
Want to create or adapt books like this? Learn more about how Pressbooks supports open publishing practices.
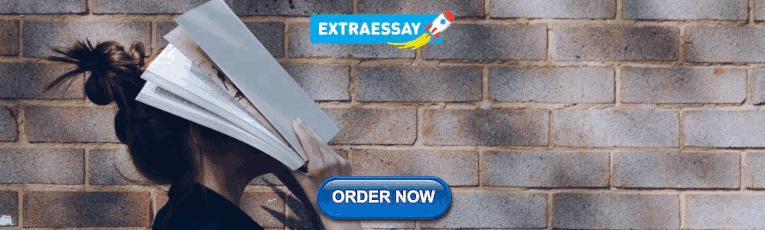
4.3 Modes of Inheritance
Usually, we are presented with a pedigree of an uncharacterized disease or trait, and one of the first tasks is to determine which modes of inheritance are possible, and then, which mode of inheritance is most likely. This information is essential in calculating the probability that the trait will be inherited in any future offspring. We will mostly consider five major types of inheritance: autosomal dominant (AD), autosomal recessive (AR), X-linked dominant (XD), X-linked recessive (XR), and Y-linked (Y) inheritance.
We generally make two assumptions in analyzing Pedigree Charts. These are as follows:
- Complete Penetrance — an individual in the pedigree will be affected (express the phenotype associated with a trait) when the individual carries at least one dominant allele of a dominant trait, or two recessive alleles of a recessive a trait.
- Rare-in-Population — generally, the trait in question is rare in the general population.
The following are some hints and clues to help us interpret Pedigree Charts:
- An unaffected individual cannot have any alleles of a dominant trait (because a single allele of a dominant trait causes an individual to be affected).
- Individuals marrying into the family are assumed to have no disease alleles – they will never be affected and can never be carriers of a recessive trait (because the trait is rare in the population).
- An unaffected individual can be a carrier (have one allele) of a recessive trait (because two alleles of a recessive trait are required for an individual to be affected).
- When a trait is X-linked, a single recessive allele is sufficient for a male to be affected (because the male is hemizygous – he only has one allele of an X-linked trait).
- A father transmits his allele of X-linked genes to his daughters, but not his sons. A mother transmits an allele of X-linked genes to both her daughters and her sons.
Take a look at the following video, Pedigree Analysis, by AK Lecture Series (2015) on YouTube, which discusses Pedigree Charts and how to analyze them .
Let us now take a look at the various modes of inheritance and typical pedigree charts which are characteristic of each mode.
Autosomal Dominant (AD)
When a disease is caused by a dominant allele of a gene, every person with that allele will show symptoms of the disease (assuming complete penetrance), and only one disease allele needs to be inherited for an individual to be affected. Thus, every affected individual must have an affected parent. A pedigree with affected individuals in every generation is typical of AD diseases. However, beware that other modes of inheritance can also show the disease in every generation, as described below. It is also possible for an affected individual with an AD disease to have a family without any affected children, if the affected parent is a heterozygote. This is particularly true in small families, where the probability of every child inheriting the normal, rather than disease allele is not extremely small. Note that AD diseases are usually rare in populations, therefore affected individuals with AD diseases tend to be heterozygotes (otherwise, both parents would have had to been affected with the same rare disease). Huntington Disease, Achondroplastic dwarfism, and Polydactyly are all examples of human conditions that may follow an AD mode of inheritance.

Example: Achondroplasia is a common form of dwarfism. FGFR3 gene at 4p16 (chromosome 4, p arm, region 1, band 6) encodes a receptor protein that negatively regulates bone development. A specific base pair substitution in the gene makes an over-active protein and this results in shortened bones. Achondroplasia is considered autosomal dominant because the defective proteins made in A / a embryos halt bone growth prematurely. A / A embryos do not make enough limb bones to survive. Most, but not all dominant mutations are also recessive lethal. In achondroplasia, the A allele shows dominant visible phenotype (shortness) and recessive lethal phenotype.
X-Linked Dominant (XD)
In X-linked dominant inheritance, the gene responsible for the disease is located on the X-chromosome, and the allele that causes the disease is dominant to the normal allele in females. Because females have twice as many X-chromosomes as males, females tend to be more frequently affected than males in the population. However, not all pedigrees provide sufficient information to distinguish XD and AD. One definitive indication that a trait is inherited as AD, and not XD, is that an affected father passes the disease to a son; this type of transmission is not possible with XD, since males inherit their X chromosome from their mothers.
Example: fragile x syndrome — The FMR1 gene at Xq21 (X chromosome, q arm, region 2, band 1) encodes a protein needed for neuron development. There is a (CGG)n repeat array in the 5’UTR (untranslated region). If there is expansion of the repeat in the germline cell the child will inherit a non-functional allele. X A / Y males have fragile X mental retardation (IQ < 50) because none of their neurons can make FMR1 proteins. Fragile X syndrome is considered X-linked dominant because only some neurons in X A / X a females can make FMR1 proteins. The severity (IQ 50 – 70) in these females depends upon the number and location of these cells within in the brain.
Autosomal Recessive (AR)
Diseases that are inherited in an autosomal recessive pattern require that both parents of an affected individual carry at least one copy of the disease allele. With AR traits, many individuals in a pedigree can be carriers, probably without knowing it. Compared to pedigrees of dominant traits, AR pedigrees tend to show fewer affected individuals and are more likely than AD or XD to “skip a generation”. Thus, the major feature that distinguishes AR from AD or XD is that unaffected individuals can have affected offspring. Attached earlobes is a human condition that may follow an AR mode of inheritance.
AR example: phenylketonuria (PKU) – Individuals with phenylketonuria (PKU) have a mutation in the PAH gene at 12q24 (chromosome 12, q arm, region 2, band 4), which encodes an enzyme that breaks down phenylalanine into tyrosine called phenylalanine hydrolase (PAH). Without PAH, the accumulation of phenylalanine and other metabolites, such as phenylpyruvic acid, disrupts brain development, typically within a year after birth, and can lead to intellectual disability. Fortunately, this condition is both easy to diagnose and can be successfully treated with a low phenylalanine diet. There are over 450 different mutant alleles of the PAH gene, so most people with PKU are compound heterozygotes . Compound heterozygotes have two different mutant alleles (different base pair changes) at a given locus, in this case the PAH gene.
X-Linked Recessive (XR)
Because males have only one X-chromosome, any male that inherits an X-linked recessive disease allele will be affected by it (assuming complete penetrance). Therefore, in XR modes of inheritance, males tend to be affected more frequently than females in a population. This contrasts with AR and AD, where both sexes tend to be affected equally, and XD, in which females are affected more frequently. Note, however, in the small sample sizes typical of human families, it is usually not possible to accurately determine whether one sex is affected more frequently than others. On the other hand, one feature of a pedigree that can be used to definitively establish that an inheritance pattern is not XR is the presence of an affected daughter from unaffected parents; because she would have had to inherit one X-chromosome from her father, he would also have been affected in XR.
XR example: hemophilia A- F8 gene at Xq28 (X chromosome, q arm, region 2, band 8) encodes blood clotting factor VIIIc. Without Factor VIIIc, internal and external bleeding can’t be stopped. Back in the 1900s, Xa / Y male’s average life expectancy was 1.4 years, but in the 2000s it has increased to 65 years with the advent of Recombinant Human Factor VIIIc. Hemophilia A is recessive because XA / Xa females have normal blood coagulation, while Xa / Xa females have hemophilia.
Only males are affected in human Y-linked inheritance (and other species with the X/Y sex determining system). There is only father-to-son transmission. This is the easiest mode of inheritance to identify, but it is one of the rarest because there are so few genes located only on the Y-chromosome.
A common, but incorrect, example of Y-linked inheritance is the hairy-ear-rim phenotype seen in some Indian families. A better example are the Y-chromosome DNA polymorphisms that have been used to follow the male lineage in large families or through ancient ancestral lineages. For example, the Y-chromosome of Mongolian ruler Genghis Khan (1162-1227 CE), and his male relatives, accounts for ~8% of the Y-chromosome lineage of men in Asia (0.5% world wide).
Media Attributions
- Figure 4.3.1 Autosomal dominant by Simon Caulton, CC BY-SA 3.0 , via Wikimedia Commons
- Figure 4.3.2 Wiki Drawing – X-Linked Dominant (1) by Madibc68, CC BY-SA 4.0 , via Wikimedia Commons
- Figure 4.3.3 Autosomal Recessive Pedigree Chart by Jerome Walker , CC BY-SA 3.0 , via Wikimedia Commons
- Figure 4.3.4 Wiki Drawing – X-Linked Recessive (1) by Madibc68, CC BY-SA 4.0 , via Wikimedia Commons
- Figure 4.3.5 Wiki Drawing – Y-Linked (1) by Madibc68, CC BY-SA 4.0 , via Wikimedia Commons
AK Lecture Series. (2015, January 13). Pedigree Analysis (video file). YouTube. https://youtu.be/Wgmgt_Ph6Ko
Long Descriptions
- Figure 4.3.1 Pedigree chart showing inheritance of an autosomal dominant trait over four generations. Affected females are shown as red-coloured circles; normal females are blue-coloured circles; affected males are red-coloured squares; normal males are blue-coloured squares. Generation I begins with a normal male and an affected female, mating to produce five offspring. Their offspring are: two affected and three unaffected. Two of these Generation II individuals mate, and their progeny is shown, along with a final Generation IV, with the characteristic pattern for autosomal dominant traits depicted. [Back to Figure 4.3.1 ]
- Figure 4.3.2 Pedigree chart showing the inheritance of a X-linked dominant trait over three generations. An affected female mates with a normal male in Generation I, to produce four offspring – one normal male, one affected male, one normal female and one affected female. The normal female mates with a normal male, and they produce two unaffected children. The affected male of Generation II mates with a normal female, and they produce four children – two affected females and two unaffected males. This pattern is characteristic for X-linked dominant inheritance. [Back to Figure 4.3.2 ]
- Figure 4.3.3 Pedigree chart showing the inheritance of an autosomal recessive trait, over three generations. Red and blue colours depict differences in the male and female. Both colours mean heterozygous, solid red colour means homozygous recessive, and solid blue color means wild type or homozygous dominant. Generation I begins with two carrier parents, who are therefore heterozygous. They produce four children, two are normal, one is a carrier and one is affected. This pattern is consistent with the inheritance of autosomal recessive traits. [Back to Figure 4.3.3 ]
- Figure 4.3.4 Pedigree chart showing the inheritance of a X-linked recessive trait over three generations. Generation I outlines one affected female and one affected male, mating to produce four children, two unaffected females and two affected males. One of the unaffected females mates with a normal man, and they produce two children: one affected male and an unaffected female. This indicates the unaffected mother was a carrier. This pattern of inheritance is typical of an X-linked recessive trait. [Back to Figure 4.3.4 ]
- Figure 4.3.5 Pedigree chart showing the inheritance of a Y-linked trait. Three generations are shown, starting with a normal female and an affected male, who produce three offspring. Offspring are: one normal female and two affected males. One affected male mates with a normal female, and their offspring comprises one normal female and two affected males. This pattern of inheritance is typical of Y-linked traits. [Back to Figure 4.3.5 ]
Introduction to Genetics Copyright © 2023 by Natasha Ramroop Singh, Thompson Rivers University is licensed under a Creative Commons Attribution-NonCommercial-ShareAlike 4.0 International License , except where otherwise noted.
Share This Book
This page has been archived and is no longer updated
Gregor Mendel and the Principles of Inheritance

Traits are passed down in families in different patterns. Pedigrees can illustrate these patterns by following the history of specific characteristics, or phenotypes, as they appear in a family. For example, the pedigree in Figure 1 shows a family in which a grandmother (generation I) has passed down a characteristic (shown in solid red) through the family tree. The inheritance pattern of this characteristic is considered dominant , because it is observable in every generation. Thus, every individual who carries the genetic code for this characteristic will show evidence of the characteristic. In contrast, Figure 2 shows a different pattern of inheritance, in which a characteristic disappears in one generation, only to reappear in a subsequent one. This pattern of inheritance, in which the parents do not show the phenotype but some of the children do, is considered recessive . But where did our knowledge of dominance and recessivity first come from?
Gregor Mendel’s Courage and Persistence
Mendel was curious about how traits were transferred from one generation to the next, so he set out to understand the principles of heredity in the mid-1860s. Peas were a good model system, because he could easily control their fertilization by transferring pollen with a small paintbrush. This pollen could come from the same flower (self-fertilization), or it could come from another plant's flowers (cross-fertilization). First, Mendel observed plant forms and their offspring for two years as they self-fertilized, or "selfed," and ensured that their outward, measurable characteristics remained constant in each generation. During this time, Mendel observed seven different characteristics in the pea plants, and each of these characteristics had two forms (Figure 3). The characteristics included height (tall or short), pod shape (inflated or constricted), seed shape (smooth or winkled), pea color (green or yellow), and so on. In the years Mendel spent letting the plants self, he verified the purity of his plants by confirming, for example, that tall plants had only tall children and grandchildren and so forth. Because the seven pea plant characteristics tracked by Mendel were consistent in generation after generation of self-fertilization, these parental lines of peas could be considered pure-breeders (or, in modern terminology, homozygous for the traits of interest). Mendel and his assistants eventually developed 22 varieties of pea plants with combinations of these consistent characteristics.
Mendel not only crossed pure-breeding parents, but he also crossed hybrid generations and crossed the hybrid progeny back to both parental lines. These crosses (which, in modern terminology, are referred to as F 1 , F 1 reciprocal, F 2 , B 1 , and B 2 ) are the classic crosses to generate genetically hybrid generations.
Understanding Dominant Traits
Understanding recessive traits.
When conducting his experiments, Mendel designated the two pure-breeding parental generations involved in a particular cross as P 1 and P 2 , and he then denoted the progeny resulting from the crossing as the filial, or F 1 , generation. Although the plants of the F 1 generation looked like one parent of the P generation, they were actually hybrids of two different parent plants. Upon observing the uniformity of the F 1 generation, Mendel wondered whether the F 1 generation could still possess the nondominant traits of the other parent in some hidden way.
To understand whether traits were hidden in the F 1 generation, Mendel returned to the method of self-fertilization. Here, he created an F 2 generation by letting an F 1 pea plant self-fertilize (F 1 x F 1 ). This way, he knew he was crossing two plants of the exact same genotype . This technique, which involves looking at a single trait, is today called a monohybrid cross . The resulting F 2 generation had seeds that were either round or wrinkled. Figure 4 shows an example of Mendel's data.
When looking at the figure, notice that for each F 1 plant, the self-fertilization resulted in more round than wrinkled seeds among the F 2 progeny. These results illustrate several important aspects of scientific data:
- Multiple trials are necessary to see patterns in experimental data.
- There is a lot of variation in the measurements of one experiment.
- A large sample size, or "N," is required to make any quantitative comparisons or conclusions.
In Figure 4, the result of Experiment 1 shows that the single characteristic of seed shape was expressed in two different forms in the F 2 generation: either round or wrinkled. Also, when Mendel averaged the relative proportion of round and wrinkled seeds across all F 2 progeny sets, he found that round was consistently three times more frequent than wrinkled. This 3:1 proportion resulting from F 1 x F 1 crosses suggested there was a hidden recessive form of the trait. Mendel recognized that this recessive trait was carried down to the F 2 generation from the earlier P generation .
Mendel and Alleles
As mentioned, Mendel's data did not support the ideas about trait blending that were popular among the biologists of his time. As there were never any semi-wrinkled seeds or greenish-yellow seeds, for example, in the F 2 generation, Mendel concluded that blending should not be the expected outcome of parental trait combinations. Mendel instead hypothesized that each parent contributes some particulate matter to the offspring. He called this heritable substance "elementen." (Remember, in 1865, Mendel did not know about DNA or genes.) Indeed, for each of the traits he examined, Mendel focused on how the elementen that determined that trait was distributed among progeny. We now know that a single gene controls seed form, while another controls color, and so on, and that elementen is actually the assembly of physical genes located on chromosomes. Multiple forms of those genes, known as alleles , represent the different traits. For example, one allele results in round seeds, and another allele specifies wrinkled seeds.
One of the most impressive things about Mendel's thinking lies in the notation that he used to represent his data. Mendel's notation of a capital and a lowercase letter ( Aa ) for the hybrid genotype actually represented what we now know as the two alleles of one gene : A and a . Moreover, as previously mentioned, in all cases, Mendel saw approximately a 3:1 ratio of one phenotype to another. When one parent carried all the dominant traits ( AA ), the F 1 hybrids were "indistinguishable" from that parent. However, even though these F 1 plants had the same phenotype as the dominant P 1 parents, they possessed a hybrid genotype ( Aa ) that carried the potential to look like the recessive P 1 parent ( aa ). After observing this potential to express a trait without showing the phenotype, Mendel put forth his second principle of inheritance: the principle of segregation . According to this principle, the "particles" (or alleles as we now know them) that determine traits are separated into gametes during meiosis , and meiosis produces equal numbers of egg or sperm cells that contain each allele (Figure 5).
Dihybrid Crosses
Mendel had thus determined what happens when two plants that are hybrid for one trait are crossed with each other, but he also wanted to determine what happens when two plants that are each hybrid for two traits are crossed. Mendel therefore decided to examine the inheritance of two characteristics at once. Based on the concept of segregation , he predicted that traits must sort into gametes separately. By extrapolating from his earlier data, Mendel also predicted that the inheritance of one characteristic did not affect the inheritance of a different characteristic.
Mendel tested this idea of trait independence with more complex crosses. First, he generated plants that were purebred for two characteristics, such as seed color (yellow and green) and seed shape (round and wrinkled). These plants would serve as the P 1 generation for the experiment. In this case, Mendel crossed the plants with wrinkled and yellow seeds ( rrYY ) with plants with round, green seeds ( RRyy ). From his earlier monohybrid crosses, Mendel knew which traits were dominant: round and yellow. So, in the F 1 generation, he expected all round, yellow seeds from crossing these purebred varieties, and that is exactly what he observed. Mendel knew that each of the F 1 progeny were dihybrids; in other words, they contained both alleles for each characteristic ( RrYy ). He then crossed individual F 1 plants (with genotypes RrYy ) with one another. This is called a dihybrid cross . Mendel's results from this cross were as follows:
- 315 plants with round, yellow seeds
- 108 plants with round, green seeds
- 101 plants with wrinkled, yellow seeds
- 32 plants with wrinkled, green seeds
Thus, the various phenotypes were present in a 9:3:3:1 ratio (Figure 6).
Next, Mendel went through his data and examined each characteristic separately. He compared the total numbers of round versus wrinkled and yellow versus green peas, as shown in Tables 1 and 2.
Table 1: Data Regarding Seed Shape
Table 2: Data Regarding Pea Color
The proportion of each trait was still approximately 3:1 for both seed shape and seed color. In other words, the resulting seed shape and seed color looked as if they had come from two parallel monohybrid crosses; even though two characteristics were involved in one cross, these traits behaved as though they had segregated independently. From these data, Mendel developed the third principle of inheritance: the principle of independent assortment . According to this principle, alleles at one locus segregate into gametes independently of alleles at other loci. Such gametes are formed in equal frequencies.
Mendel’s Legacy
More lasting than the pea data Mendel presented in 1862 has been his methodical hypothesis testing and careful application of mathematical models to the study of biological inheritance. From his first experiments with monohybrid crosses, Mendel formed statistical predictions about trait inheritance that he could test with more complex experiments of dihybrid and even trihybrid crosses. This method of developing statistical expectations about inheritance data is one of the most significant contributions Mendel made to biology.
But do all organisms pass their on genes in the same way as the garden pea plant? The answer to that question is no, but many organisms do indeed show inheritance patterns similar to the seminal ones described by Mendel in the pea. In fact, the three principles of inheritance that Mendel laid out have had far greater impact than his original data from pea plant manipulations. To this day, scientists use Mendel's principles to explain the most basic phenomena of inheritance.
References and Recommended Reading
- Add Content to Group
Article History
Flag inappropriate.


Email your Friend

- | Lead Editor: Terry McGuire

Within this Subject (29)
- Gene Linkage (5)
- Methods for Studying Inheritance Patterns (7)
- The Foundation of Inheritance Studies (11)
- Variation in Gene Expression (6)
Other Topic Rooms
- Gene Inheritance and Transmission
- Gene Expression and Regulation
- Nucleic Acid Structure and Function
- Chromosomes and Cytogenetics
- Evolutionary Genetics
- Population and Quantitative Genetics
- Genes and Disease
- Genetics and Society
- Cell Origins and Metabolism
- Proteins and Gene Expression
- Subcellular Compartments
- Cell Communication
- Cell Cycle and Cell Division

© 2014 Nature Education
- Press Room |
- Terms of Use |
- Privacy Notice |

Visual Browse
If you're seeing this message, it means we're having trouble loading external resources on our website.
If you're behind a web filter, please make sure that the domains *.kastatic.org and *.kasandbox.org are unblocked.
To log in and use all the features of Khan Academy, please enable JavaScript in your browser.
AP®︎/College Biology
Course: ap®︎/college biology > unit 5.
- Boveri-Sutton chromosome theory
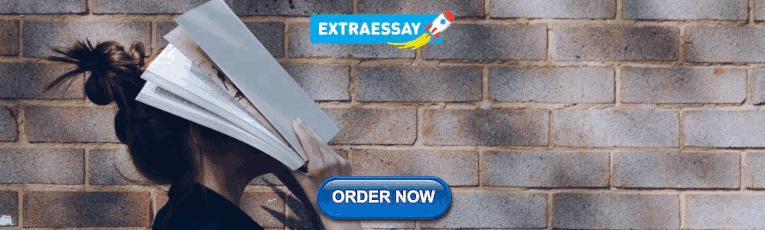
The chromosomal basis of inheritance
- Aneuploidy & chromosomal rearrangements
- Variation in a species
- Chromosomal inheritance
Key points:
- Boveri and Sutton's chromosome theory of inheritance states that genes are found at specific locations on chromosomes, and that the behavior of chromosomes during meiosis can explain Mendel’s laws of inheritance.
- Thomas Hunt Morgan, who studied fruit flies, provided the first strong confirmation of the chromosome theory.
- Morgan discovered a mutation that affected fly eye color. He observed that the mutation was inherited differently by male and female flies.
- Based on the inheritance pattern, Morgan concluded that the eye color gene must be located on the X chromosome.
Introduction
The chromosome theory of inheritance.
- Chromosomes, like Mendel's genes, come in matched (homologous) pairs in an organism. For both genes and chromosomes, one member of the pair comes from the mother and one from the father. See diagram Diagram comparing: 1) paired gene copies in an organism (Aa), one from the mother and one from the father with 2) paired homologous chromosomes in the same organism, one of which bears an A allele at a particular location, and the other of which bears an a allele at a corresponding location; one homologue came from the organism's mother, while the other came from its father
- The members of a homologous pair separate in meiosis, so each sperm or egg receives just one member. This process mirrors segregation of alleles into gametes in Mendel's law of segregation . See diagram Diagram comparing: 1) segregation of alleles into gametes: an Aa organism produces A gametes and a gametes with 2) segregation of chromosomes into gametes during meiosis. One homologous chromosome bears an A allele, while the other bears an a allele at the corresponding location. During meiosis I, the homologous chromosomes separate. During meiosis II, the chromatids of each homologous chromosome separate. Ultimately, four gametes are produced: two containing a chromosome with an A allele, and two containing a chromosome with an a allele.
- The members of different chromosome pairs are sorted into gametes independently of one another in meiosis, just like the alleles of different genes in Mendel's law of independent assortment . See diagram Diagram comparing: 1) How an AaBb individual is proposed to make four equally common gamete types, AB, Ab, aB, and ab, in Mendelian genetics with 2) The independent assortment of chromosomes in meiosis. The diagram depicts the relationship between chromosome configuration at meiosis I and homologue segregation to gametes for two pairs of homologous chromosomes. The larger chromosome pair bears the A gene and the smaller chromosome pair bears the B gene. The organism is heterozygous, so the larger homologous pair consists of one chromosome with an A allele and another with an a allele, while the smaller homologous pair consists of one chromosome with a B allele and another with a b allele. If the homologues bearing the A and B alleles are positioned on one side of the metaphase plate, the homologues bearing the a and b alleles will be positioned on the other side of the metaphase plate, and AB and ab gametes will ultimately be produced. If, on the other hand, the homologues bearing the A and b alleles are positioned on one side of the metaphase plate (and the homologues bearing the a and B alleles on the other), Ab and aB gametes will ultimately be produced.
T. H. Morgan: Fun with fruit flies
A "sex limited" inheritance pattern 6 , x marks the spot, confirming the model, attribution:, works cited:.
- Meiosis. (2015, December 6). Retrieved December 8, 2015 from Wikipedia: https://en.wikipedia.org/wiki/Meiosis .
- Hegreness, M. and Meselson, M. (2007). What did Sutton see? Thirty years of confusion over the chromosomal basis of Mendelism. Genetics , 176 (4), 1939-1944. http://dx.doi.org/10.1534/genetics.104.79723 .
- Baltzer, F. (1967). Theodor Boveri: The life of a great biologist 1862-1915. (D. Rudnick, Trans.). In Developmental biology companion website . Retrieved from http://10e.devbio.com/article.php?ch=7&id=75
- Boveri-Sutton chromosome theory. (2015, 31 October). Retrieved December 8, 2015 from Wikipedia: https://en.wikipedia.org/wiki/Boveri%E2%80%93Sutton_chromosome_theory .
- Bergmann, D. C. (2011). The chromosomal theory of heredity. In Genetics lecture notes . Biosci41, Stanford University, 21.
- Morgan, T. H. (1910). Sex-limited inheritance in Drosophila. Science 32 , 120-122 (page 1 of reprint). Retrieved from http://www.esp.org/foundations/genetics/classical/thm-10a.pdf .
- Robbins, R. J. (2000). Introduction [to ESP edition of "Sex limited inheritance in Drosophila"]. In ESP foundations reprint series , v. Electronic Scholarly Publishing Project. Retrieved from http://www.esp.org/foundations/genetics/classical/thm-10a.pdf .
- Morgan, T. H. (1910). Sex-limited inheritance in Drosophila. Science 32 , 120-122 (page 4 of reprint). Retrieved from http://www.esp.org/foundations/genetics/classical/thm-10a.pdf .
- Morgan, T. H. (1910). Sex-limited inheritance in Drosophila. Science 32 , 120-122 (page 6 of reprint). Retrieved from http://www.esp.org/foundations/genetics/classical/thm-10a.pdf .
- Robbins, R. J. (2000). Introduction [to ESP edition of "Sex limited inheritance in Drosophila"]. In ESP foundations reprint series , vi. Electronic Scholarly Publishing Project. Retrieved from http://www.esp.org/foundations/genetics/classical/thm-10a.pdf .
- Hartwell, L. H., Hood, L., Goldberg, M. L., Reynolds, A. E., Silver, L. M., and Veres, R. C. (2008). Analysis of rare mistakes in meiosis provided further support for the chromosome theory. In Genetics: From genes to genomes (3rd ed., pp. 109-110). Boston, MA: McGraw-Hill.
Additional references:
Want to join the conversation.
- Upvote Button navigates to signup page
- Downvote Button navigates to signup page
- Flag Button navigates to signup page


Understanding the Modes of Inheritance: A Simple Guide
Inheritance is the process by which genetic information is passed from parents to offspring. This genetic blueprint determines everything from eye color to susceptibility to certain diseases. Understanding the modes of inheritance is essential for geneticists and healthcare professionals. In this article, we'll explore the various modes of inheritance, providing real-world examples to illustrate each one.
Understanding Pedigrees in Genetics
A pedigree is a visual chart that geneticists use to map the inheritance of a specific trait, disorder, or gene within a family. It's akin to a family tree, but with standardized symbols and structure to represent relationships and the presence or absence of the trait being studied. In a pedigree chart, males are represented by squares, females by circles, and affected individuals are typically shaded or filled in. Lines connect family members, and each generation is usually arranged in a row, allowing for easy visualization of inheritance patterns. By analyzing a pedigree, geneticists can determine the mode of inheritance, predict the likelihood of an individual inheriting a trait, and provide valuable insights for genetic counseling and medical decision-making. Understanding pedigrees is a foundational skill in genetics, bridging the gap between genetic theory and real-world application.

Heterozygous and Homozygous Inheritance
In the context of inheritance, an individual can be heterozygous or homozygous for a particular mutation or trait. Being heterozygous means having two different alleles for a gene, and these individuals are often carriers for certain traits or disorders. Being homozygous means having two identical alleles. These genetic configurations play a crucial role in determining how traits are expressed and inherited. For example, in autosomal dominant inheritance, being heterozygous for a dominant allele is enough to express the trait, whereas in autosomal recessive inheritance, being homozygous for a recessive allele is required.

1. Autosomal Dominant Inheritance
In autosomal dominant inheritance, only one copy of a mutated gene is needed to express the trait or disorder. If one parent carries the dominant allele, there is a 50% chance that the child will inherit the trait.
Pedigree Feature: Affected individuals often appear in every generation.
Example: Huntington's disease is a well-known example of autosomal dominant inheritance. A child with one affected parent has a 50% chance of inheriting the mutated gene that causes the disease.
2. Autosomal Recessive Inheritance
Autosomal recessive inheritance requires two copies of a mutated gene (one from each parent) to express the trait or disorder. If both parents are carriers, there is a 25% chance that the child will inherit the condition.
Pedigree Feature: Affected individuals often appear in siblings, with parents being carriers. If both parents express the trait, then all their offspring should also express the trait
Example: Cystic fibrosis is an example of autosomal recessive inheritance. Both parents must carry the defective CFTR gene for a child to be affected.

3. X-Linked Dominant Inheritance
X-linked dominant inheritance occurs when a gene on the X chromosome causes a trait or disorder. Females have two X chromosomes, so they can be heterozygous or homozygous for the trait. Males, having only one X chromosome, will express the trait if they inherit the mutated gene.
Pedigree Feature: Affected males pass the trait to all daughters but no sons.
Example: Rett syndrome is an example of X-linked dominant inheritance, primarily affecting females.
4. X-Linked Recessive Inheritance
In X-linked recessive inheritance, the gene causing the trait or disorder is located on the X chromosome. Males are more frequently affected because they have only one X chromosome.
Pedigree Feature: Affected males often appear in each generation, with females being carriers.
Example: Hemophilia is a classic example of X-linked recessive inheritance. Males are more commonly affected, while females are typically carriers.

5. Y-Linked Inheritance
Y-linked inheritance, also known as holandric inheritance, involves genes found only on the Y chromosome. Only males can inherit these traits, and they pass them to all their sons.
Pedigree Feature: The trait is passed exclusively from father to son.
Example: Some forms of male infertility are linked to Y-linked inheritance.
6. Mitochondrial Inheritance
Mitochondrial inheritance, or maternal inheritance, involves genes located in the mitochondria. Since mitochondria are inherited exclusively from the mother, all children of an affected mother will inherit the trait or disorder.
Pedigree Feature: The trait is passed exclusively through the maternal line.
Example: Leber's hereditary optic neuropathy (LHON) is a condition inherited through mitochondrial DNA.

7. Multifactorial Inheritance
Multifactorial inheritance involves multiple genes and environmental factors. These complex interactions can lead to a wide range of traits and disorders.
Pedigree Feature: No specific pattern, as both genetic and environmental factors influence the trait.
Example: Height, skin color, and conditions like heart disease and diabetes are influenced by multifactorial inheritance.
The modes of inheritance are fundamental to understanding how genetic traits and disorders are passed down through generations. From simple dominant and recessive patterns to more complex multifactorial interactions, the study of inheritance provides valuable insights into human biology and medicine. Platforms like Bionl.ai are instrumental in analyzing genetic data, furthering our understanding of these inheritance patterns, and contributing to personalized healthcare solutions.
This article was co-authored with ChatGPT .

An official website of the United States government
The .gov means it's official. Federal government websites often end in .gov or .mil. Before sharing sensitive information, make sure you're on a federal government site.
The site is secure. The https:// ensures that you are connecting to the official website and that any information you provide is encrypted and transmitted securely.
- Publications
- Account settings
- Browse Titles
NCBI Bookshelf. A service of the National Library of Medicine, National Institutes of Health.
Genetic Alliance; The New York-Mid-Atlantic Consortium for Genetic and Newborn Screening Services. Understanding Genetics: A New York, Mid-Atlantic Guide for Patients and Health Professionals. Washington (DC): Genetic Alliance; 2009 Jul 8.

Understanding Genetics: A New York, Mid-Atlantic Guide for Patients and Health Professionals.
Appendix e inheritance patterns.
- Inheritance Patterns
It is important to understand the basic laws of inheritance to appreciate how conditions are passed on in a family. An accurate family health history is a valuable tool to illustrate how conditions are passed down through generations.
A person has two copies of almost every gene, one copy from mom and one copy from dad. Scientists have studied human genes to learn how they normally work and how changes in genes can change how they work. Some changes are very minor and do not affect the way a gene works. These changes are often called single nucleotide polymorphisms (SNPs, pronounced “snips”) or gene variants. Other changes, called mutations, affect how a gene works and can lead to disease.
For some conditions, family members with the same mutation may not have the same symptoms. For other conditions, individuals with different mutations can have similar characteristics. This is because gene expression is influenced by genes, as well as by the environment.
Diseases caused by mutations in a single gene are usually inherited in a simple pattern, depending on the location of the gene and whether one or two normal copies of the gene are needed. This is often referred to as Mendelian inheritance because Gregor Mendel first observed these patterns in garden pea plants. Most single gene disorders are rare; but, in total, they affect millions of people in the United States.
Several basic modes of inheritance exist for single-gene disorders: autosomal dominant, autosomal recessive, X-linked dominant, and X-linked recessive. However, not all genetic conditions will follow these patterns, and other rare forms of inheritance such as mitochondrial inheritance exist. (See table at the end of this section.)
View in own window
Dominant mutations are expressed when only one copy of that mutation is present. Therefore, anyone who inherits one dominant disease mutation such as the mutation for Huntington’s disease will have that disease. Dominantly inherited genetic diseases tend to occur in every generation of a family. Each affected person usually has one affected parent. However, dominant mutations can also happen in an individual for the first time, with no family history of the condition (spontaneous mutation).
Recessive mutations require two mutated copies for disease to develop. Recessive genetic diseases are typically not seen in every generation of an affected family. The parents of an affected person are generally carriers: unaffected people who have a copy of a mutated gene. If both parents are carriers of the same mutated gene and both pass it to the child, the child will be affected.
Inheritance patterns differ for genes on sex chromosomes (chromosomes X and Y) compared to genes located on autosomes, non-sex chromosomes (chromosomes numbers 1-22). This is due to the fact that, in general, females carry two X chromosomes (XX), while males carry one X and one Y chromosome (XY). Therefore, females carry two copies of each X-linked gene, but males carry only one copy each of X-linked and Y-linked genes. Females carry no copies of Y-linked genes.
Diseases caused by mutated genes located on the X chromosome can be inherited in either a dominant or recessive manner. Since males only have one X chromosome, any mutated gene on the X chromosome, dominant or recessive, will result in disease. Because females have two copies of X-linked genes, they will not be affected by inheriting of a single recessive mutation on an X-linked gene. For X-linked recessive diseases to occur in females, both copies of the gene must be mutated. Families with an X-linked recessive disorder often have affected males, but rarely affected females, in each generation.
For X-linked dominant diseases, however, a mutation in one copy of an X-linked gene will result in disease for both males and females. Families with an X-linked dominant disorder often have both affected males and affected females in each generation.
A striking characteristic of X-linked inheritance is that fathers cannot pass X-linked traits to their sons; fathers only pass X chromosomes to their daughters and Y chromosomes to their sons. In contrast, mothers pass X-linked genes to both sons and daughters.
- GeneTests www.genetests.org.
- Online Mendelian Inheritance in Man (OMIM) www.ncbi.nlm.nih.gov/entrez/query.fcgi?db=OMIM.
All Genetic Alliance content, except where otherwise noted, is licensed under a Creative Commons Attribution License, which permits unrestricted use, distribution, and reproduction in any medium, provided the original work is properly cited.
- Cite this Page Genetic Alliance; The New York-Mid-Atlantic Consortium for Genetic and Newborn Screening Services. Understanding Genetics: A New York, Mid-Atlantic Guide for Patients and Health Professionals. Washington (DC): Genetic Alliance; 2009 Jul 8. APPENDIX E, INHERITANCE PATTERNS.
- PDF version of this title (22M)
In this Page
Other titles in this collection.
- Genetic Alliance Monographs and Guides
Recent Activity
- INHERITANCE PATTERNS - Understanding Genetics INHERITANCE PATTERNS - Understanding Genetics
Your browsing activity is empty.
Activity recording is turned off.
Turn recording back on
Connect with NLM
National Library of Medicine 8600 Rockville Pike Bethesda, MD 20894
Web Policies FOIA HHS Vulnerability Disclosure
Help Accessibility Careers
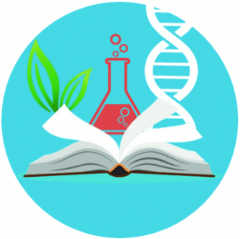
BIOLOGY JUNCTION
Test And Quizzes for Biology, Pre-AP, Or AP Biology For Teachers And Students
Lab 7 Sample 3 Fruitflies
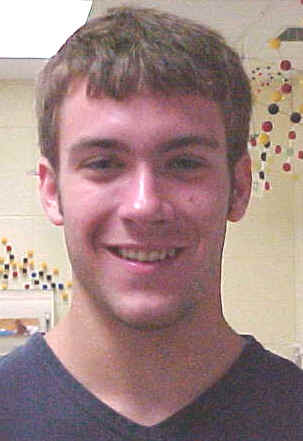
Introduction
Drosophila Melanogaster, the fruit fly, is a great organism for genetic use because it has simple food requirements, occupies little space, is hardy, completes its life cycle in 12 days, makes a large number of offspring, can be knocked out easily, and it has many types of hereditary variations that can be seen with low power microscopes. Drosophila has a small number of chromosomes, four pairs. They are easily located in the large salivary glands. The Drosophila can be obtained from many places. Research of Drosophilae has led to a lot of knowledge about many of its genes.
Many factors combine to affect the length of the Drosophila life cycle. Temperature affects the life cycle the most. At room temperature the average life cycle of the Drosophila is about 12 days. Eggs of the Drosophila are small, oval shaped, and have two filaments at one end. They are usually laid on the surface of the culture medium, and with practice, can be seen with the naked eye. After one day the eggs hatch into the larva.
The larval stage of the Drosophila eats all the time. Larvae tunnel into the culture medium when they eat. The larva will shed its skin as it increases in size. In the last of the three larval stages, the cells of the salivary glands contain giant chromosomes that can be seen under low power in a microscope.
The pupal stage. Before a larva becomes a pupa it climbs the side of the container. The last larval covering then becomes harder and darker, forming the pupal case. Through this case the later stages of metamorphosis to an adult fly can be seen. In particular, the eyes, the wings, and the legs become visible.
The adult stage. When metamorphosis is over, the adult fly emerges form the pupal case. They are fragile and light in color and their wings are not fully expanded. They get darker in about an hour. They live about a month and then die. A female refrains from mating for about 12 days after she emerges from the pupal case. After she mates her receptacles contain large amounts of sperm and she lays her eggs. Make sure that the first flies you use are virgins.
The experiment will take several weeks. You will be assigned Drosophila with well-defined mutant traits by your teacher. You will keep a close record of what happens as each of these flies mate and pass there traits off to their offspring over a few generations.
There are three types of crosses that are studied in this lab. In monohybrid crosses the mode of inheritance is determined when a single contrasting pair of characteristics is involved. In a dihybrid cross the mode of inheritance is determined when the two pairs of contrasting of characteristics are considered simultaneously. In a sex-linked cross the mode of inheritance is determined when the mutant characteristic is associated with the X chromosome.
In the sex linked cross of Drosophila Melanogaster, a phenotypic ratio of 1:1 will be obtained.
The materials used in this lab are as follows: a vile of Drosophilia with c designated trait, vials containing a medium, a refrigerator, ice packs, Petri dishes, a light microscope, a vial of wild type flies, an incubator, a pencil and paper.
Begin by obtaining a vial of wild type flies. Practice immobilizing and sexing these flies. Make sure to examine the flies and determine the characteristics of their eyes, wings, bristles, and antennae. Next, these are the steps for immobilizing the flies. Hold the vial containing the flies at an angle and place it in a refrigerator for several minutes. When the flies are immobilized, place them into a small plastic Petri dish. Then place the Petri dish on top of the icepack in order to maintain the cool temperature necessary to keep flies immobilized. Use the dissecting microscope to view the flies. Make sure to top the petri dish on when viewing the flies.
You can easily distinguish male flies from females by looking for the following characteristics: males are usually smaller than the females, males have dark blunt abdomens and females have lighter pointed abdomens. The males have sex combs, which are black bristles on the uppermost joint of the forelegs. Next, get a vial containing experimental flies. Make sure to write down the number of the vial that you have. The flies you now have are the P1 generation. The females should have laid eggs. The eggs and larvae are the F1 generation. Then after there are eggs present knock out remove the adult flies from the vial. Sex the adult flies and write down any mutations. Place the flies in the morgue that contains alcohol. Make sure to label the vial with the symbols for the mating.
After about another week has passed knock out and record characteristics of the remaining F1 flies and record the results in table 7.1. Then place the six pairs of these flies in a new vial and place the remaining flies in the morgue. Label the new vial F1, and tell the cross, date and your name.
After another week has passed, remove the F1 flies and put them in the morgue. The F2 generation are the eggs and larvae in the vial. Place the vial back into the incubator. Once again, after another week has passed remove the F2 flies and record their sex and characteristics and place the results in Table 7.2. Recording a greater number of F2 flies will make your results more accurate. Try to collect at least 200 flies. In order to analyze your data you will first have to be able to be able to complete Chi-Square Analysis.
Table 7.1 F1 Generation
1. Describe the observed mutations? In the F1 generation the males had white eyes and the females had red eyes. In the F2 generation the males and females could have had either red or white eyes.
2. Write a hypothesis which describes the mode of inheritance of the trait you studied. This is your null hypothesis ( as described in the Statistical Analysis Section). For a sex linked cross there will always be a one to one ratio of the phenotypes. In the F1 generation there will be a one to one ratio of red eyed females to the number of white eyed males. In the F2 generation there will be a one to one ratio of red eyed females to white eyed females. There will also be a one to one ratio of red eyed males to white eyed males.
3. Refer to a textbook and review Punnett squares. In the space below construct two Punnett squares to predict the expected results of both the parental and F1 crosses from your null hypothesis.
Parental cross
4. Refer to the Punnett squares above. Record the expected ratios for the genotypes and phenotypes of the F1 and F2 in the experiment below.
5. Do the actual results deviate from what was expected? If so, explain how. No my results do no deviate much from what was expected. However in the F2 generation there were 67 white females and 52 red females.
6. For the results describe your cross? My cross is a sex linked cross.
7. Are the deviations for the phenotypic ratio of the F2 generation within the limits expected by chance? To answer this question, statistically analyze the data using the Chi-Square-Analysis. Calculate the Chi-Square for the F2 generation in the chart below. Refer to the critical values of the Chi Square distribution table to determine the P value that is associated with your statistic.
(a) Calculate the Chi-Square value for these data.
1. How many degrees of freedom are there? 3 degrees of freedom
2. Chi Square=2.52
3. Referring to the critical values chart what is the probability value for these data? Greater than .05 probabilities that the null hypothesis is right.
(b) According to the probability value, can you accept or reject your null hypothesis? Explain why. I can accept the null hypothesis because my Chi-Square answer is less than the critical value form the table. I have 3 degrees of freedom and my Chi- Square answer was3.0 which is less than 7.82.
1. Why was it necessary for the females of the parental generation to be virgins? The females store sperm in their receptacles and if they were not virgins we would not be able to tell who the fathers were.
2. Why was it not necessary to isolate virgin females for the F1 cross? The females store sperm in their receptacles and if they were not virgins we would not be able to tell who the fathers were
3. Why the adult flies were removed from the vials at weeks 2 and 4? So, they are not be able to mate with the next generation.
Chi-Square Analysis
Statistics can be used to determine if differences among groups are significant, or simply the result of predictable error. The statistical test most frequently used to determine whether data obtained experimentally provide a good fit, or approximation to the expected or theoretical data is the Chi-square test. This test can be used to determine if deviations from the expected values are due to chance alone or to comeother circumstance.
To determine if the observed data fall with in acceptable limits, a Chi-Square analysis is performed to test the validity of a null hypothesis; that there is no statistically significant difference between the observed and expected data. If the Chi-Square analysis indicates that the data vary too much from the expected 3: 1 an alternative hypothesis is accepted.
The formula for Chi-square is:
X2=E(o-e)2 E
O= observed number of individuals
e= expected number of individuals
E= the sum of the values
The (df) are determined by taking the number of possible phenotypes and subtracting one from it. If the Chi- Square answer is greater than the number from the critical values chart then the null hypothesis is incorrect. The results are said to be significant at .05. This means that only 5 % of the time you would expect to see similar data if the null hypothesis were correct. The probability can also be rejected at .001. This time it means that less than 1 % of the time would you expect to see similar data.
Critical Values Chart
Practice Problem
An investigator observes that when pure-breeding long winged Drosophila are mated with pure breeding short wing flies the F1 have an intermediate wing length. When several intermediate wing length flies are allowed to interbreed the following results are obtained. 230 long wings. 510 intermediate length wings. 260 short wings.
a. What is the genotype of the F intermediate wing length flies? The genotype is Ll.
b. Write a hypothesis describing the mode of inheritance of wing length in Drosophila. There will be 333 long winged flies. 666 intermediate winged flies. There will be 33 short wing flies.
c. Complete the table
1. How many degrees of freedom are there? There are 2 degrees of freedom.
2. Chi-Square= 84.4
3. Referring to the critical values chart, what is the probability value for these data? Less than .001.
4. According to the probability value can you except or reject the null hypothesis?
I can reject the null hypothesis because the Chi-square answer is greater than the critical value from the table.
Error Analysis
Results from this lab could have been affected by many things. The constant knocking out of flies could have caused some of the larvae to not hatch therefore affecting our numbers. Also, incorrectly identifying the characteristics of the flies could have also greatly affected the results received. Improper calculation of numbers could have also caused inaccurate results. Finally, some flies could have gotten stuck in the medium and could have been identified.
From the results of the experiment I can conclude that I received results that were close to a 1:1 ratio. The Chi- Square worked from my data was accepted at a possibility greater than .05. The null hypothesis in this case can be accepted.

Bennie Hawra

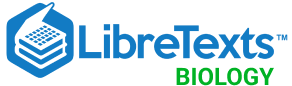
- school Campus Bookshelves
- menu_book Bookshelves
- perm_media Learning Objects
- login Login
- how_to_reg Request Instructor Account
- hub Instructor Commons
- Download Page (PDF)
- Download Full Book (PDF)
- Periodic Table
- Physics Constants
- Scientific Calculator
- Reference & Cite
- Tools expand_more
- Readability
selected template will load here
This action is not available.
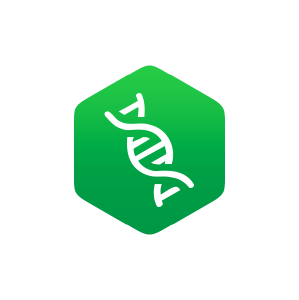
13: Modern Understandings of Inheritance
- Last updated
- Save as PDF
- Page ID 1802
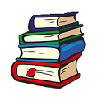
The gene is the physical unit of inheritance, and genes are arranged in a linear order on chromosomes. The behaviors and interactions of chromosomes during meiosis explain, at a cellular level, the patterns of inheritance that we observe in populations. Genetic disorders involving alterations in chromosome number or structure may have dramatic effects and can prevent a fertilized egg from developing altogether.
- 13.0: Prelude to Modern Understandings of Inheritance The gene is the physical unit of inheritance, and genes are arranged in a linear order on chromosomes. The behaviors and interactions of chromosomes during meiosis explain, at a cellular level, the patterns of inheritance that we observe in populations. Genetic disorders involving alterations in chromosome number or structure may have dramatic effects and can prevent a fertilized egg from developing altogether.
- 13.1: Chromosomal Theory and Genetic Linkage The Chromosomal Theory of inheritance, proposed by Sutton and Boveri, states that chromosomes are the vehicles of genetic heredity. Neither Mendelian genetics nor gene linkage is perfectly accurate; instead, chromosome behavior involves segregation, independent assortment, and occasionally, linkage. Sturtevant devised a method to assess recombination frequency and infer the relative positions and distances of linked genes on a chromosome on the basis of the average number of crossovers.
- 13.2: Chromosomal Basis of Inherited Disorders The number, size, shape, and banding pattern of chromosomes make them easily identifiable in a karyogram and allows for the assessment of many chromosomal abnormalities. Disorders in chromosome number, or aneuploidies, are typically lethal to the embryo, although a few trisomic genotypes are viable. Because of X inactivation, aberrations in sex chromosomes typically have milder phenotypic effects. Aneuploidies also include instances in which segments of a chromosome are duplicated or deleted.
- 13.E: Modern Understandings of Inheritance (Exercises)
Thumbnail: Chromosomes are threadlike nuclear structures consisting of DNA and proteins that serve as the repositories for genetic information. The chromosomes depicted here were isolated from a fruit fly’s salivary gland, stained with dye, and visualized under a microscope. Akin to miniature bar codes, chromosomes absorb different dyes to produce characteristic banding patterns, which allows for their routine identification. (credit: modification of work by “LPLT”/Wikimedia Commons; scale-bar data from Matt Russell)
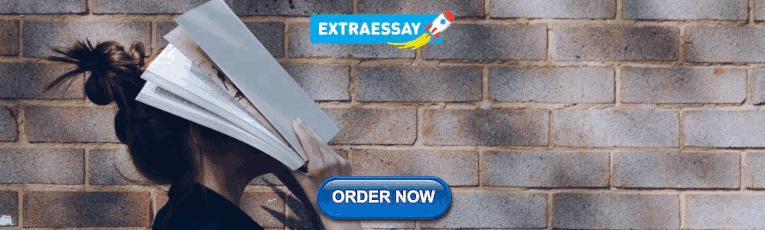
IMAGES
VIDEO
COMMENTS
One definitive indication that a trait is inherited as AD, and not XD, is that an affected father passes the disease to a son; this type of transmission is not possible with XD, since males inherit their X chromosome from their mothers. Figure 5.3.5 5.3. 5: Some types of rickets may follow an XD mode of inheritance.
From Genotype to Phenotype. Each human body cell has a full complement of DNA stored in 23 pairs of chromosomes. The image below shows the pairs in a systematic arrangement called a karyotype.Among these is one pair of chromosomes, called the sex chromosomes, that determines the sex of the individual (XX in females, XY in males).The remaining 22 chromosome pairs are called autosomal chromosomes.
This is the easiest mode of inheritance to identify, but it is one of the rarest because there are so few genes located only on the Y-chromosome. Figure 4.3.5 A Pedigree Consistent with Y-Linked Inheritance [Long description] A common, but incorrect, example of Y-linked inheritance is the hairy-ear-rim phenotype
Gregor Mendel's principles of heredity, observed through patterns of inheritance in pea plants, form the basis of modern genetics. Mendel proposed that traits were specified by "heritable elements" called genes. Genes come in different versions, or alleles, with dominant alleles being expressed over recessive alleles.
By experimenting with pea plant breeding, Mendel developed three principles of inheritance that described the transmission of genetic traits, before anyone knew genes existed. Mendel's insight ...
Boveri and Sutton's chromosome theory of inheritance states that genes are found at specific locations on chromosomes, and that the behavior of chromosomes during meiosis can explain Mendel's laws of inheritance. Thomas Hunt Morgan, who studied fruit flies, provided the first strong confirmation of the chromosome theory.
Mechanisms of inheritance generally refer to traits resulting from a single factor or gene, called unifactorial inheritance, or from the interaction of multiple factors or genes, called multifactorial inheritance. Because it is the simplest inheri-tance pattern, unifactorial inheritance is the best understood.
1. Autosomal Dominant Inheritance. In autosomal dominant inheritance, only one copy of a mutated gene is needed to express the trait or disorder. If one parent carries the dominant allele, there is a 50% chance that the child will inherit the trait. Pedigree Feature: Affected individuals often appear in every generation.
Inheritance patterns differ for genes on sex chromosomes (chromosomes X and Y) compared to genes located on autosomes, non-sex chromosomes (chromosomes numbers 1-22). This is due to the fact that, in general, females carry two X chromosomes (XX), while males carry one X and one Y chromosome (XY). Therefore, females carry two copies of each X ...
The probability of the homozygote or the heterozygote is 1/4 + 1/2 = 3/4 using the sum rule. The same probability can be obtained in the same way for each of the other genes, so that the probability of a dominant phenotype at A and B and C and D is, using the product rule, equal to 3/4 × 3/4 × 3/4 × 3/4, or 27/64.
Cross 2: Wild Type Female x Yellow Body Male Yellow body- y, Wild type-y* Predicted Genotypes: х F, Generation 533 F2 Generation 236 PHENOTYPE Wild Type Males Yellow body Males Wild Type Females Yellow body Females 0 224 486 483 0 0 Write a NULL hypothesis that describes the mode of inheritance for the trait(s) you studied: There is no difference between the observed data and the data ...
Genetic inheritance is a basic principle of genetics and explains how characteristics are passed from one generation to the next. Genetic inheritance occurs due to genetic material, in the form of DNA, being passed from parents to their offspring. When organisms reproduce, all the information for growth, survival, and reproduction for the next generation is found in the DNA passed down from ...
Write a hypothesis which describes the mode of inheritance of the trait you studied. This is your null hypothesis ( as described in the Statistical Analysis Section). ... Write a hypothesis describing the mode of inheritance of wing length in Drosophila. There will be 333 long winged flies. 666 intermediate winged flies. There will be 33 short ...
Write a NULL hypothesis that describes the mode of inheritance for the trait(s) you studied: There is no difference between the observed data and the data expected for a(n) wild type female and lobe eyes, curved wings male _____cross. I would expect this phenotypic ratio in the F 1 offspring: 3:
In 1856, he began a decade-long research pursuit involving inheritance patterns in honeybees and plants, ultimately settling on pea plants as his primary model system (a system with convenient characteristics used to study a specific biological phenomenon to be applied to other systems). In 1865, Mendel presented the results of his experiments ...
D The null hypothesis should be rejected because the critical value is greater than the calculated value. and more. ... 11 sepia eyes Which of the following best describes the likely mode of inheritance for the eye-color gene? A The eye-color gene is likely autosomal because males and females have similar phenotype ratios.
Based on these observations, Mendel formulated his first law of inheritance. This law is called the law of segregation. It states that there are two factors controlling a given characteristic, one of which dominates the other, and these factors separate and go to different gametes when a parent reproduces.
Write A Hypothesis That Describes The Mode Of Inheritance Of The Traits You Studied, Popular Biography Writing Sites For College, Professional Research Paper Writing For Hire For Mba, Contoh Soal Essay Tentang Hari Kiamat, Pollution Solution Essay, Best Research Proposal Ghostwriting Site For Masters, Drugs And Alcohol Essay
This problem has been solved! You'll get a detailed solution from a subject matter expert that helps you learn core concepts. Question: Write the null hypothesis about the mode of inheritance for the gene is X-linked recessive. The format will be If the ____ gene is X-linked recessive, then, the expected segregation would be_______. Write the ...
13.1: Chromosomal Theory and Genetic Linkage. The Chromosomal Theory of inheritance, proposed by Sutton and Boveri, states that chromosomes are the vehicles of genetic heredity. Neither Mendelian genetics nor gene linkage is perfectly accurate; instead, chromosome behavior involves segregation, independent assortment, and occasionally, linkage.