
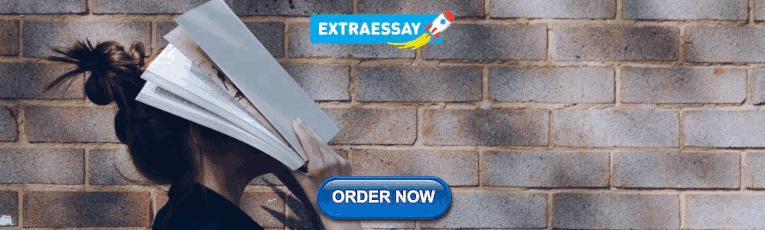
Free Fall Motion: Explanation, Review, and Examples
- The Albert Team
- Last Updated On: February 16, 2023
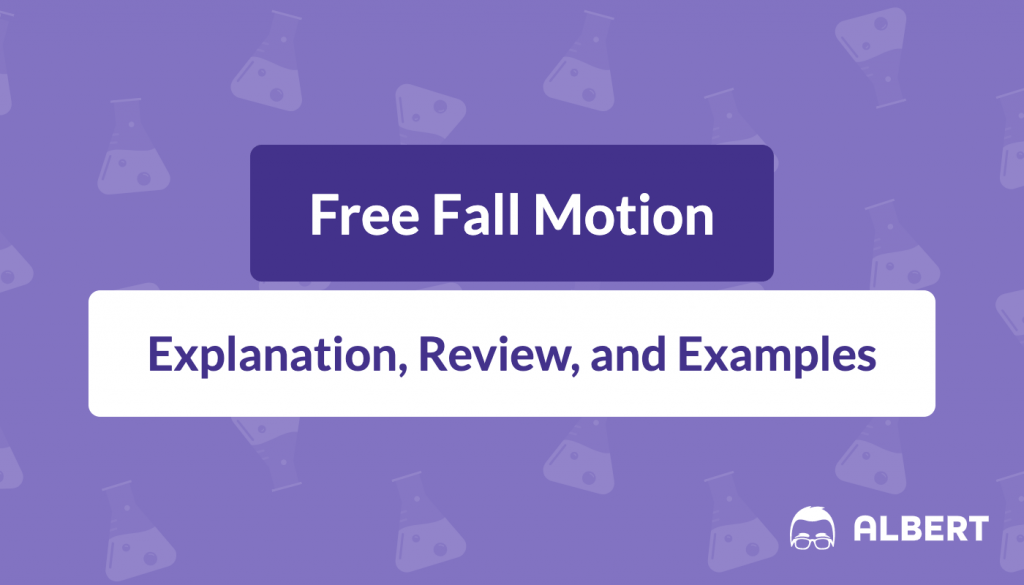
Free fall and projectile motion describe objects that are moving through the air and acted on only by gravity. In this post, we will describe this type of motion using both graphs and kinematic equations. Since projectile motion involves two dimensions, these problems can be complex. We will explain many examples so you can see how to solve different types of projectile motion.
What We Review
An object that is moving under only the influence of gravity is in free fall. In order for an object to be in free fall, wind and air resistance must be ignored. On Earth, all objects in free fall accelerate downward at the rate of gravity or 9.81\text{ m/s}^2 .
Applying Free Fall to Kinematic Equations
When analyzing free fall motion, we can apply the same kinematic equations as we did for motion on the ground. We can then use these equations to determine properties such as distance, time, and velocity.
How to Find Distance Fallen for an Object in Free Fall
If an object is in free fall, we can use kinematic equations to find the distance it falls during a certain time. You will typically use the following kinematic equation to calculate the distance fallen:
In order to use this equation, you need to know the initial velocity of the object and the time of flight. Remember that the acceleration of a free falling object is always equal to the acceleration due to gravity, 9.81\text{ m/s}^2 .
Many free fall physics problems will include scenarios where objects are dropped from rest. In this case, the initial velocity is zero and the first term of the kinematic equation above will cancel out.
If the time is not known, another method for calculating the distance fallen is to use the following kinematic equation:
In this case, you must know the final velocity v_f of the object. Then, you can solve the equation for the distance d .
How to Find Time for an Object in Free Fall
The amount of time an object is in free fall will depend on its velocity and the distance it falls. Similar to distance, there are two equations you can use to find the time, depending on what you know.
If you know the initial and final velocity of the object, then the simplest way to calculate time is using the kinematic equation:
This equation can be solved for time. Then, you’ll only need to substitute the values for the velocities and the acceleration due to gravity.
Another method to find time if you do not know the object’s final velocity is to use the equation:
Note that in this equation there are two terms that include the time t . Unless the initial velocity is zero, this can make it more challenging to solve this equation for time. If using this equation, you may need to use the quadratic formula to solve for time.
How to Find Final Velocity for an Object in Free Fall
The final velocity of an object in free fall depends on the amount of time it falls. Due to the acceleration of gravity, the velocity will increase every second by 9.81\text{ m/s} . The final velocity can be calculated using the equation:
If you do not know the amount of time the object is falling, another method for calculating the final velocity is using the kinematic equation:
This equation requires that you instead know the distance that the object falls. If you are using this equation to find the final velocity, remember that the final velocity is squared in this equation. That means you will need to take a square root as your final step to solve for the final velocity.
Examples of Free Fall
In this next section, we’ll apply the methods you just learned to solve some problems about free fall motion.
Example 1: How to Find the Distance for an Object Dropped from Rest
For example, an object is dropped from rest from the top of a tall building. It hits the ground 5\text{ s} after it is dropped. What is the height of the building?
In this scenario, we know that the object’s initial velocity is zero because it was dropped from rest. We also know that the acceleration is 9.81\text{ m/s}^2 . This problem is asking us to find the distance the object falls. This will be equal to the height of the building.
Based on this information, we can use the following kinematic equation to find the distance:
Substituting the given values produces:
Therefore, the height of the building is about 123\text{ m} .
Example 2: How to Find the Final Velocity for an Object with Initial Velocity
In another example, an object in free fall has an initial, downward velocity of 2\text{ m/s} and falls a distance of 45\text{ m} . What is the object’s final velocity?
In this scenario, we are given the object’s initial velocity, v_i and the distance d . We also know that the acceleration is 9.81\text{ m/s}^2 . Based on this information, we can use the following kinematic equation to find the final velocity:
Since the initial velocity is in the same direction as the acceleration (downward) we can use the same sign for both values.
Our last step is to eliminate the square by taking the square root:
Therefore, the final velocity of the object is about 30\text{ m/s} .
Motion Graphs for Objects in Free Fall
In addition to using physics equations, we can also represent free fall motion with motion graphs. Position-time graphs, velocity-time graphs, and acceleration-time graphs can tell us a lot about the object’s motion over time. Want a more in-depth review of motion graphs? Check out this blog post !
Position-Time Graph for an Object in Free Fall
In terms of position, many objects in free fall start at a high position, or height off the ground, and move downward. Objects in free fall accelerate due to gravity. Therefore, the position-time graph for free fall motion must be curved. This means that objects in free fall start with a slow velocity and gradually speed up which is represented by the steep downward curve of the graph.

Velocity-Time Graph for an Object in Free Fall
As an object falls, its velocity increases due to the acceleration of gravity. This means that the velocity starts slow and steadily increases in the downward direction. The graph below shows the velocity-time for an object in free fall:

Note that the slope of this graph is constant and represents the acceleration due to gravity, or -9.81\text{ m/s}^2 .
Acceleration-Time Graph for an Object in Free Fall
Free fall acceleration is constant. Throughout the entire time that an object is falling, it is accelerating at a rate equal to the acceleration due to gravity, -9.81\text{ m/s}^2 . As shown in the graph below, the acceleration-time graph is a constant negative line.

Projectile Motion
A projectile is an object that is launched or thrown into the air and then only influenced by gravity. Projectile motion has many similarities to free fall motion, however, projectiles may also travel a horizontal distance in addition to falling vertically down.
Examples of Projectile Motion
The exact trajectory, or path, a projectile will take depends on how it is launched. However, all projectiles follow a curved trajectory such as in the image shown below:
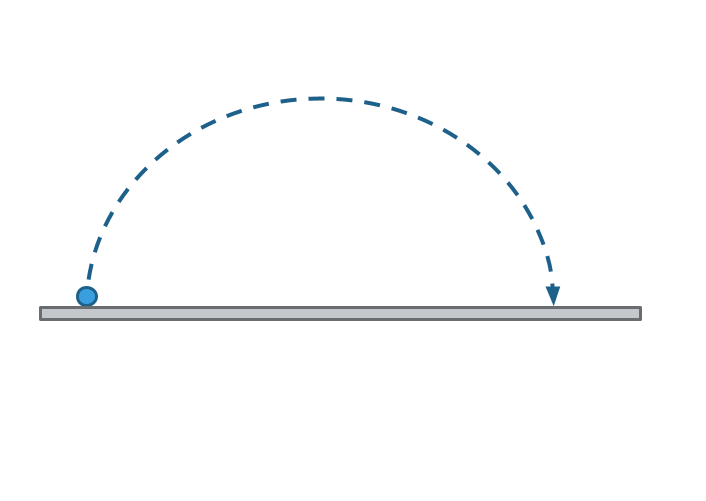
If you play or watch sports, you likely have already observed projectile motion. Projectile motion describes the arc of a basketball in a free throw, a fly ball in baseball, or a volleyball bumped over the net.
Horizontal Component of Velocity
To analyze projectile motion, we must separate the motion into horizontal and vertical components. The horizontal component of a projectile’s velocity is independent of the vertical component of velocity. Since gravity acts vertically, there are no horizontal forces acting on projectiles. This means that the horizontal component of a projectile’s velocity remains constant throughout the entire flight.
Example: Finding the Horizontal Component
For example, a projectile is launched from the ground with an initial speed of 8\text{ m/s} at a 60^{\circ} angle. What is the horizontal component of the projectile’s velocity?
We will need to use trig identities to determine the components of the velocity. We can visualize the components as a triangle where the hypotenuse is the initial velocity and the sides represent the horizontal, v_{ix} , and vertical, v_{iy} , components of the velocity.

Cosine is defined as the adjacent side of the triangle divided by the hypotenuse. Since the horizontal component is adjacent to the angle, we can use cosine to find the horizontal component of velocity:
Therefore, the horizontal component of the initial velocity is 4\text{ m/s} .
Need to review your trig identities? Try out this resource from Khan Academy .
Vertical Component of Velocity
The vertical component of a projectile’s velocity will be influenced by gravity, which acts vertically on the object causing it to accelerate downward. Therefore, the vertical component of velocity will change throughout the projectile’s flight. We can calculate the vertical component of velocity at a particular time in a method similar to calculating the horizontal component.
Example: Finding the Vertical Component
In the same example, a projectile is launched from the ground with an initial speed of 8\text{ m/s} at a 60^{\circ} angle. What is the vertical component of the projectile’s velocity?
As we visualize the velocity components, we are solving this time for the opposite side of the triangle. Sine is defined as the opposite side of the triangle divided by the hypotenuse. Therefore, the initial vertical velocity is:
Solving Projectile Motion Questions
Let’s apply what we’ve learned to some examples of projectile motion!
Example 1: Finding the Range of a Projectile
In this example, a projectile is fired horizontally with a speed of 5\text{ m/s} from a cliff with a height of 60\text{ m} . How far from the base of the cliff will the projectile land?
In this scenario, we are given the initial horizontal velocity v_{ix}=5\text{ m/s} and the vertical change in position d_y=-60\text{ m} . Since the projectile is launched horizontally, the initial vertical velocity, v_{iy} , is zero. We also always know in projectile motion that the vertical acceleration is a_y=-9.81\text{ m/s}^2 and the horizontal acceleration, a_x , is zero.
This problem is asking us to find the horizontal displacement, or d_x . This is also referred to as the range . We can use the following kinematic equation to find the projectile’s final horizontal position:
Since the horizontal acceleration of a projectile is zero, this equation can be simplified to:
Before we can solve this equation, we must first determine the time of the projectile’s flight. We can actually use this same equation in the vertical direction to solve for time:
Since the initial vertical velocity is zero, this equation can be simplified to:
Solving for t :
Substituting the given values:
Now we can use this time to calculate the horizontal displacement of the projectile:
Therefore, the projectile will land about 17.5\text{ m} from the base of the cliff.
Example 2: Finding the Maximum Height of a Projectile
As another example, a projectile is launched from the ground with an initial velocity of 25\text{ m/s} at an angle of 50^{\circ} . What is the projectile’s maximum height?
As a projectile travels upward, its vertical velocity becomes slower and slower due to the negative acceleration of gravity. At the maximum height of the trajectory, the projectile’s vertical velocity will momentarily be zero as the projectile stops and turns to move downward. Therefore, in this scenario, our final vertical velocity, v_{fy} , is zero.
We can use the following kinematic equation to solve for the maximum height, d_y :
Solving for d_y :
Before we can use this equation to calculate the height, we will need to use the sine trig identity to find the vertical component of the initial velocity:
Since the initial velocity is in the opposite direction as the acceleration, it’s really important to remember the sign here. If we define moving up as positive, then the initial velocity is positive and the acceleration is negative. Substituting this initial vertical velocity and the given values into the equation above gives:
Therefore, the projectile will reach a maximum height of about 18.7\text{ m} .
For more examples and an explanation of solving these types of projectile motion problems, check out this youtube video from Professor Dave .
Understanding free fall and projectile motion allows you to solve some of the most complex problems you will encounter in introductory physics. All projectiles are acted on only by gravity, and the vertical and horizontal components of motion are independent of each other. This allows us to apply our kinematic equations to solve for a projectile’s time of flight, velocity, and displacement in each direction.
Interested in a school license?
Popular posts.

AP® Score Calculators
Simulate how different MCQ and FRQ scores translate into AP® scores
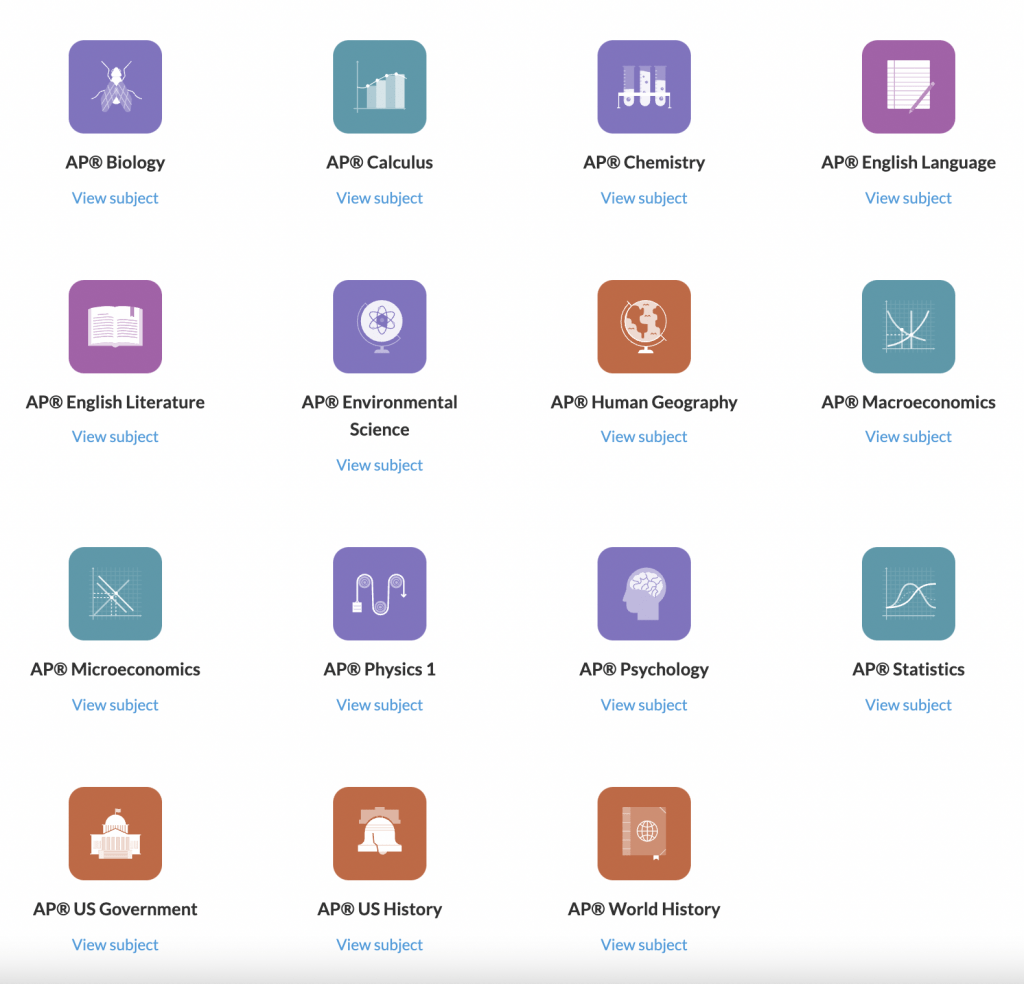
AP® Review Guides
The ultimate review guides for AP® subjects to help you plan and structure your prep.

Core Subject Review Guides
Review the most important topics in Physics and Algebra 1 .

SAT® Score Calculator
See how scores on each section impacts your overall SAT® score
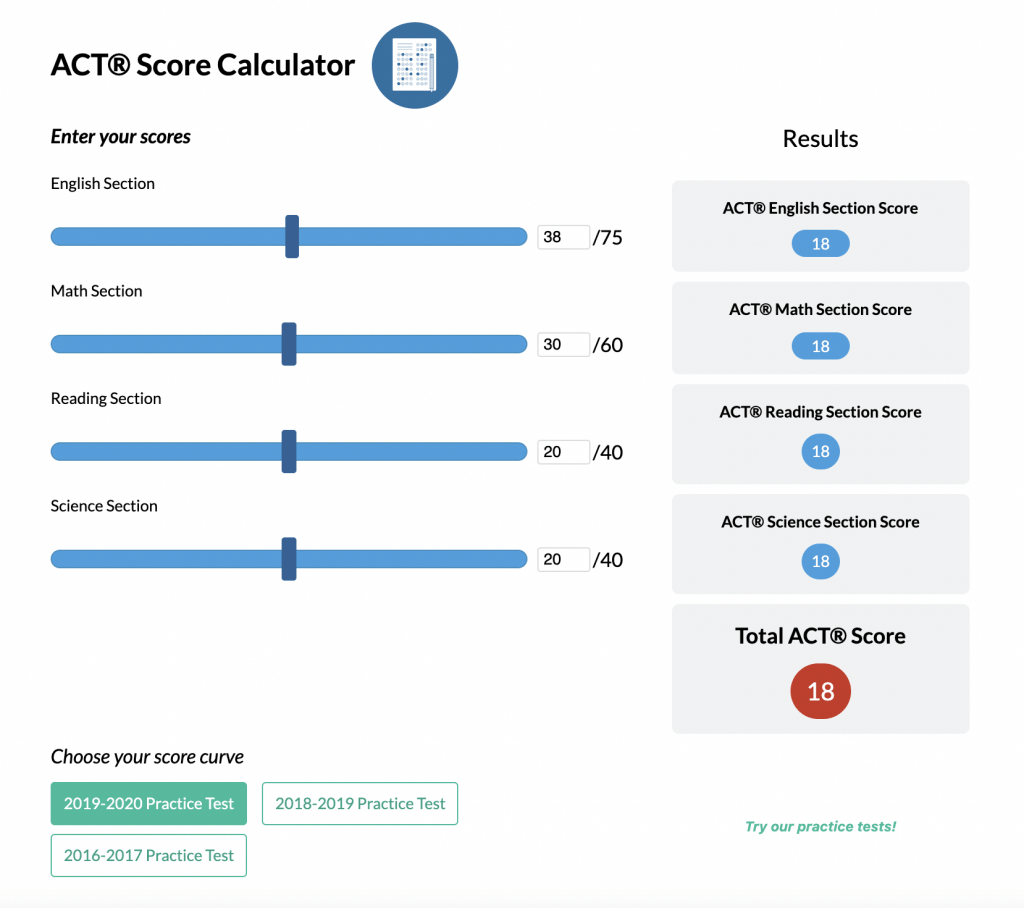
ACT® Score Calculator
See how scores on each section impacts your overall ACT® score
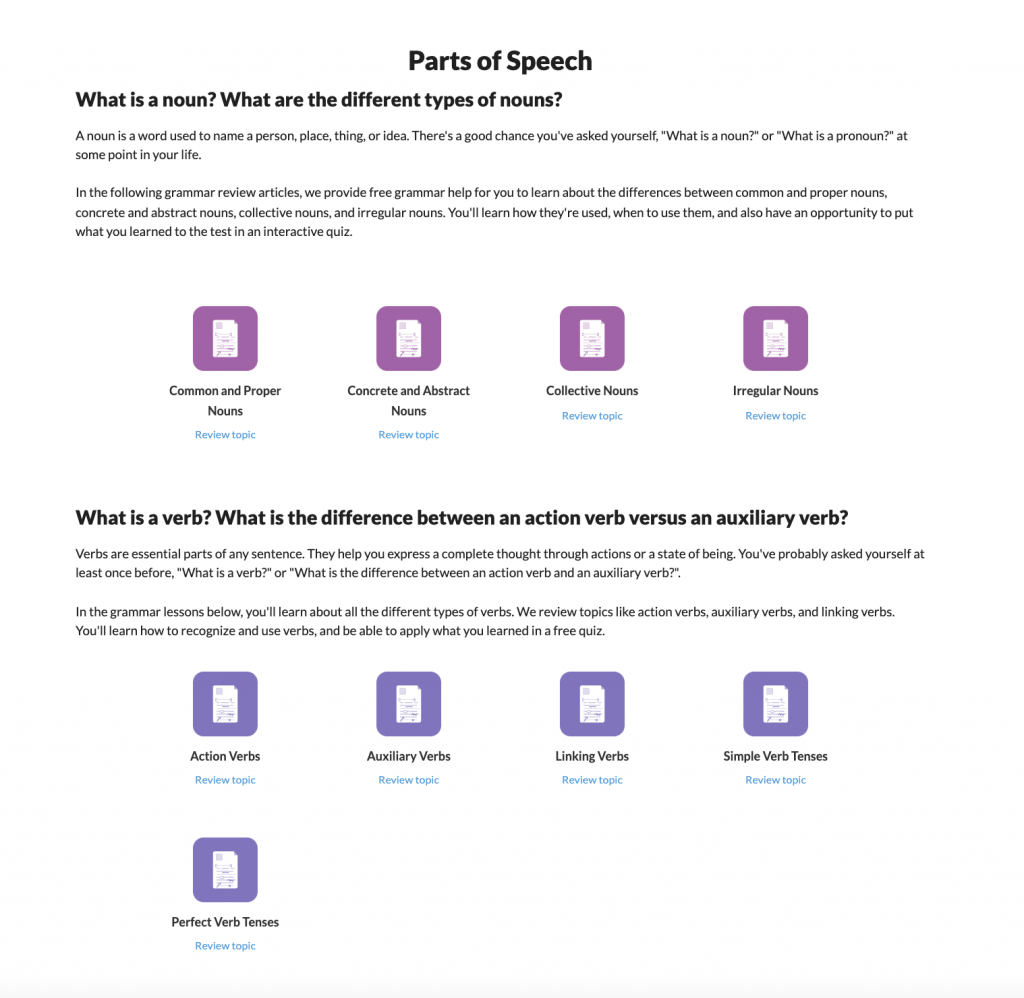
Grammar Review Hub
Comprehensive review of grammar skills
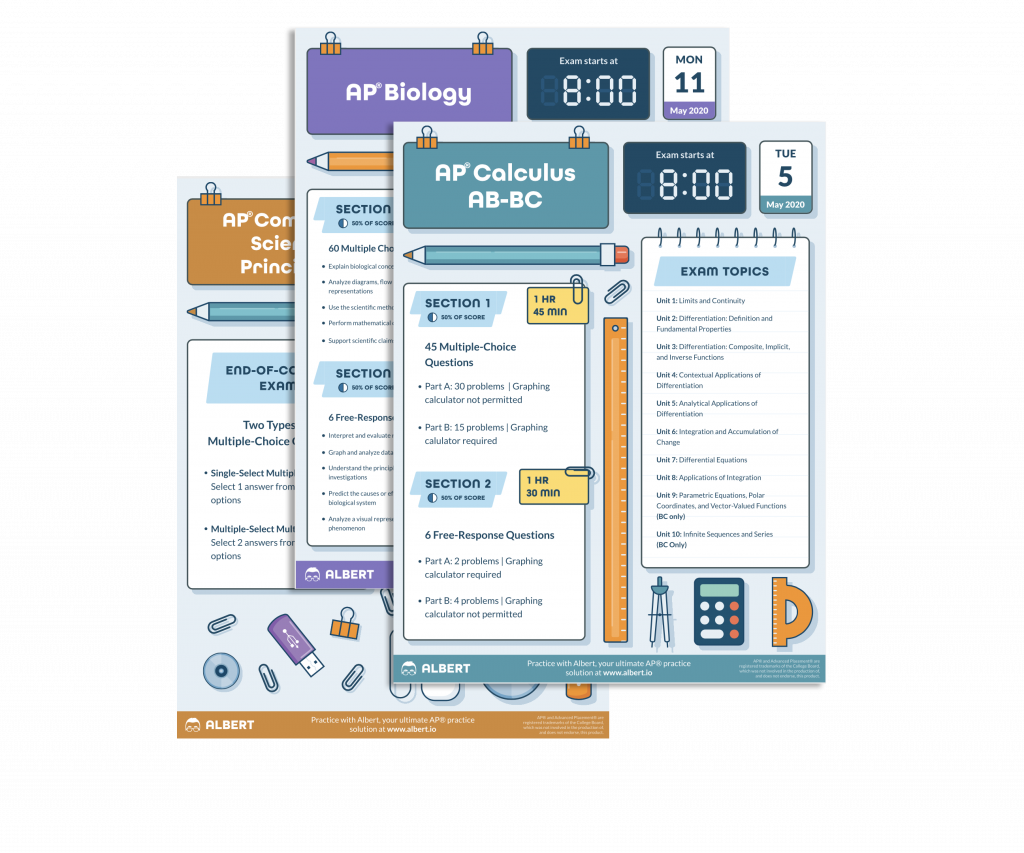
AP® Posters
Download updated posters summarizing the main topics and structure for each AP® exam.
Interested in a school license?
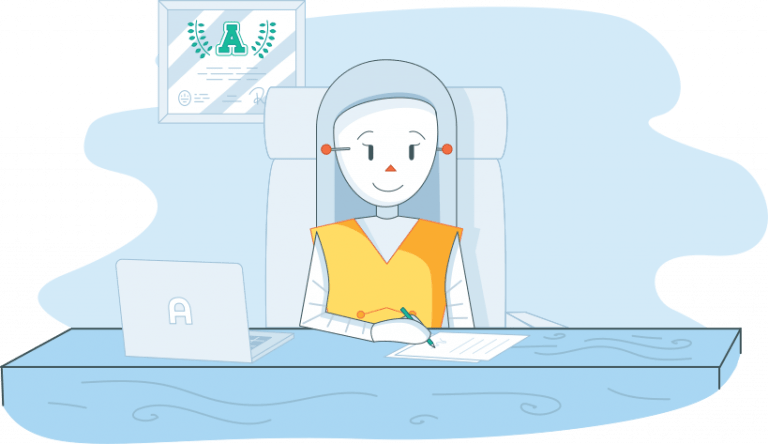
Bring Albert to your school and empower all teachers with the world's best question bank for: ➜ SAT® & ACT® ➜ AP® ➜ ELA, Math, Science, & Social Studies aligned to state standards ➜ State assessments Options for teachers, schools, and districts.
Get in touch with us
Are you sure you want to logout?
Please select your grade.
- Earth and space

Free Fall – Introduction, Explanation & Case Study
Key concepts:.
- Mass and Weight
- Weightlessness
Introduction:
The mass of an object tells us how much matter it is composed of. It is measured in kilogram. The weight of an object is the force of gravity that acts on it and is measured in Newton.
The formula of weight:
Force = Mass × Acceleration
Weight = Mass × Gravitational acceleration

Gravitational Acceleration = g
(Acceleration caused by the pull of gravitational force)
Gravitational Acceleration = g = GM / R 2
Here G = Gravitational Constant
M = Mass of the planet
R = Radius of the Planet
There are two types of forces contact forces and non-contact forces. The contact forces are frictional force , tension force, and normal reaction or support force. The non-contact forces are Gravitational force, electric force, and magnetic force.
Explanation:
We can feel contact forces but not gravity because gravity is a non-contact force,
Freefall is a kind of sensation in which the object doesn’t feel the effect of gravity in absence of contact forces. When the only force acting on an object is gravity, we say that the object is falling freely.
Weight is the force with which the Earth attracts us. We are conscious of our own weight when we are standing on a surface since the surface applies a force opposite to our weight to keep us at rest.

A man standing on floor is in equilibrium under the action of two forces : weight w and normal reaction N.
ΣF = N – W = 0
The same principle holds good when we measure the weight of an object by a spring balance hung from a fixed point like a ceiling. When we hang an object to a spring balance, the spring is pulled down a little by the gravitational pull of the object and in turn, the spring exerts a force on the object vertically upwards.

Now imagine the top end of the spring balance is no longer fixed to the ceiling both ends of the spring as well as the object move with the same acceleration g. The spring is not stretched and does not exert any upward force on the object which is moving down with acceleration g due to gravity. The reading recorded in the spring balance will be zero.

If the object were a human being, he or she will not feel his weight since there is no upward force on him. Thus, when an object is in free fall it is weightless.
In an elevator, the forces acting on an object with a mass m are:
The gravitational force (mg) in the downward direction
The reaction force N or push of the elevator floor on the object (N) in the upward direction.
Acceleration of Lift = a.
The weighing scale reading:
When the lift is at rest or in uniform motion
Acceleration = a = 0
Net force on lift = F = N – mg = 0
But N = reading of the weighting scale = W

When the lift is moving downwards with acceleration = a
Then mg – N will provide downward acceleration
Net force on lift = F = mg – N = ma
N = mg – ma
W = mg – ma

When the lift is moving upwards with acceleration = a
Then N – mg will provide upward acceleration
Net force on lift = F = N – mg = ma
N = mg + ma
W = mg + ma

When the lift is under freefall
moving downwards with acceleration = g
Then N – mg will provide downward acceleration g
Net force on lift = F = N – mg = mg
But N = rearing of the weighting scale = W

Weightlessness: A freely falling object experiences weightlessness as the only force acting on it is the force of gravity which acts downwards. The object’s acceleration is equal to the acceleration due to gravity. Hence, the net force acting on the object is zero.
The apparent weight we feel while riding a roller coaster along a curved path arises from the support we get from the floor and seat. When we ride a roller coaster towards the top, the downward acceleration of our seats is equal to the acceleration due to gravity. As a result, we experience weightlessness since there is no net force acting on us during free-fall.
Another situation when we feel weightless is during the take-off and landing of an airplane. When the airplane accelerates or decelerates at a rate equal to the acceleration due to gravity, we experience weightlessness.
Weightlessness time duration:
During High Jump or Long Jump: 1 Sec
Jumping from a Tower: 1-2 Sec
Freefall ride: 2-3 sec
During Airplane landing or take-off (Or Sky Diving): 10-30sec
- The difference between Weight and Mass s that the mass of an object tells us how much matter it is composed of whereas the weight of an object is the force of gravity that acts on it. Mass is measured in kilogram and weight is measured in Newton.
- We can feel contact forces but not gravity because gravity is a non-contact force.
- Free fall is a kind of sensation in which the object doesn’t feel the effect of gravity in absence of contact forces.
- In an elevator, the forces acting on an object with a mass m are the gravitational force(mg) in the downward direction and the reaction force or push of the elevator floor on the object (N = ma) in the upward direction. Acceleration of Lift = a.
- Weighing Scale Reading:
Case 1: When the lift is at rest or in uniform motion Acceleration = a =0, W = mg,
Case 2: When the lift is moving down with acceleration a. (a – acting downward) W = mg – ma Lightweight
Case 3: When the lf is moving upward with acceleration a. (a – acting upward) W = mg + ma Heavy Weight
Case 4: When the lift is falling freely a= g (Free Fall) W = 0 Weightlessness
- Weightlessness: A freely falling object experiences weightlessness as the only force acting on it is the force of gravity which acts downwards. The object’s acceleration is equal to the acceleration due to gravity. Hence, the net force acting on the object s zero.
- A freely falling object experiences weightlessness as the only force acting on it is the force of gravity which acts downwards. The object’s acceleration is equal to the acceleration due to gravity. Hence, the net force acting on the object is zero.
Related topics
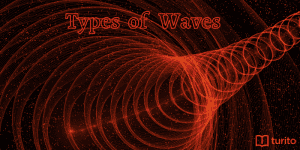
Different Types of Waves and Their Examples
Introduction: We can’t directly observe many waves like light waves and sound waves. The mechanical waves on a rope, waves on the surface of the water, and a slinky are visible to us. So, these mechanical waves can serve as a model to understand the wave phenomenon. Explanation: Types of Waves: Fig:1 Types of waves […]
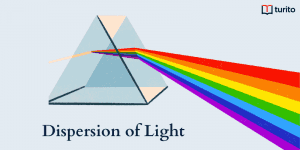
Dispersion of Light and the Formation of Rainbow
Introduction: Visible Light: Visible light from the Sun comes to Earth as white light traveling through space in the form of waves. Visible light contains a mixture of wavelengths that the human eye can detect. Visible light has wavelengths between 0.7 and 0.4 millionths of a meter. The different colors you see are electromagnetic waves […]
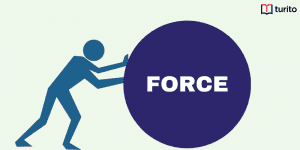
Force: Balanced and Unbalanced Forces
Introduction: In a tug of war, the one applying more force wins the game. In this session, we will calculate this force that makes one team win and one team lose. We will learn about it in terms of balanced force and unbalanced force. Explanation: Force Force is an external effort that may move a […]
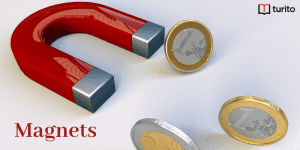
Magnets: Uses, Materials, and Their Interactions
Introduction: Nowadays magnets are widely used for many applications. In this session, we will discuss the basics of magnets and their properties, and the way they were and are used. Explanation: Magnets: Magnetic and Non-magnetic Materials: Poles of a Magnet: Fig No. 1.2: Poles of a magnet Compass: Interaction Between Magnets: The north pole of […]

Other topics

How to Find the Area of Rectangle?

How to Solve Right Triangles?

Ways to Simplify Algebraic Expressions
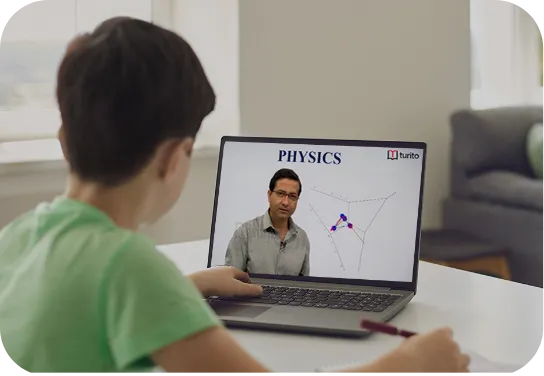
3.5 Free Fall
Learning objectives.
By the end of this section, you will be able to:
- Use the kinematic equations with the variables y and g to analyze free-fall motion.
- Describe how the values of the position, velocity, and acceleration change during a free fall.
- Solve for the position, velocity, and acceleration as functions of time when an object is in a free fall.
An interesting application of Equation 3.4 through Equation 3.14 is called free fall , which describes the motion of an object falling in a gravitational field, such as near the surface of Earth or other celestial objects of planetary size. Let’s assume the body is falling in a straight line perpendicular to the surface, so its motion is one-dimensional. For example, we can estimate the depth of a vertical mine shaft by dropping a rock into it and listening for the rock to hit the bottom. But “falling,” in the context of free fall, does not necessarily imply the body is moving from a greater height to a lesser height. If a ball is thrown upward, the equations of free fall apply equally to its ascent as well as its descent.
The most remarkable and unexpected fact about falling objects is that if air resistance and friction are negligible, then in a given location all objects fall toward the center of Earth with the same constant acceleration , independent of their mass . This experimentally determined fact is unexpected because we are so accustomed to the effects of air resistance and friction that we expect light objects to fall slower than heavy ones. Until Galileo Galilei (1564–1642) proved otherwise, people believed that a heavier object has a greater acceleration in a free fall. We now know this is not the case. In the absence of air resistance, heavy objects arrive at the ground at the same time as lighter objects when dropped from the same height Figure 3.26 .
In the real world, air resistance can cause a lighter object to fall slower than a heavier object of the same size. A tennis ball reaches the ground after a baseball dropped at the same time. (It might be difficult to observe the difference if the height is not large.) Air resistance opposes the motion of an object through the air, and friction between objects—such as between clothes and a laundry chute or between a stone and a pool into which it is dropped—also opposes motion between them.
For the ideal situations of these first few chapters, an object falling without air resistance or friction is defined to be in free fall . The force of gravity causes objects to fall toward the center of Earth. The acceleration of free-falling objects is therefore called acceleration due to gravity . Acceleration due to gravity is constant, which means we can apply the kinematic equations to any falling object where air resistance and friction are negligible. This opens to us a broad class of interesting situations.
Acceleration due to gravity is so important that its magnitude is given its own symbol, g . It is constant at any given location on Earth and has the average value
Although g varies from 9.78 m/s 2 to 9.83 m/s 2 , depending on latitude, altitude, underlying geological formations, and local topography, let’s use an average value of 9.8 m/s 2 rounded to two significant figures in this text unless specified otherwise. Neglecting these effects on the value of g as a result of position on Earth’s surface, as well as effects resulting from Earth’s rotation, we take the direction of acceleration due to gravity to be downward (toward the center of Earth). In fact, its direction defines what we call vertical. Note that whether acceleration a in the kinematic equations has the value + g or − g depends on how we define our coordinate system. If we define the upward direction as positive, then a = − g = −9.8 m/s 2 , a = − g = −9.8 m/s 2 , and if we define the downward direction as positive, then a = g = 9.8 m/s 2 a = g = 9.8 m/s 2 .
One-Dimensional Motion Involving Gravity
The best way to see the basic features of motion involving gravity is to start with the simplest situations and then progress toward more complex ones. So, we start by considering straight up-and-down motion with no air resistance or friction. These assumptions mean the velocity (if there is any) is vertical. If an object is dropped, we know the initial velocity is zero when in free fall. When the object has left contact with whatever held or threw it, the object is in free fall. When the object is thrown, it has the same initial speed in free fall as it did before it was released. When the object comes in contact with the ground or any other object, it is no longer in free fall and its acceleration of g is no longer valid. Under these circumstances, the motion is one-dimensional and has constant acceleration of magnitude g . We represent vertical displacement with the symbol y .
Kinematic Equations for Objects in Free Fall
We assume here that acceleration equals − g (with the positive direction upward).
Problem-Solving Strategy
- Decide on the sign of the acceleration of gravity. In Equation 3.15 through Equation 3.17 , acceleration g is negative, which says the positive direction is upward and the negative direction is downward. In some problems, it may be useful to have acceleration g as positive, indicating the positive direction is downward.
- Draw a sketch of the problem. This helps visualize the physics involved.
- Record the knowns and unknowns from the problem description. This helps devise a strategy for selecting the appropriate equations to solve the problem.
- Decide which of Equation 3.15 through Equation 3.17 are to be used to solve for the unknowns.
Example 3.14
Free fall of a ball.
- Substitute the given values into the equation: y = y 0 + v 0 t − 1 2 g t 2 − 98.0 m = 0 − ( 4.9 m/s ) t − 1 2 ( 9.8 m/s 2 ) t 2 . y = y 0 + v 0 t − 1 2 g t 2 − 98.0 m = 0 − ( 4.9 m/s ) t − 1 2 ( 9.8 m/s 2 ) t 2 . This simplifies to t 2 + t − 20 = 0 . t 2 + t − 20 = 0 . This is a quadratic equation with roots t = −5.0 s and t = 4.0 s t = −5.0 s and t = 4.0 s . The positive root is the one we are interested in, since time t = 0 t = 0 is the time when the ball is released at the top of the building. (The time t = −5.0 s t = −5.0 s represents the fact that a ball thrown upward from the ground would have been in the air for 5.0 s when it passed by the top of the building moving downward at 4.9 m/s.)
- Using Equation 3.15 , we have v = v 0 − g t = −4.9 m/s − ( 9.8 m/s 2 ) ( 4.0 s ) = −44.1 m/s . v = v 0 − g t = −4.9 m/s − ( 9.8 m/s 2 ) ( 4.0 s ) = −44.1 m/s .
Significance
Example 3.15, vertical motion of a baseball.
- Equation 3.16 gives y = y 0 + v 0 t − 1 2 g t 2 y = y 0 + v 0 t − 1 2 g t 2 0 = 0 + v 0 ( 5.0 s ) − 1 2 ( 9.8 m / s 2 ) ( 5.0 s ) 2 , 0 = 0 + v 0 ( 5.0 s ) − 1 2 ( 9.8 m / s 2 ) ( 5.0 s ) 2 , which gives v 0 = 24.5 m/s v 0 = 24.5 m/s .
- At the maximum height, v = 0 v = 0 . With v 0 = 24.5 m/s v 0 = 24.5 m/s , Equation 3.17 gives v 2 = v 0 2 − 2 g ( y − y 0 ) v 2 = v 0 2 − 2 g ( y − y 0 ) 0 = ( 24.5 m/s ) 2 − 2 ( 9.8 m/s 2 ) ( y − 0 ) 0 = ( 24.5 m/s ) 2 − 2 ( 9.8 m/s 2 ) ( y − 0 ) or y = 30.6 m . y = 30.6 m .
- To find the time when v = 0 v = 0 , we use Equation 3.15 : v = v 0 − g t v = v 0 − g t 0 = 24.5 m/s − ( 9.8 m/s 2 ) t . 0 = 24.5 m/s − ( 9.8 m/s 2 ) t . This gives t = 2.5 s t = 2.5 s . Since the ball rises for 2.5 s, the time to fall is 2.5 s.
- The acceleration is 9.8 m/s 2 everywhere, even when the velocity is zero at the top of the path. Although the velocity is zero at the top, it is changing at the rate of 9.8 m/s 2 downward.
- The velocity at t = 5.0 s t = 5.0 s can be determined with Equation 3.15 : v = v 0 − g t = 24.5 m/s − 9.8 m/s 2 ( 5.0 s ) = −24.5 m/s . v = v 0 − g t = 24.5 m/s − 9.8 m/s 2 ( 5.0 s ) = −24.5 m/s .
Check Your Understanding 3.7
A chunk of ice breaks off a glacier and falls 30.0 m before it hits the water. Assuming it falls freely (there is no air resistance), how long does it take to hit the water? Which quantity increases faster, the speed of the ice chunk or its distance traveled?
Example 3.16
Rocket booster.
- From Equation 3.17 , v 2 = v 0 2 − 2 g ( y − y 0 ) v 2 = v 0 2 − 2 g ( y − y 0 ) . With v = 0 and y 0 = 0 v = 0 and y 0 = 0 , we can solve for y : y = v 0 2 2 g = ( 2.0 × 10 2 m / s ) 2 2 ( 9.8 m / s 2 ) = 2040.8 m . y = v 0 2 2 g = ( 2.0 × 10 2 m / s ) 2 2 ( 9.8 m / s 2 ) = 2040.8 m . This solution gives the maximum height of the booster in our coordinate system, which has its origin at the point of release, so the maximum height of the booster is roughly 7.0 km.
- An altitude of 6.0 km corresponds to y = 1.0 × 10 3 m y = 1.0 × 10 3 m in the coordinate system we are using. The other initial conditions are y 0 = 0 , and v 0 = 200.0 m/s y 0 = 0 , and v 0 = 200.0 m/s . We have, from Equation 3.17 , v 2 = ( 200.0 m / s ) 2 − 2 ( 9.8 m / s 2 ) ( 1.0 × 10 3 m ) ⇒ v = ± 142.8 m / s . v 2 = ( 200.0 m / s ) 2 − 2 ( 9.8 m / s 2 ) ( 1.0 × 10 3 m ) ⇒ v = ± 142.8 m / s .
Interactive
Engage the Phet simulation below to learn about graphing polynomials. The shape of the curve changes as the constants are adjusted. View the curves for the individual terms (for example, y = bx ) to see how they add to generate the polynomial curve.
As an Amazon Associate we earn from qualifying purchases.
This book may not be used in the training of large language models or otherwise be ingested into large language models or generative AI offerings without OpenStax's permission.
Want to cite, share, or modify this book? This book uses the Creative Commons Attribution License and you must attribute OpenStax.
Access for free at https://openstax.org/books/university-physics-volume-1/pages/1-introduction
- Authors: William Moebs, Samuel J. Ling, Jeff Sanny
- Publisher/website: OpenStax
- Book title: University Physics Volume 1
- Publication date: Sep 19, 2016
- Location: Houston, Texas
- Book URL: https://openstax.org/books/university-physics-volume-1/pages/1-introduction
- Section URL: https://openstax.org/books/university-physics-volume-1/pages/3-5-free-fall
© Jan 19, 2024 OpenStax. Textbook content produced by OpenStax is licensed under a Creative Commons Attribution License . The OpenStax name, OpenStax logo, OpenStax book covers, OpenStax CNX name, and OpenStax CNX logo are not subject to the Creative Commons license and may not be reproduced without the prior and express written consent of Rice University.
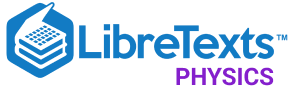
- school Campus Bookshelves
- menu_book Bookshelves
- perm_media Learning Objects
- login Login
- how_to_reg Request Instructor Account
- hub Instructor Commons
- Download Page (PDF)
- Download Full Book (PDF)
- Periodic Table
- Physics Constants
- Scientific Calculator
- Reference & Cite
- Tools expand_more
- Readability
selected template will load here
This action is not available.
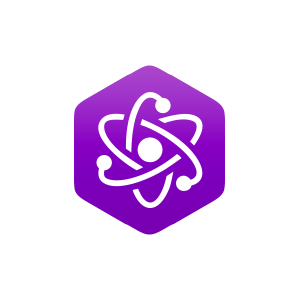
2.5: Free-Falling Objects
- Last updated
- Save as PDF
- Page ID 16932
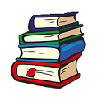
learning objectives
- Solve basic problems concerning free fall and distinguish it from other kinds of motion
The motion of falling objects is the simplest and most common example of motion with changing velocity. If a coin and a piece of paper are simultaneously dropped side by side, the paper takes much longer to hit the ground. However, if you crumple the paper into a compact ball and drop the items again, it will look like both the coin and the paper hit the floor simultaneously. This is because the amount of force acting on an object is a function of not only its mass, but also area. Free fall is the motion of a body where its weight is the only force acting on an object.
Free Fall : This clip shows an object in free fall.
Galileo also observed this phenomena and realized that it disagreed with the Aristotle principle that heavier items fall more quickly. Galileo then hypothesized that there is an upward force exerted by air in addition to the downward force of gravity. If air resistance and friction are negligible, then in a given location (because gravity changes with location), all objects fall toward the center of Earth with the same constant acceleration , independent of their mass , that constant acceleration is gravity. Air resistance opposes the motion of an object through the air, while friction opposes motion between objects and the medium through which they are traveling. The acceleration of free-falling objects is referred to as the acceleration due to gravity gg. As we said earlier, gravity varies depending on location and altitude on Earth (or any other planet), but the average acceleration due to gravity on Earth is 9.8 \(\mathrm{\frac{m}{s^2}}\). This value is also often expressed as a negative acceleration in mathematical calculations due to the downward direction of gravity.
The best way to see the basic features of motion involving gravity is to start by considering straight up and down motion with no air resistance or friction. This means that if the object is dropped, we know the initial velocity is zero. Once the object is in motion, the object is in free-fall. Under these circumstances, the motion is one-dimensional and has constant acceleration, gg. The kinematic equations for objects experiencing free fall are:
\[\begin{align} \mathrm{v} & \mathrm{=v_0−gt} \\ \mathrm{y} & \mathrm{=y_0+v_0t−\frac{1}{2}gt^2} \\ \mathrm{v^2} & \mathrm{=v^2_0−2g(y−y_0),} \end{align}\]
where \(\mathrm{v=velocity, g=gravity, t=time,}\) and \(\mathrm{y=vertical \; displacement}\).
Video \(\PageIndex{1}\) : Free Fall Motion - Describes how to calculate the time for an object to fall if given the height and the height that an object fell if given the time to fall.
Example \(\PageIndex{1}\):
Some examples of objects that are in free fall include:
- A spacecraft in continuous orbit. The free fall would end once the propulsion devices turned on.
- An stone dropped down an empty well.
- An object, in projectile motion, on its descent.
- The acceleration of free-falling objects is called the acceleration due to gravity, since objects are pulled towards the center of the earth.
- The acceleration due to gravity is constant on the surface of the Earth and has the value of 9.80 \(\mathrm{\frac{m}{s^2}}\) .
The amount by which a speed or velocity changes within a certain period of time (and so a scalar quantity or a vector quantity).
CC LICENSED CONTENT, SHARED PREVIOUSLY
If you're seeing this message, it means we're having trouble loading external resources on our website.
If you're behind a web filter, please make sure that the domains *.kastatic.org and *.kasandbox.org are unblocked.
To log in and use all the features of Khan Academy, please enable JavaScript in your browser.
Class 11 Physics (India)
Course: class 11 physics (india) > unit 6.
- Plotting projectile displacement, acceleration, and velocity
- Projectile height given time
- Impact velocity from given height
Freefall review
- Freefall: graphs and conceptual questions
- Solving freefall problems using kinematic formulas
- Advanced: Freefall problems
Analyzing motion for objects in freefall
Common mistakes and misconceptions, want to join the conversation.
- Upvote Button navigates to signup page
- Downvote Button navigates to signup page
- Flag Button navigates to signup page

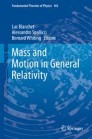
Mass and Motion in General Relativity pp 561–603 Cite as
Free Fall and Self-Force: an Historical Perspective
- Alessandro Spallicci 4
- First Online: 01 January 2010
2273 Accesses
1 Altmetric
Part of the book series: Fundamental Theories of Physics ((FTPH,volume 162))
Free fall has signed the greatest markings in the history of physics through the leaning Pisa tower, the Woolsthorpe apple tree and the Einstein lift. The perspectives offered by the capture of stars by supermassive black holes are to be cherished, because the study of the motion of falling stars will constitute a giant step forward in the understanding of gravitation in the regime of strong field. After an account on the perception of free fall in ancient times and on the behaviour of a gravitating mass in Newtonian physics, this chapter deals with last century debate on the repulsion for a Schwarzschild–Droste black hole and mentions the issue of an infalling particle velocity at the horizon. Further, black hole perturbations and numerical methods are presented, paving the way to the introduction of the self-force and other back-action related methods. The impact of the perturbations on the motion of the falling particle is computed via the tail, the back-scattered part of the perturbations, or via a radiative Green function. In the former approach, the self-force acts upon the background geodesic; in the latter, the geodesic is conceived in the total (background plus perturbations) field. Regularisation techniques (mode-sum and Riemann–Hurwitz z function) intervene to cancel divergencies coming from the infinitesimal size of the particle. An account is given on the state of the art, including the last results obtained in this most classical problem, together with a perspective encompassing future space gravitational wave interferometry and head-on particle physics experiments. As free fall is patently non-adiabatic, it requires the most sophisticated techniques for studying the evolution of the motion. In this scenario, the potential of the self-consistent approach, by means of which the background geodesic is continuously corrected by the self-force contribution, is examined.
This is a preview of subscription content, log in via an institution .
Buying options
- Available as PDF
- Read on any device
- Instant download
- Own it forever
- Available as EPUB and PDF
- Compact, lightweight edition
- Dispatched in 3 to 5 business days
- Free shipping worldwide - see info
- Durable hardcover edition
Tax calculation will be finalised at checkout
Purchases are for personal use only
Leonardo spent his final years at Amboise, nowadays part of the French Région Centre, under invitation of François I, King of France and Duke of Orléans.
His book presents the contributions by several less-known researchers in the flow of time, being a well argued and historical – but rather uncritical – account. An other limitation is the neglect of non-Western contributions to the development of physics.
Indeed, it has been stated by Synge [ 196 ] ‘...Perhaps they speak of the principle of equivalence. If so, it is my turn to have a blank mind, for I have never been able to understand this principle...’
For a review on experimental status of these fundamental laws, see Will’s classical references [ 211 , 212 ], or else Lämmerzahl’s alternative view [ 113 ], while the relation to energy conservation is analysed by Haugan [ 99 ].
For the first definition, it is worth mentioning the following observation [ 196 ] ‘...Does it mean that the effects of a gravitational field are indistinguishable from the effects of an observer’s acceleration? If so, it is false. In Einstein’s theory, either there is a gravitational field or there is none, according as the Riemann tensor does not or does vanish. This is an absolute property; it has nothing to do with any observer’s world-line. Space-time is either flat or curved...’ Patently, the converse is also far reaching: if an inertial acceleration was strictly equivalent to one produced by a gravitational field, curvature would be then associated to inertial accelerations. Rohrlich [ 173 ] stresses that the gravitational field must be static and homogeneous and thus in absence of tidal forces. But no such a gravitational field exists or even may be conceived! Furthermore, the particle internal structure has to be neglected.
The second definition is under scrutiny by numerous experimental tests compelled by modern theories as pointed out by Damour [ 46 ] and Fayet [ 79 ].
First and last two definitions are correct in the limit of a point mass. An interesting discussion is offered by Ciufolini and Wheeler [ 38 ] on the non-applicability of the concept of a locally inertial frame (indeed a spherical drop of liquid in a gravity field would be deformed by tidal forces after some time, and a state-of -the-art gradiometer may reach sensitivities such as to detect the tidal forces of a weak gravitational field in a freely falling cabin). Mathematically, locality, for which the metric tensor g μν reduces to the Minkowski metric and the first derivatives of the metric tensor are zero, is limited by the non-vanishing of the Riemann curvature tensor, as in general certain combinations of the second derivatives of g μν cannot be removed. Pragmatically, it may be concluded that violating effects on the EP may be negligible in a sufficiently small spacetime region, close to a given event.
Again, this opinion is comforted [ 196 ] ‘...the principle of equivalence performed the essential office of midwife at the birth of general relativity...I suggest that the midwife be now buried with appropriate honours...’.
The difference between fall in vacuum and in the air has been the subject of a polemics between the former French Minister of Higher Education and Research Claude Allègre and the Physics Nobel Prize Georges Charpak, solicited by the satirical weekly ‘Le Canard Enchaîné’ [ 120 ]. The Minister affirmed on French television in 1999 ‘Pick a student, ask him a simple question in physics: take a petanque and a tennis ball, release them; which one arrives first? The student would tell you ‘the petanque’. Hey no, they arrive together; and it is a fundamental problem, for which 2000 years were necessary to understand it. These are the basis that everyone should know’. The humourists wisecracked that the presence of air would indeed prove the student being right and tested their claim by means of filled and empty plastic water bottles being released from the second floor of their editorial offices … and asked the Nobel winner to compute the difference due to the air, whose influence was denied by the Minister. But in this polemics, no one drew the attention to the Newtonian back-action, also during the polemics revamped in 2003 by Allègre [ 1 ] who compared this time a heavy object and a paper ball. Such forgetfulness or misconception is best represented by the Apollo 15 display of the simultaneous fall of a feather and a hammer [ 4 ].
During the Bloomington 2009 Capra meeting, this state of affairs was presented as ‘the confusion gauge’.
Rothman [ 174 ] gives a brief historical account on Droste’s independent derivation of the same metric published by Schwarzschild, in the same year 1916. Eisenstaedt [ 76 ] mentions previous attempts by Droste [ 64 ] on the basis of the preliminary versions of general relativity by Einstein and Grossmann [ 73 ], later followed by Einstein’s works (general relativity was completed in 1915 and first systematically presented in 1916 [ 72 ]) and Hilbert’s [ 101 ]. Antoci [ 2 ] and Liebscher [ 3 ] emphasise Hilbert’s [ 102 ] and Weyl’s [ 207 ] later derivations of solutions for spherically symmetric non-rotating bodies. Incidentally, Ferraris, Francaviglia and Reina [ 80 ] point to the contributions of Einstein and Grossmann [ 74 ], Lorentz [ 126 ] and obviously Hilbert [ 101 ] to the variational formulation.
Earman and Eisenstaedt [ 69 ] describe the lack of interest of Einstein for singularities in general relativity. The debate at the Collège de France during Einstein’s visit in Paris in 1922 included a witty exchange on pression (the Hadamard ‘disaster’), see Biezunski [ 26 ].
The translation of the title and of the introduction to Section 5 of [ 77 ] serves best this paragraph ‘The impasse (or have the relativists fear of the free fall?) [..] the problem of the free fall of bodies in the frame of [..] the Schwarzschild solution. More than any other, this question gathers the optimal conditions of interest, on the technical and epistemological levels, without inducing nevertheless a focused concern by the experts. Though, is it necessary to emphasise that it is a first class problem to which classical mechanics has always showed great concern … from Galileo; which more is the reference model expressing technically the paradigm of the lift in free fall dear to Einstein? The matter is such that the case is the most elementary, most natural, an extremely simple problem … apparently, but which raises extremely delicate questions to which only the less conscious relativists believe to reply with answers [..]. Exactly the type of naive question that best experts prefer to leave in the shadow, in absence of an answer that has to be patently clear to be an answer. Without doubts, it is also the reason for which this question induces a very moderate interest among the relativists … ’
This definition is not faultless (there is no shield to gravity), but it is the most suitable to describe the debate, following Cavalieri and Spinelli [ 31 , 32 , 193 ] and Thirring [ 199 ].
Generally, the setting of the proper initial conditions may be a delicate issue e.g. when associated with an initial radiation content expressing the previous history of the motion as it will be later discussed; or, in absence of radiation, when an external (sort of third body) mechanism prompting the motion to the two body system is to be taken into account. The latter case is represented by the thought experiment conceived by Copperstock [ 39 ] aiming to criticise the quadrupole formula. The experiment consisted in two fluid balls assumed to be in static equilibrium and held apart by a strut, with membranes to contain the fluid, until time t = 0. Between t = 0 and t = t 1 , the strut and the membranes are dissolved and afterwards the balls fall freely. Due to the static initial conditions, there is a clear absence of incident radiation, but the behaviour of the fluid balls in the free fall phase depends on how the transition from the equilibrium to the free fall takes place. This initial dependence obscured the debate on the quadrupole formula.
Free fall has also been studied in other contexts. Synge [ 196 ] undertakes a detailed investigation of the problem and shows that, actually, the gravitational field (i.e. the Riemann tensor) plays an extremely small role in the phenomenon of free fall and the acceleration of 980 cm/s is, in fact, due to the curvature of the world line of the tree branch. The apple is accelerated until the stem breaks, then the world line of the apple becomes inertial until the ground collides with it.
For a critical assessment of black hole stability, see Dafermos and Rodnianski [ 44 ].
A well-organised introduction, largely based on works by Friedman [ 84 ] and Chandrasekhar [ 33 ], is presented in the already mentioned book by the latter [ 34 ]. Some selected publications geared to the finalities of this chapter are to be listed: earlier works by Mathews [ 141 ], Stachel [ 195 ], Vishveshvara [ 204 ]; the relation between odd and parity perturbations [ 35 ]; the search for a gauge invariant formalism by Martel and Poisson [ 140 ] complements a recent review on gauge invariant non-spherical metric perturbations of the SD black hole spacetimes by Nagar and Rezzolla [ 154 ]; a classic reference on multiple expansion of gravitational radiation by Thorne [ 200 ]; the derivation by computer algebra by Cruciani [ 41 , 42 ] of the wave equation governing black hole perturbations; the numerical hyperboloidal approach by Zenginonğlu [ 217 ].
Two warnings: the literature on perturbations and numerical methods is rather plagued by editorial errors (likely herein too...) and different terminologies for the same families of perturbations. Even parity waves have been named also polar or electric or magnetic, generating some confusion (see the correlation table, Table II, in [ 220 ]). Sago, Nakano and Sasaki [ 180 ] have corrected the Zerilli equations (a minus sign missing in all right-hand side terms) but introduced a wrong definition of the scalar product leading to errors in the coefficients of the energy-momentum tensor.
There is an editorial error, a numerical coefficient, in the corresponding expressions (2.16) in [ 133 ] and (2.8) in [ 134 ], which the footnote 1 at page 3 in [ 139 ] does not address.
For a starting point different from infinity or a non-null starting velocity, but not their combination, see Lousto and Price [ 133 – 135 ], Martel and Poisson [ 139 ].
The divergence in summing over all l modes is said to be taken away by considering a finite size particle [ 49 ].
There are editorial errors in the corresponding expressions (3.6) in [ 134 ] and (3.4) in [ 139 ].
There are editorial errors in the corresponding expressions (3.9) in [ 134 ] and (3.5) in [ 139 ].
The Heaviside or step distribution, like the wavefunction of the Zerilli equation, belongs to the C − 1 continuity class; the Dirac delta distribution and its derivative belong to the C − 2 and C − 3 continuity class, respectively.
A point-like mass m moves along a geodesic of the background spacetime if m → 0; if not, the motion is no longer geodesic. It is sometimes stated that the interaction of the particle with its own gravitational field gives rise to the self-force. It should be added, though, that such interaction is due to an external factor like a background curved spacetime or a force imposing an acceleration on the mass. In other words, a single and unique mass in an otherwise empty universe cannot experience any self-force. Conceptually, the self-force is thus a manifestation of non-locality in the sense of Mach’s inertia [ 136 ].
It is currently believed that the core of most galaxies host supermassive black holes on which stars and compact objects in the neighbourhood inspiral-down and plunge-in. Gravitational waves might also be detected when radiated by the Milky Way Sgr*A, the central black hole of more than 3 million solar masses [ 30 , 83 ]. The EMRIs are further characterised by a huge number of parameters that, when spanned over a large period, produce a yet unmanageable number of templates. Thus, in alternative to matched filtering, other methods based on covariance or on time and frequency analysis are investigated. If the signal from a capture is not individually detectable, it still may contribute to the statistical background [ 17 ].
In 2002 at the Capra Penn State meeting by Eric Poisson.
Given the elegance of this classic approach, the self-force expression should be rebaptised as MiSaTaQuWa-DeWh.
Detweiler and Whiting [ 59 ] refer to the contribution inside the light cone via the Hadamard expression [ 97 ] of the Green function.
The self-force being affected by the gauge choice, the EP allows to find a gauge where the self-force disappears. Again, as in Newtonian physics, such gauge will be dependent of the mass m , impeding the uniqueness of acceleration.
Apart from some editorial errors therein, α 1, 2, 6 correspond to the A , B , C coefficients in [ 129 , 130 ], which are not to be confused with the A , B , C coefficients of the mode-sum!
Supposing that the relative strengths of the perturbations and the deviations behave as:
then, the coordinate acceleration correction would be given by an expansion up to first order in perturbations and second order in deviation [ 190 ]:
In Eq. 62 : (i) solely second order terms in perturbations are not considered; (ii) the terms \({\alpha }_{2}\left (g,{\dot{z}}_{u}\right )\Delta \dot{z},{\alpha }_{3}\left (g,{\dot{z}}_{u}\right )\Delta {z}^{2},{\alpha }_{4}\left (g\right )\Delta {\dot{z}}^{2},{\alpha }_{5}\left (g,{\dot{z}}_{u}\right )\Delta z\Delta \dot{z}\) represent the background field evaluated on the perturbed trajectory at second order in deviation; (iii) α 3 − 5 tend to infinity close to the horizon, conversely to the α 1, 2 coefficients; (iv) \({\alpha }_{7}\left (h,{\dot{z}}_{u}\right )\Delta z,{\alpha }_{8}\left (h,{\dot{z}}_{u}\right )\Delta \dot{z}\) represent the perturbed field on the perturbed trajectory, and the α 7 − 8 coefficients are larger near the horizon. These last two coefficients may be regularised in l by the Riemann–Hurwitz ζ function as shown in [ 190 ].
The jump conditions were also dealt with by Sopuerta and Laguna [ 188 ].
Having suppressed the l index for clarity of notation, after visual inspection of Eq. 26 , containing a derivative of the Dirac delta distribution, it is evinced that the wavefunction Ψ is of C − 1 continuity class and thus can be written as:
where Θ 1 = Θr − z u ( t ) and Θ 2 = Θz u ( t ) − r are two Heaviside step distributions. Computing the first and second, space and time and mixed derivatives, Dirac delta distributions and derivatives are obtained of the type δ[ r − z u ( t )] and δ ′ [ r − z u ( t )], respectively. It is wished that the discontinuities of Ψ and its derivatives are such that they are canceled when combined in K , H 2 , and H 1 . After replacing Ψ and its derivatives in Eqs. 31 – 33 , continuity requires that the coefficients of Θ 1 must be equal to the coefficients of Θ 2 , while the coefficients of δ and δ ′ must vanish separately. After some tedious computing and making use of one of the Dirac delta distribution properties, f ( r )δ ′ [ r − z u ( t )] = f [ z u ( t )]δ ′ [ r − z u ( t )] − f′ ( z u ( t ))δ[ r − z u ( t )], at the position of the particle, the jump conditions for Ψ and its derivatives are found. Furthermore, the jump conditions allow a new method of integration, as shown by Aoudia and Spallicci [ 6 ].
Lousto [ 129 ] comments only this former part and not the acceleration boost taking place after the Zerilli potential peak.
In the Rapid Communication [ 18 ] there are seven citations of a yet unpublished material containing mathematical and numerical justifications of the results therein. The author acknowledges private communications by L. Barack.
The evolution of an orbit is lately getting the necessary concern. Pound and Poisson [ 162 ] apply osculating orbits to EMRI, but unfortunately their method is not applicable to plunge, for two reasons: the semi-latus rectum of the orbit, which decreases for radiation reaction, is smaller than a given quantity, considered as limit in their study case; the velocities and fields in the plunge are highly relativistic and their post-Newtonian expansion of the perturbing force becomes inaccurate. Non-applicability to plunge stands also for the work by Hinderer and Flanagan [ 104 ].
Indeed, it affirms that it is preferable to apply successively a first order expansion at x 0 and then at x 1 , x 2 , …, x m , rather then a second or higher order expansion at solely x 0 . It is evident, though, that self-consistency and perturbation order are decoupled concepts and that the former may be conceptually applicable to higher orders and more specifically, when, and if, a second order formalism will be available. In the same line of reasoning it would be preferable to apply successively a second order expansion at x 0 and then at x 1 , x 2 , …, x m , rather then a third or higher order expansion at solely x 0 .
See Levi-Civita [ 122 , 123 ], Ciufolini and Wheeler [ 38 ], Ciufolini [ 37 ].
For Mino and Brink [ 149 ], the energy and momentum radiated are computed on the assumption that the small body falls in a dynamical time scale, with respect to proper time, well short of the radiation reaction time scale and therefore the gravitational radiation back-action on the orbit is considered negligible. The particle plunges on a geodesic trajectory, incidentally starting from a circular orbit, thus at zero initial radial velocity.
For head-on collisions, Price and Pullin have surprisingly shown the applicability of perturbation theory for the computation of the radiated energy and of the waveform for two equal black holes, starting at very small separation distances [ 164 ], the so-called close-limit approximation.
A non-recent analysis by Simone, Poisson and Will [ 187 ], between pN and perturbation methods in head-on collisions, was limited to the computation of the gravitational wave energy flux.
C. Allègre, Un peu de science pour tout le monde (Fayard, Paris, 2003)
Google Scholar
S. Antoci, in Meteorological and Geophysical Fluid Dynamics (a book to commemorate the centenary of the birth of Hans Ertel) , ed. by W. Schröder (Science Edition, Bremen, 2004), p. 343
S. Antoci, D.-E. Liebscher, Astron. Nachr. 322 , 137 (2001)
MATH MathSciNet ADS Google Scholar
Apollo 15, http://nssdc.gsfc.nasa.gov/planetary/lunar/apollo.html
S. Aoudia, Capture des étoiles par les trous noirs et ondes gravitationnelles , Doctorate thesis, Dir. A.D.A.M. Spallicci, Université de Nice-Sophia Antipolis, 2008
S. Aoudia, A.D.A.M. Spallicci, in Proc. 12th Marcel Grossmann Mtg , Paris 12–18 July 2009, ed. by T. Damour, R.T. Jantzen, R. Ruffini (World Scientific, Singapore, to appear), arXiv:1003.3107v3 [gr-qc] (2010); see also S. Aoudia, A.D.A.M. Spallicci, arXiv: 1008.2507v1 [gr-qc] (2010)
S. Aoudia, A.D.A.M. Spallicci, to appear
R.F. Aranha, H.P. de Oliveira, I. Damião Soares, E.V. Tonini, Int. J. Mod. Phys. D 17 , 2049 (2008)
MATH ADS Google Scholar
L.I. Arifov, Problemy Teor. Gravitatsii i Èlement. Chastits 11 , 96 (1980)
MathSciNet ADS Google Scholar
L.I. Arifov, Izv. Vyssh. Uchebn. Zaved. Fiz. 4 , 61 (1981). English translation: Rus. Phys. J. 24 , 346 (1981)
Aristotélēs, Corpus Aristotelicum: Physica (184a), De cælo (268a), Methaphysica (980a), in Latin (in parenthesis the Bekker index). English translation: The Complete Works of Aristotle , ed. by J. Barnes (Princeton Univ. Press, Princeton, 1984)
R. Baierlein, Phys. Rev. D 8 , 4639 (1973)
ADS Google Scholar
L. Barack, Phys. Rev. D 62 , 084027 (2000)
L. Barack, Phys. Rev. D 64 , 084021 (2001)
L. Barack, Class. Q. Grav. 26 , 213001 (2009)
L. Barack, L. Burko, Phys. Rev. D 62 , 084040 (2000)
L. Barack, C. Cutler, Phys. Rev. D 70 , 122002 (2004)
L. Barack, C.O. Lousto, Phys. Rev. D 66 , 061502 (2002)
L. Barack, C.O. Lousto, Phys. Rev. D 72 , 104026 (2005)
L. Barack, A. Ori, Phys. Rev. D 61 , 061502 (2000)
L. Barack, A. Ori, Phys. Rev. D 64 , 124003 (2001)
L. Barack, Y. Mino, H. Nakano, A. Ori, M. Sasaki, Phys. Rev. Lett. 88 , 091101 (2002)
L. Barack, N. Sago, Phys. Rev. D 81 , 084021 (2010)
H. Bauer, Mathematische einführung in die gravitationstheorie Einsteins, nebst einer exakten darstellung ihrer wichtigsten ergebnisse (F. Deuticke, Leipzig and Wien, 1922)
MATH Google Scholar
S. Bernuzzi, A. Nagar, Phys. Rev. D 81 , 084056 (2010)
M. Biezunski, Einstein à Paris: le temps n’est plus (Presse Univ. de Vincennes, St. Denis, 1991)
L. Blanchet, S. Detweiler, A. Le Tiec, B.F. Whiting, Phys. Rev. D 81 , 064004 (2010)
L. Blanchet, S. Detweiler, A. Le Tiec, B.F. Whiting, Phys. Rev. D 81 , 084033 (2010)
L. Burko, Class. Q. Grav. 22 , S847 (2005)
A. Cădez̆, M. Calvani, A. Gomboc, U. Kostić, in Albert Einstein Century International Conference , ed. by J.-M. Alimi, A. Füzfa, AIP Conf. Proc. 861 (AIP, Melville, 2006) pp. 566–571
G. Cavalleri, G. Spinelli, Lett. Nuovo Cimento 6 , 5 (1973)
G. Cavalleri, G. Spinelli, Phys. Rev. D 15 , 3065 (1977)
S. Chandrasekhar, Proc. R. Soc. Lond. A 343 , 289 (1975)
S. Chandrasekhar, The Mathematical Theory of Black Holes (Oxford University Press, Oxford, 1983)
S. Chandrasekhar, S. Detweiler, Proc. R. Soc. Lond. A 344 , 441 (1975)
K.P. Chung, Nuovo Cimento B 14 , 293 (1973)
I. Ciufolini, Phys. Rev. D 34 , 1014 (1986)
I. Ciufolini, J.A. Wheeler, Gravitation and Inertia (Princeton University Press, Princeton, 1995)
F.I. Copperstock, Phys. Rev. D 10 , 3171 (1974)
P. Crawford, I. Tereno, Gen. Rel. Grav. 34 , 2075 (2002)
G. Cruciani, Nuovo Cimento B 115 , 693 (2000)
G. Cruciani, Nuovo Cimento B 120 , 1045 (2005)
C. Cutler, D. Kennefick, E. Poisson, Phys. Rev. D 50 , 3816 (1994)
M. Dafermos, I. Rodnianski, in Clay Summer School , Zurich 23 June - 18 July 2008, arXiv:0811.0354v1 [gr-qc] (2008)
T. Damour, arXiv:0910.5533v1 [gr-qc] (2009)
T. Damour, Sp. Sc. R. 148 , 191 (2009)
M. Davis, R. Ruffini, Lett. Nuovo Cimento 2 , 1165 (1972)
M. Davis, R. Ruffini, W.H. Press, R.H. Price, Phys. Rev. Lett. 27 , 1466 (1971)
M. Davis, R. Ruffini, J. Tiomno, Phys. Rev. D 5 , 2932 (1972)
T. de Donder, La gravifique Einsteinienne (Gauthier-Villars, Paris, 1921)
T. de Donder, The Mathematical Theory of Relativity (M.I.T. Press, Cambridge, 1927)
C. de Jans, Mem. Acad. R. Belgique Cl. Sci. 7 , 1 (1923)
C. de Jans, Mem. Acad. R. Belgique Cl. Sci. 7 , 1 (1924)
C. de Jans, Mem. Acad. R. Belgique Cl. Sci. 7 , 96 (1924)
S. Detweiler, Phys. Rev. Lett. 86 , 1931 (2001)
S. Detweiler, Class. Q. Grav. 22 , S681 (2005)
S. Detweiler, Phys. Rev. D 77 , 124026 (2008)
S. Detweiler, E. Poisson, Phys. Rev. D 69 , 084019 (2004)
S. Detweiler, B.F. Whiting, Phys. Rev. D 67 , 024025 (2003)
B.S. DeWitt, R.W. Brehme, Ann. Phys. (N.Y.) 9 , 220 (1960)
P.A.M. Dirac, Proc. R. Soc. Lond. A 167 , 148 (1938)
N.A. Doughty, Am. J. Phys. 49 , 412 (1981)
S. Drake, Two New Sciences/A History of Free Fall, Aristotle to Galileo (Wall and Emerson, Toronto, 2000)
J. Droste, Kon. Ak. Wetensch. Amsterdam 23 , 968 (1915). English translation: Proc. Acad. Sci. Amsterdam 17 , 998 (1915)
J. Droste, Het zwaartekrachtsveld van een of meer lichamen volgens de theorie van Einstein , Doctorate thesis, Dir. H.A. Lorentz, Rijksuniversiteit van Leiden, 1916
J. Droste, Kon. Ak. Wetensch. Amsterdam 25 , 163 (1916). English translation: Proc. Acad. Sci. Amsterdam 19 , 197 (1917)
P. Drumaux, Ann. Soc. Sci. Bruxelles 56 , 5 (1936)
I.G. Dymnikova, Yad. Fiz. 31 , 679 (1980). English translation: Sov. J. Nucl. Phys. 31 , 353 (1980)
J. Earman, J. Eisenstaedt, Stud. Hist. Phil. Mod. Phys. 30 , 185 (1999)
MathSciNet Google Scholar
A.S. Eddington, Nature 105 , 37 (1920)
A.S. Eddington, Nature 113 , 192 (1924)
A. Einstein, Ann. Phys. (Leipzig) 49 , 769 (1916). English translations: The Principle of Relativity (Methuen and Company, London, 1923; reprinted by Dover, New York, 1952); The Collected Papers of Albert Einstein: The Berlin Years: Writings: 1914–1917 , ed. by M.J. Klein, A.J. Kox, J. Renn, R. Schulmann, vol. 6 (Princeton University Press, Princeton, 1996), p. 146
A. Einstein, M. Grossmann, Entwurf einer verallgemeinerten relativitätstheorie und einer theorie der gravitation (Teubner, Leipzig, 1913); reprinted by Zeitschrift Math. Phys. 62 , 225 (1914). English translation: The Collected Papers of Albert Einstein: The Swiss Years: Writings: 1914–1917 , ed. by M.J. Klein, A.J. Kox, J. Renn, R. Schulmann, vol. 4 (Princeton University Press, Princeton, 1996), p. 151
A. Einstein, M. Grossmann, Zeitschrift Math. Phys. 63 , 215 (1914). English translation: The Collected Papers of Albert Einstein: The Berlin Years: Writings: 1914–1917 , ed. by M.J. Klein, A.J. Kox, J. Renn, R. Schulmann, vol. 6 (Princeton University Press, Princeton, 1996), p. 6
L.P. Eisenhart, Riemannian Geometry (Princeton University Press, Princeton, 1926)
J. Eisenstaedt, Arch. Hist. Exact Sci. 27 , 157 (1982)
J. Eisenstaedt, Arch. Hist. Exact Sci. 37 , 275 (1987)
E.D. Fackerell, Astrophys. J. 166 , 197 (1971)
P. Fayet, arXiv:hep-ph/0111282v1 (2001)
M. Ferraris, M. Francaviglia, C. Reina, Gen. Rel. Grav. 14 , 243 (1982)
D. Finkelstein, Phys. Rev. 110 , 965 (1958)
P. Fiziev, Class. Q. Grav. 26 , 2447 (2006)
M. Freitag, Astrophys. J. 583 , L21 (2003)
J. Friedman, Proc. R. Soc. Lond. A 335 , 163 (1973)
V. Frolov, I. Novikov, Black Hole Physics (Kluwer, Dordrecht, 1998)
G. Galilei, Letter to P. Sarpi (1604)
G. Galilei, Dialogo sopra i due massimi sistemi del mondo, Tolemaico e Copernicano (G.B. Landini, Firenze, 1632). English translations: Dialogue Concerning the Two Chief World Systems, Ptolemaic and Copernican (McMillan, New York, 1914; California University Press, Berkeley, 1967)
G. Galilei, Discorsi e dimostrazioni matematiche intorno à due nuove scienze attenenti alla mecanica e i movimenti locali (Elzevier, Leiden, 1638). English translation: see reference [63]
D.V. Gal’tsov, G. Kofinas, P. Spirin, T.N. Tomaras, arXiv:1003.2982v1 [hep-th] (2010)
D.V. Gal’tsov, G. Kofinas, P. Spirin, T.N. Tomaras, Phys. Lett. B. 683 , 331 (2010)
D.V. Gal’tsov, P. Spirin, S. Staub, in Gravitation and Astrophysics , Proc. VII Asia-Pacific Int. Conf., Chungli 23–26 November 2005, ed. by J.M. Nester, C.-M. Chen, J.-P. Hsu (World Scientific, Singapore, 2006), p. 346
S.E. Gralla, R.M. Wald, Class. Q. Grav. 25 , 205009 (2008)
G. Green, J. Reine Ang. Math. J. Crelle 39 , 73 (1850)
G. Green, J. Reine Ang. Math. J. Crelle 44 , 356 (1852)
G. Green, J. Reine Ang. Math. J. Crelle 47 , 161 (1854)
E. Guéron, R.A. Mosna, Phys. Rev. D 75 , 081501 (2007)
J. Hadamard, Lectures on Cauchy’s Problem in Linear Partial Differential Equations (Yale University Press, New Haven, 1923)
Y. Hagihara, Jap. J. Astron. Geophys. 8 , 67 (1931)
M.P. Haugan, Ann. Phys. (N.Y.) 118 , 156 (1979)
M.P. Haugan, S.L. Shapiro, I. Wasserman, Astrophys. J. 257 , 283 (1982)
D. Hilbert, Nachr. König. Ges. Wiss. Göttingen, Math. Phys. Kl. 395 (1915). English translation: in The Genesis of General Relativity, Boston Stud. Phil. Sc. , ed. by J. Renn, vol. 4 (Springer, Dordrecht, 2007)
D. Hilbert, Nachr. König. Ges. Wiss. Göttingen, Math. Phys. K1. 53 (1917). English translation: in The Genesis of General Relativity, Boston Studies Phil. Sc. , ed. by J. Renn, vol. 4 (Springer, Dordrecht, 2007)
D. Hilbert, Math. Ann. 92 , 1 (1924) (reproduction with extensive changes of [102])
T. Hinderer, É.É. Flanagan, Phys. Rev. D 78 , 064028 (2008)
J.M. Hobbs, Ann. Phys. (N.Y.) 47 , 141 (1968)
A. Hurwitz, Z. Math. Phys. 27 , 86 (1882)
J. Jaffe, I.I. Shapiro, Phys. Rev. D 6 , 405 (1972)
J. Jaffe, I.I. Shapiro, Phys. Rev. D 8 , 4642 (1973)
A.I. Janis, Phys. Rev. D 8 , 2360 (1973)
A.I. Janis, Phys. Rev. D 15 , 3068 (1977)
D. Kennefick, Traveling at the Speed of Thought: Einstein and the Quest for Gravitational Waves (Princeton University Press, Princeton, 2007)
M. Kutschera, W. Zajiczek, arXiv:0906.5088v1 [astro-ph.EP] (2009)
C. Lämmerzahl, Eur. Phys. J. Sp. Top. 163 , 255 (2008)
L.D. Landau, E.M. Lifshits, Teoriya polya (MIR, Moskva, 1941). English translation: The Classical Theory of Fields (Pergamon, Oxford, 1951)
P.S. Laplace, Exposition du système du monde , vol. 2 (Imprimerie du Cercle Social, Paris, 1796). English translation: The System of the World (Richard Phillips, London, 1809)
P.S. Laplace, Allgemeine geographische ephemeriden , vol. 4 (Im verlage des industrie-comptoirs, Weimar, 1799). English translation: H. Stephani, arXiv:gr-qc/0304087v1 (2003)
E.W. Leaver, Proc. R. Soc. Lond. A 402 , 285 (1985)
E.W. Leaver, J. Math. Phys. 27 , 1238 (1986)
E.W. Leaver, Phys. Rev. D 34 , 384 (1986)
Le Canard Enchaîné , February 24th, March 3rd, March 10th, March 17th (1999)
Leonardo da Vinci, Code A 22v , Institut de France. Original manuscript and text online, http://www.leonardodigitale.com
T. Levi-Civita, Lezioni di calcolo differenziale assoluto , compiled by E. Persico (Stock, Roma, 1925). English translation: The Absolute Differential Calculus (Blackie, Glasgow, 1927)
T. Levi-Civita, Math. Ann. 97 , 291 (1926)
MATH MathSciNet Google Scholar
LISA, http://www.esa.int/science/lisa , http://lisa.jpl.nasa.gov
A. Loinger, T. Marsico, arXiv:0904.1578v1 [physics.gen-ph] (2009)
H.A. Lorentz, Kon. Ak. Wetensch. Amsterdam 23 , 1073 (1915). English translation: Proc. Acad. Sci. Amsterdam 19 , 751 (1915)
L. Lorenz, Philos. Mag. 34 , 287 (1867)
C.O. Lousto, Mod. Phys. Lett. A 12 , 1879 (1997)
C.O. Lousto, Phys. Rev. Lett. 84 , 5251 (2000)
C.O. Lousto, Class. Q. Grav. 18 , 3989 (2001)
C.O. Lousto, Class. Q. Grav. 22 , S543 (2005)
C.O. Lousto, H. Nakano, Class. Q. Grav. 26 , 015007 (2009)
C.O. Lousto, R.H. Price, Phys. Rev. D 55 , 2124 (1997)
C.O. Lousto, R.H. Price, Phys. Rev. D 56 , 6439 (1997)
C.O. Lousto, R.H. Price, Phys. Rev. D 57 , 1073 (1998)
E. Mach, Die mechanik in ihrer entwicklung historisch-kritisch dargestellt (Brockhaus, Leipzig, 1883). English translation: The Science of Mechanics. A Critical and Historical Exposition of Its Principals (The Open Court Publishing Co., Chicago, 1893)
S. Mano, H. Suzuki, E. Takasugi, Progr. Theor. Phys. 96 , 549 (1996)
F. Markley, Am. J. Phys. 41 , 45 (1973)
K. Martel, E. Poisson, Phys. Rev. D 66 , 084001 (2002)
K. Martel, E. Poisson, Phys. Rev. D 71 , 104003 (2005)
J. Mathews, J. Soc. Ind. Appl. Math. 10 , 768 (1962)
C.H. McGruder III, Phys. Rev. D 25 , 3191 (1982)
G.C. McVittie, General Relativity and Cosmology (Chapman and Hall, London, 1956)
J. Michell, Phil. Trans. R. Soc. Lond. 74 , 35 (1784)
Y. Mino, Class. Q. Grav. 22 , S375 (2005)
Y. Mino, Class. Q. Grav. 22 , S717 (2005)
Y. Mino, Progr. Theor. Phys. 113 , 733 (2005)
Y. Mino, Progr. Theor. Phys. 115 , 43 (2006)
Y. Mino, J. Brink, Phys. Rev. D 78 , 124015 (2008)
Y. Mino, M. Sasaki, T. Tanaka, Phys. Rev. D 55 , 3457 (1997)
A. Mitra, Found. Phys. Lett. 13 , 543 (2000)
V. Moncrief, Ann. Phys. (N.Y.) 88 , 323 (1974)
T. Müller, Gen. Rel. Grav. 40 , 2185 (2008)
A. Nagar, L. Rezzolla, Class. Q. Grav. 22 , R167 (2005); Corrigendum, ibidem 23 , 4297 (2006)
T. Nakamura, K. Oohara, Y. Koijma, Progr. Theor. Phys. Suppl. 90 , 110 (1987)
I. Newton, Philosophiæ naturalis principia mathematica (S. Pepys Reg. Soc. Præses, London, 1687). English translation: The Principia, Mathematical Principles of Natural Philosophy – A New Translation (California University Press, Berkeley, 1999)
L. Page, Nature 104 , 692 (1920)
L.I. Petrich, S.L. Shapiro, I. Wasserman, Astrophys. J. Suppl. S. 58 , 297 (1985)
E. Poisson, Living Rev. Rel. 7 , URL (cited on 4 May 2010): http://www.livingreviews.org/lrr-2004-6
H. Poli de Souza, Report for the Master thesis, Dir. A.D.A.M. Spallicci, Observatoire de Paris (2008)
A. Pound, Phys. Rev. D 81 , 024023 (2010)
A. Pound, E. Poisson, Phys. Rev. D 77 , 044013 (2008)
G. Preti, Found. Phys. 39 , 1046 (2009)
R.H. Price, J. Pullin, Phys. Rev. Lett. 72 , 329 (1994)
T.C. Quinn, R.M. Wald, Phys. Rev. D 56 , 3381 (1997)
T.C. Quinn, R.M. Wald, Phys. Rev. D 60 , 064009 (1999)
T. Regge, Nuovo Cimento 5 , 325 (1957); Additional remarks and errata corrige, ibidem 6 , 1233 (1957)
T. Regge, J.A. Wheeler, Phys. Rev. 108 , 1063 (1957)
G.F.B. Riemann, Monatsber. Königl. Preuss. Akad. Wiss. Berlin , 671 (1859). English translation by H.M. Edwards, Riemann’s Zeta Function (Academic, New York, 1974)
W. Rindler, Essential Relativity , 2nd revised edn. (Springer, New York, 1979)
W. Rindler, Relativity (Oxford University Press, New York, 2006)
P. Ritter, Report for the Master thesis, Dir. A.D.A.M. Spallicci, Université de Toulouse III Paul Sabatier, 2010
F. Rohrlich, Found. Phys. 30 , 621 (2000)
T. Rothman, Gen. Rel. Grav. 34 , 1541 (2002)
R. Ruffini, in Black Holes , Les Houches 30 July–31 August 1972, ed. by C. de Witt, B. de Witt (Gordon and Breach Science, New York, 1973), p. 451
R. Ruffini, Phys. Rev. D 7 , 972 (1973)
R. Ruffini, in Physics and Astrophysics of Neutron stars and Black Holes , Proc. of the Int. School of Physics E. Fermi Course LXV, Varenna 14–26 July 1975, ed. by R. Giacconi, R. Ruffini (North-Holland, Amsterdam and Soc. It. Fisica, Bologna, 1978), p. 287
R. Ruffini, J.A. Wheeler, in Significance of Space Research for Fundamental Physics , ESRO colloquium, Interlaken 4 September 1969, ESRO-52, ed. by A.F. Moore, V. Hardy (European Space Research Organisation, Paris, 1971), p. 45
R. Ruffini, J.A. Wheeler, in The Astrophysical Aspects of the Weak Interactions , Cortona 10-12 June 1970, ANL Quaderno n. 157 (Accademia Nazionale dei Lincei, Roma, 1971), p. 165
N. Sago, H. Nakano, M. Sasaki, Phys. Rev. D 67 , 104017 (2003)
K. Schwarzschild, Sitzungsber. Preuss. Akad. Wiss., Phys. Math. Kl. 189 (1916). English translation with foreword by S. Antoci and A. Loinger, arXiv:physics/9905030v1 [physics.hist-ph] (1999)
S.L. Shapiro, S.A. Teukolsky, Black Holes, White Dwarfs, and Neutron Stars: the Physics of Compact Objects (Wiley, New York, 1983)
S.L. Shapiro, I. Wasserman, Astrophys. J. 260 , 838 (1982)
M. Shibata, T. Nakamura, Progr. Theor. Phys. 87 , 1139 (1992)
M. Shibata, H. Okawa, T. Yamamoto, Phys. Rev. D 78 , 101501 (2008)
Simplikios, De cælo commentaria . English translation: On the Heavens (Duckworth, London and Cornell University Press, Ithaca, 1987)
L.E. Simone, E. Poisson, C.M. Will, Phys. Rev. D 52 , 4481 (1995)
C.F. Sopuerta, P. Laguna, Phys. Rev. D 73 , 044028 (2006)
A.D.A.M. Spallicci, in Proc. 8th Marcel Grossmann Mtg , Jerusalem 22–28 June 1997, ed. by T. Piran, R. Ruffini (World Scientific, Singapore, 1998), p. 1107
A.D.A.M. Spallicci, S. Aoudia, Class. Q. Grav. 21 , S563 (2004)
U. Sperhake, Private communication on 18 September 2009
U. Sperhake, V. Cardoso, F. Pretorius, E. Berti, J.A. González, Phys. Rev. D 101 , 161101 (2008)
G. Spinelli, in Proc. 5th Marcel Grossmann Mtg , Western Australia 8–13 August 1988, ed. by D.G. Blair, M.J. Buckingam, R. Ruffini (World Scientific, Singapore, 1989), p. 373
K.N. Srinivasa Rao, Ann. Inst. H. Poincaré (A) Phys. Théor. 5 , 227 (1966)
J. Stachel, Nature 220 , 779 (1968)
J.L. Synge, Relativity: the General Theory (North-Holland Publishing Co., Amsterdam, 1960)
Y. Tashiro, H. Ezawa, Prog. Theor. Phys. 66 , 1612 (1981)
E.F. Taylor, J.A. Wheeler, Exploring Black Holes (Addison Wesley Longman, San Francisco, 2000)
W. Thirring, Ann. Phys. (N.Y.) 16 , 96 (1961)
K.S. Thorne, Rev. Mod. Phys. 52 , 299 (1980)
H.J. Treder, Die relativität de trägheit (Akademie Verlag, Berlin, 1972)
H.J. Treder, K. Fritze, Astron. Nachr. 296 , 109 (1975)
G. Veneziano, Seminar at the Institut d’Astrophysique de Paris on 25 January 2010
C.V. Vishveshwara, Phys. Rev. D 1 , 2870 (1970)
M. von Laue, Die relativitätstheorie. Die allgemeine relativitätstheorie , vol. 2, 1st edn. (Vieweg und Sohn, Braunschweig, 1921). French translation: La théorie de la relativité. La relativité générale et la théorie de la gravitation d’Einstein , vol. 2 (Gauthier-Villars et C ie , Paris, 1926) translation in French of the revised and integrated German 4th edn. (1924)
E. von Rabe, Astron. Nachr. 275 , 251 (1947)
H. Weyl, Ann. Phys. (Leipzig) 54 , 117 (1917)
J.A. Wheeler, Public lecture held at the Goddard Institute of Space Studies on 29 December 1967; Am. Sch. 37 , 248 (1968); Am. Sci. 56 , 1 (1968)
B.F. Whiting, S. Detweiler, Int. J. Mod. Phys. D 9 , 1709 (2003)
E.T. Whittaker, A History of the Theories of Aether and Electricity , vol. 2 (Nelson, London, 1953)
C.M. Will, Theory and Experiments in Gravitational Physics , revised edition (Cambridge University Press, Cambridge, 1993)
C.M. Will, Living Rev. Rel. 9 , URL (cited on 4 May 2010): http://www.livingreviews.org/lrr-2006-3
M. White, Isaac Newton: the Last Sorcerer (Fourth Estate Limited, London, 1997)
H. Yoshino, M. Shibata, Phys. Rev. D 9 , 084025 (2009)
Y.B. Zel’dovich, I.D. Novikov, Dokl. Akad. Nauk 155 , 1033 (1964). English translation: Sov. Phys. Doklady 9 , 246 (1964)
Y.B. Zel’dovich, I.D. Novikov, Relyativistskaya astrofyzika (Izdatel’svo Nauka Moskva, 1967). English translation (revised and enlarged): Relativistic Astrophysics (Chicago University Press, Chicago, 1971)
A. Zenginonğlu, Class. Q. Grav. 27 , 045015 (2010)
F.J. Zerilli, J. Math. Phys. 11 , 2203 (1970)
F.J. Zerilli, Phys. Rev. Lett. 24 , 737 (1970)
F.J. Zerilli, Phys. Rev. D 2 , 2141 (1970); Erratum, in Black holes , Les Houches 30 July - 31 August 1972, ed. by C. DeWitt, B. DeWitt (Gordon and Breach Science, New York, 1973)
V.I. Zhdanov, Izv. Vyssh. Uchebn. Zaved. Fiz. 9 , 34 (1979). English translation: Sov. Phys. J. 22 , 951 (1979)
Download references
Acknowledgements
Discussions throughout the years with S. Aoudia, L. Barack, S. Chandrasekhar, S. Detweiler, C. Lousto, J. Martin-Garcia, S. Gralla, E. Poisson, R. Price, R. Wald, B. Whiting are acknowledged. I would like to thank E. Vergès for the support to CNRS School on Mass and the 11th Capra conference held in June 2008 in Orléans. It was my sincere hope that both events could put together different communities working on mass and motion: part of the contributors to this book and their colleagues (Blanchet, Detweiler, Le Tiec and Whiting) have already made concrete steps towards such cooperation [ 27 , 28 ].
Author information
Authors and affiliations.
Observatoire des Sciences de l’Univers en région Centre, Université d’Orléans, LPC2E, Campus CNRS, 3A Avenue de la Recherche Scientifique, 45071, Orléans, France
Alessandro Spallicci
You can also search for this author in PubMed Google Scholar
Corresponding author
Correspondence to Alessandro Spallicci .
Editor information
Editors and affiliations.
Institut d'Astrophysique de Paris, boulevard Arago 98bis, Paris, 75014, France
Luc Blanchet
Observatoire de la Cote d'Azur, Nice, France
Dept. Physics, University of Florida, Gainesville, 32611-8440, Florida, USA
Bernard Whiting
Rights and permissions
Reprints and permissions
Copyright information
© 2009 Springer Science+Business Media B.V.
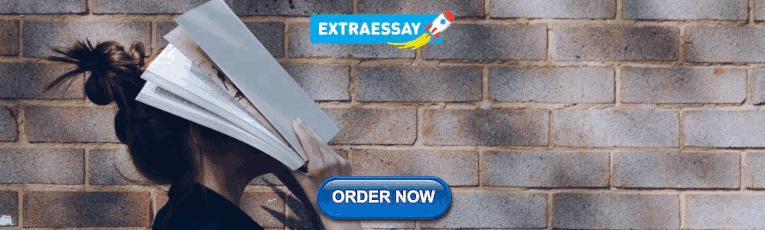
About this chapter
Cite this chapter.
Spallicci, A. (2009). Free Fall and Self-Force: an Historical Perspective. In: Blanchet, L., Spallicci, A., Whiting, B. (eds) Mass and Motion in General Relativity. Fundamental Theories of Physics, vol 162. Springer, Dordrecht. https://doi.org/10.1007/978-90-481-3015-3_20
Download citation
DOI : https://doi.org/10.1007/978-90-481-3015-3_20
Published : 07 October 2010
Publisher Name : Springer, Dordrecht
Print ISBN : 978-90-481-3014-6
Online ISBN : 978-90-481-3015-3
eBook Packages : Physics and Astronomy Physics and Astronomy (R0)
Share this chapter
Anyone you share the following link with will be able to read this content:
Sorry, a shareable link is not currently available for this article.
Provided by the Springer Nature SharedIt content-sharing initiative
- Publish with us
Policies and ethics
- Find a journal
- Track your research
Accessibility Links
- Skip to content
- Skip to search IOPscience
- Skip to Journals list
- Accessibility help
- Accessibility Help
Click here to close this panel.
Purpose-led Publishing is a coalition of three not-for-profit publishers in the field of physical sciences: AIP Publishing, the American Physical Society and IOP Publishing.
Together, as publishers that will always put purpose above profit, we have defined a set of industry standards that underpin high-quality, ethical scholarly communications.
We are proudly declaring that science is our only shareholder.
Using Tracker to understand 'toss up' and free fall motion: a case study
Loo Kang Wee 1 , Kim Kia Tan 2 , Tze Kwang Leong 3 and Ching Tan 1
Published 19 June 2015 • © 2015 IOP Publishing Ltd Physics Education , Volume 50 , Number 4 Citation Loo Kang Wee et al 2015 Phys. Educ. 50 436 DOI 10.1088/0031-9120/50/4/436
Article metrics
13006 Total downloads
Share this article
Author e-mails.
Author affiliations
1 Ministry of Education, Educational Technology Division, Singapore
2 Ministry of Education, Evergreen Secondary School, Singapore
3 Ministry of Education, Raffles Girls School, Singapore
- Received 12 January 2015
- Accepted 31 March 2015
- Published 19 June 2015
Peer review information
Method : Single-anonymous Revisions: 1 Screened for originality? No
Buy this article in print
This paper reports the use of Tracker as a computer-based learning tool to support effective learning and teaching of 'toss up' and free fall motion for beginning secondary three (15 year-old) students. The case study involved ( N = 123) students from express pure physics classes at a mainstream school in Singapore. We used eight multiple-choice questions pre- and post-test to gauge the impact on learning. The experimental group showed learning gains of d = 0.79 ± 0.23 (large effect) for Cohen's d effect size analysis, and gains with a gradient of < g > total = 0.42 ± 0.08 (medium gain) above the traditional baseline value of < g > non interactive = 0.23 for Hake's normalized gain regression analysis. This applied to all of the teachers and students who participated in this study. Our initial research findings suggest that allowing learners to relate abstract physics concepts to real life through coupling traditional video analysis with video modelling might be an innovative and effective method for teaching and learning about free fall motion.
Export citation and abstract BibTeX RIS
Content from this work may be used under the terms of the Creative Commons Attribution 3.0 licence . Any further distribution of this work must maintain attribution to the author(s) and the title of the work, journal citation and DOI.
1. Introduction
Many young students harbour misconceptions (Kavanagh and Sneider 2006 ) about free fall motion and its scientific representation, for example in graphs displaying displacement versus time ( y versus t ), velocity versus time ( v y versus t ) and acceleration versus time ( a y versus t ). When these are not well understood, it is difficult to use them to apply deductive reasoning in different scenarios, such as tossing a ball up with a higher initial speed or on the Moon's surface.
While the use of real-life examples (such as tossing a ball to demonstrate free fall) can be performed by students in class, it is nevertheless challenging for them to translate this world view into x and y coordinates in typical scientific multiple representations (Wong et al 2011 ).
Our study involves the implementation of two 70 min computer laboratory lessons using the worksheets available at stacks.iop.org/PhysED/50/436/mmedia (free fall–investigate using tracker-student 2.docx), where students fill in the blanks of printouts. There were three pairs of teachers, and each pair taught one class with a total number of students of N = 123. A conceptual eight-item multiple-choice questionnaire was used in pre-post-tests to serve as an indication of the learning gains generated after three weeks of wholly traditional and computer-laboratory-based kinematics lessons.
Although not implemented in this study, the video modelling pedagogical approach (Brown 2009 ) is also recommended for difficult-to-visualize velocity-versus-time graphs comparing two cases; for example, tossing up a ball with (a) greater force (higher initial velocity) on Earth and (b) with the same force on the Moon's surface. The free software tool Tracker 4 can be downloaded from the open source physics website (Christian et al 2011 ) and has been used by a number of Physics Education authors (Persson and Hagen 2011 , Kinchin 2012 , Wee et al 2012 , Poonyawatpornkul and Wattanakasiwich 2013 , Rodrigues and Carvalho 2013 ).
2. Installation of Tracker
Tracker is a video analysis and modelling tool built on the Open Source Physics (OSP) Java framework. Although it is possible to run it from a 5.6Mb Tracker_487.jar file, we recommend using the installers (see footnote 1), in particular to enable the Xuggle video engine, which can decode most video file formats. Installers for Tracker version 4.87 are available for Windows, Mac OS X and Linux operating systems.
3. Study design
3.1. purpose of study.
The study aims to determine the learning gains from two 75 min computer laboratory lessons (figure 1 ) that add the Tracker tool and Tracker resource 'bosstossup.trz' (available at stacks.iop.org/PhysED/50/436/mmedia ; double click the file in Tracker to launch) to existing typical Singapore school teaching practices for the topic of kinematic free fall. The gains are assessed using Cohen's d effect size and Hake's normalized (Hake 1998 ) gain regression analysis.
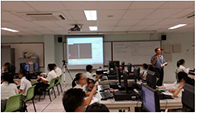
Figure 1. Typical computer laboratory lesson setup, with a teacher guiding approximately 40 students through a hands-on activity that uses Tracker to teach/learn the kinematics of free fall.
Download figure:
3.2. Methodology
3.2.1. research design..
A case study approach was adopted with the aim of providing a richly descriptive analysis of the impact of using Tracker on student learning in a normal school setting.
3.2.2. Participants.
The participants in this study are shown in table 1 . In the 2 × 75 min computer laboratory lessons the secondary-three-level (15 year-old) students were divided into classes of ~40 pupils, with a pair of teachers per class.
Table 1. Class sizes for the instructors of the experimental group. Students were taking ordinary level pure physics.
3.2.3. Lesson plan.
The plan was to use these 2 × 75 min computer laboratory lessons to allow students to gain personal experience of the physics of ball 'toss up' motion. The worksheet served as a guide to support the use of Tracker and also to prompt students to predict-observe-explain (Radovanović and Sliško 2013 ) what they were supposed to understand.
3.2.3.1. Teacher professional development.
The six teachers were introduced to Tracker approximately three months before implementation of the lessons. Teacher TKK lead the training sessions with the other secondary three physics teachers in the school as part of the Singapore schools professional learning community team effort, a 2012 initiative from the Singapore Ministry of Education. They designed the worksheet in regular fortnightly meetings and used a Google site 5 for professional development and maintenance of consistent and high-fidelity teaching practices.
3.2.3.2. Laboratory preparation.
The computer laboratory technical assistant installed Tracker on the school's computers; one difficulty encountered was the inability to launch Tracker using the Windows|Start|Programs|Tracker|Tracker icon. The solution devised was to create a shortcut directly to the computer's Tracker installed folder: C:\Program Files\Tracker\Tracker.jar. This error was fixed in subsequent releases of Tracker as a result of our feedback.
3.2.3.3. Laboratory activities.
The teachers guided the students, aided by a customized worksheet showing the steps necessary to open Tracker and load the video 'tossup.mov' using the teaching staff's computers and laboratory projectors (figure 1 ). Typically, one teacher showed and explained how to use Tracker, with one or two other teachers around to help with any issues arising from the hands-on activities.
3.3. Data collection instruments
3.3.1. pre-post-test..
An online 6 pre-post-test was constructed, which referenced the activity and worksheets to align the learning tasks with the test items.
3.3.2. Focus group discussions with students.
To gather more qualitative evidence regarding the lessons, post-lesson focus group discussions were conducted with a total of nine students from teacher TKK's class divided into three groups. The discussions also provided insights into data that the authors wished to rationalize; for example, the negative pre-post-test results registered for some students, who had answered 4–6 questions correctly out of eight (50–70%) in the pre-test.
3.3.3. Informal discussions/interviews with teachers.
The discussions revealed that some of the students and teachers were not comfortable using Tracker, as it was new to them, and that they would require further support to conduct lessons accurately according to the lesson plans. The pairs of teachers served to address this issue, as the partner teacher was able to co-teach and support the lesson.
As the worksheet was being employed in lessons for the first time, some difficulties surfaced while students used it. The newly designed worksheet benefitted from our research, for example through more appropriate scaffolds, such as more closely targeted hints.
3.4. Data collection procedures
At the beginning of the first computer-based lessons, students were told to complete the pre-test in the first 10–15 min after they had navigated to the lesson site. 7 Students were encouraged to complete the pre-test as it would give the teachers an idea of what parts to focus on later in class. Some students managed to discuss their answers, and this may have contributed to some students with 50, 62.5 and 75% correct scores at pre-test later registering lower post-test scores.
After the end of the topic on kinematics, about three weeks after the first lesson, the students were brought back to complete the same items post-test, individually and without discussions.
3.5. Results
Table 2 shows the pre- and post-test results for the eight kinematics questions, tabulated as percentages of the 123 students. The average number of correct scores pre-test was 33% and post-test it was 48%.
Table 2. Percentage of students (total number of students = 123) who selected (a)–(d) for questions Q(1)–(8). The correct response for each question is italicized. The average percentage of correct answers was 33% pre-test and 48% post-test.
3.5.1. Questions 1 to 6.
Questions 1, 2 and 3 test students' ability to recall and identify simple y versus t , v y versus t and a y versus t graphs, with each registering gains ranging from 16 to 23%.
Question 4 is a common conceptual test item that requires students to understand that the velocity and acceleration at the highest point of the vertical toss-up motion is zero and non-zero, respectively. The change for this was +10%.
Questions 5 and 6 are simple understanding test items that require students to know that when the ball moves upward and downward, the gradient of the displacement-time graph is positive and decreasing in magnitude, and negative and increasing in magnitude, respectively. The change for these was higher, at around 30%.
3.5.2. Questions 7 and 8.
Questions 7 and 8 are applications test items. In question 7 the v y versus t graph on Earth is given (green), where the higher initial velocity would result in a parallel but higher v y intercept line (blue). The change was only +4% for this. For question 8, the v y versus t graph on Earth is given (green), and here a lower gravitational acceleration would result in a line with the same intercept but a smaller gradient. This time the change was −10%.
3.6. Discussion
3.6.1. questions 1 to 6..
Questions 1 to 6 registered positive gains, which is to be expected. Interestingly, question 4 option (a) also registered a small +5% gain, from 46% to 51%, suggesting that the misconception that an object at the top of its motion suddenly has zero acceleration remains pervasive and that this is a difficult concept to understand correctly; we hope to design better lessons to address this in future.
3.6.2. Question 7 and 8.
Question 7 is almost unchanged, with a 4% gain. This is not surprising, as the teachers did not explicate this concept using Tracker's modelling pedagogy (Wee et al 2012 ), which we argue holds great potential for experiential learning and deepening understanding.
Question 8 surprisingly registered a −10% change, which, after analyzing option (b), suggests that students were 'tricked' by 'due to lower mass with higher air resistance', not realizing that it will be a terminal velocity trail when time is large, while the correct answer of (d) is a linear trail suggesting constant acceleration with no drag.
3.7. Results
Based on the scores collected for the pre = 1, post = 2 test made up of eight multiple-choice questions, all three classes ( N 1 = N 2 = 123) registered positive gains after the incorporation of two hands-on computer-based laboratories with Tracker into a traditional three-week block of lessons on the kinematics of toss up and free fall motion. Classes C (blue), I (red) and R (green) all registered gains (figure 2 ) and it is worth noting that the total scores (purple) were
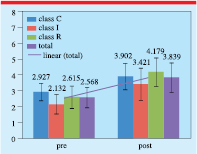
Figure 2. Bar chart of pre-post-test scores of students from class C (blue), I (red), R (green) and the combined total (purple) (left to right) showing total pre-test and post-test scores C pre, post = (2.927 ± 1.237, 3.902 ± 2.022), I pre, post = (2.132 ± 1.104, 3.421 ± 1.648), R pre, post = (2.615 ± 1.407, 4.179 ± 1.824) and TOTAL pre, post = (2.568 ± 1.298, 3.839 ± 1.868).
Using the normalized gain (Hake 1998 ) < g > in equation ( 2 ), where the post-test and pre-test scores are x 2 and x 1 , respectively, and 8 is the maximum score for the test, we analyzed the normalized learning gains in percentages across the three classes' pre-test scores as the horizontal axis:
We used only the pre-test data equal to and below 50%, as < g > is generally negative for pre-test scores of 62.5% and 75%, and there were no students with 87.5% or 100%. Our interviews with eight students suggested that the negative gains might be attributed to a longer pre-test time of 15 minutes compared to the post-test time of 10 minutes and the benefits of peer discussion the during pre-test.
The general trend was not adversely affected by neglecting scores from 62.5 and 75%, thus we chose scores of 50% and below to simplify presentation. The results from passing through (0%, 0%) linear regression using < g > data in percentage versus pre-test scores (figure 3 ) suggest that the three classes' normalized gains < g > are near the medium gain classification: < g > C = 0.40 (blue),< g > I = 0.50 (red),< g > R = 0.45 (green) and < g > total = 0.42 (purple) are in a range of gradients well above the traditional normalized gain of < g > traditional = 0.23.
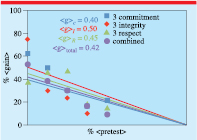
Figure 3. Gain versus pre-test for all three classes. Pre-test scores ranging from 0 marks, 0%, to 4 marks, 50%, are given on the horizontal axis and the gain in percentage is given on the vertical axis. Note that the gains range from 0.40 to 0.50, with < g > total = 0.42 suggesting that the lessons are as effective as most interactive engagement lessons, above the traditional lesson gains of 0.23, as reported in Hake ( 1998a ).
Based on the standardized mean difference, Cohen's d = 0.79 (large effect) and normalized gain < g > = 0.42 (medium gain) analysis, the evidence suggests that the students did learn about kinematics concepts more effectively than they would have with traditional passive, non-interactive lessons.
We recommend use of a design-based research (Juuti and Lavonen 2006 ) method to continually improve these Tracker-based lessons, as this is the first year that teachers have conducted them, and we believe that there is still much scope for improving their implementation and deepening the learning experience through video modelling (Brown 2012a), not elaborated in this paper.
4. Students' reflections on the Tracker lesson
To give some insights into the conditions and processes seen during the laboratory lessons, the following are some excerpts from the informal interviews with students. Words in square brackets [] have added to improve the readability of the qualitative interviews.
4.1. Tracker supports interactive and real-world physics
'We are able to see the connections between real life [video] and the [scientific] graph[s]. Tracker helps me to confirm the theory [in kinematics] I have learned.'
'The video analysis [Tracker] gives me the opportunity to check the data collected. I realized that in real-life data collection there are random errors, which was shown from the graph plotted.'
'Compared to teachers' explanations on the board, video analysis gives us more opportunity to have a real learning experience, rather than being spoon fed with content. By allowing us to use the video analysis [Tracker], we have been able to see more precisely [the relationship] between the ball and the graph plotted.'
'Perhaps we can have a practical lesson [a performance task] in the curriculum. We would be interested in trying it out ourselves, doing the experiment and recording the videos ourselves.'
4.2. Overcoming initial difficulties using Tracker
'I do not have the experience to load the video and track the video. I would like teachers to use the video Tracker to show us the scenarios in learning, so that we can strike a balance between learning effectively and not spending too much time in setting up the video [tool] Tracker.'
To address this difficulty, teacher TKK has created YouTube video tutorials 8 to help his students.
5. Teachers' reflection
Despite the relative success of the Tracker-based learning, the teachers reflected on the method and made three recommendations for improvement.
5.1. Start using Tracker for easier horizontal kinematics tasks
Start the year with an easier horizontal kinematics task, such as investigating a constant speed object moving on a frictionless track. This will serve to address the cognitive overload (Roth 1999 ) problem encountered when using Tracker and the relative complexity of the toss up and free fall motion concept for new secondary three students.
5.2. The practice of video modelling, especially for questions 7 and 8
As mentioned before, the teachers were only became aware of the video modelling approach later, and were unable to incorporate this approach across all three classes. Thus, subsequent teaching interventions will include direct instruction from teachers regarding the video modelling activities not explained in this paper.
5.3. Integration of Tracker into the teaching/learning of kinematics, dynamics and work, and energy
We also recognize that for sustained learning gains to be achieved Tracker use should be integrated into the teaching/learning of topics like kinematics, dynamics and work, and energy, so that Tracker's analytical capabilities can be employed seamlessly.
6. Conclusion
This case study involved ( N = 123) students from express pure physics classes at a mainstream school in Singapore. We used eight multiple-choice questions pre- and post-test to gauge the impact of our activities on learning. The experimental group showed learning gains of d = 0.79 ± 0.23 (large effect) for Cohen's d effect size analysis, and gains with a gradient of < g > total = 0.42 (medium gain) for Hake's normalized gain regression analysis. This applied to all of the six teachers and three classes in the study. The evidence suggests the students learned kinematics concepts more effectively than they would have with traditional passive, non-interactive lessons.
We have made three recommendations to further strengthen learning. (1) Start using Tracker for easier horizontal kinematics tasks to lower cognitive loading for novice students; (b) use the video modelling pedagogical approach (Wee et al 2012 ) to improve learning targeted at questions 7 and 8; and (c) integrate Tracker into the teaching of kinematics, dynamics and work, and energy, to promote sustainable and seamless learning with it.
Acknowledgments
We wish to acknowledge the contributions of D Brown, W Christian, M Belloni, A Cox, F Esquembre, H Gould, B Junkin, A Titus and J Tobochnik to the creation of the Tracker video analysis and modelling tool. This research was made possible thanks to eduLab project NRF2013-EDU001-EL017 'Becoming scientists through video analysis', awarded by the National Research Foundation (NRF), Singapore in collaboration with National Institute of Education (NIE), Singapore and the Ministry of Education (MOE), Singapore.
www.cabrillo.edu/~dbrown/tracker
www.tinyurl.com/evg3phy
https://docs.google.com/forms/d/1zm9NWWC7DWHkO2OYdbf150YyivJFPJeuT5vkO_KFKdI/viewform
www.youtube.com/user/kimkiatan/videos
Biographies
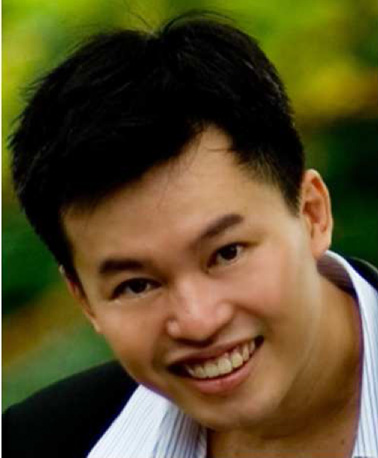
Loo Kang Wee is currently an educational technology specialist II at the Ministry of Education, Singapore. His open source physics contributions have been recognized with a number of awards, including the Public Service PS21 Distinguished Star Service Award 2014, Best Ideator 2012, Best Innovator Award 2013 and Excellent Service Award 2012.
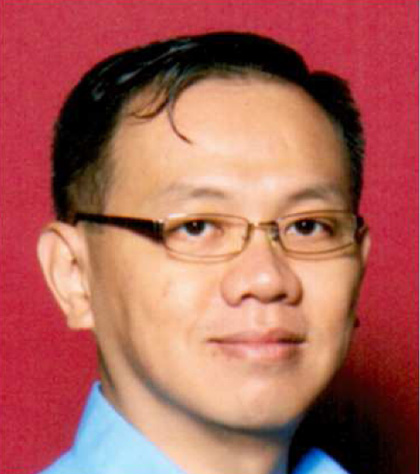
Kim Kia Tan is a physics teacher at Evergreen Secondary School, Singapore. He currently leads a professional learning team in the teaching and learning of physics using ICT at his school.
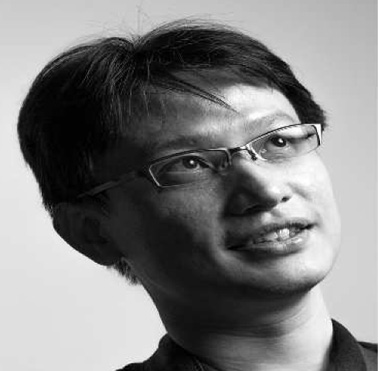
Tze Kwang Leong is currently teaching physics at Raffles Girls' School, Singapore. His interest is in demonstrations and ICT tools that augment lessons to deepen student understanding.
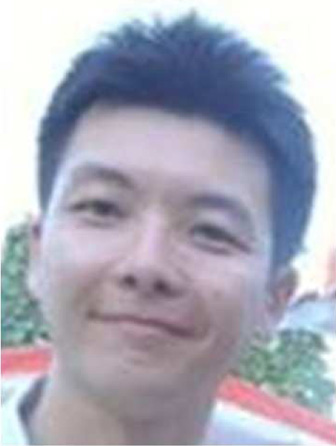
Ching Tan is an educational technology officer at the Educational Technology Division, Ministry of Education, Singapore. He is a science teacher with a keen interest in visual thinking and multiple representations.
Free fall - investigate using tracker-student 2.docx. (5.4 MB, Word doc)
bosstossup.trz. (767 kb, TRZ file)
- Open supplemental data
- Reference Manager
- Simple TEXT file
People also looked at
Original research article, associations between monitor-independent movement summary (mims) and fall risk appraisal combining fear of falling and physiological fall risk in community-dwelling older adults.
- 1 Department of Mechanical Engineering, University of Central Florida, Orlando, FL, United States
- 2 Disability, Aging and Technology Cluster, University of Central Florida, Orlando, FL, United States
- 3 College of Medicine, University of Central Florida, Orlando, FL, United States
- 4 School of Kinesiology and Rehabilitation Sciences, College of Health Professions and Sciences, University of Central Florida, Orlando, FL, United States
- 5 Department of Statistics and Data Science, University of Central Florida, Orlando, FL, United States
- 6 College of Nursing, University of Central Florida, Orlando, FL, United States
Introduction: Fall Risk Appraisal (FRA), a process that integrates perceived and objective fall risk measures, serves as a crucial component for understanding the incongruence between fear of falling (FOF) and physiological fall risk in older adults. Despite its importance, scant research has been undertaken to investigate how habitual physical activity (PA) levels, quantified in Monitor-Independent Movement Summary (MIMS), vary across FRA categories. MIMS is a device-independent acceleration summary metric that helps standardize data analysis across studies by accounting for discrepancies in raw data among research-grade and consumer devices.
Objective: This cross-sectional study explores the associations between MIMS (volume and intensity) and FRA in a sample of older adults in the United States.
Methods: We assessed FOF (Short Falls Efficacy Scale-International), physiological fall risk (balance: BTrackS Balance, leg strength: 30-s sit-to-stand test) and 7-day free-living PA (ActiGraph GT9X) in 178 community-dwelling older adults. PA volume was summarized as average daily MIMS (MIMS/day). PA intensity was calculated as peak 30-min MIMS (average of highest 30 non-consecutive MIMS minutes/day), representing a PA index of higher-intensity epochs. FRA categorized participants into following four groups: Rational (low FOF-low physiological fall risk), Irrational (high FOF-low physiological fall risk), Incongruent (low FOF-high physiological fall risk) and Congruent (high FOF-high physiological fall risk).
Results: Compared to rational group, average MIMS/day and peak 30-min MIMS were, respectively, 15.8% ( p = .025) and 14.0% ( p = .004) lower in irrational group, and 16.6% ( p = .013) and 17.5% ( p < .001) lower in congruent group. No significant differences were detected between incongruent and rational groups. Multiple regression analyses showed that, after adjusting for age, gender, and BMI (reference: rational), only irrational FRA was significantly associated with lower PA volume (β = −1,452.8 MIMS/day, p = .034); whereas irrational and congruent FRAs were significantly associated with lower “peak PA intensity” (irrational: β = −5.40 MIMS/day, p = .007; congruent: β = −5.43 MIMS/day, p = .004).
Conclusion: These findings highlight that FOF is a significant barrier for older adults to participate in high-intensity PA, regardless of their balance and strength. Therefore, PA programs for older adults should develop tailored intervention strategies (cognitive reframing, balance and strength exercises, or both) based on an individual’s FOF and physiological fall risk.
Introduction
In the United States (US), over 14 million adults aged 65 years or older fall each year ( Moreland et al., 2020 ; Kakara et al., 2023 ). According to the US Centers for Disease Control and Prevention, about 20% of falls in older adults cause serious injuries, which results in limited functional mobility, loss of independence, reduced quality of life, and premature death ( Ambrose et al., 2013 ). Fear of falling (FOF) has been recognized as an important psychological aspect associated with falls in older adults ( Jansen et al., 2021 ). However, studies report that many older adults might show a discrepancy between their FOF and physiological fall risk, known as maladaptive fall risk appraisal (FRA) ( Thiamwong et al., 2021a ), and such discrepancies can lead to adverse consequences. For example, individuals with low physiological fall risk but high FOF may overestimate their actual fall risk and restrict their daily activities, which can further lead to physical deconditioning and loss of muscle strength ( Deshpande et al., 2008 ). On the contrary, those with high physiological fall risk but low FOF may underestimate their actual fall risk and engage in unnecessary risky behavior beyond their physical capacity, making them even more vulnerable to falling ( Delbaere et al., 2010 ).
Therefore, FRA combining subjective and objective fall risk measures is important for understanding the discrepancy between FOF and physiological fall risk in older adults to inform more targeted interventions for fall prevention ( Thiamwong et al., 2020a ; Thiamwong et al., 2020b ). FRA is a two-dimensional fall risk assessment matrix that classifies older adults into four groups based on their FOF and physiological fall risk status ( Thiamwong, 2020 ). In FRA matrix, as shown in Figure 1 , two groups have their FOF level aligned with their physiological fall risk status, which are denoted as Rational (low FOF-low physiological fall risk) and Congruent (high FOF-high physiological fall risk). The other two groups show a mismatch between their FOF level and physiological fall risk status and are denoted as Incongruent (low FOF-high physiological fall risk) and Irrational (high FOF-low physiological fall risk).
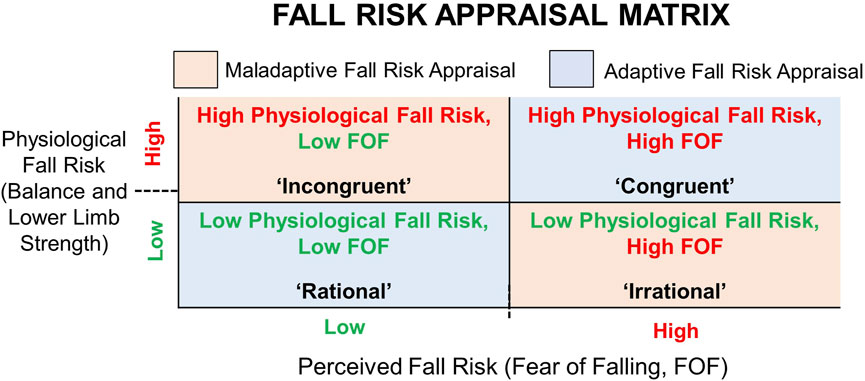
Figure 1 . Fall Risk Appraisal (FRA) based on Fear of Falling (FOF) and physiological fall risk. Maladaptive FRA = mismatch between FOF and physiological fall risk; Adaptive FRA = FOF aligned with physiological fall risk.
Prior research has mostly focused on exploring the independent associations of FOF and objective fall risk measures with physical activity (PA) participation in older adults ( Gregg et al., 2000 ; Chan et al., 2007 ; Zijlstra et al., 2007 ; Heesch et al., 2008 ; Mendes da Costa et al., 2012 ). To date, only a small number of studies have investigated the combined effects of FOF and objective fall risk on PA engagement. For example, one study examined the joint associations of FOF and objective fall risk with everyday walking activities in older adults. This study used a four-group categorization from ( Delbaere et al., 2010 ), and found that the number of steps/day in their study sample was in accordance with objective fall risk rather than FOF ( Jansen et al., 2021 ). Another study examined accelerometry-based PA levels between FRA categories using the intensity cut-point approach and found that participants with high FOF accumulated significantly less time in moderate-to-vigorous PA (MVPA) compared to those with rational FRA, regardless of their balance performance ( Thiamwong et al., 2023 ). However, there exists a lack of evidence on how habitual PA levels, expressed in Monitor-Independent Movement Summary (MIMS) units, differ between FRA categories in older adults.
MIMS is used to summarize the acceleration measurements obtained on the x-, y-, and z-axes of wrist-worn activity monitors. This PA metric was first introduced in 2019 to summarize participant-level PA data for the 2011-2012 and 2013-2014 cycles of the US National Health and Nutrition Examination Survey (NHANES) ( John et al., 2019 ). The major benefit of using MIMS is that it is generated by a nonproprietary device–independent universal algorithm, allowing us to compare the total movement across studies regardless of the heterogeneity introduced by different brands, models and device types (such as consumer vs. research-grade) ( John et al., 2019 ). Similar to other traditional PA metrics such as steps/day or daily activity counts, PA volume can be expressed as daily MIMS (i.e., total MIMS unit accumulated per day) across valid days of assessment, where larger MIMS/day indicates higher daily PA volume ( Wolff-Hughes et al., 2014 ).
Traditionally, quantification of accelerometer-measured PA intensity has been predominantly based on minutes/day (or minutes/week) spent in MVPA, using either manufacturer-specific or device-specific cut points corresponding with ≥3 Metabolic Equivalents of Task (METs) ( Troiano et al., 2008 ). Recently, to establish an intensity-based expression for MIMS units, the concept of peak 30-min MIMS has been introduced ( Zheng et al., 2023 ). It is analogous to the concept of peak 30-min cadence, i.e., the average of 30 highest cadence (steps/minutes) values within a day, representing an individual’s best efforts ( Tudor-Locke et al., 2012 ). Similar to cadence (steps/minutes), MIMS/minutes values were shown to have a strong correlation with higher PA intensity ( John et al., 2019 ). Therefore, peak 30-min MIMS (i.e., the average of the highest 30 non-consecutive MIMS [minutes/day] values within a day) can be used as a measure of higher-intensity epochs across the PA monitoring period ( Zheng et al., 2023 ). Evaluating daily MIMS (volume) and peak 30-min MIMS (intensity) can facilitate a more comprehensive assessment of PA and its relationship with FRA.
Thus, the aim of this study is to investigate the associations between wrist-worn accelerometer-measured PA (expressed as daily MIMS and peak 30-min MIMS) and FRA in a sample of community-dwelling older adults. We are particularly interested in the question: “Which of the maladaptive FRA groups, i.e., Incongruent (low FOF-high physiological fall risk) and Irrational (high FOF-low physiological fall risk), differ more from the Rational (low FOF-low physiological fall risk) group in terms of habitual PA level?.” This will allow us to understand which of the two factors—FOF or physiological fall risk—has a stronger relationship with reduced PA participation among older adults.
Materials and methods
Study design and participants.
In this cross-sectional study, purposive sampling was used to recruit 178 community-dwelling older adults from the region of Central Florida, United States, between February 2021 and March 2023. The inclusion criteria were: i) 60 years of age or older; ii) being able to walk with or without an assistive device (but without the assistance of another person); iii) no marked cognitive impairment [i.e., Memory Impairment Screen score ≥5 ( Buschke et al., 1999 )], iv) fluency in English or Spanish, and v) living in their own homes or apartments. The exclusion criteria were: i) medical conditions that prevent PA engagement (e.g., shortness of breath, tightness in the chest, dizziness, or unusual fatigue at light exertion), ii) unable to stand on the balance plate, iii) currently receiving treatment from a rehabilitation facility, and iv) having medical implants (e.g., pacemakers). This study was approved by the Institutional Review Board at the University of Central Florida (Protocol No: 2189; 10 September 2020). All subjects provided written informed consent to participate. This cross-sectional assessment required one visit to the study site during which participants completed a demographic survey and anthropometric measurements, followed by assessments of FOF and physiological fall risk. At the end of the visit, each participant was fitted with a wrist-worn accelerometer for 7-day PA monitoring in free-living conditions.
Measurements
Fear of falling (fof).
FOF was assessed using the Short Falls Efficacy Scale-International (FES-I) questionnaire ( Yardley et al., 2005 ; Kempen et al., 2008 ). It is a 7-item, self-administered tool that uses a 4-point Likert scale to measure the level of concern about falling while performing seven activities (1 = not at all concerned to 4 = very concerned). The total scores ranged from 7 to 28. Short FES-I scores of 7–10 indicated low FOF, while scores of 11–28 indicated high FOF.
Physiological fall risk
Physiological fall risk was assessed using balance test and lower limb strength assessment. BTrackS Balance System (Balance Tracking Systems, San Diego, CA, United States) was used to measure static balance. This system includes a portable BTrackS Balance Plate and BTrackS Assess Balance Software running on a computer. It has shown high test–retest reliability (intraclass correlation coefficient, ICC = 0.83) and excellent validity (Pearson’s product-moment correlations, r > 0.90) in evaluating static balance ( Levy et al., 2018 ). The test protocol included four trials (each trial taking 20 s) with less than 10 s of inter-trial delays. During the trials, participants were asked to stand still on the BTrackS Balance Plate with their eyes closed, hands on their hips, and feet placed shoulder-width apart. BTrackS balance plate is an FDA-registered, lightweight force plate that measures center of pressure (COP) excursions during the static stance. The first trial was done for familiarization only. Results from the remaining three trials were used to calculate the average COP path length (in cm) across trials. COP path length is considered as a proxy measure for postural sway magnitude; thus, the larger the COP path length, the greater the postural sway is ( Goble et al., 2017 ). COP path length of 0–30 cm was used to indicate normal balance, while ≥31 cm indicated poor balance ( Thiamwong et al., 2021b ).
Lower limb strength was assessed using the 30-s sit-to-stand (STS) test, in accordance with the established protocol ( Yee et al., 2021 ; Choudhury et al., 2023 ). Participants were instructed to keep their arms folded across their chest, rise from a seated position on a chair to a standing posture and return to the sitting position as many times as possible within 30 s. The number of chair stands completed was counted and recorded. If a participant used his/her arms to stand, the test was stopped, and the score was recorded as zero. Age- and gender-specific STS normative scores were used as cut-offs to classify participants into below-average and average STS scores, as shown in Table 1 ( Rikli and Jones, 1999 ). A below-average STS score was indicative of a higher risk of fall. Meeting both normal balance and average STS score criteria was defined as low physiological fall risk, while not meeting either or both criteria was defined as high physiological fall risk.
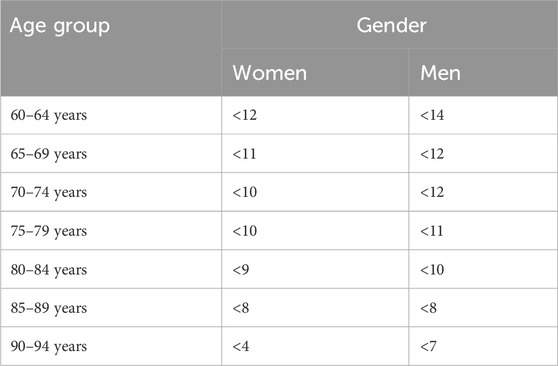
Table 1 . Age and gender-specific below average scores for 30-s sit-to-stand test.
Fall risk appraisal (FRA) matrix
The FRA matrix was obtained using a combination of FOF and physiological fall risk status. Participants were grouped into the following four categories based on their FOF and physiological fall risk according to existing literature ( Thiamwong et al., 2020a ): i) Rational (low FOF-low physiological fall risk), ii) Irrational (high FOF-low physiological fall risk), iii) Incongruent (low FOF-high physiological fall risk), and iv) Congruent (high FOF-high physiological fall risk).
Physical activity (PA)
PA was assessed using ActiGraph GT9X Link (ActiGraph LLC., Pensacola, FL, United States), which contains a triaxial accelerometer with a dynamic range of ±8 gravitational units (g). The device was initialized to record acceleration data at 30 Hz sampling frequency. Participants wore the ActiGraph on their non-dominant wrists for seven consecutive days in free-living conditions. They were given instructions to wear it during waking hours and remove it only during sleeping, showering, swimming and medical imaging tests. After 7-day of PA monitoring, ActiGraph devices were collected from participants. Participants with ≥4 valid days were included in the analysis, and a day was considered valid if participants wore the device for at least 14 h or more ( Choudhury et al., 2023 ).
Raw acceleration data were downloaded as “.csv” files using ActiLife software v6.13.4 (ActiGraph LLC, Pensacola, FL, United States) and converted into MIMS units using MIMSunit package ( John et al., 2019 ) in R statistical software (R Core Team, Vienna, Austria). The data processing steps included: i) interpolating data to a consistent sampling rate (i.e., 100 Hz ) to account for inter-device variability in sampling rate, ii) extrapolating data to extend maxed-out signals to account for inter-device variability in dynamic range, iii) band-pass filtering to remove artifacts from acceleration signals that do not pertain to voluntary human movement, and iv) aggregation of processed signals from each axis into a sum of MIMS-units that represents the total amount of movement activity [details on MIMS-unit algorithm are published elsewhere ( John et al., 2019 )].
PA volume, denoted by daily MIMS (MIMS/day), was calculated by summing up all triaxial MIMS/minutes accumulated throughout a day and averaged across all valid days. PA intensity, expressed as peak 30-min MIMS, was obtained by (a) first rank ordering a participant’s triaxial MIMS/minutes values within each valid day, (b) calculating the average of the highest 30 MIMS/minutes values within each day, and (c) finally taking the average of the resulting MIMS/minutes values across all valid wear days.
Anthropometric measurements
Height (in cm) was measured using a stadiometer. Body mass (in kilograms) was measured using a digital scale with no shoes. Body mass index (BMI) was calculated as the weight (kg) divided by the square of height (m 2 ).
Statistical analyses
All statistical analyses were performed in R statistical software (version 4.1.2, R Core Team, Vienna, Austria) with statistical significance level set at .05. Descriptive characteristics of participants were summarized as mean (standard deviation, SD) for normally distributed continuous variables, as median (Interquartile Range, IQR) for non-normally distributed continuous variables, and as frequency (percentage) for categorical variables, stratified by FRA categories. The Shapiro-Wilk test was performed to check if a continuous variable followed a normal distribution. Differences across groups were examined using one-way analysis of variance (ANOVA) for normally distributed data and Kruskal–Wallis test for non-normally distributed data, with Bonferroni adjustment for post hoc comparisons.
Multiple linear regression was conducted for each outcome variable (i.e., daily MIMS and peak 30-min MIMS) using the four FRA groups—“Rational,” “Irrational,” “Incongruent” and “Congruent”—as explanatory variables, controlled by age, gender and BMI. A priori sample size calculation for multiple linear regression revealed that the minimum number of samples for 8 explanatory variables at a statistical power level of 0.8, α = 0.05, and a medium effect size (Cohen f 2 = 0.15) would be 108; therefore, our sample size (i.e., N = 178) had sufficient statistical power for multiple regression. The rational group (i.e., low FOF-low physiological fall risk) was selected as the reference group in the regression analysis.
Among 178 participants, 163 samples were included in the analyses, after retaining only those who had at least 4 days of valid PA data and completed both FOF and physiological fall risk assessments. The mean (SD) age of participants was 75.3 (7.1) years, and 73.6% of participants were in 60–79 years of age group ( n = 120) and 26.4% were above 80 years of age ( n = 43). Figure 2 shows the scatterplot of participants’ age (years) and FOF scores, stratified by physiological fall risk status. The proportion of participants with low FOF was 71.7% ( n = 86) in the 60–79 years of age group and 48.8% ( n = 21) in the ≥80 years of age group. The median (IQR) BMI of participants was 26.6 (6.3) kg/m 2 and majority of participants were female (79.1%). The median (IQR) Short FES-I score was 9 (5) and 34.4% of participants had high FOF. The median (IQR) COP path length was 27 (15) cm , and the median (IQR) sit-to-stand score was 13 (6) reps. 38.0% of participants had poor balance, 27.0% had below average lower limb strength, and 48.5% showed both poor balance and below average lower limb strength. Finally, 37.4% of participants were screened as rational ( n = 61), 14.2% were irrational ( n = 23), 28.2% were incongruent ( n = 46) and 20.2% were congruent ( n = 37). Table 2 summarizes the characteristics of study participants according to FRA categories.
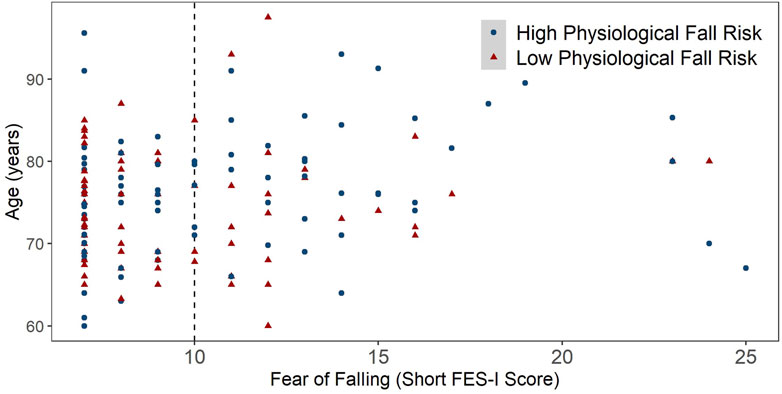
Figure 2 . Scatterplot of Age (years) across Fear of Falling scores, stratified by physiological fall risk status. Low physiological fall risk = meeting both normal static balance cut-off and average sit-to-stand score cut-off. High physiological fall risk = not meeting normal static balance cut-off or average sit-to-stand score cut-off or both.
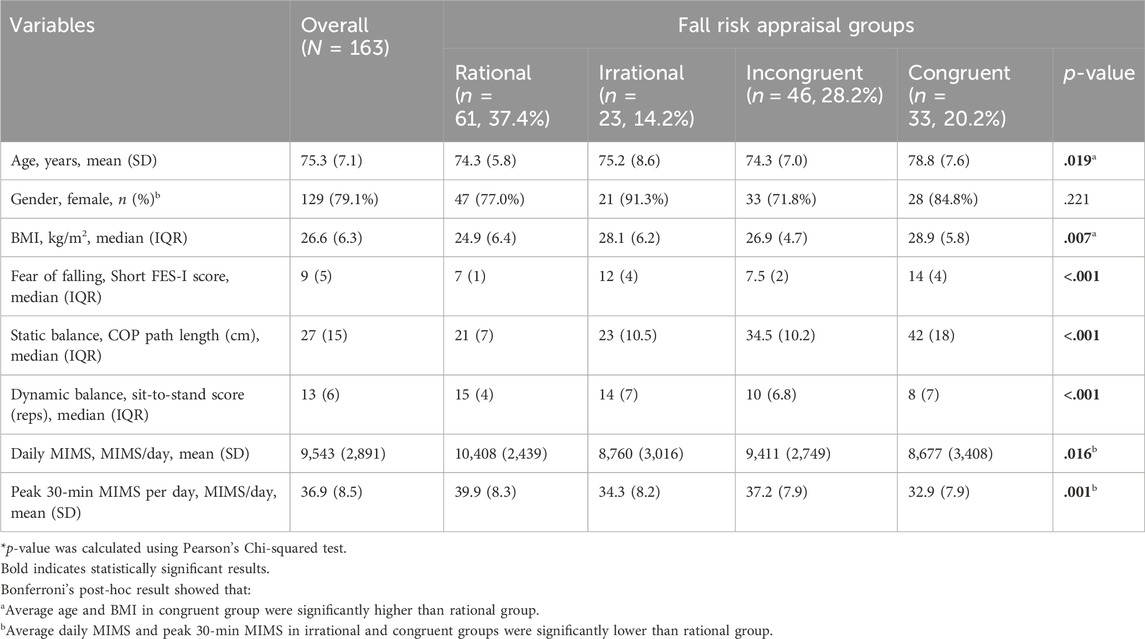
Table 2 . Participant characteristics stratified by Fall Risk Appraisal matrix.
In Figure 3A , the variations in average MIMS (MIMS/hours) over 24-h by FRA categories are shown. The average MIMS across all groups was in general low at night, then substantially increased during morning hours and gradually decreased as the day progressed and evening approached. In Figure 3B , the mean (line) and standard error (shaded area) of MIMS/hours for each FRA group is shown. Overall, rational group showed the highest average MIMS/hours across the day hours, while congruent had the lowest average MIMS/hours. Among maladaptive FRA groups, the peak was higher in incongruent group than their irrational counterparts, which indicates the potential role of FOF in limiting high-intensity PA participation.
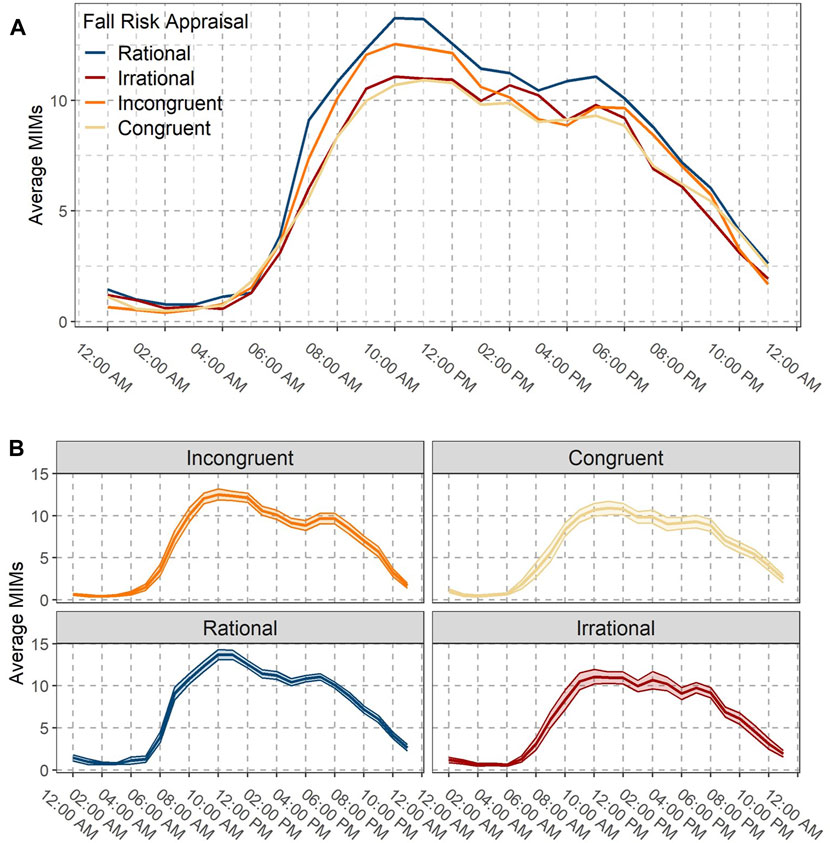
Figure 3 . (A) Daily patterns of average MIMS per hour by Fall Risk Appraisal (FRA) groups. (B) Mean (line) and standard error (shaded area) of MIMS per hour for each FRA group.
The mean (SD) age in congruent group was 78.8 (7.6) years, which was higher than both rational (74.3 [5.8] years, p = .005) and incongruent (74.3 [7.0] years, p = .010) groups, as shown in Supplementary Figure S1 . This suggests that prevalence of high FOF, irrespective of balance performance and lower limb strength, may increase with advanced age. Also, the median (IQR) BMI in congruent group (28.9 [5.8]) kg/m 2 ) was higher in comparison to rational (24.9 [6.4] kg/m 2 , p = .001) and incongruent (26.9 [4.7] kg/m 2 , p = .018) groups (shown in Supplementary Figure S2 ), indicating that higher BMI in older adults may result in high FOF. However, no significant group differences were observed between rational and irrational groups in terms of age and BMI.
The mean (SD) daily MIMS in rational group was 10,408 (2,439) MIMS/day, which was 15.8% higher than irrational ( p = .025) and 16.6% higher than congruent ( p = .013) groups, as shown in Figure 4 . Also, the mean (SD) peak 30-min MIMS in rational group was 39.9 (8.3) MIMS/day, which was 14.0% higher than irrational ( p = .004) and 17.5% higher than congruent ( p < .001) groups ( Figure 5 ). Compared to rational group, incongruent participants showed no significant differences in PA volume and intensity, despite having poor balance and below average lower limb strength.
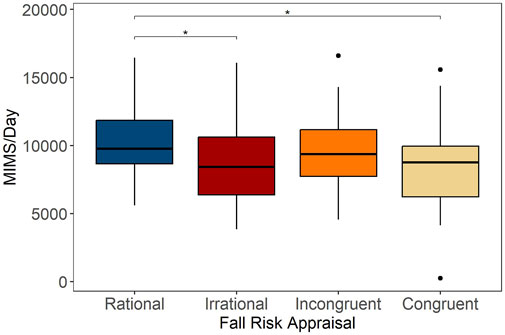
Figure 4 . Average daily MIMS (MIMS/day) across categories of Fall Risk Appraisal combining FOF and physiological fall risk, * p < .05.
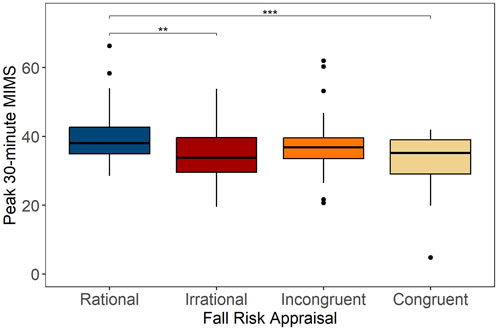
Figure 5 . Peak 30-min MIMS per day across categories of Fall Risk Appraisal combining FOF and physiological fall risk, ** p < .01, *** p < .001.
Table 2 presents the regression models for daily MIMS. In comparison to reference group (i.e., rational), lower PA volume was associated with irrational ( β [SE] = −1,463.2 [687.7] MIMS/day, p = .035) and congruent ( β [SE] = −1,579.5 [582.9] MIMS/day, p = .007) FRAs in Model 1 (unadjusted). In model 2, after adjusting for age, gender and BMI, only irrational FRA was significantly associated with lower PA volume ( β [SE] = −1,476.41 [582.26] MIMS/day, p = .025; regression coefficients of covariates are presented in Supplementary Table S1 ).
Results for regression analysis for peak 30-min MIMS are presented in Table 3 . In model 1 (unadjusted), lower ‘peak PA intensity’ was associated with irrational ( β [SE] = −5.63 [1.99] MIMS/day, p = .005) and congruent FRAs ( β [SE] = −7.06 [1.76] MIMS/day, p < .001) compared to the reference group. In Model 2 ( Table 4 ), after adjusting for age, gender and BMI, both irrational and congruent FRAs were still significantly associated with lower “peak PA intensity”(irrational: β [SE] = −5.40 [1.97] MIMS/day, p = .007; congruent: β [SE] = −5.43 [1.86] MIMS/day, p = .004; regression coefficients of covariates are presented in Supplementary Table S2 ).
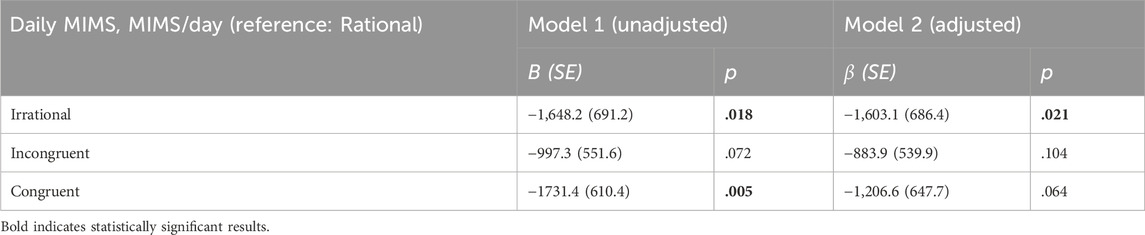
Table 3 . Association between Fall Risk Appraisal groups and average daily MIMS (MIMS/day) using linear regression. Model 2 was adjusted for age, gender and BMI.
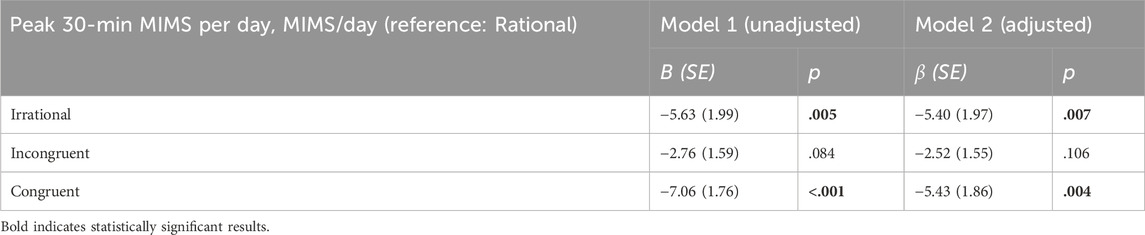
Table 4 . Association between Fall Risk Appraisal groups and peak 30-min MIMS per day (MIMS/day) using linear regression. Model 2 was adjusted for age, gender and BMI.
This is the first study, to our knowledge, to evaluate the associations of FRA with daily MIMS and peak 30-min MIMS in a sample of community-dwelling US older adults. In general, both the volume and intensity of PA were highest in the rational group and lowest in the congruent group. In maladaptive FRA groups, high FOF (i.e., irrational FRA) was associated with lower PA volume and intensity compared to the reference group (i.e., rational FRA), but no significant differences were observed for high physiological fall risk (i.e., incongruent FRA).
Prior research has shown that FOF is associated with reduced PA levels in community-dwelling older adults using objectively measured PA data ( Jefferis et al., 2014 ; Choudhury et al., 2022 ). Our results broadly agree with it, showing that total daily PA volume was significantly lower in two high FOF groups (i.e., irrational and congruent) than the rational group. This suggests that regardless of balance performance and lower limb strength, low FOF was associated with high PA volume in our study sample. In linear regression analysis, after accounting for age, gender and BMI, reduced daily MIMS was significantly associated with irrational FRA, but not with congruent FRA. It can be attributed to the fact that the average age and BMI of congruent participants were higher than all other groups, and evidence suggests that that increasing older age and higher BMI contribute to lower PA levels in older adults ( Smith et al., 2015 ).
We did not observe any significant difference between two low FOF groups (i.e., rational and incongruent) in terms of daily PA volume. This suggests that, for maladaptive FRA, high physiological fall risk (not high FOF) had a stronger association with reduced daily PA accumulation in our study sample. In contrast to our findings, a recent study found that low physiological fall risk was more strongly associated with increased walking activity (steps/day) than low perceived fall risk in a sample of community-dwelling German older adults ( n = 294) ( Jansen et al., 2021 ). However, it should be noted that Jansen et al. used multiple independent risk factors (i.e., previous falls, balance impairment, gait impairment, and multimedication) to distinguish between high and low physiological fall risk, whereas they used only one tool (Short FES-I) to assess perceived fall risk. Furthermore, participants with low FOF and high physiological fall risk in that German older adult cohort ( Jansen et al., 2021 ) were relatively older than those in our study sample [mean (SD) age: 81.6 (5.5) years vs. 74.3 (7.0) years in our study]. Previous studies indicate that the likelihood of reduced participation in PA gradually increases with advanced age, because of age-related declines in muscle mass, muscle strength, and functional fitness (i.e., the physical capacity to perform activities of daily living independently and without the early onset of fatigue) ( Milanović et al., 2013 ; Westerterp, 2018 ; Suryadinata et al., 2020 ). Therefore, future research should examine how age-related functional declines mediate the relationship between maladaptive FRA and daily PA volume in older adults.
In our study, the peak PA intensity in both high FOF groups (i.e., irrational and congruent) was significantly lower than the rational group. Despite the differences in the PA metrics, this is in general agreement with the previous findings that showed older adults with irrational and congruent FRAs were more likely to spend less time in MVPA ( Thiamwong et al., 2023 ). Interestingly, after adjusting for confounders, the decrease in peak 30-min MIMS for irrational and congruent groups was almost equivalent in our study. This suggests that older adults with high FOF may restrict their participation in high intensity PA, irrespective of their physiological fall risk status. Our findings extend the previously reported association between PA intensity and FOF in older adults ( Sawa et al., 2020 ), highlighting the need to integrate cognitive behavioral therapy to reduce FOF in fall intervention programs.
For peak 30-min MIMS, we did not find any significant difference between two low FOF groups (i.e., rational and incongruent). This suggests that, similar to total PA volume, peak PA intensity was more strongly associated with high FOF (rather than high physiological fall risk) for maladaptive FRA in our sample. Unlike MVPA cut points that exclude PA intensities ≤3 METs or equivalent, peak 30-min MIMS considers acceleration magnitudes ranging from lower to higher peak efforts within a day, enabling comparison over the whole spectrum of PA intensity levels (e.g., light vs. vigorous) ( Zheng et al., 2023 ). Further research should investigate domains of peak PA efforts across different FRA groups, so that informed strategies can be developed to promote high-intensity PA participation according to the perceived and physiological risk of fall.
Based on the findings of our study, it can be conferred that the FRA assessment may be useful in designing customized PA interventions to promote an active lifestyle in older adults. For example, to increase PA participation in older adults with irrational FRA, cognitive behavioral therapy can be integrated into PA programs to improve their self-efficacy and sense of control over falling ( Tennstedt et al., 1998 ). For incongruent FRA, PA recommendations should include exercise regimens specifically designed to reduce physiological fall risk, such as high intensity balance and strength training, in addition to aerobic activities ( Sherrington et al., 2008 ). On the other hand, older adults with congruent FRA may benefit from PA programs that combine both balance and strength exercises, and cognitive behavioral therapy ( Brouwer et al., 2003 ).
A strength of our study is the use of MIMS metric to provide a comprehensive PA assessment (volume and intensity) enabling reliable, cross-study comparisons of our findings with other MIMS-based studies regardless of the device type, model or manufacturer. Furthermore, we used evidence-based cut-off points to determine FOF level (low vs. high FOF), balance status (poor vs. normal balance) and lower limb strength (below average vs. average strength) to categorize participants into FRA groups. However, our study has several limitations. First, to determine physiological fall risk status, we didn’t use the Physiological Profile Assessment ( Delbaere et al., 2010 ) or multiple independent risk factors ( Jansen et al., 2021 ), which might have led to different group formations than those studies. Instead, we used static balance and lower limb strength as physiological fall risk indicators. While balance and strength deficits are important predictors of falls in older adults, they might not account for all aspects of physiological fall risk (such as gait impairment, visual and sensory deficits, use of multi-medications etc.) ( Fabre et al., 2010 ). Second, it is to be noted that the balance performance measure (i.e., static balance) used in this study may not capture the full spectrum of an individual’s balance capabilities. There are different measures of balance performance, including static steady-state balance (i.e., the ability to maintain a steady position while standing or sitting), dynamic steady-state balance (i.e., the ability to maintain a steady position while performing postural transitions and walking), proactive balance (i.e., the ability to anticipate and mitigate a predicted postural disturbance), and reactive balance (i.e., the ability to recover a stable position following an unexpected postural disturbance) ( Shumway-Cook and Woollacott, 2007 ). Therefore, future studies may consider using more comprehensive assessments of balance performance in older adults to define physiological fall risk in FRA. Third, our study only considered FOF as the psychological fall risk measure in FRA and did not investigate other psychological constructs such as falls efficacy or balance confidence ( Moore et al., 2011 ). FOF and falls efficacy are two major fall-related psychological constructs in preventing and managing fall risks in older adults. It is to be noted that, though FOF and falls efficacy are correlated, they represent theoretically distinct concepts ( Hadjistavropoulos et al., 2011 ). FOF is defined as “the lasting concerns about falling that leads to an individual avoiding activities that one remains capable of performing.” Some common instruments for FOF measurement include FES-I, Short FES-I, Iconographical Falls Efficacy Scale (ICON-FES), Geriatric Fear of Falling Measure (GFFM), Survey of Activities and Fear of Falling in the Elderly (SAFE), Fear of Falling Avoidance Behaviour Questionnaire (FFABQ) etc., ( Soh et al., 2021 ). On the other hand, falls efficacy is defined as the perceived confidence in one’s ability to carry out activities of daily living without experiencing a fall ( Moore and Ellis, 2008 ). Existing instruments for measuring falls efficacy include Falls Efficacy Scale (FES), modified FES (MFES), Perceived Ability to Prevent and Manage Fall Risks (PAPMFR), and Perceived Ability to Manage Risk of Falls or Actual Falls (PAMF) ( Soh et al., 2021 ). Prior research has reported that, compared to FOF, falls efficacy shows stronger relationship with measures of basic and instrumental activities of daily living (ADL-IADL), and physical and social functioning ( Tinetti et al., 1994 ). Therefore, future studies should consider exploring the combined effects of falls efficacy and physiological fall risk measures on habitual PA level to determine whether FOF or fall efficacy should be considered as a target for PA interventions in older adults. Fourth, to date, there exists no established cut-offs for the MIMS metric to categorize total PA volume and intensity that correspond to meeting national PA guidelines, and it is still unknown how well MIMS/minute can estimate energy expenditure ( Vilar-Gomez et al., 2023 ). Our study just provided a first step toward the use of a standardized metric to associate PA behavior with FRA in a community-dwelling older adult sample in the US. Future studies should examine such associations in large, nationally representative populations to establish benchmark values for daily MIMS and peak 30-min MIMS in different FRA categories. Fifth, the cross-sectional design of the study didn’t allow us to determine a causal relationship between FRA and PA, so reverse and/or bidirectional causality might still be present. Sixth, although we controlled for age, gender, and BMI in the regression analyses, there remains the possibility of additional residual confounding [such as neuropsychological constructs that have been associated with FOF, which include depression, anxiety, neuroticism, attention, and executive function ( Delbaere et al., 2010 )]. Finally, our sample size was relatively small and 79% of participants were female. The generalizability of our findings might be restricted by the small, female dominant nature of our sample.
In conclusion, compared to rational FRA, the habitual PA level (daily MIMS and peak 30-min MIMS) was lower in both high FOF groups (i.e., irrational and congruent), but not in incongruent group. This suggests that, for maladaptive FRA in our study sample, high perceived fall risk had a stronger association with reduced PA level, rather than high physiological fall risk. When controlled for covariates, decrease in peak PA intensity remained significantly associated with irrational and congruent FRAs, indicating that older adults with high FOF performed PA at lower peak efforts, irrespective of their physiological fall status. Future prospective studies should focus on identifying the optimal habitual PA level (total PA volume and peak PA intensity) in accordance with an older adult’s FOF and physiological fall risk to better inform public health policies for sustainable, effective PA framework.
Data availability statement
The raw data supporting the conclusion of this article will be made available by the authors, without undue reservation.
Ethics statement
The studies involving humans were approved by the Institutional Review Board, University of Central Florida. The studies were conducted in accordance with the local legislation and institutional requirements. The participants provided their written informed consent to participate in this study.
Author contributions
RC: Data curation, Formal Analysis, Investigation, Methodology, Software, Visualization, Writing–original draft. J-HP: Conceptualization, Funding acquisition, Investigation, Methodology, Resources, Supervision, Writing–review and editing. CB: Formal Analysis, Visualization, Writing–review and editing. MC: Data curation, Investigation, Writing–review and editing. DF: Conceptualization, Funding acquisition, Writing–review and editing. RX: Conceptualization, Funding acquisition, Writing–review and editing. JS: Conceptualization, Funding acquisition, Supervision, Writing–review and editing. LT: Conceptualization, Funding acquisition, Project administration, Resources, Supervision, Writing–review and editing.
The author(s) declare that financial support was received for the research, authorship, and/or publication of this article. The research was funded by the National Institute on Aging (R03AG06799) and the National Institute on Minority Health and Health Disparities (R01MD018025) of National Institutes of Health. This research also received financial support from the University of Central Florida CONNECT CENTRAL (Interdisciplinary research seed grant; AWD00001720 and AWD00005378).
Conflict of interest
The authors declare that the research was conducted in the absence of any commercial or financial relationships that could be construed as a potential conflict of interest.
The author(s) declared that they were an editorial board member of Frontiers, at the time of submission. This had no impact on the peer review process and the final decision.
Publisher’s note
All claims expressed in this article are solely those of the authors and do not necessarily represent those of their affiliated organizations, or those of the publisher, the editors and the reviewers. Any product that may be evaluated in this article, or claim that may be made by its manufacturer, is not guaranteed or endorsed by the publisher.
Supplementary material
The Supplementary Material for this article can be found online at: https://www.frontiersin.org/articles/10.3389/fragi.2024.1284694/full#supplementary-material
Ambrose, A. F., Paul, G., and Hausdorff, J. M. (2013). Risk factors for falls among older adults: a review of the literature. Maturitas 75 (1), 51–61. doi:10.1016/j.maturitas.2013.02.009
PubMed Abstract | CrossRef Full Text | Google Scholar
Brouwer, B. J., Walker, C., Rydahl, S. J., and Culham, E. G. (2003). Reducing fear of falling in seniors through education and activity programs: a randomized trial. J. Am. Geriatr. Soc. 51 (6), 829–834. doi:10.1046/j.1365-2389.2003.51265.x
Buschke, H., Kuslansky, G., Katz, M., Stewart, W. F., Sliwinski, M. J., Eckholdt, H. M., et al. (1999). Screening for dementia with the memory impairment screen. Neurology 52 (2), 231–238. doi:10.1212/wnl.52.2.231
Chan, B. K., Marshall, L. M., Winters, K. M., Faulkner, K. A., Schwartz, A. V., and Orwoll, E. S. (2007). Incident fall risk and physical activity and physical performance among older men: the Osteoporotic Fractures in Men Study. Am. J. Epidemiol. 165 (6), 696–703. doi:10.1093/aje/kwk050
Choudhury, R., Park, J. H., Banarjee, C., Thiamwong, L., Xie, R., and Stout, J. R. (2023). Associations of mutually exclusive categories of physical activity and sedentary behavior with body composition and fall risk in older women: a cross-sectional study. Int. J. Environ. Res. Public Health 20 (4), 3595. doi:10.3390/ijerph20043595
Choudhury, R., Park, J. H., Thiamwong, L., Xie, R., and Stout, J. R. (2022). Objectively measured physical activity levels and associated factors in older US women during the COVID-19 pandemic: cross-sectional study. JMIR Aging 5 (3), e38172. doi:10.2196/38172
Delbaere, K., Close, J. C., Brodaty, H., Sachdev, P., and Lord, S. R. (2010). Determinants of disparities between perceived and physiological risk of falling among elderly people: cohort study. Bmj 341, c4165. doi:10.1136/bmj
Deshpande, N., Metter, E. J., Lauretani, F., Bandinelli, S., Guralnik, J., and Ferrucci, L. (2008). Activity restriction induced by fear of falling and objective and subjective measures of physical function: a prospective cohort study. J. Am. Geriatr. Soc. 56 (4), 615–620. doi:10.1111/j.1532-5415.2007.01639.x
Fabre, J. M., Ellis, R., Kosma, M., and Wood, R. H. (2010). Falls risk factors and a compendium of falls risk screening instruments. J. Geriatr. Phys. Ther. 33 (4), 184–197. doi:10.1519/jpt.0b013e3181ff2a24
Goble, D. J., Hearn, M. C., and Baweja, H. S. (2017). Combination of BTrackS and Geri-Fit as a targeted approach for assessing and reducing the postural sway of older adults with high fall risk. Clin. Interv. Aging 12, 351–357. doi:10.2147/cia.S131047
Gregg, E. W., Pereira, M. A., and Caspersen, C. J. (2000). Physical activity, falls, and fractures among older adults: a review of the epidemiologic evidence. J. Am. Geriatr. Soc. 48 (8), 883–893. doi:10.1111/j.1532-5415.2000.tb06884.x
Hadjistavropoulos, T., Delbaere, K., and Fitzgerald, T. D. (2011). Reconceptualizing the role of fear of falling and balance confidence in fall risk. J. Aging Health 23 (1), 3–23. doi:10.1177/0898264310378039
Heesch, K. C., Byles, J. E., and Brown, W. J. (2008). Prospective association between physical activity and falls in community-dwelling older women. J. Epidemiol. Community Health 62 (5), 421–426. doi:10.1136/jech.2007.064147
Jansen, C. P., Klenk, J., Nerz, C., Todd, C., Labudek, S., Kramer-Gmeiner, F., et al. (2021). Association between everyday walking activity, objective and perceived risk of falling in older adults. Age Ageing 50 (5), 1586–1592. doi:10.1093/ageing/afab037
Jefferis, B. J., Iliffe, S., Kendrick, D., Kerse, N., Trost, S., Lennon, L. T., et al. (2014). How are falls and fear of falling associated with objectively measured physical activity in a cohort of community-dwelling older men? BMC Geriatr. 14, 114. doi:10.1186/1471-2318-14-114
John, D., Tang, Q., Albinali, F., and Intille, S. (2019). An open-source monitor-independent movement summary for accelerometer data processing. J. Meas. Phys. Behav. 2 (4), 268–281. doi:10.1123/jmpb.2018-0068
Kakara, R., Bergen, G., Burns, E., and Stevens, M. (2023). Nonfatal and fatal falls among adults aged ≥65 Years - United States, 2020-2021. MMWR Morb. Mortal. Wkly. Rep. 72 (35), 938–943. doi:10.15585/mmwr.mm7235a1
Kempen, G. I., Yardley, L., van Haastregt, J. C., Zijlstra, G. A., Beyer, N., Hauer, K., et al. (2008). The Short FES-I: a shortened version of the falls efficacy scale-international to assess fear of falling. Age Ageing 37 (1), 45–50. doi:10.1093/ageing/afm157
Levy, S. S., Thralls, K. J., and Kviatkovsky, S. A. (2018). Validity and reliability of a portable balance tracking system, BTrackS, in older adults. J. Geriatr. Phys. Ther. 41 (2), 102–107. doi:10.1519/jpt.0000000000000111
Mendes da Costa, E., Pepersack, T., Godin, I., Bantuelle, M., Petit, B., and Levêque, A. (2012). Fear of falling and associated activity restriction in older people. results of a cross-sectional study conducted in a Belgian town. Arch. Public Health 70 (1), 1. doi:10.1186/0778-7367-70-1
Milanović, Z., Pantelić, S., Trajković, N., Sporiš, G., Kostić, R., and James, N. (2013). Age-related decrease in physical activity and functional fitness among elderly men and women. Clin. Interv. Aging 8, 549–556. doi:10.2147/cia.S44112
Moore, D. S., and Ellis, R. (2008). Measurement of fall-related psychological constructs among independent-living older adults: a review of the research literature. Aging Ment. Health 12 (6), 684–699. doi:10.1080/13607860802148855
Moore, D. S., Ellis, R., Kosma, M., Fabre, J. M., McCarter, K. S., and Wood, R. H. (2011). Comparison of the validity of four fall-related psychological measures in a community-based falls risk screening. Res. Q. Exerc. Sport 82 (3), 545–554. doi:10.1080/02701367.2011.10599787
Moreland, B., Kakara, R., and Henry, A. (2020). Trends in nonfatal falls and fall-related injuries among adults aged ≥65 Years - United States, 2012-2018. MMWR Morb. Mortal. Wkly. Rep. 69 (27), 875–881. doi:10.15585/mmwr.mm6927a5
Rikli, R. E., and Jones, C. J. (1999). Functional fitness normative scores for community-residing older adults, ages 60-94. J. Aging Phys. Act. 7 (2), 162–181. doi:10.1123/japa.7.2.162
CrossRef Full Text | Google Scholar
Sawa, R., Asai, T., Doi, T., Misu, S., Murata, S., and Ono, R. (2020). The association between physical activity, including physical activity intensity, and fear of falling differs by fear severity in older adults living in the community. J. Gerontol. B Psychol. Sci. Soc. Sci. 75 (5), 953–960. doi:10.1093/geronb/gby103
Sherrington, C., Whitney, J. C., Lord, S. R., Herbert, R. D., Cumming, R. G., and Close, J. C. (2008). Effective exercise for the prevention of falls: a systematic review and meta-analysis. J. Am. Geriatr. Soc. 56 (12), 2234–2243. doi:10.1111/j.1532-5415.2008.02014.x
Shumway-Cook, A., and Woollacott, M. H. (2007). Motor control: translating research into clinical practice . United States: Lippincott Williams & Wilkins .
Google Scholar
Smith, L., Gardner, B., Fisher, A., and Hamer, M. (2015). Patterns and correlates of physical activity behaviour over 10 years in older adults: prospective analyses from the English Longitudinal Study of Ageing. BMJ Open 5 (4), e007423. doi:10.1136/bmjopen-2014-007423
Soh, S. L., Tan, C. W., Thomas, J. I., Tan, G., Xu, T., Ng, Y. L., et al. (2021). Falls efficacy: extending the understanding of self-efficacy in older adults towards managing falls. J. Frailty Sarcopenia Falls 6 (3), 131–138. doi:10.22540/jfsf-06-131
Suryadinata, R. V., Wirjatmadi, B., Adriani, M., and Lorensia, A. (2020). Effect of age and weight on physical activity. J. Public Health Res. 9 (2), 1840. doi:10.4081/jphr.2020.1840
Tennstedt, S., Howland, J., Lachman, M., Peterson, E., Kasten, L., and Jette, A. (1998). A randomized, controlled trial of a group intervention to reduce fear of falling and associated activity restriction in older adults. J. Gerontol. B Psychol. Sci. Soc. Sci. 53 (6), P384–P392. doi:10.1093/geronb/53b.6.p384
Thiamwong, L. (2020). A hybrid concept analysis of fall risk appraisal: integration of older adults' perspectives with an integrative literature review. Nurs. Forum 55 (2), 190–196. doi:10.1111/nuf.12415
Thiamwong, L., Huang, H. J., Ng, B. P., Yan, X., Sole, M. L., Stout, J. R., et al. (2020a). Shifting maladaptive fall risk appraisal in older adults through an in-home physio-fEedback and exercise pRogram (peer): a pilot study. Clin. Gerontol. 43 (4), 378–390. doi:10.1080/07317115.2019.1692120
Thiamwong, L., Ng, B. P., Kwan, R. Y. C., and Suwanno, J. (2021a). Maladaptive fall risk appraisal and falling in community-dwelling adults aged 60 and older: implications for screening. Clin. Gerontol. 44 (5), 552–561. doi:10.1080/07317115.2021.1950254
Thiamwong, L., Sole, M. L., Ng, B. P., Welch, G. F., Huang, H. J., and Stout, J. R. (2020b). Assessing fall risk appraisal through combined physiological and perceived fall risk measures using innovative Technology. J. Gerontol. Nurs. 46 (4), 41–47. doi:10.3928/00989134-20200302-01
Thiamwong, L., Stout, J. R., Park, J. H., and Yan, X. (2021b). Technology-based fall risk assessments for older adults in low-income settings: protocol for a cross-sectional study. JMIR Res. Protoc. 10 (4), e27381. doi:10.2196/27381
Thiamwong, L., Xie, R., Park, J. H., Choudhury, R., Malatyali, A., Li, W., et al. (2023). Levels of accelerometer-based physical activity in older adults with a mismatch between physiological fall risk and fear of falling. J. Gerontol. Nurs. 49 (6), 41–49. doi:10.3928/00989134-20230512-06
Tinetti, M. E., Mendes de Leon, C. F., Doucette, J. T., and Baker, D. I. (1994). Fear of falling and fall-related efficacy in relationship to functioning among community-living elders. J. Gerontol. 49 (3), M140–M147. doi:10.1093/geronj/49.3.m140
Troiano, R. P., Berrigan, D., Dodd, K. W., Mâsse, L. C., Tilert, T., and McDowell, M. (2008). Physical activity in the United States measured by accelerometer. Med. Sci. Sports Exerc. 40 (1), 181–188. doi:10.1249/mss.0b013e31815a51b3
Tudor-Locke, C., Brashear, M. M., Katzmarzyk, P. T., and Johnson, W. D. (2012). Peak stepping cadence in free-living adults: 2005-2006 NHANES. J. Phys. Act. Health 9 (8), 1125–1129. doi:10.1123/jpah.9.8.1125
Vilar-Gomez, E., Vuppalanchi, R., Gawrieh, S., Pike, F., Samala, N., and Chalasani, N. (2023). Significant dose-response association of physical activity and diet quality with mortality in adults with suspected NAFLD in a population study. Am. J. Gastroenterol. 118 (9), 1576–1591. doi:10.14309/ajg.0000000000002222
Westerterp, K. R. (2018). Changes in physical activity over the lifespan: impact on body composition and sarcopenic obesity. Obes. Rev. 19 (1), 8–13. doi:10.1111/obr.12781
Wolff-Hughes, D. L., Bassett, D. R., and Fitzhugh, E. C. (2014). Population-referenced percentiles for waist-worn accelerometer-derived total activity counts in U.S. youth: 2003 - 2006 NHANES. PLoS One 9 (12), e115915. doi:10.1371/journal.pone.0115915
Yardley, L., Beyer, N., Hauer, K., Kempen, G., Piot-Ziegler, C., and Todd, C. (2005). Development and initial validation of the falls efficacy scale-international (FES-I). Age Ageing 34 (6), 614–619. doi:10.1093/ageing/afi196
Yee, X. S., Ng, Y. S., Allen, J. C., Latib, A., Tay, E. L., Abu Bakar, H. M., et al. (2021). Performance on sit-to-stand tests in relation to measures of functional fitness and sarcopenia diagnosis in community-dwelling older adults. Eur. Rev. Aging Phys. Act. 18 (1), 1. doi:10.1186/s11556-020-00255-5
Zheng, P., Pleuss, J. D., Turner, D. S., Ducharme, S. W., and Aguiar, E. J. (2023). Dose-response association between physical activity (daily MIMS, peak 30-minute MIMS) and cognitive function among older adults: NHANES 2011-2014. J. Gerontol. A Biol. Sci. Med. Sci. 78 (2), 286–291. doi:10.1093/gerona/glac076
Zijlstra, G. A., van Haastregt, J. C., van Eijk, J. T., van Rossum, E., Stalenhoef, P. A., and Kempen, G. I. (2007). Prevalence and correlates of fear of falling, and associated avoidance of activity in the general population of community-living older people. Age Ageing 36 (3), 304–309. doi:10.1093/ageing/afm021
Keywords: falls, physical activity, accelerometry, aging, fear of falling, fall risk, MIMS
Citation: Choudhury R, Park J-H, Banarjee C, Coca MG, Fukuda DH, Xie R, Stout JR and Thiamwong L (2024) Associations between monitor-independent movement summary (MIMS) and fall risk appraisal combining fear of falling and physiological fall risk in community-dwelling older adults. Front. Aging 5:1284694. doi: 10.3389/fragi.2024.1284694
Received: 28 August 2023; Accepted: 20 March 2024; Published: 09 April 2024.
Reviewed by:
Copyright © 2024 Choudhury, Park, Banarjee, Coca, Fukuda, Xie, Stout and Thiamwong. This is an open-access article distributed under the terms of the Creative Commons Attribution License (CC BY). The use, distribution or reproduction in other forums is permitted, provided the original author(s) and the copyright owner(s) are credited and that the original publication in this journal is cited, in accordance with accepted academic practice. No use, distribution or reproduction is permitted which does not comply with these terms.
*Correspondence: Joon-Hyuk Park, [email protected]
This article is part of the Research Topic
Insights into Falls Efficacy and Fear of Falling
Academia.edu no longer supports Internet Explorer.
To browse Academia.edu and the wider internet faster and more securely, please take a few seconds to upgrade your browser .
Enter the email address you signed up with and we'll email you a reset link.
- We're Hiring!
- Help Center
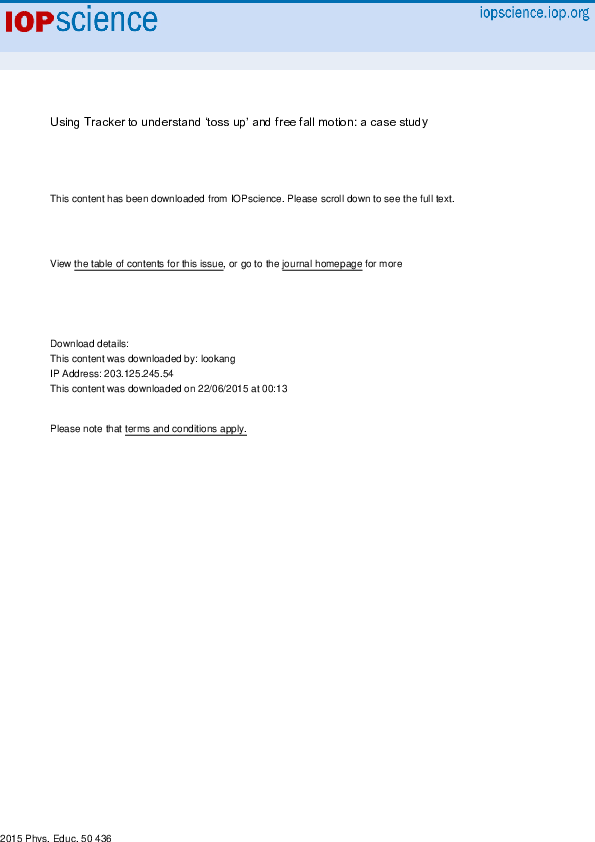
Using Tracker to understand ‘toss up’ and free fall motion: a case study

2015, Physics Education
Related Papers
Loo Kang Wee , Samuel Tan
This paper reports the use of Tracker as a pedagogical tool in the effective learning and teaching of projectile motion in physics. When computer model building learning processes is supported and driven by video analysis data, this free Open Source Physics (OSP) tool can provide opportunities for students to engage in active inquiry-based learning. We discuss the pedagogical use of Tracker to address some common misconceptions of projectile motion by allowing students to test their hypothesis by juxtaposing their mental models against the analysis of real life videos. Initial research findings suggest that allowing learners to relate abstract physics concepts to real life through coupling computer modeling with traditional video analysis could be an innovative and effective way to learn projectile motion.
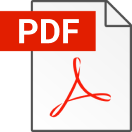
Loo Kang Wee
This workshop aims to demonstrate how the Tracker Video Analysis and Modeling Tool engages, enables and empowers teachers to be learners so that we can be leaders in our teaching practice. Through this workshop, the kinematics of a falling ball and a projectile motion are explored using video analysis and in the later video modeling. We hope to lead and inspire other teachers by facilitating their experiences with this ICT–enabled video modeling pedagogy (Brown, 2008) and free tool for facilitating students-centered active learning, thus motivate students to be more self–directed.
Nindy Ulqia
Visualization of physical phenomena through modeling and virtual experiment help students to understand the physics concepts . The concept of momentum is quite challenging to explain without the help of virtual experiments. This study aimed to analyze students' independent learning after conducting the learning process on the concept of momentum using Tracker software as a virtual experimental model. This study was quasi-experimental with the purposive sampling technique to determine the control class and the experimental class. There were 42 students involved in this research. The control class was treated by actual experiments on learning, while the experimental class use d the Tracker software as a virtual experiment model. The instrument used was questionnaires given at the end of learning to measure students’ learning independence. The data were analyzed by using the descriptive statistics method. Learning independence in the control class obtained 51.31% in the medium cate...
Physics Education
Anna Ambrosis
Exedy Lampara , Joel Maquiling
Video Analysis (VA) is an emerging technology currently used in sports, transportation, and security systems. VA is a method where an actual event is filmed, and the video is analysed and modeled using a software. The software involved generation of numerical values, equations, and graphs. This method can be applied to different experiments, such as those in Physics. However, there is a little knowledge on how this tool affects the motivation of students in learning the subject. In this study, the effect of VA on students' motivation towards learning Physics is investigated. This study was implemented in a high school for six to eight weeks and utilized two groups, the VA group and Non-VA group. Each group comprised of thirty-five Grade 9 students. The study reveals that both groups have statistically indistinguishable self-efficacy (p = 0.067) and stimulation of learning environment (p = 0.086). On the other hand, students who used VA have statistically higher active learning strategies (p = 0.004), Physics learning values (p = 0.013), performance goals (p<0.000), and achievement goals (p = 0.003). Thus, students who used VA have higher motivation towards learning Physics than students who did not use it (p <0.000).
International Journal of Advanced Computer Science and Applications
Louis Trudel
Suritno Fayanto
The purpose of this study is to determine the effectiveness of the use of discovery-assisted discovery learning models to improve physics learning outcomes in terms of students' initial knowledge. This research was conduct at Senior High School 1 Talibura in the academic year 2019/2010. This research is an experimental research that uses a quasi-experimental design consisting of a nonequivalent (pretest-posttest) control group design. Sampling uses simple random sampling so that two sample classes obtained, namely level XI MIA 1 as an experimental class and class XI MIA 2 as a control class. The first knowledge instrument and learning outcomes are subjective tests (essays) that have been tested for validity and reliability. Hypothesis testing using ANCOVA test. Based on data analysis, the results showed that there was an influence of the tracker assisted discovery hearing model on student physics learning outcomes, where F count is higher than F table (4,484 > 3,20) with the significant value obtained is smaller than the significance level (0,017 < 0,05). From this study, we can conclude that the discovery-assisted discovery learning model tracker is handy to be used in physics learning to improve student physics learning outcomes.
International Education Studies
Muhammad Nasir
We will share on the use of Tracker as a pedagogical tool in the effective learning and teaching of physics performance tasks taking root in some Singapore Grade 9 (Secondary 3) schools. We discuss the pedagogical use of Tracker help students to be like scientists in these 6 to 10 weeks where all Grade 9 students are to conduct a personal video analysis and where appropriate the 8 practices of sciences (1. ask question, 2. use models, 3. Plan and carry out investigation, 4. Analyse and interpret data, 5. Using mathematical and computational thinking, 6. Construct explanations, 7. Discuss from evidence and 8. Communicating information). We will situate our sharing on actual students work and discuss how tracker could be an effective pedagogical tool. Initial research findings suggest that allowing learners conduct performance task using Tracker, a free open source video analysis and modelling tool, guided by the 8 practices of sciences and engineering, could be an innovative and effe...
The Physics Teacher
julio benegas
Being able to facilitate effective hands-on laboratory experiences in introductory physics courses is a challenging task, even when contemporary laboratory facilities, equipment, and new technologies for data collection and analysis are available. At institutions without adequate resources, especially those in developing countries, we have found that the problem of providing effective laboratory experiences is especially daunting for at least two reasons: 1) the lack of equipment and contemporary measuring devices; and 2) even at institutions that have some laboratory equipment, students who have access to cell phones with digital timing and video capabilities or inexpensive digital cameras are bored with trying to use ``old-fashioned'' apparatus for measurements.
RELATED PAPERS
Roger Motson
Human reproduction (Oxford, England)
Japanese Journal of Religious Studies
Frank Usarski
Jurnal Ilmiah FIFO
efy yosrita
Astronomy and Astrophysics
Riccardo Smareglia
Analytical Methods
Muhammad Raihan
Ignacio Gentile
Weliton Soares
The European Physical Journal C
SCIENTIFIC JOURNAL OF TAN TRAO UNIVERSITY
Hương Thanh
J. Bahlcke und W. Korthaase (Hrsg.), Daniel Ernst Jablonski. Religion, Wissenschaft und Politik um 1700
Reimund Leicht
physica status solidi (b)
Zhiliang Yuan
Jambura Journal of Mathematics
Ruhiyat Ruhiyat
Geochimica et Cosmochimica Acta
Marc Evrard
teresa spignoli
Environmental Science & Technology
Shweta Singh
Revista Latino-Americana de Enfermagem
NATHALIA SARAIVA
SPE Economics & Management
Reidar Bratvold
Cenk Indelen
Journal of Cardiovascular Magnetic Resonance
Anselm Uebing
Istie Rahayu
The Journal of biological chemistry
Julian Whitelegge
IEEE Engineering Management Newsletter
Terrance Malkinson
Vol.2, Núm.3
ACI Avances en Ciencias e Ingenierías
Dr Muralidhar L B
RELATED TOPICS
- We're Hiring!
- Help Center
- Find new research papers in:
- Health Sciences
- Earth Sciences
- Cognitive Science
- Mathematics
- Computer Science
- Academia ©2024
Search Cornell
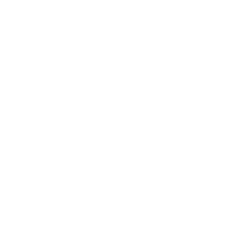
Class Roster
Section menu.
- Toggle Navigation
- Summer 2024
- Spring 2024
- Winter 2024
- Archived Rosters
Last Updated
- Schedule of Classes - April 11, 2024 7:34PM EDT
- Course Catalog - April 11, 2024 7:07PM EDT
SHUM 2437 Economy, Power, and Inequality
Course description.
Course information provided by the Courses of Study 2023-2024 . Courses of Study 2024-2025 is scheduled to publish mid-June.
How do humans organize production, distribution, exchange, and consumption? What social, political, environmental, and religious values underlie different forms of economic organization? And how do they produce racial, ethnic, class, gender, and sexual inequalities? This course uses a range of historical and contemporary case studies to address these questions, in the process introducing a range of analytic approaches including formalism, substantivism, Marxist and feminist theory, critical race studies, and science and technology studies. Course themes include gifts and commodities; the nature of money, markets, and finance; credit and debt relations; labor, property, and value; licit and illicit economies; capitalism and socialism; development and underdevelopment.
When Offered Spring.
Distribution Category (SBA-AS, SCD-AS, SSC-AS) Course Attribute (CU-SBY)
View Enrollment Information
Regular Academic Session. Choose one lecture and one discussion. Combined with: ANTHR 2437
Credits and Grading Basis
3 Credits Stdnt Opt (Letter or S/U grades)
Class Number & Section Details
19461 SHUM 2437 LEC 001
Meeting Pattern
- TR 10:10am - 11:00am To Be Assigned
- Aug 26 - Dec 7, 2024
Instructors
To be determined. There are currently no textbooks/materials listed, or no textbooks/materials required, for this section. Additional information may be found on the syllabus provided by your professor.
For the most current information about textbooks, including the timing and options for purchase, see the Cornell Store .
Additional Information
Instruction Mode: In Person
19462 SHUM 2437 DIS 201
- F 12:20pm - 1:10pm To Be Assigned
19463 SHUM 2437 DIS 202
- F 1:25pm - 2:15pm To Be Assigned
Or send this URL:
Available Syllabi
About the class roster.
The schedule of classes is maintained by the Office of the University Registrar . Current and future academic terms are updated daily . Additional detail on Cornell University's diverse academic programs and resources can be found in the Courses of Study . Visit The Cornell Store for textbook information .
Please contact [email protected] with questions or feedback.
If you have a disability and are having trouble accessing information on this website or need materials in an alternate format, contact [email protected] for assistance.
Cornell University ©2024
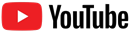
- TPC and eLearning
- Read Watch Interact
- What's NEW at TPC?
- Practice Review Test
- Teacher-Tools
- Subscription Selection
- Seat Calculator
- Ad Free Account
- Edit Profile Settings
- Classes (Version 2)
- Student Progress Edit
- Task Properties
- Export Student Progress
- Task, Activities, and Scores
- Metric Conversions Questions
- Metric System Questions
- Metric Estimation Questions
- Significant Digits Questions
- Proportional Reasoning
- Acceleration
- Distance-Displacement
- Dots and Graphs
- Graph That Motion
- Match That Graph
- Name That Motion
- Motion Diagrams
- Pos'n Time Graphs Numerical
- Pos'n Time Graphs Conceptual
- Up And Down - Questions
- Balanced vs. Unbalanced Forces
- Change of State
- Force and Motion
- Mass and Weight
- Match That Free-Body Diagram
- Net Force (and Acceleration) Ranking Tasks
- Newton's Second Law
- Normal Force Card Sort
- Recognizing Forces
- Air Resistance and Skydiving
- Solve It! with Newton's Second Law
- Which One Doesn't Belong?
- Component Addition Questions
- Head-to-Tail Vector Addition
- Projectile Mathematics
- Trajectory - Angle Launched Projectiles
- Trajectory - Horizontally Launched Projectiles
- Vector Addition
- Vector Direction
- Which One Doesn't Belong? Projectile Motion
- Forces in 2-Dimensions
- Being Impulsive About Momentum
- Explosions - Law Breakers
- Hit and Stick Collisions - Law Breakers
- Case Studies: Impulse and Force
- Impulse-Momentum Change Table
- Keeping Track of Momentum - Hit and Stick
- Keeping Track of Momentum - Hit and Bounce
- What's Up (and Down) with KE and PE?
- Energy Conservation Questions
- Energy Dissipation Questions
- Energy Ranking Tasks
- LOL Charts (a.k.a., Energy Bar Charts)
- Match That Bar Chart
- Words and Charts Questions
- Name That Energy
- Stepping Up with PE and KE Questions
- Case Studies - Circular Motion
- Circular Logic
- Forces and Free-Body Diagrams in Circular Motion
- Gravitational Field Strength
- Universal Gravitation
- Angular Position and Displacement
- Linear and Angular Velocity
- Angular Acceleration
- Rotational Inertia
- Balanced vs. Unbalanced Torques
- Getting a Handle on Torque
- Torque-ing About Rotation
- Properties of Matter
- Fluid Pressure
- Buoyant Force
- Sinking, Floating, and Hanging
- Pascal's Principle
- Flow Velocity
- Bernoulli's Principle
- Balloon Interactions
- Charge and Charging
- Charge Interactions
- Charging by Induction
- Conductors and Insulators
- Coulombs Law
- Electric Field
- Electric Field Intensity
- Polarization
- Case Studies: Electric Power
- Know Your Potential
- Light Bulb Anatomy
- I = ∆V/R Equations as a Guide to Thinking
- Parallel Circuits - ∆V = I•R Calculations
- Resistance Ranking Tasks
- Series Circuits - ∆V = I•R Calculations
- Series vs. Parallel Circuits
- Equivalent Resistance
- Period and Frequency of a Pendulum
- Pendulum Motion: Velocity and Force
- Energy of a Pendulum
- Period and Frequency of a Mass on a Spring
- Horizontal Springs: Velocity and Force
- Vertical Springs: Velocity and Force
- Energy of a Mass on a Spring
- Decibel Scale
- Frequency and Period
- Closed-End Air Columns
- Name That Harmonic: Strings
- Rocking the Boat
- Wave Basics
- Matching Pairs: Wave Characteristics
- Wave Interference
- Waves - Case Studies
- Color Addition and Subtraction
- Color Filters
- If This, Then That: Color Subtraction
- Light Intensity
- Color Pigments
- Converging Lenses
- Curved Mirror Images
- Law of Reflection
- Refraction and Lenses
- Total Internal Reflection
- Who Can See Who?
- Formulas and Atom Counting
- Atomic Models
- Bond Polarity
- Entropy Questions
- Cell Voltage Questions
- Heat of Formation Questions
- Reduction Potential Questions
- Oxidation States Questions
- Measuring the Quantity of Heat
- Hess's Law
- Oxidation-Reduction Questions
- Galvanic Cells Questions
- Thermal Stoichiometry
- Molecular Polarity
- Quantum Mechanics
- Balancing Chemical Equations
- Bronsted-Lowry Model of Acids and Bases
- Classification of Matter
- Collision Model of Reaction Rates
- Density Ranking Tasks
- Dissociation Reactions
- Complete Electron Configurations
- Elemental Measures
- Enthalpy Change Questions
- Equilibrium Concept
- Equilibrium Constant Expression
- Equilibrium Calculations - Questions
- Equilibrium ICE Table
- Ionic Bonding
- Lewis Electron Dot Structures
- Limiting Reactants
- Line Spectra Questions
- Mass Stoichiometry
- Measurement and Numbers
- Metals, Nonmetals, and Metalloids
- Metric Estimations
- Metric System
- Molarity Ranking Tasks
- Mole Conversions
- Name That Element
- Names to Formulas
- Names to Formulas 2
- Nuclear Decay
- Particles, Words, and Formulas
- Periodic Trends
- Precipitation Reactions and Net Ionic Equations
- Pressure Concepts
- Pressure-Temperature Gas Law
- Pressure-Volume Gas Law
- Chemical Reaction Types
- Significant Digits and Measurement
- States Of Matter Exercise
- Stoichiometry Law Breakers
- Stoichiometry - Math Relationships
- Subatomic Particles
- Spontaneity and Driving Forces
- Gibbs Free Energy
- Volume-Temperature Gas Law
- Acid-Base Properties
- Energy and Chemical Reactions
- Chemical and Physical Properties
- Valence Shell Electron Pair Repulsion Theory
- Writing Balanced Chemical Equations
- Mission CG1
- Mission CG10
- Mission CG2
- Mission CG3
- Mission CG4
- Mission CG5
- Mission CG6
- Mission CG7
- Mission CG8
- Mission CG9
- Mission EC1
- Mission EC10
- Mission EC11
- Mission EC12
- Mission EC2
- Mission EC3
- Mission EC4
- Mission EC5
- Mission EC6
- Mission EC7
- Mission EC8
- Mission EC9
- Mission RL1
- Mission RL2
- Mission RL3
- Mission RL4
- Mission RL5
- Mission RL6
- Mission KG7
- Mission RL8
- Mission KG9
- Mission RL10
- Mission RL11
- Mission RM1
- Mission RM2
- Mission RM3
- Mission RM4
- Mission RM5
- Mission RM6
- Mission RM8
- Mission RM10
- Mission LC1
- Mission RM11
- Mission LC2
- Mission LC3
- Mission LC4
- Mission LC5
- Mission LC6
- Mission LC8
- Mission SM1
- Mission SM2
- Mission SM3
- Mission SM4
- Mission SM5
- Mission SM6
- Mission SM8
- Mission SM10
- Mission KG10
- Mission SM11
- Mission KG2
- Mission KG3
- Mission KG4
- Mission KG5
- Mission KG6
- Mission KG8
- Mission KG11
- Mission F2D1
- Mission F2D2
- Mission F2D3
- Mission F2D4
- Mission F2D5
- Mission F2D6
- Mission KC1
- Mission KC2
- Mission KC3
- Mission KC4
- Mission KC5
- Mission KC6
- Mission KC7
- Mission KC8
- Mission AAA
- Mission SM9
- Mission LC7
- Mission LC9
- Mission NL1
- Mission NL2
- Mission NL3
- Mission NL4
- Mission NL5
- Mission NL6
- Mission NL7
- Mission NL8
- Mission NL9
- Mission NL10
- Mission NL11
- Mission NL12
- Mission MC1
- Mission MC10
- Mission MC2
- Mission MC3
- Mission MC4
- Mission MC5
- Mission MC6
- Mission MC7
- Mission MC8
- Mission MC9
- Mission RM7
- Mission RM9
- Mission RL7
- Mission RL9
- Mission SM7
- Mission SE1
- Mission SE10
- Mission SE11
- Mission SE12
- Mission SE2
- Mission SE3
- Mission SE4
- Mission SE5
- Mission SE6
- Mission SE7
- Mission SE8
- Mission SE9
- Mission VP1
- Mission VP10
- Mission VP2
- Mission VP3
- Mission VP4
- Mission VP5
- Mission VP6
- Mission VP7
- Mission VP8
- Mission VP9
- Mission WM1
- Mission WM2
- Mission WM3
- Mission WM4
- Mission WM5
- Mission WM6
- Mission WM7
- Mission WM8
- Mission WE1
- Mission WE10
- Mission WE2
- Mission WE3
- Mission WE4
- Mission WE5
- Mission WE6
- Mission WE7
- Mission WE8
- Mission WE9
- Vector Walk Interactive
- Name That Motion Interactive
- Kinematic Graphing 1 Concept Checker
- Kinematic Graphing 2 Concept Checker
- Graph That Motion Interactive
- Two Stage Rocket Interactive
- Rocket Sled Concept Checker
- Force Concept Checker
- Free-Body Diagrams Concept Checker
- Free-Body Diagrams The Sequel Concept Checker
- Skydiving Concept Checker
- Elevator Ride Concept Checker
- Vector Addition Concept Checker
- Vector Walk in Two Dimensions Interactive
- Name That Vector Interactive
- River Boat Simulator Concept Checker
- Projectile Simulator 2 Concept Checker
- Projectile Simulator 3 Concept Checker
- Hit the Target Interactive
- Turd the Target 1 Interactive
- Turd the Target 2 Interactive
- Balance It Interactive
- Go For The Gold Interactive
- Egg Drop Concept Checker
- Fish Catch Concept Checker
- Exploding Carts Concept Checker
- Collision Carts - Inelastic Collisions Concept Checker
- Its All Uphill Concept Checker
- Stopping Distance Concept Checker
- Chart That Motion Interactive
- Roller Coaster Model Concept Checker
- Uniform Circular Motion Concept Checker
- Horizontal Circle Simulation Concept Checker
- Vertical Circle Simulation Concept Checker
- Race Track Concept Checker
- Gravitational Fields Concept Checker
- Orbital Motion Concept Checker
- Angular Acceleration Concept Checker
- Balance Beam Concept Checker
- Torque Balancer Concept Checker
- Aluminum Can Polarization Concept Checker
- Charging Concept Checker
- Name That Charge Simulation
- Coulomb's Law Concept Checker
- Electric Field Lines Concept Checker
- Put the Charge in the Goal Concept Checker
- Circuit Builder Concept Checker (Series Circuits)
- Circuit Builder Concept Checker (Parallel Circuits)
- Circuit Builder Concept Checker (∆V-I-R)
- Circuit Builder Concept Checker (Voltage Drop)
- Equivalent Resistance Interactive
- Pendulum Motion Simulation Concept Checker
- Mass on a Spring Simulation Concept Checker
- Particle Wave Simulation Concept Checker
- Boundary Behavior Simulation Concept Checker
- Slinky Wave Simulator Concept Checker
- Simple Wave Simulator Concept Checker
- Wave Addition Simulation Concept Checker
- Standing Wave Maker Simulation Concept Checker
- Color Addition Concept Checker
- Painting With CMY Concept Checker
- Stage Lighting Concept Checker
- Filtering Away Concept Checker
- InterferencePatterns Concept Checker
- Young's Experiment Interactive
- Plane Mirror Images Interactive
- Who Can See Who Concept Checker
- Optics Bench (Mirrors) Concept Checker
- Name That Image (Mirrors) Interactive
- Refraction Concept Checker
- Total Internal Reflection Concept Checker
- Optics Bench (Lenses) Concept Checker
- Kinematics Preview
- Velocity Time Graphs Preview
- Moving Cart on an Inclined Plane Preview
- Stopping Distance Preview
- Cart, Bricks, and Bands Preview
- Fan Cart Study Preview
- Friction Preview
- Coffee Filter Lab Preview
- Friction, Speed, and Stopping Distance Preview
- Up and Down Preview
- Projectile Range Preview
- Ballistics Preview
- Juggling Preview
- Marshmallow Launcher Preview
- Air Bag Safety Preview
- Colliding Carts Preview
- Collisions Preview
- Engineering Safer Helmets Preview
- Push the Plow Preview
- Its All Uphill Preview
- Energy on an Incline Preview
- Modeling Roller Coasters Preview
- Hot Wheels Stopping Distance Preview
- Ball Bat Collision Preview
- Energy in Fields Preview
- Weightlessness Training Preview
- Roller Coaster Loops Preview
- Universal Gravitation Preview
- Keplers Laws Preview
- Kepler's Third Law Preview
- Charge Interactions Preview
- Sticky Tape Experiments Preview
- Wire Gauge Preview
- Voltage, Current, and Resistance Preview
- Light Bulb Resistance Preview
- Series and Parallel Circuits Preview
- Thermal Equilibrium Preview
- Linear Expansion Preview
- Heating Curves Preview
- Electricity and Magnetism - Part 1 Preview
- Electricity and Magnetism - Part 2 Preview
- Vibrating Mass on a Spring Preview
- Period of a Pendulum Preview
- Wave Speed Preview
- Slinky-Experiments Preview
- Standing Waves in a Rope Preview
- Sound as a Pressure Wave Preview
- DeciBel Scale Preview
- DeciBels, Phons, and Sones Preview
- Sound of Music Preview
- Shedding Light on Light Bulbs Preview
- Models of Light Preview
- Electromagnetic Radiation Preview
- Electromagnetic Spectrum Preview
- EM Wave Communication Preview
- Digitized Data Preview
- Light Intensity Preview
- Concave Mirrors Preview
- Object Image Relations Preview
- Snells Law Preview
- Reflection vs. Transmission Preview
- Magnification Lab Preview
- Reactivity Preview
- Ions and the Periodic Table Preview
- Periodic Trends Preview
- Intermolecular Forces Preview
- Melting Points and Boiling Points Preview
- Reaction Rates Preview
- Ammonia Factory Preview
- Stoichiometry Preview
- Gaining Teacher Access
- Tasks and Classes
- Tasks - Classic
- Subscription
- Subscription Locator
- 1-D Kinematics
- Newton's Laws
- Vectors - Motion and Forces in Two Dimensions
- Momentum and Its Conservation
- Work and Energy
- Circular Motion and Satellite Motion
- Thermal Physics
- Static Electricity
- Electric Circuits
- Vibrations and Waves
- Sound Waves and Music
- Light and Color
- Reflection and Mirrors
- About the Physics Interactives
- Task Tracker
- Usage Policy
- Newtons Laws
- Vectors and Projectiles
- Forces in 2D
- Momentum and Collisions
- Circular and Satellite Motion
- Balance and Rotation
- Electromagnetism
- Waves and Sound
- Forces in Two Dimensions
- Work, Energy, and Power
- Circular Motion and Gravitation
- Sound Waves
- 1-Dimensional Kinematics
- Circular, Satellite, and Rotational Motion
- Einstein's Theory of Special Relativity
- Waves, Sound and Light
- QuickTime Movies
- About the Concept Builders
- Pricing For Schools
- Directions for Version 2
- Measurement and Units
- Relationships and Graphs
- Rotation and Balance
- Vibrational Motion
- Reflection and Refraction
- Teacher Accounts
- Task Tracker Directions
- Kinematic Concepts
- Kinematic Graphing
- Wave Motion
- Sound and Music
- About CalcPad
- 1D Kinematics
- Vectors and Forces in 2D
- Simple Harmonic Motion
- Rotational Kinematics
- Rotation and Torque
- Rotational Dynamics
- Electric Fields, Potential, and Capacitance
- Transient RC Circuits
- Light Waves
- Units and Measurement
- Stoichiometry
- Molarity and Solutions
- Thermal Chemistry
- Acids and Bases
- Kinetics and Equilibrium
- Solution Equilibria
- Oxidation-Reduction
- Nuclear Chemistry
- NGSS Alignments
- 1D-Kinematics
- Projectiles
- Circular Motion
- Magnetism and Electromagnetism
- Graphing Practice
- About the ACT
- ACT Preparation
- For Teachers
- Other Resources
- Newton's Laws of Motion
- Work and Energy Packet
- Static Electricity Review
- Solutions Guide
- Solutions Guide Digital Download
- Motion in One Dimension
- Work, Energy and Power
- Frequently Asked Questions
- Purchasing the Download
- Purchasing the CD
- Purchasing the Digital Download
- About the NGSS Corner
- NGSS Search
- Force and Motion DCIs - High School
- Energy DCIs - High School
- Wave Applications DCIs - High School
- Force and Motion PEs - High School
- Energy PEs - High School
- Wave Applications PEs - High School
- Crosscutting Concepts
- The Practices
- Physics Topics
- NGSS Corner: Activity List
- NGSS Corner: Infographics
- About the Toolkits
- Position-Velocity-Acceleration
- Position-Time Graphs
- Velocity-Time Graphs
- Newton's First Law
- Newton's Second Law
- Newton's Third Law
- Terminal Velocity
- Projectile Motion
- Forces in 2 Dimensions
- Impulse and Momentum Change
- Momentum Conservation
- Work-Energy Fundamentals
- Work-Energy Relationship
- Roller Coaster Physics
- Satellite Motion
- Electric Fields
- Circuit Concepts
- Series Circuits
- Parallel Circuits
- Describing-Waves
- Wave Behavior Toolkit
- Standing Wave Patterns
- Resonating Air Columns
- Wave Model of Light
- Plane Mirrors
- Curved Mirrors
- Teacher Guide
- Using Lab Notebooks
- Current Electricity
- Light Waves and Color
- Reflection and Ray Model of Light
- Refraction and Ray Model of Light
- Classes (Legacy Version)
- Teacher Resources
- Subscriptions

- Newton's Laws
- Einstein's Theory of Special Relativity
- About Concept Checkers
- School Pricing
- Newton's Laws of Motion
- Newton's First Law
- Newton's Third Law
Teacher Resources
The Physics Classroom has been devoted to helping students, teachers, and classrooms since the 1990s. We are as passionate about that mission now as we have ever been. If you are a teacher of Physics or Physical Science, we encourage you to use our Video Tutorial with your students. And we also encourage you to consider the use of other resources on our website that coordinate with the video. We have listed a few below to help you get started.
Teacher Toolkits: Free Fall
Curriculum Corner: 1D Kinematics
Science Reasoning Center: 1D Kinematics: Kinematics
Concept Builder: Free Fall
Concept Builder: Up and Down
The Physics Classroom Tutorial: 1D Kinematics, Lesson 5
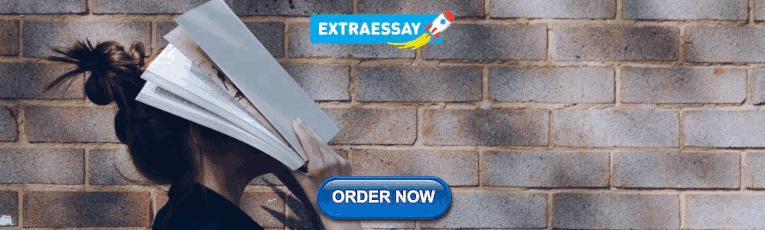
IMAGES
VIDEO
COMMENTS
Free Fall. An object that is moving under only the influence of gravity is in free fall. In order for an object to be in free fall, wind and air resistance must be ignored. On Earth, all objects in free fall accelerate downward at the rate of gravity or 9.81\text { m/s}^2 9.81 m/s2.
Case 2: When the lift is moving down with acceleration a. (a - acting downward) W = mg - ma Lightweight. Case 3: When the lf is moving upward with acceleration a. (a - acting upward) W = mg + ma Heavy Weight. Case 4: When the lift is falling freely a= g (Free Fall) W = 0 Weightlessness. Weightlessness: A freely falling object experiences ...
Free Fall. Decide on the sign of the acceleration of gravity. In Equation 3.15 through Equation 3.17, acceleration g is negative, which says the positive direction is upward and the negative direction is downward. In some problems, it may be useful to have acceleration g as positive, indicating the positive direction is downward.; Draw a sketch of the problem.
Learn about the concept and equations of free fall, a special case of motion with constant acceleration due to gravity. Explore examples and applications of free fall in physics. Physics LibreTexts provides free and open educational resources for students and teachers of physics.
A free falling object is an object that is falling under the sole influence of gravity. Any object that is being acted upon only by the force of gravity is said to be in a state of free fall. There are two important motion characteristics that are true of free-falling objects: Free-falling objects do not encounter air resistance. Because free ...
An astronaut holds a rock at 100m above the surface of Planet X. The rock is thrown upward with a speed of 15m/s. The rock reaches the ground 10s after it is thrown. The atmosphere of Planet X has a negligible effect on the rock when it is in free fall. Based on my calculations, I found that the acceleration due to gravity of the rock when it ...
'toss up' and free fall motion: a case study Loo Kang Wee1, Kim Kia Tan2, Tze Kwang Leong 3 and Ching Tan1 1 Ministry of Education, Educational Technology Division, Singapore 2 Ministry of Education, Evergreen Secondary School, Singapore
This very simple Java simulation lets students explore the motion of an object in free fall. You can set the initial height (0-20m), set an initial velocity from -20 to 20 m/s, and change the rate of gravitational acceleration from zero to 20 m/s/s. You can also launch the ball upward from any point on the line of motion.
This video tutorial lesson defines free fall and describes the changes (or lack of changes) in the position, velocity, and acceleration over the course of a free fall motion. Numerical data, dot diagrams, vector diagrams, and velocity-time graphs are presented for both falling and up-and-down motion. The video emphasizes the meaning of the free fall acceleration.
Abstract. Free fall has signed the greatest markings in the history of physics through the leaning Pisa tower, the Woolsthorpe apple tree and the Einstein lift. The perspectives offered by the capture of stars by supermassive black holes are to be cherished, because the study of the motion of falling stars will constitute a giant step forward ...
Abstract. This paper reports the use of Tracker as a computer-based learning tool to support effective learning and teaching of 'toss up' and free fall motion for beginning secondary three (15 year-old) students. The case study involved ( N = 123) students from express pure physics classes at a mainstream school in Singapore.
Falls Management Program Case Study; Discussion Guide for Inservice #1; Discussion Guide for Inservice #2; Illustration of Fall Response; Falls Management Program Case Study. Mrs. P is a 93 year old white female admitted to your facility. She has had Alzheimer's disease for approximately 7 years and has been cared for by her husband and ...
free fall motion for beginning secondary three (age 15 years old) students. This is a case study with (N=123) students of express-pure physics classes in a mainstream school in Singapore where we used a 8 multi-choice questions as a proxy to assess learning gains in pre and posttest to gauge the impact on learning.
Zago and Lacquaniti (2005) hypothesized that, differently from the cognitive system, the motor system relies on an internal representation of free fall which is consistent with physics. Implications for physics education. The results of the present study have important implications for physics education.
The case study involved (N = 123) students from express pure physics classes at a mainstream school in Singapore. We used eight multiple-choice questions pre- and post-test to gauge the impact on ...
Model test with the case study free fall lifeboat: (a) test setup and (b) body plan. Download : Download high-res image (151KB) Download : Download full-size image; Fig. 3. Velocity at two cross section locations: for the aft (dotted line, 0.3 m from stern) and the fore (solid line, 12.3 m from stern) section. Zero velocity relative to water ...
Timed Up and Go: 15 seconds with a cane on left, minimal arm swing noted. 30-Second Chair Stand Test: Able to rise from the chair 7 times without using her arms. 4-Stage Balance Test: Able to stand for 10 seconds in Position 1(feet side by side) and Position 2 (semi-tandem). However, she loses her balance after 3 seconds in Position 3 (tandem).
Therefore, this case study explores and discusses how wearable video-based data capture can be used to better inform mobility-based fall risk within free-living environments. Additionally, this case study proposes a plausible computer vision processes for use on wearable video data to enable an automated approach for better IMU-based mobility ...
implementing best practices from evidence-based studies and getting every employee involved in determining the root causes of the fall, which was the single biggest change in our practice." First Responder's Responsibilities Prior to the study, nurses were the primary staff who responded to resident falls, but that changed when all employees
Introduction: Fall Risk Appraisal (FRA), a process that integrates perceived and objective fall risk measures, serves as a crucial component for understanding the incongruence between fear of falling (FOF) and physiological fall risk in older adults. Despite its importance, scant research has been undertaken to investigate how habitual physical activity (PA) levels, quantified in Monitor ...
July 2015 Using Tracker to understand 'toss up' and free fall motion: a case study 'The video analysis [Tracker] gives me the opportunity to check the data collected. I realized that in real-life data collection there are random errors, which was shown from the graph plotted.' 'Compared to teachers' explanations on the board, video ...
A case study on a primary care practice implementing two automated screeners, one on opioid use and one on risk for falls. Keywords: opioid, use, fall, risk, screen, primary, care, patient, case, study Created Date: 12/13/2023 2:16:48 PM
The purpose of this course is to give students a broad understanding of financial statement analysis and valuation issues. The emphasis is on helping students understand the basic equity research process for use in investment and business decision applications. The course format is a combination of examples, case studies, and lectures. Potential topics include information sources, strategic ...
Kinematic Equations and Free Fall. As mentioned in Lesson 5, a free-falling object is an object that is falling under the sole influence of gravity. That is to say that any object that is moving and being acted upon only be the force of gravity is said to be "in a state of free fall ." Such an object will experience a downward acceleration of 9 ...
We will then test the applicability of these general theories to the Arab World through our examination of specific case studies. Fall 2024 - ASRC 4672 - This seminar examines the emergence of national identities, nationalist movements, and nation-states in the modern Arab world. First, we will examine various approaches to the question of ...
This course uses a range of historical and contemporary case studies to address these questions, in the process introducing a range of analytic approaches including formalism, substantivism, Marxist and feminist theory, critical race studies, and science and technology studies. ... Fall 2024 - SHUM 2437 - How do humans organize production ...
A Lack of Stacking. The report also found that fewer previous graduates returned to college to earn more credentials. The number of students with certificates and earning associate degrees fell 2.5 percent, associate degree holders earning bachelor's degrees decreased 3.3 percent and graduates with bachelor's degrees or master's degrees earning certificates declined 3.7 percent.
As Bernstein analyst Toni Sacconaghi wrote in a note to investors on Tuesday, Tesla's (TSLA 2.25%) first-quarter deliveries were "brutal." Deliveries fell about 9% year over year and 20% ...
This video tutorial lesson defines free fall and describes the changes (or lack of changes) in the position, velocity, and acceleration over the course of a free fall motion. Numerical data, dot diagrams, vector diagrams, and velocity-time graphs are presented for both falling and up-and-down motion. The video emphasizes the meaning of the free fall acceleration.
This video tutorial lesson defines free fall and describes the changes (or lack of changes) in the position, velocity, and acceleration over the course of a free fall motion. Numerical data, dot diagrams, vector diagrams, and velocity-time graphs are presented for both falling and up-and-down motion. The video emphasizes the meaning of the free fall acceleration.