Enjoy a completely custom, expertly-written dissertation. Choose from hundreds of writers, all of whom are career specialists in your subject.
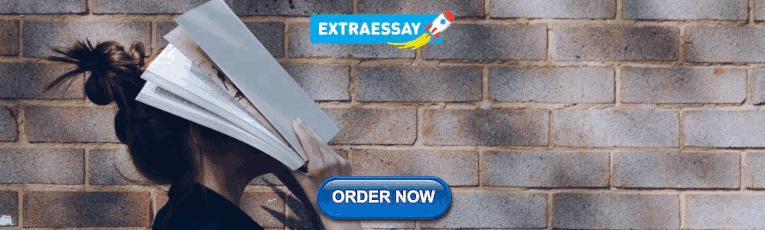
45 Biomedical Research Topics for You
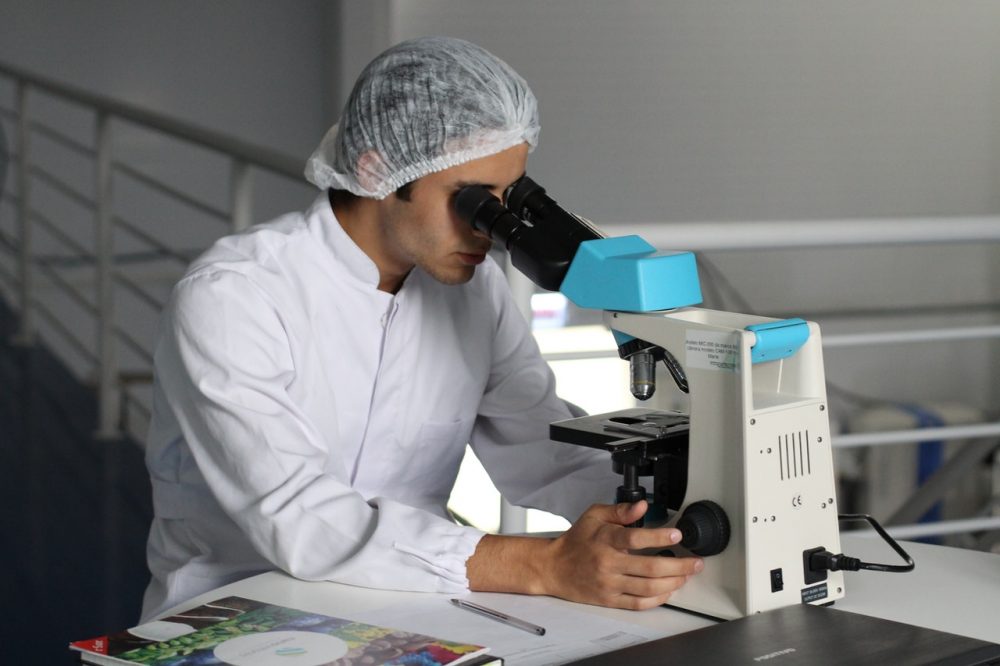
Although choosing relevant biomedical research topics is often an arduous task for many, it shouldn’t be for you. You no longer have to worry as we have provided you with a list of topics in biomedical science in this write-up.
Biomedical research is a broad aspect of science, and it is still evolving. This aspect of science involves a variety of ways to prevent and treat diseases that lead to illness and death in people.
This article contains 45 biomedical topics. The topics were carefully selected to guide you in choosing the right topics. They can be used for presentations, seminars, or research purposes, as the case may be.
So, suppose you need topics in biomedical ethics for papers or biomedical thesis topics for various purposes. In that case, you absolutely have to keep reading! Are you ready to see our list of biomedical topics? Then, let’s roll.
Biomedical Engineering Research Topics
Biomedical engineering is the branch of engineering that deals with providing solutions to problems in medicine and biology. Biomedical engineering research is an advanced area of research. Are you considering taking up research in this direction?
Research topics in this area cannot just be coined while eating pizza. It takes a lot of hard work to think out something meaningful. However, we have made a list for you! Here is a list of biomedical engineering topics!
- How to apply deep learning in biomedical engineering
- Bionics: the latest discoveries and applications
- The techniques of genetic engineering
- The relevance of medical engineering today
- How environmental engineering has affected the world
Biomedical Ethics Topics
There are ethical issues surrounding healthcare delivery, research, biotechnology, and medicine. Biomedical Ethics is fundamental to successful practice experience and is addressed by various disciplines. If you want to research this area, then you do not have to look for topics. Here’s a list of biomedical ethics for paper that you can choose from:
- The fundamentals of a physician-patient relationship
- How to handle disability issues as a health care sector
- Resource allocation and distribution
- All you need to know about coercion, consent, and or vulnerability
- Ethical treatment of subjects or animals in clinical trials
Relevant Biomedical Topics
Topics in Biomedical science are numerous, but not all are relevant today. Since biomedical science is constantly evolving, newer topics are coming up. If you desire in your topic selection, read on. Here is a list of relevant biomedical topics just for you!
- The replacement of gene therapy by gene editing
- Revolution of vaccine development by synthetic biology
- Introduction of artificial blood – the impact on the health sector
- Ten things know about artificial womb
- Transplanted reproductive organs and transgender birth
Biomedical Science Topics
Biomedical science is the aspect of scientific studies that focuses on applying biology and chemistry to health care. This field of science has a broad range of disciplines. If you intend to do research in this field, look at this list of research topics in biomedical science.
- The role of biomechanics in health care delivery
- Importance of biomaterials and regeneration engineering
- The application of cell and molecular engineering to medicine
- The evolution of medical instrumentation and devices
- Neural engineering- the latest discoveries
Seminar Topics for Biomedical Instrumentation
Biomedical science is constantly making progress, especially in the aspect of biomedical instrumentation. This makes it worthy of a seminar presentation in schools where it is taught. However, choosing a biomedical research topic for a biomedical instrumentation seminar may not come easy. This is why we have collated five brilliant topics for biomedical instrumentation just for you. They include:
- Microelectrode in neuro-transplants
- Hyperbaric chamber for oxygen therapy
- How concentric ring electrodes can be used to manage epilepsy
- How electromagnetic interference makes cochlear implants work
- Neuroprosthetics Management using Brain-computer interfaces (BCI)
Biomedical Engineering Topics for Presentation
One of the interesting aspects of biomedical science in biomedical engineering. It is the backbone that gives the biomedical science structure. Are you interested in making presentations about biomedical engineering topics? Or do you need biomedical engineering topics for paper? Get started here! We have compiled a list of biomedical engineering topics for you. Here they are:
- In-the-ear device to control stuttering: the basis of its operation
- How to implement the magnetic navigated catheterization
- Semiconductor-cell interfaces: the rudiments of its application
- The benefits of tissue engineering of muscle
- The benefits of sensitive artificial skin for prosthetic arms
Hot Topics in Biomedical Research
Biomedical research is fun because it is often relatable. As interesting as it seems, choosing a topic for research doesn’t come easy at all. Yet, there are also a lot of trending events around biomedical topics. To simplify your selection process, we have written out a few of them here.
Here are some hot biomedical research topics below.
- What is immunology, and what is the relevance today?
- Regenerative medicine- definition, importance, and application
- Myths about antibiotic resistance
- Vaccine development for COVID-19
- Infectious diseases now and before
Biomedical Research Topics
Biomedical research is an extensive process. It requires a lot of time, dedication, and resources. Getting a topic shouldn’t be added to that list. There are biomedical thesis topics and research topics in biomedical science for you here:
- Air pollution- sources, impact, and prevention
- Covid-19 vaccination- the effect on life expectancy
- Hyper insomnia- what is responsible?
- Alzheimer’s disease- newer treatment approaches
- Introduction of MRI compatible infusion pump
Biomedical Nanotechnology Topics
Biomedical research topics and areas now include nanotechnology. Nanotechnology has extended its tentacles to medicine and has been used to treat cancer successfully. This makes it a good research area. It is good for seminar presentations. Here are some biomedical nanotechnology topics below.
- The uses of functional particles and nanomaterials
- Nanoparticles based drug delivery system
- The incorporation of nanoporous membranes into biomedical devices
- Nanostructured materials for biological sensing
- Nanocrystals- imaging, transportation, and toxicity features
Seeking professional assistance to write your biomedical research or thesis? Look no further! At our reputable writing service, our experienced writers specialize in providing tailored support for the complexities of biomedical research. When you say, “ do my thesis for me ” we’re here to guide you through formulating research questions, conducting literature reviews, and analyzing data sets. Entrust the writing process to our experts while you focus on exploring the frontiers of biomedical research. Contact us today for a meticulously crafted thesis that enhances your chances of success.
We believe you have been thoroughly equipped with a list of biomedical topics. This way, you wouldn’t have to go through the stress of choosing a topic for research, seminars, or other educational purposes. Now that you have the topics at your fingertips make your choice and enjoy!
Frequently Asked Questions
Richard Ginger is a dissertation writer and freelance columnist with a wealth of knowledge and expertise in the writing industry. He handles every project he works on with precision while keeping attention to details and ensuring that every work he does is unique.
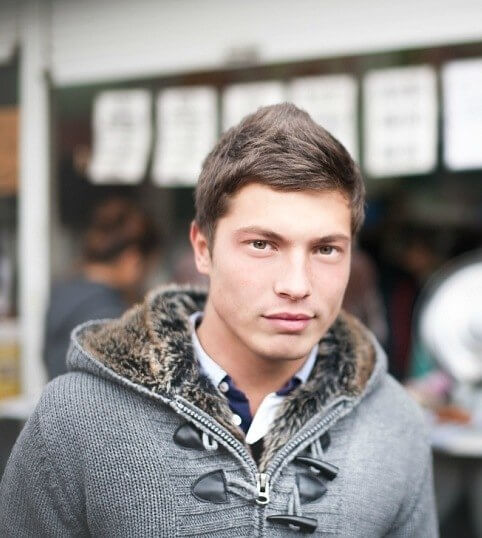
Succeed With A Perfect Dissertation
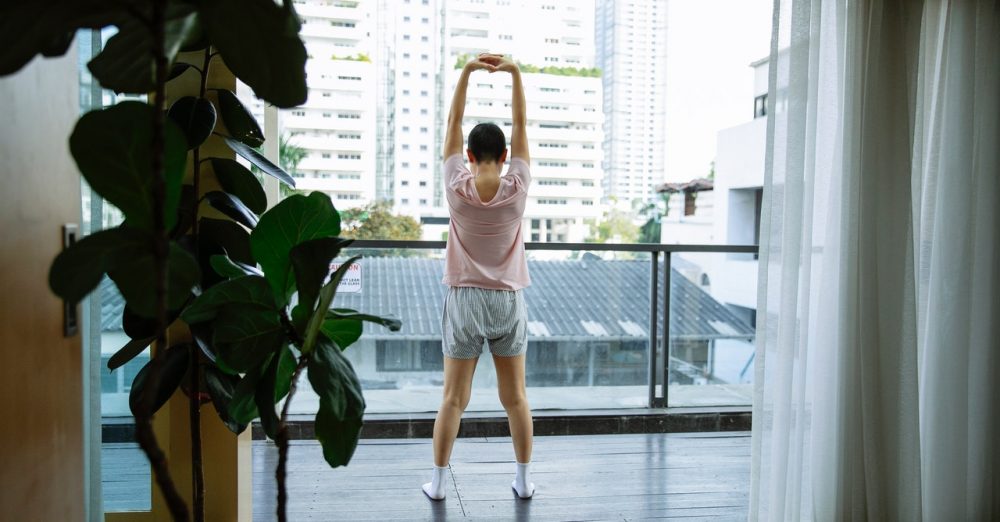
Leave a Reply Cancel reply
Your email address will not be published. Required fields are marked *
Save my name, email, and website in this browser for the next time I comment.
As Putin continues killing civilians, bombing kindergartens, and threatening WWIII, Ukraine fights for the world's peaceful future.
Ukraine Live Updates
Biomedical Research Paper Topics

This page offers students an extensive list of biomedical research paper topics , expert advice on how to choose these topics, and guidance on how to write a compelling biomedical research paper. The guide also introduces the services of iResearchNet, an academic assistance company that caters to the unique needs of each student. Offering expert writers, custom-written works, and a host of other features, iResearchNet provides the tools and support necessary for students to excel in their biomedical research papers.
100 Biomedical Research Paper Topics
Biomedical research is a vibrant field, with an extensive range of topics drawn from various sub-disciplines. It encompasses the study of biological processes, clinical medicine, and even technology and engineering applied to the domain of healthcare. Given the sheer breadth of this field, choosing a specific topic can sometimes be overwhelming. To help you navigate this rich landscape, here is a list of biomedical research paper topics, divided into ten categories, each with ten specific topics.
Academic Writing, Editing, Proofreading, And Problem Solving Services
Get 10% off with 24start discount code.
1. Genetics and Genomics
- Role of genetics in rare diseases
- Advances in gene editing: CRISPR technology
- Human genome project: findings and implications
- Genetic basis of cancer
- Personalized medicine through genomics
- Epigenetic modifications and disease progression
- Genomic data privacy and ethical implications
- Role of genetics in mental health disorders
- Prenatal genetic screening and ethical considerations
- Gene therapy in rare genetic disorders
2. Bioengineering and Biotechnology
- Tissue engineering in regenerative medicine
- Bioprinting of organs: possibilities and challenges
- Role of nanotechnology in targeted drug delivery
- Biosensors in disease diagnosis
- Bioinformatics in drug discovery
- Development and application of biomaterials
- Bioremediation and environmental cleanup
- Biotechnology in agriculture and food production
- Therapeutic applications of stem cells
- Role of biotechnology in pandemic preparedness
3. Neuroscience and Neurology
- Pathophysiology of Alzheimer’s disease
- Advances in Parkinson’s disease research
- Role of neuroimaging in mental health diagnosis
- Understanding the brain-gut axis
- Neurobiology of addiction
- Role of neuroplasticity in recovery from brain injury
- Sleep disorders and cognitive function
- Brain-computer interfaces: possibilities and ethical issues
- Neural correlates of consciousness
- Epigenetic influence on neurodevelopmental disorders
4. Immunology
- Immune response to COVID-19
- Role of immunotherapy in cancer treatment
- Autoimmune diseases: causes and treatments
- Vaccination and herd immunity
- The hygiene hypothesis and rising allergy prevalence
- Role of gut microbiota in immune function
- Immunosenescence and age-related diseases
- Role of inflammation in chronic diseases
- Advances in HIV/AIDS research
- Immunology of transplantation
5. Cardiovascular Research
- Advances in understanding and treating heart failure
- Role of lifestyle factors in cardiovascular disease
- Cardiovascular disease in women
- Hypertension: causes and treatments
- Pathophysiology of atherosclerosis
- Role of inflammation in heart disease
- Novel biomarkers for cardiovascular disease
- Personalized medicine in cardiology
- Advances in cardiac surgery
- Pediatric cardiovascular diseases
6. Infectious Diseases
- Emerging and re-emerging infectious diseases
- Role of antiviral drugs in managing viral diseases
- Antibiotic resistance: causes and solutions
- Zoonotic diseases and public health
- Role of vaccination in preventing infectious diseases
- Infectious diseases in immunocompromised individuals
- Role of genomic sequencing in tracking disease outbreaks
- HIV/AIDS: prevention and treatment
- Advances in malaria research
- Tuberculosis: challenges in prevention and treatment
7. Aging Research
- Biological mechanisms of aging
- Impact of lifestyle on healthy aging
- Age-related macular degeneration
- Role of genetics in longevity
- Aging and cognitive decline
- Social aspects of aging
- Advances in geriatric medicine
- Aging and the immune system
- Role of physical activity in aging
- Aging and mental health
8. Endocrinology
- Advances in diabetes research
- Obesity: causes and health implications
- Thyroid disorders: causes and treatments
- Role of hormones in mental health
- Endocrine disruptors and human health
- Role of insulin in metabolic syndrome
- Advances in treatment of endocrine disorders
- Hormones and cardiovascular health
- Reproductive endocrinology
- Role of endocrinology in aging
9. Mental Health Research
- Advances in understanding and treating depression
- Impact of stress on mental health
- Advances in understanding and treating schizophrenia
- Child and adolescent mental health
- Mental health in the elderly
- Impact of social media on mental health
- Suicide prevention and mental health services
- Role of psychotherapy in mental health
- Mental health disparities
10. Oncology
- Advances in cancer immunotherapy
- Role of genomics in cancer diagnosis and treatment
- Lifestyle factors and cancer risk
- Early detection and prevention of cancer
- Advances in targeted cancer therapies
- Role of radiation therapy in cancer treatment
- Cancer disparities and social determinants of health
- Pediatric oncology: challenges and advances
- Role of stem cells in cancer
- Cancer survivorship and quality of life
These biomedical research paper topics represent a wide array of studies within the field of biomedical research, providing a robust platform to delve into the intricacies of human health and disease. Each topic offers a unique opportunity to explore the remarkable advancements in biomedical research, contributing to the ongoing quest to enhance human health and wellbeing.
Choosing Biomedical Research Paper Topics
The selection of a suitable topic for your biomedical research paper is a critical initial step that will largely influence the course of your study. The right topic will not only engage your interest but will also be robust enough to contribute to the existing body of knowledge. Here are ten tips to guide you in choosing the best topic for your biomedical research paper.
- Relevance to Your Coursework and Interests: Your topic should align with the courses you have taken or are currently enrolled in. Moreover, a topic that piques your interest will motivate you to delve deeper into research, resulting in a richer, more nuanced paper.
- Feasibility: Consider the practicality of your proposed research. Do you have access to the necessary resources, including the literature, laboratories, or databases needed for your study? Ensure that your topic is one that you can manage given your resources and time constraints.
- Novelty and Originality: While it is essential to ensure your topic aligns with your coursework and is feasible, strive to select a topic that brings a new perspective or fresh insight to your field. Originality enhances the contribution of your research to the broader academic community.
- Scope: A well-defined topic helps maintain a clear focus during your research. Avoid choosing a topic too broad that it becomes unmanageable, or so narrow that it lacks depth. Balancing the scope of your research is key to a successful paper.
- Future Career Goals: Consider how your chosen topic could align with or benefit your future career goals. A topic related to your future interests can provide an early start to your career, showcasing your knowledge in that particular field.
- Available Supervision and Mentoring: If you’re in a setting where you have a mentor or supervisor, choose a topic that fits within their area of expertise. This choice will ensure you have the best possible guidance during your research process.
- Ethical Considerations: Some topics may involve ethical considerations, particularly those involving human subjects, animals, or sensitive data. Make sure your topic is ethically sound and you’re prepared to address any related ethical considerations.
- Potential Impact: Consider the potential impact of your research on the field of biomedical science. The best research often addresses a gap in the current knowledge or has the potential to bring about change in healthcare practices or policies.
- Literature Gap: Literature review can help identify gaps in the existing body of knowledge. Choosing a topic that fills in these gaps can make your research more valuable and unique.
- Flexibility: While it’s essential to start with a clear topic, remain open to slight shifts or changes as your research unfolds. Your research might reveal a different angle or a more exciting question within your chosen field, so stay flexible.
Remember, choosing a topic should be an iterative process, and your initial ideas will likely evolve as you conduct a preliminary literature review and discuss your thoughts with your mentors or peers. The ultimate goal is to choose a topic that you are passionate about, as this passion will drive your work and make the research process more enjoyable and fulfilling.
How to Write a Biomedical Research Paper
Writing a biomedical research paper can be a daunting task. However, with careful planning and strategic execution, the process can be more manageable and rewarding. Below are ten tips to help guide you through the process of writing a biomedical research paper.
- Understand Your Assignment: Before you begin your research or writing, make sure you understand the requirements of your assignment. Know the expected length, due date, formatting style, and any specific sections or components you need to include.
- Thorough Literature Review: A comprehensive literature review allows you to understand the current knowledge in your research area and identify gaps where your research can contribute. It will help you shape your research question and place your work in context.
- Clearly Define Your Research Question: A well-defined research question guides your research and keeps your writing focused. It should be clear, specific, and concise, serving as the backbone of your study.
- Prepare a Detailed Outline: An outline helps organize your thoughts and create a roadmap for your paper. It should include all the sections of your research paper, such as the introduction, methods, results, discussion, and conclusion.
- Follow the IMRaD Structure: Most biomedical research papers follow the IMRaD format—Introduction, Methods, Results, and Discussion. This structure facilitates the orderly and logical presentation of your research.
- Use Clear and Concise Language: Biomedical research papers should be written in a clear and concise manner to ensure the reader understands the research’s purpose, methods, and findings. Avoid unnecessary jargon and ensure that complex ideas are explained clearly.
- Proper Citation and Reference: Always properly cite the sources of information you use in your paper. This not only provides credit where it’s due but also allows your readers to follow your line of research. Be sure to follow the citation style specified in your assignment.
- Discuss the Implications: In your discussion, go beyond simply restating your findings. Discuss the implications of your results, how they relate to previous research, and how they contribute to the existing knowledge in the field.
- Proofread and Edit: Never underestimate the importance of proofreading and editing. Checking for grammatical errors, punctuation mistakes, and clarity of language can enhance the readability of your paper.
- Seek Feedback Before Final Submission: Before submitting your paper, seek feedback from peers, mentors, or supervisors. Fresh eyes can often spot unclear sections or errors that you may have missed.
Writing a biomedical research paper is a significant academic endeavor, but remember that every researcher started where you are right now. It’s a process that requires time, effort, and patience. Remember, the ultimate goal is not just to get a good grade but also to contribute to the vast body of biomedical knowledge.
iResearchNet’s Custom Writing Services
Navigating the process of writing a biomedical research paper can be complex and demanding. At iResearchNet, we understand these challenges and strive to offer a stress-free, seamless solution to support your academic journey. With our roster of highly skilled, degree-holding writers, we are committed to delivering top-quality, custom-written papers tailored specifically to your individual requirements and desired outcomes.
- Expert Degree-Holding Writers: iResearchNet takes pride in our team of knowledgeable and experienced writers who hold advanced degrees in diverse fields. These writers are not only academic experts but are also keenly in tune with the complex landscape of biomedical research. This breadth and depth of expertise ensure that your paper benefits from a thorough understanding of the topic, resulting in a well-informed, academically credible document.
- Custom Written Works: We appreciate the unique academic goals and distinct requirements of each student. That’s why iResearchNet specializes in providing custom-written papers. Our aim is to capture your individual academic voice and perspective, blending it with our professional acumen to create a paper that reflects your specific academic needs and aspirations.
- In-Depth Research: Every paper that we produce is founded on the bedrock of extensive and in-depth research. Our writers are committed to exploring a wide range of credible and reputable sources to enrich your paper with diverse viewpoints and comprehensive information. This dedication to rigorous research ensures that your paper is not only thoroughly informed but also accurately referenced, adding to its academic integrity.
- Custom Formatting: Academic institutions often require different formatting styles. Be it APA, MLA, Chicago/Turabian, or Harvard, our writers are adept at all these academic formatting styles. We strive to adhere strictly to your specified formatting style, contributing to the polished and professional presentation of your paper.
- Top Quality: Quality is the cornerstone of our services at iResearchNet. We believe that each paper we craft should demonstrate a high standard of scholarship. Our writers dedicate their skills and effort to ensure every aspect of your paper, from clarity of language to depth of analysis and precision of information, reflects top-quality work.
- Customized Solutions: Recognizing that each research paper brings a distinct set of challenges and requirements, we offer customized solutions. Our approach is to thoroughly understand your specific needs and shape our writing services accordingly. We ensure that every aspect of your paper, from its overarching structure to the smallest details, aligns with your expectations.
- Flexible Pricing: We believe that high-quality academic writing services should be accessible. That’s why we offer our top-quality services at competitive prices, striking a careful balance between affordability and excellence. We provide a range of pricing options designed to cater to various budget needs without compromising on the quality of our services.
- Short Deadlines: Facing a fast-approaching deadline can be nerve-wracking. But with iResearchNet, you need not worry. Our expert writers are skilled at delivering high-quality papers under tight deadlines, even as short as three hours. Despite the time pressure, we ensure that the quality of your paper remains top-notch.
- Timely Delivery: At iResearchNet, we understand the critical importance of adhering to deadlines in the academic world. We commit to the timely delivery of all orders, ensuring that you are always able to submit your work on time. With our service, you can put aside worries about late submissions.
- 24/7 Support: Academic queries or concerns can arise at any time, and we are here to assist you around the clock. We have a dedicated support team ready to answer your questions, address your concerns, or simply provide guidance about your project, at any time of the day or night.
- Absolute Privacy: Your privacy is of utmost importance to us. All personal and financial information you share with us is handled with the highest level of confidentiality and security. Our strict privacy policies ensure that your details remain safe and private.
- Easy Order Tracking: We believe in providing a seamless experience for our clients. With our user-friendly platform, you can track your order’s progress easily and stay updated on your paper’s status. This feature provides real-time status reports, giving you peace of mind and assurance about the progress of your work.
- Money Back Guarantee: Your satisfaction is our ultimate goal. We strive to meet your expectations, but if for any reason the final work falls short, we offer a money-back guarantee. This policy is a testament to our confidence in the quality of our services and our commitment to your academic success.
At iResearchNet, we strive to be more than just a writing service provider. We aspire to be a trusted academic partner, providing support and expertise to help you navigate the complexities of writing a biomedical research paper. With our combination of expert knowledge, high commitment to quality, and excellent customer service, we are the ideal choice for all your academic writing needs.
Start Your Journey to Academic Success with iResearchNet Today!
Are you ready to elevate your academic journey and achieve your full potential? At iResearchNet, we are prepared to be your trusted partner every step of the way. Our team of expert writers, experienced in biomedical research, are ready and waiting to transform your academic vision into a top-quality, custom-written biomedical research paper that meets all your requirements.
Navigating the complexities of biomedical research can be overwhelming, but with iResearchNet, you don’t have to do it alone. Our dedicated team of professionals is committed to taking the stress out of the writing process, allowing you to focus on your learning. Imagine the relief of knowing your assignment is in the hands of experienced, degree-holding experts who are passionate about your success. With our meticulous research and thorough understanding of biomedical topics, we guarantee a paper that not only meets but surpasses your expectations.
From in-depth research and custom formatting to a final product that reflects the highest academic standards, iResearchNet provides a comprehensive solution for your academic needs. And it’s not just about delivering excellent papers. Our commitment extends to providing an exceptional experience marked by 24/7 support, absolute privacy, and a transparent order tracking system.
The clock is ticking, and your academic success is just a click away. Don’t let the opportunity to excel in your biomedical research paper slip through your fingers. Reach out to us today to start your journey with iResearchNet. You bring your academic aspirations, and we’ll bring our expertise and commitment. Together, let’s make your academic dreams come true.
ORDER HIGH QUALITY CUSTOM PAPER


An official website of the United States government
The .gov means it's official. Federal government websites often end in .gov or .mil. Before sharing sensitive information, make sure you're on a federal government site.
The site is secure. The https:// ensures that you are connecting to the official website and that any information you provide is encrypted and transmitted securely.
- Publications
- Account settings
- Browse Titles
NCBI Bookshelf. A service of the National Library of Medicine, National Institutes of Health.
National Research Council (US) Committee for Monitoring the Nation's Changing Needs for Biomedical, Behavioral, and Clinical Personnel. Advancing the Nation's Health Needs: NIH Research Training Programs. Washington (DC): National Academies Press (US); 2005.

Advancing the Nation's Health Needs: NIH Research Training Programs.
- Hardcopy Version at National Academies Press
2 Basic Biomedical Sciences Research
Basic biomedical research, which addresses mechanisms that underlie the formation and function of living organisms, ranging from the study of single molecules to complex integrated functions of humans, contributes profoundly to our knowledge of how disease, trauma, or genetic defects alter normal physiological and behavioral processes. Recent advances in molecular biology techniques and characterization of the human genome, as well as the genomes of an increasing number of model organisms, have provided basic biomedical researchers with the tools to elucidate molecular-, cellular-, and systems-level processes at an unprecedented depth and rate.
Thus basic biomedical research affects clinical research and vice versa. Biomedical researchers supply many of the new ideas that can be translated into potential therapies and subsequently tested in clinical studies, while clinical researchers may suggest novel mechanisms of disease that can then be tested in basic studies using animal models.
The tools also now exist to rapidly apply insights gained from model organisms to human health and disease. For example, gene mutations known to contribute to human disease can be investigated in model organisms, whose underlying characteristics lend them to rapid assessment. Resulting treatment strategies can then be tested in mammalian species prior to the design of human clinical trials.
These and other mutually supportive systems suggest that such interactions between basic biomedical and clinical researchers not only will continue but will grow as the two domains keep expanding. But the two corresponding workforces will likely remain, for the most part, distinct.
Similarly, there is a symbiosis between basic biomedical and behavioral and social sciences research (covered in Chapter 3 ) and an obvious overlap at the interface of neuroscience, physiological psychology, and behavior. The boundary between these areas is likely to remain indistinct as genetic and environmental influences that affect brain formation and function are better understood. Consequently, such investigations will impact the study of higher cognitive functions, motivation, and other areas traditionally studied by behavioral and social scientists.
Basic biomedical research will therefore undoubtedly continue to play a central role in the discovery of novel mechanisms underlying human disease and in the elucidation of those suggested by clinical studies. As an example, although a number of genes that contribute to disorders such as Huntington's, Parkinson's, and Alzheimer's disease have been identified, the development of successful therapies will require an understanding of the role that the proteins encoded by these genes play in normal cellular processes. Similarly, realizing the potential of stem cell–based therapies for a number of disorders will require characterization of the signals that cause stem cells to differentiate into specific cell types. Thus a workforce trained in basic biomedical research will be needed to apply current knowledge and that gained in the future toward the improvement of human health. Since such research will be carried out not only in academic institutions but increasingly in industry as well, the workforce must be sufficient to supply basic biomedical researchers for large pharmaceutical companies as well as smaller biotech and bioengineering firms, thereby contributing to the economy as well as human health.
The role of the independent investigator in academe, industry, and government is crucial to this research enterprise. They provide the ideas that expand knowledge and the research that leads to discovery. The doubling of the NIH budget has increased the number of research grants and the number of investigators but not at a rate commensurate to the budget increases. Grants have become bigger and senior investigators have received more of them. While this trend has not decreased the nation's research capacity, there may be things that will affect the future pool of independent researchers, such as a sufficient number of academic faculty that can apply for research grants, an industrial workforce that is more application oriented, and most important, a decline in doctorates from U.S. institutions.
- BIOMEDICAL RESEARCH WORKFORCE
The research workforce for the biomedical sciences is broad and diverse. It is primarily composed of individuals who hold Ph.D.s, though it also includes individuals with broader educational backgrounds, such as those who have earned their M.D.s from the Medical Scientist Training Program (MSTP) or other dual-degree programs. In addition, some individuals with M.D.s but without Ph.D.s have acquired the necessary training to do basic biomedical research. But although the analysis in this report should ideally be based on the entire workforce just defined, there are no comprehensive databases that identify the research activities of M.D.s. Therefore much of the analysis will be restricted to holders of a Ph.D. in one of the fields listed in Appendix C , with the assumption that an individual's area of research is related to his or her degree field. A separate section in this chapter is devoted to M.D.s doing biomedical research, and an analysis of the clinical research M.D. workforce is given in Chapter 4 .
It should also be noted that the discussion in this chapter does not include individuals with doctorates in other professions, such as dentistry and nursing even if they hold a Ph.D. in addition to their professional degrees. However, there are important workforce issues in these two fields, and they are addressed separately in Chapters 5 and 6 of this report.
- EDUCATIONAL PROGRESSION
The major sources of Ph.D. researchers in the biomedical sciences are the U.S. research universities, but a substantial number also come from foreign institutions. These scientists, whether native or foreign born, enter the U.S. biomedical research workforce either directly into permanent assignments or via postdoctoral positions.
For most doctorates in the biomedical sciences, interest in the field begins at an early age, in high school or even grade school. In fact, almost all high school graduates (93 percent) in the class of 1998 took a biology course—a rate much greater than other science fields, for which the percentages are below 60 percent. 1 Even in the early 1980s, over 75 percent of high school graduates had taken biology, compared to about 30 percent for chemistry, which had the next-highest enrollment. This interest in biology continues into college, with 7.3 percent of the 2000 freshman science and engineering (S&E) population having declared a major in biology. This was an increase from about 6 percent of freshman majors in the early 1980s but less than the high of about 9.5 percent in the mid-1990s. Overall, the number of freshman biology majors increased from about 50,000 in the early 1980s to over 73,000 in 2000. 2 In terms of actual bachelor's degrees awarded in the biological sciences, there was a decrease from about 47,000 in 1980 to 37,000 in 1989 and then a relatively sharp rise to over 67,000 in 1998. This was followed by a slight decline to about 65,000 in 2000.
There is attrition, however, in the transition from undergraduate to graduate school. In the 1980s and 1990s only about 11,000 first-year students were enrolled at any one time in graduate school biology programs. Percentage-wise, this loss of students is greater than in other S&E fields but is understandable: many undergraduates obtain a bachelor's degree in biology as a precursor to medical school and have no intention of graduate study in biology per se. The total graduate enrollment in biomedical sciences at Ph.D.-granting institutions grew in the early 1990s and was steady at a little under 50,000 during the latter part of the decade. However, there was some growth in 2001, of about 4 percent over the 2000 level, and the growth from 2000 to 2002 was about 10 percent (see Figure 2-1 ), driven in large part by an 18.9 percent increase of temporary residents. The overall growth may not continue, however, as the first-year enrollment for this group slowed from 8.9 percent in 2001 to 3.0 percent in 2002.
First-year and total graduate enrollment in the biomedical sciences at Ph.D.-granting institutions, 1980–2002. SOURCE: National Science Foundation Survey of Graduate Students and Postdoctorates in Science and Engineering.
The tendency for graduate students to receive a doctorate in a field similar to that of their baccalaureate degree is not as strong in the biomedical sciences as it is in other fields, where it is about 85 percent. From 1993 to 2002, some 68.4 percent of the doctorates in biomedical programs received their bachelor's degree in the same field and another 8.4 percent received bachelor's degrees in chemistry. 3 This relative tendency to shift fields should not be viewed negatively, however, as doctoral students with exposure to other disciplines at the undergraduate level could provide the opportunity for greater interdisciplinary training and research.
- EARLY CAREER PROGRESSION IN THE BIOMEDICAL SCIENCES
Advances in biomedical research and health care delivery, together with a strong economy in the 1990s and increased R&D support, drove the growth of academic programs. Total academic R&D expenditures in the biological sciences, in 2001 dollars, began to rise dramatically in the early 1980s. They started from a base of about $3 billion and reached a plateau of almost $5 billion in the mid-1990s. As seen in Figure 2-2 , this increase of about $2 billion was virtually repeated in the much shorter period from the late 1990s to 2002, as the NIH budget doubled. Although the increases in R&D support during the earlier period were reflected in the increased graduate enrollments of the 1980s and mid-1990s (seen in Figure 2-1 ), the enrollments since then have not kept pace with fast-growing R&D expenditures. This disconnect between research funding and enrollment in the late 1990s is difficult to explain but could in part be due to the unsettled career prospects in the biomedical sciences. In a report 4 from the American Society for Cell Biology, the authors examined the data on enrollment and surveyed both undergraduate and graduate students and postdoctorates on their career goals and found that students were aware of and concerned about the problem young people were having in establishing an independent research career. This ASCB report, as well as in the National Research Council report, Trends in the Early Careers of Life Scientists, 5 express concern for the future of biomedical research, if the best young people pursue different career paths. This slowdown in graduate enrollment in the late 1990s might have also contributed to the expansion of the postdoctoral and non-tenure-track faculty pool of researchers, since there was an increasing need for research personnel.
Academic research and development expenditures in the biological sciences. (All dollars are in thousands.) SOURCE: National Science Foundation R&D Expenditures at Universities and Colleges, 1973–2002. Adjusted to 2002 dollars by the Biomedical (more...)
The increase in funding and enrollments in the early 1990s did lead to an increase in doctoral degrees awarded in the late 1990s, as seen in Figure 2-3 . Since the 1970s, Ph.D.s awarded by U.S. institutions in the biomedical sciences increased from roughly 3,000 then to 5,366 in 2002. Most of the increase occurred in the mid-1990s and has since remained fairly constant. The year with the largest number of doctorates was 2000, when 5,532 degrees were awarded. The number of degrees in 2000 may be an anomaly, since the number in 2001 (5,397 Ph.D.), 2002 (5,375 Ph.D.), and 2003 (5,412 Ph.D.) are more in line with the number in the late 1990s (see Appendix Table E-1 ).
Number of doctorates in the biomedical sciences, 1970–2003. SOURCE: National Science Foundation Survey of Earned Doctorates, 2001.
Increases in doctorates were seen among women, temporary residents, and underrepresented minorities. Notably, since 1986 much of the increase in the number of doctorates has come from increased participation by women. In 1970 only 16 percent of doctorates were awarded to women; by 2003 the percentage had grown to 45.2. Temporary residents earned about 10 percent of the doctorates in 1970, and although this had increased to almost one-quarter in the early 2000s, it was still lower than the percentage awarded in many other fields in the physical sciences and engineering. Participation by underrepresented minorities in 2003 stood at 9.4 percent—as in many other S&E fields, substantially below their representation in the general population.
The percentage of doctorates with definite postdoctoral study plans increased from about 50 percent in the early 1970s to a high of 79 percent in 1995. It then declined to 71 percent in 2002 but increased to 75 percent in 2003. The changes in doctorates electing postdoctoral study are reflected in those choosing employment after they received their degrees (from 20 percent in 1995 to 28 percent in 2002 and 25 percent in 2003). It is difficult to find reasons for these changes in career plans. Prior to 2003 it may be the result of more diverse and attractive employment opportunities generated by recent advances in the applied biological sciences, especially in industry, or a conscious choice not to pursue an academic research career, where postdoctoral training is required since an academic position may not be available down the road. The increase in postdoctoral appointments in 2003 and the decline in employment might be due to poor economic conditions in the early part of this decade. Whether these changes will impact the quality of the biomedical workforce and its research should be monitored.
Time to degree, age at receipt of degree, and the long training period prior to reaching R01 research status have been cited as critical issues in the career progression of biomedical researchers. 6 Graduate students are taking longer and longer periods of time to earn their Ph.Ds. The median registered time in a graduate degree program gradually increased from 5.4 years in 1970 to 6.7 years in 2003, and the median age of a newly minted degree holder in the biomedical sciences grew during the same period—from 28.9 in 1970 to 30.6 in 2003 (see Appendix E ). It should be noted that this time to degree is shorter than those of such fields as physics, computer science, and the earth sciences. Only chemistry, mathematics, and engineering have a lower median age at time of degree. While shortening the time in graduate school would reduce the age at which doctorates could become independent investigators, it may not significantly affect their career paths since postdoctoral training is required of almost all researchers in the biomedical sciences, and the time spent in these positions seems to be lengthening.
With the growth of research funding and productivity in the biomedical sciences, the postdoctoral appointment has become a normal part of research training. From the 1980s to the late 1990s, the number of postdoctoral appointments doubled for doctorates from U.S. educational institutions (see Figure 2-4 ). The rapid increase in the postdoctoral pool from 1993 to 1999 in particular appears to be the result of longer training periods for individuals and not the result of an increase in the number of individuals being trained since Table E-1 shows a decline in the number of new doctorates planning postdoctoral study and the number of doctorates has remained fairly constant over recent years.
Postdoctoral appointments in the biomedical sciences by sector, 1973–2001. SOURCE: National Science Foundation Survey of Doctorate Recipients.
The lengthening of postdoctoral training is documented by data collected in 1995 on the employment history of doctorates. 7 Of the Ph.D.s who pursued postdoctoral study after graduating in the early 1970s, about 35 percent spent less than two years and about 65 percent spent more than 2 years in a postdoctoral appointment. By contrast, of Ph.D.s who received their degrees in the late 1980s and completed postdoctorates in the 1990s, 80 percent spent more than two years and 20 percent spent less than 2 years in such appointments. More indicative of the change in postdoctoral training was the increase in the proportion that spent more than 4 years in a position, from about 20 percent to nearly 40 percent.
In 2001 the number of postdoctoral appointments actually declined across all employment sectors. This decline might be the result of lower interest by new doctorates in postdoctoral study and an academic career but is probably a response to the highlighting of issues related to postdoctoral appointments, such as the long periods of training with lack of employment benefits, the general perception that the positions are more like low-paying jobs than training experiences, and the poor prospects of a follow-up position as an independent investigator. Not only is interest in postdoctoral positions declining, there appears to be more rapid movement out of them by present incumbents. (These phenomena are more fully explored in Chapter 9 , Career Progression.)
The above discussion applies only to U.S. doctorates. There are also a large number of individuals with Ph.D.s from foreign institutions being trained in postdoctoral positions in U.S. educational institutions and other employment sectors. Data from another source are available for postdoctorates from this population at academic institutions, 8 but there is no source for data in the industrial, governmental, and nonprofit sectors other than an estimate that about half of the 4,000 intramural postdoctoral appointments at NIH are held by temporary residents. Almost all of these temporary residents have foreign doctorates. The number of temporary residents in academic institutions steadily increased through the 1980s and 1990s until 2002 when the number reached 10,000 (see Figure 2-5 ). The data also show that the rate at which temporary residents took postdoctoral positions slowed in 2002. The decline in academic appointments in 2001 for U.S. citizens and permanent residents population that was described above is also seen in this data, but that might be temporary since there was an increase in 2002. The reasons for this change may be twofold: a tighter employment market for citizens and permanent residents and immigration restrictions. However, it is still important to recognize that foreign-educated researchers hold about two-thirds of the postdoctoral positions in academic institutions. If national security policies were to limit the flow of foreign scientists into the United States, this could adversely affect the research enterprise in the biomedical sciences.
Postdoctoral appointments in academic institutions in the biomedical sciences. SOURCE: National Science Foundation Survey of Graduate Students and Postdoctorates in Science and Engineering.
- A PORTRAIT OF THE WORKFORCE
The traditional career progression for biomedical scientists after graduate school includes a postdoctoral position followed by an academic appointment, either a tenure-track or nonpermanent appointment that is often on “soft” research money. As shown in Figure 2-6 , the total population of academic biomedical sciences researchers, excluding postdoctoral positions, grew at an average annual rate of 3.1 percent from 1975 to 1989. 9
Academic positions for doctorates in the biomedical sciences, 1975–2001. SOURCE: National Science Foundation Survey of Doctorate Recipients.
Since 1995, growth slowed to about 2.5 percent, with almost all of the growth in the non-tenure-track area. From 1999 to 2001 there was actually a decline in the number of non-tenure-track positions (by a few hundred). The fastest-growing employment category since the early 1980s has been “Other Academic Appointments,” which is currently increasing at about 4.9 percent annually (see Appendix E-2 ). These jobs are essentially holding positions, filled by young researchers, coming from postdoctoral experiences, who would like to join an academic faculty on a tenure track and are willing to wait. In effect, they are gambling because institutions are restricting the number of faculty appointments in order to reduce the possible long-term commitments that come with such positions. From 1993 to 2001, the number of tenure-track appointments increased by only 13.8 percent, while those for non-tenure-track faculty and other academic appointments increased by 45.1 percent and 38.9 percent, respectively.
The longer time to independent research status is also seen by looking at the age distributions of tenure-track faculty over the past two decades (see Figure 2-7 ). By comparing age cohorts in 1985 and 2001, it is observed that doctorates entered tenure-track positions at a later age in 2001.
Age distribution of biomedical tenured and tenure-track faculty, 1985, 1993, and 2001. SOURCE: National Science Foundation Survey of Doctorate Recipients.
For example, while about 1,000 doctorates in the 33 to 34 age cohort were in faculty positions in 1985, only about half that number were similarly employed in 2001, even though the number of doctorates for that cohort was greater in the late 1990s than in the early 1980s. The age cohort data also show that the academic workforce is aging, with about 20 percent of the 2001 academic workforce over the age of 58. The constraints of a rather young biomedical academic workforce and the conservative attitudes of institutions to not expand their faculties in the tight economic times of the early 1990s may have slowed the progression of young researchers into research positions. However, this may change in the next 8 to 10 years as more faculty members retire.
Meanwhile, over 40 percent of the biomedical sciences workforce is employed in nonacademic institutions (see Figure 2-8 ). Researchers' employment in industry, the largest of these other sectors, has been growing at a 15 percent rate over the past 20 years. There was a lull in employment in the early 1990s, but growth since the mid-1990s has been strong. The increases in industrial employment may be due to the unavailability of faculty positions, but is more likely fueled by the R&D growth in pharmaceutical and other medical industries from $9.3 billion in 1992 to $24.6 in 2001 (constant 2001 dollars). 10 In 1992 almost all of this funding was from nonfederal sources, but in 2001 only 42 percent was from those sources. The result of this increase in federal funding has resulted in an increase in R&D employment, but not as large as would be expected. It is difficult to estimate the increase in biomedical doctorates in this industrial sector since they are drawn from many fields and are at different degree levels, but the total full-time equivalent R&D scientists and engineers increased 6.2 percent from 38,700 in 1992 to 41,100 in 2001. 11 However, there may be a trend toward increased employment in this sector, since a growing fraction of new doctorates are planning industrial employment (see Table E-1 ). The downturn in 2003 may be an anomaly due to the economy and not the strength of the medical industry, but data from a longer time period will be needed before definite trends in industrial employment can be determined. The government and nonprofit sectors have been fairly stable in their use of biomedical scientists, with about 8 and 4 percent growth rates, respectively, in recent years.
Employment of biomedical scientists by sector, 1973–2001. SOURCE: National Science Foundation Survey of Doctorate Recipients.
The number of underrepresented minorities in the basic biomedical sciences workforce increased from 1,066 in 1975 to 5,345 in 2001 and now accounts for 5.3 percent of the research employment in the field. Even though the annual average rate of growth for minorities in the workforce has been 15 percent over the past 10 years—more than twice the growth rate of the total workforce (6.5 percent)—the overall representation of minorities in biomedical research is still a small percentage of the overall workforce (see Appendix E-2 ). Their representation is also important from the scientific perspective, since researchers from minority groups may be better able and willing to address minority health care issues.
- PHYSICIAN-RESEARCHERS
Throughout this report Ph.D.s are considered to be researchers or potential researchers, but no such assumption is made of M.D.s because they could be practitioners. The above discussion, in particular, applies only to Ph.D.s in the fields listed in Appendix C , but it does not take into consideration physicians who are doing basic biomedical research. It is difficult to get a complete picture of this workforce because there is no database that tracks M.D.s involved in such activity, but a partial picture can be obtained from NIH files on R01 awards.
In 2001, R01 grants were awarded to 4,383 M.D.s (and to 17,505 Ph.D.s). 12 The number of R01-supported M.D. researchers has been increasing over the years (see Table 2-1 ) but has remained at about 20 percent. This means that the size of the biomedical workforce could be as much as one-fifth larger than indicated above. In fact, since NIH began to classify clinical research awards in 1996, it has become evident that both M.D.s and M.D./Ph.D.s supported by the agency are more likely to conduct nonclinical—that is, biomedical—than clinical research. Because many physician-investigators approach nonclinical research with the goal of understanding the mechanisms underlying a particular disease or disorder, their findings are likely to ultimately contribute to improvements in human health.
Number of M.D.s and Ph.D.s with Grant Support from NIH .
Some data are available from the American Medical Association on the national supply of physicians potentially in research. In 2002 there were 15,316 medical school faculty members in basic science departments and 82,623 in clinical departments. Of those in basic science, 2,255 had M.D. degrees, 11,471 had Ph.D.s, and 1,128 had combined M.D./ Ph.D. degrees. To identify the M.D.s in basic science departments who were actually doing research, the Association of American Medical Colleges Faculty Roster was linked to NIH records; it found that 1,261 M.D.s had been supported as principal investigators (PIs) on an R01 NIH grant at some point. This number is clearly an undercount of the M.D. research population, however, given that there are forms of NIH research support other than PI status and non-NIH organizations also support biomedical research.
- THE NATIONAL RESEARCH SERVICE AWARD PROGRAM AND BIOMEDICAL TRAINING SUPPORT
The National Research Service Award Program
In 1975, when the National Research Service Award (NRSA) program began, 23,968 graduate students in the basic biomedical sciences received some form of financial assistance for their studies, and about 8,000 supported their own education through loans, savings, or family funds. 13 The number of fellowships and traineeships, whether institutional or from external sources, was about 8,500 in 1975 and remained at about that level into the early 1990s, increasing only recently to 12,186 in 2002 (see Figure 2-9 ).
Mechanisms of support for full-time graduate students in the biomedical sciences, 1979–2002. SOURCE: National Science Foundation Survey of Graduate Students and Postdoctorates in Science and Engineering.
In the 1970s the majority of graduate student support came from these fellowships, traineeships, and institutional teaching assistantships. The picture began to change in the early 1980s as the prevalence of research grants grew. By 2002 it represented almost 50 percent of the support for graduate study in the biomedical sciences, and NIH's funding of this mechanism grew as well. In the early 1980s, NIH research grants formed about 40 percent of the total, and by the early 1990s this fraction grew to 64 percent and has remained at about this level through 2002 (see Figure 2-10 ). Even during the years when the NIH budget doubled, there was not a shift in this balance. In fact, from 1997 to 2002 both research grant and trainee/fellowship support from NIH increased by 14 percent. NIH in its response to the 2000 assessment of the NRSA program 14 has stated that research grants and trainee/fellowship awards are not used for the control of graduate support and that it would be inappropriate to try to do so.
FIGURE 2-10
Graduate support for NIH, 1979–2002. SOURCE: National Science Foundation Survey of Graduate Students and Postdoctorates in Science and Engineering.
The NRSA program now comprises the major part of NIH's fellowship and traineeship support. It began small in 1975—with 1,046 traineeships and 26 fellowships—but quickly expanded. By 1980 the number was nearly 5,000 for the traineeships; it remained at that level until 2001, though it dropped to a little over 4,000 in 2002 (see Table 2-2 ). (The drop in 2002 traineeships was probably an institutional reporting issue. Given that the total number of awards by NIH under the T32 15 mechanisms was about the same as in 2001, it is unlikely that the awards in the biomedical sciences would fall below the 2000 or 2001 levels.)
NRSA Predoctoral Trainee and Fellowship Support in the Basic Biomedical Sciences .
Information on funding patterns for postdoctorates in the basic biomedical sciences is not as complete as that for graduate students since academic institutions are the only sources of data. As has been the case for graduate student support, the portion of federal funds devoted to postdoctoral training grants and fellowships has diminished since the 1970s. In 1995, 1,966 (or 45.3 percent) of the 4,343 federally funded university-based postdoctorates received their training on a fellowship or traineeship. By 2002 the number had increased to 2,670 but was still only 20.3 percent of the total federal funding. The remaining 79.7 percent (or 10,514) in 2002 were supported by federal research grants. Meanwhile, the number of postdoctoral positions, funded by nonfederal institutional sources, was fairly constant at about 25 percent and grew from 1,325 in 1975 to 4,628 in 2002.
The picture for NRSA support at the postdoctoral level for the period following introduction of the NRSA program resembled that of the graduate level. However, in 2002 there was a sharp decrease in the number of postdoctoral traineeships; but, as in the case for predoctoral trainees, this may be an institutional reporting issue (see Table 2-3 ). Since the decline from 2001 to 2002 is nearly 50 percent and the decline for predoctoral trainees was only 20 percent, there may be a real decline at the postdoctoral level. The reason for this is unclear, though factors may include the limited number of individuals who can be supported under the increased stipend levels and the general decline in the number of postdoctoral research trainees eligible for NRSA support.
NRSA Postdoctoral Trainee and Fellowship Support in the Basic Biomedical Sciences .
The shift in the pattern of federal research training support, at both the graduate and postdoctoral levels, can be traced to a number of related trends. Over the past 25 years, the number of research grants awarded by the NIH and other agencies of the U.S. Department of Health and Human Services has more than doubled. 16 PIs have come to depend on graduate students and postdoctorates to carry out much of their day-to-day research work, and, as a result, the number of universities awarding Ph.D.s in the basic biomedical sciences, as well as the quantity of Ph.D.s awarded by existing programs, has grown.
Furthermore, federal funding policies have inadvertently provided universities with an incentive to appoint students and postdoctorates to research assistantships instead of training grants or fellowships. An example given in the eleventh NRSA study 17 shows that in 1999 the NIH provided almost $9,000 more to research assistants and their institutions (largely in the form of indirect cost payments to universities) than to NRSA trainees or fellows. Because the indirect cost rate for institutional training grants is generally about 7 percent compared to the 60 to 70 percent rate on research grants, it is financially advantageous for an institution to have as many research grants as possible for the support of graduate students. However, current policies at NIH have raised the NRSA predoctoral stipend levels to $19,968 and starting postdoctoral levels to $34,200. These increases might force stipends on research grants to similar levels and reduce the number of students who can be supported on research grants.
As described earlier, the number of students and postdoctorates provided with research training through NRSA training grants and fellowships has been deliberately limited over much of the past 25 years, as a control on the number of researchers entering the workforce. No similar federal effort has been undertaken thus far to ensure an adequate supply of technically prepared support staff in research, nor is there a system for regulating the number of research assistantships. As Massy and Goldman concluded in their 1995 analysis of science and engineering Ph.D. production, the size of doctoral programs is driven largely by departmental needs for research and teaching assistants rather than by the labor market for Ph.D.s. 18
In any case, NRSA training grants to institutions are highly prized and competitively sought. They confer prestige and add stability to graduate programs as they are usually for five years and allow for planning into the future. On the other hand, since the legislation that established the NRSA program allows only U.S. citizens and permanent residents to be trained through these grants and fellowships, the growing number of graduate students with temporary-resident status must be supported by other mechanisms.
Another factor in the shifting pattern of federal research training support is the type of education the students receive. Since the beginning of the NRSA program, NIH has required predoctoral training grants in the basic biomedical sciences to be “multidisciplinary” in order to expose students to a range of biomedical fields and even to other branches of science. Given that research collaborations between a wide variety of scientists have been producing significant advances, this requirement is even more important. Although the level of multidisciplinary training varies from program to program, students in training grant programs with this as part of their curriculum may better be ensured of such interdisciplinary training than those on a research assistantship. The committee considers multidisciplinary training in the biomedical sciences to be very valuable and of increasing importance. (A full discussion of these issues is presented in Chapter 8 , Emerging Fields and Interdisciplinary Studies.)
Although research grants provide an important base for training, data suggest that NRSA training grant participants complete training faster and go on to more productive research careers than do non-NRSA-supported students at their institution or doctorates from universities without NRSA training programs. This is supported by an assessment, completed in 1984, in which NRSA participants were found to complete their doctoral degrees faster and were more likely to go on to NIH-supported postdoctoral training than graduate students with other forms of support. 19 They also received a higher percentage of NIH research grants, authored more articles, and were cited more frequently by their peers.
Comparable outcomes were seen in a more recent study conducted by NIH. 20 Ph.D.s in the basic biomedical sciences who received NRSA support for at least one academic year spent less time in graduate school. About 57 percent of NRSA trainees and fellows received their doctorates by age 30, while only 39 percent of their classmates and 32 percent of graduates from departments without NRSA support similarly reached that milestone.
The study also showed that NRSA trainees and fellows were more likely to move into faculty or other research positions. Nearly 40 percent of the NRSA program participants held faculty appointments at institutions ranking in the top 100 in NIH funding, as opposed to 24 and 16 percent, respectively, for non-NRSA graduates from the same institution and graduates from non-NRSA institutions. Similarly, NRSA trainees and fellows were more likely to be successful in competing for grants and had better publication records than either of the other groups.
The NRSA program is essential to training in the biomedical sciences not only for these and other direct reasons; there are also its indirect benefits, such as establishing high standards for the entire graduate program and creating a generally improved environment for all students. Also, when students are supported by a combination of NRSA and research grant support, the NRSA funding is significantly leveraged.
The Medical Scientist Training Program
The MSTP was established at NIH in 1964 by the National Institute of General Medical Sciences (NIGMS) to support education leading to the M.D./Ph.D degree. By combining graduate training in the biomedical sciences with clinical training offered through medical schools, the program was designed to produce investigators who could better bridge the gap between basic science and clinical research. Since its inception, the Ph.D. portion of the training has been expanded to include the physical sciences, computer science, behavioral and social sciences, economics, epidemiology, public health, bioengineering, biostatistics, and bioethics, though almost all trainees receive a Ph.D. in a biomedical field.
When the MSTP began, it had only three programs, but it has since grown—in 2003 it had 41 programs involving 45 degree-granting institutions, with a total of 925 full-time training slots. This number is slightly down from the 933 slots in 2002. In addition, about 75 medical schools that do not have MSTP grants nevertheless offer opportunities for M.D./Ph.D. studies. The number of new students supported each year by MSTP funds varies from 2 or 3 at many institutions to 10 to 12 at a few exceptional ones, such as Duke University and the University of California, San Francisco. Some 170 new students nationwide are added to the program each year, with selection being highly competitive. The program provides 6 years of support for both phases of training, and institutions usually continue the awards for any additional years needed to complete the degrees. Support includes a tuition allowance, a stipend that is usually supplemented by the institution, and modest funds for travel, equipment, and supplies.
While the funds from NIH are sufficient to support only a few students in any one year of their training, institutions have been able to parlay the NIH funds by judiciously using institutional or research grant funds to support more students. A typical scenario is to support a student on MSTP funds during the first two years of medical training and again in the sixth or seventh years, when he or she returns to complete the medical degree. But during their Ph.D. studies, MSTP students are in a position to receive research grant support just like any other Ph.D. student. For example, one institution uses MSTP funds to support only 10 students during their first year and 2 during their second year in medical school, but there are 60 students in the MSTP program, with the remaining 48 receiving institutional or research grant support. This combination of funding results in the awarding of about 350 MSTP M.D./Ph.D. graduates each year. In the eyes of NIH, any student who receives MSTP funds and is supported for his or her entire course of study is considered a product of the program.
These graduates usually move on to postdoctoral, intern, and residency appointments and after completing their training tend to find academic research positions relatively easily. Another measure of the program's success is seen at the other end of the cycle—the competition among students for entry into the program. Some institutions, such as Johns Hopkins University, receive over 500 applications for the 10 or 12 available positions. Many of these students are highly qualified, and they apply for many programs simultaneously. Institutions easily fill their MSTP class, but some institutions with smaller and less well recognized programs have only a 30 percent acceptance rate. Occasionally these institutions lose students to other programs and begin the year with unfilled MSTP slots. Although not all applicants find MSTP positions, many end up pursuing a joint dual-degree program at an MSTP institution with partial or sometimes full support from non-MSTP funds. They follow the same track as the MSTP students and are indistinguishable from them.
Funding of the program is an issue at almost all MSTP institutions. While institutions are creative in the use of MSTP funds, they are unable to support many highly qualified students who have an interest in research but opt instead to attend just medical school and pursue a professional career. At a time when there is a need for more researchers with a medical background, it would be advantageous to have more M.D.s who are generally debt free and able to pursue research that requires the unique combination of biomedical and clinical training.
In addition to the advantages to biomedical and clinical research, MSTP graduates appear to have more productive research careers. In 1998 the NIGMS published a study of past recipients of MSTP support. 21 This study used résumé data of MSTP graduates with both an M.D. and a Ph.D. to compare their careers to four other groups of doctorates: MSTP-supported students who received only an M.D., Ph.D. recipients at MSTP institutions supported by NIH training grants, non-MSTP dual-degree graduates from an MSTP institution, and non-MSTP dual-degree graduates from a non-MSTP institution. The individuals in the study were divided into four 5-year cohorts from 1970 to 1995 to allow for changes over time in the educational characteristics and research environment. The cohorts and doctoral grouping were also compared on existing data from NIH files. The training and career paths of the MSTP graduates and the comparison groups were assessed from different perspectives, including time to degree, postdoctoral training, employment history, and research support and publication outcomes. By almost all measures, the MSTP-trained graduates fared better than the other groups. For example, they entered graduate training more quickly and took less time to complete the two degrees. Only the Ph.D. group applied for NIH postdoctoral fellowships at a higher rate, but the MSTP success rate was about the same as for the Ph.D. group. Depending on the cohort, between 60 and 70 percent of the MSTP graduates had a clinical fellowship and about 50 percent had both a clinical fellowship and postdoctoral training.
In terms of research activity, the NIH data showed that the MSTP graduates applied for research grant support from NIH at a greater rate and they were more successful in receiving support. The research productivity of the MSTP graduates across each of the cohorts as measured by published articles from the résumé data was about the same as that for the Ph.D. group and only slightly higher than the non-MSTP graduates from MSTP institutions. However, an examination of publications over the period from 1993 to 1995 showed that the earlier cohorts were more likely to be currently active than the Ph.D. graduates by publishing twice as many articles. The 1976–1980 non-MSTP cohorts, from MSTP institutions, also continued to be almost as active in publishing as the MSTP graduates.
The résumé analysis also provided insight into the professional and research activities of the different groups. About 83 percent of MSTP graduates in the study who were employed in 1995 had one or more academic appointments. This was higher than the M.D.- and Ph.D.-only groups and somewhat higher than the non-MSTP M.D./Ph.D.s group. Most of the dual-degree graduates in either group were in clinical departments and probably indicates some responsibility with regard to patient-oriented care. To better assess the type of research conducted by the different groups, the study classified the publications reported on the résumés into basic, clinical, and mixed type. Even though many of the dual-degree graduates are in clinical departments, they are still more likely to publish in basic journals, and this tendency is stronger in later cohorts.
The conclusions drawn from this analysis are that MSTP graduates appear to have been highly successful in establishing research careers, and their recent publication records suggest that members of all cohorts continue to be productive researchers. However, MSTP graduates appear most similar to non-MSTP M.D./Ph.D.s from the same institution; both groups are likely to be employed in academia with appointments in clinical or dual clinical and basic science departments, and both have similar publication patterns. This is not surprising, since non-MSTP-supported students at MSTP institutions follow the same program as their MSTP counterparts, complete the same degree requirements, and benefit from the MSTP-sponsored training efforts at those institutions.
- RESEARCH LABOR FORCE PROJECTIONS
The biomedical workforce with degrees from U.S. universities was estimated to be 100,262 in 2001. This included individuals in postdoctoral positions but did not count the 4,935 doctorates with degrees in biomedical fields who were unemployed or the 8,091 in positions not considered related to biomedical research (see Table 2-4 ). These three groups brought the potential workforce of U.S. doctorates to 113,288 (the only doctorates excluded were those who had retired). Table 2-4 also shows the change in this workforce over the past decade.
Potential Workforce in the Biomedical Sciences by Employment Status, 1991–2001 .
Note that in 2001 almost 80 percent of the potential workforce was employed in S&E and unemployment was less than 1 percent. Even with the inclusion of those unemployed and not seeking employment, only about 4.5 percent were unemployed.
The above figures represent only part of the total potential workforce, however, because foreign-trained doctorates also are employed in this country (and a few U.S. doctorates leave the country). Estimating this foreign component is difficult, given that no database describes the demographics of this group. Some data sources with information on foreign-trained doctorates exist, but they provide only a partial picture. 22 Based on these sources, it is estimated that about 15,500 such individuals are involved in biomedical research in the United States, though the size of this contingent could be as high as 25,000.
How the overall size of the S&E workforce might change over the next 10 years will be influenced by several factors: the number of doctorates who graduate each year, the unemployment levels in the field, the number of foreign-trained doctorates, and retirement rates. These factors can be accounted for by taking a multistate life-table approach, which models the workforce to estimate the numbers of researchers who enter and exit the workforce at various stages. It is also important to know the age of the workforce and the age at which individuals enter it, as this information determines retirement rates. What follows in this section is a short summary of the findings from this model's analysis, with full details available in Appendix D (Demographic Projections of the Research Workforce).
The largest and most relevant source of new researchers is the set of graduates from U.S. doctoral programs. The size of this group grew significantly in the 1990s but has leveled off or declined in recent years. Making projections of the numbers of future graduates, therefore, depends on which years are used to develop the model (a quadratic regression). Rather than choose just one scenario, three different scenarios for Ph.D. growth were developed. The first was a regression from 1985 to obtain a high estimate; the second was a low estimate, based on the assumption of constant growth from the 2001 level; and the third was the average of the two to represent “moderate” growth. For the high estimate the annual number of Ph.D.s grows from 5,386 in 2001 to 7,433 in 2011, and the average of this number and the one resulting from no growth yields 6,441 in 2011 (see 10-year totals in Table 2-5 ).
Projected Changes in U.S. and Foreign Doctorates Entering the Biomedical Workforce Between 2001 and 2011 .
A similar approach—with low, median, and high scenarios—was used for the inflow of foreign doctorates. However, because it is difficult to estimate the number of individuals in the current workforce with a foreign doctorate, the scenarios are based on estimates of the growth rate in the 1990s and the resulting population in 2001. Based on these estimates, it is possible to project the potential workforce in the biomedical sciences between 2001 and 2011. Using estimates of unemployment and the flow of doctorates in and out of the S&E workforce, the employed biomedical researcher population can also be estimated. Table 2-6 shows the results of the multistate life-table analysis under the medium scenario. These totals exhibit an annual growth rate in the biomedical workforce of 2 to 2.5 percent, which is comparable to the projected annual growth rate of the overall labor force.
Projected Workforce by Status for the Median Scenario, 2001–2011 .
Although these workforce projections are subject to many caveats, such as incomplete data and uncertainties in the economy and government spending, the balance between Ph.D. production and employment looks quite stable through 2011. Unemployment remains at about 1 percent, and the portion of the workforce remaining in science is about 80 percent. The committee believes this is a healthy percentage of trained people employed in science, but it has concerns about those unemployed and not seeking employment. The percentage of women in this category is significantly greater than their male counterparts, and there is a fear that some talented researchers may be lost because of the difficulty of balancing a career in science and raising a family. (This matter is considered further in Chapter 9 , Career Progression.)
The analysis in this chapter suggests that the number of researchers in basic biomedical research will remain stable for the next decade, as will employment opportunities, and the percentage of postdoctorals in holding patterns appears to be declining. Nevertheless, the committee's concern about the increased time to degree and the length of postdoctoral appointments should be noted—an infusion of young people into independent research positions, after all, is critical to the health of the research community. However, we also note that the increase in the average age of researchers parallels the aging of the general population.
“Success” is not easily quantified, but anecdotal evidence suggests that the NRSA program has successfully produced high-quality research personnel and has been important for the upgrading of research training in general. The MSTP program also merits special mention. It has been brilliantly successful at attracting outstanding physicians into basic biomedical research, much to the benefit of future health care. Given their special knowledge of human disease, physicians lend a unique perspective to such research.
The committee's recommendations for future training in the basic biomedical sciences are presented below, along with brief justifications based on the analysis described in this chapter.
- RECOMMENDATIONS
Recommendation 2-1: This committee recommends that the total number of NRSA positions awarded in the biomedical sciences should remain at least at the 2003 level. Furthermore, the committee recommends that training levels after 2003 be commensurate with the rise in the total extramural research funding in the biomedical, clinical, and behavioral and social sciences.
Although manpower models have been developed in this report, they are not particularly useful in assessing the role of NRSA support in particular, as this represents only a small fraction of the total training support in the biomedical sciences. Available information, however, suggests that the system is in reasonable balance. Stipends clearly should rise over time, but this should be accomplished by the allocation of additional funds, not by decreasing the number of trainees. The relatively low unemployment among Ph.D.s in the biomedical sciences, an almost constant number of U.S.-trained doctorates from 2001 to 2003, and the fact that the pool of postdoctorates appears to be stabilizing or declining justify the suggested level, which should not fall below that of 2003.
The year 2001 is the last one for which reasonably accurate data were available for awards specific to the biomedical sciences. However, the total number of NRSA awards continued to rise ( Figure 1-1 ) in 2002 and 2003, and it is assumed that the awards in the biomedical sciences have also increased. Using the percentage increase from 2001 to 2003 from Table 1-1 and the actual awards data for 2001 in Tables 2-2 and 2-3 , the predoctoral and postdoctoral traineeships in the biomedical sciences in 2003 are estimated to be 5,390 and 1,740, respectively. Fellowship data for 2002 appear to be more complete and show that awards at the postdoctoral level are somewhat below those of 2001. Based on the totals for NRSA predoctoral and postdoctoral training in 2001 and 2003, the estimated levels for fellowships in 2003 for the biomedical sciences are 425 and 1,450, respectively.
The primary rationale for NRSA is to attract high-quality people into specific research areas and to set the training standards for major research fields. NRSAs should be a paragon for quality training and have served this role admirably. NRSA programs are an important investment in the future to ensure the health of the research enterprise and should be made by all NIH institutes and centers.
Beyond the monetary requirements of maintaining NRSA training numbers, this committee does not recommend that support be shifted from research grants to training grants (contrary to the recommendation of the previous committee). A balance is needed between research and training grants for the productive support of students and postdoctorates. Research grants offer an alternative training venue, and students and postdoctorates are essential for accomplishing the research specified in research grants. Moreover, a variety of support mechanisms for training is desirable. The NRSA provides multiple pipelines into the research endeavor, most notably for foreign students and postdoctorates. In certain technical areas, insufficient numbers of U.S. citizens are available to train in and carry out national research efforts in critical areas. The training of foreign scientists on research grants has also significantly enriched the talent pool in this country, as they often join the workforce for extended periods of time, including permanent residence.
Although two earlier National Academies committees 23 , 24 have recommended that some NIH research funding be shifted to training grants and fellowships, our committee has concluded—based on the uncertainty about the rate of future growth in employment opportunities in industry, and perhaps other sectors, and the considerations discussed above—that the number of graduate students supported on NRSA training grants should not increase any faster than NIH research funding, which is a principal determinant of employment demand. With regard to postdoctoral support, another National Academies committee 25 has recommended that foreign scientists be permitted to receive training grant and fellowship support—thereby increasing the size of the eligible pool—and that some research funds be transferred to training budgets. However, consideration of the current restriction on supporting foreign scientists on NRSA training was outside the scope of this study and was not discussed by our committee.
At the present time, the committee does not recommend a shift in the overall proportion of training dollars spent on NRSA versus other training vehicles but does suggest that the ratios of research dollars to fellows/students be maintained in approximate alignment for the different areas and that training efforts be supported by all NIH institutes and centers. Better coordination of training efforts across institutes is needed. The committee recognizes, however, that the balance may vary from field to field and will evolve over time.
Recommendation 2-2: This committee recommends that the size of MSTP programs be expanded by at least 20 percent and that the scope be expanded to include the clinical, health services, and behavioral and social sciences.
Available evidence suggests that it is increasingly difficult for physicians to move into research because of the high cost of medical training and graduates' enormous debt load. Nevertheless, the committee believes that it is very important to attract physicians into research and that MSTP programs have done so with remarkable success; the excellent record of these programs' M.D./Ph.D.s in obtaining research grants and remaining in research is well documented. This would increase the number of trainees from the 2003 level of 933 to about 1,120.
As has been the policy, MSTP grants should be confined to institutions where high-quality medical and research training are both available. Expanding the range of disciplines should be helpful in attracting physicians into clinical and health services research but not at the expense of current MSTP support for basic biomedical training. Today's applicant pool for MSTP positions can easily accommodate a doubling of the size of the program without compromising its current quality. However, in recognition of the high cost of the MSTP program and budget constraints, the committee recommends a 20 percent increase as a significant and prudent investment.
U.S. Department of Education. 2000 .
Tabulations from the Higher Education Research Institute and the U.S. Department of Education.
Unpublished tabulation from the Survey of Earned Doctorates, 2001. Available from the National Academies.
Freeman, R. B., et. al. 2001 .
National Research Council. 1998c .
Goldman, E. and E. Marshall. 2002 .
National Science Foundation. 1997 .
National Science Foundation. 2002b .
The down turn in 1991 may be due to a change in the Survey of Doctorate Recipient data collection methods.
National Science Foundation. 2004 .
NIH Web site: http://grants.nih.gov/grants/award/research/rgbydgre01.htm . Accessed on October 22, 2004.
Unpublished tabulation from the NIH IMPAC System.
NIH Web site: NIH Statement in Response to Addressing the Nation's Changing Needs for Biomedical and Behavioral Scientists, http://grants.nih.gov/training/nas_report/NIHResponse.htm .
See Appendix B for a complete explanation of the awards.
NIH Web site. Available on http://grants.nih.gov/grants/award/research/rgbydgre01.htm . Accessed October 22, 2004.
National Research Council. 2000b .
Massy, W. F., and C. A. Goldman. 1995 .
Coggeshall, P., and P. W. Brown. 1984 .
Pion, G. M. 2000 .
National Institute of General Medical Sciences. 1998 .
Partial data are available from the Association of American Medical College's Faculty Roster and from the National Science Foundation, National Survey of College Graduates.
National Research Council. 2000a .
National Research Council. 1998c . op. cit.
National Research Council. 2005 .
- Cite this Page National Research Council (US) Committee for Monitoring the Nation's Changing Needs for Biomedical, Behavioral, and Clinical Personnel. Advancing the Nation's Health Needs: NIH Research Training Programs. Washington (DC): National Academies Press (US); 2005. 2, Basic Biomedical Sciences Research.
- PDF version of this title (1.4M)
In this Page
Other titles in this collection.
- The National Academies Collection: Reports funded by National Institutes of Health
Recent Activity
- Basic Biomedical Sciences Research - Advancing the Nation's Health Needs Basic Biomedical Sciences Research - Advancing the Nation's Health Needs
Your browsing activity is empty.
Activity recording is turned off.
Turn recording back on
Connect with NLM
National Library of Medicine 8600 Rockville Pike Bethesda, MD 20894
Web Policies FOIA HHS Vulnerability Disclosure
Help Accessibility Careers
- Search by keyword
- Search by citation
Page 1 of 32
Significance of hepatitis B virus capsid dephosphorylation via polymerase
It is generally believed that hepatitis B virus (HBV) core protein (HBc) dephosphorylation (de-P) is important for viral DNA synthesis and virion secretion. HBV polymerase contains four domains for terminal pr...
- View Full Text
Association of TRAIL receptor with phosphatase SHP-1 enables repressing T cell receptor signaling and T cell activation through inactivating Lck
T cell receptor (TCR) signaling and T cell activation are tightly regulated by gatekeepers to maintain immune tolerance and avoid autoimmunity. The TRAIL receptor (TRAIL-R) is a TNF-family death receptor that ...
Improving CRISPR–Cas9 directed faithful transgene integration outcomes by reducing unwanted random DNA integration
The field of genome editing has been revolutionized by the development of an easily programmable editing tool, the CRISPR–Cas9. Despite its promise, off-target activity of Cas9 posed a great disadvantage for g...
A matter of new life and cell death: programmed cell death in the mammalian ovary
The mammalian ovary is a unique organ that displays a distinctive feature of cyclic changes throughout the entire reproductive period. The estrous/menstrual cycles are associated with drastic functional and mo...
Engineered extracellular vesicles carrying let-7a-5p for alleviating inflammation in acute lung injury
Acute lung injury (ALI) is a life-threatening respiratory condition characterized by severe inflammation and lung tissue damage, frequently causing rapid respiratory failure and long-term complications. The mi...
The rise of big data: deep sequencing-driven computational methods are transforming the landscape of synthetic antibody design
Synthetic antibodies (Abs) represent a category of artificial proteins capable of closely emulating the functions of natural Abs. Their in vitro production eliminates the need for an immunological response, st...
Tick-borne encephalitis virus transmitted singly and in duo with Borrelia burgdorferi sensu lato and Anaplasma phagocytophilum bacteria by ticks as pathogens modifying lipid metabolism in human blood
Ticks are vectors of various pathogens, including tick-borne encephalitis virus causing TBE and bacteria such as Borrelia burgdorferi sensu lato and Anaplasma phagocytophilum causing e.g. viral-bacterial co-infec...
Integration of transcription regulation and functional genomic data reveals lncRNA SNHG6’s role in hematopoietic differentiation and leukemia
Long non-coding RNAs (lncRNAs) are pivotal players in cellular processes, and their unique cell-type specific expression patterns render them attractive biomarkers and therapeutic targets. Yet, the functional ...
Reduced interleukin-18 secretion by human monocytic cells in response to infections with hyper-virulent Streptococcus pyogenes
Streptococcus pyogenes (group A streptococcus, GAS) causes a variety of diseases ranging from mild superficial infections of the throat and skin to severe invasive infections, such as necrotizing soft tissue infe...
Metabolism-regulating non-coding RNAs in breast cancer: roles, mechanisms and clinical applications
Breast cancer is one of the most common malignancies that pose a serious threat to women's health. Reprogramming of energy metabolism is a major feature of the malignant transformation of breast cancer. Compar...
Genetic and pharmacologic p32-inhibition rescue CHCHD2-linked Parkinson’s disease phenotypes in vivo and in cell models
Mutations in CHCHD2 have been linked to Parkinson’s disease, however, their exact pathophysiologic roles are unclear. The p32 protein has been suggested to interact with CHCHD2, however, the physiological functio...
The role of pregnancy associated plasma protein-A in triple negative breast cancer: a promising target for achieving clinical benefits
Pregnancy associated plasma protein-A (PAPP-A) plays an integral role in breast cancer (BC), especially triple negative breast cancer (TNBC). This subtype accounts for the most aggressive BC, possesses high tu...
Translational research on drug development and biomarker discovery for hepatocellular carcinoma
Translational research plays a key role in drug development and biomarker discovery for hepatocellular carcinoma (HCC). However, unique challenges exist in this field because of the limited availability of hum...
Germline mutations of homologous recombination genes and clinical outcomes in pancreatic cancer: a multicenter study in Taiwan
Cancer susceptibility germline mutations are associated with pancreatic ductal adenocarcinoma (PDAC). However, the hereditary status of PDAC and its impact on survival is largely unknown in the Asian population.
Rab37 mediates trafficking and membrane presentation of PD-1 to sustain T cell exhaustion in lung cancer
Programmed cell death protein 1 (PD-1) is an immune checkpoint receptor expressed on the surface of T cells. High expression of PD-1 leads to T-cell dysfunction in the tumor microenvironment (TME). However, th...
FLT3L-induced virtual memory CD8 T cells engage the immune system against tumors
Previous research in FMS-like tyrosine kinase 3 ligands (FLT3L) has primarily focused on their potential to generate dendritic cells (DCs) from bone marrow progenitors, with a limited understanding of how thes...
Promising antibacterial efficacy of arenicin peptides against the emerging opportunistic pathogen Mycobacterium abscessus
Mycobacterium abscessus , a fast-growing non-tuberculous mycobacterium, is an emerging opportunistic pathogen responsible for chronic bronchopulmonary infections in people with respiratory diseases such as cystic ...
Targeting MDM2 in malignancies is a promising strategy for overcoming resistance to anticancer immunotherapy
MDM2 has been established as a biomarker indicating poor prognosis for individuals undergoing immune checkpoint inhibitor (ICI) treatment for different malignancies by various pancancer studies. Specifically, ...
Mechanisms and functions of SUMOylation in health and disease: a review focusing on immune cells
SUMOylation, which is a type of post-translational modification that involves covalent conjugation of small ubiquitin-like modifier (SUMO) proteins to target substrates, regulates various important molecular a...
Hesperetin activates CISD2 to attenuate senescence in human keratinocytes from an older person and rejuvenates naturally aged skin in mice
CDGSH iron-sulfur domain-containing protein 2 (CISD2), a pro-longevity gene, mediates healthspan in mammals. CISD2 is down-regulated during aging. Furthermore, a persistently high level of CISD2 promotes longe...
Plectin plays a role in the migration and volume regulation of astrocytes: a potential biomarker of glioblastoma
The expression of aquaporin 4 (AQP4) and intermediate filament (IF) proteins is altered in malignant glioblastoma (GBM), yet the expression of the major IF-based cytolinker, plectin (PLEC), and its contributio...
Modelling the complex nature of the tumor microenvironment: 3D tumor spheroids as an evolving tool
Cancer remains a serious burden in society and while the pace in the development of novel and more effective therapeutics is increasing, testing platforms that faithfully mimic the tumor microenvironment are l...
TEM1/endosialin/CD248 promotes pathologic scarring and TGF-β activity through its receptor stability in dermal fibroblasts
Pathologic scars, including keloids and hypertrophic scars, represent a common form of exaggerated cutaneous scarring that is difficult to prevent or treat effectively. Additionally, the pathobiology of pathol...
Physiology and pharmacological targeting of phase separation
Liquid–liquid phase separation (LLPS) in biology describes a process by which proteins form membraneless condensates within a cellular compartment when conditions are met, including the concentration and postt...
Inactivation of pentraxin 3 suppresses M2-like macrophage activity and immunosuppression in colon cancer
The tumor microenvironment is characterized by inflammation-like and immunosuppression situations. Although cancer-associated fibroblasts (CAFs) are among the major stromal cell types in various solid cancers,...
Engineered EVs with pathogen proteins: promising vaccine alternatives to LNP-mRNA vaccines
Extracellular vesicles (EVs) are tiny, lipid membrane-bound structures that are released by most cells. They play a vital role in facilitating intercellular communication by delivering bioactive cargoes to rec...
Attenuation of neurovirulence of chikungunya virus by a single amino acid mutation in viral E2 envelope protein
Chikungunya virus (CHIKV) has reemerged as a major public health concern, causing chikungunya fever with increasing cases and neurological complications.
Scaffold-based 3D cell culture models in cancer research
Three-dimensional (3D) cell cultures have emerged as valuable tools in cancer research, offering significant advantages over traditional two-dimensional (2D) cell culture systems. In 3D cell cultures, cancer c...

Therapeutic antibodies for the prevention and treatment of cancer
The developments of antibodies for cancer therapeutics have made remarkable success in recent years. There are multiple factors contributing to the success of the biological molecule including origin of the an...
Immune evasion in cell-based immunotherapy: unraveling challenges and novel strategies
Cell-based immunotherapies (CBIs), notably exemplified by chimeric antigen receptor (CAR)-engineered T (CAR-T) cell therapy, have emerged as groundbreaking approaches for cancer therapy. Nevertheless, akin to ...
Exploring the relationship between metabolism and immune microenvironment in osteosarcoma based on metabolic pathways
Metabolic remodeling and changes in tumor immune microenvironment (TIME) in osteosarcoma are important factors affecting prognosis and treatment. However, the relationship between metabolism and TIME needs to ...
The synergism of cytosolic acidosis and reduced NAD + /NADH ratio is responsible for lactic acidosis-induced vascular smooth muscle cell impairment in sepsis
During sepsis, serve vascular dysfunctions lead to life-threatening multiple organ failure, due to vascular smooth muscle cells (VSMC) impairments, resulting in vasoplegia, hypotension and hypoperfusion. In ad...
Localization, traffic and function of Rab34 in adipocyte lipid and endocrine functions
Excessive lipid accumulation in the adipose tissue in obesity alters the endocrine and energy storage functions of adipocytes. Adipocyte lipid droplets represent key organelles coordinating lipid storage and m...
Nano-modified viruses prime the tumor microenvironment and promote the photodynamic virotherapy in liver cancer
As of 2020, hepatocellular carcinoma (HCC), a form of liver cancer, stood as the third most prominent contributor to global cancer-related mortality. Combining immune checkpoint inhibitors (ICI) with other the...
A novel mucosal bivalent vaccine of EV-A71/EV-D68 adjuvanted with polysaccharides from Ganoderma lucidum protects mice against EV-A71 and EV-D68 lethal challenge
Human enteroviruses A71 (EV-A71) and D68 (EV-D68) are the suspected causative agents of hand-foot-and-mouth disease, aseptic meningitis, encephalitis, acute flaccid myelitis, and acute flaccid paralysis in chi...

A secreted form of chorismate mutase (Rv1885c) in Mycobacterium bovis BCG contributes to pathogenesis by inhibiting mitochondria-mediated apoptotic cell death of macrophages
Mycobacterium tuberculosis is the causative agent of tuberculosis (TB), and its pathogenicity is associated with its ability to evade the host defense system. The secretory form of the chorismate mutase of M. tub...
ARID1A loss activates MAPK signaling via DUSP4 downregulation
ARID1A , a tumor suppressor gene encoding BAF250, a protein participating in chromatin remodeling, is frequently mutated in endometrium-related malignancies, including ovarian or uterine clear cell carcinoma (CCC)...
SCEL regulates switches between pro-survival and apoptosis of the TNF-α/TNFR1/NF-κB/c-FLIP axis to control lung colonization of triple negative breast cancer
Patients with metastatic triple-negative breast cancer (mTNBC) have a higher probability of developing visceral metastasis within 5 years after the initial diagnosis. Therefore, a deeper understanding of the p...

Butterflies in the gut: the interplay between intestinal microbiota and stress
Psychological stress is a global issue that affects at least one-third of the population worldwide and increases the risk of numerous psychiatric disorders. Accumulating evidence suggests that the gut and its ...
Spatiotemporal roles of AMPK in PARP-1- and autophagy-dependent retinal pigment epithelial cell death caused by UVA
Although stimulating autophagy caused by UV has been widely demonstrated in skin cells to exert cell protection, it remains unknown the cellular events in UVA-treated retinal pigment epithelial (RPE) cells.
The ‘speck’-tacular oversight of the NLRP3-pyroptosis pathway on gastrointestinal inflammatory diseases and tumorigenesis
The NLRP3 inflammasome is an intracellular sensor and an essential component of the innate immune system involved in danger recognition. An important hallmark of inflammasome activation is the formation of a s...
Complete spectrum of adverse events associated with chimeric antigen receptor (CAR)-T cell therapies
Chimeric antigen receptor (CAR)-T cell therapies have been approved by FDA to treat relapsed or refractory hematological malignancies. However, the adverse effects of CAR-T cell therapies are complex and can b...
Small interfering RNA (siRNA)-based therapeutic applications against viruses: principles, potential, and challenges
RNA has emerged as a revolutionary and important tool in the battle against emerging infectious diseases, with roles extending beyond its applications in vaccines, in which it is used in the response to the CO...
Human ACE2 protein is a molecular switch controlling the mode of SARS-CoV-2 transmission
Human angiotensin-converting enzyme 2 (hACE2) is the receptor mediating severe acute respiratory syndrome coronavirus 2 (SARS-CoV-2) infection. hACE2 expression is low in the lungs and is upregulated after SAR...
Crosstalk between mitochondrial biogenesis and mitophagy to maintain mitochondrial homeostasis
Mitochondrial mass and quality are tightly regulated by two essential and opposing mechanisms, mitochondrial biogenesis (mitobiogenesis) and mitophagy, in response to cellular energy needs and other cellular a...
Extracellular release in the quality control of the mammalian mitochondria
Mammalian cells release a wealth of materials to their surroundings. Emerging data suggest these materials can even be mitochondria with perturbed morphology and aberrant function. These dysfunctional mitochon...
mRNA-based vaccines and therapeutics: an in-depth survey of current and upcoming clinical applications
mRNA-based drugs have tremendous potential as clinical treatments, however, a major challenge in realizing this drug class will promise to develop methods for safely delivering the bioactive agents with high e...
Clinical trials of new drugs for Alzheimer disease: a 2020–2023 update
Alzheimer's disease (AD) is the leading cause of dementia, presenting a significant unmet medical need worldwide. The pathogenesis of AD involves various pathophysiological events, including the accumulation o...
Induced pluripotent stem cells: ex vivo models for human diseases due to mitochondrial DNA mutations
Mitochondria are essential organelles for cellular metabolism and physiology in eukaryotic cells. Human mitochondria have their own genome (mtDNA), which is maternally inherited with 37 genes, encoding 13 poly...
Interaction of the AKT and β-catenin signalling pathways and the influence of photobiomodulation on cellular signalling proteins in diabetic wound healing
The induction of a cells destiny is a tightly controlled process that is regulated through communication between the matrix and cell signalling proteins. Cell signalling activates distinctive subsections of ta...
- Editorial Board
- Manuscript editing services
- Instructions for Editors
- Sign up for article alerts and news from this journal
Journal of Biomedical Science is supported by the National Science and Technology Council (NSTC) , Taiwan.
Annual Journal Metrics
2022 Citation Impact 11.0 - 2-year Impact Factor 10.9 - 5-year Impact Factor 2.367 - SNIP (Source Normalized Impact per Paper) 2.520 - SJR (SCImago Journal Rank)
2023 Speed 14 days submission to first editorial decision for all manuscripts (Median) 107 days submission to accept (Median)
2023 Usage 1,698,723 downloads 3,813 Altmetric mentions
- More about our metrics
Journal of Biomedical Science
ISSN: 1423-0127
- Submission enquiries: Access here and click Contact Us
- General enquiries: [email protected]
Help | Advanced Search
Computer Science > Computation and Language
Title: pubmedqa: a dataset for biomedical research question answering.
Abstract: We introduce PubMedQA, a novel biomedical question answering (QA) dataset collected from PubMed abstracts. The task of PubMedQA is to answer research questions with yes/no/maybe (e.g.: Do preoperative statins reduce atrial fibrillation after coronary artery bypass grafting?) using the corresponding abstracts. PubMedQA has 1k expert-annotated, 61.2k unlabeled and 211.3k artificially generated QA instances. Each PubMedQA instance is composed of (1) a question which is either an existing research article title or derived from one, (2) a context which is the corresponding abstract without its conclusion, (3) a long answer, which is the conclusion of the abstract and, presumably, answers the research question, and (4) a yes/no/maybe answer which summarizes the conclusion. PubMedQA is the first QA dataset where reasoning over biomedical research texts, especially their quantitative contents, is required to answer the questions. Our best performing model, multi-phase fine-tuning of BioBERT with long answer bag-of-word statistics as additional supervision, achieves 68.1% accuracy, compared to single human performance of 78.0% accuracy and majority-baseline of 55.2% accuracy, leaving much room for improvement. PubMedQA is publicly available at this https URL .
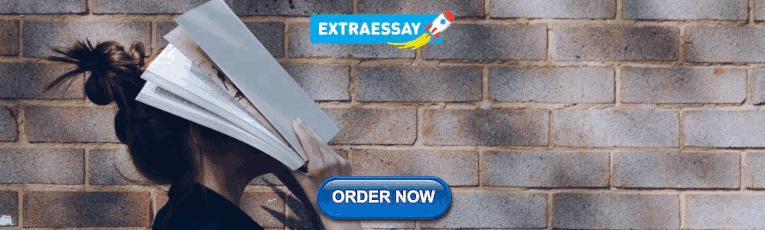
Submission history
Access paper:.
- Other Formats
References & Citations
- Google Scholar
- Semantic Scholar
DBLP - CS Bibliography
Bibtex formatted citation.

Bibliographic and Citation Tools
Code, data and media associated with this article, recommenders and search tools.
- Institution
arXivLabs: experimental projects with community collaborators
arXivLabs is a framework that allows collaborators to develop and share new arXiv features directly on our website.
Both individuals and organizations that work with arXivLabs have embraced and accepted our values of openness, community, excellence, and user data privacy. arXiv is committed to these values and only works with partners that adhere to them.
Have an idea for a project that will add value for arXiv's community? Learn more about arXivLabs .
School of Biomedical Sciences
Biomedical research themes & projects.
The School’s diverse research themes bring together researchers from the social sciences, epidemiology and public health, preventive, clinical and primary care medicine and from basic and applied sciences.
Cancer in Biomedicine
Characterising the molecular mechanisms responsible for cancer progression, predisposition and resistance to treatment.
Cardio-Respiratory
Focusing on the impact of diseases and adverse impact on cardio-respiratory function.
Cell Signalling
Researching cell signaling pathways and their importance in conveying information among cells to assist in understanding the development of a variety of human diseases.
Cellular Imaging & Structural Biology
Applying cellular imaging and structural biology expertise across a wide range of biomedical research programs. Platforms include fluorescence, live imaging, 2-photon imaging, transmission and scanning electron microscopy as well as whole body imaging and imaging mass spectroscopy.
Infection & Immunity
Focusing on the mechanisms of infection and immunity and development of new ways to control and treat infectious diseases - from molecular analysis of bacterial and viral infections to the development and function of the immune system.
Molecular Mechanisms of Disease
Modelling human diseases in experimental systems and analysing cells and tissues to gain insights into the underlying fundamental mechanisms of a range of diseases affecting the nervous system, respiratory system, muscle diseases and infectious disease.
Biomedical Neuroscience
Seeking to understand fundamental biological mechanisms in order to develop new treatments for injury and disease states, across neuroscience, cell and developmental biology, and anatomical sciences.
Characterising cellular and molecular mechanisms that underlie stem cell behaviour in development and disease, leading to the identification and characterisation of innovative biomarkers and therapeutic targets.
Systems Biology
Making quantitative measurements and computational analysis of the interacting components in systems biology using genomics, proteomics, bioinformatics, metabolomics and mass spectrometry.
Therapeutics & Translation
The translation of discoveries in Biomedical Sciences across clinical and commercial settings.
Scholarship of Teaching and Learning
Bringing together teaching specialists and academics across the School focused on improving student learning, outcomes, experiences and engagement across the biomedical sciences.
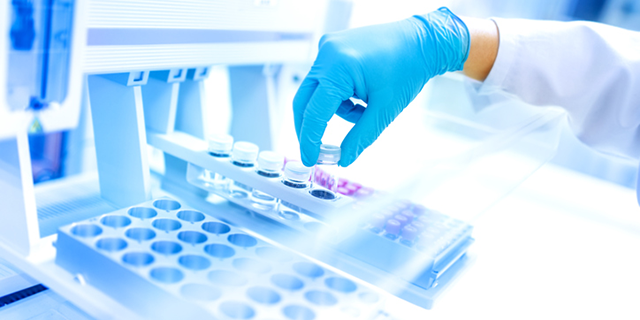
Research Projects by theme
Our researchers are undertaking world-class research across a wide range of health and social issues in a diverse range of settings.
View projects
- Faculty of Medicine, Dentistry and Health Sciences
- Department of Anatomy and Physiology
- Department of Biochemistry and Pharmacology
- Department of Microbiology and Immunology
- Australian Venom Research Unit
- Centre for Muscle Research
- Centre for Stem Cell Systems
- Lung Health Research Centre
- News & Events
- Biomedical Research Projects by Theme
- Research Groups
- Graduate Research
- The Melbourne Biomedical Precinct
- Our Degrees
- Current Students
- Honours & Masters by Coursework
- Scholarships, Bursaries & Prizes
- Head of School's Welcome
- Staff Intranet
The task of PubMedQA is to answer research questions with yes/no/maybe (e.g.: Do preoperative statins reduce atrial fibrillation after coronary artery bypass grafting? ) using the corresponding abstracts.
For more details about PubMedQA, please refer to this paper:
PubMedQA Paper (EMNLP'19)
PubMedQA has 1k expert labeled, 61.2k unlabeled and 211.3k artificially generated QA instances.
Please visit our GitHub repository to download the dataset:
PubMedQA Data & Code Repository
To submit your model, please follow the instructions in the GitHub repository.
If you use PubMedQA in your research, please cite our paper by:
Thank you for visiting nature.com. You are using a browser version with limited support for CSS. To obtain the best experience, we recommend you use a more up to date browser (or turn off compatibility mode in Internet Explorer). In the meantime, to ensure continued support, we are displaying the site without styles and JavaScript.
- View all journals
- My Account Login
- Explore content
- About the journal
- Publish with us
- Sign up for alerts
- Data Descriptor
- Open access
- Published: 27 March 2023
BioASQ-QA: A manually curated corpus for Biomedical Question Answering
- Anastasia Krithara ORCID: orcid.org/0000-0003-0491-4507 1 ,
- Anastasios Nentidis 1 , 2 ,
- Konstantinos Bougiatiotis 1 &
- Georgios Paliouras 1
Scientific Data volume 10 , Article number: 170 ( 2023 ) Cite this article
3269 Accesses
2 Citations
5 Altmetric
Metrics details
- Information technology
- Scientific data
The BioASQ question answering (QA) benchmark dataset contains questions in English, along with golden standard (reference) answers and related material. The dataset has been designed to reflect real information needs of biomedical experts and is therefore more realistic and challenging than most existing datasets. Furthermore, unlike most previous QA benchmarks that contain only exact answers, the BioASQ-QA dataset also includes ideal answers (in effect summaries), which are particularly useful for research on multi-document summarization. The dataset combines structured and unstructured data. The materials linked with each question comprise documents and snippets, which are useful for Information Retrieval and Passage Retrieval experiments, as well as concepts that are useful in concept-to-text Natural Language Generation. Researchers working on paraphrasing and textual entailment can also measure the degree to which their methods improve the performance of biomedical QA systems. Last but not least, the dataset is continuously extended, as the BioASQ challenge is running and new data are generated.
Similar content being viewed by others
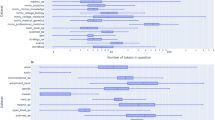
ThoughtSource: A central hub for large language model reasoning data
Simon Ott, Konstantin Hebenstreit, … Matthias Samwald
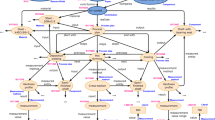
The SciQA Scientific Question Answering Benchmark for Scholarly Knowledge
Sören Auer, Dante A. C. Barone, … Eleni Tsalapati
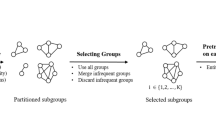
Selective UMLS knowledge infusion for biomedical question answering
Hyeryun Park, Jiye Son, … Jinwook Choi
Background & Summary
More than 2 articles are published in biomedical journals every minute, leading to MEDLINE/PubMed 1 currently comprising more than 32 million articles, while the number and size of non-textual biomedical data sources also increases rapidly. As an example, since the outbreak of the COVID-19 pandemic, there has been an explosion of new scientific literature about the disease and the virus that causes it, with about 10,000 new COVID-19 related articles added each month 2 . This wealth of new knowledge plays a central role in the progress achieved in biomedicine and its impact on public health, but it is also overwhelming for the biomedical expert. Ensuring that this knowledge is used for the benefit of the patients in a timely manner is a demanding task.
BioASQ 3 (Biomedical Semantic Indexing and Question Answering) pushes research towards highly precise biomedical information access systems through a series of evaluation campaigns, in which systems from teams around the world compete. BioASQ campaigns run annually since 2012, providing data, open-source software and a stable evaluation environment for the participating systems. In the last ten years that the challenge has been running, around 100 different universities and companies, from all continents, have participated in BioASQ, providing a competitive, but also synergetic ecosystem. The fact that the participants of the BioASQ challenges are all working on the same benchmark data, facilitates significantly the exchange and fusion of ideas and eventually accelerates progress in the field. The ultimate goal is to lead biomedical information access systems to the maturity and reliability required by biomedical researchers.
BioASQ comprises two main tasks. In Task A systems are asked to automatically assign Medical Subject Headings (MeSH) 4 terms to biomedical articles, thus assisting the indexing of biomedical literature. Task B focuses on obtaining precise and comprehensible answers to biomedical research questions. The systems that participate in Task B are given English questions that are written by biomedical experts and reflect real-life information needs. For each question, the systems are required to return relevant articles, snippets of the articles, concepts from designated ontologies, RDF triples from Linked Life Data 5 , an ‘exact’ answer (e.g., a disease or symptom), and a paragraph-sized summary answer. Hence, this task combines traditional information retrieval, with question answering from text and structured data, as well as multi-document text summarization.
One of the main tangible outcomes of BioASQ is its benchmark datasets. The BioASQ-QA dataset that is generated for Task B, contains questions in English, along with golden standard (reference) answers and supporting material. The BioASQ data are more realistic and challenging than most existing datasets for biomedical expert question answering 6 , 7 . In order to achieve this, BioASQ employs a team of trained experts, who provide annually a set of around 500 questions from their specialized field of expertise. Figure 1 provides the lifecycle of the BioASQ dataset creation, which is presented in detail in the following sections. Using this process, a set of 4721 questions and answers have been generated so far, constituting a unique resource for the development of QA systems.
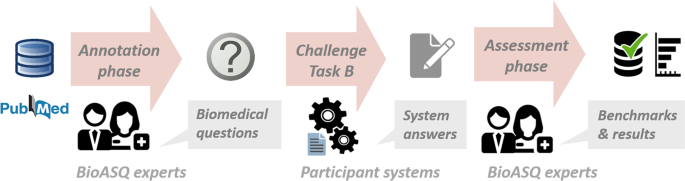
During the annotation phase of the BioASQ, the experts compose biomedical questions. The participating systems provide answers in the challenge. Finally, in the assessment phase, the experts manually assess the system responses and refine and extend the dataset.
The BioASQ infrastructure and ecosystem
Figure 2 summarises the main components of the BioASQ infrastructure, as well as key stakeholders in the related ecosystem. The BioASQ infrastructure includes tools for annotating data, tools for assessing the results of participating systems, benchmark repositories, evaluation services, etc. The infrastructure allows challenge participants to access training and test data, submit their results and be informed about the performance of their systems, in comparison to other systems. The BioASQ infrastructure is also used by the experts during the creation of the benchmark datasets and helps improve the quality of the data. In the following subsections, the different components of the BioASQ ecosystems are described.
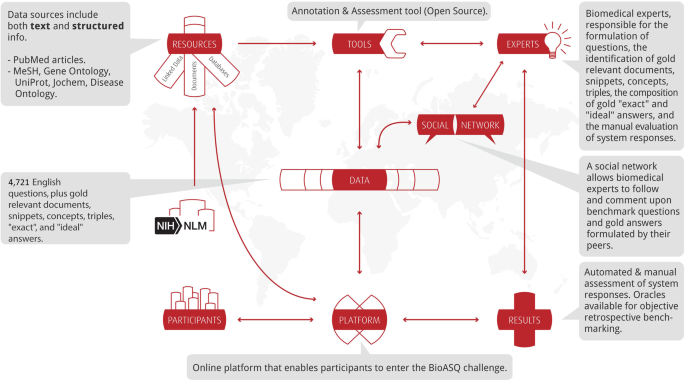
The BioASQ infrastructure and ecosystem.
Expert team
As the goal of BioASQ is to reflect real information needs of biomedical experts, their involvement was necessary in the creation of the dataset. The biomedical expert team of BioASQ was first established in 2012, but has changed through the years. Several experts were considered at that time, from a variety of institutions across Europe. The final selection of the experts was based on the need to cover the broad biomedical scientific field, representing as much as possible, medicine, biosciences and bioinformatics. The members of the biomedical team hold positions in universities, hospitals or research institutes in Europe. Their primary research interests include: cardiovascular endocrinology, psychiatry, psychophysiology, pharmacology, drug repositioning, cardiac remodeling, cardiovascular pharmacology, computational genomics, pharmacogenomics, comparative genomics, molecular evolution, proteomics, mass spectometry, protein evolution, clinical information retrieval from electronic health records, and clinical practice guidelines. In total 21 experts have contributed to the creation of the dataset, 7 of whom have been involved most actively. The main job of the biomedical expert team is the creation of the QA benchmark dataset, using an annotation tool provided by BiOASQ. With the use of the tool, the experts can set their questions and retrieve relevant documents and snippets from MEDLINE. Additionally, the biomedical expert team assesses the responses of the participating systems. In addition to scoring the systems’ answers, during this process the experts have the opportunity to enrich and modify the gold material that they have provided, thus improving the quality of the benchmark dataset.
Regular physical and virtual meetings are organised with the experts. Partly, these meetings aim to train the new members of the team and inform the existing ones about changes that have happened. In particular, the goals of the training sessions are as follows:
Familiarization with the annotation and assessment tools used during the formulation and assessment of biomedical questions respectively. This step also involves familiarization of the experts with the specific types of questions used in the challenge, i.e. factoid, yes/no, list and summary questions. At the same time, the experts provide feedback and help shaping the BioASQ tools.
Familiarization with the resources used in BioASQ, both MEDLINE and various structured sources. The aim is to help the experts understand the data provided by these source, in response to different questions they may formulate.
Resolution of issues that come up during the question composition and assessment tasks. This is a continuous process that extends beyond the training sessions. Continuous support is provided to the experts, while the experts can also interact with each other and provide feedback on the data being created.
Data selection
The QA benchmark is based primarily on documents indexed for MEDLINE . In addition, a wide range of biomedical concepts are drawn from ontologies and linked data that describe different facets of the domain. The selected resources follow commonly used drug-target-disease triangle , which defines the prime information axes for medical investigations. The main principle is shown in Figure 3 .
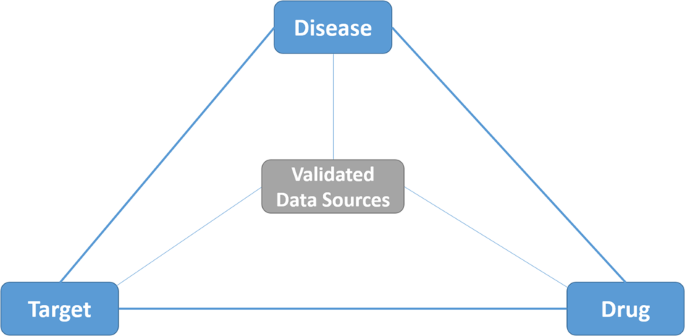
The drug-target-disease triangle, adopted in BioASQ .
This “ knowledge-triangle ” supports the conceptual linking of biomedical knowledge databases and related resources. Based on this, systems can address questions, linking natural language questions with relevant ontology concepts. In this context, the following resources have been selected for BioASQ.
Drugs: Jochem 8 , the Joint Chemical Dictionary, is a dictionary for the identification of small molecules and drugs in text, combining information from UMLS, MeSH, ChEBI, DrugBank, KEGG, HMDB, and ChemIDplus. Given the variety and the population of the different resources in it, Jochem is currently one of the largest biomedical resources for drugs and chemicals.
Targets: Gene Ontology (GO) 9 , 10 is currently the most successful case of ontology use in bioinformatics and provides a controlled vocabulary to describe functional aspects of gene products. The ontology covers three domains: cellular component, molecular function, and biological process.
Universal Protein Resource (UniProt 11 ) provides a comprehensive, high-quality and freely accessible resource of protein sequence and functional information. Its protein knowledge base consists of two sections: SwissProt, which is manually annotated and reviewed, and contains more than 500 thousand sequences, and TrEMBL, which is automatically annotated and is not reviewed, and contains a few million sequences. In BioASQ the SwissProt component of UniProt is used.
Diseases: Disease Ontology (DO) 12 contains data associating genes with human diseases, using established disease codes and terminologies. Approximately 8,000 inherited, developmental and acquired human diseases are included in the resource. The DO semantically integrates disease and medical vocabularies through extensive cross-mapping and integration of MeSH, ICD, NCI’s thesaurus, SNOMED CT and OMIM disease-specific terms and identifiers.
Document Sources: The main source of biomedical literature is NLM’s MEDLINE and is accessible through PubMed and PubMed Central. PubMed, indexes over 34 million citations, while PubMed Central (PMC) provides free access to approximately 8.5 million full-text biomedical and life-science articles.
The Medical Subject Headings Hierarchy (MeSH) is a hierarchy of terms maintained by the US National Library of Medicine (NLM) and its purpose is to provide headings (terms), which can be used to index scientific publications in the life sciences, e.g., journal articles, books, and articles in conference proceedings. The indexed publications may be searched through popular search engines, such as PubMed, using the MeSH headings to filter semantically the results. This retrieval methodology seems to be in some cases beneficial, especially when precision of the retrieved results is important 13 . The primary MeSH terms (called descriptors ) are organized into 16 trees, and are approximately 30,200. MeSH is the main resource used by PubMed to index the biomedical scientific bibliography in MEDLINE.
Linked Data: During the first few years of BioASQ, the Linked Life Data platform was used to identify subject-verb-object triples related to questions. Linked Life Data is a data warehouse that syndicates large volumes of heterogeneous biomedical knowledge in a common data model. It contains more than 10 billion statements. The statements are extracted from 25 biomedical resources, such as PubMed, UMLS, DrugBank, Diseasome, and Gene Ontology. This resource has been abandoned in recent editions of BioASQ, due to issues with the triple selection process.
Question formulation
The members of the biomedical expert team formulate English questions, reflecting real-life information needs encountered during their work (e.g., in diagnostic research). Figure 4 provides an overview of the most frequent topics covered in the questions generated so far by the experts. Each question is independent of all other questions and is associated with an answer and other supportive information, as explained below.
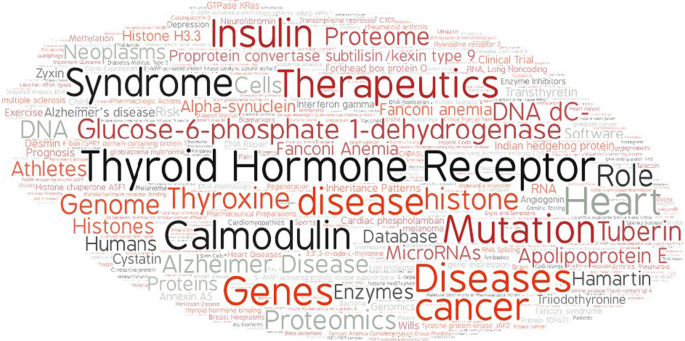
Most frequent topics in the BioASQ questions.
In addition to the training sessions mentioned above, guidelines are provided to the BioASQ experts to help them create the questions, reference answers, and other supportive information 14 . The guidelines cover the number and types of questions to be created by the experts, the information sources the experts should consider and how to use them, the types and sizes of the answers, additional supportive information the experts should provide, etc. The experts use the BioASQ annotation tool for this process, which is accessible through a Web interface.
The annotation tool provides the necessary functionality to create questions and select relevant information. The annotation tool is designed to be easy to use, adopting a simple five-step-paradigm: authenticate, search, select, annotate and store. The authentication ensures that each question created by a certain expert can be assigned to this given expert.
The annotation process comprises the following steps:
Step 1: Question formulation
The experts formulate an English stand-alone question, reflecting their information needs. Questions may belong to one of the following four categories:
Yes/no questions: These are questions that, strictly speaking, require either a “yes” or a “no” as an answer, though of course in practice a longer answer providing additional information is useful. For example, “ Do CpG islands colocalise with transcription start sites? ” is a yes/no question.
Factoid questions: These are questions that require a particular entity (e.g., a disease, drug, or gene) as an answer, though again a longer answer is useful. For example, “ Which virus is best known as the cause of infectious mononucleosis? ” is a factoid question.
List questions: These are questions that require a list of entities (e.g., a list of genes) as an answer; again, in practice additional supportive information is desirable. For example, “ Which are the Raf kinase inhibitors? ” is a list question.
Summary questions: These are questions that do not belong in any of the previous categories and can only be answered by producing a short text summarizing the most prominent relevant information. For example, “ How does dabigatran therapy affect aPTT in patients with atrial fibrillation? ” is a summary question. When formulating summary questions, the experts aimed at questions that they can answer in a satisfactory manner with a one-paragraph summary, intended to be read by other experts of the same field. In all four categories, the experts aim at questions for which a limited number of articles (min. 10, max. 60) are retrieved through PubMed queries. Questions which are controversial or that have no clear answers in the literature are avoided. Moreover, all questions are related to the biomedical domain. For example, in the case of the following two questions:
Q 1 : Which are the differences between Hidden Markov Models (HMMs) and Artificial Neural Networks (ANNs) ?
Q 2 : Which are the uses of Hidden Markov Models (HMMs) in gene prediction?
Although HMMs and ANNs are used in the biomedical domain, Q 1 is not suitable for the needs of BioASQ, since there is not a direct indication that it is related to the biomedical domain. On the other hand, Q 2 links to “gene prediction” and is appropriate.
Step 2: Relevant concepts
A set of terms that are relevant to each question is selected. The set of relevant terms may include terms that are already mentioned in the question, but it may also include synonyms of the question terms, closely related broader and narrower terms etc. For the question “ Do CpG islands colocalise with transcription start sites? ”, the set of relevant terms would most probably include the question terms “ CpG Island ” and “ transcription start site ”, but possibly also other terms, like the synonym“ Transcription Initiation Site ”.
Step 3: Information retrieval
Using the selected terms, the BioASQ annotation tool allows the experts to issue queries and retrieve relevant articles through PubMed. More than one query may be associated with each question and each query can be enriched with the advanced search tags of PubMed. The search window (Figure 5 ) allows selecting information that is necessary to answer the question. One of the main powers of the annotation tool is that it implements interfaces to different data sources of different types, i.e., unstructured, semi-structured or structured. Given that we cannot expect domain experts to be familiar with Semantic Web standards, such as RDF, the annotation tool also implements an innovative natural language generation method that converts RDF into natural language. The iterative improvement of the annotation tool has led to a framework that is widely accepted by the BioASQ biomedical expert team. Interestingly, a study of the queries used by different experts to answer the same questions made clear that indeed “many roads lead to Rome”, i.e. different experts will use different queries for the same question.
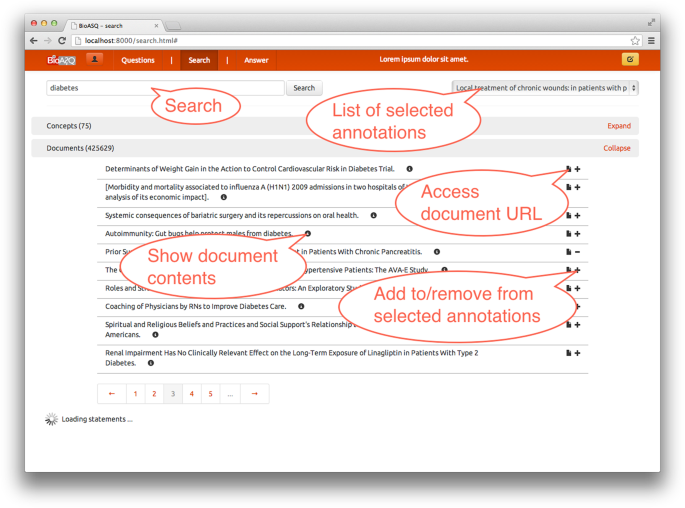
Screenshot of the annotation tool’s search and data selection screen with the section for document results expanded.
Returning to the example question “ Do CpG islands colocalise with transcription start sites? ” a query may be “ CpG Island ” AND “ transcription start site ”. Some of the articles retrieved by this query are shown in Table 1 .
Step 4: Selection of articles
Based on the results of Step 3, the experts select a set of articles that are sufficient for answering the question. Using the annotation tool, they choose among the retrieved list of articles, the ones that contain relevant information to form an answer.
Step 5: Text snippet extraction
Using the articles selected in step 4, the experts mark every text snippet (piece of text) out of the articles selected in Step 4. Snippets can be easily extracted using the annotation tool (Figure 6 ) and may answer the question either fully or partially. A text snippet should contain one or more entire and consecutive sentences. If there are multiple snippets that provide the same (or almost the same) information (in the same or in different articles), all of them are selected. Examples of relevant snippets are shown in Table 2 .
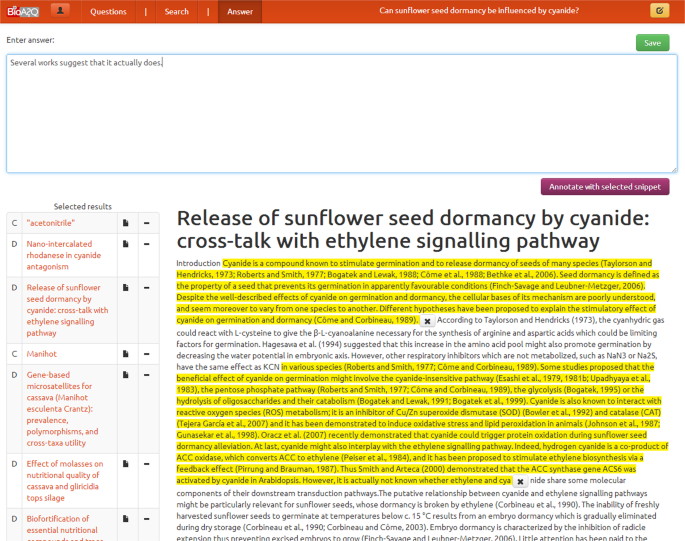
Screenshot of the annotation tool’s snippet annotation process.
Step 6: Query revision
If the expert judges that the articles and snippets gathered during steps 2 to 5 are insufficient for answering the question, the process can be repeated. The articles that the expert has already selected can be saved before performing a new search, along with the snippets the expert has already extracted. The query can be revised several times, until the expert feels that the gathered information is sufficient to answer the question. At the end, if the expert judges that the question can still not be answered adequately, the question is discarded.
Step 7: Exact answer
In steps 2 to 6, the expert identifies relevant material for answering the question. Given this material, the next step is to formulate the actual answer. For a yes/no question, the exact answer is simply “yes” or “no”. For a factoid question, the exact answer is the name of the entity (e.g., gene, disease) sought by the question; if the entity has several synonyms, the expert provides, to the extent possible, all of its synonyms. For a list question, the exact answer is a list containing the entities sought by the question; if a member of the list has several synonyms, the expert provides again as many of the synonyms as possible. For a summary question, the exact answer is left blank. The exact answers of yes/no, factoid, and list questions should be based on the information of the text snippets that the expert has selected, rather than personal experience.
Step 8: Ideal answer
At this final step, the expert formulates what we call an ideal answer for the question. The ideal answer should be a one-paragraph text that answers the question in a manner that the expert finds satisfactory. The ideal answer should be written in English, and it should be intended to be read by other experts of the same field. For the example question “ Do CpG islands colocalise with transcription start sites? ”, an ideal answer might be the one shown in Table 3 . Again, the ideal answer should be based on the information of the text snippets that the expert has selected, rather than personal experience. The experts, however, are allowed (and should) rephrase or shorten the snippets, order or combine them etc., in order to make the ideal answer more concise and easier to read.
Notice that in the example above, the ideal answer provides additional information supporting the exact answer. If the expert feels that the exact answer of a yes/no, factoid, or list question is sufficient and no additional information needs to be reported, the ideal answer can be the same as the exact answer. For summary questions, an ideal answer must always be provided.
Figure 7 presents the distribution of questions created each year of the challenge. Over the years, there is an increase in the number of factoid questions, and a decrease in the number of list questions. The possible reason is that it is more difficult to find the material (i.e. articles and snippets) that are sufficient for answering a factoid question than a list question. Table 4 presents the different versions of the BioASQ-QA dataset, including the number of questions, and the average number of documents and snippets. Each version of the training dataset enriches its previous version with the new questions created the respective year.
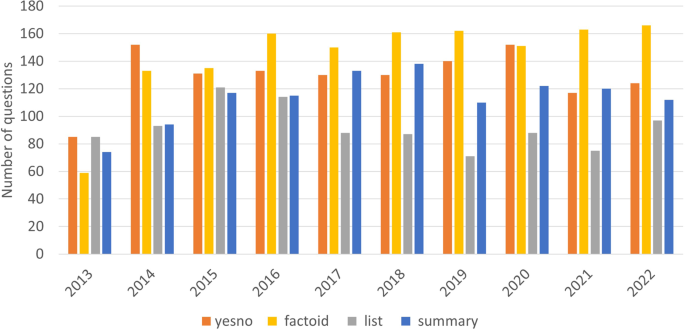
Distribution of types of questions per year.
During the years that BioASQ has been running, three significant changes have taken place, in response to feedback obtained by the experts and the challenge participants:
Since BioASQ 3 (2015), the focus of the experts is only on relevant articles and their contents. In other words, the experts do not provide relevant concepts or statements, as it was found cumbersome and led to questionable results. Nevertheless, concepts are included in the gold dataset, as they are added by the systems and assessed by the experts in the assessment phase).
Since BioSQ 4 (2016) only a sufficient set of articles, that allow the answer to be found with confidence, is requested by the experts. This is again in contrast to earlier years, where the experts were asked to identify all relevant articles; something that proved to be unrealistic. Again, if the participating systems retrieve more relevant documents, not identified in the annotation phase by the experts, these are added in the gold dataset, during the assessment phase.
In early versions of the challenge, we considered using full-text articles from PubMed Central (PMC). Given the small percentage of the overall literature that appears in PMC, since BioASQ 4 (2016) we decided to restrict the challenge to article abstracts only.
Following each round of the challenge, the answers of the participating systems are collected and assessed. Exact answers can be assessed automatically against the golden answers provided by the experts during the annotation phase. However, the ‘ideal’ answers are assessed manually by the experts. In fact each expert gets to assess the answers to the questions they have created, in terms of information recall (does the ‘ideal’ answer reports all the necessary information?), information precision (does the answer contain only relevant information?), information repetition (does the ‘ideal’ answer avoid repeating the same information multiple times? e.g., when sentences of the ‘ideal’ answer that have been extracted from different articles convey the same information), and readability (is the ‘ideal’ answer easily readable and fluent?). A 1 to 5 scale is used in all four criteria (1 for ‘very poor’, 5 for ‘excellent’).
The assessment tool is designed to be a companion to the annotation tool and is implemented by reusing most of its functionality. The tool can also be used to perform an inter-annotator agreement study. In that case, domain experts are provided with answers generated by other (anonymous) domain experts and are asked to evaluate them.
The design of the interface is such that the users can always see the answers/annotations only to questions that they are asked to review (Figure 8 ). Moreover, the interface can adapt to different question types, by showing different answering fields for each of them. Finally, all information sources that were used to answer the question can also be reviewed. By these means, domain experts can perform an informed assessment.
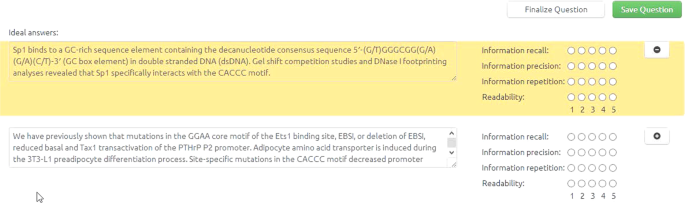
Assessment tool for evaluating system answers. The gold standard answer is at the top.
The assessment tool plays a key role in the creation of the benchmark and the quality assurance of the results generated by the experts during the BioASQ challenges. Moreover, the assessment tool allows the experts to improve their own gold answers and associated material, based on the answers provided by the systems. In particular, the experts revise the documents and snippets returned by the systems, and enrich the gold answers with material identified by the systems. This leads to an improvement of the benchmark datasets that are provided publicly.
Data Records
The dataset is available at Zenodo 15 and follows the JSON format. Specifically, it contains an array of questions, where each question (represented as an object in the JSON format) is constructed as shown in Table 5 .
Technical Validation
Improving the state-of-the-art performance.
The participation of strong research teams in the BioASQ challenge has helped to measure objectively the state-of-the-art performance in biomedical question answering 16 , 17 , 18 . During BioASQ this performance has improved (Figures 10 , 11 , 12 ). It is particularly encouraging that the BioASQ biomedical expert team have assessed the ideal answers provided by the participating systems as being of very good quality. The average manual scores are above 80% (above 4 out of 5 in Fig. 12 ). Still, there is much room for improvement and the future challenges of BioASQ, as well as the benchmark datasets that it provides, will hopefully push further towards that direction.
Based on the evaluation of the participating systems from the experts, one very interesting result is that humans seem satisfied by “imperfect” system responses. In other words, they are satisfied if the systems provide the information and answer needed, even if it is not perfectly formed.
Inter-annotation agreement
An inter-annotation agreement evaluation has been conducted in order to evaluate the consistency of the dataset. To this end, during the first six years, a small subset of the created questions, was given to other experts, in order to compare the different formulated answers. The pairs of experts answer the exact same questions. As each of them uses their own queries, they get a different list of possible relevant documents. The latter leads to the selection of different documents and snippets to answer the questions, which leads to low mean F1 score (<50%) (Figure 9a ). Nevertheless, in the formulated ideal answers, there is a high agreement between the experts (Figure 9b ). In other words, they reach the same or very similar answers, but following different paths.
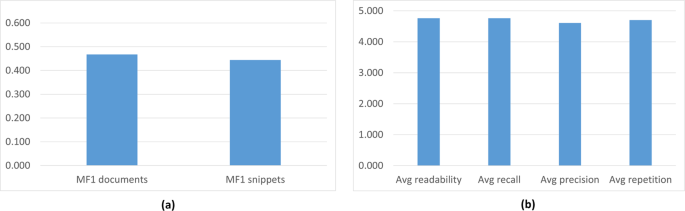
Inter-annotator agreement: ( a ) Scores of the snippets and the documents retrieved by the additional expert, compared to the original one; and ( b ) average manual scores of the expert ideal answers.
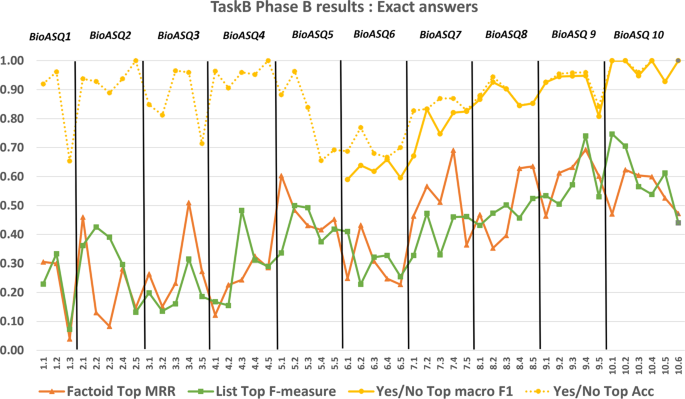
The performance achieved by systems in exact answer generation, across different years of the BioASQ challenge. For each test set the performance of the best performing system (Top) is presented based on the official evaluation measures. Since BioASQ6 the macro-averaged F1 score (macro F1) is the official measure for Yes/No questions, but accuracy (Acc), the former official measure, is also presented.
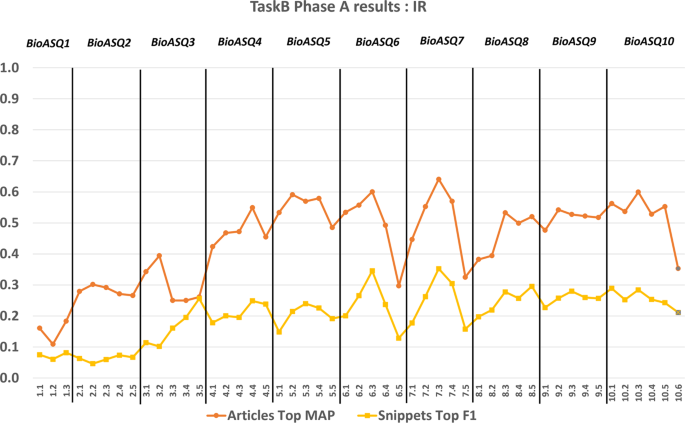
The performance achieved by systems in the information retrieval part of Task B, across different years of the BioASQ challenge. For each test set the performance of the best performing system (Top) is presented, based on the official evaluation measures.
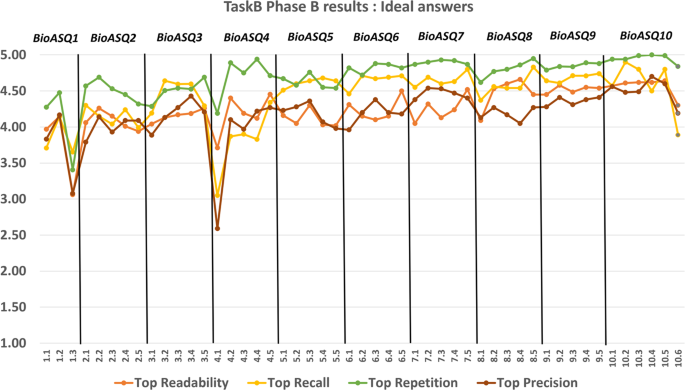
The performance achieved by systems in the ideal answer generation part of Task B, across different years of the BioASQ challenge. For each test set the performance of the best performing system (Top) is presented based on the official evaluation measures. In BioASQ 4, the performance was lower due to the fact that it was the first time BioASQ run after the end of the EU-funded project, and the top teams in ideal answer generation started participating after the second batch of the same year.
Another important point is that the BioASQ challenge, as well as the environment in which it takes place, evolve. One consequence of this is the changes that we had to make in the data generation process in response to feedback from the experts and the participants. Additionally, the evolution of vocabularies and databases cause complications. For example, each year’s data are annotated with the current version of the MeSH hierarchy, which is updated annually. In addition, only the articles of the current year of annotation are used for formulating the answers, while articles that will appear in the future may also be of relevance. These are issues that we need to handle and adapt to them, in order to have real-life, useful challenge and relevant dataset.
The BioASQ challenge will continue to run in the coming years, and the dataset will be further enriched with new interesting questions and answers.
Usage Notes
Up to date guidelines and usage examples pertaining the dataset can be found in: http://participants-area.bioasq.org/
Code availability
BioASQ has created a lively ecosystem, supported by tools and systems that facilitate the creation of the benchmarks. All software is provided with open-source licenses ( https://github.com/BioASQ ). In addition, the data produced are open to the public 15 .
National Library of Medicine. Medline pubmed production statistics. https://www.nlm.nih.gov/bsd/pmresources.html (2022).
Chen, Q., Allot, A. & Lu, Z. LitCovid: an open database of COVID-19 literature. Nucleic Acids Research 49 , D1534–D1540, https://doi.org/10.1093/nar/gkaa952 (2020).
Article CAS PubMed Central Google Scholar
Nentidis, A., Krithara, A. & Paliouras, G. BioASQ website. www.BioASQ.org (2022).
National Library of Medicine. The Medical Subject Headings (MeSH) thesaurus. https://www.nlm.nih.gov/mesh/meshhome.html (2022).
Linked Life Data (LLD). http://linkedlifedata.com/ (2012).
Wasim, M., Mahmood, D. W. & Khan, D. U. G. A survey of datasets for biomedical question answering systems. International Journal of Advanced Computer Science and Applications 8 , https://doi.org/10.14569/IJACSA.2017.080767 (2017).
Jin, Q. et al . Biomedical question answering: A survey of approaches and challenges. ACM Comput. Surv . 55 , https://doi.org/10.1145/3490238 (2022).
Hettne, K. M. et al . A dictionary to identify small molecules and drugs in free text. Bioinformatics 25 , 2983–2991, https://doi.org/10.1093/bioinformatics/btp535 (2009).
Article CAS PubMed Google Scholar
Ashburner, M. et al . Gene ontology: tool for the unification of biology. the gene ontology consortium. Nat Genet 25 , 25–29, https://doi.org/10.1038/75556 (2000).
The Gene Ontology Consortium. The Gene Ontology Resource: 20 years and still GOing strong. Nucleic Acids Research 47 , D330–D338, https://doi.org/10.1093/nar/gky1055 (2018).
The UniProt Consortium. UniProt: the Universal Protein Knowledgebase. Nucleic Acids Research 51 , D523–D531, https://doi.org/10.1093/nar/gkac1052 (2022).
Article Google Scholar
Schriml, L. M. et al . Human Disease Ontology 2018 update: classification, content and workflow expansion. Nucleic Acids Research 47 , D955–D962, https://doi.org/10.1093/nar/gky1032 (2018).
Doms, A. & Schroeder, M. GoPubMed: exploring PubMed with the Gene Ontology. Nucleic Acids Research 33 , W783–W786, https://doi.org/10.1093/nar/gki470 (2005).
Article CAS PubMed PubMed Central Google Scholar
Malakasiotis, P., Androutsopoulos, I., Almirantis, Y., Polychronopoulos, D. & Pavlopoulos, I. Tutorials and guidelines 2 http://www.bioasq.org/sites/default/files/PublicDocuments/BioASQ_D3.7-TutorialsGuidelines2ndVersion_final_0.pdf (2013).
Krithara, A., Nentidis, A., Bougiatiotis, K. & Paliouras, G. BioASQ-QA: A manually curated corpus for biomedical question answering. zenodo https://doi.org/10.5281/zenodo.7655130 (2023).
Nentidis, A. et al . Overview of BioASQ 2020: The eighth BioASQ challenge on large-scale biomedical semantic indexing and question answering. In 11th International Conference of the CLEF Association , vol. 12260 of Lecture Notes in Computer Science , 194–214, https://doi.org/10.1007/978-3-030-58219-7_16 (2020).
Nentidis, A. et al . Overview of BioASQ 2021: The Ninth BioASQ Challenge on Large-Scale Biomedical Semantic Indexing and Question Answering. In 12th International Conference of the CLEF Association , vol. 12880 of Lecture Notes in Computer Science , 239–263, https://doi.org/10.1007/978-3-030-85251-1_18 (2021).
Nentidis, A. et al . Overview of BioASQ 2022: The Tenth BioASQ Challenge on Large-Scale Biomedical Semantic Indexing and Question Answering. In 13th International Conference of the CLEF Association , vol. 13390 of Lecture Notes in Computer Science , 337–361, https://doi.org/10.1007/978-3-031-13643-6_22 (2022).
Download references
Acknowledgements
Google was a proud sponsor of the BioASQ Challenge in 2020, 2021, and 2022. BioASQ was also sponsored by Atypon Systems inc. and VISEO. BioASQ is grateful to the biomedical experts, who have created and manually curated the dataset, as well as to the participants during all these years. Also, BioASQ is grateful to NIH/NLM, who has supported the project by two conference grants (grant n.5R13LM012214-02 and 5R13LM012214-03). For the first two years, BioASQ has received funding from the European Commission’s Seventh Framework Programme (FP7/2007-2013, ICT-2011.4.4(d), Intelligent Information Management, Targeted Competition Framework) under grant agreement n. 318652.Last but not least, BioAQ is grateful to all past collaborators, namely the University of Houston (US), Transinsight GmbH (DE), Universite Joseph Fourier (FR), University Leipzig (DE), Universite Pierre et Marie Curie Paris 6 (FR), Athens University of Economics and Business – Research Centre (GR).
Author information
Authors and affiliations.
Institute of Informatics and Telecommunications, National Center for Scientific Research “Demokritos”, Athens, Greece
Anastasia Krithara, Anastasios Nentidis, Konstantinos Bougiatiotis & Georgios Paliouras
School of Informatics, Aristotle University of Thessaloniki, Thessaloniki, Greece
Anastasios Nentidis
You can also search for this author in PubMed Google Scholar
Contributions
G.P. and A.K. originated the BioASQ challenge and dataset creation. All authors participated in the collection of the data, the development of the annotation and the evaluation tools, and validated the data. A.K. and A.N. drafted the manuscript. All authors reviewed the manuscript.
Corresponding author
Correspondence to Anastasia Krithara .
Ethics declarations
Competing interests.
The authors declare no competing interests.
Additional information
Publisher’s note Springer Nature remains neutral with regard to jurisdictional claims in published maps and institutional affiliations.
Rights and permissions
Open Access This article is licensed under a Creative Commons Attribution 4.0 International License, which permits use, sharing, adaptation, distribution and reproduction in any medium or format, as long as you give appropriate credit to the original author(s) and the source, provide a link to the Creative Commons license, and indicate if changes were made. The images or other third party material in this article are included in the article’s Creative Commons license, unless indicated otherwise in a credit line to the material. If material is not included in the article’s Creative Commons license and your intended use is not permitted by statutory regulation or exceeds the permitted use, you will need to obtain permission directly from the copyright holder. To view a copy of this license, visit http://creativecommons.org/licenses/by/4.0/ .
Reprints and permissions
About this article
Cite this article.
Krithara, A., Nentidis, A., Bougiatiotis, K. et al. BioASQ-QA: A manually curated corpus for Biomedical Question Answering. Sci Data 10 , 170 (2023). https://doi.org/10.1038/s41597-023-02068-4
Download citation
Received : 19 December 2022
Accepted : 13 March 2023
Published : 27 March 2023
DOI : https://doi.org/10.1038/s41597-023-02068-4
Share this article
Anyone you share the following link with will be able to read this content:
Sorry, a shareable link is not currently available for this article.
Provided by the Springer Nature SharedIt content-sharing initiative
Quick links
- Explore articles by subject
- Guide to authors
- Editorial policies
Sign up for the Nature Briefing newsletter — what matters in science, free to your inbox daily.

- Featured News
- Artificial Intelligence
- Bioprocessing
- Drug Discovery
- Genome Editing
- Infectious Diseases
- Translational Medicine
- Browse Issues
- Learning Labs
- eBooks/Perspectives
- GEN Biotechnology
- Re:Gen Open
- New Products
- Conference Calendar
- Get GEN Magazine
- Get GEN eNewsletters

Top 10 Biomedical Issues of the Next Decade
Some biomedical trends will defuse old arguments; others will spark new disputes
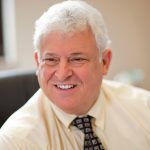
Forecasting the future is a dangerous activity. Still, even if forcasting is difficult, it is important to try to anticipate what is to come in the not-so-distant future, if only to trigger the requisite public debate about the ethics of what is perhaps likely to come. So, in the service of keeping the ethics ahead of the biomedical science, here are my top 10 picks for exciting, likely, troubling, and attention-grabbing issues for the next 10 years. I look forward to the addendums and emendations that are sure to come!
1. GMOs save crops struggling with climate change and beset by plant pests that find climate change advantageous
Anti-GMO sentiment will flip as the world utterly fails to achieve control over climate change. With temperatures rising, droughts increasing, and the climate becoming more turbulent, genetically engineered plants are increasingly the Band-Aid to maintain food supply, expand growing seasons, and battle pests. The “value” of genetic engineering becomes self-evident as farmers and fishermen struggle to maintain yields and famine threatens many parts of the world.
2. IVF clinics do vast bulk of their business making healthier babies for the fertile
A drop in the number of people seeking infertility treatment pushes clinics to offer more services to fertile persons that could help achieve “healthier” offspring. Investors own and control clinics, and they market “better” babies aggressively around the world. Richer families seeking an edge in a highly competitive world increasingly turn to these services, ignoring the protests of some who see the emphasis on “health” as code for eugenics.
3. Efforts at protecting patient privacy are abandoned as benefits from full big data meta-studies take off
More and more benefits in terms of earlier diagnoses, preventive measures, and lifestyle enhancements flow from the use of big data. Increasingly interlinked, big data takes in healthcare information around the world. Privacy is not a priority for a generation that has grown up expecting none. The older generation of civil libertarians who see privacy as vital begins to die off. Laws protecting privacy have proven utterly ineffective, especially as hacking remains widespread and bribing data managers to provide access becomes more common. Laws and legislation affirming privacy begin to be abandoned.
4. First transgender birth occurs thanks to transplanted reproductive organs
The entire female reproductive system—uterus, ovary, fallopian tubes—is now transplantable. Those involved in transitioning their gender will pay money to undergo what is essentially experimental surgery but is not in clinical trials given the commercialization of IVF working with transplant programs. Donor organs are hard to obtain, but the first baby has been born via reproductive system transplantation.
5. Gene editing begins to replace gene therapy as therapeutic modality of choice in embryos, fetuses, newborns, and young children
The safety of gene editing along with greater precision has fueled interest in eliminating—and not just treating—genetic diseases and risk factors. Embryo editing is becoming routine for many conditions, some of which are oriented toward enhancement and improvement. Gene therapies are still in use, but genetic medicine and counseling are shifting toward prevention. Some governments are taking an interest in encouraging gene editing to control the high cost of healthcare and disability services.
6. Synthetic biology revolutionalizes vaccine development
Needles and jabs are gone. Genetically edited viruses can be inhaled, opening a new route for vaccinations and helping us fend off most viral and bacterial infections. These can also be used in animals to reduce reliance on antibiotics. The same technology is beginning to be used to help clean out blood vessels clogged with fatty deposits.
7. Artificial blood is introduced, prompting blood banks to shut down and encouraging medics to stock up
The dream of artificial blood, permitting universal donation, is achieved using synthetic biology, cloning, and better bioincubation. This means safer but more expensive transfusions as viral and bacterial risks fall to zero. Blood matching is rapidly disappearing, as is the need for blood drives and blood collections. In the field, transfusion is becoming routine for a variety of needs including traumatic accidents, gunshot wounds, and injuries caused by combat and terrorism. Poorer nations are desperate to acquire the technology (as they have never been able to achieve safe, adequate blood supplies), but the price is prohibitive. The Gates, Zuckerberg, and Bezos Foundations are under enormous pressure to subsidize cost.
8. Life forms are created from scratch
The mystery of “life” has yielded to biological reductionism. Synthetic biologists are creating functioning microbes from DNA and artificial cell parts. The line between organic and inorganic is found to reside in particular genetic messages. Our understanding of how life might have evolved on earth or elsewhere is greatly enhanced.
9. Hospitals close some units as home-based care and residential care rapidly grow
Increasingly, elderly persons desire care for terminal and chronic conditions at home. Telemedicine, better implantables, and robot-assisted chronic care make this option more attractive and lower in cost than institutional care. The explosion in Alzheimer’s disease and other neurological impairments overwhelms the capacity of hospitals and nursing homes, leading to some closure of units in hospitals and nursing homes. Tax breaks and other incentives have led to the beginning of a shift to more residential/home care.
10. First artificial womb is used to deliver preemie infant at 18 weeks
Scientists and biomedical researchers, having studied artificial wombs in sheep, pigs, and primates, have begun to deploy them at a few academic medical centers for fetuses born before 23 weeks with underdeveloped lungs. The experiments require a reexamination of the concept of “viability,” stoking the ongoing battles over abortion in the United States and elsewhere. Some nations and states could pass laws mandating the use of this new, experimental technology.
Arthur L. Caplan, PhD ( [email protected] ), is the Drs. William F. and Virginia Connolly Mitty Professor of Bioethics and founding head of the Division of Medical Ethics at NYU Robert I. Grossman School of Medicine.
Single-Cell Cloning Remains a Challenge
New approach to nanopore sequencing that is sure to catch your....
- U.S. Department of Health & Human Services

- Virtual Tour
- Staff Directory
- En Español
You are here
Nih…turning discovery into health ®, the future of biomedicine.
To achieve its goal of turning discovery into health and to maintain its role as the world's premier biomedical research agency, NIH must support the best scientific ideas and brightest scientific minds. That means looking to the future and ensuring that we have a strong and diverse workforce to catalyze discoveries in all fields of biomedicine including emergent areas like data science.
tomorrow-science.jpg
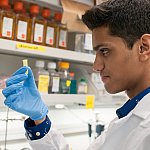
Tomorrow’s Scientists
Part of the NIH mission is supporting the next generation of scientists, funding thousands of graduate students and postdoctoral fellows across the United States.
diversity-nih.jpg
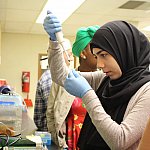
Innovation Through Diversity
Enhancing diversity in the NIH-funded workforce is urgent, given shifting U.S. demographics and the need to draw insights from all corners of America.
« Previous: A Healthy Mind Next: Transformative Technologies »
This page last reviewed on November 16, 2023
Connect with Us
- More Social Media from NIH
The Ohio State University
- BuckeyeLink
- Search Ohio State

Post Doctoral Scholar Spotlight: Mario Mendez, PhD
Get to know the people of Ohio State University's Department of Biomedical Engineering (BME) through our series of Spotlight Stories. Read what our BME folks are up to-- you might learn about our labs' latest research, our faculty and their classes, our alumni and their careers, our postdoc's research, our student's research and their plans for the future, and more.
Post Doctoral Scholar: Mario J Mendez (he/him)

A globally recognized physician-scientist who studies and treats blood cancer is joining Virginia Tech to lead cancer research in Washington, D.C., said Michael Friedlander, Virginia Tech’s vice president for health sciences and technology and executive director of the Fralin Biomedical Research Institute at VTC.
Christopher Hourigan, a senior investigator and chief of the Laboratory of Myeloid Malignancies at the National Institutes of Health, will join Virginia Tech as a professor with the institute and director of its Cancer Research Center in Washington, D.C.
“Dr. Hourigan exemplifies the prototype of a physician-scientist, integrating insights from his patient interactions directly into his fundamental and translational laboratory research,” Friedlander said. “We are extremely enthusiastic to have him join Virginia Tech and become a member of the Fralin Biomedical Research Institute team to take on this important new leadership role for our growing cancer research programs in Washington, D.C., and to further strengthen our collaborations with Carilion Clinic, Children’s National Hospital, and other health systems and universities.
The focus of the research effort, known as Fralin Biomedical Research Institute Cancer Research Center - D.C., is to bring basic, translational, clinical, and computational researchers together in the nation’s capital to focus on the shared aim of engineering cancer solutions.
“Convening top talent like Dr. Hourigan and developing deep and diverse partnerships are key to solving our most complex global challenges,” said Virginia Tech President Tim Sands. “It’s exciting to see our vision for the university advancing across the commonwealth and in the greater Washington, D.C., metro area.”
Your browser does not support iframes. Link to iframe content: https://www.youtube.com/embed/tS1TgAcvxhA?si=IuLYq7Em-QpT-w55
“I also anticipate the innovations and insights that will emerge from Dr. Hourigan and the other Virginia Tech scientists in D.C. collaborating with the institute's cancer research teams in Roanoke as well as with our computer science and engineering colleagues at Virginia Tech’s new Innovation Campus in Alexandria,” Friedlander said.
Research will involve diverse, expert teams and national and international collaborations.
“We have the opportunity to build a new cancer research center from the ground up, focusing on getting talented and highly motivated teams working in innovative new ways to reduce the burden of suffering from cancer in the United States,” Hourigan said. “It's clear we're not doing well enough for people who are dealing with cancer, and this is our chance to come up with new ways to do better.”
The addition of Hourigan will accelerate the university’s initiatives in Washington, D.C. Newly renovated research facilities on the site of the former Walter Reed Army Medical Center on the Children’s National Research & Innovation Campus already house Fralin Biomedical Research Institute cancer research scientists Jia-Ray Yu and Kathleen Mulvaney, along with teams of researchers from the Center for Genetic Medicine Research and Rare Disease Institute of Children’s National Hospital.
Precise answers to hard questions
Hourigan’s research focuses on a high-risk form of blood cancer called acute myeloid leukemia, which annually affects about 20,000 Americans. He looks at reasons for why some people survive cancer while others end up dying, even though initially they seem to have had the same response to treatment.
“The person who's newly diagnosed with cancer and has gone through treatment will ask a reasonable question, ‘Well, what about me? I want to know what my actual likelihood of surviving is going to be and are you sure I don’t need more or different treatment?’” Hourigan said. “As an oncologist, those are often hard questions to answer. You have a lot of empathy with the person in front of you and wish we could give better, more personalized, answers. We're strongly focused on the idea that if we had better diagnostic tools to allow a precision medicine approach, we could give doctors and patients a better understanding of exactly where they are now and what’s the best thing for them to do next.”
In addition to his primary appointment with the institute, Hourigan will be a professor in the Department of Internal Medicine at the Virginia Tech Carilion School of Medicine.
Hourigan received his medical degree and research doctorates from Oxford University and completed residency and oncology fellowship training at Johns Hopkins University School of Medicine, where he was subsequently a practicing physician on the acute leukemia service and faculty member. He is board certified in hematology and medical oncology.
Prior to joining Virginia Tech, Hourigan was a senior investigator, co-director of the Myeloid Malignancies Program, and chief of the Laboratory of Myeloid Malignancies at the National Institutes of Health.
He was awarded the Presidential Early Career Award for Scientists and Engineers, the National Institutes of Health Director’s Challenge Innovation Award, and was elected to Alpha Omega Alpha Medical Honor Society. He is a fellow of both the American College of Physicians and the Royal College of Physicians.
He looks forward to the task ahead.
“Virginia Tech has some key strategic advantages,” Hourigan said. “It is nationally known for engineering and computational science, which are going to be increasingly important components of cancer research. I think there's also this sense of energy, innovation, engineering, and teams working across disciplines on hard problems here. And cancer is a hard problem.”
Ultimately, he wants to put cancer researchers out of business.
“I don't want there to be a need for cancer research anymore, whether that happens in my lifetime or the lifetimes of those individuals I train,” Hourigan said. “The ultimate objective is not to have an industry of cancer research. Our focus has to be on the patient and on working hard to come up with real solutions to hard problems. It is only with research that we’ll be able to do better with cancer prevention, diagnosis, and treatment tomorrow than we currently can today. We need to have a sense of urgency and purpose because people are counting on us to come up with answers.”
(540) 526-2222
- Cancer Research Center (DC)
- Destination Areas
- Fralin Biomedical Research Institute at VTC
- Fralin Biomedical Research Institute at VTC - top news
- Fralin Biomedical--Cancer Research Group
- Friedlander News
- Hourigan Lab
Related Content
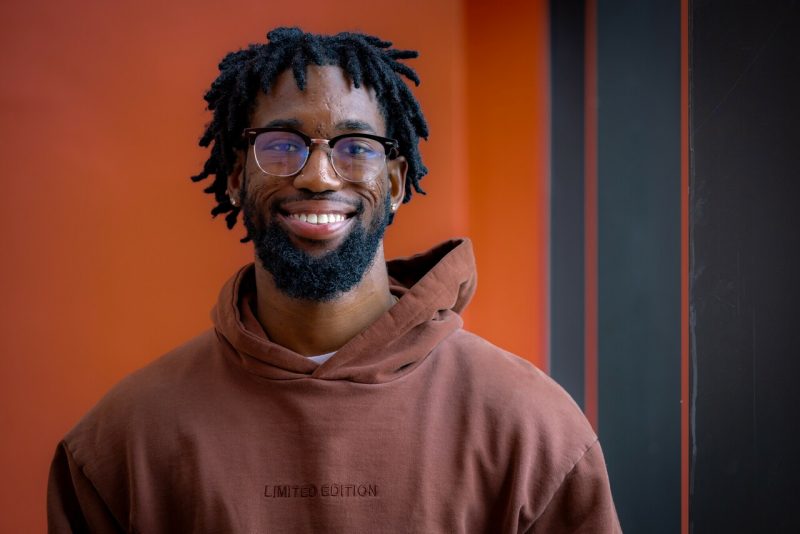
- Computer Vision
- Federated Learning
- Reinforcement Learning
- Natural Language Processing
- New Releases
- AI Dev Tools
- Advisory Board Members
- 🐝 Partnership and Promotion
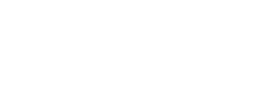
Tanya Malhotra
Tanya Malhotra is a final year undergrad from the University of Petroleum & Energy Studies, Dehradun, pursuing BTech in Computer Science Engineering with a specialization in Artificial Intelligence and Machine Learning. She is a Data Science enthusiast with good analytical and critical thinking, along with an ardent interest in acquiring new skills, leading groups, and managing work in an organized manner.
- Tanya Malhotra https://www.marktechpost.com/author/tanyamalhotra/ This AI Paper from Intel Presents a SYCL Implementation of Fully Fused Multi-Layer Perceptrons (MLPs) on Intel Data Center GPU Max
- Tanya Malhotra https://www.marktechpost.com/author/tanyamalhotra/ Alibaba Releases Qwen1.5-MoE-A2.7B: A Small MoE Model with only 2.7B Activated Parameters yet Matching the Performance of State-of-the-Art 7B models like Mistral 7B
- Tanya Malhotra https://www.marktechpost.com/author/tanyamalhotra/ Recall to Imagine (R2I): A New Machine Learning Approach that Enhances Long-Term Memory by Incorporating State Space Models into Model-based Reinforcement Learning (MBRL)
- Tanya Malhotra https://www.marktechpost.com/author/tanyamalhotra/ This AI Paper from China Proposes a Lightweight Machine Learning Method that Enhances Scalable Structural Inference and Dynamic Prediction Accuracy
RELATED ARTICLES MORE FROM AUTHOR
Naver ai lab introduces model stock: a groundbreaking fine-tuning method for machine learning model efficiency, meet opendevin: an open-source alternative to devin (an autonomous ai software engineer), tencent propose aniportrait: an audio-driven synthesis of photorealistic portrait animation, meet guide labs: an ai research startup building interpretable foundation models that can reliably explain their reasoning, alibaba researchers propose reward learning on policy (rlp): an unsupervised ai framework that refines a reward model using policy samples to keep it on-distribution, 10 artificial intelligence (ai) applications/platforms in healthcare, naver ai lab introduces model stock: a groundbreaking fine-tuning method for machine learning model..., meet guide labs: an ai research startup building interpretable foundation models that can reliably..., alibaba researchers propose reward learning on policy (rlp): an unsupervised ai framework that refines....
- AI Magazine
- Privacy & TC
- Cookie Policy
🐝 FREE AI Courses on RAG + Deployment of an Healthcare AI App + LangChain Colab Notebook all included
Thank You 🙌
Privacy Overview
H.I. Ep. 92 - Kenneth T. Kishida - Human consciousness and decision making Healthcare Insights
Kenneth T. Kishida is an Associate Professor at Wake Forest School of Medicine with appointments in the Department of Physiology and Pharmacology, the Department of Neurosurgery, and the Department of Biomedical Engineering. His research grapples with the questions of how the human brain gives rise to conscious subjective experiences and how those processes guide human behavior and decision-making. Leanr more at https://www.kishidalab.com/
- More Episodes
- All rights reserved
Top Podcasts In Education
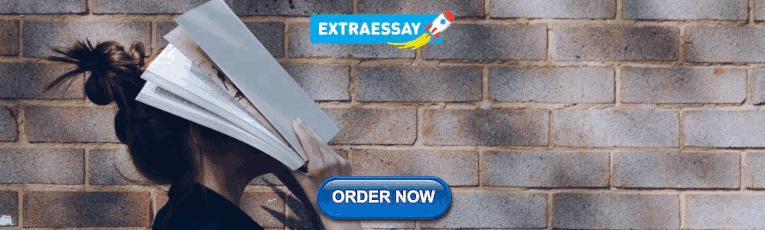
IMAGES
VIDEO
COMMENTS
Biomedical Research Topics. Biomedical research is an extensive process. It requires a lot of time, dedication, and resources. Getting a topic shouldn't be added to that list. There are biomedical thesis topics and research topics in biomedical science for you here: Air pollution- sources, impact, and prevention
Biomedical research is a vibrant field, with an extensive range of topics drawn from various sub-disciplines. It encompasses the study of biological processes, clinical medicine, and even technology and engineering applied to the domain of healthcare. Given the sheer breadth of this field, choosing a specific topic can sometimes be overwhelming.
Explore the latest questions and answers in Biomedical Research, and find Biomedical Research experts. Questions (51) Publications (34,126) Questions related to Biomedical Research.
Basic biomedical research, which addresses mechanisms that underlie the formation and function of living organisms, ranging from the study of single molecules to complex integrated functions of humans, contributes profoundly to our knowledge of how disease, trauma, or genetic defects alter normal physiological and behavioral processes. Recent advances in molecular biology techniques and ...
Biomedical researchers are at the forefront of scientific innovation, seeking answers to the world's most pressing questions in human health. For 37 years, The Pew Charitable Trusts has encouraged these pursuits by supporting promising, early-career biomedical scientists tackling these challenges and driving medical breakthroughs.
About Biomedical Research Topics. This series provides an essential introduction to the theory and practice of undertaking research in some of the most exciting and rapidly advancing areas of biomedical studies. Each volume provides a framework for understanding the scientific principles before going on to outline experimental approaches and to ...
Chimeric antigen receptor (CAR)-T cell therapies have been approved by FDA to treat relapsed or refractory hematological malignancies. However, the adverse effects of CAR-T cell therapies are complex and can b... Chieh Yang, John Nguyen and Yun Yen. Journal of Biomedical Science 2023 30 :89.
Medical research involves research in a wide range of fields, such as biology, chemistry, pharmacology and toxicology with the goal of developing new medicines or medical procedures or improving ...
Scholars and fellows in Pew's biomedical research programs were at the forefront of research in topics ranging from how vaccines work, to immunology and cell biology. As 2021 comes to a close, we look back at some of their notable endeavors. Researchers explain the science behind vaccines
4 answers. Dec 16, 2015. The current progress in biomedical science works on the basis of the identification of a gene, a protein, a biochemical function and a biological activity followed by a ...
PubMedQA is the first QA dataset where reasoning over biomedical research texts, especially their quantitative contents, is required to answer the questions. Our best performing model, multi-phase fine-tuning of BioBERT with long answer bag-of-word statistics as additional supervision, achieves 68.1% accuracy, compared to single human ...
Applying cellular imaging and structural biology expertise across a wide range of biomedical research programs. Platforms include fluorescence, live imaging, 2-photon imaging, transmission and scanning electron microscopy as well as whole body imaging and imaging mass spectroscopy.
Citation. If you use PubMedQA in your research, please cite our paper by: @inproceedings{jin2019pubmedqa, title={PubMedQA: A Dataset for Biomedical Research Question Answering}, author={Jin, Qiao and Dhingra, Bhuwan and Liu, Zhengping and Cohen, William and Lu, Xinghua}, booktitle={Proceedings of the 2019 Conference on Empirical Methods in Natural Language Processing and the 9th International ...
The findings suggest that people can learn to reduce the brain activity causing some types of chronic pain that occur in the absence of injury or persist after healing. 2021 Research Highlights — Basic Research Insights >>. NIH findings with potential for enhancing human health include new drugs and vaccines in development for COVID-19 ...
What is Biomedical Research? Biomedical research is the pursuit of answers to medical questions. These investigations lead to discoveries, which in turn lead to the development of new preventions, therapies and cures for human and veterinary health. Biomedical research generally takes two forms: basic science and applied research.
BioASQ 3 (Biomedical Semantic Indexing and Question Answering) pushes research towards highly precise biomedical information access systems through a series of evaluation campaigns, in which ...
Gene therapies are still in use, but genetic medicine and counseling are shifting toward prevention. Some governments are taking an interest in encouraging gene editing to control the high cost of ...
This page last reviewed on November 16, 2023. To achieve its goal of turning discovery into health and to maintain its role as the world's premier biomedical research agency, NIH must support the best scientific ideas and brightest scientific minds. That means looking to the future and ensuring that we have a strong and diverse workforce to ...
Post Doctoral Scholar Spotlight: Mario Mendez, PhD. Posted: March 25, 2024. Get to know the people of Ohio State University's Department of Biomedical Engineering (BME) through our series of Spotlight Stories. Read what our BME folks are up to-- you might learn about our labs' latest research, our faculty and their classes, our alumni and their ...
PubMedQA is the first QA dataset where reasoning over biomedical research texts, especially their quantitative contents, is required to answer the questions. Our best performing model, multi-phase fine-tuning of BioBERT with long answer bag-of-word statistics as additional supervision, achieves 68.1% accuracy, compared to single human ...
Learn more about our seven focus areas: Biomedical Data Science. Extract knowledge from biomedical datasets of all sizes to understand and solve health-related problems. Learn More. Computational Medicine. Generate solutions in personalized medicine by building and utilizing computational models of health and disease.
Biomedical researchers are at the heart of nearly every medical advancement. From cutting-edge cancer treatments to breakthrough discoveries about emerging viruses, these scientists use creativity and ingenuity to explore new horizons in human health and medicine. And for nearly 40 years, The Pew Charitable Trusts has supported more than 1,000 early-career researchers committed to this work.
lenging benchmark Natural Language Processing. (NLP) task for evaluating the abilities of intelli-. gent systems to understand a question, retrieve and. utilize relevant materials and finally ...
A globally recognized physician-scientist who studies and treats blood cancer is joining Virginia Tech to lead cancer research in Washington, D.C., said Michael Friedlander, Virginia Tech's vice president for health sciences and technology and executive director of the Fralin Biomedical Research Institute at VTC.
19. Tell me about a time when you had to make a difficult decision related to your research. As a biomedical scientist, you need to be able to make decisions quickly and accurately. This question is designed to assess your ability to make decisions quickly, while also considering the implications of those decisions.
These models have demonstrated potential for use in biomedical research and healthcare applications by performing well on a variety of tasks, including summarization and question-answering. Specialized models, such as Med-PaLM 2, have greatly influenced fields such as healthcare and biomedical research by enabling activities like radiological ...
Kenneth T. Kishida is an Associate Professor at Wake Forest School of Medicine with appointments in the Department of Physiology and Pharmacology, the Department of Neurosurgery, and the Department of Biomedical Engineering. His research grapples with the questions of how the human brain gives rise to conscious subjective experiences and how ...
Biomedical Engineer Interview Questions: Soft Skills. Even though much of a biomedical engineer's job is focused on the technical side of things, they must also have great communication, time management, and people skills. These questions may help assess a candidate's soft skills in a biomedical engineer interview: Describe a time when you ...