
An official website of the United States government
The .gov means it’s official. Federal government websites often end in .gov or .mil. Before sharing sensitive information, make sure you’re on a federal government site.
The site is secure. The https:// ensures that you are connecting to the official website and that any information you provide is encrypted and transmitted securely.
- Publications
- Account settings
Preview improvements coming to the PMC website in October 2024. Learn More or Try it out now .
- Advanced Search
- Journal List

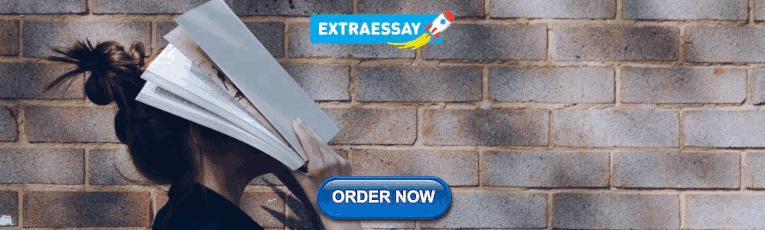
Antioxidant Activity of Deferasirox and Its Metal Complexes in Model Systems of Oxidative Damage: Comparison with Deferiprone
Viktor a. timoshnikov.
1 Institute of Chemical Kinetics & Combustion, 630090 Novosibirsk, Russia; ur.csn.scitenik@vokinhsomit (V.A.T.); moc.liamg@ahciklil (L.A.K.); [email protected] (O.Y.S.); ur.csn.scitenik@vokaylop (N.E.P.)
Lilia A. Kichigina
Olga yu. selyutina, nikolay e. polyakov, george j. kontoghiorghes.
2 Postgraduate Research Institute of Science, Technology, Environment and Medicine, Limassol CY-3021, Cyprus
Associated Data
Data is contained within the article.
Deferasirox is an orally active, lipophilic iron chelating drug used on thousands of patients worldwide for the treatment of transfusional iron overload. The essential transition metals iron and copper are the primary catalysts of reactive oxygen species and oxidative damage in biological systems. The redox effects of deferasirox and its metal complexes with iron, copper and other metals are of pharmacological, toxicological, biological and physiological importance. Several molecular model systems of oxidative damage caused by iron and copper catalysis including the oxidation of ascorbic acid, the peroxidation of linoleic acid micelles and the oxidation of dihydropyridine have been investigated in the presence of deferasirox using UV-visible and NMR spectroscopy. Deferasirox has shown antioxidant activity in all three model systems, causing substantial reduction in the rate of oxidation and oxidative damage. Deferasirox showed the greatest antioxidant activity in the oxidation of ascorbic acid with the participation of iron ions and reduced the reaction rate by about a 100 times. Overall, deferasirox appears to have lower affinity for copper in comparison to iron. Comparative studies of the antioxidant activity of deferasirox and the hydrophilic oral iron chelating drug deferiprone in the peroxidation of linoleic acid micelles showed lower efficiency of deferasirox in comparison to deferiprone.
1. Introduction
There are hundreds of thousands of iron overloaded patients belonging to different categories of inherited and other diseases, who receive regular red blood cell transfusions for the treatment of their refractory anemia [ 1 , 2 , 3 ]. Iron overload is considered as an independent adverse prognostic factor in all diseases. Excess iron from chronic transfusions is deposited in many organs causing serious damage and eventually death, unless effective chelation therapy is used for iron removal [ 4 , 5 ]. For example, most regularly transfused iron loaded thalassemia patients, not receiving chelation therapy, die before the age of 20 years because of congestive cardiac failure caused from excess, toxic iron deposition in the heart [ 1 , 4 , 5 , 6 , 7 ]. In addition, there are many other patients with normal iron stores such as Friedreich ataxia, Alzheimer’s and Parkinson’s disease patients, where localized “focal” iron deposits have been identified, by magnetic resonance imaging (MRI) techniques, as present in the brain, causing neurodegenerative damage [ 8 , 9 , 10 , 11 ]. The treatment of iron overload in regularly red-blood-cell-transfused patients and also patients with localized tissue “focal” iron deposits can only be achieved by the removal of toxic iron using chelation therapy [ 6 , 7 , 8 , 9 , 10 , 11 ].
There are currently three regulatory approved iron chelating drugs which are used daily for the treatment of transfusional iron overload, namely deferoxamine, deferiprone (L1) and deferasirox (DFRA) [ 4 , 6 , 7 , 8 , 12 ]. All three chelating drugs have different structures, physicochemical, pharmacological, toxicological and other properties [ 13 , 14 ]. Both L1 and DFRA are orally active, whereas deferoxamine is administered subcutaneously [ 4 , 6 , 7 , 8 , 12 , 13 , 14 ].
Investigations on the affinity and other interactions of the chelating drugs with essential and redox active metal ions are crucial for determining their therapeutic activity and toxicity potential [ 13 , 14 , 15 ]. Of particular interest are the interactions of chelating drugs with the essential transition and redox active metal ions iron and copper, which are present in all the cells and play vital role in physiological and biochemical pathways. Molecular studies using chelating drugs are very important because they can amongst other identify serious toxic effects including pro-oxidant effects and biomolecular damage, which under certain conditions can progress to subcellular, cellular and organ damage [ 16 , 17 ].
The molecular interactions with other metal ions in addition to iron and copper, such as zinc, and with nutrients such as ascorbic acid (Asc) and also many other natural or xenobiotic molecules, are also expected to interfere with the therapeutic and toxicity potential of iron chelating drugs as well as other drugs [ 16 , 17 , 18 , 19 , 20 , 21 , 22 ]. Furthermore, the treatment of many associated iron and copper metabolic imbalance conditions, as well as other diseases related to free radical pathology are also expected to be affected [ 23 ].
The study of the pro-oxidant/antioxidant effects of the iron chelating drugs and their iron and copper complexes is important for pharmacological/toxicological parameters affecting their mode of action and possibly their efficacy in vivo. However, the determination of the antioxidant potential of chelating drugs is also of major pharmacological interest because of possible therapeutic applications in many diseases associated with oxidative stress toxicity such as cancer, neurodegenerative, cardiac, liver, renal and other diseases [ 22 , 23 , 24 , 25 , 26 , 27 , 28 ]. Many studies have already been carried out investigating the antioxidant and other effects of L1 and deferoxamine in vitro, in vivo and in clinical models [ 29 , 30 ]. In contrast, the pro-oxidant/antioxidant effects of DFRA have not been yet been fully studied and characterized.
In this work the pro-oxidant/antioxidant effects of DFRA and its iron and copper complexes have been investigated using several molecular models of oxidative damage of linoleic acid (LA), Asc and dihydropyridine (DHP). In some of the studies L1 and Asc were used for comparison.
Linoleic acid peroxidation is widely studied in different model systems, including liposomes [ 31 ], but in most cases the rate of lipid peroxidation is estimated from the kinetics of formation of lipid peroxidation products [ 32 , 33 , 34 , 35 ]. Several other techniques have been previously used to estimate the initiation rate of lipid peroxidation, but in most cases these also relied on the detection of the products, for example, conjugated lipid hydroperoxides [ 36 ]. In the current investigation, we examined the influence of different ligands on the initial stage of lipid peroxidation, the abstraction of hydrogen atoms from bis-allylic position with formation of conjugated dienes and lipid radicals using 1 H-NMR spectroscopy.
Deferasirox is known to have high affinity for iron and copper binding. The stoichiometries of these complexes and stability constants have been measured in previous studies [ 37 , 38 , 39 , 40 ]. It was found that the complex of DFRA with Cu 2+ ions has stoichiometry 1:1, and the stoichiometry of DFRA complex with Fe 3+ ions 2:1. In the present studies we have tried to answer the question whether these complexes are redox active or not. For this purpose, the transition metal-induced oxidation of molecular model systems, namely LA, an unsaturated lipid, Asc and DHP as analogs of the nicotinamide adenine dinucleotide (NADH) molecule ( Figure 1 ), have been studied by nuclear magnetic resonance (NMR) and ultraviolet-visible (UV-Vis) spectroscopy techniques.

Chemical structures of 3-hydroxy-1,2-dimethylpyridin-4(1 H )-one (deferiprone or L1), 4-[(3 Z ,5 E )-3,5-bis(6-oxo-1-cyclohexa-2,-dienylidene)-1,2,4-triazolidin-1-yl]-benzoic acid (deferasirox or DFRA) (2R)-2-[(1S)-1,2-dihydroxyethyl]-3,4-dihydroxy-2H-furan5-one (ascorbic acid or Asc), (9 Z ,12 Z )-octadeca-9,12-dienoic acid (linoleic acid or LA) and 1,1′-(2,6-dimethyl-1,4-dihydropyridine-3,5-diyl)-di(propan-1-one) (dihydropyridine or DHP).
2.1. UV-Vis Spectroscopic Studies of the Influence of Deferasirox on the Oxidation of Ascorbic Acid by Iron and Copper Ions
The first model for studying the antioxidant activity of DFRA and its chelate complexes with iron and copper ions was the oxidation of Asc. The biological functions of Asc include antioxidant, but also chelating activities [ 41 , 42 , 43 ]. In contrast to its antioxidant effects, Asc under certain conditions can also act as a pro-oxidant and a source of free radicals [ 41 , 42 , 44 , 45 ]. The mechanism of the pro-oxidant activity of Asc is related to its ability to reduce ferric iron via chelate complex formation, which results in the formation of ferrous iron and ascorbate radical. Taking into account that ferrous iron is involved in the process of reactive oxygen species (ROS) generation via the Fenton reaction, the reduction of ferric to ferrous iron will turn on the cyclic oxidation process. This is why the interaction of Asc with other chelators and their chelate complexes with Fe 3+ and Cu 2+ ions attracts great attention [ 46 , 47 , 48 ].
A series of UV-Vis spectroscopic experiments were carried out to investigate the effect of DFRA on Asc oxidation in the presence of iron and copper ions at various ratios of the concentrations of chelator and metal ions in solution. The kinetics of changes in the optical density of the solutions were measured at 262 nm—a wavelength near the absorbance maximum in the absorption band of Asc (see Figure 2 ).

UV-Vis absorption spectra of deferasirox (DFRA, 0.1 mM), its chelate complexes with Fe 3+ (0.1 mM) and Cu 2+ (0.1 mM), FeCl 3 (0.01 mM), CuCl 2 (0.02 mM) and ascorbic acid (Asc, 0.1 mM) in ethanol solution.
The kinetics of oxidation of Asc in the presence and absence of DFRA iron and copper complexes were monitored in further UV-Vis spectroscopic studies. As it can be seen from Figure 3 , the kinetics of the oxidation of Asc which correspond to the decrease in the optical density of the solutions are different in each case. In the case of mixtures containing iron ions, iron DFRA complexes and copper DFRA complexes, the kinetics follow a mono-exponential progression, reaching a plateau, while in the mixture containing copper ions, a rapid decrease first occurs, followed by a slow decay. It can be assumed that the primary process is due to Asc oxidation, while the secondary process is due to the decomposition of Asc oxidation products. Overall, it appears that the rates of these reactions are different and in each case depend on the type of transition metal involved.

Changes in the rate of oxidation of ascorbic acid (Asc) in the presence of deferasirox (DFRA) Fe and Cu complexes. Time profile changes of the optical density of Asc at 262 nm (0.1 mM in EtOH) in the presence of FeCl 3 (0.05 mM), and CuCl 2 (0.02 mM) and various concentrations of DFRA (0, 0.05 and 0.1 mM for experiments with FeCl 3 , left ), and DFRA (0, 0.02 and 0.04 mM for experiments with CuCl 2 , right ).
When DFRA was added to the solution, the rate of Asc oxidation decreased significantly. The maximum effect of DFRA for Fe 3+ and Cu 2+ ions was achieved at a concentration ratio of DFRA:metal ion = 2:1. A further increase in the concentration of the chelator in the solution has practically no effect on the reaction rate. Based on these data, the rate constants of the reactions were calculated ( Table 1 ). The rate constants of Asc oxidation have been determined using a mono-exponential rate model in the case of the iron ions, iron DFRA complexes and DFRA copper complexes. In the case of copper ions, the initial range of kinetics was selected, corresponding to the “fast” part, which is best described by the mono-exponential decay (about 75 s).
The rate constants of the reactions of ascorbic acid (Asc) with Fe 3 + and Cu 2+ ions and their deferasirox (DFRA) complexes calculated from data from Figure 3 . The concentration of Asc in both cases was 0.1 mM.
As it can be shown from the data described in Table 1 , DFRA slows down the rate of Asc oxidation by more than 100 times in the case of Fe 3+ ions and by about 30 times in the case of Cu 2+ .
2.2. NMR Studies of the Influence of Deferasirox and Deferiprone on Lipid Peroxidation of Linoleic Acid (LA) in the Presence of Iron and Copper Ions
The antioxidant effects of DFRA have been investigated in another molecular model of oxidative damage involving LA peroxidation in the presence of iron and copper ions. In these studies peroxidative changes in LA were monitored using an NMR method [ 49 , 50 ]. The reaction leading to LA peroxidation is widely used as a model of lipid oxidation [ 31 , 33 , 34 , 35 ]. This reaction consists of several stages. The “zero stage” is the generation of ROS via the Fenton reaction with the participation of iron and copper ions (1) and (2) as follows:
In these reaction processes, OH radicals (OH · ) are formed, which, in turn, are capable of conversion to other types of ROS, including the peroxyl radical and superoxide anion. The next stage of these reaction processes is “initiation”, in which the LA radical and the LA peroxyl radical are formed (3) and (4) as follows:
Later, at the stage of “prolongation”, LA radicals are also formed, as well as other reaction products, including aldehydes, hydroperoxides, and epoxides (5) and (6) as follows:
At the “termination stage”, various LA dimers and polymers are formed (7)–(9) as follows:
It is also widely known that LA forms micellar solutions in an aqueous environment [ 51 ]. In this context and in order to study the kinetics of LA oxidation, a series of NMR experiments were carried out with the participation of LA micelles and iron and copper salts in the presence and absence of DFRA. Figure 4 for example, shows fragments of 1 H-NMR spectra of the initial LA in the presence of ferrous sulfate (FeSO 4 ) and after 24 h in the absence and in the presence of DFRA. It can be seen that after 24 h, the total signal intensity of LA protons was reduced significantly. In addition, the lines at 2.7 and 2.09 ppm, corresponding to protons near the double bonds (1 and 2), disappeared. All of the above, together with the fact that an insoluble precipitate was formed during the reaction, indicates the complete consumption of LA in the solution and the formation of polymers. In the presence of DFRA, 24 h after mixing, the overall signal level was also reduced, indicating the formation of polymers, but the signals corresponding to protons near double bonds did not completely disappear. This observation suggests that there is a decrease of the initiation rate of LA oxidation in the presence of DFRA.

Chemical structure of linoleic acid (LA) and fragments of 1 H-NMR spectra of the initial LA (3.5 mM) and the reaction mixture with H 2 O 2 (0.5 M) and FeSO 4 (0.1 mM) after 24 h in the absence and in the presence of deferasirox (DFRA, 2 mM). The studies were carried out at room temperature, in buffer solution (0.1 M) of pH 7.4. LA protons and their corresponding signals in the fragments of 1 H-NMR spectra are designated by numbers 1–4.
It is known that the reaction of LA with OH radical starts with hydrogen atom abstraction from position 1 (see Figure 4 ) [ 31 ], and this observation was used in the present study for direct measurements of the rate constant of the “initiation” stage of the reaction. On the other hand, the decrease of total integral intensity on NMR signal due to polymers formation can be used to calculate the rate constants of the “termination” stage.
This molecular model of oxidative damage monitoring using LA peroxidation has been previously used for studying the antioxidant activity of the iron chelating drug L1 in the presence of copper ions [ 50 ]. Similar conditions have been used in a number of experiments for studying the time dependences of the integral intensity of the LA protons 1 and 4 ( Figure 4 ), which were measured in the absence and presence of chelators. A typical example of a signal intensity change at 2.7 ppm is shown in Figure 5 . The integral intensity of the initial signal at 2.7 ppm was normalized to 100. Intensity is given in arbitrary units (a.u.).

Kinetics of the initiation reaction phase of the linoleic acid (LA, 3.5 mM) peroxidation induced by FeSO 4 (0.1 mM) and H 2 O 2 (0.5 M) in the presence and absence of deferasirox (DFRA, 2 mM) and deferiprone (L1, 2 mM). The studies were carried out at room temperature, in buffer solution (0.1 M) of pH 7.4. The graphs were plotted using the decay of the integral intensity signal of the LA protons at 2.7 ppm (a.u., arbitrary units).
As can be shown in Figure 5 , the rate of LA peroxidation caused by ferrous ions is significantly reduced in the presence of the chelating drugs L1 and DFRA. Furthermore, it can also be shown in this molecular model that the antioxidant activity of L1 in this LA peroxidation reaction is higher than that of DFRA.
The kinetics of the formation of polymeric products of LA during the oxidation reaction with ferrous ions, which are calculated from the changes in the signal intensity of CH 3 protons in LA, are shown in Figure 6 . The procedure for data gathering and evaluation were based on previous observations, which suggested that a decrease in the integral intensity of the signal from the methyl group corresponds to an increase in the amount of various polymers formed in the oxidation reactions (7)–(9). The intensity of polymer products was calculated as follows (10):
where I(t) is the intensity of polymer products, I CH3 (t) is the integral intensity of the signal from CH 3 -groups of LA, which corresponds to the concentration of LA. The integral intensity of the initial signal of the signal from CH 3 -groups at 0.9 ppm (I CH3 (0)) was normalized to 100. Intensity is given in arbitrary units (a.u.).

Kinetics of the termination reaction step of the linoleic acid (LA, 3.5 mM) peroxidation leading to polymeric products formation, which are induced by FeSO 4 (0.1 mM) and H 2 O 2 (0.5 M) in the absence and in the presence of deferasirox (DFRA, 2 mM) and deferiprone (L1, 2 mM). The studies were carried out at room temperature, in buffer solution (0.1 M) of pH 7.4. The graphs have been plotted using the decay of the integral intensity signal of the LA protons at 0.9 ppm (a.u., arbitrary units).
As can be shown in Figure 6 , the rate of formation of polymer products decreases significantly in the presence of L1 and DFRA chelators. In particular, in the presence of DFRA, the chain termination polymerization step is limited by the initiation step. In contrast, in the absence of a chelator, a faster reduction in the number of double bonds occurs with the formation of oxidized products that are already involved in the formation of polymers.
The experimental points in Figure 6 were approximated to fit an exponential decay, and subsequently the observed reaction rate constants were calculated from the fitting parameters. The calculated reaction rate constants are shown in Table 2 .
Initiation and termination rate constants of the reactions of linoleic acid (LA) peroxidation induced by iron and copper ions in the absence and in the presence of deferasirox (DFRA) and deferiprone (L1).
* Data obtained from [ 50 ].
The results presented in Table 2 suggest that DFRA inhibits LA peroxidation in the presence of iron and copper ions by 6 and 3 times, respectively, while the inhibitory effect of LA peroxidation by L1 under similar conditions is about 500 times. It is known that the redox potentials of DFRA and L1 chelate complexes with iron are very close [ 52 ], this is why it can be assumed that the reason of the observed antioxidant activity differences in this reaction might be due to differences in the lipophilicity of these two chelators. It is also known that L1 and its iron chelate complexes are hydrophilic and can be located outside the LA micelles. On the other hand, DFRA and its iron chelate complexes are lipophilic and can penetrate into lipid particles directly to the main reaction center (hydrophobic tail) [ 53 ].
2.3. NMR Studies of the Interaction of Deferasirox with Dihydropyridine
An additional molecular model system for studying the antioxidant activity of DFRA was the oxidation of DHP. The reactions involving DHP are often used as models of radical processes of electron and proton transfer in living systems with the participation of NADH [ 54 , 55 ]. Dihydropyridine was chosen for the studies because is capable of reacting with various radicals, including the OH radical.
The formation of radicals for the NMR studies was initiated by the Fenton reaction using both iron and copper as catalysts and under similar conditions reported in previous studies [ 50 , 56 ]. Since the DHP is not soluble in water, the reaction was carried out in methanolic solution. In this case, the OH radical first reacts with the solvent CD 3 OD producing ● CD 2 OD C-centered radical [ 57 ]. As a result of hydrogen abstraction reaction by this radical, a neutral C-centered DHP radical is formed [ 54 ]. The main reaction product 1,1′-(2,6-dimethylpyridine-3,5-diyl)-di(propan-1-one) is formed via disproportionation reaction of two DHP radicals ( Figure 7 ).

Scheme of the oxidation of 1,1′-(2,6-dimethyl-1,4-dihydropyridine-3,5-diyl)di(propan-1-one) (DHP). DHP reacts with a hydroxyl radical or ● CD 2 OD C-centered radical to form a DHP neutral radical. Subsequently two DHP radicals may be involved in the disproportionation reaction to form the initial DHP and 1,1′-(2,6-dimethylpyridine-3,5-diyl)-di(propan-1-one).
The antioxidant activity of DFRA in the molecular model of the oxidation reaction of DHP was studied in a series of 1 H-NMR experiments, which were performed in the presence of iron and copper ions. Differences were observed in the 1 H-NMR spectra of different reaction mixtures. Figure 8 for example shows fragments of the 1 H-NMR spectra of pure DHP, as well as reaction mixture of DHP with Fe 3+ ions in the presence and absence of DFRA, 15 h after the initiation of the reaction.

Fragments of 1 H-NMR spectra of the initial DHP (5 mM) and of the reaction mixture with FeCl 3 (0.2 mM) and H 2 O 2 (0.2 mM) after 15 h in the absence and in the presence of DFRA (2 mM) in CD 3 OD solution at 278 K.
It appears from Figure 8 that DFRA almost completely inhibits the oxidation reaction of DHP with free radicals, which are caused by iron ions and hydrogen peroxide. Similar experiments were carried out with the participation of copper ions and hydrogen peroxide. The samples were kept at a temperature of 278 K in the absence of light. The fractions of degraded DHP were measured 15 h and also 2.5 days following mixing and the estimated amount of oxidation was based on the changes in the integral signal intensity of DHP protons. The fraction of degraded DHP in the absence of ferric ions was also calculated for comparison. The results are shown in Table 3 .
The amount of oxidation products of DHP after the reaction with metal ions in the presence of hydrogen peroxide.
The results in Table 3 show that in the presence of DFRA, the rate of DHP decomposition induced by iron and copper ions significantly decreases. These findings suggest that in non-aqueous media there is redox inactivity of chelate complexes of DFRA with iron and copper ions in the Fenton reaction. It is also worth noting that the yield of the reaction products in the case of the DFRA-Fe complex is lower than that of DFRA-Cu complex, which may suggest that there is a higher affinity of the chelator for iron under these experimental conditions.
3. Discussion
The experimental findings of this study have several important pharmacological, biological and physiological implications, especially for understanding the influence of DFRA on various redox systems. Deferasirox manifests its antioxidant activity in all studied model systems, including oxidation of Asc, peroxidation of LA micelles, and oxidation of DHP, with reduction in the rate of oxidation by up to several tens of times. Comparative analysis of the antioxidant activity of DFRA and L1 in lipid peroxidation showed a lower efficiency of DFRA in comparison to L1. The significance of these processes is underlined below from the different experiments carried out within this study.
Taking into account that in living systems copper and iron ions are mainly in bound forms, the study of the interaction of chelating drugs with natural complexants is an important task for elucidating the mechanism of drug action. One of the main natural antioxidant with chelating properties is Asc [ 42 , 43 , 45 , 58 ]. At the moment, there are many studies, both in vivo and in vitro, devoted to the interaction of chelating drugs with Asc [ 48 , 56 ]. However, this issue has not been studied for DFRA. It is known that Asc is capable of forming short-lived chelate complexes with transition metals, such as Fe 3+ and Cu 2+ , which decompose to form Fe 2+ or Cu + , and the ascorbate radical (11) and (12) [ 58 , 59 , 60 ]:
Subsequently, ascorbate radicals are also capable of oxidizing, forming dehydroascorbate, which, in turn, undergoes the stages of hydration and decarboxylation, forming secondary reaction products [ 61 , 62 ]. In addition, the reduced forms of iron and copper ions can react with dissolved oxygen or another oxidizing agent, thereby forming additional channels for the oxidation of ascorbic acid (13) and (14) [ 52 , 63 ]:
The study of Asc oxidation was carried out in ethanol since DFRA and its complexes with iron and copper ions have much lower solubility in water. Based on Figure 3 and Table 1 , we can conclude that DFRA exhibits antioxidant activity in the reaction of Asc oxidation, with the rate constants of oxidation decreasing tens of times. In addition, it should be noted that in the case of iron ions, iron complexes, and complexes with copper, the limiting stage of the reaction is the stage of oxidation of Asc to the ascorbate radical.
Another, but no less important, effect of the antioxidant activity of chelators is the ability to inhibit lipid peroxidation. The study of this reaction is part of the solution to a wider problem, namely, the study of ferroptosis-programmed cell death. This mechanism is based on the oxidation of the cell membrane by ROS, formed in redox reactions with the participation of iron ions [ 64 , 65 ]. At this moment, the process of ferroptosis is quite well studied; however, the problems associated with radical reactions occurring in the lipid bilayer and the influence of various antioxidants, including chelators, on the reaction mechanism remain unsolved [ 66 ]. The effect of the chelator L1 on the ferroptosis process is best studied. Deferasirox and L1 are similar chelators. At the moment, these drugs are among the most widely used in clinical practice for the treatment of iron overload diseases [ 53 ]. Within this context, it was interesting to compare their effect on the system of lipid peroxidation with the participation of redox active transition metal ions.
At the moment, there are many different methods for studying lipid peroxidation, the vast majority of which are based on the detection of oxidation by-products of trichloroacetic and 2-thiobarbituric acids [ 33 , 34 , 67 , 68 ]. The NMR stands out positively in these methods, which makes it possible to follow the functional groups and determine the change in the concentrations of both the initial substances and the reaction products. It has been shown that DFRA inhibits the process of ferroptosis, as well as the reaction of lipid peroxidation in cell cultures, however, the antioxidant mechanism of action at the molecular level has not yet been described [ 64 , 69 , 70 ].
In the present study it was shown that DFRA inhibits LA peroxidation in micelles; however, the antioxidant effect of this chelator is significantly lower than that of L1. This finding can be explained by two possible mechanisms. One mechanism is that L1 oxidizes faster than DFRA, ferrous to ferric iron and forms ferric iron complex, which is not facilitating LA peroxidation. The other mechanism is related to the much higher lipophilicity of DFRA [ 53 ], which allows DFRA, as well as its chelate complexes to penetrate into micelles directly to the reaction center. Due to the reversibility of the complexation reaction, chelate complexes can decompose with the release of a free metal ion. As a result, a free metal ion is able to react with hydrogen peroxide to form a short-lived hydroxyl radical (1) and (2). In addition, it can be noted that in the case of DFRA, the rate of peroxidation is limited by the initiation stage ( Figure 6 ).
The last part of our study was to investigate the effect of DFRA on redox active transition metal induced DHP oxidation. Dihydropyridines are widely used as analogues of NADH, which is a natural substrate in metabolic reactions of electron transfer in living cells [ 71 , 72 ]. Uncontrolled oxidation of NADH can negatively affect cell homeostasis and lead to cell death. As a result of this study, it was shown that DFRA inhibits the oxidation of DHP in a reaction involving Fe 3+ and Cu 2+ ions. It can be seen that the efficiency of the antioxidant activity of DFRA is higher in the case of Fe 3+ than with Cu 2+ which is explained by the higher affinity of the chelator for iron ions than for copper ions [ 37 , 38 ].
The antioxidant activity of DFRA in all three molecular model systems, confirms similar antioxidant activity observed in these and other models of oxidative damage with the other two chelating drugs L1 and deferoxamine [ 50 , 56 , 73 , 74 , 75 ].
4. Materials and Methods
4.1. materials.
Ascorbic acid (Asc, 99%), ferric chloride (FeCl 3, 97%), ferrous sulphate (FeSO 4 , 99%), copper chloride (CuCl 2 , 99%) and H 2 O 2 (35.5%) were obtained from Sigma-Aldrich, Moscow, Russia. Deferiprone (L1; 99%) was received from LIPOMED Inc., Arlesheim, Switzerland. Deferasirox (DFRA; 99%) was obtained from Shanghai Daeyeon Chemicals Co., Ltd., Shanghai, China, DHP 1,1′-(2,6-dimethyl-1,4-dihydropyridine-3,5-diyl)-di(propan-1-one) (99%) was synthesized according to the classic Hantzsch scheme and obtained from prof. G. Duburs, Institute of Organic Synthesis, Riga [ 54 ]. All compounds were used as received. All experiments were carried out at room temperature.
4.2. Methods
4.2.1. uv-vis optical density investigation of ascorbic acid oxidation.
UV-Vis optical density spectra and kinetics were measured in ethanol solution in 1 cm quartz cuvette using a SF-2000 spectrophotometer (Saint-Petersburg, Russia). The influence of DFRA on the oxidation of Asc was studied by addition of various concentrations of chelator into the mixture of iron or copper salts (CuCl 2 and FeCl 3 ) with Asc (0.1 mM) at room temperature and by monitoring changes at the absorption spectra. The wavelength of 262 nm, which corresponds to the absorption band of Asc, was selected based on the UV-Vis spectrometry data and the analysis of the spectra. This wavelength was selected because it also corresponds to a local minimum in this region of the absorption spectra of DFRA chelate complexes with iron and copper ions. The concentrations of the reagents used in the study were selected in the way that the optical density of the solution did not exceed the detection limit (optical density = 2) of the UV-Vis spectrometer.
4.2.2. The 1 H-NMR Study of Lipid Peroxidation
The study of lipid peroxidation was carried out using a reaction mixture consisted of LA micelles (3.5 mM), H 2 O 2 (0.5 M), DFRA or L1 (1 mM), and FeSO 4 or CuCl 2 (0.1 mM) in deuterated phosphate-saline buffer (0.1 M) (pH 7.4). The reaction was initiated by addition of hydrogen peroxide. In the case of DFRA, LA was mixed with DFRA in chloroform, then the solvent was evaporated leaving behind a film, which was dissolved in deuterated phosphate-saline buffer (pH 7.4). Then FeSO 4 or CuCl 2 was added and the sample was incubated for 30 min to establish equilibrium. In the case of L1, LA was dissolved in chloroform, then the solvent was evaporated and the remaining film was dissolved in deuterated phosphate-saline buffer (pH 7.4). Then L1 and FeSO 4 or CuCl 2 was added and the sample was incubated for 30 min. The reaction was initiated by addition of hydrogen peroxide. The 1 H-NMR spectra were recorded using a Bruker AVHD-500 spectrometer (500 MHz) (Rheinstetten, Germany).
4.2.3. The Study of Dihydropyridine Oxidation
The investigations of dihydropyridine were carried out using a reaction mixture which consisted of 1,1′-(2,6-dimethyl-1,4-dihydropyridine-3,5-diyl)di(propan-1-one) (DHP) (5 mM), H 2 O 2 (0.2 M), DFRA (2 mM), and FeCl 3 or CuCl 2 (0.2 mM) in deuterated methanol. The reaction was initiated by the addition of the metal salt into the sample. Dihydropyridines are quite sensitive to external influences, such as light [ 76 ], therefore samples were incubated at a temperature of 278 K and in the absence of light in order to minimize the effect of DHP autoxidation. The 1 H-NMR spectra were also recorded using a Bruker AVHD-500 spectrometer (500 MHz).
5. Conclusions
Molecular studies including the redox effects of DFRA and its metal complexes with iron and copper are of major importance for determining the general pharmacological and toxicological properties of DFRA, as well as all other drugs. Furthermore, the findings from such studies may have therapeutic implications for patients receiving DFRA and other drugs.
In this study several molecular model systems of oxidative damage on Asc, LA micelles and DHP caused by iron and copper catalysis have been used for examining the effects of DFRA. The antioxidant activity of DFRA in the three molecular model systems of oxidative damage in this study, confirms previous findings in similar models and also the general antioxidant potential in biological systems of chelating drugs including DFRA, L1 and deferoxamine. However, differences remain between the chelating drugs on redox properties, affinity for metal ions including iron and copper, pharmacological and toxicological properties and also therapeutic effects.
Further studies are needed to investigate the antioxidant effects of DFRA and the other iron chelating drugs in different in vitro, in vivo and clinical models of oxidative damage. It is hoped that the antioxidant properties of DFRA and other chelating drugs will increase the prospects for the development of antioxidant drugs for the treatment of many different categories of free radical pathology patients, including patients with neurodegenerative diseases, cancer and cardiac, renal, liver and other organ damage.
Abbreviations
Author contributions.
Conceptualization, V.A.T., N.E.P. and G.J.K.; methodology, V.A.T., O.Y.S. and N.E.P.; validation, N.E.P., O.Y.S. and G.J.K.; formal analysis, L.A.K., V.A.T. and O.Y.S.; investigation, L.A.K., V.A.T. and O.Y.S.; resources, V.A.T.; writing—original draft preparation, V.A.T. and N.E.P.; writing—review and editing, V.A.T., N.E.P. and G.J.K.; visualization, V.A.T. and O.Y.S.; supervision, N.E.P. and G.J.K.; project administration, N.E.P.; funding acquisition, O.Y.S. and V.A.T. All authors have read and agreed to the published version of the manuscript.
This research was supported by grants from the Russian Foundation for Basic Research No. 20-33-70019. The reported research was also funded by the Russian Ministry of Science and Education (State assignments No. 0304-2017-0009) and by internal funds of the Postgraduate Research Institute of Science, Technology, Environment and Medicine of Cyprus.
Data Availability Statement
Conflicts of interest.
The authors declare no conflict of interest.
Sample Availability
Samples of the compounds are not available from the authors.
Publisher’s Note: MDPI stays neutral with regard to jurisdictional claims in published maps and institutional affiliations.
Drug Repurposing: Deferasirox Inhibits the Anti-Apoptotic Activity of Mcl-1
Affiliations.
- 1 Normandie Université, UNIROUEN, INSA de Rouen, CNRS Laboratoire COBRA (UMR 6014 & FR 3038), Rouen, 76000, France.
- 2 Normandie Univ, UNICAEN, CERMN, Caen, 14000, France.
- 3 Normandie Université, UNICAEN, Inserm U1086 ANTICIPE «Interdisciplinary Research Unit for Cancer Prevention and Treatment», Biology and Innovative Therapeutics for Ovarian Cancers Group (BioTICLA), Centre de Lutte Contre le Cancer F. Baclesse, Caen, 14076, France.
- 4 UNICANCER, Centre de Lutte Contre le Cancer F. Baclesse, Caen, 14076, France.
- 5 Sorbonne Université, École Normale Supérieure, PSL University, CNRS, Laboratoire des Biomolécules, LBM, Paris, France.
- PMID: 34949914
- PMCID: PMC8688747
- DOI: 10.2147/DDDT.S323077
Introduction: With the aim of repositioning commercially available drugs for the inhibition of the anti-apoptotic myeloid cell leukemia protein, Mcl-1, implied in various cancers, five molecules, highlighted from a published theoretical screening, were selected to experimentally validate their affinity toward Mcl-1.
Results: A detailed NMR study revealed that only two of the five tested drugs, Torsemide and Deferasirox, interacted with Mcl-1. NMR data analysis allowed the complete characterization of the binding mode of both drugs to Mcl-1, including the estimation of their affinity for Mcl-1. Biological assays evidenced that the biological activity of Torsemide was lower as compared to the Deferasirox, which was able to efficiently and selectively inhibit the anti-apoptotic activity of Mcl-1. Finally, docking and molecular dynamics led to a 3D model for the Deferasirox:Mcl-1 complex and revealed the positioning of the drug in the Mcl-1 P2/P3 pockets as well as almost all synthetic Mcl-1 inhibitors. Interestingly, contrary to known synthetic Mcl-1 inhibitors which interact through Arg263, Deferasirox, establishes a salt bridge with Lys234.
Conclusion: Deferasirox could be a potential candidate for drug repositioning as Mcl-1 inhibitor.
Keywords: Deferasirox; Mcl-1; NMR; docking; drug repurposing; dynamics.
© 2021 Bourafai-Aziez et al.
- Apoptosis Regulatory Proteins / drug effects*
- Deferasirox / chemistry
- Deferasirox / pharmacology*
- Drug Repositioning*
- Lenalidomide / chemistry
- Lenalidomide / pharmacology
- Magnetic Resonance Spectroscopy
- Molecular Docking Simulation
- Molecular Dynamics Simulation
- Molecular Structure
- Myeloid Cell Leukemia Sequence 1 Protein / antagonists & inhibitors*
- Oxcarbazepine / chemistry
- Oxcarbazepine / pharmacology
- Risperidone / chemistry
- Risperidone / pharmacology
- Torsemide / chemistry
- Torsemide / pharmacology
- Apoptosis Regulatory Proteins
- Myeloid Cell Leukemia Sequence 1 Protein
- Lenalidomide
- Risperidone
- Deferasirox
- Oxcarbazepine
Thank you for visiting nature.com. You are using a browser version with limited support for CSS. To obtain the best experience, we recommend you use a more up to date browser (or turn off compatibility mode in Internet Explorer). In the meantime, to ensure continued support, we are displaying the site without styles and JavaScript.
- View all journals
- Explore content
- About the journal
- Publish with us
- Sign up for alerts
- Review Article
- Published: 22 July 2014
Deferasirox nephrotoxicity—the knowns and unknowns
- Juan Daniel Díaz-García 1 ,
- Angel Gallegos-Villalobos 2 ,
- Liliana Gonzalez-Espinoza 2 ,
- Maria D. Sanchez-Niño 3 ,
- Jesus Villarrubia 4 &
- Alberto Ortiz 5
Nature Reviews Nephrology volume 10 , pages 574–586 ( 2014 ) Cite this article
2600 Accesses
55 Citations
6 Altmetric
Metrics details
- Acid, base, fluid, electrolyte disorders
- Adverse effects
- Fanconi syndrome
- Toxin-induced nephropathy
Deferasirox is an oral iron chelator with nephrotoxic potential that could result in decreased glomerular filtration requiring dialysis and in proximal tubular dysfunction
Although deferasirox nephrotoxicity has been reported to promote tubular cell injury, the molecular and cellular mechanisms are poorly understood
Postmarketing monitoring of potential long-term effects of chronic deferasirox treatment on kidney function is required
Careful selection and monitoring of patients treated with deferasirox might decrease the risks of serious renal adverse effects
In 2005, the oral iron chelator deferasirox was approved by the FDA for clinical use as a first-line therapy for blood-transfusion-related iron overload. Nephrotoxicity is the most serious and frequent adverse effect of deferasirox treatment. This nephrotoxicity can present as an acute or chronic decrease in glomerular filtration rate (GFR). Features of proximal tubular dysfunction might also be present. In clinical trials and observational studies, GFR is decreased in 30–100% of patients treated with deferasirox, depending on dose, method of assessment and population studied. Nephrotoxicity is usually nonprogressive and/or reversible and rapid iron depletion is one of several risk factors. Scarce data are available on the molecular mechanisms of nephrotoxicity and the reasons for the specific proximal tubular sensitivity to the drug. Although deferasirox promotes apoptosis of cultured proximal tubular cells, the trigger has not been well characterized. Observational studies are required to track current trends in deferasirox prescription, assess the epidemiology of deferasirox nephrotoxicity in routine clinical practice, explore the effect on outcomes of various monitoring and dose-adjustment protocols and elucidate the long-term consequences of the different features of nephrotoxicity. Deferasirox nephrotoxicity can be more common in the elderly; thus, specific efforts should be dedicated to investigate the effect of deferasirox use in this group of patients.
This is a preview of subscription content, access via your institution
Access options
Subscribe to this journal
Receive 12 print issues and online access
195,33 € per year
only 16,28 € per issue
Rent or buy this article
Prices vary by article type
Prices may be subject to local taxes which are calculated during checkout
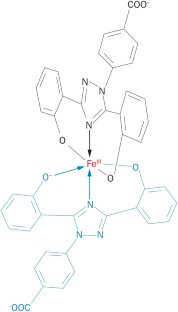
Similar content being viewed by others
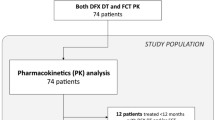
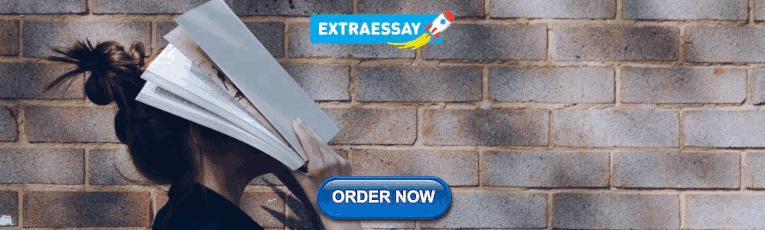
Pharmacological and clinical evaluation of deferasirox formulations for treatment tailoring
Andrea Piolatto, Paola Berchialla, … Filomena Longo
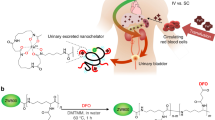
Renal clearable nanochelators for iron overload therapy
Homan Kang, Murui Han, … Jonghan Kim
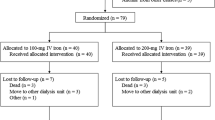
Maintenance intravenous iron in hemodialysis patients to minimize erythropoietin doses: a double-blinded, randomized controlled trial (the MAINTAIN IRON trial)
Suthiya Anumas, Aphichat Chatkrailert & Pichaya Tantiyavarong
Nick, H. et al . ICL670A: preclinical profile. Adv. Exp. Med. Biol. 509 , 185–203 (2002).
Article CAS PubMed Google Scholar
Taher, A. et al . Efficacy and safety of deferasirox, an oral iron chelator, in heavily iron-overloaded patients with β-thalassaemia: the ESCALATOR study. Eur. J. Haematol. 82 , 458–465 (2009).
Article CAS PubMed PubMed Central Google Scholar
Kwiatkowski, J. L. Real-world use of iron chelators. Hematology Am. Soc. Hematol. Educ. Program 2011 , 451–458 (2011).
Article PubMed Google Scholar
Food and Drug Administration. Drug Approval Package [online] , (2006).
The European Medicines Agency. EPAR summary for the public [online] , (2013).
Riva, A. Deferasirox's toxicity. Lancet 382 , 127–128 (2013).
The European Medicines Agency. EMEA/COMP/50/02 Rev. 2 [online] , (2007).
The European Medicines Agency. EPAR Product Information [online] , (2014).
Vichinsky, E. et al . A randomised comparison of deferasirox versus deferoxamine for the treatment of transfusional iron overload in sickle cell disease. Br. J. Haematol. 136 , 501–508 (2007).
Food and Drug Administration. Prescribing information [online] , (2013).
Greenberg, P. L. et al . Prospective assessment of effects on iron-overload parameters of deferasirox therapy in patients with myelodysplastic syndromes. Leuk. Res. 34 , 1560–1565 (2010).
Porter, J. et al . Relative response of patients with myelodysplastic syndromes and other transfusion-dependent anaemias to deferasirox (ICL670): a 1-yr prospective study. Eur. J. Haematol. 80 , 168–176 (2008).
CAS PubMed PubMed Central Google Scholar
Chirnomas, D. et al . Deferasirox pharmacokinetics in patients with adequate versus inadequate response. Blood 114 , 4009–4013 (2009).
Taher, A. et al . Efficacy and safety of deferasirox doses of >30 mg/kg per d in patients with transfusion-dependent anaemia and iron overload. Br. J. Haematol. 147 , 752–759 (2009).
Meerpohl, J. J. et al . Deferasirox for managing iron overload in people with thalassaemia. Cochrane Database of Systematic Reviews , Issue 2. Art. No.: CD007476. http://dx.doi.org/10.1002/14651858.CD007476.pub2 .
Food and Drug Administration. Important drug warning [online] , (2010).
Kontoghiorghes, G. J. Turning a blind eye to deferasirox's toxicity? Lancet 381 , 1183–1184 (2013).
Anapol Schwartz, LLC. FDA reports [online] , (2012).
eHealthMe. Review: could Exjade cause blood creatinine increased? [online] , (2014).
Food and Drug Administration. New molecular entity (NME) — early safety findings [online] , (2007).
Hirschberg, R., Bennett, W., Scheinman, J., Coppo, R. & Ponticelli, C. Acute kidney injury due to deferoxamine in a renal transplant patient. Nephrol. Dial. Transplant. 23 , 2704–2705 (2008).
Cappellini, M. D. et al . A phase 3 study of deferasirox (ICL670), a once-daily oral iron chelator, in patients with β-thalassemia. Blood 107 , 3455–3462 (2006).
Piga, A. et al . Randomized phase II trial of deferasirox (Exjade, ICL670), a once-daily, orally-administered iron chelator, in comparison to deferoxamine in thalassemia patients with transfusional iron overload. Haematologica 91 , 873–880 (2006).
CAS PubMed Google Scholar
Galanello, R. et al . Phase II clinical evaluation of deferasirox, a once-daily oral chelating agent, in pediatric patients with β-thalassemia major. Haematologica 91 , 1343–1351 (2006).
Shemesh, O., Golbetz, H., Kriss, J. P. & Myers, B. D. Limitations of creatinine as a filtration marker in glomerulopathic patients. Kidney Int. 28 , 830–838 (1985).
Kidney Disease: Improving Global Outcomes (KDIGO) Acute Kidney Injury Work Group. KDIGO clinical practice guideline for acute kidney jnjury. Kidney Int. Suppl. 2 , 1–138 (2012).
Rafat, C., Fakhouri, F., Ribeil, J. A., Delarue, R. & Le Quintrec, M. Fanconi syndrome due to deferasirox. Am. J. Kidney Dis. 54 , 931–934 (2009).
Yew, C. T. et al . Acute interstitial nephritis secondary to deferasirox causing acute renal injury needing short-term dialysis. Nephrology (Carlton) 15 , 377 (2010).
Article Google Scholar
Brosnahan, G., Gokden, N. & Swaminathan, S. Acute interstitial nephritis due to deferasirox: a case report. Nephrol. Dial. Transplant. 23 , 3356–3358 (2008).
Kidney Disease: Improving Global Outcomes (KDIGO) CKD Work Group. KDIGO 2012 clinical practice guideline for the evaluation and management of chronic kidney disease. Kidney Int. Suppl. 3 , 1–150 (2013).
Milat, F. et al . A case of hypophosphatemic osteomalacia secondary to deferasirox therapy. J. Bone Miner. Res. 27 , 219–222 (2012).
Papadopoulos, N., Vasiliki, A., Aloizos, G., Tapinis, P. & Kikilas, A. Hyperchloremic metabolic acidosis due to deferasirox in a patient with beta thalassemia major. Ann. Pharmacother. 44 , 219–221 (2010).
Baum, M. Renal Fanconi syndrome secondary to deferasirox: where there is smoke there is fire. J. Pediatr. Hematol. Oncol. 32 , 525–526 (2010).
Article PubMed PubMed Central Google Scholar
Grangé, S., Bertrand, D. M., Guerrot, D., Eas, F. & Godin, M. Acute renal failure and Fanconi syndrome due to deferasirox. Nephrol. Dial. Transplant. 25 , 2376–2378 (2010).
Even-Or, E., Becker-Cohen, R. & Miskin, H. Deferasirox treatment may be associated with reversible renal Fanconi syndrome. Am. J. Hematol. 85 , 132–134 (2010).
PubMed Google Scholar
Rheault, M. N., Bechtel, H., Neglia, J. P. & Kashtan, C. E. Reversible Fanconi syndrome in a pediatric patient on deferasirox. Pediatr. Blood Cancer 56 , 674–676 (2011).
Chueh, H. W. et al . Iron chelation treatment with deferasirox prior to high-dose chemotherapy and autologous stem cell transplantation may reduce the risk of hepatic veno-occlusive disease in children with high-risk solid tumors. Pediatr. Blood Cancer 58 , 441–447 (2012).
Dubourg, L. et al . Deferasirox-induced renal impairment in children: an increasing concern for pediatricians. Pediatr. Nephrol. 27 , 2115–2122 (2012).
Wei, H. Y., Yang, C. P., Cheng, C. H. & Lo, F. S. Fanconi syndrome in a patient with β-thalassemia major after using deferasirox for 27 months. Transfusion 51 , 949–954 (2011).
Yacobovich, J. et al . Acquired proximal renal tubular dysfunction in β-thalassemia patients treated with deferasirox. J. Pediatr. Hematol. Oncol. 32 , 564–567 (2010).
Yusuf, B., McPhedran, P. & Brewster, U. C. Hypocalcemia in a dialysis patient treated with deferasirox for iron overload. Am. J. Kidney Dis. 52 , 587–590 (2008).
European Medicines Agency. Scientific discussion [online] , (2006).
Vichinsky, E. et al . Efficacy and safety of deferasirox compared with deferoxamine in sickle cell disease: Two-year results including pharmacokinetics and concomitant hydroxyurea. Am. J. Hematol. 88 , 1068–1073 (2013).
European Medicines Agency. Assessment report [online] , (2013).
Cancado, R. et al . Two-year analysis of efficacy and safety of deferasirox treatment for transfusional iron overload in sickle cell anemia patients. Acta Haematol. 128 , 113–118 (2012).
Cappellini, M. D. et al . Iron chelation with deferasirox in adult and pediatric patients with thalassemia major: efficacy and safety during 5 years' follow-up. Blood 118 , 884–893 (2011).
Gattermann, N. et al . Deferasirox in iron-overloaded patients with transfusion-dependent myelodysplastic syndromes: Results from the large 1-year EPIC study. Leuk. Res. 34 , 1143–1150 (2010).
Kennedy, G. A. et al . A prospective phase II randomized study of deferasirox to prevent iatrogenic iron overload in patients undertaking induction/consolidation chemotherapy for acute myeloid leukaemia. Br. J. Haematol. 161 , 794–801 (2013).
Lee, J. W. et al . Iron chelation therapy with deferasirox in patients with aplastic anemia: a subgroup analysis of 116 patients from the EPIC trial. Blood 116 , 2448–2454 (2010).
List, A. F. et al . Deferasirox reduces serum ferritin and labile plasma iron in RBC transfusion-dependent patients with myelodysplastic syndrome. J. Clin. Oncol. 30 , 2134–2139 (2012).
Metzgeroth, G. et al . Deferasirox in MDS patients with transfusion-caused iron overload—a phase-II study. Ann. Hematol. 88 , 301–310 (2009).
Miyazawa, K. et al . A safety, pharmacokinetic and pharmacodynamic investigation of deferasirox (Exjade, ICL670) in patients with transfusion-dependent anemias and iron-overload: a phase I study in Japan. Int. J. Hematol. 88 , 73–81 (2008).
Nolte, F. et al . Results from a 1-year, open-label, single arm, multi-center trial evaluating the efficacy and safety of oral deferasirox in patients diagnosed with low and int-1 risk myelodysplastic syndrome (MDS) and transfusion-dependent iron overload. Ann. Hematol. 92 , 191–198 (2013).
Pennell, D. J. et al . Deferasirox for up to 3 years leads to continued improvement of myocardial T2 * in patients with β-thalassemia major. Haematologica 97 , 842–848 (2012).
Phatak, P. et al . A phase 1/2, dose-escalation trial of deferasirox for the treatment of iron overload in HFE-related hereditary hemochromatosis. Hepatology 52 , 1671–1779 (2010).
Taher, A. T. et al . Deferasirox reduces iron overload significantly in nontransfusion-dependent thalassemia: 1-year results from a prospective, randomized, double-blind, placebo-controlled study. Blood 120 , 970–977 (2012).
Vichinsky, E. et al . Long-term safety and efficacy of deferasirox (Exjade) for up to 5 years in transfusional iron-overloaded patients with sickle cell disease. Br. J. Haematol. 154 , 387–397 (2011).
Voskaridou, E. et al . Deferasirox effectively decreases iron burden in patients with double heterozygous HbS/β-thalassemia. Ann. Hematol. 90 , 11–15 (2011).
Wood, J. C. et al . The effect of deferasirox on cardiac iron in thalassemia major: impact of total body iron stores. Blood 116 , 537–543 (2010).
Al-Khabori, M. et al . Side effects of deferasirox iron chelation in patients with beta thalassemia major or intermedia. Oman. Med. J. 28 , 121–124 (2013).
Economou, M. et al . Renal dysfunction in patients with beta-thalassemia major receiving iron chelation therapy either with deferoxamine and deferiprone or with deferasirox. Acta Haematol. 123 , 148–152 (2010).
Efthimia, V. et al . Nephrolithiasis in beta thalassemia major patients treated with deferasirox: an advent or an adverse event? A single Greek center experience. Ann. Hematol. 92 , 263–265 (2013).
Tunç, B. et al . Deferasirox therapy in children with Fanconi aplastic anemia. J. Pediatr. Hematol. Oncol. 34 , 247–251 (2012).
Unal, S., Hazirolan, T., Eldem, G. & Gumruk, F. The effects of deferasirox on renal, cardiac and hepatic iron load in patients with β-thalassemia major: preliminary results. Pediatr. Hematol. Oncol. 28 , 217–221 (2011).
Cermak, J. et al . A comparative study of deferasirox and deferiprone in the treatment of iron overload in patients with myelodysplastic syndromes. Leuk. Res. 37 , 1612–1615 (2013).
Ktena, Y. P., Athanasiadou, A., Lambrou, G., Adamaki, M. & Moschovi, M. Iron chelation with deferasirox for the treatment of secondary hemosiderosis in pediatric oncology patients: a single-center experience. J. Pediatr. Hematol. Oncol. 35 , 447–450 (2013).
Karimi, M. et al . Iranian experience of deferasirox (Exjade ® ) in transfusion-dependent patients with iron overload: what is the most effective dose based on serum ferritin levels? Hematology 17 , 367–371 (2012).
Aydinok, Y. et al . Observational study comparing long-term safety and efficacy of deferasirox with desferrioxamine therapy in chelation-naïve children with transfusional iron overload. Eur. J. Haematol. 88 , 431–438 (2012).
Panigrahi, I., Vaidya, P. C., Bansal, D. & Marwaha, R. K. Efficacy of deferasirox in North Indian β-thalassemia major patients: a preliminary report. J. Pediatr. Hematol. Oncol. 34 , 51–53 (2012).
Gattermann, N. et al . Deferasirox treatment of iron-overloaded chelation-naïve and prechelated patients with myelodysplastic syndromes in medical practice: results from the observational studies eXtend and eXjange. Eur. J. Haematol. 88 , 260–268 (2012).
Eshghi, P. et al . Efficacy and safety of Iranian made deferasirox (Osveral ® ) in Iranian major thalassemic patients with transfusional iron overload: A one year prospective multicentric open-label non-comparative study. Daru 19 , 240–248 (2011).
Chang, H. H. et al . Improved efficacy and tolerability of oral deferasirox by twice-daily dosing for patients with transfusion-dependent β-thalassemia. Pediatr. Blood Cancer 56 , 420–424 (2011).
Pennell, D. J. et al . A 1-year randomized controlled trial of deferasirox versus deferoxamine for myocardial iron removal in β-thalassemia major (CORDELIA). Blood 123 , 1447–1454 (2014).
Fernandez-Fernandez, B. et al . Tenofovir nephrotoxicity: 2011 update. AIDS Res. Treat. 2011 , 354908 (2011).
PubMed PubMed Central Google Scholar
Ortiz, A. et al . Tubular cell apoptosis and cidofovir-induced acute renal failure. Antivir. Ther. 10 , 185–190 (2005).
Hider, R. C. Charge states of deferasirox-ferric iron complexes. Am. J. Kidney Dis. 55 , 614–615 (2010).
Huang, X. P., Thiessen, J. J., Spino, M. & Templeton, D. M. Transport of iron chelators and chelates across MDCK cell monolayers: implications for iron excretion during chelation therapy. Int. J. Hematol. 91 , 401–412 (2010).
Sánchez-González, P. D., López-Hernandez, F. J., Morales, A. I., Macías-Nuñez, J. F. & López-Novoa, J. M. Effects of deferasirox on renal function and renal epithelial cell death. Toxicol. Lett. 203 , 154–161 (2011).
Goldstein, D. J. et al . Cyclosporine-associated end-stage nephropathy after cardiac transplantation: incidence and progression. Transplantation 63 , 664–668 (1997).
Klein, I. H. et al . Different effects of tacrolimus and cyclosporine on renal hemodynamics and blood pressure in healthy subjects. Transplantation 73 , 732–736 (2002).
Oexle, H., Gnaiger, E. & Weiss, G. Iron-dependent changes in cellular energy metabolism: influence on citric acid cycle and oxidative phosphorylation. Biochim. Biophys. Acta 1413 , 99–107 (1999).
Gattermann, N. et al . Impact on Iron removal of dose reduction for non-progressive serum creatinine increases during treatment with the once-daily, oral iron chelator deferasirox (Exjade ® , ICL670) [abstract]. Blood 108 , a3824 (2006).
Article CAS Google Scholar
Baliga, R., Zhang, Z., Baliga, M., Ueda, N. & Shah, S. V. In vitro and in vivo evidence suggesting a role for iron in cisplatin-induced nephrotoxicity. Kidney Int. 53 , 394–401 (1998).
Dixon, S. J. et al . Ferroptosis: an iron-dependent form of nonapoptotic cell death. Cell 149 , 1060–1072 (2012).
Cianciulli, P. et al . Acute renal failure occurring during intravenous desferrioxamine therapy: recovery after haemodialysis. Haematologica 77 , 514–515 (1992).
Food and Drug Administration. Safety [online] , (2012).
Food and Drug Administration. Safety [online] , (2010).
Walker, P. D. & Shah, S. V. Evidence suggesting a role for hydroxyl radical in gentamicin-induced acute renal failure in rats. J. Clin. Invest. 81 , 334–341 (1988).
Koren, G., Kochavi-Atiya, Y., Bentur, Y. & Olivieri, N. F. The effects of subcutaneous deferoxamine administration on renal function in thalassemia major. Int. J. Hematol. 54 , 371–375 (1991).
Clajus, C. et al . Acute kidney injury due to deferoxamine in a renal transplant patient. Nephrol. Dial. Transplant. 23 , 1061–1064 (2008).
Yoon, Y. S., Byun, H. O., Cho, H., Kim, B. K. & Yoon, G. Complex II defect via down-regulation of iron-sulfur subunit induces mitochondrial dysfunction and cell cycle delay in iron chelation-induced senescence-associated growth arrest. J. Biol. Chem. 278 , 51577–51586 (2003).
Justo, P., Lorz, C., Sanz, A., Egido, J. & Ortiz, A. Intracellular mechanisms of cyclosporin A-induced tubular cell apoptosis. J. Am. Soc. Nephrol. 14 , 3072–3080 (2003).
Molitoris, B. A. et al . siRNA targeted to p53 attenuates ischemic and cisplatin-induced acute kidney injury. J. Am. Soc. Nephrol. 20 , 1754–1764 (2009).
Kelly, K. J., Plotkin, Z., Vulgamott, S. L. & Dagher, P. C. P53 mediates the apoptotic response to GTP depletion after renal ischemia-reperfusion: protective role of a p53 inhibitor. J. Am. Soc. Nephrol. 14 , 128–138 (2003).
Allon, M., Lawson, L., Eckman, J. R., Delaney, V. & Bourke, E. Effects of nonsteroidal antiinflammatory drugs on renal function in sickle cell anemia. Kidney Int. 34 , 500–506 (1988).
Lee, J. W. et al . Pharmacogenetic study of deferasirox, an iron chelating agent. PLoS One 8 , e64114 (2013).
Lai, M. E. et al . Renal function in patients with β-thalassaemia major: a long-term follow-up study. Nephrol. Dial. Transplant. 27 , 3547–3551 (2012).
Han, J. Y. et al . Comprehensive analysis of UGT1A polymorphisms predictive for pharmacokinetics and treatment outcome in patients with non-small-cell lung cancer treated with irinotecan and cisplatin. J. Clin. Oncol. 24 , 2237–2244 (2006).
Satoh, T. et al . Genotype-directed, dose-finding study of irinotecan in cancer patients with UGT1A1 * 28 and/or UGT1A1 * 6 polymorphisms. Cancer Sci. 102 , 1868–1873 (2011).
Shulman, K. et al . Clinical implications of UGT1A1 * 28 genotype testing in colorectal cancer patients. Cancer 117 , 3156–3162 (2011).
Mathijssen, R. H. et al . Clinical pharmacokinetics and metabolism of irinotecan (CPT-11). Clin. Cancer Res. 7 , 2182–2194 (2001).
Adams, R. L. & Bird, R. J. Safety and efficacy of deferasirox in the management of transfusion-dependent patients with myelodysplastic syndrome and aplastic anaemia: a perspective review. Ther. Adv. Hematol. 4 , 93–102 (2013).
Ataga, K. I. & Orringer, E. P. Renal abnormalities in sickle cell disease. Am. J. Hematol. 63 , 205–211 (2000).
McKie, K. T. et al . Prevalence, prevention, and treatment of microalbuminuria and proteinuria in children with sickle cell disease. J. Pediatr. Hematol. Oncol. 29 , 140–144 (2007).
Vichinsky, E. Clinical application of deferasirox: practical patient management. Am. J. Hematol. 83 , 398–402 (2008).
Download references
Acknowledgements
The authors' research was supported by the grants FIS PS09/00447, PI13/00047, ISCIII-RETIC REDinREN RD12/0021, Comunidad de Madrid S2010/BMD-2378, and CYTED IBERERC, by Programa Intensificación Actividad Investigadora (ISCIII) to A.O., by ERA–EDTA fellowship to L.G.E. and by Programa Interinstitucional para el Fortalecimiento de la Investigación y el Posgrado del Pacífico to J.D.D.-G.
Author information
Authors and affiliations.
Escuela Superior de Medicina del Instituto Politécnico Nacional, Avenida Salvador Díaz Mirón s/n, Ciudad de México, 11340, México
Juan Daniel Díaz-García
IIS-Fundación Jiménez Díaz-UAM, Avenida Reyes Católicos 2, Madrid, 28040, Spain
Angel Gallegos-Villalobos & Liliana Gonzalez-Espinoza
IDIPAZ, Paseo de la Castellana 261, Madrid, 28046, Spain
Maria D. Sanchez-Niño
Hospital Ramon y Cajal, Carretera de Colmenar Viejo km. 9,100, Madrid, 28034, Spain
Jesus Villarrubia
Unidad de Diálisis, Fundación Jiménez Díaz-IRSIN, Avenida Reyes Católicos 2, Madrid, 28040, Spain
Alberto Ortiz
You can also search for this author in PubMed Google Scholar
Contributions
All authors researched the data for the article, provided substantial contributions to discussions of its content, wrote the article and undertook review and/or editing of the manuscript before submission.
Corresponding author
Correspondence to Alberto Ortiz .
Ethics declarations
Competing interests.
The authors declare no competing financial interests.
Supplementary information
Supplementary table 1.
Diagnostic criteria used to diagnose proximal tubular injury secondary to deferasirox (PDF 105 kb)
Supplementary Table 2
Deferasirox nephrotoxicity in RCTs (PDF 137 kb)
Supplementary Table 3
Deferasirox nephrotoxicity in clinical practice (PDF 154 kb)
PowerPoint slides
Powerpoint slide for fig. 1, powerpoint slide for fig. 2, powerpoint slide for fig. 3, powerpoint slide for fig. 4, powerpoint slide for table 1, powerpoint slide for table 2, powerpoint slide for table 3, rights and permissions.
Reprints and permissions
About this article
Cite this article.
Díaz-García, J., Gallegos-Villalobos, A., Gonzalez-Espinoza, L. et al. Deferasirox nephrotoxicity—the knowns and unknowns. Nat Rev Nephrol 10 , 574–586 (2014). https://doi.org/10.1038/nrneph.2014.121
Download citation
Published : 22 July 2014
Issue Date : October 2014
DOI : https://doi.org/10.1038/nrneph.2014.121
Share this article
Anyone you share the following link with will be able to read this content:
Sorry, a shareable link is not currently available for this article.
Provided by the Springer Nature SharedIt content-sharing initiative
This article is cited by
Metabolic mechanisms of acute proximal tubular injury.
- Andrew M. Hall
- Sophie de Seigneux
Pflügers Archiv - European Journal of Physiology (2022)
Drug toxicity in the proximal tubule: new models, methods and mechanisms
- Francesco Trepiccione
- Robert J. Unwin
Pediatric Nephrology (2022)
The multifaceted role of iron in renal health and disease
- Rachel P. L. van Swelm
- Jack F. M. Wetzels
- Dorine. W. Swinkels
Nature Reviews Nephrology (2020)
The iron chelator Deferasirox causes severe mitochondrial swelling without depolarization due to a specific effect on inner membrane permeability
- Esther M. Gottwald
- Claus D. Schuh
Scientific Reports (2020)
Thalassemia: Common Clinical Queries in Management
- Ashutosh Lal
- Deepak Bansal
The Indian Journal of Pediatrics (2020)
Quick links
- Explore articles by subject
- Guide to authors
- Editorial policies
Sign up for the Nature Briefing newsletter — what matters in science, free to your inbox daily.

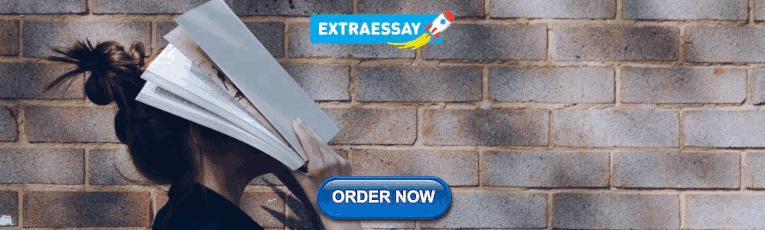
IMAGES
VIDEO
COMMENTS
The NMR assignment of Mcl-1 backbone resonances in the free form was previously obtained from the analysis of 1 H, 15 N, and 13 C triple resonance experiments.36,62. ... another residue revealed as strong perturbed in NMR study. Deferasirox established a salt bridge with Lys234, an H-bond with Arg263 and a π-π stacking interaction with Phe270
The NMR spectral data of deferasirox and Imp-1 were compared (Table 1). The 1 H NMR spectrum of deferasirox and Imp-1 showed three singlets at δ 10.05, 10.95, 13.20 and 10.25, 10.92, 13.25 ppm assigned to exchangeable hydroxyl and carboxylic protons respectively. It is apparent from the comparison of the NMR spectral data of deferasirox and ...
The 1 H NMR spectrum of deferasirox in DMSO-d 6 is shown in Fig. 6a (signal range) and in Fig. 6b (only aromatic region). A systematic numbering of the carbon atoms from C-1 to C-24 was used for ...
NMR Studies of the Interaction of Deferasirox with Dihydropyridine An additional molecular model system for studying the antioxidant activity of DFRA was the oxidation of DHP. The reactions involving DHP are often used as models of radical processes of electron and proton transfer in living systems with the participation of NADH [ 54 , 55 ].
Deferasirox is an orally active, lipophilic iron chelating drug used on thousands of patients worldwide for the treatment of transfusional iron overload. The essential transition metals iron and copper are the primary catalysts of reactive oxygen species and oxidative damage in biological systems. The redox effects of deferasirox and its metal complexes with iron, copper and other metals are ...
Unambiguous assignment of all the. 1. H NMR and. 13. C NMR signals of deferasirox was a key prerequisite to. ... NMR of deferasirox. The. 1. H NMR spectrum of deferasirox in. DMSO-d. 6.
Deferasirox is an iron-chelating drug developed by Novartis company for treatment of diseases accompanied by chronic iron overload; such as β-thalassemia or sickle cell diseases. ... (FTIR) and nuclear magnetic resonance spectroscopy (NMR) analysis for its functional groups. In addition, the selected analytical methods are summarized to aid ...
NMR data analysis allowed the complete characterization of the binding mode of both drugs to Mcl-1, including the estimation of their affinity for Mcl-1. Biological assays evidenced that the biological activity of Torsemide was lower as compared to the Deferasirox, which was able to efficiently and selectively inhibit the anti-apoptotic ...
Complexation of deferasirox (4-[3,5-bis(2-hydroxyphenyl)-1,2,4-triazol-1-yl]benzoic acid, H 3 L B) and its decarboxy derivative (H 2 L A) with Ru(III), Os(IV), Co(III) and Ga(III) was studied.These novel complexes with likely multitargeted capabilities are expected to show anticancer potential after biotransformation via both of the metal ion and the liberated ligand.
The synthetic procedure of sensor TAD was based on cyclization reaction of deferasirox hydrazide (Scheme 1).Structure of the compound TAD was confirmed by 1 H NMR, 13 C NMR and mass spectrometry techniques. In 1 H NMR analysis appearance of two characteristic signals one for NH group at 14.02 ppm as a broad singlet and the second at 5.80 ppm, a broad singlet for two protons of NH 2 group ...
Deferasirox, an FDA-approved iron chelator, has gained increasing attention for use in anticancer and antimicrobial applications. Recent efforts by our group led to the identification of this core as an easy-to-visualize aggregation-induced emission platform, or AIEgen, that provides a therapeutic effect equivalent to deferasirox (J. Am. Chem. Soc. 2021, 143, 3, 1278-1283). However, the ...
Deferasirox (DFX) is the newest among three different chelators available to treat iron overload in iron-loading anaemias, firstly released as Dispersible Tablets (DT) and more recently replaced ...
The recent X-ray structure of titanium(IV)-bound human serum transferrin (STf) exhibiting citrate as a synergistic anion reveals a difference in Ti(IV) coordination versus iron(III), the metal endogenously delivered by the protein to cells. This finding enriches our bioinspired drug design strategy for Ti(IV)-based anticancer therapeutics, which applies a family of Fe(III) chelators termed ...
Results: A detailed NMR study revealed that only two of the five tested drugs, Torsemide and Deferasirox, interacted with Mcl-1. NMR data analysis allowed the complete characterization of the ...
The search for alternatives to Gd-containing magnetic resonance imaging (MRI) contrast agents addresses the field of Fe(III)-bearing species with the expectation that the use of an essential metal ion may avoid the issues raised by the exogenous Gd. Attention is currently devoted to highly stable Fe(III) complexes with hexacoordinating ligands, although they may lack any coordinated water ...
Compound DEFERASIROXwith free spectra: 2 NMR. KnowItAll NMR Spectral Library Author: Wiley. The KnowItAll NMR Spectral Library offers a comprehensive collection of NMR reference spectra, including NMR, CNMR, and XNMR, and covers a wide range of compounds including organics, polymers, monomers, metabolites, and more.
Abstract. Deferasirox is a once-daily, oral iron chelator that is widely used in the management of patients with transfusional hemosiderosis. Several Phase II trials along with their respective extension studies as well as a Phase III trial have established the efficacy and safety of this novel agent in transfusion-dependent patients with β-thalassemia, sickle-cell disease and bone marrow ...
The general synthetic routes for the preparation of the target compounds 5a (deferasirox), 5b, and 7-64 is depicted in Scheme 1.As a key intermediate of our designed compounds, compounds 5a (deferasirox) and 5b were synthesized according to the literature [17, 18].Despite the same synthetic conditions for compounds 5a (yield: >80 %) and 5b (yield: ∼30 %), the yields differed substantially.
Since the approval of deferasirox for patients with blood-transfusion-related iron overload by the FDA in 2005, >150,000 patient-years of exposure have occurred. However, nephrotoxicity is a ...
DEFERASIROX View entire compound with spectra: 2 NMR. SpectraBase Compound ID: 2fJXs1b5Gdw: InChI: ... 13C Nuclear Magnetic Resonance (NMR) Chemical Shifts. View the Full Spectrum for FREE! View the Full Spectrum for FREE! The full spectrum can only be viewed using a FREE account.
Deferasirox. In Meyler's Side Effects of Drugs (Sixteenth Edition), 2016. Drug-drug interactions. A large list of drugs that are thought to interact with deferasirox is available on the Internet [21]; the interactions are classified as major (n = 219), moderate (n = 400), and minor (n = 5).. Aluminium salts. Deferasirox has affinity for aluminium and forms lipophilic complexes with it.
We first adjusted the carboxyl groups on the deferasirox scaffold, introduced different electron-donating and electron-withdrawing groups through the Suzuki reaction, and explored their effects on molecular properties to screen out suitable fluorescent probe precursors, as shown in Scheme 1.The fluorescence properties of the five deferasirox derivatives were investigated in a solvent system ...