
- Science Notes Posts
- Contact Science Notes
- Todd Helmenstine Biography
- Anne Helmenstine Biography
- Free Printable Periodic Tables (PDF and PNG)
- Periodic Table Wallpapers
- Interactive Periodic Table
- Periodic Table Posters
- How to Grow Crystals
- Chemistry Projects
- Fire and Flames Projects
- Holiday Science
- Chemistry Problems With Answers
- Physics Problems
- Unit Conversion Example Problems
- Chemistry Worksheets
- Biology Worksheets
- Periodic Table Worksheets
- Physical Science Worksheets
- Science Lab Worksheets
- My Amazon Books
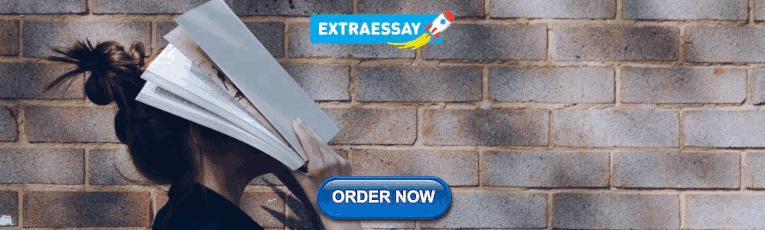
Heat Transfer – Conduction, Convection, Radiation

Heat transfer occurs when thermal energy moves from one place to another. Atoms and molecules inherently have kinetic and thermal energy, so all matter participates in heat transfer. There are three main types of heat transfer, plus other processes that move energy from high temperature to low temperature.
What Is Heat Transfer?
Heat transfer is the movement of heat due to a temperature difference between a system and its surroundings. The energy transfer is always from higher temperature to lower temperature, due to the second law of thermodynamics . The units of heat transfer are the joule (J), calorie (cal), and kilocalorie (kcal). The unit for the rate of heat transfer is the kilowatt (KW).
The Three Types of Heat Transfer With Examples
The three types of heat transfer differ according to the nature of the medium that transmits heat:
- Conduction requires contact.
- Convection requires fluid flow.
- Radiation does not require any medium.
- Conduction is heat transfer directly between neighboring atoms or molecules. Usually, it is heat transfer through a solid. For example, the metal handle of a pan on a stove becomes hot due to convection. Touching the hot pan conducts heat to your hand.
- Convection is heat transfer via the movement of a fluid, such as air or water. Heating water on a stove is a good example. The water at the top of the pot becomes hot because water near the heat source rises. Another example is the movement of air around a campfire. Hot air rises, transferring heat upward. Meanwhile, the partial vacuum left by this movement draws in cool outside air that feeds the fire with fresh oxygen.
- Radiation is the emission of electromagnetic radiation. While it occurs through a medium, it does not require one. For example, it’s warm outside on a sunny day because solar radiation crosses space and heats the atmosphere. The burner element of a stove also emits radiation. However, some heat from a burner comes from conduction between the hot element and a metal pan. Most real-life processes involve multiple forms of heat transfer.
Conduction requires that molecules touch each other, making it a slower process than convection or radiation. Atoms and molecules with a lot of energy have more kinetic energy and engage in more collisions with other matter. They are “hot.” When hot matter interacts with cold matter, some energy gets transferred during the collision. This drives conduction. Forms of matter that readily conduct heat are called thermal conductors .
Examples of Conduction
Conduction is a common process in everyday life. For example:
- Holding an ice cube immediately makes your hands feel cold. Meanwhile, the heat transferred from your skin to the ice melts it into liquid water.
- Walking barefoot on a hot road or sunny beach burns your feet because the solid material transmits heat into your foot.
- Iron clothes transfers heat from the iron to the fabric.
- The handle of a coffee cup filled with hot coffee becomes warm or even hot via conduction through the mug material.
Conduction Equation
One equation for conduction calculates heat transfer per unit of time from thermal conductivity, area, thickness of the material, and the temperature difference between two regions:
Q = [K ∙ A ∙ (T hot – T cold )] / d
- Q is heat transfer per unit time
- K is the coefficient of thermal conductivity of the substance
- A is the area of heat transfer
- T hot is the temperature of the hot region
- T cold is the temperature of the cold region
- d is the thickness of the body
Convection is the movement of fluid molecules from higher temperature to lower temperature regions. Changing the temperature of a fluid affects its density, producing convection currents. If the volume of a fluid increases, than its density decreases and it becomes buoyant.
Examples of Convection
Convection is a familiar process on Earth, primarily involving air or water. However, it applies to other fluids, such as refrigeration gases and magma. Examples of convection include:
- Boiling water undergoes convection as less dense hot molecules rise through higher density cooler molecules.
- Hot air rises and cooler air sinks and replaces it.
- Convection drives global circulation in the oceans between the equators and poles.
- A convection oven circulates hot air and cooks more evenly than one that only uses heating elements or a gas flame.
Convection Equation
The equation for the rate of convection relates area and the difference between the fluid temperature and surface temperature:
Q = h c ∙ A ∙ (T s – T f )
- Q is the heat transfer per unit time
- h c is the coefficient of convective heat transfer
- T s is the surface temperature
- T f is the fluid temperature
Radiation is the release of electromagnetic energy. Another name for thermal radiation is radiant heat. Unlike conduction or convection, radiation requires no medium for heat transfer. So, radiation occurs both within a medium (solid, liquid, gas) or through a vacuum.
Examples of Radiation
There are many examples of radiation:
- A microwave oven emits microwave radiation, which increases the thermal energy in food
- The Sun emits light (including ultraviolet radiation) and heat
- Uranium-238 emits alpha radiation as it decays into thorium-234
Radiation Equation
The Stephan-Boltzmann law describes relationship between the power and temperature of thermal radiation:
P = e ∙ σ ∙ A· (Tr – Tc) 4
- P is the net power of radiation
- A is the area of radiation
- Tr is the radiator temperature
- Tc is the surrounding temperature
- e is emissivity
- σ is Stefan’s constant (σ = 5.67 × 10 -8 Wm -2 K -4 )
More Heat Transfer – Chemical Bonds and Phase Transitions
While conduction, convection, and radiation are the three modes of heat transfer, other processes absorb and release heat. For example, atoms release energy when chemical bonds break and absorb energy in order to form bonds. Releasing energy is an exergonic process, while absorbing energy is an endergonic process. Sometimes the energy is light or sound, but most of the time it’s heat, making these processes exothermic and endothermic .
Phase transitions between the states of matter also involve the absorption or release of energy. A great example of this is evaporative cooling, where the phase transition from a liquid into a vapor absorbs thermal energy from the environment.
- Faghri, Amir; Zhang, Yuwen; Howell, John (2010). Advanced Heat and Mass Transfer . Columbia, MO: Global Digital Press. ISBN 978-0-9842760-0-4.
- Geankoplis, Christie John (2003). Transport Processes and Separation Principles (4th ed.). Prentice Hall. ISBN 0-13-101367-X.
- Peng, Z.; Doroodchi, E.; Moghtaderi, B. (2020). “Heat transfer modelling in Discrete Element Method (DEM)-based simulations of thermal processes: Theory and model development”. Progress in Energy and Combustion Science . 79: 100847. doi: 10.1016/j.pecs.2020.100847
- Welty, James R.; Wicks, Charles E.; Wilson, Robert Elliott (1976). Fundamentals of Momentum, Heat, and Mass Transfer (2nd ed.). New York: Wiley. ISBN 978-0-471-93354-0.
Related Posts
Introduction to Heat Transfer: How Does Heat Transfer?
What Heat Transfer Is and How Heat Moves From One Body to Another
- Thermodynamics
- Physics Laws, Concepts, and Principles
- Quantum Physics
- Important Physicists
- Cosmology & Astrophysics
- Weather & Climate
:max_bytes(150000):strip_icc():format(webp)/AZJFaceShot-56a72b155f9b58b7d0e783fa.jpg)
- M.S., Mathematics Education, Indiana University
- B.A., Physics, Wabash College
What is heat? How does heat transfer take place? What are the effects on matter when heat transfers from one body to another? Here's what you need to know:
Heat Transfer Definition
Heat transfer is a process by which internal energy from one substance transfers to another substance. Thermodynamics is the study of heat transfer and the changes that result from it. An understanding of heat transfer is crucial to analyzing a thermodynamic process , such as those that take place in heat engines and heat pumps.
Forms of Heat Transfer
Under the kinetic theory, the internal energy of a substance is generated from the motion of individual atoms or molecules. Heat energy is the form of energy which transfers this energy from one body or system to another. This heat transfer can take place in a number of ways:
- Conduction is when heat flows through a heated solid through a heat current moving through the material. You can observe conduction when heating a stove burner element or a bar of metal, which goes from red hot to white hot.
- Convection is when heated particles transfer heat to another substance, such as cooking something in boiling water.
- Radiation is when heat is transferred through electromagnetic waves, such as from the sun. Radiation can transfer heat through empty space, while the other two methods require some form of matter-on-matter contact for the transfer.
In order for two substances to affect each other, they must be in thermal contact with each other. If you leave your oven open while turned on and stand several feet in front of it, you are in thermal contact with the oven and can feel the heat it transfers to you (by convection through the air).
Normally, of course, you do not feel the heat from the oven when you're several feet away and that is because the oven has thermal insulation to keep the heat inside of it, thus preventing thermal contact with the outside of the oven. This is of course not perfect, so if you stand nearby you do feel some heat from the oven.
Thermal equilibrium is when two items that are in thermal contact no longer transfer heat between them.
Effects of Heat Transfer
The basic effect of heat transfer is that the particles of one substance collide with the particles of another substance. The more energetic substance will typically lose internal energy (i.e. "cool down") while the less energetic substance will gain internal energy (i.e. "heat up").
The most blatant effect of this in our day-to-day life is a phase transition, where a substance changes from one state of matter to another, such as ice melting from a solid to a liquid as it absorbs heat. The water contains more internal energy (i.e. the water molecules are moving around faster) than in the ice.
In addition, many substances go through either thermal expansion or thermal contraction as they gain and lose internal energy. Water (and other liquids) often expands as it freezes, which anyone who has put a drink with a cap in the freezer for too long has discovered.
Heat Capacity
The heat capacity of an object helps define how that object's temperature responds to absorbing or transmitting heat. Heat capacity is defined as the change in heat divided by the change in temperature.
- Laws of Thermodynamics
Heat transfer is guided by some basic principles which have become known as the laws of thermodynamics , which define how heat transfer relates to work done by a system and place some limitations on what it is possible for a system to achieve.
Edited by Anne Marie Helmenstine, Ph.D.
- An Overview of Thermodynamics
- A Scientific Way to Define Heat Energy
- What Is a Thermodynamic Process?
- What Is Conduction?
- Definition and Examples of Latent Heat
- What Is the Zeroth Law of Thermodynamics?
- What Is Isobaric Process?
- The Isochoric Process
- Thermodynamics: Adiabatic Process
- What Is an Isothermal Process in Physics?
- Calculating Heat Current
- Understanding Calorimetry to Measure Heat Transfer
- Convection and Weather
- Enthalpy Definition in Chemistry and Physics
- What Is Entropy and How to Calculate It
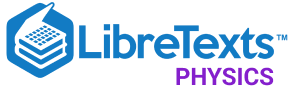
- school Campus Bookshelves
- menu_book Bookshelves
- perm_media Learning Objects
- login Login
- how_to_reg Request Instructor Account
- hub Instructor Commons
- Download Page (PDF)
- Download Full Book (PDF)
- Periodic Table
- Physics Constants
- Scientific Calculator
- Reference & Cite
- Tools expand_more
- Readability
selected template will load here
This action is not available.
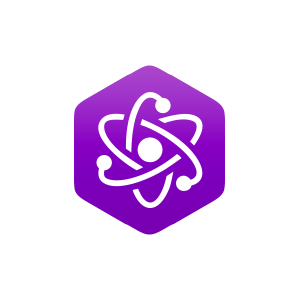
5.4: Modes of Heat Transfer
- Last updated
- Save as PDF
- Page ID 27781
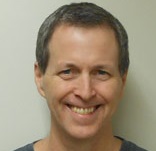
- Tom Weideman
- University of California, Davis
We know the effects of heat being transferred into or out of systems, but now we are going to take a look at the ways in which this transfer can occur. As we stated earlier, “heat” is a rather generic description of energy transfer due to a temperature difference between two systems, and we will see there are three modes through which this transfer can occur. The first is the most intuitive, and as it turns out, the one we can most easily deal with mathematically. It is called conduction .
As we saw with thermal expansion, the trick to understanding conduction is to consider what is happening on a microscopic scale. Consider a solid cylindrical object that connects two systems at different temperatures. This cylinder acts as the conduit for heat energy to flow from the hotter system to the cooler one. We model this cylinder microscopically using parallel chains of particles joined by springs.
Figure 5.4.1 – Heat Conductor Model
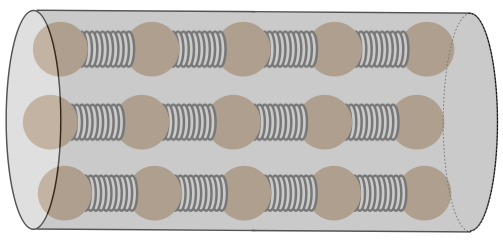
[ Technically, these particles should be attached to all of their nearest neighbors by springs, but we will only be looking at the transfer of the heat along the length of the cylinder, so we have simplified the model accordingly. ]
It should be clear from this model how heat energy can be transferred from one end of the cylinder to the other: If we vibrate the particles on one end of the cylinder, they will vibrate their nearest neighbors, and the effect will carry its way down to the other end. This is easy to see, but what is tougher to understand is that we will be considering only steady-state circumstances, which means that the particles on each end of the cylinder vibrate with amplitudes that have energies that match the systems with which they are in contact (these regions are called thermal reservoirs , because during the heat transfer process their temperatures don’t change appreciably). Every particle between the ends vibrates with an amplitude between the two extremes defined by the hot and cold reservoir.
Okay, so our task now (as it will be with all forms of heat transfer) is to determine the rate at which energy is transferred from one system to another in terms of the conditions provided. We'll do this by considering each element of this model in turn. As with any analysis of a continuously-changing phenomenon, we start with differential elements. In this case, we have two small segments of the cylinder at slightly different temperatures, across which some heat is transferred.
Figure 5.4.2 – Differential Heat Conduction

The more chains of spring-connected particles we can use, the faster the energy can be transferred. The number of chains is proportional to the cross-sectional area of the cylinder, so the rate of heat transfer is also proportional to the cross-sectional area:
\[\dfrac{dQ}{dt} \propto A\]
The next factor in determining the rate of heat flow between these two segments is the temperature difference. It should not be surprising that heat will flow faster when the difference in temperature is greater. It turns out that the rate of heat flow is directly proportional to the temperature difference. This phenomenon is often referred to as Newton's law of cooling , and works fairly well as an approximation in more general circumstances, though it is only strictly applicable to this one. We have to be careful about the sign we use; recall that the sign for \(dQ\) is positive when heat is flowing into a system, but in this case the heat is flowing out of the system with the higher temperature:
\[\dfrac{dQ}{dt} \propto -dT\]
In order to get all the energy in the first segment to be transferred into the second segment, energy in the left end of the segment has to traverse the length of that segment, \(dx\). The longer this segment is, the longer it will take, so the rate of heat transfer is inversely-proportional to that distance:
\[\dfrac{dQ}{dt} \propto \dfrac{1}{dx}\]
Different substances will be structured differently (different springs, different masses of particles, etc.), so we have to take into account the type of substance. We do that by incorporating that into the constant of proportionality ( thermal conductivity, \(k\) ) that turns the proportional relationships into an equality:
\[\dfrac{dQ}{dt} = -kA\dfrac{dT}{dx}\]
The derivative of the temperature is called the temperature gradient , and can be thought of as the steepness at which the temperature tapers-off from the origin of the heat transfer (the hotter thermal reservoir) to its destination (the cooler thermal reservoir), which in the most general cases (e.g. in non-steady-state situations) will not be constant. This is known as the heat equation , but really it is a specific example of the diffusion equation , which applies to many other phenomena as well.
It turns out that this equation is overkill for our purposes (we are not about to start solving differential equations), and in fact in all the cases we address we will deal with steady-state situations with the temperature gradient being linear. When the temperature changes linearly from the hot thermal reservoir to the cool one, the gradient is a constant, equal to simply the temperature difference of the two reservoirs (\(\Delta T\)), divided by the distance separating them (\(L\)):
\[\dfrac{dQ}{dt} = -\dfrac{kA}{L}\Delta T\]
Example \(\PageIndex{1}\)
An iron bar with a square cross-section is used to melt square holes in a slab of ice. The bar is then cut in half and the two halves are welded together on their sides to make a new shorter bar with a rectangular cross-section. If the mass of ice melted in 10 minutes by the original bar is \(M\), how much ice will be melted in 10 minutes by the new configuration? Assume the temperature on the hot end of the bar is the same in both cases.
The rate of heat conduction is proportional to the cross-sectional area and inversely proportional to the length of the material through which the heat passes, and in this case the area doubles while the length is divided in half. The material is the same (thermal conductivity is unchanged), so the rate of heat flow is quadrupled. With four times as much energy transferred into the ice in the same period of time, four times as much ice is melted, so the answer is \(4M\).
Convection is another form of heat transfer, that operates through a completely different mechanism from conduction. Rather than particles interacting with each other, the energy is transferred by simply having particles with more KE move (thanks to random motion) from the hotter region to the cooler one, while lower- KE particles take their place in the hotter region (there is no net exchange of particles), resulting in a transfer of energy.
Figure 5.4.3 – Convection Mechanism

Applying rigorous analysis to derive a mathematical model for the rate of heat flow via convection is well beyond the scope of this course. But an approximate relation based on the temperature difference of the two reservoirs is:
\[\dfrac{dQ}{dt} \propto \left[\Delta T\right]^{\frac{5}{4}}\]
Two things to note here:
- While this doesn’t quite follow “Newton's Law of Cooling” like conduction, it comes very close.
- We don’t have the constant of proportionality, or even what this constant depends upon. That doesn’t mean this isn’t useful, because we can still compare the convection rates for two scenarios where “all else is equal.”
Without being more specific about the proportionality constants for conduction and convection, it is nevertheless a safe bet to say that convection in general is a faster mode of heat transport. For example, if you have a plastic bag full of hot water and a plastic bag full of cold water, and you want two plastic bags of warm water (so you want to transfer heat from the hot bag of water to the cold bag of water), the fastest way to achieve that is by mixing the water from the bags together, rather than by bringing the bags in contact with each other.
The third way in which heat transfer can occur should become obvious when one thinks about how our Earth stays warm. After all, it is in contact with the vacuum of space (which in the absence of a nearby sun is at a temperature of \(3K\)), so heat should be transferring out of it at an alarming rate. The source of the Earth’s incoming heat transfer is of course the Sun. But the space between the Sun and Earth is not conducting heat (it’s empty space - no particles connected with springs), and the Sun isn’t firing really hot particles to mix with the Earth’s atmosphere (well actually it is, but that “convection” is not doing much to heat our atmosphere, though it puts on a nice light show at the poles). Instead, the Sun is transferring energy to the Earth via radiation .
We already know that radiation is just light waves. We also know that light waves are driven by vibrating electric charges (electrons). One source of vibrating charges is atoms in a sample with thermal energy. The hotter the sample gets, the more energetically the charges vibrate, which means more energy is sent out in the form of radiation, so we would expect the electromagnetic power output of a sample to grow as its temperature grows, but it is by no means obvious in what way the power output will mathematically depend on the temperature.
These light waves don’t know where they are going, they only know that some vibrating electrons are driving them, so it is not the temperature difference that is causing this heat transfer, but rather the absolute temperature. A fellow named Boltzmann derived the dependence of the power output on temperature, and a guy named Stefan measured it. It turns out that the power output goes as the fourth power of the absolute temperature. The actual power output also depends upon the surface area (more space for the radiation to come out of), and a property called emissivity , which measures how well the surface emits light (how rough/smooth the surface is, how it is shaped, etc.) into the region just outside the object (the surface is the border between these two regions):
\[\left|\dfrac{dQ}{dt}\right| = \sigma e A T^4\;,\;\;\;\;\;\sigma \equiv 5.67\times10^{-8}\frac{W}{m^2\;K^4}\]
The constant \(\sigma\) is called the Stefan-Boltzmann constant, \(e\) is the emissivity, and \(A\) is the surface area of the emitting body. The absolute value is included here because this equation involves the absolute temperature rather than a temperature difference. The sign we put to this equation depends upon whether we are talking about the rate at which heat that is exiting the object at temperature \(T\) (in which case the sign is negative), or the rate at which heat is entering the region surrounding the object at temperature \(T\) (in which case the sign is positive).
Nowhere here have we mentioned the frequency of the light emitted. It turns out that all of the frequencies (up to a certain maximum) are emitted, but the energy transferred is not uniform across frequencies. Remember, these thermal electrons are vibrating randomly , although that randomness has a non-uniform distribution, making some frequencies more common than others. Most of the light emitted in this manner at “everyday” temperatures (say, hundreds of kelvins) is in a part of the spectrum that we refer to as infrared , a frequency range we are unable to see with the naked eye, though we can see it with the help of special devices (e.g. infrared cameras). The part of the power output that is in the visible spectrum is too low for us to be able to see when the temperature is at “typical” temperatures in the region of 300 K . But if something gets significantly hotter, the power output of every frequency goes up, and power in the visible spectrum can reach a level that we can see – the object “glows hot.”
Recall we said that heat transfer requires a temperature difference to occur, but here we seem to be saying that heat is transferred out of an object at an absolute temperature. Well, any object that can emit light can also absorb it. So let’s consider an object sitting in an environment which is at a different temperature.
Figure 5.4.4 – Heat Transfer to/from Surroundings Via Radiation

The emissivity is a property of the boundary surface between the two realms exchanging heat (which in this case we are calling the object and its surroundings), so naturally it is the same value going in both directions (we'll see another reason that this must be the case shortly). Obviously the surface area of the boundary is also the same going both ways as well. So the only thing that makes the heat energy exiting the object different (and entering the surroundings) from the heat energy going the other way (from surroundings to object) is the difference in temperature. We can now employ the sign convention for heat and conclude that the net rate of heat entering the surroundings is:
\[\dfrac{dQ}{dt} = \sigma e A \left(T^4-T_S^4\right) \]
Once again we see that net heat flow is induced by a difference in temperature, though like convection, this mode does not obey Newton's law of cooling.
Example \(\PageIndex{2}\)
A typical red giant star is so big that it can fit about 1,000,000 stars the size of our sun inside of it (i.e. red giants occupy a volume about 1 million times greater than the volume occupied by our sun). For our sun to radiate energy at the same rate as such a star, how would their temperatures need to compare?
One million times the volume translates into 100 times the radius, which in turn translates into 10,000 times the surface area. The rate of energy transfer due to radiation is proportional to the surface area, so if the temperatures were equal, the red giant would radiate energy at a rate 10,000 times that of our sun. The rate of energy transfer due to radiation also goes as the 4th power of the temperature, so if the sun was 10 times hotter than the red giant (it turns out it is not, though it is close to twice as hot), then that would exactly compensate for the much greater surface area of the red giant.
Digression: Temperatures Near Stars
As seen in the example, a common application of the Stefan-Boltzmann law comes from the study of stars. But such cases do not involve two regions sharing a common surface border at different temperatures. That is, the space immediately outside the surface of the sun is not the same near-absolute-zero temperature that it is far outside the solar system. Intuitively it makes sense that at steady-state the temperature would gradually decrease from what it is at the surface of the sun, down to the ~3K temperature we see in deep space, but is there some way to compute this temperature gradient? It's clear it can't be linear as it is for conduction, because drawing a straight line from the 5800K temperature of our sun down to 3K many billions of light years away would mean that the earth is residing in space that has a temperature that is essentially the same temperature as the sun. Figuring out this temperature gradient is essential to finding planets around other stars that could support life, because the planets will be in approximate thermal equilibrium with the space around them, and we assume life can only be sustained within a certain temperature range (the so-called "Goldilocks zone").
To solve this problem, let's consider a star with radius \(R_o\) and surface temperature \(T_o\). Next, construct an imaginary spherical surface centered at the center of the star, with a radius \(R\). We wish to compute the temperature \(T\) evaluated at this surface. Treating the star as a blackbody, the rate at which energy is radiated from it is:
\[\frac{dQ}{dt}\left(star\right)=\sigma \left(4\pi R_o^2\right)T_o^4 \nonumber\]
Now let's imagine that we treat our imaginary surface as a radiator of energy – we don't even know about the star inside of it. Naturally it behaves like a blackbody, because none of the radiation that strikes it from outside is reflected (it is imaginary!), and a perfect absorber is exactly the definition of a blackbody. We can therefore compute the rate at which it radiates energy outward:
\[\frac{dQ}{dt}\left(sphere\right)=\sigma \left(4\pi R^2\right)T^4 \nonumber\]
But of course, all of the power that comes from the star passes through this surface, so the power emitted by the surface is the same as the power emitted by the star. Setting them equal and solving for \(T\) gives:
\[T\left(R\right)=\sqrt{\frac{R_o}{R}}T_o \nonumber\]
So we see that the temperature drops as the inverse-square-root of the distance from the star.
Video Lecture
3 videos: conduction, convection, radiation, with demos and examples
Introduction to the Study of Heat Transfer
- First Online: 11 March 2021
Cite this chapter
- S. P. Venkateshan 2
3119 Accesses
H eat transfer is an important area of study since its applications are universal. Heat transfer plays a role in all sciences and technologies. It plays an important role in both living and non-living things. The scale at which heat transfer takes place spans the smallest scales at the atomic level to very large scales at the level of the universe. The present book is an introduction to the field of “Heat Transfer”, not necessarily covering all its aspects. The present book aims to develop the subject ground up and brings it to a level sufficient to motivate the reader to look at current heat transfer literature.
This is a preview of subscription content, log in via an institution to check access.
Access this chapter
- Available as PDF
- Read on any device
- Instant download
- Own it forever
- Available as EPUB and PDF
- Compact, lightweight edition
- Dispatched in 3 to 5 business days
- Free shipping worldwide - see info
- Durable hardcover edition
Tax calculation will be finalised at checkout
Purchases are for personal use only
Institutional subscriptions
These scientists were early contributors to the field of heat transfer; between 1600 and 1950 CE. Several Non-dimensional numbers in Fluid Mechanics and Heat Transfer are named after them.
Mass transfer is not considered in the present book.
The medium is assumed to have the same property at all locations inside it.
These are in the nature of postulates or axioms used in modeling conduction heat transfer in a homogeneous medium.
Jean-Baptiste Joseph Fourier 1768–1830, French mathematician and physicist.
Jožef Stefan, 1835–1893 and Ludwig Boltzmann—1844–1906, Austrian physicists.
Isaac Newton 1643–1727, English physicist and mathematician.
Author information
Authors and affiliations.
Department of Mechanical Engineering, Indian Institute of Technology Madras, Chennai, Tamil Nadu, India
S. P. Venkateshan
You can also search for this author in PubMed Google Scholar
Corresponding author
Correspondence to S. P. Venkateshan .
Rights and permissions
Reprints and permissions
Copyright information
© 2021 The Author(s), under exclusive license to Springer Nature Switzerland AG
About this chapter
Venkateshan, S.P. (2021). Introduction to the Study of Heat Transfer. In: Heat Transfer . Springer, Cham. https://doi.org/10.1007/978-3-030-58338-5_1
Download citation
DOI : https://doi.org/10.1007/978-3-030-58338-5_1
Published : 11 March 2021
Publisher Name : Springer, Cham
Print ISBN : 978-3-030-58337-8
Online ISBN : 978-3-030-58338-5
eBook Packages : Engineering Engineering (R0)
Share this chapter
Anyone you share the following link with will be able to read this content:
Sorry, a shareable link is not currently available for this article.
Provided by the Springer Nature SharedIt content-sharing initiative
- Publish with us
Policies and ethics
- Find a journal
- Track your research
1.4 Heat Transfer, Specific Heat, and Calorimetry
Learning objectives.
By the end of this section, you will be able to:
- Explain phenomena involving heat as a form of energy transfer
- Solve problems involving heat transfer
We have seen in previous chapters that energy is one of the fundamental concepts of physics. Heat is a type of energy transfer that is caused by a temperature difference, and it can change the temperature of an object. As we learned earlier in this chapter, heat transfer is the movement of energy from one place or material to another as a result of a difference in temperature. Heat transfer is fundamental to such everyday activities as home heating and cooking, as well as many industrial processes. It also forms a basis for the topics in the remainder of this chapter.
We also introduce the concept of internal energy, which can be increased or decreased by heat transfer. We discuss another way to change the internal energy of a system, namely doing work on it. Thus, we are beginning the study of the relationship of heat and work, which is the basis of engines and refrigerators and the central topic (and origin of the name) of thermodynamics.
Internal Energy and Heat
A thermal system has internal energy , which is the sum of the microscopic energies of the system. This includes thermal energy, which is associated with the mechanical energies of its molecules and which is proportional to the system’s temperature. As we saw earlier in this chapter, if two objects at different temperatures are brought into contact with each other, energy is transferred from the hotter to the colder object until the bodies reach thermal equilibrium (that is, they are at the same temperature). No work is done by either object because no force acts through a distance (as we discussed in Work and Kinetic Energy ). These observations reveal that heat is energy transferred spontaneously due to a temperature difference. Figure 1.9 shows an example of heat transfer.
The meaning of “heat” in physics is different from its ordinary meaning. For example, in conversation, we may say “the heat was unbearable,” but in physics, we would say that the temperature was high. Heat is a form of energy flow, whereas temperature is not. Incidentally, humans are sensitive to heat flow rather than to temperature.
Since heat is a form of energy, its SI unit is the joule (J). Another common unit of energy often used for heat is the calorie (cal), defined as the energy needed to change the temperature of 1.00 g of water by 1.00 ° C 1.00 ° C —specifically, between 14.5 ° C 14.5 ° C and 15.5 ° C 15.5 ° C , since there is a slight temperature dependence. Also commonly used is the kilocalorie (kcal), which is the energy needed to change the temperature of 1.00 kg of water by 1.00 ° C 1.00 ° C . Since mass is most often specified in kilograms, the kilocalorie is convenient. Confusingly, food calories (sometimes called “big calories,” abbreviated Cal) are actually kilocalories, a fact not easily determined from package labeling.
Mechanical Equivalent of Heat
It is also possible to change the temperature of a substance by doing work, which transfers energy into or out of a system. This realization helped establish that heat is a form of energy. James Prescott Joule (1818–1889) performed many experiments to establish the mechanical equivalent of heat — the work needed to produce the same effects as heat transfer . In the units used for these two quantities, the value for this equivalence is
We consider this equation to represent the conversion between two units of energy. (Other numbers that you may see refer to calories defined for temperature ranges other than 14.5 ° C 14.5 ° C to 15.5 ° C 15.5 ° C .)
Figure 1.10 shows one of Joule’s most famous experimental setups for demonstrating that work and heat can produce the same effects and measuring the mechanical equivalent of heat. It helped establish the principle of conservation of energy. Gravitational potential energy ( U ) was converted into kinetic energy ( K ), and then randomized by viscosity and turbulence into increased average kinetic energy of atoms and molecules in the system, producing a temperature increase. Joule’s contributions to thermodynamics were so significant that the SI unit of energy was named after him.
Increasing internal energy by heat transfer gives the same result as increasing it by doing work. Therefore, although a system has a well-defined internal energy, we cannot say that it has a certain “heat content” or “work content.” A well-defined quantity that depends only on the current state of the system, rather than on the history of that system, is known as a state variable . Temperature and internal energy are state variables. To sum up this paragraph, heat and work are not state variables .
Incidentally, increasing the internal energy of a system does not necessarily increase its temperature. As we’ll see in the next section, the temperature does not change when a substance changes from one phase to another. An example is the melting of ice, which can be accomplished by adding heat or by doing frictional work, as when an ice cube is rubbed against a rough surface.
Temperature Change and Heat Capacity
We have noted that heat transfer often causes temperature change. Experiments show that with no phase change and no work done on or by the system, the transferred heat is typically directly proportional to the change in temperature and to the mass of the system, to a good approximation. (Below we show how to handle situations where the approximation is not valid.) The constant of proportionality depends on the substance and its phase, which may be gas, liquid, or solid. We omit discussion of the fourth phase, plasma, because although it is the most common phase in the universe, it is rare and short-lived on Earth.
We can understand the experimental facts by noting that the transferred heat is the change in the internal energy, which is the total energy of the molecules. Under typical conditions, the total kinetic energy of the molecules K total K total is a constant fraction of the internal energy (for reasons and with exceptions that we’ll see in the next chapter). The average kinetic energy of a molecule K ave K ave is proportional to the absolute temperature. Therefore, the change in internal energy of a system is typically proportional to the change in temperature and to the number of molecules, N . Mathematically, Δ U ∝ Δ K total = N K ave ∝ N Δ T Δ U ∝ Δ K total = N K ave ∝ N Δ T The dependence on the substance results in large part from the different masses of atoms and molecules. We are considering its heat capacity in terms of its mass, but as we will see in the next chapter, in some cases, heat capacities per molecule are similar for different substances. The dependence on substance and phase also results from differences in the potential energy associated with interactions between atoms and molecules.
Heat Transfer and Temperature Change
A practical approximation for the relationship between heat transfer and temperature change is:
where Q is the symbol for heat transfer (“quantity of heat”), m is the mass of the substance, and Δ T Δ T is the change in temperature. The symbol c stands for the specific heat (also called “ specific heat capacity ”) and depends on the material and phase. In the SI system, the specific heat is numerically equal to the amount of heat necessary to change the temperature of 1.00 1.00 kg of mass by 1.00 ° C 1.00 ° C . The SI unit for specific heat is J/ ( kg × K ) J/ ( kg × K ) or J/ ( kg × °C ) J/ ( kg × °C ) . (Recall that the temperature change Δ T Δ T is the same in units of kelvin and degrees Celsius.)
Values of specific heat must generally be measured, because there is no simple way to calculate them precisely. Table 1.3 lists representative values of specific heat for various substances. We see from this table that the specific heat of water is five times that of glass and 10 times that of iron, which means that it takes five times as much heat to raise the temperature of water a given amount as for glass, and 10 times as much as for iron. In fact, water has one of the largest specific heats of any material, which is important for sustaining life on Earth.
The specific heats of gases depend on what is maintained constant during the heating—typically either the volume or the pressure. In the table, the first specific heat value for each gas is measured at constant volume, and the second (in parentheses) is measured at constant pressure. We will return to this topic in the chapter on the kinetic theory of gases.
In general, specific heat also depends on temperature. Thus, a precise definition of c for a substance must be given in terms of an infinitesimal change in temperature. To do this, we note that c = 1 m Δ Q Δ T c = 1 m Δ Q Δ T and replace Δ Δ with d :
Except for gases, the temperature and volume dependence of the specific heat of most substances is weak at normal temperatures. Therefore, we will generally take specific heats to be constant at the values given in the table.
Example 1.5
Calculating the required heat.
- Calculate the temperature difference: Δ T = T f − T i = 60.0 ° C . Δ T = T f − T i = 60.0 ° C .
- Calculate the mass of water. Because the density of water is 1000 kg/m 3 1000 kg/m 3 , 1 L of water has a mass of 1 kg, and the mass of 0.250 L of water is m w = 0.250 kg m w = 0.250 kg .
- Calculate the heat transferred to the water. Use the specific heat of water in Table 1.3 : Q w = m w c w Δ T = ( 0.250 kg ) ( 4186 J/kg ° C ) ( 60.0 ° C ) = 62.8 kJ . Q w = m w c w Δ T = ( 0.250 kg ) ( 4186 J/kg ° C ) ( 60.0 ° C ) = 62.8 kJ .
- Calculate the heat transferred to the aluminum. Use the specific heat for aluminum in Table 1.3 : Q Al = m A1 c A1 Δ T = ( 0.500 kg ) ( 900 J/kg ° C ) ( 60.0 ° C ) = 27.0 kJ . Q Al = m A1 c A1 Δ T = ( 0.500 kg ) ( 900 J/kg ° C ) ( 60.0 ° C ) = 27.0 kJ .
- Find the total transferred heat: Q Total = Q W + Q Al = 89.8 kJ . Q Total = Q W + Q Al = 89.8 kJ .
Significance
Example 1.6 illustrates a temperature rise caused by doing work. (The result is the same as if the same amount of energy had been added with a blowtorch instead of mechanically.)
Example 1.6
Calculating the temperature increase from the work done on a substance.
Calculate the temperature increase of 10 kg of brake material with an average specific heat of 800 J/kg · °C 800 J/kg · °C if the material retains 10% of the energy from a 10,000-kg truck descending 75.0 m (in vertical displacement) at a constant speed.
Because the kinetic energy of the truck does not change, conservation of energy tells us the lost potential energy is dissipated, and we assume that 10% of it is transferred to internal energy of the brakes, so take Q = M g h / 10 Q = M g h / 10 . Then we calculate the temperature change from the heat transferred, using
where m is the mass of the brake material. Insert the given values to find
In a common kind of problem, objects at different temperatures are placed in contact with each other but isolated from everything else, and they are allowed to come into equilibrium. A container that prevents heat transfer in or out is called a calorimeter , and the use of a calorimeter to make measurements (typically of heat or specific heat capacity) is called calorimetry .
We will use the term “calorimetry problem” to refer to any problem in which the objects concerned are thermally isolated from their surroundings. An important idea in solving calorimetry problems is that during a heat transfer between objects isolated from their surroundings, the heat gained by the colder object must equal the heat lost by the hotter object, due to conservation of energy:
We express this idea by writing that the sum of the heats equals zero because the heat gained is usually considered positive; the heat lost, negative.
Example 1.7
Calculating the final temperature in calorimetry.
- Use the equation for heat transfer Q = m c Δ T Q = m c Δ T to express the heat transferred from the pan in terms of the mass of the pan, the specific heat of aluminum, the initial temperature of the pan, and the final temperature: Q hot = m A1 c A1 ( T f − 150 ° C ) . Q hot = m A1 c A1 ( T f − 150 ° C ) .
- Express the heat gained by the water in terms of the mass of the water, the specific heat of water, the initial temperature of the water, and the final temperature: Q cold = m w c w ( T f − 20.0 ° C ) . Q cold = m w c w ( T f − 20.0 ° C ) .
- Note that Q hot < 0 Q hot < 0 and Q cold > 0 Q cold > 0 and that as stated above, they must sum to zero: Q cold + Q hot = 0 Q cold = − Q hot m w c w ( T f − 20.0 ° C ) = − m A1 c A1 ( T f − 150 ° C ) . Q cold + Q hot = 0 Q cold = − Q hot m w c w ( T f − 20.0 ° C ) = − m A1 c A1 ( T f − 150 ° C ) .
- Bring all terms involving T f T f on the left hand side and all other terms on the right hand side. Solving for T f , T f , T f = m A1 c A1 ( 150 ° C ) + m w c w ( 20.0 ° C ) m A1 c A1 + m w c w , T f = m A1 c A1 ( 150 ° C ) + m w c w ( 20.0 ° C ) m A1 c A1 + m w c w , and insert the numerical values: T f = ( 0.500 kg ) ( 900 J/kg ° C ) ( 150 ° C ) + ( 0.250 kg ) ( 4186 J/kg ° C ) ( 20.0 ° C ) ( 0.500 kg ) ( 900 J/kg ° C ) + ( 0.250 kg ) ( 4186 J/kg ° C ) = 59.1 ° C . T f = ( 0.500 kg ) ( 900 J/kg ° C ) ( 150 ° C ) + ( 0.250 kg ) ( 4186 J/kg ° C ) ( 20.0 ° C ) ( 0.500 kg ) ( 900 J/kg ° C ) + ( 0.250 kg ) ( 4186 J/kg ° C ) = 59.1 ° C .
Check Your Understanding 1.3
If 25 kJ is necessary to raise the temperature of a rock from 25 °C to 30 ° C, 25 °C to 30 ° C, how much heat is necessary to heat the rock from 45 °C to 50 ° C 45 °C to 50 ° C ?
Example 1.8
Temperature-dependent heat capacity.
We solve this equation for Q by integrating both sides: Q = m ∫ T 1 T 2 c d T . Q = m ∫ T 1 T 2 c d T .
Then we substitute the given values in and evaluate the integral:
As an Amazon Associate we earn from qualifying purchases.
This book may not be used in the training of large language models or otherwise be ingested into large language models or generative AI offerings without OpenStax's permission.
Want to cite, share, or modify this book? This book uses the Creative Commons Attribution License and you must attribute OpenStax.
Access for free at https://openstax.org/books/university-physics-volume-2/pages/1-introduction
- Authors: Samuel J. Ling, William Moebs, Jeff Sanny
- Publisher/website: OpenStax
- Book title: University Physics Volume 2
- Publication date: Oct 6, 2016
- Location: Houston, Texas
- Book URL: https://openstax.org/books/university-physics-volume-2/pages/1-introduction
- Section URL: https://openstax.org/books/university-physics-volume-2/pages/1-4-heat-transfer-specific-heat-and-calorimetry
© Jan 19, 2024 OpenStax. Textbook content produced by OpenStax is licensed under a Creative Commons Attribution License . The OpenStax name, OpenStax logo, OpenStax book covers, OpenStax CNX name, and OpenStax CNX logo are not subject to the Creative Commons license and may not be reproduced without the prior and express written consent of Rice University.
If you're seeing this message, it means we're having trouble loading external resources on our website.
If you're behind a web filter, please make sure that the domains *.kastatic.org and *.kasandbox.org are unblocked.
To log in and use all the features of Khan Academy, please enable JavaScript in your browser.
Physics library
Course: physics library > unit 5.
- Introduction to work and energy
- Work and energy (part 2)
- Conservation of energy
- What are energy and work?
- What is kinetic energy?
- What is gravitational potential energy?
- What is conservation of energy?
- Work and the work-energy principle
- Work as the transfer of energy
- Work example problems
- Work as area under curve
Thermal energy from friction
What is thermal energy.
- Work/energy problem with friction
- Conservative forces
- What is power?
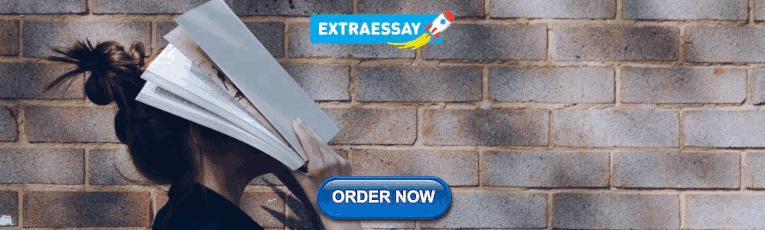
Thermal energy from drag
Want to join the conversation.
- Upvote Button navigates to signup page
- Downvote Button navigates to signup page
- Flag Button navigates to signup page

Energy Transfers and Transformations
Energy cannot be created or destroyed, but it can be transferred and transformed. There are a number of different ways energy can be changed, such as when potential energy becomes kinetic energy or when one object moves another object.
Earth Science, Physics
Water Boiling Pot
There are three types of thermal energy transfer: conduction, radiation, and convection. Convection is a cyclical process that only occurs in fluids.
Photograph by Liu Kuanxi
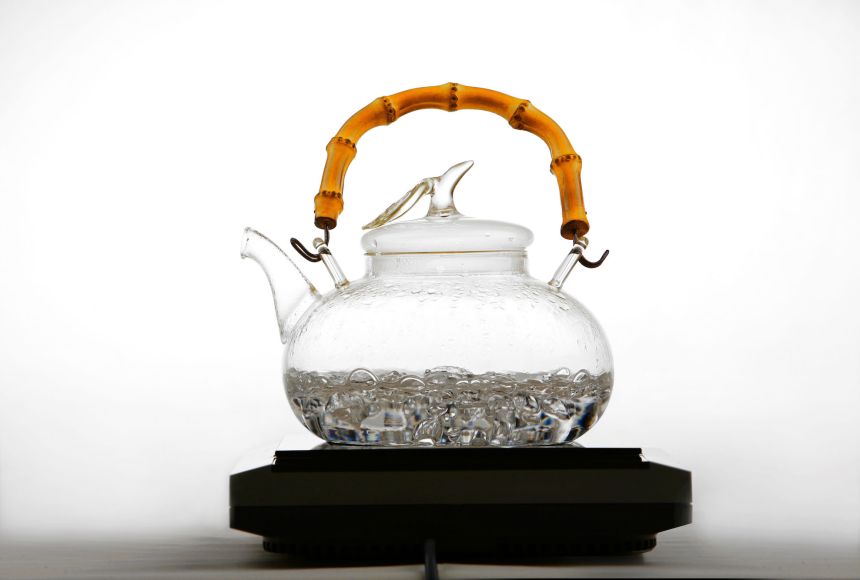
Energy cannot be created or destroyed, meaning that the total amount of energy in the universe has always been and will always be constant. However, this does not mean that energy is immutable; it can change form and even transfer between objects. A common example of energy transfer that we see in everyday life is the transfer of kinetic energy —the energy associated with motion—from one moving object to a stationary object via work. In physics, work is a measure of energy transfer and refers to the force applied by an object over a distance. When a golf club is swung and hits a stationary golf ball, some of the club’s kinetic energy transfers to the ball as the club does “work” on the ball. In an energy transfer such as this one, energy moves from one object to another, but stays in the same form. A kinetic energy transfer is easy to observe and understand, but other important transfers are not as easy to visualize. Thermal energy has to do with the internal energy of a system due to its temperature. When a substance is heated, its temperature rises because the molecules it is composed of move faster and gain thermal energy through heat transfer. Temperature is used as a measurement of the degree of “hotness” or “coldness” of an object, and the term heat is used to refer to thermal energy being transferred from a hotter system to a cooler one. Thermal energy transfers occur in three ways: through conduction , convection , and radiation . When thermal energy is transferred between neighboring molecules that are in contact with one another, this is called conduction . If a metal spoon is placed in a pot of boiling water, even the end not touching the water gets very hot. This happens because metal is an efficient conductor , meaning that heat travels through the material with ease. The vibrations of molecules at the end of the spoon touching the water spread throughout the spoon, until all the molecules are vibrating faster (i.e., the whole spoon gets hot). Some materials, such as wood and plastic, are not good conductors —heat does not easily travel through these materials—and are instead known as insulators . Convection only occurs in fluids, such as liquids and gases. When water is boiled on a stove, the water molecules at the bottom of the pot are closest to the heat source and gain thermal energy first. They begin to move faster and spread out, creating a lower density of molecules at the bottom of the pot. These molecules then rise to the top of the pot and are replaced at the bottom by cooler, denser water. The process repeats, creating a current of molecules sinking, heating up, rising, cooling down, and sinking again. The third type of heat transfer— radiation —is critical to life on Earth and is important for heating bodies of water. With radiation , a heat source does not have to touch the object being heated; radiation can transfer heat even through the vacuum of space. Nearly all thermal energy on Earth originates from the sun and radiates to the surface of our planet, traveling in the form of electromagnetic waves, such as visible light. Materials on Earth then absorb these waves to be used for energy or reflect them back into space. In an energy transformation , energy changes form. A ball sitting at the top of a hill has gravitational potential energy , which is an object’s potential to do work due to its position in a gravitational field. Generally speaking, the higher on the hill this ball is, the more gravitational potential energy it has. When a force pushes it down the hill, that potential energy transforms into kinetic energy . The ball continues losing potential energy and gaining kinetic energy until it reaches the bottom of the hill. In a frictionless universe, the ball would continue rolling forever upon reaching the bottom, since it would have only kinetic energy . On Earth, however, the ball stops at the bottom of the hill due to the kinetic energy being transformed into heat by the opposing force of friction. Just as with energy transfers , energy is conserved in transformations. In nature, energy transfers and transformations happen constantly, such as in a coastal dune environment. When thermal energy radiates from the sun, it heats both the land and ocean, but water has a specific high heat capacity, so it heats up slower than land. This temperature difference creates a convection current, which then manifests as wind. This wind possesses kinetic energy , which it can transfer to grains of sand on the beach by carrying them a short distance. If the moving sand hits an obstacle, it stops due to the friction created by the contact and its kinetic energy is then transformed into thermal energy , or heat. Once enough sand builds up over time, these collisions can create sand dunes, and possibly even an entire dune field. These newly formed sand dunes provide a unique environment for plants and animals. A plant may grow in these dunes by using light energy radiated from the sun to transform water and carbon dioxide into chemical energy , which is stored in sugar. When an animal eats the plant, it uses the energy stored in that sugar to heat its body and move around, transforming the chemical energy into kinetic and thermal energy . Though it may not always be obvious, energy transfers and transformations constantly happen all around us and are what enable life as we know it to exist.
Media Credits
The audio, illustrations, photos, and videos are credited beneath the media asset, except for promotional images, which generally link to another page that contains the media credit. The Rights Holder for media is the person or group credited.
Production Managers
Program specialists, last updated.
October 19, 2023
User Permissions
For information on user permissions, please read our Terms of Service. If you have questions about how to cite anything on our website in your project or classroom presentation, please contact your teacher. They will best know the preferred format. When you reach out to them, you will need the page title, URL, and the date you accessed the resource.
If a media asset is downloadable, a download button appears in the corner of the media viewer. If no button appears, you cannot download or save the media.
Text on this page is printable and can be used according to our Terms of Service .
Interactives
Any interactives on this page can only be played while you are visiting our website. You cannot download interactives.
Related Resources
- Thermodynamics
- Heat Transfer
Modes of Heat Transfer (Conduction Examples)
In our everyday life, it has been observed that when a pan full of water is boiled on a flame, its temperature increases, but when the flame is turned off, it slowly cools down.
Table of Contents
What are the different modes of heat transfer, recommended videos, factors affecting heat transfer.
- Frequently Asked Questions – FAQs
This is because of the phenomenon of heat transfer taking place between the pan full of water and the flame. It has been established that heat transfer takes place from hotter objects to colder objects.
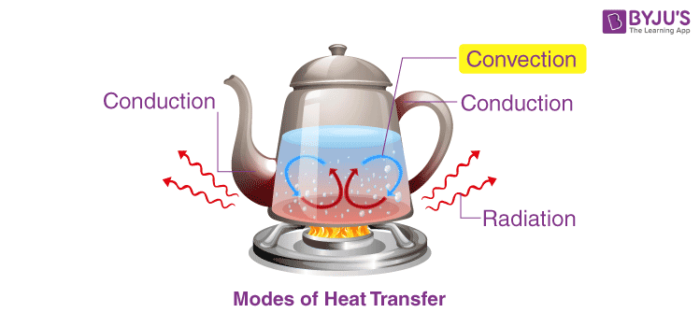
When there are objects which are at different temperatures or there is an object at a different temperature from the surroundings, then the transfer of heat takes place so that the object and the surrounding, both reach an equilibrium temperature.
There are three modes of heat transfer.
1. Conduction of Heat
Heat conduction is a process in which heat is transferred from the hotter part to the colder part in a body without involving any actual movement of the molecules of the body. Heat transfer takes place from one molecule to another molecule as a result of the vibratory motion of the molecules. Heat transfer through the process of conduction occurs in substances which are in direct contact with each other. It generally takes place in solids.
Conduction example: When frying vegetables in a pan. Heat transfer takes place from flame to the pan and then to the vegetables.
Based on the conductivity of heat , substances can be classified as conductors and insulators. Substances that conduct heat easily are known as conductors and those that do not conduct heat are known as insulators.
2. Convection of Heat
In this process, heat is transferred in the liquid and gases from a region of higher temperature to a region of lower temperature. Convection heat transfer occurs partly due to the actual movement of molecules or due to the mass transfer.
For example. Heating of milk in a pan.
3. Radiation of Heat
It is the process in which heat is transferred from one body to another body without involving the molecules of the medium. Radiation heat transfer does not depend on the medium.
For example: In a microwave, the substances are heated directly without any heating medium.
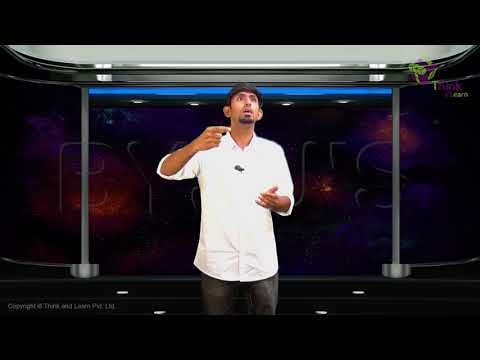
Now we will discuss the rate of heat transfer or the factors on which it depends. The rate of heat transfer depends on the following:
ΔQΔt ∝ A(T1–T2)x
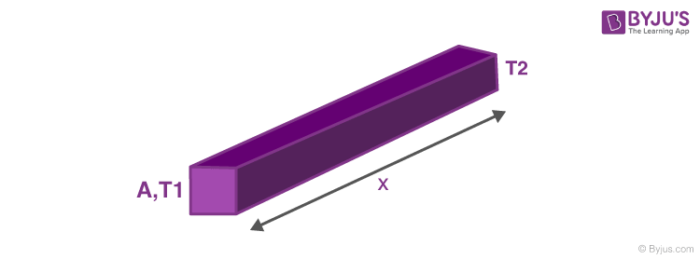
So the heat transfer equation comes out to be, ΔQΔt = K A(T1–T2)x where, K is the heat transfer coefficient. Here if heat flow is positive then we can infer T1 > T2. So heat flows from higher temperature to lower temperature. We can see that an analogy with electricity can be drawn, here temperature plays the role of potential difference and rate of heat transfer is like current while the rest of expression is like Electric Resistance . Now that we have drawn an analogy, so there must be series and parallel connections here also,
1. Heat Transfer in Series
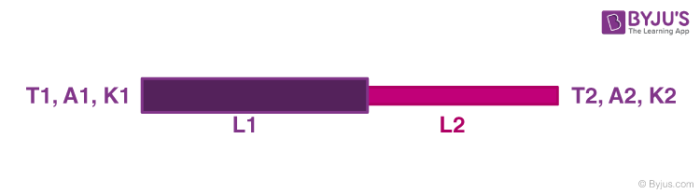
Let the temperature of the junction be T. Therefore for the first rod,
⇒ ΔQΔt = K1 A1(T1–T)L1 —- (1)
Also for the second rod,
⇒ ΔQΔt = K2 A2(T–T2)L2 —- (2)
Since the temperature of conjunction remains constant, so the rate of heat transfer in (1) and (2) must be the same. Using the equation we can find the Value of temperature ‘T′.
2. Heat Transfer in Parallel
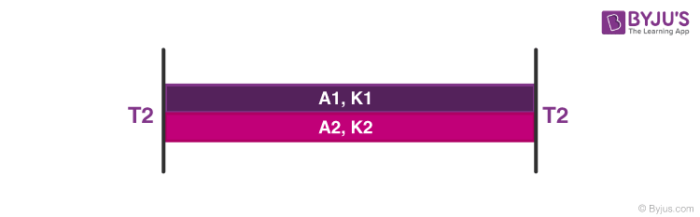
ΔQΔt = K1A1(T1–T2)L —- (3)
ΔQΔt = K2 A2(T1–T2)L —- (4)
So, net heat flow is the summation of (3) and (4). Suppose that the outside temperature is T and the depth of the lake is h. How much time will it take to freeze the entire lake? The latent heat of ice is L and thermal conductivity is K.
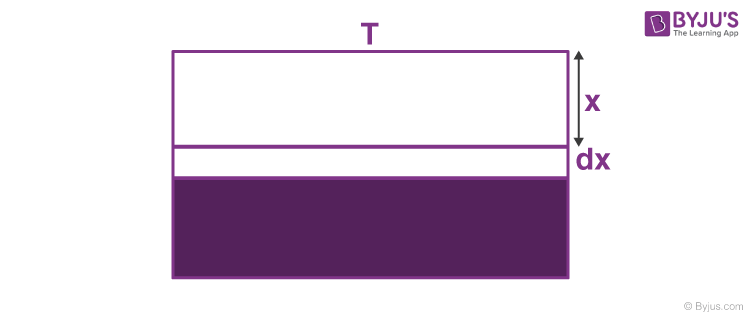
At this point, the rate of heat transfer is, ⇒ dQdt = KATx
⇒ dQ = KATx dt —- (5)
This heat is taken out and dx layer of ice is formed.
dm = ρA.dx —- (6)
Putting values from (5) and (6) we get,
KATx dt = ρA.dx.L
⇒ ∫t0 dt = ρLKT∫h0x.dx
Integrating with limits we get,
t = ρLh22KT
Frequently Asked Questions – FAQs
What are the different modes of heat transfer.
There are primarily three modes of heat transfer: Conduction, Convection and Radiation.
What is heat conduction?
Heat conduction is a process in which heat is transferred from the hotter part to the colder part of a body without involving any actual movement of the body’s molecules. Example: When frying vegetables in a pan. Heat transfer occurs from the flame to the pan and the vegetables.
What is heat convection?
Heat convection is a process in which heat is transferred in the liquid and gases from a higher temperature region to a lower temperature region. Convection heat transfer occurs partly due to the actual movement of molecules or due to the mass transfer. Example. Heating of milk in a pan.
What is heat radiation?
Heat radiation is a process in which heat is transferred from one body to another without involving the medium’s molecules. Radiation heat transfer does not depend on the medium. Example: In a microwave, the substances are heated directly without any heating medium.
What is the cause of heat transfer?
The difference in temperature is the primary cause of heat transfer.
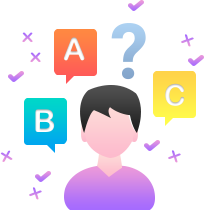
Put your understanding of this concept to test by answering a few MCQs. Click ‘Start Quiz’ to begin!
Select the correct answer and click on the “Finish” button Check your score and answers at the end of the quiz
Visit BYJU’S for all Chemistry related queries and study materials
Your result is as below
Request OTP on Voice Call
Leave a Comment Cancel reply
Your Mobile number and Email id will not be published. Required fields are marked *
Post My Comment

- Share Share
Register with BYJU'S & Download Free PDFs
Register with byju's & watch live videos.

Home — Essay Samples — Science — Energy — Thermal Conduction, Convection and Radiation

Thermal Conduction, Convection and Radiation
- Categories: Energy
About this sample

Words: 667 |
Published: Dec 5, 2018
Words: 667 | Page: 1 | 4 min read
Thermal Conduction
- exposed surface area
- conductivity
- acceleration due to gravity
Thermal Radiation
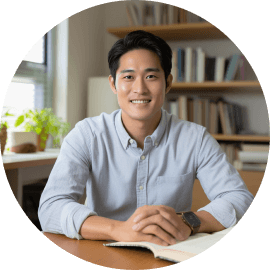
Cite this Essay
Let us write you an essay from scratch
- 450+ experts on 30 subjects ready to help
- Custom essay delivered in as few as 3 hours
Get high-quality help

Dr. Karlyna PhD
Verified writer
- Expert in: Science
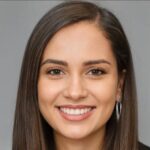
+ 120 experts online
By clicking “Check Writers’ Offers”, you agree to our terms of service and privacy policy . We’ll occasionally send you promo and account related email
No need to pay just yet!
Related Essays
3 pages / 1456 words
3 pages / 1184 words
1 pages / 596 words
2 pages / 689 words
Remember! This is just a sample.
You can get your custom paper by one of our expert writers.
121 writers online
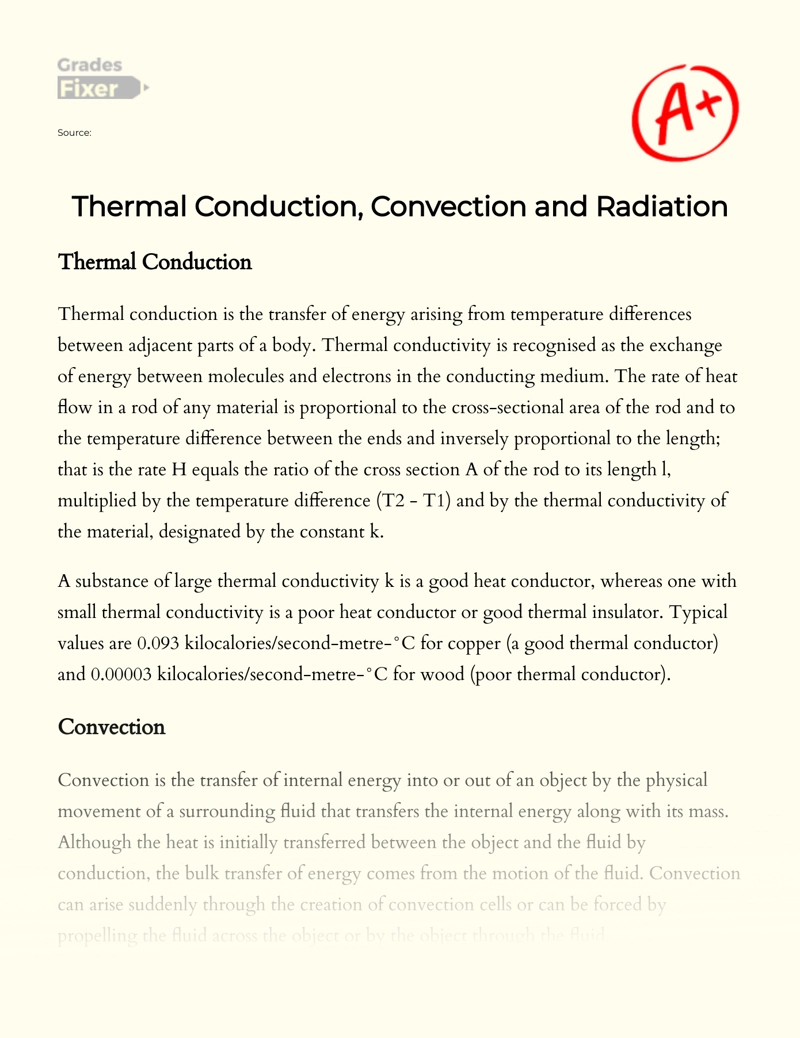
Still can’t find what you need?
Browse our vast selection of original essay samples, each expertly formatted and styled
Related Essays on Energy
Oil, coal, and natural gas are non-renewable energy sources known as fossil fuels which are currently the world’s primary source of energy. These are formed by prehistoric plants and animals that died over a million years ago [...]
Green energy comes from natural sources such as sunlight, wind, water and, biogas. These energy resources are renewable, meaning they’re naturally replenished. They are the safe way to produce energy that will not harm the world [...]
In a world driven by constant technological advancements and increasing global demands, the importance of energy cannot be overstated. From powering our homes and businesses to fueling our vehicles and industries, energy is the [...]
Upon making a move to a WFD, you might find your energy levels begin to rise. Despite the fact this diet may inadvertently decrease your caloric intake which ordinarily would diminish energy stores, studies show that people who [...]
In the last few years, a range of energy-storage device applications has expanded from portable electronics to large-scale energy storage systems, including renewable energy storage and electric transportation . To fulfill the [...]
Light arrives on our planet after a speedy trip from the Sun, 149 million km (93 million miles away). Light travels at 186,000 miles (300,000 km) per second, so the light you’re seeing now was still tucked away in the Sun about [...]
Related Topics
By clicking “Send”, you agree to our Terms of service and Privacy statement . We will occasionally send you account related emails.
Where do you want us to send this sample?
By clicking “Continue”, you agree to our terms of service and privacy policy.
Be careful. This essay is not unique
This essay was donated by a student and is likely to have been used and submitted before
Download this Sample
Free samples may contain mistakes and not unique parts
Sorry, we could not paraphrase this essay. Our professional writers can rewrite it and get you a unique paper.
Please check your inbox.
We can write you a custom essay that will follow your exact instructions and meet the deadlines. Let's fix your grades together!
Get Your Personalized Essay in 3 Hours or Less!
We use cookies to personalyze your web-site experience. By continuing we’ll assume you board with our cookie policy .
- Instructions Followed To The Letter
- Deadlines Met At Every Stage
- Unique And Plagiarism Free
- Share full article
Advertisement
Supported by
Guest Essay
We Don’t See What Climate Change Is Doing to Us

By R. Jisung Park
Dr. Park is an environmental and labor economist and assistant professor at the University of Pennsylvania and the author of “Slow Burn: The Hidden Costs of a Warming World.”
Many of us realize climate change is a threat to our well-being. But what we have not yet grasped is that the devastation wreaked by climate change comes not just from headline-grabbing catastrophes but also from the subtler accumulation of innumerable slow and unequal burns that are already underway — the nearly invisible costs that may not raise the same alarm but that, in their pervasiveness and inequality, may be much more harmful than commonly realized. Recognizing these hidden costs will be essential as we prepare ourselves for the warming that we have ahead of us.
Responsibility for mitigating climate change on the local level lies in part with public institutions not only in encouraging emissions reductions but also in facilitating adaptation. Public discourse around climate change too often misses the central role that local institutions play in this latter function, how much of the realized pain locally depends on not simply the physical phenomena of climate change per se but also how they interact with human systems — economic, educational, legal and political.
Let’s start with heat, which is killing more people than most other natural disasters combined. Research shows that record-breaking heat waves are only part of the story. Instead, it may be the far more numerous unremarkably hot days that cause the bulk of societal destruction, including through their complex and often unnoticed effects on human health and productivity. In the United States, even moderately elevated temperatures — days in the 80s or 90s Fahrenheit — are responsible for just as many excess deaths as the record triple-digit heat waves, if not more, according to my calculations based on a recent analysis of Medicare records.
In some highly exposed and physically demanding industries, like mining, a day in the 90s can increase injury risk by over 65 percent relative to a day in the 60s. While some of these incidents involve clear cases of heat illness, my colleagues and I have found that a vast majority appear to come from ostensibly unrelated accidents, like construction workers falling off ladders and manufacturing workers mishandling hazardous machinery. In California, our research shows, heat might have routinely caused 20,000 workplace injuries per year, only a tiny fraction of which were officially recorded as heat-related.
A growing body of literature links temperature to cognitive performance and decision making. Research shows that hotter days lead to more mistakes, including among professional athletes ; more local crime ; and more violence in prisons , according to working papers. They also correspond with more use of profanity on social media , suggesting that even an incrementally hotter world is likely to be a nontrivially more irritable, error-prone and conflictual one.
Children are not immune. In research using over four million student test scores from New York City, I found that, from 1999 to 2011, students who took their high school Regents exams on a 90-degree day were 10 percent less likely to pass their subjects relative to a day in the 60s. In other research, my colleagues Joshua Goodman, Michael Hurwitz and Jonathan Smith and I found that across the country, hotter school years led to slower gains on standardized exams like the Preliminary SAT exams. It may not seem a huge effect, on average: roughly 1 percent of learning lost per one-degree-hotter school year temperatures. Probably hardly noticeable in any given year. But because these learning effects are cumulative, they may have significant consequences.
And that’s just heat. Researchers are bringing to light the more subtle yet cumulatively damaging effects of increased wildfires and other natural disasters. The hidden consequences of wildfire smoke may cut even deeper than the more visible death and destruction caused by the flames. Tallying the downstream economic and health costs of smoke exposure, researchers have estimated in a not-yet-published paper that increased wildfire smoke due to climate change may cause more than 20,000 additional deaths per year nationwide by 2050. Very few of these will be officially categorized as having been caused by wildfires, because they will have been the result of the cumulative influence of worsened air quality and weakened health over the course of many weeks and months. Research now suggests that wildfire smoke can adversely affect fetal health , student learning and workers’ earnings as well.
Since even noncatastrophic climate change may be more subtly damaging and inequality amplifying than we used to think, local interventions are essential to help us prepare for the warming that is to come.
At present, our social and economic systems are not well prepared to adjust to the accumulating damage wreaked by climate change, even though much of what determines whether climate change hurts us depends on the choices we make as individuals and as a society. Whether a hot day leads to mild discomfort or widespread mortality comes down to human decisions — individual decisions such as whether to install and operate air-conditioning and collective decisions around the pricing and availability of insurance, the allocation of hospital beds or the procedures and norms governing how and when people work.
Recent research indicates that how temperature affects human health depends greatly on the adaptations that happen to be at play locally. For instance, a day above 85 degrees in the coldest U.S. ZIP codes has nearly 10 times the effect on elderly mortality relative to in the warmest ZIP codes. In other words, a string of such days in a place like Seattle will lead to a much higher increase in the mortality rate than in a place like Houston, even though both places have similar income levels. In rural India , institutional factors like access to banking may affect how many lives are ultimately lost because of heat; heat can reduce crop yields, leaving subsistence farmers dependent on financing sources to keep them afloat.
In our research of heat and learning , we found that the adverse effects of a one-degree-hotter school year are two to three times as large for Black and Hispanic students, who are less likely to have working air-conditioning at school or at home even within a given city, and are virtually nonexistent in schools and neighborhoods with high levels of home and school air-conditioning. We estimate that hotter temperatures may already be responsible for 5 percent of racial academic achievement gaps. Without remedial investments, climate change is likely to widen these gaps. With a shift in focus to these subtler social costs, we can devise and carry out more effective strategies. But right now, adaptation efforts remain highly fragmented and are often focused on more visibly salient climate hazards, like storm surges .
And, of course, an empirically nuanced understanding of climate damages makes it even clearer that reducing emissions aggressively makes cost-benefit sense not only because we want to insure against total ecological breakdown (cue “extinction rebellion” and “tipping points”) but also because the economic costs of even noncatastrophic warming may be considerable. Recent Environmental Protection Agency estimates that incorporate just some of these cumulative impacts suggest that a single ton of carbon dioxide sets in motion $190 worth of future social costs, which means that technologies that can reduce such emissions at a lower per-ton cost are most likely worth pursuing.
Climate change is a complex phenomenon whose ultimate costs will depend not only on how quickly we transition away from fossil fuels but also on how well we adapt our social and economic systems to the warming we have in store. A proactive stance toward adaptation and resilience may be useful from the standpoint of safeguarding one’s own physical and financial security, whether as a homeowner or the head of a Fortune 500 company. It may be vital for ensuring that the ladders of economic opportunity are not deteriorating for those attempting to climb its lower rungs.
R. Jisung Park is an environmental and labor economist and assistant professor at the University of Pennsylvania and the author of “Slow Burn: The Hidden Costs of a Warming World.”
The Times is committed to publishing a diversity of letters to the editor. We’d like to hear what you think about this or any of our articles. Here are some tips . And here’s our email: [email protected] .
Follow the New York Times Opinion section on Facebook , Instagram , TikTok , WhatsApp , X and Threads .
The Unbearable Heat of Prisons in Summer
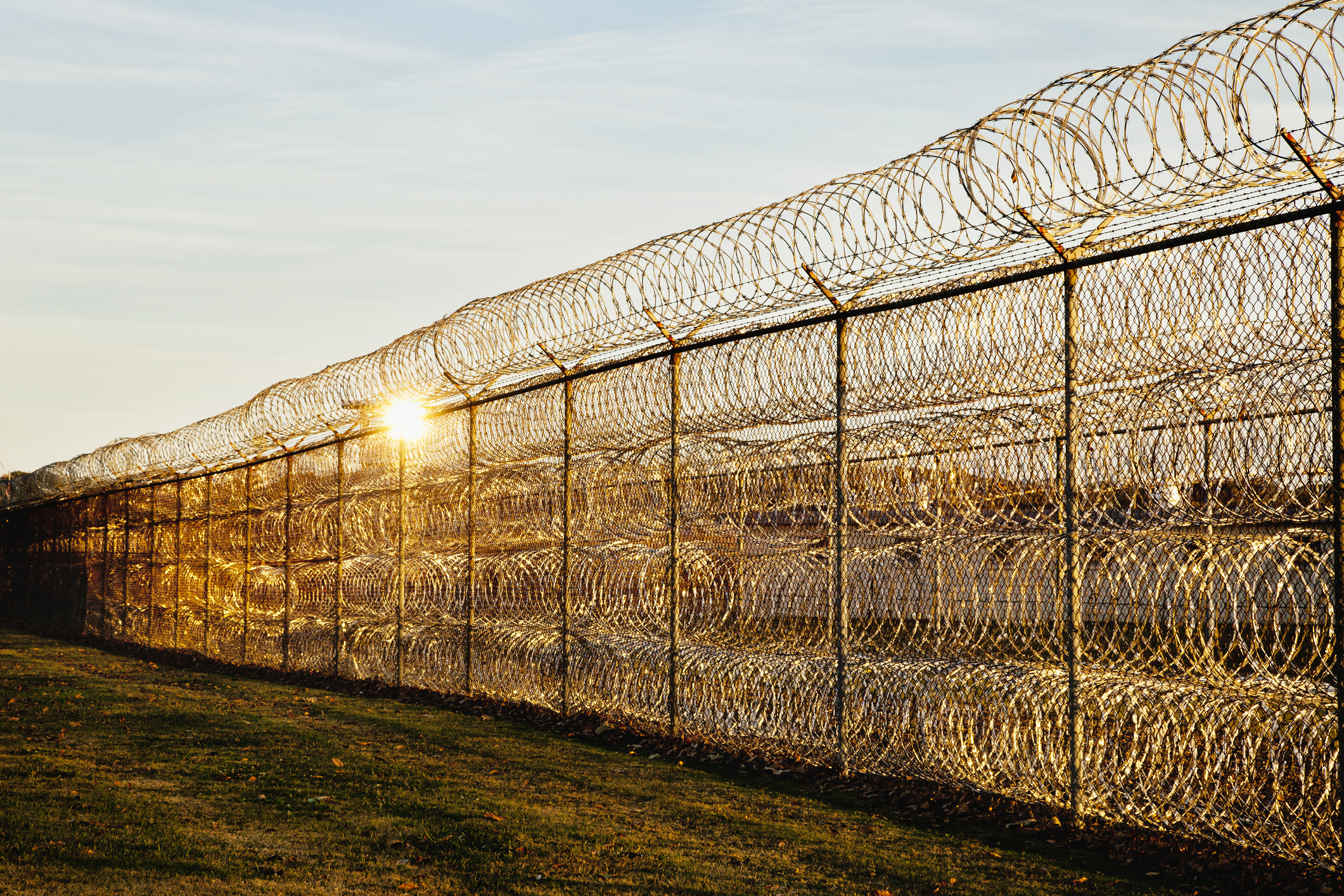
L ike many people, I knew nothing about the heat crisis in U.S. prisons for most of my life. I first learned of the crisis in early 2016, when I started teaching at a maximum security prison in South Texas. I taught creative writing there for almost seven years, and every summer my students would write about the daily torture they endured, locked in a facility without air conditioning, where temperatures often topped 110 degrees Fahrenheit. The heat gave them searing headaches and blurred vision, dizziness and nausea, as well as a despair that their society could allow such cruel treatment, ignoring their basic human rights. It was not just a physical torture, but also a psychological punishment, a message that they were subhuman. Such temperatures would violate animal cruelty laws , but they didn’t deserve this dignity.
My students were far from alone in this suffering. Every summer, people in many U.S. prisons are forced to take drastic measures: flooding their toilets to lie down in the slightly cooler water, wrapping wet rags around their limbs, spending their meager prison wages (usually less than a dollar an hour) to buy fans that actually increase the problem, circulating rather than cooling the stifling air. Many stop taking their medications, as this would put added strain on their hearts, which can prove lethal if added to the coronary strain of the heat. Now and then people do die, usually of cardiac incidents, though prison authorities often deny this is related to the heat torture. As a 2023 study from academic journal PLOS ONE has shown , prison suicide rates increase after heatwaves as well. One might think the temperature in the cells would lower at night, but it often barely goes down .
My purpose here is not to dwell on the basic facts of the prison heat crisis, which have been reported so well elsewhere . Though opinions differ (often by political party) on what counts as an “ethical” prison, there’s no denying that high temperatures can pose health risks . Some hard-right cynics might still deny the reality of global warming, but you only need a thermometer to know that our prisons get hotter every year. Around 44 states lack universal air conditioning in their prisons, many the hottest states in the nation; in Texas prisons alone, according to a 2022 study by Brown University researchers, there are an average of 14 heat-related deaths per year. No jury ever sentences a defendant to heat torture, but this is the punishment that hundreds of thousands must face. Some have received a death sentence for as little as cashing a bad check .
With its rising number of deaths, Texas prisons have received a specific censure from the U.N. Convention Against Torture. The U.N. called the prisons “unbearably hot” and pressed for “urgent measures.” Very few have yet been taken. Nor is this issue confined to Southern states: many Northeastern prisons lack air conditioning, and a 2023 study of their state prisons by the Prison Policy Initiative found that deaths increased around 21% from two-day heat waves.
Read More: Air Conditioning is a Human Right.’ Heat-Related Prison Deaths Are Rising Due to Climate Change
These facts are tragic enough, but there are wider implications to the crisis. In her groundbreaking book, The New Jim Crow: Mass Incarceration in the Age of Colorblindness , legal scholar Michelle Alexander wrote about the troubling demographics of U.S. prisons, which incarcerate minorities at a much higher rate than the white population. “The United States imprisons a larger percentage of its black population than South Africa did at the height of apartheid,” Alexander explained. “These stark racial disparities cannot be explained by rates of drug crime. Studies show that people of all colors use and sell illegal drugs at remarkably similar rates. …In some states, black men have been admitted to prison on drug charges at rates 20 to 50 times greater than those of white men.”
This excessive imprisonment of minorities began in the 1860s, just after the purported “end of slavery.” In Tennessee, for instance, the prison population under slavery was less than 5% Black; a year after the Civil War, that number had jumped to 52%; by 1891, it was 75% . Numbers like this could be seen across the South, and the purpose was not only punitive: the state governments colluded with business and landowners in a practice called “ convict leasing ,” which consigned large segments of the Black population to a slavery in all but name .
It is common for white Americans (often raised on simplistic history texts) to think that injustices like this stopped in the 1960s, with the victories of the Civil Rights Movement. In fact, the most shocking prison statistics come from the 1980s-2000s, when the “War on Drugs” swelled our penal population from around 300,000 to over 2 million, the highest in the world. Not surprisingly, much of that rise came from minority drug convictions. For Black men born in 1981, 1 in 3 can expect incarceration; in 2001, 1 in 5. Despite reforms, Black Americans are still imprisoned at around five times the rate of whites, and over nine times in supposedly liberal states like California, Connecticut, Maine, and New Jersey. Once released, they then face legalized discrimination in voting, housing, employment, education, and public benefits--the restricted rights of “second-class” citizens, not unsimilar to life in an apartheid state. As of December 2022, the U.S. imprisoned 1.67 million people , just 15 thousand less than the world leader, China, whose population is over a billion larger than ours. As Alexander wrote, “The American penal system has emerged as a system of social control unparalleled in world history.”
These racial statistics should reframe the discussion of the prison heat crisis. Yes, we should install air conditioning in every U.S. prison; yes, we should pass federal laws for prison temperature standards. But we must also examine the insidious patterns of racism in our nation, where we not only incarcerate a disproportionate number of minority citizens, but then submit them to month after month of literal torture, living conditions that would qualify as war crimes and violate articles of the Geneva Convention. In the words of one union president for Texas correctional officers, “We’re supposed to run prisons, not concentration camps.”
Over the past few years, we have decried the suffering of Palestinian and Ukrainian citizens; we have criticized Russia and Israel for their human rights abuses. Without question, this outcry is necessary and just. But at the same time, we have ignored the hundreds of thousands who suffer from heat torture in our prisons, many from minority groups that have been targeted and oppressed for all of U.S. history.
That this causes no real scandal is a flagrant sign of our racism. Every year, there is a trickle of news stories about the crisis (John Oliver ran a notable segment two years ago) but our politicians do little to oppose the torture of the systematically oppressed. President Biden’s Build Back Better Bill—rightly lauded as a major achievement—never included a single dollar to support the upgrade of jails and detention centers, even before conservatives slashed its budget. This was not even a talking point for most progressives. As a nation, it’s clear we like to pick and choose which Amendments matter to us. The Second Amendment is a rallying cry for many, but the Eighth—against cruel and unusual punishment—can barely find a voice. The reason for this is simple: the victims of torture rarely finance political campaigns.
In a moment like this one, where human rights abuses fill our newsfeeds, nearly all of us feel powerless and overwhelmed. But we are not as voiceless as those who suffer in our prisons, and prison reform is possible with enough pressure on our politicians. After a sanction from the Justice Department, Mississippi began last year to install air conditioning in all its prisons; in Texas this year, the state House passed a bill to spend $545 million on prison air conditioning (though this was then gutted by the State Senate). With the many issues that press upon us, it is easy to forget the most vulnerable in our society, especially when these people are behind bars, kept out of sight. But I ask you to remember the words of Tona Southards Naranjo, whose son, Jon Southards, died in a Texas prison this summer from a heat-related heart attack: “We need desperate change, and we need it today, this hour. They’re cooking our babies alive.”
More Must-Reads From TIME
- The 100 Most Influential People of 2024
- The Revolution of Yulia Navalnaya
- 6 Compliments That Land Every Time
- What's the Deal With the Bitcoin Halving?
- If You're Dating Right Now , You're Brave: Column
- The AI That Could Heal a Divided Internet
- Fallout Is a Brilliant Model for the Future of Video Game Adaptations
- Want Weekly Recs on What to Watch, Read, and More? Sign Up for Worth Your Time
Contact us at [email protected]
- FanNation FanNation FanNation
- SI.COM SI.COM SI.COM
- SI SWIMSUIT SI SWIMSUIT SI SWIMSUIT
- SI Sportsbook SI Sportsbook SI Sportsbook
- SI Tickets SI Tickets SI Tickets
- SI Showcase SI Showcase SI Showcase
- SI Resorts SI Resorts SI Resorts
© Ehsan Kassim/Tallahassee Democrat / USA
Former FSU Football Defender And Son Of Seminole Legend Enters NCAA Transfer Portal
Jaden Floyd is the son of William Floyd, a fullback for the 1993 national championship team.
- Author: Jackson Bakich
In this story:
Former Florida State football defensive back Jaden Floyd has entered the transfer portal. The redshirt junior will have two years of eligibility remaining. Jaden is the son of FSU football legend and color commentator for Seminole IMG Sports Network, William Floyd.
He released a statement announcing his intention to enter the portal.
“First off, I would just like to start out by thanking the Most High for blessing me with this opportunity. Ever since I was 5 years old, a good amount of my life revolved around Florida State. You can ask anyone that has been around me throughout my journey, from running around Doak as a kid to running out of the tunnel for the first time against Notre Dame my freshman year, I knew that I wanted to be a part of such a legendary program and make my presence felt during my time here. But I’m a firm believer that the Lord guides you into these journeys to lead you to the next big thing. With that being said, after long talks with God, my family, and careful thought and consideration, I will be entering the transfer portal with two years of eligibility,” Floyd wrote.
“Thank you to my family for continuing to be there for me through everything. Thank you coach Norvell, coach Fuller, coach Surtain, and coach Woodson for giving me the opportunity to play for the Noles," Floyd continued. "Thank you to everyone involved with FSU athletics as well for the endless love and relationships … And lastly, to my teammates, thank you for pushing me each and everyday, and becoming my lifelong brothers which made this decision hard to make. Continue to climb and to get that 1% each and every day. I will forever bleed Garnet & Gold, and I will forever be grateful to forever call myself a Florida State Seminole. Doak boy for life! J Floyd out – Love 39.”
READ MORE: Expected Recruiting Visitor List For FSU Football's Garnet & Gold Spring Showcase
As a walk-on and scout team player at FSU, Jaden Floyd appeared in the second game of the 2023 season against Southern Mississippi. He recorded a tackle and the Seminoles won 66-13.
In 2022, he was one of 6 recipients of the team’s Bill McGrotha Award, presented by the Tallahassee QB Club to the top humanitarians on the squad.
The elder Floyd was a fullback at Florida State for three years including the 1993 national championship season, his last with the program. He played in 35 games and recorded 933 scrimmage yards and scored 20 touchdowns for the Seminoles.
William Floyd was selected 28th overall in the 1994 NFL Draft by the San Francisco 49ers and helped them win a Super Bowl in 1995. He played in 90 NFL games over seven seasons and scored 25 touchdowns.
As of now, Florida State has had two scholarship players enter the portal this spring; wide receiver Joshua Burrell and cornerback Greedy Vance. The program still has to shed a fair amount of scholarship players to get down to the limit of 85 scholarships.
The Seminoles saw quarterback Tate Rodemaker (Southern Miss), quarterback AJ Duffy (San Diego State), running back Rodney Hill (Arkansas), running back CJ Campbell (FAU), wide receiver Goldie Lawrence (?), tight end Markeston Douglas (Arizona State), tight end Preston Daniel (Miami-Ohio), offensive lineman Thomas Shrader (Appalachian State), offensive lineman Bless Harris (TCU), offensive lineman Daughtry Richardson (FAU), offensive lineman Qae'shon Sapp (?), defensive end Gilber Edmond (South Carolina), defensive lineman Dennis Briggs (Illinois), defensive tackle Malcolm Ray (Rutgers), defensive tackle Ayobami Tifase (?), linebacker Dylan Brown-Turner (UTEP), and kicker Tyler Keltner (Oklahoma) enter the portal during the winter transfer portal window that closed on January 4.
READ MORE: FSU Football Quarterbacks Set to Debut Turquoise Heritage Jerseys at Spring Showcase
Stick with NoleGameday for more coverage of Florida State football throughout the spring
Follow NoleGameday on and Twitter , Facebook , Instagram , and TikTok
Latest Noles News
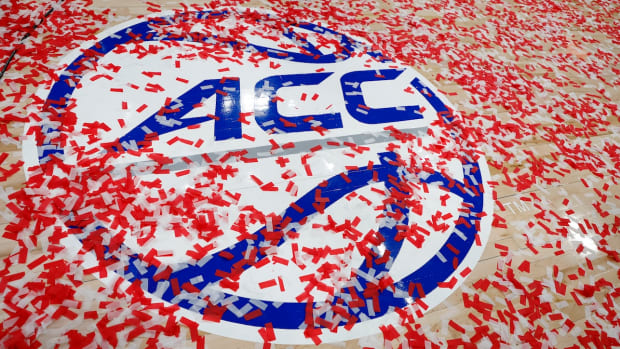
Everyone Is to Blame for Slowly Killing College Sports
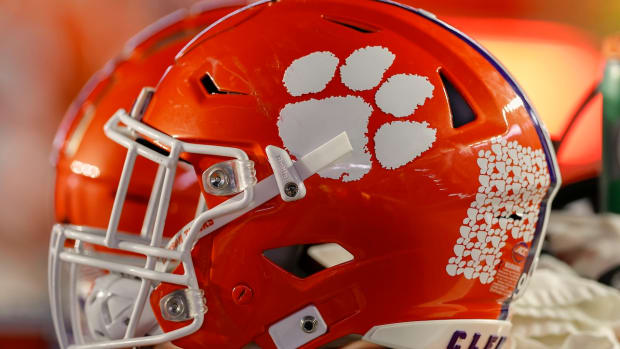
Clemson’s Lawsuit Turns Up the Heat on an ACC Already in Hot Water
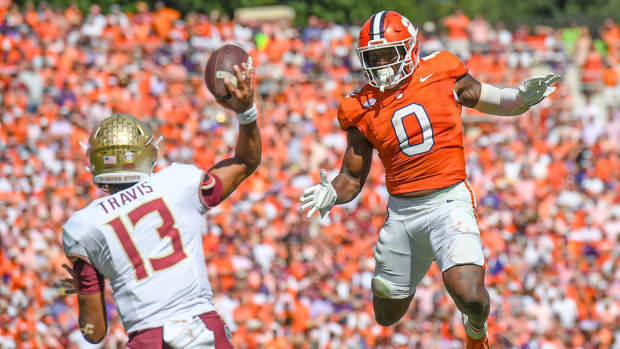
Clemson Takes Aim at ACC in Lawsuit, Following FSU’s Lead
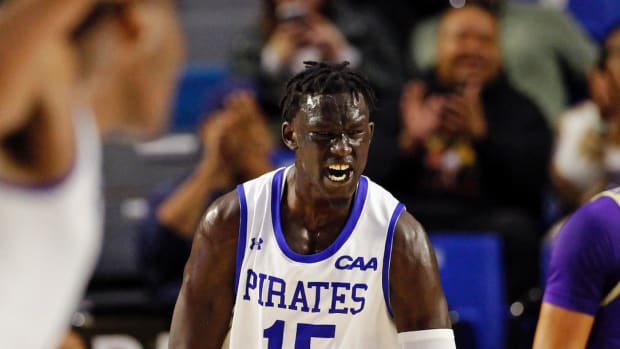
FSU Basketball Contacts Transfer From Hampton
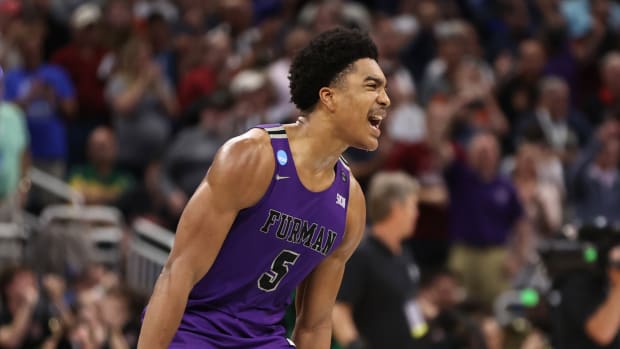
FSU Basketball In Contact With All-Conference Transfer From Furman
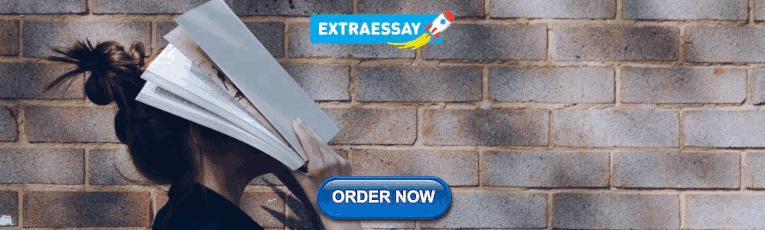
COMMENTS
The three types of heat transfer differ according to the nature of the medium that transmits heat: Conduction requires contact. Convection requires fluid flow. Radiation does not require any medium. Conduction is heat transfer directly between neighboring atoms or molecules. Usually, it is heat transfer through a solid.
According to thermodynamic systems, heat transfer is defined as. "The movement of heat across the border of the system due to a difference in temperature between the system and its surroundings.". Interestingly, the difference in temperature is said to be a 'potential' that causes the transfer of heat from one point to another. 2,48,152.
Heat transfer is fundamental to such everyday activities as home heating and cooking, as well as many industrial processes. It also forms a basis for the topics in the remainder of this chapter. 1.6: Phase Changes Phase transitions play an important theoretical and practical role in the study of heat flow. In melting (or "fusion"), a solid ...
About. Transcript. There are three forms of thermal energy transfer: conduction, convection, and radiation. Conduction involves molecules transferring kinetic energy to one another through collisions. Convection occurs when hot air rises, allowing cooler air to come in and be heated. Thermal radiation happens when accelerated charged particles ...
Heat transfer, any or all of several kinds of phenomena, considered as mechanisms, that convey energy and entropy from one location to another. The specific mechanisms are usually referred to as convection, thermal radiation, and conduction. Transfer of heat usually involves all these processes.
Example 13.4.1 13.4. 1: Calculating Heat Transfer by Convection: Convection of Air Through the Walls of a House. Most houses are not airtight: air goes in and out around doors and windows, through cracks and crevices, following wiring to switches and outlets, and so on. The air in a typical house is completely replaced in less than an hour.
Heat Transfer Definition. Heat transfer is a process by which internal energy from one substance transfers to another substance. Thermodynamics is the study of heat transfer and the changes that result from it. An understanding of heat transfer is crucial to analyzing a thermodynamic process, such as those that take place in heat engines and ...
Heat transfer is a discipline of thermal engineering that concerns the generation, use, conversion, and exchange of thermal energy between physical systems. Heat transfer is classified into various mechanisms, such as thermal conduction, thermal convection, thermal radiation, and transfer of energy by phase changes. Engineers also consider the ...
Figure 5.4.2 - Differential Heat Conduction. The more chains of spring-connected particles we can use, the faster the energy can be transferred. The number of chains is proportional to the cross-sectional area of the cylinder, so the rate of heat transfer is also proportional to the cross-sectional area: dQ dt ∝ A (5.4.1) (5.4.1) d Q d t ∝ A.
2. Irreversible heat transfer. Heat transfer is a flow of energy (q) that proceeds by itself in one direction, from a high temperature (T H) to a lower temperature (T L).This feature of the flow is illustrated in figure 1a [19,20].The thermodynamic system is traversed by q.The system occupies the square space shown between T H and T L.Said another way, the system is embedded in an environment ...
Q = mcΔT, Q = m c Δ T, 11.7. where m is the mass of the substance and Δ T is the change in its temperature, in units of Celsius or Kelvin. The symbol c stands for specific heat, and depends on the material and phase. The specific heat is the amount of heat necessary to change the temperature of 1.00 kg of mass by 1.00 ºC.
1.1 Introduction. Heat transfer or the lack of it and its consequences are important in most day-to-day activities in industry and human life. Most engineering design involves, at some stage or the other, a consideration of heat transfer and its prediction for reliable operation of the designed system or product.
We consider this equation to represent the conversion between two units of energy. (Other numbers that you may see refer to calories defined for temperature ranges other than 14.5 ° C 14.5 ° C to 15.5 ° C 15.5 ° C.). Figure 1.10 shows one of Joule's most famous experimental setups for demonstrating that work and heat can produce the same effects and measuring the mechanical equivalent of ...
Heat is the flow of thermal energy. A whole branch of physics, thermodynamics, deals with how heat is transferred between different systems and how work is done in the process (see the 1ˢᵗ law of thermodynamics ). In the context of mechanics problems, we are usually interested in the role thermal energy plays in ensuring conservation of energy.
A kinetic energy transfer is easy to observe and understand, but other important transfers are not as easy to visualize. Thermal energy has to do with the internal energy of a system due to its temperature. When a substance is heated, its temperature rises because the molecules it is composed of move faster and gain thermal energy through heat ...
Convection heat transfer occurs partly due to the actual movement of molecules or due to the mass transfer. For example. Heating of milk in a pan. 3. Radiation of Heat. It is the process in which heat is transferred from one body to another body without involving the molecules of the medium.
Thermal Conduction. Thermal conduction is the transfer of energy arising from temperature differences between adjacent parts of a body. Thermal conductivity is recognised as the exchange of energy between molecules and electrons in the conducting medium. The rate of heat flow in a rod of any material is proportional to the cross-sectional area ...
2511. Heat transfer, a phenomenon known by various names such as heat flow, heat exchange, or simply heat, constitutes the transfer of thermal energy from one region of matter or a physical system to another. It is a ubiquitous process wherein an object at a different temperature from its surroundings undergoes spontaneous heat transfer until ...
756 Words4 Pages. Chapter one. Introduction. Heat transfer. Heat is defined in physics as the transfer of thermal energy across a well-defined boundary around a thermodynamic system. Three modes of heat transfer are considered. Conduction is the transfer of energy between objects that are in physical contact.
Insulation can just refer to the insulation materials employed to slow heat loss, such as: cellulose, glass wool, rock wool, polystyrene, urethane foam, vermiculite (type of chemical insulation), and earth or soil. But it can also involve a range of designs and techniques to address the main modes of heat transfer - conduction, radiation and ...
touch a hot surface, your fingers get burned because of the heat transfer. For example, when ironing, heat from the iron moves directly onto the clothing. Convection. is the transfer of heat energy in liquids and gases by the movement of molecules. As a gas or liquid is heated the heat causes the molecules to move up, carrying heat to the area
This essay shows that heat is very important to nearly everything in life and how it is used nearly everywhere. 12/12/11. "Heat energy (or just heat) is a form of energy which transfers among particles in a substance (or system) by means of kinetic energy of those particles. In other words, under kinetic theory, the heat is transferred by ...
DOI: 10.2307/3106026 Corpus ID: 110778822; History of heat transfer : essays in honor of the 50th anniversary of the ASME Heat Transfer Division @article{Layton1991HistoryOH, title={History of heat transfer : essays in honor of the 50th anniversary of the ASME Heat Transfer Division}, author={Edwin T. Layton and John H. Lienhard}, journal={Technology and Culture}, year={1991}, volume={32 ...
Semantic Scholar extracted view of "On conjugate heat transfer in microchannel heat sinks" by N. Fathi et al. ... Search 217,973,379 papers from all fields of science. Search. Sign In Create Free Account. DOI: 10.1016/j.ijft.2024.100658; Corpus ID: 269208577; On conjugate heat transfer in microchannel heat sinks
The present study elucidates the experimental investigations on a novel flexible heat transfer device that can be used in a wide range of modern electronic device cooling applications which demand flexibility. The objective of the present is to address the challenges encountered by current flexible heat transfer devices, including concerns related to out-gassing and the permeation of non ...
Climate change is a complex phenomenon whose ultimate costs will depend not only on how quickly we transition away from fossil fuels but also on how well we adapt our social and economic systems ...
In fact, the most shocking prison statistics come from the 1980s-2000s, when the "War on Drugs" swelled our penal population from around 300,000 to over 2 million, the highest in the world ...
fsu seminoles. Florida State had a somewhat surprising portal entry earlier this month when redshirt senior cornerback Greedy Vance elected to move on from the program. At the time, Vance was in ...
The 6'3'', 288-pound lineman out of Pace, FL, appeared in 14 games for the Seminoles throughout his five-year tenure in Tallahassee, making his college debut in Florida State's 59-3 win over the ...
NFL Draft. Former Florida State football defensive back Jaden Floyd has entered the transfer portal. The redshirt junior will have two years of eligibility remaining. Jaden is the son of FSU ...