that make up an atom. The story doesn’t end there either; physicists have since discovered that the protons and neutrons that make up the nucleus are themselves divisible into particles called quarks – but that’s beyond the scope of this post! At any rate, the atom gives us a great example of how scientific models can change over time and shows how new evidence can lead to new models.
Enjoyed this post & graphic? Consider supporting Compound Interest on Patreon!
The graphic in this article is licensed under a Creative Commons Attribution-NonCommercial-NoDerivatives 4.0 International License . See the site’s content usage guidelines .
References & Further Reading
- The development of the atomic model – R Allain, Wired
- Models of the atom – M Fowler
- History and philosophy of science through models: some challenges in the case of the atom (£) – R Justi and J Gilbert
Antonio Balordi
Why bohr’s model couldn’t explain obsvervations of heavier elements?
Justin Mason
Because the Bohr model assumes that electrons are just added as though we were just adding more and more hydrogen. This is incorrect, because things get very complicated after hydrogen, with electron orbitals, the uncertainty principle and wave functions of electrons and many other things.
- Trackback: Atomic Theory | Mrs. Petersen's Site
- Trackback: Atomes | Pearltrees
- Trackback: Atomes et molécules | Pearltrees
- Trackback: Whewell’s Gazette: Year 3, Vol. #09 | Whewell's Ghost
- Trackback: A Brief History of Atomic Theory - Relatively Interesting
Comments are closed.
You May Also Like

A Visual Guide to Chemistry Glassware

Sir Harry Kroto & Buckminsterfullerene

A Guide to Chemical Hazard Symbols
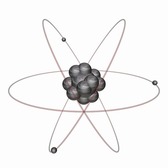
- Number of visits 103
- Number of saves 4
Models of the Atom
- Report this resource
Description

Wyoming Science Content and Performance Standards
Learning Domain: Matter and Its Interactions
Standard: Use the periodic table as a model to predict the relative properties of elements based on the patterns of electrons in the outermost energy level of atoms.
Degree of Alignment: Not Rated (0 users)
Next Generation Science Standards
Science Domain: Physical Sciences
Topic: Structure and Properties of Matter
Standard: Use the periodic table as a model to predict the relative properties of elements based on the patterns of electrons in the outermost energy level of atoms. [Clarification Statement: Examples of properties that could be predicted from patterns could include reactivity of metals, types of bonds formed, numbers of bonds formed, and reactions with oxygen.] [Assessment Boundary: Assessment is limited to main group elements. Assessment does not include quantitative understanding of ionization energy beyond relative trends.]
Evaluations
No evaluations yet.
- Atomic Structure
- Bohr Model of the Atom
- History of the Atom
- Iowa K- 12 E-Curriculum
Version History
Review Criteria
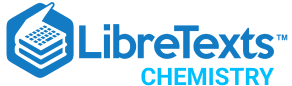
- school Campus Bookshelves
- menu_book Bookshelves
- perm_media Learning Objects
- login Login
- how_to_reg Request Instructor Account
- hub Instructor Commons
- Download Page (PDF)
- Download Full Book (PDF)
- Periodic Table
- Physics Constants
- Scientific Calculator
- Reference & Cite
- Tools expand_more
- Readability
selected template will load here
This action is not available.
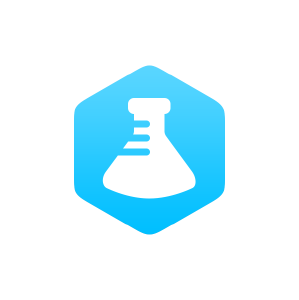
2.7.1: Atomic Models of the Twentieth Century
- Last updated
- Save as PDF
- Page ID 208942
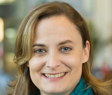
- Elizabeth Gordon
- Furman University
Learning Objectives
- Compare different theories regarding electron arrangement within the atom.
- Understand the concept of quantized energy.
- Draw modified Bohr models for every element up through atomic number 20.
- Identify the valence electrons in a Bohr model.
- Realize the Roman numeral group numbers on the periodic table represent valence electrons.
Plum Pudding and Bohr Models
In the early twentieth century, scientists attempted to construct plausible models of the atom. One of first theories was proposed by J.J. Thomson, the physicist who discovered the electron. Thomson believed that the atom contained both positive and negative charges. He was not aware of the existence of a third neutrally charged particle (neutron). Using a plum pudding (today's comparison could be chocolate chip cookie dough) dessert for a visual representation, Thomson stated that the positive mass could be represented by the actual pudding component of the dessert. The negative species inside each atom would be scattered randomly like the plums in pudding (or chocolate chips in cookie dough).

By 1911, Ernest Rutherford discovered a centrally located positive core of the atom. Rutherford's nucleus would house the positive protons while the negative electrons would remain outside this core. Chemists and physicists immediately abandoned the plum pudding model and looked to describe electron placement in more detail. In 1915, the Danish physicist Niels Bohr proposed a new model of the atom that involved electrons orbiting the nucleus. Stationary states or energy levels would be fixed distances from the nucleus (see below). In this model, these energy levels or shells would be represented by the letter "n."
This planetary model of the atom was attractive to scientists because it was similar to something with which they were already familiar, namely the solar system. Unfortunately, there was a serious flaw in the planetary model. It was already known that when a charged particle (such as an electron) moves in a curved path, it gives off some form of light and loses energy in doing so. This is, after all, how we produce TV signals. If the electron circling the nucleus in an atom loses energy, it would necessarily have to move closer to the nucleus as it loses energy and would eventually crash into the nucleus. These difficulties cast a shadow on the planetary model and indicated that, eventually, it would have to be replaced.

Energy Levels
Bohr's electrons reside in energy levels or shells. These levels are denoted with the letter "n" and are associated with a precise energy value (units would be in Joules). Shells (or levels) closer to the nucleus would require smaller amounts of energy for an electron while orbits farther from the nucleus would require the electrons to have a greater amount of energy.
Bohr hypothesized that the only way electrons could gain or lose energy would be to move from one energy level to another, thus gaining or losing precise amounts of energy. The energy levels are quantized , meaning that only specific amounts are possible. To imagine this phenomenon, note the ladder in the image below. Ladders contain rungs that are spaced at specified distances from the ground. On the ladder, an individual must remain on one of the rungs. He or she cannot step between the rungs and remain on the ladder successfully. The lowest rung required the least amount of energy for a person to exert. Moving to higher levels on the ladder would involve inputting more energy or effort.

In the Bohr atom, the rungs represent energy levels. Electrons remain in these levels until they absorb or emit a quantum of energy. When an electron gains energy, then it will move further away from the nucleus to a higher energy level. Upon emission of energy (see the red arrow in the image below), the electron will fall back to a lower energy level. The energy absorbed or emitted during these transitions is quantized.
Drawing Bohr Models
Although not completely correct, the Bohr model can give us a visual representation of electronic levels in atoms. Bohr used the formula 2n 2 to calculate maximum number of electrons that could be housed in an energy level or shell. In this formula, "n" represents the particular energy level. When substituting an integer above the number zero, one can obtain maximum electrons for each shell. For example, the first energy level would be n =1 and this level could hold 2(1) 2 = 2 maximum electrons. The second energy level could accommodate 2(2) 2 = 8 electrons. In drawing Bohr diagrams, different amounts of shells are required to store a specific atom's electrons. Looking at a Bohr diagram for the carbon atom, one can see that two energy levels/shells are required for its six electrons.
Bohr diagrams for a variety of elements are illustrated in chart below . When determining the number of electrons of an atom, remember to refer to the atomic number (the whole number) on the periodic table.

In this course, we will be using a modified Bohr model . In other words, we will limit each shell after n=1 to a maximum of eight total electrons. We will also be limited in drawing Bohr diagrams of only the first twenty elements on the periodic table. This process will allow us to identify the valence or active electrons of these particular elements. Watch this video of your professor drawing Bohr models and identifying valence electrons.
Example \(\PageIndex{1}\): Drawing BOhr Diagrams
Draw modified Bohr diagrams for
commons.wikimedia.org/wiki/E...-_no_label.svg
Bohr model of nitrogen: (CC BY-SA 2.0 uk; Greg Robson);
Bohr model of calcium: (CC BY-SA 2.0 uk; Greg Robson):
Bohr model of sulfur: (CC BY-SA 2.0 uk; Greg Robson).
Valence electrons are located in the highest energy level of an atom. When drawing a Bohr diagram, the valence electrons would be present in the outermost electronic level/shell (furthest away from the nucleus). An atom can have from one to eight valence electrons. In the bonding process, atoms will either gain, lose, or share valence electrons to become more stable. A quick method of checking valence electrons is to refer to an element's group number (in Roman numerals) on the periodic table. Even if you cannot draw a Bohr diagram (Bohr's are limited to elements with atomic numbers (Z)< 21), you can still determine valence electrons by looking to the group number.
Example \(\PageIndex{2}\): Identifying Valence Electrons
From the Bohr Diagrams constructed in Example \(\PageIndex{1}\), how many valence electrons are on each atom.
Quantum Theory
The Bohr Theory failed to explain why the electron did not spiral into the nucleus after a certain amount of revolutions around the nucleus. In addition, this theory had difficulty diagramming multi-electron systems (atoms or ions with more than one electron). For these reasons, scientists turned to the quantum mechanical theory. Since 1927, this has been the working theory that most chemistry courses use ( we will not use this theory in our course ). For a more detailed explanation of quantum mechanics, click here .
- The Bohr model postulates that electrons orbit the nucleus at fixed energy levels.
- Orbits further from the nucleus exist at higher energy levels.
- When electrons return to a lower energy level, they emit energy in the form of light.
- Bohr's model suggests each atom has a set of unchangeable energy levels and electrons in the electron cloud of that atom must be in one of those energy levels..
- Energy levels: Possible orbits that an electron can have in the electron cloud of an atom.
- Quantized: requiring or possessing specific amounts of energy.
Contributors and Attributions
Elizabeth R. Gordon (Furman University)
Isabella Quiros (Furman University)
Models of the atom - physics quiz
Part of Physics (Single Science) Atomic structure
Take the physics models of the atom quiz
The questions in this quiz are suitable for GCSE physics students studying developing the atom, Rutherford and the nucleus and further developments to the atomic model.
If you struggled with the quiz, don't panic - we've got you! You can models of the atom stores by clicking the links below.
Revise models of the atom
To brush up on your GCSE physics models of the atom knowledge, click on the link to your awarding body specific revision:
More GCSE physics quizzes
Click the links below to try our GCSE physics exam-style or quick-fire quizzes and test your skills and knowledge.
GCSE Physics
GCSE Physics: exam-style questions
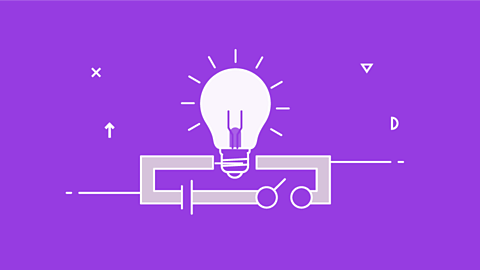
GCSE Physics quick-fire
GCSE Physics: quick-fire questions
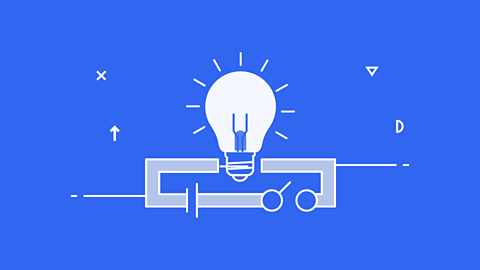
GCSE exam-style quizzes
Improve your exam skills
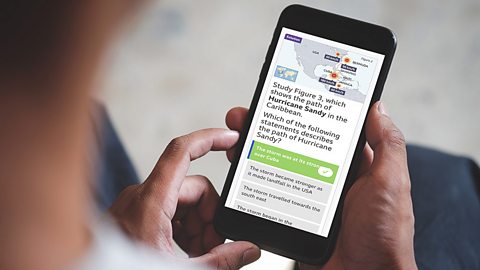
How to Build a Model Atom.
Most recent answer: 10/22/2007
(published on 10/22/2007)
Follow-Up #1: models of atoms
Follow-up #2: what is an atom.
(published on 11/04/2007)
Follow-Up #3: cloudy models
(published on 12/10/2007)
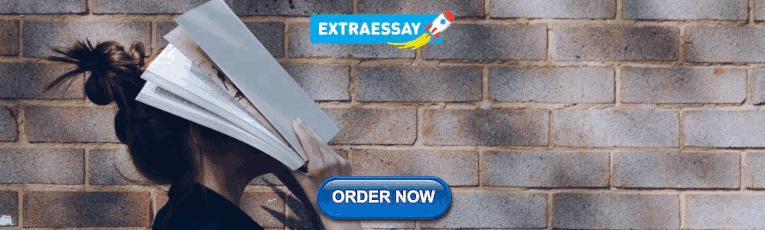
Follow-Up #4: How to make an atom?
(published on 06/26/2008)
Follow-Up #5: picturing atoms?
(published on 09/08/2008)
Follow-Up #6: another atom model view
(published on 10/03/2008)
Follow-Up #7: atom models
(published on 03/24/2009)
Follow-Up #8: atoms and MM
(published on 04/24/2009)
Follow-Up #9: back to Bohr
(published on 06/05/2009)
Follow-Up #10: A comment on atom model building
(published on 06/09/2009)
Follow-Up #11: atom models redux
(published on 08/31/2009)
Follow-Up #12: atom model
(published on 09/04/2009)
Follow-Up #13: back to models
(published on 09/22/2009)
Follow-Up #14: atom models redux
(published on 09/27/2009)
Follow-Up #15: electrons, etc.
(published on 10/04/2009)
Follow-Up #16: atom models redux
(published on 10/06/2009)
Follow-Up #17: atom models redux
(published on 10/12/2009)
Follow-Up #18: atomic teaching
(published on 10/20/2009)
Follow-Up #19: philosophy of teaching
(published on 10/22/2009)
Follow-Up #20: atom models again
(published on 11/01/2009)
Follow-Up #21: thoughts on education
(published on 11/05/2009)
Follow-Up #22: Bohr model
(published on 12/08/2009)
Follow-Up #23: atoms and art
(published on 12/10/2009)
Follow-Up #24: atom models with balloons
(published on 01/12/2010)
Follow-Up #25: atoms
(published on 01/27/2010)
Follow-Up #26: freezing atom in time?
(published on 01/30/2010)
Follow-Up #27: Bohr and quantum chemistry
(published on 02/24/2010)
Follow-Up #28: atom models
(published on 02/25/2010)
Follow-Up #29: atom models
Follow-up #30: model details.
(published on 02/27/2010)
Follow-Up #31: going atomic
(published on 03/06/2010)
Follow-Up #32: emotional support
(published on 03/09/2010)
Follow-Up #33: teaching about the atom
(published on 08/12/2010)
Follow-Up #34: my defense
(published on 09/22/2010)
Follow-Up #35: ancient china etc.
(published on 10/06/2010)
Follow-Up #36: Where is the electron, really?
(published on 11/22/2010)
Follow-Up #37: confusing?
(published on 12/02/2010)
Follow-Up #38: specific model-building ideas
(published on 01/03/2011)
Follow-Up #39: atom frustration
(published on 01/09/2011)
Follow-Up #40: joke?
Follow-up #41: further atom thoughts.
(published on 01/10/2011)
Follow-Up #42: old questions
(published on 01/17/2011)
Follow-Up #43: home-schooling quantum physics
(published on 02/08/2011)
Follow-Up #44: How to teach about atoms?
(published on 02/23/2011)
Follow-Up #45: Kerri from Knoxville
(published on 03/10/2011)
Follow-Up #46: slave labor
Follow-up #47: bigger atom models.
(published on 04/09/2011)
Follow-Up #48: arsenic models
(published on 04/10/2011)
Follow-Up #49: Bromine model
(published on 06/10/2011)
Follow-Up #50: On teaching science
(published on 09/03/2011)
Follow-Up #51: on science education
(published on 11/19/2011)
Follow-Up #52: edible atoms
(published on 01/31/2012)
Follow-Up #53: against quantum mechanics
(published on 02/01/2012)
Follow-Up #54: Bohr for the masses
(published on 02/10/2012)
Follow-Up #55: atoms in quantum field theory
(published on 02/28/2012)
Follow-Up #56: relativistic atoms
(published on 03/02/2012)
Follow-Up #57: more on relativistic atoms
Hi Haley- Yes, our question reader deletes all sorts of control characters, I think as a precaution against malware. It often leads to hassles for formatted inputs. The Lamb shift does indeed come from photon effects, the quantization of the electromagnetic field. You can think of this as coming from a vacuum cloud of photon possibilities. The reason those effects are measurable rather directly in atoms but not the effects from the background cloud of electron-positron pairs is that the electron-positron pair has significant rest mass. It's not cheap to create a pair, unlike photons which have no rest mass. Just to try to find something I know that's dimly related to your question, the electron-positron pair creation does become prominent when a heavy nucleus is bare, stripped of electrons. Then its electric field is so big that it causes sparking of the vacuum- pulling electrons out of nothing and spitting out positrons. That quickly reduces the field and the process stops. In an atom, the electrons are already present, suppressing such effects. Mike W.
p.s. I finally remembered to get some help from a colleague on this. The positron density even in uranium is "very low". The reason is that it costs so much energy (1 MeV) to make an electron-positron pair. My colleague (Eduardo Fradkin) say that the math for calculating the positron density is similar to the math for calculating the carrier density in an intrinsic semiconductor, with that 1 MeV playing a role similar to the band gap. The concentration of positrons falls off exponentially with the size of that gap. He says it's much less than 1% even for uranium. For higher atomic numbers the field gets larger near the nucleus and the energy scale goes up , so you start getting the sparking we described above, and actually make real electron-photon pairs.
(published on 03/03/2012)
Follow-Up #58: malicious atom models
Whoops, this slipped between the cracks some time ago. It's a relief to see readers with even worse attitudes than ourr own. Needless to say, this approach would be risky to a student's grade.
(published on 04/26/2012)
Follow-Up #59: a student's perspective on atom models
Your note helps remind us of how things look from a student's perspective.
(published on 10/10/2012)
Follow-Up #60: What do electrons really do?
(published on 03/02/2013)
Follow-Up #61: model of modern quantum theory of atom
(published on 03/27/2013)
Follow-Up #62: tips on atom models
(published on 04/15/2013)
Follow-Up #63: looking back on atom models
John- Thanks for your kind note. It's alwauys encouraging to hear that some of the old answers are still being read with real interest.
The atom model question is just one little piece, not the most important one, of the whole issue of how best to teach science and math. My wife teaches a massive elementary statistics course, perhaps the most important technical topic for most people who aren't going on in specialized fields. There's a dispute there between teaching a big set of formulas and teaching somewhat less but with more thorough understanding. It's an interesting case, because, unlike the quantum atom, the deeper way of teaching doesn't rely on students having special preparation. She's on the side of understanding, which turns out to be popular with most students, but is not the way of most statistics teachers.
(published on 11/29/2013)
Follow-Up #64: Is answering physics questions boring?
Lee and I and the other volunteers here aren't paid. So we only answer what we want when we want. So it seems we do enjoy it.
We're delighted that you found it useful. That adds to the enjoyment.
(published on 12/01/2014)
Follow-Up #65: atomic weirdness?
Let's blame it on the atoms. They started the weirdness.
(published on 10/31/2015)
Follow-Up #66: model of strontium
Wow, that's not easy. I guess you can put 38 sparkles into some sort of gel. That captures some of the flavor, but not the structure of those wave functions. Even with a 3-D printer, those overlapping states aren't so easy. Printers don't do negative ψ!
It's interesting that strontium was picked as the one to do. Since it's got filled shells the overall state is spherically symmetric. I wonder if the teacher would accept that overall symmetry and allow a spherical model?
(published on 11/02/2015)
Follow-up on this answer
Related Questions
- electrons and elements
- Proton proton forces
- atomic shells
- What are the advantages of the plum pudding model?
- 8 states in atomic shell
- atom models
- Minimum and maximum masses of nucleii
- color of nuclei?
- why are atoms stable?
- Is charge quantized?
Still Curious?
Expore Q&As in related categories
- Inside the Atom
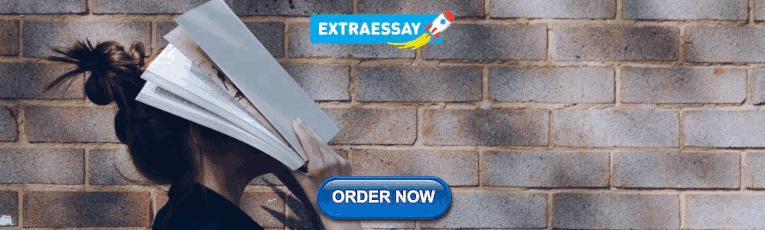
IMAGES
VIDEO
COMMENTS
In this activity, you will learn about classic models of the atom and how views of atomic structure changed over time as scientists did more experiments and made new discoveries. You will also learn
An atom is mostly empty space. Almost all of the mass of the atom is concentrated in the nucleus. The protons and neutrons in the nucleus are very tightly packed. The number of protons and neutrons is always the same in the neutral atom. All of the above statements (A-D) are true.
Figure 2.2.1 2.2. 1: The Structure of the Atom. Atoms have protons and neutrons in the center, making the nucleus, while the electrons orbit the nucleus. The modern atomic theory states that atoms of one element are the same, while atoms of different elements are different.
As a result of Rutherford's work, it became clear that an α particle contains two protons and neutrons, and is therefore the nucleus of a helium atom. 2.4. 9. Figure \(\PageIndex{12}\): The Evolution of Atomic Theory, as Illustrated by Models of the Oxygen Atom. Bohr's model and the current model are described in Chapter 6, "The Structure ...
Learn. Quantum Wavefunction. The quantum mechanical model of the atom. Quantum numbers. Quantum numbers for the first four shells. Shells, subshells, and orbitals. The periodic table, electron shells, and orbitals.
Dig into atomic models. Download Student Worksheet. Purpose: Students will work in groups to research, create and present different models of the atom that show the evolution of physicists ...
This page contains materials for the session on the atomic models of Rutherford and Bohr. It features a 1-hour lecture video, and also presents the prerequisites, learning objectives, reading assignment, lecture slides, homework with solutions, and resources for further study.
An atom is mostly empty space. Almost all of the mass of the atom is concentrated in the nucleus. The protons and neutrons in the nucleus are very tightly packed. The number of protons and neutrons is always the same in the neutral atom. All of the above statements (A-D) are true.
Discuss the mass number in relation to an atom's protons and neutrons. Understand the law of conservation of matter. Visualize how orbitals are superimposed upon one another within an atom. Explain the meaning of conservation of energy. Describe that when energy is added to an atom its electron(s) are able to temporarily move to an excited state.
Helpful visual aid: Bohr's Model of the atom. Assignment: BASICS. Demonstration: The teacher calls on 6 volunteers to the front of the classroom and hands a "proton" to 2 students, a "neutron" to 2 students, and 2 "electrons" to the last student. The "protons" and "neutrons" stand together, while the "electrons ...
This assignment can be found in Google Docs: ... Niels Bohr developed a quantum model for the hydrogen atom. The lowest possible energy state of a molecule or atom is called its ground state. A gamma ray of wavelength 1.00 × 10 -8 cm has enough energy to remove an electron from a hydrogen atom.
This graphic takes a look at the key models proposed for the atom, and how they changed over time. Though our graphic starts in the 1800s, the idea of atoms was around long before. In fact, we have to go all the way back to Ancient Greece to find its genesis. The word 'atom' actually comes from Ancient Greek and roughly translates as ...
Atomic Models: Homeschool Assignment. Instructor Megan Sarkisian. Cite this lesson. In a short essay, students will describe the creation and features of an atomic model, as well as the advances ...
5: Models of the Atom. Page ID. 68587. An introductory chemistry Libretexts Textmap organized around the textbook. Introductory Chemistry: Concepts & Critical Thinking. by Charles Corwin. I II III IV V VI VII VIII IX X XI XII XIII XIV XV XVI XVII XVIII XIX XX Homework.
The now outdated solar system model of the atom allows us to visualize the meaning of the potential energy levels. The main energy levels, also called shells, are given by the main quantum number n. nucleus + n = 1 n = 2 n = 3 n = 4 The potential energy increases as the distance from the nucleus increases
Primarily, the atomic structure of matter is made up of protons, electrons and neutrons. The protons and neutrons make up the nucleus of the atom, which is surrounded by the electrons belonging to the atom. The atomic number of an element describes the total number of protons in its nucleus. Neutral atoms have equal numbers of protons and ...
Atomic Model (Atomic Theory) - An atom is the smallest unit of ordinary matter that forms a chemical element.Atoms are made of fundamental particles called protons, neutrons and electrons. To learn more about the Atomic Theory, Structure, number, Mass and Atomic species with examples and FAQs, visit BYJU'S
• An atom is the particle of an that still has the identity and properties of the element. particles (particles that are smaller than atoms). and • Atoms are made up of • These particles are , Summary of the Parts of an Atom: Name Symbol Charge Location Relative Mass The Nucleus • At the of every atom is a
Atoms are made of electrons, Protons, and Neutrons. Protons and Neutrons reside in the nucleus of the atom and electrons orbit the nucleus. Atoms always have an equal number of protons and electrons and the number of neutrons and protons is usually the same as well. Protons and Neutrons are held together in the nucleus by a strong force called ...
To draw the models, cards are provided with examples for students to draw. Students can use the PhET Simulation: Build an Atom to check their answers. Remix of: AEA Template for Building Lessons Subject: Chemistry Level: High School Material Type: Homework/Assignment, Lesson, Simulation Author: Julie Buerman Date Added: 07/30/2020
Figure 2.7.1.3 2.7.1. 3: The Bohr model of the atom illustrating levels of electrons. This planetary model of the atom was attractive to scientists because it was similar to something with which they were already familiar, namely the solar system. Unfortunately, there was a serious flaw in the planetary model.
Revise models of the atom for your physics GCSE foundation and higher triple science exams with Bitesize interactive practice quizzes covering feedback and common errors.
Study with Quizlet and memorize flashcards containing terms like Write a brief passage describing a neutral atom of nitrogen-14 (N-14). Describe the number of protons, neutrons, and electrons in the atom, where each type of particle is located, and how the terms atomic number, mass number, and atomic mass are related to the particles. Use the periodic table to help you., An atom of sodium-23 ...
Would it be a good idea to use the concept of malicious compliance to complete the atom model assignment? All That is required is a nucleus model including an appropriate number of good sized balls of two different colors. Perhaps the teacher won't understand so include a note that points out that the nucleus is about 99.97% of the atom's mass.