Subscribe to the PwC Newsletter
Join the community, trending research, mora: enabling generalist video generation via a multi-agent framework.
lichao-sun/mora • 20 Mar 2024
Sora is the first large-scale generalist video generation model that garnered significant attention across society.
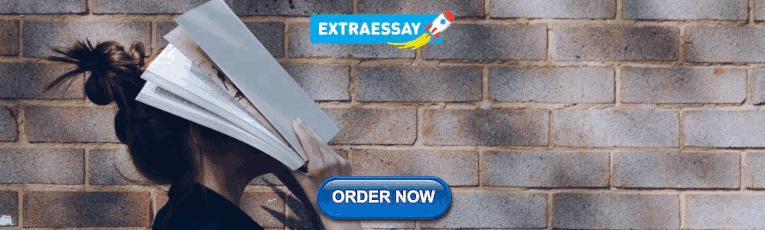
SDXS: Real-Time One-Step Latent Diffusion Models with Image Conditions
IDKiro/sdxs • 25 Mar 2024
Recent advancements in diffusion models have positioned them at the forefront of image generation.

StreamingT2V: Consistent, Dynamic, and Extendable Long Video Generation from Text
picsart-ai-research/streamingt2v • 21 Mar 2024
To overcome these limitations, we introduce StreamingT2V, an autoregressive approach for long video generation of 80, 240, 600, 1200 or more frames with smooth transitions.
Evolutionary Optimization of Model Merging Recipes

Surprisingly, our Japanese Math LLM achieved state-of-the-art performance on a variety of established Japanese LLM benchmarks, even surpassing models with significantly more parameters, despite not being explicitly trained for such tasks.
T-Rex2: Towards Generic Object Detection via Text-Visual Prompt Synergy
idea-research/t-rex • 21 Mar 2024
Recognizing the complementary strengths and weaknesses of both text and visual prompts, we introduce T-Rex2 that synergizes both prompts within a single model through contrastive learning.

FeatUp: A Model-Agnostic Framework for Features at Any Resolution
Deep features are a cornerstone of computer vision research, capturing image semantics and enabling the community to solve downstream tasks even in the zero- or few-shot regime.

Analyzing and Improving the Training Dynamics of Diffusion Models
Diffusion models currently dominate the field of data-driven image synthesis with their unparalleled scaling to large datasets.

General Object Foundation Model for Images and Videos at Scale
We present GLEE in this work, an object-level foundation model for locating and identifying objects in images and videos.

One-Step Image Translation with Text-to-Image Models
In this work, we address two limitations of existing conditional diffusion models: their slow inference speed due to the iterative denoising process and their reliance on paired data for model fine-tuning.

LLMLingua-2: Data Distillation for Efficient and Faithful Task-Agnostic Prompt Compression
The challenge is that information entropy may be a suboptimal compression metric: (i) it only leverages unidirectional context and may fail to capture all essential information needed for prompt compression; (ii) it is not aligned with the prompt compression objective.

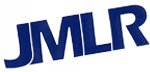
Frequently Asked Questions
JMLR Papers
Select a volume number to see its table of contents with links to the papers.
Volume 25 (January 2024 - Present)
Volume 24 (January 2023 - December 2023)
Volume 23 (January 2022 - December 2022)
Volume 22 (January 2021 - December 2021)
Volume 21 (January 2020 - December 2020)
Volume 20 (January 2019 - December 2019)
Volume 19 (August 2018 - December 2018)
Volume 18 (February 2017 - August 2018)
Volume 17 (January 2016 - January 2017)
Volume 16 (January 2015 - December 2015)
Volume 15 (January 2014 - December 2014)
Volume 14 (January 2013 - December 2013)
Volume 13 (January 2012 - December 2012)
Volume 12 (January 2011 - December 2011)
Volume 11 (January 2010 - December 2010)
Volume 10 (January 2009 - December 2009)
Volume 9 (January 2008 - December 2008)
Volume 8 (January 2007 - December 2007)
Volume 7 (January 2006 - December 2006)
Volume 6 (January 2005 - December 2005)
Volume 5 (December 2003 - December 2004)
Volume 4 (Apr 2003 - December 2003)
Volume 3 (Jul 2002 - Mar 2003)
Volume 2 (Oct 2001 - Mar 2002)
Volume 1 (Oct 2000 - Sep 2001)
Special Topics
Bayesian Optimization
Learning from Electronic Health Data (December 2016)
Gesture Recognition (May 2012 - present)
Large Scale Learning (Jul 2009 - present)
Mining and Learning with Graphs and Relations (February 2009 - present)
Grammar Induction, Representation of Language and Language Learning (Nov 2010 - Apr 2011)
Causality (Sep 2007 - May 2010)
Model Selection (Apr 2007 - Jul 2010)
Conference on Learning Theory 2005 (February 2007 - Jul 2007)
Machine Learning for Computer Security (December 2006)
Machine Learning and Large Scale Optimization (Jul 2006 - Oct 2006)
Approaches and Applications of Inductive Programming (February 2006 - Mar 2006)
Learning Theory (Jun 2004 - Aug 2004)
Special Issues
In Memory of Alexey Chervonenkis (Sep 2015)
Independent Components Analysis (December 2003)
Learning Theory (Oct 2003)
Inductive Logic Programming (Aug 2003)
Fusion of Domain Knowledge with Data for Decision Support (Jul 2003)
Variable and Feature Selection (Mar 2003)
Machine Learning Methods for Text and Images (February 2003)
Eighteenth International Conference on Machine Learning (ICML2001) (December 2002)
Computational Learning Theory (Nov 2002)
Shallow Parsing (Mar 2002)
Kernel Methods (December 2001)

Analytics Insight
Top 10 Machine Learning Research Papers of 2021

Machine learning research papers showcasing the transformation of the technology
Unbiased gradient estimation in unrolled computation graphs with persistent evolution, solving high-dimensional parabolic pdes using the tensor train format.
- TOP 10 MACHINE LEARNING TOOLS 2021
- TOP COMPANIES USING MACHINE LEARNING IN A PROFITABLE WAY
- MACHINE LEARNING GUIDE: DIFFERENCES BETWEEN PYTHON AND JAVA
Oops I took a gradient: Scalable sampling for discrete distributions
Optimal complexity in decentralized training, understanding self-supervised learning dynamics without contrastive pairs, how transferable are featured in deep neural networks, do we need hundreds of classifiers to solve real-world classification problems, knowledge vault: a web-scale approach to probabilistic knowledge fusion, scalable nearest neighbor algorithms for high dimensional data, trends in extreme learning machines.

Disclaimer: Any financial and crypto market information given on Analytics Insight are sponsored articles, written for informational purpose only and is not an investment advice. The readers are further advised that Crypto products and NFTs are unregulated and can be highly risky. There may be no regulatory recourse for any loss from such transactions. Conduct your own research by contacting financial experts before making any investment decisions. The decision to read hereinafter is purely a matter of choice and shall be construed as an express undertaking/guarantee in favour of Analytics Insight of being absolved from any/ all potential legal action, or enforceable claims. We do not represent nor own any cryptocurrency, any complaints, abuse or concerns with regards to the information provided shall be immediately informed here .
You May Also Like

BREAKING: BNB Slows Down, Borroe.Finance Keeps Up The Pace With 100 Million Tokens Sold

Internship Programs for Engineers in the US: Explore the List

Shiba Inu’s Next Stop Could Be US$0.001 Only If The V-trend Continues

How can the Implementation of AI help the Indian Government?

Analytics Insight® is an influential platform dedicated to insights, trends, and opinion from the world of data-driven technologies. It monitors developments, recognition, and achievements made by Artificial Intelligence, Big Data and Analytics companies across the globe.

- Select Language:
- Privacy Policy
- Content Licensing
- Terms & Conditions
- Submit an Interview
Special Editions
- Dec – Crypto Weekly Vol-1
- 40 Under 40 Innovators
- Women In Technology
- Market Reports
- AI Glossary
- Infographics
Latest Issue

Disclaimer: Any financial and crypto market information given on Analytics Insight is written for informational purpose only and is not an investment advice. Conduct your own research by contacting financial experts before making any investment decisions, more information here .
Second Menu

machine learning Recently Published Documents
Total documents.
- Latest Documents
- Most Cited Documents
- Contributed Authors
- Related Sources
- Related Keywords
An explainable machine learning model for identifying geographical origins of sea cucumber Apostichopus japonicus based on multi-element profile
A comparison of machine learning- and regression-based models for predicting ductility ratio of rc beam-column joints, alexa, is this a historical record.
Digital transformation in government has brought an increase in the scale, variety, and complexity of records and greater levels of disorganised data. Current practices for selecting records for transfer to The National Archives (TNA) were developed to deal with paper records and are struggling to deal with this shift. This article examines the background to the problem and outlines a project that TNA undertook to research the feasibility of using commercially available artificial intelligence tools to aid selection. The project AI for Selection evaluated a range of commercial solutions varying from off-the-shelf products to cloud-hosted machine learning platforms, as well as a benchmarking tool developed in-house. Suitability of tools depended on several factors, including requirements and skills of transferring bodies as well as the tools’ usability and configurability. This article also explores questions around trust and explainability of decisions made when using AI for sensitive tasks such as selection.
Automated Text Classification of Maintenance Data of Higher Education Buildings Using Text Mining and Machine Learning Techniques
Data-driven analysis and machine learning for energy prediction in distributed photovoltaic generation plants: a case study in queensland, australia, modeling nutrient removal by membrane bioreactor at a sewage treatment plant using machine learning models, big five personality prediction based in indonesian tweets using machine learning methods.
<span lang="EN-US">The popularity of social media has drawn the attention of researchers who have conducted cross-disciplinary studies examining the relationship between personality traits and behavior on social media. Most current work focuses on personality prediction analysis of English texts, but Indonesian has received scant attention. Therefore, this research aims to predict user’s personalities based on Indonesian text from social media using machine learning techniques. This paper evaluates several machine learning techniques, including <a name="_Hlk87278444"></a>naive Bayes (NB), K-nearest neighbors (KNN), and support vector machine (SVM), based on semantic features including emotion, sentiment, and publicly available Twitter profile. We predict the personality based on the big five personality model, the most appropriate model for predicting user personality in social media. We examine the relationships between the semantic features and the Big Five personality dimensions. The experimental results indicate that the Big Five personality exhibit distinct emotional, sentimental, and social characteristics and that SVM outperformed NB and KNN for Indonesian. In addition, we observe several terms in Indonesian that specifically refer to each personality type, each of which has distinct emotional, sentimental, and social features.</span>
Compressive strength of concrete with recycled aggregate; a machine learning-based evaluation
Temperature prediction of flat steel box girders of long-span bridges utilizing in situ environmental parameters and machine learning, computer-assisted cohort identification in practice.
The standard approach to expert-in-the-loop machine learning is active learning, where, repeatedly, an expert is asked to annotate one or more records and the machine finds a classifier that respects all annotations made until that point. We propose an alternative approach, IQRef , in which the expert iteratively designs a classifier and the machine helps him or her to determine how well it is performing and, importantly, when to stop, by reporting statistics on a fixed, hold-out sample of annotated records. We justify our approach based on prior work giving a theoretical model of how to re-use hold-out data. We compare the two approaches in the context of identifying a cohort of EHRs and examine their strengths and weaknesses through a case study arising from an optometric research problem. We conclude that both approaches are complementary, and we recommend that they both be employed in conjunction to address the problem of cohort identification in health research.
Export Citation Format
Share document.
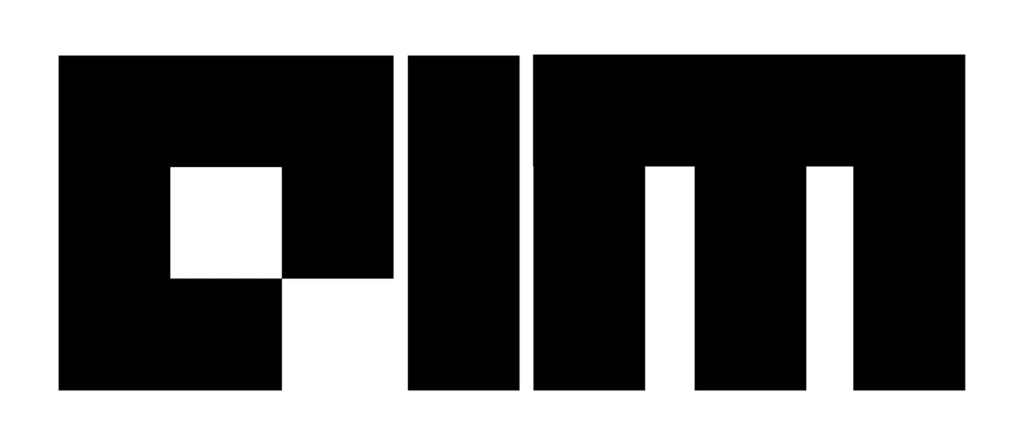
- Last updated November 18, 2021
- In AI Origins & Evolution
Top Machine Learning Research Papers Released In 2021
- Published on November 18, 2021
- by Dr. Nivash Jeevanandam
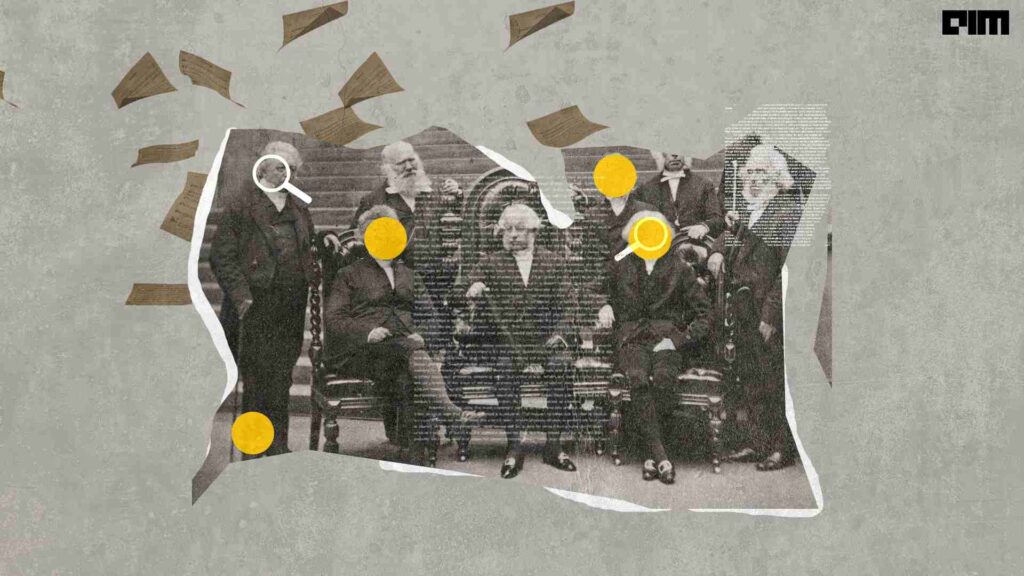
Advances in machine learning and deep learning research are reshaping our technology. Machine learning and deep learning have accomplished various astounding feats this year in 2021, and key research articles have resulted in technical advances used by billions of people. The research in this sector is advancing at a breakneck pace and assisting you to keep up. Here is a collection of the most important recent scientific study papers.
Rebooting ACGAN: Auxiliary Classifier GANs with Stable Training
The authors of this work examined why ACGAN training becomes unstable as the number of classes in the dataset grows. The researchers revealed that the unstable training occurs due to a gradient explosion problem caused by the unboundedness of the input feature vectors and the classifier’s poor classification capabilities during the early training stage. The researchers presented the Data-to-Data Cross-Entropy loss (D2D-CE) and the Rebooted Auxiliary Classifier Generative Adversarial Network to alleviate the instability and reinforce ACGAN (ReACGAN). Additionally, extensive tests of ReACGAN demonstrate that it is resistant to hyperparameter selection and is compatible with a variety of architectures and differentiable augmentations.
This article is ranked #1 on CIFAR-10 for Conditional Image Generation.
For the research paper, read here .
For code, see here .
Subscribe to our Newsletter
Join our editors every weekday evening as they steer you through the most significant news of the day, introduce you to fresh perspectives, and provide unexpected moments of joy, your newsletter subscriptions are subject to aim privacy policy and terms and conditions., dense unsupervised learning for video segmentation.
The authors presented a straightforward and computationally fast unsupervised strategy for learning dense spacetime representations from unlabeled films in this study. The approach demonstrates rapid convergence of training and a high degree of data efficiency. Furthermore, the researchers obtain VOS accuracy superior to previous results despite employing a fraction of the previously necessary training data. The researchers acknowledge that the research findings may be utilised maliciously, such as for unlawful surveillance, and that they are excited to investigate how this skill might be used to better learn a broader spectrum of invariances by exploiting larger temporal windows in movies with complex (ego-)motion, which is more prone to disocclusions.
This study is ranked #1 on DAVIS 2017 for Unsupervised Video Object Segmentation (val).
Temporally-Consistent Surface Reconstruction using Metrically-Consistent Atlases
The authors offer an atlas-based technique for producing unsupervised temporally consistent surface reconstructions by requiring a point on the canonical shape representation to translate to metrically consistent 3D locations on the reconstructed surfaces. Finally, the researchers envisage a plethora of potential applications for the method. For example, by substituting an image-based loss for the Chamfer distance, one may apply the method to RGB video sequences, which the researchers feel will spur development in video-based 3D reconstruction.
This article is ranked #1 on ANIM in the category of Surface Reconstruction.
EdgeFlow: Achieving Practical Interactive Segmentation with Edge-Guided Flow
The researchers propose a revolutionary interactive architecture called EdgeFlow that uses user interaction data without resorting to post-processing or iterative optimisation. The suggested technique achieves state-of-the-art performance on common benchmarks due to its coarse-to-fine network design. Additionally, the researchers create an effective interactive segmentation tool that enables the user to improve the segmentation result through flexible options incrementally.
This paper is ranked #1 on Interactive Segmentation on PASCAL VOC
Learning Transferable Visual Models From Natural Language Supervision
The authors of this work examined whether it is possible to transfer the success of task-agnostic web-scale pre-training in natural language processing to another domain. The findings indicate that adopting this formula resulted in the emergence of similar behaviours in the field of computer vision, and the authors examine the social ramifications of this line of research. CLIP models learn to accomplish a range of tasks during pre-training to optimise their training objective. Using natural language prompting, CLIP can then use this task learning to enable zero-shot transfer to many existing datasets. When applied at a large scale, this technique can compete with task-specific supervised models, while there is still much space for improvement.
This research is ranked #1 on Zero-Shot Transfer Image Classification on SUN
CoAtNet: Marrying Convolution and Attention for All Data Sizes
The researchers in this article conduct a thorough examination of the features of convolutions and transformers, resulting in a principled approach for combining them into a new family of models dubbed CoAtNet. Extensive experiments demonstrate that CoAtNet combines the advantages of ConvNets and Transformers, achieving state-of-the-art performance across a range of data sizes and compute budgets. Take note that this article is currently concentrating on ImageNet classification for model construction. However, the researchers believe their approach is relevant to a broader range of applications, such as object detection and semantic segmentation.
This paper is ranked #1 on Image Classification on ImageNet (using extra training data).
SwinIR: Image Restoration Using Swin Transformer
The authors of this article suggest the SwinIR image restoration model, which is based on the Swin Transformer . The model comprises three modules: shallow feature extraction, deep feature extraction, and human-recognition reconstruction. For deep feature extraction, the researchers employ a stack of residual Swin Transformer blocks (RSTB), each formed of Swin Transformer layers, a convolution layer, and a residual connection.
This research article is ranked #1 on Image Super-Resolution on Manga109 – 4x upscaling.
Access all our open Survey & Awards Nomination forms in one place >>

Dr. Nivash Jeevanandam
Download our mobile app.

CORPORATE TRAINING PROGRAMS ON GENERATIVE AI
Generative ai skilling for enterprises, our customized corporate training program on generative ai provides a unique opportunity to empower, retain, and advance your talent., 3 ways to join our community, telegram group.
Discover special offers, top stories, upcoming events, and more.
Discord Server
Stay Connected with a larger ecosystem of data science and ML Professionals
Subscribe to our Daily newsletter
Get our daily awesome stories & videos in your inbox, recent stories.
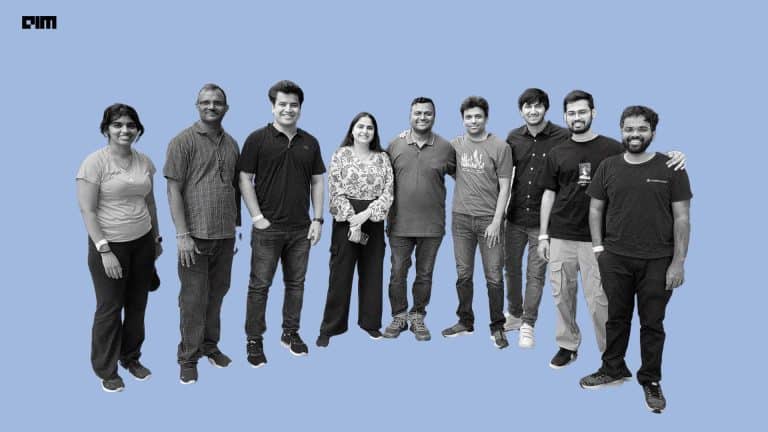
Data Science Hiring Process at Confluent
The company is seeking data scientists and engineers to further bolster its tech team.
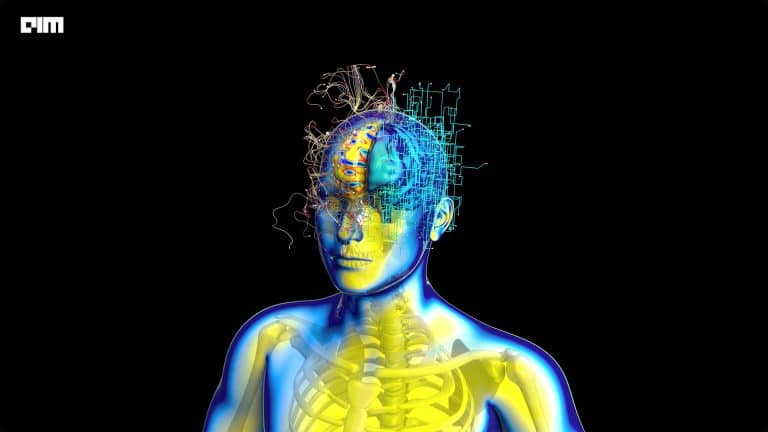
Neuralink would Need up to a Million Electrodes to Make Humans Immortal
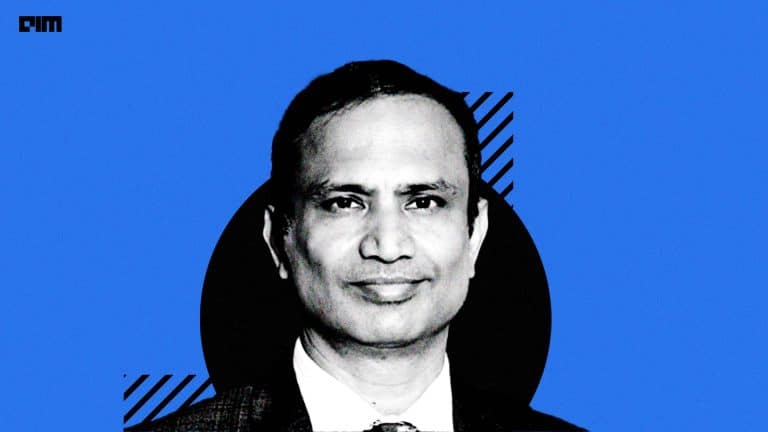
LatentView Analytics Acquires Decision Point Analytics for Generative AI Solutions
Language is the os of the future.
AI experts agree more on the topic than they wish to accept.

AWS Teams Up with Minfy for Cloud and AI Boost through Global Expansion
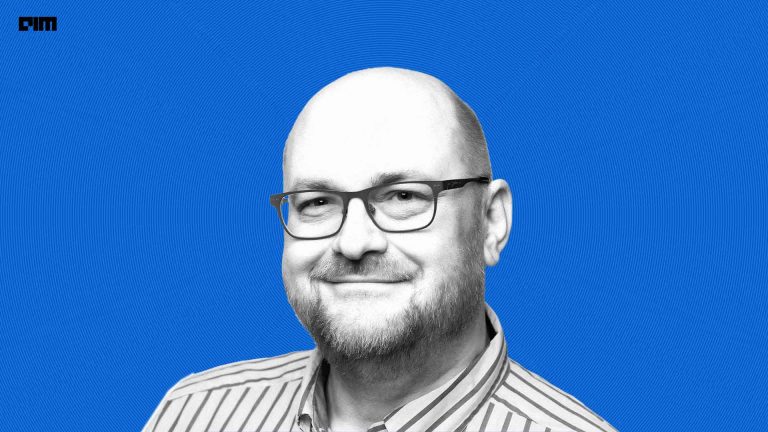
MongoDB has Over 3,000 Customers in India and Growing
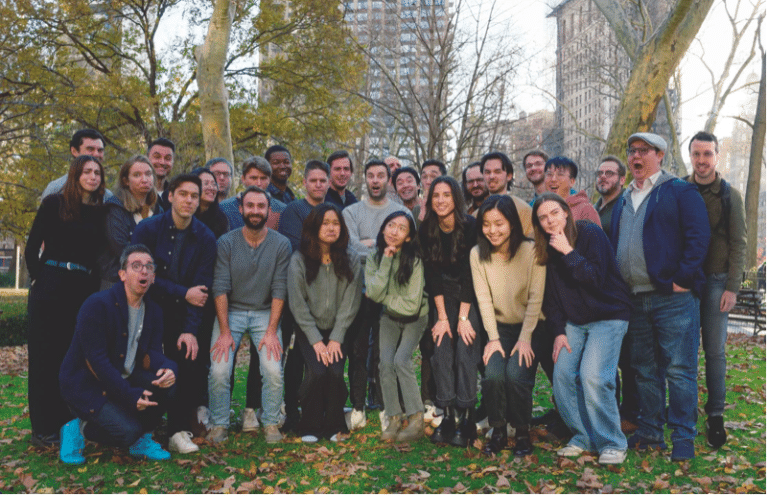
Forget OpenAI’s ChatGPT, Hume AI’s Empathetic Voice Interface (EVI) Might Be the Next Big Thing in AI!
Almost feels like you are talking to a human
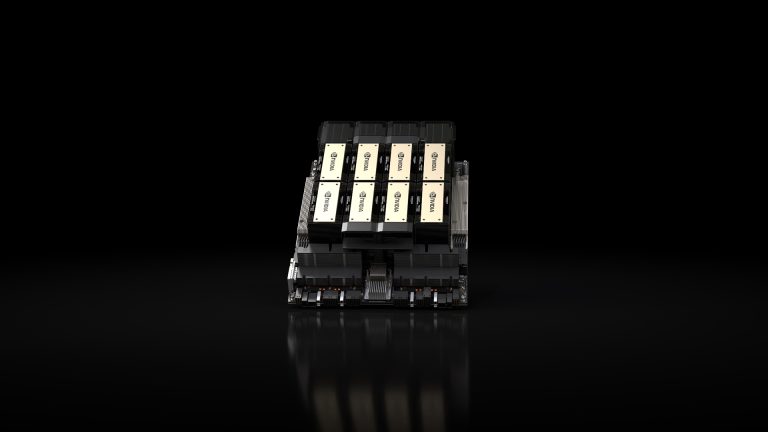
NVIDIA Hopper Leads in Generative AI Inference at MLPerf Results
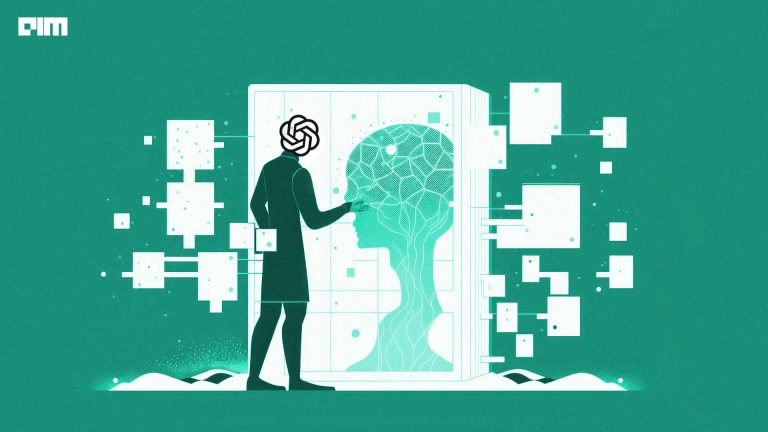
OpenAI Kick Starts its GPT Earnings Programme in the US
OpenAI launched the GPT Store on March 12 for ChatGPT Plus, Team, and Enterprise users.
Our mission is to bring about better-informed and more conscious decisions about technology through authoritative, influential, and trustworthy journalism.
Shape the future of ai.
© Analytics India Magazine Pvt Ltd & AIM Media House LLC 2024
- Terms of use
- Privacy Policy
Subscribe to Our Newsletter
The Belamy, our weekly Newsletter is a rage. Just enter your email below.
Advertisement
Deep Learning: A Comprehensive Overview on Techniques, Taxonomy, Applications and Research Directions
- Review Article
- Published: 18 August 2021
- Volume 2 , article number 420 , ( 2021 )
Cite this article
- Iqbal H. Sarker ORCID: orcid.org/0000-0003-1740-5517 1 , 2
179k Accesses
600 Citations
24 Altmetric
Explore all metrics
Deep learning (DL), a branch of machine learning (ML) and artificial intelligence (AI) is nowadays considered as a core technology of today’s Fourth Industrial Revolution (4IR or Industry 4.0). Due to its learning capabilities from data, DL technology originated from artificial neural network (ANN), has become a hot topic in the context of computing, and is widely applied in various application areas like healthcare, visual recognition, text analytics, cybersecurity, and many more. However, building an appropriate DL model is a challenging task, due to the dynamic nature and variations in real-world problems and data. Moreover, the lack of core understanding turns DL methods into black-box machines that hamper development at the standard level. This article presents a structured and comprehensive view on DL techniques including a taxonomy considering various types of real-world tasks like supervised or unsupervised. In our taxonomy, we take into account deep networks for supervised or discriminative learning , unsupervised or generative learning as well as hybrid learning and relevant others. We also summarize real-world application areas where deep learning techniques can be used. Finally, we point out ten potential aspects for future generation DL modeling with research directions . Overall, this article aims to draw a big picture on DL modeling that can be used as a reference guide for both academia and industry professionals.
Similar content being viewed by others
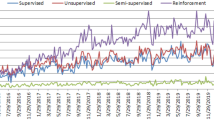
Machine Learning: Algorithms, Real-World Applications and Research Directions
Iqbal H. Sarker
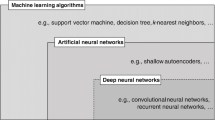
Machine learning and deep learning
Christian Janiesch, Patrick Zschech & Kai Heinrich
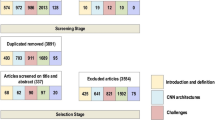
Review of deep learning: concepts, CNN architectures, challenges, applications, future directions
Laith Alzubaidi, Jinglan Zhang, … Laith Farhan
Avoid common mistakes on your manuscript.
Introduction
In the late 1980s, neural networks became a prevalent topic in the area of Machine Learning (ML) as well as Artificial Intelligence (AI), due to the invention of various efficient learning methods and network structures [ 52 ]. Multilayer perceptron networks trained by “Backpropagation” type algorithms, self-organizing maps, and radial basis function networks were such innovative methods [ 26 , 36 , 37 ]. While neural networks are successfully used in many applications, the interest in researching this topic decreased later on. After that, in 2006, “Deep Learning” (DL) was introduced by Hinton et al. [ 41 ], which was based on the concept of artificial neural network (ANN). Deep learning became a prominent topic after that, resulting in a rebirth in neural network research, hence, some times referred to as “new-generation neural networks”. This is because deep networks, when properly trained, have produced significant success in a variety of classification and regression challenges [ 52 ].
Nowadays, DL technology is considered as one of the hot topics within the area of machine learning, artificial intelligence as well as data science and analytics, due to its learning capabilities from the given data. Many corporations including Google, Microsoft, Nokia, etc., study it actively as it can provide significant results in different classification and regression problems and datasets [ 52 ]. In terms of working domain, DL is considered as a subset of ML and AI, and thus DL can be seen as an AI function that mimics the human brain’s processing of data. The worldwide popularity of “Deep learning” is increasing day by day, which is shown in our earlier paper [ 96 ] based on the historical data collected from Google trends [ 33 ]. Deep learning differs from standard machine learning in terms of efficiency as the volume of data increases, discussed briefly in Section “ Why Deep Learning in Today's Research and Applications? ”. DL technology uses multiple layers to represent the abstractions of data to build computational models. While deep learning takes a long time to train a model due to a large number of parameters, it takes a short amount of time to run during testing as compared to other machine learning algorithms [ 127 ].
While today’s Fourth Industrial Revolution (4IR or Industry 4.0) is typically focusing on technology-driven “automation, smart and intelligent systems”, DL technology, which is originated from ANN, has become one of the core technologies to achieve the goal [ 103 , 114 ]. A typical neural network is mainly composed of many simple, connected processing elements or processors called neurons, each of which generates a series of real-valued activations for the target outcome. Figure 1 shows a schematic representation of the mathematical model of an artificial neuron, i.e., processing element, highlighting input ( \(X_i\) ), weight ( w ), bias ( b ), summation function ( \(\sum\) ), activation function ( f ) and corresponding output signal ( y ). Neural network-based DL technology is now widely applied in many fields and research areas such as healthcare, sentiment analysis, natural language processing, visual recognition, business intelligence, cybersecurity, and many more that have been summarized in the latter part of this paper.
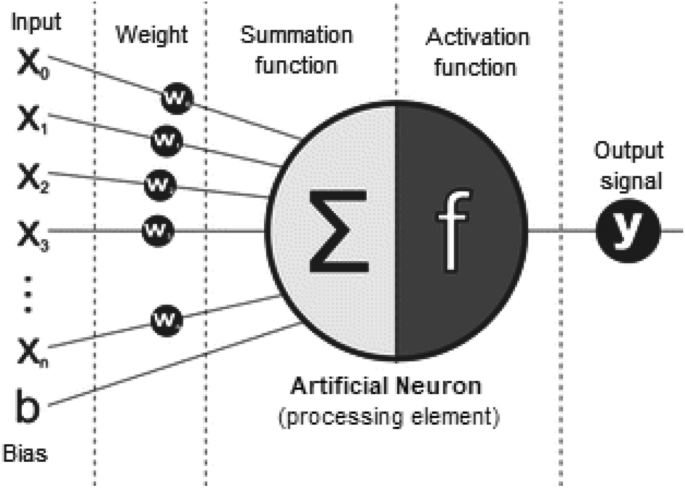
Schematic representation of the mathematical model of an artificial neuron (processing element), highlighting input ( \(X_i\) ), weight ( w ), bias ( b ), summation function ( \(\sum\) ), activation function ( f ) and output signal ( y )
Although DL models are successfully applied in various application areas, mentioned above, building an appropriate model of deep learning is a challenging task, due to the dynamic nature and variations of real-world problems and data. Moreover, DL models are typically considered as “black-box” machines that hamper the standard development of deep learning research and applications. Thus for clear understanding, in this paper, we present a structured and comprehensive view on DL techniques considering the variations in real-world problems and tasks. To achieve our goal, we briefly discuss various DL techniques and present a taxonomy by taking into account three major categories: (i) deep networks for supervised or discriminative learning that is utilized to provide a discriminative function in supervised deep learning or classification applications; (ii) deep networks for unsupervised or generative learning that are used to characterize the high-order correlation properties or features for pattern analysis or synthesis, thus can be used as preprocessing for the supervised algorithm; and (ii) deep networks for hybrid learning that is an integration of both supervised and unsupervised model and relevant others. We take into account such categories based on the nature and learning capabilities of different DL techniques and how they are used to solve problems in real-world applications [ 97 ]. Moreover, identifying key research issues and prospects including effective data representation, new algorithm design, data-driven hyper-parameter learning, and model optimization, integrating domain knowledge, adapting resource-constrained devices, etc. is one of the key targets of this study, which can lead to “Future Generation DL-Modeling”. Thus the goal of this paper is set to assist those in academia and industry as a reference guide, who want to research and develop data-driven smart and intelligent systems based on DL techniques.
The overall contribution of this paper is summarized as follows:
This article focuses on different aspects of deep learning modeling, i.e., the learning capabilities of DL techniques in different dimensions such as supervised or unsupervised tasks, to function in an automated and intelligent manner, which can play as a core technology of today’s Fourth Industrial Revolution (Industry 4.0).
We explore a variety of prominent DL techniques and present a taxonomy by taking into account the variations in deep learning tasks and how they are used for different purposes. In our taxonomy, we divide the techniques into three major categories such as deep networks for supervised or discriminative learning, unsupervised or generative learning, as well as deep networks for hybrid learning, and relevant others.
We have summarized several potential real-world application areas of deep learning, to assist developers as well as researchers in broadening their perspectives on DL techniques. Different categories of DL techniques highlighted in our taxonomy can be used to solve various issues accordingly.
Finally, we point out and discuss ten potential aspects with research directions for future generation DL modeling in terms of conducting future research and system development.
This paper is organized as follows. Section “ Why Deep Learning in Today's Research and Applications? ” motivates why deep learning is important to build data-driven intelligent systems. In Section“ Deep Learning Techniques and Applications ”, we present our DL taxonomy by taking into account the variations of deep learning tasks and how they are used in solving real-world issues and briefly discuss the techniques with summarizing the potential application areas. In Section “ Research Directions and Future Aspects ”, we discuss various research issues of deep learning-based modeling and highlight the promising topics for future research within the scope of our study. Finally, Section “ Concluding Remarks ” concludes this paper.
Why Deep Learning in Today’s Research and Applications?
The main focus of today’s Fourth Industrial Revolution (Industry 4.0) is typically technology-driven automation, smart and intelligent systems, in various application areas including smart healthcare, business intelligence, smart cities, cybersecurity intelligence, and many more [ 95 ]. Deep learning approaches have grown dramatically in terms of performance in a wide range of applications considering security technologies, particularly, as an excellent solution for uncovering complex architecture in high-dimensional data. Thus, DL techniques can play a key role in building intelligent data-driven systems according to today’s needs, because of their excellent learning capabilities from historical data. Consequently, DL can change the world as well as humans’ everyday life through its automation power and learning from experience. DL technology is therefore relevant to artificial intelligence [ 103 ], machine learning [ 97 ] and data science with advanced analytics [ 95 ] that are well-known areas in computer science, particularly, today’s intelligent computing. In the following, we first discuss regarding the position of deep learning in AI, or how DL technology is related to these areas of computing.
The Position of Deep Learning in AI
Nowadays, artificial intelligence (AI), machine learning (ML), and deep learning (DL) are three popular terms that are sometimes used interchangeably to describe systems or software that behaves intelligently. In Fig. 2 , we illustrate the position of deep Learning, comparing with machine learning and artificial intelligence. According to Fig. 2 , DL is a part of ML as well as a part of the broad area AI. In general, AI incorporates human behavior and intelligence to machines or systems [ 103 ], while ML is the method to learn from data or experience [ 97 ], which automates analytical model building. DL also represents learning methods from data where the computation is done through multi-layer neural networks and processing. The term “Deep” in the deep learning methodology refers to the concept of multiple levels or stages through which data is processed for building a data-driven model.
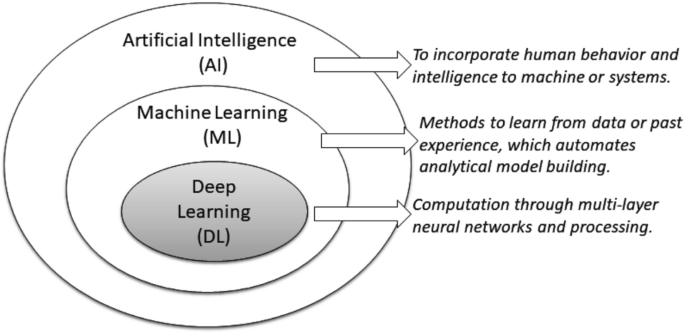
An illustration of the position of deep learning (DL), comparing with machine learning (ML) and artificial intelligence (AI)
Thus, DL can be considered as one of the core technology of AI, a frontier for artificial intelligence, which can be used for building intelligent systems and automation. More importantly, it pushes AI to a new level, termed “Smarter AI”. As DL are capable of learning from data, there is a strong relation of deep learning with “Data Science” [ 95 ] as well. Typically, data science represents the entire process of finding meaning or insights in data in a particular problem domain, where DL methods can play a key role for advanced analytics and intelligent decision-making [ 104 , 106 ]. Overall, we can conclude that DL technology is capable to change the current world, particularly, in terms of a powerful computational engine and contribute to technology-driven automation, smart and intelligent systems accordingly, and meets the goal of Industry 4.0.
Understanding Various Forms of Data
As DL models learn from data, an in-depth understanding and representation of data are important to build a data-driven intelligent system in a particular application area. In the real world, data can be in various forms, which typically can be represented as below for deep learning modeling:
Sequential Data Sequential data is any kind of data where the order matters, i,e., a set of sequences. It needs to explicitly account for the sequential nature of input data while building the model. Text streams, audio fragments, video clips, time-series data, are some examples of sequential data.
Image or 2D Data A digital image is made up of a matrix, which is a rectangular array of numbers, symbols, or expressions arranged in rows and columns in a 2D array of numbers. Matrix, pixels, voxels, and bit depth are the four essential characteristics or fundamental parameters of a digital image.
Tabular Data A tabular dataset consists primarily of rows and columns. Thus tabular datasets contain data in a columnar format as in a database table. Each column (field) must have a name and each column may only contain data of the defined type. Overall, it is a logical and systematic arrangement of data in the form of rows and columns that are based on data properties or features. Deep learning models can learn efficiently on tabular data and allow us to build data-driven intelligent systems.
The above-discussed data forms are common in the real-world application areas of deep learning. Different categories of DL techniques perform differently depending on the nature and characteristics of data, discussed briefly in Section “ Deep Learning Techniques and Applications ” with a taxonomy presentation. However, in many real-world application areas, the standard machine learning techniques, particularly, logic-rule or tree-based techniques [ 93 , 101 ] perform significantly depending on the application nature. Figure 3 also shows the performance comparison of DL and ML modeling considering the amount of data. In the following, we highlight several cases, where deep learning is useful to solve real-world problems, according to our main focus in this paper.
DL Properties and Dependencies
A DL model typically follows the same processing stages as machine learning modeling. In Fig. 4 , we have shown a deep learning workflow to solve real-world problems, which consists of three processing steps, such as data understanding and preprocessing, DL model building, and training, and validation and interpretation. However, unlike the ML modeling [ 98 , 108 ], feature extraction in the DL model is automated rather than manual. K-nearest neighbor, support vector machines, decision tree, random forest, naive Bayes, linear regression, association rules, k-means clustering, are some examples of machine learning techniques that are commonly used in various application areas [ 97 ]. On the other hand, the DL model includes convolution neural network, recurrent neural network, autoencoder, deep belief network, and many more, discussed briefly with their potential application areas in Section 3 . In the following, we discuss the key properties and dependencies of DL techniques, that are needed to take into account before started working on DL modeling for real-world applications.
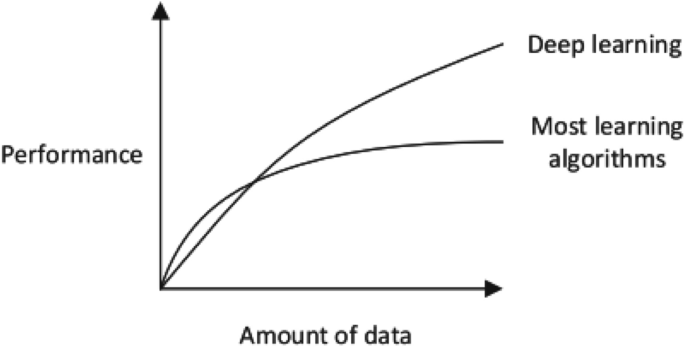
An illustration of the performance comparison between deep learning (DL) and other machine learning (ML) algorithms, where DL modeling from large amounts of data can increase the performance
Data Dependencies Deep learning is typically dependent on a large amount of data to build a data-driven model for a particular problem domain. The reason is that when the data volume is small, deep learning algorithms often perform poorly [ 64 ]. In such circumstances, however, the performance of the standard machine-learning algorithms will be improved if the specified rules are used [ 64 , 107 ].
Hardware Dependencies The DL algorithms require large computational operations while training a model with large datasets. As the larger the computations, the more the advantage of a GPU over a CPU, the GPU is mostly used to optimize the operations efficiently. Thus, to work properly with the deep learning training, GPU hardware is necessary. Therefore, DL relies more on high-performance machines with GPUs than standard machine learning methods [ 19 , 127 ].
Feature Engineering Process Feature engineering is the process of extracting features (characteristics, properties, and attributes) from raw data using domain knowledge. A fundamental distinction between DL and other machine-learning techniques is the attempt to extract high-level characteristics directly from data [ 22 , 97 ]. Thus, DL decreases the time and effort required to construct a feature extractor for each problem.
Model Training and Execution time In general, training a deep learning algorithm takes a long time due to a large number of parameters in the DL algorithm; thus, the model training process takes longer. For instance, the DL models can take more than one week to complete a training session, whereas training with ML algorithms takes relatively little time, only seconds to hours [ 107 , 127 ]. During testing, deep learning algorithms take extremely little time to run [ 127 ], when compared to certain machine learning methods.
Black-box Perception and Interpretability Interpretability is an important factor when comparing DL with ML. It’s difficult to explain how a deep learning result was obtained, i.e., “black-box”. On the other hand, the machine-learning algorithms, particularly, rule-based machine learning techniques [ 97 ] provide explicit logic rules (IF-THEN) for making decisions that are easily interpretable for humans. For instance, in our earlier works, we have presented several machines learning rule-based techniques [ 100 , 102 , 105 ], where the extracted rules are human-understandable and easier to interpret, update or delete according to the target applications.
The most significant distinction between deep learning and regular machine learning is how well it performs when data grows exponentially. An illustration of the performance comparison between DL and standard ML algorithms has been shown in Fig. 3 , where DL modeling can increase the performance with the amount of data. Thus, DL modeling is extremely useful when dealing with a large amount of data because of its capacity to process vast amounts of features to build an effective data-driven model. In terms of developing and training DL models, it relies on parallelized matrix and tensor operations as well as computing gradients and optimization. Several, DL libraries and resources [ 30 ] such as PyTorch [ 82 ] (with a high-level API called Lightning) and TensorFlow [ 1 ] (which also offers Keras as a high-level API) offers these core utilities including many pre-trained models, as well as many other necessary functions for implementation and DL model building.
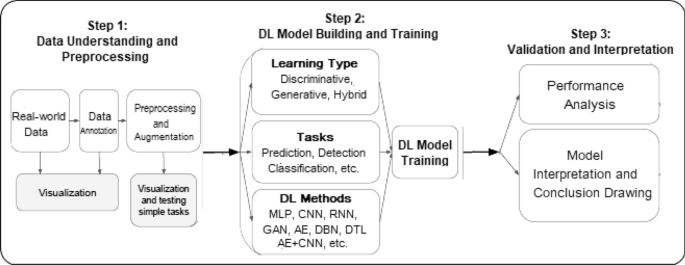
A typical DL workflow to solve real-world problems, which consists of three sequential stages (i) data understanding and preprocessing (ii) DL model building and training (iii) validation and interpretation
Deep Learning Techniques and Applications
In this section, we go through the various types of deep neural network techniques, which typically consider several layers of information-processing stages in hierarchical structures to learn. A typical deep neural network contains multiple hidden layers including input and output layers. Figure 5 shows a general structure of a deep neural network ( \(hidden \; layer=N\) and N \(\ge\) 2) comparing with a shallow network ( \(hidden \; layer=1\) ). We also present our taxonomy on DL techniques based on how they are used to solve various problems, in this section. However, before exploring the details of the DL techniques, it’s useful to review various types of learning tasks such as (i) Supervised: a task-driven approach that uses labeled training data, (ii) Unsupervised: a data-driven process that analyzes unlabeled datasets, (iii) Semi-supervised: a hybridization of both the supervised and unsupervised methods, and (iv) Reinforcement: an environment driven approach, discussed briefly in our earlier paper [ 97 ]. Thus, to present our taxonomy, we divide DL techniques broadly into three major categories: (i) deep networks for supervised or discriminative learning; (ii) deep networks for unsupervised or generative learning; and (ii) deep networks for hybrid learning combing both and relevant others, as shown in Fig. 6 . In the following, we briefly discuss each of these techniques that can be used to solve real-world problems in various application areas according to their learning capabilities.
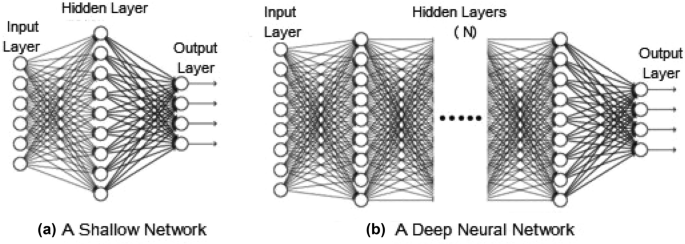
A general architecture of a a shallow network with one hidden layer and b a deep neural network with multiple hidden layers
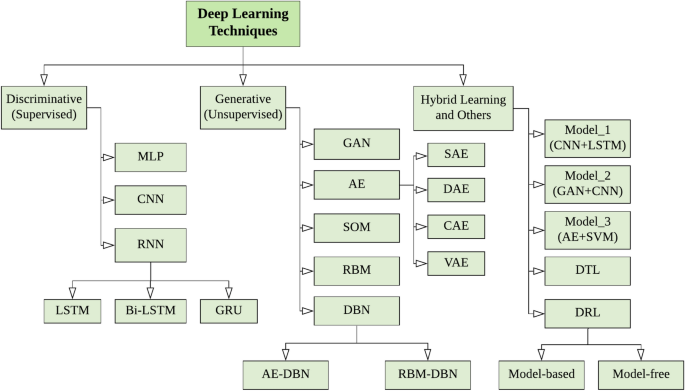
A taxonomy of DL techniques, broadly divided into three major categories (i) deep networks for supervised or discriminative learning, (ii) deep networks for unsupervised or generative learning, and (ii) deep networks for hybrid learning and relevant others
Deep Networks for Supervised or Discriminative Learning
This category of DL techniques is utilized to provide a discriminative function in supervised or classification applications. Discriminative deep architectures are typically designed to give discriminative power for pattern classification by describing the posterior distributions of classes conditioned on visible data [ 21 ]. Discriminative architectures mainly include Multi-Layer Perceptron (MLP), Convolutional Neural Networks (CNN or ConvNet), Recurrent Neural Networks (RNN), along with their variants. In the following, we briefly discuss these techniques.
Multi-layer Perceptron (MLP)
Multi-layer Perceptron (MLP), a supervised learning approach [ 83 ], is a type of feedforward artificial neural network (ANN). It is also known as the foundation architecture of deep neural networks (DNN) or deep learning. A typical MLP is a fully connected network that consists of an input layer that receives input data, an output layer that makes a decision or prediction about the input signal, and one or more hidden layers between these two that are considered as the network’s computational engine [ 36 , 103 ]. The output of an MLP network is determined using a variety of activation functions, also known as transfer functions, such as ReLU (Rectified Linear Unit), Tanh, Sigmoid, and Softmax [ 83 , 96 ]. To train MLP employs the most extensively used algorithm “Backpropagation” [ 36 ], a supervised learning technique, which is also known as the most basic building block of a neural network. During the training process, various optimization approaches such as Stochastic Gradient Descent (SGD), Limited Memory BFGS (L-BFGS), and Adaptive Moment Estimation (Adam) are applied. MLP requires tuning of several hyperparameters such as the number of hidden layers, neurons, and iterations, which could make solving a complicated model computationally expensive. However, through partial fit, MLP offers the advantage of learning non-linear models in real-time or online [ 83 ].
Convolutional Neural Network (CNN or ConvNet)
The Convolutional Neural Network (CNN or ConvNet) [ 65 ] is a popular discriminative deep learning architecture that learns directly from the input without the need for human feature extraction. Figure 7 shows an example of a CNN including multiple convolutions and pooling layers. As a result, the CNN enhances the design of traditional ANN like regularized MLP networks. Each layer in CNN takes into account optimum parameters for a meaningful output as well as reduces model complexity. CNN also uses a ‘dropout’ [ 30 ] that can deal with the problem of over-fitting, which may occur in a traditional network.
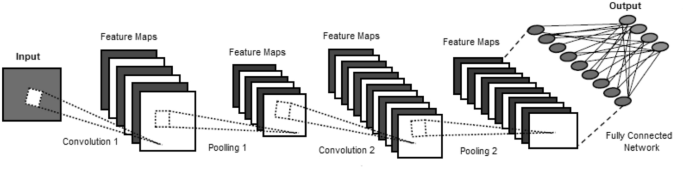
An example of a convolutional neural network (CNN or ConvNet) including multiple convolution and pooling layers
CNNs are specifically intended to deal with a variety of 2D shapes and are thus widely employed in visual recognition, medical image analysis, image segmentation, natural language processing, and many more [ 65 , 96 ]. The capability of automatically discovering essential features from the input without the need for human intervention makes it more powerful than a traditional network. Several variants of CNN are exist in the area that includes visual geometry group (VGG) [ 38 ], AlexNet [ 62 ], Xception [ 17 ], Inception [ 116 ], ResNet [ 39 ], etc. that can be used in various application domains according to their learning capabilities.
Recurrent Neural Network (RNN) and its Variants
A Recurrent Neural Network (RNN) is another popular neural network, which employs sequential or time-series data and feeds the output from the previous step as input to the current stage [ 27 , 74 ]. Like feedforward and CNN, recurrent networks learn from training input, however, distinguish by their “memory”, which allows them to impact current input and output through using information from previous inputs. Unlike typical DNN, which assumes that inputs and outputs are independent of one another, the output of RNN is reliant on prior elements within the sequence. However, standard recurrent networks have the issue of vanishing gradients, which makes learning long data sequences challenging. In the following, we discuss several popular variants of the recurrent network that minimizes the issues and perform well in many real-world application domains.
Long short-term memory (LSTM) This is a popular form of RNN architecture that uses special units to deal with the vanishing gradient problem, which was introduced by Hochreiter et al. [ 42 ]. A memory cell in an LSTM unit can store data for long periods and the flow of information into and out of the cell is managed by three gates. For instance, the ‘Forget Gate’ determines what information from the previous state cell will be memorized and what information will be removed that is no longer useful, while the ‘Input Gate’ determines which information should enter the cell state and the ‘Output Gate’ determines and controls the outputs. As it solves the issues of training a recurrent network, the LSTM network is considered one of the most successful RNN.
Bidirectional RNN/LSTM Bidirectional RNNs connect two hidden layers that run in opposite directions to a single output, allowing them to accept data from both the past and future. Bidirectional RNNs, unlike traditional recurrent networks, are trained to predict both positive and negative time directions at the same time. A Bidirectional LSTM, often known as a BiLSTM, is an extension of the standard LSTM that can increase model performance on sequence classification issues [ 113 ]. It is a sequence processing model comprising of two LSTMs: one takes the input forward and the other takes it backward. Bidirectional LSTM in particular is a popular choice in natural language processing tasks.
Gated recurrent units (GRUs) A Gated Recurrent Unit (GRU) is another popular variant of the recurrent network that uses gating methods to control and manage information flow between cells in the neural network, introduced by Cho et al. [ 16 ]. The GRU is like an LSTM, however, has fewer parameters, as it has a reset gate and an update gate but lacks the output gate, as shown in Fig. 8 . Thus, the key difference between a GRU and an LSTM is that a GRU has two gates (reset and update gates) whereas an LSTM has three gates (namely input, output and forget gates). The GRU’s structure enables it to capture dependencies from large sequences of data in an adaptive manner, without discarding information from earlier parts of the sequence. Thus GRU is a slightly more streamlined variant that often offers comparable performance and is significantly faster to compute [ 18 ]. Although GRUs have been shown to exhibit better performance on certain smaller and less frequent datasets [ 18 , 34 ], both variants of RNN have proven their effectiveness while producing the outcome.
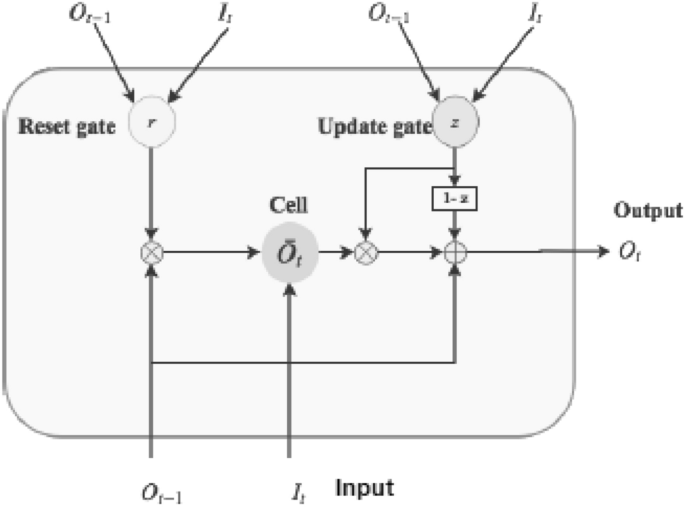
Basic structure of a gated recurrent unit (GRU) cell consisting of reset and update gates
Overall, the basic property of a recurrent network is that it has at least one feedback connection, which enables activations to loop. This allows the networks to do temporal processing and sequence learning, such as sequence recognition or reproduction, temporal association or prediction, etc. Following are some popular application areas of recurrent networks such as prediction problems, machine translation, natural language processing, text summarization, speech recognition, and many more.
Deep Networks for Generative or Unsupervised Learning
This category of DL techniques is typically used to characterize the high-order correlation properties or features for pattern analysis or synthesis, as well as the joint statistical distributions of the visible data and their associated classes [ 21 ]. The key idea of generative deep architectures is that during the learning process, precise supervisory information such as target class labels is not of concern. As a result, the methods under this category are essentially applied for unsupervised learning as the methods are typically used for feature learning or data generating and representation [ 20 , 21 ]. Thus generative modeling can be used as preprocessing for the supervised learning tasks as well, which ensures the discriminative model accuracy. Commonly used deep neural network techniques for unsupervised or generative learning are Generative Adversarial Network (GAN), Autoencoder (AE), Restricted Boltzmann Machine (RBM), Self-Organizing Map (SOM), and Deep Belief Network (DBN) along with their variants.
Generative Adversarial Network (GAN)
A Generative Adversarial Network (GAN), designed by Ian Goodfellow [ 32 ], is a type of neural network architecture for generative modeling to create new plausible samples on demand. It involves automatically discovering and learning regularities or patterns in input data so that the model may be used to generate or output new examples from the original dataset. As shown in Fig. 9 , GANs are composed of two neural networks, a generator G that creates new data having properties similar to the original data, and a discriminator D that predicts the likelihood of a subsequent sample being drawn from actual data rather than data provided by the generator. Thus in GAN modeling, both the generator and discriminator are trained to compete with each other. While the generator tries to fool and confuse the discriminator by creating more realistic data, the discriminator tries to distinguish the genuine data from the fake data generated by G .
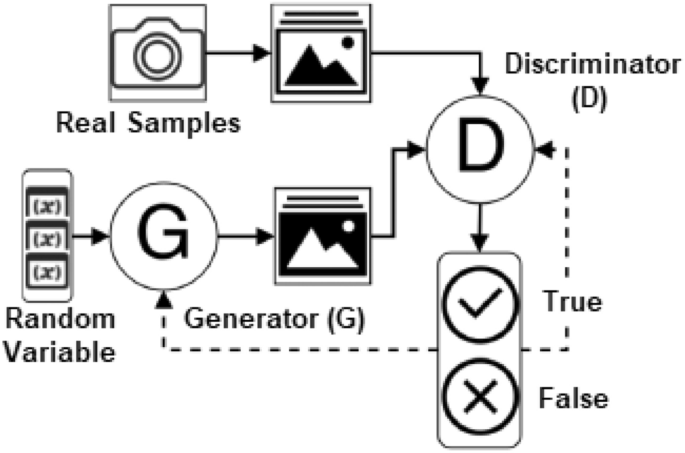
Schematic structure of a standard generative adversarial network (GAN)
Generally, GAN network deployment is designed for unsupervised learning tasks, but it has also proven to be a better solution for semi-supervised and reinforcement learning as well depending on the task [ 3 ]. GANs are also used in state-of-the-art transfer learning research to enforce the alignment of the latent feature space [ 66 ]. Inverse models, such as Bidirectional GAN (BiGAN) [ 25 ] can also learn a mapping from data to the latent space, similar to how the standard GAN model learns a mapping from a latent space to the data distribution. The potential application areas of GAN networks are healthcare, image analysis, data augmentation, video generation, voice generation, pandemics, traffic control, cybersecurity, and many more, which are increasing rapidly. Overall, GANs have established themselves as a comprehensive domain of independent data expansion and as a solution to problems requiring a generative solution.
Auto-Encoder (AE) and Its Variants
An auto-encoder (AE) [ 31 ] is a popular unsupervised learning technique in which neural networks are used to learn representations. Typically, auto-encoders are used to work with high-dimensional data, and dimensionality reduction explains how a set of data is represented. Encoder, code, and decoder are the three parts of an autoencoder. The encoder compresses the input and generates the code, which the decoder subsequently uses to reconstruct the input. The AEs have recently been used to learn generative data models [ 69 ]. The auto-encoder is widely used in many unsupervised learning tasks, e.g., dimensionality reduction, feature extraction, efficient coding, generative modeling, denoising, anomaly or outlier detection, etc. [ 31 , 132 ]. Principal component analysis (PCA) [ 99 ], which is also used to reduce the dimensionality of huge data sets, is essentially similar to a single-layered AE with a linear activation function. Regularized autoencoders such as sparse, denoising, and contractive are useful for learning representations for later classification tasks [ 119 ], while variational autoencoders can be used as generative models [ 56 ], discussed below.
Sparse Autoencoder (SAE) A sparse autoencoder [ 73 ] has a sparsity penalty on the coding layer as a part of its training requirement. SAEs may have more hidden units than inputs, but only a small number of hidden units are permitted to be active at the same time, resulting in a sparse model. Figure 10 shows a schematic structure of a sparse autoencoder with several active units in the hidden layer. This model is thus obliged to respond to the unique statistical features of the training data following its constraints.
Denoising Autoencoder (DAE) A denoising autoencoder is a variant on the basic autoencoder that attempts to improve representation (to extract useful features) by altering the reconstruction criterion, and thus reduces the risk of learning the identity function [ 31 , 119 ]. In other words, it receives a corrupted data point as input and is trained to recover the original undistorted input as its output through minimizing the average reconstruction error over the training data, i.e, cleaning the corrupted input, or denoising. Thus, in the context of computing, DAEs can be considered as very powerful filters that can be utilized for automatic pre-processing. A denoising autoencoder, for example, could be used to automatically pre-process an image, thereby boosting its quality for recognition accuracy.
Contractive Autoencoder (CAE) The idea behind a contractive autoencoder, proposed by Rifai et al. [ 90 ], is to make the autoencoders robust of small changes in the training dataset. In its objective function, a CAE includes an explicit regularizer that forces the model to learn an encoding that is robust to small changes in input values. As a result, the learned representation’s sensitivity to the training input is reduced. While DAEs encourage the robustness of reconstruction as discussed above, CAEs encourage the robustness of representation.
Variational Autoencoder (VAE) A variational autoencoder [ 55 ] has a fundamentally unique property that distinguishes it from the classical autoencoder discussed above, which makes this so effective for generative modeling. VAEs, unlike the traditional autoencoders which map the input onto a latent vector, map the input data into the parameters of a probability distribution, such as the mean and variance of a Gaussian distribution. A VAE assumes that the source data has an underlying probability distribution and then tries to discover the distribution’s parameters. Although this approach was initially designed for unsupervised learning, its use has been demonstrated in other domains such as semi-supervised learning [ 128 ] and supervised learning [ 51 ].
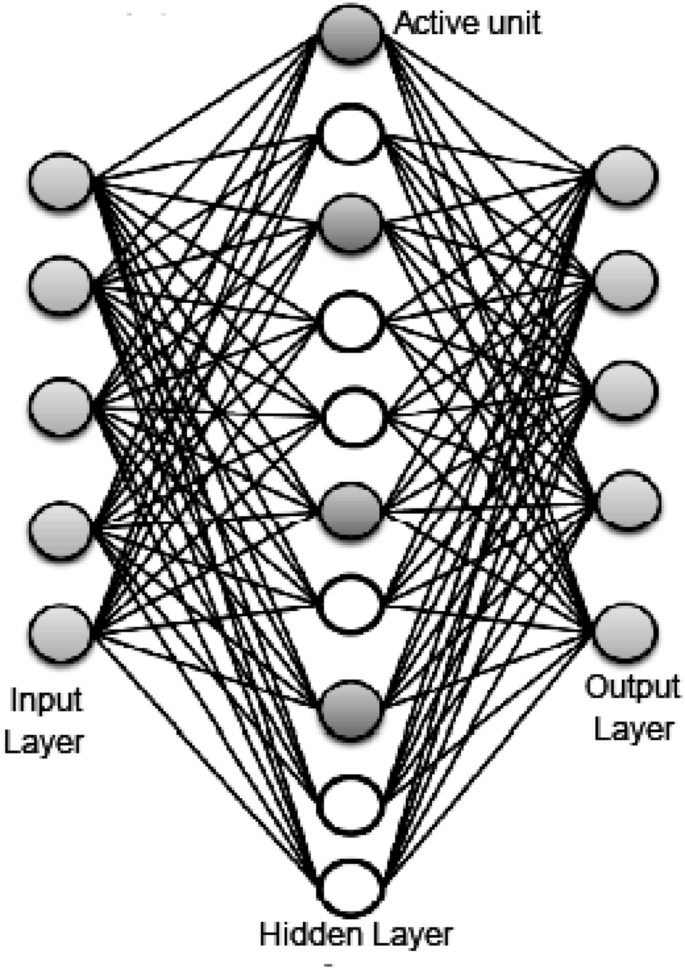
Schematic structure of a sparse autoencoder (SAE) with several active units (filled circle) in the hidden layer
Although, the earlier concept of AE was typically for dimensionality reduction or feature learning mentioned above, recently, AEs have been brought to the forefront of generative modeling, even the generative adversarial network is one of the popular methods in the area. The AEs have been effectively employed in a variety of domains, including healthcare, computer vision, speech recognition, cybersecurity, natural language processing, and many more. Overall, we can conclude that auto-encoder and its variants can play a significant role as unsupervised feature learning with neural network architecture.
Kohonen Map or Self-Organizing Map (SOM)
A Self-Organizing Map (SOM) or Kohonen Map [ 59 ] is another form of unsupervised learning technique for creating a low-dimensional (usually two-dimensional) representation of a higher-dimensional data set while maintaining the topological structure of the data. SOM is also known as a neural network-based dimensionality reduction algorithm that is commonly used for clustering [ 118 ]. A SOM adapts to the topological form of a dataset by repeatedly moving its neurons closer to the data points, allowing us to visualize enormous datasets and find probable clusters. The first layer of a SOM is the input layer, and the second layer is the output layer or feature map. Unlike other neural networks that use error-correction learning, such as backpropagation with gradient descent [ 36 ], SOMs employ competitive learning, which uses a neighborhood function to retain the input space’s topological features. SOM is widely utilized in a variety of applications, including pattern identification, health or medical diagnosis, anomaly detection, and virus or worm attack detection [ 60 , 87 ]. The primary benefit of employing a SOM is that this can make high-dimensional data easier to visualize and analyze to understand the patterns. The reduction of dimensionality and grid clustering makes it easy to observe similarities in the data. As a result, SOMs can play a vital role in developing a data-driven effective model for a particular problem domain, depending on the data characteristics.
Restricted Boltzmann Machine (RBM)
A Restricted Boltzmann Machine (RBM) [ 75 ] is also a generative stochastic neural network capable of learning a probability distribution across its inputs. Boltzmann machines typically consist of visible and hidden nodes and each node is connected to every other node, which helps us understand irregularities by learning how the system works in normal circumstances. RBMs are a subset of Boltzmann machines that have a limit on the number of connections between the visible and hidden layers [ 77 ]. This restriction permits training algorithms like the gradient-based contrastive divergence algorithm to be more efficient than those for Boltzmann machines in general [ 41 ]. RBMs have found applications in dimensionality reduction, classification, regression, collaborative filtering, feature learning, topic modeling, and many others. In the area of deep learning modeling, they can be trained either supervised or unsupervised, depending on the task. Overall, the RBMs can recognize patterns in data automatically and develop probabilistic or stochastic models, which are utilized for feature selection or extraction, as well as forming a deep belief network.
Deep Belief Network (DBN)
A Deep Belief Network (DBN) [ 40 ] is a multi-layer generative graphical model of stacking several individual unsupervised networks such as AEs or RBMs, that use each network’s hidden layer as the input for the next layer, i.e, connected sequentially. Thus, we can divide a DBN into (i) AE-DBN which is known as stacked AE, and (ii) RBM-DBN that is known as stacked RBM, where AE-DBN is composed of autoencoders and RBM-DBN is composed of restricted Boltzmann machines, discussed earlier. The ultimate goal is to develop a faster-unsupervised training technique for each sub-network that depends on contrastive divergence [ 41 ]. DBN can capture a hierarchical representation of input data based on its deep structure. The primary idea behind DBN is to train unsupervised feed-forward neural networks with unlabeled data before fine-tuning the network with labeled input. One of the most important advantages of DBN, as opposed to typical shallow learning networks, is that it permits the detection of deep patterns, which allows for reasoning abilities and the capture of the deep difference between normal and erroneous data [ 89 ]. A continuous DBN is simply an extension of a standard DBN that allows a continuous range of decimals instead of binary data. Overall, the DBN model can play a key role in a wide range of high-dimensional data applications due to its strong feature extraction and classification capabilities and become one of the significant topics in the field of neural networks.
In summary, the generative learning techniques discussed above typically allow us to generate a new representation of data through exploratory analysis. As a result, these deep generative networks can be utilized as preprocessing for supervised or discriminative learning tasks, as well as ensuring model accuracy, where unsupervised representation learning can allow for improved classifier generalization.
Deep Networks for Hybrid Learning and Other Approaches
In addition to the above-discussed deep learning categories, hybrid deep networks and several other approaches such as deep transfer learning (DTL) and deep reinforcement learning (DRL) are popular, which are discussed in the following.
Hybrid Deep Neural Networks
Generative models are adaptable, with the capacity to learn from both labeled and unlabeled data. Discriminative models, on the other hand, are unable to learn from unlabeled data yet outperform their generative counterparts in supervised tasks. A framework for training both deep generative and discriminative models simultaneously can enjoy the benefits of both models, which motivates hybrid networks.
Hybrid deep learning models are typically composed of multiple (two or more) deep basic learning models, where the basic model is a discriminative or generative deep learning model discussed earlier. Based on the integration of different basic generative or discriminative models, the below three categories of hybrid deep learning models might be useful for solving real-world problems. These are as follows:
Hybrid \(Model\_1\) : An integration of different generative or discriminative models to extract more meaningful and robust features. Examples could be CNN+LSTM, AE+GAN, and so on.
Hybrid \(Model\_2\) : An integration of generative model followed by a discriminative model. Examples could be DBN+MLP, GAN+CNN, AE+CNN, and so on.
Hybrid \(Model\_3\) : An integration of generative or discriminative model followed by a non-deep learning classifier. Examples could be AE+SVM, CNN+SVM, and so on.
Thus, in a broad sense, we can conclude that hybrid models can be either classification-focused or non-classification depending on the target use. However, most of the hybrid learning-related studies in the area of deep learning are classification-focused or supervised learning tasks, summarized in Table 1 . The unsupervised generative models with meaningful representations are employed to enhance the discriminative models. The generative models with useful representation can provide more informative and low-dimensional features for discrimination, and they can also enable to enhance the training data quality and quantity, providing additional information for classification.
Deep Transfer Learning (DTL)
Transfer Learning is a technique for effectively using previously learned model knowledge to solve a new task with minimum training or fine-tuning. In comparison to typical machine learning techniques [ 97 ], DL takes a large amount of training data. As a result, the need for a substantial volume of labeled data is a significant barrier to address some essential domain-specific tasks, particularly, in the medical sector, where creating large-scale, high-quality annotated medical or health datasets is both difficult and costly. Furthermore, the standard DL model demands a lot of computational resources, such as a GPU-enabled server, even though researchers are working hard to improve it. As a result, Deep Transfer Learning (DTL), a DL-based transfer learning method, might be helpful to address this issue. Figure 11 shows a general structure of the transfer learning process, where knowledge from the pre-trained model is transferred into a new DL model. It’s especially popular in deep learning right now since it allows to train deep neural networks with very little data [ 126 ].
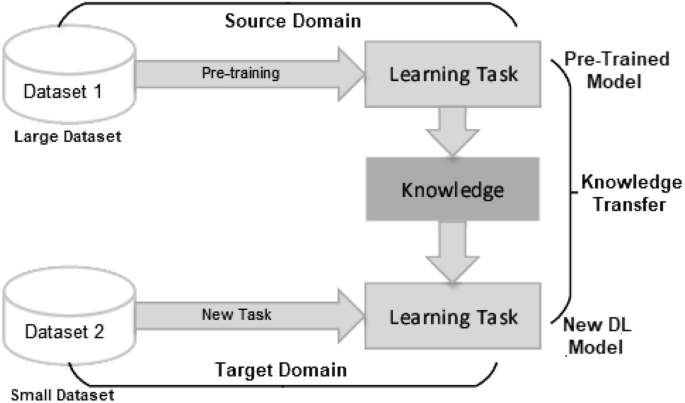
A general structure of transfer learning process, where knowledge from pre-trained model is transferred into new DL model
Transfer learning is a two-stage approach for training a DL model that consists of a pre-training step and a fine-tuning step in which the model is trained on the target task. Since deep neural networks have gained popularity in a variety of fields, a large number of DTL methods have been presented, making it crucial to categorize and summarize them. Based on the techniques used in the literature, DTL can be classified into four categories [ 117 ]. These are (i) instances-based deep transfer learning that utilizes instances in source domain by appropriate weight, (ii) mapping-based deep transfer learning that maps instances from two domains into a new data space with better similarity, (iii) network-based deep transfer learning that reuses the partial of network pre-trained in the source domain, and (iv) adversarial based deep transfer learning that uses adversarial technology to find transferable features that both suitable for two domains. Due to its high effectiveness and practicality, adversarial-based deep transfer learning has exploded in popularity in recent years. Transfer learning can also be classified into inductive, transductive, and unsupervised transfer learning depending on the circumstances between the source and target domains and activities [ 81 ]. While most current research focuses on supervised learning, how deep neural networks can transfer knowledge in unsupervised or semi-supervised learning may gain further interest in the future. DTL techniques are useful in a variety of fields including natural language processing, sentiment classification, visual recognition, speech recognition, spam filtering, and relevant others.
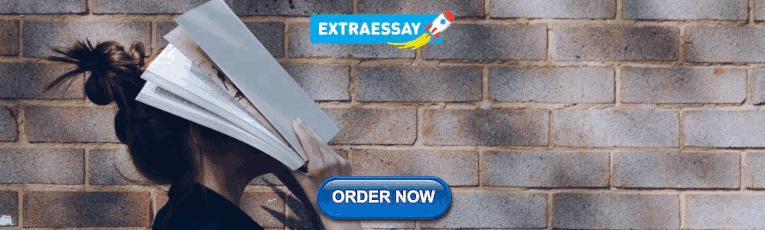
Deep Reinforcement Learning (DRL)
Reinforcement learning takes a different approach to solving the sequential decision-making problem than other approaches we have discussed so far. The concepts of an environment and an agent are often introduced first in reinforcement learning. The agent can perform a series of actions in the environment, each of which has an impact on the environment’s state and can result in possible rewards (feedback) - “positive” for good sequences of actions that result in a “good” state, and “negative” for bad sequences of actions that result in a “bad” state. The purpose of reinforcement learning is to learn good action sequences through interaction with the environment, typically referred to as a policy.
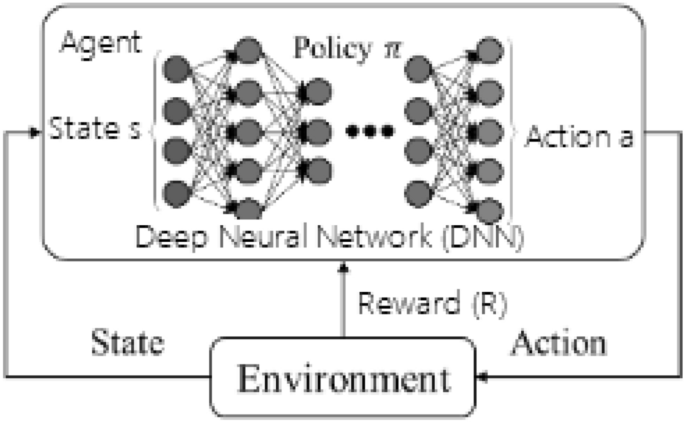
Schematic structure of deep reinforcement learning (DRL) highlighting a deep neural network
Deep reinforcement learning (DRL or deep RL) [ 9 ] integrates neural networks with a reinforcement learning architecture to allow the agents to learn the appropriate actions in a virtual environment, as shown in Fig. 12 . In the area of reinforcement learning, model-based RL is based on learning a transition model that enables for modeling of the environment without interacting with it directly, whereas model-free RL methods learn directly from interactions with the environment. Q-learning is a popular model-free RL technique for determining the best action-selection policy for any (finite) Markov Decision Process (MDP) [ 86 , 97 ]. MDP is a mathematical framework for modeling decisions based on state, action, and rewards [ 86 ]. In addition, Deep Q-Networks, Double DQN, Bi-directional Learning, Monte Carlo Control, etc. are used in the area [ 50 , 97 ]. In DRL methods it incorporates DL models, e.g. Deep Neural Networks (DNN), based on MDP principle [ 71 ], as policy and/or value function approximators. CNN for example can be used as a component of RL agents to learn directly from raw, high-dimensional visual inputs. In the real world, DRL-based solutions can be used in several application areas including robotics, video games, natural language processing, computer vision, and relevant others.
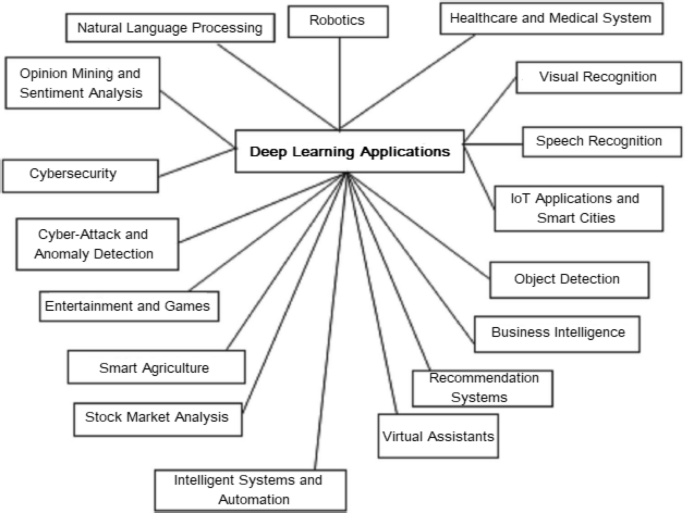
Several potential real-world application areas of deep learning
Deep Learning Application Summary
During the past few years, deep learning has been successfully applied to numerous problems in many application areas. These include natural language processing, sentiment analysis, cybersecurity, business, virtual assistants, visual recognition, healthcare, robotics, and many more. In Fig. 13 , we have summarized several potential real-world application areas of deep learning. Various deep learning techniques according to our presented taxonomy in Fig. 6 that includes discriminative learning, generative learning, as well as hybrid models, discussed earlier, are employed in these application areas. In Table 1 , we have also summarized various deep learning tasks and techniques that are used to solve the relevant tasks in several real-world applications areas. Overall, from Fig. 13 and Table 1 , we can conclude that the future prospects of deep learning modeling in real-world application areas are huge and there are lots of scopes to work. In the next section, we also summarize the research issues in deep learning modeling and point out the potential aspects for future generation DL modeling.
Research Directions and Future Aspects
While existing methods have established a solid foundation for deep learning systems and research, this section outlines the below ten potential future research directions based on our study.
Automation in Data Annotation According to the existing literature, discussed in Section 3 , most of the deep learning models are trained through publicly available datasets that are annotated. However, to build a system for a new problem domain or recent data-driven system, raw data from relevant sources are needed to collect. Thus, data annotation, e.g., categorization, tagging, or labeling of a large amount of raw data, is important for building discriminative deep learning models or supervised tasks, which is challenging. A technique with the capability of automatic and dynamic data annotation, rather than manual annotation or hiring annotators, particularly, for large datasets, could be more effective for supervised learning as well as minimizing human effort. Therefore, a more in-depth investigation of data collection and annotation methods, or designing an unsupervised learning-based solution could be one of the primary research directions in the area of deep learning modeling.
Data Preparation for Ensuring Data Quality As discussed earlier throughout the paper, the deep learning algorithms highly impact data quality, and availability for training, and consequently on the resultant model for a particular problem domain. Thus, deep learning models may become worthless or yield decreased accuracy if the data is bad, such as data sparsity, non-representative, poor-quality, ambiguous values, noise, data imbalance, irrelevant features, data inconsistency, insufficient quantity, and so on for training. Consequently, such issues in data can lead to poor processing and inaccurate findings, which is a major problem while discovering insights from data. Thus deep learning models also need to adapt to such rising issues in data, to capture approximated information from observations. Therefore, effective data pre-processing techniques are needed to design according to the nature of the data problem and characteristics, to handling such emerging challenges, which could be another research direction in the area.
Black-box Perception and Proper DL/ML Algorithm Selection In general, it’s difficult to explain how a deep learning result is obtained or how they get the ultimate decisions for a particular model. Although DL models achieve significant performance while learning from large datasets, as discussed in Section 2 , this “black-box” perception of DL modeling typically represents weak statistical interpretability that could be a major issue in the area. On the other hand, ML algorithms, particularly, rule-based machine learning techniques provide explicit logic rules (IF-THEN) for making decisions that are easier to interpret, update or delete according to the target applications [ 97 , 100 , 105 ]. If the wrong learning algorithm is chosen, unanticipated results may occur, resulting in a loss of effort as well as the model’s efficacy and accuracy. Thus by taking into account the performance, complexity, model accuracy, and applicability, selecting an appropriate model for the target application is challenging, and in-depth analysis is needed for better understanding and decision making.
Deep Networks for Supervised or Discriminative Learning: According to our designed taxonomy of deep learning techniques, as shown in Fig. 6 , discriminative architectures mainly include MLP, CNN, and RNN, along with their variants that are applied widely in various application domains. However, designing new techniques or their variants of such discriminative techniques by taking into account model optimization, accuracy, and applicability, according to the target real-world application and the nature of the data, could be a novel contribution, which can also be considered as a major future aspect in the area of supervised or discriminative learning.
Deep Networks for Unsupervised or Generative Learning As discussed in Section 3 , unsupervised learning or generative deep learning modeling is one of the major tasks in the area, as it allows us to characterize the high-order correlation properties or features in data, or generating a new representation of data through exploratory analysis. Moreover, unlike supervised learning [ 97 ], it does not require labeled data due to its capability to derive insights directly from the data as well as data-driven decision making. Consequently, it thus can be used as preprocessing for supervised learning or discriminative modeling as well as semi-supervised learning tasks, which ensure learning accuracy and model efficiency. According to our designed taxonomy of deep learning techniques, as shown in Fig. 6 , generative techniques mainly include GAN, AE, SOM, RBM, DBN, and their variants. Thus, designing new techniques or their variants for an effective data modeling or representation according to the target real-world application could be a novel contribution, which can also be considered as a major future aspect in the area of unsupervised or generative learning.
Hybrid/Ensemble Modeling and Uncertainty Handling According to our designed taxonomy of DL techniques, as shown in Fig 6 , this is considered as another major category in deep learning tasks. As hybrid modeling enjoys the benefits of both generative and discriminative learning, an effective hybridization can outperform others in terms of performance as well as uncertainty handling in high-risk applications. In Section 3 , we have summarized various types of hybridization, e.g., AE+CNN/SVM. Since a group of neural networks is trained with distinct parameters or with separate sub-sampling training datasets, hybridization or ensembles of such techniques, i.e., DL with DL/ML, can play a key role in the area. Thus designing effective blended discriminative and generative models accordingly rather than naive method, could be an important research opportunity to solve various real-world issues including semi-supervised learning tasks and model uncertainty.
Dynamism in Selecting Threshold/ Hyper-parameters Values, and Network Structures with Computational Efficiency In general, the relationship among performance, model complexity, and computational requirements is a key issue in deep learning modeling and applications. A combination of algorithmic advancements with improved accuracy as well as maintaining computational efficiency, i.e., achieving the maximum throughput while consuming the least amount of resources, without significant information loss, can lead to a breakthrough in the effectiveness of deep learning modeling in future real-world applications. The concept of incremental approaches or recency-based learning [ 100 ] might be effective in several cases depending on the nature of target applications. Moreover, assuming the network structures with a static number of nodes and layers, hyper-parameters values or threshold settings, or selecting them by the trial-and-error process may not be effective in many cases, as it can be changed due to the changes in data. Thus, a data-driven approach to select them dynamically could be more effective while building a deep learning model in terms of both performance and real-world applicability. Such type of data-driven automation can lead to future generation deep learning modeling with additional intelligence, which could be a significant future aspect in the area as well as an important research direction to contribute.
Lightweight Deep Learning Modeling for Next-Generation Smart Devices and Applications: In recent years, the Internet of Things (IoT) consisting of billions of intelligent and communicating things and mobile communications technologies have become popular to detect and gather human and environmental information (e.g. geo-information, weather data, bio-data, human behaviors, and so on) for a variety of intelligent services and applications. Every day, these ubiquitous smart things or devices generate large amounts of data, requiring rapid data processing on a variety of smart mobile devices [ 72 ]. Deep learning technologies can be incorporate to discover underlying properties and to effectively handle such large amounts of sensor data for a variety of IoT applications including health monitoring and disease analysis, smart cities, traffic flow prediction, and monitoring, smart transportation, manufacture inspection, fault assessment, smart industry or Industry 4.0, and many more. Although deep learning techniques discussed in Section 3 are considered as powerful tools for processing big data, lightweight modeling is important for resource-constrained devices, due to their high computational cost and considerable memory overhead. Thus several techniques such as optimization, simplification, compression, pruning, generalization, important feature extraction, etc. might be helpful in several cases. Therefore, constructing the lightweight deep learning techniques based on a baseline network architecture to adapt the DL model for next-generation mobile, IoT, or resource-constrained devices and applications, could be considered as a significant future aspect in the area.
Incorporating Domain Knowledge into Deep Learning Modeling Domain knowledge, as opposed to general knowledge or domain-independent knowledge, is knowledge of a specific, specialized topic or field. For instance, in terms of natural language processing, the properties of the English language typically differ from other languages like Bengali, Arabic, French, etc. Thus integrating domain-based constraints into the deep learning model could produce better results for such particular purpose. For instance, a task-specific feature extractor considering domain knowledge in smart manufacturing for fault diagnosis can resolve the issues in traditional deep-learning-based methods [ 28 ]. Similarly, domain knowledge in medical image analysis [ 58 ], financial sentiment analysis [ 49 ], cybersecurity analytics [ 94 , 103 ] as well as conceptual data model in which semantic information, (i.e., meaningful for a system, rather than merely correlational) [ 45 , 121 , 131 ] is included, can play a vital role in the area. Transfer learning could be an effective way to get started on a new challenge with domain knowledge. Moreover, contextual information such as spatial, temporal, social, environmental contexts [ 92 , 104 , 108 ] can also play an important role to incorporate context-aware computing with domain knowledge for smart decision making as well as building adaptive and intelligent context-aware systems. Therefore understanding domain knowledge and effectively incorporating them into the deep learning model could be another research direction.
Designing General Deep Learning Framework for Target Application Domains One promising research direction for deep learning-based solutions is to develop a general framework that can handle data diversity, dimensions, stimulation types, etc. The general framework would require two key capabilities: the attention mechanism that focuses on the most valuable parts of input signals, and the ability to capture latent feature that enables the framework to capture the distinctive and informative features. Attention models have been a popular research topic because of their intuition, versatility, and interpretability, and employed in various application areas like computer vision, natural language processing, text or image classification, sentiment analysis, recommender systems, user profiling, etc [ 13 , 80 ]. Attention mechanism can be implemented based on learning algorithms such as reinforcement learning that is capable of finding the most useful part through a policy search [ 133 , 134 ]. Similarly, CNN can be integrated with suitable attention mechanisms to form a general classification framework, where CNN can be used as a feature learning tool for capturing features in various levels and ranges. Thus, designing a general deep learning framework considering attention as well as a latent feature for target application domains could be another area to contribute.
To summarize, deep learning is a fairly open topic to which academics can contribute by developing new methods or improving existing methods to handle the above-mentioned concerns and tackle real-world problems in a variety of application areas. This can also help the researchers conduct a thorough analysis of the application’s hidden and unexpected challenges to produce more reliable and realistic outcomes. Overall, we can conclude that addressing the above-mentioned issues and contributing to proposing effective and efficient techniques could lead to “Future Generation DL” modeling as well as more intelligent and automated applications.
Concluding Remarks
In this article, we have presented a structured and comprehensive view of deep learning technology, which is considered a core part of artificial intelligence as well as data science. It starts with a history of artificial neural networks and moves to recent deep learning techniques and breakthroughs in different applications. Then, the key algorithms in this area, as well as deep neural network modeling in various dimensions are explored. For this, we have also presented a taxonomy considering the variations of deep learning tasks and how they are used for different purposes. In our comprehensive study, we have taken into account not only the deep networks for supervised or discriminative learning but also the deep networks for unsupervised or generative learning, and hybrid learning that can be used to solve a variety of real-world issues according to the nature of problems.
Deep learning, unlike traditional machine learning and data mining algorithms, can produce extremely high-level data representations from enormous amounts of raw data. As a result, it has provided an excellent solution to a variety of real-world problems. A successful deep learning technique must possess the relevant data-driven modeling depending on the characteristics of raw data. The sophisticated learning algorithms then need to be trained through the collected data and knowledge related to the target application before the system can assist with intelligent decision-making. Deep learning has shown to be useful in a wide range of applications and research areas such as healthcare, sentiment analysis, visual recognition, business intelligence, cybersecurity, and many more that are summarized in the paper.
Finally, we have summarized and discussed the challenges faced and the potential research directions, and future aspects in the area. Although deep learning is considered a black-box solution for many applications due to its poor reasoning and interpretability, addressing the challenges or future aspects that are identified could lead to future generation deep learning modeling and smarter systems. This can also help the researchers for in-depth analysis to produce more reliable and realistic outcomes. Overall, we believe that our study on neural networks and deep learning-based advanced analytics points in a promising path and can be utilized as a reference guide for future research and implementations in relevant application domains by both academic and industry professionals.
Abadi M, Barham P, Chen J, Chen Z, Davis A, Dean J, Devin Ma, Ghemawat S, Irving G, Isard M, et al. Tensorflow: a system for large-scale machine learning. In: 12th { USENIX } Symposium on operating systems design and implementation ({ OSDI } 16), 2016; p. 265–283.
Abdel-Basset M, Hawash H, Chakrabortty RK, Ryan M. Energy-net: a deep learning approach for smart energy management in iot-based smart cities. IEEE Internet of Things J. 2021.
Aggarwal A, Mittal M, Battineni G. Generative adversarial network: an overview of theory and applications. Int J Inf Manag Data Insights. 2021; p. 100004.
Al-Qatf M, Lasheng Y, Al-Habib M, Al-Sabahi K. Deep learning approach combining sparse autoencoder with svm for network intrusion detection. IEEE Access. 2018;6:52843–56.
Article Google Scholar
Ale L, Sheta A, Li L, Wang Y, Zhang N. Deep learning based plant disease detection for smart agriculture. In: 2019 IEEE Globecom Workshops (GC Wkshps), 2019; p. 1–6. IEEE.
Amarbayasgalan T, Lee JY, Kim KR, Ryu KH. Deep autoencoder based neural networks for coronary heart disease risk prediction. In: Heterogeneous data management, polystores, and analytics for healthcare. Springer; 2019. p. 237–48.
Anuradha J, et al. Big data based stock trend prediction using deep cnn with reinforcement-lstm model. Int J Syst Assur Eng Manag. 2021; p. 1–11.
Aqib M, Mehmood R, Albeshri A, Alzahrani A. Disaster management in smart cities by forecasting traffic plan using deep learning and gpus. In: International Conference on smart cities, infrastructure, technologies and applications. Springer; 2017. p. 139–54.
Arulkumaran K, Deisenroth MP, Brundage M, Bharath AA. Deep reinforcement learning: a brief survey. IEEE Signal Process Mag. 2017;34(6):26–38.
Aslan MF, Unlersen MF, Sabanci K, Durdu A. Cnn-based transfer learning-bilstm network: a novel approach for covid-19 infection detection. Appl Soft Comput. 2021;98:106912.
Bu F, Wang X. A smart agriculture iot system based on deep reinforcement learning. Futur Gener Comput Syst. 2019;99:500–7.
Chang W-J, Chen L-B, Hsu C-H, Lin C-P, Yang T-C. A deep learning-based intelligent medicine recognition system for chronic patients. IEEE Access. 2019;7:44441–58.
Chaudhari S, Mithal V, Polatkan Gu, Ramanath R. An attentive survey of attention models. arXiv preprint arXiv:1904.02874, 2019.
Chaudhuri N, Gupta G, Vamsi V, Bose I. On the platform but will they buy? predicting customers’ purchase behavior using deep learning. Decis Support Syst. 2021; p. 113622.
Chen D, Wawrzynski P, Lv Z. Cyber security in smart cities: a review of deep learning-based applications and case studies. Sustain Cities Soc. 2020; p. 102655.
Cho K, Van MB, Gulcehre C, Bahdanau D, Bougares F, Schwenk H, Bengio Y. Learning phrase representations using rnn encoder-decoder for statistical machine translation. arXiv preprint arXiv:1406.1078, 2014.
Chollet F. Xception: Deep learning with depthwise separable convolutions. In: Proceedings of the IEEE Conference on computer vision and pattern recognition, 2017; p. 1251–258.
Chung J, Gulcehre C, Cho KH, Bengio Y. Empirical evaluation of gated recurrent neural networks on sequence modeling. arXiv preprint arXiv:1412.3555, 2014.
Coelho IM, Coelho VN, da Eduardo J, Luz S, Ochi LS, Guimarães FG, Rios E. A gpu deep learning metaheuristic based model for time series forecasting. Appl Energy. 2017;201:412–8.
Da'u A, Salim N. Recommendation system based on deep learning methods: a systematic review and new directions. Artif Intel Rev. 2020;53(4):2709–48.
Deng L. A tutorial survey of architectures, algorithms, and applications for deep learning. APSIPA Trans Signal Inf Process. 2014; p. 3.
Deng L, Dong Yu. Deep learning: methods and applications. Found Trends Signal Process. 2014;7(3–4):197–387.
Article MathSciNet MATH Google Scholar
Deng S, Li R, Jin Y, He H. Cnn-based feature cross and classifier for loan default prediction. In: 2020 International Conference on image, video processing and artificial intelligence, volume 11584, page 115841K. International Society for Optics and Photonics, 2020.
Dhyani M, Kumar R. An intelligent chatbot using deep learning with bidirectional rnn and attention model. Mater Today Proc. 2021;34:817–24.
Donahue J, Krähenbühl P, Darrell T. Adversarial feature learning. arXiv preprint arXiv:1605.09782, 2016.
Du K-L, Swamy MNS. Neural networks and statistical learning. Berlin: Springer Science & Business Media; 2013.
MATH Google Scholar
Dupond S. A thorough review on the current advance of neural network structures. Annu Rev Control. 2019;14:200–30.
Google Scholar
Feng J, Yao Y, Lu S, Liu Y. Domain knowledge-based deep-broad learning framework for fault diagnosis. IEEE Trans Ind Electron. 2020;68(4):3454–64.
Garg S, Kaur K, Kumar N, Rodrigues JJPC. Hybrid deep-learning-based anomaly detection scheme for suspicious flow detection in sdn: a social multimedia perspective. IEEE Trans Multimed. 2019;21(3):566–78.
Géron A. Hands-on machine learning with Scikit-Learn, Keras. In: and TensorFlow: concepts, tools, and techniques to build intelligent systems. O’Reilly Media; 2019.
Goodfellow I, Bengio Y, Courville A, Bengio Y. Deep learning, vol. 1. Cambridge: MIT Press; 2016.
Goodfellow I, Pouget-Abadie J, Mirza M, Xu B, Warde-Farley D, Ozair S, Courville A, Bengio Y. Generative adversarial nets. In: Advances in neural information processing systems. 2014; p. 2672–680.
Google trends. 2021. https://trends.google.com/trends/ .
Gruber N, Jockisch A. Are gru cells more specific and lstm cells more sensitive in motive classification of text? Front Artif Intell. 2020;3:40.
Gu B, Ge R, Chen Y, Luo L, Coatrieux G. Automatic and robust object detection in x-ray baggage inspection using deep convolutional neural networks. IEEE Trans Ind Electron. 2020.
Han J, Pei J, Kamber M. Data mining: concepts and techniques. Amsterdam: Elsevier; 2011.
Haykin S. Neural networks and learning machines, 3/E. London: Pearson Education; 2010.
He K, Zhang X, Ren S, Sun J. Spatial pyramid pooling in deep convolutional networks for visual recognition. IEEE Trans Pattern Anal Mach Intell. 2015;37(9):1904–16.
He K, Zhang X, Ren S, Sun J. Deep residual learning for image recognition. In: Proceedings of the IEEE Conference on computer vision and pattern recognition, 2016; p. 770–78.
Hinton GE. Deep belief networks. Scholarpedia. 2009;4(5):5947.
Hinton GE, Osindero S, Teh Y-W. A fast learning algorithm for deep belief nets. Neural Comput. 2006;18(7):1527–54.
Hochreiter S, Schmidhuber J. Long short-term memory. Neural Comput. 1997;9(8):1735–80.
Huang C-J, Kuo P-H. A deep cnn-lstm model for particulate matter (pm2. 5) forecasting in smart cities. Sensors. 2018;18(7):2220.
Huang H-H, Fukuda M, Nishida T. Toward rnn based micro non-verbal behavior generation for virtual listener agents. In: International Conference on human-computer interaction, 2019; p. 53–63. Springer.
Hulsebos M, Hu K, Bakker M, Zgraggen E, Satyanarayan A, Kraska T, Demiralp Ça, Hidalgo C. Sherlock: a deep learning approach to semantic data type detection. In: Proceedings of the 25th ACM SIGKDD International Conference on knowledge discovery & data mining, 2019; p. 1500–508.
Imamverdiyev Y, Abdullayeva F. Deep learning method for denial of service attack detection based on restricted Boltzmann machine. Big Data. 2018;6(2):159–69.
Islam MZ, Islam MM, Asraf A. A combined deep cnn-lstm network for the detection of novel coronavirus (covid-19) using x-ray images. Inf Med Unlock. 2020;20:100412.
Ismail WN, Hassan MM, Alsalamah HA, Fortino G. Cnn-based health model for regular health factors analysis in internet-of-medical things environment. IEEE. Access. 2020;8:52541–9.
Jangid H, Singhal S, Shah RR, Zimmermann R. Aspect-based financial sentiment analysis using deep learning. In: Companion Proceedings of the The Web Conference 2018, 2018; p. 1961–966.
Kaelbling LP, Littman ML, Moore AW. Reinforcement learning: a survey. J Artif Intell Res. 1996;4:237–85.
Kameoka H, Li L, Inoue S, Makino S. Supervised determined source separation with multichannel variational autoencoder. Neural Comput. 2019;31(9):1891–914.
Karhunen J, Raiko T, Cho KH. Unsupervised deep learning: a short review. In: Advances in independent component analysis and learning machines. 2015; p. 125–42.
Kawde P, Verma GK. Deep belief network based affect recognition from physiological signals. In: 2017 4th IEEE Uttar Pradesh Section International Conference on electrical, computer and electronics (UPCON), 2017; p. 587–92. IEEE.
Kim J-Y, Seok-Jun B, Cho S-B. Zero-day malware detection using transferred generative adversarial networks based on deep autoencoders. Inf Sci. 2018;460:83–102.
Kingma DP, Welling M. Auto-encoding variational bayes. arXiv preprint arXiv:1312.6114, 2013.
Kingma DP, Welling M. An introduction to variational autoencoders. arXiv preprint arXiv:1906.02691, 2019.
Kiran PKR, Bhasker B. Dnnrec: a novel deep learning based hybrid recommender system. Expert Syst Appl. 2020.
Kloenne M, Niehaus S, Lampe L, Merola A, Reinelt J, Roeder I, Scherf N. Domain-specific cues improve robustness of deep learning-based segmentation of ct volumes. Sci Rep. 2020;10(1):1–9.
Kohonen T. The self-organizing map. Proc IEEE. 1990;78(9):1464–80.
Kohonen T. Essentials of the self-organizing map. Neural Netw. 2013;37:52–65.
Kök İ, Şimşek MU, Özdemir S. A deep learning model for air quality prediction in smart cities. In: 2017 IEEE International Conference on Big Data (Big Data), 2017; p. 1983–990. IEEE.
Krizhevsky A, Sutskever I, Hinton GE. Imagenet classification with deep convolutional neural networks. In: Advances in neural information processing systems. 2012; p. 1097–105.
Latif S, Rana R, Younis S, Qadir J, Epps J. Transfer learning for improving speech emotion classification accuracy. arXiv preprint arXiv:1801.06353, 2018.
LeCun Y, Bengio Y, Hinton G. Deep learning. Nature. 2015;521(7553):436–44.
LeCun Y, Bottou L, Bengio Y, Haffner P. Gradient-based learning applied to document recognition. Proc IEEE. 1998;86(11):2278–324.
Li B, François-Lavet V, Doan T, Pineau J. Domain adversarial reinforcement learning. arXiv preprint arXiv:2102.07097, 2021.
Li T-HS, Kuo P-H, Tsai T-N, Luan P-C. Cnn and lstm based facial expression analysis model for a humanoid robot. IEEE Access. 2019;7:93998–4011.
Liu C, Cao Y, Luo Y, Chen G, Vokkarane V, Yunsheng M, Chen S, Hou P. A new deep learning-based food recognition system for dietary assessment on an edge computing service infrastructure. IEEE Trans Serv Comput. 2017;11(2):249–61.
Liu W, Wang Z, Liu X, Zeng N, Liu Y, Alsaadi FE. A survey of deep neural network architectures and their applications. Neurocomputing. 2017;234:11–26.
López AU, Mateo F, Navío-Marco J, Martínez-Martínez JM, Gómez-Sanchís J, Vila-Francés J, Serrano-López AJ. Analysis of computer user behavior, security incidents and fraud using self-organizing maps. Comput Secur. 2019;83:38–51.
Lopez-Martin M, Carro B, Sanchez-Esguevillas A. Application of deep reinforcement learning to intrusion detection for supervised problems. Expert Syst Appl. 2020;141:112963.
Ma X, Yao T, Menglan H, Dong Y, Liu W, Wang F, Liu J. A survey on deep learning empowered iot applications. IEEE Access. 2019;7:181721–32.
Makhzani A, Frey B. K-sparse autoencoders. arXiv preprint arXiv:1312.5663, 2013.
Mandic D, Chambers J. Recurrent neural networks for prediction: learning algorithms, architectures and stability. Hoboken: Wiley; 2001.
Book Google Scholar
Marlin B, Swersky K, Chen B, Freitas N. Inductive principles for restricted boltzmann machine learning. In: Proceedings of the Thirteenth International Conference on artificial intelligence and statistics, p. 509–16. JMLR Workshop and Conference Proceedings, 2010.
Masud M, Muhammad G, Alhumyani H, Alshamrani SS, Cheikhrouhou O, Ibrahim S, Hossain MS. Deep learning-based intelligent face recognition in iot-cloud environment. Comput Commun. 2020;152:215–22.
Memisevic R, Hinton GE. Learning to represent spatial transformations with factored higher-order boltzmann machines. Neural Comput. 2010;22(6):1473–92.
Article MATH Google Scholar
Minaee S, Azimi E, Abdolrashidi AA. Deep-sentiment: sentiment analysis using ensemble of cnn and bi-lstm models. arXiv preprint arXiv:1904.04206, 2019.
Naeem M, Paragliola G, Coronato A. A reinforcement learning and deep learning based intelligent system for the support of impaired patients in home treatment. Expert Syst Appl. 2021;168:114285.
Niu Z, Zhong G, Hui Yu. A review on the attention mechanism of deep learning. Neurocomputing. 2021;452:48–62.
Pan SJ, Yang Q. A survey on transfer learning. IEEE Trans Knowl Data Eng. 2009;22(10):1345–59.
Paszke A, Gross S, Massa F, Lerer A, Bradbury J, Chanan G, Killeen T, Lin Z, Gimelshein N, Antiga L, et al. Pytorch: An imperative style, high-performance deep learning library. Adv Neural Inf Process Syst. 2019;32:8026–37.
Pedregosa F, Varoquaux G, Gramfort A, Michel V, Thirion B, Grisel O, Blondel M, Prettenhofer P, Weiss R, Dubourg V, et al. Scikit-learn: machine learning in python. J Mach Learn Res. 2011;12:2825–30.
MathSciNet MATH Google Scholar
Pi Y, Nath ND, Behzadan AH. Convolutional neural networks for object detection in aerial imagery for disaster response and recovery. Adv Eng Inf. 2020;43:101009.
Piccialli F, Giampaolo F, Prezioso E, Crisci D, Cuomo S. Predictive analytics for smart parking: A deep learning approach in forecasting of iot data. ACM Trans Internet Technol (TOIT). 2021;21(3):1–21.
Puterman ML. Markov decision processes: discrete stochastic dynamic programming. Hoboken: Wiley; 2014.
Qu X, Lin Y, Kai G, Linru M, Meng S, Mingxing K, Mu L, editors. A survey on the development of self-organizing maps for unsupervised intrusion detection. Mob Netw Appl. 2019; p. 1–22.
Rahman MW, Tashfia SS, Islam R, Hasan MM, Sultan SI, Mia S, Rahman MM. The architectural design of smart blind assistant using iot with deep learning paradigm. Internet of Things. 2021;13:100344.
Ren J, Green M, Huang X. From traditional to deep learning: fault diagnosis for autonomous vehicles. In: Learning control. Elsevier. 2021; p. 205–19.
Rifai S, Vincent P, Muller X, Glorot X, Bengio Y. Contractive auto-encoders: Explicit invariance during feature extraction. In: Icml, 2011.
Rosa RL, Schwartz GM, Ruggiero WV, Rodríguez DZ. A knowledge-based recommendation system that includes sentiment analysis and deep learning. IEEE Trans Ind Inf. 2018;15(4):2124–35.
Sarker IH. Context-aware rule learning from smartphone data: survey, challenges and future directions. J Big Data. 2019;6(1):1–25.
Article MathSciNet Google Scholar
Sarker IH. A machine learning based robust prediction model for real-life mobile phone data. Internet of Things. 2019;5:180–93.
Sarker IH. Cyberlearning: effectiveness analysis of machine learning security modeling to detect cyber-anomalies and multi-attacks. Internet of Things. 2021;14:100393.
Sarker IH. Data science and analytics: an overview from data-driven smart computing, decision-making and applications perspective. SN Comput Sci. 2021.
Sarker IH. Deep cybersecurity: a comprehensive overview from neural network and deep learning perspective. SN Computer. Science. 2021;2(3):1–16.
MathSciNet Google Scholar
Sarker IH. Machine learning: Algorithms, real-world applications and research directions. SN Computer. Science. 2021;2(3):1–21.
Sarker IH, Abushark YB, Alsolami F, Khan AI. Intrudtree: a machine learning based cyber security intrusion detection model. Symmetry. 2020;12(5):754.
Sarker IH, Abushark YB, Khan AI. Contextpca: Predicting context-aware smartphone apps usage based on machine learning techniques. Symmetry. 2020;12(4):499.
Sarker IH, Colman A, Han J. Recencyminer: mining recency-based personalized behavior from contextual smartphone data. J Big Data. 2019;6(1):1–21.
Sarker IH, Colman A, Han J, Khan AI, Abushark YB, Salah K. Behavdt: a behavioral decision tree learning to build user-centric context-aware predictive model. Mob Netw Appl. 2020;25(3):1151–61.
Sarker IH, Colman A, Kabir MA, Han J. Individualized time-series segmentation for mining mobile phone user behavior. Comput J. 2018;61(3):349–68.
Sarker IH, Furhad MH, Nowrozy R. Ai-driven cybersecurity: an overview, security intelligence modeling and research directions. SN Computer. Science. 2021;2(3):1–18.
Sarker IH, Hoque MM, Uddin MK. Mobile data science and intelligent apps: concepts, ai-based modeling and research directions. Mob Netw Appl. 2021;26(1):285–303.
Sarker IH, Kayes ASM. Abc-ruleminer: User behavioral rule-based machine learning method for context-aware intelligent services. J Netw Comput Appl. 2020;168:102762.
Sarker IH, Kayes ASM, Badsha S, Alqahtani H, Watters P, Ng A. Cybersecurity data science: an overview from machine learning perspective. J Big data. 2020;7(1):1–29.
Sarker IH, Kayes ASM, Watters P. Effectiveness analysis of machine learning classification models for predicting personalized context-aware smartphone usage. J Big Data. 2019;6(1):1–28.
Sarker IH, Salah K. Appspred: predicting context-aware smartphone apps using random forest learning. Internet of Things. 2019;8:100106.
Satt A, Rozenberg S, Hoory R. Efficient emotion recognition from speech using deep learning on spectrograms. In: Interspeec, 2017; p. 1089–1093.
Sevakula RK, Singh V, Verma NK, Kumar C, Cui Y. Transfer learning for molecular cancer classification using deep neural networks. IEEE/ACM Trans Comput Biol Bioinf. 2018;16(6):2089–100.
Sujay Narumanchi H, Ananya Pramod Kompalli Shankar A, Devashish CK. Deep learning based large scale visual recommendation and search for e-commerce. arXiv preprint arXiv:1703.02344, 2017.
Shao X, Kim CS. Multi-step short-term power consumption forecasting using multi-channel lstm with time location considering customer behavior. IEEE Access. 2020;8:125263–73.
Siami-Namini S, Tavakoli N, Namin AS. The performance of lstm and bilstm in forecasting time series. In: 2019 IEEE International Conference on Big Data (Big Data), 2019; p. 3285–292. IEEE.
Ślusarczyk B. Industry 4.0: are we ready? Pol J Manag Stud. 2018; p. 17
Sumathi P, Subramanian R, Karthikeyan VV, Karthik S. Soil monitoring and evaluation system using edl-asqe: enhanced deep learning model for ioi smart agriculture network. Int J Commun Syst. 2021; p. e4859.
Szegedy C, Liu W, Jia Y, Sermanet P, Reed S, Anguelov D, Erhan D, Vanhoucke V, Rabinovich A. Going deeper with convolutions. In: Proceedings of the IEEE Conference on computer vision and pattern recognition, 2015; p. 1–9.
Tan C, Sun F, Kong T, Zhang W, Yang C, Liu C. A survey on deep transfer learning. In: International Conference on artificial neural networks, 2018; p. 270–279. Springer.
Vesanto J, Alhoniemi E. Clustering of the self-organizing map. IEEE Trans Neural Netw. 2000;11(3):586–600.
Vincent P, Larochelle H, Lajoie I, Bengio Y, Manzagol P-A, Bottou L. Stacked denoising autoencoders: Learning useful representations in a deep network with a local denoising criterion. J Mach Learn Res. 2010;11(12).
Wang J, Liang-Chih Yu, Robert Lai K, Zhang X. Tree-structured regional cnn-lstm model for dimensional sentiment analysis. IEEE/ACM Trans Audio Speech Lang Process. 2019;28:581–91.
Wang S, Wan J, Li D, Liu C. Knowledge reasoning with semantic data for real-time data processing in smart factory. Sensors. 2018;18(2):471.
Wang W, Zhao M, Wang J. Effective android malware detection with a hybrid model based on deep autoencoder and convolutional neural network. J Ambient Intell Humaniz Comput. 2019;10(8):3035–43.
Wang X, Liu J, Qiu T, Chaoxu M, Chen C, Zhou P. A real-time collision prediction mechanism with deep learning for intelligent transportation system. IEEE Trans Veh Technol. 2020;69(9):9497–508.
Wang Y, Huang M, Zhu X, Zhao L. Attention-based lstm for aspect-level sentiment classification. In: Proceedings of the 2016 Conference on empirical methods in natural language processing, 2016; p. 606–615.
Wei P, Li Y, Zhang Z, Tao H, Li Z, Liu D. An optimization method for intrusion detection classification model based on deep belief network. IEEE Access. 2019;7:87593–605.
Weiss K, Khoshgoftaar TM, Wang DD. A survey of transfer learning. J Big data. 2016;3(1):9.
Xin Y, Kong L, Liu Z, Chen Y, Li Y, Zhu H, Gao M, Hou H, Wang C. Machine learning and deep learning methods for cybersecurity. Ieee access. 2018;6:35365–81.
Xu W, Sun H, Deng C, Tan Y. Variational autoencoder for semi-supervised text classification. In: Thirty-First AAAI Conference on artificial intelligence, 2017.
Xue Q, Chuah MC. New attacks on rnn based healthcare learning system and their detections. Smart Health. 2018;9:144–57.
Yousefi-Azar M, Hamey L. Text summarization using unsupervised deep learning. Expert Syst Appl. 2017;68:93–105.
Yuan X, Shi J, Gu L. A review of deep learning methods for semantic segmentation of remote sensing imagery. Expert Syst Appl. 2020;p. 114417.
Zhang G, Liu Y, Jin X. A survey of autoencoder-based recommender systems. Front Comput Sci. 2020;14(2):430–50.
Zhang X, Yao L, Huang C, Wang S, Tan M, Long Gu, Wang C. Multi-modality sensor data classification with selective attention. arXiv preprint arXiv:1804.05493, 2018.
Zhang X, Yao L, Wang X, Monaghan J, Mcalpine D, Zhang Y. A survey on deep learning based brain computer interface: recent advances and new frontiers. arXiv preprint arXiv:1905.04149, 2019; p. 66.
Zhang Y, Zhang P, Yan Y. Attention-based lstm with multi-task learning for distant speech recognition. In: Interspeech, 2017; p. 3857–861.
Download references
Author information
Authors and affiliations.
Swinburne University of Technology, Melbourne, VIC, 3122, Australia
Chittagong University of Engineering & Technology, Chittagong, 4349, Bangladesh
You can also search for this author in PubMed Google Scholar
Corresponding author
Correspondence to Iqbal H. Sarker .
Ethics declarations
Conflict of interest.
The author declares no conflict of interest.
Additional information
Publisher's note.
Springer Nature remains neutral with regard to jurisdictional claims in published maps and institutional affiliations.
This article is part of the topical collection “Advances in Computational Approaches for Artificial Intelligence, Image Processing, IoT and Cloud Applications” guest edited by Bhanu Prakash K. N. and M. Shivakumar.
Rights and permissions
Reprints and permissions
About this article
Sarker, I.H. Deep Learning: A Comprehensive Overview on Techniques, Taxonomy, Applications and Research Directions. SN COMPUT. SCI. 2 , 420 (2021). https://doi.org/10.1007/s42979-021-00815-1
Download citation
Received : 29 May 2021
Accepted : 07 August 2021
Published : 18 August 2021
DOI : https://doi.org/10.1007/s42979-021-00815-1
Share this article
Anyone you share the following link with will be able to read this content:
Sorry, a shareable link is not currently available for this article.
Provided by the Springer Nature SharedIt content-sharing initiative
- Deep learning
- Artificial neural network
- Artificial intelligence
- Discriminative learning
- Generative learning
- Hybrid learning
- Intelligent systems
- Find a journal
- Publish with us
- Track your research

An official website of the United States government
The .gov means it’s official. Federal government websites often end in .gov or .mil. Before sharing sensitive information, make sure you’re on a federal government site.
The site is secure. The https:// ensures that you are connecting to the official website and that any information you provide is encrypted and transmitted securely.
- Publications
- Account settings
Preview improvements coming to the PMC website in October 2024. Learn More or Try it out now .
- Advanced Search
- Journal List
- Vis Comput Ind Biomed Art
- v.4; 2021 Dec

A comprehensive review of machine learning techniques on diabetes detection
Toshita sharma.
1 Department of Electronics and Communication Technology, Nirma University, 382481 Ahmedabad, Gujarat India
2 Department of Chemical Engineering, School of Technology, Pandit Deendayal Energy University, 382426 Gandhinagar, Gujarat India
Associated Data
All relevant data and material are presented in the main paper.
Diabetes mellitus has been an increasing concern owing to its high morbidity, and the average age of individual affected by of individual affected by this disease has now decreased to mid-twenties. Given the high prevalence, it is necessary to address with this problem effectively. Many researchers and doctors have now developed detection techniques based on artificial intelligence to better approach problems that are missed due to human errors. Data mining techniques with algorithms such as - density-based spatial clustering of applications with noise and ordering points to identify the cluster structure, the use of machine vision systems to learn data on facial images, gain better features for model training, and diagnosis via presentation of iridocyclitis for detection of the disease through iris patterns have been deployed by various practitioners. Machine learning classifiers such as support vector machines, logistic regression, and decision trees, have been comparative discussed various authors. Deep learning models such as artificial neural networks and recurrent neural networks have been considered, with primary focus on long short-term memory and convolutional neural network architectures in comparison with other machine learning models. Various parameters such as the root-mean-square error, mean absolute errors, area under curves, and graphs with varying criteria are commonly used. In this study, challenges pertaining to data inadequacy and model deployment are discussed. The future scope of such methods has also been discussed, and new methods are expected to enhance the performance of existing models, allowing them to attain greater insight into the conditions on which the prevalence of the disease depends.
Introduction
Given the growing population, it is necessary to develop systems to augment health and mitigate increasing concerns around the world. As scientific research continues to advance, the development of such system is becoming more efficient. Healthcare systems are designed to provide people with the requirements for good health and perform the detection and diagnosis of disease and conditions correctly with greater efficiency, as proposed in the conventional methods. In general patients are often highly concerned as to the quality of healthcare system and facilities available to provide treatment. The benefits of improvements in healthcare systems tend to affect people who have prevailing ailments more directly, and this group comprises the majority of the group of people affected by many diseases such as diabetes, blood sugar, and blood pressure issues [ 1 ]. According to the National Diabetes Statistics Report 2020, every 1 in 10 people in United States have diabetes, and new cases of diabetes 1 and 2 have significantly increased among young people. As health and healthcare form a critical pillars of a healthy society, it is necessary to use the capabilities of computational methods and artificial intelligence [ 2 ] to develop new methods for application in healthcare systems to promote a healthier society and reduce the risk of such diseases in our generations, further increasing the quality of life.
There has been a huge impact in the medical world with the advancement of technology. Health outcomes may depend on a matter of seconds for individuals who may not be able to reach a hospital or receive emergency treatment. Technology bridges this gap in distance and resources for all people to whom its benefits are extended. Various technologies have been developed using magnetic resonance imaging machines in video technology. Internet-based applications can provide patients with customizable services. After a few clinical visits, the remainder of the work can be fulfilled through high-tech services such as telehealth. Clinicians can communicate with patients through the Internet to better serve their needs [ 3 ]. One example of the use of video technology involves the provision of such mechanization in case of emergency to patients in trauma in rural and urban areas where clinical care may be unavailable [ 4 ]. Technology has the capacity to enable home healthcare with better productivity and security [ 5 ]. Data accuracy and availability have been proposed as among the most significant problems faced by hospitals, which involve maintaining and further processing patient data. Various algorithms in the field of machine learning and deep learning have been beneficially applied in practice to medical treatments. Some state-of-the-art ideas emerge from the massive implementation of technologies such as the creation of matching algorithms and natural language processing [ 6 ]. Data mining can be used to extract data directly, instead of relying on expert knowledge. These methods are considered to produce unique and distinctive patterns to create personalized plans for each hospital [ 7 ].
Diabetes mellitus (DM) is one of the most archetypal diseases worldwide. It is a disease that implies that a person’s body systems are unable to work efficiently to use energy from food. There are four major types of diabetes known, including type-1, type-2, gestational, and other forms, with the most common being type-1 and type-2 [ 8 ]. Type-1 diabetes usually occurs in the young age group of 30–40 and is insulin dependent. Patients are required to take in doses of insulin for entire lives. In contrast type-2, predominates among the over-40 age cohort and is often related patients’ weight. Type-2 diabetes is known to have greater prevalence globally, accounting for more than 90 % of cases [ 9 ]. Because this disease has long been near the top of global rankings listing serious of diseases, many researchers and doctors have proposed algorithms and methods for its treatment and detection. The implementation of these algorithms is rooted in the disciplines of deep learning and machine learning. With predictive analysis supported by neural networks, specifically convolutional neural networks (CNNs) and recurrent neural networks (RNNs), such methods have the ability to determine sentiments and learn high model features automatically [ 10 , 11 ]. Some researchers have implemented the use of machine learning through algorithms such as gradient boosted trees, which can be used to create predictive models of the progression from prediabetes to diabetes, and have aimed to provide early diagnosis for better treatment and to reduce further risks [ 12 ]. Another study applied a modified support vector machine (SVM) algorithm as an efficient method for both linear and non-linear data [ 13 ].
Since the advent of artificial intelligence and related technologies, such computational methods have been applied to real-time detection models in almost every field. The use of data mining, machine learning, deep learning, and computer vision has drastically reduced the difficulty of studying newer techniques that can significantly improvement of existing methods. In the next section, the algorithms and methods are surveyed.
Application of latest technology in diabetes detection
The algorithms used in data mining, machine learning, or any field of artificial intelligence perform predictive modeling, that is, the use of data and statistics to predict future outcomes based on historical data. The most common symptoms of diabetes include abnormal metabolism, hyperglycemia, and an associated risk for specific complications affecting the eyes, kidneys, and nervous system, which are major parts of the body. Such symptoms are used to gather data, and then the modeling is performed based on age and gender categories. One such algorithm is ordering points to identify cluster structure (OPTICS), which is set of ordering points to identify clustering structures. OPTICS is an advanced version of density based spatial clustering of applications (DBSCAN) with noise, and it eliminates all negative aspects of DBSCAN. The data clustering method used in this algorithm is a balanced iterative reducing and clustering algorithm using hierarchies (BIRCH) that selects the most suitable data for further analysis. Thus, the naïve Bayes (NB) data mining technique is used, and BIRCH and OPTICS are used for clustering similar types of data and used for identification of the correct algorithm for better accuracy [ 14 ]. Apache Spark is among the fastest growing platforms for health analysis. It operates more rapidly than the Hadoop platform, making it more easily usable and applicable to clinical practice [ 11 ].
Another such application in the field of OpenCV is the use of the computer-assisted non-invasive DM detection system. This system provided immediate results from facial images. The model examined four health blocks of the skin: forehead, left cheek, right cheek, and nose bridge. Then, feature extraction was performed using a local binary pattern and then classification using the k -nearest neighbor (KNN) clustering and a SVM. All these features were connected with software and show the results in real time [ 15 ].
Islam et al. [ 16 ] used different algorithms for a diabetes symptom dataset, include NB, random forest (RF), logistic regression (LR), and a decision tree (DT). First, the dataset with the patient system is entered into the system on which predictive algorithms are applied. After this, the dataset is input to the database and the performance or accuracy achieved by the model is observed. The most suitable algorithm is commonly selected based on the highest accuracy and best performance. The user’s data is again taken as the input for the algorithm for further training and evaluation to increase the accuracy of the model in real time.
Irido-diagnosis is a predictive system in which the disease is detected through iris patterns. This was used for the detection of diabetes via the following method. Data were gathered from diabetic and non-diabetic patients on which pre-processing was performed. The next part is called image segmentation, wherein the iris of the eye is separated from the image in the dataset. Normalization is performed wherein the circular iris is converted to a rectangle using polar mapping. Feature extraction is then performed on this final image. A gray-level co-occurrence matrix is used to characterize images, which is a process for examining the texture. This process assigns numbers from 1 to 8 to images areas by calculating how often pairs of pixels with specific values and in a specified spatial relationship occur in the image. In this matrix, features such as contrast, correlation, dissimilarity, homogeneity, variance, and entropy are the features are pertinent to provide high-quality data.
These are just some of the authors among many who have contributed to the literature in this field. Below, a detailed survey of the methodology used by other researchers in this field is provided.
Machine learning in diabetes detection
Machine learning is a method by which a computational system learns the features of input data. Such methods haves proven effective for the detection of diabetes. Many machine learning algorithms have been developed, including supervised, unsupervised, and reinforcement learning methods. This is evidently practical because machine learning methods are driven by data. With such massive amounts of data fed into the database, machine learning can save considerable human effort. Models are trained on this data and provide the most suitable output based on the input data. The models can be trained on any parameters that are feasible for practicality and medical requirements. Some might examine facial features, while others look for blood report data obtained from patients. Because there are many symptoms of the disease, the parameters vary accordingly. With many proposed methods, researchers have probed various algorithms and tweaked numerous hyperparameters to obtain results that seem most suitable for real-life applications.
Choudhury and Gupta [ 17 ] used different algorithms to classify people into two categories: high- and low-risk individuals. They used a SVM to establish a hyperplane for categorization, a KNN classification technique for clustering new data into groups, DTs, RF and NB classifiers, and the binary classifier method called LR. On comparing the accuracies for this classification in the form of a confusion matrix, as shown in Fig. 1 , the LR algorithm was found to be the most efficient and accurate, while the DT algorithm, achieved the lowest accuracy.

Classification results of SVM, KNN, NB, DTs and LR in form of true positive (TP), false positive (FP), true negative (TN) and false negative (FN) which are the parameters of confusion matrix [ 17 ]
Shukla [ 18 ] used a LR algorithm, took out a dataset that showed the maximum accuracy would be yielded if parameters such as glucose, body mass index (BMI), and pregnancies, were used, which were represented in the form of a bar chart, as shown in Fig. 2 . The author also attempted to showcase that the disease predominantly depends on those features that seem meager to us but have noted by doctors as relevant in possibly leading to a higher risk of diseases later. The LR model trained with the dominant features showed an accuracy of 82.92%. For the model forecasting, 0.458 was the probability of class zero and 0.572 for class one, which estimates the probability of a person being diabetic.

The weight of each of the features which yield the result variable [ 18 ]
Dalakleidi et al. [ 19 ] used two datasets named PID, Case 1 and Hippokrateion, which is Case 2 from that the PID is split into 50% for training and 50% for testing, whereas the Hippokrateion has a bifurcation of 70% for training and the other 30% for testing. They used binary logistic regression (BLM), logistic model tree algorithm (LMT), which is a combination of LR and DT learning in simple models. The model’s performance was measured using classification accuracy (ACC) and area under the curve (AUC). BLM achieved an ACC of 80.47 and AUC of 0.85, whereas the LMT achieved an ACC of 77.6 and AUC of 0.84 in Case 1. In Case 2, the BLM outperformed LMT with an ACC of 93.45, whereas the LMT had an ACC of 92.86.
Islam et al. [ 20 ] used several algorithms to analyze a dataset using the NB and LR algorithms as well as the RF algorithm, after applying 10-fold cross-validation and percentage split evaluation techniques. Figure 3 shows their proposed architecture. The dataset contained records of 520 people who were asked for possible reasons for diabetes. After data pre-processing, there were a total of 314 positive values and 186 negative values. Positive values represent the person being diabetic, and negative implies that they were not. The best result was achieved using the RF algorithm with an accuracy of 99%. Thus, it is an effective algorithm for a newly created dataset. Figure 4 shows exactly how each algorithm performed on modelling and prediction.

Proposed architecture of the detection system [ 16 ]

Performance of each algorithm on the newly acquired dataset [ 20 ]
Harris et al. [ 21 ] performed clinical diagnosis for the detection of non-insulin dependent diabetes mellitus (NIDDM) using weighted linear regression. The relationship between the prevalence of retinopathy and duration of NIDDM was determined according to individual years of duration and assessed using weighted linear regression with weights for each year’s data being inversely proportional to the binomial variance. The author stated that the retinopathy condition is an important parameter for the early diagnosis of the disease. It typically appears almost 4–7 years earlier than the clinical diagnosis of the disease. Figure 5 provides an accurate graph of the obtained results.

Depicts how at the clinical diagnosis of NIDDM, the patients had the prevalent condition of diabetic retinopathy
Ameena and Ashadevi [ 22 ] used the R language to build a model on SVM, DTs, RF, and LR. They used a dataset of 768 women, all of whom were older than 20 years. They used the following features: BMI, blood sugar, number of pregnancies, and diabetes pedigree function. They are defined two classes: 1, which affirmed diabetes and 0 for negation. On a comparison of the accuracies, the author concluded that the RF algorithm showed the maximum correct estimations, with an accuracy of almost 77% compared to the other models.
Daanouni et al. [ 23 ] used KNN and the DT algorithm on two datasets, with the first one having 2000 instances and the second having 768. They used eight features or attributes to train the model, such as BMI, glucose, blood sugar, and pregnancy. The authors used 80% for training and the remaining 20% for testing. They used optimized hyperparameters to reduce the loss. The results are plotted on two types of data: pre-processing and without. The comparison of results was performed using a receiver operating curve (ROC). The author concluded that KNN has a maximum accuracy of 97.53% and an AUC of 0.9689. Table 1 shows a comparison table for the accuracies obtained for training the model using the KNN classifier.
Comparison table obtained for the KNN and the DT model on pre-processed dataset and other without pre-processing [ 23 ]
Sisodia D and Sisodia DS [ 24 ] used three classifiers, including SVM, NB, and DT. The classification is performed on PIMA Indian diabetes dataset, which is the PIMA Indian diabetes dataset taken from the UCI. To measure the accuracy, internal cross-validation was 10-folds. Accuracy, F-measure, recall, precision, and ROC measures were used. The attributes used were glucose concentration, blood pressure, BMI, age, skin fold thickness, number of pregnancies, 2-h insulin concentration, pedigree function, and class 0 or 1. On modeling, the authors computed that NB showed the maximum accuracy with 586 correctly identifying instances. The following Fig. 6 shows the different types of classifiers used along with number of classified instances.

Different classification algorithms result on prediction modelling where NB outgrows the other two
Ahuja et al. [ 25 ] used the dataset from the UCI containing 768 records of women in which 500 were diabetic, while the remaining 268 were not. The authors used eight features for classification and applied a feature selection technique, which is linear discriminant analysis (LDA), to extract the important features required for classification. They used five types of classifiers for machine learning, including SVM, DT, LR, RF, and a multilayer perceptron. The authors used four parameters for evaluation, including accuracy, precision, recall, and F score. Based on these parameters, the authors concluded that multilayer perceptron yields the best results. Table 2 mentions the results using different values of k -fold validation.
Accuracy results of different classifiers at different values of k -fold validation (%)
Alehegn et al. [ 26 ] used the PIMA Indian diabetes dataset with eight features to train on and the 130 D hospital dataset with a larger number of values. There were four classification methods used, including RF, KNN, NB, and J48-DT algorithm. J48 is an upgraded version of the Iterative Dichotomiser 3 (ID3) classification algorithm. A 10 K cross-validation was used for 90% training and 10% testing. The author built a hybrid model consisting of all of the above algorithms. The author concluded that NB and J48 are good for large data computations, and the KNN classifier is better for smaller datasets. Figure 7 shows the different algorithms used along with the correctly and incorrectly identified instances.

Accuracy of the classification algorithms on the PIMA Indian diabetes dataset. The proposed hybrid model shows the maximum accuracy
Some more work done by other researchers which has been mentioned in Table 3 . It contains a study of the machine learning algorithms used in the methods.
A comprehensive study of the machine learning methods done by some researchers
Deep learning in diabetes detection
Deep learning is a computational field that is usually involved where high computational power is required. Deep learning focuses on neural networks, their types, training epochs, layers of hidden, input, and output. The input layer is the first layer, and the hidden layers are responsible for all the calculations and manipulations, such as convolutions and pooling. The output layer determines the number of classes for the classification. Because of data augmentation, which means tweaking the data to increase accuracy, is also available in deep learning, it finds many applications with image training. The more layers the network has, the more it is capable of classification. Because of the many advantages, it has been widely used in the medical field to compute results with high accuracy. There are different types of networks with the most proficient artificial neural networks (ANNs), deep neural networks (DNNs), CNNs, and RNNs. Many researchers who work for the detection of a disease compare machine learning and deep learning algorithms to analyze which provides maximum accuracy.
Daanouni et al. [ 23 ] used ANNs and DNNs on two datasets of 2000 and 768 instances. They included eight attributes with the label of output as 1 for positive and 0 for negative results. The network was trained on two types of data: pre-processed and non-pre-processed. The DNN model seems to achieve high accuracy on both the data obtained, with an accuracy of 98% for the pre-processed dataset and 99.5% for dataset 1. On dataset 2, the non-pre-processed data had an accuracy of 80.99% and the other 96.35%. Hence, the authors concluded that DNN is an optimal classifier for diabetes detection.
Rakshit et al. [ 40 ] used R, SQL, and Python in a Microsoft Azure machine learning studio environment with the PIMA diabetes dataset, in which 80% was used for training and the other 20% for testing. This dataset is primarily concerned with diabetes in women. This contains eight attributes that are important for model building for a class – 2 neural network. Figure 8 shows the general representation of the neural network. The hidden layer had 100 nodes, with the output layer connected to the nth hidden layer. With the model trained for over 1000 epochs with a learning rate of 0.01, they achieved an accuracy of 83.3% on a dataset with 262 negative cases and 131 positive cases.

General representation of a neural network, x m shows the input weights and y p is the output weights
Sapon et al. [ 41 ] presented diabetes prediction using supervised ANNs. The dataset comprises approximately 250 patients with 27 variables or features,, where exactly 50% was used for training, while the remaining 50% was used for testing with the MATLAB tool. The gradient algorithms used included the Fletcher-Powell conjugate gradient, Polak-Ribiére conjugate gradient, and scaled conjugate gradient. These algorithms were used to train the model and then analyzed using the correlation coefficient (CC) R. The results of these algorithms are plotted at different epochs against the mean square error. Based on a comparison of the values of R, the authors conclude that the scaled conjugate gradient confirms the highest accuracy with a value of 0.88, followed by the Fletcher-Powell conjugate gradient with a value of 0.097219 and, finally, the Polak-Ribiére conjugate gradient with 0.056466.
Refs. [ 31 , 42 ] both performed detection using an ANN. Ref. [ 42 ] used the PIMA Indian population dataset for women in Phoenix, while [ 31 ] performed this algorithm on a questionnaire model that contained data of 1487 people with positive and negative results. The features that remained common for modeling were BMI, age, weight, marital status, pregnancies, and ref. [ 31 ] collected a large number of variables, such as consumption of alcohol, meat, cigarette smoking count, beverage variety, and their counts and routine for exercise and sleep. Considering the structure of the ANN, ref. [ 31 ] had approximately 15 hidden nodes, while the same varied from 0 to 5 in ref. [ 42 ]. Ref. [ 31 ] achieved a ACC of 73.52% against ref. [ 42 ], who achieved an accuracy of 80.21% on the test data.
Ref. [ 43 ] conducted a comparative study of neural networks in diabetes detection. Using the PIMA Indian diabetes set again, they used the eight common features required for model preparation. With the first 576 cases used for testing, they used a 10-fold cross-validation technique too estimate the results. The author used a multilayer neural network (MLNN) with 50 neurons for each hidden layer and an output layer using the non-sigmoid activation function and a PNN, which is a probabilistic neural network with a single hidden layer. The author concluded his results on accuracy, showing that the MLNN model achieved an accuracy of 79.62, and the PNN achieved an accuracy of 78.65.
Ref. [ 44 ] used the PIMA diabetes set and computed the model using an ANN and used eight features for modeling. The author explained the different functions used for pre-processing and model training. The activation function was a sigmoid function, and backpropagation is used to calculate the gradient of the loss function. The error function computed the final error to be approximately 8% at the end of the model building. The results were validated on ROC and RMSE. The author achieved an ROC area of 0.88 and RMSE equal to 0.39, which is a FAIR classifier. Figure 9 shows the results as plotted in form of lines.

The graphs plotted between actual value shown by the blue line and predicted values shown by the red line
Ref. [ 29 ] used an ANN for his model trained over a dataset consisting of over 30000 instances and 11 features to train on. With the hidden layers equal to 12, the values of the layers were calculated using the sigmoid function. Bagging and boosting methods were implemented. Bagging was also set to reduce the variance in the model, and boosting was performed to reduce the error. The neural network with bagging achieved an accuracy of 85.324%, followed by the ANN model with boosting with an accuracy of 84.815%, and then the ANN with 84.532%. Finally, the author validates the final comparison using an ROC.
Ref. [ 45 ] used machine learning and deep learning techniques to detect DM. The dataset used here was the PIMA Indian diabetes dataset consisting of 768 features and eight features to train on with a total of 500 instances belonging to the non-diabetic class and the remaining 268, which are diabetic class. Sixty percent of the data was selected for training and the remaining 40% for testing; a CNN was used. They are composed of three layers, with the classification is performed by the output layer. The prediction accuracy achieved using the model was 76.81%.
Some more work done by other researchers which has been mentioned in Table 4 . It contains a study of the deep learning algorithms used in the methods.
A comprehensive study of the deep learning methods done by some researchers
Challenges and future scope
Although there many methods and algorithms which have been proposed in the field of machine learning or deep learning, many challenges remain, as mentioned by the authors in their works. The first concern that comes to mind when building a model is data. Refs. [ 32 , 33 , 49 ] were some of the authors that referenced the problem. One of the biggest problems encountered during the survey of the papers was finding articles and papers that did not relate to the popular PIMA Indian dataset. This dataset was chosen to ensure that the models provided good results because of the establishment of the dataset. The datasets are either too small or inadequate, or they lack real-time data. Small datasets pose a problem of overfitting on the model, which shows higher accuracy, but they are not able to deal with newer testing data. Hence, the model is not feasible for real-time implementation. Some authors dealt with CGM data, which was real-time, but the model training with that data was not efficient. The datasets were also selected from particular regions that are not representative of a common system. Different regions have different people and lifestyles. Generally, researchers spend 80% of their time cleaning and managing data for model training. Hence, data complexity leads to higher cost and maintenance charges. The next step is feature selection. While some authors neglected some of the features, some grouped them for feasible training. Every dataset poses the problem of having appropriate features to cater to the needs of a single algorithm. After all the data are made available, the technical stacks are finalized. Many tools are available to construct machine learning models, but choosing a model to optimize performance is also necessary. The next challenge is debugging. This becomes easy if tools such as Jupyter Notebooks are used where the code is divided into cells. This becomes difficult when the model runs on automation batch processes. In addition, as there are only a few diabetes datasets available on the Internet; more public data should be available for research. More research should be performed using heart rate, as it requires less bandwidth, and its computational complexity is also low. They can also be used in cloud or mobile devices. HR signals should also be used to detect other cardiac diseases. In some cases, authors required a time-series dataset. Since they are not available across any online resource, it is difficult to replicate such work. Such special models require extensive tuning and large datasets for both training and testing.
The next part is the construction of an actual model. To achieve perfect accuracy, many parameters must be adjusted. Random states, kernel, number of trees, hyperparameter tuning, and various others are considered while creating a model. Selecting a correct algorithm with suitable hyperparameters should also be performed precisely. Some classification models will only train on a single parameter, which results in a decreased accuracy for the model in real-time detection. It is evident from the analysis of these schemes in all classes that most of them suffer from either a single data input parameter or the feature selection is not optimal. Along with such restrictions on parameters, few classification-based schemes are purely dependent on kinds of hardware devices, which increases the difficulty of availability and adaptability of these schemes.
A healthcare-based machine learning model is only useful if it can be used for the benefit of people. Here, the model deployment in practical applications is critical. Many authors have proposed deploying models on mobile platforms. In real-life implementations, only engineers with background and experience with cloud servers and DevOps can deploy models. In this ongoing process, many issues need to be considered, such as how frequently the predictions are required to be displayed or the number of applications that are required for model processing. Although considerable precautions were taken to ensure there were no discrepancies in the study, no study could claim to be perfect and there is always scope for improvement. The development of more inquisitive study providing deeper insights into aspects that enable the predictive power of models rather than only pre-defined parameters such as accuracy, precision, F1 score, ROC, and AUC would be beneficial in the future. For classification, to distinguish between diabetic and normal profiles, clustering-based schemes provide accurate results. However, most of the clustering algorithms struggle with plug-n-play problems, which means that they usually contain human intervention during classification and analysis, which involves the possibility of error.
Considering all the above challenges, it can still be considered that they can be overcome in the future. Scholars and clinicians will continue to work toward the construction of larger and better datasets and design more efficient models and algorithms for better classification and accuracy. Any of the diseases occurring on a wide scale, such as diabetes, can be controlled through artificial intelligence techniques and automation. One can create state-of-the-art efficient models based on studies that provide early detection of diabetes and can help people to further change their lifestyle. Because deep learning performs better on most datasets, it should be combined with different algorithms to achieve better accuracy and performance. Hybrid schemes play an important role in improving the performance of the models. Through early detection, patients can be treated much earlier to avoid further risks of heart problems in cases of diabetes. Any model that can be deployed on mobile platforms should cater to the masses for their help and be representative. An implication of this survey is that ML models that have yielded efficient results that can be utilized by future researchers to further polish and improve as well as create a pipeline or an ensemble of correct and efficient models to increase the chances of predicting the disease with even more probability. Such models can be further improvised to automate the system created so that it can deal with newer data without problems.
Conclusions
Diabetes can be devastating after a certain period if not detected or diagnosed correctly. Many machine learning methods have been discussed, starting from different basic algorithms such as the LR, SVM, DTs, to further classification including the ID3, C4.5, C5.0, J48 and CART and NB. Ensemble methods, such as bagging, boosting, and RF regressors, are further used to enhance the accuracy and performance of models. These techniques have been implemented on all types of platforms such as Python or MATLAB, and the models have been analyzed using different parameters such as area under curve or confusion matrices or mathematical terms such as the RMSE or MAE. Machine learning has been introduced in medical diagnosis systems as it has proven to be accurate in detection, successful in application to treatments, and is more cost effective. Although the above are very strong classifiers, we believe that deep learning, which is a subset of machine learning, can learn large amounts of unstructured and unlabeled data. Deep learning models are more complex and accurate. Different models for deep learning start from the most basic ANNs to convolutional nets to further RNNs, including LSTM and Bi-LSTM. Temporal and deep belief networks have also been discussed. In contrast, deep learning involves some shortcomings such as increased computational time, resources, and frequent adjustment of the parameters. Deep learning performs better on image datasets; therefore, for diabetes diagnosis, images would be better. Most researchers have implemented several algorithms in both machine and deep learning to compare their performance on the data, while others have combined two or three methods to gain more accuracy on a single system.
Researchers, clinical practitioners, and people in the industry widely believe that artificial intelligence has the power to alter the ongoing situations of late medication and detection due to human errors. Automation has the capability to construct efficient and reliable medical detection systems. Machine learning, by means of its powerful predictive and classification models, plays an important role in helping to achieve this.
Acknowledgements
The authors are grateful to Department of Electronics and Communication Engineering, Nirma University and Department of Chemical Engineering School of Technology, Pandit Deendayal Energy University for the permission to publish this research.
Abbreviations
Authors’ contributions.
All the authors make substantial contributions to this manuscript; TS and MS participated in drafting the manuscript; TS wrote the main manuscript; all the authors discussed the results and implication on the manuscript at all stages The authors read and approved the final manuscript.
Not applicable.
Availability of data and materials
Declarations.
The authors declare that they have no competing interests.
Publisher’s Note
Springer Nature remains neutral with regard to jurisdictional claims in published maps and institutional affiliations.
Thank you for visiting nature.com. You are using a browser version with limited support for CSS. To obtain the best experience, we recommend you use a more up to date browser (or turn off compatibility mode in Internet Explorer). In the meantime, to ensure continued support, we are displaying the site without styles and JavaScript.
- View all journals
- My Account Login
- Explore content
- About the journal
- Publish with us
- Sign up for alerts
- Open access
- Published: 26 March 2024
Predicting and improving complex beer flavor through machine learning
- Michiel Schreurs ORCID: orcid.org/0000-0002-9449-5619 1 , 2 , 3 na1 ,
- Supinya Piampongsant 1 , 2 , 3 na1 ,
- Miguel Roncoroni ORCID: orcid.org/0000-0001-7461-1427 1 , 2 , 3 na1 ,
- Lloyd Cool ORCID: orcid.org/0000-0001-9936-3124 1 , 2 , 3 , 4 ,
- Beatriz Herrera-Malaver ORCID: orcid.org/0000-0002-5096-9974 1 , 2 , 3 ,
- Christophe Vanderaa ORCID: orcid.org/0000-0001-7443-5427 4 ,
- Florian A. Theßeling 1 , 2 , 3 ,
- Łukasz Kreft ORCID: orcid.org/0000-0001-7620-4657 5 ,
- Alexander Botzki ORCID: orcid.org/0000-0001-6691-4233 5 ,
- Philippe Malcorps 6 ,
- Luk Daenen 6 ,
- Tom Wenseleers ORCID: orcid.org/0000-0002-1434-861X 4 &
- Kevin J. Verstrepen ORCID: orcid.org/0000-0002-3077-6219 1 , 2 , 3
Nature Communications volume 15 , Article number: 2368 ( 2024 ) Cite this article
542 Altmetric
Metrics details
- Chemical engineering
- Gas chromatography
- Machine learning
- Metabolomics
- Taste receptors
The perception and appreciation of food flavor depends on many interacting chemical compounds and external factors, and therefore proves challenging to understand and predict. Here, we combine extensive chemical and sensory analyses of 250 different beers to train machine learning models that allow predicting flavor and consumer appreciation. For each beer, we measure over 200 chemical properties, perform quantitative descriptive sensory analysis with a trained tasting panel and map data from over 180,000 consumer reviews to train 10 different machine learning models. The best-performing algorithm, Gradient Boosting, yields models that significantly outperform predictions based on conventional statistics and accurately predict complex food features and consumer appreciation from chemical profiles. Model dissection allows identifying specific and unexpected compounds as drivers of beer flavor and appreciation. Adding these compounds results in variants of commercial alcoholic and non-alcoholic beers with improved consumer appreciation. Together, our study reveals how big data and machine learning uncover complex links between food chemistry, flavor and consumer perception, and lays the foundation to develop novel, tailored foods with superior flavors.
Similar content being viewed by others
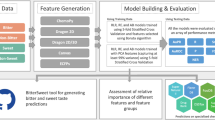
BitterSweet: Building machine learning models for predicting the bitter and sweet taste of small molecules
Rudraksh Tuwani, Somin Wadhwa & Ganesh Bagler
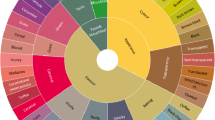
Sensory lexicon and aroma volatiles analysis of brewing malt
Xiaoxia Su, Miao Yu, … Tianyi Du
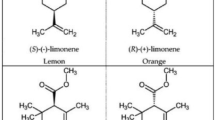
Predicting odor from molecular structure: a multi-label classification approach
Kushagra Saini & Venkatnarayan Ramanathan
Introduction
Predicting and understanding food perception and appreciation is one of the major challenges in food science. Accurate modeling of food flavor and appreciation could yield important opportunities for both producers and consumers, including quality control, product fingerprinting, counterfeit detection, spoilage detection, and the development of new products and product combinations (food pairing) 1 , 2 , 3 , 4 , 5 , 6 . Accurate models for flavor and consumer appreciation would contribute greatly to our scientific understanding of how humans perceive and appreciate flavor. Moreover, accurate predictive models would also facilitate and standardize existing food assessment methods and could supplement or replace assessments by trained and consumer tasting panels, which are variable, expensive and time-consuming 7 , 8 , 9 . Lastly, apart from providing objective, quantitative, accurate and contextual information that can help producers, models can also guide consumers in understanding their personal preferences 10 .
Despite the myriad of applications, predicting food flavor and appreciation from its chemical properties remains a largely elusive goal in sensory science, especially for complex food and beverages 11 , 12 . A key obstacle is the immense number of flavor-active chemicals underlying food flavor. Flavor compounds can vary widely in chemical structure and concentration, making them technically challenging and labor-intensive to quantify, even in the face of innovations in metabolomics, such as non-targeted metabolic fingerprinting 13 , 14 . Moreover, sensory analysis is perhaps even more complicated. Flavor perception is highly complex, resulting from hundreds of different molecules interacting at the physiochemical and sensorial level. Sensory perception is often non-linear, characterized by complex and concentration-dependent synergistic and antagonistic effects 15 , 16 , 17 , 18 , 19 , 20 , 21 that are further convoluted by the genetics, environment, culture and psychology of consumers 22 , 23 , 24 . Perceived flavor is therefore difficult to measure, with problems of sensitivity, accuracy, and reproducibility that can only be resolved by gathering sufficiently large datasets 25 . Trained tasting panels are considered the prime source of quality sensory data, but require meticulous training, are low throughput and high cost. Public databases containing consumer reviews of food products could provide a valuable alternative, especially for studying appreciation scores, which do not require formal training 25 . Public databases offer the advantage of amassing large amounts of data, increasing the statistical power to identify potential drivers of appreciation. However, public datasets suffer from biases, including a bias in the volunteers that contribute to the database, as well as confounding factors such as price, cult status and psychological conformity towards previous ratings of the product.
Classical multivariate statistics and machine learning methods have been used to predict flavor of specific compounds by, for example, linking structural properties of a compound to its potential biological activities or linking concentrations of specific compounds to sensory profiles 1 , 26 . Importantly, most previous studies focused on predicting organoleptic properties of single compounds (often based on their chemical structure) 27 , 28 , 29 , 30 , 31 , 32 , 33 , thus ignoring the fact that these compounds are present in a complex matrix in food or beverages and excluding complex interactions between compounds. Moreover, the classical statistics commonly used in sensory science 34 , 35 , 36 , 37 , 38 , 39 require a large sample size and sufficient variance amongst predictors to create accurate models. They are not fit for studying an extensive set of hundreds of interacting flavor compounds, since they are sensitive to outliers, have a high tendency to overfit and are less suited for non-linear and discontinuous relationships 40 .
In this study, we combine extensive chemical analyses and sensory data of a set of different commercial beers with machine learning approaches to develop models that predict taste, smell, mouthfeel and appreciation from compound concentrations. Beer is particularly suited to model the relationship between chemistry, flavor and appreciation. First, beer is a complex product, consisting of thousands of flavor compounds that partake in complex sensory interactions 41 , 42 , 43 . This chemical diversity arises from the raw materials (malt, yeast, hops, water and spices) and biochemical conversions during the brewing process (kilning, mashing, boiling, fermentation, maturation and aging) 44 , 45 . Second, the advent of the internet saw beer consumers embrace online review platforms, such as RateBeer (ZX Ventures, Anheuser-Busch InBev SA/NV) and BeerAdvocate (Next Glass, inc.). In this way, the beer community provides massive data sets of beer flavor and appreciation scores, creating extraordinarily large sensory databases to complement the analyses of our professional sensory panel. Specifically, we characterize over 200 chemical properties of 250 commercial beers, spread across 22 beer styles, and link these to the descriptive sensory profiling data of a 16-person in-house trained tasting panel and data acquired from over 180,000 public consumer reviews. These unique and extensive datasets enable us to train a suite of machine learning models to predict flavor and appreciation from a beer’s chemical profile. Dissection of the best-performing models allows us to pinpoint specific compounds as potential drivers of beer flavor and appreciation. Follow-up experiments confirm the importance of these compounds and ultimately allow us to significantly improve the flavor and appreciation of selected commercial beers. Together, our study represents a significant step towards understanding complex flavors and reinforces the value of machine learning to develop and refine complex foods. In this way, it represents a stepping stone for further computer-aided food engineering applications 46 .
To generate a comprehensive dataset on beer flavor, we selected 250 commercial Belgian beers across 22 different beer styles (Supplementary Fig. S1 ). Beers with ≤ 4.2% alcohol by volume (ABV) were classified as non-alcoholic and low-alcoholic. Blonds and Tripels constitute a significant portion of the dataset (12.4% and 11.2%, respectively) reflecting their presence on the Belgian beer market and the heterogeneity of beers within these styles. By contrast, lager beers are less diverse and dominated by a handful of brands. Rare styles such as Brut or Faro make up only a small fraction of the dataset (2% and 1%, respectively) because fewer of these beers are produced and because they are dominated by distinct characteristics in terms of flavor and chemical composition.
Extensive analysis identifies relationships between chemical compounds in beer
For each beer, we measured 226 different chemical properties, including common brewing parameters such as alcohol content, iso-alpha acids, pH, sugar concentration 47 , and over 200 flavor compounds (Methods, Supplementary Table S1 ). A large portion (37.2%) are terpenoids arising from hopping, responsible for herbal and fruity flavors 16 , 48 . A second major category are yeast metabolites, such as esters and alcohols, that result in fruity and solvent notes 48 , 49 , 50 . Other measured compounds are primarily derived from malt, or other microbes such as non- Saccharomyces yeasts and bacteria (‘wild flora’). Compounds that arise from spices or staling are labeled under ‘Others’. Five attributes (caloric value, total acids and total ester, hop aroma and sulfur compounds) are calculated from multiple individually measured compounds.
As a first step in identifying relationships between chemical properties, we determined correlations between the concentrations of the compounds (Fig. 1 , upper panel, Supplementary Data 1 and 2 , and Supplementary Fig. S2 . For the sake of clarity, only a subset of the measured compounds is shown in Fig. 1 ). Compounds of the same origin typically show a positive correlation, while absence of correlation hints at parameters varying independently. For example, the hop aroma compounds citronellol, and alpha-terpineol show moderate correlations with each other (Spearman’s rho=0.39 and 0.57), but not with the bittering hop component iso-alpha acids (Spearman’s rho=0.16 and −0.07). This illustrates how brewers can independently modify hop aroma and bitterness by selecting hop varieties and dosage time. If hops are added early in the boiling phase, chemical conversions increase bitterness while aromas evaporate, conversely, late addition of hops preserves aroma but limits bitterness 51 . Similarly, hop-derived iso-alpha acids show a strong anti-correlation with lactic acid and acetic acid, likely reflecting growth inhibition of lactic acid and acetic acid bacteria, or the consequent use of fewer hops in sour beer styles, such as West Flanders ales and Fruit beers, that rely on these bacteria for their distinct flavors 52 . Finally, yeast-derived esters (ethyl acetate, ethyl decanoate, ethyl hexanoate, ethyl octanoate) and alcohols (ethanol, isoamyl alcohol, isobutanol, and glycerol), correlate with Spearman coefficients above 0.5, suggesting that these secondary metabolites are correlated with the yeast genetic background and/or fermentation parameters and may be difficult to influence individually, although the choice of yeast strain may offer some control 53 .
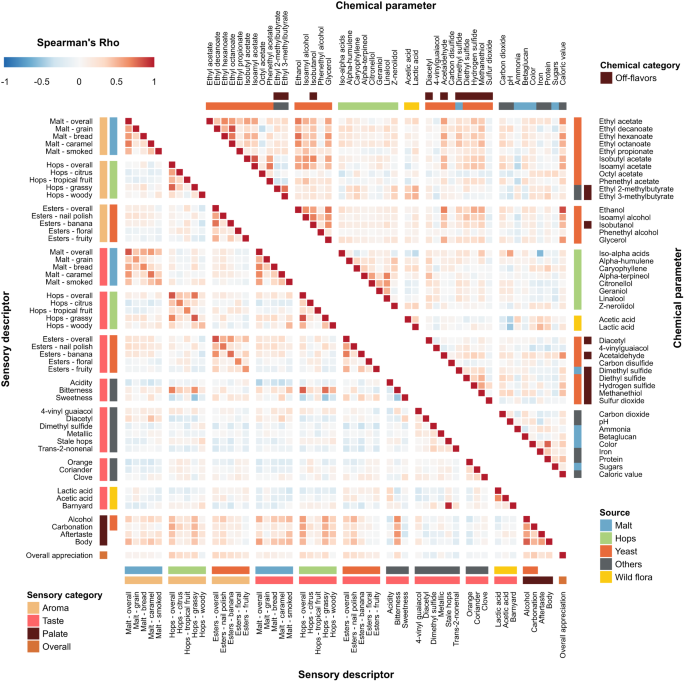
Spearman rank correlations are shown. Descriptors are grouped according to their origin (malt (blue), hops (green), yeast (red), wild flora (yellow), Others (black)), and sensory aspect (aroma, taste, palate, and overall appreciation). Please note that for the chemical compounds, for the sake of clarity, only a subset of the total number of measured compounds is shown, with an emphasis on the key compounds for each source. For more details, see the main text and Methods section. Chemical data can be found in Supplementary Data 1 , correlations between all chemical compounds are depicted in Supplementary Fig. S2 and correlation values can be found in Supplementary Data 2 . See Supplementary Data 4 for sensory panel assessments and Supplementary Data 5 for correlation values between all sensory descriptors.
Interestingly, different beer styles show distinct patterns for some flavor compounds (Supplementary Fig. S3 ). These observations agree with expectations for key beer styles, and serve as a control for our measurements. For instance, Stouts generally show high values for color (darker), while hoppy beers contain elevated levels of iso-alpha acids, compounds associated with bitter hop taste. Acetic and lactic acid are not prevalent in most beers, with notable exceptions such as Kriek, Lambic, Faro, West Flanders ales and Flanders Old Brown, which use acid-producing bacteria ( Lactobacillus and Pediococcus ) or unconventional yeast ( Brettanomyces ) 54 , 55 . Glycerol, ethanol and esters show similar distributions across all beer styles, reflecting their common origin as products of yeast metabolism during fermentation 45 , 53 . Finally, low/no-alcohol beers contain low concentrations of glycerol and esters. This is in line with the production process for most of the low/no-alcohol beers in our dataset, which are produced through limiting fermentation or by stripping away alcohol via evaporation or dialysis, with both methods having the unintended side-effect of reducing the amount of flavor compounds in the final beer 56 , 57 .
Besides expected associations, our data also reveals less trivial associations between beer styles and specific parameters. For example, geraniol and citronellol, two monoterpenoids responsible for citrus, floral and rose flavors and characteristic of Citra hops, are found in relatively high amounts in Christmas, Saison, and Brett/co-fermented beers, where they may originate from terpenoid-rich spices such as coriander seeds instead of hops 58 .
Tasting panel assessments reveal sensorial relationships in beer
To assess the sensory profile of each beer, a trained tasting panel evaluated each of the 250 beers for 50 sensory attributes, including different hop, malt and yeast flavors, off-flavors and spices. Panelists used a tasting sheet (Supplementary Data 3 ) to score the different attributes. Panel consistency was evaluated by repeating 12 samples across different sessions and performing ANOVA. In 95% of cases no significant difference was found across sessions ( p > 0.05), indicating good panel consistency (Supplementary Table S2 ).
Aroma and taste perception reported by the trained panel are often linked (Fig. 1 , bottom left panel and Supplementary Data 4 and 5 ), with high correlations between hops aroma and taste (Spearman’s rho=0.83). Bitter taste was found to correlate with hop aroma and taste in general (Spearman’s rho=0.80 and 0.69), and particularly with “grassy” noble hops (Spearman’s rho=0.75). Barnyard flavor, most often associated with sour beers, is identified together with stale hops (Spearman’s rho=0.97) that are used in these beers. Lactic and acetic acid, which often co-occur, are correlated (Spearman’s rho=0.66). Interestingly, sweetness and bitterness are anti-correlated (Spearman’s rho = −0.48), confirming the hypothesis that they mask each other 59 , 60 . Beer body is highly correlated with alcohol (Spearman’s rho = 0.79), and overall appreciation is found to correlate with multiple aspects that describe beer mouthfeel (alcohol, carbonation; Spearman’s rho= 0.32, 0.39), as well as with hop and ester aroma intensity (Spearman’s rho=0.39 and 0.35).
Similar to the chemical analyses, sensorial analyses confirmed typical features of specific beer styles (Supplementary Fig. S4 ). For example, sour beers (Faro, Flanders Old Brown, Fruit beer, Kriek, Lambic, West Flanders ale) were rated acidic, with flavors of both acetic and lactic acid. Hoppy beers were found to be bitter and showed hop-associated aromas like citrus and tropical fruit. Malt taste is most detected among scotch, stout/porters, and strong ales, while low/no-alcohol beers, which often have a reputation for being ‘worty’ (reminiscent of unfermented, sweet malt extract) appear in the middle. Unsurprisingly, hop aromas are most strongly detected among hoppy beers. Like its chemical counterpart (Supplementary Fig. S3 ), acidity shows a right-skewed distribution, with the most acidic beers being Krieks, Lambics, and West Flanders ales.
Tasting panel assessments of specific flavors correlate with chemical composition
We find that the concentrations of several chemical compounds strongly correlate with specific aroma or taste, as evaluated by the tasting panel (Fig. 2 , Supplementary Fig. S5 , Supplementary Data 6 ). In some cases, these correlations confirm expectations and serve as a useful control for data quality. For example, iso-alpha acids, the bittering compounds in hops, strongly correlate with bitterness (Spearman’s rho=0.68), while ethanol and glycerol correlate with tasters’ perceptions of alcohol and body, the mouthfeel sensation of fullness (Spearman’s rho=0.82/0.62 and 0.72/0.57 respectively) and darker color from roasted malts is a good indication of malt perception (Spearman’s rho=0.54).
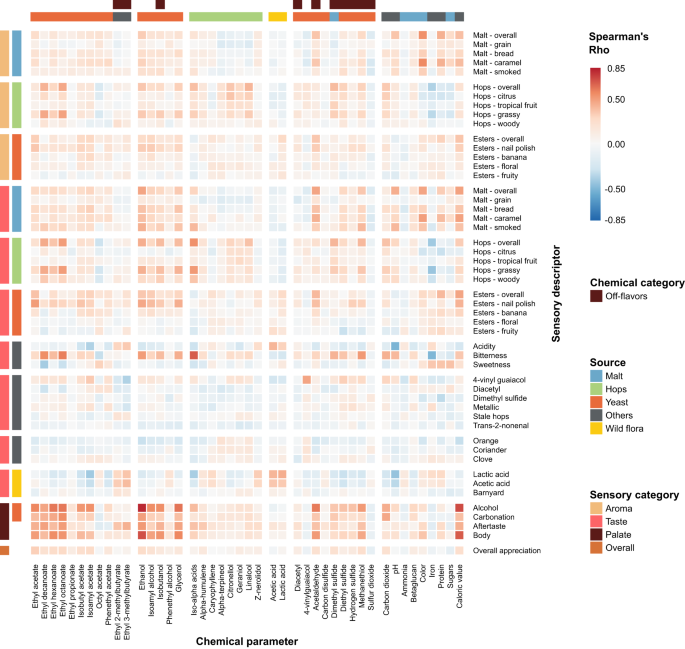
Heatmap colors indicate Spearman’s Rho. Axes are organized according to sensory categories (aroma, taste, mouthfeel, overall), chemical categories and chemical sources in beer (malt (blue), hops (green), yeast (red), wild flora (yellow), Others (black)). See Supplementary Data 6 for all correlation values.
Interestingly, for some relationships between chemical compounds and perceived flavor, correlations are weaker than expected. For example, the rose-smelling phenethyl acetate only weakly correlates with floral aroma. This hints at more complex relationships and interactions between compounds and suggests a need for a more complex model than simple correlations. Lastly, we uncovered unexpected correlations. For instance, the esters ethyl decanoate and ethyl octanoate appear to correlate slightly with hop perception and bitterness, possibly due to their fruity flavor. Iron is anti-correlated with hop aromas and bitterness, most likely because it is also anti-correlated with iso-alpha acids. This could be a sign of metal chelation of hop acids 61 , given that our analyses measure unbound hop acids and total iron content, or could result from the higher iron content in dark and Fruit beers, which typically have less hoppy and bitter flavors 62 .
Public consumer reviews complement expert panel data
To complement and expand the sensory data of our trained tasting panel, we collected 180,000 reviews of our 250 beers from the online consumer review platform RateBeer. This provided numerical scores for beer appearance, aroma, taste, palate, overall quality as well as the average overall score.
Public datasets are known to suffer from biases, such as price, cult status and psychological conformity towards previous ratings of a product. For example, prices correlate with appreciation scores for these online consumer reviews (rho=0.49, Supplementary Fig. S6 ), but not for our trained tasting panel (rho=0.19). This suggests that prices affect consumer appreciation, which has been reported in wine 63 , while blind tastings are unaffected. Moreover, we observe that some beer styles, like lagers and non-alcoholic beers, generally receive lower scores, reflecting that online reviewers are mostly beer aficionados with a preference for specialty beers over lager beers. In general, we find a modest correlation between our trained panel’s overall appreciation score and the online consumer appreciation scores (Fig. 3 , rho=0.29). Apart from the aforementioned biases in the online datasets, serving temperature, sample freshness and surroundings, which are all tightly controlled during the tasting panel sessions, can vary tremendously across online consumers and can further contribute to (among others, appreciation) differences between the two categories of tasters. Importantly, in contrast to the overall appreciation scores, for many sensory aspects the results from the professional panel correlated well with results obtained from RateBeer reviews. Correlations were highest for features that are relatively easy to recognize even for untrained tasters, like bitterness, sweetness, alcohol and malt aroma (Fig. 3 and below).
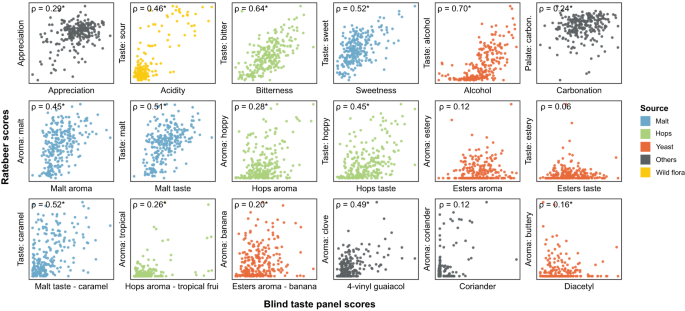
RateBeer text mining results can be found in Supplementary Data 7 . Rho values shown are Spearman correlation values, with asterisks indicating significant correlations ( p < 0.05, two-sided). All p values were smaller than 0.001, except for Esters aroma (0.0553), Esters taste (0.3275), Esters aroma—banana (0.0019), Coriander (0.0508) and Diacetyl (0.0134).
Besides collecting consumer appreciation from these online reviews, we developed automated text analysis tools to gather additional data from review texts (Supplementary Data 7 ). Processing review texts on the RateBeer database yielded comparable results to the scores given by the trained panel for many common sensory aspects, including acidity, bitterness, sweetness, alcohol, malt, and hop tastes (Fig. 3 ). This is in line with what would be expected, since these attributes require less training for accurate assessment and are less influenced by environmental factors such as temperature, serving glass and odors in the environment. Consumer reviews also correlate well with our trained panel for 4-vinyl guaiacol, a compound associated with a very characteristic aroma. By contrast, correlations for more specific aromas like ester, coriander or diacetyl are underrepresented in the online reviews, underscoring the importance of using a trained tasting panel and standardized tasting sheets with explicit factors to be scored for evaluating specific aspects of a beer. Taken together, our results suggest that public reviews are trustworthy for some, but not all, flavor features and can complement or substitute taste panel data for these sensory aspects.
Models can predict beer sensory profiles from chemical data
The rich datasets of chemical analyses, tasting panel assessments and public reviews gathered in the first part of this study provided us with a unique opportunity to develop predictive models that link chemical data to sensorial features. Given the complexity of beer flavor, basic statistical tools such as correlations or linear regression may not always be the most suitable for making accurate predictions. Instead, we applied different machine learning models that can model both simple linear and complex interactive relationships. Specifically, we constructed a set of regression models to predict (a) trained panel scores for beer flavor and quality and (b) public reviews’ appreciation scores from beer chemical profiles. We trained and tested 10 different models (Methods), 3 linear regression-based models (simple linear regression with first-order interactions (LR), lasso regression with first-order interactions (Lasso), partial least squares regressor (PLSR)), 5 decision tree models (AdaBoost regressor (ABR), extra trees (ET), gradient boosting regressor (GBR), random forest (RF) and XGBoost regressor (XGBR)), 1 support vector regression (SVR), and 1 artificial neural network (ANN) model.
To compare the performance of our machine learning models, the dataset was randomly split into a training and test set, stratified by beer style. After a model was trained on data in the training set, its performance was evaluated on its ability to predict the test dataset obtained from multi-output models (based on the coefficient of determination, see Methods). Additionally, individual-attribute models were ranked per descriptor and the average rank was calculated, as proposed by Korneva et al. 64 . Importantly, both ways of evaluating the models’ performance agreed in general. Performance of the different models varied (Table 1 ). It should be noted that all models perform better at predicting RateBeer results than results from our trained tasting panel. One reason could be that sensory data is inherently variable, and this variability is averaged out with the large number of public reviews from RateBeer. Additionally, all tree-based models perform better at predicting taste than aroma. Linear models (LR) performed particularly poorly, with negative R 2 values, due to severe overfitting (training set R 2 = 1). Overfitting is a common issue in linear models with many parameters and limited samples, especially with interaction terms further amplifying the number of parameters. L1 regularization (Lasso) successfully overcomes this overfitting, out-competing multiple tree-based models on the RateBeer dataset. Similarly, the dimensionality reduction of PLSR avoids overfitting and improves performance, to some extent. Still, tree-based models (ABR, ET, GBR, RF and XGBR) show the best performance, out-competing the linear models (LR, Lasso, PLSR) commonly used in sensory science 65 .
GBR models showed the best overall performance in predicting sensory responses from chemical information, with R 2 values up to 0.75 depending on the predicted sensory feature (Supplementary Table S4 ). The GBR models predict consumer appreciation (RateBeer) better than our trained panel’s appreciation (R 2 value of 0.67 compared to R 2 value of 0.09) (Supplementary Table S3 and Supplementary Table S4 ). ANN models showed intermediate performance, likely because neural networks typically perform best with larger datasets 66 . The SVR shows intermediate performance, mostly due to the weak predictions of specific attributes that lower the overall performance (Supplementary Table S4 ).
Model dissection identifies specific, unexpected compounds as drivers of consumer appreciation
Next, we leveraged our models to infer important contributors to sensory perception and consumer appreciation. Consumer preference is a crucial sensory aspects, because a product that shows low consumer appreciation scores often does not succeed commercially 25 . Additionally, the requirement for a large number of representative evaluators makes consumer trials one of the more costly and time-consuming aspects of product development. Hence, a model for predicting chemical drivers of overall appreciation would be a welcome addition to the available toolbox for food development and optimization.
Since GBR models on our RateBeer dataset showed the best overall performance, we focused on these models. Specifically, we used two approaches to identify important contributors. First, rankings of the most important predictors for each sensorial trait in the GBR models were obtained based on impurity-based feature importance (mean decrease in impurity). High-ranked parameters were hypothesized to be either the true causal chemical properties underlying the trait, to correlate with the actual causal properties, or to take part in sensory interactions affecting the trait 67 (Fig. 4A ). In a second approach, we used SHAP 68 to determine which parameters contributed most to the model for making predictions of consumer appreciation (Fig. 4B ). SHAP calculates parameter contributions to model predictions on a per-sample basis, which can be aggregated into an importance score.
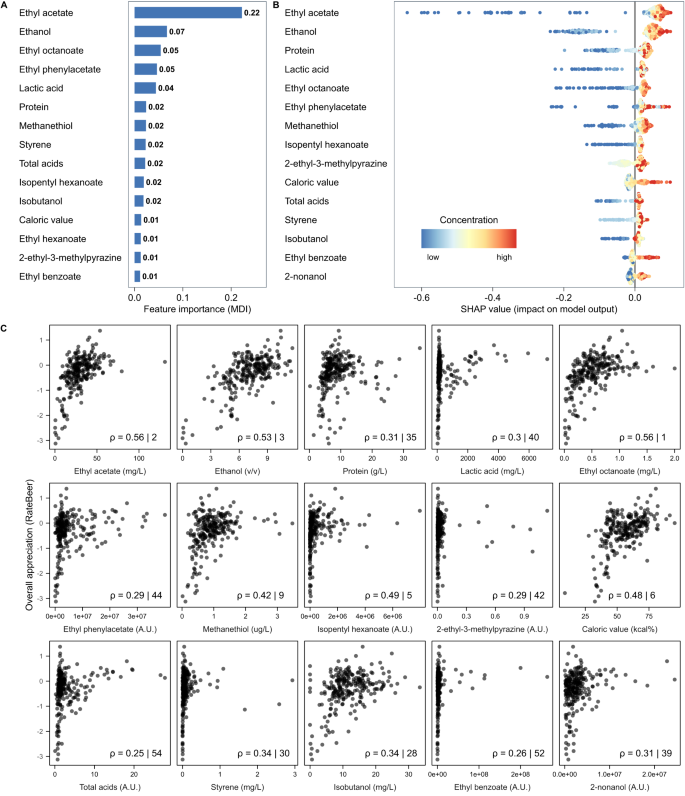
A The impurity-based feature importance (mean deviance in impurity, MDI) calculated from the Gradient Boosting Regression (GBR) model predicting RateBeer appreciation scores. The top 15 highest ranked chemical properties are shown. B SHAP summary plot for the top 15 parameters contributing to our GBR model. Each point on the graph represents a sample from our dataset. The color represents the concentration of that parameter, with bluer colors representing low values and redder colors representing higher values. Greater absolute values on the horizontal axis indicate a higher impact of the parameter on the prediction of the model. C Spearman correlations between the 15 most important chemical properties and consumer overall appreciation. Numbers indicate the Spearman Rho correlation coefficient, and the rank of this correlation compared to all other correlations. The top 15 important compounds were determined using SHAP (panel B).
Both approaches identified ethyl acetate as the most predictive parameter for beer appreciation (Fig. 4 ). Ethyl acetate is the most abundant ester in beer with a typical ‘fruity’, ‘solvent’ and ‘alcoholic’ flavor, but is often considered less important than other esters like isoamyl acetate. The second most important parameter identified by SHAP is ethanol, the most abundant beer compound after water. Apart from directly contributing to beer flavor and mouthfeel, ethanol drastically influences the physical properties of beer, dictating how easily volatile compounds escape the beer matrix to contribute to beer aroma 69 . Importantly, it should also be noted that the importance of ethanol for appreciation is likely inflated by the very low appreciation scores of non-alcoholic beers (Supplementary Fig. S4 ). Despite not often being considered a driver of beer appreciation, protein level also ranks highly in both approaches, possibly due to its effect on mouthfeel and body 70 . Lactic acid, which contributes to the tart taste of sour beers, is the fourth most important parameter identified by SHAP, possibly due to the generally high appreciation of sour beers in our dataset.
Interestingly, some of the most important predictive parameters for our model are not well-established as beer flavors or are even commonly regarded as being negative for beer quality. For example, our models identify methanethiol and ethyl phenyl acetate, an ester commonly linked to beer staling 71 , as a key factor contributing to beer appreciation. Although there is no doubt that high concentrations of these compounds are considered unpleasant, the positive effects of modest concentrations are not yet known 72 , 73 .
To compare our approach to conventional statistics, we evaluated how well the 15 most important SHAP-derived parameters correlate with consumer appreciation (Fig. 4C ). Interestingly, only 6 of the properties derived by SHAP rank amongst the top 15 most correlated parameters. For some chemical compounds, the correlations are so low that they would have likely been considered unimportant. For example, lactic acid, the fourth most important parameter, shows a bimodal distribution for appreciation, with sour beers forming a separate cluster, that is missed entirely by the Spearman correlation. Additionally, the correlation plots reveal outliers, emphasizing the need for robust analysis tools. Together, this highlights the need for alternative models, like the Gradient Boosting model, that better grasp the complexity of (beer) flavor.
Finally, to observe the relationships between these chemical properties and their predicted targets, partial dependence plots were constructed for the six most important predictors of consumer appreciation 74 , 75 , 76 (Supplementary Fig. S7 ). One-way partial dependence plots show how a change in concentration affects the predicted appreciation. These plots reveal an important limitation of our models: appreciation predictions remain constant at ever-increasing concentrations. This implies that once a threshold concentration is reached, further increasing the concentration does not affect appreciation. This is false, as it is well-documented that certain compounds become unpleasant at high concentrations, including ethyl acetate (‘nail polish’) 77 and methanethiol (‘sulfury’ and ‘rotten cabbage’) 78 . The inability of our models to grasp that flavor compounds have optimal levels, above which they become negative, is a consequence of working with commercial beer brands where (off-)flavors are rarely too high to negatively impact the product. The two-way partial dependence plots show how changing the concentration of two compounds influences predicted appreciation, visualizing their interactions (Supplementary Fig. S7 ). In our case, the top 5 parameters are dominated by additive or synergistic interactions, with high concentrations for both compounds resulting in the highest predicted appreciation.
To assess the robustness of our best-performing models and model predictions, we performed 100 iterations of the GBR, RF and ET models. In general, all iterations of the models yielded similar performance (Supplementary Fig. S8 ). Moreover, the main predictors (including the top predictors ethanol and ethyl acetate) remained virtually the same, especially for GBR and RF. For the iterations of the ET model, we did observe more variation in the top predictors, which is likely a consequence of the model’s inherent random architecture in combination with co-correlations between certain predictors. However, even in this case, several of the top predictors (ethanol and ethyl acetate) remain unchanged, although their rank in importance changes (Supplementary Fig. S8 ).
Next, we investigated if a combination of RateBeer and trained panel data into one consolidated dataset would lead to stronger models, under the hypothesis that such a model would suffer less from bias in the datasets. A GBR model was trained to predict appreciation on the combined dataset. This model underperformed compared to the RateBeer model, both in the native case and when including a dataset identifier (R 2 = 0.67, 0.26 and 0.42 respectively). For the latter, the dataset identifier is the most important feature (Supplementary Fig. S9 ), while most of the feature importance remains unchanged, with ethyl acetate and ethanol ranking highest, like in the original model trained only on RateBeer data. It seems that the large variation in the panel dataset introduces noise, weakening the models’ performances and reliability. In addition, it seems reasonable to assume that both datasets are fundamentally different, with the panel dataset obtained by blind tastings by a trained professional panel.
Lastly, we evaluated whether beer style identifiers would further enhance the model’s performance. A GBR model was trained with parameters that explicitly encoded the styles of the samples. This did not improve model performance (R2 = 0.66 with style information vs R2 = 0.67). The most important chemical features are consistent with the model trained without style information (eg. ethanol and ethyl acetate), and with the exception of the most preferred (strong ale) and least preferred (low/no-alcohol) styles, none of the styles were among the most important features (Supplementary Fig. S9 , Supplementary Table S5 and S6 ). This is likely due to a combination of style-specific chemical signatures, such as iso-alpha acids and lactic acid, that implicitly convey style information to the original models, as well as the low number of samples belonging to some styles, making it difficult for the model to learn style-specific patterns. Moreover, beer styles are not rigorously defined, with some styles overlapping in features and some beers being misattributed to a specific style, all of which leads to more noise in models that use style parameters.
Model validation
To test if our predictive models give insight into beer appreciation, we set up experiments aimed at improving existing commercial beers. We specifically selected overall appreciation as the trait to be examined because of its complexity and commercial relevance. Beer flavor comprises a complex bouquet rather than single aromas and tastes 53 . Hence, adding a single compound to the extent that a difference is noticeable may lead to an unbalanced, artificial flavor. Therefore, we evaluated the effect of combinations of compounds. Because Blond beers represent the most extensive style in our dataset, we selected a beer from this style as the starting material for these experiments (Beer 64 in Supplementary Data 1 ).
In the first set of experiments, we adjusted the concentrations of compounds that made up the most important predictors of overall appreciation (ethyl acetate, ethanol, lactic acid, ethyl phenyl acetate) together with correlated compounds (ethyl hexanoate, isoamyl acetate, glycerol), bringing them up to 95 th percentile ethanol-normalized concentrations (Methods) within the Blond group (‘Spiked’ concentration in Fig. 5A ). Compared to controls, the spiked beers were found to have significantly improved overall appreciation among trained panelists, with panelist noting increased intensity of ester flavors, sweetness, alcohol, and body fullness (Fig. 5B ). To disentangle the contribution of ethanol to these results, a second experiment was performed without the addition of ethanol. This resulted in a similar outcome, including increased perception of alcohol and overall appreciation.
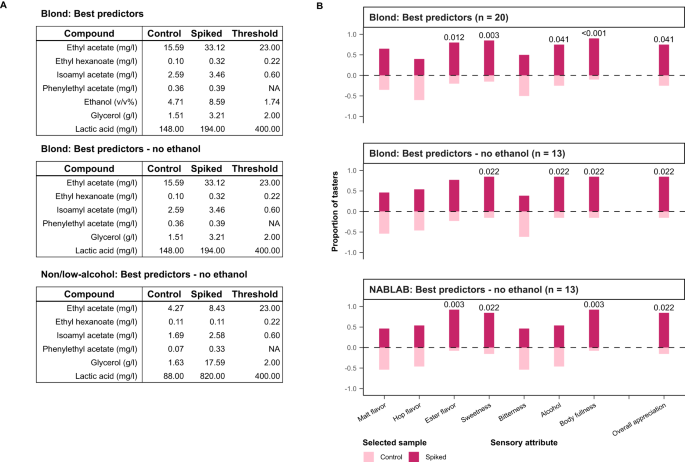
Adding the top chemical compounds, identified as best predictors of appreciation by our model, into poorly appreciated beers results in increased appreciation from our trained panel. Results of sensory tests between base beers and those spiked with compounds identified as the best predictors by the model. A Blond and Non/Low-alcohol (0.0% ABV) base beers were brought up to 95th-percentile ethanol-normalized concentrations within each style. B For each sensory attribute, tasters indicated the more intense sample and selected the sample they preferred. The numbers above the bars correspond to the p values that indicate significant changes in perceived flavor (two-sided binomial test: alpha 0.05, n = 20 or 13).
In a last experiment, we tested whether using the model’s predictions can boost the appreciation of a non-alcoholic beer (beer 223 in Supplementary Data 1 ). Again, the addition of a mixture of predicted compounds (omitting ethanol, in this case) resulted in a significant increase in appreciation, body, ester flavor and sweetness.
Predicting flavor and consumer appreciation from chemical composition is one of the ultimate goals of sensory science. A reliable, systematic and unbiased way to link chemical profiles to flavor and food appreciation would be a significant asset to the food and beverage industry. Such tools would substantially aid in quality control and recipe development, offer an efficient and cost-effective alternative to pilot studies and consumer trials and would ultimately allow food manufacturers to produce superior, tailor-made products that better meet the demands of specific consumer groups more efficiently.
A limited set of studies have previously tried, to varying degrees of success, to predict beer flavor and beer popularity based on (a limited set of) chemical compounds and flavors 79 , 80 . Current sensitive, high-throughput technologies allow measuring an unprecedented number of chemical compounds and properties in a large set of samples, yielding a dataset that can train models that help close the gaps between chemistry and flavor, even for a complex natural product like beer. To our knowledge, no previous research gathered data at this scale (250 samples, 226 chemical parameters, 50 sensory attributes and 5 consumer scores) to disentangle and validate the chemical aspects driving beer preference using various machine-learning techniques. We find that modern machine learning models outperform conventional statistical tools, such as correlations and linear models, and can successfully predict flavor appreciation from chemical composition. This could be attributed to the natural incorporation of interactions and non-linear or discontinuous effects in machine learning models, which are not easily grasped by the linear model architecture. While linear models and partial least squares regression represent the most widespread statistical approaches in sensory science, in part because they allow interpretation 65 , 81 , 82 , modern machine learning methods allow for building better predictive models while preserving the possibility to dissect and exploit the underlying patterns. Of the 10 different models we trained, tree-based models, such as our best performing GBR, showed the best overall performance in predicting sensory responses from chemical information, outcompeting artificial neural networks. This agrees with previous reports for models trained on tabular data 83 . Our results are in line with the findings of Colantonio et al. who also identified the gradient boosting architecture as performing best at predicting appreciation and flavor (of tomatoes and blueberries, in their specific study) 26 . Importantly, besides our larger experimental scale, we were able to directly confirm our models’ predictions in vivo.
Our study confirms that flavor compound concentration does not always correlate with perception, suggesting complex interactions that are often missed by more conventional statistics and simple models. Specifically, we find that tree-based algorithms may perform best in developing models that link complex food chemistry with aroma. Furthermore, we show that massive datasets of untrained consumer reviews provide a valuable source of data, that can complement or even replace trained tasting panels, especially for appreciation and basic flavors, such as sweetness and bitterness. This holds despite biases that are known to occur in such datasets, such as price or conformity bias. Moreover, GBR models predict taste better than aroma. This is likely because taste (e.g. bitterness) often directly relates to the corresponding chemical measurements (e.g., iso-alpha acids), whereas such a link is less clear for aromas, which often result from the interplay between multiple volatile compounds. We also find that our models are best at predicting acidity and alcohol, likely because there is a direct relation between the measured chemical compounds (acids and ethanol) and the corresponding perceived sensorial attribute (acidity and alcohol), and because even untrained consumers are generally able to recognize these flavors and aromas.
The predictions of our final models, trained on review data, hold even for blind tastings with small groups of trained tasters, as demonstrated by our ability to validate specific compounds as drivers of beer flavor and appreciation. Since adding a single compound to the extent of a noticeable difference may result in an unbalanced flavor profile, we specifically tested our identified key drivers as a combination of compounds. While this approach does not allow us to validate if a particular single compound would affect flavor and/or appreciation, our experiments do show that this combination of compounds increases consumer appreciation.
It is important to stress that, while it represents an important step forward, our approach still has several major limitations. A key weakness of the GBR model architecture is that amongst co-correlating variables, the largest main effect is consistently preferred for model building. As a result, co-correlating variables often have artificially low importance scores, both for impurity and SHAP-based methods, like we observed in the comparison to the more randomized Extra Trees models. This implies that chemicals identified as key drivers of a specific sensory feature by GBR might not be the true causative compounds, but rather co-correlate with the actual causative chemical. For example, the high importance of ethyl acetate could be (partially) attributed to the total ester content, ethanol or ethyl hexanoate (rho=0.77, rho=0.72 and rho=0.68), while ethyl phenylacetate could hide the importance of prenyl isobutyrate and ethyl benzoate (rho=0.77 and rho=0.76). Expanding our GBR model to include beer style as a parameter did not yield additional power or insight. This is likely due to style-specific chemical signatures, such as iso-alpha acids and lactic acid, that implicitly convey style information to the original model, as well as the smaller sample size per style, limiting the power to uncover style-specific patterns. This can be partly attributed to the curse of dimensionality, where the high number of parameters results in the models mainly incorporating single parameter effects, rather than complex interactions such as style-dependent effects 67 . A larger number of samples may overcome some of these limitations and offer more insight into style-specific effects. On the other hand, beer style is not a rigid scientific classification, and beers within one style often differ a lot, which further complicates the analysis of style as a model factor.
Our study is limited to beers from Belgian breweries. Although these beers cover a large portion of the beer styles available globally, some beer styles and consumer patterns may be missing, while other features might be overrepresented. For example, many Belgian ales exhibit yeast-driven flavor profiles, which is reflected in the chemical drivers of appreciation discovered by this study. In future work, expanding the scope to include diverse markets and beer styles could lead to the identification of even more drivers of appreciation and better models for special niche products that were not present in our beer set.
In addition to inherent limitations of GBR models, there are also some limitations associated with studying food aroma. Even if our chemical analyses measured most of the known aroma compounds, the total number of flavor compounds in complex foods like beer is still larger than the subset we were able to measure in this study. For example, hop-derived thiols, that influence flavor at very low concentrations, are notoriously difficult to measure in a high-throughput experiment. Moreover, consumer perception remains subjective and prone to biases that are difficult to avoid. It is also important to stress that the models are still immature and that more extensive datasets will be crucial for developing more complete models in the future. Besides more samples and parameters, our dataset does not include any demographic information about the tasters. Including such data could lead to better models that grasp external factors like age and culture. Another limitation is that our set of beers consists of high-quality end-products and lacks beers that are unfit for sale, which limits the current model in accurately predicting products that are appreciated very badly. Finally, while models could be readily applied in quality control, their use in sensory science and product development is restrained by their inability to discern causal relationships. Given that the models cannot distinguish compounds that genuinely drive consumer perception from those that merely correlate, validation experiments are essential to identify true causative compounds.
Despite the inherent limitations, dissection of our models enabled us to pinpoint specific molecules as potential drivers of beer aroma and consumer appreciation, including compounds that were unexpected and would not have been identified using standard approaches. Important drivers of beer appreciation uncovered by our models include protein levels, ethyl acetate, ethyl phenyl acetate and lactic acid. Currently, many brewers already use lactic acid to acidify their brewing water and ensure optimal pH for enzymatic activity during the mashing process. Our results suggest that adding lactic acid can also improve beer appreciation, although its individual effect remains to be tested. Interestingly, ethanol appears to be unnecessary to improve beer appreciation, both for blond beer and alcohol-free beer. Given the growing consumer interest in alcohol-free beer, with a predicted annual market growth of >7% 84 , it is relevant for brewers to know what compounds can further increase consumer appreciation of these beers. Hence, our model may readily provide avenues to further improve the flavor and consumer appreciation of both alcoholic and non-alcoholic beers, which is generally considered one of the key challenges for future beer production.
Whereas we see a direct implementation of our results for the development of superior alcohol-free beverages and other food products, our study can also serve as a stepping stone for the development of novel alcohol-containing beverages. We want to echo the growing body of scientific evidence for the negative effects of alcohol consumption, both on the individual level by the mutagenic, teratogenic and carcinogenic effects of ethanol 85 , 86 , as well as the burden on society caused by alcohol abuse and addiction. We encourage the use of our results for the production of healthier, tastier products, including novel and improved beverages with lower alcohol contents. Furthermore, we strongly discourage the use of these technologies to improve the appreciation or addictive properties of harmful substances.
The present work demonstrates that despite some important remaining hurdles, combining the latest developments in chemical analyses, sensory analysis and modern machine learning methods offers exciting avenues for food chemistry and engineering. Soon, these tools may provide solutions in quality control and recipe development, as well as new approaches to sensory science and flavor research.
Beer selection
250 commercial Belgian beers were selected to cover the broad diversity of beer styles and corresponding diversity in chemical composition and aroma. See Supplementary Fig. S1 .
Chemical dataset
Sample preparation.
Beers within their expiration date were purchased from commercial retailers. Samples were prepared in biological duplicates at room temperature, unless explicitly stated otherwise. Bottle pressure was measured with a manual pressure device (Steinfurth Mess-Systeme GmbH) and used to calculate CO 2 concentration. The beer was poured through two filter papers (Macherey-Nagel, 500713032 MN 713 ¼) to remove carbon dioxide and prevent spontaneous foaming. Samples were then prepared for measurements by targeted Headspace-Gas Chromatography-Flame Ionization Detector/Flame Photometric Detector (HS-GC-FID/FPD), Headspace-Solid Phase Microextraction-Gas Chromatography-Mass Spectrometry (HS-SPME-GC-MS), colorimetric analysis, enzymatic analysis, Near-Infrared (NIR) analysis, as described in the sections below. The mean values of biological duplicates are reported for each compound.
HS-GC-FID/FPD
HS-GC-FID/FPD (Shimadzu GC 2010 Plus) was used to measure higher alcohols, acetaldehyde, esters, 4-vinyl guaicol, and sulfur compounds. Each measurement comprised 5 ml of sample pipetted into a 20 ml glass vial containing 1.75 g NaCl (VWR, 27810.295). 100 µl of 2-heptanol (Sigma-Aldrich, H3003) (internal standard) solution in ethanol (Fisher Chemical, E/0650DF/C17) was added for a final concentration of 2.44 mg/L. Samples were flushed with nitrogen for 10 s, sealed with a silicone septum, stored at −80 °C and analyzed in batches of 20.
The GC was equipped with a DB-WAXetr column (length, 30 m; internal diameter, 0.32 mm; layer thickness, 0.50 µm; Agilent Technologies, Santa Clara, CA, USA) to the FID and an HP-5 column (length, 30 m; internal diameter, 0.25 mm; layer thickness, 0.25 µm; Agilent Technologies, Santa Clara, CA, USA) to the FPD. N 2 was used as the carrier gas. Samples were incubated for 20 min at 70 °C in the headspace autosampler (Flow rate, 35 cm/s; Injection volume, 1000 µL; Injection mode, split; Combi PAL autosampler, CTC analytics, Switzerland). The injector, FID and FPD temperatures were kept at 250 °C. The GC oven temperature was first held at 50 °C for 5 min and then allowed to rise to 80 °C at a rate of 5 °C/min, followed by a second ramp of 4 °C/min until 200 °C kept for 3 min and a final ramp of (4 °C/min) until 230 °C for 1 min. Results were analyzed with the GCSolution software version 2.4 (Shimadzu, Kyoto, Japan). The GC was calibrated with a 5% EtOH solution (VWR International) containing the volatiles under study (Supplementary Table S7 ).
HS-SPME-GC-MS
HS-SPME-GC-MS (Shimadzu GCMS-QP-2010 Ultra) was used to measure additional volatile compounds, mainly comprising terpenoids and esters. Samples were analyzed by HS-SPME using a triphase DVB/Carboxen/PDMS 50/30 μm SPME fiber (Supelco Co., Bellefonte, PA, USA) followed by gas chromatography (Thermo Fisher Scientific Trace 1300 series, USA) coupled to a mass spectrometer (Thermo Fisher Scientific ISQ series MS) equipped with a TriPlus RSH autosampler. 5 ml of degassed beer sample was placed in 20 ml vials containing 1.75 g NaCl (VWR, 27810.295). 5 µl internal standard mix was added, containing 2-heptanol (1 g/L) (Sigma-Aldrich, H3003), 4-fluorobenzaldehyde (1 g/L) (Sigma-Aldrich, 128376), 2,3-hexanedione (1 g/L) (Sigma-Aldrich, 144169) and guaiacol (1 g/L) (Sigma-Aldrich, W253200) in ethanol (Fisher Chemical, E/0650DF/C17). Each sample was incubated at 60 °C in the autosampler oven with constant agitation. After 5 min equilibration, the SPME fiber was exposed to the sample headspace for 30 min. The compounds trapped on the fiber were thermally desorbed in the injection port of the chromatograph by heating the fiber for 15 min at 270 °C.
The GC-MS was equipped with a low polarity RXi-5Sil MS column (length, 20 m; internal diameter, 0.18 mm; layer thickness, 0.18 µm; Restek, Bellefonte, PA, USA). Injection was performed in splitless mode at 320 °C, a split flow of 9 ml/min, a purge flow of 5 ml/min and an open valve time of 3 min. To obtain a pulsed injection, a programmed gas flow was used whereby the helium gas flow was set at 2.7 mL/min for 0.1 min, followed by a decrease in flow of 20 ml/min to the normal 0.9 mL/min. The temperature was first held at 30 °C for 3 min and then allowed to rise to 80 °C at a rate of 7 °C/min, followed by a second ramp of 2 °C/min till 125 °C and a final ramp of 8 °C/min with a final temperature of 270 °C.
Mass acquisition range was 33 to 550 amu at a scan rate of 5 scans/s. Electron impact ionization energy was 70 eV. The interface and ion source were kept at 275 °C and 250 °C, respectively. A mix of linear n-alkanes (from C7 to C40, Supelco Co.) was injected into the GC-MS under identical conditions to serve as external retention index markers. Identification and quantification of the compounds were performed using an in-house developed R script as described in Goelen et al. and Reher et al. 87 , 88 (for package information, see Supplementary Table S8 ). Briefly, chromatograms were analyzed using AMDIS (v2.71) 89 to separate overlapping peaks and obtain pure compound spectra. The NIST MS Search software (v2.0 g) in combination with the NIST2017, FFNSC3 and Adams4 libraries were used to manually identify the empirical spectra, taking into account the expected retention time. After background subtraction and correcting for retention time shifts between samples run on different days based on alkane ladders, compound elution profiles were extracted and integrated using a file with 284 target compounds of interest, which were either recovered in our identified AMDIS list of spectra or were known to occur in beer. Compound elution profiles were estimated for every peak in every chromatogram over a time-restricted window using weighted non-negative least square analysis after which peak areas were integrated 87 , 88 . Batch effect correction was performed by normalizing against the most stable internal standard compound, 4-fluorobenzaldehyde. Out of all 284 target compounds that were analyzed, 167 were visually judged to have reliable elution profiles and were used for final analysis.
Discrete photometric and enzymatic analysis
Discrete photometric and enzymatic analysis (Thermo Scientific TM Gallery TM Plus Beermaster Discrete Analyzer) was used to measure acetic acid, ammonia, beta-glucan, iso-alpha acids, color, sugars, glycerol, iron, pH, protein, and sulfite. 2 ml of sample volume was used for the analyses. Information regarding the reagents and standard solutions used for analyses and calibrations is included in Supplementary Table S7 and Supplementary Table S9 .
NIR analyses
NIR analysis (Anton Paar Alcolyzer Beer ME System) was used to measure ethanol. Measurements comprised 50 ml of sample, and a 10% EtOH solution was used for calibration.
Correlation calculations
Pairwise Spearman Rank correlations were calculated between all chemical properties.
Sensory dataset
Trained panel.
Our trained tasting panel consisted of volunteers who gave prior verbal informed consent. All compounds used for the validation experiment were of food-grade quality. The tasting sessions were approved by the Social and Societal Ethics Committee of the KU Leuven (G-2022-5677-R2(MAR)). All online reviewers agreed to the Terms and Conditions of the RateBeer website.
Sensory analysis was performed according to the American Society of Brewing Chemists (ASBC) Sensory Analysis Methods 90 . 30 volunteers were screened through a series of triangle tests. The sixteen most sensitive and consistent tasters were retained as taste panel members. The resulting panel was diverse in age [22–42, mean: 29], sex [56% male] and nationality [7 different countries]. The panel developed a consensus vocabulary to describe beer aroma, taste and mouthfeel. Panelists were trained to identify and score 50 different attributes, using a 7-point scale to rate attributes’ intensity. The scoring sheet is included as Supplementary Data 3 . Sensory assessments took place between 10–12 a.m. The beers were served in black-colored glasses. Per session, between 5 and 12 beers of the same style were tasted at 12 °C to 16 °C. Two reference beers were added to each set and indicated as ‘Reference 1 & 2’, allowing panel members to calibrate their ratings. Not all panelists were present at every tasting. Scores were scaled by standard deviation and mean-centered per taster. Values are represented as z-scores and clustered by Euclidean distance. Pairwise Spearman correlations were calculated between taste and aroma sensory attributes. Panel consistency was evaluated by repeating samples on different sessions and performing ANOVA to identify differences, using the ‘stats’ package (v4.2.2) in R (for package information, see Supplementary Table S8 ).
Online reviews from a public database
The ‘scrapy’ package in Python (v3.6) (for package information, see Supplementary Table S8 ). was used to collect 232,288 online reviews (mean=922, min=6, max=5343) from RateBeer, an online beer review database. Each review entry comprised 5 numerical scores (appearance, aroma, taste, palate and overall quality) and an optional review text. The total number of reviews per reviewer was collected separately. Numerical scores were scaled and centered per rater, and mean scores were calculated per beer.
For the review texts, the language was estimated using the packages ‘langdetect’ and ‘langid’ in Python. Reviews that were classified as English by both packages were kept. Reviewers with fewer than 100 entries overall were discarded. 181,025 reviews from >6000 reviewers from >40 countries remained. Text processing was done using the ‘nltk’ package in Python. Texts were corrected for slang and misspellings; proper nouns and rare words that are relevant to the beer context were specified and kept as-is (‘Chimay’,’Lambic’, etc.). A dictionary of semantically similar sensorial terms, for example ‘floral’ and ‘flower’, was created and collapsed together into one term. Words were stemmed and lemmatized to avoid identifying words such as ‘acid’ and ‘acidity’ as separate terms. Numbers and punctuation were removed.
Sentences from up to 50 randomly chosen reviews per beer were manually categorized according to the aspect of beer they describe (appearance, aroma, taste, palate, overall quality—not to be confused with the 5 numerical scores described above) or flagged as irrelevant if they contained no useful information. If a beer contained fewer than 50 reviews, all reviews were manually classified. This labeled data set was used to train a model that classified the rest of the sentences for all beers 91 . Sentences describing taste and aroma were extracted, and term frequency–inverse document frequency (TFIDF) was implemented to calculate enrichment scores for sensorial words per beer.
The sex of the tasting subject was not considered when building our sensory database. Instead, results from different panelists were averaged, both for our trained panel (56% male, 44% female) and the RateBeer reviews (70% male, 30% female for RateBeer as a whole).
Beer price collection and processing
Beer prices were collected from the following stores: Colruyt, Delhaize, Total Wine, BeerHawk, The Belgian Beer Shop, The Belgian Shop, and Beer of Belgium. Where applicable, prices were converted to Euros and normalized per liter. Spearman correlations were calculated between these prices and mean overall appreciation scores from RateBeer and the taste panel, respectively.
Pairwise Spearman Rank correlations were calculated between all sensory properties.
Machine learning models
Predictive modeling of sensory profiles from chemical data.
Regression models were constructed to predict (a) trained panel scores for beer flavors and quality from beer chemical profiles and (b) public reviews’ appreciation scores from beer chemical profiles. Z-scores were used to represent sensory attributes in both data sets. Chemical properties with log-normal distributions (Shapiro-Wilk test, p < 0.05 ) were log-transformed. Missing chemical measurements (0.1% of all data) were replaced with mean values per attribute. Observations from 250 beers were randomly separated into a training set (70%, 175 beers) and a test set (30%, 75 beers), stratified per beer style. Chemical measurements (p = 231) were normalized based on the training set average and standard deviation. In total, three linear regression-based models: linear regression with first-order interaction terms (LR), lasso regression with first-order interaction terms (Lasso) and partial least squares regression (PLSR); five decision tree models, Adaboost regressor (ABR), Extra Trees (ET), Gradient Boosting regressor (GBR), Random Forest (RF) and XGBoost regressor (XGBR); one support vector machine model (SVR) and one artificial neural network model (ANN) were trained. The models were implemented using the ‘scikit-learn’ package (v1.2.2) and ‘xgboost’ package (v1.7.3) in Python (v3.9.16). Models were trained, and hyperparameters optimized, using five-fold cross-validated grid search with the coefficient of determination (R 2 ) as the evaluation metric. The ANN (scikit-learn’s MLPRegressor) was optimized using Bayesian Tree-Structured Parzen Estimator optimization with the ‘Optuna’ Python package (v3.2.0). Individual models were trained per attribute, and a multi-output model was trained on all attributes simultaneously.
Model dissection
GBR was found to outperform other methods, resulting in models with the highest average R 2 values in both trained panel and public review data sets. Impurity-based rankings of the most important predictors for each predicted sensorial trait were obtained using the ‘scikit-learn’ package. To observe the relationships between these chemical properties and their predicted targets, partial dependence plots (PDP) were constructed for the six most important predictors of consumer appreciation 74 , 75 .
The ‘SHAP’ package in Python (v0.41.0) was implemented to provide an alternative ranking of predictor importance and to visualize the predictors’ effects as a function of their concentration 68 .
Validation of causal chemical properties
To validate the effects of the most important model features on predicted sensory attributes, beers were spiked with the chemical compounds identified by the models and descriptive sensory analyses were carried out according to the American Society of Brewing Chemists (ASBC) protocol 90 .
Compound spiking was done 30 min before tasting. Compounds were spiked into fresh beer bottles, that were immediately resealed and inverted three times. Fresh bottles of beer were opened for the same duration, resealed, and inverted thrice, to serve as controls. Pairs of spiked samples and controls were served simultaneously, chilled and in dark glasses as outlined in the Trained panel section above. Tasters were instructed to select the glass with the higher flavor intensity for each attribute (directional difference test 92 ) and to select the glass they prefer.
The final concentration after spiking was equal to the within-style average, after normalizing by ethanol concentration. This was done to ensure balanced flavor profiles in the final spiked beer. The same methods were applied to improve a non-alcoholic beer. Compounds were the following: ethyl acetate (Merck KGaA, W241415), ethyl hexanoate (Merck KGaA, W243906), isoamyl acetate (Merck KGaA, W205508), phenethyl acetate (Merck KGaA, W285706), ethanol (96%, Colruyt), glycerol (Merck KGaA, W252506), lactic acid (Merck KGaA, 261106).
Significant differences in preference or perceived intensity were determined by performing the two-sided binomial test on each attribute.
Reporting summary
Further information on research design is available in the Nature Portfolio Reporting Summary linked to this article.
Data availability
The data that support the findings of this work are available in the Supplementary Data files and have been deposited to Zenodo under accession code 10653704 93 . The RateBeer scores data are under restricted access, they are not publicly available as they are property of RateBeer (ZX Ventures, USA). Access can be obtained from the authors upon reasonable request and with permission of RateBeer (ZX Ventures, USA). Source data are provided with this paper.
Code availability
The code for training the machine learning models, analyzing the models, and generating the figures has been deposited to Zenodo under accession code 10653704 93 .
Tieman, D. et al. A chemical genetic roadmap to improved tomato flavor. Science 355 , 391–394 (2017).
Article ADS CAS PubMed Google Scholar
Plutowska, B. & Wardencki, W. Application of gas chromatography–olfactometry (GC–O) in analysis and quality assessment of alcoholic beverages – A review. Food Chem. 107 , 449–463 (2008).
Article CAS Google Scholar
Legin, A., Rudnitskaya, A., Seleznev, B. & Vlasov, Y. Electronic tongue for quality assessment of ethanol, vodka and eau-de-vie. Anal. Chim. Acta 534 , 129–135 (2005).
Loutfi, A., Coradeschi, S., Mani, G. K., Shankar, P. & Rayappan, J. B. B. Electronic noses for food quality: A review. J. Food Eng. 144 , 103–111 (2015).
Ahn, Y.-Y., Ahnert, S. E., Bagrow, J. P. & Barabási, A.-L. Flavor network and the principles of food pairing. Sci. Rep. 1 , 196 (2011).
Article CAS PubMed PubMed Central Google Scholar
Bartoshuk, L. M. & Klee, H. J. Better fruits and vegetables through sensory analysis. Curr. Biol. 23 , R374–R378 (2013).
Article CAS PubMed Google Scholar
Piggott, J. R. Design questions in sensory and consumer science. Food Qual. Prefer. 3293 , 217–220 (1995).
Article Google Scholar
Kermit, M. & Lengard, V. Assessing the performance of a sensory panel-panellist monitoring and tracking. J. Chemom. 19 , 154–161 (2005).
Cook, D. J., Hollowood, T. A., Linforth, R. S. T. & Taylor, A. J. Correlating instrumental measurements of texture and flavour release with human perception. Int. J. Food Sci. Technol. 40 , 631–641 (2005).
Chinchanachokchai, S., Thontirawong, P. & Chinchanachokchai, P. A tale of two recommender systems: The moderating role of consumer expertise on artificial intelligence based product recommendations. J. Retail. Consum. Serv. 61 , 1–12 (2021).
Ross, C. F. Sensory science at the human-machine interface. Trends Food Sci. Technol. 20 , 63–72 (2009).
Chambers, E. IV & Koppel, K. Associations of volatile compounds with sensory aroma and flavor: The complex nature of flavor. Molecules 18 , 4887–4905 (2013).
Pinu, F. R. Metabolomics—The new frontier in food safety and quality research. Food Res. Int. 72 , 80–81 (2015).
Danezis, G. P., Tsagkaris, A. S., Brusic, V. & Georgiou, C. A. Food authentication: state of the art and prospects. Curr. Opin. Food Sci. 10 , 22–31 (2016).
Shepherd, G. M. Smell images and the flavour system in the human brain. Nature 444 , 316–321 (2006).
Meilgaard, M. C. Prediction of flavor differences between beers from their chemical composition. J. Agric. Food Chem. 30 , 1009–1017 (1982).
Xu, L. et al. Widespread receptor-driven modulation in peripheral olfactory coding. Science 368 , eaaz5390 (2020).
Kupferschmidt, K. Following the flavor. Science 340 , 808–809 (2013).
Billesbølle, C. B. et al. Structural basis of odorant recognition by a human odorant receptor. Nature 615 , 742–749 (2023).
Article ADS PubMed PubMed Central Google Scholar
Smith, B. Perspective: Complexities of flavour. Nature 486 , S6–S6 (2012).
Pfister, P. et al. Odorant receptor inhibition is fundamental to odor encoding. Curr. Biol. 30 , 2574–2587 (2020).
Moskowitz, H. W., Kumaraiah, V., Sharma, K. N., Jacobs, H. L. & Sharma, S. D. Cross-cultural differences in simple taste preferences. Science 190 , 1217–1218 (1975).
Eriksson, N. et al. A genetic variant near olfactory receptor genes influences cilantro preference. Flavour 1 , 22 (2012).
Ferdenzi, C. et al. Variability of affective responses to odors: Culture, gender, and olfactory knowledge. Chem. Senses 38 , 175–186 (2013).
Article PubMed Google Scholar
Lawless, H. T. & Heymann, H. Sensory evaluation of food: Principles and practices. (Springer, New York, NY). https://doi.org/10.1007/978-1-4419-6488-5 (2010).
Colantonio, V. et al. Metabolomic selection for enhanced fruit flavor. Proc. Natl. Acad. Sci. 119 , e2115865119 (2022).
Fritz, F., Preissner, R. & Banerjee, P. VirtualTaste: a web server for the prediction of organoleptic properties of chemical compounds. Nucleic Acids Res 49 , W679–W684 (2021).
Tuwani, R., Wadhwa, S. & Bagler, G. BitterSweet: Building machine learning models for predicting the bitter and sweet taste of small molecules. Sci. Rep. 9 , 1–13 (2019).
Dagan-Wiener, A. et al. Bitter or not? BitterPredict, a tool for predicting taste from chemical structure. Sci. Rep. 7 , 1–13 (2017).
Pallante, L. et al. Toward a general and interpretable umami taste predictor using a multi-objective machine learning approach. Sci. Rep. 12 , 1–11 (2022).
Malavolta, M. et al. A survey on computational taste predictors. Eur. Food Res. Technol. 248 , 2215–2235 (2022).
Lee, B. K. et al. A principal odor map unifies diverse tasks in olfactory perception. Science 381 , 999–1006 (2023).
Mayhew, E. J. et al. Transport features predict if a molecule is odorous. Proc. Natl. Acad. Sci. 119 , e2116576119 (2022).
Niu, Y. et al. Sensory evaluation of the synergism among ester odorants in light aroma-type liquor by odor threshold, aroma intensity and flash GC electronic nose. Food Res. Int. 113 , 102–114 (2018).
Yu, P., Low, M. Y. & Zhou, W. Design of experiments and regression modelling in food flavour and sensory analysis: A review. Trends Food Sci. Technol. 71 , 202–215 (2018).
Oladokun, O. et al. The impact of hop bitter acid and polyphenol profiles on the perceived bitterness of beer. Food Chem. 205 , 212–220 (2016).
Linforth, R., Cabannes, M., Hewson, L., Yang, N. & Taylor, A. Effect of fat content on flavor delivery during consumption: An in vivo model. J. Agric. Food Chem. 58 , 6905–6911 (2010).
Guo, S., Na Jom, K. & Ge, Y. Influence of roasting condition on flavor profile of sunflower seeds: A flavoromics approach. Sci. Rep. 9 , 11295 (2019).
Ren, Q. et al. The changes of microbial community and flavor compound in the fermentation process of Chinese rice wine using Fagopyrum tataricum grain as feedstock. Sci. Rep. 9 , 3365 (2019).
Hastie, T., Friedman, J. & Tibshirani, R. The Elements of Statistical Learning. (Springer, New York, NY). https://doi.org/10.1007/978-0-387-21606-5 (2001).
Dietz, C., Cook, D., Huismann, M., Wilson, C. & Ford, R. The multisensory perception of hop essential oil: a review. J. Inst. Brew. 126 , 320–342 (2020).
CAS Google Scholar
Roncoroni, Miguel & Verstrepen, Kevin Joan. Belgian Beer: Tested and Tasted. (Lannoo, 2018).
Meilgaard, M. Flavor chemistry of beer: Part II: Flavor and threshold of 239 aroma volatiles. in (1975).
Bokulich, N. A. & Bamforth, C. W. The microbiology of malting and brewing. Microbiol. Mol. Biol. Rev. MMBR 77 , 157–172 (2013).
Dzialo, M. C., Park, R., Steensels, J., Lievens, B. & Verstrepen, K. J. Physiology, ecology and industrial applications of aroma formation in yeast. FEMS Microbiol. Rev. 41 , S95–S128 (2017).
Article PubMed PubMed Central Google Scholar
Datta, A. et al. Computer-aided food engineering. Nat. Food 3 , 894–904 (2022).
American Society of Brewing Chemists. Beer Methods. (American Society of Brewing Chemists, St. Paul, MN, U.S.A.).
Olaniran, A. O., Hiralal, L., Mokoena, M. P. & Pillay, B. Flavour-active volatile compounds in beer: production, regulation and control. J. Inst. Brew. 123 , 13–23 (2017).
Verstrepen, K. J. et al. Flavor-active esters: Adding fruitiness to beer. J. Biosci. Bioeng. 96 , 110–118 (2003).
Meilgaard, M. C. Flavour chemistry of beer. part I: flavour interaction between principal volatiles. Master Brew. Assoc. Am. Tech. Q 12 , 107–117 (1975).
Briggs, D. E., Boulton, C. A., Brookes, P. A. & Stevens, R. Brewing 227–254. (Woodhead Publishing). https://doi.org/10.1533/9781855739062.227 (2004).
Bossaert, S., Crauwels, S., De Rouck, G. & Lievens, B. The power of sour - A review: Old traditions, new opportunities. BrewingScience 72 , 78–88 (2019).
Google Scholar
Verstrepen, K. J. et al. Flavor active esters: Adding fruitiness to beer. J. Biosci. Bioeng. 96 , 110–118 (2003).
Snauwaert, I. et al. Microbial diversity and metabolite composition of Belgian red-brown acidic ales. Int. J. Food Microbiol. 221 , 1–11 (2016).
Spitaels, F. et al. The microbial diversity of traditional spontaneously fermented lambic beer. PLoS ONE 9 , e95384 (2014).
Blanco, C. A., Andrés-Iglesias, C. & Montero, O. Low-alcohol Beers: Flavor Compounds, Defects, and Improvement Strategies. Crit. Rev. Food Sci. Nutr. 56 , 1379–1388 (2016).
Jackowski, M. & Trusek, A. Non-Alcohol. beer Prod. – Overv. 20 , 32–38 (2018).
Takoi, K. et al. The contribution of geraniol metabolism to the citrus flavour of beer: Synergy of geraniol and β-citronellol under coexistence with excess linalool. J. Inst. Brew. 116 , 251–260 (2010).
Kroeze, J. H. & Bartoshuk, L. M. Bitterness suppression as revealed by split-tongue taste stimulation in humans. Physiol. Behav. 35 , 779–783 (1985).
Mennella, J. A. et al. A spoonful of sugar helps the medicine go down”: Bitter masking bysucrose among children and adults. Chem. Senses 40 , 17–25 (2015).
Wietstock, P., Kunz, T., Perreira, F. & Methner, F.-J. Metal chelation behavior of hop acids in buffered model systems. BrewingScience 69 , 56–63 (2016).
Sancho, D., Blanco, C. A., Caballero, I. & Pascual, A. Free iron in pale, dark and alcohol-free commercial lager beers. J. Sci. Food Agric. 91 , 1142–1147 (2011).
Rodrigues, H. & Parr, W. V. Contribution of cross-cultural studies to understanding wine appreciation: A review. Food Res. Int. 115 , 251–258 (2019).
Korneva, E. & Blockeel, H. Towards better evaluation of multi-target regression models. in ECML PKDD 2020 Workshops (eds. Koprinska, I. et al.) 353–362 (Springer International Publishing, Cham, 2020). https://doi.org/10.1007/978-3-030-65965-3_23 .
Gastón Ares. Mathematical and Statistical Methods in Food Science and Technology. (Wiley, 2013).
Grinsztajn, L., Oyallon, E. & Varoquaux, G. Why do tree-based models still outperform deep learning on tabular data? Preprint at http://arxiv.org/abs/2207.08815 (2022).
Gries, S. T. Statistics for Linguistics with R: A Practical Introduction. in Statistics for Linguistics with R (De Gruyter Mouton, 2021). https://doi.org/10.1515/9783110718256 .
Lundberg, S. M. et al. From local explanations to global understanding with explainable AI for trees. Nat. Mach. Intell. 2 , 56–67 (2020).
Ickes, C. M. & Cadwallader, K. R. Effects of ethanol on flavor perception in alcoholic beverages. Chemosens. Percept. 10 , 119–134 (2017).
Kato, M. et al. Influence of high molecular weight polypeptides on the mouthfeel of commercial beer. J. Inst. Brew. 127 , 27–40 (2021).
Wauters, R. et al. Novel Saccharomyces cerevisiae variants slow down the accumulation of staling aldehydes and improve beer shelf-life. Food Chem. 398 , 1–11 (2023).
Li, H., Jia, S. & Zhang, W. Rapid determination of low-level sulfur compounds in beer by headspace gas chromatography with a pulsed flame photometric detector. J. Am. Soc. Brew. Chem. 66 , 188–191 (2008).
Dercksen, A., Laurens, J., Torline, P., Axcell, B. C. & Rohwer, E. Quantitative analysis of volatile sulfur compounds in beer using a membrane extraction interface. J. Am. Soc. Brew. Chem. 54 , 228–233 (1996).
Molnar, C. Interpretable Machine Learning: A Guide for Making Black-Box Models Interpretable. (2020).
Zhao, Q. & Hastie, T. Causal interpretations of black-box models. J. Bus. Econ. Stat. Publ. Am. Stat. Assoc. 39 , 272–281 (2019).
Article MathSciNet Google Scholar
Hastie, T., Tibshirani, R. & Friedman, J. The Elements of Statistical Learning. (Springer, 2019).
Labrado, D. et al. Identification by NMR of key compounds present in beer distillates and residual phases after dealcoholization by vacuum distillation. J. Sci. Food Agric. 100 , 3971–3978 (2020).
Lusk, L. T., Kay, S. B., Porubcan, A. & Ryder, D. S. Key olfactory cues for beer oxidation. J. Am. Soc. Brew. Chem. 70 , 257–261 (2012).
Gonzalez Viejo, C., Torrico, D. D., Dunshea, F. R. & Fuentes, S. Development of artificial neural network models to assess beer acceptability based on sensory properties using a robotic pourer: A comparative model approach to achieve an artificial intelligence system. Beverages 5 , 33 (2019).
Gonzalez Viejo, C., Fuentes, S., Torrico, D. D., Godbole, A. & Dunshea, F. R. Chemical characterization of aromas in beer and their effect on consumers liking. Food Chem. 293 , 479–485 (2019).
Gilbert, J. L. et al. Identifying breeding priorities for blueberry flavor using biochemical, sensory, and genotype by environment analyses. PLOS ONE 10 , 1–21 (2015).
Goulet, C. et al. Role of an esterase in flavor volatile variation within the tomato clade. Proc. Natl. Acad. Sci. 109 , 19009–19014 (2012).
Article ADS CAS PubMed PubMed Central Google Scholar
Borisov, V. et al. Deep Neural Networks and Tabular Data: A Survey. IEEE Trans. Neural Netw. Learn. Syst. 1–21 https://doi.org/10.1109/TNNLS.2022.3229161 (2022).
Statista. Statista Consumer Market Outlook: Beer - Worldwide.
Seitz, H. K. & Stickel, F. Molecular mechanisms of alcoholmediated carcinogenesis. Nat. Rev. Cancer 7 , 599–612 (2007).
Voordeckers, K. et al. Ethanol exposure increases mutation rate through error-prone polymerases. Nat. Commun. 11 , 3664 (2020).
Goelen, T. et al. Bacterial phylogeny predicts volatile organic compound composition and olfactory response of an aphid parasitoid. Oikos 129 , 1415–1428 (2020).
Article ADS Google Scholar
Reher, T. et al. Evaluation of hop (Humulus lupulus) as a repellent for the management of Drosophila suzukii. Crop Prot. 124 , 104839 (2019).
Stein, S. E. An integrated method for spectrum extraction and compound identification from gas chromatography/mass spectrometry data. J. Am. Soc. Mass Spectrom. 10 , 770–781 (1999).
American Society of Brewing Chemists. Sensory Analysis Methods. (American Society of Brewing Chemists, St. Paul, MN, U.S.A., 1992).
McAuley, J., Leskovec, J. & Jurafsky, D. Learning Attitudes and Attributes from Multi-Aspect Reviews. Preprint at https://doi.org/10.48550/arXiv.1210.3926 (2012).
Meilgaard, M. C., Carr, B. T. & Carr, B. T. Sensory Evaluation Techniques. (CRC Press, Boca Raton). https://doi.org/10.1201/b16452 (2014).
Schreurs, M. et al. Data from: Predicting and improving complex beer flavor through machine learning. Zenodo https://doi.org/10.5281/zenodo.10653704 (2024).
Download references
Acknowledgements
We thank all lab members for their discussions and thank all tasting panel members for their contributions. Special thanks go out to Dr. Karin Voordeckers for her tremendous help in proofreading and improving the manuscript. M.S. was supported by a Baillet-Latour fellowship, L.C. acknowledges financial support from KU Leuven (C16/17/006), F.A.T. was supported by a PhD fellowship from FWO (1S08821N). Research in the lab of K.J.V. is supported by KU Leuven, FWO, VIB, VLAIO and the Brewing Science Serves Health Fund. Research in the lab of T.W. is supported by FWO (G.0A51.15) and KU Leuven (C16/17/006).
Author information
These authors contributed equally: Michiel Schreurs, Supinya Piampongsant, Miguel Roncoroni.
Authors and Affiliations
VIB—KU Leuven Center for Microbiology, Gaston Geenslaan 1, B-3001, Leuven, Belgium
Michiel Schreurs, Supinya Piampongsant, Miguel Roncoroni, Lloyd Cool, Beatriz Herrera-Malaver, Florian A. Theßeling & Kevin J. Verstrepen
CMPG Laboratory of Genetics and Genomics, KU Leuven, Gaston Geenslaan 1, B-3001, Leuven, Belgium
Leuven Institute for Beer Research (LIBR), Gaston Geenslaan 1, B-3001, Leuven, Belgium
Laboratory of Socioecology and Social Evolution, KU Leuven, Naamsestraat 59, B-3000, Leuven, Belgium
Lloyd Cool, Christophe Vanderaa & Tom Wenseleers
VIB Bioinformatics Core, VIB, Rijvisschestraat 120, B-9052, Ghent, Belgium
Łukasz Kreft & Alexander Botzki
AB InBev SA/NV, Brouwerijplein 1, B-3000, Leuven, Belgium
Philippe Malcorps & Luk Daenen
You can also search for this author in PubMed Google Scholar
Contributions
S.P., M.S. and K.J.V. conceived the experiments. S.P., M.S. and K.J.V. designed the experiments. S.P., M.S., M.R., B.H. and F.A.T. performed the experiments. S.P., M.S., L.C., C.V., L.K., A.B., P.M., L.D., T.W. and K.J.V. contributed analysis ideas. S.P., M.S., L.C., C.V., T.W. and K.J.V. analyzed the data. All authors contributed to writing the manuscript.
Corresponding author
Correspondence to Kevin J. Verstrepen .
Ethics declarations
Competing interests.
K.J.V. is affiliated with bar.on. The other authors declare no competing interests.
Peer review
Peer review information.
Nature Communications thanks Florian Bauer, Andrew John Macintosh and the other, anonymous, reviewer(s) for their contribution to the peer review of this work. A peer review file is available.
Additional information
Publisher’s note Springer Nature remains neutral with regard to jurisdictional claims in published maps and institutional affiliations.
Supplementary information
Supplementary information, peer review file, description of additional supplementary files, supplementary data 1, supplementary data 2, supplementary data 3, supplementary data 4, supplementary data 5, supplementary data 6, supplementary data 7, reporting summary, source data, source data, rights and permissions.
Open Access This article is licensed under a Creative Commons Attribution 4.0 International License, which permits use, sharing, adaptation, distribution and reproduction in any medium or format, as long as you give appropriate credit to the original author(s) and the source, provide a link to the Creative Commons licence, and indicate if changes were made. The images or other third party material in this article are included in the article’s Creative Commons licence, unless indicated otherwise in a credit line to the material. If material is not included in the article’s Creative Commons licence and your intended use is not permitted by statutory regulation or exceeds the permitted use, you will need to obtain permission directly from the copyright holder. To view a copy of this licence, visit http://creativecommons.org/licenses/by/4.0/ .
Reprints and permissions
About this article
Cite this article.
Schreurs, M., Piampongsant, S., Roncoroni, M. et al. Predicting and improving complex beer flavor through machine learning. Nat Commun 15 , 2368 (2024). https://doi.org/10.1038/s41467-024-46346-0
Download citation
Received : 30 October 2023
Accepted : 21 February 2024
Published : 26 March 2024
DOI : https://doi.org/10.1038/s41467-024-46346-0
Share this article
Anyone you share the following link with will be able to read this content:
Sorry, a shareable link is not currently available for this article.
Provided by the Springer Nature SharedIt content-sharing initiative
By submitting a comment you agree to abide by our Terms and Community Guidelines . If you find something abusive or that does not comply with our terms or guidelines please flag it as inappropriate.
Quick links
- Explore articles by subject
- Guide to authors
- Editorial policies
Sign up for the Nature Briefing: Translational Research newsletter — top stories in biotechnology, drug discovery and pharma.

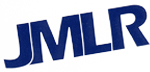
Frequently Asked Questions
JMLR Papers
Select a volume number to see its table of contents with links to the papers.
Volume 23 (January 2022 - Present)
Volume 22 (January 2021 - December 2021)
Volume 21 (January 2020 - December 2020)
Volume 20 (January 2019 - December 2019)
Volume 19 (August 2018 - December 2018)
Volume 18 (February 2017 - August 2018)
Volume 17 (January 2016 - January 2017)
Volume 16 (January 2015 - December 2015)
Volume 15 (January 2014 - December 2014)
Volume 14 (January 2013 - December 2013)
Volume 13 (January 2012 - December 2012)
Volume 12 (January 2011 - December 2011)
Volume 11 (January 2010 - December 2010)
Volume 10 (January 2009 - December 2009)
Volume 9 (January 2008 - December 2008)
Volume 8 (January 2007 - December 2007)
Volume 7 (January 2006 - December 2006)
Volume 6 (January 2005 - December 2005)
Volume 5 (December 2003 - December 2004)
Volume 4 (Apr 2003 - December 2003)
Volume 3 (Jul 2002 - Mar 2003)
Volume 2 (Oct 2001 - Mar 2002)
Volume 1 (Oct 2000 - Sep 2001)
Special Topics
Bayesian Optimization
Learning from Electronic Health Data (December 2016)
Gesture Recognition (May 2012 - present)
Large Scale Learning (Jul 2009 - present)
Mining and Learning with Graphs and Relations (February 2009 - present)
Grammar Induction, Representation of Language and Language Learning (Nov 2010 - Apr 2011)
Causality (Sep 2007 - May 2010)
Model Selection (Apr 2007 - Jul 2010)
Conference on Learning Theory 2005 (February 2007 - Jul 2007)
Machine Learning for Computer Security (December 2006)
Machine Learning and Large Scale Optimization (Jul 2006 - Oct 2006)
Approaches and Applications of Inductive Programming (February 2006 - Mar 2006)
Learning Theory (Jun 2004 - Aug 2004)
Special Issues
In Memory of Alexey Chervonenkis (Sep 2015)
Independent Components Analysis (December 2003)
Learning Theory (Oct 2003)
Inductive Logic Programming (Aug 2003)
Fusion of Domain Knowledge with Data for Decision Support (Jul 2003)
Variable and Feature Selection (Mar 2003)
Machine Learning Methods for Text and Images (February 2003)
Eighteenth International Conference on Machine Learning (ICML2001) (December 2002)
Computational Learning Theory (Nov 2002)
Shallow Parsing (Mar 2002)
Kernel Methods (December 2001)
Help | Advanced Search
Computer Science > Computer Vision and Pattern Recognition
Title: deep learning for robust and explainable models in computer vision.
Abstract: Recent breakthroughs in machine and deep learning (ML and DL) research have provided excellent tools for leveraging enormous amounts of data and optimizing huge models with millions of parameters to obtain accurate networks for image processing. These developments open up tremendous opportunities for using artificial intelligence (AI) in the automation and human assisted AI industry. However, as more and more models are deployed and used in practice, many challenges have emerged. This thesis presents various approaches that address robustness and explainability challenges for using ML and DL in practice. Robustness and reliability are the critical components of any model before certification and deployment in practice. Deep convolutional neural networks (CNNs) exhibit vulnerability to transformations of their inputs, such as rotation and scaling, or intentional manipulations as described in the adversarial attack literature. In addition, building trust in AI-based models requires a better understanding of current models and developing methods that are more explainable and interpretable a priori. This thesis presents developments in computer vision models' robustness and explainability. Furthermore, this thesis offers an example of using vision models' feature response visualization (models' interpretations) to improve robustness despite interpretability and robustness being seemingly unrelated in the related research. Besides methodological developments for robust and explainable vision models, a key message of this thesis is introducing model interpretation techniques as a tool for understanding vision models and improving their design and robustness. In addition to the theoretical developments, this thesis demonstrates several applications of ML and DL in different contexts, such as medical imaging and affective computing.
Submission history
Access paper:.
- HTML (experimental)
- Other Formats

References & Citations
- Google Scholar
- Semantic Scholar
BibTeX formatted citation

Bibliographic and Citation Tools
Code, data and media associated with this article, recommenders and search tools.
- Institution
arXivLabs: experimental projects with community collaborators
arXivLabs is a framework that allows collaborators to develop and share new arXiv features directly on our website.
Both individuals and organizations that work with arXivLabs have embraced and accepted our values of openness, community, excellence, and user data privacy. arXiv is committed to these values and only works with partners that adhere to them.
Have an idea for a project that will add value for arXiv's community? Learn more about arXivLabs .
Main navigation
- Researchers
Courtney Y. Paquette receives 2024 Alfred P. Sloan Research Fellowship

- Tweet Widget
It is a great pleasure to announce that our colleague Courtney Paquette has been named a 2024 Alfred P. Sloan Research Fellow in Computer Science.
Machine learning relies heavily on probabilistic optimization algorithms.
While machine learning papers are written at a staggering rate, there is much less foundational mathematical work that addresses the success of machine learning.
Since her PhD, Courtney has been at the forefront of this foundational work.
More recently, with her partner in crime Elliot Paquette, she has developed a pioneering program blending random matrices, high dimensional probability and optimization theory.
Please join me in congratulating Courtney on this huge, highly deserved honour.
Rustum Choksi
Professor and Department Chair
Department of Mathematics and Statistics
McGill University
Related Links
- Dept. of Mathematics and Statistics
- Faculty of Science
Department and University Information
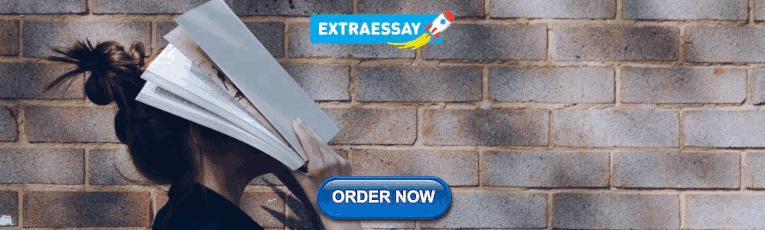
IMAGES
VIDEO
COMMENTS
Papers With Code highlights trending Machine Learning research and the code to implement it. Browse State-of-the-Art Datasets ; Methods; More ... Subscribe to the PwC Newsletter ×. Stay informed on the latest trending ML papers with code, research developments, libraries, methods, and datasets. Read previous issues. Subscribe.
To discuss the applicability of machine learning-based solutions in various real-world application domains. To highlight and summarize the potential research directions within the scope of our study for intelligent data analysis and services. The rest of the paper is organized as follows.
Accelerating protein sensor optimization with machine learning. A recent study introduces a machine learning approach to investigate the effects of mutations on protein sensors commonly employed ...
The Journal of Machine Learning Research (JMLR) provides an international forum for the electronic and paper publication of high-quality scholarly articles in all areas of machine learning.JMLR seeks previously unpublished papers that contain:new algorithms with empirical, theoretical, psychological, or biological justification; experimental and/or theoretical studies yielding new insight into ...
The Journal of Machine Learning Research (JMLR), established in 2000, provides an international forum for the electronic and paper publication of high-quality scholarly articles in all areas of machine learning. All published papers are freely available online. JMLR has a commitment to rigorous yet rapid reviewing. Final versions are published ...
XuranMeng, JeffYao; (28):1−40, 2023. (Machine Learning Open Source Software Paper) HiClass: a Python Library for Local Hierarchical Classification Compatible with Scikit-learn. Fábio M. Miranda, Niklas Köhnecke, Bernhard Y. Renard; (29):1−17, 2023.
Since ML appeared in the 1990s, all published documents (i.e., journal papers, reviews, conference papers, preprints, code repositories and more) related to this field from 1990 to 2020 have been selected, and specifically, within the search fields, the following keywords were used: "machine learning" OR "machine learning-based approach" OR ...
JMLR Papers. Select a volume number to see its table of contents with links to the papers. Volume 25 (January 2024 - Present) ... Machine Learning and Large Scale Optimization (Jul 2006 - Oct 2006) Approaches and Applications of Inductive Programming (February 2006 - Mar 2006)
Today, intelligent systems that offer artificial intelligence capabilities often rely on machine learning. Machine learning describes the capacity of systems to learn from problem-specific training data to automate the process of analytical model building and solve associated tasks. Deep learning is a machine learning concept based on artificial neural networks. For many applications, deep ...
Machine Learning is an international forum focusing on computational approaches to learning. ... Improves how machine learning research is conducted. Prioritizes verifiable and replicable supporting evidence in all published papers. Editor-in-Chief. Hendrik Blockeel; Impact factor 7.5 (2022) 5 year impact factor
A variety of innovative topics are included in the agenda of the published papers in this special issue including topics such as: Stock market Prediction using Machine learning. Detection of Apple Diseases and Pests based on Multi-Model LSTM-based Convolutional Neural Networks. ML for Searching. Machine Learning for Learning Automata
Read the latest Research articles in Machine learning from Nature. Skip to main content. ... She hadn't — but her paper was rejected anyway. E. M. Wolkovich; News | 05 February 2024.
Challenges and Research Directions. Our study on machine learning algorithms for intelligent data analysis and applications opens several research issues in the area. Thus, in this section, we summarize and discuss the challenges faced and the potential research opportunities and future directions.
Machine learning, especially its subfield of Deep Learning, had many amazing advances in the recent years, and important research papers may lead to breakthroughs in technology that get used by billio. ns of people. The research in this field is developing very quickly and to help our readers monitor the progress we present the list of most ...
Simplicity Bias of Transformers to Learn Low Sensitivity Functions. Bhavya Vasudeva, Deqing Fu, Tianyi Zhou, Elliott Kau, Youqi Huang, Vatsal Sharan. Subjects: Machine Learning (cs.LG); Artificial Intelligence (cs.AI); Computation and Language (cs.CL); Machine Learning (stat.ML)
Machine learning research papers showcasing the transformation of the technology In 2021, machine learning and deep learning had many amazing advances and important research papers may lead to breakthroughs in technology that get used by billions of people. The research in this field is developing very quickly and to help you monitor the progress here is the list of most important recent ...
This article examines the background to the problem and outlines a project that TNA undertook to research the feasibility of using commercially available artificial intelligence tools to aid selection. ... this research aims to predict user's personalities based on Indonesian text from social media using machine learning techniques. This ...
Top Machine Learning Research Papers Released In 2021. Advances in the machine and deep learning in 2021 could lead to new technologies utilised by billions of people worldwide. Published on November 18, 2021. by Dr. Nivash Jeevanandam. Advances in machine learning and deep learning research are reshaping our technology.
a few innovative research works and their applications in real-world, such as stock trading, medical and healthcare systems, and software automation. The chapters in ... Machine learning (ML) is the ability of a system to automatically acquire, integrate, and then develop knowledge from large-scale data, and then expand the acquired ...
The paper aims at reviewing machine learning techniques and algorithms. The research methodology is based on qualitative analysis where various literatures is being reviewed based on machine ...
Deep learning (DL), a branch of machine learning (ML) and artificial intelligence (AI) is nowadays considered as a core technology of today's Fourth Industrial Revolution (4IR or Industry 4.0). Due to its learning capabilities from data, DL technology originated from artificial neural network (ANN), has become a hot topic in the context of computing, and is widely applied in various ...
Machine learning is a method by which a computational system learns the features of input data. Such methods haves proven effective for the detection of diabetes. Many machine learning algorithms have been developed, including supervised, unsupervised, and reinforcement learning methods.
To our knowledge, no previous research gathered data at this scale (250 samples, 226 chemical parameters, 50 sensory attributes and 5 consumer scores) to disentangle and validate the chemical ...
The application of machine learning in causal inference has garnered significant attention from researchers. A particular focus lies in the integration of machine learning into instrumental variable (IV) regression, a cornerstone methodology for causal inference. IV regression typically employs a two-stage least squares (2SLS) approach.
Geological mapping, as the fundamental core of geological research and investigation, provides indispensable basic understanding and exploration data for mineral prospecting, disaster prevention and control, and other related fields. The emergence of big data algorithms and models, such as machine learning and deep learning, has brought new assistance to the task of geological mapping. By ...
JMLR Papers. Select a volume number to see its table of contents with links to the papers. Volume 23 (January 2022 - Present) . Volume 22 (January 2021 - December 2021) . Volume 21 (January 2020 - December 2020) . Volume 20 (January 2019 - December 2019) . Volume 19 (August 2018 - December 2018) . Volume 18 (February 2017 - August 2018) . Volume 17 (January 2016 - January 2017)
Recent breakthroughs in machine and deep learning (ML and DL) research have provided excellent tools for leveraging enormous amounts of data and optimizing huge models with millions of parameters to obtain accurate networks for image processing. These developments open up tremendous opportunities for using artificial intelligence (AI) in the automation and human assisted AI industry. However ...
The census and management of hazard-bearing entities, along with the integrity of data quality, form crucial foundations for disaster risk assessment and zoning. By addressing the challenge of feature confusion, prevalent in single remotely sensed image recognition methods, this paper introduces a novel method, Spatially Constrained Deep Learning (SCDL), that combines deep learning with ...
It is a great pleasure to announce that our colleague Courtney Paquette has been named a 2024 Alfred P. Sloan Research Fellow in Computer Science.. Machine learning relies heavily on probabilistic optimization algorithms. While machine learning papers are written at a staggering rate, there is much less foundational mathematical work that addresses the success of machine learning.