
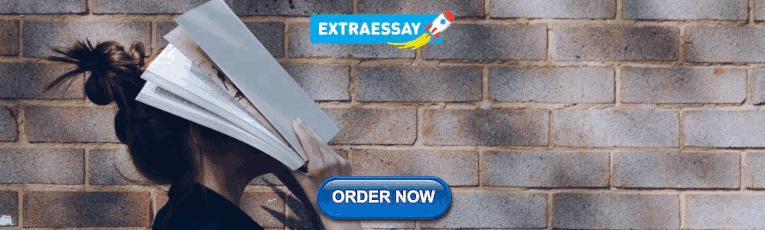
Ecological Impacts of Climate Change (2008)
Chapter: 1 introduction.
Below is the uncorrected machine-read text of this chapter, intended to provide our own search engines and external engines with highly rich, chapter-representative searchable text of each book. Because it is UNCORRECTED material, please consider the following text as a useful but insufficient proxy for the authoritative book pages.
1 Introduction The worldâs climate is changing, and it will continue to change throughout the 21st century and beyond. Rising temperatures, new precipitation patterns, and other changes are already affecting many aspects of human society and the natural world. Climate change is transforming ecosystems at extraordinary rates and scales. As each species responds to its changing environment, its interactions with the physical world and the creatures around it changeâtriggering a cascade of impacts throughout the ecosystem, such as expansion into new areas, the intermingling of formerly non-overlapping species, and even species extinctions Climate change is happening on a global scale, but the ecological impacts are often local and vary from place to place. To illuminate how climate change has affected specific species and ecosystems, this document presents a series of examples of ecological impacts of climate change that have already been observed across the United States. Human actions have been a primary cause of the climate changes observed today, but humans are capable of changing our behavior in ways that reduce the rate of future climate change. Human actions are also needed to help wild species adapt to climate changes that cannot be avoided. Our approaches to energy, agriculture, water management, fishing, biological conservation, and many other activities will all affect the ways and extent to which climate change will alter the natural worldâand the ecosystems on which we depend. What are ecosystems and why are they important? Humans share Earth with a vast diversity of animals, plants, and microorganisms. Virtually every part of the planetââthe continents, the oceans, and the atmosphereââteems with life. Even the deepest parts of the ocean and rock formations hundreds of meters below the surface are populated with organisms adapted to cope with the unique challenges each environment presents. In our era organisms almost everywhere are facing a new set of challenges; specifically, the challenges presented by rapid climate change. How have plants, animals, and microorganisms coped with the climate changes that have already occurred, and how might they cope with future changes? To explore these questions we start with a discussion of how plants, animals, and microorganisms fit together in ecosystems and the role of climate in those relationships. Earth has a great diversity of habitats. These differ in climate, of course, but also in soils, day length, elevation, water sources, chemistry, and many other factors, and consequently, in the kinds of organisms that inhabit them. The animals, plants, and microorganisms that live in one place, along with the water, soils, and landforms, make an ecosystem. When we attempt to understand the impacts of climate change, thinking about ecosystemsââand not just individual speciesââcan be helpful because each ecosystem depends on a wide array of interactions among individuals. Some of these involve competition. For example, some plants shade others or several animals compete for the same scarce food. Some involve relationships between animals and their prey. Others involve decomposition, the process of decay that returns minerals and organic matter to the soil. And some interactions are beneficial to both partners, for example, bees that obtain food from flowers while pollinating them. Climate influences ecosystems and the species that inhabit them in many ways. In general, each type of ecosystem is consistently associated with a particular combination of climate characteristics (Walter 1968). Warm tropical lands with year-round rain typically support
2 Introduction tall forests with evergreen broadleaved trees. Midlatitude lands with cold winters and moist summers usually support deciduous forests, while drier areas are covered in grasslands, shrublands, or conifer forests. In a similar fashion shallow tropical-ocean waters harbor coral reefs on rocky bottoms and mangrove forests along muddy shores, whereas temperate shores are characterized by kelp forests on rocky bottoms and seagrasses or salt marshes on sediment- covered bottoms. These major vegetation types or biomes can cover vast areas. Within these areas a wide range of subtly different ecosystems utilize sites with different soils, topography, land-use history, ocean currents, or climate details. Humans are an important part of most ecosystems, and many ecosystems have been heavily modified by humans. A plot of intensively managed farmland, a fish pond, and a grazed grassland are just as much ecosystems as is a pristine tropical forest. All are influenced by climate, all depend on a wide variety of interactions, and all provide essential benefits to people. The lives of animals, plants, and microorganisms are strongly attuned to changes in climate, such as variation in temperatures; the amount, timing, or form of precipitation; or changes in ocean currents. Some are more sensitive and vulnerable to climate fluctuations than others. If the climate change is modest and slow, the majority of species will most likely adapt successfully. If the climate change is large or rapid, more and more species will face ecological changes to which they may not be able to adapt. But as we will see later, even modest impacts of climate change can cause a range of significant responses, even if the changes are not so harsh that the organism dies. Organisms may react to a shift in temperature or precipitation by altering the timing of an event like migration or leaf emergence, which in turn has effects that ripple out to other parts of the ecosystem. For example, such timing changes may alter the interactions between predator and prey, or plants (including many crops) and the insects that pollinate their flowers. Ultimately we want to understand how climate change alters the overall functioning of the ecosystem and in particular how it alters the ability of the ecosystem to provide valuable services for humans. Ecosystems play a central role in sustaining humans (Figure 1) (Daily 1997; Millennium Ecosystem Assessment 2005). Ecosystems provide products directly consumed by people. This includes food and fiber from agricultural, marine, and forest ecosystems, plus fuel, including wood, grass, and even waste from some agricultural crops, and medicines (from plants, animals and seaweeds). Our supply and quality of fresh water also depends on ecosystems, as they play a critical role in circulating, cleaning, and replenishing water supplies. Ecosystems also regulate our environment; for example, forests, floodplains, and streamside vegetation can be critically important in controlling risks from floods; likewise, mangroves, kelp forests, and coral reefs dampen the impact of storms on coastal communities. Ecosystems provide cultural services that improve our quality of life in ways that range from the sense of awe many feel when looking up at a towering sequoia tree to educational and recreational opportunities. Ecosystems also provide natureâs support structure; without ecosystems there would be no soil to support plants, nor all the microorganisms and animals that depend on plants. In the oceans, ecosystems sustain the nutrient cycling that supports marine plankton, which in turn supply food for the fish and other seafood humans eat. Algae in ocean ecosystems produce much of the oxygen that we breathe. In general, we do not pay for the services we get from ecosystems, even though we could not live without them and would have to pay a high price to provide artificially.
Introduction 3 FIGURE 1 Ecosystem services. SOURCE: Millennium Ecosystem Assessment (2005). Ecosystem services rely on complex interactions among many species, so in most environments it is critical that they contain a diverse array of organisms. Even those services that appear to depend on a single species, like the production of honey, actually depend on the interactions of many species, sometimes many hundreds or thousands. Honey comes from honeybees, but the bees depend on pollen and nectar from the plants they pollinate. These plants depend not only on the bees but also on the worms and other soil animals that aerate the soil, the microorganisms that release nutrients, and the predatory insects that limit populations of plant- eating insects. Scientists are still at the early stages of understanding exactly how diversity contributes to ecosystem resilienceâthe ability of an ecosystem to withstand stresses like pollution or a hurricane without it resulting in a major shift in the ecosystemâs type or the services it provides (Schulze and Mooney 1993; Chapin et al. 1997; Tilman et al. 2006; Worm et al. 2006). But we are already certain about one thing. Each species is a unique solution to a challenge posed by nature and each speciesâ DNA is a unique and complex blueprint. Once a species goes extinct, we canât get it back. Therefore, as we look at the impacts of climate change on ecosystems, it is critical to remember that some kinds of impactsâlosses of biological diversityâare irreversible. What do we know about current climate change? Over the last 20 years the worldâs governments have requested a series of authoritative assessments of scientific knowledge about climate change, its impacts, and possible approaches
4 Introduction for dealing with climate change. These assessments are conducted by a unique organization, the Intergovernmental Panel on Climate Change (IPCC). Every five to seven years, the IPCC uses volunteer input from thousands of scientists to synthesize available knowledge. The IPCC conclusions undergo intense additional review and evaluation by both the scientific community and the worldâs governments, resulting in final reports that all countries officially accept (Bolin 2007). The information in the IPCC reports has thus been through multiple reviews and is the most authoritative synthesis of the state of the science on climate change. Earthâs average temperature is increasing In 2007 the IPCC reported that Earthâs average temperature is unequivocally warming (IPCC 2007b). Multiple lines of scientific evidence show that Earthâs global average surface temperature has risen some 0.75°C (1.3°F) since 1850 (the starting point for a useful global network of thermometers). Not every part of the planetâs surface is warming at the same rate. Some parts are warming more rapidly, particularly over land, and a few parts (in Antarctica, for example) have cooled slightly (Figure 2). But vastly more areas are warming than cooling. In the United States average temperatures have risen overall, with the change in temperature generally much higher in the northwest, especially in Alaska, than in the south (Figure 3). The eight warmest years in the last 100 years, according to NASA's Goddard Institute for Space Studies, have all occurred since 1998 (http://www.giss.nasa.gov/research/news/20080116/). During the second half of the 20th century, oceans have also become warmer. Warmer ocean waters cause sea ice to melt, trigger bleaching of corals, result in many species shifting their geographic ranges, stress many other species that cannot move elsewhere, contribute to sea- level rise (see below), and hold less oxygen and carbon dioxide.
Introduction 5 FIGURE 2 Global trends in temperature. The upper map shows the average change in temperature per decade from 1870 to 2005. Areas in orange have seen temperatures rise between 0.1-0.2oC per decade, so that they average 1.35 to 2.7oC warmer in 2005 than in 1870. The lower map shows the average change in temperature per decade from 1950 to 2005. Areas in deep red have seen temperatures rise on average more than 0.4oC per decade, so that they average more than 2oC warmer in 2005 than in 1950. SOURCE: Joint Institute for the Study of the Atmosphere and Ocean, University of Washington.
6 Introduction FIGURE 3 Temperature trends in North America, 1955 to 2005. The darker areas have experienced greater changes in temperature. For example, the Pacific Northwest had average temperatures about 1oC higher in 2005 than in 1955, while Alaskaâs average temperature had risen by over 2oC. SOURCE: Created with data from Goddard Institute for Space Studies. Sea levels are rising Climate change also means that sea levels are rising. Not only do warmer temperatures cause glaciers and land ice to melt (adding more volume to oceans), but seawater also expands in volume as it warms. The global average sea level rose by just under 2 mm/yr (0.08in/yr) during the 20th century, but since satellite measurements began in 1992, the rate has been 3.1 mm/year (0.12in/yr)(IPCC 2007a). Along some parts of the U.S. coast, tide gauge records show that sea level rose even faster (up to 10 mm/yr, 0.39in/yr) because the land is also subsiding. As sea level rises, shoreline retreat has been taking place along most of the nationâs sandy or muddy shorelines, and substantial coastal wetlands have been lost due to the combined effects of sea- level rise and direct human activities. In Louisiana alone, 4900 km2 (1900 mi2) of wetlands have been lost since 1900 as a result of high rates of relative sea-level rise together with curtailment of the supply of riverborne sediments needed to build wetland soils. The loss of these wetlands has diminished the ability of that region to provide many ecosystem services, including commercial fisheries, recreational hunting and fishing, and habitats for rare, threatened, and migratory species, as well as weakening the regionâs capacity to absorb storm surges like those caused by Hurricane Katrina (Day et al. 2007). Higher sea levels can also change the salinity and water circulation patterns of coastal estuaries and bays, with varying consequences for the mix of species that can thrive there.
Introduction 7 Other effects are being seen Water Cycle Climate change is linked to a number of other changes that already can be seen around the world. These include earlier spring snowmelt and peak stream flow, melting mountain glaciers, a dramatic decrease in sea ice during the arctic summer, and increasing frequency of extreme weather events, including the most intense hurricanes (IPCC 2007b). Changes in average annual precipitation have varied from place to place in the United States (Figure 4). Climate dynamics and the cycling of water between land, rivers and lakes, and clouds and oceans are closely connected. Climate change to date has produced complicated effects on water balances, supply, demand, and quality. When winter precipitation falls as rain instead of snow and as mountain snowpacks melt earlier, less water is âstoredâ in the form of snow for slow release throughout the summer (Mote 2003), when it is needed by the wildlife in and around streams and rivers and for agriculture and domestic uses. Even if the amount of precipitation does not change, warmer temperatures mean that moisture evaporates more quickly, so that the amount of moisture available to plants declines. The complex interaction between temperature and water demand and availability means that climate change can have many different kinds of effects on ecosystems. FIGURE 4 Trends in precipitation from 1901 to 2006 in the United States. Areas in red are averaging some 30 percent less precipitation per year now than they received early in the 1900s. Dark blue areas are averaging 50 percent more precipitation per year. SOURCE: Backlund 2008. Created with data from the USGS and NOAA/NCDC. Extreme Events The character of extreme weather and climate events is also changing on a global scale. The number of frost days in midlatitude regions is decreasing, while the number of days with extreme warm temperatures is increasing. Many land regions have experienced an increase in days with very heavy rain, but the recent CCSP report on climate extremes concluded that âthere are recent
8 Introduction regional tendencies toward more severe droughts in the southwestern U.S., parts of Canada and Alaska, and Mexicoâ (Kunkel et al. 2008, Dai et al. 2004; Seager et al., 2007). These seemingly contradictory changes are consistent with a climate in which a greater input of heat energy is leading to a more active water cycle. In addition, warmer ocean temperatures are associated with the recent increase in the fraction of hurricanes that grow to the most destructive categories 4 and 5 (Emanuel 2005; Webster et al. 2005). Arctic Sea Ice Every year the area covered by sea ice in the Arctic Ocean expands in the winter and contracts in the summer. In the first half of the 20th century the annual minimum sea-ice area in the Arctic was usually in the range of 10 to 11 million km2 (3.86 to 4.25 million mi2) (ACIA 2005). In September 2007 sea-ice area hit a single-day minimum of 4.1 million km2 (1.64 million mi2), a loss of about half since the 1950s (Serreze et al. 2007). The decrease in area is matched by a dramatic decrease in thickness. From 1975 to 2000 the average thickness of Arctic sea ice decreased by 33 percent, from 3.7 to 2.5 m (12.3 to 8.3 ft) (Rothrock et al. 2008). Ocean Acidification About one-third of the carbon dioxide emitted by human activity has already been taken up by the oceans, thus moderating the increase of carbon dioxide concentration in the atmosphere and global warming. But, as the carbon dioxide dissolves in sea water, carbonic acid is formed, which has the effect of acidifying, or lowering the pH, of the ocean (Orr et al. 2005). Although not caused by warming, acidification is a result of the increase of carbon dioxide, the same major greenhouse gas that causes warming. Ocean acidification has many impacts on marine ecosystems. To date, laboratory experiments have shown that although ocean acidification may be beneficial to a few species, it will likely be highly detrimental to a substantial number of species ranging from corals to lobsters and from sea urchins to mollusks (Raven et al. 2005; Doney et al. 2008; Fabry et al. 2008). Causes of climate change Both natural variability and human activities are contributing to observed global and regional warming, and both will contribute to future climate trends. It is very likely that most of the observed warming for the last 50 years has been due to the increase in greenhouse gases related to human activities (in IPCC reports, âvery likelyâ specifically means that scientists believe the statement is at least 90 percent likely to be true; âlikelyâ specifically means about two-thirds to 90 percent likely to be true [IPCC 2007b]). While debate over details is an important part of the scientific process, the climate science community is virtually unanimous on this conclusion. The physical processes that cause climate change are scientifically well documented. The basic physics of the way greenhouse gases warm the climate were well established by Tyndall, Ahrrenius, and others in the 19th century (Bolin 2007). The conclusions that human actions have very likely caused most of the recent warming and will likely cause more in the future are based on the vast preponderance of accumulated scientific evidence from many different kinds of observations (IPCC 2007b). Since the beginning of the Industrial Revolution, human activities that clear land or burn fossil fuels have been injecting rapidly increasing amounts of greenhouse gases such as carbon dioxide (CO2) and methane (CH4) into the atmosphere. In 2006 emissions of CO2 were about 36 billion metric tons (39.6 billion English tons), or about 5.5 metric tons (6.0 English tons) for every human being (Raupach et al. 2007). In the United States average CO2
Introduction 9 emissions in 2006 were approximately 55 kg (120 lb) per person per day. As a consequence of these emissions, atmospheric CO2 has increased by about 35 percent since 1850. Scientists know that the increases in carbon dioxide in the atmosphere are due to human activities, not natural processes, because they can fingerprint carbon dioxide (for example, by the mix of carbon isotopes it contains, its spatial pattern, and trends in concentration over time) and identify the sources. Concentrations of other greenhouse gases have also increased, some even more than CO2 in percentage terms (Figure 5). Methane, which is 25 times more effective per molecule at trapping heat than CO2, has increased by 150 percent. Nitrous oxide (N2O), which is nearly 300 times more effective per molecule than CO2 at trapping heat, has increased by over 20 percent (Forster et al. 2007). Scientific knowledge of climate is far from complete. Much remains to be learned about the factors that control the sensitivity of climate to increases in greenhouse gases, rates of change, and the regional outcomes of the global changes. These uncertainties, however, concern the details and not the core mechanisms that give scientists high confidence in their basic conclusions.
10 Introduction Atmospheric concentrations of CO2, CH4 and N2O over the last 10,000 years (large panels) and since 1750 (inset panels). Measurements are shown from ice cores (symbols with different colors for different studies) and atmospheric samples (red lines). The corresponding radiative forcings (amount of energy trapped per unit area) relative to 1750 are shown on the right hand axes of the large panels. Source: IPCC 2007d. FIGURE 5: Historical concentrations of greenhouse gasses CO2, CH4, and N2O over the past 10,000 years. For each of these greenhouse gases, the characteristic âhockey stickâ shape of the
Introduction 11 curve is the result of large increases in the concentrations of these gases very recently, compared to their relatively stable levels over the past 10,000 years. SOURCE: IPCC 2007d. What do we expect from future climate change? Evidence of rising atmospheric and ocean temperatures, changing precipitation patterns, rising sea levels, and decreasing sea ice is already clear. Average temperatures will almost certainly be warmer in the future. The amount of future climate change depends on human actions. A large number of experiments with climate models indicate that if the world continues to emphasize rapid economic development powered by fossil fuels, it will probably experience dramatic warming during the 21st century. For this kind of âbusiness as usualâ future the IPCC (IPCC 2007b) projects a likely range of global warming over 1990 levels of 2.4-6.4ºC (4.3-11.5ºF) by 2100 (Figure 6, scenario A1F1). If greenhouse gas emissions grow more slowly, peak around the year 2050, and then fall, scientists project a likely warming over 1990 levels of 1.1-2.9ºC (2.0- 5.2ºF) by 2100 (Figure 6, scenario B1).5 Temperature increases at the high end of the range of possibilities are very likely to exceed many climate thresholds. Warming of 6°C (10.8°F) or more (the upper end of the projections that the 2007 IPCC rates as âlikelyâ) would probably have catastrophic consequences for lifestyles, ecosystems, agriculture, and other livelihoods, especially in the regions and populations with the least resources to invest in adaptationâthat is, the strategies and infrastructure for coping with the climate changes. Warming to the high end of the range would also entail a global average rate of temperature change that, for the next century or two, would dramatically exceed the average rates of the last 20,000 years, and possibly much further into the past. Mean seawater temperatures in some U.S. coastal regions have increased by as much as 1.1°C (2°F) during the last half of the 20th century and, based on IPCC model projections of air temperature, are likely to increase by as much as 2.2-4.4°C (4-8°F) during the present century. âBusiness as usualâ emissions through 2100 would likely lead to oceans with surface temperatures that are 2-4ºC (3.6-7.2ºF) higher than now and surface waters so acidified that only a few isolated locations would support the growth of corals (Cao et al. 2007). Most marine animals, especially sedentary ones, and plants are expected to be significantly stressed by these changes (Hoegh-Guldberg et al. 2007). Some may be able to cope with either increased temperatures or more acidic waters, but adjusting to both may not be feasible for many species. 5 Projections of warming are given as a range of temperatures for three reasons. First, gaps in the scientific understanding of climate limit the accuracy of projections for any specific concentration of greenhouse gases. Changes in wind and clouds can increase or decrease the warming that occurs in response to an increase in the concentration of greenhouse gases. Loss of ice on the sea or snow on land increases the amount of the incoming sunlight that is absorbed, amplifying the warming from greenhouse gases. Second, the pattern of future emissions and the mix of compounds released to the atmosphere cannot be predicted with high confidence. Some kinds of compounds that produce warming remain in the atmosphere only a few days (Ramanathan et al. 2007). Others, like CO2, remain for centuries and longer (Matthews and Caldeira 2008). Still other compounds tend to produce aerosols or tiny droplets or particles that reflect sunlight, cooling the climate. Third, there is substantial uncertainty about the future role of the oceans and ecosystems on land. In the past, oceans and land ecosystems have stored, at least temporarily, about half of the carbon emitted to the atmosphere by human actions. If the rate of storage increases, atmospheric CO2 will rise more slowly. If it decreases, then atmospheric CO2 will rise more rapidly (Field et al. 2007).
12 Introduction Continued emissions under the âbusiness as usualâ scenario could lead by 2100 to 0.6 m (2 ft) or more of sea-level rise. Continuation of recent increases in loss of the ice caps that cover Greenland and West Antarctica could eventually escalate the rate of sea-level rise by a factor of 2 (Overpeck et al. 2006; Meehl et al. 2007; Alley et al. 2005; Gregory and Huybrechts 2006; Rahmstorf 2007). There will also be hotter extreme temperatures and fewer extreme cold events. An increase in climate variability, projected in some models, will entail more frequent conditions of extreme heat, drought, and heavy precipitation. A warmer world will experience more precipitation at the global scale, but the changes will not be the same everywhere. In general, the projections indicate that dry areas, especially in the latitude band just outside the tropics (for example, the southwestern United States), will tend to get drier on average (IPCC 2007b; Kunkel et al. 2008). Areas that are already wet, especially in the tropics and closer to the poles, will tend to get wetter on average. Increased climate variability and increased evaporation in a warmer world could both increase the risk and likely intensity of future droughts. Changes in the frequency or intensity of El Niño events forecast by climate models are not consistent (IPCC 2007b). El Niños are important because they are often associated with large-scale drought and floods in the tropics and heavy rains just outside the tropics, but projecting how the interaction between climate change and El Niño events will affect precipitation patterns is difficult. Another example of inconsistent results from models is that model simulations indicate that future hurricane frequency and average intensity could either increase or decrease (Emanuel et al. 2008), but it is likely that rainfall and top wind speeds in general will increase in a world of warmed ocean temperatures. For all of these different factorsââtemperature, precipitation patterns, sea-level rise and extreme eventsââboth the magnitude and speed of change are important. For both ecosystems and human activities, a rapid rate of climate change presents challenges that are different from, but no less serious than, the challenges from a large amount of change (Schneider and Root 2001).
Introduction 13 Solid lines are multi-model global averages of surface warming for scenarios A2, A1B and B1, shown as continuations of the 20th-century simulations. These projections also take into account emissions of short-lived GHGs and aerosols. The pink line is not a scenario, but is for Atmosphere-Ocean General Circulation Model (AOGCM) simulations where atmospheric concentrations are held constant at year 2000 values. The bars at the right of the figure indicate the best estimate (solid line within each bar) and the likely range assessed for the six SRES marker scenarios at 2090-2099. All temperatures are relative to the period 1980-1999. SOURCE IPCC 2007b. FIGURE 6 Projected future temperatures. This figure shows projected trends of average global surface temperature, based on output from all of the major climate models, shown as continuations of the 20th century observations (with the average for 1980-1999 plotted as 0). The pink line represents what would happen if CO2 concentrations could be held constant at year 2000 levels. Scenarios B1, A1B and A2 represent alternative possible futures. A1B and B1 are futures with modest population growth, rapid economic growth, and a globally integrated economy, with A1B focusing on manufacturing and B1 focusing on service industries. A2 is a world with more rapid population growth but slower economic growth and less economic integration. The bars to the right of the graph represent the likely range of average global temperature from the same models in the years 2090-2099 for a wider range of possible futures, with the horizontal bar in the middle indicating the average across the models. As of 2006, actual CO2 emissions were higher than those in the A2 scenario, making the full range of scenarios look like underestimates, at least for the first years of the 21st century. (IPCC 2007b, Raupach et al. 2007).
14 Introduction Climate change can impact ecosystems in many ways Hundreds of studies have documented responses of ecosystems, plants, and animals to the climate changes that have already occurred (Parmesan 2006; Rosenzweig et al. 2007). These studies demonstrate many direct and indirect effects of climate change on ecosystems. Changes in temperature, for example, have been shown to affect ecosystems directly: the date when some plants bloom is occurring earlier in response to warmer temperatures and earlier springs. Extreme temperatures, both hot and cold, can be important causes of mortality, and small changes in extremes can sometimes determine whether a plant or animal survives and reproduces in a given location. Changes in temperature, especially when combined with changes in precipitation, can have indirect effects as well. For many plants and animals soil moisture is critically important for many life processes; changes in precipitation and in the rate of evaporation interact to determine whether moisture levels remain at a level suitable for various organisms. For fish and other aquatic organisms both water temperature and water flow are important and influenced by the combined effects of altered air temperatures and precipitation. For example, warmer, drier years in the northwestern United States, often associated with El Niño events and anticipated to be more common under many climate scenarios, have historically been associated with below- average snowpack, stream flow, and salmon survival (Mote 2003). Some salmon populations are especially sensitive to summer temperatures; others are sensitive to low stream-flow volumes in the fall (Crozier and Zabel 2006). The fact that climate change leads to rising seas means that organisms and ecosystems located in coastal zones between the ocean and terrestrial habitats are squeezed, especially when the coastal land is occupied by buildings or crops. The ecological impacts of climate change are not inherently beneficial or detrimental for an ecosystem. The concept that a change is beneficial or detrimental has meaning mainly from the human perspective. For an ecosystem, responses to climate change are simply shifts away from the state prior to human-caused climate change. Measured by particular ecosystem services, some changes could be beneficial; for example, warmer temperatures extend the growing season in some latitudes, and higher CO2 levels increase the growth of some land plants, with higher potential yields of food and forestry products (Nemani et al. 2003). Others are detrimental, for example, western mountain areas with a longer snow-free season are experiencing increased wildfires, reduced potential wood harvests, and loss of some recreational opportunities (Westerling et al. 2006). In some settings uncertainty about future ecosystem services may be a cost in itself, motivating investments that may not turn out to be necessary or that may be insufficient to effectively address changing needs. To date, many species have responded to the effects of climate change by extending their range boundaries both toward the poles (for example, northward in the U.S.) and up in elevation, and by shifting the timing of spring and autumn events. Plants and animals needing to move but prevented from doing so, for example, because appropriate habitat is not present at higher elevations, are at greater risk of extinction. Shifting species ranges, changes in the timing of biological events, and a greater risk of extinction all affect the ability of ecosystems to provide the critical servicesâproducts, regulation of the environment, enhanced human quality of life, and natural infrastructureâthey have been providing.
Introduction 15 Ecosystems can adjust to changeâover time Ecosystems are not static. They are collections of living organisms that grow and interact and die. Ecosystems encounter an ever changing landscape of weather conditions and various kinds of disturbances, both subtle and severe. Whatever conditions an ecosystem encounters, the individual organisms and species react to the changes in different ways. Ecosystems themselves do not move, individuals and species do; some species can move farther and faster than others, but some may not be able to move at all. For example, a long-lived tree species may take decades to spread to a new range, while an insect with many hatches per year could move quickly. A species that already lives on mountaintops may have nowhere else to retreat. Rapid and extreme disturbances can have major and long-lasting ecological impacts. For example, a severe drought, wildfire, or hurricane can fundamentally reshape an area, often for many decades. In one of the most dramatic examples the impact of an asteroid 65 million years ago is believed to have so radically changed conditions on Earth that the dominant animals, the dinosaurs, died off and were supplanted by mammals (Alvarez et al. 1990). On longer time scales, most places on Earth have experienced substantial climate changes. During the peak of the last ice age, approximately 21,000 years ago, most of Canada and the northern United States were under thousands of feet of ice (Jansen et al. 2007). Arctic vegetation thrived in Kentucky, and sea levels were about 120 m (400 ft) lower than at present. Over the past million years Earth has experienced a series of ice ages, separated by warmer conditions. Global average temperatures during these ice ages were about 4-7°C (7.2-12.6°F) cooler than present, with the cooling and warming occurring over many thousands of years (Jansen et al. 2007). These ice ages triggered extensive ecological responses, including large shifts in the distributions of plants and animals, as well as extinctions. The massive changes during past ice ages certainly pushed ecosystems off large swaths of Earthâs surface as ice- dominated landscapes advanced. However, these changes were generally slow enough that surviving species could move and reassemble into novel, as well as familiar-looking, ecosystems as the ice retreated (Pitelka et al. 1997; Overpeck et al. 2003). The 10,000 years since the last ice age have seen substantial regional and local climate variation, but on a global scale climate was relatively stable, and these regional climate changes did not drive species to extinction nor result in the scale of global ecosystem change seen during glacial-to-interglacial transitions. Even when the global climate is not changing noticeably, regional climate variability (droughts, storms, and heat waves) can have dramatic regional (often short-term) impacts. In a period of climate change it is important to remember that this climate variability will continue to occur on top of the more long-term human-caused climate changes. Data on ecosystem responses to disturbances in the distant past can provide valuable information about likely responses to current and future climate change. But it is important to recognize that the current rate of increase of CO2 in Earthâs atmosphere is faster than at any time measured in the past, indicating that human-caused global climate change in the current era is likely to be exceedingly rapid, many times faster than the long-term global changes associated with onset and termination of the ice ages (Jansen et al. 2007). One of the big concerns about the future is that climate changes in some places may be too fast for organisms to respond in the ways that have helped sustain ecosystem services in response to natural changes in the past. Understanding how quickly ecosystems can and cannot adjust is one of the key challenges in climate change research.
16 Introduction Climate change, other stresses, and the limits of ecosystem resilience Climate change is not the only way humans are affecting ecosystems. Humans have a large and pervasive influence on the planet. We use a substantial portion of the land for agriculture and the oceans for fishing (Worm et al. 2006; Ellis and Ramankutty 2008). Many rivers are dammed to provide water for crops or people, or they are polluted with fertilizer or other chemicals. Chemical residues and the by-products of industrial activity, from acid precipitation to ozone, affect plant growth. Human activities, especially land and ocean use, limit some opportunities for species migrations while opening routes for other species. Globally humans have moved many non-native species from one ecosystem to another. Ecosystems operate in a context of multiple human influences and interacting factors. Earthâs ecosystems are generally resilient to some range of changes in climate. A resilient ecosystem is one that can withstand a stress like pollution or rebuild after a major disturbance like a serious storm. A resilient ecosystem can cope with a drought or an unusually hot summer in ways that alter some aspects of ecosystem function but do not lead to a major shift in the type of ecosystem or the services it provides. Thus, a resilient ecosystem may not appear to be affected by modest or slow climate changes. But this resilience has limits. When a change exceeds those limits, or is coupled with other simultaneous changes that cause stress, the ecosystem undergoes a major change, often shifting to a fundamentally different ecosystem type. There is a threshold point when dramatic ecosystem transformations may occur (Gunderson and Pritchard 2002). These thresholds are like the top of a levee as the water level rises. As long as the water level is even slightly below the top of the levee, function is normal. But once it rises above the levee, there is a flood. This kind of threshold response is common in ecosystems, where extreme events like heat waves often serve as triggers for an irreversible transition of the ecosystem to a new state. Currently plants and animals are responding to rapid climate change while simultaneously coping with other human-created stresses such as habitat loss and fragmentation due to development, pollution, invasive species, and overharvesting. How do we know climate change itself is causing major changes in ecosystems? First, species changing their ranges in the Northern Hemisphere are almost uniformly moving their ranges northward and up in elevation in search of cooler temperatures (Parmesan and Yohe 2003; Parmesan 2006; Rosenzweig et al. 2007). If any or all of the other stressors were the major cause of ecosystem changes, plants and animals would move in many directions in addition to north, and to lower as well as higher elevations. Second, when we look at the association over time of changes between species ranges and temperatures modeled using only natural variation in climate, such as sunspots and volcanic dust in the stratosphere, the relationship is poor. When temperatures are modeled using natural variability as well as human-caused drivers, such as emission of CO2 and methane, the association is very strong. Consequently, humans are very likely causing changes in regional temperatures to which in turn the plants and animals are responding (Root et al. 2005).
The world's climate is changing, and it will continue to change throughout the 21st century and beyond. Rising temperatures, new precipitation patterns, and other changes are already affecting many aspects of human society and the natural world.
In this book, the National Research Council provides a broad overview of the ecological impacts of climate change, and a series of examples of impacts of different kinds. The book was written as a basis for a forthcoming illustrated booklet, designed to provide the public with accurate scientific information on this important subject.
READ FREE ONLINE
Welcome to OpenBook!
You're looking at OpenBook, NAP.edu's online reading room since 1999. Based on feedback from you, our users, we've made some improvements that make it easier than ever to read thousands of publications on our website.
Do you want to take a quick tour of the OpenBook's features?
Show this book's table of contents , where you can jump to any chapter by name.
...or use these buttons to go back to the previous chapter or skip to the next one.
Jump up to the previous page or down to the next one. Also, you can type in a page number and press Enter to go directly to that page in the book.
To search the entire text of this book, type in your search term here and press Enter .
Share a link to this book page on your preferred social network or via email.
View our suggested citation for this chapter.
Ready to take your reading offline? Click here to buy this book in print or download it as a free PDF, if available.
Get Email Updates
Do you enjoy reading reports from the Academies online for free ? Sign up for email notifications and we'll let you know about new publications in your areas of interest when they're released.
- Climate Change - A Global Issue
- Dag Hammarskjöld Library
- Research Guides
- A Global Issue
- At the United Nations
- Major Reports
- Books & Journals
- Consulting the Experts
- Keeping up to date
- Data & Statistics

This site contains links and references to third-party databases, web sites, books and articles. It does not imply the endorsement of the content by the United Nations.
What is climate change?
- Background Climate change is an urgent global challenge with long-term implications for the sustainable development of all countries.

Exploring the Topic
- Climate.gov NOAA (National Oceanic and Atmospheric Administration) maintains this gateway to peer-reviewed information on climate change for various audiences, from the layperson to teachers to scientists to planners and policy makers. Provides access to relevant data sets from a number of agencies, including the National Climatic Data Center and the NOAA Climate Prediction Center.
- Eldis resource guide on climate change (IDS) The Eldis website is maintained by the Institute of Development Studies at the University of Sussex. It facilitates the sharing of information on development issues by aggregating information materials from reputable sources into the resource guide on climate change. It offers tools to create online communities for development practitioners; several such communities exist to discuss specific aspects of climate change. Eldis topic editors compile email newsletters, so-called reporters, including the “Climate Change and Development” reporter.
- IIED - International Institute for Environment and Development Well-established policy research institute that offers an online library of information materials on climate change and related topics, such as energy, biodiversity and forests. Publicizes its research output through email newsletters and on various social media channels.
- IISD - International Institute for Sustainable Development IISD offers a searchable and browsable knowledge base of its publications and video on climate change. IISDs LINKAGES reporting services closely monitor major international climate change meetings, including those of the IPCC and under the UNFCCC. IISD publishes the Earth Negotiations Bulletin, hosts the climate-l electronic mailing list and publicizes its work on twitter and Facebook.
Climate Change 2022: Mitigation of Climate Change
Watch this video by the Intergovernmental Panel on Climate Change to learn more about what is at stake and what actions need to be taken to mitigate the impact of climate change globally.
More videos on other aspects of climate change can be found on the IPCC's YouTube page .
Climate Change FAQs
Check out what questions others have asked about climate change and related issues in Ask DAG , our FAQ database.
Related Research Guides
UN Research Guides on issues related to environment:
- Climate Change - A Global Issue by Dag Hammarskjöld Library Last Updated Jan 5, 2024 7927 views this year
- UN Documentation: Environment by Dag Hammarskjöld Library Last Updated Dec 29, 2023 6039 views this year
Other resource guides on climate change and related topics:
- Peace Palace Library: Environmental Law Starting point for research in the field of International Environmental Law provided by the Peace Palace Library in The Hague.
- Next: At the United Nations >>
- Last Updated: Jan 5, 2024 5:23 PM
- URL: https://research.un.org/en/climate-change

An official website of the United States government
The .gov means it’s official. Federal government websites often end in .gov or .mil. Before sharing sensitive information, make sure you’re on a federal government site.
The site is secure. The https:// ensures that you are connecting to the official website and that any information you provide is encrypted and transmitted securely.
- Publications
- Account settings
Preview improvements coming to the PMC website in October 2024. Learn More or Try it out now .
- Advanced Search
- Journal List
- Springer Nature - PMC COVID-19 Collection

Heat waves: a hot topic in climate change research
Werner marx.
1 Max Planck Institute for Solid State Research, Heisenbergstr. 1, 70569 Stuttgart, Germany
Robin Haunschild
Lutz bornmann.
2 Science Policy and Strategy Department, Max Planck Society, Administrative Headquarters, Hofgartenstr. 8, 80539 Munich, Germany
Associated Data
Not applicable.
Research on heat waves (periods of excessively hot weather, which may be accompanied by high humidity) is a newly emerging research topic within the field of climate change research with high relevance for the whole of society. In this study, we analyzed the rapidly growing scientific literature dealing with heat waves. No summarizing overview has been published on this literature hitherto. We developed a suitable search query to retrieve the relevant literature covered by the Web of Science (WoS) as complete as possible and to exclude irrelevant literature ( n = 8,011 papers). The time evolution of the publications shows that research dealing with heat waves is a highly dynamic research topic, doubling within about 5 years. An analysis of the thematic content reveals the most severe heat wave events within the recent decades (1995 and 2003), the cities and countries/regions affected (USA, Europe, and Australia), and the ecological and medical impacts (drought, urban heat islands, excess hospital admissions, and mortality). An alarming finding is that the limit for survivability may be reached at the end of the twenty-first century in many regions of the world due to the fatal combination of rising temperatures and humidity levels measured as “wet-bulb temperature” (WBT). Risk estimation and future strategies for adaptation to hot weather are major political issues. We identified 104 citation classics, which include fundamental early works of research on heat waves and more recent works (which are characterized by a relatively strong connection to climate change).
Introduction
As a consequence of the well-documented phenomenon of global warming, climate change has become a major research field in the natural and medical sciences, and more recently also in the social and political sciences. The scientific community has contributed extensively to a comprehensive understanding of the earth’s climate system, providing various data and projections on the future climate as well as on the effects and risks of anticipated global warming (IPCC 2014; CSSR 2017; NCA4 2018; and the multitude of references cited therein). During recent decades, climate change has also become a major political, economic, and environmental issue and a central theme in political and public debates.
One consequence of global warming is the increase of extreme weather events such as heat waves, droughts, floods, cyclones, and wildfires. Some severe heat waves occurring within the last few decades made heat waves a hot topic in climate change research, with “hot” having a dual meaning: high temperature and high scientific activity. “More intense, more frequent, and longer lasting heat waves in the twenty-first century” is the title of a highly cited paper published 2004 in Science (Meehl and Tebaldi 2004 ). This title summarizes in short what most climate researchers anticipate for the future. But what are heat waves (formerly also referred to as “heatwaves”)? In general, a heat wave is a period of excessively hot weather, which may be accompanied by high humidity. Since heat waves vary according to region, there is no universal definition, but only definitions relative to the usual weather in the area and relative to normal temperatures for the season. The World Meteorological Organization (WMO) defines a heat wave as 5 or more consecutive days of prolonged heat in which the daily maximum temperature is higher than the average maximum temperature by 5 °C (9 °F) or more ( https://www.britannica.com/science/heat-wave-meteorology ).
Europe, for example, has suffered from a series of intense heat waves since the beginning of the twenty-first century. According to the World Health Organization (WHO) and various national reports, the extreme 2003 heat wave caused about 70,000 excess deaths, primarily in France and Italy. The 2010 heat wave in Russia caused extensive crop loss, numerous wildfires, and about 55,000 excess deaths (many in the city of Moscow). Heat waves typically occur when high pressure systems become stationary and the winds on their rear side continuously pump hot and humid air northeastward, resulting in extreme weather conditions. The more intense and more frequently occurring heat waves cannot be explained solely by natural climate variations and without human-made climate change (IPCC 2014; CSSR 2017; NCA4 2018). Scientists discuss a weakening of the polar jet stream caused by global warming as a possible reason for an increasing probability for the occurrence of stationary weather, resulting in heavy rain falls or heat waves (Broennimann et al. 2009 ; Coumou et al. 2015 ; Mann 2019 ). This jet stream is one of the most important factors for the weather in the middle latitude regions of North America, Europe, and Asia.
Until the end of the twentieth century, heat waves were predominantly seen as a recurrent meteorological fact with major attention to drought, being almost independent from human activities and unpredictable like earthquakes. However, since about 1950, distinct changes in extreme climate and weather events have been increasingly observed. Meanwhile, climate change research has revealed that these changes are clearly linked to the human influence on the content of greenhouse gases in the earth’s atmosphere. Climate-related extremes, such as heat waves, droughts, floods, cyclones, and wildfires, reveal significant vulnerability to climate change as a result of global warming.
In recent years, research on heat waves has been established as an emerging research topic within the large field of current climate change research. Bibliometric analyses are very suitable in order to have a systematic and quantitative overview of the literature that can be assigned to an emerging topic such as research dealing with heat waves (e.g., Haunschild et al. 2016 ). No summarizing overview on the entire body of heat wave literature has been published until now. However, a bibliometric analysis of research on urban heat islands as a more specific topic in connection with heat waves has been performed (Huang and Lu 2018 ).
In this study, we analyzed the publications dealing with heat waves using appropriate bibliometric methods and tools. First, we determined the amount and time evolution of the scientific literature dealing with heat waves. The countries contributing the most papers are presented. Second, we analyzed the thematic content of the publications via keywords assigned by the WoS. Third, we identified the most important (influential) publications (and also the historical roots). We identified 104 citation classics, which include fundamental early works and more recent works with a stronger connection to climate change.
Heat waves as a research topic
The status of the current knowledge on climate change is summarized in the Synthesis Report of the Fifth Assessment Report (AR5) of the Intergovernmental Panel on Climate Change (IPCC) (IPCC 2014, https://www.ipcc.ch/report/ar5/syr/ ). This panel is the United Nations body for assessing the science related to climate change. The Synthesis Report is based on the reports of the three IPCC Working Groups , including relevant Special Reports . In its Summary for Policymakers , it provides an integrated view of climate change as the final part of the Fifth Assessment Report (IPCC 2014, https://www.ipcc.ch/site/assets/uploads/2018/02/AR5_SYR_FINAL_SPM.pdf ).
In the chapter Extreme Events , it is stated that “changes in many extreme weather and climate events have been observed since about 1950. Some of these changes have been linked to human influences, including a decrease in cold temperature extremes, an increase in warm temperature extremes, an increase in extreme high sea levels and an increase in the number of heavy precipitation events in a number of regions … It is very likely that the number of cold days and nights has decreased and the number of warm days and nights has increased on the global scale. It is likely that the frequency of heat waves has increased in large parts of Europe, Asia and Australia. It is very likely that human influence has contributed to the observed global scale changes in the frequency and intensity of daily temperature extremes since the mid-twentieth century. It is likely that human influence has more than doubled the probability of occurrence of heat waves in some locations” (p. 7–8). Under Projected Changes , the document summarizes as follows: “Surface temperature is projected to rise over the twenty-first century under all assessed emission scenarios. It is very likely that heat waves will occur more often and last longer, and that extreme precipitation events will become more intense and frequent in many regions” (p. 10).
With regard to the USA, the Climate Science Special Report of the U.S. Global Change Research Program (CSSR 2017, https://science2017.globalchange.gov/ ) mentions quite similar observations and states unambiguously in its Fourth National Climate Assessment (Volume I) report ( https://science2017.globalchange.gov/downloads/CSSR2017_FullReport.pdf ) under Observed Changes in Extremes that “the frequency of cold waves has decreased since the early 1900s, and the frequency of heat waves has increased since the mid-1960s (very high confidence). The frequency and intensity of extreme heat and heavy precipitation events are increasing in most continental regions of the world (very high confidence). These trends are consistent with expected physical responses to a warming climate [p. 19]. Heavy precipitation events in most parts of the United States have increased in both intensity and frequency since 1901 (high confidence) [p. 20]. There are important regional differences in trends, with the largest increases occurring in the northeastern United States (high confidence). Recent droughts and associated heat waves have reached record intensity in some regions of the United States … (very high confidence) [p. 21]. Confidence in attribution findings of anthropogenic influence is greatest for extreme events that are related to an aspect of temperature” (p. 123).
Among the key findings in the chapter on temperature changes in the USA, the report states that “there have been marked changes in temperature extremes across the contiguous United States. The frequency of cold waves has decreased since the early 1900s, and the frequency of heat waves has increased since the mid-1960s (very high confidence). Extreme temperatures in the contiguous United States are projected to increase even more than average temperatures. The temperatures of extremely cold days and extremely warm days are both expected to increase. Cold waves are projected to become less intense while heat waves will become more intense (very high confidence) [p. 185]. Most of this methodology as applied to extreme weather and climate event attribution, has evolved since the European heat wave study of Stott et al.” (p. 128).
Heat waves are also discussed in the Fourth National Climate Assessment (Volume II) report (NCA4 2018, https://nca2018.globalchange.gov/ ). The Report-in-Brief ( https://nca2018.globalchange.gov/downloads/NCA4_Report-in-Brief.pdf ) for example states: “More frequent and severe heat waves and other extreme events in many parts of the United States are expected [p. 38]. Heat waves and heavy rainfalls are expected to increase in frequency and intensity [p. 93]. The season length of heat waves in many U.S. cities has increased by over 40 days since the 1960s [p. 30]. Cities across the Southeast are experiencing more and longer summer heat waves [p. 123]. Exposure to hotter temperatures and heat waves already leads to heat-associated deaths in Arizona and California. Mortality risk during a heat wave is amplified on days with high levels of ground-level ozone or particulate air pollution” (p. 150).
In summary, climate change research expects more frequent and more severe heat wave events as a consequence of global warming. It is likely that the more frequent and longer lasting heat waves will significantly increase excess mortality, particularly in urban regions with high air pollution. Therefore, research around heat waves will become increasingly important and is much more than a temporary research fashion.
Methodology
Dataset used.
This analysis is based on the relevant literature retrieved from the following databases accessible under the Web of Science (WoS) of Clarivate Analytics: Web of Science Core Collection: Citation Indexes, Science Citation Index Expanded (SCI-EXPANDED), Social Sciences Citation Index (SSCI), Arts & Humanities Citation Index (A&HCI), Conference Proceedings Citation Index—Science (CPCI-S), Conference Proceedings Citation Index—Social Science & Humanities (CPCI-SSH), Book Citation Index—Science (BKCI-S), Book Citation Index—Social Sciences & Humanities (BKCI-SSH), Emerging Sources Citation Index (ESCI).
We applied the search query given in Appendix 1 to cover the relevant literature as completely as possible and to exclude irrelevant literature. We practiced an iterative query optimization by identifying and excluding the WoS subject categories with most of the non-relevant papers. For example, heat waves are also mentioned in the field of materials science but have nothing to do with climate and weather phenomena. Unfortunately, WoS obviously assigned some heat wave papers related to climate to materials science-related subject categories. Therefore, these subject categories were not excluded. By excluding the other non-relevant subject categories, 597 out of 8,568 papers have been removed, resulting in a preliminary publication set of 7,971 papers (#2 of the search query). But this is no safe method, since the excluded categories may well include some relevant papers. Therefore, we have combined these 597 papers with search terms related to climate or weather and retrieved 62 relevant papers in addition, which we added to our preliminary paper subset, eventually receiving 8,033 publications (#3 to #5 of the search query).
Commonly, publication sets for bibliometric analyses are limited to articles, reviews, and conference proceedings as the most relevant document types and are restricted to complete publication years. In this study, however, we have included all relevant WoS document types for a better literature coverage of the research topic analyzed. For example, conference meetings and early access papers may well be interesting for the content analysis of the literature under study. Such literature often anticipates important results, which are published later as regular articles. Furthermore, we have included the literature until the date of search for considering the recent rapid growth of the field. Our search retrieved a final publication set of 8,011 papers indexed in WoS until the date of search (July 1, 2021) and dealing with heat waves (#6 of the search query). We have combined this publication set with climate change-related search terms from a well-proven search query (Haunschild et al. 2016 ) resulting in 4,588 papers dealing with heat waves in connection with climate change or global warming (# 11 of the search query). Also, we have selected a subset of 2,373 papers dealing with heat waves and mortality (#13 of the search query). The complete WoS search query is given in Appendix 1.
The final publication set of 8,011 papers dealing with heat waves still contains some non-relevant papers primarily published during the first half of the twentieth century, such as some Nature papers within the WoS category Multidisciplinary Sciences . Since these papers are assigned only to this broad subject category and have no abstracts and no keywords included, they cannot be excluded using the WoS search and refinement functions. We do not expect any bias through these papers, because their keywords do not appear in our maps. Also, they normally contain very few (if any) cited references, which could bias/impact our reference analysis.
We used the VOSviewer software (Van Eck and Waltman 2010 ) to map co-authorship with regard to the countries of authors (88 countries considered) of the papers dealing with heat waves ( www.vosviewer.com ). The map of the cooperating countries presented is based on the number of joint publications. The distance between two nodes is proportionate to the number of co-authored papers. Hence, largely cooperating countries are positioned closer to each other. The size of the nodes is proportionate to the number of papers published by authors of the specific countries.
The method that we used for revealing the thematic content of the publication set retrieved from the WoS is based on the analysis of keywords. For better standardization, we chose the keywords allocated by the database producer (keywords plus) rather than the author keywords. We also used the VOSviewer for mapping the thematic content of the 104 key papers selected by reference analysis. This map is also based on keywords plus.
The term maps (keywords plus) are based on co-occurrence for positioning the nodes on the maps. The distance between two nodes is proportionate to the co-occurrence of the terms. The size of the nodes is proportionate to the number of papers with a specific keyword. The nodes on the map are assigned by VOSviewer to clusters based on a specific cluster algorithm (the clusters are highlighted in different colors). These clusters identify closely related (frequently co-occurring) nodes, where each node is assigned to only one cluster.
Reference Publication Year Spectroscopy
A bibliometric method called “Reference Publication Year Spectroscopy” (RPYS, Marx et al. 2014 ) in combination with the tool CRExplorer ( http://www.crexplorer.net , Thor et al. 2016a , b ) has proven useful for exploring the cited references within a specific publication set, in order to detect the most important publications of the relevant research field (and also the historical roots). In recent years, several studies have been published, in which the RPYS method was basically described and applied (Marx et al. 2014 ; Marx and Bornmann 2016 ; Comins and Hussey 2015 ). In previous studies, Marx et al. have analyzed the roots of research on global warming (Marx et al. 2017a ), the emergence of climate change research in combination with viticulture (Marx et al. 2017b ), and tea production (Marx et al. 2017c ) from a quantitative (bibliometric) perspective. In this study, we determined which references have been most frequently cited by the papers dealing with heat waves.
RPYS is based on the assumption that peers produce a useful database by their publications, in particular by the references cited therein. This database can be analyzed statistically with regard to the works most important for their specific research field. Whereas individual scientists judge their research field more or less subjectively, the overall community can deliver a more objective picture (based on the principle of “the wisdom of the crowds”). The peers effectively “vote” via their cited references on which works turned out to be most important for their research field (Bornmann and Marx 2013 ). RPYS implies a normalization of citation counts (here: reference counts) with regard to the research area and the time of publication, which both impact the probability to be cited frequently. Basically, the citing and cited papers analyzed were published in the same research field and the reference counts are compared with each other only within the same publication year.
RPYS relies on the following observation: the analysis of the publication years of the references cited by all the papers in a specific research topic shows that publication years are not equally represented. Some years occur particularly frequently among the cited references. Such years appear as distinct peaks in the distribution of the reference publication years (i.e., the RPYS spectrogram). The pronounced peaks are frequently based on a few references that are more frequently cited than other references published in the same year. The frequently cited references are—as a rule—of specific significance to the research topic in question (here: heat waves) and the earlier references among them represent its origins and intellectual roots (Marx et al. 2014 ).
The RPYS changes the perspective of citation analysis from a times cited to a cited reference analysis (Marx and Bornmann 2016 ). RPYS does not identify the most highly cited papers of the publication set being studied (as is usually done by bibliometric analyses in research evaluation). RPYS aims to mirror the knowledge base of research (here: on heat waves).
With time, the body of scientific literature of many research fields is growing rapidly, particularly in climate change research (Haunschild et al. 2016 ). The growth rate of highly dynamic research topics such as research related to heat waves is even larger. As a consequence, the number of potentially citable papers is growing substantially. Toward the present, the peaks of individual publications lie over a broad continuum of newer publications and are less numerous and less pronounced. Due to the many publications cited in the more recent years, the proportion of individual highly cited publications in specific reference publication years falls steadily. Therefore, the distinct peaks in an RPYS spectrogram reveal only the most highly cited papers, in particular the earlier references comprising the historical roots. Further inspection and establishing a more entire and representative list of highly cited works requires consulting the reference table provided by the CRExplorer. The most important references within a specific reference publication year can be identified by sorting the cited references according to the reference publication year (RPY) and subsequently according to the number of cited references (N_CR) in a particular publication year.
The selection of important references in RPYS requires the consideration of two opposing trends: (1) the strongly growing number of references per reference publication year and (2) the fall off near present due to the fact that the newest papers had not sufficient time to accumulate higher citation counts. Therefore, we decided to set different limits for the minimum number of cited references for different periods of reference publication years (1950–1999: N_CR ≥ 50, 2000–2014: N_CR ≥ 150, 2015–2020: N_CR ≥ 100). This is somewhat arbitrary, but is helpful in order to adapt and limit the number of cited references to be presented and discussed.
In order to apply RPYS, all cited references ( n = 408,247) of 216,932 unique reference variants have been imported from the papers of our publication set on heat waves ( n = 8,011). The cited reference publication years range from 1473 to 2021. We removed all references (297 different cited reference variants) with reference publication years prior to 1900. Due to the very low output of heat wave-related papers published before 1990, no relevant literature published already in the nineteenth century can be expected. Also, global warming was no issue before 1900 since the Little Ice Age (a medieval cold period) lasted until the nineteenth century. The references were sorted according to RPY and N_CR for further inspection.
The CRExplorer offers the possibility to cluster and merge variants of the same cited reference (Thor et al. 2016a , b ). We clustered and merged the associated reference variants in our dataset (which are mainly caused by misspelled references) using the corresponding CRExplorer module, clustering the reference variants via volume and page numbers and subsequently merging aggregated 374 cited references (for more information on using the CRExplorer see “guide and datasets” at www.crexplorer.net ).
After clustering and merging, we applied a further cutback: to focus the RPYS on the most pronounced peaks, we removed all references ( n = 212,324) with reference counts below 10 (resulting in a final number of 3,937 cited references) for the detection of the most frequently cited works. A minimum reference count of 10 has proved to be reasonable, in particular for early references (Marx et al. 2014 ). The cited reference publication years now range from 1932 to 2020.
In this study, we have considered all relevant WoS document types for a preferably comprehensive coverage of the literature of the research topic analyzed. The vast majority of the papers of our publication set, however, have been assigned to the document types “article” ( n = 6.738, 84.1%), “proceedings paper” ( n = 485, 6.1%), and “review” ( n = 395 papers, 4.9%). Note that some papers belong to more than one document type.
Time evolution of literature
In Fig. 1 , the time evolution between 1990 and 2020 of the publications dealing with heat waves is shown (there are only 109 pre-1990 publications dealing with heat waves and covered by the WoS).

Time evolution of the overall number of heat wave publications, of heat wave publications in connection with climate change, and of heat wave publications in connection with mortality, each between 1990 and 2020. For comparison, the overall number of publications (scaled down) in the field of climate change research and the total number of publications covered by the WoS database (scaled down, too) are included
According to Fig. 1 , research dealing with heat waves is a highly dynamic research topic, currently doubling within about 5 years. The number of papers published per year shows a strong increase: since around 2000, the publication output increased by a factor of more than thirty, whereas in the same period, the overall number of papers covered by the WoS increased only by a factor of around three. Also, the portion of heat wave papers dealing with climate change increased substantially: from 16.1 in the period 1990–1999 to 25.7% in 2000, reaching 66.9% in 2020. The distinct decrease of the overall number of papers covered by the WoS between 2019 and 2020 might be a result of the Covid-19 pandemic.
With regard to the various impacts of heat waves, excess mortality is one of the most frequently analyzed and discussed issues in the scientific literature (see below). Whereas the subject specific literature on heat waves increased from 2000 to 2020 by a factor of 33.6, literature on heat waves dealing with mortality increased from 2000 to 2020 by a factor of 51.5. The dynamics of the research topic dealing with heat waves is mirrored by the WoS Citation Report , which shows the time evolution of the overall citation impact of the papers of the publication set (not presented). The citation report curve shows no notable citation impact before 2005, corresponding to the increase of the publication rate since about 2003 as shown in Fig. 1 .
Countries of authors
In Table Table1, 1 , the number of papers assigned to the countries of authors with more than 100 publications dealing with heat waves is presented, showing the national part of research activities on this research topic. For comparative purposes, the percentage of overall papers in WoS of each country is shown. As a comparison with the overall WoS, we only considered WoS papers published between 2000 and 2020, because the heat wave literature started to grow substantially around 2000.
Top countries of authors with more than 100 papers dealing with heat waves up to the date of the search
The country-specific percentages from Table Table1 1 are visualized in Fig. 2 . Selected countries are labeled. Countries with a higher relative percentage of more than two percentage points in heat wave research than in WoS overall output are marked blue (blue circle). Countries with a relative percentage at least twice as high in heat wave research than in overall WoS output are marked green (green cross), whereas countries with a relative percentage at most half as much in heat wave research than in overall WoS output are marked with a yellow cross. Only Japan has a much lower output in heat wave research than in WoS overall output, as indicated by the red circle and yellow cross. Most countries are clustered around the bisecting line and are marked gray (gray circle). China and the USA are outside of the plot region. Both countries are rather close to the bisecting line. Some European countries show a much larger activity in heat wave research than in overall WoS output. Australia shows the largest difference and ratio in output percentages as shown by the blue circle and green cross.

Publication percentages of countries in Table Table1. 1 . Countries with large deviations between heat wave output and overall WoS output are labeled. Countries with an absolute percentage of more than two percentage points higher (lower) in heat wave research than in overall WoS output are marked blue (red). Countries with a relative percentage at least twice as high (at most half as much) in heat wave research than in overall WoS output are marked green (yellow)
The results mainly follow the expectations of such bibliometric analyses, with one distinct exception: Australia increasingly suffers from extreme heat waves and is comparatively active in heat wave research—compared with its proportion of scientific papers in general. The growth factor of the Australian publication output since 2010 is 8.5, compared to 5.3 for the USA and 3.3 for Germany.
Figure 3 shows the co-authorship network with regard to the countries of authors of the papers dealing with heat waves using the VOSviewer software.

Co-authorship overlay map with regard to the countries of authors and their average publication years from the 8,011 papers dealing with heat waves. The minimum number of co-authored publications of a country is 5; papers with more than 25 contributing countries are neglected; of the 135 countries, 89 meet the threshold, and 88 out of 89 countries are connected and are considered (one country, Armenia, that is disconnected from the network has been removed). The co-authorship network of a single country can be depicted by clicking on the corresponding node in the interactive map. Readers interested in an in-depth analysis can use VOSviewer interactively and zoom into the map via the following URL: https://tinyurl.com/3ywkwv8t
According to Fig. 3 and in accordance with Table Table1, 1 , the USA is most productive in heat wave research. This is not unexpected, because the US publication output is at the top for most research fields. However, this aside, the USA has been heavily affected by heat wave events and is leading with regard to the emergence of the topic. Australia appears as another major player and is strongly connected with the US publications within the co-authorship network and thus appears as a large node near the US node in the map. Next, the leading European countries England, France, Germany, Italy, and Spain appear.
The overlay version of the map includes the time evolution of the research activity in the form of coloring of the nodes. The map shows the mean publication year of the publications for each specific author country. As a consequence, the time span of the mean publication years ranges only from 2014 to 2018. Nevertheless, the early activity in France and the USA and the comparatively recent activity in Australia and China, with the European countries in between, become clearly visible.
Topics of the heat wave literature
Figure 4 shows the keywords (keywords plus) map for revealing the thematic content of our publication set using the VOSviewer software. This analysis is based on the complete publication set ( n = 8,011). The minimum number of occurrences of keywords is 10; of the 10,964 keywords, 718 keywords met the threshold. For each of the 718 keywords, the total strength of the co-occurrence links with other keywords was calculated. The keywords with the greatest total link strength were selected for presentation in the map.

Co-occurrence network map of the keywords plus from the 8,011 papers dealing with heat waves for a rough analysis of the thematic content. The minimum number of occurrences of keywords is 10; of the 10,964 keywords, 718 meet the threshold. Readers interested in an in-depth analysis can use VOSviewer interactively and zoom into the map via the following URL: https://tinyurl.com/enrdbw
According to Fig. 4 , the major keywords are the following: climate change, temperature, mortality, impact, heat waves (searched), and variability. The colored clusters identify closely related (frequently co-occurring) nodes. The keywords marked red roughly originate from fundamental climate change research focused on the hydrological cycle (particularly on drought), the keywords of the green cluster are around heat waves and moisture or precipitation, the keywords marked blue result from research concerning impacts of heat waves on health, the keywords marked yellow are focused on the various other impacts of heat waves, and the keywords of the magenta cluster are around adaptation and vulnerability in connection with heat waves.
The clustering by the VOSviewer algorithm provides basic categorizations, but many related keywords also appear in different clusters. For example, severe heat wave events are marked in different colors. For a better overview of the thematic content of the publications dealing with heat waves, we have assigned the keywords of Fig. 4 (with a minimum number of occurrences of 50) to ten subject categories (each arranged in the order of occurrence):
- Countries/regions: United-States, Europe, France, China, Pacific, Australia, London, England
- Cities: cities, city, US cities, Chicago, communities
- Events: 2003 heat-wave, 1995 heat-wave
- Impacts: impact, impacts, air-pollution, drought, soil-moisture, exposure, heat-island, urban, islands, photosynthesis, pollution, heat-island, air-quality, environment, precipitation extremes, biodiversity, emissions
- Politics: risk, responses, vulnerability, adaptation, management, mitigation, risk-factors, scenarios
- Biology: vegetation, forest, diversity, stomatal conductance
- Medicine: mortality, health, stress, deaths, morbidity, hospital admissions, public-health, thermal comfort, population, heat, sensitivity, human health, disease, excess mortality, heat-stress, heat-related mortality, comfort, behavior, death, stroke
- Climate research: climate change, temperature, climate, model, simulation, energy, projections, simulations, cmip5, ozone, el-nino, parametrization, elevated CO 2 , models, climate variability, carbon, carbon-dioxide
- Meteorology: heat waves, variability, precipitation, summer, heat-wave, weather, ambient-temperature, waves, extremes, wave, cold, water, rainfall, circulation, heat, air-temperature, extreme heat, climate extremes, heatwaves, temperature extremes, temperatures, temperature variability, high-temperature, ocean, extreme temperatures, atmospheric circulation, interannual variability, sea-surface temperature, oscillation, surface temperature, surface
- Broader terms (multi-meaning): trends, events, patterns, growth, performance, time-series, indexes, system, dynamics, association, index, tolerance, productivity, ensemble, resilience, increase, quality, prediction, frequency, particulate matter, future, framework, 20 th -century, time, reanalysis, systems
Although allocated by the database provider, the keywords are not coherent. For example, the same keyword may appear as singular or plural, and complex keywords are written with and without hyphens.
In order to compare the thematic content of the complete publication set with the earlier literature on heat waves, we have analyzed the pre-2000 publications ( n = 297) separately. Figure 5 shows the keywords (keywords plus) map for revealing the thematic content of the pre-2000 papers.

Co-occurrence network map of the keywords plus from the 297 pre-2000 papers dealing with heat waves for a rough analysis of the thematic content. The minimum number of occurrences of keywords is 1; of the 389 keywords, 277 keywords are connected, and all items are shown. Readers interested in an in-depth analysis can use VOSviewer interactively and zoom into the map via the following URL: https://tinyurl.com/u2zzr399
The major nodes in Fig. 5 are heat waves (searched), temperature, United States, and mortality, with climate change appearing only as a smaller node here. Obviously, the connection between heat waves and climate change was not yet pronounced, which can also be seen from Fig. 1 . Compared with Fig. 4 , the thematic content of the clusters is less clear and the clusters presented in Fig. 5 can hardly be assigned to specific research areas. For a better overview of the thematic content of the early publications dealing with heat waves, we have assigned the connected keywords of Fig. 5 to seven subject categories:
- Countries/regions: United-States, Great-Plains
- Cities: St-Louis, Athens, Chicago
- Events: 1980 heat-wave, 1995 heat-wave
- Impacts: impacts, responses, drought, precipitation, comfort, sultriness
- Climate research: climate, climate change, model, temperature, variability
- Medicine: cardiovascular deaths, mortality, air pollution
- Meteorology: atmospheric flow, weather, heat, humidity index
Important publications
Figures 6 – 8 show the results of the RPYS analysis performed with the CRExplorer and present the distribution of the number of cited references across the reference publication years. Figure 6 shows the RPYS spectrogram of the full range of reference publication years since 1925. Figure 7 presents the spectrogram for the reference publication year period 1950–2000 for better resolving the historical roots. Figure 8 shows the spectrogram for the period 2000–2020, comprising the cited references from the bulk of the publication set analyzed.

Annual distribution of cited references throughout the time period 1925–2020, which have been cited in heat wave-related papers (published between 1964 and 2020). Only references with a minimum reference count of 10 are considered

Annual distribution of cited references throughout the time period 1950–2000, which have been cited in heat wave-related papers (published between 1972 and 2020). Only references with a minimum reference count of 10 are considered

Annual distribution of cited references throughout the time period 2000–2020, which have been cited in heat wave-related papers (published between 2000 and 2020). Only references with a minimum reference count of 10 are considered
The gray bars (Fig. 6 ) and red lines (Figs. (Figs.7 7 – 8 ) in the graphs visualize the number of cited references per reference publication year. In order to identify those publication years with significantly more cited references than other years, the (absolute) deviation of the number of cited references in each year from the median of the number of cited references in the two previous, the current, and the two following years (t − 2; t − 1; t; t + 1; t + 2) is also visualized (blue lines). This deviation from the 5-year median provides a curve smoother than the one in terms of absolute numbers. We inspected both curves for the identification of the peak papers.
Which papers are most important for the scientific community performing research on heat waves? We use the number of cited references (N_CR) as a measure of the citation impact within the topic-specific literature of our publication set. N_CR should not be confused with the overall number of citations of the papers as given by the WoS citation counts (times cited). These citation counts are based on all citing papers covered by the complete database (rather than a topic-specific publication set) and are usually much higher.
Applying the selection criteria mentioned above (minimum number of cited references between 50 and 150 in three different periods), 104 references have been selected as key papers (important papers most frequently referenced within the research topic analyzed) and are presented in Table Table2 2 in Appendix 2. The peak papers corresponding to reference publication years below about 2000 can be seen as the historical roots of the research topic analyzed. Since around 2000, the number of references with the same publication year becomes increasingly numerous, usually with more than one highly referenced (cited) paper at the top. Although there are comparatively fewer distinct peaks visible in the RPYS spectrogram of Fig. 8 , the most frequently referenced papers can easily be identified via the CRE reference listing. Depending on the specific skills and needs (i.e., the expert knowledge and the intended depth of the analysis), the number of top-referenced papers considered key papers can be defined individually.
Listing of the key papers ( n = 104) revealed by RPYS via CRE ( RPY reference publication year, N_CR number of cited references, Title title of the cited reference, DOI allows easily to retrieve the full paper via WoS or Internet)
*N_TOP10 > 9; the N_TOP10 indicator is the number of reference publication years in which a focal cited reference belongs to the 10% most referenced publications.
Table Table2 2 lists the first authors and titles of the 104 key papers selected, their number of cited references (N_CR), and the DOIs for easy access. Some N_CR values are marked by an asterisk, indicating a high value of the N_TOP10 indicator implemented in the CRExplorer. The N_TOP10 indicator value is the number of reference publication years in which a focal cited reference belongs to the 10% most referenced publications. In the case of about half of the cited references in Table Table2 2 ( n = 58), the N_TOP10 value exceeded a value of 9. The three highest values in our dataset are 24, 21, and 20.
Out of the 104 key papers from Table Table2, 2 , 101 have a DOI of which we found 101 papers in the WoS. Three papers have no DOI but could be retrieved from WoS. The altogether 104 papers were exported and their keywords (keywords plus) were displayed in Fig. 9 for revealing the thematic content of the key papers from the RPYS analysis at a glance.

Co-occurrence network map of the keywords plus of the 104 key papers dealing with heat waves selected applying RPYS via CRE software and listed in Table Table2. 2 . The minimum number of occurrences of keywords is 2; of the 310 keywords, 91 meet the threshold. Readers interested in an in-depth analysis can use VOSviewer interactively and zoom into the map via the following URL: https://tinyurl.com/4vwpc4t2
Overall, the keywords mapped in Fig. 9 are rather similar to the keywords presented in Fig. 4 . Besides climate change, temperature, weather, and air-pollution, the keywords deaths, health, mortality, and United-States appear as the most pronounced terms.
The key papers presented in Table Table2 2 can be categorized as follows: (1) papers dealing with specific heat wave events, (2) the impact of heat waves on human health, (3) heat wave-related excess mortality and implications for prevention, (4) the interaction between air pollution and high temperature, (5) circulation pattern and the meteorological basis, (6) future perspectives and risks, and (7) climate models, indicators, and statistics.
Today, the hypothesis of a human-induced climate change is no longer abstract but has become a clear fact, at least for the vast majority of the scientific community (IPCC 2014; CSSR 2017; NCA4 2018; and the multitude of references cited therein). The consequences of a warmer climate are already obvious. The rapidly growing knowledge regarding the earth’s climate system has revealed the connection between global warming and extreme weather events. Heat waves impact people directly and tangibly and many people are pushing for political actions. Research on heat waves came up with the occurrence of some severe events in the second half of the twentieth century and was much stimulated by the more numerous, more intense, and longer lasting heat waves that have occurred since the beginning of the twenty-first century.
As already mentioned in Sect. 1 , the more intense and more frequently occurring heat waves cannot be explained solely by natural climate variations but only with human-made climate change. As a consequence, research on heat waves has become embedded into meteorology and climate change research and has aimed to understand the specific connection with global warming. Scientists discuss a weakening of the polar jet stream as a possible reason for an increasing probability for the occurrence of heat waves (e.g., Broennimann et al. 2009 ; Coumou et al. 2015 ; Mann 2019 ). Climate models are used for projections of temperature and rainfall variability in the future, based on various scenarios of greenhouse gas emissions. As a result, the corresponding keywords appear in the maps of Figs. Figs.4 4 and and9. 9 . Also, the application of statistics plays a major role in the papers of our publication set; some of the most highly referenced (early) papers in Table Table2 2 primarily deal with statistical methods. These methods provide the basis for research on heat waves.
Our analysis shows that research on heat waves has become extremely important in the medical area, since severe heat waves have caused significant excess mortality (e.g., Kalkstein and Davis 1989 ; Fouillet et al. 2006 ; Anderson and Bell 2009 , 2011 ). The most alarming is that the limit for survivability may be reached at the end of the twenty-first century in many regions of the world due to the fatal combination of rising temperatures and humidity levels (e.g., Pal and Eltahir 2016 ; Im et al. 2017 ; Kang and Eltahir 2018 ). The combination of heat and humidity is measured as the “wet-bulb temperature” (WBT), which is the lowest temperature that can be reached under current ambient conditions by the evaporation of water. At 100% relative humidity, the wet-bulb temperature is equal to the air temperature and is different at lower humidity levels. For example, an ambient temperature of 46 °C and a relative humidity of 50% correspond to 35 °C WBT, which is the upper limit that can kill even healthy people within hours. By now, the limit of survivability has almost been reached in some places. However, if global warming is not seriously tackled, deadly heat waves are anticipated for many regions that have contributed little to climate change.
According to high-resolution climate change simulations, North China and South Asia are particularly at risk, because the annual monsoon brings hot and humid air to these regions (Im et al. 2017 ; Kang and Eltahir 2018 ). The fertile plain of North China has experienced vast expansion of irrigated agriculture, which enhances the intensity of heat waves. South Asia, a region inhabited by about one-fifth of the global human population, is likely to approach the critical threshold by the late twenty-first century, if greenhouse gas emissions are not lowered significantly. In particular, the densely populated agricultural regions of the Ganges and Indus river basins are likely to be affected by extreme future heat waves. Also, the Arabic-speaking desert countries of the Gulf Region in the Middle East and the French-speaking parts of Africa are expected to suffer from heat waves beyond the limit of human survival. But to date, only 12 papers have been published on heat waves in connection with wet-bulb temperature (#15 of the search query); no paper was published before 2016. Some papers report excess hospital admissions during heat wave events (e.g., Semenza et al. 1999 ; Knowlton et al. 2009 ), with the danger of a temporary capacity overload of local medical systems in the future. Presumably, this will be an increasingly important issue in the future, when more and larger urban areas are affected by heat waves beyond the limit of human survival indicated by wet-bulb temperatures above 35° C.
The importance of heat waves for the medical area is underlined by the large portion of papers discussing excess hospital admissions and excess mortality during intense heat wave events, particularly in urban areas with a high population density. As was the case during the boom phase of the Covid-19 pandemic, local medical health care systems may become overstressed by long-lasting heat wave events and thus adaptation strategies are presented and discussed. Finally, the analysis of the keywords in this study reveals the connection of heat wave events with air pollution in urban regions. There seems to be evidence of an interaction between air pollution and high temperatures in the causation of excess mortality (e.g., Katsouyanni et al. 1993 ). Two more recent papers discuss the global risk of deadly heat (Mora et al. 2017 ) and the dramatically increasing chance of extremely hot summers since the 2003 European heat wave (Christidis et al. 2015 ).
Another important topic of the heat wave papers is related to the consequences for agriculture and forestry. Reduced precipitation and soil moisture result in crop failure and put food supplies at risk. Unfortunately, large regions of the world that contribute least to the emission of greenhouse gases are affected most by drought, poor harvests, and hunger. Some more recent papers discuss the increasing probability of marine heat waves (Oliver et al. 2018 ) and the consequences for the marine ecosystem (Smale et al. 2019 ).
The results of this study should be interpreted in terms of its limitations:
- We tried to include in our bibliometric analyses all relevant heat wave papers covered by the database. Our long-standing experience in professional information retrieval has shown, however, that it is sheer impossible to get complete and clean results by search queries against the backdrop of the search functions provided by literature databases like WoS or others. Also, the transition from relevant to non-relevant literature is blurred and is a question of the specific needs. In this study, we used bibliometric methods that are relatively robust with regard to the completeness and precision of the publication sets analyzed. For example, it is an advantage of RPYS that a comparatively small portion of relevant publications (i.e., an incomplete publication set) contains a large amount of the relevant literature as cited references. The number of cited references is indeed lowered as a consequence of an incomplete publication set. However, this does not significantly affect the results, since the reference counts are only used as a relative measure within specific publication years.
Two other limitations of this study refer to the RPYS of the heat wave paper set:
- There are numerous rather highly cited references retrieved by RPYS via CRExplorer but not considered in the listing of Table Table2 2 due to the selection criteria applied. Many of these non-selected papers have N_CR values just below the limits that we have set. Therefore, papers not included in our listing are not per se qualified as much less important or even unimportant.
- In the interpretation of cited references counts, one should have in mind that they rely on the “popularity” of a publication being cited in subsequent research. The counts measure impact but not scientific importance or accuracy (Tahamtan and Bornmann 2019 ). Note that there are many reasons why authors cite publications (Tahamtan and Bornmann 2018 ), thus introducing a lot of “noise” in the data (this is why RPYS focuses on the cited reference peaks).
Our suggestions for future empirical analysis refer to the impact of the scientific heat wave discourse on social networks and funding of basic research on heat waves around topics driven by political pressure. Whereas this paper focuses on the scientific discourse around heat waves, it would be interesting if future studies were to address the policy relevance of the heat waves research.
Appendix 1 1)
WoS search query (date of search: July 1, 2021)
Table Table2 2
Author contribution
All authors contributed to the study conception and design. Material preparation, data collection, and analysis were performed by Werner Marx, Robin Haunschild, and Lutz Bornmann. The first draft of the manuscript was written by Werner Marx and all authors commented on previous versions of the manuscript. All authors read and approved the final manuscript.
Open Access funding enabled and organized by Projekt DEAL.
Data availability
Code availability, declarations.
The authors declare no competing interests.
Publisher's Note
Springer Nature remains neutral with regard to jurisdictional claims in published maps and institutional affiliations.
Change history
The original version of this paper was updated to add the missing compact agreement Open Access funding note.
Contributor Information
Werner Marx, Email: [email protected] .
Robin Haunschild, Email: [email protected] .
Lutz Bornmann, Email: [email protected] , Email: ed.gpm.vg@nnamnrob .
- Anderson BG, Bell ML (2009) Weather-related mortality how heat, cold, and heat waves affect mortality in the United States. Epidemiology 20(2):205–213. 10.1097/EDE.0b013e318190ee08 [ PMC free article ] [ PubMed ]
- Anderson GB, Bell ML. Heat waves in the United States: mortality risk during heat waves and effect modification by heat wave characteristics in 43 U.S. communities. Enviro Health Perspect. 2011; 119 (2):210–218. doi: 10.1289/ehp.1002313. [ PMC free article ] [ PubMed ] [ CrossRef ] [ Google Scholar ]
- Bornmann L, Marx W. The wisdom of citing scientists. J Am Soc Inf Sci. 2013; 65 (6):1288–1292. doi: 10.1002/asi.23100. [ CrossRef ] [ Google Scholar ]
- Broennimann S, Stickler A, Griesser T, Ewen T, Grant AN, Fischer AM, Schraner M, Peter T, Rozanov E, Ross T (2009) Exceptional atmospheric circulation during the "Dust Bowl". Geophysical Research Letters 36: article number L08802. 10.1029/2009GL037612
- Christidis N, Jones G, Stott P. Dramatically increasing chance of extremely hot summers since the 2003 European heatwave. Nat Clim Chang. 2015; 5 :46–50. doi: 10.1038/nclimate2468. [ CrossRef ] [ Google Scholar ]
- Comins JA, Hussey TW. Detecting seminal research contributions to the development and use of the global positioning system by Reference Publication Year Spectroscopy. Scientometrics. 2015; 104 :575–580. doi: 10.1007/s11192-015-1598-2. [ CrossRef ] [ Google Scholar ]
- Coumou D, Lehmann J, Beckmann J. The weakening summer circulation in the Northern Hemisphere mid-latitudes. Science. 2015; 348 :324–327. doi: 10.1126/science.1261768. [ PubMed ] [ CrossRef ] [ Google Scholar ]
- CSSR 2017: Climate Science Special Report: Fourth National Climate Assessment, Volume I [Wuebbles, D.J., D.W. Fahey, K.A. Hibbard, D.J. Dokken, B.C. Stewart, and T.K. Maycock (eds.)]. U.S. Global Change Research Program, Washington, DC, USA, 470, 10.7930/J0J964J6, https://science2017.globalchange.gov/downloads/CSSR2017_FullReport.pdf
- Fouillet A, Rey G, Laurent F, Pavillon G, Bellec S, Guihenneuc-Jouyaux C, Clavel J, Jougla E, Hemon D. Excess mortality related to the August 2003 heat wave in France. Int Arch Occup Environ Health. 2006; 80 (1):16–24. doi: 10.1007/s00420-006-0089-4. [ PMC free article ] [ PubMed ] [ CrossRef ] [ Google Scholar ]
- Haunschild R, Bornmann L, Marx W. Climate change research in view of bibliometrics. PLoS ONE. 2016; 11 :e0160393. doi: 10.1371/journal.pone.0160393. [ PMC free article ] [ PubMed ] [ CrossRef ] [ Google Scholar ]
- Huang QF, Lu YQ. Urban heat island research from 1991 to 2015: a bibliometric analysis. Theoret Appl Climatol. 2018; 131 (3–4):1055–1067. doi: 10.1007/s00704-016-2025-1. [ CrossRef ] [ Google Scholar ]
- Im ES, Pal JS, Eltahir EAB (2017) Deadly heat waves projected in the densely populated agricultural regions of South Asia. Science Advances 3(8): article number e1603322. 10.1126/sciadv.1603322 [ PMC free article ] [ PubMed ]
- IPCC 2014: Climate change, Synthesis Report. Contribution of Working Groups I, II and III to the Fifth Assessment Report of the Intergovernmental Panel on Climate Change [Core Writing Team, R.K. Pachauri and L.A. Meyer (eds.)]. IPCC, Geneva, Switzerland, 151 https://www.ipcc.ch/report/ar5/syr/
- IPCC 2014: Climate Change, Summary for Policymakers. In: IPCC, 2014: Climate Change 2014: Synthesis Report. Contribution of Working Groups I, II and III to the Fifth Assessment Report of the Intergovernmental Panel on Climate Change [Core Writing Team, R.K. Pachauri and L.A. Meyer (eds.)]. IPCC, Geneva, Switzerland, 151 https://www.ipcc.ch/site/assets/uploads/2018/02/AR5_SYR_FINAL_SPM.pdf
- Kalkstein LS, Davis RE. Weather and human mortality – an evaluation of demographic and interregional responses in the United-States. Ann Assoc Am Geogr. 1989; 79 (1):44–64. doi: 10.1111/j.1467-8306.1989.tb00249.x. [ CrossRef ] [ Google Scholar ]
- Kang S, Eltahir EAB (2018) North China Plain threatened by deadly heatwaves due to climate change and irrigation. Nature Communications 9: article number 2894. 10.1038/s41467-018-05252-y [ PMC free article ] [ PubMed ]
- Katsouyanni K, Pantazopoulou A, Touloumi G, Tselepidaki I, Moustris K, Asimakopoulos D, Poulopoulou G, Trichopoulos D. Evidence for interaction between air-pollution and high-temperature in the causation of excess mortality. Arch Environ Health. 1993; 48 (4):235–242. doi: 10.1080/00039896.1993.9940365. [ PubMed ] [ CrossRef ] [ Google Scholar ]
- Knowlton K, Rotkin-Ellman M, King G, Margolis HG, Smith D, Solomon G, Trent R, English P. The 2006 California heat wave: impacts on hospitalizations and emergency department visits. Environ Health Perspect. 2009; 117 (1):61–67. doi: 10.1289/ehp.11594. [ PMC free article ] [ PubMed ] [ CrossRef ] [ Google Scholar ]
- Mann ME. The weather amplifier: strange waves in the jet stream foretell a future full of heat waves and floods. Sci Am. 2019; 320 (3):43–49. [ Google Scholar ]
- Marx W, Bornmann L, Barth A, Leydesdorff L. Detecting the historical roots of research fields by Reference Publication Year Spectroscopy (RPYS) J Am Soc Inf Sci. 2014; 65 :751–764. doi: 10.1002/asi.23089. [ CrossRef ] [ Google Scholar ]
- Marx W, Bornmann L. On the causes of subject-specific citation rates in Web of Science. Scientometrics. 2015; 102 (2):1823–1827. doi: 10.1007/s11192-014-1499-9. [ CrossRef ] [ Google Scholar ]
- Marx W, Bornmann L. Change of perspective: Bibliometrics from the point of view of cited references. A literature overview on approaches to the evaluation of cited references in bibliometrics. Scientometrics. 2016; 109 (2):1397–1415. doi: 10.1007/s11192-016-2111-2. [ PMC free article ] [ PubMed ] [ CrossRef ] [ Google Scholar ]
- Marx W, Haunschild R, Thor A, Bornmann L (2017a) Which early works are cited most frequently in climate change research literature? A bibliometric approach based on reference publication year spectroscopy. Scientometrics 110(1):335–353. 10.1007/s11192-016-2177-x [ PMC free article ] [ PubMed ]
- Marx W, Haunschild R, Bornmann L. Climate change and viticulture—a quantitative analysis of a highly dynamic research field. Vitis. 2017; 56 :35–43. doi: 10.5073/vitis.2017.56.35-43. [ CrossRef ] [ Google Scholar ]
- Marx W, Haunschild R, Bornmann L (2017c) Global warming and tea production – the bibliometric view on a newly emerging research topic. Climate 5(3): article number 46. 10.3390/cli5030046
- Meehl GA, Tebaldi C. More intense, more frequent, and longer lasting heat waves in the 21 st century. Science. 2004; 305 :994–997. doi: 10.1126/science.1098704. [ PubMed ] [ CrossRef ] [ Google Scholar ]
- Mora C, Dousset B, Caldwell I, et al. Global risk of deadly heat. Nat Clim Chang. 2017; 7 :501–506. doi: 10.1038/nclimate3322. [ CrossRef ] [ Google Scholar ]
- NCA4 2018: Fourth National Climate Assessment, Volume II: impacts, risks, and adaptation in the United States. https://nca2018.globalchange.gov/ NCA 2018 Report-in-Brief: https://nca2018.globalchange.gov/downloads/NCA4_Report-in-Brief.pdf
- Oliver ECJ, Donat MG, Burrows MT, et al. Longer and more frequent marine heatwaves over the past century. Nat Commun. 2018; 9 :1324. doi: 10.1038/s41467-018-03732-9. [ PMC free article ] [ PubMed ] [ CrossRef ] [ Google Scholar ]
- Pal JS, Eltahir EAB. Future temperature in southwest Asia projected to exceed a threshold for human adaptability. Nat Clim Chang. 2016; 6 (2):197–200. doi: 10.1038/NCLIMATE2833. [ CrossRef ] [ Google Scholar ]
- Semenza JC, McCullough JE, Flanders WD, McGeehin MA, Lumpkin JR. Excess hospital admissions during the July 1995 heat wave in Chicago. Am J Prev Med. 1999; 16 (4):269–277. doi: 10.1016/S0749-3797(99)00025-2. [ PubMed ] [ CrossRef ] [ Google Scholar ]
- Smale DA, Wernberg T, Oliver ECJ, et al. Marine heatwaves threaten global biodiversity and the provision of ecosystem services. Nat Clim Chang. 2019; 9 :306–312. doi: 10.1038/s41558-019-0412-1. [ CrossRef ] [ Google Scholar ]
- Tahamtan I, Bornmann L. Core elements in the process of citing publications: Conceptual overview of the literature. J Informet. 2018; 12 :203–216. doi: 10.1016/j.joi.2018.01.002. [ CrossRef ] [ Google Scholar ]
- Tahamtan I, Bornmann L. What do citation counts measure? An updated review of studies on citations in scientific documents published between 2006 and 2018. Scientometrics. 2019; 121 :1635–1684. doi: 10.1007/s11192-019-03243-4. [ CrossRef ] [ Google Scholar ]
- Thor A, Marx W, Leydesdorff L, Bornmann L. Introducing CitedReferencesExplorer (CRExplorer): a program for Reference Publication Year Spectroscopy with cited references disambiguation. J Informet. 2016; 10 :503–515. doi: 10.1016/j.joi.2016.02.005. [ CrossRef ] [ Google Scholar ]
- Thor A, Marx W, Leydesdorff L, Bornmann L. New features of CitedReferencesExplorer (CRExplorer) Scientometrics. 2016; 109 :2049–2051. doi: 10.1007/s11192-016-2082-3. [ CrossRef ] [ Google Scholar ]
- Thor A, Bornmann L, Marx W, Mutz R. Identifying single influential publications in a research field: new analysis opportunities of the CRExplorer. Scientometrics. 2018; 116 (1):591–608. doi: 10.1007/s11192-018-2733-7. [ CrossRef ] [ Google Scholar ]
- Van Eck NJ, Waltman L. Software survey: VOSviewer, a computer program for bibliometric mapping. Scientometrics. 2010; 84 (2):523–538. doi: 10.1007/s11192-009-0146-3. [ PMC free article ] [ PubMed ] [ CrossRef ] [ Google Scholar ]

Search the United Nations
- What Is Climate Change
- Myth Busters
- Renewable Energy
- Finance & Justice
- Initiatives
- Sustainable Development Goals
- Paris Agreement
- Climate Ambition Summit 2023
- Climate Conferences
- Press Material
- Communications Tips
What Is Climate Change?
Climate change refers to long-term shifts in temperatures and weather patterns. Such shifts can be natural, due to changes in the sun’s activity or large volcanic eruptions. But since the 1800s, human activities have been the main driver of climate change , primarily due to the burning of fossil fuels like coal, oil and gas.
Burning fossil fuels generates greenhouse gas emissions that act like a blanket wrapped around the Earth, trapping the sun’s heat and raising temperatures.
The main greenhouse gases that are causing climate change include carbon dioxide and methane. These come from using gasoline for driving a car or coal for heating a building, for example. Clearing land and cutting down forests can also release carbon dioxide. Agriculture, oil and gas operations are major sources of methane emissions. Energy, industry, transport, buildings, agriculture and land use are among the main sectors causing greenhouse gases.
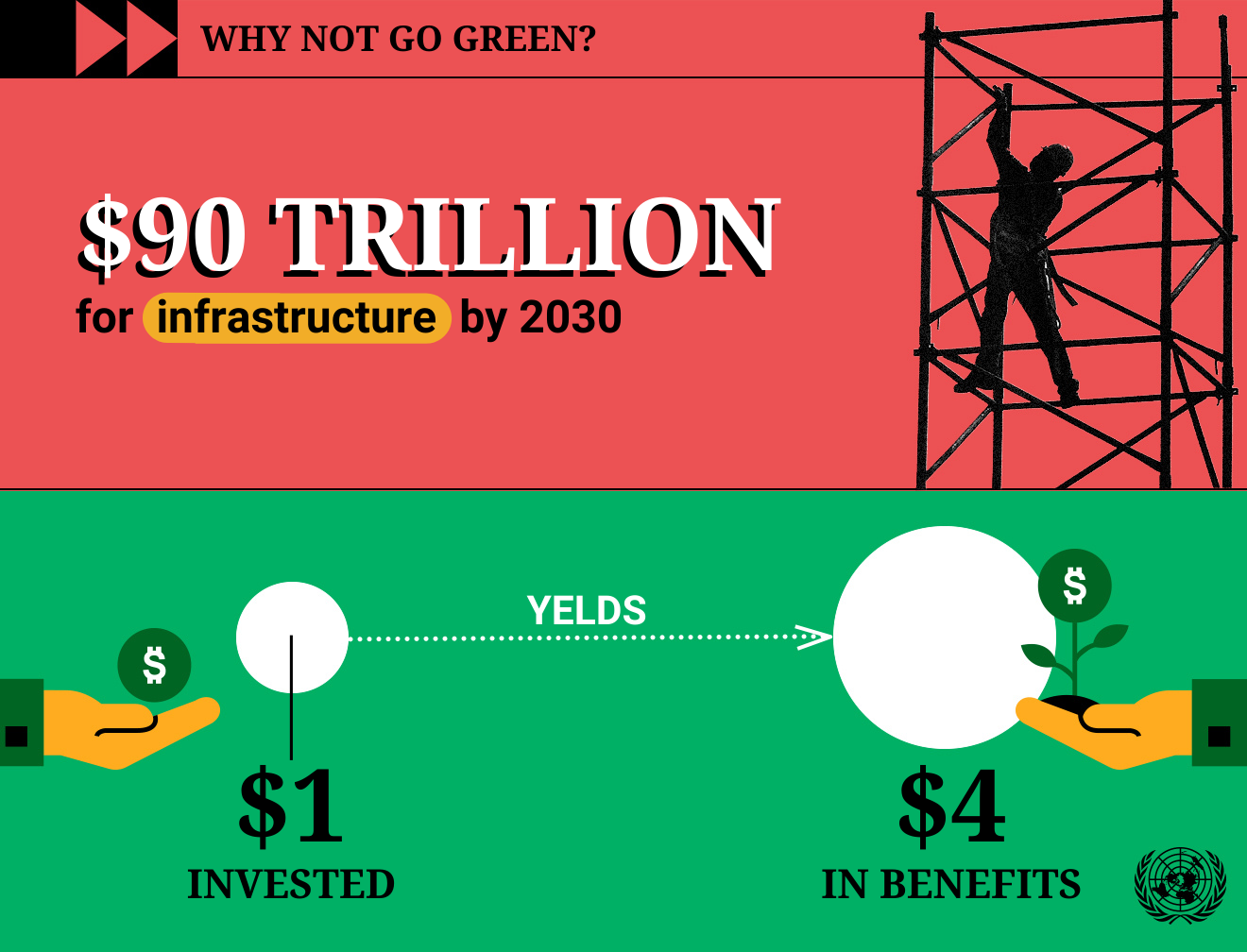
Humans are responsible for global warming
Climate scientists have showed that humans are responsible for virtually all global heating over the last 200 years. Human activities like the ones mentioned above are causing greenhouse gases that are warming the world faster than at any time in at least the last two thousand years.
The average temperature of the Earth’s surface is now about 1.1°C warmer than it was in the late 1800s (before the industrial revolution) and warmer than at any time in the last 100,000 years. The last decade (2011-2020) was the warmest on record , and each of the last four decades has been warmer than any previous decade since 1850.
Many people think climate change mainly means warmer temperatures. But temperature rise is only the beginning of the story. Because the Earth is a system, where everything is connected, changes in one area can influence changes in all others.
The consequences of climate change now include, among others, intense droughts, water scarcity, severe fires, rising sea levels, flooding, melting polar ice, catastrophic storms and declining biodiversity.
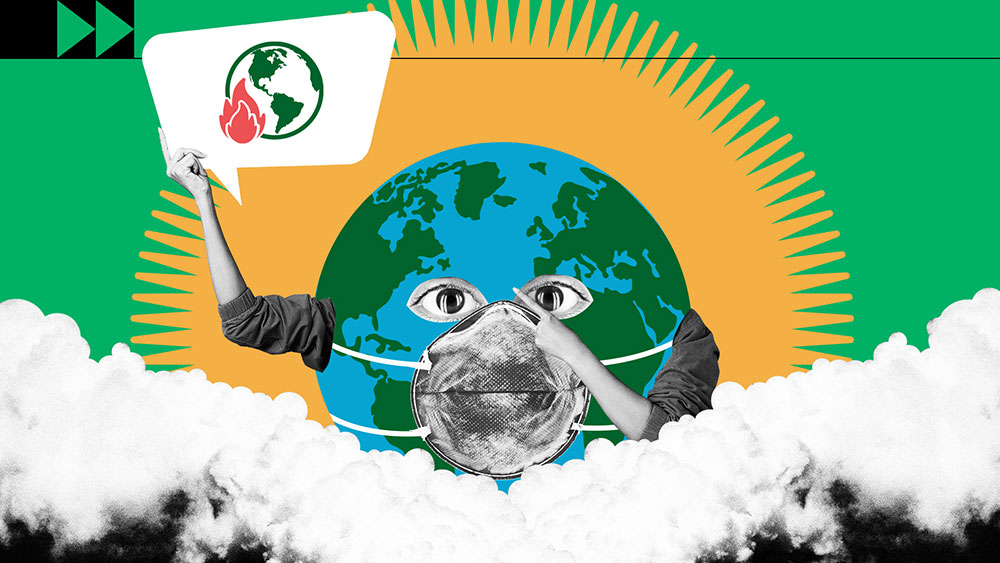
People are experiencing climate change in diverse ways
Climate change can affect our health , ability to grow food, housing, safety and work. Some of us are already more vulnerable to climate impacts, such as people living in small island nations and other developing countries. Conditions like sea-level rise and saltwater intrusion have advanced to the point where whole communities have had to relocate, and protracted droughts are putting people at risk of famine. In the future, the number of people displaced by weather-related events is expected to rise.
Every increase in global warming matters
In a series of UN reports , thousands of scientists and government reviewers agreed that limiting global temperature rise to no more than 1.5°C would help us avoid the worst climate impacts and maintain a livable climate. Yet policies currently in place point to a 3°C temperature rise by the end of the century.
The emissions that cause climate change come from every part of the world and affect everyone, but some countries produce much more than others .The seven biggest emitters alone (China, the United States of America, India, the European Union, Indonesia, the Russian Federation, and Brazil) accounted for about half of all global greenhouse gas emissions in 2020.
Everyone must take climate action, but people and countries creating more of the problem have a greater responsibility to act first.
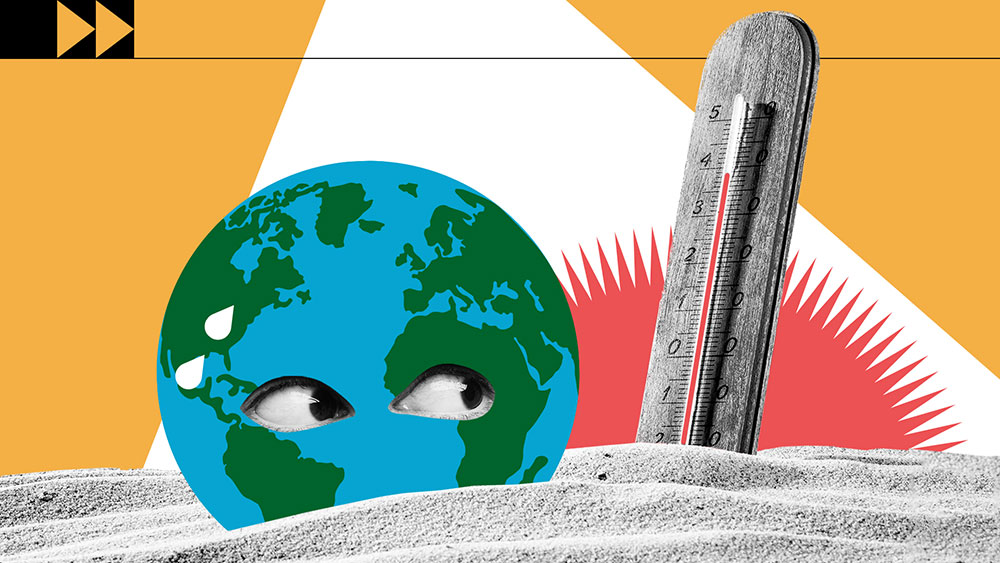
We face a huge challenge but already know many solutions
Many climate change solutions can deliver economic benefits while improving our lives and protecting the environment. We also have global frameworks and agreements to guide progress, such as the Sustainable Development Goals , the UN Framework Convention on Climate Change and the Paris Agreement . Three broad categories of action are: cutting emissions, adapting to climate impacts and financing required adjustments.
Switching energy systems from fossil fuels to renewables like solar or wind will reduce the emissions driving climate change. But we have to act now. While a growing number of countries is committing to net zero emissions by 2050, emissions must be cut in half by 2030 to keep warming below 1.5°C. Achieving this means huge declines in the use of coal, oil and gas: over two-thirds of today’s proven reserves of fossil fuels need to be kept in the ground by 2050 in order to prevent catastrophic levels of climate change.
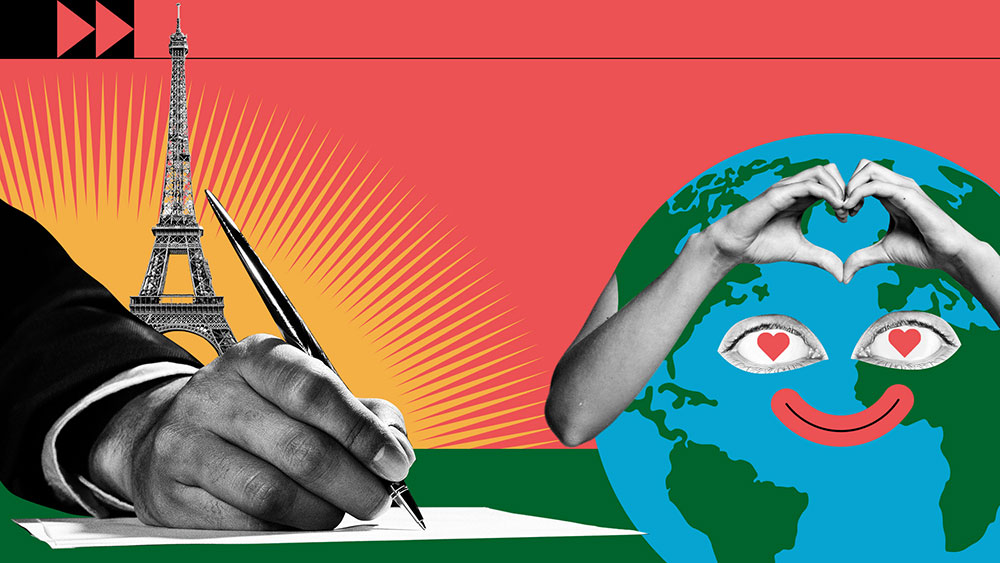
Adapting to climate consequences protects people, homes, businesses, livelihoods, infrastructure and natural ecosystems. It covers current impacts and those likely in the future. Adaptation will be required everywhere, but must be prioritized now for the most vulnerable people with the fewest resources to cope with climate hazards. The rate of return can be high. Early warning systems for disasters, for instance, save lives and property, and can deliver benefits up to 10 times the initial cost.
We can pay the bill now, or pay dearly in the future
Climate action requires significant financial investments by governments and businesses. But climate inaction is vastly more expensive. One critical step is for industrialized countries to fulfil their commitment to provide $100 billion a year to developing countries so they can adapt and move towards greener economies.
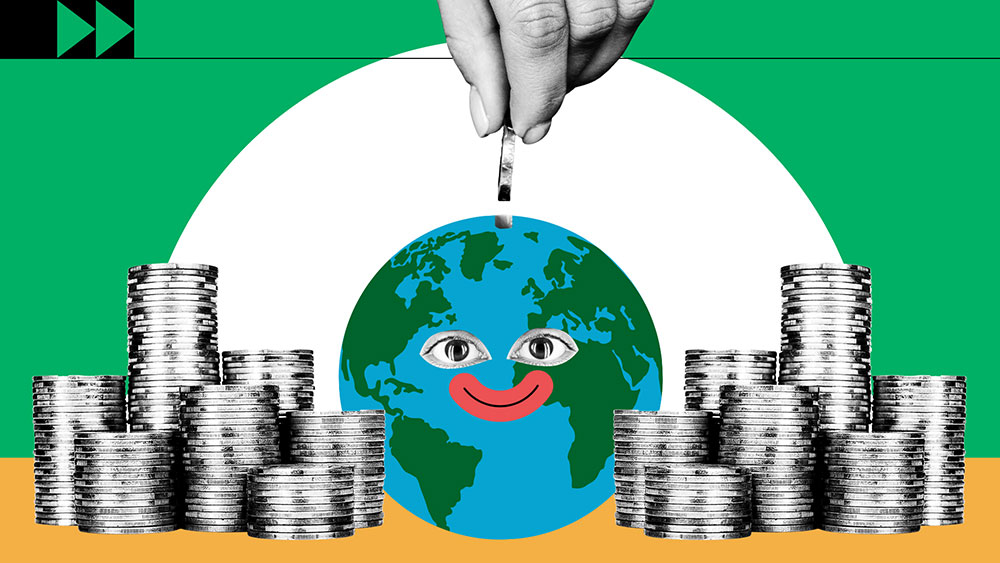
To get familiar with some of the more technical terms used in connection with climate change, consult the Climate Dictionary .
Learn more about…
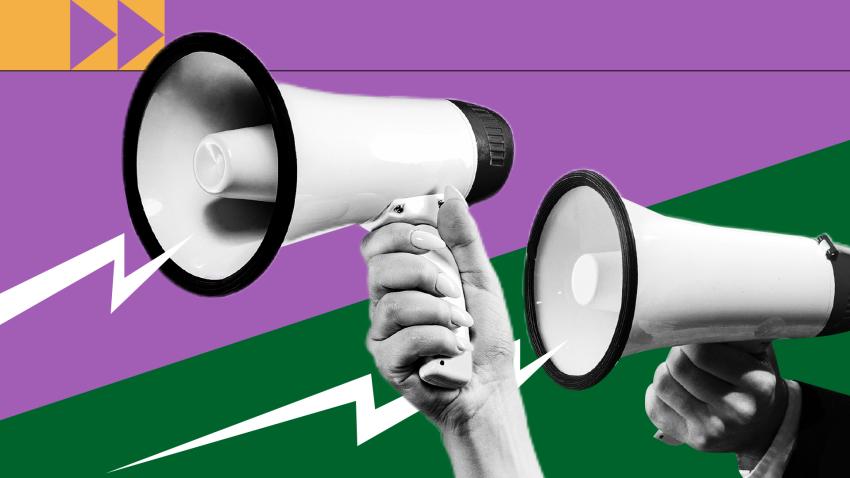
The facts on climate and energy
Climate change is a hot topic – with myths and falsehoods circulating widely. Find some essential facts here .
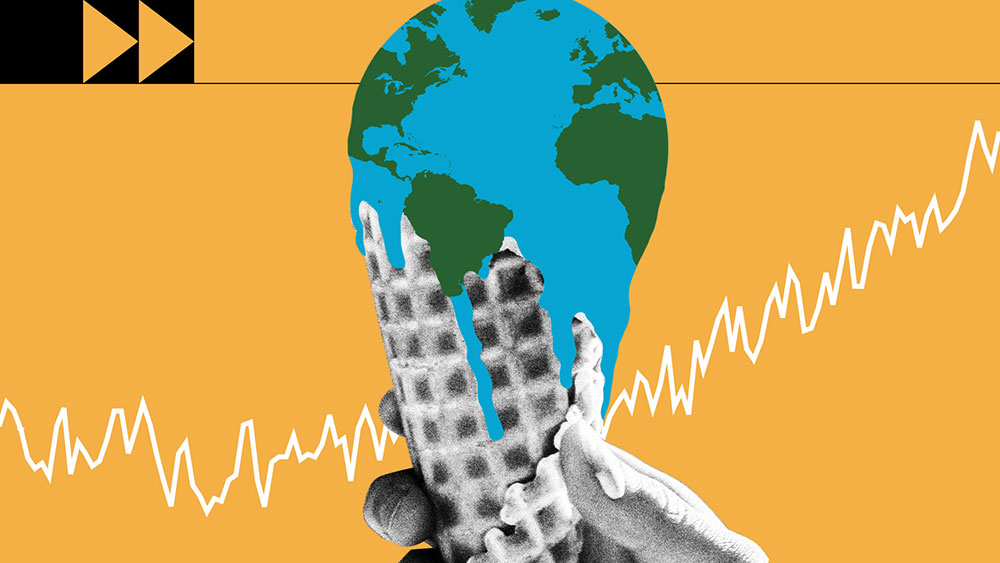
The science
See the latest climate reports from the United Nations as well as climate action facts .
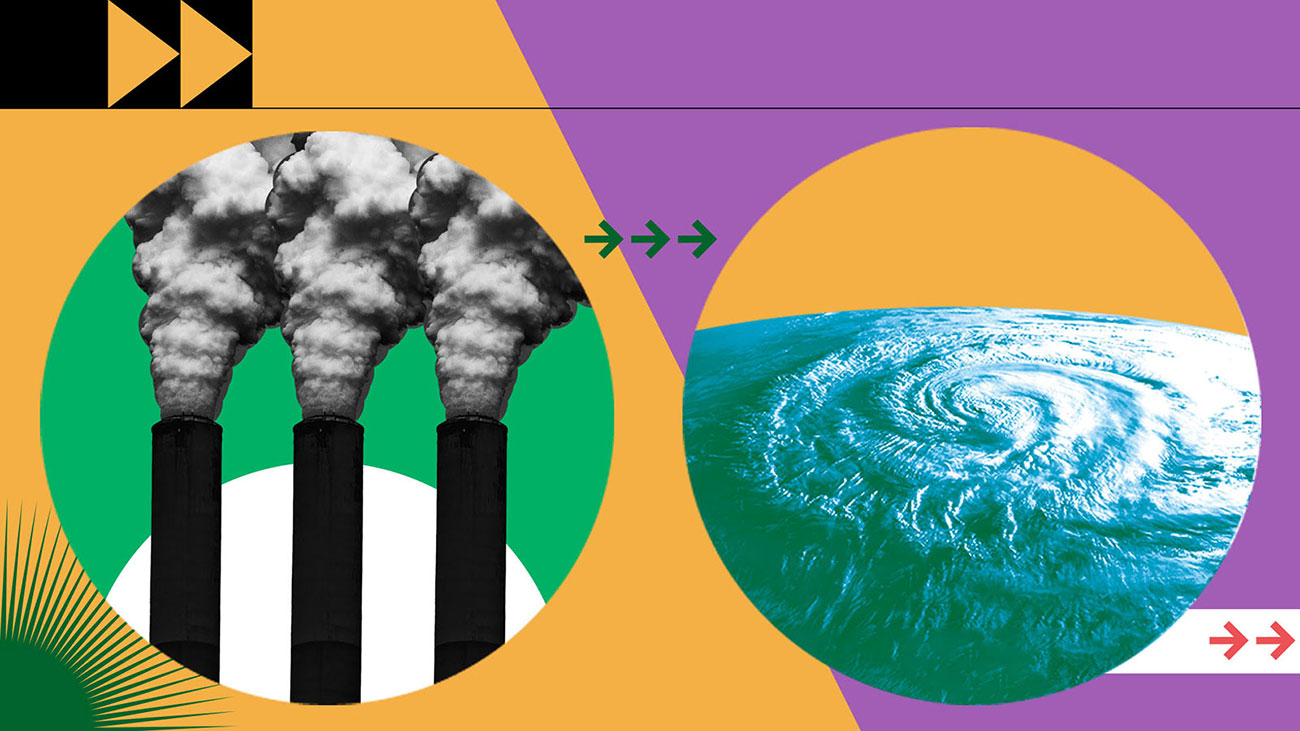
Causes and Effects
Fossil fuels are by far the largest contributor to the greenhouse gas emissions that cause climate change, which poses many risks to all forms of life on Earth. Learn more .
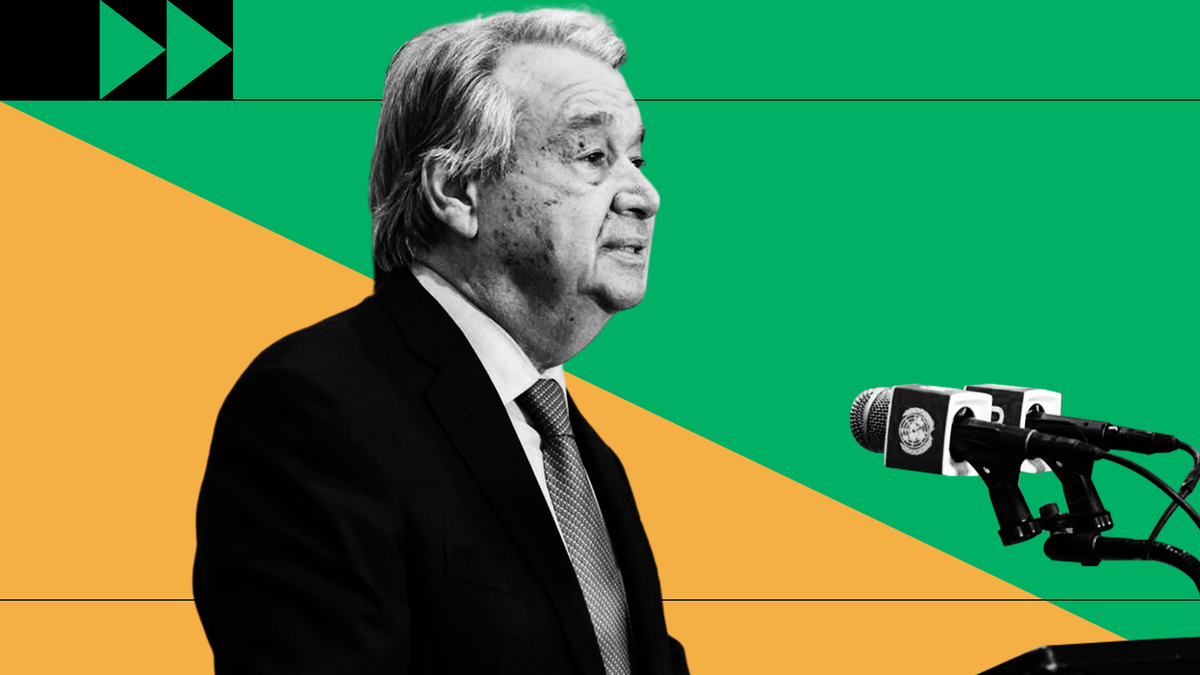
From the Secretary-General
Read the UN Chief’s latest statements on climate action.
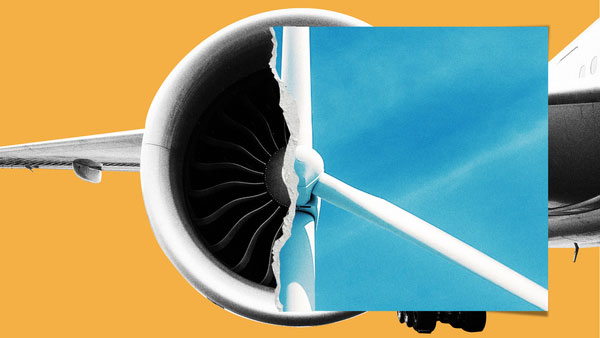
What is net zero? Why is it important? Our net-zero page explains why we need steep emissions cuts now and what efforts are underway.
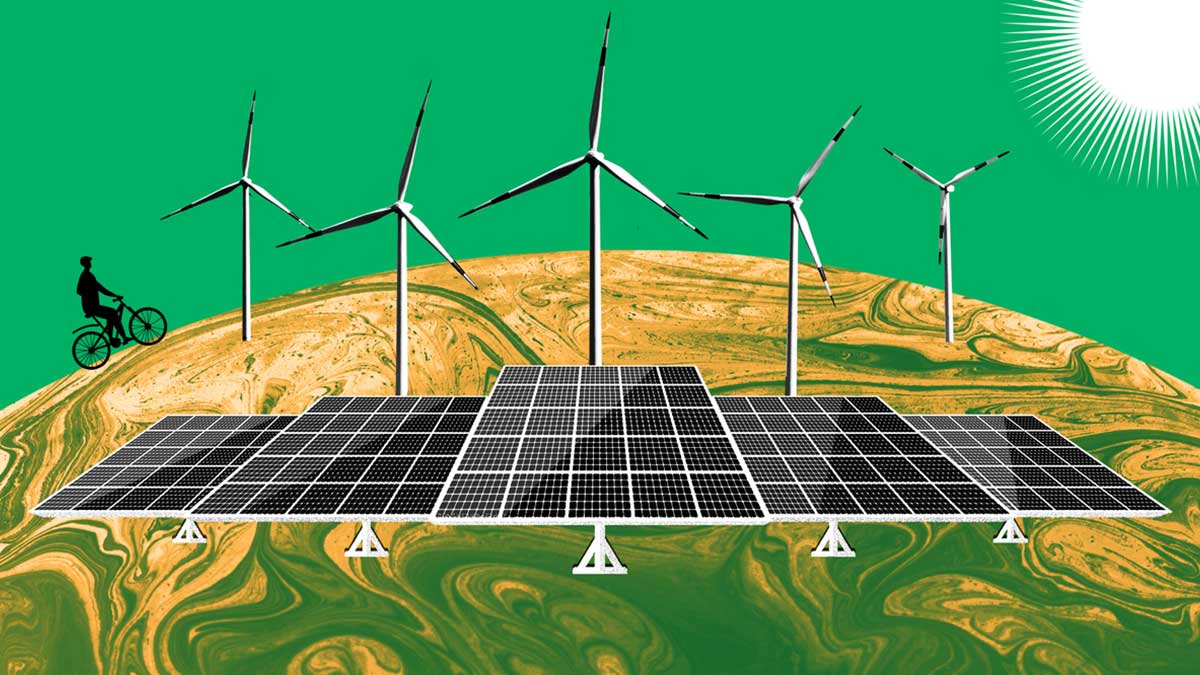
Renewable energy – powering a safer future
What is renewable energy and why does it matter? Learn more about why the shift to renewables is our only hope for a brighter and safer world.
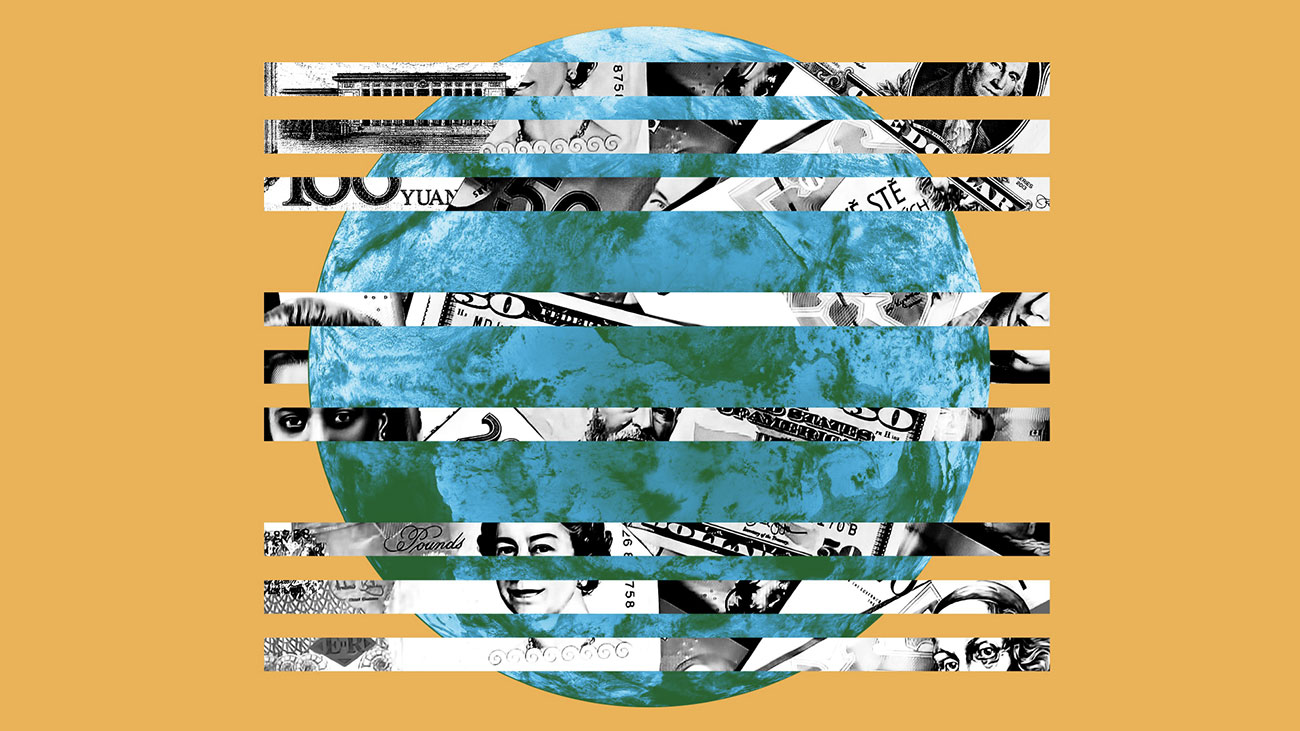
How will the world foot the bill? We explain the issues and the value of financing climate action.

What is climate adaptation? Why is it so important for every country? Find out how we can protect lives and livelihoods as the climate changes.
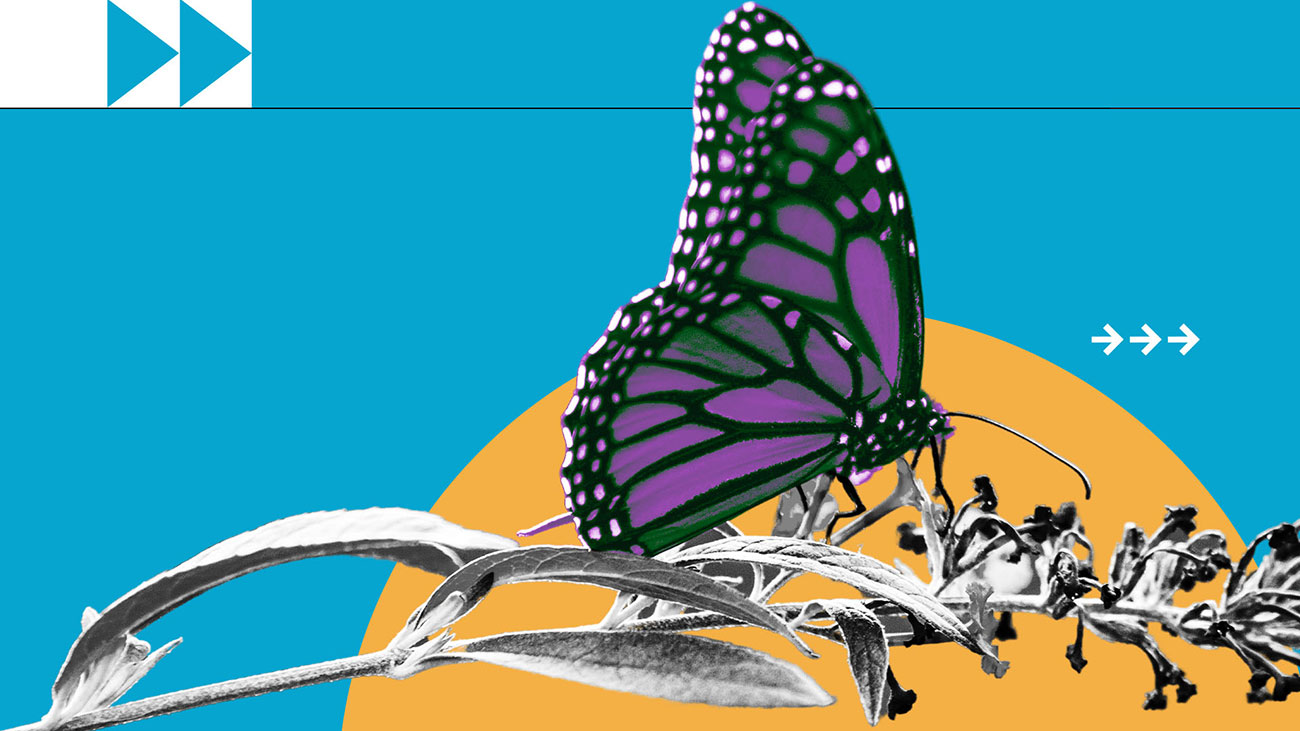
Climate Issues
Learn more about how climate change impacts are felt across different sectors and ecosystems.
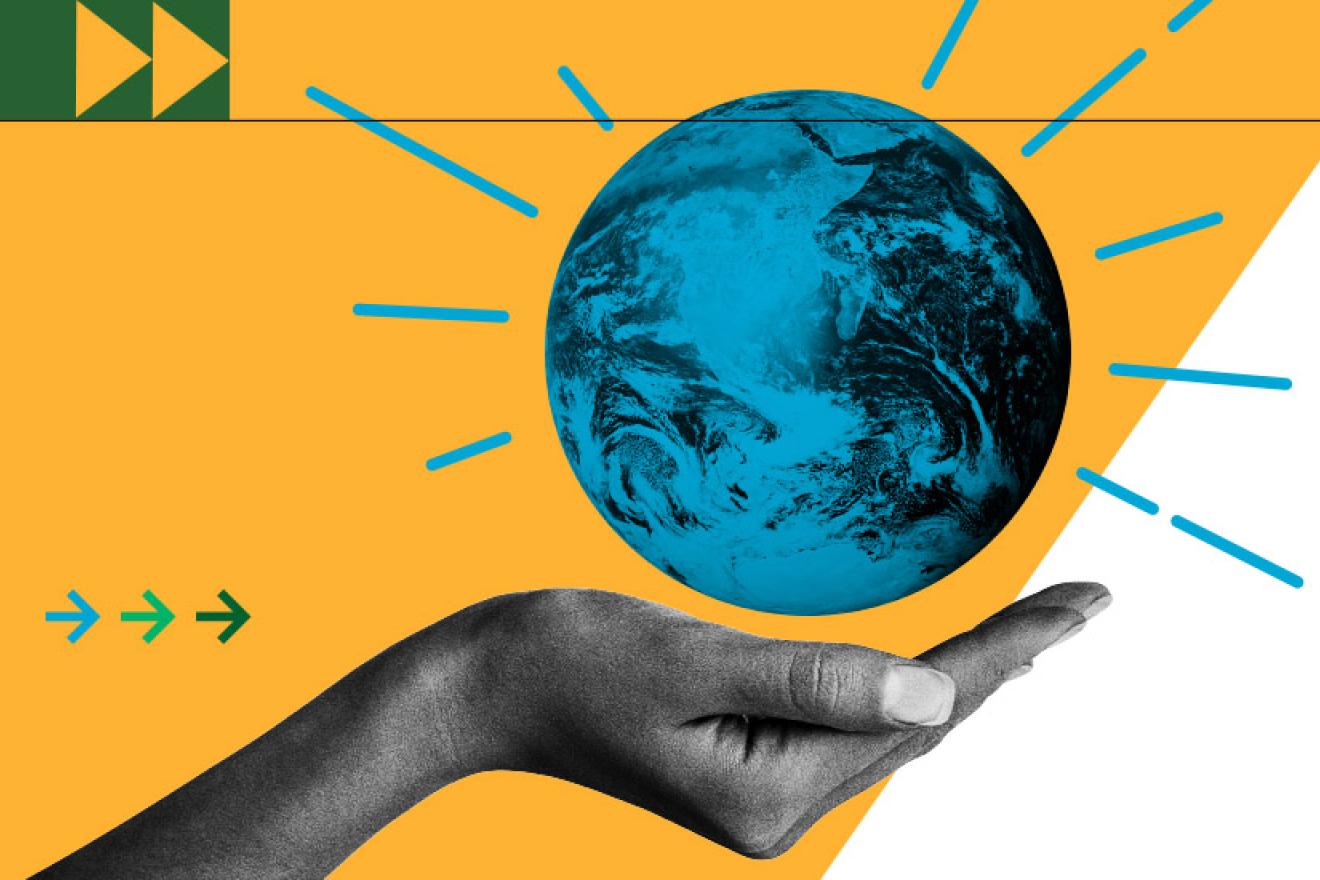
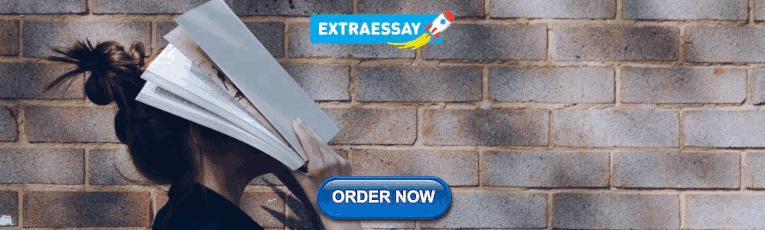
Why women are key to climate action
Women and girls are on the frontlines of the climate crisis and uniquely situated to drive action. Find out why it’s time to invest in women.
Facts and figures
- What is climate change?
- Causes and effects
- Myth busters
Cutting emissions
- Explaining net zero
- High-level expert group on net zero
- Checklists for credibility of net-zero pledges
- Greenwashing
- What you can do
Clean energy
- Renewable energy – key to a safer future
- What is renewable energy
- Five ways to speed up the energy transition
- Why invest in renewable energy
- Clean energy stories
- A just transition
Adapting to climate change
- Climate adaptation
- Early warnings for all
- Youth voices
Financing climate action
- Finance and justice
- Loss and damage
- $100 billion commitment
- Why finance climate action
- Biodiversity
- Human Security
International cooperation
- What are Nationally Determined Contributions
- Acceleration Agenda
- Climate Ambition Summit
- Climate conferences (COPs)
- Youth Advisory Group
- Action initiatives
- Secretary-General’s speeches
- Press material
- Fact sheets
- Communications tips
We use cookies. Read more about them in our Privacy Policy .
- Accept site cookies
- Reject site cookies
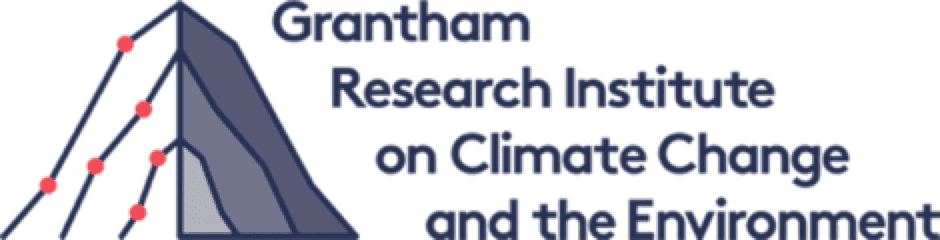
Enhancing heatwave resilience in the UK: insights and strategies from stakeholders
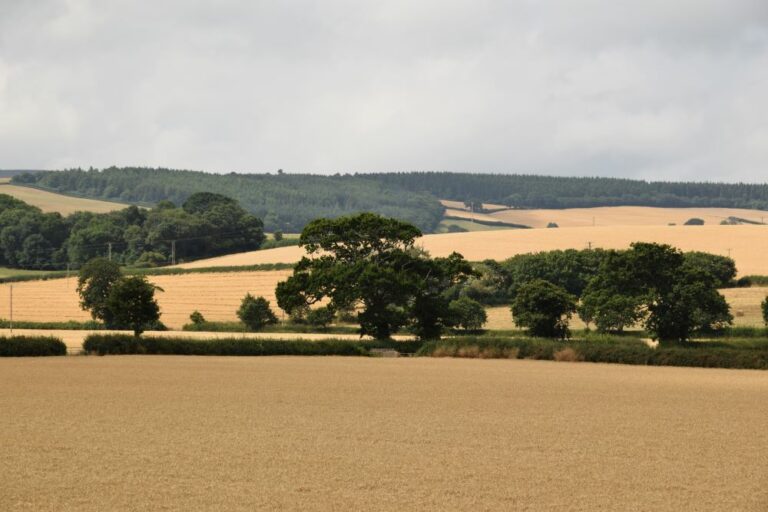
Heatwave events are on the rise in the UK and Europe, with a projected increase in frequency, intensity and persistence. Despite the escalating risk, responses and adaptation strategies are lagging behind, exacerbated by a lack of comprehensive understanding of heat-related risks and effective measures.
To address this gap in understanding, this paper analyses the UK’s response to the heatwaves of summer 2022 which claimed 3,000 lives. It is informed by stakeholders involved in the response. Overall, the findings emphasise the need for proactive and integrated approaches to heatwave preparedness, including both short-term emergency responses and long-term mitigation strategies.
Key points for decision-makers
- This paper applies a combination of Forensic Disaster Analysis and Fuzzy Cognitive Mapping (FCM) to analyse the UK’s response to the heatwaves of summer 2022.
- The authors interviewed 38 stakeholders from various sectors involved in the response to the heatwaves, developing cognitive maps to capture local knowledge and perceptions regarding the causes, impacts and actions taken before, during and afterwards.
- The study identifies cascading effects of heatwaves and factors amplifying negative impacts, along with effective and missed mitigating measures.
- Stakeholders identified primary impacts such as health issues, secondary hazards and disruption to transport, with further analysis revealing the interdependencies between these factors, resulting in cascading effects.
- Socioeconomic vulnerability, inadequate building standards and behavioural factors like non-adaptive behaviour were identified as the most significant exacerbators of the heatwave impacts.
- Stakeholders’ combined fuzzy cognitive maps indicated the importance of public messaging and awareness campaigns to improve heat risk communication and community engagement.
- Effective mitigation measures, including forecasting and early-warning systems, were highlighted as important to the 2022 heatwave response, but gaps in public awareness and learning from past responses remain challenges for future preparedness.
- Concerns were also raised about resource adequacy for prolonged heatwaves, particularly regarding building retrofitting and heat vulnerability assessments.
- The study highlights important variations in perspectives, preferences and priorities, which have implications for heat adaptation policy design.
- The findings contribute to enhancing understanding of heatwave risks and the actions that must be taken in preparation for future heatwaves in the UK, informing more robust and holistic policymaking for heat risk reduction.
Sign up to our newsletter

- News Releases
A third of China’s urban population at risk of city sinking, new satellite data shows
University of East Anglia
Land subsidence is overlooked as a hazard in cities, according to scientists from the University of East Anglia (UEA) and Virginia Tech.
Writing in the journal Science, Prof Robert Nicholls of the Tyndall Centre for Climate Change Research at UEA and Prof Manoochehr Shirzaei of Virginia Tech and United Nations University for Water, Environment and Health, Ontario, highlight the importance of a new research paper analysing satellite data that accurately and consistently maps land movement across China.
While they say in their comment article that consistently measuring subsidence is a great achievement, they argue it is only the start of finding solutions. Predicting future subsidence requires models that consider all drivers, including human activities and climate change, and how they might change with time.
The research paper, published in the same issue, considers 82 cities with a collective population of nearly 700 million people. The results show that 45% of the urban areas that were analysed are sinking, with 16% falling at a rate of 10mm a year or more.
Nationally, roughly 270 million urban residents are estimated to be affected, with nearly 70 million experiencing rapid subsidence of 10mm a year or more. Hotspots include Beijing and Tianjin.
Coastal cities such as Tianjin are especially affected as sinking land reinforces climate change and sea-level rise. The sinking of sea defences is one reason why Hurricane Katrina’s flooding brought such devastation and death-toll to New Orleans in 2005.
Shanghai – China’s biggest city – has subsided up to 3m over the past century and continues to subside today. When subsidence is combined with sea-level rise, the urban area in China below sea level could triple in size by 2120, affecting 55 to 128 million residents. This could be catastrophic without a strong societal response.
"Subsidence jeopardises the structural integrity of buildings and critical infrastructure and exacerbates the impacts of climate change in terms of flooding, particularly in coastal cities where it reinforces sea-level rise," said Prof Nicholls, who was not involved in the study, but whose research focusses on sea-level rise, coastal erosion and flooding, and how communities can adapt to these changes.
The subsidence is mainly caused by human action in the cities. Groundwater withdrawal, that lowers the water table is considered the most important driver of subsidence, combined with geology and weight of buildings.
In Osaka and Tokyo, groundwater withdrawal was stopped in the 1970s and city subsidence has ceased or greatly reduced showing this is an effective mitigation strategy. Traffic vibration and tunnelling is potentially also a local contributing factor - Beijing has sinking of 45mm a year near subways and highways. Natural upward or downward land movement also occurs but is generally much smaller than human induced changes.
While human-induced subsidence was known in China before this study, Profs Nicholls and Shirzaei say these new results reinforce the need for a national response. This problem happens in susceptible cities outside China and is a widespread problem across the world.
They call for the research community to move from measurement to understanding implications and supporting responses. The new satellite measurements are delivering new detailed subsidence data but the methods to use this information to work with city planners to address these problems needs much more development. Affected coastal cities in China and more widely need particular attention.
“Many cities and areas worldwide are developing strategies for managing the risks of climate change and sea-level rise,” said Prof Nicholls. “We need to learn from this experience to also address the threat of subsidence which is more common than currently recognised.”
10.1126/science.ado9986
Method of Research
Commentary/editorial
Article Title
Earth’s sinking surface: China’s major cities show considerable subsidence from human activities
Article Publication Date
18-Apr-2024
Disclaimer: AAAS and EurekAlert! are not responsible for the accuracy of news releases posted to EurekAlert! by contributing institutions or for the use of any information through the EurekAlert system.
Thank you for visiting nature.com. You are using a browser version with limited support for CSS. To obtain the best experience, we recommend you use a more up to date browser (or turn off compatibility mode in Internet Explorer). In the meantime, to ensure continued support, we are displaying the site without styles and JavaScript.
- View all journals
- My Account Login
- Explore content
- About the journal
- Publish with us
- Sign up for alerts
- Open access
- Published: 17 April 2024
Diversity-dependent speciation and extinction in hominins
- Laura A. van Holstein ORCID: orcid.org/0000-0003-1222-9593 1 &
- Robert A. Foley ORCID: orcid.org/0000-0003-0479-3039 1
Nature Ecology & Evolution ( 2024 ) Cite this article
134 Altmetric
Metrics details
- Biological anthropology
The search for drivers of hominin speciation and extinction has tended to focus on the impact of climate change. Far less attention has been paid to the role of interspecific competition. However, research across vertebrates more broadly has shown that both processes are often correlated with species diversity, suggesting an important role for interspecific competition. Here we ask whether hominin speciation and extinction conform to the expected patterns of negative and positive diversity dependence, respectively. We estimate speciation and extinction rates from fossil occurrence data with preservation variability priors in a validated Bayesian framework and test whether these rates are correlated with species diversity. We supplement these analyses with calculations of speciation rate across a phylogeny, again testing whether these are correlated with diversity. Our results are consistent with clade-wide diversity limits that governed speciation in hominins overall but that were not quite reached by the Australopithecus and Paranthropus subclade before its extinction. Extinction was not correlated with species diversity within the Australopithecus and Paranthropus subclade or within hominins overall; this is concordant with climate playing a greater part in hominin extinction than speciation. By contrast, Homo is characterized by positively diversity-dependent speciation and negatively diversity-dependent extinction—both exceedingly rare patterns across all forms of life. The genus Homo expands the set of reported associations between diversity and macroevolution in vertebrates, underscoring that the relationship between diversity and macroevolution is complex. These results indicate an important, previously underappreciated and comparatively unusual role of biotic interactions in Homo macroevolution, and speciation in particular. The unusual and unexpected patterns of diversity dependence in Homo speciation and extinction may be a consequence of repeated Homo range expansions driven by interspecific competition and made possible by recurrent innovations in ecological strategies. Exploring how hominin macroevolution fits into the general vertebrate macroevolutionary landscape has the potential to offer new perspectives on longstanding questions in vertebrate evolution and shed new light on evolutionary processes within our own lineage.
Similar content being viewed by others
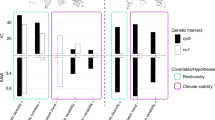
Evolutionary history and past climate change shape the distribution of genetic diversity in terrestrial mammals
Spyros Theodoridis, Damien A. Fordham, … David Nogues-Bravo
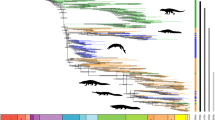
Decoupling speciation and extinction reveals both abiotic and biotic drivers shaped 250 million years of diversity in crocodile-line archosaurs
Alexander R. D. Payne, Philip D. Mannion, … Katie E. Davis
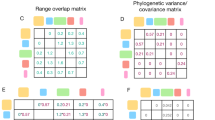
Clade density and the evolution of diversity-dependent diversification
Marcio R. Pie, Raquel Divieso & Fernanda S. Caron
The diversification of a lineage is the net output of speciation minus extinction. A theme that runs through much research into human evolution is whether the determinants of hominin diversification conform to or diverge from those seen in other taxa. At one extreme lie ideas such as Wolpoff’s ‘single species hypothesis’ 1 , which suggested that there can be no speciation in the hominin lineage, as its niche is ‘culture’. Culture, in Wolpoff’s view, is uniquely human and prevents boundaries between populations from occurring; hence, speciation was prohibited in hominins, but not in other clades. At the other extreme are interpretations that emphasize commonalities between patterns of hominin speciation and extinction and those of other clades 2 . Within this group, research interest has primarily been devoted to examining the role of climate in shaping hominin diversification 2 , 3 , 4 , 5 , 6 , 7 . What has received far less attention as a potential driver of hominin diversification than climate, however, is competition.
Competition occurs across taxonomic scales, from interindividual competition within populations 8 to intergroup competition within species 9 and interspecific competition 10 . Competition at each of these levels has been shown to act as an important driver of evolution at equal or higher scales 11 , 12 , 13 . Here we focus on interspecific competition for niches (hereafter ‘competition’) and its consequences above the species level. Although the concept of ‘niche’ has only rarely been formally defined in previous work on diversity-dependent speciation 14 , 15 , 16 , its implicit definition in previous work is that of a Hutchinsonian ecological niche 17 —an n -dimensional hypervolume describing all environmental resources and conditions required for species persistence. We adopt this conventional definition throughout this paper. The consequences of competition can include three processes: speciation, extinction and morphological change through, for example, character displacement 13 , 18 . There is some indirect evidence that competition resulted in morphological evolution in our lineage: competition between Homo and Paranthropus in East Africa probably led to character displacement in the mandibular premolar morphology of these two groups 19 . However, much less work has been devoted to exploring the effects of competition on hominin speciation or extinction.
Ecological competition with large carnivores is thought to have exerted a strong effect on hominin ranging patterns 20 , 21 , hunting behaviour 22 and, of particular interest at a macroevolutionary scale, geographic dispersals 23 , 24 . Although it is unknown whether competition with large carnivores had direct effects on hominin speciation or extinction, the link between dispersals and these macroevolutionary processes is well established 25 . Compared with competition between hominins and non-hominins, the dynamics and effects of competition between hominin species have received comparatively little attention. Although competition may have contributed to a pulse of hominin extinction around 1.5 million years ago 26 , and some recent reviews have used evidence for hominin sympatry in East and South Africa to suggest the possibility of competition 27 , 28 , an explicit investigation of the extent to which competition drove hominin diversification is lacking.
Competition has probably had a major role in animal diversification, however, leaving signals in correlations between species diversity, on the one hand, and speciation and extinction on the other 14 , 16 , 29 , 30 , 31 . Speciation can be both positively or negatively diversity dependent or occur independently of a clade’s own diversity. Under positive diversity dependence, speciation rates rise as a function of the novel evolutionary opportunities and interactions created by other species 32 . This pattern is exceptionally rare among all forms of life, however, having been reported only in island-dwelling beetles 33 , plants and arthropods 34 , and this latter case is contentious 35 , 36 . Instead, if a relationship exists between vertebrate speciation and diversity, this is usually negative 14 , 16 , 30 , 31 . There are two processes by which speciation may be negatively controlled by diversity: competition for (1) niche space, or (2) geographic space 15 . In both, speciation is regulated by bounded ecological opportunities. In classical Darwinian diversity dependence 16 , 37 , speciation into a niche occupied by a closely related species is prohibited, producing a negative relationship. At a higher level of taxonomic organization, models of asymptotic diversity predict slowdowns in speciation as a finite number of niches within an adaptive grade, or a finite number of ranges within bounded space, become occupied by closely related species as a clade grows 25 , 38 . However, findings of diversity-independent speciation in some clades has led to intense debate about whether negative diversity dependence is universal across vertebrates; the same is true for the related question of whether absolute limits to niches or geographic ranges even exist 39 , 40 .
The relationship between extinction and diversity has received less explicit empirical attention than that between speciation and diversity. However, when a relationship is reported, extinction is typically positively diversity dependent 41 , 42 . These patterns align with expectations based on theory. Under Darwinian diversity dependence, competition between ecologically similar species should result in extinction of outcompeted species 37 even in the absence of absolute limits to species diversity. Models of asymptotic diversity 16 , 43 predict increased rates of extinction as species diversity approaches an explicitly predicted diversity limit. Asymptotic diversity dynamics have been reported for multiple vertebrate clades 44 , 45 , although other studies have suggested that these trends are unclear among terrestrial vertebrates 32 . As is the case for speciation, then, there is some empirical evidence for a typical direction of the relationship between diversity and extinction—in this case, positive—but the universality of this pattern among vertebrates, too, remains an open question.
Hominin evolution is represented by a well-studied and rich fossil record and occurs across temporal and spatial scales that sit squarely at the expected intersection of climatic and competitive processes 46 . Therefore, exploring how hominin macroevolution fits into the general vertebrate macroevolutionary landscape has the potential to offer new perspectives on longstanding questions in vertebrate evolution, as well as addressing the comparative dearth of explicit research on diversity-dependent macroevolution in the hominin lineage.
Here we ask whether hominins also follow the pattern of diversity-dependent diversification that characterizes many other vertebrate clades. More specifically, we ask: were hominins characterized by negative diversity-dependent speciation and positive diversity-dependent extinction?
At which taxonomic level should these patterns be expected? Negative diversity-dependent speciation and positive diversity-dependent extinction at the level of the hominin clade as a whole would imply either that hominins were characterized by species’ inabilities to diverge ecologically from each other, as in Darwinian diversity dependence—and in an extension of Wolpoff’s ‘culture’ argument—or that hominins occupy a bounded set of niches in broader ecological context, as in asymptotic diversity dependence. One possibility is that hominin diversification is not diversity dependent, either because hominin speciation and extinction are purely climate-driven and not determined by diversity-mediated competitive dynamics 2 , 3 , 4 , 5 , 6 , 7 , or because the lineage was characterized by consistent ecological divergence, or because a limit to species diversity did not exist or was not reached. A second possibility is that hominins, overall, conform to the expected patterns. This would indicate a powerful and underappreciated role for interspecific competition in hominin evolution.
However, Darwinian diversity dependence 16 , 37 predicts stronger signals of diversity-dependent dynamics within and not across adaptive grades 15 , 16 , 37 , as species within adaptive grades should be more ecologically similar to each other. Given that there is strong support for Homo having occupied an adaptive grade distinct from earlier hominins 47 , 48 , we contrast the patterns found between Homo and Plio-Pleistocene non- Homo species ( Australopithecus and Paranthropus ). In addition to the two possible patterns described above, this comparison presents a third possibility: conflicting patterns between adaptive grades. Such a pattern will have resonance with the major issue of how far hominin evolution conforms to general evolutionary patterns, and why it might diverge.
Analyses based on speciation and extinction times
To explore whether species diversity predicts species origination and extinction, we ran birth–death models in a validated Bayesian framework 49 , 50 on five datasets of estimated times of species origination and extinction. The first dataset comprised published first and last appearance dates (FADs and LADs), which are conventionally used as proxies for times of species origination and extinction without accounting for variability in fossil preservation rates. The subsequent four datasets were based on our database of hominin fossil occurrences, recorded at two operational definitions of localities (at the finest-grained occurrence level available ( n = 385 occurrences) and at the broadest occurrence level (that in which all occurrences at a site complex were merged into a single occurrence; n = 267 occurrences)). We applied two sets of explicit fossil preservation rate priors (time-based variability and within-lifetime variability; both models also included between-lineage variability) to these two occurrence datasets, generating four new sets of times of species origination and extinction. As there were no differences in the direction of inferred relationships between these datasets, we report results for both models of preservation from the most fine-grained occurrence level here. Results for the broadest occurrence level are provided in Supplementary Table 1 .
Times of speciation and extinction estimated for fine-grained occurrences under (1) lineage- and time-based variability and (2) lineage- and within-species lifetime variability in fossil preservation rates are presented in Table 1 . Those for the same analyses across the broadest occurrence level are reported in Supplementary Table 2 . Speciation times were significantly different between the new dates estimated under both preservation rate priors and between dates estimated under preservation rate priors and the published FADs and LADs of fossil species (pairwise paired t -tests with Bonferroni correction, P < 0.05). Extinction times did not differ significantly between dates estimated under both preservation rate priors, but both of these did differ significantly from the published FADs and LADs (pairwise paired t -tests with Bonferroni correction, P < 0.05). Compared to published FADs, estimates of speciation and extinction times that account fossil preservation extended species’ lifespans. These models estimate that species originated, on average, 0.49 million years earlier (within-lifetime variability) and 0.37 million years earlier (time-based variability) than published dates suggest, and that they went extinct 0.27 million years later (within-lifetime variability) and 0.15 million years later (time-based variability) than published dates suggest (Fig. 1a and Table 1 ).
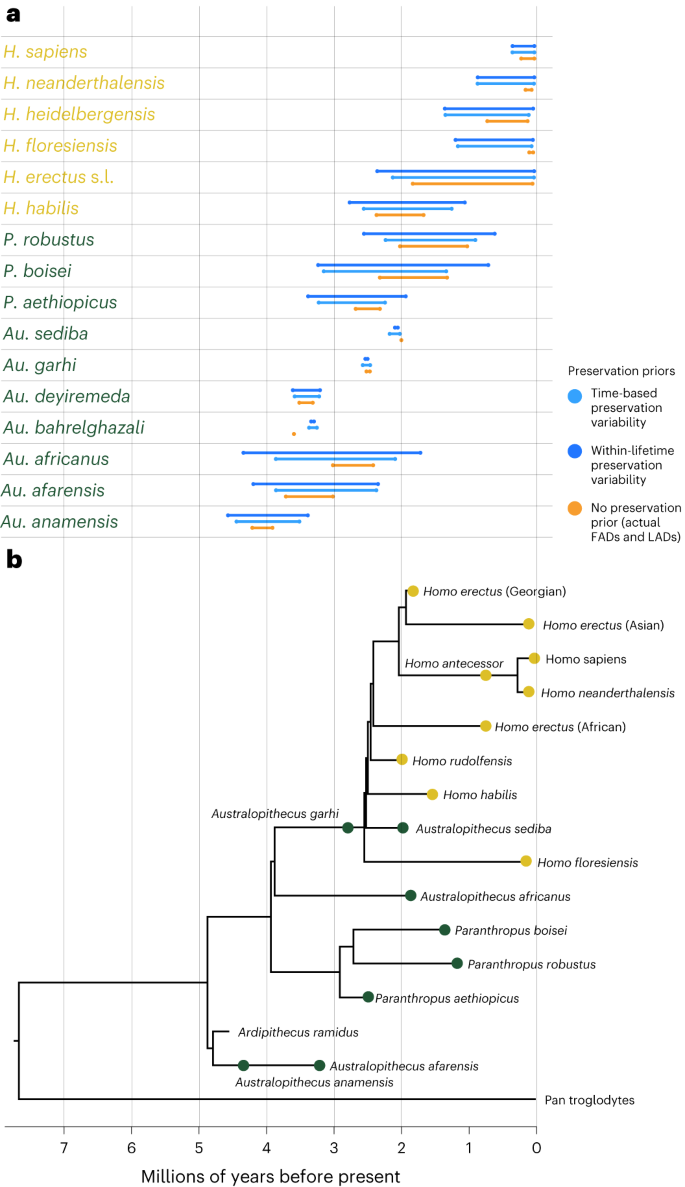
a , Species lifespans, comprising the time between speciation and extinction dates based on three datasets. Orange: published fossil FADs and LADs estimated without taking fossil preservation into account. Light blue: speciation and extinction dates estimated in a Bayesian framework incorporating time-based variability in fossil preservation rates. Dark blue: speciation and extinction dates estimated in a Bayesian framework incorporating within-lineage variability in fossil preservation rates. Note that these taxa are those the published dates and our new database have in common; actual analyses incorporated Homo ergaster in the no-preservation-prior dataset and Homo rudolfensis in the preservation prior datasets. Homo erectus s.l. refers to Homo erectus sensu lato. b , The Parins-Fukuchi et al. 87 phylogeny used in this study, with species coloured by taxonomic grouping (yellow: Homo; green: non- Homo ).
Across each of the three datasets, the results suggest at reasonable confidence that speciation was a negatively diversity-dependent process across the hominin clade as a whole (Fig. 2a and Table 2 ). The 50% credible intervals did not overlap with 0, although the 95% credible intervals did. In two models, >75% of the posterior distribution was negative. For non- Homo species, the signal was more diffuse. Although mean correlation parameter estimates were negative, the 50% credible interval overlapped with 0 in all cases (Fig. 2a ). By contrast, the results suggest at reasonable confidence that speciation in Homo was positively diversity-dependent across all three datasets (Fig. 2a and Table 2 ). In the model with no preservation priors, the 50% credible interval overlapped with 0, but the correlation parameter was positive in 67.9% of the iterations. In models incorporating fossil preservation variability, the 50% credible intervals did not overlap with 0, although the 95% credible intervals did (Fig. 2a ). In these models, the correlation parameter was positive in 78% and 83.8% of the iterations of the time-based preservation variability model and the within-lifetime preservation variability models, respectively.
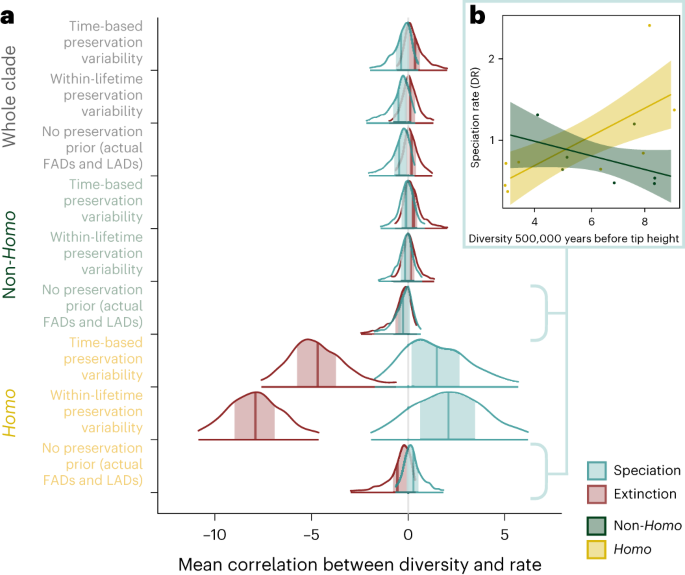
a , Results from PyRate birth–death models, run across three datasets with different fossil preservation priors (no preservation priors: published FADs and LADs; time-based preservation variability, where preservation is allowed to vary every 1 million years—prior applied to fossil occurrence data from three databases; and within-lifetime preservation variability, where preservation rate is allowed to vary across a species’ lifespan—prior applied to fossil occurrence data from three databases). In the latter two models, the preservation rate was also allowed to vary between lineages. The posterior distribution of the correlation parameter is shown, with the 50% credible interval shaded and the 95% credible interval indicated by the outline. The mean correlation parameter is indicated by a thick line. Results for speciation are indicated in blue; those for extinction are shown in red. b , The relationship between diversity 500,000 years before tip height and speciation rate (tip DR) across the Parins-Fukuchi et al. 87 phylogeny. Shaded area indicates 95% confidence interval.
The strongest signal across both processes was that extinction in Homo was unexpectedly negatively diversity dependent. For all three models, >75% of the posterior distribution of the diversity correlation parameter was negative, and the 50% credible intervals did not overlap with 0; and in the two models incorporating fossil preservation variability, >99% of the distribution was negative (Fig. 2a and Table 2 ). The pattern in Homo stands in stark contrast to the lack of a strong signal within non- Homo or the hominin clade as a whole. Although the mean of the posterior distribution of the correlation parameter was positive in all but one model (non- Homo , no preservation priors), <75% of the posterior distributions for these models was positive, and the 50% and 95% credible intervals overlapped with 0 in all cases (Fig. 2a and Table 2 ).
Phylogeny-based analyses of speciation rate
To explore support for the results from models incorporating variability in fossil preservation versus those from the model without, we developed a complementary but independent analytical approach and calculated the speciation rate across a phylogeny. There was a statistically significant difference in the relationship between diversity and speciation between Homo and non- Homo groups (phylogenetic generalized least squares (GLS): difference between Homo and non- Homo regression slope, P < 0.05). In non- Homo species, speciation rate decreased as a function of diversity, consistent with a negative diversity-dependent speciation regime, whereas Homo was characterized by a significant positive relationship between speciation rate and diversity (Fig. 1b ). Model outputs are provided in Supplementary Table 3 .
The differences between Homo and non- Homo are unlikely to be the consequence of higher undersampling of non- Homo species richness: the same result was obtained across 99% of trees with 12.5% increased non- Homo species richness and 98%, 95% and 94% of trees with 25%, 37.5% and 50% increased non- Homo species richness, respectively.
The phylogeny-based method performs reasonably well across small datasets; across the small trees simulated under a diversity-dependent process, it correctly inferred negative diversity dependence across 73% of the trees, and this remained 73% when up to 40% species were randomly removed from the phylogenies to simulate incomplete sampling. The method falsely identified a relationship between diversity and speciation across 31% of the simulated constant-rate birth–death trees, and this rose to 41% when up to 40% species were randomly removed from the phylogenies. In the incorrect sample of 31%, negative diversity dependence was inferred across nearly all trees (97%), and this did not change across the phylogenies across which incomplete sampling was simulated. Positive diversity-dependent speciation was incorrectly inferred across 0.02% of the complete simulated constant-rate birth–death trees and 0.3% of the trees across which incomplete sampling was simulated. Taken together, these results suggest that there can be reasonable but not total confidence that non- Homo speciation was characterized by negative diversity dependence; there is a 69% chance that it was not a false positive result across a tree generated under a non-diversity-dependent process. There is only a 0.02% chance that the positive diversity-dependent speciation of Homo is a methodological artefact.
We investigated whether hominin speciation and extinction are correlated with species diversity, as they are across many—but not all—vertebrate clades. Our results across the clade as a whole suggest that speciation was probably negatively regulated by diversity, and that this was also true for the group comprising Australopithecus and Paranthropus , although the signal was weaker in this subclade. By contrast, there is reasonably strong evidence that the relationship between speciation and diversity in the genus Homo diverges from that in other hominins and many other vertebrates: across two analytical approaches, Homo speciation was positively diversity dependent. Homo extinction, furthermore, showed a very strongly negatively diversity-dependent pattern, which differs from the lack of a relationship between extinction and diversity found both across hominins as a whole and in the Australopithecus and Paranthropus subgroup.
From a broader vertebrate perspective, the reasonably strong evidence for negative diversity-dependent speciation across the clade as a whole (mean 79% and 77% of the posterior distributions <0 for all Bayesian models and Bayesian models accounting for fossil preservation bias, respectively; Table 1 ), paired with the much more diffuse signal of negative diversity dependence in non- Homo (mean 62.8% and 60.5% of the posterior distributions <0 for all Bayesian models and Bayesian models accounting for fossil preservation bias, respectively, and a 69% chance that the patterns across the phylogeny were not false positives) echoes theoretical uncertainty about the taxonomic scale across which diversity dependence should operate 16 . Previous work in higher taxonomic groupings within birds and squamates found evidence of negative diversity-dependent speciation 14 , 29 , 31 , whereas work within terrestrial vertebrate orders, including Primates, recovered no such relationship 39 . Although our analyses were conducted at a lower scale, we recovered a corresponding pattern, with stronger evidence for diversity-dependent speciation at higher taxonomic scales. Turning to broader questions about whether ecological limits to species diversity are even to be expected 51 , the comparison between signal strength at the two scales we report here suggests that limits to diversity should exist at higher taxonomic levels. One potential explanation is that if limits to ecologically similar species diversity exist, subclades may often not reach these individually before extinction begins to outpace speciation. Through a Darwinian diversity-dependent lens, this ‘limit’ may simply reflect the point at which species are no longer able to diverge ecologically from one another 16 and so has more to do with the evolvability of the clade itself, whereas an asymptotic view holds that there is a set number of niches or limited geographic space to speciate into a priori. We cannot distinguish between these alternatives based on the models we present here, but our results are consistent with clade-wide diversity limits that governed speciation overall but were not quite reached by the non- Homo subclade before its extinction.
Notably, neither non- Homo subclade extinction nor that across the clade as a whole carried a strong signal of diversity dependence (Table 1 ). This aligns with previous work reporting an absence of diversity-dependent extinction—for example, the lack of empirical evidence for extinctions in ‘saturated’ communities following species invasions 52 . Previous work has made a strong case that hominin extinction is more closely linked to climate than hominin speciation 53 , and climate-driven extinction before a theoretical cap on diversity—whether Darwinian or asymptotic—was reached can explain these results.
Contrary to expectations, we found reasonably strong evidence for positively diversity-dependent speciation in Homo (mean 76.6% and 80.9% of the posterior distributions >0 for all Bayesian models and Bayesian models accounting for fossil preservation bias, respectively, and a 0.02% chance that the patterns across the phylogeny were false positives). This pattern is much rarer than negatively diversity-dependent speciation and non-diversity-related speciation across all forms of life, having been reported for only a few groups, including island-dwelling beetles 33 , plants and arthropods 34 , with this last case being contentious 35 , 36 . In the vertebrate context, then, our results not only expand the set of reported associations between diversity and speciation but, crucially, underscore previous findings that the relationship between speciation and diversity is complex 39 , 52 , 54 .
This complexity is no doubt at least in part the consequence of the fact that both extrinsic factors and intrinsic traits modify any feedback loop between species diversity and speciation 18 , 55 . One explanation that incorporates both is repeated dispersals. Homo is the only hominin genus to expand its range outside of Africa 56 , 57 , and recurrent expansions into new habitats that promote new adaptations, and therefore speciation, while source populations persist, will result in a positive correlation between speciation rate and diversity. This correlation may reflect no causal relationship between competition and speciation at all, but a complementary model is that high levels of competition between closely related and ecologically similar taxa in the ‘source’ location drove dispersal in the first place 58 , 59 . For example, Carbonell and colleagues 60 suggested that the earliest European hominins were competitively displaced from Africa by populations that developed the Acheulian.
A second and non-mutually exclusive explanation is that ‘diversity begets diversity’ 32 —that is, that existing species provide evolutionary pressure and opportunities for (the evolution of) new species 61 , 62 , 63 . Increased species diversity may produce interactions that can promote speciation and can extend species’ lifespans providing that the activities of the species upon which the niche of another is built persist. Species may create novel ecological opportunities for new species to exploit through ecosystem modification 64 , 65 , 66 . Ecosystem modification is particularly likely to lead to evolutionary consequences if it increases structural and resource heterogeneity 64 . Although the time-averaged nature of the fossil record makes it difficult to reconstruct the ecological effects of hominin behaviour, it is not unlikely that hominins, particularly those belonging to the genus Homo , were ecosystem engineers 67 , 68 , and that the ecological opportunities afforded by their behaviour promoted the appearance of novel hominin species. Behaviours that may have contributed particularly strongly to such dynamics are the use of fire 69 , which can cause widespread landscape modification, and the adoption of active and intensive hunting, which will have exerted new pressures on the distribution and population sizes of hominin prey 68 .
An adaptive ‘trait’ potentially critical to repeated geographic expansions—whether caused by competition or not—as well as creating ecological opportunities for new species and exploiting ecological opportunities afforded by other hominins, is technology 70 , 71 . Of course, lithic technology predates Homo 72 , but what can be said with some certainty is that neither Australopithecus and Paranthropus , nor other vertebrates, rely on stone tool technology to the same degree as Homo 73 . The suggestion that repeated and ratcheted technological innovation promoted speciation stands in stark contrast to Wolpoff’s single species hypothesis 1 .
However, it may be that Homo speciation merely appears to be positively diversity dependent because non- Homo taxa were present at the time of Homo species origination, and that it is not necessarily the presence of these taxa that resulted in Homo speciation. What is incongruous about this explanation, in the context of the evidence for negative diversity dependent speciation across the clade as a whole, is why the presence of these other species did not restrict Homo speciation as predicted. This overlap hints at competitive displacement from occupied niches, rather than the opportunistic replacement documented in, for example, carnivores 74 .
Homo is characterized, finally, by negative diversity-dependent extinction (mean 92.3% and 99.7% of the posterior distributions <0 for all Bayesian models and Bayesian models accounting for fossil preservation bias, respectively). To the best of our knowledge, this pattern has not been documented in other clades. It may be the case that negative diversity-dependent extinction is a statistical artefact of coeval extinction events unrelated to diversity—presumably precipitated by climate change 53 . Although we cannot rule this out, because we did not explicitly contrast the effects of climate and diversity in our models, there are problems with a climate-only explanation for this pattern. Given that Homo evolution occurred during a period of increased climatic deterioration and change 5 , 75 , 76 , and that estimated extinction times did not occur at exactly the same time, and both before and after episodes of major cooling (Table 1 ), it is difficult to pinpoint a single climatic event that underlies Homo extinctions. We propose two alternative explanations for this pattern. First, it may be the case that repeated innovations in adaptive strategies in Homo resulted in coeval competitive replacement of a number of species by a single innovator species. For instance, shifts in life history and dietary strategy within Homo erectus sensu lato 77 may have allowed this taxon to replace competitively early forms of Homo , and the same may have been true for the cognitive and behavioural innovations of Homo sapiens relative to Late Pleistocene hominins 78 . This echoes a recent point made by Bokma and colleagues 79 . A related explanation for late Pleistocene replacement by Homo sapiens is that most roughly coeval hominin extinctions are caused by climate, but that the extreme generalism of Homo sapiens prevents late-surviving forms from speciating 16 . These mechanisms, which may interact with climatic and environmental shifts which stimulated the adaptive novelties, would produce a negative diversity signal.
Accounting for variability in fossil preservation rates within and between lineages, and across time, resulted in longer estimated species lifespans (Fig. 1a ) than those based on published FADs and LADs, and these extended lifespans are more in line with the mammalian average of 1 million years 80 , 81 . That actual hominin fossil FADs and LADs do not fully represent species’ lifespans aligns with findings that hominin fossils are comparatively rare within mammal assemblages 82 . Further implications of these results relate to phylogeny: there are three sets of conventionally hypothesized ancestor–descendant relationships that our new origination and extinction times suggest cannot have occurred unless in the context of non-Hennigian speciation 83 , in which the ancestral species persists alongside its daughter species. Paranthropus boisei originated ~0.1 million years after its putative ancestor Paranthropus aethiopicus 84 , and they overlapped temporally for >0.5 million years. This latter pattern is also true for Homo heidelbergensis and Homo neanderthalensis , and for Australopithecus anamensis and Australopithecus afarensis 85 . In the case of Au. anamensis and Au. afarensis , our results echo recent evidence for the contemporaneity of the two species 86 . Further, the earlier origination date of Homo floresiensis aligns with that inferred across the Parins-Fukuchi et al. 87 phylogeny. Overall, these new datasets underscore previous calls 82 to account for incomplete sampling in analyses of hominin macroevolution. Our results suggest that using conventional FADs and LADs underestimates species’ temporal ranges, with attendant problems for the validity of conclusions.
Evolution is clearly a pluralistic process, with the attendant expectation that climatic processes and competition both influence vertebrate macroevolution. Disentangling their relative roles is an area of ongoing research 13 , 88 , 89 , and the first step in doing so in hominins was addressing the comparative dearth of explicit research on diversity-dependent macroevolution. The evidence for negative diversity dependence in speciation across the clade overall and the strong and unexpected evidence for the part that interspecific competition may have played in both speciation and extinction in the genus Homo are difficult to reconcile with conventional models that place exclusive emphasis on the role of climate in hominin macroevolution. Ultimately, the climatic ‘Court Jester’ must set the stage upon which the ‘Red Queen’ of interspecific competition ‘dances’ 90 , and our results point to a need to further explore the relationship between climate and competition, and how this relationship drove macroevolution, in our own lineage. Finally, an important effect of the inferred longer species lifespans is notably extended periods of temporal overlap between sympatric species, such as P. boisei and early Homo in East Africa 19 as well as Paranthropus robustus and Australopithecus africanus in South Africa. Extended periods of sympatry provide the context for interspecific competition at smaller scales, with effects at equivalent scales, such as microevolutionary morphological evolution driven by competition-mediated niche separation 10 , 13 , 91 . Our results, then, point to the need to further explore the possible effects of interspecific competition at all scales 16 .
In vertebrates, speciation is often negatively diversity dependent, and extinction is expected to show positive diversity dependence. Our results are consistent with diversity limits at the level of the hominin tribe that governed speciation in hominins overall, and with these not being quite reached by the Australopithecus and Paranthropus subclade before its extinction. There was no signal of diversity dependence in Australopithecus and Paranthropus subclade extinction, or that of hominins overall: this is concordant with climate playing a bigger part in hominin extinction than speciation. Homo emerged as an evolutionary outlier amongst its hominin and vertebrate relatives. There is strong evidence that Homo extinction was negatively diversity dependent. Whether this reflects a process of repeated replacements of numerous older forms by more modern species of Homo or simply a correlation with pulsed climatic events, or a more complex relationship between climate and diversity, are new questions raised by these results. Finally, speciation in Homo was found to be relatively robustly positively diversity dependent across two analytical approaches. We argue that the comparatively unusual pattern of positive diversity-dependent speciation we report is concordant with a set of underappreciated and non-mutually exclusive drivers of speciation in Homo : interspecific competition, repeated geographic expansions potentially driven by interspecific competition, and ecosystem engineering by other members of Homo opening up new niches. Whatever the exact processes driving these patterns, the results presented here suggest that Homo was characterized by comparatively unusual and unexpected macroevolutionary dynamics.
Data collection
Occurrence data and fads and lads.
Fossil occurrence data were obtained in November 2023 from the Paleobiology Database ( https://paleobiodb.org/#/ ), using a taxon search for ‘hominin’; the NOW Database 92 ; and the ROCEEH ROAD Database 93 . The taxonomy of the Paleobiology Database occurrence data was checked for spelling errors using the PyRate ‘check_names’ function 49 and manually for synonyms. Occurrence data from the three databases were merged. Duplicates were identified manually, and records with most up-to-date age estimates were retained. If occurrences did not have a specified accession number, duplicates were identified based on location (geological formation and/or member, latitude and longitude) in combination with inspection of specified source publications (if available). To account for differences in the three databases’ approach to defining occurrence localities (for example, Au. afarensis at Laetoli comprises two entries in the Paleobiology Database, both of which are composites of more than two find spots, whereas all find spots are separate entries in the NOW database), we took a hierarchical approach to recording occurrences, recording ‘Site complex’ (for example, the Woranso-Mille palaeoanthropological research area), ‘Site’ (for example, Taung), ‘Subsite’ (for example, localities or surface find spots within a ‘site’; subsite ‘type’ was also recorded), ‘Formation’ (for example, Koobi Fora) and ‘Stratigraphic unit’ (for example, Member 4). Not all occurrences had information for all variables: for example, the Mauer site is not part of a larger ‘Site’ complex. We supplemented and updated the merged database with occurrence information obtained from literature reviews of papers published after 2016 and cross-checked our database with occurrence information supplied in published overviews of research where available 84 , 94 , 95 .
Species’ published FADs and LADs, which are conventionally taken as speciation and extinction ‘times’, were taken from Wood and Boyle 96 and supplemented with dates of more recently published species in the manner described by van Holstein and Foley 97 .
We used the phylogeny with the best Akaike information criterion score from Parins-Fukuchi et al. 87 . In contrast to other hominin phylogenies 98 , 99 , 100 , this phylogeny combines probabilistic models of morphological evolution and fossil preservation to recover anagenetic relationships between hominin species. It therefore uniquely recovers ancestor–descendant relationships that are (1) likely to be more realistic than those on phylogenies that do not incorporate them, and (2) broadly accepted based on morphological evidence alone (for example, between Au. anamensis and Au. afarensis 85 ).
The species diversification rate, tip DR, of Jetz et al. 101 , which calculates the tip-specific speciation rate, was calculated for every tip using R code from Upham et al. 102 :
where DR is the tip DR for species i , N i is the number of edges between species I and the root, and l is the length of edge j (with j = 1 being the edge closest to the extant tip). For each tip, the number of extant species at 500,000 years before the tip height was obtained using the ‘getExtant’ function in the phytools package 103 .
To determine whether speciation and extinction times were correlated with species diversity, we ran birth–death models, with diversity as predictor, in a validated Bayesian framework 49 , 50 on five datasets with estimated times of species origination and extinction. The first dataset was based on the conservative FADs and LADs estimated by Wood and Boyle 96 , with additions from van Holstein and Foley 97 , and thus incorporates no variability in fossil preservation rates. The subsequent four datasets were based on our new hominin occurrence database. From these data, we estimated four new sets of times of speciation and extinction with two sets of explicit fossil preservation rate priors and two operational definitions of localities (at the finest-grained occurrence level available ( n = 385 occurrences) and at the broadest occurrence level (that is, with all occurrences at a site complex merged into a single occurrence; n = 267 occurrences). As there were no differences in the direction of inferred relationships between these datasets, we report results for both models of preservation from the most fine-grained occurrence level; results for the broadest occurrence level are provided Supplementary Table 1 .
In the first dataset, we modelled fossil preservation as a function of a time-variable Poisson process. Preservation rates were allowed to vary every 1 million years. In the second dataset, we allowed fossil preservation to vary over the course of a species’ lifespan by modelling it with a non-homogeneous Poisson process of preservation 49 . This allowed us to take into account that fossils are less likely to form at the start and end of a species’ lifespan, as the number of individuals belonging to a species is low. In both datasets, fossil preservation was also allowed to vary between lineages by incorporating a gamma model of rate heterogeneity 49 . We generated ten replicates of estimated times of species origination and extinction for both preservation regimes using the Reversible Jump MCMC algorithm in the python programme PyRate 49 , to incorporate dating uncertainty into the results 104 . All analyses described below were then performed on the ten replicates, and results were joined into a single posterior sample.
We generated lineage-through-time estimates for all three datasets in PyRate. We then applied a PyRate birth–death model in which Homo and non- Homo speciation and extinction rates were determined by an exponential correlation to a time-variable predictor, in this case clade-wide lineage-through-time estimates for each set of times of origination and extinction. To compare these results with the pattern across the whole clade, we ran an exponential diversity-dependent birth–death model, in which the whole clade’s own diversity was used as the predictor variable. As the sample size was inevitably relatively small, we ran each model for 2,000,000 iterations, sampling every 1,000 iterations.
Analyses based on phylogeny
These analyses were performed in R 4.01 (ref. 105 ). To explore further the relationship between speciation and diversity—and, in particular, the difference between Homo and non- Homo species—we ran phylogenetic GLS regressions to determine whether there were differences between Homo and non- Homo in the relationship between speciation rates and previous clade-wide diversity:
where DR is the tip DR and SD is the phylogeny-based species diversity at 500,000 years before the tip.
The phylogenetic correlation structure of residual error in the phylogenetic GLS was accounted for in the nlme ‘correlation’ argument 106 . The model assumed a Brownian motion model for residual error structure, following previous work on regressions including speciation rates 101 , 107 , 108 . Non-contemporaneity of tips was represented in the nlme argument ‘weights’.
To test the ability of the phylogeny-based approach described above to correctly distinguish between diversity-dependent and non-diversity-dependent speciation, we simulated 1,000 phylogenies under a constant-rate birth–death process using the ‘pbtree’ function in the phytools package 103 , preserving extinct tips, and repeated the analyses described above for equation ( 2 ) to estimate how often diversity-dependent speciation was erroneously inferred across non-ultrametric trees generated under a non-diversity-dependent process. We then generated 1,000 phylogenies simulated under a diversity-dependent regime using the ‘ddsim’ function in the DDD package 109 . These phylogenies were simulated with birth and death rates and carrying capacities randomly drawn from a normal distribution with means that produced trees with similar tip numbers to those of the Parins-Fukuchi et al. 87 phylogeny in a trial run, and with a maximum tree height of 7, so as to produce similarly small phylogenies to the Parins-Fukuchi et al. 87 tree. We then randomly removed up to 40% of tips and repeated the analyses to investigate the sensitivity of results to incomplete sampling.
We also tested the sensitivity of the results of phylogeny-based analyses using equation (2) to the increased probability of species discovery towards the present 82 , which could have resulted in underrepresentation of non- Homo species relative to the younger Homo species included in the analyses. To do so, we generated 4,000 phylogenies with up to +50% non- Homo species added in random locations and with random tip heights to the original Parins-Fukuchi et al. 87 phylogeny, using the ‘bind_tip’ function in the phytools package 103 . We repeated the analyses described above for equation (2) and calculated the proportion of trees across which the original results were maintained.
Reporting summary
Further information on research design is available in the Nature Portfolio Reporting Summary linked to this article.
Data availability
All data are available on figshare: https://figshare.com/s/46fe37e09047513e31b0 (ref. 110 ).
Code availability
All code is available on figshare: https://figshare.com/s/46fe37e09047513e31b0 (ref. 110 ).
Wolpoff, M. H. Competitive exclusion among Lower Pleistocene hominids: the single species hypothesis. Man 6 , 601 (1971).
Article Google Scholar
Vrba, E. S. Paleoclimate and Evolution, with Emphasis on Human Origins (Yale Univ. Press, 1995).
Vrba, E. S. Turnover-pulses, the Red Queen, and related topics. Am. J. Sci. 293 A , 418–452 (1993).
Faith, J. T. & Behrensmeyer, A. K. Climate Change and faunal turnover: testing the mechanics of the turnover-pulse hypothesis with South African fossil data. Paleobiology 39 , 609–627 (2013).
Potts, R. Environmental hypotheses of hominin evolution. Yearb. Phys. Anthropol. 107 , 93–136 (1998).
Potts, R. Variability selection in hominid evolution. Evol. Anthropol. https://doi.org/10.1002/(SICI)1520-6505(1998)7:3<81::AID-EVAN3>3.0.CO;2-A (1998).
Potts, R. & Faith, J. T. Alternating high and low climate variability: the context of natural selection and speciation in Plio-Pleistocene hominin evolution. J. Hum. Evol. 87 , 5–20 (2015).
Article PubMed Google Scholar
West-Eberhard, M. Sexual selection, social competition, and evolution. Proc. Am. Philos. Soc. 123 , 222–234 (1979).
Google Scholar
Wrangham, R. W. An ecological model of female-bonded primate groups. Behaviour 75 , 262–300 (1980).
Schreier, B. M., Harcourt, A. H., Coppeto, S. A. & Somi, M. F. Interspecific competition and niche separation in primates: a global analysis. Biotropica 41 , 283–291 (2009).
Clutton-Brock, T. H. & Huchard, E. Social competition and selection in males and females. Philos. Trans. R. Soc. Lond. B Biol. Sci. 368 , 20130074 (2013).
Article CAS PubMed PubMed Central Google Scholar
Stockley, P. & Bro-Jørgensen, J. Female competition and its evolutionary consequences in mammals. Biol. Rev. 86 , 341–366 (2011).
Drury, J. P. et al. Contrasting impacts of competition on ecological and social trait evolution in songbirds. PLoS Biol. 16 , e2003563 (2018).
Article PubMed PubMed Central Google Scholar
Phillimore, A. B. & Price, T. D. Density-dependent cladogenesis in birds. PLoS Biol. 6 , e71 (2008).
Moen, D. & Morlon, H. Why does diversification slow down? Trends Ecol. Evol. 29 , 190–197 (2014).
Rabosky, D. L. Diversity-dependence, ecological speciation, and the role of competition in macroevolution. Annu Rev. Ecol. Evol. Syst. 44 , 481–502 (2013).
Hutchinson, G. E. & MacArthur, R. H. A theoretical ecological model of size distributions among species of animals. Am. Nat. 93 , 117–125 (1959).
Schluter, D. Ecological character displacement in adaptive radiation. Am. Nat. 156 , S4–S16 (2000).
Schroer, K. & Wood, B. The role of character displacement in the molarization of hominin mandibular premolars. Evolution 69 , 1630–1642 (2015).
Egeland, C. P. Taphonomic estimates of competition and the role of carnivore avoidance in hominin site use within the Early Pleistocene Olduvai Basin. Quat. Int. 322-323 , 95–106 (2014).
Starkovich, B. M. & Conard, N. J. in Human Behavioural Adaptations to Interglacial Lakeshore Environments (eds García-Moreno, A. et al.) Ch. 6 (Propylaeum, 2020).
Stiner, M. C. Carnivory, coevolution, and the geographic spread of the genus Homo. J. Archaeol. Res. 10 , 1–63 (2002).
Rodríguez-Gómez, G., Rodríguez, J., Martín-González, J. A. & Mateos, A. Evaluating the impact of Homo-carnivore competition in European human settlements during the early to middle Pleistocene. Quat. Res. 88 , 129–151 (2017).
Brantingham, P. J. Hominid-carnivore coevolution and invasion of the predatory guild. J. Anthropol. Archaeol. 17 , 327–353 (1998).
Gavrilets, S. & Vose, A. Dynamic patterns of adaptive radiation. Proc. Natl Acad. Sci. USA 102 , 18040–18045 (2005).
Grove, M. Amplitudes of orbitally induced climatic cycles and patterns of hominin speciation. J. Archaeol. Sci. 39 , 3085–3094 (2012).
Maslin, M. A. et al. East African climate pulses and early human evolution. Quat. Sci. Rev. 101 , 1–17 (2014).
Maslin, M. A., Shultz, S. & Trauth, M. H. A synthesis of the theories and concepts of early human evolution. Philos. Trans. R. Soc. B Biol. Sci. 370 , 20140064 (2015).
Rabosky, D. L. & Lovette, I. J. Density-dependent diversification in North American wood warblers. Proc. R. Soc. B Biol. Sci. 275 , 2363–2371 (2008).
Etienne, R. S. et al. Diversity-dependence brings molecular phylogenies closer to agreement with the fossil record. Proc. R. Soc. B Biol. Sci. 279 , 1300–1309 (2012).
Weir, J. T. & Mursleen, S. Diversity-dependent cladogenesis and trait evolution in the adaptive radiation of the auks (Aves: Alcidae). Evolution 67 , 403–416 (2013).
Benton, M. J. & Emerson, B. C. How did life become so diverse? The dynamics of diversification according to the fossil record and molecular phylogenetics. Palaeontology 50 , 23–40 (2007).
Emerson, B. C. & Oromi, P. Diversification of the forest beetle genus Tarphius on the Canary Islands, and the evolutionary origins of island endemics. Evolution 59 , 586–598 (2005).
PubMed Google Scholar
Emerson, B. C. & Kolm, N. Species diversity can drive speciation. Nature 434 , 1015–1017 (2005).
Article CAS PubMed Google Scholar
Kiflawi, M., Belmaker, J., Brokovich, E., Einbinder, S. & Holzman, R. Species diversity can drive speciation: comment. Ecology 88 , 2132–2135 (2007).
Emerson, B. C. & Kolm, N. Response to comments on Species diversity can drive speciation. Ecography 30 , 334–338 (2007).
Darwin, C. On the Origin of Species (John Murray, 1859).
Schluter, D. Ecological causes of adaptive radiation. Am. Nat. 148 , S40–S64 (1996)
Pie, M. R., Divieso, R. & Caron, F. S. Clade density and the evolution of diversity-dependent diversification. Nat. Commun. 14 , 4576 (2023).
Shoemaker, L. & Clauset, A. Body mass evolution and diversification within horses (family Equidae). Ecol. Lett. 17 , 211–220 (2014).
Aguilée, R., Gascuel, F., Lambert, A. & Ferriere, R. Clade diversification dynamics and the biotic and abiotic controls of speciation and extinction rates. Nat. Commun. 9 , 3013 (2018).
Quental, T. B. & Marshall, C. R. How the Red Queen drives terrestrial mammals to extinction. Science 341 , 290–292 (2013).
Alroy, J. Geographical, environmental and intrinsic biotic controls on Phanerozoic marine diversification. Palaeontology 53 , 1211–1235 (2010).
Barry, J. C. et al. Faunal and environmental change in the late Miocene Siwaliks of northern Pakistan. Paleobiology 28 , 1–71 (2002).
Alroy, J. Speciation and extinction in the fossil record of North American mammals. Ecol. Rev. 2009 , 301–323 (2009).
Benton, M. J. The Red Queen and the Court Jester: species diversity and the role of biotic and abiotic factors through time. Science 323 , 728–732 (2009).
Collard, M. & Wood, B. in Handbook of Paleoanthropology 2nd edn (eds Henke, W. & Tattersall, I.) 2107–2144 (Springer, 2015).
Foley, R. A. Mosaic evolution and the pattern of transitions in the hominin lineage. Philos. Trans. R. Soc. B Biol. Sci. 371 , 20150244 (2016).
Silvestro, D., Salamin, N. & Schnitzler, J. PyRate: a new program to estimate speciation and extinction rates from incomplete fossil data. Methods Ecol. Evol. 5 , 1126–1131 (2014).
Silvestro, D., Salamin, N., Antonelli, A. & Meyer, X. Improved estimation of macroevolutionary rates from fossil data using a Bayesian framework. Paleobiology 45 , 546–570 (2019).
Harmon, L. J. & Harrison, S. Species diversity is dynamic and unbounded at local and continental scales. Am. Nat. 185 , 584–593 (2015).
Sax, D. F. et al. Ecological and evolutionary insights from species invasions. Trends Ecol. Evol. 22 , 465–471 (2007).
Foley, R. A. Speciation, extinction and climatic change in hominid evolution. J. Hum. Evol. 26 , 275–289 (1994).
Alroy, J. Dynamics of origination and extinction in the marine fossil record. Proc. Natl Acad. Sci. USA 105 , 11536–11542 (2008).
Heard, S. B. & Hauser, D. L. Key evolutionary innovations and their ecological mechanisms. Hist. Biol. 10 , 151–173 (1995).
Lordkipanidze, D. et al. A complete skull from Dmanisi, Georgia, and the evolutionary biology of early Homo . Science 342 , 326–331 (2013).
Scardia, G., Neves, W. A., Tattersall, I. & Blumrich, L. What kind of hominin first left Africa? Evol. Anthropol. 30 , 122–127 (2021).
Metz, J. A. J. & Gyllenberg, M. How should we define fitness in structured metapopulation models? Including an application to the calculation of evolutionarily stable dispersal strategies. Proc. R. Soc. B Biol. Sci. 268 , 499–508 (2001).
Article CAS Google Scholar
De Meester, N., Derycke, S., Rigaux, A. & Moens, T. Active dispersal is differentially affected by inter- and intraspecific competition in closely related nematode species. Oikos 124 , 561–570 (2015).
Carbonell, E., Mosquera, M., Rodríguez, X. P. & Sala, R. The first human settlement of Europe. J. Anthropol. Res 52 , 107–114 (1996).
Nosil, P. & Crespi, B. J. Experimental evidence that predation promotes divergence in adaptive radiation. Proc. Natl Acad. Sci. USA 103 , 9090–9095 (2006).
Tokeshi, M. Species Coexistence: Ecological and Evolutionary Perspectives (Blackwell, 1999).
Erwin, D. H. Seeds of diversity. Science 308 , 1752–1753 (2005).
Erwin, D. Macroevolution of ecosystem engineering, niche construction and diversity. Trends Ecol. Evol. 23 , 304–310 (2008).
Wright, J. P. & Jones, C. G. The concept of organisms as ecosystem engineers ten years on: progress, limitations, and challenges. BioScience 56 , 203–209 (2006).
Jones, C. G., Lawton, J. H. & Shachak, M. Positive and negative effects of organisms as physical ecosystem engineers. Ecology 78 , 1946–1957 (1997).
Braun, D. R. et al. Ecosystem engineering in the Quaternary of the west coast of South Africa. Evol. Anthropol. 30 , 50–62 (2021).
Root‐Bernstein, M. & Ladle, R. Ecology of a widespread large omnivore, Homo sapiens , and its impacts on ecosystem processes. Ecol. Evol. 9 , 10874–10894 (2019).
Roebroeks, W. & Villa, P. On the earliest evidence for habitual use of fire in Europe. Proc. Natl Acad. Sci. USA 108 , 5209–5214 (2011).
Bird, D. W. & O’Connell, J. F. Behavioral ecology and archaeology. J. Archaeol. Res. 14 , 143–188 (2006).
Blumenschine, R. J. & Pobiner, B. in Evolution of the Human Diet: The Known, the Unknown, and the Unknowable (ed. Ungar, P. S.) Ch. 10 (Oxford Univ. Press, 2007).
Harmand, S. et al. 3.3-million-year-old stone tools from Lomekwi 3, West Turkana, Kenya. Nature 521 , 310–315 (2015).
Shea, J. J. Occasional, obligatory, and habitual stone tool use in hominin evolution. Evol. Anthropol. 26 , 200–217 (2017).
Van Valkenburgh, B. Major patterns in the history of carnivorous mammals. Annu. Rev. Earth Planet Sci. 27 , 463–493 (1999).
Potts, R. Hominin evolution in settings of strong environmental variability. Quat. Sci. Rev. 73 , 1–13 (2013).
Potts, R. Evolution and climate variability. Science https://doi.org/10.1126/science.273.5277.922 (1996).
Antón, S. C. Natural history of Homo erectus . Am. J. Phys. Anthropol. 122 , 126–170 (2003).
Timmermann, A. Quantifying the potential causes of Neanderthal extinction: abrupt climate change versus competition and interbreeding. Quat. Sci. Rev. 238 , 106331 (2020).
Bokma, F., van den Brink, V. & Stadler, T. Unexpectedly many extinct hominins. Evolution 66 , 2969–2974 (2012).
Rosenzweig, M. L. Species Diversity in Space and Time (Cambridge Univ. Press, 1995).
Rosenblum, E. B. et al. Goldilocks meets Santa Rosalia: an ephemeral speciation model explains patterns of diversification across time scales. Evol. Biol. 39 , 255–261 (2012).
Maxwell, S. J., Hopley, P. J., Upchurch, P. & Soligo, C. Sporadic sampling, not climatic forcing, drives observed early hominin diversity. Proc. Natl Acad. Sci. USA 115 , 4891–4896 (2018).
Ezard, T. H. G., Pearson, P. N., Aze, T. & Purvis, A. The meaning of birth and death (in macroevolutionary birth-death models). Biol. Lett. 8 , 139–142 (2012).
Wood, B. & Schroer, K. in Human Paleontology and Prehistory (eds Marom, A. & Hovers, E.) Ch. 8 (Springer, 2017); https://doi.org/10.1007/978-3-319-46646-0_8
Kimbel, W. et al. Was Australopithecus anamensis ancestral to A. afarensis ? A case of anagenesis in the hominin fossil record. J. Hum. Evol. 51 , 134–152 (2006).
Haile-Selassie, Y., Melillo, S. M., Vazzana, A., Benazzi, S. & Ryan, T. M. A 3.8-million-year-old hominin cranium from Woranso-Mille, Ethiopia. Nature 573 , 214–219 (2019).
Parins-Fukuchi, C., Greiner, E., MacLatchy, L. M. & Fisher, D. C. Phylogeny, ancestors, and anagenesis in the hominin fossil record. Paleobiology 45 , 378–393 (2019).
Drury, J., Clavel, J., Manceau, M. & Morlon, H. Estimating the effect of competition on trait evolution using maximum likelihood inference. Syst. Biol. 65 , 700–710 (2016).
Clavel, J. & Morlon, H. Accelerated body size evolution during cold climatic periods in the Cenozoic. Proc. Natl Acad. Sci. USA 114 , 4183–4188 (2017).
Barnosky, A. D. Distinguishing the effects of the Red Queen and Court Jester on Miocene mammal evolution in the northern Rocky. J. Vertebr. Paleontol. 21 , 172–185 (2001).
Legault, G., Bitters, M. E., Hastings, A. & Melbourne, B. A. Interspecific competition slows range expansion and shapes range boundaries. Proc. Natl Acad. Sci. USA 117 , 26854–26860 (2020).
Žliobaitė, I. et al. in Evolution of Cenozoic Land Mammal Faunas and Ecosystems (eds Casanovas-Vilar, I. et al.) 33–42 (Springer, 2023).
Kandel, A. W. et al. The ROCEEH Out of Africa Database (ROAD): a large-scale research database serves as an indispensable tool for human evolutionary studies. PLoS ONE 18 , e0289513 (2023).
Bobe, R. & Wood, B. Estimating origination times from the early hominin fossil record. Evol. Anthropol. 31 , 92–102 (2022).
Kimbel, W. H. & Delezene, L. K. ‘Lucy’ redux: a review of research on Australopithecus afarensis . Am. J. Phys. Anthropol. 140 , 2–48 (2009).
Wood, B. & K. Boyle, E. Hominin taxic diversity: fact or fantasy? Am. J. Phys. Anthropol. 159 , 37–78 (2016).
van Holstein, L. A. & Foley, R. A. A process‐based approach to hominin taxonomy provides new perspectives on hominin speciation. Evol. Anthropol. 31 , 166–174 (2022).
Dembo, M. et al. The evolutionary relationships and age of Homo naledi : an assessment using dated Bayesian phylogenetic methods. J. Hum. Evol. 97 , 17–26 (2016).
Püschel, H. P., Bertrand, O. C., O’Reilly, J. E., Bobe, R. & Püschel, T. A. Divergence-time estimates for hominins provide insight into encephalization and body mass trends in human evolution. Nat. Ecol. Evol. 5 , 808–819 (2021).
Mongle, C. S., Strait, D. S. & Grine, F. E. Expanded character sampling underscores phylogenetic stability of Ardipithecus ramidus as a basal hominin. J. Hum. Evol. 131 , 28–39 (2019).
Jetz, W., Thomas, G. H., Joy, J. B., Hartmann, K. & Mooers, A. O. The global diversity of birds in space and time. Nature 491 , 444–448 (2012).
Upham, N. S., Esselstyn, J. A. & Jetz, W. Inferring the mammal tree: species-level sets of phylogenies for questions in ecology, evolution, and conservation. PLoS Biol. 17 , e3000494 (2019).
Revell, L. J. phytools: an R package for phylogenetic comparative biology (and other things). Methods Ecol. Evol. 3 , 217–223 (2012).
Pimiento, C. et al. Selective extinction against redundant species buffers functional diversity. Proc. R. Soc. B Biol. Sci. 287 , 20201162 (2020).
R Development Core Team. R: A Language and Environment for Statistical Computing (R Foundation for Statistical Computing, 2016).
Pinheiro, J., Bates, D., DebRoy, S., Sarkar, D. & R Core Team. nlme: linear and nonlinear mixed effects models, version 3.1 – 164 (R Foundation for Statistical Computing, 2020).
Freckleton, R. P., Phillimore, A. B. & Pagel, M. Relating traits to diversification: a simple test. Am. Nat. 172 , 102–115 (2008).
Harvey, M. G. & Rabosky, D. L. Continuous traits and speciation rates: alternatives to state-dependent diversification models. Methods Ecol. Evol. 9 , 984–993 (2018).
Etienne, R. S. et al. Diversity-dependence brings molecular phylogenies closer toagreement with the fossil record. Proc. Biol. Sci. 279 ,1300–1309 (2012).
van Holstein, L. A. Diversity-dependent speciation and extinction in hominins. figshare https://doi.org/10.6084/m9.figshare.24183417 (2024).
Download references
Acknowledgements
We thank J. Saers and M. Mirazón-Lahr for insightful discussion of this manuscript. This work was supported by a Clare College (University of Cambridge) Junior Research Fellowship (2022-2025) awarded to L.v.H.
Author information
Authors and affiliations.
Leverhulme Centre for Human Evolutionary Studies, Department of Archaeology, University of Cambridge, Cambridge, UK
Laura A. van Holstein & Robert A. Foley
You can also search for this author in PubMed Google Scholar
Contributions
L.v.H. conceived the study, collected data, performed analyses, interpreted results and wrote the manuscript. R.A.F. contributed to study conceptualization and interpretation of results and critically edited the manuscript.
Corresponding author
Correspondence to Laura A. van Holstein .
Ethics declarations
Competing interests.
The authors declare no competing interests.
Peer review
Peer review information.
Nature Ecology & Evolution thanks the anonymous reviewers for their contribution to the peer review of this work. Peer reviewer reports are available.
Additional information
Publisher’s note Springer Nature remains neutral with regard to jurisdictional claims in published maps and institutional affiliations.
Supplementary information
Supplementary information.
Supplementary Tables 1–3.
Reporting Summary
Peer review file, rights and permissions.
Open Access This article is licensed under a Creative Commons Attribution 4.0 International License, which permits use, sharing, adaptation, distribution and reproduction in any medium or format, as long as you give appropriate credit to the original author(s) and the source, provide a link to the Creative Commons licence, and indicate if changes were made. The images or other third party material in this article are included in the article’s Creative Commons licence, unless indicated otherwise in a credit line to the material. If material is not included in the article’s Creative Commons licence and your intended use is not permitted by statutory regulation or exceeds the permitted use, you will need to obtain permission directly from the copyright holder. To view a copy of this licence, visit http://creativecommons.org/licenses/by/4.0/ .
Reprints and permissions
About this article
Cite this article.
van Holstein, L.A., Foley, R.A. Diversity-dependent speciation and extinction in hominins. Nat Ecol Evol (2024). https://doi.org/10.1038/s41559-024-02390-z
Download citation
Received : 08 July 2022
Accepted : 08 March 2024
Published : 17 April 2024
DOI : https://doi.org/10.1038/s41559-024-02390-z
Share this article
Anyone you share the following link with will be able to read this content:
Sorry, a shareable link is not currently available for this article.
Provided by the Springer Nature SharedIt content-sharing initiative
Quick links
- Explore articles by subject
- Guide to authors
- Editorial policies
Sign up for the Nature Briefing newsletter — what matters in science, free to your inbox daily.

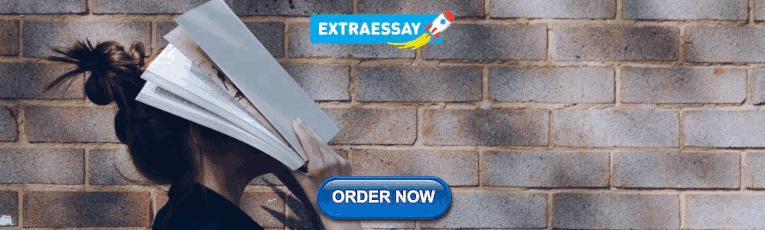
IMAGES
VIDEO
COMMENTS
climate change and it drives other changes in the climate, such as changes in. rainfall patterns and the frequency and distribution of weather events such as. droughts, storms, floods and heat ...
Abstract. Climate change is a long-lasting change in the weather arrays across tropics to polls. It is a global threat that has embarked on to put stress on various sectors. This study is aimed to conceptually engineer how climate variability is deteriorating the sustainability of diverse sectors worldwide.
This paper introduces a thematic issue dedicated to the interaction between climate change and the biosphere. ... explore the science and potential of a specific and somewhat unconventional but striking megafaunal approach to climate change mitigation: the introduction of grazing and browsing megafauna (horses, bison, cattle) to Arctic high ...
This paper describes an interdisciplinary research framework organized around three "Science Challenges" that directly respond to the needs of environmental protection organizations. ... Introduction. Climate change is a central concern of environmental protection because it poses substantial risks to societal interests in protecting—and ...
Keywords: Climate action, Climate change adaptation, Climate change mitigation, Research agenda Introduction Numerous publications in various scientific disciplines have confirmed climate change ...
CHAPTER ONE Introduction. T he idea that Earth's climate could abruptly change in a drastic manner has been around for several decades. Early studies of ice cores showed that very large changes in climate could happen in a matter of a few decades or even years, for example, local to regional temperature changes of a dozen degrees or more, doubling or halving of precipitation rates, and dust ...
C ONCLUSION. This document explains that there are well-understood physical mechanisms by which changes in the amounts of greenhouse gases cause climate changes. It discusses the evidence that the concentrations of these gases in the atmosphere have increased and are still increasing rapidly, that climate change is occurring, and that most of ...
Introduction 3 2. Climate change, drivers of risk, & challenges for sustainable development ... This argument derives from a review of recent progress in understanding climate impacts. The paper assesses recent meta-reviews of scientific literature including the 2012 Intergovernmental ... Research has documented evidence on the extent of human ...
1 Introduction The worldâ s climate is changing, and it will continue to change throughout the 21st century and beyond. Rising temperatures, new precipitation patterns, and other changes are already affecting many aspects of human society and the natural world. Climate change is transforming ecosystems at extraordinary rates and scales.
Meanwhile, climate researchers may wish to engage with the policy community and to increase the practical impact of their research. Nature Climate Change has published many papers that have been ...
Climate change is an urgent global challenge with long-term implications for the sustainable development of all countries. Exploring the Topic. Climate.gov. NOAA (National Oceanic and Atmospheric Administration) maintains this gateway to peer-reviewed information on climate change for various audiences, from the layperson to teachers to ...
This book outlines the impact of climate change in four developing country regions: Africa, Asia, Latin America and small island developing States; the vulnerability of these regions to future climate change; current adaptation plans, strategies and actions; and future adaptation options and needs.
Keywords: Climate change communication, climate change, systematic review, environmental communication, communication research trends 1. Introduction Earth's surface is at substantial risk of changes due to increased greenhouse gas emissions (Nolan et al, 2019) and in many places, climate change is already happening, seen
Certainties, uncertainties, probabilities. The natural greenhouse effect already warms us by 30°C. Man's activities increased CO2 & other greenhouse gases. More greenhouse gases will warm us further. The earth has warmed by about 0.7°C over last 100y. Most of the last 50y warming is likely due to Man's activities.
Climate change refers to a statistically defined change in the average and/or variability of the climate system, this includes the atmosphere, the water cycle, the land surface, ice and the living ...
This paper will discuss urban sustainability in the context of. climate change and address the following research objectives: (1) to examine how. climate change is affecting urban areas; (2) to assess how cities can enhance urban. sustainability by addressing climate change; (3) to discuss resources available for city.
Research Handbook on Communicating Climate Change. Communicating climate change in the twenty-first century has never been more pressing. The science is in, the solutions known, but the communication is not nearly adequate to the gravity of the issue, and current emissions pathways are rapidly hurtling towards a climate ever more dangerous for ...
Abstract. Research on heat waves (periods of excessively hot weather, which may be accompanied by high humidity) is a newly emerging research topic within the field of climate change research with high relevance for the whole of society. In this study, we analyzed the rapidly growing scientific literature dealing with heat waves.
Climate Explained, a part of Yale Climate Connections, is an essay collection that addresses an array of climate change questions and topics, including why it's cold outside if global warming is real, how we know that humans are responsible for global warming, and the relationship between climate change and national security.
Climate Explained is a collection of short primers that answer diverse climate change questions, including why it's cold outside if global warming is real, how we know that humans are responsible for global warming, and the relationship between climate change and national security. Image 1. Example Climate Explained essays on the Yale Climate ...
INTRODUCTION TO CLIMATE CHANGE. The word climatemeans the long-term weather patterns for a particular area. On Earth we have different climates depending on how far away you are from the equator and other factors like the movement of the ocean and the Earth's tilt. Regions closest to the equator tend to have very hot climates whereas regions ...
Arid zone ecosystems, integral to terrestrial systems, exhibit relatively low stability and are prone to influences from human activities and climate change. To elucidate the influence on the ecological environment of the arid zone by climate change and human activities, the paper takes normalized difference vegetation index (NDVI) as an evaluation index of the ecosystem and uses trend ...
When climate policy emerged in the 1990s, market-based ideas dominated the set of available policy solutions 1. This was reflected in the Kyoto Protocol's focus on market mechanisms and the rise ...
Climate change refers to long-term shifts in temperatures and weather patterns. Such shifts can be natural, due to changes in the sun's activity or large volcanic eruptions. But since the 1800s ...
This paper applies a combination of Forensic Disaster Analysis and Fuzzy Cognitive Mapping (FCM) to analyse the UK's response to the heatwaves of summer 2022. The authors interviewed 38 stakeholders from various sectors involved in the response to the heatwaves, developing cognitive maps to capture local knowledge and perceptions regarding ...
Writing in the journal Science, Prof Robert Nicholls of the Tyndall Centre for Climate Change Research at UEA and Prof Manoochehr ... and how they might change with time. The research paper ...
Within this group, research interest has primarily been devoted to examining the role of climate in shaping hominin diversification 2,3,4,5,6,7. What has received far less attention as a potential ...