- Age: 14-16 MYP Individuals and Societies
- Age: 14-16 GCSE / IGCSE Geography
- Natural Environments
- Economic Development
- IGCSE Geography Revision Question Bank
- 2.1 Earthquakes and volcanoes
- 2.4 Weather
- 2.5 Climate and natural vegetation
- Distribution
- Plate Tectonics
- Plate Boundaries | Plate Margins
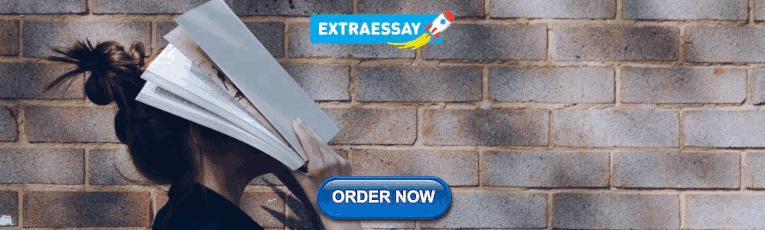
Volcano case study - Mount Etna (2002-2003), Italy
- Volcano case study - Mount Nyiragongo, Democratic Republic of Congo
- Volcanic hazard management - Mount Rainier, USA
- Earthquakes
- Earthquake case study - 2005 Kashmir
- Earthquake case study - Chuetsu Offshore Earthquake - 2007
- Why was the Haitian Earthquake so deadly?
- Earthquakes - Managing the hazard
Can you describe the location of Mount Etna? Could you draw a sketch map to locate Mount Etna?

Case study task
Use the resources and links that can be found on this page to produce a detailed case study of the 2002-2003 eruption of Mount Etna. You should use the 'Five W's" subheadings to give your case study structure.
What happened?
The Guardian - Sicilian city blanketed in ash [28 October 2002]
When did it happen?
Immediately before midnight on 26 October 2002 (local time=GMT+1), a new flank eruption began on Mount Etna. The eruption ended after three months and two days, on 28 January 2003.
Where did it happen?
The eruption occurred from fissures on two sides of the volcano: at about 2750 m on the southern flank and at elevations between 2500 and 1850 m on the northeastern flank.

Why did it happen?
Mount Etna is a volcano. The reasons why Mount Etna is located where it is are complex. Here are some of the theories:
- One theory envisages a hot spot or mantle-plume origin for this volcano, like those that produce the volcanoes in Hawaii.
- Another theory involves the subduction of the African plate under the Eurasian plate.
- Another group of scientists believes that rifting along the eastern coast of Sicily allows the uprise of magma.
Who was affected by it happening?
- The Italian Government declared a state of emergency in parts of Sicily, after a series of earthquakes accompanying the eruption of forced about 1,000 people flee their homes.
- A ship equipped with a medical clinic aboard was positioned off Catania - to the south of the volcano - to be ready in case of emergency.
- Emergency workers dug channels in the earth in an attempt to divert the northern flow away from the town of Linguaglossa.
- Schools in the town have been shut down, although the church has remained open for people to pray.
- Villagers also continued their tradition of parading their patron saint through the streets to the railway station, to try to ward off the lava flow.
- Civil protection officials in Catania, Sicily's second-biggest city, which sits in the shadow of Etna, surveyed the mountain by helicopter and were ready to send water-carrying planes into the skies to fight the fires.
- The tourist complex and skiing areas of Piano Provenzana were nearly completely devastated by the lava flows that issued from the NE Rift vents on the first day of the eruption.
- Heavy tephra falls caused by the activity on the southern flank occurred mostly in areas to the south of the volcano and nearly paralyzed public life in Catania and nearby towns.
- For more than two weeks the International Airport of Catania, Fontanarossa, had to be closed due to ash on the runways.
- Strong seismicity and ground deformation accompanied the eruption; a particularly strong shock (magnitude 4.4) on 29 October destroyed and damaged numerous buildings on the lower southeastern flank, in the area of Santa Venerina.
- Lava flows from the southern flank vents seriously threatened the tourist facilities around the Rifugio Sapienza between 23 and 25 November, and a few days later destroyed a section of forest on the southwestern flank.
- The eruption brought a heightened awareness of volcanic and seismic hazards to the Sicilian public, especially because it occurred only one year and three months after the previous eruption that was strongly featured in the information media.
Look at this video clip from an eruption on Mount Etna in November 2007. What sort of eruption is it?
There is no commentary on the video - could you add your own explaining what is happening and why?
You should be able to use the knowledge and understanding you have gained about 2002-2003 eruption of Mount Etna to answer the following exam-style question:
In many parts of the world, the natural environment presents hazards to people. Choose an example of one of the following: a volcanic eruption, an earthquake, or a drought. For a named area, describe the causes of the example which you have chosen and its impacts on the people living there. [7 marks]
- Comment on Twitter
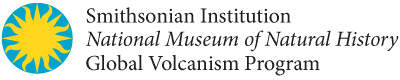
- Current Eruptions
- Smithsonian / USGS Weekly Volcanic Activity Report
- Bulletin of the Global Volcanism Network
- Weekly Report 20th Anniversary
- Holocene Volcano List
- Pleistocene Volcano List
- Country Volcano Lists
- Volcano Search
- Eruption Search
- Deformation Search
- Emission Search
- Webservices
- Database Information
- Image Collections
- Video Collections
- Theme Collections
- Keyword Collections
- St. Helens 40th Anniversary
- Frequent Questions
- Information Sources
- Google Earth Placemarks
- This Dynamic Planet
- Eruptions, Earthquakes & Emissions Application
- Volcano Numbers
- Volcano Naming
- How to Cite
- Terms of Use
Report on Etna (Italy) — November 2002
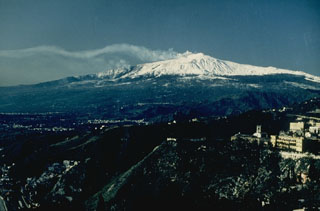
Bulletin of the Global Volcanism Network, vol. 27, no. 11 (November 2002) Managing Editor: Richard Wunderman. Etna (Italy) Witnesses saw N- and S-flank eruptions begin at around 0200 on 27 October
Please cite this report as: Global Volcanism Program, 2002. Report on Etna (Italy) (Wunderman, R., ed.). Bulletin of the Global Volcanism Network , 27:11. Smithsonian Institution. https://doi.org/10.5479/si.GVP.BGVN200211-211060
37.748°N, 14.999°E; summit elev. 3357 m
All times are local (unless otherwise noted).
After the violent flank eruption of July-August 2001, Mount Etna was rather calm for more than 10 months, except for usual fumes from the four summit craters [and minor ash emissions]. In the first days of July 2002 weak magmatic activity resumed sporadically at the NE Crater with ejection of bombs that fell on the outer slopes of the cone. On 12 September explosions occurred every 2 or 3 minutes and were violent enough to throw large spatter as far as the northern rim of the Voragine (Central Crater). However, there were many days without explosive activity and, at other times, the NE Crater emitted large clouds of brownish ash. Although a magnitude 3.7 earthquake had struck the northern flank of the volcano on 22 September, subsequent days were so calm that, to these contributors, the following events came as quite a surprise.
As the National Institute of Geophysics and Volcanology (INGV) previously reported ( BGVN 27:10), a seismic swarm began to shake Etna late during the evening of 26 October 2002. One observer, Maurice Aubert, happened to be in a hotel on the northern flank (at Piano Provenzana, 1,816 m elevation). There the seismic shocks were distinctly felt after midnight and rapidly reached hazardous levels. Hours later, at 0205 on 27 October, lava fountains began to play along a fissure 1-2 km up slope, but decreased at 0220 when lava flows expanded downwards.
The seismic intensity of earthquakes felt the night of the 26th ranged from II to VII or perhaps VIII. The approximate timing and seismic intensity was recorded as follows at 0030, II; at 0140, VI; at 0200, VI; at 0320, VII; and at 0343, VII or VIII. Maurice Aubert and his group hastily retreated shortly after 0320, exiting while cracks were developing through the mountain road. The last of the above-reported intensities was felt during their departure, when a strong earthquake shook their car.
Vents at ~2,700 m elevation on the southern flank (on the Piano del Lago) are here called the S2700 vents. These new S-flank vents lay just SE of the ancient cone of Monte Frumento Supino and ~800 m NW of the Laghetto cone, which appeared in 2001.
Watching the S2700 vents, Giuseppe Scarpinati saw two lava fountains develop after 0200, together with a large ash plume that drifted S. The eruptive phenomena were accompanied by strong detonations and rumblings together with continuous earthquakes that were felt in Acireale, a town at Etna's southeastern foot.
Lava flows from the northern vents invaded and over ran the flat area containing tourist facilities at Piano Provenzana and proceeded as two branches downwards through the pine trees towards Linguaglossa, a village ~10 km to the NE. The greatest damage was not the loss of all tourist facilities at Piano Provenzana, but was instead due to heavy ashfall S of the volcano, which led to closing of the Catania airport on the afternoon of 27 October.
On the morning of 28 October the S fissure had developed at least three explosive vents. A 100-to-200-m-high lava fountain, ~200 m downslope, fed lava flows that extended by more than 2 km toward the uninhabited area of Monte Nero degli Zappini (figures 97 and 98). During the day, however, the effusive activity significantly decreased, and on 29 October the lava fronts virtually stopped on the southern side, although violent degassing at the upper end of the fissure continued unabated. Sustained release of high pressure gas fed a voluminous SE-directed ash plume that reached to more than 5 km altitude. At the same time on the 29th, a large plume of white vapor was emitted at the summit from the central crater vents (Bocca Nuova, Voragine) and the NE Crater. The SE crater, the main site of the 2001 eruption, remained entirely calm.
Strong earthquakes on 29 October caused damage on the lower E flank of the mountain, particularly at Santa Venerina where some 1,000 people were left homeless. The main shock was recorded by Jean-Claude Tanguy in the SE region of the volcano (Trecastagni) at 17 seconds after 1102 (± 5 sec). Horizontal ground motions there lasted 7 to 8 seconds. The INGV reported the seismic event as M 4.4, located 8-9 km beneath Santa Venerina. Other strong shocks at 1739 and 1814 (M 4.0 and 4.1) caused walls to collapse along the road between Zafferana and Milo.
On 30 October soon after midday the Bocca Nuova vent began to emit large clouds of brownish ash. This activity culminated between 1310 and 1320, and the ash cloud merged into the still large, dark ash plume from the southern lateral vents. However, Strombolian explosive activity was still vigorous at the main explosive center, which included a group of about six vents near 2,000 m elevation (called the N2000 vents). These vents, which produced photogenic activity into the night (figure 99), lie just to the E of an old cinder cone known as Monte Ponte di Ferro (at 2,040 m elevation). Here the accumulation of pyroclasts had built a spatter rampart ~200 m long and 30 to 40 m high, the upper part of which reached 2,035 m elevation (± 5 m, measured from Mt. Ponte di Ferro using both altimeter and inclinometer).
On 31 October the wind gradually shifted from the N to the W and then SW, so that ashfall from S2700 vents affected localities NE of the volcano including Reggio di Calabria, whose airport also had to be closed. At the northern vents the lava effusion was on a waning stage, but violent explosions from the two upper vents of the N2000 group threw blocks of ancient material amid juvenile tephra (figure 100).
On 1 November all activity ceased on the northern side except for very small residual lava flows, but the S2700 upper vent appeared to enter a phase of sustained explosive activity resembling a small subplinian column that continued to cause disruptions around the volcano. It was not until 12 November at 1340 that the activity abruptly changed to typical Strombolian explosions of liquid lava clots with loud detonations. On 13 November at about 1600 a small lava flow began to trickle from the lower base of the S2700 cone. The lava effusion increased on 14 November, expanding downwards along the 27-28 October flows. Meanwhile ash emission recommenced at the S2700 crater.
This kind of eruption style is quite unusual at Mount Etna. The authors suggest that it could indicate that a considerable amount of magma has intruded into the S rift zone, which would account for strong degassing without any significant lava effusion between 2 and 13 November.
Geological Summary. Mount Etna, towering above Catania on the island of Sicily, has one of the world's longest documented records of volcanism, dating back to 1500 BCE. Historical lava flows of basaltic composition cover much of the surface of this massive volcano, whose edifice is the highest and most voluminous in Italy. The Mongibello stratovolcano, truncated by several small calderas, was constructed during the late Pleistocene and Holocene over an older shield volcano. The most prominent morphological feature of Etna is the Valle del Bove, a 5 x 10 km caldera open to the east. Two styles of eruptive activity typically occur, sometimes simultaneously. Persistent explosive eruptions, sometimes with minor lava emissions, take place from one or more summit craters. Flank vents, typically with higher effusion rates, are less frequently active and originate from fissures that open progressively downward from near the summit (usually accompanied by Strombolian eruptions at the upper end). Cinder cones are commonly constructed over the vents of lower-flank lava flows. Lava flows extend to the foot of the volcano on all sides and have reached the sea over a broad area on the SE flank.
Information Contacts: Jean-Claude Tanguy , University of Paris 6 & Institut de Physique du Globe, 94107 St. Maur des Fossés, France; Maurice Aubert , University of Clermont-Ferrand, Department of Geology, 63038 Clermont-Ferrand, France; Roberto Clocchiatti , CNRS-CEN Saclay, Lab. Pierre Süe, 91191 Gif sur Yvette, France; Santo La Delfa and Giuseppe Patané , University of Catania, Department of Geological Sciences, Corso Italia 55, 95129 Catania, Italy; Giuseppe Scarpinati ,via Muggia 7, 95024 Acireale, Italy.

A multi-disciplinary study of the 2002-03 Etna eruption: insights into a complex plumbing system
Research output : Contribution to journal › Article › peer-review
- multi-disciplinary study
- 2002-03 eruption
- eccentric eruptions
- flank activity
- etna feeding system
- volcanic processes
- pernicana fault
Access to Document
- 10.1007/s00445-004-0372-8
- http://download.springer.com/static/pdf/9/art%253A10.1007%252Fs00445-004-0372-8.pdf?originUrl=http%3A%2F%2Flink.springer.com%2Farticle%2F10.1007%2Fs00445-004-0372-8&token2=exp=1440429750~acl=%2Fstatic%2Fpdf%2F9%2Fart%25253A10.1007%25252Fs00445-004-0372-8.pdf%3ForiginUrl%3Dhttp%253A%252F%252Flink.springer.com%252Farticle%252F10.1007%252Fs00445-004-0372-8*~hmac=1f8108d7e5c19089f8bcdfae7dce3e3ca4e498e63d754cf52dd2d8f1a213d6e1
Fingerprint
- Investigation Earth and Planetary Sciences 100%
- Volcanic Eruption Earth and Planetary Sciences 100%
- Magma Earth and Planetary Sciences 41%
- Lava Flow Earth and Planetary Sciences 25%
- Fissure Earth and Planetary Sciences 25%
- Output Earth and Planetary Sciences 16%
- Fraction Earth and Planetary Sciences 16%
- Petrology Earth and Planetary Sciences 8%
T1 - A multi-disciplinary study of the 2002-03 Etna eruption: insights into a complex plumbing system
AU - Andronico, D
AU - Branca, S
AU - Calvari, S
AU - Burton, M
AU - Caltabiano, T
AU - Corsaro, R A
AU - Del Carlo, P
AU - Garfi, G
AU - Lodato, L
AU - Miraglia, L
AU - Mure, F
AU - Neri, M
AU - Pecora, E
AU - Pompilio, M
AU - Salerno, G
AU - Spampinato, L
N1 - 914kk Times Cited:171 Cited References Count:54
N2 - The 2002 - 03 Mt Etna flank eruption began on 26 October 2002 and finished on 28 January 2003, after three months of continuous explosive activity and discontinuous lava flow output. The eruption involved the opening of eruptive fissures on the NE and S flanks of the volcano, with lava flow output and fire fountaining until 5 November. After this date, the eruption continued exclusively on the S flank, with continuous explosive activity and lava flows active between 13 November and 28 January 2003. Multi-disciplinary data collected during the eruption ( petrology, analyses of ash components, gas geochemistry, field surveys, thermal mapping and structural surveys) allowed us to analyse the dynamics of the eruption. The eruption was triggered either by (i) accumulation and eventual ascent of magma from depth or (ii) depressurisation of the edifice due to spreading of the eastern flank of the volcano. The extraordinary explosivity makes the 2002 - 03 eruption a unique event in the last 300 years, comparable only with La Montagnola 1763 and the 2001 Lower Vents eruptions. A notable feature of the eruption was also the simultaneous effusion of lavas with different composition and emplacement features. Magma erupted from the NE fissure represented the partially degassed magma fraction normally residing within the central conduits and the shallow plumbing system. The magma that erupted from the S fissure was the relatively undegassed, volatile-rich, buoyant fraction which drained the deep feeding system, bypassing the central conduits. This is typical of most Etnean eccentric eruptions. We believe that there is a high probability that Mount Etna has entered a new eruptive phase, with magma being supplied to a deep reservoir independent from the central conduit, that could periodically produce sufficient overpressure to propagate a dyke to the surface and generate further flank eruptions.
AB - The 2002 - 03 Mt Etna flank eruption began on 26 October 2002 and finished on 28 January 2003, after three months of continuous explosive activity and discontinuous lava flow output. The eruption involved the opening of eruptive fissures on the NE and S flanks of the volcano, with lava flow output and fire fountaining until 5 November. After this date, the eruption continued exclusively on the S flank, with continuous explosive activity and lava flows active between 13 November and 28 January 2003. Multi-disciplinary data collected during the eruption ( petrology, analyses of ash components, gas geochemistry, field surveys, thermal mapping and structural surveys) allowed us to analyse the dynamics of the eruption. The eruption was triggered either by (i) accumulation and eventual ascent of magma from depth or (ii) depressurisation of the edifice due to spreading of the eastern flank of the volcano. The extraordinary explosivity makes the 2002 - 03 eruption a unique event in the last 300 years, comparable only with La Montagnola 1763 and the 2001 Lower Vents eruptions. A notable feature of the eruption was also the simultaneous effusion of lavas with different composition and emplacement features. Magma erupted from the NE fissure represented the partially degassed magma fraction normally residing within the central conduits and the shallow plumbing system. The magma that erupted from the S fissure was the relatively undegassed, volatile-rich, buoyant fraction which drained the deep feeding system, bypassing the central conduits. This is typical of most Etnean eccentric eruptions. We believe that there is a high probability that Mount Etna has entered a new eruptive phase, with magma being supplied to a deep reservoir independent from the central conduit, that could periodically produce sufficient overpressure to propagate a dyke to the surface and generate further flank eruptions.
KW - multi-disciplinary study
KW - mount etna
KW - 2002-03 eruption
KW - eccentric eruptions
KW - flank activity
KW - etna feeding system
KW - volcanic processes
KW - mount-etna
KW - mt.-etna
KW - pernicana fault
KW - mechanisms
KW - ascent
U2 - 10.1007/s00445-004-0372-8
DO - 10.1007/s00445-004-0372-8
M3 - Article
SN - 1432-0819
JO - Bulletin of Volcanology
JF - Bulletin of Volcanology
- Search Menu
- Volume 237, Issue 3, June 2024 (In Progress)
- Volume 237, Issue 2, May 2024
- Advance Access
- Applied and Marine Geophysics
- General Geophysical Methods
- Geodynamics and Tectonics
- Gravity, Geodesy and Tides
- Heat Flow and Volcanology
- Magnetic and Electrical Fields
- Rock and Mineral Physics, Rheology
- 100 Influential Papers
- Advance Articles
- Express Letters
- Hunga Volcano Special Issue
- East Anatolia Fault Special Issue
- Special Issues
- Why Publish
- Author Guidelines
- Submission Site
- Read & Publish
- Developing Countries Initiative
- Author Resources
- Self-Archiving policy
- Rights and Permissions
- About Geophysical Journal International
- Editorial Board
- About the Royal Astronomical Society
- About the DGG
- Journals on Oxford Academic
- Books on Oxford Academic

Article Contents
1 the 2002 ne-rift eruption, 2 network and surveys, 3 gps data: presentation and modelling, 4 gravity data: presentation and modelling, 5 discussion, 6 concluding remarks.
- < Previous
Intrusive mechanism of the 2002 NE-rift eruption at Mt Etna (Italy) modelled using GPS and gravity data
- Article contents
- Figures & tables
- Supplementary Data
Alessandro Bonforte, Daniele Carbone, Filippo Greco, Mimmo Palano, Intrusive mechanism of the 2002 NE-rift eruption at Mt Etna (Italy) modelled using GPS and gravity data, Geophysical Journal International , Volume 169, Issue 1, April 2007, Pages 339–347, https://doi.org/10.1111/j.1365-246X.2006.03249.x
- Permissions Icon Permissions
Microgravity and GPS data collected at Mt Etna during a 1-yr time interval encompassing the 2002 NE-rift eruption are analysed. The common GPS-gravity profile traverses the summit area of Mt Etna, between the summit craters (about 3000 m) and the northernmost tip of the eruptive fractures (2500 m). Displacements (up to 2 m in both the horizontal and vertical directions) and gravity variations (up to 350 μGal, after having removed the effect of elevation changes) observed during this period are among the largest ever recorded at Etna.
Displacements and gravity changes have been modelled separately, assuming a magma influx from the summit feeder pipe to the NE-rift. Models obtained through surface deformation data partially overlap with those explaining the gravity data but in general are narrower and extend to a greater depth. The discrepancies found between gravity and deformation models are significant and can be regarded as due to the different structural features encountered by the intruding magma during its downslope propagation along the NE-rift. In particular, on the grounds of our result, we infer that both the eastward sliding of the east flank of the volcano and the curved shape of the NE-rift influenced the 2002 intrusive process.
The NE-rift, one of the main intrusion zones of Etna, covers a 2-km wide and 7-km long area which stretches from 2500 to 1700 m a.s.l. ( Fig. 1 ). It consists of subparallel eruptive fissures with azimuth values between 42°E and 62°E ( Kieffer 1975 ). The NE-rift is affected by strong extensional tectonics which several authors consider to be controlled by the gravitational collapse of Etna's eastern flank towards ESE ( Borgia et al. 1992 ; Lo Giudice & Rasà 1992 ; Froger et al. 2001 ). During the last 300 yr, several eruptive events occurred from fissure systems which formed from the base of the summit craters down to the NE-rift zone ( Romano & Sturiale 1982 ; Branca & Del Carlo 2003 ).

Schematic map showing the position of the GPS and gravity benchmarks and the area covered by the lava flows from Etna's 2002 NE-rift eruption. Inset legend: (1) fault; (2) eruptive fissure; (3) lava flow; (4) gravity benchmark and (5) GPS benchmark.
The 2002 NE-rift eruption was heralded by a seismic swarm beginning at about 20:12 GMT on October 26 ( Andronico et al. 2005 ). In the early morning of October 27, a set of extensional fractures formed in the Piano delle Concazze area, (2800 m a.s.l.; Fig. 1 ) and during the following hours, the fissure system propagated downslope along the eastern border of the NE-rift, until 1900 m a.s.l. ( Fig. 1 ), producing both explosive and effusive activity.
Lava was first erupted (October 27) from vents located at 2400 and 2250 m a.s.l. Afterwards, a new vent located at 2150 m a.s.l. started outpouring lava at a high effusion rate. It flowed northeastwards to the Piano Provenzana area ( Fig. 1 ) and then eastward. In the early morning of the 28th, another vent opened at the lower tip of the eruptive fissure system at 1920–1900 m a.s.l. and two lava flows were emitted, northeastwards and eastwards. During the following days the effusion rate from all the vents gradually decreased and by November the eruption came to an end. About 10 × 10 6 m 3 of lava was erupted from these NE fractures ( Andronico et al. 2005 ).
In the present study, GPS and gravity surveys, carried out on Mt Etna along a common profile on the summit area of the volcano ( Fig. 1 ) and during a time interval (2002 June–2003 July) encompassing the 2002 NE-rift eruption, are analysed. The profile includes 15 gravity and 13 GPS stations (11 in common, i.e. within 200 m from each other), which lie between the summit craters zone (about 3000 m a.s.l.) and the Piano Provenzana area (about 1770 m a.s.l.; Fig. 1 ).
Along this profile measurements are usually performed when the snow cover does not prevent the stations from being accessed by car (measurements can usually be accomplished between June and October).
GPS measurements along this profile are usually carried out by the semi-kinematic method, which allows great spatial detail to be achieved in less time than the static method, used to measure the other stations on the volcano ( Bonforte & Puglisi 2003 ; Bonforte et al. 2004 ) and with an acceptable accuracy. The semi-kinematic sessions are carried out using at least three static stations of the Mt Etna GPS monitoring network as reference, while a roving receiver moves from one benchmark to another along the NS profile. The benchmarks are occupied in sequence, the profile being remeasured two times. Exceptionally, during the July 2003 survey, the above profile was surveyed by the static method, and thus a better accuracy could be achieved.
Dual frequency Trimble GPS receivers (4000 SSI and SSE models), equipped with Choke Ring or microcentred L1/L2 (with ground plane) antennas were used to accomplish all the GPS measurements.
The gravity stations are also measured in sequence and the profile is traversed two or three times for each survey (profile method; Torge 1989 ). Gravity measurements presented here were performed using a Scintrex CG-3M gravimeter (serial # 9310234; Budetta & Carbone 1997 ).
GPS data collected during the surveys carried out in 2002 July and 2003 July along the profile described in the previous section were processed together with those from stations outside the volcanic edifice, in order to link Etna's network to the ITRF system ( Bonforte & Puglisi 2003 ). Trimble Geomatics Office software package (release 1.5) and precise ephemerides were utilized to process the data. In order to improve the precision of the antenna phase centre location, antenna calibration models by NGS ( http://www.ngs.noaa.gov/ANTCAL/ ) were introduced. The data were processed using both L1 and L2 GPS frequencies. The ionosphere-free observable (L3) was also used for baselines longer than 10 km, for which ionospheric noise could become significant. The two-step method described by Puglisi et al. (2001) was followed to adjust the baseline solutions. Furthermore, in order to achieve a better accuracy in the stop-and-go initialization, a free (inner constraint) adjustment was performed before processing the kinematic data. Typically, the static positioning is affected by uncertainties of 3–4 and 6–7 mm on the horizontal and vertical components, respectively. The use of self-centring benchmarks ( Puglisi et al. 2001 ), allowing station set-up errors to be avoided, improves the precision on the kinematic positioning. It is affected by typical uncertainties of 6–8 mm on the horizontal component and twice that value on the vertical component ( Bonforte & Puglisi 2003 ; Bonforte et al. 2004 ). The error on displacements along the summit profile during the 2002 July–2003 July period is estimated at 16 and 8 mm, for the vertical and horizontal component, respectively.
As shown in Fig. 2 , the 2002 July–2003 July dislocations measured at the GPS stations along the summit profile reach an amplitude of up to 2 m along both the horizontal ( Fig. 2a ) and vertical ( Fig. 2b ) directions.

Displacements in the horizontal (a) and vertical (b) directions and gravity changes (c) observed between June 2002 and July 2003 along the common GPS-gravity profile on the summit area of Mt Etna. Inset legend in (a): (1) gravity benchmark; (2) GPS benchmark; (3) trace of the ABC profile and (4) scale of horizontal displacements.
The observed deformation pattern, with horizontal displacement vectors spanning a wide range of azimuths along the profile ( Fig. 2a ), indicates that a complex framework of sources activated during the 2002 NE-rift eruption. In particular, a cluster of four stations on the higher part of the profile (NS11 to PLU) shows an homogeneous WNW-ward displacement; moving downwards, the further five stations (NS08–NS05) present a more disturbed ground deformation pattern, with more widely spread azimuths (NNE–ENE) and higher magnitude of the horizontal displacements; finally, the remaining lowermost stations show opposite W-to-NWward and E-to-SEward displacements.
Points NS05–NS08 reveal a narrow subsiding area, elongated NNE–SSW on the uppermost part of the NE-rift. This local ground deformation is clearly controlled by the dry fracture field present at Piano delle Concazze area, as testified by the abrupt disappearing of the subsidence a few hundreds metres eastwards, across the dry fractures, at OBS station.
We can assume that the most important source of ground deformation follows the track of the eruptive fracture on the surface. The different ground deformation patterns evidenced by GPS data and discussed above suggest that the dynamics of the intrusion along the rift underwent some changes. This particular feature, together with the change of azimuth of the fractures, from almost NS, in the summit zone, to ENE, at lower altitudes (see Fig. 1 ), compelled us to consider two different planar sources along the NE-rift. Furthermore, the WNW-ward displacement on the upper part of the volcano suggests that a further tensile source activated beneath the summit craters area.
In order to model the structural framework and define the dynamics of the ground deformation sources, a data inversion was performed through the Okada (1985) dislocation model and a least-squares algorithm (LSA) approach. The use of a LSA implies an appropriate set of starting values to be assumed for each parameter of the source. The Okada model implies 10 parameters to be inverted. We fix the position and the orientation of the three dislocation planes based on field observations (position of dry and eruptive fractures). Thus, three parameters (easting, northing and azimuth; see Table 1 ) for each model-source are fixed. Furthermore, relying on the homogeneous WNW-ward displacement observed at the southernmost stations of the profile (see Fig. 2 ), we assume GPS source 1 to be a pure tensional fracture, and hold both its dip-slip and strike-slip fixed at zero (see Table 1 ). In conclusion, 19 parameters are to be inverted against 42 observations (three-component deformation measured at 14 stations).

Parameter of the sources modelled through surface deformation data. Model numbers refer to Fig. 4 . Coordinates are in UTM projection. Fixed parameters are marked with italic style letters.
The solutions, referred in the local UTM (zone 33N) frame, are reported in Table 1 . The resulting composite model consists of one vertical tensile structure located beneath the summit area and two normal faults dipping eastwards (the projection of the modelled sources onto the horizontal plane is shown in Fig. 3a ). This framework matches the scenario hypothesized by Del Negro et al. (2004) who analysed data from two magnetic stations (within 1 km from the NE-rift), and assumed the magnetic changes to be due to stress redistributions within the edifice.

Comparison between displacements (a and b) and gravity changes (c) observed during the June2002–July 2003 period on the summit area of Mt Etna and the effect of the best-fitting model sources discussed in the text. Inset legend in (a): (1) gravity benchmark; (2) GPS benchmark; (3) trace of the ABC profile; (4) source modelled through GPS data; (5) source modelled through gravity data; (6) scale of observed horizontal displacements and (7) scale of calculated horizontal displacements.
Comparison between observed and calculated horizontal and vertical deformations is presented in Figs 3(a) and (b) , respectively. To assess the quality of the model a paired t -test is performed ( Goulden 1956 ). It tests the hypothesis that the mean of the differences between the observed deformations (horizontal deformations are split into east and north components) and the corresponding calculated effect of the model is equal to zero. The p -value obtained (0.55) indicates that there are 55 in 100 chances that the hypothesized zero mean difference would occur by chance. The above result indicates that, in spite of the minimization performed by the LSA algorithm, the misfit remains quite high, especially in the vertical direction, along which only the overall shape of the observed changes could be reproduced ( Fig. 3b ). That may be due to the following reasons:
During the 2002–2003 eruption the kinematic response of the eastern flank of Etna was controlled by a complex interaction between volcanic and tectonic processes, as inferred by Barberi et al. (2004) on the grounds of syn-eruptive seismic data, and thus the observed dislocations are likely to be the effect of various, contemporaneously acting deformation mechanisms, not entirely explainable by simple elastic models.
The 2002–2003 eruptive fracture field formed over a medium already weakened by the eastward sliding ( Borgia et al. 1992 ; Lo Giudice & Rasà 1992 ; Froger et al. 2001 ), which could have locally reacted in a non-elastic manner, producing inhomogeneities in the overall ground deformation pattern [e.g. a set of extensional N–S-trending dry fractures formed in the Piano delle Concazze area ( Branca et al. 2003 ; see Section 1 .) and strongly affected the deformation data from NS07 and NS08].
A significant contribution to the misfit found between observed and calculated ground deformation is made by the vertical displacements at NS05 to NS08 benchmarks that are located inside a graben-like structure (between the northernmost tip of the eruptive fracture and the dry fractures at Piano delle Concazze), where a local ground deformation pattern occurs.
Our data come from comparison of two surveys spanning a 1-yr period and thus, beside reflecting the processes which occurred during the 2002 NE-rift eruption, other minor effects which took place before and/or after it could also affect the final difference.
It is worth stressing that a significant ground deformation was observed by GPS, EDM, levelling, tilt and DInSAR data on the NE flank of the volcano one month before the eruption onset ( Bonforte et al. , 2007 ), revealing an acceleration of the slip rate along the Pernicana fault (from the usual value of about 3 cm yr −1 to about 10 cm yr −1 ) and an extension of the EDM lines crossing the Provenzana fault (1–3 cm). Subsequently, until the summer of 2003, measurements at the summit zone of Etna were not possible because of the volcanic activity and, lately, because of the snow cover. However, daily GPS measurements carried out during the 2002 eruption, along the lower portion of the Pernicana fault, revealed exceptional values of the slip-rates (up to about 800 cm yr −1 ). The slip rate gradually decreased during the last part of the eruption and soon after it, but it reached its usual value only 1 yr after the end of the eruption ( Palano et al. 2007 ).
Thus, even though within the summit deformation observed between 2002 July and 2003 July it is not possible to distinguish the syn- from the pre- and post-eruptive deformation, the observed higher slip rates along the lower portion of the Pernicana fault are likely to indicate the accommodation of anomalous large-scale movements of the eastern flank of Etna during a period spanning the eruption and lasting more than 1 yr. These movements are in turn expected to increase the residuals between the observed 2002 July and 2003 July summit deformation and the corresponding deformation calculated through a model which takes into account only the elastic behaviour of the medium in response to the intrusive process (Okada model).
During the 2002 June–2003 July period, gravity changes among the strongest ever observed at Mt Etna took place along the profile under study ( Fig. 2 ). The data were reduced for tidal effect in the field using a suitable software loaded into the memory of the digital Scintrex CG3-M gravimeter ( Scintrex Ltd 1992 ). This software is based on Longman's formula (1959) and allows a standard earth tide correction to be generated. All measurements were then corrected for instrumental drift ( Rymer 1989 ) and referred to a station (BEL; see Fig. 1 ), located about 16 km south of the eruption site, where it is assumed that the gravity field does not change over time.

Stations MC, PPR, RPP and MAR, on the northern edge of the profile ( Fig. 1 ), lack the elevation control. Accordingly, the first three stations were not taken into account when calculating the model for the 2002 NE-rift intrusion, while the datum from MAR (a quasi-zero value on the northernmost edge of the profile), was considered to better constrain the calculation. It is worth stressing that most of the gravity changes observed at stations PPR and RPP are likely to be the effect of the nearby emplacement of the 2002 lava flow field ( Fig. 1 ). Residual gravity changes, which reflect mass redistributions under the surface, reach the maximum amplitude (around 350 μGal) at stations DP and PZ ( Fig. 3c ). Moving towards south, the amplitude of the gravity change decreases sharply, being almost within the error bar at benchmarks CO, PG, LZ and DG and than rises again (up to about 60 μGal) over the four southernmost benchmarks of the profile (CT, PL, BS and MG), which lay in the summit crater zone.
The high amplitude of the residual variations of the gravity field (after reduction for the free-air effect) suggests that an important mass redistribution, not directly associated to the ensuing displacement, occurred. Accordingly, the residual gravity changes are not inverted through elastic modelling. Rather, they are assumed to be due to underground mass changes. To assess the geometrical characteristic of the best-fitting model and the amount of mass involved in the redistribution process, a 3-D program, able to calculate at any observation point (in its actual position on the topographic surface) the effect of buried masses, is utilized. This program is called GRAVERSE and was designed on-purpose under the LabVIEW ® environment ( Carbone 2001 ). The program simulates the effect of the buried homogeneous mass by representing it as a lattice of point masses (in the case of a parallelepiped-shaped body (dyke), the program utilizes 1000 nodes). The program calculates the vertical component of the gravity effect due to each node of the lattice at the observation points. The effects due to the nodes in the lattice are then added, to calculate the total effect of the body.
The above described characteristic of the residual change, with two separate positive anomalies, suggest that two distinct sources activated, one close to the active summit craters of Etna and the other close to the southernmost edge of the eruptive fractures. This view is in keeping with the results of the previous analysis over the GPS data (at least as for the two southernmost source bodies) and indicate that, even though the observed gravity changes and deformation are not due to the same mechanism, they result from the same overall (intrusive) process.
The ease and functionality of the GRAVERSE interface (possibility of changing all the model parameters through graphical controls; charts showing the projection onto profiles of the observed and calculated gravity changes to check in real-time the overall goodness of the fit) make it possible to solve the inverse problem through a trial-and-error procedure. We started from the model parameters obtained through the above analysis over the GPS data and refined them until a satisfactory fit was assessed.
The residual June 2002–July 2003 gravity changes were found to be best modelled ( Fig. 3c ) using two quasi-vertical sheets, whose projections onto the horizontal plane are shown in Fig. 3(a) (for their characteristics see Table 2 ). The mass increase is 1.59 × 10 10 and 1.57 × 10 10 kg for gravity source 1 and 2, respectively.

Parameter of the sources modelled through surface gravity data. Model numbers refer to Fig. 4 . Coordinates are in UTM projection.
It is important to remind that the opening and the density change of the gravity sources do not appear in Table 2 since they are not sensitive parameters, that is, provided that their product remain the same, relative changes of these two parameters do not influence the effect of the model source. Thus an a priori assumption must be made on one of these parameters, to assess the value of the other one. For example, if the density of the intruding magma is set to 2700 kg m −3 , with a porosity of the medium of 0.3, the opening of gravity source 1 results equal to 6 m, while the opening of gravity source 2 results equal to 13 m
In keeping with the good fit obtained ( Fig. 3c ), the paired t -test returns a high probability (greater than 95 per cent) of 0-difference between observed and calculated data.
A further point that is worth stressing is that a lateral intrusion along the NE-rift implies a mass decrease within the volume where the intruding magma comes from. The eruptive dynamics of the 2002 NE-rift eruption suggests that the intruding magma was supplied by the fast empting of the central conduit ( Branca et al. 2003 ). A 3-D calculation performed with GRAVERSE (Cylinder Kernel; Carbone 2001 ) shows that a mass decrease, comparable with the increase along the NE-rift and occurring within a cylinder-shaped source whose projection on the horizontal plane roughly coincides with the position of the summit craters and whose top depth and width coincide with those of gravity source 1 ( Table 2 ), has a significant effect only at stations CT and PL, the closest stations to the summit craters ( Fig. 1 ). The negative gravity effect at those stations would imply that the calculated mass increase within gravity source 1 ( Table 2 ) is overestimated by about 40 per cent (gravity source 1 would be shorter and its centre would be shifted towards north).
Mt Etna is nowadays one of the best monitored volcanoes in the world. The large amount of geophysical and volcanological data available makes the 2002–2003 Etna eruption a very thoroughly studied one. Accordingly, various papers focusing on that event can be found in the literature ( Aloisi et al. 2003 ; Branca et al. 2003 ; Neri et al. 2003 ; Andronico et al. 2005 ; Barberi et al. 2004 ; Del Negro et al. 2004 ; Gambino et al. 2004 ). Most of the available geophysical papers deal with continuous data sequences, through which the Authors were able to set strict constraints on the timing of the paroxysmal events. Nevertheless, in the case of both Del Negro et al. (2004) , who analyse geomagnetic data from two continuous stations, and Branca et al. (2003) , who deal with continuous gravity data from only one station, geometrical constraints on the source models could not be defined and the Authors are only able to verify the compatibility of the variations they observe with models built from seismological and volcanological data.
Data from a continuously recording gravity meter working at a site (OBS in Fig. 1 ) very close to the eruptive fissures along the NE-rift, evidenced a marked decrease (about 400 μGal in less than 1 hr) about 4 hr before lava was first emitted ( Branca et al. 2003 ). This anomaly reversed soon afterward at a high rate (roughly 100 μGal hr −1 ), until the gravity signal returned to the mean level it had before the anomaly took place. Accordingly, the change of the gravity field observed at PG (the benchmark of the discrete gravity array closer to the site where the continuous gravity station is installed) between July 2002 and July 2003 is negligible (within 15 μGal). The lack of continuous GPS data, at a rate suitable to reduce the continuous gravity sequence, prevents strict constraints from being set on the sin-intrusive mechanism leading to the observed 2-hr-lasting gravity anomaly ( Branca et al. 2003 ). As a consequence, it is difficult to infer which kind of connection exists between the mechanism leading to the temporary gravity change detected by the continuous gravity station and the ‘permanent’ gravity increase detected along the summit profile through the repeated measurements.
Using the data collected between 2002 October 26 and 27, from permanent tilt and GPS stations, Aloisi et al. (2003) modelled a single 6.6 km long radial intrusion in the NE flank of the volcano whose projection on the horizontal plane roughly coincide with the projection of the models we found. The model by Aloisi et al. (2003) is placed at depths between 1500 m a.s.l. and 3100 m b.s.l. and thus is both larger and deeper than the models calculated in the present paper ( Table 1 and Fig. 4 ).

3-D perspective showing the sources modelled through surface ground deformation and gravity data.
This discrepancy can be explained by a combination of two factors:
Aloisi et al. (2003) inverted a one-day-long continuous data set, while we utilize discrete GPS data spanning a period of 1 yr. Likely, while only the effect of the radial intrusion leading to the eruption is present in the one-day-lasting sequence, other phenomena altering the deformation pattern at the surface could have occurred during the 1-yr period between the two GPS/microgravity campaigns, that is, structural readjustments after the main intrusive event such as closing of the deeper part of the intrusive path by lithostatic pressure.
The data of Aloisi et al. (2003) , come from stations at elevations below 2000 m and thus the large width of the model they found could result from the marked eastward sliding of the east flank ( Bonforte & Puglisi 2003 ) which amplified the horizontal displacements recorded from the GPS stations located in the low eastern sector. Our data come from stations on the summit zone of the volcano which are supposed to be less affected by the movement of the east flank.
It is to be stressed that, since the data we present here comes from the comparison between two discrete gravity/GPS surveys spanning a 1-yr period, we cannot set any constraints on the timing of the events discussed. However, relying on (i) a dense network in the summit northeastern zone of the volcano and (ii) the availability of contemporaneous gravity and GPS data, we can deliver a detailed model as for both geometry and mechanism of the intrusive process, at least for the shallower part of the intrusive path.
The models which best fit our surface deformation data partially overlap with those explaining our gravity data but are in general both narrower and deeper ( Tables 1 and 2 ; Fig. 4 ). The agreement between the structures modelled through deformation data and those obtained through gravity data as for position, azimuth and horizontal length is better in the summit crater zone than further north, along the NE-rift. In spite of the limitations of (i) the approach followed here (separate inversion of gravity and GPS data) and (ii) the above discussed misfit between observed and calculated surface deformation data, we think that the discrepancies found are significant. In our view, the facts that (1) along the southernmost part of the rift, at shallower depths a process causing mass changes with negligible deformation is predominant while, at greater depth, processes leading to surface dislocation are increasingly more important and (2) along the northernmost portion of the NE-rift only deformation processes occur, both result from structural features of the rift itself. The NE-rift has a curved shape in plan-view, with its azimuth changing gradually from NS, in the highest part, to NNE, and then to ENE, at its northeastern termination ( Figs 1 and 5 ; Tibaldi & Groppelli 2002 ). Coupled with the extensional tectonics affecting the NE-rift along the ESE direction, and produced by the sliding of the eastern flank of Etna ( Bonforte 2002 ; Bonforte & Puglisi 2003 ; Palano 2003 ; Puglisi & Bonforte 2004 ), this curved shape results in different kinematics between the northern and southern parts of the rift: pure extension, with the maximum fissuring dilation, occurs in the southernmost and central segments, while a transcurrent, left-lateral component characterizes the NE segment ( Fig. 5 ). In other words, a kinematic S–N readjustment leads to the replacement of the extensional tectonics by the transtensional movements typical of the Pernicana fault ( Tibaldi & Groppelli 2002 ).

Simplified block diagram (not to scale) illustrating the geometrical relationships between the 2002 magma influx from the central conduit and the curved Etna NE-rift, undergoing an ESE gravitational sliding.
Therefore, during the intrusion leading to the 2002 eruption, the magma, moving from the central conduit towards lower portions of the NE-rift, encountered changeable structural features in both the horizontal and vertical directions ( Fig. 5 ). Our analysis shows that a ‘pure’ mass increase (i.e. not accompanied by an overpressure within the space(s) hosting the new mass), a phenomenon likely due to filling of pre-existing voids, occurred at shallow depths along the southernmost part of the rift. The position of the modelled gravity sources in the area where the maximum fissuring dilation occurs is in keeping with this interpretation. Following the calculation in the previous section, one can conclude that open voids along the southernmost and central segments of the NE-rift are more abundant within the top 1–1.5 km below the surface. Open voids become progressively less abundant (1) at greater depths along the southernmost and central portions of the NE-rift, likely because of the lithostatic loading and (2) at any depth, towards the northeastern edge of the NE-rift where, as stated before, because of the morphology of the rift itself, extensional forces are progressively less effective ( Fig. 5 ). Within those portions of the NE-rift where open voids are less abundant, the intruding magma had to push its way forward. Therefore, it produced a significant displacement on the surface on one hand and a negligible gravity effect on the other, given (1) the less effective contribution in generating gravity variations of the magma/host-rocks density contrast with respect to the magma/voids density contrast (in the case of void-filling) and (b) the relatively small opening of the forceful dykes.
In this paper, new evidence on the intrusive mechanism of the 2002 NE-rift eruption at Mt Etna is provided through the analysis of ground deformation and gravity data from a common network. Displacement and gravity changes measured over the summit NE sector of the volcano between July 2002 and July 2003 are among the largest ever observed on Mt Etna.
Gravity data were reduced for the effect of elevation changes and inverted separately from ground deformation data. Results provide complementary information and indicate a composite intrusive mechanism with the magma moving passively through the upper part of the intrusive path and more forcefully with depth and towards the northeastern segment of the NE-rift, as open voids are progressively less abundant.
Unfortunately, the shortcomings discussed in Section 3 , which affect our ground deformation data, especially over the central part of the profile of common stations, prevent the accuracy in the modelling that could be achieved through our local array from being assessed.
The present work represents a further evidence of what many authors have already concluded ( Sanderson et al. 1983 ; Rymer et al. 1993 ; Budetta & Carbone 1998 ; Carbone et al. 2003 ): that in a structural framework such as Etna's rift zones ( Acocella & Neri 2003 ; Lanzafame et al. 2003 ), where tectonic movements and gravitational collapses are likely to take place together with volcanic processes ( Neri et al. 2003 ; Barberi et al. 2004 ), the synergistic use of deformation and gravity data can allow a more complete picture of the dynamics of any intrusive processes to be gained, as ‘passive’ intrusions (gravity changes occur without deformation) and forceful intrusions of relatively small magma bodies (measurable surface deformations accompanied by negligible changes of the gravity field) can be recognized.
Acknowledgments
This study was performed with financial support from the ETNA project (DPC–INGV 2004–2006 contract) and the VOLUME project (European Commission FP6–2004-Global-3). Thanks are due to the technicians of INGV (section of Catania) for their unflinching help in collecting the data in the field.
Acocella V. Neri M. , 2003 . What controls flank eruptions? The 2001 Etna eruption and the possible triggering mechanisms , Bull. Volcanol. , 65 , 517 – 529 .
Google Scholar
Aloisi M. Bonaccorso A. Gambino S. Mattia M. Puglisi G. , 2003 . Etna 2002 eruption imaged from continuous tilt and GPS data , Geophys. Res. Lett. , 30 , 2214 – 2218 .
Andronico D. et al. , 2005 . A multi-disciplinary study of the 2002–03 Etna eruption: insights into a complex plumbing system , Bull. Volcanol. , 67 , 314 – 330 .
Barberi G. Cocina O. Maiolino V. Musumeci C. Privitera E. , 2004 . Insight into Mt. Etna (Italy) kinematics during 2002–2003 eruption as inferred by seismic stress and strain tensors inversion , Geophys. Res. Lett. , 31 , L2164 , doi: arXiv .
Bonforte A. , 2002 . Study of the north-eastern sector of the hyblean plateau and of the eastern flank of Mt. Etna by GPS spatial techniques , PhD thesis Università degli Studi di Catania , Italy .
Bonforte A. Puglisi G. , 2003 . Magma uprising and flank dynamics on Mt. Etna volcano, studied by GPS data (1994–1995) , J. geophys. Res. , 108 ( B3 ), 2153 – 2162 .
Bonforte A. Guglielmino F. Palano M. Puglisi G. , 2004 . A syn-eruptive ground deformation episode measured by GPS, during the 2001 eruption on the upper southern flank of Mt. Etna , Bull. Volcanol. , 66 , 336 – 341 .
Bonforte A. Gambino S. Guglielmino F. Obrizzo F. Palano M. Puglisi G. , 2007 . Ground deformation modeling of flank dynamics prior to the 2002 eruption of Mt. Etna , Bull. Volcanol. , DOI: arXiv .
Borgia A. Ferrari L. Pasquaré G. , 1992 . Importance of gravitational spreading in the tectonic and volcanic evolution of Mount Etna , Nature , 357 , 231 – 235 .
Branca S. Del Carlo P. , 2003 . Eruptions of Mt. Etna during the past 3,200 years: a revised compilation integrating the historical and stratigraphic records , in Etna Volcano Laboratory , pp. 1 – 27 , eds Calvari S. Bonaccorso A. Coltelli M. Del Negro C. Falsaperla S. , Geophys. Monogr. Ser, AGU (Geophysical monograph series).
Google Preview
Branca S. Carbone D. Greco F. , 2003 . Intrusive mechanism of the 2002 NE-Rift eruption at Mt. Etna (Italy) inferred through continuous microgravity data and volcanological evidences , Geophys. Res. Lett. , 30 ( 20 ), 2077 , doi: arXiv .
Budetta G. Carbone D. , 1997 . Potential application of the Scintrex CG-3M gravimeter for monitoring volcanic activity: results of field trials on Mt. Etna, Sicily , J. Volc. Geotherm. Res. , 66 , 199 – 214 .
Budetta G. Carbone D. , 1998 . Temporal variations in gravity at Mt Etna (Italy) associated with the 1989 and 1991 eruptions , Bull. Volcanol. , 59 , 311 – 326 .
Carbone D. , 2001 . Gravity monitoring of Mount Etna (Italy) through discrete and continuous measurements , PhD thesis The Open University , Milton Keynes, UK .
Carbone D. Budetta G. Greco F. , 2003 . Possible mechanisms of magma redistribution under Mt Etna during the 1994–1999 period detected through microgravity measurements , Geophys. J. Int. , 153 , 187 – 200 .
Del Negro C. Currenti G. Napoli R. Vicari A. , 2004 . Volcanomagnetic changes accompanying the onset of the 2002–2003 eruption of Mt. Etna (Italy) , Earth planet. Sci. Lett. , 229 ( 1-2 ), 1 – 14 , doi: arXiv .
Froger J.L. Merle O. Briole P. , 2001 . Active spreading and regional extension at Mount Etna imaged by SAR interferometry , Earth planet. Sci. Lett. , 187 , 245 – 258 .
Gambino S. Mostaccio A. Patanè D. Scarfì L. Ursino A. , 2004 . High-precision locations of the microseismicity preceding the 2002–2003 Mt. Etna eruption , Geophys. Res. Lett. , 31 , L18604 , doi: arXiv .
Goulden C.H. , 1956 . Methods of Statistical Analysis , 2nd ed., Wiley , New York , p. 443 .
Kieffer G. , 1975 . Sur l'existence d'une “Rift zone”à L'Etna , C.R. Acad. Sci. Paris D. 280 , 236 – 266 .
Lanzafame G. Neri M. Acocella V. Billi A. Funiciello R. Giordano G. , 2003 . Structural features of the July-August 2001 Mount Etna eruption: evidence for a complex magma supply system , J. Geol. Soc. Lond. , 160 , 531 – 544 .
Lo Giudice E. Rasà R. , 1992 . Very shallow earthquakes and brittle deformation in active volcanic areas: the Etnean region as an example , Tectonophysics , 202 , 257 – 268 .
Longman I.M. , 1959 . Formulas for computing the tidal accelerations due to the Moon and the Sun , J. geophys. Res. , 64 ( 12 ), 2351 – 2355 .
Neri M. Acocella V. Behncke B. , 2003 . The role of the Pernicana Fault System in the spreading of Mt. Etna (Italy) during the 2002–2003 eruption , Bull. Volcanol. , 66 , doi: arXiv .
Okada Y. , 1985 . Surface deformation due to shear and tensile fault in half-space , Bull. seism. Soc. Am. , 75 , 1135 – 1154 .
Palano M. , 2003 . InSar techniques in structural geology: some application to Mt. Etna volcano , PhD thesis Università degli Studi di Catania , Italy .
Palano M. et al. , 2007 . Kinematics and strain analyses of the eastern segment of the Pernicana fault (Mt. Etna, Italy) derived from geodetic techniques (1997–2005) , Ann. Geophys. , in press.
Puglisi G. Bonforte A. , 2004 . Dynamics of Mt Etna volcano inferred from static and kinematic GPS measurements , J. geophys. Res. , 109 ( B11 ), B11404 , doi: arXiv .
Puglisi G. Bonforte A. Maugeri S.R. , 2001 . Ground deformation patterns on Mt. Etna, between 1992 and 1994, inferred from GPS data , Bull. Volcanol. , 62 , 371 – 384 .
Romano R. Sturiale C. , 1982 . The historical eruptions of Mt. Etna (Volcanological data) , Mem. Soc. Geol. It. , 23 , 75 – 97 .
Rymer H. , 1989 . A contribution to precision microgravity data analysis using LaCoste and Romberg gravity meters , Geophys. J. , 97 , 311 – 322 .
Rymer H. Murray J.B. Brown G.C. Ferrucci F. McGuire J. , 1993 . Mechanisms of magma eruption and emplacement at Mt Etna between 1989 and 1992 , Nature , 361 , 439 – 441 .
Sanderson T.J.O. Berrino G. Corrado G. Grimaldi M. , 1983 . Ground deformation and gravity changes accompanying the March 1981 eruption of Mount Etna , J. Volc. Geotherm. Res. , 16 , 299 – 315 .
Scintrex Limited Autograv , 1992 . Operator manual version 4.4 .
Tibaldi A. Groppelli G. , 2002 . Volcano-tectonic activity along structures of the unstable NE flank of Mt. Etna (Italy) and their possible origin , J. Volc. Geotherm. Res. , 115 , 277 – 302 .
Torge W. , 1989 . Gravimetry , de Gruyter , Berlin .
Email alerts
Astrophysics data system, citing articles via.
- Recommend to your Library
- Advertising and Corporate Services
- Journals Career Network
Affiliations
- Online ISSN 1365-246X
- Print ISSN 0956-540X
- Copyright © 2024 The Royal Astronomical Society
- About Oxford Academic
- Publish journals with us
- University press partners
- What we publish
- New features
- Open access
- Institutional account management
- Rights and permissions
- Get help with access
- Accessibility
- Advertising
- Media enquiries
- Oxford University Press
- Oxford Languages
- University of Oxford
Oxford University Press is a department of the University of Oxford. It furthers the University's objective of excellence in research, scholarship, and education by publishing worldwide
- Copyright © 2024 Oxford University Press
- Cookie settings
- Cookie policy
- Privacy policy
- Legal notice
This Feature Is Available To Subscribers Only
Sign In or Create an Account
This PDF is available to Subscribers Only
For full access to this pdf, sign in to an existing account, or purchase an annual subscription.

An official website of the United States government
The .gov means it’s official. Federal government websites often end in .gov or .mil. Before sharing sensitive information, make sure you’re on a federal government site.
The site is secure. The https:// ensures that you are connecting to the official website and that any information you provide is encrypted and transmitted securely.
- Publications
- Account settings
Preview improvements coming to the PMC website in October 2024. Learn More or Try it out now .
- Advanced Search
- Journal List
- Multidiscip Respir Med
- v.8(1); 2013

A retrospective study on acute health effects due to volcanic ash exposure during the eruption of Mount Etna (Sicily) in 2002
Daniele lombardo.
1 Department of Clinical and Molecular Biomedicine, University of Catania, Catania, Italy
Nicola Ciancio
2 Pulmonology Unit, A.O.U. Policlinico-Vittorio Emanuele, Catania, Italy
Raffaele Campisi
Annalisa di maria, laura bivona.
3 Department of Diseases of the Thorax, Pulmonology Unit, Pierantoni-Morgagni Hospital, Forlì, Italy
Venerino Poletti
Antonio mistretta.
4 Department of Hygiene and Public Health “G.F. Ingrassia”, University of Catania, Catania, Italy
Annibale Biggeri
5 Department of Statistics, Informatics and Applications “G. Parenti”, University of Florence, Florence, Italy
6 Biostatistics Unit ISPO Cancer Prevention and Research Institute, Florence, Italy
Giuseppe Di Maria
Mount Etna, located in the eastern part of Sicily (Italy), is the highest and most active volcano in Europe. During the sustained eruption that occurred in October-November 2002 huge amounts of volcanic ash fell on a densely populated area south-east of Mount Etna in Catania province. The volcanic ash fall caused extensive damage to infrastructure utilities and distress in the exposed population. This retrospective study evaluates whether or not there was an association between ash fall and acute health effects in exposed local communities.
We collected the number and type of visits to the emergency department (ED) for diseases that could be related to volcanic ash exposure in public hospitals of the Province of Catania between October 20 and November 7, 2002. We compared the magnitude of differences in ED visits between the ash exposure period in 2002 and the same period of the previous year 2001.
We observed a significant increase of ED visits for acute respiratory and cardiovascular diseases, and ocular disturbances during the ash exposure time period.
Conclusions
There was a positive association between exposure to volcanic ash from the 2002 eruption of Mount Etna and acute health effects in the Catania residents. This study documents the need for public health preparedness and response initiatives to protect nearby populations from exposure to ash fall from future eruptions of Mount Etna.
There are a number of active volcanoes throughout the world and many of them are close to urban settings and major cities. At times, they are responsible for pyroclastic emissions, consisting of a mixture of gases, vapours, aerosols, and particulate matter. These emissions have the potential to cause massive environmental pollution and impact on climate, surface infrastructures and human activities, thus resulting in a significant economic burden for both the community and the individuals [ 1 ]. Among the adverse effects of pyroclastic emissions (ash fall), those related to human health raise significant concern in both exposed populations and health service administrators [ 1 , 2 ].
Previous observations report that although volcanic ash may not be acutely toxic, its exposure has been associated with a variety of health effects - spanning from sudden, asphyxia-induced death due to acute respiratory tract irritation and symptoms either in subjects with existing respiratory disorders like asthma or healthy individuals [ 3 - 6 ]. Adverse respiratory effects from exposure to volcanic ash have been documented during the eruption of Montserrat, British West Indies, in 1995 [ 7 , 8 ], and from Eyjafjallajökull volcano, Iceland, in 2010 [ 9 , 10 ]. In addition, repeated exposure to ash fall from Mount Sakurajima, Japan, was associated with increased mortality due to respiratory diseases including lung cancer and chronic obstructive pulmonary disease (COPD) [ 11 ].
Despite the fact that volcanic eruptions are common throughout the world, and millions of people are exposed to their health hazards, few studies have been published that assess acute and chronic health effects thereby shaping prevention and measures of intervention for local communities.
Mount Etna, located in the eastern part of Sicily (Italy), is the most active volcano in Europe. In 2002 an intense eruption activity began in October and ended up November 2002 [ 12 ]. This long lasting eruption produced explosions and jets of lava from two newly formed fissures in the proximity of the volcano’s summit, and it was also characterized by the emission of pyroclastic flows that were more intense during the first two weeks of activity [ 12 ]. Local winds carried the emissions for kilometers and subsequent ash fall affected a largely populated area extending south of Mount Etna, which includes Catania, a major city with nearly 315,000 inhabitants (Figure 1 ). During the eruption the exposed population complained of health disturbances such as eye and airway irritation, cough, or acute exacerbation of chronic respiratory disorders. However, a subsequent study investigating the rate of hospital admissions and causes of death only found an increase in cardiovascular diseases along with a decrease in mortality for respiratory diseases during the eruption period in the same area [ 13 , 14 ].

Area interested by the eruption and ashfall from satellite, with indication of the Hospital (H) reported in the study. Local winds carried the emissions for kilometers and affected a largely populated district extending south of Mount Etna.
To ascertain whether or not there was an association between the Mount Etna's ash fall and acute health hazards in the exposed communities, we carried out a retrospective study to investigate the number and type of visits to the emergency departments (ED) of hospitals near Mount Etna during the 2002 eruption.
To test the hypothesis that there is a putative association between acute health effects reported by the exposed population and the volcanic ash fall, we collected the number and type of visits to the ED for various acute diseases in public hospitals (see end-note) near the volcano in the Province of Catania between October 20 and November 7, 2002. We compared these results with the number of ED visits during the corresponding period in 2001. Disease conditions were classified according to previous surveillance activity that was conducted during the eruption as required by the Prefecture of Catania.The surveillance activity was aimed to detect and monitor acute health effects in order to provide prompt intervention to exposed residents. These conditions included acute respiratory disorders of both the upper and lower respiratory tract, cardiovascular diseases such as heart attack, coronary and cerebrovascular diseases, arterial hypertension and cardiac arrhythmias, as well as ocular disturbances, neuropsychiatric disorders, skin diseases and traumatic events. The outcome measure was the proportion of ED visits for those conditions among the total ED visits, whereas the effect measure was the ratio between the proportion in the exposed time period during 2002 to the proportion in same yet unexposed time period during 2001. Whereas the population denominators were constant for the two considered periods, the total number of ED visits changed because of a change in policies of the healthcare system in Italy. We therefore used the proportion instead of the rate of ED visits for the selected disease states [ 15 ].
In addition to health monitoring, levels of particulate matter ≤10 μm (PM 10 ), and 2.5 μm (PM 2.5 ) in diameter were also recorded in the same geographic area near Mount Etna [ 13 , 14 ].
The chemical composition of volcanic ash and the amount of each surface component were analyzed by mass spectrometry.
Statistical analysis was performed to quantify and verify the relevance of differences between the two study periods. Statistical tests used were the “Chi-Square (χ 2 )” and the “Z test” with a two-sided α =0.05. We also applied multinomial logistic regression to adjust for hospital of admission on a restricted sample of 73% of ED visits with complete information. This model produced exposure Odds Ratio (OR) estimates. The reference category was the number of ED visits for all other diseases. For example, an exposure OR of 1.30 for acute respiratory disease would be interpreted as an increase of 30% in the odds of having a ED visit for acute respiratory disease versus the odds of having an ED visit for a condition not related to volcanic ash exposure.
We first summarize the results of chemical analysis of volcanic ash sampled in Catania from this eruption. Whereas, in the second part we report the results of acute health effects during the intense ash fall period.
Analysis of volcanic ash
The Protezione Civile, the Italian organization for disaster management, estimated that four days after the beginning of eruption, 9 kg of ash per square meter had fallen in Nicolosi (a small town 15 km south of the volcano), and 2.5 kg per square meter in Catania (24 km south-east) [ 7 ].
The chemical analysis carried out by mass spectrometry on ash samples of October 28, 2002, indicates that the fine particles of volcanic ash were mainly composed of ferrous ions (Fe ++ ) and a great presence of free crystalline silica in large particles.
Acute health effects
The results of this retrospective study are presented in Table 1 , which shows the frequency and level of statistical significance in the proportion of ED visits to the Hospitals in the Province of Catania - for disease states and health effects that may be related to the exposure to volcanic ash emission. The difference in the proportion of selected health effects varied among the different hospitals for each selected disease category. There was a significant increase in the number of ED visit for selected disease states in the three main hospitals serving the municipality of Catania, i.e. Vittorio Emanuele, Cannizzaro and Garibaldi Hospital. These hospitals were located in the area that received the plume of ash fall (Figure 1 ). Taking into account the data obtained from the EDs of these three main hospitals only, the cumulative number of total visits in 2002 was less than the corresponding number in 2001 (10,337 and 12,230, respectively). Despite this, the proportion of ED visits for the selected categories was significantly higher with the exclusion of neuropsychiatric disorders and skin diseases (Table 2 ). Therefore, a significant increase in the number of visits for acute diseases of upper and lower respiratory tract, cardiovascular diseases and ocular symptoms was observed between October 20 and November 7 2002 compared to the corresponding period of 2001.
Level of significance and difference in the frequency, in ED visits of the hospitals in the province of Catania, for some acute diseases
p < 0,01 highly significant difference.
p < 0,05 statistically significant difference.
p > 0,05 not significant difference.
The period is between October 20 and November 7, 2002. The results obtained were compared with those of the same period in 2001. Table 2 contains more detailed information in relation to the three major hospitals in Catania.
Number and frequency of visits, to ER of the Hospitals Vittorio Emanuele, Cannizzaro and Garibaldi in Catania, for some acute affections
We also analyzed the frequency of visits to ED of the Hospitals in Catania through multinomial logistic regression adjusting for hospital of admission, but we found no difference from previous analyses (Table 3 ).
OR trough polytomic multinomial logistic regression
Number of observations = 16.069.
According to current knowledge volcanic ash creates minor health problems such as eye and upper airway irritation, and may exacerbate pre-existing respiratory diseases including asthma and chronic bronchitis. In this study we found that during the sustained pyroclastic emission of volcanic ash due to Mount Etna eruption in 2002, ED visits for acute health disorders of respiratory tract and cardiovascular system increased significantly. This increase suggests that volcanic ash fall is associated with acute health effects on exposed local communities, and confirms the results of previous studies showing that short-term exposure to volcanic emission is associated with acute respiratory morbidity, asthma attacks, and increased hospital visits for respiratory illness [ 3 - 6 , 9 ], as well as for cardiovascular symptoms and morbidity [ 14 , 16 ].
Due to the lack of information on time relationship between daily levels of particulate matter and daily rate of ED visits we were unable to perform time-series analysis of our data. Consequently, we cannot conclude that a causal relationship exists between exposure to volcanic ash and health effects observed in our study. The existence of an association, however, is further supported by the evidence that unusually high levels of respirable sized particulate matter, along with a nearly threefold increase in PM 10 and fourfold increase in sulfur dioxide, were recorded during the same period in the same area as compared to the previous year [ 13 ]. Contrary to the results reported by Fano and coworkers [ 13 , 14 ] of an increase in hospital admissions for cardiovascular morbidity along with a decrease in the rate of admission for respiratory diseases, we found a significantly higher frequency of ED visits for both cardiovascular and respiratory diseases in the three main hospitals of Catania in 2002 as compared to the same period of the previous year. By taking into account that hospital admissions represent a higher intensity of care than an ED visit, a reason for this discrepancy could rely upon a precautionary attitude of ED doctors towards the hospital admission of patients with cardiovascular symptoms rather than of those presenting with respiratory symptoms. On the other hand, the inclusion in our selection of minor respiratory problems, such as the upper airway irritation, and the higher number of hospitals considered for the rate of hospitalization might represent further explanation for the different behavior of hospital admissions and ED visits for acute respiratory diseases. In addition, our data do not provide crude figures of mortality, nor they allow for the stratification of age range, gender, smoking habit, occupational risks, and pre-existing disease states.
Volcanic eruptions are relatively frequent throughout the world, yet few other observational studies have been carried out on this topic. In addition, besides the scarcity of published studies, researchers have investigated ash fall and associated health effects by using varied approaches thus making the broad interpretation of findings difficult or not fully comparable. For example, some studies analyzed hospital admissions or ED visits, rather than outpatient or GP visits. In Alaska during the volcanic activity of Mount Spurr in 1992, located 60 miles west of the city of Anchorage, an increase in PM 10 along with an increase for outpatients visits for bronchial asthma and upper respiratory diseases was observed [ 17 ]. Few investigations with conflicting data are available on mortality rate during or after eruptions, as well as on the long-term effects of volcanic ash exposure. No effects on overall and cause-specific mortality were observed during the eruption of Mount Etna in 2002, as reported by a different group of investigators [ 13 , 14 ]. By contrast, residents in Sakurajima-Tarumizu area located near Mt. Sakurajima in Japan, over the long term (>30 years) experienced a relatively high mortality for respiratory diseases, which included lung cancer and COPD [ 11 ]. In 6–9 months after the end of Eyjafjallajökull eruption, residents from exposed areas reported several medical problems including increased wheezing, cough and phlegm, as well as eye and skin irritation [ 10 ].
Another problem is to determine whether or not some working categories, such as local police, firefighters or people involved in extensive ash-removal operations, have a higher level of exposure and maybe a higher risk for developing acute health effects. During the eruption of Mount St. Helens, on May 18, 1980 respiratory symptoms and ocular problems were significantly higher among loggers who were regularly employed outdoors in areas with heavy ash exposure, than among other groups of workers who weren’t assigned to clean up after volcanic eruptions. Loggers inhaled higher concentration of ash showing a significant, short-lived reduction in lung function. This transitory functional impairment was directly proportional to the intensity of exposure to volcanic ash [ 2 - 4 ]. One reason for the observed higher risk of respiratory illness among population involved in ash-removal operations may be due to the “re-suspension” of fine ash particles and their chemical components. However, pragmatically, this distinction may not make much difference. A proportion of silica particles detected during Mount Etna’s eruption were small enough to penetrate deeply into the lungs of exposed subjects. It is conceivable that re-suspension caused by atmospheric factors or human activity such as road traffic and ash removal may have re-suspended particles with smaller diameter and more likely to be inhaled, thus determining an increase in exposure. Soundly, this is not trivial and suggests the opportunity to adopt strategies avoiding the phenomenon of re-suspension.
Little information is available about the relevance of age, sex, and more importantly the possible presence of pre-existing chronic respiratory diseases in patients that presented to EDs during the 2002 eruptive event at Mount Etna. During the July 1995 eruption in Montserrat, a study was performed with the aim to evaluate whether ash fall had any effect on the respiratory health of children. The results were that volcanic ash emission adversely affected the respiratory health and produced exercise-induced bronchoconstriction in the Montserrat children [ 7 ]. The health effects of volcanic ash on the respiratory system might depend on size and composition of the inhaled particles. Their diameter is the most important determinant of penetration into the peripheral airways. The size of 10 μm is considered to be the cut-off point for the aerodynamic diameter of respirable particles. However, penetration to the respiratory bronchioles and alveolar spaces is limited to particles with diameter of 2.5 μm or less.
By reaching the small airways, it is thought that volcanic ash causes airway inflammation, and it has been reported that patients with chronic obstructive pulmonary disease and bronchial asthma, are more prone than healthy people [ 3 ].
Referring to traumatic injuries, a further analysis of our data showed that they were significantly reduced in the main Hospital located in the downtown area of Catania. This may be explained by the advice issued by local authorities, that was not extended to neighboring communities, about risks of driving motorcycles on ash-laddened roads in downtown Catania city.
Other adverse effects of volcanic ash include the irritation of upper respiratory tract causing nasopharyngitis, with transient hyperemia, as well as eye irritation causing conjunctivitis. Our data are in agreement with those reported in previous studies [ 9 , 10 ].
Finally, it is important to take into account the composition of volcanic ash, and evaluate the possible tumorigenic effects of some ash components. In our experience, during the eruption of Mount Etna in 2002, analysis of large particles performed with a mass spectrometer showed a relevant proportion of free crystalline silica, which upon long-term exposure to particularly high concentrations, could represent a risk factor for the development of pneumoconiosis [ 18 ]. Furthermore, the presence of ferrous ions on the surface of fine particles, was registered with the same method. It has been reported that removable divalent iron (Fe ++ ), can trigger inflammatory processes causing the release of free radicals in the lung tissue [ 6 ]. An in vitro study has shown that biochemical processes induced by exposure to high levels of PM 10 could produce cytotoxicity and DNA alterations [ 19 ]. In summary, these observations are highly suggestive that volcanic ash exposure could represent a hazard for developing lung cancer. The existing data on this issue, however, are not conclusive and further studies are needed.
In conclusion, in this study we found an association between exposure to volcanic ash fall and acute health effects in the resident population of Catania at Mount Etna. Peculiar evolution of the eruption in 2002 and the volcanic and geographical characteristic of the volcano do not provide an “ideal-model” to study health hazards of ash producing eruptions. However, the information provided by this study is useful to assist the Province of Catania in planning environmental and public health measures that could limit exposure and protect the population. Indeed much work remains to determine the specific short-term and long-term health risks volcanoes pose to humans. In the future, it may be necessary to design and implement longitudinal epidemiological observations in areas at high risk of volcanic emissions, to answer the questions still left open by the present and other investigations.
Denomination of the Hospital cited in the article. The main hospital in the town of Catania are the first three listed.
1) Azienda Ospedali Vittorio Emanuele, Ferrarotto e S. Bambino,
2) Azienda Ospedaliera Cannizzaro,
3) Azienda Ospedali Garibaldi, S. Luigi-Currò, Ascoli-Tomaselli,
4) Presidio Ospedaliero S. Giovanni di Dio e S. Isidoro di Giarre,
5) Presidio Ospedaliero di Militello Val di Catania,
6) Presidio Ospedaliero S. Marta e S. Venera di Acireale,
7) Presidio Ospedaliero SS. Salvatore di Paternò,
8) Presidio Ospedaliero di Biancavilla,
9) Presidio Ospedaliero di Bronte.
Competing interest
All authors have no conflicts of interest in this issue.
- Self S. The effects and consequences of very large explosive volcanic eruptions. Phil Trans R Soc A. 2006; 364 :2073–2097. doi: 10.1098/rsta.2006.1814. [ PubMed ] [ CrossRef ] [ Google Scholar ]
- Bernstein RS, Baxter PJ, Falk H, Ing R, Foster L, Frost F. Immediate public health concerns and actions in volcanic eruptions: lessons from the Mount St. Helens eruptions, May 18-October 18, 1980. Am J Public Health. 1986; 76 :25–37. doi: 10.2105/AJPH.76.Suppl.25. [ PMC free article ] [ PubMed ] [ CrossRef ] [ Google Scholar ]
- Baxter PJ, Ing R, Falk H, French J, Stein GF, Bernstein RS, Merchant JA, Allard J. Mount St. Helens eruptions, May 18 to June 12, 1980. An overview of the acute health impact. JAMA. 1981; 246 :2585–2589. doi: 10.1001/jama.1981.03320220035021. [ PubMed ] [ CrossRef ] [ Google Scholar ]
- Baxter PJ, Ing R, Falk H, Plikaytis B. Mount St. Helens eruptions: the acute respiratory effects of volcanic ash in a North American community. Arch Environ Health. 1983; 38 :138–143. doi: 10.1080/00039896.1983.10543994. [ PubMed ] [ CrossRef ] [ Google Scholar ]
- Buist AS, Bernstein RS, Johnson LR, Vollmer WM. Evaluation of physical health effects due to volcanic hazards: human studies. Am J Public Health. 1986; 76 (3):66–75. [ PMC free article ] [ PubMed ] [ Google Scholar ]
- Hansell AL, Horwell CJ, Oppenheimer C. The health hazards of volcanoes and geothermal areas. Occup Environ Med. 2006; 63 :149–156. doi: 10.1136/oem.2005.022459. [ PMC free article ] [ PubMed ] [ CrossRef ] [ Google Scholar ]
- Forbes L, Jarvis D, Potts J, Baxter PJ. Volcanic ash and respiratory symptoms in children on the Island of Montserrat, British West Indies. Occup Environ Med. 2003; 60 :207–211. doi: 10.1136/oem.60.3.207. [ PMC free article ] [ PubMed ] [ CrossRef ] [ Google Scholar ]
- Horwell CJ, Fenoglio I, Ragnarsdottir KV, Sparks RS, Fubini B. Surface reactivity of volcanic ash from the eruption of Soufriere Hills volcano, Montserrat, West Indies with implications for health hazards. Environ Res. 2003; 93 :202–215. doi: 10.1016/S0013-9351(03)00044-6. [ PubMed ] [ CrossRef ] [ Google Scholar ]
- Carlsen HK, Hauksdottir A, Valdimarsdottir UA, Gíslason T, Einarsdottir G, Runolfsson H, Briem H, Finnbjornsdottir RG, Gudmundsson S, Kolbeinsson TB, Thorsteinsson T, Pétursdóttir G. Health effects following the Eyjafjallajokull volcanic eruption: a cohort study. BMJ Open. 2012; 2 :e001851. doi: 10.1136/bmjopen-2012-001851. [ PMC free article ] [ PubMed ] [ CrossRef ] [ Google Scholar ]
- Carlsen HK, Gislason T, Benediktsdottir B, Kolbeinsson TB, Hauksdottir A, Thorsteinsson T, Briem H. A survey of early health effects of the Eyjafjallajokull 2010 eruption in Iceland: a population-based study. BMJ Open. 2012; 2 :e000343. doi: 10.1136/bmjopen-2011-000343. [ PMC free article ] [ PubMed ] [ CrossRef ] [ Google Scholar ]
- Higuchi K, Koriyama C, Akiba S. Increased mortality of respiratory diseases, including lung cancer, in the area with large amount of ashfall from Mount Sakurajima volcano. J Environ Public Health. 2012. article ID 257831. [ PMC free article ] [ PubMed ] [ CrossRef ]
- http://www.protezionecivile.gov.it/jcms/it/emergenza_etna_2002_2003.wp (accessed February 2013)
- Fano V, Cernigliaro A, Scondotto S, Cuccia M, Forastiere F, Nicolosi A, Oliveri C, Scillieri R, Distefano P, Perrucci CA. Health effects of environmental contamination due to volcanic ash of Mount Etna in autumn 2002. Epidemiol Prev. 2005; 29 :180–187. [ PubMed ] [ Google Scholar ]
- Fano V, Cernigliaro A, Scondotto S, Perucci CA, Forastiere F. The fear of volcano: short-term health effects after Mount Etna’s eruption in 2002. Eur Respir J. 2010; 36 :1216–1218. doi: 10.1183/09031936.00078910. [ PubMed ] [ CrossRef ] [ Google Scholar ]
- VanEenwyk J. Guidelines for using and developing rates for public health assessment. Washington State Department of Health; 2012. http://www.doh.wa.gov/DataandStatisticalReports/DataGuidelines.aspx . [ Google Scholar ]
- Longo BM, Rossignol A, Green JB. Cardiorespiratory health effects associated with sulphurous volcanic air pollution. Public Health. 2008; 122 :809–820. doi: 10.1016/j.puhe.2007.09.017. [ PubMed ] [ CrossRef ] [ Google Scholar ]
- Gordian ME, Ozkaynak H, Xue J, Morris J, Spengler J. Particulate air pollution and respiratory disease in Anchorage, Alaska. Environ Health Perspect. 1996; 104 :290–297. doi: 10.1289/ehp.96104290. [ PMC free article ] [ PubMed ] [ CrossRef ] [ Google Scholar ]
- Martin TR, Wehner AP, Butler J. Evaluation of physical health effects due to volcanic hazards: the use of experimental systems to estimate the pulmonary toxicity of volcanic ash. Am J Public Health. 1986; 76 (Suppl):59–65. doi: 10.2105/AJPH.76.Suppl.59. [ PMC free article ] [ PubMed ] [ CrossRef ] [ Google Scholar ]
- Garcia Cuellar C, Alfaro Moreno E, Martinez Romero F, Ponce De Leon Rosales S, Rosas I, Peres Cardenas E, Osornio Vargas AR. DNA Damage Induced by PM 10 from Different Zones of Mexico City. Ann Occup Hyg. 2002; 46 (1):425–428. [ Google Scholar ]
A multi-disciplinary study of the 2002–03 Etna eruption: insights into a complex plumbing system
- Research article
- Published: 07 July 2004
- Volume 67 , pages 314–330, ( 2005 )
Cite this article
- Daniele Andronico 1 ,
- Stefano Branca 1 ,
- Sonia Calvari 1 ,
- Michael Burton 1 ,
- Tommaso Caltabiano 1 ,
- Rosa Anna Corsaro 1 ,
- Paola Del Carlo 1 ,
- Gaetano Garfì 1 ,
- Luigi Lodato 1 ,
- Lusia Miraglia 1 ,
- Filippo Murè 1 ,
- Marco Neri 1 ,
- Emilio Pecora 1 ,
- Massimo Pompilio 1 ,
- Guiseppe Salerno 1 &
- Letizia Spampinato 1
2075 Accesses
261 Citations
8 Altmetric
Explore all metrics
The 2002–03 Mt Etna flank eruption began on 26 October 2002 and finished on 28 January 2003, after three months of continuous explosive activity and discontinuous lava flow output. The eruption involved the opening of eruptive fissures on the NE and S flanks of the volcano, with lava flow output and fire fountaining until 5 November. After this date, the eruption continued exclusively on the S flank, with continuous explosive activity and lava flows active between 13 November and 28 January 2003. Multi-disciplinary data collected during the eruption (petrology, analyses of ash components, gas geochemistry, field surveys, thermal mapping and structural surveys) allowed us to analyse the dynamics of the eruption. The eruption was triggered either by (i) accumulation and eventual ascent of magma from depth or (ii) depressurisation of the edifice due to spreading of the eastern flank of the volcano. The extraordinary explosivity makes the 2002–03 eruption a unique event in the last 300 years, comparable only with La Montagnola 1763 and the 2001 Lower Vents eruptions. A notable feature of the eruption was also the simultaneous effusion of lavas with different composition and emplacement features. Magma erupted from the NE fissure represented the partially degassed magma fraction normally residing within the central conduits and the shallow plumbing system. The magma that erupted from the S fissure was the relatively undegassed, volatile-rich, buoyant fraction which drained the deep feeding system, bypassing the central conduits. This is typical of most Etnean eccentric eruptions. We believe that there is a high probability that Mount Etna has entered a new eruptive phase, with magma being supplied to a deep reservoir independent from the central conduit, that could periodically produce sufficient overpressure to propagate a dyke to the surface and generate further flank eruptions.
This is a preview of subscription content, log in via an institution to check access.
Access this article
Price includes VAT (Russian Federation)
Instant access to the full article PDF.
Rent this article via DeepDyve
Institutional subscriptions
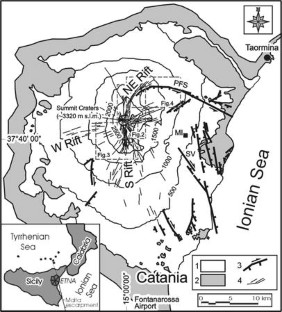
Similar content being viewed by others
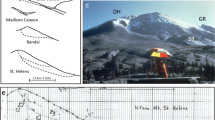
Lateral edifice collapse and volcanic debris avalanches: a post-1980 Mount St. Helens perspective
Lee Siebert & Mark E. Reid
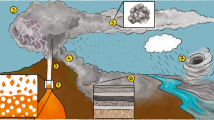
The lifecycle of volcanic ash: advances and ongoing challenges
Joali Paredes-Mariño, Pablo Forte, … Ulrich Kueppers
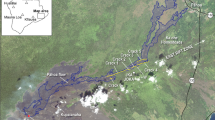
Pre-existing ground cracks as lava flow pathways at Kīlauea in 2014
Tim R. Orr, Edward W. Llewellin, … Matthew R. Patrick
Acocella V, Neri M (2003) What controls flank eruptions: the 2001 Etna eruption and the possible triggering mechanisms. Bull Volcanol 65:517–529 (DOI: 10.1007/s00445–003–0280–3)
Google Scholar
Acocella V, Behncke B, Neri M, D’Amico S (2003) Link between major flank slip and eruptions at Mt. Etna (Italy). J Geophys Res 30(24):2286 (DOI:10.1029/2003GL018642)
Alparone S, Andronico D, Lodato L, Sgroi T (2003) Relationship between tremor and volcanic activity during the Southeast Crater eruption on Mount Etna in early 2000. J Geophys Res 108(B5):2241 (DOI: 10.1029/2002JB001866)
Andronico D, Coltelli M, Cristalli A, Del Carlo P, Scollo S (2003a) The 2002–2003 Etna ash fallout and its effects. Proc Cities on Volcanoes 3 Conf, Hilo Hawai’i, USA, 14–18 July 2003
Andronico D, Cristaldi A, Del Carlo P, Taddeucci J (2003b) Monitoring the ashes from the 2002–2003 flank eruption of Mount Etna (Italy). EAE03-A-06783, EAE03-A-06783, EGS-AGU-EUG Joint Assembly, Nice, France, April 2003
Armienti P, Innocenti F, Petrini R, Pompilio M, Villari L (1988) Sub-aphyric alkali basalt from Mt. Etna: inferences on the depth and composition of the source magma. Rend Soc It Mineral Petrol 43:877–891
Azzaro R, Branca S, Giammanco S, Gurrieri S, Rasà R, Valenza M (1998) New evidence for the form and extend of the Pernicana Fault System (Mt. Etna) from structural and soil-gas surveying. J Volcanol Geoth Res 84:143–152
Article CAS Google Scholar
Behncke B, Neri M (2003a) The July-August 2001 eruption of Mt. Etna (Sicily). Bull Volcanol 65:461–476 (DOI: 10.1007/s00445–003–0274–1)
Behncke B, Neri M (2003b) Cycles and trends in the recent eruptive behaviour of Mount Etna (Italy). Can J Earth Sci 40:1405–1411 (DOI: 10.1139/E03–052)
Billi A, Acocella V, Funiciello R, Giordano G, Lanzafame G, Neri M (2003) Mechanisms for ground-surface fracturing and incipient slope failure associated to the July-August 2001 eruption of Mt. Etna, Italy: analysis of ephemeral field data. J Volcanol Geoth Res 122:281–294
Blaserna P, Silvestri O, Gemmellaro C (1879) L’eruzione dell’Etna del 26 Maggio 1879. Relazione della Commissione nominata dai Ministri di Agricoltura Industria e Commercio e della Pubblica Istruzione, Tipografia Eredi Botta, Roma, Italia, pp 1–13
Branca S, Del Carlo P (2004) Eruptions of Mt Etna during the past 3,200 years: a revised compilation integrating the historical and stratigraphic records. In: Bonaccorso A, Calvari S, Coltelli M, Del Negro C, Falsaperla S (eds) Etna volcano laboratory. AGU (Geophysical monograph series), Washington, DC 143:384
Branca S, Carbone D, Greco F (2003) Intrusive mechanism of the 2002 NE-Rift eruption at Mt. Etna (Italy) inferred through continuous microgravity data and volcanological evidences. Geophys Res Lett 30(20):2077 (DOI:10.1029/2003GL018250)
Bruno N, Caltabiano T, Romano R (1999) SO 2 emissions at Mt. Etna with particular reference to the period 1993–1995. Bull Volcanol 60:405–411
Article Google Scholar
Bruno N, Caltabiano T, Giammanco S, Romano R (2001) Degassing of SO 2 and CO 2 at Mount Etna (Sicily) as indicator of pre-eruptive ascent and shallow emplacement of magma. J Volcanol Geoth Res 110:137–153
Burton M, Allard P, Murè F, Oppenheimer C (2003) FTIR remote sensing of fractional magma degassing at Mt. Etna, Sicily. In: Oppenheimer C, Pyle DM, Barclay J (eds) Volcanic degassing. Geological Society (Special Publications), London, 213:281–293
Caltabiano T, Romano R (1988) Messa a punto di metodologie di misura con apparecchiatura COSPEC del flusso di SO 2 da vulcani attivi italiani. Boll GNV IV:133–145
Caltabiano T, Romano R, Budetta G (1994) SO 2 measurements at Mount Etna, Sicily. J Geophys Res 99:12809–12811
Calvari S, Pinkerton H (2002) Instabilities in the summit region of Mount Etna during the 1999 eruption. Bull Volcanol 63:526–535
Calvari S, Pinkerton H (2004) Birth, growth and morphologic evolution of the “Laghetto” cinder cone during the 2001 Etna eruption. J Volcanol Geoth Res (in press)
Calvari S, Coltelli M, Neri M, Pompilio M, Scribano V (1994) The 1991–1993 Etna eruption: chronology and lava flow-field evolution. Acta Vulcanol 4:1–14
Calvari S, and the whole scientific staff of INGV – Sezione di Catania (2001) Multidisciplinary approach yields insight into Mt. Etna 2001 eruption. EOS Transactions, AGU 82:653–656
Calvari S, Neri M, Pinkerton H (2002) Effusion rate estimations during the 1999 summit eruption on Mt. Etna, and growth of two distinct lava flow fields. J Volcanol Geoth Res 119:107–123
Calvari S, Lodato L, Burton M, Andronico D (2003) Dike emplacement at Mount Etna before the 2002 flank eruption revealed by surveys with a portable thermal camera. EGS-AGU-EUG Joint Assembly, Nice, France, April 2003, Geophys Res Abstr 5:05329
Coltelli M, Pompilio M, Del Carlo P, Calvari S, Pannucci S, Scribano V (1998) Mt. Etna 1993–95 Eruptive activity. Acta Vulcanol 10:141–148
Coltelli M, Del Carlo P, Pompilio M (2000a) Etna: Eruptive activity in 1996. Acta Vulcanol 12(1):63–67
Coltelli M, Del Carlo P, Vezzoli L (2000b) Stratigraphic constraints for explosive activity in the past 100 ka at Etna Volcano, Italy. Int J Earth Sci 89:665–677
Corsaro RA, Pompilio M (2004a) Magmatic processes in the shallow plumbing system of Mt. Etna as recorded by compositional variations in volcanics of recent summit activity (1995–1999). J Volcanol Geoth Res (in press)
Corsaro RA, Pompilio M (2004b) Magma dynamics at Mount Etna. In: Bonaccorso A, Calvari S, Coltelli M, Del Negro C, Falsaperla S (eds) Etna volcano laboratory. AGU (Geophysical monograph series), Washington, DC 143:384
Cucuzza Silvestri S (1949) L’eruzione dell’Etna del 1947. Bull Volcanol II, IX:81–111
Cumin G (1947) Dati e considerazioni sulla recente eruzione. “Vie economiche” del 15 Marzo 1947, Catania
Cumin G (1950) L’eruzione laterale etnea del dicembre 1949. Boll Acc Gioenia Sci Nat Catania IV:1–7
Del Carlo P, Branca S (1998) Tephrostratigraphic dating of the pre-1300 AD SE flank eruptions of Mt Etna. Acta Vulcanol 10:33–37
Francis P, Burton MR, Oppenheimer C (1998) Remote measurements of volcanic gas compositions by solar occultation spectroscopy. Nature 396:567–570
Garduño VH, Neri M, Pasquarè G, Borgia A, Tibaldi A (1997) Geology of the NE-Rift of Mount Etna (Sicily, Italy). Acta Vulcanol 9:91–100
Groppelli G, Tibaldi A (1999) Control of rock reology on deformation style and slip-rate along the active Pernicana Fault, Mt. Etna, Italy. Tectonophysics 305:521–537
Harris AJL, Neri M (2002) Volumetric observations during paroxysmal eruptions at Mount Etna: pressurized drainage of a shallow chamber or pulsed supply? J Volcanol Geoth Res 116:79–95
Kieffer G (1975) Sur l’existence d’une “Rift zone” à L’Etna. CR Acad Sci Paris D 280:236–266
Lanzafame G, Neri M, Acocella V, Billi A, Funiciello R, Giordano G (2003) Structural features of the July-August 2001 Mount Etna eruption: evidence for a complex magma supply system. J Geol Soc London 160:531–544
Le Maitre RW (1989) A classification of igneous rocks and glossary of terms. Recommendations of the IUGS Subcommission on the Systematics of Igneous rocks. Blackwell Scientific, London, pp 193
McGuire WJ, Pullen AD (1989) Location and orientation of eruptive fissures and feeder-dykes at Mount Etna: influence of gravitational and regional tectonic stress regimes. J Volcanol Geoth Res 38:352–344
Neri M, Acocella V, Behncke B (2004) The role of the Pernicana Fault System in the spreading of Mount Etna (Italy) during the 2002–2003 eruption. Bull Volcanol (in press, DOI: 10.1007/s00445–003–0322)
Patanè D (2002) Aggiornamento delle attività di monitoraggio sismico all’Etna. http://www.ct.ingv.it/report/Rapporto_eruzione20021030.pdf
Patanè D, De Gori P, Chiarabba C, Bonaccorso A (2003) Magma ascent and the pressurization of Mount Etna’s volcanic system. Science 299:2061–2063
Article PubMed Google Scholar
Pyle DM (1989) The thickness, volume and grain size of tephra fall deposits. Bull Volcanol 51:1–15
Pompilio M, Coltelli M, Del Carlo P, Vezzoli L (1995) How do basaltic magmas, feeding explosive eruptions, rise and differentiate at Mt. Etna? Period Mineralogia 64:253-254
Pompilio M, Corsaro RA, Freda C, Miraglia L, Scarlato P, Taddeucci J (2001) Petrological evidence of a complex plumbing system feeding the July-August 2001 eruption of Mt. Etna. EOS, AGU Trans – Fall Meeting Suppl Abstr 82(47):F1412
Ponte G (1948) L’eruzione dell’Etna del Febbraio-Marzo 1947. Ann Geofis 1:56–68
Recupero G (1815) Storia naturale e generale dell’Etna. Edizioni Dafni, Tringali Editore, Catania, 1970
Rittmann A (1965) Notizie sull’Etna. Nuovo Ciment I 3:1117–1123
Romano R, Sturiale C (1982) The historical eruptions of Mt. Etna (Volcanological data). Mem Soc Geol It 23:75–97
CAS Google Scholar
Salerno G, Caltabiano T, Bruno N, Longo V (2003) Anomalous SO 2 emissions from Mt. Etna between 2001 and 2002–2003 eruptions. EGS-AGU-EUG Joint Assembly, Nice, France, April 2003, Geophysical Research Abstracts 5:11953
Silvestri O (1879) Sulla doppia eruzione scoppiata il 26 Maggio 1879. Rapporto alle LL.EE. i Ministri dell’Interno dell’Istruzione Pubblica, e di Agricoltura, Industria e Commercio. Tipografia Galatolà, Catania, pp 1–19
Taddeucci J, Pompilio M, Scarlato P (2002) Monitoring the explosive activity of the July-August 2001 eruption of Mt. Etna (Italy) by ash characterization. Geophys Res Lett 29:1029–1032
Tonarini S, Armienti P, D’Orazio M, Innocenti F, Pompilio M, Petrini R (1995) Geochemical and isotopic monitoring of Mt. Etna 1989–93. Eruptive activity: bearing on the shallow feeding system. J Volcanol Geoth Res 64:95–115
Download references
Acknowledgements
We wish to thank G. Bertolaso and the Civil Protection for their substantial support of our activities; the helicopter pilots of Civil Protection, Air Walser, and the Fire Service, whose expertise has allowed us to collect a huge amount of data; colleagues of INGV from Catania, Napoli, Roma and Pisa, and from the Universities of Milan, Bologna, Cosenza and Catania who helped during our monitoring efforts, and E. Boschi who strongly encouraged this work. The paper benefited from meticulous and thoughtful reviews by M. Edmonds and C. Heliker.
Author information
Authors and affiliations.
Istituto Nazionale di Geofisica e Vulcanologia – Sezione di Catania, Piazza Roma 2, 95123, Catania, Italy
Daniele Andronico, Stefano Branca, Sonia Calvari, Michael Burton, Tommaso Caltabiano, Rosa Anna Corsaro, Paola Del Carlo, Gaetano Garfì, Luigi Lodato, Lusia Miraglia, Filippo Murè, Marco Neri, Emilio Pecora, Massimo Pompilio, Guiseppe Salerno & Letizia Spampinato
You can also search for this author in PubMed Google Scholar
Corresponding author
Correspondence to Daniele Andronico .
Additional information
Editorial responsibility: J. Donnelly-Nolan
Rights and permissions
Reprints and permissions
About this article
Andronico, D., Branca, S., Calvari, S. et al. A multi-disciplinary study of the 2002–03 Etna eruption: insights into a complex plumbing system. Bull Volcanol 67 , 314–330 (2005). https://doi.org/10.1007/s00445-004-0372-8
Download citation
Received : 07 July 2003
Accepted : 02 April 2004
Published : 07 July 2004
Issue Date : April 2005
DOI : https://doi.org/10.1007/s00445-004-0372-8
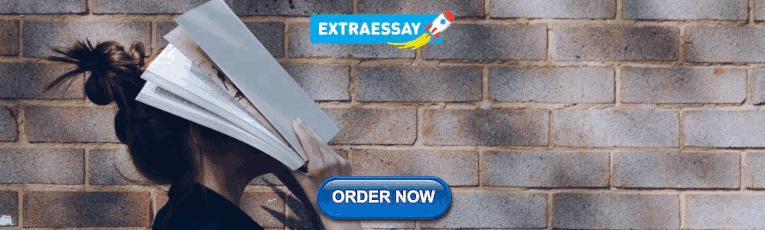
Share this article
Anyone you share the following link with will be able to read this content:
Sorry, a shareable link is not currently available for this article.
Provided by the Springer Nature SharedIt content-sharing initiative
- Multi-disciplinary study
- 2002–03 eruption
- Eccentric eruptions
- Flank activity
- Etna feeding system
- Volcanic processes
- Find a journal
- Publish with us
- Track your research
Thank you for visiting nature.com. You are using a browser version with limited support for CSS. To obtain the best experience, we recommend you use a more up to date browser (or turn off compatibility mode in Internet Explorer). In the meantime, to ensure continued support, we are displaying the site without styles and JavaScript.
- View all journals
- My Account Login
- Explore content
- About the journal
- Publish with us
- Sign up for alerts
- Open access
- Published: 13 November 2023
Assessment of eruption source parameters using infrasound and plume modelling: a case study from the 2021 eruption of Mt. Etna, Italy
- Silvio De Angelis ORCID: orcid.org/0000-0003-2636-3056 1 , 2 ,
- Luciano Zuccarello ORCID: orcid.org/0000-0003-0094-9577 1 nAff2 ,
- Simona Scollo ORCID: orcid.org/0000-0001-8704-8629 3 &
- Luigi Mereu ORCID: orcid.org/0000-0003-0303-1171 4 , 5
Scientific Reports volume 13 , Article number: 19857 ( 2023 ) Cite this article
698 Accesses
Metrics details
- Natural hazards
- Volcanology
Atmospheric injection of volcanic ash during eruptions is a threat to aviation. Reliable forecast of airborne ash dispersal relies on empirical and numerical models. Key inputs into these models are so-called eruption source parameters such as the rate at which pyroclastic material is ejected from the vent and the maximum height of eruptive columns. Here, we use infrasound data recorded during eruptive activity in June 2021 at Mt. Etna, Italy, to demonstrate its potential for assessment of eruption rates in near-real time. We calculate a time series of flow velocity at the vent using data corrected for topographic scattering, and the effect of vent geometry on the acoustic source radiation. We obtain values of flow velocity of 50–125 m/s during a period of sustained, paroxysmal, activity. We use independent estimates from other ground-based remote sensing data to validate our results. Further, we use the infrasound-derived flow velocities as input into a 1D plume model to estimate the maximum height of the eruption column. Our results suggest that infrasound technology holds promise to assess eruption rates and inform modelling of volcanic plumes. We anticipate that implementation of real-time operational workflows based on infrasound data and plume modelling will impact decision-making and risk mitigation at active volcanoes.
Similar content being viewed by others
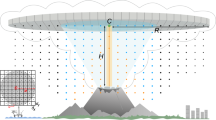
The radius of the umbrella cloud helps characterize large explosive volcanic eruptions
Robert Constantinescu, Aurelian Hopulele-Gligor, … Alain C. M. Volentik
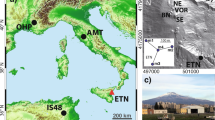
Long range infrasound monitoring of Etna volcano
E. Marchetti, M. Ripepe, … P. Husson
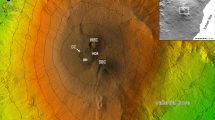
Assessing impending hazards from summit eruptions: the new probabilistic map for lava flow inundation at Mt. Etna
Francesco Zuccarello, Giuseppe Bilotta, … Annalisa Cappello
Introduction
Challenges in monitoring volcanic plumes and ash transport.
Explosive eruptions frequently release significant amounts of fine rock particles and glass fragments, referred to as volcanic ash, into the atmosphere. The emission of volcanic ash, owing to its highly abrasive nature and the low melting point of its glass component, can cause severe damage to aircrafts 1 , 2 , 3 . Nine Volcanic Ash Advisory Centres (VAACs) are deputed to evaluate the presence and extent of volcanic ash in the atmosphere, and to issue ash-cloud warnings to the aviation community around the world 1 . The ability of these operational centres to forecast the movement of ash in the atmosphere, and thus identify at-risk regions, is underpinned by complex numerical models. Such models require a set of input parameters that represent the meteorology of the atmosphere at the time of eruption (e.g., wind speed and direction, vertical temperature profile) and the nature of the eruptive source (e.g., source location, time and duration of eruption, vent geometry, height of the plume, mass eruption rate, particle size distribution, ash density and shape) 4 . Constraining the extensive parametrization of atmospheric ash transport models is challenging, and thus, their outputs are affected by substantial uncertainty. Meteorological parameters are usually well-constrained by data and models with temporal and spatial resolutions of the order of hours and few kilometers, respectively (e.g., the Met Office Unified Model 5 used by the London VAAC 4 ). Conversely, larger uncertainties are linked to estimates of eruption source parameters 6 , 7 (ESP), in particular mass or volume eruption rates (MER or VER, respectively). Commonly, VER is estimated through inversion of so-called 0D eruption plume models (EPMs), that is empirical scaling relationships between VER and the height reached by the plume above the vent. The simplest, and most widely used, family of EPMs takes the general form \(H=C{V}^{n}\) , where \(H\) is the maximum height reached by the plume above the vent, \(V\) is VER (measured as \({\mathrm{m}}^{3}\) of dense-rock equivalent per second), \(C\) and \(n\) are constants 1 , 8 . For the purpose of informing volcanic ash transport modelling, 1D integral EPMs have also been increasingly tested owing to their ability to include processes such as the entrainment of atmospheric air into the eruption column, and to account for the effect of wind speed and direction on plume rise 4 , 9 . The main challenge in the use of EPMs for assessment of VER is that they require validation, which depends on the availability of independent measurements of both plume heights and MER/VER 1 , 10 , 11 . Independent column height estimates are available from either satellite and ground-based (e.g., camera, radar, lidar) measurements albeit with limitations posed by data latency, logistics and weather conditions. The majority of independent VER estimates are average measurements of the ratio between the total volume of erupted tephra and the duration of the eruptive event. These data can exhibit significant scatter 4 , 12 , which arises from differences in the techniques employed to evaluate the volume of tephra deposits and the duration of eruptions, and factors such as the impact of wind speed and direction on the rise of volcanic plumes. Therefore, the need for new methods to quantify volcanic emissions has emerged as a critical step towards improving our capacity to assess and mitigate hazards from volcanic ash.
Measurements of volume eruption rate
In recent years, a series of studies have proposed methods to estimate VER/MER from analyses of ground-based radar, thermal infrared (IR), and acoustic data. Gouhier and Donnadieu 13 and Freret-Lorgeril et al. 14 used echo power data from Doppler Radar measurements to estimate MER at Stromboli and Mt. Etna (Italy), respectively; Marzano et al. 15 proposed a workflow to measure MER using microwave weather radar and infrasound array data collected during eruptions at Eyjafjallajökull (Iceland, 2010), Grímsvötn (Iceland, 2011) and Mt. Etna (2013); Ripepe et al. 16 employed a combination of thermal camera imagery and infrasound array data to evaluate plume exit velocity during the 2010 Eyjafjallajökull eruption; Calvari et al. 17 calculated the exit velocity of the eruptive jet from thermal IR data during paroxysmal activity at Mt. Etna in 2020–2021. Among these methods, infrasound has emerged as a promising tool to quantify VER/MER owing to the comparatively low costs associated with sensor installation and maintenance, data availability in real-time, and its suitability for automated data processing. A substantial body of research has informed continuous development of methods for inversion of acoustic waveforms aimed at quantifying volcanic emissions. Caplan-Auerbach e al. 18 , Lamb et al. 19 , Ripepe et al. 16 used scaling laws that link the power radiated by acoustic sources to gas velocity during flow from a volcanic vent 20 . Johnson et al. 21 and Johnson and Miller 22 used a monopole source model, that is a compact source that radiates acoustic waves hemispherically, to quantify volcanic emissions at Mt. Erebus (Antarctica) and Sakurajima volcano (Japan), respectively. Kim et al. 23 introduced a waveform inversion method to calculate VER at Tungurahua volcano (Ecuador) based on the Green’s Function solution to the inhomogeneous Helmoltz wave equation in a half-space; the method, based on the representation of the acoustic pressure wavefield as a combination of monopole and dipole terms, was also applied by De Angelis et al. 24 at Santiaguito volcano (Guatemala). Progress in numerical modelling of the acoustic wavefield generated by compact volcanic sources 25 , 26 underpinned additional studies focussed on retrieval of VER via full waveform inversion of infrasound signals using 3D numerical Green’s Functions 27 , 28 , 29 , 30 . A comprehensive review of these methods and their underlying theoretical frameworks can be found in De Angelis et al. 31 . More recently Hantusch et al. 32 and Freret-lorgeril et al. 33 have shown that VER can be calculated from direct integration of the acoustic pressure wavefield after applying corrections for scattering from topography, wavefield directivity (controlled by vent geometry and the acoustic wavenumber) and reflectance at the conduit outlet. Hantusch et al. 32 accounted for topographic scattering of the acoustic wavefield at Copahue volcano (Argentina) via the insertion loss (IL) parameter in the screen diffraction approximation. Freret-Lorgeril et al. 33 assumed that topographic scattering at Mt. Etna was negligible as the position of the infrasound array used was in line-of-sight with the erupting vent. Maher et al. 34 demonstrated that the screen diffraction approximation is only appropriate under specific conditions and suggested that numerical simulations are the best tool to estimate IL; a workflow for calculating maps of IL based on numerical modelling of acoustic wave propagation over volcanic topography was proposed by Lacanna and Ripepe 26 .
Here, we build on this extensive body of research and demonstrate a methodology to obtain independent estimates of VER from the integration of attenuation-corrected infrasound data recorded at Mt. Etna, Italy. We benchmark our results with independent estimates of flow velocity at the vent obtained from analyses of ground-based thermal IR imagery. Finally, we show how VER can be used as a direct input into 1D plume models for rapid assessment of maximum column height, thus, providing key monitoring information when other observations may not be available. The results of plume modelling are further validated using ground-based X-band radar data and satellite imagery gathered during eruption.
Eruptive activity at Mt. Etna: June 2021
Mt. Etna, Italy, is one of the most active volcanoes in the world. One of its distinctive features is the frequent occurrence of so-called paroxysms, that is episodes of intense explosive activity lasting from tens of minutes to many hours 35 . Paroxysms typically occur in clusters within eruptive periods that can last from few weeks to months 36 . Between December 2020 and February 2022 Mt. Etna produced 66 paroxysms 17 . In this study, we focus on an episode on 20 June, 2021, part of a sequence of paroxysms that occurred, with striking regularity, during the second half of June 2021. These events had durations of up to few hours and all followed the characteristic pattern of paroxysmal activity at Mt. Etna. Initial rapid-fire Strombolian explosions evolved into nearly continuous lava fountaining, feeding lava flows and volcanic plumes with heights of up to several kilometers above the vent 37 . Infrasound and thermal IR data recorded during the paroxysmal activity on 20 June are shown in Fig. 1 a–f. Figure 1 c, d illustrate early Strombolian activity, consisting of discrete explosions clearly distinguishable in both the waveform (Fig. 1 c) and spectrogram (Fig. 1 d). During the later stages of the paroxysm, the acoustic signal evolves into nearly continuous tremor (Fig. 1 e) and individual explosions are only occasionally discerned (Fig. 1 f). Figure 1 b shows thermal IR images tracking the change from discrete explosions to lava fountains feeding a sustained volcanic plume.
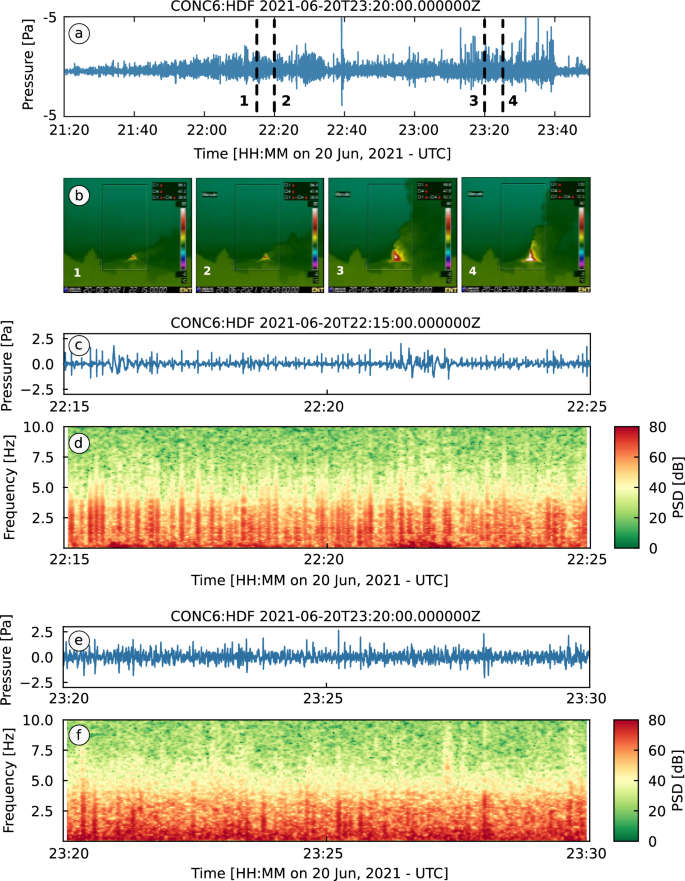
Infrasound and thermal IR data recorded during the paroxysmal activity at Etna on 20 June, 2021. ( a ) Pressure waveform recorded through a paroxysmal event on 20 June, 2021. Infrasound data are recorded by the central sensor of a temporary 6-element infrasound array at approximately 6 km from the active vent (see Fig. 2 a–c); ( b ) Four images recorded by a thermal IR camera of the Istituto Nazionale di Geofisica e Vulcanologia, Osservatorio Etneo (INGV-OE), located approximately 15 km South of the Etna summit craters (see Fig. 2 a). The images correspond to times 1–4 marked by dashed lines in ( a ); ( c ) Zoomed waveform showing 15 min of acoustic data during Strombolian activity; ( d ) Spectrogram of the signal in ( c ). The spectrogram is calculated as the Power Spectral Density of the pressure time series over a sliding window of 10.24 s with 50% overlap; ( e ) Zoomed waveform showing 10 min of acoustic data during lava fountaining activity; ( f ) Spectrogram of the signal in e) calculated with the same parameters as in ( d ).
Infrasound array
For this study, we used acoustic data recorded by a temporary 6-element infrasound array deployed at Mt. Etna in June 2021. The array was installed and operated by the University of Liverpool and the Istituto Nazionale di Geofisica e Vulcanologia (INGV sezione di Pisa and INGV-Osservatorio Etneo) at the Mt. Conca site (Fig. 2 a, CONC), approximately 6 km from the active vent within the South East Crater (SEC) area (Fig. 2 b). The array had an aperture (i.e., largest distance between any two sensors) of approximately 70 m (Fig. 2 c) and was instrumented with IST2018 infrasound microphones 38 (full-scale range of 480 Pa peak-to-peak, flat response between 0.1 and 40 Hz). Data were recorded on-site with a sampling frequency of 100 Hz and 24-bit resolution.
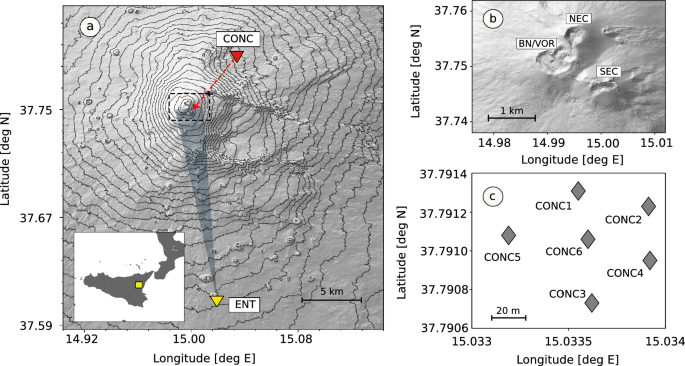
Map of Mt. Etna (digital elevation model data source: https://tinitaly.pi.ingv.it/ ) and location of infrasound and thermal IR sensors used in this study. ( a ) Map showing the locations of temporary infrasound array CONC (red triangle) and thermal IR ENT camera (yellow triangle). CONC was installed at the site Monte Conca, approximately 6 km from the active vent; ENT operated by the Istituto Nazionale di Geofisica e Vulcanologia, Osservatorio Etneo, and installed in the town of Nicolosi, approximately 15 km South of the active vent. The inset plot shows the location of Mt. Etna (yellow square) in Sicily (Italy). The dashed box identifies the summit area. The red dashed arrow indicates the backazimuth between array CONC and the active vent within the South East Crater area; ( b ) Detail of the summit area and craters at Mt. Etna in June 2021 obtained from an Uncrewed Aerial Vehicle survey of the summit area: Bocca Nuova/Voragine (BN/VOR), North East Crater (NEC) and South East Crater (SEC); c) Configuration of the 6-element infrasound array CONC. All maps were produced with the Python packages Matplotlib v3.8 ( https://matplotlib.org/ ) and GDAL v3.7.0 ( https://gdal.org/index.html ).
Mt. Etna is routinely monitored by INGV-Osservatorio Etneo (INGV-OE) with an extensive multi-parameter sensor network, including thermal IR cameras. Here, we used thermal imagery of the ENT camera recorded at the site located in Nicolosi (Fig. 2 a), approximately 15 km from the active summit craters. The site is equipped with a Flir A40M camera recording IR images in the \(7.5 - 13\,\upmu {\text{m}}\) band, with a field of view of 640 × 480 pixels and thermal sensitivity of \(80\;{\text{mK}}\) at \(25^\circ\) 17 , 39 . The camera records images with a sampling rate of \(0.5\;{\text{Hz}}\) , and provides a spatial resolution of 1.3 μrad ( \(\sim 15\;{\text{m}}\) at the ENT site). We chose this site as it provides the best trade-off between data availability, spatial and temporal resolution, and the ability to track the development of the eruptive plume during paroxysmal activity.
X-band radar
Volcanic plumes from Etna are also observed using a dual polarimetric X-band radar, managed by the Italian Department of the Civil Protection, part of the national weather radar network 40 . The X-band dual-polarization radar, located at the international airport of Catania (distance of about 32 km from the SEC), has a wavelength of 3.1 cm (9.6 GHz), peak power of 50 kW and half-power beam width of 1.3°. The radar scans a volume defined by an area of 160 × 160 km 2 and a height of 20 km, recording data along 12 elevation angles every 10 min.
Here, we have investigated an episode of paroxysmal activity that occurred on 20 June, 2021 starting at approximately 21:30 (UTC), lasting for about 2 h (Fig. 3 a). For this event, INGV-OE issued two Volcano Observatory Notice for Aviation (VONA) with color code Red (the highest level of concern) at 22:01 and 22:19 UTC 41 , 42 ; INGV-OE reported lava fountaining at the SEC and a plume drifting towards the East-South East. However, the height of the plume could not be estimated in real-time during the paroxysm.
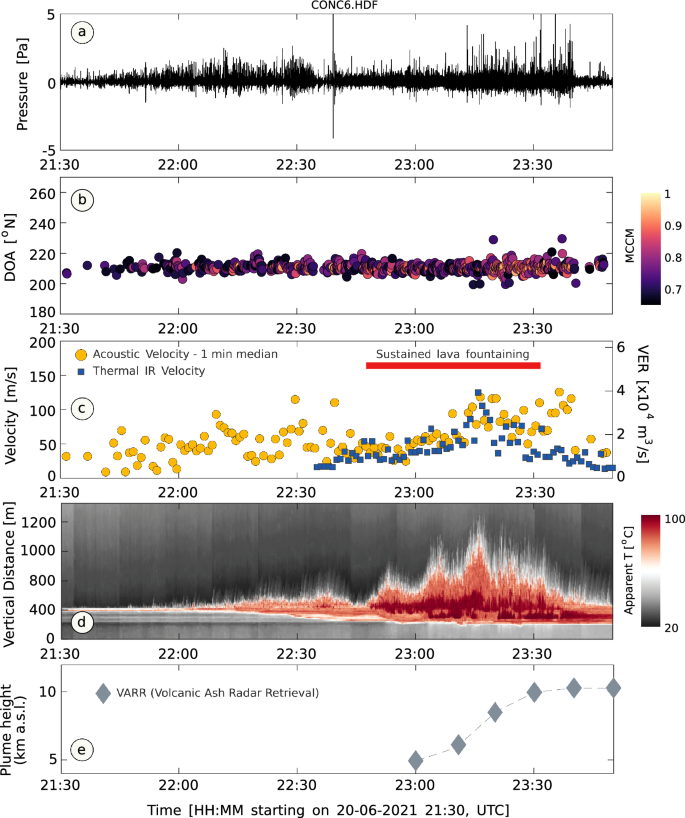
Time series of VER and flow velocity at the vent (infrasound array and thermal IR), and plume height (X-band radar data) during paroxysmal activity on 20 June, 2021. ( a ) Infrasound signal through the paroxysm, recorded by the central sensor of the a temporary 6-element infrasound array at approximately 6 km from the active vent (Fig. 2 a, c); ( b ) Time series of high-quality (MCMM > 0.65) Direction of Arrival estimates throughout the paroxysm; ( c ) Time series of flow velocity at the vent estimated from integration of infrasound data (yellow circles) and analysis of thermal IR data (blue squares); ( d ) time series of thermal IR data from the ENT site (Fig. 2 a). The plot is produced from stacks across a vertical section (through the crater area) of each time-lapse thermal IR image (one image every 2 s); ( e ) Time evolution of plume height estimated from X-band radar reflectivity data (uncertainty on plume height measurements is +/− 300 m).
VER and flow velocity from infrasound array data analyses
We analysed infrasound data recorded at the CONC site (Fig. 2 a, c) during paroxysmal activity on 20 June, 2021. Infrasound arrays allow to identify windows of coherent signal and the direction of their source region, providing an effective tool for discrimination of eruptive activity from other signals. We processed the CONC data using slowness inversion 43 to obtain a time series of Direction of Arrival (DOA), that is the direction from which infrasound energy travels as it crosses the array. The results, in Fig. 3 b, show coherent acoustic energy crossing the array from a stable backazimuth in the direction of the active SEC (~ 205°–215° N). We selected data windows corresponding to high quality array locations (MCMM > 0.65) and integrated the pressure signal recorded at the CONC6 microphone (Fig. 2 c) to calculate a time series of VER (Fig. 3 c). Finally, we used a vent radius of 10 m 44 to convert VER into flow velocity and calculated its 1-min median, which is shown in Fig. 3 c (yellow circles). We also performed calculations for a second paroxysm that occurred on 24 June, 2021 (supplementary material, Fig. S1 ).
Flow velocity from thermal IR data analyses
Figure 3 c shows a time series of measurements of jet velocity at the vent (blue squares) obtained from analyses of thermal IR data recorded at the ENT site (Fig. 2 a) during paroxysmal activity on 20 June, 2021. The measurements, performed with a time step of 1 min, can be compared with the 1-min median jet velocity obtained from acoustic data (Fig. 3 c, yellow circles); the two independent time series show excellent agreement throughout the phase of sustained lava fountaining (~ 22:45–23:30). After 23:30, concurrent with waning of lava fountaining, thermal IR estimates of velocity decrease while infrasound-derived jet velocities remain high as acoustic sensors continue detecting individual explosions with no significant associated lava fountaining. Figure 3 d shows the thermal IR data from the ENT camera plotted as a time series. The figure, produced by plotting stacks across a vertical section (through the crater area) of each time-lapse thermal IR image (one image every 2 s), allows to track the evolution of the paroxysm in space and time, clearly showing its onset (~ 22:00), peak (22:45–23:40) and waning (Fig. 3 c) phases.
Radar-derived height of the plume and plume model
We retrospectively analysed X-band radar data to estimate the temporal evolution of plume height during paroxysmal activity. Figure 3 e shows radar detection of a ~ 5 km ash plume starting from 23:00 increasing to a maximum height of 10 km at 23:30. The plume top was estimated at 10 km until ~ 00:00 (21 June, 2021) when it started to progressively wane during the late stages of the paroxysm (supplementary material, Fig. S2 ). We also used flow velocity estimates from the analysis of infrasound data as input into a 1D model of plume rise 9 . The model output, displayed in Fig. 4 for values of flow velocities of 50, 75 and 125 m/s (VER of \(1.6\, \times \,10^{4}\) , \(2.3\, \times \,10^{4}\) , and \(3.9\, \times \,10^{4} \,{\text{m}}^{3} {\text{/s}}\) considering a vent radius of \(10\,{\text{m}}\) ) shows the plume drifting towards the East (Fig. 4 a), extending vertically to a maximum height of 8–10 km above sea level (Fig. 4 b). These results agree with both the radar-derived maximum height (Fig. 3 e) and observations of the plume drifting eastwards (Fig. 4 c) from satellite thermal imagery collected by the Spinning Enhanced Visible and InfraRed Imager (SEVIRI, 8.7, 10.8, and 12 μm wavelenghts).
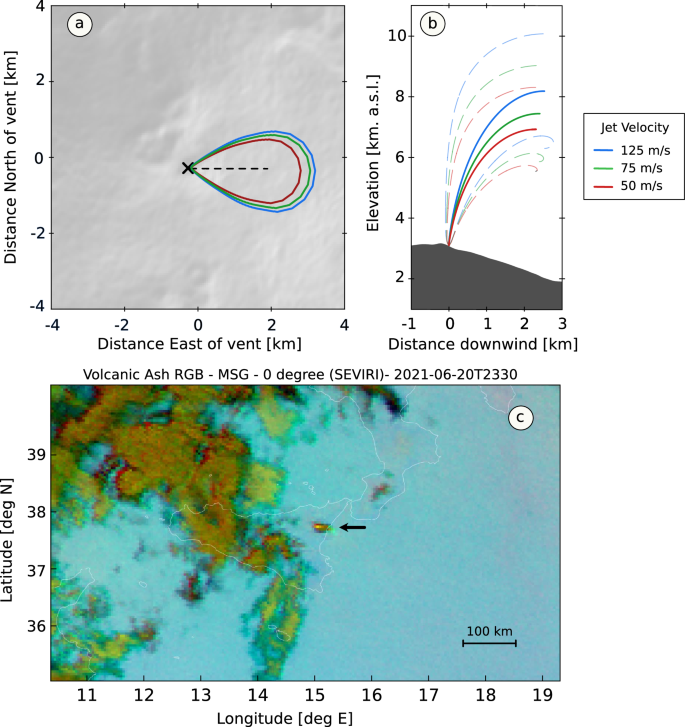
Model and satellite image of the ash plume during paroxysmal activity at Mt. Etna on 20 June, 2023. ( a ) Map view of the modelled plume showing ash drifting towards the East; ( b ) Cross-section (West–East) view of the modelled plume for values of flow velocity of 50, 75 and 125 m/s. Solid lines are represent the height of the plume at its centre and the dashed lines are lower and upper height of the plume as defined by its Gaussian width; ( c ) Composite thermal IR (8.7, 10.8, 12 \(\mathrm{\mu m}\) wavelengths) satellite image from the Spinning Enhanced Visible and InfraRed Imager (SEVIRI) at 23:30 on 20 June, 2021 (UTC time). The black arrow indicates the eruptive plume drifting towards the East. Panels ( a ) and ( b ) were generated by the PlumeRise model web interface ( https://www.plumerise.bris.ac.uk ). Panel ( c ) contains EUMETSAT Meteosat Volcanic Ash RGB—MSG—0 degree product derived from SEVIRI data. SEVIRI data were downloaded from the EUMETSAT data portal ( https://view.eumetsat.int/productviewer?v=default ). The map was produced with the Python packages Matplotlib v3.8 ( https://matplotlib.org/ ) and GDAL v3.7.0 ( https://gdal.org/index.html ).
Discussion and concluding remarks
The potential impact of airborne volcanic ash on aviation and infrastructure is well-known. Mitigation of the associated risks depends critically on the ability to issue timely forecasts of atmospheric ash dispersion, and thus, characterize ESP in real- or near real-time. In this study, we have demonstrated an infrasound-based methodology to obtain measurements of VER that holds potential for real-time implementation. We have analysed acoustic data gathered during a paroxysmal event at Mt. Etna on 20 June, 2021, and have benchmarked infrasound-derived measurements of VER (transformed into flow velocity assuming a vent with circular cross-section) with independent estimates of flow velocity at the vent from thermal IR imagery, one of the tools routinely employed by INGV-OE to detect the onset of eruptions, track their evolution and investigate the height of lava fountains during paroxysms.
Flow velocity at the vent and VER are important parameters to constrain eruption dynamics and to evaluate the height of the eruptive plume. Infrasound is a widely adopted tool for volcano monitoring and provides an effective means to measure VER. The main advantages of infrasound over other methods with comparable resolution, such as Doppler radar, are its low installation costs, and simple and rapid data processing that can easily be automated for real-time implementation. Unlike other methods such as those based on analyses of optical or thermal IR imagers, infrasound is not affected by cloud cover. Strong wind can, however, increase noise levels and deteriorate infrasound signal quality although its effects at the recording site can be mitigated through careful sensor installation. Strong directional winds can still severely affect along-path acoustic propagation and obscure signal; installation of multiple arrays at different azimuths with respect to the position of the active vent/s would provide effective mitigation of such adverse wind effects. We also stress that, for application of the methods presented in this study, acoustic arrays are preferred to larger aperture deployments of individual microphones in network configuration owing to their superior capabilities to separate coherent eruption signals from noise. Owing to its characteristics, infrasound is used for operational eruption early warning at Mt. Etna 45 and worldwide. Implementation of the methodology presented here for VER measurements would be a straightforward addition and a valuable complement to the existing monitoring systems. The main limitation of using infrasound to measure VER is that it requires a well-constrained source mechanism, as well as knowledge of topographic scattering and true signal attenuation, and reflectivity effects at the vent-atmosphere interface. In the case study presented here, as well as other volcanoes 32 , 33 , the acoustic source is well-represented by an isotropically radiating monopole (see “ Methods ” section for details). We adopted the formulation of Lacanna and Ripepe 46 to estimate VER, which provides a tool to characterize and correct the pressure wavefield for reflectivity at the vent-atmosphere interface in terms of signal frequency and the characteristic source dimension. Their formulation also provides a means to identify non-isotropic radiation patterns in terms of the product of two measurable parameters, that is the characteristic dimension of the source (i.e., the vent radius, a) and the acoustic wavenumber ( k ). The assumption of a pure isotropically radiating source (i.e., an acoustic monopole) holds for values of \(ka\le 0.43\) ; for values of \(ka>0.43\) additional corrections accounting for wavefield directivity are required. Finally, correction for signal attenuation from topographic scattering represented by IL is included; IL is evaluated from numerical modelling of acoustic wave propagation (see “ Methods ” section for additional details).
The acoustic and thermal IR flow velocity time series shown in Fig. 3 c show good agreement until approximately 23:15 on 20 June, 2021. After that time the acoustic data continues tracking eruption while the thermal IR signal decreases abruptly. Infrasound waveforms and array DOAs (Fig. 3 a, b) confirm that eruptive activity was still ongoing until approximately 23:45; at the same time the X-band radar detected a well-established plume (Fig. 3 e). A plausible explanation for the divergence between acoustic and thermal IR data starting from 23:15 is a migration of the fragmentation front deeper into the conduit towards the final stages of paroxysmal activity. In this scenario explosive activity would still be ongoing at depth into the conduit, as detected by infrasound data (Fig. 3 a, b), but it would only produce low-level lava fountaining at the surface (which would not be efficiently detected by the ENT camera) while still feeding an airborne ash plume. Infrasound activity declined at ~ 23:45 marking the waning of the paroxysm. A plume between 8 and 10 km persisted until at least 00:30 (supplementary material Fig. S2 ).
Finally, we have shown that infrasound-derived flow velocities can be used as input into a 1D plume rise model to obtain realistic estimates of the maximum height of the ash column. It should be noted that flow velocities ( \({v}_{jet}\) ) were calculated from the infrasound-derived VER and the cross-sectional area of the vent, \(S\) , as \({v}_{jet}=VER/S=VER/\pi\) , thus making the conduit radius, \(a\) , an important parameter. For example, for values of VER of the order of \(10^{4} \;{\text{m}}^{3} /{\text{s}}\) , thus similar to those reported in this study, an increase in conduit radius from \(10\) to \(20\;{\text{m}}\) would result in a decrease in calculated flow velocity of a factor of \(\sim 4\) . We suggest that a realistic vent radius is used for these calculations, or multiple plume simulations are conducted to assess the sensitivity of plume height to a range of plausible flow velocities at the vent. At Mt. Etna, and many other volcanoes, these issues are increasingly mitigated by the frequent availability of updated digital terrain models. These models, mostly obtained from Uncrewed Aerial Vehicle surveys, allow estimating vent dimensions with very high (< 1 m) spatial resolution e.g., 44 .
We stress that PlumeRise 9 , the 1D model used in this study, was capable of producing results for a single model in < 1 s, showing its suitability and potential for use in real-time. The results of plume modelling were benchmarked with data from a ground-based X-band radar (Fig. 3 e) and the Spinning Enhanced Visible and InfraRed Imager (SEVIRI) satellite (Fig. 4 c), which confirmed the maximum height (~ 10 km) and direction (East) of the ash plume, respectively. We note that the one of the objectives of this study was to provide a proof-of-concept for the combined use of infrasound data and plume rise modelling to assess ESP; a full sensitivity analysis of the plume model employed here to its parametrization was beyond the objectives of this work. Plume height is a key ESP as it defines the spreading height of the volcanic cloud in atmospheric ash dispersal models 33 and it is also often used for assessment of eruption rates. At Mt. Etna plume height is presently derived from analyses of optical images 47 , ground-based radar 40 and satellite data 48 ; successful application of these methods depends on whether specific conditions are met. Methods based on visible cameras are contingent on favorable meteorological conditions (e.g., no cloud cover), can only be used during daytime, and the retrievable height of volcanic plumes is dependent on source-camera distance and the camera field-of-view. Radar data are not affected by meteorological conditions and can be used during both daytime and nighttime; however, detection capabilities depend on source-receiver distance and direction of plume dispersion. Satellite-based measurements of plume height are generally delayed compared to other methods as they require a fully developed (opaque) plume to produce reliable height estimates 47 . Numerical modelling (or alternatively selection of a scenario from an ensemble of pre-computed models) informed by real-time analysis of acoustic data offers a valuable complement to these methods for rapid assessment of plume height.
This study aimed at introducing an alternative methodology for real-time assessment of ESP during eruptions, in particular VER and the top height of the eruptive plume. A number of methods already exist for measurement of ESP; some have briefly been discussed in this manuscript, including advantages and limitations. We have introduced a complementary strategy that relaxes some of the assumptions and simplifications underpinning widely used 0D EPM and overcomes the limitations of other existing techniques. We have demonstrated how analysis of infrasound data allows reliable estimates of VER that can be used to inform 1D plume models, thus, providing real-time estimates of the height of volcanic plumes. These methods would prove especially valuable in the early stages of a volcanic crisis when other measurements are typically not available. At Mt. Etna, for example, rapid information on plume height would be of great importance when issuing warnings to aviation authorities (e.g., VONA), and as a preliminary tool to assess plume dynamics and identify the most likely ash dispersal scenario.
We envision that infrasound-based methods for assessment of ESP, along with numerical modelling, will play an increasingly important role in volcano monitoring operations, and suggest that the implementation of our methodology into an operational workflow will be transformative in influencing decision making and risk mitigation during future volcanic crises.
Thermal IR estimates of jet velocity
Thermal IR images at the ENT site (Fig. 2 a) were processed using the algorithm of Mereu et al. 49 ; they provide a time series of brightness temperature over the course of a paroxysmal event. The Incandescent Jet Region (IJR), that is the near-vent jet that feeds the volcanic plume, is identified from thermal images as the saturated portion within each frame by evaluating the gradient of the brightness temperature as a function of a given threshold. As a first-order approximation, the height of the IJR is assumed to correspond to the height of the lava fountain 39 , \(H_{lf}\) , and the jet velocity at the vent ( \(v_{jet}\) ) is then calculated as:
where \(g\) is the acceleration of gravity. Detection of the IJR and calculation of \(v_{jet}\) are performed for each thermal IR frame and the results averaged every minute (Fig. 3 c). The validity of this method depends on considering the IJR a region of non-viscous ballistic flow, a reasonable assumption for volcanic jets near the vent. Additional limitations of the method are that: (1) the correct identification of the IJR can be affected by atmospheric conditions causing variable levels of image saturation; (2) the IJR may be higher or lower than the lava fountain owing to the presence of bombs and lapilli at higher elevation than the lava fountain, or to some of the activity obscured by ash cover 49 .
Infrasound array processing
Infrasound array data were processed following the methodology of De Angelis et al. 43 . The processing workflow implements least-squares beamforming to evaluate the DOA of coherent energy and the propagation velocity of acoustic waves across the array. The method implements a simple inversion problem:
where \({\mathbf{d}}\) is a data vector of time delay measurements between each pair of sensors within the array, \({\mathbf{m}} = (s_{x} ,s_{y} )^{T} = (sin\theta /v,cos\theta /v)^{T}\) is a model vector of slowness in the East–West and North–South directions, \({\mathbf{G}}\) is a \(N \times 2\) matrix of distances between each pair of sensors ( \(N\) is the number of sensors, \(\theta\) is the DOA, \(v\) is the acoustic wave velocity across the array). Delay times for each data window analysed are measured using cross-correlation between all pairs of sensors within the array. The results in Fig. 3 b were obtained by processing 10-s sliding windows with 50% overlap; a preliminary bandpass filter (0.7–3 Hz, Butterworth, 2-pole) was applied to the data. Only high-quality DOA estimates were selected for plotting, that is those corresponding to a median value of the cross-correlation maximum (MCCM) greater than 0.65.
Infrasound estimates of VER
We estimated VER by direct integration of the infrasound pressure time series recorded at station CONC6, at central element of the CONC array, (Fig. 2 c) following the formulation of Lacanna and Ripepe 46 :
where \(r\) is the source-receiver distance ( \(5690\,{\text{m}}\) ), \(\rho\) is atmospheric density ( \(1.1\;{\text{kg/m}}^{3}\) ), \(R\) is reflectance at the vent, \(\alpha\) is wavefield directivity, \(IL\) is insertion loss, and \(\Delta P\) is the recorded pressure time series.
We used values of \(R = 0.95\) and \(\alpha = 1\) (i.e., isotropic radiation) for a vent radius of \(10m\) , sound velocity of \(330\;{\text{m/s}}\) and a dominant frequency of the acoustic waves of \(2\;{\text{Hz}}\) 26 , 32 . \(IL\) represents scattering effect of topography on the wavefield, beyond simple geometric attenuation, which can be calculated from numerical modelling of acoustic wave propagation. \(IL\) can be expressed as:
that is, the ratio between the infrasound pressure calculated at a given position from numerical modelling ( \(P_{topo}\) ) and the expected pressure considering only the effect of geometrical spreading ( \(P_{geo}\) ). Figure 5 shows a map of IL at Mt. Etna obtained from 3D Finite Difference Time Domain modelling using the software package infraFDTD 28 , 29 . InfraFDTD is a 3D linear acoustic wave propagation model over topography with an absorbing boundary (implemented using perfect matched layers) to prevent spurious reflections at the solid interface between the atmosphere and the ground. We note that there are several alternatives to finite difference modelling for acoustic wave propagation over topography, including the family of open source spectral element codes SPECFEM 50 . Similar to Diaz-Moreno et al. 29 we implement a Blackman-Harris source function with an amplitude of 10000 kg/m 3 and a cutoff frequency of 4.5 Hz to include the dominant frequency range of the infrasound recorded during the paroxysm (0.7–3 Hz). The topography of Mt. Etna was derived merging a 0.55 m resolution digital elevation model of the SEC area from UAV surveys conducted in March 2021 44 with a regional 30-m Advanced Spaceborne Thermal Emission and Reflection Radiometer DEM for the rest of the study area 29 ; the resulting area was resampled into a grid of 5 × 5 m cells and the acoustic pressure wavefield was propagated over the topography using 20 grid points per wavelength to ensure numerical stability of the procedures 51 . At each grid point we applied Eq. (3) to produce a map of \(IL\) for the study area. We calculated a value of \(IL = - 9.4\;{\text{dB}}\) at the CONC site. We note that assessment of VER using infrasound requires estimates of IL along a single or a limited number of source-receiver paths. In light of this, 3D modelling could be replaced with 2D modeling, which would greatly increase computational efficiency (and only requires readily accessible computational resources) at the comparatively minor cost of neglecting second-order out-of-plane effects on wavefield propagation. One additional key consideration on IL mapping, is that significant topographic changes, such as major edifice collapses, would require updating IL maps.
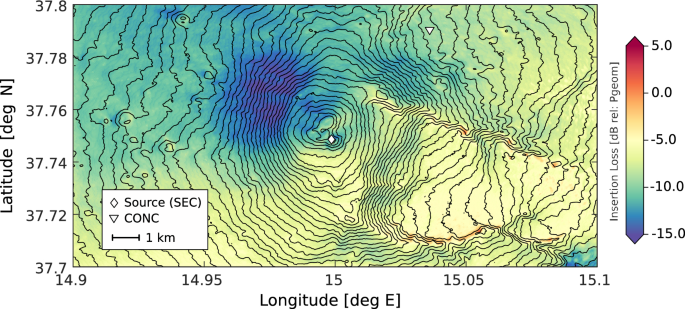
Map of insertion loss at Mt. Etna for a 4.5 Hz source positioned in the SEC area (white diamond). Insertion Loss evaluated from Finite Difference Time Domain numerical modelling of the acoustic wavefield (see “Methods” section of this manuscript). The inverted white triangle shows the position of the CONC array used in this study (IL = − 9.4 dB). Note high signal attenuation (IL ~ − 5 dB) in the area behind BN/VOR and NEC, and the effect of constructive interference from reflections of the acoustic wavefield along the edges of Valle del Bove (IL > 0).
Plume model and atmospheric data
We used the PlumeRise model of Woodhouse et al. 9 to estimate the maximum height reached by the volcanic plume. The model is a 3-dimensional description of the rise of volcanic ash columns based on the fluid dynamics of turbulent buoyant plumes in a windy atmosphere. This is achieved by combining an integral model of pure plumes in a horizontal wind field 52 with an integral model of volcanic columns in a static atmosphere 53 . PlumeRise also accounts for the thermodynamics of heat transfer between hot pyroclasts and the surrounding atmosphere and the effects of a variable atmosphere on the rise of volcanic plumes. Additional details and the full model formulation can be found in Ref. 9 . In this study, we used a model of the atmosphere obtained from the Reanalysis v5 (ERA5) dataset. ERA5, produced by the of the European Centre for Medium-Range Weather Forecasts of the Copernicus Climate Change Service, provides hourly estimates of many atmospheric, land and oceanic climate variables. These data cover the Earth on a 30 km grid and resolve the atmosphere using 137 levels from the surface up to a height of 80 km 54 ; we used data corresponding to the grid node that is closest to Mt. Etna, at 23:00 on 20 June, 2021. Values for all additional parameters required by the model are shown in the supplementary material (Table S1 ) and are based on previous work on plume modelling at Mt. Etna 47 .
Plume height measurements with X-band radar
We applied the VARR (Volcanic Ash Radar Retrieval) algorithm to the measured co-polar radar reflectivity Zhh (dBZ) to obtain information on the nature and height of the volcanic plume 7 , 55 . The algorithm performs tephra classification using a maximum a-posteriori probability criterion and estimates tephra features such as its mass concentration and number-weighted mean diameter. The top height of the plume (HTP) is estimated using a threshold algorithm on the measured Zhh of the probed plume and on the retrieved Ct. This estimate equals the central point of the range bin volume. The distance between the radar and the summit craters and the half-power beam width of the radar are used to reconstruct the radar beam cone; an uncertainty on HTP of ± 300 m is evaluated as the half-aperture (radius) with respect to the axis of the truncated cone in the proximal area above the crater 56 .
Data availability
The infrasound data and related metadata used in this study are available at https://zenodo.org/record/8207343 . The software used for infrasound array processing is hosted at: https://github.com/silvioda/Infrasound-Array-Processing-Matlab . The PlumeRise model is freely available at https://www.plumerise.bris.ac.uk/ . The infraFDTD software is available via request to the author, K. Kim ([email protected]). Thermal IR (Fig. 3 d), radar (Fig. 3 e) and high-resolution UAV digital elevation model data (Fig. 2 b) are available via reasonable request to the corresponding authors. All other digital elevation model data used in this study are available at: https://tinitaly.pi.ingv.it/ . The EUMETSAT Meteosat Volcanic Ash RGB—MSG—0 degree product derived from SEVIRI data is freely available, for research and education use, from the EUMETSAT data portal ( https://view.eumetsat.int/productviewer?v=default ) after user registration. The EUMETSAT data used in this study were obtained by SDA under EUMETSAT licenses 99999992, 99999993, 99999997, 99999999.
Mastin, L. G. et al. A multidisciplinary effort to assign realistic source parameters to models of volcanic ash-cloud transport and dispersion during eruptions. J. Volcanol. Geotherm. Res. 186 , 10–21 (2009).
Article CAS ADS Google Scholar
Dürig, T. et al. Mass eruption rates in pulsating eruptions estimated from video analysis of the gas thrust-buoyancy transitiona case study of the 2010 eruption of Eyjafjallajökull Iceland. Earth Planets Space 67 , 1–17 (2015).
Article Google Scholar
Dioguardi, F., Beckett, F., Dürig, T. & Stevenson, J. A. The impact of eruption source parameter uncertainties on ash dispersion forecasts during explosive volcanic eruptions. J. Geophys. Res. Atmos. 125 , e2020JD032717 (2020).
Article ADS Google Scholar
Witham, C. et al. The Current Volcanic Ash Modelling Setup at the London VAAC . https://www.metoffice.gov.uk/binaries/content/assets/metofficegovuk/pdf/services/transport/aviation/vaac/london_vaac_current_modelling_setup.pdf (2019).
Met Office UK. Unified Model . https://www.metoffice.gov.uk/research/approach/modelling-systems/unified-model .
Plu, M. et al. An ensemble of state-of-the-art ash dispersion models: Towards probabilistic forecasts to increase the resilience of air traffic against volcanic eruptions. Nat. Hazard 21 , 2973–2992 (2021).
Mereu, L. et al. Ground-based remote sensing and uncertainty analysis of the mass eruption rate associated with the 35 December 2015 paroxysms of Mt. Etna. IEEE J. Sel. Top. Appl. Earth Obs. Remote Sens. 15 , 504–518 (2022).
Wilson, L. & Walker, G. P. L. Explosive volcanic eruptions—VI. Ejecta dispersal in plinian eruptions: The control of eruption conditions and atmospheric properties. Geophys. J. Int. 89 , 657–679 (1987).
Woodhouse, M. J., Hogg, A. J., Phillips, J. C. & Sparks, R. S. J. Interaction between volcanic plumes and wind during the 2010 Eyjafjallajökull eruption Iceland. J. Geophys. Res. Solid Earth 118 , 92–109 (2013).
Aubry, T. J. et al. The Independent Volcanic Eruption Source Parameter Archive (IVESPA version 1.0): A new observational database to support explosive eruptive column model validation and development. J. Volcanol. Geotherm. Res. 417 , 107295 (2021).
Article CAS Google Scholar
Wilson, L., Sparks, R. S. J., Huang, T. C. & Watkins, N. D. The control of volcanic column heights by eruption energetics and dynamics. J. Geophys. Res. Solid Earth 83 , 1829–1836 (1978).
Garver, L. et al. Eruption Data for Ash-Cloud Model Validation . https://theghub.org/resources/2431 (2013).
Gouhier, M. & Donnadieu, F. Mass estimations of ejecta from Strombolian explosions by inversion of Doppler radar measurements. J. Geophys. Res. 113 , B10202 (2008).
Freret-Lorgeril, V. et al. Mass eruption rates of tephra plumes during the 2011–2015 lava fountain paroxysms at Mt. Etna from Doppler radar retrievals. Front. Earth Sci. 6 , 73 (2018).
Marzano, F. S. et al. Near-real-time detection of tephra eruption onset and mass flow rate using microwave weather radar and infrasonic arrays. IEEE Trans. Geosci. Remote Sens. 54 , 6292–6306 (2016).
Ripepe, M. et al. Ash-plume dynamics and eruption source parameters by infrasound and thermal imagery: The 2010 Eyjafjallajökull eruption. Earth Planet. Sci. Lett. 366 , 112–121 (2013).
Calvari, S. et al. Explosive paroxysmal events at Etna volcano of different magnitude and intensity explored through a multidisciplinary monitoring system. Remote Sens. 14 , 4006 (2022).
Caplan-Auerbach, J., Bellesiles, A. & Fernandes, J. K. Estimates of eruption velocity and plume height from infrasonic recordings of the 2006 eruption of Augustine Volcano Alaska. J. Volcanol. Geotherm. Res. 189 , 12–18 (2010).
Lamb, O. D., Angelis, S. D. & Lavallée, Y. Using infrasound to constrain ash plume rise. J. Appl. Volcanol. 4 , 1–9 (2015).
Woulff, G. & McGetchin, T. R. Acoustic noise from volcanoes: Theory and experiment. Geophys. J. Int. 45 , 601–616 (1976).
Johnson, J., Aster, R., Jones, K. R., Kyle, P. & McIntosh, B. Acoustic source characterization of impulsive Strombolian eruptions from the Mount Erebus lava lake. J. Volcanol. Geotherm. Res. 177 , 673–686 (2008).
Johnson, J. B. & Miller, A. J. C. Application of the monopole source to quantify explosive flux during vulcanian explosions at Sakurajima Volcano (Japan). Seismol. Res. Lett. 85 , 1163–1176 (2014).
Kim, K., Lees, J. M. & Ruiz, M. Acoustic multipole source model for volcanic explosions and inversion for source parameters. Geophys. J. Int. 191 , 1192–1204 (2012).
De Angelis, S. et al. Characterization of moderate ash-and-gas explosions at Santiaguito volcano Guatemala, from infrasound waveform inversion and thermal infrared measurements. Geophys. Res. Lett. 43 , 6220–6227 (2016).
Article PubMed PubMed Central ADS Google Scholar
Kim, K. & Lees, J. M. Local volcano infrasound and source localization investigated by 3D simulation. Seismol. Res. Lett. 85 , 1177–1186 (2014).
Lacanna, G. & Ripepe, M. Influence of near-source volcano topography on the acoustic wavefield and implication for source modeling. J. Volcanol. Geotherm. Res. 250 , 9–18 (2013).
Fee, D. et al. Eruption mass estimation using infrasound waveform inversion and ash and gas measurements: Evaluation at Sakurajima Volcano Japan. Earth Planet. Sci. Lett. 480 , 42–52 (2017).
Kim, K., Fee, D., Yokoo, A. & Lees, J. M. Acoustic source inversion to estimate volume flux from volcanic explosions. Geophys. Res. Lett. 42 , 5243–5249 (2015).
Diaz-Moreno, A. et al. Volume flow rate estimation for small explosions at Mt. Etna Italy, from acoustic waveform inversion. Geophys. Res. Lett. 46 , 11071–11079 (2019).
Iezzi, A. M., Fee, D., Kim, K., Jolly, A. D. & Matoza, R. S. Three-dimensional acoustic multipole waveform inversion at Yasur Volcano, Vanuatu. J. Geophys. Res. Solid Earth 124 , 8679–8703 (2019).
De Angelis, S., Diaz-Moreno, A. & Zuccarello, L. Recent developments and applications of acoustic infrasound to monitor volcanic emissions. Remote Sens. 11 , 1302 (2019).
Hantusch, M. et al. Low-energy fragmentation dynamics at Copahue Volcano (Argentina) as revealed by an infrasonic array and ash characteristics. Front. Earth Sci. 9 , 578437 (2021).
Freret-Lorgeril, V. et al. Examples of multi-sensor determination of eruptive source parameters of explosive events at Mount Etna. Remote Sens. 13 , 2097 (2021).
Maher, S., Matoza, R., de Groot-Hedlin, C., Kim, K. & Gee, K. Evaluating the applicability of a screen diffraction approximation to local volcano infrasound. Volcanica https://doi.org/10.30909/vol.04.01.6785 (2021).
Harris, A. J. L. & Neri, M. Volumetric observations during paroxysmal eruptions at Mount Etna: Pressurized drainage of a shallow chamber or pulsed supply?. J. Volcanol. Geotherm. Res. 116 , 79–95 (2002).
Andronico, D., Cannata, A., Grazia, G. D. & Ferrari, F. The 1986–2021 paroxysmal episodes at the summit craters of Mt. Etna: Insights into volcano dynamics and hazard. Earth-Sci. Rev. 220 , 103686 (2021).
Bollettino Settimanale sul monitoraggio vulcano, geochimico e sismico del vulcano Etna del 22/06/2021. https://www.ct.ingv.it/index.php/monitoraggio-e-sorveglianza/prodotti-del-monitoraggio/bollettini-settimanali-multidisciplinari .
Grangeon, J. & Lesage, P. A robust low-cost and well-calibrated infrasound sensor for volcano monitoring. J. Volcanol. Geotherm. Res. 387 , 106668 (2019).
Mereu, L. et al. Automatic early warning to derive eruption source parameters of paroxysmal activity at Mt. Etna (Italy). Remote Sens. 15 , 3501 (2023).
Marzano, F. S., Mereu, L., Scollo, S., Donnadieu, F. & Bonadonna, C. Tephra mass eruption rate from ground-based X-band and L-band microwave radars during the November 23 2013, Etna Paroxysm. IEEE Trans. Geosci. Remote Sens. 58 , 3314–3327 (2020).
Volcano Observatory Notice for Aviation, Etna, 2021/06/20 22:01. https://www.ct.ingv.it/index.php/monitoraggio-e-sorveglianza/prodotti-del-monitoraggio/comunicati-vona .
Volcano Observatory Notice for Aviation, Etna, 2021/06/20 22:19. https://www.ct.ingv.it/index.php/monitoraggio-e-sorveglianza/prodotti-del-monitoraggio/comunicati-vona .
De Angelis, S. et al. Uncertainty in detection of volcanic activity using infrasound arrays: Examples from Mt. Etna, Italy. Front. Earth Sci. 8 , 169 (2020).
Sciotto, M. et al. Infrasonic gliding reflects a rising magma column at Mount Etna (Italy). Sci. Rep. 12 , 16954 (2022).
Article CAS PubMed PubMed Central ADS Google Scholar
Ripepe, M. et al. Infrasonic early warning system for explosive eruptions. J. Geophys. Res. Solid Earth 123 , 9570–9585 (2018).
Lacanna, G. & Ripepe, M. Modeling the acoustic flux inside the magmatic conduit by 3D-FDTD simulation. J. Geophys. Res. Solid Earth 125 , e18849 (2020).
Scollo, S. et al. Near-real-time tephra fallout assessment at Mt. Etna, Italy. Remote Sens. 11 , 2987 (2019).
Corradini, S. et al. Proximal monitoring of the 2011–2015 Etna lava fountains using MSG-SEVIRI data. Geosciences 8 , 140 (2018).
Mereu, L., Scollo, S., Bonadonna, C., Freret-Lorgeril, V. & Marzano, F. S. Multisensor characterization of the incandescent jet region of lava fountain-fed tephra plumes. Remote Sens. 12 , 3629 (2020).
SPECFEM. A Family of Open-Source Spectral-Element Method Software Codes for Computational Seismology . https://specfem.org .
Wang, S. Finite-difference time-domain approach to underwater acoustic scattering problems. J. Acoust. Soc. Am. 99 , 1924–1931 (1996).
Hewett, T. A., Fay, J. A. & Hoult, D. P. Laboratory experiments of smokestack plumes in a stable atmosphere. Atmos. Environ. 1967 (5), 767–789 (1971).
Woods, A. W. The fluid dynamics and thermodynamics of eruption columns. Bull. Volcanol. 50 , 169–193 (1988).
ECMWF Reanalysis v5. https://www.ecmwf.int/en/forecasts/dataset/ecmwf-reanalysis-v5 .
Marzano, F. S., Picciotti, E., Vulpiani, G. & Montopoli, M. Synthetic signatures of volcanic ash cloud particles from X-band dual-polarization radar. IEEE Trans. Geosci. Remote Sens. 50 , 193–211 (2012).
Mereu, L. et al. A new radar-based statistical model to quantify mass eruption rate of volcanic plumes. Geophys. Res. Lett. 50 , e2022GL100596 (2023).
Download references
Acknowledgements
SDA was supported by by NERC Grant NE/W004771/1 and project SINFONIA, progetto Bando Ricerca Libera 2021- Delibera 214/2021-INGV. LZ was supported by project SINFONIA, progetto Bando Ricerca Libera 2021—Delibera 214/2021-INGV and by the INGV-MIUR project Pianeta Dinamico—“Working Earth”—Sub-project VT—DYNAMO—2023. We thank all INGV-OE technicians who are involved in the maintenance of the thermal IR and visibile cameras operated by INGV-OE.
Author information
Luciano Zuccarello
Present address: Istituto Nazionale di Geofisica e Vulcanologia, Sezione di Pisa, Via Cesare Battisti, 53, 56125, Pisa, Italy
Authors and Affiliations
School of Environmental Sciences, University of Liverpool, 4 Brownlow Street, Liverpool, L69 3GP, UK
Silvio De Angelis & Luciano Zuccarello
Istituto Nazionale di Geofisica e Vulcanologia, Sezione di Pisa, Via Cesare Battisti, 53, 56125, Pisa, Italy
Silvio De Angelis
Istituto Nazionale di Geofisica e Vulcanologia, Sezione di Catania - Osservatorio Etneo, Piazza Roma, 2, 95125, Catania, Italy
Simona Scollo
Istituto Nazionale di Geofisica e Vulcanologia, Sezione di Bologna, Bologna, Italy
Luigi Mereu
CETEMPS Center of Excellence, University of L’Aquila, L’Aquila, Italy
You can also search for this author in PubMed Google Scholar
Contributions
S.D.A. and L.Z. wrote the proposal that funded installation and maintenance of the infrasound array, designed the field experiment and installed all equipment. S.D.A. and L.Z. jointly designed the study, performed analyses of infrasound data, numerical modelling of acoustic wavefield and plume rise, and prepared all figures. S.S. and L.M. analysed thermal I.R. and radar data. S.D.A. wrote the initial draft of the manuscript and all authors contributed to the final version.
Corresponding author
Correspondence to Silvio De Angelis .
Ethics declarations
Competing interests.
The authors declare no competing interests.
Additional information
Publisher's note.
Springer Nature remains neutral with regard to jurisdictional claims in published maps and institutional affiliations.
Supplementary Information
Supplementary information., rights and permissions.
Open Access This article is licensed under a Creative Commons Attribution 4.0 International License, which permits use, sharing, adaptation, distribution and reproduction in any medium or format, as long as you give appropriate credit to the original author(s) and the source, provide a link to the Creative Commons licence, and indicate if changes were made. The images or other third party material in this article are included in the article's Creative Commons licence, unless indicated otherwise in a credit line to the material. If material is not included in the article's Creative Commons licence and your intended use is not permitted by statutory regulation or exceeds the permitted use, you will need to obtain permission directly from the copyright holder. To view a copy of this licence, visit http://creativecommons.org/licenses/by/4.0/ .
Reprints and permissions
About this article
Cite this article.
De Angelis, S., Zuccarello, L., Scollo, S. et al. Assessment of eruption source parameters using infrasound and plume modelling: a case study from the 2021 eruption of Mt. Etna, Italy. Sci Rep 13 , 19857 (2023). https://doi.org/10.1038/s41598-023-46160-6
Download citation
Received : 07 August 2023
Accepted : 28 October 2023
Published : 13 November 2023
DOI : https://doi.org/10.1038/s41598-023-46160-6
Share this article
Anyone you share the following link with will be able to read this content:
Sorry, a shareable link is not currently available for this article.
Provided by the Springer Nature SharedIt content-sharing initiative
By submitting a comment you agree to abide by our Terms and Community Guidelines . If you find something abusive or that does not comply with our terms or guidelines please flag it as inappropriate.
Quick links
- Explore articles by subject
- Guide to authors
- Editorial policies
Sign up for the Nature Briefing newsletter — what matters in science, free to your inbox daily.

- International
- Schools directory
- Resources Jobs Schools directory News Search
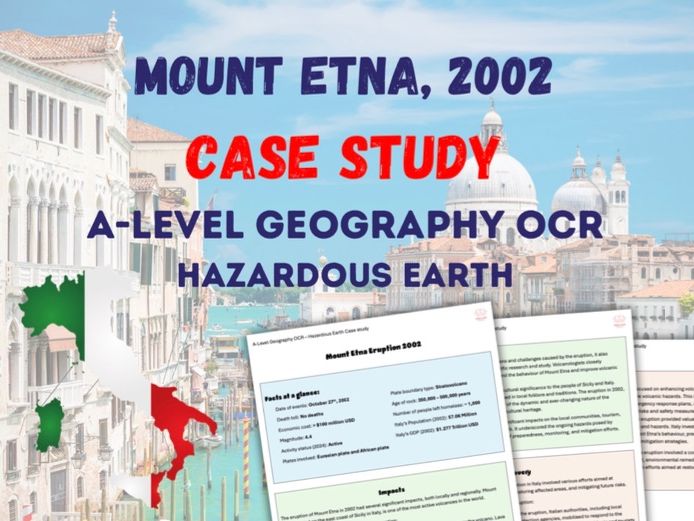
Mount Etna 2002 Case Study A-Level Geography OCR
Subject: Geography
Age range: 16+
Resource type: Lesson (complete)

Last updated
2 April 2024
- Share through email
- Share through twitter
- Share through linkedin
- Share through facebook
- Share through pinterest
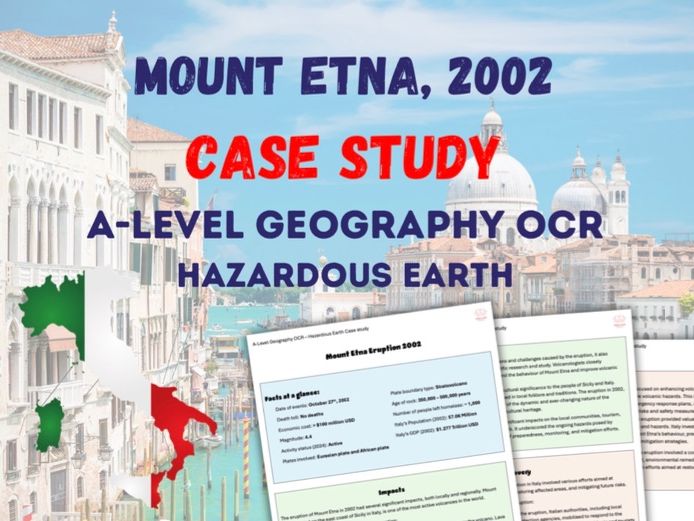
This 3 page case study consists of three sections (facts at a glance, impacts, and recovery), all of which are valuable knowledge to students studying A-Level Geography OCR. This particular Mount Etna case study is for the Hazard Earth topic.
This case study will help students get an understanding of the different ways in which volcanic eruptions can impact an area as well as the effects eruptions have socially, environmentally, and economically. This knowledge will be valuable to students in the summer exam series.
Italy’s Mount Etna volcano, which erupted in 2002 had many widespread impacts on the local economy as well as on the environment also, so acts as a good case study for students to learn about as part of the hazardous earth topic of the OCR specification.
A-Level geography is a great subject for A-Level students to learn. It teaches them a range of topics from different landscape systems, understanding food around the world, migration patterns, and more. All of which will be used by students throughout their careers and beyond.
Who are Phillips Resources?
At Phillips Resources we use your feedback to create the highest quality and most desired resources so that students can get the grades they desire and teachers are trusting in the resources they use and also are left stress free from not having to create their own resources as often.
Tes paid licence How can I reuse this?
Get this resource as part of a bundle and save up to 35%
A bundle is a package of resources grouped together to teach a particular topic, or a series of lessons, in one place.
6x Hazardous Earth Case Studies OCR A-Level Geography
Included is 6 case studies made for the hazardous earth topic of the A-Level Geography OCR specification. The six case studies include: - Chile Earthquake 2010 - China Earthquake 2008 - Haiti Earthquake 2010 - Iceland Volcanic eruption 2010 - Japan Earthquake and Tsunami 2011 - Mount Etna Eruption 2002 Each case study covers elements which would be useful for students to know for each case study that can be applied in the exam. Examples include recovery methods, reasons for vulnerability, impacts, causes, and more. Each case study is 2/3 pages and they all include a short header section which includes a list of facts for students to memorize to gain extra marks in the exam. A-Level geography is a great subject for A-Level students to learn. It teaches them a range of topics from different landscape systems, understanding food around the world, migration patterns, and more. All of which will be used by students throughout their careers and beyond. Who are Phillips Resources? At Phillips Resources we use your feedback to create the highest quality and most desired resources so that students can get the grades they desire and teachers are trusting in the resources they use and also are left stress free from not having to create their own resources as often.
Your rating is required to reflect your happiness.
It's good to leave some feedback.
Something went wrong, please try again later.
This resource hasn't been reviewed yet
To ensure quality for our reviews, only customers who have purchased this resource can review it
Report this resource to let us know if it violates our terms and conditions. Our customer service team will review your report and will be in touch.
Not quite what you were looking for? Search by keyword to find the right resource:
- 0 Shopping Cart
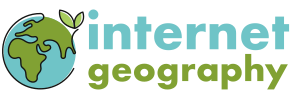
Europe’s most active volcano , Mount Etna, has been hitting the headlines recently after a series of spectacular eruptions. In Etna’s first eruption of 2021, explosive lava fountains reached over 1500 m in one of the most amazing eruptions in decades.
Mount Etna, located on the island of Sicily, has been largely dormant for the past two years. The stratovolcano (composite) dominates the skyline of the Italian island, where it sits on the eastern coast.
Located between the cities of Messina and Catania, it is the highest active volcano in Europe outside the Caucasus – a region between the Black Sea and the Caspian Sea – and the highest peak in Italy south of the Alps.
The recent activity is typical of a strombolian eruption among the normal activities of the more than 3,300-metre-high volcano. The recent eruption is the strongest explosion in the southern crater since it was discovered in 1971.
On Monday 22nd February 2021, at around 11 pm, the lava fountains, surrounded by gigantic clouds of smoke, exceeded 1,500 metres (4,900ft) in height, while thousands of rock fragments, some the size of fridges, were thrown from the crater into the sky for several kilometres.
Etna is a hyperactive volcano with over 3,500 years of historically documented eruptions. The volcano has been erupting on and off since September 2013. Since September 2019, it’s been erupting from its various summit craters virtually continuously. In December 2020, Etna’s explosive activity and lava output began to spike, and in February 2021, it has been launching fluid lava skywards.
Etna is an unusual volcano in that it can produce explosive eruptions of runny lava and release slower flowing, thick lava flows. Scientists are still trying to work out why this is the case.
The magma from the latest eruption appears to be coming up from deep within the mantle . Extremely hot, fluid magma is rapidly rising through the network of conduits within and below the volcano. However, there is another factor that is contributing to the current explosive eruptions.
There are high quantities of water vapour in Etna’s magma, which makes it explosive. The water does not cool the magma. As the molten magma approaches the surface, the pressure drops, and the bubble of water vapour expands violently, leading to lava being ejected out of the volcano.
Following each explosive lava fountain , less gassy magma lingers just below the vent. This is then cleared when a new volley of gassy magma rises from below. These explosive eruptions are known as volcanic paroxysms.
Authorities have reported no danger to the nearby towns, however, local airports have been temporarily closed, as has the airspace around the volcano. Etna’s last major eruption was in 1992. Despite the explosive nature of the recent eruption, there is no risk to the population, other than from the ash that covers buildings and smoke that can, after a few hours, cause breathing problems. In March 2017 vulcanologists, tourists and a BBC film crew were injured during an eruption when a flow of lava ran into snow, producing superheated steam that sent fragments of rock flying in all directions.
Further reading For a Volcanologist Living on Mount Etna, the Latest Eruption Is a Delight – Advisory – this article contains expletives (swear words).
In Pictures: Mount Etna eruption lights up Sicily’s night sky
Mount Etna: BBC crew caught up in volcano blast
Mount Etna illuminates night sky with 1,500-metre lava fountain
Share this:
- Click to share on Twitter (Opens in new window)
- Click to share on Facebook (Opens in new window)
- Click to share on Pinterest (Opens in new window)
- Click to email a link to a friend (Opens in new window)
- Click to share on WhatsApp (Opens in new window)
- Click to print (Opens in new window)
Leave a Reply
Leave a reply cancel reply.
Your email address will not be published. Required fields are marked *
Save my name, email, and website in this browser for the next time I comment.
I agree to the terms and conditions laid out in the Privacy Policy
Notify me of follow-up comments by email.
Notify me of new posts by email.
This site uses Akismet to reduce spam. Learn how your comment data is processed .
Please Support Internet Geography
If you've found the resources on this site useful please consider making a secure donation via PayPal to support the development of the site. The site is self-funded and your support is really appreciated.
Search Internet Geography
Top posts and pages.

Latest Blog Entries

Pin It on Pinterest
- Click to share
- Print Friendly
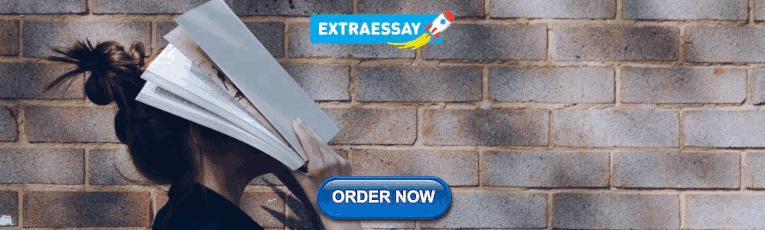
IMAGES
VIDEO
COMMENTS
Case study task. Use the resources and links that can be found on this page to produce a detailed case study of the 2002-2003 eruption of Mount Etna. You should use the 'Five W's" subheadings to give your case study structure. What happened? The Guardian - Sicilian city blanketed in ash [28 October 2002] When did it happen?
After the violent flank eruption of July-August 2001, Mount Etna was rather calm for more than 10 months, except for usual fumes from the four summit craters [and minor ash emissions]. In the first days of July 2002 weak magmatic activity resumed sporadically at the NE Crater with ejection of bombs that fell on the outer slopes of the cone.
[1] The onset of Mt. Etna's 2002-03 eruption was marked by intense explosive activity beginning on 27 October 2002 and persisting until 30 December. This phase of activity produced abundant ash emission that impacted the local economy and air traffic. Thereafter, explosive activity declined with the eruption ceasing on 28 January 2003.
After 2000 the upper flanks were affected by two long-lasting explosive/effusive eruptions in 2001 and 2002-2003 (Behncke and Neri, 2003, Andronico et al., 2005 and 2008), ... Monitoring ash emission episodes at Mt. Etna: the 16 November 2006 case study. J. Volcanol. Geotherm. Res., 180 (2-4) (2009), pp. 123-134, 10.1016/j.jvolgeores.2008. ...
The 2002 - 03 Mt Etna flank eruption began on 26 October 2002 and finished on 28 January 2003, after three months of continuous explosive activity and discontinuous lava flow output. The eruption involved the opening of eruptive fissures on the NE and S flanks of the volcano, with lava flow output and fire fountaining until 5 November.
Etna Volcano - Italy, 2002. CATANIA, Sicily, October 2002 - Fountains of ash and sand continued raining down from Mount Etna for a second day, as at least two rivers of lava poured down its slopes and more cracks opened up. However, the lava did not go past an altitude of about 2,200 meters, posing no threats to towns or villages, the National ...
Depending on their intensity and position, volcanic eruptions at Mt. Etna (Sicily, Italy, Fig. 1) have the potential to cause significant social and economic damage.Etna is characterized by ...
1 The 2002 Ne-Rift Eruption. The NE-rift, one of the main intrusion zones of Etna, covers a 2-km wide and 7-km long area which stretches from 2500 to 1700 m a.s.l. ().It consists of subparallel eruptive fissures with azimuth values between 42°E and 62°E (Kieffer 1975).The NE-rift is affected by strong extensional tectonics which several authors consider to be controlled by the gravitational ...
Abstract. After a period of deflation during the 1991-1993 flank eruption, Mount Etna underwent a rapid inflation. Seismicity and ground deformation show that since 1994, a huge volume of magma intruded beneath the volcano, producing from 1998 onward a series of eruptions at the summit and on the flank of the volcano.
The 2002-03 Mt Etna flank eruption began on 26 October 2002 and finished on 28 January 2003, after three months of continuous explosive activity and discontinuous lava flow output.
earthquakes at Mt. Etna (Sicily, Italy): A case study from the 1981. and 2001 events, Geophys. Res. ... Seismic activity linked to the 2002-03 Mt. Etna eruption was investigated by analyzing the ...
The case of the 1981 eruption of Mount Etna, an. ... case studies from Etna, Krafla, Cerro Negro, Fogo, ... 2002- 3 Mt. Etna eruption inferred from thermal infra-
Identifying pre-seismic ionospheric disturbances using space geodesy: A case study of the 2011 Lorca earthquake (Mw 5.1), Spain ... Acocella, V., et al., Contrasting Triggering Mechanisms of the 2001 and 2002-2003 Eruptions of Mount Etna (Italy), J. Volcanol. Geotherm. Res., 2005, vol. 144, pp. 235-255. Article Google Scholar
1. Introduction [2] Earthquakes and eruptions are often coupled. In fact, earthquakes may trigger eruptions by modifying the state of stress in magma systems and, conversely, large magma intrusions change stress conditions in the volcanic edifice, eventually inducing earthquakes [Hill et al., 2002; Stein, 2003; Walter and Amelung, 2004].[3] Different studies show a correlation between sectors ...
In our experience, during the eruption of Mount Etna in 2002, analysis of large particles performed with a mass spectrometer showed a relevant proportion of free crystalline silica, ... Pétursdóttir G. Health effects following the Eyjafjallajokull volcanic eruption: a cohort study. BMJ Open. 2012; 2:e001851. doi: 10.1136/bmjopen-2012-001851.
The 2002-03 Mt Etna flank eruption began on 26 October 2002 and finished on 28 January 2003, after three months of continuous explosive activity and discontinuous lava flow output. The eruption involved the opening of eruptive fissures on the NE and S flanks of the volcano, with lava flow output and fire fountaining until 5 November. After this date, the eruption continued exclusively on the ...
Assessment of eruption source parameters using infrasound and plume modelling: a case study from the 2021 eruption of Mt. Etna, Italy
Disasters. 1996. TLDR. The 1928 eruption of Mount Etna demonstrates that lava can reach the lower flanks of the volcano within a short period after the onset of an eruption, and provides an opportunity for the fascist government of the time to demonstrate efficient centralised planning. Expand.
The October 2002 Pernicana seismic swarm was directly correlated to the dyke-forming intrusions occurring during the 2002-2003 Mt. Etna eruption (Pulvirenti et al., 2016). As in our case, there was not always a direct link between volcanic and seismic activity. ... 2010 earthquake along the Pernicana fault (Mt. Etna − Italy) case study ...
Case studies all in one ... Mont Etna 2002 volcano - No deaths - The Italian Government declared a 'state of emergency' in the eastern half of Sicily, after a series of earthquakes accompanying the eruption caused thousands of people to flee their homes.
This particular Mount Etna case study is for the Hazard Earth topic. ... - China Earthquake 2008 - Haiti Earthquake 2010 - Iceland Volcanic eruption 2010 - Japan Earthquake and Tsunami 2011 - Mount Etna Eruption 2002 Each case study covers elements which would be useful for students to know for each case study that can be applied in the exam ...
The 2018 December Mt. Etna eruption shows that the eccentric upraise of a large magma body carries a potential energy on structurally complex volcanoes that can be released by activating peripheral faults; this is the case, in particular, of lateral spreading volcanoes (Poland, Peltier, Bonforte, & Puglisi, 2017). The fault slip discharges the ...
Mount Etna is Europe's most active volcano. It is a composite volcano (pointy). Etna has the longest documented record of eruptions of any volcano in the world. The volcano's fertile and dangerous slopes are home to over 900,000 people. The main eruption occurred in 2002
Europe's most active volcano, Mount Etna, has been hitting the headlines recently after a series of spectacular eruptions. In Etna's first eruption of 2021, explosive lava fountains reached over 1500 m in one of the most amazing eruptions in decades. Mount Etna, located on the island of Sicily, has been largely dormant for the past two years.