
An official website of the United States government
The .gov means it's official. Federal government websites often end in .gov or .mil. Before sharing sensitive information, make sure you're on a federal government site.
The site is secure. The https:// ensures that you are connecting to the official website and that any information you provide is encrypted and transmitted securely.
- Publications
- Account settings
- Browse Titles
NCBI Bookshelf. A service of the National Library of Medicine, National Institutes of Health.
National Research Council (US) Committee for Monitoring the Nation's Changing Needs for Biomedical, Behavioral, and Clinical Personnel. Advancing the Nation's Health Needs: NIH Research Training Programs. Washington (DC): National Academies Press (US); 2005.

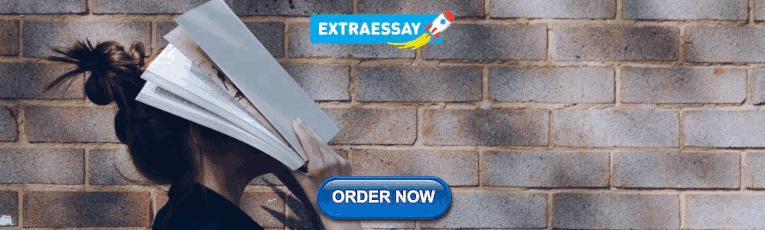
Advancing the Nation's Health Needs: NIH Research Training Programs.
- Hardcopy Version at National Academies Press
2 Basic Biomedical Sciences Research
Basic biomedical research, which addresses mechanisms that underlie the formation and function of living organisms, ranging from the study of single molecules to complex integrated functions of humans, contributes profoundly to our knowledge of how disease, trauma, or genetic defects alter normal physiological and behavioral processes. Recent advances in molecular biology techniques and characterization of the human genome, as well as the genomes of an increasing number of model organisms, have provided basic biomedical researchers with the tools to elucidate molecular-, cellular-, and systems-level processes at an unprecedented depth and rate.
Thus basic biomedical research affects clinical research and vice versa. Biomedical researchers supply many of the new ideas that can be translated into potential therapies and subsequently tested in clinical studies, while clinical researchers may suggest novel mechanisms of disease that can then be tested in basic studies using animal models.
The tools also now exist to rapidly apply insights gained from model organisms to human health and disease. For example, gene mutations known to contribute to human disease can be investigated in model organisms, whose underlying characteristics lend them to rapid assessment. Resulting treatment strategies can then be tested in mammalian species prior to the design of human clinical trials.
These and other mutually supportive systems suggest that such interactions between basic biomedical and clinical researchers not only will continue but will grow as the two domains keep expanding. But the two corresponding workforces will likely remain, for the most part, distinct.
Similarly, there is a symbiosis between basic biomedical and behavioral and social sciences research (covered in Chapter 3 ) and an obvious overlap at the interface of neuroscience, physiological psychology, and behavior. The boundary between these areas is likely to remain indistinct as genetic and environmental influences that affect brain formation and function are better understood. Consequently, such investigations will impact the study of higher cognitive functions, motivation, and other areas traditionally studied by behavioral and social scientists.
Basic biomedical research will therefore undoubtedly continue to play a central role in the discovery of novel mechanisms underlying human disease and in the elucidation of those suggested by clinical studies. As an example, although a number of genes that contribute to disorders such as Huntington's, Parkinson's, and Alzheimer's disease have been identified, the development of successful therapies will require an understanding of the role that the proteins encoded by these genes play in normal cellular processes. Similarly, realizing the potential of stem cell–based therapies for a number of disorders will require characterization of the signals that cause stem cells to differentiate into specific cell types. Thus a workforce trained in basic biomedical research will be needed to apply current knowledge and that gained in the future toward the improvement of human health. Since such research will be carried out not only in academic institutions but increasingly in industry as well, the workforce must be sufficient to supply basic biomedical researchers for large pharmaceutical companies as well as smaller biotech and bioengineering firms, thereby contributing to the economy as well as human health.
The role of the independent investigator in academe, industry, and government is crucial to this research enterprise. They provide the ideas that expand knowledge and the research that leads to discovery. The doubling of the NIH budget has increased the number of research grants and the number of investigators but not at a rate commensurate to the budget increases. Grants have become bigger and senior investigators have received more of them. While this trend has not decreased the nation's research capacity, there may be things that will affect the future pool of independent researchers, such as a sufficient number of academic faculty that can apply for research grants, an industrial workforce that is more application oriented, and most important, a decline in doctorates from U.S. institutions.
- BIOMEDICAL RESEARCH WORKFORCE
The research workforce for the biomedical sciences is broad and diverse. It is primarily composed of individuals who hold Ph.D.s, though it also includes individuals with broader educational backgrounds, such as those who have earned their M.D.s from the Medical Scientist Training Program (MSTP) or other dual-degree programs. In addition, some individuals with M.D.s but without Ph.D.s have acquired the necessary training to do basic biomedical research. But although the analysis in this report should ideally be based on the entire workforce just defined, there are no comprehensive databases that identify the research activities of M.D.s. Therefore much of the analysis will be restricted to holders of a Ph.D. in one of the fields listed in Appendix C , with the assumption that an individual's area of research is related to his or her degree field. A separate section in this chapter is devoted to M.D.s doing biomedical research, and an analysis of the clinical research M.D. workforce is given in Chapter 4 .
It should also be noted that the discussion in this chapter does not include individuals with doctorates in other professions, such as dentistry and nursing even if they hold a Ph.D. in addition to their professional degrees. However, there are important workforce issues in these two fields, and they are addressed separately in Chapters 5 and 6 of this report.
- EDUCATIONAL PROGRESSION
The major sources of Ph.D. researchers in the biomedical sciences are the U.S. research universities, but a substantial number also come from foreign institutions. These scientists, whether native or foreign born, enter the U.S. biomedical research workforce either directly into permanent assignments or via postdoctoral positions.
For most doctorates in the biomedical sciences, interest in the field begins at an early age, in high school or even grade school. In fact, almost all high school graduates (93 percent) in the class of 1998 took a biology course—a rate much greater than other science fields, for which the percentages are below 60 percent. 1 Even in the early 1980s, over 75 percent of high school graduates had taken biology, compared to about 30 percent for chemistry, which had the next-highest enrollment. This interest in biology continues into college, with 7.3 percent of the 2000 freshman science and engineering (S&E) population having declared a major in biology. This was an increase from about 6 percent of freshman majors in the early 1980s but less than the high of about 9.5 percent in the mid-1990s. Overall, the number of freshman biology majors increased from about 50,000 in the early 1980s to over 73,000 in 2000. 2 In terms of actual bachelor's degrees awarded in the biological sciences, there was a decrease from about 47,000 in 1980 to 37,000 in 1989 and then a relatively sharp rise to over 67,000 in 1998. This was followed by a slight decline to about 65,000 in 2000.
There is attrition, however, in the transition from undergraduate to graduate school. In the 1980s and 1990s only about 11,000 first-year students were enrolled at any one time in graduate school biology programs. Percentage-wise, this loss of students is greater than in other S&E fields but is understandable: many undergraduates obtain a bachelor's degree in biology as a precursor to medical school and have no intention of graduate study in biology per se. The total graduate enrollment in biomedical sciences at Ph.D.-granting institutions grew in the early 1990s and was steady at a little under 50,000 during the latter part of the decade. However, there was some growth in 2001, of about 4 percent over the 2000 level, and the growth from 2000 to 2002 was about 10 percent (see Figure 2-1 ), driven in large part by an 18.9 percent increase of temporary residents. The overall growth may not continue, however, as the first-year enrollment for this group slowed from 8.9 percent in 2001 to 3.0 percent in 2002.
First-year and total graduate enrollment in the biomedical sciences at Ph.D.-granting institutions, 1980–2002. SOURCE: National Science Foundation Survey of Graduate Students and Postdoctorates in Science and Engineering.
The tendency for graduate students to receive a doctorate in a field similar to that of their baccalaureate degree is not as strong in the biomedical sciences as it is in other fields, where it is about 85 percent. From 1993 to 2002, some 68.4 percent of the doctorates in biomedical programs received their bachelor's degree in the same field and another 8.4 percent received bachelor's degrees in chemistry. 3 This relative tendency to shift fields should not be viewed negatively, however, as doctoral students with exposure to other disciplines at the undergraduate level could provide the opportunity for greater interdisciplinary training and research.
- EARLY CAREER PROGRESSION IN THE BIOMEDICAL SCIENCES
Advances in biomedical research and health care delivery, together with a strong economy in the 1990s and increased R&D support, drove the growth of academic programs. Total academic R&D expenditures in the biological sciences, in 2001 dollars, began to rise dramatically in the early 1980s. They started from a base of about $3 billion and reached a plateau of almost $5 billion in the mid-1990s. As seen in Figure 2-2 , this increase of about $2 billion was virtually repeated in the much shorter period from the late 1990s to 2002, as the NIH budget doubled. Although the increases in R&D support during the earlier period were reflected in the increased graduate enrollments of the 1980s and mid-1990s (seen in Figure 2-1 ), the enrollments since then have not kept pace with fast-growing R&D expenditures. This disconnect between research funding and enrollment in the late 1990s is difficult to explain but could in part be due to the unsettled career prospects in the biomedical sciences. In a report 4 from the American Society for Cell Biology, the authors examined the data on enrollment and surveyed both undergraduate and graduate students and postdoctorates on their career goals and found that students were aware of and concerned about the problem young people were having in establishing an independent research career. This ASCB report, as well as in the National Research Council report, Trends in the Early Careers of Life Scientists, 5 express concern for the future of biomedical research, if the best young people pursue different career paths. This slowdown in graduate enrollment in the late 1990s might have also contributed to the expansion of the postdoctoral and non-tenure-track faculty pool of researchers, since there was an increasing need for research personnel.
Academic research and development expenditures in the biological sciences. (All dollars are in thousands.) SOURCE: National Science Foundation R&D Expenditures at Universities and Colleges, 1973–2002. Adjusted to 2002 dollars by the Biomedical (more...)
The increase in funding and enrollments in the early 1990s did lead to an increase in doctoral degrees awarded in the late 1990s, as seen in Figure 2-3 . Since the 1970s, Ph.D.s awarded by U.S. institutions in the biomedical sciences increased from roughly 3,000 then to 5,366 in 2002. Most of the increase occurred in the mid-1990s and has since remained fairly constant. The year with the largest number of doctorates was 2000, when 5,532 degrees were awarded. The number of degrees in 2000 may be an anomaly, since the number in 2001 (5,397 Ph.D.), 2002 (5,375 Ph.D.), and 2003 (5,412 Ph.D.) are more in line with the number in the late 1990s (see Appendix Table E-1 ).
Number of doctorates in the biomedical sciences, 1970–2003. SOURCE: National Science Foundation Survey of Earned Doctorates, 2001.
Increases in doctorates were seen among women, temporary residents, and underrepresented minorities. Notably, since 1986 much of the increase in the number of doctorates has come from increased participation by women. In 1970 only 16 percent of doctorates were awarded to women; by 2003 the percentage had grown to 45.2. Temporary residents earned about 10 percent of the doctorates in 1970, and although this had increased to almost one-quarter in the early 2000s, it was still lower than the percentage awarded in many other fields in the physical sciences and engineering. Participation by underrepresented minorities in 2003 stood at 9.4 percent—as in many other S&E fields, substantially below their representation in the general population.
The percentage of doctorates with definite postdoctoral study plans increased from about 50 percent in the early 1970s to a high of 79 percent in 1995. It then declined to 71 percent in 2002 but increased to 75 percent in 2003. The changes in doctorates electing postdoctoral study are reflected in those choosing employment after they received their degrees (from 20 percent in 1995 to 28 percent in 2002 and 25 percent in 2003). It is difficult to find reasons for these changes in career plans. Prior to 2003 it may be the result of more diverse and attractive employment opportunities generated by recent advances in the applied biological sciences, especially in industry, or a conscious choice not to pursue an academic research career, where postdoctoral training is required since an academic position may not be available down the road. The increase in postdoctoral appointments in 2003 and the decline in employment might be due to poor economic conditions in the early part of this decade. Whether these changes will impact the quality of the biomedical workforce and its research should be monitored.
Time to degree, age at receipt of degree, and the long training period prior to reaching R01 research status have been cited as critical issues in the career progression of biomedical researchers. 6 Graduate students are taking longer and longer periods of time to earn their Ph.Ds. The median registered time in a graduate degree program gradually increased from 5.4 years in 1970 to 6.7 years in 2003, and the median age of a newly minted degree holder in the biomedical sciences grew during the same period—from 28.9 in 1970 to 30.6 in 2003 (see Appendix E ). It should be noted that this time to degree is shorter than those of such fields as physics, computer science, and the earth sciences. Only chemistry, mathematics, and engineering have a lower median age at time of degree. While shortening the time in graduate school would reduce the age at which doctorates could become independent investigators, it may not significantly affect their career paths since postdoctoral training is required of almost all researchers in the biomedical sciences, and the time spent in these positions seems to be lengthening.
With the growth of research funding and productivity in the biomedical sciences, the postdoctoral appointment has become a normal part of research training. From the 1980s to the late 1990s, the number of postdoctoral appointments doubled for doctorates from U.S. educational institutions (see Figure 2-4 ). The rapid increase in the postdoctoral pool from 1993 to 1999 in particular appears to be the result of longer training periods for individuals and not the result of an increase in the number of individuals being trained since Table E-1 shows a decline in the number of new doctorates planning postdoctoral study and the number of doctorates has remained fairly constant over recent years.
Postdoctoral appointments in the biomedical sciences by sector, 1973–2001. SOURCE: National Science Foundation Survey of Doctorate Recipients.
The lengthening of postdoctoral training is documented by data collected in 1995 on the employment history of doctorates. 7 Of the Ph.D.s who pursued postdoctoral study after graduating in the early 1970s, about 35 percent spent less than two years and about 65 percent spent more than 2 years in a postdoctoral appointment. By contrast, of Ph.D.s who received their degrees in the late 1980s and completed postdoctorates in the 1990s, 80 percent spent more than two years and 20 percent spent less than 2 years in such appointments. More indicative of the change in postdoctoral training was the increase in the proportion that spent more than 4 years in a position, from about 20 percent to nearly 40 percent.
In 2001 the number of postdoctoral appointments actually declined across all employment sectors. This decline might be the result of lower interest by new doctorates in postdoctoral study and an academic career but is probably a response to the highlighting of issues related to postdoctoral appointments, such as the long periods of training with lack of employment benefits, the general perception that the positions are more like low-paying jobs than training experiences, and the poor prospects of a follow-up position as an independent investigator. Not only is interest in postdoctoral positions declining, there appears to be more rapid movement out of them by present incumbents. (These phenomena are more fully explored in Chapter 9 , Career Progression.)
The above discussion applies only to U.S. doctorates. There are also a large number of individuals with Ph.D.s from foreign institutions being trained in postdoctoral positions in U.S. educational institutions and other employment sectors. Data from another source are available for postdoctorates from this population at academic institutions, 8 but there is no source for data in the industrial, governmental, and nonprofit sectors other than an estimate that about half of the 4,000 intramural postdoctoral appointments at NIH are held by temporary residents. Almost all of these temporary residents have foreign doctorates. The number of temporary residents in academic institutions steadily increased through the 1980s and 1990s until 2002 when the number reached 10,000 (see Figure 2-5 ). The data also show that the rate at which temporary residents took postdoctoral positions slowed in 2002. The decline in academic appointments in 2001 for U.S. citizens and permanent residents population that was described above is also seen in this data, but that might be temporary since there was an increase in 2002. The reasons for this change may be twofold: a tighter employment market for citizens and permanent residents and immigration restrictions. However, it is still important to recognize that foreign-educated researchers hold about two-thirds of the postdoctoral positions in academic institutions. If national security policies were to limit the flow of foreign scientists into the United States, this could adversely affect the research enterprise in the biomedical sciences.
Postdoctoral appointments in academic institutions in the biomedical sciences. SOURCE: National Science Foundation Survey of Graduate Students and Postdoctorates in Science and Engineering.
- A PORTRAIT OF THE WORKFORCE
The traditional career progression for biomedical scientists after graduate school includes a postdoctoral position followed by an academic appointment, either a tenure-track or nonpermanent appointment that is often on “soft” research money. As shown in Figure 2-6 , the total population of academic biomedical sciences researchers, excluding postdoctoral positions, grew at an average annual rate of 3.1 percent from 1975 to 1989. 9
Academic positions for doctorates in the biomedical sciences, 1975–2001. SOURCE: National Science Foundation Survey of Doctorate Recipients.
Since 1995, growth slowed to about 2.5 percent, with almost all of the growth in the non-tenure-track area. From 1999 to 2001 there was actually a decline in the number of non-tenure-track positions (by a few hundred). The fastest-growing employment category since the early 1980s has been “Other Academic Appointments,” which is currently increasing at about 4.9 percent annually (see Appendix E-2 ). These jobs are essentially holding positions, filled by young researchers, coming from postdoctoral experiences, who would like to join an academic faculty on a tenure track and are willing to wait. In effect, they are gambling because institutions are restricting the number of faculty appointments in order to reduce the possible long-term commitments that come with such positions. From 1993 to 2001, the number of tenure-track appointments increased by only 13.8 percent, while those for non-tenure-track faculty and other academic appointments increased by 45.1 percent and 38.9 percent, respectively.
The longer time to independent research status is also seen by looking at the age distributions of tenure-track faculty over the past two decades (see Figure 2-7 ). By comparing age cohorts in 1985 and 2001, it is observed that doctorates entered tenure-track positions at a later age in 2001.
Age distribution of biomedical tenured and tenure-track faculty, 1985, 1993, and 2001. SOURCE: National Science Foundation Survey of Doctorate Recipients.
For example, while about 1,000 doctorates in the 33 to 34 age cohort were in faculty positions in 1985, only about half that number were similarly employed in 2001, even though the number of doctorates for that cohort was greater in the late 1990s than in the early 1980s. The age cohort data also show that the academic workforce is aging, with about 20 percent of the 2001 academic workforce over the age of 58. The constraints of a rather young biomedical academic workforce and the conservative attitudes of institutions to not expand their faculties in the tight economic times of the early 1990s may have slowed the progression of young researchers into research positions. However, this may change in the next 8 to 10 years as more faculty members retire.
Meanwhile, over 40 percent of the biomedical sciences workforce is employed in nonacademic institutions (see Figure 2-8 ). Researchers' employment in industry, the largest of these other sectors, has been growing at a 15 percent rate over the past 20 years. There was a lull in employment in the early 1990s, but growth since the mid-1990s has been strong. The increases in industrial employment may be due to the unavailability of faculty positions, but is more likely fueled by the R&D growth in pharmaceutical and other medical industries from $9.3 billion in 1992 to $24.6 in 2001 (constant 2001 dollars). 10 In 1992 almost all of this funding was from nonfederal sources, but in 2001 only 42 percent was from those sources. The result of this increase in federal funding has resulted in an increase in R&D employment, but not as large as would be expected. It is difficult to estimate the increase in biomedical doctorates in this industrial sector since they are drawn from many fields and are at different degree levels, but the total full-time equivalent R&D scientists and engineers increased 6.2 percent from 38,700 in 1992 to 41,100 in 2001. 11 However, there may be a trend toward increased employment in this sector, since a growing fraction of new doctorates are planning industrial employment (see Table E-1 ). The downturn in 2003 may be an anomaly due to the economy and not the strength of the medical industry, but data from a longer time period will be needed before definite trends in industrial employment can be determined. The government and nonprofit sectors have been fairly stable in their use of biomedical scientists, with about 8 and 4 percent growth rates, respectively, in recent years.
Employment of biomedical scientists by sector, 1973–2001. SOURCE: National Science Foundation Survey of Doctorate Recipients.
The number of underrepresented minorities in the basic biomedical sciences workforce increased from 1,066 in 1975 to 5,345 in 2001 and now accounts for 5.3 percent of the research employment in the field. Even though the annual average rate of growth for minorities in the workforce has been 15 percent over the past 10 years—more than twice the growth rate of the total workforce (6.5 percent)—the overall representation of minorities in biomedical research is still a small percentage of the overall workforce (see Appendix E-2 ). Their representation is also important from the scientific perspective, since researchers from minority groups may be better able and willing to address minority health care issues.
- PHYSICIAN-RESEARCHERS
Throughout this report Ph.D.s are considered to be researchers or potential researchers, but no such assumption is made of M.D.s because they could be practitioners. The above discussion, in particular, applies only to Ph.D.s in the fields listed in Appendix C , but it does not take into consideration physicians who are doing basic biomedical research. It is difficult to get a complete picture of this workforce because there is no database that tracks M.D.s involved in such activity, but a partial picture can be obtained from NIH files on R01 awards.
In 2001, R01 grants were awarded to 4,383 M.D.s (and to 17,505 Ph.D.s). 12 The number of R01-supported M.D. researchers has been increasing over the years (see Table 2-1 ) but has remained at about 20 percent. This means that the size of the biomedical workforce could be as much as one-fifth larger than indicated above. In fact, since NIH began to classify clinical research awards in 1996, it has become evident that both M.D.s and M.D./Ph.D.s supported by the agency are more likely to conduct nonclinical—that is, biomedical—than clinical research. Because many physician-investigators approach nonclinical research with the goal of understanding the mechanisms underlying a particular disease or disorder, their findings are likely to ultimately contribute to improvements in human health.
Number of M.D.s and Ph.D.s with Grant Support from NIH .
Some data are available from the American Medical Association on the national supply of physicians potentially in research. In 2002 there were 15,316 medical school faculty members in basic science departments and 82,623 in clinical departments. Of those in basic science, 2,255 had M.D. degrees, 11,471 had Ph.D.s, and 1,128 had combined M.D./ Ph.D. degrees. To identify the M.D.s in basic science departments who were actually doing research, the Association of American Medical Colleges Faculty Roster was linked to NIH records; it found that 1,261 M.D.s had been supported as principal investigators (PIs) on an R01 NIH grant at some point. This number is clearly an undercount of the M.D. research population, however, given that there are forms of NIH research support other than PI status and non-NIH organizations also support biomedical research.
- THE NATIONAL RESEARCH SERVICE AWARD PROGRAM AND BIOMEDICAL TRAINING SUPPORT
The National Research Service Award Program
In 1975, when the National Research Service Award (NRSA) program began, 23,968 graduate students in the basic biomedical sciences received some form of financial assistance for their studies, and about 8,000 supported their own education through loans, savings, or family funds. 13 The number of fellowships and traineeships, whether institutional or from external sources, was about 8,500 in 1975 and remained at about that level into the early 1990s, increasing only recently to 12,186 in 2002 (see Figure 2-9 ).
Mechanisms of support for full-time graduate students in the biomedical sciences, 1979–2002. SOURCE: National Science Foundation Survey of Graduate Students and Postdoctorates in Science and Engineering.
In the 1970s the majority of graduate student support came from these fellowships, traineeships, and institutional teaching assistantships. The picture began to change in the early 1980s as the prevalence of research grants grew. By 2002 it represented almost 50 percent of the support for graduate study in the biomedical sciences, and NIH's funding of this mechanism grew as well. In the early 1980s, NIH research grants formed about 40 percent of the total, and by the early 1990s this fraction grew to 64 percent and has remained at about this level through 2002 (see Figure 2-10 ). Even during the years when the NIH budget doubled, there was not a shift in this balance. In fact, from 1997 to 2002 both research grant and trainee/fellowship support from NIH increased by 14 percent. NIH in its response to the 2000 assessment of the NRSA program 14 has stated that research grants and trainee/fellowship awards are not used for the control of graduate support and that it would be inappropriate to try to do so.
FIGURE 2-10
Graduate support for NIH, 1979–2002. SOURCE: National Science Foundation Survey of Graduate Students and Postdoctorates in Science and Engineering.
The NRSA program now comprises the major part of NIH's fellowship and traineeship support. It began small in 1975—with 1,046 traineeships and 26 fellowships—but quickly expanded. By 1980 the number was nearly 5,000 for the traineeships; it remained at that level until 2001, though it dropped to a little over 4,000 in 2002 (see Table 2-2 ). (The drop in 2002 traineeships was probably an institutional reporting issue. Given that the total number of awards by NIH under the T32 15 mechanisms was about the same as in 2001, it is unlikely that the awards in the biomedical sciences would fall below the 2000 or 2001 levels.)
NRSA Predoctoral Trainee and Fellowship Support in the Basic Biomedical Sciences .
Information on funding patterns for postdoctorates in the basic biomedical sciences is not as complete as that for graduate students since academic institutions are the only sources of data. As has been the case for graduate student support, the portion of federal funds devoted to postdoctoral training grants and fellowships has diminished since the 1970s. In 1995, 1,966 (or 45.3 percent) of the 4,343 federally funded university-based postdoctorates received their training on a fellowship or traineeship. By 2002 the number had increased to 2,670 but was still only 20.3 percent of the total federal funding. The remaining 79.7 percent (or 10,514) in 2002 were supported by federal research grants. Meanwhile, the number of postdoctoral positions, funded by nonfederal institutional sources, was fairly constant at about 25 percent and grew from 1,325 in 1975 to 4,628 in 2002.
The picture for NRSA support at the postdoctoral level for the period following introduction of the NRSA program resembled that of the graduate level. However, in 2002 there was a sharp decrease in the number of postdoctoral traineeships; but, as in the case for predoctoral trainees, this may be an institutional reporting issue (see Table 2-3 ). Since the decline from 2001 to 2002 is nearly 50 percent and the decline for predoctoral trainees was only 20 percent, there may be a real decline at the postdoctoral level. The reason for this is unclear, though factors may include the limited number of individuals who can be supported under the increased stipend levels and the general decline in the number of postdoctoral research trainees eligible for NRSA support.
NRSA Postdoctoral Trainee and Fellowship Support in the Basic Biomedical Sciences .
The shift in the pattern of federal research training support, at both the graduate and postdoctoral levels, can be traced to a number of related trends. Over the past 25 years, the number of research grants awarded by the NIH and other agencies of the U.S. Department of Health and Human Services has more than doubled. 16 PIs have come to depend on graduate students and postdoctorates to carry out much of their day-to-day research work, and, as a result, the number of universities awarding Ph.D.s in the basic biomedical sciences, as well as the quantity of Ph.D.s awarded by existing programs, has grown.
Furthermore, federal funding policies have inadvertently provided universities with an incentive to appoint students and postdoctorates to research assistantships instead of training grants or fellowships. An example given in the eleventh NRSA study 17 shows that in 1999 the NIH provided almost $9,000 more to research assistants and their institutions (largely in the form of indirect cost payments to universities) than to NRSA trainees or fellows. Because the indirect cost rate for institutional training grants is generally about 7 percent compared to the 60 to 70 percent rate on research grants, it is financially advantageous for an institution to have as many research grants as possible for the support of graduate students. However, current policies at NIH have raised the NRSA predoctoral stipend levels to $19,968 and starting postdoctoral levels to $34,200. These increases might force stipends on research grants to similar levels and reduce the number of students who can be supported on research grants.
As described earlier, the number of students and postdoctorates provided with research training through NRSA training grants and fellowships has been deliberately limited over much of the past 25 years, as a control on the number of researchers entering the workforce. No similar federal effort has been undertaken thus far to ensure an adequate supply of technically prepared support staff in research, nor is there a system for regulating the number of research assistantships. As Massy and Goldman concluded in their 1995 analysis of science and engineering Ph.D. production, the size of doctoral programs is driven largely by departmental needs for research and teaching assistants rather than by the labor market for Ph.D.s. 18
In any case, NRSA training grants to institutions are highly prized and competitively sought. They confer prestige and add stability to graduate programs as they are usually for five years and allow for planning into the future. On the other hand, since the legislation that established the NRSA program allows only U.S. citizens and permanent residents to be trained through these grants and fellowships, the growing number of graduate students with temporary-resident status must be supported by other mechanisms.
Another factor in the shifting pattern of federal research training support is the type of education the students receive. Since the beginning of the NRSA program, NIH has required predoctoral training grants in the basic biomedical sciences to be “multidisciplinary” in order to expose students to a range of biomedical fields and even to other branches of science. Given that research collaborations between a wide variety of scientists have been producing significant advances, this requirement is even more important. Although the level of multidisciplinary training varies from program to program, students in training grant programs with this as part of their curriculum may better be ensured of such interdisciplinary training than those on a research assistantship. The committee considers multidisciplinary training in the biomedical sciences to be very valuable and of increasing importance. (A full discussion of these issues is presented in Chapter 8 , Emerging Fields and Interdisciplinary Studies.)
Although research grants provide an important base for training, data suggest that NRSA training grant participants complete training faster and go on to more productive research careers than do non-NRSA-supported students at their institution or doctorates from universities without NRSA training programs. This is supported by an assessment, completed in 1984, in which NRSA participants were found to complete their doctoral degrees faster and were more likely to go on to NIH-supported postdoctoral training than graduate students with other forms of support. 19 They also received a higher percentage of NIH research grants, authored more articles, and were cited more frequently by their peers.
Comparable outcomes were seen in a more recent study conducted by NIH. 20 Ph.D.s in the basic biomedical sciences who received NRSA support for at least one academic year spent less time in graduate school. About 57 percent of NRSA trainees and fellows received their doctorates by age 30, while only 39 percent of their classmates and 32 percent of graduates from departments without NRSA support similarly reached that milestone.
The study also showed that NRSA trainees and fellows were more likely to move into faculty or other research positions. Nearly 40 percent of the NRSA program participants held faculty appointments at institutions ranking in the top 100 in NIH funding, as opposed to 24 and 16 percent, respectively, for non-NRSA graduates from the same institution and graduates from non-NRSA institutions. Similarly, NRSA trainees and fellows were more likely to be successful in competing for grants and had better publication records than either of the other groups.
The NRSA program is essential to training in the biomedical sciences not only for these and other direct reasons; there are also its indirect benefits, such as establishing high standards for the entire graduate program and creating a generally improved environment for all students. Also, when students are supported by a combination of NRSA and research grant support, the NRSA funding is significantly leveraged.
The Medical Scientist Training Program
The MSTP was established at NIH in 1964 by the National Institute of General Medical Sciences (NIGMS) to support education leading to the M.D./Ph.D degree. By combining graduate training in the biomedical sciences with clinical training offered through medical schools, the program was designed to produce investigators who could better bridge the gap between basic science and clinical research. Since its inception, the Ph.D. portion of the training has been expanded to include the physical sciences, computer science, behavioral and social sciences, economics, epidemiology, public health, bioengineering, biostatistics, and bioethics, though almost all trainees receive a Ph.D. in a biomedical field.
When the MSTP began, it had only three programs, but it has since grown—in 2003 it had 41 programs involving 45 degree-granting institutions, with a total of 925 full-time training slots. This number is slightly down from the 933 slots in 2002. In addition, about 75 medical schools that do not have MSTP grants nevertheless offer opportunities for M.D./Ph.D. studies. The number of new students supported each year by MSTP funds varies from 2 or 3 at many institutions to 10 to 12 at a few exceptional ones, such as Duke University and the University of California, San Francisco. Some 170 new students nationwide are added to the program each year, with selection being highly competitive. The program provides 6 years of support for both phases of training, and institutions usually continue the awards for any additional years needed to complete the degrees. Support includes a tuition allowance, a stipend that is usually supplemented by the institution, and modest funds for travel, equipment, and supplies.
While the funds from NIH are sufficient to support only a few students in any one year of their training, institutions have been able to parlay the NIH funds by judiciously using institutional or research grant funds to support more students. A typical scenario is to support a student on MSTP funds during the first two years of medical training and again in the sixth or seventh years, when he or she returns to complete the medical degree. But during their Ph.D. studies, MSTP students are in a position to receive research grant support just like any other Ph.D. student. For example, one institution uses MSTP funds to support only 10 students during their first year and 2 during their second year in medical school, but there are 60 students in the MSTP program, with the remaining 48 receiving institutional or research grant support. This combination of funding results in the awarding of about 350 MSTP M.D./Ph.D. graduates each year. In the eyes of NIH, any student who receives MSTP funds and is supported for his or her entire course of study is considered a product of the program.
These graduates usually move on to postdoctoral, intern, and residency appointments and after completing their training tend to find academic research positions relatively easily. Another measure of the program's success is seen at the other end of the cycle—the competition among students for entry into the program. Some institutions, such as Johns Hopkins University, receive over 500 applications for the 10 or 12 available positions. Many of these students are highly qualified, and they apply for many programs simultaneously. Institutions easily fill their MSTP class, but some institutions with smaller and less well recognized programs have only a 30 percent acceptance rate. Occasionally these institutions lose students to other programs and begin the year with unfilled MSTP slots. Although not all applicants find MSTP positions, many end up pursuing a joint dual-degree program at an MSTP institution with partial or sometimes full support from non-MSTP funds. They follow the same track as the MSTP students and are indistinguishable from them.
Funding of the program is an issue at almost all MSTP institutions. While institutions are creative in the use of MSTP funds, they are unable to support many highly qualified students who have an interest in research but opt instead to attend just medical school and pursue a professional career. At a time when there is a need for more researchers with a medical background, it would be advantageous to have more M.D.s who are generally debt free and able to pursue research that requires the unique combination of biomedical and clinical training.
In addition to the advantages to biomedical and clinical research, MSTP graduates appear to have more productive research careers. In 1998 the NIGMS published a study of past recipients of MSTP support. 21 This study used résumé data of MSTP graduates with both an M.D. and a Ph.D. to compare their careers to four other groups of doctorates: MSTP-supported students who received only an M.D., Ph.D. recipients at MSTP institutions supported by NIH training grants, non-MSTP dual-degree graduates from an MSTP institution, and non-MSTP dual-degree graduates from a non-MSTP institution. The individuals in the study were divided into four 5-year cohorts from 1970 to 1995 to allow for changes over time in the educational characteristics and research environment. The cohorts and doctoral grouping were also compared on existing data from NIH files. The training and career paths of the MSTP graduates and the comparison groups were assessed from different perspectives, including time to degree, postdoctoral training, employment history, and research support and publication outcomes. By almost all measures, the MSTP-trained graduates fared better than the other groups. For example, they entered graduate training more quickly and took less time to complete the two degrees. Only the Ph.D. group applied for NIH postdoctoral fellowships at a higher rate, but the MSTP success rate was about the same as for the Ph.D. group. Depending on the cohort, between 60 and 70 percent of the MSTP graduates had a clinical fellowship and about 50 percent had both a clinical fellowship and postdoctoral training.
In terms of research activity, the NIH data showed that the MSTP graduates applied for research grant support from NIH at a greater rate and they were more successful in receiving support. The research productivity of the MSTP graduates across each of the cohorts as measured by published articles from the résumé data was about the same as that for the Ph.D. group and only slightly higher than the non-MSTP graduates from MSTP institutions. However, an examination of publications over the period from 1993 to 1995 showed that the earlier cohorts were more likely to be currently active than the Ph.D. graduates by publishing twice as many articles. The 1976–1980 non-MSTP cohorts, from MSTP institutions, also continued to be almost as active in publishing as the MSTP graduates.
The résumé analysis also provided insight into the professional and research activities of the different groups. About 83 percent of MSTP graduates in the study who were employed in 1995 had one or more academic appointments. This was higher than the M.D.- and Ph.D.-only groups and somewhat higher than the non-MSTP M.D./Ph.D.s group. Most of the dual-degree graduates in either group were in clinical departments and probably indicates some responsibility with regard to patient-oriented care. To better assess the type of research conducted by the different groups, the study classified the publications reported on the résumés into basic, clinical, and mixed type. Even though many of the dual-degree graduates are in clinical departments, they are still more likely to publish in basic journals, and this tendency is stronger in later cohorts.
The conclusions drawn from this analysis are that MSTP graduates appear to have been highly successful in establishing research careers, and their recent publication records suggest that members of all cohorts continue to be productive researchers. However, MSTP graduates appear most similar to non-MSTP M.D./Ph.D.s from the same institution; both groups are likely to be employed in academia with appointments in clinical or dual clinical and basic science departments, and both have similar publication patterns. This is not surprising, since non-MSTP-supported students at MSTP institutions follow the same program as their MSTP counterparts, complete the same degree requirements, and benefit from the MSTP-sponsored training efforts at those institutions.
- RESEARCH LABOR FORCE PROJECTIONS
The biomedical workforce with degrees from U.S. universities was estimated to be 100,262 in 2001. This included individuals in postdoctoral positions but did not count the 4,935 doctorates with degrees in biomedical fields who were unemployed or the 8,091 in positions not considered related to biomedical research (see Table 2-4 ). These three groups brought the potential workforce of U.S. doctorates to 113,288 (the only doctorates excluded were those who had retired). Table 2-4 also shows the change in this workforce over the past decade.
Potential Workforce in the Biomedical Sciences by Employment Status, 1991–2001 .
Note that in 2001 almost 80 percent of the potential workforce was employed in S&E and unemployment was less than 1 percent. Even with the inclusion of those unemployed and not seeking employment, only about 4.5 percent were unemployed.
The above figures represent only part of the total potential workforce, however, because foreign-trained doctorates also are employed in this country (and a few U.S. doctorates leave the country). Estimating this foreign component is difficult, given that no database describes the demographics of this group. Some data sources with information on foreign-trained doctorates exist, but they provide only a partial picture. 22 Based on these sources, it is estimated that about 15,500 such individuals are involved in biomedical research in the United States, though the size of this contingent could be as high as 25,000.
How the overall size of the S&E workforce might change over the next 10 years will be influenced by several factors: the number of doctorates who graduate each year, the unemployment levels in the field, the number of foreign-trained doctorates, and retirement rates. These factors can be accounted for by taking a multistate life-table approach, which models the workforce to estimate the numbers of researchers who enter and exit the workforce at various stages. It is also important to know the age of the workforce and the age at which individuals enter it, as this information determines retirement rates. What follows in this section is a short summary of the findings from this model's analysis, with full details available in Appendix D (Demographic Projections of the Research Workforce).
The largest and most relevant source of new researchers is the set of graduates from U.S. doctoral programs. The size of this group grew significantly in the 1990s but has leveled off or declined in recent years. Making projections of the numbers of future graduates, therefore, depends on which years are used to develop the model (a quadratic regression). Rather than choose just one scenario, three different scenarios for Ph.D. growth were developed. The first was a regression from 1985 to obtain a high estimate; the second was a low estimate, based on the assumption of constant growth from the 2001 level; and the third was the average of the two to represent “moderate” growth. For the high estimate the annual number of Ph.D.s grows from 5,386 in 2001 to 7,433 in 2011, and the average of this number and the one resulting from no growth yields 6,441 in 2011 (see 10-year totals in Table 2-5 ).
Projected Changes in U.S. and Foreign Doctorates Entering the Biomedical Workforce Between 2001 and 2011 .
A similar approach—with low, median, and high scenarios—was used for the inflow of foreign doctorates. However, because it is difficult to estimate the number of individuals in the current workforce with a foreign doctorate, the scenarios are based on estimates of the growth rate in the 1990s and the resulting population in 2001. Based on these estimates, it is possible to project the potential workforce in the biomedical sciences between 2001 and 2011. Using estimates of unemployment and the flow of doctorates in and out of the S&E workforce, the employed biomedical researcher population can also be estimated. Table 2-6 shows the results of the multistate life-table analysis under the medium scenario. These totals exhibit an annual growth rate in the biomedical workforce of 2 to 2.5 percent, which is comparable to the projected annual growth rate of the overall labor force.
Projected Workforce by Status for the Median Scenario, 2001–2011 .
Although these workforce projections are subject to many caveats, such as incomplete data and uncertainties in the economy and government spending, the balance between Ph.D. production and employment looks quite stable through 2011. Unemployment remains at about 1 percent, and the portion of the workforce remaining in science is about 80 percent. The committee believes this is a healthy percentage of trained people employed in science, but it has concerns about those unemployed and not seeking employment. The percentage of women in this category is significantly greater than their male counterparts, and there is a fear that some talented researchers may be lost because of the difficulty of balancing a career in science and raising a family. (This matter is considered further in Chapter 9 , Career Progression.)
The analysis in this chapter suggests that the number of researchers in basic biomedical research will remain stable for the next decade, as will employment opportunities, and the percentage of postdoctorals in holding patterns appears to be declining. Nevertheless, the committee's concern about the increased time to degree and the length of postdoctoral appointments should be noted—an infusion of young people into independent research positions, after all, is critical to the health of the research community. However, we also note that the increase in the average age of researchers parallels the aging of the general population.
“Success” is not easily quantified, but anecdotal evidence suggests that the NRSA program has successfully produced high-quality research personnel and has been important for the upgrading of research training in general. The MSTP program also merits special mention. It has been brilliantly successful at attracting outstanding physicians into basic biomedical research, much to the benefit of future health care. Given their special knowledge of human disease, physicians lend a unique perspective to such research.
The committee's recommendations for future training in the basic biomedical sciences are presented below, along with brief justifications based on the analysis described in this chapter.
- RECOMMENDATIONS
Recommendation 2-1: This committee recommends that the total number of NRSA positions awarded in the biomedical sciences should remain at least at the 2003 level. Furthermore, the committee recommends that training levels after 2003 be commensurate with the rise in the total extramural research funding in the biomedical, clinical, and behavioral and social sciences.
Although manpower models have been developed in this report, they are not particularly useful in assessing the role of NRSA support in particular, as this represents only a small fraction of the total training support in the biomedical sciences. Available information, however, suggests that the system is in reasonable balance. Stipends clearly should rise over time, but this should be accomplished by the allocation of additional funds, not by decreasing the number of trainees. The relatively low unemployment among Ph.D.s in the biomedical sciences, an almost constant number of U.S.-trained doctorates from 2001 to 2003, and the fact that the pool of postdoctorates appears to be stabilizing or declining justify the suggested level, which should not fall below that of 2003.
The year 2001 is the last one for which reasonably accurate data were available for awards specific to the biomedical sciences. However, the total number of NRSA awards continued to rise ( Figure 1-1 ) in 2002 and 2003, and it is assumed that the awards in the biomedical sciences have also increased. Using the percentage increase from 2001 to 2003 from Table 1-1 and the actual awards data for 2001 in Tables 2-2 and 2-3 , the predoctoral and postdoctoral traineeships in the biomedical sciences in 2003 are estimated to be 5,390 and 1,740, respectively. Fellowship data for 2002 appear to be more complete and show that awards at the postdoctoral level are somewhat below those of 2001. Based on the totals for NRSA predoctoral and postdoctoral training in 2001 and 2003, the estimated levels for fellowships in 2003 for the biomedical sciences are 425 and 1,450, respectively.
The primary rationale for NRSA is to attract high-quality people into specific research areas and to set the training standards for major research fields. NRSAs should be a paragon for quality training and have served this role admirably. NRSA programs are an important investment in the future to ensure the health of the research enterprise and should be made by all NIH institutes and centers.
Beyond the monetary requirements of maintaining NRSA training numbers, this committee does not recommend that support be shifted from research grants to training grants (contrary to the recommendation of the previous committee). A balance is needed between research and training grants for the productive support of students and postdoctorates. Research grants offer an alternative training venue, and students and postdoctorates are essential for accomplishing the research specified in research grants. Moreover, a variety of support mechanisms for training is desirable. The NRSA provides multiple pipelines into the research endeavor, most notably for foreign students and postdoctorates. In certain technical areas, insufficient numbers of U.S. citizens are available to train in and carry out national research efforts in critical areas. The training of foreign scientists on research grants has also significantly enriched the talent pool in this country, as they often join the workforce for extended periods of time, including permanent residence.
Although two earlier National Academies committees 23 , 24 have recommended that some NIH research funding be shifted to training grants and fellowships, our committee has concluded—based on the uncertainty about the rate of future growth in employment opportunities in industry, and perhaps other sectors, and the considerations discussed above—that the number of graduate students supported on NRSA training grants should not increase any faster than NIH research funding, which is a principal determinant of employment demand. With regard to postdoctoral support, another National Academies committee 25 has recommended that foreign scientists be permitted to receive training grant and fellowship support—thereby increasing the size of the eligible pool—and that some research funds be transferred to training budgets. However, consideration of the current restriction on supporting foreign scientists on NRSA training was outside the scope of this study and was not discussed by our committee.
At the present time, the committee does not recommend a shift in the overall proportion of training dollars spent on NRSA versus other training vehicles but does suggest that the ratios of research dollars to fellows/students be maintained in approximate alignment for the different areas and that training efforts be supported by all NIH institutes and centers. Better coordination of training efforts across institutes is needed. The committee recognizes, however, that the balance may vary from field to field and will evolve over time.
Recommendation 2-2: This committee recommends that the size of MSTP programs be expanded by at least 20 percent and that the scope be expanded to include the clinical, health services, and behavioral and social sciences.
Available evidence suggests that it is increasingly difficult for physicians to move into research because of the high cost of medical training and graduates' enormous debt load. Nevertheless, the committee believes that it is very important to attract physicians into research and that MSTP programs have done so with remarkable success; the excellent record of these programs' M.D./Ph.D.s in obtaining research grants and remaining in research is well documented. This would increase the number of trainees from the 2003 level of 933 to about 1,120.
As has been the policy, MSTP grants should be confined to institutions where high-quality medical and research training are both available. Expanding the range of disciplines should be helpful in attracting physicians into clinical and health services research but not at the expense of current MSTP support for basic biomedical training. Today's applicant pool for MSTP positions can easily accommodate a doubling of the size of the program without compromising its current quality. However, in recognition of the high cost of the MSTP program and budget constraints, the committee recommends a 20 percent increase as a significant and prudent investment.
U.S. Department of Education. 2000 .
Tabulations from the Higher Education Research Institute and the U.S. Department of Education.
Unpublished tabulation from the Survey of Earned Doctorates, 2001. Available from the National Academies.
Freeman, R. B., et. al. 2001 .
National Research Council. 1998c .
Goldman, E. and E. Marshall. 2002 .
National Science Foundation. 1997 .
National Science Foundation. 2002b .
The down turn in 1991 may be due to a change in the Survey of Doctorate Recipient data collection methods.
National Science Foundation. 2004 .
NIH Web site: http://grants.nih.gov/grants/award/research/rgbydgre01.htm . Accessed on October 22, 2004.
Unpublished tabulation from the NIH IMPAC System.
NIH Web site: NIH Statement in Response to Addressing the Nation's Changing Needs for Biomedical and Behavioral Scientists, http://grants.nih.gov/training/nas_report/NIHResponse.htm .
See Appendix B for a complete explanation of the awards.
NIH Web site. Available on http://grants.nih.gov/grants/award/research/rgbydgre01.htm . Accessed October 22, 2004.
National Research Council. 2000b .
Massy, W. F., and C. A. Goldman. 1995 .
Coggeshall, P., and P. W. Brown. 1984 .
Pion, G. M. 2000 .
National Institute of General Medical Sciences. 1998 .
Partial data are available from the Association of American Medical College's Faculty Roster and from the National Science Foundation, National Survey of College Graduates.
National Research Council. 2000a .
National Research Council. 1998c . op. cit.
National Research Council. 2005 .
- Cite this Page National Research Council (US) Committee for Monitoring the Nation's Changing Needs for Biomedical, Behavioral, and Clinical Personnel. Advancing the Nation's Health Needs: NIH Research Training Programs. Washington (DC): National Academies Press (US); 2005. 2, Basic Biomedical Sciences Research.
- PDF version of this title (1.4M)
In this Page
Other titles in this collection.
- The National Academies Collection: Reports funded by National Institutes of Health
Recent Activity
- Basic Biomedical Sciences Research - Advancing the Nation's Health Needs Basic Biomedical Sciences Research - Advancing the Nation's Health Needs
Your browsing activity is empty.
Activity recording is turned off.
Turn recording back on
Connect with NLM
National Library of Medicine 8600 Rockville Pike Bethesda, MD 20894
Web Policies FOIA HHS Vulnerability Disclosure
Help Accessibility Careers
Office: 919.785.1304 | Fax: 919.785.1306
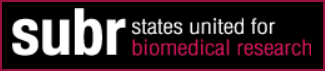
- Donate to SUBR
- Copyright and Privacy Statements
- Why Are Animals Needed in Research?
- What About Using Fewer Animals or No Animals?
- Product and Environmental Safety Research
- What is Biomedical Research?
- Biomedical Research Definitions
- Research Options
- How Animals Help
- Member Organizations
WHAT IS BIOMEDICAL RESEARCH?
Biomedical research is the broad area of science that looks for ways to prevent and treat diseases that cause illness and death in people and in animals. This general field of research includes many areas of both the life and physical sciences.
Utilizing biotechnology techniques, biomedical researchers study biological processes and diseases with the ultimate goal of developing effective treatments and cures. Biomedical research is an evolutionary process requiring careful experimentation by many scientists, including biologists and chemists. Discovery of new medicines and therapies requires careful scientific experimentation, development, and evaluation.
Why are Animals Used in Biomedical Research?
The use of animals in some types of research is essential to the development of new and more effective methods for diagnosing and treating diseases that affect both humans and animals. Scientists use animals to learn more about health problems, and to assure the safety of new medical treatments. Medical researchers need to understand health problems before they can develop ways to treat them. Some diseases and health problems involve processes that can only be studied in living organisms. Animals are necessary to medical research because it is impractical or unethical to use humans.
Animals make good research subjects for a variety of reasons. Animals are biologically similar to humans. They are susceptible to many of the same health problems, and they have short life-cycles so they can easily be studied throughout their whole life-span or across several generations. In addition, scientists can easily control the environment around animals (diet, temperature, lighting), which would be difficult to do with people. Finally, a primary reason why animals are used is that most people feel it would be wrong to deliberately expose human beings to health risks in order to observe the course of a disease.
Animals are used in research to develop drugs and medical procedures to treat diseases. Scientists may discover such drugs and procedures using alternative research methods that do not involve animals. If the new therapy seems promising, it is tested in animals to see whether it seems to be safe and effective. If the results of the animal studies are good, then human volunteers are asked to participate in a clinical trial. The animal studies are conducted first to give medical researchers a better idea of what benefits and complications they are likely to see in humans.
A variety of animals provide very useful models for the study of diseases afflicting both animals and humans. However, approximately 95 percent of research animals in the United States are rats, mice, and other rodents bred specifically for laboratory research. Dogs, cats, and primates account for less than one percent of all the animals used in research.
Those working in the field of biomedical research have a duty to conduct research in a manner that is humane, appropriate, and judicious. CBRA supports adherence to standards of care developed by scientific and professional organizations, and compliance with governmental regulations for the use of animals in research.
Scientists continue to look for ways to reduce the numbers of animals needed to obtain valid results, refine experimental techniques, and replace animals with other research methods whenever feasible.
© California Biomedical Research Association
- U.S. Department of Health & Human Services

- Virtual Tour
- Staff Directory
- En Español
You are here
Biomedical research leads science’s 2021 breakthroughs, nih blog post date, connect with us.
- More Social Media from NIH

An official website of the United States government
Here’s how you know
Official websites use .gov A .gov website belongs to an official government organization in the United States.
Secure .gov websites use HTTPS A lock ( Lock A locked padlock ) or https:// means you’ve safely connected to the .gov website. Share sensitive information only on official, secure websites.

History of Medicine
About | Collections | Exhibitions | Research Tools | Copyright | Get Involved | Visit | Contact

- Introduction
- Health Care for Seamen
- Fighting the Spread of Epidemic Diseases
- The Beginnings of Organized Biomedical Research
- Notable Contributions to Biomedical Research
- Current Research
- Pure Food and Drugs
- Mental Health and Drug Abuse
- Health Care Delivery
- International Health
- Index of Images
Biomedical Research
Current research.
Following World War II, federal support for biomedical research was greatly expanded and so was the role of the National Institute of Health, which was renamed the National Institutes of Health to reflect the growth of research functions. Today NIH encompasses seventeen research institutes, two research divisions, the world's largest research hospital, the National Library of Medicine, the National Center for Human Genome Research, the National Center for Research Resources, and the Fogarty International Center. Its operating budget in 1990 was 7.6 billion dollars.
NIH conducts research on its Bethesda, Maryland campus and supports research throughout the United States by means of a competitive grant system. This research deals with every aspect of human biology and almost every disease and disability.
Some of the major topics of research today include: oncogenes and the question of how a normal cell becomes a cancer; the workings of immune cells and how they help to defend the body against cancer; the isolation of genes, including those for blood clotting factors; brain changes in Alzheimer's disease; the application of genetic engineering techniques to vaccine production; the discovery and isolation of bone growth factors; the study of neurotransmitters and modulation in the brain; cancer risk from passive smoking; new uses of the laser for the treatment of eye diseases; the construction of artificial chromosomes; the development and refinement of new body imaging technologies, such as magnetic resonance imaging (MRI) and, of course, the study of AIDS.
In 1968, Dr. Marshall W. Nirenberg of the National Heart, Lung, and Blood Institute became the first of four NIH Nobel laureates to date. He won the Nobel Prize in Physiology or Medicine for his work in translating the genetic code and its function in protein synthesis; he is shown here with his molecular models.
The National Heart, Lung, and Blood Institute has been a leader in conducting and supporting clinical studies of heart disease, including open heart surgery and artificial hearts, as well as educating the public about the prevention of heart attacks through control of high blood pressure, control of high levels of blood cholesterol, and cessation of smoking. Research advances and lifestyle changes have helped lower the death rate from heart disease by 43 percent since 1972.
Researcher at the National Heart, Lung, and Blood Institute's Laboratory of Kidney and Electrolyte Metabolism uses fluorescence microscopy to study kidney physiology, specifically kidney tubule transport. Other important research work on kidney function, such as improving the prevention and management of end-stage renal disease and improving dialysis techniques, is done at the National Institute of Diabetes and Digestive and Kidney Diseases.
Researcher at the National Heart, Lung, and Blood Institute engaged in the study of sickle cell anemia, a genetic blood disease which, in the United States, affects primarily Afro-Americans and is caused by an abnormal hemoglobin molecule. A new test, known as chorionic villus biopsy, promises to advance the prenatal diagnosis of genetic blood diseases, including sickle cell anemia, from the second trimester of pregnancy to the first. Tissue from the chorionic villi, hairlike projections of the membrane that surrounds the early embryo, can be removed before the 10th week of pregnancy and analyzed immediately for chromosomal or biochemical defects.
The National Institute of Diabetes and Digestive and Kidney Diseases (NIDDK) has pioneered in the study and treatment of diabetes, the fifth leading cause of death in the United States. The NIDDK supported research which led to the development of the insulin pump. A physician instructs a patient in its use.
Dr. John Klippel of the National Institute of Arthritis and Musculoskeletal and Skin Diseases (NIAMS) examines and measures the extension of the fingers of a patient with lupus, a disease characterized by eruption of scarred lesions and inflamed joints. Researchers at the NIAMS elaborated the underlying immunologic mechanisms in systemic lupus erythematosus (SLE), developed more effective treatments, and dramatically improved survival.
The development and testing of new and promising experimental drugs or vaccines for a variety of ailments is one of the key functions of the National Institutes of Health researchers.
Increasingly sophisticated instrumentation such as this fast atomic bombardment mass spectrometer, which separates chemical components, is being used at the FDA's Center for Biologics Evaluation and Research to determine the purity and potency of biologics and other products.
Laser technology is being applied to biomedical research by scientists at the FDA's Center for Biologics Evaluation and Research. Here a laser cell separation machine is separating a line of cells, such as T-cells, for immunology studies.
A computed tomography (CT) scan, a noninvasive method of getting good cross-sectional images of the body, is being done on a patient at the NIH's Warren U. Magnuson Clinical Center. The Clinical Center is the world's largest hospital devoted solely to biomedical research where physicians from all the different NIH institutes, together with the Center's staff, pursue clinical and laboratory studies related to patient care.
Legionnaires' disease was first recognized in July 1976, when a sudden outbreak of pneumonia, resulting in several deaths, occurred mostly in persons who had attended an American Legion convention in Philadelphia. Researchers Charles C. Shepard (1914-85) and Joseph E. McDade of the Centers for Disease Control and Prevention were the first in 1977 to identify the disease-causing bacterium -- Legionella pneumophilis, which is pictured here. Since then more than 20 species in the Legionella genus have been identified and the mystery surrounding many illnesses associated with them solved, in the grand tradition of the microbe hunters of the late 19th and early 20th centuries. Many of these modern day microbe hunters or epidemiologists were trained in the Centers for Disease Control and Prevention's Epidemic Intelligence Service, which was established by Dr. Alexander Laugmuir in 1951.
Researchers, such as this man in the Centers for Disease Control and Prevention's new maximum containment virology laboratory, use the most advanced technology available to study dangerous organisms like the Lassa, Machupo, Ebola, and AIDS viruses that cause deadly diseases for which no cure or vaccine exists. Statistics about these and other diseases are published in the Morbidity and Mortality Weekly Report (MMWR), a widely read publication around the world. Weekly reporting of morbidity and mortality statistics to the Public Health Service began in 1893. Various bureaus of the Service have published these reports. Since 1961, the Center for Disease Control and Prevention's Epidemiology Program Office has been responsible for publishing the MMWR.
Researcher at the National Eye Institute (NEI) tests the peripheral and central visual fields of a patient to find areas of visual loss. The NEI has helped pioneer the use of lasers in preventing visual loss from diabetes and other eye diseases, and is testing new medicines that may he able to prevent diabetes-induced damage to the retina and other tissues.
Researcher at the National Institute of Dental Research (NIDR) uses computers to measure very small changes in teeth and their surrounding tissues that are not detectable with conventional dental X-rays. Since its creation in 1948, the NIDR has been the primary sponsor of dental research in the United States. Much of its work was focused on preventing tooth decay, through such programs as community water fluoridation.
Dr. Robert C. Gallo, since 1972 chief of the National Cancer Institute's Laboratory of Tumor Cell Biology, is an internationally prominent investigator of human viruses and tumor cells. He played a leading role in isolating and characterizing the family of human viruses to which the AIDS causing virus, HIV (human immunodeficiency virus), belongs. Gallo and his colleagues are also responsible for the development of a blood test to detect HIV antibodies in blood collected for transfusions.
Vice-President George Bush addresses the AIDS Executive Committee during the centennial year of the National Institutes of Health. As the NIH enters its second century it faces one of its greatest research challenges -- a cure and vaccine against the deadly viral disease AIDS (acquired immunodeficiency syndrome), which has become the major scourge of the late twentieth century. To the left of the Vice-President is Dr. James B. Wyngaarden, director of the NIH, and to the right of the Vice-President is Dr. Anthony Fauci, director of the NIH's National Institute of Allergy and Infectious Diseases and coordinator of NIH research on AIDS.
The National Library of Medicine was established in 1836 as the Library of the Army Surgeon General's Office. The Armed Forces Institute of Pathology and its Medical Museum were founded in 1862 as the Army Medical Museum. Throughout their history the Army Medical Library and the Army Medical Museum often shared quarters. This red-brick building was built on the Mall in Washington, D.C., in 1887 for these two institutions. It was torn down in the late 1960s to make room for the Hirshorn Museum of Art. By an act of Congress in 1936 the Library collection was transferred from the Department of Defense to the Public Health Service of the Department of Health, Education and Welfare and renamed the National Library of Medicine. The Library moved to its current quarters in Bethesda, Maryland, on the campus of the National Institutes of Health in 1962.
Under the very able leadership of Dr. John Shaw Billings (1838-1913), a Civil War surgeon who served as director from 1865 to 1895, the Library increased in size from about 2,000 volumes to over 100,000 volumes of books and bound serials and expanded from serving primarily military medical officers to serving all physicians. It soon became the leading medical library in the United States and then the world. The first issue of Index Medicus, a comprehensive monthly medical bibliography with a subject and author index to articles published in medical journals around the world, was published in 1879.
The National Library of Medicine is now the world's largest medical research library. Its holdings include more than 3.5 million books, journals, technical reports, theses, pamphlets, photographs, manuscripts and audiovisual materials covering more than 40 biomedical areas and related subjects. The Library also houses one of the world's finest historical collections of rare medical texts and manuscripts. The 10-story Lister Hill Center was built in 1980 to house the Library's expanding computer facilities, audiovisual studios, and research and development laboratories. With materials in 70 languages and international exchange capabilities the Library can serve health professionals worldwide.
In order to keep up with the ever-increasing volume of biomedical literature that had to be included in the Index Medicus bibliography, the Library turned to computers in the early 1960s. An extensive computerized literature retrieval system, known as MEDLARS, became totally operational in 1964. It performed thousands of searches before on-line searching capabilities and data bases, such as MEDLINE, became available in 1971. Through MEDLINE, health professionals and other interested individuals have immediate access to more than 3 million journal article references accumulated since 1965 and growing at a rate of over 300,000 a year.
| Table of Contents | Next >

An official website of the United States government
The .gov means it’s official. Federal government websites often end in .gov or .mil. Before sharing sensitive information, make sure you’re on a federal government site.
The site is secure. The https:// ensures that you are connecting to the official website and that any information you provide is encrypted and transmitted securely.
- Publications
- Account settings
Trending Articles
- Depleting myeloid-biased haematopoietic stem cells rejuvenates aged immunity. Ross JB, et al. Nature. 2024. PMID: 38538791
- Transketolase promotes MAFLD by limiting inosine-induced mitochondrial activity. Tong L, et al. Cell Metab. 2024. PMID: 38547864
- Formation of memory assemblies through the DNA-sensing TLR9 pathway. Jovasevic V, et al. Nature. 2024. PMID: 38538785
- Embracing cancer complexity: Hallmarks of systemic disease. Swanton C, et al. Cell. 2024. PMID: 38552609 Review.
- Oxygen imaging of hypoxic pockets in the mouse cerebral cortex. Beinlich FRM, et al. Science. 2024. PMID: 38547288
Latest Literature
- Cancer Res (6)
- J Biol Chem (7)
- J Clin Endocrinol Metab (2)
- J Immunol (6)
- Lancet (10)
- PLoS One (78)
- Pediatrics (1)
- Proc Natl Acad Sci U S A (25)
NCBI Literature Resources
MeSH PMC Bookshelf Disclaimer
The PubMed wordmark and PubMed logo are registered trademarks of the U.S. Department of Health and Human Services (HHS). Unauthorized use of these marks is strictly prohibited.
- Skip to primary navigation
- Skip to main content
- Skip to footer
Human Research Protection Program
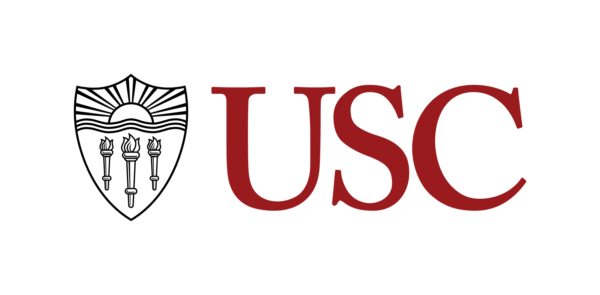
Biomedical Research
What is biomedical research.
Biomedical scientists study human physiology and the treatment or understanding of disease. Biomedical research applies the principles of the physical sciences to medicine. Most biomedical research is conducted by physicians or biomedical scientists, but many studies are conducted by biologists, chemists, physicists, and other medical and scientific professionals.
Most biomedical research involves clinical trials, which are phased studies using human volunteers, designed to answer safety and efficacy questions about biologics, devices, pharmaceuticals, new therapies or new ways of using known treatments. Trials are often conducted in small group initially but expanding in later stages once safety and efficacy are demonstrated. Most clinical trials are FDA regulated, but there are some exceptions.
Types and Methods
- research on therapies ( e.g. , drugs, exercise, surgical interventions, or medical devices)
- diagnostic procedures ( e.g. , CAT scans, prenatal diagnosis through amniocentesis)
- preventive measures ( e.g. , vaccines, diet, or fluoridated toothpaste)
- studies of the human body while exercising, fasting, feeding, sleeping, or learning
- responding to such things as stress or sensory stimulation
- Studies comparing the functioning of a particular physiological system at different stages of development ( e.g. , infancy, childhood, adolescence, adulthood, or old age)
- Studies defining normal childhood development so that deviations from normal can be identified
- Records research – often used to develop and refine hypotheses
- research on the biochemical changes associated with AIDS
- research on the neurological changes associated with senile dementia
- Research on the human genome and genetic markers – for the purpose of creating new avenues for understanding disease processes and their eventual control
- research with animals
- research on preexisting samples of materials (tissue, blood, or urine) collected for other purposes, where the information is recorded by the investigator in such a manner that subjects cannot be identified, directly or through identifiers linked to the subjects
- research based on records, when the data are recorded in such a manner that the individuals to whom the records pertain cannot be identified, either directly or through identifiers linked to them
Risk is the probability of harm or injury (physical, psychological, social, or economic) occurring as the result of participation in a research study. Biomedical researchers must consider the following risks when conducting their study:
- Social, psychological, or economic harm (See Social Behavioral Research for details)
- exercise-induced or repetition-exacerbated physical harm, such as carpal tunnel syndrome, stress fractures, asthma attacks, or heart attacks
- exposure to minor pain, discomfort (e.g. dizziness), or injury from invasive medical procedures
- possible side effects of drugs
Although most of the adverse effects that result from medical procedures or drugs are temporary, investigators must be aware of the potential for harm. The IRB will want to know how such outcomes will be minimized or addressed and is responsible for conducting a risk/benefit assessment.
When submitting an application in iStar you will see the following in section 1.5.
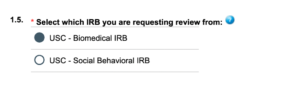
Genomic Data Sharing (GDS)/Stem Cell Research
- USC Stem Cell Regenerative Medicine Initiative
- USC Stem Cell Research Oversight Committee (SCRO)
- NIH Genomic Data Sharing Policy (formerly ‘GWAS’)
- California Institute for Regenerative Medicine (CIRM)
- Genetics and Public Policy Center
- NIH: Stem Cell Research
- U.K. Human Fertilisation and Embryological Authority
- The Hinxton Group: An International Consortium on Stem Cells, Ethics and Law
- The International Society for Stem Cell Research (ISSCR)
- Human Genome Project: Educational Kit
3720 S. Flower Street, Suite 325 Los Angeles, CA 90089
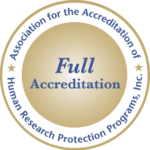
- Announcements
- Getting Started
- Internal Staff Webpage
- Education & Certification
- HRPP Performance & Metrics
- Policies and Procedures
- Post Approval Monitoring (PAM)
- Youtube Channel
- Biospecimen & Data Repositories
- Investigational Drugs and Devices
- Investigator-Initiated Trials
- Emergency Research
- SBIRB Social Behavioral Research
- Student Researchers
- Requesting USC to Rely on an External IRB
- Requesting USC IRB to Act as the sIRB
- Starting a Research Trial: the Basics
- Forms and Templates
- FWA and IRB Registration Numbers
- IRB Member Toolbox
- IRB Review: How to
- IRB Submission Guidelines
- Levels of IRB Review
- Not Human Subjects Research (NHSR)
- Post IRB Review and Approval
- Privacy, Confidentiality, and Anonymity in Human Subjects Research
- Urgent Review
Eminent cancer physician-scientist joins Virginia Tech to lead research in Washington, D.C.
Christopher Hourigan will spearhead innovative cancer solutions at the Fralin Biomedical Research Institute Cancer Research Center in the nation's capital.
John Pastor
- Share on Facebook
- Share on Twitter
- Copy address link to clipboard
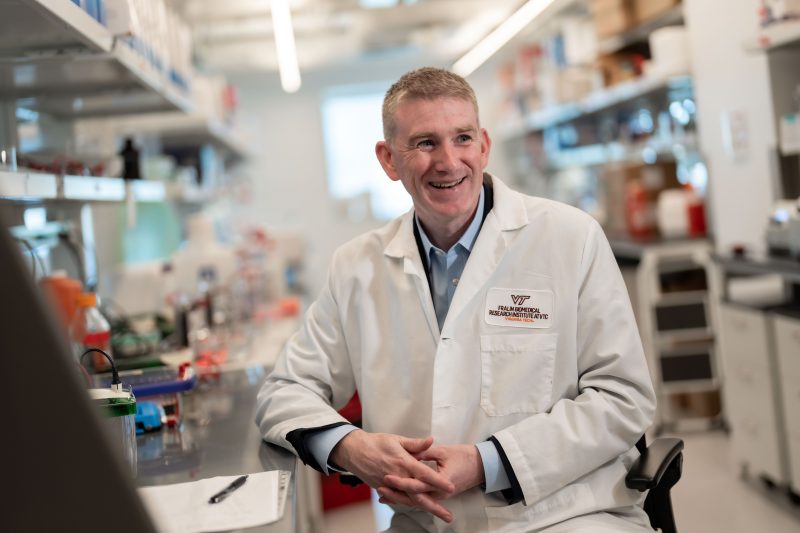
A globally recognized physician-scientist who studies and treats blood cancer is joining Virginia Tech to lead cancer research in Washington, D.C., said Michael Friedlander, Virginia Tech’s vice president for health sciences and technology and executive director of the Fralin Biomedical Research Institute at VTC.
Christopher Hourigan , a senior investigator and chief of the Laboratory of Myeloid Malignancies at the National Institutes of Health, will join Virginia Tech as a professor with the institute and director of its Cancer Research Center in Washington, D.C.
“Dr. Hourigan exemplifies the prototype of a physician-scientist, integrating insights from his patient interactions directly into his fundamental and translational laboratory research,” Friedlander said. “We are extremely enthusiastic to have him join Virginia Tech and become a member of the Fralin Biomedical Research Institute team to take on this important new leadership role for our growing cancer research programs in Washington, D.C., and to further strengthen our collaborations with Carilion Clinic, Children’s National Hospital, and other health systems and universities.
The focus of the research effort, known as Fralin Biomedical Research Institute Cancer Research Center - D.C., is to bring basic, translational, clinical, and computational researchers together in the nation’s capital to focus on the shared aim of engineering cancer solutions.
“Convening top talent like Dr. Hourigan and developing deep and diverse partnerships are key to solving our most complex global challenges,” said Virginia Tech President Tim Sands. “It’s exciting to see our vision for the university advancing across the commonwealth and in the greater Washington, D.C., metro area.”
Your browser does not support iframes. Link to iframe content: https://www.youtube.com/embed/tS1TgAcvxhA?si=IuLYq7Em-QpT-w55
“I also anticipate the innovations and insights that will emerge from Dr. Hourigan and the other Virginia Tech scientists in D.C. collaborating with the institute's cancer research teams in Roanoke as well as with our computer science and engineering colleagues at Virginia Tech’s new Innovation Campus in Alexandria,” Friedlander said.
Research will involve diverse, expert teams and national and international collaborations.
“We have the opportunity to build a new cancer research center from the ground up, focusing on getting talented and highly motivated teams working in innovative new ways to reduce the burden of suffering from cancer in the United States,” Hourigan said. “It's clear we're not doing well enough for people who are dealing with cancer, and this is our chance to come up with new ways to do better.”
The addition of Hourigan will accelerate the university’s initiatives in Washington, D.C. Newly renovated research facilities on the site of the former Walter Reed Army Medical Center on the Children’s National Research & Innovation Campus already house Fralin Biomedical Research Institute cancer research scientists Jia-Ray Yu and Kathleen Mulvaney , along with teams of researchers from the Center for Genetic Medicine Research and Rare Disease Institute of Children’s National Hospital.
Precise answers to hard questions
Hourigan’s research focuses on a high-risk form of blood cancer called acute myeloid leukemia, which annually affects about 20,000 Americans. He looks at reasons for why some people survive cancer while others end up dying, even though initially they seem to have had the same response to treatment.
“The person who's newly diagnosed with cancer and has gone through treatment will ask a reasonable question, ‘Well, what about me? I want to know what my actual likelihood of surviving is going to be and are you sure I don’t need more or different treatment?’” Hourigan said. “As an oncologist, those are often hard questions to answer. You have a lot of empathy with the person in front of you and wish we could give better, more personalized, answers. We're strongly focused on the idea that if we had better diagnostic tools to allow a precision medicine approach, we could give doctors and patients a better understanding of exactly where they are now and what’s the best thing for them to do next.”
In addition to his primary appointment with the institute, Hourigan will be a professor in the Department of Internal Medicine at the Virginia Tech Carilion School of Medicine .
Hourigan received his medical degree and research doctorates from Oxford University and completed residency and oncology fellowship training at Johns Hopkins University School of Medicine, where he was subsequently a practicing physician on the acute leukemia service and faculty member. He is board certified in hematology and medical oncology.
Prior to joining Virginia Tech, Hourigan was a senior investigator, co-director of the Myeloid Malignancies Program, and chief of the Laboratory of Myeloid Malignancies at the National Institutes of Health.
He was awarded the Presidential Early Career Award for Scientists and Engineers, the National Institutes of Health Director’s Challenge Innovation Award, and was elected to Alpha Omega Alpha Medical Honor Society. He is a fellow of both the American College of Physicians and the Royal College of Physicians.
He looks forward to the task ahead.
“Virginia Tech has some key strategic advantages,” Hourigan said. “It is nationally known for engineering and computational science, which are going to be increasingly important components of cancer research. I think there's also this sense of energy, innovation, engineering, and teams working across disciplines on hard problems here. And cancer is a hard problem.”
Ultimately, he wants to put cancer researchers out of business.
“I don't want there to be a need for cancer research anymore, whether that happens in my lifetime or the lifetimes of those individuals I train,” Hourigan said. “The ultimate objective is not to have an industry of cancer research. Our focus has to be on the patient and on working hard to come up with real solutions to hard problems. It is only with research that we’ll be able to do better with cancer prevention, diagnosis, and treatment tomorrow than we currently can today. We need to have a sense of urgency and purpose because people are counting on us to come up with answers.”
(540) 526-2222
- Cancer Research Center (DC)
- Destination Areas
- Fralin Biomedical Research Institute at VTC
- Fralin Biomedical Research Institute at VTC - top news
- Fralin Biomedical--Cancer Research Group
- Friedlander News
- Hourigan Lab
- Virginia Tech Carilion School of Medicine
Related Content
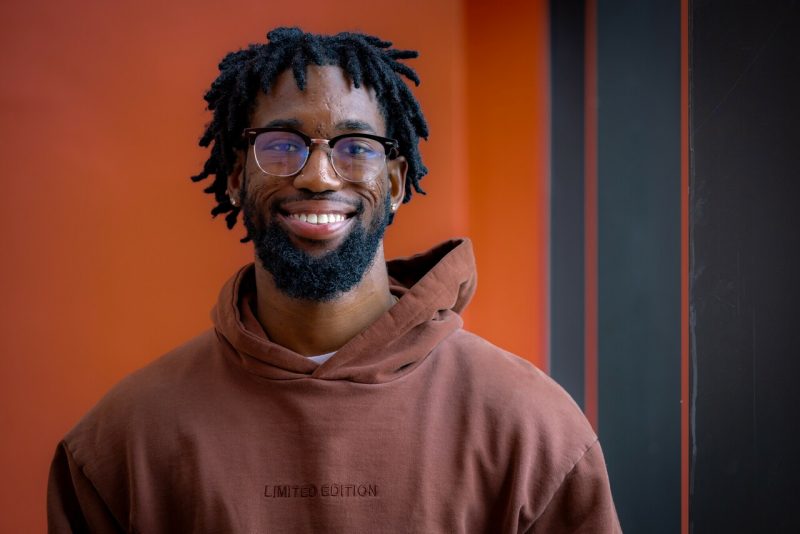
Librarians/Admins
- EBSCOhost Collection Manager
- EBSCO Experience Manager
- EBSCO Connect
- Start your research
- EBSCO Mobile App
Clinical Decisions Users
- DynaMed Decisions
- Dynamic Health
- Waiting Rooms
- NoveList Blog
MEDLINE Ultimate for Biomedical Research
Medical Students and biomedical researchers can depend on MEDLINE Ultimate for all their research questions. This video highlights the benefits of the leading biomedical database that covers more than 500 topics and includes the leading biomedical journals, ahead of print content and MeSH subject headings.
For More Information: ebsco.com/products/research-databases/medline-ultimate
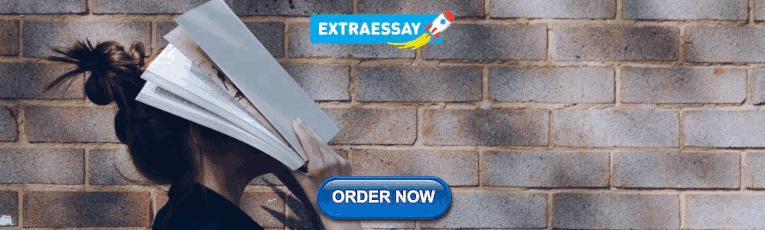
Transcript | Download
Would you like to give medical students and biomedical researchers the ultimate advantage?
Give them access to MEDLINE Ultimate.
Comprehensive in scope, MEDLINE Ultimate delivers everything necessary for research success.
Covering more than 500 topics, it is the largest collection of full text biomedical journals.
Ahead-of-print content representing the most current biomedical research.
Thousands of international journals providing global context to research.
MEDLINE subject headings aligned with MeSH established by the National Library of Medicine.
Easy linking from PubMed Index to the full text in MEDLINE Ultimate with a simple link resolver.
With all this and more, it’s no wonder that MEDLINE Ultimate is a trusted resource for researchers around the world.
MEDLINE Ultimate. For the ultimate biomedical research experience.
You might also be interested in
Cinahl ultimate for nursing and allied health research, public library solutions from ebsco, ebsco resources for english and language arts curricula.
Cookie Notice
This website uses cookies. Contact us for questions or requests.
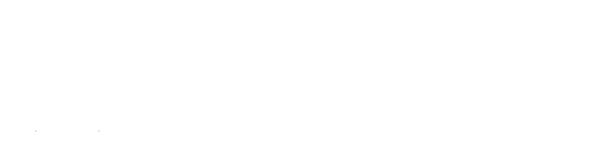
Information for:
- Current Students
- Faculty & Staff
- Degrees & Programs
- Campus Maps
- Jobs & Careers
- Campus Shuttles
- Student Life
- Giving
- Augusta University
- Centers & Institutes
- Resources for principle investigators
Resources for principle investigators: Quality and productivity improvement in biomedical research
Our study collected information about the most frequent quality defects in biomedical research. analyzed their prevelance an highlighted opportunities for prevention. Self-check your research and do not let journal reviewer catch weaknesses in your study. The below slides provide the error specifications and their associated frequancies. APA citation to the reporting article is also provided.
Institute for Public and Preventive Health
Health Sciences Campus
Pavilion III
706-721-1104
BMRI recommended data sources for analysis or research performance
With the rapidly expanding scientific enterprise scientific enterprise and the great interest in growing research, an unprecedented need for effectiveness studies is emerging. Informed decision-making needs wide ranging data analyses from a variety of sources. The BMRI laboratory team compiled a list of sources with description and access links.
Featured Clinical Reviews
- Screening for Atrial Fibrillation: US Preventive Services Task Force Recommendation Statement JAMA Recommendation Statement January 25, 2022
- Evaluating the Patient With a Pulmonary Nodule: A Review JAMA Review January 18, 2022
- Download PDF
- Share X Facebook Email LinkedIn
- Permissions
Clinical, Biomarker, and Research Tests Among US Government Personnel and Their Family Members Involved in Anomalous Health Incidents
- 1 Rehabilitation Medicine Department, Clinical Center, National Institutes of Health, Bethesda, Maryland
- 2 The Military Traumatic Brain Injury Initiative, Bethesda, Maryland
- 3 National Institute of Neurological Disorders and Stroke, National Institutes of Health, Bethesda, Maryland
- 4 National Institute on Deafness and Other Communication Disorders, National Institutes of Health, Bethesda, Maryland
- 5 National Intrepid Center of Excellence Walter Reed National Military Medical Center, Bethesda, Maryland
- 6 Uniformed Services University of the Health Sciences, Bethesda, Maryland
- 7 National Eye Institute, National Institutes of Health, Bethesda, Maryland
- 8 Henry M. Jackson Foundation for the Advancement of Military Medicine Inc, Bethesda, Maryland
- 9 Laboratory on Quantitative Medical Imaging, National Institute of Biomedical Imaging and Bioengineering, Bethesda, Maryland
- Editorial Neurological Illness and National Security David A. Relman, MD JAMA
- Preliminary Communication Clinical Findings and Outcomes in US Government Personnel Reporting Directional Sensory Phenomena in Cuba Randel L. Swanson II, DO, PhD; Stephen Hampton, MD; Judith Green-McKenzie, MD, MPH; Ramon Diaz-Arrastia, MD, PhD; M. Sean Grady, MD; Ragini Verma, PhD; Rosette Biester, PhD; Diana Duda, PT, DPT; Ronald L. Wolf, MD, PhD; Douglas H. Smith, MD JAMA
- Original Investigation Neuroimaging Findings in US Government Personnel With Possible Exposure to Directional Phenomena in Cuba Ragini Verma, PhD; Randel L. Swanson, DO, PhD; Drew Parker, BS; Abdol Aziz Ould Ismail, MD; Russell T. Shinohara, PhD; Jacob A. Alappatt, BTech; Jimit Doshi, MS; Christos Davatzikos, PhD; Michael Gallaway, OD; Diana Duda, PT, DPT; H. Isaac Chen, MD; Junghoon J. Kim, PhD; Ruben C. Gur, PhD; Ronald L. Wolf, MD, PhD; M. Sean Grady, MD; Stephen Hampton, MD; Ramon Diaz-Arrastia, MD, PhD; Douglas H. Smith, MD JAMA
- Original Investigation Neuroimaging Findings in Individuals Involved in Anomalous Health Incidents Carlo Pierpaoli, MD, PhD; Amritha Nayak, ME; Rakibul Hafiz, PhD; M. Okan Irfanoglu, PhD; Gang Chen, PhD; Paul Taylor, PhD; Mark Hallett, MD; Michael Hoa, MD; Dzung Pham, PhD; Yi-Yu Chou, MS; Anita D. Moses, MSN; André J. van der Merwe, BS; Sara M. Lippa, PhD; Carmen C. Brewer, PhD; Chris K. Zalewski, PhD; Cris Zampieri, PhD; L. Christine Turtzo, MD, PhD; Pashtun Shahim, MD, PhD; Leighton Chan, MD, MPH; NIH AHI Intramural Research Program Team; Brian Moore, DMSc, MPH; Lauren Stamps, BS; Spencer Flynn, BA; Julia Fontana, BS; Swathi Tata, BS; Jessica Lo, BS; Mirella A. Fernandez, BS; Annie Lori-Joseph, BS; Jesse Matsubara, DPT; Julie Goldberg, MA; Thuy-Tien D. Nguyen, MS; Noa Sasson, BS; Justine Lely, BS; Bryan Smith, MD; Kelly A. King, AuD, PhD; Jennifer Chisholm, AuD; Julie Christensen, MS; M. Teresa Magone, MD; Chantal Cousineau-Krieger, MD; Louis M. French, PsyD; Simge Yonter, MD; Sanaz Attaripour, MD; Chen Lai, PhD JAMA
Questions Do US government officials and their family members involved in anomalous health incidents (AHIs) differ from control participants with respect to clinical, biomarker, and research assessments?
Findings In this exploratory study that included 86 participants reporting AHIs and 30 vocationally matched control participants, there were no significant differences in most tests of auditory, vestibular, cognitive, visual function, or blood biomarkers between the groups. Participants with AHIs performed significantly worse on self-reported and objective measures of balance, and had significantly increased symptoms of fatigue, posttraumatic stress disorder, and depression compared with the control participants; 24 participants (28%) with AHIs presented with functional neurological disorders.
Meaning In this exploratory study, there were no significant differences between individuals reporting AHIs and matched control participants with respect to most clinical, research, and biomarker measures, except for self-reported and objective measures of imbalance; symptoms of fatigue, posttraumatic stress, and depression; and the development of functional neurological disorders in some.
Importance Since 2015, US government and related personnel have reported dizziness, pain, visual problems, and cognitive dysfunction after experiencing intrusive sounds and head pressure. The US government has labeled these anomalous health incidents (AHIs).
Objective To assess whether participants with AHIs differ significantly from US government control participants with respect to clinical, research, and biomarker assessments.
Design, Setting, and Participants Exploratory study conducted between June 2018 and July 2022 at the National Institutes of Health Clinical Center, involving 86 US government staff and family members with AHIs from Cuba, Austria, China, and other locations as well as 30 US government control participants.
Exposures AHIs.
Main Outcomes and Measures Participants were assessed with extensive clinical, auditory, vestibular, balance, visual, neuropsychological, and blood biomarkers (glial fibrillary acidic protein and neurofilament light) testing. The patients were analyzed based on the risk characteristics of the AHI identifying concerning cases as well as geographic location.
Results Eighty-six participants with AHIs (42 women and 44 men; mean [SD] age, 42.1 [9.1] years) and 30 vocationally matched government control participants (11 women and 19 men; mean [SD] age, 43.8 [10.1] years) were included in the analyses. Participants with AHIs were evaluated a median of 76 days (IQR, 30-537) from the most recent incident. In general, there were no significant differences between participants with AHIs and control participants in most tests of auditory, vestibular, cognitive, or visual function as well as levels of the blood biomarkers. Participants with AHIs had significantly increased fatigue, depression, posttraumatic stress, imbalance, and neurobehavioral symptoms compared with the control participants. There were no differences in these findings based on the risk characteristics of the incident or geographic location of the AHIs. Twenty-four patients (28%) with AHI presented with functional neurological disorders.
Conclusions and Relevance In this exploratory study, there were no significant differences between individuals reporting AHIs and matched control participants with respect to most clinical, research, and biomarker measures, except for objective and self-reported measures of imbalance and symptoms of fatigue, posttraumatic stress, and depression. This study did not replicate the findings of previous studies, although differences in the populations included and the timing of assessments limit direct comparisons.
- Editorial Neurological Illness and National Security JAMA
Read More About
Chan L , Hallett M , Zalewski CK, et al. Clinical, Biomarker, and Research Tests Among US Government Personnel and Their Family Members Involved in Anomalous Health Incidents. JAMA. Published online March 18, 2024. doi:10.1001/jama.2024.2413
Manage citations:
© 2024
Artificial Intelligence Resource Center
Cardiology in JAMA : Read the Latest
Browse and subscribe to JAMA Network podcasts!
Others Also Liked
Select your interests.
Customize your JAMA Network experience by selecting one or more topics from the list below.
- Academic Medicine
- Acid Base, Electrolytes, Fluids
- Allergy and Clinical Immunology
- American Indian or Alaska Natives
- Anesthesiology
- Anticoagulation
- Art and Images in Psychiatry
- Artificial Intelligence
- Assisted Reproduction
- Bleeding and Transfusion
- Caring for the Critically Ill Patient
- Challenges in Clinical Electrocardiography
- Climate and Health
- Climate Change
- Clinical Challenge
- Clinical Decision Support
- Clinical Implications of Basic Neuroscience
- Clinical Pharmacy and Pharmacology
- Complementary and Alternative Medicine
- Consensus Statements
- Coronavirus (COVID-19)
- Critical Care Medicine
- Cultural Competency
- Dental Medicine
- Dermatology
- Diabetes and Endocrinology
- Diagnostic Test Interpretation
- Drug Development
- Electronic Health Records
- Emergency Medicine
- End of Life, Hospice, Palliative Care
- Environmental Health
- Equity, Diversity, and Inclusion
- Facial Plastic Surgery
- Gastroenterology and Hepatology
- Genetics and Genomics
- Genomics and Precision Health
- Global Health
- Guide to Statistics and Methods
- Hair Disorders
- Health Care Delivery Models
- Health Care Economics, Insurance, Payment
- Health Care Quality
- Health Care Reform
- Health Care Safety
- Health Care Workforce
- Health Disparities
- Health Inequities
- Health Policy
- Health Systems Science
- History of Medicine
- Hypertension
- Images in Neurology
- Implementation Science
- Infectious Diseases
- Innovations in Health Care Delivery
- JAMA Infographic
- Law and Medicine
- Leading Change
- Less is More
- LGBTQIA Medicine
- Lifestyle Behaviors
- Medical Coding
- Medical Devices and Equipment
- Medical Education
- Medical Education and Training
- Medical Journals and Publishing
- Mobile Health and Telemedicine
- Narrative Medicine
- Neuroscience and Psychiatry
- Notable Notes
- Nutrition, Obesity, Exercise
- Obstetrics and Gynecology
- Occupational Health
- Ophthalmology
- Orthopedics
- Otolaryngology
- Pain Medicine
- Palliative Care
- Pathology and Laboratory Medicine
- Patient Care
- Patient Information
- Performance Improvement
- Performance Measures
- Perioperative Care and Consultation
- Pharmacoeconomics
- Pharmacoepidemiology
- Pharmacogenetics
- Pharmacy and Clinical Pharmacology
- Physical Medicine and Rehabilitation
- Physical Therapy
- Physician Leadership
- Population Health
- Primary Care
- Professional Well-being
- Professionalism
- Psychiatry and Behavioral Health
- Public Health
- Pulmonary Medicine
- Regulatory Agencies
- Reproductive Health
- Research, Methods, Statistics
- Resuscitation
- Rheumatology
- Risk Management
- Scientific Discovery and the Future of Medicine
- Shared Decision Making and Communication
- Sleep Medicine
- Sports Medicine
- Stem Cell Transplantation
- Substance Use and Addiction Medicine
- Surgical Innovation
- Surgical Pearls
- Teachable Moment
- Technology and Finance
- The Art of JAMA
- The Arts and Medicine
- The Rational Clinical Examination
- Tobacco and e-Cigarettes
- Translational Medicine
- Trauma and Injury
- Treatment Adherence
- Ultrasonography
- Users' Guide to the Medical Literature
- Vaccination
- Venous Thromboembolism
- Veterans Health
- Women's Health
- Workflow and Process
- Wound Care, Infection, Healing
- Register for email alerts with links to free full-text articles
- Access PDFs of free articles
- Manage your interests
- Save searches and receive search alerts
Helping Texans make the best real estate decisions since 1971.
- TG Magazine
How can we help you?
As the state’s population grows, so does the need for more housing. Here are the data and tools you need to keep up with housing market trends in your area.
How Natural Disasters Affect Homebuying Decisions
- Texas Housing Insight
- MSA Housing Reports
- Monthly Housing Data
- Texas Housing Affordability Index
- Home Price Index
- Building Permits
- 2024 Texas Real Estate Forecast
- A Towering Task
- All in the Family
- Austin Home Price Illusion
- Austin’s Power
Whether you’re talking about DFW’s financial services industry, Austin’s tech sector, Houston’s energy corridor, or the medical hub that is San Antonio, commercial real estate is big business in Texas.
- Thinking Outside the Grid
- Texas Quarterly Commercial Report
- 2024 Texas Real Estate Forecast UPDATE
- Mechanic’s & Materialman’s Liens
- What’s My Line?
- A Bumpy Ride
Mineral rights. Water issues. Wildlife management and conservation. Eminent domain. The number of factors driving Texas land markets is as big as the state itself. Here’s information that can help.
Post-Pandemic Land Prognosis
- Land Occupier’s Liability Guide
- On the Trail of ESG
- A Nagging Question
- Texas Small Rural Land
Texas is a large, diversified state boasting one of the biggest economies in the world. Our reports and articles help you understand why.
- Outlook for the Texas Economy
- Texas Border Economy
- Employment BLS
- Employment TWC
- Take Me to Texas
- Go With the Flow
Data & Reports
Center research is fueled by accurate, high-quality, up-to-date data acquired from such sources as Texas MLSs, the U.S. Bureau of Labor Statistics, and the U.S. Census Bureau. Data and reports included here are free.
- Housing Activity
- Housing Affordability
- Housing Reports By MSA
- Data Gallery
Stay current on the latest happenings around the Center and the state with our news releases, NewsTalk Texas online searchable news database, and more.
Texas’ mortgage market showing signs of stabilizing, survey shows
- 12-story condo tower being developed in Rice Village
- Tesla supplier next to sign on to Robinson Business Park
- New plans for former Rackspace headquarters
- PID approval comes with 1,400 new homes in Austin
- Partnership acquires 621K-sf industrial portfolio in northwest Houston
- Financing secured for Addison industrial redevelopment
- Press Releases
Conferences & Courses
We offer a number of educational opportunities throughout the year, including our popular Outlook for Texas Land Markets conference. Check here for updates.
Outlook for Texas Land Markets
- Broker Responsibility Instructor Training
- Legal Update I & II Instructor Training
Established in 1971, the Texas Real Estate Research Center is the nation’s largest publicly funded organization devoted to real estate research. Learn more about our history here and meet our team.
- Our History
- Advisory Committee
- Collaborations
- Our Gallery
Texas Real Estate Research Center 2115 TAMU College Station, TX 77843
Groundbreaking for biomedical research: TMC3

The 1.5 million-sf facility will be located just south of Brays Bayou and north of Old Spanish Trail in the Texas Medical Center footprint.
The groundbreaking project will generate a $5.2 billion stimulus to Texas and create 30,000 new jobs.
The project will break ground in 2019 with estimated completion in 2022.
TMC3 will be shared by the Texas Medical Center, Baylor College of Medicine, Texas A&M University Health Science Center, The University of Texas Health Science Center at Houston, and The University of Texas MD Anderson Cancer Center.
In This Article
‘amenities gone wild’ at bryan tower, 248-unit denton multifamily community opens, kansas logistics firm snags 900k-sf distribution building lease, you might also like.
Check out the latest issue of our flagship publication.
Publications
Receive our economic and housing reports and newsletters for free.
You are leaving this website
You are now being directed to an external page. Please note that we are not responsible for the content or security of the linked website.
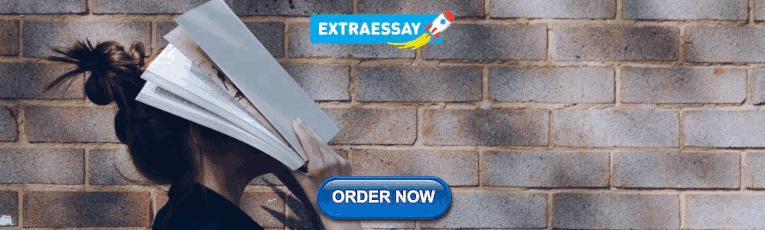
COMMENTS
Basic biomedical research, which addresses mechanisms that underlie the formation and function of living organisms, ranging from the study of single molecules to complex integrated functions of humans, contributes profoundly to our knowledge of how disease, trauma, or genetic defects alter normal physiological and behavioral processes. Recent advances in molecular biology techniques and ...
The Working Group decided to broaden the scope of the 2002 Guidelines from "biomedical research" to "health-related research". The Working Group considered biomedical research too narrow since that term would not cover research with health-related data, for example. At the same time, the Working
The University of Florida Cancer and Genetics Research Complex is an integrated medical research facility. Medical research (or biomedical research ), also known as health research, refers to the process of using scientific methods with the aim to produce knowledge about human diseases, the prevention and treatment of illness, and the promotion ...
Biomedical research is the broad area of science that looks for ways to prevent and treat diseases that cause illness and death in people and in animals. This general field of research includes many areas of both the life and physical sciences. Utilizing biotechnology techniques, biomedical researchers study biological processes and diseases ...
The past 200 years have seen rapid advances in western biomedicine. A model arising from western Europe and North America, current biomedical science is largely driven by efforts to prevent or cure diseases. It uses hierarchies of evidence generated from observational and experimental research,1 and is arguably driven by the interests of scientists who hold this underlying philosophy, with ...
Basic biomedical research refers to the use of fundamental scientific principles in medi-cal and biological research directed towards developing tools to detect, prevent or treat human disease. Basic biomedical research is commonly encountered in the discovery and exploratory stages of product/drug development. This type of research is not yet
That changed in 2021 with success using monoclonal antibodies against infections with SARS-CoV-2 as well as respiratory syncytial virus (RSV), human immunodeficiency virus (HIV), and other infectious diseases. This earned them a prominent spot among Science 's breakthroughs of 2021. Monoclonal antibodies delivered via intravenous infusions ...
Biomedical sciences. A biochemist engaged in bench research. Biomedical sciences are a set of sciences applying portions of natural science or formal science, or both, to develop knowledge, interventions, or technology that are of use in healthcare or public health. [1] Such disciplines as medical microbiology, clinical virology, clinical ...
Having doubled between 1998 and 2003, the NIH budget is expected to be $28.6 billion for fiscal year 2007, a 0.1 percent decrease from last year, 1 or a 3.8 percent decrease after adjustment for ...
Biomedical Research Current research. Following World War II, federal support for biomedical research was greatly expanded and so was the role of the National Institute of Health, which was renamed the National Institutes of Health to reflect the growth of research functions. Today NIH encompasses seventeen research institutes, two research ...
Biomedical Research. Few topics in biomedical research, other than research funding and the JIF, the latter of which is a direct output of the peer review process, have galvanized opinion in biomedical researchers to the same degree as peer review with numerous erudite and often emotive statements that reflect on the innate bias of reviewers ...
What is Biomedical Research? Biomedical research is the pursuit of answers to medical questions. These investigations lead to discoveries, which in turn lead to the development of new preventions, therapies and cures for human and veterinary health. Biomedical research generally takes two forms: basic science and applied research.
PubMed is a comprehensive database of biomedical literature from various sources, including MEDLINE, life science journals, and online books. You can search for citations, access full text content, and explore topics related to health, medicine, and biology. PubMed also provides advanced search options and tools for researchers and clinicians.
Biomedical research applies the principles of the physical sciences to medicine. Most biomedical research is conducted by physicians or biomedical scientists, but many studies are conducted by biologists, chemists, physicists, and other medical and scientific professionals. Most biomedical research involves clinical trials, which are phased ...
Biomedical research jobs may focus on any area of the health care system, from pharmaceutical research to community health. Philanthropic organizations, professional societies as well as the federal government may provide research grants for a broad range of biomedical projects. A biomedical scientist could work as part of a team studying ...
A globally recognized physician-scientist who studies and treats blood cancer is joining Virginia Tech to lead cancer research in Washington, D.C., said Michael Friedlander, Virginia Tech's vice president for health sciences and technology and executive director of the Fralin Biomedical Research Institute at VTC.. Christopher Hourigan, a senior investigator and chief of the Laboratory of ...
Get new journal Tables of Contents sent right to your email inbox Get New Issue Alerts
Comprehensive in scope, MEDLINE Ultimate delivers everything necessary for research success. Covering more than 500 topics, it is the largest collection of full text biomedical journals. Ahead-of-print content representing the most current biomedical research. Thousands of international journals providing global context to research.
Biomedical research is such unique platform to collect and disseminate state-of-the-art scientific understanding on biomedicine and related discipline. This multidisciplinary open access journal is rendering a global podium for the academicians, researchers and students of the relevant disciplines to share their scientific excellence in the ...
Recognition and prevention of quality defects in basic biomedical research. Our study collected information about the most frequent quality defects in biomedical research. analyzed their prevelance an highlighted opportunities for prevention. Self-check your research and do not let journal reviewer catch weaknesses in your study.
Key Points. Questions Do US government officials and their family members involved in anomalous health incidents (AHIs) differ from control participants with respect to clinical, biomarker, and research assessments?. Findings In this exploratory study that included 86 participants reporting AHIs and 30 vocationally matched control participants, there were no significant differences in most ...
HOUSTON - A new 30-acre research center dubbed Texas Medical Center 3 (TMC3) is expected to establish Houston as an international hub for biomedical research. The 1.5 million-sf facility will be located just south of Brays Bayou and north of Old Spanish Trail in the Texas Medical Center footprint.
Sponsored by the Office for Research, the Busch Biomedical Grant program is designed to enhance biomedical research at the university and to strengthen the competitive position of faculty members who seek external research funds. The program is funded by the interest income from the Charles and Johanna Busch bequest to reflect the wishes of ...