- Jacobs School of Medicine and Biomedical Sciences
- UB Directory
- Pediatrics >
- Education & Training >
- Medical Student Education >
- Research Opportunities >
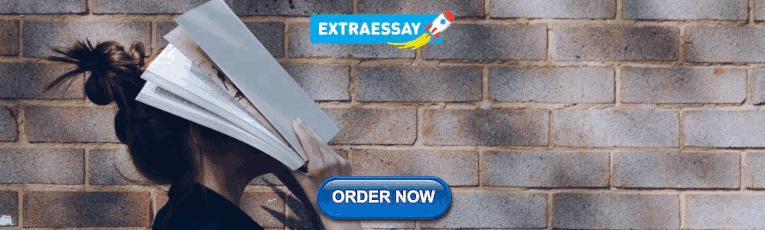
Summer Pediatrics Internship in Research
The Summer Pediatric Internship in Research is a 7-week summer program combining research and clinical aspects of pediatrics and/or pediatric subspecialties.
The Summer Pediatric Internship in Research is a mentored program designed to provide research experience to first-year Jacobs School medical students. In our program, you will be paired with a faculty mentor to complete a pediatric or pediatric specialty research project.
In addition to conducting research, you will attend departmental meetings to gain exposure to the clinical aspects of the subspecialty. Expectation will be to work on plan of research with mentor prior to starting project. At the end of the 7-week summer internship, you will be expected to have minimally completed data collection. Work may be needed to complete analysis and prepare your presentation during the winter break. You will have the opportunity to present your work to faculty and peers in the department, at the Pediatrics Department Research Forum, and at the schoolwide Medical Student Research Forum .
Our faculty mentors encourage our student researchers to publish study results and present at national meetings.
Current projects focus on:
- Kawasaki disease
- B cell immunology in children
Internships take place on the Buffalo Niagara Medical Campus (Mark Hicar, MD, PhD).
Number of Positions
We accept 1-4 students per year, depending on student/mentor fit and funding.
Eligibility
First-year Jacobs School medical students in good standing are eligible.
Program Dates
This is a 7-week internship during the summer between your first and second year.
May 28 - July 19, 2024
Prior Recipients
- Devin Decotes, MD '26
- Karlie Gambino, MD '26
- Callista Zayatz, MD '26

Michelle Smith, MD ’16 Jacobs School of Medicine and Biomedical Sciences

Rachel Engelberg, MD ’18 Jacobs School of Medicine and Biomedical Sciences
How to Apply
Please complete this form to apply.
Application Deadline
February 20th
Award Notification
Applications will be reviewed and interviews conducted on a rolling basis, with the goal to finalize offers by March.
If you have questions about this research opportunity, please contact:
Mark Hicar, MD, PhD, FPIDS, FIDSA
Associate Professor; Interim Chief, Pediatric Infectious Diseases; Program Director, Pediatric Infectious Diseases Fellowship
UBMD Pediatrics Division of Infectious Diseases 1001 Main Street Buffalo, NY 14203
Phone: (716) 323-0150; Fax: (716) 323-0296
Email: [email protected]
- B cell immunology in children
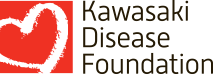
KD Research Centers
Kawasaki disease research centers, researchers worldwide are working to solve the kawasaki disease puzzle. understanding the disease can lead to improved care and treatment for kd patients. learn about the different research studies taking place and help accelerate breakthroughs in kd treatment by participating in research..
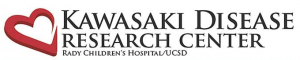
University of California San Diego Kawasaki Disease Research Center
The Kawasaki Disease Research Center is a collaboration between the Departments of Pediatrics, Medicine, and Family and Preventive Medicine and Sociology at UC San Diego, the Scripps Institute of Oceanography, and Rady Children’s Hospital San Diego.
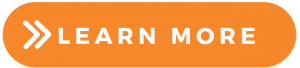
Portman Research Group at Seattle Children’s Hospital
The Portman Research Group is developing innovative ways to protect children’s hearts from damage related to heart surgery and is improving how the medical community understands and treats Kawasaki disease.
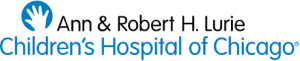
Kawasaki Disease Research at Ann & Robert H. Lurie Children’s Hospital
Lurie Children’s has been a leader in clinical research on Kawasaki disease dating back to the treatment trials in the 1980s and early 1990s. Our clinician scientists are continuing their research efforts in finding the cause of this illness, improving treatment and identifying factors that determine susceptibility.

Kawasaki Disease Research at Boston Children’s Hospital
Dr. Newburger designed an NIH-funded multicenter randomized trial comparing treatments for Kawasaki disease and directed the coordinating center for the study. The first phase of that trial compared high-dose intravenous gamma globulin plus aspirin to aspirin alone during the acute stage of the disease; the second phase compared the effects of two dose regimens of intravenous gamma globulin. Her other prospective studies in Kawasaki disease have focused on its epidemiology and long-term effects.
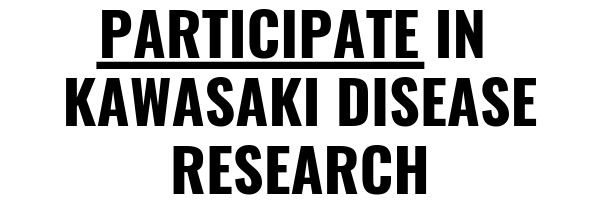
Find a Research Study>>
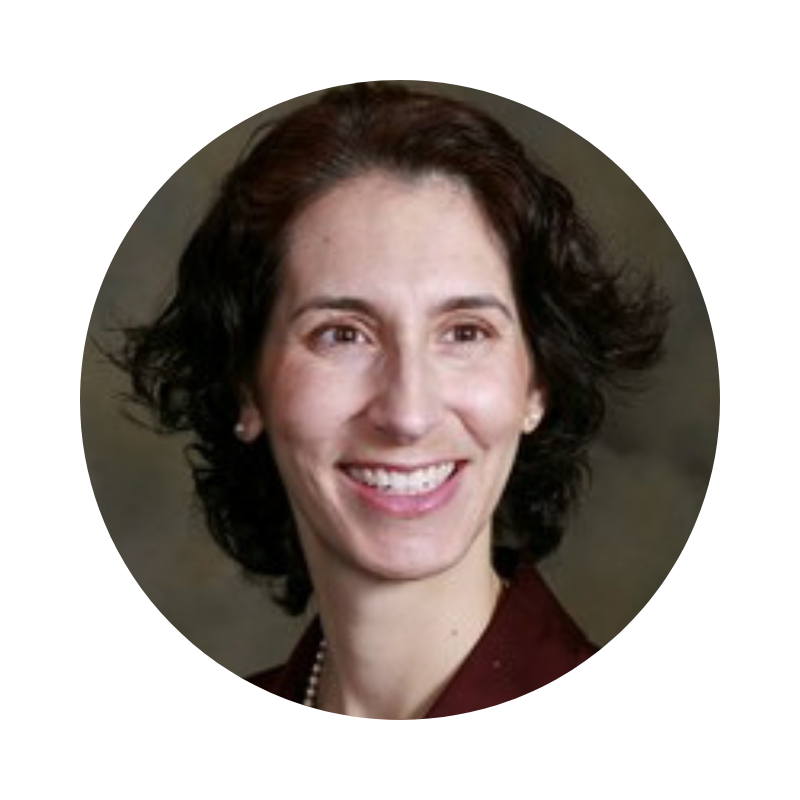
I’m blessed every day by the families I get to meet, by the team members with whom I work and by the colleagues I have met in a variety of fields around the world. As I say often, I look forward to the day when we figure out the cause(s) of KD and eradicate it. I’ll be happy to find another calling then. Until then though, I’ll be here.
- School of Medicine Home
- Administration
COVID-19 Updates
Visit UC San Diego's Coronavirus portal for the latest information for the campus community.
View Details
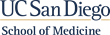
- Hospital & Clinic Locations
- Find a Provider
- Medical Services
- MyUCSDChart Login
- Clinical Trials
- Refer a Patient
- School of Medicine
- Academic Departments
- Skaggs School of Pharmacy
- Continuing Medical Education
- Altman Clinical and Translational Research Institute
- Moores Cancer Center
- Research Centers
- Research Labs
- Volunteering
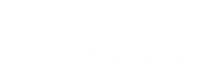
- Adult KD Study
- Past Research Studies
- Active Clinical Trials
- Kawasaki Disease Symposium
- Long Term Effects of KD
- Kawasaki Disease Clinic
- Information on Kawasaki Disease
- Kawasaki Disease Signs
- For Physicians

- People Currently selected
- UC San Diego School of Medicine
- 9500 Gilman Drive

- About School of Medicine

- Policy Notices
Medical Research Internship For Kawasaki Disease
- London ( Greater London )
Job description
Are you passionate about making a real difference in saving lives? Are you looking for the opportunity to gain valuable work experience to help bolster your CV? Are you hardworking and enthusiastic? We are offering unpaid internship opportunities for recent graduates or students currently studying in Biology, Biomedical Sciences, Medicine or related disciplines for a period of 1-6 months. Interested? Well, as our intern you will be working under the guidance of Dr Qian Xu, who is an Emergency Medicine Doctor, and get a wide-range of hands on experiences that will include data analysis and research on Kawasaki Disease, day-to-day liaising and integrating with the core team to complete key priorities and a chance to help develop awareness of Kawasaki Disease and to make a real difference! You will have communication and correspondence with world renowned specialists from Imperial College and the Kawasaki Fund in Australia, and Dr. Kawasaki himself. The Kawasaki Fund internship is an excellent opportunity for ambitious graduates to contribute to developing awareness and statistical data regarding this obscure condition and the impact it has on lives. We are looking for team players and people who want to work in a vibrant energetic environment. We are looking for individuals who are highly organised, self-starters with a proactive and professional attitude and outstanding interpersonal skills. Those who complete the internship successfully and impress will gain the opportunity for a permanent, paid job. Personal requirements Very strong written and spoken English: additional language is a plus. Ability to work in the UK is required. Poised decision making skills and the capability to prioritise workload effectively Competency in Word, PowerPoint and other Office programs essential A genuine interest and passion for Charitable work Available immediately for a minimum of 3 months (ideally 6 months) Interviews will be held on a rolling basis Company information Kawasaki Fund is a charity with the mission to save lives by promoting prompt diagnosis and raising awareness of the classic symptoms of an infection that triggers the heart disease, the leading cause of paediatric heart condition. The Kawasaki Fund internship is an excellent opportunity for ambitious students to learn about charity work and to gain some valuable work experience to bolster your CV.
Desired profile
- Recent graduate (no more than 1-2 years post graduate work experience) or current student at a leading school with discipline in Biology, Biomedical Sciences, Medicine or any related disciplines. - Excellent academic record and a high level of medical understanding and statistical familiarity.
- Job directory
- Business directory
Clinical Research of Kawasaki Disease
- First Online: 23 August 2022
Cite this chapter
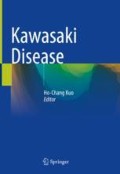
- Hsin Chi 2
296 Accesses
Kawasaki disease (KD) is an acute systemic inflammation illness. The diagnostic five clinical manifestations which notable in acute phase are bilateral nonexudative bulbar conjunctivitis which sparing the limbal area, dry fissured lips accompanied with hyperemia in oropharyngeal mucosa or strawberry tongue, unilateral anterior cervical lymphadenopathy, changes in the peripheral extremities, and polymorphous skin rash. Recognition of nonspecific clinical features observed in incomplete KD is key for prompt diagnosis. Kawasaki disease shock syndrome is a potentially life-threatening type of KD. Refractory KD patients are IVIG-resistant and at higher risk of developing coronary artery lesions (CALs). Infants under 1 year of age and adolescent with KD may have different manifestations and high risk of developing CALs.
- Coronary artery lesions
- Kawasaki disease
This is a preview of subscription content, log in via an institution to check access.
Access this chapter
- Available as PDF
- Read on any device
- Instant download
- Own it forever
- Available as EPUB and PDF
- Compact, lightweight edition
- Dispatched in 3 to 5 business days
- Free shipping worldwide - see info
- Durable hardcover edition
Tax calculation will be finalised at checkout
Purchases are for personal use only
Institutional subscriptions
Singh S, Vignesh P, Burgner D. The epidemiology of Kawasaki disease: a global update. Arch Dis Child. 2015;100(11):1084–8.
Article PubMed Google Scholar
Lue HC, Chen LR, Lin MT, Chang LY, Wang JK, Lee CY, et al. Estimation of the incidence of Kawasaki disease in Taiwan. A comparison of two data sources: nationwide hospital survey and national health insurance claims. Pediatr Neonatol. 2014;55(2):97–100.
Alexoudi I, Kanakis M, Kapsimali V, Vaiopoulos G. Kawasaki disease: current aspects on aetiopathogenesis and therapeutic management. Autoimmun Rev. 2011;10(9):544–7.
McCrindle BW, Rowley AH, Newburger JW, Burns JC, Bolger AF, Gewitz M, et al. Diagnosis, treatment, and long-term management of Kawasaki disease: a scientific statement for health professionals from the American Heart Association. Circulation. 2017;135(17):e927–e99.
Newburger JW, Takahashi M, Gerber MA, Gewitz MH, Tani LY, Burns JC, et al. Diagnosis, treatment, and long-term management of Kawasaki disease: a statement for health professionals from the Committee on Rheumatic Fever, Endocarditis and Kawasaki Disease, Council on Cardiovascular Disease in the Young, American Heart Association. Circulation. 2004;110(17):2747–71.
Greco A, De Virgilio A, Rizzo MI, Tombolini M, Gallo A, Fusconi M, et al. Kawasaki disease: an evolving paradigm. Autoimmun Rev. 2015;14(8):703–9.
El-Radhi AS, Carroll J, Klein N. Fever in non-infectious diseases. In: Clinical manual of fever in children. Berlin: Springer; 2009. p. 137–73.
Chapter Google Scholar
Golshevsky D, Cheung M, Burgner D. Kawasaki disease—the importance of prompt recognition and early referral. Aust Fam Physician. 2013;42:473–6.
PubMed Google Scholar
Salo E, Pelkonen P, Pettay O. Outbreak of Kawasaki syndrome in Finland. Acta Paediatr Scand. 1986;75(1):75–80.
Article CAS PubMed Google Scholar
Jaggi P, Wang W, Dvorchik I, Printz B, Berry E, Kovalchin JP, et al. Patterns of fever in children after primary treatment for Kawasaki disease. Pediatr Infect Dis J. 2015;34(12):1315–8.
Article PubMed PubMed Central Google Scholar
Kobayashi T, Ayusawa M, Suzuki H, Abe J, Ito S, Kato T, et al. Revision of diagnostic guidelines for Kawasaki disease (6th revised edition). Pediatr Int. 2020;62(10):1135–8.
Son MBF, Newburger JW. Kawasaki disease. Pediatr Rev. 2018;39(2):78–90.
Cunningham ET Jr. Uveitis in children. Ocul Immunol Inflamm. 2000;8(4):251–61.
Madhusudan S, Singh S, Suri D, Gupta A, Gupta A. Acute anterior uveitis as the presenting feature of Kawasaki disease. Indian J Pediatr. 2014;81(4):415.
Burke MJ, Rennebohm RM, Crowe W, Levinson JE. Follow-up ophthalmologic examinations in children with Kawasaki’s disease. Am J Ophthalmol. 1981;91(4):537–9.
Shiari R, Jari M, Karimi S, Salehpour O, Rahmani K, Hassas Yeganeh M, et al. Relationship between ocular involvement and clinical manifestations, laboratory findings, and coronary artery dilatation in Kawasaki disease. Eye (Lond). 2020;34(10):1883–7.
Article CAS Google Scholar
Choi HS, Lee SB, Kwon JH, Kim HS, Sohn S, Hong YM. Uveitis as an important ocular sign to help early diagnosis in Kawasaki disease. Korean J Pediatr. 2015;58(10):374–9.
Article CAS PubMed PubMed Central Google Scholar
Smith LB, Newburger JW, Burns JC. Kawasaki syndrome and the eye. Pediatr Infect Dis J. 1989;8(2):116–8.
CAS PubMed Google Scholar
Burke MJ, Rennebohm RM. Eye involvement in Kawasaki disease. J Pediatr Ophthalmol Strabismus. 1981;18(5):7–11.
Burns JC, Joffe L, Sargent RA, Glode MP. Anterior uveitis associated with Kawasaki syndrome. Pediatr Infect Dis. 1985;4(3):258–61.
Chang LY, Lu CY, Shao PL, Lee PI, Lin MT, Fan TY, et al. Viral infections associated with Kawasaki disease. J Formos Med Assoc. 2014;113(3):148–54.
Cavallo F, Chiarelli F. An outbreak of Kawasaki-like disease in children during SARS-CoV-2 epidemic: no surprise? Acta Biomed. 2020;91(3):e2020015.
CAS PubMed PubMed Central Google Scholar
Singh A, Jindal AK, Pilania RK, Indla RT, Suri D, Singh S. Severe lip involvement in Kawasaki disease: a clinical oddity. J Clin Rheumatol. 2020;26(5):e149–e50.
Cakan M, Aktay Ayaz N, Keskindemirci G, Onan SH, Akoz Saydam F. A case of Kawasaki disease with severe lip and oral mucosa involvement complicated with microstomia and corrected with surgery. Arch Rheumatol. 2018;33(2):238–40.
Falcini F, Ricci L, Poggi GM, Simonini G, Calabri GB, de Martino M. Severe cutaneous manifestations in a child with refractory Kawasaki disease. Rheumatology (Oxford, England). 2006;45(11):1444–5.
Banday AZ, Neelam H, Singh MP, Sudhakar M, Jindal AK. Severe lip excoriation in Kawasaki disease: beware of herpes simplex virus. Rheumatology (Oxford, England). 2020; https://doi.org/10.1093/rheumatology/keaa081 .
Shimizu A, Ebara Y, Nomura S, Yamada Y. Chronological changes in strawberry tongue in toxic shock syndrome toxin-1-mediated exanthematous disease. J Gen Fam Med. 2020;21(6):280–1.
Li X, Chen Y, Tang Y, Ding Y, Xu Q, Sun L, et al. Predictors of intravenous immunoglobulin-resistant Kawasaki disease in children: a meta-analysis of 4442 cases. Eur J Pediatr. 2018;177(8):1279–92.
Isidori C, Sebastiani L, Esposito S. A case of incomplete and atypical Kawasaki disease presenting with retropharyngeal involvement. Int J Environ Res Public Health. 2019;16(18):3262.
Article PubMed Central Google Scholar
Fukushige J, Takahashi N, Ueda Y, Ueda K. Incidence and clinical features of incomplete Kawasaki disease. Acta Paediatr. 1994;83(10):1057–60.
Tashiro N, Matsubara T, Uchida M, Katayama K, Ichiyama T, Furukawa S. Ultrasonographic evaluation of cervical lymph nodes in Kawasaki disease. Pediatrics. 2002;109(5):E77.
Kanegaye JT, Van Cott E, Tremoulet AH, Salgado A, Shimizu C, Kruk P, et al. Lymph-node-first presentation of Kawasaki disease compared with bacterial cervical adenitis and typical Kawasaki disease. J Pediatr. 2013;162(6):1259–63, 63.e1–2.
Jun WY, Ann YK, Kim JY, Son JS, Kim SJ, Yang HS, et al. Kawasaki disease with fever and cervical lymphadenopathy as the sole initial presentation. Korean Circ J. 2017;47(1):107–14.
Piram M, Burns JC. Kawasaki disease for the pediatric dermatologist: skin manifestations and new insights into the pathophysiology. Clin Exp Dermatol. 2021;46(3):503–9.
Piram M, Darce Bello M, Tellier S, Di Filippo S, Boralevi F, Madhi F, et al. Defining the risk of first intravenous immunoglobulin unresponsiveness in non-Asian patients with Kawasaki disease. Sci Rep. 2020;10(1):3125.
Okada S, Hirano R, Okazaki-Emi S, Fukunaga S, Ishikawa Y. Erythema multiforme- and not erythema multiforme-like rashes in Kawasaki disease. Pediatr Int. 2019;61(12):1272–4.
Kamath S, Gurnee EA, Schenck OL, Chamlin SL, Mancini AJ. Pustular eruption in Kawasaki disease. J Pediatr. 2019;213:241–e1.
Haddock ES, Calame A, Shimizu C, Tremoulet AH, Burns JC, Tom WL. Psoriasiform eruptions during Kawasaki disease (KD): a distinct phenotype. J Am Acad Dermatol. 2016;75(1):69–76.e2.
Demir F, Abul MH, Dilber E, Orhan F, Kalyoncu M. A case of Kawasaki disease presenting with atypical cutaneous involvement and mimicking Stevens–Johnson syndrome. North Clin Istanb. 2020;7(1):67–70.
Bayers S, Shulman ST, Paller AS. Kawasaki disease: part I. Diagnosis, clinical features, and pathogenesis. J Am Acad Dermatol. 2013;69(4):501.e1–11; quiz 11–2.
Article Google Scholar
Vignesh P, Singh S. Perianal skin peeling: an important clinical pointer toward Kawasaki disease. Eur J Rheumatol. 2018;5(1):81–2.
Uehara R, Igarashi H, Yashiro M, Nakamura Y, Yanagawa H. Kawasaki disease patients with redness or crust formation at the Bacille Calmette–Guerin inoculation site. Pediatr Infect Dis J. 2010;29(5):430–3.
Garrido-Garcia LM, Castillo-Moguel A, Vazquez-Rivera M, Cravioto P, Fernando G. Reaction of the BCG scar in the acute phase of Kawasaki disease in Mexican children. Pediatr Infect Dis J. 2017;36(10):e237–e41.
Araki T, Kodera A, Kitada K, Fujiwara M, Muraoka M, Abe Y, et al. Analysis of factors associated with development of Bacille Calmette–Guerin inoculation site change in patients with Kawasaki disease. J Int Med Res. 2018;46(4):1640–8.
Wang S, Best BM, Burns JC. Periungual desquamation in patients with Kawasaki disease. Pediatr Infect Dis J. 2009;28(6):538–9.
Mohsin M, Akhtar S. Kawasaki disease complicated by peripheral gangrene and a ventricular septal defect: an unusual association. Turk J Pediatr. 2019;61(2):301–4.
Malekzadeh I, Ziaee V, Sadrosadat T, Moardinejad MH, Sayadpour-Zanjani K. Kawasaki disease and peripheral gangrene in infancy. Iran J Pediatr. 2015;25(6):e3309.
Durall AL, Phillips JR, Weisse ME, Mullett CJ. Infantile Kawasaki disease and peripheral gangrene. J Pediatr. 2006;149(1):131–3.
Yamazaki-Nakashimada MA, Deguchi K, Gamez-Gonzalez B, Ulloa-Gutierrez R, Hamada H. Orange–brown chromonychia: a valid sign in Kawasaki disease in children of different ethnicities. Int J Rheum Dis. 2019;22(6):1160–1.
Thapa R, Pal P. Transverse orange–brown chromonychia in Kawasaki disease. Int J Dermatol. 2010;49(2):227–8.
Pal P, Giri PP. Orange–brown chromonychia, a novel finding in Kawasaki disease. Rheumatol Int. 2013;33(5):1207–9.
Parish LC. Hurwitz clinical pediatric dermatology: a textbook of skin disorders of childhood and adolescence. JAMA. 2012;307(15):1646.
Leung AKC, Leong KF, Lam JM. Onychomadesis in a 20-month-old child with Kawasaki disease. Case Rep Pediatr. 2019;2019:3156736.
PubMed PubMed Central Google Scholar
Jindal AK, Bishnoi A, Dogra S, Singh S. A unique combination of nail changes in a boy with Kawasaki disease. J Clin Rheumatol. 2020;26(6):e210–e1.
Chang F-Y, Hwang B, Chen S-J, Lee P-C, Meng CCL, Lu J-H. Characteristics of Kawasaki disease in infants younger than six months of age. Pediatr Infect Dis J. 2006;25(3):241–4.
Chuang CH, Hsiao MH, Chiu CH, Huang YC, Lin TY. Kawasaki disease in infants three months of age or younger. J Microbiol Immunol Infect. 2006;39(5):387–91.
Sonobe T, Kiyosawa N, Tsuchiya K, Aso S, Imada Y, Imai Y, et al. Prevalence of coronary artery abnormality in incomplete Kawasaki disease. Pediatr Int. 2007;49(4):421–6.
Yu JJ. Diagnosis of incomplete Kawasaki disease. Korean J Pediatr. 2012;55(3):83.
Perrin L, Letierce A, Guitton C, Tran T-A, Lambert V, Koné-Paut I. Comparative study of complete versus incomplete Kawasaki disease in 59 pediatric patients. Joint Bone Spine. 2009;76(5):481–5.
Manlhiot C, Christie E, McCrindle BW, Rosenberg H, Chahal N, Yeung RS. Complete and incomplete Kawasaki disease: two sides of the same coin. Eur J Pediatr. 2012;171(4):657–62.
Yellen ES, Gauvreau K, Takahashi M, Burns JC, Shulman S, Baker AL, et al. Performance of 2004 American Heart Association recommendations for treatment of Kawasaki disease. Pediatrics. 2010;125(2):e234–e41.
Ghelani SJ, Sable C, Wiedermann BL, Spurney CF. Increased incidence of incomplete Kawasaki disease at a pediatric hospital after publication of the 2004 American Heart Association guidelines. Pediatr Cardiol. 2012;33(7):1097–103.
Verdoni L, Mazza A, Gervasoni A, Martelli L, Ruggeri M, Ciuffreda M, et al. An outbreak of severe Kawasaki-like disease at the Italian epicentre of the SARS-CoV-2 epidemic: an observational cohort study. Lancet. 2020;395(10239):1771–8.
Toubiana J, Poirault C, Corsia A, Bajolle F, Fourgeaud J, Angoulvant F, et al. Kawasaki-like multisystem inflammatory syndrome in children during the covid-19 pandemic in Paris, France: prospective observational study. BMJ. 2020;369:m2094.
Riphagen S, Gomez X, Gonzalez-Martinez C, Wilkinson N, Theocharis P. Hyperinflammatory shock in children during COVID-19 pandemic. Lancet. 2020;395(10237):1607–8.
Kanegaye JT, Wilder MS, Molkara D, Frazer JR, Pancheri J, Tremoulet AH, et al. Recognition of a Kawasaki disease shock syndrome. Pediatrics. 2009;123(5):e783–e9.
Chen PS, Chi H, Huang FY, Peng CC, Chen MR, Chiu NC. Clinical manifestations of Kawasaki disease shock syndrome: a case-control study. J Microbiol Immunol Infect. 2015;48(1):43–50.
Lin MT, Fu CM, Huang SK, Huang SC, Wu MH. Population-based study of Kawasaki disease shock syndrome in Taiwan. Pediatr Infect Dis J. 2013;32(12):1384–6.
Dominguez SR, Friedman K, Seewald R, Anderson MS, Willis L, Glodé MP. Kawasaki disease in a pediatric intensive care unit: a case-control study. Pediatrics. 2008;122(4):e786–e90.
Li Y, Zheng Q, Zou L, Wu J, Guo L, Teng L, et al. Kawasaki disease shock syndrome: clinical characteristics and possible use of IL-6, IL-10 and IFN-gamma as biomarkers for early recognition. Pediatr Rheumatol Online J. 2019;17(1):1.
Lin YJ, Cheng MC, Lo MH, Chien SJ. Early differentiation of Kawasaki disease shock syndrome and toxic shock syndrome in a pediatric intensive care unit. Pediatr Infect Dis J. 2015;34(11):1163–7.
Gamez-Gonzalez LB, Moribe-Quintero I, Cisneros-Castolo M, Varela-Ortiz J, Munoz-Ramirez M, Garrido-Garcia M, et al. Kawasaki disease shock syndrome: unique and severe subtype of Kawasaki disease. Pediatr Int. 2018;60(9):781–90.
Yilmazer MM, Ozdemir R, Mese T, Kucuk M, Oner T, Devrim I, et al. Kawasaki disease in Turkish children: a single center experience with emphasis on intravenous immunoglobulin resistance and giant coronary aneurysms. Turk J Pediatr. 2019;61(5):648–56.
Kobayashi T, Inoue Y, Takeuchi K, Okada Y, Tamura K, Tomomasa T, et al. Prediction of intravenous immunoglobulin unresponsiveness in patients with Kawasaki disease. Circulation. 2006;113(22):2606–12.
Egami K, Muta H, Ishii M, Suda K, Sugahara Y, Iemura M, et al. Prediction of resistance to intravenous immunoglobulin treatment in patients with Kawasaki disease. J Pediatr. 2006;149(2):237–40.
Sano T, Kurotobi S, Matsuzaki K, Yamamoto T, Maki I, Miki K, et al. Prediction of non-responsiveness to standard high-dose gamma-globulin therapy in patients with acute Kawasaki disease before starting initial treatment. Eur J Pediatr. 2007;166(2):131–7.
Kobayashi T, Saji T, Otani T, Takeuchi K, Nakamura T, Arakawa H, et al. Efficacy of immunoglobulin plus prednisolone for prevention of coronary artery abnormalities in severe Kawasaki disease (RAISE study): a randomised, open-label, blinded-endpoints trial. Lancet. 2012;379(9826):1613–20.
Ogata S, Ogihara Y, Honda T, Kon S, Akiyama K, Ishii M. Corticosteroid pulse combination therapy for refractory Kawasaki disease: a randomized trial. Pediatrics. 2012;129(1):e17–23.
Lin MT, Chang CH, Sun LC, Liu HM, Chang HW, Chen CA, et al. Risk factors and derived formosa score for intravenous immunoglobulin unresponsiveness in Taiwanese children with Kawasaki disease. J Formos Med Assoc. 2015;115(5):350–5.
Article PubMed CAS Google Scholar
Acet H, Ertas F, Akil MA, Ozyurtlu F, Polat N, Bilik MZ, et al. Relationship between hematologic indices and global registry of acute coronary events risk score in patients with ST-segment elevation myocardial infarction. Clin Appl Thromb Hemost. 2016;22(1):60–8.
Kawamura Y, Takeshita S, Kanai T, Yoshida Y, Nonoyama S. The combined usefulness of the neutrophil-to-lymphocyte and platelet-to-lymphocyte ratios in predicting intravenous immunoglobulin resistance with Kawasaki disease. J Pediatr. 2016;178:281–4.e1.
Hangai M, Kubota Y, Kagawa J, Yashiro M, Uehara R, Nakamura Y, et al. Neonatal Kawasaki disease: case report and data from nationwide survey in Japan. Eur J Pediatr. 2014;173(11):1533–6.
Rowley AH, Baker SC, Shulman ST, Garcia FL, Guzman-Cottrill JA, Chou P, et al. Detection of antigen in bronchial epithelium and macrophages in acute Kawasaki disease by use of synthetic antibody. J Infect Dis. 2004;190(4):856–65.
Nomura Y, Yoshinaga M, Masuda K, Takei S, Miyata K. Maternal antibody against toxic shock syndrome toxin-1 may protect infants younger than 6 months of age from developing Kawasaki syndrome. J Infect Dis. 2002;185(11):1677–80.
Singh S, Agarwal S, Bhattad S, Gupta A, Suri D, Rawat A, et al. Kawasaki disease in infants below 6 months: a clinical conundrum? Int J Rheum Dis. 2016;19(9):924–8.
Liu H-C, Lo C-W, Hwang B, Lee P-C. Clinical manifestations vary with different age spectrums in infants with Kawasaki disease. Sci World J. 2012;2012:210382.
Google Scholar
Manlhiot C, Yeung RS, Clarizia NA, Chahal N, McCrindle BW. Kawasaki disease at the extremes of the age spectrum. Pediatrics. 2009;124(3):e410–e5.
Shi L, Li J, Qie D, Hua X, Pan J, Shi X, et al. Clinical manifestations of Kawasaki disease in different age groups: retrospective data from Southwest China. Clin Rheumatol. 2020;39(10):3027–32.
Mastrangelo G, Cimaz R, Calabri GB, Simonini G, Lasagni D, Resti M, et al. Kawasaki disease in infants less than one year of age: an Italian cohort from a single center. BMC Pediatr. 2019;19(1):1–7.
Yeo Y, Kim T, Ha K, Jang G, Lee J, Lee K, et al. Incomplete Kawasaki disease in patients younger than 1 year of age: a possible inherent risk factor. Eur J Pediatr. 2009;168(2):157–62.
Uehara R, Igarashi H, Yashiro M, Nakamura Y, Yanagawa H. Kawasaki disease patients with redness or crust formation at the Bacille Calmette–Guérin inoculation site. Pediatr Infect Dis J. 2010;29(5):430–3.
Kang JH, Hong SJ, Seo IA, Kwak MH, Cho SM, Kim DK, et al. Early detection of Kawasaki disease in infants. Korean Circ J. 2015;45(6):510.
Seo JH, Yu JJ, Ko HK, Choi HS, Kim Y-H, Ko J-K. Diagnosis of incomplete Kawasaki disease in infants based on an inflammation at the Bacille Calmette-Guérin inoculation site. Korean Circ J. 2012;42(12):823.
Zhao QM, Huang M, Huang MR, Chen S, Liu F, Huang GY, et al. Characteristics and trends in diagnosis of Kawasaki disease outside the usual age range. Clin Rheumatol. 2021;40(4):1515–23.
Cai Z, Zuo R, Liu Y. Characteristics of Kawasaki disease in older children. Clin Pediatr. 2011;50(10):952–6.
Advani N, Santoso LA, Sastroasmoro S. Profile of Kawasaki disease in adolescents: is it different. Acta Med Indones. 2019;51(1):42–6.
No SJ, Kim DO, Choi KM, Eun LY. Do predictors of incomplete Kawasaki disease exist for infants? Pediatr Cardiol. 2013;34(2):286–90.
Hwang JY, Lee KY, Rhim JW, Youn YS, Oh JH, Han JW, et al. Assessment of intravenous immunoglobulin non-responders in Kawasaki disease. Arch Dis Child. 2011;96(11):1088–90.
Seo YM, Kang HM, Lee SC, Yu JW, Kil HR, Rhim JW, et al. Clinical implications in laboratory parameter values in acute Kawasaki disease for early diagnosis and proper treatment. Korean J Pediatr. 2018;61(5):160–6.
Download references
Author information
Authors and affiliations.
Mackay Children’s Hospital, Taipei, Taiwan
You can also search for this author in PubMed Google Scholar
Corresponding author
Correspondence to Hsin Chi .
Editor information
Editors and affiliations.
Kawasaki Disease Center, Department of Pediatrics and College of Medicine, Kaohsiung Chang Gung Memorial Hospital and Chang Gung University, Kaohsiung, Taiwan
Ho-Chang Kuo
Rights and permissions
Reprints and permissions
Copyright information
© 2022 The Author(s), under exclusive license to Springer Nature Singapore Pte Ltd.
About this chapter
Chi, H. (2022). Clinical Research of Kawasaki Disease. In: Kuo, HC. (eds) Kawasaki Disease. Springer, Singapore. https://doi.org/10.1007/978-981-19-2944-1_9
Download citation
DOI : https://doi.org/10.1007/978-981-19-2944-1_9
Published : 23 August 2022
Publisher Name : Springer, Singapore
Print ISBN : 978-981-19-2943-4
Online ISBN : 978-981-19-2944-1
eBook Packages : Medicine Medicine (R0)
Share this chapter
Anyone you share the following link with will be able to read this content:
Sorry, a shareable link is not currently available for this article.
Provided by the Springer Nature SharedIt content-sharing initiative
- Publish with us
Policies and ethics
- Find a journal
- Track your research
Thank you for visiting nature.com. You are using a browser version with limited support for CSS. To obtain the best experience, we recommend you use a more up to date browser (or turn off compatibility mode in Internet Explorer). In the meantime, to ensure continued support, we are displaying the site without styles and JavaScript.
- View all journals
- Explore content
- About the journal
- Publish with us
- Sign up for alerts
- Review Article
- Published: 26 May 2020
Kawasaki disease: pathophysiology and insights from mouse models
- Magali Noval Rivas ORCID: orcid.org/0000-0001-5570-8928 1 , 2 &
- Moshe Arditi ORCID: orcid.org/0000-0001-9042-2909 1 , 2 , 3
Nature Reviews Rheumatology volume 16 , pages 391–405 ( 2020 ) Cite this article
45k Accesses
132 Citations
56 Altmetric
Metrics details
- Experimental models of disease
- Immunopathogenesis
- Inflammation
- Vasculitis syndromes
Kawasaki disease is an acute febrile illness and systemic vasculitis of unknown aetiology that predominantly afflicts young children, causes coronary artery aneurysms and can result in long-term cardiovascular sequelae. Kawasaki disease is the leading cause of acquired heart disease among children in the USA. Coronary artery aneurysms develop in some untreated children with Kawasaki disease, leading to ischaemic heart disease and myocardial infarction. Although intravenous immunoglobulin (IVIG) treatment reduces the risk of development of coronary artery aneurysms, some children have IVIG-resistant Kawasaki disease and are at increased risk of developing coronary artery damage. In addition, the lack of specific diagnostic tests and biomarkers for Kawasaki disease make early diagnosis and treatment challenging. The use of experimental mouse models of Kawasaki disease vasculitis has considerably improved our understanding of the pathology of the disease and helped characterize the cellular and molecular immune mechanisms contributing to cardiovascular complications, in turn leading to the development of innovative therapeutic approaches. Here, we outline the pathophysiology of Kawasaki disease and summarize and discuss the progress gained from experimental mouse models and their potential therapeutic translation to human disease.
Kawasaki disease is a childhood systemic vasculitis leading to the development of coronary artery aneurysms; it is the leading cause of acquired heart disease in children in developed countries.
The cause of Kawasaki disease is unknown, although it is suspected to be triggered by an unidentified infectious pathogen in genetically predisposed children.
Kawasaki disease might not be a normal immune response to an unusual environmental stimulus, but rather a genetically determined unusual and uncontrolled immune response to a common stimulus.
Although the aetiological agent in humans is unknown, mouse models of Kawasaki disease vasculitis demonstrate similar pathological features and have substantially accelerated discoveries in the field.
Genetic and transcriptomic analysis of blood samples from patients with Kawasaki disease and experimental evidence generated using mouse models have demonstrated the critical role of IL-1β in the pathogenesis of this disease and the therapeutic potential of targeting this pathway (currently under investigation in clinical trials).
Similar content being viewed by others
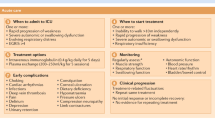
Diagnosis and management of Guillain–Barré syndrome in ten steps
Sonja E. Leonhard, Melissa R. Mandarakas, … Bart C. Jacobs
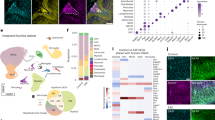
Myeloid cell replacement is neuroprotective in chronic experimental autoimmune encephalomyelitis
Marius Marc-Daniel Mader, Alan Napole, … Marius Wernig
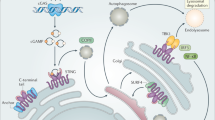
The cGAS–STING pathway as a therapeutic target in inflammatory diseases
Alexiane Decout, Jason D. Katz, … Andrea Ablasser
Introduction
Kawasaki disease is a systemic vasculitis that affects infants and young children 1 , 2 , 3 . Kawasaki disease is now the leading cause of acquired heart disease among children in North America, Europe and Japan 4 , 5 . The cardiovascular sequelae resulting from childhood Kawasaki disease are increasingly recognized to extend into adulthood, and the disease is no longer considered self-limiting 6 , 7 , 8 , 9 . The triggering agents for Kawasaki disease remain unidentified; however, results from our laboratory 10 , 11 and others 12 , 13 are consistent with the interpretation that a conventional antigen is probably responsible. Coronary arteritis and predominantly coronary artery aneurysms (CAAs) occur in up to 30% of untreated children, although this rate is reduced to 5–7% in children treated with high-dose intravenous immunoglobulin (IVIG) 3 , 14 , 15 . IVIG treatment leads to CAA regression in 60–75% of patients with Kawasaki disease 16 , 17 . However, the exact mechanisms by which IVIG reduces the rate of cardiovascular complications are unknown 18 . Up to 15–20% of patients with Kawasaki disease do not respond to IVIG treatment, and these individuals have an increased rate of CAA development 3 , 15 , 19 , 20 , 21 .
Kawasaki disease is associated with infiltration of the coronary artery wall by a broad variety of innate and adaptive immune cells. Immunohistochemical analysis of human post-mortem tissues shows accumulation in the arterial wall of monocytes, macrophages and neutrophils 22 , 23 , and the presence of activated CD8 + T cells 24 as well as IgA + plasma cells 25 , 26 . The release of pro-inflammatory cytokines, such as TNF and IL-1β, by infiltrating immune cells promotes vascular endothelial cell damage and the development of CAAs 27 , 28 .
However, understanding of Kawasaki disease pathophysiology is limited by the low availability of human tissues of the disease, failure to identify specific aetiological agents triggering the disease, and incomplete understanding of the molecular and cellular mechanisms leading to cardiovascular sequelae. Therefore, experimental animal models mimicking the human features of Kawasaki disease and their translational utility have been invaluable to investigation of this disease. In this Review, we discuss advances from human and mouse studies that have contributed to an improved understanding of Kawasaki disease pathophysiology and the cellular and molecular circuitries involved in disease development. We also outline how evidence obtained from experimental mouse models of Kawasaki disease vasculitis has paved the way for the development of new efficient therapeutics to treat human Kawasaki disease.
Aetiological agents
The causative agents initiating the disease have still not been identified >50 years after the first description of Kawasaki disease. However, the trigger is suspected to be of viral origin and to enter the body through the mucosal surfaces in the lung 29 (Fig. 1 ). This hypothesis is supported by the seasonality of Kawasaki disease outbreaks, which is similar to that of other respiratory infections. In Japan, two seasonal peaks have been observed, one in winter and another in summer, whereas in the USA, the incidence peaks are observed during spring and winter 30 . Development of Kawasaki disease is age specific, with children from 6 months to 5 years of age at greatest risk 3 , 30 , 31 , which suggests a protective maternal passive immunity against the causative agent from birth to 6 months of age and the importance of immune system maturation in children ≥6 years of age 29 .
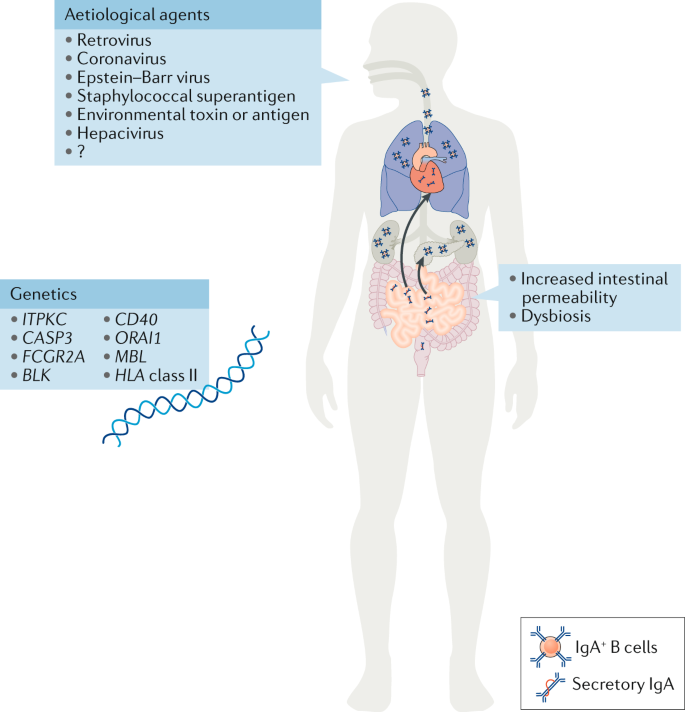
Different aetiological agents, from viruses to environmental toxins, have been proposed as triggering agents for Kawasaki disease; however, none has been corroborated, and the aetiological agent remains unidentified. Increased numbers of IgA + plasma cells have been detected in the pancreas, the kidneys, the coronary artery wall and the respiratory tract of patients with Kawasaki disease. Patients with Kawasaki disease have increased concentrations of secretory IgA in their serum, indicative of defective intestinal barrier function and increased intestinal permeability. Changes in the gut microbiota composition (dysbiosis) have also been suggested to have a role in the development of Kawasaki disease. Single nucleotide polymorphisms in the genes listed have been associated with susceptibility to Kawasaki disease and disease severity. The current understanding is that Kawasaki disease is triggered in genetically predisposed children by a ubiquitous environmental stimulus that typically would not result in an uncontrolled immune response and development of vasculitis.
The clinical features of Kawasaki disease, such as high fever, skin rash and peeling, conjunctivitis and intense release of pro-inflammatory cytokines, are reminiscent of other infectious diseases such as staphylococcal and streptococcal toxic shock syndromes 32 . Some studies have shown that, compared with healthy control individuals, patients with Kawasaki disease have a skewed Vβ T cell repertoire and increased frequencies of circulating Vβ2 + and Vβ8.1 + T cells, leading to the early suggestion that a superantigen toxin might have a role in triggering Kawasaki disease 33 , 34 , 35 . However, similar results were not reproduced in later studies 36 , 37 , leading to the more generalized hypothesis that the development of Kawasaki disease might be triggered by multiple conventional antigens.
Several early studies showed reduced prevalence of antibodies to the Epstein–Barr virus (EBV) capsid antigen in Japanese children with Kawasaki disease compared with age and sex-matched control patients 38 , 39 , 40 , suggesting the involvement of an abnormal immune response to EBV in disease development. However, this difference in EBV antibody seropositivity could not be reproduced in other studies 41 , 42 , 43 . A human coronavirus was detected more frequently in respiratory secretions of patients with Kawasaki disease than in control individuals 44 , although, again, other studies could not replicate this finding 45 , 46 , indicating that the original association might have been coincidental. The possibility that a retrovirus is the triggering agent for Kawasaki disease has also been proposed, owing to detection of retrovirus-specific reverse transcriptase activity in the co-culture supernatant of peripheral blood mononuclear cells (PBMCs) from patients with Kawasaki disease but not controls 47 , 48 . However, this result could not be replicated in later studies 49 , 50 , 51 . A peptide recognized by antibodies produced during the acute phase of Kawasaki disease has been identified in 2020 (ref. 52 ). Although the protein epitopes seem similar to hepaciviruses 53 , further studies are required to determine the specific gene sequence from which this peptide emerges.
Altogether, the absence of consistent and reproducible studies pinpointing a specific aetiological agent suggests that Kawasaki disease is caused not by one but by multiple infectious agents. Acute Kawasaki disease is associated with infiltration of IgA + plasma cells in the respiratory tract, implying that the upper airways act as a portal of entry 25 , 26 . One suggestion is that the triggering agent might be an environmental toxin or antigen transported by wind currents 54 ; however, this possibility cannot be rigorously assessed until precise identification of the aetiological agents is achieved 29 .
SNPs influencing susceptibility
Although Kawasaki disease has been observed around the world and in multiple ethnic groups, geographical differences exist in incidence. The highest incidence is in Asian countries such as Korea and Japan, where it has increased over the past decades and is now 10–20 times more prevalent than in North America and Europe 30 . This increased susceptibility in Asian children, as well as in children with Asian ancestry living in North America 31 , indicates that genetic components predispose to disease susceptibility. In Japan, siblings of children with Kawasaki disease are at increased risk of developing the disease 55 . Single nucleotide polymorphisms (SNPs) in multiple genes have been associated with increased susceptibility to Kawasaki disease (Fig. 1 ); however, mechanisms linking those SNPs with Kawasaki disease progression are not yet well understood and require more investigation.
Calcium signalling pathway
Inositol 1,4,5-trisphosphate 3-kinase C (ITPKC), a kinase that phosphorylates inositol 1,4,5-triphosphate (IP 3 ), is involved in many signalling processes in a wide array of cells. In T cells, IP 3 is released after T cell receptor stimulation, thus increasing levels of intracellular Ca 2+ through IP 3 receptors expressed on the endoplasmic reticulum and leading to nuclear translocation of nuclear factor of activated T cells (NFAT), IL-2 production and T cell activation 56 . By blocking the interaction of IP 3 with its receptor, ITPKC negatively regulates T cell activation. A functional SNP in ITPKC has been associated with increased risk of coronary artery lesions in Taiwanese 57 , Japanese and American patients with Kawasaki disease 58 . Mechanistically, this ITPKC polymorphism might directly contribute to T cell hyperactivity, and more importantly, it might promote NLRP3 inflammasome activation and increase production of IL-1β and IL-18 (ref. 59 ). ORAI1 is a membrane-bound Ca 2+ channel protein encoded by ORAI1 that is involved in the Ca 2+ –calcineurin–NFAT signalling pathway. Although no significant association between ORAI1 polymorphisms and Kawasaki disease susceptibility or IVIG treatment response was initially reported in the Taiwanese population 60 , an SNP in exon 2 of ORAI1 is associated with Kawasaki disease susceptibility in the Japanese population 61 , and interestingly this SNP is 20 times more frequent in the general Japanese population than in the general European population 61 . Another SNP in SLC8A1 , which encodes the Na + –Ca 2+ exchanger, is also associated with susceptibility to Kawasaki disease and aneurysm formation 62 , further highlighting the critical role of calcium signalling pathways in development of Kawasaki disease. Crucially, the Ca 2+ –NFAT signalling pathway is also key to intracellular Ca 2+ regulation and therefore to NLRP3 inflammasome activation and IL-1β production 63 , 64 .
CD40 ligand
CD40 ligand (CD40L) is a protein expressed by a large array of cells including activated T cells, B cells, monocytes and platelets. CD40L receptor, CD40, is expressed by antigen-presenting cells as well as endothelial cells 65 . CD40 engagement is associated with cell survival, activation, proliferation and cytokine production 65 . Compared with control patients with other febrile illnesses, patients with Kawasaki disease have increased CD40L expression on CD4 + T cells and platelets, which correlates with increased development of coronary artery lesions and is reduced by IVIG treatment 66 . An SNP in CD40L has been reported in Japanese patients with Kawasaki disease and is more frequent in male patients with coronary artery lesions than in female patients 67 . This polymorphism was not observed in a cohort of Taiwanese patients 68 ; however, another SNP in the CD40 gene has been reported in an independent cohort of Taiwanese patients and is associated with increased susceptibility to Kawasaki disease and development of coronary artery lesions 69 . These results indicate a role of the CD40–CD40L pathway in the development and severity of Kawasaki disease and highlight this pathway as a potential therapeutic target.
Mannose-binding lectin
Mannose-binding lectin (MBL), a pattern recognition molecule of the innate immune system, binds the surface of pathogenic organisms and activates the complement pathway 70 . A polymorphism in MBL2 was found to be an age-related risk factor for development of coronary artery lesions in a Dutch cohort of patients 71 , 72 . Another study in a cohort of Japanese patients with Kawasaki disease showed that codon 54 variants in MBL2 are significantly associated with susceptibility to Kawasaki disease 73 . Interestingly, in the Candida albicans water-soluble fraction (CAWS) mouse model of Kawasaki disease vasculitis, MBL-A and MBL-C deposition are observed in the aortic root, suggesting involvement of the MBL-dependent lectin pathway in this experimental model 74 . However, further studies are required to understand the pathogenic roles of those two proteins as well as their potential as therapeutic targets.
Fcγ receptors
Polymorphisms in genes encoding the receptors for the Fc portion of immunoglobulins, Fcγ receptors (FcγRs), have been associated with the development of autoimmune and infectious diseases 75 , 76 , 77 . As Kawasaki disease is considered an infectious disorder, several studies have investigated the potential association of FcγR SNPs with Kawasaki disease susceptibility and the development of coronary artery lesions. In a cohort of Dutch patients, no difference in FcγR SNP distribution was observed between healthy individuals and patients with Kawasaki disease, and no association was noted between SNPs in FcγR genes and Kawasaki disease susceptibility 78 . However, a later study with >2,000 patients with Kawasaki disease and 9,000 control patients from multiple independent cohorts across different populations highlighted a Kawasaki disease-associated polymorphism in the FCGR2A locus, which encodes FcγRIIA (CD32a), a member of the family of IgG receptors 79 . This polymorphism has important implications as the standard of care for Kawasaki disease is IVIG, a pool of plasma IgG that interacts with FcγRs on immune cells. Interestingly, 15–20% of patients with Kawasaki disease have IVIG-resistant disease and require another round of IVIG treatment or the use of adjunctive therapies 15 , 19 , 20 , 80 . The exact mechanisms by which IVIG mediates its therapeutic effect and how IVIG resistance develops remain unknown, and the potential involvement of this FcγRIIA polymorphism in IVIG resistance requires further investigation.
Pathophysiology of Kawasaki disease
The innate immune response.
The immune response associated with Kawasaki disease is complex and involves the activation and infiltration of the coronary artery wall by both innate and adaptive immune cells (Fig. 2 ). On the basis of studies of post-mortem tissue from patients with Kawasaki disease, Kawasaki disease vascular pathology has been classified into three sequential linked pathological processes 81 . Necrotizing arteritis develops in the first 2 weeks of the disease and is associated with neutrophilic infiltrations, which gradually destroy the coronary artery intima, media and some portions of the adventitia. Alarmins from the S100 protein family, which are present in the cytoplasm of neutrophils, monocytes and macrophages 82 , also participate in this inflammatory process. Concentrations of circulating S100A8/A9 heterodimers (calprotectin) and S100A12 are substantially higher in patients with Kawasaki disease during the acute phase than in control patients with other febrile illnesses and decline after IVIG treatment 83 , 84 , 85 . After the acute phase of Kawasaki disease, plasma concentrations of S100A8/A9 heterodimers only remain elevated in patients with giant CAAs 84 , highlighting its potential utility as a biomarker to monitor long-term persistence of inflammation. S100A12 also contributes to the acute inflammatory response by directly stimulating monocytes to produce IL-1β, which in turn activates coronary endothelial cells 85 . Necrotizing arteritis might result in the formation of CAAs and is followed by two other processes, subacute or chronic vasculitis and luminal myofibroblast proliferation (LMP), which occur simultaneously and might be observed for months to years after disease onset 81 . The inflammatory infiltrates are composed of CD8 + T cells, IgA + plasma cells, eosinophils and macrophages, which release pro-inflammatory cytokines contributing to cardiovascular pathology. Meanwhile, myofibroblasts, mainly derived from smooth muscle cells, and their matrix products progressively obstruct the coronary lumen 81 (Fig. 2 ). Persistent subacute and chronic vasculitis and LMP can lead to stenosis and thrombosis after acute illness 6 , 9 .
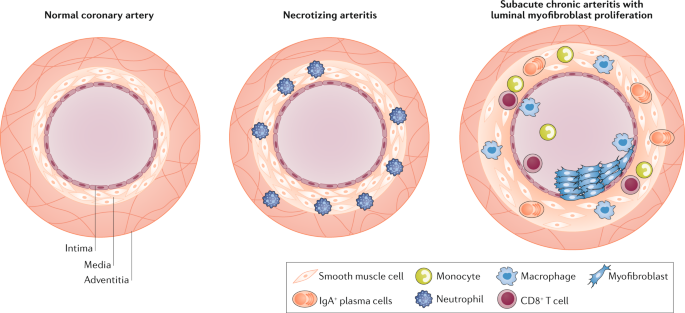
The normal coronary artery is composed of three general layers: the tunica intima, tunica media and tunica adventitia. The intima is mainly composed of endothelial cells, the media of smooth muscle cells and the adventitia of loose connective tissue. In Kawasaki disease, necrotizing arteritis develops in the first 2 weeks of the disease and is associated with neutrophilic infiltration, which gradually destroys the intima, media and some portions of the adventitia of the coronary artery. CD8 + T cells, IgA + plasma cells, monocytes and macrophages compose the inflammatory infiltrate during subacute chronic arteritis. These cells release pro-inflammatory cytokines such as IL-1β and TNF, which contribute to luminal myofibroblast proliferation, in which myofibroblasts, mainly derived from smooth muscle cells, and their matrix products progressively obstruct the coronary lumen.
Matrix metalloproteinases
Matrix metalloproteinases (MMPs; zinc-dependent endopeptidases that degrade extracellular matrix components) are known to have an important role in both inflammation and tissue remodelling processes 86 . Increased expression and activity of a diverse set of MMPs has been demonstrated in acute Kawasaki disease 87 , 88 , 89 . The expression levels of MMP3 and MMP9, both known to mediate vascular smooth muscle cell migration and neointimal formation 90 , are increased in patients with Kawasaki disease 91 , and the circulating levels of these MMPs correlate with the development of CAAs in these patients 92 . MMP3 SNPs are also associated with the development of CAAs 88 , and this protease is considered to be a driving factor allowing IL-1-induced signalling to lead to migration of vascular smooth muscle cells and their transition to proliferating myofibroblasts 93 , 94 , 95 . Whereas MMP9 has been studied and implicated in elastin breakdown in the Lactobacillus casei cell wall extract (LCWE)-induced Kawasaki disease mouse model 96 , 97 , information about the role of MMP3 in this mouse model is lacking.
MicroRNAs (miRNAs; a class of small non-coding RNAs that regulate mRNA expression) are emerging as critical gene regulators in a host of cellular processes, including inflammation 98 . Of human coding genes, 60–70% are estimated to be regulated by miRNAs 99 . Several studies attempting to discover Kawasaki disease biomarkers have found that the miRNA profiles of serum exosome or coronary artery tissues are associated with acute Kawasaki disease 100 , 101 , 102 , 103 , 104 . These miRNAs include miR-23a 100 , 101 , 102 , 103 , miR-27b 100 , miR-223 (refs 100 , 101 , 102 , 103 ) and miR-145 (ref. 103 ). These miRNAs might provide clues as to the molecular mechanisms involved in the development of the cardiovascular lesions associated with Kawasaki disease. For example, miR-145 is highly expressed in vascular smooth muscle cells and has been reported to promote their switching to neointimal proliferating cells 105 , 106 and to regulate the transforming growth factor-β signalling pathway 103 . Increased levels of miR-23a contribute to cardiomyocyte apoptosis and may promote inflammatory responses by blocking macrophage autophagy activity 107 , 108 . However, improved understanding and characterization of the molecular and cellular mechanisms underlying the different roles of miRs during Kawasaki disease require further studies with animal models.
Myocarditis
Most attention in Kawasaki disease research and clinical practice has focused on the development of CAAs and long-term complications of coronary artery stenosis and ischaemia 109 . However, the subacute and chronic inflammation of Kawasaki disease is also associated with the development of myocarditis 3 , 6 , 110 , 111 , 112 . Myocarditis has been described as the ‘hidden face of the moon’ in Kawasaki disease 110 . Reports indicate that myocarditis occurs frequently during acute Kawasaki disease 111 , and serial myocardial biopsy studies have documented that histological myocarditis develops in the majority of patients with Kawasaki disease, even in the absence of coronary aneurysms 113 , 114 . More recent data indicate that myocardial inflammation can be documented in 50–70% of patients using gallium citrate ( 67 Ga) scans and technetium-99 ( 99m Tc)-labelled white blood cell scans 115 . Another study has shown that myocardial inflammatory changes and myocardial oedema in Kawasaki disease occur even before coronary artery abnormalities and without concurrent ischaemic damage 112 .
Myocarditis in Kawasaki disease tends to develop early, and acute left ventricular dysfunction is generally transient and responds readily to anti-inflammatory treatment 116 . However, Kawasaki disease myocarditis might be associated with fatal arrhythmias in infants, and in certain cases might lead to long-term complications including myocardial fibrosis 81 , 117 . Therefore, myocarditis during Kawasaki disease and its potential consequences deserve serious investigation, and long-term studies into late adulthood are needed.
Complement and immune complexes
Kawasaki disease affects small and medium sized vessels, particularly the coronary arteries; however, dilatations and aneurysms can occur systemically, including in the axillary, subclavian, brachial, renal and iliac arteries as well as the abdominal aorta 23 , 118 , 119 , 120 . Post-mortem findings have revealed that 73% of patients with Kawasaki disease have renal artery involvement and acute kidney injury 121 involving glomerulonephritis with intracapillary changes and deposition of immune complex composed of IgA and complement component 3 (C3) 22 , 122 , 123 . These findings are comparable to those in two other human vasculitis diseases, IgA vasculitis (IgAV) and IgA nephropathy (IgAN), which are similarly characterized by IgA immune complexes with C3 deposition in kidney glomeruli (see below). Increased concentrations of circulating IgA and secretory IgA (sIgA) have been reported in the serum of children with Kawasaki disease during the acute phase 124 . IgA + plasma cells are present in the coronary artery wall and in non-vascular tissues, such as the kidney, trachea and pancreas of patients with Kawasaki disease 25 , 26 . This IgA response is oligoclonal, seems to be antigen driven and might be caused by Kawasaki disease-triggering agents 125 , 126 .
The IL-1 signalling pathway
Evidence from mouse models of Kawasaki disease 11 , 127 , 128 , as well as transcriptome analysis performed on whole blood of patients with Kawasaki disease during the acute or convalescent phase 129 , 130 , demonstrate the involvement of innate immune cells and inflammasome overactivation throughout the acute phase of the disease. In vitro cultured PBMCs isolated from patients with Kawasaki disease spontaneously release IL-1β into the supernatant, and this process is substantially reduced after IVIG treatment 28 . Serum concentrations of both IL-1β and IL-18 are also higher in children with acute Kawasaki disease than in control patients with other febrile illnesses, and markedly decrease during the convalescent phase 59 , supporting the concept of activation of the NLRP3 inflammasome complex. Similarly, IL-1 and NLRP3-related gene transcripts are upregulated in PBMCs from patients with acute Kawasaki disease and are decreased during the convalescent phase of the disease 59 , and an IL1B -gene-related signature is associated with acute phase disease and IVIG resistance 130 . Furthermore, a study has shown that differential expression of IL-1β and related signalling genes might have a role in mediating the sex-based differences seen in patients with Kawasaki disease 131 . In the LCWE mouse model of Kawasaki disease, the activation of caspase 1, IL-1α and IL-1β is key to the development of coronary arteritis, aneurysms, myocarditis and abdominal aorta aneurysms 127 , 128 , 132 . IL-1 has the capacity to expand and promote the differentiation of antigen-specific CD8 + T cells 133 , and indeed the frequencies of circulating CD4 + and CD8 + T cells are increased in patients with Kawasaki disease 134 . Infiltrations of mature dendritic cells as well as activated cytotoxic CD8 + T cells have been reported in arterial layers of coronary aneurysms 24 , 135 . Therefore, blocking the NLRP3–IL-1β pathway seems to be a valid therapeutic option in Kawasaki disease.
Role of the gastrointestinal tract
Intestinal permeability.
The intestinal barrier has a critical role in maintaining intestinal homeostasis and health by preventing harmful organisms and luminal antigens from entering the circulation. A dysfunctional intestinal barrier, characterized by increased intestinal permeability, is recognized as a pathogenic factor in many inflammatory diseases 136 . In Kawasaki disease, abdominal pain, diarrhoea and vomiting are often observed at the onset of acute illness, affecting up to 60% of diagnosed patients and indicating that the gastrointestinal tract is also affected 4 , 137 , 138 , 139 , 140 . A multicentre study of >300 patients revealed that gastrointestinal manifestations at onset of disease complicate diagnosis, delay adequate treatment and correlate with IVIG resistance and severity of CAAs 141 . Immunohistochemical studies have revealed higher numbers of activated CD4 + T cells and macrophages along with lower numbers of CD8 + T cells in the jejunum lamina propria in patients with Kawasaki disease than in control patients with diarrhoea from cows’ milk protein intolerance 142 . However, these cellular abnormalities are specific to the acute phase of the disease and return to normal during the convalescent phase 142 . IgA + plasma cells have also been observed in a variety of different vascular and non-vascular tissues in patients with Kawasaki disease 26 , and patients with Kawasaki disease also have increased concentrations of sIgA, which is produced at the intestinal mucosal surface, in their serum 124 . These studies indicate that the gastrointestinal tract is affected during Kawasaki disease and that mucosal immune activation might compensate and protect from defective intestinal barriers.
The role of gut-related immunity in the induction of inflammation in organ systems distant from the gut has been the subject of intensive investigation. We have observed increased intestinal permeability and a dysregulated intestinal immune response characterized by increased numbers of IgA + B cells in the Peyer’s patches in the LCWE-induced mouse model of Kawasaki disease 143 (Fig. 3 ). In this model, the excessive IL-1β release associated with LCWE injection acts on intestinal epithelial cells to open tight junctions, and administration of IVIG or pharmacological agents that block intestinal permeability significantly reduces disease development 143 . Altogether, these observations link increased intestinal permeability and defective intestinal barrier function with systemic IL-1β release in Kawasaki disease.
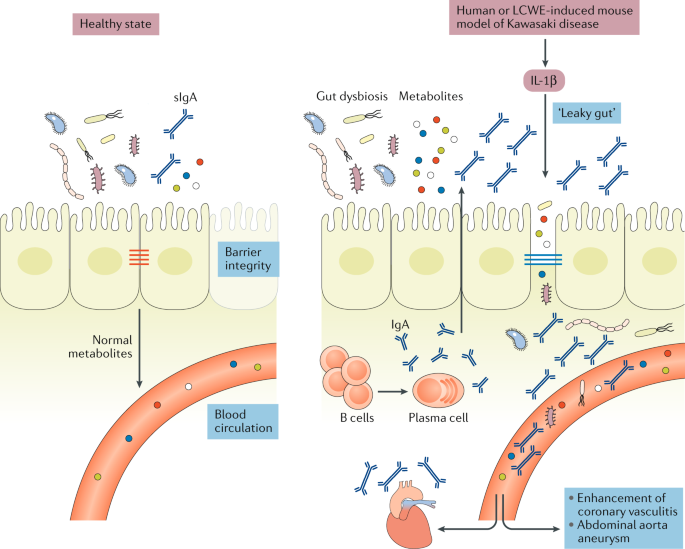
In healthy individuals, intestinal epithelial cells are sealed together by intestinal tight junctions, and the intestinal epithelium acts as a barrier that prevents the passage of commensal bacteria and pathogens while permitting intercellular flux of ions, molecules and metabolites. Lactobacillus casei cell wall extract (LCWE)-induced Kawasaki disease vasculitis and human Kawasaki disease are associated with increased IL-1β production, which leads to decreased expression of intestinal tight junctions, resulting in increased intestinal permeability. Differences in intestinal microbiota composition have been observed in patients with Kawasaki disease, and intestinal dysbiosis might contribute further to the inflammatory process. LCWE injection is also associated with a dysregulated intestinal immune response characterized by increased numbers of IgA + B cells in the gastrointestinal tract and elevated secretory IgA (sIgA) concentrations. Intestinal barrier dysfunction results in sIgA leakage to the systemic circulation and pathogenic IgA–C3 immune complex deposition in the vascular tissues.
The intestinal microbiome
Despite the strong connection between the intestinal microbiome and development of cardiovascular diseases 144 , 145 , only a few studies have investigated the role of the intestinal microbiome during development of Kawasaki disease or treatment resistance. Microbiological culture-based methods demonstrated that, compared with healthy control individuals, patients with Kawasaki disease have a different intestinal microbiota composition characterized by a lower incidence of the Lactobacillus genus 146 , 147 and increased Streptococcus and Staphylococcus 148 species. Lactobacilli have been reported to prevent diarrhoeal disorders 149 , 150 and to improve intestinal barrier function by increasing the expression of intestinal tight junctions 151 , 152 , enhancing the intestinal mucus layer 153 and modulating the intestinal microbiota composition 154 . Lactobacilli have also been shown to boost innate and immune functions against a variety of bacterial infections 155 , 156 , 157 , and their disappearance during acute Kawasaki disease might lead to the blooming of other bacterial pathogens, which might further promote intestinal barrier dysfunction and inflammation. Intriguingly, a retrospective study of 364 patients with Kawasaki disease showed that children who received microbiome-altering antibiotics in the week before Kawasaki disease diagnosis were substantially more likely to have IVIG-resistant disease than those who did not receive antibiotics 158 . Antibiotics alter the abundance, taxonomic richness and diversity of the bacterial 159 , 160 as well as fungal 161 intestinal microbiome, and those alterations might persist from weeks to years after treatment discontinuation 159 , 160 , 162 . A longitudinal metagenomic study of faecal samples derived from patients with Kawasaki disease showed a marked increase of five Streptococcus spp. during the acute phase of Kawasaki disease 163 ; however, all patients in that study were treated with antibiotics in the early stage of disease, therefore this observation might be reflective of antibiotic-induced dysbiosis and not Kawasaki disease itself. Nonetheless, how this intestinal dysbiosis occurs and how its effect on intestinal permeability affects the development of cardiovascular lesions during Kawasaki disease vasculitis remains unknown and under-appreciated.
Link with IgA vasculitis
IgAV, or Henoch-Schönlein purpura, is an IgA-mediated necrotizing vasculitis resulting in fibrinoid destruction of the affected small vessels. Renal involvement, characterized by IgA deposition in the kidney glomeruli, is also observed in IgAV 164 . IgAV nephritis is closely related to another glomerular disease, IgAN, wherein accumulation and deposition of IgA and IgA immune complexes in the kidney glomerular mesangium drive glomerular inflammation 165 . As IgA is mainly found at mucosal surfaces, a ‘gut–kidney axis’, influenced by a mix of genetic, microbial and dietary factors, has been suggested to be involved in the development of both IgAN 166 and IgAV in paediatric and adult patients 167 . We have demonstrated that the LCWE-induced mouse model of Kawasaki disease vasculitis is associated with the deposition of IgA and IgA–C3 immune complexes in vascular tissues, such as the inflamed coronary artery and abdominal aorta 143 . Deposited IgA and IgA–C3 immune complexes might result in overactivation of the immune cells present in the cardiovascular lesions and subsequent amplification of inflammation 143 . Substantial evidence indicates that immune complexes might promote vascular damage during human Kawasaki disease through the activation and aggregation of platelets, the release of vasoactive mediators, and the subsequent recruitment of neutrophils and leukocytes to the site of inflammation (reviewed elsewhere 168 ).
Interestingly, we have also observed IgA and C3 deposition in the kidney glomeruli of LCWE-injected mice developing Kawasaki disease 143 , and immune complex-mediated nephropathy has also been observed in Kawasaki disease 123 . However, to date IgA deposition has not been reported in CAAs of patients with Kawasaki disease. Given that availability of human tissue samples is limited, and those that are available are usually collected at the end stage of the disease, they might not be representative of active Kawasaki disease pathological features, and further studies are warranted. Like Kawasaki disease, IgAV develops mostly in children, affects males more than females, is more predominant in Asian countries such as Japan and Korea, and is also associated with abdominal pain, diarrhoea, skin rash and IgA deposition in the affected small vessels 169 . IgAN also shares pathological features with Kawasaki disease, such as increased intestinal permeability, low to moderate intestinal inflammation associated with activation of inflammatory cells in the small intestinal mucosa and colocalization of sIgA-complement in the glomerular mesangium 165 , 170 . Moreover, a polymorphism in the promoter of the lipopolysaccharide (LPS) receptor CD14 (CD14/159) is associated with coronary artery abnormalities in patients with Kawasaki disease 171 and has been linked to progression of IgAN to more severe renal disease 172 . IL-1β has a key pathogenic role during Kawasaki disease and also seems to be implicated in renal complications related to IgAV 173 and IgAN 174 . Altogether, given that Kawasaki disease shares clinical features and pathological mechanisms with both IgAV and IgAN, it is possible that Kawasaki disease is a form of IgAV. Similarly, treatments that have shown efficacy in Kawasaki disease, such as anakinra and IVIG, might be suitable and useful for treating IgAV 175 and IgAN.
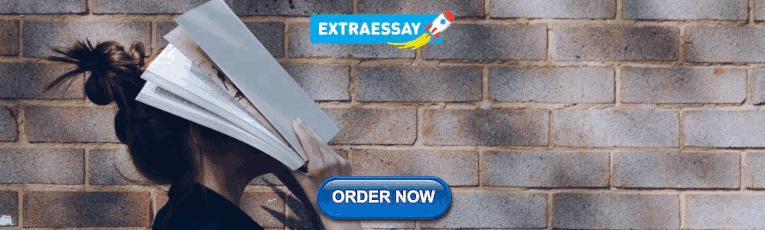
Mouse models of Kawasaki disease
The lack of identification of specific aetiological agents and incomplete understanding of the molecular mechanisms involved in Kawasaki disease cardiovascular pathology have delayed the development of targeted and effective treatment options for this disease. In addition, the limited availability of tissue samples from patients with Kawasaki disease has considerably impeded progress in understanding the pathogenesis of the disease, making the availability of relevant animal models of Kawasaki disease extremely valuable. Kawasaki disease vasculitis can be induced in mice by injection of cell wall components from L. casei 176 , C. albicans 177 or nucleotide-binding oligomerization domain containing 1 (Nod1) ligand 178 (Table 1 ). These mouse models of Kawasaki disease have accelerated research and have enhanced understanding of the pathogenesis of this disease. However, no animal model perfectly recapitulates human disease. Particularly in the context of Kawasaki disease, given that the aetiology remains unknown, researchers must exercise caution in interpreting results based on experimental models and confirm findings in patient cohorts. Nevertheless, even though the extrapolation of preclinical mouse data to humans is far from straightforward, mouse models are still invaluable tools to study certain pathological aspects of human inflammatory diseases and gain mechanistic insights.
The LCWE mouse model
L. casei is a Gram-positive bacteria that colonizes the gastrointestinal and urogenital tracts of both human and animals 179 . More than 35 years ago, Lehman et al. 180 demonstrated that a single intraperitoneal injection of LCWE induces a dose-dependent and chronic polyarthritis in rats. However, when injected into mice, LCWE induces instead a focal coronary arteritis 176 . How and which element of LCWE triggers Kawasaki disease vasculitis is unknown. LCWE is mainly composed of peptidoglycans, contains high levels of rhamnose and is resistant to lysozyme degradation 176 .
The cardiovascular lesions induced in mice by LCWE are histologically similar to those observed in human disease. LCWE-induced Kawasaki disease vasculitis is characterized by infiltration of inflammatory cells in the aortic root, development of necrotizing arteritis in the coronary artery followed by luminal obstruction due to LMP that can lead to complete coronary artery stenosis 181 , recapitulating the three pathological processes of human Kawasaki disease described above (Fig. 4a – d ). In children with Kawasaki disease, thrombotic occlusion of the inflamed coronary artery leads to ischaemic heart disease 23 , 120 , and similarly, occluding organizing thrombus in the coronary artery can be observed in LCWE-injected mice (Fig. 4e ). Acute myocarditis and chronic scarring of the coronary arteries with the formation of stenotic fragments are also observed in LCWE-induced Kawasaki disease vasculitis (Fig. 4f ), even long after the acute phase 182 , which is similar to the fibrotic lesions that might lead children with Kawasaki disease to develop long-term cardiovascular sequelae in adulthood 8 , 9 . MRI and echocardiography in LCWE-injected mice demonstrate the presence of electrocardiographic changes (as observed in human Kawasaki disease) and myocardial dysfunction, which are responsive to anakinra therapy 183 , 184 .
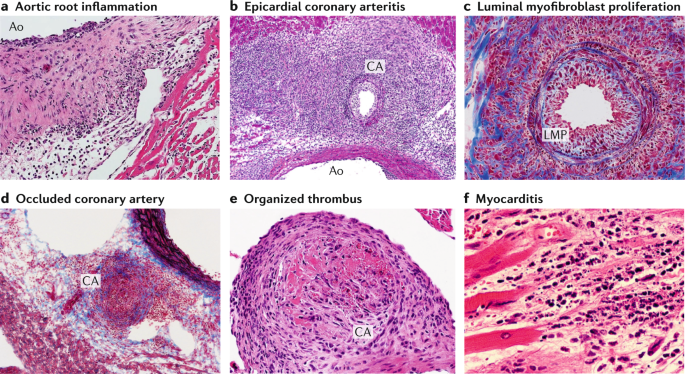
Wild-type mice underwent intraperitoneal injection with Lactobacillus casei cell wall extract (LCWE), and heart tissues were harvested 2 weeks later. Haematoxylin and eosin (H&E) and trichrome staining were performed on heart sections. a | Inflammatory cell infiltration in the aortic route (H&E staining; ×40). b | Arteritis development in epicardial muscular coronary artery (H&E staining; ×20). c | Luminal myofibroblast proliferation (LMP) and non-specific neointimal proliferation injury to the arterial wall (trichrome staining; ×200). d | Complete occlusion of the coronary artery by LMP (trichrome staining; ×20). e | Organized thrombus in the coronary artery (H&E staining; ×200). f | Myocarditis (H&E staining; ×200). Ao; aorta, CA; coronary artery.
The LCWE-induced Kawasaki disease vasculitis in mice is dependent on intact TLR2 and MyD88 signalling and the subsequent release of pro-inflammatory cytokines, including IL-1β, IL-6 and TNF 10 . Genetic depletion of the TNF receptor or pharmacological blockade of the TNF signalling pathway (with infliximab (monoclonal antibodies to TNF) or etanercept (soluble TNF receptors)) protects mice from LCWE-induced Kawasaki disease vasculitis 132 , 185 . This model is also T cell dependent, as Rag1 –/– mice develop fewer cardiovascular lesions 11 . CD8 + T cells are specifically required for LCWE-induced Kawasaki disease vasculitis as treatment of LCWE-injected mice with an anti-CD8-depleting antibody prevents the development of vasculitis 181 . This finding correlates with human disease, in which infiltrations of CD3 + T cells 135 , and particularly CD8 + T cells, are detected in the CAAs 24 . The LCWE model has also confirmed the importance of the ITPKC pathway in Kawasaki disease development and demonstrated that ITPKC deficiency is associated with increased Ca 2+ flux and levels of IL-1β in vitro 59 . Interestingly, the relatively mild development of coronary arteritis in LCWE-injected CBA/N mice — which are characterized by a defective B cell maturation process and poor humoral immune responses — suggests that the humoral immune response might participate in amplification of the disease 186 . IgA + plasma cells infiltrate vascular and non-vascular tissues during the acute phase of Kawasaki disease 25 , 26 , resulting in the development of an oligoclonal IgA response in the coronary artery 125 , 126 . Interestingly, we have observed increased numbers of IgA + plasmablasts in the spleen, Peyer’s patches and abdominal aorta draining lymph nodes of LCWE-injected mice, as well as increased concentrations of circulating IgA and IgA deposition in heart tissues, abdominal aorta and kidney glomureli 143 .
Mouse models also provide a useful opportunity to evaluate the efficacy of therapeutic regimens on the development and healing of cardiovascular lesions. When given up to 5 days after LCWE injection, IVIG substantially decreases the severity of cardiovascular lesions in mice 187 , mirroring the effects of IVIG treatment in humans. As described above, IL-1β signalling is higher in patients with Kawasaki disease than in age-matched control patients with other febrile illnesses 91 , 188 , and studies using the LCWE model helped lead to the discovery of the importance of this pathway in the pathogenesis of the disease and the therapeutic potential of IL-1 blockade. Depletion of macrophages or blocking the IL-1 pathway either genetically using IL1R −/− , IL1α −/− or IL1β −/− mice or with antibodies targeting IL-1α or IL-1β, or anakinra (IL1Ra), strongly reduces cardiovascular lesion development as well as myocardial dysfunction in LCWE-injected mice 128 , 132 , 184 .
The CAWS mouse model
C. albicans is a harmless commensal fungus normally present in the human gastrointestinal tract that can transition into a pathogen capable of inducing inflammation in immune-impaired hosts. In 1979, Murata demonstrated that an alkaline extract made from C. albicans isolated from faeces from a patient with Kawasaki disease induced coronary arteritis in mice 177 . CAWS is composed of polysaccharides, mainly β-glucans and α-mannan proteins of the yeast cell wall 189 , and needs to be injected intraperitoneally for five consecutive days in the first week of the disease to induce vasculitis in the aortic valves and the coronary arteries 189 , 190 . In this model, recognition of α-mannan proteins by the dectin-2 receptor seems to be essential, as CAWS-injected Dectin-2 −/− mice do not develop vasculitis 191 .
The CAWS model shares some histological similarities with human Kawasaki disease pathology in that inflammation affects both the aortic root and the proximal region of the coronary arteries 190 . Inflammation can also affect non-coronary artery sites in 25% of CAWS-injected mice and can be observed in the lymph nodes, the kidneys and the liver 190 , 192 . CAWS-induced coronary artery lesions resemble those of human Kawasaki disease and are typically proliferative, granulomatous and characterized by intimal thickening with destruction of the elastic lamina and media 190 . Echocardiography in CAWS-injected mice indicates a marked decrease of cardiac function, which can be restored by IL-10 supplementation 193 . IL-10 is a potent anti-inflammatory cytokine that might improve the outcome of CAWS-induced vasculitis by inhibiting the release of pro-inflammatory mediators, such as TNF and IL-1β, from tissue-infiltrating innate immune cells 194 . Interestingly, CAWS-induced Kawasaki disease vasculitis is also strain dependent, as CAWS injections lead to a high incidence of vasculitis in CD-1, C3H/HeN, DBA/2 and C57BL/6N mice, but the CBA/JN strain is resistant to coronary arteritis 190 , 195 . The DBA/2 strain is the most sensitive, with the highest mortality rate resulting from a more intense coronary arteritis 195 . The sensitivity of DBA/2 mice is associated with increased production of the pro-inflammatory cytokines TNF, IL-6 and IFNγ 195 , 196 , whereas resistance of CBA/JN mice is explained by increased levels of IL-10 production in that strain 197 .
Despite the presence of T cell and B cell infiltration in the inflamed coronary artery, mice lacking T cells still develop moderate to typical cardiac inflammation, indicating that T cells might not be required in the development of Kawasaki disease vasculitis in this particular model 198 , 199 . Absence of both T cells and B cells in Rag1 −/− mice leads to lower incidence of CAWS-induced Kawasaki disease vasculitis; reconstitution of Rag1 −/− mice with wild-type, but not CCR2 −/− , T cells and B cells restores cardiovascular lesions, suggesting roles for both T cells and B cells and the modulation of disease development by CCR2 expression 200 . The innate immune response also participates in vasculitis development; resident macrophages recognize the CAWS antigens through the dectin-2 receptor, leading to their activation, release of CCL2, and recruitment of neutrophils and inflammatory monocytes producing IL-1β in the aortic root 201 .
CAWS-induced vasculitis is also associated with the rapid production of granulocyte–monocyte colony-stimulating factor in the heart, which subsequently drives inflammatory myocarditis by activating tissue macrophages and promoting recruitment of neutrophils and monocytes 199 . TNF is also produced during the acute phase of CAWS-induced Kawasaki disease vasculitis and is essential for the development of acute myocarditis, as TNF receptor-deficient mice are protected from the development of CAWS vasculitis 202 . IVIG administration substantially reduces CAWS-induced heart vessel inflammation 203 . Like the LCWE model, the CAWS model is also dependent on the IL-1 pathway, as IL1R −/− , IL1β −/− , Asc −/− and Nlrp3 −/− mice are protected from induction of vasculitis, and treatment with anti-IL-1β agents substantially attenuates CAWS vasculitis 202 , 204 , 205 .
The Nod1 ligand mouse model
Endothelial cells are equipped to sense microbial components through Toll-like receptors and nucleotide-binding oligomerization domain-containing protein like receptors. Subcutaneous injection or oral delivery of FK565, a specific synthetic Nod1 ligand, in mice primed with LPS results in a diffuse cellular inflammation of the aortic root and transmural infiltration of inflammatory cells in the coronary artery wall 178 , 206 . Other arteries, such as the iliac and renal arteries, also show signs of inflammation associated with a thickening of the intima 206 .
The mechanisms by which FK565 induces coronary arteritis in mice remain unknown. When administered orally, FK565 does not induce intestinal mucosa inflammation, but specifically activates vascular cells to produce a diverse array of pro-inflammatory cytokines, including IL-1β 206 , and chemokines such as CCL2, resulting in the recruitment of inflammatory cells in the tissues 178 . This model seems to be independent of T cells, B cells and natural killer T cells, as LPS-primed Rag-1 −/− mice still develop aortitis and coronary arteritis after FK565 injection 207 . The inflammatory infiltrates observed around the inflamed aortic root and coronary arteries mainly comprise neutrophils and CD11c + cardiac macrophages; their specific depletion considerably reduces the development of FK565-induced Kawasaki disease vasculitis 178 , 207 . The concentration of circulating IL-1β is substantially increased in the serum of FK565-injected mice compared with control or CAWS-injected animals, and higher IL-1β levels correlate with a larger inflammation area 206 . However, specific studies further investigating the role of IL-1β in this model are needed.
Treatment of Kawasaki disease
Traditional and novel therapies in humans.
The current standard of care for Kawasaki disease is the use of high-dose IVIG together with aspirin. If given during the first 10 days of the disease, IVIG reduces the risk of development of coronary arteritis and aneurysms from about 30% to 5–7% 14 , 15 . The mechanisms by which IVIG treatment reduces the inflammatory responses are still unknown; however, IVIG is suspected to have a wide spectrum of action targeting multiple arms of the immune response 18 . IVIG has been shown to inhibit IL-1β production from in vitro stimulated macrophages and to stimulate the production of IL-1Ra 208 , 209 . During Kawasaki disease, IVIG reduces production of inflammatory cytokines and chemokines, and decreases the activation and number of circulating neutrophils, monocytes, macrophages and activated T cells by saturating Fc receptors 18 . The majority of patients with Kawasaki disease who are treated with IVIG improve and do not develop coronary artery damage; however, up to 20% of children with Kawasaki disease do not respond to treatment or have fever recurrence after initial IVIG treatment, and these patients are at the highest risk of developing coronary artery lesions 3 , 20 , 210 .
The involvement of pro-inflammatory cytokines in the acute phase of Kawasaki disease suggests that combinational therapy, composed of IVIG associated with TNF inhibitors, steroids, calcineurin inhibitors or anakinra, might be useful to treat patients with IVIG-resistant disease. The use of TNF inhibitors in combination with IVIG has had mixed results thus far. Infliximab was associated with decreased fever duration and reduced markers of inflammation (C-reactive protein and neutrophil counts), suggesting a possible improvement of coronary artery outcomes 211 ; however, etanercept treatment resulted in a substantial reduction in IVIG resistance only in patients >1 year old 212 .
An important area of research is the use of biomarkers to predict IVIG resistance in Kawasaki disease. The Kobayashi scoring system, based on a combination of laboratory test results (for example, C-reactive protein levels, neutrophil percentages, platelets counts and levels of aspartate and alanine aminotransferase) and demographic variables (sex, age and number of days of illness before the start of the treatment) has been successfully used to predict IVIG-resistance in Japanese patients 213 , but not in North American children with Kawasaki disease 214 . The combination of prednisolone and IVIG to treat Japanese patients with Kawasaki disease predicted to have IVIG-resistant disease according to the Kobayashi score (RAISE study) resulted in more rapid fever resolution, reduced development of CAAs and lower incidence of additional rescue treatment 215 compared with IVIG alone.
As discussed above, Kawasaki disease susceptibility and increased coronary artery lesion risk are associated with an SNP in ITPKC 58 that results in a lack of NFAT regulation and activation of the T cell compartment owing to increased IL-2 production 216 . CD8 + cytotoxic T cells are present in the inflamed arterial wall during Kawasaki disease 24 , 135 ; therefore, targeting T cell expansion might be an efficient approach to preventing CAAs during Kawasaki disease. A combination treatment of IVIG and ciclosporin, a calcineurin inhibitor that suppresses IL-2 production and T cell activation, was tested in a clinical trial in Japanese patients with Kawasaki disease predicted to have IVIG-resistant disease based on the Kobayashi score (KAICA trial) 217 . In this trial, the combination treatment was shown to be safe and associated with a lower incidence of CAAs; however, treatment was linked with increased risk of relapse 217 . Furthermore, the scoring system used to identify IVIG-non-responders is poorly predictive in European children with Kawasaki disease, limiting the conclusions of this study.
The important role of the IL-1β–IL-1 receptor pathway in Kawasaki disease development has been demonstrated in both human patients 27 , 28 , 129 , 130 and mouse models 127 , 132 , 202 , 204 . Therefore, clinical trials investigating IL-1 pathway inhibition by using anakinra, which blocks both IL-1α and IL-1β, have been initiated in North America (ANAKID; ClinicalTrials.gov identifier NCT02179853) 218 and Europe (Kawakinra; European Clinical Trials number 2014-002715-4) 219 . Already, multiple case reports exist of the successful use of anakinra to treat patients with IVIG-resistant Kawasaki disease 220 , 221 , 222 , 223 , 224 , indicating the promise of this second-line therapy.
Therapeutic insights from mouse models
Although no animal model can fully mimic human disease, the LCWE-induced Kawasaki disease mouse model has been accepted by many in the research community as a reliable experimental model providing novel insights that can be tested in patients. For example, IVIG efficiently prevents coronary arteritis development in LCWE-injected mice 187 as well as in the CAWS mouse model of Kawasaki disease 203 .
The effects of the calcineurin inhibitors ciclosporin and tacrolimus have been investigated in the Nod1 ligand-induced mouse model of Kawasaki disease vasculitis 225 . This approach was rational given the established role of T cells and calcium signalling in Kawasaki disease. However, contrary to the expected outcome, these inhibitors exacerbated the coronary arteritis 225 . Notably, however, this result was probably related to the choice of mouse model, as the Nod1 ligand-mediated mouse model of Kawasaki disease vasculitis has previously been shown to be T cell-independent 207 . Indeed, in an independent study using the CAWS mouse model, which is T cell dependent, ciclosporin suppressed CAWS-induced vasculitis 226 , emphasizing the importance of model selection in preclinical studies. Most importantly, results in human studies bear out the therapeutic potential of calcineurin inhibition, as the Japanese phase III trial (KAICA trial) showed that adding ciclosporin to IVIG in patients with Kawasaki disease who were at high risk of IVIG resistance was beneficial in diminishing overall incidence of CAAs 217 .
The role of TNF has been investigated in both the LCWE and the CAWS mouse models of Kawasaki disease vasculitis 185 . Initially, etanercept treatment or genetic deletion of TNF receptor 1 was shown to protect mice from LCWE-induced coronary arteritis 185 , 202 . Infliximab treatment also prevented the development of both LCWE-induced coronary arteritis and myocarditis 132 . Similar results were obtained in the CAWS mouse model of Kawasaki disease vasculitis, in which etanercept 226 , 227 suppressed the incidence and decreased the severity of vasculitis. Mechanistically, TNF has been proposed to be produced by myeloid cells in the acute phase and to promote myocarditis and recruitment of immune cells by acting on cardiac stromal cells 202 . However, infliximab and etanercept might not directly target the TNF signalling pathway, and their observed effects might be indirect. Indeed, infliximab is not able to bind mouse TNF 227 , 228 ; therefore, the anti-inflammatory effect of infliximab might be attributable to the binding of Fc receptors at the surface of activated cells 229 , 230 .
The overwhelming evidence for the critical role of IL-1β in promoting LCWE-induced Kawasaki disease vasculitis in mice 127 , 128 , 132 led to the initiation of clinical trials testing the effect of anakinra for blocking IL-1β as a second therapy option to treat children with IVIG-resistant Kawasaki disease. Multiple case reports now outline the successful use of anakinra to treat patients with IVIG-resistant Kawasaki disease 221 , 222 , 223 , 224 . Alternatively, direct inhibition of the NLRP3 inflammasome might be a more targeted therapeutic strategy to treat Kawasaki disease, as it would affect several pathways beyond IL-1β, including IL-1α and IL-18. Several NLRP3 inhibitors have been identified 231 and tested in mouse models of inflammatory diseases, such as experimental autoimmune encephalomyelitis and cryopyrin-associated periodic syndrome 232 . It would be interesting to determine if such drugs could be used to prevent and reduce the cardiovascular complications in mouse models of Kawasaki disease vasculitis.
Conclusions
Over the past 40 years, research has improved our understanding of Kawasaki disease pathology and the development of coronary vasculitis. However, some questions still remain unanswered, such as the identification of the aetiological agents, how the disease is triggered, and the specific immune pathways associated with coronary vasculitis development and IVIG resistance. Owing to the rarity of human tissues from patients with Kawasaki disease, the use of animal models reproducing human Kawasaki disease features is invaluable. Many advances have been made over the decades by combining biological observations in human samples with mechanistic insights from experimental animal models. This ‘bench to bedside’ approach successfully led to the identification of the critical role of IL-1β in Kawasaki disease and resulted in the development of clinical trials in which anakinra is being used to treat children with IVIG-resistant Kawasaki disease.
LCWE-injected mice exhibit a dysfunctional intestinal barrier, and the increased IgA response and elevated sIgA levels in both LCWE-injected mice and children with Kawasaki disease reveal the existence of a ‘gut–vascular’ axis 143 . In evaluating this model system and the role of IgA, it should not be forgotten that injection of identically prepared LCWE induces chronic polyarthritis in selected inbred rat strains 180 , 233 . This observation implies that a common immunogenetic pathway might underlie a variety of autoimmune illnesses, with disease expression moderated not by the inducing agent, but rather by host genetics. The fact that cell wall fragments of common gut bacteria can produce varying disease manifestations in the face of inflammation-induced increased gut permeability suggests that some autoimmune diseases might not in fact be induced by the normal response to an unusual agent, but rather an unusual response to a common agent. Similarly, we hypothesize that vasculitic diseases, including Kawasaki disease, are not a usual response to an unusual environmental stimulus, but rather an unusual response (genetically determined) to a common environmental stimulus. This hypothesis has major implications for understanding the aetiology and pathogenesis of not only Kawasaki disease but also IgA-mediated diseases and perhaps others. In addition, it strongly suggests that inhibition of IL-1β might be effective for the many chronic inflammatory diseases in which IgA deposition is a key finding.
Kawasaki, T., Kosaki, F., Okawa, S., Shigematsu, I. & Yanagawa, H. A new infantile acute febrile mucocutaneous lymph node syndrome (MLNS) prevailing in Japan. Pediatrics 54 , 271–276 (1974).
CAS PubMed Google Scholar
Newburger, J. W., Takahashi, M. & Burns, J. C. Kawasaki disease. J. Am. Coll. Cardiol. 67 , 1738–1749 (2016).
Article PubMed Google Scholar
McCrindle, B. W. et al. Diagnosis, treatment, and long-term management of Kawasaki disease: a scientific statement for health professionals from the American Heart Association. Circulation 135 , e927–e999 (2017).
Newburger, J. W. et al. Diagnosis, treatment, and long-term management of Kawasaki disease: a statement for health professionals from the Committee on Rheumatic Fever, Endocarditis and Kawasaki Disease, Council on Cardiovascular Disease in the Young, American Heart Association. Circulation 110 , 2747–2771 (2004).
Singh, S., Vignesh, P. & Burgner, D. The epidemiology of Kawasaki disease: a global update. Arch. Dis. Child. 100 , 1084–1088 (2015).
Gordon, J. B., Kahn, A. M. & Burns, J. C. When children with Kawasaki disease grow up: myocardial and vascular complications in adulthood. J. Am. Coll. Cardiol. 54 , 1911–1920 (2009).
Article PubMed PubMed Central Google Scholar
Daniels, L. B. et al. Prevalence of Kawasaki disease in young adults with suspected myocardial ischemia. Circulation 125 , 2447–2453 (2012).
Rizk, S. R. et al. Acute myocardial ischemia in adults secondary to missed Kawasaki disease in childhood. Am. J. Cardiol. 115 , 423–427 (2015).
Gordon, J. B. et al. The spectrum of cardiovascular lesions requiring intervention in adults after Kawasaki disease. JACC Cardiovasc. Interv. 9 , 687–696 (2016).
Rosenkranz, M. E. et al. TLR2 and MyD88 contribute to Lactobacillus casei extract-induced focal coronary arteritis in a mouse model of Kawasaki disease. Circulation 112 , 2966–2973 (2005).
Article CAS PubMed Google Scholar
Schulte, D. J. et al. Involvement of innate and adaptive immunity in a murine model of coronary arteritis mimicking Kawasaki disease. J. Immunol. 183 , 5311–5318 (2009).
Article CAS PubMed PubMed Central Google Scholar
Rowley, A. H., Baker, S. C., Orenstein, J. M. & Shulman, S. T. Searching for the cause of Kawasaki disease–cytoplasmic inclusion bodies provide new insight. Nat. Rev. Microbiol. 6 , 394–401 (2008).
Rowley, A. H. et al. Ultrastructural, immunofluorescence, and RNA evidence support the hypothesis of a new virus associated with Kawasaki disease. J. Infect. Dis. 203 , 1021–1030 (2011).
Burns, J. C., Capparelli, E. V., Brown, J. A., Newburger, J. W. & Glode, M. P. Intravenous gamma-globulin treatment and retreatment in Kawasaki disease. US/Canadian Kawasaki Syndrome Study Group. Pediatr. Infect. Dis. J. 17 , 1144–1148 (1998).
Tremoulet, A. H. et al. Resistance to intravenous immunoglobulin in children with Kawasaki disease. J. Pediatr. 153 , 117–121 (2008).
Kato, H. et al. Long-term consequences of Kawasaki disease. A 10- to 21-year follow-up study of 594 patients. Circulation 94 , 1379–1385 (1996).
Friedman, K. G. et al. Coronary artery aneurysms in Kawasaki disease: risk factors for progressive disease and adverse cardiac events in the US population. J. Am. Heart Assoc. 5 , e003289 (2016).
Article PubMed PubMed Central CAS Google Scholar
Burns, J. C. & Franco, A. The immunomodulatory effects of intravenous immunoglobulin therapy in Kawasaki disease. Expert. Rev. Clin. Immunol. 11 , 819–825 (2015).
Moffett, B. S., Syblik, D., Denfield, S., Altman, C. & Tejtel-Sexson, K. Epidemiology of immunoglobulin resistant Kawasaki disease: results from a large, national database. Pediatr. Cardiol. 36 , 374–378 (2015).
Skochko, S. M. et al. Kawasaki disease outcomes and response to therapy in a multiethnic community: a 10-year experience. J. Pediatr. 203 , 408–415 (2018).
Makino, N. et al. Nationwide epidemiologic survey of Kawasaki disease in Japan, 2015–2016. Pediatr. Int. 61 , 397–403 (2019).
Takahashi, K., Oharaseki, T., Yokouchi, Y., Hiruta, N. & Naoe, S. Kawasaki disease as a systemic vasculitis in childhood. Ann. Vasc. Dis. 3 , 173–181 (2010).
Takahashi, K., Oharaseki, T. & Yokouchi, Y. Histopathological aspects of cardiovascular lesions in Kawasaki disease. Int. J. Rheum. Dis. 21 , 31–35 (2017).
Article PubMed CAS Google Scholar
Brown, T. J. et al. CD8 T lymphocytes and macrophages infiltrate coronary artery aneurysms in acute Kawasaki disease. J. Infect. Dis. 184 , 940–943 (2001).
Rowley, A. H., Eckerley, C. A., Jack, H. M., Shulman, S. T. & Baker, S. C. IgA plasma cells in vascular tissue of patients with Kawasaki syndrome. J. Immunol. 159 , 5946–5955 (1997).
Rowley, A. H. et al. IgA plasma cell infiltration of proximal respiratory tract, pancreas, kidney, and coronary artery in acute Kawasaki disease. J. Infect. Dis. 182 , 1183–1191 (2000).
Leung, D. Y. et al. Two monokines, interleukin 1 and tumor necrosis factor, render cultured vascular endothelial cells susceptible to lysis by antibodies circulating during Kawasaki syndrome. J. Exp. Med. 164 , 1958–1972 (1986).
Leung, D. Y. et al. Endothelial cell activation and high interleukin-1 secretion in the pathogenesis of acute Kawasaki disease. Lancet 2 , 1298–1302 (1989).
Rowley, A. H. Is Kawasaki disease an infectious disorder? Int. J. Rheum. Dis. 21 , 20–25 (2018).
Uehara, R. & Belay, E. D. Epidemiology of Kawasaki disease in Asia, Europe, and the United States. J. Epidemiol. 22 , 79–85 (2012).
Holman, R. C. et al. Hospitalizations for Kawasaki syndrome among children in the United States, 1997–2007. Pediatr. Infect. Dis. J. 29 , 483–488 (2010).
PubMed Google Scholar
Faulkner, L., Cooper, A., Fantino, C., Altmann, D. M. & Sriskandan, S. The mechanism of superantigen-mediated toxic shock: not a simple Th1 cytokine storm. J. Immunol. 175 , 6870–6877 (2005).
Abe, J. et al. Selective expansion of T cells expressing T-cell receptor variable regions V beta 2 and V beta 8 in Kawasaki disease. Proc. Natl Acad. Sci. USA 89 , 4066–4070 (1992).
Abe, J. et al. Characterization of T cell repertoire changes in acute Kawasaki disease. J. Exp. Med. 177 , 791–796 (1993).
Curtis, N., Zheng, R., Lamb, J. R. & Levin, M. Evidence for a superantigen mediated process in Kawasaki disease. Arch. Dis. Child. 72 , 308–311 (1995).
Mancia, L. et al. Characterization of the T-cell receptor V-beta repertoire in Kawasaki disease. Scand. J. Immunol. 48 , 443–449 (1998).
Pietra, B. A., De Inocencio, J., Giannini, E. H. & Hirsch, R. TCR V beta family repertoire and T cell activation markers in Kawasaki disease. J. Immunol. 153 , 1881–1888 (1994).
Iwanaga, M. et al. Kawasaki disease and Epstein-Barr virus. Lancet 317 , 938–939 (1981).
Article Google Scholar
Kikuta, H. et al. Recurrence of Kawasaki disease and Epstein-Barr virus infection. J. Infect. Dis. 162 , 1215–1215 (1990).
Kikuta, H. et al. Epstein-Barr virus genome-positive T lymphocytes in a boy with chronic active EBV infection associated with Kawasaki-like disease. Nature 333 , 455–457 (1988).
Marchette, N. J. et al. Epstein-Barr virus and other herpesvirus infections in Kawasaki syndrome. J. Infect. Dis. 161 , 680–684 (1990).
Fuse, S. et al. Children with Kawasaki disease are not infected with Epstein-Barr virus. Pediatr. Infect. Dis. J. 29 , 286–287 (2010).
Lee, S. J., Lee, K. Y., Han, J. W., Lee, J. S. & Whang, K. T. Epstein-Barr virus antibodies in Kawasaki disease. Yonsei Med. J. 47 , 475–479 (2006).
Esper, F. et al. Association between a novel human coronavirus and Kawasaki disease. J. Infect. Dis. 191 , 499–502 (2005).
Ebihara, T., Endo, R., Ma, X., Ishiguro, N. & Kikuta, H. Lack of association between New Haven coronavirus and Kawasaki disease. J. Infect. Dis. 192 , 351–352 (2005).
Belay, E. D. et al. Kawasaki disease and human coronavirus. J. Infect. Dis. 192 , 352–353; author reply 353 (2005).
Shulman, S. T. & Rowley, A. H. Does Kawasaki disease have a retroviral aetiology? Lancet 2 , 545–546 (1986).
Burns, J. C. et al. Polymerase activity in lymphocyte culture supernatants from patients with Kawasaki disease. Nature 323 , 814–816 (1986).
Nigro, G. & Midulla, M. Retrovirus and Kawasaki disease. Lancet 2 , 1045 (1986).
Rauch, A. M., Fultz, P. N. & Kalyanaraman, V. S. Retrovirus serology and Kawasaki syndrome. Lancet 329 , 1431 (1987).
Okamoto, T. et al. Lack of evidence of retroviral involvement in Kawasaki disease. Pediatrics 81 , 599 (1988).
Rowley, A. H. et al. A protein epitope targeted by the antibody response to Kawasaki disease. J. Infect. Dis. https://doi.org/10.1093/infdis/jiaa066 (2020).
Rowley, A. H. et al. Monoclonal antibodies from children with Kawasaki disease (KD) recognize hepacivirus peptides [abstract]. Presented at the 2019 Pediatric Academic Societies Meeting https://www.xcdsystem.com/pas2019/program/2019/index.cfm?pgid=156&sid=1060 (2019).
Rodo, X. et al. Tropospheric winds from northeastern China carry the etiologic agent of Kawasaki disease from its source to Japan. Proc. Natl Acad. Sci. USA 111 , 7952–7957 (2014).
Fujita, Y. et al. Kawasaki disease in families. Pediatrics 84 , 666–669 (1989).
Jayaraman, T., Ondriasova, E., Ondrias, K., Harnick, D. J. & Marks, A. R. The inositol 1,4,5-trisphosphate receptor is essential for T-cell receptor signaling. Proc. Natl Acad. Sci. USA 92 , 6007–6011 (1995).
Kuo, H. C. et al. ITPKC single nucleotide polymorphism associated with the Kawasaki disease in a Taiwanese population. PLoS One 6 , e17370 (2011).
Onouchi, Y. et al. ITPKC functional polymorphism associated with Kawasaki disease susceptibility and formation of coronary artery aneurysms. Nat. Genet. 40 , 35–42 (2008).
Alphonse, M. P. et al. Inositol-triphosphate 3-kinase C mediates inflammasome activation and treatment response in Kawasaki disease. J. Immunol. 197 , 3481–3489 (2016).
Kuo, H. C. et al. Lack of association between ORAI1/CRACM1 gene polymorphisms and Kawasaki disease in the Taiwanese children. J. Clin. Immunol. 31 , 650–655 (2011).
Onouchi, Y. et al. Variations in ORAI1 gene associated with Kawasaki disease. PLoS One 11 , e0145486 (2016).
Shimizu, C. et al. Genetic variation in the SLC8A1 calcium signaling pathway is associated with susceptibility to Kawasaki disease and coronary artery abnormalities. Circ. Cardiovasc. Genet. 9 , 559–568 (2016).
Murakami, T. et al. Critical role for calcium mobilization in activation of the NLRP3 inflammasome. Proc. Natl Acad. Sci. USA 109 , 11282–11287 (2012).
Rossol, M. et al. Extracellular Ca2+ is a danger signal activating the NLRP3 inflammasome through G protein-coupled calcium sensing receptors. Nat. Commun. 3 , 1329 (2012).
Elgueta, R. et al. Molecular mechanism and function of CD40/CD40L engagement in the immune system. Immunol. Rev. 229 , 152–172 (2009).
Wang, C. L. et al. Expression of CD40 ligand on CD4+ T-cells and platelets correlated to the coronary artery lesion and disease progress in Kawasaki disease. Pediatrics 111 , E140–E147 (2003).
Onouchi, Y. et al. CD40 ligand gene and Kawasaki disease. Eur. J. Hum. Genet. 12 , 1062–1068 (2004).
Huang, F. Y. et al. Genetic polymorphisms in the CD40 ligand gene and Kawasaki disease. J. Clin. Immunol. 28 , 405–410 (2008).
Kuo, H.-C. et al. CD40 gene polymorphisms associated with susceptibility and coronary artery lesions of Kawasaki disease in the Taiwanese population. ScientificWorldJournal 2012 , 520865 (2012).
Turner, M. W. The role of mannose-binding lectin in health and disease. Mol. Immunol. 40 , 423–429 (2003).
Biezeveld, M. H. et al. Polymorphisms in the mannose-binding lectin gene as determinants of age-defined risk of coronary artery lesions in Kawasaki disease. Arthritis Rheum. 54 , 369–376 (2006).
Biezeveld, M. H. et al. Association of mannose-binding lectin genotype with cardiovascular abnormalities in Kawasaki disease. Lancet 361 , 1268–1270 (2003).
Sato, S. et al. Association of mannose-binding lectin gene polymorphisms with Kawasaki disease in the Japanese. Int. J. Rheum. Dis. 12 , 307–310 (2009).
Nakamura, A. et al. Involvement of mannose-binding lectin in the pathogenesis of Kawasaki disease-like murine vasculitis. Clin. Immunol. 153 , 64–72 (2014).
Manger, K. et al. Fcgamma receptor IIa polymorphism in Caucasian patients with systemic lupus erythematosus: association with clinical symptoms. Arthritis Rheum. 41 , 1181–1189 (1998).
Brun, J. G., Madland, T. M. & Vedeler, C. A. Immunoglobulin G fc-receptor (FcgammaR) IIA, IIIA, and IIIB polymorphisms related to disease severity in rheumatoid arthritis. J. Rheumatol. 29 , 1135–1140 (2002).
Bredius, R. G. et al. Fc gamma receptor IIa (CD32) polymorphism in fulminant meningococcal septic shock in children. J. Infect. Dis. 170 , 848–853 (1994).
Biezeveld, M. et al. The involvement of Fc gamma receptor gene polymorphisms in Kawasaki disease. Clin. Exp. Immunol. 147 , 106–111 (2007).
CAS PubMed PubMed Central Google Scholar
Khor, C. C. et al. Genome-wide association study identifies FCGR2A as a susceptibility locus for Kawasaki disease. Nat. Genet. 43 , 1241–1246 (2011).
Tremoulet, A. H. Adjunctive therapies in Kawasaki disease. Int. J. Rheum. Dis. 21 , 76–79 (2018).
Orenstein, J. M. et al. Three linked vasculopathic processes characterize Kawasaki disease: a light and transmission electron microscopic study. PLoS One 7 , e38998 (2012).
Austermann, J., Spiekermann, C. & Roth, J. S100 proteins in rheumatic diseases. Nat. Rev. Rheumatol. 14 , 528–541 (2018).
Abe, J. et al. Gene expression profiling of the effect of high-dose intravenous Ig in patients with Kawasaki disease. J. Immunol. 174 , 5837–5845 (2005).
Lech, M. et al. Circulating markers of inflammation persist in children and adults with giant aneurysms after Kawasaki disease. Circ. Genom. Precis. Med. 12 , e002433 (2019).
Armaroli, G. et al. Monocyte-derived interleukin-1β as the driver of S100A12-induced sterile inflammatory activation of human coronary artery endothelial cells: implications for the pathogenesis of Kawasaki disease. Arthritis Rheumatol. 71 , 792–804 (2019).
Siefert, S. A. & Sarkar, R. Matrix metalloproteinases in vascular physiology and disease. Vascular 20 , 210–216 (2012).
Senzaki, H. The pathophysiology of coronary artery aneurysms in Kawasaki disease: role of matrix metalloproteinases. Arch. Dis. Child. 91 , 847–851 (2006).
Shimizu, C. et al. Matrix metalloproteinase haplotypes associated with coronary artery aneurysm formation in patients with Kawasaki disease. J. Hum. Genet. 55 , 779–784 (2010).
Korematsu, S. et al. Cell distribution differences of matrix metalloproteinase-9 and tissue inhibitor of matrix metalloproteinase-1 in patients with Kawasaki disease. Pediatr. Infect. Dis. J. 31 , 973–974 (2012).
Johnson, J. L., Dwivedi, A., Somerville, M., George, S. J. & Newby, A. C. Matrix metalloproteinase (MMP)-3 activates MMP-9 mediated vascular smooth muscle cell migration and neointima formation in mice. Arterioscler. Thromb. Vasc. Biol. 31 , e35–e44 (2011).
Popper, S. J. et al. Gene-expression patterns reveal underlying biological processes in Kawasaki disease. Genome Biol. 8 , R261 (2007).
Matsuyama, T. Tissue inhibitor of metalloproteinases-1 and matrix metalloproteinase-3 in Japanese healthy children and in Kawasaki disease and their clinical usefulness in juvenile rheumatoid arthritis. Pediatr. Int. 41 , 239–245 (1999).
Bonin, P. D., Fici, G. J. & Singh, J. P. Interleukin-1 promotes proliferation of vascular smooth muscle cells in coordination with PDGF or a monocyte derived growth factor. Exp. Cell Res. 181 , 475–482 (1989).
Sasu, S. & Beasley, D. Essential roles of IκB kinases α and β in serum- and IL-1-induced human VSMC proliferation. Am. J. Physiol. Heart Circ. Physiol. 278 , H1823–H1831 (2000).
Alexander, M. R. et al. Genetic inactivation of IL-1 signaling enhances atherosclerotic plaque instability and reduces outward vessel remodeling in advanced atherosclerosis in mice. J. Clin. Invest. 122 , 70–79 (2012).
Lau, A. C., Duong, T. T., Ito, S. & Yeung, R. S. Matrix metalloproteinase 9 activity leads to elastin breakdown in an animal model of Kawasaki disease. Arthritis Rheum. 58 , 854–863 (2008).
Lau, A. C., Duong, T. T., Ito, S., Wilson, G. J. & Yeung, R. S. Inhibition of matrix metalloproteinase-9 activity improves coronary outcome in an animal model of Kawasaki disease. Clin. Exp. Immunol. 157 , 300–309 (2009).
Tahamtan, A., Teymoori-Rad, M., Nakstad, B. & Salimi, V. Anti-inflammatory microRNAs and their potential for inflammatory diseases treatment. Front. Immunol. 9 , 1377 (2018).
van Rooij, E. The art of microRNA research. Circ. Res. 108 , 219–234 (2011).
Chu, M. et al. Bone marrow-derived microRNA-223 works as an endocrine genetic signal in vascular endothelial cells and participates in vascular injury from Kawasaki disease. J. Am. Heart Assoc. 6 , e004878 (2017).
Kuo, H.-C. et al. Next-generation sequencing identifies micro-RNA-based biomarker panel for Kawasaki disease. J. Allergy Clin. Immunol. 138 , 1227–1230 (2016).
Rowley, A. H. et al. A study of cardiovascular miRNA biomarkers for Kawasaki disease. Pediatr. Infect. Dis. J. 33 , 1296–1299 (2014).
Shimizu, C. et al. Differential expression of miR-145 in children with Kawasaki disease. PLoS One 8 , e58159 (2013).
He, M. et al. miR-483 targeting of CTGF suppresses endothelial-to-mesenchymal transition: therapeutic implications in Kawasaki disease. Circ. Res. 120 , 354–365 (2017).
Rangrez, A. Y., Massy, Z. A., Meuth, V. M.-L. & Metzinger, L. miR-143 and miR-145: molecular keys to switch the phenotype of vascular smooth muscle cells. Circ. Cardiovasc. Genet. 4 , 197–205 (2011).
Parmacek, M. S. MicroRNA-modulated targeting of vascular smooth muscle cells. J. Clin. Invest. 119 , 2526–2528 (2009).
Si, X. et al. miR‑23a downregulation modulates the inflammatory response by targeting ATG12‑mediated autophagy. Mol. Med. Rep. 18 , 1524–1530 (2018).
Long, B., Gan, T.-Y., Zhang, R.-C. & Zhang, Y.-H. miR-23a regulates cardiomyocyte apoptosis by targeting manganese superoxide dismutase. Mol. Cells 40 , 542–549 (2017).
Suzuki, A. et al. Active remodeling of the coronary arterial lesions in the late phase of Kawasaki disease: immunohistochemical study. Circulation 101 , 2935–2941 (2000).
Dahdah, N. Not just coronary arteritis, Kawasaki disease is a myocarditis, too. J. Am. Coll. Cardiol. 55 , 1507 (2010).
Dionne, A. & Dahdah, N. Myocarditis and Kawasaki disease. Int. J. Rheum. Dis. 21 , 45–49 (2017).
Harada, M. et al. Histopathological characteristics of myocarditis in acute-phase Kawasaki disease. Histopathology 61 , 1156–1167 (2012).
Yutani, C. et al. Cardiac biopsy of Kawasaki disease. Arch. Pathol. Lab. Med. 105 , 470–473 (1981).
Takahashi, M. Myocarditis in Kawasaki syndrome. A minor villain? Circulation 79 , 1398–1400 (1989).
Kao, C. H., Hsieh, K. S., Wang, Y. L., Wang, S. J. & Yeh, S. H. The detection of ventricular dysfunction and carditis in children with Kawasaki disease using equilibrium multigated blood pooling ventriculography and 99Tcm-HMPAO-labelled WBC heart scans. Nucl. Med. Commun. 14 , 539–543 (1993).
Printz, B. F. et al. Noncoronary cardiac abnormalities are associated with coronary artery dilation and with laboratory inflammatory markers in acute Kawasaki disease. J. Am. Coll. Cardiol. 57 , 86–92 (2011).
Burns, J. C. Kawasaki disease update. Indian. J. Pediatr. 76 , 71–76 (2009).
Miyake, T., Yokoyama, T., Shinohara, T., Seto, S. & Oiki, M. Transient dilatation of the abdominal aorta in an infant with Kawasaki disease associated with thrombocytopenia. Acta Paediatr. Jpn. 37 , 521–525 (1995).
Canter, C. E., Bower, R. J. & Strauss, A. W. Atypical Kawasaki disease with aortic aneurysm. Pediatrics 68 , 885–888 (1981).
Amano, S. et al. General pathology of Kawasaki disease. On the morphological alterations corresponding to the clinical manifestations. Acta Pathol. Jpn. 30 , 681–694 (1980).
Watanabe, T. Kidney and urinary tract involvement in Kawasaki disease. Int. J. Pediatr. 2013 , 831834 (2013).
Watanabe, T. Clinical features of acute kidney injury in patients with Kawasaki disease. World J. Clin. Pediatr. 7 , 83–88 (2018).
Ohshio, G. et al. High levels of IgA-containing circulating immune complex and secretory IgA in Kawasaki disease. Microbiol. Immunol. 31 , 891–898 (1987).
Rowley, A. H. et al. Cloning the arterial IgA antibody response during acute Kawasaki disease. J. Immunol. 175 , 8386–8391 (2005).
Rowley, A. H., Shulman, S. T., Spike, B. T., Mask, C. A. & Baker, S. C. Oligoclonal IgA response in the vascular wall in acute Kawasaki disease. J. Immunol. 166 , 1334–1343 (2001).
Lee, Y. et al. IL-1 signaling is critically required in stromal cells in Kawasaki disease vasculitis mouse model: role of both IL-1α and IL-1β. Arterioscler. Thromb. Vasc. Biol. 35 , 2605–2616 (2015).
Wakita, D. et al. Role of interleukin-1 signaling in a mouse model of Kawasaki disease-associated abdominal aortic aneurysm. Arterioscler. Thromb. Vasc. Biol. 36 , 886–897 (2016).
Hoang, L. T. et al. Global gene expression profiling identifies new therapeutic targets in acute Kawasaki disease. Genome Med. 6 , 541 (2014).
Fury, W. et al. Transcript abundance patterns in Kawasaki disease patients with intravenous immunoglobulin resistance. Hum. Immunol. 71 , 865–873 (2010).
Porritt, R. A. et al. Iinterleukin-1-mediated sex differences in Kawasaki disease vasculitis development and response to treatment. Arterioscler. Thromb. Vasc. Biol. 40 , 802–818 (2020).
Lee, Y. et al. Interleukin-1β is crucial for the induction of coronary artery inflammation in a mouse model of Kawasaki disease. Circulation 125 , 1542–1550 (2012).
Ben-Sasson, S. Z. et al. IL-1 enhances expansion, effector function, tissue localization, and memory response of antigen-specific CD8 T cells. J. Exp. Med. 210 , 491–502 (2013).
Hirao, J. & Sugita, K. Circulating CD4+CD8+ T lymphocytes in patients with Kawasaki disease. Clin. Exp. Immunol. 111 , 397–401 (1998).
Yilmaz, A. et al. Activated myeloid dendritic cells accumulate and co-localize with CD3+ T cells in coronary artery lesions in patients with Kawasaki disease. Exp. Mol. Pathol. 83 , 93–103 (2007).
Bischoff, S. C. et al. Intestinal permeability–a new target for disease prevention and therapy. BMC Gastroenterol. 14 , 189 (2014).
Miyake, T. et al. Small bowel pseudo-obstruction in Kawasaki disease. Pediatr. Radiol. 17 , 383–386 (1987).
Yaniv, L., Jaffe, M. & Shaoul, R. The surgical manifestations of the intestinal tract in Kawasaki disease. J. Pediatr. Surg. 40 , e1–e4 (2005).
Baker, A. L. et al. Associated symptoms in the ten days before diagnosis of Kawasaki disease. J. Pediatr. 154 , 592–595 (2009).
Colomba, C. et al. Intestinal involvement in Kawasaki disease. J. Pediatr. 202 , 186–193 (2018).
Fabi, M. et al. Gastrointestinal presentation of Kawasaki disease: a red flag for severe disease? PLoS One 13 , e0202658 (2018).
Nagata, S., Yamashiro, Y., Maeda, M., Ohtsuka, Y. & Yabuta, K. Immunohistochemical studies on small intestinal mucosa in Kawasaki disease. Pediatr. Res. 33 , 557–563 (1993).
Noval Rivas, M. et al. Intestinal permeability and IgA provoke immune vasculitis linked to cardiovascular inflammation. Immunity 51 , 508–521 (2019).
Tang, W. H. W. & Hazen, S. L. The contributory role of gut microbiota in cardiovascular disease. J. Clin. Invest. 124 , 4204–4211 (2014).
Tang, W. H. W. & Hazen, S. L. The gut microbiome and its role in cardiovascular diseases. Circulation 135 , 1008–1010 (2017).
Takeshita, S., Kobayashi, I., Kawamura, Y., Tokutomi, T. & Sekine, I. Characteristic profile of intestinal microflora in Kawasaki disease. Acta Paediatr. 91 , 783–788 (2002).
Yamashiro, Y., Nagata, S., Ohtsuka, Y., Oguchi, S. & Shimizu, T. Microbiologic studies on the small intestine in Kawasaki disease. Pediatr. Res. 39 , 622–624 (1996).
Nagata, S. et al. Heat shock proteins and superantigenic properties of bacteria from the gastrointestinal tract of patients with Kawasaki disease. Immunology 128 , 511–520 (2009).
Goldenberg, J. Z. et al. Probiotics for the prevention of pediatric antibiotic-associated diarrhea. Cochrane Database Syst. Rev. 12 , CD004827 (2015).
Google Scholar
Allen, S. J., Martinez, E. G., Gregorio, G. V. & Dans, L. F. Probiotics for treating acute infectious diarrhoea. Cochrane Database Syst. Rev. 11 , CD003048 (2010).
Karczewski, J. et al. Regulation of human epithelial tight junction proteins by Lactobacillus plantarum in vivo and protective effects on the epithelial barrier. Am. J. Physiol. Gastrointest. Liver Physiol. 298 , G851–G859 (2010).
Han, X. et al. Lactobacillus rhamnosus GG prevents epithelial barrier dysfunction induced by interferon-gamma and fecal supernatants from irritable bowel syndrome patients in human intestinal enteroids and colonoids. Gut Microbes 10 , 59–76 (2019).
Mack, D. R., Michail, S., Wei, S., McDougall, L. & Hollingsworth, M. A. Probiotics inhibit enteropathogenic E. coli adherence in vitro by inducing intestinal mucin gene expression. Am. J. Physiol. 276 , G941–G950 (1999).
van Baarlen, P., Wells, J. M. & Kleerebezem, M. Regulation of intestinal homeostasis and immunity with probiotic lactobacilli. Trends Immunol. 34 , 208–215 (2013).
Belkacem, N. et al. Lactobacillus paracasei feeding improves immune control of influenza infection in mice. PLoS One 12 , e0184976 (2017).
Belkacem, N., Bourdet-Sicard, R. & Taha, M. K. Lactobacillus paracasei feeding improves the control of secondary experimental meningococcal infection in flu-infected mice. BMC Infect. Dis. 18 , 167 (2018).
Gebremariam, H. G. et al. Lactobacillus gasseri suppresses the production of proinflammatory cytokines in Helicobacter pylori-infected macrophages by inhibiting the expression of ADAM17. Front. Immunol. 10 , 2326 (2019).
Downie, M. L. et al. Variability in response to intravenous immunoglobulin in the treatment of Kawasaki disease. J. Pediatr. 179 , 124–130 (2016).
Dethlefsen, L. & Relman, D. A. Incomplete recovery and individualized responses of the human distal gut microbiota to repeated antibiotic perturbation. Proc. Natl Acad. Sci. USA 108 (Suppl. 1), 4554–4561 (2011).
Modi, S. R., Collins, J. J. & Relman, D. A. Antibiotics and the gut microbiota. J. Clin. Invest. 124 , 4212–4218 (2014).
Wheeler, M. L. et al. Immunological consequences of intestinal fungal dysbiosis. Cell Host Microbe 19 , 865–873 (2016).
Jernberg, C., Löfmark, S., Edlund, C. & Jansson, J. K. Long-term ecological impacts of antibiotic administration on the human intestinal microbiota. ISME J. 1 , 56–66 (2007).
Kinumaki, A. et al. Characterization of the gut microbiota of Kawasaki disease patients by metagenomic analysis. Front. Microbiol. 6 , 824 (2015).
Audemard-Verger, A., Pillebout, E., Guillevin, L., Thervet, E. & Terrier, B. IgA vasculitis (Henoch-Shönlein purpura) in adults: diagnostic and therapeutic aspects. Autoimmun. Rev. 14 , 579–585 (2015).
Floege, J. & Feehally, J. The mucosa-kidney axis in IgA nephropathy. Nat. Rev. Nephrol. 12 , 147–156 (2016).
Coppo, R. The gut-kidney axis in IgA nephropathy: role of microbiota and diet on genetic predisposition. Pediatr. Nephrol. 33 , 53–61 (2018).
Moja, P. et al. Is there IgA from gut mucosal origin in the serum of children with Henoch-Schönlein purpura? Clin. Immunol. Immunopathol. 86 , 290–297 (1998).
Menikou, S., Langford, P. R. & Levin, M. Kawasaki disease: the role of immune complexes revisited. Front. Immunol. 10 , 1156 (2019).
Oni, L. & Sampath, S. Childhood IgA vasculitis (Henoch Schonlein Purpura)–advances and knowledge gaps. Front. Pediatr. 7 , 257 (2019).
Oortwijn, B. D. et al. A pathogenic role for secretory IgA in IgA nephropathy. Kidney Int. 69 , 1131–1138 (2006).
Nishimura, S. et al. A polymorphism in the promoter of the CD14 gene (CD14/-159) is associated with the development of coronary artery lesions in patients with Kawasaki disease. J. Pediatr. 143 , 357–362 (2003).
Yoon, H. J. et al. Association of the CD14 gene -159C polymorphism with progression of IgA nephropathy. J. Med. Genet. 40 , 104–108 (2003).
Boyer, E. M., Turman, M. & O’Neil, K. M. Partial response to anakinra in life-threatening Henoch-Schönlein purpura: case report. Pediatr. Rheumatol. Online J. 9 , 21 (2011).
Chun, J. et al. NLRP3 localizes to the tubular epithelium in human kidney and correlates with outcome in IgA nephropathy. Sci. Rep. 6 , 24667 (2016).
Meinzer, U. et al. Interleukin-1 targeting drugs in familial Mediterranean fever: a case series and a review of the literature. Semin. Arthritis Rheum. 41 , 265–271 (2011).
Lehman, T. J., Walker, S. M., Mahnovski, V. & McCurdy, D. Coronary arteritis in mice following the systemic injection of group B Lactobacillus casei cell walls in aqueous suspension. Arthritis Rheum. 28 , 652–659 (1985).
Murata, H. Experimental candida-induced arteritis in mice. Relation to arteritis in the mucocutaneous lymph node syndrome. Microbiol. Immunol. 23 , 825–831 (1979).
Nishio, H. et al. Nod1 ligands induce site-specific vascular inflammation. Arterioscler. Thromb. Vasc. Biol. 31 , 1093–1099 (2011).
Hill, D. et al. The Lactobacillus casei group: history and health related applications. Front. Microbiol. 9 , 2107 (2018).
Lehman, T. J., Allen, J. B., Plotz, P. H. & Wilder, R. L. Polyarthritis in rats following the systemic injection of Lactobacillus casei cell walls in aqueous suspension. Arthritis Rheum. 26 , 1259–1265 (1983).
Noval Rivas, M. et al. CD8+ T cells contribute to the development of coronary arteritis in the Lactobacillus casei cell wall extract-induced murine model of Kawasaki disease. Arthritis Rheumatol. 69 , 410–421 (2017).
Matundan, H. H. et al. Myocardial fibrosis after adrenergic stimulation as a long-term sequela in a mouse model of Kawasaki disease vasculitis. JCI Insight 4 , e126279 (2019).
Article PubMed Central Google Scholar
Abe, M. et al. IL-1-dependent electrophysiological changes and cardiac neural remodeling in a mouse model of Kawasaki disease vasculitis. Clin. Exp. Immunol. 199 , 303–313 (2019).
Gorelik, M. et al. IL-1 receptor antagonist, anakinra, prevents myocardial dysfunction in a mouse model of Kawasaki disease vasculitis and myocarditis. Clin. Exp. Immunol. 198 , 101–110 (2019).
Hui-Yuen, J. S., Duong, T. T. & Yeung, R. S. M. TNF-α is necessary for induction of coronary artery inflammation and aneurysm formation in an animal model of Kawasaki disease. J. Immunol. 176 , 6294–6301 (2006).
Lehman, T. J., Warren, R., Gietl, D., Mahnovski, V. & Prescott, M. Variable expression of Lactobacillus casei cell wall-induced coronary arteritis: an animal model of Kawasaki’s disease in selected inbred mouse strains. Clin. Immunol. Immunopathol. 48 , 108–118 (1988).
Myones, B., Bathoria, J., Lehman, T. & Shulman, S. in Kawasaki Disease: Proceedings of the 5th International Kawasaki Disease Symposium (ed. Kato, H et al.) 252–256 (Elsevier, 1995).
Maury, C. P., Salo, E. & Pelkonen, P. Circulating interleukin-1β in patients with Kawasaki disease. N. Engl. J. Med. 319 , 1670–1671 (1988).
Ohno, N. Chemistry and biology of angiitis inducer, Candida albicans water-soluble mannoprotein-β-glucan complex (CAWS). Microbiol. Immunol. 47 , 479–490 (2003).
Takahashi, K. et al. Histopathological features of murine systemic vasculitis caused by Candida albicans extract–an animal model of Kawasaki disease. Inflamm. Res. 53 , 72–77 (2004).
Oharaseki, T. et al. Recognition of alpha-mannan by dectin 2 is essential for onset of Kawasaki disease-like murine vasculitis induced by Candida albicans cell-wall polysaccharide. Mod. Rheumatol. 30 , 350–357 (2020).
Yoshikane, Y. et al. JNK is critical for the development of Candida albicans-induced vascular lesions in a mouse model of Kawasaki disease. Cardiovasc. Pathol. 24 , 33–40 (2015).
Nakamura, J. et al. Adeno-associated virus vector-mediated interleukin-10 induction prevents vascular inflammation in a murine model of Kawasaki disease. Sci. Rep. 8 , 7601 (2018).
Sabat, R. et al. Biology of interleukin-10. Cytokine Growth Factor. Rev. 21 , 331–344 (2010).
Ohno, N. Murine model of Kawasaki disease induced by mannoprotein-beta-glucan complex, CAWS, obtained from Candida albicans. Jpn. J. Infect. Dis. 57 , S9–S10 (2004).
Miura, N. N. et al. IL-10 is a negative regulatory factor of CAWS-vasculitis in CBA/J mice as assessed by comparison with Bruton’s tyrosine kinase-deficient CBA/N mice. J. Immunol. 183 , 3417–3424 (2009).
Ohno, N. A murine model of vasculitis induced by fungal polysaccharide. Cardiovasc. Hematol. Agents Med. Chem. 6 , 44–52 (2008).
Nagi-Miura, N., Adachi, Y. & Ohno, N. Coronary arteritis induced by CAWS (Candida albicans water-soluble fraction) in various strains of mice. Nihon Ishinkin Gakkai Zasshi 49 , 287–292 (2008).
Stock, A. T., Hansen, J. A., Sleeman, M. A., McKenzie, B. S. & Wicks, I. P. GM-CSF primes cardiac inflammation in a mouse model of Kawasaki disease. J. Exp. Med. 213 , 1983–1998 (2016).
Martinez, H. G. et al. Important role of CCR2 in a murine model of coronary vasculitis. BMC Immunol. 13 , 56 (2012).
Miyabe, C. et al. Dectin-2-induced CCL2 production in tissue-resident macrophages ignites cardiac arteritis. J. Clin. Invest. 130 , 3610–3624 (2019).
Stock, A. T., Jama, H. A., Hansen, J. A. & Wicks, I. P. TNF and IL-1 play essential but temporally distinct roles in driving cardiac inflammation in a murine model of Kawasaki disease. J. Immunol. 202 , 3151–3160 (2019).
Takahashi, K. et al. Administration of human immunoglobulin suppresses development of murine systemic vasculitis induced with Candida albicans water-soluble fraction: an animal model of Kawasaki disease. Mod. Rheumatol. 20 , 160–167 (2010).
Hashimoto, Y. et al. Interleukin-1beta inhibition attenuates vasculitis in a mouse model of Kawasaki disease. J. Nippon. Med. Sch. 86 , 108–116 (2019).
Anzai, F. et al. Crucial role of NLRP3 inflammasome in a murine model of Kawasaki disease. J. Mol. Cell Cardiol. 138 , 185–196 (2019).
Ohashi, R. et al. Characterization of a murine model with arteritis induced by Nod1 ligand, FK565: a comparative study with a CAWS-induced model. Mod. Rheumatol. 27 , 1024–1030 (2017).
Motomura, Y. et al. Identification of pathogenic cardiac CD11c+ macrophages in Nod1-mediated acute coronary arteritis. Arterioscler. Thromb. Vasc. Biol. 35 , 1423–1433 (2015).
Iwata, M., Shimozato, T., Tokiwa, H. & Tsubura, E. Antipyretic activity of a human immunoglobulin preparation for intravenous use in an experimental model of fever in rabbits. Infect. Immun. 55 , 547–554 (1987).
Ruiz de Souza, V. et al. Selective induction of interleukin-1 receptor antagonist and interleukin-8 in human monocytes by normal polyspecific IgG (intravenous immunoglobulin). Eur. J. Immunol. 25 , 1267–1273 (1995).
Uehara, R. et al. Analysis of potential risk factors associated with nonresponse to initial intravenous immunoglobulin treatment among Kawasaki disease patients in Japan. Pediatr. Infect. Dis. J. 27 , 155–160 (2008).
Tremoulet, A. H. et al. Infliximab for intensification of primary therapy for Kawasaki disease: a phase 3 randomised, double-blind, placebo-controlled trial. Lancet 383 , 1731–1738 (2014).
Portman, M. A. et al. Etanercept with IVIg for acute Kawasaki disease: a randomized controlled trial. Pediatrics 143 , e20183675 (2019).
Kobayashi, T. et al. Prediction of intravenous immunoglobulin unresponsiveness in patients with Kawasaki disease. Circulation 113 , 2606–2612 (2006).
Sleeper, L. A. et al. Evaluation of Kawasaki disease risk-scoring systems for intravenous immunoglobulin resistance. J. Pediatr. 158 , 831–835 (2011).
Kobayashi, T. et al. Efficacy of immunoglobulin plus prednisolone for prevention of coronary artery abnormalities in severe Kawasaki disease (RAISE study): a randomised, open-label, blinded-endpoints trial. Lancet 379 , 1613–1620 (2012).
Lee, J., Kim, L. & Choi, J. Revisiting the concept of targeting NFAT to control T cell immunity and autoimmune diseases. Front. Immunol. 9 , 2747–2747 (2018).
Hamada, H. et al. Efficacy of primary treatment with immunoglobulin plus ciclosporin for prevention of coronary artery abnormalities in patients with Kawasaki disease predicted to be at increased risk of non-response to intravenous immunoglobulin (KAICA): a randomised controlled, open-label, blinded-endpoints, phase 3 trial. Lancet 393 , 1128–1137 (2019).
Tremoulet, A. H. et al. Rationale and study design for a phase I/IIa trial of anakinra in children with Kawasaki disease and early coronary artery abnormalities (the ANAKID trial). Contemp. Clin. Trials 48 , 70–75 (2016).
Koné-Paut, I. et al. KAWAKINRA: a phase IIA multicenter trial to assess the efficacy, and safety of anakinra in patients with intravenous immunoglobulin-resistant Kawasaki disease [abstract OP0147]. Ann. Rheum. Dis. 78 (Suppl. 2), 149 (2019).
Cohen, S. et al. A child with severe relapsing Kawasaki disease rescued by IL-1 receptor blockade and extracorporeal membrane oxygenation. Ann. Rheum. Dis. 71 , 2059–2061 (2012).
Shafferman, A., Birmingham, J. D. & Cron, R. Q. High dose anakinra for treatment of severe neonatal Kawasaki disease: a case report. Pediatr. Rheumatol. Online J. 12 , 26 (2014).
Guillaume, M. P., Reumaux, H. & Dubos, F. Usefulness and safety of anakinra in refractory Kawasaki disease complicated by coronary artery aneurysm. Cardiol. Young 28 , 739–742 (2018).
Sánchez-Manubens, J. et al. A child with resistant Kawasaki disease successfully treated with anakinra: a case report. BMC Pediatr. 17 , 102 (2017).
Blonz, G. et al. Severe late-onset Kawasaki disease successfully treated with anakinra. J. Clin. Rheumatol. 26 , e42–e43 (2020).
Murata, K. et al. Calcineurin inhibitors exacerbate coronary arteritis via the MyD88 signalling pathway in a murine model of Kawasaki disease. Clin. Exp. Immunol. 190 , 54–67 (2017).
Ohashi, R. et al. Etanercept suppresses arteritis in a murine model of Kawasaki disease: a comparative study involving different biological agents. Int. J. Vasc. Med. 2013 , 543141 (2013).
PubMed PubMed Central Google Scholar
Oharaseki, T. et al. The role of TNF-α in a murine model of Kawasaki disease arteritis induced with a Candida albicans cell wall polysaccharide. Mod. Rheumatol. 24 , 120–128 (2014).
Assas, B. M. et al. Anti-inflammatory effects of infliximab in mice are independent of tumour necrosis factor α neutralization. Clin. Exp. Immunol. 187 , 225–233 (2017).
Arora, T. et al. Differences in binding and effector functions between classes of TNF antagonists. Cytokine 45 , 124–131 (2009).
Vos, A. C. et al. Anti-tumor necrosis factor-α antibodies induce regulatory macrophages in an Fc region-dependent manner. Gastroenterology 140 , 221–230 (2011).
Mangan, M. S. J. et al. Targeting the NLRP3 inflammasome in inflammatory diseases. Nat. Rev. Drug. Discov. 17 , 688 (2018).
Coll, R. C. et al. A small-molecule inhibitor of the NLRP3 inflammasome for the treatment of inflammatory diseases. Nat. Med. 21 , 248–255 (2015).
Lehman, T. J., Allen, J. B., Plotz, P. H. & Wilder, R. L. Lactobacillus casei cell wall-induced arthritis in rats: cell wall fragment distribution and persistence in chronic arthritis-susceptible LEW/N and -resistant F344/N rats. Arthritis Rheum. 27 , 939–942 (1984).
Download references
Acknowledgements
The work of M.A. is supported by the NIH Grant R01 AI072726 and M.N.R. is supported by the NIH grant R01 HL139766.
Author information
Authors and affiliations.
Departments of Pediatrics, Division of Infectious Diseases and Immunology, and Infectious and Immunologic Diseases Research Center (IIDRC), Department of Biomedical Sciences, Cedars-Sinai Medical Center, Los Angeles, CA, USA
Magali Noval Rivas & Moshe Arditi
Department of Pediatrics, David Geffen School of Medicine at UCLA, Los Angeles, CA, USA
Cedars-Sinai Smidt Heart Institute, Cedars-Sinai Medical Center, Los Angeles, CA, USA
Moshe Arditi
You can also search for this author in PubMed Google Scholar
Contributions
Both authors contributed equally to all aspects of the manuscript.
Corresponding authors
Correspondence to Magali Noval Rivas or Moshe Arditi .
Ethics declarations
Competing interests.
The authors declare no competing interests.
Additional information
Peer review information.
Nature Reviews Rheumatology thanks Ho-Chang Kuo, Isabelle Kone-Paut and the other, anonymous, reviewer(s) for their contribution to the peer review of this work.
Publisher’s note
Springer Nature remains neutral with regard to jurisdictional claims in published maps and institutional affiliations.
Rights and permissions
Reprints and permissions
About this article
Cite this article.
Noval Rivas, M., Arditi, M. Kawasaki disease: pathophysiology and insights from mouse models. Nat Rev Rheumatol 16 , 391–405 (2020). https://doi.org/10.1038/s41584-020-0426-0
Download citation
Accepted : 23 April 2020
Published : 26 May 2020
Issue Date : July 2020
DOI : https://doi.org/10.1038/s41584-020-0426-0
Share this article
Anyone you share the following link with will be able to read this content:
Sorry, a shareable link is not currently available for this article.
Provided by the Springer Nature SharedIt content-sharing initiative
This article is cited by
Development of a prediction model for progression of coronary artery lesions in kawasaki disease.
- Ye-Shi Chen
- Xiao-Hui Li
Pediatric Research (2024)
Immunopathology of and potential therapeutics for secondary hemophagocytic lymphohistiocytosis/macrophage activation syndrome: a translational perspective
- Tram T. T. Nguyen
- Yoon Tae Kim
Experimental & Molecular Medicine (2024)
Markers of Endothelial Dysfunction in Kawasaki Disease: An Update
- Rajni Kumrah
- Surjit Singh
Clinical Reviews in Allergy & Immunology (2024)
[18F]FDG PET-MR characterization of aortitis in the IL1rn−/− mouse model of giant-cell arteritis
- Samuel Deshayes
- Caroline Baugé
- Achille Aouba
EJNMMI Research (2023)
Kawasaki disease: ubiquitin-specific protease 5 promotes endothelial inflammation via TNFα-mediated signaling
- Chengcheng Huang
Pediatric Research (2023)
Quick links
- Explore articles by subject
- Guide to authors
- Editorial policies
Sign up for the Nature Briefing newsletter — what matters in science, free to your inbox daily.

- Patient Care & Health Information
- Diseases & Conditions
- Kawasaki disease
Kawasaki disease causes swelling, called inflammation, in the walls of small to medium-sized blood vessels that carry blood throughout the body. Kawasaki disease most often affects the heart arteries in children. Those arteries supply oxygen-rich blood to the heart.
Kawasaki disease is sometimes called mucocutaneous lymph node syndrome. That's because it also causes swelling in glands, called lymph nodes, and mucous membranes inside the mouth, nose, eyes and throat.
Children with Kawasaki disease might have high fever, swollen hands and feet with skin peeling, and red eyes and tongue. But Kawasaki disease is often treatable. With early treatment, most children get better and have no long-lasting problems.
Products & Services
- A Book: Mayo Clinic Family Health Book, 5th Edition
- Newsletter: Mayo Clinic Health Letter — Digital Edition
Symptoms of Kawasaki disease include a fever greater than 102.2 degrees Fahrenheit (39 degrees Celsius) for five or more days. And the child has at least four of the following symptoms.
- A rash on the main part of the body or in the genital area.
- An enlarged lymph node in the neck.
- Very red eyes without a thick discharge.
- Red, dry, cracked lips and a red, swollen tongue.
- Swollen, red skin on the palms of the hands and the soles of the feet. Later the skin on fingers and toes peels.
The symptoms might not happen at the same time. Let your child's healthcare professional know about a symptom that has gone away.
Other symptoms might include:
- Belly pain.
- Joint pain.
Some children get a high fever for five or more days but have fewer than four of the symptoms needed for a diagnosis of Kawasaki disease. They might have what's called incomplete Kawasaki disease. Children with incomplete Kawasaki disease are still at risk of damage to the heart arteries. They still need treatment within 10 days of when symptoms appear.
Kawasaki disease can have symptoms like those of a condition called multisystem inflammatory syndrome in children. The syndrome happens in children with COVID-19.
When to see a doctor
If your child has a fever that lasts more than three days, contact your child's healthcare professional. Treating Kawasaki disease within 10 days of when it began may reduce the chances of lasting damage to the arteries that supply the heart.
There is a problem with information submitted for this request. Review/update the information highlighted below and resubmit the form.
From Mayo Clinic to your inbox
Sign up for free and stay up to date on research advancements, health tips, current health topics, and expertise on managing health. Click here for an email preview.
Error Email field is required
Error Include a valid email address
To provide you with the most relevant and helpful information, and understand which information is beneficial, we may combine your email and website usage information with other information we have about you. If you are a Mayo Clinic patient, this could include protected health information. If we combine this information with your protected health information, we will treat all of that information as protected health information and will only use or disclose that information as set forth in our notice of privacy practices. You may opt-out of email communications at any time by clicking on the unsubscribe link in the e-mail.
Thank you for subscribing!
You'll soon start receiving the latest Mayo Clinic health information you requested in your inbox.
Sorry something went wrong with your subscription
Please, try again in a couple of minutes
No one knows what causes Kawasaki disease. But experts don't believe the disease spreads from person to person. Some think that Kawasaki disease happens after a bacterial or viral infection, or that it's linked to factors in the environment. Certain genes might make children more likely to get Kawasaki disease.
Risk factors
Three things are known to increase a child's risk of developing Kawasaki disease.
- Age. Children under 5 years old are at highest risk of Kawasaki disease.
- Sex. Children who are assigned male at birth are slightly more likely to get Kawasaki disease.
- Ethnicity. Children of Asian or Pacific Islander descent have higher rates of Kawasaki disease.
Kawasaki disease tends to occur seasonally. In North America and countries with like climates, it most often happens in the winter and early spring.
Complications
Kawasaki disease is a leading cause of heart disease in children who live in developed countries. But, with treatment, few children have lasting damage.
Heart complications include:
- Swelling of blood vessels, most often the arteries that send blood to the heart.
- Swelling of the heart muscle.
- Heart valve problems.
Any of these complications can damage the heart. Swelling of the heart arteries can weaken them and cause a bulge in the artery wall, called an aneurysm. Aneurysms raise the risk of blood clots. These can lead to a heart attack or cause bleeding inside the body.
Rarely, for children who get heart artery problems, Kawasaki disease can cause death.
Kawasaki disease care at Mayo Clinic
- Ferri FF. Kawasaki disease. In: Ferri's Clinical Advisor 2022. Elsevier; 2022. https://www.clinicalkey.com. Accessed Sept. 3, 2021.
- Elsevier Point of Care. Clinical overview: Kawasaki disease. https://www.clinicalkey.com. Accessed Aug. 10, 2023.
- AskMayoExpert. Kawasaki disease (child). Mayo Clinic; 2023.
- Sundel R. Kawasaki disease: Clinical features and diagnosis. https://www.uptodate.com/contents/search. Accessed Aug. 10, 2023.
- Sundel R. Kawasaki disease: Initial treatment and prognosis. https://www.uptodate.com/contents/search. Accessed Aug. 10, 2023.
- Rife E, et al. Kawasaki disease: An update. Current Rheumatology Reports. 2020; doi:10.1007/s11926-020-00941-4.
Associated Procedures
- Complete blood count (CBC)
- Echocardiogram
- Electrocardiogram (ECG or EKG)
Mayo Clinic in Rochester, Minnesota, has been recognized as one of the top Cardiology & Heart Surgery hospitals in the nation for 2023-2024 by U.S. News & World Report.
- Symptoms & causes
- Diagnosis & treatment
- Doctors & departments
- Care at Mayo Clinic
Mayo Clinic does not endorse companies or products. Advertising revenue supports our not-for-profit mission.
- Opportunities
Mayo Clinic Press
Check out these best-sellers and special offers on books and newsletters from Mayo Clinic Press .
- Mayo Clinic on Incontinence - Mayo Clinic Press Mayo Clinic on Incontinence
- The Essential Diabetes Book - Mayo Clinic Press The Essential Diabetes Book
- Mayo Clinic on Hearing and Balance - Mayo Clinic Press Mayo Clinic on Hearing and Balance
- FREE Mayo Clinic Diet Assessment - Mayo Clinic Press FREE Mayo Clinic Diet Assessment
- Mayo Clinic Health Letter - FREE book - Mayo Clinic Press Mayo Clinic Health Letter - FREE book
Your gift holds great power – donate today!
Make your tax-deductible gift and be a part of the cutting-edge research and care that's changing medicine.

An official website of the United States government
The .gov means it’s official. Federal government websites often end in .gov or .mil. Before sharing sensitive information, make sure you’re on a federal government site.
The site is secure. The https:// ensures that you are connecting to the official website and that any information you provide is encrypted and transmitted securely.
- Publications
- Account settings
Preview improvements coming to the PMC website in October 2024. Learn More or Try it out now .
- Advanced Search
- Journal List
- Arch Med Sci Atheroscler Dis

Kawasaki disease: a comprehensive review
Kamleshun ramphul.
1 Department of Pediatrics, Shanghai Xin Hua Hospital affiliated to the Shanghai Jiao Tong University, School of Medicine, Shanghai, China
Stephanie Gonzalez Mejias
2 Department of Pediatrics, Robert Reid Cabral Children’s Hospital affiliated to the University Iberoamericana UNIBE, School of Medicine, Santo Domingo, Dominican Republic
Kawasaki disease is an acute systemic vasculitis that was first reported in 1961. Over the last 5 decades multiple papers have been published to further understand this disease. The diagnosis of Kawasaki disease is made based on the clinical findings. Atypical Kawasaki disease includes patients who do not meet all the criteria for diagnosis. The main complication of Kawasaki disease is coronary aneurysm, and the treatment is intravenous immunoglobulin and aspirin. A second dose of immunoglobulin is given if the patient does not improve, and several other treatment options have been proposed over the last few years as second and third line options.
Introduction
Kawasaki disease (KD) is characterized as an acute systemic vascular disease that affects mostly medium sized and small vessels [ 1 ]. It is generally self-limited and the highest incidence occurs in children under five years of age. Multiple theories have been proposed for the pathophysiology of the condition but the etiology of KD remains poorly understood. Proper diagnosis based on clinical findings, proper investigations and follow-ups are important to treat and prevent associated complications of the disease.
History of Kawasaki disease
In January 1961, Dr. Tomisaku Kawasaki encountered his first case of Kawasaki disease [ 2 ]. A 4-year-old male patient presented with high fever for 2 weeks, bilateral conjunctival hyperemia, fissured, dried, reddish and bleeding lips, strawberry tongue, and diffuse erythema of the oral cavity and mucous membrane. The patient also had initial left then subsequent right cervical lymphadenopathy. Red palms and soles as well as polymorphous erythema were present all over his body. It was followed by membranous desquamation of the hands and feet over the next 2 weeks.
When the case was presented at the pediatric department meeting of the hospital, the differential diagnosis of atypical scarlet fever and milder forms of Steven-Johnson syndrome was suggested, but no proper diagnosis was confirmed on discharge. Dr. Kawasaki then encountered a second case a year later in 1962 in a 2-year-old male patient presenting with similar symptoms, and by October that year he had come across another 5 cases. He reported these 7 cases in a paper at the Chiba Prefecture Pediatric meeting; however there was no positive feedback at that time.
Over the next 5 years following the initial case, Dr. Kawasaki observed 50 cases with similar presentations, following which he published a paper entitled ‘Acute febrile musculocutaneous lymph node syndrome: clinical observation of 50 cases’ in the Japanese Journal of Allergy in 1967. His paper received proper recognition 35 years later from the western side of the world when Dr. Jane Burns from the University of California San Diego presented the paper in English in the Pediatric Infectious Diseases Journal. From 1970, Dr Kawasaki managed to obtain proper funding to study the disease and the initial diagnostic guidelines were established. The committee also came across cases resulting in death and the autopsy showed presence of coronary aneurysm. Coronary angiography was performed to confirm the presence of coronary aneurysm in some of the patients and the introduction of better 2D echocardiography in 1976 allowed better progress to be made in the field [ 3 ].
Epidemiology
Kawasaki disease has a universal distribution and has been found in children of different ethnicities worldwide. The prevalence of KD is higher in Asian countries such as Japan, where the annual incidence rate rose from 239.6 per 100 000 children in 2010 [ 4 ] to 264 per 100,000 children recently [ 5 ]. It has been reported that the incidence of KD had peaks in January and June/July and was lowest in October. The incidence of the disease was also at its peak in the US during winter as well as early spring [ 6 ]. There is also a higher risk among Korean and Taiwanese children, where 134 and 66, respectively, out of 100 000 children under 5 years of age have the disease [ 7 , 8 ]. The prevalence is significantly lower in non-Asian countries; 8.39 per 100 000 children in England [ 9 ], and 9.34 per 100 000 children under 5 years in Australia [ 10 ].
Etiology and pathophysiology
Multiple theories have been hypothesized for the etiology of KD. It is currently believed that there is a genetic predisposition to the development of KD and the interaction of an unknown infective cause can also predispose to KD [ 11 ].
Current evidence strongly suggests that genetic factors have a more important role in the occurrence of KD. Polymorphisms of the IgG receptor can increase the susceptibility of children to KD and increase the risk of developing coronary artery aneurysm. The higher prevalence of KD among Asian countries such as Japan, Korea and Taiwan compared to non-Asian countries also suggests a strong genetic susceptibility based on ethnicity. Japanese American children in Hawaii had a higher incidence rate of 210 per 100 000 compared to 13 per 100 000 in Caucasian American children living in the same area in Hawaii [ 12 ]. Various genome-wide association studies in KD have also been reported [ 13 – 17 ].
An infective cause was also suggested for the incidence of KD. The major epidemics of KD in Japan have shown origination in specific areas and peak incidence in specific months [ 18 ]. The acute onset of the disease mimics viral and bacterial infection patterns. In 2013 Jaggi et al. suggested that there could be a relationship between an infection with adenovirus and the onset of KD. Other studies have suggested that a virus could have been inhaled and later engulfed by tissue macrophages leading to activation of the innate immune response. Further activation of the adaptive immune responses can lead to antigen specific T lymphocytes and plasma cell activation. The presence of the infection in coronary tissue leads to secretion of multiple growth factors such as vascular endothelial growth factors, tumor necrosis factor-α (TNF-α), and metalloproteinase 9, leading to destruction of the intima, and fragmentation of the internal and external elastic lamina of the coronary artery. This results in the development of a coronary artery aneurysm [ 19 ] Furthermore, it has also been suggested that KD antibodies can identify intra-cytoplasmic inclusion bodies in tissues. Other studies have also reported several inclusion bodies corresponding to aggregates of viral RNA and proteins that are yet to be identified [ 20 ].
The diagnosis of KD relies mostly on clinical signs and symptoms. The criteria in the US require the presence of fever for at least 5 days along with four of five other clinical features listed in Table I .
Diagnostic criteria for Kawasaki disease. Four of these five plus a fever of more than 5 days confirms the diagnosis of Kawasaki disease
An algorithm was created by the American Heart Association (AHA) in 2004 to help identify patients at risk [ 21 ]. The statement suggests the use of supportive laboratory testing and echocardiography to help detect patients who are at risk and do not meet all the criteria for diagnosis. These were termed “incomplete” KD or “atypical” KD patients, but they are still at risk for developing KD. An Australian study suggested that this occurs in up to 9.6% of cases of KD [ 10 ]. Suggested laboratory testing included markers of inflammation, presence of anemia, leukocytosis, thrombocytosis, hypoalbuminemia, elevated liver enzymes and possible sterile pyuria. An echocardiogram can also help rule out the presence of any early coronary artery abnormalities or involvement.
The multiple differential diagnosis to rule out KD includes drug hypersensitivity, Stevens-Johnson syndrome, staphylococcal scalded skin syndrome, juvenile idiopathic arthritis, toxic shock syndrome, viral infection, and streptococcal scarlet fever [ 21 ].
Kawasaki disease is treated with intravenous immunoglobulin (IVIG) and high dose aspirin, although the use of aspirin in the management has been controversial in some studies [ 22 – 24 ]. A meta-analysis showed that the use of corticosteroids with IVIG as initial treatment has a better effect on reducing the risk of coronary artery abnormalities compared to the use of IVIG only [ 25 ]. It is highly recommended to start the treatment immediately if the patient meets the clinical criteria for KD.
The IVIG can prevent the development of coronary artery aneurysms. The use and dose of IVIG have been supported by multiple randomized controlled trials [ 26 – 28 ]. It has a dose-dependent effect; a single dose of 2 g/kg is administered within 10 days of illness or later if the patient has signs of inflammation, persistent fever or aneurysm on echocardiography. It can lower the risk of giant aneurysm to 1% and coronary artery aneurysms from 25% to less than 5% [ 21 , 22 ]. The IVIG can influence the T cell activity and reduce the synthesis of antibodies and cytokines causing the symptoms of KD [ 21 , 29 – 32 ].
Aspirin is believed to modify the inflammatory state present in KD and prevent the risk of thrombosis. Some studies have however questioned the impact of aspirin use in KD to prevent the development of coronary aneurysm [ 21 , 22 , 24 , 33 ]. The AHA recommends administration of a high dose of aspirin of 80 to 100 mg/day divided into 4 doses until the patient is afebrile for 48 to 72 h. The dose is then reduced to 3 to 5 mg/kg/day as a single dose for 6 to 8 weeks after the onset of the disease. It is also recommended to continue the use of aspirin if there are any persisting coronary abnormalities [ 21 , 24 ]. Children on long courses of aspirin are recommended to receive the influenza vaccine to prevent the risk of Reye syndrome.
If the patient does not respond to the initial therapy, a second infusion of IVIG at 2 g/kg is administered. Kobayashi et al. conducted a retrospective study in 2013 and found that the use of corticosteroids along with IVIG for patients with refractory KD showed better improvement and also lowered the risk of coronary aneurysms [ 34 ]. The AHA has also suggested the use of TNF-α antagonists in patients who do not respond to IVIG. The use of infliximab had faster resolution compared to IVIG retreatment in some studies, while other studies have shown that the use of infliximab helped decrease the duration of fever but did not provide an improvement in treatment over IVIG and aspirin when used alone [ 35 ]. Patients with refractory KD and mild to moderate aneurysm can be treated with aspirin alone or along with antiplatelet agents. Larger aneurysms can be treated with heparin and warfarin as required [ 21 ].
Other possible treatment options that have been proposed include the use of cyclosporine A and methotrexate. Cyclosporine A is a calcineurin inhibitor that targets the Ca 2+ /NFAT signaling pathway. It leads to a decrease in inflammatory response [ 13 , 36 ]. It has been used as a third line of treatment in some cases of KD in Japan [ 37 , 38 ]. Methotrexate can lead to the accumulation of polyglutamate metabolites in the cells [ 39 ]. Since the mechanism of action and its role in KD are not fully understood, the AHA does not have any recommendations for its use in KD [ 40 ].
Prognostic factors
Some prognostic factors for the development of coronary aneurysm in KD patients identified in some studies that involved Japanese children are male sex, C-reactive protein (CRP) > 200 mg/dl, age less than 12 months old or more than 8 years old, albumin < 35 g/l, platelet count < 35 × 10 4 /mm 3 , delay of initiation of IVIG, lower dose of IVIG and recurrent KD [ 41 , 42 ]. One study suggested a score system to identify patients at risk of IVIG resistance as shown in Table II . A combined score of 0 to 3 predicted a low risk while a score of more than 4 suggested a higher risk of having resistance to IVIG therapy [ 43 , 44 ].
Predicting the risk of intravenous immunoglobulin (IVIG) resistance in Kawasaki disease
Long-term follow-up
The AHA recommends a baseline echocardiography examination during the acute phase of the illness. Repeated echocardiography is also recommended at week 2 and weeks 6–8 after the illness [ 21 ]. If there is evidence of higher risk factors or poor recovery, more frequent imaging is recommended.
The AHA also advised that patients who are at low risk and with no detectable coronary abnormalities should be followed for at least 10 to 20 years after being diagnosed with KD, even in the absence of any abnormalities. For moderate-risk patients if there is regression of the aneurysm at weeks 6 to 8, they can be treated as low-risk patients. Regular cardiac assessments every 3 to 5 years are recommended. High-risk patients have a higher likelihood of having progression to coronary artery stenosis. The long-term use of anti-platelet therapy and warfarin or heparin is strongly recommended. β-Blockers can be added to decrease the myocardial oxygen demand. A thorough cardiac examination, electrocardiogram and echocardiogram should be performed at least twice a year. It is also advised to do a yearly stress test and perfusion study. Patients should be advised to limit physical activities based on their conditions and their risks of bleeding. More invasive tests such as angiography can be performed as needed per case [ 45 ].
Conclusions
While Kawasaki disease remains a poorly understood condition, it can be managed with proper treatment and follow-up. A rapid diagnosis and treatment can immensely help improve the prognosis and lower the associated complications of KD. Recent advances in the treatment and detection of KD such as the discovery of NT-proBNP as a biomarker for early detection of the disease show a promising future to combat KD. More expert consensus along with multiple published clinical experiences will continue to help in guiding the proper management of KD.
Conflict of interest
The authors declare no conflict of interest.
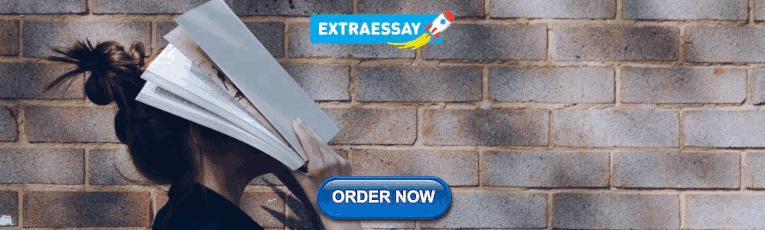
IMAGES
VIDEO
COMMENTS
The Kawasaki Disease Research Center is a joint collaboration between the Departments of Pediatrics, Medicine, and Family and Preventive Medicine and Sociology at UCSD, the Scripps Institute of Oceanography, and Rady Children's Hospital San Diego. The Program was created to help foster excellence in care for patients with Kawasaki Disease (KD ...
The Kawasaki Disease Foundation is a 501 (c) (3) nonprofit organization. The Foundation's tax exemption number and federal tax ID are 17053137024021 and 04-3536123, respectively. KDF is supported by donations and volunteers. We have no paid staff, and our operating costs are minimal.
The Kawasaki Fund internship is an excellent opportunity for ambitious students to learn about charity work and to gain some valuable work experience to bolster your CV. Desired profile - Recent graduate (no more than 1-2 years post graduate work experience) or current student at a leading school with discipline in Biology, Biomedical Sciences ...
The Summer Pediatric Internship in Research is a 7-week summer program combining research and clinical aspects of pediatrics and/or pediatric subspecialties. The Summer Pediatric Internship in Research is a mentored program designed to provide research experience to first-year Jacobs School medical students. ... Kawasaki disease; B cell ...
Lurie Children's has been a leader in clinical research on Kawasaki disease dating back to the treatment trials in the 1980s and early 1990s. Our clinician scientists are continuing their research efforts in finding the cause of this illness, improving treatment and identifying factors that determine susceptibility. Anne H. Rowley, MD>>.
She was Chief of the Division of Allergy, Immunology, and Rheumatology from 2000 to 2013. Currently, Dr. Burns is Director of the Kawasaki Disease Research Center at UCSD/Rady Children's Hospital where she leads a multidisciplinary team that cares for 90-100 new Kawasaki Disease patients each year and follows over 1,300 families in the KD Clinic.
The team is recruiting patients for a clinical trial to evaluate the treatment of children with acute Kawasaki disease and coronary artery disease with atorvastatin. If you are a parent of a child with Kawasaki dsease and would like to learn more about this study, please call the Hospital operator at 858-576-1700 and ask for the Kawasaki ...
KAWASAKI FUND CHARITY REG:1064518. Interns invited! Exciting opportunity for a bright medical research student to intern with us at Kawasaki Disease UK. Our office is in West London. If you are ...
Kawasaki Disease Research. Lurie Children's has been a leader in clinical research on Kawasaki disease dating back to the treatment trials in the 1980s and early 1990s. Our clinician scientists are continuing their research efforts in finding the cause of this illness, improving treatment and identifying factors that determine susceptibility.
Pathology of Kawasaki disease: I: pathology and morphogenesis of the vascular changes. Jpn Circ J. 1979; 43:633-643. Crossref Medline Google Scholar; 75. Naoe S, Takahashi K, Masuda H, Tanaka N. Kawasaki disease: with particular emphasis on arterial lesions. Acta Pathol Jpn. 1991; 41:785-797. Medline Google Scholar; 76. Landing BH, Larson EJ.
The efficacy of other agents (atorvastatin, doxycycline) is currently being investigated. MIS-C is a clinically distinct entity from KD with broad clinical manifestations and multiorgan involvement (cardiac, GI, hematologic, dermatologic, respiratory, renal). MIS-C with Kawasaki manifestations is more commonly seen in children < 5 years of age.
Abstract. Kawasaki disease is a necrotising small-to-medium vessel vasculitis affecting children between age groups of 6 months and 5 years. Following the first description in Japanese infants, it has been recognised as the single most common cause of non-infectious vasculitis in children worldwide. Presentation in adult age groups, although ...
Well, as our intern you will be working under the guidance of Dr Qian Xu, who is an Emergency Medicine Doctor, and get a wide-range of hands on experiences that will include data analysis and research on Kawasaki Disease, day-to-day liaising and integrating with the core team to complete key priorities and a chance to help develop awareness of ...
Kawasaki disease (KD) is an acute systemic inflammation illness primarily affecting children [1, 2].The diagnosis of KD is made up of fever of at least 5 days' duration in addition to four of five signs of mucocutaneous inflammation, which included bilateral conjunctival injection, erythema of the lips and oral cavity, cervical lymphadenopathy, changes in the distal extremities, and rash [3 ...
Summary. Kawasaki disease (KD) is a rare systemic inflammatory disease that predominately affects children less than 5 years of age. Pathogenesis of KD remains unknown; the leading theory is that an unknown stimulus triggers an immune-mediated inflammatory cascade in a genetically susceptible child. Classic KD is a clinical diagnosis based on ...
Kawasaki disease is an acute febrile illness and systemic vasculitis of unknown aetiology that predominantly afflicts young children, causes coronary artery aneurysms and can result in long-term ...
Owners: Shaila Siraj, MD; Meghan Martin, MD;; Juan Dumois, MD; Ashish Shah, MD; Courtney Titus, PA-C Updated July 2021
Kawasaki disease (KD) is an acute, self-limiting systemic vasculitis that predominately affects children aged 6 months to 5 years. ... Adolescent Kawasaki disease with uveitis. Intern Med J 2002; 32: 421-422. [Google Scholar] 19. ... Articles from The Journal of International Medical Research are provided here courtesy of SAGE Publications ...
Epidemiology. In the past, the illness may have masqueraded in various guises, and old reports on infantile polyarteritis nodosa in Western countries describe pathological findings identical to those of fatal KD. 4-8 First described in Japan, KD has now been described worldwide. 9-17 However, the disease is markedly more prevalent in children in Japan, where the annual incidence was 243.1 ...
Abstract. Kawasaki disease (KD) is characterized with acute systemic vasculitis, occurs predominantly in children between 6 months to 5 years of age. Patients with this disease recover well and the disease is self-limited in most cases. Since it can lead to devastating cardiovascular complications, KD needs special attention.
Symptoms. Symptoms of Kawasaki disease include a fever greater than 102.2 degrees Fahrenheit (39 degrees Celsius) for five or more days. And the child has at least four of the following symptoms. A rash on the main part of the body or in the genital area. An enlarged lymph node in the neck.
Abstract. Kawasaki disease is an acute systemic vasculitis that was first reported in 1961. Over the last 5 decades multiple papers have been published to further understand this disease. The diagnosis of Kawasaki disease is made based on the clinical findings. Atypical Kawasaki disease includes patients who do not meet all the criteria for ...