Thank you for visiting nature.com. You are using a browser version with limited support for CSS. To obtain the best experience, we recommend you use a more up to date browser (or turn off compatibility mode in Internet Explorer). In the meantime, to ensure continued support, we are displaying the site without styles and JavaScript.
- View all journals
- My Account Login
- Explore content
- About the journal
- Publish with us
- Sign up for alerts
- Open access
- Published: 21 February 2022
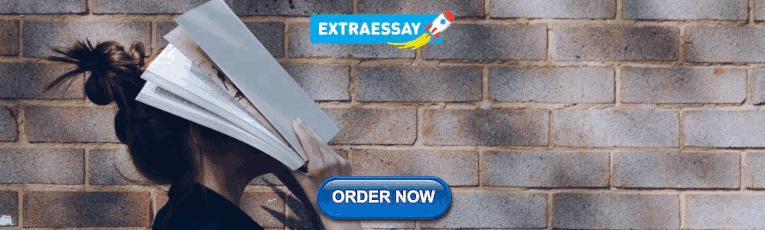
Physicochemical and biological analysis of river Yamuna at Palla station from 2009 to 2019
- Pankaj Joshi 1 ,
- Akshansha Chauhan 2 ,
- Piyush Dua 3 ,
- Sudheer Malik 4 &
- Yuei-An Liou 2 , 5
Scientific Reports volume 12 , Article number: 2870 ( 2022 ) Cite this article
6857 Accesses
9 Citations
2 Altmetric
Metrics details
- Environmental sciences
Yamuna is one of the main tributaries of the river Ganga and passes through Delhi, the national capital of India. In the last few years, it is considered one of the most polluted rivers of India. We carried out the analysis for the physiochemical and biological conditions of the river Yamuna based on measurements acquired at Palla station, Delhi during 2009–19. For our analysis, we considered various physicochemical and biological parameters (Dissolved Oxygen (DO) Saturation, Biological Oxygen Demand (BOD), Chemical Oxygen Demand (COD), Total Alkalinity, Total Dissolved Solids (TDS), and Total Coliform. The water stats of river Yamuna at Palla station were matched with Water Standards of India, United Nations Economic Commission for Europe (UNECE), and World Health Organization (WHO). Maximum changes are observed in DO saturation and total coliform, while BOD and COD values are also seen higher than the upper limits. Total alkalinity rarely meets the minimum standards. TDS is found to be satisfactory as per the standard limit. The river quality falls under Class D or E (IS2296), Class III or IV (UNECE), and fails to fulfill WHO standards for water. After spending more than 130 million USD for the establishment of a large number of effluent treatment plants, sewage treatment plants, and common effluent treatment plants, increasing discharges of untreated sewage, partially treated industrial effluents and reduced discharge of freshwater from Hathnikund are causing deterioration in water quality and no major improvements are seen in water quality of river Yamuna.
Similar content being viewed by others
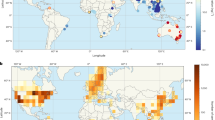
Underestimated burden of per- and polyfluoroalkyl substances in global surface waters and groundwaters
Diana Ackerman Grunfeld, Daniel Gilbert, … Denis M. O’Carroll
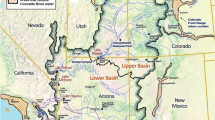
New water accounting reveals why the Colorado River no longer reaches the sea
Brian D. Richter, Gambhir Lamsal, … John C. Schmidt
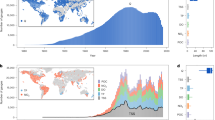
Deep learning for water quality
Wei Zhi, Alison P. Appling, … Li Li
Introduction
Water is the main need of human life. The majority of ancient civilizations were developed on the bank of major rivers across the world. Rivers fulfill the major demands of the freshwater supply from drinking to agriculture. In the northern parts of India, the Yamuna River basin is ranked the second largest basin after the Ganga River. It is the second-largest tributary of the river Ganga (the longest river of India) with a total catchment area of 345,848 km 2 and it originates at Yamunotri Glacier, Uttrakhand, India at a height of 6387 m 1 . It covers a total distance of 1376 km through four major states of India: Uttarakhand, Haryana, Delhi, and Uttar Pradesh, and finally confluences with river Ganga at Triveni Sangam, Prayagraj, Uttar Pradesh. Although it does not flow through Himachal Pradesh but receives water via the river Tons (which originates in Himachal Pradesh). The other tributaries of the river Yamuna are Chambal, Sindh, Betwa, and Ken 2 , 3 . The water abstracted from the river is mostly used for irrigation (about 94%), while 4% for domestic water supply and the remaining 2% for industrial and other uses 4 .
During the last few decades, Yamuna has been considered one of the most polluted rivers of India. Discharge from industries, partially or untreated sewage, and agricultural waste are the main sources for the river Yamuna degradation 5 , 6 , 7 . Almost 85% of the total pollution in the river Yamuna is due to domestic sources mainly from urban cities Sonipat, Panipat, Delhi, Ghaziabad, Mathura, Agra, Etawah, and Prayagraj. Industrial zones at various places like Yamunanagar, Panipat, Ghaziabad, Delhi, Noida, Faridabad, and Baghpat which are in the upper Yamuna basin (Fig. 1 ), comprise industries like Oil refineries, distilleries, pulp, pharmaceutical, chemical, electroplating, weaving, and sugar, and contribute to the degradation of Yamuna water quality significantly 8 , 9 . According to Kumar et al. 10 , Delhi leads the list of cities with 79% pollution load in river Yamuna followed by Agra and Mathura with a contribution of 9% and 4%, respectively, whereas a pollution load of 2% by Sonipat and Baghpat. The annual mixing of sewage from domestic and industrial sources in the Yamuna River basin is about 9.63 km 3 , 11 . In the last few decades, a sudden rise in the built-up and cropland areas is observed in the Yamuna River basin (Fig. 2 ). Kumar et al. 10 suggested a rise of 100% in the urbanization in Haryana and Rajasthan states and significant fall is observed in wetland, grassland, water bodies and forest areas of Yamuna River basin. The green revolution in India helped rise in the productivity of various crops, but the major water supply to the crop depends on the groundwater. The DO level of water in Delhi stretch shows a sudden fall due to high carbon level so that most of the time the river can not sustain fishery.
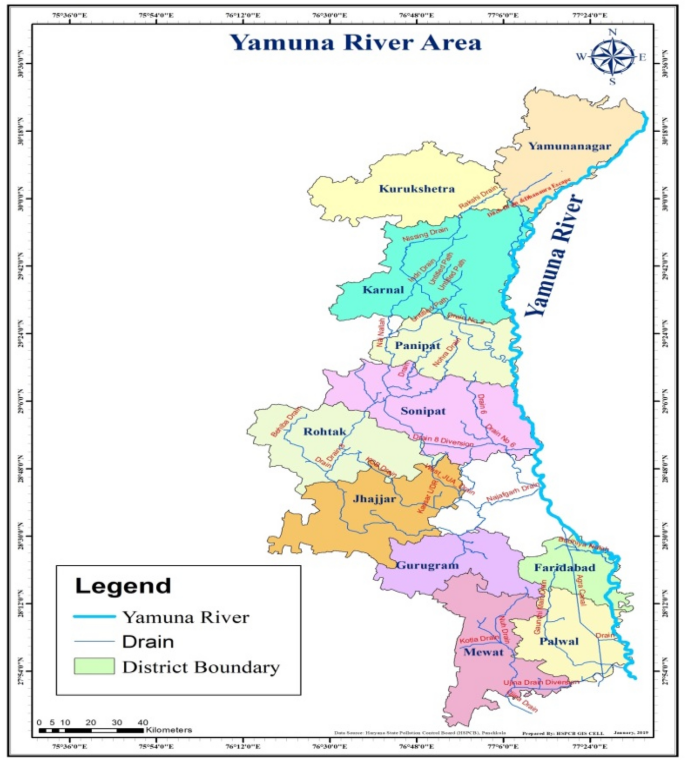
( Source : HSPCB).
River Yamuna in Haryana.
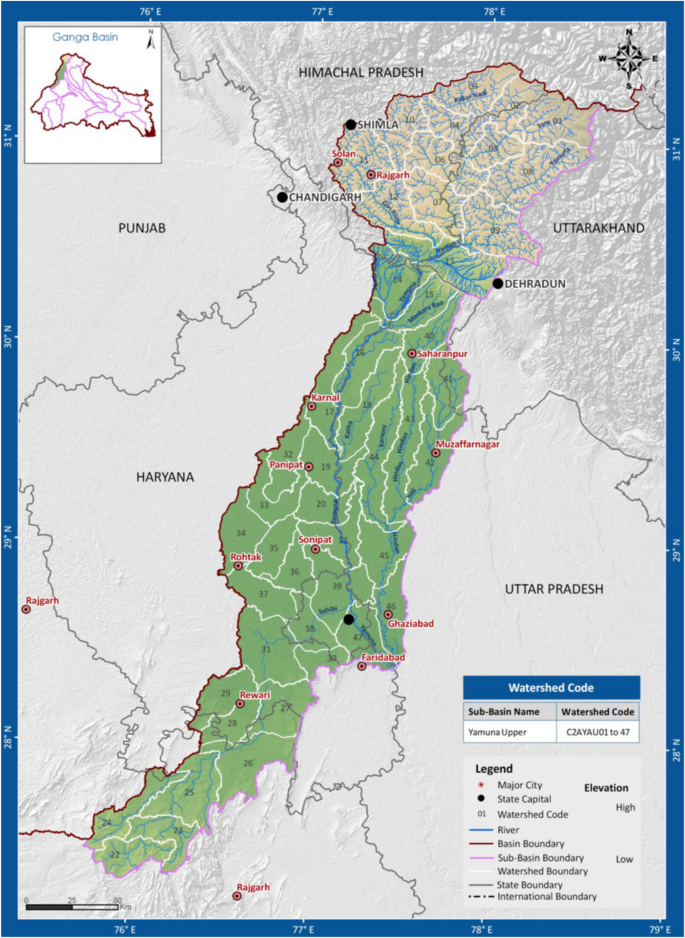
( Source : WRIS).
Upper river Yamuna basin area.
Parween et al. 12 showed the positive rise in the potassium and nitrate that affected the Yamuna River basin. Domestic waste consists of mainly organic matter and micro-organisms along with detergents, grease and total salts mixed in river Yamuna through various drainages in National Capital region. Lokhande and Tare 3 have shown rise in the flow rate of Yamuna during non-monsoon months due to the sewage water. Industrial effluents are the main source of heavy metal pollution like Cd, As, Cr, Fe and Zn with other inorganic and organic wastes adding to pollutant inventory 10 . According to National Capital Region Planning Board (NCRPB) report, sewage generation in Haryana was 374 MLD in 2001, and 599 MLD in 2011, whereas the sewage treatment capacity was 164 MLD in 2001 and 199 MLD in 2011. State monitoring committee appointed by National Green Tribunal (NGT) 2019 suggested that Haryana discharged 1140 MLD of untreated or partially treated sewage per day into river Yamuna, also 1268 industrial units discharged 138.75 MLD partially treated and another 827 units discharged 48.319 MLD of treated effluents per day in Yamuna River 13 .
Central Pollution Control Board (CPCB) is responsible for controlling the various sources of pollution in India and also monitoring water quality of the rivers with the State Pollution Control Board (SPCB) 4 , 5 , 14 , 15 . CPCB started national water quality monitoring in 1978 under Global Environmental Monitoring System (GEMS), followed by the Monitoring of Indian National Aquatic Resources (MINARS) in 1984, and helped reduce river pollution via National Water Quality Monitoring Programme (NWMP) 16 . Central Water Commission (CWE) is monitoring the water quality of all the major river basins in India through 519 water quality sites and 33 water sampling stations. According to CWC, the water quality of river Yamuna is monitored at 18 different stations of which 12 are manual and 6 are telemetry stations, starting from Naugaon (N-30.78, E-78.13) at Uttarakhand as the first river point station to the last river point station Pratappur (N-25.37, E-81.67) Uttar Pradesh.
Due to degradation in the water quality of the Yamuna River, Yamuna Action Plan (YAP-I) was launched in 1993 by the Ministry of Environment and Forests (MoEF), India to rejuvenate the Yamuna River especially in the Delhi segment having maximum pollution load. Haryana and Uttar Pradesh were also included along with Delhi in YAP-II in 2003. YAP-III, with an estimated cost of Rs.1656 crore, was launched in 2018 as an integrated component of the Namami Gange Mission 17 . The river Yamuna is analyzed monthly, seasonally, and yearly for its physical, chemical, and biological properties in previous research for various states and cities influenced by it or small stretches of river 18 , 19 , 20 , 21 . A sufficient amount of literature is available for the water quality of Yamuna and most of the analysis focused on the Delhi and stations lie after the Delhi’s. Kumar et al. 10 discussed the variability of various water quality parameters from 1999 to 2005 to investigate the relationship between environmental parameters and pollution sources. Kaur et al. 22 discussed the impact of industrial development and land use/land cover changes over the Yamuna water quality in Panipat, which is located between the Hathnikund and Baghpat stretch. The sampling was carried out during February, July, October, and December, 2018 to observe the variations in the quality of water and the impact of pollutants on the river Yamuna. Patel et al. 23 carried out the water quality analysis over the Yamuna River using satellite and remote sensing during the lockdown period and compared it with the water quality parameter before the lockdown. The analysis was carried out from January 2020 to April 2021. The impact of lockdown on the water quality was discussed assuming that the industrial waste and the other pollutants sources were reduced during the time of lockdown. Paliwal et al. 24 carried out the modeling analysis of the Yamuna water quality for the Delhi stretch. They emphasize the inflow of untreated water and effluents from various drains in the Yamuna in the Delhi stretch using QUAL2E-UNCAS before 2007. They also highlighted the need for common treatment plants and a rise in freshwater supply. Krishan et al. 25 conducted the groundwater study near the bank of the Yamuna in the Agra and Mathura districts. The study area is located downstream of Delhi and affected by severe falls in water quality. The change in the water quality at Agra and Mathura was discussed and the assessment of the treated water after the filtration was discussed. Kaur et al. 26 investigated the Yamuna water quality at the Delhi stretch and one site located near the Yamunotri. The analyses were implemented during March and October for 2017 and 2018. They have discussed the impact of the inflow of pollutants from the Delhi and NCR regions in the Yamuna. Jaiswal et al. 21 carried out the multivariate study of the Yamuna river water to study the river water quality across the whole stretch. The samples were collected from July to October and November to June for 2013 and 2014. The analysis suggested that the water quality of Palla was suitable for drinking during the study period. We found that these analyses were mostly carried out for a short period in recent years. Some long-term analyses were carried out before 2007. So, there is a dire need for long-term analysis in recent years. In recent times, Lokhande and Tare 3 performed the first long-term analysis of various water quality parameters of the river Yamuna and discussed the trends of various parameters. Due to classified data, Lokhande and Tare 3 were not able to quantify the monthly variations of various water parameters. Hence, these analyses, lack the long-term variability discussion and quantitative changes. In the current study, we conducted monthly and annual mean analysis of various physical and biological parameters, including Biological Oxygen Demand (BOD), Chemical Oxygen demand (COD), Dissolved Oxygen (DO) Saturation, Total Alkalinity, Total Dissolved Solids (TDS), Total Coliform and average rainfall from 2009 to 2019 at Palla station located at northwestern Delhi. We have shown the quantitative change in the physiochemical and biological parameters of the river Yamuna at the Palla station, which is mostly affected by the pollutants load of the watershed of Haryana. We compared the results with the water quality guidelines of national and international standards given in Table 1 to figure out the changes in water quality of river Yamuna at Palla station in the last 11 years. The current water quality at Palla is not suitable for drinking and sometimes not good for agricultural purposes due to the high influx of water pollutants.
The river Yamuna enters National Capital Territory (NCT) at approximately 1.5 km before village Palla, which is 23 km upstream of the Wazirabad barrage. Palla station (N-28.82 and E-77.22) is a manual type station with zero gauges at 206 m. Before entering NCT at Palla, the river Yamuna traveled about 393 km from its source and about 220 km from the Hatnikund barrage. According to Haryana State Pollution Control Board (HSPCB), the numbers of industries in Yamunanagar, Kernal, Panipat, and Sonipat are 142, 9, 346, and 503, generating effluent of 16,420.90, 26.00, 65,696.97, and 15,668.50 KLD, respectively, up to August 2019. As in Fig. 3 , several drains of Haryana state including 3 major drains at Dhanaura escape, Main Drain No.2, and Drain No. 8 also fallout in river Yamuna before reaching Palla 28 (Figs. 3 , 11 ).
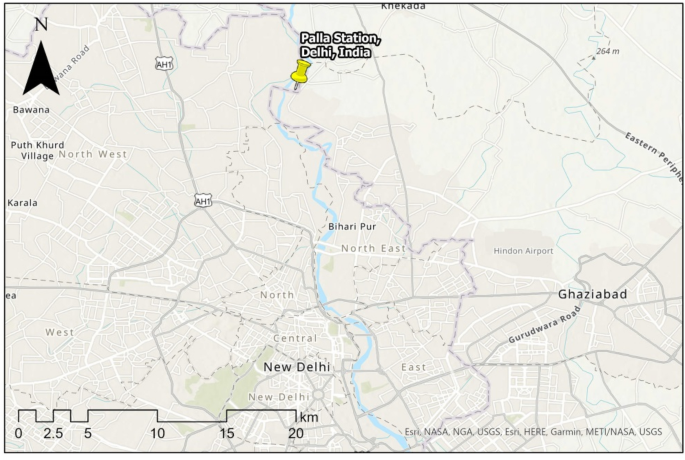
Location of Palla station. The base image is provided by ESRI and projection is done using ArcGIS Pro.
Data retrieval
Water quality data of Yamuna River at Palla station was obtained from Water Resources and Information System (WRIS), India, which is a centralized platform, acting as a database related to all water resources at the national or state level. It was initiated by CWC along with the Ministry of Water Resources, Ministry of Jal Shakti, and Indian Space Research Organization (ISRO) in 2008 to provide a single-window solution to all water resources data and information in a standardized national GIS framework 29 . Depending upon the availability of monthly average data, the parameters BOD, COD, DO saturation, total alkalinity, TDS, and total coliform at Palla station were analyzed during the period from January 2009 to December 2019. The rainfall data was procured from the Modern-Era Retrospective analysis for Research and Applications, Version 2 (MERRA-2) and available on the website http://www.soda-pro.com/web-services/meteo-data/merra . The spatial resolution of data is 0.50° × 0.625° and the time step ranges from 1 min to 1 month 30 , 31 . We also included the water discharge data in the current study of three locations Hathnikund Barrage, Baghpat, and Delhi Railway Bridge (DRB) stations. The freshwater in river Yamuna reached to the Palla station is controlled at the Hathnikund Barrage. Baghpat station is located just before the Palla station (no data is available at Palla station) and DRB is located after the Palla station. These stations are chosen based on the availability of data and the locations to show the water discharge in the Yamuna. The water discharge data of this location is taken from the Central Pollution Control Board of India ( https://yamuna-revival.nic.in/wp-content/uploads/2020/07/Final-Report-of-YMC-29.06.2020.pdf ).
Ethics approval and consent to participate
The paper did not involve any human participants.
Result and discussion
Analysis of various parameters related to the water quality of river Yamuna at Palla station was carried out.
The annual mean variation in rainfall at the Palla region (Fig. 4 a) suggested that the highest annual mean rainfall (95.8 mm) occurred during the year 2010. After 2010, the yearly average rainfall continuously declined every year till 2014 with a mean value of 17.9 mm. Although from 2014 to 2018, a rise in average rainfall was observed each year and during 2018, the average rainfall was calculated as 53.1 mm, but the linear trend illustrated a sharp decline in average rainfall between 2009 and 2019 as shown in Fig. 4 a.
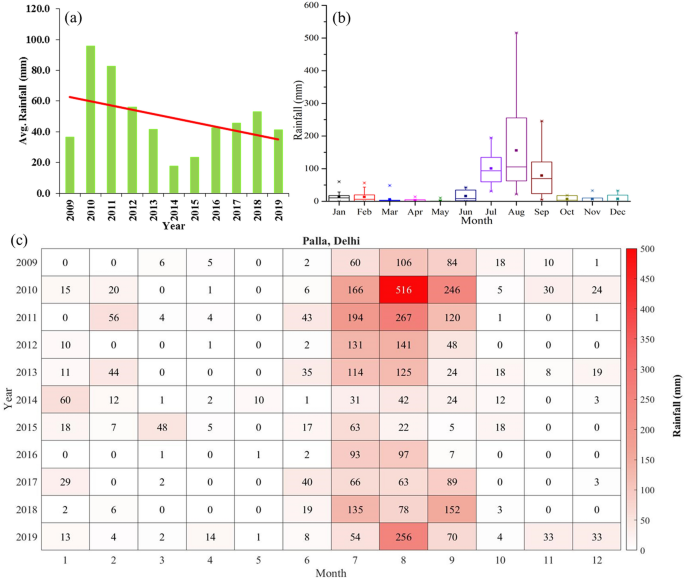
( a ) Yearly mean rainfall at Palla station and adjacent region from 2009 to 2019. The red line shows a linear trend of rainfall. ( b ) Rainfall monthly distribution at Palla station from 2009 to 2019. ( c ) Monthly mean variation of rainfall from 2009 to 2019.
The box plot in Fig. 4 b shows the rainfall data of each month during 2009–2019. The distribution suggested that the monthly mean rainfall variations were highest during August along with July and September. May was an almost driest month along with April and March as the rainfall was minimum during these months. The highest values of average rainfall for August, September, and July were 515.28, 233.45, 207.99 mm respectively, whereas these months’ minimum average rainfalls were 101.74, 61.95, and 72.76 mm, respectively, during 2009 and 2019. The median values of rainfall during March, April, October, and November were below 10 mm and, during January, February, June, and December, the median values were between 10 and 20 mm, while the median values for July, August, and September were above 60 mm.
The monthly mean variation of rainfall (2009–19) further elaborates the stats of rainfall in the Palla region. From 2009 to 2019 rainfall data showed various ups and downs in monthly rainfall (Fig. 4 c). In India, the onset of monsoon is observed during the start of June each year in the coastal part of India, which lasts approximately for a period of four months (June to September) each year. The northern parts of India receive major rainfall during this period, which is known as the monsoon season. During June, the average rainfall remained lower than the decadal mean during 2014–2019 except for 2017. During July, we observed the same deficit of rainfall during 2014–2019 except in 2018. The major deficit in rainfall was observed for August from 2012 to 2018 whereas, during August 2019, the rainfall remained higher than the decadal mean value. During September, the rainfall deficit was observed from 2012 to 2016. Hence, during 2012–2017, we observed a significant fall in the monsoon rainfall. The winter rainfall is also observed during January and February in northern parts of India. We also observed a significant fall in the winter rainfall for January and February since 2014. Yamuna River before Palla station receives runoff water from cities like Sonipat, Panipat, Karnal, and Yamunanagar. The rainfall statistics of Haryana analyzed by India Meteorological Department (IMD) between 1989 and 2018 showed annual rainfalls of 1053.5, 578.8, 573.9, and 495.3 mm at Yamunanagar, Sonipat, Karnal, and Panipat districts, respectively 32 . During the monsoon season, sudden changes in physicochemical and biological parameters were observed by various researchers. The addition of monsoon runoff in rivers dilutes the industrial effluent and sewage, causing the decline in parameters like BOD, COD, alkalinity, pH, and conductivity 33 , 34 , 35 , 36 . The catchment area of Yamuna is the smallest in Delhi. The size of the catchment area of the river is an important factor for the dilution of anthropogenic waste in river water. The small catchment area increases soil components in the river and makes difficult the dilution process of anthropogenic waste 37 . These conditions can further affect the river water quality. Hence, we further analyzed the variations in the various physical and biological parameters for each month from 2009 to 2019.
Dissolved oxygen (DO) saturation
Dissolved Oxygen (DO) saturation is a vibrant parameter for aquatic system health determination. The pollutants like sewage, soil, agricultural runoff, and other organic pollutants can reduce the DO saturation of water 38 and low DO saturation can impact the life of major aquatic organisms. The water having DO < 5% of saturation lies in an extremely severe pollution region; DO between 5 and 10% of saturation lies in severe pollution; DO in a range of 10–70% represents moderate pollution, whereas DO above 70% indicates slightly or no pollution condition. Heavy pollution load due to untreated sewage and industrial effluents are the main causes of decreasing DO concentration 39 . Value of DO saturation is also affected by the change in water salinity (chlorine), temperature, and air pressure.
Increasing pollution in the Yamuna River caused a decrease in DO saturation concentration along with an increase in temperature and salinity of water 3 . Figure 5 shows the yearly variation of DO saturation, and monthly distribution and mean variation of DO saturation at Palla station from 2009 to 2019. In recent years, the annual mean value of DO saturation during 2017–19 was found to be 4.81%. From 2009 to 2011, the mean DO saturation was found to be 69.49% with a maximum value (81.58%) in 2010. From 2012 to 2015, the yearly mean DO saturation was 86.48%. During this period, a small decrease was seen between 2013 and 2015. In recent years, the DO saturation reached critically low values to the average value before 2015 as demonstrated in Fig. 5 a. These conditions indicate the rise in pollutants in the river Yamuna.
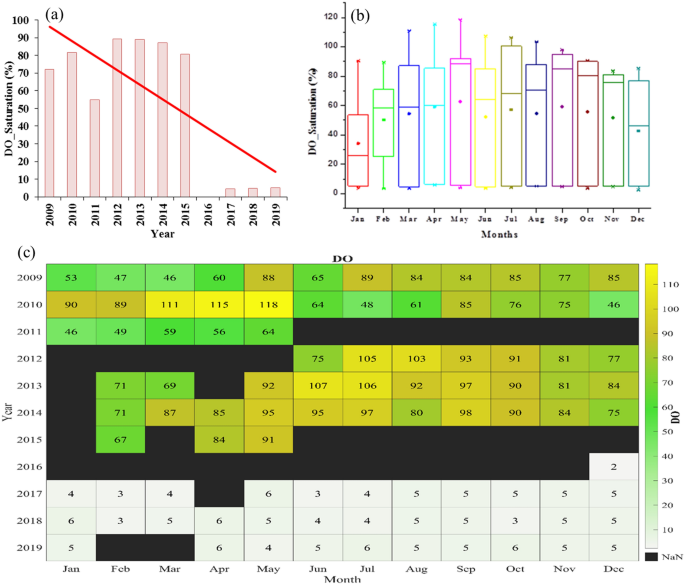
( a ) Yearly variation of DO saturation from 2009 to 2019. The red line shows a linear trend of DO saturation. ( b ) Monthly distribution of DO saturation at Palla station from 2009 to 2019. ( c ) Monthly mean variation of DO (%) saturation from 2009 to 2019.
The monthly distribution of DO saturation during 2009–2019 is shown in Fig. 5 b with boxes ranging from 25 to 75%. During May, the maximum median value was 88%, whereas in January minimum median value was about 25%. Similarly, the maximum monthly mean value was 62.7% during May, while the minimum mean value was 34.1% during January. Similarly, maximum DO saturation of 118% was perceived for May, while a minimum of 2.4% was observed for December. The monthly mean distribution suggested that the mean DO saturation plunged between 50 and 60% with a slight growth in trend from January to December. We further showed the monthly mean values of DO saturation in Fig. 5 c. From 2009 to 2015, the values suggested no major changes with monthly values well above 50, but just after 2017, a sudden fall was observed in the DO saturation each month. We observed 10 times fall in DO saturation just after 2017. During 2009–14, the DO saturation of Yamuna River at Palla station was Class I category of international standards for surface water of UNECE, but during 2016–19 its quality degraded to Class IV due to regular fall in the DO values of the river water. The lack of fresh water and rising carbon concentration have affected the DO concentration significantly.
Biological oxygen demand (BOD)
Biological Oxygen Demand (BOD) is one of the methods to assess the quality of water by calculating the oxygen requirement for decomposition of its organic matter. The yearly variations in BOD at Palla station during 2009–19 are shown in Fig. 6 a. The yearly mean BODs in 2009 and 2010 were estimated to be around 6 mg/l, while, during 2013 and 2012, the least values of BOD (1.4 and 2.7 mg/l, respectively) were found. The year 2015 showed the highest yearly mean BOD value of around 12 mg/l followed by years 2014 and 2016 with a value of about 9.5 mg/l. The decline in yearly mean BOD was observed from 2015 to 2019. In particular, a BOD of 3.5 mg/l was perceived for years 2018 and 2019. The monthly variation of BOD during 2009–19 was shown in Fig. 6 b, where the interquartile range for each month was between 25 and 75 percentiles. The first quartile for all months lay below the range between 0 and 5 mg/l, with outliers for months May, July, October, and December with BOD above 20 mg/l. January and February had the highest median value of BOD at 6.7 mg/l, while September had the least median value of 2.1 mg/l.
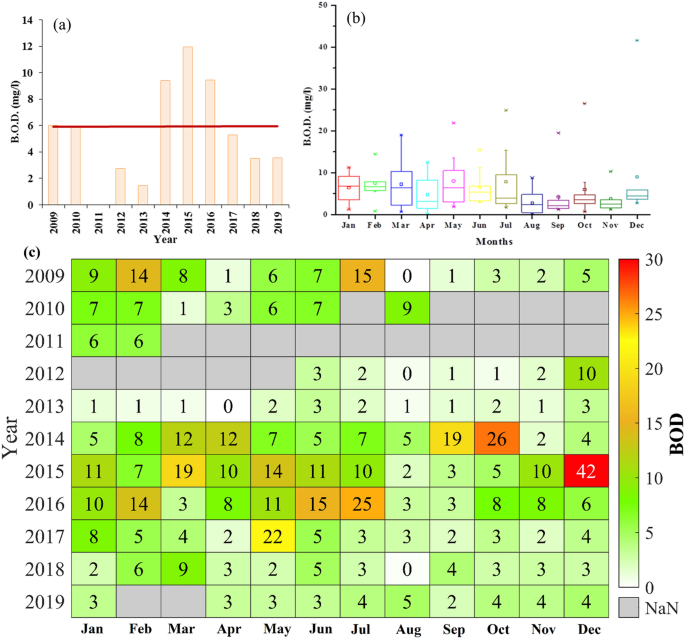
( a ) Temporal variation of yearly mean BOD from 2009 to 2019. The red line shows a linear trend of BOD. ( b ) Monthly distribution of BOD at Palla station from 2009 to 2019. ( c ) Monthly mean variation of BOD from 2009 to 2019.
The median value for April, June, July, October, and December lay between 3 and 5 mg/l. The monthly mean BOD during 2009–19 represented that BOD was maximum in December (8.9 mg/l) followed by the second highest mean value of 8 mg/l in May. The minimum mean value of 2.7 mg/l was found in August, while in April, September, October, and November, the mean BOD lay between 3 and 6 mg/l. Mean BOD from January to March and in July was in the range of 6–7.5 mg/l.
The fluctuation in BOD was observed during 2009–19 in Fig. 6 c. In January, February, and March, a decrease in BOD occurred during 2009–13, while in May, June, and July increase in BOD was seen during 2013–16. In August, September, and October, BOD mostly fell below 5 mg/l throughout the study period. The highest BOD values were measured in December 2015, October 2014, and July 2016 (41.6, 26.5, and 24.8 mg/l, respectively). The decreasing trend was observed for January, February, and July, with a rising trend for March, April, May, and November. From 2014 to 2016, the BOD values were found to be several times higher than the acceptable limits. In March and April, BOD values were less than 4, and else BOD was higher than 4 even in monsoon months. In years 2018–19, BOD is observed to be mostly ≤ 4 mg/l. However, the overall BOD values suggest that the water quality of Yamuna mostly lay beyond the C category (BIS) as the BOD values are mostly > 4 mg/l. To attain river quality standard it has to be ≤ 3 mg/l.
Chemical oxygen demand (COD)
Chemical Oxygen Demand (COD) determines the amount of oxygen required for the oxidation of organic matter present in water. We have shown the changes in COD at Palla station in Fig. 7 . Yearly mean COD varied indistinctly during 2009–19 even though the linear trend has moved upward with increasing year as shown in Fig. 7 a. The yearly mean COD increased from 15.9 to 30.8 mg/l during 2010–2012 and from 11.5 to 44.3 mg/l during 2013- 2015. Mean COD for the years 2018 and 2019 was 15.1 and 20.4 mg/l, respectively. The highest yearly mean COD was 44.33 mg/l for the year 2015 followed by the year 2012 with 30.8 mg/l, while the least mean COD value was observed in 2013 as 11.5 mg/l. A sharp decline in yearly mean COD was noted during the years 2012–13 and 2015–16. The monthly data of each month during 11 years period is shown in Fig. 7 b. The width between the first and third quartiles for January, April, and May indicated maximum variation in COD values. The September quartile indicated less variation in COD as compared with the other months. The CODs for January and May had the highest median values of 27 and 24.5 mg/l, respectively, whereas April had the least median value of 8 mg/l. The median CODs for March, August, September, October, and November lay in the range of 11–16 mg/l. We found that the monthly mean CODs of May and November had maximum and minimum values of 33.9 and 14.2 mg/l, respectively.
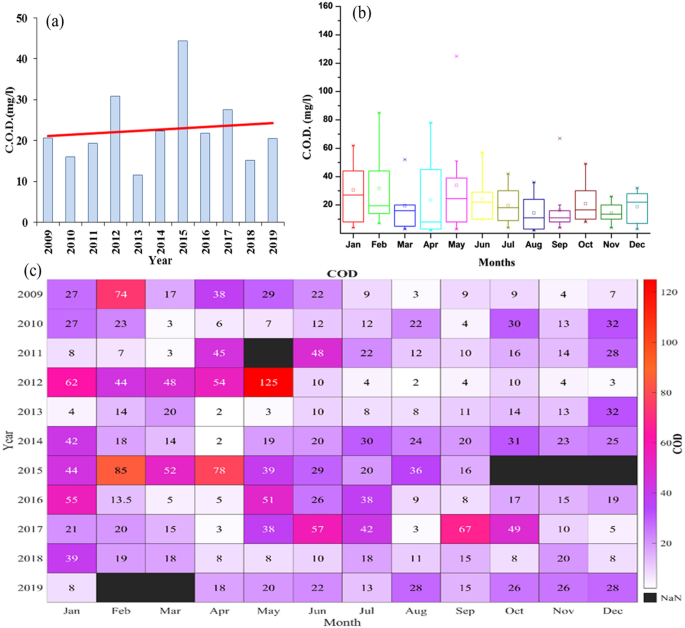
( a ) Yearly variation of COD from 2009 to 2019. The red line shows a linear trend of COD. ( b ) Monthly distribution of COD saturation at Palla station from 2009 to 2019. ( c ) Monthly mean variation of COD from 2009 to 2019.
The trend of COD followed a decreasing path moving from January to December. The monthly mean CODs of January, February, and May were found to be higher than 30 mg/l whereas for April, June, and October they lay between 20 and 30 mg/l, and for the remaining months mean COD was found to be lesser than 20 mg/l. A decline in the mean COD was observed from May to August, with a sharp increase in mean COD from March to May.
Figure 7 c shows changing COD for each month as the year preceding. From 2009 to 2012, the COD values for January, February, March, and April were higher than 30 mg/l, whereas, since 2017, the COD values were seen well below 30 mg/l during these months. In May, an exceptional high COD of 125 mg/l was observed and for the same month, a continuous increase in COD was noted from the years 2013 to 2016. Also, the rise in COD was seen for June, August, October, and December during 2012–15. Excluding May, February, April, and September had maximum CODs of 85, 78, and 67 mg/l, respectively. During 2009–19, January and June to November showed a growing linear trend, whereas the rest of the months had a declining trend. Compared with the international standards, the annual and monthly mean CODs exceeded the WHO guideline and were classified as Classes III-V of UNECE standards. Also, the values were found well above the threshold value. During the years 2009, 2012, and 2013, the monsoon period showed COD value in Class II, while, during the overall monsoon period, water quality lay in Class III. During the year 2015, Yamuna, in terms of COD, was in the worst condition as it lied in Class V. Monthly variation COD for 2018–19 rarely plunged under WHO standards and represented to be in Classes III and IV.
Total alkalinity
Total alkalinity is mostly due to calcium carbonate (CaCO 3 ) and also important for sustaining aquatic life. The yearly mean variation of total alkalinity during 2009–19 is shown in Fig. 8 a. Total alkalinity was found to be the highest during 2015 followed by 2011 with values of 187.37 and 164.6 mg/l, respectively, while, for the rest of the year, the annual means were in the range of 110–150 mg/l. The linear trend for the yearly mean for the entire period remained constant. With these values of total alkalinity, the quality of river water lay in category II (UNECE 1994).
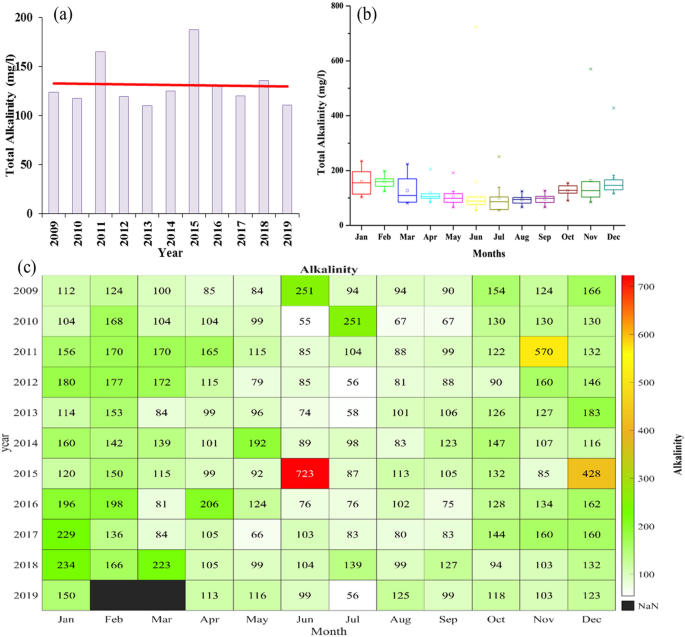
( a ) Yearly variation of total alkalinity from 2009 to 2019. The red line shows a linear trend of total alkalinity. ( b ) Monthly variation of total alkalinity from 2009 to 2019. ( c ) Monthly mean variation of total alkalinity from 2009 to 2019.
We have shown the monthly distribution of total alkalinity in Fig. 8 b with the third quartile of each month lying below the mark of 200 mg/l. The median values dropped from January to July and then raised from July to December. The monthly mean total alkalinities in February and January showed the first and second-highest median values of 159.8 and 155.5 mg/l, respectively, while July and June showed the lowest median values of 86.5 and 88.9 mg/l, respectively. Median values during May, August, and September lay in the range of 90–100 mg/l, while for March, October, November, and December they were between 100 and 150 mg/l. The linear trend for monthly mean total alkalinity also followed the same pattern as the yearly mean. The total alkalinity declined from January to May from 159.4 to 105.5 mg/l and, then from August to December, it raised from 93.8 to 170.7 mg/l. August and December showed the lowest and highest values of total alkalinity, respectively.
The monthly variation in total alkalinity for each year during 2009–19 is shown in Fig. 8 c. June (2015), November (2011), and December (2015) were the months with the highest total alkalinities of 723.3, 569.9, and 428.1 mg/l, respectively. During 2009–19, total alkalinities for February, March, May, July to September remained within 200 mg/l. The lowest total alkalinity of around 55 mg/l was noticed for June (2010) and July (2012). Although the linear trend for monthly total alkalinity showed almost the same slope except for January, July, and November. A wave pattern in total alkalinity for August, September, and October was noticed with an increasing trend during 2009–19. In 2019, the total alkalinity was below 150 mg/l throughout the year 2019. For maintaining WHO and BIS standards, the minimum total alkalinity must be 200 mg/l, while it was not possible to fulfill the standards for both monthly and yearly aspects in the study areas of concern. There were only a few months when total alkalinity was found to be higher than 200. Comparing with UNECE standard, 11 out of 12 monthly mean alkalinities lay in Class II and the remaining one month in Class III category. March 2018 was the last month since 2018 when Yamuna's total alkalinity was well above-mentioned the water standards.
Total dissolved solids (TDS)
Total Dissolved Solids (TDS) define the presence of inorganic compounds along with organic matter in small concentrations originated by naturally, household, and industrial sources. The data was available from 2013 onwards. The yearly mean TDS was found to be highest in 2015 (447 mg/l) followed by 2017 and 2018 with 421 mg/l (Fig. 9 a). The lowest yearly mean TDS was observed as 256 mg/l in 2010, while the recent value of 272 mg/l was observed in 2019. Although the linear trend for yearly TDS indicated the rise in overall TDS. A maximum drop in yearly TDS was observed with a fall of 36% during 2018–19.
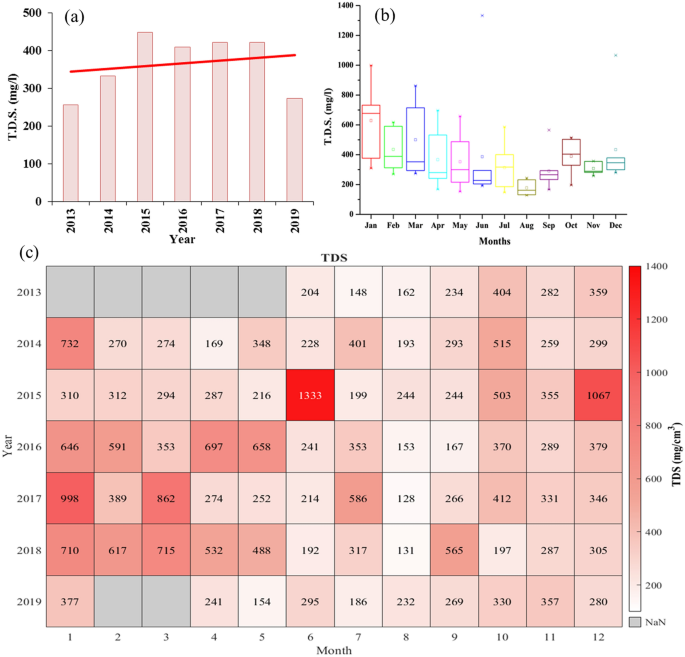
( a ) Yearly variation of TDS from 2013 to 2019. The Red line shows a linear trend of TDS. ( b ) Monthly variation of TDS from 2013 to 2019. ( c ) Monthly mean variation of TDS from 2013 to 2019.
The 25 to 75 percentiles of interquartile range of all twelve months for TDS are shown in Fig. 9 b. Although March had the maximum width of interquartile range, the maximum median value of TDS was 678 mg/l for January. Except for August and October, the median TDS values for the rest of the months lay in the range of 200–400 mg/l. August had the lowest median TDS of 162 mg/l and October had 404 mg/l. A sharp decline in the trend of monthly mean TDS was observed during 2013–19, but mean TDS fluctuated throughout the year. Similarly, with median TDS, the monthly mean TDS for January and August had maximum-minimum mean values of 628.3 and 177.57 mg/l, respectively. Except for August, the monthly mean values of TDS were above the mark of 200 mg/l. From April to July, they lay in the range of 300–400 mg/l, while in February, March, and December, they ranged between 400 and 500 mg/l.
In February and March, TDS had a higher magnitude of rising, while it appeared to be almost constant in August as shown in Fig. 9 c. In January, TDS was measured as 732 mg/l in 2014 and dropped to its lowest point of 310 mg/l in the next year, but then it reached 998 mg/l in 2017. The TDS values during June were well below the mark of 300 mg/l from 2009 to 2019, but during June 2015, the monthly mean TDS was found to be 1333 mg/l. This was the maximum value of TDS during the whole study period. For the same year, the second-highest TDS of 1067 mg/l was also observed in December. TDS remained mostly below 300 mg/l for August and September (mostly during monsoon months) with the lowest TDS of 128 mg/l in August 2017. In 2019, the TDS value mostly ranged between 200 and 300 mg/l. Yearly and monthly mean values of TDS were observed almost under WHO standards and in the Class A category of Indian standards. The TDS value was found below 500 mg/l during each monsoon season. During winter, summer, and post-monsoon months, the TDS of river water never exceeded the Class C category as per BIS standard and during January, the monthly mean average remained higher in comparison to other months.
Total coliform
Human and animal discharges are the main source of fecal coliform bacteria whose excessive presence in water degrades the water quality. During 2009–19, there was an exponential rise in total coliform as shown in Fig. 10 a. The yearly mean in 2009 was 177 MPN/100 ml, which in the decade reached 139,200 MPN/100 ml in 2019. The difference of yearly mean for two periods of 2009–13 and 2016–19 was more than 100 times the value at its starting period. The year 2018 was observed with the highest yearly mean of 490,818 MPN/100 ml, which was reduced in 2019, with the lowest total coliform count of 136.7 MPN/100 ml in 2010. Note that the years 2014–15 were excluded from comparison for this case due to less availability of data for the whole year.
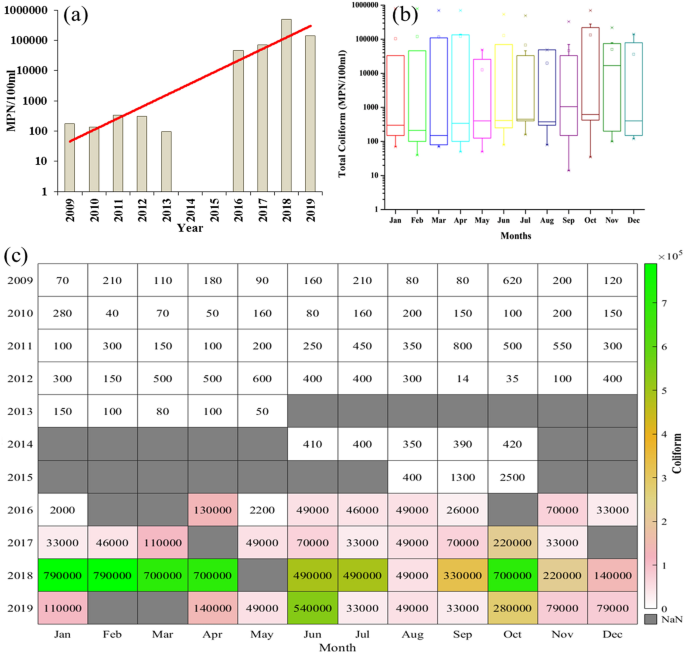
( a ) Yearly variation of total coliform from 2009 to 2019. Redline shows an exponential trend of total coliform. ( b ) Monthly variation of total coliform from 2009 to 2019. ( c ) Monthly mean variation of total coliform from 2009 to 2019.
Figure 10 b shows a large monthly variation in total coliform in each month during 2009–19. The median of most of the months was below 500 MPN/100 ml, whereas median values were 1050, 620, and 16,775 MPN/100 ml for September, October, and November, respectively. The monthly mean for January, February, March, April, June, and October was above 100,000 MPN/100 ml. Maximum and minimum monthly means were observed for October (133,797 MPN/100 ml) and May (12,663 MPN/100 ml), respectively.
Figure 10 c indicates the exponential rise in the trend of total coliform in each month since 2009. Total coliform counts during 2009–14 were well below the 500 MPN/100 ml mark, while some months also showed counts near 800 MPN/100 ml. However, from 2016 onwards, the counts crossed a sustainable mark of 5000 MPN/100 ml for every month of each year. From 2009 to 2013, the total coliform counts fell mostly in Class B but exceeded all limits of Indian water quality standards to a great extent during 2016–19. The monthly mean was not even close to the maximum total coliform limit of 5000 MPN/100 ml, which made water quality in Class D and E categories. WHO standards nullify the presence of fecal coliform in water, whereas the Yamuna River was found to be in its alarming situation for this particular parameter.
Water discharge
The freshwater supply and inflow of wastewater in the river may affect the water quality. To uncover the influence, we analyzed the water discharge in the river Yamuna from 2013 to 2018 (the data is available for this period only). In Fig. 11 , we show the major locations of water abstraction and confluence in the Yamuna river starting from Yamunotri to Faridabad stretch. Hathnikund barrage was constructed to regulate the Yamuna water supply to Haryana, Uttar Pradesh, and Delhi for agricultural and domestic purposes and it was also decided by the Government to maintain 10 cumes of water in the Yamuna downstream to maintain the aqua life in river. In Fig. 12 , we show the water discharge data of Hathnikund, Baghpat, and DRB. From 2013 to 2018, a significant fall in the water discharge is observed especially at Hathnikund, which allows the upstream water to reach Palla. The mean discharge in the river was found to be 123.7 cumes during the whole study period and the minimum was found much lower than 10 cumes (as suggested by the Government of India). Also, a significant fall is observed in recent years. The mean water discharge values at Baghpat are found to be 225 cumes and sometimes they reached far below than 5 cumes.
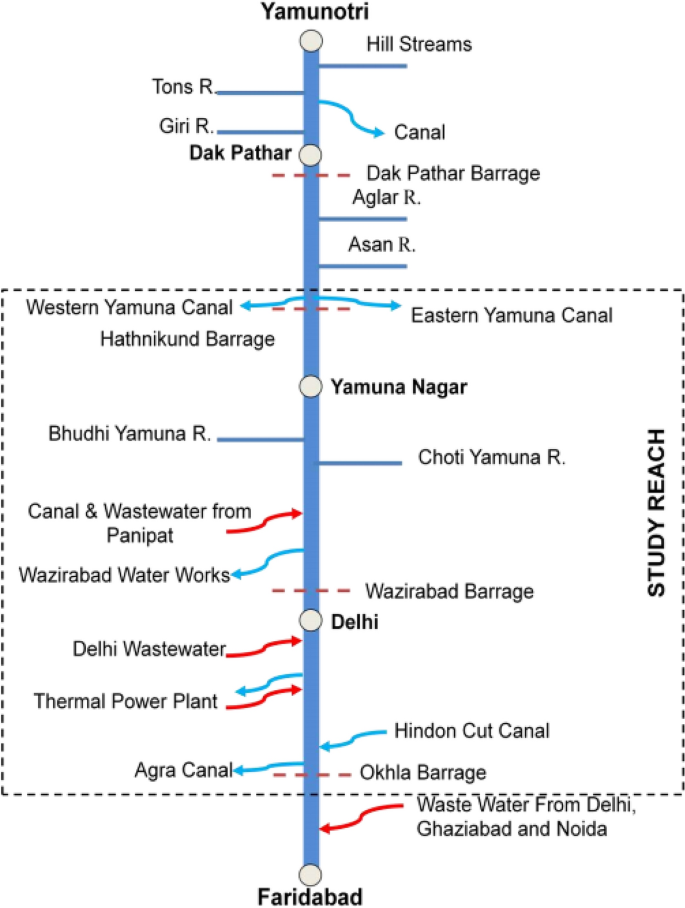
( Source : https://yamuna-revival.nic.in/wp-content/uploads/2020/07/Final-Report-of-YMC-29.06.2020.pdf ).
Points of water abstraction and additions in Yamuna river.
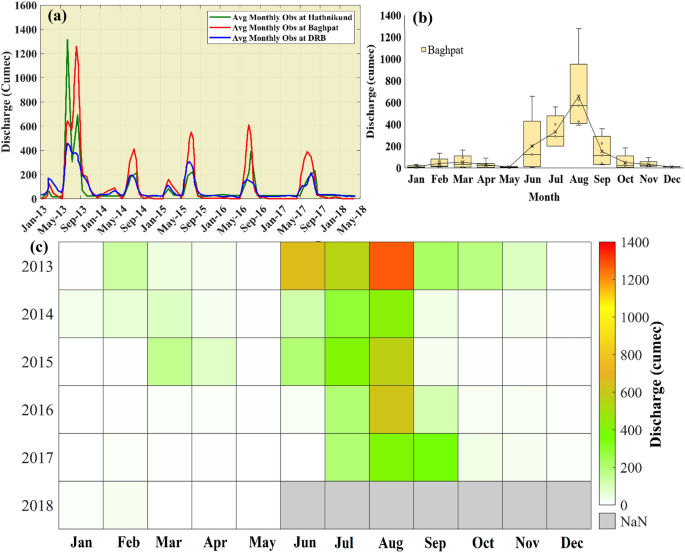
( a ) Temporal variation of water discharge at Hathnikund, Baghpat, and Delhi Railway Bridge (DRB) stations during Jan 2013 to May 2018. ( b ) The distribution of monthly mean water discharge during Jan 2013 to May 2018 at Baghpat. ( c ) Monthly mean variation of discharge from 2013 to 2018.
At DRB, the mean discharge value is found to be 107 cumes. During monsoon months, the water discharge at Baghpat is higher than that at the Hathnikund so that the watershed of the Yamuna also helps in the rise of the water discharge due to rainfall and also major drains confluence in the Yamuna. We can see that during August, the water discharge is the highest followed by July and September. During January, May, and December, the water discharge values reached far below the prescribed limit, and hence sometimes during these seasons, the Yamuna almost dried up and only the sewage and the industrial wastes water flow during these months (Fig. 12 c). After 2015, a significant fall in water discharge is observed during the summer and winter months. With the abstraction of fresh water at Hathnikund barrage and inflow of the drains, the water quality parameters are affected by a large extent in river Yamuna. Therefore, for further analysis of the impact of water discharge and rainfall, we also analyzed the relationship of monthly mean discharge, rainfall, DO saturation, BOD, and COD as shown by the polar plots (Figs. 13 , 14 ).
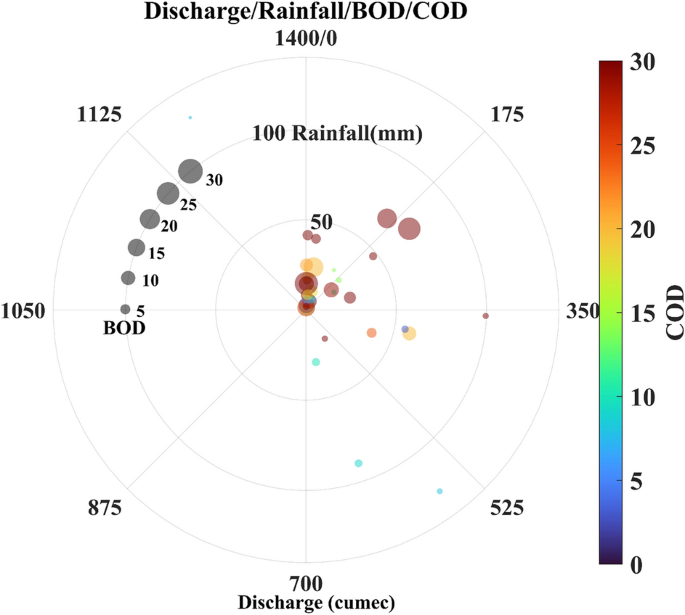
The relationship between the water discharge, Rainfall, BOD, and COD.
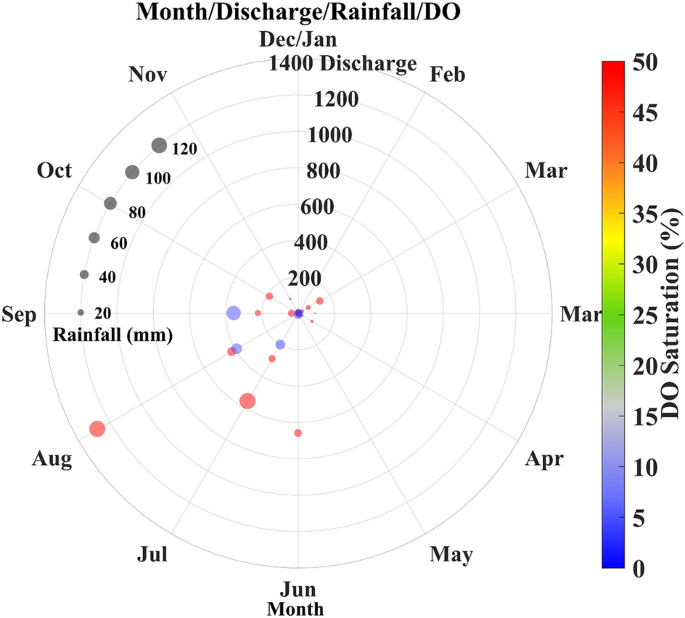
The monthly relationship between the water discharge, Rainfall and DO saturation.
Variability of DO, BOD, and COD
Water quality can be affected by various factors. Hence, we investigated the variability of the DO, BOD, and COD with water discharge and rainfall. In Fig. 13 , we plot the relationship of monthly mean water discharge at Baghpat, rainfall, BOD, and COD. The rainfall mostly affected the discharge values, but, sometimes, with low rainfall high discharge is observed. COD is mostly higher during the whole study period whereas BOD values have shown a fall in recent years. The impact of rainfall and high discharge is visible and low BOD and COD are observed. When the discharge is more than 525 cumes, the COD and BOD values are lower, and also more than 30 mm of rainfall is observed at that time. Further, we investigated the monthly relationship of the Discharge, rainfall, and DO saturation. Further analysis (Fig. 14 ) clearly shows that most rainfall occurred from June to September and caused a rise in the water discharge. During this time, the DO saturation values are more than 50%. The low DO saturation values are mostly observed during the time of low discharge and low rainfall (< 10%). We also found that during July to September with discharge between 200 to 400 cumes and rainfall more than 40 mm, the DO saturation remains lower than 10%. Further, Drain No. 6 having a catchment area of Samalkha Ganur and Sonipat, carries around 49 MLD of sewage in the year 2017 and rises to 210 MLD in the year 2018. Drain No. 8 crosses Drain No. 6 at Akbarpur, Sonipat, and meets river Yamuna just upstream of Palla village. There is a huge increase in the flow of Drain No. 8 between 2017 and 2018 from 196 to 2590 MLD. Drain No. 6 lined separately flow inside Drain No. 8 for 10 KM. Effluents from both drains mix with each other during the rainy season and due to accidental breach ( https://sandrp.in/2015/04/13/blow-by-blow-how-pollution-kills-the-yamuna-river-a-field-trip-report/ ). Similarly, sewage flow of Drain No. 2, which meets river Yamuna 100 km upstream of Delhi is increased from 62 to 2092 MLD between 2017 and 2018 by Central Pollution Control Board of India. As per Haryana State Pollution Control Board (HSPCB), the capacities of Sewage treatment plants (STP) for Drains No. 2, 6, and 8 were 72, 104.5, 125.3 MLD, respectively, till 2018. Also, the capacities of the common effluent treatment plant (CETP) for Drains No. 2, 6, and 8 were 21, 33.2, and 10 MLD, respectively. Since the total capacity for treating wastewater was far beyond sewage generation during 2017–18 and hence the untreated water mixed with the river water. This rise in wastewater generation from industrial and urban areas has caused a drastic decrease in DO saturation and an increase in Total Coliform. As per CPCB 2018 report ( https://yamuna-revival.nic.in/wp-content/uploads/2020/07/Final-Report-of-YMC-29.06.2020.pdf ), 7-day average discharge of the 10-year return period (7Q10) does not meet the habitat requirements of the indicator fish species. These conditions show the impact of rapid urbanization and industrialization along the river bank with high carbon concentration. With the recent development in industrial regions, change in land use/land cover and rapid urbanization in the Haryana, the watershed of Yamuna suffered a lot and hence the water quality of the river. One of the major causes of the sudden fall in the DO saturation in recent years is the fall in the freshwater discharge at the Hathnikund and also the fall in the rainfall in recent years. Also, the deteriorating water quality of Yamuna is a major concern for the Government and mostly this is affected in the stretch between Hathnikund and Palla due to rise in inflow of untreated drains water supply. In recent years, the National Green Tribunal (NGT) of India also requested the states Government to take necessary actions to combat present situation of river Yamuna by installation of more STEPs, ETPs and maintaining the treatment capacity of present treatment plants and channelizing the sewage network to reach treatment plants properly. However, due to lack of adequate fresh water supply and mixing of untreated sewage through regulated and unregulated drains, the quality of the Yamuna river lies in critical conditions.
Although National Capital Territory (NCT) is held responsible for most polluting river Yamuna, the study reveals that the quality of the river it receives is not admirable. The study of physiochemical and biological parameters shows variation in its monthly and yearly values during 2009–19. The effect of monsoon season can be easily seen on parameters like BOD, COD, total alkalinity, TDS and total coliform as their values declined, while DO saturation % showed a significant rise. DO saturation declined by more than 85% during this period. The BOD values improved during the last two years (2018–2019), but were still slightly higher than the permissible limit, while the COD value always remained quite higher than the permissible limits. In 2015, the worst condition was observed in terms of BOD and COD. Total alkalinity also remained low and below the prescribed standards, but TDS is the only parameter whose value was mostly in desired limits throughout the period. An exponential rise was observed in the total coliform count, which was 100–1000 times the maximum limit of IS:2296. Increasing discharge of partially treated industrial effluent and untreated sewage into the Yamuna in the past decade is considered to be the primary cause of the deterioration of water quality. Even after completion of YAP phases I and II, and ongoing phase III, the river still falls in the category of Class D or E under BIS specification, Class III or IV of UNECE standards, and does not fulfill the WHO guideline for water quality at Palla station.
Data availability
All the data used in the present study is freely available in the public domain and the web addresses are discussed in the manuscript, however, we will provide data to all the interested scientists.
Abbreviations
Bureau of Indian Standards
Biological oxygen demand
Common effluent treatment plants
Chemical oxygen demand
Central Pollution Control Board
Central Water Commission
Dissolved oxygen
Effluent treatment plant
Global Environmental Monitoring System
Haryana State Pollution Control Board
India Meteorological Department
Indian Space Research Organization
Kiloliters per day
Modern-Era Retrospective analysis for Research and Applications, Version 2
Monitoring of Indian National Aquatic Resources
Millions of litres per day
Ministry of Environment and Forests
Most probable number
National Capital Region Planning Board
National Capital Territory
National Green Tribunal
National Water Quality Monitoring Programme
State Pollution Control Board
Total dissolved solid
United Nations Economic Commission for Europe
World Health Organization
Water Resources and Information System
Yamuna Action Plan
CPCB 1980-81, The Ganga River Part I The Yamuna basin ADSORBS/22 . Central Pollution Control Board, Delhi, India (1981).
Jain, C. K. Metal fractionation study on bed sediments of River Yamuna, India. Water Res. 38 , 569–578. https://doi.org/10.1016/j.watres.2003.10.042 (2004).
Article CAS PubMed Google Scholar
Lokhande, S. & Tare, V. Spatio-temporal trends in the flow and water quality: Response of river Yamuna to urbanization. Environ. Monit. Assess. https://doi.org/10.1007/s10661-021-08873-x (2021).
Article PubMed Google Scholar
CPCB (2006) Water Quality Status Of Yamuna River (1999 – 2005). Central Pollution Control Board, Ministry Of Environment & Forests, Assessment and Development of River Basin Series: ADSORBS/41/2006-07.
CPCB (2003) Annual Report 2002–2003,Water Quality Status of River Yamuna in Delhi.
Misra, A. K. A river about to die: Yamuna. J. Water Resour. Prot. 02 , 489–500. https://doi.org/10.4236/jwarp.2010.25056 (2010).
Article CAS Google Scholar
Sharma, D., Kansal, A. & Pelletier, G. Water quality modeling for urban reach of Yamuna river, India (1999–2009), using QUAL2Kw. Appl. Water Sci. 7 , 1535–1559. https://doi.org/10.1007/s13201-015-0311-1 (2017).
Article ADS CAS Google Scholar
Manju, S. & Smita, C. Assessment of ground water quality in vicinity of industries and along Yamuna River in Yamuna Nagar, Haryana, India. Asian J. Sci. Technol. 4 , 54–61 (2013).
Google Scholar
Sharma, S. & Chhabra, M. S. Understanding the chemical metamorphosis of Yamuna River due to pollution load and human use. Int. Res. J. Environ. Sci. 4 , 2319–1414 (2015).
Kumar, B., Singh, U. K. & Ojha, S. N. Evaluation of geochemical data of Yamuna River using WQI and multivariate statistical analyses: A case study. Int. J. River Basin Manag. 17 , 143–155. https://doi.org/10.1080/15715124.2018.1437743 (2019).
Article Google Scholar
Pachamuthu Muthaiyah, N. Rejuvenating Yamuna river by wastewater treatment and management. Int. J. Energy Environ. Sci. 5 , 14. https://doi.org/10.11648/j.ijees.20200501.13 (2020).
Parween, M., Ramanathan, A. & Raju, N. J. Waste water management and water quality of river Yamuna in the megacity of Delhi. Int. J. Environ. Sci. Technol. 14 (10), 2109–2124. https://doi.org/10.1007/s13762-017-1280-8 (2017).
Arora, D. P. With most STPs out of order, Haryana continues to pollute the Yamuna [WWW Document]. Hindustan Times, Gurugram (2019). https://www.hindustantimes.com/gurugram/with-most-stps-out-of-order-haryana-continues-to-pollute-the-yamuna/story-4XTWZ9ErsWZrHvk17gWv0M.html . Accessed 16 Feb 2020.
CPCB 1982–1983 (1983) Assimilation capacity of point pollution load, CUPS/12
CPCB 1999–2000 (2000) Water quality status of Yamuna River, ADSORBS/32
Bhardwaj RM (2005) Water quality monitoring in India-achievements and constraints. IWG-Env International Work Session on Water Statistics 1–12
Sharma, A. Here We Go Again: Yamuna Action Plan Phase III [WWW Document]. Delhi Greens (2018). https://delhigreens.com/2018/12/29/here-we-go-again-yamuna-action-plan-phase-iii/ . Accessed 4 Oct 2020.
Anand, C., Akolkar, P. & Chakrabarti, R. Bacteriological water quality status of River Yamuna in Delhi. J. Environ. Biol. 27 , 97–101 (2006).
PubMed Google Scholar
Hassan, T., Parveen, S., Nabi, B. & Ahmad, U. Seasonal variations in water quality parameters of river Yamuna, India. Int. J. Curr. Microbiol. Appl. Sci 6 , 694–712. https://doi.org/10.20546/ijcmas.2017.605.079 (2017).
Mutiyar, P. K., Gupta, S. K. & Mittal, A. K. Fate of pharmaceutical active compounds (PhACs) from River Yamuna, India: An ecotoxicological risk assessment approach. Ecotoxicol. Environ. Saf. 15 (150), 297–304. https://doi.org/10.1016/j.ecoenv.2017.12.041 (2018).
Jaiswal, M., Hussain, J., Gupta, S. K., Nasr, M. & Nema, A. K. Comprehensive evaluation of water quality status for entire stretch of Yamuna River, India. Environ. Monit. Assess. 191 , 208. https://doi.org/10.1007/s10661-019-7312-8 (2019).
Kaur, L., Rishi, M. S. & Arora, N. K. Deciphering pollution vulnerability zones of River Yamuna in relation to existing land use land cover in Panipat, Haryana, India. Environ. Monit. Assess. 193 , 1–22. https://doi.org/10.1007/s10661-020-08832-y (2021).
Patel, P. P., Mondal, S. & Ghosh, K. G. Some respite for India’s dirtiest river? Examining the Yamuna’s water quality at Delhi during the COVID-19 lockdown period. Sci. Total Environ. 744 , 140851. https://doi.org/10.1016/j.scitotenv.2020.140851 (2020).
Article ADS CAS PubMed PubMed Central Google Scholar
Paliwal, R., Sharma, P. & Kansal, A. Water quality modelling of the river Yamuna (India) using QUAL2E-UNCAS. J. Environ. Manag. 83 , 131–144. https://doi.org/10.1016/j.jenvman.2006.02.003 (2007).
Krishan, G., Singh, S., Sharma, A., Sandhu, C., Grischek, T. et al. Assessment of river quality for river bank filtration along Yamuna River in Agra-Mathura districts of Uttar Pradesh. In Proceedings of National Conference on Monitoring and Management of Drinking Water Quality (MMDWQ) & XXVIII Annual Conference of National Environment Science Academy during 21–23 December, 2015 at UCOST, Dehradun (2015).
Kaur, H., Warren, A. & Kamra, K. Spatial variation in ciliate communities with respect to water quality in the Delhi NCR stretch of River Yamuna, India. Eur. J. Protistol. 79 , 125793. https://doi.org/10.1016/j.ejop.2021.125793 (2021).
UNECE (1994) Standard Statistical Classification of Surface Freshwater Quality for the Maintenance of Aquatic Life. In Readings in International Environment Statistics . United Nations Economic Commission for Europe, United Nations, New York and Geneva.
HSPCB (2018) Action Plan for River Yamuna November 2018
Satyanarayana, P. & Sharma, J. R. Web Enabled Water Resources Information System for India . ARC India News (2009).
Global Modeling and Assimilation Office (GMAO). MERRA-2 tavg1_2d_slv_Nx: 2d,1-Hourly,Time-Averaged,Single-Level,Assimilation,Single-Level Diagnostics V5.12.4, Greenbelt, MD, USA, Goddard Earth Sciences Data and Information Services Center (GES DISC) [WWW Document] (2015). https://doi.org/10.5067/VJAFPLI1CSIV . Accessed 8 Oct 2020.
Gelaro, R. et al. The modern-era retrospective analysis for research and applications, version 2 (MERRA-2). J. Clim. 30 , 5419–5454. https://doi.org/10.1175/JCLI-D-16-0758.1 (2017).
Article ADS PubMed Google Scholar
Guhathakurta, P., Narkhede, N., Menon, P., Prasad, A. K. & Sangwan, N. (2020) Observed Rainfall Variability and Changes over Haryana State. Report on ESSO/IMD/HS/Rainfall Variability/09(2020)/33.
Servais, P., Seidl, M. & Mouchel, J. M. Comparison of parameters characterizing organic matter in a combined sewer during rainfall events and dry weather. Water Environ. Res 71 , 408–417. https://doi.org/10.2175/106143097X122112 (1999).
Khan, M. Y. A., Gani, K. M. & Chakrapani, G. J. Spatial and temporal variations of physicochemical and heavy metal pollution in Ramganga River—A tributary of River Ganges, India. Environ. Earth Sci. 76 , 231. https://doi.org/10.1007/s12665-017-6547-3 (2017).
Kumar, V., Sharma, A., Thukral, A. K. & Bhardwaj, R. Water quality of River Beas, India. Curr. Sci. 112 , 1138–1157. https://doi.org/10.18520/cs/v112/i06/1138-1157 (2017).
Liu, J. et al. Spatial scale and seasonal dependence of land use impacts on riverine water quality in the Huai River basin, China. Environ. Sci. Pollut. Res. 24 , 20995–21010. https://doi.org/10.1007/s11356-017-9733-7 (2017).
Singh, K. P., Malik, A., Mohan, D. & Sinha, S. Multivariate statistical techniques for the evaluation of spatial and temporal variations in water quality of Gomti River (India)—A case study. Water Res. 38 , 3980–3992. https://doi.org/10.1016/j.watres.2004.06.011 (2004).
Prasad, B. S. R. V., Srinivasu, P. D. N., Varma, P. S., Raman, A. V. & Ray, S. Dynamics of dissolved oxygen in relation to saturation and health of an aquatic body: A case for Chilka Lagoon, India. J Ecosyst 2014 , 1–17. https://doi.org/10.1155/2014/526245 (2014).
Ravindra, K., Meenakshi, A., Rani, M. & Kaushik, A. Seasonal variations in physico-chemical characteristics of River Yamuna in Haryana and its ecological best-designated use. J. Environ. Monit. 5 , 419–426. https://doi.org/10.1039/b301723k (2003).
Download references
Acknowledgements
We would like to thank the National Water Informatics Centre (NWIC), a unit of the Ministry of Jal Shakti for providing updated data on water resources through a ‘Single Window’ source. We also thank research center O.I.E of Mines Paris Tech and ARMINES for providing meteorological data. The data used in the current study were freely available and their links are mentioned in their respective places. We express a great sense of gratitude towards the Central Pollution Control Board of India and other agencies for making data available.
This research was financially supported by the Ministry of Science and Technology (MOST) of Taiwan under the codes MOST 109-2923-E-008-004-MY2 and MOST 110-2111-M-008-008.
Author information
Authors and affiliations.
Department of Civil Engineering, SRM IST, Delhi-NCR Campus, Ghaziabad, India
Pankaj Joshi
Centre for Space and Remote Sensing Research, National Central University, Taoyuan City, Taiwan
Akshansha Chauhan & Yuei-An Liou
Department of Engineering, University of Technology and Applied Sciences, Suhar, 311, Sultanate of Oman
Department of Chemistry, SRM IST, Delhi-NCR Campus, Ghaziabad, India
Sudheer Malik
National Museum Marine Science and Technology (NMMST), No. 367, Beining Rd., Zhongzheng Dist., Keelung City, 202010, Taiwan
Yuei-An Liou
You can also search for this author in PubMed Google Scholar
Contributions
Conceptualization—A.C. and Y.-A.L., Data analysis: P.J. and A.C., Methodology: P.J., S.M., Y.-A.L., Writing—original draft by P.J., A.C., S.M., P.D.; Review and editing—A.C., P.D., and Y.-A.L.
Corresponding author
Correspondence to Yuei-An Liou .
Ethics declarations
Competing interests.
The authors declare no competing interests.
Additional information
Publisher's note.
Springer Nature remains neutral with regard to jurisdictional claims in published maps and institutional affiliations.
Rights and permissions
Open Access This article is licensed under a Creative Commons Attribution 4.0 International License, which permits use, sharing, adaptation, distribution and reproduction in any medium or format, as long as you give appropriate credit to the original author(s) and the source, provide a link to the Creative Commons licence, and indicate if changes were made. The images or other third party material in this article are included in the article's Creative Commons licence, unless indicated otherwise in a credit line to the material. If material is not included in the article's Creative Commons licence and your intended use is not permitted by statutory regulation or exceeds the permitted use, you will need to obtain permission directly from the copyright holder. To view a copy of this licence, visit http://creativecommons.org/licenses/by/4.0/ .
Reprints and permissions
About this article
Cite this article.
Joshi, P., Chauhan, A., Dua, P. et al. Physicochemical and biological analysis of river Yamuna at Palla station from 2009 to 2019. Sci Rep 12 , 2870 (2022). https://doi.org/10.1038/s41598-022-06900-6
Download citation
Received : 08 December 2021
Accepted : 07 February 2022
Published : 21 February 2022
DOI : https://doi.org/10.1038/s41598-022-06900-6
Share this article
Anyone you share the following link with will be able to read this content:
Sorry, a shareable link is not currently available for this article.
Provided by the Springer Nature SharedIt content-sharing initiative
This article is cited by
Water quality modeling-based assessment for the scope of wastewater treatment of the urban reach of river yamuna at delhi, india.
- Nibedita Verma
- Geeta Singh
- Naved Ahsan
Environmental Monitoring and Assessment (2024)
By submitting a comment you agree to abide by our Terms and Community Guidelines . If you find something abusive or that does not comply with our terms or guidelines please flag it as inappropriate.
Quick links
- Explore articles by subject
- Guide to authors
- Editorial policies
Sign up for the Nature Briefing newsletter — what matters in science, free to your inbox daily.

- Reference Manager
- Simple TEXT file
People also looked at
Original research article, analysis of water pollution using different physicochemical parameters: a study of yamuna river.
- 1 Department of Electronics and Communication Engineering, Faculty of Engineering and Technology, SRM Institute of Science and Technology, Delhi- NCR Campus, Ghaziabad, India
- 2 Department of Computer Science and Engineering, Gandhi Institute of Engineering and Technology University, Gunupur, India
- 3 School of Computer Engineering, Kalinga Institute of Industrial Technology Deemed to Be University, Bhubaneswar, India
- 4 Department of Civil, Environmental and Natural Resources Engineering, Lulea University of Technology, Lulea, Sweden
- 5 Department of Electronics and Communication Engineering, Krishna Institute of Engineering and Technology, Group of Institutions, Ghaziabad, India
- 6 Department of Computer Science, SRM Institute of Science and Technology, NCR Campus, Ghaziabad, India
- 7 Department of Applied Sciences and Humanities, Teerthankar Mahaveer University, Moradabad, India
- 8 Institute of Research and Development, Duy Tan University, Da Nang, Vietnam
- 9 Geotechnical Engineering and Artificial Intelligence Research Group (GEOAI), University of Transport Technology, Hanoi, Vietnam
The Yamuna river has become one of the most polluted rivers in India as well as in the world because of the high-density population growth and speedy industrialization. The Yamuna river is severely polluted and needs urgent revival. The Yamuna river in Dehradun is polluted due to exceptional tourist activity, poor sewage facilities, and insufficient wastewater management amenities. The measurement of the quality can be done by water quality assessment. In this study, the water quality index has been calculated for the Yamuna river at Dehradun using monthly measurements of 12 physicochemical parameters. Trend forecasting for river water pollution has been performed using different parameters for the years 2020–2024 at Dehradun. The study shows that the values of four parameters namely, Temperature, Total Coliform, TDS, and Hardness are increasing yearly, whereas the values of pH and DO are not rising heavily. The considered physicochemical parameters for the study are TDS, Chlorides, Alkalinity, DO, Temperature, COD, BOD, pH, Magnesium, Hardness, Total Coliform, and Calcium. As per the results and trend analysis, the value of total coliform, temperature, and hardness are rising year by year, which is a matter of concern. The values of the considered physicochemical parameters have been monitored using various monitoring stations installed by the Central Pollution Control Board (CPCB), India.
Introduction
Due to historical, geographical, religious, political, and sociocultural reasons, India has a unique place in the world Agarwal et al., 2016 . Pollution-causing activities have caused severe changes in aquatic environments over the last few decades. Serious questions have been raised in context to the safe use of river water for drinking and other purposes in recent times. Numerous contaminants are playing a major role in polluting the river water. It is one of the main concerns for most of the metropolitan cities of developing nations. Rivers play a vital role in shaping up the natural, cultural, and economic aspects of any country ( Rafiq, 2016 ). The Yamuna river is one such river. The Yamuna river provides sustenance to ecology and is therefore considered holy by the people of India. It derives from the glacier called Yamunotri in the Himalayan ranges. States through which the Yamuna river flows are the Uttarakhand, Himachal Pradesh, Uttar Pradesh, Haryana, and Delhi. The Yamuna river is also divided into several tributaries such as the Hindon, Tons, Giri, Rishiganga, Hanuman Ganga, Sasur Khaderi, Chambal, Betwa, Ken, Sindh, and Baghain as it is flowing through several cities. These cities are the Yamuna Nagar, Delhi, Faridabad, Mathura, Agra, Etawah, and Prayagraj. It is a tributary of the river Ganges in India. Two of them together have had substantial importance in shaping up the history and geography of our country. The river on which our research primarily focuses is the Yamuna river. It passes through several states such as Uttar Pradesh, Himachal Pradesh, Uttarakhand, Haryana, and Delhi. It has a length of approximately 1,380 km. More than 600 lakh people are dependent on their living and income on this river ( Census Reports of India 2001 , 1971–1991 ). Such is the greatness of this river. Our research is based on the Yamuna river in Dehradun in Uttarakhand.
The process, in which the people from rustic areas shift to the town areas in search of a brighter future, thus resulting in a drastic increment in the population of people living in cities, is called urbanization. As a result, the number of cities and towns increases exponentially. There is an atrocious amount of stress on the weakening natural resources. As it is, the natural resources are facing major deterioration issues considering the unthoughtful plundering by the people. In the last few decades, the rate of spread in various segments of the world has been unprecedented and unimaginable. The proportion of the rate of infrastructure expansion has not been able to match up to the pace of urbanization in most cities. The amplified requirement of water, deficiency of sewage facilities, and scarce wastewater treatment facilities rigorously affect the water resources, and change the environment and ecology. Agricultural lands, rural unpaved areas, and natural wetlands are converted into paved and impervious urban areas, during urbanization. Augmented impervious land surface in urbanized areas leads to severe and radical changes in the natural order of things ( Ahmad et al., 2017 ). There has been a drastic decline in the Yamuna river water quality since the last few years. The water is highly polluted, and it is a joint responsibility of the government and all the citizens to make sure that the Yamuna river is clean again. The primary step toward understanding and deliberating about the sorts of water pollution and developing effective reduction strategies is monitoring ( Marale, 2012 ). Physical, chemical, and biological compositions determine the quality of water ( Allee and Johnson, 1999 ). The substances such as heavy metals, pesticides, detergents, and petroleum form the chemical composition ( Tiwari et al., 2020 ). Turbidity, color, and temperature comprise the physical composition, whereas the biological arrangement includes pigments and planktons. Observation and analysis of these water quality parameters need sampling from extensively distributed locations, which is time consuming and requires a lot of field and lab efforts to come up with statistical results ( Wang et al., 2004 ; Icaga, 2007 ; Kazi et al., 2009 ; Amandeep, 2011 ; Duong, 2012 ; Singh et al., 2013 ; Nazeer and Nichol, 2015 ; Shi et al., 2018 ).
Conventionally, monitoring-based methods are used to find out the water quality parameters. They involve wide-ranging field sampling and expensive lab analysis, which is time inefficient and can only be accomplished for areas that are smaller ( Song et al., 2012 ). Hence, these restraints and drawbacks make the conventional methods challenging for continuous water quality prediction at spatial scales ( Panwar et al., 2015 ; Chabuk et al., 2017 ). For observing and analyzing water quality parameters, such as turbidity, chlorophyll, temperature, and suspended inorganic materials, techniques, such as optical remote sensing, are being used ( Pattiaratchi et al., 1994 ; Fraser, 1998 ; Kondratyev et al., 1998 ). To calculate the measure of solar irradiance at varied wavelength bands reflected by the surface water, remote sensing satellite sensors are used ( Zhang et al., 2003 ; Dwivedi and Pathak, 2007 ; Girgin et al., 2010 ; Ronghang et al., 2019 ). Amplified demand for water, poor sewage facility, and insufficient wastewater management amenities, relentlessly affect the resources of water resources. Models such as hydrological models have been used to evaluate the effect of numerous factors in rain-related procedures of the cosmopolitan areas ( Trombadore et al., 2020 ). Knowledge and information about interconnections between climate, population, and ecology are essential for understanding and promoting sustainable development ( Sharma et al., 2020 ). It also requires better knowledge of equipment and methodical planning. Proper management will reduce the degradation of rivers ( Shukla et al., 2018 ). In this study, we focus on trying to find out contaminants in the river, finding the water pollution index, and subsequently enforcing measures to curb water pollution.
Contribution of the Study:
1. In the present study, water samples were collected every year from the Yamuna river canal in Dehradun, Uttarakhand, India.
2. The samples have been analyzed for 12 different physicochemical attributes like ph, BOD, COD, Total Coliform, Temp, DO, Alkalinity, Chlorides, Calcium, Magnesium, and Hardness as Calcium Carbonate and TDS.
3. The measurement of the water quality index has been taken into consideration for the years 2017, 2018, and 2019.
4. Forecasting the pollution trend for the Yamuna river water from 2016 to 2024.
Materials and Methods
Mathematical model.
In this research paper, the water sample of the Yamuna river is considered for analysis. The 12 physicochemical parameters in the water are studied and analyzed. The water sample of the Holy River called the Yamuna river is considered for a certain period. The ratio of water components mainly Temperature, Total Coliform, TDS, and Hardness are varied irregularly at various locations of India. Due to the abrupt changes in the water component, the water quality is also changed. In this research paper, a sampling distribution-based analytical model called Equipoise Evaluator (EE) is proposed for the discrete parameter value of the water components. The EE model is suitable to analyze random discrete parameters. The EE model can be applied for any kind of sample analysis where the analysis is based on sample molecules. To analyze the discrete sample in the form of the symmetric normal distribution for a particular location, the EE model is applied. In this research paper, the water sample varies based on the molecules of e water components. This EE model is also applicable for the analysis of metallurgy to detect the impurity of the metal. In this research paper, the EE model is deployed for the water sample of the Yamuna river.
Sampling distribution is proposed to transform the variable at different levels.
As per the linear transformation
Now, by applying the Jacobian transformation on a non-singular matrix M,
From Equation (2), the relational equation for all connected differential elements is defined as Equation (3)
As M is considered as an orthogonal matrix, hence Z = MW, which transformed into a quadratic form of preserving from the standard value.
To determine the dissimilarity distance from a standard sample value, the partitioning matrix is deployed.
Assume that matrix M is partitioned into qth numbers, then M i M j ′ = 0 ∀ i ≠ j .
As per the partitioning matrix, all q sub-matrices are orthogonal to each other except orthogonal themselves. Now, Equation (1) is rewritten as
where, M 1 ,…., M q are an exclusive subset of the tested variables.
Applying transformation in Equation (7)
where, B i = ( M i M i ′ ) - 1 a n d η i = M i μ
Equation (8) determines the transformation of each partition into quadratic form with exclusive subsets of tested parameters. In this analysis, M is considered as fully orthogonal, with each row orthogonal to every other row. The result of transforming all the variables to test bed data variables of D is then,
It is considered that the water molecules of the sample water have symmetric normally distributed for a particular location. The mean of the water molecules is z ¯ = 1 q ∑ i = 1 q w i .
As per orthogonal transformation
where u 1 = q z ̄ and σ = u 1 2 + u 2 2 + . . . . + u q 2 .
u 1 and σ are independently distributed. The sample mean and sample variance of the experimented sample water are independently distributed.
Water Quality Index and Trend Analysis
The primary focus of this study is to measure and analyze the drastic changes in the Yamuna river water quality at Dehradun, Uttarakhand. Standardized and the universally accepted water quality index (WQI) has been adopted to measure the variation in water quality of the Yamuna river at the prime location of the study—Dehradun over 3 years. The standard method has been used to examine and evaluate the water quality for 12 Physicochemical parameters (TDS, Chlorides, Alkalinity, DO, Temperature, COD, BOD, pH, Magnesium, Hardness, Total Coliform, and Calcium). In this study, the water quality index has been calculated using the different Physicochemical parameters documented and verified from the monitored locations. The water quality index (WQI) is stated by
where Ii signifies the ith water quality parameters, the weight associated and related to the parameters is denoted by Wi, and p notifies us about the number of water quality parameters. This WQI is based on the index introduced by the NSF (National Sanitation Foundation) ( Bhutiani et al., 2016 ). This index is established by the Central Pollution Control board with different advancements in terms of water quality criteria. The Water quality index is supported and developed by the National Sanitation Foundation (NSF) ( Brown et al., 1970 ). It is also known as NSF-WQI. This water quality index is denoted as
where P denotes the ith parameter measured values, quality rating is denoted by q i , and the relative weight of the ith parameters is denoted by w i .
The water quality index arithmetic index was presented ( Cude, 2001 ). It is a very popular and standard method used by many investors and researchers in their studies ( Ramakrishniah et al., 2009 ; Ahmad et al., 2012 ).
In this study, the quality rating can be calculated using the following equation:
where q i signifies the ith parameter quality rating for n water quality parameters, water.
The quality parameters' actual and definite value is denoted by V actual , the parameters ideal value is symbolized by V ideal , and the standard value of the parameters, which is suggested by the WHO, is denoted by V standard . The ideal values for DO and pH are 14.6 and 7 mg/L, whereas for the other parameters, it is equal to zero. After the calculation of quality rating, (relative weight), Wi has to be calculated by inversing the standard value of the parameter. Finally, the following equation was used to calculate the overall water quality index (WQI):
Here, signifying the relative weight and quality rating is symbolized by Wi and qi .
Trend Analysis
In this study, to forecast the pollution trend analysis, the linear regression model has been used. According to the linear regression model, the relationship between the two variables a and b can be expressed as:
Where x and y are the model parameters, which are known as regression coefficients, and B is the dependent variable. A is known as an independent variable, and € is the error variable. For making a prediction using a linear regression model is
The parameters x and y are calculated using the following equations:
Methodology
The flow chart for the methodology used is shown in Figure 1 . The water quality index is calculated using the weight arithmetic water quality index method, which has been discussed in the Water Quality Index and Trend Analysis section.
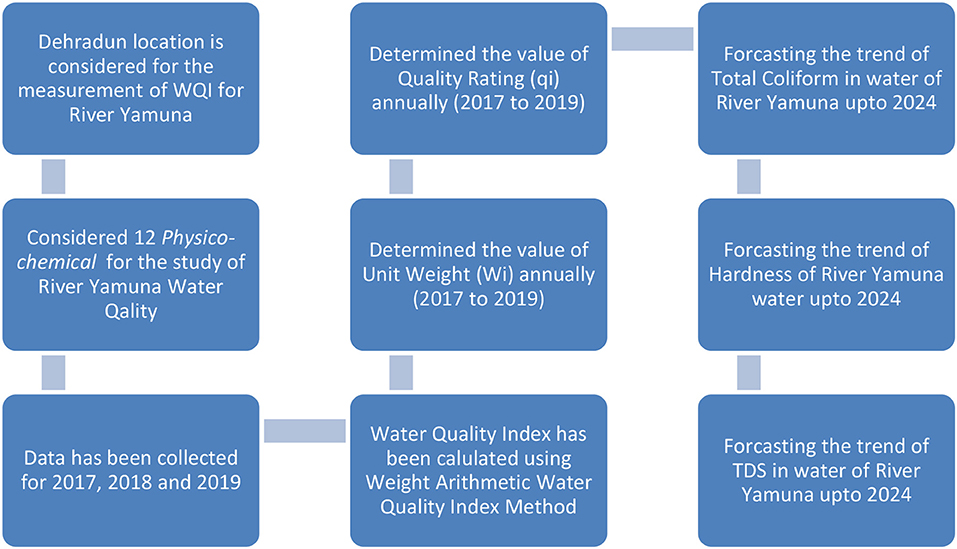
Figure 1 . Flow chart for the methodology used.
The value of the water quality index has been compared with the standard values of WQI, which is shown in Table 1 . The water quality rating is divided into five categories. The range from 0 to 25 is coming under (A) grading with excellent water quality, the range from 26 to 50 is for grading (B) with good water quality, and respectively, (C), (D), and (E) gradings are categorized for different WQI values ( Chauhan and Singh, 2010 ).
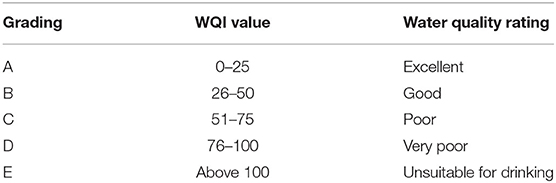
Table 1 . The standard values of water quality index (WQI) using weight arithmetic water quality index method.
Dataset Collection
The most populous city of Uttarakhand is Dehradun also spelled Dear Doon. It is the capital of Uttrakhand, which is one among the 28 states in India ( Figure 2 ). It is famous for its Doon Basmati Rice. Dehradun city has famous institutions like IMA (Indian Military Academy) regarded as one of the best officer training academies in India, Forest Research Institute, Indian Institute of Petroleum, and the famous ONGC training institute. This city is also famous among the tourists. It has many adventurous activities like rafting, bungee jumping, paragliding, etc. ( Rafiq, 2016 ). The city is located about 255 km from New Delhi and 168 km from Chandigarh. The climate condition of Dehradun is humid, subtropical, and a summer temperature can reach a maximum of 44°C. This city is also located very close to Nainital, which has the famous Jim Corbett National Park attracting many tourists ( Bhutiani et al., 2015 ).
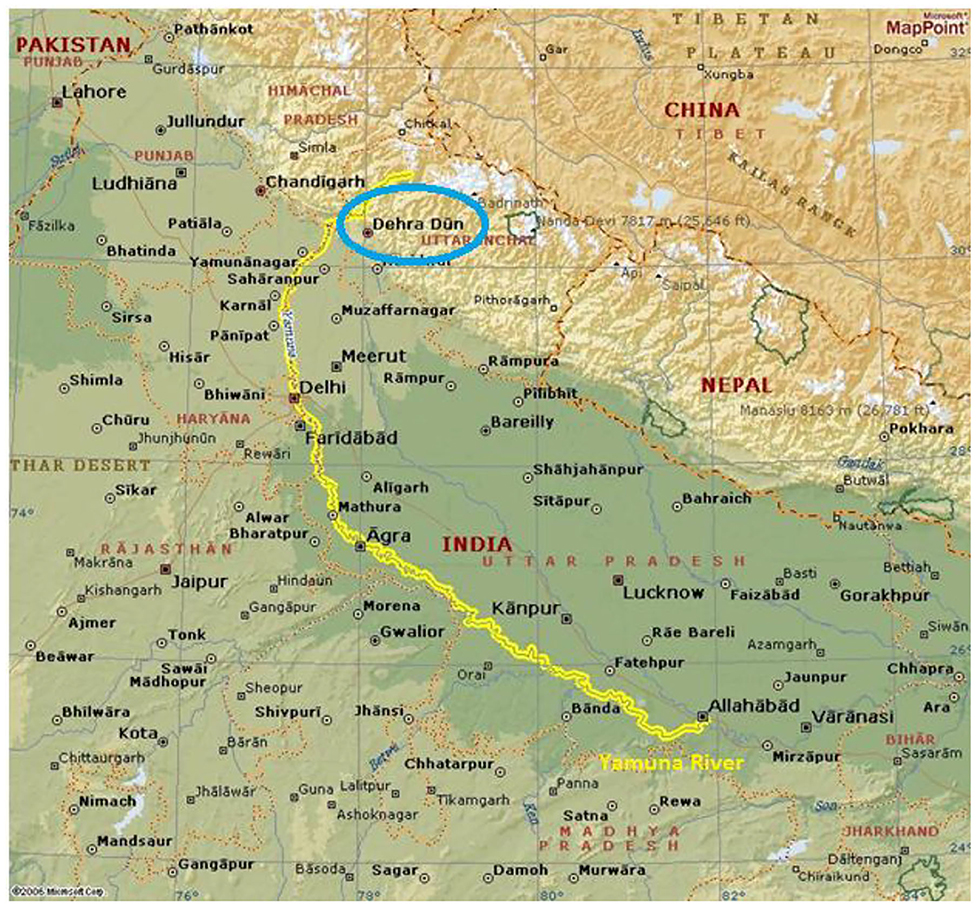
Figure 2 . Map for considering location for Yamuna, Dehradun ( https://www.bcmtouring.com/forums/thread ).
The present study was undertaken for a period of 3 years from 2017 to 2019 to check the water quality analysis for the physicochemical attributes below. In the present study, water samples were collected on a yearly basis from the Yamuna river canal in Dehradun, Uttarakhand, India. The samples were analyzed for 12 different physicochemical attributes like ph, BOD, COD, Total Coliform, Temp, DO, Alkalinity, Chlorides, Calcium, Magnesium, and Hardness as Calcium Carbonate, and TDS ( Tyagi et al., 2020 ). The Yamuna river plays a very crucial role in Dehradun's geography. The Yamuna river is severely polluted and needs urgent revival. The river passes through Uttarakhand. Uttarakhand has always been a tourist spot and experiences heavy tourists perennially, and Dehradun, being the capital city, also bears the brunt. The Yamuna river in Dehradun is polluted due to the exceptional tourist activities. Dehradun is also famous for the Kumaoni Holi, Jhanda Fair, Tapkeshwar Mela, and Bissu Mela. A lot of waste materials are dumped into the Yamuna river, and they contaminate the river. Water might be untreated for long spans of time. Also, a lot of industries–primarily biotechnology and food processing, are set up in Dehradun; they also mindlessly dump their waste in the Yamuna river. Industrial waste is not fully responsible for the pollution, but some poor sewage systems and human activities are also responsible for it ( Bhutiani and Khanna, 2007 ).
Dehradun is a home to many agricultural and horticulture activities such as rice, litchi, and tea plantations. Agricultural waste also plays a major role in polluting the Yamuna river in Dehradun. The pollution is also increased by the excessive usage of insecticides and pesticides ( Tiwari et al., 2020 ). There are also people who wash their clothes, utensils, and defecate in or around the river, thus leading to pollution. The stretch of the Yamuna river in Dehradun thus has a lot of coliform bacteria. Government projects such as road construction might also be responsible for dumping waste, although rules have been drastically upgraded in the last two decades or so. Some cattle washing activities and religious activities also polluted the Yamuna river ( Bhutiani et al., 2018 ).
Results and Discussion
The study aims to examine the alteration in the quality of water of the Yamuna river at Dehradun in the year 2017. Water quality index (WQI) is going to be used in the study so that the changes and variations in the quality of water of the Yamuna river can be measured. The conventional method by which inspection can be done for the water quality has 12 physicochemical parameters (TDS, Chlorides, Alkalinity, DO, Temperature, COD, BOD, pH, Magnesium, Hardness, Total Coliform, and Calcium). These parameters will be measured carefully, and their respective value will be found. So, the standard value and observed value will be compared with each other, and the variation is going to be measured between them. By this variation, identification of the quality of water can be done.
Measurement of Physicochemical Parameters at Dehradun for 2017
Water samples have been taken at different months for the year 2017 ( Table 2 ). The mean and standard deviation for the measured values have been also calculated. The mean is the number found by summing every data point and dividing by the number of data points. It is also called average. The standard deviation is defined as the number that is going to tell about the measurements for a group that is spread out from the mean or expected value. A low standard deviation signifies that many numbers are very close to the mean ( Bisht et al., 2017 ). A high standard deviation signifies that the numbers are very much spread out. So, the accurate value for the quality of water can be found out easily using this.
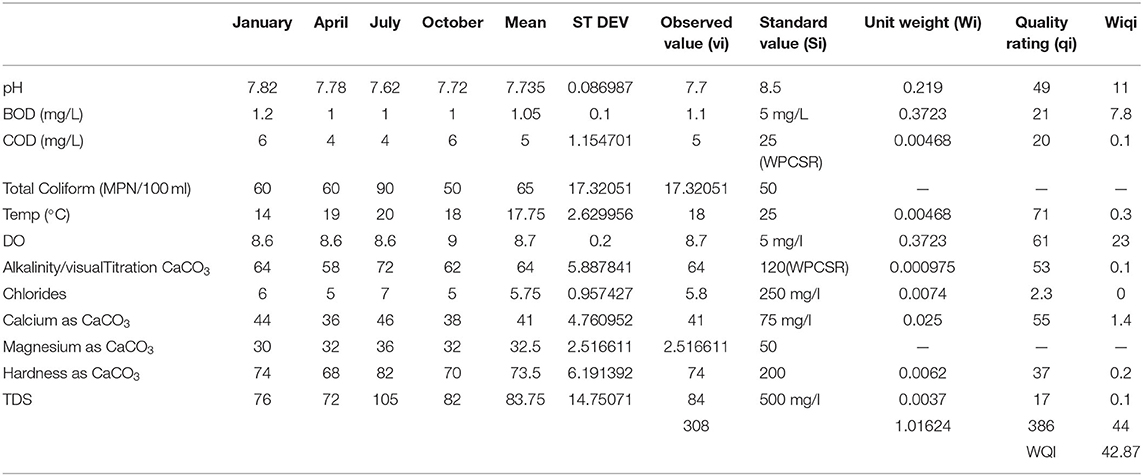
Table 2 . Physicochemical parameters and water quality analysis at Dehradun for 2017.
The maximum value of pH is in the month of January when the water is a little more basic, and the minimum value is in July when it is less basic. The mean pH is 7.735, and the standard deviation is 0.086986589. The maximum value of the biochemical oxygen demand (BOD) is in January, which indicates more polluted water, and the minimum value is in the months of April, July, and October, which indicates less polluted water. The mean of BOD is 1.05, and the standard deviation is 0.1. The maximum value of COD is in January and October, which indicates a large quantity of oxidizable organic materials in the sample, and the minimum value is in April and July which indicates a lesser quantity of oxidizable organic materials in the sample. The mean COD is 5 and the standard deviation is 1.154700538.
The maximum value of Total Coliform is in July, which indicates that the water-borne illness is increased, and the minimum value is in October which indicates that the water-borne illness is decreased. The mean of Total Coliform is 65, and the standard deviation is 17.32050808. The maximum value of Temp is in July, which indicates increased chemical reactions generally, and the minimum value is in January, which indicates decreased chemical reactions. The mean of Temp is 17.75, and the standard deviation is 2.62995564. The maximum value of DO is in October, and the minimum value is in January, April, and July. The mean of DO is 8.7, and the standard deviation is 0.2. The maximum value of Alkalinity/visual titration CaCO 3 is in July, which indicates greater buffering capacity against pH changes, and the minimum value is in April, which indicates lesser buffering capacity against pH changes. The mean of Alkalinity/visual titration CaCO 3 is 64, and the standard deviation is 5.887840578. The maximum value of Chlorides is in July, which indicates body-related diseases, and the minimum value is in April and October. The mean of Chlorides is 5.75, and the standard deviation is 0.9574271078. The maximum value of Calcium as CaCO 3 is in July, which has a positive effect on the body, and the minimum value is in April, which has a lesser positive effect on the body. The mean of CaCO 3 is 41, and the standard deviation is 4.760952286. The maximum value of Magnesium as CaCO 3 is in July, which has a positive effect on the body, and the minimum value is in January, which has a lesser positive effect on the body. The mean of Magnesium as CaCO 3 is 32.5, and the standard deviation is 2.516611478. The maximum value of Hardness as CaCO 3 is in July, which has a good effect on the body, and the minimum value is in April. The mean of Hardness as CaCO 3 is 73.5, and the standard deviation is 6.191391874. The maximum value of TDS is in July, which specifies more toxic minerals, and the minimum value is in April, which specifies less toxic minerals. The mean of TDS is 83.75, and the standard deviation is 14.7507062. Water quality index (WQI) was used for the evaluation of the variation in the water quality of the Yamuna river at Dehradun over 3 years. The standard and prescribed methods have been used to analyze the water quality for 12 physicochemical parameters (TDS, Chlorides, Alkalinity, DO, Temperature, COD, BOD, pH, Magnesium, Hardness, Total Coliform, and Calcium). Calculations have been performed using the standardized formula and mathematical models. Detailed calculations and methodology have been used to find the water quality index as accurately as possible. The WQI of the Yamuna river in Dehradun for the year 2017 was 42.87 ( Table 2 ). According to WHO, the WQI should be below 60 for its quality to be at least fair. Here, it can be easily concluded that the Yamuna river is polluted, but it is still revivable. Developmental and maintaining efforts can be adopted to make the Yamuna river clean again and improve the WQI drastically.
Total coliform is positively correlated with CaCO 3 , chlorides, and hardness of CaCO 3 .Temp is positively correlated with the magnesium of CaCO 3 and TDS and negatively correlated with pH, BOD, and COD. DO is positively correlated with COD and negatively correlated with chlorides. Alkalinity is positively correlated with chlorides, TDS, hardness, and the magnesium of CaCO 3 and negatively correlated with pH. Chlorides are positively correlated with calcium and hardness of CaCO 3 and negatively correlated with pH and DO. Magnesium (CaCO 3 ) is positively correlated with hardness and TDS, and negative with pH, BOD, and COD. Hardness (CaCO 3 ) is positive for TDS, Chlorides, Magnesium, and negative for pH. TDS is negative for pH and positive for all. The dendrogram and graphical representation for physicochemical parameters at Dehradun for 2017 are plotted between the months (January, April, July, and October) and the parameters [TDS, Total Coliform (MPN/100 ml), Alkalinity/visual titration CaCO 3 , Hardness as CaCO 3 , Calcium as CaCO 3 , Magnesium as CaCO 3 , Temp, BOD, pH, DO, COD, and Chlorides] ( Figures 3 , 4 ).
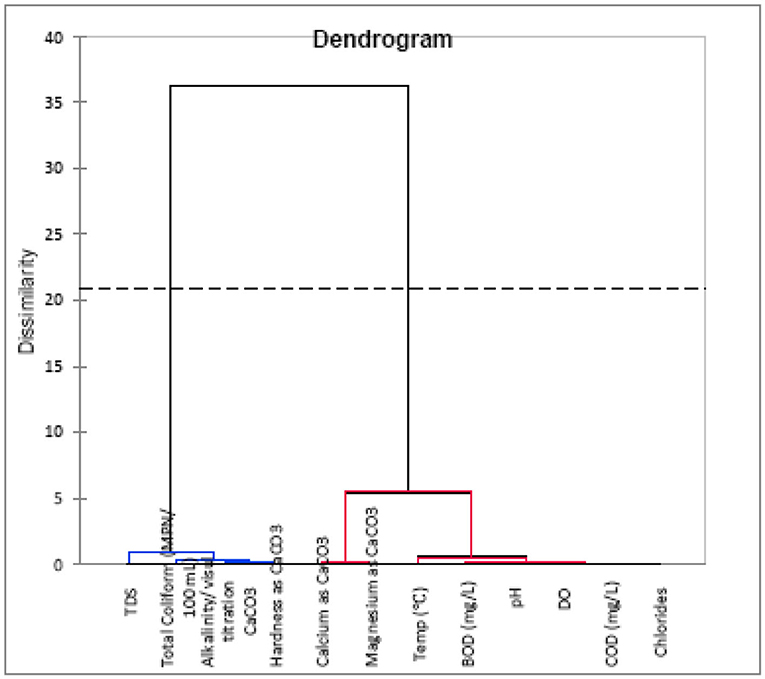
Figure 3 . Dendrogram for physicochemical parameters at Dehradun for 2017.
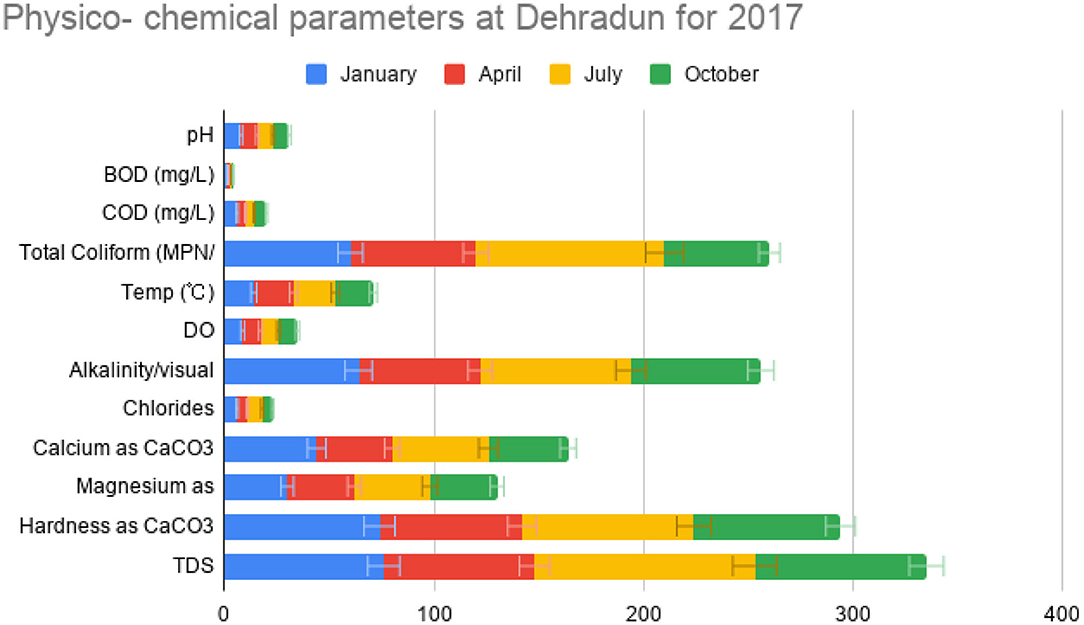
Figure 4 . Graphical representation of physicochemical parameters at Dehradun for 2017.
Cluster 1 (blue) represents lightly polluted, and the parameters include TDS, Total Coliform (MPN/100 ml), Alkalinity/visual titration CaCO 3 , and Hardness as CaCO 3 . Cluster 2 (red) represents moderately polluted, and the parameters include Calcium as CaCO 3 , Magnesium as CaCO 3 , Temp, BOD, pH, DO, COD, and Chlorides. Cluster 3 (black) represents heavily polluted and the parameters include Total Coliform (MPN/100 ml), Alkalinity/visual titration CaCO 3 , Hardness as CaCO 3 , Calcium as CaCO 3 , Magnesium as CaCO 3 , and Temp.
Measurement of Physicochemical Parameters at Dehradun for 2018
Water samples have been taken in different months for the year 2018 ( Table 3 ). Mean and standard deviation for the measured values have been also calculated. The maximum value of pH is in October so the water is a little more basic, and the minimum value is in January, which means the water is less basic. The mean pH is 7.6325, and the standard deviation is 0.420585. The maximum value and minimum value of BOD are equal every month. The mean of BOD is 1, and the standard deviation is 0.
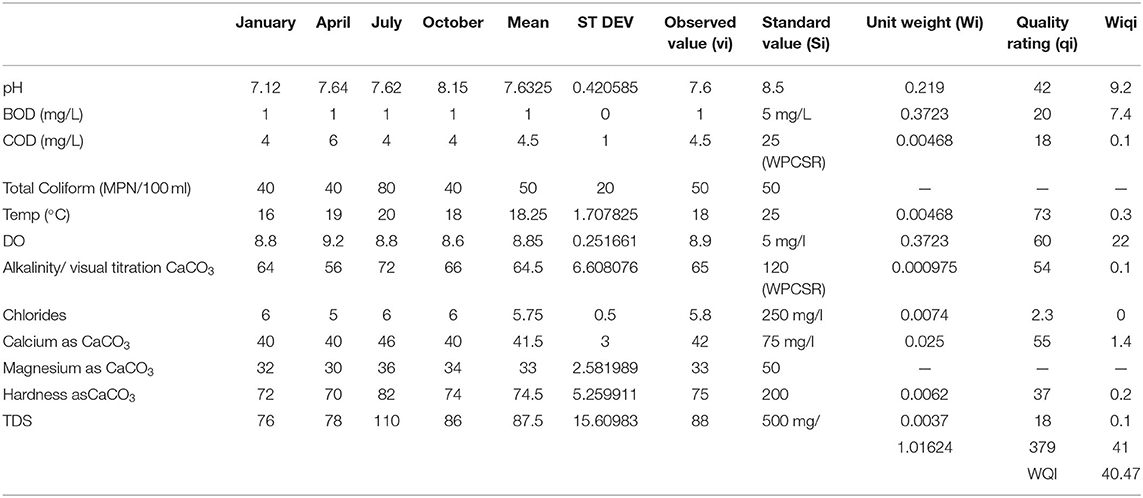
Table 3 . Physicochemical parameters and water quality analysis at Dehradun for 2018.
The maximum value of COD is in April indicating a large quantity of oxidizable organic material in the sample, and the minimum value is in January, July, and October indicating a lesser quantity of oxidizable organic materials in the sample. The mean of COD is 4.5, and the standard deviation is 1. The maximum value of Total Coliform is in July indicating the water-borne illness is increased, and the minimum value is in January, April, and October indicating the water-borne illness is decreased. The mean of Total Coliform is 50, and the standard deviation is 20. The maximum value of Temp is in July indicating increased chemical reactions generally, and the minimum value is in January indicating decreased chemical reactions. The mean of Temp is 18.25, and the standard deviation is 1.707825. The maximum value of DO is in April, and the minimum value is in January and July. The mean of DO is 8.85, and the standard deviation is 0.251661. The maximum value of Alkalinity/visual titration CaCO 3 is in July indicating higher buffering capacity against pH changes, and the minimum value is in April indicating lower buffering capacity against pH changes. The mean of Alkalinity/visual titration CaCO 3 is 64.5, and the standard deviation is 6.608076.
The maximum value of Chlorides is in January, July, and October indicating body-related diseases, and the minimum value is in April. The mean of Chlorides is 5.75, and the standard deviation is 0.5. The maximum value of Calcium as CaCO 3 is in July, which has a positive effect on the body, and the minimum value is in January, April, and October, which has a less positive effect on the body. The mean of CaCO 3 is 41.5, and the standard deviation is 3. The maximum value of Magnesium as CaCO 3 is in July, which has a positive effect on the body, and the minimum value is in April, which has a less positive effect on the body. The mean of Magnesium as CaCO 3 is 33, and the standard deviation is 2.581989. The maximum value of Hardness as CaCO 3 is in July, which has a good effect on the body, and the minimum value is in April. The mean of Hardness as CaCO 3 is 74.5, and the standard deviation is 5.259911. The maximum value of TDS is in July specifying the presence of toxic minerals, and the minimum value is in January specifying the presence of less toxic minerals. The mean of TDS is 87.5, and the standard deviation is 15.60983.
Water quality index (WQI) was used in the evaluation of the variation in water quality of the Yamuna river at Dehradun over 3 years. The standard and prescribed method has been used to analyze the water quality for the 12 physicochemical parameters (TDS, Chlorides, Alkalinity, DO, Temperature, COD, BOD, pH, Magnesium, Hardness, Total Coliform, and Calcium). Calculations have been performed using the standardized formula and mathematical models. Detailed calculations and methodology have been used to find the water quality index as accurately as possible. The WQI of the Yamuna river in Dehradun for the year 2018 was 40.47 ( Table 3 ). According to WHO, the WQI should be below 60 for its quality to be at least fair. Here, it can be easily concluded that the Yamuna river is polluted, but it is still revivable. Developmental and maintaining efforts can be adopted to make the Yamuna river clean again and improve the WQI severely. Moreover, it is a positive sign that the WQI of the Yamuna river has improved greatly for the year 2018 compared to the year 2017.
The dendrogram and graphical representation for the physicochemical parameters at Dehradun for 2018 are plotted between the months (January, April, July, and October) and also the parameters [TDS, Total Coliform (MPN/100 ml), Alkalinity/visual titration CaCO 3 , Hardness as CaCO 3 , Calcium as CaCO 3 , Magnesium as CaCO 3 , Temp, BOD, pH, DO, COD, and Chlorides] ( Figures 5 , 6 ). Cluster 1 (blue) represents lightly polluted and the parameters include Temp, BOD, pH, DO, COD, and Chlorides.
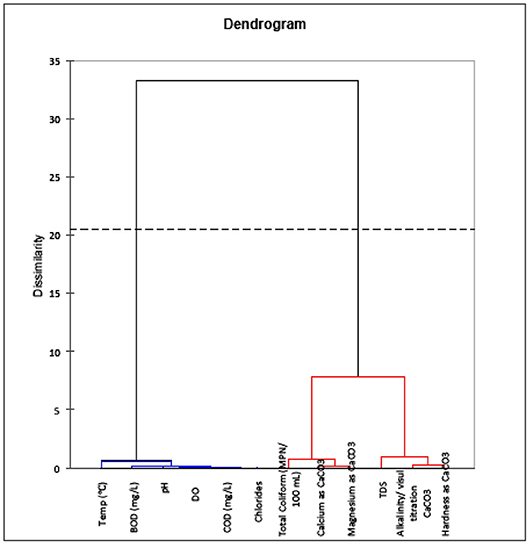
Figure 5 . Dendrogram for physicochemical parameters at Dehradun for 2018.
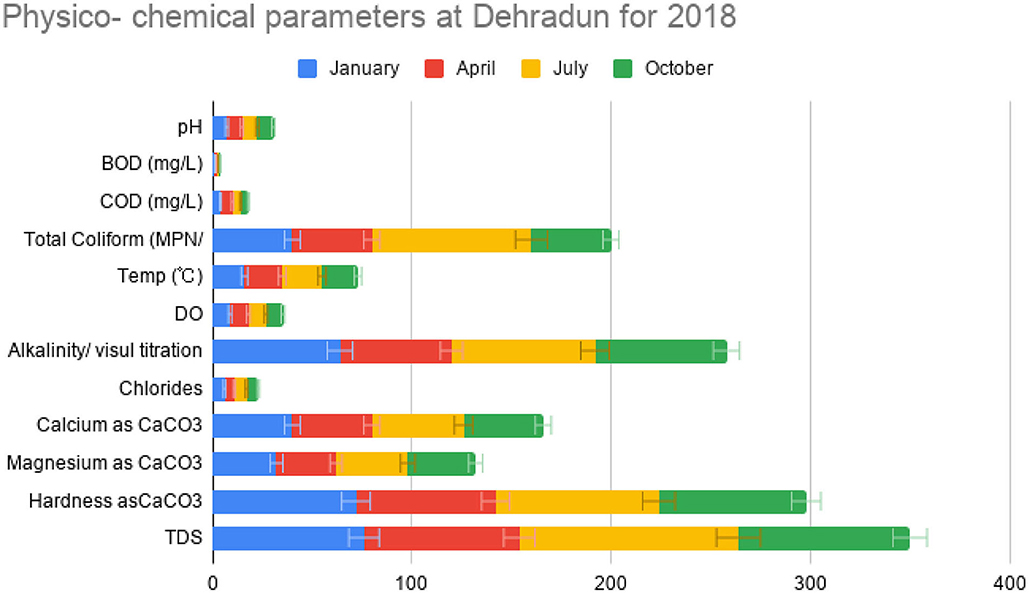
Figure 6 . Graphical representation of physicochemical parameters at Dehradun for 2018.
Cluster 2 (red) represents moderately polluted, and the parameters include Total Coliform (MPN/100 ml), Calcium as CaCO 3 , Magnesium as CaCO 3 , TDS, Alkalinity/visual titration CaCO 3 , Hardness as CaCO 3 . Cluster 3 (black) represents heavily polluted, and the parameters include BOD, pH, DO, COD, Chlorides, Total Coliform (MPN/100 ml), Alkalinity/visual titration CaCO 3 , Hardness as CaCO 3 , Calcium as CaCO 3 , and Magnesium as CaCO 3 .
Measurement of Physicochemical Parameters at Dehradun for 2019
Water samples have been taken in different months for the year 2019 ( Table 4 ). The mean and standard deviation for the measured values have been also calculated. The mean is the number found by summing every data point and dividing by the number of data points. Standard deviation is defined as the number that is going to tell about the measurements for a group that is spread out from the mean or expected value. Comparing the values of this year with those of the previous years leads to the outcomes being observed.
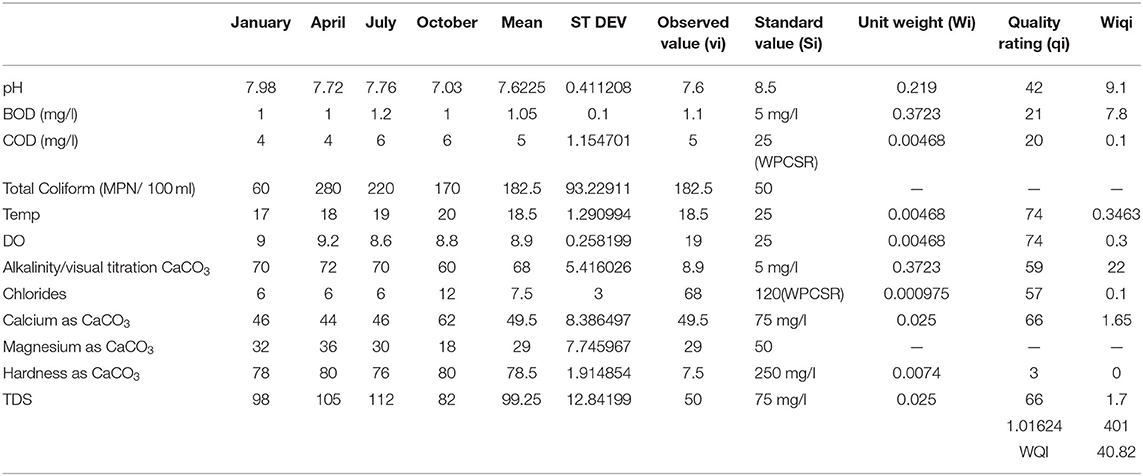
Table 4 . Physicochemical parameters and water quality analysis at Dehradun for 2019.
The maximum value of pH is in the month of January when the, water is a little more basic and the minimum value is in the month of October when the water is less basic. The mean pH is 7.6225, and the standard deviation is 0.411208. The maximum value of BOD is in July when there is a large quantity of polluted water, and the minimum value is in January, April, and October when there is less quantity of polluted water. The mean of BOD is 1.05, and the standard deviation is 0.1. The maximum value of COD is in July and October indicating a greater amount of oxidizable organic materials in the sample, and the minimum value is in January and April indicating a lesser amount of oxidizable organic materials in the sample. The mean of COD is 5, and the standard deviation is 1.154701. The maximum value of Total Coliform is in April indicating that water-borne illness is increased, and the minimum value is in January indicating that water-borne illness is decreased. The mean of Total Coliform is 182.5, and the standard deviation is 93.22911. The maximum value of Temp is in October indicating increased chemical reactions generally, and the minimum value is in January indicating decreased chemical reactions. The mean of Temp is 18.5, and the standard deviation is 1.290994. The maximum value of DO is in April, and the minimum value is in July. The mean of DO is 8.9, and the standard deviation is 0.258199. The maximum value of Alkalinity/visual titration CaCO 3 is in April indicating higher buffering capacity against pH changes, and the minimum value is in October indicating lower buffering capacity against pH changes. The mean of Alkalinity/visual titration CaCO 3 is 68, and the standard deviation is 5.416026.
The maximum value of Chlorides is in October indicating body-related diseases, and the minimum value is in January, July, and April. The mean of Chlorides is 7.5, and the standard deviation is 3. The maximum value of Calcium as CaCO 3 is in October, which has a positive effect on the body, and the minimum value is in April, the month which has a less positive effect on the body. The mean of CaCO 3 is 49.5, and the standard deviation is 8.386497. The maximum value of Magnesium as CaCO 3 is in April, which has a positive effect on the body, and the minimum value is in October, which has a less positive effect on the body. The mean of Magnesium as CaCO 3 is 29, and the standard deviation is 7.745967. The maximum value of Hardness as CaCO 3 is in April and October, which has a good effect on the body, and the minimum value is in July. The mean of Hardness as CaCO 3 is 78.5, and the standard deviation is 1.914854. The maximum value of TDS is in July specifying the presence of toxic minerals, and the minimum value is in October specifying the presence of less toxic minerals. The mean of TDS is 99.25, and the standard deviation is 12.84199.
Water quality index (WQI) was used to evaluate the variation in water quality of the Yamuna river at Dehradun over 3 years. The standard and prescribed method has been used to analyze the water quality for 12 physiochemical parameters (TDS, Chlorides, Alkalinity, DO, Temperature, COD, BOD, pH, Magnesium, Hardness, Total Coliform, and Calcium). Calculations have been performed using the standardized formula and mathematical models. Detailed calculations and methodology have been used to find the water quality index as accurately as possible. The WQI of the Yamuna river in Dehradun for the year 2019 was 40.82 ( Table 4 ). According to WHO, the WQI should be below 60 for its quality to be at least fair. Here, it can be easily concluded that the Yamuna river is polluted, but it is still revivable. Developmental and maintaining efforts can be adopted to make the Yamuna river clean again and improve the WQI drastically. Moreover, it is a positive sign that the WQI of the Yamuna river has improved for year 2019 compared to the year 2017, whereas the WQI has increased again in 2019 compared to 2018. It can be documented that the Yamuna river was the cleanest in the year 2018, and its water quality in 2019 has improved in collation to the year 2017.
The correlation coefficients between the inspected parameters of the Yamuna river water at Dehradun in the year 2019 are shown in Table 5 . Ph is positive for TDS, alkalinity, and Magnesium (CaCO 3 ) and negative for COD, Temp, and Calcium (CaCO 3 ). BOD is positive for COD and TDS and negative for DO and hardness (CaCO 3 ). COD is positive for Temp, Chlorides, and Calcium and negative for DO, magnesium (CaCO 3 ), and alkalinity. Temp is positive for calcium (CaCO 3 ) and negative for alkalinity. DO is positive for Magnesium (CaCO 3 ). Alkalinity is positive for TDS and negative for Calcium, Magnesium, and hardness of CaCO 3 . The dendrogram and graphical representation for physicochemical parameters at Dehradun for 2017 are plotted between months (January, April, July, and October) and parameters (TDS, Total Coliform (MPN/100 ml), Alkalinity/visual titration CaCO 3 , Hardness as CaCO 3 , Calcium as CaCO 3 , Magnesium as CaCO 3 , Temp, BOD, pH, DO, COD, and Chlorides) ( Figures 7 , 8 ).
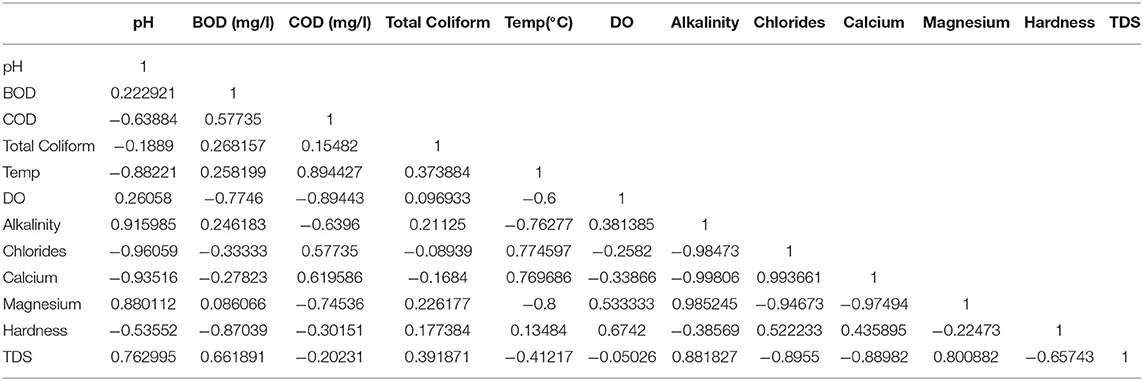
Table 5 . Correlation table for physicochemical parameters at Dehradun for 2019.
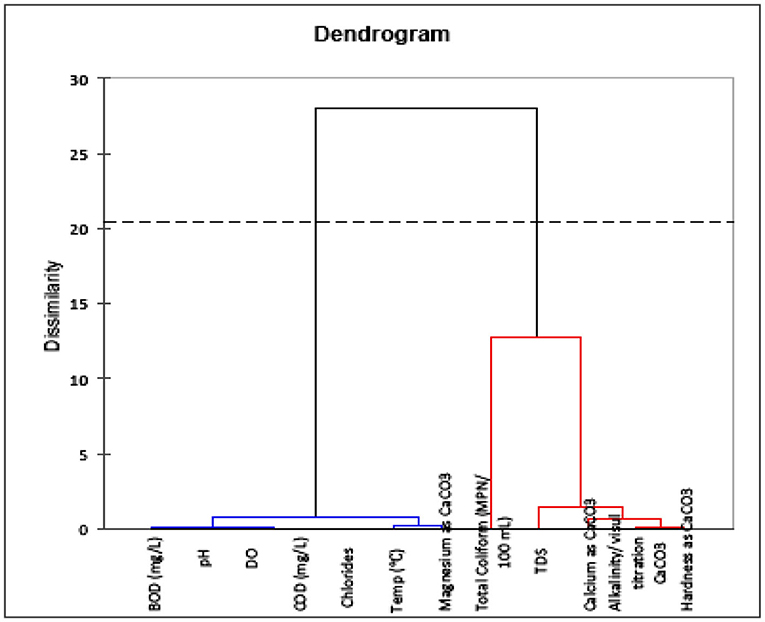
Figure 7 . Dendrogram for physicochemical parameters at Dehradun for 2019.
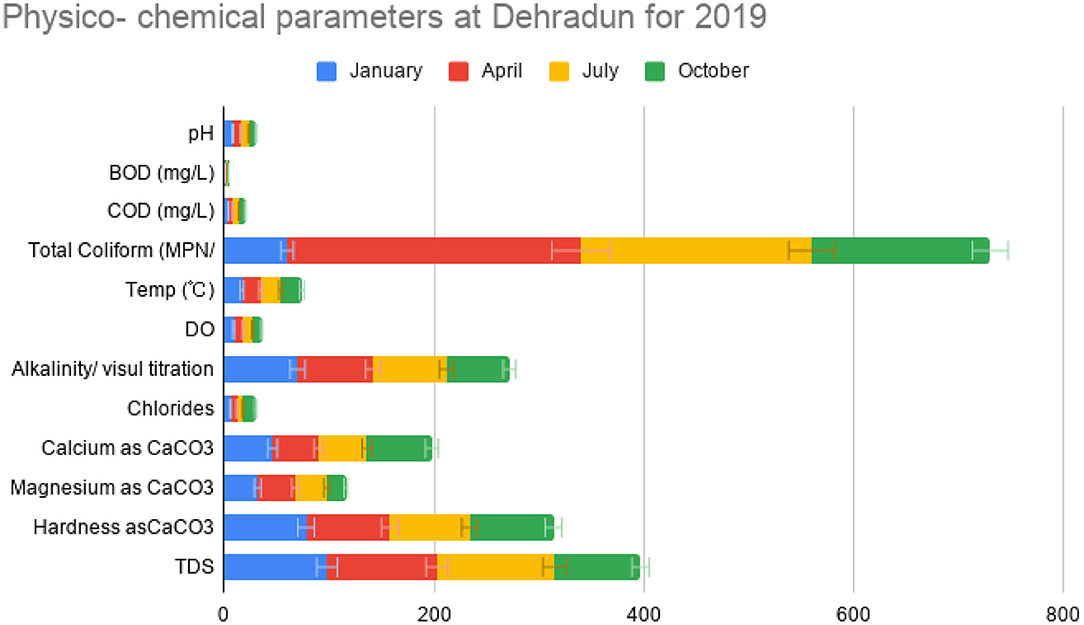
Figure 8 . Graphical representation of physicochemical parameters at Dehradun for 2019.
Cluster 1 (blue) represents lightly polluted, and the parameters include BOD, pH, DO, COD, Chlorides, Temp, and Magnesium as CaCO 3 . Cluster 2 (red) represents moderately polluted, and the parameters include Total Coliform (MPN/100 ml), TDS, Calcium as CaCO 3 , Alkalinity/visual titration CaCO 3 , and Hardness as CaCO 3 . Cluster 3 (black) represents heavily polluted, and the parameters include COD, Chlorides, Temp, Magnesium as CaCO 3 , Total Coliform (MPN/100 ml), and TDS.
In Table 6 , the variations in the 12 physicochemical parameter values for Yamuna water at Dehradun for 2017, 2018, and 2019 are shown.
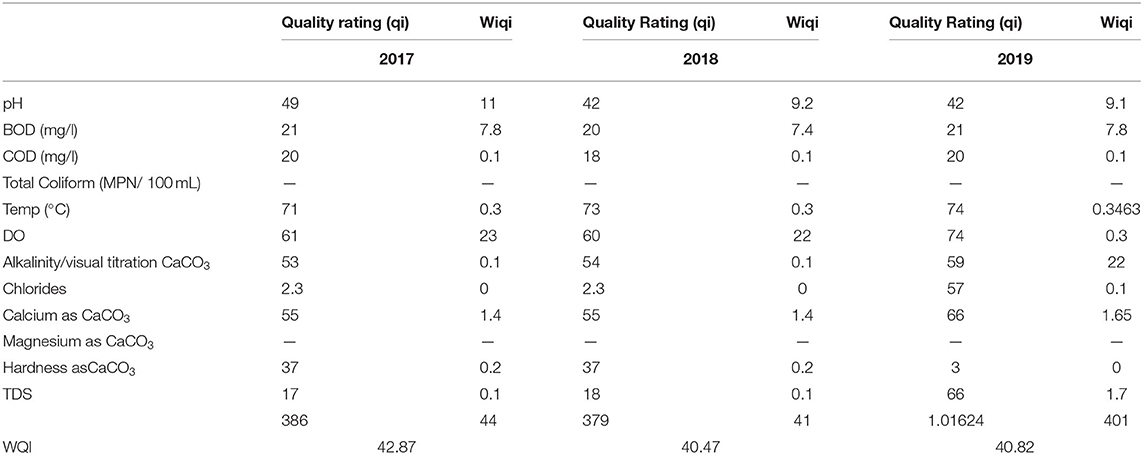
Table 6 . Water quality index (WQI) for Yamuna river at Dehradun for 2017, 2018, and 2019.
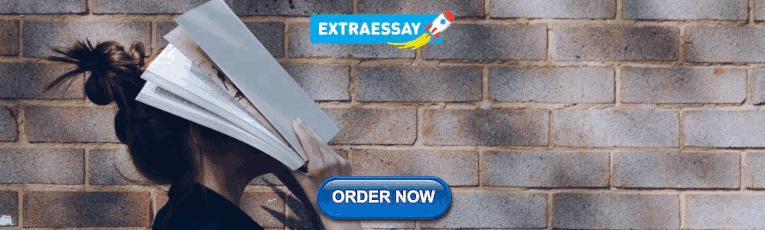
Trend Forecasting
This section is briefing about the Yamuna river water pollution trend in the next 4 years. The study demonstrates the trend of six physicochemical parameters for the years 2020 to 2024. The considered parameters for calculating the trend forecasting are Temp, Total Coliform, TDS, Hardness, pH, and DO. The forecasting for the said parameters are shown in Figures 9 – 11 .
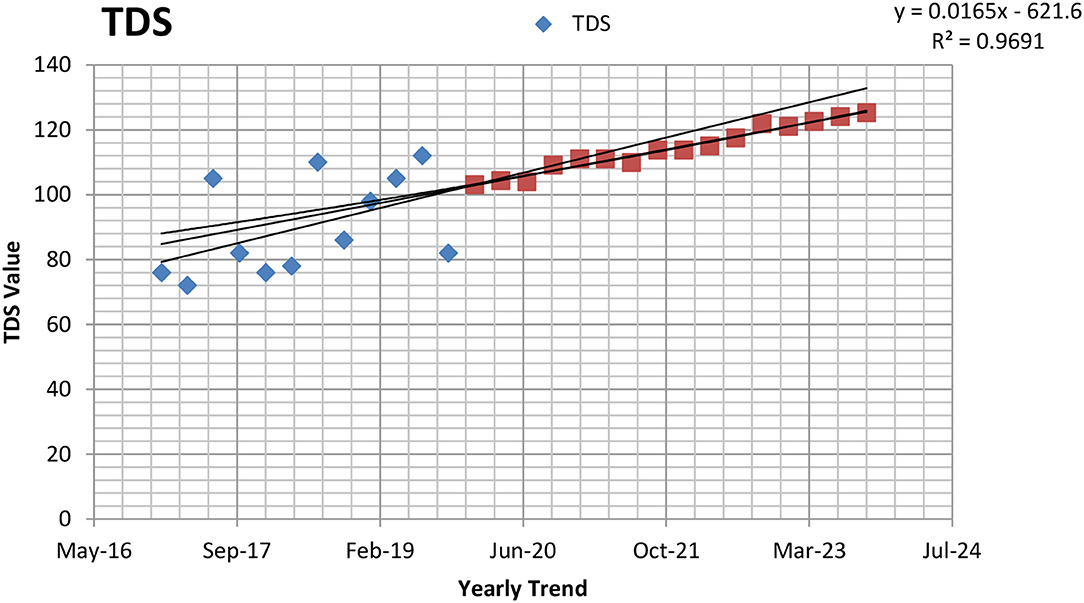
Figure 9 . Trend forecasting of TDS at Dehradun for the years from 2020 to 2024.
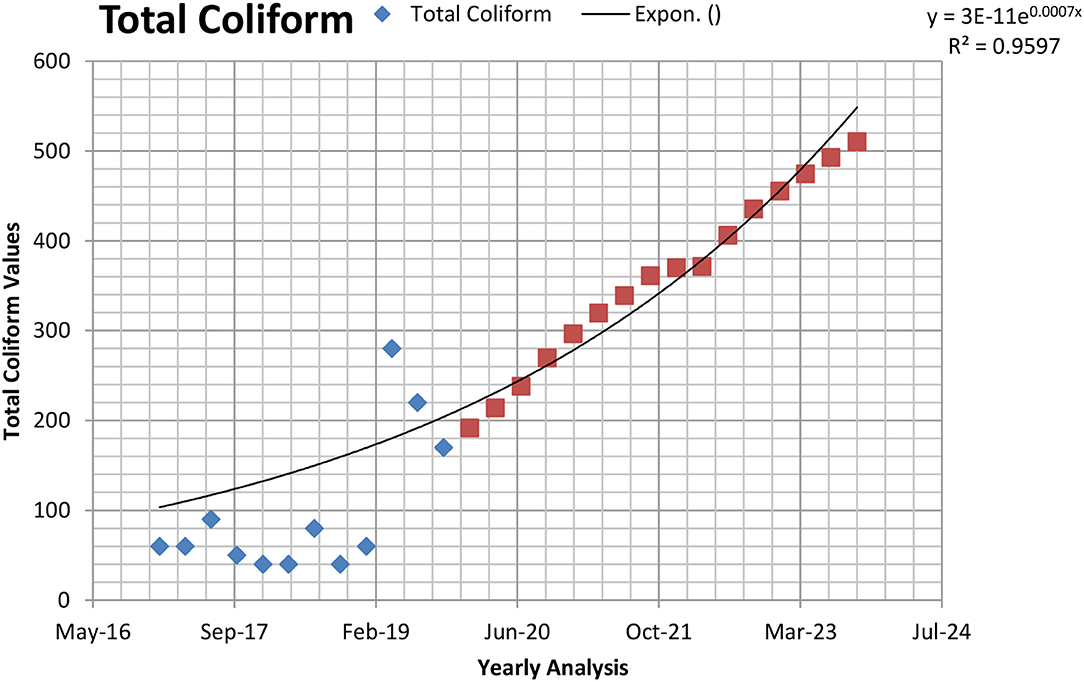
Figure 10 . Trend forecasting of total Coliform at Dehradun for the years from 2020 to 2024.
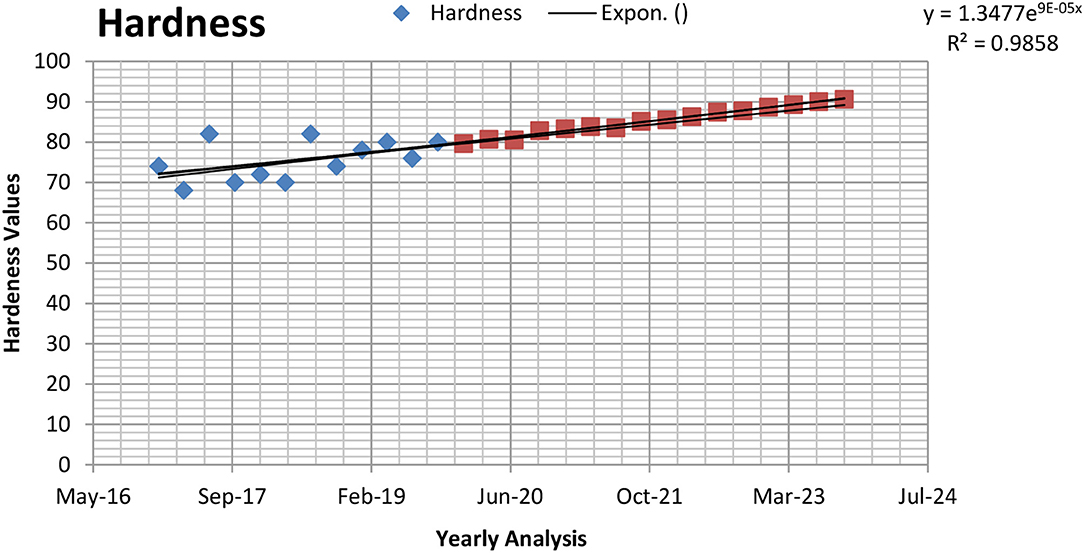
Figure 11 . Trend forecasting of hardness at Dehradun for the years from 2020 to 2024.
According to the trend analysis, the values of four parameters named Temperature, Total Coliform, TDS, and Hardness are increasing yearly, whereas the values of pH and DO are not rising year by year. The trend forecasting is verifying whether the exceptional tourist activity, poor sewage facility, and insufficient wastewater management amenities, is degrading the water of the Yamuna river at Dehradun year by year.
The dendrogram of the mean is plotted between years (2017, 2018, and 2019) and parameters [TDS, Total Coliform (MPN/100 ml), Alkalinity/visual titration CaCO 3 , Hardness as CaCO 3 , Calcium as CaCO 3 , Magnesium as CaCO 3 , Temp, BOD, pH, DO, COD, and Chlorides] ( Figure 12 ). Cluster 1 (blue) represents lightly polluted, and the parameters include Total Coliform (MPN/100 ml), TDS, Alkalinity/visual titration CaCO 3 , and Hardness as CaCO 3 . Cluster 2 (red) represents moderately polluted, and the parameters include Calcium as CaCO 3 and Magnesium as CaCO 3 . Cluster 3 (black) represents heavily polluted, and the parameters include TDS, Alkalinity/visual titration CaCO 3 , Hardness as CaCO 3 , Calcium as CaCO 3 , Magnesium as CaCO 3 , and Temp. Cluster 4 (green) represents equal parameters, and it includes Temp, BOD, pH, DO, COD, and Chlorides.
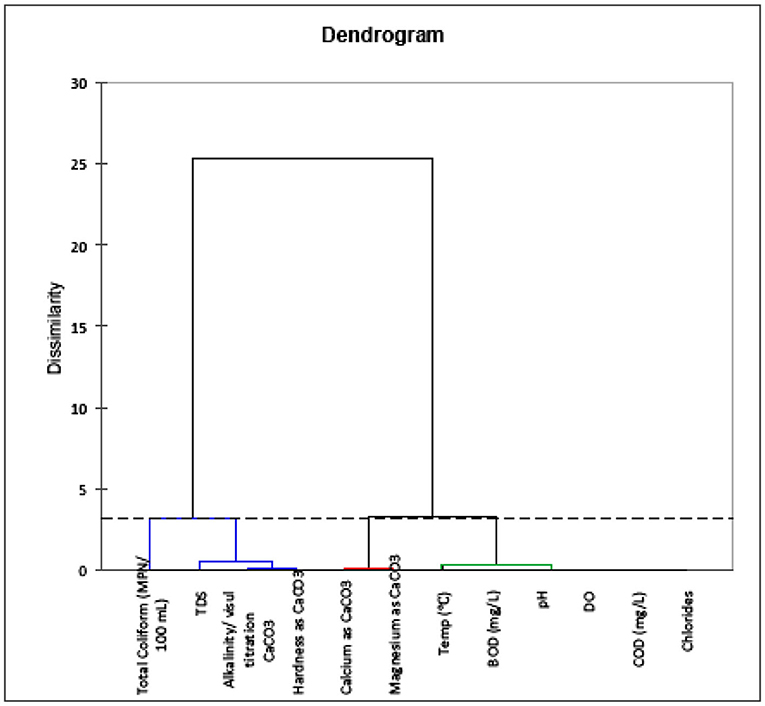
Figure 12 . Dendrogram for physicochemical parameter mean values for 2017, 2018, and 2019.
The variation in observed values, quality rating, and Wiqi can be analyzed using Tables 2 , 4 , 6 .
According to WHO, the WQI should be below 60 for its quality to be at least fair. If it is more than 60, then the quality of the water is surely poor. If the WQI is <30, then the water quality is good. The WQI of the Yamuna river in Dehradun for the year 2017 was 42.87. It can be easily said that the Yamuna river was quite polluted back then. Developmental and maintaining plans were implemented to make the Yamuna river clean again and improve the WQI drastically. The WQI of the Yamuna river in 2017 was the highest in collation to the subsequent years. This must have set the alarm bells ringing for the government and the citizens. The government has introduced many measures to curb water pollution and revive the Yamuna river as quickly as possible. It is a positive sign that the WQI of the Yamuna river has improved significantly for the years 2018 to 40.47. It was a marked difference in comparison to that of the year 2017. Joint efforts and collaboration by the government and the citizens ensured that the Yamuna river is much cleaner than before, although in 2019, the WQI rose by a small margin to 40.82. It is a sign of relief that it is still much better than the quality of the water in the year 2017. If the measures of the government and corporation by the citizens continue to go hand in hand, the results will be for everyone to see. Even regions in the west would emulate the policies adopted to revive the rivers. Policies included a big budget for the revival project, strict norms for the industries, and appropriate penalties for the defaulters. A common concern for the degrading water quality index of the Yamuna river resulted in some swift actions from the citizens as well as from those who became more aware and conscious. It can be easily and comfortably said that the Yamuna river would be much cleaner and in a much-improved condition by the year 2025.
A comparative analysis is shown in Table 7 . A comparison in description and limitations with previously published approaches are organized in this table.
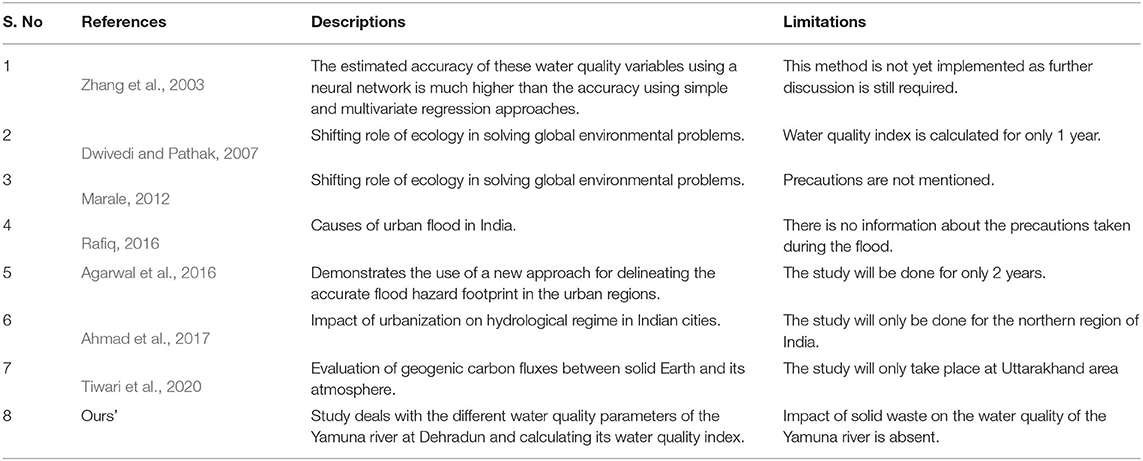
Table 7 . Comparative analysis with previous work done.
Due to historical, geographical, religious, political, and sociocultural reasons, India has a unique place in the world. Pollution-causing activities have caused severe changes in aquatic environments over the last few decades. This paper aims to calculate the water quality index of the Yamuna river in Uttarakhand using 12 physicochemical parameters for a while for 3 years from 2017 to 2019. The values of the considered physicochemical parameters have been monitored using the various examining stations installed by the Central Pollution Control Board (CPCB), India. According to WHO, the WQI should be below 60 for its quality to be at least fair. If it is more than 60, then the quality of the water is surely poor. If the WQI is <30, then the water quality is good. The WQI of the Yamuna river in Dehradun for the year 2017 was 42.87. It can be easily said that the Yamuna river was quite polluted back then. Developmental and maintaining plans were implemented to make the Yamuna river clean again and improve the WQI drastically. The WQI of the Yamuna river in 2017 was the highest in collation to the subsequent years. This must have set the alarm bells ringing for the government and the citizens.
According to the trend analysis, the values of four parameters named Temperature, Total Coliform, TDS, and Hardness are increasing yearly, whereas the values of pH and DO are not rising year by year. The trend forecasting verifies whether the exceptional tourist activity, poor sewage facilities, and insufficient wastewater management amenities is degrading the water of the Yamuna river at Dehradun year by year. It is a positive sign that the WQI of the Yamuna river has improved significantly for the years 2018 to 40.47. It was a marked difference in comparison to the year 2017. Joint efforts and collaboration by the government and the citizens ensured that the Yamuna river is much cleaner than before, although in 2019, the WQI raised by a small margin to 40.82. It is a sign of relief that it is still much better than the quality of the water in the year 2017. If the measures of the government and corporation by the citizens continue to go hand in hand, the results will be for everyone to see. Even regions in the west would emulate the policies adopted to revive the rivers. A common concern for the degrading water quality index of the Yamuna river resulted in some swift actions from the citizens as well as those who became more aware and conscious.
Data Availability Statement
The datasets presented in this study can be found in online repositories. The names of the repository/repositories and accession number(s) can be found in the article/supplementary material.
Author Contributions
Data curation was done by RS and RK. Formal analysis was made by RS, RK, and SS. The investigation was done by RS, RK, and KS. The methodology was done by RS, NA-A, and RM. Project administration was performed by HL and AA. BP was in charge of the resources and software. BP and NA-A supervised the study. Visualization was done by BP and NA-A. RS, AA, RM, and BP wrote the original draft. All authors contributed to the article and approved the submitted version.
Conflict of Interest
The authors declare that the research was conducted in the absence of any commercial or financial relationships that could be construed as a potential conflict of interest.
Agarwal, A., Rafique, F., Rajesh, E., and Ahmed, S. (2016). Urban flood hazard mapping using change detection on wetness transformed images. Hydrol. Sci. J. 61, 816–825. doi: 10.1080/02626667.2014.952638
CrossRef Full Text | Google Scholar
Ahmad, I. K., Salih, N. M., and Nzar, Y. H. (2012). Determination of water quality index (WQI) for Qalyasan stream in Sulaimani city/Kurdistan region of Iraq. Int. J. Plant Anim. Environ. Sci . 2, 148–157.
Google Scholar
Ahmad, S., Farooq, S., Zahoor-Ul-Islam Khan Md., A., Zaidiand, W. A., and Matloob, H. (2017). Impact of urbanization on hydro logical regime in Indian cities. J. Environ. Res. Develop. 2, 594–604.
Allee, R. J., and Johnson, J. E. (1999). Use of satellite imagery to estimate surface chlorophyll a and Secchi disc depth of Bull Shoals Reservoir, Arkansas, USA. Int. J. Remote Sens . 20, 1057–1072. doi: 10.1080/014311699212849
Amandeep, V. (2011). Identification of land and water regions in a satellite image: a texture based approach. Int. J. Comput. Sci. Eng. Technol. 1, 361–365.
Bhutiani, R., Ahamad, F., Tyagi, V., and Ram, K. (2018). Evaluation of water quality of River Malin using water quality index (WQI). Environ. Conserv. J . 19, 191–201. doi: 10.36953/ECJ.2018.191228
Bhutiani, R., and Khanna, D. R. (2007). Ecological status of river Suswa: modelling DO and BOD. Environ. Monit. Assess . 125, 183–195. doi: 10.1007/s10661-006-9251-4
PubMed Abstract | CrossRef Full Text | Google Scholar
Bhutiani, R., Khanna, D. R., Kulkarni, D. B., and Ruhela, M. (2016). Assessment of Ganga river ecosystem at Haridwar, Uttarakhand, India with reference to water quality indices. Appl. Water Sci. 6, 107–113. doi: 10.1007/s13201-014-0206-6
Bhutiani, R., Khanna, D. R., Tyagi, B., Tyagi, P. K., and Kulkarni, D. B. (2015). Assessing environmental contamination of River Ganga using correlation and multivariate analysis. Pollution 1, 265–273.
Bisht, A. K., Singh, R., Bhutiani, R., Bhatt, A., and Kumar, K. (2017). Water quality modelling of the River Ganga using artificial neural network with reference to the various training functions. Environ. Conserv. J . 18, 41–48. doi: 10.36953/ECJ.2017.181206
Brown, R. M., McClelland, N. I., Deininger, R. A., and Tozer, R. G. (1970). Water quality index-do we dare? Water Sew. Works 117, 339–343.
Census Reports of India 2001. (1971–1991). Available online at: https://censusindia.gov.in/
Chabuk, A., Al-Ansari, N., Hussain, H. M., Knutsson, S., Pusch, R., and Laue, J. (2017). Combining GIS applications and method of multi-criteriadecision-making (AHP) for landfill siting in Al-HashimiyahQadhaa, Babylon, Iraq. Sustainability 9, 19–32. doi: 10.3390/su9111932
Chauhan, A., and Singh, S. (2010). Evaluation of Ganga water for drinking purpose by water quality index at Rishikesh, Uttarakhand, India. Rep. Opin. 2, 53–61.
Cude, C. G. (2001). Oregon water quality index: a tool for evaluating water quality management effectiveness. J. Am. Water Resour. Assoc . 37, 125–137. doi: 10.1111/j.1752-1688.2001.tb05480.x
Duong, D. N. (2012). Water body extraction from multi spectral image by spectral pattern analysis. J. Photogramm Remote Sens. Spat. Inf. Sci. Melb. XXXIX-B 8, 248–259. doi: 10.5194/isprsarchives-XXXIX-B8-181-2012
Dwivedi, S. L., and Pathak, V. A. (2007). Preliminary assignment of water quality index to Mandakini river, Chitrakoot. Indian J. Environ. Prot. 27, 1036–1038.
Fraser, R. N. (1998). Multispectral remote sensing of turbidity among Nebraska Sand Hills Lakes. Int. J. Remote Sens . 19, 3011–3016. doi: 10.1080/014311698214406
Girgin, S., Kazanci, N., and Dügel, M. (2010). Relationship between aquatic insects and heavy metals in an urban stream using multivariate techniques. Int. J. Environ. Sci. Technol . 7, 653–664. doi: 10.1007/BF03326175
Icaga, Y. (2007). Fuzzy evaluation of water quality classification. Ecol. Indic J. Elsevier 7, 710–718. doi: 10.1016/j.ecolind.2006.08.002
Kazi, T. G., Arain, M. B., Jamali, M. K., Jalbani, N., Afridi, H. I., Sarfraz, R. A., et al. (2009). Assessment of water quality of polluted lake using multivariate statistical techniques: a case study. Ecotoxicol. Environ. Saf. J. 72, 301–309. doi: 10.1016/j.ecoenv.2008.02.024
Kondratyev, K. Y., Pozdnyakov, D. V., and Pettersson, L. H. (1998). Water quality remote sensing in the visible spectrum. Int. J. Remote Sens . 19, 957–979. doi: 10.1080/014311698215810
Marale, S. (2012). Shifting role of ecology in solving global environmental problems: selected practical tools. Environ. Develop. Sustain . 14, 869–884. doi: 10.1007/s10668-012-9362-8
Nazeer, M., and Nichol, J. E. (2015). Combininglandsat TM/ETM + and HJ-1 A/B CCD sensors for monitoring coastal water quality in Hong Kong. IEEE Geosci. Remote Sens. Lett . 12, 1898–1902. doi: 10.1109/LGRS.2015.2436899
Panwar, A., Bartwal, S., Dangwal, S., Aswal, A., Bhandari, A., and Rawat, S. (2015). Water quality assessment of River Ganga using remote sensing and GIS technique. Int. J. Adv. Remote Sens. GIS 4, 1253–1261. doi: 10.23953/cloud.ijarsg.116
Pattiaratchi, C. B., Lavery, P., Wyllie, A., and Hick, P. (1994). Estimates of water-quality in coastal waters using multi-date Landsat Thematic Mapper data. Int. J. Remote Sens . 15, 84–1571. doi: 10.1080/01431169408954192
Rafiq, F. (2016). Urban floods in India. Int. J. Sci. Eng. Res . 7, 721–734.
Ramakrishniah, C. R., Sadashivaiah, C., and Ranganna, G. (2009). Assessment of water quality index for the groundwater in Tumkur Taluk. E-J Chem . 6, 523–530. doi: 10.1155/2009/757424
Ronghang, M., Gupta, A., Mehrotra, I., Kumar, P., Patwal, P., Kumar, S., et al. (2019). Riverbank filtration: a case study of four sites in the hilly regions of Uttarakhand, India. Sustain. Water Resour. Manag . 5, 831–845. doi: 10.1007/s40899-018-0255-3
Sharma, P., Sood, S., and Mishra, S. K. (2020). Development of multiple linear regression model for biochemical oxygen demand (BOD) removal efficiency of different sewage treatment technologies in Delhi, India. Sustain. Water Resour. Manag . 6:29. doi: 10.1007/s40899-020-00377-9
Shi, L., Mao, Z., and Wang, Z. (2018). Retrieval of total suspended matter concentrations from high resolution WorldView-2 imagery: a case study of inland rivers. IOP Conf. Ser. Earth Environ. Sci. 121:032036. doi: 10.1088/1755-1315/121/3/032036
Shukla, S., Khire, M. V., and Godan, S. S. (2018). Effects of urbanization on surface and subsurface hydrologic variables of upper bhima river basin, Maharashtra, India. Model. Earth Syst. Environ. 4, 699–728. doi: 10.1007/s40808-018-0446-9
Singh, A., Jakubowski, A. R., Chidister, I., and Townsend, P. A. (2013). A MODIS approach to predicting stream water quality in Wisconsin. Remote Sens. Environ . 128, 74–86. doi: 10.1016/j.rse.2012.10.001
Song, K. S., Li, L., Li, S., Tedesco, L., Hall, B., and Li, L. H. (2012). Hyperspectral remote sensing of total phosphorus (TP) in three central Indiana water supply reservoirs. J. Water Air Soil Pollut . 223, 1481–1502. doi: 10.1007/s11270-011-0959-6
Tiwari, S. K., Gupta, A. K., and Asthana, A. K. L. (2020). Evaluating CO 2 flux and recharge source in geothermal springs, Garhwal Himalaya, India: stable isotope systematics and geochemical proxies. Environ. Sci. Pollut. Res . 27, 14818–14835. doi: 10.1007/s11356-020-07922-1
Trombadore, O., Nandi, I., and Shah, K. (2020). Effective data convergence, mapping, and pollution categorization of ghats at Ganga River Front in Varanasi. Environ. Sci. Pollut. Res . 27, 15912–15924. doi: 10.1007/s11356-019-06526-8
Tyagi, S., Dubey, R. C., Bhutiani, R., and Ahamad, F. (2020). Multivariate Statistical analysis of river ganga water at Rishikesh and Haridwar, India. Anal. Chem. Lett . 10, 195–213. doi: 10.1080/22297928.2020.1756405
Wang, Y. P., Xia, H., Fu, J. M., and Sheng, G. Y. (2004). Water quality change in reservoirs of Shenzhen, China: detection using LANDSAT/TM data. Sci. Total Environ . 328, 195–206. doi: 10.1016/j.scitotenv.2004.02.020
Zhang, Y., Pulliainen, J. T., Koponen, S. S., and Hallikainen, M. T. (2003). Water quality retrievals from combined landsat TM Data and ERS-2 SAR data in the Gulf of Finland. IEEE Trans. Geosci. Remote Sens . 41, 622–629. doi: 10.1109/TGRS.2003.808906
Keywords: water quality index, Yamuna river, physico-chemical parameters, water pollution, Dehradun city
Citation: Sharma R, Kumar R, Satapathy SC, Al-Ansari N, Singh KK, Mahapatra RP, Agarwal AK, Le HV and Pham BT (2020) Analysis of Water Pollution Using Different Physicochemical Parameters: A Study of Yamuna River. Front. Environ. Sci. 8:581591. doi: 10.3389/fenvs.2020.581591
Received: 09 July 2020; Accepted: 11 November 2020; Published: 11 December 2020.
Reviewed by:
Copyright © 2020 Sharma, Kumar, Satapathy, Al-Ansari, Singh, Mahapatra, Agarwal, Le and Pham. This is an open-access article distributed under the terms of the Creative Commons Attribution License (CC BY) . The use, distribution or reproduction in other forums is permitted, provided the original author(s) and the copyright owner(s) are credited and that the original publication in this journal is cited, in accordance with accepted academic practice. No use, distribution or reproduction is permitted which does not comply with these terms.
*Correspondence: Nadhir Al-Ansari, nadhir.alansari@ltu.se ; Hiep Van Le, levanhiep2@duytan.edu.vn ; Binh Thai Pham, binhpt@utt.edu.vn
This article is part of the Research Topic
The Environmental Hazards of Toxic Metals Pollution
Natural and sustainable filtration of polluted water of River Yamuna for municipal use
- Original Article
- Published: 10 April 2023
- Volume 9 , article number 67 , ( 2023 )
Cite this article
- Soma Mishra ORCID: orcid.org/0000-0001-7781-6938 1 ,
- Pradeep Kumar 1 nAff2 &
- Indu Mehrotra 1
173 Accesses
Explore all metrics
River Yamuna in Delhi is facing severe degradation due to insufficiently treated wastewater entering into it from industries and local communities. Despite the efforts being made to restore the river water quality through the Yamuna Action Plan phase I and II (YAP), river water quality has not been improved. The objective of the present study was to assess the changes in water quality during natural and sustainable bank filtration of contaminated river water. To meet the objective, water samples from the Yamuna River and eight Ranney wells (RWs) were analysed in the non-monsoon and monsoon seasons. The proportion of the bank filtrate drawn by the RWs was determined by monitoring δ 18 O and δ 2 H, the stable isotopes of the water samples. An attempt was made to establish the hydraulic connectivity between the river and RWs using δ 18 O data. Despite turbidity of > 1500 NTU in river water, particularly during the monsoon, the goal of achieving a prescribed limit of turbidity (< 5 NTU) through riverbank filtration (RBF) is attainable. RBF was very useful for removal of organics. Even in case of source waters affected by wastewaters (non-monsoon average DOC > 15 mg/L), attenuation of DOC < 2 mg/L in RWs is possible. The same trend of removal was followed by ultraviolet absorption at 254 nm (UV-254) and specific UV absorbance (SUVA). E.coli and total coliform were reduced by > 4-log (base 10). However, in the case of source waters rich in organics, production of coliform-free filtrate does not seem to be a realistic target. Post-treatment of filtrate is a must. The study concludes that even in the case of polluted raw water sources, the value of RBF as an effective natural pre-treatment step cannot be undermined.
Graphical abstract
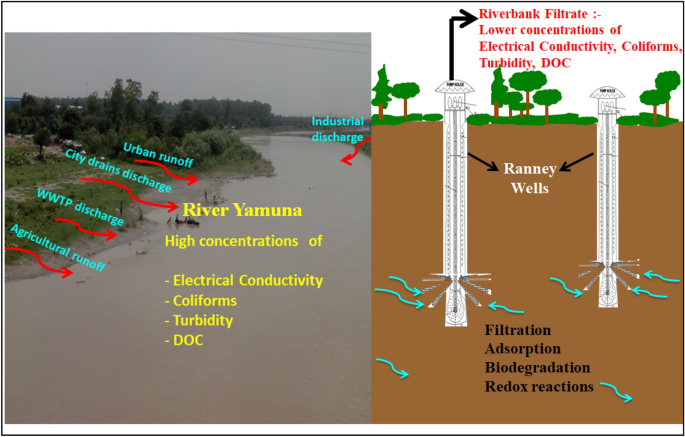
Similar content being viewed by others
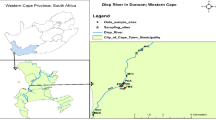
The impact of informal settlement on water quality of Diep River in Dunoon
Babalwa Gqomfa, Thabang Maphanga & Karabo Shale
Nature-Based Solutions for Domestic Wastewater Treatment in the Philippines
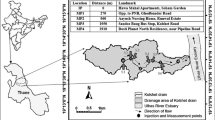
Water quality monitoring and modeling for an urban storm drainage channel in Thane, India
Kuldeep Swarnkar, Kapil Gupta & Vinay Nikam
Avoid common mistakes on your manuscript.
Introduction
In natural or riverbank filtration (RBF), the river water travels through the riverbed and bank that serve as a natural filter and contaminants are removed mainly by physical and biogeochemical processes (filtration, adsorption, biodegradation, chemical precipitation). The dilution of the filtrate with the local groundwater further alters the quality of the filtrate (Hiscock and Grischek 2001 ). The turbidity, bacteria, organic impurities, etc. present in the polluted river water are removed during natural filtration. RBF is either a complete treatment as practised in many places in Europe or a pre-treatment as prevalent in the USA. It is a well-accepted simple and a low-cost treatment (or pre-treatment) technique, in use since the 1870s along the rivers Rhine, Elbe and Danube (Grischek et al. 1998 ; Kühn and Müller 2000 ).
The RBF production wells are of different types. There are vertical wells, horizontal collector wells with radials, large-diameter dug wells and small devices such as Uttaranchal koops (Koops mean wells) (Dash 2011 ). For water supply, Ranney wells (RWs), also known as radial or horizontal collector wells, are in use in India since the early 1970s. The range of variation in the dimensions of the RWs is given in Table 1 . Globally, RWs have been placed in three different ways: (a) wells some distance away from the river with their laterals fully extended within the aquifer as practised in European countries (Hunt et al. 2003 ), (b) wells constructed close enough to the bank such that some of the laterals are partially below the bed as used in the USA (Grischek et al. 2003 ; Hunt et al. 2003 ) and (c) wells placed within the river as popular in India (Singh et al. 2010 ; Kumar et al. 2012 ).
In India, RBF wells have been installed on the banks of many rivers/lakes in the past five decades (Dash et al. 2008 , 2010 ; Sprenger et al. 2008 ; Lorenzen et al. 2010 ; Singh et al. 2010 ; Cady 2011 ; Kumar et al. 2012 ; Cady et al. 2013 ; Gupta et al. 2015 ). However, in the last five decades, pollution load in several rivers has increased gradually due to rapid population growth, urbanization, industrialization, excessive use of chemicals in agricultural fields and inadequate treatment facilities for both domestic and industrial wastewaters. It has resulted in the deterioration of the quality of the bank filtrate at many places (Singh et al. 2010 ; Kumar et al. 2012 ). Natural filtration systems originally conceived for the attenuation of turbidity and microbes are now facing challenges due to the presence of higher concentrations and a wider variety of contaminants in source waters. In few cases, this has resulted in bank filtrate not conforming to drinking water quality standards (Singh et al. 2010 ; Glorian et al. 2018 ; Sandhu et al. 2019 ) and closure of RBF well (Saph Pani 2012 ; Groeschke et al. 2016 ) in recent times.
There are only a few reports on the treatment of polluted surface waters by natural filtration or RBF compared to significant number of research publications on application of RBF along clean surface waters. Cosovic et al. ( 1996 ) conducted the study on the polluted Gorjak stream, a tributary of the Sava River in Croatia. The river receives industrial effluent from baker’s yeast and pharmaceutical production. The COD of the river water was found to vary from 870 to 5330 mg/L. The COD of the water from the well at 5 m from the river was 3–5 mg/L. The residual organics were identified as fulvic and humic substances. Miettinen et al. ( 1998 ) showed that high molecular weight organic fraction of the water of Lake Kallavesi, Finland, was reduced during bank filtration. Attenuation of organic fraction reduced the formation of halogenated compounds during chlorination. Reduction of the organic carbon content of the source water was found to be strongly dependent on the filtration distance. Wu et al. ( 2007 ) studied the effectiveness of RBF of heavily polluted water of Kuihe River in China. The biochemical processes in the saturated percolation zone could remove about 95% of nitrogen present in the river water.
Water quality improvement during bed filtration of the polluted water of the River Yamuna was studied by Singh et al. ( 2010 ) and Kumar et al. ( 2012 ) at Mathura, India. Coliforms, colour and organics measured as DOC and UV-254 of the river water were reduced by more than 50%. It was concluded that RBF could eliminate the need for a pre-chlorination step and thereby decrease the formation of adsorbable organic halogens (AOX) and disinfection by-products (DBPs). The performance of an RBF system on polluted River Kali, in Karnataka, south India was studied by Cady ( 2011 ) and Cady et al. ( 2013 ). Results showed 88% to more than 99% removal of bacteria in the water from RBF well. Heavy metal concentrations were also found to decrease during RBF. Maeng and Lee ( 2019 ) found that biodegradable organic matter in Nakdong River, South Korea, was reduced by more than 40% during soil passage.
RBF has been in use around Akshardham Temple in Central Delhi for approximately five decades. Over this period, the water quality of River Yamuna has gradually degraded. Yamuna River has been extensively studied over several decades for its quality and sources of pollution (Karn and Harada 2001 ; CPCB 2006 , 2012 ; Kaushik et al. 2008 ; Sprenger et al. 2008 ; Mandal et al. 2010 ; Lorenzen et al. 2010 ; Mutiyar et al. 2011 ; Sehgal et al. 2012 ). As per the report from the Central Pollution Control Board (CPCB 2012 ), only ~ 1558 ML/d of sewage was being treated in Delhi in 2012. The remaining ~ 2242 ML/d of untreated sewage was discharged into the River Yamuna. Karn and Harada ( 2001 ) estimated discharges from the industries in Delhi as 300 ML/d. These discharges lead to high amount of pollutants in River Yamuna. During two of the European Union (EU) funded projects, Techneau (duration 2006–10) and Saph Pani (duration 2011–2014), studies were carried out close to Akshardham Temple by drilling water quality monitoring wells on the flood plain of Yamuna. Lorenzen et al. ( 2010 ) indicated that losing stream conditions prevail. Groeschke et al. ( 2016 , 2017a ) found elevated and fluctuating concentrations of ammonium (NH 4 + ) as high as 35 mg/L in abstracted groundwater. As wells draw high amount of bank filtrate, the NH 4 + concentrations in water pumped from some of the wells exceed the desirable value of 0.5 mg/L ammonia-N (IS 10500 2012). It is suggested that sewage contaminated water of Yamuna infiltrates into approximately 16 m-thick shallow floodplain aquifer and serves as the main source of NH 4 + plume. A 1–2 m-thick layer of calcareous nodules, which is locally known as kankar, overlain by medium sand lies within aquifer. Kankar layer is reported to have higher hydraulic conductivity (3.7 \(\times {10}^{-3} \mathrm{m}/\mathrm{s}\) ) compared to sand (3.5 \(\times {10}^{-4} \mathrm{m}/\mathrm{s}\) ) and provides preferential pathway to infiltrating river water (Groeschke et al. 2017a ). Due to losing river conditions, good hydraulic conductivity and high pumping rate, it is believed that RWs located on floodplain draw a high share of bank filtrate. The results of 2D numerical modelling carried out by Groeschke et al. ( 2017b ) indicated that infiltrating river water is not diluted by the groundwater. They also suggested that large drinking water production wells located in the alluvial aquifer draw high share of bank filtrate. The same needs to be verified and fraction of river water in water abstracted through RWs has to be assessed quantitatively. Secondly, although the water quality of River Yamuna has been studied extensively along with samples from observation wells installed in the flood plain close to Akshardam Temple, a comprehensive study of water quality of bank filtrate collected from RWs is lacking. Characterization of the bank filtrate water is essential as it is supplied and used by the community for drinking purpose.
The present study, therefore, is limited (i) to the analysis of the proportion of riverbank filtrate in the water being pumped from RWs (based on stable isotope δ 18 O and δ 2 H data) and (ii) to the assessment of the improvement in the quality of river water after bank filtration. To investigate these two aspects, water quality of the River Yamuna and RWs was evaluated. Water samples were collected in non-monsoon and monsoon months of the year 2013–2014 and analysed for stable isotopes δ 18 O and δ 2 H, bacteriological indicators, turbidity, DOC and UV absorbance at 254 nm (UV-254).
Site description
The present study was carried out in a well field, having nine RWs in Central Delhi located on the left bank of the River Yamuna around Akshardham Temple (Fig. 1 ). A typical cross section of the well field, across RWs: P-3, P-4, P-5 and P-6, is shown in Fig. 2 . As the River Yamuna enters Delhi, its water is diverted to water treatment plants. Surplus water is stored by closing the gates of the Wazirabad Barrage. Gates remain closed during non-monsoon (October–middle of July) and are opened during monsoon (Middle of July–September) to release the flood water consisting of snow melt and rainfall in the catchment. Around 80% of the water flows through Delhi stretch of the river in the short monsoon period and results in considerable dilution of sewage flowing in the river. During non-monsoon, the stretch in Delhi, downstream of Wazirabad barrage, is mostly fed by the discharge of partially treated and untreated domestic and industrial waste waters by 22 major wastewater drains. The 22-km stretch of the Yamuna in Delhi, which is less than even 2% of the total length of the river (1376 km), gets over 80% of the total pollution load (CSE 2007 ). The entire stretch of the River Yamuna has been monitored at 19 locations for 6 years from 1999 to 2005 (CPCB 2006 ). The data reveals that compared to the other sites, Yamuna has the highest level of pollution at Nizamuddin (28°35'N, 77°17'E), very close to the Akshardham Temple. Well field around Akshardham Temple is very unique because at this site the Yamuna is reported to be the most contaminated having BOD as high as 51 mg/L (CPCB 2012 ). This well field site has many RWs installed, out of which nine operating RWs continuously working for water supply to the community. An unconfined aquifer having good hydraulic connection exists between the river and RWs. The design parameters of the RWs in the well field are compiled in Table 2 .
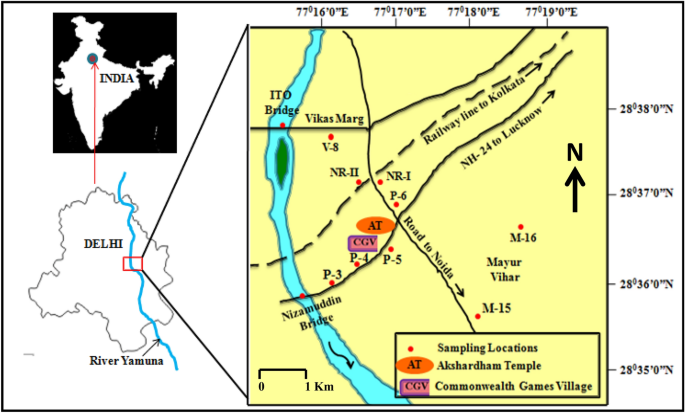
Well field around the Akshardham Temple lying between longitude 28°35' and 28°38'N, and latitude 77°15' and 77°19'E on the left bank of the River Yamuna. Only important landmarks, e.g. bridges, railway line and roads are shown to identify sampling locations, two on River Yamuna and nine RWs
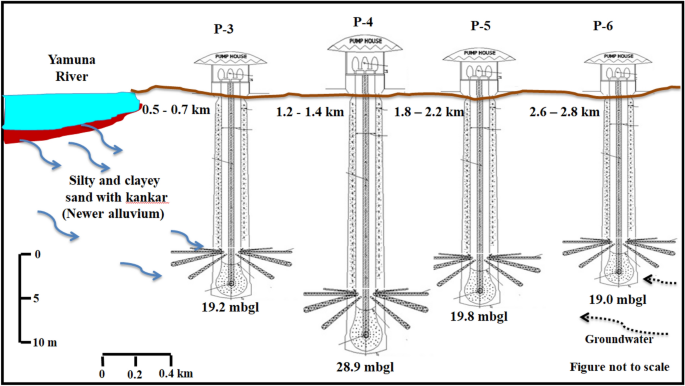
Cross section of the well field, across RWs P-3, P-4, P-5 and P-6. Horizontal distances are the shortest distances from the bank measured from the Google Earth in non-monsoon. Figure is representation only and not to the scale as horizontal distances are in km, while vertical distances are in m. ( mbgl meter below ground level)
The Yamuna flood plain of Delhi comprises younger alluvium overlying older alluvium. The composition of the younger alluvium is mainly a mixture of medium-to-coarse sand, silt, clay and kankar (local name of small calcareous concretions mixed with small gravels) (Chatterjee et al. 2009 ). The major water-bearing horizon is fine- to coarse-grained sand with kankar (CGWB 2006 ).
Materials and methodology
Water samples from the River Yamuna (at Nizamuddin and ITO Bridges) and all the RWs (Fig. 1 ) were collected between August 2013 and August 2014. Due to 8–9 mg/L of NH 4 + in water, the operation of the RW: P-3 was stopped in November 2013 (Saph Pani 2012 ). Samples of water from P-3 could not be collected beyond November 2013. Therefore, data of P-3 collected from August to November 2013 is not reported. A total of 16 sampling campaigns were organized, 7 in the monsoon (2.5 months, mid-July–September) and 9 in non-monsoon (9.5 months, October–mid-July). If any of the RWs was not already in operation at the time of the sampling campaign, it was purged for at least 20 min prior to the sampling. Samples were collected in glass bottles for organics and polypropylene bottles for other physicochemical water quality parameters. Samples for bacteriological analysis were collected in sterilized glass bottles. For stable isotope analysis, samples were collected in polypropylene bottles of 15 mL capacity, ensuring that there was no air entrapped in the bottles. Electrical conductivity (EC), pH and temperature were measured on-site with a portable multi-parameter probe (HQ40d, Hach, Loveland, USA). Water samples were transported within 8 h to the Environmental Engineering Laboratory, IIT Roorkee, for the analysis of bacteria, turbidity and organics. Total coliform (TC) and E. coli were measured using IDEXX Colilert -18 and Quanti-Tray method. For all other parameters, samples were stored at 4 °C and analysis was completed within 48 h after sampling. Parameters were analysed as per the methods prescribed in the Standard Methods (APHA 2005 ).
For the determination of DOC and UV-254, samples were filtered through 0.45 µm filter paper and analysed using TOC-VCSN total organic carbon analyser (Shimadzu) and Hach DR-5000 UV–VIS spectrophotometer with 10 mm quartz cell, respectively. Specific UV absorbance (SUVA) was calculated as the ratio of UV-254 (1/m) to DOC (mg/L). Turbidity was measured using Hach 2100 AN turbidity meter.
Stable isotopes (δ 18 O and δ 2 H) were determined using a dual inlet isotope ratio mass spectrometer (GV Instruments, Isoprime) at the National Institute of Hydrology, Roorkee. Samples were equilibrated with Pt–H 2 and CO 2 to measure δ 18 O and δ 2 H, respectively (Epstein and Mayeda 1953 ; Brenninkmeijer and Morrison 1987 ). The isotopes are reported as a per mil difference (δ ‰) between the isotopic ratios of the sample and standard relative to the international Vienna Standard Mean Ocean Water (VSMOW). The precisions in the measurement of δ 18 O and δ 2 H were ± 0.1 ‰ and ± 1 ‰, respectively.
Results and discussions
- Stable isotopes
Seasonal variation
The temporal variation of δ 18 O for water from the river and RWs is presented in Fig. 3 . The perusal of the data indicates a significant difference between the values of δ 18 O of the monsoon and non-monsoon river water. The average values of δ 18 O of the monsoon and non-monsoon river water were − 9.25 and − 7.28, respectively. The monsoon values are related to the rainfall. Higher the rainfall, lesser is the value. The δ 18 O of − 11.09 of the river water sample in August 2013 corresponds to the flood conditions. Dalai et al. ( 2002 ) have also found the depleted δ 18 O values of the River Yamuna water by 0.5–1.5 ‰ in the monsoon compared to the summer months. The seasonal variations in δ 18 O of the well waters are in narrow range (Figs. 3 and 4 ).
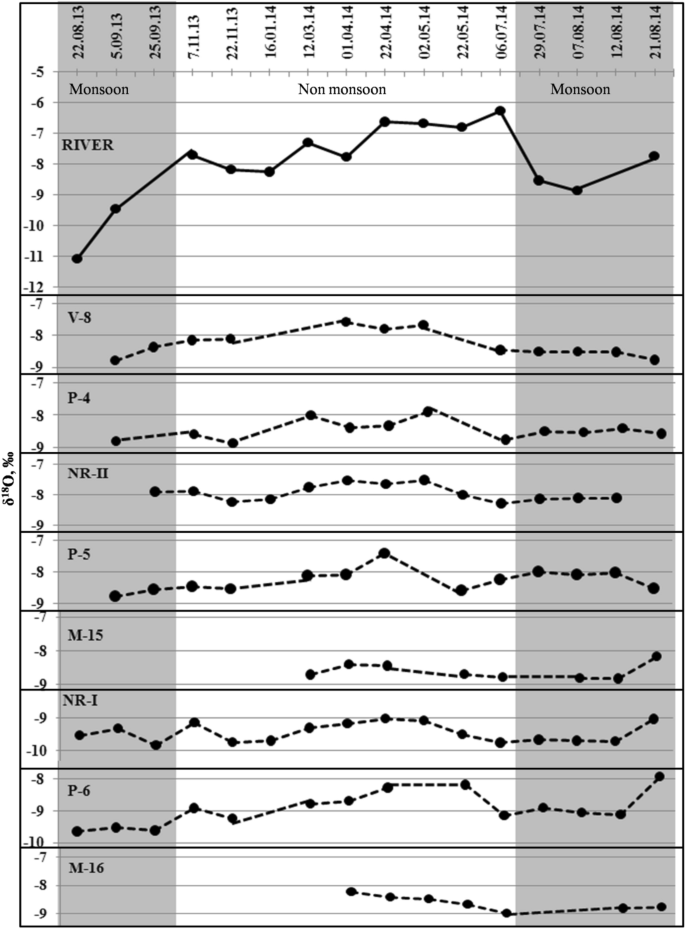
River Yamuna and Ranney wells: temporal variation of δ 18 O. Points marked on the horizontal axis are dates of sampling. Since the interval between the dates of sampling varied, points on the x-axis are not to the scale and trends shown are not true representation
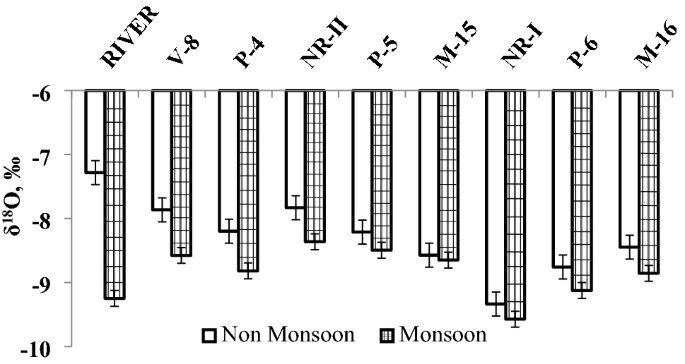
River Yamuna and Ranney wells: average values of δ. 18 O with standard error. [Non-monsoon ( n , number of samples) = 9, monsoon ( n ) = 7]
Also, δ 18 O and δ 2 H of the river water in the monsoon months coincide with the local meteoric water line (LMWL) (Fig. 5 ). The river is fed primarily by the rainwater. The non-monsoon δ 18 O and δ 2 H of Yamuna water are shifted towards the heavier side of the LMWL. The high values could be due to the evaporative enrichment and/or different source water. The physicochemical analysis indicates high electrical conductivity and substantial organic pollution of the Yamuna water during non-monsoon (Figs. 6 and 8 ). The conductivity of the river water in non-monsoon is three to four times the monsoon water conductivity (Fig. 6 ). The high conductivity and organic pollution of the river water coupled with the isotopic signatures (Fig. 5 ) point towards the different source water rather than the evaporative enrichment.
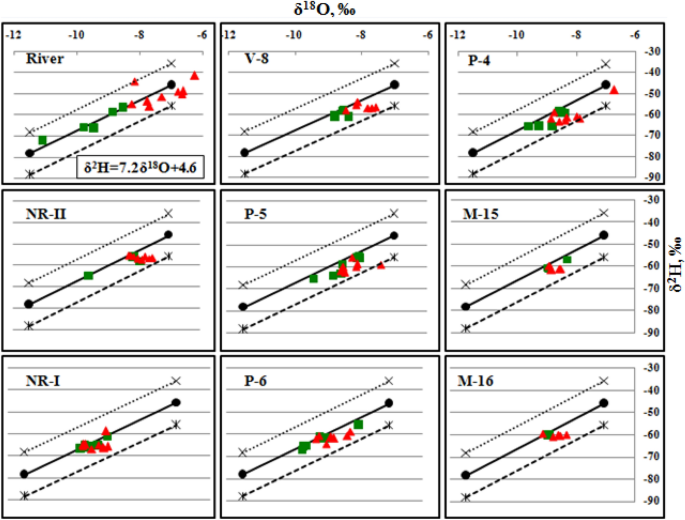
River Yamuna and RWs: average EC with standard error in non-monsoon ( n = 9) and monsoon ( n = 7). Details of each RWs is mentioned in Table 2
The water quality data is in conformity with the operation of the water supply schemes upstream of the Akshardham well field. The glacial melt feeding the Yamuna in non-monsoon is diverted to the Wazirabad and Chandrawal water works through the Wazirabad barrage that is located 15 km upstream of the Akshardham well field. Also, 22 wastewater drains join the river between the Wazirabad barrage and the Akshardham well field. The river in this stretch is polluted particularly during non-monsoon as it mainly carries the wastewater released by the drains.
Spatial variation
Average values with standard errors of δ 18 O of the water samples from the river and RWs are presented in Fig. 4 . The data suggest that during non-monsoon, the water from all the RWs is (i) lighter than the river water and (ii) heavier than the corresponding monsoon water. In an RBF field, the well water is generally heavier than the river water. The observations during non-monsoon for the present well field, however, are unusual.
The maximum difference between the monsoon and non-monsoon values of δ 18 O has been observed for the river water. As far as the wells are concerned, the difference in the case of water from the RWs: V-8, P-4 and NR-II is more than the other well waters. These three wells are at a distance of less than 2 km from the river, whereas the other wells are at a distance ranging from 2 to 4 km (Table 2 ). It, therefore, suggests that the RWs close to the river receive more river water than the wells away from the river. Water in the wells that are away from the river is in equilibrium with the aquifer. Also, the river discharge during non-monsoon is small as most of the water upstream of the well field is diverted to the waterworks.
Among the wells, the water from the NR-I is lighter than the other well water. The NR-I is at a distance of 2–2.2 km from the river. The NR-I may receive rainwater through infiltration and/or river water through the sub-surface flow. The observed isotopic composition of the water from the other wells could be due to the mixing of the river water and NR-I water as well as travel time.
Mixing ratios
The mixing ratios in the wells, therefore, have been calculated from the δ 18 O of the NR-I and the river water using the mass balance method (Eq. 1 ). The values of the mixing ratio for different seasons are presented in Table 3 .
where Q river / Q t = fraction of the river water in the well water. δ 18 O RW = average δ 18 O of the RW water for which mixing ratio is to be calculated. δ 18 O river = average δ 18 O value of river water.δ 18 O NR-I = average δ 18 O value of the NR-I water.
Calculations indicate more than the 100% of the river water in a few RWs in the monsoon season (Table 3 ). One of the implications of this observation could be that these wells receive river water through the sub-surface flow and/or rainwater through infiltration. The signature of the water, however, changes due to evaporative enrichment or mixing of some other water. The evaporative enrichment could be due to the long travel time, which has not been estimated in the present study. The fraction of the river water in the wells has been calculated by taking values of δ 18 O of the river water, NR-I water and the RW water for the same months. The monsoon lasts for 2–3 months, and travel time is more than the monsoon period. Therefore, the effect of the same monsoon water on the RWs water may not simultaneously appear in the same year. The non-monsoon RWs water being lighter than the river water could also be because of the long travel time. Since these wells are in an unconfined aquifer, the mixing of rainwater through infiltration cannot be ruled out.
Deuterium excess (d)
According to Dansgaard ( 1964 ), the deuterium excess (d), on the basis of the global meteoric water line (GMWL) is defined by Eq. 2 . However, d-excess values, as well as the slope of correlation for local lines, are different. The d value (from Eq. 2 ) for the Yamuna water in the Himalayan ranges varies from 5.2 to 17.3 ‰ (Dalai et al. 2002 ). The long-term average d value for the precipitation in New Delhi from Eq. 2 is about 8.5‰, and during non-monsoon (October) it is > 10‰ (Araguas et al. 1998 ). The values of slope and d-excess depend on the humidity conditions. Evaporative trends have a slope between 2 and 5 depending on the relative humidity. The higher value of d in the precipitation is the consequence of low humidity conditions (Clark and Fritz 1997 ).
LMWL for Delhi is given by Eq. 3 :
Equation 3 provides the baseline to compare the isotopic composition of the river (surface water) and RWs water (sub-surface water). The δ 18 O and δ 2 H data shown in Fig. 5 indicate the following:
The monsoon data for the river and RWs water are close to the LMWL for Delhi.
The LMWL can describe the river data in the monsoon except the outlier corresponding to the flood event of Aug. 2013.
The non-monsoon data is within − 10‰ of the LMWL, i.e. d value from the Eq. 3 ranges between 4.6 to and 5.4 ‰ (+ 4.6 – 10 = –5.4).
Most of the data points other than the river in monsoon, and a few RW data exhibit oxygen enrichment.
The δ 18 O and δ 2 H for the individual RW water were not found to be correlated due to oxygen enrichment as well as mixing. Since the non-monsoon river water is a mixture of the glacier melt and the wastewater, it is not described by the LMWL. All the data show oxygen enrichment, which results in a decrease in d-excess from + 4.6 to a minimum of –5.4 (Fig. 5 ). The d-excess for the water decreases with the oxygen enrichment provided the slope does not change. The range of d-excess calculated from the data shown in Fig. 5 using Eq. 3 is given in Table 4 . The minimum values of d intercept for all the non-monsoon samples are negative, i.e. oxygen enrichment is significant enough to decrease d value more than 4.6 (Eq. 3 , LMWL). The oxygen enrichment for the monsoon samples is less than the non-monsoon. Higher the oxygen enrichment lesser is the d-excess. In fact, the maximum value of d-excess for the monsoon RWs water is mostly less than the LMWL value of 4.6. The oxygen enrichment could be due to the evaporation of water or mixing of water that is rich in δ 18 O. The negative value of d-excess has been reported for lake water (Yuan et al. 2011 ).
On-site measurement
Temperature and ph.
The temperature of the Yamuna River water during non-monsoon was observed in the range of 15.2 (winter) to 32.5 °C (summer), whereas during monsoon the temperature varied from 29.2 to 32.1 °C. However, a wide range of variation from winter to summer was not observed for RWs water. The temperature of the water from the RWs ranged from 26 to 30 °C. The pH of the river water ranged from 7.4 to 8.4 and for RWs from 6.7 to 8.4.
Electrical conductivity (EC)
The electrical conductivity of the river water is significantly different in the monsoon and non-monsoon seasons. The information extracted from the EC data is as follows:
The EC values of the monsoon and non-monsoon river water are around 400 and 1400 µs/ cm, respectively.
The EC of the monsoon RW water is more than the river water. It may be due to the mineralization of the river water during the sub-surface flow (Dash et al. 2010 ).
There is not much difference in the magnitude of EC of the monsoon and non-monsoon well water except that the scatter in data is large for the water from the RWs P-4 and P-5 (Table 5 and Fig. 6 ).
Scatter may be due to the connectivity to the river. The analysis of the isotope data suggests the connectivity of the other wells, along with P-4 and P-5 (Table 5 ). EC, however, of NR-I, NR-II, M-15, M-16 and P-6 is stable, i.e. data is not scattered. The water is in equilibrium with the aquifer material. The observed difference in the response of water samples to isotope and EC could be due to the difference in travel time or mixing of the river water with the water other than the NR-I water. With the limited data available, it is difficult to comment on this aspect.
Removal of coliform, turbidity and organics
Box plots of total coliform and E. coli in water samples collected from the river and RWs during non-monsoon and monsoon were included in an earlier publication (Kumar et al. 2018 ). Figure 7 is reproduced from Kumar et al. ( 2018 ). The coliform count of the river water was found to be between (i) log 6 and 7 in the non-monsoon and (ii) log 5 and 6 in the monsoon. The coliform counts in the monsoon in the river water were found to be lower by around 1 log, mainly due to the dilution. In non-monsoon, river primarily carries treated and untreated wastewaters. Water samples from RWs were also found to be bacteriological contaminated in both the seasons. However, compared to river water, counts of coliform were significantly low. Bacterial attenuation, as noticed in the water from the RWs was more than log 5 and log 4 during non-monsoon and monsoon, respectively. Like total coliform, average E. coli counts were also found one log less in river water in monsoon compared to non-monsoon (Fig. 7 ). In the water from RWs, decrease in E. coli was more than 5 log both in non-monsoon and monsoon seasons. Despite 4–5 log removal of coliform, the water from the RWs cannot be classified as safe for drinking. Disinfection is essential.
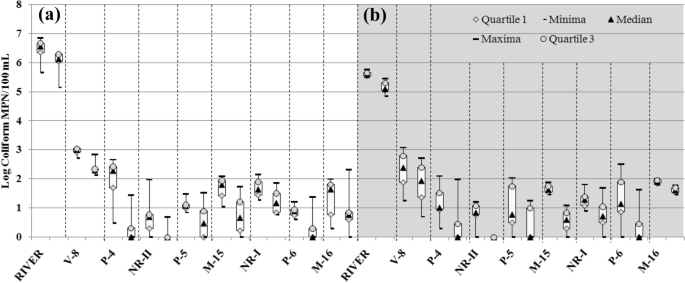
River Yamuna and RWs: total coliform and E. coli for ( a ) non-monsoon ( n = 9) and ( b ) monsoon ( n = 7) (in each vertical column the first box plot represents total coliform and the second one is for E. coli) (Kumar et al. 2018 ). Details of each RWs is mentioned in Table 2
Turbidity of the river water exhibits a broad range of variation. Ranney well water turbidity is mostly less than 2 (Table 6 ). The turbidity of the well water complies with the drinking water standard IS: 10500 ( 2012 ).
Aqueous organics
Aqueous organics have been monitored by measuring DOC and UV-254. Organics have further been characterized by estimating SUVA from DOC and UV-254. The perusal of the data indicates the following:
River water
DOC has been found to vary from about 2 to 66 mg/L.
DOC of the monsoon samples is between 3 and 8 mg/L. Non-monsoon samples except January 2014 sample had DOC ranging from 5 to 15 mg/L. The DOC of the January sample was 66 mg/L.
The numerical values of UV-254 (m −1 ) for all the samples except the January sample are more than the corresponding DOC (mg/L).
SUVA values (L/mg m) of the (i) monsoon samples are around one and (ii) non-monsoon samples are greater than 2.
It is evident that the nature of the aqueous organics is different in the monsoon and non-monsoon samples. It is quite evident as the river carries rainwater in the monsoon and substantial amount of wastewater in the non-monsoon. It corroborates the observations and conclusion derived from the isotope and EC data. The SUVA value greater than two indicates the presence of hydrophobic aromatic compounds in water (Table 7 ). These compounds are removed during bank filtration. The SUVA of the filtered water is less than 2.
Ranney wells water
The water from the NR-I is different than the water from the other wells.
DOC of the NR-I is in the range from 6 to 14 mg/L.
The average DOC of the non-monsoon NR-I samples is more than the average DOC of the monsoon samples (Fig. 8 ).
UV-254 of all the NR-I samples except one monsoon sample is less than two m −1 , and SUVA is less than one. The residual organics in NR-I water are hydrophilic in nature as the hydrophobic organics would be sorbed by the aquifer material (Ruffino and Zanetti 2009 ).
The DOC of the water from the other wells is mostly less than 2 mg/L. There are three out of about fifty observations that have recorded DOC greater than 2 mg/L.
UV-254 of the water has been found to vary from 0.1 to less than 4 m −1 .
There is no definite trend in SUVA values. SUVA values are from 0.04 to about 2 L/mg m.
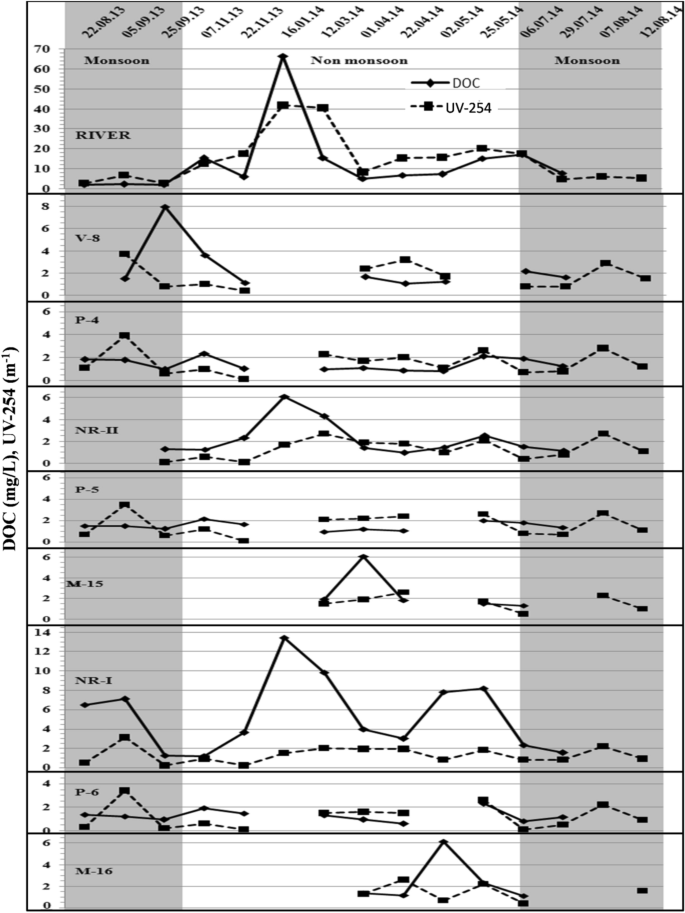
River Yamuna and Ranney wells: temporal DOC and UV-254 plots for non-monsoon and monsoon. Points marked on the horizontal axis are dates of sampling. Since interval between dates of sampling varied, points on the x-axis are not to the scale and trends shown are not true representation
The water from the NR-I has to be treated for DOC removal prior to use for domestic consumption. Another RW on the bed of the River Yamuna is located at Mathura, 155 km downstream of Delhi. The DOC of the river water is reduced by about 59–78% during bed filtration. The filtrate DOC of 1.65–6.30 mg/L has been reported (Kumar et al. 2012 ). At both places, river water is heavily polluted, and filtrate needs post-treatment to consistently get filtrate of DOC less than 2 mg/L.
Conclusions
The non-monsoon river water is polluted. It is isotopically heavier than the monsoon river water as well as water from the RWs. The non-monsoon river water has a significant wastewater discharge from the drains. The effect of the monsoon river water on the water from the RWs is not seen due to the long travel time. The d-excess (from LMWL Eq. 3 ) decreases from + 4.6 to − 5.4 with oxygen enrichment.
The water from RWs away from the river is in equilibrium with the aquifer. These wells do not respond to seasonal fluctuations in the river water. Despite the considerable amount of turbidity in the source water during monsoon (> 1500 NTU), the goal of achieving prescribed limits of turbidity for drinking water through RBF is attainable. Even in case of source waters consistently infected by wastewaters (non-monsoon average DOC > 15 mg/L), attenuation of organics in terms of DOC < 2 mg/L through RBF is possible. However, in case of contaminated source water (rich in organics), coliform survive over long distances in the aquifer. Production of coliform free filtrate does not seem to be a realistic target. Post-treatment of filtrate is a must.
Overall, it could be summarized that in the case of contaminated surface waters, natural filtration alone is not adequate for the production of guaranteed safe and wholesome drinking water. An increase in the hardness of filtered water and the presence of non-degradable and non-adsorbable compounds make further treatment of riverbank filtrate mandatory. In some cases, bank filtration is not efficient in removing heavy metals such as ammonium, arsenic and iron. Although, results suggest that even in the case of a highly polluted source of water, the value of RBF as an effective pre-treatment step could not be undermined.
Data availability
The data that support the findings of this study are available from the corresponding author upon reasonable request.
APHA, AWWA, WEF (2005) Standard Methods for the Examination of Water and Wastewater, 21st ed. American Public Health Association, Washington DC
Araguas L, Froehlich K, Rozanski K (1998) Stable isotope composition of precipitation over southeast Asia. J of Geophy Res 103(28):721–742
Google Scholar
Brenninkmeijer CAM, Morrison PD (1987) An automated system for isotopic equilibration of CO 2 and H 2 O for 18 O analysis of water. Chem Geol 66(1–2):21–26
Cady P (2011) A riverbank filtration demonstration project on the Kali River, Dandeli, Karnataka, India. Dissertation. Available online: http://digitalcommons.uri.edu/cgi/viewcontent.cgi?article=1068&context=theses . Accessed Mar 2020
Cady P, Boving TB, Choudri BS, Cording A, Patil K, Reddy V (2013) Attenuation of bacteria at riverbank filtration site in rural India. Water Environ Res 85(11):2164–2174
Article Google Scholar
CGWB (2006) Ground Water Year Book 2005–2006, National Capital Territory, Delhi. Central Ground Water Board, Govt. of India, New Delhi.
Chatterjee R, Gupta B, Mohiddin S, Singh P, Shekhar S, Rajaram P (2009) Dynamic groundwater resources of National Capital Territory, Delhi: assessment, development and management options. Environ Earth Sci 59(3):669–686
Clark I, Fritz P (1997) Environmental Isotopes in Hydrogeology. Lewis Publishers, New York, p 328
Cosovic B, Hrsak D, Vojvodic V, Krznaric D (1996) Transformation of organic matter and bank filtration from a polluted stream. Water Res 30(12):2921–2928
CPCB (2006) Water Quality Status of Yamuna River 1995–2005. Central Pollution Control Board, Ministry of Environment and Forests, Govt. of India, New Delhi.
CPCB (2012) River Yamuna Wastewater Plan in Delhi. Central Pollution Control Board, Ministry of Environment and Forests, Govt. of India, New Delhi.
CSE (2007) Sewage Canal- How to Clean up the Yamuna. Centre for Science and Environment, New Delhi.
Dalai TK, Bhattacharya SK, Krishnaswami S (2002) Stable isotopes in the source waters of the Yamuna and its tributaries: seasonal and altitudinal variations and relation to major cations. Hydrol Process 16(17):3345–3364
Dansgaard W (1964) Stable isotopes in precipitation. Tellus 16:436–468
Dash RR (2011) Water purification through natural filtration. Ph. D. Dissertation, Department of Civil Engineering, Indian Institute of Technology Roorkee, India.
Dash RR, Mehrotra I, Kumar P, Grischek T (2008) Lake Bank filtration at Nainital, India: water-quality evaluation. Hydrogeol J 16(6):1089–1099
Dash RR, Prakash EVPB, Kumar P, Mehrotra I, Sandhu C, Grischek T (2010) River bank filtration in Haridwar, India: removal of turbidity, organics and bacteria. Hydrogeol J 18(4):973–983
Epstein S, Mayeda T (1953) Variation of 18 O content of waters from natural sources. Geochimica Et Cos-Mochimica Acta 4:213–224
Glorian H, Bornick H, Sandhu C, Grischek T (2018) Water quality monitoring in northern India for an evaluation of the efficiency of bank filtration sites. Water 10:1804
Grischek T, Hiscock KM, Metschies T, Dennis PF, Nestler W (1998) Factors affecting denitrification during infiltration of river water into a sand and gravel aquifer in Saxony. Germany Water Res 32(2):450–460
Grischek T, Schoenheinz D, Ray C (2003) Siting and design issues for riverbank filtration schemes. In: Ray C, Melin G, Linsky RB (eds) Riverbank filtration: improving source-water quality. Kluwer Academic Publishers, Dordrecht, pp 291–302
Chapter Google Scholar
Groeschke M, Kumar P, Winkler A, Grützmacher G (2016) The role of agricultural activity for ammonium contamination at a riverbank filtration site in central Delhi (India). Environ Earth Sci 75:129
Groeschke M, Frommen T, Taute T, Schneider M (2017a) The impact of sewage-contaminated river water on groundwater ammonium and arsenic concentrations at a riverbank filtration site in Central Delhi, India. Hydrogeol J 25:2185–2198
Groeschke M, Frommen T, Winkler A, Schneider M (2017b) Sewage-borne ammonium at a river bank filtration site in Central Delhi, India: Simplified flow and reactive transport modelling to support decision-making about water management strategies. Geosciences 7(48):1–16
Gupta A, Singh H, Ahmed F, Mehrotra I, Kumar P, Kumar S, Grischek T, Sandhu C (2015) Lake Bank filtration in landslide debris: irregular hydrology with effective filtration. Sustain Water Resour Manag 1(1):15–26
Hiscock KM, Grischek T (2001) Attenuation of groundwater pollution by bank filtration. J Hydrol 266:139–144
Hunt H, Schubert J, Ray C (2003) Conceptual design of riverbank filtration systems. In: Ray C, Melin G, Linsky RB (eds) Riverbank filtration-improving source-water quality, vol 43. Kluwer Academic Publishers, Dordrecht, pp 19–27
IS: 10500 (2012) Indian Standard: Drinking Water Specifications. Bureau of Indian Standards, New Delhi
Karn SK, Harada H (2001) Surface water pollution in three urban territories of Nepal, India, and Bangladesh. Environ Manag 28(4):483–496
Kaushik CP, Sharma HR, Jain S, Dawra J, Kaushik A (2008) Pesticide residues in river Yamuna and its canals in Haryana and Delhi, India. Environ Monit Assess 144:329–340
Kühn W, Müller U (2000) Riverbank filtration: an overview. J AWWA 92(12):60–69
Kumar P, Mehrotra I, Boernick H, Schmalz V, Worch E, Schmidt W, Grischek T (2012) Riverbank filtration: an alternative to pre-chlorination. J. Indian Water Works Association, Special Issue on Riverbank Filtration, 50–58.
Kumar P, Mehrotra I, Gupta A, Kumari S (2018) Riverbank filtration: a sustainable process to attenuate contaminants during drinking water production. J Sustain Dev Energy Water Environ Syst 6(1):150–161
Lorenzen G, Sprenger C, Taute T, Pekdeger A, Mittal A, Massmann G (2010) Assessment of the potential for bank filtration in a water-stressed megacity (Delhi, India). Environ Earth Sci 61:1419–1434
Maeng SK, Lee K-H (2019) Riverbank filtration for the water supply on the Nakdong River, South Korea. Water 11(1):129. https://doi.org/10.3390/w11010129
Mandal P, Upadhyay R, Hasan A (2010) Seasonal and spatial variation of Yamuna River water quality in Delhi, India. Environ Monit Assess 170:661–670
Miettinen IT, Martikainen PJ, Vartiainen T (1998) Mutagenicity and amount of chloroform after chlorination of bank filtered lake water. Sci Total Environ 215(1–2):9–17
Mutiyar PK, Mittal AK, Pekdeger A (2011) Status of organochlorine pesticides in the drinking water well-field located in the Delhi region of the flood plains of river Yamuna. Drink Water Engg Sci 4:51–60
Ruffino B, Zanetti M (2009) Adsorption study of several hydrophobic organic contaminants on an aquifer material. Am J Environ Sci 5(4):507–515
Sandhu C, Grischek T, Bornick H, Feller J, Sharma SK (2019) A water quality appraisal of some existing and potential riverbank filtration sites in India. Water 11(215):1–17
Saph Pani (2012) Enhancement of natural water systems and treatment methods for safe and sustainable water supply in India. D 1.1 Database of relevant pollutants in urban areas and their attenuation at RBF sites. Available online: http://www.saphpani.eu/fileadmin/uploads/Administrator/Deliverables/D1_1_Database_of_relevant_pollutants.pdf . Accessed Jan 2019
Sehgal M, Garg A, Suresh R, Dagar P (2012) Heavy metal contamination in the Delhi segment of Yamuna basin. Environ Monit Assess 184:1181–1196
Singh P, Kumar P, Mehrotra I, Grischek T (2010) Impact of riverbank filtration on treatment of polluted river water. J of Env Manag 91:1055–1062
Sprenger C, Lorengen G, Pekdeger A (2008) Occurrence and fate of microbial pathogens and organic trace compounds at riverbank filtration sites in Delhi, India. TECHNEAU integrated project: deliverable D 5.2.6. Available online: http://www.techneau.org ; accessed: July 2015.
Wu Y, Hui L, Wang H, Li Y, Zeng R (2007) Effectiveness of riverbank filtration for removal of nitrogen from heavily polluted rivers: a case study of Kuihe River, Xuzhou, Jiangsu, China. Environ Geol 52:19–25
Yuan F, Sheng Y, Yao T, Fan C, Li J, Zhao H, Lei Y (2011) Evaporative enrichment of oxygen-18 and deuterium in lake waters on the Tibetan Plateau. J Paleolimnol 46:291–307
Download references
This work was funded by the European Commission, 282911.
Author information
Pradeep Kumar
Present address: Department of Civil Engineering, SET, Sharda University, Greater Noida, 201310, India
Authors and Affiliations
Department of Civil Engineering, Indian Institute of Technology Roorkee, Roorkee, 247667, India
Soma Mishra, Pradeep Kumar & Indu Mehrotra
You can also search for this author in PubMed Google Scholar
Corresponding author
Correspondence to Soma Mishra .
Ethics declarations
Conflict of interest.
All authors have participated in analysis and interpretation of data and approval of final version. The authors have no affiliation with any organization with a direct or indirect financial interest in the subject matter discussed in the manuscript. The authors declare no conflict of interest.
Additional information
Publisher's note.
Springer Nature remains neutral with regard to jurisdictional claims in published maps and institutional affiliations.
Rights and permissions
Springer Nature or its licensor (e.g. a society or other partner) holds exclusive rights to this article under a publishing agreement with the author(s) or other rightsholder(s); author self-archiving of the accepted manuscript version of this article is solely governed by the terms of such publishing agreement and applicable law.
Reprints and permissions
About this article
Mishra, S., Kumar, P. & Mehrotra, I. Natural and sustainable filtration of polluted water of River Yamuna for municipal use. Sustain. Water Resour. Manag. 9 , 67 (2023). https://doi.org/10.1007/s40899-023-00846-x
Download citation
Received : 04 October 2021
Accepted : 31 March 2023
Published : 10 April 2023
DOI : https://doi.org/10.1007/s40899-023-00846-x
Share this article
Anyone you share the following link with will be able to read this content:
Sorry, a shareable link is not currently available for this article.
Provided by the Springer Nature SharedIt content-sharing initiative
- Riverbank filtration
- Polluted River Yamuna water
- Find a journal
- Publish with us
- Track your research
Make a presentation on pollution of Ganga and Yamuna river effects on air pollution on Taj Mahal.
Pollution of ganga river the main causes of water pollution in the ganges river are the increase in the population density, various human activities such as bathing, washing clothes, bathing of animals, and dumping of various harmful industrial waste into the rivers. pollution of yamuna river the most polluted river the yamuna. ... despite such an esteem status, rivers are being polluted due to open sewage drains, lack of sufficient sewage treatment plants, soil erosion, and by dumping plastic garbage in river water, etc. effect of air pollution on taj mahal taj mahal is slowly decaying due to air pollution. dense smog created by human activities is slowly discoloring the marble and turning it to sickly yellowish-brown color. now the question arises who is to be blamed. there are several factors: heavy traffic, wood-burning crematoriums, smoke from neighbouring factories, and agra’s growing population, the last of which demands more and more water. as the yamuna river dries up, it risks sliding the taj mahal off its picturesque banks into a sea of mud. the burning of municipal solid waste in the open results in thick toxic smog which is the main reason for the discoloration of the taj mahal. these wastes should be properly deposited in landfalls that do not happen and the reason is bureaucratic incompetence. the supreme court of india has ordered that all the wood-burning crematoriums be replaced by an electric one. the up govt has banned the burning of cow dung which serves as a cheap source of fuel but at the same time, it produces brown carbon the same type which is turning the taj mahal into yellowish-brown color. it is also the responsibility of every citizen of agra, delhi and its neighbouring states to see that activities that lead to producing of toxic smog should be stopped. we need a bold and effective solution asap if we want our future generation to see the beautiful taj mahal in its former glory..
Write a note on the pollution of Ganga and Yamuna rivers and the effect of air pollution on Taj Mahal?
Taj Mahal is one of the Seven Wonders of the world. It is situated on the banks of river Yamuna.________ pollution has damaged the marble of the monument.
How is the Taj Mahal affected by air pollution?
THE TAJ MAHAL IS MADE UP OF WHITE MARBLE.WHAT IS EFFECT OF POLLUTION ON TAJ MAHAL.HOW CAN WE PROTECT FROM IT FROM POLLUTION.
Write a note on the pollution of the Taj mahal.
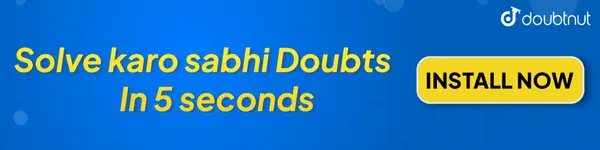
- Online Class
- Ask Doubt on Whatsapp
- Search Doubtnut
Make a presentation on pollution of Ganga and Yamuna Rivers and effects of air pollution on Taj Mahal.
I. river ganga and yamuna are polluted due to various human activities like bathing, washing clothes , dumping of various harmful industrial wastes, etc., near river bank. ii. primary air pollutants like carbon containing particles and secondary pollutants like hazardous chemicals in acid rains are causing discoloration of taj mahal..
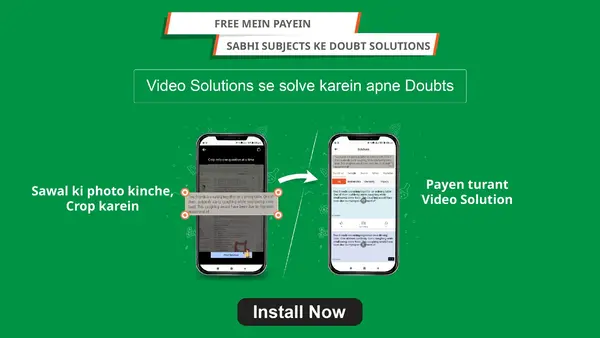
Related Playlists
ENVIRONMENTAL MANAGEMENT
EFFECTS OF ELECTRIC CURRENT
GRAVITATION
Similar Questions
Pollution Of Ganga River
Describe the causes and effects of air pollution.
What are causes of pollution of Ganga ?
Which of these is not an effect of air pollution?
Harmful effects of air pollutant is dependent on
Discuss the effect of air pollutants on plants.
What are the cause of pollution of river Ganga ?
TARGET PUBLICATION - ENVIRONMENTAL MANAGEMENT - APPLY OUR KNOWLEDGE
Which are different trophic levels in food chain ?
What is energy pyramid ?
A bird builing nest on a tree feeds upon the fishes in nearby pond. ...
What is environment?
Which cycles are operated in environment ?What is their importance ?
Which are the types of pollution ?
What do we mean by natural and artificial pollution ?
How do butterflies contribute to environment balance ?
Collect the information about Chipko Movement and discuss between two ...
Internet is my friend. i. Noise Pollution ( Regulation and Control ...
Most harmful effect of environmental pollution occurs on the living or...
We are not above to see some specific animals about which we had list...
Describe about the role among conservator, organizer, guide, plant fri...
Where are sacred groves frond in Maharashtra ? Make a list and visit ...
Collect more information about the work of voluntary and international...
Attempts at various levels are performed for conserving environment. R...
Collect the names of extinct birds and animals of India and tell those...
Surveys the plants and animals in your area. Maintain a record about t...
What will happen in future if animal species continue to go extinct ?
Make a presentation on pollution of Ganga and Yamuna Rivers and effect...
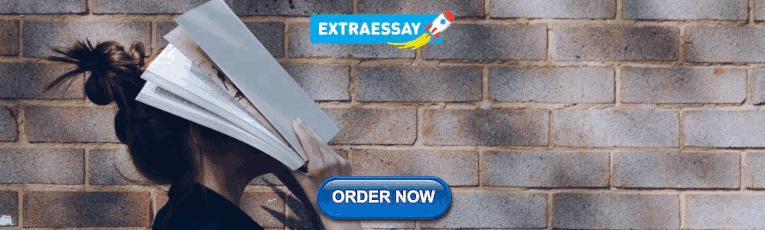
IMAGES
VIDEO
COMMENTS
River Yamuna is one of the most polluted rivers of the India. It originates from Yamunotri glaciers in the. lower Himalayas at an elevation of approximately 6387 meters. The barrages formed on the ...
The main pollution segment i.e., Delhi segment is the thing majorly focused on. Yamuna (only after treatment) which is claimed as polluted 2. Segments by several agencies a DO Yamuna is divided into fivesegments depending on several hydrological and ecological parameters. The Himalayan Segment, the Upper Segment, the Delhi Segment, the
Figure 1: Diagrammatic presentation of the segments of the Yamuna River Source: CPCB,2006-2007 Table 2: Major Water Quality Segments of the Yamuna River ... 4.Pollution in the Yamuna River and its major Sources The Yamuna River is one of the mostcontaminated rivers of India (CPCB 2010; Misra, A.K.,2010). Untreated or
The main stream of the river Yamuna originates from the Yamunotri glacier near Bandar Punch (38. o. 59' N 78. o. 27' E) in the Mussourie range of the lower Himalayas at an elevation of about 6320 meter above mean sea level in the district Uttarkashi (Uttranchal). The catchment (table 1&2) of the Yamuna river system covers parts of the states of
water quality c riteria with respect to pH only throughout its length. The pH value of river at Delhi varies from. 7.2 to 7.9, dissolved oxygen (DO) from 0.4 to 7.9 mg/l, biochemical oxygen demand ...
According to Kumar et al. 10, Delhi leads the list of cities with 79% pollution load in river Yamuna followed by Agra and Mathura with a contribution of 9% and 4%, respectively, whereas a ...
The Yamuna River in Delhi is a prevalent epitome of the depleted ecosystem that has transformed into a sewage drain due to intense pollution and blooming anthropogenic pressure.
5. Heavy metal Pollution sources of River Yamuna As a result of ecological stress created by humans on the aquatic environment, the pollution levels have significantly increased. Numerous studies have been conducted for testing the presence of heavy metals in the river Yamuna. In a study for determination of heavy metals in fish species (Sen et ...
The Yamuna river has become one of the most polluted rivers in India as well as in the world because of the high-density population growth and speedy industrialization. The Yamuna river is severely polluted and needs urgent revival. The Yamuna river in Dehradun is polluted due to exceptional tourist activity, poor sewage facilities, and insufficient wastewater management amenities. The ...
Polluted Yamuna, not industrial emission, main reason behind Taj Mahal decay: study. Yamuna pollution was identified as a threat to the Taj five years ago, blaming the formation of phosphorous in the river water for the breeding of insects whose excreta was leaving patches on the marbles. Now, a new study offers a different perspective ...
River Yamuna is the largest tributary of river Ganges and has been acclaimed as a heavenly waterway in Indian mythology. However, 22-km segment of river Yamuna passing through Delhi from downstream of Wazirabad barrage up to Okhla barrage is considered as the filthiest stretch having been rendered into a sewer drain. The present study employs high-resolution GeoEye-2 imagery for mapping and ...
The research analyses the causes of water pollution and its. impact on the quality of river water. The Yamuna river basin is a. part of Great Ganga basin in India. During the last two decades. the cities and towns in the basin have undergone rapid. urbanization and industrialization, leading to rise in water. pollution.
The present study was conducted on the river Yamuna, which passes through Delhi-NCR from Baghpat to Chhainssa, a distance of about 125 km, at six sampling locations to evaluate the concentrations of heavy metals in surface water using heavy metal pollution index (HPI) approach. The river serves both urban-industrial and rural areas in the study area; hence, domestic, industrial, and ...
Rivers Ganga and Yamuna have been subjected to massive degradation and pollution due to the vast amount of wastewater entering the river during the Kumbh Mela. The Kumbh Mela, the largest religious congregation, was organized at Prayagraj, India, from January to March 2019. Despite the efforts, the river quality during Kumbh Mela was not suitable for public use, and even after Kumbh Mela, it ...
formed the National Ganga River Basin Body (NGRBA) in 2009 as an empowered planning, funding, monitoring, and coordinating authority for the Ganga River to achieve suc-cessful pollution abatement and river conservation through a comprehensive strategy. On the 13th of May, 2015, the Cabinet approved the Namami Gange policy as a holistic
The Yamuna river in Dehradun is polluted due to exceptional tourist activity, poor sewage facilities, and insufficient wastewater management amenities. The measurement of the quality can be done ...
The Ganga and Yamuna rivers are two of India's most important rivers but are also two of its most polluted due to waste and sewage being dumped into their waters from various domestic and industrial sources. Attempts to clean the rivers have failed. Air pollution is also damaging the Taj Mahal, causing the white marble to discolor, putting the 17th century monument at risk unless measures are ...
Yamuna Action Plan - Free download as Powerpoint Presentation (.ppt / .pptx), PDF File (.pdf), Text File (.txt) or view presentation slides online. The document discusses the Yamuna Action Plan which aims to address pollution in the Yamuna River. It provides background on the Yamuna River and sources of pollution such as industrial and domestic waste, agriculture, and solid waste.
Yamuna ppt - Download as a PDF or view online for free. Yamuna ppt - Download as a PDF or view online for free ... Presentation on case study of uttarakhand flood Ajay Sharma ... , which dumps about 57% of its waste into the river (Central Pollution Control Board, 2005) 3. Representation of the SCenario 4.
The present study was carried out in a well field, having nine RWs in Central Delhi located on the left bank of the River Yamuna around Akshardham Temple (Fig. 1). A typical cross section of the well field, across RWs: P-3, P-4, P-5 and P-6, is shown in Fig. 2. As the River Yamuna enters Delhi, its water is diverted to water treatment plants.
Despite such an esteem status, rivers are being polluted due to open sewage drains, lack of sufficient sewage treatment plants, soil erosion, and by dumping plastic garbage in river water, etc. EFFECT OF AIR POLLUTION ON TAJ MAHAL. Taj Mahal is slowly decaying due to air pollution. Dense smog created by human activities is slowly discoloring ...
The National Green. proper facilities and human resources for monitoring and. GANGA AND YAMUNA: THROUGH THE LENS OF NA. water supplies were severely degraded be yond repair, with a. total of 40 ...
\nMake a presentation on pollution of Ganga and Yamuna Rivers and effects of air pollution on Tajmahal\nClass: 12Subject: BIOLOGYChapter: WEB QUESTIONSBoard:...
Text Solution. i. River Ganga and Yamuna are polluted due to various human activities like bathing, washing clothes , dumping of various harmful industrial wastes, etc., near river bank. ii. Primary air pollutants like carbon containing particles and secondary pollutants like hazardous chemicals in acid rains are causing discoloration of Taj Mahal.