
An official website of the United States government
The .gov means it’s official. Federal government websites often end in .gov or .mil. Before sharing sensitive information, make sure you’re on a federal government site.
The site is secure. The https:// ensures that you are connecting to the official website and that any information you provide is encrypted and transmitted securely.
- Publications
- Account settings
Preview improvements coming to the PMC website in October 2024. Learn More or Try it out now .
- Advanced Search
- Journal List
- Biomolecules

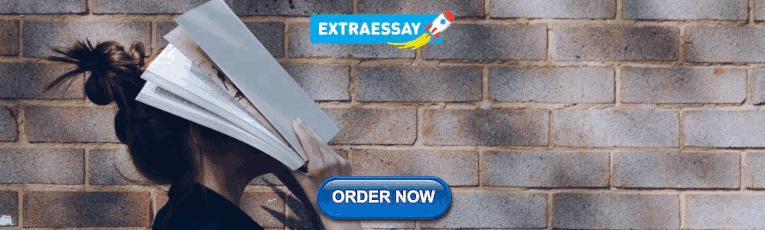
Plant-Based Green Synthesis of Nanoparticles: Production, Characterization and Applications
Christophe hano.
1 Laboratoire de Biologie des Ligneux et des Grandes Cultures (LBLGC), INRAE USC1328, Eure et Loir Campus, Université d’Orléans, 28000 Chartres, France
Bilal Haider Abbasi
2 Department of Biotechnology, Quaid-i-Azam University, Islamabad 45320, Pakistan
Associated Data
All the data are included in the present study.
1. Introduction
Nanotechnology is a fast-expanding and multidisciplinary field with many applications in science and technology [ 1 , 2 , 3 , 4 , 5 , 6 , 7 , 8 , 9 , 10 , 11 , 12 , 13 ]. This field combines key concepts from a variety of disciplines, including chemistry, engineering, physics, and biology, in order to provide novel methods for controlling and generating nanoparticles (NPs). These NPs are particles with at least one dimension ranging from 1–100 nm. Nanotechnology deals with the synthesis, characterization, and applications of a variety of NPs. Noble metals, such as gold, silver, or platinum, are commonly used to synthesize NPs by a variety of chemical and physical techniques; however, these processes are not ecologically friendly [ 1 , 4 , 9 , 14 , 15 , 16 ]. There is a pressing need to develop a non-toxic, environmentally friendly NPs production technology. Several safe, easy, cost-effective, reproducible, and scalable green synthesis approaches for NPs have been developed in recent years, inspired by the safety-by-design concept. As a result, several biological systems, such as yeast, fungus, bacteria, and plant extracts, are currently extensively employed in green synthesis approaches for the generation of NPs [ 1 , 9 ]. Plant-based NP green synthesis is now regarded as a gold standard among these green biological techniques owing to its ease of use and the diversity of plants. This work serves both as editorial for the present Special Issue, composed of two reviews and sixteen research articles, as well as a brief overview of current trends in green synthesis, characterization, and applications of a range of plant-derived NPs.
2. Green Synthesis and Characterization of Plant-Derived NPs
During the last decade, the concept of “Green Chemistry” for “Sustainable Development” has been widely investigated [ 17 ]. Sustainable development is described as development that meets the current demands while also balancing the ability of future generations to satisfy their needs [ 18 ]. Due to its concern with the evidence of pollution and the indiscriminate use of natural resources, sustainable development is especially important for various chemistry-based sectors [ 19 ]. The selection of a green or environmentally friendly solvent (the most widely used are water, ethanol, and their mixtures), a suitable non-toxic reducing agent, and a safe substance for stabilization are the three most important requirements for the green synthesis of NPs. Indeed, extensive synthetic pathways have been used to produce nanoparticles, with physical, chemical, and biosynthetic routes being the most popular. Chemical procedures are generally excessively costly and involve the use of toxic and hazardous chemicals that entail a variety of environmental risks [ 20 ]. In contrast, green synthesis is a safe, biocompatible, and environmentally friendly green method of synthesizing NPs for various applications, including biomedical uses [ 21 ]. Fungi, algae, bacteria, and plants have been used to carry out this green synthesis. However, plant components, including leaves, fruits, roots, stems, and seeds, have been widely utilized to synthesize different NPs [ 22 ]. Indeed, plant extracts have the ability to produce NPs with defined size, shape, and composition. Furthermore, the presence of a wide array of phytochemicals in their extract may function as natural stabilizing and/or reducing agents for NPs production. It is accepted that plant-derived NPs are also less likely to cause harmful side effects in humans when compared to chemically synthesized NPs, and exhibit a high biological potential with applications in agriculture, food science and technology, bioengineering, cosmetic or nanomedicine, and human health protection.
It is essential that these NPs be precisely and thoroughly characterized in order to ensure reproducibility in their production, biological activity, and safety. For this purpose, a wide range of physicochemical methods are used to very precisely characterized the synthesized NPs including ultraviolet-visible spectroscopy, Fourier transform infrared spectroscopy (FTIR), attenuated total reflection (ATR), Raman spectroscopy, photoluminescence analysis (PL), dynamic light scattering (DLS), UV-visible diffuse reflectance spectroscopy (UV-DRS), transmission electron microscopy (TEM), scanning electron microscopy (SEM), atomic force microscopy (AFM), field emission scanning electron microscopy (FE-SEM), X-ray diffractometer (XRD), X-ray photoelectron microscopy (XPS), energy dispersion analysis of X-ray (EDAX), thermal gravimetric differential thermal analysis (TG-DTA), or nuclear magnetic resonance (NMR) [ 23 , 24 , 25 , 26 , 27 , 28 , 29 , 30 , 31 , 32 , 33 , 34 , 35 , 36 , 37 , 38 ].
3. An Overview of the Different Types of Plant-Derived NPs
Different types of plant-derived NPs are presented, and their synthesis, characterization, and applications are discussed and published in this Special Issue.
Plant-based silver nanoparticles (AgNPs) are among the easiest to prepare [ 24 , 26 , 27 , 30 , 35 , 38 ]. For the green synthesis of silver nanoparticles, a silver metal ion solution and a reducing biological agent are required. The easiest and least expensive method for producing AgNPs is to reduce and stabilize Ag ions using a mixture of biomolecules, such as polysaccharides, vitamins, amino acids, proteins, phenolics, saponins, alkaloids, and/or terpenes [ 39 ]. Almost all plants have the potential to be exploited to prepare AgNPs.
Gold nanoparticles (AuNPs) have received tremendous attention because of their facile synthesis, easy surface functionalization [ 40 ], and unique characteristics, such as their high potential for use in medicine [ 41 ], low toxicity [ 42 ], and highly biocompatible nature [ 43 ]. Various chemical moieties in biogenic complexes operate as reducing agents in the production of gold nanoparticles, resulting in the reduction of gold metal ions and the formation of nanoparticles. Some studies have revealed that biomolecules, such as flavonoids, phenols, protein, and others, have an important role in the reduction of metal ions and the topping of gold nanoparticles in plant extracts [ 24 , 37 ].
Zinc oxide nanoparticles (ZnONPs) have received considerable attention over the last years because of their wide array of potential applications in biomedicine, cosmetic, optics, and electronics. Thus far, several investigations on the synthesis and utilization of ZnONPs by plants, microorganisms, and other species have been reported. Many studies have raised interest in their low-cost, safe, and simple synthesis. ZnONPs may be made from a variety of plant components, including flowers, roots, seeds, and leaves. Remarkably, these nanoparticles exhibit a high exciton binding energy of 60 meV and a huge bandgap of 3.37 eV, giving them a wide range of semiconducting properties [ 28 , 31 , 32 , 36 ].
Copper (Cu) is a comparatively low-cost metal that is more cost-effective than Au and Ag, and CuNPs have been synthesized by the reduction of aqueous Cu ions by different plant extracts [ 8 , 33 ]. The existence of a 578-nm peak on a UV-visible spectrometer, in particular, confirms their formation [ 44 ]. However, numerous questions about their biosafety persist [ 8 ].
Other metals, such as nickel (Ni) [ 29 ] or manganese (Mn) [ 25 ], are also presented. Note that some additional metals, such as titanium (Ti), palladium (Pd), cerium (Ce), or platinum (Pt), have lately been employed to prepare plant-based NPs with various biomedical or industrial applications [ 1 , 9 , 45 ].
4. Applications of Plant-Derived NPs
4.1. an overview of the potential applications of plant-derived nps.
NPs are currently in high demand commercially due to their wide range of applications in industries, electronics, environment, energy, and more particularly in biomedical fields. NPs, such as the most commonly known Ag and Au NPs, have been widely explored in this sector and are of tremendous interest for biological applications. In general, plant-derived green NPs are also less likely to cause severe side effects in humans when compared to chemically synthesized NPs, and have a great application potential with applications in a variety of areas, including but not limited to:
- - Nanomedicine and human health protection (antimicrobial, antiparasitic, antiproliferative, pro-apoptotic, pro- or anti-oxidative depending on the context, anti-inflammatory activities, etc.) [ 2 , 4 , 10 , 13 , 45 ];
- - Agriculture (precision farming with controlled release of agrochemicals, target-specific delivery of biomolecules, more efficient nutrients absorption, detection and control of plant diseases, etc.) [ 3 , 46 ];
- - Food science and technology (processing, storage, and packaging processes), in bioengineering (biocatalysts, photocatalysts, biosensors, etc.) [ 5 ];
- - Cosmetics (sunscreen, anti-aging, hair growth, bioactive compounds delivery, nano-emulsion, etc.) [ 7 ].
With two review papers dealing with algae-based NPs synthesis and CuNPs, the current Special Issue sheds light on two less investigated tools and methodologies of green plant-based nanotechnology [ 6 ].
Algae are definitely ideal candidates for the green synthesis of NPs because they are rich in secondary metabolites that act as reducing and capping agents. Many potential applications have been already described including antimicrobial or anticancer actions, but also as antifouling, bioremediation or biosensing agents. However, unlike terrestrial medicinal and aromatic plants, algae were underutilized in the beginning of studies on the green synthesis of NPs using plant extracts. As this sector is still in its onset, scaling up for commercial applications is still challenging [ 6 ].
Cu is a relatively low-cost metal that is for example more cost-effective than Au and Ag. CuNPs have been produced via the reduction of aqueous Cu ions by various plant extracts. The review by Letchumanan et al. [ 8 ] provides a very comprehensive overview and current update of plant-mediated Cu/CuO (Cu oxide) NPs, covering their synthesis, therapeutic uses, and mechanisms. Although Cu/CuO NPs have a variety of therapeutic benefits, their toxicity to normal cells and important organs in humans might have significant adverse effects. As a result, prior to the use of these NPs in medicine, this potential toxic issue should be extensively examined. The toxicity of these NPs, as well as their effectiveness in comparison to commercial NPs in both in vitro and in vivo research, are reviewed and discussed [ 8 ]. This review also sheds light on the future prospects for producing plant-based Cu/CuO NPs as a therapeutic agent for a variety of diseases (including microbial infection, cancer, wounding, or inflammation) [ 8 ].
4.2. Anti-Cancer Potential
Nanomedicine is the use of nanotechnology in the treatment, screening, and diagnosis of a variety of diseases, including cancer [ 10 , 11 , 12 , 47 ]. It adds complete procedures and effective approaches against cancer through cancer prediction and diagnostics, prevention and medication, as well as possible individualized therapy [ 10 , 12 ].
Many plant-derived NPs have shown some potential against cancer cells. ZnONPs produced from a Cassia auriculata leaf extract, in particular, has shown tumoricidal activity against MCF-7 breast cancer cells while having no detrimental effect on normal MCF-12A human breast cells [ 34 ].
Similarly, green AuNPs produced from a Trachyspermum ammi seed extract inhibited cellular growth in HepG2 cancer cell lines in a concentration-dependent manner, which was linked to a reactive oxygen species (ROS)-driven apoptosis [ 37 ]. This mechanism has recently been reported to be potentially connected to mitochondrial action via ROS-induced Caspase-3 gene expression and enzyme activity following mitochondrial membrane potential disruption caused by plant-based NPs [ 11 , 47 ].
However, in addition to a deeper understanding of the molecular mechanism of action of NPs against cancer cells, there is also a need to properly understand the fate of NPs. These questions include how long NPs stay in the body, what conditions influence the duration of NP degradation, how to make NPs stay for longer or shorter periods, what are the long-term and short-term effects of NPs, how the body behaves towards these outsider entities on a micro and macro level, and how we can standardize NPs to ensure experiment reproducibility. These should be solved before introducing nanotechnologies into the healthcare industry. Aside from this, there are several questions that require further research and testing. In order to avoid any unanticipated consequences, we must also determine the possible risks linked with these nanomaterials. Furthermore, in order to obtain the safest and most successful therapy regimen, the numerous nanomedicines and nanoformulations targeting specific cancer cells must be thoroughly constructed. We conclude with the hope that nanotechnology will propel the development of more viable medicines to treat cancer, as well as offer researchers with powerful tools to overcome several bottlenecks in this health sector.
4.3. Anti-Leishmanial Potential
Leishmaniasis is a protozoan vector-borne illness that affects almost 350 million people worldwide. Chemotherapeutic medicines were initially used to treat leishmaniasis, but they had adverse side effects. Due to their unique properties, such as bioavailability, reduced toxicity, targeted drug delivery, and biodegradability, a variety of nanotechnology-based techniques and products have emerged as anti-leishmanial drugs, including liposomes, lipid nano-capsules, metal and metallic oxide nanoparticles, polymeric nanoparticles, nanotubes, and nanovaccines [ 2 ]. AgNPs containing xylan (also known as nanoxylan) synthesized in a green synthesis route with corncob xylan as a reducing and stabilizing agent demonstrated effective inhibitory activity against Leishmania amazonensis promastigote viability, whereas xylan alone had no effect [ 35 ]. This work nicely illustrates the potential of the nanoxylan as a promising new type of antiparasitic agent [ 35 ].
4.4. Antimicrobial Potential
Antibiotic resistance is one of the most pressing issues of recent years, and it is only going to become worse. Bacteria have developed resistance to antimicrobial agents as a result of the rapid evolution of the bacterial genome. Thus, in the search for a new therapy, biogenic NPs have shown encouraging results in the treatment of multidrug-resistant bacteria and might be a potential choice in the fight against such resistant pathogenesis [ 1 ]. To improve the antimicrobial response, NPs and other conjugates have been combined with different organic and inorganic compounds.
Ag has long been known for its antibacterial properties against a variety of bacterial strains. In particular, green AgNPs prepared from a Carissa carandas leaf extract demonstrated antibacterial efficacy against a variety of human pathogenic bacteria, with Gram-negative bacteria, particularly Shigella flexineri responsible for shigellosis, being more likely to be inhibited [ 27 ]. Similarly, bimetallic nanostructures coated with reduced graphene oxide generated from a stevia leaf extract, such as Pd-Ag nanostructures, can limit the development of Gram-negative bacteria Escherichia coli [ 23 ]. AgNPs obtained from the Saudi Arabian desert plant Sisymbrium irio showed potent inhibition potential against multidrug-resistant Pseudomonas aeruginosa and Acinetobacter baumanii that are responsible for ventilator-associated pneumonia [ 38 ]. Furthermore, antifungal activity of nanoxylan derived from corncob xylan against Candida albicans , Candida parapsilosis , and Cryptococcus neoformans has been described [ 35 ], whereas AgNPs obtained from the leaf extract of Clerodendrum inerme showed a dual antibacterial and antifungal actions against a wide range of human pathogenic strains [ 24 ].
Interestingly, AuNPs produced from the same C. inerme extract also showed very similar inhibition capacity [ 24 ]. The authors concluded that these NPs may have improved antimicrobial activity due to the synergistic effect of biologically active absorbed phytochemicals from this plant [ 24 ]. Antibiofilm action of AuNPs produced from a T. amni seed extract was also observed against Listeria monocytogenes and Serratia marcescens , most likely as a result of intracellular ROS production [ 37 ].
ZnONPs also showed potential antimicrobial activity as evidenced by the action of ZnONPs derived from a Cinnamomum verum bark extract against E. coli and Staphylococcus aureus [ 13 ]. Similarly, ZnONPs derived from a C. auriculata leaf extract exhibited antibacterial activity due to direct cell contact, which disrupted bacterial cell integrity [ 34 ].
Other metallic NPs, such as CuONPs derived from Cymbopogon citratus , can exhibit significant antimicrobial activity, including antibiofilm properties [ 33 ]. Interestingly, these authors noted a variation in antibiofilm activity, which they suspect is due to differences in the cell wall compositions of the examined bacterial strains [ 33 ]. MnONPs derived from an Abutilon indicum leaf extract demonstrated potent antibacterial activity against both Gram-negative and Gram-positive bacteria [ 25 ], whereas NiONPs deriving from stevia leaf extract were more effective against Gram-negative bacteria [ 29 ]. This shows that antimicrobial activity is influenced by the type of NPs produced, but also the composition of the coated phytochemicals on their surfaces, which is affected by the plant extract used for NPs synthesis.
Cell wall disruption, cell membrane disintegration, massive free radical production, specific (targeted) and/or specific actions against proteins, DNA fragmentation, vital enzyme inhibition, loss of cellular fluids, and disruption in electron transport have all been proposed as possible mechanisms for NPs antibacterial activity [ 1 ]. Bio-mediated NPs might also have an antifungal effect by causing excessive ROS generation. However, few studies have focused only on fungus as of yet [ 1 ]. Despite advances in understanding of the antimicrobial efficacy of plant-based NPs, much remains unclear regarding their specific mechanism of action, toxicity, and possible environmental issues.
4.5. Agricultural Applications
When agricultural pathogens are targeted, the antimicrobial activity outlined in the previous Section may be effective for crop protection. In particular, ZnONPs have demonstrated their wide agricultural interest showing an anti-phytopathogenic action against both bacteria as evidenced by ZnONPs derived from lemon fruit against soft rot bacteria pathogen Dickeya dadantii [ 31 ], and fungi as illustrated by the fungicidal activity of ZnONPs produced using a Eucalyptus globules extract against major pathogens of apple orchards [ 32 ]. It is noteworthy that TiO 2 NPs produced from lemon fruit showed antibacterial activity comparable to ZnONPs against D. dadantii [ 31 ].
Through modifying abscisic acid concentration, ion homeostasis, and defense mechanisms comprising both enzymatic and non-enzymatic antioxidants, AgNPs synthesized from a wheat extract significantly contributed to alleviate the negative effects of salinity stress in wheat [ 30 ]. Interestingly, ZnONPs exhibited low toxicity and the capacity to stimulate the antioxidant response of flax seedlings as well [ 28 ].
4.6. Antioxidant Action
Excessive oxidative stress generated by the action of mitochondria and other internal or external sources may result in oxidative damages to various cell macromolecules (membrane lipids, proteins, and DNA), leading to functional declines, degenerative diseases, and aging [ 48 ]. Antioxidants may be able to reverse this detrimental process and may be used to treat aging and age-related diseases. Some green plant-derived NPs have been described for their antioxidant potential as shown for AgNPs produced from a C. carandas leaf extract [ 27 ], AuNPs and AgNPs deriving from a C. inerme leaf extract [ 24 ], or NiONPs prepared from a stevia leaf extract [ 29 ]. The phytochemicals coated on the NPs surface have certainly a prominent influence in the observed antioxidant action. Commonly, just one in vitro assay, such as the DPPH (2,2-diphenyl-1-picrylhydrazyl) assay, is performed. However, due to the complex nature of phytochemicals, and in particular, because the determination of antioxidant activity is significantly reliant on the reaction mechanism involved, antioxidant activity of should not be measured using a single approach [ 49 ]. Therefore, the validity of the results from in vitro cell-free antioxidant tests must be restricted to the interpretation in terms of chemical reactivity, but in vivo (cellular) validation is strongly required.
4.7. Other Applications
Other potential applications, such as (photo)catalytic and/or absorption potential applications, are also described. AgNPs produced by Matricaria chamomilla showed effective catalytic activity against Rhodamine B under UV light, which could make it a promising material for wastewater treatment [ 26 ]. MnONPs produced from an Abutilon indicum leaf extract has shown efficient absorption activity against the heavy metal CrVI as well as strong photocatalytic activity, indicating the potential to remediate various organic and inorganic contaminants [ 25 ]. Finally, the photocatalytic H 2 production, mediated by Pd-Ag bimetallic nanostructures coated with reduced graphene oxide produced from a stevia leaf extract, can be noted [ 23 ].
5. Conclusions and Future Directions
The growing demand for green chemistry and nanotechnology has pushed for the development of green synthetic methods for the production of nanomaterials using plants, microbes, and other natural resources. Researchers have been focusing on the green synthesis of NPs, using an environmentally favorable technique. Due to their cost-effectiveness, nontoxic approach, simple availability, and ecofriendly nature, considerable research has been conducted on plant extract-mediated NPs production and their prospective uses in numerous industries. Plants have a variety of unique compounds that help in the synthesis process and accelerate the synthesis kinetic. The use of plants for green nanoparticle synthesis is an interesting and emerging aspect of nanotechnology that has a significant impact on the environment and contributes to nanoscience’s long-term sustainability and progress. Catalysis, medicine, cosmetic, agriculture, food packaging, water treatment, dye degradation, textile engineering, bioengineering sciences, sensors, imaging, biotechnology, electronics, optics, and other biological sectors are just some of the potential applications of these green plant-based NPs. These NPs might be the future impetus for the biomedical field in the drug delivery system. These green NPs might be also employed in a variety of ways, including phytopathogen treatment in agriculture or water disinfection for environmental cleanup. This green approach of NPs synthesis is becoming more popular and is expected to develop exponentially in the future; nevertheless, long-term impacts on animals and humans, as well as the accumulation of these NPs in the environment and their influence, must be addressed in the future. This Special Issue gathered cutting-edge research and review articles on the plant-based green synthesis of NPs, their production, characterization, and applications, with the goal of providing the most comprehensive overview of all these features and future challenges.
Acknowledgments
C.H. and B.H.A. gratefully acknowledge the support of Le Studium-Institute for Advanced Studies (Loire Valley, Orléans, France), Campus France (Peridot program), Ligue contre le Cancer (Comité d’Eure et Loir), and Conseil Départemental d’Eure et Loir.
Author Contributions
Conceptualization, writing—original draft preparation, review and editing, project administration, funding acquisition, C.H. and B.H.A. All authors have read and agreed to the published version of the manuscript.
This research was supported by Cosmetosciences, a global training and research program dedicated to the cosmetic industry. Located in the heart of the Cosmetic Valley, this program led by University of Orléans is funded by the Région Centre-Val de Loire (projects VALBIOCOSM, ACTI-LIN and INNOCOSM). This research was supported by ARD2020 Biomedicaments, a biopharmaceutical program of the Région Centre-Val de Loire.
Institutional Review Board Statement
Not applicable.
Informed Consent Statement
Data availability statement, conflicts of interest.
The authors declare no conflict of interest.
Publisher’s Note: MDPI stays neutral with regard to jurisdictional claims in published maps and institutional affiliations.
Open Access is an initiative that aims to make scientific research freely available to all. To date our community has made over 100 million downloads. It’s based on principles of collaboration, unobstructed discovery, and, most importantly, scientific progression. As PhD students, we found it difficult to access the research we needed, so we decided to create a new Open Access publisher that levels the playing field for scientists across the world. How? By making research easy to access, and puts the academic needs of the researchers before the business interests of publishers.
We are a community of more than 103,000 authors and editors from 3,291 institutions spanning 160 countries, including Nobel Prize winners and some of the world’s most-cited researchers. Publishing on IntechOpen allows authors to earn citations and find new collaborators, meaning more people see your work not only from your own field of study, but from other related fields too.
Brief introduction to this section that descibes Open Access especially from an IntechOpen perspective
Want to get in touch? Contact our London head office or media team here
Our team is growing all the time, so we’re always on the lookout for smart people who want to help us reshape the world of scientific publishing.
Home > Books > Green Chemistry for Environmental Sustainability - Prevention-Assurance-Sustainability (P-A-S) Approach
Green Synthesis of Nanoparticles: A Biological Approach
Submitted: 29 May 2023 Reviewed: 05 June 2023 Published: 11 August 2023
DOI: 10.5772/intechopen.1002203
Cite this chapter
There are two ways to cite this chapter:
From the Edited Volume
Green Chemistry for Environmental Sustainability - Prevention-Assurance-Sustainability (P-A-S) Approach
Kinjal J. Shah
To purchase hard copies of this book, please contact the representative in India: CBS Publishers & Distributors Pvt. Ltd. www.cbspd.com | [email protected]
Chapter metrics overview
1,009 Chapter Downloads
Impact of this chapter
Total Chapter Downloads on intechopen.com

Total Chapter Views on intechopen.com
Nanoparticles are often associated with their small size and numerous applications. However, the synthesis process is equally important as it determines the size and properties of the nanoparticles. While traditional nanoparticle synthesis methods require the use of hazardous chemicals and high-energy consumption, green synthesis offers a sustainable, cost-effective, and environmentally friendly alternative. This approach utilizes natural resources and biologically active compounds that can act as reducing, stabilizing, or capping agents in the one-step synthesis of nanoparticles. Green synthesis offers numerous advantages, including the development of processes with minimal environmental impact and improved safety for nanoparticle synthesis. Overall, the synthesis of nanoparticles using green chemistry is a promising approach for sustainable and efficient production. This chapter provides a general overview of nanoparticles, their applications, and green synthesis, and highlights the various biological resources used in these processes and the factors affecting their synthesis.
- green synthesis
- nanoparticles
- plant extract
- microorganisms
- phytochemicals
Author Information
Rafael álvarez-chimal *.
- Laboratory 113 Synthesis of Magnetic Nanomaterials, Condensed Matter Department, Institute of Physic, National Autonomous University of Mexico, Ciudad Universitaria, Mexico City, Coyoacán, Mexico
Jesús Ángel Arenas-Alatorre
*Address all correspondence to: [email protected]
1. Introduction
Nanoparticles are small particles with sizes ranging from 1 to 100 nanometers. These materials have gained importance and interest in recent years owing to their large number of applications, because the matter at this scale presents a more compact arrangement of atoms and molecules, generating phenomena and acquiring or enhancing mechanical [ 1 ], electrical [ 2 ], magnetic [ 3 ], optical [ 4 ], catalytic [ 5 ], and antibacterial [ 6 , 7 ] properties that are completely different from those of their macroscopic counterparts [ 8 ]. They can be classified based on their composition, shape, and size. The most common types of nanoparticles are metals, metal oxides, carbon-based, and quantum dots. Owing to their unique sizes and properties, nanoparticles have attracted significant attention in various fields including medicine, electronics, energy, and environmental science [ 9 , 10 ]. By reducing their size, nanoparticles can have a higher surface-to-volume ratio, enabling a greater number of atoms or molecules per volume, which means that less material is needed to obtain the same activity and exhibit other properties ( Figure 1 ) [ 11 ].
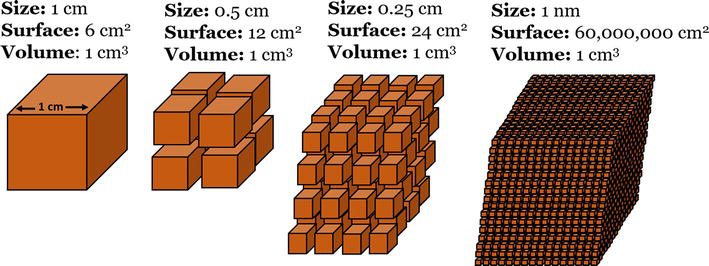
Surface-to-volume ratio of nanoparticles compared with that of bulk materials.
Nanoparticles have many potential benefits for the environment. For example, nanoparticles can be used to improve the efficiency of water treatment, air filtration, and soil remediation; reduce pollution, and develop new types of renewable energy technologies [ 12 ]. In medicine, nanoparticles have shown potential for drug delivery, imaging, and cancer therapy. They can be functionalized with targeting moieties, making them capable of selectively targeting cancer cells, while sparing normal cells. Additionally, nanoparticles can enhance the efficacy of chemotherapy by improving drug delivery to the tumor site and reducing systemic toxicity [ 13 ]. In electronics, nanoparticles are used to fabricate high-performance devices such as sensors, transistors, and solar cells [ 14 ]. Nanoparticles have potential applications in fuel cells, hydrogen storage, and catalysis [ 15 ].
However, it is also important to address the environmental impact of the nanoparticles. Some studies have shown that nanoparticles can harm plants, animals, and humans, but it depends on many factors, such as concentration, size, and time of exposure [ 16 , 17 ]. Nanoparticles can easily be released into the environment through various sources, such as industrial emissions, consumer products, and medical procedures. Once released into the environment, nanoparticles can be difficult to control and monitor. There is potential for long-term accumulation. Nanoparticles can accumulate in the environment, and they may be able to persist for long periods. This raises concerns about the potential for nanoparticles to cause long-term harm to the environment and human health [ 17 , 18 ]. However, one of the alternatives for reducing their environmental impact is to control the synthesis process.
There are many methods for synthesizing nanoparticles, including physical, chemical, and biological processes [ 19 ]. Green synthesis, which refers to the eco-friendly and sustainable production of nanoparticles without the use of hazardous chemicals or toxic solvents, has gained attention in recent years within biological processes. Natural sources, such as plants and microorganisms, are popular green synthesis approaches [ 20 ]. This method has several advantages over traditional synthesis methods, including low cost, scalability, and reduction of hazardous waste. Moreover, green synthesis can produce nanoparticles with unique shapes, sizes, and surface properties tailored for specific applications [ 21 ]. The biological sources used for the green synthesis of nanoparticles contain biologically active compounds, such as enzymes, proteins, polyphenols, flavonoids, and terpenoids, which can act as catalyzing, reducing, stabilizing, or capping agents for one-step synthesis [ 20 , 21 ].
In summary, this chapter provides a general overview of nanoparticles, their properties and applications, and how green synthesis is used to synthesize them. This chapter also discusses the different biological resources used for green synthesis, the factors that participate, and the mechanisms involved in their production.
2. Traditional nanoparticle synthesis methods
Chemical reduction: This method involves the reduction of metal ions in solution using chemical reagents such as sodium borohydride or sodium hydroxide to form nanoparticles [ 22 ].
Coprecipitation: Synthesis involves mixing two or more solutions containing metal ions. When the solutions are mixed, metal ions precipitate out of the solution and form nanoparticles [ 23 ].
Sol-gel: The process requires mixing a metal salt with a solvent and gelling agent. The solvent is evaporated leaving behind the gel. The gel is then heated, causing it to solidify and form nanoparticles [ 24 ].
Microemulsion: This method needs surfactants, water-soluble compounds, and oil-soluble compounds. The mixture forms small droplets that contain the metal ions. When droplets are heated, metal ions precipitate out of the solution and form nanoparticles [ 25 ].
Solvothermal/hydrothermal synthesis: This reaction involves heating a solution of metal ions in water or an organic solvent under high pressure. High pressure and temperature cause metal ions to precipitate out of the solution and form nanoparticles [ 26 ].
Sonochemical/electrochemical synthesis: This process uses ultrasound or an electrical current to break down metal salts into nanoparticles [ 27 ].
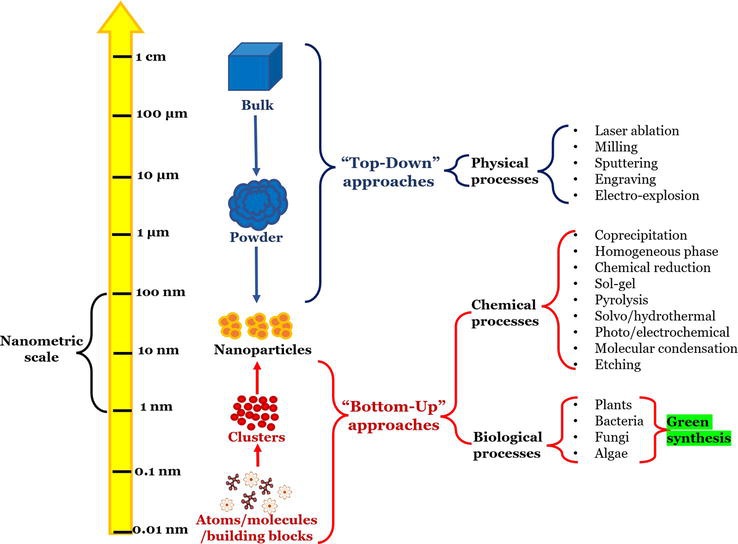
Nanometric scale and different approaches to nanoparticle synthesis.
In addition, there are physical processes, such as laser ablation, milling, and sputtering, where the material is reduced to nanoparticles by the mechanical action of the equipment used [ 28 ].
The choice of method depends on the type of nanoparticles being synthesized, the desired size and shape, and the availability of equipment and reagents.
2.1 Environmental limitations in nanoparticle synthesis
Traditional methods for synthesizing nanoparticles have several limitations.
Using organic reagents can harm the environment, humans, and animals, causing illnesses, such as liver damage [ 18 ]. In addition, wastewater generated from nanoparticle synthesis can contain harmful chemicals [ 29 ].
The low yield is another disadvantage: only a small percentage of the starting materials is converted into nanoparticles, generating raw material waste. The high cost of the starting materials, equipment, labor required, long-time synthesis, and the inability to control the size and shape can limit their applications [ 30 , 31 ].
2.2 Strategies to overcome barriers to nanoparticle synthesis
Several strategies can be used to overcome the disadvantages of nanoparticle synthesis, such as the use of environmentally friendly solvents, reagents, and processes. Using water, ionic liquids, and supercritical fluids are examples of eco-friendly solvents [ 21 , 32 ] or we can even perform solvent-free synthesis, eliminating the need for hazardous chemicals and reducing the environmental impact of nanoparticle synthesis [ 33 ].
Many nanoparticle synthesis methods are not scalable, which limits their application. Therefore, it is necessary to develop cost-effective and efficient processes to obtain large quantities of nanoparticles [ 8 ].
Multipurpose nanoparticles can be used to improve their performance in a variety of applications and fields. For example, biocompatible nanoparticles are used in biomedicine or as stable nanoparticles for long-term applications [ 34 ].
The characterization of nanoparticles is important for understanding their size, shape, surface properties, and chemical composition. This information can be used to understand how nanoparticles interact with their environment and ensure they are safe [ 35 ].
Strategies to overcome these barriers in nanoparticle synthesis are still under study to develop more innovative, efficient, cost-effective, and environmentally friendly methods.
3. Green synthesis of nanoparticles: an overview
Green synthesis aims to promote innovative chemical technologies to reduce or eliminate the use and production of hazardous substances in the design, manufacture, and use of chemical products. This involves minimizing or, if possible, eliminating the pollution produced in the synthesis processes, avoiding the consumption and wastage of nonrenewable raw materials, using hazardous or polluting materials in product manufacturing, and reducing the synthesis time. Paul J. Anastas, considered the father of green chemistry, defined it as “a work philosophy that involves the use of alternative tools and pathways to prevent pollution,” referring to both the design of the synthetic strategy and the treatment of possible secondary products originating from that route [ 36 , 37 ].
Two approaches can be used to generate nanoparticles [ 37 , 38 ] ( Figure 2 ).
“Top-down” approach: In which nanoparticles are produced using physical techniques such as grinding or abrasion of a material.
Chemical synthesis: The method of producing molecules or particles by the reaction of substances used as raw materials.
Self-assembly: A technique in which atoms or molecules self-order through physical and/or chemical interactions.
Positional assembly: The atoms, molecules, and aggregates are deliberately manipulated and positioned individually. However, this method is extremely laborious and unsuitable for industrial applications.
The “bottom-up” approach is preferred over the “top-down” approach because specialized equipment is not required and the time to obtain nanoparticles is shorter. Green synthesis is gaining relevance in producing nanoparticles within the “bottom-up” approach [ 37 ].
The use of plant species, algae, or microorganisms such as bacteria or fungi is one of the most commonly used resources for this procedure. Various compounds from plants or microorganisms, including terpenes, polyphenols, alkaloids, carbohydrates, proteins, and genetic materials, play an important role in the synthesis of nanoparticles by acting together [ 39 , 40 ].
In addition to the biological resources used to perform the synthesis (plants, algae, or microorganisms), other factors influence the shape and size of nanoparticles, such as the concentration of the metal ion, pH, reaction time, and temperature [ 39 , 41 ].
Initial phase: Obtaining the reaction medium, which is the aqueous extract of one or several parts of the plant species or the culture media for the growth of microorganisms, in addition to the precursor salt, which is the source of metal ions.
Activation phase: Chemical reduction of metal ions and generation of nucleation centers occur where nanoparticles emerge and grow.
Growth phase: Small adjacent nanoparticles spontaneously fuse into larger particles, forming aggregates, which are influenced by factors such as temperature, concentration, and type of compounds, pH, and reaction time.
Termination phase: The final shape of the nanoparticles is determined, and the compounds that participate in the reaction help stabilize and enhance their properties.
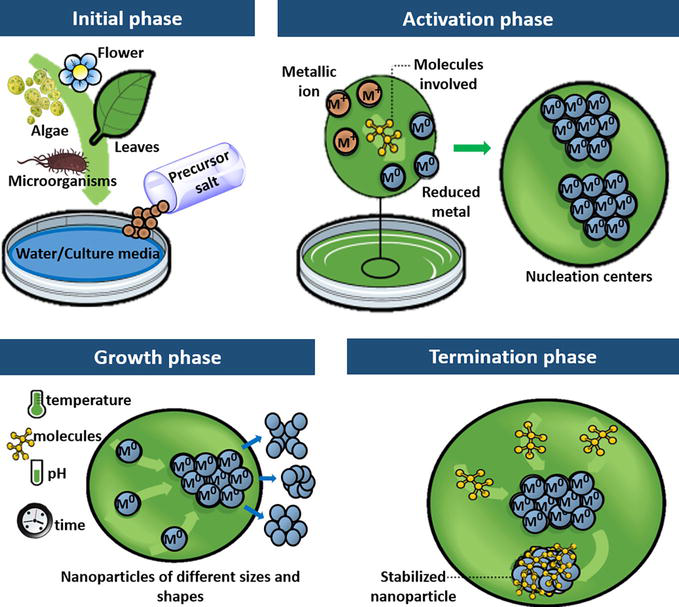
Phases involved in the green synthesis of nanoparticles.
3.1 Biological resources for the green synthesis of nanoparticles
As stated previously, nanoparticles have attracted attention in the fields of biology, medicine, and electronics in recent years, owing to their remarkable applications ( Figure 4 ). Numerous nanoparticle synthesis techniques have been developed; however, these may involve the use of toxic compounds and high-energy physical processes. An alternative is the use of biological methods to circumvent these obstacles. Bacteria, fungi, algae, and plant species are some of the most commonly used biological resources for the green synthesis of nanoparticles ( Figure 4 ). This biological approach has provided a method that is reliable, straightforward, benign, and environmentally beneficial [ 40 , 42 ].
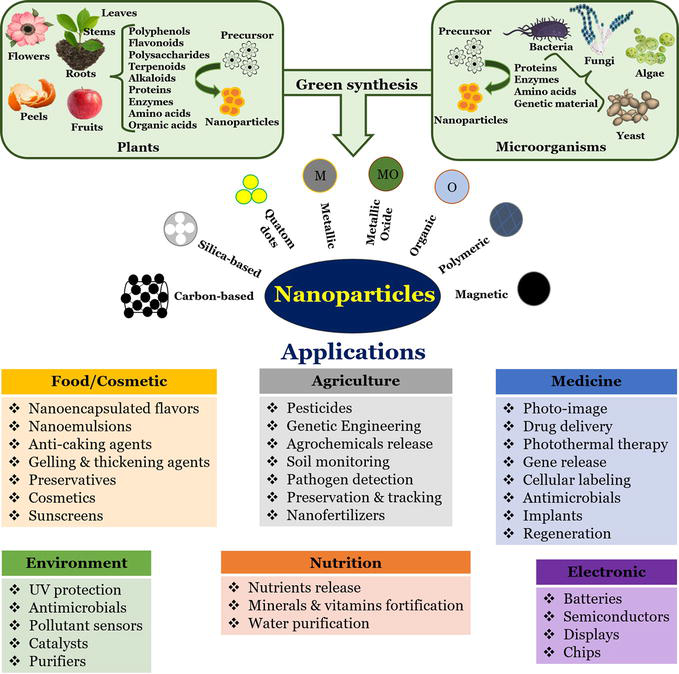
Biological resources and compounds used for the green synthesis of nanoparticles and some of their applications [ 9 ].
3.1.1 Bacteria
Nanoparticle synthesis using bacteria is performed both extracellularly and intracellularly [ 38 ].
Intracellular: The synthesis is carried out inside the living microorganism, using its growth conditions to favor synthesis, known as “nanoparticle micro-factories.” To recover nanoparticles, bacteria must be destroyed [ 43 ].
Extracellular: The components released by the bacteria after lysis are used. The synthesis is performed by adding a metal salt precursor to the medium in which these components are located. Extracellular synthesis has the advantage of being faster because it does not require additional steps to recover nanoparticles from microorganisms [ 43 , 44 ].
Enzymes, such as reductases, which catalyze the reduction of metal ions into nanoparticles, participate in the synthesis. Even components of the genetic material participate in this process [ 45 , 46 ].
3.1.2 Fungi
Fungi contain active biomolecules, such as proteins or enzymes, that participate in nanoparticle synthesis, improving their yields and stability [ 47 ].
Some fungal species can synthesize nanoparticles using extracellular amino acids. For example, glutamic and aspartic acids on the surface of yeast or the reductase enzyme in the cytosol of fungi reduce metal ions to form nanoparticles. This is facilitated by the presence of hydroxyl groups in the mycelium, which donate electrons to the metal ion and reduce it to form nanoparticles. Aliphatic and aromatic amines or some proteins act as coating agents to stabilize them [ 48 , 49 ].
3.1.3 Algae
Algae are used in nanotechnology because of their low toxicity and their ability to bioaccumulate and reduce metals [ 50 ].
Nanoparticle synthesis can be intracellular, with the metal ion entering the alga, or extracellular, and involves compounds such as polysaccharides, proteins, and pigments that direct the reduction of metal ions and coat the newly formed nanoparticles. These particles are subsequently released from the cell in the form of colloids [ 51 ].
3.1.4 Plant species
The use of plants in nanoparticle synthesis is one of the most widely used methods because of its environmentally friendly nature, as it avoids the use of toxic or harmful substances. It is also one of the fastest and most economical methods because it involves fewer steps [ 39 , 40 ]. This makes it highly efficient in the nanoparticle production process compared to synthesis using microorganisms.
Plants contain several compounds (terpenes, flavonoids, polyphenols, alkaloids, proteins, etc.) that reduce metal ions and stabilize the resulting nanoparticles [ 52 ].
This type of synthesis can be performed using intracellular, extracellular, and phytochemical-mediated methods [ 53 ].
Intracellular: The synthesis is carried out inside the plant cell, and the nanoparticles are recovered by breaking down the structure, which is very similar to the intracellular method using microorganisms. Control of the growth factors of plant species is required so that they do not interfere with synthesis [ 54 ].
Extracellular: This method is the most commonly used because of its ease and speed. The process begins by obtaining a plant extract, which is generally water-based, to which a metal salt precursor is added. Owing to the action of the different compounds present in the extract, nanoparticles are generated and stabilized in a single step [ 54 , 55 ].
Phytochemically mediated: This is based on the extracellular method, but with the difference that isolated phytochemical compounds are used and other substances are added to stabilize the nanoparticles. There is greater control over the synthesis, but more components and steps are involved [ 53 ].
3.2 Factors involved in the green synthesis of nanoparticles
As in any synthesis process, reaction conditions, such as temperature, pH, and reaction time, play an important role in the shape, size, and yield of the synthesized nanoparticles [ 39 , 40 , 41 ] ( Figure 3 ).
Temperature: This is one of the most influential factors, as the shape (spherical, prismatic, flakes, triangular, octahedral, etc.), size, and synthesis depend on temperature. As the temperature increases, the reaction rate and the formation of nucleation centers increase, resulting in higher yields. Different temperatures promote different interactions between the reactants, giving rise to various shapes; the larger the temperature increase, the larger the size of the nanoparticles [ 56 , 57 ].
pH: This influences the nucleation centers, generating more centers at higher pH values. Another important influence of pH is that some nanoparticles can only be synthesized in acidic or alkaline media. For example, magnetic nanoparticles are synthesized at an alkaline pH, and metal oxide nanoparticles are generally synthesized at an acidic or neutral pH [ 58 ].
Time: This parameter plays an important role in defining the size of the nanoparticles. It has been observed that longer reaction times favor an increase in the size of the nanoparticles and higher yields, owing to the prolonged interaction time between reactants [ 59 ].
3.3 The mechanism involved in the green synthesis of nanoparticles
The plant extract or organism used for the synthesis is an important factor that influences the morphology and size of nanoparticles because different concentrations of metabolites or cellular components give rise to differences in the nanoparticles [ 40 , 60 ] ( Figure 5 ).
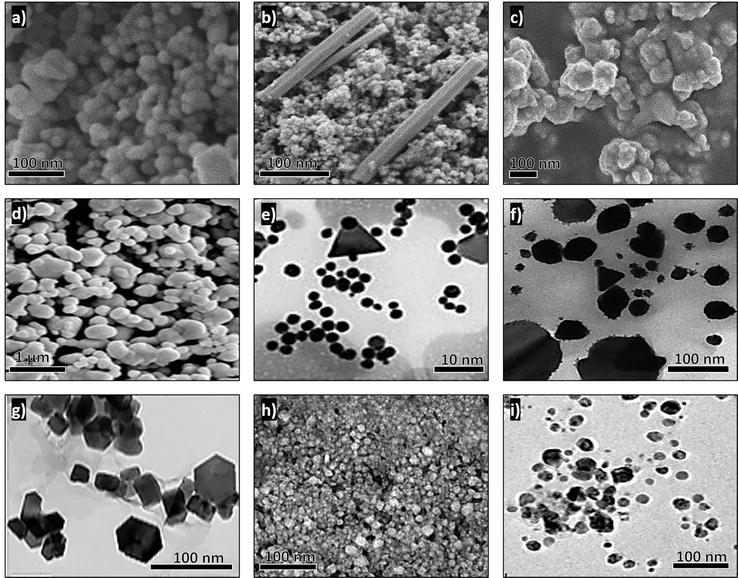
Green-synthesized nanoparticles. (a) Spherical ZnO nanoparticles using the leaves of Dysphania ambrosioides (plant). (b) Prismatic ZnO nanoparticles using the stems and leaves of Dysphania ambrosioides (plant). (c) Quasi-spherical Fe 3 O 4 nanoparticles using the leaves of Datura innoxia (plant). (d) Quasi-spherical Ag nanoparticles using stems of Aloe vera (plant) [ 61 ]. (e) Spherical and triangular Au nanoparticles using Lentinula edodes (fungus) [ 43 ]. (f) Irregular Ag and triangular Au nanoparticles using Ganoderma lucidum (fungus) [ 43 ]. (g) Hexagonal MgO nanoparticles using the flowers of Saussurea costus (plant) [ 62 ]. (h) Irregular Cu nanoparticles using Salmonella typhimurium (bacterium) [ 63 ]. (i) Quasi-spherical Ag nanoparticles using Dunaliella salina (alga) [ 64 ].
Proteins and enzymes facilitate the formation of nanoparticles from metal ions. Because of their high reducing activity, proteins and enzymes can attract metal ions to specific regions of a molecule responsible for reduction, facilitating the formation of nanoparticles; however, their chelating activity is not excessive. The amino acids of a protein can greatly influence the size, morphology, and quantity of nanoparticles generated, thus playing a very important role in determining their shape and yield. Removing a proton from amino acids or other molecules results in the formation of resonant structures capable of further oxidation. This process is accompanied by the active reduction of metal ions followed by the formation of nanoparticles [ 39 ].
Flavonoids are a large group of polyphenolic compounds that can actively chelate and reduce metal ions because they contain multiple functional groups capable of forming these structures. Structural transformations of flavonoids also generate protons that reduce metal ions to form nanoparticles; therefore, they are involved in the nucleation stage, their formation, and further aggregation. Saccharides can also play a role in nanoparticle formation. Monosaccharides, such as glucose, can act as reducing agents, as the aldehyde group of the sugar is oxidized to a carboxyl group through the addition of hydroxyl groups, which in turn leads to the reduction of metal ions and the synthesis of nanoparticles [ 39 ].
The mechanism of green synthesis of nanoparticles has been associated with the action of polyphenols, which act as ligands. Metal ions form coordination compounds, in which the fundamental structural unit is the central metal ion surrounded by coordinated groups arranged spatially at the corners of a regular tetrahedron. The aromatic hydroxyl groups in polyphenols bind to metal ions and form stable coordinated complexes. This system undergoes direct decomposition at high temperatures, releasing nanoparticles from the complex system [ 65 ].
Flavonoids, amino acids, proteins, terpenoids, tannins, and reducing sugars have hydroxyl groups that surround the metal ions to form complexes. After this process, the hydroxyl ions are oxidized to carbonyl groups, which stabilize the nanoparticles. Synthesis is favored if the participating molecules have at least two hydroxyl groups at the ortho- and para-positions [ 52 , 65 ].
Amino acids influence the size, morphology, and yield of nanoparticles generated [ 23 ], depending on the specific amino acids present in the extract and their concentration, along with the reaction conditions that give rise to nanoparticles with different shapes [ 65 ].
4. Confirming that the biological approach of nanoparticle synthesis is a green chemistry method
To corroborate that the processes of nanoparticle synthesis using biological resources are “green synthesis methods,” the 12 principles mentioned above are revisited [ 66 , 67 , 68 ] ( Table 1 ).
The 12 principles of green synthesis are fulfilled with the biological approach to produce nanoparticles.
Considering the above, the 12 principles of green synthesis are fulfilled using biological resources, such as plants, bacteria, fungi, and algae, to synthesize nanoparticles [ 69 , 70 , 71 ].
Finally, green synthesis of nanoparticles is a sustainable and environmentally friendly alternative to traditional methods of nanoparticle synthesis. Traditional methods often take long periods of time, use toxic chemicals and solvents, or generate waste products that can pollute the environment and pose health risks to humans and animals. In contrast, the green synthesis method uses renewable natural resources, such as plant extracts and microorganisms, which are less damaging and can be replenished over time. In addition, these methods are often more cost-effective and faster than traditional procedures because they do not require expensive chemicals or equipment and are considered one-step syntheses, which contribute to energy savings [ 72 ].
In furtherance of these advantages, green synthesis methods are still being developed to improve their efficiency and scalability, leading to the potential benefits of green synthesis of nanoparticles or even their application to the synthesis of other molecules as drugs or nutraceuticals.
5. Conclusion
Nanoparticles have emerged as a versatile and promising class of materials with unique properties that can be harnessed for various applications. The use of green synthesis utilizing natural resources and biologically active compounds to produce nanoparticles is an area of continuous research to improve processes, reduce environmental damage, and meet the increasing demand for the application of these nanostructures. Utilizing biological resources, the synthesis of nanoparticles is inexpensive, faster, and considered a one-step synthesis while preserving or even improving the physical and chemical properties of the nanoparticles. With the great potential of this method and the sustainable and efficient production of nanoparticles, different sizes and shapes can be obtained, which makes it a very attractive option not only for the synthesis of nanostructures, but also for the application of this technique in the synthesis of other compounds.
Acknowledgments
The authors acknowledge Dr. Samuel Tehuacanero Cuapa, Physicist. Roberto Hernández Reyes, and Arq. Diego Quiterio Vargas for their technical support.
Thanks to the Consejo Nacional de Humanidades, Ciencias y Tecnologías (CONAHCYT) for the scholarship granted to Rafael Álvarez-Chimal with the CVU number: 579637.
Funding was provided by the UNAM-DGAPA- PAPIIT project IN112422.
Conflict of interest
The authors declare no conflicts of interest.
- 1. Wu Q , Shou MW, Du ZY, Jun GH, Hui D. Mechanical properties of nanomaterials: A review. Nanotechnology Reviews. 2020; 9 (1):259-273. DOI: 10.1515/ntrev-2020-0021
- 2. Min SH, Lee TH, Lee S, Song JH, Lee GY, Zontar D, et al. Simulation of electrical conductivity for nanoparticles and nanotubes composite sensor according to geometrical properties of nanomaterials. Composites Part B: Engineering. 2019; 174 :107003. DOI: 10.1016/j.compositesb.2019.107003
- 3. Martínez-Mera I, Espinosa-Pesqueira ME, Pérez-Hernández R, Arenas-Alatorre J. Synthesis of magnetite (Fe 3 O 4 ) nanoparticles without surfactants at room temperature. Materials Letters. 2007; 61 (23-24):4447-4451. DOI: 10.1016/j.matlet.2007.02.018
- 4. Kelly KL, Coronado E, Zhao LL, Schatz GC. The optical properties of metal nanoparticles: The influence of size, shape, and dielectric environment. The Journal of Physical Chemistry. B. 2003; 107 (3):668-677. DOI: 10.1021/jp026731y
- 5. Zeng J, Zhang Q , Chen J, Xia Y. A comparison study of the catalytic properties of Au-based nanocages, nanoboxes, and nanoparticles. Nano Letters. 2010; 10 (1):30-35. DOI: 10.1021/nl903062e
- 6. Álvarez-Chimal R, García-Pérez VI, Álvarez-Pérez MA, Arenas-Alatorre JÁ. Green synthesis of ZnO nanoparticles using a Dysphania ambrosioides extract. Structural characterization and antibacterial properties. Materials Science and Engineering: C. 2021; 118 :111540. DOI: 10.1016/j.msec.2020.111540
- 7. Reyes-Carmona L, Camps E, Campos-González E, Mercado-Celis G, Cervantes-Garduño A, Pérez-Ibarra EA, et al. Antimicrobial evaluation of bismuth subsalicylate nanoparticles synthesized by laser ablation against clinical oral microorganisms. Optics and Laser Technology. 2023; 158 :108930. DOI: 10.1016/j.optlastec.2022.108930
- 8. Baig N, Kammakakam I, Falath W. Nanomaterials: A review of synthesis methods, properties, recent progress, and challenges. Materials Advances. 2021; 2 (6):1821-1871. DOI: 10.1039/D0MA00807A
- 9. Chaudhry N, Dwivedi S, Chaudhry V, Singh A, Saquib Q , Azam A, et al. Bio-inspired nanomaterials in agriculture and food: Current status, foreseen applications and challenges. Microbial Pathogenesis. 2018; 123 :196-200. DOI: 10.1016/j.micpath.2018.07.013
- 10. Kolahalam LA, Kasi Viswanath IV, Diwakar BS, Govindh B, Reddy V, Murthy YLN. Review on nanomaterials: Synthesis and applications. Materials Today: Proceedings. 2019; 18 :2182-2190. DOI: 10.1016/j.matpr.2019.07.371
- 11. Khan I, Saeed K, Khan I. Nanoparticles: Properties, applications and toxicities. Arabian Journal of Chemistry. 2019; 12 (7):908-931. DOI: 10.1016/j.arabjc.2017.05.011
- 12. Lu AH, Salabas EL, Schüth F. Magnetic nanoparticles: Synthesis, protection, functionalization, and application. Angewandte Chemie, International Edition. 2007; 46 (8):1222-1244. DOI: 10.1002/anie.200602866
- 13. Zhang L, Gu F, Chan J, Wang A, Langer R, Farokhzad O. Nanoparticles in medicine: Therapeutic applications and developments. Clinical Pharmacology and Therapeutics. 2008; 83 (5):761-769. DOI: 10.1038/sj.clpt.6100400
- 14. Gao J, Gu H, Xu B. Multifunctional magnetic nanoparticles: Design, synthesis, and biomedical applications. Accounts of Chemical Research. 2009; 42 (8):1097-1107. DOI: 10.1021/ar9000026
- 15. Singh R, Altaee A, Gautam S. Nanomaterials in the advancement of hydrogen energy storage. Heliyon. 2020; 6 (7):e04487. DOI: 10.1016/j.heliyon.2020.e04487
- 16. Yao D, Chen Z, Zhao K, Yang Q , Zhang W. Limitation and challenge faced to the researches on environmental risk of nanotechnology. Procedia Environmental Sciences. 2013; 18 :149-156. DOI: 10.1016/j.proenv.2013.04.020
- 17. Wilson N. Nanoparticles: Environmental problems or problem solvers? Bioscience. 2018; 68 (4):241-246. DOI: 10.1093/biosci/biy015
- 18. Ray PC, Yu H, Fu PP. Toxicity and environmental risks of nanomaterials: Challenges and future needs. Journal of Environmental Science and Health, Part C. 2009; 27 (1):1-35. DOI: 10.108010590500802708267/
- 19. Iravani S, Korbekandi H, Mirmohammadi SV, Zolfaghari B. Synthesis of silver nanoparticles: Chemical, physical and biological methods. Research in Pharmaceutical Sciences. 2014; 9 (6):385-406
- 20. Mustapha T, Misni N, Ithnin NR, Daskum AM, Unyah NZ. A review on plants and microorganisms mediated synthesis of silver nanoparticles, role of plants metabolites and applications. International Journal of Environmental Research and Public Health. 2022; 19 (2):674. DOI: 10.3390/ijerph19020674
- 21. Shafey AME. Green synthesis of metal and metal oxide nanoparticles from plant leaf extracts and their applications: A review. Green Processing and Synthesis. 2020; 9 (1):304-339. DOI: 10.1515/gps-2020-0031
- 22. Manikam VR, Cheong KY, Razak KA. Chemical reduction methods for synthesizing Ag and Al nanoparticles and their respective nanoalloys. Materials Science and Engineering B. 2011; 176 (3):187-203. DOI: 10.1016/j.mseb.2010.11.006
- 23. Nam NH, Luong NH. Nanoparticles: Synthesis and applications. In: Materials for Biomedical Engineering. Amsterdam, Netherlands: Elsevier; 2019. pp. 211-240. DOI: 10.1016/B978-0-08-102814-8.00008-1
- 24. Bokov D, Turki Jalil A, Chupradit S, Suksatan W, Javed Ansari M, Shewael IH, et al. Nanomaterial by sol-gel method: Synthesis and application. Advances in Materials Science and Engineering. 2021; 2021 :1-21. DOI: 10.1155/2021/5102014
- 25. Cid A. Synthesis of NPs by microemulsion method. In: Microemulsion - A Chemical Nanoreactor. London, UK: IntechOpen; 2018. DOI: 10.5772/intechopen.80633
- 26. Ndlwana L, Raleie N, Dimpe KM, Ogutu HF, Oseghe EO, Motsa MM, et al. Sustainable hydrothermal and solvothermal synthesis of advanced carbon materials in multidimensional applications: A review. Materials. 2021; 14 (17):5094. DOI: 10.3390/ma14175094
- 27. Ashassi-Sorkhabi H, Rezaei-moghadam B, Bagheri R, Abdoli L, Asghari E. Synthesis of Au nanoparticles by thermal, sonochemical and electrochemical methods: Optimization and characterization. Polymerase Chain Reaction. 2015; 3 (1):24-34. DOI: 10.22036/pcr.2015.7311
- 28. Krishnia L, Thakur P, Thakur A. Synthesis of Nanoparticles by Physical Route. Synthesis and Applications of Nanoparticles. Singapore: Springer Nature Singapore; 2022. pp. 45-59. DOI: 10.1007/978-981-16-6819-7_3
- 29. Khoshnamvand M, Hao Z, Fadare OO, Hanachi P, Chen Y, Liu J. Toxicity of biosynthesized silver nanoparticles to aquatic organisms of different trophic levels. Chemosphere. 2020; 258 :127346. DOI: 10.1016/j.chemosphere.2020.127346
- 30. Rahimi HR, Doostmohammadi M. Nanoparticle synthesis, applications, and toxicity. In: Applications of Nanobiotechnology. London, UK: IntechOpen; 2020. DOI: 10.5772/intechopen.87973
- 31. Jamkhande PG, Ghule NW, Bamer AH, Kalaskar MG. Metal nanoparticles synthesis: An overview on methods of preparation, advantages and disadvantages, and applications. Journal of Drug Delivery Science and Technology. 2019; 53 :101174. DOI: 10.1016/j.jddst.2019.101174
- 32. Ying S, Guan Z, Ofoegbu PC, Clubb P, Rico C, He F, et al. Green synthesis of nanoparticles: Current developments and limitations. Environmental Technology and Innovation. 2022; 26 :102336. DOI: 10.1016/j.eti.2022.102336
- 33. Landge S, Ghosh D, Aiken K. Solvent-Free Synthesis of Nanoparticles, Green Chemistry. Amsterdam, Netherlands: Elsevier; 2018. pp. 609-646. DOI: 10.1016/B978-0-12-809270-5.00022-4
- 34. Kim D, Shin K, Kwon SG, Hyeon T. Synthesis and biomedical applications of multifunctional nanoparticles. Advanced Materials. 2018; 30 (49):1802309. DOI: 10.1002/adma.201802309
- 35. Halamoda-Kenzaoui B, Vandebriel RJ, Howarth A, Siccardi M, David CAW, Liptrott NJ, et al. Methodological needs in the quality and safety characterisation of nanotechnology-based health products: Priorities for method development and standardisation. Journal of Controlled Release. 2021; 336 :192-206. DOI: 10.1016/j.jconrel.2021.06.016
- 36. Anastas PT, Warner JC. Green Chemistry: Theory and Practice. Oxford [England], New York: Oxford University Press; 1998. p. 135
- 37. Singh J, Dutta T, Kim KH, Rawat M, Samddar P, Kumar P. ‘Green’ synthesis of metals and their oxide nanoparticles: Applications for environmental remediation. Journal of Nanbiotechnology. 2018; 16 (1):84. DOI: 10.1186/s12951-018-0408-4
- 38. Singh A, Gautam PK, Verma A, Singh V, Shivapriya PM, Shivalkar S, et al. Green synthesis of metallic nanoparticles as effective alternatives to treat antibiotics resistant bacterial infections: A review. Biotechnology Reports. 2020; 25 :e00427. DOI: 10.1016/j.btre.2020.e00427
- 39. Makarov VV, Love AJ, Sinitsyna OV, Makarova SS, Yaminsky IV, Taliansky ME, et al. “Green” nanotechnologies: Synthesis of metal nanoparticles using plants. Acta Naturae. 2014; 6 (1):35-44. DOI: 10.32607/20758251-2014-6-1-35-44
- 40. Hebbalalu D, Lalley J, Nadagouda MN, Varma RS. Greener techniques for the synthesis of silver nanoparticles using plant extracts, enzymes, bacteria, biodegradable polymers, and microwaves. ACS Sustainable Chemistry & Engineering. 2013; 1 (7):703-712. DOI: 10.1021/sc4000362
- 41. Agarwal H, Venkat Kumar S, Rajeshkumar S. A review on green synthesis of zinc oxide nanoparticles – An eco-friendly approach. Resource-Efficient Technologies. 2017; 3 (4):406-413. DOI: 10.1016/j.reffit.2017.03.002
- 42. Velusamy P, Kumar GV, Jeyanthi V, Das J, Pachaiappan R. Bio-inspired green nanoparticles: Synthesis, mechanism, and antibacterial application. Toxicological Research. 2016; 32 (2):95-102. DOI: 10.5487/TR.2016.32.2.095
- 43. Vetchinkina E, Loshchinina E, Kupryashina M, Burov A, Pylaev T, Nikitina V. Green synthesis of nanoparticles with extracellular and intracellular extracts of basidiomycetes. PeerJ. 2018; 6 :e5237. DOI: 10.7717/peerj.5237
- 44. Das VL, Thomas R, Varghese RT, Soniya EV, Mathew J, Radhakrishnan EK. Extracellular synthesis of silver nanoparticles by the Bacillus strain CS 11 isolated from industrialized area. 3 Biotechnology. 2014; 4 (2):121-126. DOI: 10.1007/s13205-013-0130-8
- 45. Singh P, Kim YJ, Zhang D, Yang DC. Biological synthesis of nanoparticles from plants and microorganisms. Trends in Biotechnology. 2016; 34 (7):588-599. DOI: 10.1016/j.tibtech.2016.02.006
- 46. Messaoudi O, Bendahou M. Biological synthesis of nanoparticles using endophytic microorganisms: Current development. In: Nanotechnology and the Environment. London, UK: IntechOpen; 2020. DOI: 10.5772/intechopen.93734
- 47. Mukherjee P, Ahmad A, Mandal D, Senapati S, Sainkar SR, Khan MI, et al. Fungus-mediated synthesis of silver nanoparticles and their immobilization in the mycelial matrix: A novel biological approach to nanoparticle synthesis. Nano Letters. 2001; 1 (10):515-519. DOI: 10.1021/nl0155274
- 48. Syed A, Ahmad A. Extracellular biosynthesis of platinum nanoparticles using the fungus fusarium oxysporum . Colloids and Surfaces. B, Biointerfaces. 2012; 97 :27-31. DOI: 10.1016/j.colsurfb.2012.03.026
- 49. Riddin TL, Gericke M, Whiteley CG. Analysis of the inter- and extracellular formation of platinum nanoparticles by fusarium oxysporum f. sp. lycopersici using response surface methodology. Nanotechnology. 2006; 17 (14):3482-3489. DOI: 10.1088/0957-4484/17/14/021
- 50. Rana A, Yadav K, Jagadevan S. A comprehensive review on green synthesis of nature-inspired metal nanoparticles: Mechanism, application and toxicity. Journal of Cleaner Production. 2020; 272 :122880. DOI: 10.1016/j.jclepro.2020.122880
- 51. Dahoumane SA, Yéprémian C, Djédiat C, Couté A, Fiévet F, Coradin T, et al. A global approach of the mechanism involved in the biosynthesis of gold colloids using micro-algae. Journal of Nanoparticle Research. 2014; 16 (10):2607. DOI: 10.1007/s11051-014-2607-8
- 52. Carrillo-López LM, Soto-Hernández RM, Zavaleta-Mancera HA, Vilchis-Néstor AR. Study of the performance of the organic extracts of Chenopodium ambrosioides for Ag nanoparticle synthesis. Journal of Nanomaterials. 2016; 2016 :1-13. DOI: 10.1155/2016/4714162
- 53. Dauthal P, Mukhopadhyay M. Noble metal nanoparticles: Plant-mediated synthesis, mechanistic aspects of synthesis, and applications. Industrial and Engineering Chemistry Research. 2016; 55 (36):9557-9577. DOI: 10.1021/acs.iecr.6b00861
- 54. Saim AK, Kumah FN, Oppong MN. Extracellular and intracellular synthesis of gold and silver nanoparticles by living plants: A review. Nanotechnology for Environmental Engineering. 2021; 6 (1):1. DOI: 10.1007/s41204-020-00095-9
- 55. Naikoo GA, Mustaqeem M, Hassan IU, Awan T, Arshad F, Salim H, et al. Bioinspired and green synthesis of nanoparticles from plant extracts with antiviral and antimicrobial properties: A critical review. Journal of Saudi Chemical Society. 2021; 25 (9):101304. DOI: 10.1016/j.jscs.2021.101304
- 56. Álvarez-Chimal R, García-Pérez VI, Álvarez-Pérez MA, Tavera-Hernández R, Reyes-Carmona L, Martínez-Hernández M, et al. Influence of the particle size on the antibacterial activity of green synthesized zinc oxide nanoparticles using Dysphania ambrosioides extract, supported by molecular docking analysis. Arabian Journal of Chemistry. 2022; 15 (6):103804. DOI: 10.1016/j.arabjc.2022.103804
- 57. Thanh NTK, Maclean N, Mahiddine S. Mechanisms of nucleation and growth of nanoparticles in solution. Chemical Reviews. 2014; 114 (15):7610-7630. DOI: 10.1021/cr400544s
- 58. Handayani W, Ningrum AS, Imawan C. The role of pH in synthesis silver nanoparticles using Pometia pinnata (Matoa) leaves extract as bioreductor. Journal of Physics: Conference Series. 2020; 1428 (1):012021. DOI: 10.1088/1742-6596/1428/1/012021
- 59. De Oliveira RC, Amoresi RAC, Marana NL, Zaghete MA, Ponce M, Chiquito AJ, et al. Influence of synthesis time on the morphology and properties of CeO 2 nanoparticles: An experimental–Theoretical study. Crystal Growth and Design. 2020; 20 (8):5031-5042. DOI: 10.1021/acs.cgd.0c00165
- 60. Kuppusamy P, Yusoff MM, Maniam GP, Govindan N. Biosynthesis of metallic nanoparticles using plant derivatives and their new avenues in pharmacological applications – An updated report. Saudi Pharmaceutical Journal. 2016; 24 (4):473-484. DOI: 10.1016/j.jsps.2014.11.013. DOI: 10.1016/j.molstruc.2016.12.069
- 61. Tippayawat P, Phromviyo N, Boueroy P, Chompoosor A. Green synthesis of silver nanoparticles in aloe vera plant extract prepared by a hydrothermal method and their synergistic antibacterial activity. PeerJ. 2016; 4 . DOI: 10.7717/peerj.2589
- 62. Amina M, Al Musayeib NM, Alarfaj NA, El-Tohamy MF, Oraby HF, Al Hamoud GA, et al. Biogenic green synthesis of MgO nanoparticles using Saussurea costus biomasses for a comprehensive detection of their antimicrobial, cytotoxicity against MCF-7 breast cancer cells and photocatalysis potentials. PLoS One. 2020; 15 (8). DOI: 10.1371/journal.pone.0237567
- 63. Ghorbani HR. Extracellular synthesis of copper nanoparticles using culture supernatants of salmonella typhimurium . Oriental Journal of Chemistry. 2015; 31 (1):527-529. DOI: 10.13005/ojc/310165
- 64. Singh AK, Tiwari R, Kumar V, Singh P, Riyazat Khadim SK, Tiwari A, et al. Photo-induced biosynthesis of silver nanoparticles from aqueous extract of Dunaliella salina and their anticancer potential. Journal of Photochemistry and Photobiology, B: Biology. 2017; 166 :202-211. DOI: 10.1016/j.jphotobiol.2016.11.020
- 65. Nava OJ, Luque PA, Gómez-Gutiérrez CM, Vilchis-Nestor AR, Castro-Beltrán A, Mota-González ML, et al. Influence of Camellia sinensis extract on zinc oxide nanoparticle green synthesis. Journal of Molecular Structure. 2017; 1134 :121-125
- 66. Duan H, Wang D, Li Y. Green chemistry for nanoparticle synthesis. Chemical Society Reviews. 2015; 44 (16):5778-5792. DOI: 10.1039/C4CS00363B
- 67. Razavi M, Salahinejad E, Fahmy M, Yazdimamaghani M, Vashaee D, Tayebi L. Green Chemical and Biological Synthesis of Nanoparticles and their Biomedical Applications. Green Processes for Nanotechnology. Cham: Springer International Publishing; 2015. pp. 207-235. DOI: 10.1007/978-3-319-15461-9_7
- 68. Badria F, AbouHabieb M, Bar FA. Synthesis of Nanoparticles Using Green Chemistry Green Synthesis. 1. Auflage ed. München: Grin Verlag; 2019
- 69. Chopra H, Bibi S, Singh I, Hasan MM, Khan MS, Yousafi Q , et al. Green metallic nanoparticles: Biosynthesis to applications. Frontiers in Bioengineering and Biotechnology. 2022; 10 :874742. DOI: 10.3389/fbioe.2022.874742
- 70. Michael A, Singh A, Roy A, Islam MR. Fungal- and algal-derived synthesis of various nanoparticles and their applications. Bioinorganic Chemistry and Applications. 2022; 2022 :1-14. DOI: 10.1155/2022/3142674
- 71. Raj S, Trivedi R, Soni V. Biogenic synthesis of silver nanoparticles, characterization and their applications—A review. Surfaces. 2021; 5 (1):67-90. DOI: 10.3390/surfaces5010003
- 72. Kebede MA, Wubieneh TA, Yohannes YB, Shah KJ. Green synthesis of zinc oxide from aqueous fruit extract of Dovyalis abyssinica (Koshem) and application for water purification. Ethiopian Journal of Science and Technology. 2023; 16 (1):1-12. DOI: 10.4314/ejst.v16i1.1
© The Author(s). Licensee IntechOpen. This chapter is distributed under the terms of the Creative Commons Attribution 3.0 License , which permits unrestricted use, distribution, and reproduction in any medium, provided the original work is properly cited.
Continue reading from the same book
Advances in green chemistry.
Edited by Kinjal Shah
Published: 31 January 2024
By Faisal Nawaz, Irum Jamil and Bushra Shakoor
84 downloads
By Anja Verbič, Gregor Primc, Martin Šala and Marija ...
48 downloads
195 downloads
Green Synthesis of Nanoparticles Using Different Plant Extracts and Their Characterizations
- First Online: 23 July 2021
Cite this chapter
- Lina M. Alnaddaf 4 ,
- Abdulsalam K. Almuhammady 5 ,
- Khaled F. M. Salem 6 nAff7 ,
- Maysaa T. Alloosh 8 ,
- Maysoun M. Saleh 9 &
- Jameel M. Al-Khayri 10
1329 Accesses
5 Citations
The green nanoparticles synthesis is a modern field that currently resonates compared to other preparation methods due to its characteristics that make it used in all fields. This chapter briefly explained traditional and biological methods for preparing nanomaterials and mentioned the advantage and disadvantage to these methods, then explained in more detail the phytofabrication of nanoparticles from different parts of the plant, which are considered a good source for biological molecules that act as reducing agents and modifies metal ions into nanoparticles that have unique properties. It also illustrates the green methods for preparing nanoparticles such as silver, zinc oxide and copper in some detail and their reaction conditions which influence the size, shape and structure of NPs. In addition to mechanisms of their formation and the different biomolecules that contribute to its synthesis.
This is a preview of subscription content, log in via an institution to check access.
Access this chapter
- Available as PDF
- Read on any device
- Instant download
- Own it forever
- Available as EPUB and PDF
- Compact, lightweight edition
- Dispatched in 3 to 5 business days
- Free shipping worldwide - see info
- Durable hardcover edition
Tax calculation will be finalised at checkout
Purchases are for personal use only
Institutional subscriptions
Abdullah FH, Bakar NA, Bakar MA (2020) Low-temperature biosynthesis of crystalline zinc oxide nanoparticles from Musa acuminata peel extract for visible-light degradation of methylene blue. Optik 206:164279
Article CAS Google Scholar
Ahmed S, Saifullah Ahmad M et al (2016) Green synthesis of silver nanoparticles using Azadirachta indica aqueous leaf extract. J Radiat Res Appl Sci 9:1–7. https://doi.org/10.1016/j.jrras.2015.06.006
Ahmad S, Munir S, Zeb N et al (2019) Green nanotechnology: a review on green synthesis of silver nanoparticles-an ecofriendly approach. Inter J Nanomedicine 14:5087–5107
Aina DA, Owolo O, Lateef A et al (2019) Biomedical applications of Chasmanthera dependens stem extract mediated silver nanoparticles as antimicrobial, antioxidant, anticoagulant, thrombolytic and Larvicidal agents. Karbala Int J Mod Sci 5:70–80. https://doi.org/10.33640/2405-609X.1018
Article Google Scholar
Ajitha B, Reddy YAK, Reddy PS (2014) Biogenic nano-scale silver particles by Tephrosia purpurea leaf extract and their inborn antimicrobial activity. Spectrochimica Acta A 121:164–172. https://doi.org/10.1016/j.saa.2013.10.077
Al Banna LS, Salem NM, Jaleel GA et al (2020) Green synthesis of sulfur nanoparticles using Rosmarinus officinalis leaves extract and nematicidal activity against Meloidogyne javanica . Chem Int 6:137–143
CAS Google Scholar
Alagarasi A (2011) Introduction to nanomaterials. In: Viswanathan B (ed) Nanomaterials. Narosa Publishing House pp 1–76
Google Scholar
Alishah H, Seyedi SP, Ebrahimipour SY et al (2016) A green approach for the synthesis of silver nanoparticles using root extract of Chelidonium majus : characterization and antibacterial evaluation. J Clust Sci 27:421–429
Al-Othman MR, Abeer AE (2019) Effect of green synthesis silver nanoparticles from five fruits peel on protein capped and anti-fungal properties. Int J Adv Res Biol Sci 6(2):156–165
Alqadi MK, Abo Noqtah OA, Alzoubi FY et al (2014) pH effect on the aggregation of silver nanoparticles synthesized by chemical reduction. Mater Sci Pol 32:107–111. https://doi.org/10.2478/s13536-013-0166-9
Armendariz V, Herrera I, Peralta-Videa JR et al (2004) Size controlled gold nanoparticle formation by Avena sativa biomass: use of plants in nanobiotechnology. J Nanopart Res 6:377–382. https://doi.org/10.1007/s11051-004-0741-4
Arokiyaraj S, Vincent S, Saravanan M et al (2017) Green synthesis of silver nanoparticles using Rheum palmatum root extract and their antibacterial activity against Staphylococcus aureus and Pseudomonas aeruginosa . Artif Cell Nanomed Biotechnol 45:372–379
Aswini R, Murugesan S, Kannan K (2020) Bio-engineered TiO 2 nanoparticles using Ledebouria revoluta extract: Larvicidal, histopathological, antibacterial and anticancer activity. Int J Environ Anal Chem 1–11 https://doi.org/10.1080/03067319.2020.1718668
Awwad AM, Amer MW (2020) Biosynthesis of copper oxide nanoparticles using Ailanthus altissima leaf extract and antibacterial activity. Chem Int 6:210–217
Awwad AM, Amer MW, Salem NM et al (2020) Green synthesis of zinc oxide nanoparticles (ZnO-NPs) using Ailanthus altissima fruit extracts and antibacterial activity. Chem Int 6:151–159
Aygün A, Gülbağça F, Nas MS et al (2020) Biological synthesis of silver nanoparticles using Rheum ribes and evaluation of their anticarcinogenic and antimicrobial potential: a novel approach in phytonanotechnology. J Pharm Biomed Anal 179:1–24. https://doi.org/10.1016/j.jpba.2019.113012
Azarbani F, Shiravand S (2020) Green synthesis of silver nanoparticles by Ferulago macrocarpa flowers extract and their antibacterial, antifungal and toxic effects. Green Chem Lett Rev 13:41–49
Backx BP, Santana JCS (2018) Green synthesis of polymer blend impregnated with silver nanoparticles in Euterpe Oleracea dispersive medium. Inter J Green Herbal Chem 7:424–429. https://doi.org/10.24214/IJGHC/GC/7/2/42429
Balasubramanian S, Jeyapaul U, Kala SMJ (2019) Antibacterial activity of silver nanoparticles using Jasminum auriculatum stem extract. Int J Nanosci 18:1850011
Baer DR (2011) Surface characterization of nanoparticles: critical needs and significant challenges. J Surf Anal 17(3):163–169. https://doi.org/10.1384/jsa.17.163
Article CAS PubMed PubMed Central Google Scholar
Barman K, Chowdhury D, Baruah PK (2020) Bio-synthesized silver nanoparticles using Zingiber officinale rhizome extract as efficient catalyst for the degradation of environmental pollutants. Inorg Nano-Met Chem 50:57–65. https://doi.org/10.1080/24701556.1661468
Bartolucci C, Antonacci A, Arduini F et al (2020) Green nanomaterials fostering agrifood sustainability. TrAC Trend Anal Chem 125: https://doi.org/10.1016/j.trac.2020.115840
Baruwati B, Varma RS (2009) High value products from waste: grape pomace extract-a three-in-one package for the synthesis of metal nanoparticles. Chem Sus Chem 2:1041–1044
Behravan M, Panahi AH, Naghizadeh A et al (2019) Facile green synthesis of silver nanoparticles using Berberis vulgaris leaf and root aqueous extract and its antibacterial activity. Int J Biol Macromol 124:148–154
Article CAS PubMed Google Scholar
Bharathi D, Vasantharaj S, Bhuvaneshwari V (2018) Green synthesis of silver nanoparticles using Cordia dichotoma fruit extract and its enhanced antibacterial, anti-biofilm and photo catalytic activity. Mater Res Express 5:1–24
Biasiolo M, MTD C, Tornadore N (2004) Micromorphological characterization of ten mulberry cultivars ( Moru s spp). Econ Bot 58:639–646. https://doi.org/10.1663/00130001
Bibi I, Nazar N, Ata S et al (2019) Green synthesis of iron oxide nanoparticles using pomegranate seeds extract and photocatalytic activity evaluation for the degradation of textile dye. J Mater Res Technol 8:6115–6124
Bindhu MR, Umadevi M, Esmail GA et al (2020) Green synthesis and characterization of silver nanoparticles from Moringa oleifera flower and assessment of antimicrobial and sensing properties. J Photochem Photobiol B:1–29
Bin-Jumah M, Monera A-A, Albasher G et al (2020) Effects of green silver nanoparticles on apoptosis and oxidative stress in normal and cancerous human hepatic cells in vitro. Int J Nanomedicine 15:1537–1548
Blank DE, Justen D, Fraga S et al (2018) Chemical composition and antioxidant activity of Bunchosia glandulifera fruit at different ripening stages. Food Nutr Sci 9:1147–1159. https://doi.org/10.4236/fns.2018.910083
Buazar F, Sweidi S, Badri M et al (2019) Biofabrication of highly pure copper oxide nanoparticles using wheat seed extract and their catalytic activity: a mechanistic approach. Green Process Synth 8:691–702
Chinnappan S, Kandasamy S, Arumugam S et al (2017) Biomimetic synthesis of silver nanoparticles using flower extract of Bauhinia purpurea and its antibacterial activity against clinical pathogens. Environ Sci Pollut Res:1–7. https://doi.org/10.1007/s11356-017-0841-1
Chokkalingam M, Singh P, Huo Y et al (2019) Facile synthesis of Au and Ag nanoparticles using fruit extract of Lycium chinense and their anticancer activity. J Drug Deliv Sci Technol 49:308–315
Dhand C, Dwivedi N, Jun Loh X et al (2015) Methods and strategies for the synthesis of diverse nanoparticles and their applications: a comprehensive overview. RSC Adv 5:105003–105037. https://doi.org/10.1039/c5ra19388e
Danbature WL, Shehu Z, Yoro M, Adam MM (2020) Nanolarvicidal effect of green synthesized Ag-Co bimetallic nanoparticles on Culex quinquefasciatus Mosquito. Adv Biol Chem 10:16
Darroudi M, Ahmad MB, Zamiri R et al (2011) Time-dependent effect in green synthesis of silver nanoparticles. Int J Nanomed 6:677–681. https://doi.org/10.2147/IJN.S17669
Dash SS, Samanta S, Dey S et al (2020) Rapid green synthesis of biogenic silver nanoparticles using Cinnamomum tamala leaf extract and its potential antimicrobial application against clinically isolated multidrug-resistant bacterial strains. Biol Trace Elem Res. https://doi.org/10.1007/s12011-020-02107-w
Article PubMed Google Scholar
Dawodu FA, Onuh CU, Akpomie KG et al (2019) Synthesis of silver nanoparticle from Vigna unguiculata stem as adsorbent for malachite green in a batch system. SN Appl Sci 1:346. https://doi.org/10.1007/s42452-019-0353-3
Dudhane AA, Waghmode SR, Dama LB et al (2019) Synthesis and characterization of gold nanoparticles using plant extract of Terminalia arjuna with antibacterial activity. Int J Nanosci Nanotechnol 15:75–82
Ezealisiji KM, Noundou XS, Ukwueze SE (2017) Green synthesis and characterization of monodispersed silver nanoparticles using root bark aqueous extract of Annona muricata Linn and their antimicrobial activity. Appl Nanosci 7:905–911
Gajendran B, Durai P, Varier KM et al (2019) Green synthesis of silver nanoparticle from Datura inoxia flower extract and its cytotoxic activity. BioNanoSci 9:564–572
Gamez G, Gardea-Torresdey JL, Tiemann KJ et al (2003) Recovery of gold (III) from multi-elemental solutions by alfalfa biomass. Adv Environ Res 7(2):563–571. https://doi.org/10.1016/S1093-0191(02)00021-7
Gan PP, Li SF (2012) Potential of plant as a biological factory to synthesize gold and silver nanoparticles and their applications. Rev Environ Sci Bio 11(2):169–206. https://doi.org/10.1007/s11157-012-9278-7
Gebremedhn K, Kahsay MH, Aklilu M (2019) Green synthesis of CuO nanoparticles using leaf extract of Catha edulis and its antibacterial activity. J Pharm Pharmacol 7:327–342. https://doi.org/10.17265/2328-2150/2019.06.007
Gnanasangeetha D, Thambavani DS (2013) Biogenic production of zinc oxide nanoparticles using Acalypha indica . J Chem Biol Phys Sci 4(1):238–246
Gomathi AC, Rajarathinam SX, Sadiq AM et al (2020) Anticancer activity of silver nanoparticles synthesized using aqueous fruit shell extract of Tamarindus indica on MCF-7 human breast cancer cell line. J Drug Deliv Sci Technol 55:101376
Gonnelli C, Cacioppo F, Giordano C et al (2015) Cucurbita pepo L. extracts as a versatile hydrotropic source for the synthesis of gold nanoparticles with different shapes. Green Chem Lett Rev 8:1 39–47
Gour A, Jain NK (2019) Advances in green synthesis of nanoparticles. Artif Cells Nanomed Biotechnol 47:844–851. https://doi.org/10.1080/21691401.2019.1577878
Gupta M, Tomar RS, Mishra RK (2020) Factors affecting biosynthesis of green nanoparticles. Our Heritage 68:10535–10555
Haider A, Ijaz M, Ali S et al (2020) Green synthesized phytochemically ( Zingiber officinale and Allium sativum ) reduced nickel oxide nanoparticles confirmed bactericidal and catalytic potential. Nanoscale Res Lett 15:1–11
Hassan KH, Saadi SK, Jarullah AA et al (2018) Green synthesis and structural characterisation of CuO nanoparticles prepared by using fig leaves extract. Pak J Sci Ind Res Ser Phys Sci 61:59–65
Hemmati S, Rashtiani A, Zangeneh MM et al (2019) Green synthesis and characterization of silver nanoparticles using fritillaria flower extract and their antibacterial activity against some human pathogens. Polyhedron 158:8–14
Hussain I, Singh NB, Singh A et al (2016) Green synthesis of nanoparticles and its potential application. Biotechnol Lett 38:545–560
Irshad S, Riaz M, Anjum AA et al (2020) Biosynthesis of ZnO nanoparticles using Ocimum Basilicum and determination of its antimicrobial activity. J Anim Plant Sci 30:185–191
Ismail MIM (2020) Green synthesis and characterizations of copper nanoparticles. Mater Chem Phys 240: https://doi.org/10.1016/j.matchemphys.2019.122283
Jagtap UB, Bapat VA (2013) Green synthesis of silver nanoparticles using Artocarpus heterophyllus Lam. seed extract and its antibacterial activity. Ind Crops Prod 46:132–137. https://doi.org/10.1016/j.indcrop.2013.01.019
Jayaprakash N, Vijaya JJ, Kaviyarasu K et al (2017) Green synthesis of Ag nanoparticles using Tamarind fruit extract for the antibacterial studies. J Photochem Photobiol, B 169:178–185
Jebril S, Dridi C (2020) Green synthesis of silver nanoparticles using Melia azedarach leaf extract and their antifungal activities: in vitro and in vivo . Mater Chem Phys 122898
Kalaiselvi D, Mohankumar A, Shanmugam G et al (2019) Green synthesis of silver nanoparticles using latex extract of Euphorbia tirucalli : a novel approach for the management of root-knot nematode, Meloidogyne incognita . Crop Prot 117:108–114
Kanniah P, Radhamani J, Chelliah P et al (2020) Green synthesis of multifaceted silver nanoparticles using the flower extract of Aerva lanata and evaluation of its biological and environmental applications. Chem Select 5:2322–2331
Karpagavinayagam P, Vedhi C (2019) Green synthesis of iron oxide nanoparticles using Avicennia marina flower extract. Vacuum 160:286–292
Khan MM, Saadah NH, Khan ME et al (2019) Phytogenic synthesis of band gap-narrowed ZnO nanoparticles using the bulb extract of Costus woodsonii . BioNanoSci 9:334–344
Kharissova OV, Dias HR, Kharisov BI et al (2013) The greener synthesis of nanoparticles. Trends Biotechnol 31(4):240–248
Kuchibhatla SV, Karakoti AS, Baer DR et al (2012) Influence of aging and environment on nanoparticle chemistry: implication to confinement effects in nanoceria. J Phys Chem C 116(26):14108–14114
Lakshmanan G, Sathiyaseelan A, Kalaichelvan PT et al (2018) Plant-mediated synthesis of silver nanoparticles using fruit extract of Cleome viscosa L.: assessment of their antibacterial and anticancer activity. Karbala Int J Mod Sci 4:61–68
Liu D, Liu L, Yao L et al (2020) Synthesis of ZnO nanoparticles using radish root extract for effective wound dressing agents for diabetic foot ulcers in nursing care. J Drug Deliv Sci Technol 55:
Makarov V, Love AJ, Makarova SS, Sinitsyna OV (2014) Biosynthesis of stable iron oxide nanoparticles in aqueous extracts of Hordeum vulgare and Rumex acetosa plants. Langmuir 30(20):5982–5988. https://doi.org/10.1021/la5011924
Matijevic E (1977) The role of chemical complexing in the formation and stability of colloidal dispersions. J Colloid Interf Sci 58(2):374–389
Matijevic E (1985) Production of monodispersed colloidal particles. Annu Rev Mater Sci 15(1):483–516
Manjari G, Saran S, Arun T et al (2017) Catalytic and recyclability properties of phytogenic copper oxide nanoparticles derived from Aglaia elaeagnoidea flower extract. J Saudi Chem Soc 21:610–618. https://doi.org/10.1016/j.jscs.2017.02.004
Marchiol L, Mattiello A, Pošćić F et al (2014) In vivo synthesis of nanomaterials in plants: location of silver nanoparticles and plant metabolism. Nanoscale Res Lett 9:101. https://doi.org/10.1186/1556-276x-9-101
Article PubMed PubMed Central Google Scholar
Mari A, Vincent MV, Mookkaiah R et al (2020) Catharanthus roseus leaf extract mediated facile green synthesis of copper oxide nanoparticles and its photocatalytic activity. Chem Method 424–436
Martínez-Fernández D, Barroso D, Komárek M (2016) Root water transport of Helianthus annuus L. under iron oxide nanoparticle exposure. Environ Sci Pollut Res 23(2):1732–1741
Maurya IC, Singh S, Senapati S et al (2019) Green synthesis of TiO 2 nanoparticles using Bixa orellana seed extract and its application for solar cells. J Sol Energy 194:952–958
Mohammadi F, Yousefi M, Ghahremanzadeh R (2019) Green synthesis, characterization and antimicrobial activity of silver nanoparticles (AgNPs) using leaves and stems extract of some plants. Adv J Chem A 2:266–275
Mohammed AE (2016) Arta ( Calligonum Comosum , L’Her.) shoot extracts: bio-mediator in silver nanoparticles formation and antimycotic potential. Nano Biomed Eng 8:128–135
Mohseni MS, Khalilzadeh MA, Mohseni M et al (2020) Green synthesis of Ag nanoparticles from pomegranate seeds extract and synthesis of Ag-Starch nanocomposite and characterization of mechanical properties of the films. Biocatal Agric Biotechnol 101569:1–31. https://doi.org/10.1016/j.bcab.2020.101569
Mondal A, Hajra A, Shaikh WA et al (2019) Synthesis of silver nanoparticle with Colocasia esculenta (L.) stem and its larvicidal activity against Culex quinquefasciatus and Chironomus sp. Asian Pac J Trop Biomed 9:510–517
Mudunkotuwa IA, Pettibone JM, Grassian VH (2012) Environmental implications of nanoparticle aging in the processing and fate of copper-based nanomaterials. Environ Sci Technol 46(13):7001–7010. https://doi.org/10.1021/es203851d
Mukha I, Vityuk N, Severynovska O et al (2016) The pH-dependent stucture and properties of Au and Ag nanoparticles produced by tryptophan reduction. Nanoscale Res Lett 11:101. https://doi.org/10.1186/s11671-016-1318-8
Nadagouda MN, Varma RS (2008) Green synthesis of silver and palladium nanoparticles at room temperature using coffee and tea extract. Green Chem 10:859–862
Nagajyothi PC, Cha SJ, Yang IJ et al (2015) Antioxidant and anti-inflammatory activities of zinc oxide nanoparticles synthesized using Polygala tenuifolia root extract. J Photochem Photobiol, B 146:10–17
Nasseri MA, Shahabi M, Allahresani A et al (2019) Eco-friendly biosynthesis of silver nanoparticles using aqueous solution of Spartium junceum flower extract. Asian J Green Chem 3:382–390
Nnadozie EC, Ajibade PA (2020) Green synthesis and characterization of magnetite (Fe 3 O 4 ) nanoparticles using Chromolaena odorata root extract for smart nanocomposite. Mater Lett 263:127145
Nordin NR, Shamsuddin M (2019) Biosynthesis of copper (II) oxide nanoparticles using Murayya koeniggi aqueous leaf extract and its catalytic activity in 4-nitrophenol reduction. Malays J Fundam Appl Sci 15:218–224
Odeniyi MA, Okumah VC, Adebayo-Tayo BC et al (2020) Green synthesis and cream formulations of silver nanoparticles of Nauclea latifolia (African peach) fruit extracts and evaluation of antimicrobial and antioxidant activities. Sustain Chem Pharm 15:100197
Oza G, Reyes-Calderón A, Mewada A et al (2020) Plant-based metal and metal alloy nanoparticle synthesis: a comprehensive mechanistic approach. J Mater Sci 55:1309–1330. https://doi.org/10.1007/s10853-019-04121-3
Pansambal S, Gavande S, Ghotekar S et al (2017) Green synthesis of CuO nanoparticles using Ziziphus Mauritiana L. extract and its characterizations. Int J Sci Res Sci Tech 3:1388–1392
Park J, Kwon SG, Jun SW et al (2012) Large-scale synthesis of ultra-small-sized silver nanoparticles. Chem Phys Chem 13:2540–2543
Patil MP, Singh RD, Koli PB et al (2018) Antibacterial potential of silver nanoparticles synthesized using Madhuca longifolia flower extract as a green resource. Microb Pathog 121:184–189. https://doi.org/10.1016/j.micpath.2018.05.040
Pethakamsetty L, Kothapenta K, Nammi HR et al (2017) Green synthesis, characterization and antimicrobial activity of silver nanoparticles using methanolic root extracts of Diospyros sylvatica . J Environ Sci 55:157–163
Pirtarighat S, Ghannadnia M, Baghshahi S (2019) Green synthesis of silver nanoparticles using the plant extract of Salvia spinosa grown in vitro and their antibacterial activity assessment. J Nanostructure Chem 9:1–9. https://doi.org/10.1007/s40097-018-0291-4
Ponvel KM, Narayanaraja T, Prabakaran J (2015) Biosynthesis of silver nanoparticles using root extract of the medicinal plant Justicia adhatoda : characterization, electrochemical behavior and applications. Int J Nano Dimens 6(4):339–349
Poojary MM, Passamonti P, Adhikari AV (2016) Green synthesis of silver and gold nanoparticles using root bark extract of Mammea suriga : characterization, process optimization and their antibacterial activity. BioNanoSci 6:110–120
Prachi AN, Negi DS (2019) Plant mediated synthesis of zinc oxide nanoparticles using leaf and twig extract of Premna Barbata : a comparative approach on its physical characteristic and antibacterial property. Plant Arch 19:2469–2475
Soni N, Prakash NS (2011) Factors affecting the geometry of silver nanoparticles synthesis in Chrysosporium tropicum and Fusarium oxysporum . Am J Nanotechnol 2:112–121. https://doi.org/10.3844/ajnsp.2011.112.121
Rafique M, Tahir R, Gillani SSA et al (2020) Plant-mediated green synthesis of zinc oxide nanoparticles from Syzygium Cumini for seed germination and wastewater purification. Int J Environ Anal Chem. https://doi.org/10.1080/03067319.2020.1715379
Rai A, Singh A, Ahmad A, Sastry M (2006) Role of halide ions and temperature on the morphology of biologically synthesized gold nanotriangles. Langmuir 22(2):736–741
Rajagopal T, Jemimah IAA, Ponmanickam P et al (2015) Synthesis of silver nanoparticles using Catharanthus roseus root extract and its larvicidal effects. J Environ Biol 36:1283
CAS PubMed Google Scholar
Ramesh R, Catherine G, John Sundaram S et al (2020) Synthesis of Mn 3 O 4 nano complex using aqueous extract of Helianthus annuus seed cake and its effect on biological growth of Vigna radiata . Mater Today Proc:8. https://doi.org/10.1016/j.matpr.2020.02.883
Rao NH, Lakshmidevi N, Pammi SVN et al (2016) Green synthesis of silver nanoparticles using methanolic root extracts of Diospyros paniculata and their antimicrobial activities. Mater Sci Eng, C 62:553–557
Rashmi TS, Divyashree K, Gagan KK (2018) Bioactive compound profiling and green synthesis of silver nanoparticle; its characterization using bamboo shoot extract. Int J Biol Med Res 9:6235–6244
Ratchagar V, Jagannathan K (2016) Effect of pH on magnetic, thermal and dielectric properties of SnO 2 nanomaterials. J Alloys Compd 689:1088–1095
Rawat A, Kumar R, Bhatt B, Ram P (2018) Nanotechnology in agriculture—a review. Int J Curr Microbiol App Sci 7(8):969–978
Rostamizadeh E, Iranbakhsh A, Majd A et al (2020) Green synthesis of Fe 2 O 3 nanoparticles using fruit extract of Cornus mas L. and its growth-promoting roles in barley. J Nanostruct Chem 10:125–130. https://doi.org/10.1007/s40097-020-00335-z
Salari S, Bahabadi SE, Samzadeh-Kermani A et al (2019) In-vitro evaluation of antioxidant and antibacterial potential of green synthesized silver nanoparticles using Prosopis farcta fruit extract. Iran J Pharm Res 18(1):430–455
CAS PubMed PubMed Central Google Scholar
Sandhya J, Kalaiselvam S (2020) Biogenic synthesis of magnetic iron oxide nanoparticles using inedible borassus flabellifer seed coat: characterization, antimicrobial, antioxidant activity and in vitro cytotoxicity analysis. Mater Res Express 7:015045. https://doi.org/10.1088/2053-1591/ab6642
Sankaranarayanan A, Munivel G, Karunakaran G et al (2017) Green synthesis of silver nanoparticles using Arachis hypogaea (ground nut) root extract for antibacterial and clinical applications. J Clust Sci 28:995–1008
Sathishkumar G, Logeshwaran V, Sarathbabu S et al (2018) Green synthesis of magnetic Fe 3 O 4 nanoparticles using Couroupita guianensis Aubl. fruit extract for their antibacterial and cytotoxicity activities. Artif Cells Nanomed Biotechnol 46:589–598
Shaik AM, Raju MD, Reddy DR (2020) Green synthesis of zinc oxide nanoparticles using aqueous root extract of Sphagneticola trilobata L. in and investigate its role in toxic metal removal, sowing germination and fostering of plant growth. Inorg Nano-Metal Chem 50:569–579. https://doi.org/10.1080/24701556.2020.1722694
Shaikh NS, Shaikh RS, Kashid S (2020) In vitro biosynthesis of silver nanoparticles using flower extract of parasitic plant Cascuta reflexa and evaluation of its biological properties. Asian J Nanosci Mater 3:121–130
Shaikh R, Zainuddin Syed I, Bhende P (2019) Green synthesis of silver nanoparticles using root extracts of Cassia toral L. and its antimicrobial activities. Asian J Green Chem 3:70–81
Shameli K, Ahmad M, Zamanian A et al (2012) Green biosynthesis of silver nanoparticles using Curcuma longa tuber powder. Int J Nanomedicine 7:5603–5610
Sharma SK, Khan AU, Khan M et al (2020) Biosynthesis of MgO nanoparticles using Annona squamosa seeds and its catalytic activity and antibacterial screening. Micro Nano Lett 15:30–34
Shobana S, Veena S, Sameer SSM et al (2020) Green synthesis of silver nanoparticles using Artocarpus hirsutus seed extract and its antibacterial activity. Curr Pharm Biotechnol. https://doi.org/10.2174/1389201021666200107115849
Shreema K, Priyadharshini K, Mathammal R et al (2020) Green synthesis of zinc oxide nanoparticles using leaf extract of Salvia officinalis . Stud Indian Place Names 40:1175–1187
Santos DSM, Santos DLAO, Filho AS et al (2019) Can green synthesis of nanoparticles be efficient all year long? Nanomater Chem Technol 1:32–36
Singh A, Singh NB, Hussain I et al (2016) Green synthesis of nano zinc oxide and evaluation of its impact on germination and metabolic activity of Solanum lycopersicum . J Biotechnol 233:84–94
Singh J, Dutta T, Kim KH et al (2018) Green synthesis of metals and their oxide nanoparticles: applications for environmental remediation. Nanobiotechnol 16:84. https://doi.org/10.1186/s12951-018-0408-4
Singh J, Kumar V, Kim KH et al (2019) Biogenic synthesis of copper oxide nanoparticles using plant extract and its prodigious potential for photocatalytic degradation of dyes. Environ Res 177:108569. https://doi.org/10.1016/j.envres.2019.108569
Sorbiun M, Mehr ES, Ramazani A et al (2018) Green synthesis of zinc oxide and copper oxide nanoparticles using aqueous extract of oak fruit hull (jaft) and comparing their photocatalytic degradation of basic violet 3. Int J Environ Res 12:29–37
Soto KM, Quezada-Cervantes CT, Hernández-Iturriaga M et al (2019) Fruit peels waste for the green synthesis of silver nanoparticles with antimicrobial activity against foodborne pathogens. LWT 103:293–300
Sukumar S, Rudrasenan A, Nambiar DP (2020) Green-synthesized rice-shaped copper oxide nanoparticles using Caesalpinia bonducella seed extract and their applications. ACS Omega 5:1040–1051. https://doi.org/10.1021/acsomega.9b02857
Sundrarajan M, Bama K, Bhavani M et al (2017) Obtaining titanium dioxide nanoparticles with spherical shape and antimicrobial properties using M. citrifolia leaves extract by hydrothermal method. J Photochem Photobiol, B 171:117–124
Taghizadeh S, Taghizadeh SM, Ghasemi Y et al (2018) Living plant-mediated synthesis of nanoparticles. J Adv Med Sci Appl Technol 4:1–6
Tahir K, Nazir S, Li B et al (2015) An efficient photo catalytic activity of green synthesized silver nanoparticles using Salvadora persica stem extract. Sep Purif Technol 150:316–324
Tanase C, Berta L, Mare A et al (2020) Biosynthesis of silver nanoparticles using aqueous bark extract of Picea abies L. and their antibacterial activity. Europ J Wood Prod 78:281–291
Thunugunta T, Reddy A, Reddy Reddy L (2015) Green synthesis of nanoparticles: current prospectus. Nanotechnol Rev 4(4):303–323
Trotta F, Mele A (2019) Nanomaterials: classification and properties. In: Trotta F, Mele A (eds) Nanosponges: synthesis and applications, 1st edn. Wiley-VCH Verlag GmbH & Co KGaA
Vadlapudi V, Kaladhar DSVGK (2014) Review: green synthesis of silver and gold nanoparticles. Middle East J Sci Res 19(6):834–842
Valsalam S, Agastian P, Esmail GA et al (2019) Biosynthesis of silver and gold nanoparticles using Musa acuminata colla flower and its pharmaceutical activity against bacteria and anticancer efficacy. J Photochem Photobiol, B 201:111670. https://doi.org/10.1016/j.jphotobiol.2019.111670
Varadavenkatesan T, Selvaraj R, Vinayagam R (2019) Dye degradation and antibacterial activity of green synthesized silver nanoparticles using Ipomoea digitata Linn. flower extract. Int J Environ Sci Technol 16:2395–2404
Vidovix TB, Quesada HB, Januário EFD et al (2019) Green synthesis of copper oxide nanoparticles using Punica granatum leaf extract applied to the removal of methylene blue. Mater Lett 257:126685. https://doi.org/10.1016/j.matlet.2019.126685
Vijaya JJ, Jayaprakash N, Kombaiah K et al (2017) Bioreduction potentials of dried root of Zingiber officinale for a simple green synthesis of silver nanoparticles: antibacterial studies. J Photochem Photobiol, B 177:62–68
Vijayan R, Joseph S, Mathew B (2019) Anticancer, antimicrobial, antioxidant, and catalytic activities of green-synthesized silver and gold nanoparticles using Bauhinia purpurea leaf extract. Bioprocess Biosyst Eng 42:305–319
Vishwasrao C, Momin B, Ananthanarayan L (2019) Green synthesis of silver nanoparticles using sapota fruit waste and evaluation of their antimicrobial activity. Waste Biomass Valorization 10:2353–2363
Wang D, Markus J, Wang C et al (2017) Green synthesis of gold and silver nanoparticles using aqueous extract of Cibotium barometz root. Artif Cells Nanomedicine Biotechnol 45:1548–1555
Wang Z, Que B, Gan J et al (2020) Zinc oxide nanoparticles synthesized from Fraxinus rhynchophylla extract by green route method attenuates the chemical and heat induced neurogenic and inflammatory pain models in mice. J Photochem Photobiol, B 202:111668
Yang N, Li F, Jian T et al (2017) Biogenic synthesis of silver nanoparticles using ginger ( Zingiber officinale ) extract and their antibacterial properties against aquatic pathogens. Acta Oceanol Sin 36:95–100. https://doi.org/10.1007/s13131-017-1099-7
Zhang T, Dang M, Zhang W, Lin X (2020) Gold nanoparticles synthesized from Euphorbia fischeriana root by green route method alleviates the isoprenaline hydrochloride induced myocardial infarction in rats. J Photochem Photobiol, B 202:111705. https://doi.org/10.1016/j.jphotobiol.2019.111705
Download references
Author information
Khaled F. M. Salem
Present address: Department of Biology, College of Science and Humanitarian Studies, Shaqra University, Qwaieah, Saudi Arabia
Authors and Affiliations
Biotechnology and Molecular Biology, Faculty of Agriculture, Al-Baath University, Homs, Syria
Lina M. Alnaddaf
Arab Center for Nanotechnology, Cairo, Egypt
Abdulsalam K. Almuhammady
Department of Plant Biotechnology, Genetic Engineering and Biotechnology Research Institute (GEBRI), University of Sadat City, Sadat City, Egypt
Faculty of Pharmacy, Department of Biochemistry and Microbiology, Al-Baath University, Homs, Syria
Maysaa T. Alloosh
Genetic Resources Department, General Commission for Scientific Agricultural Research (GCSAR), Damascus, Syria
Maysoun M. Saleh
Department of Agricultural Biotechnology, College of Agriculture and Food Sciences, King Faisal University, Al-Ahsa, Saudi Arabia
Jameel M. Al-Khayri
You can also search for this author in PubMed Google Scholar
Corresponding author
Correspondence to Lina M. Alnaddaf .
Editor information
Editors and affiliations.
Department of Agricultural Biotechnology, King Faisal University, Al-Ahsa, Saudi Arabia
Department of Botany, University of Lucknow, Lucknow, Uttar Pradesh, India
Mohammad Israil Ansari
Department of Biotechnology, School of Life Sciences, Mahatma Gandhi Central University, Motihari, Bihar, India
Akhilesh Kumar Singh
Rights and permissions
Reprints and permissions
Copyright information
© 2021 Springer Nature Switzerland AG
About this chapter
Alnaddaf, L.M., Almuhammady, A.K., Salem, K.F.M., Alloosh, M.T., Saleh, M.M., Al-Khayri, J.M. (2021). Green Synthesis of Nanoparticles Using Different Plant Extracts and Their Characterizations. In: Al-Khayri, J.M., Ansari, M.I., Singh, A.K. (eds) Nanobiotechnology . Springer, Cham. https://doi.org/10.1007/978-3-030-73606-4_8
Download citation
DOI : https://doi.org/10.1007/978-3-030-73606-4_8
Published : 23 July 2021
Publisher Name : Springer, Cham
Print ISBN : 978-3-030-73605-7
Online ISBN : 978-3-030-73606-4
eBook Packages : Biomedical and Life Sciences Biomedical and Life Sciences (R0)
Share this chapter
Anyone you share the following link with will be able to read this content:
Sorry, a shareable link is not currently available for this article.
Provided by the Springer Nature SharedIt content-sharing initiative
- Publish with us
Policies and ethics
- Find a journal
- Track your research
Thank you for visiting nature.com. You are using a browser version with limited support for CSS. To obtain the best experience, we recommend you use a more up to date browser (or turn off compatibility mode in Internet Explorer). In the meantime, to ensure continued support, we are displaying the site without styles and JavaScript.
- View all journals
- My Account Login
- Explore content
- About the journal
- Publish with us
- Sign up for alerts
- Open access
- Published: 19 February 2024
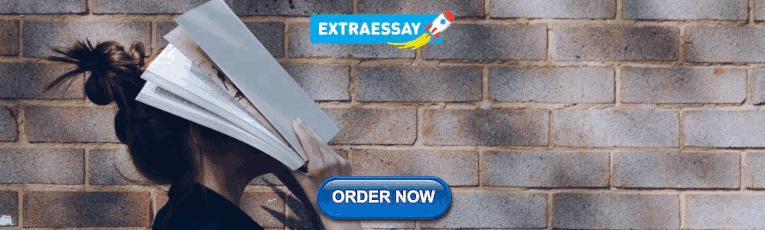
Process optimization for green synthesis of silver nanoparticles using Rubus discolor leaves extract and its biological activities against multi-drug resistant bacteria and cancer cells
- Saeed Ghasemi 1 ,
- Sara Dabirian 2 ,
- Faezeh Kariminejad 3 ,
- Diba Eghbali Koohi 3 ,
- Mehran Nemattalab 4 ,
- Sina Majidimoghadam 3 ,
- Ehsan Zamani 5 &
- Fatemeh Yousefbeyk 3
Scientific Reports volume 14 , Article number: 4130 ( 2024 ) Cite this article
1574 Accesses
3 Citations
Metrics details
- Drug screening
- Infectious diseases
Multi-drug resistant (MDR) bacteria are considered a serious public health threat. Also, increasing rate of resistance to anticancer drugs, as well as their toxicity, is another point of concern. Therefore, the new antibacterial and anticancer agents are always needed. The synthesizing silver nanoparticles (AgNPs) using medicinal plants, is an effective approach for developing novel antibacterial and anticancer agents. Rubus discolor , a native species of the Caucasus region, produces leaves that are typically discarded as a by-product of raspberry production. The present study has focused on optimizing the green synthesis of AgNPs using R. discolor leaves extract through response surface methodology. The optimal values for AgNPs synthesis were an AgNO 3 concentration of 7.11 mM, a time of 17.83 h, a temperature of 56.51 °C, and an extract percentage of 29.22. The production of AgNPs was confirmed using UV–visible spectroscopy (λ max at 456.01 nm). TEM analysis revealed well-dispersed AgNPs (an average size of 37 nm). The XRD analysis confirmed the crystalline structure. The EDX detected a strong peak at 3 keV corresponded to Ag. The zeta potential value (− 44.2 mV) indicated the stability of nanoparticles. FT-IR spectra showed the presence of various functional groups from plant compounds, which play an important role in the capping and bio-reduction processes. The AgNPs revealed impressive antibacterial activities against MDR Escherichia coli and Pseudomonas aeruginosa (MIC ranging from 0.93 to 3.75 mg ml −1 ). The phytochemical analysis indicated the presence of phenolics, tannins, and flavonoids on the surface of AgNPs. They also showed significant cytotoxic effects on A431, MCF-7, and HepG2 cells (IC 50 values ranging from 11 to 49.1 µg ml −l ).
Similar content being viewed by others
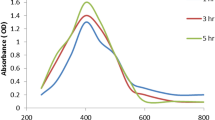
Eco friendly silver nanoparticles synthesis by Brassica oleracea and its antibacterial, anticancer and antioxidant properties
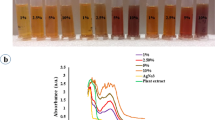
Comparative assessment of the biological activity of the green synthesized silver nanoparticles and aqueous leaf extract of Perilla frutescens (L.)
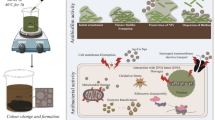
Phytofabricated bimetallic synthesis of silver-copper nanoparticles using Aerva lanata extract to evaluate their potential cytotoxic and antimicrobial activities
Introduction.
The study and application of nanostructured materials, with sizes ranging from 1 to 100 nm, have been highlighted recently because they are widely applicable in many multidisciplinary fields 1 , 2 , 3 , 4 , 5 . Compared to bulk materials, nanoparticles (NPs) have a larger surface area to volume ratio and ultra-small size, which give them unique thermal, physiochemical, and biological properties 6 , 7 . These characteristics make them attractive candidates for developing countless applications in agriculture, biology, chemical engineering, biomedicine, and pharmaceutical industry 8 . According to their shapes, sizes, and properties, NPs have been classified into several groups, including carbon-based nanoparticles, polymeric nanoparticles, ceramic nanoparticles, and metal nanoparticles 9 . Among them, metal nanoparticles comprise gold, silver, copper, magnetic (cobalt, iron, and nickel), and semiconducting materials 2 , 10 . Silver nanoparticles (AgNPs) have gained significant attention based on various biological activities, including, antibacterial, antifungal, antiviral, anticancer, anti-inflammatory, and wound healing properties 11 , 12 . They have been used in food processing, cosmetics, home cleaning, catalytic and garment production, and pharmaceutical industry 13 .
There are various mechanical and chemical methods for producing nanomaterial that have several disadvantages, such as high cost, low yield, and being relatively complicated. Also, they are not environmentally friendly due to the application of toxic chemicals and solvents, and the production of dangerous by-products 6 , 9 , 14 . However, the green approaches for NPs production are eco-friendly, easy to apply, and safe for the environment, human beings, and living organisms 11 , 15 . Therefore, there is an increasing demand for developing safe methods to produce nanomaterials, using fungi, bacteria, or plants 6 , 16 , 17 , 18 , 19 . The plant extracts are preferred over other natural materials. Many natural compounds have been discovered in medicinal plants that play a crucial role in the preparation of nanoparticles 12 . The plant’s secondary metabolites, such as phenolic compounds, tannins, flavonoids, anthraquinones, carbohydrates, alkaloids, alkynes, allylic benzenes, ascorbic acids, alcoholic compounds, sugars, amides, amino acid residues, carotenes, steroids, saponins, and triterpenoids have proven to be able to reduce silver nitrate to AgNPs 7 , 12 , 20 . Recent investigations have revealed that NPs synthesized by bioactive phytochemicals possess more beneficial and effective properties than traditional herbal drugs 12 .
The World Health Organization (WHO) has recently declared antibacterial resistance as one of the three main risks to human health 21 . Drug-resistant pathogens kill about 700,000 people worldwide each year, and this number could increase to 10 million deaths a year by 2050 22 . Presently, the rapid development of drug-resistant strains of microorganisms as well as the serious lack of effective antibiotics, have revealed that the discovery and development of novel antimicrobial agents are logically necessary 22 , 23 . Silver is an inorganic antibacterial agent, which is nontoxic and safe. It is effective against 650 types of pathogenic microorganisms 24 . Studies showed that AgNPs can exert significant antibacterial activity against MDR (multi-drug resistant) bacteria by several mechanisms, such as inhibition of the cell respiration chain, disrupting the cellular signal transduction pathways, and generating reactive oxygen species (ROS), which causes toxicity in cells 12 , 25 , 26 , 27 .
Moreover, cancer is the second leading cause of death universally after cardiovascular diseases 28 . The American Cancer Society predicted that the worldwide burden of cancer will surge to 21.7 million fresh cases by the year 2030 29 . Today, there is growing attention to discovering inexpensive and more cost-effective drugs using natural resources like medicinal plants. The plants have been provided various new approaches for the cancer treatment, including green synthesis of AgNPs 29 .
Blackberries, Rubus spp., Rosaceae family, are widely distributed and cultivated worldwide and are of growing commercial relevance 30 . This genus comprises over 750 species in the world. The sweet taste fruits of many species are popular as a healthy and nutritious food, containing various phenolic compounds, dietary fiber, vitamin C, α-tocopherol, and carotenoids 31 , 32 . Also, blackberry leaves have been used traditionally, in form of tea or as a mouthwash, and gargle solution. It is reported that blackberry leaves have important bioactive components, such as phenols, flavonoids, tannins, terpenoids, and other anti-aging and antioxidant compounds, and can serve as a potential source for use in the food, pharmaceutical industry, and cosmetic 32 , 33 . In the flora of Iran, eight species of blackberry have been reported 34 . Rubus discolor Weihe & Nees., commonly known as Himalayan blackberry, is a native species of the Caucasus region of Eurasia 35 . It is widely distributed in the North and Northwest of Iran as a common weed 36 . Since the Rubus leaves are significantly consumed less than fruits, a large number of leaves are disposed of as a by-product of raspberry production 32 .Currently, the assessment of bioactive phytochemicals in the eliminated plant material has attracted great interest, because these by-products have high levels of constituents with biological properties 37 . As a result, the ecofriendly synthesis of AgNPs from the leaves which have potential biological activities could be of interest.
In the present study, the aqueous extract of Rubus discolor leaves was used for the biosynthesis of the AgNPs. The synthesis condition was optimized by response surface methodology (RSM). The nanoparticles were characterized by UV–Vis and FT-IR spectroscopic methods, as well as XRD, DLS, SEM–EDX, and TEM methods. The preliminary phytochemical investigations and the determination of total phenolic, tannin, and flavonoid contents were performed. The antibacterial activity was tested against two ATCC Gram-positive ( Streptococcus aureus and Bacillus subtilis ) and two ATCC Gram-negative bacteria ( Escherichia coli and Pseudomonas aeruginosa ). Also, ten MDR isolates of E. coli and P. aeruginosa were tested to investigate their susceptibility to the synthesized AgNPs. The cytotoxic activities of AgNPs and extract were investigated against three cancerous cell lines, including MCF-7 (breast cancer), A431 (epidermoid carcinoma), and HepG2 (liver hepatocellular carcinoma) as well as a normal cell line (HU02) by MTT assay.
Results and discussion
Phytochemical investigation of the aqueous extract and agnps.
The preliminary phytochemical analysis of the aqueous extract of leaves revealed the presence of flavonoids, tannins, steroids, and carbohydrates (Table 1 ).
The total phenolic content (TPC) of the aqueous extract and AgNPs were calculated based on the gallic acid standard curve equation (y = 0.000899x − 0.0355, R 2 = 0.999), using the Folin-Ciocalteu method. Also, the total flavonoid contents (TFC) were measured, based on the quercetin standard curve (y = 0.0192x − 0.0198, R 2 = 0.995). The total tannin contents (TTC) were measured using the following standard curve plotted for tannic acid (as standard compound): y = 0.005x + 0.0281; R 2 = 0.995. All the results are depicted in Table 2 . According to the results, AgNPs showed lower TPC, TTC, and TFC than the aqueous leave extract. The reduction in polyphenolic contents was also reported in other studies 7 , 38 , 39 . The suggested reason is that the phenolic, tannin, and flavonoid compounds in the extract were consumed in the reduction process in the green synthesis of the AgNPs. Moreover, the concentration of these secondary metabolites determines the kinetics of the reaction, shape, and size of AgNPs 38 .
Statistical process optimization of green synthesis AgNPs using RSM
The results of the central composite design (CCD) for optimizing Ag synthesis conditions, including AgNO 3 concentration, time, temperature, and the extract percent, were represented in Table 3 .
A set of 30 runs based on the formula 2 N + 2 N + X was conducted, where N is the number of selected factors with 2 N factorial (16 runs), 2N axial (8), and X center points repetitions (6 runs). The Eq. 1 shows the correlation between the absorbance at 456 nm (as an indicator of SPR) and the four studied parameters in coded terms:
where Y is the absorbance at 456 nm; A is AgNO 3 concentration; B is the time of reaction; C is temperature; and D is the extract percentage. The analysis of variance (ANOVA) was carried out to investigate the suitability of the obtained model (Table 4 ).
Based on the statistics, a quadratic model was suggested to relate the experimental factors and their combinations and the response. The high F-value (30.34) and the low p value ( p < 0.0001, only 0.01% chance of noise) showed that the obtained model is significant and acceptable. The variables A, B, C, D, AD, A 2 , B 2 , C 2 , and D 2 were the significant parameters on the basis of p value (p < 0.05). The F-value lack of fit was 3.38 with a p value of 0.1723 (chance of noise 17.23%), which shows the model is valid.
Three parameters, including the calculated determination coefficient (R 2 and adjusted R 2 ) and adequate precision, were used to evaluate the model’s efficacy of R 2 and adjusted R 2 values of 0.9703 and 0.9383, respectively, showed that the model has high efficacy and can properly explain the variability. Adequate precision (AP) of 19.305 (AP > 4 is desirable), which shows the signal-to -noise ratio, indicated adequate signal-to-noise. The Predicted R 2 of 0.7957 showed a high correlation between predicted and observed responses. It should be in reasonable agreement with adjusted R 2 (within the range of 0.2 adjusted R 2 ).
For evaluation of the best condition for each factor to obtain the maximum AgNPs yield, the 3D surface and contour plots were used (Fig. 1 ). These plots were on the basis of the corresponding interactions of two factors, while the third parameter was fixed at the optimum condition. The shape of the 3D contour plot shows the interaction significance.
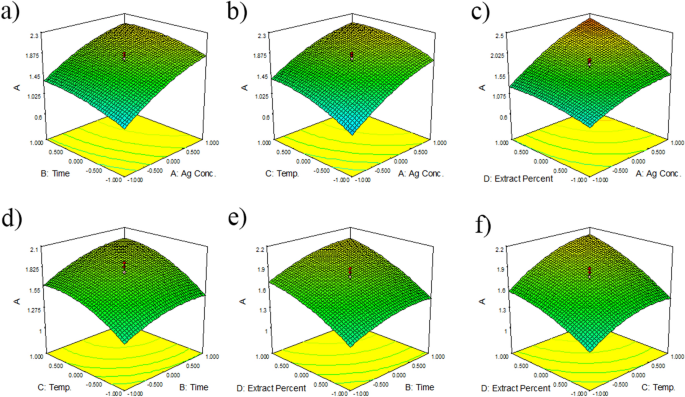
Three dimensions surface plots of AgNPs biosynthesis ( a – f ): Interaction effects of AgNO 3 concentration ( A ), time ( B ), temperature ( C ), and extract percent ( D ) on the maximum absorbance value (Amax).
Figure. 1 a–c shows that AgNO 3 concentration (A) had a significant effect on the AgNPs synthesis. When AgNO 3 concentration increased, the yield of AgNPs increased depending on the second parameter. Othman et al. reported that AgNO 3 concentration strongly affected the yield of AgNPs synthesis when interacting with other factors such as reaction pH value 40 . Likewise, El-Rafie showed that increasing the silver nitrate concentration dramatically increased the absorbance intensity 41 . Figures 1a, e, and f show that the time of the reaction (B) had a lesser momentous influence on yield of AgNPs synthesis in the interaction with AgNO 3 concentration (A), temperature (C), and extract percent (D). When the reaction time increased, the AgNPs biosynthesis increased by the interaction of the second factor, including A, C, or D. Figures 1b, d, and f prove that temperature (C) had a stronger effect on the AgNPs biosynthesis than the time of reaction. Also, in a study, the AgNPs were synthesized using Plantago major extract, and it was showed that temperature had higher effect on the absorbance in comparison with time 42 . Figures 1c, e, and f explain that extract percent (D) had the second rank in the AgNPs synthesis after AgNO 3 concentration. The yield of AgNPs biosynthesis increased with the increase in extract percent due to higher reducing agents in the reaction mixture 43 . A strong interaction was observed between A and D, and other interactions were not significant. The 3D surface plots showed that the effects of the four studied parameters on the AgNPs green synthesis were not equal, and the order of factors was as follows: A > D > C > B, respectively. In the optimized condition, the selected experimental model was tested using AgNO 3 concentration of 7.11 mM, time of 17.83 h, temperature of 56.51, and extract percent of 29.22. The predicted absorbance at 456 nm was 1.92, which is close to the experimental value (2.12) that indicates the validity of the models. The yield of the reaction, in optimized condition was 53.31%.
Characterization of AgNPs
Optical properties and uv–vis spectroscopy of the synthesized agnps.
The formation of AgNPs was first characterized by the observation of color change from pale yellow to dark brown, that revealed the Ag + reduction into Ag 0 nanoparticles. The color transformation is due to AgNPs’ optical properties and known as the localized surface plasmon resonance (SPR) 44 . Various factors like particle type, size, shape, morphology, dielectric environment, and composition have an impact on SPR. Also, UV–Vis spectroscopy is a common characterization tool to detect the SPR absorption peak of NPs and demonstrate their formation 45 . As depicted in Fig. 2 , the UV–V is spectrum of AgNPs showed a SPR at 456.01 nm. In a study, Said et al. reported that the UV–vis spectrum of the AgNPs they produced was observed at 460 nm 16 . Additionally, Patra et al. revealed that their AgNPs had a maximum absorbance peak at 456 nm 46 . These findings are consistent with our study.
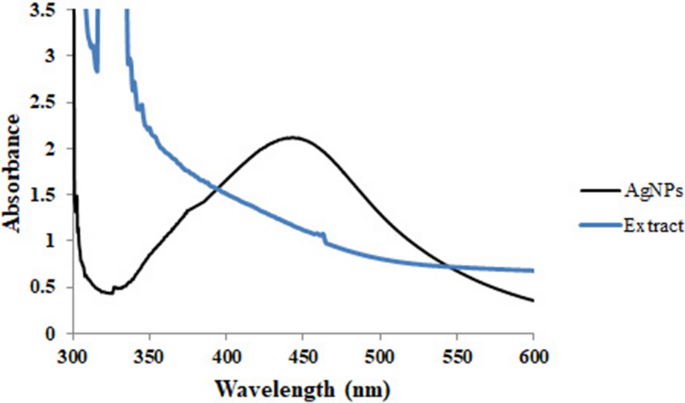
UV–Vis spectrum of the R. discolor extract and AgNPs.
The numerous phytochemicals present in the aqueous extract of R. discolor could be responsible for the rapid bioreduction and capping of synthesized AgNPs. Typically, the bioactive compounds such as vitamins, flavonoids, tannins, phenolic acids, proteins, etc., are responsible for the fast reduction of Ag + , and control the size distribution and morphology of synthesized NPs 47 . According to the results of this study, the leaves extract contained tannins, flavonoids, steroids, and carbohydrates, which can act as reducing agents.
TEM analysis
The AgNPs were evaluated by the transmission electron microscopes (TEM) for elucidation of the size, shape, and morphology. The microphotographs displayed that the nanoparticles were well-distributed and roughly spherical, with polydispersity, and without agglomeration. The size of the most particles ranged between 20 and 50 nm, with an average size of 37 nm (Fig. 3 ). It can be suggested that during the reaction, the content of reducing agent in plant extract deceased gradually, which led to the formation of AgNPs in different sizes. Also, careful observation of TEM images revealed no direct connection among AgNPs, even within the aggregates, presenting that AgNPs were surrounded by a thin layer of natural phytochemicals like amino acids and phenolic compounds 7 , 38 . In a study, Mariadoss et al. reported that the morphology of the AgNPs synthesized by the extract of Malus domestica was spherical, with polydispersity and a size ranging from 40 to 100 nm 48 . Also, Yassin et al. synthesized AgNPs from Origanum majorana , which showed polydispersity, with a size range from 10 to 60 nm 8 .
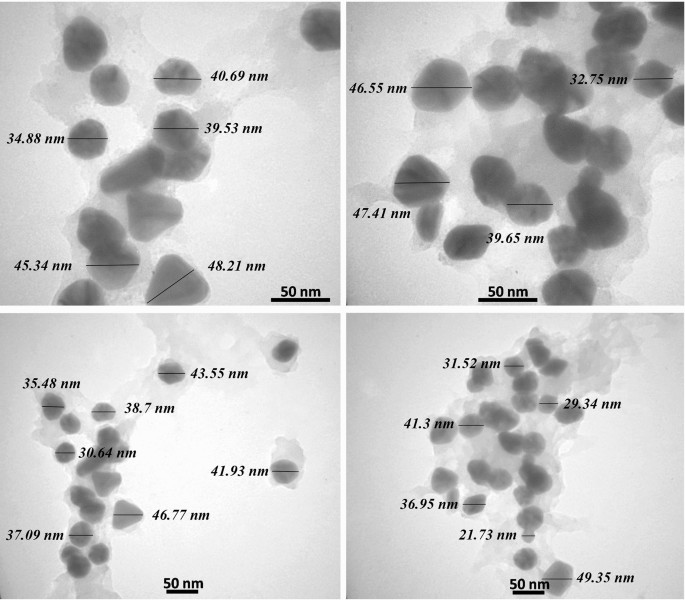
TEM images of AgNPs at optimized condition.
SEM–EDX analysis
The surface nature and the elemental configuration of the AgNPs were determined by Scanning Electron Microscopy (SEM) with Energy Dispersive X-Ray (EDX) analysis. The SEM image displayed that AgNPs were polydisperse, about 38 nm in size, and predominantly spherical in shape (Fig. 4 ). The EDS analysis of the AgNPs was conducted to study the elemental composition of AgNPs. EDX analysis displayed a strong signal at 3.0 keV, which is characteristic of metallic Ag because of surface plasmon resonance, associated with the Ag-L a line 49 . Additionally, the profile exhibited peaks for oxygen and carbon which could be attributed to the phytoconstituents attached to the surface of the AgNPs. Our results were in accordance with those of Okaiyeto et al., which produced AgNPs using aqueous extract of Oedera genistifolia , showing the presence of intense peak of silver element at 3.0 keV 50 . Moreover, Patra et al. synthesized AgNPs from Pisum sativum . The EDX spectra of their study showed the elemental composition of the AgNPs, with a strong peak at 3 keV that corresponded to Ag 46 .
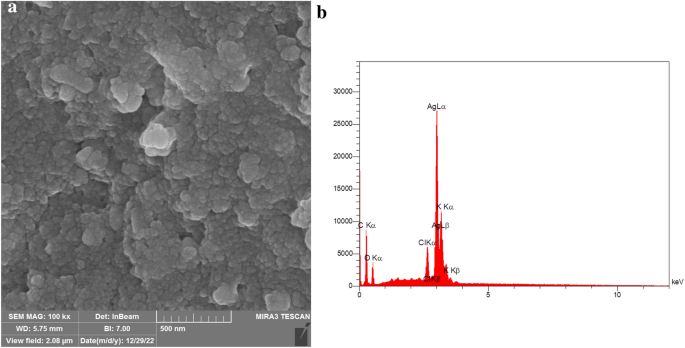
SEM image ( a ) and EDX spectrum ( b ) of biosynthesized AgNPs.
Zeta potential and DLS analysis
The particle size distribution of biosynthesized AgNPs was determined using a dynamic light scattering (DLS). The DLS determines the hydrodynamic size of colloids, and can estimate the average size of the nanoparticles in the mixture, approximately 51 . The results of this study indicated that the particle size and poly-dispersity index (PDI) values of AgNPs were 151.7 nm and 0.25, respectively (Fig. 5 ). As reported in previous studies, PDI (also known as heterogeneity index) shows the non-uniformity of particles in a colloidal solution. This value is unitless and is considered between 0.05 and 0.7. A PDI value close to 0.05 indicates that the particles are monodispersed, while colloidal solutions with PDI values close to 0.7 are heterogenous 51 , 52 . In this study, the reported PDI value of 0.25 is acceptable. On the other hand, the zeta potential (ζ) is one of the important factors for the characterization of the stability of nanoparticles in a solution. Nanoparticles with zeta potentials larger than + 30 mV and less than − 30 mV show considerable stability for colloidal dispersions 51 . The value of AgNPs zeta potential was − 44.2 mV. The highly negative value of ζ proved that the synthesized AgNPs had high stability 53 . Since, in this study, the external stabilizers were not used, meaning that the plant phytochemicals acted not only as the reducing agents of the Ag + to Ag 0 , but also stabilized the synthesized nanoparticles.
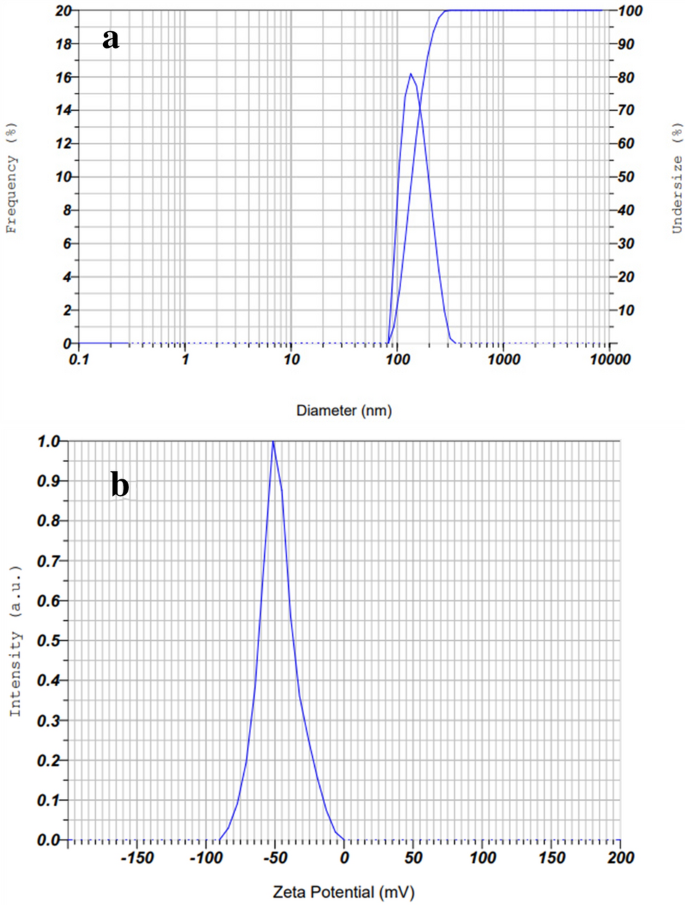
Size ( a ) and zeta potential value ( b ) of AgNPs prepared by R. discolor.
It is obvious that the particle size in the DLS analysis is larger than in the TEM test. The DLS measurement is carried out in a fluid phase. This means that the AgNPs particles are in constant movement because of Brownian motion. Also, AgNPs have a charge on their surfaces, and consequently, they can interact with other ions, molecules, and surfaces, which contributes to the creation of adsorbed layers on the surface of the nanoparticles 14 . Therefore, the DLS shows the hydrodynamic diameter of the biomolecules surrounding AgNPs and the intensity-weighted average particle size 44 . However, the TEM image is taken in a dry state. Thus, the results of DLS and TEM cannot be in line with each other, and the results of DLS showed a normally larger size than those of TEM 14 .
X‑Ray Diffraction Spectroscopy
The X-ray diffraction (XRD) pattern of AgNPs synthesized using aqueous extract of R. discolor is shown in Fig. 6 . The peaks at 2 theta (θ) degrees of 38.1°, 44.2°, 64.5°, and 77.6° could be related to (111), (200), (220), and (311) facets, respectively, which corresponded to the database of the Joint Committee on Powder Diffraction Standards (JCPDS), file No. 00-004-0783. Debye–Scherrer formula (Eq. 2 ) was used to calculate the size of AgNPs, as follow:
where D is the average crystallin size of AgNPs, λ is the wavelength of X-ray which is 0.1546 nm, β is the width at half maximum of the peak in radians, and θ is Braggs angle in degrees 54 .Similar to previous studies, it shows that synthesized AgNPs are face-centered cubic. Additional peaks on the XRD spectrum could be correlated to the crystallization of the plant phytochemicals coating the AgNPs 38 , 44 . The average size of AgNPs was 18 nm, which complied with the result from TEM images. These findings were consistent with previous study by Said et al. that reported the formation of AgNPs by detecting diffraction peaks at 2θ degrees of 38.1°, 44.2°, 64.4°, and 77.2°, which are corresponded to the planes (111), (200), (220), and (311), respectively. Similarly, Yassin et al. reported AgNPs, with the face-centered cubic structure and diffraction peaks at 2θ degrees of 38.18◦, 44.36◦, 64.35◦, and 77.54◦, which are related to reflection planes of (111), (200), (220), (311), respectively 8 .
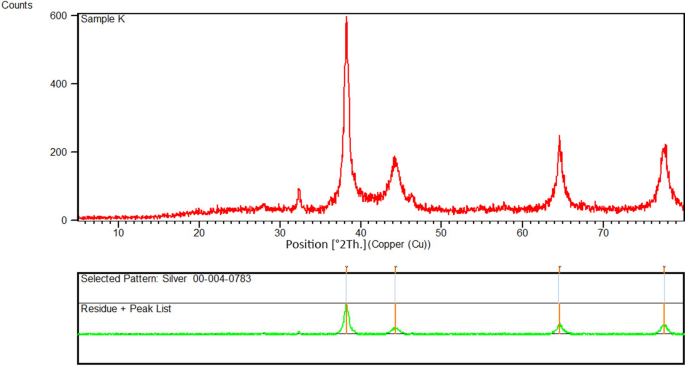
XRD pattern of green synthesized AgNPs.
FT-IR analysis
FT-IR analysis was performed to determine the structure of phytochemicals, exciting in aqueous leaves extract of R. discolor , which are responsible for surface coating and stabilization of the AgNPs. Figure 7 shows the IR spectra of the aqueous extract and the synthesized AgNPs.
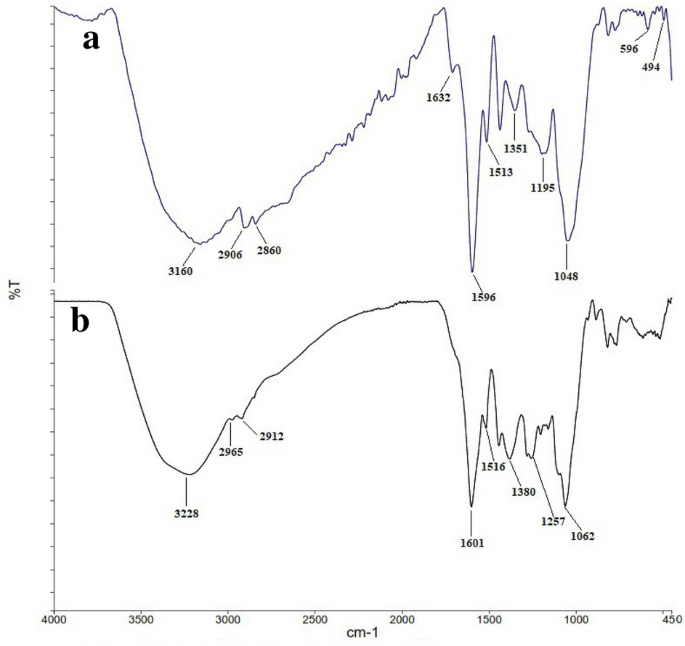
FTIR spectra of ( a ) AgNPs and ( b ) extract.
The wavenumbers of different functional groups are summarized in Table 5 . The characteristic peaks are determined by comparing peaks with the FT-IR results of other studies that have biosynthesized AgNPs using green methods.
By comparison the FT-IR spectra of AgNPs and the leaves aqueous extract, it was demonstrated that some peaks were shifted. Also, the intensity of some peaks reduced or increased, and the appearance of several new peaks changed significantly. For example, peaks at 3228, 1601, 1516, and 1380 cm −1 , corresponding to O–H and N–H stretching vibrations, shifted to 3160, 1596, 1513, and 3151 cm −1 , respectively. That could be due to some electrostatic interactions among the AgNPs and functional groups of capping agents. Moreover, in the FT-IR spectrum of AgNPs, the appearance of a peak at 1632 cm -1 , as well as increasing the intensity of the peak at 1436 cm -1 , which are attributed to carbonyl vibrations, designated that the reduction of the silver ions is due to the oxidation of the hydroxyl groups to the carbonyl groups in the plant extract. The reduced peak intensity at 3160 cm −1 revealed the important role of OH and N–H in the reduction and binding mechanism 7 . Finally, new peaks at 596 and 494 cm −1 may be attributed to the bonding of AgNPs with phytochemicals in the extract. Similar to our study, Said et al. reported peaks at 775 to 540 cm −1 which were correspondent to the bonding of AgNPs to functional groups in the extract 16 . Also, Aref et al. suggested that the peaks at 488 and 407 cm −1 may refer to the binding of AgNPs to phytochemical groups 55 . Moreover, Yassin et al. showed that the AgNPs synthesized by Origanum majorana had characteristic peaks for functional groups such as phenolic, amines, hydroxyl, and alkyl groups.
Suggested mechanism of formation of AgNPs
The plant extract contains various molecules such as polyphenols, terpene derivatives, saccharides, alkaloids, etc. These molecules are responsible for the reduction of AgNO 3 to Ag 0 . The probable mechanism of AgNPs synthesis is depicted in Fig. 8 . Generally, the functional groups such as hydroxyl (–OH) of these biomolecules interact with AgNO 3 . When AgNO 3 dissolves in water, it dissociates into two ions, Ag + and NO 3 − . The acidic nature of OH groups of phytochemicals resulted in donation of H + ions and acquisition of a negative charge. The negative functional groups like O − of phenols interact electrostatically with Ag + . This process leads to the reduction of Ag + ions. The NO 3 − ions accept H + from phenolic OH resulted in the formation of HNO 3 . Ag remains in a free metallic state (Ag 0 ) to form AgNPs 50 .
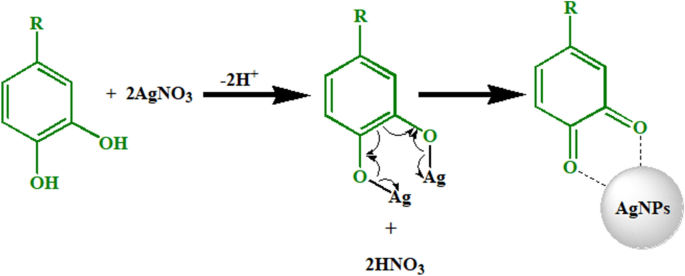
Suggested reduction mechanism of Ag + to Ag 0 .
Antibacterial activity
Green synthesized AgNPs (at concentration of 1 mg ml −1 ) displayed significant antibacterial activity against ATCC gram-negative bacteria, including P. aeruginosa and E. coli , with inhibition zone of 18 and 16.5 mm, respectively. However, the AgNPs did not show any antibacterial activity against gram-positive pathogens (Fig. 9 ). The aqueous extract of R. discolor exhibited no antibacterial effect even in a high concentration (300 mg ml −1 ). Also, the best MIC value was for P. aeruginosa ATCC (0.83 mg ml −1 ) (Table 6 ).
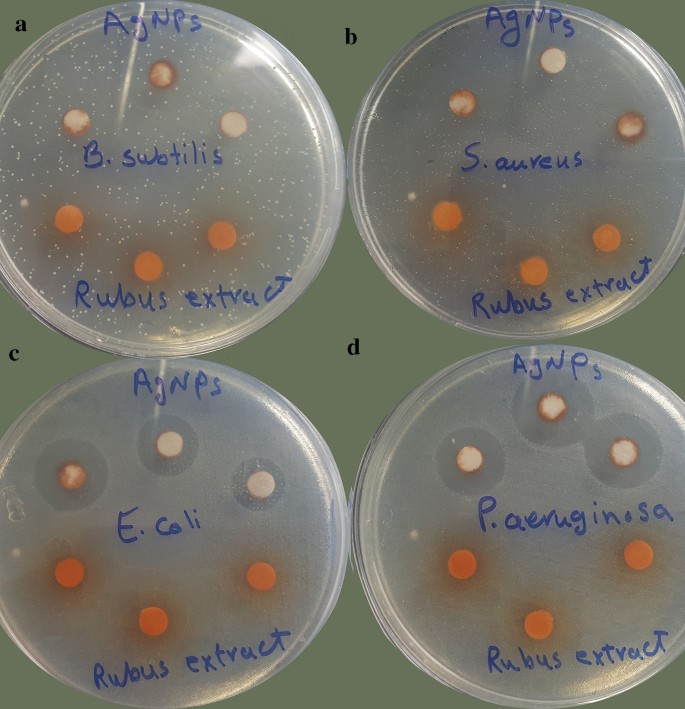
The zones of inhibition formed by the AgNPs and R. discolor leaves extract against ( a ) B. subtilis, ( b ) S. aureus , ( c ) E. coli , (d) P. aeruginosa .
Also, the antibacterial activity was measured against MDR E. coli and P. aeruginosa isolated. Results of the antibiogram susceptibility test of eight antibiotics against ten isolates are depicted in Table 7 .
Also, Fig. 10 describes the zone of inhibition (mm) for eleven antibiotics and AgNPs against isolate number 5 of MRD E. coli and P. aeruginosa . As is presented in Table 8 , the AgNPs showed antibacterial activity against MDR E. coli with MIC values ranged from 1.87 to 3.75 mg ml −1 . The MBCs were 5 mg ml −1 for all of the MDR E. coli isolates . Moreover, the MIC values against MDR P. aeruginosa isolated ranged from 0.93 to 1.87 mg ml −1 , and the MBC values were 2.5–5 mg ml −1 . The aqueous extract did not have any antibacterial activity against tested MDR isolates.
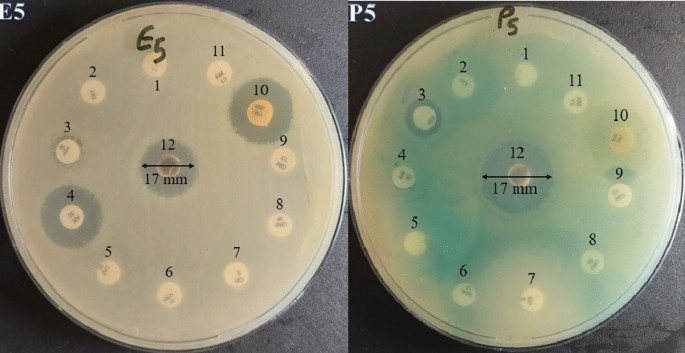
The zone of inhibition (mm) for eleven antibiotics (No. 1–11) and AgNPs (No. 12) against isolate number 5 of MRD E. coli and P. aeruginosa (E5 and P5).
AgNPs had ultra-small size and uniform distribution that led to significant antibacterial activity 7 . It is proposed that AgNPs release Ag + that attach to the negative charge of the microbial cell wall, denaturing the membrane proteins. Also, AgNPs have potent affinity for the sulfur-containing proteins in the cell wall, leading to changes in the morphological structure of the cell membrane. This irreversible damage increases the permeability of the cell membrane, thereby disrupting the cell ability to regulate normal activity. This can lead to the loss or leakage of cellular contents such as, proteins, cytoplasm, ions, and cellular energy sources 57 . After crossing the cell membrane, AgNPs disturb the bacteria’s metabolic pathways. They cause several intracellular changes like enzyme inhibition, interaction with bacterial DNA resulting in denaturation of DNA, interruption of the bacteria growth, and inducing electrolyte imbalance 7 , 15 , 47 . Another mechanism of action is increasing oxidative stress by inducing overproduction of ROS (reactive oxygen species). ROS can oxidate macromolecules like lipids, DNA, and proteins and therefore, cause the bacterial death (Fig. 11 ).
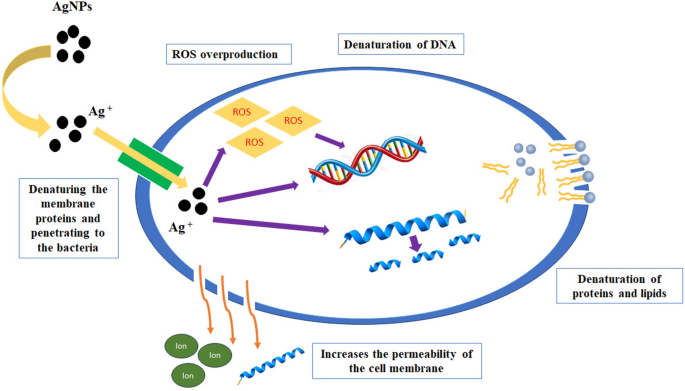
The antibacterial mechanism of action of AgNPs.
Studies have been shown that AgNPs are more effective against gram-negative bacteria strains than gram-positive ones. The suggested reason is that the gram-positive bacteria consist of one cytoplasmic membrane and a relatively thick cell wall that include numerous peptidoglycan layers (thickness between 20 and 80 nm). In contrast, gram-negative strains, there is an external layer of lipopolysaccharide (LPS) as well as one thin layer of peptidoglycan and an internal plasma membrane 58 . Our results are in consistence with previous reports.
Cytotoxic assay
The anti-proliferative effects of silver NPs and the leaves aqueous extract were investigated against three human cancerous cell lines and a healthy cell line. The IC 50 of AgNPs on selected cancerous cell lines ranged from 11.2 to 49.1 µg ml −1 (Fig. 12 ). The silver NPs exhibited more cytotoxic activities on MCF-7 and A431 cells than on HepG2 cells. Also, AgNPs showed more potent anti-proliferative activity than the aqueous extract on all cancerous cell line, particularly on HepG2 that the cytotoxicity of AgNPs was 2.5 times more than crude extract. Furthermore, the cytotoxic effect of AgNPs was investigated on HU02 (a noncancerous cell line). It was revealed that AgNPs had much less cytotoxic activity against the normal cell line (IC 50 of 158 µg ml −1 ) in comparison with the extract.
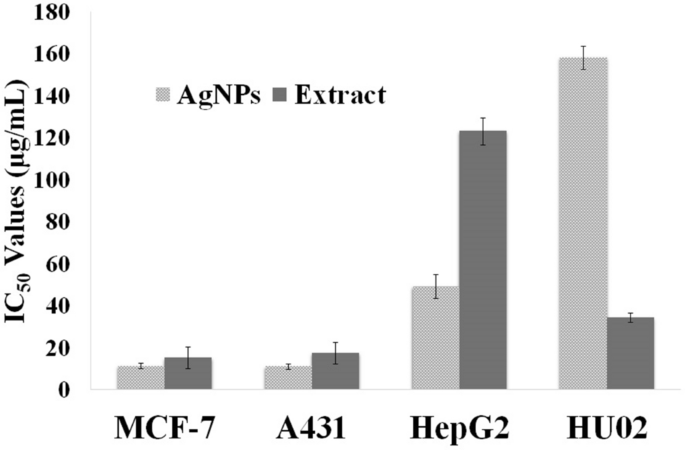
Cytotoxic activities (IC 50 values) of AgNPs and R. discolor leaves extract against MCF-7, A431, HepG2, and HU02; The results are the mean ± SD.
Recently, AgNPs have attracted great attention for their possible use as an anticancer therapeutic agent because of their significant cytotoxic effect on cancerous cell lines, while they are less toxic on normal cell lines 7 , 38 . It is suggested that the Ag + , released from AgNPs, can directly bind to RNA polymerase, disturbing its activity. Another main proposed mechanism of cytotoxicity is the generation of ROS, which leads to intracellular oxidative stress and consequently cell death. It has been observed that the cytotoxicity of AgNPs is size-dependent. The smaller AgNPs can more easily penetrate the cell membrane and interact with different cell parts. Also, it has been reported that the AgNPs, with higher surface area, can sustainably release more concentration of silver cations 38 , 59 . It has been revealed that the green synthesized AgNPs can carry numerous plant secondary metabolites on their surface that enhance the effectiveness of AgNPs 7 , 38 . Table 9 summarizes the IC 50 values of AgNPs prepared from several plant extracts against the same cancerous cell lines as our study. As is presented, the IC 50 values of AgNPs synthesizes from leaves of R. discolor was in the range of previous studies. Also, AgNPs from R. discolor had stronger cytotoxic activities against MCF-7, and A431 compared to the AgNPs that are prepared from other mentioned plant extracts in Table 9 .
In current study, the biosynthesized AgNPs were characterized using UV–Vis spectroscopy, FT-IR analysis, DLS, TEM, SEM–EDX, and XRD. All these characterizations confirmed the synthesis of AgNPs with average size of 37 nm. The results of the FTIR spectra showed that the phytochemicals present in R. discolor extract play a key role in the production of AgNPs. Phytochemical analysis showed that the leaves of R. discolor are a good source of phytochemicals, including phenolics, tannins, and flavonoids. Besides, having health-beneficial effects, these compounds have the ability to reduce silver ions, along with surface coating and stabilization of the AgNPs. The study also aimed to optimize the physical parameters and discover the interaction relations between variables affecting AgNPs biosynthesis, using RSM. The experimental results exhibited that all the factors studied were significant for the variable responses. The optimized condition was found to be an AgNO 3 concentration of 7.11 mM, a time of 17.83 h, a temperature of 56.51 °C, and an extract percentage of 29.22, with a yield of 53.31%. Considering the surge in antibiotic resistance, the AgNPs prepared from R. discolor can be potentially used as an antibacterial agent against MDR E. coli and P. aeruginosa pathogens (MIC 0.93–3.75 mg ml −1 ). The AgNPs depicted significant cytotoxicity against A431, MCF7, and HepG2 (IC 50 11.2–49.1 µg ml −1 ), while no significant toxicity against normal cell line was observed. This optimized, low-cost, and environmentally-friendly method is a valuable approach for producing bioactive silver NPs with high yield and small size.
Material and method
Mueller Hinton broth (MHB), Mueller Hinton Agar (MHA), Aluminum trichloride (AlCl 3 ), silver nitrate (AgNO 3 ), sodium bicarbonate (NaHCO 3 ), 3-(4,5-dimethylthiazol-2-yl)-2,5-diphenyl-2 H -tetrazolium bromide (MTT), and Folin–Ciocalteu’s reagent were obtained from Merk, Germany. Standard compounds, including gallic acid, tannic acid, and quercetin were bought from Sigma Chemical Company (USA). The MCF7, HePG2, and A431 cancerous cell lines were obtained from the Iranian Biological Resource Center (Iran). The normal cell line (Hu02) was purchased from the National Cell Bank of Pasteur Institute (Tehran, Iran). Dulbecco's Modified Eagle Medium (DMEM) was obtained from Gibco. The fetal bovine serum was got from Invitrogen. All other reagents and solvents used were of analytical grade.
Plant materials and extraction
The aerial parts of R. discolor were collected from Fuman-Saravan Road, Guilan province, in the North of Iran, in May 2021 (Fig. 13 ). The voucher specimen (113 HGUM) was kept in the herbarium of the faculty of pharmacy, Guilan University of Medical Sciences, Rasht, Iran. The plant's leaves were separated from the stems. Then, leaves were shade-dried at room temperature for two weeks and powdered with a mixer grinder. Consequently, 100 g of powder were added to 600 ml of deionized water (DW) and boiled for 15 min. After that, the mixture was cooled and filtered through the Whatman filter paper 7 . Lastly, the solvent was evaporated using a rotary vacuum evaporator (Heidolph, Germany) at 45 °C to obtain 4.1 g of dried extract. It was kept in the refrigerator at 4ºC until required.
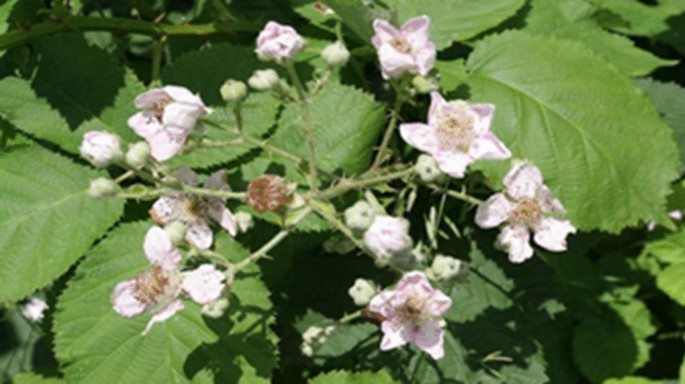
Rubus discolor Weihe & Nees.
Preliminary phytochemical tests
The preliminary qualitative phytochemical assays were carried out to identify the presence of secondary metabolites in the extract, including flavonoids, tannins, anthraquinones, steroids, carbohydrates, coumarins, and alkaloids, using the standard protocols described by Saeidnia & Ghohari 67 .
Determination of total phenolic content (TPC)
The Folin-Ciocalteu method was used to measure the total phenolic contents in the extract and AgNPs 68 . In this test, 1 ml of each sample (1 mg ml −1 ) was added to 5 ml of freshly prepared Folin-Ciocalteu reagent (diluted tenfold with distilled water). Then, the mixtures were incubated for 10 min at room temperature before mixing with 4 ml sodium bicarbonate solution (75 g l −1 ). They were incubated for 30 min in the dark. Lastly, the absorbance was obtained at 765 nm using a UV/Vis spectrophotometer. All the experiments were repeated three times. The gallic acid (GA) was used as the reference standard in different concentrations (10, 25, 50, 100, and 150 µg ml −1 ), and the calibration curve was plotted. The total phenolic contents were expressed as mg of gallic acid equivalents (GAE)/g extract.
Determination of total flavonoid content (TFC)
The measurement of the flavonoid was carried out by the Dowd method 67 , 68 . First, aluminum trichloride (AlCl 3 ) (2%) was prepared in methanol. Then, 5 ml of AlCl 3 solution was added to 5 ml of each sample (2 mg ml −1 ). The mixtures were incubated for 10 min at room temperature. Finally, the absorbance was measured at 415 nm using a UV/Vis spectrophotometer 7 . All the experiments were repeated three times. The quercetin was used as the standard compound with five known concentrations (10, 25, 50, 75, and 100 µg ml −1 ). Finally, the total flavonoid content was expressed as mg of quercetin as equivalents (QE)/g of extract.
Determination of total tannin content (TTC)
The aqueous extract and synthesized AgNPs were examined for the total tannin contents by a colorimetric method using polyvinylpolypyrrolidone (PVPP) 69 , 70 , 71 . In this assay, PVPP binds to tannins and precipitates them. Different concentrations of tannic acid (20, 40, 60, 80, 100, 150, and 200 μg ml −1 ) were used for plotting the calibration curve. This method involved two steps. In the first step, 1 ml of each sample (1 mg ml −1 ) was combined with 0.5 ml Folin-Ciocalteu reagent (1 N). Next, sodium carbonate solution (2.5 ml, 20%) was added to each mixture. After 40 min, the absorbance was read at 725 nm. The amounts of total phenols as tannic acid equivalent (X) were calculated using the calibration curve. In the second step, the tannins were removed from tannin-containing samples by adding PVPP (100 mg of PVPP is adequate to bind 2 mg of total phenols). The samples were vigorously shaken (5 min) and kept at 4 °C (15 min). After that, the samples were centrifuged at 4000 g (20 min), and the supernatants were collected. The supernatant only contained simple phenols other than tannins. The phenolic contents of the supernatants were measured, as explained in the first step. The contents of non-tannin phenols (Y) were determined. Lastly, X–Y showed mg of tannin as tannic acid equivalent (TAE)/g extracts.
Green synthesis of AgNPs
In a typical reaction procedure, different amount of aqueous extract was added to five different concentrations of AgNO 3 solution (50 ml, 1–10 mM), based on CCD described in the next section. The mixtures were stirred on a magnetic stirrer (Heidolph, Germany) at a constant rate (500 rpm) at different times and temperatures. Next, the mixtures were centrifuged for 15 min at 10,000 rpm using a centrifuge machine 7 . Finally, the sediments were washed three times with deionized water, and dried in a vacuum oven (45 °C). The yield of the AgNPs formation was calculated in optimized condition.
Experimental design and optimization of AgNPs synthesis by RSM
Previous studies showed that different parameters like concentration of extract and AgNO 3 , time, and temperature have great influence on the size and yield of synthesized AgNPs 72 . In this study, a central composite design (CCD) under Response Surface Methodology (RSM) was employed for the optimization of the most prominent parameters and also for the identification of their cooperative interactions using Design-Expert 7.0 (Stat-Ease, Inc., USA software). Four independent variables were selected, including reaction time (h), reaction temperature (°C), AgNO 3 concentration, and percentage of extract (%). Each variable was evaluated at five coded levels (− 2, − 1, 0, 1, 2) (Table 10 ). The total experimental runs were calculated using the following equation: 2 k + 2k + x 0 , where k is a variable number and x 0 is the repetition number of experiments at the center point 40 .
The color change from yellowish-green to dark brown was a confirmatory sign for NP formation. Consequently, small sample of the synthesized AgNPs was dispersed in the distilled water and the absorption was measured in the wavelength range of 200–800 nm using a UV–Vis spectrophotometer (PerkinElmer, USA). Distilled water was used as a blank 8 . FT-IR spectroscopy was performed to analyze the surface chemistry and the molecular vibrations of the synthesized AgNPs. The synthesized AgNPs and the extract were screened with a Spectrum Two FT-IR spectrometer with UATR accessory (PerkinElmer, USA) in 400–4000 cm −1 6 , 8 . The TEM was used to determine the morphology (size and shape) of the AgNPs. The microphotographs were obtained using a Zeiss—EM10C—100 kV instrument (Germany). In order to confirm the surface morphology and elemental composition of green-synthesized AgNPs, SEM–EDX instrument was used. A MIRA3 FE-SEM from TESCAN was employed to get SEM images 49 . The preparation of the sample was performed by following and abiding by the manufacturer’s instructions. The size distribution and ζ-potential of NPs was measured by a DLS and Zeta potential analyzer (Nanopartica SZ-100; HORIBA Ltd, Kyoto, Japan). The obtained spectrum provides the hydrodynamic size, distribution, and PDI. For determining the crystalline structure, XRD analysis was carried out using the Bruker AXS model D8 Advance powder X-ray diffractometer ranging from 5° to 80° 7 .
Antibacterial assay
For investigation of the antibacterial activity of AgNPs and plant extract, two gram-positive ( Staphylococcus aureus ATCC 6538, Bacillus subtilis ATCC 9634) and two gram-negative ( Escherichia coli ATCC 8739, and Pseudomonas aeruginosa ATCC 9027) bacteria were used. In addition, 10 MDR E. coli and P. aeruginosa isolates, resistant to 8 antibiotics (Gentamicin, Trimethoprim-Sulfamethoxazole, Ceftazidime, Ampicillin, Amikacin, Cefepime, Ceftriaxone, and Ciprofloxacin), obtained from the 17 Shahrivar Children’s Hospital (Rasht, Iran), were used for further investigation of antibacterial properties of AgNPs 73 . The zone of inhibition was measured by the disc diffusion method. Also, minimum inhibitory concentration (MIC) and minimum bactericidal concentration (MBC) were measured by the broth microdilution method using 96 U-shaped well plates 74 .
Bacterial cultures of all bacteria were grown for 24 h in nutrient broth. In agar disc diffusion test, the petri dishes, containing 25 ml of Mueller Hinton Agar (MHA) were used. The agar plates were swabbed with broth cultures standardized with 0.5 McFarland standard solution (1.5 × 10 8 CFU ml −1 ) of each strain. The sterile discs were located in the agar, and each sample (10 μl) was placed on each disc. The plates were incubated at 37 °C for 48 h. Lastly, the plates were evaluated for the inhibition zones (mm) 75 . All experiments were conducted in triplicate.
For the MIC assay, a stock solution from each sample was prepared in distilled water. Then, a doubling dilution of each sample’s stock solution (100 μl) was prepared in wells using Mueller Hinton broth (MHB). The serial dilutions of samples (extract: 150–0.84 mg ml −1 , and AgNPs: 10–0.005 mg ml −1 ) were prepared in microplates. Each bacteria inoculum (1.5 × 10 8 CFU ml −1 ) was diluted in 0.9% saline to give 10 7 CFU ml −1 . The plates were spot-inoculated with 100 μl of each prepared bacterial suspension (10 5 CFU/spot). The bacteria were incubated at 37 °C for 48 h. The plates were tested for the absence or presence of visible growth compared to the negative control wells. The endpoint of MIC was the lowest concentration of the compounds in which there was no visible growth 75 , 76 . The MBC values were determined by culturing 100 μl of no-growth wells in Petri dishes, contained MHA and incubating at 37 °C for 24 h. The MBCs were reported as the lowest concentration that killed 99.9% of bacterial cells 73 .
Cytotoxicity assay
Biosynthesized AgNPs and aqueous extract of R. discolor were evaluated for anti-proliferative activities at different concentrations in MCF-7, A431, and HU02 cell lines using the MTT assay. Cell lines were cultured in Dulbecco’s Modified Eagles Medium (DMEM). For this reason, 5 × 10 3 cells per well were seeded in a 96-well plate in complete DMEM and incubated at 37 °C for 24 h in a humidified atmosphere, containing 5% CO 2 . Next, non-adherent cells were removed, and adherent cells were treated with the following concentrations of samples (prepared by serial dilution): 1000, 500, 250, 125, 62.5, 31.2, 15.6, 7.8, and 3.9 μg ml −1 . The samples were incubated for 48 h. Next, the MTT solution (20 µl, 5 mg ml −1 in PBS) and DMEM (180 µl) were added to seeded cells and incubated for 4 h. Then, the supernatants were removed. For dissolving formazan crystals, DMSO (150 µl) was added to each well and shaken for 10 min 75 , 77 . The optical density (OD) was read at 490 nm using an absorbance microplate reader (BioTek) (the reference wavelength was 630 nm). Each test was repeated three times. The percentage of viable cells was calculated using the following equation:
IC 50 of samples (the concentration in which 50% of cells were alive) was calculated using GraphPad Prism (Version 8, GraphPad Software, USA) 77 , 78 .
Statistical analysis
Every experiment was carried out in triplicates. All the results are expressed as mean ± standard deviation (SD). The calculation of IC 50 values (the concentration required for 50% inhibitory activity) was made by nonlinear regression with the normalized fitted dose–response curve (GraphPad Prism Software., version 5, Inc. San Diego, USA) 77 .
Ethics approval and consent to participate
This study was approved by the Ethical Committee of Guilan University of Medical Sciences (IR.GUMS.REC.1400.093). The collection and use of plant material complies with relevant institutional and national guideline and regulations of plant protection.
Data availability
The datasets used and/or analyzed during the current study are available from the corresponding author upon reasonable request.
Vanin dos Santos Lima, M. et al. Green synthesis of silver nanoparticles using Ilex paraguariensis extracts: Antimicrobial activity and acetilcolinesterase modulation in rat brain tissue. Green Chem. Lett. Rev. 15 (1), 128–138 (2022).
Article Google Scholar
Alharbi, N. S., Alsubhi, N. S. & Felimban, A. I. Green synthesis of silver nanoparticles using medicinal plants: Characterization and application. J. Radiat. Res. Appl. Sci. 15 (3), 109–124 (2022).
CAS Google Scholar
Salem, S. S. & Fouda, A. Green synthesis of metallic nanoparticles and their prospective biotechnological applications: An overview. Biol. Trace Elem. Res. 199 (1), 344–370 (2021).
Article CAS PubMed Google Scholar
Salem, S. S. A mini review on green nanotechnology and its development in biological effects. Arch. Microbiol. 205 (4), 128 (2023).
Article CAS PubMed PubMed Central Google Scholar
Salem, S. S., Hammad, E. N., Mohamed, A. A. & El-Dougdoug, W. A comprehensive review of nanomaterials: Types, synthesis, characterization, and applications. Biointerface Res. Appl. Chem. 13 (1), 41 (2022).
Hawar, S. N. et al. Green synthesis of silver nanoparticles from Alhagi graecorum leaf extract and evaluation of their cytotoxicity and antifungal activity. J. Nanomater. 2022 , 1–8 (2022).
Yousefbeyk, F. et al. Green synthesis of silver nanoparticles from Stachys byzantina K. Koch: Characterization, antioxidant, antibacterial, and cytotoxic activity. Part. Sci. Technol. 40 (2), 219–232 (2022).
Article CAS Google Scholar
Yassin, M. T., Mostafa, A.A.-F., Al-Askar, A. A. & Al-Otibi, F. O. Facile green synthesis of silver nanoparticles using aqueous leaf extract of Origanum majorana with potential bioactivity against multidrug resistant bacterial strains. Crystals 12 (5), 603 (2022).
Mustapha, T., Misni, N., Ithnin, N. R., Daskum, A. M. & Unyah, N. Z. A review on plants and microorganisms mediated synthesis of silver nanoparticles, role of plants metabolites and applications. Int. J. Environ. Res. Public Health 19 (2), 674 (2022).
Ahmed, H. M., El-khateeb, M. A., Sobhy, N. A., Hefny, M. M. & Abdel-Haleem, F. M. Green synthesis of magnetite nanoparticles using waste natural materials and its application for wastewater treatment. Environ. Sci. Proc. 25 (1), 99 (2023).
Google Scholar
Hashemi, Z., Mizwari, Z. M., Mohammadi-Aghdam, S., Mortazavi-Derazkola, S. & Ebrahimzadeh, M. A. Sustainable green synthesis of silver nanoparticles using Sambucus ebulus phenolic extract (AgNPs@ SEE): Optimization and assessment of photocatalytic degradation of methyl orange and their in vitro antibacterial and anticancer activity. Arab. J. Chem. 15 (1), 103525 (2022).
Habeeb rahuman, H. B. et al. Medicinal plants mediated the green synthesis of silver nanoparticles and their biomedical applications. IET Nanobiotechnol. 16 (4), 115–144 (2022).
Article PubMed PubMed Central Google Scholar
Dua, T. K. et al. Green synthesis of silver nanoparticles using Eupatorium adenophorum leaf extract: Characterizations, antioxidant, antibacterial and photocatalytic activities. Chem. Pap. https://doi.org/10.1007/s11696-023-02676-9 (2023).
Rizwana, H., Alwhibi, M. S., Al-Judaie, R. A., Aldehaish, H. A. & Alsaggabi, N. S. Sunlight-mediated green synthesis of silver nanoparticles using the berries of Ribes rubrum (Red Currants): Characterisation and evaluation of their antifungal and antibacterial activities. Molecules 27 (7), 2186 (2022).
Khan, M. et al. Green synthesis of silver nanoparticles using Juniperus procera extract: Their characterization, and biological activity. Crystals 12 (3), 420 (2022).
Said, A., Abu-Elghait, M., Atta, H. M. & Salem, S. S. Antibacterial activity of green synthesized silver nanoparticles using Lawsonia inermis against common pathogens from urinary tract infection. Appl. Biochem. Biotechnol. 196 (1), 85–98 (2024).
Salem, S. S., Ali, O. M., Reyad, A. M., Abd-Elsalam, K. A. & Hashem, A. H. Pseudomonas indica -mediated silver nanoparticles: Antifungal and antioxidant biogenic tool for suppressing Mucormycosis fungi. J. Fungi 8 (2), 126 (2022).
Salem, S. S. Baker’s yeast-mediated silver nanoparticles: Characterisation and antimicrobial biogenic tool for suppressing pathogenic microbes. BioNanoScience 12 (4), 1220–1229 (2022).
Soliman, M. K. Y., Salem, S. S., Abu-Elghait, M. & Azab, M. S. Biosynthesis of silver and gold nanoparticles and their efficacy towards antibacterial, antibiofilm, cytotoxicity, and antioxidant activities. Appl. Biochem. Biotechnol. 195 (2), 1158–1183 (2023).
Priya, S., Murali, A., Preeth, D. R., Dharanibalaji, K. C. & Jeyajothi, G. Green synthesis of silver nanoparticle-embedded poly(methyl methacrylate-co-methacrylic acid) copolymer for fungal-free leathers. Polym. Bull. 79 (7), 4607–4626 (2022).
Talank, N. et al. Bioengineering of green-synthesized silver nanoparticles: In vitro physicochemical, antibacterial, biofilm inhibitory, anticoagulant, and antioxidant performance. Talanta 243 , 123374 (2022).
Cui, X., Lü, Y. & Yue, C. Development and research progress of anti-drug resistant bacteria drugs. Infect. Drug Res. 14 , 5575–5593 (2021).
Mortezagholi, B. et al. Plant-mediated synthesis of silver-doped zinc oxide nanoparticles and evaluation of their antimicrobial activity against bacteria cause tooth decay. Microsc. Res. Tech. 85 (11), 3553–3564 (2022).
Widatalla, H. A. et al. Green synthesis of silver nanoparticles using green tea leaf extract, characterization and evaluation of antimicrobial activity. Nanoscale Adv. 4 (3), 911–915 (2022).
Article ADS CAS PubMed PubMed Central Google Scholar
Al-Rajhi, A. M. H., Salem, S. S., Alharbi, A. A. & Abdelghany, T. M. Ecofriendly synthesis of silver nanoparticles using Kei-apple ( Dovyalis caffra ) fruit and their efficacy against cancer cells and clinical pathogenic microorganisms. Arab. J. Chem. 15 (7), 103927 (2022).
Soliman, M. K. Y., Abu-Elghait, M., Salem, S. S. & Azab, M. S. Multifunctional properties of silver and gold nanoparticles synthesis by Fusarium pseudonygamai . Biomass Convers. Biorefinery https://doi.org/10.1007/s13399-022-03507-9 (2022).
Elakraa, A. A., Salem, S. S., El-Sayyad, G. S. & Attia, M. S. Cefotaxime incorporated bimetallic silver-selenium nanoparticles: Promising antimicrobial synergism, antibiofilm activity, and bacterial membrane leakage reaction mechanism. RSC Adv. 12 (41), 26603–26619 (2022).
Nagai, H. & Kim, Y. H. Cancer prevention from the perspective of global cancer burden patterns. J. Thorac. Dis. 9 (3), 448 (2017).
Ovais, M. et al. Green synthesis of silver nanoparticles via plant extracts: Beginning a new era in cancer theranostics. Nanomedicine 12 (23), 3157–3177 (2016).
Robinson, J. A., Bierwirth, J. E., Greenspan, P. & Pegg, R. B. Blackberry polyphenols: Review of composition, quantity, and health impacts from in vitro and in vivo studies. J. Food Bioact. https://doi.org/10.31665/JFB.2020.9217 (2020).
Lee, J., Dossett, M. & Finn, C. E. Rubus fruit phenolic research: The good, the bad, and the confusing. Food Chem. 130 (4), 785–796 (2012).
Zhang, S. et al. Comparative study of three raspberry cultivar ( Rubus idaeus L.) leaves metabolites: Metabolome profiling and antioxidant activities. Appl. Sci. 12 (3), 990 (2022).
Article MathSciNet Google Scholar
Oszmiański, J. et al. Determination of phenolic compounds and antioxidant activity in leaves from wild Rubus L. species. Molecules 20 (3), 4951–4966 (2015).
Kasalkheh, R., Jorjani, E., Sabouri, H., Habibi, M. & Sattarian, A. Chromosome numbers and karyotypes of four species Rubus L. from North of Iran. J. Genet. Resour. 5 (1), 59–64 (2019).
Caplan, J. S. & Yeakley, J. A. Rubus armeniacus (Himalayan blackberry) occurrence and growth in relation to soil and light conditions in western oregon. Northwest Sci. 80 (1), 9 (2006).
Sedighi, E. & Rahimmalek, M. Evaluation of genetic diversity of Rubus hyrcanus using inter simple sequence repeat (ISSR) and morphological markers. Biologia 70 , 339–348 (2015).
Nunes, A. R., Goncalves, A. C., Falcao, A., Alves, G. & Silva, L. R. Prunus avium L. (Sweet Cherry) by-products: A source of phenolic compounds with antioxidant and anti-hyperglycemic properties—A review. Appl. Sci. 11 (18), 8516 (2021).
Jalilian, F., Chahardoli, A., Sadrjavadi, K., Fattahi, A. & Shokoohinia, Y. Green synthesized silver nanoparticle from Allium ampeloprasum aqueous extract: Characterization, antioxidant activities, antibacterial and cytotoxicity effects. Adv. Powder Technol. 31 (3), 1323–1332 (2020).
Vitta, Y., Figueroa, M., Calderon, M. & Ciangherotti, C. Synthesis of iron nanoparticles from aqueous extract of Eucalyptus robusta Sm and evaluation of antioxidant and antimicrobial activity. Mater. Sci. Energy Technol. 3 , 97–103 (2020).
Othman, A. M., Elsayed, M. A., Elshafei, A. M. & Hassan, M. M. Application of response surface methodology to optimize the extracellular fungal mediated nanosilver green synthesis. J. Genet. Eng. Biotechnol. 15 (2), 497–504 (2017).
El-Rafie, M., Shaheen, T. I., Mohamed, A. & Hebeish, A. Bio-synthesis and applications of silver nanoparticles onto cotton fabrics. Carbohydr. Polym. 90 (2), 915–920 (2012).
Nikaeen, G., Yousefinejad, S., Rahmdel, S., Samari, F. & Mahdavinia, S. Central composite design for optimizing the biosynthesis of silver nanoparticles using plantago major extract and investigating antibacterial, antifungal and antioxidant activity. Sci. Rep. 10 (1), 9642 (2020).
Khammar, Z. et al. Optimization of biosynthesis of stabilized silver nanoparticles using bitter orange peel by-products and glycerol. Biocatal. Agric. Biotechnol. 43 , 102425 (2022).
Yousefbeyk, F. et al. Phytochemical analysis and antioxidant activity of eight cultivars of tea ( Camellia sinensis ) and rapid discrimination with FTIR spectroscopy and pattern recognition techniques. Pharm. Sci. 29 (1), 100–110 (2022).
Palithya, S. et al. Green synthesis of silver nanoparticles using flower extracts of Aerva lanata and their biomedical applications. Part. Sci. Technol. 40 (1), 84–96 (2022).
Patra, J. K., Das, G. & Shin, H.-S. Facile green biosynthesis of silver nanoparticles using Pisum sativum L. outer peel aqueous extract and its antidiabetic, cytotoxicity, antioxidant, and antibacterial activity. Int. J. Nanomed. 14 , 6679–6690 (2019).
Barabadi, H. et al. Green synthesis, characterization, antibacterial and biofilm inhibitory activity of silver nanoparticles compared to commercial silver nanoparticles. Inorg. Chem. Commun. 129 , 108647 (2021).
Mariadoss, A. V. A. et al. Green synthesis, characterization and antibacterial activity of silver nanoparticles by Malus domestica and its cytotoxic effect on (MCF-7) cell line. Microb. Pathog. 135 , 103609 (2019).
Kaviya, S., Santhanalakshmi, J., Viswanathan, B., Muthumary, J. & Srinivasan, K. Biosynthesis of silver nanoparticles using Citrus sinensis peel extract and its antibacterial activity. Spectrochim. Acta Part A Mol. Biomol. Spectrosc. 79 (3), 594–598 (2011).
Article ADS CAS Google Scholar
Okaiyeto, K., Ojemaye, M. O., Hoppe, H., Mabinya, L. V. & Okoh, A. I. Phytofabrication of silver/silver chloride nanoparticles using aqueous leaf extract of Oedera genistifolia : Characterization and antibacterial potential. Molecules 24 (23), 4382 (2019).
Badeggi, U. M. et al. Characterization and toxicity of hypoxoside capped silver nanoparticles. Plants 11 (8), 1037 (2022).
Khane, Y. et al. Green synthesis of silver nanoparticles using aqueous Citrus limon zest extract: Characterization and evaluation of their antioxidant and antimicrobial properties. Nanomaterials 12 (12), 2013 (2022).
Kokila, T., Ramesh, P. S. & Geetha, D. Biosynthesis of AgNPs using Carica papaya peel extract and evaluation of its antioxidant and antimicrobial activities. Ecotoxicol. Environ. Saf. 134 , 467–473 (2016).
Gecer, E. N., Erenler, R., Temiz, C., Genc, N. & Yildiz, I. Green synthesis of silver nanoparticles from Echinacea purpurea (L.) Moench with antioxidant profile. Part. Sci. Technol. 40 (1), 50–57 (2022).
Aref, M. S. & Salem, S. S. Bio-callus synthesis of silver nanoparticles, characterization, and antibacterial activities via Cinnamomum camphora callus culture. Biocatal. Agric. Biotechnol. 27 , 101689 (2020).
Abdel-Raouf, N., Al-Enazi, N. M., Ibraheem, I. B. M., Alharbi, R. M. & Alkhulaifi, M. M. Biosynthesis of silver nanoparticles by using of the marine brown alga Padina pavonia and their characterization. Saudi J. Biol. Sci. 26 (6), 1207–1215 (2019).
Salleh, A. et al. The potential of silver nanoparticles for antiviral and antibacterial applications: A mechanism of action. Nanomaterials 10 (8), 1566 (2020).
Liao, C., Li, Y. & Tjong, S. C. Bactericidal and cytotoxic properties of silver nanoparticles. Int. J. Mol. Sci. 20 (2), 449 (2019).
Qing, Y. A. et al. Potential antibacterial mechanism of silver nanoparticles and the optimization of orthopedic implants by advanced modification technologies. Int. J. Nanomed. 13 , 3311–3327 (2018).
Usmani, A., Mishra, A., Jafri, A., Arshad, M. & Siddiqui, M. A. Green synthesis of silver nanocomposites of Nigella sativa seeds extract for hepatocellular carcinoma. Curr. Nanomater. 4 (3), 191–200 (2019).
Gajendran, B. et al. Green synthesis of silver nanoparticle from Datura inoxia flower extract and its cytotoxic activity. BioNanoScience 9 (3), 564–572 (2019).
Vivek, R. et al. Green biosynthesis of silver nanoparticles from Annona squamosa leaf extract and its in vitro cytotoxic effect on MCF-7 cells. Process Biochem. 47 (12), 2405–2410 (2012).
Saratale, R. G. et al. Exploiting antidiabetic activity of silver nanoparticles synthesized using Punica granatum leaves and anticancer potential against human liver cancer cells (HepG2). Artif. Cells Nanomed. Biotechnol. 46 (1), 211–222 (2018).
Nayak, D., Pradhan, S., Ashe, S., Rauta, P. R. & Nayak, B. Biologically synthesised silver nanoparticles from three diverse family of plant extracts and their anticancer activity against epidermoid A431 carcinoma. J. Colloid Interface Sci. 457 , 329–338 (2015).
Article ADS CAS PubMed Google Scholar
Pathak, M. et al. Green Synthesis of Silver Nanoparticles Using Scindapsus officinalis (Gajpipli): In-Vitro Cytotoxic Activity Against HepG-2 & MCF-7 Cancer Cell Lines . Preprints: Preprints; 2019.
Huo, Y. et al. Biological synthesis of gold and silver chloride nanoparticles by Glycyrrhiza uralensis and in vitro applications. Artif. Cells Nanomed. Biotechnol. 46 (2), 303–312 (2018).
Saeidnia, S. & Gohari, A. R. Pharmacognosy and Molecular Pharmacognosy in Practice: A Laboratory Desk Reference of Pharmacognosy for Researchers and Students (LAP Lambert Academic Publishing, 2012).
Yousfbeyk, F. et al. Antioxidant activity, total phenol and total anthocyanin contents of Cornus sanguinea L. subsp. australis . (CA Mey.) Jáv. (2014).
FOOD, O. N. T. I. Quantification of Tannins in Tree Foliage (2000).
Manayi, A. et al. Biological activity and microscopic characterization of Lythrum salicaria L. DARU J. Pharm. Sci. 21 , 1–7 (2013).
Saeidnia, S., Nikan, M., Mirnezami, T., Gohari, A. R. & Manayi, A. Micromorphological characterizations and phytochemicals contents of some Phlomis species from Iran. Int. J. Pharm. Pharm. Sci. 8 (1), 157–161 (2015).
Sarkar, M., Denrah, S., Das, M. & Das, M. Statistical optimization of bio-mediated silver nanoparticles synthesis for use in catalytic degradation of some azo dyes. Chem. Phys. Impact 3 , 100053 (2021).
Nemattalab, M., Rohani, M., Evazalipour, M. & Hesari, Z. Formulation of Cinnamon ( Cinnamomum verum ) oil loaded solid lipid nanoparticles and evaluation of its antibacterial activity against multi-drug resistant Escherichia coli . BMC Complement. Med. Ther. 22 (1), 289 (2022).
Kiehlbauch, J. A. et al. Use of the National Committee for Clinical Laboratory Standards guidelines for disk diffusion susceptibility testing in New York state laboratories. J. Clin. Microbiol. 38 (9), 3341–3348 (2000).
Yousefbeyk, F. et al. Phytochemical analysis, antioxidant, antibacterial, and cytotoxic activities of leaves and roots of Rubus hyrcanus Juz. Eur. Food Res. Technol. 248 (1), 141–152 (2022).
Yousefbeyk, F. et al. Chemical composition and antimicrobial activity of essential oils from different parts of Daucus littoralis Smith subsp. hyrcanicus Rech. f. J. Essent. Oil Bear. Plants 17 (4), 570–576 (2014).
Azmian Moghadam, F., Kefayati, H., Evazalipour, M. & Ghasemi, S. Design, synthesis, biological evaluation, and docking study of novel 4-anilinoquinazolines derivatives as anticancer agents. Iran. J. Chem. Chem. Eng. 41 (2), 353–367 (2022).
Moghadam, F. A., Evazalipour, M., Kefayati, H. & Ghasemi, S. 6, 7-disubstituted-4-anilinoquinazoline: Design, synthesis and anticancer activity as a novel series of potent anticancer agents. Pharm. Sci. 27 (2), 209–218 (2020).
Download references
Acknowledgements
This work was supported by Guilan University of Medical Sciences (99121804).
This work was supported by Guilan University of Medical Sciences (Grant Number 99121804).
Author information
Authors and affiliations.
Department of Medicinal Chemistry, School of Pharmacy, Guilan University of Medical Sciences, Rasht, Iran
Saeed Ghasemi
Department of Pharmaceutical Biotechnology, School of Pharmacy, Guilan University of Medical Sciences, Rasht, Iran
Sara Dabirian
Department of Pharmacognosy, School of Pharmacy, Guilan University of Medical Sciences, Rasht, Iran
Faezeh Kariminejad, Diba Eghbali Koohi, Sina Majidimoghadam & Fatemeh Yousefbeyk
Department of Pharmaceutics, School of Pharmacy, Guilan University of Medical Sciences, Rasht, Iran
Mehran Nemattalab
Department of Pharmacology and Toxicology, School of Pharmacy, Guilan University of Medical Sciences, Rasht, Iran
Ehsan Zamani
You can also search for this author in PubMed Google Scholar
Contributions
S.G. and F.Y. supervised the study process and participated in the research design and formal analysis, S.D., F.K., D.E.K, and M.N., participated in the performance of research and data acquisition, S.M., and E.Z. contributed to data collection. F.Y. wrote original draft. All authors reviewed the manuscript and agreed to the published version of the manuscript.
Corresponding author
Correspondence to Fatemeh Yousefbeyk .
Ethics declarations
Competing interests.
The authors declare no competing interests.
Additional information
Publisher's note.
Springer Nature remains neutral with regard to jurisdictional claims in published maps and institutional affiliations.
Rights and permissions
Open Access This article is licensed under a Creative Commons Attribution 4.0 International License, which permits use, sharing, adaptation, distribution and reproduction in any medium or format, as long as you give appropriate credit to the original author(s) and the source, provide a link to the Creative Commons licence, and indicate if changes were made. The images or other third party material in this article are included in the article's Creative Commons licence, unless indicated otherwise in a credit line to the material. If material is not included in the article's Creative Commons licence and your intended use is not permitted by statutory regulation or exceeds the permitted use, you will need to obtain permission directly from the copyright holder. To view a copy of this licence, visit http://creativecommons.org/licenses/by/4.0/ .
Reprints and permissions
About this article
Cite this article.
Ghasemi, S., Dabirian, S., Kariminejad, F. et al. Process optimization for green synthesis of silver nanoparticles using Rubus discolor leaves extract and its biological activities against multi-drug resistant bacteria and cancer cells. Sci Rep 14 , 4130 (2024). https://doi.org/10.1038/s41598-024-54702-9
Download citation
Received : 13 November 2023
Accepted : 15 February 2024
Published : 19 February 2024
DOI : https://doi.org/10.1038/s41598-024-54702-9
Share this article
Anyone you share the following link with will be able to read this content:
Sorry, a shareable link is not currently available for this article.
Provided by the Springer Nature SharedIt content-sharing initiative
- Himalayan blackberry
- Total phenols
- Multidrug-resistant bacteria
- Cancerous cell lines
This article is cited by
Synthesis and characterization of variable-sized silver nanoparticles using pistacia palaestina leaf extract.
- Rahaf Mousa
Plasmonics (2024)
By submitting a comment you agree to abide by our Terms and Community Guidelines . If you find something abusive or that does not comply with our terms or guidelines please flag it as inappropriate.
Quick links
- Explore articles by subject
- Guide to authors
- Editorial policies
Sign up for the Nature Briefing: Cancer newsletter — what matters in cancer research, free to your inbox weekly.

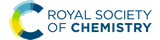
Synthesis of green and pure copper oxide nanoparticles using two plant resources via solid-state route and their phytotoxicity assessment

First published on 15th January 2021
Among the conventional methods in synthesizing nanoparticles, the methods that use biological resources, as reducing and stabilizing agents, can be considered eco-friendly methods. In this study, the leaf tissue of green tea ( Camellia sinensis L.) and lavender ( Lavandula anguistifolia ) were utilized by the solid-state method as a one-step and low-cost method for the biosynthesis of copper oxide nanoparticles (CuO NPs). The results of the X-ray Diffraction (XRD), field emission scanning electron spectroscopy (FESEM) and transmission electron microscopy (TEM) showed that lavender is more productive in the synthesis of pure and uniform CuO NPs (50 nm). Comparing biogenic synthesized CuO NPs with chemically synthesized CuO NPs in terms of induction of phytotoxicity, exposed in treatments with concentrations of 40, 400 and 4000 μg ml −1 , green CuO NPs had less inhibitory effects on the seed germination factors ( i.e. , germination percentage, germination rate, shoot and root length, etc. ) of lettuce ( Lactuca sativa L.), and tomato ( Solanum lycopersicum L.) seeds. However, both green/chemically synthesized CuO NPs at their lowest concentrations (4 μg ml −1 ), had an effective role in root and shoot expansion of lettuce and tomato seedlings.
Introduction
Various methods were employed in the past to synthesize different copper-based nanoparticles, such as chemical synthesis, electrochemical synthesis, solvothermal route and phytosynthesis. 14–17 In this regard, several studies have reported the usage of herbal extracts in the green synthesis of Cu NPs such as Garcinia mangostana leaf extract, Rubus glaucus Benth, fruit and leaf extract, extract of seedless dates, leaves extract of Ocimum sanctum L., Punica granatum peel extract, root and leaf extract of Asparagus adscendens Roxb., fruit extract of Ziziphus spina-christi L. and leaf aqueous extract of Nerium oleander . 2,13,18–23 In particular, the green synthesis of CuO NPs and their various applications in the biomedical, agricultural and environmental fields have been recently reviewed. 24,25
Considering this background into account, in this work, we investigate the formation of CuO NPs using Lavandula anguistifolia and Camellia sinensis L. under solid-phase procedures as a very facile, inexpensive and eco-friendly method. Although no report has been published on the synthesis of CuO NPs in the solid-phase procedures, successful reports of the use of this method in the synthesis of Ag NPs have persuaded us to use this method in the synthesis of CuO NPs. 26,27
Lavender ( Lavandula anguistifolia ), also known as medicinal lavender, is a shrub of the family Lamiaceae , native to the Mediterranean region, but is grown in many other climates of the world. 28 This plant is a natural source of organic compounds such as coumarin, coumaric acid, ursolic acid, valeric acid, anthocyanins, essential oils, tannins, phytosterols, herniarin and glycolic acid. 29 Tea ( Camellia sinensis L.), belongs to the family Theacea, is the oldest caffeine-containing non-alcoholic beverage in the world. 30 The biochemical compositions of tea leaves, such as alkaloids (theobromine, caffeine, etc. ) and polyphenols (catechins and flavonoids), polysaccharides, volatile oils, vitamins and inorganic elements, have antimicrobial, antioxidant, anti-inflammatory and antiallergic activity. 31 According to previous reports, the use of lavender in the biosynthesis of CuO NPs in this study is innovative and the only use of the tea plant in the biosynthesis of copper nanoparticles has been just in the aqueous phase, not in the solid phase. 4,32–34 In the following, through phytotoxicity assessment, green synthesized CuO NPs were evaluated in comparison with the chemically synthesized CuO NPs. Hence, evaluating the phytotoxicity effectes of both green and chemically synthesized nanoparticles was performed using both seed germination and seedling growth assay of two plant species lettuce ( Lactuca sativa L.), and tomato ( Solanum lycopersicum L.).
Materials and methods
Plant materials, synthesis of cuo nps, characterization of cuo nps, phytotoxicity study of cuo nps, statistical analysis, results and discussion, characterization of green as-synthesized cuo nps.
Moreover, for better clarification of the morphology of CuO NPs, TEM analysis was utilized. Based on the XRD and SEM results, Cu–L2.5 sample was selected for TEM analysis. The TEM images of this sample confirmed that CuO NPs were well-formed using lavender leaf powder through solid-state route ( Fig. 3 ). It could be evidently seen uniform and spherical CuO NPs which were aggregated to some extent.
Phytotoxicity of green and chemically synthesized CuO NPs
Germination percentage (gp), germination rate, abnormal seedling, shoot and root length.
The effect of nanoparticles on plants can be very diverse based on NPs characteristics such as type, size, concentration, chemical and physical properties as well as plant-specific features. 37 Some reports indicated an influential role of metallic nanoparticles in damaging living organism's DNA, but with unknown molecular mechanisms. 38 The most prominent observations in this study were the lower phytotoxic effects of green synthesized CuO NPs compared to the chemically synthesized CuO NPs, on the germination indices. In the last two decades, a number of reports have also presented the inhibitory effects of copper nanoparticles on plant growth factors; For example, the results of a study revealed the growth of Triticum aestivum and Phaseolus radiatus seedlings was limited under treatments of 200–1000 mg l −1 of Cu NPs. 39 The 15 nm sized Cu NPs synthesized by polyols process method reduced stem and root elongation in Eruca sativa plant under the treatment of Murashige and Skoog (MS) medium containing 30 μg ml −1 Cu NPs. 40 In another study, the root and shoot elongation of two important plant species, Glycine max and Cicer arietinum , significantly decreased in concentration of >100 ppm of copper oxide nanoparticles. 41 According to the copper oxide NPs phytotoxicity results, Glycine max seedlings were negatively affected by concentrations more than 50 mg l −1 . However, the roots showed superior sensitivity to copper oxide NPs toxicity compared with the shoots. 42
Despite the reports of the mentioned researches, comparing the toxic effect of green and chemically synthesized CuO NPs on plant growth characteristics has not been reported so far.
Similar to the results of this study, there are reports of the positive effect of nanoparticles on the growth attributes of some plant species. In a recent study, 25 and 50 mg l −1 copper nanoparticles treatments significantly stimulated the soybean primary roots elongation. 43 Furthermore, it has been reported that concentrations of less than 1 ppm (0.2–1.0 ppm) of copper nanoparticles in wheat seedling medium led to a significant effect on increasing root dry weight and leaf area. 44 However, the massive accumulation of nanoparticles in shoots and roots could lead to phytotoxicity, the lower concentration of nanoparticles induced activity of antioxidant enzyme system, rubisco and chloroplast; therefore, the activity of these biosynthetic systems under low concentrations of nanoparticles may be effective in stimulating plant growth. 44–47
In general, the toxicity of a nanoparticle can be attributed to the properties of the nanoparticle (size, surface area and intrinsic catalytic activity) and the reducing and stabilizing agents used in its synthesis and since the green nanoparticles at least lacks potential toxic reducing and stabilizing agents, these behave more gently in inducing phytotoxicity. Comparing green CuO NPs to chemically synthesized CuO NPs, some research reported that the green NPs induced less phytotoxicity whereas the chemically synthesized NPs showed more severe inhibitory effects. The results of trial using green and chemically synthesized Ag NPs with concentrations of 0, 100, 200, 400 and 600 ppm on the seed germination percentage and seedling length of Matricaria chamomilla and Ocimum basilicum revealed that, the green Ag NPs was significantly less toxic than chemically synthesized Ag NPs. 26 According to another report, the green Ag NPs nanoparticles synthesized by Laminaria japonica algal extract had no significant effect on the germination of Triticum aestivum and Phaseolus mungo seeds at the concentration of 0–80 ppm, whereas the non-toxic effect of nanoparticles on shoot and root elongation was factual at concentrations of below 30 ppm. 48
Generally, CuO NPs have potentially inhibitory effects on germination features ( e.g. , germination percentage, shoot and root elongation, etc. ). Nevertheless, depending on their synthesis process whether green or chemically synthesized, different intensities of induced toxicity can be observed. Thus, green CuO NPs with the effects of toxicity much less than the chemically synthesized CuO NPs could be recognized as more eco-friendly NPs. Interestingly, CuO NPs can have a dual performance depending on their concentration, a nanotoxicant at high concentrations or a nanonutrient at low concentrations. At a constant concentration, each of the germination indices compared to the other exhibits various responses from inattention to sensitivity or stimulation. Different plant species could show variability in response patterns in the same CuO NPs treatments.
Conclusions
Conflicts of interest, acknowledgements.
- E. K. Elumalai, T. N. Prasad, J. Hemachandran, S. V. Therasa, T. Thirumalai and E. David, J Pharm Sci Res , 2010, 2 , 549–554 CAS .
- Y. T. Prabhu, K. V. Rao, V. S. Sai and T. Pavani, J. Saudi Chem. Soc. , 2017, 21 , 180–185 CrossRef CAS .
- S. Sukumar, A. Rudrasenan and D. Padmanabhan Nambiar, ACS Omega , 2020, 5 , 1040–1051 CrossRef CAS .
- J. Sarkar, N. Chakraborty, A. Chatterjee, A. Bhattacharjee, D. Dasgupta and K. Acharya, Nanomaterials , 2020, 10 , 312 CrossRef CAS .
- S. Shende, A. P. Ingle, A. Gade and M. Rai, World J. Microbiol. Biotechnol. , 2015, 31 , 865–873 CrossRef CAS .
- R. Zhou, X. Wu, X. Hao, F. Zhou, H. Li and W. Rao, Nucl. Instrum. Methods Phys. Res. Sect. B Beam Interact. Mater. Atoms , 2008, 266 , 599–603 CrossRef CAS .
- Q. M. LIU, D. B. ZHOU, Y. Yamamoto, R. Ichino and M. Okido, Trans. Nonferrous Metals Soc. China , 2012, 22 , 117–123 CrossRef CAS .
- X. Su, J. Zhao, H. Bala, Y. Zhu, Y. Gao, S. Ma and Z. Wang, J. Phys. Chem. C , 2007, 111 , 14689–14693 CrossRef CAS .
- R. Cuevas, N. Durán, M. C. Diez, G. R. Tortella and O. Rubilar, J. Nanomater. , 2015, 2015 Search PubMed .
- A. K. Mittal, Y. Chisti and U. C. Banerjee, Biotechnol. Adv. , 2013, 31 , 346–356 CrossRef CAS .
- K. Vithiya and S. Sen, Int. J. Pharm. Sci. Res. , 2011, 2 , 2781 CAS .
- P. Parikh, D. Zala and B. A. Makwana, Open Access Library , 2014, 1 , 1–15 Search PubMed .
- M. Gopinath, R. Subbaiya, M. M. Selvam and D. Suresh, Int. J. Curr. Microbiol. Appl. Sci. , 2014, 3 , 814–818 Search PubMed .
- S. P. Mondal, K. Das, A. Dhar and S. K. Ray, Nanotechnology , 2007, 18 , 095606 CrossRef .
- D. Mo, J. Liu, H. J. Yao, J. L. Duan, M. D. Hou, Y. M. Sun, Y. F. Chen, Z. H. Xue and L. Zhang, J. Cryst. Growth , 2008, 310 , 612–616 CrossRef CAS .
- J. S. Jang, U. A. Joshi and J. S. Lee, J. Phys. Chem. C , 2007, 111 , 13280–13287 CrossRef CAS .
- R. C. Kasana, N. R. Panwar, R. K. Kaul and P. Kumar, Environ. Chem. Lett. , 2017, 15 , 233–240 CrossRef CAS .
- B. Kumar, K. Smita, L. Cumbal, A. Debut and Y. Angulo, J. Saudi Chem. Soc. , 2017, 21 , S475–S480 CrossRef CAS .
- E. A. Mohamed, Heliyon , 2020, 6 , e03123 CrossRef .
- S. Usha, K. T. Ramappa, S. Hiregoudar, G. D. Vasanthkumar and D. S. Aswathanarayana, Int. J. Curr. Microbiol. Appl. Sci. , 2017, 6 , 2219–2228 CrossRef .
- P. Kaur, R. Thakur and A. Chaudhury, Green Chem. Lett. Rev. , 2016, 9 , 33–38 CrossRef CAS .
- S. Thakur, S. Sharma, S. Thakur and R. Rai, Int. J. Curr. Microbiol. Appl. Sci. , 2018, 7 , 683–694 CrossRef .
- R. Khani, B. Roostaei, G. Bagherzade and M. Moudi, J. Mol. Liq. , 2018, 255 , 541–549 CrossRef CAS .
- S. A. Akintelu, A. S. Folorunso, F. A. Folorunso and A. K. Oyebamiji, Heliyon , 2020, 6 , e04508 CrossRef .
- K. S. Siddiqi and A. Husen, Biomater. Res. , 2020, 24 , 1–15 CrossRef .
- J. Nasiri, M. Rahimi, Z. Hamezadeh, E. Motamedi and M. R. Naghavi, J. Cleaner Prod. , 2018, 192 , 514–530 CrossRef CAS .
- M. R. Moghadas, E. Motamedi, J. Nasiri, M. R. Naghavi and M. Sabokdast, Heliyon , 2020, 6 , e04730 CrossRef .
- R. Prusinowska and K. B. Śmigielski, Herba Pol. , 2014, 60 , 56–66 Search PubMed .
- K. B. Śmigielski, R. Prusinowska, K. Krosowiak and M. Sikora, J. Essent. Oil Res. , 2013, 25 , 291–299 CrossRef .
- Y. S. Lin, Y. J. Tsai, J. S. Tsay and J. K. Lin, J. Agric. Food Chem. , 2003, 51 , 1864–1873 CrossRef CAS .
- A. B. Sharangi, Food Res. Int. , 2009, 42 , 529–535 CrossRef CAS .
- M. I. Din, F. Arshad, Z. Hussain and M. Mukhtar, Nanoscale Res. Lett. , 2017, 12 , 638 CrossRef .
- P. Sutradhar, M. Saha and D. Maiti, J. Nanostruct. Chem. , 2014, 4 , 86 CrossRef .
- A. H. Keihan, H. Veisi and H. Veasi, Appl. Organomet. Chem. , 2017, 31 , e3642 CrossRef .
- R. Borah, E. Saikia, S. J. Bora and B. Chetia, RSC Adv. , 2016, 6 , 100443–100447 RSC .
- F. Nishino, M. Jeem, L. Zhang, K. Okamoto, S. Okabe and S. Watanabe, Sci. Rep. , 2017, 7 , 1–11 CrossRef CAS .
- T. A. Shalaby, Y. Bayoumi, N. Abdalla, H. Taha, T. Alshaal, S. Shehata, M. Amer, É. Domokos-Szabolcsy and H. El-Ramady, Nanoscience in Food and Agriculture , 2016, 1 , 283–312 CrossRef .
- D. H. Atha, H. Wang, E. J. Petersen, D. Cleveland, R. D. Holbrook, P. Jaruga, M. Dizdaroglu, B. Xing and B. C. Nelson, Environ. Sci. Technol. , 2012, 46 , 1819–1827 CrossRef CAS .
- W. M. Lee, Y. J. An, H. Yoon and H. S. Kweon, Environ. Toxicol. Chem. , 2008, 27 , 1915–1921 CrossRef CAS .
- M. Zaka, B. H. Abbasi, L. U. Rahman, A. Shah and M. Zia, IET Nanobiotechnol. , 2016, 10 , 134–140 CrossRef .
- T. Adhikari, S. Kundu, A. K. Biswas, J. C. Tarafdar and A. S. Rao, J. Agric. Sci. Technol. A , 2012, 2 , 815 CAS .
- P. M. G. Nair and I. M. Chung, Biol. Trace Elem. Res. , 2014, 162 , 342–352 CrossRef CAS .
- T. H. Pham, C. M. Nguyen, L. Le Quynh, K. B. Ninh, V. M. Chau, H. C. Nguyen, Q. B. Ngo, T. H. Dao, T. V. Nguyen, T. H. Le Thi and M. L. Tran, Int. J. Agric. Biol. , 2018, 20 , 1562–1568 Search PubMed .
- A. Hafeez, A. Razzaq, T. Mahmood and H. M. Jhanzab, J Nanosci Adv Technol , 2015, 1 , 6–11 Search PubMed .
- G. F. Nekrasova, O. S. Ushakova, A. E. Ermakov, M. A. Uimin and I. V. Byzov, Russ. J. Ecol. , 2011, 42 , 458 CrossRef CAS .
- F. Gao, F. Hong, C. Liu, L. Zheng, M. Su, X. Wu, F. Yang, C. Wu and P. Yang, Biol. Trace Elem. Res. , 2006, 111 , 239–253 CrossRef CAS .
- F. Hong, J. Zhou, C. Liu, F. Yang, C. Wu, L. Zheng and P. Yang, Biol. Trace Elem. Res. , 2005, 105 , 269–279 CrossRef CAS .
- D. Y. Kim, R. G. Saratale, S. Shinde, A. Syed, F. Ameen and G. Ghodake, J. Cleaner Prod. , 2018, 172 , 2910–2918 CrossRef CAS .
- My Shodhganga
- Receive email updates
- Edit Profile
Shodhganga : a reservoir of Indian theses @ INFLIBNET
- Shodhganga@INFLIBNET
- Hemwati Nandan Bahuguna Garhwal University
- Department of English
Items in Shodhganga are licensed under Creative Commons Licence Attribution-NonCommercial-ShareAlike 4.0 International (CC BY-NC-SA 4.0).

- Architecture and Design
- Asian and Pacific Studies
- Business and Economics
- Classical and Ancient Near Eastern Studies
- Computer Sciences
- Cultural Studies
- Engineering
- General Interest
- Geosciences
- Industrial Chemistry
- Islamic and Middle Eastern Studies
- Jewish Studies
- Library and Information Science, Book Studies
- Life Sciences
- Linguistics and Semiotics
- Literary Studies
- Materials Sciences
- Mathematics
- Social Sciences
- Sports and Recreation
- Theology and Religion
- Publish your article
- The role of authors
- Promoting your article
- Abstracting & indexing
- Publishing Ethics
- Why publish with De Gruyter
- How to publish with De Gruyter
- Our book series
- Our subject areas
- Your digital product at De Gruyter
- Contribute to our reference works
- Product information
- Tools & resources
- Product Information
- Promotional Materials
- Orders and Inquiries
- FAQ for Library Suppliers and Book Sellers
- Repository Policy
- Free access policy
- Open Access agreements
- Database portals
- For Authors
- Customer service
- People + Culture
- Journal Management
- How to join us
- Working at De Gruyter
- Mission & Vision
- De Gruyter Foundation
- De Gruyter Ebound
- Our Responsibility
- Partner publishers

Your purchase has been completed. Your documents are now available to view.
Green synthesis of nanoparticles: current prospectus
Tejaswi Thunugunta, DST INSPIRE fellow at the Indian Institute of Horticultural Research, Bangalore, India, is working on nanobiotechnology. Her major area of interest is to study the effect of nanoparticles on plants. She has published two papers on nanoparticle synthesis and their antimicrobial activity in international journals, during her postgraduation project work.
Anand C. Reddy is a research scholar at the Indian Institute of Horticultural Research and a postgraduate of Nottingham Trent University, UK. He has a keen interest in molecular biology and nanotechnology. He is currently working on gene delivery mechanisms in plants, mediated by nanoparticles. He is also working on molecular marker-assisted selection (MAS) in plants.
Lakshmana Reddy D.C. has a MS in Agriculture with a specialization in Plant Biotechnology, and a PhD in Agricultural Biotechnology. He has 8 years’ experience as a scientist in the Agricultural Research Service, Indian Council of Agricultural Research (ICAR). He has published in more than 25 national and international journals. Currently, he is working on molecular marker development, utilization in various horticulture crops for trait improvement, and nanobiotechnology applications for the improvement of horticultural crop production and productivity.
In the past few years, nanoparticles have been applied in various fields of science and technology, ranging from material science to biotechnology. Thus, the synthesis of nanoparticles can be considered as a dynamic area in research and application of nanoparticles. The different methods of nanoparticle synthesis include physical, chemical, and biological methods. Of these methods, the biological synthesis is to be comparatively widely used due to its advantages of being low cost, nontoxic and environmental friendly. Bio-applications of nanoparticles have pawed way for green synthesis of nanoparticles. In this review, we have provided brief information on various biological agents used for the synthesis of nanoparticles.
About the authors

Acknowledgments
This work is supported by the Department of Science and Technology, Government of India.
Conflict of interests: There is no competing interest on this review.
[1] Tadeusz M, Waldemar K, Marek F, Jussi K, Pavel Z. Human Factors of a Global Society, A System of Systems Perspective, Technology and Engineering , CRC Press, London, June 2, 2014, pp. 108. Search in Google Scholar
[2] Fendler JH, Ed., Nanoparticles and Nanostructured Films, Preparation, Characterization and Applications , John Wiley & Son: New York, NY, USA, 1998. Search in Google Scholar
[3] Parashar UK, Saxena PS, Srivastava A. Bioinspired synthesis of silver nanoparticles. Dig. J. Nanomater. Biostruct . 2009, 41, 159–166. Search in Google Scholar
[4] Schmidt-Ott A. New approaches to in situ characterization of ultrafine agglomerates. J. Aerosol. Sci . 1988, 195, 553–557, 559–563. Search in Google Scholar
[5] Håkansson G, Hultman L, Sundgren JE. Microstructures of TiN films grown by various physical vapour deposition techniques. Surf. Coat. Technol . 1991, 481, 51–67. Search in Google Scholar
[6] Sun Y, Fuge GM, Ashfold MNR, Growth of aligned ZnO nanorod arrays by catalyst-free pulsed laser deposition methods. Chem. Phys. Lett . 2004, 3961–3963, 21–26. Search in Google Scholar
[7] Zhu J, Palchik O, Chen S, Gedanken A. Microwave assisted preparation of CdSe, PbSe, and Cu 2- x Se nanoparticles. J. Phys. Chem. B . 2000, 10431, 7344–7347. Search in Google Scholar
[8] Chen Y, Fitz Gerald J, Williams JS, Bulcock S. Synthesis of boron nitride nanotubes at low temperatures using reactive ball milling. Chem. Phys. Lett . 1999, 2993–2994, 260–264. Search in Google Scholar
[9] Bikiaris DN, Papageorgiou GZ, Pavlidou E, Vouroutzis N, Palatzoglou P, Karayannidis GP. Preparation by melt mixing and characterization of isotactic polypropylene/SiO 2 nanocomposites containing untreated and surface-treated nanoparticles. J. Appl. Polym. Sci. 2006, 1004, 2684–2696. Search in Google Scholar
[10] Mafuné F, Kohno J, Takeda Y, Kondow T. Structure and stability of silver nanoparticles in aqueous solution produced by laser ablation. J. Phys. Chem. B . 2000, 10435, 8333–8337. Search in Google Scholar
[11] Stepanov AL, Hole DE, Townsend PD. Formation of silver nanoparticles in soda-lime silicate glass by ion implantation near room temperature. J. Non Cryst. Solids 1999, 2601–2602, 65–74. Search in Google Scholar
[12] Ayyub P, Chandra R, Taneja P, Sharma AK, Pinto R. Synthesis of nanocrystalline material by sputtering and laser ablation at low temperatures. Appl. Phys. A. 2001, 731, 67–73. Search in Google Scholar
[13] Journet C, Maser WK, Bernier P, Loiseau A, Lamy de la Chapelle M, Lefrant S, Deniard P, Lee R, Fischer JE, Large-scale production of single-walled carbon nanotubes by the electric-arc technique. Nature 1997, 388, 756–758. Search in Google Scholar
[14] Park J, Kwon SG, Jun SW, Kim BH, Hyeon T. Large-scale synthesis of ultra-small-sized silver nanoparticles. Chem. Phys. Chem. 2012, 13, 2540–2543. Search in Google Scholar
[15] Chen D-H, He X-R. Synthesis of nickel ferrite nanoparticles by sol-gel method. Mater. Res. Bull . 2001, 36, 1369–1377. Search in Google Scholar
[16] Kim YI, Kim D, Lee CS. Synthesis and characterization of CoFe 2 O 4 magnetic nanoparticles prepared by temperature-controlled coprecipitation method. Physica B: Condens. Matter 2003, 337, 42–51. Search in Google Scholar
[17] Cassell AM, Raymakers JA, Kong J, Dai H. Large scale CVD synthesis of single-walled carbon nanotubes. J. Phys. Chem. B . 1999, 103, 6484–6492. Search in Google Scholar
[18] Li J-G, Ikeda M, Ye R, Moriyoshi Y, Ishigaki T. Control of particle size and phase formation of TiO 2 nanoparticles synthesized in RF induction plasma. J. Phys. D: Appl. Phys. 2007, 40, 2348. Search in Google Scholar
[19] Li B, Xie Y, Huang J, Qian Y. Synthesis by a solvothermal route and characterization of CuInSe 2 nanowhiskers and nanoparticles. Adv. Mater . 1999, 11, 1456–1459. Search in Google Scholar
[20] Singh AK, Viswanath V, Janu VC. Synthesis, effect of capping agents, structural, optical and photoluminescence properties of ZnO nanoparticles. India J. Lumin. 2009, 1298, 874–878. Search in Google Scholar
[21] Mohanpuri P, Rana NK, Yadav SK. Biosynthesis of nanoparticles, technological concepts and future applications. J. Nanopart. Res. 2008, 10, 507–517. Search in Google Scholar
[22] Jang E, Ryu BH, Shim HW, Ju H, Kim DW, Kim TD. Adsorption of microbial esterases on Bacillus subtilis -templated cobalt oxide nanoparticles. Int. J. Biol. Macromol . 2014, 65, 188–192. Search in Google Scholar
[23] Ahmad A, Mukherjee P, Mandal D, Senapati S, Khan MI, Kumar R, Sastry M. Enzyme mediated extracellular synthesis of CdS nanoparticles by the fungus, Fusarium oxysporum . J. Am. Chem. Soc. 2002, 124, 12108–12109. Search in Google Scholar
[24] Shukla R, Nune SK, Chanda N, Katti K, Mekapothula S, Kulkarni RR, Welshons WV, Kannan R, Katti KV. Soybeans as a phytochemical reservoir for the production and stabilization of biocompatible gold nanoparticles. Small 2008, 4, 1425–1436. Search in Google Scholar
[25] Lee J, Park EY, Lee J. Non-toxic nanoparticles from phytochemicals, preparation and biomedical application. Bioproc. Biosyst. Eng . 2014, 376, 983–989. Search in Google Scholar
[26] Raveendran P, Fu J, Wallen SL. Completely green synthesis and stabilization of metal nanoparticles. J. Am. Chem. Soc. 2003, 12546, 13940–13941. Search in Google Scholar
[27] Inbakandan D, Venkatesan R, Khan SA. Biosynthesis of gold nanoparticles utilizing marine sponge Acanthella elongata . Colloid. Surface B 2010, 812, 634–639. Search in Google Scholar
[28] Nellorea J, Paulineb PC, Amarnath K. Biogenic synthesis by S phearanthus amaranthoids towards the efficient production of the biocompatible gold nanoparticles. Dig. J. Nanomater. Bios. 2012, 7, 123–133. Search in Google Scholar
[29] Tejaswi T, Venkateshwara Rao K, Shilpa C. Sliver nanoparticles synthesis and stabilization by different species of ocimum and characterization for its antimicrobial activity. Int. J. Curr. Eng. Tech. 2013, 32, 501–506. Search in Google Scholar
[30] Klaus T, Joerger R, Olsson E, Granqvist CG. Silver-based crystalline nanoparticles, microbially fabricated. Proc. Natl. Acad. Sci. USA 1999, 9624, 13611–13614. Search in Google Scholar
[31] Mohseniazar M, Barin M, Zarredar H, Alizadeh S, Shanehbandi D. Potential of microalgae and lactobacilli in biosynthesis of silver nanoparticles. BioImpacts 2011, 1, 149–152. Search in Google Scholar
[32] Gericke M, Pinches A. Biological synthesis of metal nanoparticles. Hydrometallurgy 2006, 83, 132–140. Search in Google Scholar
[33] Husseiny MI, EI-Aziz MA, Badr Y, Mahmoud MA. Biosynthesis of gold nanoparticles using Pseudomonas aeruginosa. Spectrochim. Acta Part A 2007, 673–674, 1003–1006. Search in Google Scholar
[34] Fu JZ, Liu YY, Gu PY, Shang DL, Lin ZY, Yao BX, Weng SZ. Spectroscopic characterization on the biosorption and bioreduction of AgI by Lactobacillus sp. A09. Acta Physico. Chim. Sin. 2000, 169, 770–782. Search in Google Scholar
[35] Joerger R, Klaus T, Granqvist CG. Biologically produced Ag-C composite materials for optically functional thin film coatings. Adv. Mat. 2000, 12, 407–409. Search in Google Scholar
[36] Mukherjee P, Ahmad A, Mandal D. Bioreduction of AuCl4- ions by the fungus, Verticillium sp. and surface trapping of the gold nanoparticles formed. Angew. Chem. Int. Ed. Engl. 2001, 40, 3585–3588. Search in Google Scholar
[37] Shankar SS, Rai A, Ahmad A, Sastry M. Rapid synthesis of Au, Ag and bimetallic Au core- Ag shell nanoparticles using neem Azadirachtaindica leaf broth. J. Colloid Interf. Sci . 2004, 275, 496–502. Search in Google Scholar
[38] Ganesh Babu MM, Gunasekaran P. Production and structural characterization of crystalline silver nanoparticles from Bacillus cereus isolate. Colloid. Surface B 2009, 74, 191–195. Search in Google Scholar
[39] Jain D, Kachhwaha S, Jain R, Srivastava G, Kothari SL. Novel microbial route to synthesize silver nanoparticles using spore crystal mixture of Bacillus thuringiensis . Indian J. Exp. Biol. 2010, 48, 1152–1156. Search in Google Scholar
[40] Gurunathan S, Kalishwaralal K, Vaidyanathan R, Venkataraman D, Pandian SR, Muniyandi J, Hariharan N, Eom SH. Biosynthesis, purification and characterization of silver nanoparticles using Escherichia coli. Colloid. Surface B 2009, 74, 328–335. Search in Google Scholar
[41] Sweeney RY, Mao C, Gao X, Burt JL, Belcher AM, Georgiou G, Iverson BL. Bacterial biosynthesis of cadmium sulfide nanocrystals. Chem. Biol. 2004, 11, 1553–1559. Search in Google Scholar
[42] Sintubin L, De Windt W, Dick J, Mast J, van der Ha D, Verstraete W, Boon N. Lactic acid bacteria as reducing and capping agent for the fast and efficient production of silver nanoparticles. Appl. Microbiol. Biotechnol. 2009, 84, 741–749. Search in Google Scholar
[43] Zhang H, Li Q, Lu Y, Sun D, Lin X, Deng X, He N, Zheng S. Biosorption and bioreduction of diamine silver complex by Corynebacterium. J. Chem. Technol. Biotechnol. 2005, 80, 285–290. Search in Google Scholar
[44] Nanda A, Saravanan M. Biosynthesis of silver nanoparticles from Staphylococcus aureus and its antimicrobial activity against MRSA and MRSE. Nanomedicine. 2009, 5, 452–456. Search in Google Scholar
[45] Juibari MM, Abbasalizadeh S, Jouzani GS, Noruzi M. Intensified biosynthesis of silver nanoparticles using a native extremophilic Ureibacillus thermosphaericus strain. Mater. Lett. 2011, 65, 1014–1017. Search in Google Scholar
[46] Fu JK, Zhang WD, Liu YY, Lin ZY, Yao BX, Weng SZ, Zeng JL. Characterization of adsorption and reduction of noble metal ions by bacteria. Chem. J. Chinese U. 1999, 20, 1452–1454. Search in Google Scholar
[47] Parikh RP, Singh S, Prasad BLV, Patole MS, Sastry M, Shouche YS. Extracellular synthesis of crystalline silver nanoparticles and molecular evidence of silver resistance from Morganella sp towards understanding biochemical synthesis mechanism. Chembiochem. 2008, 9, 1415–1422. Search in Google Scholar
[48] Nair B, Pradeep T. Coalescence of nanoclusters and formation of submicron crystallites assisted by Lactobacillus strains. Cryst. Growth Des. 2002, 2, 293–298. Search in Google Scholar
[49] Lengke M, Fleet M, Southam G. Biosynthesis of silver nanoparticles by filamentous cyanobacteria from a silver I nitrate complex. Langmuir 2006, 10, 1021–1030. Search in Google Scholar
[50] Cunningham DP, Lundie LL. Precipitation of cadmium by Clostridium thermoaceticum. Appl. Environ. Microbiol. 1993, 9, 7–14. Search in Google Scholar
[51] Bharde A, Wani A, Shouche Y, Pattayil A, Bhagavatula L, Sastry M. Bacterial aerobic synthesis of nanocrystalline magnetite. JACS 2005, 127, 9326–9327. Search in Google Scholar
[52] Konishi Y, Ohno K, Saitoh N, Nomura T, Nagamine S. Microbial synthesis of gold nanoparticles by metal reducing bacterium. Trans. Mater. Res. Soc. Jpn. 2004, 29, 2341–2343. Search in Google Scholar
[53] Shiying H, Zhirui G, Zhanga Y, Zhanga S, Wanga J, Ning G. Biosynthesis of gold nanoparticles using the bacteria Rhodopseudomonas capsulata. Mater. Lett. 2007, 61, 3984–3987. Search in Google Scholar
[54] Liangwei D, Hong J, Xiaohua L, Erkang W. Biosynthesis of gold nanoparticles assisted by Escherichia coli DH5α and its application on direct electrochemistry of hemoglobin. Electrochem. Commun. 2007, 9, 1165–1170. Search in Google Scholar
[55] Ahmad A, Senapati S, Khan MI, Kumar R, Sastry M. Extracellular biosynthesis of monodisperse gold nanoparticles by a novel extremophilic actinomycete, Thermomonospora sp. Langmuir 2003, 19, 3550–3553. Search in Google Scholar
[56] Ahmad A, Senapati S, Khan MI, Ramani R, Srinivas V, Sastry M. Intracellular synthesis of gold nanoparticles by a novel alkalotolerant actinomycete, Rhodococcus species. Nanotechnology 2003, 14, 824–828. Search in Google Scholar
[57] Shahverdi AR, Fakhimi A, Shahverdi HR, Minaian S. Synthesis and effect of silver nanoparticles on the antibacterial activity of different antibiotics against Staphylococcus aureus and Escherichia coli. Nanomedicine 2007, 3, 168–171. Search in Google Scholar
[58] Husseiney MI, Abd El-Aziz M, Badr Y, Mahmoud MA. Biosynthesis of gold nanoparticles using Pseudomonas aeruginosa. Spectrochim. Acta A. 2007, 67, 1003–1006. Search in Google Scholar
[59] Marshall M, Beliaev A, Dohnalkova A, David W, Shi L, Wang Z, Boyanov MI, Lai B, Kemner KM, McLean JS, Reed SB, Culley DE, Bailey VL, Simonson CJ, Saffarini DA, Romine MF, Zachara JM, Fredrickson JK. c-Type cytochrome-dependent formation of UIV nanoparticles by Shewanella oneidensis. PLoS Biol. 2007, 8, 1324–1333. Search in Google Scholar
[60] Vijayakumar PS, Prasad BLV. Intracellular biogenic silver nanoparticles for the generation of carbon supported antiviral and sustained bactericidal agents. Langmuir 2009, 25, 11741–11747. Search in Google Scholar
[61] Kalimuthu K, Suresh Babu R, Venkataraman D, Bilal M, Gurunathan S. Biosynthesis of silver nanocrystals by Bacillus licheniformis. Colloid. Surface B 2008, 65, 150–153. Search in Google Scholar
[62] Shahverdi AR, Minaeian S, Shahverdi HR, Jamalifar H, Nohi AA. Rapid synthesis of silver nanoparticles using culture supernatants of Enterobacteriaceae: a novel biological process. Biochem. 2007, 42, 919–923. Search in Google Scholar
[63] Varshney R, Bhadauria S, Gaur MS, Pasricha R. Copper nanoparticles synthesis from electroplating industry effluent. Nano. Biomed. Eng. 2011, 3, 115–119. Search in Google Scholar
[64] Balagurunathan R, Radhakrishnan M, Babu Rajendranand R, Velmurugan D. Biosynthesis of gold nanoparticles by actinomycetes Streptomyces viridogens strain HM10. Indian J. Biochem. Biophys. 2011, 48, 331–335. Search in Google Scholar
[65] Sharma N, Pinnaka AK, Raje M, Fnu A, Bhattacharyya MS, Choudhury AR. Exploitation of marine bacteria for production of gold nanoparticles. Microb. Cell Fact. 2012, 11, 86. Search in Google Scholar
[66] Yeary LW, Moon JW, Love LJ, Thompson JR, Rawn CJ, Phelps TJ. Magnetic properties of biosynthesized magnetite nanoparticles. IEEE T. Magn. 2005, 41, 4384–4389. Search in Google Scholar
[67] Yeary LW, Moon JW, Rawn CJ, Love LJ, Rondinone AJ, Thompson JR, Chakoumakos BC, Phelps TJ. Magnetic properties of bio-synthesized zinc ferrite nanoparticles. J. Magn. Magn. Mater . 2011, 323, 3043–3048. Search in Google Scholar
[68] Sundaram PA, Augustine R, Kannan M. Extracellular biosynthesis of iron oxide nanoparticles by Bacillus subtilis strains isolated from rhizosphere soil. Biotechnol. Bioprocess Eng. 2012, 4, 835–840. Search in Google Scholar
[69] Malarkodi C, Rajeshkumar S, Paulkumar K, Vanaja M, Jobitha GDG, Annadurai G. Bactericidal activity of bio mediated silver nanoparticles synthesized by Serratia nematodiphila. Drug. Invention Today 2013, 5, 119–125. Search in Google Scholar
[70] Lee JH, Roh Y, Hur HG. Microbial production and characterization of superparamagnetic magnetite nanoparticles by Shewanella sp. HN-41. J. Microbiol. Biotechn. 2008, 18, 1572–1577. Search in Google Scholar
[71] Du L, Jiang H, Liu X, Wang E. Biosynthesis of gold nanoparticles assisted by Escherichia coli DH5α and its application on direct electrochemistry of hemoglobin. Electrochem. Commun. 2007, 9, 1165–1170. Search in Google Scholar
[72] He S, Guo Z, Zhang Y, Zhang S, Wang J, Gu N. Biosynthesis of gold nanoparticles using the bacteria Rhodopseudomonas capsulate. Mater. Lett. 2007, 61, 3984–3987. Search in Google Scholar
[73] Kalishwaralal K, Deepak V, Ram Kumar Pandian S, Kottaisamy M, BarathmaniKanth S, Kartikeyan B, Gurunathan S. Biosynthesis of silver and gold nanoparticles using Brevibacterium casei. Colloid. Surface B 2010, 77, 257–262. Search in Google Scholar
[74] Sinha A, Khare SK. Mercury bioaccumulation and simultaneous nanoparticle synthesis by Enterobacter sp. Cells. Bioresource Technol. 2011, 102, 4281–4284. Search in Google Scholar
[75] Bao H, Lu Z, Cui X, Qiao Y, Guo J, Anderson JM, Li CM. Extracellular microbial synthesis of biocompatible CdTe quantum dots. Acta Biomaterialia. 2010, 6, 3534–3541. Search in Google Scholar
[76] Kashefi K, Lovley DR. Reduction of FeIII, MnIV, and toxic metals at 100°C by Pyrobaculum islandicum. Appl. Environ. Microbiol . 2000, 66, 1050–1056. Search in Google Scholar
[77] Lloyd JR, Yong P, Macaskie LE. Enzymatic recovery of elemental palladium by using sulfate-reducing bacteria. Appl. Environ. Microbiol . 1998, 64, 4607–4609. Search in Google Scholar
[78] Amemiya Y, Arakaki A, Staniland SS, Tanaka T, Matsunaga T. Controlled formation of magnetite crystal by partial oxidation of ferrous hydroxide in the presence of recombinant magnetotactic bacterial protein Mms6. Biomaterials . 2007, 28, 5381–5389. Search in Google Scholar
[79] Li W, Yu L, Zhou P, Zhu M. A Magnetospirillum strain WM-1 from a freshwater sediment with intracellular magnetosomes. World J. Microbiol. Biotechn. 2007, 23, 1489–1492. Search in Google Scholar
[80] Jha AK, Prasad K. Ferroelectric BaTiO3 nanoparticles, biosynthesis and characterization. Colloid. Surface B 2010, 75, 330–334. Search in Google Scholar
[81] Jha AK, Prasad K, Kulkarni AR. Synthesis of TiO2 nanoparticles using microorganisms. Colloid. Surface B 2009, 71, 226–229. Search in Google Scholar
[82] Zhu K, Pan H, Li J, Yu-Zhang K, Zhang SD, Zhang WY, Zhou K, Yue H, Pan Y, Xiao T, Wu LF. Isolation and characterization of a marine magnetotactic spirillum axenic culture QH-2 from an intertidal zone of the China Sea. Res. Microbiol . 2010, 161, 276–283. Search in Google Scholar
[83] Arakaki A, Shibusawa M, Hosokawa M, Matsunaga T. Preparation of genomic DNA from a single species of uncultured magnetotactic bacterium by multiple-displacement amplification. Appl. Environ. Microbiol . 2010, 76, 1480–1485. Search in Google Scholar
[84] Bai HJ, Zhang ZM, Guo Y, Yang GE. Biosynthesis of cadmium sulfide nanoparticles by photosynthetic bacteria Rhodopseudomonas palustris. Colloid. Surface B 2009, 70, 142–146. Search in Google Scholar
[85] Prasad K, Jha AK. Biosynthesis of CdS nanoparticles, an improved green and rapid procedure. J. Colloid Interf. Sci . 2010, 342, 68–72. Search in Google Scholar
[86] Bai HJ, Zhang ZM, Gong J. Biological synthesis of semiconductor zinc sulfide nanoparticles by immobilized Rhodobacter sphaeroides . Biotechnol. Lett . 2006, 28, 1135–1139. Search in Google Scholar
[87] Labrenz M, Druschel GK, Thomsen-Ebert T, Gilbert B, Welch SA, Kemner KM, Logan GA, Summons RE, De Stasio G, Bond PL, Lai B, Kelly SD, Banfield JF. Formation of sphalerite ZnS deposits in natural biofilms of sulfate-reducing bacteria. Science 2000, 290, 1744–1747. Search in Google Scholar
[88] Bai HJ, Zhang ZM. Microbial synthesis of semiconductor lead sulfide nanoparticles using immobilized Rhodobacter sphaeroides. Mater. Lett. 2009, 63, 764–766. Search in Google Scholar
[89] Watson JHP, Ellwood DC, Soper AK, Charnock J. Nanosized strongly-magnetic bacterially-produced iron sulfide materials. J. Magn. Magn. Mater . 1999, 203, 69–72. Search in Google Scholar
[90] Pandian SRK, Deepak V, Kalishwaralal K, Muniyandi J, Rameshkumar N, Gurunathan S. Synthesis of PHB nanoparticles from optimized medium utilizing dairy industrial waste using Brevibacterium casei SRKP2, a green chemistry approach. Colloid. Surface B 2009, 74, 266–273. Search in Google Scholar
[91] Sastry M, Ahmad A, Khan MI, Kumar R. Biosynthesis of metal nanoparticles using fungi and actinomycete. Curr. Sci. 2003, 852, 162–170. Search in Google Scholar
[92] Gade AK, Bonde PP, Ingle AP, Marcato P, Duran N, Rai MK. Exploitation of Aspergillus niger for synthesis of silver nanoparticles. J. Biobased Mater. Bio . 2008, 2, 1–5. Search in Google Scholar
[93] Binupriya AR, Sathishkumar M, Yun SI. Myco-crystallization of silver ions to nanosized particles by live and dead cell filtrates of Aspergillus oryzae var. viridis and its bactericidal activity toward Staphylococcus aureus KCCM 12256. Ind Eng Chem Res . 2010, 49, 852–858. Search in Google Scholar
[94] Duran N, Marcato PD, De Souza GIH, Alves OL, Esposito E. Antibacterial effect of silver nanoparticles produced by fungal process on textile fabrics and their effluent treatment. J. Biomed. Nanotechnol . 2007, 3, 203–208. Search in Google Scholar
[95] Ingle A, Rai M, Gade A, Bawaskar M. Fusarium solani, A novel biological agent for the extracellular synthesis of silver nanoparticles. J. Nanopart. Res . 2009, 11, 2079–2085. Search in Google Scholar
[96] Nithya R, Ragunathan R. Synthesis of silver nanoparticles using Pleurotus sajor caju and its antimicrobial study. Dig. J. Nanomater. Bios. 2009, 4, 623–629. Search in Google Scholar
[97] Thakkar KN, Mhatre SS, Parikh RY. Biological synthesis of metallic nanoparticles. Nanomedicine . 2010, 6, 257–262. Search in Google Scholar
[98] FesharakiI PJ, NazariI P, ShakibaieI M, RezaieII S, Banoee M, AbdollahiM, ShahverdiAR. Biosynthesis of selenium nanoparticles using Klebsiella pneumoniae and their recovery by a simple sterilization process. Braz. J. Microbiol . 2010, 41, 461–466. Search in Google Scholar
[99] Chen JC, Lin ZH, Ma XX. Evidence of the production of silver nanoparticles via pretreatment of Phoma sp.3.2883 with silver nitrate. Lett. Appl. Microbiol . 2003, 37, 105–108. Search in Google Scholar
[100] Ahmad A, Mukherjee P, Senapati S, Mandal D, Khan MI, Kumar R, Sastry M. Extracellular biosynthesis of silver nanoparticles using the fungus Fusarium oxysporum . Colloid. Surface B 2003, 28, 313–318. Search in Google Scholar
[101] Mukherjee P, Ahmad A, Mandal D, Senapati S, Sainkar SR, Khan MI, Parishcha R, Ajaykumar PV, Alam M, Kumar R, Sastry M. Fungus mediated synthesis of silver nanoparticles and their immobilization in the mycelial matrix, a novel biological approach to nanoparticle synthesis. Nano Lett . 2001, 1, 515–519. Search in Google Scholar
[102] Bhainsa KC, D’Souza SF. Extracellular biosynthesis of silver nanoparticle using the fungus Aspergillus fumigates. Colloid. Surface B 2006, 47, 160–164. Search in Google Scholar
[103] Mukherjee P, Roy M, Mandal B, Dey G, Mukherjee P, Ghatak J, Tyagi AK, Kale SP. Green synthesis of highly stabilized nanocrystalline silver particles by a non-pathogenic and agriculturally important fungus T. asperellum . Nanotechnology 2008, 19, 75103–75110. Search in Google Scholar
[104] Vigneshwaran N, Kathe AA, Varadarajan PV, Nachane RP, Balasubramanya RH. Biomimetics of Ag nanoparticles by white rot fungus, Phaenerochaete chrysosporium . Colloid. Surface B 2006, 53, 55–59. Search in Google Scholar
[105] Bharde A, Rautaray D, Bansal V, Ahmad A, Sarkar I, Yusuf SM, Sanyal M, Sastry M. Extracellular biosynthesis of magnetite using fungi. Small 2006, 21, 135–141. Search in Google Scholar
[106] Vigneshwaran N, Ashtaputre NM, Varadarajan PV, Nachane RP, Paralikar KM, Balasubramanya RH. Biological synthesis of silver nanoparticles using the fungus Aspergillus flavus . Mater. Lett. 2007, 61, 1413–1418. Search in Google Scholar
[107] Ingle A, Gade A, Pierrat S, Sonnichsen C, Rai M. Mycosynthesis of silver nanoparticles using the fungus Fusarium acuminatum and its activity against some human pathogenic bacteria. Current Nanoscience . 2008, 4, 141–144. Search in Google Scholar
[108] Basavaraja SS, Balaji SD, Lagashetty AK, Rajasab AH, Venkataraman A. Extracellular biosynthesis of silver nanoparticles using the fungus Fusarium semitectum . Mater. Res. Bull . 2008, 43, 1164–1170. Search in Google Scholar
[109] Li G, He D, Qian Y, Guan B, Gao S, Cui Y, Yokoyama K, Wang L. Fungus-mediated green synthesis of silver nanoparticles using Aspergil lusterreus . Int. J. Mol. Sci. 2012, 13, 466–476. Search in Google Scholar
[110] Bansal V, Rautaray D, Ahmad A, Sastry M. Biosynthesis of zirconia nanoparticles using the fungus Fusarium oxysporum. J. Mater. Chem . 2004, 14, 3303–3305. Search in Google Scholar
[111] Tarafdar JC, Raliya R. Rapid, low-cost, and ecofriendly approach for iron nanoparticle synthesis using Aspergillus oryzae TFR9. J. Nanopart. 2013, 4 pages. Search in Google Scholar
[112] Agnihotri M, Joshi S, Kumar AR, Zinjarde S, Kulkarni S. Biosynthesis of gold nanoparticles by the tropical marine yeast Yarrowia lipolytica NCIM 3589. Mater. Lett. 2009, 63, 1231–1234. Search in Google Scholar
[113] Castro-Longoria E, Vilchis-Nestor AR, Avalos Borja M. Biosynthesis of silver, gold and bimetallic nanoparticles using the filamentous fungus Neurospora crassa . Colloid. Surface B 2011, 83, 42–48. Search in Google Scholar
[114] Bansal V, Rautaray D, Bharde A, Ahire K, Sanyal A, Ahmad A, Sastry M. Fungus-mediated biosynthesis of silica and titania particles. J. Mater. Chem . 2005, 15, 2583–2589. Search in Google Scholar
[115] Bansal V, Poddar P, Ahmad A, Sastry M. Room temperature biosynthesis of ferroelectric barium titanate nanoparticles. J. Am. Chem. Soc . 2006, 128, 11958–11963. Search in Google Scholar
[116] Sanghi R, Verma P. A facile green extracellular biosynthesis of CdS nanoparticles by immobilized fungus. Chem. Eng. J . 2009, 155, 886–891. Search in Google Scholar
[117] Kowshik M, Deshmuke N, Vogal W, Urban J, Kulkarni SK, Paknikar KM. Microbial synthesis of semiconductor CdS nanoparticles, their characterization, and their use in the fabrication of an ideal diode. Biotechnol Bioeng. 2002, 78, 583–588. Search in Google Scholar
[118] Dameron CT, Reese RN, Mehra RK, Kortan AR, Carroll PJ, Steigerwald ML, Brus LE, Winge DR. Biosynthesis of cadmium sulphide quantum semiconductor crystallites. Nature 1989, 338, 596–597. Search in Google Scholar
[119] Sanyal A, Rautaray D, Bansal V, Ahmad A, Sastry M. Heavy-metal remediation by a fungus as a means of production of lead and cadmium carbonate crystals. Langmuir 2005, 21, 7220–7224. Search in Google Scholar
[120] Rautaray D, Sanyal A, Adyanthaya SD, Ahmad A, Sastry M. Biological synthesis of strontium carbonate crystals using the fungus Fusarium oxysporum . Langmuir 2004, 20, 6827–6833. Search in Google Scholar
[121] Kumar SA, Ansary AA, Abroad A, Khan MI. Extracellular biosynthesis of CdSe quantum dots by the fungus, Fusarium oxysporum . J. Biomed. Nanotechnol . 2007, 32, 190–194. Search in Google Scholar
[122] Sanghi R, Verma P. Biomimetic synthesis and characterization of protein capped silver nanoparticles. Bioresource Technol. 2009, 100, 501–504. Search in Google Scholar
[123] Balaji DS, Basavaraja S, Deshpande R, Mahesh DB, Prabhakar BK, Venkataraman A. Extracellular biosynthesis of functionalized silver nanoparticles by strains of Cladosporium cladosporioides fungus. Colloid. Surface B 2009, 68, 88–92. Search in Google Scholar
[124] Kowshik M, Ashtaputre S, Kharrazi S, Vogel W, Urban J, Kulkarni SK, Paknikar KM. Extracellular synthesis of silver nanoparticles by a silver-tolerant yeast strain MKY3. Nanotechnology 2003, 14, 95–100. Search in Google Scholar
[125] Mourato A, Gadanho M, Lino AR, Tenreiro R. Biosynthesis of crystalline silver and gold nanoparticles by extremophilic yeasts. Bioinorg Chem Appl . 2011, 546074. Search in Google Scholar
[126] Seshadri S, Saranya K, Kowshik M. Green synthesis of lead sulfide nanoparticles by the lead resistant marine yeast, Rhodosporidium diobovatum . Biotechnol. Progr. 2011, 27, 1464–1469. Search in Google Scholar
[127] Zhou W, He W, Zhong S, Wang Y, ZhaoH, Li Z, Yan S. Biosynthesis and magnetic properties of mesoporous Fe3O4 composites. J. Magn. Magn. Mater . 2009, 321, 1025–1028. Search in Google Scholar
[128] Zhou W, He W, Zhang X, Yan S, Sun X, Tian X, Han X. Biosynthesis of iron phosphate nanopowders. Powder Technol. 2009, 194, 106–108. Search in Google Scholar
[129] Jha AK, Prasad K. A green low-cost biosynthesis of Sb2O3 nanoparticles. Biochem. Eng. J . 2009, 43, 303–306. Search in Google Scholar
[130] Yan S, He W, Sun C, Zhang X, Zhao H, Li Z, Zhou W, Tian X, Sun X, Han X. The biomimetic synthesis of zinc phosphate nanoparticles. Dyes Pigments 2009, 80, 254–258. Search in Google Scholar
[131] Thirumalairaj VK, Vijayan MP, Durairaj G, Shanmugaasokan L, Yesudas R, Gunasekara S. Potential antibacterial activity of crude extracts and silver nanoparticles synthesized from Sargassum wightii. Int. Curr. Pharm. J. 2014, 3, 322–325. Search in Google Scholar
[132] Luna C, Ilyn M, Vega V, Prida VM, González J, Mendoza-Reséndez R. Size distribution and frustrated antiferromagnetic coupling effects on the magnetic behavior of ultrafine akaganéite (β-FeOOH) nanoparticles. J. Phys. Chem. C , 2014, 118, 21128–21139. Search in Google Scholar
[133] Dhas TS, Kumar VG, Karthick V, Angel KJ, Govindaraju K. Facile synthesis of silver chloride nanoparticles using marine alga and its antibacterial efficacy. Spectrochim. Acta A 2014, 120, 416–420. Search in Google Scholar
[134] Singaravelu G, Arockiamary JS, Ganesh Kumar V, Govindaraju K. A novel extracellular synthesis of monodisperse gold nanoparticles using marine alga, Sargassum wightii Greville. Colloid. Surface B 2007, 57, 97–101. Search in Google Scholar
[135] Lengke MF, Fleet ME, Southam G. Morphology of gold nanoparticles synthesized by filamentous cyanobacteria from goldI-thiosulfate and goldIII-chloride complexes. Langmuir 2006, 22, 2780–2787. Search in Google Scholar
[136] Lengke MF, Ravel B, Fleet ME, Wanger G, Gordon RA, Southam G. Mechanisms of gold bioaccumulation by filamentous cyanobacteria from goldIII-chloride complex. Environ. Sci. Technol . 2006, 40, 6304–6309. Search in Google Scholar
[137] Konishi Y, Tsukiyama T, Tachimi T, Saitoh N, Nomura T, Nagamine S. Microbial deposition of gold nanoparticles by the metal-reducing bacterium Shewanella algae. Electrochim. Acta 2007, 53, 186–192. Search in Google Scholar
[138] Konishi Y, Ohno K, Saitoh N, Nomura T, Nagamine S, Hishida H, Takahashi Y, Uruga T. Bioreductive deposition of platinum nanoparticles on the bacterium Shewanella algae. J. Biotechnol . 2007, 128, 648–653. Search in Google Scholar
[139] Lee JH, Han J, Choi H, Hur HG. Effects of temperature and dissolved oxygen on SeIV removal and SeO precipitation by Shewanella sp. HN-41. Chemosphere 2007, 68, 1898–1905. Search in Google Scholar
[140] Perez-Gonzalez T, Jimenez-Lopez C, Neal AL, Rull-Perez F, Rodriguez-Navarro A, Fernandez-Vivas A, Iañez-Pareja E. Magnetite biomineralization induced by Shewanella oneidensis. Geochim. Cosmochim. Ac . 2010, 74, 967–979. Search in Google Scholar
[141] Bose S, Hochella MF, Gorby YA, Kennedy DW, McCready DE, Madden AS, Lower BH. Bioreduction of hematite nanoparticles by the dissimilatory iron reducing bacterium Shewanella oneidensis MR-1. Geochim. Cosmochim. Ac . 2009, 73, 962–976. Search in Google Scholar
[142] Lefèvre CT, Abreu F, Schmidt ML, Lins U, Frankel RB, Hedlund BP, Bazylinski DA Moderately thermophilic magnetotactic bacteria from hot springs in Nevada. Appl. Environ. Microbiol . 2010, 76, 3740–3743. Search in Google Scholar
[143] Huang J, Li Q, Sun D, Lu Y, Su Y, Yang X, Wang H, Wang Y, Shao W, He N, Hong J, Chen C. Biosynthesis of silver and gold nanoparticles by novel sundried Cinnamomum camphora leaf. Nanotechnology 2007, 18, 11–15. Search in Google Scholar
[144] Leela A, Vivekanandan M. Tapping the unexploited plant resources for the synthesis of silver nanoparticles. Afr. J. Biotechnol. 2007, 7, 3162–3165. Search in Google Scholar
[145] Manimekalai G, Selvakumar S, Gopikrishnan V, Radhakrishnan M, Balagurunathan R. Antibacterial effect of medicinal plants Leucas aspera Linn and Ocimum basilicum Linn. Int. J. Adv. Pharmaceut. Res . 2011, 26, 276–280. Search in Google Scholar
[146] Ahmad A, Senapati S, Khan MI, Kumar R, Ramani R, Srinivas V, Sastry M. Intracellular synthesis of gold nanoparticles by a novel alkalotolerantactinomycete, Rhodococcus species. Nanotechnology 2003, 14, 824–828. Search in Google Scholar
[147] Torresday JLG, Parsons JG, Gomez E, Videa JP, Troiani HE, Santiago P, Yacaman MJ. Formation and growth of Au nanoparticles inside live alfa alfa plants. Nanoletters 2002, 24, 397–401. Search in Google Scholar
[148] Chandran SP, Chaudhary M, Pasricha R, Ahmad A, Sastry M. Synthesis of gold nanotriangles and silver nanoparticles using Aloe vera plant extract. Biotechnol. Prog. 2006, 22, 577–583. Search in Google Scholar
[149] Vijayaraghavan K, Kamala Nalini SP, Udaya P, Rakash N, Madhankumar D. Biomimetic synthesis of silver nanoparticles by aqueous extract of Syzygium aromaticum. Mater. Lett. 2012, 75, 33–35. Search in Google Scholar
[150] Christensen L, Vivekanandhan S, Misra M, Mohanty A. Biosynthesis of silver nanoparticles using Murraya koenigii curry leaf. An investigation on the effect of broth concentration in reduction mechanism and particle size. Adv. Mat. Lett. 2011, 2, 429–434. Search in Google Scholar
[151] Patil CD, Patil SV, Borase HP, Salunke BK, Salunkhe RB. Larvicidal activity of silver nanoparticles synthesised using Plumeria rubra plant latex against Aedes aegypti and Anopheles stephensi. Parasitol. Res. 2012, 110, 1815–1822. Search in Google Scholar
[152] Pala R, Pathipati UR, Bojja S. Qualitative assessment of silver and gold nanoparticle synthesis in various plants. A photobiological approach. J. Nanopart. Res. 2010, 12, 1711–1721. Search in Google Scholar
[153] Shankar SS, Rai A, Ahmad A, Sastry M. Biosynthesis of silver and gold nanoparticles from extracts of different parts of the Geranium plant. Applications Nanotechnology 2004, 1, 69–77. Search in Google Scholar
[154] Daizy P. Green synthesis of gold and silver nanoparticles using Hibiscus rosa sinensis. Physica E . 2010, 42, 1417–1424. Search in Google Scholar
[155] Shankar S, Rai A, Ankamwar B, Singh A, Ahmad A, Sastry M. Biological synthesis of triangular gold nanoprisms. Nat. Mater. 2004, 3, 482–488. Search in Google Scholar
[156] Armendariz V, Herrera I, Peralta-Videa JR, Jose-Yacaman M, Troiani H, Santiago P, Gardea-Torresdey JL. Size controlled gold nanoparticles formation by Avena sativa biomass, use of plants in nanobiotechnology. J. Nanopart. Res. 2004, 6, 377–82. Search in Google Scholar
[157] Torresdey JL, Gomez E, Peralta-Videa JR, Parsons JG, Troiani H, Jose-Yacaman M. Alfalfa sprouts, a natural source for the synthesis of silver nanoparticles. Langmuir 2003, 19, 1357–1361. Search in Google Scholar
[158] Parida UK, Bindhani BK, Nayak P. Green synthesis and characterization of gold nanoparticles using onion Allium cepa extract. World J. NanoSci. Eng. 2011, 1, 93–98. Search in Google Scholar
[159] Von White II G, Kerscher P, Brown RM, Morella JD, McAllister W, Dean D, Kitchens CL. Green synthesis of robust, biocompatible silver nanoparticles using garlic extract. J. Nanomater . 2012, 12, 730746-58. Search in Google Scholar
[160] Kalidasan M, Yogamoorthi A. Biosynthesis of silver nanoparticles using Achyranthus aspera and its characterization. Int. J. Nanomater. Biostructures 2014, 41, 5–11. Search in Google Scholar
[161] Sheny DS, Mathew J, Philip D. Phytosynthesis of Au, Ag and Au-Ag bimetallic nanoparticles using aqueous extract and dried leaf of Anacardium occidentale. Spectrochim Acta A Mol Biomol Spectrosc . Int. Res. J. Biological Sci . 2013, 26, 66–76. Search in Google Scholar
[162] Sulochana S, Krishnamoorthy P, Sivaranjani K. Synthesis of silver nanoparticles using leaf extract of Andrographis paniculata. J. Pharmacol. Toxicol. 2012, 7, 251–258. Search in Google Scholar
[163] Kora AJ, Arunachala J. Green fabrication of silver nanoparticles by gum Tragacanth Astragalus gummifer . A dual functional reductant and stabilizer. J. Nanomater . 2012, 8 pages, Article ID 869765, doi, 10.1155/2012/869765. Search in Google Scholar
[164] Thirumurugan A, Jiflin GJ, Rajagomathi G, Tomy NA, Ramachandran S, Jaiganesh R. Biotechnological synthesis of gold nanoparticles of Azadirachta indica leaf extract. Int. J. Biol. Tech . 2010, 1, 75–77. Search in Google Scholar
[165] Boruah SK, Boruah PK, Sarma P, Medhi C, Medhi OK. Green synthesis of gold nanoparticles using Camellia sinensis and kinetics of the reaction. Adv. Mat. Lett . 2012, 3, 481–486. Search in Google Scholar
[166] Jain D, Daima HK, Kachhwaha S, Kothari SL. Synthesis of plant-mediated silver nanoparticles using papaya fruit extract and evaluation of their antimicrobial activities. Dig. J. Nanomater. Bios . 2009, 4, 557–563. Search in Google Scholar
[167] Palaniselvam K, Velanganni AAJ, Govindan SN, Karthi. Leaf assisted bioreduction of silver ions using leaves of Centella asiatica L. and its bioactivity. E-J. Life Sci . 2012, 1, 46–49. Search in Google Scholar
[168] Dwivedi AD, Gopal K. Plant- mediated biosynthesis of silver and gold nanoparticles. J. Biomed. Nanotechnol . 2011, 7, 163–164. Search in Google Scholar
[169] Vanaja M, Annadurai G. Coleus aromaticus leaf extract mediated synthesis of silver nanoparticles and its bactericidal activity. Appl. Nanosci. 2013, 3, 217–223. Search in Google Scholar
[170] Sathishkumar M, Sneha K, Kwak IS, Mao J, Tripathy SJ, Yun YS. Phyto-crystallization of palladium through reduction process using Cinnamom zeylanicum bark extract. J. Hazard Mater . 2009, 171, 400–404. Search in Google Scholar
[171] Xin Y, Qingbiao L, Huixuan W, Jiale H, Liqin L, Wenta W, Daohua S, Yuanbo S, James O, Luwei H, Yuanpeng W, Ning H, Lishan J. Green synthesis of palladium nanoparticles using broth of Cinnamomum camphora leaf. J. Nanopart. Res. 2010, 12, 1589–1598. Search in Google Scholar
[172] Satyavani K, Ramanathan T, Gurudeeban S. Plant mediated synthesis of biomedical silver nanoparticles by using leaf extract of Citrullus colocynthis. J. Nanosci. Nanotechno. 2011, 1, 95–101. Search in Google Scholar
[173] Kesharwani J, Yoon KY, Hwang J, Rai M. Phytofabrication of silver nanoparticles by leaf extract of Datura metel , hypothetical mechanism involved in synthesis. J. Bionanosci . 2009, 3, 39–44. Search in Google Scholar
[174] Ahmad N, Sharma S, Singh VN, Shamsi SF, Fatma A, Mehta BR. Biosynthesis of silver nanoparticles from Desmodium triflorum . A novel approach towards weed utilization. Biotechnol. Res. Int . 2011, Article ID 454090, 8 pages. doi, 10.4061/2011/454090. Search in Google Scholar
[175] Song JY, Kwon EY, Kim BS. Biological synthesis of platinum nanoparticles using Diopyros kaki leaf extract. Bioprocess. Biosyst. Eng. 2010, 33, 159–164. Search in Google Scholar
[176] Ghosh S, Patil S, Ahire M, Kitture R, Kale S, Pardesi K, Cameotra SS, Bellare J, Dhavale DD, Jabgunde A, Chopade AB. Synthesis of silver nanoparticles using Dioscorea bulbifera tuber extract and evaluation of its synergistic potential in combination with antimicrobial agents. Int. J. Nanomed. 2012, 7, 483–496. Search in Google Scholar
[177] Maheswari RU, Prabha AL, Nandagopalan V, Anburaja V. Green synthesis of silver nanoparticles by using rhizome extract of Dioscorea oppositifolia L. and their anti microbial activity against human pathogens. IOSR J. Pharma. Biol. Sci. 2012, 1, 38–42. Search in Google Scholar
[178] Gnanajobitha G, Annadurai G, Kannan C. Green synthesis of silver nanoparticles using Elettaria cardamomom and assessment of its antimicrobial activity. Int. J. Pharma. Sci. Res. 2012, 3, 323–330. Search in Google Scholar
[179] Jia L, Zhang Q, Li Q, Song H. The biosynthesis of palladium nanoparticles by antioxidants in Gardenia jasminoides Ellis, long lifetime nanocatalysts for pnitrotoluene hydrogenation. Nanotech. 20. 2012, doi, 10.1088/0957-4484/20/38/385601. Search in Google Scholar
[180] Dinesh S, Karthikeyan S, Arumugam P. Biosynthesis of silver nanoparticles from Glycyrrhiza glabra root extract. Arch. Appl. Sci. Res. 2012, 4, 178–187. Search in Google Scholar
[181] Bindhu MR, Umadevi M. Synthesis of monodispersed silver nanoparticles using Hibiscus cannabinus leaf extract and its antimicrobial activity. Spectrochim Acta A Mol. Biomol. Spectrosc. 2012, 101, 184–190. Search in Google Scholar
[182] Sable N, Gaikwad S, Bonde S, Gade A, Rai MM. Phytofabrication of silver nanoparticles by using aquatic plant Hydrilla verticilata. Nus. Biosci. 2012, 4, 45–49. Search in Google Scholar
[183] Hudlikar M, Joglekar S, Dhaygude M, Kodam K. Latex-mediated synthesis of ZnS nanoparticles, green synthesis approach. J. Nano. Res. 2012, 14, 1–6. Search in Google Scholar
[184] Fazaludeena MF, Manickamb C, Ashankytyc MAI, Ahmedd MQ, Beg QZ. Synthesis and characterizations of gold nanoparticles by Justicia gendarussa Burm F leaf extract. J. Microbiol. Biotech. Res. 2012, 2, 23–34. Search in Google Scholar
[185] Sivakumar P, Nethradevi C, Renganathan S. Synthesis of silver nanoparticles using Lantana camara fruit extract and its effect on pathogens. Asian J. Pharm. Clin. Res. 2012, 5, 97–101. Search in Google Scholar
[186] Im AR, Han L, Kim ER, Kim J, Kim YS, Park Y. Enhanced antibacterial activities of Leonuri herba extracts containing silver nanoparticles. Phytother. Res. 2012, 26, 1249–1255. Search in Google Scholar
[187] Aromal SA, Vidhu VK, Philip D. Green synthesis of well-dispersed gold nanoparticles using Macrotyloma uniflorum. Spectrochim Acta A Mol. Biomol. Spectrosc. 2012, 85, 99–104. Search in Google Scholar
[188] Ali DM, Thajuddin N, Jeganathan K, Gunasekaran M. Plant extract mediated synthesis of silver and gold nanoparticles and its antibacterial activity against clinically isolated pathogens. Colloid. Surface B 2011, 85, 360–365. Search in Google Scholar
[189] Vankar PS, Bajpai D. Preparation of gold nanoparticles from Mirabilis jalapa flowers. Indian J. Biochem. Biophys. 2010, 47, 157–160. Search in Google Scholar
[190] Mary EJ, Inbathamizh L. Green synthesis and characterization of nano silver using leaf extract of Morinda pubescens. Asian J. Pharm. Clin. Res. 2012, 5, 159–162. Search in Google Scholar
[191] Ahmad N, Sharma S, Alam MK, Singh VN, Shamsi SF, Mehta BR, Fatma A. Rapid synthesis of silver nanoparticles using dried medicinal plant of basil. Colloid. Surface B 2010, 81, 81–86. Search in Google Scholar
[192] Ashok kumar D. Rapid and green synthesis of silver nanoparticles using the leaf extracts of Parthenium hysterophorus . A novel biological approach. Int. Res. J. Pharma. 2012, 3, 169–173. Search in Google Scholar
[193] Sundaravadivelan C, Nalini M. Biolarvicidal effect of phyto-synthesized silver nanoparticles using Pedilanthus tithymaloides L. Poit stem extract against the dengue vector Aedes aegypti L. diptera. Culicidae. Asian Pac. J. Trop Biomed. 2011, 1–8. Search in Google Scholar
[194] Mallikarjuna K, Dillip GR, Narashima G, Sushma NJ, Raju BDP. Phyto-fabrication and characterization of silver nanoparticles from Piper betel broth. Res. J. Nanosci. Nanotech. 2012, 2, 17–23. Search in Google Scholar
[195] Garg S. Rapid biogenic synthesis of silver nanoparticles using black pepper Piper nigrum corn extract. Int. J. Innov. Biol. Chem. Sci. 2012, 3, 5–10. Search in Google Scholar
[196] Nabikhan A, Kandasamy K, Raj A, Alikunhi MN. Synthesis of antimicrobial silver nanoparticles by callus and leaf extracts from saltmarsh plant, Sesuvium portulacastrum L. Colloid. Surface B 2010, 79, 2488–2493. Search in Google Scholar
[197] Amin M, Anwar F, Janjua MRSA, Iqbal MA, Rashid U. Green synthesis of silver nanoparticles through reduction with Solanum xanthocarpum L. berry extract, characterization, antimicrobial and urease inhibitory activities against Helicobacter pylori. Int. J. Mol. Sci. 2012, 13, 9923–9941. Search in Google Scholar
[198] Njagi EC, Huang H, Stafford L, Genuino H, Galindo MH, Collins BJ, Hoag EG, Suib LS. Biosynthesis of iron and silver nanoparticles at room temperature using aqueous sorghum bran extracts. Langmuir 2011, 27, 264–271. Search in Google Scholar
[199] Petla RK, Vivekanandhan S, Misra M, Mohanty AK, Satyanarayana N. Soybean glycine max leaf extract based green synthesis of palladium nanoparticles. J. Biomater. Nanobiotech. 2012, 3, 14–19. Search in Google Scholar
[200] Mondal S, Roy N, Laskar RA, Sk I, Basu S, Mandal D, Begum NA. Biogenic synthesis of Ag, Au and bimetallic Au/Ag alloy nanoparticles using aqueous extract of mahogany Swietenia mahogani JACQ. leaves. Colloid. Surface B 2011, 82, 497–504. Search in Google Scholar
[201] Deshpande R, Bedre DM, Basavaraja S, Sawle B, Manjunath SY, Venkataraman A. Rapid biosynthesis of irregular shaped gold nanoparticles from macerated aqueous extracellular dried clove buds Syzygium aromaticum solution. Colloid. Surface B 2010, 79, 235–240. Search in Google Scholar
[202] Ankamwar B. Biosynthesis of gold nanoparticles (green-gold) using leaf extract of Terminalia catappa. E-J. Chem. 2010, 7, 1334–1339. Search in Google Scholar
[203] Geethalakshmi R, Sarada DVL. Synthesis of plantmediated silver nanoparticles using Trianthema decandra extract and evaluation of their anti microbial activities. Int. J. Eng. Sci. Technol. 2010, 2, 970–975. Search in Google Scholar
[204] Gopalakrishnan K, Ramesh C, Ragunathan V, Thamilselvan M. Antibacterial activity of copperoxide nanoparticles on E. coli synthesized from Tridax procumbens leaf extract and surface coating with polyaniline. Dig. J. Nanomater. Bios. 2012, 7, 833–839. Search in Google Scholar
[205] Pavani KV, Swati T, Snehika V, Sravya K, Sirisha M. Phytofabrication of lead nanoparticles using grape skin extract. Int. J. Eng. Sci. Tech. 2012, 4, 3376–3380. Search in Google Scholar
[206] Singh C, Sharma V, Naik KRP, Khandelwal V, Singh H. A green biogenic approach for synthesis of gold and silver nanoparticles using Zingiber officinale. Dig. J. Nanomater. Bios. 2011, 6, 535–542. Search in Google Scholar
[207] Dhuper S, Panda D, Nayak PL. Green synthesis and characterization of zero valent iron nanoparticles from the leaf extract of Mangifera indica. Nano Trends, A J. Nanotechnology and Its Applications 2012, 13, 16–22. Search in Google Scholar
[208] Pattanayak M, Mohapatra D, Nayak PL. Green synthesis and characterization of zero valent iron nanoparticles from the leaf extract of Syzygium aromaticum clove. Middle-E. J. Sci. Res. 2013, 18, 623–626. Search in Google Scholar
[209] Naseem T, Farrukh MA. Antibacterial activity of green synthesis of iron nanoparticles using Lawsonia inermis and Gardenia Jasminoides leaves extract. J. Chem. 2015, 7pages. Search in Google Scholar
[210] Weng X, Huang L, Chen Z, Megharaj M, Naidu R. Synthesis of iron-based nanoparticles by green tea extract and their degradation of malachite. Ind. Crop. Prod. 2013, 51, 342–347. Search in Google Scholar
[211] Pattanayak M, Nayak PL. Ecofriendly green synthesis of iron nanoparticles from various plants and spices extract. Int. J. Pl., An. Env. Sci. 2013, 3, 68–78. Search in Google Scholar
[212] Mohanraj S, Kodhaiyolii S, Rengasamy M, Pugalenthi V. Green synthesized iron oxide nanoparticles effect on fermentative hydrogen production by Clostridium acetobutylicum. Appl. Biochem. Biotech. 2014, 173, 318–331. Search in Google Scholar
[213] Makarov VV, Makarova SS, Love AJ, Sinitsyna OV, Dudnik AO, Yaminsky IV, Taliansky ME, Kalinina NO. Biosynthesis of stable iron oxide nanoparticles in aqueous extracts of Hordeum vulgare and Rumex acetosa plants. Langmuir 2014, 30, 5982–5988. Search in Google Scholar
[214] AbdelHamid AA, Al-Ghobashy MA, Fawzy M, Mohamed MB, Abdel Mottaleb MMSA. Phytosynthesis of Au, Ag, and Au-Ag bimetallic nanoparticles using aqueous extract of sago pondweed Potamogeton pectinatus L. ACS Sustainable Chem. Eng. 2013, 1, 1520–1529. Search in Google Scholar
[215] Kulkarni AA, Bhanage BM. Ag@AgCl nanomaterial synthesis using sugar cane juice and its application in degradation of azo dyes. ACS Sustainable Chem. Eng. 2014, 2, 1007–1013. Search in Google Scholar
[216] Patel VK, Bhattacharya S. High-performance nanothermite composites based on aloevera-directed CuO nanorods. ACS Appl. Mater. Interfaces. 2013, 5, 13364–13374. Search in Google Scholar
[217] Wang Z, Fang C, Megharaj M. Characterization of iron-polyphenol nanoparticles synthesized by three plant extracts and their fenton oxidation of azo dye. ACS Sustainable Chem. Eng. 2014, 2, 1022–1025. Search in Google Scholar
[218] Ashokkumar S, Ravi S, Velmuruganb S. Green synthesis of silver nanoparticles from Gloriosa superba L. leaf extract and their catalytic activity. Spectrochim. Acta A 2013, 115, 388–392. Search in Google Scholar
[219] Jagtap UB, Bapat VA. Biosynthesis, characterization and antibacterial activity of silver nanoparticles by aqueous Annona squamosa L. leaf extract at room temperature. J. Plant Biochem. Biot. 2013, 22, 434–440. Search in Google Scholar
[220] Haldar KM, Haldar B, Chandra G. Fabrication, characterization and mosquito larvicidal bioassay of silver nanoparticles synthesized from aqueous fruit extract of putranjiva, Drypetes roxburghii (Wall). Parasitol. Res. 2013, 112, 1451–1459. Search in Google Scholar
[221] Jha AK, Prasad K, Prasad K, Kulkarni AR. Plant system: nature’s nanofactory. Colloid. Surface B 2009, 73, 219–223. Search in Google Scholar
[222] Subhankari I, Nayak PL. Synthesis of copper nanoparticles using Syzygium aromaticum (cloves) aqueous extract by using green chemistry. World J. Nanosci. Nanotech. 2013, 2, 14–17. Search in Google Scholar
[223] Logeswari P, Silambarasan S, Abraham J. Synthesis of silver nanoparticles using plants extract and analysis of their antimicrobial property. Journal of Saudi Chemical Society 2015, 19, 311–317. Search in Google Scholar
[224] Jayaseelan C, Ramkumar R, Rahuman AA, Perumal P. Green synthesis of gold nanoparticles using seed aqueous extract of Abelmoschus esculentus and its antifungal activity. Ind. Crop. Prod. 2013, 45, 423–429. Search in Google Scholar
[225] Priya MM, Selvia KB, Paul JJA. Green synthesis of silver nanoparticles from the leaf extracts of Euphorbia hirta and Nerium indicum. Dig. J. Nanomater. Bios. 2011, 6, 869–877. Search in Google Scholar
[226] Das RK, Gogoi N, Bora U. Green synthesis of gold nanoparticles using Nyctanthes arbortristis flower extract. Bioprocess. Biosyst. Eng. 2011, 34, 615–619. Search in Google Scholar
[227] Forough M, Farhadi K. Biological and green synthesis of silver nanoparticles. Turkish J. Eng. Environ. Sci. 2010, 34, 281–287. Search in Google Scholar
[228] Raghunandan D, Basavaraja S, Mahesh B, Balaji S, Manjunath S, Venkataraman A. Biosynthesis of stable polyshaped gold nanoparticles from microwave-exposed aqueous extracellular anti-malignant guava Psidium guajava leaf extract. Nanobiotechnol. 2009, 5, 34–41. Search in Google Scholar
[229] Venkateswarlu S, Rao YS, Balaji T, Prathima B, Jyothi NVV. Biogenic synthesis of Fe3O4 magnetic nanoparticles using plantain peel extract. Mater. Lett. 2013, 100, 241–244. Search in Google Scholar
[230] Bankar A, Joshi B, Ravi Kumar A, Zinjarde S. Banana peel extract mediated novel route for the synthesis of palladium nanoparticles. Mater. Lett. 2010, 64, 1951–1953. Search in Google Scholar
[231] Venkateswarlu S, Natesh Kumar B, Prathima B, Anitha K, Jyothi NVV. A novel green synthesis of Fe3O4-Ag core shell recyclable nanoparticles using Vitis vinifera stem extract and its enhanced antibacterial performance. Physica B: Condensed Matter. 2015, 457, 30–35. Search in Google Scholar
[232] Nasrollahzadeh M, Sajadi SM, Rostami-Vartooni A, Khalaj M. Green synthesis of Pd/Fe3O4 nanoparticles using Euphorbia condylocarpa M. bieb root extract and their catalytic applications as magnetically recoverable and stable recyclable catalysts for the phosphine-free Sonogashira and Suzuki coupling reactions. J. Mol. Catal. A: Chem. 2015, 396, 31–39. Search in Google Scholar
[233] Otari SV, Patil RM, Ghosh SJ, Pawar SH. Green phytosynthesis of silver nanoparticles using aqueous extract of Manilkara zapota L. seeds and its inhibitory action against Candida species. Mater. Lett. 2014, 116, 367–369. Search in Google Scholar
[234] Ashokkumar S, Ravi S, Kathiravan V, Velmurugan S. Synthesis, characterization and catalytic activity of silver nanoparticles using Tribulus terrestris leaf extract. Spectrochim. Acta A 2014, 121, 88–93. Search in Google Scholar
[235] Seralathan J, Stevenson P, Subramaniam S, Raghavan R, Pemaiah B, Sivasubramanian A, Veerappan A. Spectroscopy investigation on chemo-catalytic, free radical scavenging and bactericidal properties of biogenic silver nanoparticles synthesized using Salicornia brachiata aqueous extract. Spectrochim. Acta A 2014, 118, 349–355. Search in Google Scholar
[236] Basavegowda N, Lee YR. Synthesis of silver nanoparticles using satsuma mandarin Citrus unshiu peel extract: a novel approach towards waste utilization. Mater. Lett. 2013, 109, 31–33. Search in Google Scholar
[237] Yallappa S, Manjanna J, Sindhe MA, Satyanarayan ND, Pramod SN, Nagaraja K. Microwave assisted rapid synthesis and biological evaluation of stable copper nanoparticles using T. arjuna bark extract. Spectrochim. Acta A 2013, 110, 108–115. Search in Google Scholar
[238] Yudha SS, Notriawan D, Angasa E, Suharto TE, Hendri J, Nishina Y. Green synthesis of silver nanoparticles using aqueous rinds extract of Brucea javanica L. Merr at ambient temperature. Mater. Lett. 2013, 97, 181–183. Search in Google Scholar
[239] Edison TJ, Sethuraman MG. Biogenic robust synthesis of silver nanoparticles using Punica granatum peel and its application as a green catalyst for the reduction of an anthropogenic pollutant 4-nitrophenol. Spectrochim. Acta A 2013, 104, 262–264. Search in Google Scholar
[240] Gopinath V, Priyadarshini S, Meera Priyadharsshini N, Pandian K, Velusamy P. Biogenic synthesis of antibacterial silver chloride nanoparticles using leaf extracts of Cissus quadrangularis Linn. Mater. Lett. 2013, 91, 224–227. Search in Google Scholar
[241] Cai Y, Shen Y, Xie A, Li S, Wang X. Green synthesis of soya bean sprouts-mediated superparamagnetic Fe3O4 nanoparticles. J. Magn. Magn. Mater. 2010, 322, 2938–2943. Search in Google Scholar
[242] Kumar B, Smita K, Cumbal L, Debut A. Biogenic synthesis of iron oxide nanoparticles for 2-arylbenzimidazole fabrication. J. Saudi Chem. Soc. 2014, 18, 364–369. Search in Google Scholar
[243] Basavegowda N, Somai Magar KB, Mishra K, Lee YR. Green fabrication of ferromagnetic Fe3O4 nanoparticles and their novel catalytic applications for the synthesis of biologically interesting benzoxazinone and benzthioxazinone derivatives. N. J. Chem. 2014, 38, 5415–5420. Search in Google Scholar
[244] Bonde S. A biogenic approach for green synthesis of silver nanoparticles using extract of Foeniculum vulgare and its activity against Staphylococcus aureus and Escherichia coli. Nusantara Biosci. 2011, 3, 59–63. Search in Google Scholar
[245] Shekhawat MS, Kannan N, Manokari M. Biogenesis of silver nanoparticles using leaf extract of Turnera ulmifolia Linn. and screening of their antimicrobial activity. J. Ecobiotechnol. 2012, 4, 54–57. Search in Google Scholar
[246] Govindaraju K, Tamilselvan S, Kiruthiga V, Singaravelu G. Biogenic silver nanoparticles by Solanum torvum and their promising antimicrobial activity. J. Biopestic . 2010, 3, 394–399. Search in Google Scholar
[247] Basu S, Maji P, Ganguly J. Rapid green synthesis of silver nanoparticles by aqueous extract of seeds of Nyctanthes arbor-tristis . Appl. Nanosci. 2015, DOI 10.1007/s13204-015-0407-9. Search in Google Scholar
[248] Sasikala A, Savithramma N. Biological synthesis of silver nanoparticles from Cochlospermum religiosum and their antibacterial efficacy. J. Pharm Sci. Res. 2012, 4, 1836–1839. Search in Google Scholar
[249] Yogalakshmi M, Sumalatha D, Madhan R. Biosynthesis of silver nanoparticles using xtracts of Polyalthia longifolia leaves and their antimicrobial properties. J. Biological Inform. Sci. 2012, 1, 21–24. Search in Google Scholar
[250] Sivakumar J, Premkumar C, Santhanam P, Saraswathi N. Biosynthesis of silver nanoparticles using Calotropis gigantean leaf. Afr. J. Basic Appl. Sci. 2011, 3, 265–270. Search in Google Scholar
[251] Shukla VK, Singh RP, Pandey AC. Black pepper assisted biomimetic synthesis of silver nanoparticles. J. Alloys Compd. 2010, 507, L13–L16. Search in Google Scholar
[252] Zargar M, Hamid AA, Bakar FA, Shamsudin MN. Green synthesis and antibacterialeffect of silver nanoparticles using Vitex negundo L. Molecules 2011, 16, 6667–6676. Search in Google Scholar
[253] Awwad AM, Salem NM. Green synthesis of silver nanoparticles by mulberry leaves extract. Nanosci. Nanotechnol . 2012, 2, 125–128. Search in Google Scholar
[254] Korbekandi H, Asghari G, Jalayer SS, Jalayer MS, Bandegani M. Nanosilver particle production using Juglans Regia L. (walnut) leaf extract. J. Nat. Pharm. Prod. 2013, 8, 20–26. Search in Google Scholar
[255] Kulkarni AP, Srivastava AA, Harpale PM, Zunjarrao RS. Plant mediated synthesis of silver nanoparticles – tapping the unexploited sources. J. Nat. Prod. Plant Resour . 2011, 1, 100–107. Search in Google Scholar
[256] Bali R, Razak N, Lumb A, Harris AT. The synthesis of metal nanoparticles inside live plants. International Conference on Nanoscience and Nanotechnology, IEEE Xplore . 2006, DOI 10.1109/ICONN.2006.340592 2006. Search in Google Scholar
[257] Parashar V, Parashar R, Sharma B, Pandey AC. Parthenium leaf extract mediated synthesis of silver nanoparticles, a novel approach towards weed utilization. Dig. J. Nanomater. Bios. 2009, 4, 45–50. Search in Google Scholar
[258] Roy N, Barik A. Green Synthesis of silver nanoparticles from the unexploited weed resources. Int. J. Nanotechnol. App. 2010, 42, 95–101. Search in Google Scholar
©2015 by De Gruyter
- X / Twitter
Supplementary Materials
Please login or register with De Gruyter to order this product.
Journal and Issue
Articles in the same issue.
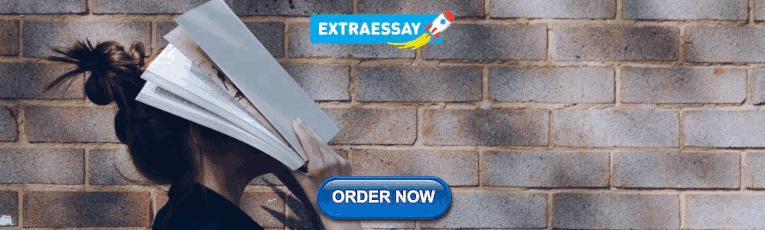
IMAGES
VIDEO
COMMENTS
Green synthesis method; provides a faster metallic nanoparticle production by offering an environmentally friendly, simple, economical and reproducible approach. Given the wide range of ...
Introduction. Nanoscience has gained remarkable attention due to its diverse applications in multiple fields. The cornerstone of nanoscience is the cost-effective synthesis of nanoparticles (NPs) with unique physical, chemical, optical, electrochemical, and thermal properties [1, 2].The applications of NPs in the environment sector have good potential to overcome the challenges of ...
Green synthesis of nanoparticles Dissertation zur Erlangung des akademischen Grades Doktor der Ingenieurwissenschaften (Dr.-Ing.) ... In this thesis we focus on two different green synthesis techniques. In our first technique, silver/gold plasmonic bionanocomposites (BNCs) as well as 3D bio-shells decorated with BNCs ...
So, as an alternative to all aforementioned techniques the use of green synthesis metal nanoparticles is quite well and suitable against several types of pathogens (human and plant infected) 17,18.
peel extract. After addition of the reducing agent the gradual generation of silver nanoparticles. started which is indicated by the increase in the absorbance intensity at 400-410nm in UV. spectra.The Orange peel extract showing absorption bands at 324 and around 284 nm Figure 3.
The use of plants for green nanoparticle synthesis is an interesting and emerging aspect of nanotechnology that has a significant impact on the environment and contributes to nanoscience's long-term sustainability and progress. Catalysis, medicine, cosmetic, agriculture, food packaging, water treatment, dye degradation, textile engineering ...
In the arena of material sciences, one of the burning topics for research has been biogenically synthesizing nanoparticles (NPs) from plant derivatives and studying their applicability to be used as sustainable catalysts. The contemporary work happening on nanocatalysts focuses on the scope of application of green catalysts. For devising a technology that is ecofriendly as well as rapid, it ...
Green synthesis of nanoparticles has many potential applications in environmental and biomedical fields. Green synthesis aims in particular at decreasing the usage of toxic chemicals. For instance, the use of biological materials such as plants is usually safe. Plants also contain reducing and capping agents. Here we present the principles of green chemistry, and we review plant-mediated ...
Vidyasagar is a PhD student at Banaras Hindu University, India. He is pursuing his PhD in Chemistry under the supervision of Dr Meenakshi Singh. The area of his research is modern organic synthesis and incorporation of nanoparticles in the medical field. He completed his bachelor's degree at Dr Ram Manohar Lohia Avadh University, Faizabad and his
Green synthesis of Au NPs, NZVI, iron oxide nanoparticles, and Cu NPs has also tried to make full use of local plants. Fenugreek, used in the synthesis of Au NPs, is widely distributed in China and the east coast of the Mediterranean, while peppermint is native to Central Europe and West Asia ( Aswathy Aromal and Philip, 2012 ).
Nanoparticles are often associated with their small size and numerous applications. However, the synthesis process is equally important as it determines the size and properties of the nanoparticles. While traditional nanoparticle synthesis methods require the use of hazardous chemicals and high-energy consumption, green synthesis offers a sustainable, cost-effective, and environmentally ...
Green synthesis is a modern technology used to prepare nanomaterials (NM) that lead to a new era that reveals the plant potential in synthesizing stable nanoparticles (NPs) and increases the life of NPs (Ahmad et al. 2019).Constraints on chemical and physical methods are also overcome, as the first depends primarily on chemical reactions with its risks and side effects.
Green synthesis processes help in reducing the chances of environmental toxification thus, making the environment living worth. In the present study, a systematic investigation has been carried out to synthesize highly oriented and uniform zinc oxide (ZnO) and silver doped zinc oxide (AgZnO) nanoparticles using the extract of Moringa oleifera ...
Synthesis of metal nanoparticles using plant extracts is one of the most simple, convenient, economical, and environmentally friendly methods that mitigate the involvement of toxic chemicals. Hence, in recent years, several eco-friendly processes for the rapid synthesis of silver nanoparticles have been reported us 2021 Reviews in RSC Advances Chemistry in the battle against infections ...
We also provide an overview of the biomolecules that were found to be suitable for NP synthesis. This work is meant to be a support for researchers who intend to develop new green approaches for the synthesis of NPs. Keywords: metal nanoparticle; metal oxide nanoparticle; green synthesis; biological method. 1.
Widatalla, H. A. et al. Green synthesis of silver nanoparticles using green tea leaf extract, characterization and evaluation of antimicrobial activity. Nanoscale Adv. 4 (3), 911-915 (2022).
Nanoparticles (NPs) are elements derived from a cluster of atoms with one or more dimensions in the nanometer scale in the range of 1-100 nm. The bio nanofabrication of metallic NPs is now an important dynamic area of research, with major significance in applied research. Biogenic synthesis of NPs is more desirable than physical and chemical synthesis due to its eco-friendliness, non ...
The nanoparticles (NPs) can be synthesized from agro-waste like Cocos nucifera coir, corn cob, fruit seeds and peels, wheat and rice bran, palm oil, etc. These compounds are rich in biomolecules like flavonoids, phenolic and proteins that could act as reductive agent for the synthesis of NPs [ 12 ].
Synthesis of green and pure copper oxide nanoparticles using two plant resources via solid-state route and their phytotoxicity assessment. Iman Khaldari a, Mohammad Reza Naghavi * a and Elaheh Motamedi * b a Division of Biotechnology, Department of Agronomy and Plant Breeding, Agricultural and Natural Resources College, University of Tehran, Karaj, Iran. E-mail: [email protected] b Department ...
Green synthesis of metal nanoparticles and assessment of their biological activity: Researcher: Prachi: Guide(s): Negi, D.S. Keywords: Biological Activity Chemistry Chemistry Multidisciplinary Green Synthesis Metal Nanoparticles Physical Sciences: University: Hemwati Nandan Bahuguna Garhwal University: Completed Date: 2019: Abstract:
In the past few years, nanoparticles have been applied in various fields of science and technology, ranging from material science to biotechnology. Thus, the synthesis of nanoparticles can be considered as a dynamic area in research and application of nanoparticles. The different methods of nanoparticle synthesis include physical, chemical, and biological methods. Of these methods, the ...
nanoparticles (NP), and their application on pathogenic bacteria were investigated. ZnO NP were synthesized by chemical reduction method using starch as capping agent and silver NP was prepared by green synthesis process from AgNO3 solution through the extract of Citrus sinensis (sweet lime). The detail characterization of the nanoparticles was ...