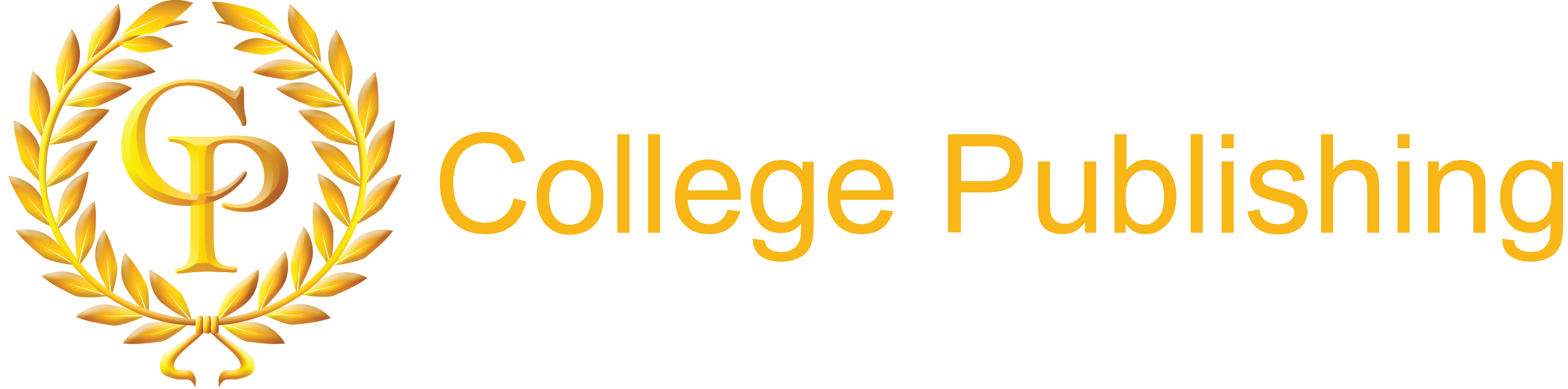
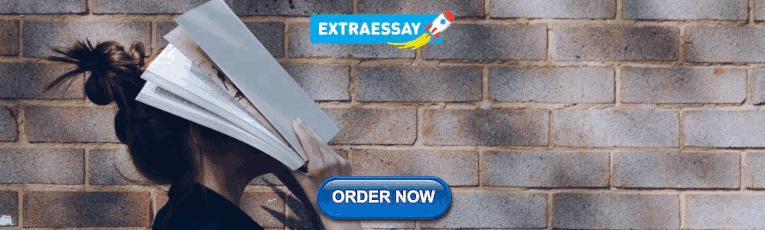
Co-Editors-in-Chief
Simi Hoque & Jian Zuo
eISSN: 1943-4618 ISSN: 1552-6100
About the Journal of Green Building
The purpose of the Journal of Green Building is to present the very best peer-reviewed research for the sustainable built environment at the building, community, neighborhood and urban scale. As such, the journal’s coverage includes green building design and construction... Read more about the Journal

- JGB Twitter
Open Access Volumes
Issue 1 to end of volume.
Volume 1, Issues 1-4 ( One , Two , Three , Four ) Volume 2, Issues 1-4 ( One , Two , Three , Four ) Volume 3, Issues 1-4 ( One , Two , Three , Four ) Volume 4, Issues 1-4 ( One , Two , Three , Four ) Volume 5, Issues 1-4 ( One , Two , Three , Four ) Volume 6, Issues 1-4 ( One , Two , Three , Four ) Volume 7, Issues 1-4 ( One , Two , Three , Four ) Volume 8, Issues 1-4 ( One , Two , Three , Four ) Volume 9, Issues 1-4 ( One , Two , Three , Four ) Volume 10, Issues 1-4 ( One , Two , Three , Four ) Volume 11, Issues 1-4 ( One , Two , Three , Four ) Volume 12, Issues 1-4 ( One , Two , Three , Four ) Volume 13, Issues 1-4 ( One , Two , Three , Four ) Volume 14, Issues 1-4 ( One , Two , Three , Four ) Volume 15, Issues 1-4 ( One , Two , Three , Four )
Journal Citation Indicator Rank (JCI) 2022 / Architecture JCI Ranking : 4th out of 95 JCI Percentile : 96.1 JCI Quartile : Q1
Get an E-mail Alert when a new issue is posted.
Not Yet Registered ? Benefits of Registration Include:
- A Unique User Profile that will allow you to manage your current subscriptions (including online access)
- The ability to customize email alerts to receive specific notifications about the topics you care most about and special offers
Features & Information
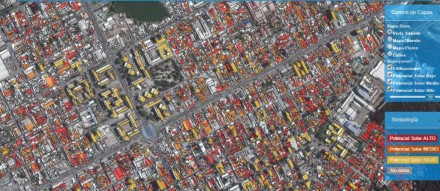
ABSTRACTED AND INDEXED BY:
Scopus, Clarivate’s Arts & Humanities Citation Index (AHCI), Web of Science (WoS), ScienceOpen, Columbia’s Avery Index to Architectural Periodicals, CNPIEC, J-Gate, Construction & Building Abstracts (CBA) and Google Scholar
SCIMAGO’S JOURNAL & COUNTRY RANK
Featured Article
TEACHING VISUAL SCRIPTING IN BIM: A CASE STUDY USING A PANEL CONTROLLED BY SOLAR ANGLES by Karen M. Kensek, University of Southern California School of Architecture
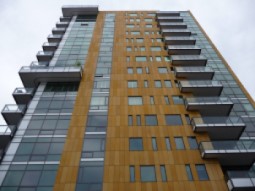
Open Access (OA) and Institutional Repository (IR) Guidelines
*To arrange Open Access (OA), the author, their institution, or funding agency pays an Article Publication Charge (APC) of $1,800 USD. The terms of this Open Access are according to the Creative Commons license CC BY-NC (Attribution-NonCommercial; https://creativecommons.org/licenses/ ).
**Authors may post an Institutional Repository (IR) copy of their paper with their university/company repository or archive. The IR copy is the same version of the paper as published on the journal’s website.
- About This Journal
- Editorial Guidelines
- Submission Guidelines
- Permissions
Affiliations
- eISSN 1943-4618
- ISSN 1552-6100
- Privacy Policy
- Get Adobe Acrobat Reader
This Feature Is Available To Subscribers Only
Sign In or Create an Account

An official website of the United States government
The .gov means it’s official. Federal government websites often end in .gov or .mil. Before sharing sensitive information, make sure you’re on a federal government site.
The site is secure. The https:// ensures that you are connecting to the official website and that any information you provide is encrypted and transmitted securely.
- Publications
- Account settings
Preview improvements coming to the PMC website in October 2024. Learn More or Try it out now .
- Advanced Search
- Journal List
- Springer Nature - PMC COVID-19 Collection

A comprehensive review on green buildings research: bibliometric analysis during 1998–2018
1 School of Environmental Science and Engineering, Tianjin University, No. 135 Yaguan Road, Tianjin, 300350 China
2 Tianjin University Research Institute of Architectural Design and Urban Planning Co., Ltd, Tianjin, 300072 China
3 Center for Green Buildings and Sponge Cities, Georgia Tech Tianjin University Shenzhen Institute, Shenzhen, 518071 Guangdong China
Umme Marium Ahmad
Xiaotong wang.
4 School of Architecture & Built Environment, The University of Adelaide, Adelaide, Australia
Associated Data
Buildings account for nearly 2/5ths of global energy expenditure. Due to this figure, the 90s witnessed the rise of green buildings (GBs) that were designed with the purpose of lowering the demand for energy, water, and materials resources while enhancing environmental protection efforts and human well-being over time. This paper examines recent studies and technologies related to the design, construction, and overall operation of GBs and determines potential future research directions in this area of study. This global review of green building development in the last two decades is conducted through bibliometric analysis on the Web of Science, via the Science Citation Index and Social Sciences Citation Index databases. Publication performance, countries’ characteristics, and identification of key areas of green building development and popular technologies were conducted via social network analysis, big data method, and S-curve predictions. A total of 5246 articles were evaluated on the basis of subject categories, journals’ performance, general publication outputs, and other publication characteristics. Further analysis was made on dominant issues through keyword co-occurrence, green building technologies by patent analysis, and S-curve predictions. The USA, China, and the UK are ranked the top three countries where the majority of publications come from. Australia and China had the closest relationship in the global network cooperation. Global trends of the top 5 countries showed different country characteristics. China had a steady and consistent growth in green building publications each year. The total publications on different cities had a high correlation with cities’ GDP by Baidu Search Index. Also, barriers and contradictions such as cost, occupant comfort, and energy consumption were discussed in developed and developing countries. Green buildings, sustainability, and energy efficiency were the top three hotspots identified through the whole research period by the cluster analysis. Additionally, green building energy technologies, including building structures, materials, and energy systems, were the most prevalent technologies of interest determined by the Derwent Innovations Index prediction analysis. This review reveals hotspots and emerging trends in green building research and development and suggests routes for future research. Bibliometric analysis, combined with other useful tools, can quantitatively measure research activities from the past and present, thus bridging the historical gap and predicting the future of green building development.
Supplementary Information
The online version contains supplementary material available at 10.1007/s11356-021-12739-7.
Introduction
Rapid urban development has resulted in buildings becoming a massive consumer of energy (Yuan et al. 2013 ), liable for 39% of global energy expenditure and 68% of total electricity consumption in the USA (building). In recent years, green buildings (GBs) have become an alternative solution, rousing widespread attention. Also referred to as sustainable buildings, low energy buildings, and eco-buildings, GBs are designed to reduce the strain on environmental resources as well as curb negative effects on human health by efficiently using natural resources, reducing garbage, and ensuring the residents’ well-being through improved living conditions ( Agency USEP Indoor Air Quality ; Building, n.d ). As a strategy to improve the sustainability of the construction industry, GBs have been widely recognized by governments globally, as a necessary step towards a sustainable construction industry (Shen et al. 2017 ).
Zuo and Zhao ( 2014 ) reviewed the current research status and future development direction of GBs, focusing on connotation and research scope, the benefit-difference between GBs and traditional buildings, and various ways to achieve green building development. Zhao et al. ( 2019 ) presented a bibliometric report of studies on GBs between 2000 and 2016, identifying hot research topics and knowledge gaps. The verification of the true performance of sustainable buildings, the application of ICT, health and safety hazards in the development of green projects, and the corporate social responsibility were detected as future agenda. A scientometrics review of research papers on GB sources from 14 architectural journals between 1992 and 2018 was also presented (Wuni et al. 2019a ). The study reported that 44% of the world participated in research focusing on green building implementation; stakeholder management; attitude assessment; regulations and policies; energy efficiency assessment; sustainability performance assessment; green building certification, etc.
With the transmission of the COVID-19 virus, society is now aware of the importance of healthy buildings. In fact, in the past 20 years, the relationship between the built environment and health has aroused increasing research interest in the field of building science. Public spaces and dispersion of buildings in mixed-use neighborhoods are promoted. Furthermore, telecommuting has become a trend since the COVID-19 pandemic, making indoor air quality even more important in buildings, now (Fezi 2020 ).
The system for evaluating the sustainability of buildings has been established for nearly two decades. But, systems dedicated to identifying whether buildings are healthy have only recently appeared (McArthur and Powell 2020 ). People are paying more and more attention to health factors in the built environment. This is reflected in the substantial increase in related academic papers and the increase in health building certification systems such as WELE and Fitwel (McArthur and Powell 2020 ).
Taking the above into consideration, the aim of this study is to examine the stages of development of GBs worldwide and find the barriers and the hotpots in global trends. This study may be beneficial to foreign governments interested in promoting green building and research in their own nations.
Methodology
Overall description of research design.
Since it is difficult to investigate historical data and predict global trends of GBs, literature research was conducted to analyze their development. The number of published reports on a topic in a particular country may influence the level of industrial development in that certain area (Zhang et al. 2017 ). The bibliometric analysis allows for a quantitative assessment of the development and advancement of research related to GBs and where they are from. Furthermore, it has been shown that useful data has been gathered through bibliometrics and patent analysis (Daim et al. 2006 ).
In this report, the bibliometric method, social network analysis (SNA), CiteSpace, big data method, patent analysis, and S-curve analysis are used to assess data.
Bibliometrics analysis
Bibliometrics, a class of scientometrics, is a tool developed in 1969 for library and information science. It has since been adopted by other fields of study that require a quantitative assessment of academic articles to determine trends and predict future research scenarios by compiling output and type of publication, title, keyword, author, institution, and countries data (Ho 2008 ; Li et al. 2017 ).
Social network analysis
Social network analysis (SNA) is applied to studies by modeling network maps using mathematics and statistics (Mclinden 2013 ; Ye et al. 2013 ). In the SNA, nodes represent social actors, while connections between actors stand for their relationships (Zhang et al. 2017 ). Correlations between two actors are determined by their distance from each other. There is a variety of software for the visualization of SNA such as Gephi, Vosviewer, and Pajek. In this research, “Pajek” was used to model the sequence of and relationships between the objects in the map (Du et al. 2015 ).
CiteSpace is an open-source Java application that maps and analyzes trends in publication statistics gathered from the ISI-Thomson Reuters Scientific database and produces graphic representations of this data (Chen 2006 ; Li et al. 2017 ). Among its many functions, it can determine critical moments in the evolution of research in a particular field, find patterns and hotspots, locate areas of rapid growth, and breakdown the network into categorized clusters (Chen 2006 ).
Big data method
The big data method, with its 3V characters (volume, velocity, and variety), can give useful and accurate information. Enormous amounts of data, which could not be collected or computed manually through conventional methods, can now be collected through public data website. Based on large databases and machine learning, the big data method can be used to design, operate, and evaluate energy efficiency and other index combined with other technologies (Mehmood et al. 2019 ). The primary benefit of big data is that the data is gathered from entire populations as opposed to a small sample of people (Chen et al. 2018 ; Ho 2008 ). It has been widely used in many research areas. In this research, we use the “Baidu Index” to form a general idea of the trends in specific areas based on user interests. The popularity of the keywords could imply the user’s behavior, user’s demand, user’s portrait, etc. Thus, we can analyze the products or events to help with developing strategies. However, it must be noted that although big data can quantitatively represent human behavior, it cannot determine what motivates it. With the convergence of big data and technology, there are unprecedented applications in the field of green building for the improved indoor living environment and controlled energy consumption (Marinakis 2020 ).
Patent analysis
Bibliometrics, combined with patent analysis, bridges gaps that may exist in historical data when predicting future technologies (Daim et al. 2006 ). It is a trusted form of technical analysis as it is supported by abundant sources and commercial awareness of patents (Guozhu et al. 2018 ; Yoon and Park 2004 ). Therefore, we used patent analysis from the Derwent patent database to conduct an initial analysis and forecast GB technologies.
There are a variety of methods to predict the future development prospects of a technology. Since many technologies are developed in accordance with the S-curve trend, researchers use the S-curve to observe and predict the future trend of technologies (Bengisu and Nekhili 2006 ; Du et al. 2019 ; Liu and Wang 2010 ). The evolution of technical systems generally goes through four stages: emerging, growth, maturity, and decay (saturation) (Ernst 1997 ). We use the logistics model (performed in Loglet Lab 4 software developed by Rockefeller University) to simulate the S-curve of GB-related patents to predict its future development space.
Data collection
The Web of Science (WOS) core collection database is made up of trustworthy and highly ranked journals. It is considered the leading data portal for publications in many fields (Pouris and Pouris 2011 ). Furthermore, the WOS has been cited as the main data source in many recent bibliometric reviews on buildings (Li et al. 2017 ).
Access to all publications used in this paper was attained through the Science Citation Index-Expanded and the Social Sciences Citation Index databases. Because there is no relevant data in WOS before 1998, our examination focuses on 1998 to 2018. With consideration of synonyms, we set a series of green building-related words (see Appendix ) in titles, abstracts, and keywords for bibliometric analysis. For example, sustainable, low energy, zero energy, and low carbon can be substituted for green; housing, construction, and architecture can be a substitute for building (Zuo and Zhao 2014 ).
Analytical procedure
The study was conducted in three stages; data extraction was the first step where all the GB-related words were screened in WOS. Afterwards, some initial analysis was done to get a complete idea of GB research. Then, we made a further analysis on countries’ characteristics, dominant issues, and detected technology hotspots via patent analysis (Fig. (Fig.1 1 ).

Analytical procedure of the article
Results and analysis
General results.
Of the 6140 publications searched in the database, 88.67% were articles, followed by reviews (6.80%), papers (3.72%), and others (such as editorial materials, news, book reviews). Most articles were written in English (96.78%), followed by German (1.77%), Spanish (0.91%), and other European languages. Therefore, we will only make a further analysis of the types of articles in English publications.
The subject categories and their distribution
The SCI-E and SSCI database determined 155 subjects from the pool of 5246 articles reviewed, such as building technology, energy and fuels, civil engineering, environmental, material science, and thermodynamics, which suggests green building is a cross-disciplinary area of research. The top 3 research areas of green buildings are Construction & Building Technology (36.98%), Energy & Fuels (30.39%), and Engineering Civil (29.49%), which account for over half of the total categories.
The journals’ performance
The top 10 journals contained 38.8% of the 5246 publications, and the distribution of their publications is shown in Fig. Fig.2. 2 . Impact factors qualitatively indicate the standard of journals, the research papers they publish, and researchers associated with those papers (Huibin et al. 2015 ). Below, we used 2017 impact factors in Journal Citation Reports (JCR) to determine the journal standards.

The performance of top10 most productive journals
Publications on green building have appeared in a variety of titles, including energy, building, environment, materials, sustainability, indoor built environment, and thermal engineering. Energy and Buildings, with its impact factor 4.457, was the most productive journal apparently from 2009 to 2017. Sustainability (IF = 2.075) and Journal of Cleaner Production (IF = 5.651) rose to significance rapidly since 2015 and ranked top two journals in 2018.
Publication output
The total publication trends from 1998 to 2018 are shown in Fig. Fig.3, 3 , which shows a staggering increase across the 10 years. Since there was no relevant data before 1998, the starting year is 1998. Before 2004, the number of articles published per year fluctuated. The increasing rate reached 75% and 68% in 2004 and 2007, respectively, which are distinguished in Fig. Fig.3 3 that leads us to believe that there are internal forces at work, such as appropriate policy creation and enforcement by concerned governments. There was a constant and steady growth in publications after 2007 in the worldwide view.

The number of articles published yearly, between 1998 and 2018
The characteristics of the countries
Global distribution and global network were analyzed to illustrate countries’ characteristics. Many tools such as ArcGIS, Bibexcel, Pajek, and Baidu index were used in this part (Fig. (Fig.4 4 ).

Analysis procedure of countries’ characteristics
Global distribution of publications
By extracting the authors’ addresses (Mao et al. 2015 ), the number of publications from each place was shown in Fig. Fig.5 5 and Table Table1. 1 . Apparently, the USA was the most productive country accounting for 14.98% of all the publications. China (including Hong Kong and Taiwan) and the UK followed next by 13.29% and 8.27% separately. European countries such as Italy, Spain, and Germany also did a lot of work on green building development.

Global geographical distribution of the top 20 publications based on authors’ locations
Global research network
Global networks illustrate cooperation between countries through the analysis of social networks. Academic partnerships among the 10 most productive countries are shown in Fig. Fig.6. 6 . Collaboration is determined by the affiliation of the co-authors, and if a publication is a collaborative research, all countries or institutions will benefit from it (Bozeman et al. 2013 ). Every node denotes a country and their size indicates the amount of publications from that country. The lines linking the nodes denote relationships between countries and their thickness indicates the level of collaboration (Mao et al. 2015 ).

The top 10 most productive countries had close academic collaborative relationships
It was obvious that China and Australia had the strongest linking strength. Secondly, China and the USA, China, and the UK also had close cooperation with each other. Then, the USA with Canada and South Korea followed. The results indicated that cooperation in green building research was worldwide. At the same time, such partnerships could help countries increase individual productivity.
Global trend of publications
The time-trend analysis of academic inputs to green building from the most active countries is shown in Fig. Fig.7 7 .

The publication trends of the top five countriesbetween 1998 and 2018 countries areshown in Fig 7.
Before 2007, these countries showed little growth per year. However, they have had a different, growing trend since 2007. The USA had the greatest proportion of publications from 2007, which rose obviously each year, reaching its peak in 2016 then declined. The number of articles from China was at 13 in 2007, close to the USA. Afterwards, there was a steady growth in China. Not until 2013 did China have a quick rise from 41 publications to 171 in 2018. The UK and Italy had a similar growth trend before 2016 but declined in the last 2 years.
Further analysis on China, the USA, and the UK
Green building development in china, policy implementation in china.
Green building design started in China with the primary goal of energy conservation. In September 2004, the award of “national green building innovation” of the Ministry of Construction was launched, which kicked off the substantive development of GB in China. As we can see from Fig. Fig.7, 7 , there were few publications before 2004 in China. In 2004, there were only 4 publications on GB.
The Ministry of Construction, along with the Ministry of Science and Technology, in 2005, published “The Technical Guidelines for Green Buildings,” proposing the development of GBs (Zhang et al. 2018 ). In June 2006, China had implemented the first “Evaluation Standard for Green Building” (GB/T 50378-2006), which promoted the study of the green building field. In 2007, the demonstration of “100 projects of green building and 100 projects of low-energy building” was launched. In August 2007, the Ministry of Construction issued the “Green Building Assessment Technical Regulations (try out)” and the “Green Building Evaluation Management,” following Beijing, Tianjin, Chongqing, and Shanghai, more than 20 provinces and cities issued the local green building standards, which promoted GBs in large areas in China.
At the beginning of 2013, the State Council issued the “Green Building Action Plan,” so the governments at all levels continuously issued incentive policies for the development of green buildings (Ye et al. 2015 ). The number of certified green buildings has shown a blowout growth trend throughout the country, which implied that China had arrived at a new chapter of development.
In August 2016, the Evaluation Standard for Green Renovation of Existing Buildings was released, encouraging the rise of residential GB research. Retrofitting an existing building is often more cost-effective than building a new facility. Designing significant renovations and alterations to existing buildings, including sustainability measures, will reduce operating costs and environmental impacts and improve the building’s adaptability, durability, and resilience.
At the same time, a number of green ecological urban areas have emerged (Zhang et al. 2018 ). For instance, the Sino-Singapore Tianjin eco-city is a major collaborative project between the two governments. Located in the north of Tianjin Binhai New Area, the eco-city is characterized by salinization of land, lack of freshwater, and serious pollution, which can highlight the importance of eco-city construction. The construction of eco-cities has changed the way cities develop and has provided a demonstration of similar areas.
China has many emerging areas and old centers, so erecting new, energy efficiency buildings and refurbishing existing buildings are the best steps towards saving energy.
Baidu Search Index of “green building”
In order to know the difference in performance among cities in China, this study employs the big data method “Baidu Index” for a smart diagnosis and assessment on green building at finer levels. “Baidu Index” is not equal to the number of searches but is positively related to the number of searches, which is calculated by the statistical model. Based on the keyword search of “green building” in the Baidu Index from 2013 to 2018, the top 10 provinces or cities were identified (Fig. (Fig.8 8 ).

Baidu Search Index of green building in China 2013–2018 from high to low
The top 10 search index distributes the east part and middle part of China, most of which are the high GDP provinces (Fig. (Fig.9). 9 ). Economically developed cities in China already have a relatively mature green building market. Many green building projects with local characteristics have been established (Zhang et al. 2018 ).

TP GDP & Search Index were highly related
We compared the city search index (2013–2018) with the total publications of different cities by the authors’ address and the GDP in 2018. The correlation coefficient between the TP and the search index was 0.9, which means the two variables are highly related. The correlation coefficient between the TP and GDP was 0.73, which also represented a strong relationship. We inferred that cities with higher GDP had more intention of implementation on green buildings. The stronger the local GDP, the more relevant the economic policies that can be implemented to stimulate the development of green buildings (Hong et al. 2017 ). Local economic status (Yang et al. 2018 ), property developer’s ability, and effective government financial incentives are the three most critical factors for green building implementation (Huang et al. 2018 ). However, Wang et al. ( 2017 ) compared the existing green building design standards and found that they rarely consider the regional economy. Aiming at cities at different economic development phases, the green building design standards for sustainable construction can effectively promote the implementation of green buildings. Liu et al. ( 2020 ) mainly discussed the impact of sustainable construction on GDP. According to the data, there is a strong correlation between the percentage of GDP increments in China and the amount of sustainable infrastructure (Liu et al. 2020 ). The construction of infrastructure can create jobs and improve people’s living standards, increasing GDP as a result (Liu et al. 2020 ).
Green building development in the USA and the UK
The sign that GBs were about to take-off occurred in 1993—the formation of the United States Green Building Council (USGBC), an independent agency. The promulgation of the Energy Policy Act 2005 in the USA was the key point in the development of GBs. The Energy Policy Act 2005 paid great attention to green building energy saving, which also inspired publications on GBs.
Leadership in Energy and Environmental Design (LEED), a popular metric for sustainable buildings and homes (Jalaei and Jrade 2015 ), has become a thriving business model for green building development. It is a widely used measure of how buildings affect the environment.
Another phenomenon worth discussion, combined with Fig. Fig.7, 7 , the increasing rate peaked at 75% in 2004 and 68% in 2007 while the publications of the UK reached the peak in 2004 and 2007. The UK Green Building Council (UKGBC), a United Kingdom membership organization, created in 2007 with regard to the 2004 Sustainable Building Task Group Report: Better Buildings - Better Lives, intends to “radically transform,” all facets of current and future built environment in the UK. It is predicted that the establishment of the UKGBC promoted research on green buildings.
From the China, the USA, and the UK experience, it is predicted that the foundation of a GB council or the particular projects from the government will promote research in this area.
Barriers and contradicts of green building implement
On the other hand, it is obvious that the USA, the UK, and Italian publications have been declining since 2016. There might be some barriers and contradicts on the adoption of green buildings for developed countries. Some articles studied the different barriers to green building in developed and developing countries (Chan et al. 2018 ) (Table (Table2). 2 ). Because the fraction of energy end-uses is different, the concerns for GBs in the USA, China, and the European Union are also different (Cao et al. 2016 ).
Top Barriers for Green building in US, UK and China
It is regarded that higher cost is the most deterring barrier to GB development across the globe (Nguyen et al. 2017 ). Other aspects such as lack of market demand and knowledge were also main considerations of green building implementation.
As for market demand, occupant satisfaction is an important factor. Numerous GB post-occupancy investigations on occupant satisfaction in various communities have been conducted.
Paul and Taylor ( 2008 ) surveyed personnel ratings of their work environment with regard to ambience, tranquility, lighting, sound, ventilation, heat, humidity, and overall satisfaction. Personnel working in GBs and traditional buildings did not differ in these assessments. Khoshbakht et al. ( 2018 ) identified two global contexts in spite of the inconclusiveness: in the west (mainly the USA and Britain), users experienced no significant differences in satisfaction between green and traditional buildings, whereas, in the east (mainly China and South Korea), GB user satisfaction is significantly higher than traditional building users.
Dominant issues
The dominant issues on different stages.
Bibliometric data was imported to CiteSpace where a three-stage analysis was conducted based on development trends: 1998–2007 initial development; 2008–2015 quick development; 2016–2018 differentiation phase (Fig. (Fig.10 10 ).

Analysis procedure of dominant issues
CiteSpace was used for word frequency and co-word analysis. The basic principle of co-word analysis is to count a group of words appearing at the same time in a document and measure the close relationship between them by the number of co-occurrences. The top 50 levels of most cited or occurred items from each slice (1998 to 2007; 2008 to 2015; 2016 to 2018) per year were selected. After merging the similar words (singular or plural form), the final keyword knowledge maps were generated as follows.
Initial phase (1998–2007)
In the early stage (Fig. (Fig.11), 11 ), “green building” and “sustainability” were the main two clusters. Economics and “environmental assessment method” both had high betweenness centrality of 0.34 which were identified as pivotal points. Purple rings denote pivotal points in the network. The relationships in GB were simple at the initial stage of development.

Co-word analysis from 1998–2007
Sustainable construction is further enabled with tools that can evaluate the entire life cycle, site preparation and management, materials and their reusability, and the reduction of resource and energy consumption. Environmental building assessment methods were incorporated to achieve sustainable development, especially at the initial project appraisal stage (Ding 2008 ). Green Building Challenge (GBC) is an exceptional international research, development, and dissemination effort for developing building environmental performance assessments, primarily to help researchers and practitioners in dealing with difficult obstacles in assessing performance (Todd et al. 2001 ).
Quick development (2008–2015)
In the rapid growing stage (Fig. (Fig.12), 12 ), pivot nodes and cluster centers were more complicated. Besides “green building” and “sustainability,” “energy efficiency” was the third hotspot word. The emergence of new vocabulary in the keyword network indicated that the research had made progress during 2008 – 2015. Energy performance, energy consumption, natural ventilation, thermal comfort, renewable energy, and embodied energy were all energy related. Energy becomes the most attractive field in achieving sustainability and green building. Other aspects such as “life cycle assessment,” “LEED,” and “thermal comfort” became attractive to researchers.

Co-word analysis from 2008–2015
The life cycle assessment (LCA) is a popular technique for the analysis of the technical side of GBs. LCA was developed from environmental assessment and economic analysis which could be a useful method to evaluate building energy efficiency from production and use to end-use (Chwieduk 2003 ). Much attention has been paid to LCA because people began to focus more on the actual performance of the GBs. Essentially, LCA simplifies buildings into systems, monitoring, and calculating mass flow and energy consumption over different stages in their life cycle.
Leadership in Energy and Environmental Design (LEED) was founded by the USGBC and began in the early twenty-first century (Doan et al. 2017 ). LEED is a not-for-profit project based on consumer demand and consensus that offers an impartial GB certification. LEED is the preferred building rating tool globally, with its shares growing rapidly. Meanwhile, UK’s Building Research Establishment Assessment Method (BREEAM) and Japan’s Comprehensive Assessment System for Building Environmental Efficiency (CASBEE) have been in use since the beginning of the twenty-first century, while New Zealand’s Green Star is still in its earlier stages. GBs around the world are made to suit regional climate concerns and need.
In practice, not all certified green buildings are necessarily performing well. Newsham et al. ( 2009 ) gathered energy-use information from 100 LEED-certified non-residential buildings. Results indicated that 28–35% of LEED structures actually consumed higher amounts of energy than the non-LEED structures. There was little connection in its actual energy consumption to its certification grade, meaning that further improvements are required for establishing a comprehensive GB rating metric to ensure consistent performance standards.
Thermal comfort was related to many aspects, such as materials, design scheme, monitoring system, and human behaviors. Materials have been a focus area for improving thermal comfort and reducing energy consumption. Wall (Schossig et al. 2005 ), floor (Ansuini et al. 2011 ), ceiling (Hu et al. 2018 ), window, and shading structures (Shen and Li 2016 ) were building envelopes which had been paid attention to over the years. Windows were important envelopes to improve thermal comfort. For existing and new buildings, rational use of windows and shading structures can enhance the ambient conditions of buildings (Mcleod et al. 2013 ). It was found that redesigning windows could reduce the air temperature by 2.5% (Elshafei et al. 2017 ), thus improving thermal comfort through passive features and reducing the use of active air conditioners (Perez-Fargallo et al. 2018 ). The monitoring of air conditioners’ performance could also prevent overheating of buildings (Ruellan and Park 2016 ).
Differentiation phase (2016–2018)
In the years from 2016 to 2018 (Fig. (Fig.13), 13 ), “green building,” ”sustainability,” and “energy efficiency” were still the top three hotspots in GB research.

Co-word analysis from 2016–2018
Zero-energy building (ZEB) became a substitute for low energy building in this stage. ZEB was first introduced in 2000 (Cao et al. 2016 ) and was believed to be the solution to the potential ramifications of future energy consumption by buildings (Liu et al. 2019 ). The EU has been using ZEB standards in all of its new building development projects to date (Communuties 2002 ). The USA passed the Energy Independence and Security Act of 2007, aiming for zero net energy consumption of 1 out of every 2 commercial buildings that are yet to be built by 2040 and for all by 2050 (Sartori et al. 2012 ). Energy consumption became the most important factor in new building construction.
Renewable energy was a key element of sustainable development for mankind and nature (Zhang et al. 2013 ). Using renewable energy was an important feature of ZEBs (Cao et al. 2016 ; Pulselli et al. 2007 ). Renewable energy, in the form of solar, wind, geothermal, clean bioenergy, and marine can be used in GBs. Solar energy has been widely used in recent years while wind energy is used locally because of its randomness and unpredictable features. Geothermal energy is mainly utilized by ground source heat pump (GSHP), which has been lauded as a powerful energy system for buildings (Cao et al. 2016 ). Bioenergy has gained much popularity as an alternative source of energy around the globe because it is more stable and accessible than other forms of energy (Zhang et al. 2015 ). There is relatively little use of marine energy, yet this may potentially change depending on future technological developments (Ellabban et al. 2014 ).
Residential buildings receive more attention because people spend 90% of their time inside. Contrary to popular belief, the concentration of contaminants found indoors is more than the concentration outside, sometimes up to 10 times or even 100 times more (agency). The renovation of existing buildings can save energy, upgrade thermal comfort, and improve people’s living conditions.
Energy is a substantial and widely recognized cost of building operations that can be reduced through energy-saving and green building design. Nevertheless, a consensus has been reached by academics and those in building-related fields that GBs are significantly more energy efficient than traditional buildings if designed, constructed, and operated with meticulousness (Wuni et al. 2019b ). The drive to reduce energy consumption from buildings has acted as a catalyst in developing new technologies.
Compared with the article analysis, patents can better reflect the practical technological application to a certain extent. We extracted the information of green building energy-related patent records between 1998 and 2018 from the Derwent Innovations Index database. The development of a technique follows a path: precursor–invention–development–maturity. This is commonly known as an S-type growth (Mao et al. 2018 ). Two thousand six hundred thirty-eight patents were found which were classified into “Derwent Manual Code,” which is the most distinct feature just like “keywords” in the Derwent Innovations Index. Manual codes refer to specific inventions, technological innovations, and unique codes for their applications. According to the top 20 Derwent Manual Code which accounted for more than 80% of the total patents, we classified the hotspots patents into three fields for further S-curve analysis, which are “structure,” “material,” and “energy systems” (Table (Table3 3 ).
Top 20 keywords in classified patents
Sustainable structural design (SSD) has gained a lot of research attention from 2006 to 2016 (Pongiglione and Calderini 2016 ). The S-curve of structure* (Fig. (Fig.14) 14 ) has just entered the later period of the growth stage, accounting for 50% of the total saturation in 2018. Due to its effectiveness and impact, SSD has overtime gained recognition and is now considered by experts to be a prominent tool in attaining sustainability goals (Pongiglione and Calderini 2016 ).

The S-curves of different Structure types from patents
Passive design is important in energy saving which is achieved by appropriately orientating buildings and carefully designing the building envelope. Building envelopes, which are key parts of the energy exchange between the building and the external environment, include walls, roofs, windows, and floors. The EU increased the efficiency of its heat-regulating systems by revamping building envelopes as a primary energy-saving task during 2006 to 2016 (Cao et al. 2016 ).
We analyzed the building envelope separately. According to the S-curve (Fig. (Fig.14), 14 ), the number of patents related to GB envelops are in the growth stage. At present, building envelops such as walls, roofs, windows, and even doors have not reached 50% of the saturated quantity. Walls and roofs are two of the most important building envelops. The patent contents of walls mainly include wall materials and manufacturing methods, modular wall components, and wall coatings while technologies about roofs mainly focus on roof materials, the combination of roof and solar energy, and roof structures. Green roofs are relatively new sustainable construction systems because of its esthetic and environmental benefits (Wei et al. 2015 ).
The material resources used in the building industry consume massive quantities of natural and energy resources consumptions (Wang et al. 2018 ). The energy-saving building material is economical and environmentally friendly, has low coefficient heat conductivity, fast curing speed, high production efficacy, wide raw material source and flame, and wear resistance properties (Zhang et al. 2014 ). Honeycomb structures were used for insulating sustainable buildings. They are lightweight and conserve energy making them eco-friendly and ideal for construction (Miao et al. 2011 ).
According to the S-curve (Fig. (Fig.15), 15 ), it can be seen that the number of patents on the GB “material” is in the growth stage. It is expected that the number of patents will reach 50% of the total saturation in 2022.

The S-curves of a different material from patents
Building material popularly used comprised of cement, concrete, gypsum, mortar compositions, and boards. Cement is widely used in building material because of its easy availability, strong hardness, excellent waterproof and fireproof performance, and low cost. The S-curve of cement is in the later period of the growth stage, which will reach 90% of the total saturation in 2028. Composite materials like Bamcrete (bamboo-concrete composite) and natural local materials like Rammed Earth had better thermal performance compared with energy-intensive materials like bricks and cement (Kandya and Mohan 2018 ). Novel bricks synthesized from fly ash and coal gangue have better advantages of energy saving in brick production phases compared with that of conventional types of bricks (Zhang et al. 2014 ). For other materials like gypsum or mortar, the numbers of patents are not enough for S-curve analysis. New-type green building materials offer an alternative way to realize energy-saving for sustainable constructions.
Energy system
The energy system mainly included a heating system and ventilation system according to the patent analysis. So, we analyzed solar power systems and air conditioning systems separately. Heat* included heat collecting panels and a fluid heating system.
The results indicated that heat*-, solar-, and ventilation-related technologies were in the growth stage which would reach 50% of the total saturation in 2022 (Fig. (Fig.16). 16 ). Photovoltaic technology is of great importance in solar energy application (Khan and Arsalan 2016 ).

The S-curves of energy systems from patents
On the contrary, air conditioning technologies had entered into the mature stage after a decade of development. It is worth mentioning that the design of the fresh air system of buildings after the COVID-19 outbreak is much more important. With people spending the majority of their time inside (Liu et al. 2019 ), volatile organic compounds, formaldehyde, and carbon dioxide received the most attention worldwide (Wei et al. 2015 ). Due to health problems like sick building syndrome, and more recently since the COVID-19 outbreak, the supply of fresh air can drastically ameliorate indoor air quality (IAQ) (Liu et al. 2019 ). Regulating emissions from materials, enhanced ventilation, and monitoring air indoors are the main methods used in GBs for maintaining IAQ (Wei et al. 2015 ). Air circulation frequency and improved air filtration can reduce the risk of spreading certain diseases, while controlling the airflow between rooms can also prevent cross-infections. Poor indoor air quality and ventilation provide ideal conditions for the breeding and spreading of viruses by air (Chen et al. 2019 ). A diverse range of air filters coupled with a fresh air supply system should be studied. A crucial step forward is to create a cost-effective, energy-efficient, intelligent fresh air supply system (Liu et al. 2017 ) to monitor, filter outdoor PM2.5 (Chen et al. 2017 ), and saving building energy (Liu and Liu 2005 ). Earth-air heat exchanger system (EAHE) is a novel technology that supplies fresh air using underground soil heat (Chen et al. 2019 ).
A total of 5246 journal articles in English from the SCI and SSCI databases published in 1998–2018 were reviewed and analyzed. The study revealed that the literature on green buildings has grown rapidly over the past 20 years. The findings and results are summarized:
Data analysis revealed that GB research is distributed across various subject categories. Energy and Buildings, Building and Environment, Journal of Cleaner Production, and Sustainability were the top journals to publish papers on green buildings.
Global distribution was done to see the green building study worldwide, showing that the USA, China, and the UK ranked the top three countries, accounting for 14.98%, 13.29%, and 8.27% of all the publications respectively. Australia and China had the closest relationship on green building research cooperation worldwide.
Further analysis was made on countries’ characteristics, dominant issues through keyword co-occurrence, green building technology by patent analysis, and S-curve prediction. Global trends of the top 5 countries showed different characteristics. China had a steady and consistent growth in publications each year while the USA, the UK, and Italy were on a decline from 2016. The big data method was used to see the city performance in China, finding that the total publications had a high correlation with the city’s GDP and Baidu Search Index. Policies were regarded as the stimulation for green building development, either in China or the UK. Also, barriers and contradictions such as cost, occupants’ comfort, and energy consumption were discussed about the developed and developing countries.
Cluster and content analysis via CiteSpace identified popular and trending research topics at different stages of development; the top three hotspots were green buildings, sustainability, and energy efficiency throughout the whole research period. Energy efficiency has shifted from low to zero energy buildings or even beyond it in recent years. Energy efficiency was the most important drive to achieve green buildings while LCA and LEED were the two potential ways to evaluate building performance. Thermal comfort and natural ventilation of residential buildings became a topic of interest to the public.
Then, we combined the keywords with “energy” to make further patent analysis in Derwent Innovations Index. “Structure,” “material,” and “energy systems” were three of the most important types of green building technologies. According to S-curve analysis, most of the technologies of energy-saving buildings were on the fast-growing trend, and even though there were conflicts and doubts in different countries on GB adoption, it is still a promising field.
Future directions
An establishment of professional institutes or a series of policies and regulations on green building promulgated by government departments will promote research development (as described in the “Further Analysis on China, the USA, and the UK” section). Thus, a policy enacted by a formal department is of great importance in this particular field.
Passive design is important in energy saving which is ensured by strategically positioning buildings and precisely engineering the building envelope, i.e., roof, walls, windows, and floors. A quality, the passive-design house is crucial to achieving sustained thermal comfort, low-carbon footprint, and a reduced gas bill. The new insulation material is a promising field for reducing building heat loss and energy consumed. Healthy residential buildings have become a focus of future development due to people’s pursuit of a healthy life. A fresh air supply system is important for better indoor air quality and reduces the risk of transmission of several diseases. A 2020 study showed the COVID-19 virus remains viable for only 4 hours on copper compared to 24 h on cardboard. So, antiviral materials will be further studied for healthy buildings (Fezi 2020 ).
With the quick development of big data method and intelligent algorithms, artificial intelligence (AI) green buildings will be a trend. The core purpose of AI buildings is to achieve optimal operating conditions through the accurate analysis of data, collected by sensors built into green buildings. “Smart buildings” and “Connected Buildings” of the future, fitted with meters and sensors, can collect and share massive amounts of information regarding energy use, water use, indoor air quality, etc. Analyzing this data can determine relationships and patterns, and optimize the operation of buildings to save energy without compromising the quality of the indoor environment (Lazarova-Molnar and Mohamed 2019 ).
The major components of green buildings, such as building envelope, windows, and skylines, should be adjustable and versatile in order to get full use of AI. A digital control system can give self-awareness to buildings, adjusting room temperature, indoor air quality, and air cooling/heating conditions to control power consumption, and make it sustainable (Mehmood et al. 2019 ).
Concerns do exist, for example, occupant privacy, data security, robustness of design, and modeling of the AI building (Maasoumy and Sangiovanni-Vincentelli 2016 ). However, with increased data sources and highly adaptable infrastructure, AI green buildings are the future.
This examination of research conducted on green buildings between the years 1998 and 2018, through bibliometric analysis combined with other useful tools, offers a quantitative representation of studies and data conducted in the past and present, bridging historical gaps and forecasting the future of green buildings—providing valuable insight for academicians, researchers, and policy-makers alike.
(DOCX 176 kb)
Availability of data and materials
The datasets generated and analyzed throughout the current study are available in the Web of Science Core Collection.
(From Web of Science Core Collection)
Topic: (“bioclimatic architect*” or “bioclimatic build*” or “bioclimatic construct*” or “bioclimatic hous*” or “eco-architect*” or “eco-build*” or “eco-home*” or “eco-hous*” or “eco-friendly build*” or “ecological architect*” or “ecological build*” or “ecological hous*” or “energy efficient architect*” or “energy efficient build*” or “energy efficient construct*” or “energy efficient home*” or “energy efficient hous*” or “energy efficient struct*” or “energy saving architect*” or “energy saving build*” or “energy saving construct*” or “energy saving home*” or “energy saving hous*” or “energy saving struct*” or “green architect*” or “green build*” or “green construct*” or “green home*” or “low carbon architect*” or “low carbon build*” or “low carbon construct*” or “low carbon home*” or “low carbon hous*” or “low energy architect*” or “low energy build*” or “low energy construct*” or “low energy home*” or “low energy hous*” or “sustainable architect*” or “sustainable build*” or “sustainable construct*” or “sustainable home*” or “sustainable hous*” or “zero energy build*” or “zero energy home*” or “zero energy hous*” or “net zero energy build*” or “net zero energy home*” or “net zero energy hous*” or “zero-carbon build*” or “zero-carbon home*” or “zero-carbon hous*” or “carbon neutral build*” or “carbon neutral construct*” or “carbon neutral hous*” or “high performance architect*” or “high performance build*” or “high performance construct*” or “high performance home*” or “high performance hous*”)
Time span: 1998-2018。 Index: SCI-EXPANDED, SSCI。
Author contributions
Ying Li conceived the frame of the paper and wrote the manuscript. Yanyu Rong made the data figures and participated in writing the manuscript. Umme Marium Ahmad helped with revising the language. Xiaotong Wang consulted related literature for the manuscript. Jian Zuo contributed significantly to provide the keywords list. Guozhu Mao helped with constructive suggestions.
This study was supported by The National Natural Science Foundation of China (No.51808385).
Declarations
This manuscript is ethical.
Not applicable.
The authors declare no competing interest.
Publisher’s Note
Springer Nature remains neutral with regard to jurisdictional claims in published maps and institutional affiliations.
- Agency USEP Indoor Air Quality https://www.epa.gov/indoor-air-quality-iaq.
- Ansuini R, Larghetti R, Giretti A, Lemma M. Radiant floors integrated with PCM for indoor temperature control. Energy Build. 2011; 43 :3019–3026. doi: 10.1016/j.enbuild.2011.07.018. [ CrossRef ] [ Google Scholar ]
- Bengisu M, Nekhili R. Forecasting emerging technologies with the aid of science and technology databases. Technol Forecast Soc Chang. 2006; 73 :835–844. doi: 10.1016/j.techfore.2005.09.001. [ CrossRef ] [ Google Scholar ]
- Bozeman B, Fay D, Slade CP. Research collaboration in universities and academic entrepreneurship: the-state-of-the-art. J Technol Transf. 2013; 38 :1–67. doi: 10.1007/s10961-012-9281-8. [ CrossRef ] [ Google Scholar ]
- Building AG Importance of Green Building. https://www.greenbuilt.org/about/importance-of-green-building
- Cao XD, Dai XL, Liu JJ. Building energy-consumption status worldwide and the state-of-the-art technologies for zero-energy buildings during the past decade. Energy Build. 2016; 128 :198–213. doi: 10.1016/j.enbuild.2016.06.089. [ CrossRef ] [ Google Scholar ]
- Chan APC, Darko A, Olanipekun AO, Ameyaw EE. Critical barriers to green building technologies adoption in developing countries: the case of Ghana. J Clean Prod. 2018; 172 :1067–1079. doi: 10.1016/j.jclepro.2017.10.235. [ CrossRef ] [ Google Scholar ]
- Chen CC, Lo TH, Tsay YS, Lee CY, Liu KS. Application of a novel formaldehyde sensor with MEMS (micro electro mechanical systems) in indoor air quality test and improvement in medical spaces. Appl Ecol Environ Res. 2017; 15 :81–89. doi: 10.15666/aeer/1502_081089. [ CrossRef ] [ Google Scholar ]
- Chen CM. CiteSpace II: detecting and visualizing emerging trends and transient patterns in scientific literature. J Am Soc Inf Sci Technol. 2006; 57 :359–377. doi: 10.1002/asi.20317. [ CrossRef ] [ Google Scholar ]
- Chen X, Lu WS, Xue F, Xu JY. A cost-benefit analysis of green buildings with respect to construction waste minimization using big data in Hong Kong. J Green Build. 2018; 13 :61–76. doi: 10.3992/1943-4618.13.4.61. [ CrossRef ] [ Google Scholar ]
- Chen XY, Niu RP, Lv LN, Kuang DQ, LOP (2019) Discussion on existing problems of fresh air system. In: 4th International Conference on Advances in Energy Resources and Environment Engineering, vol 237. IOP Conference Series-Earth and Environmental Science. Iop Publishing Ltd, Bristol. doi:10.1088/1755-1315/237/4/042030
- Chwieduk D. Towards sustainable-energy buildings. Appl Energy. 2003; 76 :211–217. doi: 10.1016/s0306-2619(03)00059-x. [ CrossRef ] [ Google Scholar ]
- Communuties CotE (2002) Directive of the European parliament and the council.
- Daim TU, Rueda G, Martin H, Gerdsri P. Forecasting emerging technologies: use of bibliometrics and patent analysis. Technol Forecast Soc Chang. 2006; 73 :981–1012. doi: 10.1016/j.techfore.2006.04.004. [ CrossRef ] [ Google Scholar ]
- Ding GKC. Sustainable construction - the role of environmental assessment tools. J Environ Manag. 2008; 86 :451–464. doi: 10.1016/j.jenvman.2006.12.025. [ PubMed ] [ CrossRef ] [ Google Scholar ]
- Doan DT, Ghaffarianhoseini A, Naismith N, Zhang TR, Ghaffarianhoseini A, Tookey J. A critical comparison of green building rating systems. Build Environ. 2017; 123 :243–260. doi: 10.1016/j.buildenv.2017.07.007. [ CrossRef ] [ Google Scholar ]
- Du HB, Li BL, Brown MA, Mao GZ, Rameezdeen R, Chen H. Expanding and shifting trends in carbon market research: a quantitative bibliometric study. J Clean Prod. 2015; 103 :104–111. doi: 10.1016/j.jclepro.2014.05.094. [ CrossRef ] [ Google Scholar ]
- Du HB, Liu DY, Lu ZM, Crittenden J, Mao GZ, Wang S, Zou HY. Research development on sustainable urban infrastructure from 1991 to 2017: a bibliometric analysis to inform future innovations. Earth Future. 2019; 7 :718–733. doi: 10.1029/2018ef001117. [ CrossRef ] [ Google Scholar ]
- Ellabban O, Abu-Rub H, Blaabjerg F. Renewable energy resources: current status, future prospects and their enabling technology. Renew Sust Energ Rev. 2014; 39 :748–764. doi: 10.1016/j.rser.2014.07.113. [ CrossRef ] [ Google Scholar ]
- Elshafei G, Negm A, Bady M, Suzuki M, Ibrahim MG. Numerical and experimental investigations of the impacts of window parameters on indoor natural ventilation in a residential building. Energy Build. 2017; 141 :321–332. doi: 10.1016/j.enbuild.2017.02.055. [ CrossRef ] [ Google Scholar ]
- Ernst H. The use of patent data for technological forecasting: the diffusion of CNC-technology in the machine tool industry. Small Bus Econ. 1997; 9 :361–381. doi: 10.1023/A:1007921808138. [ CrossRef ] [ Google Scholar ]
- Fezi BA. Health engaged architecture in the context of COVID-19. J Green Build. 2020; 15 :185–212. doi: 10.3992/1943-4618.15.2.185. [ CrossRef ] [ Google Scholar ]
- Guozhu, et al. Bibliometric analysis of insights into soil remediation. J Soils Sediments. 2018; 18 :2520–2534. doi: 10.1007/s11368-018-1932-4. [ CrossRef ] [ Google Scholar ]
- Ho S-Y. Bibliometric analysis of biosorption technology in water treatment research from 1991 to 2004. Int J Environ Pollut. 2008; 34 :1–13. doi: 10.1504/ijep.2008.020778. [ CrossRef ] [ Google Scholar ]
- Hong WX, Jiang ZY, Yang Z, (2017) Iop (2017) Analysis on the restriction factors of the green building scale promotion based on DEMATEL. In: 2nd International Conference on Advances in Energy Resources and Environment Engineering, vol 59. IOP Conference Series-Earth and Environmental Science. Iop Publishing Ltd, Bristol. doi:10.1088/1755-1315/59/1/012064
- Hu J, Kawaguchi KI, Ma JJB. Retractable membrane ceiling on indoor thermal environment of residential buildings. Build Environ. 2018; 146 :289–298. doi: 10.1016/j.buildenv.2018.09.035. [ CrossRef ] [ Google Scholar ]
- Huang N, Bai LB, Wang HL, Du Q, Shao L, Li JT. Social network analysis of factors influencing green building development in China. Int J Environ Res Public Health. 2018; 15 :16. doi: 10.3390/ijerph15122684. [ PMC free article ] [ PubMed ] [ CrossRef ] [ Google Scholar ]
- Huibin D, Guozhu M, Xi L, Jian Z, Linyuan W. Way forward for alternative energy research: a bibliometric analysis during 1994-2013. Sustain Energy Rev. 2015; 48 :276–286. doi: 10.1016/j.rser.2015.03.094. [ CrossRef ] [ Google Scholar ]
- Jalaei F, Jrade A. Integrating building information modeling (BIM) and LEED system at the conceptual design stage of sustainable buildings. Sustain Cities Soc. 2015; 18 (95-10718):95–107. doi: 10.1016/j.scs.2015.06.007. [ CrossRef ] [ Google Scholar ]
- Kandya A, Mohan M. Mitigating the urban heat island effect through building envelope modifications. Energy Build. 2018; 164 :266–277. doi: 10.1016/j.enbuild.2018.01.014. [ CrossRef ] [ Google Scholar ]
- Khan J, Arsalan MH. Solar power technologies for sustainable electricity generation - a review. Renew Sust Energ Rev. 2016; 55 :414–425. doi: 10.1016/j.rser.2015.10.135. [ CrossRef ] [ Google Scholar ]
- Khoshbakht, et al. Are green buildings more satisfactory? A review of global evidence. Habitat Int. 2018; 74 :57–65. doi: 10.1016/j.habitatint.2018.02.005. [ CrossRef ] [ Google Scholar ]
- Lazarova-Molnar S, Mohamed N. Collaborative data analytics for smart buildings: opportunities and models. Clust Comput. 2019; 22 :1065–1077. doi: 10.1007/s10586-017-1362-x. [ CrossRef ] [ Google Scholar ]
- Li X, Wu P, Shen GQ, Wang X, Teng Y. Mapping the knowledge domains of building information modeling (BIM): a bibliometric approach. Autom Constr. 2017; 84 :195–206. doi: 10.1016/j.autcon.2017.09.011. [ CrossRef ] [ Google Scholar ]
- Liu CY, Wang JC. Forecasting the development of the biped robot walking technique in Japan through S-curve model analysis. Scientometrics. 2010; 82 :21–36. doi: 10.1007/s11192-009-0055-5. [ CrossRef ] [ Google Scholar ]
- Liu GL, et al. A review of air filtration technologies for sustainable and healthy building ventilation. Sustain Cities Soc. 2017; 32 :375–396. doi: 10.1016/j.scs.2017.04.011. [ CrossRef ] [ Google Scholar ]
- Liu J, Liu GQ. Some indoor air quality problems and measures to control them in China Indoor. Built Environ. 2005; 14 :75–81. doi: 10.1177/1420326x05050362. [ CrossRef ] [ Google Scholar ]
- Liu ZB, Li WJ, Chen YZ, Luo YQ, Zhang L. Review of energy conservation technologies for fresh air supply in zero energy buildings. Appl Therm Eng. 2019; 148 :544–556. doi: 10.1016/j.applthermaleng.2018.11.085. [ CrossRef ] [ Google Scholar ]
- Liu ZJ, Pyplacz P, Ermakova M, Konev P. Sustainable construction as a competitive advantage. Sustainability. 2020; 12 :13. doi: 10.3390/su12155946. [ CrossRef ] [ Google Scholar ]
- Maasoumy M, Sangiovanni-Vincentelli A. Smart connected buildings design automation: foundations and trends found trends. Electron Des Autom. 2016; 10 :1–3. doi: 10.1561/1000000043. [ CrossRef ] [ Google Scholar ]
- Mao G, Zou H, Chen G, Du H, Zuo J. Past, current and future of biomass energy research: a bibliometric analysis. Sustain Energy Rev. 2015; 52 :1823–1833. doi: 10.1016/j.rser.2015.07.141. [ CrossRef ] [ Google Scholar ]
- Mao GZ, Shi TT, Zhang S, Crittenden J, Guo SY, Du HB. Bibliometric analysis of insights into soil remediation. J Soils Sediments. 2018; 18 :2520–2534. doi: 10.1007/s11368-018-1932-4. [ CrossRef ] [ Google Scholar ]
- Marinakis V. Big data for energy management and energy-efficient buildings. Energies. 2020; 13 :18. doi: 10.3390/en13071555. [ CrossRef ] [ Google Scholar ]
- McArthur JJ, Powell C. Health and wellness in commercial buildings: systematic review of sustainable building rating systems and alignment with contemporary research. Build Environ. 2020; 171 :18. doi: 10.1016/j.buildenv.2019.106635. [ CrossRef ] [ Google Scholar ]
- Mcleod RS, Hopfe CJ, Kwan A. An investigation into future performance and overheating risks in Passivhaus dwellings. Build Environ. 2013; 70 :189–209. doi: 10.1016/j.buildenv.2013.08.024. [ CrossRef ] [ Google Scholar ]
- Mclinden D. Concept maps as network data: analysis of a concept map using the methods of social network analysis. Eval Program Plann. 2013; 36 :40–48. doi: 10.1016/j.evalprogplan.2012.05.001. [ PubMed ] [ CrossRef ] [ Google Scholar ]
- Mehmood MU, Chun D, Zeeshan, Han H, Jeon G, Chen K. A review of the applications of artificial intelligence and big data to buildings for energy-efficiency and a comfortable indoor living environment. Energy Build. 2019; 202 :13. doi: 10.1016/j.enbuild.2019.109383. [ CrossRef ] [ Google Scholar ]
- Miao XL, Yao Y, Wang Y, Chu YP. Experimental research on the sound insulation property of lightweight composite paper honeycomb core wallboard. In: Jiang ZY, Li SQ, Zeng JM, Liao XP, Yang DG, editors. Manufacturing Process Technology, Pts 1-5. Stafa-Zurich: Advanced Materials Research. Trans Tech Publications Ltd; 2011. pp. 1334–1339. [ Google Scholar ]
- Newsham GR, Mancini S, Energy BJ. Do LEED-certified buildings save energy? Yes, but… Energy Build. 2009; 41 :897–905. doi: 10.1016/j.enbuild.2009.03.014. [ CrossRef ] [ Google Scholar ]
- Nguyen HT, Skitmore M, Gray M, Zhang X, Olanipekun AO. Will green building development take off? An exploratory study of barriers to green building in Vietnam. Resour Conserv Recycl. 2017; 127 :8–20. doi: 10.1016/j.resconrec.2017.08.012. [ CrossRef ] [ Google Scholar ]
- Paul WL, Taylor PA. A comparison of occupant comfort and satisfaction between a green building and a conventional building. Build Environ. 2008; 43 :1858–1870. doi: 10.1016/j.buildenv.2007.11.006. [ CrossRef ] [ Google Scholar ]
- Perez-Fargallo A, Rubio-Bellido C, Pulido-Arcas JA, Gallego-Maya I, Guevara-Garcia FJ. Influence of adaptive comfort models on energy improvement for housing in cold areas. Sustainability. 2018; 10 :15. doi: 10.3390/su10030859. [ CrossRef ] [ Google Scholar ]
- Pongiglione M, Calderini C. Sustainable structural design: comprehensive literature review. J Struct Eng. 2016; 142 :15. doi: 10.1061/(asce)st.1943-541x.0001621. [ CrossRef ] [ Google Scholar ]
- Pouris A, Pouris A. Scientometrics of a pandemic: HIV/AIDS research in South Africa and the World. Scientometrics. 2011; 86 :541–552. doi: 10.1007/s11192-010-0277-6. [ CrossRef ] [ Google Scholar ]
- Pulselli RM, Simoncini E, Pulselli FM, Bastianoni S. Emergy analysis of building manufacturing, maintenance and use: Em-building indices to evaluate housing sustainability. Energy Build. 2007; 39 :620–628. doi: 10.1016/j.enbuild.2006.10.004. [ CrossRef ] [ Google Scholar ]
- Ruellan M, Park H, Bennacer R. Residential building energy demand and thermal comfort: thermal dynamics of electrical appliances and their impact. Energy Build. 2016; 130 :46–54. doi: 10.1016/j.enbuild.2016.07.029. [ CrossRef ] [ Google Scholar ]
- Sartori I, Napolitano A, Voss K. Net zero energy buildings: a consistent definition framework. Energy Build. 2012; 48 :220–232. doi: 10.1016/j.enbuild.2012.01.032. [ CrossRef ] [ Google Scholar ]
- Schossig P, Henning HM, Gschwander S, Haussmann T (2005) Micro-encapsulated phase-change materials integrated into construction materials. Solar Energy Mater Solar Cells 89:297-306
- Shen C, Li XT. Solar heat gain reduction of double glazing window with cooling pipes embedded in venetian blinds by utilizing natural cooling. Energy Build. 2016; 112 :173–183. doi: 10.1016/j.enbuild.2015.11.073. [ CrossRef ] [ Google Scholar ]
- Shen L, Yan H, Fan H, Wu Y, Zhang Y. An integrated system of text mining technique and case-based reasoning (TM-CBR) for supporting green building design. Build Environ. 2017; 124 :388–401. doi: 10.1016/j.buildenv.2017.08.026. [ CrossRef ] [ Google Scholar ]
- Todd JA, Crawley D, Geissler S, Lindsey G. Comparative assessment of environmental performance tools and the role of the Green Building Challenge. Build Res Inf. 2001; 29 :324–335. doi: 10.1080/09613210110064268. [ CrossRef ] [ Google Scholar ]
- Wang H, Chiang PC, Cai Y, Li C, Wang X, Chen TL, Wei S, Huang Q. Application of wall and insulation materials on green building: a review. Sustainability. 2018; 10 :21. doi: 10.3390/su10093331. [ CrossRef ] [ Google Scholar ]
- Wang J, Liu Y, Ren J, Cho S, (2017) Iop (2017) A brief comparison of existing regional green building design standards in China. In: 2nd International Conference on Advances in Energy Resources and Environment Engineering, vol 59. IOP Conference Series-Earth and Environmental Science. Iop Publishing Ltd, Bristol. doi:10.1088/1755-1315/59/1/012013
- Wei WJ, Ramalho O, Mandin C. Indoor air quality requirements in green building certifications. Build Environ. 2015; 92 :10–19. doi: 10.1016/j.buildenv.2015.03.035. [ CrossRef ] [ Google Scholar ]
- Wuni IY, Shen GQP, Osei-Kyei R. Scientometric review of global research trends on green buildings in construction journals from 1992 to 2018. Energy Build. 2019; 190 :69–85. doi: 10.1016/j.enbuild.2019.02.010. [ CrossRef ] [ Google Scholar ]
- Wuni IY, Shen GQP, Osei RO (2019b) Scientometric review of global research trends on green buildings in construction journals from 1992 to 2018 Energy Build 190:69-85 doi:10.1016/j.enbuild.2019.02.010
- Yang XD, Zhang JY, Zhao XB. Factors affecting green residential building development: social network analysis. Sustainability. 2018; 10 :21. doi: 10.3390/su10051389. [ CrossRef ] [ Google Scholar ]
- Ye L, Cheng Z, Wang Q, Lin H, Lin C, Liu B. Developments of Green Building Standards in China. Renew Energy. 2015; 73 :115–122. doi: 10.1016/j.renene.2014.05.014. [ CrossRef ] [ Google Scholar ]
- Ye Q, Li T, Law R. A coauthorship network analysis of tourism and hospitality research collaboration. J Hosp Tour Res. 2013; 37 :51–76. doi: 10.1177/1096348011425500. [ CrossRef ] [ Google Scholar ]
- Yoon B, Park Y. A text-mining-based patent network: analytical tool for high-technology trend. J High Technol Manag Res. 2004; 15 :37–50. doi: 10.1016/j.hitech.2003.09.003. [ CrossRef ] [ Google Scholar ]
- Yuan XL, Wang XJ, Zuo J. Renewable energy in buildings in China-a review. Renew Sust Energ Rev. 2013; 24 :1–8. doi: 10.1016/j.rser.2013.03.022. [ CrossRef ] [ Google Scholar ]
- Zhang HT, Hu D, Wang RS, Zhang Y. Urban energy saving and carbon reduction potential of new-types of building materials by recycling coal mining wastes. Environ Eng Manag J. 2014; 13 :135–144. doi: 10.30638/eemj.2014.017. [ CrossRef ] [ Google Scholar ]
- Zhang HZ, Li H, Huang BR, Destech Publicat I (2015) Development of biogas industry in Beijing. 2015 4th International Conference on Energy and Environmental Protection. Destech Publications, Inc, Lancaster
- Zhang SF, Andrews-Speed P, Zhao XL, He YX. Interactions between renewable energy policy and renewable energy industrial policy: a critical analysis of China’s policy approach to renewable energies. Energy Policy. 2013; 62 :342–353. doi: 10.1016/j.enpol.2013.07.063. [ CrossRef ] [ Google Scholar ]
- Zhang Y, Huang K, Yu YJ, Yang BB. Mapping of water footprint research: a bibliometric analysis during 2006-2015. J Clean Prod. 2017; 149 :70–79. doi: 10.1016/j.jclepro.2017.02.067. [ CrossRef ] [ Google Scholar ]
- Zhang Y, Kang J, Jin H. A review of Green Building Development in China from the perspective of energy saving. Energies. 2018; 11 :18. doi: 10.3390/en11020334. [ CrossRef ] [ Google Scholar ]
- Zhao XB, Zuo J, Wu GD, Huang C. A bibliometric review of green building research 2000-2016. Archit Sci Rev. 2019; 62 :74–88. doi: 10.1080/00038628.2018.1485548. [ CrossRef ] [ Google Scholar ]
- Zuo J, Zhao ZY. Green building research-current status and future agenda: a review. Renew Sust Energ Rev. 2014; 30 :271–281. doi: 10.1016/j.rser.2013.10.021. [ CrossRef ] [ Google Scholar ]
Advertisement
Rammed earth, as a sustainable and structurally safe green building: a housing solution in the era of global warming and climate change
- Original Paper
- Published: 30 October 2019
- Volume 21 , pages 119–136, ( 2020 )
Cite this article
- Binod Khadka ORCID: orcid.org/0000-0002-5974-929X 1 , 2 , 3
1795 Accesses
15 Citations
Explore all metrics
Today with rapidly increasing population and their housing demands, the whole world has been victimized by critical consequences of global warming and climate change. From simple to complex construction, materials being used not only consume massive energy and resources during their life cycle but also deteriorate the environment releasing an enormous amount of dust, solid waste, and greenhouse gases. With an intention to provide immediate solution in the form of sustainable alternative materials, rammed earth is chosen in this study where, natural sub-soil is used as primary constituent, i.e. clay (15–25%), sand (50–60%), gravel (15–20%) with/without small percentage of stabilizer like cement (3–5%), and minimum water (8–12%), and finally tamping the mix in required formwork using simple methods/tools, have clearly justified this construction as low consumer of resources and energy compared to other conventional building materials like brick and cement. This paper outlines environmental impacts of building construction and justifies suitability of rammed earth as an ideal sustainable housing both in terms of environmental and structural stability, based on results obtained from lab tests (compressive strength: 1.5–6.5 MPa, water resistivity, optimum water content) of 100 mm stabilized and un-stabilized cube samples (with variation in moisture content, soil and stabilizer types), site investigation for around 6 years, constructing and demonstrating two small-scaled stabilized and un-stabilized rammed earth houses, discussion with local people, builders and masons. Comparatively, cement-stabilized (5% cement) samples had better overall results than un-stabilized and dung-stabilized samples. In addition, cost comparison showed that 360-mm-thick rammed earth wall was 10–15% cheaper than 230-mm-thick brick wall. Similarly, further verifying and comparing the obtained findings with previous-detailed studies is one of the highlighting parts of this paper.
This is a preview of subscription content, log in via an institution to check access.
Access this article
Price includes VAT (Russian Federation)
Instant access to the full article PDF.
Rent this article via DeepDyve
Institutional subscriptions
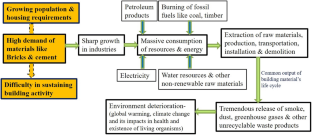
Similar content being viewed by others
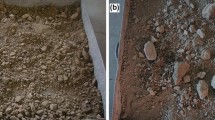
Comparative compressive strength of stabilized and un-stabilized rammed earth
Natural and Recycled Stabilizers for Rammed Earth Material Optimization
Recent Innovations in Stabilized Earthen Construction
Adam, E., & Agib, A. (2001). Compressed stabilised earth block manufacture in Sudan . France: Printed by Graphoprint for UNESCO.
Google Scholar
Arezoumandi, M., Volz, J. S., Ortega, C. A., & Myers, J. J. (2013). Effect of total cementitious content on shear strength of high-volume fly ash concrete beams. Materials and Design, 46, 301–309.
Article Google Scholar
Australia Standard. (2002). Australian earth building handbook . Sydney: Google Scholar.
Bahar, R., Benazzoug, M., & Kenai, S. (2004). Performance of compacted cement-stabilised soil. Cement and Concrete Composites, 26 (7), 811–820.
Barbosa, N., Swamy, N., & Mattone, R. (2007). Potentialities of earth construction in Latin-America. In: International symposium on earthen structures , vol. 1, pp. 29–37.
Berge, B. (2001). Ecology of building materials: Beyond 2000. Discovery channel . Oxford: Architectural Press.
Berge, B. (2009). The ecology of building materials . Abingdon: Routledge.
Book Google Scholar
BS 5628:Part 1. (1992). Code of practice for use of masonry . United Kingdom: British Standards Institute.
Bureau I. (2009). Draft reference document on best available techniques in the cement, lime and magnesium oxide manufacturing industries . Seville: European IPPC Bureau.
Burroughs, S. (2008). Soil property criteria for rammed earth stabilization. Journal of Materials in Civil Engineering, 20 (3), 264–273.
Cassell, R. (1993). A traditional research paper: Rammed earth construction, the compaction of successive layers of earth between forms to build a wall.
Dakwale, V. A., & Ralegaonkar, R. V. (2011). Review of carbon emission through buildings: Threats, causes and solution. International Journal of Low Carbon Technologies, 7 (2), 143–148.
Dixon, W. (2010). The impacts of construction and the built environment. Briefing notes . Letchworth Garden City: Willmott-Dixon Group.
Downton P (2013) Rammed earth.
Edwards, B. (2005). Rough guide to sustainability (2nd ed.). London: RIBA Enterprises Ltd.
Guettala, A., Abibsi, A., & Houari , H. (2006). Durability study of stabilized earth concrete under both laboratory and climatic conditions exposure. Construction and Building Materials, 20 (3), 119–127.
Guillaud, H. (2008). Characterization of earthen materials. Terra Literature Review, 2008, 21.
Hadjri, K., Osmani, M., Baiche, B., & Chifunda, C. (2007). (2007). Attitudes towards earth building for Zambian housing provision.
Hall, M., Najim, K., & Dehdezi, P. K. (2012). Soil stabilisation and earth construction: Materials, properties and techniques. In M. Hall (Ed.), Modern earth buildings (pp. 222–255). Oxford: Elsevier.
Chapter Google Scholar
Houben, H., Rigassi, V., & Garnier, P. (1994). Compressed earth blocks: Production equipment (Technologies (CDI) No. 5) . Brussels: Centre Devt Industry (CDI).
Howieson, S. (2005). Housing and asthma . Routledge: Taylor & Francis.
IPCC. (2007). Climate change 2007: The physical science basis . Geneva: IPCC.
IPCC. (2014). Climate change 2014: Mitigation of climate change . Geneva: Intergovernmental Panel on Climate Change.
Kadoshin, S., Nishiyama, T., & Ito, T. (2000). The trend in current and near future energy consumption from a statistical perspective. Applied Energy, 67 (4), 407–417.
Kasotia, P. (2007). The health effects of global warming: Developing countries are the most vulnerable . UN Chronicle.
Kateregga, J. (1983). Improvement and use of earth construction products for low cost housing. Appropriate Building Materials For Low Cost Housing African Region, 1983, 23–60.
Kaveh, A., & Vazirinia, Y. (2019). Smart-home electrical energy scheduling system using multi-objective ant lion optimizer and evidential reasoning. Scientia Iranica .
Khadka, B., & Shakya, M. (2016). Comparative compressive strength of stabilized and un-stabilized rammed earth. Materials and Structures, 49 (9), 3945–3955.
Khanzadi, M., Kaveh, A., Moghaddam, M. R., & Pourbagheri, S. M. (2018). Optimization of building components with sustainability aspects in BIM environment. Periodica Polytechnica Civil Engineering . https://doi.org/10.3311/PPci.12551 .
King, B. (1996). Buildings of earth and straw: Structural design for rammed earth and straw-bale architecture . New York: Ecological Design Press.
Kneifel, J. (2010). Life-cycle carbon and cost analysis of energy efficiency measures in new commercial buildings. Energy and buildings, 42 (3), 333–340.
Lal, A. K. (1995). Hand book of low cost housing . New Delhi: New Age International.
Maini, S. (2005). Earthen architecture for sustainable habitat and compressed stabilised earth block technology . Auroville: The Auroville Earth Institute, Auroville Building Center-India.
Middleton, G. F., & Lawrence, M. S. (1987). Bulletin 5 earth-wall construction (4th ed.). Australia: National Building Technology Centre.
Morton, T. (2007). Towards the development of contemporary Earth Construction in the UK: Drivers and benefits of Earth Masonry as a Sustainable Mainstream Construction Technique. In International Symposium on Earthen Structures, Indian Institute of Science, Bangalore (Vol. 22, p. 24).
Morton, T. (2008). Earth masonry. Design and Construction Guidelines .
Ngowi, A. (1997). Improving the traditional earth construction: A case study of Botswana. Construction and Building Materials, 11 (1), 1–7.
NZS 4297. (1998). Engineering design of earth buildings . Wellington: Standard New Zealand.
Outlook, A. E. (2008). Energy information administration . Official Energy Statistics from the US Government. http://www.eia.doe.gov .
Pacheco-Torgal, F., & Jalali, S. (2012). Earth construction: Lessons from the past for future eco-efficient construction. Construction and Building Materials, 29, 512–519.
Pérez-Lombard, L., Ortiz, J., & Pout, C. (2008). A review on buildings energy consumption information. Energy and Buildings, 40 (3), 394–398.
Prairie Village, K. (1998). Characterization of building-related construction and demolition debris in the United States. The US Environmental Protection Agency Municipal and Industrial Solid Waste Division Office of Solid Waste .
Price, L., Can, S. D. L. R. D., Sinton, J., Worrell, E., Nan, Z., Sathaye, J., et al. (2006). Sectoral trends in global energy use and greenhouse gas emissions . Berkeley: Environmental Energy Technologies Division, Ernest Orlando Lawrence Berkeley National Laboratory.
Raut, A. (2003). Brick Kilns in Kathmandu Valley: Current status, environmental impacts and future options. Himalayan Journal of Sciences, 1 (1), 59–61.
Reddy, V. B. (2007). Indian standard code of practice for manufacture and use of stabilised mud blocks for masonry.
Roodman, D. M., Lenssen, N. K., & Peterson, J. A. (1995). A building revolution: How ecology and health concerns are transforming construction . Washington, DC: Worldwatch Institute.
Sethna, Z. (2008). A sustainability approach to standards for rammed earth construction in Bhutan . Cambridge: University of Cambridge, Fourth-year undergraduate project.
Stajanča, M., & Eštoková, A. (2012). Environmental impacts of cement production.
UN Green Building Council. (2009). Green building by the numbers . Washington, DC: UN Green Building Council.
United Nations (2017). World Population 2017 (P. D. Department of Economic and Social Affairs, Trans.). United Nations.
USGS. (2005). US geological survey: Estimated use of water in the United States in 2000 . Reston: US Geological Service.
Venkatarama Reddy, B. (2009). Sustainable materials for low carbon buildings. International Journal of Low-Carbon Technologies, 4 (3), 175–181.
Vroomen, R. R. (2007). Gypsum stabilised earth: Research on the properties of cast Gypsum - stabilised earth and its suitability for low cost housing construction in developing countries . Final thesis for Msc Architecture, Building and Planning, Eindhoven University of Technology, The Netherlands.
Walker, P., Keable, R., Martin, J., & Maniatidis, V. (2005). Rammed earth: Design and construction guidelines.
Zami, M. S., & Lee, A. (2009). Use of stabilised earth in the construction of low cost sustainable housing in Africa—an energy solution in the era of climate change. International Journal of Architectural Research: ArchNet-IJAR, 3 (2), 51–65.
Download references
Acknowledgements
The author wants to thank all the team members (B.E. Civil batch 2010, Khwopa Engineering College) who participated in model preparation of rammed earth house and the respective faculties. Similarly, the author also wanted to thank Dr. Manjip Shakya and Santosh Shrestha for their valuable suggestions. The suitable working environment provided by Khwopa Engineering College and Rammed Earth Solution Pvt. ltd. is gratefully acknowledged.
Author information
Authors and affiliations.
Department of Disaster Mitigation for Structures, College of Civil Engineering, Tongji University, Shanghai, China
Binod Khadka
State Key Laboratory of Disaster Reduction in Civil Engineering, Tongji University, Shanghai, 200092, China
Department of Civil Engineering, Khwopa Engineering College, Bhaktapur, Nepal
You can also search for this author in PubMed Google Scholar
Corresponding author
Correspondence to Binod Khadka .
Ethics declarations
Conflict of interest.
The corresponding author confirms that there are no conflicts of interest associated with this publication.
Additional information
Publisher's note.
Springer Nature remains neutral with regard to jurisdictional claims in published maps and institutional affiliations.
Rights and permissions
Reprints and permissions
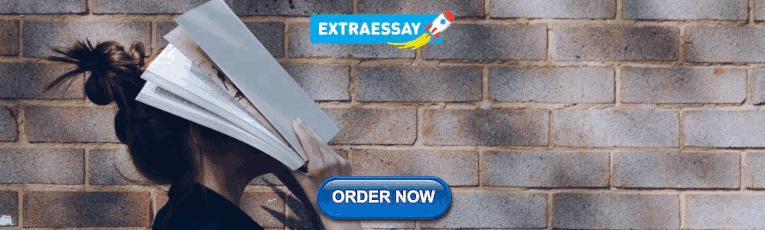
About this article
Khadka, B. Rammed earth, as a sustainable and structurally safe green building: a housing solution in the era of global warming and climate change. Asian J Civ Eng 21 , 119–136 (2020). https://doi.org/10.1007/s42107-019-00202-5
Download citation
Received : 25 June 2019
Accepted : 07 October 2019
Published : 30 October 2019
Issue Date : February 2020
DOI : https://doi.org/10.1007/s42107-019-00202-5
Share this article
Anyone you share the following link with will be able to read this content:
Sorry, a shareable link is not currently available for this article.
Provided by the Springer Nature SharedIt content-sharing initiative
- Rammed earth construction
- Building materials
- Green building
- Global warming
- Sustainability
- Housing demand
- Stabilization
- Find a journal
- Publish with us
- Track your research
- Open access
- Published: 29 May 2019
Green building literacy: a framework for advancing green building education
- Laura B. Cole ORCID: orcid.org/0000-0001-5730-1881 1
International Journal of STEM Education volume 6 , Article number: 18 ( 2019 ) Cite this article
29k Accesses
37 Citations
2 Altmetric
Metrics details
Despite the increasing square footage of green buildings worldwide, green building expertise remains largely in the domain of building industry professionals. However, the performance of and advocacy for green buildings would benefit from a green building literate general public. Green building education is an expanding frontier for STEM education and can create opportunities to integrate science and environmental literacies into the study of everyday environments. Few resources exist, however, to help STEM educators incorporate green building themes into the science classroom. The work here developed educational tools for connecting green buildings and science education through a multi-step process. An interdisciplinary literature review yielded a series of frameworks that were improved through two focus groups with science and environmental educators and built environment professionals.
The result of this process is a toolbox of conceptual frameworks for educators interested in using a systems-based approach to teach about green buildings as sites for complex interactions between human activity and Earth systems. The work here first leverages the broad definition of environmental literacy (knowledge, skills, affect, and behavior) to advance a working definition for “green building literacy.” Next, major domains of green building knowledge are developed and linked to the Next Generation Science Standards.
Conclusions
Green building literacy has been an ill-defined term and green building themes have not been rigorously connected to science and environmental education. The work here provides a foundation for promoting green building literacy through K-12 STEM education. The educational tools developed through this process can be used as a starting point for lesson planning to catalyze green building education in a variety of formal and informal settings.
Introduction
The overarching goals of building “green” are to reduce the social and environmental impacts of the built environment while improving the quality of life for occupants within buildings. In the USA, residential and commercial buildings consume 40% of total energy consumption and 75% of all electricity produced (U.S. Energy Information Administration, 2012 ). The average American family uses 300 gal of water a day, where 70% of that water use occurs indoors (U.S. Environmental Protection Agency, n.d. ). The contemporary green building movement promotes buildings that lessen these environmental impacts through better building construction (e.g., less construction waste), building operation and maintenance (e.g., water and energy conservation and better indoor air quality), and lifecycle considerations (e.g., recycling and deconstruction at the end of a building’s life) (International Living Future Institute, n.d. ; USGBC, n.d. ). However, the problem remains that few people outside the building industry understand the myriad benefits of building green (Cole, 2013 ).
Public green building education matters for a variety of reasons. To begin, people are life-long building consumers and occupants within buildings can be crucial agents of change for resource conservation measures such as energy efficiency and material recycling (e.g., Gill, Tierney, Pegg, & Allan, 2010 ; Wu, DiGiacomo, Lenkic, Wong, & Kingstone, 2016 ). A building’s design and resultant ecological performance may depend on occupant behaviors such as minimizing space heaters under the desk, turning off lights, and knowing when to close or open a window. Additionally, for residential structures, many people will at some point in their lives own and maintain, or rent and seek to improve, their own homes. Adults will increasingly need to engage with tax incentives for green home features and learn how to do home renovations or consult professionals for upgrades like better insulation, water efficient fixtures, or solar panel installation. Gaining expertise in green building strategies is therefore comparable to other basic skills in the home economy such as cooking nutritious meals or balancing finances. Finally, energy and atmosphere issues dominate the U.S. Labor Industry’s working definition of a green workforce (SEED Center, n.d. ). Green buildings can make significant contributions to solving energy and atmosphere challenges and will require an increasingly knowledgeable workforce to design, build, and maintain. These foundations for green building literacy can begin in the K-12 classroom.
Green building literacy (GBL) is the term used here to describe the hoped-for outcome of green building education [which falls within the larger movement for public “built environment education” (e.g., Portillo & Rey-Barreau, 1995 )]. To craft green building education programs, a framework is needed to understand prospects for GBL. The conceptual framework for GBL presented below builds off the four core themes integrated into frameworks for environmental literacy over time. Despite much variation in terminology, these four dimensions are knowledge, skills, affect, and behavior (terms used in the McBride, Brewer, Berkowitz, & Borrie, 2013 overview of environmental literacy frameworks). To date, little effort has been made to stitch together these domains with the topic of green building design. The current author presented the “ Major features of green building literacy ” in previous reporting as a theoretical background for empirical work in green schools (Cole, 2015 ). This work utilized the Marcinkowski ( 2010 ) matrix for “Major Features of Environmental Literacy,” a framework chosen for its clear distillation of themes and for its practical emphasis on identifying issues and solving problems. Previous publications on GBL, however, have not clearly identified the multiple dimensions of GBL from a theoretical point of view or aligned outcomes to STEM education. Doing so can inform a range of practices (from curriculum to building design) for various age groups given the broad nature of the foundational categories for environmental literacy presented by McBride et al. ( 2013 ).
Previous work on GBL was additionally conducted through a research project entitled “Green Building Technology Education,” funded by the National Science Council in Taiwan, which focused on green building education at the college level (Shiao, Lin, & Sung, 2013 ). Scholars in this group used the Roth ( 1992 ) work on environmental literacy to build curriculum and develop an evaluation tool for GBL (Jan, Lin, Shiao, Wei, Huang, & Sung, 2012 ). Their study involved a curricular intervention in an undergraduate general education course with pre- and post-course surveys measuring GBL, where they found significant increases in knowledge, attitudes, and behaviors from pre- to post-course (Shiao et al., 2013 ). They also identified a gap between positive attitudes and actual behaviors related to green buildings, which they attributed to the lack of green building skills (Jan et al., 2012 ). The work here expands the scholarship from Taiwan in several key ways. First, the “ Major features of green building literacy ” framework presented here offers a broader spectrum of pedagogical approaches to green building education compared to work by Shiao et al. ( 2013 ). The framework presented here incorporates multiple dimensions of green building knowledge (factual, conceptual, and procedural), where previous work has not considered various knowledge domains (i.e., those inspired by Bloom, Engelhart, Furst, Hill, & Krathwohl, 1956 ) and how these different kinds of knowledge relate to green building education. Second, this paper outlines a larger array of green building learning content. The scholarship in Taiwan was based on the Taiwan Green Building Label rating system (Tawain Green Building Label, n.d. ), which was developed specifically for tropical climates. This paper integrates international green building rating systems to offer 14 green building knowledge categories. This paper additionally provides educators with an integrative framework that places green buildings within infrastructural, ecological, and social contexts in alignment with the Next Generation Science Standards (NGSS) (NGSS Lead States, 2013 ) (Additional file 1 ). In doing so, the hope is that educators can increasingly teach about green buildings as dynamically interconnected with surrounding social and physical contexts while meeting stringent standards for science education. Finally, work in Taiwan was crafted specifically for the green building education of college students. The work here seeks to inform K-12 educators and curriculum developers who aspire to increase GBL for youth.
Improving the definition of GBL has implications for theories of teaching and learning. First, crafting a framework for GBL promotes exciting future directions for educators interested in experiential and place-based education (Barr, Dunbar, & Schiller, 2012 ). Learners can, of course, engage with green buildings themes by reading and watching educational media. However, green building knowledge can also be gained through hands-on lessons in the home, school building, public buildings, and beyond in community infrastructure (Cole, McPhearson, Herzog, & Kudryavtsev, 2017 ). As Sobel ( 2004 ) defines it, place-based education is “the process of using the local community and environment as a starting point to teach concepts in language arts, mathematics, social studies, science and other subjects across the curriculum” (p. 6). The study of buildings within infrastructure and ecology aligns well with Sobel’s vision of treating the surrounding community as an extension of the classroom and complement to textbook learning (Sobel, 2004 ).
The sections to follow address each the practical and theoretical aspects of GBL. First, GBL is theoretically positioned within the larger discourses of environmental literacy and science literacy. Second, the “ Major features of green building literacy ” are presented as a set of frameworks that can be used by educators and curriculum developers to integrate green building themes into STEM education.
Theorizing green building literacy
While green building themes can be viewed through numerous disciplinary lenses, the current work examines green building design as nested within the broader topics of environmental literacy and science literacy. A green building literate citizen will benefit from foundational knowledge from environmental/sustainability education and science education to understand both the what and why of green building design and ultimately how to engage in transformative green buildings practices.
Just as the term “environmental literacy” has been the subject of much debate (e.g., McBride et al., 2013 ), the term “science literacy” has been similarly elusive to define (e.g., DeBoer, 2000 ; Roberts, 2007 ). Both types of literacy, however, share the challenge of blurred boundaries between the physical sciences and socio-cultural themes. Environmental literacy is conceptualized as a combination of social and ecological forces, or an overlap of ecological literacy with civics literacy (Berkowitz, Ford, & Brewer, 2005 ; McBride et al., 2013 ), that attempts to thread together the complex relationships between human activity and ecosystem health. Likewise, for science literacy, the needs to place science within applied contexts necessitates some level of systems thinking that engages disciplines outside the physical sciences, which stands in contrast to a formulation of science literacy that stays “within science” (a distinction well-articulated by the Roberts, 2007 notion of Visions I and II for science/scientific literacy). Science standards for K-12 education, such as the NGSS, additionally include guidelines for teaching at the intersections of Earth systems and human activity (NGSS Lead States, 2013 ). Architectural environments, commonly infused with scientific advancements, are potent and very tangible manifestations of how humans interface with ecology. Green building design is thus uniquely positioned at the intersection of a variety of socio-cultural, technological, and ecological themes. However, educators need not expand to dimensions beyond science to engage in green building education. Green building design is fundamentally based on scientific concepts and can be viewed through a purely scientific lens. While the topic of green buildings is malleable to a variety of conceptualizations within the broader ideas of science literacy and environmental literacy, the frameworks introduced here were created with a mind toward the potential for interdisciplinarity.
Adding the notion of green building literacy (GBL) to the crowded field of “literacies” is not an exercise to take lightly. A more in-depth justification for why literacy is the appropriate terminology for advancing green building education is warranted. Stables and Bishop ( 2001 ) warn that “the term ‘literacy’ has been degraded as a result of its indiscriminate application” (p. 90). They argue that the application of the term to a variety of domains (e.g., environmental, technological, and computer literacies) has not been sufficiently grounded in the linguistic and literary origins of the notion of “literacy.” In their argument for a “strong conception of environmental literacy,” they advocate for an expansive conceptualization of the term “environmental literacy,” where literacy is not restricted to textual literacy, but understood as a broader engagement with the biophysical environment. Their work draws on work by de Saussure ( 1966 ) on semiology, the study of both linguistic and non-linguistic communication via the use of “signs” that are open to a variety of interpretations. Stables and Bishop ( 2001 ) argue for an understanding of environmental literacy as a semiotic engagement with our surrounding environment where the biophysical environment can be thought of a text that we both “read” (understand) and “write” (act on). In this view, the environment is not only an ecological reality but also open to a variety of scientific, historical, and esthetic interpretations (Stables & Bishop, 2001 , p. 93). McBride et al. ( 2013 ) also addressed the need to understand the term “literacy” beyond textual literacy by stating that “… expectations for a literate citizenry have been extended to include the ability to understand, make informed decisions, and act with respect to complex topics and issues facing society today” (McBride et al., 2013 , p. 2).
Conceptualizations of GBL can meet the Stables and Bishop ( 2001 ) criteria of being “strong.” Like the biophysical environment, buildings too can be read and written. This is a particularly interesting question in school buildings where the building can act as one stream of messaging among many others (Cole, 2018 ; Higgs & McMillan, 2006 ; Shapiro, 2015 ). Aligning with Stables and Bishop’s ( 2001 ) conceptualization, buildings may fit the same role as the biophysical environment, offering a palette of signs open to interpretation by building occupants and therefore providing a unique medium for environmental education. Buildings, perhaps even more than natural settings, are open to a multitude of interpretations with diverse layers—socio-cultural, biophysical, technical, historic, etc.—to comprehend. The term “green building literacy,” as used here, thus shares the strong conception of environmental literacy envisioned by Stables and Bishop ( 2001 ) as a fluid outcome rooted in time and context.
Like textual literacy and the Stables ( 1998 ) model for environmental literacy, GBL can also be understood as variously functional, cultural, and critical. The framework for GBL presented here lays the groundwork for functional GBL (the basic ability to “read” a green building) by outlining the diverse domains of green building knowledge. These knowledge domains are not simply about buildings as objects, however; the framework conceives green buildings as cultural artifacts that intersect with themes of economy, social justice, and esthetics. Green building education, therefore, can integrate cultural GBL (understanding the significance of green building practices) by encouraging learners to decode the kinds of socio-cultural messages that buildings impart. Better yet, green building education can foster critical GBL, where learners critically engage with green buildings to question the cultural, social, and political forces that both shape—and are shaped by—buildings. Stables ( 1998 ) argues that functional and cultural literacy are required for critical literacy, and effective environmental action requires critical environmental literacy. This may be true in the arena of green building design, where a basic understanding of green buildings is the foundation for active and effective participation in the green building movement.
Methods: green building literacy framework development
To develop this provisional framework for green building literacy (GBL), the guiding question was: what are the core qualities of a green building literate citizen ? This study used a simplified and qualitative Delphi technique (e.g., Murry & Hammons, 1995 ) to create, present, and revise the frameworks presented here. First, an interdisciplinary review of literature across environmental education and built environment studies yielded a series of diagrams and tables that convey the major tenants of GBL. Second, these intellectual resources were shared with an expert panel of practitioners and scholars in both education and architecture in two web-based focus group settings. Finally, insights from the focus groups were used to improve the frameworks including the creation of additional tools to connect green building knowledge domains with current standards for science education.
Interdisciplinary literature review
The foundation of the framework begins with the core dimensions of environmental literacy (knowledge, skills, affect, and behaviors) (McBride et al., 2013 ) which are then adapted to the topic of green building design (Table 1 ). Frameworks from the realms of education and architectural studies are then used to build each of these dimensions outward. First, the domains of knowledge and skills in this framework are informed by a revised version of Bloom’s Taxonomy (Krathwohl, 2002 ). The dimension of “skills” is here identified as “procedural knowledge” and combined with the other dimensions of knowledge to illustrate a continuum of knowledge from understanding (factual and conceptual knowledge) to action (procedural knowledge). Next, frameworks for green building design are used to establish a series of categories for green building knowledge (Table 2 ). The section on “ Green building knowledge and skills ” additionally includes a review of key crossover themes between green buildings and the NGSS to identify the strong potential for curricular integration. Finally, the themes of affect and behavior within green building education are discussed. The result is a provisional framework for GBL that can be used to both create and evaluate green building curriculum for the K-12 classroom.
Focus groups
Following ethics approval and consent of the participants, the first iterations of Tables 1 and 2 were shared with professionals in two focus group settings. One focus group was comprised of professionals in the realm of environmental and science education ( n = 5), hereafter called the “Educator Focus Group.” The second focus group engaged built environment (BE) professionals across interior design and architecture who all had experience in the area of green building design ( n = 7), hereafter called the “BE Focus Group.” Both groups were comprised of a mix of practitioners and academic scholars. A convenience sampling technique followed by a snowball sampling technique were both used to identify and recruit focus group participants. The researcher invited contacts in her own network and requested that those contacts help to identify other professionals who could offer valuable perspective on the topic of green building education. This sampling resulted in a group of experts who are all in North America and mostly located in regions across the USA. The Educator focus group included one West coast educator, a Midwest scholar, a Midwest sustainability coordinator originally from India, an East coast educator, and an East coast non-profit manager. The BE focus group included one scholar from the Mountain West, an architect from the Midwest, a scholar/architect from Turkey who resides in the Midwest, two scholars from the Southern US, a scholar from the East coast, and a scholar from Canada. Both focus group sessions were 60-min long, conducted online, and included a 10-min presentation of the frameworks by the researcher followed by a structured conversation that focused on obtaining expert feedback. Consensus was not derived through successive quantitative surveys, as is common in Delphi panels. Instead, points of contention were discussed as they arose in the focus group setting and the researcher ensured that all points of view were registered before changing topics.
The transcripts from each focus group were imported into qualitative analysis software and analyzed by the researcher in a two-step coding process that first identified topics of discussion through open coding then a second examination of the data to coalesce topics into broader themes. The final Tables 1 and 2 frameworks presented here are the result of integrating feedback across the professional and disciplinary perspectives. The “ Major features of green building literacy ” are presented in the next section followed by a summary of the three major themes that arose in the focus group settings in reaction to the frameworks.
Major features of green building literacy
Green building knowledge and skills.
What kind of knowledge might a green building literate citizen possess? The sections below unpack the multiple dimensions of green building knowledge. The Taxonomy table from the Krathwohl ( 2002 ) adaptation to Bloom’s Taxonomy (Bloom et al., 1956 ) is a framework commonly employed by environmental education scholars (e.g., Iozzi, Laveault, & Marcinkowski, 1990 ; Monroe, Andrews, & Biedenweg, 2008 ). The framework posits a six-step cognitive process dimension (remember, understand, apply, analyze, evaluate, create) and draws it across four different kinds of knowledge (factual, conceptual, procedural, metacognitive). Green building lesson plans can incorporate the six cognitive processes. Further, green building knowledge can fall along this spectrum of knowledge types. The sections below take the first three knowledge types as a starting point to define a typology for green building knowledge.
Factual green building knowledge
The factual information that underlies green building design is vast. Green buildings intersect with a wide set of environmental issues (materials, energy, water, etc.). However, numerous existing frameworks are used to organize and measure what it means for a building to be green. These tools can be used to organize content areas for green building lesson planning. Table 2 collects themes in one place, where categories are derived from previous GBL frameworks (Shiao et al., 2013 ), green building rating systems (CHPS, 2014 ; International Living Future Institute, n.d. ; USGBC, 2008 ), and green school award programs (Pastorius & Marcinkowski, 2013 ) and focus group feedback. Further inspiration was drawn from the McLennan ( 2004 ) compendium on philosophies of sustainable design. The five key categories most commonly found across green building rating systems include sustainable sites, location and transportation, energy and atmosphere, water, materials, and indoor air quality. The category of “shape of building” has been added to this framework given the importance of building orientation on the site and building size relative to the number of occupants. The Living Building Challenge, the most stringent guideline in North America, additionally includes categories of social equity and beauty to argue that green buildings not only perform well ecologically, but also socially with enduring esthetics (International Living Future Institute, n.d. ). The study of green buildings can also include economic analyses since various building features add costs, save costs, and sometimes pay for themselves over time. The concept of lifecycle analysis is especially pertinent for studying building materials, where the Braungart, McDonough, and Bollinger ( 2007 ) notion of “cradle to cradle” products (products designed to avoid the landfill) can be taught. The rating system dedicated specifically to schools in the USA, the Collaborative for High Performing Schools (CHPS), additionally includes the category “operations and metrics,” which addresses themes such as green cleaning, ongoing maintenance, and the monitoring of building performance of the building over time (CHPS, 2014 ). The category of “local and healthy food” is included because green building and landscape design can offer infrastructure for sustainable food production and consumption. This category may be especially pertinent for K-12 educators who already introduce lesson plans on local food systems and wish to intersect these themes with built environment education. Finally, the category of “policy” was added to the framework based on focus group feedback that stressed the importance of the political context for green building design. Addressing the broader social systems within which green buildings are created is yet another lens for understanding human impacts on ecosystems.
Conceptual green building knowledge
Taken together, the categories in Table 2 outline the foundation for an increasingly sophisticated understanding of green buildings. Beyond a grasp of individual building elements (factual knowledge) is the understanding of the complex interrelationships between building elements, and the ways in which these built features interact with the local communities and local ecologies—the human, air, water, plant, and animal life that are affected by the building (conceptual knowledge) (Fig. 1 ). Conceptual knowledge may include, for example, making the connection between a light bulb, functional illumination in the room, and the building energy that comes from a nearby coal power plant, which is then connected to air quality. Another example of conceptual understanding would be making the connection between an exotic hardwood and the cultural and ecological effects of deforestation in another country, a lesson that would highlight themes of building materiality, biodiversity loss, and social equity. Thus, while factual information within the categories described above can be taught and tested, a more advanced curriculum is needed to help students to connect factual knowledge into a systems-level understanding of green building themes.
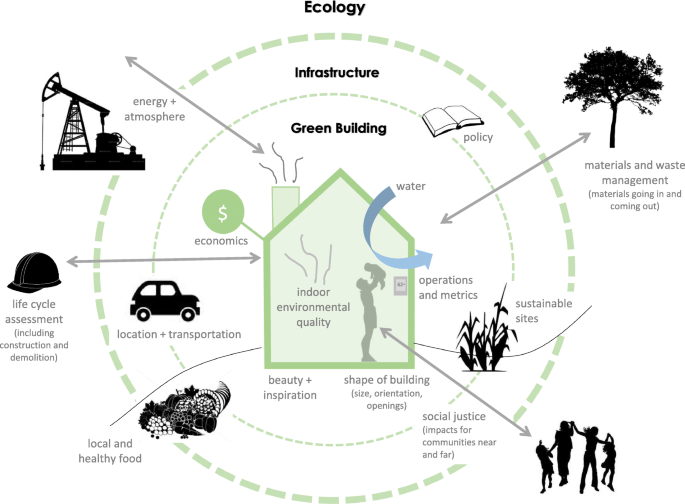
Factual and conceptual green building knowledge. This diagram shows the many ways that green building themes can be connected to broader social and ecological systems
Procedural green building knowledge (skills)
Beyond increasing factual and conceptual knowledge of green buildings, increasing procedural knowledge of green building issues moves students from understanding into action. Procedural knowledge relative to green buildings involves an expansive array of skill sets. Table 2 offers examples of procedural green building knowledge for each factual knowledge domain. Procedural knowledge in green buildings can draw on various disciplines. It can involve research on building materials, mathematical calculations on energy or financial savings, or hands-on activities such as building furniture from salvaged materials or installing a rain barrel. Procedural knowledge also spans across the life of a built environment—from designing and constructing to inhabiting and maintaining.
Green building knowledge and the NGSS
Factual, conceptual, and procedural green building knowledge can be acquired in standards-aligned green building education programs. An in-depth examination of the Next Generation Science Standards (NGSS) reveals the many ways that green building design themes can help educators to meet a variety of performance expectations (PEs) within standards across grade levels (NGSS Lead States, 2013 ). Additional file 1 illustrates a provisional overlapping of the NGSS standards and PEs with the 14 domains of green building knowledge (from Table 2 ). While isolated opportunities exist across the NGSS framework in areas such as energy, matter, and Earth’s systems, the areas with the highest potential are (1) Earth and Human Activity (ESS3) and (2) Engineering Design (ETS1).
Green building education can align quite well with the Earth and Human Activity (ESS3) PEs from Kindergarten through 12th grade. Beginning in Kindergarten with standards that require students to “communicate solutions that will reduce the impact of humans on the land, water, air, and/or other living things in the local environment” (K-ESS3-3) to standards such as the fifth grade PE to “obtain and combine information about ways individual communities use science ideas to protect the Earth’s resources and environment” (5-ESS3-1). Green building themes can advance through the upper grades with middle school requirements such as “apply scientific principles to design a method for monitoring and minimizing a human impact on the environment” (MS-ESS3-3) and high school PEs such as “use a computational representation to illustrate the relationships among Earth systems and how those relationships are being modified due to human activity” (HS-ESS3-6).
The engineering design standards (ETS1) within the NGSS additionally present a clear opportunity for green building education. The PEs for these standards were written quite broadly around the idea of “design process,” which can connect to a variety of disciplines such as architecture, engineering, product design, and well beyond. The PEs in ETS1 additionally require rich overlaps between technical, social, and environmental domains such as the middle school Standard MS-ETS1-1:
Define the criteria and constraints of a design problem with sufficient precision to ensure a successful solution, taking into account relevant scientific principles and potential impacts on people and the natural environment that may limit possible solutions (MS-ETS1-1).
The ETS1 standards in high school provide similar, and more complex, guidance:
Evaluate a solution to a complex real-world problem based on prioritized criteria and tradeoffs that account for a range of constraints, including cost, safety, reliability, and aesthetics, as well as possible social, cultural and environmental impacts (HS-ETS1-3).
Standards such as these could engage students, for example, in the design process for a piece of furniture for their classroom that meets a set of given functional, social, and environmental criteria. Beyond encouraging technical skills, these projects additionally provide avenues to make connections to themes of esthetics and social justice. In route, students could engage with additional NGSS standards such as MS-PS1-3 that encourages exploration of how “synthetic materials come from natural resources and impact society” and MS-LS2-3 which requires students to “develop a model to describe the cycling of matter and flow of energy among living and non-living parts of an ecosystem.” This is an example of how a single green building theme like furniture design can overlap with the physical and life sciences as students engage in a design process guided by the ETS1 engineering design standards.
Affective dispositions and green buildings
Just as the “environmentally literate individual has a well-developed set of environmental values or morals” (McBride et al., 2013 , p.7), so too does the green building literate citizen. Beyond having knowledge, a green building literate student may also have attitudes and values that shape knowledge and become a basis for environmental action. Within the Table 1 framework, affective dispositions include a person’s environmental sensitivity, environmental concern, self-efficacy, feelings of personal responsibility, and willingness to take action. Affective depositions such as these have a dual role as both an outcome to which we might aspire (e.g., environmentally sensitive citizens) and a predictor of other positive outcomes (e.g., environmentally responsible behaviors). Scholars across disciplines have taken an interest in affect for its potential importance in the process of learning (e.g., Picard et al., 2004 ) and for the links to pro-environmental action (e.g., Ajzen, 1991 ; Hines, Hungerford, & Tomera, 1987 ; Stern, 2000 ). It should be noted, however, that environmentally responsible behaviors are often multi-determined by an array of predictors where attitudes have been shown to be an unstable predictor across studies (Kollmuss & Agyeman, 2002 ). Within the Hungerford and Volk ( 1990 ) “Environmental Behavior Model,” affective dispositions occupy every dimension of the framework—from entry-level to ownership to empowerment variables—that all lead toward citizenship behavior. This is all to say, the relationship between affect and behavior is complex and rarely linear. Research further shows that attitudinal factors, such as environmental sensitivity, are outcomes that typically occur for individuals over many years and are influenced by factors such as role models and time in nature (Chawla, 1998 ; Marcinkowski, 1998 ; Tanner, 1980 ).
Despite the growing body of research on affect in fields of education, environmental education, and conservation psychology, the study of affect in green buildings is yet in the nascent stages. In the realm of green building literature, we have much yet to understand about how affect is influenced by green building design, and conversely, how green building design practices are advanced by people with positive affective dispositions. Each of these angles—alternatively viewing affective dispositions resulting from and contributing to green building practices—merits further elaboration.
First, previous research illustrates that context matters for fostering environmental sensitivity. Chawla ( 1998 ) found numerous pathways to environmental careers that included influences such as frequent contact in nature and solitude in nature, both experiences that can be fostered by green landscape design. On the other hand, and more specific to building interiors, McCunn and Gifford ( 2012 ) conducted one of the earliest studies on how a green office environment impacts employee environmental attitudes. Their results were surprising in that employee attitudes dropped as green building features increased. The researchers suggest that dissatisfaction with the building design, and perhaps faded novelty of the green building, were potential reasons for the negative attitudes. It appears to make a difference who occupies the green office building, however. A study in Australia found that office building occupants with higher levels of environmental concern were more likely to forgive a green building’s shortcomings (Deuble & de Dear, 2012 ).
Second, it is possible that positive emotions about green buildings will bring about positive outcomes for green buildings, where positive affect becomes a building block toward action. Sung et al. ( 2014 ) present pioneering quantitative work on GBL in a study of over 1000 Taiwanese college students. They found that attitudes about green buildings were an essential link between knowledge and behaviors. In fact, they found that “[w]ithout attitudes and responsibility as mediators, greater knowledge indicated poorer behavior” (Sung et al., 2014 , p. 173). Taken together, the research thus far suggests that green building attitudes can work for and against the pursuit of building green. The study of affect and green buildings is complex and potentially fertile area for future research. Research is especially lacking for youth in the K-12 school environment.
Despite the need for better understanding about affect in and for green buildings, there are several key takeaways regarding “affect” for practitioners interested in increasing GBL. The first is that attitudes and values about green buildings are not likely to change rapidly for building users. Green buildings are one potentially positive force for engendering environmental sensitivity along with other factors like access to nature and environmental role models, and these are influences that work over time. Further, practitioners designing curricula and interventions for green building education may want to understand the initial affective dispositions of learners relative to environmental themes to create the appropriate starting point for lesson planning. Finally, affect plays a role in the process of green building education just as it does in any educational process. Fredrickson ( 2001 ) argued that positive emotions allow people to “broaden” their scope of attention and “build” intellectual resources. Therefore, green building education that is infused with positive learning experiences may help learners to open up to novel experiences and revise their mental models of what the built environment can be.
Behaviors and green buildings
The ultimate goal of environmental education is to bring about change not only in people’s minds but in tangible benefits to our natural and built environment (e.g., Hines et al., 1987 ). The work here aligns well with the Sung et al. ( 2014 ) view of green building actions that focuses first on involvement and decision-making relative to green design and then expands to encompass more general environmentally responsible actions. Thus, within a framework of GBL, two distinct types of behaviors can be examined: actions that (1) advocate for green building practices, and (2) occur in and around green buildings.
First, a major goal of green building education is to inspire action that advances the green building movement. Marcinkowski ( 2010 ) conceptualizes behavior as multi-faceted, including actions taken individually and collectively on levels local, national, and global. These many forms of action are applicable to the topic of GBL. For example, consider the many ways a student could take action on energy issues. At the level of the building, a student can help turn off lights and shut down computers. The same student could work with peers in an environmental club to advocate for energy efficiency on their school campus. Further reach beyond the school building might include trying behaviors at home or writing local legislators about energy issues in public buildings. In this way, green building education can provide a link to planning and policy conversations in the classroom given the broader social systems (such as building codes, regulations, and guidelines) that either hinder or support innovative building design.
Second, consider the occupant actions within buildings that impact the performance of a green building. The repertoire of actions possible within a green building are largely determined by the opportunities a building affords such as recycling, composting, adjusting thermostats, and so on. Schools promoting green building education can align opportunities for environmentally friendly practices within the building with educational programming. The lessons for students are twofold. First, students can build awareness about how the physical built environment is structured to either hinder or support environmental action. Second, students can learn how informed and active building occupants can make a difference for their own school building’s environmental performance.
Focus group results and discussion
Education and built environment (BE) professionals provided input on the frameworks in Tables 1 and 2 . These tables were improved and Additional file 1 was created as a result of participant feedback. The three broad themes addressed by experts (each given a pseudonym) are summarized below.
Theme 1: framing green building literacy
The Table 1 framework for GBL resonated across groups and participants. Various professionals, however, recommended different ways to frame the importance of GBL. Numerous experts wanted to see more clear links between green building design and the realm of policy and planning given that the political and city planning context is a critical set of factors that can limit or give rise to innovative green building design (e.g., Simons, Choi, & Simons, 2009 ). BE professionals further expressed concern that building occupant political views will shape how individuals experience green buildings and respond to green building education programs, a notion that has some potential connection to the broader discourse on political consumerism (e.g., Wirt, 2017 ). The theme of policy was thus added to the framework. Claire discussed the complexity by noting that “there’s kind of a transactional thing that happens there with the attitudes that people bring into the building” that can either promote or deter environmentally friendly actions in buildings. The Table 1 “affective dimensions” category was split into two sub-themes as a result of this discussion. Stephen, an expert in using green schools as teaching tools, further advocated for stronger ways to frame green buildings for educators. He recommended framing green buildings as physical manifestations and microcosms of the larger environmental values that many educators already seek to foster in students. In sum, conversations across groups revealed the variety of lenses through which green buildings can be viewed. The work here examines green buildings for STEM education and maintains a dominant focus on the building itself and immediate landscape (the set of decisions that are largely within the power of school districts and architectural designers to make). However, educators, curriculum developers, and designers have vast options to tailor green building themes to their unique educational contexts and purposes.
Theme 2: green building knowledge categories
Numerous focus group participants indicated that Table 2 with “green building knowledge categories” was one of the key contributions of this body of work. As James, a public school curriculum coordinator, expressed:
Table two jumps out as a very effective set of principles and illustrations that dovetails very well into the sort of work that public schools are looking towards when it comes to the meaningful integration of sustainability practices. It's one thing to build the building, but … the practices are everything (James).
Discussion around the specific Table 2 categories comprised the major portion of both focus group sessions. The green building knowledge categories were thus impacted and refined as a result of the focus group feedback. Participants recommended that the titles of the knowledge categories maintain alignment with the prominent standards for green building design, which may be especially helpful for curriculum within schools with certified green buildings. Key points of conversation (in order of appearance in the framework) included:
Location and transportation: This category was originally included within “sustainable sites” but was extracted and given its own category as recommended by members of the BE focus group. This choice also reflects the latest changes within the LEED ® Green Building Rating System (USGBC, n.d. ).
Social justice: Educator focus group participants debated the inclusion of the “social justice” category. Stephen questioned if the theme deviated to far from the core topic of green buildings and Janice additionally commented that the theme could be difficult to address in lower elementary classes. However, three other participants vigorously defended the importance of keeping social justice in the framework, with one educator noting that “It is so front and center” (Sara) for the work that she does in public schools. James further emphasized the point commenting that “one cannot separate equity from environmental and sustainability focuses. It’s essential for kids.” These latter perspectives synchronize with the choice of the developers of the rigorous “Living Building Challenge” standards for ecologically friendly buildings, which include social justice as a core set of guidelines for living buildings (International Living Future Institute, n.d. ).
Local and healthy food: The Collaborative for High Performing Schools (CHPS) includes “school gardens” as a credit within the sites category (CHPS, 2014 ). Given that food system themes largely occur outside the building, the researcher asked participants if this category cohered with the other content in Table 2 . Educators and BE professionals overwhelmingly agreed that the built environment plays an important supporting role in sustainable farm-to-table food production and that this category fits well with current health food initiatives already happening at many schools. James summarized the group sentiments:
It [local and healthy food] is an authentic daily practice-driven integration that capitalizes upon required and normal school function, a critical one inside our schools, but we have found that integrating our outdoor garden as well as our hydroponic garden alongside recycling, composting and food donation has been an extraordinarily effective vehicle for all age levels. So I’m glad to see that represented here. “I think it’s low hanging fruit that you are wise to include” (James).
Two members of the Educator focus group did express some concern that “there is going to be a lot of red tape” (Janice) and logistical issues (Sara) in terms of connecting school gardens to school cafeterias. Public health concerns, student allergies, and pre-existing contracts with food vendors were several of the potential issues highlighted.
Policy: As mentioned previously, the topic of politics and policy was noted by BE focus group members as a potentially important issue to include. Inclusion of this category could encourage educators to engage social studies or civics themes into green building lesson planning. This theme could also inspire teaching about the green building rating systems themselves as guidelines that could be adopted into policy in the future.
Theme 3: implementation within schools
A central theme in the Educator focus group was the ways in which frameworks for GBL can be useful to educators and curriculum developers. The conversation began with James noting his frustration with identifying useful frameworks to inform practice. His comments highlighted the challenge of providing frameworks as tools for curriculum development, and particularly the challenge of striking a balance between providing overly broad versus excessively specific guidance. Janice suggested, and the educators all agreed, that alignment between GBL and science standards is a critical missing piece for promoting adoption of green building education in K-12 classrooms. Additional file 1 was created in response to this concern, and the tables therein reveal a multitude of connections between green building themes and the NGSS standards.
Numerous professionals asked clarifying questions about what types of school buildings, and school systems broadly, are the target audience for GBL frameworks. Within this conversation, the group discussed the ways that GBL can be promoted in schools both with and without green buildings—and for a spectrum of green buildings from partial renovations to entire new construction buildings. Educators additionally emphasized the importance of ensuring that these themes are not only pursued within special private and charter schools, but also within public school systems that may have less access to resources for green building design. The frameworks presented here are broad enough to apply to both green certified and non-green buildings across both school types and age groups. They are tools for educators and curriculum developers to use as a catalyst for connecting their unique contexts to green building design to advance a great variety of learning outcomes in K-12 science classrooms.
Overall, the group of professionals confirmed and expanded the conceptualizations of green building knowledge, contributing to the overarching question guiding this inquiry, which sought to define key qualities of a green building literate citizen. Despite having a variety of professional perspectives among participants, a key limitation to the focus groups was the sampling frame that began with the author’s own network and expanded outward. Three participants were foreign born; however, the dominant perspectives are US-centric and may need adaptation to other settings.
Green building education, while prominent in architectural and engineering professions, is scarce for the general public. Green building education can begin in K-12 schooling to enhance science education amid increasing calls to teach students about human impacts on nature (NGSS Lead States, 2013 ). If advances are to be made for public green building education, a framework for outlining the diverse educational content and outcomes could provide a useful starting point for curricula that are formal, informal, or even non-formal in nature. The “ Major features of green building literacy ” matrix builds on previous work to propose a framework for green building literacy. The major features discussed were knowledge (factual, conceptual, and procedural), affect, and behavior. This work calls for green building education that is not only factual in nature but also interweaves complex topics into a more conceptual understanding of green buildings and scaffolds toward skills and actions. However, a “strong conception” (Stables & Bishop, 2001 ) of green building literacy calls for building occupants who are both “reading” and “writing” green buildings. Building occupants are not only passive dwellers of buildings, but individuals who are an active part of a green building’s performance and have the capability to advocate for better building practices.
Abbreviations
Built environment
The Collaborative for High Performing Schools
- Green building literacy
Indoor environmental quality
Leadership in Energy and Environmental Design
Next Generation Science Standards
Performance expectation
United States Green Building Council
Ajzen, I. (1991). The theory of planned behavior. Organizational Behavior and Human Decision Processes, 50 (2), 179–211. https://doi.org/10.1016/0749-5978(91)90020-T .
Article Google Scholar
Barr, S., Dunbar, B., & Schiller, C. (2012). Sustainability in schools: Why green buildings have become a catalyst. Educational Facility Planner, 46 (1), 19–22.
Google Scholar
Berkowitz, A. R., Ford, M. E., & Brewer, C. A. (2005). A framework for integrating ecological literacy, civics literacy, and environmental citizenship in environmental education. In E. Johnson & M. Mappin (Eds.), Environmental education and advocacy: Changing perspectives of ecology and education (pp. 227–266). Cambridge: Cambridge University Press. https://doi.org/10.2980/1195-6860.2006.13/423:eeaacp/2.0.co.2 .
Chapter Google Scholar
Bloom, B. S., Engelhart, M. D., Furst, E. J., Hill, W. H., & Krathwohl, D. R. (1956). Taxonomy of educational objectives: The classification of educational goals. Handbook 1: Cognitive domain . New York: David McKay.
Braungart, M., McDonough, W., & Bollinger, A. (2007). Cradle-to-cradle design: Creating healthy emissions—a strategy for eco-effective product and system design. Journal of Cleaner Production, 15 (13), 1337–1348. https://doi.org/10.1016/j.jclepro.2006.08.003 .
Chawla, L. (1998). Significant life experiences revisited: A review of research on sources of environmental sensitivity. The Journal of Environmental Education, 29 (3), 11–21. https://doi.org/10.1080/00958969809599114 .
CHPS (2014). Project scorecard: 2014 U.S. collaborative for high performing school (CHPS) criteria. https://chps.net/sites/default/files/2014_US-CHPS-Scorecard.pdf . Accessed 1 Feb 2019.
Cole, L. B. (2013). The green building as medium for environmental education. The Michigan Journal of Sustainability, 1 (1), 161–169. https://doi.org/10.3998/mjs.12333712.0001.012 .
Cole, L. B. (2015). Fostering green building literacy in the school building: A study of five middle schools in the United States. Children & Youth Environments, 25 (3), 145–174. https://doi.org/10.7721/chilyoutenvi.25.3.0145 .
Cole, L. B. (2018). The teaching green building: Five theoretical perspectives. In F. W. Leal, R. Marans, & J. Callewaert (Eds.), Handbook of sustainability and social science research. World sustainability series (pp. 107–125). Cham: Springer. https://doi.org/10.1007/978-3-319-67122-2_6 .
Cole, L. B., McPhearson, T., Herzog, C., & Kudryavtsev, A. (2017). Green infrastructure. In A. Russ & M. E. Krasny (Eds.), Urban environmental education review (pp. 261–270). Ithaca: Cornell Press.
de Saussure, F. (1966). Course in general linguistics . New York: McGraw Hill.
DeBoer, G. E. (2000). Scientific literacy: Another look at its historical and contemporary meanings and its relationship to science education reform. Journal of Research in Science Teaching, 37 (6), 582–601. https://doi.org/10.1002/1098-2736(200008)37:6<582::AID-TEA5>3.0.CO;2-L .
Deuble, M. P., & de Dear, R. J. (2012). Green occupants for green buildings: The missing link? Building and Environment, 56 , 21–27. https://doi.org/10.1016/j.buildenv.2012.02.029 .
Fredrickson, B. L. (2001). The role of positive emotions in positive psychology: The broaden-and-build theory of positive emotions. American Psychologist, 56 (3), 218. https://doi.org/10.1037/0003-066X.56.3.218 .
Gill, Z. M., Tierney, M. J., Pegg, I. M., & Allan, N. (2010). Low-energy dwellings: The contribution of behaviours to actual performance. Building Research & Information, 38 (5), 491–508. https://doi.org/10.1080/09613218.2010.505371 .
Higgs, A. L., & McMillan, V. M. (2006). Teaching through modeling: Four schools’ experiences in sustainability education. The Journal of Environmental Education, 38 (1), 39–53. https://doi.org/10.3200/joee.38.1.39-53 .
Hines, J., Hungerford, H., & Tomera, A. (1987). Analysis and synthesis of research on responsible environmental behavior: A meta-analysis. Journal of Environmental Education, 18 (2), 1–8. https://doi.org/10.1080/00958964.1987.9943482 .
Hungerford, H. R., & Volk, T. L. (1990). Changing learner behavior through environmental education. The Journal of Environmental Education, 21 (3), 8–21. https://doi.org/10.1080/00958964.1990.10753743 .
International Living Future Institute. (n.d.). The living building challenge 3.1: A visionary path to a regenerative future. http://living-future.org/lbc . Accessed 1 Feb 2019.
Iozzi, L., Laveault, D., & Marcinkowski, T. (1990). Assessment of learning outcomes in environmental education. In Methods and techniques for evaluating environmental education . Paris: UNESCO.
Jan, Y.-L., Lin, M.-L., Shiao, K.-Y., Wei, C.-C., Huang, L.-T., & Sung, Q.-C. (2012). Development of an evaluation instrument for green building literacy among college students in Taiwan. International Journal of Technology and Human Interaction, 8 (3), 31–45. https://doi.org/10.4018/jthi.2012070104 .
Kollmuss, A., & Agyeman, J. (2002). Mind the gap: Why do people act environmentally and what are the barriers to pro-environmental behavior? Environmental Education Research, 8 (3), 239–260. https://doi.org/10.1080/13504620220145401 .
Krathwohl, D. R. (2002). A revision of Bloom’s taxonomy: An overview. Theory Into Practice, 41 (4), 212–218. https://doi.org/10.1207/s15430421tip4104_2 .
Marcinkowski, T. (1998). Predictors of responsible environmental behavior: A review of three dissertation studies. In H. R. Hungerford, W. Bluhm, T. Volk, & J. Ramsey (Eds.), Essential readings in environmental education (pp. 247–276). Champaign: Stipes.
Marcinkowski, T. (2010). Major features of environmental literacy . Melbourne: Department of Science and Mathematics Education, Florida Institute of Technology.
McBride, B., Brewer, C., Berkowitz, A., & Borrie, W. (2013). Environmental literacy, ecological literacy, ecoliteracy: What do we mean and how did we get here? Ecosphere, 4 (5), 1–20. https://doi.org/10.1890/ES13-00075.1 .
McCunn, L. J., & Gifford, R. (2012). Do green offices affect employee engagement and environmental attitudes? Architectural Science Review, 55 (2), 128–134. https://doi.org/10.1080/00038628.2012.667939 .
McLennan, J. F. (2004). The philosophy of sustainable design: The future of architecture . Kansas City: Ecotone Publishing.
Monroe, M. C., Andrews, E., & Biedenweg, K. (2008). A framework for environmental education strategies. Applied Environmental Education & Communication, 6 (3–4), 205–216. https://doi.org/10.1080/15330150801944416 .
Murry, J. W., Jr., & Hammons, J. O. (1995). Delphi: A versatile methodology for conducting qualitative research. The Review of Higher Education, 18 (4), 423–436. https://doi.org/10.1353/rhe.1995.0008 .
NGSS Lead States. (2013). Next generation science standards: For states, by states . Washington, D.C.: National Academies Press.
Pastorius, E., & Marcinkowski, T. (2013). A comparative analysis of federal and selected state green school frameworks. In Paper presented at the green schools national conference, west Palm Beach, Florida .
Picard, R. W., Papert, S., Bender, W., Blumberg, B., Breazeal, C., Cavallo, D., et al. (2004). Affective learning—A manifesto. BT Technology Journal, 22 (4), 253–269. https://doi.org/10.1023/B:BTTJ.0000047603.37042.33 .
Portillo, M., & Rey-Barreau, J. A. (1995). The place of interior design in K-12 dducation and the built environment education movement. Journal of Interior Design, 21 (1), 39–43. https://doi.org/10.1111/j.1939-1668.1995.tb00207.x .
Roberts, D. (2007). Scientific literacy/science literacy. In S. K. Abell & N. G. Lederman (Eds.), Handbook of research on science education (pp. 729–780). Mahwah: Lawrence Erlbaum Associates.
Roth, C. E. (1992). Environmental literacy: Its roots, evolution and directions in the 1990s . Columbus: ERIC Clearinghouse for Science, Mathematics, and Environmental Education.
SEED Center. (n.d.). Defining the Green Workforce. http://theseedcenter.org/Resources/SEED-Toolkits/Defining-the-Green-Workforce . Accessed 1 Sept 2018.
Shapiro, B. (2015). Structures that teach: Using a semiotic framework to study the environmental messages of learning settings. Eco-thinking, 1 (1), 5–17.
Shiao, K. Y., Lin, M. L., & Sung, Q. C. (2013). Curriculum innovation for fostering green building literacy in general education. Applied Mechanics and Materials, 284-287 , 1290–1294. https://doi.org/10.4028/www.scientific.net/amm.284-287.1290 .
Simons, R., Choi, E., & Simons, D. (2009). The effect of state and city green policies on the market penetration of green commercial buildings. Journal of Sustainable Real Estate, 1 (1), 139–166.
Sobel, D. (2004). Place-based education: Connecting classroom and community. Nature and Listening, 4 , 1–7.
Stables, A. (1998). Environmental literacy: Functional, cultural, critical. The case of the SCAA guidelines. Environmental Education Research, 4 (2), 155–164. https://doi.org/10.1080/1350462980040203 .
Stables, A., & Bishop, K. (2001). Weak and strong conceptions of environmental literacy: Implications for environmental education. Environmental Education Research, 7 (1), 89–97. https://doi.org/10.1080/13504620125643 .
Stern, P. C. (2000). Toward a coherent theory of environmentally significant behavior. Journal of Social Issues, 56 , 407–424. https://doi.org/10.1111/0022-4537.00175 .
Sung, Q.-C., Lin, M.-L., Shiao, K.-Y., Wei, C.-C., Jan, Y.-L., & Huang, L.-T. (2014). Changing behaviors: Does knowledge matter? A structural equation modeling study on green building literacy of undergraduates in Taiwan. Sustainable Environment Research, 24 (3), 173-183.
Tanner, R. T. (1980). Significant life experiences: A new research area in environmental education. The Journal of Environmental Education, 11 (4), 20–24. https://doi.org/10.1080/00958964.1980.9941386 .
Tawain Green Building Label. (n.d.). About Green Building Labeling. http://twgbqanda.com/english/e_about.php?Type=1&menu=e_about_class&pic_dir_list=0# . Accessed 1 Feb 2019.
U.S. Energy Information Administration. (2012). Annual energy outlook 2012: With projections to 2035 . Washington, DC: Government Printing Office.
U.S. Environmental Protection Agency. (n.d.). Water Use Today. https://www3.epa.gov/watersense/our_water/water_use_today.html . Accessed 1 Feb 2019.
USGBC. (2008). LEED 2009 for Schools New Construction and Major Renovations. http://www.usgbc.org/ShowFile.aspx?DocumentID=5547 . Accessed 1 Feb 2019.
USGBC. (n.d.). United States Green Building Council. http://www.usgbc.org/ . Accessed 1 Feb 2019.
Wirt, F. M. (2017). Politics, products, and markets: Exploring political consumerism past and present . New York: Routledge.
Book Google Scholar
Wu, D. W. L., DiGiacomo, A., Lenkic, P. J., Wong, V. K., & Kingstone, A. (2016). Being in a “green” building elicits “greener” recycling, but not necessarily “better” recycling. PLoS One, 11 (1), e0145737. https://doi.org/10.1371/journal.pone.0145737 .
Download references
Acknowledgements
The author would like to thank the panel of experts for their time and insight in the development of the frameworks presented in this study. The author would additionally like to thank Dr. Michaela Zint and Dr. Laura Zangori for their assistance with early drafts of this work. Additional gratitude is extended to the peer reviewers whose constructive feedback contributed greatly to this piece.
This open-access publication is supported by the National Institute of Food and Agriculture federal agricultural experiment station capacity grants (project no. MO-HANC0001) from the United States Department of Agriculture.
Availability of data and materials
The datasets used and/or analyzed during the current study are available from the corresponding author on reasonable request.
Author information
Authors and affiliations.
University of Missouri, Columbia, USA
Laura B. Cole
You can also search for this author in PubMed Google Scholar
Contributions
The author made 100% of the contributions to the current work. The author read and approved the final manuscript.
Corresponding author
Correspondence to Laura B. Cole .
Ethics declarations
Authors’ information.
Dr. Laura Cole is an interior design educator and architectural studies scholar who has been involved in the green building movement in various capacities for over 15 years. She worked as a designer in the global architecture firm of Perkins + Will where she co-lead the sustainability team and mentored junior designers on their pathways toward becoming LEED accredited professionals. Her Ph.D. work was in the combined areas of Architecture and Natural Resources and Environment. She is now an educator at the University of Missouri where she teaches sustainable design and works on interdisciplinary research teams to advance green building education in theory and practice.
Competing interests
The author declares that she has no competing interests.
Publisher’s Note
Springer Nature remains neutral with regard to jurisdictional claims in published maps and institutional affiliations.
Additional file
Additional file 1:.
Provisional Alignments between the NGSS and Green Building Knowledge Categories. (DOCX 38 kb)
Rights and permissions
Open Access This article is distributed under the terms of the Creative Commons Attribution 4.0 International License ( http://creativecommons.org/licenses/by/4.0/ ), which permits unrestricted use, distribution, and reproduction in any medium, provided you give appropriate credit to the original author(s) and the source, provide a link to the Creative Commons license, and indicate if changes were made.
Reprints and permissions
About this article
Cite this article.
Cole, L.B. Green building literacy: a framework for advancing green building education. IJ STEM Ed 6 , 18 (2019). https://doi.org/10.1186/s40594-019-0171-6
Download citation
Received : 07 September 2018
Accepted : 22 April 2019
Published : 29 May 2019
DOI : https://doi.org/10.1186/s40594-019-0171-6
Share this article
Anyone you share the following link with will be able to read this content:
Sorry, a shareable link is not currently available for this article.
Provided by the Springer Nature SharedIt content-sharing initiative
- Green building education
- Environmental literacy
- Science literacy
- Curriculum design
- Next generation science standards
- Reference Manager
- Simple TEXT file
People also looked at
Original research article, rapid carbon emission measurement during the building operation phase based on pso–svm: electric big data perspective.

- 1 State Grid Sichuan Electric Power Research Institute, Chengdu, China
- 2 State Grid Sichuan Electric Power Company, Chengdu, China
- 3 Sichuan Provincial Construction Engineering Fire and Survey Design Technology Center, Chengdu, China
With the rapid development of urbanization in China, urban energy consumption increases rapidly, leading to energy shortages and environmental pollution, of which building operational energy consumption carbon emissions (BECCE) account for a large proportion. It has a vital impact on global warming and urban green and sustainable development. Chengdu city in Sichuan Province is taken as the research area in this paper. First, basic information and power data on four types of single buildings, including large-sized buildings, small- and medium-sized buildings, government agencies, and residential buildings, are collected. Second, the characteristics of the four types of buildings are extracted, and the calculation model of BECCE (“electricity-carbon” model) based on particle swarm optimization algorithm–support vector machine (PSO–SVM) is constructed, and the model is trained and verified using the method of five-fold cross-validation. Then, according to the mean absolute error (MAE), root mean square error (RMSE), and R 2 evaluation indicators, the constructed “electricity-carbon” model is compared and evaluated. Finally, the generalization ability of the “electricity-carbon” model is verified. The research results show that (1) the “electricity-carbon” model constructed in this paper has a high accuracy rate, and the fitting ability of the PSO–SVM model is significantly better than that of the support vector regression (SVR) model; (2) in the testing stage, the fitting situation of large buildings is the best, and MAE, RMSE, and R 2 are 858.7, 1108.6, and 0.91, respectively; and (3) the spatial distribution map of regional BECCE can be quickly obtained using the “electricity-carbon” model constructed in this paper. The “electricity-carbon” model constructed in this paper can provide a scientific reference for building emission reduction.
1 Introduction
With the deepening of urbanization, carbon emissions in the construction sector also increase ( Ahmed et al., 2020 ; Zhao et al., 2022 ). According to the United Nations Environmental Program and IEA, (2017) and the United Nations Intergovernmental Panel on Climate Change (IPCC), 40% of the total energy use is consumed by the building sector, which accounts for a third of energy-related carbon emissions ( IPCC, 2014 , europa. eu). In 2018, 9.7 Gt CO 2 was emitted from the energy use of buildings in their entire life cycle, accounting for 39% of the total global energy-related CO 2 emissions. In addition, the current rapid economic development will further lead to a sharp consumption of energy and a large amount of CO 2 emissions in the future. In this context, greenhouse gas emission reduction targets have been proposed by many countries. China’s goal is to achieve a carbon peak in 2030, achieve carbon neutrality in 2060, and finally achieve net-zero CO 2 emissions ( Wei, 2015 ; Michael et al., 2016 ; Mallapaty, 2020 ).
In the whole life cycle of buildings, building operational energy consumption carbon emissions (BECCE) account for 70% of the carbon emissions, and because the energy consumption of buildings is still increasing, this value is expected to continue to rise ( Energy Information Administration, 2020 ). In order to adjust building energy conservation and emission reduction policies in a timely manner, the rapid and accurate calculation of BECCE has gradually attracted people’s attention ( Sztubecka et al., 2020 ). Nowadays, the methods for estimating BECCE can be divided into two categories: “bottom-up” and “top-down” ( Zhao et al., 2021 ), as it is difficult to extract relevant structural characteristics and detailed energy consumption data from a single building. Therefore, most of the previous studies used the “top-down” method to estimate BECCE ( Liu et al., 2020 ; Zhao et al., 2021 ), but the data collected by this method have a time lag, and BECCE can only be estimated with a certain error, making it difficult to quickly and accurately measure BECCE. So far, there is no universally accepted real-time calculation method for BECCE, which has gradually become an area of exploration for scholars today.
Electricity is the main energy consumed in the building operation phase ( Roeck et al., 2020 ) because its consumption data are real-time data with a short release time lag and small statistical errors, and it has been gradually applied to research related to carbon emission calculations in Han (2022) . In recent years, with the rapid development of artificial intelligence in the field of building carbon emissions, for example, Moraliyage et al. (2022) constructed a repeatable and transparent method for building energy-saving estimation, which uses artificial intelligence (AI) algorithms to improve the performance of the measurement and validation (M&V) protocol. It has been demonstrated to provide a robust and explainable framework for the M&V protocol aimed at achieving net-zero carbon emissions. In the same year, an explainable artificial intelligence model was proposed, which successfully predicted energy use and greenhouse gas emissions of residential buildings and identified the most influential variables as urban form, building geometry, total building area, and natural gas ( Zhang et al., 2023 ). As a highly robust machine learning method, support vector machine (SVM) can solve nonlinear problems well ( Seyedzadeh et al., 2020 ) and is widely used in building energy performance and greenhouse gas emissions. In the SVM model, the selection of the penalty and kernel functions has a great influence on its generalization ability and prediction accuracy. Considering that the particle swarm optimization (PSO) algorithm has the advantages of simple structure and fast convergence, it can be used to optimize the parameters in the SVM ( Eseye et al., 2017 ; Tang et al., 2018 ; Cuong-Le et al., 2021 ). To solve the classification prediction problem, Liang et al. (2023) used PSO–SVM to predict low-index media outbursts to improve coal mining efficiency, with a high prediction accuracy of about 90%. Zhang et al. (2023) accurately identified faults in wind turbine power converters using PSO–SVM, and the accuracy of test samples was as high as 98%. Huang et al. (2023) used the PSO–SVM model to solve the fault prediction problem of relay protection equipment, and the results showed that the prediction accuracy of the model for three different devices was at least 91%. At the same time, it was also extensively used in regression problems. Sandeep et al. (2023) compared the superiority of PSO–SVM, SVM, backpropagation neural network (BPNN), and other models in monthly flood flow prediction. Liu et al. (2023) studied the mechanical behavior of carbonate gravel using a mixed PSO–SVM model instead of a large-scale test method, and the results showed the relatively high accuracy of the model.
Based on the above literature, this paper mainly contributes to the following two aspects: (1) obtaining real-time energy consumption data for building operations poses challenges due to inherent delays. Similarly, measuring the BECCE of individual buildings in real time using the carbon emission factor method is complex. Taking into account the unique advantages of easy access and real-time power big data, the carbon emission characteristics of four types of buildings combined with the physical characteristics of buildings are hereby described. (2) The non-linear mapping relationship between power big data, building physical characteristics, and BECCE of a single building was established by the machine learning method, and the “electron-carbon” model was constructed to achieve rapid and accurate BECCE calculation. In this paper, a total of 2,526 individual buildings in Chengdu, including large public buildings, small- and medium-sized public buildings, government offices, and residential buildings, were taken as research objects, and a BECCE rapid measurement method (“electron-carbon “model) based on PSO–SVM was constructed. The structure of this paper is as follows: Section 1 outlines the advantages of power big data and machine learning methods for BECCE measurement; Section 2 mainly collects the power data and building form data (building floor and building area) of 2,526 buildings in Chengdu, Sichuan Province, and discusses the characteristics of different types of buildings; Section 3 constructs the “electricity-carbon” model based on PSO–SVM; Section 4 draws the research conclusion. Then, the discussion and conclusion are presented in Section 5 and Section 6 , respectively.
2 Research framework construction
Based on the perspective of building emission reduction, this paper proposes an accurate and rapid BECCE measurement method for real-time detection of carbon emissions during building operation. First, the traditional method (carbon emission factor method) is adopted to calculate the building carbon emissions according to the carbon emission coefficient ( Eggleston et al., 2006 ). Second, a building carbon emission measurement index system is constructed from two aspects of power data and building characteristics, and an “electricity-carbon model” is established by combining machine learning methods. Finally, BECCE is calculated according to the “electricity-carbon” model, and the model results are analyzed. The research framework is shown in Figure 1 . The specific steps are as follows:
(1) The traditional method is used to measure carbon emissions. The consumption of five energy sources (coal, natural gas, liquefied petroleum gas, artificial gas, and electricity) in Chengdu’s large buildings, small- and medium-sized public buildings, government agencies, and residential buildings is collected, and based on the carbon emission coefficient of each energy source, the BECCE of each building are calculated.
(2) An “electricity-carbon” model is constructed based on PSO–SVM. The three influencing factors of building electricity consumption, building area, and building floor are extracted as indicators; these indicators are combined with the PSO–SVM algorithm to establish the “electricity-carbon” model; a training set and a verification set are constructed; and the model is evaluated according to the evaluation indicators.
(3) The generalization ability of the “electricity-carbon” model is verified. Based on the building sample data in the test set, the “electricity-carbon” model is used to measure carbon emissions and compared with the actual data to verify the effectiveness of the “electricity-carbon” model.
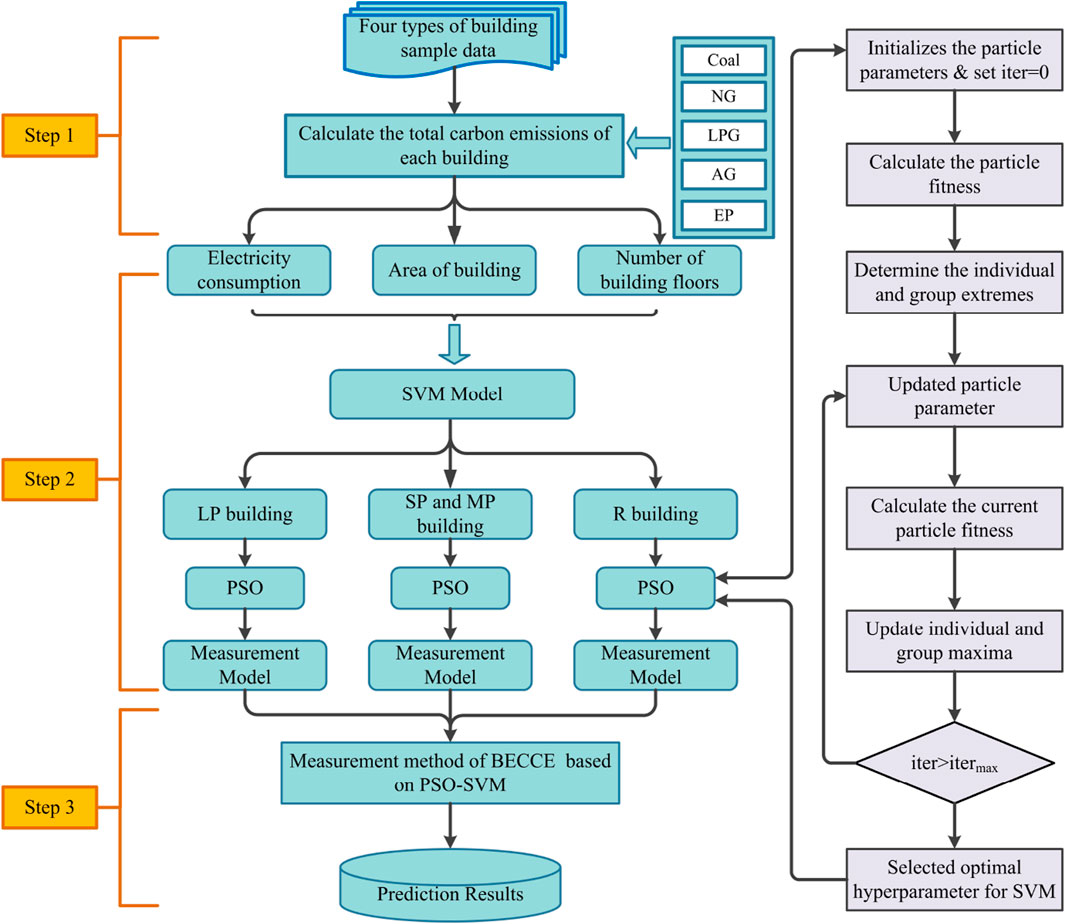
Figure 1 . Research framework.
2.1 Study area
Chengdu (102°54'∼104°53′E, 30°05'∼31°26′N) is the capital of Sichuan Province and the political and economic core of southwest China ( Figure 2 ). With a total area of 12121 × 10 4 square kilometers and a resident population of 21.192 million, its GDP exceeded 273.8 billion U.S. dollars in 2020. Since the 1980s, Chengdu’s urbanization has accelerated. The urban population has increased from 8,225,400 in 1980 to 20.947 million in 2020, while the built-up area has expanded from 60 square kilometers to 977.12 square kilometers in 2021. Building energy consumption is mainly electricity, natural gas, and liquefied petroleum gas. Chengdu’s climate is warm and humid. Since the 1980s, the climate in Chengdu has shown a trend of increasing temperature and drying ( Oleson et al., 2013 ). This indicates that the greenhouse effect in Chengdu has been more obvious. The increase in CO 2 emissions in Chengdu is expected to cause more serious climate problems due to the city’s dense population and the increase in the number of buildings. Therefore, it is necessary to make real-time carbon emissions in the building operation stage transparent to reasonably implement the building emission reduction policy. It is of great significance to study the BECCE of the rapid measurement method of regional buildings.
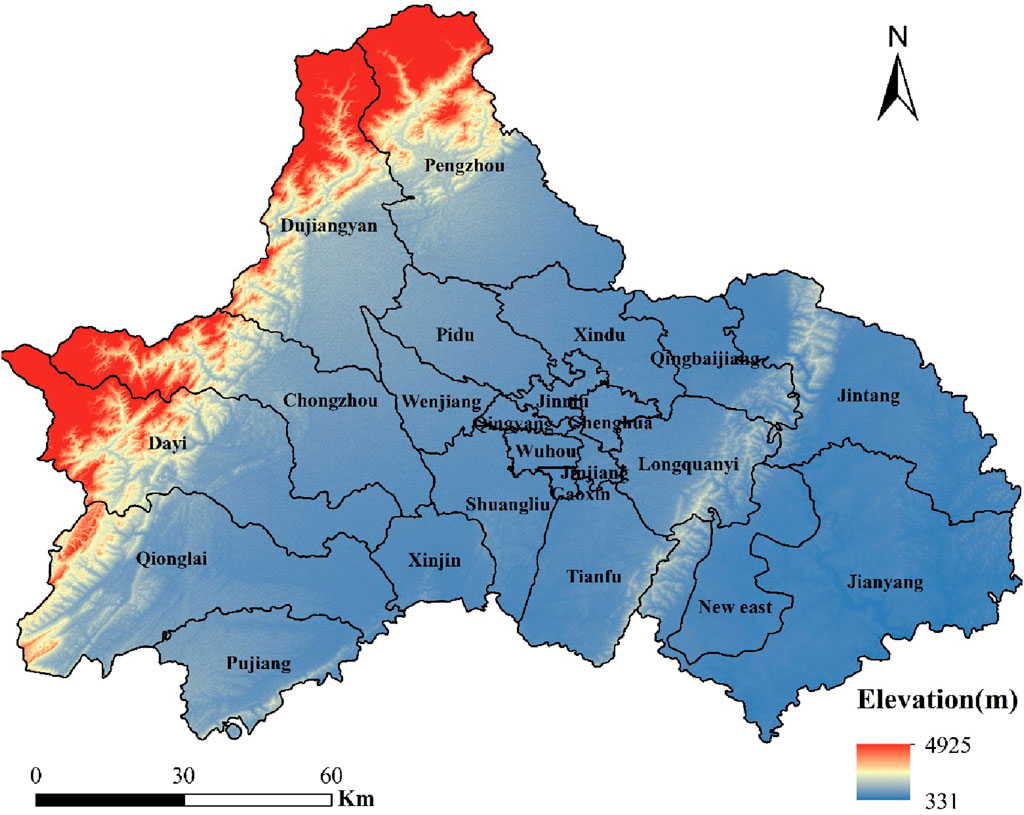
Figure 2 . Geographical distribution of Chengdu.
Considering the actual distribution of the four types of buildings in Chengdu, a total of 2,526 building samples were hereby collected from 23 residential areas, including 199 large buildings, 969 small- and medium-sized public buildings, 374 government organs, and 984 residential buildings. Each sample included the building name, address, administrative division, consumption of five types of energy in 2021, floor area, and number of floors. The detailed statistics are shown in Table 1 .
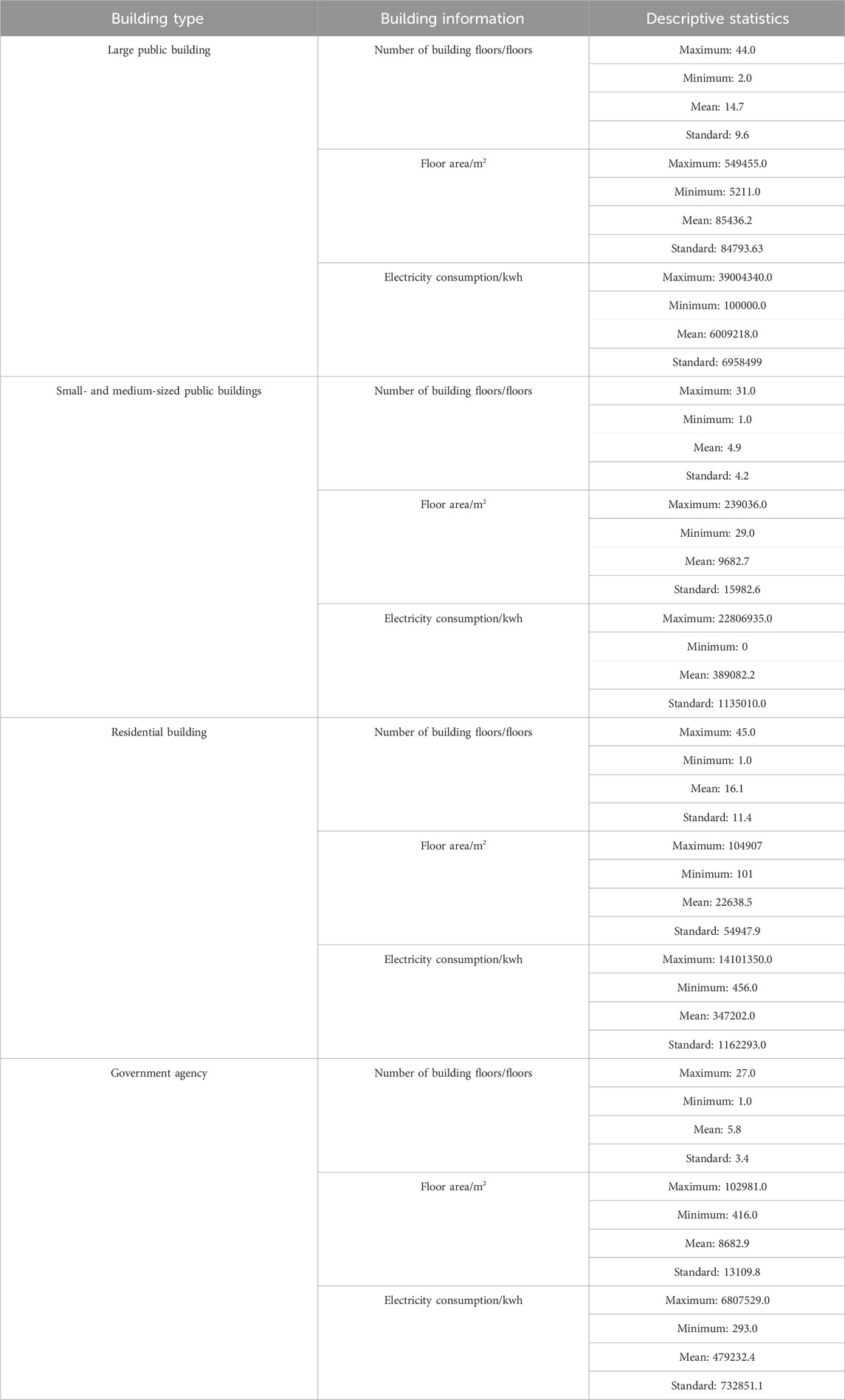
Table 1 . Statistical description of building features.
2.2 Calculating district-level building operational energy consumption carbon emissions from different sources
First, BECCE of four types of large buildings, small- and medium-sized buildings, state organ buildings, and residential buildings in each district of Chengdu are calculated according to the IPCC National Greenhouse Gas Inventory guidelines. Given that there is no central heating in Sichuan and the total carbon sink of building green space is small, the calculation formula for the carbon emissions of a specific building in Sichuan within a period of operation (not considering the full life cycle of the operation stage) is as follows:
where C is the operating carbon emissions of buildings in Chengdu within a period of time; C 1 is the direct carbon emissions during the operation of buildings in Chengdu in the current year, which are mainly caused by the direct consumption of fossil energy during the operation of buildings; and C 2 is the indirect carbon emissions during the operation of buildings in Chengdu in the current year, which are mainly caused by the use of electricity during the operation of buildings.
In China, BECCE are mainly produced from primary energy, heating power, and electric power sources ( Department of Energy Statistics, 2016 ). In this paper, five energy sources, including coal, natural gas, liquefied petroleum gas, artificial gas, and electricity, are selected to calculate BECCE in buildings based on the use of the energy structure system in Chengdu. The composition of BECCE can be expressed as follows:
where C T O T A L , i represents the BECCE of the i th type building in Chengdu. C C O A L , i , C N G , i , C L P G , i , C A G , i , and C E P , i represent, respectively, carbon dioxide emissions from coal, natural gas, liquefied petroleum gas, artificial gas, and electricity generated by i th type buildings in Chengdu.
The carbon emissions generated by energy use in buildings are calculated as follows:
where C j , i represents the carbon emissions generated by the use of the j th-type energy in the building operation stage of the i th-type building. N j is the consumption of energy in j th type, and F j is the carbon emission coefficient of j th-type energy. The specific carbon emission coefficient of each energy source is shown in Table 2 :
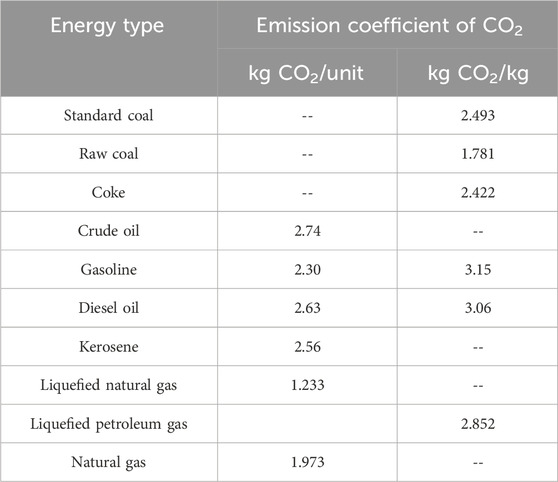
Table 2 . Carbon emission coefficient of fossil energy consumption.
The carbon emission coefficient of electric energy is related to the fossil energy used in local mainstream power generation. The carbon emission coefficient of electric energy obtained by different energy types in different regions is also different ( Table 3 ). For example, the carbon emissions of coal-fired power plants using fuel are much higher than those of hydroelectric power plants. According to different energy structures in different regions, the power carbon emission coefficient adopts the average carbon emission factor of the regional power grid published by the state in 2012 (which has not been updated since 2012), as shown in Table 2 ( Bai, 2019 ). The research area in this paper is Chengdu (Sichuan province), and 0.5257 is selected as the carbon emission factor of electric energy.
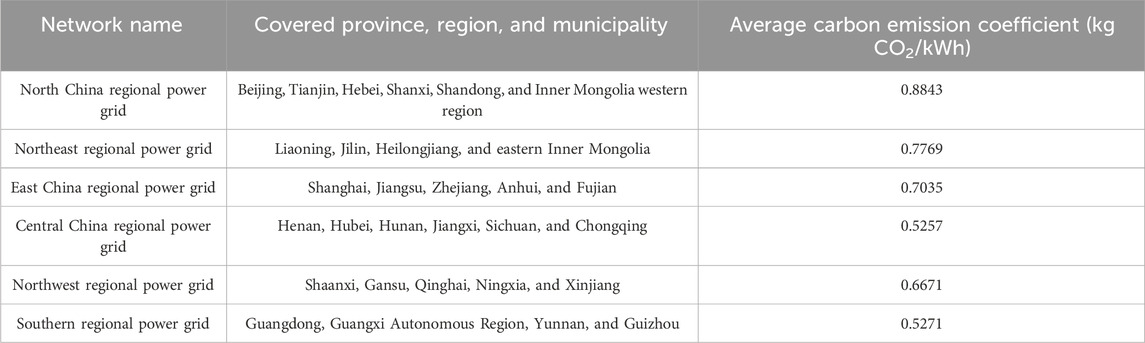
Table 3 . Average carbon emission coefficients of the regional power grid in China.
2.3 Selection of predictors for building operational energy consumption carbon emissions
The plane distribution of four different types of buildings in the city was drawn based on a total of 2,526 individual building samples collected in Chengdu, including 23 districts. Figure 3 presents the number of building types in the five major individual building sample collection areas. Jinniu District, as the main central city of Chengdu, has a relatively uniform distribution of large public buildings, small- and medium-sized public buildings, residential buildings, and government offices, thereby making the sample of all types of buildings in this paper more balanced. Compared with Jinniu District, the sampling balance of the other four districts was poor, especially for large public buildings. Given that the energy consumption data of large buildings in the operation stage of the surrounding urban areas were relatively difficult to calculate, there were only four large public buildings in Dujiangyan District and one in Qingbaijiang District.
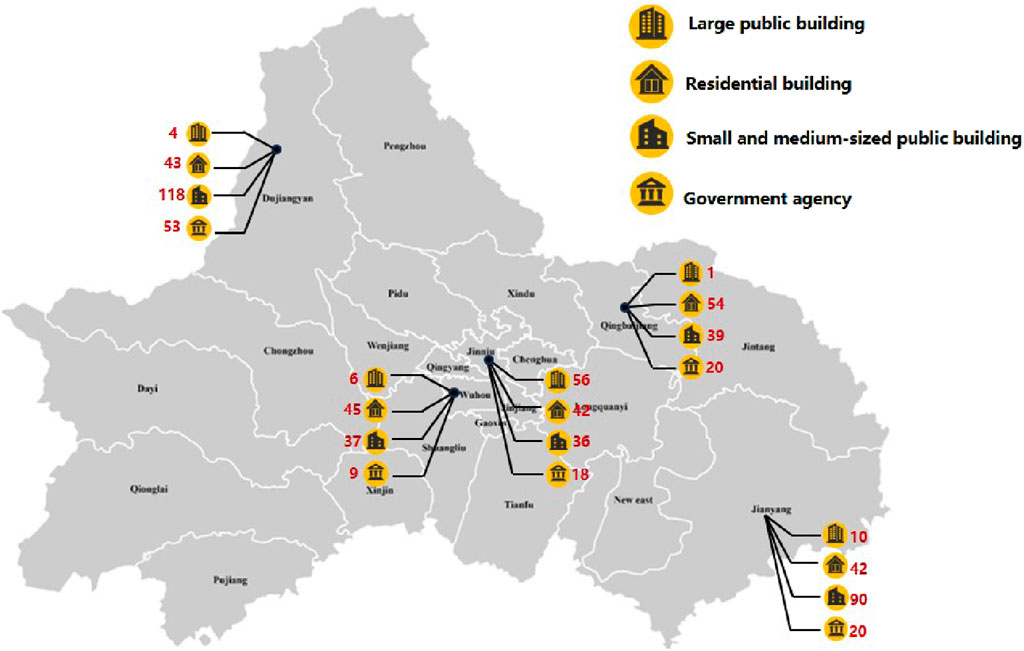
Figure 3 . Distribution of individual building samples in Chengdu.
Considering the actual distribution of the four types of buildings in Chengdu, a total of 2,526 samples of various types of buildings in 23 districts are collected in this paper, including 199 large buildings, 969 small- and medium-sized public buildings, 374 state agencies, and 984 residential buildings. Each sample includes the building name, address, administrative division, and the consumption of five kinds of energy in 2021 as well as the building area and the number of floors. The total electricity consumption data and carbon emissions of 2,526 buildings are shown in Figure 4 .
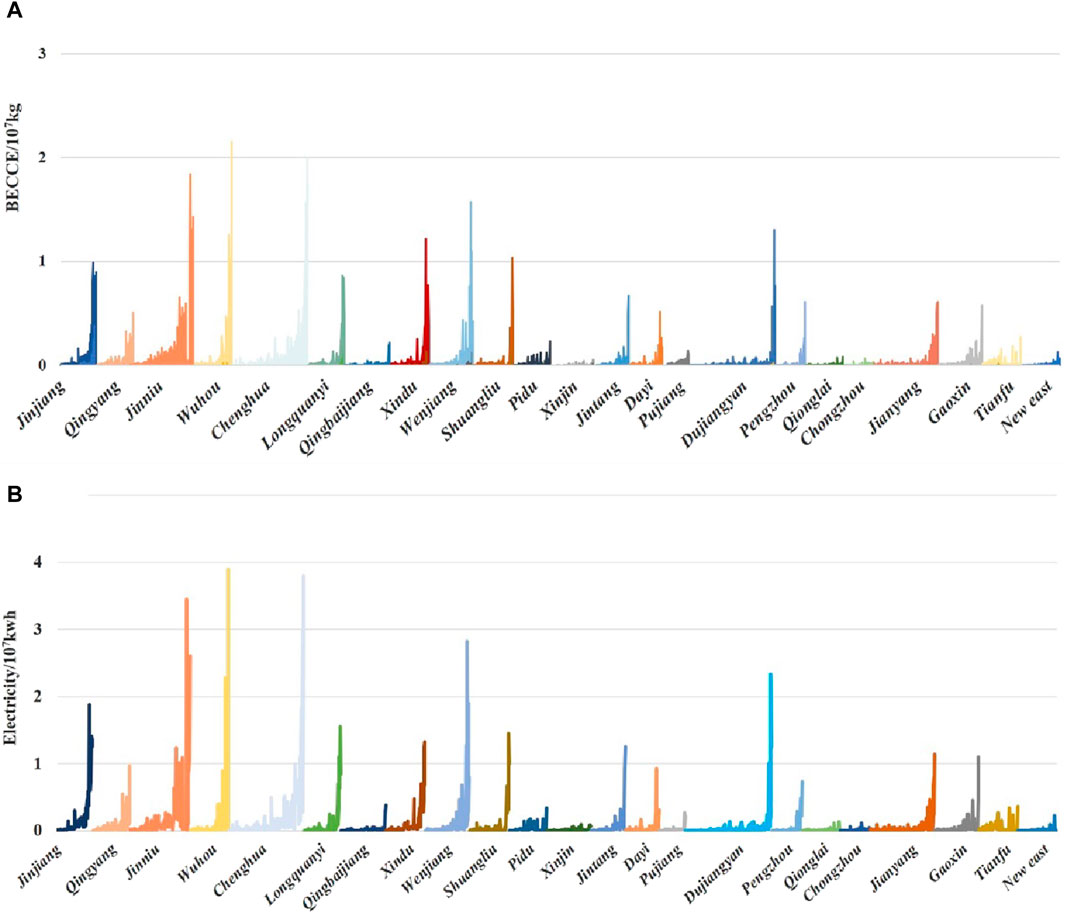
Figure 4 . BECCE and power data of buildings in 23 districts of Chengdu. (A) Building operational energy consumption carbon emissions; (B) Electricity consumption.
It can be seen from Figure 3 that the trends of carbon emissions and electricity consumption of each building are basically the same. It can be observed that there is a significant relationship between building electricity consumption and carbon emissions. Among them, Jinniu District, Wuhou District, Chenghua District, Wenjiang District and Dujiangyan generally exhibit high carbon emissions, and the corresponding electricity consumption is also relatively high. Then, according to the carbon emissions of the five energy sources, the carbon emission proportion data of each energy source of the four types of buildings are plotted using a stacked column chart, and Figure 5 is obtained.
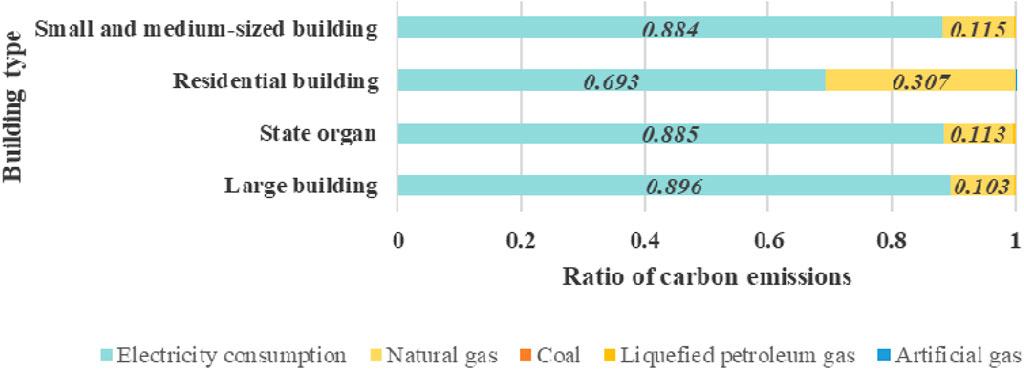
Figure 5 . Structure of energy carbon emissions of four types of buildings.
It can be seen from Figure 5 that in addition to the carbon emissions generated by electricity consumption in residential buildings accounting for 69.3%, the carbon emissions generated by electricity consumption in large buildings, small- and medium-sized buildings, and state agencies accounted for 89.6%, 88.4%, and 88.5%, respectively, which are significantly greater than 80%. Except for natural gas, the proportions of coal, liquefied petroleum gas, and artificial gas are relatively low for the other four energy sources. By analyzing the stacked histograms of the four types of building carbon emissions, it can be found that most of the BECCE in Chengdu come from CO 2 from electricity and natural gas use.
It can be seen from Figure 4 that the degree of electrification of various buildings in Chengdu is relatively high during the operation phase, but the carbon emissions of other energy sources, such as natural gas, still occupy a certain proportion. It is time-consuming and labor-intensive to conduct real-time and accurate statistics on consumption, so the carbon emissions of buildings cannot be quickly calculated. In order to achieve fast and accurate calculation of BECCE of different types of buildings, according to Figure 3 , it can be seen that there is a significant correlation between electricity consumption and BECCE. This paper is based on the power data of Chengdu’s building operation phase, taking into account the different building shapes of different building types, extracting building power data, effective area, and building floors, and a total of three indicators as characteristic variables. The BECCE of the calculation model of different building types based on machine learning is constructed, the complex mapping relationship between building power consumption, building area, building floors, and BECCE during this period is obtained, and the fast and accurate calculation of BECCE through power data is realized.
3.1 Support vector machine
The PSO–SVM model has been widely used in the field of carbon emission prediction due to its excellent fitting ability ( Li, 2020 ; AlKheder and Almusalam, 2022 ), such as in sewage treatment plants ( Szeląg et al., 2023 ), building carbon emissions ( Mao et al., 2019 ; Gao et al., 2023 ), transportation industry ( Li et al., 2022 ), and other related CO 2 emissions, and has achieved a relatively significant effect.
SVM is a novel small-sample learning method. The specific steps are as follows:
Assuming that the training set consists of N data samples, the regression model associated with SVM can be described as follows:
where ψ x is the mapping function and ω and b are the weight and deviation, respectively. The calculation of the SVM model can be obtained using the insensitive loss function ε to solve the following convex optimization problems:
where c represents the positive parameter used to control the empirical error and ξ i and ξ i * represent the relaxation variables used to control the training error. x i and y i represent input and output variables, respectively. N represents the number of samples.
3.2 Particle swarm optimization algorithm–support vector machine
Given the impact of the optimal penalty parameter c , kernel function parameter g , and kernel function ∂ on regression results, the particle swarm optimization algorithm ( Kennedy and Eberhart, 1995 ) was used to optimize SVM. As a random search method, PSO can realize individual optimization in space. The position and velocity of the ith particle are expressed using Eqs 6 , 7 , respectively.
where ω , c 1 , and c 2 , respectively, represent inertia weight, acceleration factor 1, and acceleration factor 2. r 1 and r 2 are random numbers distributed in [0,1]. k is the number of current iterations; V i is the velocity of the ith particle; P i k is the global optimal position at the kth update; and g k is the global optimal position at the kth update. The specific steps of the “electricity-carbon " model are shown in Figure 6 . PSO parameters are shown in Table 4 .
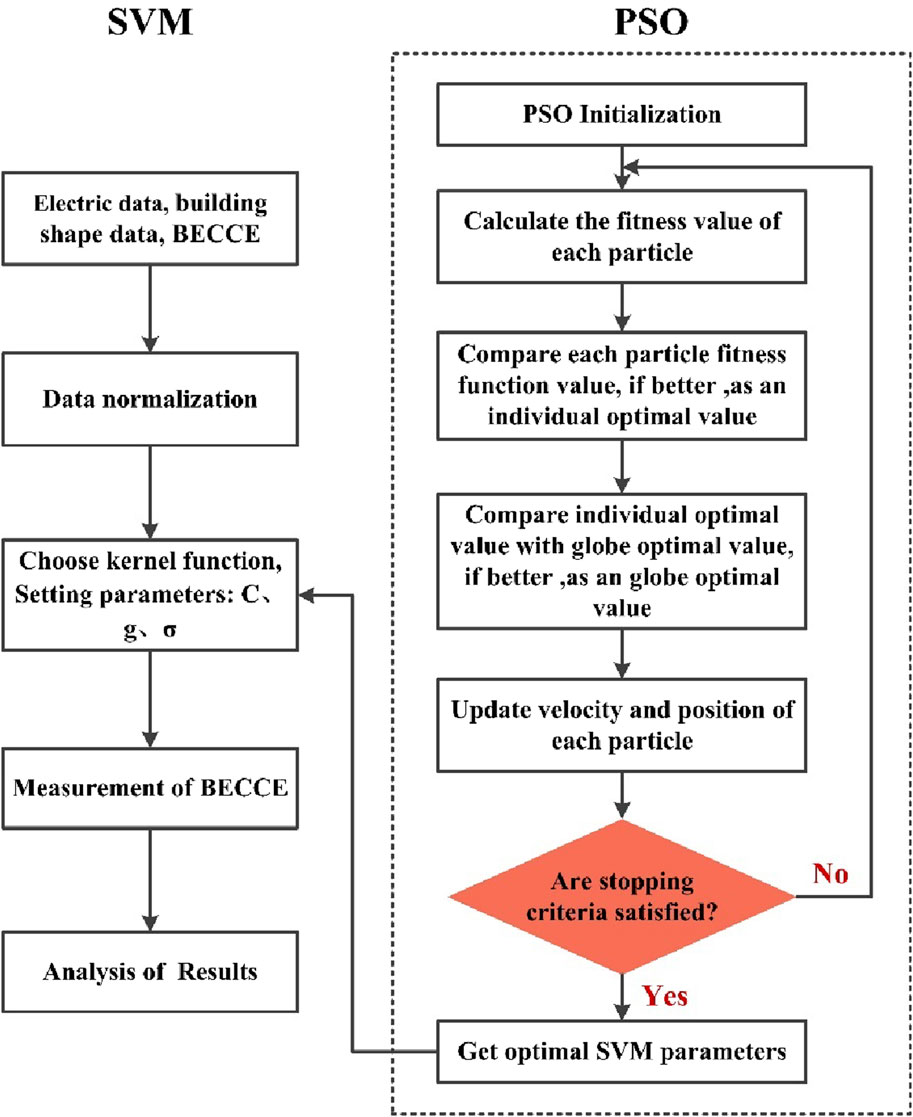
Figure 6 . Measurement model of BECCE based on PSO–SVM.
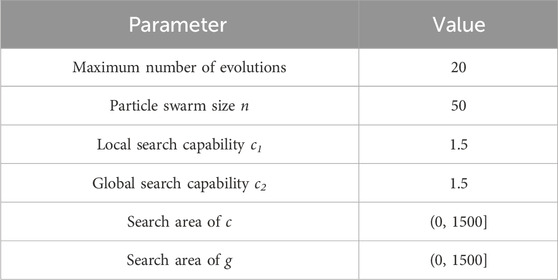
Table 4 . PSO parameter settings.
3.3 Evaluation of model performance
Evaluation of model performance is also very important as part of the test of model usability. When judging the degree of model prediction, at least one statistical error indicator and one goodness-of-fit measure should be applied ( Nourani et al., 2019 ). In this study, MAE, RMSE, and R 2 were thus used to evaluate the model performance. Among them, MAE can quantify the difference between the expected and observed values, especially ignoring the negative effect. The error of the RMSE measurement model was analyzed in quantitative prediction; R 2 quantified the correlation or collinearity between the expected and actual values. MSE is used as the fitness function of PSO. The specific calculation formula is shown in Table 5 .
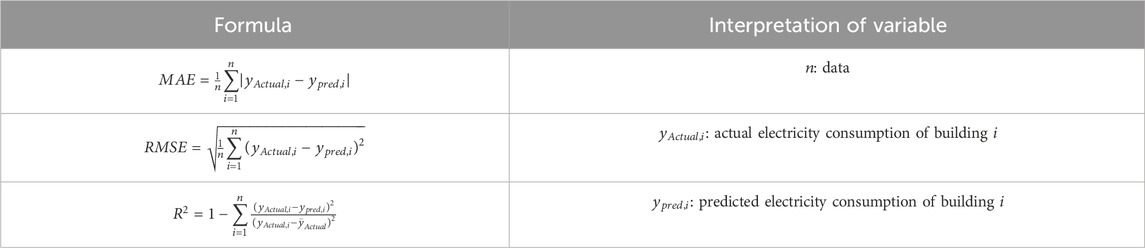
Table 5 . Specific model evaluation.
4.1 Building volume characteristics of different types of buildings
Due to differences in building types, building shapes (building floor and building area) are inevitably different, which will naturally lead to differences in carbon emissions. In order to improve the accuracy of measurement of building carbon emissions, a more targeted measurement of building carbon emissions is carried out, and the relationship between building shapes and total emissions of four types of buildings is studied. The quadrilateral method is adopted to conduct statistical analysis on the boxplot of building floors, building areas, and carbon emissions of large buildings, government agencies, residential buildings, and small- and medium-sized buildings, as shown in Figure 7 .
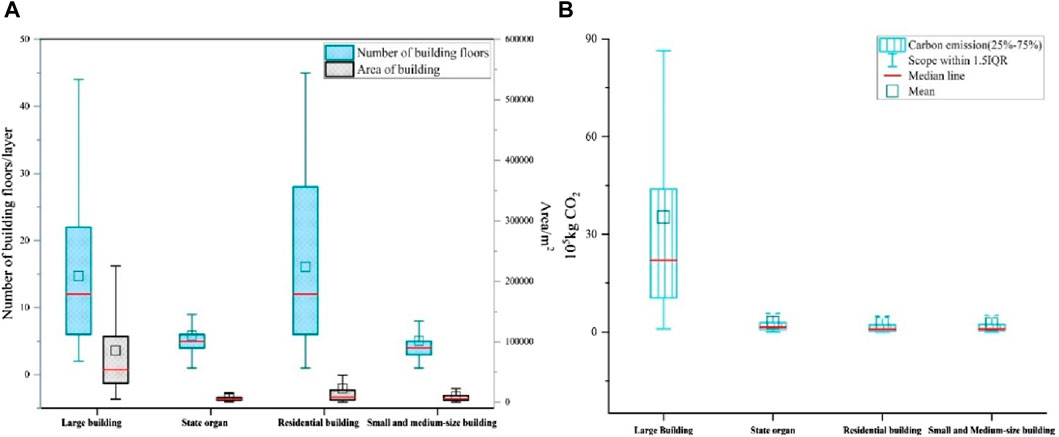
Figure 7 . Analysis of the shape and carbon emission characteristics of four types of buildings: (A) boxplot of architectural forms of four kinds of buildings and (B) boxplot of BECCE for four types of buildings.
As shown in Figure 7A , for building floors, the maximum number of floors in large and residential buildings is basically the same. Meanwhile, according to the positions of the lower quartile and median line as well as the upper quartile, large and residential buildings are concentrated on the 6–11th floor, but the floor distribution of residential buildings is more discrete, while the distribution of government agencies and small- and medium-sized buildings is concentrated. For the building area, the large building area is significantly larger than the three types of buildings, mainly distributed between 31258 m 2 and 108927 m 2 . For the other three types of buildings, the residential building area is relatively large. In Figure 7B , it can be clearly seen that the carbon emissions of large buildings are significantly higher than those of the other three types of buildings, while there is no significant difference in the carbon emissions of government agencies, residential buildings, and small- and medium-sized buildings.
Compared with Figures 7A, B , the number of floors and floor area of large buildings, government agencies, and small- and medium-sized buildings can clearly reflect the change in carbon emissions of these three types of buildings. For residential buildings, there is no significant relationship between the building floor and carbon emissions. At the same time, it can be further seen that government agencies and small- and medium-sized buildings have similar architectural shapes, and their structure of energy carbon emissions is basically the same as shown in Figure 5 . In this paper, government agencies are included in small- and medium-sized buildings for research.
In order to avoid the generality and inaccuracy of using the same model to predict the carbon emissions of buildings, this paper establishes different “electricity-carbon” models for large and residential buildings. For government agencies and small- and medium-sized buildings, the same “electricity-carbon” model can be established.
4.2 Measurement of building operational energy consumption carbon emissions of different types of buildings based on particle swarm optimization algorithm–support vector machine
Before the BECCE calculation model is established, there are big differences in the digital scale among the three parameters of building floor, building area, and electricity consumption. Therefore, this paper first carries out normalization processing on the dataset to eliminate the impact of dimension and avoid the problem of low accuracy in model construction.
4.2.1 Optimization process
In order to ensure the overall effect of the model, the PSO algorithm is used to find the optimal values of three hyperparameters, such as kernel functions, g and c. In the stage of model training, five-fold cross-validation is used to train and verify the model. A total of 80% of the data in the dataset is used as the model training hyperparameter, and the remaining 20% is used as the verification set to verify the model performance. Finally, the PSO algorithm selects the optimal hyperparameter set according to the effect of the verification set. The dataset partitioning process of the five-fold cross-validation is shown in Figure 8 .
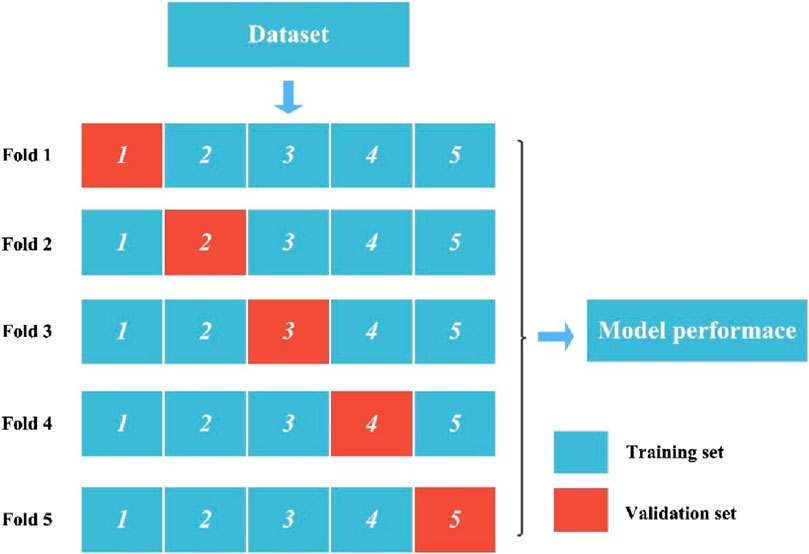
Figure 8 . Data division process of five-fold cross-validation.
In the process of PSO hyperparameter optimization, this paper takes the MSE of the verification set as the standard to save the optimal model. The hyperparameter optimization process of the “electricity-carbon” model of three types of buildings, namely, large buildings, residential buildings, and small- and medium-sized buildings, is shown in Figure 9 . In the calculation model of large buildings, when the evolution reaches the 14th generation, the fitness function decreases to the minimum, and the model reaches the best state. The combination of hyperparameters of kernel functions, c and g is (linear kernel function, 690.0, 803.2). In the calculation model of small- and medium-sized buildings, the combination of hyperparameters is (linear kernel function, 715.6, 910.6) when the evolution reaches the 13th generation. In the calculation model of residential buildings, the combination of hyperparameters is (polynomial kernel function, 402.4, 588.3) when it evolves to 12 generations.
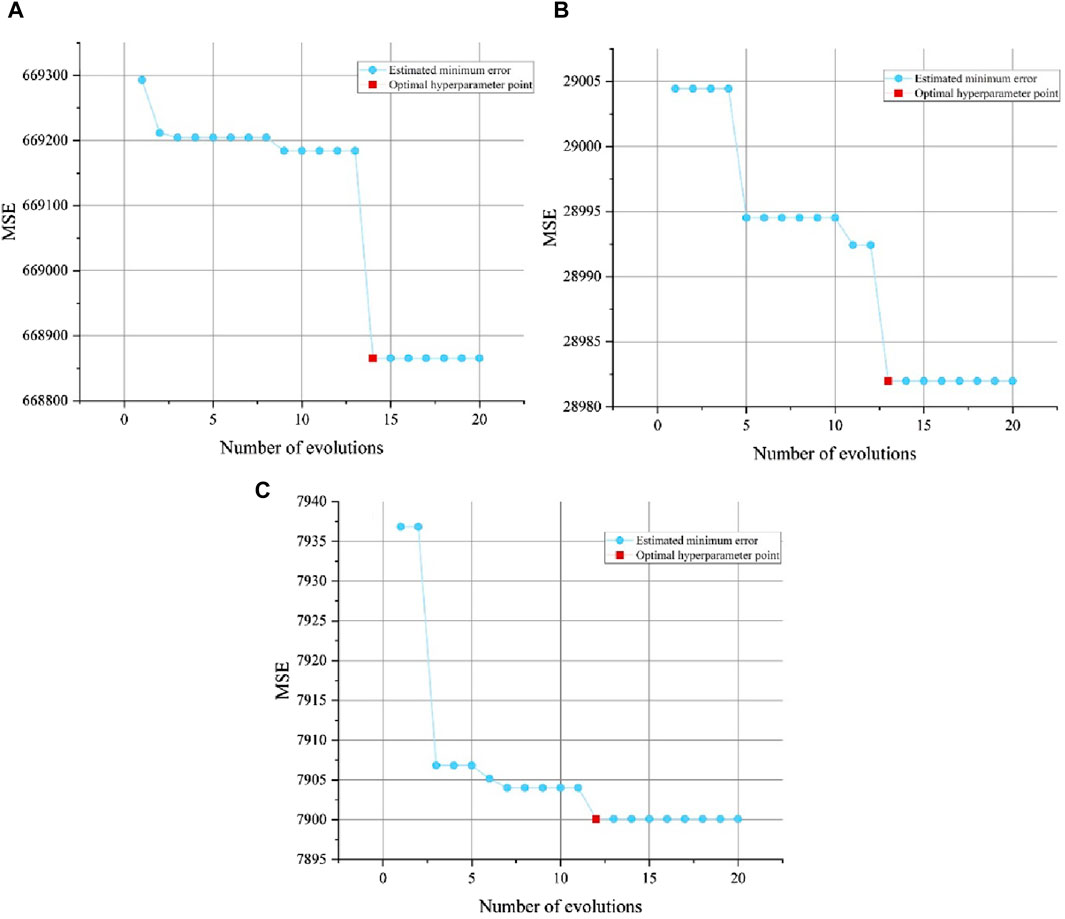
Figure 9 . Iterative process of optimal parameters of the model: (A) large buildings; (B) government agencies and small- and medium-sized buildings; and (C) residential buildings.
4.2.2 Performance comparison of support vector regression and particle swarm optimization algorithm–support vector machine models
According to the optimal “electricity-carbon” model of large buildings, small- and medium-sized buildings, and residential buildings, the fitting between the predicted results of their respective verification sets and the actual observed values is visualized, as shown in Figure 10 . Then, according to MAE, MSE, R 2 , and the other three model evaluation indexes, PSO–SVM is compared with support vector regression (SVR) without parameter optimization to verify the reliability of the model.
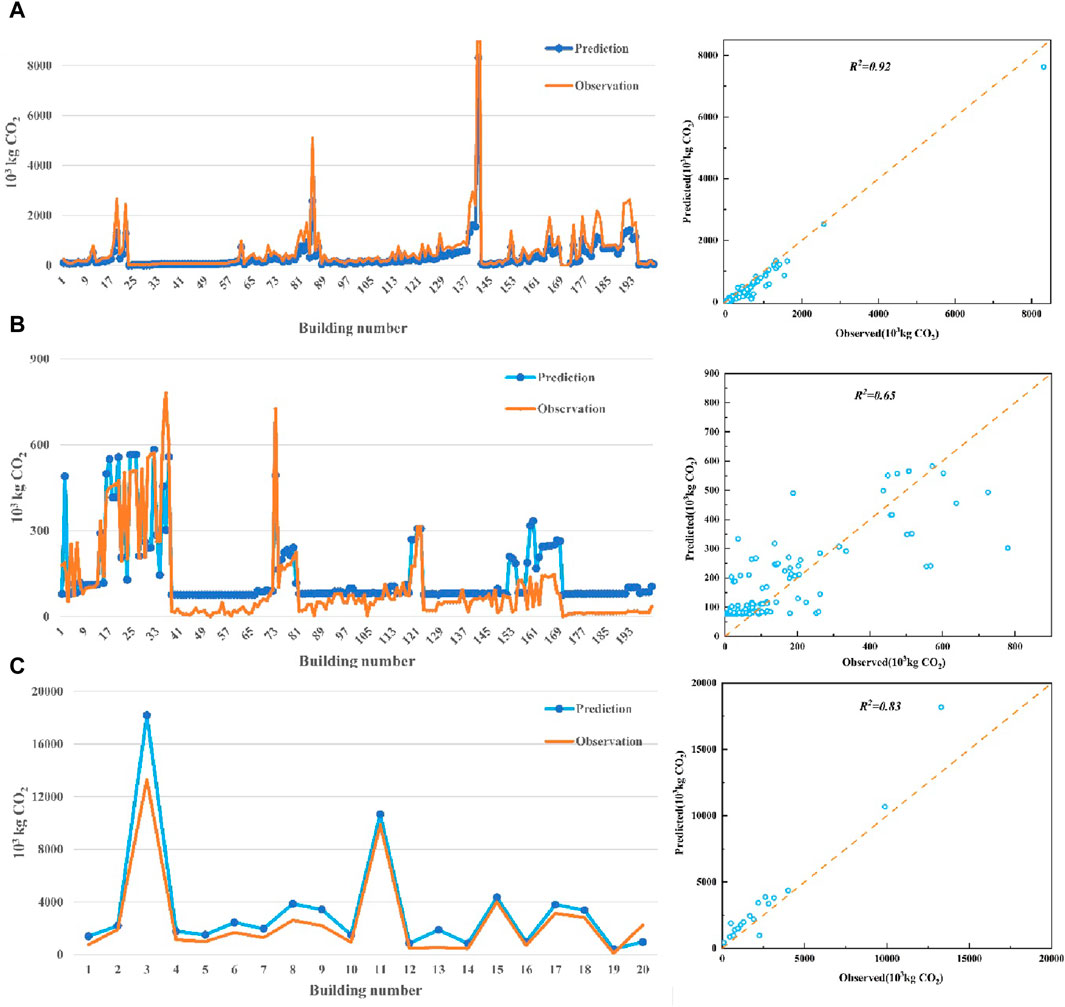
Figure 10 . Verification results of the “electricity-carbon” model for four types of buildings: (A) government agencies and small- and medium-sized buildings; (B) residential buildings; and (C) large buildings.
Figure 10 shows that the “electricity-carbon” model has a good effect on the BECCE measurement of small- and medium-sized buildings. The measured value of BECCE is basically consistent with the actual observed value, and R 2 = 0.92 also indicates that there is a significant correlation between the predicted and actual observed data. The model also has a good prediction effect on large buildings, and the trend of the line graph between the predicted and actual observed values is basically the same. The prediction effect of the model for residential buildings is relatively poor, R 2 = 0.65. It can be seen from Figure 5 ; Figure 7 that the actual situation of residential buildings is relatively complex, and it is difficult to make a good prediction. However, it can be seen from Figure 8 that the measured values of the “electricity-carbon” model are roughly consistent with the trend of the actual observed values. The evaluation results of the performance indexes of the PSO–SVM and SVR models are shown in Table 6 .
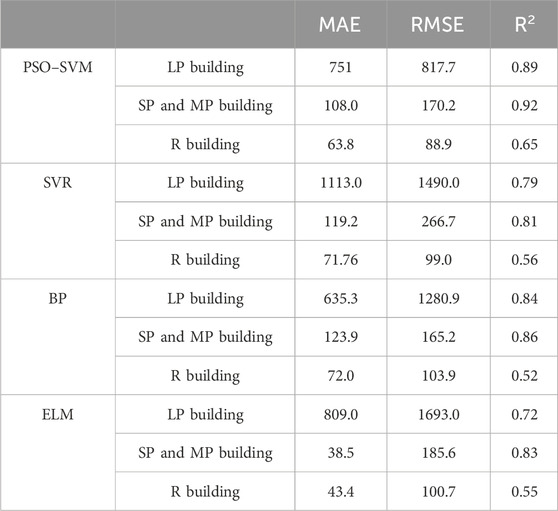
Table 6 . Model performance evaluation results.
In this paper, the performance of the PSO–SVM model was compared with that of SVR, BP, and ELM, and the index evaluation results of the four models are shown in Table 6 . Table 6 demonstrates PSO optimization as an essential part of model performance improvement. After PSO optimization, two indicators, MAE and RMSE, were significantly reduced compared to their values before optimization, and R 2 of each type of building was increased by 0.1 on average. This indicated a significant improvement in the correlation between the measured BECCE and actual values. At the same time, compared with BP and ELM, the PSO–SVM model was found to have the advantages of small-sample learning, thereby being furnished with better fitting ability and thus being more suitable for constructing the “electricity-carbon” model.
4.3 Rapid measurement of building operational energy consumption carbon emissions with different types of buildings
According to the optimal hyperparameter of the three models determined in Section 4.2.1 , the “electricity-carbon” model of large buildings, small- and medium-sized buildings, and residential buildings is constructed, respectively. By comparing the SVR model without optimization, PSO–SVM shows good model performance, but the model generalization ability is still difficult to determine. In order to test whether the “electricity-carbon” model can calculate BECCE quickly and accurately, this paper re-extracts the data of various types of buildings, including 21 large buildings, 100 small- and medium-sized buildings, and 100 residential buildings. The above data are input into the constructed “electricity-carbon” model to measure BECCE, and the measured and actual values are compared, as shown in Figure 11 .
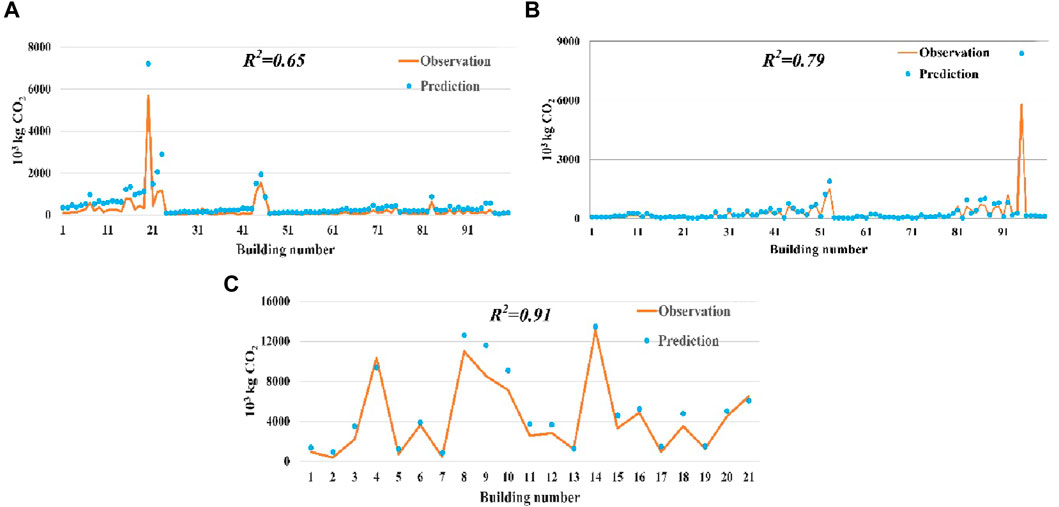
Figure 11 . Measurement results of BECCE for four types of buildings: (A) government agencies and small- and medium-sized buildings; (B) residential buildings; and (C) large buildings.
Figure 11 clearly shows the distribution area between the measured and actual values, which can help judge whether the measured result is close to the actual result. According to Figures 11A-C , the distribution area between the measured and actual values is basically consistent. Second, the R 2 values of the estimated and real values of small- and medium-sized buildings, residential buildings, and large buildings are 0.65, 0.79, and 0.91, respectively, indicating that the “electricity-carbon” model has good generalization ability.
As can be seen from Table 7 , according to MAE and RMSE evaluation results and the analysis of Figure 10 , the error of calculation results is also within an acceptable range, so it is feasible.
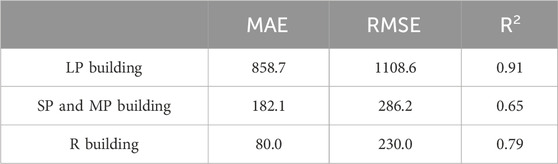
Table 7 . Evaluation results of generalization ability.
4.4 Spatial distribution of building operational energy consumption carbon emissions
The calculation results of the “electricity-carbon” model are imported into ArcGIS software to generate the BECCE spatial distribution map, as shown in Figure 12 . The central area of Chengdu (Pidu District, Wenjiang District, Chenghua District, Jinniu District, and Wuhou District) has a high carbon emission of buildings, while the surrounding areas have relatively low carbon emissions. Many large shopping malls, office buildings, and other large buildings are located in downtown Chengdu, which may result in BECCE being relatively concentrated in the central part of Chengdu, indicating that the “electricity-carbon” model has a certain reliability in the rapid measurement of BECCE results.
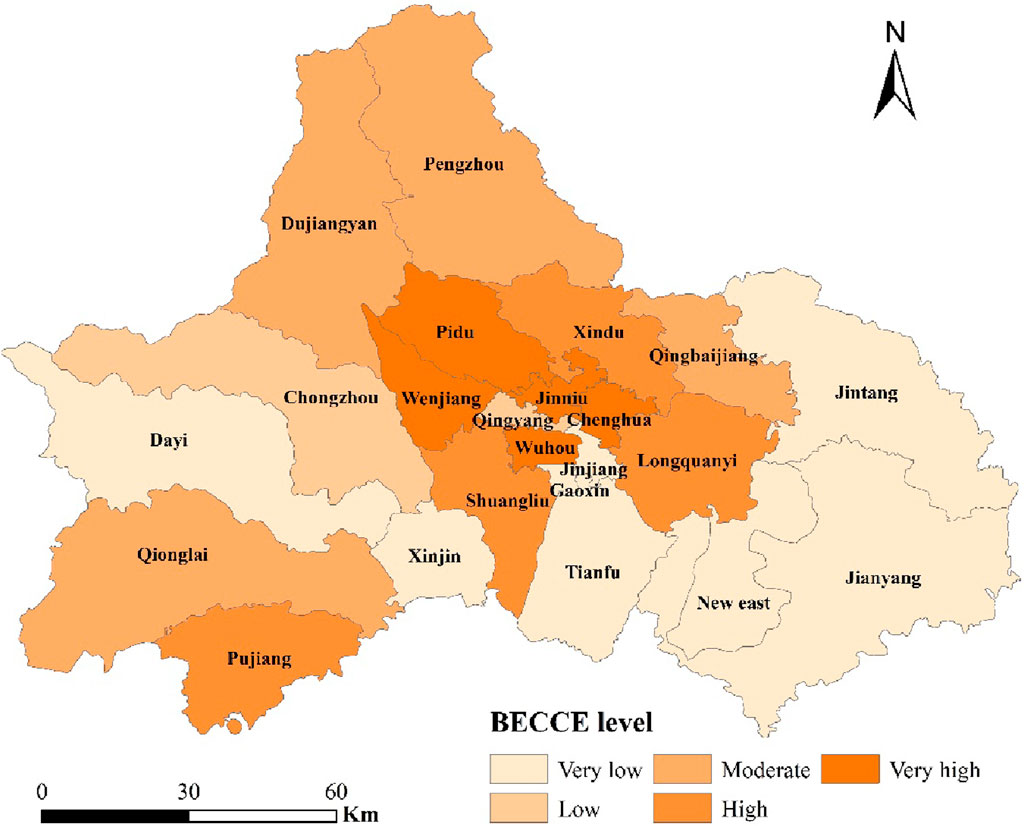
Figure 12 . Spatial distribution map of BECCE in Chengdu.
5 Discussion
The rapid development of urbanization in China is accompanied by massive energy consumption, leading to massive emissions of greenhouse gases, among which BECCE accounts for 70% of the carbon emissions in the whole life cycle of buildings ( Fenner et al., 2018 ), which is very important for global warming and sustainable urban development. BECCE’s accurate calculation as the basis of building energy conservation and emission reduction has attracted more and more attention. In 2013, the State Council issued ten measures in the Action Plan for the Prevention and Control of Air Pollution, and the energy consumption structure in the building operation stage gradually became the key to the control of air pollution in China ( Li, 2016 ; Hu et al., 2022 ). To this end, a series of “coal to gas” and “coal to electricity” policies implemented in China have contributed to the reduction in air pollutants from building operations ( Chen and Chen, 2019 ). Against such a favorable background, the concept of the “electricity-carbon” model is proposed in this paper. This model can measure the carbon emissions of individual buildings quickly and efficiently through electricity data. Taking 2,526 buildings in Chengdu as the research object, the BECCE calculation model was constructed for large public buildings, small- and medium-sized public buildings, government agencies, and residential buildings, respectively, and satisfactory results were obtained. However, in the research process, it was found that the calculation results of the “electron-carbon” model for three types of buildings, including large public buildings, small- and medium-sized public buildings, and government agencies, presented little difference from the actual results, while the BECCE calculation results for residential buildings were relatively poor. The energy carbon emission structure of various buildings ( Figure 3 ) revealed that the energy carbon emission structure of residential buildings during the operation was relatively complex, in which carbon emissions generated by electricity accounted for only 69.3% and carbon emissions generated by natural gas were as high as 30.7%, which might have a certain impact on the performance of the “electricity-carbon” model ( Huang et al., 2024 ). However, the “14th Five-Year Plan” Building Energy Conservation and Green Building Development Plan was issued, which could further promote the implementation of building energy replacement action, improve the degree of building electrification, and monitor the carbon emissions of single buildings through power data to be more effective. This could also improve the problem of poor measurement accuracy in the “electricity-carbon” model for single buildings. It also demonstrated the good popularization prospects of the “electricity-carbon” model.
In the research process, the carbon emission factors used in this paper had limitations in time, causing certain differences in the carbon emission measurement results. Therefore, further attempts will be made to extract the latest updated carbon emission factor database of relevant departments to improve the universality of the application of the “electricity-carbon” model. At the same time, there were relatively few architectural form features extracted in this paper, and the depiction of single buildings was relatively poor, which exerted a certain impact on the final calculation results. In order to further improve the accuracy of the “electricity-carbon” model, more abundant architectural form indicators will be extracted in the future. Finally, the “electricity-carbon” model proposed in this paper is more suitable for buildings with a high degree of electrification. However, with the gradual improvement of future electrification projects and the construction of new building power systems, the “electricity-carbon” model holds better application space in the future.
6 Conclusion
The rapid development of urbanization in China has further promoted a large amount of energy consumption in the building industry, resulting in a large amount of greenhouse gases, among which carbon emissions in the building operation stage account for the main proportion. Accurate measurement of carbon emissions in the operation stage of a single building has a crucial impact on the green and sustainable development of a city. In this paper, based on the research of 2,526 buildings in Chengdu, including large buildings, small- and medium-sized public buildings, government institutions, and residential buildings, this paper proposes a BECCE rapid measurement model (“electricity-carbon “model) for single buildings based on electricity consumption and building physical characteristics and draws specific conclusions.
According to the statistical comparison between the electricity consumption of 2,526 buildings of various types in Chengdu and their BECCE, there is a significant relationship between the electricity consumption of buildings and their BECCE. Combined with the carbon emission structure of various buildings, the electric-carbon emission ratio of public buildings, small- and medium-sized buildings, government agencies, and residential buildings is 0.90, 0.88, 0.89, and 0.69, respectively. Except for residential buildings, the carbon emission structure of other buildings is basically the same.
Combined with the building shapes and carbon emission structure analysis of all kinds of buildings, the characteristics of government agencies and small- and medium-sized buildings are similar in all aspects, so the two types of buildings can be studied as a whole. In this paper, using building electricity consumption, building floor, and building area as the index system, combined with the PSO–SVM algorithm, the “electricity-carbon” model for large buildings, government agencies, small- and medium-sized buildings, and residential buildings was put forward and compared with the unoptimized SVR model. MAE (751, 108.0, and 63.8), RMSE (817.7, 170.2, and 88.9), and R2 (0.89, 0.92, and 0.65) of the PSO–SVM model were significantly better than those of SVR (1113, 119.2, 71.76), RMSE (1490, 266.7, 99.0), and R2 (0.79, 0.81, 0.56), indicating that PSO–SVM is more reliable and more accurate. To verify the generalization ability of the model, data on various types of buildings were collected: 21 large buildings, 100 small- and medium-sized buildings, and 100 residential buildings, and the data were input into the “electricity-carbon” model. The R2 values between the estimated and actual values of large buildings, small- and medium-sized buildings, and residential buildings are 0.91, 0.65, and 0.79, respectively.
All the above conclusions show that the “electricity-carbon” model proposed in this paper has certain validity. However, the “electricity-carbon” model proposed in this paper is relatively poor in the calculation of residential buildings, indicating that the model is more suitable for buildings with a high degree of electrification. With the gradual improvement in future electrification projects, the “electricity-carbon” model has a large application space in the future and good prospects for popularization.
Data availability statement
The raw data supporting the conclusion of this article will be made available by the authors, without undue reservation.
Author contributions
YW: conceptualization, data curation, and writing–original draft. ZC: conceptualization, investigation, and writing–original draft. PH: data curation, methodology, and writing–original draft. HL: formal analysis, methodology, and writing–original draft. FL: conceptualization, project administration, and writing–original draft. YC: formal analysis, project administration, and writing–original draft.
The author(s) declare that financial support was received for the research, authorship, and/or publication of this article. This work was sponsored by the Sponsored by Natural Science Foundation of Sichuan, China (2023NSFSC1987).
Conflict of interest
Authors ZC and FL was employed by State Grid Sichuan Electric Power Company.
The remaining authors declare that the research was conducted in the absence of any commercial or financial relationships that could be construed as a potential conflict of interest.
Publisher’s note
All claims expressed in this article are solely those of the authors and do not necessarily represent those of their affiliated organizations, or those of the publisher, the editors, and the reviewers. Any product that may be evaluated in this article, or claim that may be made by its manufacturer, is not guaranteed or endorsed by the publisher.
Ahmed, A. K., Ahmad, M. I., and Yusup, Y. (2020). Issues. Impacts, and mitigations of carbon dioxide emissions in the building sector. Sustainability 12 (18), 7427. doi:10.3390/su12187427
CrossRef Full Text | Google Scholar
AlKheder, S., and Almusalam, A. (2022). Forecasting of carbon dioxide emissions from power plants in Kuwait using United States Environmental Protection Agency, Intergovernmental panel on climate change, and machine learning methods. Renew. Energy Int. J. 191, 819–827. doi:10.1016/j.renene.2022.04.023
Bai, L. H. (2019). Research on the whole life cycle carbon emission prediction model of public buildings . Doctoral dissertation. Tianjin University . (In Chinese). doi:10.27356/d.cnki.gtjdu.2019.004755
Chen, H., and Chen, W. Y. (2019). Potential impact of shifting coal to gas and electricity for building sectors in 28 major northern cities of China. Appl. Energy. 236, 1049–1061. doi:10.1016/j.apenergy.2018.12.051
Cuong-Le, T., Nghia-Nguyen, T., Khatir, S., Trong-Nguyen, P., Mirjalili, S., and Nguyen, K. D. (2021). An efficient approach for damage identification based on improved machine learning using pso-svm. Eng. Comput. 38, 3069–3084. doi:10.1007/s00366-021-01299-6
Department of Energy Statistics (2016). National bureau of statistics, people’s Republic of China. China energy statistical Yearbook 2016 . Beijing, China: China Statistics Press . (stats.gov.cn).
Google Scholar
Eggleston, H., Buendia, L., Miwa, K., Ngara, T., and Tanabe, K. (2006). 2006 IPCC Guidelines for national Greenhouse gas inventories ; IPCC national greenhouse gas inventories , 1–20. Programme: Washington, DC, USA.
Energy Information Administration (2020). GlobalABC roadmap for buildings and construction 2020–2050 . Washington, DC, USA: Federal Ministry of Economic Affairs and Energy .
Eseye, A. T., Zhang, J., and Zheng, D. (2017). Short-term photovoltaic solar power forecasting using a hybrid wavelet-pso-svm model based on scada and meteorological information. Renew. Energy 118 (APR), 357–367. doi:10.1016/j.renene.2017.11.011
Fenner, A. E., Kibert, C. J., Woo, J., Morque, S., Razkenari, H., Hakim, M., et al. (2018). The carbon footprint of buildings: A review of methodologies and applications. Renewable and Sustainable Energy Reviews 94, 1142–1152.
Gao, H., Wang, X. K., Wu, K., Zheng, Y. R., Wang, Q. Z., Shi, W., et al. (2023). A review of building carbon emission accounting and prediction models. Buildings 13, 1617. doi:10.3390/buildings13071617
Han, L. Y. (2022). Carbon emission Measurement based on high-frequency power data: a case study of Qinghai Province. Technol. Mark. 29, 41–43. (In Chinese). doi:10.3969/j.issn.1006-8554.2022.09.012
Hu, S., Zhang, Y., Yang, Z. Y., Yan, D., and Jiang, Y. (2022). Challenges and opportunities for carbon neutrality in China's building sector-Modelling and data. Build. Simulat. 15, 1899–1921. doi:10.1007/s12273-022-0912-1
Huang, R. G., Zhang, X. C., and Liu, K. H. (2024). Assessment of operational carbon emissions for residential buildings comparing different machine learning approaches: a study of 34 cities in China. Build. Environ. 250, 111176. doi:10.1016/j.buildenv.2024.111176
Huang, Y. M., Luo, J. H., Ma, Z. G., Bing, T., Keqi, Z., and Jianyong, Z. (2023). On combined PSO-SVM models in fault prediction of relay protection equipment. Circuits Syst. Signal Process 42, 875–891. doi:10.1007/s00034-022-02056-w
IPCC (2014). “Climate change 2014 mitigation of climate change (fifth report),” in AR5 climate change ( Mitigation of Climate Change — IPCC ). Available at: https://www.ipcc.ch/report/ar5/wg3/
Kennedy, J., and Eberhart, R. (1995). . “Particle swarm optimization,” in Proceeding of ICNN’95-international Conference on Neural Networks IEEE , 1942–1948.
Li, J. (2016). Energy performance heterogeneity in China's buildings sector: a data-driven investigation. Renew. Sustain. Energy Rev. 58, 1587–1600. doi:10.1016/j.rser.2015.12.326
Li, X. D., Ren, A., and Li, Q. (2022). Exploring patterns of transportation-related CO2 emissions using machine learning methods. Sustainability 14, 4588. doi:10.3390/su14084588
Li, Y. (2020). Forecasting Chinese carbon emissions based on a novel time series prediction method. Energy Sci. Eng. 8 (7), 2274–2285. doi:10.1002/ese3.662
Liang, Y., Mao, S., Zheng, M., Li, Q., Li, X., Li, J., et al. (2023). Study on the prediction of low-index coal and gas outburst based on PSO-SVM. Energies 16 (16), 5990. doi:10.3390/en16165990
Liu, Q. B., Li, S., Yin, J. S., Li, T. T., and Han, M. L. (2023). Simulation of mechanical behavior of carbonate gravel with hybrid PSO–SVR algorithm. Mar. Georesources Geotechnol. 41 (4), 446–459. doi:10.1080/1064119x.2022.2057261
Liu, Z., Ciais, P., Deng, Z., Lei, R., Davis, S. J., Feng, S., et al. (2020). Author Correction: near-real-time monitoring of global CO2 emissions reveals the effects of the COVID-19 pandemic. Nat. Commun. 11, 6292–6312. doi:10.1038/s41467-020-20254-5
PubMed Abstract | CrossRef Full Text | Google Scholar
Mallapaty, S. (2020). How China could be carbon neutral by mid-century. Nature 586, 482–483. doi:10.1038/d41586-020-02927-9
Mao, X. K., Wang, L. X., Li, J. W., Quan, X. L., and Wu, T. Y. (2019). Comparison of regression models for estimation of carbon emissions during building’s lifecycle using designing factors: a case study of residential buildings in Tianjin, China. Energy and Build. 204, 109519. N.PAG. doi:10.1016/j.enbuild.2019.109519
Roeck, M., Saade, M. R. M., Balouktsi, M., Rasmussen, F. N., Birgisdottir, H., Frischknecht, R., et al. (2020). Embodied ghg emissions of buildings - the hidden challenge for effective climate change mitigation. Appl. Energy 258 (Jan.15), 1–12. doi:10.1016/j.apenergy.2019.114107
Michael, A. M. N., Wei, F., Stephane de la Rue du, C., Nina, Z. K., Jing, K., and Nan, Z. (2016). Energy efficiency outlook in China’s urban buildings sector through 2030. Energy Policy 97, 532–539. doi:10.1016/j.enpol.2016.07.033
Moraliyage, H., Dahanayake, S., De Silva, D., Mills, N., Rathnayaka, P., Nguyen, S., et al. (2022). A robust artificial intelligence approach with explainability for measurement and verification of energy efficient infrastructure for net zero carbon emissions. Sensors 22 (23), 9503. doi:10.3390/s22239503
Nourani, V., Elkiran, G., and Abdullahi, J. (2019). Multi-station artificial intelligence based ensemble modeling of reference evapotranspiration using pan evaporation measurements. J. Hydrol. 577, 123958. doi:10.1016/j.jhydrol.2019.123958
Oleson, K. W., Monaghan, A., Wilhelmi, O., Barlage, M., Brunsell, N., Feddema, J., et al. (2013). Interactions between urbanization, heat stress, and climate change. Clim. Change 129 (3), 525–541. doi:10.1007/s10584-013-0936-8
Sandeep, S., Abinash, S., and Agnihotri, A. (2023). Prediction of flood discharge using hybrid PSO-SVM algorithm in barak river basin. MethodsX 10, 102060. doi:10.1016/j.mex.2023.102060
Seyedzadeh, A., Maroufpoor, S., Maroufpoor, E., Shiri, J., Bozorg-Haddad, O., and Gavazi, F. (2020). Artificial intelligence approach to estimate discharge of drip tape irrigation based on temperature and pressure. Agric. Water Manag. 228, 105905. doi:10.1016/j.agwat.2019.105905
Szeląg, B., Zaborowska, E., and Mąkinia, J. (2023). An algorithm for selecting a machine learning method for predicting nitrous oxide emissions in municipal wastewater treatment plants. J. Water Process Eng. 54, 103939. doi:10.1016/j.jwpe.2023.103939
Sztubecka, M., Skiba, M., Mrówczyńska, M., and Bazan-Krzywoszańska, A. (2020). An innovative decision support system to improve the energy efficiency of buildings in urban areas. Remote Sens. 12 (2), 259. doi:10.3390/rs12020259
Tang, X., Xie, X., Fan, B., and Sun, Y. (2018). A fault-tolerant flow measuring method based on PSO-SVM with transit-time multipath ultrasonic gas flowmeters. IEEE Trans. Instrum. Meas. 67, 992–1005. doi:10.1109/tim.2018.2795298
United Nations Environmental Program (UNEP) and IEA. (2017). Global status report 2017. Content has moved. Energy (europa.eu).
Wei, S. (2015). Enhanced actions on climate change: China’s intended nationally determined contributions .
Zhang, H., Guo, X., and Zhang, P. (2023). Improved PSO-SVM-Based fault diagnosis algorithm for wind power converter. IEEE Trans. Industry Appl. , 3341059. doi:10.1109/SPIES55999.2022.10082014
Zhang, Y., Teoh, B. L., Wu, M. Z., Chen, J. Y., and Zhang, L. M. (2023). Data-driven estimation of building energy consumption and GHG emissions using explainable artificial intelligence. Energy 262, 125468. doi:10.1016/j.energy.2022.125468
Zhao, M., Wang, S., Kang, W. B., Li, Y., and Meng, X. Z. (2022). A review of carbon emission accounting in the building sector. HVAC 11, 13–22. (In Chinese). doi:10.19991/j.hvac1971.2022.11.02
Zhao, Z., Yang, X., Yan, H., Huang, Y., Zhang, G., Lin, T., et al. (2021). Downscaling building energy consumption carbon emissions by machine learning. Remote Sens. 13, 4346. doi:10.3390/rs13214346
Keywords: electric big data, single buildings, building operational energy consumption carbon emissions, “electricity-carbon” model, particle swarm optimization–support vector machine
Citation: Wei Y, Chang Z, Hu P, Liu H, Li F and Chen Y (2024) Rapid carbon emission measurement during the building operation phase based on PSO–SVM: electric big data perspective. Front. Energy Res. 12:1329942. doi: 10.3389/fenrg.2024.1329942
Received: 30 October 2023; Accepted: 29 March 2024; Published: 25 April 2024.
Reviewed by:
Copyright © 2024 Wei, Chang, Hu, Liu, Li and Chen. This is an open-access article distributed under the terms of the Creative Commons Attribution License (CC BY). The use, distribution or reproduction in other forums is permitted, provided the original author(s) and the copyright owner(s) are credited and that the original publication in this journal is cited, in accordance with accepted academic practice. No use, distribution or reproduction is permitted which does not comply with these terms.
*Correspondence: Zhengwei Chang, [email protected]
- Find a Program
- Find A Contractor

- 2024 Announcements
- 2023 Announcements
- 2022 Announcements
- 2021 Announcements
- Social Media
- Press Release Email List
Steps Forward Announced to Support New York's Clean Energy Industry
Next phase of the state’s supply chain activities released in the form of $200 million supportive manufacturing and logistics rfp.
April 23, 2024
Governor Kathy Hochul today announced definitive steps in support of the growing clean energy industry in New York and the State’s 10-Point Action Plan . As part of the next phase of the State’s $500 million commitment to offshore wind supply chain activities, a $200 million Supportive Manufacturing and Logistics Request for Proposals is now open to support investments in infrastructure and manufacturing to grow the domestic offshore wind supply chain in New York. In addition, a Request for Information (RFI) will be issued to inform the development of New York’s fifth offshore wind project solicitation (NY5) and design of a coordinated $300 million RFP focusing on major component offshore wind supply chain investments. Today’s announcements support continued progress toward achieving New York’s Climate Leadership and Community Protection Act goal to develop 9,000 megawatts of offshore wind by 2035.
“New York is solidifying its leadership role in the offshore wind industry, building the supply chain right here in the State to build the industry and drive down costs,” Governor Hochul said . “This commitment and momentum will help us realize a future where clean energy powers our grid, creates long-term jobs, and boosts our economy.”
The 10-point Action Plan released in October 2023 offered insight and direction for the State’s plans to overcome recent macroeconomic and inflationary challenges that have impacted the renewable energy sector and outlines a comprehensive set of actions being taken to lay the foundation for a sustainable future for all New Yorkers through the expansion of the State’s growing clean energy economy and renewable energy sector.
Proposers to the supply chain RFP will be required to demonstrate how State funding will leverage significant private investments in supply chain projects and are encouraged to demonstrate that the funds awarded by the state are the final funds needed to develop a project. Proposals are due by 3:00 p.m. ET on August 13, 2024 . Solicitation details and associated documents are available on NYSERDA’s website .
New York State Energy Research and Development Authority President and CEO Doreen M. Harris said , “With the issuance of today’s supply chain funding opportunity and requests for input on our next offshore wind solicitations, we continue to demonstrate a steadfast commitment to moving large-scale renewable energy forward on a competitive basis despite macroeconomic headwinds facing the industry. We are undaunted in our efforts to build this industry with a thoughtful, collaborative approach that is responsive to market needs and inclusive of robust stakeholder engagement to ensure a solid foundation for success.”
NY5 Solicitation Following the RFI mentioned above, NYSERDA plans to launch the fifth offshore wind solicitation in summer of 2024. Once launched, the NY5 solicitation will run in parallel to a $300 million Major Component Supply Chain solicitation. The Offshore Wind Renewable Energy Certificate solicitation originally planned for release late in 2024 in coordination with the New York City Public Policy Transmission Needs (PPTN) process will be launched in 2025.
$300 Million Major Component Supply Chain Solicitation NYSERDA anticipates issuing a $300 million Major Component Supply Chain solicitation simultaneously with launching NY5 to allow offshore wind proposers to negotiate the use of localized supply chain components in their bids. The design of the solicitation will be informed by comments received through the RFI issued today and aimed at attracting investments in major offshore wind supply chain components, including major port upgrades to accommodate major offshore wind component manufacturing.
Center for Economic Growth Chief Economic Development Officer Katie Newcombe said , “The Center for Economic Growth and its partners have spent years building a robust offshore wind ecosystem, and we will continue to actively pursue manufacturing, workforce development, and supply chain opportunities. Albany/NY’s Capital Region is home to the best shovel ready port sites in the United States. We stand ready to work with developers, suppliers, and NYSERDA through this and future opportunities to realize our collective vision of advancing offshore wind in New York State and bringing economic benefit to our communities.”
New York State Building Trades President Gary LaBarbera said , "Offshore wind is a game changer for New York as it works to meet its climate goals, and for the tradesmen and tradeswomen who will hold the thousands of good-paying union jobs it creates, bolstering our middle class and stimulating our local economies. We’re thankful that Governor Hochul and NYSERDA are advancing New York’s commitment to building this important, new energy sector while ensuring strong safety standards, family-sustaining wages, and good benefits.”
New York State AFL-CIO President Mario Cilento said , “We applaud the Governor for her commitment to ensuring that New York's clean energy future is manufactured, constructed, operated, and maintained by union members. While NY3 and associated manufacturing grant funding included the most robust labor standards of any offshore wind project to date, we will continue to work with the Governor to ensure the solicitations announced today include even more robust standards and protections for union workers.”
New York City Central Labor Council, AFL-CIO President Vincent Alvarez said , “With today's announcement, Governor Hochul and NYSERDA are taking proactive steps to navigate a dynamic and evolving market. We look forward to building a New York-based supply chain with strong labor standards, and collaborating with NYSERDA, New York State, and all of the stakeholders to ensure that as we transition to a clean energy economy, we’re also creating family-sustaining union jobs and a resilient economy for all New Yorkers.”
Long Island Federation of Labor, AFL-CIO, President John Durso said , “Starts and stops are sometimes necessary and provide new opportunities for us to make sure we are delivering the best possible outcome for New Yorkers. Long Island’s working families remain committed to addressing the climate crisis in a way that creates good union jobs in the process. We look forward to working with New York State, the offshore wind industry, and all other stakeholders who are interested in a brighter future with good family sustaining wages.”
Nassau and Suffolk County Building Trades President Matt Aracich said , “It is imperative that we continue to move the offshore wind industry forward here in NY. Governor Hochul and her team at NYSERDA are effectively adapting to the constantly evolving landscape of this emerging industry. The thousands of local Building Trades Union jobs associated with the NY3 solicitation represent real family sustaining wages and benefits here on Long Island. Our Island’s economic future is tied to the success of the offshore wind industry, so it is important to get this right.”
New York Offshore Wind Alliance Director Fred Zalcman said , “Through Governor Hochul’s leadership, New York is continuing to put in place the fundamental building blocks of a robust and responsible offshore wind industry. As today’s announcement demonstrates, despite recent setbacks New York will continue to swing for the fences in seeking to localize the offshore wind supply chain. With smart policy and sustained commitment reflected in today’s actions, the Empire State is positioning itself to hit many economic development singles and doubles – and indeed home runs – in the very near future.”
New York League of Conservation Voters President Julie Tighe said , “The transition to a clean energy future is not a straight line—any step backward compels us to take two steps forward. By quickly soliciting information to advance a fifth offshore wind solicitation and putting out RFPs on the manufacturing and logistics that will shore up the supply chain, Governor Hochul is making clear that the state remains committed to greening the grid and realizing the economic promise of this new industry.”
Citizens Campaign for the Environment Executive Director Adrienne Esposito said , “New York is on the front lines of climate change, and we are already experiencing the adverse impacts. The need to transition from polluting fossil fuels is an urgent matter. Transitioning to renewable energy and updating our energy infrastructure must be given the highest priority that is why we are thrilled that Governor Hochul is continuing to advance offshore wind and is making substantial investments in the supply chain to grow our emerging ‘wind-ustry’ in New York. We are already beginning to see the environmental, health, and economic benefits offshore wind and the creation of local jobs for New Yorkers. We applaud New York’s continued leadership.”
Today’s announcements further demonstrate New York’s unwavering commitment to clean energy development, sustainability, and economic growth and supports the State’s progress towards achieving the Climate Act goals, including obtaining 70 percent of the State's electricity from renewable sources by 2030. This includes advancing an accelerated timeline for offshore wind procurements that resulted in recent conditional awards to Empire Wind 1 and Sunrise Wind under the fourth solicitation that will begin delivering power in 2026.
New York State's Nation-Leading Climate Plan
New York State's climate agenda calls for an orderly and just transition that creates family-sustaining jobs, continues to foster a green economy across all sectors and ensures that at least 35 percent, with a goal of 40 percent, of the benefits of clean energy investments are directed to disadvantaged communities. Guided by some of the nation’s most aggressive climate and clean energy initiatives, New York is advancing a suite of efforts – including the New York Cap-and-Invest program (NYCI) and other complementary policies – to reduce greenhouse gas emissions 40 percent by 2030 and 85 percent by 2050 from 1990 levels. New York is also on a path to achieving a zero-emission electricity sector by 2040, including 70 percent renewable energy generation by 2030, and economywide carbon neutrality by mid-century. A cornerstone of this transition is New York's unprecedented clean energy investments, including more than $28 billion in 61 large-scale renewable and transmission projects across the State, $6.8 billion to reduce building emissions, $3.3 billion to scale up solar, nearly $3 billion for clean transportation initiatives and over $2 billion in NY Green Bank commitments. These and other investments are supporting more than 170,000 jobs in New York’s clean energy sector as of 2022 and over 3,000 percent growth in the distributed solar sector since 2011. To reduce greenhouse gas emissions and improve air quality, New York also adopted zero-emission vehicle regulations, including requiring all new passenger cars and light-duty trucks sold in the State be zero emission by 2035. Partnerships are continuing to advance New York’s climate action with more than 400 registered and more than 130 certified Climate Smart Communities, nearly 500 Clean Energy Communities, and the State’s largest community air monitoring initiative in 10 disadvantaged communities across the State to help target air pollution and combat climate change.
Media Inquiries
- Executive Chamber, Phone: 518-474-8418, Ext. n/a Email: [email protected]
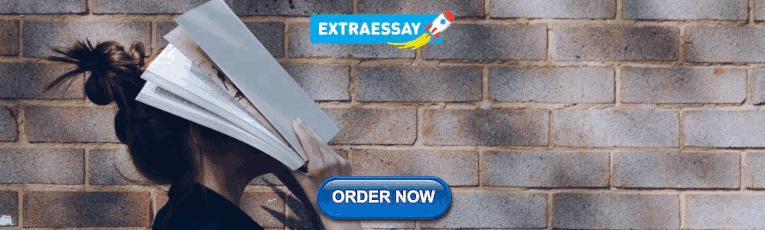
IMAGES
VIDEO
COMMENTS
Buildings account for nearly 2/5ths of global energy expenditure. Due to this figure, the 90s witnessed the rise of green buildings (GBs) that were designed with the purpose of lowering the demand for energy, water, and materials resources while enhancing environmental protection efforts and human well-being over time. This paper examines recent studies and technologies related to the design ...
This paper reports a critical review of the existing body of knowledge of researches related to green building. The common research themes and methodology were identified. These common themes are the definition and scope of green building; quantification of benefits of green buildings compared to conventional buildings; and various approaches ...
The concept of green building has gradually formed with the increase in public awareness of environmental protection, which also covers a wide range of elements. The green building is the fundamental platform of sustainable development. This review paper provides solutions for the multi-dimensional and balanced development of green building. Since green building is the development trend of the ...
The Concept of Green Architecture, also known as "sustainable architecture" or "green building," is the theory, science and style of buildings designed and constructed in accordance with environmentally friendly principles. Green architecture strives to minimize the number of resources consumed in the building's construction, use and ...
43210 Columbus Ohio, USA. Received 18 Nov 2013; accepted 25 Nov 2013. Abstract. Sustainable building design has become a wide and multidisciplinary research endeavor including mechanical ...
The purpose of the Journal of Green Building is to present the very best peer-reviewed research for the sustainable built environment at the building, community, neighborhood and urban scale. As such, the journal's coverage includes green building design and construction... Read more about the Journal. Subscribe. JGB Twitter.
This article examines outstanding "sustainable" skyscrapers that received international recognition, including LEED certification. It identifies vital green features in each building and summarizes the prominent elements for informing future projects. Overall, this research is significant because, given the mega-scale of skyscrapers, any improvement in their design, engineering, and ...
This research used papers published on three scientific and reliable databases—Web of Science, ScienceDirect, and IEEE Xplore-from 2014 to May 23, 2021. ... The methodological aspects of this research on green and sustainable building with energy efficiency are analyzed to determine the limitations of previous studies and inform future ...
Energy and Buildings, Building and Environment, Journal of Cleaner Production, and Sustainability were the top journals to publish papers on green buildings. Global distribution was done to see the green building study worldwide, showing that the USA, China, and the UK ranked the top three countries, accounting for 14.98%, 13.29%, and 8.27% of ...
Although the number of green building research papers has been increasing over the decades, there is still a research gap in the study of green building. Many research papers ... tions for sustainable development of green buildings, the research process of this review paper is divided into five main steps, as shown in Figure1. Firstly, the WoS ...
The research aims to assess, develop, and adjust the path of green architecture rating systems depending on sustainability through a practical design strategy is based on generating, evaluating, developing, and optimizing sustainable environmental concepts and thoughts; maximizing their role; and, clarifying the designer's vision.
This paper reports a critical review of the existing body of knowledge of researches related to green building. The common research themes and methodology were identified. These common themes are the definition and scope of green building; quantification of benefits of green buildings compared to conventional buildings; and various approaches ...
This paper outlines environmental impacts of building construction and justifies suitability of rammed earth as an ideal sustainable housing both in terms of environmental and structural stability, based on results obtained from lab tests (compressive strength: 1.5-6.5 MPa, water resistivity, optimum water content) of 100 mm stabilized and un ...
The purpose of this paper is to highlight how sustainable building material can contribute to lessen the impact of environmental degradation, and generate healthy buildings which can be ...
Sustainable construction plays a significant role in developing countries. However, the adoption of sustainable buildings has faced diverse challenges. Therefore, this research investigates the benefits and challenges of adopting the Green Mark in green building projects. After a literature review and a pilot study with construction experts, an industry-wide survey was conducted to collect 148 ...
paper examinesways of creating sustainable buildings, building design and construction. (green buildings) which are structurally stable, functional and healthy for people to live and. work in and ...
The papers in this Special Issue focus on emerging techniques and the most recent developments in sustainable building materials. These papers describe original work on both practical and theoretical works. Research areas may include (but not limited to) the following: Eco-friendly building materials ; Smart sustainable cities and buildings
Sustainable development encompasses numerous development goals and strategies, with green buildings (GBs) being among the implementations of this concept. The development of GBs is a topic of increasing interest due to the massive development of conventional infrastructure that has the major limitation of environmental degradation, a fact also proven by the research of the scientific ...
Accordingly, "sustainable" buildings can be more friendly with environment and human, and use key resources, such as, energy, water, and materials more optimal than the conventional buildings. Furthermore, the study was to address the benefits of developing sustainability in buildings on different perspectives, based on the review and ...
Background Despite the increasing square footage of green buildings worldwide, green building expertise remains largely in the domain of building industry professionals. However, the performance of and advocacy for green buildings would benefit from a green building literate general public. Green building education is an expanding frontier for STEM education and can create opportunities to ...
The green building movement has gained significant traction as a means to address the environmental impact of buildings and promote sustainable development. This research paper examines the application of green building practices in the context of sustainable development in India. Buildings in India account for a
1090 papers on sustainable building renovation were gathered. Fig. 1 shows the number of publications in each five years from 1990 to 2020. In general, there was an increasing trend in publication number. Before 2000, building renovation research was in its infancy that very limited attention was given to the field and the number of annual publications was less than 11.
With the rapid development of urbanization in China, urban energy consumption increases rapidly, leading to energy shortages and environmental pollution, of which building operational energy consumption carbon emissions (BECCE) account for a large proportion. It has a vital impact on global warming and urban green and sustainable development. Chengdu city in Sichuan Province is taken as the ...
As a conclusion, we can find out how important sustainable materials are and the benefit of a community self-provided material. The use of bamboo as a building façade (source: Teras Cibiru ...
Background. In line with the urgent demand for ensuring sustainable development and abiding sustainability requirements, energy analysis of buildings has become a global interesting and attractive application for governments, energy-related businesses and even consumers [Citation 1-19].Since the building industry is responsible for 20-40% of worldwide energy use [Citation 14], the overall ...
Therefore, this research investigates how historical public space revitalization and community activity contribute to Sustainable Development Goals (SDGs). This research uses the descriptive qualitative method using case study that examines the community activities using place-centered mapping and changes made by the revitalization effort.
In addition, this paper presents the main research areas found in the studies according to the Web of Science - Main Collection database, ... Green Building Index (GBI): it assesses the relationship between the environment and construction projects' performance in Malaysia. It aims to achieve and incorporate ecological issues related to ...
A cornerstone of this transition is New York's unprecedented clean energy investments, including more than $28 billion in 61 large-scale renewable and transmission projects across the State, $6.8 billion to reduce building emissions, $3.3 billion to scale up solar, nearly $3 billion for clean transportation initiatives and over $2 billion in NY ...
Green roofs have become a popular sustainable solution in urban areas, and in recent years, shipping containers have gained popularity as a sustainable alternative for housing. A promising proposal is to combine these two solutions. This research aims to analyze the thermal behavior of experimental modules of scale constructions. Four modules were constructed with different substrate ...
Sustainable design, which aims to reduce energy consumption and mitigate climate change, is a primary concern of developing countries. Hence, it reduces CO2 emissions. Residential buildings in North Iraq account for approximately 69% of all electricity consumed. To mitigate this issue, this article investigates the design of a sustainable model by considering the local climate, building design ...