- - Google Chrome
Intended for healthcare professionals
- Access provided by Google Indexer
- My email alerts
- BMA member login
- Username * Password * Forgot your log in details? Need to activate BMA Member Log In Log in via OpenAthens Log in via your institution

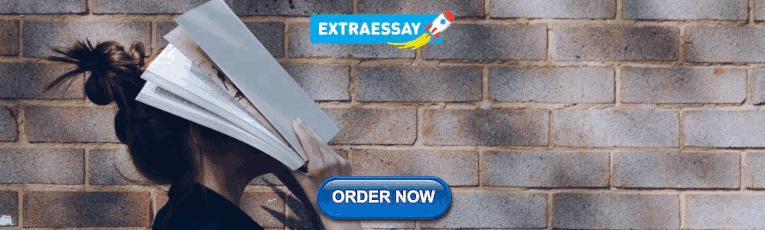
Search form
- Advanced search
- Search responses
- Search blogs
- Management of...
Management of pneumonia in critically ill patients
- Related content
- Peer review
- Catia Cillóniz , professor, senior researcher, applied research on respiratory disease 1 2 3 4 ,
- Antoni Torres , professor medicine (pulmonology), senior consultant of the respiratory and intensive care unit 1 2 3 4 ,
- Michael S Niederman , professor of clinical medicine 5
- 1 Department of pneumology, Hospital Clinic of Barcelona, Spain
- 2 August Pi i Sunyer Biomedical Research Institute (IDIBAPS), Barcelona, Spain
- 3 University of Barcelona, Barcelona, Spain
- 4 Biomedical Research Networking Centers in Respiratory Diseases (CIBERES), Barcelona, Spain
- 5 Weill Cornell Medical College, Department of Pulmonary Critical Care Medicine, New York, NY, USA
- Correspondence to MS Niederman msn9004{at}med.cornell.edu
Severe pneumonia is associated with high mortality (short and long term), as well as pulmonary and extrapulmonary complications. Appropriate diagnosis and early initiation of adequate antimicrobial treatment for severe pneumonia are crucial in improving survival among critically ill patients. Identifying the underlying causative pathogen is also critical for antimicrobial stewardship. However, establishing an etiological diagnosis is challenging in most patients, especially in those with chronic underlying disease; those who received previous antibiotic treatment; and those treated with mechanical ventilation. Furthermore, as antimicrobial therapy must be empiric, national and international guidelines recommend initial antimicrobial treatment according to the location’s epidemiology; for patients admitted to the intensive care unit, specific recommendations on disease management are available. Adherence to pneumonia guidelines is associated with better outcomes in severe pneumonia. Yet, the continuing and necessary research on severe pneumonia is expansive, inviting different perspectives on host immunological responses, assessment of illness severity, microbial causes, risk factors for multidrug resistant pathogens, diagnostic tests, and therapeutic options.
Introduction
Pneumonia is a major health problem, being associated with high morbidity and short and long term mortality. It is also the leading infectious disease cause of mortality among all ages worldwide. 1 2 Pneumonia in critically ill patients may present as pneumonia acquired in the community (community acquired pneumonia, CAP); pneumonia acquired in the hospital (hospital acquired pneumonia, HAP); or pneumonia related to mechanical ventilation (ventilator associated pneumonia, VAP). Severe pneumonia is associated with high short and long term mortality, and those who survive often have important sequelae such as alterations of lung function, reduction in mental and cognitive functions, weakness and reduction of motor function, and reduced functional autonomy. 3 4 Appropriate diagnosis of severe pneumonia is crucial to improve survival of critically ill patients. Identifying a pathogen is critical for antimicrobial stewardship in critically ill patients with severe pneumonia. However, in most patients, identifying the cause is challenging, especially in those with chronic underlying disease, those who received previous antibiotic therapy, and those treated with mechanical ventilation. Prompt and adequate antimicrobial treatment is crucial for the best outcomes in critically ill patients with severe pneumonia, and is a key focus of international guidelines for the management of pneumonia. 5 6 7
In this article, we review current knowledge on the management of pneumonia in critically ill patients, including CAP and HAP, focusing on epidemiology, microbial etiology, pathogenesis, treatment, and prevention.
Sources and selection criteria
We searched databases from 2000 to 2021, including PubMed, Medline, the Cochrane Database of Systematic Reviews, and the Central Register of Controlled Trials (clinicaltrials.gov). We used both keywords and keyword combinations, including “pneumonia”, “severe respiratory infection”, “severe pneumonia”, “pneumonia in critically ill”, “community acquired pneumonia”, “intensive care pneumonia”, “hospital acquired pneumonia”, “ventilator associated pneumonia”, “management of pneumonia”, “diagnosis of pneumonia”, and “pneumonia therapy”. We reviewed relevant titles and abstracts of this search and prioritized studies in English, considering meta-analyses, systematic reviews, international guidelines, randomized controlled trials (RCTs), and large, descriptive and observational studies. We include some additional, relevant articles published before 2000.
Defining severe pneumonia and its epidemiology
Severe community acquired pneumonia (scap).
Early identification of patients with SCAP is critical to provide rapid, definitive treatment and to avoid delay in providing intensive care treatment, in an effort to reduce mortality. 8 9 Multiple scoring systems provide support in deciding the site of care, but most of these tools predict mortality risk, and not specifically the need for intensive care admission. Proper site of initial care is important, since both length of hospital stay and mortality of patients first admitted to the general ward and then transferred to the intensive care unit (ICU) are higher than for patients directly admitted to the ICU. 10 11
The most widely accepted criteria for defining severe CAP are from the 2007 Infectious Disease Society of America/American Thoracic Society (ATS/IDSA) consensus guidelines on the management of CAP in adults 12 ( box 1 ). ICU care is needed for those who require mechanical ventilation or vasopressor support of shock, or the presence of three of nine minor criteria. Several validation studies 13 14 15 16 17 of the minor criteria found that they were accurate for predicting need for ICU admission.
ATS/IDSA criteria for severe CAP
Minor criteria.
Respiratory rate ≥30 breaths/min
PaO 2 /FiO 2 ratio ≤250
Multilobar infiltrates
Confusion/disorientation
Uremia (blood urea nitrogen level ≥20 mg/dL)
Leukopenia (white blood cell count <4 ×10 9 /L)
Thrombocytopenia (platelet count <100 ×10 9 /L )
Hypothermia (core temperature <36°C)
Hypotension requiring aggressive fluid resuscitation
Major criteria
Invasive mechanical ventilation
Septic shock with the need for vasopressors
The number of patients requiring intensive care is growing, along with the rising number of individuals who are older, immunocompromised, or with underlying serious comorbidities. In 2018, a cross sectional study 18 showed a substantial increase in hospitalization for acute respiratory infections throughout the period 2003-15. The rise was more evident in the older population: ICU admission was reported to be 3.3 times higher in patients aged 85-89 and 5.8 times higher in patients ≥90, compared with younger populations.
A secondary analysis of a prospective, population based cohort study on hospitalized patients with CAP in the US found that 23% needed ICU admission, of whom 24% required invasive mechanical ventilation and 20% required non-invasive mechanical ventilation. The authors reported an incidence of CAP in the ICU of 145 cases per 100 000 adults per year. 19
CAP is also a major cause of sepsis. In 2016, the third international consensus definition (Sepsis-3) defined sepsis as an increase in the Sequential Organ Failure Assessment (SOFA) score of two points or more, and recommended the use of the quick Sequential Organ Failure Assessment (qSOFA) score to identify patients at high risk of death and prolonged ICU stay among those with suspected infection, such as CAP. 20 Several studies evaluated the performance of qSOFA in a CAP population, 21 22 23 24 25 26 27 and showed that it was more accurate than other scores, such as CRB (confusion, respiratory rate, blood pressure), and SOFA in predicting mortality and ICU admission. In spite of early recognition and treatment of SCAP, short and long term mortality remain high (27-50%). 19
Hospital acquired pneumonia (HAP)
HAP has an estimated annual incidence of five to 10 cases per 1000 hospital admissions globally, and is considered the second most common hospital acquired infection. 7 One subgroup, ventilator associated pneumonia (VAP), which occurs in patients on mechanical ventilation for at least 48 hours, affects 10-25% of all ventilated patients, with the risk being highest in the first 5-7 days of mechanical ventilation. 5 7 VAP remains a frequent nosocomial infection in the ICU, and causes significant morbidity. However, debate continues on attributable mortality rates, varying widely and being affected by diverse confounders. 28 29 With the implementation of recent, effective prevention strategies, however, incidence and mortality have been decreasing. 30 31 32 33 In addition to VAP, some patients with severe HAP deteriorate and then require mechanical ventilation for management, and this population is referred to as ventilated HAP ( fig 1 ).

Spectrum of hospital acquired pneumonia
- Download figure
- Open in new tab
- Download powerpoint
HAP develops after ≥48 hours of hospitalization and is classified as HAP in the ward, with a mortality rate of 13-28%. HAP in the ICU (non-ventilated ICU HAP) has a mortality of 15%, and VAP and ventilated HAP have a mortality rate of up to 28%.
In epidemiological studies, the average rate of VAP in the US is four cases per 1000 days of mechanical ventilation, 5 34 which represents half the rate reported in Europe: 9.5 cases per 1000 days of mechanical ventilation. 35 In recent years, the incidence of VAP has gradually declined in the US and in Europe, possibly in relation to the implementation of preventive care bundles. 36 37 However, the use of surveillance definitions developed by the Centers for Disease Control and Prevention (CDC) resulted in concern about the accuracy of the VAP definition, especially as rates dropped but mortality did not. These VAP definitions have recently been supplemented by ventilator associated event (VAE) reporting. The updated definitions include any condition that is associated with a decline in oxygenation. Incidence of VAP is greater than with the previous definition, although VAE may not always be the result of infection, and is of unclear significance as a measure of ICU quality of care. Furthermore, it is not widely used in Europe. 38
In the US, some quality initiatives have a goal of “zero ventilator associated pneumonia” (ZERO-VAP) 38 ; however, concerns about the ZERO-VAP target have been raised. 39 First, it is difficult to quantify and determine the eradication of a preventable disease without accurate and reliable diagnostic methods, and no gold standard exists for diagnosing VAP. Second, the CDC VAP definition can be manipulated, leading to an underestimation of occurrence of VAP. Third, monitoring adherence to long term prevention measures poses a notable challenge. To determine the efficacy of interventions in reducing VAP, innovative diagnostic markers and the application of improved research methods are needed.
HAP and VAP are considered leading causes of death from nosocomial infection. 4 28 40 41 Mortality in the US is approximately 13%, 5 but a recent prospective and multicenter observational study 42 indicated that 30 day mortality caused by VAP was 30% in Europe. Similarly, after analyzing data of 14 212 patients admitted to the ICU for more than 48 hours, a separate French observational study reported that the risk of 30 day mortality increased by 82% in patients with HAP and 38% in patients with VAP. 43 Compounding these findings is an analysis of non-inferiority endpoints by Talbot et al 44 that examined seven bacterial HAP/VAP datasets and reported that 28 day all-cause mortality was higher in ventilated HAP (28%), followed by VAP (18%) and non-ventilated HAP (15%). These results highlight the influence of patient related factors on outcomes, as reported in a previous, prospective observational study, 45 and underpin the impact of preventive measures.
Antibiotic resistance is a major concern in HAP/VAP globally, especially because of its association with prolonged length of hospital stay and higher mortality. Gram negative microorganisms are the pathogens that most frequently cause HAP/VAP. 46 In an observational, 24 hour point prevalence study 46 of 15 202 ICU patients from 1155 centers across 88 countries, infections caused by Enterococcus resistant to vancomycin, Klebsiella resistant to β-lactam antibiotics—including third generation cephalosporins and carbapenems, or Acinetobacter species resistant to carbapenem—were independently associated with a higher risk of death. These findings emphasize the importance of knowing local epidemiology and risk factors for multidrug resistant pathogens to target the most likely etiologic pathogens in patients with HAP/VAP.
New concepts in pneumonia pathogenesis
Cap and cardiovascular disease.
In addition to its short term sequelae, CAP has long term implications for mortality that may be related to heart disease as a complication of pneumonia. 47 Approximately 8-25% of patients with CAP have been reported to experience at least one cardiac event during hospitalization, 48 49 50 including acute coronary syndrome, new or worsening heart failure, new or worsening arrhythmias, and acute stroke. 51 52 Such events are more commonly reported in patients with chronic cardiovascular disease, in severe cases of pneumonia, and in conditions related to pathogens, such as pneumococcus and influenza virus, 53 54 55 but they can also occur in patients without chronic cardiovascular disease. 56
The pathogenesis of cardiac dysfunction in pneumonia is multifactorial, involving host factors, pathogens, and medication. 51 57 58 A great proportion of hospitalized CAP patients are older adults with comorbidities (chronic pulmonary disease, chronic cardiovascular disease, diabetes mellitus), who may have chronic endothelial dysfunction. 59 60 61 During the acute phase of pneumonia, inflammation occurs in the lungs, but a systemic inflammatory response also occurs that augments the release of pro-inflammatory cytokines, which could directly or indirectly injure the myocardium. 62
Streptococcus pneumoniae (pneumococcus) is the most common bacterial pathogen in CAP, and clinical and animal data have shown that the organism can invade the myocardium directly and cause cardiac damage. 63 Pneumolysin, the most important virulence factor of pneumococcus, can also cause cardiomyocyte necroptosis 64 (a programmed mode of cell death that increases inflammation), which may result in cardiac damage. 65 Similarly, pneumococcal bacteremia is known to be a risk factor for cardiac events, 66 with the risk lasting for up to 10 years after the pneumonia episode. 62 66 Shenoy et al, 67 in an experimental study of pneumococcus, reported that cardiac damage depends on the ability of a clinical isolate to cause high grade bacteremia, and pathological damage may depend on the pneumococcal strain. Respiratory viruses, identified using new molecular testing, are frequently reported as a cause of CAP, and they too can cause cardiac injury. A self-controlled case series reported an increase in the risk of myocardial infarction during the week after laboratory confirmed infection with influenza virus. This risk was six times higher when compared with the risk present during the year before or after infection. Importantly, an elevated incidence of acute myocardial infarction after influenza infection was observed in adults aged ≥65. 68 Compounding these findings is a time series analysis of English hospital admissions, in which a small yet strongly significant correlation was noted between weekly occurrence of new acute myocardial infarction or ischemic stroke hospitalization in older adults, especially in patients aged ≥65 with positive laboratory results for respiratory viruses (including influenza, para-influenza, respiratory syncytial virus, human metapneumovirus, rhinovirus, and adenovirus). 69 Replication of respiratory viruses within the myocardium may lead to a cascade of inflammatory processes that causes fibrosis and, thereby, cardiac necrosis. It may also lead to alterations in lipid metabolism, by which the course of atherosclerosis would be accelerated, and serve as a possible mechanism for cardiac damage. 70 71 Several mechanisms have been suggested to help explain cardiac effects as sequelae of viral infection; however, we do not yet have complete understanding of the exact mechanisms, or the ideal approach to prevention.
VAP and the endotracheal tube
Endotracheal intubation allows pathogens to enter directly into the lower respiratory tract, interfering with normal lung defense mechanisms, with the endotracheal tube (ETT) becoming a reservoir for pathogenic microorganisms. 72 Biofilm formation on the tube has two important implications: (1) it causes intra-luminal narrowing that may impede weaning from the ventilator, and (2) bacterial pathogens grow embedded and protected in an extracellular polymeric substance or matrix that makes them more resistant to antimicrobials, 73 and they serve as a nidus of endobronchial infection 74 that is difficult to eliminate. 75 Biofilm is detectable in approximately 95% of endotracheal tubes in ventilated patients, occurring within hours of endotracheal intubation. 76 The most frequent biofilm pathogens are Acinetobacter baumannii , Pseudomonas aeruginosa , Staphylococcus aureus , and Klebsiella pneumoniae, and colonization may be the first step toward VAP. 77 78 An observational study 79 reported that biofilm formation, after at least 24 hours, was less in both silicone coated polyvinyl chloride (PVC) and noble metal coated PVC ETTs than in uncoated PVC ETTs.
The role of the microbiome in pneumonia pathogenesis
Multiple bacterial species are present in the healthy lung, which is not, as previously thought, sterile, and they comprise the “lung microbiome.” 80 Prevotella , Veillonella , Streptococcus , Fusobacterium, and Hemophilus species are the most frequently reported bacterial pathogens in this microbiome, which is a dynamic community, with a constant equilibrium among species and in an interaction with lung immunity. In the healthy state, the microbiome comprises a diverse population of organisms, and they are present in a relatively low microbial load.
Acute infection and chronic pulmonary diseases, such as pneumonia, non-cystic fibrosis bronchiectasis, 81 chronic pulmonary obstructive disease (COPD), 82 or asthma 83 disturb the equilibrium of the healthy lung microbiome. This dysbiosis results in changes in microbial communities, often leading to less diversity and an increase in the microbial load. 84 The risk factors for dysbiosis that lead to bacteria such as pneumococcus to cause disease are only partially understood. 85
In a longitudinal analysis of the lung microbiome in critically ill intubated patients, the authors observed that the biomass of the lung microbiome increased over time. 86 A progressive change also took place in community diversity, as bacterial taxa in the healthy lung are replaced with a less diverse group of organisms, including Staphylococcus spp, and Acinetobacter spp in patients with pneumonia. 87 In addition, a relation exists between the lung microbiome and lung immunity, 88 89 which shows that the enrichment of the lung microbiome with oral taxa was associated with inflammation in the lung (increased alveolar concentration of inflammatory cytokines, increased alveolar lymphocytes) and a distinct metabolomic profile.
Recently, it was proposed 88 that HAP is not only an acute condition caused by contamination of the lung by exogenous microorganisms, but also the result of a dysbiosis between the lung microbiome and mucosal immunity. This approach could lead to the implementation of new preventive methods to restore immune function, and novel therapies that allow for the manipulation of the lung microbiome instead of its elimination by antibiotics.
Diagnosis of pneumonia
Suspicion and clinical diagnosis of pneumonia in patients coming from the community rely on the presence of acute symptoms (≤7 days) of a lower respiratory tract infection that include cough, expectoration, fever, chills, and dyspnea, together with the presence of a new infiltrate on chest radiograph. 90 Typically, pneumonia symptoms may be less evident in older people, in whom the absence of fever was reported in approximately 30% of patients. 91 Similarly, individuals treated with antibiotics, steroids, and non-steroidal anti-inflammatory drugs may also present with less evident symptoms of lung infection. 92 Other conditions, such as pulmonary embolism, pulmonary edema, and lung cancer, may mimic pneumonia and present with fever and pulmonary infiltrates. 93 It is helpful and important to review prior chest radiographs, if possible, to rule out other diseases. Rarely, after a negative chest radiograph, patients may receive a diagnosis of pneumonia by more sensitive methods such as a chest computed tomography (CT) scan.
VAP is usually suspected when patients have symptoms of fever or hypothermia, leukocytosis or leukopenia, and evidence of purulent secretions in an ETT or tracheostomy aspirate, in the setting of a new or progressive lung infiltrate. Data on clinical, radiological, and laboratory parameters often provide the basis for clinicians to initiate antimicrobial treatment in patients with VAP. Interestingly, a systematic review and meta-analysis (including 25 studies and data of 1639 patients) 94 that investigated performance of several diagnostic tests for VAP reported that sensitivity and specificity of physical examination findings for VAP were poor: fever (66.4% [95% confidence interval (CI) 40.7 to 85.0], 53.9% [95% CI 34.5 to 72.2]) and purulent secretions (77.0% [95% CI 64 to 85.9], 39.0% [95% CI 25.8 to 54.0]). The presence of infiltrates on chest radiographs had a sensitivity of 88.9% (95% CI 73.9 to 95.8%) and specificity of 26.1% (95% CI 15.1 to 41.4%); the endotracheal aspirate had a sensitivity of 75.7% (95% CI 51.5 to 90.1) and specificity of 67.9% (95% CI 40.5 to 86.8); and the clinical pulmonary infection score (CPIS) >6 had a sensitivity of 73.8% (95% CI 50.6 to 88.5) and specificity of 66.4% (95% CI 43.9 to 83.3). The study found poor specificity for the classic clinical indicators for VAP diagnosis, highlighting the need for a new diagnostic tool for this condition.
For pneumonia diagnosis, radiographic confirmation is essential. It provides important information on the site, extent, and associated features of pneumonia. The presence of pleural effusion or multilobar involvement serves as an indicator of severity. 6 Reported sensitivity and specificity of chest radiographs in CAP were 66% and 77%, respectively. 95 A standard chest radiograph for CAP consists of posteroanterior and lateral views. However, diagnostic performance of chest radiographs increases with the use of lateral projection images. Chest radiographs have some limitations, as shown by results obtained in a prospective and multicenter interventional study 96 that included 319 patients diagnosed with CAP who had a chest radiograph and thoracic CT scan at admission. In this study, no evidence of pneumonia was present on CT scans in 30% of patients diagnosed with CAP based on the clinical presentation and chest radiograph. Conversely, the CT scan identified up to 35% of pneumonia cases, which had not initially been caught by chest radiographs. These results underpin the potential importance of CT scans as a complementary tool to chest radiographs in diagnosing pneumonia. Further, CT scans could prove helpful in cases with non-specific radiographic findings; pulmonary complications such as empyema or cavitation; clinical suspicion of an underlying lesion such as lung carcinoma; and recurrent or non-resolving pneumonia. 97
In VAP, clinicians often use chest radiographs to determine evidence of new infiltrates. Radiography is reported as the most widely employed imaging technique in diagnosing this condition. 98 However, chest radiographs are not sensitive or specific for VAP diagnosis. 99 100 Interestingly, lung ultrasound is a promising, non-invasive imaging tool for such diagnoses. A systematic review and meta-analysis 101 that included 12 studies with data of 1515 patients found that lung ultrasounds have a sensitivity and specificity of 88% and 89%, respectively, with an area under the curve (AUC) of 0.95. A separate systematic review and meta-analysis 102 showed that the emergence of sub-pleural consolidations on lung ultrasound in anterior lung regions had a specificity of 95% (95% CI 79% to 99%) on the eve of the day when clinical suspicion was present. On the day when clinical suspicion was present, the emergence of lobar/sub-lobar consolidations in anterior lung regions had a specificity of 100% (95% CI 87% to 100%) while the emergence of lobar/sub-lobar consolidations with dynamic air bronchograms had a specificity of 96% (95% CI 81% to 99%). These data suggest that lung ultrasounds are highly accurate in diagnosing VAP. However, applying this technique requires considerable expertise and practice, especially in mechanically ventilated patients.
Guideline recommendations 6 for microbiological diagnosis in patients with severe CAP include obtaining good quality sputum, blood, and pharyngeal swab samples to detect respiratory viruses in patients with severe illness. Molecular detection through polymerase chain reaction (PCR) is the gold standard for virus diagnosis. 103 Detecting viruses through antigen based assays is limited owing to low sensitivity (40-70%); in cases of negative results, although specificity ranges between 90% and 95%, testing cannot rule out a viral infection diagnosis. 103 Sputum is recommended to be collected before starting antibiotic treatment. Sensitivity for Gram staining of a sputum sample is approximately 80% in cases of pneumococcal pneumonia and 78% in pneumonia caused by Staphylococcus ; specificity is 93-96%. 104 105 Results of respiratory samples must be interpreted with caution: the detected microorganism may form part of the normal flora or may be present as a result of colonization in the patient. 103 Also, urinary antigen detection testing of S pneumoniae and L pneumophila has good sensitivity and specificity, and should be performed in those with severe CAP. Finally, bronchoscopic samples such as bronchoalveolar lavage in intubated patients are easy to retrieve and provide information about organisms in the lower respiratory tract.
Debate continues about the best respiratory sampling method and the most accurate diagnostic approach for nosocomial pneumonia. The latest US guidelines 5 recommend non-invasive sampling (endotracheal aspiration) with semiquantitative cultures to diagnose VAP and non-VAP. However, with the aim of reducing antibiotic exposure, the latest European guidelines 7 recommend obtaining distal quantitative cultures before antibiotic treatment in clinically stable patients with suspected VAP. Similarly, guidelines also recommend obtaining a sample from the lower respiratory tract (eg, distal or proximal quantitative or qualitative culture), even in patients with HAP, to narrow the initial spectrum of empirical antibiotic treatment. The recommendation includes retrieving lower respiratory samples before any change in antimicrobial treatment; as such a change significantly reduces the sensitivity and specificity of both qualitative and quantitative samples.
Molecular diagnostic methods that allow for the detection of multiple pathogens and antimicrobial resistance in a single sample could greatly facilitate VAP management, especially in patients with risk factors for multi-drug resistant (MDR) bacteria. These methods are highly sensitive, and if a suspected MDR pathogen is absent with this testing, it is not likely to be present on culture, and antibiotic coverage of that pathogen can be stopped. For example, an RCT 106 investigated whether rapid automated polymerase chain reaction (rPCR) assays that detect meticillin resistant S aureus (MRSA) in bronchoalveolar lavage (BAL) samples could safely decrease the use of vancomycin or linezolid for suspected cases of MRSA pneumonia in mechanically ventilated patients (22 patients received antibiotic management based on the rPCR result and 23 other patients continued with routine care). It found that duration of anti-MRSA treatment for the initial suspected episode of MRSA pneumonia was significantly shorter in the intervention group (32 h v 72 h, P<0.001). Furthermore, hospital mortality was reported to be 14% and 39% in the intervention and routine care groups, respectively (P=0.06). Similarly, a separate experimental study sought to detect S aureus in endotracheal aspirate samples from mechanically ventilated patients using GeneXpert MRSA/ S aureus endotracheal aspirate (ETA) assay in comparison with two PCR based methods (including GeneXpert) and three culture based methods. It found that the assays had both a sensitivity and specificity of 100% when compared with the other assays, underpinning the value of these new diagnostic techniques in the management of pneumonia. Nonetheless, clinical implementation of these techniques in routine clinical practice is difficult partly because of high costs and a lack of standardization. Also, because of the highly sensitive nature of these techniques, they cannot always distinguish colonization from infection.
The changing etiology of pneumonia
Severe cap: the emerging role of viruses, community acquired mrsa, and selected gram negative bacteria.
In severe CAP, pneumococcus remains the most commonly identified bacterial pathogen. 59 107 108 109 However, the past decade has seen an increase in the use of molecular diagnostic techniques that facilitate detection of multiple viruses in one respiratory sample. As a result, respiratory viruses in severe CAP have been reported with increasing frequency. 110 111 112 Additionally, an increase in the age of patients hospitalized for pneumonia is important, as this population is more susceptible to severe viral infection. 60 113 114 Data from a systematic review and meta-analysis from Europe have shown a prevalence of respiratory viruses of between 20% and 25% of CAP cases 115 116 ; these percentages are similar to those reported in studies from the US 110 and Asia. 111
The US multicenter study, 110 which included 2259 adults with radiographic evidence of pneumonia and specimens available for etiological diagnosis, found that a viral cause was detected in 23% of cases, with rhinovirus and influenza virus being the most common. Bacterial pathogens were detected in 11%, while mixed viral and bacterial pathogens were in 3%. Among the 482 ICU patients included in this study, the most common group of microorganisms included respiratory viruses (22%), followed by bacterial pathogens (19%), and bacterial-viral co-infection (4%). A prospective, multicenter observational study 111 found that, of the 2649 non-immunocompromised adult patients with CAP, viruses were identified in 915 of the 1177 with an etiological diagnosis. Influenza was present in 581 patients, and non-influenza virus in 240. ICU admission occurred in 8.3% and 5.4% owing to influenza virus and non-influenza virus, respectively. Also, the 90 day mortality rates were 3.8% and 1.7%, respectively, with no significant differences.
Similarly, a prospective cohort study that compared the outcomes of hospitalized patients with CAP and influenza with those with and without bacterial co-infection 117 found that those with viral and bacterial co-infection had acute respiratory distress syndrome (ARDS) more frequently than those with pure viral CAP or bacterial CAP (21% viral and bacterial co-infection; 19% viral CAP; and 10% bacterial CAP; P<0.001). They also required ICU admission more often (32% viral and bacterial co-infection; 32% viral CAP; and 13% bacterial CAP; P<0.001) than those with bacterial pneumonia. Interestingly, 30 day mortality did not differ among groups (4% in viral and bacterial co-infection; 3% viral CAP; and 6% bacterial CAP [P=0.232]).
In the ICU, viruses often are present in combination with bacterial pathogens. In one observational study of 49 mechanically ventilated patients with CAP, 118 49% of cases had a viral etiology, pure or mixed, with rhinovirus and adenovirus the most frequently detected. Sepsis can also complicate viral infections. A retrospective observational study 113 reported that 26% of ICU patients presented with viral sepsis and had an associated ICU mortality of 8%. Moreover, ARDS is another frequent complication in viral pneumonia. 119 One recent study found that respiratory viruses were the causal agent in 11% of mechanically ventilated patients with CAP and ARDS. 120 A prospective observational study 121 investigated the incidence of respiratory viruses in patients admitted to 16 Italian ICUs during the 2014-15 influenza season. It reported that the influenza A virus was the most frequently detected (83%), followed by human rhinovirus (12%), and RSV and influenza B virus (3% each). A prospective cohort study 122 from Australia and New Zealand reported an increase in ICU admissions resulting in pneumonia and sepsis during the winter and spring of 2017, with influenza virus strain H3N2 being predominant. This rise in ICU admissions was also higher than during the 2009 H1N1 pandemic.
Bacteria such as S pneumoniae, H influenzae, and S aureus constantly colonize the upper airways, with at least one of these bacteria colonizing approximately 20-50% of healthy individuals. Colonization can also synergize with viral infections to add to poor outcomes. 123 124 In influenza infections, colonization by S pneumonia e has been associated with an increased risk of ICU admission and mortality. Colonization by S aureus has been associated with an increased risk of mortality in adults, and MRSA co-infection has been associated with severe disease and mortality. 125 126
However, it is uncertain whether co-detection represents either true co-infection (two or more pathogens that infect the patient at the same time and run the same time course) or sequential infection instead (eg, a preceding viral infection with some residual viral shedding and a secondary bacterial infection). Both scenarios pose a challenge in routine clinical practice. A prior influenza infection is well known to damage epithelial cells, thereby leading to a compromised barrier function of the airway and exposed surface receptors, and favors adhesion of bacteria. An experimental study 127 found that a prior influenza infection increased pneumococcal colonization of the murine nasopharynx and promoted a spread of bacteria into the lungs. It observed that influenza accelerated bacterial replication in vivo, and sialic acid was identified as a host derived metabolite stimulating pneumococcal proliferation. Recent findings regarding innate and adaptive immune system responses during co-infection with influenza virus and S pneumoniae or S aureus showed that the presence of both microorganisms resulted in dysregulated cytokine and chemokine production. This type of dysregulated production occurred after pattern recognition receptors recognized the pathogen and triggered dynamic changes in immune cell recruitment and activation. 128 A hyperactivated signaling pathway elicits a massive recruitment of immune cells and an overshooting of inflammatory processes, leading to severe lung damage. As a result of such injury, pathogens can penetrate more deeply into the tissue and uncontrolled replication of viral and bacterial pathogens can occur. 128 Also, suppressed immune reactions can take place, owing to lower pathogen clearance which occurs as a result of an inhibition of immune cells or signaling cascades by pathogens. All of these mechanism can explain increased morbidity and mortality in cases of influenza virus and bacterial co-infection. 128 129
Community acquired MRSA (CA-MRSA) infections are reported globally and are associated with severe outcomes. The estimated incidence is approximately 0.51-0.64 cases per 100 000 inhabitants. 130 Clinical presentation of CA-MRSA includes necrotizing pneumonia, a severe form of pneumonia associated with abscess and cavity formation, and sometimes empyema. CA-MRSA has been reported in healthy, young adults with CAP and no exposure to healthcare settings and leads to poor clinical outcomes and high mortality, especially in those with post-influenza infection. Severe, necrotizing CA-MRSA pneumonia is thought to be caused by the exotoxin Panton-Valentine leukocidin (PVL). 131 PVL is a toxin that causes leukocyte destruction and tissue necrosis.
P aeruginosa , extended spectrum β-lactamase producing Enterobacteriaceae and MRSA (PES pathogens) form part of another important microorganism group that causes CAP. Such pathogens account for up to 6% of hospitalized patients with CAP and a microbiological diagnosis; however, current empirical therapy recommendations in guidelines do not target this pathogen group. In 2015, a prospective observational study 132 proposed the PES score to assess the risk of pneumonia owing to PES pathogens. The score showed modest accuracy in validation studies in the general population (AUC 0.81; 95% CI 0.74 to 0.88), ICU population (AUC 0.73; 95% CI 0.61 to 0.86) and in very old patients with CAP (AUC 0.64; 95% CI 0.58 to 0.71). 133 Other scores assessing the potential risk of MDR pathogens in patients with CAP have been published in the past 10 years 134 135 136 137 ( fig 2 ).

Prediction of multidrug resistant pathogens in CAP
A prospective, multicenter cohort study 138 assessed an antibiotic strategy based on risk factors for PES pathogens, independent of the site of pneumonia acquisition. Risk factors for PES pathogens included antibiotic therapy in the past 180 days; poor functional status (Barthel index <50 or performance status ≥3); hospitalization for >2 days within the past 90 days; occurrence of pneumonia ≥5 days after admission to an acute hospital; requirement for hemodialysis; and immunosuppression. In a multicenter cohort of 1089 patients (656 CAP, 238 healthcare associated pneumonia, 140 HAP and 55 VAP), patients with 0-1 risk factors for PES pathogens were to receive treatment with standard therapy (a β-lactam plus a macrolide), whereas patients with ≥2 risk factors for PES pathogens were proposed to receive appropriate therapy for HAP (a two or three drug regimen combining an antipseudomonal β-lactam with a quinolone or aminoglycoside plus optional linezolid or vancomycin). Approximately 83% of patients received treatment according to the proposed algorithm, and only 4% had inappropriate treatment. Basing the algorithm on risk factors for PES pathogens and disease severity instead of the site of pneumonia acquisition appeared to simplify antimicrobial treatment and improve the accuracy of empirical treatment. However, this algorithm requires validation in a large population. Table 1 describes the recommendations for therapy of HAP/VAP proposed by current US and European guidelines, but the principles extend to patients with severe CAP as well, and were used in the algorithm cited above.
Empiric treatment according to US And European guidelines
- View inline
It is important to recognize that in patients with CAP, PES pathogens do not have a universal distribution. A limited number of centers have reported this group of pathogens, 132 133 139 140 141 142 so clinicians should consider local etiology and resistance patterns.
VAP—the role of MRSA and drug resistant Gram negative pathogens
In the past decade, studies from the US and Europe have reported an increase in the prevalence of MDR pathogens in VAP. 143 144 145 The most frequent MDR Gram negative pathogens were P aeruginosa, Acinetobacter spp, and extended spectrum β-lactamase producing Enterobacteriaceae. In addition, MRSA is the most frequent MDR Gram positive pathogen. These pathogen groups are associated with poor clinical outcomes, especially because of inappropriate or delayed initial antimicrobial treatment. 146 147 148
Current guidelines from ATS/IDSA 5 and the European Respiratory Society, European Society of Intensive Care Medicine, European Society of Clinical Microbiology and Infectious Diseases, and Asociación Latinoamericana del Tórax 7 highlight the importance of administering prompt and adequate empirical treatments based on a patient’s risk stratification for MDR pathogens and on local microbiological and antibiotic resistance data ( table 2 ).
High risk of MDR pathogens in HAP/VAP
US guidelines 5 identify five risk factors associated with MDR pathogens: previous intravenous antibiotic treatment within 90 days; hospitalization for ≥5 days before the occurrence of VAP; septic shock at the time of VAP; ARDS preceding VAP; and the need for renal replacement treatment before VAP onset. Interestingly, a prospective cohort study 149 found that in using these risk factors for MDR pathogens, sensitivity was high, yet specificity was very low and overall performance poor, which could lead to excessive broad spectrum empirical antimicrobial treatment. Among the five risk factors, only antibiotic use in the past 90 days (negative predictive value of 79%) and ≥5 days of hospitalization (negative predictive value of 80%) before pneumonia were strongly associated with the presence of MDR pathogens. The presence of ARDS preceding VAP had a negative predictive value of 71% for the presence of MDR pathogens. European guidelines 7 do not include renal replacement treatment or ARDS in the definition of patients at high risk for MDR microorganisms. For this reason, in addition to individual patient risk factors, European guidelines include hospital settings with high rates of MDR microorganisms (>25% of all ICU pathogens are MDR), local patterns of resistance, and prior colonization by MDR microorganisms as determinants of risk for such pathogens.
CAP guideline recommendations
The ATS/IDSA CAP guidelines were published in 2019 6 and included changes from previous recommendations. 12 Most notably, the guidelines recommended eliminating the category of healthcare associated pneumonia (HCAP), to avoid overuse of empiric broad spectrum treatment. The incidence of resistant pathogens was low enough, even among those with severe CAP, and administering broad spectrum therapy for all those with HCAP led to its overuse. Patients with HCAP who faced high CAP mortality generally had clinical features and multiple comorbidities that affected survival, rather than the mere presence of risk factors for resistant pathogens. Furthermore, reports about adverse outcomes, such as an increase in infections related to Clostridium difficile , was associated with the overuse of broad spectrum antibiotics. 135 150 151
Initial choice of antibiotic
For most patients with severe CAP, initial treatment includes the combination of a β-lactam plus a macrolide or fluroquinolone. For patients without MRSA and pseudomonal risk factors, the β-lactam could be cefotaxime, ceftriaxone, ampicillin-sulbactam, or ceftaroline. 6 For those with pseudomonal risk factors, pathogen coverage could be achieved with a β-lactam such as piperacillin/tazobactam, cefepime, ceftazidime, imipenem, or meropenem. In patients who have a β-lactam allergy, aztreonam is an alternative. Newer β-lactams with activity against more resistant Gram negative pathogens are discussed below.
How to select broad spectrum antibiotics
In severe CAP, evidence suggests that the combination of β-lactam plus macrolide significantly improves the prognosis of patients, compared with non-macrolide regimens. 152 153 154 155 A retrospective observational study reported that patients with severe pneumonia had lower rates of 14 day (8% v 27%, P=0.02) and 30 day (18% v 37%, P=0.05) mortality with combination therapy of β-lactam plus macrolides than with flouroquinolones. 154 Similarly, 153 a prospective multicenter observational study assessed 257 intubated patients with severe CAP, in which 20% of patients received monotherapy and the remaining 80% combination therapy. After the authors adjusted for severity, the use of macrolides was associated with lower ICU mortality (hazard ratio 0.48, CI 95% 0.23 to 0.97, P=0.04) when compared with patients receiving fluoroquinolones. The authors recommended combination therapy with macrolides in intubated patients with severe CAP. A meta-analysis that comprised 9850 critically ill patients with CAP 155 observed that combination therapy with a macrolide and β-lactam (21% v 24%; risk ratio 0.82; 95% CI 0.70 to 0.97; P=0.02) was associated with reduced mortality when compared with other regimens. A prospective cohort study that included 1131 patients with CAP 152 found that combination treatment based on pneumonia severity index (PSI) and CURB-65 score 12 22 23 did not significantly reduce 30 day mortality in either group. However, combination therapy based on the ATS/IDSA criteria significantly reduced 30 day mortality in patients with severe pneumonia (odds ratio (OR) 0.12, 95% CI 0.007 to 0.57), albeit not in cases of non-severe pneumonia (OR 1.85, 95% CI 0.51 to 5.40).
An advantage of combination therapy is its ability to cover atypical pathogens, as well as a potential benefit from the immunomodulatory effect of macrolides to attenuate bacterial virulence factors and an excessive systemic inflammatory response. An experimental study 156 found that macrolides inhibit pneumolysin production, even in macrolide resistant strains of pneumococcus. Pneumolysin promotes the extra-pulmonary spread of pneumococcus, which can, in turn, cause cardiac damage. 57 Furthermore, when used as a part of combination therapy, 157 macrolides reduced mortality in patients with severe CAP, especially in those with pneumococcal bacteremia. 158 159 A prospective, multicenter and international study analyzing 844 cases of pneumococcal bacteremia 159 found that combination therapy was associated with a lower 14 day mortality rate (23% v 55%, P=0.015).
Optimal treatment for MRSA
Unlike nosocomial MRSA, CA-MRSA is susceptible to clindamycin, TMP-SMX, and doxycycline. 160 Clindamycin and linezolid can inhibit toxin production, which blocks bacterial protein synthesis. 160 According to the ATS/IDSA guideline recommendations, 6 antimicrobial therapy for MRSA should only be used when patients have risk factors for MRSA (prior respiratory isolation of MRSA; recent hospitalization [in the last 90 days], and use of parenteral antibiotics [in the last 90 days], and locally validated risk factors for MRSA). Clinicians should also obtain samples for cultures and perform nasal PCR testing to guide decisions regarding whether to de-escalate or continue treatment. 161 162
Options for empirical therapy for MRSA include vancomycin 15 mg/kg/12 h (adjustments made based on levels) or linezolid 600 mg/12 h. In patients with severe necrotizing CAP, it may be necessary to add an anti-toxin to treatment, such as adding clindamycin to vancomycin or using linezolid (which also has anti-toxin activity) alone. Linezolid penetrates the lung better than vancomycin, but clinicians more commonly administer it in HAP and VAP than in CAP.
Antiviral agents
Viral pneumonia is recognized as a common cause of severe CAP, and many patients have mixed viral and bacterial infections. The ATS/ISDA guidelines recommend using anti-influenza treatment (oseltamivir) in all patients with documented severe influenza, regardless of symptom duration, although the benefit is greatest if given within the first 48 hours. 6 In addition, because of a high incidence of bacterial co-infections, antibiotic treatment is recommended in confirmed influenza infections, especially to cover for pneumococcus and S aureus . However, de-escalation of antibiotics is recommended in patients who show no evidence of bacterial co-infection and show clinical stability after 48-72 hours of antibiotic treatment. 6
In patients with covid-19, bacterial pneumonia has sometimes been observed alongside viral pneumonia. 163 164 Most patients with lung infiltrates initially receive antibiotics, with discontinuation of such treatment occurring based on clinical assessment and serial measurements of biomarkers such as procalcitonin. 165 166 For hospitalized patients with covid-19, the antiviral agent remdesivir has shown some benefit, particularly during the early course of the disease, as have monoclonal antibodies (especially in early stage disease and outpatients). 167 Data from two large randomized controlled trials on the use of remdesivir in covid-19 showed varying results. The ACT-1 trial 168 found that remdesivir was superior to placebo in shortening the time to recovery in hospitalized adults with covid-19, while the SOLIDARITY trial 169 reported no mortality benefit. Currently, remdesivir is recommended for patients with severe covid-19 who require oxygen therapy, excluding mechanical ventilation or extracorporeal membrane oxygenation. 170
For other viral pneumonias in immune competent hosts, antiviral agents are not routinely administered.
Corticosteroids
Corticosteroids have immunomodulatory properties and are frequently used as adjunctive therapy in severe pneumonia because of their anti-inflammatory effects. Controversy still surrounds their use, but data from some studies have shown that the use of glucocorticoids reduces mortality in patients with severe CAP, especially in those with a high level of systemic inflammation. 171 172 However, this effect has yet to be seen in patients with non-severe pneumonia.
In a multicenter, randomized, double blind and placebo controlled trial study investigating the effect of corticosteroids on treatment failure in 120 patients with severe CAP (61 received methylprednisolone and 59 were control cases) and a high inflammatory response (initial levels of C reactive protein (CRP) >15 mg/dL), treatment failure was reported to be less frequent in the group receiving corticosteroids (13%) when compared with the placebo group (31%) (P=0.02). However, in-hospital mortality was similar between both groups (10% v 15%; P=0.37). The study concluded that the use of low dose corticosteroids (0.5 mg/kg every 12 hours for five days) decreased treatment failure in patients with severe CAP and a high inflammatory response, when compared with placebo. 171
Another systematic review and meta-analysis, 172 including data from 1506 patients (748 patients were randomized to corticosteroids and 758 patients to placebo) from six trials reported that the use of corticosteroids in hospitalized patients with CAP reduced the time to clinical stability (adjusted difference, -1.03 days; 95% CI -1.62 to -0.43 days; P=0.001) and length of hospital stay (-1.15 days; 95% CI -1.75 to -0.55 days; P<0.001) by approximately one day without any effect on mortality (OR, 0.75; 95% CI 0.46 to 1.21; P=0.24). However, the authors also reported an increased risk for CAP related re-hospitalization (OR 1.85, 95% CI 1.03 to 3.32, P=0.04) and hyperglycemia (OR 2.15; 95% CI 1.60 to 2.90, P<0.001).
Data from two randomized clinical trials 173 174 support the use of hydrocortisone as adjunctive therapy in patients with septic shock. The randomized Adjunctive Corticosteroid Treatment in Critically Ill Patients with Septic Shock trial 173 included 3658 patients with septic shock undergoing mechanical ventilation (1832 patients randomized to hydrocortisone group and 1826 to the placebo group). The participants received either hydrocortisone at a dose of 200 mg per day or placebo for seven days or until death or ICU discharge. The authors reported that the use of hydrocortisone in this population did not result in a lower 90 day mortality when compared with patients who received placebo (OR, 0.95; 95% CI 0.82 to 1.10; P=0.50). A multicenter, double blind and randomized trial 174 evaluating the effect of hydrocortisone plus fludrocortisone treatment against a placebo in 1241 patients with septic shock (614 randomized to hydrocortisone plus fludrocortisone and 627 to placebo) reported that 90 day all-cause mortality was lower in patients who received hydrocortisone plus fludrocortisones (43% v 49%, P=0.03).
Conversely, several studies observed that the use of corticosteroids in influenza may be associated with a significantly higher mortality rate possibly resulting from superinfection. 175 176 177 Despite these concerns, recent studies of severe covid-19 pneumonia with respiratory failure have shown a mortality benefit, particularly in the controlled, open label Randomized Evaluation of COVID-19 Therapy trials, which included the administration of 6 mg of dexamethasone daily for 10 days 178 in patients on oxygen or mechanical ventilation.
The ESCAPe trial ( NCT01283009 ) investigated the use of methylprednisolone (20 days of treatment: seven days (40 mg/day), seven days (20 mg/day), and six days (12 mg/day and 4 mg/day) versus placebo in 583 critically ill adult patients with CAP, with the primary study outcome being 60 day mortality. The mean age of patients was 68.8 years and mean PSI was 124 patients. Of these, 96% were male and 33% were on ventilation. With respect to 60 day mortality, the results showed no differences in superiority between methylprednisolone and placebo (OR 0.90; 95% CI 0.58 to 1.40, P=0.635). 179
Current recommendations in international guidelines on the use of corticosteroids as adjunctive therapy are to not administer them routinely in patients with either non-severe CAP or severe influenza pneumonia. However, corticosteroids may prove valuable in patients with severe pneumonia with refractory septic shock or a high systemic inflammatory response, as well as in those with pneumococcal CAP with meningitis. 6
Immunoglobulin treatment
Some studies have shown lower levels of circulating immunoglobulins in patients admitted to ICU with severe pneumonia when compared with non-ICU patients, as well as an association between these lower levels of immunoglobulins and increased mortality. 180 181 These data suggest a possible role of immunoglobulins in adjunctive treatment for severe CAP.
In a single center observational study 182 investigating the role of immunoglobulin levels (IgG, IgA, IgM) in 362 patients with CAP (172 ward and 190 ICU) and their impact on outcomes, IgG2 levels <301 mg/dL were associated with worse prognosis. Furthermore, low concentrations of IgG2 were an independent marker of ICU admission and mortality. Similarly, a separate single center observational study 181 reported that IgM concentrations were inversely associated with severity and a protective factor against mortality in cases of severe influenza CAP.
The efficacy of immunoglobulins was investigated as an adjunctive therapy in mechanically ventilated patients with severe CAP and septic shock. 183 In an observational study of 1324 patients receiving immunoglobulins as adjunctive therapy and 6940 controls, no significant association was seen between adjunctive therapy and mortality. Similarly, another post hoc subgroup analysis of data from a retrospective cohort study including 960 patients with sepsis and septic shock found no association between the use of low dose IgG immunoglobulins as adjunctive therapy and decreased ICU (21% v 18%, P=0.185) or in-hospital mortality (34% v 31%, P=0.066). 184
Additionally, a double blind phase II study 185 published data on 160 patients with severe pneumonia and requiring invasive mechanical ventilation, who were randomized to trimodulin (a polyclonal antibody preparation containing IgM, IgA, and IgG) or placebo for five consecutive days. Treatment with trimodulin did not increase ventilator-free days when compared with patients in the placebo group (median days 11 v 8, P=0.173). Interestingly, the authors observed that a subset of patients with elevated CRP and/or lower IgM levels had reduced mortality and an increase in ventilator-free days, with trimodulin treatment.
VAP guideline recommendations
In the past decade, the increased incidence of MDR pathogens reported in VAP has complicated management, and infection caused by these organisms has been associated with worse outcomes. 147 148 Local epidemiology and susceptibility information should guide empiric treatment for VAP, in conjunction with a careful assessment of patients’ risk factors for MDR pathogens. 5 Risk factors for MDR pathogens differ between US 5 and European 7 guidelines; however, when considering empiric therapy, local bacteriology and resistance patterns remain important, as does prior antibiotic treatment ( table 2 ).
Combination therapy—why and when
In general, local antibiotic susceptibility patterns and the likelihood of MDR, Gram negative, or mixed Gram positive and Gram negative infections guide the choice between monotherapy and combination therapy.
According to US guidelines, 5 if the patient has no risk factors for specific MDR pathogens, such as P aeruginosa or MRSA, and receives treatment in an ICU with a low prevalence of MDR microorganisms (<10%), the use of a single, narrow spectrum antibiotic with activity against non-resistant Gram negative microorganisms is recommended (weak recommendation, low quality evidence). Following European guidelines, the use of narrow spectrum antibiotics active against non-resistant Gram negative microorganisms is recommended for patients with a low risk of both MDR microorganisms and mortality who receive treatment in an ICU with a low prevalence of MDR microorganisms (<25%) (weak recommendation, very low quality evidence). 7
The use of dual antibiotic treatment is recommended in patients presenting with a high risk of MDR pathogens. A systematic review and meta-analysis 186 compared monotherapy and combination therapy as empiric treatment for patients with VAP. The study included data of 41 trials and 7015 patients. No significant differences were noted in mortality between both regimens. The authors reported that the ceftazidime-aminoglycoside combination was inferior to meropenem (relative risk (RR) 0.70; 95% CI 0.53 to 0.93). Mortality and treatment failure rates for monotherapy and combination regimen were similar (RR for mortality with monotherapy, 0.94; 95% CI 0.76 to 1.16; and RR for treatment failure with monotherapy 0.88; 95% CI 0.72 to 1.07). The authors highlighted the small proportion of VAP cases caused by MDR pathogens as possibly explaining the lack of benefits of combination therapy. A randomized controlled trial 187 comparing empiric monotherapy with meropenem versus ciprofloxacin plus meropenem for suspected VAP showed no significant differences in 28 day mortality between groups (RR 1.05, 95% CI, 0.78 to 1.42, P=0.74). Furthermore, no differences were noted regarding length of ICU and hospital stay, treatment response, and emergence of antibiotic resistant bacteria. However, the study excluded patients known to be colonized or infected with Pseudomonas or MRSA. Interestingly, the authors analyzed cases (n=56) that had infection caused by Pseudomonas species, Acinetobacter species, and MDR Gram negative bacilli. They observed a higher likelihood of adequate initial therapy (84.2% v 18.8%, P=0.001) and microbiological clearance (64.1% v 29.4%, P=0.05) when a combination regimen of meropenem plus ciprofloxacin was administered to those with resistant organisms, compared with monotherapy. Similarly, a systematic review, 188 including data from 12 randomized controlled trials and 3571 patients with VAP, found no significant difference in all-cause mortality (OR 0.97, 95% CI 0.73 to 1.30), clinical recovery (OR 0.88, 95% CI 0.56 to 1.36), and length of ICU stay (mean difference 0.65, 95% CI 0.007 to 1.23) between monotherapy and combination therapy. However, the authors acknowledged that these data may not be generalizable to all patient groups, given that the study did not identify patients with an increased risk of MDR bacteria. Additionally, in cases caused by MDR bacteria, results from observational studies 189 190 191 192 showed combination regimens including broad spectrum β-lactam with an aminoglycoside increased the percentage of appropriately treated cases when compared with monotherapy or a combination regimen of a β-lactam and fluoroquinolone.
US guidelines recommend the use of dual antibiotic treatment against Gram negative microorganisms for patients with a high risk of an MDR microorganism; those with lung disease; and those treated in an ICU with either an unknown or high prevalence of MDR pathogens (>10%) (weak recommendation, low quality evidence). If the patient is also at risk for an MRSA infection, treatment for this pathogen is added. Conversely, European guidelines recommend a broader spectrum approach in empiric antibiotic treatment if the patient is in an ICU with a high prevalence of MDR microorganisms (>25%) and/or at high risk of MDR microorganisms and mortality. Specific choices are then guided by the hemodynamic status of the patient. In individuals with no septic shock at diagnosis, monotherapy is considered adequate, provided that the agent is active against >90% of common Gram negative organisms in the ICU setting. Broad spectrum, multidrug treatment is recommended for patients with septic shock. However, this therapy should provide coverage for P aeruginosa , Enterobacteriaceae with extended spectrum β-lactamases, and A baumannii (if highly prevalent in the ICU) (strong recommendation, low quality evidence). The rationale for combination therapy is to provide sufficiently broad spectrum coverage to make appropriate treatment more likely than with monotherapy. In addition, combination therapy could eradicate organisms more quickly than monotherapy, being associated with a survival advantage over monotherapy for patients with septic shock and a mortality risk >25%. 7 193
Treatment for MDR pathogens: MRSA, Pseudomonas, Acinetobacter
Use of empiric anti-MRSA antimicrobials should be decided based on epidemiological data. The ATS/ISDA guidelines 5 suggest their use if >10-20% of S aureus isolates are MRSA, whereas European guidelines 7 suggest use if >25% of S aureus isolates are MRSA. For patients with a high risk of MDR pathogens or those treated in an ICU setting with >10% of Gram negative pathogens resistant to the best monotherapy option, European guidelines 7 suggest the use of two anti-pseudomonal agents from two separate classes.
The US guidelines 5 divide high risk patients into two main groups: (1) patients with a high risk of MDR pathogens and no septic shock who can receive a single broad spectrum agent active against >90% of Gram negative-likely microorganisms, and (2) patients with a high risk of MDR pathogens and septic shock who should receive a dual anti-pseudomonal regimen, with coverage for Acinetobacter spp and ESBL-producing Enterobacteriaceae if such pathogens are prevalent in the local antibiogram.
In recent years, new therapeutic options have become available for HAP/VAP, especially targeting MDR pathogens:
1. Ceftazidime-avibactam (CEF/AVI) is a combination of a third generation cephalosporin with a non-β-lactam β-lactamase inhibitor. CEF/AVI is active against a variety of β-lactamases, including Ambler Class A ( K pneumoniae carbapenemases and ESBL-type enzymes), Ambler Class C, and some Ambler-class D serine enzymes (eg, oxacillinase oxa-48). However, it is not active against metallo-β-lactamases or Acinetobacter oxa-like carbapenemases. Ceftazidime-avibactam received approval by the Food and Drug Administration (FDA) and European Medicines Agency (EMA) for use in HAP/VAP. The combination regimen was shown to be non-inferior to meropenem in a randomized controlled trial of carbapenem resistant, non- Enterobacteriaceae pneumonia. 194
2. Ceftolozane-tazobactam (CEF/TAZ) is a cephalosporin and β-lactamase inhibitor with in vitro activity against multidrug resistant P aeruginosa and ESBL-producing Enterobacteriaceae . Results from a randomized controlled double blind, non-inferiority trial including 726 mechanically ventilated patients with Gram negative nosocomial pneumonia 195 compared CEF/TAZ (2 g ceftolozane plus 1 g tazobactam infused for 1 hour every 8 hours) (362 subjects in the ceftolozane-tazobactam group) with meropenem (1 g infused for 1 hour every 8 hours) (364 subjects in the meropenem group). In the study, both drugs were equivalent and well tolerated in mechanically ventilated patients with Gram negative nosocomial pneumonia. However, CEF/TAZ had a mortality advantage in those with ventilated HAP and those with previously unsuccessful antibiotic treatment for the current nosocomial pneumonia episode. This new antibiotic has received approval by the FDA and EMA for HAP treatment. 196 197
3. Meropenem-vaborbactam is a combination agent containing an existing β-lactam antibiotic (meropenem) with a cyclic boronate non-β-lactamase inhibitor (vaborbactam) and is active against Gram negative microorganisms, including those with extended spectrum β-lactamases and K pneumoniae carbapenemases. However, it is not active against metallo-β-lactamase and oxacillinase producing strains. Although not approved for VAP, meropenem-vaborbactam showed an advantage for clinical recovery, when compared with the best available therapy (usually colistin), in a trial that included mechanically ventilated patients with carbapenem resistant Enterobacteriaceae . 198
4. Imipenem-relebactam (IR) is a combination agent containing an existing β-lactam antibiotic (imipenem-cilastatin) with non-β-lactam β-lactamase inhibitor (relebactam) and is active against Gram negative microorganisms, including Ambler class C, extended spectrum β-lactamases and K pneumoniae carbapenemases. However, it is not active against metallo-β-lactamase and oxacillinase producing strains. In a phase III trial that compared IR with pipercillin-tazobactam in 537 patients with nosocomial pneumonia, with half of the patients being mechanically ventilated, both therapies were equivalent in clinical recovery and mortality. 199 Survival was, however, higher in the subgroup of those mechanically ventilated at baseline when they received IR. 199
5. Cefiderocol is a siderophore cephalosporin that binds to iron and enters the bacterial cell, using the iron transport system. It is active against a wide range of carbapenem resistant pathogens including Enterobacteriaceae, P aeruginosa, and Acinetobacter baumanii . It is also active against Stenotrophomonas maltophilia . Cefiderocol is approved for nosocomial pneumonia, including VAP, but has shown no survival advantage or higher recovery rates when compared with meropenem. In a separate study, patients with VAP had a lower survival than with the best available therapy. 200 201
Using pharmacokinetic/pharmacodynamic principles to optimize dosing
Applying pharmacokinetic/pharmacodynamic (PK/PD) principles to antibiotic dosing and delivery regimens may improve the outcomes of patients with pneumonia. Drugs that kill bacteria in a concentration dependent fashion (aminoglycosides) have their efficacy maximized in relation to how high a high peak concentration they achieve, relative to minimum inhibitory concentration (MIC) of the target pathogen, in the serum and at the site of infection. This can be optimized when the entire 24 hour dose is administered as a single infusion. 185 194 Conversely, β-lactams (penicillins, cephalosporins, carbapenems) reach their optimal bactericidal effect in relation to how long the concentration stays above the MIC of the target pathogen and this can be optimized by using a prolonged or continuous infusion. 131 195 For example, continuous infusion of vancomycin was associated with better outcomes, 202 203 while higher doses and a continuous infusion of linezolid led to improved outcomes, especially in patients with ARDS and infections caused by resistant pathogens. 204 205
Aerosolized antibiotics
Aerosolized antibiotics can deliver higher antibiotic concentrations in the lung parenchyma and have less systemic toxicity than intravenous treatment, yet no recommendations call for their use as routine adjunctive therapy in VAP. Three randomized trials have investigated the use of adjunctive nebulized antibiotics in patients with VAP 206 207 208 209 : the IASIS trial (aerosolized adjunctive amikacin and fosfomycin in patients with VAP and suspected MDR, Gram negative bacteria); the INHALE trial (inhaled amikacin as adjunctive therapy in patients with VAP and suspected MDR, Gram negative bacteria); and the VAPORISE trial (inhaled tobramycin as adjunctive therapy in patients with VAP). All three studies showed negative results for improved clinical outcomes or mortality.
In 2019, results from a meta-analysis by the ATS/IDSA HAP guideline committee, which included nine studies of inhaled antibiotics for VAP treatment, 210 showed that the use of inhaled antibiotics was beneficial in treating VAP caused by difficult-to-treat microorganisms. The ATS/IDSA guidelines 5 therefore recommend adding inhaled antibiotics to systemic antibiotics in cases of Gram negative pneumonia caused by MDR microorganisms. Inhaled colistin should be used instead of polymyxin B. Inhaled antibiotics are also recommended as a last resort for patients with VAP and sensitive or resistant microorganisms who are not responding to treatment. European guidelines 7 do not recommend the use of inhaled antibiotics until more data have become available.
Treatment duration for CAP and HAP
Data from two meta-analyses showed that short course antibiotic therapy (five to seven days) may be adequate in treating patients with CAP. 211 212 In the first meta-analysis of 19 randomized controlled trials including data of 4861 patients and irrespective of severity of pneumonia, the authors did not find any differences regarding clinical recovery rates between short (≤6 days) and long course treatments (≥7 days). 211 Furthermore, short course treatment was associated with fewer, serious adverse events (RR=0.73; 95 CI 0.55 to 0.97) and potentially lower mortality than long course treatment (RR=0.52; 95% CI 0.33 to 0.82). A second meta-analysis analyzing data from seven randomized controlled trials including 3021 patients 212 showed that short course antibiotic treatment (five days) in adults with bacterial CAP achieved clinical responses similar to those observed in patients receiving long course antibiotic therapy (7 to 14 days). The authors also reported that all-cause mortality did not differ in relation to duration of antibiotic treatment, and short course antibiotic therapy was associated with a lower rate of adverse effects.
According to the ATS/IDSA guidelines, 6 a patient’s clinical stability should guide duration of antibiotic treatment, with it being no less than five days. The guidelines also state that longer courses of antibiotics are recommended in cases of pneumonia complicated by meningitis, endocarditis, and other deep seated infections. In cases of infection by other, less common pathogens, guidelines do not specify duration of treatment. 6
For patients with VAP, US guidelines 5 recommend a seven day course of antibiotic treatment. Depending on the rate of improvement of clinical, radiological, and laboratory parameters; however, some patients may require a shorter or longer duration of antibiotics. European guidelines 7 recommend a 7-8-day course of antibiotic treatment in patients who are not immunodeficient, do not present with cystic fibrosis or other pulmonary complications (empyema, lung abscess, cavitation, or necrotizing pneumonia); received appropriate treatment initially; do not have a highly resistant pathogen ( P aeruginosa, carbapenem resistant Acinetobacter spp, carbapenem resistant Enterobacteriaceae) ; and respond well to antibiotic treatment.
Biomarkers provide information about host response to infection and pharmacological intervention; however, heterogeneous immunological and inflammatory responses in patients with pneumonia make their universal use challenging. 213 214 Biomarkers, such as (CRP), procalcitonin (PCT), lymphocytes, red blood cell distribution, interleukin-6, pro-adrenomedullin, N-terminal pro-B-type natriuretic peptide, soluble triggering receptor expressed on myeloid cells-1, co-peptin, and soluble form of urokinase-type plasminogen activator receptor have been studied. 214 215 216 217 218 219 220 221 222
In clinical practice, CRP, PCT, and, more recently, lymphocytes are the most frequently used biomarkers to guide duration of antibiotic treatment and prognosis in pneumonia.
CRP is a major acute phase protein produced by macrophages in response to any type of inflammation, including bacterial and viral infections. It is released between four and six hours after acute injury, peaking at around 36-48 hours. However, CRP has low specificity in pneumonia diagnosis, as levels can be high owing to other clinical causes, such as neoplasia or autoimmune diseases. 223
PCT is a peptide precursor of the hormone calcitonin that is synthesized in the thyroid gland. It increases during inflammatory and infectious diseases, primarily as an acute phase reactant produced in the liver. In healthy individuals, plasma concentrations of PCT are very low (<0.1 ng/mL); however, levels rise with bacterial infections. 223
Although no PCT threshold has been found to discriminate specifically between viral and bacterial infection for adults hospitalized with CAP, 224 higher levels suggest an increased probability of bacterial infection.
Interestingly, results from secondary analyses of data from two prospective longitudinal cohorts 225 reported that the time from symptom onset in pneumonia to initial healthcare presentation may affect levels of CRP and PCT. The study included 541 patients with CAP, divided into two groups based on the time to symptoms: early presenters (<3 days since symptom onset) and late presenters (>3 days). In the study, CRP and PCT were lower in early presenters, suggesting that the time to presentation may influence the interpretation of these biomarkers. The authors also propose considering CRP as possibly a more useful biomarker in patients with a longer duration of symptoms; in patients with symptoms lasting ≤48 hours, PCT might hold better utility.
In patients with CAP, PCT has been studied as a tool to reduce duration of antibiotic treatment. Several RCTs have used serial PCT measurements to determine duration of antibiotic treatment, showing reduced treatment duration. However, control group participants who received standard treatment had antibiotic treatment courses lasting much longer than seven days 226 227 228 229 and exceeding guideline recommendations.
Current international guidelines recommend initiating antimicrobial treatment based on clinical suspicion in radiographically confirmed CAP and not on the basis of PCT levels. 6 Interestingly, studies have reported a correlation between higher levels of CRP and PCT and an increased risk of complications, admission to ICU, and short term mortality. 216 230 231 232
A large meta-analysis of 26 studies on duration of antibiotic treatment in respiratory infections (CAP, HAP, VAP, exacerbations of COPD, and bronchitis) 233 found that treatment duration guided by PCT was associated with a reduction of 2.4 days of treatment (5.7 v 8.1 days, P<0.001). Further, lower mortality was observed in the group that received PCT guided antibiotic treatment (OR 0.83, 95% CI 0.70 to 0.99, P=0.037). These benefits applied to patients with both CAP and VAP in the ICU. The recommendation set forth in European HAP/VAP guidelines 7 is to use serial PCT measurements in conjunction with clinical assessment to shorten duration of antibiotic treatment in pneumonia cases that required a prolonged course of treatment.
More recently, a study 213 investigated the value of lymphocytes as a biomarker of severity and found that lymphopenia conferred an increased risk of severity in patients with CAP, and that adding lymphocyte count to the CURB-65 score improved predictions of 30 day mortality. Another study from China 234 reported that the combination of PO 2 /FiO 2 and lymphocyte count predicted mortality and ICU admission in hospitalized patients with influenza pneumonia. The authors found that the likelihood of severe influenza pneumonia was high in patients with PO 2 /FiO 2 ≤250 or peripheral blood lymphocyte count <0.8×10 9 /L. Lymphocyte count was also studied as a biomarker in ICU acquired pneumonia (ICU-AP). A study from Spain of 473 patients reported that lymphocytopenia was an independent predictor of 90 day mortality in non-immunocompromised patients. 235 Lymphocyte count is simple and a non-costly biomarker that could prove useful, especially in hospitals where other biomarkers are not available; utility of this biomarker has been shown in severe CAP and in immunocompromised patients with ICU-AP.
Despite the promising value of CRP and PCT, limitations remain to biomarker use in daily practice. Biomarkers should be viewed as an adjunctive tool in clinical evaluations and decision making processes related to site of care and treatment duration.
Pneumonia prevention
CAP prevention focuses on vaccinations, and not so much on those at risk of severe pneumonia. This discussion will center on VAP and measures aimed at reducing the incidence of infection and improving the clinical course, with emphasis on interrupting disease pathogenesis. Currently, preventing colonization with pathogenic bacteria and modification of aspiration remain at the forefront of VAP prevention measures. Some of the most studied preventive measures in VAP include head-of-bed elevation, decreased duration or avoidance of mechanical ventilation, early mobilization, endotracheal tube cuff design, aspiration of subglottic secretions, oral care, and the use of probiotics.
Ventilated patients in the supine position (head elevation between 0° and 10°) have an increased risk of aspiring gastric contents when compared with patients in a semi-recumbent position (head of bed elevation between 30° and 45°). 236 237 238 In 2016, a review and meta-analysis 238 including eight RCTs comprising 759 patients compared a supine position with a semi-recumbent position. The authors found that the semi-recumbent position significantly reduced the risk of suspected VAP when compared with supine position (14% v 40%, RR 0.36; 95% CI 0.25 to 0.50). However, no significant difference was found in the occurrence of microbiologically confirmed VAP. A retrospective cohort study including 5539 patients undergoing mechanical ventilation for at least three days 239 reported that head-of-bed elevation, as well as measures such as sedative infusion interruptions, spontaneous breathing trials, and thromboprophylaxis, was associated with less time to extubation. The lateral Trendelenburg and semi-recumbent position were compared in a RCT 240 that included 395 patients (194 patients in the Trendelenburg position and 201 in the semi-recumbent position). The study showed that the semi-recumbent position was associated with a higher incidence of VAP than the lateral Trendelenburg position (4% v 0.5%; RR 0.13; 95% CI 0.02 to 1.03; P=0.04). No differences were seen in microbiologically confirmed VAP and mortality. However, this trial was stopped early due to adverse events in patients randomized in the lateral Trendelenburg position.
Head-of-bed elevation to a semi-recumbent position is a frequent intervention practiced as a preventive measure against VAP. 241 242
Micro-aspiration of oropharyngeal secretions owing to invasive mechanical ventilation is the main risk factor for VAP. 243 244 245 Avoiding mechanical ventilation, 246 247 248 249 decreasing the time of intubation, 250 and spontaneous breathing trials 251 252 are effective measures in preventing VAP. Various investigators have evaluated the benefits of modifying shapes and materials of the endotracheal tube cuff as it relates to minimizing fluid leakage into the lungs via the cuff and preventing VAP. Cuffs made from materials such as polyurethane have undergone testing and are shown to fit the shape of the trachea better and thus reduce the flow of fluids to the lungs. In contrast with conventional cylindrical cuffs, newer cuffs are conical in shape and approach the tracheal wall evenly and uniformly at the point of maximum cuff diameter. Nonetheless, results from RCTs and meta-analysis do not support the conclusion that these innovations prevent VAP better than traditional materials and forms. 253 254 255 256
Suctioning of subglottic secretions that pool above the endotracheal tube cuff is associated with lower VAP rates; however, controversy exists about its value in decreasing duration of mechanical ventilation, length of ICU stay, and ventilator associated events. 257 258 259 260 261 262 A recent systematic review and meta-analysis 263 that included 20 studies (nine systematic reviews and meta-analyses and 20 RCTs) found that drainage of subglottic secretions significantly reduced the incidence of VAP (RR 0.56; 95% CI 0.48 to 0.63, I 2 =0%, P=0.841) and mortality (RR 0.88; 95% CI 0:80 to 0:97, I 2 =0%, P=0.888). More high quality studies are needed to elucidate the potential contribution of this intervention in ventilated patients. Interestingly, a meta-analysis that investigated the influence of subglottic secretion drainage on the causative microorganisms of VAP found a significant association between subglottic secretion drainage and decreased VAP caused by Gram positive cocci and Hemophilus influenzae (OR 0.29, 95% CI 0.18 to 0.48; P<0.001). However, no significant differences were seen in VAP caused by non-fermentative bacteria and enterobacteria. 264
Selective digestive decontamination (SDD) is a prophylactic antibiotic strategy that controls the overgrowth of pathogens in the gut, especially those that are Gram negative and multidrug resistant, using topical oral and gastric antibiotics such as tobramycin, polymyxins, and amphotericin B, along with initial intravenous antibiotics. Previous studies 265 266 of ICU settings with low levels of antibiotic resistance observed that SDD and selective oropharyngeal decontamination (SOD) were associated with improved clinical outcomes. One study 267 reported that SDD was more effective than SOD for preventing infection.
The main concerns surrounding the use of SDD is the increased risk of antibiotic resistance 268 and the effect that antibiotic use may have on patients without bacterial infections. A study 269 showed that the use of SDD resulted in the selection of four resistance genes. It concluded that these results showed a limited risk of SDD in antibiotic resistance. Further, in ICU settings where moderate-to-high prevalence rates of antibiotic resistance pathogens exist, the use of SDD was not observed to be associated with decreased infection rates. 270 In a randomized clinical trial of decontamination strategies for mechanically ventilated patients in the ICU, the SDD strategy was reported not to add more benefit than standard care in ICU settings with a high prevalence of antibiotic resistant microorganisms. 270
In ICU settings with a low prevalence of antibiotic resistant microorganisms, the SDD strategy was associated with less antibiotic resistance and with improved clinical outcomes. More studies are required in ICU settings with a higher prevalence of resistant microorganisms.
Additionally, the use of chlorhexidine for oral hygiene in patients under mechanical ventilation has been reported to reduce the risk of VAP. 271 272 273 274 An RCT 275 found that use of chlorhexidine 2%, in comparison with chlorhexidine 0.2%, was more effective as a preventive measure for VAP and in reducing oropharyngeal colonization. A Cochrane systematic review and meta-analysis reported that more evidence was needed regarding the use of chlorhexidine and its relation with reducing infection, mortality, and length of stay in the ICU. 274 Also, results from observational studies and a meta-analysis of RCTs showed an association between the use of chlorhexidine and increased mortality risk. 239 276 277 278 The micro-aspiration of chlorhexidine may cause ARDS 273 279 and could explain this harmful association. The use of chlorhexidine was also reported to be associated with adverse effects such as damage to oral mucosa (ie, erosive oral lesions, ulcerations, white or yellow plaques, and bleeding mucosa) 280 and hypersensitivity effects. 281 282 Additionally, a reported decrease in susceptibility to chlorhexidine is concerning. 283
Finally, as part of SDD, short term and prophylactic systemic antibiotic treatment may be effective alone in patients who are urgently intubated after cardiac arrest or neurological injury. The use of 24-48 hour systemic antibiotic treatment may eradicate organisms that are aspirated during the intubation process and prevent the onset of VAP in the ensuing 48 hours. 284 285
Emerging approaches to management
Investigations in metagenomics based on next generation sequencing (NGS) may significantly improve the diagnosis and treatment of pneumonia, especially in severe cases. 286 287 288 NGS allow for more accurate and rapid detection of multiple pathogens in a single assay. Plasma microRNA signatures have also emerged as a tool capable of predicting disease severity, being recently reported as a good marker of severity and deterioration of ICU patients with a diagnosis of covid-19. 289 Indeed, this technology may help clinicians provide more personalized management 290 in severe cases of pneumonia. Furthermore, evidence is growing for the value of technology, such as the Filmarray pneumonia test. This is a multiplex PCR assay that can detect the most commonly identified pathogens in pneumonia and their resistance patterns. Data on pathogen resistance have been recently published in a sub-study of the PROGRESS trial (a prospective and multicenter randomized trial). 291 The study included 56 patients with CAP and no risk factors for MDR pathogens, as well as another 34 patients with risk factors for MDR pathogens. Specifically, the pneumonia assay had a detection rate of 72%, whereas conventional microbiological testing had a rate of 10% (P<0.001). These results underpin the value of this new diagnostic test and its potential implementation in clinical practice in the future.
In formulating this review, we considered guidelines on the management of CAP, HAP, and VAP from the Infectious Diseases Society of America and the American Thoracic Society, 5 6 the European Respiratory Society, European Society of Intensive Care Medicine, European Society of Clinical Microbiology and Infectious Diseases, and Asociacion Latinoamericana del Torax. 5 6 7 12 Key recommendations are summarized above, and include likely pathogens, recommended diagnostic testing, choice of initial antimicrobial treatment, duration of treatment, use of adjunctive therapy, and prevention of pneumonia.
In patients with severe CAP, initial treatment is with the combination of a macrolide or fluoroquinolone, with a β-lactam. The β-lactam is chosen based on the presence of pseudomonal risk factors, and additional coverage for MRSA is also decided based on the presence of specific risk factors. Patients with documented influenza should receive oseltamivir, with the addition of antibiotics. Treatment is for a minimum of 5-7 days and duration may be guided by biomarkers such as procalcitonin. Adjunctive therapy with corticosteroids is not routinely recommended, but may have benefit for selected patients. Prevention is focused on vaccination of at-risk populations.
HAP/VAP remains a diagnostic challenge, and existing guidelines differ on the value of bronchoscopic samples, cultured quantitatively. Recommendations for therapy are to treat all patients for P aeruginosa and other Gram negatives pathogens, with coverage for MDR pathogens and MRSA, based on individual risk factors and the frequency of these pathogens in a given ICU. Newer agents and inhaled antibiotics may have a role for some highly resistant pathogens. Most patients will need combination treatment, and duration of therapy can be as short as seven days if initial therapy is accurate, the pathogen is not highly resistant, and the patient has responded well to therapy. Prevention is focused on available interventions such as head-of-bed elevation, prevention of aspiration, and a focus on decreased duration or avoidance of mechanical ventilation, early mobilization, and endotracheal tube cuff design.
Conclusions
Despite advances in diagnosis, management, antimicrobial therapy, and prevention, pneumonia continues to have a major impact on healthcare systems worldwide. The emergence of multidrug resistant pathogens, the increasing age of the population, the increased number of patients with multiple comorbidities and polypharmacy are some of the challenges that clinicians face in the management of critically ill patients with pneumonia. Despite some differences in the international guidelines for the management of CAP and HAP/VAP, following these recommendations will ensure better outcomes for patients admitted to the ICU with pneumonia.
Questions for future research
What is the value of molecular diagnostic testing in the definitive microbial diagnosis of severe pneumonia? What is its role in de-escalating antimicrobial treatment?
How do we identify severe cases of pneumonia, early in the course of illness?
How do we complement the combination of biomarkers and severity scores with clinical judgment to improve the identification of severe pneumonia?
What are the characteristics of patients with severe CAP who might benefit from corticosteroids or other anti-inflammatory therapies?
Can new antimicrobial agents help us more effectively manage patients who are infected with MDR pathogens?
Is short course antibiotic treatment necessary in patients with severe viral pneumonia?
Series explanation: State of the Art Reviews are commissioned on the basis of their relevance to academics and specialists in the US and internationally. For this reason they are written predominantly by US authors
Patient involvement: no patients were directly involved in the creation of this article.
Contributors: CC, AT, and MN contributed equally to the design, development, and writing of this manuscript and guarantee authenticity of its authorship.
Provenance and peer review: commissioned; externally peer reviewed.
Competing interests: We have read and understood the BMJ policy on declaration of interests and declare the following interests: AT has participated in advisory boards for Pfizer. MN has participated in advisory boards for Pfizer, Merck, Bayer, Gilead, and Abbvie.
- GBD 2019 Diseases and Injuries Collaborators
- Cilloniz C ,
- Niederman MS ,
- Weber-Carstens S ,
- Apfelbacher C
- Legouis D ,
- Marti P-E ,
- Metersky ML ,
- Klompas M ,
- Metlay JP ,
- Waterer GW ,
- Chastre J ,
- Woodhead M ,
- Harrison DA ,
- Bellingan G ,
- Ngerng WJ ,
- Restrepo MI ,
- Mortensen EM ,
- Mandell LA ,
- Wunderink RG ,
- Anzueto A ,
- Infectious Diseases Society of America ,
- American Thoracic Society
- Liapikou A ,
- Polverino E ,
- Jephson AR ,
- Infectious Disease Society of America/American Thoracic Society 2007
- Chalmers JD ,
- Taylor JK ,
- Laporte L ,
- Hermetet C ,
- Cavallazzi R ,
- Furmanek S ,
- Arnold FW ,
- Deutschman CS ,
- Seymour CW ,
- Ranzani OT ,
- Menéndez R ,
- Kolditz M ,
- Scherag A ,
- CAPNETZ Study Group
- Tokioka F ,
- Okamoto H ,
- Yamazaki A ,
- Guignard V ,
- Schefold JC ,
- Leichtle AB ,
- Exadaktylos AK ,
- Pfortmueller CA
- Melsen WG ,
- Rovers MM ,
- Groenwold RHH ,
- Forel J-M ,
- Voillet F ,
- Markota A ,
- Dudeck MA ,
- Edwards JR ,
- Allen-Bridson K ,
- Eckenrode S ,
- Bakullari A ,
- Giuliano KK ,
- ↵ Healthcare-associated infections in intensive care units—Annual Epidemiological Report for 2017. 2017; https://www.ecdc.europa.eu/en/publications-data/healthcare-associated-infections-intensive-care-units-annual-epidemiological-1
- Álvarez-Lerma F ,
- Palomar-Martínez M ,
- Sánchez-García M ,
- Pileggi C ,
- Mascaro V ,
- Nobile CGA ,
- Álvarez Lerma F ,
- Sánchez García M ,
- Lorente L ,
- Sociedad Española de Medicina Intensiva ,
- Sociedad Española de Enfermería Intensiva
- Colombo SM ,
- Palomeque AC ,
- Magill SS ,
- Bamberg W ,
- Emerging Infections Program Healthcare-Associated Infections and Antimicrobial Use Prevalence Survey Team
- Hampton N ,
- Doubravska L ,
- Ibn Saied W ,
- Mourvillier B ,
- OUTCOMEREA Study Group
- Talbot GH ,
- Foundation for the National Institutes of Health Biomarkers Consortium HABP/VABP Project Team
- Esperatti M ,
- Vincent J-L ,
- EPIC III Investigators
- Corrales-Medina VF ,
- Musher DM ,
- Chirinos JA ,
- Garcia-Vidal C ,
- Manresa F ,
- Carratalà J
- Grupo NEUMONAC ,
- Anexo. Grupo NEUMONAC
- Tralhão A ,
- Cangemi R ,
- Falcone M ,
- SIXTUS (Thrombosis-Related Extrapulmonary Outcomes in Pneumonia) Study Group
- Aliberti S ,
- Corrales-Medina VF
- Taljaard M ,
- Anderson R ,
- Postma DF ,
- Spitoni C ,
- van Werkhoven CH ,
- van Elden LJR ,
- Oosterheert JJ ,
- Cillóniz C ,
- Dominedò C ,
- Bermejo-Martin JF ,
- Martín-Fernandez M ,
- López-Mestanza C ,
- Shachkina S ,
- Chirinos JA
- Millett ERC ,
- Orihuela CJ
- Pasparakis M ,
- Vandenabeele P
- Riegler AN ,
- Gilley RP ,
- Eurich DT ,
- Marrie TJ ,
- Minhas-Sandhu JK ,
- Majumdar SR
- Shenoy AT ,
- Brissac T ,
- Schwartz KL ,
- Campitelli MA ,
- Blackburn R ,
- Hayward A ,
- Warren-Gash C
- Sellers SA ,
- Hayden FG ,
- Fischer WA 2nd .
- Naghavi M ,
- Litovsky S ,
- Sommerstein R ,
- Kraemer JG ,
- Marschall J ,
- Tolker-Nielsen T
- Souza LCD ,
- Mota VBRD ,
- Carvalho AVDSZ ,
- Corrêa RDGCF ,
- Libério SA ,
- Fernández-Barat L ,
- Panigada M ,
- Feldman C ,
- Cantrell J ,
- Danin P-E ,
- Legrand P ,
- Thorarinsdottir HR ,
- Holmberg A ,
- Petronis S ,
- de Steenhuijsen Piters WAA ,
- Heirali AA ,
- Maschera B ,
- Naureckas ET ,
- Martin-Loeches I ,
- Dickson R ,
- Bittinger K ,
- Zakharkina T ,
- Matamoros S ,
- Roquilly A ,
- Villadangos JA ,
- Clemente JC ,
- Tsay J-CJ ,
- Antoni Torres CC
- Ceccato A ,
- San Jose A ,
- Fernando SM ,
- Laursen CB ,
- Lambrechtsen J ,
- Claessens Y-E ,
- Debray M-P ,
- Ferreira-Coimbra J ,
- Ardanuy C ,
- Zhang Z-Y ,
- Biscaro RRM ,
- Van der Eerden M
- Montoya R ,
- Fukuyama H ,
- Yamashiro S ,
- Paonessa JR ,
- Pickens CI ,
- Johnson HL ,
- Nonyane BAS ,
- AGEDD Adult Pneumococcal Burden Study Team
- Walden AP ,
- Clarke GM ,
- McKechnie S ,
- ESICM/ECCRN GenOSept Investigators
- Martín-Loeches I ,
- CDC EPIC Study Team
- CAP-China Network
- Magdaleno D ,
- Gabarrús A ,
- Lansbury L ,
- Leonardi-Bee J ,
- Nguyen-Van-Tam JS
- El-Kersh K ,
- Wiemken T ,
- Ramirez J ,
- Cavallazzi R
- Abelenda-Alonso G ,
- Rombauts A ,
- Ala-Kokko TI ,
- Vuorinen T ,
- Ohtonen P ,
- Kroeze EJBV ,
- Fouchier RAM ,
- Piralla A ,
- Mariani B ,
- Burrell A ,
- Huckson S ,
- Pilcher DV ,
- Civljak R ,
- Nicolini A ,
- Almeida ST ,
- de Lencastre H ,
- Palacios G ,
- Cisterna D ,
- Centers for Disease Control and Prevention (CDC)
- Siegel SJ ,
- Wilden JJ ,
- Ehrhardt C ,
- Boergeling Y
- Aguilera ER ,
- Vardakas KZ ,
- Matthaiou DK ,
- Wunderink RG
- Dominedo C ,
- Peroni HJ ,
- Zilberberg MD ,
- Di Pasquale M ,
- Zanaboni AM ,
- Kobayashi D ,
- Maruyama T ,
- Fujisawa T ,
- Kollef MH ,
- Deitelzweig SB ,
- Sherman BM ,
- ↵ Watkins RR, Van Duin D. Current trends in the treatment of pneumonia due to multidrug-resistant Gram negative bacteria. F1000Res. 2019 Jan 30;8:F1000 Faculty Rev-121.
- Magiorakos A-P ,
- Srinivasan A ,
- Bassetti M ,
- Graziano E ,
- Sorensen J ,
- Jephson A ,
- Tachibana H ,
- Tokumasu H ,
- Rodriguez A ,
- Lodise TP ,
- Tjosvold L ,
- Cockeran R ,
- Baddour LM ,
- Klugman KP ,
- International Pneumococcal Study Group
- Siddiqui AH ,
- Sheperd LA ,
- Woolever NL ,
- Schomberg RJ ,
- Dierkhising RA ,
- Dababneh AS ,
- Musuuza JS ,
- Parmasad V ,
- Putman-Buehler N ,
- Christensen L ,
- Gatheral T ,
- Drewett GP ,
- Smibert OC ,
- Holmes NE ,
- Trubiano JA
- ↵ National Institutes of Health. Information on covid-19 treatment, prevention and research. Covid-19 treatment guidelines . https://www.covid19treatmentguidelines.nih.gov/
- Beigel JH ,
- Tomashek KM ,
- ACTT-1 Study Group Members
- Henao-Restrepo AM ,
- WHO Solidarity Trial Consortium
- ↵ Remdesivir. https://www.idsociety.org/covid-19-real-time-learning-network/therapeutics-and-interventions/remdesivir/
- Spoorenberg SMC ,
- Snijders D ,
- Ovidius Study Group ,
- Capisce Study Group ,
- STEP Study Group
- Venkatesh B ,
- ADRENAL Trial Investigators and the Australian–New Zealand Intensive Care Society Clinical Trials Group
- Renault A ,
- Brun-Buisson C ,
- CRICS-TRIGGERSEP Network
- Rodrigo C ,
- Nguyen-Van-Tam J ,
- Rodríguez A ,
- GETGAG Study Group
- Emberson JR ,
- RECOVERY Collaborative Group
- ↵ VA Office of Research and Development. CSP #574 - Evaluate the safety and efficacy of methylprednisolone in hospitalized veterans with severe community-acquired pneumonia. clinicaltrials.gov; 2020. https://clinicaltrials.gov/ct2/show/results/NCT01283009
- de la Torre MC ,
- Serra-Prat M ,
- Almansa R ,
- Palomera E ,
- Fushimi K ,
- Sasabuchi Y ,
- Dellinger RP ,
- Aarts M-AW ,
- Hancock JN ,
- Heyland D ,
- McLeod RS ,
- Marshall JC
- Heyland DK ,
- Muscedere J ,
- Canadian Critical Care Trials Group
- Arthur LE ,
- van Driel ML ,
- Boffi El Amari E ,
- Van Delden C
- Garnacho-Montero J ,
- Sa-Borges M ,
- Sole-Violan J ,
- Martínez JA ,
- Cobos-Trigueros N ,
- Soriano A ,
- Kethireddy S ,
- Melnick D ,
- Nováček M ,
- Kivistik Ü ,
- Approval Letter ZERBAXA
- ↵ Approval letter ZERBAXA. https://www.ema.europa.eu/en/documents/variation-report/zerbaxa-h-c-3772-ii-0020-epar-assessment-report-variation_en.pdf
- Giamarellos-Bourboulis EJ ,
- ↵ Titov I, Wunderink RG, Roquilly A, et al. A randomized, double-blind, multicenter trial comparing efficacy and safety of imipenem/cilastatin/relebactam versus piperacillin/tazobactam in adults with hospital-acquired or ventilator-associated bacterial pneumonia (RESTORE-IMI 2 Study). Clin Infect Dis 2020 Aug 12:ciaa803 doi: 10.1093/cid/ciaa803 .
- Matsunaga Y ,
- Ariyasu M ,
- Flannery AH ,
- Bissell BD ,
- Bastin MT ,
- Morris PE ,
- Soraluce A ,
- Barrasa H ,
- Asín-Prieto E ,
- Taubert M ,
- Ricard J-D ,
- Corkery K ,
- Stokker J ,
- Gommers D ,
- van der Eerden M
- Sweeney DA ,
- Tansarli GS ,
- Mylonakis E
- Chang S-P ,
- NEUMONAC group
- Martin-Fernandez M ,
- Menendez R ,
- Legramante JM ,
- Mastropasqua M ,
- Julián-Jiménez A ,
- González Del Castillo J ,
- Keramat F ,
- Ghasemi Basir HR ,
- Shafiei Aghdam A ,
- Poorolajal J
- Vestjens SMT ,
- Ovidius study group
- Restrepo M ,
- Martin-Loeches I
- Grijalva CG ,
- Christ-Crain M ,
- Bingisser R ,
- Schuetz P ,
- Thomann R ,
- ProHOSP Study Group
- Wakamatsu K ,
- Singanayagam A ,
- Cavalcanti M ,
- McCluskey SM ,
- Panagiotarakou M ,
- Serra-Batlles J ,
- Drakulovic MB ,
- Nicolas JM ,
- Kleinman K ,
- Szumita PM ,
- Li Bassi G ,
- Gravity-VAP Network
- Greene MT ,
- Apisarnthanarak A ,
- Fowler KE ,
- Koulenti D ,
- Dimopoulos G ,
- EU-VAP Study Investigators
- Thille AW ,
- FLORALI Study Group ,
- REVA Network
- Monro-Somerville T ,
- Burns KEA ,
- Adhikari NKJ
- Vaschetto R ,
- Longhini F ,
- Persona P ,
- Pohlman AS ,
- O’Connor MF ,
- Girard TD ,
- Philippart F ,
- Quinquis L ,
- TOP-Cuff Study Group
- Maruyama K ,
- Hoshijima H ,
- Hirabayashi G ,
- Jaillette E ,
- Girault C ,
- BestCuff Study Group and the BoRéal Network
- Maertens B ,
- Caroff DA ,
- McKechnie K ,
- Laporta D ,
- Barrio JM ,
- Dezfulian C ,
- Shojania K ,
- Collard HR ,
- Matthay MA ,
- Alexandrou E ,
- Pozuelo-Carrascosa DP ,
- Herráiz-Adillo Á ,
- Alvarez-Bueno C ,
- Martínez-Vizcaíno V ,
- Cavero-Redondo I
- de Jonge E ,
- Schultz MJ ,
- Spanjaard L ,
- de Smet AMGA ,
- Kluytmans JAJW ,
- Cooper BS ,
- Plantinga NL ,
- Oostdijk EAN ,
- Bhalodi AA ,
- van Engelen TSR ,
- Wiersinga WJ
- Bello González TDJ ,
- Fuentes S ,
- Wittekamp BH ,
- Worthington HV ,
- Labeau SO ,
- Van de Vyver K ,
- Brusselaers N ,
- Vogelaers D ,
- Furness S ,
- Mansouri P ,
- Dehghanrad F ,
- Bahrani M ,
- Deschepper M ,
- Waegeman W ,
- Eeckloo K ,
- Howell MD ,
- Greene LR ,
- Berenholtz SM
- MacLennan G ,
- SuDDICU Collaboration
- Wittekamp BHJ ,
- Chiewchalermsri C ,
- Sompornrattanaphan M ,
- Thongngarm T
- Odedra KM ,
- La Combe B ,
- Bleibtreu A ,
- Messika J ,
- do Brasil PEA ,
- Bouadma L ,
- Bouhemad B ,
- Gonzalo-Calvo D ,
- Benítez ID ,
- Pinilla L ,
- Bonneau E ,
- Kostantin E ,
- Tsongalis GJ ,
- Kyriazopoulou E ,
- Karageorgos A ,
- Liaskou-Antoniou L ,
- Open access
- Published: 10 September 2022
Unmet needs in pneumonia research: a comprehensive approach by the CAPNETZ study group
- Mathias W. Pletz 1 , 2 na1 ,
- Andreas Vestergaard Jensen 3 na1 ,
- Christina Bahrs 1 , 4 ,
- Claudia Davenport 7 ,
- Jan Rupp 2 , 5 ,
- Martin Witzenrath 2 , 6 , 12 ,
- Grit Barten-Neiner 2 , 7 ,
- Martin Kolditz 8 ,
- Sabine Dettmer 7 , 9 ,
- James D. Chalmers 10 ,
- Daiana Stolz 11 , 12 ,
- Norbert Suttorp 6 , 13 ,
- Stefano Aliberti 14 , 15 ,
- Wolfgang M. Kuebler 16 &
- Gernot Rohde 2 , 7 , 17
Respiratory Research volume 23 , Article number: 239 ( 2022 ) Cite this article
4392 Accesses
13 Citations
6 Altmetric
Metrics details
Introduction
Despite improvements in medical science and public health, mortality of community-acquired pneumonia (CAP) has barely changed throughout the last 15 years. The current SARS-CoV-2 pandemic has once again highlighted the central importance of acute respiratory infections to human health. The “network of excellence on Community Acquired Pneumonia” (CAPNETZ) hosts the most comprehensive CAP database worldwide including more than 12,000 patients. CAPNETZ connects physicians, microbiologists, virologists, epidemiologists, and computer scientists throughout Europe. Our aim was to summarize the current situation in CAP research and identify the most pressing unmet needs in CAP research.
To identify areas of future CAP research, CAPNETZ followed a multiple-step procedure. First, research members of CAPNETZ were individually asked to identify unmet needs. Second, the top 100 experts in the field of CAP research were asked for their insights about the unmet needs in CAP (Delphi approach). Third, internal and external experts discussed unmet needs in CAP at a scientific retreat.
Eleven topics for future CAP research were identified: detection of causative pathogens, next generation sequencing for antimicrobial treatment guidance, imaging diagnostics, biomarkers, risk stratification, antiviral and antibiotic treatment, adjunctive therapy, vaccines and prevention, systemic and local immune response, comorbidities, and long-term cardio-vascular complications.
Pneumonia is a complex disease where the interplay between pathogens, immune system and comorbidities not only impose an immediate risk of mortality but also affect the patients’ risk of developing comorbidities as well as mortality for up to a decade after pneumonia has resolved. Our review of unmet needs in CAP research has shown that there are still major shortcomings in our knowledge of CAP.
In 1918, Sir William Osler observed that pneumonia had replaced tuberculosis as the leading cause of death in Europe and described pneumonia as the ‘‘Captain of the men of death’’ [ 1 ].
Even one century later, pneumonia remains a major health concern and lower respiratory tract infections continue to be the leading infectious cause of death with more than 2.3 million deaths and more than 91 million years of life lost in 2016 [ 2 ]. With the emergence of the SARS-CoV-2 pandemic the central importance of acute respiratory infections to human health has once again been highlighted.
Pneumonia is defined as an acute infection of the pulmonary parenchyma and it is commonly classified by the “pneumonia triad” into community-acquired pneumonia (CAP) as the most common form of acquisition, hospital-acquired pneumonia (HAP) including ventilator-associated pneumonia (VAP) and pneumonia in the immunocompromised host [ 3 , 4 ]. The clinical presentation and severity of pneumonia is diverse and usually categorized as mild, moderate or severe in major guidelines [ 5 , 6 , 7 ].
The annual incidence of CAP requiring hospitalization ranges from 1.1 to 8.9 per 1000 inhabitants [ 8 , 9 , 10 , 11 ] and the 30-day readmission rate ranges from 12 to 25% [ 12 , 13 , 14 , 15 ]. In patients with CAP who do not need hospital admission the mortality rate is ≤ 1% [ 16 , 17 , 18 ] while in hospitalized patients CAP the short-term mortality ranges from 8 to 14% [ 8 , 10 , 11 , 19 , 20 , 21 , 22 , 23 ]. Further, several studies have shown that long-term mortality is excessive after CAP [ 24 , 25 , 26 , 27 , 28 , 29 ]. This underlines that CAP imposes a significant impact on the healthcare system and because of the aging population and the associated increase in comorbidities predisposing for CAP, it is likely that CAP will continue to be a major health issue in the years to come.
In 1999, the German Ministry of Education and Research published a call to initiate an excellence competition for competence centres in infectious diseases. Through this call the “network of excellence on Community Acquired Pneumonia” (CAPNETZ) was founded in 2001 [ 30 ]. CAPNETZ connects physicians, microbiologists, virologists, epidemiologists and computer scientists within clinical centres in Germany, Switzerland, Austria, The Netherlands, Denmark and Italy [ 31 ]. Today CAPNETZ offers the most comprehensive CAP database worldwide with an associated biobank for samples and pathogens including more than 12,000 patients [ 31 ]. Currently, the CAPNETZ database contains information on adult patients and consequently the pneumonia research within CAPNETZ has been focusing on adults. Pneumonia in children is also a major issue but an entity of its mown due to different pathogens, less burden of comorbidities etc. Recognizing the significance of pneumonia in children CAPNETZ has expanded their collaboration and begun inclusion of children into the pedCAPNETZ cohort [ 32 ]. The pedCAPNETZ cohort is still under construction and therefore the focus of this study is on adults only.
To discuss and identify which future research areas should have the highest priority in adult CAP research, CAPNETZ invited both internal and external CAP experts to participate in a 2-day scientific retreat. This paper summarizes the main research topics discussed, and highlights areas of future research, which deserve high priority to improve the understanding of CAP in order to develop novel prophylactic and therapeutic measures and ultimately reduce the burden of CAP.
The identification of unmet needs in CAP research followed a four-step procedure as depicted in Fig. 1 . In brief, research members of CAPNETZ were asked to identify important issues to be addressed within basic, clinical and translational research. Then the top 100 experts in the field of CAP research were asked for their insights about the unmet needs in CAP research (Delphi approach). The top 100 experts were determined by CAPNETZ as the researchers with the most publications in the web of knowledge database over the past 5 years marked with “community-acquired pneumonia”. Only original publications, reviews and editorials were taken into consideration. Thirty-three of the 100 experts contributed with their views on the unmet needs in CAP research. Finally, the identified unmet needs in CAP research were discussed at a 2-day scientific retreat.
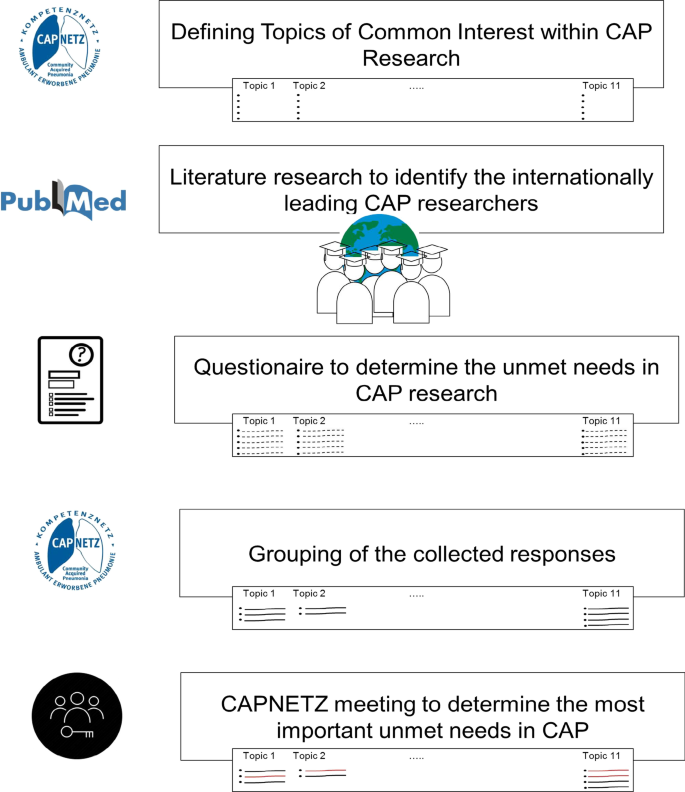
Flowchart for the identification of the unmet needs in community-acquired pneumonia research
Since the SARS-CoV-2 pandemic emerged after the scientific retreat was held no unmet needs where identified prior to the meeting. However, due to the significant impact of the SARS-CoV-2 pandemic, sections on the Covid-19 disease have been added post hoc.
Identified topics covering unmet needs in CAP research
Detection of causative pathogens, current setting.
Defining the causative pathogen in patients with pneumonia is relevant for ensuring the best-suited antimicrobial treatment. However, microbial diagnosis of pneumonia is still based on culture in most hospitals worldwide and a causative pathogen is only detected in the minority of patients. In a recent CAPNETZ study, bacteraemia rates in CAP were 0.5% in outpatients, 7.8% in hospitalized patients, 12.4% in patients admitted to an intensive care unit (ICU), and a relevant proportion of patients with bacteraemia and CAP (34.6%) was afebrile [ 33 ]. With the rapid emergence of novel diagnostic techniques (e.g. isothermic multiplex-PCRs for point of care testing, microbiome analysis, host transcriptomics and metagenomics), the question arises if these techniques will provide an added value to the currently available and established diagnostic methods and whether they will change management.
Unmet needs
Define an improved gold standard for microbial diagnosis of community-acquired pneumonia: Currently, microscopy and culture of lower respiratory tract specimens as well as blood cultures, detection of urine antigen detection and serology are routinely used to complement thorax imaging results in diagnosing pneumonia. However, most tests (particularly serology) lack sensitivity and specificity, while tests with a high sensitivity, such as PCR for non-influenza respiratory viruses may currently not properly translate into clinical relevance [ 34 , 35 ]. No internationally accepted standard for microbiological tests in CAP has been defined, both in terms of including novel PCR-based techniques but also regarding the quality of the required respiratory samples [ 36 ]. There is a need for a consented diagnostic standard that also serves as comparison for new diagnostic techniques [ 37 ].
Next generation sequencing for guidance of antimicrobial treatment
With the emergence of resistant or multidrug resistant (MDR) pathogens and increasing knowledge about potentially harmful or protective microbiomes [ 38 ], antibiotic management is becoming more complex [ 39 ]. Antibiotic stewardship is, therefore, a key priority [ 40 ] fostering pathogen directed treatment and avoiding excessive empiric broad spectrum antimicrobial therapy that increases the risk of complications, antibiotic resistance and mortality.
Metagenomics offers a potential solution. Most studies utilizing “microbiome” or metagenomics techniques to date have used target gene approaches such as sequencing amplicons of the 16s rRNA subunit [ 41 ]. As a more rapid technique, nanopore sequencing allows a much more in-depth data acquisition and analysis of viral and bacterial pathogens in real time [ 42 ]. The INHALE study from the UK showed that nanopore sequencing could provide results within 6 h of sampling from sputum with 96.6% sensitivity compared to culture [ 42 ]. This method also presented data on antibiotic resistance genes. Currently, methods to comprehensively describe bacterial, fungal and viral pathogens as well as the underlying microbiome in the same sample are still limited but technology is advancing rapidly.
Metagenomic research has, to date, been conducted in relatively small studies in limited geographical locations [ 42 ]. Much larger studies are required to fully understand the limitations and added value of metagenomic approaches.
Studies show that molecular techniques out-perform culture in identification of bacterial pathogens [ 38 , 42 ], but studies to demonstrate that implementation of these techniques improves clinical practice are lacking. There are remarkably few studies in which antibiotic choice, antibiotic duration or antibiotic spectrum are reduced or improved by the implementation of such techniques. Randomized controlled trials should be conducted to demonstrate the value of new techniques to improve CAP management.
Clinicians are not bioinformatics-scientists. Therefore, automated informatics tools that can rapidly analyse sequencing data and provide results to clinicians in a usable format are required. Antibiotic resistance genes may be detected by sequencing but their relationship to phenotypic resistance in bacteria is not always known, particularly in Gram-negatives.
Imaging diagnostics
The primary role of imaging in CAP is to confirm the clinical diagnosis. The major diagnostic challenges are the correct identification of opacities and consolidations as being evoked by infectious versus non-infectious differential diagnoses. Different methods are available with chest radiography being the most abundantly used [ 43 ]. However, its low sensitivity and specificity leads to a high false negative and false positive rate, respectively, depending on the infiltrate’s position [ 44 , 45 ]. Computer tomography (CT), therefore, is a useful addition to conventional radiography because of three-dimensional imaging with enhanced spatial resolution [ 44 , 45 , 46 , 47 , 48 ]. Due to higher radiation exposure and costs, however, CT is currently only used in selected cases [ 43 , 49 ]. Further, lung ultrasonography may be an adjuvant resource to accurately diagnose CAP [ 50 ].
Pathogen prediction through imaging analysis: Although radiographic findings do currently not allow the diagnosis of a specific pathogen, a differential diagnosis may be possible using radiological pattern recognition. Deep learning approaches may help to identify patterns that are indicative of specific viral or bacterial CAP. Integrating clinical data available at time of diagnosis may further improve such an approach.
Pre-test probability for general practitioners for chest radiography: Although chest radiographs are routinely used, the efficacy as a diagnostic tool has not been determined. The reduction of unnecessary chest radiographs particularly in the outpatient setting would decrease healthcare costs, and radiation exposure. However, even in the “Prospective Cough Complication Cohort Study (3C)”, where patients with acute cough received chest radiographs, the positive predictive value of at least one of four clinical signs of CAP (temperature > 37.8 °C, crackles on auscultation, oxygen saturation < 95%, heart rate > 100/min) for a radiography-confirmed pneumonia was low at around 20% [ 51 ]. In another European multicentre study including more than 3000 patients with acute cough, the addition of C-reactive protein > 30 mg/l to clinical signs of pneumonia (absence of runny nose and presence of breathlessness, crackles and diminished breath sounds on auscultation, tachycardia, and fever) improved the diagnostic classification [ 52 ]. However, further enhancing the pre-test probability for CAP would be necessary to provide a more reliable guide to general practitioners to identify patients with respiratory tract infections other than CAP where radiographs are not necessary.
Biomarkers may be an effective way to identify patients with severe infection and to monitor and eventually predict the course of infection. In CAP, biomarkers may be indicators of inflammation (local or systemic), may be released specifically after lung injury due to infection or originate from the genome of either the host or the pathogens. Numerous biomarkers have been investigated including pro-atrial natriuretic peptide [ 53 ], pro-vasopressin [ 53 ], cortisol [ 54 ], glucose [ 55 ], glycaemic gap [ 56 ], neutrophil extracellular traps [ 57 ], proadrenomedullin [ 58 ], Angiopoietin [ 59 ] etc. [ 60 , 61 ] but C-reactive protein and procalcitonin remain the most widely used biomarkers [ 60 ] even though they both have well known shortcomings [ 60 ]. Additionally, cardiac troponins, biomarkers for cardiac injury, may identify patients with cardiac complications of CAP that need special attention during follow-up [ 62 , 63 ]. Since coronary artery calcium (CAC), a marker of coronary atherosclerotic burden, is higher in patients already at increased atherosclerotic cardiovascular risk after pneumonia as compared to similar patients without a pneumonia event, assessment of CAC by CT may also be of predictive value [ 64 ].
As the research on immune-modulatory therapies intensifies, so will the search for the appropriate biomarkers for severity and treatment response [ 65 ]. However, the heterogeneity among patients with CAP in terms of onset of symptoms, clinical presentation, severity of disease, causative pathogen(s), comorbidities, genetic disposition etc. is vast. The discovery of a single common biomarker for CAP is therefore unlikely. Instead, combining the different sources of “biomarkers” into a systems-medicine approach reflects the complexity of CAP and could provide new insights, accurate clinical diagnosis, prediction of the severity of disease and help targeting specific adjuvant treatments. The recent advances in the field of metabolomics, genomics, epigenomics, transcriptomics, proteomics and microbiomics offer respective opportunities [ 60 , 66 ].
Risk stratification
Identifying low risk-patients that can be treated in an outpatient setting enhances patient satisfaction and reduces costs. CRB-65 is frequently used as a first step but should be supplemented by oxygenation status, assessment of instable comorbidities and functional parameters [ 67 ]. Identifying high-risk patients allows early application of intensified management, prevents organ failure and, thereby, improves prognosis. ATS/IDSA minor criteria supplemented by lactate are recommended tools [ 68 , 69 ]. Recommendations for early high-risk stratification are available but have not yet been fully implemented. Although there is a five percent risk of death within the first post-discharge weeks [ 70 ], there is only limited data available on risk stratification to predict post-discharge complications.
Harmonization with sepsis risk stratification and implementation of prediction tools: Sepsis is a major complication in CAP. Current sepsis recommendations employ a risk stratification strategy with similarities to CAP risk stratification by using a simple screening score (quickSOFA, qSOFA) and a more specific high-risk prediction score (SOFA) [ 71 ]. However, the scores and respective breakpoints (e.g. respiratory rate in qSOFA vs. CRB-65) are different from those recommended for CAP [ 72 ]. Moreover, the qSOFA with a cut off of ≥ 2 misses more than half of CAP patients whose condition will deteriorate during the course of the disease [ 73 ]. For optimal implementation of risk stratification concepts in busy emergency departments a harmonization of both concepts is needed, and prospective (cluster-randomized) clinical studies to implement recommendations with clinical outcome endpoints are necessary.
Individualization of management-based risk stratification: Today, medicine is heading towards the broad application of state-of-the-art medical technologies including “omics” approaches, which might allow more tailored treatment approaches based on a better understanding of a patient’s individual state of health [ 74 ]. In addition, machine learning could provide algorithms that predict the patient’s individual risk to deteriorate and will allow monitoring treatment responses based on routinely measured markers [ 75 ].
Risk factors for and management strategies against early post-discharge complications: Studies show high re-admission or even early death rate after discharge from hospital because of CAP [ 12 , 76 ]. So far, only little information is available on risk factors for post-discharge events, including infection- and non-infection-related complications, re-hospitalization or death. There is the need to determine the different affected patient groups and to define target groups that require interventions.
Stratification strategy for the coverage of multidrug resistant pathogens: In Europe, MDR pathogens are rare in CAP, but still can complicate the choice of empiric antibiotic therapies and may result in poor outcomes [ 77 ]. However, optimal individual MDR risk prediction is not known as existing scores lack accuracy and external validation.
Antiviral and antibiotic treatment
The early initiation of antibiotic therapy has been shown to provide a survival benefit [ 78 ]. Treatment is typically started empirically, prior to the identification of the causative pathogen. Bacteria are considered to be the primary causative pathogens in CAP and antibiotics are the mainstay of CAP treatment.
Treatment guidelines differ for outpatients and only little information is available on CAP-outpatients. In the hospital, either antibiotic monotherapy or combination therapy is applied but up to now no significant differences in outcome apart from adverse effects have been observed [ 79 ]. Most antibiotics are administered systemically, either through intravenous or oral application. The role of aerosolized antibiotics has so far been only investigated for hospital-acquired (HAP) and ventilator-associated pneumonia, but not for CAP [ 80 ].
Viral pathogens are increasingly recognized as a cause of pneumonia, especially among immunocompromised patients. Although more than 20 viruses have been linked with CAP, antiviral drugs were only available for the treatment of influenza or respiratory syncytial virus (RSV) pneumonia [ 81 ] before COVID-19. Since the occurrence of SARS-CoV-2 existing antiviral drugs such as Remdesivir and lopinavir–ritonavir have been evaluated for the treatment of Covid-19 [ 82 ]. However, lopinavir–ritonavir has not shown any beneficial effect in treating Covid-19 and the effect of Remdesivir in treating Covid-19 is still questionable [ 82 ]. The specifically developed antiviral Paxlovid may hold promise but peer-reviewed data are currently lacking [ 83 ].
Secondary bacterial pneumonia is frequently observed in viral pneumonia [ 84 , 85 ] and influenza patients often receive preventive antibiotic treatment [ 86 ]. However, the pathophysiologic mechanisms promoting a co-infection with bacterial and viral pathogens are not fully understood. Notably, the frequency of bacterial co-infections in Covid-19 patients appears to be low [ 87 ].
Monitoring of ß-lactam antibiotic and macrolide antibiotic treatment and development of resistance: ß-lactams have been the “antibiotic backbone” of antimicrobial therapy of pneumonia for decades [ 88 ]. However, increasing resistance rates are beginning to limit the utility of this antibiotic class. Drug-resistant Gram-negative pathogens associated with pneumonia have been identified with increasing frequency and a variety of resistance patterns. Among the “atypical” agents there is also several reports of increasing macrolide resistance in M. pneumoniae infections throughout the world [ 89 , 90 , 91 ]. Antibiotic resistances show a broad variety between regions. Treatment guidelines, therefore, need to be updated and validated based on local epidemiological data [ 92 ].
Development of new antibiotics vs. improved usage of available antibiotics: Although the number of newly approved antibiotics has tripled in the past 6 years after a 90% decrease between 1983 and 2012, MDR bacteria pose an increasing threat [ 93 ]. Mis- and overuse of empirically prescribed broad-spectrum antibiotics have led to a significant increase in resistance although a great diversity has been observed in different countries [ 94 ]. The timely differentiation between bacterial and viral pneumonia may support the physician in avoiding medically not indicated antibiotic administration. In CAP, development of new antibiotics and improving diagnostic-guided therapy is warranted.
Overview of the treatment of CAP in an outpatient setting: A high proportion of CAP patients is treated in an outpatient setting [ 52 , 70 ]. A comprehensive overview of existing real-life principles that guide treatment in an outpatient setting will allow the formulation of improved guidelines taking the medical environment and available methods into account.
Adjunctive therapy
An excessive inflammatory response seems to be partly responsible for treatment failure in some patients and has been associated with poor clinical outcomes [ 95 ]. Different immunomodulatory and barrier-enhancing agents have been discussed and tested for potential adjunctive therapy to antimicrobial agents in the treatment of CAP. Classical approaches have focused on corticosteroids and immunoglobulins [ 95 ], while the effects of adrenomedullin and angiopoietin-1 have been investigated in more experimental approaches [ 59 , 96 ]. Especially corticosteroids have been the subject of potential adjuncts to conventional CAP treatment [ 95 ]. They are the most used anti-inflammatory drugs and modulate a wide range of physiological processes, but their efficacy has been discussed controversially in CAP treatment [ 97 ]. In hospitalized patients with Covid-19 and who requires oxygen, corticosteroid treatment improves survival [ 98 ] and is therefore recommended [ 82 ].
Further, due to the hyperinflammation state seen in some patients with Covid-19 several immunomodulatory agents, with the intend to block the inflammatory pathway, have been evaluated [ 82 , 99 ]. Fare from all has proven effective but in hospitalized patients with Covid-19 and in need of oxygen treatment, IL-6 inhibitors may reduce the risk of mechanical ventilation or death [ 82 ]. Likewise treatment with Janus kinase (JAK) inhibitors may reduce the risk of respiratory failure and death [ 100 , 101 ]. Inhibition of the IL-1 pathway may also be associated with reduced mortality in patients with Covid-19 [ 102 ] although conflicting results exists [ 103 , 104 ].
As the understanding of the underlying pathophysiology improves, specifically tailored agents for immunomodulatory therapy will likely help to avoid adverse outcomes for specific patient groups.
Identification of patients that benefit most from macrolides: In addition to antimicrobial effects, macrolides also have an immunomodulatory effect [ 105 , 106 ]. Several studies have suggested a benefit of adding macrolides to a β-lactam in the empirical treatment, although the existing literature is conflicting [ 105 , 107 , 108 , 109 ]. It is largely unclear, which patient groups benefit most from an adjunctive macrolide therapy since the effects of macrolides appear to be influenced by the presence of bacteria [ 106 , 110 ] and by the susceptibility of the host to develop cardiac side effects associated with macrolide treatment [ 111 ].
A recent CAPNETZ study has used a machine learning approach to identify patients who benefit most from macrolide treatment [ 112 ]. Such a personalized approach is important for improved management and disease outcome. However, results need to be confirmed in a randomized controlled trial.
Immunoglobulins: Immunoglobulins have been proposed as a promising adjunctive therapy option for severe sepsis, but have only been investigated in small studies [ 113 ]. Both, IgG and IgM levels have been shown to be higher during convalescence in pneumonia [ 114 ]. Additionally, patients with severe CAP admitted to ICU showed lower levels of immunoglobulins than non-ICU patients [ 115 ]. Since therapeutic formulations of immunoglobulins are available further insights into the changes of serum levels of immunoglobulins and IgG subclasses during the course of the disease are of scientific and therapeutic interest.
Pathogen-directed strategies: In addition to antibiotic treatment, a pathogen-directed strategy may include blockers of pathogenicity, virulence, or toxins. These may be small molecule inhibitors or monoclonal antibodies [ 116 ]. Additional use of adoptive cell therapy might be possible as well as the use of phages or phage products. In the ongoing pandemic of Covid-19 treatment with monoclonal antibodies against the SARS-CoV-2 spike protein may reduce the risk of Covid-19-related hospitalization and death in ambulatory patients [ 117 ] and in hospitalized patients with Covid-19 and who are seronegative, treatment with monoclonal antibodies may reduce mortality [ 118 ].
Host-directed strategies: Alternative strategies comprise of specifically stimulating early local immune responses against pathogens, dampening particular components of the local and systemic immune responses to avoid tissue injury, increasing tissue resilience and improving resolution of inflammation and tissue repair.
Vaccines and prevention
Vaccines against pneumococci and influenza virus, the most frequent bacterial and viral causes of CAP, are available. Influenza vaccination has been shown to reduce the number of severe CAP cases and improved overall long-term survival in patients with CAP during influenza seasons [ 119 ]. The known mechanistic link between cardiovascular events and pneumonia may be the cause for the reported cardioprotective effect of vaccines against influenza and pneumococci [ 120 , 121 , 122 ].
Recently, a quadrivalent influenza vaccine that includes both influenza B lines, i.e. Yamagata and Victoria, has been made available for clinical use [ 123 ]. It has already been recommended as the primary influenza vaccine instead of the trivalent influenza vaccines in several countries. The investigational universal influenza vaccine candidate, FLU-v, has entered phase 3 clinical trials [ 124 ] after demonstrating immunogenicity and safety in a recent phase 2b study [ 125 ].
Two types of pneumococcal vaccines are used: pneumococcal conjugate vaccines (PCVs) and pneumococcal polysaccharide vaccines (PPVs). Although PPV has been shown to prevent pneumococcal bacteraemia, its protective effect against non-invasive pneumococcal pneumonia and its effectiveness in the immune-compromised host are limited [ 126 , 127 , 128 ]. Infant vaccination programs with PCV have substantially decreased the contained serotypes by herd protection effects [ 129 ]. However, the decrease of vaccine serotypes was compensated by non-vaccine serotypes (replacement effect), that comprised sometimes even the same pneumococcal clone, which has switched its capsule to another serotype to evade the selective pressure of the vaccine [ 130 ]. Furthermore, strong herd protection effects in invasive and non-invasive pneumococcal CAP seen after the global implementation of the 7-valent conjugate vaccine (PCV7) [ 129 , 131 ] were not reproduced after substitution of PCV7 by the 13-valent conjugate vaccine (PCV13) [ 132 , 133 ]. Particularly, serotype 3, which was included into PCV13, seems not to be affected by herd protection effects. Serotype 3 has been associated with disease severity, i.e. higher rate of patients with hospital admission and oxygen support, and has become one of the leading serotypes in adult CAP [ 133 , 134 ]. The decreased efficacy against serotype 3 as well as the lack of herd protection of this major serotype is not completely understood. One plausible hypothesis includes capsular shedding after binding of antibodies [ 135 ].
Optimal use for pneumococcal polysaccharide vaccines and pneumococcal conjugate vaccines: Although the two vaccines PPV23 and PCV13 share some serotypes they generate different immune responses [ 136 ]. While both vaccines generate antibodies against pneumococcal capsular antigens, only PCV13 induces a T-cell-dependent response. The broad landscape of different pneumococcal vaccination recommendation worldwide (i.e. PPV or PCV or “sequential vaccination” with PCV followed by PPV) reflects uncertainty on the optimal use of these vaccines. Furthermore, simultaneous application of both pneumococcal vaccines (PCV and PPV) is currently investigated for the first time in an ongoing randomized controlled trial to improve immunological response (pneumococcal serotype specific B-cells and humoral immune response) in the elderly [ 137 ]. More research is needed on the topic to provide vaccination recommendations especially for patients with certain underlying medical conditions such as respiratory, hepatic or renal comorbidities and immunosuppression as well as the optimal time for re-vaccination [ 138 ].
Novel vaccines: Like a universal influenza vaccine, a serotype independent pneumococcal vaccine would decrease the global CAP burden tremendously. Currently, a 15-valent PCV was announced in the US and a 20-valent PCV is close to market license [ 139 ]. However, experience with PCV7 and PCV13 have shown that pneumococcal evolution is highly dynamic and that non-vaccine serotypes will emerge and fill the niche created by PCV-induced reduction of vaccine serotypes. Since the inclusion of even more serotypes into a novel vaccine is limited, a serotype independent vaccine targeting e.g. surface proteins would represent a major breakthrough [ 140 ]. Also, a more effective—even singular—vaccine against serotype 3 would decrease the burden of CAP tremendously.
The progress in microbiological diagnostics—and also the recent SARS-CoV-2 pandemics- has uncovered the burden of non-influenza respiratory viruses in CAP. Particularly, RSV seems to expose a substantial burden on adult CAP. Therefore, a RSV vaccine is highly desirable but remains a challenge [ 141 ], since earlier RSV vaccines had no efficacy and even seemed to aggravate disease [ 142 ].
Systemic and local immune response
In order to facilitate a rapid and efficient immune response against invading microbial pathogens, the lung combines different defence strategies including anatomic, mechanical, humoral, and cellular mechanisms aiming towards the rapid expulsion of pathogens [ 143 ]. The lung is a fragile organ that is finely designed for gas exchange, so that an excessive immune response may itself be damaging and lead to irreparable tissue damage that might be lethal [ 144 , 145 ]. Many anti-inflammatory strategies have failed to improve survival in pneumonia [ 146 ]. It seems that more specific anti-inflammatory strategies, subphenotyping of patients, and precise timing are crucial to achieve beneficial modulation of the inflammatory response. Differences in immune responses may result from genetic predispositions that influence immunomodulation [ 147 , 148 ]. Deciphering the mechanisms of inflammatory response in respiratory infection would allow the identification of different inflammatory phenotypes.
Determination of different inflammatory phenotypes for personalized medicine: Despite sharing the same underlying pathogen, in some patients CAP manifests as a serious disease while in others the course of disease is mild. The susceptibility to infection as well as CAP severity is most likely a phenotypical trait determined by uncountable pathogen- and host-specific factors, including polymorphisms in many collaborating genes in otherwise healthy persons [ 149 ], immunosenescence, pregnancy, lung diseases, immunodeficiency, and specific therapies for preceding diseases or the CAP itself, to name a few. Therefore, specific conditions need specific strategies for promising personalized adjunctive immunomodulatory therapy.
Pulmonary long-term consequences of CAP: While most patients return to normal lung function following pneumonia within weeks to months, some fail to recover ad integrum due to pleura-involvement or parenchymal alteration, and some may be at an increased risk of developing chronic non-infectious lung inflammation including cryptogenic organizing pneumonia (COP) and idiopathic pulmonary fibrosis (IPF) after an episode of pneumonia. The early identification of these patients would allow taking timely countermeasures. For this, specific markers (of clinical course, lung function, imaging, biomarkers, etc.) and therapeutic strategies need to be identified. Therefore, a deeper patho-mechanistic understanding of the pulmonary long-term sequelae of CAP is needed.
Extra-pulmonary long-term consequences of CAP (see also “ Long-term cardio-vascular complications ”): Severe pulmonary and systemic inflammation upon lung infection may result in long-term sequelae regarding organ dysfunction, vascular pathology, and neuromuscular function. Some patho-mechanistic links have been proposed, e.g. direct cardiac damage by bacterial invasion into the myocardium and formation of microscopic lesions finally leading to cardiac scarring [ 150 ] and a causal relationship between pulmonary inflammation and atherosclerotic plaque formation in systemic arteries [ 151 ]. However, many key patho-mechanisms by which pneumonia may trigger or promote subsequent organ dysfunction remain unclear. The complexity of the interplay between pulmonary inflammation and distant organ pathology and the relatively long timeframes render preclinical as well as clinical investigations in this field challenging. Nevertheless, the emerging evidence for the relevance of long-term sequelae for patient outcome and the probability for potentially effective secondary prophylactic measures warrant intense joint scientific efforts.
Comorbidities
Pre-existing comorbidities including chronic respiratory, cardiovascular diseases and diabetes mellitus are frequent in the elderly population and increase the risk of CAP as well as mortality [ 152 , 153 ]. Immunosenescence and therapies with immunosuppressive agents increase the number of immunosuppressed patients. Immunosuppression has been recognized as an independent risk factor for CAP [ 9 ]. Although the prevalence of different comorbidities in CAP patients has been evaluated across several studies, data on their impact on the course of the disease as well as their management during CAP are limited. Furthermore, most of the international guidelines on CAP management clearly state that the proposed recommendations do not apply to patients with immunosuppression [ 5 ]. International and observational studies on immunocompromised patients are limited or consider only a single specific risk factor. A direct implication of this scenario is the possible underestimation of the real prevalence of immunosuppression with a higher rate of treatment failure or an overestimation and overuse of wide-spectrum antibiotics.
Treatment guidelines for immunocompromised patients: Up to 29% of hospitalized patients with CAP have some level of immunosuppression [ 154 ] and it is foreseeable that this population will expand in the following years. These patients may be at risk of both the “core” CAP pathogens and opportunistic microorganisms. Unfortunately, such patients are often excluded from pneumonia studies resulting in a marked knowledge gap concerning causative pathogens, performance of existing prognostic risk scores and performance of advanced diagnostic such as metagenomics among others. Immunocompromised patients do not form a homogeneous group in terms of underlying disease, treatment and severity of immunosuppression and the different immunosuppression states in the context of CAP need to be defined. It is possible that severely immunocompromised patients with CAP may benefit from adjunctive therapies to enhance specific functions of the immune system. Therefore, future studies need to focus on patients with risk factors for immunodeficiency in order to provide clinicians with recommendations for the management of immunocompromised patients with CAP.
Treatment guidelines for patients on special medication (i.e. patients on chronic steroids, biological drugs and cancer patients): Patients suffering from pre-existing lung diseases such as asthma or COPD are often treated with either inhaled or systemic corticosteroids [ 155 ]. Although these drugs reduce inflammation and might prevent exacerbations, the impact of the routine use of corticosteroids on CAP patients has not been investigated to full extent [ 156 ]. Biological drugs targeting tumour immune evasive pathways for the treatment of lung cancer [ 157 ] may guide different treatment regimens of CAP due to a changed immune status in these patients. With the ever-increasing number of patients suffering from pre-existing lung disease, it will be necessary to develop adjusted guidelines and treatment recommendations.
Influence of CAP on dementia and neurological damage: As in sepsis, during the acute phase of pneumonia, confusion is frequently observed [ 158 , 159 ]. Evidence suggests that delirium may hasten cognitive deterioration in people with pre-existing dementia. Since pneumonia is primarily a disease of older patients there is the possibility that an episode of pneumonia will cause neurological damage and that the early onset of worsening of pre-existing dementia is a long-term consequence of CAP that has been overlooked so far.
Long-term cardio-vascular complications
Hospitalized CAP patients have an increased risk of major acute and long-term cardiovascular complications (i.e. new/worsening heart failure, new/worsening arrhythmias, myocardial infarctions and/or strokes) [ 151 , 160 ], which are associated with a 60% increase in short-term mortality [ 161 ]. Mortality remains increased even in long-term survivors of pneumonia [ 27 , 162 ], an effect that has at least in part been attributed to an increased risk for cardiovascular diseases such as heart failure or atherosclerosis [ 151 , 163 , 164 ]. As a result of both acute and long-term effects, the ensuing risk for cardiovascular events associated with pneumonia is similar to or higher as compared to classic cardiovascular risk factors such as smoking or diabetes [ 165 ]. The underlying pathophysiological processes, however, are so far largely unclear.
Spectrum, incidence and outcome of cardio-vascular disease after pneumonia: While there is clearly emerging evidence from epidemiological and preclinical studies for the association of pneumonia with both short- and long-term cardiovascular events, a comprehensive analysis of the various clinical manifestations (e.g. systolic or diastolic heart failure, arrhythmias, atherosclerotic and ischemic events) of cardiovascular disease (CVD), their incidence at different time points following a pneumonia event, and their association with outcome is as yet lacking, but would be critical to develop better diagnostic tools and ultimately tailor interventional trials.
Patients at increased risk of developing cardio-vascular disease after pneumonia: Patients with pre-existing chronic CVD have the strongest risk, followed by patients with common comorbidities including chronic obstructive pulmonary disease (COPD), ischemic heart disease, and diabetes [ 160 ]. The influence of these risk factors prior to the event of pneumonia, however, has not been stratified. Similarly, genetic traits associated with an increased risk for CVD after pneumonia have so far not been assessed. Furthermore, most studies focus so far on short-term outcomes of CAP. Cardiovascular complications associated with CAP may occur up to 10 years or more after the event of pneumonia. It would be helpful to stratify known cardiovascular risk factors to identify patients at an increased risk of developing CVD as early as possible and to develop therapeutic interventions to reduce the incidence of cardiac complications following CAP. Similarly, it would be helpful to identify microbial characteristics, and/or markers of pneumonia severity and host response that may predict association with CVD and CVD-related mortality [ 166 ].
Prevention of cardio-vascular disease after pneumonia: At present, our understanding of the patho-mechanisms that drive acute and chronic CVD following pneumonia is at best rudimentary. Discussed mechanisms include but are not limited to invasion of microbes such as S. pneumoniae into the myocardium with formation of microscopic lesions [ 150 ], or dissemination of inflammatory cells or pro-inflammatory mediators and extracellular vesicles driving inflammatory cardiomyopathic syndromes or formation and destabilization of atherosclerotic plaques [ 151 ]. A detailed in-depth understanding of these mechanisms by comparative systems medicine approaches in patient cohorts and preclinical models is required to inform the development and testing of targeted interventions to prevent CVD in patients-at-risk and/or in specific responder subgroups and as such, to create personalized therapeutic approaches.
Pneumonia has been known as the leading infectious cause of death for more than a century and many attempts have been made to change this fact. However, the mortality of CAP has barely changed in the last 50 years and the Covid-19 pandemic has once again highlighted the central importance of acute respiratory infections to human health. Pneumonia is not just an infection of the lung but a complex disease where the interplay between the pathogen(s), immune system and comorbidities not only impose an immediate risk of mortality but also affect the patients’ risk of developing comorbidities as well as mortality for up to a decade after the pneumonia has resolved. Despite the importance of pneumonia on human health and the fact that many of the identified topics have been focus points for several years, our review of unmet needs in CAP research has shown that there are still major shortcomings in our knowledge of CAP. The poor evidence base that exists for most clinical decisions in acute respiratory infections can no longer be considered acceptable and a co-ordinated focus and investment into research on acute respiratory infections is now needed.
Take home message
Unmet needs have been identified for diagnostics, risk stratification, treatment, adjunctive therapy, and prevention. Major knowledge gaps include immune response, role of comorbidities, and long-term cardio-vascular complications.
Availability of data and materials
Not applicable.
Abbreviations
Cardiovascular disease
Community-acquired pneumonia
Computer tomography
Coronary artery calcium
Chronic obstructive pulmonary disease
Cryptogenic organizing pneumonia
Hospital-acquired pneumonia
Idiopathic pulmonary fibrosis
Intensive care unit
Janus kinase
Multidrug resistant
Network of excellence on Community Acquired Pneumonia
Pneumococcal conjugate vaccines
Pneumococcal polysaccharide vaccines
Respiratory syncytial virus
Ventilator-associated pneumonia
7-Valent conjugate vaccine
13-Valent conjugate vaccine
Osler W, McCrae T. The principles and practice of medicine. New York, London: D. Appleton and company; 1920. http://archive.org/details/principlesandpr00mccrgoog . Accessed 15 May 2019.
Wang H, Abajobir AA, Abate KH, Abbafati C, Abbas KM, Abd-Allah F, et al. Global, regional, and national under-5 mortality, adult mortality, age-specific mortality, and life expectancy, 1970–2016: a systematic analysis for the Global Burden of Disease Study 2016. Lancet. 2017;390:1084–150.
Article Google Scholar
Chalmers JD, Pletz MW, Aliberti S. European respiratory monograph community-acquired pneumonia. European Respiratory Society; 2014.
Aliberti S, Cruz CSD, Amati F, Sotgiu G, Restrepo MI. Community-acquired pneumonia. Lancet. 2021;398:906–19.
Article PubMed Google Scholar
Metlay JP, Waterer GW, Long AC, Anzueto A, Brozek J, Crothers K, et al. Diagnosis and treatment of adults with community-acquired pneumonia. An official clinical practice guideline of the American Thoracic Society and Infectious Diseases Society of America. Am J Respir Crit Care Med. 2019;200:e45–67.
Article PubMed PubMed Central Google Scholar
Lim WS, Baudouin SV, George RC, Hill AT, Jamieson C, Jeune IL, et al. BTS guidelines for the management of community acquired pneumonia in adults: update 2009. Thorax. 2009;64:iii1–55.
Ewig S, Höffken G, Kern W, Rohde G, Flick H, Krause R, et al. Behandlung von erwachsenen Patienten mit ambulant erworbener Pneumonie und Prävention—Update 2016. Pneumologie. 2016;70:151–200.
Article CAS PubMed Google Scholar
Egelund GB, Jensen AV, Andersen SB, Petersen PT, Lindhardt BØ, von Plessen C, et al. Penicillin treatment for patients with community-acquired pneumonia in Denmark: a retrospective cohort study. BMC Pulm Med. 2017;17:66.
Article PubMed PubMed Central CAS Google Scholar
Torres A, Peetermans WE, Viegi G, Blasi F. Risk factors for community-acquired pneumonia in adults in Europe: a literature review. Thorax. 2013;68:1057–65.
Ewig S, Birkner N, Strauss R, Schaefer E, Pauletzki J, Bischoff H, et al. New perspectives on community-acquired pneumonia in 388 406 patients. Results from a nationwide mandatory performance measurement programme in healthcare quality. Thorax. 2009;64:1062–9.
Søgaard M, Nielsen RB, Schønheyder HC, Nørgaard M, Thomsen RW. Nationwide trends in pneumonia hospitalization rates and mortality, Denmark 1997–2011. Respir Med. 2014;108(8):1214–22.
Petersen PT, Egelund GB, Jensen AV, Andersen SB, Pedersen MF, Rohde G, et al. Associations between biomarkers at discharge and co-morbidities and risk of readmission after community-acquired pneumonia: a retrospective cohort study. Eur J Clin Microbiol Infect Dis. 2018;37(6):1–9.
Article CAS Google Scholar
Jasti H, Mortensen EM, Obrosky DS, Kapoor WN, Fine MJ. Causes and risk factors for rehospitalization of patients hospitalized with community-acquired pneumonia. Clin Infect Dis. 2008;46:550–6.
Shorr AF, Zilberberg MD, Reichley R, Kan J, Hoban A, Hoffman J, et al. Readmission following hospitalization for pneumonia: the impact of pneumonia type and its implication for hospitals. Clin Infect Dis. 2013;57:362–7.
Micek ST, Lang A, Fuller BM, Hampton NB, Kollef MH. Clinical implications for patients treated inappropriately for community-acquired pneumonia in the emergency department. BMC Infect Dis. 2014;14:61.
Fine MJ, Smith MA, Carson CA, et al. Prognosis and outcomes of patients with community-acquired pneumonia: a meta-analysis. JAMA. 1996;275:134–41.
Carratala J, Fernández-Sabé N, Ortega L, Castellsagué X, Rosón B, Dorca J, et al. Outpatient care compared with hospitalization for community-acquired pneumonia: a randomized trial in low-risk patients. Ann Intern Med. 2005;142:165–72.
Lim WS, van der Eerden MM, Laing R, Boersma WG, Karalus N, Town GI, et al. Defining community acquired pneumonia severity on presentation to hospital: an international derivation and validation study. Thorax. 2003;58:377–82.
Article CAS PubMed PubMed Central Google Scholar
Arnold FW, Wiemken TL, Peyrani P, Ramirez JA, Brock GN. Mortality differences among hospitalized patients with community-acquired pneumonia in three world regions: results from the community-acquired pneumonia organization (CAPO) international cohort study. Respir Med. 2013;107:1101–11.
Metersky ML, Waterer G, Nsa W, Bratzler DW. Predictors of in-hospital vs postdischarge mortality in pneumonia. Chest. 2012;142:476–81.
Fine MJ, Stone RA, Singer DE, Coley CM, Marrie TJ, Lave JR, et al. Processes and outcomes of care for patients with community-acquired pneumonia: results from the pneumonia patient outcomes research team (PORT) cohort study. Arch Intern Med. 1999;159:970–80.
Lindenauer PK, Lagu T, Shieh M-S, Pekow PS, Rothberg MB. Association of diagnostic coding with trends in hospitalizations and mortality of patients with pneumonia, 2003–2009. JAMA. 2012;307:1405–13.
Ramirez JA, Wiemken TL, Peyrani P, Arnold FW, Kelley R, Mattingly WA, et al. Adults hospitalized with pneumonia in the united states: incidence, epidemiology, and mortality. Clin Infect Dis. 2017;65:1806–12.
Bruns AHW, Oosterheert JJ, Cucciolillo MC, El Moussaoui R, Groenwold RHH, Prins JM, et al. Cause-specific long-term mortality rates in patients recovered from community-acquired pneumonia as compared with the general Dutch population. Clin Microbiol Infect. 2011;17:763–8.
Koivula I, Stén M, Mäkelä P. Prognosis after community-acquired pneumonia in the elderly: a population-based 12-year follow-up study. Arch Intern Med. 1999;159:1550–5.
Brancati FL, Chow JW, Wagener MM, Vacarello SJ, Yu VL. Is pneumonia really the old man’s friend? Two-year prognosis after community-acquired pneumonia. Lancet. 1993;342:30–3.
Mortensen EM, Kapoor WN, Chang C-CH, Fine MJ. Assessment of mortality after long-term follow-up of patients with community-acquired pneumonia. Clin Infect Dis. 2003;37:1617–24.
Yende S, D’Angelo G, Kellum JA, Weissfeld L, Fine J, Welch RD, et al. Inflammatory markers at hospital discharge predict subsequent mortality after pneumonia and sepsis. Am J Respir Crit Care Med. 2008;177:1242–7.
Johnstone J, Eurich DT, Majumdar SR, Jin Y, Marrie TJ. Long-term morbidity and mortality after hospitalization with community-acquired pneumonia: a population-based cohort study. Medicine. 2008;87:329–34.
Welte T, Suttorp N, Marre R. CAPNETZ—community-acquired pneumonia competence network. Infection. 2004;32:234–8.
CAPNETZ. https://www.capnetz.de/html/capnetz/lccs . Accessed 29 Nov 2017.
pedCAPNETZ. http://www.capnetz.de/html/pedcapnetz/project . Accessed 18 May 2022.
Forstner C, Patchev V, Rohde G, Rupp J, Witzenrath M, Welte T, et al. Rate and predictors of bacteremia in afebrile community-acquired pneumonia. Chest. 2019;157(3):529–39.
Lutfiyya MN, Henley E, Chang LF, Reyburn SW. Diagnosis and treatment of community-acquired pneumonia. AFP. 2006;73:442–50.
Google Scholar
Self WH, Williams DJ, Zhu Y, Ampofo K, Pavia AT, Chappell JD, et al. Respiratory viral detection in children and adults: comparing asymptomatic controls and patients with community-acquired pneumonia. J Infect Dis. 2016;213:584–91.
Prendki V, Huttner B, Marti C, Mamin A, Fubini PE, Meynet MP, et al. Accuracy of comprehensive PCR analysis of nasopharyngeal and oropharyngeal swabs for CT-scan-confirmed pneumonia in elderly patients: a prospective cohort study. Clin Microbiol Infect. 2019;25:1114–9.
File TM Jr. New diagnostic tests for pneumonia: what is their role in clinical practice? Clin Chest Med. 2011;32:417–30.
Torres A, Lee N, Cilloniz C, Vila J, der Eerden MV. Laboratory diagnosis of pneumonia in the molecular age. Eur Respir J. 2016;48:1764–78.
Chalmers JD, Rother C, Salih W, Ewig S. Healthcare-associated pneumonia does not accurately identify potentially resistant pathogens: a systematic review and meta-analysis. Clin Infect Dis. 2014;58:330–9.
Aliberti S, Cilloniz C, Chalmers JD, Zanaboni AM, Cosentini R, Tarsia P, et al. Multidrug-resistant pathogens in hospitalised patients coming from the community with pneumonia: a European perspective. Thorax. 2013;68:997–9.
Faner R, Sibila O, Agustí A, Bernasconi E, Chalmers JD, Huffnagle GB, et al. The microbiome in respiratory medicine: current challenges and future perspectives. Eur Respir J. 2017;49:1602086.
Charalampous T, Kay GL, Richardson H, Aydin A, Baldan R, Jeanes C, et al. Nanopore metagenomics enables rapid clinical diagnosis of bacterial lower respiratory infection. Nat Biotechnol. 2019;37:783–92.
Franquet T. Imaging of pneumonia: trends and algorithms. Eur Respir J. 2001;18:196–208.
Self WH, Courtney DM, McNaughton CD, Wunderink RG, Kline JA. High discordance of chest X-ray and CT for detection of pulmonary opacities in ED patients: implications for diagnosing pneumonia. Am J Emerg Med. 2013;31:401–5.
Claessens Y-E, Debray M-P, Tubach F, Brun A-L, Rammaert B, Hausfater P, et al. Early chest computed tomography scan to assist diagnosis and guide treatment decision for suspected community-acquired pneumonia. Am J Respir Crit Care Med. 2015;192:974–82.
Haga T, Fukuoka M, Morita M, Cho K, Tatsumi K. Computed tomography for the diagnosis and evaluation of the severity of community-acquired pneumonia in the elderly. Intern Med. 2016;55:437–41.
Nazerian P, Volpicelli G, Vanni S, Gigli C, Betti L, Bartolucci M, et al. Accuracy of lung ultrasound for the diagnosis of consolidations when compared to chest computed tomography. Am J Emerg Med. 2015;33:620–5.
Reissig A, Copetti R, Mathis G, Mempel C, Schuler A, Zechner P, et al. Lung ultrasound in the diagnosis and follow-up of community-acquired pneumonia: a prospective, multicenter, diagnostic accuracy study. Chest. 2012;142:965–72.
Simpson S, Kay FU, Abbara S, Bhalla S, Chung JH, Chung M, et al. Radiological Society of North America expert consensus statement on reporting chest CT findings related to COVID-19. Endorsed by the Society of Thoracic Radiology, the American College of Radiology, and RSNA. J Thorac Imaging. 2020. https://doi.org/10.1097/RTI.0000000000000524 .
Llamas-Álvarez AM, Tenza-Lozano EM, Latour-Pérez J. Diaphragm and lung ultrasound to predict weaning outcome: systematic review and meta-analysis. Chest. 2017;152:1140–50.
Moore M, Stuart B, Little P, Smith S, Thompson MJ, Knox K, et al. Predictors of pneumonia in lower respiratory tract infections: 3C prospective cough complication cohort study. Eur Respir J. 2017;50:1700434.
van Vugt SF, Broekhuizen BDL, Lammens C, Zuithoff NPA, de Jong PA, Coenen S, et al. Use of serum C reactive protein and procalcitonin concentrations in addition to symptoms and signs to predict pneumonia in patients presenting to primary care with acute cough: diagnostic study. BMJ. 2013. https://doi.org/10.1136/bmj.f2450 .
Krüger S, Papassotiriou J, Marre R, Richter K, Schumann C, von Baum H, et al. Pro-atrial natriuretic peptide and pro-vasopressin to predict severity and prognosis in community-acquired pneumonia. Intensive Care Med. 2007;33:2069–78.
Article PubMed CAS Google Scholar
Kolditz M, Höffken G, Martus P, Rohde G, Schütte H, Bals R, et al. Serum cortisol predicts death and critical disease independently of CRB-65 score in community-acquired pneumonia: a prospective observational cohort study. BMC Infect Dis. 2012;12:90.
Lepper PM, Ott S, Nuesch E, von Eynatten M, Schumann C, Pletz MW, et al. Serum glucose levels for predicting death in patients admitted to hospital for community acquired pneumonia: prospective cohort study. BMJ. 2012;344: e3397.
Jensen AV, BaunbækEgelund G, Bang Andersen S, Petersen PT, Benfield T, Witzenrath M, et al. The glycemic gap and 90-day mortality in community-acquired pneumonia. A prospective cohort study. Ann ATS. 2019;16:1518–26.
Ebrahimi F, Giaglis S, Hahn S, Blum CA, Baumgartner C, Kutz A, et al. Markers of neutrophil extracellular traps predict adverse outcome in community-acquired pneumonia: secondary analysis of a randomised controlled trial. Eur Respir J. 2018;51:1701389.
Krüger S, Ewig S, Giersdorf S, Hartmann O, Suttorp N, Welte T. Cardiovascular and inflammatory biomarkers to predict short- and long-term survival in community-acquired pneumonia. Am J Respir Crit Care Med. 2010;182:1426–34.
Gutbier B, Neuhauß A-K, Reppe K, Ehrler C, Santel A, Kaufmann J, et al. Prognostic and pathogenic role of angiopoietin-1 and -2 in pneumonia. Am J Respir Crit Care Med. 2018;198:220–31.
Karakioulaki M, Stolz D. Biomarkers in pneumonia—beyond procalcitonin. Int J Mol Sci. 2019;20:2004.
Article CAS PubMed Central Google Scholar
Holub M, Džupová O, Růžková M, Stráníková A, Bartáková E, Máca J, et al. Selected biomarkers correlate with the origin and severity of sepsis. Mediat Inflamm. 2018;2018:7028267.
Vestjens SMT, Spoorenberg SMC, Rijkers GT, Grutters JC, Ten Berg JM, Noordzij PG, et al. High-sensitivity cardiac troponin T predicts mortality after hospitalization for community-acquired pneumonia. Respirology. 2017;22(5):1000–6.
Frencken JF, van Baal L, Kappen TH, Donker DW, Horn J, van der Poll T, et al. Myocardial injury in critically ill patients with community-acquired pneumonia. A cohort study. Ann Am Thorac Soc. 2019;16:606–12.
Corrales-Medina VF, Dwivedi G, Taljaard M, Petrcich W, Lima JA, Yende S, et al. Coronary artery calcium before and after hospitalization with pneumonia: the MESA study. PLoS ONE. 2018;13: e0191750.
Kox WJ, Volk T, Kox SN, Volk H-D. Immunomodulatory therapies in sepsis. Intensive Care Med. 2000;26:S124–8.
Waterer G. Community-acquired pneumonia: genomics, epigenomics, transcriptomics, proteomics, and metabolomics. Semin Respir Crit Care Med. 2012;33:257–65.
Kolditz M, Ewig S, Schütte H, Suttorp N, Welte T, Rohde G. Assessment of oxygenation and comorbidities improves outcome prediction in patients with community-acquired pneumonia with a low CRB-65 score. J Intern Med. 2015;278:193–202.
Lim WS, Smith DL, Wise MP, Welham SA. British thoracic society community acquired pneumonia guideline and the NICE pneumonia guideline: how they fit together. Thorax. 2015;70:698–700.
Frenzen FS, Kutschan U, Meiswinkel N, Schulte-Hubbert B, Ewig S, Kolditz M. Admission lactate predicts poor prognosis independently of the CRB/CURB-65 scores in community-acquired pneumonia. Clin Microbiol Infect. 2018;24:306.e1-306.e6.
Kolditz M, Tesch F, Mocke L, Höffken G, Ewig S, Schmitt J. Burden and risk factors of ambulatory or hospitalized CAP: a population based cohort study. Respir Med. 2016;121:32–8.
Singer M, Deutschman CS, Seymour CW, Shankar-Hari M, Annane D, Bauer M, et al. The third international consensus definitions for sepsis and septic shock (sepsis-3). JAMA. 2016;315:801–10.
Kolditz M, Scherag A, Rohde G, Ewig S, Welte T, Pletz M, et al. Comparison of the qSOFA and CRB-65 for risk prediction in patients with community-acquired pneumonia. Intensive Care Med. 2016;42:2108–10.
Jiang J, Yang J, Jin Y, Cao J, Lu Y. Role of qSOFA in predicting mortality of pneumonia. Medicine (Baltimore). 2018;97: e12634.
Antcliffe DB, Burnham KL, Al-Beidh F, Santhakumaran S, Brett SJ, Hinds CJ, et al. Transcriptomic signatures in sepsis and a differential response to steroids. From the VANISH randomized trial. Am J Respir Crit Care Med. 2019;199:980–6.
Delahanty RJ, Alvarez J, Flynn LM, Sherwin RL, Jones SS. Development and evaluation of a machine learning model for the early identification of patients at risk for sepsis. Ann Emerg Med. 2019;73:334–44.
Wadhera RK, Joynt Maddox KE, Wasfy JH, Haneuse S, Shen C, Yeh RW. Association of the hospital readmissions reduction program with mortality among medicare beneficiaries hospitalized for heart failure, acute myocardial infarction, and pneumonia. JAMA. 2018;320:2542–52.
Ewig S, Kolditz M, Pletz MW, Chalmers J. Healthcare-associated pneumonia: is there any reason to continue to utilize this label in 2019? Clin Microbiol Infect. 2019;25:1173–9.
Lee JS, Giesler DL, Gellad WF, Fine MJ. Antibiotic therapy for adults hospitalized with community-acquired pneumonia: a systematic review. JAMA. 2016;315:593–602.
Pakhale S, Mulpuru S, Verheij TJ, Kochen MM, Rohde GG, Bjerre LM. Antibiotics for community-acquired pneumonia in adult outpatients. Cochrane Database Syst Rev. 2014. https://doi.org/10.1002/14651858.CD002109.pub4 .
Schreiber MP, Shorr AF. Inhaled antibiotics for the treatment of pneumonia. Curr Opin Pulm Med. 2019;25:289–93.
Dandachi D, Rodriguez-Barradas MC. Viral pneumonia: etiologies and treatment. J Investig Med. 2018;66:957–65.
Chalmers JD, Crichton ML, Goeminne PC, Cao B, Humbert M, Shteinberg M, et al. Management of hospitalised adults with coronavirus disease 2019 (COVID-19): a European Respiratory Society living guideline. Eur Respir J. 2021;57:2100048.
Graham F. Daily briefing: Pfizer’s COVID pill looks promising. Nature. 2021. https://doi.org/10.1038/d41586-021-03379-5 .
Martin-Loeches I, van Someren GF, Schultz MJ. Bacterial pneumonia as an influenza complication. Curr Opin Infect Dis. 2017;30:201–7.
Deinhardt-Emmer S, Haupt KF, Garcia-Moreno M, Geraci J, Forstner C, Pletz M, et al. Staphylococcus aureus pneumonia: preceding influenza infection paves the way for low-virulent strains. Toxins (Basel). 2019;11:734.
Cawcutt K, Kalil AC. Pneumonia with bacterial and viral coinfection. Curr Opin Crit Care. 2017;23:385–90.
Langford BJ, So M, Raybardhan S, Leung V, Westwood D, MacFadden DR, et al. Bacterial co-infection and secondary infection in patients with COVID-19: a living rapid review and meta-analysis. Clin Microbiol Infect. 2020;26:1622–9.
Bell BG, Schellevis F, Stobberingh E, Goossens H, Pringle M. A systematic review and meta-analysis of the effects of antibiotic consumption on antibiotic resistance. BMC Infect Dis. 2014;14:13.
Yamazaki T, Kenri T. Epidemiology of Mycoplasma pneumoniae infections in Japan and therapeutic strategies for macrolide-resistant M. pneumoniae . Front Microbiol. 2016;7:693.
Peuchant O, Ménard A, Renaudin H, Morozumi M, Ubukata K, Bébéar CM, et al. Increased macrolide resistance of Mycoplasma pneumoniae in France directly detected in clinical specimens by real-time PCR and melting curve analysis. J Antimicrob Chemother. 2009;64:52–8.
Diaz MH, Benitez AJ, Winchell JM. Investigations of Mycoplasma pneumoniae infections in the United States: trends in molecular typing and macrolide resistance from 2006 to 2013. J Clin Microbiol. 2015;53:124–30.
Bassetti M, Welte T, Wunderink RG. Treatment of Gram-negative pneumonia in the critical care setting: is the beta-lactam antibiotic backbone broken beyond repair? Crit Care. 2016;20:1–9.
Nielsen TB, Brass EP, Gilbert DN, Bartlett JG, Spellberg B. Sustainable discovery and development of antibiotics—is a nonprofit approach the future? N Engl J Med. 2019;381:503–5.
Arancibia F, Ruiz M. Risk factors for drug-resistant cap in immunocompetent patients. Curr Infect Dis Rep. 2017;19:11.
Sibila O, Rodrigo-Troyano A, Torres A. Nonantibiotic adjunctive therapies for community-acquired pneumonia (corticosteroids and beyond): where are we with them? Semin Respir Crit Care Med. 2016;37:913–22.
Stefan H, Martin W, Bernd S, Andreas H, Mathias K, Matthias K, et al. Adrenomedullin reduces endothelial hyperpermeability. Circ Res. 2002;91:618–25.
Chalmers JD. Corticosteroids for community-acquired pneumonia: a critical view of the evidence. Eur Respir J. 2016;48:984–6.
Sterne JAC, Murthy S, Diaz JV, Slutsky AS, Villar J, Angus DC, et al. Association between administration of systemic corticosteroids and mortality among critically ill patients with COVID-19. JAMA. 2020;324:1–13.
PubMed Central Google Scholar
COVID-19: management in hospitalized adults—UpToDate. https://www.uptodate.com/contents/covid-19-management-in-hospitalized-adults?search=Interleukin%201%20antagonists%20covid§ionRank=1&usage_type=default&anchor=H3001451751&source=machineLearning&selectedTitle=3~150&display_rank=3#H3001451751 . Accessed 6 Jan 2022.
Marconi VC, Ramanan AV, de Bono S, Kartman CE, Krishnan V, Liao R, et al. Efficacy and safety of baricitinib for the treatment of hospitalised adults with COVID-19 (COV-BARRIER): a randomised, double-blind, parallel-group, placebo-controlled phase 3 trial. Lancet Respir Med. 2021;9:1407–18.
Guimarães PO, Quirk D, Furtado RH, Maia LN, Saraiva JF, Antunes MO, et al. Tofacitinib in patients hospitalized with Covid-19 pneumonia. N Engl J Med. 2021;385:406–15.
Kyriazopoulou E, Poulakou G, Milionis H, Metallidis S, Adamis G, Tsiakos K, et al. Early treatment of COVID-19 with anakinra guided by soluble urokinase plasminogen receptor plasma levels: a double-blind, randomized controlled phase 3 trial. Nat Med. 2021;27:1752–60.
Caricchio R, Abbate A, Gordeev I, Meng J, Hsue PY, Neogi T, et al. Effect of canakinumab vs placebo on survival without invasive mechanical ventilation in patients hospitalized with severe COVID-19: a randomized clinical trial. JAMA. 2021;326:230–9.
Tharaux PL, Pialoux G, Pavot A, Mariette X, Hermine O, Resche-Rigon M, Porcher R, Ravaud P, Bureau S, Dougados M, Tibi A. Effect of anakinra versus usual care in adults in hospital with COVID-19 and mild-to-moderate pneumonia (CORIMUNO-ANA-1): a randomised controlled trial. Lancet Respir Med. 2021;9:295–304.
Aliberti S, Chalmers JD, Pletz MW. Anti-infectives and the lung. 2017. https://doi.org/10.1183/2312508X.erm7517 . Accessed 25 May 2018.
Kanoh S, Rubin BK. Mechanisms of action and clinical application of macrolides as immunomodulatory medications. Clin Microbiol Rev. 2010;23:590–615.
Postma DF, van Werkhoven CH, van Elden LJR, Thijsen SFT, Hoepelman AIM, Kluytmans JAJW, et al. Antibiotic treatment strategies for community-acquired pneumonia in adults. N Engl J Med. 2015;372:1312–23.
Ito A, Ishida T, Tachibana H, Tokumasu H, Yamazaki A, Washio Y. Azithromycin combination therapy for community-acquired pneumonia: propensity score analysis. Sci Rep. 2019;9:1–8.
Okumura J, Shindo Y, Takahashi K, Sano M, Sugino Y, Yagi T, et al. Mortality in patients with community-onset pneumonia at low risk of drug-resistant pathogens: impact of β-lactam plus macrolide combination therapy. Respirology. 2018;23:526–34.
Wunderink RG, Mandell L. Adjunctive therapy in community-acquired pneumonia. Semin Respir Crit Care Med. 2012;33:311–8.
Postma DF, Spitoni C, van Werkhoven CH, van Elden LJR, Oosterheert JJ, Bonten MJM. Cardiac events after macrolides or fluoroquinolones in patients hospitalized for community-acquired pneumonia: post-hoc analysis of a cluster-randomized trial. BMC Infect Dis. 2019;19:1–12.
König R, Cao X, Oswald M, Forstner C, Rohde G, Rupp J, et al. Macrolide combination therapy for hospitalised CAP patients? An individualised approach supported by machine learning. Eur Respir J. 2019;54:1900824.
Welte T, Dellinger RP, Ebelt H, Ferrer M, Opal SM, Singer M, et al. Efficacy and safety of trimodulin, a novel polyclonal antibody preparation, in patients with severe community-acquired pneumonia: a randomized, placebo-controlled, double-blind, multicenter, phase II trial (CIGMA study). Intensive Care Med. 2018;44:438–48.
de la Torre MC, Bolíbar I, Vendrell M, de Gracia J, Vendrell E, Rodrigo MJ, et al. Serum immunoglobulins in the infected and convalescent phases in community-acquired pneumonia. Respir Med. 2013;107:2038–45.
de la Torre MC, Torán P, Serra-Prat M, Palomera E, Güell E, Vendrell E, et al. Serum levels of immunoglobulins and severity of community-acquired pneumonia. BMJ Open Respir Res. 2016;3(1): e000152.
Wienhold S-M, Lienau J, Witzenrath M. Towards inhaled phage therapy in western Europe. Viruses. 2019;11:295.
Dougan M, Nirula A, Azizad M, Mocherla B, Gottlieb RL, Chen P, et al. Bamlanivimab plus etesevimab in mild or moderate Covid-19. N Engl J Med. 2021;385:1382–92.
Group RC, Horby PW, Mafham M, Peto L, Campbell M, Pessoa-Amorim G, et al. Casirivimab and imdevimab in patients admitted to hospital with COVID-19 (RECOVERY): a randomised, controlled, open-label, platform trial. Lancet. 2021. https://doi.org/10.1101/2021.06.15.21258542v1 .
Tessmer A, Welte T, Schmidt-Ott R, Eberle S, Barten G, Suttorp N, et al. Influenza vaccination is associated with reduced severity of community-acquired pneumonia. Eur Respir J. 2011;38:147–53.
Udell JA, Zawi R, Bhatt DL, Keshtkar-Jahromi M, Gaughran F, Phrommintikul A, et al. Association between influenza vaccination and cardiovascular outcomes in high-risk patients: a meta-analysis. JAMA. 2013;310:1711–20.
Chiang M-H, Wu H-H, Shih C-J, Chen Y-T, Kuo S-C, Chen T-L. Association between influenza vaccination and reduced risks of major adverse cardiovascular events in elderly patients. Am Heart J. 2017;193:1–7.
Fröbert O, Götberg M, Erlinge D, Akhtar Z, Christiansen EH, MacIntyre CR, et al. Influenza vaccination after myocardial infarction: a randomized, double-blind, placebo-controlled, multicenter trial circulation. Am Heart Assoc. 2021;144:1476–84.
Pletz MW, Rohde GG, Welte T, Kolditz M, Ott S. Advances in the prevention, management, and treatment of community-acquired pneumonia. Research. 2016. https://doi.org/10.12688/f1000research.7657.1 .
Abbasi J. FLU-v, a universal flu vaccine candidate, advances in trial. JAMA. 2020;323:1336.
PubMed Google Scholar
Pleguezuelos O, Dille J, de Groen S, Oftung F, Niesters HGM, Islam MA, et al. Immunogenicity, safety, and efficacy of a standalone universal influenza vaccine, FLU-v, in healthy adults. Ann Intern Med. 2020;172:453–62.
Moberley S, Holden J, Tatham DP, Andrews RM. Vaccines for preventing pneumococcal infection in adults. Cochrane Database Syst Rev. 2013;2013:CD000422.
Suzuki M, Dhoubhadel BG, Ishifuji T, Yasunami M, Yaegashi M, Asoh N, et al. Serotype-specific effectiveness of 23-valent pneumococcal polysaccharide vaccine against pneumococcal pneumonia in adults aged 65 years or older: a multicentre, prospective, test-negative design study. Lancet Infect Dis. 2017;17:313–21.
French N, Nakiyingi J, Carpenter LM, Lugada E, Watera C, Moi K, et al. 23–valent pneumococcal polysaccharide vaccine in HIV-1-infected Ugandan adults: double-blind, randomised and placebo controlled trial. Lancet. 2000;355:2106–11.
Pilishvili T, Lexau C, Farley MM, Hadler J, Harrison LH, Bennett NM, et al. Sustained reductions in invasive pneumococcal disease in the era of conjugate vaccine. J Infect Dis. 2010;201:32–41.
Makarewicz O, Lucas M, Brandt C, Herrmann L, Albersmeier A, Rückert C, et al. Whole genome sequencing of 39 invasive Streptococcus pneumoniae sequence type 199 isolates revealed switches from serotype 19A to 15B. PLoS ONE. 2017;12: e0169370.
Pletz MW, Ewig S, Rohde G, Schuette H, Rupp J, Welte T, et al. Impact of pneumococcal vaccination in children on serotype distribution in adult community-acquired pneumonia using the serotype-specific multiplex urinary antigen detection assay. Vaccine. 2016;34:2342–8.
Rodrigo C, Bewick T, Sheppard C, Greenwood S, Mckeever TM, Trotter CL, et al. Impact of infant 13-valent pneumococcal conjugate vaccine on serotypes in adult pneumonia. Eur Respir J. 2015;45:1632–41.
Forstner C, Kolditz M, Kesselmeier M, Ewig S, Rohde G, Barten-Neiner G, et al. Pneumococcal conjugate serotype distribution and predominating role of serotype 3 in German adults with community-acquired pneumonia. Vaccine. 2019;38(5):1129–36.
LeBlanc JJ, ElSherif M, Ye L, MacKinnon-Cameron D, Ambrose A, Hatchette TF, et al. Streptococcus pneumoniae serotype 3 is masking PCV13-mediated herd immunity in Canadian adults hospitalized with community acquired pneumonia: a study from the serious outcomes surveillance (SOS) network of the Canadian immunization research network (CIRN). Vaccine. 2019;37:5466–73.
Azarian T, Mitchell PK, Georgieva M, Thompson CM, Ghouila A, Pollard AJ, et al. Global emergence and population dynamics of divergent serotype 3 CC180 pneumococci. PLoS Pathog. 2018;14: e1007438.
Pletz MW, Maus U, Krug N, Welte T, Lode H. Pneumococcal vaccines: mechanism of action, impact on epidemiology and adaption of the species. Int J Antimicrob Agents. 2008;32:199–206.
Pletz M. Sequential versus simultaneous vaccination with pneumococcal conjugate vaccine (prevenar 13) and pneumococcal polysaccharide vaccine (pneumovax 23) in elderly: immunological memory and antibody levels. clinicaltrials.gov; 2021. Report No. NCT02637583. https://clinicaltrials.gov/ct2/show/NCT02637583 .
Green C, Moore CA, Mahajan A, Bajaj K. A simple approach to pneumococcal vaccination in adults. J Glob Infect Dis. 2018;10:159–62.
Oligbu G. Higher valent pneumococcal conjugate vaccines: is it a roller coaster? AIMS Public Health. 2020;7:29–32.
Lagousi T, Basdeki P, Routsias J, Spoulou V. Novel protein-based pneumococcal vaccines: assessing the use of distinct protein fragments instead of full-length proteins as vaccine antigens. Vaccines (Basel). 2019;7:E9.
Green CA, Drysdale SB, Pollard AJ, Sande CJ. Vaccination against respiratory syncytial virus. Vaccines Older Adults Curr Pract Future Oppor. 2020;43:182–92.
Ponnuraj EM, Springer J, Hayward AR, Wilson H, Simoes EAF. Antibody-dependent enhancement, a possible mechanism in augmented pulmonary disease of respiratory syncytial virus in the bonnet monkey model. J Infect Dis. 2003;187:1257–63.
Boyton RJ, Openshaw PJ. Pulmonary defences to acute respiratory infection. Br Med Bull. 2002;61:1–12.
Sun X, Wang T, Cai D, Hu Z, Chen J, Liao H, et al. Cytokine storm intervention in the early stages of COVID-19 pneumonia. Cytokine Growth Factor Rev. 2020;53:38–42.
Crane MJ, Lee KM, FitzGerald ES, Jamieson AM. Surviving deadly lung infections: innate host tolerance mechanisms in the pulmonary system. Front Immunol. 2018;9:1421.
Müller-Redetzky H, Lienau J, Suttorp N, Witzenrath M. Therapeutic strategies in pneumonia: going beyond antibiotics. Eur Respir Rev. 2015;24:516–24.
Smelaya TV, Belopolskaya OB, Smirnova SV, Kuzovlev AN, Moroz VV, Golubev AM, et al. Genetic dissection of host immune response in pneumonia development and progression. Sci Rep. 2016;6:1–12.
Wang X, Guo J, Wang Y, Xiao Y, Wang L, Hua S. Genetic variants of interferon regulatory factor 5 associated with the risk of community-acquired pneumonia. Gene. 2018;679:73–80.
Kloek AT, Brouwer MC, van de Beek D. Host genetic variability and pneumococcal disease: a systematic review and meta-analysis. BMC Med Genom. 2019;12:130.
Reyes LF, Restrepo MI, Hinojosa CA, Soni NJ, Anzueto A, Babu BL, et al. Severe pneumococcal pneumonia causes acute cardiac toxicity and subsequent cardiac remodeling. Am J Respir Crit Care Med. 2017;196:609–20.
Brack MC, Lienau J, Kuebler WM, Witzenrath M. Cardiovascular sequelae of pneumonia. Curr Opin Pulm Med. 2019;25:257–62.
Aliberti S, Ramirez J, Cosentini R, Valenti V, Voza A, Rossi P, et al. Acute myocardial infarction versus other cardiovascular events in community-acquired pneumonia. ERJ Open Res. 2015. https://doi.org/10.1183/23120541.00020-2015 .
Jensen AV, Faurholt-Jepsen D, Egelund GB, Andersen SB, Petersen PT, Benfield T, et al. Undiagnosed diabetes mellitus in community-acquired pneumonia: a prospective cohort study. Clin Infect Dis. 2017;65:2091–8.
Aliberti S, Di Pasquale M, Zanaboni AM, Cosentini R, Brambilla AM, Seghezzi S, et al. Stratifying risk factors for multidrug-resistant pathogens in hospitalized patients coming from the community with pneumonia. Clin Infect Dis. 2012;54:470–8.
Di Pasquale MF, Sotgiu G, Gramegna A, Radovanovic D, Terraneo S, Reyes LF, et al. Prevalence and etiology of community-acquired pneumonia in immunocompromised patients. Clin Infect Dis. 2019;68:1482–93.
Scholl T, Kiser TH, Vondracek SF. Evaluation of systemic corticosteroids in patients with an acute exacerbation of COPD and a diagnosis of pneumonia. Chronic Obstr Pulm Dis. 2018;5:57–65.
PubMed PubMed Central Google Scholar
Zugazagoitia J, Molina-Pinelo S, Lopez-Rios F, Paz-Ares L. Biological therapies in nonsmall cell lung cancer. Eur Respir J. 2017;49:1601520.
Faverio P, Aliberti S, Bellelli G, Suigo G, Lonni S, Pesci A, et al. The management of community-acquired pneumonia in the elderly. Eur J Intern Med. 2014;25:312–9.
Aliberti S, Bellelli G, Belotti M, Morandi A, Messinesi G, Annoni G, et al. Delirium symptoms during hospitalization predict long-term mortality in patients with severe pneumonia. Aging Clin Exp Res. 2015;27:523–31.
Restrepo MI, Reyes LF. Pneumonia as a cardiovascular disease. Respirology. 2018;23:250–9.
Corrales-Medina VF, Musher DM, Shachkina S, Chirinos JA. Acute pneumonia and the cardiovascular system. Lancet. 2013;381:496–505.
Eurich DT, Marrie TJ, Minhas-Sandhu JK, Majumdar SR. Ten-year mortality after community-acquired pneumonia. A prospective cohort. Am J Respir Crit Care Med. 2015;192:597–604.
Eurich DT, Marrie TJ, Minhas-Sandhu JK, Majumdar SR. Risk of heart failure after community acquired pneumonia: prospective controlled study with 10 years of follow-up. BMJ. 2017;356: j413.
Jaw JE, Tsuruta M, Oh Y, Schipilow J, Hirano Y, Ngan DA, et al. Lung exposure to lipopolysaccharide causes atherosclerotic plaque destabilisation. Eur Respir J. 2016;48:205–15.
Corrales-Medina VF, Alvarez KN, Weissfeld LA, Angus DC, Chirinos JA, Chang C-CH, et al. Association between hospitalization for pneumonia and subsequent risk of cardiovascular disease. JAMA. 2015;313:264–74.
Dela Cruz CS, Wunderink RG, Christiani DC, Cormier SA, Crothers K, Doerschuk CM, et al. Future research directions in pneumonia. NHLBI working group report. Am J Respir Crit Care Med. 2018;198:256–63.
Download references
Acknowledgements
CAPNETZ is a multidisciplinary approach to better understand and treat patients with community-acquired pneumonia. The network has only been made possible by the contribution of many investigators. We are especially indebted to the work of the investigators in the local clinical centres (LCC) that established and maintained contact with all practitioners, physicians, and respiratory specialists cooperating within the network. The members of the CAPNETZ study group are as follows:
M. Dreher, C. Cornelissen (Aachen); W. Knüppel (Bad Arolsen); D. Stolz (Basel, Switzerland); N. Suttorp, M. Niebank, A. Mikolajewska, M. Witzenrath, W. Pankow, S. Gläser, D. Thiemig (Berlin); M. Prediger, S. Schmager (Cottbus); M. Kolditz, B. Schulte-Hubbert, S. Langner (Dresden); G. Rohde, C. Bellinghausen (Frankfurt); M. Panning (Freiburg); C. Hoffmann (Hamburg); T. Welte, J. Freise, G. Barten, W. Kröner, M. Nawrocki, J. Naim, T. Illig, N. Klopp (Hannover); M. Pletz, C. Kroegel, B. Schleenvoigt, C. Forstner, A. Moeser (Jena); D. Drömann, P. Parschke, K. Franzen, J. Rupp, N. Käding (Lübeck); M. Wouters, K. Walraven, D. Braeken (Maastricht, The Netherlands); C. Spinner (Munich); A. Zaruchas (Paderborn); Schaberg, D. Heigener, I. Hering (Rotenburg/Wümme); W. Albrich, F. Waldeck, F. Rassouli, S. Baldesberger (St. Gallen, Switzerland); S. Stenger, M. Wallner (Ulm); H. Burgmann, L. Traby (Vienna); and all study nurses.
We sincerely thank the international CAP experts for their important and invaluable feedback. Without their contribution, this work would not have been possible.
Open Access funding enabled and organized by Projekt DEAL. CAPNETZ was funded by a German Federal Ministry of Education and Research grant (01KI07145) 2001–2011 and is supported by the German Center for Lung Research (DZL).
Author information
Mathias W. Pletz and Andreas Vestergaard Jensen have contributed equally to this publication
Authors and Affiliations
Institute of Infectious Diseases and Infection Control, Jena University Hospital - Friedrich Schiller University Jena, Jena, Germany
Mathias W. Pletz & Christina Bahrs
CAPNETZ STIFTUNG, Hannover, Germany
Mathias W. Pletz, Jan Rupp, Martin Witzenrath, Grit Barten-Neiner & Gernot Rohde
Department of Pulmonary and Infectious Diseases, Nordsjællands Hospital, Hillerød, Denmark
Andreas Vestergaard Jensen
Division of Infectious Diseases and Tropical Medicine, Department of Medicine I, Medical University of Vienna, Vienna, Austria
Christina Bahrs
Department of Infectious Diseases and Microbiology, University Hospital Schleswig-Holstein, German Center for Infection Research (DZIF), Partner Site Hamburg-Lübeck-Borstel-Riems, Lübeck, Germany
Department of Infectious Diseases and Respiratory Medicine, Charité - Universitätsmedizin Berlin, Berlin, Germany
Martin Witzenrath & Norbert Suttorp
Biomedical Research in Endstage and Obstructive Lung Disease Hannover (BREATH), German Center for Lung Research (DZL), Hannover, Germany
Claudia Davenport, Grit Barten-Neiner, Sabine Dettmer & Gernot Rohde
Division of Pulmonology, Medical Department I, University Hospital Carl Gustav Carus, Dresden, Germany
Martin Kolditz
Department of Diagnostic and Interventional Radiology, Hannover Medical School, Hannover, Germany
Sabine Dettmer
Scottish Centre for Respiratory Research, Ninewells Hospital and Medical School, University of Dundee, Dundee, DD1 9SY, UK
James D. Chalmers
Clinic of Respiratory Medicine and Pulmonary Cell Research, University Hospital, Basel, Switzerland
Daiana Stolz
Department of Pneumology, University Medical Center, Freiburg, Germany
Martin Witzenrath & Daiana Stolz
German Center for Lung Research (DZL), Berlin, Germany
Norbert Suttorp
Department of Biomedical Sciences, Humanitas University, Via Rita Levi Montalcini 4, 20072, Pieve Emanuele, Milan, Italy
Stefano Aliberti
IRCCS Humanitas Research Hospital, Respiratory Unit, Via Manzoni 56, 20089, Rozzano, Milan, Italy
Institute of Physiology, Charité – Universitätsmedizin Berlin, Berlin, Germany
Wolfgang M. Kuebler
Department of Respiratory Medicine, Medical Clinic I, University hospital, Goethe University Frankfurt, Theodor-Stern-Kai 7, 60596, Frankfurt/Main, Germany
Gernot Rohde
You can also search for this author in PubMed Google Scholar
Contributions
GBN, as part of CAPNETZ, was responsible for organizing the meeting. AVJ was responsible for grouping the views from the invited researchers into headlines for discussion at the scientific retreat. CD took minutes during the scientific retreat which was used for drafting the individual sections. The individual sections were then drafted by one of the participants of the scientific retreat as follows; Detection of causative pathogens (JR), Next generation sequencing for guidance of antimicrobial treatment (JDC), Imaging diagnostics (SD), Biomarkers (DS). Risk stratification (MK), Antiviral and antibiotic treatment (GR), Adjunctive therapy (NS), Vaccines and prevention (MWP, CB), Systemic and local immune response (MW), Comorbidities (SA), Long-term cardio-vascular complications (WMK). AVJ and MWP reviewed the individual sections and prepared the first draft. All authors reviewed the draft manuscript. All authors were responsible for the decision to publish the manuscript. All authors read and approved the final manuscript.
Corresponding author
Correspondence to Gernot Rohde .
Ethics declarations
Ethics approval and consent to participate, consent for publication, competing interests.
The authors declare no conflicts of interests relevant to the content of the article.
Additional information
Publisher's note.
Springer Nature remains neutral with regard to jurisdictional claims in published maps and institutional affiliations.
Rights and permissions
Open Access This article is licensed under a Creative Commons Attribution 4.0 International License, which permits use, sharing, adaptation, distribution and reproduction in any medium or format, as long as you give appropriate credit to the original author(s) and the source, provide a link to the Creative Commons licence, and indicate if changes were made. The images or other third party material in this article are included in the article's Creative Commons licence, unless indicated otherwise in a credit line to the material. If material is not included in the article's Creative Commons licence and your intended use is not permitted by statutory regulation or exceeds the permitted use, you will need to obtain permission directly from the copyright holder. To view a copy of this licence, visit http://creativecommons.org/licenses/by/4.0/ . The Creative Commons Public Domain Dedication waiver ( http://creativecommons.org/publicdomain/zero/1.0/ ) applies to the data made available in this article, unless otherwise stated in a credit line to the data.
Reprints and permissions
About this article
Cite this article.
Pletz, M.W., Jensen, A.V., Bahrs, C. et al. Unmet needs in pneumonia research: a comprehensive approach by the CAPNETZ study group. Respir Res 23 , 239 (2022). https://doi.org/10.1186/s12931-022-02117-3
Download citation
Received : 20 January 2022
Accepted : 15 July 2022
Published : 10 September 2022
DOI : https://doi.org/10.1186/s12931-022-02117-3
Share this article
Anyone you share the following link with will be able to read this content:
Sorry, a shareable link is not currently available for this article.
Provided by the Springer Nature SharedIt content-sharing initiative
- CAP pathogens
- Next generation sequencing
- Pneumococcal vaccines
- Universal influenza vaccine
- Translational research
- Personalized medicine
- Immune-compromised host
- Machine learning
- The “omics” approach
Respiratory Research
ISSN: 1465-993X
- General enquiries: [email protected]
Featured article: Innate immune responses in pneumonia
Read the article
Which children still die from pneumonia
Edited by: Grant Austin Mackenzie: Medical Research Council, Gambia Submission Status: Open until 30 June 2024
- Most accessed
Sex-dependent vulnerability for Wistar rats model following intranasal instillation with Klebsiella pneumoniae ATCC 43816 causing lobar pneumonia
Authors: Patrick Hervé Diboue Betote, Esther Del Florence Ndedi Moni, Sonia Raïssa Gayap Matchuenkam, Sandrine Suzanne Bayengue Beack, Rodrigue Fifen, Raogo Ouedraogo, Gabriel A. Agbor, Rasmané Semde, Nga Nnanga and Maximilienne Ascension Nyegue
Combination of a multiplex pneumonia panel and Gram staining for antimicrobial selection to treat lower respiratory tract infection
Authors: Hiroshi Matsuura, Koudai Arimoto, Yoshihito Takahashi and Masafumi Kishimoto
Safety and efficacy of favipiravir in COVID-19 patients with pneumonia. A randomized, double-blind, placebo-controlled study (FAVID)
Authors: Juan P. Horcajada, Rebeca Aldonza, Mónica Real, Silvia Castañeda-Espinosa, Elena Sendra, Joan Gomez-Junyent, Inmaculada López-Montesinos, Silvia Gómez-Zorrilla, Silvia Briansó, Montserrat Duran-Taberna, Andrés Fernández, Cristina Tarragó and Teresa Auguet-Quintillá
The effectiveness of glucocorticoid treatment in post-COVID-19 pulmonary involvement
Authors: Jan Mizera, Samuel Genzor, Milan Sova, Ladislav Stanke, Radim Burget, Petr Jakubec, Martin Vykopal, Pavol Pobeha and Jana Zapletalová
Serum soluble toll-like receptor 4 and risk for clinical severity in COVID-19 patients
Authors: Maha E. Houssen, Marwa O. Elmaria, Dina Badr, Rasha El-Mahdy, Mayada A. Ghannam, Shaimaa El-Ashwah, May Denewer and Metwaly Ibrahim Mortada
Most recent articles RSS
View all articles
Long-term effects of pneumonia in young children
Authors: Keith Grimwood and Anne B. Chang
The role of co-infections and secondary infections in patients with COVID-19
Authors: Charles Feldman and Ronald Anderson
The definition and classification of pneumonia
Authors: Grant Mackenzie
The diagnosis of pneumonia requires a chest radiograph (x-ray)—yes, no or sometimes?
Authors: Dan Wootton and Charles Feldman
The radiological diagnosis of pneumonia in children
Authors: Kerry-Ann F. O’Grady, Paul J. Torzillo, Kieran Frawley and Anne B. Chang
Most accessed articles RSS
Sign up to receive article alerts
Pneumonia is published continuously online-only. We encourage you to sign up to receive free email alerts to keep up to date with all of the latest articles by registering here .
Aims and scope
Pneumonia is the only journal to focus exclusively on pneumonia. Publishing original research, case reports, reviews, commentaries and correspondence, Pneumonia provides an international forum for the exchange of knowledge by scientists and clinicians involved in studying the etiology and pathogenesis of pneumonia, as well as its diagnosis, epidemiology, treatment and prevention. The journal's scope extends to research on lung infections and diagnosis, inflammation and immunity, microbial pathogenesis and viral-bacterial interactions.
Cutting-edge research, insightful reviews and dedication to the community make Pneumonia an essential resource for clinicians, researchers, respirologists and allied professionals involved with infectious diseases.
Pneumonia archive
Pneumonia transferred to BioMed Central from Griffith University ePress in January 2016. The archive of the journal has been transferred to a new platform and all articles previously published in the journal can be accessed here .
Latest Tweets
Your browser needs to have JavaScript enabled to view this timeline
Editors' profiles
Giovanni sotgiu.
- Editorial Board
- Manuscript editing services
- Instructions for Editors
- Sign up for article alerts and news from this journal
- Follow us on Twitter
Annual Journal Metrics
2022 Citation Impact 6.8 - 2-year Impact Factor 5.4 - 5-year Impact Factor
2023 Speed 13 days submission to first editorial decision for all manuscripts (Median) 122 days submission to accept (Median)
2023 Usage 363,579 downloads 274 Altmetric mentions
- More about our metrics
ISSN: 2200-6133
- General enquiries: [email protected]
Click through the PLOS taxonomy to find articles in your field.
For more information about PLOS Subject Areas, click here .
Loading metrics
Open Access
Peer-reviewed
Research Article
Improved treatment of community-acquired pneumonia through tailored interventions: Results from a controlled, multicentre quality improvement project
Roles Conceptualization, Data curation, Formal analysis, Project administration, Supervision, Writing – original draft
* E-mail: [email protected]
Affiliation Department of Internal Medicine, Section for Pulmonary Diseases, Herlev Gentofte Hospital, Hellerup, Denmark

Roles Conceptualization, Methodology, Supervision, Validation, Writing – review & editing
Affiliations Unisanté, Lausanne, Switzerland, Department of Clinical Research, University of Southern Denmark, Odense, Denmark
Roles Data curation, Formal analysis, Methodology, Software, Supervision, Validation, Writing – review & editing
Affiliation Centre of Diagnostic Investigation, Rigshospitalet, Copenhagen, Denmark
Roles Conceptualization, Supervision, Writing – review & editing
Affiliation Department of Infectious Diseases, Amager Hvidovre Hospital, Hvidovre, Denmark
Affiliation Diagnostic Centre, Silkeborg Regional Hospital, Silkeborg, Denmark
Roles Conceptualization, Data curation, Investigation, Project administration, Writing – review & editing
Affiliation Department of Respiratory and Infectious Diseases, Nordsjaellands Hospital, Hillerød, Denmark
Roles Resources, Supervision, Writing – review & editing
Roles Data curation, Investigation, Writing – review & editing
Roles Data curation, Investigation, Software, Writing – review & editing
Roles Conceptualization, Funding acquisition, Project administration, Supervision, Writing – original draft, Writing – review & editing
Affiliation Department of Internal Medicine, Section for Infectious Diseases, Herlev Gentofte Hospital, Hellerup, Denmark
- Markus Fally,
- Christian von Plessen,
- Jacob Anhøj,
- Thomas Benfield,
- Britta Tarp,
- Lise Notander Clausen,
- Lilian Kolte,
- Emma Diernaes,
- Line Molzen,
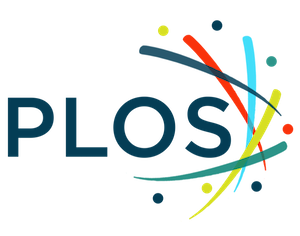
- Published: June 11, 2020
- https://doi.org/10.1371/journal.pone.0234308
- Reader Comments
Community-acquired pneumonia (CAP) is one of the leading causes of healthcare utilisation and death worldwide. Treatment according to evidence-based clinical guidelines can reduce mortality, antibiotic exposure and length of hospital stay related to CAP.
Local problem
Several studies, including a pilot study from one of our sites, indicate that physicians show a low grade of guideline adherence when managing patients with CAP.
To improve the guideline-based treatment of patients with CAP admitted to hospital, we designed a quality improvement study. Four process indicators were combined in a CAP care bundle: chest X-ray, CURB-65 severity score, lower respiratory tract samples and antibiotics within 8 hours from admission. After a 4-month baseline period, we applied multiple interventions at three hospitals during 8 months. Progression in our process indicators was measured continuously and compared with a control site without interventions. After the 8-month intervention period, we continued with a 4-month follow-up period to assess the sustainability of the improvements.
The care bundle utilisation rate within 8 hours increased from 11% at baseline to 41% in the follow-up period at the intervention sites, whereas it remained below 3% at the control site. The most considerable improvements have been observed regarding documentation of CURB-65 (34% at baseline, 68% at follow-up) and the collection of lower respiratory tract samples (43% at baseline, 63% at follow-up).
Our study has demonstrated poor adherence to CAP guidelines at all sites at baseline. After implementing multiple tailored interventions, guideline adherence increased substantially. In conclusion, we recommend that CAP guidelines should be actively adapted in order to be followed in a daily routine.
Citation: Fally M, von Plessen C, Anhøj J, Benfield T, Tarp B, Clausen LN, et al. (2020) Improved treatment of community-acquired pneumonia through tailored interventions: Results from a controlled, multicentre quality improvement project. PLoS ONE 15(6): e0234308. https://doi.org/10.1371/journal.pone.0234308
Editor: Vineet Gupta, University of California San Diego, UNITED STATES
Received: November 20, 2019; Accepted: May 24, 2020; Published: June 11, 2020
Copyright: © 2020 Fally et al. This is an open access article distributed under the terms of the Creative Commons Attribution License , which permits unrestricted use, distribution, and reproduction in any medium, provided the original author and source are credited.
Data Availability: All anonymized data as well as the R code to reproduce the figures and numbers used in the manuscript are included in the Supporting Information.
Funding: The study was funded by The Danish Ministry of Health and by the participating hospitals. The funders had no role in study design, data collection and analysis, decision to publish, or preparation of the manuscript.
Competing interests: The authors have declared that no competing interests exist.
Introduction
Community-acquired pneumonia (CAP) is a common disease and causes significant morbidity and mortality, particularly among the elderly and patients with comorbidity [ 1 ]. In Denmark, CAP accounts for approximately 45.000 hospitalisations annually, with a 30-day mortality of 10–15% [ 2 , 3 ].
In recent decades, professional societies have developed evidence-based guidelines to assist clinicians in treating patients with CAP [ 4 – 7 ]. Adherence to these guidelines can reduce antibiotic exposure, length of stay, mortality and health care costs without negatively affecting patient outcomes [ 8 – 11 ].
Generally, adherence to guidelines is highly variable and differs depending on the local context, disease and outcome of interest [ 12 – 14 ]. In line with these findings, unpublished data from a pilot study at a Danish regional hospital indicated low adherence to CAP guidelines, including the infrequent collection of microbiological samples and delayed and protracted use of antibiotics (Ravn et al., personal communication). Such unsatisfactory adherence to CAP guidelines can be attributed to numerous factors, including physicians’ knowledge, beliefs and preferences, and inefficient health care processes, as well as the heterogeneity of the manifestations of CAP [ 15 ].
One strategy to increase evidence-based patient management is the implementation of care bundles [ 16 ]. A systematic review on the general effect of care bundles concluded that they may reduce the risk of negative outcomes; however, the quality of evidence was very low [ 17 ].
The care bundle approach has been successful in the prevention of ventilator-associated pneumonia (VAP), reducing VAP incidence, length of stay and mortality [ 17 – 22 ]. Few studies have explored the effect of care bundles on the quality of care for CAP and those were not conclusive. One recently published study did not identify any outcome benefits of implementing a care bundle for CAP patients [ 23 ]. However, this may be due to the study’s small size and the inclusion of heterogeneous and controversial elements of CAP care, such as corticosteroid treatment [ 23 ]. Two larger studies with 2819 [ 24 ] and 23315 [ 25 ] patients, respectively, reported that the implementation of a CAP care bundle led to a higher proportion of patients receiving antibiotics within 4 hours as well as a reduction in mortality rate.
To enhance patient care in CAP, our project aimed to increase adherence to a care bundle at three clinical sites in Denmark. A fourth site served as a control site without any interventions.
Project design and local context
The Optimising Treatment of Community-Acquired Pneumonia (optiCAP) project was designed as a 16-month, prospective, open, interventional, controlled, multicentre quality improvement study, evaluating a change programme by applying statistical process control (SPC) [ 26 ].
The study was conducted at four regional hospitals in Denmark: Nordsjaellands Hospital (Site 1), Gentofte Hospital (Site 2), Silkeborg Regional Hospital (Site 3) and Hvidovre Hospital (Site 4). At each hospital, emergency departments (EDs), as well as departments of respiratory medicine and infectious diseases, served as study sites. S1 Fig in the S1 File presents an overview of the study sites, with further details available in S1 File .
Based on a baseline audit (November 2017 until February 2018), interventions were scheduled to take place from March until October 2018 at Sites 1–3. This included both technical and non-technical interventions based on the model for improvement, including Plan-Do-Study-Act (PDSA) cycles [ 27 ]. Site 4 served as control site without any interventions, and thus without deliberate attempts to improve the quality of CAP care.
During a follow-up period from November 2018 until February 2019, we evaluated the sustainability of the improvements.
Study population
The present study included adult patients admitted to our study sites (age ≥ 18 years) with CAP, treated with antibiotics. The diagnostic criteria used to define CAP are generally very heterogeneous [ 28 ]. In our study, we used one of the common definitions. Hence, CAP was defined by the presence of a new infiltrate on chest X-ray and at least one of the following signs and symptoms: cough, sputum production, dyspnoea, core body temperature >38.0°C and auscultatory findings of rales.
Exclusion criteria were hospital admission during the last 28 days, active tuberculosis and immunosuppression. Patients were classified as immunosuppressed if they had received treatment with corticosteroids (≥20 mg prednisolone-equivalent/day > 14 days), were HIV positive, had received chemotherapy during the last 28 days, had neutropenia (< 1000/μl), were an organ transplant recipient or received biological response modifier therapy.
Based on current evidence, patients admitted with CAP should be diagnosed and receive antibiotic treatment within 8 hours from admission, as this reduces mortality [ 29 ]. Furthermore, the collection of lower respiratory tract samples is recommended for all patients admitted with CAP in Denmark. As the Scandinavian countries continue to use narrow-spectrum antibiotics for most CAP patients, the microbiological results may eventually be used for altering the antibiotic therapy [ 4 , 30 , 31 ]. In Denmark, as in other countries, the CURB-65 score is used to assess CAP severity and ultimately to determine which type of empiric antibiotic to prescribe [ 4 – 6 ].
Based on these facts, we defined the following key indicators of adequate care for CAP:
- Chest X-ray
- Collection of lower respiratory tract samples (LRTS)
- Documentation of mortality risk assessment by CURB-65 score (confusion, plasma urea, respiration frequency, blood pressure, age 65 or older) [ 32 ]
- Administration of an antibiotic
- Outcome measure [ 27 ]: Care-bundle treatment, i.e. proportion of patients who received all four elements as described in the previous point
Data collection
At each site, data were collected weekly by auditing electronic health records. An audit of 5 to 10 health records per site per week was considered appropriate, and these numbers could be reached by including all CAP patients at out study sites [ 27 ]. Local teams collected the data, which were transferred and stored in Research Electronic Data Capture (REDCap, version 9.3) software [ 33 ]. Raw data were subsequently cleansed using tidyverse (version 1.2.1) for R (version 3.6.0, R Core Team 2019). This implicated the detection and correction of missing values, negative values and outliers (values below the first and above the third quartile).
Baseline results and theory of change
Baseline data indicated variation in performance at the different sites, but also room for improvement regarding all of the defined process measures. Median time (interquartile range [IQR]) to chest X-ray was 2.3 (1.2–4.5) hours, corresponding to a completion rate of 88.7% within 8 hours. LRTS were collected in 60.6% of cases; the completion rate was 39.6% within 8 hours. CURB-65 score was documented in 31.2% of cases at a completion rate of 26.9% within 8 hours. The median time to administration of the first antibiotic (IQR) was 5.2 (3.4–8.0) hours, corresponding to a completion rate of 75.1% within 8 hours. The complete care bundle was delivered within 8 hours in 7.1% of cases.
Non-adherence to most of the given recommendations was an issue at all sites. To develop solutions and improve adherence to CAP guidelines, our team defined the following explanatory theories over the course of several seminars and meetings [ 34 ]:
- Lack of information regarding the disease as well as how and why to manage it as recommended by the guidelines
- Lack of skills regarding acquisition of LRTS
- Ineffective processes and unclear responsibilities
- Lack of effective electronic health record system (EHRS) tools to guide clinicians
Through the following approaches, we expected that we could reach our aim of achieving sustainable changes [ 34 ]:
- Provide more and easily accessible information on the disease, current guidelines and evidence-based practices, clearly explaining why they should be applied
- Provide feedback to clinicians when care delivery problems are discovered during the audit process
- Change ineffective processes using an integrative approach (feedback from clinicians)
- Streamline information and guideline content
The drivers and detailed change ideas are provided in the driver diagram in Fig 1 .
- PPT PowerPoint slide
- PNG larger image
- TIFF original image
CAP: community-acquired pneumonia, CURB-65: pneumonia mortality risk score (confusion, urea, respiration frequency, blood pressure, age 65 or older) [ 32 ], MD: medical doctors.
https://doi.org/10.1371/journal.pone.0234308.g001
Interventions
The interventions included both non-technical and technical activities ( Table 1 ), which were derived after a baseline workflow assessment using optiCAP team members as observers. Furthermore, staff members were interviewed to identify limitations of the use of CAP guidelines and to gather suggestions for improvement.
https://doi.org/10.1371/journal.pone.0234308.t001
The technical interventions were designed to increase the amount of LRTS and consisted of hands-on training for physicians and nurses. Training in tracheal suction consisted of repeated sessions 10–20 minutes in duration with 5–15 participants. A senior physician explained and performed the procedure on a dummy. The training was offered to physicians (Sites 2 and 3) and nurses (Sites 1 and 3). After these sessions, participants were encouraged to continue with bed side training under the supervision of more experienced colleagues. At Site 2, an experienced senior nurse designed and implemented hands-on training in sputum induction, which consisted of a 5-10-minute theoretical introduction followed by bed-side training for 1–3 nurses per session.
Regarding the non-technical interventions, we divided the activities into (1) educational activities, (2) the design and distribution of educational material and (3) improvement of CAP patient flow.
The education of nurses and physicians involved repeated presentations lasting 15–45 minutes. Presentation topics included CAP pathogenesis, epidemiology, and guideline-based assessment and treatment. Later, during the intervention period, the results of the ongoing audit were presented and discussed. These educational sessions were held by an optiCAP team member, often as a part of the morning report or other regular teaching sessions involving 10–40 participants. Additionally, physicians received both confirming and corrective feedback from the study team during the health record audit. Feedback was always provided personally, either face-to-face, via secure work email or via the message system in the electronic health record system.
Educational material on the most important aspects of CAP included slide presentations for education sessions, newsletters to all physicians, and posters in all staff offices of the departments involved in the project, as well as pocket cards related to CAP. This material provided information on important steps in the assessment and treatment of CAP. The newsletters and presentations also included audit results and figures on the overall progress of the project.
Process changes involved authorising nurses to order X-rays and LRTS based on triage information at the ED, as well as an agreement with the Department of Clinical Microbiology to perform both standard microscopy, culture and sensitivity analysis (MCS) along with PCR for atypical bacteria from a single LRTS. Previously, this testing required two samples, and physicians often had to choose which test to perform. Furthermore, we developed several tools for the electronic health record systems (EHRS; Epic EMR and MidtEPJ) to assist physicians in treating CAP patients. These tools offered standard phrases for CURB-65 documentation as well as order sets consisting of guideline-based tests and antibiotic treatment packages.
Study of interventions and data analysis
For effect evaluation, we produced run charts with qicharts2 (version 0.6.0) for R [ 35 ]. Run charts are point-and-line graphs used to distinguish random from special cause (non-random) variation in time series data. The R code to reproduce the run charts and the final database are attached in the Supporting Information. For interpretation, we applied the Anhoej rules with the median as process centre [ 36 ]. The Anhoej rules are two tests for special cause variation: (1) unusually long runs of consecutive data points on the same side of the centre line, and (2) unusually few crossings of the centre line. Critical values for run length and number of crossings depend on the number of available data points and may either be calculated or found in statistical tables [ 36 – 38 ]. For example, in a run chart with 24–26 data points, a run of more than 8 data points or fewer than 8 crossings would indicate the presence of special cause variation. In the initial analysis, we used the median from the baseline period, including data from 12 ten-day periods each representing 12–23 patients at the control centre and 31–48 at the intervention sites as reference. With 49 data points in total, special cause variation was declared if any run exceeded 9 data points in length or if the curve crossed the centre line fewer than 19 times. Next, we recalculated the process centre (medians) for four periods: the baseline period, early intervention period (March 2018 to July 2018), late intervention period (August 2018 to October 2018) and the follow-up period.
Ethical considerations
The presented project was designed as a clinical audit and quality improvement project without direct patient contact. Therefore, the national authorities determined that ethical approval was not required to conduct this project. Local approval was granted by the respective hospital boards. Data for analysis were anonymized and handled according to the national regulations of the Danish Data Protection Agency (registration number HGH-2017-039).
Patient characteristics
In total, we audited health records of 2015 patients with CAP who were admitted at the study sites ( Table 2 ). The cohort had a median age of 75 years and a balanced gender distribution. Chronic obstructive pulmonary disease (COPD) was the most common respiratory comorbidity among 30% of patients, followed by asthma in 9%, interstitial lung disease in 3%, lung cancer in 2% and bronchiectasis in 2%. Over half of all patients were former or current smokers; only 18% were never smokers. Smoking history was not documented in nearly one-third of the patient files.
https://doi.org/10.1371/journal.pone.0234308.t002
More than 50% of all patients had a CURB-65 score of 0 or 1, corresponding to a very low risk of pneumonia-related mortality [ 32 ]. Overall in-hospital mortality was 7% (range 5–8%). There were no statistically significant demographical differences between the sites.
CAP care bundle utilisation over time
Since the beginning of the intervention period in March 2018, we noted a steady increase in care bundle utilisation, with special cause variation caused by a sustained shift at all the intervention sites ( Fig 2 , S2.1 and S2.2 Figs in S1 File ) At the control site, we only observed random variation.
Each dot represents 12–48 cases of CAP. The vertical, grey, dashed line marks the beginning of the intervention period. The vertical, black, solid line denotes the beginning of the follow-up period. The process centre (horizontal line representing the median) was frozen after the baseline period. Special cause variation can be identified by a red, dashed process centre (sustained shift) [ 36 ]. See S2.1 Fig in S1 File for run charts for the individual intervention sites along with information on the timing of our interventions.
https://doi.org/10.1371/journal.pone.0234308.g002
In the follow-up period, the care bundle was completed in 41% of cases as compared to 11% at baseline at the intervention sites, indicating sustained improvements (S2.2 Fig in S1 File ). Meanwhile, the completion rate remained below 3% at the control site.
The detailed, site-specific run charts including information on the implementation schedule are presented in S2.1 Fig in S1 File .
Utilisation of the individual elements of the care bundle over time
At the intervention sites, we observed special cause variation for all the elements of the care bundle: chest X-ray, LRTS, CURB-65 score and antibiotics within 8 hours from admission ( Fig 3 ). At the control site, we saw random variation without substantial improvements regarding all bundle elements throughout the study period.
Each dot represents 12–48 cases of CAP. The vertical, grey, dashed line denotes the beginning of the intervention period. The vertical, black, solid line marks the beginning of the follow-up period. The process centre (horizontal line representing the median) is frozen after the baseline period. Special cause variation can be identified by a red, dashed process centre (sustained shift) [ 36 ]. See S3.1–3.8 Figs in S1 File for run charts for the individual intervention sites along with information on the timing of our interventions.
https://doi.org/10.1371/journal.pone.0234308.g003
Utilisation of chest X-ray within 8 hours.
In general, all sites had a high utilisation rate of chest X-ray within 8 hours (S3.2 Fig in S1 File ). While we observed a more stable process regarding the acquisition of X-rays within 8 hours at Site 3 during the baseline period, the other sites displayed more pronounced variation (S3.1 Fig in S1 File ). At the intervention sites, we detected special cause variation after the end of the baseline period due to a sustained moderate shift, both in the run charts for the individual sites and the combined chart. Site 2 exhibited a more stable process towards 100% after authorizing nurses to order chest X-rays, the only intervention specifically targeting this element of the care bundle (S3.1 Fig in S1 File ).
Acquisition of LRTS within 8 hours.
Regarding the acquisition of LRTS, we observed special cause variation with an increase in LRTI samples taken within 8 hours at the intervention sites after baseline ( Fig 3 ). The proportion of patients from whom LRTS were taken fluctuated and seemed to increase almost every time after performing the hands-on training in tracheal suction (S3.3 Fig in S1 File ). The effect lasted about one month at the larger intervention sites (Sites 1 and 2). The proportion of LRTS taken improved from 43% in the baseline to 63% in the follow-up period at the intervention centres, whereas it decreased from 40% to 30% at the control centre (S3.4 Fig in S1 File ).
Documentation of CURB-65 within 8 hours.
The documentation of CURB-65 at baseline was generally low but highly variable amongst the study sites, ranging from 14% at Site 4 to 60% at Site 3. During the intervention period, a steady improvement in CURB-65 utilisation was observed at the intervention sites, whereas random variation continued at the control site ( Fig 3 ). The most distinct improvements were seen at Sites 1 and 2 after the implementation of a CURB-65 tool for the EHRS (S3.5 Fig in S1 File ). The documentation of CURB-65 increased from 34% in the baseline to 68% in the follow-up period at the intervention centres, while remaining stable at 14% at the control centre (S3.6 Fig in S1 File ).
Administration of antibiotics within 8 hours.
Regarding the final element of the care bundle—the administration of antibiotics within 8 hours from admission—we observed a high utilisation rate at all centres of 65% to 85% at baseline (S3.7 Fig in S1 File ). Improvements with special cause variation after baseline were once again seen at the intervention sites, whereas random variation continued at the control site ( Fig 3 ). The improvements were mainly driven by a moderate sustained shift at the larger intervention sites (Site 1 and 2; S3.7 Fig in S1 File ).
Main results
Our project aimed to improve patient care by increasing adherence to CAP guidelines through the implementation of tailored interventions. Throughout the study, changes in key process measures following the interventions were subsequently assessed and summarised in a CAP care bundle. Overall, we achieved sustained improvement in the use of the bundle comprised of chest X-ray, CURB-65, LRTS and antibiotics administered within 8 hours from hospital admission.
At baseline, completion rates for all defined indicators varied, and the study sites faced different challenges regarding the management of CAP patients. This information highlights the necessity of focusing on the local challenges when designing improvement studies, and to tailor the interventions to these challenges [ 27 ].
Only approximately one-third of all patients were scored with CURB-65 or had an LRTS taken within 8 hours from admission at the hospital. Antibiotics were administered within the recommended 8 hours in 75% of all cases. Chest X-ray within 8 hours was the only component that reached a completion rate of 89% at baseline.
Throughout the intervention period, we observed significant improvements with special cause variation at the intervention sites, especially regarding the documentation of CURB-65, but also in the acquisition of LRTS and, to a lesser degree, chest X-rays and antibiotic administration within 8 hours. The last two measures were those with the highest completion rates at the start of the study. Thus, it seems that the improvement potential was higher for the other two elements of the care bundle.
Our outcome measure was an all-or-none indicator, combining the key process measures in a care bundle [ 16 ]. Thus, the system we studied reflected the effectiveness of the health care system, as opposed to patient outcomes [ 27 ]. We chose this approach based on evidence from larger clinical studies clearly documenting that guideline-based treatment, also with the application of care bundles, improves outcomes in CAP [ 24 , 25 , 29 , 39 – 41 ].
Care bundle completion rates within 8 hours increased considerably at our intervention sites from 11% at baseline to 41% in the follow-up period.
The finding that only 41% of all CAP patients received assessments and treatments conforming to established guidelines might seem concerning. However, given that the care bundle relies on four independent elements, we believe that this result still is remarkable when compared to other studies on CAP care bundles reporting compliance rates of 20–29% [ 16 , 23 , 42 ]. LRTS collection can be challenging to improve in CAP, but it is regarded as crucial in the Nordics for potentially altering the often narrow-spectred empiric antibiotic treatment [ 4 , 30 , 31 , 43 ]. Omitting this element of the care bundle from our analysis yielded a completion rate of over 70%.
We used a range of interventions during the intervention period, all of which likely contributed to the observed improvements. We believe that the repeated educational activities with reiterations every 1–2 months were crucial for increasing and sustaining care bundle completion [ 44 ]. This is also reflected in the run charts, where compliance with the care bundle increased almost every time an educational activity took place. Beyond that, process changes, such as authorising nurses to order tests (i.e. chest X-rays and LRTS) and providing standard phrases and order sets in the EHRS to guide clinicians also seemed to be effective. In this context, a thorough review of local processes was vital to understanding the local difficulties and needs for advancement, as is generally recommended in quality improvement [ 27 ].
Since all of our interventions were reasonably simple, dedicated clinicians should be able to integrate them in their daily routine at little expense if they are given time to do so. Apart from human resources, the only intervention incurring additional costs was the pocket cards on CAP treatment.
The most time-consuming aspects of our project were the screening for CAP patients via the X-ray systems and the health record audit. Approximately 15 hours per week per site were spent on this task throughout the study period. We chose this approach because of the well-documented inaccuracy of the International Classification of Diseases (ICD)-10 diagnosis of pneumonia. In a recent Danish study, more than one fourth of patients diagnosed with pneumonia did not fulfil the criterion of a new infiltrate on chest X-ray [ 31 ].
To ensure a consistent and streamlined audit and reliable data across all sites, clinicians were not permitted to audit the health records. A simpler and more feasible audit approach should be implemented in a daily routine and could involve continuous monitoring of CAP cases by a team of dedicated clinical staff members.
The greatest strengths of our study are its size as well as the participation of one control and three intervention sites. The use of a control site is unusual in quality improvement, and the finding of improvements at the intervention sites together with a lack of change at the control site strengthens our conclusions [ 45 ]. In addition, we included a representative study population from study sites covering regions that serve approximately 15% of the Danish population. Hence, we think comparable programs could be designed throughout the country leading to comparable results.
Moreover, the study population was a real-life CAP cohort that is demographically comparable to another large Danish CAP cohort [ 31 ].
Limitations
The main limitation of our study is the reliance on the audit of health records that only contain the information that health professionals document. We could not identify a study investigating the quality of documentation in the EHRS used at our study sites; however, based on our experiences, it is generally high. Nevertheless, we cannot be sure whether a certain proportion of patients did not meet the bundle care criteria due to a lack of documentation.
Finally, the limited follow-up period of only four months does not allow an assessment of sustainability. This question will be the focus of a follow-up study starting in November 2019 and continuing until February 2020.
Conclusions
During the intervention period of our quality improvement project, we observed significant enhancement of patient care for CAP at the intervention sites with a simultaneous lack of improvement at the control site. Additionally, the standard of care remained much higher than at baseline during four months of follow-up. Our study emphasizes that the existence of guidelines alone does not assure high-quality patient care. We found that achieving improvements in CAP patient care required a combination of focus on the disease, locally tailored interventions and changes to key processes.
Supporting information
https://doi.org/10.1371/journal.pone.0234308.s001
https://doi.org/10.1371/journal.pone.0234308.s002
https://doi.org/10.1371/journal.pone.0234308.s003
Acknowledgments
The authors want to thank the rest of the optiCAP group: Susanne Nielsen, RN (Silkeborg Regional Hospital), Susanne Rasmussen, RN (Silkeborg Regional Hospital), Gunhild Agnete Hansen, RN (Gentofte Hospital), Lea Normand Nielsen, RN (Nordsjaellands Hospital), as well as medical students Magnus Søltoft Lindhardt and Malte Schmücker (both Hvidovre Hospital). Furthermore, we want to acknowledge Bjarne Ørskov (Hvidovre Hospital) for initiating this project.
Finally, we are grateful for the cooperation of the participating hospitals’ managers and department leaders.
- View Article
- PubMed/NCBI
- Google Scholar
- 2. Danmarks Statistikbank [Internet]. [cited 2017 Sep 23]. Available from: http://www.statistikbanken.dk
- 4. Athlin S, Lidman C, Lundqvist A, Naucler P, Nilsson AC, Spindler C, et al. Management of community-acquired pneumonia in immunocompetent adults: updated Swedish guidelines 2017 [Internet]. Vol. 50, Infectious Diseases. Taylor & Francis; 2018 [cited 2019 Jun 18]. p. 247–72. Available from: https://www.tandfonline.com/doi/full/10.1080/23744235.2017.1399316 pmid:29119848
- 6. National Institute for Health and Care Excellence. Diagnosis and Management of Community- and Hospital-Acquired Pneumonia in Adults. 2014.
- 27. Langley GJ. The Improvement Guide: A Practical Approach to Enhancing Organizational Performance. 2. Edition. John Wiley and Sons Ltd; 2009.
Thank you for visiting nature.com. You are using a browser version with limited support for CSS. To obtain the best experience, we recommend you use a more up to date browser (or turn off compatibility mode in Internet Explorer). In the meantime, to ensure continued support, we are displaying the site without styles and JavaScript.
- View all journals
- My Account Login
- Explore content
- About the journal
- Publish with us
- Sign up for alerts
- Open access
- Published: 12 June 2020
Association between oral health and incidence of pneumonia: a population-based cohort study from Korea
- Minkook Son 1 ,
- Sangyong Jo 2 ,
- Ji Sung Lee 3 &
- Dong Hyun Lee 4
Scientific Reports volume 10 , Article number: 9576 ( 2020 ) Cite this article
12k Accesses
19 Citations
15 Altmetric
Metrics details
- Health care
- Medical research
Pneumonia is related to oral health of the elderly and intensive care unit patients. However, studies on the relationship between overall oral health and pneumonia in the general population have been limited. The purpose of this study was to investigate the association between oral health and pneumonia using a nationwide population-based Korean cohort database. Data from 122,251 participants who underwent health screening and oral examinations in 2004 or 2005 were analyzed. Cox proportional hazard regression analysis was performed to evaluate the association between oral health and pneumonia. The risk of pneumonia increased significantly in groups with a higher number of dental caries and missing teeth, with respective adjusted hazard ratios (HRs) and 95% confidence interval (CI) of 1.265 (1.086–1.473; p = 0.0025) and 1.218 (1.113–1.332; p < 0.0001), and decreased significantly in frequent tooth brushing and regular professional dental cleaning groups, with respective adjusted HRs and 95% CI of 0.853 (0.786–0.926; p = 0.0001) and 0.920 (0.855–0.990; p = 0.0255). In addition, regardless of age and comorbidities, oral health status and oral hygiene behaviors were associated with pneumonia. The results indicate that improved oral health may reduce the risk of pneumonia in the general population.
Similar content being viewed by others
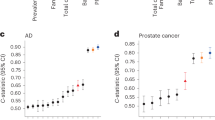
Integration of polygenic and gut metagenomic risk prediction for common diseases
Yang Liu, Scott C. Ritchie, … Michael Inouye
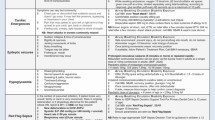
Medical emergencies in the dental practice poster: revised and updated
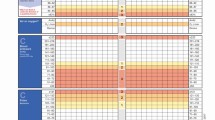
Using National Early Warning Score (NEWS) 2 to help manage medical emergencies in the dental practice
Phil Jevon & Shaam Shamsi
Introduction
Pneumonia is a debilitating disease that can result in death in elderly individuals 1 and has been reported to be related to oral health 2 . The oral cavity is a complex environment for multiple microorganisms and cytokines 3 . In particular, aspiration of microorganisms and biological mediators such as cytokines and hydrolytic enzymes from the oral cavity can provoke inflammation and cause infections 4 , 5 . The association between pneumonia and oral health has been examined in nursing homes, and the incidence of pneumonia has been reported to be lower in elderly populations receiving oral care 6 . Besides, several studies have suggested that hospitalized intensive care unit (ICU) patients appear to benefit from daily oral cleansing 7 , 8 .
Oral hygiene can be improved through individual-based interventions 9 . Daily personal oral hygiene behaviors such as tooth brushing are fundamental in the prevention of periodontal disease and bacterial plaque, which can respectively lead to tooth loss and dental caries 10 , 11 . In addition, professional dental cleaning has been proven to reduce periodontal disease, dental caries, and tooth loss 12 . Dentists recommend tooth brushing at least twice daily as well as regular dental visits for professional dental cleaning 13 .
While the link between pneumonia and oral health has been gaining increased attention, studies evaluating the relationship between overall oral health and pneumonia in the general population have been limited. The purpose of this study was to investigate the association between oral health and pneumonia using a nationwide population-based Korean cohort from the National Health Insurance Service-Health Screening (NHIS-HealS) database.
Data source
This study used the NHIS-HealS database 14 . The NHIS database is registered with 97% of all Koreans and includes all insurance claims data. We analyzed the cohort data, which was extracted from a random sampling of approximately 10% of the 5 million people (age ≥40 years) who underwent health screening in 2002 or 2003. Information about demographic data (age, sex, and socioeconomics), clinical data (medical service use, disease diagnosis, and treatment), and health screening data (physical examination, laboratory tests, and questionnaires on lifestyle and medical histories) is included in the NHIS database accessible to researchers. Registrants in the NHIS database are recommended to receive a standardized health screening at least every 2 years. A detailed description of the data can be found on the relevant website 14 , 15 .
Study population
The first two years (2002–2003) were considered as a washout period. Among the 514,866 people in the NHIS-HealS database, the participants included in this study were limited to those who underwent health screening with oral examinations in 2004 or 2005. We excluded participants with a pneumonia diagnosis before the day of health screening. Participants with one or more missing values were excluded. Finally, 122,251 participants were included in our study (Fig. 1 ).
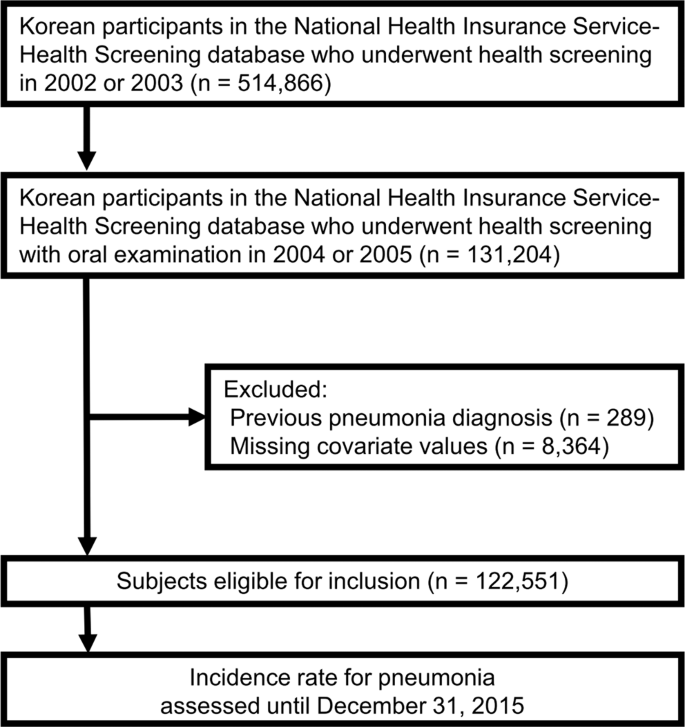
Flow of study participants.
Oral health status and oral hygiene behaviors
The oral health screening program was provided to all registrants. The program was composed of oral examinations conducted by professional dentists and self-reported questionnaires. Registrants were inspected by dentists for periodontal status and for the number of decayed and missing teeth. In this study, periodontal disease was categorized as either absent or present. The number of dental caries and missing teeth was categorized as 0, 1–4, and ≥5. Oral health questionnaires included dental visits, dental symptoms, and oral hygiene behaviors. Based on the questionnaires, gum bleeding was classified as absent or present. The number of tooth brushing sessions per day was classified as 0–1, 2, and ≥3. Professional dental cleaning was classified as <1 or ≥1 time per year.
The main study outcome was the incidence of pneumonia 16 , 17 . We used 3 definitions for pneumonia (Supplementary Fig. S1 ). The first definition of pneumonia (Def. 1) was defined based on the 10 th revision of the International Statistical Classification of Diseases and Related Health Problems (ICD-10) codes for pneumonia (J10-J18) with any prescription of antibiotics (J01). In addition to Def. 1, the second definition of pneumonia (Def. 2) includes hospitalization for 2 days or more, and the third definition of pneumonia (Def. 3) includes hospitalization for 7 days or more. The study participants were followed up on from the day of health screening at index year to the day of incidence of pneumonia, the day of death, or December 31, 2015, whichever came first (Supplementary Fig. S2 ).
Age, sex, body mass index (BMI), hypertension, diabetes, dyslipidemia, Charlson comorbidity index (CCI) category, smoking, drinking, exercise, income, periodontal disease, dental caries, missing teeth, gum bleeding, tooth brushing, and professional dental cleaning were considered as covariates. BMI was calculated by dividing body weight (kg) by the square of the height in meters (m 2 ). Hypertension, diabetes mellitus, and dyslipidemia were defined using criteria from previous studies 18 , 19 , 20 . The presence of hypertension was defined according to the following criteria: (1) ICD-10 codes for hypertension (I10, I11) with at least one claim per year for prescription of an antihypertensive drug, or (2) systolic blood pressure ≥ 140 mmHg or diastolic blood pressure ≥90 mmHg. The presence of diabetes was defined according to the following criteria: (1) ICD-10 codes for diabetes (E10-E14) with at least one claim per year for the prescription of an anti-diabetic drug, or (2) fasting glucose level ≥ 126 mg/dL. The presence of dyslipidemia was defined per the following criteria: (1) ICD-10 codes (E78) for dyslipidemia with at least one claim per year for prescription of lipid-lowering agents or (2) total cholesterol level ≥240 mg/dL. The CCI was calculated based on preexisting diseases, including myocardial infarction, congestive heart failure, peripheral vascular disease, cerebrovascular disease, dementia, chronic pulmonary disease, connective tissue disease, peptic ulcer, mild liver disease, diabetes with and without complications, paraplegia or hemiplegia, renal disease, any or metastatic cancer, moderate or severe liver disease, and acquired immune deficiency syndrome before the start of the follow-up period 21 , 22 . Information on smoking status, drinking status, and exercise status was obtained using questionnaires. For smoking status, participants were classified as non-smokers, ex-smokers, or smokers. To identify drinking status, participants were categorized into non-drinking or drinking groups. Regular exercise was defined as at least five exercise sessions per week. Income was divided into two groups based on the tenth percentile in the income distribution.
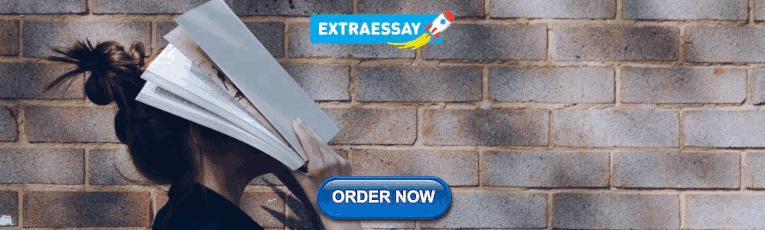
Statistical analysis
Baseline characteristics were presented as mean with standard deviation for continuous variables except age and as number with percentage (%) for categorical variables. Ages were presented as medians with interquartile ranges. The incidence rate of pneumonia was calculated by dividing the number of pneumonia cases by the total follow-up duration (person-years). The graphs for the incidence rate of pneumonia according to the oral health and oral hygiene behaviors were calculated using Kaplan-Meier curves and evaluated by the log-rank test and Gray’s test for competing risk 23 . The hazard ratio (HR) and 95% confidence interval (CI) for the incidence of pneumonia were evaluated using the Cox proportional hazards model. The proportional hazard assumption was evaluated by the Schoenfeld residuals test with the logarithm of cumulative hazards function based on Kaplan-Meier estimates. There was no interference with the assumption of proportional hazard risk over time. The multivariable-adjusted cox proportional hazard models were adjusted for age, sex, BMI, hypertension, diabetes, dyslipidemia, CCI category, smoking, drinking, exercise, income, periodontal disease, dental caries, missing teeth, gum bleeding, tooth brushing, and professional dental cleaning. Besdies, the Fine and Gray model for competing risk with death was applied to evaluate the HR and 95% CI for incidence of pneumonia 23 . Subgroup analyses according to age and comorbidities were performed. In the subgroup analysis, ages were divided into under and over 60 years categories. The presence of comorbidities was defined as the case where CCI was not 0. Statistical analyses were performed using SAS version 9.4 (SAS Institute Inc., Cary, NC, USA) and all figures were produced using R 3.6.0 ( https://www.r-project.org/ ). A two-sided p-value < 0.05 was considered statistically significant.
Ethical consideration
This study was approved by the Institutional Review Board of the Dong-A University Hospital (DAUHIRB-EXP-20-006), which waived the requirement for informed consent in regard to the anonymized data analyzed retrospectively.
Baseline characteristics
The study population with Def. 3 comprised 122,551 healthy participants with no history of pneumonia (Table 1 ). The mean age of participants was 52.4 years and 64.0% were male. The mean systolic blood pressure and fasting glucose were 125.5 mmHg and 96.7 mg/dL, respectively. 49.6% of participants had periodontal disease. 2.7% and 6.0% of participants had five or more dental caries and missing teeth, respectively. According to the self-reported questionnaires, 45.0% of participants brushed their teeth three times or more per day, and 26.0% replied having professional dental cleaning at least once per year. Supplementary Tables S1 – S2 show the baseline characteristics of the study participants according to the definition of pneumonia. Supplementary Tables S3 – S7 compare the baseline characteristics of participants according to oral health status and oral hygiene behaviors in Def. 3 of pneumonia.
Oral health and risk of pneumonia
A total of 4,681 pneumonia cases per Def. 3 (3.8%) were identified over a median follow-up period of 10.6 ± 1.1 years. Figure 2 shows the incidence rate of pneumonia according to oral health status and oral hygiene behaviors. The risk of pneumonia was higher in groups with a higher number of dental caries and missing teeth. In contrast, the risk of pneumonia was lower in the frequent tooth brushing group and the regular professional dental cleaning group. There was no significant difference in the risk of pneumonia between groups with and without periodontal disease (p = 0.1904). The number of dental caries and missing teeth, and the frequency of tooth brushing and professional dental cleaning, were associated with the incidence of pneumonia according to multivariable-adjusted Cox proportional hazard models with Def. 3 (Table 2 ). The risk of pneumonia was significantly higher in the group with a higher number of dental caries (adjusted HR 1.265, 95% CI: 1.086–1.473; p = 0.0025) and the group with more missing teeth (adjusted HR 1.218, 95% CI: 1.113–1.332; p < 0.0001). The risk of pneumonia decreased significantly in the frequent tooth brushing group (adjusted HR 0.853,; 95% CI, 0.786–0.926; p = 0.0001) and in the regular professional dental cleaning group (adjusted HR 0.920,; 95% CI, −0.855–0.990; p = 0.0255). In addition, most of the results using the Fine and Gray model for competing risk were consistent with our main finding (Supplementary Table S8 ).
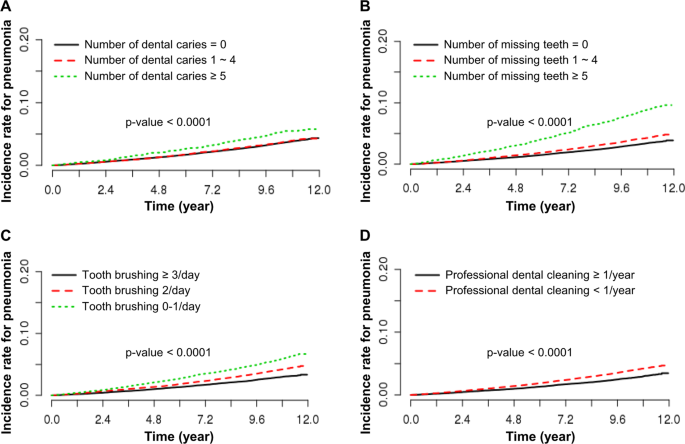
Kaplan-Meier curves for the incidence of pneumonia associated with oral health status and oral hygiene behaviors. ( A ) Number of dental caries, ( B ) number of missing teeth, ( C ) frequency of tooth brushing, and ( D ) frequency of professional dental cleaning. p-value for the log-rank test.
Comparison of hazard ratios and 95% confidence intervals between definitions of pneumonia
Figure 3 shows the HR and 95% CI for the incidence of pneumonia according to oral health status and oral hygiene behaviors, with different definitions of pneumonia (Def. 1-Def. 3). With Def. 2 and Def. 3, it showed a prominent and significantly adjusted HR and 95% CI compared to Def. 1. The adjusted HR and 95% CI for the incidence of pneumonia with other definitions according to oral health status and oral hygiene behaviors are shown in Supplementary Tables S9 – S11 .
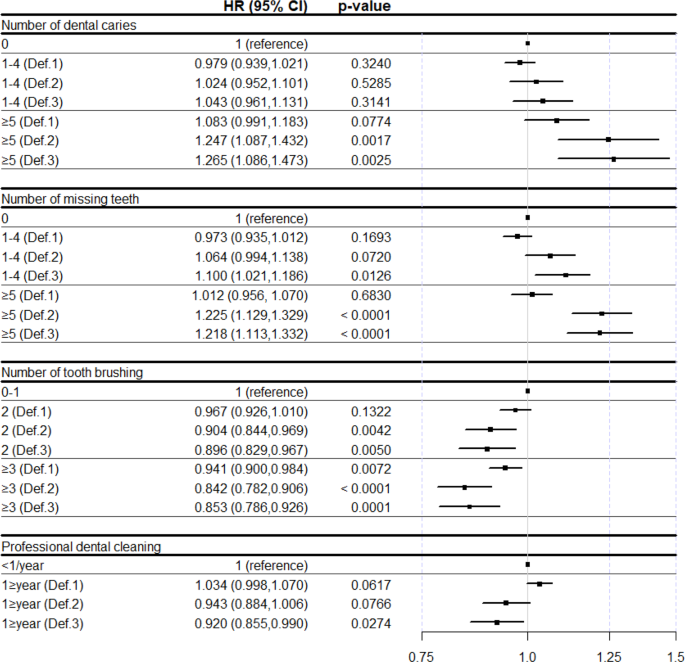
Hazard ratio and 95% confidence interval for incidence of pneumonia according to oral health status and oral hygiene behaviors with different definitions of pneumonia. HR, hazard ratio; CI, confidence interval.
Subgroup analysis according to age and comorbidities
Figure 4 shows the subgroup analysis for Def. 3 according to age and comorbidities. Regardless of age, the number of dental caries and missing teeth were associated with an increased incidence of pneumonia. In the frequent tooth brushing group under 60 years and the regular professional dental cleaning group over 60 years, the risk of pneumonia significantly decreased. Regardless of the underlying disease, the number of missing teeth and the frequency of tooth brushing were associated with the incidence rate of pneumonia.
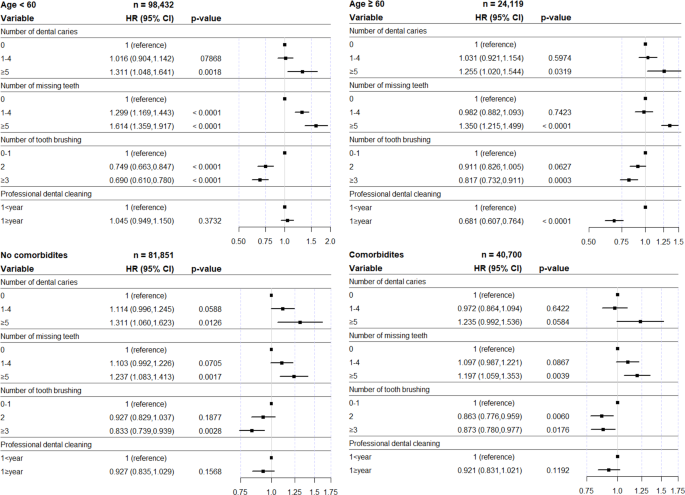
Hazard ratio and 95% confidence interval for incidence of pneumonia in subgroup analysis according to oral health status and oral hygiene behaviors. HR, hazard ratio; CI, confidence interval.
This study analyzed data from a nationwide population-based Korean cohort from the NHIS-HealS database with a long-term follow-up period, and described the relationship between oral health and the incidence of pneumonia. Oral health status and oral hygiene behaviors were associated with the incidence of pneumonia in the general population. We found that an increased number of dental caries and missing teeth were associated with a higher incidence of pneumonia, and that better oral hygiene care, such as frequent tooth brushing and regular professional dental cleaning, was associated with a lower incidence of pneumonia (Table 2 ). The HR and 95% CI remained significant after adjustment for covariates including age, sex, BMI, and CCI category. In particular, the link between oral health and pneumonia was more prominent in pneumonia combined with longer hospitalization (Fig. 3 ). The adjusted HR and 95% CI for the incidence of pneumonia compared to the number of dental caries and tooth brushing sessions per day with the reference group were significant based on Def. 2. For the number of missing teeth and the presence of professional dental cleaning, the HR and 95% CI were significant for Def. 3. Since length of stay is associated with the pneumonia severity index 24 , 25 , it could be assumed that oral health is related to the severity of pneumonia.
Several studies have investigated the relationship between oral hygiene and pneumonia. More plaque, the presence of bacteria in saliva, and colonization in the oropharynx appear to be associated with pneumonia in hospitalized and ICU patients 26 , 27 . Manger et al . also reported that pneumonia is associated with dental caries and the presence of oral plaque 28 . Suma et al . reported that high levels of tooth loss may indicate an increased risk of mortality from pneumonia in the general population 29 . In this study, oral health issues such as dental caries and missing teeth remained significant after adjusting for possible covariates. In addition, Nasiriani et al . reported that tooth brushing twice daily in ICU patients can reduce the incidence of ventilator-associated pneumonia 30 . Our results describe that better oral hygiene management, including tooth brushing and professional dental cleaning, remained significant factors after adjusting for covariates.
One remarkable finding in this study was that the association between periodontal disease and pneumonia was not statistically significant. Indeed, in several studies, the results between these two factors were inconsistent. Some epidemiologic studies found no clear relationship between periodontal hygiene and acute respiratory disease 31 , 32 . However, some studies have reported the association between periodontal disease and pneumonia 33 , 34 , 35 , and Yang et al . reported that patients with periodontal treatment exhibited a decreased risk of pneumonia 36 . In this study, NHIS-HealS data did not include the severity and treatment of periodontal disease; therefore, the two factors could potentially be found to be unrelated. Besides, the diagnosis and classification of periodontal disease is the summation of information from medical and dental histories, combined with the findings of clinical and radiographic examinations 37 . Therefore, it is difficult to perform an accurate analysis based simply on information about the presence of periodontal disease obtained only by dental examination, and further research may be necessary.
We performed subgroup analysis, categorized by age and comorbidities in Fig. 4 . Many studies have reported an association between oral health and pneumonia in elderly and ICU patients 6 , 7 , 8 , but we confirmed regardless of age and comorbidities, oral health status, and oral hygiene behaviors, especially frequent tooth brushing, was significantly related to the incidence of pneumonia. In fact, tooth brushing by critically ill patients has been advocated by several studies 30 , 38 , but based on these results, it can be emphasized to the general population.
Several mechanisms have been proposed to explain these results. First, there can be biological plausibility for a causal relationship between oral health and pulmonary disease. An increase in cytokines induced by inflammation of periodontal disease, which is associated with multiple tooth loss and dental caries 39 , 40 , may promote systemic inflammation, including the lung 41 . Without effective oral care, initial plaque formation can occur within 48 hours and the clump of oropharyngeal flora, which becomes more colonized by gram-negative pathogens, may be transported to the lung, causing pulmonary infection 42 . Second, tooth loss and dental caries are associated with swallowing 43 , 44 . The loss of processing efficiency for food increases the risk of aspiration into the airways and changes food selection towards decreased consumption of vegetables and fruits 45 , 46 , causing malnutrition, which is independently associated with pneumonia 47 .
Some limitations should be acknowledged in this study. First, there could be inconsistencies in the diagnostic criteria between dental specialties and general practitioners. In this study, the presence of periodontal diseases was not determined by objective measures such as radiographic examination 37 . In addition, there was a lack of information regarding the severity and treatment of periodontal disease. Second, the number of tooth brushing sessions per day and professional dental cleaning were evaluated using self-reporting questionnaires. Third, there was a possibility that unadjusted covariates could not be excluded. Participants with better oral status and hygiene may have better lifestyles, which can reduce the risk of pneumonia. Finally, there was a possibility that the state of variables would change, which could not guarantee temporality. Future research is required to overcome these limitations.
In conclusion, the increased number of dental caries and missing teeth was associated with an increased incidence of pneumonia. In contrast, improved oral hygiene care, such as frequent tooth brushing and regular professional dental cleaning, was associated with a reduced incidence of pneumonia.
Henig, O. & Kaye, K. S. Bacterial Pneumonia in Older Adults. Infect. Dis. Clin. North Am. 31 , 689–713, https://doi.org/10.1016/j.idc.2017.07.015 (2017).
Article PubMed PubMed Central Google Scholar
Wise, M. P., Williams, D. W., Lewis, M. A., Thomas, J. G. & Frost, P. J. Impact of poor dental health on pneumonia. Eur. Respir. J. 32 , 1123–1124, https://doi.org/10.1183/09031936.00096808 (2008).
Article CAS PubMed Google Scholar
Terpenning, M. Geriatric oral health and pneumonia risk. Clin. Infect. Dis. 40 , 1807–1810, https://doi.org/10.1086/430603 (2005).
Article PubMed Google Scholar
Terpenning, M. S. et al . Aspiration pneumonia: dental and oral risk factors in an older veteran population. J. Am. Geriat. Soc. 49 , 557–563, https://doi.org/10.1046/j.1532-5415.2001.49113.x (2001).
Paju, S. & Scannapieco, F. A. Oral biofilms, periodontitis, and pulmonary infections. Oral Dis. 13 , 508–512, https://doi.org/10.1111/j.1601-0825.2007.01410a.x (2007).
Article CAS PubMed PubMed Central Google Scholar
Yoneyama, T., Yoshida, M., Matsui, T. & Sasaki, H. Oral care and pneumonia. Oral Care Working Group. Lancet 354 , 515, https://doi.org/10.1016/s0140-6736(05)75550-1 (1999).
Genuit, T., Bochicchio, G., Napolitano, L. M., McCarter, R. J. & Roghman, M. C. Prophylactic chlorhexidine oral rinse decreases ventilator-associated pneumonia in surgical ICU patients. Surg. Infect. 2 , 5–18, https://doi.org/10.1089/109629601750185316 (2001).
Article CAS Google Scholar
Mori, H. et al . Oral care reduces incidence of ventilator-associated pneumonia in ICU populations. Intensive Care Med. 32 , 230–236, https://doi.org/10.1007/s00134-005-0014-4 (2006).
Jepsen, S. et al . Prevention and control of dental caries and periodontal diseases at individual and population level: consensus report of group 3 of joint EFP/ORCA workshop on the boundaries between caries and periodontal diseases. J. Clin. Periodontol. 44 , S85–S93, https://doi.org/10.1111/jcpe.12687 (2017).
Chapple, I. L. et al . Primary prevention of periodontitis: managing gingivitis. J. Clin. Periodontol. 42 (Suppl 16), S71–76, https://doi.org/10.1111/jcpe.12366 (2015).
van der Weijden, F. & Slot, D. E. Oral hygiene in the prevention of periodontal diseases: the evidence. Periodontology 2000 55 , 104–123, https://doi.org/10.1111/j.1600-0757.2009.00337.x (2011).
Axelsson, P., Nystrom, B. & Lindhe, J. The long-term effect of a plaque control program on tooth mortality, caries and periodontal disease in adults. Results after 30 years of maintenance. J. Clin. Periodontol. 31 , 749–757, https://doi.org/10.1111/j.1600-051x.2004.00563.x (2004).
Davies, G. M. & Davies, R. M. Delivering better oral health–an evidence-based toolkit for prevention: a review. Dent. Update 35 , 460–462, 464, https://doi.org/10.12968/denu.2008.35.7.460 (2008).
Seong, S. C. et al . Cohort profile: the National Health Insurance Service-National Health Screening Cohort (NHIS-HEALS) in Korea. BMJ Open 7 , e016640, https://doi.org/10.1136/bmjopen-2017-016640 (2017).
National Health Insurance Sharing Service , https://nhiss.nhis.or.kr/bd/ab/bdaba000eng.do (2020).
Song, T. J. & Kim, J. Risk of post-stroke pneumonia with proton pump inhibitors, H2 receptor antagonists and mucoprotective agents: A retrospective nationwide cohort study. PloS ONE 14 , e0216750, https://doi.org/10.1371/journal.pone.0216750 (2019).
Kim, B., Kim, J., Jo, Y. H., Lee, J. H. & Hwang, J. E. The change in age distribution of CAP population in Korea with an estimation of clinical implications of increasing age threshold of current CURB65 and CRB65 scoring system. PloS ONE 14 , e0219367, https://doi.org/10.1371/journal.pone.0219367 (2019).
Kim, J. A. et al . Impact of Visit-to-Visit Fasting Plasma Glucose Variability on the Development of Type 2 Diabetes: A Nationwide Population-Based Cohort Study. Diabetes Care 41 , 2610–2616, https://doi.org/10.2337/dc18-0802 (2018).
Kim, M. K. et al . Effects of Variability in Blood Pressure, Glucose, and Cholesterol Concentrations, and Body Mass Index on End-Stage Renal Disease in the General Population of Korea. J. Clin. Med . 8 , https://doi.org/10.3390/jcm8050755 (2019).
Kim, M. K. et al . Cholesterol variability and the risk of mortality, myocardial infarction, and stroke: a nationwide population-based study. Eur. Heart J. 38 , 3560–3566, https://doi.org/10.1093/eurheartj/ehx585 (2017).
Kim, K. H. [Comparative study on three algorithms of the ICD-10 Charlson comorbidity index with myocardial infarction patients]. J. Prev. Med. Public Health 43 , 42–49, https://doi.org/10.3961/jpmph.2010.43.1.42 (2010).
de Groot, V., Beckerman, H., Lankhorst, G. J. & Bouter, L. M. How to measure comorbidity. a critical review of available methods. J. Clin. Epidemiol. 56 , 221–229, https://doi.org/10.1016/s0895-4356(02)00585-1 (2003).
Fine, J. P. & Gray, R. J. A Proportional Hazards Model for the Subdistribution of a Competing. Risk. J. Am. Stat. Assoc. 94 , 496–509, https://doi.org/10.2307/2670170 (1999).
Article MathSciNet MATH Google Scholar
Menendez, R., Ferrando, D., Valles, J. M., Martinez, E. & Perpina, M. Initial risk class and length of hospital stay in community-acquired pneumonia. Eur. Respir. J. 18 , 151–156, https://doi.org/10.1183/09031936.01.00090001 (2001).
Garau, J. et al . Factors impacting on length of stay and mortality of community-acquired pneumonia. Clin. Microbiol. Infect. 14 , 322–329, https://doi.org/10.1111/j.1469-0691.2007.01915.x (2008).
El-Solh, A. A. et al . Colonization of dental plaques: a reservoir of respiratory pathogens for hospital-acquired pneumonia in institutionalized elders. Chest 126 , 1575–1582, https://doi.org/10.1378/chest.126.5.1575 (2004).
Bonten, M. J. et al . Risk factors for pneumonia, and colonization of respiratory tract and stomach in mechanically ventilated ICU patients. Am . J. Respir. Crit. Care Med. 154 , 1339–1346, https://doi.org/10.1164/ajrccm.154.5.8912745 (1996).
Manger, D. et al . Evidence summary: the relationship between oral health and pulmonary disease. Brit. Dent. J. 222 , 527–533, https://doi.org/10.1038/sj.bdj.2017.315 (2017).
Suma, S. et al . Tooth loss and pneumonia mortality: A cohort study of Japanese dentists. PloS ONE 13 , e0195813, https://doi.org/10.1371/journal.pone.0195813 (2018).
Nasiriani, K., Torki, F., Jarahzadeh, M. H. & Rashidi Maybodi, F. The Effect of Brushing with a Soft Toothbrush and Distilled Water on the Incidence of Ventilator-Associated Pneumonia in the Intensive Care Unit. Tanaffos 15 , 101–107 (2016).
PubMed PubMed Central Google Scholar
Scannapieco, F. A., Papandonatos, G. D. & Dunford, R. G. Associations between oral conditions and respiratory disease in a national sample survey population. Ann. Periodontol. 3 , 251–256, https://doi.org/10.1902/annals.1998.3.1.251 (1998).
Treloar, D. M. & Stechmiller, J. K. Use of a clinical assessment tool for orally intubated patients. Am. J. Crit. Care 4 , 355–360 (1995).
Iwasaki, M. et al . Periodontal disease and pneumonia mortality in haemodialysis patients: A 7-year cohort study. J. Clin. Periodontol. 45 , 38–45, https://doi.org/10.1111/jcpe.12828 (2018).
de Melo Neto, J. P. et al . Periodontal infections and community-acquired pneumonia: a case-control study. Eur. J. Clin. Microbiol. Infect. Dis. 32 , 27–32, https://doi.org/10.1007/s10096-012-1710-y (2013).
Gomes-Filho, I. S. et al . Influence of periodontitis in the development of nosocomial pneumonia: a case control study. Journal of periodontology 85 , e82–90, https://doi.org/10.1902/jop.2013.130369 (2014).
Yang, L.-C. et al . The Association of Periodontal Treatment and Decreased Pneumonia: A Nationwide Population-Based Cohort Study. Int. J. Environ. Res. Public Health 17 , 356, https://doi.org/10.3390/ijerph17010356 (2020).
Article CAS PubMed Central Google Scholar
Preshaw, P. M. Detection and diagnosis of periodontal conditions amenable to prevention. BMC Oral Health 15 , S5, https://doi.org/10.1186/1472-6831-15-S1-S5 (2015).
Ames, N. J. Evidence to support tooth brushing in critically ill patients. Am. J. Crit. Care 20 , 242–250, https://doi.org/10.4037/ajcc2011120 (2011).
Merchant, A. T. Periodontitis and dental caries occur together. J. Evid. Based Dent. Pract. 12 , 18–19, https://doi.org/10.1016/s1532-3382(12)70005-2 (2012).
Nilsson, H., Sanmartin Berglund, J. & Renvert, S. Longitudinal evaluation of periodontitis and tooth loss among older adults. J. Clin. Periodontol. 46 , 1041–1049, https://doi.org/10.1111/jcpe.13167 (2019).
Scannapieco, F. A., Wang, B. & Shiau, H. J. Oral bacteria and respiratory infection: effects on respiratory pathogen adhesion and epithelial cell proinflammatory cytokine production. Ann. Periodontol. 6 , 78–86, https://doi.org/10.1902/annals.2001.6.1.78 (2001).
Scannapieco, F. A. Role of oral bacteria in respiratory infection. J. Periodontol. 70 , 793–802, https://doi.org/10.1902/jop.1999.70.7.793 (1999).
Okamoto, N. et al . Relationship between swallowing problems and tooth loss in community-dwelling independent elderly adults: the Fujiwara-kyo study. J. Am. Geriat. Soc. 60 , 849–853, https://doi.org/10.1111/j.1532-5415.2012.03935.x (2012).
Furuta, M. & Yamashita, Y. Oral Health and Swallowing Problems. Curr. Phys. Med. Rehabil. Rep. 1 , 216–222, https://doi.org/10.1007/s40141-013-0026-x (2013).
Quandt, S. A. et al . Food avoidance and food modification practices of older rural adults: association with oral health status and implications for service provision. Gerontologist 50 , 100–111, https://doi.org/10.1093/geront/gnp096 (2010).
Savoca, M. R. et al . Association between dietary quality of rural older adults and self-reported food avoidance and food modification due to oral health problems. J. Am. Geriat. Soc. 58 , 1225–1232, https://doi.org/10.1111/j.1532-5415.2010.02909.x (2010).
Loeb, M. & High, K. The effect of malnutrition on risk and outcome of community-acquired pneumonia. Respir. Care Clin. N. Am. 11 , 99–108, https://doi.org/10.1016/j.rcc.2004.10.002 (2005).
Download references
Acknowledgements
We would like to thank Editage for English language editing. This study used the National Health Information Database of the National Health Insurance Service (NHIS). This work was supported by the Dong-A University Research Fund. The funders had no role in the study design, data collection and analysis, decision to publish, or preparation of the manuscript.
Author information
Authors and affiliations.
Department of Biomedical Science and Engineering, Gwangju Institute of Science and Technology, Gwangju, Korea
Minkook Son
Department of Internal Medicine, Air Force 3rd Flying Training Wing, Sacheon, Korea
Sangyong Jo
Clinical Research Center, Asan Medical Center, Ulsan University College of Medicine, Seoul, Korea
Ji Sung Lee
Departement of Pulmonology and Intensive Care Medicine, Dong-A University, College of Medicine, Busan, Korea
Dong Hyun Lee
You can also search for this author in PubMed Google Scholar
Contributions
All authors contributed to the study conception and design. M.S., S.J. and J.S.L. contributed to the analysis and interpretation of data. M.S. and D.H.L. contributed to drafting of the manuscript. J.S.L and D.H.L. contributed to critical revision of the manuscript for important intellectual content and statistical analysis. D.H.L. contributed to obtained funding, administrative, technical, material support and study supervision. All authors reviewed the manuscript and approved it for publication.
Corresponding author
Correspondence to Dong Hyun Lee .
Ethics declarations
Competing interests.
The authors declare no competing interests.
Additional information
Publisher’s note Springer Nature remains neutral with regard to jurisdictional claims in published maps and institutional affiliations.
Supplementary information
Supplementary information., rights and permissions.
Open Access This article is licensed under a Creative Commons Attribution 4.0 International License, which permits use, sharing, adaptation, distribution and reproduction in any medium or format, as long as you give appropriate credit to the original author(s) and the source, provide a link to the Creative Commons license, and indicate if changes were made. The images or other third party material in this article are included in the article’s Creative Commons license, unless indicated otherwise in a credit line to the material. If material is not included in the article’s Creative Commons license and your intended use is not permitted by statutory regulation or exceeds the permitted use, you will need to obtain permission directly from the copyright holder. To view a copy of this license, visit http://creativecommons.org/licenses/by/4.0/ .
Reprints and permissions
About this article
Cite this article.
Son, M., Jo, S., Lee, J.S. et al. Association between oral health and incidence of pneumonia: a population-based cohort study from Korea. Sci Rep 10 , 9576 (2020). https://doi.org/10.1038/s41598-020-66312-2
Download citation
Received : 16 January 2020
Accepted : 18 May 2020
Published : 12 June 2020
DOI : https://doi.org/10.1038/s41598-020-66312-2
Share this article
Anyone you share the following link with will be able to read this content:
Sorry, a shareable link is not currently available for this article.
Provided by the Springer Nature SharedIt content-sharing initiative
This article is cited by
Modeling longitudinal oral health status and pneumonia risk: secondary data analyses of an integrated dental-medical cohort.
- Richard L. Berg
- Ingrid Glurich
- Amit Acharya
BMC Oral Health (2023)
Periodontal disease does not increase the risk of subsequent psoriasis
- Yoo Sang Baek
- Eun-Jung Kwak
- Jiehyun Jeon
Scientific Reports (2023)
Temporal dynamics of oropharyngeal microbiome among SARS-CoV-2 patients reveals continued dysbiosis even after Viral Clearance
- Suman Kalyan Paine
- Usha Kiran Rout
- Analabha Basu
npj Biofilms and Microbiomes (2022)
Pulmonary disease and periodontal health: a meta-analysis
- ZhiDong Guo
Sleep and Breathing (2022)
By submitting a comment you agree to abide by our Terms and Community Guidelines . If you find something abusive or that does not comply with our terms or guidelines please flag it as inappropriate.
Quick links
- Explore articles by subject
- Guide to authors
- Editorial policies
Sign up for the Nature Briefing newsletter — what matters in science, free to your inbox daily.

- Research article
- Open access
- Published: 27 May 2020
Prevalence of pneumonia and its associated factors among under-five children in East Africa: a systematic review and meta-analysis
- Biruk Beletew 1 ,
- Melaku Bimerew 1 ,
- Ayelign Mengesha 1 ,
- Mesfin Wudu 1 &
- Molla Azmeraw 1
BMC Pediatrics volume 20 , Article number: 254 ( 2020 ) Cite this article
40k Accesses
12 Citations
Metrics details
Pneumonia is defined as an acute inflammation of the Lungs’ parenchymal structure. It is a major public health problem and the leading cause of morbidity and mortality in under-five children especially in developing countries. In 2015, it was estimated that about 102 million cases of pneumonia occurred in under-five children, of which 0.7 million were end up with death. Different primary studies in Eastern Africa showed the burden of pneumonia. However, inconsistency among those studies was seen and no review has been conducted to report the amalgamated magnitude and associated factors. Therefore, this review aimed to estimate the national prevalence and associated factors of pneumonia in Eastern Africa
Using PRISMA guideline, we systematically reviewed and meta-analyzed studies that examined the prevalence and associated factors of pneumonia from PubMed, Cochrane library, and Google Scholar. Heterogeneity across the studies was evaluated using the Q and the I 2 test. A weighted inverse variance random-effects model was applied to estimate the national prevalence and the effect size of associated factors. The subgroup analysis was conducted by country, study design, and year of publication. A funnel plot and Egger’s regression test were used to see publication bias. Sensitivity analysis was also done to identify the impact of studies.
A total of 34 studies with 87, 984 participants were used for analysis. The pooled prevalence of pneumonia in East Africa was 34% (95% CI; 23.80–44.21). Use of wood as fuel source (AOR = 1.53; 95% CI:1.30–1.77; I 2 = 0.0%; P = 0.465), cook food in living room (AOR = 1.47;95% CI:1.16–1.79; I 2 = 0.0%; P = 0.58), caring of a child on mother during cooking (AOR = 3.26; 95% CI:1.80–4.72; I 2 = 22.5%; P = 0.26), Being unvaccinated (AOR = 2.41; 95% CI:2.00–2.81; I 2 = 51.4%; P = 0.055), Child history of Acute Respiratory Tract Infection (ARTI) (AOR = 2.62; 95% CI:1.68–3.56; I 2 = 11.7%; P = 0.337) were identified factors of pneumonia.
The prevalence of pneumonia in Eastern Africa remains high. This review will help policy-makers and program officers to design pneumonia preventive interventions.
Peer Review reports
Pneumonia is defined as an acute inflammation of the Lungs’ parenchymal structure. It can be classified based on place of acquisition: as community acquired or hospital acquired; based on its causative agents/ mechanism as bacterial, viral, fungal, Aspiration, or ventilator-associated pneumonia; based on the anatomy of the lungs involved as lobar pneumonia, bronchial pneumonia or acute interstitial pneumonia; and on the basis of its clinical severity as “no pneumonia”, “pneumonia” or “severe pneumonia” [ 1 , 2 , 3 ].
Under-five children are more vulnerable to pneumonia and pneumonia remains the leading cause of morbidity and mortality in those children [ 4 ]. According to a global estimate made in 2000, approximately 156 million cases of pneumonia had occurred each year in under-five children, of which 151 million episodes were in the developing countries and about 1.2 million of them were end up in death. South-east Asia and Africa were the two continents with high magnitude of childhood pneumonia, having an estimated of 61 million and 35 million annual cases of pneumonia in under-five children respectively [ 5 ]. The magnitude of under-five pneumonia was decreased to 120 million (with 0.88 million deaths) in 2010 and to 102 million (with 0.7 million deaths) in 2015 globally. These decrement was due to decrease in the magnitude of its key risk factors, increasing socioeconomic development and preventive interventions, improved access to care, and quality of care in hospitals. Despite this progress, pneumonia is still a major public health problem for children especially in developing countries [ 4 ].
Globally, many researches had been conducted to identify risk factors of pneumonia. Despite the inconsistency of findings, low birth weight, malnutrition, indoor air pollution, parental smoking, being unvaccinated, overcrowding, lack of separate kitchen, being not on exclusive breast feeding, and maternal education were identified as factors associated with occurrence of pneumonia in under-five children [ 6 , 7 , 8 , 9 ].
Besides, in East African countries different researchers had tried to investigate the magnitude of pneumonia in under-five children and have reported a prevalence ranges from 5.5% [ 10 ] up to 89.8% [ 11 ]. They had also identified risk factors for pneumonia among under-five children. But, reported finding lack consistency and as per the investigators knowledge there is no a systematic review and meta-analysis conducted to address these inconsistent findings reported from East African countries. Moreover, assessing the magnitude of pneumonia and identifying its associated factors for risk based diagnosis of pneumonia contribute in better interventions and helps to reduce the higher burden of pneumonia in under-five children. Hence, this systematic review and meta-analysis was conducted to assess the magnitude of pneumonia and its associated factors among under-five children in East Africa.
The results of this review were reported based on the Preferred Reporting Items for Systematic Review and Meta-Analysis statement (PRISMA) guideline ( Supplementary file-PRISMA checklist ) and, it is registered in the Prospero database: (PROSPERO 2019: CRD42019136707) Available from https://www.crd.york.ac.uk/PROSPERO/#myprosperoID = CRD42019136707.
Searching strategy and information sources
We identified studies providing data on the prevalence of and potential risk factors of pneumonia among under-five children, with the search focused on Eastern Africa from PubMed, Cochrane library, and Google Scholar. The search included MeSH terms and keywords, combinations, and snowball searching in references list of papers found through the data base search to retrieve additional articles. Articles with incomplete reported data were handled through contacting corresponding authors. Unpublished studies were retrieved from the official websites of international and local organizations and universities. The search was performed by keywords, medical subject headings (MeSH) terms. We used the search terms independently and/or in combination using “OR” or “AND”. The core search terms and phrases were “under five”, “children”, “child”, “infant”, and “pneumonia”, “respiratory infection”, causes, risk factors, determinants, associated factors, predictors and Eastern Africa. The search strategies were developed using different Boolean operators. Remarkably, to fit advanced PubMed database, the following search strategy was applied: (prevalence OR magnitude OR epidemiology) AND (causes OR determinants OR associated factors OR predictors OR risk factors) AND (children [MeSH Terms] OR under five OR child OR childhood) AND (pneumonia [MeSH Terms] OR respiratory tract infection) AND Eastern Africa. We also screened at the reference lists of the remaining papers to identify additional relevant studies to this review.
Study selection / eligibility criteria
Retrieved studies were exported to reference manager software, Endnote version 8 to remove duplicate studies. Two investigators (BB and AM) independently screened the selected studies using their titles and abstracts before retrieval of full-text papers. We used pre-specified inclusion criteria to further screen the full-text articles. Disagreements were discussed during a consensus meeting with other reviewers (MW and MB) for the final selection of studies to be included in the systematic review and meta-analysis.
Inclusion and exclusion criteria
All observational studies (cross-sectional, case-control, and cohort studies) were included. Those studies had reported the prevalence and/or at least one associated factors of pneumonia among under-five children and published in English language from 2000 up to 2019 in Eastern Africa were considered. A consideration was extended to unpublished work among children under five were also considered. Citations without abstract and/or full-text, anonymous reports, editorials, and qualitative studies were excluded from the analysis. Furthermore, researches which did not report our results of interest were excluded. Regarding inclusion and exclusion criteria of included studies, children below 59 months of age with mother / care giver visiting out patients department during data collection period were included. Severely sick child need life treating intervention and whose mother / care givers refused were excluded from the study.
Quality assessment
Duplicate articles were removed using Endnote (version X8) after combining the Database search results. The Joanna Briggs Institute (JBI) quality appraisal checklist was used [ 12 , 13 ] . Four independent authors appraised the quality of the studies. The appraisal was repeated by exchanging with each other. Thus, one paper was appraised by two Authors. Any disagreement between the reviewers was solved by taking the mean score of the two reviewers. Studies were considered as low risk or good quality when it scored 5 and above for all designs (cross sectional, case control, and cohort) and were included [ 12 , 13 ] whereas the score was 4 and below the studies considered as high risk or poor quality and was not included.
Data extraction
The authors developed data extraction form on the excel sheet which includes author name, year of publication, study country, study design, sample size, prevalence of pneumonia, and categories of factors reported. The data extraction sheet was piloted using 4 papers randomly. The extraction form was adjusted after piloted the template. Two of the authors extracted the data using the extraction form in collaboration. The third and fourth authors check the correctness of the data independently. Any disagreements between reviewers were resolved through discussions with a third reviewer and fourth reviewer if required. The mistyping of data was resolved through crosschecking with the included papers. If we got incomplete data, we excluded the study after two attempts were made to contact the corresponding author by email.
Outcome measurement
Pneumonia was considered when under five children with cough and/or difficulty of breathing, have fast breathing and/or chest indrawing and suggestive X-ray findings [ 14 , 15 ].
Statistical analysis
After the data was extracted using Microsoft Excel format we imported the data to STATA version 14.0 statistical software for further analysis. Using the binomial distribution formula, Standard error was calculated for each study. We pooled the overall magnitude estimates of pneumonia by a random effect meta-analysis [ 16 ]. The pooled prevalence of pneumonia with 95% CI was presented using forest plots and Odds ratio (OR) with 95% CI was also presented in forest plot to show the associated factors of pneumonia. We examined the heterogeneity between the studies using Cochrane’s Q statistics (Chi-square), invers variance (I2) and p -values [ 17 ].
In this study, the I 2 statistic value of zero indicates true homogeneity, whereas the value 25, 50, and 75% represented low, moderate and high heterogeneity respectively [ 18 , 19 ]. For the data identified as heterogeneous, we conducted our analysis by random-effects model analysis. In addition subgroup analysis was done by the study country, design, and year of publication. When statistical pooling is not possible, non-pooled data was presented in table form. Sensitivity analysis was employed to see the effect of a single study on the overall estimation. Publication bias was checked by funnel plot and more objectively through Egger’s regression test [ 20 ].
Study selection
A total of 6879 studies were identified using electronic searches (through Databases searching ( n = 6867)) and other sources ( n = 12)) that were conducted from 2000 up to 2019. After duplication removed, a total of 3150 articles remained (3729 duplicated). Finally, 200 studies were screened for full-text review and, 34 articles with ( n = 87,984 patients) were selected for the prevalence and/ or associated factors analysis (Fig. 1 ).
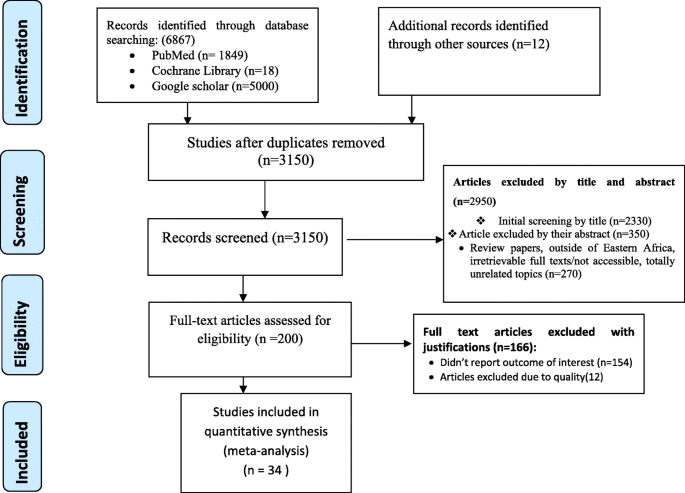
PRISMA flow diagram showed the results of the search and reasons for exclusion
Characteristics of included studies
Table 1 summarizes the characteristics of the 34 included studies in the systematic review and meta-analysis [ 10 , 11 , 21 , 22 , 23 , 24 , 25 , 26 , 27 , 28 , 29 , 30 , 31 , 32 , 33 , 34 , 35 , 36 , 37 , 39 , 40 , 41 , 42 , 43 , 44 , 45 , 46 , 47 , 48 , 49 , 50 , 51 , 52 ]. 16 studies were found in Ethiopia [ 10 , 22 , 23 , 24 , 25 , 26 , 27 , 28 , 29 , 30 , 31 , 32 , 33 , 34 , 35 , 36 ], 8 in Kenya [ 11 , 37 , 39 , 40 , 41 , 42 , 43 ], 2 in Uganda [ 51 , 52 ],1 Eritrea [ 21 ], 1 in Somali [ 44 ],4 Sudan [ 45 , 46 , 47 , 48 ],2 Tanzania [ 49 , 50 ].
23 studies were cross-sectional, while the others used either case-control ( n = 9) or cohort ( n = 2) study design. Most of the studies 23/34(70.5%) were published between 2015 and 2019. The studies included participants, ranging from 40 [ 45 ] to 73,778 [ 44 ] (Table 1 ).
Meta-analysis
Prevalence of pneumonia among fewer than five children in ethiopia.
Most of the studies ( n = 23) had reported the prevalence of pneumonia [ 10 , 11 , 21 , 22 , 23 , 24 , 25 , 28 , 29 , 33 , 34 , 35 , 36 , 41 , 42 , 43 , 44 , 45 , 46 , 47 , 50 , 51 , 52 ]. The prevalence of pneumonia were ranged from 5.5% [ 10 ] up to 89.8% [ 11 ]. The random-effects model analysis from those studies revealed that, the pooled prevalence of pneumonia in East Africa was found to be 34% (95%CI; 23.80–44.21; I 2 = 99.4%; p < 0.001) (Fig. 2 ).
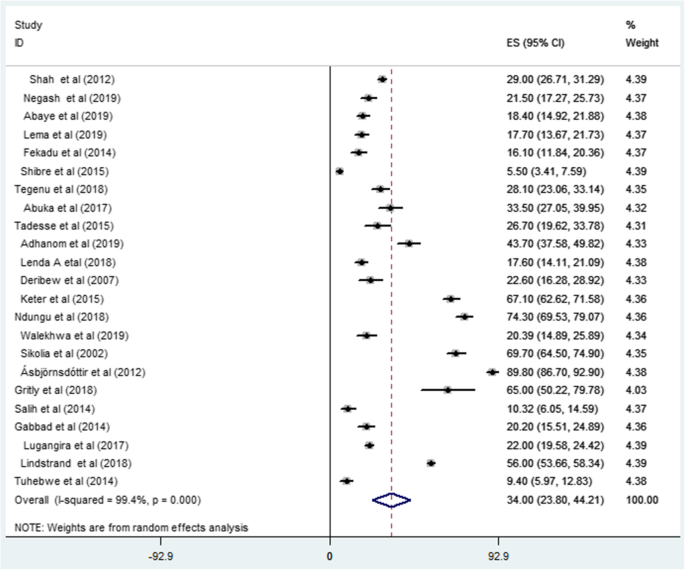
Forest plot showing the pooled prevalence of pneumonia among under-five children in Eastern Africa from 2000 up to 2019
Subgroup analysis of the prevalence of pneumonia in eastern Africa
The subgroup analysis was done through stratified by country, study design, and year of publication. Based on this, the prevalence of pneumonia among under five children was found to be 29 in Eritrea, 22.62 in Ethiopia, 64.3 in Kenya, 29.71 in Sudan, 22 in Tanzania, and 32.72 in Uganda (Supplementary Fig. 1 and Table 2 ). Based on the study design, the prevalence of pneumonia was found to be 32.33 in cross-sectional studies, 55.68% in cohort studies and 22.6 in case control studies (Supplementary Fig. 2 and Table 2 ). Based on the year of publication, the prevalence of pneumonia was found to be 33.4 from 2000 to 2015, while it was 34.29 from studies conducted from 2016 to 2019(Supplementary Fig. 3 , Table 2 ).
Sensitivity analysis
We employed a leave-one-out sensitivity analysis to identify the potential source of heterogeneity in the analysis of the prevalence of pneumonia in Eastern Africa. The results of this sensitivity analysis showed that our findings were not dependent on a single study. Our pooled estimated prevalence of pneumonia varied between 31.38(22.93–39.83) [ 11 ] and 35.3(25.13–45.49) [ 10 ] after deletion of a single study (Supplementary Fig. 4 ).
Publication Bias
We have also checked publication bias and a funnel plot showed symmetrical distribution. Egger’s regression test p -value was 0.63, which indicated the absence of publication bias (Supplementary Fig. 5 ).
Factors associated with pneumonia
Out of the total included studies 18 studies [ 10 , 22 , 23 , 24 , 25 , 26 , 27 , 28 , 30 , 31 , 32 , 33 , 34 , 35 , 37 , 39 , 40 , 43 ] revealed the factors associated with pneumonia among under five children in Eastern Africa (Table 3 ).
Use of wood as fuel source
Eight studies found significant association between use of wood as fuel source and pneumonia among under five children. Of these the highest risk factor, AOR = 7.41 (95% CI: 2.75, 19.95), Fekadu et al [ 25 ] and lowest risk factor AOR = 1.15(0.47,1.88),Negash et al [ 22 ] compared to those who use non wood items as a source of fuel (Table 3 ). Regarding heterogeneity test, Galbraith plot showed homogeneity and combining the result of eight studies, the forest plot showed the overall estimate of AOR of using wood as fuel source was 1.53(95%C I: 1.30, 1.77;I 2 = 0.0%; P = 0.465). I-Squared (I 2 ) and P -value also showed homogeneity (Supplementary Fig. 6 ). Regarding publication bias, a funnel plot showed a symmetrical distribution. During the Egger’s regression test, the p -value was 0.176, which indicated the absence of publication bias (Supplementary Fig. 7 ).
We employed a leave-one-out sensitivity analysis to identify the potential source of heterogeneity in the analysis of the pooled estimate of using wood as fuel source as a risk factor of pneumonia in Eastern Africa. The results of this sensitivity analysis showed that our findings were not dependent on a single study. Our pooled estimate of using wood as fuel source varied between 1.409(95% CI, 1.122–1.696) and 1.664 (95% CI, 1.321–2.008) after deletion of a single study (Supplementary Fig. 8 ).
Cooking food in living room
Six studies found significant association between cooking food at living room and pneumonia among under five children. Of these the highest risk factors, AOR = 3.27(1.4, 7.9) Tegenu et al [ 28 ] and lowest risk factor AOR = 1.35(0.3,0.99) Sikolia et al [ 43 ] compared to those who cook food at kitchen (Table 3 ). Regarding heterogeneity test for cooking food at in living room, Galbraith plot showed homogeneity and combining the result of six studies the forest plot showed the overall estimate of AOR of cooking food in living room was 1.47(95%CI: 1.16–1.79;I 2 = 0.0%; P = 0.58).I-Squared (I 2 ) and P -value also showed homogeneity (Supplementary Fig. 9 ). Regarding publication of bias for cooking food at home, the funnel plot analysis showed asymmetrical distribution. During the Egger’s regression test, the p -value was 0.026, which indicated the presence of publication bias (Supplementary Fig. 10 ). Trim and fill analysis was done, and 3 study were added and the total number of studies become 9. The pooled estimate of AOR of preterm becomes 1.406 (Supplementary Fig. 11 ). We employed a leave-one-out sensitivity analysis to identify the potential source of heterogeneity in the analysis of the pooled estimate of cooking food in living room as a risk factor of pneumonia in Eastern Africa. The results of this sensitivity analysis showed that our findings were not dependent on a single study. Our pooled estimate of cooking food in living room varied between 1.428(95%CI, 1.102–1.755) and 2.09(95%CI, 1.314–2.875) after deletion of a single study (Supplementary Fig. 12 ).
Caring of the child on mothers during cooking
Seven studies found significant association between putting a child at the back during cooking and pneumonia among under five children. Of these the highest risk factors, AOR = 11.76(4.6, 30.08) Lema et al [ 24 ] and lowest risk factor AOR = 1.37(0.24,7.83) Abuka et al [ 29 ] compared to those who didn’t put their baby at their back (Table 3 ). Regarding heterogeneity test, Galbraith plot showed homogeneity and combining the result of seven studies the forest plot showed the overall estimate of AOR of pneumonia was 3.26(95%CI: 1.80–4.72;I 2 = 22.5%; P = 0.258).I-Squared (I 2 ) and P -value also showed homogeneity (Supplementary Fig. 13 ). Regarding test of publication bias a funnel plot showed a symmetrical distribution. Egger’s regression test p -value was 0.074, which indicated the presence of publication bias (Supplementary Fig. 14 ). We employed a leave-one-out sensitivity analysis to identify the potential source of heterogeneity in the analysis of the pooled estimate of putting a child at the back during cooking as a risk factor of pneumonia in Eastern Africa. The results of this sensitivity analysis showed that our findings were not dependent on a single study. Our pooled estimate of putting a child at the back during cooking varied between 2.87(95% CI, 1.329–4.426) and 3.59(95% CI, 1.828–5.355) after deletion of a single study (Supplementary Fig. 16 ).
Being unvaccinated
Seven studies found significant association between being unvaccinated and pneumonia among under five children. Of these the highest risk factors, AOR = 4.62(2.64, 11) Tegenu et al [ 28 ] and lowest risk factor AOR = 1.6(0.9,2.9) Geleta et al [ 27 ] compared to those who have been vaccinated (Table 3 ). Regarding heterogeneity test, Galbraith plot showed homogeneity and combining the result of seven studies, the forest plot showed the overall estimate of AOR of not being vaccinated was 2.41(95%C I: 2.00–2.81;I 2 = 51.4%; P = 0.055).I-Squared (I 2 ) and P -value also showed homogeneity (Supplementary Fig. 17 ). Regarding publication bias, a funnel plot showed a symmetrical distribution. During the Egger’s regression test, the p -value was 0.177, which indicated the absence of publication bias (Supplementary Fig. 18 ). We employed a leave-one-out sensitivity analysis to identify the potential source of heterogeneity in the analysis of the pooled estimate of being unvaccinated as a risk factor of pneumonia in Eastern Africa. The results of this sensitivity analysis showed that our findings were not dependent on a single study. Our pooled estimate of being unvaccinated varied between 2.4(95%CI, 2.07–2.72) and 2.71(95%CI, 2.55–2.86) after deletion of a single study (Supplementary Fig. 19 ).
Non-exclusive breast feeding
Eleven studies found significant association between non-exclusive breast feeding and pneumonia among under five children. Of these the highest risk factors, AOR = 8.33(2.6.3,10.50) Gedefaw et al [ 32 ] and lowest risk factor AOR = 1.51(0.88,2.58) Negash et al [ 22 ] compared to those who breast feed exclusively (Table 3 ). Regarding heterogeneity test, Galbraith plot showed heterogeneity and combining the result of eleven studies, the forest plot showed the overall estimate of AOR of non-exclusive breast feeding was 2.47(95%C I: 1.79, 3.16;I 2 = 65.0%; P = 0.01).I-Squared (I 2 ) and P -value also showed heterogeneity (Supplementary Fig. 20 ). Regarding publication bias, a funnel plot showed an asymmetrical distribution. During the Egger’s regression test, the p -value was 0.016, which indicated the presence of publication bias (Supplementary Fig. 21 ). Due to presence of publication bias trim and fill analysis was done and 5 studies were added, and the total number of studies becomes 16. The pooled estimate of AOR of non-exclusive breast feeding was found to be 2.05 (Supplementary Fig. 22 ). We employed a leave-one-out sensitivity analysis to identify the potential source of heterogeneity in the analysis of the pooled estimate of being non-exclusive breast feeding as a risk factor of pneumonia in Eastern Africa. The results of this sensitivity analysis showed that our findings were not dependent on a single study. Our pooled estimate of being for non-exclusive breast feeding is found to be between 1.757(95%CI, 1.49–2.01) and 1.936(95%CI, 1.70–2.17) after deletion of a single study (Supplementary Fig. 23 ).
History acuter respiratory tract infection (ARTI)
History ARTI was considered when a child has history of ARTI with in the 2 weeks before being diagnosed for pneumonia. Nine studies found significant association between history ARTI and pneumonia among under five children. Of these the highest risk factors, AOR = 17.13(5.01,60.26) Muthumbi et al [ 40 ] and lowest risk factor AOR = 1.36(0.26,7.21) Abaye et al [ 23 ] compared to those who use non wood item as a source of fuel (Table 3 ). Regarding heterogeneity test, Galbraith plot showed homogeneity and combining the result of nine studies, the forest plot showed the overall estimate of AOR of history ARTI was considered was 2.62(95%C I: 1.68, 3.56;I 2 = 11.7%; P = 0.337).I-Squared (I 2 ) and P -value also showed homogeneity (Supplementary Fig. 24 ). Regarding publication bias, a funnel plot showed an asymmetrical distribution. During the Egger’s regression test, the p -value was 0.024, which indicated the presence of publication bias (Supplementary Fig. 25 ). Due to presence of publication bias trim and fill analysis was done and 5 studies were added, and the total number of studies becomes 14. The pooled estimate of AOR of history of ARTI was found to be 1.958(Supplementary Fig. 26 ).
We employed a leave-one-out sensitivity analysis to identify the potential source of heterogeneity in the analysis of the pooled estimate of being history of ARTI as a risk factor of pneumonia in Eastern Africa. The results of this sensitivity analysis showed that our findings were not dependent on a single study. Our pooled estimate of having history of ARTI ranges between 2.195(95%CI, 1.36–3.02) and 3.28(95%CI, 2.153–4.417) after deletion of a single study (Supplementary Fig. 27 ).
This systematic review and meta-analysis was conducted to assess the magnitude of pneumonia and its associated factors among under-five children in East Africa. Thirty-four studies were included for the final analysis. Twenty-two studies had reported the prevalence of pneumonia and the pooled prevalence of pneumonia in under-five children was found to be 34% with 95% CI of (23.8–44.21%). This result was higher than a study conducted in Dibrugarh, India which had reported the prevalence of pneumonia in under-five children to be 16.34% [ 9 ]. This might be due to socioeconomic and seasonal discrepancies as countries in East Africa are less developed than India. A study conducted in Nigeria had revealed the prevalence of pneumonia in under-five children to be 31.6% which was consistence with the findings of this systematic review [ 53 ]. This consistency might be due to similarities in socio-economic status as Nigeria is an African country probably having comparable socio-economic status with east African countries. In addition the discrepancy might be due to difference in case definition of pneumonia.
This finding is higher than other studies done in Austria (4.1%) [ 54 ], in Mali (6.7%) [ 55 ], and in Bangladesh (21.3%) [ 56 ]. This variation might be due to socio-economic and socio-demographic vitiations, the variation in the study setting, seasonal variation, unreachability and provision of Vitamin A supplementation and immunization, lack of confirmatory laboratories and imaging investigations.
This systematic review and meta-analysis had also revealed using woods as a source of fuel, cooking foods living rooms, holding children on back while cooking foods, being unvaccinated, history of being not on exclusive breast feeding, history of upper respiratory tract infection and parental smoking as a significant risk factors for increased prevalence of pneumonia among under-five children in East Africa.
Higher odds of pneumonia were observed in under-five children whose family uses wood as a source of fuel. This result was in line with studies conducted in India [ 57 ], and Sri Lanka [ 58 ]; and with systematic reviews conducted in Low and Middle income countries [ 59 ], and Africa, China and Latin America [ 60 ]. It was also consistent with a global review conducted by Jackson et al. [ 61 ]. The association between using wood as a source of fuel and pneumonia in under-five children might be due to the fact that using woods as a source of fuel results in release of wood smokes containing major air pollutants like carbon monoxide and particulate matters which causes indoor air pollution [ 62 ]. Indoor air pollution and inhaling wood smoke in turn impairs the function of pulmonary alveolar macrophages and epithelial cells which will increase the likelihood of pulmonary infections including pneumonia [ 62 , 63 ].
According to this systematic review and meta-analysis, cooking foods in living rooms was found to be significantly associated with occurrence of pneumonia in under-five children as higher odds of pneumonia was exhibited among children living in families who cooks food at living rooms than children living in families who cooks food in kitchen. Holding children on back while cooking foods was another factor found to be significantly associated with pneumonia. This association might be due to the reason that cooking foods in living rooms will cause indoor air pollution and holding a child on back while cooking foods can increase the probability of inhaling smokes and food vapors (steams) which in turn will increase the risk of acquiring pneumonia by altering the structure and function of the respiratory tract [ 58 , 63 ].
In this systematic review children with history of Acute Respiratory Tract Infections (ARTIs) were found to be at increased risk to acquire pneumonia; as the odds of pneumonia among children who had history of ARTIs was higher than children without history of ARTIs. The reason behind this association might be due to the fact that ARTIs will alter the structure and function of the respiratory tract and can cause Lower Respiratory Infections (LRTIs) including pneumonia in two ways— by increasing invasion of the Lower respiratory tract (LRT) with other microorganisms which cause secondary infections or by progressive invasion of LRT with the same microorganism causing the ARTIs (Primary infections) [ 64 ].
The risk of acquiring pneumonia in unvaccinated children was found to be higher than vaccinated children. This result was similar with studies conducted in Brazil [ 65 ], Bellary [ 7 ], and India [ 66 ]. A systematic review conducted by Jackson et al. [ 61 ] was also in line with this result. Similarly, children who were not on exclusive breast feeding were at higher risk to develop pneumonia than children who were on exclusive breast feeding for the first 6 months of age. This result was consistent with different studies conducted across the world [ 7 , 61 , 67 , 68 ]. The reason behind this association might be due to low or weak immunity. Because exclusive breast feeding and vaccination are strategies used to increase the immunity of children and prevent childhood infections. So, children who were not on Exclusive breast feeding and/ or unvaccinated will have weak immunity and increased probability of acquiring infections including pneumonia [ 69 ].
Strength and limitations
This study has several strengths: First, we used a pre-specified protocol for search strategy and data abstraction and used internationally accepted tools for a critical appraisal system for quality assessment of individual studies. Second, we employed subgroup and sensitivity analysis based on study country, study design, and publication year to identify the small study effect and the risk of heterogeneity. Nevertheless, this review had some limitations: There may be publication bias because not all grey literature was included and language biases since all included studies are published in English.
Conclusion and recommendation
The prevalence of pneumonia among under-five children in Eastern Africa remains high. Use of wood as fuel source, cooking food in living room, caring of a child on mother during cooking, being unvaccinated, on-exclusive breast feeding,child history of ARTI, and parental smoking were independent potential predictors of under-five pneumonia in Eastern Africa. Hence, appropriate intervention on potential determinates such as health education on exclusive breastfeeding, place of food cooking, increase vaccination coverage and early control of respiratory tract infection was recommended to prevent those risk factors.
Availability of data and materials
The datasets analyzed during the current study are available from the corresponding author upon reasonable request.
Abbreviations
Confidence Interval
Under Five Mortalities
World Health Organization
Demographic and Health Surveys
Ethiopian Demographic and Health Survey
Adjusted odds ratio
Acute Respiratory Tract Infections
Mackenzie G. The definition and classification of pneumonia. Pneumonia. 2016;8(1):14.
Article PubMed PubMed Central Google Scholar
WHO. Revised WHO classification and treatment of childhood pneumonia at health facilities. WHO. 2014.
Kalil AC, Metersky ML, Klompas M, Muscedere J, Sweeney DA, Palmer LB, et al. Management of adults with hospital-acquired and ventilator-associated pneumonia: 2016 clinical practice guidelines by the Infectious Diseases Society of America and the American Thoracic Society. Clin Infect Dis. 2016;63(5):e61–e111.
McAllister DA, Liu L, Shi T, Chu Y, Reed C, Burrows J, et al. Global, regional, and national estimates of pneumonia morbidity and mortality in children younger than 5 years between 2000 and 2015: a systematic analysis. Lancet Glob Health. 2019;7(1):e47–57.
Article PubMed Google Scholar
Rudan I, Boschi-Pinto C, Biloglav Z, Mulholland K, Campbell H. Epidemiology and etiology of childhood pneumonia. Bull World Health Organ. 2008;86:408–16B.
Ramezani M, Aemmi SZ, Emami MZ. Factors affecting the rate of pediatric pneumonia in developing countries: a review and literature study. Int J Pediatr. 2015;3(6.2):1173–81.
Google Scholar
Hemagiri K, Sameena A, Aravind K, Khan W, Vasanta S. Risk factors for severe pneumonia in under five children—a hospital based study. Int J Res Health Sci. 2014;2(1):47–57.
Htar MTT, Stuurman AL, Ferreira G, Alicino C, Bollaerts K, Paganino C, et al. Effectiveness of pneumococcal vaccines in preventing pneumonia in adults, a systematic review and meta-analyses of observational studies. PloS One. 2017;12(5).
Nirmolia N, Mahanta TG, Boruah M, Rasaily R, Kotoky RP, Bora R. Prevalence and risk factors of pneumonia in under five children living in slums of Dibrugarh town. Clin Epidemiol Glob Health. 2018;6(1):1–4.
Article Google Scholar
Shibre G. Assessment of the Prevalence and associated factors of pneumonia in children 2to 59 months old, Debreberhan District, North East Ethiopia: Addis Abeba University; 2015.
Ásbjörnsdóttir KH, Slyker JA, Weiss NS, Mbori-Ngacha D, Maleche-Obimbo E, Wamalwa D, et al. Breastfeeding is associated with decreased pneumonia incidence among HIV-exposed, uninfected Kenyan infants. AIDS (London, England). 2013;27(17):2809.
Peters MD, Godfrey CM, McInerney P, Soares CB, Khalil H, Parker D. The Joanna Briggs institute reviewers' manual 2015: methodology for JBI scoping reviews. 2015.
Institute JB. Meta-analysis of statistics: assessment and review instrument (JBI mastari). Adelaide. 2006;20032007.
Elfving K, Shakely D, Andersson M, Baltzell K, Ali AS, Bachelard M, et al. Acute uncomplicated febrile illness in children aged 2–59 months in Zanzibar–Aetiologies, antibiotic treatment and outcome. PloS One. 2016;11(1).
Article PubMed PubMed Central CAS Google Scholar
Jiskani FB, Shaikh F, Syal AR, Nizamani MA, Shaikh MA. X-ray CHEST findings in children aged 2 months to 59 months classified as pneumonia ACCORDING to IMNCI guidelines. Pak Armed Forces Med J. 2017;67(5):779–82.
Borenstein M, Hedges LV, Higgins JP, Rothstein HR. A basic introduction to fixed-effect and random-effects models for meta-analysis. Res Synth Methods. 2010;1(2):97–111.
Higgins JP, Thompson SG, Deeks JJ, Altman DG. Measuring inconsistency in meta-analyses. BMJ. 2003;327(7414):557–60.
Ioannidis JP. Interpretation of tests of heterogeneity and bias in meta-analysis. J Eval Clin Pract. 2008;14(5):951–7.
Higgins JP, Thompson SG. Quantifying heterogeneity in a meta-analysis. Stat Med. 2002;21(11):1539–58.
Egger M, Smith GD, Schneider M, Minder C. Bias in meta-analysis detected by a simple, graphical test. BMJ. 1997;315(7109):629–34.
Article CAS PubMed PubMed Central Google Scholar
Shah S, Zemichael O, Meng HD. Factors associated with mortality and length of stay in hospitalised neonates in Eritrea, Africa: a cross-sectional study. BMJ Open. 2012;2(5):e000792.
Negash AA, Asrat D, Abebe W, Hailemariam T, Hailu T, Aseffa A, et al., editors. Bacteremic community-acquired pneumonia in Ethiopian children: etiology, antibiotic resistance, risk factors, and clinical outcome. Open forum infectious diseases; 2019: Oxford University Press US.
Abaye G, Fekadu H, Haji K, Alemu D, Anjulo AA, Yadate DT. Prevalence and risk factors of pneumococcal nasopharyngeal carriage in healthy children attending kindergarten, in district of Arsi zone, south east, Ethiopia. BMC Res Notes. 2019;12(1):253.
Lema B, Seyoum K, Atlaw D. Prevalence of community acquired pneumonia among children 2 to 59 months old and its associated factors in Munesa District, Arsi zone, Oromia region. Ethiop Clin Mother Child Health. 2019;16:334.
Fekadu GA, Terefe MW, Alemie GA. Prevalence of pneumonia among under-five children in Este town and the surrounding rural Kebeles, Northwest Ethiopia: a community based cross sectional study. Sci J Public Health. 2014;2(3):150–5.
Dadi AF, Kebede Y, Birhanu Z. Determinants of pneumonia in children aged two months to five years in urban areas of Oromia zone, Amhara region. Ethiop Open Access Library J. 2014;1(08):1.
Geleta D, Tessema F, Ewnetu H. Determinants of community acquired pneumonia among children in Kersa District, Southwest Ethiopia: facility based case control study. J Pediatr Neonatal Care. 2016;5(2):00179.
Tegenu K. Prevalence and associated factors of pneumonia among under-five children at public hospitals in Jimma zone, South West of Ethiopia, 2018: Addis Ababa Universty; 2018.
Abuka T. Prevalence of pneumonia and factors associated among children 2–59 months old in Wondo genet district, Sidama zone, SNNPR, Ethiopia. Current Pediatric Research. 2017.
Workineh Y, Hailu D, Gultie T. Determinants of pneumonia among under two children in southern Ethiopia: A case control study 2016. Curr Pediatr Res. 2017;21(4).
Markos Y, Dadi AF, Demisse AG, Ayanaw Habitu Y, Derseh BT, Debalkie G. Determinants of under-five pneumonia at Gondar University hospital, Northwest Ethiopia: an unmatched case-control study. J Environ Public Health. 2019;2019.
Gedefaw M, Berhe R. Determinates of childhood pneumonia and diarrhea with special emphasis to exclusive breastfeeding in north Achefer district, Northwest Ethiopia: a case control study. Open J Epidemiol. 2015;5(02):107.
Tadesse R. Household biomass fuel use and acute respiratory infections among younger children: an exposure assessment in Anilemo Woreda. Southern Ethiopia: Addis Ababa University; 2015.
Adhanom G, Gebreegziabiher D, Weldu Y, Gebreyesus Wasihun A, Araya T, Legese H, et al. Species, risk factors, and antimicrobial susceptibility profiles of bacterial isolates from HIV-infected patients suspected to have pneumonia in Mekelle zone, Tigray, northern Ethiopia. Biomed Res Int 2019;2019.
Lenda A, Demena M, Mengistie B. Prevalence of pneumonia and associated factors among under-five children in Boloso bombe Woreda, southern Ethiopia: a community based study: Haramaya University; 2018.
Deribew A, Tessema F, Girma B. Determinants of under-five mortality in Gilgel gibe field research center, Southwest Ethiopia. Ethiop J Health Dev. 2007;21(2):117–24.
MANYA AS. Risk factors for pneumonia in children under five years of age, HOSPITALIZED IN A RURAL DISTRICT HOSPITAL OF WESTERN KENYA, 2005.
Keter PKK. Knowledge, attitudes and practices of mothers in relation to childhood pneumonia and factors associated with pneumonia and seeking health Care in Kapsabet District Hospital in Nandi County. Kenya: JKUAT; 2015.
Onyango D, Kikuvi G, Amukoye E, Omolo J. Risk factors of severe pneumonia among children aged 2–59 months in western Kenya: a case control study. Pan Afr Med J. 2012;13(1).
Muthumbi E, Lowe BS, Muyodi C, Getambu E, Gleeson F, Scott JAG. Risk factors for community-acquired pneumonia among adults in Kenya: a case–control study. Pneumonia. 2017;9(1):17.
Ndungu EW, Okwara FN, Oyore JP. Cross sectional survey of care seeking for acute respiratory illness in children under 5 years in rural Kenya. Am J Pediatr. 2018;4(3):69–79.
Walekhwa M, Muturi M, Revathi Gunturu EK, Kabera B. Streptococcus pneumoniae serotype epidemiology among PCV-10 vaccinated and unvaccinated children at Gertrude’s Children’s Hospital, Nairobi County: a cross-sectional study. F1000Research. 2018;7.
角井, 信弘. The prevalence of acute respiratory infections and the associated risk factors: a study of children under five years of age in Kibera Lindi village, Nairobi, Kenya. J Natl Inst Public Health. 2002;51:1.
Kinyoki DK, Manda SO, Moloney GM, Odundo EO, Berkley JA, Noor AM, et al. Modelling the ecological comorbidity of acute respiratory infection, diarrhoea and stunting among children under the age of 5 years in Somalia. Int Stat Rev. 2017;85(1):164–76.
Gritly SM, Elamin MO, Rahimtullah H, Ali AYH, Dhiblaw A, Mohamed EA, et al. Risk factors of pneumonia among children under 5 years at a pediatric hospital in Sudan. Int J Med Res Health Sci. 2018;7(4):60–8.
Salih KEM, Bilal JA, Alfadeel MA, Hamid Y, Eldouch W, Elsammani E, et al. Poor adherence to the World Health Organization guidelines of treatment of severe pneumonia in children at Khartoum, Sudan. BMC Res Notes. 2014;7(1):531.
Gabbad AA, Alrahman GMA, Elawad MA. Childhood pneumonia at Omdurman paediatric hospital, Khartoum, Sudan. Int J of Multidisciplinary and Current research. 2014.
Deng AA. Risk factors for acute lower respiratory tract infections in children under five years of age in juba. Southern Sudan: JKUAT-COHES; 2019.
Ndosa A, Kidenya BR, Mushi MF, Mirambo MM, Hokororo A, Mshana SE. Factors associated with colonization of Streptococcus pneumoniae among under-fives attending clinic in Mwanza City, Tanzania. Tanzan J Health Res. 2015;17(1).
Lugangira K, Kalokola F. Morbidity and mortality of children aged 2–59 months admitted in the Tanzania Lake Zone’s public hospitals: a cross-sectional study. BMC Res Notes. 2017;10(1):502.
Lindstrand A, Kalyango J, Alfven T, Darenberg J, Kadobera D, Bwanga F, et al. Pneumococcal carriage in children under five years in Uganda-will present pneumococcal conjugate vaccines be appropriate? PloS one. 2016;11(11).
Tuhebwe D, Tumushabe E, Leontsini E, Wanyenze RK. Pneumonia among children under five in Uganda: symptom recognition and actions taken by caretakers. Afr Health Sci. 2014;14(4):993–1000.
Ujunwa F, Ezeonu C. Risk factors for acute respiratory tract infections in under-five children in Enugu Southeast Nigeria. Ann Med Health Sci Res. 2014;4(1):95–9.
Kurz H GH, Huber K, Krugluger W, Asbott F, Wabnegger L, Apfalter P, Sebesta, C. Spectrum of pathogens of in-patient children and youths with community acquired pneumonia: a 3 year survey of a community hospital in Vienna, Austria. Wiener klinische Wochenschrift 2013, 125(21–22):674–679. 2013.
Bénet T SM, Messaoudi M, Picot VS, Telles J-N, Diakite A-A, Komurian-Pradel F,, Endtz H DS, Paranhos-Baccalà G. Etiology and factors associated with pneumonia in children under 5 years of age in Mali: a prospective casecontrol study. PloS one 2015, 10(12):e0145447. 2015.
KMAK A. Risk factors for acute respiratory infections (ARI) among under five children in Bangladesh. J Sci Res 2009, 1(1):72–81. 2009.
Kankaria A, Nongkynrih B, Gupta SK. Indoor air pollution in India: implications on health and its control. Ind J Commun Med. 2014;39(4):203.
Ranathunga N, Perera P, Nandasena S, Sathiakumar N, Kasturiratne A, Wickremasinghe R. Effect of household air pollution due to solid fuel combustion on childhood respiratory diseases in a semi urban population in Sri Lanka. BMC Pediatr. 2019;19(1):306.
Adaji EE, Ekezie W, Clifford M, Phalkey R. Understanding the effect of indoor air pollution on pneumonia in children under 5 in low-and middle-income countries: a systematic review of evidence. Environ Sci Pollut Res. 2019;26(4):3208–25.
Article CAS Google Scholar
Dherani M, Pope D, Mascarenhas M, Smith KR, Weber M, Bruce N. Indoor air pollution from unprocessed solid fuel use and pneumonia risk in children aged under five years: a systematic review and meta-analysis. Bull World Health Organ. 2008;86:390–8C.
Jackson S, Mathews KH, Pulanić D, Falconer R, Rudan I, Campbell H, et al. Risk factors for severe acute lower respiratory infections in children–a systematic review and meta-analysis. Croatian Med J. 2013;54(2):110–21.
Chafe Z, Brauer M, Héroux M-E, Klimont Z, Lanki T, Salonen RO, et al. Residential heating with wood and coal: health impacts and policy options in Europe and North America. 2015.
Cardani A, Boulton A, Kim TS, Braciale TJ. Alveolar macrophages prevent lethal influenza pneumonia by inhibiting infection of type-1 alveolar epithelial cells. PLoS Pathog. 2017;13(1):e1006140.
Thomas M, Bomar PA. Upper respiratory tract infection. StatPearls [Internet]: StatPearls Publishing; 2019.
da Fonseca Lima EJ, Mello MJG, Lopes MIL, Serra GHC, Lima DEP, Correia JB. Risk factors for community-acquired pneumonia in children under five years of age in the post-pneumococcal conjugate vaccine era in Brazil: a case control study. BMC Pediatr. 2016;16(1):157.
Gothankar J, Doke P, Dhumale G, Pore P, Lalwani S, Quraishi S, et al. Reported incidence and risk factors of childhood pneumonia in India: a community-based cross-sectional study. BMC Public Health. 2018;18(1):1–11.
Horta BL, Victora CG, Organization WH. Short-term effects of breastfeeding: a systematic review on the benefits of breastfeeding on diarrhoea and pneumonia mortality. 2013.
Lamberti LM, Zakarija-Grković I, Walker CLF, Theodoratou E, Nair H, Campbell H, et al. Breastfeeding for reducing the risk of pneumonia morbidity and mortality in children under two: a systematic literature review and meta-analysis. BMC Public Health. 2013;13(S3):S18.
Alasil S, Kutty P. Breastfeeding as a tool that empowers infant immunity through maternal vaccination. J Vaccines Vaccin. 2015;6(271):2.
Download references
Acknowledgments
We would like to thank the primary authors of the included studies.
No funding was obtained for this study.
Author information
Authors and affiliations.
Department of Nursing, College of Health Sciences, Woldia University, P.O.Box 400, Woldia, Ethiopia
Biruk Beletew, Melaku Bimerew, Ayelign Mengesha, Mesfin Wudu & Molla Azmeraw
You can also search for this author in PubMed Google Scholar
Contributions
BB, MB, MA, AM and MW: developed the study design and protocol, literature review, selection of studies, quality assessment, data extraction, statistical analysis, interpretation of the data and developing the initial drafts of the manuscript and prepared the final draft of the manuscript. All authors read and approved the final manuscript.
Corresponding author
Correspondence to Biruk Beletew .
Ethics declarations
Ethics approval and consent to participate.
Not applicable.
Consent for publication
Competing interests.
We have confirmed that we have no competing interests.
Additional information
Publisher’s note.
Springer Nature remains neutral with regard to jurisdictional claims in published maps and institutional affiliations.
Supplementary information
Additional file 1..
PRISMA 2009 Checklist
Additional file 2 Supplementary Figure 1
. Forest plot showing subgroup analysis (by country) of pooled prevalence of pneumonia among under-five children in Ethiopia from2002 up to 2019. Supplementary Figure 2 . Forest plot showing subgroup analysis (by study design) of pooled prevalence of pneumonia among under-five children in Ethiopia from2002 up to 2019. Supplementary Figure 3 . Forest plot showing subgroup analysis (by country) of pooled prevalence of pneumonia among under-five children in Ethiopia from2002 up to 2019. Supplementary Figure 4 . sensitivity of pooled prevalence of pneumonia among under-five children in Ethiopia from2002 up to 2019. Supplementary Figure 5 . publication bias of pooled prevalence of pneumonia among under-five children in Ethiopia from2002 up to 2019. Supplementary Figure 6 . Forest plot showing of pooled estimate of AOR for using wood as fuel source as a predictor of pneumonia among under-five children in Ethiopia from2002 up to 2019. Supplementary Figure 7 . publication bias of pooled estimate of AOR for using wood as fuel source as a predictor of pneumonia among under-five children in Ethiopia from2002 up to 2019. Supplementary Figure 8 . sensitivity analysis of pooled estimate of AOR for using wood as fuel source as a predictor of pneumonia among under-five children in Ethiopia from2002 up to 2019. Supplementary Figure 9 : Forest plot showing the pooled estimate of AOR for cooking food at home as a predictor of pneumonia among under-five children in Ethiopia from2002 up to 2019. Supplementary Figure 10 . publication bias for pooled estimate of AOR for cooking food at home as a predictor of pneumonia among under-five children in Ethiopia from2002 up to 2019. Supplementary Figure 11 . Trim and fill analysis for pooled estimate of AOR for cooking food at home as a predictor of pneumonia among under-five children in Ethiopia from2002 up to 2019. Supplementary Figure 12 . Sensitivity analysis for pooled estimate of AOR for cooking food at home as a predictor of pneumonia among under-five children in Ethiopia from2002 up to 2019. Supplementary Figure 13. Forest plot showing estimate of AOR for caring of the child on mothers during cooking as a predictor of pneumonia among under-five children in Ethiopia from2002 up to 2019. Supplementary Figure 14 . publication bias for estimate of AOR for caring of the child on mothers during cooking as a predictor of pneumonia among under-five children in Ethiopia from2002 up to 2019. Supplementary Figure 15 . trim and fill analysis for estimate of AOR for caring of the child on mothers during cooking as a predictor of pneumonia among under-five children in Ethiopia from2002 up to 2019. Supplementary Figure 16 . sensitivity analysis for estimate of AOR for caring of the child on mothers during cooking as a predictor of pneumonia among under-five children in Ethiopia from2002 up to 2019. Supplementary Figure 17 . Forest plot showing the pooled estimate of AOR for being unvaccinated as a predictor of pneumonia among under-five children in Ethiopia from2002 up to 2019. Supplementary Figure 18 . publication bias for pooled estimate of AOR for being unvaccinated as a predictor of pneumonia among under-five children in Ethiopia from2002 up to 2019. Supplementary Figure 19 . sensitivity analysis for pooled estimate of AOR for being unvaccinated as a predictor of pneumonia among under-five children in Ethiopia from2002 up to 2019. Supplementary Figure 20 . Forest plot showing the pooled estimate of AOR for non-exclusive breast feeding as a predictor of pneumonia among under-five children in Ethiopia from 2002 up to 2019. Supplementary Figure 21 . publication bias for the pooled estimate of AOR for non-exclusive breast feeding as a predictor of pneumonia among under-five children in Ethiopia from 2002 up to 2019. Supplementary Figure 22 . Trim and fill analysis for the pooled estimate of AOR for non-exclusive breast feeding as a predictor of pneumonia among under-five children in Ethiopia from 2002 up to 2019. Supplementary Figure 23 . Sensitivity analysis for the pooled estimate of AOR for non-exclusive breast feeding as a predictor of pneumonia among under-five children in Ethiopia from 2002 up to 2019. Supplementary Figure 24 . Forest plot showing the pooled estimate of AOR for history of ARTI as a predictor of pneumonia among under-five children in Ethiopia from 2002 up to 2019. Supplementary Figure 25 . Publication bias for the pooled estimate of AOR for history of ARTI as a predictor of pneumonia among under-five children in Ethiopia from 2002 up to 2019. Supplementary Figure 26 . Trim and fill analysis for the pooled estimate of AOR for history of ARTI as a predictor of pneumonia among under-five children in Ethiopia from 2002 up to 2019. Supplementary Figure 27 . sensitivity analysis for the pooled estimate of AOR for history of ARTI as a predictor of pneumonia among under-five children in Ethiopia from 2002 up to 2019
Additional file 3 Table S1
. Search strategy used for one of the databases
Additional file 4 Table S2
. Quality appraisal result of included studies in East Africa, from 2002 to 2019. Using Joanna Briggs Institute (JBI) quality appraisal checklist
Additional file 5 Table S3
. Adjusted confounders and main findings extracted from included studies in East Africa
Rights and permissions
Open Access This article is licensed under a Creative Commons Attribution 4.0 International License, which permits use, sharing, adaptation, distribution and reproduction in any medium or format, as long as you give appropriate credit to the original author(s) and the source, provide a link to the Creative Commons licence, and indicate if changes were made. The images or other third party material in this article are included in the article's Creative Commons licence, unless indicated otherwise in a credit line to the material. If material is not included in the article's Creative Commons licence and your intended use is not permitted by statutory regulation or exceeds the permitted use, you will need to obtain permission directly from the copyright holder. To view a copy of this licence, visit http://creativecommons.org/licenses/by/4.0/ . The Creative Commons Public Domain Dedication waiver ( http://creativecommons.org/publicdomain/zero/1.0/ ) applies to the data made available in this article, unless otherwise stated in a credit line to the data.
Reprints and permissions
About this article
Cite this article.
Beletew, B., Bimerew, M., Mengesha, A. et al. Prevalence of pneumonia and its associated factors among under-five children in East Africa: a systematic review and meta-analysis. BMC Pediatr 20 , 254 (2020). https://doi.org/10.1186/s12887-020-02083-z
Download citation
Received : 05 February 2020
Accepted : 13 April 2020
Published : 27 May 2020
DOI : https://doi.org/10.1186/s12887-020-02083-z
Share this article
Anyone you share the following link with will be able to read this content:
Sorry, a shareable link is not currently available for this article.
Provided by the Springer Nature SharedIt content-sharing initiative
- Eastern-Africa
- Under five children
- Indicator Cluster Surveys (MICS) Child Health/Pneumonia.2017
BMC Pediatrics
ISSN: 1471-2431
- Submission enquiries: [email protected]
- General enquiries: [email protected]
- Introduction
- Conclusions
- Article Information
A full course of antibiotic treatment was defined as more than 3 days of therapy, and a brief empirical course was defined as 3 or fewer days of therapy. Each bar represents a hospital (N = 48), and the dashed line indicates the mean proportion of inappropriate diagnosis across all hospitals in the collaborative over the study period.
Respiratory viral panel (both negative and positive) was compared with no test or missing data, and 2 or more systemic inflammatory response syndrome (SIRS) criteria plus end organ dysfunction was compared with fewer than 2 SIRS criteria. AOR indicates adjusted odds ratio.
eTable 1. Patient Flow
eTable 2. Hospital Characteristics, n=48
eTable 3. Characteristics of Patients Treated for Community-Acquired Pneumonia by Antibiotic Duration, bivariable analysis
eTable 4. Characteristics Associated with Full Course Antibiotic Therapy vs. Brief, Empiric Therapy among those Inappropriately Diagnosed with Community-Acquired Pneumonia, multivariable model
Data Sharing Statement
See More About
Select your interests.
Customize your JAMA Network experience by selecting one or more topics from the list below.
- Academic Medicine
- Acid Base, Electrolytes, Fluids
- Allergy and Clinical Immunology
- American Indian or Alaska Natives
- Anesthesiology
- Anticoagulation
- Art and Images in Psychiatry
- Artificial Intelligence
- Assisted Reproduction
- Bleeding and Transfusion
- Caring for the Critically Ill Patient
- Challenges in Clinical Electrocardiography
- Climate and Health
- Climate Change
- Clinical Challenge
- Clinical Decision Support
- Clinical Implications of Basic Neuroscience
- Clinical Pharmacy and Pharmacology
- Complementary and Alternative Medicine
- Consensus Statements
- Coronavirus (COVID-19)
- Critical Care Medicine
- Cultural Competency
- Dental Medicine
- Dermatology
- Diabetes and Endocrinology
- Diagnostic Test Interpretation
- Drug Development
- Electronic Health Records
- Emergency Medicine
- End of Life, Hospice, Palliative Care
- Environmental Health
- Equity, Diversity, and Inclusion
- Facial Plastic Surgery
- Gastroenterology and Hepatology
- Genetics and Genomics
- Genomics and Precision Health
- Global Health
- Guide to Statistics and Methods
- Hair Disorders
- Health Care Delivery Models
- Health Care Economics, Insurance, Payment
- Health Care Quality
- Health Care Reform
- Health Care Safety
- Health Care Workforce
- Health Disparities
- Health Inequities
- Health Policy
- Health Systems Science
- History of Medicine
- Hypertension
- Images in Neurology
- Implementation Science
- Infectious Diseases
- Innovations in Health Care Delivery
- JAMA Infographic
- Law and Medicine
- Leading Change
- Less is More
- LGBTQIA Medicine
- Lifestyle Behaviors
- Medical Coding
- Medical Devices and Equipment
- Medical Education
- Medical Education and Training
- Medical Journals and Publishing
- Mobile Health and Telemedicine
- Narrative Medicine
- Neuroscience and Psychiatry
- Notable Notes
- Nutrition, Obesity, Exercise
- Obstetrics and Gynecology
- Occupational Health
- Ophthalmology
- Orthopedics
- Otolaryngology
- Pain Medicine
- Palliative Care
- Pathology and Laboratory Medicine
- Patient Care
- Patient Information
- Performance Improvement
- Performance Measures
- Perioperative Care and Consultation
- Pharmacoeconomics
- Pharmacoepidemiology
- Pharmacogenetics
- Pharmacy and Clinical Pharmacology
- Physical Medicine and Rehabilitation
- Physical Therapy
- Physician Leadership
- Population Health
- Primary Care
- Professional Well-being
- Professionalism
- Psychiatry and Behavioral Health
- Public Health
- Pulmonary Medicine
- Regulatory Agencies
- Reproductive Health
- Research, Methods, Statistics
- Resuscitation
- Rheumatology
- Risk Management
- Scientific Discovery and the Future of Medicine
- Shared Decision Making and Communication
- Sleep Medicine
- Sports Medicine
- Stem Cell Transplantation
- Substance Use and Addiction Medicine
- Surgical Innovation
- Surgical Pearls
- Teachable Moment
- Technology and Finance
- The Art of JAMA
- The Arts and Medicine
- The Rational Clinical Examination
- Tobacco and e-Cigarettes
- Translational Medicine
- Trauma and Injury
- Treatment Adherence
- Ultrasonography
- Users' Guide to the Medical Literature
- Vaccination
- Venous Thromboembolism
- Veterans Health
- Women's Health
- Workflow and Process
- Wound Care, Infection, Healing
Others Also Liked
- Download PDF
- X Facebook More LinkedIn
Gupta AB , Flanders SA , Petty LA, et al. Inappropriate Diagnosis of Pneumonia Among Hospitalized Adults. JAMA Intern Med. Published online March 25, 2024. doi:10.1001/jamainternmed.2024.0077
Manage citations:
© 2024
- Permissions
Inappropriate Diagnosis of Pneumonia Among Hospitalized Adults
- 1 Medicine Service, VA Ann Arbor Healthcare System, Ann Arbor, Michigan
- 2 Division of Hospital Medicine, Department of Internal Medicine, University of Michigan Medical School, Ann Arbor
- 3 Division of Infectious Diseases, Department of Internal Medicine, University of Michigan Medical School, Ann Arbor
- 4 Department of Emergency Medicine, University of Wisconsin School of Medicine and Public Health, Madison
- 5 Veterans Affairs Center for Clinical Management Research, Ann Arbor, Michigan
- 6 Division of General Medicine, Department of Internal Medicine, University of Michigan Medical School, Ann Arbor
- 7 Section of Infectious Diseases, Trinity Health Michigan, Ann Arbor
- 8 Intermountain Healthcare, Salt Lake City, Utah
- 9 Department of Medicine, University of Colorado School of Medicine, Aurora
- 10 Division of General Internal Medicine, Department of Internal Medicine, University of Utah School of Medicine, Salt Lake City
- 11 Division of Health System Innovation & Research, Department of Population Health Sciences, University of Utah School of Medicine, Salt Lake City
Question In hospitalized patients diagnosed with pneumonia, what is the incidence of and factors associated with inappropriate diagnosis?
Findings In this cohort study of 17 290 hospitalized adults treated for pneumonia in 48 Michigan hospitals, 12.0% were inappropriately diagnosed. Older patients, those with dementia, and those presenting with altered mental status had the highest risk of being inappropriately diagnosed, and for those inappropriately diagnosed, receipt of a full antibiotic duration was associated with antibiotic-associated adverse events.
Meaning Inappropriate diagnosis of pneumonia among hospitalized adults is common, particularly among older adults with geriatric syndromes, and may be harmful.
Importance Little is known about incidence of, risk factors for, and harms associated with inappropriate diagnosis of community-acquired pneumonia (CAP).
Objective To characterize inappropriate diagnosis of CAP in hospitalized patients.
Design, Setting, and Participants This prospective cohort study, including medical record review and patient telephone calls, took place across 48 Michigan hospitals. Trained abstractors retrospectively assessed hospitalized patients treated for CAP between July 1, 2017, and March 31, 2020. Patients were eligible for inclusion if they were adults admitted to general care with a discharge diagnostic code of pneumonia who received antibiotics on day 1 or 2 of hospitalization. Data were analyzed from February to December 2023.
Main Outcomes and Measures Inappropriate diagnosis of CAP was defined using a National Quality Forum–endorsed metric as CAP-directed antibiotic therapy in patients with fewer than 2 signs or symptoms of CAP or negative chest imaging. Risk factors for inappropriate diagnosis were assessed and, for those inappropriately diagnosed, 30-day composite outcomes (mortality, readmission, emergency department visit, Clostridioides difficile infection, and antibiotic-associated adverse events) were documented and stratified by full course (>3 days) vs brief (≤3 days) antibiotic treatment using generalized estimating equation models adjusting for confounders and propensity for treatment.
Results Of the 17 290 hospitalized patients treated for CAP, 2079 (12.0%) met criteria for inappropriate diagnosis (median [IQR] age, 71.8 [60.1-82.8] years; 1045 [50.3%] female), of whom 1821 (87.6%) received full antibiotic courses. Compared with patients with CAP, patients inappropriately diagnosed were older (adjusted odds ratio [AOR], 1.08; 95% CI, 1.05-1.11 per decade) and more likely to have dementia (AOR, 1.79; 95% CI, 1.55-2.08) or altered mental status on presentation (AOR, 1.75; 95% CI, 1.39-2.19). Among those inappropriately diagnosed, 30-day composite outcomes for full vs brief treatment did not differ (25.8% vs 25.6%; AOR, 0.98; 95% CI, 0.79-1.23). Full vs brief duration of antibiotic treatment among patients was associated with antibiotic-associated adverse events (31 of 1821 [2.1%] vs 1 of 258 [0.4%]; P = .03).
Conclusions and Relevance In this cohort study, inappropriate diagnosis of CAP among hospitalized adults was common, particularly among older adults, those with dementia, and those presenting with altered mental status. Full-course antibiotic treatment of those inappropriately diagnosed with CAP may be harmful.
Lower respiratory tract infection, including community-acquired pneumonia (CAP), is the fourth most common cause of medical hospitalization 1 and most common infectious cause of hospitalization in the US. 2 , 3 While many hospitalized patients treated for pneumonia have an infection, inaccurate or inappropriate diagnosis of pneumonia (ie, pneumonia diagnosis when pneumonia is not present) is common. 4 , 5
While some inappropriate diagnosis of CAP is unavoidable due to diagnostic uncertainty when patients are first hospitalized, many patients remain inappropriately diagnosed even on hospital discharge. 6 Inappropriate diagnosis of CAP may harm patients through delayed recognition and treatment of acute (eg, exacerbations of congestive heart failure 7 ), chronic (eg, pulmonary cancer 8 ), or novel diagnoses (eg, pulmonary cancer 8 ) and may lead to unnecessary antibiotic use, 9 , 10 adverse effects, and antibiotic resistance. 11 - 13
Accurately quantifying the proportion of patients treated for CAP who are inappropriately diagnosed has been challenging due to lack of validated definitions. In 2022, we devised a metric to quantify inappropriate diagnosis of CAP that was validated and subsequently endorsed by the National Quality Forum (NQF). 14 - 16 Herein, we apply this metric to a cohort of hospitalized patients treated for CAP in 48 Michigan hospitals to understand epidemiology and outcomes associated with inappropriate CAP diagnosis.
The Michigan Hospital Medicine Safety Consortium (HMS) is a multi-institutional collaborative quality initiative developed to improve care for hospitalized patients. As of January 2023, 69 of 92 (75%) noncritical access, nonfederal hospitals in Michigan participate in HMS and share data on antibiotic use. This study includes 48 hospitals participating during the entire study period.
Because the purpose of HMS is to improve the quality of current care practices, this study received a status designation of not regulated by the University of Michigan Medical School’s institutional review board, which also waived the requirement for informed consent. We followed Strengthening the Reporting of Observational Studies in Epidemiology ( STROBE ) reporting guidelines. 17
As in prior HMS studies, 10 , 18 trained abstractors retrospectively assessed patients hospitalized and treated for pneumonia between July 1, 2017, and March 31, 2020. Patients were eligible for inclusion if they were adults admitted to general care with a discharge diagnostic code of pneumonia 19 who received antibiotics on day 1 or 2 of hospitalization (to specify community-onset infection; see eligible International Statistical Classification of Diseases and Related Health Problems, Tenth Revision, codes in the eAppendix in Supplement 1 ). Patients who had documentation of treatment for an additional infection unrelated to pneumonia, were severely immunocompromised, were pregnant, were admitted for comfort measures, or who left against medical advice were ineligible. Abstractors screened consecutive patients via medical record review 30 days after discharge and included the first eligible patient daily. We excluded patients who received care in an intensive care unit or who received ventilation at any point during the hospitalization, were admitted to hospitals with fewer than 10 qualifying patients, had unknown or missing antibiotic treatment data, received more than 14 days of antibiotic treatment (ie, likely to have an infectious complication), or met all eligibility criteria but had a documented chronic obstructive pulmonary disease (COPD) exacerbation treated with azithromycin or doxycycline alone (ie, no additional pneumonia treatment). See the eAppendix in Supplement 1 for detailed definitions and protocols.
HMS’s data collection and quality assurance procedures have been previously described. 10 , 18 Briefly, trained abstractors entered data from the medical record into a central deidentified repository. Patient data, including demographics, comorbid and concurrent conditions, laboratory studies, vital signs, antibiotic type and duration, and outcomes, were collected from 90 days preceding hospitalization through 30 days following hospitalization. To enable assessment of inappropriate diagnosis, abstractors abstracted (1) any signs (eg, leukocytosis) or symptoms (eg, new/increased cough, sputum production, dyspnea) potentially associated with pneumonia documented in clinician and nursing notes through hospital day 3, including documentation associated with the symptom (eg, “patient coughing,” “family-reported increased cough”) ( Table 1 and eAppendix in Supplement 1 ); (2) radiographic findings (eg, pulmonary consolidation) from chest imaging reports through day 4 of the hospitalization, including details (eg, single lobe, multifocal), and markers of uncertainty (eg, abstraction tool in the eAppendix in Supplement 1 ); and (3) antibiotic administration, including in the emergency department or prescribed at hospital discharge.
To assess associations between antibiotic use and patient outcomes, abstractors collected data from the medical record through 30 days postdischarge. Outcomes included 30-day mortality, readmission, emergency department visit, Clostridioides difficile infection, and/or in-hospital antibiotic-associated adverse events documented by a physician. To reduce risk of missing important outcomes, patients were also called 30 days postdischarge to obtain additional outcome data. Patients discharged to inpatient hospice, an extended care facility, or prison were ineligible for follow-up telephone calls.
The primary outcome of interest was inappropriate diagnosis of CAP. To determine this, we used an NQF-endorsed definition of inappropriate diagnosis of CAP metric, which defines inappropriate diagnosis of CAP as any antibiotic treatment of CAP in a patient with fewer than 2 signs or symptoms of pneumonia or who lacked radiographic findings consistent with pneumonia (eAppendix in Supplement 1 ). 14 - 16 This metric underwent substantial validity and reliability testing, including comparison with physician medical record review and evaluation by a national expert panel. In contrast with the NQF definition, we considered patients not meeting criteria for pneumonia who had bacteremia (n = 25) or positive streptococcal (n = 17) or legionella (n = 10) urine antigen tests to be appropriately diagnosed (2 patients had multiple positive tests).
The secondary outcomes were a composite of 30-day patient outcomes including 30-day all-cause postdischarge mortality, hospital readmission, emergency department visit, C difficile infection, and/or physician-documented antibiotic-associated adverse event. Mortality, readmission, emergency department visit, and C difficile infection included events abstracted from the medical records and patient-reported events obtained from the 30-day postdischarge telephone call. Outcomes were also assessed independently. To assess the association of antibiotic treatment and patient outcomes in patients inappropriately diagnosed, we compared outcomes in patients who received full (>3 days) vs brief (≤3 days) empirical antibiotic treatment. We used 3 days as a cutoff because multiple guidelines recommend reassessing antibiotic necessity in hospitalized patients treated empirically with antibiotics and stopping antibiotics within 48 to 72 hours in the absence of evidence of infection. 20 , 21
Descriptive statistics were used to describe prevalence of inappropriate diagnosis of CAP by hospital, variation of inappropriate diagnosis across hospitals, and characteristics of patients inappropriately diagnosed vs those meeting criteria for CAP. To determine patient characteristics associated with inappropriate diagnosis of CAP, we first compared characteristics of patients with inappropriate diagnosis of CAP vs those meeting criteria for CAP using χ 2 tests for categorical variables or Wilcoxon rank-sum tests for continuous variables. Next, we created a multivariable logit generalized estimating equation (GEE) model with exchangeable correlation structure to account for the clustering of patients within hospitals. To identify variables retained in the final model, we used a stepwise selection procedure based on the Schwarz criterion, 22 starting with all variables with P < .10 in the bivariable analysis. Variables having collinearity (ie, variables between which a strong correlation exists, making it difficult or impossible to estimate their individual regression coefficients reliably [eg, Pneumonia Severity Index and age, altered mental status]) were removed. Variables known to be associated with diagnosis (eg, age, home oxygen) were forced into the final model.
To characterize the associations of antibiotic treatment with 30-day patient outcomes, we first calculated unadjusted odds ratios (ORs) comparing outcomes between full vs brief empirical antibiotic treatment. Next, we identified characteristics of full vs brief empirical antibiotic treatment among patients inappropriately diagnosed with CAP. Finally, we used logit GEE models with exchangeable correlation to account for clustering of patients within hospitals to compare the composite 30-day postdischarge patient outcome (and each individual 30-day postdischarge outcome) in patients inappropriately diagnosed with CAP who received full vs brief empirical antibiotic therapy. Final GEE models were adjusted for baseline covariates known to be associated with outcomes or found to be associated with full vs brief empirical treatment (covariates listed in Table 2 ). Because missing data were minimal among patients (insurance, 3.3%; race, 0.3%; and body mass index, 1.0%), we did not impute missing variables. Results of all multivariable models were expressed as adjusted odds ratios (AORs) with 95% CIs, and P < .05 was considered statistically significant. Analyses were performed in SAS, version 9.4 (SAS Institute). Because disparities related to patient demographics may be present, we report data on sex and race and ethnicity obtained from the medical records (eAppendix in Supplement 1 ).
Between July 1, 2017, and March 1, 2020, 17 290 patients treated for pneumonia were included from 48 Michigan hospitals (eTables 1 and 2 in Supplement 1 ); 2079 patients (12.0%) met NQF criteria for inappropriate diagnosis. The mean (SD) percentage of patients treated for CAP who were inappropriately diagnosed varied by hospital (12.8% [5.4%]), with 30 of 48 hospitals (62.5%) inappropriately diagnosing 10% or more of patients with CAP ( Figure 1 ).
Of the 2079 patients inappropriately diagnosed with CAP, 1531 (73.6%) lacked radiographic criteria, 507 (24.4%) had fewer than 2 signs or symptoms of pneumonia, and 41 (2.0%) met neither criterion. Chest computed tomography was obtained in 42.1% of all patients. Like patients with CAP, those inappropriately diagnosed most often presented with dyspnea and/or cough ( Table 1 ). There was no difference in median length of stay for patients meeting vs not meeting criteria for CAP. On bivariable analysis, compared with patients with CAP, patients inappropriately diagnosed with CAP were older (eg, ≥75 years old) and more likely to have public insurance, present with altered mental status, have decreased mobility (eg, bedridden, wheelchair bound) on admission, or have had an inpatient hospitalization within the previous 90 days ( Table 3 ). Regardless of living arrangement on admission (eg, home vs skilled nursing facility), patients inappropriately diagnosed with CAP were more often discharged to a skilled nursing facility. On multivariable analysis, compared with patients with CAP, inappropriately diagnosed patients were older (AOR, 1.08; 95% CI, 1.05-1.11; P < .001 per decade) and more likely to have dementia (AOR, 1.79; 95% CI, 1.55-2.08; P < .001) or present with altered mental status without dementia (AOR, 1.75; 95% CI, 1.39-2.19; P < .001) ( Figure 2 ).
Patients inappropriately diagnosed with CAP received a median (IQR) of 7.0 (5.0-9.0) days of antibiotics, including 4.0 (3.0-5.0) inpatient days. The majority of inappropriately diagnosed patients (1821 of 2079 [87.6%]) received a full course of antibiotics. On bivariable analysis, patients treated with full vs brief, empirical antibiotics were more likely to be White (75.0% vs 66.7%; P = .004), have a history of COPD (44.6% vs 35.3%; P = .005), or present with a concurrent COPD exacerbation (28.2% vs 15.1%; P < .001) (eTable 3 in Supplement 1 ). On multivariable analysis, concurrent COPD exacerbation was associated with a full vs brief course of antibiotics (AOR, 1.74; 95% CI, 1.13-2.68; P = .01), while hemodialysis and negative procalcitonin (vs those not tested) were associated with brief courses (AOR, 0.29; 95% CI, 0.20-0.41; P < .001; and AOR, 0.47; 95% CI, 0.32-0.68; P < .001, respectively) (eTable 4 in ( Supplement 1 ).
The composite outcome of any 30-day postdischarge adverse event occurred in 536 of 2079 patients (25.8%) inappropriately diagnosed with CAP. Full vs brief empirical antibiotic therapy in patients inappropriately diagnosed with CAP was not associated with 30-day composite outcomes in either unadjusted or adjusted analyses (AOR, 1.00; 95% CI, 0.78-1.29; P = .99; Table 2 ). For individual 30-day outcomes, only physician-documented antibiotic-associated adverse events were associated with full vs brief therapy on unadjusted (31 of 1821 [2.1%] vs 1 of 258 [0.4%]) and adjusted (AOR, 7.23; 95% CI, 1.18-44.35; P = .03) analyses.
We applied a novel, validated metric to a unique dataset of manually collected data from hospitalized patients treated for CAP and found that approximately 1 in 8 patients were inappropriately diagnosed. Most hospitals inappropriately diagnosed more than 10% of patients. Patients at highest risk of inappropriate diagnosis were older, had dementia, or presented with altered mental status. Overall, nearly 88% of patients inappropriately diagnosed with CAP received a full antibiotic course, which was associated with physician-documented antibiotic-associated adverse events.
Why might physicians inaccurately diagnose CAP? First, because CAP is common, physicians are at high risk for cognitive biases such as availability bias (ie, the tendency to make decisions based on information that comes most readily to mind). 23 , 24 Second, CAP symptoms are nonspecific and may overlap with other cardiopulmonary diseases (eg, congestive heart failure exacerbation), making diagnosis difficult. Given poor outcomes associated with CAP, in the setting of uncertainty, health care professionals may favor overtreatment rather than potentially missing a CAP diagnosis. 25 - 27 Third, historical quality metrics imposed by organizations such as The Joint Commission (eg, requiring antibiotics within 6 hours of presentation) may have unintentionally led to more inappropriate diagnoses of CAP. 28 , 29 These measures, in place in the 2000s and 2010s, may continue to affect health care professional practice behaviors related to diagnosis. Finally, previously published data show a correlation between inappropriate diagnosis of CAP and inappropriate diagnosis of urinary tract infection at the hospital level, 6 suggesting that local policies, procedures, or culture may affect accurate diagnosis.
Unsurprisingly, we found that older patients, particularly those presenting with impaired cognition, had higher odds of inappropriate diagnosis. In one study of more than 45 000 patients, the rate of CAP ranged from 18.2 cases per 1000 patient-years among those aged 65 to 69 years to 52.3 cases per 1000 patient-years among those 85 years and older. 30 The high underlying prevalence of CAP in older populations likely fuels previously discussed cognitive biases. Additionally, patients with cognitive impairment may have difficulty communicating. As a result, physicians may anchor on nonspecific data (eg, white blood cell count, fever in isolation) to make the diagnosis of CAP. Older adults—particularly those with dementia or altered mental status—are also more likely to be inappropriately diagnosed with other conditions, such as urinary tract infection (ie, asymptomatic bacteriuria). 31 While altered mental status may be a sign of infection, including severe infection, it has a broad differential diagnosis (eg, polypharmacy, pain, dehydration), and anchoring on CAP may delay proper diagnosis and management. Finally, because older patients with CAP typically experience worse health outcomes, 32 there may be an increased tendency to rapidly diagnose and treat presumed CAP, leading to higher rates of inappropriate diagnosis.
For patients at high risk of poor outcomes from delayed treatment of CAP, it may be pertinent to empirically prescribe antibiotics while finishing diagnostic evaluation. In these populations, guidelines recommend reconsideration, de-escalation, and cessation of antibiotics within 48 to 72 hours once infection has been ruled out. 20 , 21 In the present study, we found little evidence of antibiotic cessation. Rather, patients empirically receiving antibiotic therapy for presumed CAP typically received a full antibiotic course. Compared with brief empirical antibiotics, we found that full courses were associated with antibiotic-associated adverse events. Making causal inferences about this association is complicated by the fact that length of treatment is not a baseline characteristic at the time of inappropriate diagnosis but a postdiagnosis observation and is thus endogenous (or likely to be determined in part by other predictors in the model). Nevertheless, longer durations of antibiotics are known to be associated with increased morbidity 9 and delayed diagnosis of underlying conditions. Particularly, older patients who may have more comorbid diseases, or who are more likely to be taking medications that interact with antibiotics, are at high risk of harm from antibiotics and delayed diagnosis. 33 - 35
This study has limitations. First, because the assessment of diagnostic error relied on medical record review with limitations associated with documentation of alternative causes for signs, symptoms, or radiographic findings, we likely underestimate inappropriate diagnosis of CAP. Including inappropriately diagnosed patients within the CAP group may bias the risk factor analysis toward the null. Also, omissions in documentation of patient-documented symptoms could have resulted in misclassification of patients with appropriate diagnosis of CAP as inappropriate. 36 , 37 Second, as mentioned previously, the finding that duration was associated with physician-documented antibiotic-associated adverse events does not prove causation, and, because few patients received brief antibiotic durations, the statistical power was limited, resulting in wide confidence intervals. Furthermore, documentation of antibiotic-associated adverse events may have influenced outcomes by lack of physician documentation or lack of attribution of symptoms to antibiotics, especially for those receiving a short duration of therapy. Third, bias from unmeasured confounders may exist. Fourth, we were unable to assess outcomes related to missed or delayed diagnosis or capture alternative diagnoses for those inappropriately diagnosed. Fifth, the ideal acceptable amount of inappropriate diagnosis of CAP is unclear. As the metric was designed to favor specificity over sensitivity (ie, ensuring a case classified as “inappropriate diagnosis” was inappropriately diagnosed), we believe that the proportion of patients inappropriately diagnosed should be close to, but not exactly, zero. Finally, diagnostic criteria for nonspecific CAP and findings such as fatigue or altered mental status may be attributed as symptoms of CAP. Although we used the best definition of CAP available, and included many objective criteria, inability of patients with altered mental status to convey symptom data remains a limitation.
This study also has several strengths. First, the diversity of hospitals improves generalizability. Second, abstractors underwent extensive, centralized training to ensure standardized data collection, and data were audited at routine intervals to ensure data validity. Third, the definition of inappropriate diagnosis was tested and subsequently endorsed by the NQF, increasing the validity of findings.
This cohort study has important clinical and policy implications. Because hospitalizations for CAP are common, so too are inappropriate diagnoses of CAP. Risks of inappropriate diagnosis are not uniform across populations—already highly vulnerable groups are at highest risk of inappropriate diagnosis. These same vulnerable populations are also most likely to be affected by antibiotic-associated adverse events and resulting morbidity. Thus, balancing harms of underdiagnosis and overdiagnosis of CAP remains essential.
Accepted for Publication: January 4, 2024.
Published Online: March 25, 2024. doi:10.1001/jamainternmed.2024.0077
Open Access: This is an open access article distributed under the terms of the CC-BY License . © 2024 Gupta AB et al. JAMA Internal Medicine .
Corresponding Author: Ashwin B. Gupta, MD, Medicine Service, VA Ann Arbor Healthcare System, 2215 Fuller Rd, Mail Code 111, Ann Arbor, MI 48105 ( [email protected] ).
Author Contributions: Mr Ratz had full access to all of the data in the study and takes responsibility for the integrity of the data and the accuracy of the data analysis.
Concept and design: Gupta, Malani, Patel, Chopra, Vaughn.
Acquisition, analysis, or interpretation of data: Gupta, Flanders, Petty, Gandhi, Pulia, Horowitz, Ratz, Bernstein, Malani, Hofer, Basu, Vaughn.
Drafting of the manuscript: Gupta, Horowitz, Ratz, Hofer, Vaughn.
Critical review of the manuscript for important intellectual content: Gupta, Flanders, Petty, Gandhi, Pulia, Horowitz, Bernstein, Malani, Patel, Hofer, Basu, Chopra, Vaughn.
Statistical analysis: Petty, Ratz, Hofer, Basu.
Obtained funding: Gupta, Flanders, Chopra, Vaughn.
Administrative, technical, or material support: Horowitz.
Supervision: Flanders, Gandhi, Malani, Chopra, Vaughn.
Conflict of Interest Disclosures: Dr Bernstein reported grants from the Agency for Healthcare Research and Quality and the Centers for Disease Control and Prevention outside the submitted work. No other disclosures were reported.
Funding/Support: This research was funded in part by the Gordon and Betty Moore Foundation through grants GBMF8839 and 8839.01 to Drs Gupta and Vaughn. This project was also supported by grant funding from the Claude D. Pepper Older Americans Independence Center and the Blue Cross Blue Shield of Michigan and Blue Care Network as part of the Blue Cross Blue Shield of Michigan Value Partnerships program. Dr Vaughn is supported by grant number K08HS026530 from the Agency for Healthcare Research and Quality.
Role of the Funder/Sponsor: The funders had no role in the design and conduct of the study; collection, management, analysis, and interpretation of the data; preparation, review, or approval of the manuscript; and decision to submit the manuscript for publication.
Disclaimer: The authors are solely responsible for this article’s contents, findings, and conclusions, which do not necessarily represent the views of the Agency for Healthcare Research and Quality or the US Department of Veterans Affairs.
Data Sharing Statement: See Supplement 2 .
- Register for email alerts with links to free full-text articles
- Access PDFs of free articles
- Manage your interests
- Save searches and receive search alerts

An official website of the United States government
The .gov means it’s official. Federal government websites often end in .gov or .mil. Before sharing sensitive information, make sure you’re on a federal government site.
The site is secure. The https:// ensures that you are connecting to the official website and that any information you provide is encrypted and transmitted securely.
- Publications
- Account settings
Preview improvements coming to the PMC website in October 2024. Learn More or Try it out now .
- Advanced Search
- Journal List
- Rev Esp Quimioter
- v.35(Suppl 1); 2022

Top-ten papers in pneumonia (2020-2021)
Despite the fact that the last year has been marked by the SARS-CoV-2 pandemic, there have been many articles published on non-COVID pneumonia. Making the selection has not been easy, having based on those articles that we think can bring us some novelty and help in clinical practice. We have divided the selection into seven sections: patient severity, diagnosis, treatment, ventilation, novelties in the guidelines, fungal infection and organ donation.
INTRODUCTION
In a year marked by the SARS-CoV-2 pandemic, we thought that publications on non-COVID-19 pneumonia would be scarce, however, after a first review on severe pneumonia, nosocomial pneumonia and ventilator associated pneumonia (VAP), we found more than 3000 articles. We have made the selection based on those articles that we think may provide us with something new and that may help us in our healthcare practice, and, obviously, it does not have to coincide with what any of our readers would have made.
We have structured the selection in 7 parts:
- Patient severity
- Ventilation
- What´s new in the guides
- Fungal infection
- Organ donation
PATIENT SEVERITY
We begin the review from the arrival of the patient to the emergency room, assessing the severity of the patient and the predictors of mortality, to decide where we admit the patient.
In the first article, Carmo et al. [ 1 ] assess whether pneumonia severity scores adequately predict mortality in critically ill patients admitted with pneumonia. To do this, they conduct a three-year prospective observational cohort study (2015-2018) in which they study both the intensive care unit (ICU) severity scores (SAPS 3, qSOFA) and the pneumonia severity scores (CURB-65 and CRB-65). With the variables related to mortality in the multivariate analysis, they elaborate a prognostic score, the pneumonia shock score (PSS) ( table 1 ), so that a PSS ≥ 3 carries a mortality > 26%. They compare this score with SAPS 3, CURB-65, CRB-65 and qSOFA, and observe that it is the one with the best sensitivity, and a higher specificity than pneumonia severity scores. They then use an external validation cohort where they get the same results. The authors conclude that the PSS is a new tool that can help select patients who should be admitted to the ICU, and offers an alternative prognostic tool with a great performance in predicting mortality.
Pneumonia Shock Score
In the letter to the editor about this article, Reyes et al [ 2 ] study this new score as a long-term predictor, and note that PSS is superior to SAPS-3, CURB-65, CRB-65 and qSOFA in predicting hospital mortality, but that as a long-term predictor its sensitivity and specificity decreases dramatically.
In the following article, Gautam et al [ 3 ] consider whether procalcitonin (PCT) should be used as a marker of bacterial co-infection during a viral respiratory infection. They conducted an 18-month retrospective cohort study comparing patients with pure viral respiratory infection with patients with bacterial co-infection observing that the latter have a higher PCT, greater severity, and higher mortality. However, when both cohorts are matched by severity, the specificity of PCT for bacterial coinfection decreases (from 72% to 61%). They then develop a murine model where they infect mice with influenza viruses and see that PCT rises in relation to markers of severity. The authors conclude that PCT is elevated during pure viral infection in proportion to disease severity. Data from the study suggest that PCT is a better indicator of disease severity than bacterial co-infection during viral respiratory infection.
On the basis that there is no gold standard for the diagnosis of nosocomial pneumonia, the authors of this review [ 4 ] review all the tools we have. They begin with imaging techniques, where the low sensitivity and specificity of the chest X-ray stands out, the greater sensitivity of the thoracic computed tomography (CT) and the fact that their findings are not specific, ending the section with lung ultrasound (LUS), which have a greater sensitivity (95%) and a high specificity (91,3%) when they are performed serially.
Within the microbiological techniques we have those based on culture (highlighting the importance of colony counting to distinguish between colonization and infection), and molecular diagnostic techniques, highlighting their speed and their high sensitivity and specificity, differentiating between the different existing platforms.
Finally, the authors comment on some biomarkers remarking that they are not recommended in the nosocomial pneumonia guidelines due to their lack of precision; and name metabolomics and artificial intelligence as the immediate future in diagnosis.
Given the current importance of LUS as a point-of-care, the following article is a study conducted by Haaksma et al. [ 5 ] whose objectives were to determine the diagnostic accuracy of dynamic air bronchogram and color Doppler imaging for the diagnosis of pneumonia in patients with consolidation on chest radiograph. This is a prospective diagnostic accuracy study carried out in two periods (September 2018 – January 2020 and September 2020 – December 2020) in which patients with chest X-ray with some consolidations are included, performing a LUS within 24 hours of the X-ray, and another follow-up at 72h., and with the results obtained they elaborate a decision tree. They observe that the air bronchogram has a specificity of 99% and a positive predictive value (PPV) of 96%, with a low sensitivity, on the contrary, the color Doppler has a sensitivity of 90%, with a negative predictive value (NPV) of 90%, while the elaborate decision tree presents a sensitivity and specificity of 86%, and a NPV of 90%. When comparing their results with the BLUE protocol, and with the sCPIS and lusCPIS scores, they conclude that in ICU patients with pulmonary consolidation on chest X-ray, an extended lung ultra-sound protocol based on the evaluation of air bronchograms and measurements of pulsatile flow is an accurate and directly bedside available tool to differentiate pneumonia and atelectasis. It outperforms standard lung ultrasound and clinical scores.
Within the section of treatment, the first work selected is that of Mahmood and Shorr [ 6 ] in which they review the pharmacokinetics and pharmacodynamics of antibiotics. Within the pharmacokinetics, the importance of the penetration of the drug into the lung in the case of pneumonia stands out, a fact that we lack in most antibiotics of routine use. Regarding pharmacodynamics, they emphasize the increased renal clearance, defined as GRF > 130 ml/min/ 1.73 m 2 , which appears in more than 30% of critical patients, and in which the estimation of glomerular filtration rate may be underestimated. In patients with increased renal clearance, antibiotics with renal metabolism are eliminated more quickly, so we are underdosing antibiotics (especially β-lactams, carbapenems and vancomycin).
We are not yet clear whether we should use corticosteroids in the treatment of pneumonia. To try to shed some light on the subject we have chosen the article by Póvoa et al [ 7 ] whose objective is to evaluate the evidence and recommendations of the prescription of corticosteroids as an adjuvant treatment in severe community acquired pneumonia (CAP). They conclude that only in moderate-severe Pneumocistys jiroveci pneumonia in HIV patients have corticosteroids been shown to decrease mortality, and in varicella pneumonia they have a positive influence on prognosis. In the case of other pathogens, corticosteroids have been shown to increase mortality (influenza pneumonia), or a clear effect on prognosis has not been defined. With the available evidence its use in severe CAP is not recommended in the latest published guidelines. In addition, it is necessary to improve the characterization of corticosteroids in terms of type and efficacy, dose, route of administration, duration of treatment, and possible interactions with other treatments administered such as macrolides.
VENTILATION
In recent years, there has been a great development of both non-invasive ventilation and the use of high flow, in ICUs and in hospitalization wards. Cutuli et al. [ 8 ] reviews these two types of oxygen therapy in CAP. In this work they make an interesting review about the pathophysiology of acute respiratory failure (hypoxemic and hypercapnic) indicating that they can provide us in each of them with these types of ventilation and when we should use each of them. Highlight the importance of early identification of treatment failure with non-invasive respiratory support, to prevent delayed orotracheal intubation and protective invasive mechanical ventilation.
WHAT´S NEW IN THE GUIDES
Although in the last year most management and treatment publications and guidelines have focused on SARSCoV-2, some guidelines on CAP and nosocomial pneumonia have been published. In this paper, Martin-Loeches and Torres [ 9 ] highlight recent advances in guidelines for the treatment of severe CAP. Regarding the etiology, they emphasize the importance of their knowledge through molecular techniques with the main objective of adjusting antibiotic treatment in order to reduce treatment failure and overuse of antimicrobials. They emphasize the importance of prognostic scores to decide the location of the patient, so that the best score to decide hospital admission is the PSI, while for admission to the ICU the major and lower criteria of the IDSA/ATS should be used. Regarding the duration of antibiotic treatment, a balance should be made between clinical success and the need to avoid the development of antibiotic resistance. Finally, biomarkers should be a mainstay in the management of patients with CAP, especially in severe forms, to decrease treatment failure.
FUNGAL INFECTION
Within a review on severe pneumonia, we cannot forget about fungal pneumonias. In this section we highlight the work carried out by Loughlin et al. [ 10 ], whose objective was to estimate the prevalence of Aspergillus infection in ventilated patients, not neutropenic, with suspected pneumonia associated with mechanical ventilation. To this end, they carried out 2 multicenter prospective studies between February 2012 and September 2016, in patients from whom they obtained serum and BAL mycological samples, diagnosing them with probable aspergillosis according to clinical, radiological and mycological criteria. Of a total of 194 patients, they identified 12.4% who met criteria for probable aspergillosis, with higher mortality in the ICU than those who did not meet the diagnostic criteria, and with a longer stay in the ICU. As discussed in the editorial [ 11 ], aspergillus is a more frequent cause of VAP in non-immunosuppressed patients than we think, and by applying non-culture-based diagnostic methods such as galactomannan in BAL and serum, some additional cases can be diagnosed.
ORGAN DONATION
Initially, choosing one last article among the many that have been published this last year on other topics such as pneumonia in immunocompromised, prevention, microbiota, omics, artificial intelligence, etc. was not easy, until we found the next work because of the importance we consider it may have. The work of Poignant et al. [ 12 ] is the only one we have found on pneumonia and organ donation. This is a 4-year multicenter observational retrospective cohort study (January 2013 - December 2016) whose objectives were to describe the clinical and microbiological characteristics of bacterial pneumonia in brain-dead patients and to assess the impact of pneumonia on lung suitability for extraction in patients without initial contraindication to donation. The results show that among the patients proposed for lung donation, 27,4% presented aspiration pneumonia, and 8,2% had early VAP. In the multivariate analysis, the independent predictors of pneumonia in brain-dead patients were age, anoxic brain damage, aspiration before or during tracheal intubation, and no antimicrobial use at day 1. Among the authors’ conclusions, it stands out that the initiation of antibiotic prophylaxis on the first day of stay in the ICU in comatose patients with severe brain damage could increase the current pool of lung donors.
CONFLICTS OF INTEREST
Authors declare no conflicts of interest
Challenges for a broad international implementation of the current severe community-acquired pneumonia guidelines
Affiliations.
- 1 D'Or Institute for Research and Education (IDOR), Rio de Janeiro, RJ, Brazil. [email protected].
- 2 Postgraduate Program of Internal Medicine, Federal University of Rio de Janeiro, Rio de Janeiro, UFRJ, Brazil. [email protected].
- 3 NOVA Medical School, CHRC, New University of Lisbon, Lisbon, Portugal.
- 4 Research Unit of Clinical Epidemiology, Institute of Clinical Research, Odense University Hospital, University of Southern Denmark Centre for Clinical Epidemiology, Odense, Denmark.
- 5 Department of Intensive Care, Hospital de São Francisco Xavier, CHLO, Lisbon, Portugal.
- 6 Pandemic Science Hub and Centre for Inflammation Research, University of Edinburgh, Edinburgh, UK.
- 7 NICS-MORU, Colombo, Sri Lanka.
- 8 Division of Infectious Diseases, Department of Internal Medicine, University of Nebraska Medical Center, Omaha, NE, USA.
- 9 Anesthesia and Critical Care, Makerere University College of Health Sciences, P.O. Box 7072, Kampala, Uganda.
- 10 Division of Pulmonary, Critical Care and Sleep Medicine, Department of Medicine, University of California, La Jolla, San Diego, CA, USA.
- 11 Department of Intensive Care, Alfred Health, Commercial Road3004, Prahran, VIC, Australia.
- 12 The Australian and New Zealand Intensive Care Society (ANZICS) Centre for Outcome and Resource Evaluation, Camberwell, Australia.
- 13 Pneumology Department, Hospital Universitari Vall d'Hebron, Vall d'Hebron Institut de Recerca (VHIR), Vall d'Hebron Barcelona Hospital Campus, CIBERES, Barcelona, Spain.
- 14 Division of Infectious Diseases, Department of Diagnostics and Public Health, University of Verona, Verona, Italy.
- 15 Hospital Interzonal de Agudos General San Martín, Servicio de Terapia Intensiva, Buenos Aires, Argentina.
- 16 CHU de Poitiers, Médecine Intensive Réanimation, Poitiers, France.
- 17 INSERM, CIC-1402, IS-ALIVE, Faculté de Médecine Et de Pharmacie de Poitiers, Université de Poitiers, Poitiers, France.
- 18 Norton Infectious Diseases Institute, Norton Healthcare, Louisville, KY, USA.
- 19 University of Louisville, Louisville, KY, USA.
- 20 Unisabana Center for Translational Science, School of Medicine, Universidad de La Sabana, Chia, Colombia.
- 21 Pandemic Sciences Institute, University of Oxford, Oxford, UK.
- 22 Servei de Medicina Intensiva, Parc Taulí Hospital Universitari, Institut de Recerca Part Taulí - I3PT, Parc del Taulí 1, 08028, Sabadell, Spain.
- 23 Departament de Medicina, Universitat Autònoma de Barcelona, Bellaterra, Spain.
- 24 Centre de Réanimation, CHU de Lille, 59000, Lille, France.
- 25 Team Fungal Associated Invasive and Inflammatory Diseases, Lille Inflammation Research International Center, Université de Lille, INSERM U995, Lille, France.
- 26 Department of Internal Medicine, Medical School and University Hospital, Universidade Federal de Minas Gerais, Belo Horizonte, Brazil.
- 27 Critical Care Department, Programa de Pós-Graduação em Ciencias Pneumologicas, Hospital de Clinicas de Porto Alegre, Universidade Federal do Rio Grande do Sul, Porto Alegre, Brazil.
- 28 Department of Intensive Care Medicine, Multidisciplinary Intensive Care Research Organization, St. James's University Hospital, Trinity Centre for Health Sciences, Dublin, Ireland.
- PMID: 38546855
- DOI: 10.1007/s00134-024-07381-z
Severe community-acquired pneumonia (sCAP) remains one of the leading causes of admission to the intensive care unit, thus consuming a large share of resources and is associated with high mortality rates worldwide. The evidence generated by clinical studies in the last decade was translated into recommendations according to the first published guidelines focusing on severe community-acquired pneumonia. Despite the advances proposed by the present guidelines, several challenges preclude the prompt implementation of these diagnostic and therapeutic measures. The present article discusses the challenges for the broad implementation of the sCAP guidelines and proposes solutions when applicable.
Keywords: Acute respiratory failure; Guidelines; ICU; Lower respiratory tract infection; Sepsis; Severe community-acquired pneumonia.
© 2024. Springer-Verlag GmbH Germany, part of Springer Nature.
Publication types
- New Terms of Use
- New Privacy Policy
- Your Privacy Choices
- Closed Caption Policy
- Accessibility Statement
This material may not be published, broadcast, rewritten, or redistributed. ©2024 FOX News Network, LLC. All rights reserved. Quotes displayed in real-time or delayed by at least 15 minutes. Market data provided by Factset . Powered and implemented by FactSet Digital Solutions . Legal Statement . Mutual Fund and ETF data provided by Refinitiv Lipper .
Pneumonia misdiagnoses are common among hospitalized adults, study finds: There are 'implications'
Experts warn of the dangers of unnecessary antibiotic treatments: ‘adverse effects’.

Fox News Flash top headlines for March 31
Fox News Flash top headlines are here. Check out what's clicking on Foxnews.com.
Adults who are admitted to the hospital are often inappropriately diagnosed with — and treated for — pneumonia, new research suggests.
These misdiagnosed adults almost always receive a full course of antibiotics that may not be necessary, according to a new study published in JAMA Internal Medicine.
Among older adults who were treated for community-acquired pneumonia in the hospital, 12% were misdiagnosed, researchers found.
ANTIBIOTIC RESISTANCE IS ON THE RISE, DOCTOR WARNS: ‘THIS IS AN ENORMOUS PROBLEM’
Among those patients who were misdiagnosed, 88% received a full antibiotic treatment course — with 2% of those experiencing adverse side effects from the medication, the study also found.
The Centers for Disease Control and Prevention (CDC) estimates that about 47 million courses of antibiotics are prescribed annually in the U.S. for infections that don’t require that course of action.
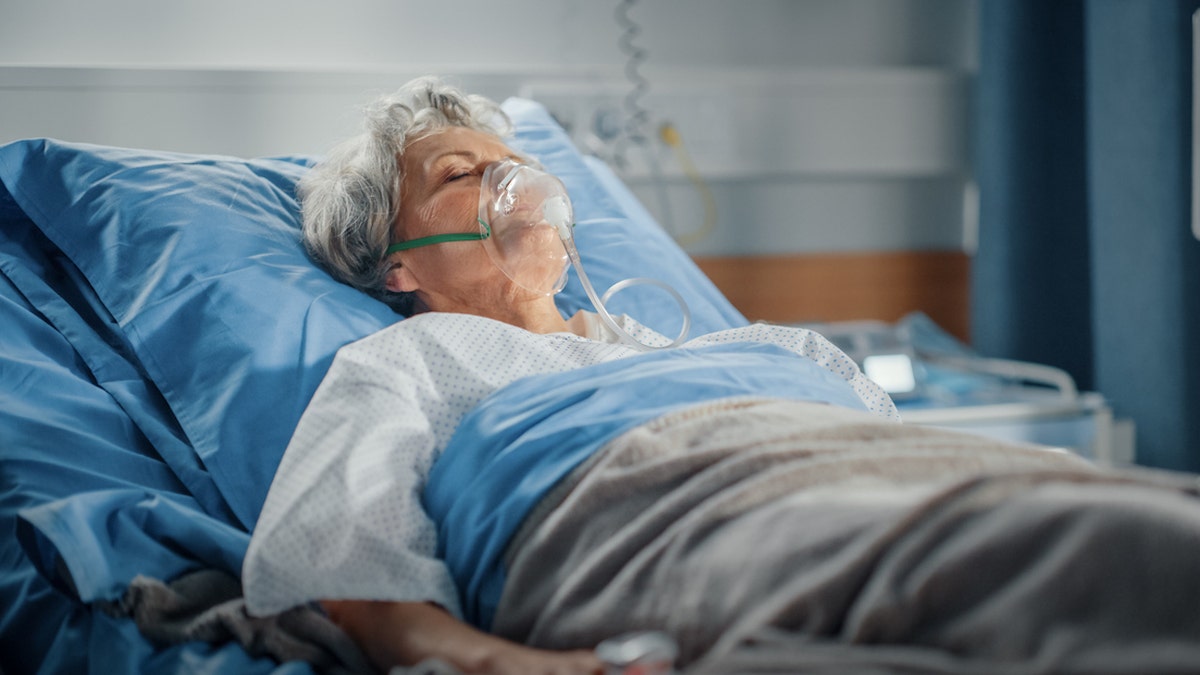
Among older adults who were treated for community-acquired pneumonia in the hospital, 12% were misdiagnosed, researchers found. (iStock)
Overuse of antibiotics can lead to resistance, meaning that the bacteria becomes strong enough to withstand the medication.
Each year, over 23,000 deaths occur in the U.S. due to antibiotic resistance, per the CDC.
This can limit treatment options and make it more difficult for doctors to treat and cure infections.
Main types of pneumonia
There are two primary types of pneumonia: community-acquired and hospital-acquired.
Community-acquired pneumonia (CAP) describes a type of pneumonia in a patient who has not recently been hospitalized.
In these scenarios, patients acquire the lung infection while in a community setting, according to the National Institutes of Health (NIH).
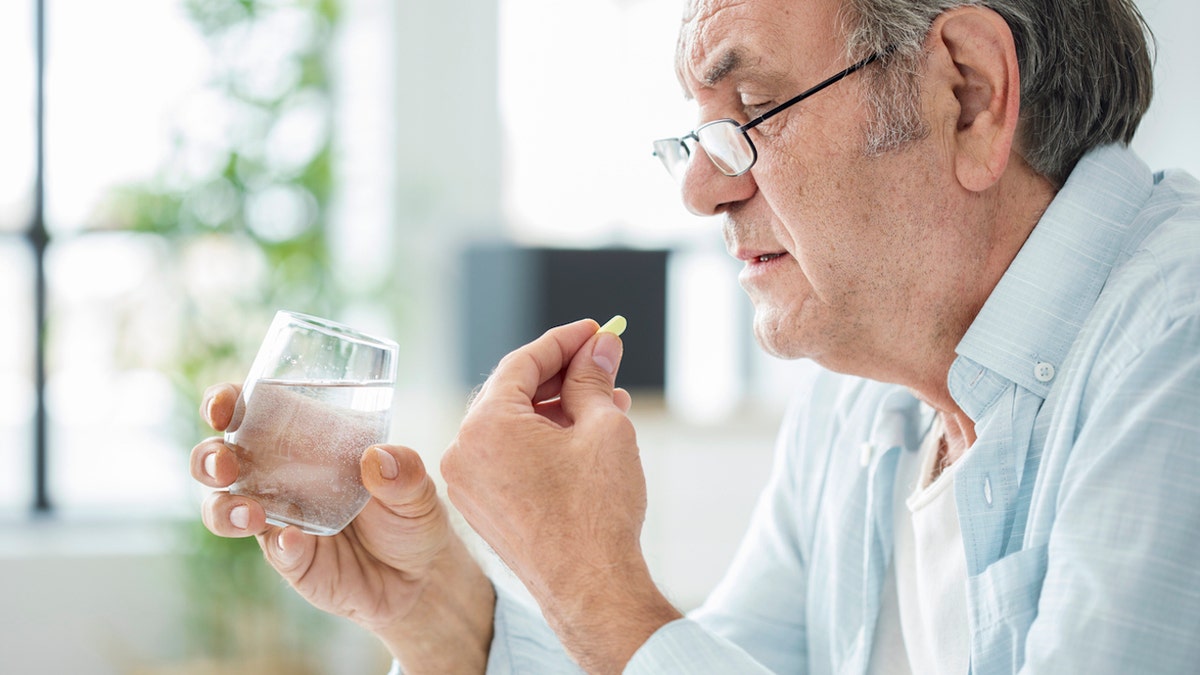
Overuse of antibiotics can lead to resistance, meaning the bacteria becomes strong enough to withstand the medication. (iStock)
Hospital-acquired pneumonia occurs after patients are admitted to the hospital.
They don’t have the infection when they're first admitted, but acquire it later after being exposed to bacteria in the hospital setting.
Many patients with community-acquired pneumonia are treated without needing to be hospitalized.
AMID CHILDHOOD PNEUMONIA OUTBREAKS, INFECTIOUS DISEASES EXPERT REVEALS KEY FACTS ABOUT ‘WHITE LUNG SYNDROME’
Certain individuals with CAP, however, may require hospitalization if they have serious medical problems, severe symptoms, are unable to eat or drink, are over age 65, or are taking antibiotics but not improving, the NIH noted.
CAP is one of the most common causes of hospitalization in the U.S., according to the CDC.
What the study found
Researchers at the Veterans Affairs Ann Arbor Healthcare System and the University of Michigan Medical School – led by lead researcher Ashwin B. Gupta, M.D., clinical associate professor at the University of Michigan Health – examined patient records from the Michigan Hospital Medicine Safety Consortium, an initiative to improve the hospitalized care of patients .
Patients in the study were admitted to the hospital for general care and later were diagnosed with pneumonia and were treated with antibiotics on the first or second day of admission.
They analyzed approximately 17,000 adults who were hospitalized between July 1, 2017, and March 31, 2020, at 48 hospitals in Michigan.
DAILY MARIJUANA SMOKERS FACE HIGHER RISK OF HEART ATTACK, STROKE, SAYS AMERICAN HEART ASSOCIATION STUDY
Participants were considered "inappropriately diagnosed" if they had fewer than two symptoms of pneumonia or if their chest X-rays tested negative for the infection. (In other words, if they got the diagnosis but didn't have two symptoms or positive X-rays, the diagnosis was wrong.)
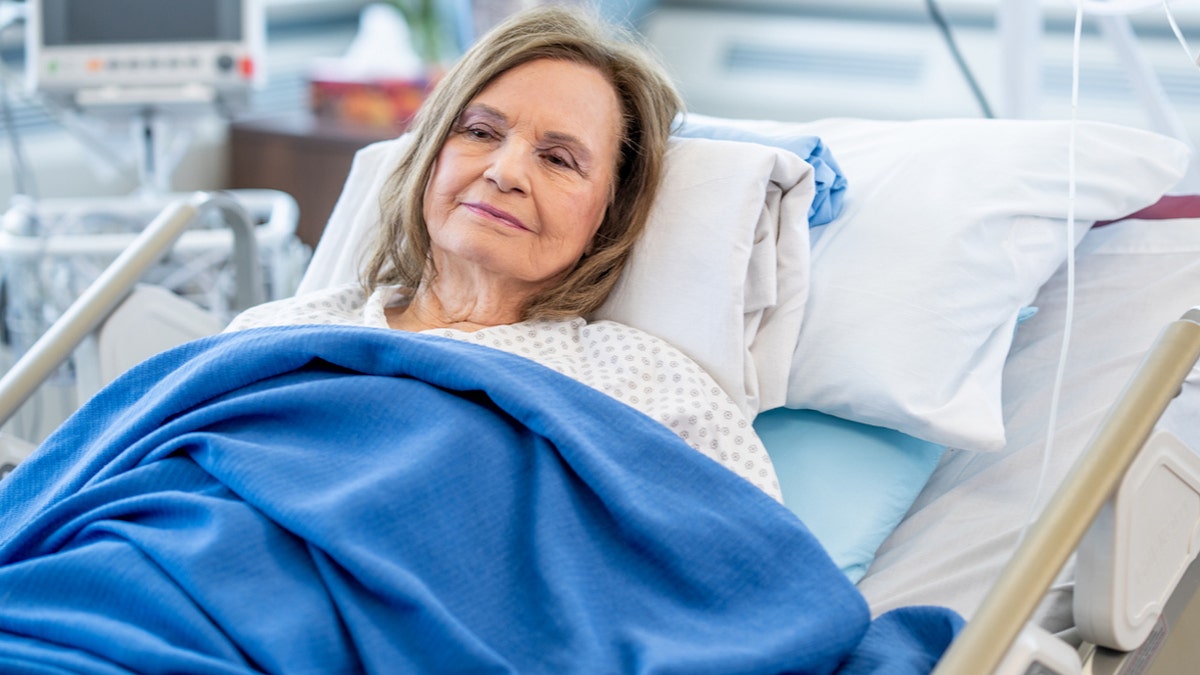
Patients in the study were admitted to the hospital for general care and later were diagnosed with pneumonia and were treated with antibiotics on the first or second day of admission. (iStock)
The study found that an inappropriate diagnosis of community-acquired pneumonia among hospitalized patients is common – especially among certain groups, such as older adults or those with dementia and altered mental status.
Those who were inappropriately diagnosed almost always received a full course of antibiotics, the researchers noted.
Common symptoms
A classic diagnosis of pneumonia involves typical symptoms — such as cough, fever, chills and shortness of breath — combined with radiographic evidence on a chest X-ray , according to experts.
HEALTH CARE IS ‘OVERWHELMINGLY COMPLEX’ FOR OLDER ADULTS, EXPERTS SAY: ‘EVER-INCREASING HURDLE’
The X-ray is necessary because many symptoms can overlap with other conditions.
"When you present to the hospital with cough or shortness of breath, for example, the list of potential etiologies is quite broad," lead researcher Gupta told Fox News Digital via email.
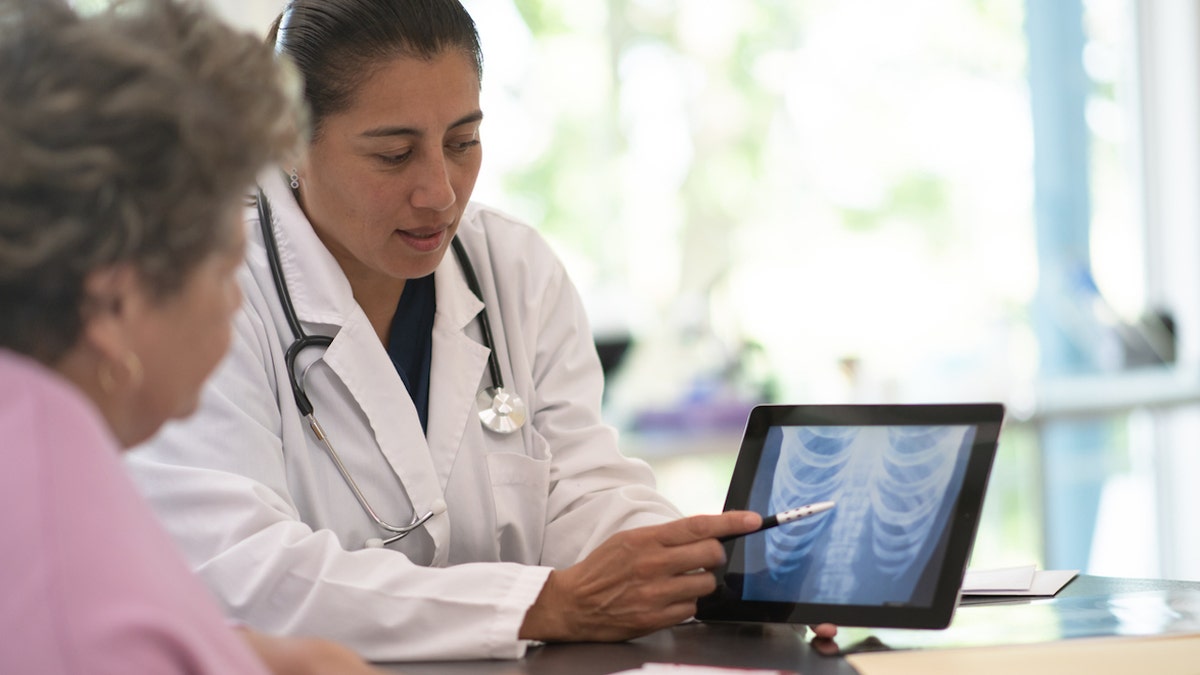
A classic diagnosis of pneumonia involves typical symptoms — such as cough, fever, chills and shortness of breath — combined with radiographic evidence on a chest X-ray. (iStock)
"We found that often, those who likely have an underlying non-pneumonia etiology (e.g., congestive heart failure ) are being classified as having pneumonia and are treated as such," he continued.
"Inappropriate diagnosis and treatment of pneumonia may have implications, such as delays in understanding the actual problem or adverse effects related to antibiotic treatment."
Dangers of unnecessary antibiotics
"Inappropriate diagnosis is not benign," the lead researcher warned.
"It means that the understanding of the underlying cause of a patient’s illness may be delayed or missed altogether."
He added, "Antibiotic therapy is not benign, either — our study demonstrated that full durations of antibiotic therapy in this population are associated with antibiotic-associated adverse events."
"Inappropriate diagnosis … means the underlying cause of a patient’s illness may be delayed or missed altogether."
Outside experts also warned of the risk.
"I think the study is alarming in that many patients (one out of eight, or over 10%) are receiving inappropriate diagnoses of pneumonia and being given full treatment courses," Dr. Scott Roberts, associate medical director of infection prevention at Yale School of Medicine in New Haven, Connecticut, told Fox News Digital.
He was not involved in the study.
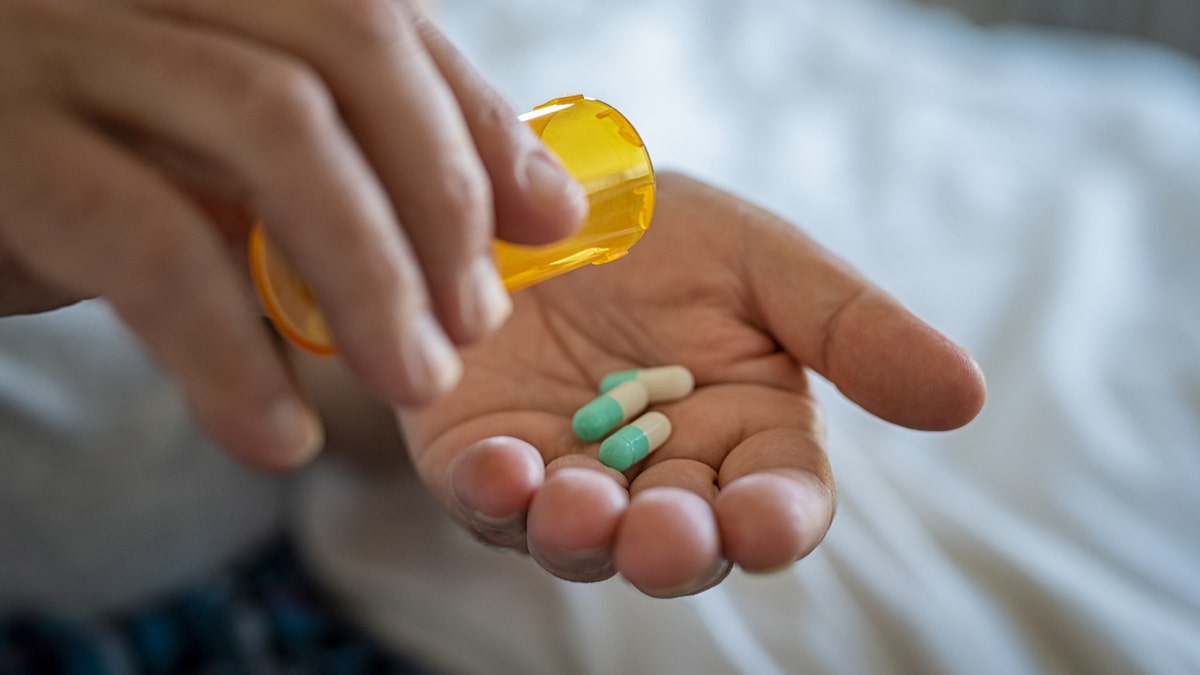
Common side effects of antibiotic use include an itchy, full-body rash; nausea and diarrhea; and yeast infections, according to the CDC. (iStock)
"This is especially problematic because the patient population where this is occurring are the ones most at risk for the adverse events associated with antibiotic use."
Roberts emphasized the need for providers to diagnose conditions accurately before subjecting patients to full courses of antibiotics , which may be unnecessary and could lead to adverse side effects.
CLICK HERE TO SIGN UP FOR OUR HEALTH NEWSLETTER
Common side effects of antibiotics include an itchy, full-body rash; nausea and diarrhea; and yeast infections, according to the CDC.
Serious side effects may include life-threatening allergic reactions and a bacterial infection called C. diff (Clostridioides difficile), which can lead to severe colon damage and sometimes death.
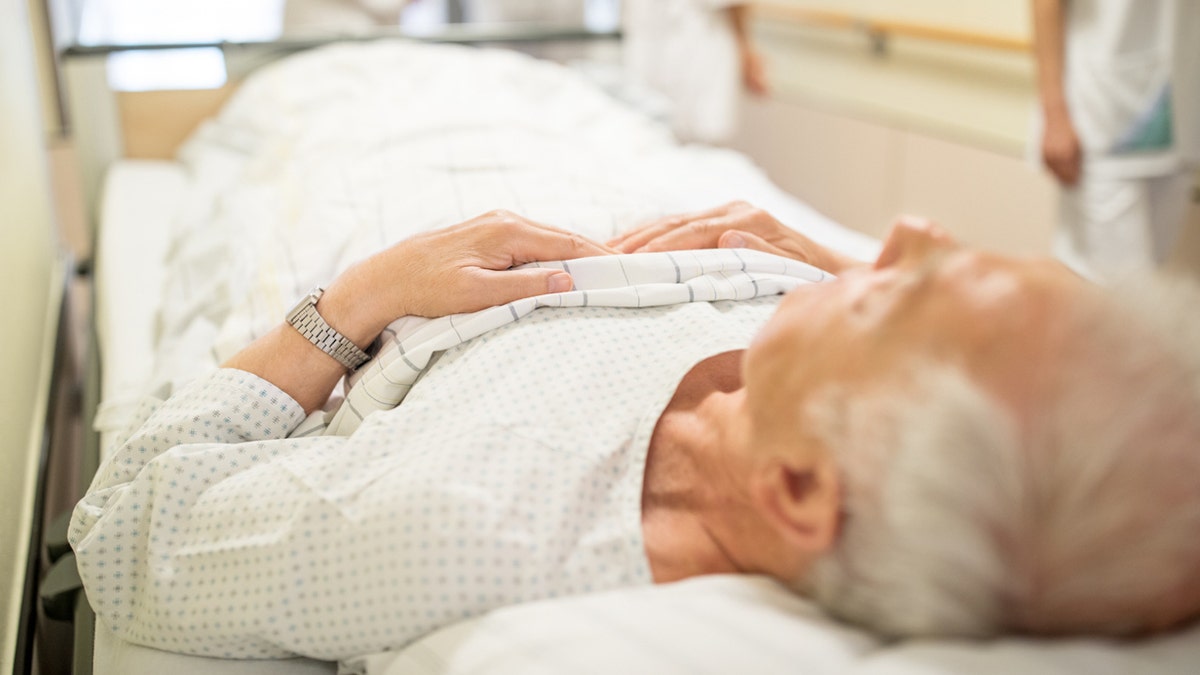
A doctor emphasized the need for providers to diagnose conditions accurately before subjecting patients to full courses of antibiotics. (iStock)
"Diagnosis is challenging, and by and large, providers are trying to best care for their patients," Gupta noted.
"We often think about problems related to underdiagnosis of conditions (e.g., missed infection), though there are real risks to inappropriate diagnosis as well," he said.
Study limitations
The researchers likely underestimated the number of patients who were inappropriately diagnosed, as the signs and symptoms of pneumonia have significant overlap with other conditions, the researchers noted.
The study was also not designed to assess "causation," Gupta pointed out.
CLICK HERE TO GET THE FOX NEWS APP
"So, while we can say that there is an association between a full duration of antibiotics and antibiotic-associated adverse events, we cannot say that a full duration of antibiotics caused the antibiotic-associated adverse events," he continued.
He said he hoped that "this work will provide a framework on which providers, hospitals and health systems can calibrate the accuracy of their pneumonia diagnoses among hospitalized patients."
For more Health articles, visit www.foxnews.com/health .
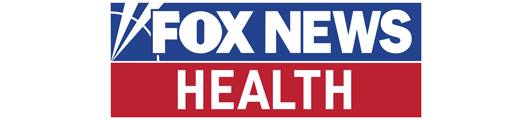
Stay up-to-date on the biggest health and wellness news with our weekly recap.
You've successfully subscribed to this newsletter!
- International edition
- Australia edition
- Europe edition
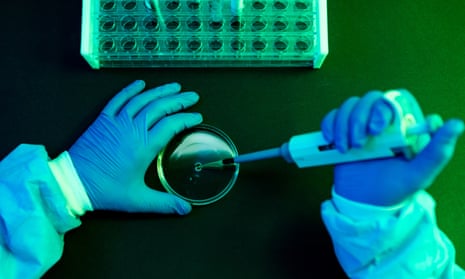
Cancer signs could be spotted years before symptoms, says new research institute
Tests that can identify early changes in cells would give doctors more time to offer treatment, say Cambridge researchers
Scientists at a recently opened cancer institute at Cambridge University have begun work that is pinpointing changes in cells many years before they develop into tumours. The research should help design radically new ways to treat cancer, they say.
The Early Cancer Institute – which has just received £11m from an anonymous donor – is focused on finding ways to tackle tumours before they produce symptoms. The research will exploit recent discoveries which have shown that many people develop precancerous conditions that lie in abeyance for long periods.
“The latency for a cancer to develop can go on for years, sometimes for a decade or two, before the condition abruptly manifests itself to patients,” said Prof Rebecca Fitzgerald, the institute’s director.
“Then doctors find they are struggling to treat a tumour which, by then, has spread through a patient’s body. We need a different approach, one that can detect a person at risk of cancer early on using tests that can be given to large numbers of people.”
One example of this is the cytosponge – a sponge on a string – which has been developed by Fitzgerald and her team. It is swallowed like a pill, expands in the stomach into a sponge and is then pulled up the gullet collecting oesophagus cells on the way. Those cells that contain a protein, called TFF3 – which is found only in precancerous cells – then provide an early warning that a patient is at risk of oesophageal cancer and needs to be monitored. Crucially, this test can be administered simply and on a wide scale.
This contrasts with current approaches to other cancers, added Fitzgerald. “At present, we are detecting many cancers late and are having to come up with medicines, which have become incrementally more expensive. We are often extending life by a few weeks at a cost of tens of thousands of pounds. We need to look at this from a different perspective.”
One approach being taken by the institute – which is to be renamed the Li Ka-shing Early Cancer Institute after the Hong Kong philanthropist who has supported other Cambridge cancer research – focuses on blood samples. Provided by women as part of past screening services for ovarian cancer and kept in special stores, these samples have now been repurposed by the institute. “We have around 200,000 such samples and they are a goldmine,” said Jamie Blundell, a research group leader at the institute.
Using these samples, researchers have identified changes that differentiate those donors who have subsequently been diagnosed with a blood cancer 10 or even 20 years after they provided samples, with those who did not develop such conditions.
“We are finding that there are clear genetic changes in a person’s blood more than a decade before they start to display symptoms of leukaemia,” said Blundell. “That shows there is a long window of opportunity that you could use to intervene and give treatments that will reduce the odds of going on to get cancer.”
Cancers grow in stages and by spotting those with cells that have taken an early step on this ladder, it should be possible to block or hamper further developments. The crucial point is that at this early stage there is time for doctors to take action and avoid them having to deal with a cancer at a late stage when it has spread.
A similar strategy is being taken by Harveer Dev, another group leader, who has investigated men who have had their prostates removed. His team are now developing biomarkers that will provide better ways to pinpoint those who are likely to suffer poor outcomes from prostate cancer, one of the most common tumours in the UK.
“Our pilot data suggests that these tests may be much better than existing PSA tests and will be crucial in spotting those who with prostate cancer that is likely to progress,” said Dev.
Pinpointing those at risk of cancer – for example, people from families who have an inherited predisposition to tumours – will form a key part of the institute’s strategy. In addition, it will focus on finding ways to reduce cancer risks, as well as ensuring treatments can be widely administered.
A woman had, in her 80s, decided to leave the university £1m for cancer research, Fitzgerald said. “However, she lived until she was over 100 and only died recently, so we only just got that donation. We want to understand what makes some live into very old age while others get cancer, so more people can live as long as she did.”
- Cancer research
- The Observer
- Medical research
- Health policy
- Prostate cancer
- University of Cambridge
Most viewed

- SUGGESTED TOPICS
- The Magazine
- Newsletters
- Managing Yourself
- Managing Teams
- Work-life Balance
- The Big Idea
- Data & Visuals
- Reading Lists
- Case Selections
- HBR Learning
- Topic Feeds
- Account Settings
- Email Preferences
Research: How Different Fields Are Using GenAI to Redefine Roles
- Maryam Alavi
Examples from customer support, management consulting, professional writing, legal analysis, and software and technology.
The interactive, conversational, analytical, and generative features of GenAI offer support for creativity, problem-solving, and processing and digestion of large bodies of information. Therefore, these features can act as cognitive resources for knowledge workers. Moreover, the capabilities of GenAI can mitigate various hindrances to effective performance that knowledge workers may encounter in their jobs, including time pressure, gaps in knowledge and skills, and negative feelings (such as boredom stemming from repetitive tasks or frustration arising from interactions with dissatisfied customers). Empirical research and field observations have already begun to reveal the value of GenAI capabilities and their potential for job crafting.
There is an expectation that implementing new and emerging Generative AI (GenAI) tools enhances the effectiveness and competitiveness of organizations. This belief is evidenced by current and planned investments in GenAI tools, especially by firms in knowledge-intensive industries such as finance, healthcare, and entertainment, among others. According to forecasts, enterprise spending on GenAI will increase by two-fold in 2024 and grow to $151.1 billion by 2027 .
- Maryam Alavi is the Elizabeth D. & Thomas M. Holder Chair & Professor of IT Management, Scheller College of Business, Georgia Institute of Technology .
Partner Center
- See us on facebook
- See us on twitter
- See us on youtube
- See us on linkedin
- See us on instagram
Pilot study shows ketogenic diet improves severe mental illness
A small clinical trial led by Stanford Medicine found that the metabolic effects of a ketogenic diet may help stabilize the brain.
April 1, 2024 - By Nina Bai

A study led by researchers at Stanford Medicine showed that diet can help those with serious mental illness. nishihata
For people living with serious mental illness like schizophrenia or bipolar disorder, standard treatment with antipsychotic medications can be a double-edged sword. While these drugs help regulate brain chemistry, they often cause metabolic side effects such as insulin resistance and obesity, which are distressing enough that many patients stop taking the medications.
Now, a pilot study led by Stanford Medicine researchers has found that a ketogenic diet not only restores metabolic health in these patients as they continue their medications, but it further improves their psychiatric conditions. The results, published March 27 in Psychiatry Research , suggest that a dietary intervention can be a powerful aid in treating mental illness.
“It’s very promising and very encouraging that you can take back control of your illness in some way, aside from the usual standard of care,” said Shebani Sethi , MD, associate professor of psychiatry and behavioral sciences and the first author of the new paper.
Making the connection
Sethi, who is board certified in obesity and psychiatry, remembers when she first noticed the connection. As a medical student working in an obesity clinic, she saw a patient with treatment-resistant schizophrenia whose auditory hallucinations quieted on a ketogenic diet.
That prompted her to dig into the medical literature. There were only a few, decades-old case reports on using the ketogenic diet to treat schizophrenia, but there was a long track record of success in using ketogenic diets to treat epileptic seizures.
“The ketogenic diet has been proven to be effective for treatment-resistant epileptic seizures by reducing the excitability of neurons in the brain,” Sethi said. “We thought it would be worth exploring this treatment in psychiatric conditions.”
A few years later, Sethi coined the term metabolic psychiatry, a new field that approaches mental health from an energy conversion perspective.
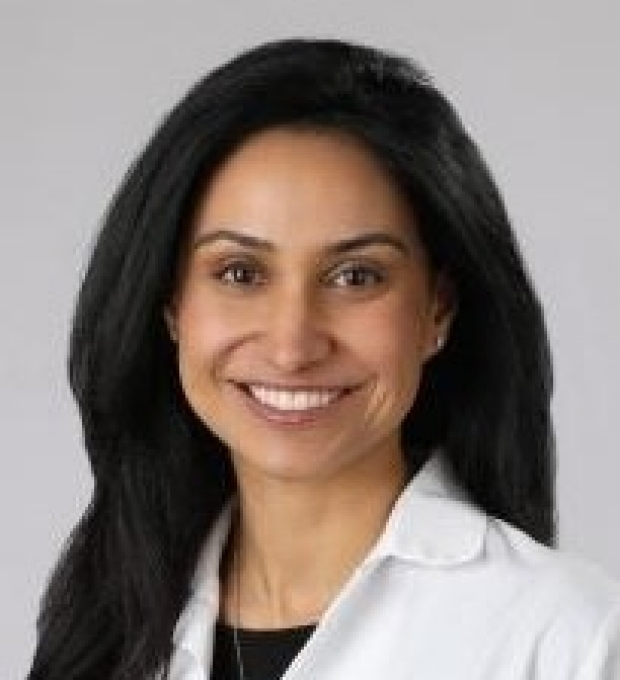
Shebani Sethi
In the four-month pilot trial, Sethi’s team followed 21 adult participants who were diagnosed with schizophrenia or bipolar disorder, taking antipsychotic medications, and had a metabolic abnormality — such as weight gain, insulin resistance, hypertriglyceridemia, dyslipidemia or impaired glucose tolerance. The participants were instructed to follow a ketogenic diet, with approximately 10% of the calories from carbohydrates, 30% from protein and 60% from fat. They were not told to count calories.
“The focus of eating is on whole non-processed foods including protein and non-starchy vegetables, and not restricting fats,” said Sethi, who shared keto-friendly meal ideas with the participants. They were also given keto cookbooks and access to a health coach.
The research team tracked how well the participants followed the diet through weekly measures of blood ketone levels. (Ketones are acids produced when the body breaks down fat — instead of glucose — for energy.) By the end of the trial, 14 patients had been fully adherent, six were semi-adherent and only one was non-adherent.
The participants underwent a variety of psychiatric and metabolic assessments throughout the trial.
Before the trial, 29% of the participants met the criteria for metabolic syndrome, defined as having at least three of five conditions: abdominal obesity, elevated triglycerides, low HDL cholesterol, elevated blood pressure and elevated fasting glucose levels. After four months on a ketogenic diet, none of the participants had metabolic syndrome.
On average, the participants lost 10% of their body weight; reduced their waist circumference by 11% percent; and had lower blood pressure, body mass index, triglycerides, blood sugar levels and insulin resistance.
“We’re seeing huge changes,” Sethi said. “Even if you’re on antipsychotic drugs, we can still reverse the obesity, the metabolic syndrome, the insulin resistance. I think that’s very encouraging for patients.”
The participants reported improvements in their energy, sleep, mood and quality of life.
The psychiatric benefits were also striking. On average, the participants improved 31% on a psychiatrist rating of mental illness known as the clinical global impressions scale, with three-quarters of the group showing clinically meaningful improvement. Overall, the participants also reported better sleep and greater life satisfaction.
“The participants reported improvements in their energy, sleep, mood and quality of life,” Sethi said. “They feel healthier and more hopeful.”
The researchers were impressed that most of the participants stuck with the diet. “We saw more benefit with the adherent group compared with the semi-adherent group, indicating a potential dose-response relationship,” Sethi said.
Alternative fuel for the brain
There is increasing evidence that psychiatric diseases such as schizophrenia and bipolar disorder stem from metabolic deficits in the brain, which affect the excitability of neurons, Sethi said.
The researchers hypothesize that just as a ketogenic diet improves the rest of the body’s metabolism, it also improves the brain’s metabolism.
“Anything that improves metabolic health in general is probably going to improve brain health anyway,” Sethi said. “But the ketogenic diet can provide ketones as an alternative fuel to glucose for a brain with energy dysfunction.”
Likely there are multiple mechanisms at work, she added, and the main purpose of the small pilot trial is to help researchers detect signals that will guide the design of larger, more robust studies.
As a physician, Sethi cares for many patients with both serious mental illness and obesity or metabolic syndrome, but few studies have focused on this undertreated population.
She is the founder and director of the metabolic psychiatry clinic at Stanford Medicine.
“Many of my patients suffer from both illnesses, so my desire was to see if metabolic interventions could help them,” she said. “They are seeking more help. They are looking to just feel better.”
Researchers from the University of Michigan; the University of California, San Francisco; and Duke University contributed to the study.
The study was supported by Baszucki Group Research Fund, Keun Lau Fund and the Obesity Treatment Foundation.
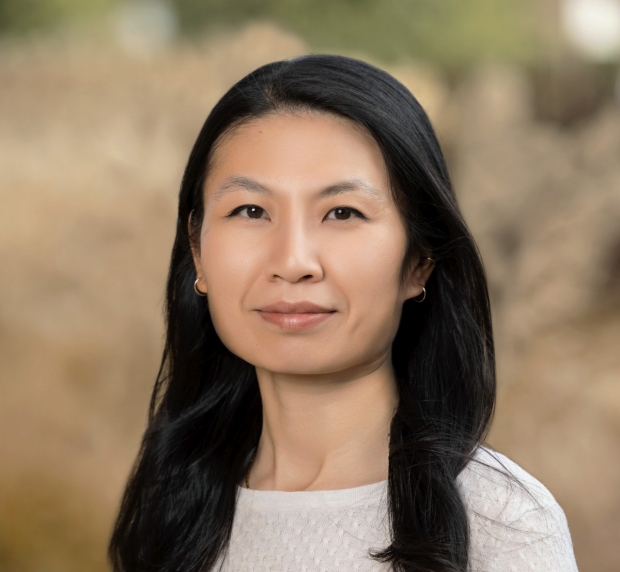
About Stanford Medicine
Stanford Medicine is an integrated academic health system comprising the Stanford School of Medicine and adult and pediatric health care delivery systems. Together, they harness the full potential of biomedicine through collaborative research, education and clinical care for patients. For more information, please visit med.stanford.edu .
Artificial intelligence
Exploring ways AI is applied to health care

- Share full article
Advertisement
Supported by
More Studies by Columbia Cancer Researchers Are Retracted
The studies, pulled because of copied data, illustrate the sluggishness of scientific publishers to address serious errors, experts said.

By Benjamin Mueller
Scientists in a prominent cancer lab at Columbia University have now had four studies retracted and a stern note added to a fifth accusing it of “severe abuse of the scientific publishing system,” the latest fallout from research misconduct allegations recently leveled against several leading cancer scientists.
A scientific sleuth in Britain last year uncovered discrepancies in data published by the Columbia lab, including the reuse of photos and other images across different papers. The New York Times reported last month that a medical journal in 2022 had quietly taken down a stomach cancer study by the researchers after an internal inquiry by the journal found ethics violations.
Despite that study’s removal, the researchers — Dr. Sam Yoon, chief of a cancer surgery division at Columbia University’s medical center, and Changhwan Yoon, a more junior biologist there — continued publishing studies with suspicious data. Since 2008, the two scientists have collaborated with other researchers on 26 articles that the sleuth, Sholto David, publicly flagged for misrepresenting experiments’ results.
One of those articles was retracted last month after The Times asked publishers about the allegations. In recent weeks, medical journals have retracted three additional studies, which described new strategies for treating cancers of the stomach, head and neck. Other labs had cited the articles in roughly 90 papers.
A major scientific publisher also appended a blunt note to the article that it had originally taken down without explanation in 2022. “This reuse (and in part, misrepresentation) of data without appropriate attribution represents a severe abuse of the scientific publishing system,” it said .
Still, those measures addressed only a small fraction of the lab’s suspect papers. Experts said the episode illustrated not only the extent of unreliable research by top labs, but also the tendency of scientific publishers to respond slowly, if at all, to significant problems once they are detected. As a result, other labs keep relying on questionable work as they pour federal research money into studies, allowing errors to accumulate in the scientific record.
“For every one paper that is retracted, there are probably 10 that should be,” said Dr. Ivan Oransky, co-founder of Retraction Watch, which keeps a database of 47,000-plus retracted studies. “Journals are not particularly interested in correcting the record.”
Columbia’s medical center declined to comment on allegations facing Dr. Yoon’s lab. It said the two scientists remained at Columbia and the hospital “is fully committed to upholding the highest standards of ethics and to rigorously maintaining the integrity of our research.”
The lab’s web page was recently taken offline. Columbia declined to say why. Neither Dr. Yoon nor Changhwan Yoon could be reached for comment. (They are not related.)
Memorial Sloan Kettering Cancer Center, where the scientists worked when much of the research was done, is investigating their work.
The Columbia scientists’ retractions come amid growing attention to the suspicious data that undergirds some medical research. Since late February, medical journals have retracted seven papers by scientists at Harvard’s Dana-Farber Cancer Institute . That followed investigations into data problems publicized by Dr. David , an independent molecular biologist who looks for irregularities in published images of cells, tumors and mice, sometimes with help from A.I. software.
The spate of misconduct allegations has drawn attention to the pressures on academic scientists — even those, like Dr. Yoon, who also work as doctors — to produce heaps of research.
Strong images of experiments’ results are often needed for those studies. Publishing them helps scientists win prestigious academic appointments and attract federal research grants that can pay dividends for themselves and their universities.
Dr. Yoon, a robotic surgery specialist noted for his treatment of stomach cancers, has helped bring in nearly $5 million in federal research money over his career.
The latest retractions from his lab included articles from 2020 and 2021 that Dr. David said contained glaring irregularities . Their results appeared to include identical images of tumor-stricken mice, despite those mice supposedly having been subjected to different experiments involving separate treatments and types of cancer cells.
The medical journal Cell Death & Disease retracted two of the latest studies, and Oncogene retracted the third. The journals found that the studies had also reused other images, like identical pictures of constellations of cancer cells.
The studies Dr. David flagged as containing image problems were largely overseen by the more senior Dr. Yoon. Changhwan Yoon, an associate research scientist who has worked alongside Dr. Yoon for a decade, was often a first author, which generally designates the scientist who ran the bulk of the experiments.
Kun Huang, a scientist in China who oversaw one of the recently retracted studies, a 2020 paper that did not include the more senior Dr. Yoon, attributed that study’s problematic sections to Changhwan Yoon. Dr. Huang, who made those comments this month on PubPeer, a website where scientists post about studies, did not respond to an email seeking comment.
But the more senior Dr. Yoon has long been made aware of problems in research he published alongside Changhwan Yoon: The two scientists were notified of the removal in January 2022 of their stomach cancer study that was found to have violated ethics guidelines.
Research misconduct is often pinned on the more junior researchers who conduct experiments. Other scientists, though, assign greater responsibility to the senior researchers who run labs and oversee studies, even as they juggle jobs as doctors or administrators.
“The research world’s coming to realize that with great power comes great responsibility and, in fact, you are responsible not just for what one of your direct reports in the lab has done, but for the environment you create,” Dr. Oransky said.
In their latest public retraction notices, medical journals said that they had lost faith in the results and conclusions. Imaging experts said some irregularities identified by Dr. David bore signs of deliberate manipulation, like flipped or rotated images, while others could have been sloppy copy-and-paste errors.
The little-noticed removal by a journal of the stomach cancer study in January 2022 highlighted some scientific publishers’ policy of not disclosing the reasons for withdrawing papers as long as they have not yet formally appeared in print. That study had appeared only online.
Roland Herzog, the editor of the journal Molecular Therapy, said that editors had drafted an explanation that they intended to publish at the time of the article’s removal. But Elsevier, the journal’s parent publisher, advised them that such a note was unnecessary, he said.
Only after the Times article last month did Elsevier agree to explain the article’s removal publicly with the stern note. In an editorial this week , the Molecular Therapy editors said that in the future, they would explain the removal of any articles that had been published only online.
But Elsevier said in a statement that it did not consider online articles “to be the final published articles of record.” As a result, company policy continues to advise that such articles be removed without an explanation when they are found to contain problems. The company said it allowed editors to provide additional information where needed.
Elsevier, which publishes nearly 3,000 journals and generates billions of dollars in annual revenue , has long been criticized for its opaque removals of online articles.
Articles by the Columbia scientists with data discrepancies that remain unaddressed were largely distributed by three major publishers: Elsevier, Springer Nature and the American Association for Cancer Research. Dr. David alerted many journals to the data discrepancies in October.
Each publisher said it was investigating the concerns. Springer Nature said investigations take time because they can involve consulting experts, waiting for author responses and analyzing raw data.
Dr. David has also raised concerns about studies published independently by scientists who collaborated with the Columbia researchers on some of their recently retracted papers. For example, Sandra Ryeom, an associate professor of surgical sciences at Columbia, published an article in 2003 while at Harvard that Dr. David said contained a duplicated image . As of 2021, she was married to the more senior Dr. Yoon, according to a mortgage document from that year.
A medical journal appended a formal notice to the article last week saying “appropriate editorial action will be taken” once data concerns had been resolved. Dr. Ryeom said in a statement that she was working with the paper’s senior author on “correcting the error.”
Columbia has sought to reinforce the importance of sound research practices. Hours after the Times article appeared last month, Dr. Michael Shelanski, the medical school’s senior vice dean for research, sent an email to faculty members titled “Research Fraud Accusations — How to Protect Yourself.” It warned that such allegations, whatever their merits, could take a toll on the university.
“In the months that it can take to investigate an allegation,” Dr. Shelanski wrote, “funding can be suspended, and donors can feel that their trust has been betrayed.”
Benjamin Mueller reports on health and medicine. He was previously a U.K. correspondent in London and a police reporter in New York. More about Benjamin Mueller
Eminent cancer physician-scientist joins Virginia Tech to lead research in Washington, D.C.
Christopher Hourigan will spearhead innovative cancer solutions at the Fralin Biomedical Research Institute Cancer Research Center in the nation's capital.
John Pastor
- Share on Facebook
- Share on Twitter
- Copy address link to clipboard
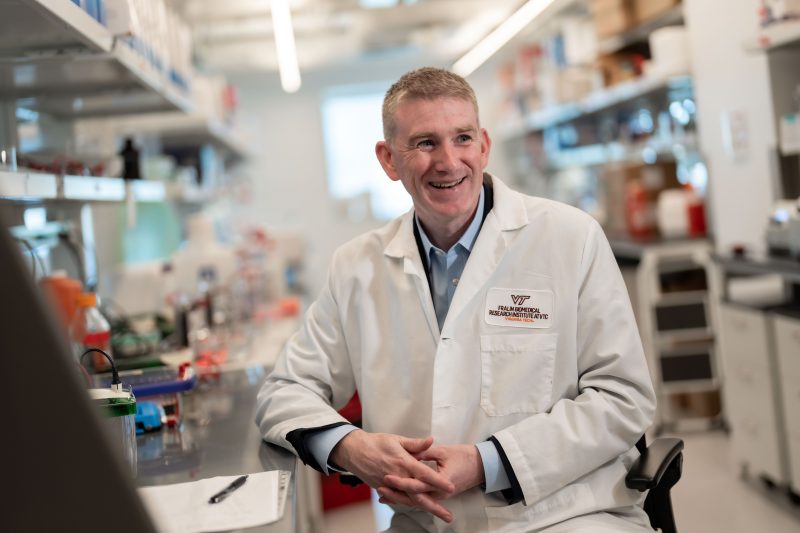
A globally recognized physician-scientist who studies and treats blood cancer is joining Virginia Tech to lead cancer research in Washington, D.C., said Michael Friedlander, Virginia Tech’s vice president for health sciences and technology and executive director of the Fralin Biomedical Research Institute at VTC.
Christopher Hourigan , a senior investigator and chief of the Laboratory of Myeloid Malignancies at the National Institutes of Health, will join Virginia Tech as a professor with the institute and director of its Cancer Research Center in Washington, D.C.
“Dr. Hourigan exemplifies the prototype of a physician-scientist, integrating insights from his patient interactions directly into his fundamental and translational laboratory research,” Friedlander said. “We are extremely enthusiastic to have him join Virginia Tech and become a member of the Fralin Biomedical Research Institute team to take on this important new leadership role for our growing cancer research programs in Washington, D.C., and to further strengthen our collaborations with Carilion Clinic, Children’s National Hospital, and other health systems and universities.
The focus of the research effort, known as Fralin Biomedical Research Institute Cancer Research Center - D.C., is to bring basic, translational, clinical, and computational researchers together in the nation’s capital to focus on the shared aim of engineering cancer solutions.
“Convening top talent like Dr. Hourigan and developing deep and diverse partnerships are key to solving our most complex global challenges,” said Virginia Tech President Tim Sands. “It’s exciting to see our vision for the university advancing across the commonwealth and in the greater Washington, D.C., metro area.”
Your browser does not support iframes. Link to iframe content: https://www.youtube.com/embed/tS1TgAcvxhA?si=IuLYq7Em-QpT-w55
“I also anticipate the innovations and insights that will emerge from Dr. Hourigan and the other Virginia Tech scientists in D.C. collaborating with the institute's cancer research teams in Roanoke as well as with our computer science and engineering colleagues at Virginia Tech’s new Innovation Campus in Alexandria,” Friedlander said.
Research will involve diverse, expert teams and national and international collaborations.
“We have the opportunity to build a new cancer research center from the ground up, focusing on getting talented and highly motivated teams working in innovative new ways to reduce the burden of suffering from cancer in the United States,” Hourigan said. “It's clear we're not doing well enough for people who are dealing with cancer, and this is our chance to come up with new ways to do better.”
The addition of Hourigan will accelerate the university’s initiatives in Washington, D.C. Newly renovated research facilities on the site of the former Walter Reed Army Medical Center on the Children’s National Research & Innovation Campus already house Fralin Biomedical Research Institute cancer research scientists Jia-Ray Yu and Kathleen Mulvaney , along with teams of researchers from the Center for Genetic Medicine Research and Rare Disease Institute of Children’s National Hospital.
Precise answers to hard questions
Hourigan’s research focuses on a high-risk form of blood cancer called acute myeloid leukemia, which annually affects about 20,000 Americans. He looks at reasons for why some people survive cancer while others end up dying, even though initially they seem to have had the same response to treatment.
“The person who's newly diagnosed with cancer and has gone through treatment will ask a reasonable question, ‘Well, what about me? I want to know what my actual likelihood of surviving is going to be and are you sure I don’t need more or different treatment?’” Hourigan said. “As an oncologist, those are often hard questions to answer. You have a lot of empathy with the person in front of you and wish we could give better, more personalized, answers. We're strongly focused on the idea that if we had better diagnostic tools to allow a precision medicine approach, we could give doctors and patients a better understanding of exactly where they are now and what’s the best thing for them to do next.”
In addition to his primary appointment with the institute, Hourigan will be a professor in the Department of Internal Medicine at the Virginia Tech Carilion School of Medicine .
Hourigan received his medical degree and research doctorates from Oxford University and completed residency and oncology fellowship training at Johns Hopkins University School of Medicine, where he was subsequently a practicing physician on the acute leukemia service and faculty member. He is board certified in hematology and medical oncology.
Prior to joining Virginia Tech, Hourigan was a senior investigator, co-director of the Myeloid Malignancies Program, and chief of the Laboratory of Myeloid Malignancies at the National Institutes of Health.
He was awarded the Presidential Early Career Award for Scientists and Engineers, the National Institutes of Health Director’s Challenge Innovation Award, and was elected to Alpha Omega Alpha Medical Honor Society. He is a fellow of both the American College of Physicians and the Royal College of Physicians.
He looks forward to the task ahead.
“Virginia Tech has some key strategic advantages,” Hourigan said. “It is nationally known for engineering and computational science, which are going to be increasingly important components of cancer research. I think there's also this sense of energy, innovation, engineering, and teams working across disciplines on hard problems here. And cancer is a hard problem.”
Ultimately, he wants to put cancer researchers out of business.
“I don't want there to be a need for cancer research anymore, whether that happens in my lifetime or the lifetimes of those individuals I train,” Hourigan said. “The ultimate objective is not to have an industry of cancer research. Our focus has to be on the patient and on working hard to come up with real solutions to hard problems. It is only with research that we’ll be able to do better with cancer prevention, diagnosis, and treatment tomorrow than we currently can today. We need to have a sense of urgency and purpose because people are counting on us to come up with answers.”
(540) 526-2222
- Cancer Research Center (DC)
- Destination Areas
- Fralin Biomedical Research Institute at VTC
- Fralin Biomedical Research Institute at VTC - top news
- Fralin Biomedical--Cancer Research Group
- Friedlander News
- Hourigan Lab
- Virginia Tech Carilion School of Medicine
Related Content
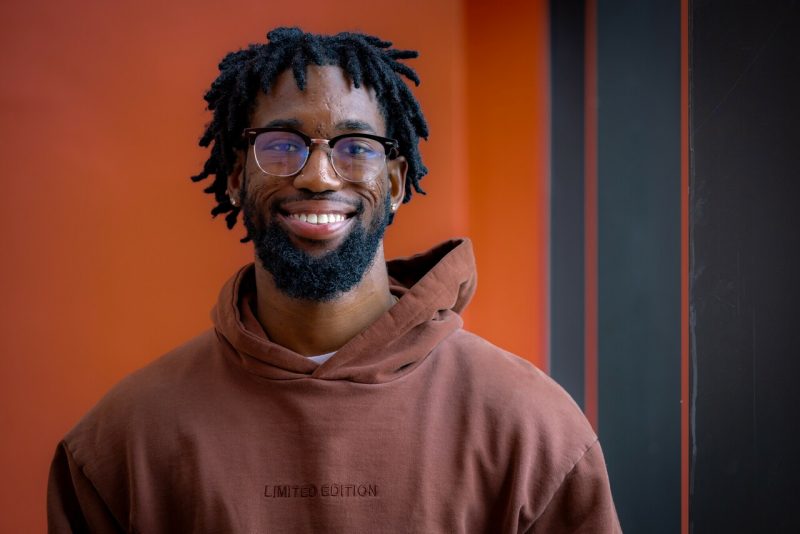
Industries Overview
Latest articles, the big answers: banking & payments quiz, industry kpis: youtube and x emerge as leaders for social media advertising attention, uk finfluencers face prison time for false or misleading financial product promotions there, generative ai’s actual use isn’t meeting its perceived marketing potential—yet, retailers criticize mastercard and visa’s swipe fee settlement—here’s our take, teads and lg ads extend ctv advertising partnership across europe and apac, 2024 us ad market to hit $369 billion, buoyed by politics, says magna, linear, streaming, avod, and beyond: what do common tv terms mean, social media is a core part of young people’s lives, walmart connect’s enhances network, leverages store footprint, emerging brands, about emarketer, the digital healthcare consumer 2023, what providers and marketers need to know about the digital patient journey.
- Executive Summary
Digital healthcare usage was fast-tracked by the pandemic. But now, with so many tools in the market, providers and marketers must deliver differentiated experiences to stay competitive. They’ll need to know how and why patients use these tools—and how to resolve the pain points.
Key Question: Which digital health products and services are US consumers using, and how does usage differ across populations?
KEY STAT: About 46% of US consumers—an estimated 119 million patients—engage with their healthcare providers using a combination of patient portals, telehealth appointments, apps, and traditional in-person visits, per PYMNTS.
Here’s what’s in the full report
Exportable files for easy reading, analysis and sharing.
Reliable data in simple displays for presentations and quick decision making.
Table of Contents
- Consumer adoption of digital health tools is still growing.
- Who is the healthcare tech user?
- Patients demand an omnichannel experience.
- The patient healthcare journey often starts online.
- Patients are using social media to find health information.
- Patients believe digital health tools lead to better health.
- Consumers want doctors to embrace digital health tools.
- Healthcare is falling short on delivering a quality digital experience.
- Providers are at risk of losing patients if they don’t go digital.
- How can providers and marketers keep pace with the digital healthcare consumer?
- Media Gallery
Charts in This Report
Rajiv leventhal, contributors, access all charts and data.
Gain access to reliable data presented in clear and intelligible displays for quick understanding and decision making on the most important topics related to your industry
Industries →
Advertising & marketing.
- Social Media
- Content Marketing
- Email Marketing
- Browse All →
- Value-Based Care
- Digital Therapeutics
- Online Pharmacy
Ecommerce & Retail
- Ecommerce Sales
- Retail Sales
- Social Commerce
- Connected Devices
- Artificial Intelligence (AI)
Financial Services
- Wealth Management
More Industries
- Real Estate
- Customer Experience
- Small Business (SMB)
Geographies
- Asia-Pacific
- Central & Eastern Europe
- Latin America
- Middle East & Africa
- North America
- Western Europe
- Data Partnerships
Media Services
- Advertising & Sponsorship Opportunities
Free Content
- Newsletters
Contact Us →
Worldwide hq.
One Liberty Plaza 9th Floor New York, NY 10006 1-800-405-0844
Sales Inquiries
1-800-405-0844 [email protected]
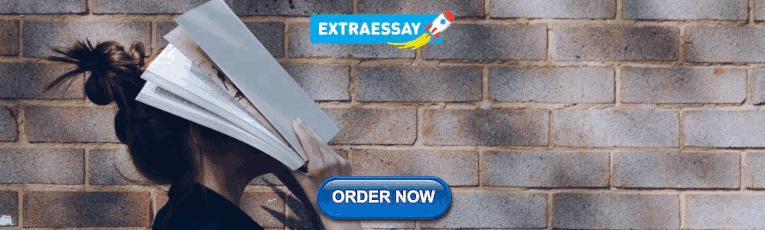
IMAGES
COMMENTS
Pneumonia is a leading cause of hospitalization among both adults and children in the United States, accounting for more than 800,000 hospitalizations and more than 400,000 emergency department visits in 2014.1, ... As this is an area of research, multiple recent systematic reviews and meta-analyses have been published, some with conflicting ...
Abstract. Pneumonia is a common acute respiratory infection that affects the alveoli and distal airways; it is a major health problem and associated with high morbidity and short-term and long ...
Abstract. Pneumonia is very common and continues to exact a high burden on health. The Global Burden of Disease Study 2015 found lower respiratory infections (LRIs) were the leading infectious cause of death and the fifth leading cause of death overall. Pneumococcal pneumonia caused 55% of LRI deaths in all ages (1.5 million deaths).
Abstract. Pneumonia is a common acute respiratory infection that affects the alveoli and distal airways; it is a major health problem and associated with high morbidity and short-term and long-term mortality in all age groups worldwide. Pneumonia is broadly divided into community-acquired pneumonia or hospital-acquired pneumonia.
The annual incidence of pneumonia was estimated at 1.07-1.2 cases per 1,000 persons per year in Europe and 16.9 cases per 1,000 persons per year in Asia 2. Diagnosis of pneumonia in adults presenting with signs of lower respiratory tract infection is important because it requires specific treatment and follow up.
Determinants of community acquired pneumonia among 2 to 59 months of age children in Northeast Ethiopia: a case-control study. Pneumonia is the leading cause of mortality and morbidity in under-five children. Regardless of this fact, efforts to identify determinants of pneumonia have been limited in the study area.
Pneumonia remains a leading cause of death worldwide without substantial improvements in mortality since the introduction of antibiotics over 50 years ago. Approximately 1 million adults in the USA attend hospital for the treatment of pneumonia each year, and 50 000 die from the disease, with the majority being older than 65 years. Community-acquired pneumonia (CAP) is responsible for most of ...
Although community-acquired pneumonia has traditionally been viewed as an acute disease of the lungs, the current understanding is that it is a multisystem disease that can result in acute and ...
Despite decades of advances in clinical management protocols and new antibiotics, pneumonia continues to be a leading cause of morbidity and mortality worldwide. The 2019 Global Burden of Disease Study indicated that lower respiratory infections, including pneumonia, were the fourth leading cause of disability-adjusted life-years across all ages.1 People at the extremes of age, specifically ...
Pneumonia is a persistent, pervasive, and difficult pulmonary disease. Lower respiratory tract infection is the most common infectious cause of death in the world, responsible for almost 2.7 million deaths yearly and 103 million disability-adjusted life-years lost in 2015 ().The burden of disease consistently ranks first or second largest worldwide, with economic costs in the United States ...
Introduction. Pneumonia is a major health problem, being associated with high morbidity and short and long term mortality. It is also the leading infectious disease cause of mortality among all ages worldwide.1 2 Pneumonia in critically ill patients may present as pneumonia acquired in the community (community acquired pneumonia, CAP); pneumonia acquired in the hospital (hospital acquired ...
Introduction Despite improvements in medical science and public health, mortality of community-acquired pneumonia (CAP) has barely changed throughout the last 15 years. The current SARS-CoV-2 pandemic has once again highlighted the central importance of acute respiratory infections to human health. The "network of excellence on Community Acquired Pneumonia" (CAPNETZ) hosts the most ...
Community-Acquired Pneumonia. Long recognized as a major cause of death, pneumonia has been studied intensively since the late 1800s, the results of which led to many formative insights in modern ...
Pneumonia is the only journal to focus exclusively on pneumonia. Publishing original research, case reports, reviews, commentaries and correspondence, Pneumonia provides an international forum for the exchange of knowledge by scientists and clinicians involved in studying the etiology and pathogenesis of pneumonia, as well as its diagnosis, epidemiology, treatment and prevention.
Patients for community-acquired pneumonia incurred a substantial annual economic burden with high cost of hospitalisation. 14. An episode of community-acquired pneumonia managed in the hospital is associated with a mean all-cause total health-care cost of US$11 148 in uncomplicated cases and $51 219 in complicated cases. 15.
Background Community-acquired pneumonia (CAP) is one of the leading causes of healthcare utilisation and death worldwide. Treatment according to evidence-based clinical guidelines can reduce mortality, antibiotic exposure and length of hospital stay related to CAP. Local problem Several studies, including a pilot study from one of our sites, indicate that physicians show a low grade of ...
Pneumonia is a debilitating disease that can result in death in elderly individuals 1 and has been reported to be related to oral health 2.The oral cavity is a complex environment for multiple ...
Background Pneumonia is defined as an acute inflammation of the Lungs' parenchymal structure. It is a major public health problem and the leading cause of morbidity and mortality in under-five children especially in developing countries. In 2015, it was estimated that about 102 million cases of pneumonia occurred in under-five children, of which 0.7 million were end up with death. Different ...
Pneumonia has been defined as an infection of the lung parenchyma. Rather than looking at it as a single disease, health care professionals must remember that pneumonia is an umbrella term for a group of syndromes caused by a variety of organisms resulting in varied manifestations and sequelae. There have been many attempts to classify ...
Key Points. Question In hospitalized patients diagnosed with pneumonia, what is the incidence of and factors associated with inappropriate diagnosis?. Findings In this cohort study of 17 290 hospitalized adults treated for pneumonia in 48 Michigan hospitals, 12.0% were inappropriately diagnosed. Older patients, those with dementia, and those presenting with altered mental status had the ...
Pneumonia is an umbrella term for a group of syndromes caused by a variety of organisms that result in infection of the lung parenchyma. Classification schemata have helped establish the common organisms responsible for each type of pneumonia and helped to formulate treatment guidelines for efficient management, in both inpatient and outpatient ...
In the letter to the editor about this article, Reyes et al [] study this new score as a long-term predictor, and note that PSS is superior to SAPS-3, CURB-65, CRB-65 and qSOFA in predicting hospital mortality, but that as a long-term predictor its sensitivity and specificity decreases dramatically.In the following article, Gautam et al [] consider whether procalcitonin (PCT) should be used as ...
Severe community-acquired pneumonia (sCAP) remains one of the leading causes of admission to the intensive care unit, thus consuming a large share of resources and is associated with high mortality rates worldwide. ... 4 Research Unit of Clinical Epidemiology, Institute of Clinical Research, Odense University Hospital, University of Southern ...
Adults who are admitted to the hospital are often inappropriately diagnosed with — and treated for — pneumonia, new research suggests. Experts discuss the risks of unnecessary treatment.
The research should help design radically new ways to treat cancer, they say. The Early Cancer Institute - which has just received £11m from an anonymous donor - is focused on finding ways to ...
The interactive, conversational, analytical, and generative features of GenAI offer support for creativity, problem-solving, and processing and digestion of large bodies of information. Therefore ...
The research team tracked how well the participants followed the diet through weekly measures of blood ketone levels. (Ketones are acids produced when the body breaks down fat — instead of glucose — for energy.) By the end of the trial, 14 patients had been fully adherent, six were semi-adherent and only one was non-adherent.
March 20, 2024. Scientists in a prominent cancer lab at Columbia University have now had four studies retracted and a stern note added to a fifth accusing it of "severe abuse of the scientific ...
A globally recognized physician-scientist who studies and treats blood cancer is joining Virginia Tech to lead cancer research in Washington, D.C., said Michael Friedlander, Virginia Tech's vice president for health sciences and technology and executive director of the Fralin Biomedical Research Institute at VTC.. Christopher Hourigan, a senior investigator and chief of the Laboratory of ...
KEY STAT: About 46% of US consumers—an estimated 119 million patients—engage with their healthcare providers using a combination of patient portals, telehealth appointments, apps, and traditional in-person visits, per PYMNTS.