Multiregional Hypothesis: Human Evolutionary Theory
A Now-Discredited Theory of Human Evolution
- Ancient Civilizations
- Excavations
- History of Animal and Plant Domestication
- M.A., Anthropology, University of Iowa
- B.Ed., Illinois State University
The Multiregional Hypothesis model of human evolution (abbreviated MRE and known alternatively as Regional Continuity or Polycentric model) argues that our earliest hominid ancestors (specifically Homo erectus ) evolved in Africa and then radiated out into the world. Based on paleoanthropological data rather than genetic evidence, the theory says that after H. erectus arrived in the various regions in the world hundreds of thousands of years ago, they slowly evolved into modern humans. Homo sapiens , so MRE posits, evolved from several different groups of Homo erectus in several places throughout the world.
However, genetic and paleoanthropological evidence gathered since the 1980s has shown conclusively that that simply cannot be the case: Homo sapiens evolved in Africa and dispersed out into the world, somewhere between 50,000-62,000 years ago. What happened then is quite interesting.
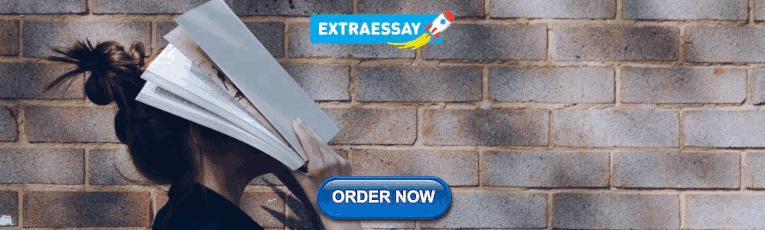
Background: How Did the Idea of MRE Arise?
In the mid-19th century, when Darwin wrote Origin of Species , the only lines of evidence of human evolution he had were comparative anatomy and a few fossils. The only hominin (ancient human) fossils known in the 19th century were Neanderthals , early modern humans , and H. erectus . A lot of those early scholars didn't even think those fossils were humans or related to us at all.
When in the early 20th century numerous hominins with robust large-brained skulls and heavy brow ridges (now usually characterized as H. heidelbergensis ) were discovered, scholars started to develop a wide variety of scenarios about how we were related to these new hominins, as well as Neanderthals and H. erectus . These arguments still had to be tied directly to the growing fossil record: again, no genetic data was available. The predominant theory then was that H. erectus gave rise to Neanderthals and then modern humans in Europe; and in Asia, modern humans evolved separately directly from H. erectus .
Fossil Discoveries
As more and more distantly-related fossil hominins were identified in the 1920s and 1930s, such as Australopithecus , it became clear that human evolution was much older than previously considered and much more varied. In the 1950s and 60s, numerous hominins of these and other older lineages were found in East and South Africa: Paranthropus , H. habilis , and H. rudolfensis . The predominant theory then (although it varied greatly from scholar to scholar), was that there were nearly independent origins of modern humans within the various regions of the world out of H. erectus and/or one of these various regional archaic humans.
Don't kid yourself: that original hardline theory was never really tenable -- modern humans are simply too much alike to be evolved from different Homo erectus groups, but more reasonable models such as those put forward by paleoanthropologist Milford H. Wolpoff and his colleagues argued that you could account for the similarities in human beings on our planet because there was lots of gene flow between these independently evolved groups.
In the 1970s, paleontologist W.W. Howells proposed an alternate theory: the first Recent African Origin model (RAO), called the "Noah's Ark" hypothesis. Howells argued that H. sapiens evolved solely in Africa. By the 1980s, growing data from human genetics led Stringer and Andrews to develop a model that said that the very earliest anatomically modern humans arose in Africa about 100,000 years ago and archaic populations found throughout Eurasia might be descendants of H. erectus and later archaic types but they were not related to modern humans.
The differences were stark and testable: if MRE was right, there would be various levels of ancient genetics ( alleles ) found in modern people in scattered regions of the world and transitional fossil forms and levels of morphological continuity. If RAO was right, there should be very few alleles older than the origins of anatomically modern humans in Eurasia, and a decrease in genetic diversity as you get away from Africa.
Between the 1980's and today, over 18,000 whole human mtDNA genomes have been published from people all over the world, and they all coalesce within the last 200,000 years and all the non-African lineages only 50,000-60,000 years old or younger. Any hominin lineage that branched off from the modern human species prior to 200,000 years ago did not leave any mtDNA in modern humans.
An Admixture of Humans With Regional Archaics
Today, paleontologists are convinced that humans evolved in Africa and that the bulk of modern non-African diversity is recently derived from an African source. The exact timing and pathways outside of Africa are still under debate, perhaps out of East Africa, perhaps along with a southern route from South Africa.
The most startling news from a human evolution sense is some evidence for mixing between Neanderthals and Eurasians. Evidence for this is that between 1 to 4% of genomes in people who are non-Africans are derived from Neanderthals. That was never predicted by either the RAO or the MRE. The discovery of a completely new species called the Denisovans threw another stone in the pot: even though we have very little evidence of Denisovan existence, some of their DNA has survived in some human populations.
Identifying Genetic Diversity in Human Kind
It is now clear that before we can understand the diversity in archaic humans, we have to understand the diversity in modern humans. Although MRE has not been seriously considered for decades, now it seems possible that modern African migrants hybridized with local archaics in different regions of the world. Genetic data demonstrate that such introgression did occur, but it is likely to have been minimal.
Neither Neanderthals nor Denisovans survived into the modern period, except as a handful of genes, perhaps because they were unable to adapt to the unstable climates in the world or competition with H. sapiens .
- Disotell TR. 2012. Archaic human genomics. American Journal of Physical Anthropology 149(S55):24-39.
- Ermini L, Der Sarkissian C, Willerslev E, and Orlando L. 2015. Major transitions in human evolution revisited: A tribute to ancient DNA. Journal of Human Evolution 79:4-20.
- Gamble C. 2013. In: Mock CJ, editor. Encyclopedia of Quaternary Science (Second Edition). Amsterdam: Elsevier. p 49-58.
- Hawks JD, and Wolpoff MH. 2001. The four faces of Eve: hypothesis compatibility and human origins . Quaternary International 75:41-50.
- Stringer C. 2014. Why we are not all multiregionalists now. Trends in Ecology & Evolution 29(5):248-251 .
- Did Humans First Evolve in Africa?
- Why Don't We Call Them 'Cro-Magnon' Anymore?
- Complete Guide to the Denisovans, a Newer Hominid Species
- Southern Dispersal Route: When Did Early Modern Humans Leave Africa?
- Dmanisi (Georgia)
- A Beginner's Guide to the Paleolithic Period or Stone Age
- Acheulean Tradition
- Neanderthals - Study Guide
- Vindija Cave (Croatia)
- Klasies River Caves
- Lower Paleolithic: The Changes Marked by the Early Stone Age
- What Is a Hominin?
- Zhoukoudian Cave
- Sima de los Huesos, the Pit of Bones
- Introduction to Bipedal Locomotion
- Introduction to the Middle Paleolithic
Thank you for visiting nature.com. You are using a browser version with limited support for CSS. To obtain the best experience, we recommend you use a more up to date browser (or turn off compatibility mode in Internet Explorer). In the meantime, to ensure continued support, we are displaying the site without styles and JavaScript.
- View all journals
- Explore content
- About the journal
- Publish with us
- Sign up for alerts
- Short Review
- Published: 05 March 2008
Genetic evidence and the modern human origins debate
- J H Relethford 1
Heredity volume 100 , pages 555–563 ( 2008 ) Cite this article
74k Accesses
49 Citations
50 Altmetric
Metrics details
A continued debate in anthropology concerns the evolutionary origin of ‘anatomically modern humans’ ( Homo sapiens sapiens ). Different models have been proposed to examine the related questions of (1) where and when anatomically modern humans first appeared and (2) the genetic and evolutionary relationship between modern humans and earlier human populations. Genetic data have been increasingly used to address these questions. Genetic data on living human populations have been used to reconstruct the evolutionary history of the human species by considering how global patterns of human variation could be produced given different evolutionary scenarios. Of particular interest are gene trees that reconstruct the time and place of the most recent common ancestor of humanity for a given haplotype and the analysis of regional differences in genetic diversity. Ancient DNA has also allowed a direct assessment of genetic variation in European Neandertals. Together with the fossil record, genetic data provide insight into the origin of modern humans. The evidence points to an African origin of modern humans dating back to 200 000 years followed by later expansions of moderns out of Africa across the Old World. What is less clear is what happened when these early modern humans met preexisting ‘archaic human’ populations outside of Africa. At present, it is difficult to distinguish between a model of total genetic replacement and a model that includes some degree of genetic mixture.
Similar content being viewed by others
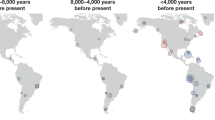
Peopling of the Americas as inferred from ancient genomics
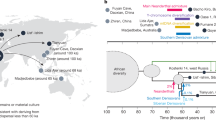
Origins of modern human ancestry
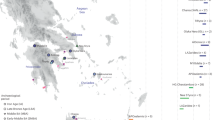
Ancient DNA reveals admixture history and endogamy in the prehistoric Aegean
Introduction.
A long-standing debate in anthropology concerns our own origins as anatomically modern humans ( Homo sapiens sapiens ). The qualifying term ‘anatomically modern’ is used to identify our early ancestors that were physically much the same as living humans, but also to distinguish them from earlier hominins that could be called ‘human’ at some level based on features such as an increased brain size relative to body size and the possession of a material culture (including stone tools). The fossil record of the past 2 Myr shows modern humans evolving from earlier humans, often referred to as ‘archaic humans’, a broad group that includes the species H. heidelbergensis as well as the Neandertals of Europe and the Middle East. What is less clear is the evolutionary relationship of modern humans to the various archaic human populations, as well as to earlier ancestors. Did modern humans evolve via anagenesis from a single archaic species across the Old World, or did they first arise in Africa? If the latter, then did modern populations expanding out of Africa replace the archaic human populations that lived outside of Africa, or did they interbreed with them? Were the Neandertals a separate species from modern humans and, if so, did any hybridization take place?
These and other questions fall under what has been termed the ‘modern human origins debate’. Although this debate is often focused on the fossil and archeological records, studies of genetic variation have become increasingly important as a source of insight. Much of the work in this area has consisted of detailed analyses of patterns of genetic variation in living human populations. The strategy here is based on the realization that whatever our species' evolutionary past, it has left visible signatures on our genome. Expectations of current genetic variation under different evolutionary scenarios are compared with observed genetic variation in our species in order to test various origin models. In addition, the last decade has also seen an increase in the analysis of ancient DNA, such that mitochondrial and nuclear DNA sequences are now available for the Neandertals, an archaic human group. The purpose of this review is to highlight some of the major findings of genetic analysis (using both living and ancient DNA) and their use (and misuse) in the modern human origins debate. The focus here is primarily on recent findings and the status of the debate as I perceive it.
The fossil record of evolution in the genus Homo
In order to understand the contributions of genetic research to the modern human origins debate, it is first necessary to provide a brief review of the fossil record for human evolution over the past 2 Myr. Only a brief review is given here; more detail is available in many current texts on human evolution (e.g., Conroy, 2005 ; Stringer and Andrews, 2005 ). Molecular evidence suggests that the hominin and African ape lines diverged about 6–7 Myr ago. The fossil record of the first possible bipeds dates back over 6 Myr ago in Africa. By 4.2 Myr ago, there is definite evidence of bipedal hominins in Africa ( Australopithecus anamensis ). These early hominins walked upright (at least on the ground), had ape-sized brains and larger protruding faces and teeth. The species H. erectus appeared in Africa 1.8 Myr ago and is characterized by modern limb proportions, increased brain size, reduction in the size of the teeth and developments in stone tool technology. Until this point in time, hominin evolution had taken place exclusively in Africa, but populations of H. erectus dispersed to Eastern Europe and Southeast Asia about 1.7 Myr ago (note: some anthropologists refer to the initial African population as the species H. ergaster and reserve the name H. erectus for the Southeast Asian populations). Some populations of Southeast Asian H. erectus may have survived until 27 000–54 000 years ago, and perhaps are related to the newly named dwarf species H. floresiensis (although others consider the type specimen to be a pathological modern human).
The descendants of H. ergaster / H. erectus have often been referred to broadly as archaic humans, a label that bridges the gap between early humans ( H. ergaster / H. erectus ) and modern humans ( H. sapiens sapiens ). The archaic humans have, on average, a brain size approaching that of modern humans, but with a lower and differently shaped skull and larger face and brow ridges compared with modern humans. Although it had been common for some time to refer to the archaics as archaic H. sapiens , there is growing sentiment to classify these forms into two (or more) species. A number of these specimens are classified as H. heidelbergensis , a species that lived in parts of Africa, Europe and possibly Asia from about 800 000–200 000 years ago. A somewhat different archaic form, the Neandertals, lived in Europe and the Middle East from about 130 000–28 000 years ago, and has a number of unique craniofacial traits that distinguish them from H. heidelbergensis and H. sapiens . Classification of the Neandertals has always been a contentious issue, with some anthropologists proposing that they should be classified as a subspecies of H. sapiens ( H. sapiens neanderthalensis ) and others arguing that they be considered a separate species ( H. neanderthalensis ).
Models of modern human origins
The core question in the modern human origins debate is what is the evolutionary relationship between archaic and modern humans? It has been common to read many articles and books that describe the debate in terms of two opposing camps—the ‘out of Africa’ model and the multiregional evolution model. In reality, this dichotomy is a bit of an oversimplification, because there is overlap between some variants of these models, and the two models are often misrepresented. Adding to possible confusion is the fact that there are two separate but related questions regarding modern human origins ( Relethford, 2001b , 2007 ). The first of these questions concerns the time and place of the transition from archaic to modern humans. Did modern human anatomy emerge first in Africa followed by dispersal across the rest of the Old World, or did modern humans instead emerge because of the mixing of different evolutionary changes taking place in different parts of the Old World? The second question concerns the evolutionary relationship between archaic and modern forms. Were they separate species that arose through cladogenesis with little if any hybridization, or were they part of a single evolutionary lineage (anagenesis)? A variety of models, some only subtly different from others, have been generated through different answers to these questions. Some models share answers to one question, but differ on the other. Consequently, there is often disagreement over what models are supported by fossil and genetic observations. Thus, it is useful to examine briefly a few of the basic models.
The African replacement model is one form of what is referred to in general terms as an out of Africa model. According to the African replacement model, anatomically modern humans arose as a new species ( H. sapiens ) in Africa between 150 000 and 200 000 years ago. By 100 000 years ago or so, populations of this new species began expanding throughout the Old World, replacing preexisting archaic human species outside of Africa (such as the Neandertals of Europe). Under this model, there was virtually no genetic input from these archaic populations. All living humans thus can trace all of their ancestry 200 000 years ago to Africa.
The multiregional evolution model presents a different explanation for the origin of modern humans. The multiregional model was first developed to explain how some traits, such as increased cranial capacity and reduction of the face, could evolve across the Old World while at the same time other traits could retain regional distinctiveness over time, such as the high prevalence of shovel-shaped incisors in past and present Asian populations. The seeming conflict between similarity between populations and regional continuity over time was explained by a balance of gene flow, selection and genetic drift ( Wolpoff et al., 1984 ; Relethford, 2001b ). According to some proponents of the multiregional model, there was no single time or place associated with the origin of modern humans. Indeed, some have argued that the distinction between archaics and moderns is arbitrary and difficult to define. Instead, this view of multiregional evolution posits that the anatomic and genetic changes leading to modern humans took place piecemeal across the Old World, and modern humans eventually resulted from the regional coalescence of these changes because of gene flow between populations. All of these changes took place within a single evolutionary lineage. Contrary to some representations of the multiregional model, it does not claim that the appearance of modern humans was due to independent or parallel evolution in different parts of the Old World ( Wolpoff et al., 2000 ; Relethford, 2001b ).
Although the modern human origins debate is frequently discussed in terms of the more extreme views regarding out of Africa and multiregional models described above, in reality a number of anthropologists have argued for models that combine an initial African origin of modern humans with varying degrees of gene flow taking place between modern humans dispersing out of Africa and preexisting human populations outside of Africa. Elsewhere ( Relethford, 2001b , 2007 ) I have labeled such models ‘primary African origin’ models, characterized by the hypothesis that modern human anatomy did emerge first in Africa (in common with the African replacement model), but that there was some degree of genetic mixture with preexisting archaic populations outside of Africa (in common with the multiregional model). One example of a primary African origin model is Smith's ‘assimilation model’, a variant of the multiregional model that allows for an initial African origin ( Smith et al., 2005 ).
The fossil evidence
It may seem strange to have a section about the fossil evidence in a paper focusing on the genetic evidence written in a genetics journal. Although brief, this section is vital to any understanding and evaluation of the genetic evidence for modern human origins. Both the fossil and genetic evidence must be considered in any assessment of the debate, as both sorts of data inform each other, and any proposed answers must be compatible with both. Arguments over which sort of data is ‘best’ have no place in the debate. Each type of data has its own advantages and disadvantages, and therefore both must be examined.
One of the more obvious inferences that can be made from the fossil record concerns the question of the time and place for the emergence of modern humans. Where and when in the fossil record do we see the first appearance of modern humans? According to a strict multiregional view that there was a gradual transition between archaic and modern anatomy, it will be difficult if not impossible to pinpoint a single point of origin. On the other hand, both the African replacement model and the assimilation model predict that anatomically modern humans appeared first in Africa. Complicating the debate is disagreement over anatomical definitions of modernity and over geologic dates. For many years, there was suggestive evidence of an early appearance of modern humans in Africa as early as 130 000 years ago, although the anatomy and dating was debated. More recently, the picture seems clearer, with evidence of early moderns in Africa at 160 000 years ago, classified by the discoverers as H. sapiens idaltu ( White et al., 2003 ). In addition, modern humans from the Omo site in Ethiopia have recently been redated to 195 000 years ago ( McDougall et al., 2005 ). Compared with these early dates, the first appearance of modern humans outside of Africa is later in time, with dates around 92 000 years ago in the Middle East, 60 000–40 000 years ago in Australia and 40 000–30 000 years ago in Europe. Given the fossil record as currently exists, it seems clear that the prediction of modern humans appearing first in Africa is supported and the type of regional coalescence predicted by some variants of multiregional evolution is less likely. As noted later in this paper, an initial African origin is also compatible with the genetic evidence. This does not mean, however, that the evidence necessarily rules in favor of the African replacement model. Instead, the debate has shifted for many to one of replacement versus admixture. In other words, what happened to the archaic populations that lived outside of Africa when they encountered modern populations from Africa? Did they become extinct, or was there some genetic contact? If there was gene flow, then was it the consequence of gene flow between local populations and/or long-range dispersal within a species, or hybridization between species, or perhaps different forms of admixture in different parts of the Old World?
There is suggestive fossil evidence for genetic mixture of archaics and moderns. Some anatomical traits are persistent over time, tending to occur more often in certain geographic populations both past and present. This regional continuity is frequently cited as evidence for a genetic contribution from archaic populations ( Wolpoff et al., 1984 ; Smith et al., 2005 ), although others have argued that it might represent the retention of traits from a shared ancestor of archaics and moderns. Other fossil evidence supporting genetic contact includes the discovery of a 4-year old anatomically modern child from Portugal that dates back 25 000 years ago that has some Neandertal traits suggesting partial Neandertal ancestry ( Duarte et al., 1999 ), although others have argued that these traits are not of Neandertals ( Tattersall and Schwartz, 1999 ).
The genetic evidence: gene trees
The rapid development of molecular genetics and the emergence of coalescent theory in population genetics have provided valuable tools for the construction and analysis of genetic genealogies known as gene/haplotype trees. Coalescent theory makes use of the fact that genetic drift over time will result in the extinction of lineages, which in turn means that when looking backward from the present-day generation, any sample of DNA markers will coalesce to a common ancestor. The application of analytic methods based on coalescent theory means that, given a sample of genetic markers, it is possible to identify characteristics regarding that sample's most recent common ancestor (MRCA), specifically the time back to the MRCA and the geographic place that the MRCA lived. If the sample of individuals includes adequate representation from across all of humanity, then these inferences tell us about the MRCA of our species. It is important to keep in mind that such methods work back only as far as the MRCA. Because of the coalescent process, all genetic variation coalesces to the MRCA and thus no information is available for population history before this individual ( Templeton, 2005 ).
Early work on gene trees focused on mitochondrial DNA (mtDNA), inherited strictly from one's mother. The maternal haploid inheritance of mtDNA means that recombination is not a problem and one can reconstruct the gene tree. The pioneering application to the modern human origins debate was analysis of mtDNA by Cann et al. (1987) , where they found evidence that the MRCA lived in Africa roughly 200 000 years ago. The idea of a common female ancestor of humanity led, perhaps inevitably, to this ancestor being given the name ‘Eve’. Although there were some methodological concerns with their analyses, later analyses confirmed the recent African origin of humanity's most recent common mitochondrial ancestor ( Relethford, 2001b ).
Both the location (Africa) and the date (200 000 years ago) of our common mtDNA ancestor have been argued as support for the African replacement model of modern human origins. The date was considered too recent to be compatible with the multiregional model, which argued for a common ancestor dating back to Africa close to 2 Myr ago. Although the observation of a recent mtDNA ancestor is certainly compatible with the African replacement model, compatibility does not automatically imply proof ( Relethford, 2001b ; Templeton, 2007 ). Compatibility with a given model only constitutes proof of a model when the observed data are incompatible with all other models. In this regard, it is important to keep in mind that any gene tree tells us only about the MRCA for that particular DNA marker. Different DNA markers will have different MRCAs, a reason why the ancestral female detected from the mitochondrial analyses is often referred to by the qualifying name ‘mitochondrial Eve’. She tells us about our common mitochondrial ancestor, and nothing about other parts of our genome. Because of difference in mutation rates and genetic drift, different DNA markers may have different evolutionary histories. As such, evolutionary inferences based on one DNA marker do not necessarily apply to others, and multiple loci must be examined in order to begin to build a picture of a population's history ( Templeton, 2005 ). In addition, potential problems such as recurrent mutation make an exclusive focus on mtDNA alone problematic. More loci are needed.
In addition to mtDNA, gene trees have now been constructed using Y-chromosome DNA (thus sampling paternal ancestry) and a number of nuclear autosomal DNA regions that show little or no recombination. The results vary, with some showing recent African ancestry and some showing more ancient African ancestry. Most, but not all, gene trees show an African root ( Takahata et al., 2001 ). In recent years, more effort has been made to look at the question of modern human origins using multiple-locus comparisons. The most comprehensive of these analyses has been performed by Templeton (2005 , 2007) who examined 25 DNA regions: mtDNA, Y chromosome DNA, 11 X-linked markers and 12 autosomal markers using a 6-Myr-old date for the human–chimpanzee divergence for calibration. Using a method known as nested-clade phylogeographic analysis, Templeton found that 15 of these markers showed evidence of geographic expansion. The estimated ages of range expansion vary significantly across these markers and do not fit a model of a single expansion, but instead cluster into three groups: (1) an expansion out of Africa 1.9 Myr ago (95% CI=0.99–3.10 Myr), (2) an expansion out of Africa 650 000 years ago (95% CI=390 000–970 000 years ago) and (3) an expansion out of Africa 130 000 years ago (95% CI=9600–169 000 years ago).
The three out-of-Africa expansions detected in Templeton's multilocus analysis correlate temporally in an interesting way with the fossil record ( Relethford, 2007 ). The earliest range expansion corresponds to the initial appearance and dispersal out of Africa of H. erectus . The second range expansion corresponds to a rapid increase in cranial capacity that took place about 700 000 years ago and overlaps with the appearance of H. heidelbergensis . The third and most recent out-of-Africa expansion corresponds to dates suggested for the dispersal of anatomically modern humans. Given the large confidence intervals typical of coalescent analysis, this correspondence should be taken as suggestive and not conclusive, but the apparent congruence of the fossil and genetic records is interesting and deserves continued attention, particularly as data on more low-recombination DNA regions become available.
The genetic evidence for three out-of-Africa expansions, each possibly associated with events in the fossil record corresponding to the appearance of a new species, might be taken as support for a broader picture of speciation within the genus Homo , of which the origin of anatomically modern humans is but the latest speciation event. However, although the most recent expansion (169 000 years ago) is similar to those often reported in the literature in support of an African replacement model, Templeton argues that the genetic evidence of earlier expansions rejects the hypothesis of a total replacement. Gene trees based on living humans will only pick up evidence from the ancestors of living humans, and if we are all descended from a single recent (<200 000 years) expansion out of Africa, then there should be no evidence of any earlier expansions. In other words, speciation and complete replacement should erase any genetic evidence for earlier speciation ( Templeton, 2005 , 2007 ). If confirmed, evidence of multiple expansions might point to a model of human evolution where the transition between one paleospecies and the next occurred through anagenesis. The first expansion, associated with H. erectus , established hominins outside of Africa for the first time. Subsequent expansions may have involved some level of gene flow between new populations and preexisting non-African populations. Of course, the level of gene flow is likely to have varied from time to time and from place to place, and it seems likely that some populations were replaced, but unlikely that this occurred to all populations.
Not everyone agrees with these interpretations. For example, Satta and Takahata (2002) note that although Templeton's results might reflect an African origin with subsequent interbreeding, they might also reflect population structuring within Africa with some populations being more isolated from Eurasia, which could account for high haplotype diversity within Africa as well as the large proportion of gene trees that have African roots. I do not view this possibility as necessarily being incompatible with multiple African expansions and subsequent interbreeding. It is possible that a variety of different models could fit observed patterns of genetic variation.
The genetic evidence: regional differences in genetic diversity
Not all living human populations show the same average level of genetic variability, and these differences in present-day diversity can provide us with inferences about our evolutionary history. DNA markers typically show higher levels of genetic diversity (heterozygosity and nucleotide diversity) in sub-Saharan African populations. This observation has been made for mtDNA ( Cann et al., 1987 ), nuclear microsatellite DNA ( Relethford and Jorde, 1999 ) and Alu insertion markers ( Watkins et al., 2001 ). The same observation has been made on measures of variation from phenotypic traits; within-group variances are highest in sub-Saharan African populations for both craniometric measures ( Relethford and Harpending, 1994 ; Manica et al., 2007 ) and skin color ( Relethford, 2000 ).
Why would one geographic region consistently show higher levels of genetic and phenotypic diversity? One possibility is greater time depth for the accumulation of mutations. The longer a population has been in existence, the greater the number of mutations that will accumulate. Under an African origin model, mutations would accumulate longer in Africa, as any populations dispersing out of Africa would likely be small, and the subsequent founder effect would effectively ‘reset’ the accumulation of mutations in the non-African populations. Thus, a model of an initial African origin followed by dispersals out of Africa at a later point in time would generate the regional differences in genetic and phenotypic diversity that we see today. If correct, the observation of higher African diversity supports the other genetic (and fossil) evidence for an African origin for modern humans, but does not distinguish between an African origin with replacement and an African origin with admixture outside of Africa except to say that if there was any admixture it was not of sufficient magnitude to erase the genetic signature of an African origin.
Furthermore, the fact that the model of accumulated mutations is compatible with the observed genetic data does not mean that it is correct if there are other reasonable interpretations that are also compatible. In the case of genetic diversity, another possible explanation is regional differences in population size, because expected diversity is proportionally related to effective population size. Smaller populations experience more genetic drift and are therefore lower levels of diversity. If the long-term effective population size of Africa were larger throughout most of recent human evolution, then diversity would be greater in Africa than elsewhere, again consistent with our observations of present-day variation. Analyses of craniometric data and microsatellite DNA support this hypothesis ( Relethford and Harpending, 1994 ; Relethford and Jorde, 1999 ). A larger African population is also consistent with archeological and ecological inferences ( Relethford, 2001b ; Eller et al., 2004 ). If higher levels of genetic diversity in sub-Saharan Africa are due to a larger long-term effective population size, then the observation of higher diversity does not provide any resolution about the modern human origins debate. All of the models proposed to date can easily accommodate a larger African population. In this case, genetic data may be telling us more about the demographic, rather than phylogenetic, history of our species.
The genetic evidence: a cline in genetic diversity
Inferences about modern human origins can also be made from the observation that in addition to sub-Saharan Africa having the highest levels of genetic diversity, there is also a geographic pattern in regional diversity. Specifically, genetic diversity outside of Africa tends to be a subset of the diversity within Africa ( Tishkoff et al., 1996 ; Watkins et al., 2001 ). In addition, global analyses of microsatellite DNA markers ( Prugnolle et al., 2005 ; Ramachandran et al., 2005 ) and craniometric traits ( Manica et al., 2007 ) have shown clear patterns of genetic and phenotypic diversity decreasing as the distance from East Africa increases.
This geographic cline in diversity is consistent with an African origin and subsequent dispersal of modern humans out of Africa. The nature of this dispersal is still being debated. For example, Ramachandran et al. (2005) argue that the diversity cline is consistent with a model of serial founding effects out of Africa, whereas Liu et al. (2006) suggest that a model of colonization events with gene flow is more appropriate. Further work also needs to consider the suggestion discussed above for larger African population sizes and, more generally, alternative demographic scenarios that might apply. It is possible that a number of different models can all fit the diversity cline equally well. Furthermore, the fundamental issue of the fate of archaic populations outside of Africa remains. At present, the diversity cline might reflect a genetic signature of an expansion out of Africa, but in my view does not resolve the debate over replacement versus assimilation. Although many analyses have used the diversity cline in support of a replacement model, Eswaran et al. (2005) have provided an expansion model that incorporates assimilation from archaic populations.
The genetic evidence: the estimated number of human ancestors
The relationship between genetic diversity and population size has meant that it is possible to estimate the effective population size of a species. When applied to data from living human populations, such estimates typically suggest a long-term average of 10 000 individuals of reproductive age ( Relethford, 2001b ). Because long-term population size reflects a harmonic mean over time, this figure further suggests that humans have recently expanded from a relatively small number of ancestors, a view that fit with many conceptions of an African replacement model. The reasoning here is that such a small number of individuals could not have been spread out throughout the Old World and still be connected via gene flow, making any sort of multiregional evolution unlikely ( Harpending et al., 1993 ). A small species effective size is thus considered more likely explained in terms of an expansion from a small initial population in Africa. The situation is not that simple, however, as the genetic estimates of population size are effective population sizes that can differ greatly from actual census size and the latter is not a proxy for the former. Effective size can be considerably less than census size. Eller et al. (2004) show that a model of extinction and recolonization of local populations with reasonable parameters could result in a long-term census size of several hundred thousand individuals and still produce an effective population size of about 10 000. In another example, Eswaran et al. (2005) have shown how a wave-of-advance model could also explain low estimates of effective species size even given a larger census size. Of course, the compatibility of a model and data does not necessarily prove the model, but it does show that the initial view of the small species effective size supporting an African replacement is also not necessarily correct.
The genetic evidence: ancient DNA and the fate of the Neandertals
The debate over replacement versus assimilation is nowhere more intense than in continuing discussions of the evolutionary status and ultimate fate of the Neandertals. Questions regarding whether Neandertals should be considered a subspecies of H. sapiens or a separate species ultimately boil down to the question of how much of our ancestry derives from them. Even if they were a biologically separate species, the question of possible genetic ancestry remains because modern humans and Neandertals could have been allotaxa that could hybridize ( Smith et al., 2005 ). What genetic evidence exists for some potential Neandertal ancestry in modern humans?
Although the question of Neandertal ancestry has long been addressed using the fossil record, the past decade has also seen the rapid development of Neandertal genomics as methods of ancient DNA analysis have been applied to Neandertal fossils. The first example of this approach was the pioneering work of Krings et al. (1997) extracting a 378-bp sequence of mtDNA from the original Neandertal specimen. Subsequent analyses of ancient DNA have shown that the results from this study were not a fluke, and short mtDNA sequences have now been extracted from 11 other European Neandertal fossils ( Green et al., 2006 ). As a group, the Neandertal mtDNA sequences are more similar to each other than to living humans. Although it was thought initially that Neandertal mtDNA exhibited relatively low levels of variation ( Krings et al., 2000 ), the accumulation of more specimens suggests their mtDNA diversity might have been greater ( Caramelli et al., 2006 ; Orlando et al., 2006 ).
The differences between Neandertal and living human mtDNA have often been interpreted as support for the view that Neandertals were a separate species that diverged about half a million years ago. This conclusion has not been universally accepted. Although the number of sequence differences between Neandertals and living humans is greater than found among living humans today, this difference is still within the range seen between chimpanzee subspecies, consistent with the view of some that Neandertals were a separate subspecies rather than a separate species ( Relethford, 2001b ).
Regional comparisons of mtDNA have also been used to support the claim that Neandertals were a separate species with little if any hybridization. It has been argued that if there was a genetic contribution of European Neandertals to living Europeans, then the mtDNA of Neandertals should be more similar to mtDNA from living Europeans than to mtDNA from living humans in other geographic regions. Instead, Neandertal mtDNA is genetically equidistant from living humans across the world, an observation taken as evidence that Neandertals were a separate species ( Krings et al., 1997 ). The accuracy of the underlying assumption of this argument, however, depends on rates of gene flow and the age of the Neandertal specimens, and it is possible for Neandertals to have contributed more genetically to living Europeans, but over time this regional difference could have diminished because of interregional gene flow ( Relethford, 2001a ).
The major finding from Neandertal mtDNA that supports the hypothesis that the Neandertals were not part of our ancestry is the fact that no Neandertal mtDNA sequences have been found among living humans. In addition, studies to date of ancient DNA from anatomically modern humans show genetic differences from Neandertal mtDNA ( Caramelli et al., 2003 ; Serre et al., 2004 ). This noticeable genetic difference can be explained in two ways. First, it is possible that there are no surviving Neandertal mtDNA sequences in our species because the Neandertals became extinct and did not contribute to our ancestry. A second possibility is that the Neandertals were part of our ancestry but their specific mitochondrial haplotypes were lost over time due to genetic drift. The question of Neandertal ancestry has now become a question of the probability of haplotype survival, and as such is dependent on the parameters used to determine these probabilities, including population sizes, rates of gene flow and duration of contact between Neandertals and modern humans in Europe. Studies to date have suggested that the total possible amount of Neandertal ancestry for living humans was not very large, but it has not yet been possible to distinguish between a model of some limited Neandertal ancestry and a model of no Neandertal ancestry ( Currat and Excoffier, 2004 ; Serre et al., 2004 ).
Although samples of Neandertal mtDNA are accumulating, there are obvious limitations on exactly how much we can learn from mtDNA, which essentially represents but a single locus. One of the most exciting developments in recent months has been technological advances that have allowed the sequencing of nuclear DNA. Two different methods were applied to a Neandertal fossil from Vindija Cave, Croatia that dates back to 38 000 years ago. One study ( Noonan et al., 2006 ) sequenced 62 500 base pairs and the other study ( Green et al., 2006 ) sequenced over one million base pairs. These initial studies confirm the genetic distinctiveness of Neandertals relative to living humans and suggest that the lineages diverged about 500 000–700 000 years ago. The question of gene flow and ancestry remains uncertain at present. Noonan et al. (2006) looked for derived alleles at low frequencies in living Europeans that matched the derived alleles found in the Neandertal sequence in order to estimate the amount of Neandertal ancestry in living humans. Their maximum-likelihood estimate was 0% suggesting complete extinction of the Neandertals, but had a wide enough confidence interval (0–20%) so that some limited admixture could not be ruled out. The analysis of Green et al. (2006) used a different approach by comparing the DNA sequences of the Neandertal specimen, living humans and chimpanzees, with chimpanzees representing the ancestral state of different single nucleotide polymorphisms (SNPs) and living humans representing the derived state. Derived alleles found in both living humans and the Neandertal specimen represent alleles that existed in the last common ancestor of modern humans and Neandertals. They found that the Neandertal sequence had the derived allele in over 30% of the SNPs, a figure they argue is too large to be compatible with a simple model where the lineages leading to Neandertals and modern humans diverged and then remained completely isolated, thus arguing for some subsequent gene flow between them.
In addition to the need for larger samples, the problem of possible contamination from modern humans in ancient DNA analysis needs to be considered. For example, Wall and Kim (2007) investigated differences between the Green et al. and Noonan et al. studies, and suggested a possibility that these differences may have been due to errors in the former. Thus, results from nuclear DNA analyses should be considered tentative and suggestive rather than conclusive. The nuclear DNA data at present do not convincingly argue for or against admixture between Neandertals and modern humans. Further analysis, combined with the possibility of a complete Neandertal genome in the near future ( Dalton, 2006 ) may provide more definitive answers regarding possible Neandertal admixture.
The evidence from ancient DNA, both mitochondrial and nuclear, must be considered alongside the fossil evidence regarding the fate of the Neandertals. As a group, the Neandertals are extinct and have been perhaps as long as 28 000 years. The real question is the nature of their disappearance. Although the idea of replacement by modern humans, presumably better adapted biologically and/or culturally, has been a popular conclusion, closer examination of the fossil evidence, combined with genetic analysis, suggests that the situation may not be that straight forward. The earliest modern humans in Europe show the presence (though at reduced frequency) of unique Neandertal traits, a pattern that is not expected under a model of complete replacement ( Smith et al., 2005 ). These traits become less common over time, and are often absent in living Europeans, suggesting that over time the Neandertals become extinct through ‘swamping’ genetically of larger population of modern humans moving into Europe ( Relethford, 2001b ; Smith et al., 2005 ). Under this model, the Neandertal gene pool was assimilated rather than replaced. Therefore, the overall ancestral contribution of Neandertals to living modern humans may be very small, a suggestion consistent with analyses of both mtDNA ( Serre et al., 2004 ), as well as patterns of linkage disequilibrium in living humans ( Plagnol and Wall, 2006 ). A continuing challenge is to develop methods capable of distinguishing between a model of very low Neandertal ancestry and a model of no Neandertal ancestry.
The genetic evidence: adaptive genetic introgression
Both the fossil and genetic evidence support an initial origin of anatomically modern humans in Africa 200 000 years ago followed by dispersal across the Old World. The primary debate at this point is the extent to which earlier archaic humans living outside of Africa contributed to our species' ancestry. The discussion this far has been on the total amount of archaic ancestry as inferred from neutral loci and traits, and thus reflects the genetic impact of genetic mixture. At least in the case of the Neandertals of Europe, DNA analysis suggests that this mixture was very low and possibly zero. Consequently, it is tempting to suggest that the debate over very low mixture or no mixture is of little import because even if present in small amounts, such mixture would have had little evolutionary impact ( Currat and Excoffier, 2004 ). Although this is certainly the case for neutral loci, the situation could be rather different when considering the additional impact of natural selection.
Genetic introgression occurs when an allele is introduced from one group (a species or subspecies, for example) into another at a low initial frequency. Introgression can have a significant impact if the newly introduced allele is favored through natural selection. Hawks and Cochran (2006) review examples of such adaptive introgression in plants and animals and make the case that the genetic introgression of ‘archaic’ alleles into the gene pool of an expanding modern human population can explain why some analyses (both on fossils and genetic data) show evidence of ancient mixture and others do not. It is possible that an expanding modern human population demographically and genetically ‘swamped’ the contributions from archaic populations outside of Africa for the most part, but some alleles persisted because of natural selection. Hawks and Cochran (2006) argue that adaptive introgression might have had a major impact on the evolution of modern humans, and outline some possible avenues for future research to test this hypothesis.
One possible example has been suggested by Evans et al. (2006) , who proposed that the microcephalin ( MCPH1 ) gene is an example of adaptive introgression in our species from an archaic human source. They found that haplogroup D of this locus has a high frequency in living humans (70%) but a fairly recent coalescent date of roughly 37 000 years ago. Their analysis further shows support for the following scenario. Two lineages of the genus Homo diverged roughly 1.1 Myr ago, and the lineage leading to H. sapiens experienced fixation of the non-D haplogroup and the D haplogroup became fixed in the other lineage. At about 37 000 years ago, the D haplogroup was introduced through an interbreeding event, and the frequency of this introgressed allele increased rapidly because of positive selection, perhaps relating to changes in brain size or function. Evans et al. argue that the only other demographic scenario that could explain the genetic history of MCPH1 gene, the complete mixture of the two initially diverged lineages, should result in similar genetic histories across the genome, which does not appear to be the case.
There are several problems with the suggestion that the MCPH1 gene is an example of adaptive introgression. Studies have found no association between the normal variants of the gene and brain size ( Woods et al., 2006 ) or intelligence test scores ( Mekel-Bobrov et al., 2007 ). In addition, Currat et al. (2006) have suggested that the patterns of variation in MCPH1 can instead be explained by a demographic model of African origin and expansion rather than adaptive introgression. Although the MCPH1 gene may not prove to be a good example, adaptive introgression is still a viable explanation for suggestions of archaic influence on the modern genome ( Hawks and Cochran, 2006 ) and is a worthwhile area for further research. What will be needed in such research is demonstration that selection accounts for patterns of diversity, rather than a neutral model, and demonstration of a selective advantage for the introgressed allele.
Conclusions
Although the modern human origins debate continues, there are signs of agreement on some general points, and it is clear that genetic data, from both living populations and from ancient DNA, have played a major role. Both geneticists and paleoanthropologists have increasingly accepted an initial African origin of modern humans. At present, the fossil evidence points to an earlier appearance of modernity in Africa than elsewhere, and a variety of genetic analyses also support an initial African origin, although this pattern is not as clear because of the strong possibility of a larger long-term effective population size in Africa and its subsequent effects on patterns of genetic variation. Although debate continues, there is also growing realization that while Africa may have been the primary source of our ancestry, it might not be the only one ( Templeton, 2005 , 2007 ). Elsewhere I have suggested that modern human ancestry can be described as mostly, but not exclusively, out of Africa ( Relethford, 2001b ). Much recent research has been directed at the quantification of how much non-African archaic ancestry exists. Some recent syntheses of the fossil record and genetic evidence make a strong case for the assimilation of non-African archaic genes into the modern human gene pool ( Smith et al., 2005 ). Although the analysis of ancient mtDNA has suggested that there was little genetic input in Europe (the Neandertals), it is possible that some genes might reflect greater archaic ancestry because of adaptive introgression. These populations became extinct, but may have left some genetic legacy behind. Continued analysis of the human genome for evidence of recent selection and the possibility of a Neandertal genome, combined with continued improvements in the fossil record, promise to make the coming decades an exciting time for modern human origins research.
Cann RL, Stoneking M, Wilson AC (1987). Mitochondrial DNA and human evolution. Nature 325 : 31–36.
Article CAS Google Scholar
Caramelli D, Lalueza-Fox C, Condemi S, Longo L, Milani L, Manfredini A et al . (2006). A highly divergent mtDNA sequence in a Neandertal individual from Italy. Curr Biol 16 : R630–R632.
Caramelli D, Lalueza-Fox C, Vernes C, Lari M, Casoli A, Mallegni F et al . (2003). Evidence for a genetic discontinuity between Neandertals and 24 000-year-old anatomically modern Europeans. Proc Natl Acad Sci USA 100 : 6593–6597.
Conroy GC (2005). Reconstructing Human Origins , 2nd edn WW Norton: New York.
Google Scholar
Currat M, Excoffier L (2004). Modern humans did not admix with Neanderthals during their range expansion into Europe. PLoS Biol 2 : e421.
Article Google Scholar
Currat M, Excoffier L, Maddison W, Otto SP, Ray N, Whitlock MC et al . (2006). Comment on ‘Ongoing adaptive evolution of ASPM , a brain size determinant in Homo sapiens ’ and ‘ Microcephalin , a gene regulating brain size, continues to evolve adaptively in humans’. Science 313 : 172a.
Dalton R (2006). Neanderthal genome sees first light. Nature 444 : 254.
Duarte C, Maurício J, Pettitt PB, Souto P, Trinkaus E, van der Plicht H et al . (1999). The early Upper Paleolithic human skeleton from the Abrigo do Lagar Velho (Portugal) and modern human emergence in Iberia. Proc Natl Acad Sci USA 96 : 7604–7609.
Eller E, Hawks J, Relethford JH (2004). Local extinction and recolonization, species effective population size, and modern human origins. Hum Biol 76 : 689–709.
Eswaran V, Harpending H, Rogers AR (2005). Genomics refutes an exclusively African origin of humans. J Hum Evol 49 : 1–18.
Evans PD, Mekel-Brown N, Vallender EJ, Hudson RR, Lahn BT (2006). Evidence that the adaptive allele of the brain size gene microcephalin introgressed into Homo sapiens from an archaic Homo lineage. Proc Natl Acad Sci USA 103 : 18178–18183.
Green RE, Krause J, Ptak SE, Briggs AW, Ronan MT, Simons JF et al . (2006). Analysis of one million base pairs of Neanderthal DNA. Nature 444 : 330–336.
Harpending HC, Sherry ST, Rogers AR, Stoneking M (1993). The genetic structure of ancient human populations. Curr Anthropol 34 : 483–496.
Hawks J, Cochran G (2006). Dynamics of adaptive introgression from archaic to modern humans. PaleoAnthropology 2006 : 101–115.
Krings M, Capelli C, Tschentscher F, Geisert H, Meyer S, von Haessler A et al . (2000). A view of Neandertal genetic diversity. Nat Genet 26 : 144–146.
Krings M, Stone A, Schmitz RW, Krainitzki H, Stoneking M, Pääbo S (1997). Neandertal DNA sequences and the origin of modern humans. Cell 90 : 19–30.
Liu H, Prugnolle F, Manica A, Balloux F (2006). A geographically explicit genetic model of worldwide human-settlement history. Am J Hum Genet 79 : 230–237.
Manica A, Amos W, Balloux F, Hanihara T (2007). The effect of ancient population bottlenecks on human phenotypic variation. Nature 448 : 346–348.
McDougall I, Brown FH, Fleagle JG (2005). Stratigraphic placement and age of modern humans from Kibish, Ethiopia. Nature 443 : 733–736.
Mekel-Bobrov N, Posthuma D, Gilbert SL, Lind P, Gosso MF, Luciano M et al . (2007). The ongoing adaptive evolution of ASPM and Microcephalin is not explained by increased intelligence. Hum Mol Genet 16 : 600–608.
Noonan JP, Coop G, Kudaravalli S, Smith D, Krause J, Alessi J et al . (2006). Sequencing and analysis of Neandertal genomic DNA. Science 314 : 1113–1118.
Orlando L, Darlu P, Toussaint M, Bonjean D, Otte M, Hänni C (2006). Revisiting Neandertal diversity with a 100 000 year old mtDNA sequence. Curr Biol 16 : R400–R402.
Plagnol V, Wall JD (2006). Possible ancestral structure in human populations. PLoS Genet 2 : e105.
Prugnolle R, Manica A, Balloux F (2005). Geography predicts neutral genetic diversity of human populations. Curr Biol 15 : R159–R160.
Ramachandran S, Deshpande O, Roseman CC, Rosenberg NA, Feldman MW, Cavalli-Sforza LL (2005). Support for the relationship of genetic and geographic distance in human populations for a serial founder effect originating in Africa. Proc Natl Acad Sci USA 102 : 15942–15947.
Relethford JH (2000). Human skin color diversity is highest in sub-Saharan African populations. Hum Biol 72 : 773–780.
CAS PubMed Google Scholar
Relethford JH (2001a). Absence of regional affinities of Neandertal DNA with living humans does not reject multiregional evolution. Am J Phys Anthropol 115 : 95–98.
Relethford JH (2001b). Genetics and the Search for Modern Human Origins . Wiley-Liss: New York.
Relethford JH (2007). Population genetics and paleoanthropology. In: Henke W, Tattersall I (eds). Handbook of Paleoanthropology Principles, Methods and Approaches vol. 1 , Springer-Verlag: Berlin, pp 621–641.
Chapter Google Scholar
Relethford JH, Harpending HC (1994). Craniometric variation, genetic theory, and modern human origins. Am J Phys Anthropol 95 : 249–270.
Relethford JH, Jorde LB (1999). Genetic evidence for larger African population size during recent human evolution. Am J Phys Anthropol 108 : 251–260.
Satta Y, Takahata N (2002). Out of Africa with regional interbreeding? Modern human origins. BioEssays 24 : 871–875.
Serre D, Langaney A, Chech M, Teschler-Nicola M, Paunovic M, Mennecier P et al . (2004). No evidence of NEANDERTAL mtDNA contribution to early modern humans. PLoS Biol 2 : 313–317.
Smith FH, Jankoviæ I, Karavaniæ I (2005). The assimilation model, modern human origins in Europe, and the extinction of the Neandertals. Quaternary Intern 137 : 7–19.
Stringer C, Andrews P (2005). The Complete World of Human Evolution . Thames & Hudson: London.
Takahata N, Lee S-H, Satta Y (2001). Testing multiregionality of modern human origins. Mol Biol Evol 18 : 172–183.
Tattersall I, Schwartz JH (1999). Hominids and hybrids: the place of Neanderthals in human evolution. Proc Natl Acad Sci USA 96 : 7117–7119.
Templeton AR (2005). Haplotype trees and modern human origins. Yrbk Phys Anthropol 48 : 33–59.
Templeton AR (2007). Genetics and recent human evolution. Evolution 61 : 1507–1519.
Tishkoff SA, Dietzsch E, Speed W, Pakstis AJ, Kidd JR, Cheung K et al . (1996). Global patterns of linkage disequilibrium at the CD4 locus and modern human origins. Science 271 : 1380–1387.
Wall JD, Kim SK (2007). Inconsistencies in Neanderthal genomic DNA sequences. PLoS Genet 3 : e175.
Watkins WS, Ricker CE, Bamshad MJ, Carroll ML, Nguyen SV, Batzer MA et al . (2001). Patterns of ancestral human diversity: an analysis of Alu -insertion and restriction-site polymorphisms. Am J Hum Genet 68 : 738–752.
White TD, Asfaw B, DeGusta D, Gilbert H, Richards GD, Suwa G et al . (2003). Pleistocene Homo sapiens from Middle Awash, Ethiopia. Nature 423 : 742–747.
Wolpoff MH, Hawks J, Caspari R (2000). Multiregional, not multiple origins. Am J Phys Anthropol 112 : 129–136.
Wolpoff MH, Wu X, Thorne AG (1984). Modern Homo sapiens origins: a general theory of hominid evolution involving the fossil evidence from East Asia. In: Smith FH, Spencer F (eds). The Origins of Modern Humans: A World Survey of the Fossil Evidence . Alan Liss: New York. pp 411–483.
Woods RP, Freimer NB, De Young JA, Fears SC, Sicotte NL, Service SK et al . (2006). Normal variants of Microcephalin and ASPM do not account for brain size variability. Hum Mol Genet 15 : 2025–2029.
Download references
Author information
Authors and affiliations.
Department of Anthropology, State University of New York College at Oneonta, Oneonta, NY, USA
J H Relethford
You can also search for this author in PubMed Google Scholar
Corresponding author
Correspondence to J H Relethford .
Rights and permissions
Reprints and permissions
About this article
Cite this article.
Relethford, J. Genetic evidence and the modern human origins debate. Heredity 100 , 555–563 (2008). https://doi.org/10.1038/hdy.2008.14
Download citation
Received : 05 September 2007
Revised : 25 January 2008
Accepted : 01 February 2008
Published : 05 March 2008
Issue Date : June 2008
DOI : https://doi.org/10.1038/hdy.2008.14
Share this article
Anyone you share the following link with will be able to read this content:
Sorry, a shareable link is not currently available for this article.
Provided by the Springer Nature SharedIt content-sharing initiative
- modern human origins
- population genetics
- paleoanthropology
This article is cited by
Species are, at the same time, kinds and individuals: a causal argument based on an empirical approach to species identity.
- Elena Casetta
- Davide Vecchi
Synthese (2021)
RETRACTED ARTICLE: Early Pioneers of the Americas: The Role of the Olmecs in Urban Education and Social Studies Curriculum
- Greg Wiggan
- Annette Teasdell
- Sheikia Talley-Matthews
The Urban Review (2020)
Syncope in Malaysian populations
- Robert S. Sheldon
Clinical Autonomic Research (2020)
Relationship between mitochondrial haplogroup and seasonal changes of physiological responses to cold
- Takayuki Nishimura
- Shigeki Watanuki
Journal of Physiological Anthropology (2014)
Comparing models on the genealogical relationships among Neandertal, Cro-Magnoid and modern Europeans by serial coalescent simulations
- E M S Belle
- G Barbujani
Heredity (2009)
Quick links
- Explore articles by subject
- Guide to authors
- Editorial policies

April 1, 1992
The Multiregional Evolution of Humans
Both fossil and genetic evidence argues that various human groups arose where they are found today
By Alan G. Thorne & Milford H. Wolpoff
- Search Menu
- Advance Articles
- Virtual Issues
- High-Impact Research Collection
- Celebrate 40 years of MBE
- Perspectives
- Discoveries
- Cover Archive
- Brief Communications
- Submission site
- Author guidelines
- Open access
- Self-archiving policy
- Reasons to submit
- About Molecular Biology and Evolution
- About the Society for Molecular Biology and Evolution
- Editorial Board
- Advertising and Corporate Services
- Journals Career Network
- Journals on Oxford Academic
- Books on Oxford Academic
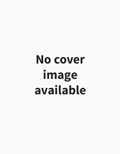
Article Contents
Introduction, materials and methods, literature cited.
- < Previous
Testing Multiregionality of Modern Human Origins
- Article contents
- Figures & tables
- Supplementary Data
Naoyuki Takahata, Sang-Hee Lee, Yoko Satta, Testing Multiregionality of Modern Human Origins, Molecular Biology and Evolution , Volume 18, Issue 2, February 2001, Pages 172–183, https://doi.org/10.1093/oxfordjournals.molbev.a003791
- Permissions Icon Permissions
In order to examine the possibility of multiple founding populations of anatomically modern Homo sapiens, we collected DNA sequence data from 10 X-chromosomal regions, 5 autosomal regions, and 1 Y-chromosomal region, in addition to mitochondrial DNA. Except for five regions which are genealogically uninformative and two other regions for which chimpanzee orthologs are not available, the ancestral sequence and population for each of the remaining regions were successfully inferred. Of these 10 ancestral sequences, 9 occurred in Africa and only 1 occurred in Asia during the Pleistocene. Computer simulation was carried out to quantify the multiregional hypothesis based solely on the premise that there was more than one founding population in the Pleistocene. Allowing the breeding size to vary among the founding populations, the hypothesis may account for the observed African ancestry in 90% of the genomic regions. However, it is required that the founding population in Africa was much larger than that outside Africa. Likelihood estimates of the breeding sizes in the founding populations were more than 9,000 in Africa and less than 1,000 in outside of Africa, although these estimates can be much less biased at the 1% significance level. If the number of African ancestral sequences further increases as more data accumulate in other genomic regions, the conclusion of a single founding population of modern H. sapiens is inevitable.
The tempo and mode of evolution of Homo are in sharp contrast to those of earlier hominids in Africa (for a review, see Tattersall 2000 ). Evolution of australopithecines appears to be relatively slow and gradual. For example, the cranial capacity increased only slightly during the 3-Myr history of australopithecines. Something unusual happened in hominids in the Late Pliocene, about 2 MYA. Cranial capacity dramatically increased from 475 cm 3 to 860 cm 3 , and body weight was doubled. There were other interrelated modifications. Almost immediately afterward, the first hominid of essentially modern body form emerged ( Hawks et al. 2000 ). It is represented by 1.9-Myr-old Homo ergaster (African Homo erectus ) KNM-ER 3228 and WT 15000 in Eastern Africa, whose cranial capacity was about 1,000 cm 3 . It is their direct descendants who first migrated out of Africa 1–2 MYA and populated Europe, ex-Soviet Georgia, Java, China, and perhaps Japan by 500,000 years ago.
It is generally accepted that anatomically modern Homo sapiens appeared 130,000–150,000 years ago. However, the origin and evolution of H. sapiens have not been fully recovered by the fossil record and have attracted much attention, particularly since the pioneer work on human mitochondrial (mt) DNAs and the proposal of the replacement hypothesis by Cann, Stoneking, and Wilson (1987) . A number of population genetics analyses have been carried out, including sequence analyses of mtDNA ( Vigilant et al. 1991 ; Horai et al. 1995 ; Krings et al. 1997, 1999 ) and genomic regions of the haploid Y chromosome ( Jobling 1994 ; Hammer 1995 ; Ruiz Linares et al. 1996 ; Hammer et al. 1997, 1998 ; Quintana-Murci et al. 1999 ), as well as those of the haplo-diploid X chromosome ( Nachman et al. 1998 ; Zietkiewicz et al. 1998 ; Anagnostopoulos et al. 1999 ; Harris and Hey 1999 ) and diploid autosomes ( Harding et al. 1997 ; Clark et al. 1998 ; Nickerson et al. 1998 ; Jin et al. 1999 ). The gene genealogy inferred from these genetic data generally exceeds the tenure of modern H. sapiens and goes back to the Pleistocene ( Takahata 1993 ; Hammer 1995 ). Although all of these DNA sequence data appear to favor the uniregionality (single-origin) of modern H. sapiens ( Takahata 1995 ; Jorde, Bamshad, and Rogers 1998 ; Jorde et al. 2000 ; Przeworski, Hudson, and Di Rienzo 2000 for reviews), no consensus has yet been reached ( Harris and Hey 1999 ; Hawks et al. 2000 ; Yu and Li 2000 ).
The purpose of this paper is to quantify an alternative hypothesis for the origin of modern H. sapiens, the multiregional hypothesis proposed by Wolpoff, Wu, and Thorne (1984) . This hypothesis is an extension of that of Weidenreich (1946) , which allows gene exchanges among multiple founding populations of modern H. sapiens. However, it has been difficult to test the multiregional hypothesis because of ambiguity in the assumed population structure ( Takahata and Klein 1998 ). In this paper, we draw several predictions solely from the very premise of multiple founding populations and examine whether these predictions are compatible with the pattern and degree of DNA polymorphism observed in various genomic regions. We collected all nucleotide sequences relevant to this end. To make our argument quantitative, we performed computer simulation based on a population genetics model for the multiregional origin of modern H. sapiens.
Predictions
The multiregional hypothesis posits that anatomically modern H. sapiens emerged in multiple founding populations that had existed worldwide since hominids first migrated out of Africa ( fig. 1 a ). We assume that these founding populations were established 1–2 MYA and had given rise to anatomically modern H. sapiens by 130,000–150,000 years ago. We designate by T d the period of the founding populations between 130,000–150,000 years ago and 1–2 MYA. We also assume that these founding populations evolved as a single evolutionary unit and at the same time independently developed regional continuity in some morphological characters. For regional continuity to develop, individual founding populations must be somehow genetically isolated from each other. However, in the complete absence of gene exchange (gene migration or gene flow) among founding populations, the whole population cannot be a single evolutionary unit in the long run. On the other hand, in the presence of frequent gene flow, it would be difficult to maintain regional continuity. This apparent contradiction may be reconciled in the following way. Because these two features are likely represented by different morphological characters, we assume that underlying genes can be different from each other and subject to different forms of natural selection. Of particular interest are two kinds of genes under natural selection. One kind comprises genes that are responsible for the single evolutionary unit and can produce advantageous mutations irrespective of regionality ( Takahata 1993 ; Nei 1995 ). They are globally advantageous. The other kind comprises genes that are responsible for regional continuity and can produce mutations adaptive to particular founding populations, but are deleterious elsewhere. Were they not deleterious but selectively neutral elsewhere, locally advantageous mutations would be fixed in the original founding population and continue to propagate copy genes to neighboring populations by migration. The end result would be fixation of such mutations in the whole population, and this could not contribute to regional continuity. Locally advantageous mutations might be exemplified by genes involved in skin pigmentation ( Cavalli-Sforza, Menozzi, and Piazza 1994 ). A third kind comprises genes that are selectively neutral everywhere.
The assumption that globally advantageous mutations are so rare that independent parallel occurrences are hardly expected seems reasonable. If this is not the case, we can invoke parallelism among the founding populations as assumed by the candelabra hypothesis ( Coon 1962 ). Globally advantageous mutations can occur in any founding population and spread out by gene flow. Fixation within founding populations is a rapid process. However, the spread over the whole population is regulated by gene flow, so the level should not be extremely low. In this situation, Wright's (1931) fixation index F ST to measure local differentiation becomes so small that there may be little or no variation within and between current populations. If such an advantageous mutation occurred relatively recently, the allelic genealogy would be correspondingly short ( fig. 2 a ), and the population of the most recent common ancestor (MRCA) would be the birthplace of the mutation. An important point is that the birthplace can be any of the founding populations. It is conceivable that the birthplace is determined by the relative breeding size, since the number of new mutations per generation in a population is in proportion to its size. Even under these circumstances, there is no reason to think that the MRCA is restricted to only one particular founding population.
Locally advantageous mutations may also be rare, but it is assumed that they can gradually accumulate in founding populations to establish regional continuity. Obviously, these mutations should occur independently in individual founding populations. A noticeable difference between globally and locally advantageous mutations is that since migrants of locally advantageous mutations are deleterious in recipient founding populations, the direct lines of descent of each mutation tend to be restricted in the birthplace. Conversely, the allelic genealogy is traced back to before the occurrence of a locally advantageous mutation. There are two situations for such an ancestral gene lineage. Either the ancestral gene is already locally adapted to some extent, or it is still nonadaptive. In the former situation, the ancestral gene lineage in one founding population must have been prevented from coalescing with gene lineages in other founding populations. In this situation, the MRCA may well go back to the ancestral population that was common to all founding populations. In the latter situation, migrants are selectively neutral, so coalescence is expected to be faster than that in the former situation. Nonetheless, such coalescence must be slower than that of unconditionally neutral genes, so the MRCA tends to be found also in the ancestral population ( fig. 2 b ). As a result, locally advantageous mutations show deep allelic genealogies and large F ST values.
With gene flow, purely neutral genes may not show extensive local differentiations. The F ST value would be moderately small, and the gene genealogy would be intermediate in its timescale compared with that expected for globally and locally advantageous genes ( fig. 2 c ). Again, the birthplace of the MRCA of neutral genes is not unique. We do not know how often or where in the genome these three types of mutations occur. However, under multiregionality, we can make some important predictions largely irrespective of the kind of genes. Most importantly, the MRCA that is found during the T d period should not be restricted to any particular founding population. Second, the time back to the MRCA (TMRCA) may vary more greatly from locus to locus than that expected under a single randomly mating population. Third, the shorter the TMRCA, the smaller the F ST .
By definition, the uniregional, or replacement, hypothesis posits that only one population during the T d period founded modern humans ( fig. 1 b ). Advantageous genes transformed the founding population of H. ergaster or H. erectus to modern H. sapiens via archaic Homo. Local adaptation in modern H. sapiens is assumed to have occurred during its tenure. As a result, the MRCA of locally advantageous genes is comparably recent. The genealogy of selectively neutral genes is even shorter than that of locally advantageous genes under the multiregional hypothesis, but it is deeper than that of globally advantageous genes. Importantly, the MRCA under the uniregional hypothesis must always be traced back to the single founding population, although this trace may be somewhat obscured by random distribution of the ancestral polymorphism into descendant populations, as well as gene flow between them, if present. In general, we do not know a priori which current local populations are more representative of the founding population or how polymorphism in the founding population was sorted out in individual local populations. Nevertheless, should we find such a population specificity in the genealogy at a number of loci, the uniregionality of founding populations appears to be an inevitable conclusion.
Multiregionality may be untenable to population-specific genealogy. If everything is equal regarding the founding populations, there should not be any population specificity. The multiregionality becomes tenable only if one founding population had predominated over the others in terms of the number of breeding individuals. However, emphasizing the overwhelming genetic contribution of only one founding population is equivalent to uniregionality. To be concrete and quantitative, we supposed that during T d ( fig. 1 a ), there were three founding populations, with the relative population sizes being p 1 : p 2 : p 3 , where Σ 3 j =1 p j = 1. If there are n independent genealogies, the probability that we find n 1 , n 2 , and n 3 MRCAs (Σ 3 j =1 n j = n ) would be multinomially distributed with parameters p j . The most likely proportion of the three founding population sizes is given by p̂ j = n j / n ( j = 1, 2, and 3). For n 1 ≥ 1, n 2 ≥ 1, and n 3 = 0, the probability distribution is binomial, and the maximum-likelihood ratio becomes L = ( p 1 / p̂ 1 ) n 1 {(1 − p 1 )/ p̂ 2 } n 2 , since p̂ 3 = 0. The L ratio can be used to set a lower confidence limit for p 1 . For instance, if n 1 = 9 and n 2 = 1, p̂ 1 = 9/10 = 0.9, and the condition of L > 0.01 leads to 0.45 < p 1 . For n 1 = 19 and n 2 = 1, p̂ 1 = 19/20 = 0.95, and the 1% lower confidence limit for p 1 is 0.68. The more MRCAs are found in only one founding population, the stronger the required asymmetry in founding population sizes.
In the following, we focus on three quantities; the time (TMRCA) and place (PMRCA) of the MRCA, and F ST . We estimated TMRCA based on the nucleotide differences within humans relative to those between humans and chimpanzees and assuming their 5 Myr old divergence. This simple method provided estimates similar to those reported in some literature which used the coalescence method of Griffiths and Tavaré (1994 a, 1994 b ). F ST may be estimated according to Wright (1931) or Nei (1973) . We have redefined it as 1 − d w / d , where d w is the average maximum nucleotide difference within local populations, and d is the maximum nucleotide difference in the whole sample taken from all local populations. This redefined F ST value is necessarily nonnegative and has properties similar to those of F ST in Slatkin (1991) .
To infer PMRCA, we first tried the cladistic method of Slatkin and Maddison (1989) . The principle is simple: if we label the sampled sequences in a reconstructed tree by their localities, we may determine the character state in a node by the majority rule. However, it is possible that this method might not work at all if a demography such as that in figure 1 a is not at equilibrium. In the case of little or no gene flow, three population-specific ancestral lineages remain distinct during T d and go back to the common ancestral population. In this situation, the method necessarily predicts that the PMRCA can be any founding population. Only with moderate or high levels of gene flow can the MRCA occur during T d so that the method may identify PMRCA. Another difficulty arises from the fact that the method assumes an accurate phylogeny reconstructed from DNA sequence differences. Actually, this requirement was hardly met in our data, which showed low levels of polymorphism and the presence of recombination or sequence convergence. In a few regions in our data set, the number of phylogenetically informative sites was discouragingly small, and the phylogenetic relationships among sequences became unreliable.
Instead, we used a parsimony method to infer the ancestral state for each nucleotide. If a particular nucleotide of a sample of human sequences was the same as the one in the chimpanzee, the nucleotide was assumed to have remained unchanged after the species divergence and to be the ancestral type. We determined the MRCA sequence as being the closest to the chimpanzee sequence and defined the population that contained this MRCA sequence as the PMRCA. In reality, it is possible that these MRCA sequences are shared by different populations. There are indeed three such genomic regions, as discussed below. In such case, we used the frequency data. Rare haplotypes in a population are likely to be migrants. If there was a large discrepancy in haplotype frequencies between populations, we assumed that the PMRCA was the population that exhibited the higher haplotype frequency.
Computer Simulation
To confirm the predictions in the preceding section, we carried out computer simulation. The simulation model ( fig. 1 a ) assumes that there was a single ancestral population in Africa that gave rise to three descendant populations, which founded modern H. sapiens. These founding populations are assumed to have evolved into the current African, European, and Asian populations. Each founding population may consist of local mating groups being connected by gene flow or undergoing extinction or recolonization. Further subdivision within a founding population increases the effective size, whereas extinction and recolonization by groups within the same founding population decrease the effective size ( Takahata 1993, 1994 ). However, since these effects of within-population subdivision are less important than those among founding populations ( Takahata and Klein 1998 ), we assume that each of the three founding populations is approximately panmictic. There are N j breeding individuals in population j ( j = 1, 2, and 3), and Σ 3 j =1 N j = N T in the entire population. Unless specified, we consider genes in an autosomal region, so there are 2 N j genes in founding population j.
In simulation, we measured time in units of generations. If one generation spans 20 years, T d would be 50,000–100,000 generations. Migration occurs from founding population i to population j with a per-generation rate of m ij . In order to keep N j constant throughout generations, we imposed the condition of N i m ij = N j m ji ; the numbers of immigrants and emigrants between a pair of founding populations were assumed to be the same. We further assumed that N 1 ≥ N 2 = N 3 , where founding populations 1, 2, and 3 are the African, European, and Asian populations, respectively. The African founding population thus assumed to have been no smaller than the European or Asian founding population. Because of these assumptions, there were only three independent parameters among the six m ij s: m 21 = m 31 , m 23 = m 32 , and m 12 = m 13 . In the following simulation, we further assume that m 21 = m 23 , so that when the ratio of N 1 to N 2 and N 3 is specified, only one migration parameter is free to vary. If we designate m 12 = m 13 by m, m 21 = m 31 = m 23 = m 32 = N 1 m / N 2 (= N 1 m / N 3 ). First, we examined the genealogy of k j neutral genes sampled from the current population of breeding size N j . Simulation (with 1,000 repeats) was done backward in time to trace the ancestral lines of the sampled genes under genetic drift and migration. Mutations were not superimposed on the genealogical tree. The FORTRAN simulation program (available on request) is a slight extension of that of Takahata (1989) with respect to migration.
Second, based on the infinite-alleles model ( Kimura and Crow 1964 ), we developed forward simulation programs for globally and locally advantageous mutations (also available on request). For globally advantageous mutations, we recorded when and in which population new mutations occurred. A new mutation always had relative fitness 1 + s over its parent, (1 + s ) 2 over its grandparent, and so on. Mutant offspring of the same parent were assumed to be equally advantageous but mutually neutral. Since fitness exceeded 1, we divided individual fitness by the maximum fitness found within each population at a generation and used the results as relative probabilities for 2 N j genes to be chosen for the next generation. This scheme of selection and genetic drift was applied to all three founding populations. A similar but slightly different fitness scheme can be used for locally advantageous mutations. As long as they remain in the birthplace, they are advantageous in exactly the same way as for globally advantageous mutations. However, once locally advantageous mutations move out by migration, they are assumed to become disadvantageous. For an individual gene lineage within each population, we counted the number of mutations which occurred in that population ( a ), as well as the number of mutations which occurred in the other populations ( b ). The relative fitness of this gene lineage was determined to be (1 + s ) a (1 − s ) b . The relative probability was then computed for simulating selection and genetic drift for the next generation. The selected mutation rate was designated u per gene per generation. To save computer time, we assumed relatively small N j and correspondingly large s, m ij , and u. Because of this scaling, T d was also shortened, but T d = 10 N T was kept throughout to imitate a plausible situation. Each simulation starting with three monomorphic populations was repeated 100 times. As under neutrality, we measured TMRCA, PMRCA, and F ST , where T d = 10 N T generations.
Neutral Mutations
When the number of breeding individuals ( N j ) was the same among the three founding populations ( r = N 1 /( N 2 + N 3 ) = 0.5 in table 1 ) and the migration rate was high ( N 1 m = 10), TMRCA was about 4 N T = 4 Σ 3 j =1 N j, PMRCA was evenly distributed among the three founding populations, and the redefined F ST value was generally greater than that expected from the well-known formula 1/(1 + 4 N 1 m ) ( Wright 1931 ). However, TMRCA was sensitive to N 1 m , and PMRCA was sensitive to r. When N 1 m = 0.1, TMRCA became longer than the history of the founding populations (with the probability of TMRCA > T d being 0.88; table 1 ), so that the MRCA tended to be found in the ancestral population. From this viewpoint, the migration rate under the multiregional hypothesis cannot be as small as N 1 m = 0.1 ( Nei 1995 ; Takahata 1993 ). Only when N 1 m was as large as 1 did the MRCA tend to occur in the T d time period (with the probability of TMRCA ≤ T d being between 0.46 and 0.94). The effect of uneven population sizes on PMRCA became visible when the African founding population ( N 1 ) was twice or three times as large as the European and Asian founding populations ( N 2 + N 3 ). To expect that the MRCA was found exclusively in Africa, the number of breeding individuals in Africa had to be much larger than that in Europe and Asia ( fig. 3 ). Interestingly, however, the sampling effect on PMRCA was small. A small sampling effect on TMRCA is well known in a panmictic population ( Griffiths and Tavaré 1994 a, 1994 b ), but little study on PMRCA has been done in relation to population structure. The present finding suggests that even small samples are useful as long as both TMRCA and PMRCA are taken into account.
Advantageous Mutations
Under the condition N 1 m > 0.1, globally advantageous mutations can sweep out the whole population, with the rate being mainly determined by the mutation rate ( u ) and the selection coefficient ( s ). During T d , there should be approximately 4 N T usT d mutations that can be fixed in the whole population of breeding size N T ( Haldane 1927 ; Kimura 1962 ). We simulated various cases of 4 N T usT d > 1 and 0.1 ≤ N 1 m ≤ 10.0. The result ( fig. 4 a and table 1 ) confirmed our predictions about TMRCA, PMRCA, and F ST . TMRCA was short compared with T d and with that of neutral genes ( table 1 ). F ST was smaller than the corresponding value under neutrality, and PMRCA occurred in proportion to the relative breeding size. Throughout this simulation, we assumed that N T = 600, u = 0.0001, and s = 0.05.
Locally advantageous mutations of N j s > 1 tend to be restricted in the birthplace even under frequent migration. We simulated cases of 4 N j usT d ≫ 1 and 0.1 ≤ N 1 m ≤ 10.0. TMRCA tended to be longer than T d , and F ST was large ( fig. 4 b and table 1 ). Thus, the MRCA almost always went back to the ancestral population. Because of the long separation time among the ancestral gene lineages in different founding populations, a number of mutations can accumulate along each ancestral allelic lineage. This also holds true in tightly linked neutral regions. Throughout this simulation, we assumed N T = 600, u = 0.001, and s = 0.05.
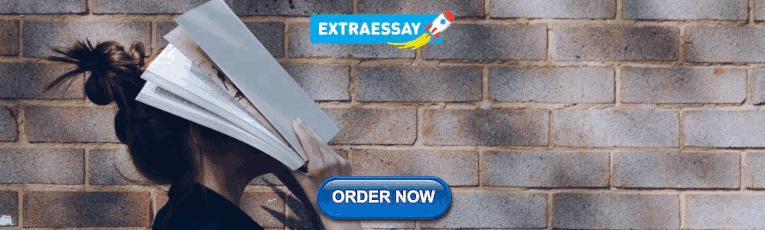
Data Analyses
We examined the currently available DNA sequence data for 10 X-linked and 5 autosomal regions ( table 2 ): Zfx ( Jaruzelska et al. 1999 ), dys44 ( Zietkiewicz et al. 1997, 1998 ; Nachman et al. 1998 ), Pdha1 ( Nachman et al. 1998 ; Harris and Hey 1999 ), Xq13.3 ( Kaessmann et al. 1999 ), Gk, Il2rg, Plp, Hprt, and Ids ( Nachman et al. 1998 ), Xq22 ( Anagnostopoulos et al. 1999 ), Ace ( Rieder et al. 1999 ), a small intergenic region near Mx1 ( Jin et al. 1999 ), Lpl ( Clark et al. 1998 ; Nickerson et al. 1998 ), β-globin ( Harding et al. 1997 ), and Mc1r ( Rana et al. 1999 ). We also included a Y-linked region ( Hammer 1995 ) and an mtDNA control region ( Vigilant et al. 1991 ). Table 2 lists all of these regions, estimates of TMRCA and PMRCA wherever possible, the pattern and degree of nucleotide differences, and F ST .
First, assuming the rate constancy of silent substitutions, we computed the average height of the MRCA within a human sample in terms of nucleotide differences, as well as one half of nucleotide differences over all pairs between human and chimpanzee sequences. The ratio of the former to the latter was the height of the TMRCA relative to the sequence divergence since the human lineage branched off. We multiplied the ratio by the species divergence time of 5 Myr to date the MRCA. The estimates ranged from 0.41 MYA to 1.59 MYA for the 10 X-linked regions ( table 2 ). The estimate was 1.36 MYA for the β-globin region. The estimates for the mtDNA and Y-linked regions were taken from Vigilant et al. (1991) and Hammer (1995) , respectively. Thus, the MRCA for all these regions appears to have existed during the T d period. The estimate of F ST varied from region to region. This variation was in good agreement with the haplo-diploid nature of the regions: lowest for autosomal regions, moderate for X-linked regions, and highest for haploid regions ( Takahata 1993 ). Overall, either gene flow among the founding populations or relatively recent population subdivision must have played significant roles in shaping genetic architecture in the current human population.
Second, based on the parsimony method, which allows polychotomy, we inferred the ancestral sequences and the PMRCAs. There were seven regions to which this method was not applied. Both Il2rg and Ids were monomorphic, Ace and Mx1 lacked chimpanzee orthologs, and the Xq22 sequences were not available. For these five regions, we could not infer the PMRCAs. There were two other regions for which we failed to determine the PMRCAs. In the Hprt region, all three ethnic groups possessed the ancestral-type sequences in similar frequencies, and in the Lpl region, recombination occurred too frequently ( Templeton et al. 2000 ). Figure 5 shows 10 parsimony trees for 9 other regions in which the ancestral sequences (boxed) could be inferred. The PMRCAs at all but Gk loci were determined as Africa. In Gk, there was only one informative site, and the ancestral nucleotide at this site was shared by two Asians and the chimpanzee. We therefore assigned Asia as its PMRCA. For Xq13.3, sequence A (four from Africans and four from Asians) and sequence S (from Biaka Pygmy) were the closest to the chimpanzee sequence, but they differed from each other. Since sequence S branched off earlier than sequence A ( fig. 5 ), we assigned Africa as the PMRCA. The MRCA-type sequences for the Plp, β-globin, and Mc1r regions occurred in more than one population. As mentioned, we used the haplotype frequency data. In each case, the highest frequency (80%, 49%, and 42%) was observed for Africans, so these three PMRCAs were assigned as Africa.
The two data sets for the Pdha1 region by Nachman et al. (1998 ) and Harris and Hey (1999) result in conflicting inferences about the PMRCA. Since the latter encompasses the former, and a larger data set is preferable, we used Harris and Hey (1999) and regarded the Pdha1 region as evidence for the African PMRCA. Here, we would like to mention one issue concerning this region. The sequence analysis showed that there are two distinct lineages, one giving rise to an African-specific haplotype and the other giving rise to the ancestral lineage leading to African as well as non-African variants—some 200,000 years ago. This observation was taken as evidence for multiregionality or as a challenge of uniregionality ( Pennisi 1999 ). As suggested by this, as well as other data ( Yu and Li 2000 ), there might indeed be a strong population subdivision in Africa. However, this is not the issue here. The real issue is whether any non-African population that existed before modern H. sapiens emerged made a significant contribution of genes to the current human population. Unless this is demonstrated, multiregionality is not supported, and the Pdha1 data alone cannot give such evidence.
In any event, there were six X-linked regions ( Plp, Gk, Pdha1, Dys44, Zfx, and Xq13.3), two autosomal regions (β-globin, Mc1r ), and one Y-linked region, as well as mtDNA, in which PMRCAs could be inferred. For these 10 regions, there were 9 African PMRCAs and 1 Asian PMRCA ( table 2 ). If we ignored the Gk region because it contained only one informative site, then all 9 regions favored the African PMRCA, and the 1% confidence limit of p 1 was 0.6. This was significantly greater than 0.45 when the Gk region was included. To be conservative, however, we considered all 10 regions.
The observation of 90% African PMRCAs ( table 2 ) is not easily explained by multiregionality unless the hypothesis assumes that the African founding population ( N 1 ) was much larger than the European and Asian founding populations ( N 2 + N 3 ). With a strong asymmetry in breeding sizes (e.g., r = N 1 /( N 2 + N 3 ) = 50), the F ST value for neutral genes is expected to be large even when the extent of gene flow is sufficiently high ( table 1 ). F ST values as large as >50% are rarely observed ( table 2 ). More importantly, with this strong asymmetry in relative breeding sizes, the genetic contribution of non-African founding populations becomes considerably small. Whereas this conclusion does not deny the existence of multiple founding populations over the world, it does deny that they all made significant genetic contributions to the evolution of modern H. sapiens. This holds true particularly for both globally and locally advantageous genes. In small populations, advantageous mutations become rare, and the effect of natural selection is counterbalanced by that of genetic drift unless selection is strong enough. Thus, neither the single evolutionary unity nor the regional continuity of modern H. sapiens during the T d period is guaranteed if the breeding sizes of some of the founding populations are substantially small.
Assuming neutrality, we carried out fairly extensive simulation to set a lower bound of the African population size. Excluding the cases in which PMRCA went back to the ancestral population ( fig. 1 ), simulation yielded the probability of African PMRCAs for various ratios of r. We then compared this probability with the observed value of 9/10 = 0.9. The probability of 90% African PMRCA is as low as 0.01 even for r = 1.5 and becomes nearly 0.4 for r = 10–50 ( fig. 6 ). These values are slightly different from those discussed based on the binomial distribution. We note two points regarding the simulation. First, when r > 0.5 or N 1 > N 2 and N 3 , migration becomes asymmetrical, and the ancestral lineages in smaller founding populations tend to come from a larger founding population. Second, simulation is based on fig. 1 a, so there are quite a few cases in which the MRCA occurs in the ancestral population, whereas the previous argument, based on the binomial distribution, assumes no such case.
Thus, N e is always greater than N T in a structured population without extinction and recolonization ( Nei and Takahata 1993 ; Takahata 1993, 1995 ). Since the estimated N e in humans is on the order of 10,000, and F ST is about 10% ( Takahata 1993 ), the total number of breeding individuals is also on this order. For simplicity, we assume that F ST = 0.1, as observed for three major ethnic groups ( Nei and Roychoudhury 1974 ), and N e = 11,000. We then have N T = 10,000 from the above formula. Therefore, when r = 1.5, we obtain N 1 = 6,000 and N 2 + N 3 = 4,000. On the other hand, for a more likely value of r = 10, N 1 is 9,000 and N 2 + N 3 is only 1,000. Whatever the cause of this small population size in Europe and Asia is, the extent of DNA polymorphism in these geographic areas becomes extremely low, such that it seems difficult to establish regional continuity.
According to the uniregional hypothesis, the transition from H. erectus to anatomically modern H. sapiens had completed in a single founding population by the end of the T d period ( fig. 1 b ). This transition may have stemmed from globally advantageous mutations. Concerning local differentiation, the hypothesis assumes that a rapid process occurred after modern H. sapiens evolved. Local differentiation might result from locally advantageous mutations, and this process might be facilitated by limited amounts of gene flow and population expansion. The MRCA of those mutations must have occurred in the single founding population from which modern H. sapiens emerged. This also applies to neutral genes in the case of a short tenure of modern H. sapiens relative to the coalescence time. The population-specific pattern of allelic lineages is thus in better agreement with uniregionality than with multiregionality.
Uniregionality does not imply that there was only one population at a given time during T d , but it assumes that one population predominated and the rest played minor roles in the evolution of anatomically modern H. sapiens. Regarding this assumption, it would be of particular interest to compare current human DNA sequences with those extracted from extinct Homo species. Hypervariable regions I and II of the Neanderthal mtDNA were sequenced and compared with the homologous sequences of 689 humans as well as 7 chimpanzees and 2 bonobos ( Krings et al. 1997, 1999 ). Although some contemporary human sequences are more divergent from each other than from the Neanderthal, the phylogenetic analysis shows that the Neanderthal sequence diverged before the MRCA in the modern human mtDNA pool, followed by divergences of two distinct African lineages. It appears that the Neanderthal and modern human mtDNA lineages separated 465,000 years ago and the MRCA of the present-day human mtDNA occurred 163,000 years ago. The recent analysis of the second Neanderthal specimen from Caucasus also shows an isolated clade of the two Neanderthal mtDNAs ( Ovchinnikov et al. 2000 ). The long coexistence of the Neanderthal and modern humans has raised questions about the absence of admixture or the possibility that these two species were really reproductively isolated. It turns out that the conditions for detecting admixture are stringent (Nordborg 2001). It is necessary that the population divergence is ancient and the time of admixture is recent. The power of detection of admixture also depends on the extent of admixture. If extensive admixture between Neanderthals and modern human ancestors occurred 30,000 years ago, close to the extinction time of the Neanderthals, and if they diverged 450,000 years ago, close to the estimate of the Neanderthal mtDNA divergence, it may be possible to detect admixture in a sample of current human mtDNAs. However, our inability to detect ancient admixture results largely from single-locus information, and many independent regions are needed to improve the power. This is only possible with autosomal regions, but unfortunately, they may not work at all for the Neanderthals. TMRCA at autosomal regions is on average four times as old as that of mtDNA ( table 2 ). Because of weaker genetic drift for autosomal regions than for mtDNA, the time of admixture can be as ancient as 100,000 years ago. Yet, the population divergence time must be as long as >1 MYA in order to obtain the same power of detection of ancient admixture. Whereas this is feasible for H. erectus, it is not feasible for Neanderthals.
Finally, we would like to discuss one evolutionary feature of 10 X-chromosomal regions. The mean amount of sequence differences between humans and chimpanzees over the regions was 0.87%. This is nearly one half of either 1.58% of the three autosomal regions examined here or 1.75% over 37 autosomal regions of about 6,500 silent sites (N.T. at Dual Congress 1998, South Africa). Concerning the X-chromosomal regions, we examined the extent of polymorphism in the ancestral population between humans and chimpanzees. It turns out that of the average 0.87% sequence differences, 0.17% were attributed to the ancestral polymorphism, and the remaining 0.68% were attributed to the sequence differences during the past 5 Myr. On the other hand, the 37 autosomal regions divided the 1.75% sequence differences into 0.45% and 1.32%, respectively. Thus, both quantities in the X-chromosomal regions were one half of those in the 37 autosomal regions. One possible cause is a reduction in mutation rates in X chromosomes. The ratio of the X-chromosomal to autosomal sequence differences, however, is even smaller than that expected under the male-driven mutation hypothesis ( Miyata et al. 1987 ). Although the small extent of ancestral polymorphism at the X-chromosomal regions may be accounted for by a smaller effective size than for the autosomal regions, the reduced substitution rate remains to be elucidated. Despite this, most regions have supported the concept of the uniregionality of modern H. sapiens.
Naruya Saitou, Reviewing Editor
Keywords: Homo sapiens, human evolution multiregional evolution recent African origin population genetics
Address for correspondence and reprints: Naoyuki Takahata, Department of Biosystems Science, Graduate University for Advanced Studies, Hayama, Kanagawa 240-0193, Japan. E-mail: [email protected].
Table 1 Simulation Results for Time Back to the Most Recent Common Ancestor (TMRCA) Birthplace of the Most Recent Common Ancestor (PMRCA), F ST
Table 2 Polymorphism in Worldwide DNA Sequence Data, F ST Time Back to the Most Recent Common Ancestor (TMRCA) and Birthplace of the Most Recent Common Ancestor (PMRCA)
Fig. 1.—Two models for the origin of modern Homo sapiens . In the multiregional model ( a ), only three founding populations during the Pleistocene are considered throughout. The founding populations are connected by gene flow. In the uniregional model ( b ), the two founding populations in Europe and Asia are replaced by newcomers from Africa. The coexistence with Neanderthals is ignored. The breeding size difference among populations is emphasized in a.
Fig. 2.—Conceptual allelic genealogy for globally and locally advantageous mutations ( a and b ) and genealogy of neutral genes ( c ). The numbers 1–3 represent populations. Thin lines represent genealogies of samples from three populations. Gene flow is assumed but is not explicitly depicted. Hatched areas indicate ancestral populations. The timescale is shown along each section
Fig. 3.—Probability of occurrence of African PMRCAs under different founding population sizes (1,000 repeats). It is assumed that N 1 (African) ≥ N 2 + N 3 (non-African) and N 2 = N 3 . The abscissa represents r = N 1 /( N 2 + N 3 ). The sample size was five for each population. The cases in which PMRCAs were found in the ancestor population are excluded. Under this condition, the probability does not change for different values of N 1 m , where m is the per-generation immigration rate per gene from Africa
Fig. 4.—Allelic genealogy under globally ( a ) or locally ( b ) advantageous mutations. It is assumed that N j = N = 100 and that Nu = 0.02, Nm = 1.0, and s = 0.05, where u is the mutation rate per gene, m is the migration rate between any pair of founding populations, and s is the selection coefficient. Simulation always started from a monomorphic state, and the allelic genealogy was recorded during the 10 N generations. A lightly hatched circle indicates a new mutation, and a circle shows the time at which the mutation occurred. A rectangle shows the time at which an allele migrated, and the arrow indicates the direction. The darkly hatched circle shows the MRCA
Fig. 5.—Gene genealogies of individual loci. A bar on each branch indicates a mutation that was inferred from comparison with chimpanzee orthologs (see text). There are two parallel mutations in the Pdha1 tree and one in the β-globin tree. These parallel changes are denoted by three different types of circles. The ancestral type sequences are boxed. Haplotype frequencies are given in parentheses (X, Y, and Z represent African, Asian, and European populations, respectively). The substitutions in the lineage leading to the chimpanzee are suppressed
Fig. 6.—Probability of African PMRCAs under different African and non-African founding population sizes when TMRCA is shorter than 10 N 1 (10,000 repeats). The probability is based on the number of cases in which 9 African PMRCAs are found in a sample of 10 unlinked regions. Each sample consists of five sequences from each of the three populations. As in figure 3 , N 1 m = 1 and the abscissa represents r = N 1 /( N 2 + N 3 ). For large r values, the probability of all 10 African PMRCAs also increases, but because N 1 m = 1, there are cases of more than one non-African PMRCA
This work is supported by Monbusho grants 08404052 and 12304046.
Anagnostopoulos, T., P. M. Green, G. Rowley, C. M. Lewis, and F. Giannelli. 1999 . DNA variation in a 5-Mb region of the X chromosome and estimates of sex-specific/type-specific mutation rates. Am. J. Hum. Genet. 64 : 508 –517.
Cann, R. L., M. Stoneking, and A. C. Wilson. 1987 . Mitochondrial DNA and human evolution. Nature 325 :31–36.
Cavalli-Sforza, L. L., P. Menozzi, and A. Piazza. 1994 . The history and geography of human genes. Princeton University Press, Princeton, N.J.
Clark, A. G., K. M. Weiss, D. A. Nickerson et al. (11 co-authors). 1998 . Haplotype structure and population genetic inferences from nucleotide-sequence variation in human lipoprotein lipase. Am. J. Hum. Genet. 63 : 595 –612.
Coon, C. S. 1962 . The origin of races. Knopf, New York.
Griffiths, R. C., and S. Tavaré. 1994 a. Ancestral inference in population genetics. Stat. Sci. 9 :307–319.
———. 1994 b. Sampling theory for neutral alleles in a varying environment. Philos. Trans. R. Soc. Lond. B Biol. Sci. 344 :403–410.
Haldane, J. B. S. 1927 . A mathematical theory of natural and artificial selection. Part V: Selection and mutation. Proc. Cambr. Philos. Soc. 23 : 838 –844.
Hammer, M. F. 1995 . A recent common ancestry for human Y chromosomes. Nature 378 :376–378.
Hammer, M. F., T. Karafet, A. Rasanayagam, E. T. Wood, T. K. Altheide, T. Jenkins, R. C. Griffiths, A. R. Templeton, and S. L. Zegura. 1998 . Out of Africa and back again: nested cladistic analysis of human Y chromosome variation. Mol. Biol. Evol. 15 : 427 –441.
Hammer, M. F., A. B. Spurdle, T. Karafet et al. (11 co-authors). 1997 . The geographic distribution of human Y chromosome variation. Genetics 145 :787–805.
Harding, R. M., S. M. Fullerton, R. C. Griffiths, J. Bond, M. J. Cox, J. A. Schneider, D. S. Moulin, and J. B. Clegg. 1997 . Archaic African and Asian lineages in the genetic ancestry of modern humans. Am. J. Hum. Genet. 60 : 772 –789.
Harris, E. E., and J. Hey. 1999 . X chromosome evidence for ancient human histories. Proc. Natl. Acad. Sci. USA 96 :3320–3324.
Hawks, J. D., K. Hunley, S.-H. Lee, and M. H. Wolpoff. 2000 . Population bottlenecks and Pleistocene human evolution. Mol. Biol. Evol. 17 : 2 –22.
Horai, S., K. Hayasaka, R. Kondo, K. Tsugane, and N. Takahata. 1995 . The recent African origin of modern humans revealed by complete sequences of hominoid mitochondrial DNAs. Proc. Natl. Acad. Sci. USA 92 :532–536.
Jaruzelska, J., E. Zietkiewicz, M. Batzer, D. E. C. Cole, J.-P. Moisan, R. Scozzari, S. Tavaré, and D. Labuda. 1999 . Spatial and temporal distribution of the neutral polymorphisms in the last ZFX intron: analysis of the haplotype structure and genealogy. Genetics 152 :1091–1101.
Jin, L., P. A. Underhill, V. Doctor, R. W. Davis, P. Shen, L. L. Cavalli-Sforza, and P. J. Oefner. 1999 . Distribution of haplotypes from a chromosome 21 region distinguishes multiple prehistoric human migrations. Proc. Natl. Acad. Sci. USA 96 :3796–3800.
Jobling, M. A. 1994 . A survey of long-range DNA polymorphisms on the human Y chromosome. Hum. Mol. Genet. 3 : 107 –114.
Jorde, L. B., M. Bamshad, and A. R. Rogers. 1998 . Using mitochondrial and nuclear DNA markers to reconstruct human evolution. BioEssays 20 :126–136.
Jorde, L. B., W. S. Watkins, M. J. Bamshad, M. E. Dixon, C. E. L. Ricker, M. T. Seielstad, and M. A. Batzer. 2000 . The distribution of human genetic diversity: a comparison of mitochondrial, autosomal, and Y-chromosome data. Am. J. Hum. Genet. 66 : 979 –988.
Kaessmann, H., F. Heißig, A. von Haeseler, and S. Pääbo. 1999 . DNA sequence variation in a non-coding region of low recombination on the human X chromosome. Nat. Genet. 22 : 78 –81.
Kimura, M. 1962 . On the probability of fixation of mutant genes in a population. Genetics 47 :713–719.
Kimura, M., and J. F. Crow. 1964 . The number of alleles that can be maintained in a finite population. Genetics 49 :725–738.
Krings, M., H. Geisert, R. W. Schmitz, H. Krainitzki, and S. Pääbo. 1999 . DNA sequence of the mitochondrial hypervariable region II from the Neandertal type specimen. Proc. Natl. Acad. Sci. USA 96 :5581–5585.
Krings, M., A. Stone, R. W. Schmitz, H. Krainitzki, M. Stoneking, and S. Pääbo. 1997 . Neandertal DNA sequences and the origin of modern humans. Cell 90 :19–30.
Miyata, T., H. Hayashida, K. Kuma, K. Mitsuyasu, and T. Yasunaga. 1987 . Male-driven molecular evolution: a model and nucleotide sequence analysis. Cold Spring Harb. Symp. Quant. Biol. 70 : 863 –867.
Nachman, M. W., V. L. Bauer, S. L. Crowell, and C. F. Aquadro. 1998 . DNA variability and recombination rates at X-linked loci in humans. Genetics 150 :1133–1141.
Nei, M. 1973 . Analysis of gene diversity in subdivided populations. Proc. Natl. Acad. Sci. USA 70 :3321–3323.
———. 1995 . Genetic support for the out-of-Africa theory of human evolution. Proc. Natl. Acad. Sci. USA 92 :6720–6722.
Nei, M., and A. K. Roychoudhury. 1974 . Genetic variation within and between the three major races of man, Caucasoids, Negroids, and Mongoloids. Am. J. Hum. Genet. 26 : 421 –443.
Nei, M., and N. Takahata. 1993 . Effective population size, genetic diversity, and coalescence time in subdivided populations. J. Mol. Evol. 37 : 240 –244.
Nickerson, D. A., S. L. Taylor, K. M. Weiss, A. G. Clark, R. G. Hutchinson, J. Stengård, V. Salomaa, E. Vartiainen, E. Boerwinkle, and C. F. Sing. 1998 . DNA sequence diversity in a 9.7-kb region of the human lipoprotein lipase gene. Nat. Genet. 19 : 233 –240.
Nordborg, M. 2001 . On detecting ancient admixture. In P. Donnelly, ed. Genes, fossils, and behaviour: an integrated approach to human evolution. NATO science series: life sciences. Vol. . IOS Press, Amsterdam (in press).
Ovchinnikov, I. V., A. Götherström, G. P. Romanova, V. M. Kharitonov, K. Lidén, and W. Goodwin. 2000 . Molecular analysis of Neanderthal DNA from the northern Caucasus. Nature 404 :490–493.
Pennisi, E. 1999 . Genetic study shakes up out of Africa theory. Science 283 :1828.
Przeworski, M., R. R. Hudson, and A. Di Rienzo. 2000 . Adjusting the focus on human variation. Trends Genet. 16 : 296 –302.
Quintana-Murci, L., O. Semino, E. S. Poloni et al. (14 co-authors). 1999 . Y-chromosome specific YCAII, DYS19 and YAP polymorphisms in human populations: a comparative study. Ann. Hum. Genet. 63 : 153 –166.
Rana, B. K., D. Hewett-Emmett, L. Jin et al. (12 co-authors). 1999 . High polymorphism at the human melanocortin I receptor locus. Genetics 151 :1547–1557.
Rieder, M. J., S. L. Taylor, A. G. Clark, and D. A. Nickerson. 1999 . Sequence variation in the human angiotensin converting enzyme. Nat. Genet. 22 : 59 –62.
Ruiz Linares, A., K. Nayar, D. B. Goldstein, J. M. Hebert, M. T. Seielstad, P. A. Underhill, A. A. Lin, M. W. Feldman, and L. L. Cavalli Sforza. 1996 . Geographic clustering of human Y-chromosome haplotypes. Ann. Hum. Genet. 60 : 401 –408.
Slatkin, M. 1991 . Inbreeding coefficients and coalescence times. Genet. Res. 58 : 167 –175.
Slatkin, M., and W. P. Maddison. 1989 . A cladistic measure of gene flow inferred from the phylogenies of alleles. Genetics 123 :603–613.
Takahata, N. 1989 . Gene genealogy in three related populations: consistency probability between gene and population trees. Genetics 122 :957–966.
———. 1993 . Allelic genealogy and human evolution. Mol. Biol. Evol. 10 : 2 –22.
———. 1994 . Repeated failures that led to the eventual success in human evolution. Mol. Biol. Evol. 11 : 803 –805.
———. 1995 . A genetic perspective on the origin and history of humans. Annu. Rev. Ecol. Syst. 26 : 343 –372.
Takahata, N., and J. Klein. 1998 . Multiregional or uniregional origin of modern humans: what do genetic data indicate? Pp. 42–53 in K. Omoto and P. V. Tobias, eds. Recent advances in human biology, Vol. . Origins and past of modern humans: towards reconciliation. World Scientific, Singapore.
Tattersall, I. 2000 . Once we were not alone. Sci. Am. 282 : 38 –44.
Templeton, A. R., A. G. Clark, K. M. Weiss, D. A. Nickerson, E. Boerwinkle, and C. F. Sing. 2000 . Recombination and mutational hotspots within the human lipoprotein lipase gene. Am. J. Hum. Genet. 66 : 69 –83.
Vigilant, L., M. Stoneking, H. Harpending, K. Hawkes, and A. C. Wilson. 1991 . African populations and the evolution of human mitochondrial DNA. Science 253 :1503–1507.
Weidenreich, F. 1946 . Apes, giants, and man. University of Chicago Press, Chicago.
Wolpoff, M. H., X. Wu, and A. G. Thorne. 1984 . Modern Homo sapiens origins: a general theory of hominid evolution involving the fossil evidence from East Asia. Pp. 411–483 in F. H. Smith and F. Spencer, eds. The origins of modern humans. Alan R. Liss, London.
Wright, S. 1931 . Evolution in Mendelian populations. Genetics 16 :97–159.
———. 1943 . Isolation by distance. Genetics 28 :114–138.
Yu, N., and W.-H. Li. 2000 . No fixed nucleotide difference between Africans and non-Africans at the pyruvate dehydrogenase E1 α-subunit locus. Genetics 155 :1481–1483.
Zietkiewicz, E., V. Yotova, M. Jarnik et al. (11 co-authors). 1997 . Nuclear DNA diversity in worldwide distributed human populations. Gene 205 :161–171.
———. 1998 . Genetic structure of the ancestral population of modern humans. J. Mol. Evol. 47 : 146 –155.
Email alerts
Citing articles via.
- Author Guidelines
Affiliations
- Online ISSN 1537-1719
- Copyright © 2024 Society for Molecular Biology and Evolution
- About Oxford Academic
- Publish journals with us
- University press partners
- What we publish
- New features
- Institutional account management
- Rights and permissions
- Get help with access
- Accessibility
- Advertising
- Media enquiries
- Oxford University Press
- Oxford Languages
- University of Oxford
Oxford University Press is a department of the University of Oxford. It furthers the University's objective of excellence in research, scholarship, and education by publishing worldwide
- Copyright © 2024 Oxford University Press
- Cookie settings
- Cookie policy
- Privacy policy
- Legal notice
This Feature Is Available To Subscribers Only
Sign In or Create an Account
This PDF is available to Subscribers Only
For full access to this pdf, sign in to an existing account, or purchase an annual subscription.
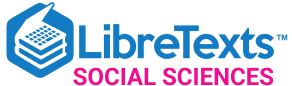
- school Campus Bookshelves
- menu_book Bookshelves
- perm_media Learning Objects
- login Login
- how_to_reg Request Instructor Account
- hub Instructor Commons
- Download Page (PDF)
- Download Full Book (PDF)
- Periodic Table
- Physics Constants
- Scientific Calculator
- Reference & Cite
- Tools expand_more
- Readability
selected template will load here
This action is not available.
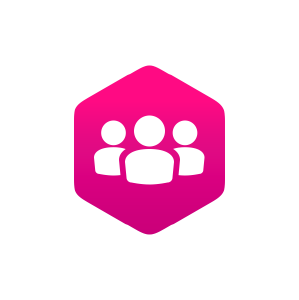
2.3: The Multiregional Origin Hypothesis
- Last updated
- Save as PDF
- Page ID 39128
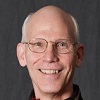
- Utah State University via Rebus Community
Human Evolution: Multiregional Origins
- Reference work entry
- pp 3514–3520
- Cite this reference work entry
- Milford H. Wolpoff 2
219 Accesses
1 Citations
Introduction
Understanding human phylogeny has always been contentious. For more than a century after Darwin, human phylogeny usually reflected current racial taxonomy. Through the end of the nineteenth century and into the twentieth, most paleoanthropologists and paleontologists were evolutionary polygenists who essentially described human races (=subspecies) as having independently evolved from different primate species, some ancient and others more recently (Wolpoff & Caspari 1997 ). In the twentieth century, evolutionary polygenism had come to focus on the independent evolution of human races (=subspecies) from a single prehuman hominid ancestor. The key element all variations of evolutionary polygenism had in common was the independent evolution of human races for so long that the races acquired their humanity separately (Wolpoff & Caspari 2000 ).
Even in its heyday evolutionary polygenism was not accepted by all paleoanthropologists, and after the end of the Second World War, as...
This is a preview of subscription content, log in via an institution to check access.
Access this chapter
- Available as PDF
- Read on any device
- Instant download
- Own it forever
- Available as EPUB and PDF
Tax calculation will be finalised at checkout
Purchases are for personal use only
Institutional subscriptions
Bräuer, G. 1984 . The "Afro-European sapiens hypothesis" and hominid evolution in East Asia during the late middle and upper Pleistocene, in P. Andrews & J.L. Franzen (ed.) The early evolution of man, with special emphasis on Southeast Asia and Africa . Courier Forschungsinstitut Senckenberg 69: 145-65.
Google Scholar
Caspari, R. 2010 . Deconstructing race: racial thinking, geographic variation, and implications for biological anthropology, in C. S. Larsen (ed.) A companion to biological anthropology : 104-23. Chichester, West Sussex: Wiley-Blackwell.
d'Errico, F. & C.B. Stringer. 2011 . Evolution, revolution or saltation scenario for the emergence of modern cultures. Philosophical Transactions of the Royal Society B 366: 1060-9.
Eller, E., J. Hawks & J.H. Relethford. 2004 . Local extinction and recolonization, species effective population size, and modern human origins. Human Biology 76(5): 689-709.
Garcia-Ramos, G. & M. Kirkpatrick. 1997 . Genetic models of adaptation and gene flow in peripheral populations. Evolution 51: 21–8.
Green, R.E., J. Krause, A.W. Briggs, T. Maricic, U. Stenzel, M. Kircher, N. Patterson, Heng Li, Weiwei Zhai, M. Hsi-Yang Fritz, N.F. Hansen, E.Y. Durand, A-S. Malaspinas, J.D. Jensen, T. Marques-Bonet, C. Alkan, K. Pr ü fer, M. Meyer, H.A. Burbano, J.M. Good, R. Schultz, A. Aximu-Petri, A. Butthof, B. Höber, B. Höffner, M. Siegemund, A. Weihmann, C. Nusbaum, E.S. Lander, C. Russ, N. Novod, Jason Affourtit, M. Egholm, C. Verna, P. Rudan, D. Brajkovic, Ž. Kucan, I. Gušic, V.B. Doronichev, L.V. Golovanova, C. Lalueza-Fox, M. de la Rasilla, J. Fortea, A. Rosas, R. W. Schmitz, P.L.F. Johnson, E.E. Eichler, D. Falush, E. Birney, J. C. Mullikin, M. Slatkin, R. Nielsen, J. Kelso, M. Lachmann, D. Reich & S. Pääbo. 2010 . A draft sequence of the Neandertal genome. Science 328: 710-22.
Hawks, J. 2011 . Centre and edge: a foundational concept in human population dynamics. Before Farming 1.
Hawks, J. & M.H. Wolpoff. 2001 . The accretion model of Neandertal evolution. Evolution 55: 1474-85.
Hawks, J., G. Cochran, H.C. Harpending & B.T. Lahn. 2008 . A genetic legacy from archaic Homo . Trends in Genetics 24(1): 19-23.
Hawks, J., E.T. Wang, G.M. Cochran, H.C. Harpending & R.K. Moyzis . 2007. Recent acceleration of human adaptive evolution. Proceedings of the National Academy of Sciences USA 104(52): 20753-8
Moore, J.H. 1994 . Putting anthropology back together again: the ethnogenetic critique of cladistic theory. American Anthropologist 96(4): 925–48.
Reich, D., R.E. Green, M. Kircher, J. Krause, N. Patterson, E.Y. Durand, B. Viola, A. W. Briggs, U, Stenzel, P.L.F. Johnson, T. Maricic, J.M. Good, T. Marques-Bonet, C. Alkan, Fu Qiaomei, S. Mallick, Li Heng, M. Meyer, E.E. Eichler, M. Stoneking, M. Richards, S. Talamo, M.V. Shunkov, A.P. Derevianko, J-J. Hublin, J. Kelso, M. Slatkin & S. Pääbo. 2010 . Genetic history of an archaic hominin group from Denisova Cave in Siberia. Nature 468: 1053-60.
Skoglund, P. & M. Jakobsson. 2011 . Archaic human ancestry in East Asia. Proceedings of the National Academy of Sciences USA 108(45): 18301-6.
Smith F.H., I. Jankovic & I. Karavanic. 2005 . The assimilation model, modern human origins in Europe, and the extinction of Neandertals. Quaternary International 137: 7-19.
Templeton, A.R . 2007. Genetics and recent human evolution. Evolution 61: 1507-19.
Thorne, A.G. & M.H. Wolpoff. 1992 . The multiregional evolution of humans. Scientific American 266(4): 76-83.
Trinkaus, E. 2007 . European early modern humans and the fate of the Neandertals. Proceedings of the National Academy of Sciences USA 104: 7367-72.
Wolpoff, M.H. 1989 . Multiregional evolution: the fossil alternative to Eden, in P. Mellars & C.B. Stringer (ed.) The human revolution: behavioural and biological perspectives on the origins of modern humans : 62-108. Edinburgh: Edinburgh University Press.
Wolpoff, M. H. & R. Caspari . 1997. Race and human evolution . New York: Simon and Schuster.
- 2000. The many species of humanity. Przegląd Antropologiczny ( Anthropological Review ) 63(1): 3-17.
Wolpoff, M.H., X. Wu & A.G. Thorne.1984 . Modern Homo sapiens origins: a general theory of hominid evolution involving the fossil evidence from East Asia, in F.H. Smith & F. Spencer (ed.) The origins of modern humans: a world survey of the fossil evidence : 411–83. New York: Alan R. Liss.
Wolpoff, M.H., A.G. Thorne, J. Jelínek & Y. Zhang. 1994 . The case for sinking Homo erectus : 100 years of Pithecanthropus is enough! Courier Forschungsinstitut Senckenberg 171: 341-61.
Wolpoff, M.H., J. Hawks & R. Caspari. 2000 Multiregional, not multiple origins. American Journal of Physical Anthropology 112(1): 129-36.
Wolpoff, M.H., J.D. Hawks, D.W. Frayer & K. Hunley. 2001 . Modern human ancestry at the peripheries: a test of the replacement theory. Science 291: 293-7.
Wu, X. 1998 . Origin of modern humans of China viewed from cranio-dental characteristics of late Homo sapiens in China. Acta Anthropologica Sinica 17(4): 276-82.
Further Reading
Crummett, T.L. 1995 . The three dimensions of shovel-shaping, in J. Moggi-Cecchi (ed.) Proceedings of the Ninth International Symposium on Dental Anthropology : 305-13. Florence: International Institute for the Study of Man, Angelo Pontecorboli Editore.
Eswaran, V. 2002 . A diffusion wave out of Africa. Current Anthropology 43(5): 749-74.
Etler, D.A. 1996 . The fossil evidence for human evolution in Asia. Annual Review of Anthropology 25: 275-301.
Frayer, D.W . 1997. Perspectives on Neanderthals as ancestors, in G.A. Clark & C.M. Willermet (ed.) Conceptual issues in modern human origins research : 220-34, 437-92. New York: Aldine de Gruyter.
Kramer, A. 1991 . Modern human origins in Australasia: replacement or evolution? American Journal of Physical Anthropology 86(4): 455-73.
Kramer, A., T.L. Crummett & M.H. Wolpoff. 2001 . Out of Africa and into the Levant: replacement or admixture in Western Asia? Quaternary International 75(1): 51-63.
Larnach, S.L. & N.W.G Macintosh. 1974 . A comparative study of Solo and Australian aboriginal crania, in A.P Elkin & N.W.G. Macintosh (ed.) Grafton Elliot Smith: the man and his work : 95-102. Sidney: Sidney University Press.
Pope, G.G. 1992 . Replacement versus regional continuity models: the paleobehavioral and fossil evidence from East Asia, in T. Akazawa, K. Aoki & T. Kimura (ed.) The evolution and dispersal of modern humans in Asia : 3-14. Tokyo: Hokusen-sha.
Smith, F.H., I. Jankovic & I. Karavanic. 2005 . The assimilation model, modern human origins in Europe, and the extinction of Neandertals. Quaternary International 137: 7-19.
Templeton, A.R. 1998 . Human races: a genetic and evolutionary perspective. American Anthropologist 100(3): 632-50.
Wildman, D.E., M. Uddin, Guozhen Liu, L. I. Grossman & M. Goodman. 2003 . Implications of natural selection in shaping 99.4 % nonsynonymous DNA identity between humans and chimpanzees: enlarging genus Homo . Proceedings of the National Academy of Sciences, USA 100(12): 7181-8.
Wu, X. 1998 . Continuity or replacement: viewed from the source of certain features of modern humans in China, in K. Omoto & P.V. Tobias (ed.) Origins and past of modern humans: towards reconciliation : 139-44. Singapore: World Scientific Press.
Download references
Author information
Authors and affiliations.
Department of Anthropology, University of Michigan, Ann Arbor, MI, 48109-1092, USA
Milford H. Wolpoff
You can also search for this author in PubMed Google Scholar
Corresponding author
Correspondence to Milford H. Wolpoff .
Editor information
Editors and affiliations.
Department of Archaeology, Flinders University, Adelaide, SA, Australia
Claire Smith
Rights and permissions
Reprints and permissions
Copyright information
© 2014 Springer Science+Business Media New York
About this entry
Cite this entry.
Wolpoff, M.H. (2014). Human Evolution: Multiregional Origins. In: Smith, C. (eds) Encyclopedia of Global Archaeology. Springer, New York, NY. https://doi.org/10.1007/978-1-4419-0465-2_672
Download citation
DOI : https://doi.org/10.1007/978-1-4419-0465-2_672
Publisher Name : Springer, New York, NY
Print ISBN : 978-1-4419-0426-3
Online ISBN : 978-1-4419-0465-2
eBook Packages : Humanities, Social Sciences and Law
Share this entry
Anyone you share the following link with will be able to read this content:
Sorry, a shareable link is not currently available for this article.
Provided by the Springer Nature SharedIt content-sharing initiative
- Publish with us
Policies and ethics
- Find a journal
- Track your research

Multiregional hypothesis
The multiregional origin hypothesis of human species holds that some, or all, of the genetic variation between the contemporary human races is attributable to genetic inheritance from either Homo sapiens subspecies, or even other hominid species.
- Human Evolution
- Early Humans
- Anthropology
- Charles Darwin
- Origin of Life
- Recent single-origin hypothesis
- Human evolution
- Timeline of human evolution
- Homo ergaster
- Homo (genus)
Fossils & Ruins News
Latest headlines.
- Oldest Evidence of Earth's Magnetic Field
- Bioluminescence in Animals 540 Million Years Ago
- Fossil Frogs Share Their Skincare Secrets
- Everest Mountaineer's Letters Digitized
- 'Forgotten City'
- Two Species Interbreeding Created New Butterfly
- RNA's Hidden Potential: Future Bioengineering
- Worst-Case Warming Less Likely, Analysis Shows
- Remarkable Memories of Mountain Chickadees
- Predicting Future Marine Extinctions
- Humans and Earth's Deep Subsurface Fluid Flow
- Holographic Displays: An Immersive Future
- Harvesting Energy Where River Meets Sea
- Making Diamonds at Ambient Pressure
- Eruption of Mega-Magnetic Star
- Clean Fuel Generation With Simple Twist
- Fussy Eater? Most Parents Play Short Order Cook
- Precise Time Measurement: Superradiant Atoms
Trending Topics
Strange & offbeat stories.
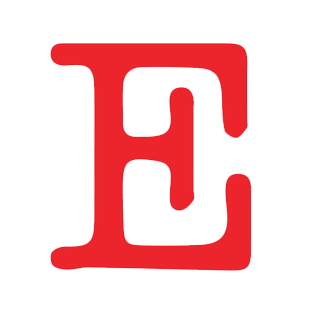
- Scholarly Community Encyclopedia
- Log in/Sign up

Video Upload Options
- MDPI and ACS Style
- Chicago Style
The multiregional hypothesis is a scientific model that provides an explanation for the pattern of human evolution. The hypothesis holds that humans first arose near the beginning of the Pleistocene two million years ago and subsequent human evolution has been within a single, continuous human species. This species encompasses archaic human forms such as Homo erectus and Neanderthals as well as modern forms, and evolved worldwide to the diverse populations of modern Homo sapiens sapiens. The theory contends that humans evolve through a combination of adaptation within various regions of the world and gene flow between those regions. Proponents of multiregional origin point to fossil and genomic data and continuity of archaeological cultures as support for their hypothesis. The primary alternative hypothesis is recent African origin of modern humans, which contends that modern humans arose in Africa around 100-200,000 years ago, moving out of Africa around 50-60,000 years ago to replace archaic human forms without interbreeding.
1. Overview
The term "multiregional hypothesis" was coined in the early 1980s by Milford H. Wolpoff and colleagues, who used the theory to explain regional similarities between archaic humans and modern humans in various regions, in what they called regional continuity . [ 1 ] [ 2 ] Wolpoff proposed that the mechanism of clinal variation allowed for the necessary balance between both local selection and overall evolution as a global species, with Homo erectus , Neanderthals, Homo sapiens and other human forms as subspecies. This species arose in Africa two million years ago as H. erectus and then spread out over the world, developing adaptations to regional conditions. Some populations became isolated for periods of time, developing in different directions, but through continuous interbreeding, replacement, genetic drift and selection, adaptations that were an advantage anywhere on earth would spread, keeping the development of the species in the same overall direction while maintaining adaptations to regional factors. By these mechanisms, surviving local varieties of the species evolved into modern humans, retaining some regional adaptations but with many features common to all regions. [ 2 ]
1.1. History
The idea of regional human evolution has its roots in Schwalbe's 1904, Gorjanović-Kramberger's 1906 and Hrdlička's 1927 theory that archaic Neanderthals were the direct ancestors of modern humans. [ 3 ] [ 4 ] [ 5 ] [ 6 ] This led Reginald Ruggles Gates to theorise in 1929 that Neanderthals were regionally ancestral to modern Europeans. [ 7 ] Java Man was discovered in 1891, and Klaatsch in 1908, Dubois in 1922 and Oppenoorth in 1932 proposed that Java man was ancestral regionally to modern Australian aborigines based on what they saw as similar features in the crania. [ 8 ] [ 9 ]
Franz Weidenreich published a paper in 1939 proposing a sequence from archaic human Peking man fossils to modern humans in China. [ 10 ] [ 11 ] [ 12 ] This theory was based on similarities between the archaic soloensis fossils and modern Chinese, such as facial flatness and shovel shaped incisor teeth. [ 11 ] [ 12 ] In 1940, Weidenreich extended his theory to Neanderthals and modern Europeans, [ 13 ] [ 14 ] [ 15 ] and in 1943 to Java Man and modern Australian Aborigines, seeing a continuation of regional archaic morphological traits in these modern races or populations, along with substantial interregional gene flow. [ 16 ] [ 17 ] [ 18 ] While Weidenreich used the term "polycentric" rather than "multiregional" to describe his evolution model, his work had a strong influence on Wolpoff. [ 19 ] Other theories, such as those of Carleton Coon, also adopted modified forms of Weidenreich's polycentric origin of the races. [ 20 ] [ 21 ]
2. Fossil Evidence
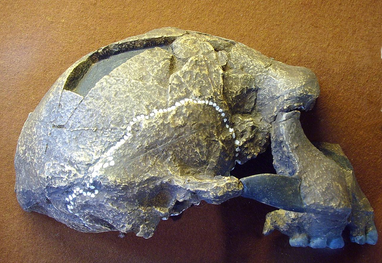
Proponents of the multiregional hypothesis see regional continuity of certain morphological traits from archaic humans to modern humans, demonstrating regional genetic continuity, even as changes in other traits occur in parallel over time across all regions, demonstrating lateral genetic exchange. [ 22 ] For example, in 2001 Wolpoff and colleagues published an analysis of character traits of the skulls of early modern human fossils in Australia and central Europe. They concluded that the diversity of these recent humans could not "result exclusively from a single late Pleistocene dispersal", and implied dual ancestry from Javan Homo erectus for Australia and from Neanderthals for Central Europe. [ 23 ] [ 24 ]
2.1. Southeast Asia
Alan Thorne held that there was regional continuity in the human fossils in southeast Asia. Wolpoff, initially skeptical, became convinced when reconstructing the Sangiran 17 Homo erectus skull from Indonesia, when he was surprised that the skull's face to vault angle matched that of the Australian modern human Kow Swamp 1 skull. Wolpoff had expected the skull to match that of the Homo erectus specimens from China like the Dali skull, but instead, the face to vault angle seemed to be retained regionally over time, even while the fossils in the two regions showed parallel increases in brain case size and parallel reductions in masticatory structures over the intervening approximately 750,000 years. [ 22 ]
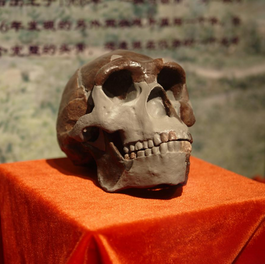
Franz Weidenreich, who oversaw the excavations of numerous "Peking man" Homo erectus fossils at Zhoukoudian in the early 20th century, believed the fossil record demonstrated certain unique features linking prehistoric and modern human populations in China. Many subsequent Chinese paleoanthropologists, such as Wu Xinzhi, were also disposed to favor the multiregional hypothesis for the same reason. [ 12 ]
More recent finds provide additional support for regional human continuity in China. The Tianyuan 1 specimen unearthed in 2003 in Tianyuan Cave, Zhoukoudian, and Carbon 14 dated to 42-39 kya exhibits a series of typical modern human features such as a distinct chin. However, the skeleton also has archaic traits such as low anterior to posterior dental proportions indicating relatively large molars and certain leg bone proportions typical of archaic forms such as Neanderthals. Shang et al. (1999) conclude that this combination of modern and archaic traits "implies that a simple spread of modern humans from Africa is unlikely." [ 25 ] A jaw bone found in 2008 and dated to 110 kya may also exhibit a mixture of archaic and modern human traits. [ 26 ]
2.3. Europe
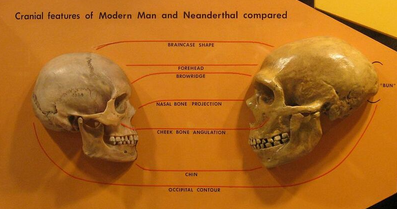
Proponents of the multiregional hypothesis argue for regional continuity in Europe on the basis of skeletal anatomy, morphology and genetics of speech, and the archaeology of the middle to upper paleolithic transition, which they believe to be inconsistent with the possibility of complete replacement of the Neanderthals in Europe without interbreeding. [ 27 ]
Some detractors of the theory have argued, in contrast, that the morphological differences between Neanderthals and early and modern humans indicate that they are different species, based on skull differences more distinct than between any subspecies pairs examined except for the two subspecies of gorilla, implying limited or no interbreeding. [ 28 ] [ 29 ]
Many of the multiregional claims regarding skeletal morphology in Europe center on forms with both archaic Neanderthal traits and modern traits, to provide evidence of interbreeding rather than replacement. Examples include the Lapedo child found in Portugal [ 30 ] and the Oase 1 mandible from Peştera cu Oase, Romania, [ 31 ] though the Lapedo child example is disputed by some. [ 32 ] In a 2007 paper examining numerous samples from European early modern humans, later European humans from the Gravettian period, and the earlier Neanderthal and east African populations from whom the first two populations could have descended, Erik Trinkaus identified numerous features in the later European samples which were absent from the African sample, but present in the Neanderthal sample. These features included various aspects of skull and mandible shape, tooth shape and size, and shapes and proportions of other bones. Trinkaus concluded that early modern Europeans had predominant African ancestry with a substantial degree of admixture from the Neanderthals then indigenous to Europe. [ 33 ] [ 34 ]
3. Genetic Evidence
Genetic evidence from the late 1980s on the mitochondrial genome indicated that all living women can trace their maternal line of descent to a single female living in Africa about 200,000 years ago, the so-called Mitochondrial Eve. This led to a hypothesis that Homo sapiens evolved in Africa, with a small founder population of humans leaving Africa and eventually replacing all archaic humans then living elsewhere without interbreeding. Recent analyses of DNA taken directly from Neanderthal specimens, however, indicates that they contributed to the genome of all humans outside of Africa. The Homo sapiens who populated the world outside Africa all have Neanderthals among their descendents. Denisova hominins also contributed to the DNA of Melanesians and Australians.
3.1. Mitochondrial DNA
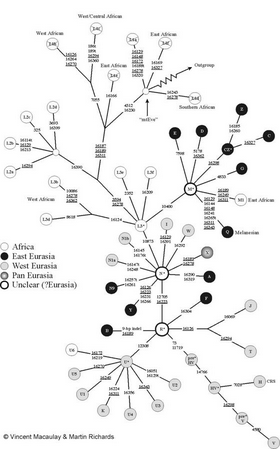
A 1987 analysis of mitochondrial DNA from 147 people from around the world indicated that their mitochondrial lineages all coalesced in a common ancestor in Africa about 200,000 years ago. The analysis suggested that this reflected the worldwide expansion of modern humans as a new species, replacing rather than mixing with local archaic humans. [ 35 ] Later analysis of mitochondrial DNA from neanderthals and from the denisova hominin indicated that those mitochondrial strains had diverged from the living human mitochondrial line long before 200,000 years ago, consistent with lack of interbreeding between early modern and archaic humans. [ 36 ] [ 37 ]
The original mitochondrial DNA results and the resulting recent African replacement theory posed a serious challenge to the multiregional hypothesis. [ 38 ] Mitochondrial DNA alone, however, could not entirely rule out interbreeding between early modern and archaic humans, since archaic human mitochondrial strains from such interbreeding could have been lost due to genetic drift or a selective sweep. [ 39 ] [ 40 ]
3.2. Nuclear DNA
Initial analysis of Y chromosome DNA, which like mitochondrial DNA is inherited from only one parent, was consistent with a recent African replacement model. However, the mitochondrial and Y chromosome data could not be explained by the same modern human expansion out of Africa; the Y chromosome expansion would have involved genetic mixing that retained regionally local mitochondrial lines. In addition, the Y chromosome data indicated a later expansion back into Africa from Asia, demonstrating that gene flow between regions was not unidirectional. [ 41 ]
An early analysis of 15 noncoding sites on the X chromosome found additional inconsistencies with the recent African replacement hypothesis. The analysis found a multimodal distribution of coalescence times to the most recent common ancestor for those sites, contrary to the predictions for recent African replacement; in particular, there were more coalescence times near 2 million years ago (mya) than expected, suggesting an ancient population split around the time humans first emerged from Africa as Homo erectus , rather than more recently as suggested by the mitochondrial data. While most of these X chromosome sites showed greater diversity in Africa, consistent with African origins, a few of the sites showed greater diversity in Asia rather than Africa. For four of the 15 gene sites that did show greater diversity in Africa, the sites' varying diversity by region could not be explained by simple expansion from Africa, as would be required by the recent African replacement hypothesis. [ 42 ]
Later analyses of X chromosome and autosomal DNA continued to find sites with deep coalescence times inconsistent with a single origin of modern humans, [ 43 ] [ 44 ] [ 45 ] [ 46 ] [ 47 ] [ 48 ] diversity patterns inconsistent with a recent expansion from Africa, [ 49 ] or both. [ 50 ] [ 51 ] For example, analyses of a region of RRM2P4 (ribonucleotide reductase M2 subunit pseudogene 4) showed a coalescence time of about 2 Mya, with a clear root in Asia, [ 52 ] [ 53 ] while the MAPT locus at 17q21.31 is split into two deep genetic lineages, one of which is common in and largely confined to the present European population, suggesting inheritance from Neanderthals. [ 54 ] [ 55 ] [ 56 ] [ 57 ] In the case of the Microcephalin D allele, evidence for rapid recent expansion indicated introgression from an archaic population. [ 58 ] [ 59 ] [ 60 ] [ 61 ]
In a 2005 review and analysis of the genetic lineages of 25 chromosomal regions, Alan Templeton found evidence of more than 34 occurrences of gene flow between Africa and Eurasia. Of these occurrences, 19 were associated with continuous restricted gene exchange through at least 1.46 million years ago; only 5 were associated with a recent expansion from Africa to Eurasia. Three were associated with the original expansion of Homo erectus out of Africa around 2 million years ago, 7 with an intermediate expansion out of Africa at a date consistent with the expansion of Acheulean tool technology, and a few others with other gene flows such as an expansion out of Eurasia and back into Africa subsequent to the most recent expansion out of Africa. Templeton rejected a hypothesis of recent African replacement with greater than 99% certainty (p < 10 −17 ). [ 62 ]
3.3. Ancient DNA
By 2006, extraction of DNA directly from some archaic human samples was becoming possible. The earliest analyses were of Neanderthal DNA, and indicated that the Neanderthal contribution to modern human genetic diversity was no more than 20%, with a most likely value of 0%. [ 63 ] By 2010, however, detailed DNA sequencing of the Neanderthal specimens from Europe indicated that the contribution was nonzero, with Neanderthals sharing 1-4% more genetic variants with living non-Africans than with living humans in sub-Saharan Africa. [ 64 ] [ 65 ] In late 2010, a recently discovered non-Neanderthal archaic human, the Denisova hominin from southern Siberia, was found to share 4-6% more of its genome with living Melanesian humans than with any other living group, supporting lateral gene transfer between two regions outside of Africa. [ 66 ] [ 67 ] In August 2011, human leukocyte antigen (HLA) alleles from the archaic Denisovan and Neanderthal genomes were found to show patterns in the modern human population demonstrating origins from these nonafrican populations; the ancestry from these archaic alleles at the HLA-A site was more than 50% for modern Europeans, 70% for Asians, and 95% for Papua New Guineans. [ 68 ] The combination of regional continuity inside and outside of Africa and lateral gene transfer between various regions around the world supports the multiregional hypothesis.
4. Recent African Replacement
The primary competing scientific hypothesis is currently recent African origin of modern humans, which proposes that modern humans arose as a new species in Africa around 100-200,000 years ago, moving out of Africa around 50-60,000 years ago to replace existing human species such as Homo erectus and the Neanderthals without interbreeding. [ 69 ] [ 70 ] [ 71 ] [ 72 ] This differs from the multiregional hypothesis in that the multiregional model predicts interbreeding with preexisting local human populations in any such migration. [ 72 ]
- 3.0.CO;2-K. PMID 10766948. http://www3.interscience.wiley.com/journal/71008905/abstract. " id="ref_1">Wolpoff, MH; Hawks J, Caspari R (2000). "Multiregional, not multiple origins". Am J Phys Anthropol 112 (1): 129–36. doi:10.1002/(SICI)1096-8644(200005)112:1 3.0.CO;2-K. PMID 10766948. http://www3.interscience.wiley.com/journal/71008905/abstract.
- Wolpoff, MH; JN Spuhler, FH Smith, J Radovcic, G Pope, DW Frayer, R Eckhardt, and G Clark (1988). "Modern Human Origins". Science 241 (4867): 772–4. http://dx.doi.org/10.1126%2Fscience.3136545
- Human evolution: an illustrated introduction, Roger Lewin, Oxford: Blackwell, 2005, p. 162.
- Schwalbe, G., 1904. Die Vorgeschichte des Menschen. Vieweg, Braunschweig.
- Hrdlicka, A., 1927. The Neanderthal phase of man. Journal of the Royal Anthropological Institute 57, 249–274.
- Gorjanovic´-Kramberger, D., 1906. Der diluviale Mensch von Krapina in Kroatien. Ein Beitrag zur Pala¨ oanthropologie. Kriedel, Wiesbaden.
- "Heredity in Man", Constable & company ltd., 1929, pp. 302ff
- Dubios, The Proto-Australian fossil man of Wadjak, Java, Koninklijke Akademie van Wetenschappen, Amsterdam, 23 (7) (1922), pp. 1013–1051.
- Oppenoorth, Ein neuer divulialer Urmensch von Java, Natur und Museum, 62 (1932), pp. 269–280.
- "Six lectures on Sinanthropus pekinensis and related problems", Bull. Geol. Soc. China. 19, 1-110)
- Wolpoff, MH; JN Spuhler, FH Smith, J Radovcic, G Pope, DW Frayer, R Eckhardt, and G Clark (1988). "Modern human origins". Science 241 (4867): 772–774. doi:10.1126/science.3136545. PMID 3136545. Bibcode: 1988Sci...241..772W. http://www.sciencemag.org/cgi/pdf_extract/241/4867/772.
- Wolpoff, Milford, and Caspari, Rachel (1997). Race and Human Evolution. Simon & Schuster. pp. 28–29.
- The "Neanderthal Man" and the Ancestors of "Homo sapiens"Franz Weidenreich, American Anthropologist, New Series, Vol. 45, No. 1, Jan. - Mar., 1943, pp. 39-48.
- The people’s Peking man, popular science and human identity in twentieth-century China, Sigrid, Schmalzer, 2008 p. 252 ISBN 0226738604
- Apes, Giants, and Man, Weidenreich, 1946 ISBN 0226881474
- Lahr, M.M. (1994) The Multiregional Model of Modern Human Origins: A Reassessment of its Morphological Basis. Journal of Human Evolution, 26: 23-56.
- "Multiregional Evolution", R. B. Eckhardt, M. H. Wolpoff and A. G. Thorne, Science, New Series, Vol. 262, No. 5136, Nov. 12, 1993, p.974.
- Coon's Theory on "The Origin of Races", Bruce G. Trigger, Anthropologica, New Series, Vol. 7, No. 2 (1965), pp. 179-187.
- Coon, Carleton S., Origin of Races (1962)
- Wolpoff, Milford, and Caspari, Rachel (1997). Race and Human Evolution. Simon & Schuster. pp. 25–26.
- Wolpoff, Milford H; John Hawks, David W Frayer, Keith Hunley (2001). "Modern Human Ancestry at the Peripheries: A Test of the Replacement Theory". Science (AAAS) 291 (5502): 293–297. doi:10.1126/science.291.5502.293. PMID 11209077. Bibcode: 2001Sci...291..293W. http://www.sciencemag.org/cgi/content/abstract/291/5502/293.
- Analysis of the full brain case shape confirms the idea that dispersal from a single population could not explain the early modern human variability, but does not confirm ties to regional archaic humans. Gunz, P., et. al. (2009-04-14). "Early modern human diversity suggests subdivided population structure and a complex out-of-Africa scenario". Proceedings of the National Academy of Sciences of the United States of America 106 (15): 6094–6098. doi:10.1073/pnas.0808160106. PMID 19307568. PMC 2669363. Bibcode: 2009PNAS..106.6094G. http://www.pnas.org/content/106/15/6094.abstract.
- Shang et al.; Tong, H; Zhang, S; Chen, F; Trinkaus, E (1999). "An early modern human from Tianyuan Cave, Zhoukoudian, China" (Free full text). Proceedings of the National Academy of Sciences of the United States of America 104 (16): 6573–8. doi:10.1073/pnas.0702169104. ISSN 0027-8424. PMID 17416672. PMC 1871827. Bibcode: 2007PNAS..104.6573S. http://www.pnas.org/cgi/pmidlookup?view=long&pmid=17416672.
- Phil McKenna, "Chinese challenge to 'out of Africa' theory", New Scientist, Nov 2009 http://www.newscientist.com/article/dn18093-chinese-challenge-to-out-of-africa-theory.html
- Wolpoff, Milford; Bruce Mannheim, Alan Mann, John Hawks, Rachel Caspari, Karen R. Rosenberg, David W. Frayer, George W. Gill and Geoffrey Clark (2004). "Why not the Neandertals?". World Archaeology 36 (4): 527. doi:10.1080/0043824042000303700. https://dx.doi.org/10.1080%2F0043824042000303700
- Harvati, Katerina; Stephen R Frost and Kieran P McNulty (2004). "Neanderthal taxonomy reconsidered: Implications of 3D primate models of intra- and interspecific differences". PNAS 101 (5): 1147–1152. doi:10.1073/pnas.0308085100. PMID 14745010. PMC 337021. Bibcode: 2004PNAS..101.1147H. http://www.pnas.org/content/101/5/1147.full.
- Pearson, Osbjorn M (2004). "Has the Combination of Genetic and Fossil Evidence Solved the Riddle of Modern Human Origins?". Evolutionary Anthropology 13 (4): 145–159. doi:10.1002/evan.20017. https://dx.doi.org/10.1002%2Fevan.20017
- Duarte C, 2. Maurício J, Pettitt P, Souto P, Trinkaus E, van der Plicht H, Zilhão J (1999). "The early Upper Paleolithic human skeleton from the Abrigo do Lagar Velho (Portugal) and modern human emergence in Iberia". Proc Natl Acad Sci USA 96 (13): 7604–7609. doi:10.1073/pnas.96.13.7604. PMID 10377462. PMC 22133. Bibcode: 1999PNAS...96.7604D. http://www.pnas.org/content/96/13/7604.abstract?ijkey=9335ab52731624a02b5f7f426c4a8c2147934993&keytype2=tf_ipsecsha.
- Trinkaus, E; Moldovan, O; Milota, S; Bîlgăr, A; Sarcina, L; Athreya, S; Bailey, Se; Rodrigo, R; Mircea, G; Higham, T; Ramsey, Cb; Van, Der, Plicht, J (Sep 2003). "An early modern human from the Peştera cu Oase, Romania" (free full text). Proceedings of the National Academy of Sciences of the United States of America 100 (20): 11231–11236. doi:10.1073/pnas.2035108100. ISSN 0027-8424. PMID 14504393. PMC 208740. Bibcode: 2003PNAS..10011231T. http://www.pnas.org/cgi/pmidlookup?view=long&pmid=14504393. "When multiple measurements are undertaken, the mean result can be determined through averaging the activity ratios. For Oase 1, this provides a weighted average activity ratio of 〈14a〉 = 1.29 ± 0.15%, resulting in a combined OxA-GrA 14C age of 34,950, +990, and –890 B.P.".
- Tattersall, Ian, and Schwartz, Jeffrey H. (1999). "Hominids and hybrids: The place of Neanderthals in human evolution". Proceedings of the National Academy of Sciences of the United States of America 96 (13): 7117–7119. doi:10.1073/pnas.96.13.7117. PMID 10377375. PMC 33580. Bibcode: 1999PNAS...96.7117T. http://www.pnas.org/cgi/content/full/96/13/7117.
- Trinkaus, E (May 2007). "European early modern humans and the fate of the Neandertals" (Free full text). Proceedings of the National Academy of Sciences of the United States of America 104 (18): 7367–72. doi:10.1073/pnas.0702214104. ISSN 0027-8424. PMID 17452632. PMC 1863481. Bibcode: 2007PNAS..104.7367T. http://www.pnas.org/cgi/pmidlookup?view=long&pmid=17452632.
- http://www.sciencedaily.com/releases/2007/04/070423185434.htm The Emerging Fate Of The Neandertals
- Cann, Rebecca L., Stoneking, Mark, and Wilson, Allan C. (1987-01-01). "Mitochondrial DNA and human evolution". Nature 325 (6099): 31–36. doi:10.1038/325031a0. PMID 3025745. Bibcode: 1987Natur.325...31C. http://artsci.wustl.edu/~landc/html/cann/.
- Hodgson, JA; Disotell TR (2008). "No evidence of a Neanderthal contribution to modern human diversity". Genome Biology (BioMed Central) 9 (2): 206. doi:10.1186/gb-2008-9-2-206. PMID 18304371. PMC 2374707. http://genomebiology.com/2008/9/2/206.
- Krause, Johannes, et al (2010-03-24). "The complete mitochondrial DNA genome of an unknown hominin from southern Siberia". Nature 464 (7290): 894–897. doi:10.1038/nature08976. PMID 20336068. Bibcode: 2010Natur.464..894K. https://dx.doi.org/10.1038%2Fnature08976
- Wolpoff, Milford, and Caspari, Rachel (1997). Race and Human Evolution. Simon & Schuster. pp. 30.
- Relethford, JH (2008-03-05). "Genetic evidence and the modern human origins debate". Heredity (Macmillan) 100 (6): 555–63. doi:10.1038/hdy.2008.14. PMID 18322457. https://dx.doi.org/10.1038%2Fhdy.2008.14
- "Selection, nuclear genetic variation, and mtDNA". john hawks weblog. http://johnhawks.net/weblog/reviews/neandertals/neandertal_dna/weaver_roseman_2005.html. Retrieved 2011-01-05.
- Hammer, M. F., et. al." (1998). "Out of Africa and Back Again: Nested Cladistic Analysis of Human Y Chromosome Variation". Molecular Biology and Evolution 15 (4): 427–441. PMID 9549093. http://mbe.oxfordjournals.org/content/15/4/427.short.
- Hammer, Mf; Garrigan, D; Wood, E; Wilder, Ja; Mobasher, Z; Bigham, A; Krenz, Jg; Nachman, Mw (Aug 2004). "Heterogeneous patterns of variation among multiple human x-linked Loci: the possible role of diversity-reducing selection in non-africans" (Free full text). Genetics 167 (4): 1841–53. doi:10.1534/genetics.103.025361. ISSN 0016-6731. PMID 15342522. PMC 1470985. http://www.genetics.org/cgi/content/abstract/167/4/1841?ijkey=cb14a3724516d1a584feb8454d2c49cd72e003ee&keytype2=tf_ipsecsha. Additional discussion of these results is available in a video of a presentation given by Hammer at http://www.youtube.com/watch?v=Ff0jwWaPlnU (video) from about 40 to 50 minutes into the video.
- The CMP-N-acetylneuraminic acid hydroxylase CMAH pseudogene shows 2.9 Mya coalescence time. Hayakawa, T; Aki, I; Varki, A; Satta, Y; Takahata, N (Feb 2006). "Fixation of the Human-Specific CMP-N-Acetylneuraminic Acid Hydroxylase Pseudogene and Implications of Haplotype Diversity for Human Evolution". Genetics 172 (2): 1139–46. doi:10.1534/genetics.105.046995. ISSN 0016-6731. PMID 16272417. http://www.pubmedcentral.nih.gov/articlerender.fcgi?tool=pmcentrez&artid=1456212
- The ALMS1 site suggests ancient and complex evolutionary history with a coalescence time of about 2 Mya. Scheinfeldt, Lb; Biswas, S; Madeoy, J; Connelly, Cf; Schadt, Ee; Akey, Jm (Jun 2009). "Population Genomic Analysis of ALMS1 in Humans Reveals a Surprisingly Complex Evolutionary History". Molecular Biology and Evolution 26 (6): 1357–67. doi:10.1093/molbev/msp045. ISSN 0737-4038. PMID 19279085. http://www.pubmedcentral.nih.gov/articlerender.fcgi?tool=pmcentrez&artid=2734137
- The PDHA1 (pyruvate dehydrogenase) locus on the X chromosome has an estimated coalescence time of 1.86 Mya, inconsistent with a recent species origin, although the worldwide lineage pattern is unlike other autosomal sites and may be consistent with recent dispersal from Africa. Rosalind M. Harding (March 16, 1999). "More on the X files". Proceedings of the National Academy of Sciences 96 (6): 2582–2584. doi:10.1073/pnas.96.6.2582. Bibcode: 1999PNAS...96.2582H. http://www.pnas.org/cgi/content/full/96/6/2582.
- A second group finds the same ancient origin for PDHA1, but finds no evidence of a recent expansion, consistent with other autosomal and X chromosome sites and contrary to mitochondrial data. Harris, E. E.; Jody Hey (1999). "X chromosome evidence for ancient human histories". Proceedings of the National Academy of Sciences 96 (6): 3320–4. doi:10.1073/pnas.96.6.3320. PMID 10077682. PMC 15940. Bibcode: 1999PNAS...96.3320H. http://www.pnas.org/content/96/6/3320.full.
- The ASAH1 gene has two recently differentiated lineages with a coalescence time 2.4±.4 Mya not explainable by balancing selection. The V lineage shows evidence of recent positive selection. The lineage pattern may be the result of hybridization during a recent range expansion from Africa with the V lineage tracing to archaic humans from outside Africa, though it is also consistent with a mixture of two long isolated groups within Africa; it is not consistent with a recent origination of a modern human species that replaced archaic forms without interbreeding. Kim, Hl; Satta, Y (Mar 2008). "Population Genetic Analysis of the N-Acylsphingosine Amidohydrolase Gene Associated With Mental Activity in Humans" (Free full text). Genetics 178 (3): 1505–15. doi:10.1534/genetics.107.083691. ISSN 0016-6731. PMID 18245333. PMC 2278054. http://www.genetics.org/cgi/pmidlookup?view=long&pmid=18245333. ""It is speculated that, when modern humans dispersed from Africa, admixture of the distinct V and M lineages occurred and the V lineage has since spread in the entire population by possible positive selection."".
- Daniel Garrigan, Zahra Mobasher, Sarah B. Kingan, Jason A. Wilder and Michael F. Hammer (Aug 2005). "Deep Haplotype Divergence and Long-Range Linkage Disequilibrium at Xp21.1 Provide Evidence That Humans Descend From a Structured Ancestral Population". Genetics 170 (4): 1849–1856. doi:10.1534/genetics.105.041095. PMID 15937130. PMC 1449746. http://www.genetics.org/cgi/content/abstract/170/4/1849.
- NAT2 SNPs lineages cluster in sub-Saharan Africa, Europe, and East Asia, with genetic distances scaling with geographic distances. Sabbagh, A; Langaney, A; Darlu, P; Gérard, N; Krishnamoorthy, R; Poloni, Es (Feb 2008). "Worldwide distribution of NAT2 diversity: Implications for NAT2 evolutionary history" (Free full text). BMC genetics 9: 21. doi:10.1186/1471-2156-9-21. PMID 18304320. PMC 2292740. http://www.biomedcentral.com/1471-2156/9/21. Also see map; may resize browser window.
- The NAT1 lineage tree is rooted in Eurasia with a coalescence time of 2.0 Mya that cannot be explained by balancing selection and with the NAT1*11A haplotype absent from subsaharan Africa. Patin, E; Barreiro, Lb; Sabeti, Pc; Austerlitz, F; Luca, F; Sajantila, A; Behar, Dm; Semino, O; Sakuntabhai, A; Guiso, N; Gicquel, B; Mcelreavey, K; Harding, Rm; Heyer, E; Quintana-Murci, L (Mar 2006). "Deciphering the Ancient and Complex Evolutionary History of Human Arylamine N-Acetyltransferase Genes". American journal of human genetics 78 (3): 423–36. doi:10.1086/500614. ISSN 0002-9297. PMID 16416399. http://www.pubmedcentral.nih.gov/articlerender.fcgi?tool=pmcentrez&artid=1380286
- johnhawks.net "Variation in NAT1 and NAT2". john hawks weblog. http://johnhawks.net/weblog/reviews/genetics/diet/nat1_nat2_patin_2006_selection.html johnhawks.net. Retrieved 2011-01.04.
- Garrigan, D; Mobasher, Z; Severson, T; Wilder, Ja; Hammer, Mf (Feb 2005). "Evidence for archaic Asian ancestry on the human X chromosome" (Free full text). Molecular Biology and Evolution 22 (2): 189–92. doi:10.1093/molbev/msi013. ISSN 0737-4038. PMID 15483323. http://mbe.oxfordjournals.org/cgi/pmidlookup?view=long&pmid=15483323.
- Cox, Mp; Mendez, Fl; Karafet, Tm; Pilkington, Mm; Kingan, Sb; Destro-Bisol, G; Strassmann, Bi; Hammer, Mf (Jan 2008). "Testing for Archaic Hominin Admixture on the X Chromosome: Model Likelihoods for the Modern Human RRM2P4 Region From Summaries of Genealogical Topology Under the Structured Coalescent" (Free full text). Genetics 178 (1): 427–37. doi:10.1534/genetics.107.080432. ISSN 0016-6731. PMID 18202385. PMC 2206091. http://www.genetics.org/cgi/pmidlookup?view=long&pmid=18202385.
- J. Hardy, A. Pittman, A. Myers, K. Gwinn-Hardy, H.C. Fung, R. de Silva, M. Hutton and J. Duckworth (2005). "Evidence suggesting that Homo neanderthalensis contributed the H2 MAPT haplotype to Homo sapiens". Biochemical Society Transactions 33, part 4: 582–585. http://www.biochemsoctrans.org/bst/033/0582/0330582.pdf.
- Zody, Mc; Jiang, Z; Fung, Hc; Antonacci, F; Hillier, Lw; Cardone, Mf; Graves, Ta; Kidd, Jm; Cheng, Z; Abouelleil, A; Chen, L; Wallis, J; Glasscock, J; Wilson, Rk; Reily, Ad; Duckworth, J; Ventura, M; Hardy, J; Warren, Wc; Eichler, Ee (Aug 2008). "Evolutionary Toggling of the MAPT 17q21.31 Inversion Region". Nature Genetics 40 (9): 1076–83. doi:10.1038/ng.193. ISSN 1061-4036. PMID 19165922. http://www.pubmedcentral.nih.gov/articlerender.fcgi?tool=pmcentrez&artid=2684794
- Almos, Pz; Horváth, S; Czibula, A; Raskó, I; Sipos, B; Bihari, P; Béres, J; Juhász, A; Janka, Z; Kálmán, J (Nov 2008). "H1 tau haplotype-related genomic variation at 17q21.3 as an Asian heritage of the European Gypsy population". Heredity 101 (5): 416–9. doi:10.1038/hdy.2008.70. ISSN 0018-067X. PMID 18648385. https://dx.doi.org/10.1038%2Fhdy.2008.70
- Stefansson H, Helgason A, Thorleifsson G, Steinthorsdottir V, Masson G, Barnard J, Baker A, Jonasdottir A, Ingason A, Gudnadottir VG, et. al. (2005-01-16). "A common inversion under selection in Europeans". Nature Genetics 37 (2): 129–137. doi:10.1038/ng1508. PMID 15654335. http://www.genetics.org/cgi/content/abstract/170/4/1849.
- Evans, Pd; Mekel-Bobrov, N; Vallender, Ej; Hudson, Rr; Lahn, Bt (Nov 2006). "Evidence that the adaptive allele of the brain size gene microcephalin introgressed into Homo sapiens from an archaic Homo lineage" (Free full text). Proceedings of the National Academy of Sciences of the United States of America 103 (48): 18178–83. doi:10.1073/pnas.0606966103. ISSN 0027-8424. PMID 17090677. PMC 1635020. Bibcode: 2006PNAS..10318178E. http://www.pnas.org/cgi/pmidlookup?view=long&pmid=17090677.
- Evans, Pd; Gilbert, Sl; Mekel-Bobrov, N; Vallender, Ej; Anderson, Jr; Vaez-Azizi, Lm; Tishkoff, Sa; Hudson, Rr; Lahn, Bt (Sep 2005). "Microcephalin, a gene regulating brain size, continues to evolve adaptively in humans". Science 309 (5741): 1717–20. doi:10.1126/science.1113722. ISSN 0036-8075. PMID 16151009. Bibcode: 2005Sci...309.1717E. https://dx.doi.org/10.1126%2Fscience.1113722
- "Introgression and microcephalin FAQ". john hawks weblog. http://johnhawks.net/weblog/reviews/neandertals/neandertal_dna/introgression_faq_2006.html. Retrieved 2011-01-05.
- Templeton, Alan R. (2005). "Haplotype Trees and Modern Human Origins". Yearbook of Physical Anthropology 48 (S41): 33–59. doi:10.1002/ajpa.20351. http://esa.ipb.pt/pdf/28.pdf.
- Noonan, James P., et. al. (2006-11-17). "Sequencing and Analysis of Neanderthal Genomic DNA". Science 314 (5802): 1113–1118. doi:10.1126/science.1131412. PMID 17110569. PMC 2583069. Bibcode: 2006Sci...314.1113N. http://www.sciencemag.org/content/328/5979/710.
- Green, Richard E., et. al. (2010-05-07). "A Draft Sequence of the Neandertal Genome". Science 328 (5979): 710–722. doi:10.1126/science.1188021. PMID 20448178. Bibcode: 2010Sci...328..710G. http://www.sciencemag.org/content/328/5979/710.
- "NEANDERTALS LIVE!". john hawks weblog. http://johnhawks.net/weblog/reviews/neandertals/neandertal_dna/neandertals-live-genome-sequencing-2010.html. Retrieved 2010-12-31.
- Reich, David, et al (2010-12-23). "Genetic history of an archaic hominin group from Denisova Cave in Siberia". Nature 468 (7327): 1053–1060. doi:10.1038/nature09710. PMID 21179161. Bibcode: 2010Natur.468.1053R. http://www.nature.com/nature/journal/v468/n7327/full/nature09710.html.
- "The Denisova genome FAQ". john hawks weblog. http://johnhawks.net/weblog/reviews/neandertals/neandertal_dna/denisova-nuclear-genome-reich-2010.html. Retrieved 2010-12-31.
- Laurent Abi-Rached, et. al. (2011-08-25). "The Shaping of Modern Human Immune Systems by Multiregional Admixture with Archaic Humans". Science 334 (6052): 89–94. doi:10.1126/science.1209202. PMID 21868630. Archived from the original on Aug 2011. http://digitalcommons.unl.edu/cgi/viewcontent.cgi?article=1122&context=publichealthresources.
- Hua Liu, et al. (2006). "A Geographically Explicit Genetic Model of Worldwide Human-Settlement History". American Journal of Human Genetics 79 (2): 230–237. doi:10.1086/505436. PMID 16826514. http://www.pubmedcentral.nih.gov/articlerender.fcgi?tool=pmcentrez&artid=1559480
- Weaver, Timothy D; Charles C. Roseman (2008). "New developments in the genetic evidence for modern human origins". Evolutionary Anthropology: Issues, News, and Reviews (Wiley-Liss) 17 (1): 69–80. doi:10.1002/evan.20161. http://www3.interscience.wiley.com/journal/117921411/abstract.
- Fagundes, NJ; Ray N, Beaumont M, Neuenschwander S, Salzano FM, Bonatto SL, Excoffier L. (2007). "Statistical evaluation of alternative models of human evolution". Proc Natl Acad Sci USA 104 (45): 17614–9. doi:10.1073/pnas.0708280104. PMID 17978179. PMC 2077041. Bibcode: 2007PNAS..10417614F. http://www.pnas.org/content/104/45/17614.long.
- Wolpoff, Milford, and Caspari, Rachel (1997). Race and Human Evolution. Simon & Schuster. p. 42.
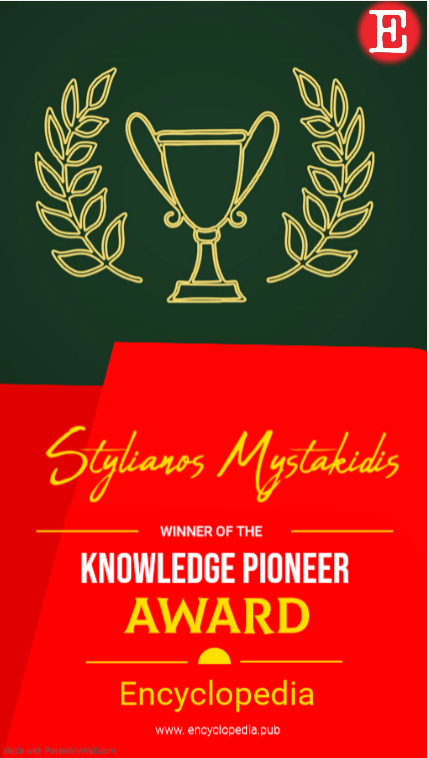
- Terms and Conditions
- Privacy Policy
- Advisory Board

- To save this word, you'll need to log in. Log In
multiregional
Definition of multiregional
Examples of multiregional in a sentence.
These examples are programmatically compiled from various online sources to illustrate current usage of the word 'multiregional.' Any opinions expressed in the examples do not represent those of Merriam-Webster or its editors. Send us feedback about these examples.
Word History
1901, in the meaning defined above
Dictionary Entries Near multiregional
multireligious
Cite this Entry
“Multiregional.” Merriam-Webster.com Dictionary , Merriam-Webster, https://www.merriam-webster.com/dictionary/multiregional. Accessed 25 Apr. 2024.
Subscribe to America's largest dictionary and get thousands more definitions and advanced search—ad free!
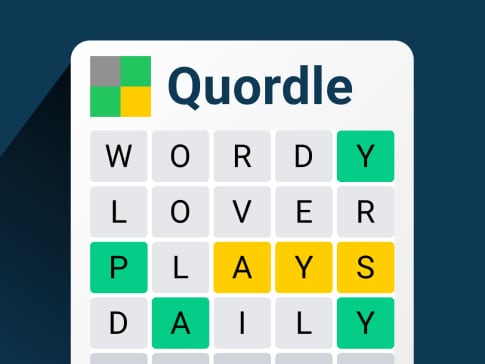
Can you solve 4 words at once?
Word of the day, tendentious.
See Definitions and Examples »
Get Word of the Day daily email!
Popular in Grammar & Usage
More commonly misspelled words, commonly misspelled words, how to use em dashes (—), en dashes (–) , and hyphens (-), absent letters that are heard anyway, how to use accents and diacritical marks, popular in wordplay, the words of the week - apr. 19, 10 words from taylor swift songs (merriam's version), 9 superb owl words, 10 words for lesser-known games and sports, your favorite band is in the dictionary, games & quizzes.
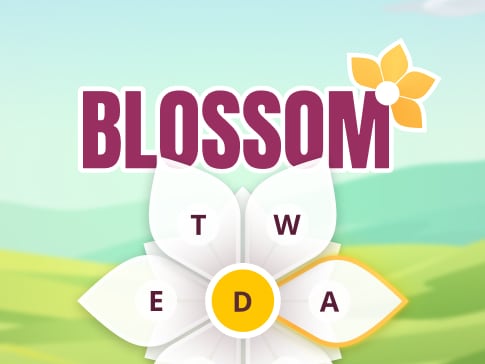
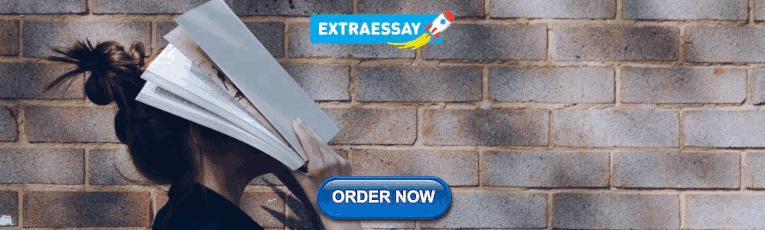
IMAGES
VIDEO
COMMENTS
The multiregional hypothesis, multiregional evolution ( MRE ), or polycentric hypothesis, is a scientific model that provides an alternative explanation to the more widely accepted "Out of Africa" model of monogenesis for the pattern of human evolution . Multiregional evolution holds that the human species first arose around two million years ...
The Multiregional Hypothesis model of human evolution (abbreviated MRE and known alternatively as Regional Continuity or Polycentric model) argues that our earliest hominid ancestors (specifically Homo erectus) evolved in Africa and then radiated out into the world.Based on paleoanthropological data rather than genetic evidence, the theory says that after H. erectus arrived in the various ...
The multiregional hypothesis states that independent multiple origins (Model D) or shared multiregional evolution with continuous gene flow between continental populations (Model C) occurred in ...
Other articles where multiregional evolution is discussed: Homo erectus: Theories of gradual change: …core of the so-called "multiregional" hypothesis (see human evolution), in which it is theorized that H. erectus evolved into Homo sapiens not once but several times as each subspecies of H. erectus, living in its own territory, passed some postulated critical threshold.
porters of the multiregional hypothesis" (Cavalli-Sforza, 1998, p. 11502). This is a misrepresentation of the term "continuity" as explicitly employed in the multiregional model, by confusing the continuity of fea-tures with a claim of unique descent,5 and as noted above, most modern human origins explanations agree about where the major-
Definition. Multiregional Evolution describes the pattern of Pleistocene human evolution (Thorne and Wolpoff 1992). It describes a long-term evolution of central (African) ... If correct, the Eve replacement theory would be the disproof of Multiregional Evolution, and the question of whether this disproof is valid is the most important recent ...
In the 1940s, Weidenreich proposed the multiregional hypothesis (Fig. 5.1B) as an alternative to the classic model (Weidenreich, 1946).The multiregional model posits that human populations living in both Africa and Eurasia evolved into their modern forms as a single species, with gene flow interconnecting them throughout the process.
Multi-Regional Hypothesis. This theory proposes that heidelbergensis, the Neanderthals, the Denisovans and archaic sapiens were all members of the same species. For instance, it proposes that the Neanderthals should be classified as Homo sapiens neanderthalensis -a sub species of Homo sapiens. The theory argues that these groups represent ...
The past half century has seen a move from a multiregionalist view of human origins to widespread acceptance that modern humans emerged in Africa. Here the authors argue that a simple out-of ...
The Multiregional Model of modern human origins predicts that a group of features, recognized as characterizing the evolution of regional populations from their archaic regional ancestors, will consistently show higher incidence in those regions. ... The definition of these two anatomical features and their expression and variation among ...
The multiregional model was first developed to explain how some traits, such as increased cranial capacity and reduction of the face, could evolve across the Old World while at the same time other ...
This article was originally published with the title "The Multiregional Evolution of Humans" in Scientific American Magazine Vol. 266 No. 4 (April 1992), p. 76. doi:10.1038 ...
The Multiregional Model of modern human origins predicts that a group of features, recognized as characterizing the evolution of regional populations from their archaic regional ancestors, will consistently show higher incidence in those regions. This model also predicts that regional morphological patterns are stable, reflecting absence of ...
MULTIREGIONAL EVOLUTION COMPLETE REPLACEMENT-THEEVE THEORY 179 The replacement theory proposes that modern humans are a new species that arose as recently as between 50,000 and 500,000 years ago (Stoneking and Cann 1989), a range usually reduced to a single date of 200,000years ago. This Eve theory postulates a unique African origin for all
Different interpretations of the multiregional hypothesis also state that genetic exchange between the populations of the world was limited (Thorne & Wolpoff 2003). According to this model, the ...
This hypothesis is an extension of that of Weidenreich (1946), which allows gene exchanges among multiple founding populations of modern H. sapiens. However, it has been difficult to test the multiregional hypothesis because of ambiguity in the assumed population structure (Takahata and Klein 1998). In this paper, we draw several predictions ...
This page titled 2.3: The Multiregional Origin Hypothesis is shared under a CC BY-NC 4.0 license and was authored, remixed, and/or curated by Nolan Weil (Rebus Community) via source content that was edited to the style and standards of the LibreTexts platform; a detailed edit history is available upon request.
Definition. Multiregional Evolution describes the pattern of Pleistocene human evolution (Thorne & Wolpoff 1992). It describes a long-term evolution of central (African) ... If correct, the Eve replacement theory would be the disproof of Multiregional Evolution, and the question of whether this disproof is valid is the most important recent ...
One theory, developed by Milford H. Wolpoff, Alan Thorne and Xinzhi Wu, that explains this transformation is called the multiregional hypothesis. Homo erectus is known to have evolved in Africa ...
A current debate opposes two theories of the origin of modern man. One view is that modernHomo sapiensemerged from Africa relatively recently, most probably within the last two or three hundred thousand years (Wilson & Cann, 1992,Sci. Am.266(4),22-27).The opposing view is that modern man has resulted from parallel evolution in different regions, producing convergent modernization of local ...
The multiregional origin hypothesis of human species holds that some, or all, of the genetic variation between the contemporary human races is attributable to genetic inheritance from either Homo ...
The multiregional hypothesis is a scientific model that provides an explanation for the pattern of human evolution. The hypothesis holds that humans first arose near the beginning of the Pleistocene two million years ago and subsequent human evolution has been within a single, continuous human species. This species encompasses archaic human forms such as Homo erectus and Neanderthals as well ...
The meaning of MULTIREGIONAL is involving, relating to, or operating in more than one region. How to use multiregional in a sentence.