Thank you for visiting nature.com. You are using a browser version with limited support for CSS. To obtain the best experience, we recommend you use a more up to date browser (or turn off compatibility mode in Internet Explorer). In the meantime, to ensure continued support, we are displaying the site without styles and JavaScript.
- View all journals
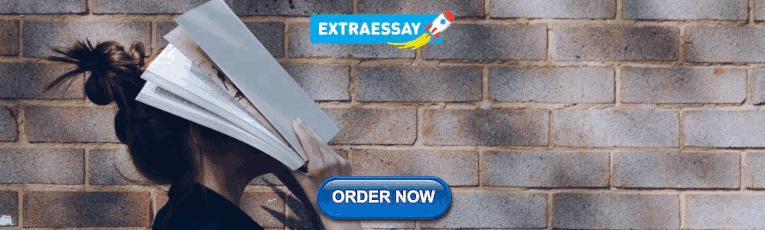
Nanoparticles articles from across Nature Portfolio
Nanoparticles are particles that exist on a nanometre scale (i.e., below 100 nm in at least one dimension). They can possess physical properties such as uniformity, conductance or special optical properties that make them desirable in materials science and biology.
Latest Research and Reviews
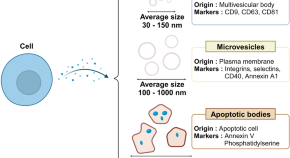
Recent advances in extracellular vesicles for therapeutic cargo delivery
- Jinbong Park
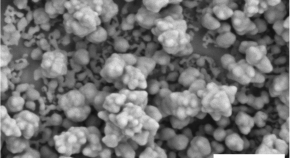
Quantification and description of photothermal heating effects in plasmon-assisted electrochemistry
Highly energetic charge carriers generated in plasmon-assisted electrocatalysis can increase reaction rates and impart novel selectivity trends, but a simple protocol to differentiate between thermal and nonthermal plasmonic contributions is lacking. Here, the authors use cyclic voltammetry and finite element simulations to show direct interband excitation of gold by visible light exclusively enhances current density via photothermal heating, while plasmon excitation leads to photothermal and nonthermal enhancements.
- Md. Al-Amin
- Johann V. Hemmer
- Andrew J. Wilson
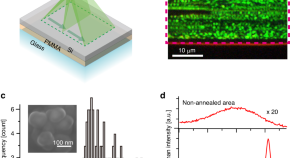
Fabrication of Mie-resonant silicon nanoparticles using laser annealing for surface-enhanced fluorescence spectroscopy
- Tatsuya Fukuta
- Taka-aki Yano
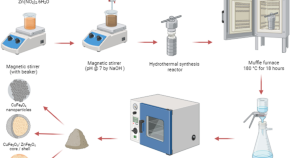
Enhancement of magnetization and optical properties of CuFe 2 O 4 /ZnFe 2 O 4 core/shell nanostructure
- A. M. Faramawy
- H. M. El-Sayed
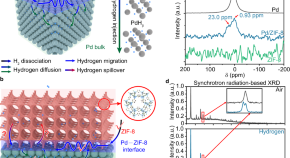
Spectroscopic visualization of reversible hydrogen spillover between palladium and metal–organic frameworks toward catalytic semihydrogenation
The authors report an in situ investigation of interfacial hydrogen spillover in Pd/ZIF-8 structures with X-ray and Raman techniques. A mechanistic picture of how Pd/ZIF-8 catalyst mediate alkyne semihydrogenation to alkynes is described.
- Yujie Xiong
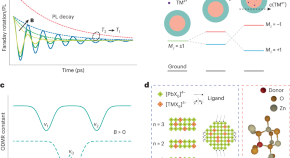
Engineering colloidal semiconductor nanocrystals for quantum information processing
This Review highlights the current potential for colloidal quantum dots for applications in quantum sensing and quantum circuits.
- Jawaher Almutlaq
- Edward H. Sargent
News and Comment
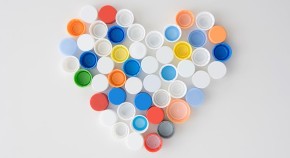
Microplastics at heart of the problem
Patients with carotid artery plaque that contain microplastics and nanoplastics were found to be at higher risk of cardiovascular events and mortality than those in whom the particles were not detected.
- Sonia Muliyil
It may all come down to the mechanisms of nanoparticle delivery
A long-standing nanoparticle delivery paradigm in cancer, that is, the enhanced permeability and retention effect, has been challenged, shifting the focus to active delivery mechanisms, which may provide a new mechanistic foundation for nanoparticle design.
Ultrabright near-infrared light
- Andries Meijerink
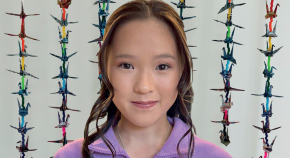
The potential for academics to inspire the next generation
Programmes to inspire the next generation of researchers can have a huge impact, as Rachel Lou explains in the recounting of her own experience.
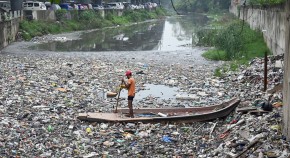
Landmark study links microplastics to serious health problems
People who had tiny plastic particles lodged in a key blood vessel were more likely to experience heart attack, stroke or death during a three-year study.
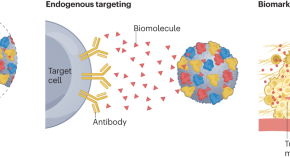
In vivo biomolecule corona and the transformation of a foe into an ally for nanomedicine
Nanoparticles (NPs) administered in the human body will undergo rapid surface modification upon contact with biological fluids driven by their interfacial interaction with a diverse range of biomolecules. Such spontaneous self-assembly and adsorption of proteins and other biomolecules onto the NP surface constitute what is commonly known as the protein or biomolecule corona. This surface biotransformation of the NPs modulates their biological interactions and impact on physiological systems and can influence their overall pharmacological profile. Here, we comment on how the initially considered ‘nuisance’ of the in vivo corona formation can now be considered a nanoparticle engineering tool for biomedical use, such as in endogenous tissue targeting, personalized biomarker discovery and immunomodulation.
- Marilena Hadjidemetriou
- Morteza Mahmoudi
- Kostas Kostarelos
Quick links
- Explore articles by subject
- Guide to authors
- Editorial policies

Journal of Nanoparticle Research
An Interdisciplinary Forum for Nanoscale Science and Technology
- Covered topics include synthesis, assembly, transport, reactivity, and stability of nanoscale structures.
- Features applications, structures, and devices with novel functions via precursor nanoparticles.
- Fosters interdisciplinary dissemination of knowledge across multiple disciplines
- Offers free online Perspective articles evaluating research, industrial and societal trends in nanotechnology.
- High author satisfaction with 96% of surveyed authors indicating they would publish again in the journal.
- Mihail C. Roco
- Nicola Pinna
Latest articles
Antibiofilm activity of silver nanoparticles-halloysite nanocomposite in serratia marcescens.
- Yuliya Cherednichenko
- Svetlana Batasheva
- Elvira Rozhina
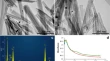
Carbon quantum dots derived from marine Eucheuma denticulatum for the efficient and sensitive detection of tetracycline
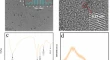
Defect-engineered Ag/ZnO and Ag 2 O/ZnO nanomaterials prepared with nanoparticles synthesized by a sustainable sol–gel method and their biological responses
- Mário R. P. da Silva
- Robert S. Matos
- Luis E. Almeida
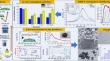
Novel pH-sensitive triptolide-loaded micelles: a potential approach to increase anti-tumor activity of the diterpenoid epoxide
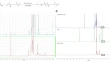
Risk assessment of occupational exposure to engineered and incidental nanomaterials: differences and challenges
- Soqrat Omari Shekaftik
- Neda Mehrparvar
- Seyed Jamaledin Shahtaheri
Journal updates
Call for papers: nanomanufacturing.
The twenty-first century has ushered in the era of nanomanufacturing.
Topical Collections in the Journal of Nanoparticle Research
Read curated collections of articles highlighting a certain topic or event.
Journal of Nanoparticle Research's Gallery of Covers
As of January 2022, each issue of the Journal of Nanoparticle Research features a visually striking figure or image on its cover. To further highlight this, we will be collecting them here.
Journal information
- Astrophysics Data System (ADS)
- Chemical Abstracts Service (CAS)
- Current Contents/Physical, Chemical and Earth Sciences
- EI Compendex
- Google Scholar
- INIS Atomindex
- Japanese Science and Technology Agency (JST)
- OCLC WorldCat Discovery Service
- Science Citation Index Expanded (SCIE)
- Semantic Scholar
- TD Net Discovery Service
- UGC-CARE List (India)
Rights and permissions
Springer policies
© Springer Nature B.V.
- Find a journal
- Publish with us
- Track your research
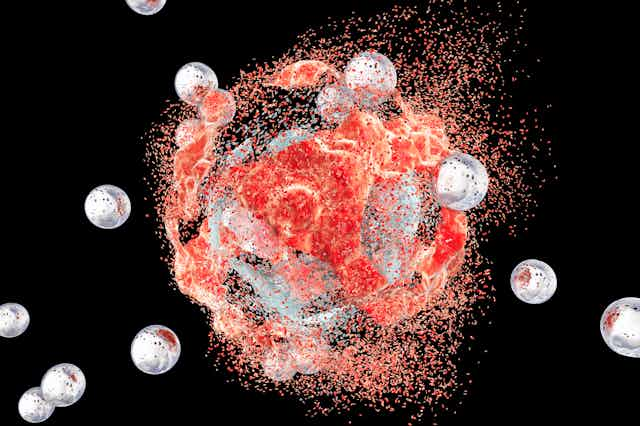
Nanoparticles are the future of medicine – researchers are experimenting with new ways to design tiny particle treatments for cancer
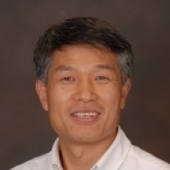
Professor of Pharmaceutical Sciences, University of Michigan
Disclosure statement
Duxin Sun receives funding from NIH, FDA and Pharmaceutical Industries for his lab research at The University of Michigan.
University of Michigan provides funding as a founding partner of The Conversation US.
View all partners
When you hear the word “nanomedicine,” it might call to mind scenarios like those in the 1966 movie “ Fantastic Voyage .” The film portrays a medical team shrunken down to ride a microscopic robotic ship through a man’s body to clear a blood clot in his brain.
Nanomedicine has not reached that level of sophistication yet. Although scientists can generate nanomaterials smaller then several nanometers – the “nano” indicating one-billionth of a meter – today’s nanotechnology has not been able to generate functional electronic robotics tiny enough to inject safely into the bloodstream. But since the concept of nanotechnology was first introduced in the 1970s, it has made its mark in many everyday products, including electronics, fabrics, food, water and air treatment processes, cosmetics and drugs. Given these successes across different fields, many medical researchers were eager to use nanotechnology to diagnose and treat disease.
I am a pharmaceutical scientist who was inspired by the promise of nanomedicine. My lab has worked on developing cancer treatments using nanomaterials over the past 20 years. While nanomedicine has seen many successes, some researchers like me have been disappointed by its underwhelming overall performance in cancer. To better translate success in the lab to treatments in the clinic, we proposed a new way to design cancer drugs using nanomaterials. Using this strategy, we developed a treatment that was able to achieve full remission in mice with metastatic breast cancer.
What is nanomedicine?
Nanomedicine refers to the use of materials at the nanoscale to diagnose and treat disease. Some researchers define nanomedicine as encompassing any medical products using nanomaterials smaller than 1,000 nanometers. Others more narrowly use the term to refer to injectable drugs using nanoparticles smaller than 200 nanometers. Anything larger may not be safe to inject into the bloodstream.
Several nanomaterials have been successfully used in vaccines. The most well-known examples today are the Pfizer-BioNTech and Moderna COVID-19 mRNA vaccines . These vaccines used a nanoparticle made of of lipids, or fatty acids, that helps carry the mRNA to where it needs to go in the body to trigger an immune response.
Researchers have also successfully used nanomaterials in diagnostics and medical imaging. Rapid COVID-19 tests and pregnancy tests use gold nanoparticles to form the colored band that designates a positive result. Magnetic resonance imaging, or MRI , often uses nanoparticles as contrast agents that help make an image more visible.
Several nanoparticle-based drugs have been approved for cancer treatment. Doxil (doxorubicin) and Abraxane (paclitaxel) are chemotherapy drugs that use nanomaterials as a delivery mechanism to improve treatment efficacy and reduce side effects.
Cancer and nanomedicine
The potential of nanomedicine to improve a drug’s effectiveness and reduce its toxicity is attractive for cancer researchers working with anti-cancer drugs that often have strong side effects. Indeed, 65% of clinical trials using nanoparticles are focused on cancer.
The idea is that nanoparticle cancer drugs could act like biological missiles that destroy tumors while minimizing damage to healthy organs. Because tumors have leaky blood vessels, researchers believe this would allow nanoparticles to accumulate in tumors . Conversely, because nanoparticles can circulate in the bloodstream longer than traditional cancer treatments, they could accumulate less in healthy organs and reduce toxicity.
Although these design strategies have been successful in mouse models, most nanoparticle cancer drugs have not been shown to be more effective than other cancer drugs. Furthermore, while some nanoparticle-based drugs can reduce toxicity to certain organs, they may increase toxicity in others. For example, while the nanoparticle-based Doxil decreases damage to the heart compared with other chemotherapy options, it can increase the risk of developing hand-foot syndrome .
Improving nanoparticle-based cancer drugs
To investigate ways to improve how nanoparticle-based cancer drugs are designed, my research team and I examined how well five approved nanoparticle-based cancer drugs accumulate in tumors and avoid healthy cells compared with the same cancer drugs without nanoparticles. Based on the findings of our lab study, we proposed that designing nanoparticles to be more specific to their intended target could improve their translation from animal models to people. This includes creating nanoparticles that address the shortcomings of a particular drug – such as common side effects – and home in on the types of cells they should be targeting in each particular cancer type.
[ Get fascinating science, health and technology news. Sign up for The Conversation’s weekly science newsletter .]
Using these criteria, we designed a nanoparticle-based immunotherapy for metastatic breast cancer. We first identified that breast cancer has a type of immune cell that suppresses immune response, helping the cancer become resistant to treatments that stimulate the immune system to attack tumors. We hypothesized that while drugs could overcome this resistance, they are unable to sufficiently accumulate in these cells to succeed. So we designed nanoparticles made of a common protein called albumin that could deliver cancer drugs directly to where these immune-suppressing cells are located.
When we tested our nanoparticle-based treatment on mice genetically modified to have breast cancer, we were able to eliminate the tumor and achieve complete remission. All of the mice were still alive 200 days after birth. We’re hopeful it will eventually translate from animal models to cancer patients.
Nanomedicine’s bright but realistic future
The success of some drugs that use nanoparticles, such as the COVID-19 mRNA vaccines , has prompted excitement among researchers and the public about their potential use in treating various other diseases, including talks about a future cancer vaccine . However, a vaccine for an infectious disease is not the same as a vaccine for cancer. Cancer vaccines may require different strategies to overcome treatment resistance. Injecting a nanoparticle-based vaccine into the bloodstream also has different design challenges than injecting into muscle.
While the field of nanomedicine has made good progress in getting drugs or diagnostics out of the lab and into the clinic, it still has a long road ahead. Learning from past successes and failures can help researchers develop breakthroughs that allow nanomedicine to live up to its promise.
- Nanotechnology
- Nanoparticles
- Drug design
- Cancer treatment
- Nanomedicine
- Nanomaterials
- Translational science
- COVID-19 vaccines
- mRNA vaccines

Lecturer (Hindi-Urdu)

Initiative Tech Lead, Digital Products COE

Director, Defence and Security

Opportunities with the new CIEHF

School of Social Sciences – Public Policy and International Relations opportunities
- Open access
- Published: 03 June 2023
Human and environmental impacts of nanoparticles: a scoping review of the current literature
- Elizabeth Adjoa Kumah 1 ,
- Raoul Djou Fopa 2 ,
- Saeed Harati 2 ,
- Paul Boadu 3 ,
- Fatemeh Vida Zohoori 4 &
- Tannaz Pak 2
BMC Public Health volume 23 , Article number: 1059 ( 2023 ) Cite this article
14k Accesses
31 Citations
5 Altmetric
Metrics details
Use of nanoparticles have established benefits in a wide range of applications, however, the effects of exposure to nanoparticles on health and the environmental risks associated with the production and use of nanoparticles are less well-established. The present study addresses this gap in knowledge by examining, through a scoping review of the current literature, the effects of nanoparticles on human health and the environment. We searched relevant databases including Medline, Web of Science, ScienceDirect, Scopus, CINAHL, Embase, and SAGE journals, as well as Google, Google Scholar, and grey literature from June 2021 to July 2021. After removing duplicate articles, the title and abstracts of 1495 articles were first screened followed by the full-texts of 249 studies, and this resulted in the inclusion of 117 studies in the presented review.
In this contribution we conclude that while nanoparticles offer distinct benefits in a range of applications, they pose significant threats to humans and the environment. Using several biological models and biomarkers, the included studies revealed the toxic effects of nanoparticles (mainly zinc oxide, silicon dioxide, titanium dioxide, silver, and carbon nanotubes) to include cell death, production of oxidative stress, DNA damage, apoptosis, and induction of inflammatory responses. Most of the included studies (65.81%) investigated inorganic-based nanoparticles. In terms of biomarkers, most studies (76.9%) used immortalised cell lines, whiles 18.8% used primary cells as the biomarker for assessing human health effect of nanoparticles. Biomarkers that were used for assessing environmental impact of nanoparticles included soil samples and soybean seeds, zebrafish larvae, fish, and Daphnia magna neonates.
From the studies included in this work the United States recorded the highest number of publications ( n = 30, 25.64%), followed by China, India, and Saudi Arabia recording the same number of publications ( n = 8 each), with 95.75% of the studies published from the year 2009. The majority of the included studies (93.16%) assessed impact of nanoparticles on human health, and 95.7% used experimental study design. This shows a clear gap exists in examining the impact of nanoparticles on the environment.
• While nanoparticles are beneficial in a range of applications, they pose significant threats to humans and the environment.
• Immortalised cell lines are mostly used as biomarkers to assess human health effect of nanoparticles.
• Biomarkers such as soil samples and zebrafish larvae are used to investigate the environmental effect of nanoparticles.
• This work has revealed the toxic effects of nanoparticles to include production of oxidative stress, DNA damage, apoptosis, cell death, and induction of inflammatory responses.
Peer Review reports
Introduction
Importance and meaning.
Nanoparticles are small particles ranging from 1 to 100 nm (nm) in size [ 1 ]. They are used in a wide range of applications and can be grouped into four types: 1) inorganic-based nanoparticles, 2) carbon-based nanoparticles, 3) organic/polymer nanoparticles, and 4) composite-based nanoparticles [ 2 ]. Inorganic-based nanoparticles are made up of different metal and metal oxides. Examples of metal-based inorganic nanoparticles include aluminium, silver, gold, zinc, lead, iron, cadmium, and copper, whereas examples of metal oxide-based inorganic nanoparticles include aluminium oxide, copper oxide, iron oxide, silica, zinc oxide, titanium oxide, and magnesium aluminium oxide. Carbon-based nanoparticles include fullerene, graphene, multi- and single-walled carbon nanotubes, carbon black, and carbon fibres. Organic-based nanoparticles are derived from organic materials without carbon, for example, liposome, dendrimers, cyclodextrin, and micelle, whereas composite nanoparticles are made from combinations of metal oxide-based, metal-based, organic-based, and/or carbon-based nanoparticles.
In recent years, nanoparticles have gained increasing attention due to their use in consumer products, medicine, soil, and aquatic environments. For example, nanoparticles have been used for textiles [ 3 ], water treatment [ 4 ], environmental remediation [ 5 , 6 , 7 ], cancer therapy [ 8 ], radiology [ 9 ], and cosmetics [ 10 ]. This growing attention and extensive usage of nanoparticles is due to specific novel characteristics exhibited by such particles, which results from their small size and large surface area [ 11 ]. These unique qualities, while advantageous, pose certain risks to living organisms.
The harmful effects of nanoparticles
The small sizes of nanoparticles give them the ability to permeate physiological barriers of living organisms, causing harmful biological reactions. Nanoparticles are known to enter the human body through the lung, intestinal tract, or skin, and can be toxic to the brain, cause lung inflammation and cardiac problems [ 12 ]. In fact, certain nanoparticles have been found to cause permanent cell damage through organ injury and oxidative stress, due to their size and composition. In a study by Magrez et al. [ 13 ] to assess the toxic effect of carbon-based nanoparticles on lung cancer cells, the authors reported findings suggesting that carbon-based nanoparticles cause size-dependent cytotoxicity. The level of toxicity of nanoparticles is suggested to be dependent on factors such as composition of the nanoparticle, size, surface functionality, crystallinity, and aggregation [ 14 ]. Moreover, the toxicity of a nanoparticle in an individual is dependent on the genetic make-up of that individual, which is determined by the individual's ability to adapt and respond to toxic substances.
The gaps of previous studies
There are growing concerns regarding the toxicologic effects of nanoparticles, and frequent exposure to nanoparticles is regarded as a public health threat [ 15 ]. While there is extensive evidence about the benefits of nanoparticles, as well as the potential health and environmental risks associated with its production and use, current understanding of the impact of nanoparticles exposure to human health and the environment is limited. The current review seeks to explore, through a scoping review of the current literature, the effects of nanoparticles on human health and the environment. This review is unique as it adopts a systematic scoping approach to explore the current literature on the health risks posed by the manufacture, distribution, and use of nanoparticles. Published studies in this area have mainly used a narrative literature review approach [ 2 , 16 , 17 ].
Objective and research questions
The objective of this review is to map the distribution of the current literature on the human and environmental impacts of nanotoxicity. Specifically, this scoping review will be guided by the following research questions:
What is the relative distribution of the current literature on the human and environmental impact of nanotoxicity?
Which exposure pathways and nanoparticles have been researched and which have not?
What biomarkers have been used in assessing the human and environmental impact of exposure to nanoparticles?
This scoping review was conducted and reported in accordance with the Joanna Briggs Institute Reviewers Manual [ 18 ]. The following steps were followed:
Defining and aligning the objectives and research questions
Developing and aligning the inclusion criteria with the objectives and research questions
Describing the planned approach to evidence searching and selection
Searching for the evidence
Extracting the evidence
Charting the evidence
Summarising the evidence in relation to the objectives and research questions
The Preferred Reporting of Items for Systematic Reviews and Meta-Analysis (PRISMA) statement was used to summarise the screening process. The protocol of this review has been registered with the Open Science Framework [ 19 ].
Search strategy
The aim of the search strategy was to find both published and unpublished studies that have examined the effect of nanotoxicity on human health and the environment. Search terms consisted of a combination of key terms and concepts in the objective and research questions, using the Boolean operators, 'AND', and 'OR' as follows:
(nanomaterials OR nanoparticles OR nanostructures) AND (toxicity OR health) AND (“biomarker* of exposure” OR biomarker OR exposure) AND (human OR environment).
The search was limited to peer-reviewed articles published from the year 2000. This was to enable us to study the current literature (research conducted over the last 2 decades). The search was limited to primary studies published in the English language due to difficulties with language translation.
Table 1 below presents a list of the databases, grey literature, and search engines that were searched for eligible papers. The reference list of all included papers was also searched for additional papers on the subject matter.
For the database searches, a master search strategy was first developed using the Medline database, this was then modified for the other databases. The supplementary material file presents the Medline search history. The literature search was conducted between 1 st June 2021 and 31 st July 2021.
Reference management
All search results were imported into an Endnote library to help manage references and to remove duplicate articles. Once duplicates were removed, the search results were exported from Endnote into Covidence (a web-based software platform that streamlines the production of scoping/systematic reviews) for screening. The Covidence software was also useful in identifying and deduplicating articles that could not be identified by Endnote.
Selection criteria
The following criteria were used to identify eligible articles for inclusion in the review.
Inclusion criteria
Types of participants.
Studies that have assessed the human and environmental impacts of nanotoxicity were considered for inclusion in this review. Human participants included children and/or adults of any age, gender, or ethnicity. Studies involving the use of animals as biomarkers for assessing the environmental impact of nanotoxicity were also considered for inclusion.
Studies that have examined the impacts of nanotoxicity as well as the biomarkers for assessing exposure to nanoparticles were eligible for inclusion in this review. While all types of nanoparticles were considered for inclusion, attention was given to studies involving metallic (oxides, pure metal) and carbonaceous (fullerenes, carbon nanotubes, and graphene) nanoparticles. This is mainly due to these particles being widely produced and used [ 20 ], therefore, they are considered the most relevant for public health.
Studies from any geographical location aimed at assessing the human and/or environmental impact of nanotoxicity were considered eligible for inclusion. Studies whose full texts were in a language other than English were excluded because there were no available translators.
Study types
We included all original primary research (both quantitative and qualitative), including, but not limited to randomised controlled studies, quasi-experimental studies, surveys, retrospective and prospective cohort studies, case studies, and phenomenological studies.
Exclusion criteria
The following exclusion criteria were applied to the title and abstract, as well as the full-text review stage:
Irrelevant problem/focus: studies that have not examined the human and/or environmental impact of nanotoxicity, or the biomarkers for assessing exposure to nanoparticles
Irrelevant type of study: review reports or studies that did not contain any original research
Selection of studies
We employed a two-step screening process to assess search results for eligible studies. The first level involved screening of the titles and abstracts and was done independently by two reviewers (EK and RF). The next step was carried out independently by three reviewers (EK, RF, and SH) and involved screening of the full-texts of potentially eligible papers. Disagreements between reviewers were resolved through discussions and consensus. Where disagreements persisted, a third reviewer (TP or FVZ) was consulted.
Data charting
We developed a standardised data extraction form in the Covidence software for data extraction. The form was designed to collect the following information from included studies: year of publication, aim/objective of study, study design, country, type of nanoparticle, application of the nanoparticle, major exposure route(s), biomarker/model used, how biomarker was obtained, and study outcome(s).
The developed data extraction form was pilot-tested using 10% of the included articles before beginning the actual data extraction. Data extraction was done by one reviewer (PB, RF, or SH) and verified by another (EK, TP, or FVZ), using the Covidence software.
Data synthesis
The extracted data was first exported into Excel for editing and to check for accuracy. The edited data was then exported from Excel into SPSS (version 26) to aid with data synthesis. Descriptive statistics was used to report included studies by their characteristics and outcome measures, described below.
Characteristics of included studies
Year of publication: studies were grouped based on their year of publication . As stated earlier, this included studies published from the year 2000 to July 2021 (the date of completion of literature searches).
Country in which study was conducted : to assess the distribution of the current literature on human and environmental impact of nanotoxicity, the countries in which eligible studies were conducted were classified into six regions based on the World Bank’s classification of countries. This included: East Asia and Pacific, Europe and Central Asia, Latin America and Caribbean, Middle East and North Africa, North America, South Asia, and Sub-Saharan Africa (World Bank Group, 2018).
Study design : randomised controlled trial, non-randomised controlled trial, cohort study, experimental study, case control study, longitudinal study, uncontrolled before and after studies.
Impact/effect assessed : human health and/or environment
Outcome measures
Type of nanoparticle : This was divided into four groups: 1) inorganic-based nanoparticles, 2) carbon-based nanoparticles, 3) organic nanoparticles, and 4) composite-based nanoparticles two groups, metallic (oxides, pure metal) and carbonaceous (fullerenes and carbon nanotubes) particles.
Biomarker or model used in assessing human and/or environmental exposure : primary cell or immortalised cell line
Effect/impact on human health and/or the environment
A narrative synthesis was then used to further explore findings.
Search results
The database searches resulted in 1553 papers (presented in Fig. 1 ): Medline ( n = 1,381); ScienceDirect ( n = 0); Sage Journals Online ( n = 50); Campbell Collaboration ( n = 0); Cochrane Collaboration ( n = 0); Embase ( n = 5); Scopus ( n = 6); Web of Science ( n = 50); CINAHL ( n = 61). Google and Google Scholar searches yielded 100 results, and no article was obtained from grey literature searches. Following removal of duplicate articles, the titles and abstracts of 1495 articles were screened to assess their eligibility for inclusion, which resulted in the exclusion of a total of 1246 articles as they did not meet the inclusion criteria. As such, the full texts of 249 articles were assessed for eligibility. Following this stage, a total of 132 articles were excluded for several reasons (see Fig. 1 ), whereas 117 studies qualified for inclusion in the review.
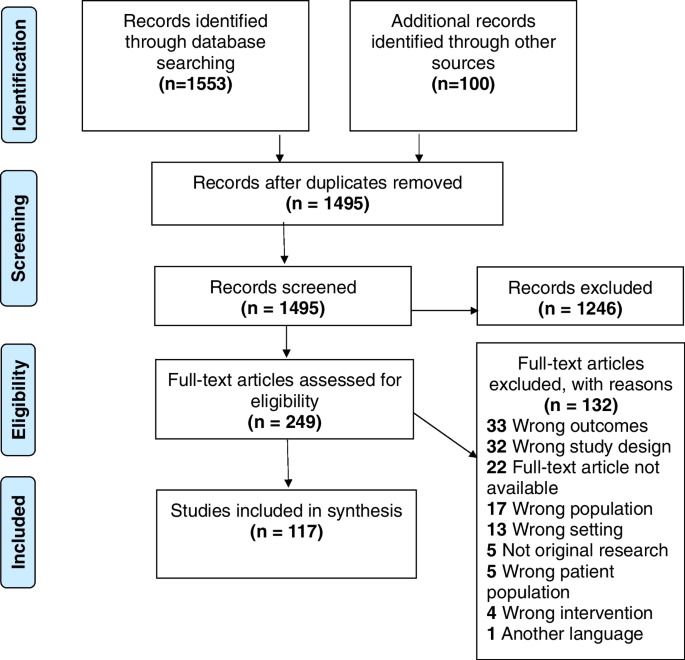
Study flow diagram (adapted from Moher et al., 2009)
The studies included in this review originated from 23 countries across several continents, with the majority of the studies originating from Europe and Central Asia ( n = 50). Nevertheless, the United Sates recorded the highest number of publications ( n = 30), followed by China, India, and Saudi Arabia recording the same number of publications ( n = 8). The lowest number of studies ( n = 1 each) originated from Argentina, Czech Republic, Egypt, Mexico, Pakistan, Poland, and Russia. There were no studies recorded from Sub-Saharan Africa. Figure 2 presents a classification of the included studies by region.
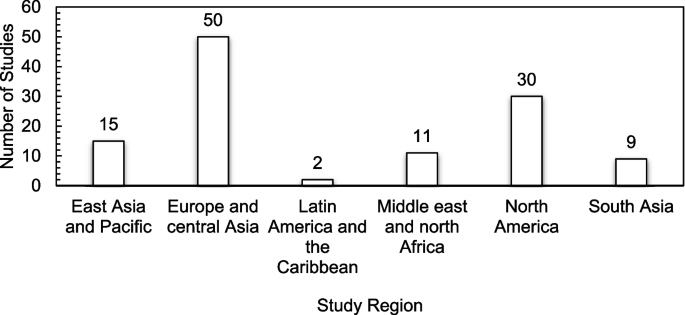
Classification of Studies by Region
Included studies were published between the year 2006 and 2021, with a high proportion of the articles (95.75%) published from the year 2009. However, the year 2020 recorded the highest number of publications ( n = 15; 12.82%), followed by 2016 ( n = 14; 11.97%). Table 2 below presents the number of publications per year.
The majority of the studies used an experimental study design ( n = 112, 95.7%), with only 5 (4.3%) studies employing a cross-sectional design. Regarding the type of impact/effect of nanoparticle assessed, a vast majority of the studies assessed impact on human health ( n = 109), 5 of the studies assessed effects on the environment, with only 3 studies assessing both human and environmental health impact (Fig. 3 ).
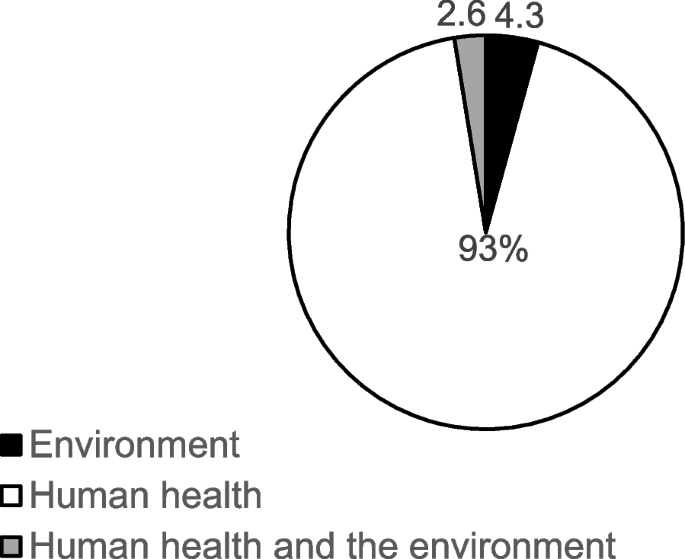
Effect/impact of nanoparticles on human/environmental health
Just over 65% ( n = 77) of the included studies investigated the human and/or environmental effect of inorganic-based nanoparticles. The inorganic-based nanoparticles that were investigated include, but not limited to, bismuth oxide (Bi 2 O 3 ), silicon dioxide (SiO 2 ), copper oxide (CuO), zinc oxide (ZnO), titanium dioxide (TiO 2 ), silver (Ag), gold (Au), platinum (Pt), iron oxide (Fe 2 O 3 ), cerium oxide (CeO 2 ), cobalt oxide (Co 3 O 4 ), aluminium oxide (Al 2 O 3 ), molybdenum trioxide (MoO3), magnesium oxide (MgO), nickel oxide (NiO), chromium oxide (Cr 2 O 3 ), tungsten oxide (WO 3 ), yttrium oxide (Y 2 O 3 ), and manganese oxide (Mn 2 O 3 ).
Thirty-five (29.9%) studies reported on carbon-based nanoparticles (including single and multi-walled carbon nanotubes (SWCNTs/MWCNTs), graphene oxide (GO), and graphene nanoplatelets, GNP). Three studies [ 21 , 22 , 23 ] reported on both inorganic- and carbon-based nanoparticles; one study [ 24 ] reported on both inorganic-based and polymer nanoparticles (i.e., Titanium dioxide, terbium-doped gadolinium, and polylactic-co-glycolic acid, PLGA), whereas another study [ 25 ] investigated the effect of Poly lactic-co-glycolic acid (a polymer) nanoparticle on the environment.
The most investigated nanoparticles were ZnO ( n = 25), followed by MWCNTs ( n = 20), TiO 2 ( n = 16), CeO ( n = 15), SWCNTs and Fe 2 O 3 ( n = 14), and SiO 2 ( n = 12). The least studied nanoparticles include Pt, Au, MgO, MoO 3 , WO 3 , Carbon Black (CB), and GNP with only one report available.
A significant number ( n = 90, 76.9%) of the included studies used immortalised cell lines as the biomarker for assessing the human health effect of nanoparticles. Examples of the immortalised cell lines that were used include the human hepatocarcinoma cell line (HepG2), human (alveolar) epithelial A549 cell line with human monocyte-derived dendritic cells (MDDCs) and macrophages (MDMs), Melanoma cells and human foreskin fibroblasts, human airway epithelial (BEAS-2B) cells, human bronchial epithelium (BEAS-2B) cells, human neuroblastoma SHSY5Y cell line, human keratinocyte (HaCaT) cell line, and MCF-7 cell line, which is a human breast cancer cell line with oestrogen, progesterone and glucocorticoid receptors. Immortalised cell lines were mostly purchased/obtained from organisations such as the American Type Culture Collection (ATCC, Manassas, VA, USA).
Twenty-two studies used primary cells obtained from study participants/volunteers. Examples of the primary cells that were used as biomarkers by included studies are human bone marrow mesenchymal stem cells (hBMMSCs) taken from the iliac crest of human donors, human lymphocytes (blood), and human dermal fibroblasts which were isolated by the outgrowth method using infant foreskins obtained after circumcision. Workplace air samples have also been used to investigate workplace exposures to graphene nanoplatelets [ 26 ]. Five studies [ 25 , 27 , 28 , 29 , 30 ] that reported on the environmental effect of nanoparticles used a variety of biomarkers, including soil samples and soybean seeds, Allium cepa bulbs, zebrafish larvae, seedlings of buckwheat, Nitrosomonas europaea KCTC 12270 bacterium (an ammonia-oxidizing bacterium) and Nitrospira moscoviensis (a nitrite-oxidizing bacterium), as well as aquatic species including Daphnia magna neonates, fish, and Carp (Cyprius carpio). The studies included in this review reported several toxicities associated with the production and application of nanoparticles. The most reported health impact of nanoparticles was found to be decreased cell viability and/or cell death (observed by twenty-nine studies). Twenty-eight studies also noted reactive oxygen species generation as a result of exposure to nanoparticles, especially to CNT ( n = 7), ZnO ( n = 7), SiO 2 ( n = 5), and TiO 2 ( n = 4). The third commonly observed health impact was dose-dependent oxidative stress in the biomarkers ( n = 25), particularly, in cases of exposure to SiO 2 ( n = 5), ZnO ( n = 5), Fe 3 O 4 ( n = 4), CeO 2 ( n = 3), and CuO ( n = 3). In addition, there were sixteen reports regarding DNA damage after exposure to nanoparticles, mainly for ZnO ( n = 4) and MWCNTs ( n = 3). Table 3 presents a comprehensive outline of the effects (human health and the environment) reported by each of the included studies. These are further explored in the ensuing section.
The objective of this scoping review was to ascertain the distribution of the current literature on the human and environmental impacts of nanoparticles. Specifically, in this review, we synthesised evidence regarding the exposure pathways and types of nanoparticles that have been researched and the ones that have not, as well as the biomarkers that have been used in assessing human and environmental impact of exposure to nanoparticles.
While the majority of studies originated from Europe and Central Asia, the United States of America (USA) alone recorded the highest number of publications. This finding is not surprising, as the USA has continuously fostered the development of nanotechnology through significant investments in research and development in this area. In 2016, the USA was projected to account for almost one-third of total global nanotechnology research funding [ 136 ]. Moreover, the USA and the European Union have over the years taken a committed approach towards enhancing the health and safety of nanoparticles [ 137 ]. As part of this commitment, annual meetings are held, where researchers discuss topics relating to nano-safety, as well as funding priorities and research needs.
While there have been some investments in nanotechnology research in African countries (including Egypt and South Africa), a recent publication by the United Nations Economic Commission for Africa (UNECA) indicates that the African continent, relative to other continents, is lagging behind with regards to nanotechnology research [ 138 ]. This assertion is consistent with the findings of this review, which found only one study originating from North Africa (Egypt), with no study conducted in Sub-Saharan Africa.
Over the past two decades, there have been increasing public awareness of nanotechnology and a growing concern about its commercial applications [ 139 ]. This has led to rapidly increasing scientific publications in this field, especially from early 2000s [ 140 ]. It is, therefore, not surprising that the studies included in this scoping review were published from the year 2006. Indeed, a literature search of nanotechnology publications by Huang et al. [ 140 ] revealed over 50,000 publications for the year 2006.
Although the included studies investigated a wide range of nanoparticles, most of them focused on inorganic-based nanoparticles (e.g., zinc oxide, titanium dioxide, copper oxide, and silica), followed by carbon-based nanoparticles (e.g., carbon-nanotubes, fullerenes, and graphene) (Table 3 ). This finding is consistent with previous reviews that have reported extensive investigation into the impact of inorganic-based and/or carbon-based nanoparticles [ 141 , 142 ]. These nanoparticles may have gained attention due to their extensive production and usage. In addition to their use for cancer treatment, inorganic and carbon-based nanoparticles provide significant benefits in photothermal therapy, diagnosis, tissue engineering, imaging contrast agents, and sensing applications [ 143 ]. This is due to their unique physical and chemical properties (such as electrical, thermal, structural, mechanical, and optical diversity), which make them stronger, flexible, and more electrically conductible towards several biological entities [ 141 , 144 ]. The advantages of, for example inorganic-based nanoparticles, including their high reactivity, small size and good capacity have been found to induce adverse harmful effects in both humans and the environment.
In this review, a number of approaches were used by included studies to assess the toxicity of nanoparticles. However, the majority of the studies applied the in vitro method, perhaps because in vitro studies are time saving and cost-effective. Nonetheless, the in vitro approach has been criticised by researchers (e.g., Bahadar et al. [ 145 ]) for producing varying results in different laboratories.
The included studies used differing methods in assessing cytotoxicity and genotoxicity: cell membrane integrity was assessed with Lactate dehydrogenase (LDH) assays [ 44 , 57 , 116 ]; cell viability was assessed using tetrazolium reduction assays [ 82 , 83 , 90 , 116 ]; apoptosis was assessed using immunohistochemistry biomarkers [ 60 , 65 , 86 ]; electron microscopy was used to assess intracellular localisation of nanoparticles [ 34 , 106 ]; and cell inflammation was estimated using chemokines biomarkers (i.e., IL-8, TNF- α, and IL-6) [ 146 ]. Compounds such as MTT, XTT, MTS, and WST-1 are used to detect viable cells [ 147 ]. However, in the current review, most of the studies employed MTT tetrazolium assays for investigating cell toxicity [ 47 , 49 , 50 , 58 , 116 ]. Similar findings have been reported by Bahadar et al. [ 145 ] who conducted a review on the toxicity of nanoparticles.
The human impact of nanoparticles
Most of the studies in this review focused on assessing the characteristics of nanoparticles, as well as the impact of nanoparticles on, particularly, human health. In recent years, there have been promising results from the application of nanoparticles to human health, especially in cancer treatment. This is due to the potential of nanoparticles to provide innovative solutions to curb the limitations of traditional treatment methods, including radiotherapy and chemotherapy [ 148 ]. Relative to conventional cancer treatment methods, nanoparticle-based drug delivery systems have been shown to have significant advantages in a) drug resistance, b) correctly targeting tumour cells, c) having good pharmacokinetics, and d) reduction of treatment side effects [ 149 ]. Notwithstanding these benefits, however, nanoparticles have potential harmful effects, and there are controversies about their safe use in humans [ 139 ]. This has undoubtedly led to the rapidly growing number of studies investigating the human health impact of nanoparticles, as was revealed in this review.
The majority of the studies (n = 90) in this review used immortalised cell lines as the biomarker for assessing human health impact of nanoparticles, and only 22 studies used primary cells as biomarkers. Immortalised cell lines have mostly been used for nano-safety studies because, relative to primary cells, they are generally less expensive, readily accessible, and easier to cultivate [ 150 ]. However, the type of cell that is used as biomarker for nano-safety studies is of great importance since this may have an impact on the general outcome of studies [ 151 ]. Cancer cell lines, for example, have a disturbed anti-apoptotic balance, and have undergone transformation in metabolism, which impacts their ability to sustain their high rate of proliferation [ 152 ]. As such, using these cells may have an impact on study findings. Nonetheless, the use of primary cells in nano-safety studies, are not without limitations. Primary cells have limited lifespan in vitro and can suffer from clonal changes.
In using immortalised cell lines, several studies [ 153 , 154 ]) have reported variations in findings regarding nanoparticle-induced effects in cell lines obtained from different species or tissues. For example, Zhang et al. [ 153 ] and Mukherjee et al. [ 154 ] investigated the effect of exposure to silver nanoparticle on mammalian cells. Zhang et al. [ 153 ] used epithelial cells and microphages, and Mukherjee et al. [ 154 ] used the human dermal and cervical cell lines as biomarkers. Mukherjee et al. [ 154 ] reported nanoparticle-induced cytotoxicity such as elevated levels of oxidative stress, cell membrane damage, and glutathione depletion, whereas Zhang et al. [ 153 ] reported effects including changes in antioxidant defence and metallothionein. Moreover, while Ekstrand-Hammarstrom et al. [ 155 ] and Kermanizadeh et al. [ 156 ] have compared the effect of nanoparticles on immortalised cell lines versus primary cells of the same species and tissues, available data regarding the relative effectiveness of these two types of cells are unclear. Therefore, it is difficult to make explicit conclusions as to which of these two types of cells can be used as a reliable biomarker for nano-safety studies.
This review has revealed that humans are exposed to nanoparticles through inhalation, ingestion, or dermal route. After their exposure, nanoparticles induce toxic effects such as production of oxidative stress at the exposure site, inflammation, DNA damage, and cell death [ 87 , 88 ]. For instance, exposure of human neuroblastoma (Sh-sy5y) cells to inorganic nanoparticles, such as titanium dioxide, silica dioxide, and silver are associated with induction of neurotoxicity, membrane damage, reaction oxygen specie formation, decrease in cell viability, and autophagy dysfunction [ 40 ]. Similarly, exposure to carbon-based nanoparticles such as single and multi-walled carbon nanotubes reduce cell viability, as well as induce changes in cell structure, cell cycle, and cell-to-cell interactions in human lung epithelial cells (BEAS-2B) [ 107 ].
The environmental impact of nanoparticles
The findings of this scoping review indicate a gap in the literature regarding environmental impact of nanoparticles. Out of the 117 included studies, only 5 had assessed the environmental impact of exposure to nanoparticles. This significant gap in the scientific literature has been highlighted by authors such as Bundschuh et al. [ 157 ]. The growing production and usage of nanoparticles has undoubtedly led to a diversification of emission sources into both the aquatic and soil environment. Nanoparticles enter the environment mainly through three emission scenarios: a) released during production of nano-enabled products and raw materials, b) during application, and c) following disposal of products containing nanoparticles [ 158 ]. These emissions occur either indirectly through systems such as landfills or wastewater treatment plants, or directly to the environment. Nonetheless, nanoparticles are mostly released during the application phase and following disposal [ 159 ]. Indeed, during production, only about 2% of the production volume is emitted [ 160 ]. The studies in this review used biomarkers such as soil samples and soybean seeds, zebrafish larvae, fish, and Daphnia magna neonates. This finding is in line with a previous review by Bundschuh et al. [ 157 ], which explored the effects of nanoparticles on the environment.
Limitations of the review
In this review, every effort was made to reduce bias. The search strategy was developed by experts of the review team with many years of experience in conducting systematic/scoping reviews. A comprehensive search of multiple relevant databases and other resources was conducted by one review author (EAK) and a rerun of the searches was done after 4 weeks of the initial search. Two authors (EAK and RF or PB and SH) independently screened the search results, and disagreements between reviewers were resolved by FVZ or TP.
The main limitation of this review is that the searches were limited to studies published in the English language. This may have led to the exclusion of potentially relevant papers published in other languages. Also, searches were restricted to studies published from the year 2000, which may have led to the omission of potentially relevant papers.
Conclusions
This review has provided an extensive synthesis of the current literature on the effects of nanoparticles on human health and the environment. The review has shown that while nanoparticles are beneficial in a range of applications, they pose significant threats to humans and the environment. Through the use of several biological models and biomarkers (e.g., human bronchial epithelial cells (Beas-2), soil samples, and soybean seeds), the included studies revealed the toxic effects of nanoparticles, with the most investigated nanoparticles being Zinc Oxide, MWCNTs, Titanium Dioxide, Cerium Oxide, SWCNTs, Ferric Oxide, and Silicon Dioxide. The main health impacts of nanoparticles identified in this review are decreased cell viability, cell death, reactive oxygen species generation, production of oxidative stress (dose-dependent), DNA damage, apoptosis, and induction of inflammatory responses.
This review has revealed a significant gap in the scientific literature regarding environmental impact of nanoparticles of all types. Future studies should be directed at investigating the impact of the various types of nanoparticles on the aquatic, terrestrial, and soil environment. The findings from this review have also shown limited data regarding the relative effectiveness of immortalised cell lines and primary cells as biomarkers in nano-safety studies. Future research should focus on evaluating the effectiveness of these two types of cells, in order to determine the cell that can be used as a reliable biomarker for nano-safety studies. There is also the need for future studies in this area to focus on exploring the toxic effects of Platinum, Gold, Magnesium Oxide, Molybdenum Trioxide, Tungsten trioxide, and Carbon Black nanoparticles, as findings from this review has shown that these nanoparticles are least researched. The findings of this review will be useful to policy makers and stakeholders in assessing the potential effects of nanoparticles.
Availability of data and materials
All data generated or analysed during this study are included in this published article and in the presented supplementary material file.
Jiao H, Barakat N. Balanced depth and breadth in a new interdisciplinary nanotechnology course. J Educ Technol Syst. 2012;40(1):75–87.
Article Google Scholar
Khan I, Saeed K, Khan I. Nanoparticles: properties, applications and toxicities. Arab J Chem. 2017;12(7):908–31.
Som C, Wick P, Krug H, Nowack B. Environmental and health effects of nanomaterials in nanotextiles and facade coatings. Environ Int. 2011;37:1131–42.
Article CAS PubMed Google Scholar
Bottero JY, Rose J, Wiesner MR. Nanotechnologies: Tools for sustainability in a new wave of water treatment processes. Integr Environ Assess Manag. 2006;2:391–5.
Pak T, Archilha NL, de Lima Luz, LF. Nanotechnology-Based Remediation of Groundwater. In: Kumar C, editor. Nanotechnology Characterization Tools for Environment, Health, and Safety. Berlin, Heidelberg: Springer; 2019. p. 145–65.
Pak T, Archilha NL, Al-Imari R. Application of nanotechnology in removal of NAPLs from contaminated aquifers: a source clean-up experimental study. Clean Technol Environ Pol. 2018;20:427–33.
Article CAS Google Scholar
Pak T, Luz LFDL, Tosco T, Costa GSR, Rosa PRR, Archilha NL. Pore-scale investigation of the use of reactive nanoparticles for in situ remediation of contaminated groundwater source. Proc Natl Acad Sci. 2020;117(24):13366–73.
Article CAS PubMed PubMed Central Google Scholar
Lammers T, Hennink WE, Storm G. Tumour-targeted nanomedicines: Principles and practice. Br J Cancer. 2008;99:392–7.
Gupta AK, Gupta M. Synthesis and surface engineering of iron oxide nanoparticles for biomedical applications. Biomaterials. 2006;26:3995–4021.
Botta C, Labille J, Auffan M, Borschneck D, Miche H, Cabie M, et al. TiO2-based nanoparticles released in water from commercialized sunscreens in a life-cycle perspective: structures and quantities. Environ Pollut. 2011;159:1543–50.
Auffan M, Bottero JY, Chaneac C, Rose J. Inorganic manufactured nanoparticles: How their physicochemical properties influence their biological effects in aqueous environments. Nanomed Nanotechnol Biol Med. 2010;5:999–1007.
CAS Google Scholar
Oberdorster G, Oberdorster E, Oberdorster J. Nanotoxicology: an emerging discipline evolving from studies of ultrafine particles. Environ Health Perspect. 2005;113:823–39.
Magrez A, Kasas S, Salicio V, Pasquier N, Seo JW, Celio M, et al. Cellular toxicity of carbon-based nanomaterials. Nano Lett. 2006;6(6):1121–5.
Mancuso L, Cao G. Acute toxicity test of CuO nanoparticles using human mesenchymal stem cells. Toxicol Mech Methods. 2014;24(7):449–54.
Yokel RA, MacPhail RC. Engineered nanomaterials: exposures, hazards, and risk prevention. J Occup Med Toxicol. 2011;6:7.
Jeevanandam J, Barhoum A, Chan YS, Dufresne A, Danquah MK. Review on nanoparticles and nanostructured materials: history, sources, toxicity and regulation. Beilstein J Nanotechnol. 2018;9:908–31.
Sahu SC, Hayes AW. Toxicity of nanomaterials found in human environment: a literature review. Toxicol Res Appl. 2017;1:2397847317726352.
Google Scholar
Aromataris E, Munn Z (Editors). JBI Manual for Evidence Synthesis. Joanna Briggs Institute. 2020. Available from: https://doi.org/10.46658/JBIMES-20-01
Kumah EA, Zohoori V, Pak T, Harati S, Raoul FD, Boadu P. Effects of nanotechnology: A scoping review of the current literature. OSF; 2021. Available from: osf.io/w67m9.
Boczkowski J, Hoet P. What’s new in nanotechnology? Implications for public health from a brief review of the 2008 literature. Nanotoxicology. 2010;4(1):1–14.
Phuyal S, Kasem M, Rubio L, Karlsson HL, Marcos R, Skaug V, et al. Effects on human bronchial epithelial cells following low-dose chronic exposure to nanomaterials: A 6-month transformation study. Toxicol Vitro : Int J Publishe Assoc BIBRA. 2017;44:230–40.
Shalini D, Senthilkumar S, Rajaguru P. Effect of size and shape on toxicity of zinc oxide (ZnO) nanomaterials in human peripheral blood lymphocytes. Toxicol Mech Methods. 2018;28(2):87–94.
Simon-Deckers A, Gouget B, Mayne-L’hermite M, Herlin-Boime N, Reynaud C, Carrière M. In vitro investigation of oxide nanoparticle and carbon nanotube toxicity and intracellular accumulation in A549 human pneumocytes. Toxicology. 2008;253(1–3):137–46.
Setyawati MI, Khoo PKS, Eng BH, Xiong S, Zhao X, Das GK, et al. Cytotoxic and genotoxic characterization of titanium dioxide, gadolinium oxide, and poly(lactic-co-glycolic acid) nanoparticles in human fibroblasts. J Biomed Mater Res, Part A. 2013;101(3):633–40.
Nishu SD, Park S, Ji Y, Han I, Key J, Lee TK. The effect of engineered PLGA nanoparticles on nitrifying bacteria in the soil environment. J Ind Eng Chem. 2020;84:297–304.
Lee JH, Han JH, Kim JH, Kim B, Bello D, Kim JK, et al. Exposure monitoring of graphene nanoplatelets manufacturing workplaces. Inhalation Toxicol. 2016;28(6):281–91.
Gaiser BK, Fernandes TF, Jepson M, Lead JR, Tyler CR, Stone V, et al. Assessing exposure, uptake and toxicity of silver and cerium dioxide nanoparticles from contaminated environments. Environ Health. 2009;8(1):S2.
Article PubMed PubMed Central Google Scholar
Ghosh M, Bhadra S, Adegoke A, Bandyopadhyay M, Mukherjee A. MWCNT uptake in Allium cepa root cells induces cytotoxic and genotoxic responses and results in DNA hyper-methylation. Mutat Res. 2015;774:49–58.
Lee S, Kim S, Kim S, Lee I. Effects of soil-plant interactive system on response to exposure to ZnO nanoparticles. J Microbiol Biotechnol. 2012;22(9):1264–70.
Yusefi-Tanha E, Fallah S, Rostamnejadi A, Pokhrel LR. Zinc oxide nanoparticles (ZnONPs) as a novel nanofertilizer: Influence on seed yield and antioxidant defense system in soil grown soybean (Glycine max cv. Kowsar). Sci Total Enviro. 2020;738:140240.
Ahamed M, Akhtar MJ, Khan MAM, Alrokayan SA, Alhadlaq HA. Oxidative stress mediated cytotoxicity and apoptosis response of bismuth oxide (Bi2O3) nanoparticles in human breast cancer (MCF-7) cells. Chemosphere. 2019;216:823–31.
Eom H-J, Choi J. Oxidative stress of silica nanoparticles in human bronchial epithelial cell, Beas-2B. Toxicol In Vitro. 2009;23(7):1326–32.
Bengalli R, Colantuoni A, Perelshtein I, Gedanken A, Collini M, Mantecca P, et al. In vitro skin toxicity of CuO and ZnO nanoparticles: Application in the safety assessment of antimicrobial coated textiles. NanoImpact. 2021;21: 100282.
Ferraro SA, Domingo MG, Etcheverrito A, Olmedo DG, Tasat DR. Neurotoxicity mediated by oxidative stress caused by titanium dioxide nanoparticles in human neuroblastoma (SH-SY5Y) cells. J Trace Elem Med Biol. 2020;57: 126413.
Article PubMed Google Scholar
Gambardella C, Morgana S, Bari GD, Ramoino P, Bramini M, Diaspro A, et al. Multidisciplinary screening of toxicity induced by silica nanoparticles during sea urchin development. Chemosphere. 2015;139:486–95.
Oh JH, Son MY, Choi MS, Kim S, Choi AY, Lee HA, Kim KS, Kim J, Song CW, Yoon S. Integrative analysis of genes and miRNA alterations in human embryonic stem cells-derived neural cells after exposure to silver nanoparticles. Toxicol Appl Pharmacol. 2016;299:8-23. https://doi.org/10.1016/j.taap.2015.11.004 .
Zhao L, Yu C, Lv J, Cui Y, Wang Y, Hou C, et al. Fluoride exposure, dopamine relative gene polymorphism and intelligence: A cross-sectional study in China. Ecotoxicol Environ Saf. 2021;209: 111826.
Ying E, Hwang H-M. In vitro evaluation of the cytotoxicity of iron oxide nanoparticles with different coatings and different sizes in A3 human T lymphocytes. Sci Total Environ. 2010;408(20):4475–81.
Jing X, Park JH, Peters TM, Thorne PS. Toxicity of copper oxide nanoparticles in lung epithelial cells exposed at the air–liquid interface compared with in vivo assessment. Toxicol In Vitro. 2015;29(3):502–11.
Lojk J, Repas J, Veranič P, Bregar VB, Pavlin M. Toxicity mechanisms of selected engineered nanoparticles on human neural cells in vitro. Toxicology. 2020;432: 152364.
Tsai S-M, Duran-Robles E, Goshia T, Mesina M, Garcia C, Young J, et al. CeO2 nanoparticles attenuate airway mucus secretion induced by TiO2 nanoparticles. Sci Total Environ. 2018;631–632:262–9.
Sramkova M, Kozics K, Masanova V, Uhnakova I, Razga F, Nemethova V, et al. Kidney nanotoxicity studied in human renal proximal tubule epithelial cell line TH1. Mut Res/Gen Toxicol Environ Mutagen. 2019;845: 403017.
Bell KJ, Lansakara TI, Crawford R, Monroe TB, Tivanski AV, Salem AK, et al. Mechanical cues protect against silica nanoparticle exposure in SH-SY5Y neuroblastoma. Toxicol In Vitro. 2021;70: 105031.
Akhtar MJ, Ahamed M, Kumar S, Siddiqui H, Patil G, Ashquin M, et al. Nanotoxicity of pure silica mediated through oxidant generation rather than glutathione depletion in human lung epithelial cells. Toxicology. 2010;276(2):95–102.
Dankers ACA, Kuper CF, Boumeester AJ, Fabriek BO, Kooter IM, Gröllers-Mulderij M, et al. A practical approach to assess inhalation toxicity of metal oxide nanoparticles in vitro. J Appl Toxicol : JAT. 2018;38(2):160–71.
Chen R, Huo L, Shi X, Bai R, Zhang Z, Zhao Y, et al. Endoplasmic reticulum stress induced by zinc oxide nanoparticles is an earlier biomarker for nanotoxicological evaluation. ACS Nano. 2014;8(3):2562–74.
Patil NA, Gade WN, Deobagkar DD. Epigenetic modulation upon exposure of lung fibroblasts to TiO 2 and ZnO nanoparticles: alterations in DNA methylation. Int J Nanomed. 2016;11:4509–19.
Mohamed BM, Verma NK, Prina-Mello A, Williams Y, Davies AM, Bakos G, et al. Activation of stress-related signalling pathway in human cells upon SiO2 nanoparticles exposure as an early indicator of cytotoxicity. J Nanobiotechnol. 2011;9:29.
Alshatwi AA, Subbarayan PV, Ramesh E, Al-Hazzani AA, Alsaif MA, Alwarthan AA. Aluminium oxide nanoparticles induce mitochondrial-mediated oxidative stress and alter the expression of antioxidant enzymes in human mesenchymal stem cells. Food Addit Contam Part A Chem Anal Control Expo Risk Assess. 2013;30(1):1–10.
Baber O, Jang M, Barber D, Powers K. Amorphous silica coatings on magnetic nanoparticles enhance stability and reduce toxicity to in vitro BEAS-2B cells. Inhalation Toxicol. 2011;23(9):532–43.
Corbalan JJ, Medina C, Jacoby A, Malinski T, Radomski MW. Amorphous silica nanoparticles aggregate human platelets: potential implications for vascular homeostasis. Int J Nanomed. 2012;7:631–9.
Branica G, Mladinić M, Omanović D, Želježić D. An alternative approach to studying the effects of ZnO nanoparticles in cultured human lymphocytes: combining electrochemistry and genotoxicity tests. Arh Hig Rada Toksikol. 2016;67(4):277–88.
Gurunathan S, Jeyaraj M, La H, Yoo H, Choi Y, Do JT, et al. Anisotropic platinum nanoparticle-induced cytotoxicity, apoptosis, inflammatory response, and transcriptomic and molecular pathways in human acute monocytic leukemia cells. Int J Mol Sci. 2020;21(2):440.
Hussain S, Al-Nsour F, Rice AB, Marshburn J, Ji Z, Zink JI, et al. Cerium dioxide nanoparticles do not modulate the lipopolysaccharide-induced inflammatory response in human monocytes. Int J Nanomed. 2012;7:1387–97.
Zerboni A, Bengalli R, Baeri G, Fiandra L, Catelani T, Mantecca P. Mixture effects of diesel exhaust and metal oxide nanoparticles in human lung a549 cells. Nanomaterials (Basel, Switzerland). 2019;9(9):1302.
Zielinska E, Tukaj C, Radomski MW, Inkielewicz-Stepniak I. Molecular mechanism of silver nanoparticles-induced human osteoblast cell death: protective effect of inducible nitric oxide synthase inhibitor. PLoS ONE. 2016;11(10): e0164137.
Rajiv S, Jerobin J, Saranya V, Nainawat M, Sharma A, Makwana P, et al. Comparative cytotoxicity and genotoxicity of cobalt (II, III) oxide, iron (III) oxide, silicon dioxide, and aluminum oxide nanoparticles on human lymphocytes in vitro. Hum Exp Toxicol. 2016;35(2):170–83.
Alarifi S, Ali D, Verma A, Alakhtani S, Ali BA. Cytotoxicity and genotoxicity of copper oxide nanoparticles in human skin keratinocytes cells. Int J Toxicol. 2013;32(4):296–307.
Sun J, Wang S, Zhao D, Hun FH, Weng L, Liu H. Cytotoxicity, permeability, and inflammation of metal oxide nanoparticles in human cardiac microvascular endothelial cells: cytotoxicity, permeability, and inflammation of metal oxide nanoparticles. Cell Biol Toxicol. 2011;27(5):333–42.
Tolliver LM, Holl NJ, Hou FYS, Lee H-J, Cambre MH, Huang Y-W. Differential cytotoxicity induced by transition metal oxide nanoparticles is a function of cell killing and suppression of cell proliferation. Int J Mol Sci. 2020;21(5):1731.
Rothen-Rutishauser B, Grass RN, Blank F, Limbach LK, Mühlfeld C, Brandenberger C, et al. Direct combination of nanoparticle fabrication and exposure to lung cell cultures in a closed setup as a method to simulate accidental nanoparticle exposure of humans. Environ Sci Technol. 2009;43(7):2634–40.
Benameur L, Auffan M, Cassien M, Liu W, Culcasi M, Rahmouni H, et al. DNA damage and oxidative stress induced by CeO2 nanoparticles in human dermal fibroblasts: Evidence of a clastogenic effect as a mechanism of genotoxicity. Nanotoxicology. 2015;9(6):696–705.
Gojova A, Lee J-T, Jung HS, Guo B, Barakat AI, Kennedy IM. Effect of cerium oxide nanoparticles on inflammation in vascular endothelial cells. Inhalation Toxicol. 2009;21(Suppl 1):123–30.
Hildebrand H, Kühnel D, Potthoff A, Mackenzie K, Springer A, Schirmer K. Evaluating the cytotoxicity of palladium/magnetite nano-catalysts intended for wastewater treatment. Environ Pollut (Barking, Essex 1987). 2010;158(1):65–73.
Lai JCK, Lai MB, Jandhyam S, Dukhande VV, Bhushan A, Daniels CK, et al. Exposure to titanium dioxide and other metallic oxide nanoparticles induces cytotoxicity on human neural cells and fibroblasts. Int J Nanomed. 2008;3(4):533–45.
Ahamed M, Siddiqui MA, Akhtar MJ, Ahmad I, Pant AB, Alhadlaq HA. Genotoxic potential of copper oxide nanoparticles in human lung epithelial cells. Biochem Biophys Res Commun. 2010;396(2):578–83.
Vergaro V, Aldieri E, Fenoglio I, Marucco A, Carlucci C, Ciccarella G. Surface reactivity and in vitro toxicity on human bronchial epithelial cells (BEAS-2B) of nanomaterials intermediates of the production of titania-based composites. Toxicol Vitro : Int J Publish Assoc BIBRA. 2016;34:171–8.
Dávila-Grana Á, Diego-González L, González-Fernández Á, Simón-Vázquez R. Synergistic effect of metal oxide nanoparticles on cell viability and activation of MAP Kinases and NFκB. Int J Mol Sci. 2018;19(1):246.
Pierscionek BK, Li Y, Schachar RA, Chen W. The effect of high concentration and exposure duration of nanoceria on human lens epithelial cells. Nanomed Nanotechnol Biol Med. 2012;8(3):383–90.
Rafieepour A, Azari MR, Khodagholi F, Jaktaji JP, Mehrabi Y, Peirovi H. The effect of single and combined exposures to magnetite and polymorphous silicon dioxide nanoparticles on the human A 549 cell line: in vitro study. Environ Sci Pollut Res Int. 2019;26(31):31752–62.
Ickrath P, Wagner M, Scherzad A, Gehrke T, Burghartz M, Hagen R, et al. Time-dependent toxic and genotoxic effects of zinc oxide nanoparticles after long-term and repetitive exposure to human mesenchymal stem cells. Int J Environ Res Public Health. 2017;14(12):1590.
Radeloff K, Radeloff A, Ramos Tirado M, Scherzad A, Hagen R, Kleinsasser NH, et al. Toxicity and Functional Impairment in Human Adipose Tissue-Derived Stromal Cells (hASCs) following long-term exposure to Very Small Iron Oxide Particles (VSOPs). Nanomaterials (Basel, Switzerland). 2020;10(4):741.
Jin M, Li N, Sheng W, Ji X, Liang X, Kong B, et al. Toxicity of different zinc oxide nanomaterials and dose-dependent onset and development of Parkinson’s disease-like symptoms induced by zinc oxide nanorods. Environ Int. 2021;146: 106179.
Kumari M, Singh SP, Chinde S, Rahman MF, Mahboob M, Grover P. Toxicity study of cerium oxide nanoparticles in human neuroblastoma cells. Int J Toxicol. 2014;33(2):86–97.
Fernández-Bertólez N, Costa C, Brandão F, Kiliç G, Duarte JA, Teixeira JP, et al. Toxicological assessment of silica-coated iron oxide nanoparticles in human astrocytes. Food Chem Toxicol : Int J Publish Bri Industr Biol Res Assoc. 2018;118:13–23.
Gliga AR, Di Bucchianico S, Åkerlund E, Karlsson HL. Transcriptome profiling and toxicity following long-term, low dose exposure of human lung cells to Ni and NiO nanoparticles-comparison with NiCl 2. Nanomaterials (Basel, Switzerland). 2020;10(4):649.
Kennedy IM, Wilson D, Barakat AI. Uptake and inflammatory effects of nanoparticles in a human vascular endothelial cell line. Res Rep (Health Effects Institute). 2009;136:3–32.
Schanen BC, Das S, Reilly CM, Warren WL, Self WT, Seal S, et al. Immunomodulation and T helper TH 1 /TH 2 response polarization by CeO 2 and TiO 2 nanoparticles. PLoS ONE. 2013;8(5): e62816.
Seker S, Elçin AE, Yumak T, Sınağ A, Elçin YM. In vitro cytotoxicity of hydrothermally synthesized ZnO nanoparticles on human periodontal ligament fibroblast and mouse dermal fibroblast cells. Toxicol Vitro : Int J Publish Assoc BIBRA. 2014;28(8):1349–58.
Könen-Adıgüzel S, Ergene S. In vitro evaluation of the genotoxicity of CeO 2 nanoparticles in human peripheral blood lymphocytes using cytokinesis-block micronucleus test, comet assay, and gamma H2AX. Toxicol Ind Health. 2018;34(5):293–300.
Henson TE, Navratilova J, Tennant AH, Bradham KD, Rogers KR, Hughes MF. In vitro intestinal toxicity of copper oxide nanoparticles in rat and human cell models. Nanotoxicology. 2019;13(6):795–811.
Gojova A, Guo B, Kota RS, Rutledge JC, Kennedy IM, Barakat AI. Induction of inflammation in vascular endothelial cells by metal oxide nanoparticles: effect of particle composition. Environ Health Perspect. 2007;115(3):403–9.
Alarifi S, Ali D, Alkahtani S, Verma A, Ahamed M, Ahmed M, et al. Induction of oxidative stress, DNA damage, and apoptosis in a malignant human skin melanoma cell line after exposure to zinc oxide nanoparticles. Int J Nanomed. 2013;8:983–93.
Åkerlund E, Islam MS, McCarrick S, Alfaro-Moreno E, Karlsson HL. Inflammation and (secondary) genotoxicity of Ni and NiO nanoparticles. Nanotoxicology. 2019;13(8):1060–72.
Jiménez-Chávez A, Solorio-Rodríguez A, Escamilla-Rivera V, Leseman D, Morales-Rubio R, Uribe-Ramírez M, et al. Inflammatory response in human alveolar epithelial cells after TiO 2 NPs or ZnO NPs exposure: Inhibition of surfactant protein A expression as an indicator for loss of lung function. Environ Toxicol Pharmacol. 2021;86: 103654.
Hussain S, Garantziotis S. Interplay between apoptotic and autophagy pathways after exposure to cerium dioxide nanoparticles in human monocytes. Autophagy. 2013;9(1):101–3.
Abudayyak M, Öztaş E, Arici M, Özhan G. Investigation of the toxicity of bismuth oxide nanoparticles in various cell lines. Chemosphere. 2017;169:117–23.
Fahmy HM, Ebrahim NM, Gaber MH. In-vitro evaluation of copper/copper oxide nanoparticles cytotoxicity and genotoxicity in normal and cancer lung cell lines. J Trace Elem Med Biol. 2020;60: 126481.
Božinović K, Nestić D, Centa UG, Ambriović-Ristov A, Dekanić A, de Bisschop L, et al. In-vitro toxicity of molybdenum trioxide nanoparticles on human keratinocytes. Toxicology. 2020;444: 152564.
Ahamed M, Alhadlaq HA, Alam J, Khan MAM, Ali D, Alarafi S. Iron oxide nanoparticle-induced oxidative stress and genotoxicity in human skin epithelial and lung epithelial cell lines. Curr Pharm Des. 2013;19(37):6681–90.
Pelclova D, Zdimal V, Kacer P, Fenclova Z, Vlckova S, Komarc M, et al. Leukotrienes in exhaled breath condensate and fractional exhaled nitric oxide in workers exposed to TiO2 nanoparticles. J Breath Res. 2016;10(3): 036004.
Valdiglesias V, Costa C, Kiliç G, Costa S, Pásaro E, Laffon B, et al. Neuronal cytotoxicity and genotoxicity induced by zinc oxide nanoparticles. Environ Int. 2013;55:92–100.
Verdon R, Gillies SL, Brown DM, Henry T, Tran L, Tyler CR, et al. Neutrophil activation by nanomaterials in vitro : comparing strengths and limitations of primary human cells with those of an immortalized (HL-60) cell line. Nanotoxicology. 2021;15(1):1–20.
Siddiqui MA, Ahamed M, Ahmad J, Majeed Khan MA, Musarrat J, Al-Khedhairy AA, et al. Nickel oxide nanoparticles induce cytotoxicity, oxidative stress and apoptosis in cultured human cells that is abrogated by the dietary antioxidant curcumin. Food Chem Toxicol : Int J Publish Bri Industr Biol Res Assoc. 2012;50(3–4):641–7.
Park E-J, Choi J, Park Y-K, Park K. Oxidative stress induced by cerium oxide nanoparticles in cultured BEAS-2B cells. Toxicology. 2008;245(1–2):90–100.
Fakhar-e-Alam M, Kishwar S, Willander M. Photodynamic effects of zinc oxide nanowires in skin cancer and fibroblast. Lasers Med Sci. 2014;29(3):1189–94.
Hackenberg S, Zimmermann F-Z, Scherzed A, Friehs G, Froelich K, Ginzkey C, et al. Repetitive exposure to zinc oxide nanoparticles induces dna damage in human nasal mucosa mini organ cultures. Environ Mol Mutagen. 2011;52(7):582–9.
Andujar P, Simon-Deckers A, Galateau-Sallé F, Fayard B, Beaune G, Clin B, et al. Role of metal oxide nanoparticles in histopathological changes observed in the lung of welders. Part Fibre Toxicol. 2014;11:23.
Sharma V, Anderson D, Dhawan A. Zinc oxide nanoparticles induce oxidative DNA damage and ROS-triggered mitochondria mediated apoptosis in human liver cells (HepG2). Apoptosis. 2012;17(8):852–70.
Senapati VA, Kumar A, Gupta GS, Pandey AK, Dhawan A. ZnO nanoparticles induced inflammatory response and genotoxicity in human blood cells: a mechanistic approach. Food Chem Toxicol. 2015;85:61–70.
Vlaanderen J, Pronk A, Rothman N, Hildesheim A, Silverman D, Hosgood HD, et al. A cross-sectional study of changes in markers of immunological effects and lung health due to exposure to multi-walled carbon nanotubes. Nanotoxicology. 2017;11(3):395–404.
Asghar W, Shafiee H, Velasco V, Sah VR, Guo S, El Assal R, et al. Toxicology study of single-walled carbon nanotubes and reduced graphene oxide in human sperm. Sci Rep. 2016;6:30270.
Periasamy VS, Athinarayanan J, Alfawaz MA, Alshatwi AA. Carbon nanoparticle induced cytotoxicity in human mesenchymal stem cells through upregulation of TNF3, NFKBIA and BCL2L1 genes. Chemosphere. 2016;144:275–84.
Beard JD, Erdely A, Dahm MM, de Perio MA, Birch ME, Evans DE, et al. Carbon nanotube and nanofiber exposure and sputum and blood biomarkers of early effect among U.S. workers. Environ Int. 2018;116:214–28.
Zhang W, Zhang D, Tan J, Cong H. Carbon nanotube exposure sensitize human ovarian cancer cells to paclitaxel. J Nanosci Nanotechnol. 2012;12(9):7211–4.
de Gabory L, Bareille R, Daculsi R, L’Azou B, Flahaut E, Bordenave L. Carbon nanotubes have a deleterious effect on the nose: the first in vitro data. Rhinology. 2011;49(4):445–52.
Eldawud R, Wagner A, Dong C, Stueckle TA, Rojanasakul Y, Dinu CZ. Carbon nanotubes physicochemical properties influence the overall cellular behavior and fate. NanoImpact. 2018;9:72–84.
Pacurari M, Qian Y, Fu W, Schwegler-Berry D, Ding M, Castranova V, et al. Cell permeability, migration, and reactive oxygen species induced by multiwalled carbon nanotubes in human microvascular endothelial cells. J Toxicol Environ Health A. 2012;75(2):112–28.
Reamon-Buettner SM, Hackbarth A, Leonhardt A, Braun A, Ziemann C. Cellular senescence as a response to multiwalled carbon nanotube (MWCNT) exposure in human mesothelial cells. Mech Ageing Dev. 2021;193: 111412.
Phuyal S, Kasem M, Knittelfelder O, Sharma A, Fonseca DDM, Vebraite V, et al. Characterization of the proteome and lipidome profiles of human lung cells after low dose and chronic exposure to multiwalled carbon nanotubes. Nanotoxicology. 2018;12(2):138–52.
Witzmann FA, Monteiro-Riviere NA. Multi-walled carbon nanotube exposure alters protein expression in human keratinocytes. Nanomedicine. 2006;2(3):158–68.
Snyder RJ, Hussain S, Rice AB, Garantziotis S. Multiwalled carbon nanotubes induce altered morphology and loss of barrier function in human bronchial epithelium at noncytotoxic doses. Int J Nanomed. 2014;9:4093–105.
Snyder RJ, Verhein KC, Vellers HL, Burkholder AB, Garantziotis S, Kleeberger SR. Multi-walled carbon nanotubes upregulate mitochondrial gene expression and trigger mitochondrial dysfunction in primary human bronchial epithelial cells. Nanotoxicology. 2019;13(10):1344–61.
Yu M, Chen R, Jia Z, Chen J, Lou J, Tang S, et al. MWCNTs Induce ROS Generation, ERK Phosphorylation, and SOD-2 expression in human mesothelial cells. Int J Toxicol. 2016;35(1):17–26.
Rizk MZ, Ali SA, Kadry MO, Fouad GI, Kamel NN, Younis EA, et al. C-reactive protein signaling and chromosomal abnormalities in nanotoxicity induced via different doses of TiO 2 (80 nm) boost liver function. Biol Trace Elem Res. 2020;198(1):157–67.
Jos A, Pichardo S, Puerto M, Sánchez E, Grilo A, Cameán AM. Cytotoxicity of carboxylic acid functionalized single wall carbon nanotubes on the human intestinal cell line Caco-2. Toxicol Vitro : Int J Published Assoc BIBRA. 2009;23(8):1491–6.
Vankoningsloo S, Piret J-P, Saout C, Noel F, Mejia J, Zouboulis CC, et al. Cytotoxicity of multi-walled carbon nanotubes in three skin cellular models: effects of sonication, dispersive agents and corneous layer of reconstructed epidermis. Nanotoxicology. 2010;4(1):84–97.
Herzog E, Byrne HJ, Davoren M, Casey A, Duschl A, Oostingh GJ. Dispersion medium modulates oxidative stress response of human lung epithelial cells upon exposure to carbon nanomaterial samples. Toxicol Appl Pharmacol. 2009;236(3):276–81.
Müller L, Riediker M, Wick P, Mohr M, Gehr P, Rothen-Rutishauser B. Oxidative stress and inflammation response after nanoparticle exposure: differences between human lung cell monocultures and an advanced three-dimensional model of the human epithelial airways. J R Soc Interface. 2010;7(Suppl 1):S27–40.
PubMed Google Scholar
Basak SC, Vracko M, Witzmann FA. Mathematical nanotoxicoproteomics: quantitative characterization of effects of Multi-walled Carbon Nanotubes (MWCNT) and TiO2 Nanobelts (TiO2-NB) on protein expression patterns in human intestinal cells. Curr Comput Aided Drug Des. 2016;12(4):259–64.
Patlolla A, Patlolla B, Tchounwou P. Evaluation of cell viability, DNA damage, and cell death in normal human dermal fibroblast cells induced by functionalized multiwalled carbon nanotube. Mol Cell Biochem. 2010;338(1–2):225–32.
Dahm MM, Schubauer-Berigan MK, Evans DE, Birch ME, Bertke S, Beard JD, et al. Exposure assessments for a cross-sectional epidemiologic study of US carbon nanotube and nanofiber workers. Int J Hyg Environ Health. 2018;221(3):429–40.
Fatkhutdinova LM, Khaliullin TO, Vasil’yeva OL, Zalyalov RR, Mustafin IG, Kisin ER, et al. Fibrosis biomarkers in workers exposed to MWCNTs. Toxicol Appl Pharmacol. 2016;299:125–31.
Zhao X, Chang S, Long J, Li J, Li X, Cao Y. The toxicity of multi-walled carbon nanotubes (MWCNTs) to human endothelial cells: The influence of diameters of MWCNTs. Food Chem Toxicol : Int J Publish Bri Industr Biol Res Assoc. 2019;126:169–77.
Öner D, Ghosh M, Coorens R, Bové H, Moisse M, Lambrechts D, et al. Induction and recovery of CpG site specific methylation changes in human bronchial cells after long-term exposure to carbon nanotubes and asbestos. Environ Int. 2020;137: 105530.
Luanpitpong S, Wang L, Castranova V, Rojanasakul Y. Induction of stem-like cells with malignant properties by chronic exposure of human lung epithelial cells to single-walled carbon nanotubes. Part Fibre Toxicol. 2014;11:22.
Shvedova AA, Yanamala N, Kisin ER, Khailullin TO, Birch ME, Fatkhutdinova LM. Integrated analysis of dysregulated ncRNA and mRNA expression profiles in humans exposed to carbon nanotubes. PLoS ONE. 2016;11(3): e0150628.
Domenech J, Hernández A, Demir E, Marcos R, Cortés C. Interactions of graphene oxide and graphene nanoplatelets with the in vitro Caco-2/HT29 model of intestinal barrier. Sci Rep. 2020;10(1):2793.
Wang L, Stueckle TA, Mishra A, Derk R, Meighan T, Castranova V, et al. Neoplastic-like transformation effect of single-walled and multi-walled carbon nanotubes compared to asbestos on human lung small airway epithelial cells. Nanotoxicology. 2014;8(5):485–507.
Mukherjee SP, Gupta G, Klöditz K, Wang J, Rodrigues AF, Kostarelos K, et al. Next-generation sequencing reveals differential responses to acute versus long-term exposures to graphene oxide in human lung cells. Small. 2020;16(21): e1907686.
Pérez RF, Soto Fernández AY, Bousquets Muñoz P, Sierra MI, Tejedor JR, Morales-Sánchez P, et al. No genome-wide DNA methylation changes found associated with medium-term reduced graphene oxide exposure in human lung epithelial cells. Epigenetics. 2020;15(3):283–93.
Xu Y, Luo Z, Li S, Li W, Zhang X, Zuo YY, et al. Perturbation of the pulmonary surfactant monolayer by single-walled carbon nanotubes: a molecular dynamics study. Nanoscale. 2017;9(29):10193–204.
Gasser M, Wick P, Clift MJD, Blank F, Diener L, Yan B, et al. Pulmonary surfactant coating of multi-walled carbon nanotubes (MWCNTs) influences their oxidative and pro-inflammatory potential in vitro. Part Fibre Toxicol. 2012;9:17.
Di Cristo L, Grimaldi B, Catelani T, Vázquez E, Pompa PP, Sabella S. Repeated exposure to aerosolized graphene oxide mediates autophagy inhibition and inflammation in a three-dimensional human airway model. Materials Today Bio. 2020;6: 100050.
Chortarea S, Clift MJD, Vanhecke D, Endes C, Wick P, Petri-Fink A, et al. Repeated exposure to carbon nanotube-based aerosols does not affect the functional properties of a 3D human epithelial airway model. Nanotoxicology. 2015;9(8):983–93.
Sargent JF. Nanotechnology: a policy primer. Congres Res Serv. 2011;4(3/4):191–203.
Duschl A, Windgasse G. A survey on the state of nanosafety research in the European Union and the United States. J Nanoparticles Res. 2018;20(12):335.
UNECA. Towards African nanotechnology future: trends, impacts and opportunities. Addis Ababa: United Nations. Economic Commission for Africa; 2020. Available from: https://archive.uneca.org/publications/towards-african-nanotechnology-future-trendsimpacts-and-opportunities .
Bayda S, Adeel M, Tuccinardi T, Cordani M, Rizzolio F. The history of nanoscience and nanotechnology: from chemical-physical applications to nanomedicine. Molecules. 2019;25(1):112.
Huang Q, Yu H, Ru Q. Bioavailability and delivery of nutraceuticals using nanotechnology. J Food Sci. 2010;75(1):R50–7.
Maiti D, Tong X, Mou X, Yang K. Carbon-based nanomaterials for biomedical applications: a recent study. Front Pharmacol. 2019;9:1401.
Pandey P, Dahiya M. A brief review on inorganic nanoparticles. J Crit Rev. 2016;3(3):18.
Lohse SE, Murphy CJ. Applications of colloidal inorganic nanoparticles: from medicine to energy. J Am Chem Soc. 2012;134(38):15607–20.
Wang P, Lombi E, Zhao FJ, Kopittke PM. Nanotechnology: a new opportunity in plant sciences. Trends Plant Sci. 2016;21(8):699–712.
Bahadar H, Maqbool F, Niaz K, Abdollahi M. Toxicity of nanoparticles and an overview of current experimental models. Iran Biomed J. 2016;20(1):1–11.
PubMed PubMed Central Google Scholar
Baktur R, Patel H, Kwon S. Effect of exposure conditions on SWCNT-induced inflammatory response in human alveolar epithelial cells. Toxicol Vitro : Int J Published Assoc BIBRA. 2011;25(5):1153–60.
Liu G, Gao J, Ai H, Chen X. Applications and potential toxicity of magnetic iron oxide nanoparticles. Small. 2013;9–10:1533–45.
Yao Y, Zhou Y, Liu L, Xu Y, Chen Q, Wang Y, et al. Nanoparticle-based drug delivery in cancer therapy and its role in overcoming drug resistance. Front Mol Biosci. 2020;7:193.
Dadwal A, Baldi A, Narang RK. Nanoparticles as carriers for drug delivery in cancer. Artificial Cells, Nanomed Biotechnol. 2018;46(Sup2):295–305.
Kermanizadeh A, Lohr M, Roursgaard M, Messner S, Gunness P, Kelm JM, et al. Hepatic toxicology following single and multiple exposure of engineered nanomaterials utilising a novel primary human 3D liver microtissue model. Part Fibre Toxicol. 2014;11:56.
Joris F, Valdepérez D, Pelaz B, Soenen SJ, Manshian BB, Parak WJ, et al. The impact of species and cell type on the nanosafety profile of iron oxide nanoparticles in neural cells. J Nanobiotechnol. 2016;14:69.
Wang Y, Chen S, Yan Z, Pei M. A prospect of cell immortalization combined with matrix microenvironmental optimization strategy for tissue engineering and regeneration. Cell Biosci. 2019;9:7.
Zhang S, Zhang X, Liu H, Qu W, Guan Z, Zeng Q, et al. Modifying effect of COMT gene polymorphism and a predictive role for proteomics analysis in children’s intelligence in endemic fluorosis area in Tianjin, China. Toxicol Sci J Soc Toxicol. 2015;144(2):238–45.
Mukherjee SG, O’Claonadh N, Casey A, Chambers G. Comparative in vitro cytotoxicity study of silver nanoparticle on two mammalian cell lines. Toxicol In Vitro. 2012;26(2):238–51.
Ekstrand-Hammarström B, Akfur CM, Andersson PO, Lejon C, Österlund L, Bucht A. Human primary bronchial epithelial cells respond differently to titanium dioxide nanoparticles than the lung epithelial cell lines A549 and BEAS-2B. Nanotoxicology. 2011;6(6):623–34.
Kermanizadeh A, Pojana G, Gaiser BK, Birkedal R, Bilanicová D, Wallin H, et al. In vitro assessment of engineered nanomaterials using a hepatocyte cell line: cytotoxicity, pro-inflammatory cytokines and functional markers. Nanotoxicology. 2013;7(3):301–13.
Bundschuh M, Filser J, Lüderwald S, McKee MS, Metreveli G, Schaumann GE, et al. Nanoparticles in the environment: where do we come from, where do we go to? Environ Sci Eur. 2018;30(1):6.
Tolaymat T, Genaidy A, Abdelraheem W, Dionysiou D, Andersen C. The effects of metallic engineered nanoparticles upon plant systems: An analytic examination of scientific evidence. Sci Total Environ. 2017;579(1):93–106.
Keller AA, McFerran S, Lazareva A, Suh S. Global life cycle releases of engineered nanomaterials. J Nanopart Res. 2013;15:1692.
Gottschalka F, Nowack B. The release of engineered nanomaterials to the environment. J Environ Monitor. 2011;13:1145–55.
Download references
Acknowledgements
We would like to thank Daphne Silva Pino and Romana Petry for their contribution at the initial stages of this research.
This research was funded through the GRUN project (Towards the first implementation of groundwater remediation using nanotechnology in Brazil) as part of UKRI’s Global Challenges Research Fund (GCRF) and a grant from the São Paulo Research Foundation (FAPESP) (Grant 17/20308–0).
Author information
Authors and affiliations.
Depeartment of International Public Health, Liverpool School of Tropical Medicine, Liverpool, UK
Elizabeth Adjoa Kumah
School of Computing, Engineering & Digital Technologies, Teesside University, Middlesbrough, TS1 3BX, UK
Raoul Djou Fopa, Saeed Harati & Tannaz Pak
Department of Health Services Research and Policy, London School of Hygiene and Tropical Medicine, London, UK
School of Health and Life Sciences, Teesside University, Middlesbrough, UK
Fatemeh Vida Zohoori
You can also search for this author in PubMed Google Scholar
Contributions
Elizabeth Adjoa Kumah: Conceptualization, Methodology, Investigation, Formal analysis, Validation, Supervision, Writing—Original Draft, Writing—Review & Editing, Raoul Djou Fopa: Investigation, Data Curation, Saeed Harati: Investigation, Data Curation, Paul Boadu: Investigation, Data Curation, Formal analysis, Fatemeh Vida Zohoori: Conceptualization, Methodology, Validation, Supervision, Writing—Review & Editing, Funding acquisition, Tannaz Pak: Conceptualization, Methodology, Validation, Supervision, Writing—Review & Editing, Funding acquisition. The author(s) read and approved the final manuscript.
Corresponding author
Correspondence to Tannaz Pak .
Ethics declarations
Ethics approval and consent to participate.
The research presented in this article is a scoping review, as such, no ethical approval was needed.
Consent for publication
Not applicable.
Competing interests
The authors declare no competing interests.
Additional information
Publisher’s note.
Springer Nature remains neutral with regard to jurisdictional claims in published maps and institutional affiliations.
Supplementary Information
Additional file 1..
MEDLINE Search History.
Rights and permissions
Open Access This article is licensed under a Creative Commons Attribution 4.0 International License, which permits use, sharing, adaptation, distribution and reproduction in any medium or format, as long as you give appropriate credit to the original author(s) and the source, provide a link to the Creative Commons licence, and indicate if changes were made. The images or other third party material in this article are included in the article's Creative Commons licence, unless indicated otherwise in a credit line to the material. If material is not included in the article's Creative Commons licence and your intended use is not permitted by statutory regulation or exceeds the permitted use, you will need to obtain permission directly from the copyright holder. To view a copy of this licence, visit http://creativecommons.org/licenses/by/4.0/ . The Creative Commons Public Domain Dedication waiver ( http://creativecommons.org/publicdomain/zero/1.0/ ) applies to the data made available in this article, unless otherwise stated in a credit line to the data.
Reprints and permissions
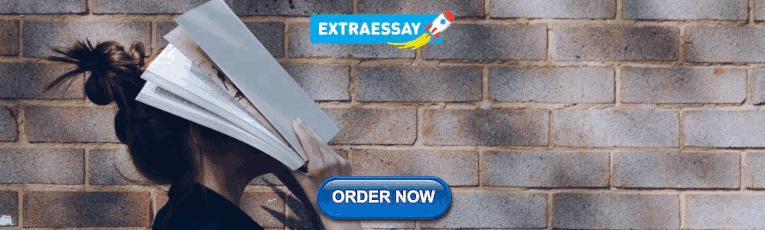
About this article
Cite this article.
Kumah, E.A., Fopa, R.D., Harati, S. et al. Human and environmental impacts of nanoparticles: a scoping review of the current literature. BMC Public Health 23 , 1059 (2023). https://doi.org/10.1186/s12889-023-15958-4
Download citation
Received : 15 August 2022
Accepted : 22 May 2023
Published : 03 June 2023
DOI : https://doi.org/10.1186/s12889-023-15958-4
Share this article
Anyone you share the following link with will be able to read this content:
Sorry, a shareable link is not currently available for this article.
Provided by the Springer Nature SharedIt content-sharing initiative
- Cytotoxicity; Environmental health
- Genotoxicity
- Human health
- Nanoparticles
- Scoping review
BMC Public Health
ISSN: 1471-2458
- Submission enquiries: [email protected]
- General enquiries: [email protected]

Chemical Society Reviews
Synthesis of metallic high-entropy alloy nanoparticles.

* Corresponding authors
a College of Energy and Mechanical Engineering, Dezhou University, Dezhou, Shandong, P. R. China
b Department of Chemistry, Temple University, 1901 North 13th Street, Philadelphia, Pennsylvania, USA E-mail: [email protected]
The theoretically infinite compositional space of high-entropy alloys (HEAs) and their novel properties and applications have attracted significant attention from a broader research community. The successful synthesis of high-quality single-phase HEA nanoparticles represents a crucial step in fully unlocking the potential of this new class of materials to drive innovations. This review analyzes the various methods reported in the literature to identify their commonalities and dissimilarities, which allows categorizing these methods into five general strategies. Physical minimization of HEA metals into HEA nanoparticles through cryo-milling represents the typical top–down strategy. The counter bottom–up strategy requires the simultaneous generation and precipitation of metal atoms of different elements on growing nanoparticles. Depending on the metal atom generation process, there are four synthesis strategies: vaporization of metals, burst reduction of metal precursors, thermal shock-induced reduction of metal precursors, and solvothermal reduction of metal precursors. Comparisons among the methods within each strategy, along with discussions, provide insights and guidance for achieving the robust synthesis of HEA nanoparticles.
- This article is part of the themed collection: Celebrating the scientific accomplishments of RSC Fellows
Article information
Download citation, permissions.

X. Sun and Y. Sun, Chem. Soc. Rev. , 2024, Advance Article , DOI: 10.1039/D3CS00954H
To request permission to reproduce material from this article, please go to the Copyright Clearance Center request page .
If you are an author contributing to an RSC publication, you do not need to request permission provided correct acknowledgement is given.
If you are the author of this article, you do not need to request permission to reproduce figures and diagrams provided correct acknowledgement is given. If you want to reproduce the whole article in a third-party publication (excluding your thesis/dissertation for which permission is not required) please go to the Copyright Clearance Center request page .
Read more about how to correctly acknowledge RSC content .
Social activity
Search articles by author.
This article has not yet been cited.
Advertisements
Find Info For
- Current Students
- Prospective Students
- Research and Partnerships
- Entrepreneurship and Commercialization
Quick Links
- Health and Life Sciences
- Info Security and AI
- Transformative Education
- Purdue Today
- Purdue Global
- Purdue in the News
March 27, 2024
Purdue researchers create biocompatible nanoparticles to enhance systemic delivery of cancer immunotherapy
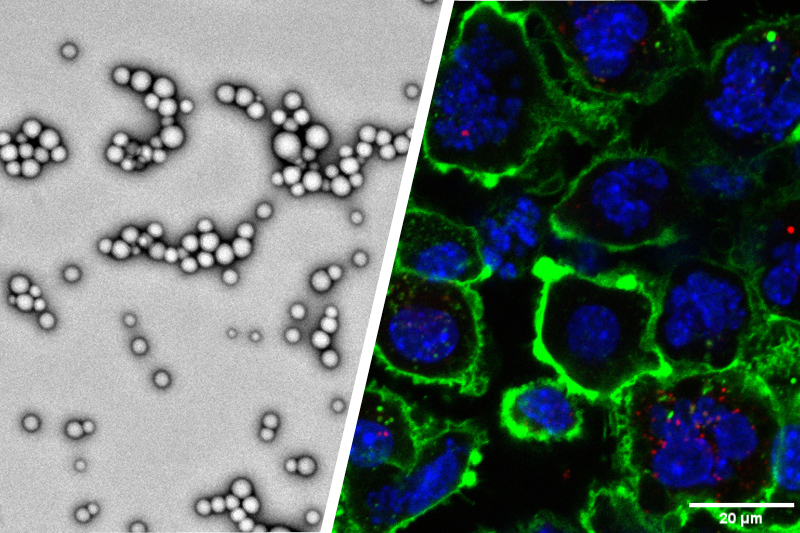
Purdue University researchers are developing and validating patent-pending nanoparticles (left) to enhance immunotherapy effects against tumors. The nanoparticles are modified with adenosine triphosphate, or ATP, to recruit dendritic cells (right), which are immune cells that recognize tumor antigens and bring specialized immune cells to fight off tumors. (Images provided by Yoon Yeo)
PLGA nanoparticles modified with ATP slowly release anti-cancer drugs and recruit immune cells to fight tumors
WEST LAFAYETTE, Ind. — Purdue University researchers are developing and validating patent-pending poly (lactic-co-glycolic acid), or PLGA, nanoparticles modified with adenosine triphosphate, or ATP, to enhance immunotherapy effects against malignant tumors.
The nanoparticles slowly release drugs that induce immunogenic cell death, or ICD, in tumors. ICD generates tumor antigens and other molecules to bring immune cells to a tumor’s microenvironment. The researchers have attached ATP to the nanoparticles, which also recruits immune cells to the tumor to initiate anti-tumor immune responses.
Yoon Yeo leads a team of researchers from the College of Pharmacy , the Metabolite Profiling Facility in the Bindley Bioscience Center , and the Purdue Institute for Cancer Research to develop the nanoparticles. Yeo is the associate department head and Lillian Barboul Thomas Professor of Industrial and Molecular Pharmaceutics and Biomedical Engineering; she is also a member of the Purdue Institute for Drug Discovery and the Purdue Institute for Cancer Research.
The researchers validated their work using paclitaxel, a chemotherapy drug used to treat several types of cancers. They found that tumors grew slower in mice treated with paclitaxel enclosed within ATP-modified nanoparticles than in mice treated with paclitaxel in non-modified nanoparticles.
“When combined with an existing immunotherapy drug, the ATP-modified, paclitaxel-loaded nanoparticles eliminated tumors in mice and protected them from rechallenge with tumor cells,” Yeo said.
The research has been published in the peer-reviewed journal ACS Nano .
Challenges to systemic immunotherapy delivery
Immunotherapy is a promising approach to fighting cancer, but Yeo said it does not benefit a large population of patients because they do not have the powerful immune cells needed to combat tumors.
“Pharmacological agents to activate immune cells can directly be given to tumors,” Yeo said. “Then the immune system can fight not only the treated tumors but also nontreated tumors in distant locations as the activated immune cells circulate in the bloodstream.”
However, Yeo said most tumors with poor prognoses are not always locatable or accessible. Therefore, they may not be effectively treated by local therapy. She and her team envisioned systemic delivery of immunotherapy, but there are challenges.
“For successful systemic administration, active ingredients that stimulate anti-tumor immune responses need to be simultaneously present in tumors to exert concerted effects on the target,” Yeo said. “The ingredients also must maintain their activity until they reach tumors, but not cause toxic off-target effects. Moreover, the carriers traditionally used in local drug delivery offer limited utility in systemic application because they may not be compatible with blood components.”
Yeo and her colleagues used biocompatible polymeric nanoparticles to deliver immunotherapy compounds and modified them to safely activate the immune system.
“We employed poly (lactic-co-glycolic acid), or PLGA, nanoparticles based on the strong track record of the polymer in FDA-approved products and its routine use in the systemic delivery of poorly water-soluble drugs,” Yeo said.
Tests verified the ATP-modified PLGA nanoparticles were well tolerated in mice upon multiple systemic injections. They were able to recruit dendritic cells, the immune cells that recognize tumor antigens and bring specialized immune cells to fight off tumors.
“Moreover, the nanoparticles were shown to control the release of paclitaxel to minimize its systemic toxicity,” Yeo said.
The next development steps
Yeo and her colleagues will continue their work on the ATP-modified nanoparticles.
“We are currently working on improving the delivery of the nanoparticles to tumors and combining them with other treatments that will circumvent the resistance to the nanoparticle-delivered immunotherapy,” Yeo said. “To finance these efforts, we will apply for continued support from the National Institutes of Health. We are also open to industry partnerships to take this technology to the clinic.”
Yeo disclosed the nanoparticles innovation to the Purdue Innovates Office of Technology Commercialization , which has applied for a patent from the U.S. Patent and Trademark Office to protect the intellectual property. Industry partners interested in developing the compound or commercializing it for the marketplace should contact Joe Kasper, assistant director of business development and licensing — life sciences, at [email protected] , about track code 69546 .
Yeo and the research team received funding from the National Institutes of Health, the National Center for Advancing Translational Sciences, the Indiana Clinical and Translational Sciences Institute, and the Purdue Institute for Cancer Research.
About Purdue University
Purdue University is a public research institution demonstrating excellence at scale. Ranked among top 10 public universities and with two colleges in the top four in the United States, Purdue discovers and disseminates knowledge with a quality and at a scale second to none. More than 105,000 students study at Purdue across modalities and locations, including nearly 50,000 in person on the West Lafayette campus. Committed to affordability and accessibility, Purdue’s main campus has frozen tuition 13 years in a row. See how Purdue never stops in the persistent pursuit of the next giant leap — including its first comprehensive urban campus in Indianapolis, the new Mitchell E. Daniels, Jr. School of Business, and Purdue Computes — at https://www.purdue.edu/president/strategic-initiatives .
About Purdue Innovates Office of Technology Commercialization
The Purdue Innovates Office of Technology Commercialization operates one of the most comprehensive technology transfer programs among leading research universities in the U.S. Services provided by this office support the economic development initiatives of Purdue University and benefit the university’s academic activities through commercializing, licensing and protecting Purdue intellectual property. In fiscal year 2023, the office reported 150 deals finalized with 203 technologies signed, 400 disclosures received and 218 issued U.S. patents. The office is managed by the Purdue Research Foundation, which received the 2019 Innovation & Economic Prosperity Universities Award for Place from the Association of Public and Land-grant Universities. In 2020, IPWatchdog Institute ranked Purdue third nationally in startup creation and in the top 20 for patents. The Purdue Research Foundation is a private, nonprofit foundation created to advance the mission of Purdue University. Contact [email protected] for more information.
Writer/Media contact: Steve Martin, [email protected]
Source: Yoon Yeo, [email protected]
Research Foundation News
Communication.
- OneCampus Portal
- Brightspace
- BoilerConnect
- Faculty and Staff
- Human Resources
- Colleges and Schools
Info for Staff
- Purdue Moves
- Board of Trustees
- University Senate
- Center for Healthy Living
- Information Technology
- Ethics & Compliance
- Campus Disruptions
Purdue University, 610 Purdue Mall, West Lafayette, IN 47907, (765) 494-4600
© 2015-24 Purdue University | An equal access/equal opportunity university | Copyright Complaints | Maintained by Office of Strategic Communications
Trouble with this page? Disability-related accessibility issue? Please contact News Service at [email protected] .
Celebrating 20 Years of Research: Highlights From NIOSH’s Nanotechnology Research Center’s Field Studies Team
As the National Institute for Occupational Safety and Health (NIOSH) Nanotechnology Research Center (NTRC) marks its 20th anniversary, we celebrate the groundbreaking work of the Field Studies Team. Organized in 2006, the team began by evaluating potential workplace exposures to engineered nanomaterials. Engineered nanomaterials (those created on purpose and not incidentally) have at least one dimension between 1 and 100 nanometers. One nanometer is extremely small—one millionth of a millimeter. Materials at this tiny scale can have unique properties.
The NTRC Field Studies Team is composed of industrial hygienists and engineers and has performed more than 140 on-site exposure assessments. They evaluate advanced materials and processes in many workplaces ranging from large manufacturers, research and development labs, and government facilities, to schools, libraries, and other non-industrial settings. Their efforts contribute to understanding health and safety risks in emerging industries that use nanomaterials.
Early Challenges and Growth
In the early years, the team faced daunting challenges. At that time, there were no proven sampling or analysis methods for nanomaterials. To improve worker safety and health programs, the team had to gain the trust of companies to allow site visits while protecting trade secrets. The team members had to interpret exposure data and communicate risks without guidance from exposure limits, which did not yet exist for any nanomaterials.
Over time, the NTRC Field Studies Team gained experience through fieldwork and partnerships. Team members explained and demonstrated how NIOSH protects intellectual property. The team compared techniques across materials and settings, allowing team members to standardize assessment approaches. These assessment strategies are documented in resources like the Nanoparticle Emission Assessment Technique (NEAT) and the Nanoparticle Exposure Assessment Technique (NEAT 2.0) .
An Expanded Focus
Over the years, the team’s focus shifted from mainly carbon nanotubes and nanofibers to other materials like titanium dioxide and silver nanomaterials. Lately, the team has been studying some newly developed materials:
- Nanocellulose
- Quantum dots
- Boron nitride nanotubes
- Thermoplastic filaments with nanomaterial additives
- Photocatalytic liquid resins
- Powders used in 3D printing
The team has been asked to evaluate these materials in diverse settings, from 3-person labs to massive aerospace companies.
What Happens During a Field Study
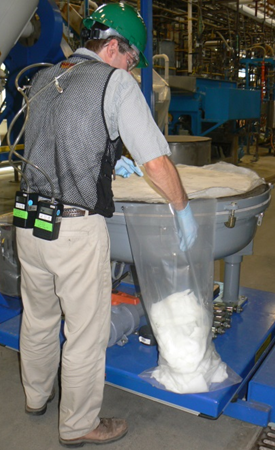
When the NTRC Field Studies Team visits a workplace, researchers assess potential exposures from nano-related processes and materials. The team also evaluates control technologies to reduce exposures. A field study can involve these activities:
- Measuring to find out if workers are exposed to harmful vapors, fumes, or particles. The team uses many kinds of tools to do this.
- Evaluating and suggesting ways to keep workers safe from exposure.
- Looking carefully at how work is done to make sure it’s safe.
- Checking the personal protective equipment workers wear, like respirators, to see if it is used correctly.
Benefits to Companies
Companies that ask for a site visit receive on-site expertise from NIOSH free of charge. NIOSH experts evaluate exposures and controls. Companies receive a confidential report with exposure results, control evaluations, and recommendations to improve worker health and safety. Workers who volunteer for personal air sampling get their personal air sampling results confidentially. The final report includes these results, but it does not identify individual workers.
Companies can use these reports to implement best practices and cost-effective controls. For example, one company had multiple team visits as they expanded production and relocated twice. The team gave exposure control recommendations that were incorporated as the company grew. This protected workers throughout the changes.
Benefits to NIOSH
NIOSH and the industry benefit from early hazard identification, risk assessments, and best practices. Here are some examples:
- Collect workplace exposure data which informs lab studies so that they are realistic and applicable to actual working conditions.
- Design and improve controls that prevent and minimize worker exposures.
- Improve risk assessment knowledge and techniques.
Looking Ahead
Materials science is expanding rapidly, outpacing occupational health research. For this reason, the team has extended its reach from focusing on nanomaterials to include any advanced materials and related manufacturing processes. NIOSH describes advanced materials as new or modified substances designed to perform better in at least one way. Although all nanomaterials are advanced, not all advanced materials are nanomaterials. This expanded scope allows the team to evaluate emerging manufacturing processes and products such as biomanufacturing and lithium-ion batteries.
The overall goal is to assist, from an occupational health and safety standpoint, in the timely and responsible development of these emerging technologies.
How to Ask for Help from NIOSH
As the nanotechnology landscape constantly evolves, the NTRC Field Studies Team seeks new partners for assessments. NIOSH welcomes opportunities to provide assistance and protect worker health alongside business goals. Companies interested in a free workplace assessment of nanomaterials or advanced materials can email [email protected] . To learn more, visit our website: Nanotechnology Field Studies Effort .
CDR Kevin L. Dunn, MS, CIH REHS/RS, DAAS, is senior industrial hygienist of the NTRC Field Studies Team in the Hazard Evaluations and Technical Assistance Branch, Division of Field Studies and Engineering.
Eric Glassford, MS, CIH, is an industrial hygienist on the NTRC Field Studies Team in the Hazard Evaluations and Technical Assistance Branch, Division of Field Studies and Engineering.
Lilia Chen, MS, CIH, is the NTRC coordinator and deputy branch chief of the Emerging Technologies Branch, Division of Science Integration.
This blog is part of a series to commemorate the 20 th anniversary of the Nanotechnology Research Center. Click here for additional blogs in the series and on other nanotechnology topics.
Information gained from these site visits has resulted in products such as tools, guidance documents, informational fact sheets, peer-reviewed publications, and the establishment of recommended exposure limits. A selection of products is listed below:
Dunn KL, Dunn KH, Hammond D, Lo S . [2020]. Three-dimensional printer emissions and employee exposures to ultrafine particles during the printing of thermoplastic filaments containing carbon nanotubes or carbon nanofibers. J Nanopart Res 22 (46), https://doi.org/10.1007/s11051-020-4750-8 .
Dunn KL, Hammond D, Menchaca K, Roth F, Dunn KH [2020]. Reducing ultrafine particulate emission from multiple 3D printers in an office environment using a prototype engineering control. J Nanopart Res 22 (112), https://doi.org/10.1007/s11051-020-04844-4 .
Glassford E, Neu-Baker NM, Dunn KL, Dunn KH [2020]. Exposures during wet production and use processes of nanomaterials: a summary of 11 worksite evaluations. Ind Health 58 :467–478, https://doi.org/10.2486/indhealth.2019-0169 .
NIOSH [2018]. Characterizing 3D printing emissions and controls in an office environment. NIOSH Science Blog. By Dunn KL, Hammond D, Tyraswski J, Duling M. August 16, https://blogs.cdc.gov/niosh-science-blog/2018/08/16/3d-printing .
NIOSH [2018]. Controlling health hazards when working with nanomaterials: Questions to ask before you start. Poster. By Glassford E, Dunn KL, Dunn KH. Cincinnati, OH: U.S. Department of Health and Human Services, Centers for Disease Control and Prevention, National Institute for Occupational Safety and Health, DHHS (NIOSH) Publication No. 2018-103, https://doi.org/10.26616/NIOSHPUB2018103 .
NIOSH [2020]. 3D printing with filaments: Health and safety questions to ask. Poster. By Glassford E, Dunn KL, Dunn KH, Hammond D, Tyrawski J. Cincinnati, OH: U.S. Department of Health and Human Services, Centers for Disease Control and Prevention, National Institute for Occupational Safety and Health, DHHS (NIOSH) Publication No. 2020-115, https://www.cdc.gov/niosh/docs/2020-115 .
NIOSH [2020]. 3D printing with metal powders: Health and safety questions to ask. Poster. By Glassford E, Dunn KL, Dunn KH, Hammond D, Tyrawski J. Cincinnati, OH: U.S. Department of Health and Human Services, Centers for Disease Control and Prevention, National Institute for Occupational Safety and Health, DHHS (NIOSH) Publication No. 2020-114. https://doi.org/10.26616/NIOSHPUB2020114 .
NIOSH [2021]. Current Intelligence Bulletin 70: Health effects of occupational exposure to silver nanomaterials. By Kuempel E, Roberts JR, Roth G, Dunn KL, Zumwalde R, Drew N, Hubbs A, Trout D, Holdsworth G. Cincinnati, OH: U.S. Department of Health and Human Services, Centers for Disease Control and Prevention, National Institute for Occupational Safety and Health, DHHS (NIOSH) Publication No. 2021-112, https://doi.org/10.26616/NIOSHPUB2021112 .
Vamsi K, Roberts J, Glassford E, Gill R, Friend S, Dunn K, Erdely A [2022]. Understanding toxicity associated with boron nitride nanotubes: Review of toxicity studies, exposure assessment at manufacturing facilities, and read-across. JMR 37 (24): 4620–4638, https://doi.org/10.1557/s43578-022-00796-8 .
Xin X, Barger M, Roach A, Bowers L, Stefaniak A, Kodali V, Glassford E, Dunn KL, Dunn KH, Wolfarth M, Friend S, Leonard S, Kashon M, Porter D, Erdely A, Roberts R [2020]. Toxicity evaluation following pulmonary exposure to an as-manufactured dispersed boron nitride nanotube (BNNT) material in vivo. NanoImpact 19 , https://doi.org/10.1016/j.impact.2020.100235 .
Post a Comment
Cancel reply.
Your email address will not be published. Required fields are marked *
- 50th Anniversary Blog Series
- Additive Manufacturing
- Aging Workers
- Agriculture
- Artificial Intelligence
- Back Injury
- Bloodborne pathogens
- Cardiovascular Disease
- cold stress
- commercial fishing
- Communication
- Construction
- Cross Cultural Communication
- Dermal Exposure
- Education and Research Centers
- Electrical Safety
- Emergency Response/Public Sector
- Engineering Control
- Environment/Green Jobs
- Epidemiology
- Fire Fighting
- Food Service
- Future of Work and OSH
- Healthy Work Design
- Hearing Loss
- Heat Stress
- Holiday Themes
- Hydraulic Fracturing
- Infectious Disease Resources
- International
- Landscaping
- Law Enforcement
- Manufacturing
- Manufacturing Mondays Series
- Mental Health
- Motor Vehicle Safety
- Musculoskeletal Disorders
- Nanotechnology
- National Occupational Research Agenda
- Needlestick Prevention
- NIOSH-funded Research
- Nonstandard Work Arrangements
- Observances
- Occupational Health Equity
- Oil and Gas
- Outdoor Work
- Partnership
- Personal Protective Equipment
- Physical activity
- Policy and Programs
- Prevention Through Design
- Prioritizing Research
- Reproductive Health
- Research to practice r2p
- Researcher Spotlights
- Respirators
- Respiratory Health
- Risk Assessment
- Safety and Health Data
- Service Sector
- Small Business
- Social Determinants of Health
- Spanish translations
- Sports and Entertainment
- Strategic Foresight
- Struck-by injuries
- Student Training
- Substance Use Disorder
- Surveillance
- Synthetic Biology
- Systematic review
- Take Home Exposures
- Teachers/School Workers
- Temporary/Contingent Workers
- Total Worker Health
- Translations (other than Spanish)
- Transportation
- Uncategorized
- Veterinarians
- Wearable Technologies
- Wholesale and Retail Trade
- Work Schedules
- Workers' Compensation
- Workplace Medical Mystery
- Workplace Supported Recovery
- World Trade Center Health Program
- Young Workers
To receive email updates about this page, enter your email address:
Exit Notification / Disclaimer Policy
- The Centers for Disease Control and Prevention (CDC) cannot attest to the accuracy of a non-federal website.
- Linking to a non-federal website does not constitute an endorsement by CDC or any of its employees of the sponsors or the information and products presented on the website.
- You will be subject to the destination website's privacy policy when you follow the link.
- CDC is not responsible for Section 508 compliance (accessibility) on other federal or private website.

Physics-based predictive tool will speed up battery and superconductor research
From lithium-ion batteries to next-generation superconductors, the functionality of many modern, advanced technologies depends on the physical property known as intercalation. Unfortunately, it's difficult to identify in advance which of the many possible intercalated materials are stable, which necessitates a lot of trial-and-error lab work in product development.
Now, in a study recently published in ACS Physical Chemistry Au , researchers from the Institute of Industrial Science, The University of Tokyo, and collaborating partners have devised a straightforward equation that correctly predicts the stability of intercalated materials. The systematic design guidelines enabled by this work will speed up the development of upcoming high-performance electronics and energy-storage devices.
To appreciate the research team's achievement, we need to understand the context of this research. Intercalation is the reversible insertion of guests (atoms or molecules) into hosts (for example, 2D-layered materials). The purpose of intercalation is commonly to modify the host's properties or structure for improved device performance, as seen in, for example, commercial lithium-ion batteries. Although many synthetic methods are available for preparing intercalated materials, researchers have had no reliable means of predicting which host-guest combinations are stable. Therefore, much lab work has been needed to devise new intercalated materials for imparting next-generation device functionalities. Minimizing this lab work by proposing a straightforward predictive tool for host-guest stability was the goal of the research team's study.
"We are the first to develop accurate predictive tools for host-guest intercalation energies, and the stability of intercalated compounds," explains Naoto Kawaguchi, lead author of the study. "Our analysis, based on a database of 9,000 compounds, uses straightforward principles from undergraduate first-year chemistry."
A particular highlight of the work is that only two guest properties and eight host-derived descriptors were necessary for the researchers' energy and stability calculations. In other words, initial 'best guesses' weren't necessary; only the underlying physics of the host-guest systems. Furthermore, the researchers validated their model against nearly 200 sets of regression coefficients.
"We're excited because our regression model formulation is straightforward and physically reasonable," says Teruyasu Mizoguchi, senior author. "Other computational models in the literature lack a physical basis or validation against unknown intercalated compounds."
This work is an important step forward in minimizing the laborious lab work that's typically required to prepare intercalated materials. Given that many current and upcoming energy storage and electronic devices depend on such materials, the time and expense required for corresponding research and development will be minimized. Consequently, products with advanced functionalities will reach the market faster than what has been previously possible.
- Materials Science
- Thermodynamics
- Computer Modeling
- Spintronics Research
- Mathematical Modeling
- Quantum number
- Materials science
- Supercomputer
- Introduction to quantum mechanics
- Security engineering
Story Source:
Materials provided by Institute of Industrial Science, The University of Tokyo . Note: Content may be edited for style and length.
Journal Reference :
- Naoto Kawaguchi, Kiyou Shibata, Teruyasu Mizoguchi. Unraveling the Stability of Layered Intercalation Compounds through First-Principles Calculations: Establishing a Linear Free Energy Relationship with Aqueous Ions . ACS Physical Chemistry Au , 2024; DOI: 10.1021/acsphyschemau.3c00063
Cite This Page :
Explore More
- Extremely Fast Wound Healing: New Treatment
- Micro-Lisa! Novel Nano-Scale Laser Writing
- Simple Brain-Computer Link: Gaming With Thoughts
- Clinical Reasoning: Chatbot Vs Physicians
- Understanding People Who Can't Visualize
- Illuminating Oxygen's Journey in the Brain
- DNA Study IDs Descendants of George Washington
- Heart Disease Risk: More Than One Drink a Day
- Unlocking Supernova Stardust Secrets
- Why Do Some Memories Become Longterm?
Trending Topics
Strange & offbeat.
Catherine Murphy part of Quadrennial Review of the National Nanotechnology Initiative
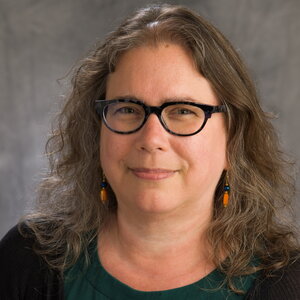
By Samantha Jones Toal
Interim Director of the Beckman Institute for Advanced Science and Technology Catherine Murphy was recently appointed to a National Academies of Sciences, Engineering, and Medicine ad hoc committee to conduct a quadrennial review of the National Nanotechnology Initiative.
Nanotechnology is the branch of science and engineering devoted to designing, producing and using structures, devices and systems by manipulating atoms and molecules at nanoscale — a scale that’s challenging to conceptualize given the average sheet of paper is 100,000 nanometers thick. Yet these microscopic alterations are responsible for applications such as the semiconductor chips that enable current computer technologies and the efficient delivery of breast cancer medicines.
“The national focus on nanotechnology began in 2003 when the Nanotechnology Research and Development Act was put into effect, allocating significant resources to the field,” Murphy said. “This year’s review [of the national technology initiative] is going to be very important. When they’ve met in the past the initiative was like a toddler, and then a teenager, and now it’s transitioned to adulthood.”
Aside from acting as interim director at Beckman, Murphy is a University of Illinois Urbana-Champaign faculty member researching materials chemistry, inorganic chemistry and biophysical chemistry alongside nanotechnology. She is a professor and the Larry R. Faulkner Endowed Chair in Chemistry; a professor in the Departments of Bioengineering, Materials Science and Engineering, and Biomedical and Translational Sciences; and an affiliate of the Materials Research Lab, Holonyak Micro & Nanotechnology Lab, and Center for Advanced Study.
“As someone in academia, it’s fun to be a part of a big picture initiative,” Murphy said. “It’s also a benefit to meet peers you wouldn’t come into contact with otherwise. Oftentimes, it’s these unique opportunities that enable scientific collaboration.”
Murphy will be joined on the committee by esteemed academics and nanotechnology industry experts from across the country, as a committee reviews the National Nanotechnology Initiative once every four years. Murphy and the rest of the committee will meet on a weekly basis to analyze the current nanotechnology trends, opportunities and emerging use cases, as well as determine the state of nanotechnology infrastructure.
The team will report on a variety of factors such as job opportunities for nanotechnology experts, as well as the global impact on U.S. nanotechnology research. The committee will author a report to share with the Nanoscale Science, Engineering, and Technology Subcommittee of the White House National Science and Technology Council and the National Nanotechnology Coordination Office aimed at improving research and development strategy and using research findings to bolster the economy and national security.
“I joined the committee because it could be that we determine best practices on training, staffing or infrastructure requirements that have a significant impact down the line,” Murphy said. “But more than anything, I’m excited to have a say in the future. The committee is all volunteer-based and will be putting in significant work this year to support and expand the field.”
Related People
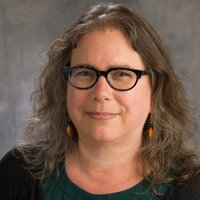
- Awards and Honors
- Women in Chemistry
- Analytical Chemistry
- Inorganic Chemistry
- Materials Chemistry
More Department News
- Catherine Murphy part of Quadrennial Review of the National Nanotechnology Initiative The Interim Director of the Beckman Institute for Advanced Science and Technology, chemistry Prof. Catherine Murphy was recently appointed to a National Academies of Sciences, Engineering, and Medicine ad hoc committee to conduct a quadrennial review of the National Nanotechnology Initiative. Read full story
- Chemistry students receive Stoesser Lecture Poster Session Awards Three graduate students in the Department of Chemistr received Stoesser Lecture Poster Session Awards. Suritra Bandyopadhyay, a PhD student in Prof. Jeff Chan’s lab, received the Top Presentation Award. And PhD students Amy Wahlmeier and Citlali Gutierrez from Prof. Liviu Mirica’s lab were runners... Read full story
- Painting a molecular portrait of the brain with mass spectrometry and deep learning Beckman researchers led by bioengineering Prof. Fan Lam, chemistry Prof. Jonathan Sweedler, and Beckman Institute Graduate Fellow Richard Xie used a biochemical imaging framework integrated with deep learning to create 3D molecular maps with cell specificity to better understand how the brain... Read full story

Nanotechnology
How to Reduce Exposures When 3D Printing with Plastic Filament
Technical Report: Occupational Exposure Sampling for Engineered Nanomaterials
Current Intelligence Bulletin 70: Health Effects of Occupational Exposure to Silver Nanomaterials
3D Printing with Filaments: Health and Safety Questions to Ask
3D Printing with Metal Powders: Health and Safety Questions to Ask
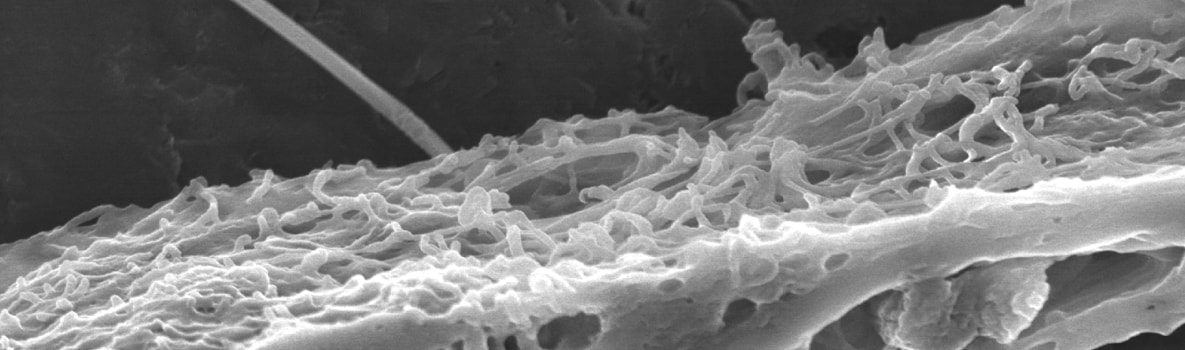
What is nanotechnology?
Nanotechnology is the manipulation of matter on a near-atomic scale to produce new structures, materials and devices. The technology promises scientific advancement in many sectors such as medicine, consumer products, energy, materials, and manufacturing. Nanotechnology refers to engineered structures, devices, and systems. Nanomaterials have a length scale between 1 and 100 nanometers. At this size, materials begin to exhibit unique properties that affect physical, chemical, and biological behavior. Researching, developing, and utilizing these properties is at the heart of new technology.
What are the risks to workers?
Workers within nanotechnology-related industries may be exposed to uniquely engineered materials. This includes materials with new sizes, shapes, and physical and chemical properties. Occupational health risks associated with manufacturing and using nanomaterials are not yet clearly understood. More research is needed to understand the impact of nanotechnology on health, and to determine appropriate exposure monitoring and control strategies. At this time, the limited evidence available suggests caution when potential exposures to free–unbound nanoparticles may occur.
What is Known?
Studies have indicated that low solubility nanoparticles are more toxic than larger particles on a mass for mass basis. Particle surface area and surface chemistry are strong indicators for observed responses in cell cultures and animals. Studies suggests that some nanoparticles can move from the respiratory system to other organs. Research is continuing to understand how these unique properties may lead to specific health effects.
The NIOSH Effort: The Nanotechnology Research Center
NIOSH, through its Nanotechnology Research Center (NTRC) , conducts research and provides guidance on the occupational safety and health implications and applications of advanced materials and nanotechnology. The Center is supported by a diverse group of scientists from across NIOSH.
The NTRC focuses on:
- Increasing the understanding of hazards and health risks to nanomaterial workers.
- Preventing occupational exposures to advanced materials such as nanomaterials through a better understanding of control and protective technologies.
NTRC activities include:
- Creating and implementing a strategic plan for investigating critical issues.
- Coordinating the NIOSH research effort.
- Developing research partnerships.
- Disseminating information and guidance.
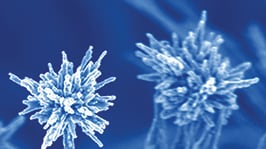
Access NIOSH nanotechnology publications, reports, and guidance.

Learn more about NIOSH efforts in 10 areas of nanotechnology.
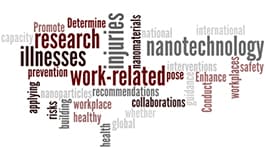
Get answers to frequently asked questions about nanotechnology and worker health.
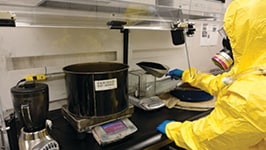
Learn more about how to partner with NIOSH for an on-site assessment.
Exit Notification / Disclaimer Policy
- The Centers for Disease Control and Prevention (CDC) cannot attest to the accuracy of a non-federal website.
- Linking to a non-federal website does not constitute an endorsement by CDC or any of its employees of the sponsors or the information and products presented on the website.
- You will be subject to the destination website's privacy policy when you follow the link.
- CDC is not responsible for Section 508 compliance (accessibility) on other federal or private website.
- Frontiers in Pharmacology
- Ethnopharmacology
- Research Topics
Natural Products and Nanoparticles in Cancer Treatment: Is There a Light at the End of the Tunnel?
Total Downloads
Total Views and Downloads
About this Research Topic
Cancer is a complex disease that affects millions of people worldwide. Despite significant advances in cancer treatment, the search for effective and safe therapies continues. Natural products have been used for centuries to treat various diseases, including cancer. These products are derived from plants, ...
Keywords : multidrug resistance, anticancer, natural products, nanoparticles, herbal medicines, synergistic effects
Important Note : All contributions to this Research Topic must be within the scope of the section and journal to which they are submitted, as defined in their mission statements. Frontiers reserves the right to guide an out-of-scope manuscript to a more suitable section or journal at any stage of peer review.
Topic Editors
Topic coordinators, recent articles, submission deadlines, participating journals.
Manuscripts can be submitted to this Research Topic via the following journals:
total views
- Demographics
No records found
total views article views downloads topic views
Top countries
Top referring sites, about frontiers research topics.
With their unique mixes of varied contributions from Original Research to Review Articles, Research Topics unify the most influential researchers, the latest key findings and historical advances in a hot research area! Find out more on how to host your own Frontiers Research Topic or contribute to one as an author.

- SUGGESTED TOPICS
- The Magazine
- Newsletters
- Managing Yourself
- Managing Teams
- Work-life Balance
- The Big Idea
- Data & Visuals
- Reading Lists
- Case Selections
- HBR Learning
- Topic Feeds
- Account Settings
- Email Preferences
Research: How Different Fields Are Using GenAI to Redefine Roles
- Maryam Alavi
Examples from customer support, management consulting, professional writing, legal analysis, and software and technology.
The interactive, conversational, analytical, and generative features of GenAI offer support for creativity, problem-solving, and processing and digestion of large bodies of information. Therefore, these features can act as cognitive resources for knowledge workers. Moreover, the capabilities of GenAI can mitigate various hindrances to effective performance that knowledge workers may encounter in their jobs, including time pressure, gaps in knowledge and skills, and negative feelings (such as boredom stemming from repetitive tasks or frustration arising from interactions with dissatisfied customers). Empirical research and field observations have already begun to reveal the value of GenAI capabilities and their potential for job crafting.
There is an expectation that implementing new and emerging Generative AI (GenAI) tools enhances the effectiveness and competitiveness of organizations. This belief is evidenced by current and planned investments in GenAI tools, especially by firms in knowledge-intensive industries such as finance, healthcare, and entertainment, among others. According to forecasts, enterprise spending on GenAI will increase by two-fold in 2024 and grow to $151.1 billion by 2027 .
- Maryam Alavi is the Elizabeth D. & Thomas M. Holder Chair & Professor of IT Management, Scheller College of Business, Georgia Institute of Technology .
Partner Center

An official website of the United States government
The .gov means it’s official. Federal government websites often end in .gov or .mil. Before sharing sensitive information, make sure you’re on a federal government site.
The site is secure. The https:// ensures that you are connecting to the official website and that any information you provide is encrypted and transmitted securely.
- Publications
- Account settings
Preview improvements coming to the PMC website in October 2024. Learn More or Try it out now .
- Advanced Search
- Journal List

Nanotechnology: A Revolution in Modern Industry
Shiza malik.
1 Bridging Health Foundation, Rawalpindi 46000, Pakistan
Khalid Muhammad
2 Department of Biology, College of Science, UAE University, Al Ain 15551, United Arab Emirates
Yasir Waheed
3 Office of Research, Innovation, and Commercialization (ORIC), Shaheed Zulfiqar Ali Bhutto Medical University (SZABMU), Islamabad 44000, Pakistan
4 Gilbert and Rose-Marie Chagoury School of Medicine, Lebanese American University, Byblos 1401, Lebanon
Associated Data
Not applicable.
Nanotechnology, contrary to its name, has massively revolutionized industries around the world. This paper predominantly deals with data regarding the applications of nanotechnology in the modernization of several industries. A comprehensive research strategy is adopted to incorporate the latest data driven from major science platforms. Resultantly, a broad-spectrum overview is presented which comprises the diverse applications of nanotechnology in modern industries. This study reveals that nanotechnology is not limited to research labs or small-scale manufacturing units of nanomedicine, but instead has taken a major share in different industries. Companies around the world are now trying to make their innovations more efficient in terms of structuring, working, and designing outlook and productivity by taking advantage of nanotechnology. From small-scale manufacturing and processing units such as those in agriculture, food, and medicine industries to larger-scale production units such as those operating in industries of automobiles, civil engineering, and environmental management, nanotechnology has manifested the modernization of almost every industrial domain on a global scale. With pronounced cooperation among researchers, industrialists, scientists, technologists, environmentalists, and educationists, the more sustainable development of nano-based industries can be predicted in the future.
1. Introduction
Nanotechnology has slowly yet deeply taken over different industries worldwide. This rapid pace of technological revolution can especially be seen in the developed world, where nano-scale markets have taken over rapidly in the past decade. Nanotechnology is not a new concept since it has now become a general-purpose technology. Four generations of nanomaterials have emerged on the surface and are used in interdisciplinary scientific fields; these are active and passive nanoassemblies, general nanosystems, and small-scale molecular nanosystems [ 1 ].
This rapid development of nanoscience is proof that, soon, nano-scale manufacturing will be incorporated into almost every domain of science and technology. This review article will cover the recent advanced applications of nanotechnology in different industries, mainly agriculture, food, cosmetics, medicine, healthcare, automotive, oil and gas industries, chemical, and mechanical industries [ 2 , 3 ]. Moreover, a brief glimpse of the drawbacks of nanotechnology will be highlighted for each industry to help the scientific community become aware of the ills and benefits of nanotechnology side by side. Nanotechnology is a process that combines the basic attributes of biological, physical, and chemical sciences. These processes occur at the minute scale of nanometers. Physically, the size is reduced; chemically, new bonds and chemical properties are governed; and biological actions are produced at the nano scale, such as drug bonding and delivery at particular sites [ 4 , 5 ].
Nanotechnology provides a link between classical and quantum mechanics in a gray area called a mesoscopic system. This mesoscopic system is being used to manufacture nanoassemblies of nature such as agricultural products, nanomedicine, and nanotools for treatment and diagnostic purposes in the medical industry [ 6 ]. Diseases that were previously untreatable are now being curtailed via nano-based medications and diagnostic kits. This technology has greatly affected bulk industrial manufacturing and production as well. Instead of manufacturing materials by cutting down on massive amounts of material, nanotechnology uses the reverse engineering principle, which operates in nature. It allows the manufacturing of products at the nano scale, such as atoms, and then develops products to work at a deeper scale [ 7 ].
Worldwide, millions and billions of dollars and euros are being spent in nanotechnology to utilize the great potential of this new science, especially in the developed world in Europe, China, and America [ 8 ]. However, developing nations are still lagging behind as they are not even able to meet the industrial progression of the previous decade [ 9 ]. This lag is mainly because these countries are still fighting economically, and they need some time to walk down the road of nanotechnology. However, it is pertinent to say that both the developed and developing world’s scientific communities agree that nanotechnology will be the next step in technological generation [ 10 ]. This will make further industrial upgrading and investment in the field of nanotechnology indispensable in the coming years.
With advances in science and technology, the scientific community adopts technologies and products that are relatively cheap, safe, and cleaner than previous technologies. Moreover, they are concerned about the financial standing of technologies, as natural resources in the world are shrinking excessively [ 11 ]. Nanotechnology thus provides a gateway to this problem. This technology is clear, cleaner, and more affordable compared to previous mass bulking and heavy machinery. Moreover, nanotechnology holds the potential to be implemented in every aspect of life. This will mainly include nanomaterial sciences, nanoelectronics, and nanomedicine, being inculcated in all dimensions of chemistry and the physical and biological world [ 12 ]. Thus, it is not wrong to predict that nanotechnology will become a compulsory field of study for future generations [ 13 ]. This review inculcates the basic applications of nanotechnology in vital industries worldwide and their implications for future industrial progress [ 14 ].
2. Nanotechnology Applications
2.1. applications of nanotechnology in different industries.
After thorough and careful analyses, a wide range of industries—in which nanotechnology is producing remarkable applications—have been studied, reviewed, and selected to be made part of this review. It should be notified that multiple subcategories of industrial links may be discussed under one heading to elaborate upon the wide-scale applications of nanotechnology in different industries. A graphical abstract at the beginning of this article indicates the different industries in which nanotechnology is imparting remarkable implications, details of which are briefly discussed under different headings in the next session.
2.2. Nanotechnology and Computer Industry
Nanotechnology has taken its origins from microengineering concepts in physics and material sciences [ 15 ]. Nanoscaling is not a new concept in the computer industry, as technologists and technicians have been working for a long time to design such modified forms of computer-based technologies that require minimum space for the most efficient work. Resultantly, the usage of nanotubes instead of silicon chips is being increasingly experimented upon in computer devices. Feynman and Drexler’s work has greatly inspired computer scientists to design revolutionary nanocomputers from which wide-scale advantages could be attained [ 13 ]. A few years ago, it was an unimaginable to consider laptops, mobiles, and other handy gadgets as thin as we have today, and it is impossible for even the common man to think that with the passage of time, more advanced, sophisticated, and lighter computer devices will be commonly used. Nanotechnology holds the potential to make this possible [ 16 ].
Energy-efficient, sustainable, and urbanized technologies have been emerging since the beginning of the 21st century. The improvement via nanotechnology in information and communication technology (ICT) is noteworthy in terms of the improvements achieved in interconnected communities, economic competitiveness, environmental stability during demographic shifts, and global development [ 17 ]. The major implications of renewable technology incorporate the roles of ICT and nanotechnology as enablers of environmental sustainability. The traditional methods of product resizing, re-functioning, and enhanced computational capabilities, due to their expensiveness and complicated manufacturing traits, have slowly been replaced by nanotechnological renovations. Novel technologies such as smart sensors logic elements, nanochips, memory storage nanodevices, optoelectronics, quantum computing, and lab-on-a-chip technologies are important in this regard [ 18 ].
Both private and public spending are increasing in the field of nanocomputing. The growth of marketing and industrialization in the biotechnology and computer industries are running in parallel, and their expected growth rates for the coming years are far higher. Researchers and technologists believe that by linking the advanced field of nanotechnology and informatics and computational industries, various problems in human society such as basic need fulfillment can be easily accomplished in line with the establishment of sustainable goals by the end of this decade [ 19 ]. The fourth industrial revolution is based upon the supporting pillars derived from hyperphysical systems including artificial intelligence, machine learning, the internet of things, robots, drones, cloud computing, fast internet technologies (5G and 6G), 3D printing, and block chain technologies [ 20 ].
Most of these technologies have a set basis in computing, nanotechnology, biotechnology, material science renovations, and satellite technologies. Nanotechnology offers useful alterations in the physiochemical, mechanical, magnetic, electrical, and optical properties of computing materials which enable innovative and newer products [ 21 ]. Thus, nanotechnology is providing a pathway for another broad-spectrum revolution in the field of automotive, aerospace, renewable energy, information technology, bioinformatics, and environmental management, all of which have root origins from nanotechnological improvements in computers. Sensors involved in software and data algorithms employ nanomaterials to induce greater sensitivity and processabilities with minimal margin-to-machine errors [ 22 ]. Nanomaterials provide better characteristics and robustness to sensor technologies which mean they are chemically inert, corrosion-resistant, and have greater tolerance profiles toward temperature and alkalinity [ 22 ].
Moreover, the use of semiconductor nanomaterials in the field of quantum computing has increased overall processing speeds with better accuracy and transmissibility. These technologies offer the creation of different components and communication protocols at the nano level, which is often called the internet of nano things [ 23 ]. This area is still in a continuous development and improvement phase with the potential for telecommunication, industrial, and medical applications. This field has taken its origin from the internet of things, which is a hyperphysical world of sensors, software, and other related technologies which allow broad-scale communication via internet operating devices [ 17 ]. The applications of these technologies range from being on the simple home scale to being on the complex industrial scale. The internet of things is mainly capable of gathering and distributing large-scale data via internet-based equipment and modern gadgets. In short, the internet of nano things is applicable to software, hardware, and network connection which could be used for data manipulation, collection, and sharing across the globe [ 24 ].
Another application of nanotechnology in the computer and information industry comes in the form of artificial intelligence, machine learning, and big data platforms which have set the basis for the fourth industrial revolution. Vast amounts of raw data are collected through interconnected robotic devices, sensors, and machines which have properties of nanomaterials [ 18 ]. After wide-scale data gathering, the next step is the amalgamation of the internet of things and the internet of people to prepare a greater analysis, understanding, and utilization of the gathered information for human benefit [ 4 ]. Such data complications can be easily understood through the use of big data in the medical industry, in which epidemiological data provide benefits for disease management [ 2 ]. Yet another example is the applications in business, where sales and retail-related data help to elucidate the target markets, sales industry, and consumer behavioral inferences for greater market consumption patterns [ 19 ].
Similarly, an important dimension of nanotechnology and computer combination comes in the form of drone and robotics technology. These technologies have a rising number of applications in maintenance, inspections, transportation, deliverability, and data inspection [ 25 ]. Drones, robots, and the internet of things are being perfectly amalgamated with the industrial sector to achieve greater goals. Drones tend to be more mobile but rely more on human control as compared to robots, which are less mobile but have larger potential for self-operation [ 26 ]. However, now, more mobile drones with better autonomous profiles are being developed to help out in the domain of manufacturing industries. These devices intensify and increase the pace of automation and precision in industries along with providing the benefits of lower costs and fewer errors [ 24 ]. The integrated fields of robotics, the internet of things, and nanotechnology are often called the internet of robotics and nano things. This field of nanorobotics is increasing the flexibility and dexterity in manufacturing processes compared to traditional robotics [ 25 ].
Drones, on the contrary, help to manage tasks that are otherwise difficult or dangerous to be managed by humans, such as working from a far distance or in dangerous regions. Nanosensors help to equip drones with the qualities of improved detection and sensation more precisely than previous sensor technologies [ 21 , 27 ]. Moreover, the over-potential of working hours, battery, and maintenance have also been improved with the operationalization of nano-based sensors in drone technology. These drones are inclusively used for various purposes such as maintaining operations, employing safety profiling, security surveys, and mapping areas [ 18 ]. However, limitations such as high speed, legal and ethical limitations, safety concerns, and greater automobility are some of the drawbacks of aerial and robotic drone technologies [ 26 ].
Three-dimensional printing is yet another important application of the nanocomputer industry, in which an integrated modus operandi works to help in production management [ 28 ]. Nanotechnology-based 3D printing offers the benefits of an autonomous, integrated, intelligent exchange network of information which enables wide-scale production benefits. These technologies have enabled a lesser need for industrial infrastructure, minimized post-processing operations, reduced waste material generation, and reduced need for human presence for overall industrial management [ 28 , 29 ]. Moreover, the benefits of 3D printing and similar technologies have potentially increased flexibility in terms of customized items, minimal environmental impacts, and sustainable practices with lower resource and energy consumption. The use of nano-scale and processed resins, metallic raw material, and thermoplastics along with other raw materials allow for customized properties of 3D printing technology [ 29 ].
The application of nanotechnology in computers cannot be distinguished from other industrial applications, because everything in modern industries is controlled by a systemic network in association with a network of computers and similar technologies. Thus, the fields of electronics, manufacturing, processing, and packaging, among several others, are interlinked with nanocomputer science [ 11 , 15 ]. Silicon tubes have had immense applications that revolutionized the industrial revolution in the 20th century; now, the industrial revolution is in yet another revolutionary phase based on nanostructures [ 16 ]. Silicon tubes have been slowly replaced with nanotubes, which are allowing a great deal of improvement and efficiency in computing technology. Similarly, lab-on-a-chip technology and memory chips are being formulated at nano scales to lessen the storage space but increase the storage volume within a small, flexible, and easily workable chip in computers for their subsequent applications in multiple other industries.
Hundreds of nanotechnology computer-related products have been marketed in the last 20 years of the nanotechnological revolution [ 30 ]. Modern industries such as textiles, automotive, civil engineering, construction, solar technologies, environmental applications, medicine, transportation agriculture, and food processing, among others are largely reaping the benefits of nano-scale computer chips and other devices. In simple terms, everything out there in nanoindustrial applications has something to do with computer-based applications in the nanoindustry [ 31 , 32 , 33 ]. Thus, all the applications discussed in this review more or less originate from nanocomputers. These applications are enabling considerable improvement and positive reports within the industrial sector. Having said that, it is hoped that computer scientists will remain engaged and will keep on collaborating with scientists in other fields to further explore the opportunities associated with nanocomputer sciences.
2.3. Nanotechnology and Bioprocessing Industries
Scientific and engineering rigor is being carried out to the link fields of nanotechnology with contributions to the bioprocessing industry. Researchers are interested in how the basics of nanomaterials could be used for the high-quality manufacturing of food and other biomaterials [ 15 , 34 ]. Pathogenic identification, food monitoring, biosensor devices, and smart packaging materials, especially those that are reusable and biodegradable, and the nanoencapsulation of active food compounds are only a few nanotechnological applications which have been the prime focus of the research community in recent years. Eventually, societal acceptability and dealing with social, cultural, and ethical concerns will allow the successful delivery of nano-based bio-processed products into the common markets for public usage [ 20 , 35 ].
With the increasing population worldwide, food requirements are increasing in addition to the concerns regarding the production of safe, healthy, and recurring food options. Sensors and diagnostic devices will help improve the sensitivity in food quality monitoring [ 36 ]. Moreover, the fake industrial application of food products could be easily scanned out of a system with the application of nanotechnology which could control brand protection throughout bio-processing [ 6 ]. The power usage in food production might also be controlled after a total nanotechnological application in the food industry. The decrease in power consumption would ultimately be positive for the environment. This could directly bring in the interplay of environment, food, and nanotechnology and would help to reduce environmental concerns in future [ 37 ].
One of the important implications of nanotechnology in bioprocessing industries can be accustomed to fermentation processes; these technologies are under usage for greater industrial demand and improved biomolecule production at a very low cost, unlike traditional fermentation processes [ 35 ]. The successful implementation and integration of fermentation and nanotechnology have allowed the development of biocompatible, safe, and nontoxic substances and nanostructures with wide-scale application in the field of food, bioprocessing, and winemaking industries [ 38 ]. Another important application is in the food monitoring and food supply chain management, present in various subsectors such as production, storage, distribution, and toxicity management. Nanodevices and nanomaterials are incorporated into chemical and biological sensor technologies to improve overall analytical performance with regard to parameters such as response time, sensitivity, selectivity, accuracy, and reliability [ 39 ]. The conventional methods of food monitoring are slowly being replaced with modern nano-based materials such as nanowires, nanocomposites, nanotubes, nanorods, nanosheets, and other materials that function to immobilize and label components [ 40 ]. These methods are either electrochemically or optically managed. For food monitoring, several assays are proposed and implemented with their roots in nano-based technologies; they may include molecular and diagnostic assays, immunological assays, and electrochemical and optical assays such as surface-enhanced Raman scattering and colorimetry technologies [ 34 ]. Materials ranging from heavy materials to microorganisms, pesticides, allergens, and antibiotics are easily monitored during commercial processing and bioprocessing in industries.
Additionally, nanotechnology has presented marvelous transformations in bio-composting materials. With the rising demand for biodegradable composites worldwide to reduce the environmental impact and increase the efficiency of industrial output, there is an increasing need for sustainable technologies [ 41 ]. Nanocomposites are thus being formulated with valuable mechanical properties better than conventional polymers, thus establishing their applicability in industries. The improved properties include optical, mechanical, catalytic, electrochemical, and electrical ones [ 42 ]. These biodegradable polymers are not only used in bioprocessing industries to create food products with relevant benefits but are also being deployed in the biomedical field, therapeutic industries, biotechnology base tissue engineering field, packing, sensor industries, drug delivery technology, water remediation, food industries, and cosmetics industries as well [ 2 , 24 , 34 , 43 ]. These nanocomposites have outstanding characteristics of biocompatibility, lower toxicities, antimicrobial activity, thermal resistance, and overall improved biodegradation properties which make them worthy of applications in products [ 44 ]. However, it is still imperative to conduct wide-scale toxicity and safety profiling for these and other nanomaterials to ensure the safety requirements, customer satisfaction, and public benefit are met [ 44 ].
Moreover, the advancement of nanotechnology has also been conferred to the development of functional food items. The exposure and integration of nanotechnology and the food industry have resulted in larger quantities of sustainable, safer, and healthier food products for human consumption, which is a growing need for the rising population worldwide [ 45 ]. The overall positive impact of nanotechnology in food processing, manufacturing, packing, pathogenic detection, monitoring, and production profiles necessitates the wide-scale application of this technology in the food industry worldwide [ 4 , 41 ]. Recent research has shown how the delivery of bioactive compounds and essential ingredients is and can be improved by the application of nanomaterials (nanoencapsulation) in food products [ 46 ]. These technologies improve the protection performance and sensitivity of bioactive ingredients while preventing unnecessary interaction with other constituents of foods, thus establishing clear-cut improved bioactivity and solubility profiles of nanofoods, thereby improving human health benefits. However, it should be kept in mind that the safety regards of these food should be carefully regulated with safety profiling, as they directly interact with human bodies [ 47 ].
2.4. Nanotechnology and Agri-Industries
Agriculture is the backbone of the economies of various nations around the globe. It is a major contributing factor to the world economy in general and plays a critical role in population maintenance by providing nutritional needs to them. As global weather patterns are changing owing to the dramatic changes caused by global warming, it is accepted that agriculture will be greatly affected [ 48 ]. Under this scenario, it is always better to take proactive measures to make agricultural practices more secure and sustainable than before. Modern technology is thus being employed worldwide. Nanotechnology has also come to play an effective role in this interplay of sustainable technologies. It plays an important role during the production, processing, storing, packaging, and transport of agricultural industrial products [ 49 ].
Nanotechnology has introduced certain precision farming techniques to enhance plant nutrients’ absorbance, alongside better pathogenic detection against agricultural diseases. Fertilizers are being improved by the application of nanoclays and zeolites which play effective roles in soil nutrient broths and in the restoration soil fertility [ 49 ]. Modern concepts of smart seeds and seed banks are also programmed to germinate under favorable conditions for their survival; nanopolymeric mixtures are used for coating in these scenarios [ 50 ]. Herbicides, pesticides, fungicides, and insecticides are also being revolutionized through nanotechnology applications. It has also been considered to upgrade linked fields of poultry and animal husbandry via the application of nanotechnology in treatment and disinfection practices.
2.5. Nanotechnology and Food Industry
The applications of nanotechnology in the food industry are immense and include food manufacturing, packaging, safety measures, drug delivery to specific sites [ 51 ], smart diets, and other modern preservatives, as summarized in Figure 1 . Nanomaterials such as polymer/clay nanocomposites are used in packing materials due to their high barrier properties against environmental impacts [ 52 ]. Similarly, nanoparticle mixtures are used as antimicrobial agents to protect stored food products against rapid microbial decay, especially in canned products. Similarly, several nanosensor and nano-assembly-based assays are used for microbial detection processes in food storage and manufacturing industries [ 53 ].

Nanotechnology applications in food and interconnected industries.
Nanoassemblies hold the potential to detect small gasses and organic and inorganic residues alongside microscopic pathogenic entities [ 54 ]. It should, however, be kept in mind that most of these nanoparticles are not directly added to food species because of the risk of toxicity that may be attached to such metallic nanoparticles. Work is being carried out to predict the toxicity attached, so that in the future, these products’ market acceptability could be increased [ 55 ]. With this, it is pertinent to say that nanotechnology is rapidly taking steps into the food industry for packing, sensing, storage, and antimicrobial applications [ 56 ].
Nanotechnology is also revolutionizing the dairy industry worldwide [ 57 ]. An outline of potential applications of nanotechnology in the dairy industry may include: improved processing methods, improved food contact and mixing, better yields, the increased shelf life and safety of dairy-based products, improved packaging, and antimicrobial resistance [ 58 ]. Additionally, nanocarriers are increasingly applied to transfer biologically active substances, drugs, enhanced flavors, colors, odors, and other food characteristics to dairy products [ 59 ].
These compounds exhibit higher delivery, solubility, and absorption properties to their targeted system. However, the problem of public acceptability due to the fear of unknown or potential side effects associated with nano-based dairy and food products needs to be addressed for the wider-scale commercialization of these products [ 60 ].
2.5.1. Nanotechnology, Poultry and Meat Industry
The poultry industry is a big chunk of the food industry and contributes millions of dollars every year to food industries around the world. Various commercial food chains are running throughout the world, the bases of which start from healthy poultry industries. The incidence of widespread foodborne diseases that originate from poultry, milk, and meat farms is a great concern for the food industry. Nanobiotechnology is certainly playing a productive role in tackling food pathogens such as those which procreate from Salmonella and Campylobacter infections by allowing increased poultry consumption while maintaining the affordability and safety of manufactured chicken products [ 61 ]. Several nano-based tools and materials such as nano-enabled disinfectants, surface biocides, protective clothing, air and water filters, packaging materials, biosensors, and detective devices are being used to confirm the authenticity and traceability of poultry products [ 62 ]. Moreover, nano-based materials are used to reduce foodborne pathogens and spoilage organisms before the food becomes part of the supply chain [ 63 ].
2.5.2. Nanotechnology—Fruit and Vegetable Industry
As already described, nanotechnology has made its way far ahead in the food industry. The agricultural, medicinal, and fruit and vegetable industries cannot remain unaffected under this scenario. Scientists are trying to increase the shelf life of fresh organic products to fulfill the nutritional needs of a growing population. From horticulture to food processing, packaging, and pathogenic detection technology, nanotechnology plays a vital role in the safety and production of vegetables and fruits [ 64 ].
Conventional technologies are now being replaced with nanotechnology due to their benefits of cost-effectiveness, satisfactory results, and overall shelf life improvement compared to past practices. Although some risks may be attached, nanotechnology has not yet reported high-grade toxicity to organic fresh green products. These technologies serve the purpose of providing safe and sufficient food sources to customers while reducing postharvest wastage, which is a major concern in developing nations [ 55 ]. Nanopackaging provides the benefits of lower humidity, oxygen passage, and optimal water vapor transmission rates. Hence, in the longer run, the shelf life of such products is increased to the desired level using nanotechnology [ 65 ].
2.5.3. Nanotechnology and Winemaking Industry
The winemaking industry is a big commercial application of the food industry worldwide. The usage of nanotechnology is also expanding in this industry. Nanotechnology serves the purpose of sensing technology through employment as nanoelectronics, nanoelectrochemical, and biological, amperometric, or fluorimetric sensors. These nanomaterials help to analyze the wine components, including polyphenols, organic acids, biogenic amines, or sulfur dioxide, and ensure they are at appropriate levels during the production of wine and complete processing [ 66 ].
Efforts are being made to further improve sensing nanotechnology to increase the accuracy, selectivity, sensitivity, and rapid response rate for wine sampling, production, and treatment procedures [ 53 ]. Specific nanoassemblies that are used in winemaking industries include carbon nanorods, nanodots, nanotubes, and metallic nanoparticles such as gold, silver, zinc oxide, iron oxide, and other types of nanocomposites. Recent research studies have introduced the concept of electronic tongues, nanoliquid chromatography, mesoporous silica, and applications of magnetic nanoparticles in winemaking products [ 67 ]. An elaborative account of these nanomaterials is out of the scope of the present study; however, on a broader scale, it is not wrong to say that nanotechnology is successfully reaping in the field of enology.
2.6. Nanotechnology and Packaging Industries
The packaging industry is continuously under improvement since the issue of environmentalism has been raised around the globe. Several different concerns are linked to the packaging industry; primarily, packaging should provide food safety to deliver the best quality to the consumer end. In addition, packaging needs to be environmentally friendly to reduce the food-waste-related pollution concern and to make the industrial processes more sustainable. Trials are being carried out to reduce the burden by replacing non-biodegradable plastic packaging materials with eco-friendly organic biopolymer-based materials which are processed at the nano scale to incur the beneficial properties of nanotechnology [ 68 ].
The nanomanufacturing of packaging biomaterials has proven effective in food packaging industries, as nanomanufacturing not only contributes to increasing food safety and production but also tackles environmental issues [ 69 ]. Some examples of these packaging nanomaterials may include anticaking agents, nanoadditives, delivery systems for nutraceuticals, and many more. The nanocompositions of packing materials are formed by mixing nanofillers and biopolymers to enhance packaging’s functionality [ 70 ]. Nanomaterials with antimicrobial properties are preferred in these cases, and they are mixed with a polymer to prevent the contamination of the packaged material. It is important to mention here that this technology is not only limited to food packaging; instead, packaging nanotechnology is now also being introduced in certain other industries such as textile, leather, and cosmetic industries in which it is providing large benefits to those industries [ 64 ].
2.7. Nanotechnology and Construction Industry and Civil Engineering
Efficient construction is the new normal application for sustainable development. The incorporation of nanomaterials in the construction industry is increasing to further the sustainability concern [ 71 ]. Nanomaterials are added to act as binding agents in cement. These nanoparticles enhance the chemical and physical properties of strength, durability, and workability for the long-lasting potential of the construction industry. Materials such as silicon dioxide which were previously also in use are now manufactured at the nano scale [ 71 ]. These nanostructures along with polymeric additives increase the density and stability of construction suspension [ 72 ]. The aspect of sustainable development is being applied to the manufacture of modern technologies coupled with beneficial applications of nanotechnology. This concept has produced novel isolative and smart window technologies which have driven roots in nanoengineering, such as vacuum insulation panels (VIPs) and phase change materials (PCMs), which provide thermal insulation effects and thus save energy and improve indoor air quality in homes [ 73 ].
A few of the unique properties of nanomaterials in construction include light structure, strengthened structural composition, low maintenance requirements, resistant coatings, improved pipe and bridge joining materials, improved cementitious materials, extensive fire resistance, sound absorption, and insulation properties, as well as the enhanced reflectivity of glass surfaces [ 74 ]. As elaborated under the heading of civil engineering applications, concrete’s properties are the most commonly discussed and widely changing in the construction industry because of concrete’s minute structure, which can be easily converted to the nano scale [ 75 ]. More specifically, the combination of nano-SiO 2 in cement could improve its performance in terms of compressiveness, large volumes with increased compressiveness, improved pore size distribution, and texture strength [ 76 ].
Moreover, some studies are also being carried out to improve the cracking properties of concrete by the application of microencapsulated healing polymers, which reduce the cracking properties of cement [ 77 ]. Moreover, some other construction materials, such as steel, are undergoing research to change their structural composites through nano-scale manufacturing. This nanoscaling improves steel’s properties such as improved corrosion resistance, increased weldability, the ease of handling for designing building materials, and construction work [ 78 ]. Additionally, coating materials have been improved by being manufactured at the nano scale. This has led to different improved coating properties such as functional improvement; anticorrosive action; high-temperature, fire, scratch, and abrasion resistance; antibacterial and antifouling self-healing capabilities; and self-assembly, among other useful applications [ 79 ].
Nanotechnology improves the compressive flexural properties of cement and reduces its porosity, making it absorb less water compared to traditional cementation preparations. This is because of the high surface-to-volume ratio of nanosized particles. Such an approach helps in reducing the amount of cement in concrete, making it more cost-effective, more strengthening, and eco-friendly, known as ‘green concrete’. Besides concrete, the revolutionary characteristics of nanotechnology are now also being adopted in other construction materials such as steel, glass, paper, wood, and multiple other engineering materials to upgrade the construction industry [ 80 ].
Similarly, carbon nanotubes, nanorods, and nanofibers are rapidly replacing steel constructions. These nanostructures along with nanoclay formations increase the mechanical properties and thus have paved the way for a new branch of civil engineering in terms of nanoengineering [ 80 ]. Apart from cement formulations, nanoparticles are included in repair mortars and concrete with healing properties that help in crack recovery in buildings. Furthermore, nanostructures, titanium dioxide, zinc, and other metallic oxides are being employed for the production of photocatalytic products with antipathogenic, self-cleaning, and water- and germ-repellent built-in technologies [ 33 ]. Similarly, quantum dot technologies are progressively employed for solar energy generation (a concept discussed later). These photovoltaic cells contribute to saving the maximum amount of solar energy [ 81 ].
2.8. Nanotechnology and Textiles Industry
The textile industry achieved glory in the 21st century with enormous outgrowth through social media platforms. Large brands have taken over the market worldwide, and millions are earned every year through textile industries. With the passing of time, nanotechnology is being slowly incorporated into the textile fiber industry owing to its unique and valuable properties. Previously, fabrics manufactured via conventional methods often curtailed the temporary effects of durability and quality [ 82 ]. However, the age of nanotechnology has allowed these fabric industries to employ nanotechnology to provide high durability, flexibility, and quality to clothes which is not lost upon laundering and wearing. The high surface-to-volume ratio of nanomaterials keeps high surface energy and thus provides better affinity to their fabrics, leading to long-term durability [ 82 ]. Moreover, a thin layering and coating of nanoparticles on the fabric make them breathable and make them smooth to the touch. This layering is carried out by processes such as printing, washing, padding, rinsing, drying, and curing to attach nanoparticles on the fabric surface. These processes are carried out to impart the properties of water repellence, soil resistance, flame resistance, hydrophobicity, wrinkle resistance, antibacterial and antistatic properties, and increased dyeability to the clothes [ 83 ].
The unique properties of nanomaterials in textile industries have attracted large-scale businesses for the financial benefits attached to their application. For this reason, competitors are increasing in nanotextile industry speedily, which may make the conventional textile industry sidelined in the near future [ 84 ]. Some benefits associated with nanotextile engineering and industry may include: improved cleaning surfaces, soil, wrinkle, stain, and color damage resistance, higher wettability and strike-through characteristics, malodor- and soil-removal abilities, abrasion resistance, a modified version of surface friction, and color enhancement through nanomaterials [ 85 ].
These characteristics have hugely improved the functionality and performance characteristics of textile and fiber materials [ 86 ]. Based upon the numerous advantages, nanotextile technology is increasingly being used in various inter-related fields, including in medical clothes, geotextiles, shock-resistant textiles, and fire-resistant and water-resistant textiles [ 87 ]. These textiles and fibers help overcome severe environmental conditions in special industries where high temperatures, pressure, and other conditions are adjusted for manufacturing purposes. These textiles are now increasingly called smart clothes due to renewed nanotechnological application to traditional methods [ 88 ].
The increasing demand for durable, appealing, and functionally outstanding textile products with a couple of factors of sustainability has allowed science to incorporate nanotechnology in the textile sector. These nano-based materials offer textile properties such as stain-repellent, wrinkle-free textures and fibers’ electrical conductivity alongside guaranteeing comfort and flexibility in clothing [ 82 ]. The characteristics of nanomaterials are also exhibited in the form of connected garments creation that undergo sensations to respond to external stimuli through electrical, colorant, or physiological signals. Thus, a kind of interconnection develops between the fields of photonic, electrical, textile and nanotechnologies [ 89 ]. Their interconnected applications confer the properties of high-scale performance, lasting durability, and connectivity in textile fibers. However, the concerns of nanotoxicity, the chances of the release of nanomaterials during washing, and the overall environmental impact of nanotextiles are important challenges that need to be ascertained and dealt with successfully in the coming years to ensure wide-scale acceptance and the global broad-spectrum application of nanotextiles [ 90 ].
The global market for the textile industry is constantly on the rise; with so many new brands, the competition is rising in regard to pricing, material, product outlook, and market exposure. Under this scenario, nanotechnology has contributed in terms of value addition to textiles by contributing the properties of water repellence, self-cleaning, and protection from radiation and UV light, along with safety against flames and microorganisms [ 82 ]. A whole new market of smart clothes is slowly taking our international markets along with improvements in textile machinery and economic standing. These advances have effectively established the sustainable character of the textile industry and have created grounds to meet the customer’s demand [ 91 ]. Some important examples of smart clothing originating from the nanotextile industry can be seen in products such as bulletproof jackets, fabric coatings, and advanced nanofibers. Fabric coatings and pressure pads can exhibit characteristics of invisibility and entail a silver, nickel, or gold nanoparticle-based material with inherent antimicrobial properties [ 92 ]. Such materials are effectively being utilized and introduced into the medical industry for bandages, dressings, etc. [ 92 ].
Similarly, woven optical fibers are already making progress in the textile and IT industry. With the incorporation of nanomaterials, optical fibers are being utilized for a range of purposes such as light transmission, sensing technologies, deformation, improved formational characteristic detection, and long-range data transmission. These optical fibers with phase-changing material properties can also be utilized for thermostability maintenance in the fiber industry. Thus, these fibers have combined applications in the computer, IT, and textile sectors [ 93 ]. In addition, the nano cellulosic material that is naturally obtained from plants confers properties of stiffness, strength, durability, and large surface area to volume ratios, which is acquired through the large number of surface hydroxyl groups embedded in nanocellulose particles [ 94 ]. Moreover, the characteristics of high resistance, lower weight, cost-effectiveness, and electrical conductivity are some additional benefits which are also linked to these nanocellulosic fibers [ 93 ]. The aforementioned technologies will allow industrialists to manufacture fabrics based on nanomaterials through a variety of chemical, physical, and biological processes. The scope of improvement in the textile properties, cost, and production methods is making the nanotextile industry a strong field of interest for future industrial investments.
2.9. Nanotechnology and Transport and Automobile Industry
The automotive industry is always improving its production. Nanotechnology is one such tool that could impart the automotive industry with a totally new approach to manufacturing. Automobile shaping could be improved greatly without any changes to the raw materials used. The replacement of conventional fabrication procedures with advanced nanomanufacturing is required to achieve the required outcome. Nanotechnology intends to partly renovate the automobile industry by enhancing the technical performance and reducing production costs excessively. However, there is a gap in fully harnessing the potential of nanomaterials in the automotive industry. Industrialists who were previously strict about automotive industrial principles are ready to employ novelties attached to nanotechnology to create successful applications to automobiles in the future [ 95 ]. Nanotechnology could provide assistance in manufacturing methods with an impartment of extended life properties. Cars that have been manufactured with nanotechnology applications have shown lower failure rates and enhanced self-repairing properties. Although the initial investment in the nanoautomated industry is high, the outcomes are enormous.
The concept of sustainable transport could also be applied to the manufacturing of such nano-based technology which is CO 2 free and imparts safe driving and quiet, clean, and wider-screen cars, which, in the future, may be called nanocars. The major interplay of nanotechnology and the automotive industry comes in the manufacturing of car parts, engines, paints, coating materials, suspensions, breaks, lubrication, and exhaust systems [ 32 ]. These properties are largely imparted via carbon nanotubes and carbon black, which renders new functionalities to automobiles. These products were previously in use, but nanoscaling and nanocoating allow for enhanced environmental, thermal, and mechanical stability to be imparted to the new generation of automobiles. In simple terms, automobiles manufactured with principal nanonovelties could result in cars with less wearing risk, better gliding potential, thinner coating lubrication requirements, and long service bodies with weight reductions [ 31 ]. These properties will ultimately reduce costs and will impart more space for improved automobile manufacturing in the future. Similarly, the development of electric cars and cars built on super capacitor technology is increasingly based on nanotechnology. The implications of nanotechnology in the form of rubber fillers, body frames made of light alloys, nanoelectronic components, nanocoatings of the interior and exterior of cars, self-repairing materials against external pressure, nanotextiles for interiors, and nanosensors are some of the nanotechnological-based implications of the automotive industry [ 96 ]. Owing to these properties, nanotechnology ventures are rapidly progressing in the automobile industry. It is expected that, soon, the automobile industry will commercialize nanotechnological perspectives on their branding strategies.
2.10. Nanotechnology, Healthcare, and Medical Industry
The genesis of nanomedicine simply cannot be ignored when we talk about the large fields of biological sciences, biotechnology, and medicine. Nanotechnology is already making its way beyond the imagination in the broader vision of nanobiotechnology. The quality of human life is continuously improved by the successful applications of nanotechnology in medicine, and resultantly, the entire new field of nanomedicine has come to the surface, which has allowed scientists to create upgraded versions of diagnostics, treatment, screening, sequencing, disease prevention, and proactive actions for healthcare [ 97 ]. These practices may also involve drug manufacturing, designing, conjugation, and efficient delivery options with advances in nano-based genomics, tissue engineering, and gene therapy. With this, it could be predicted that soon, nanomedicine will be the foremost research interest for the coming generation of biologists to study the useful impacts and risks that might be associated with them [ 98 ]. As illustrated in Figure 2 , we summarized the applications of nanotechnology in different subfields of the medical industry.

Nanotechnology applications in medical industry. Nanotechnology has a broad range of applications in various diagnostics and treatments using nanorobotics and drug delivery systems.
In various medical procedures, scientists are exploring the potential benefits of nanotechnology. In the field of medical tools, various robotic characters have been applied which have their origins in nano-scale computers, such as diagnostic surfaces, sensor technologies, and sample purification kits [ 99 ]. Similarly, some modifications are being accepted in diagnostics with the development of devices that are capable of working, responding, and modifying within the human body with the sole purpose of early diagnosis and treatment. Regenerative medicine has led to nanomanufacturing applications in addition to cell therapy and tissue engineering [ 100 ]. Similarly, some latest technologies in the form of ‘lab-on-a-chip’, as elaborated upon earlier, are being introduced with large implications in different fields such as nanomedicine, diagnostics, dentistry, and cosmetics industries [ 101 ]. Some updated nanotechnology applications in genomics and proteomics fields have developed molecular insights into antimicrobial diseases. Moreover, medicine, programming, nanoengineering, and biotechnology are being merged to create applications such as surgical nanorobotics, nanobioelectrics, and drug delivery methods [ 102 ]. All of these together help scientists and clinicians to better understand the pathophysiology of diseases and to bring about better treatment solutions in the future.
Specifically, the field of nanocomputers and linked devices help to control activation responses and their rates in mechanical procedures [ 2 ]. Through these mechanical devices, specific actions of medical and dental procedures are executed accurately. Moreover, programmed nanomachines and nanorobots allow medical practitioners to carry out medical procedures precisely at even sub-cellular levels [ 4 ]. In diagnostics fields, the use of such nanodevices is expanding rapidly, which allows predictions to be made about disease etiology and helps to regulate treatment options [ 103 ]. The use of in vitro diagnosis allows increased efficiency in disease apprehension. Meanwhile, in in vivo diagnoses, such devices have been made which carry out the screening of diseased states and respond to any kind of toxicities or carcinogenic or pathological irregularities that the body faces [ 104 ].
Similarly, the field of regenerative medicine is employing nanomaterials in various medical procedures such as cell therapy, tissue engineering, and gene sequencing for the greater outlook of treatment and reparation of cells, tissues, and organs. Nanoassemblies have been recorded in research for applications in powerful tissue regeneration technologies with properties of cell adhesion, migration, and cellular differentiation [ 102 ]. Additionally, nanotechnology is being applied in antimicrobial (antibacterial and antiviral) fields. The microscopic abilities of these pathogens are determined through nano-scale technologies [ 100 ]. Greek medicinal practices have long been using metals to cure pathogenic diseases, but the field of nanotechnology has presented a new method to improve such traditional medical practices; for example, nanosized silver nanomaterials are being used to cure burn wounds owing to the easy penetration of nanomaterials at the cellular level [ 102 , 105 ].
In the field of bioinformatics and computational biology, genomic and proteomic technologies are elucidating molecular insights into disease management [ 106 ]. The scope of targeted and personalized therapies related to pathogenic and pathophysiological diseases have greatly provided spaces for nanotechnological innovative technologies [ 107 , 108 ]. They also incorporate the benefits of cost-effectiveness and time saving [ 109 ]. Similarly, nanosensors and nanomicrobivores are utilized for military purposes such as the detection of airborne chemical agents which could cause serious toxic outcomes otherwise [ 102 ]. Some nanosensors also serve a purpose similar to phagocytes to clear toxic pathogens from the bloodstream without causing septic shock conditions, especially due to the inhalation of prohibited drugs and banned substances [ 100 , 105 , 110 ]. These technologies are also used for dose specifications and to neutralize overdosing incidences [ 110 ] Nano-scale molecules work as anticancer and antiviral nucleoside analogs with or without other adjuvants [ 21 ].
Another application of nanotechnology in the medical industry is in bone regeneration technology. Scientists are working on bone graft technology for bone reformation and muscular re-structuring [ 111 , 112 ]. Principle investigations of biomineralization, collagen mimic coatings, collagen fibers, and artificial muscles and joints are being conducted to revolutionize the field of osteology and bone tissue engineering [ 113 , 114 ]. Similarly, drug delivery technologies are excessively considering nanoscaling options to improve drug delivery stability and pharmacodynamic and pharmacokinetic profiles at a large scale [ 110 ]. The use of nanorobots is an important step that allows drugs to travel across the circulatory system and deliver drug entities to specifically targeted sites [ 99 , 115 ]. Scientists are even working on nanorobots-based wireless intracellular and intra-nucleolar nano-scale surgeries for multiple malignancies, which otherwise remain incurable [ 102 ]. These nanorobotics can work at such a minute level that they can even cut a single neuronic dendrite without causing harm to complex neuronal networks [ 116 ].
Another important application of nanotechnology in the medical field is oncology. Nanotechnology is providing a good opportunity for researchers to develop such nanoagents, fluorescent materials, molecular diagnostics kits, and specific targeted drugs that may help to diagnose and cure carcinogenesis [ 104 ]. Scientists are trying various protocols of adjoining already-available drugs with nanoparticulate conjugation to enhance drug specificity and targeting in organs [ 104 , 107 , 117 ]. Nanomedicine acts as the carrier of hundreds of specific anticancerous molecules that could be projected at tumor sites; moreover, the tumor imaging and immunotherapy approaches linked with nanomedicine are also a potential field of interest when it comes to cancer treatment management [ 112 , 117 ]. A focus is also being drawn toward lessening the impact of chemotherapeutic drugs by increasing their tumor-targeting efficiency and improving their pharmacokinetic and pharmacodynamic properties [ 112 ]. Similarly, heat-induced ablation treatment against cancer cells alongside gene therapy protocols is also being coupled with nanorobotics [ 99 , 118 ]. Anticancerous drugs may utilize the Enhanced Permeation and Retention Effect (EPR effect) by applications of nano assemblies such as liposomes, albumin nanospheres, micelles, and gold nanoparticles, which confirms effective treatment strategies against cancer [ 119 ]. Such advances in nanomedicine will bring about a more calculated, outlined, and technically programmed field of nanomedicine through association and cooperation between physicians, clinicians, researchers, and technologies.
2.10.1. Nanoindustry and Dentistry
Nanodentistry is yet another subfield of nanomedicine that involves broad-scale applications of nanotechnology ranging from diagnosis, prevention, cure, prognosis, and treatment options for dental care [ 120 ]. Some important applications in oral nanotechnology include dentition denaturalization, hypersensitivity cure, orthodontic realignment problems, and modernized enameling options for the maintenance of oral health [ 2 , 121 ]. Similarly, mechanical dentifrobots work to sensitize nerve impulse traffic at the core of a tooth in real-time calculation and hence could regulate tooth tissue penetration and maintenance for normal functioning [ 122 ]. The functioning is coupled with programmed nanocomputers to execute an action from external stimuli via connection with localized internal nerve stimuli. Similarly, there are other broad-range applications of nanotechnology in tooth repair, hypersensitivity treatment, tooth repositioning, and denaturalization technologies [ 4 , 118 , 120 , 121 ]. Some of the applications of nanotechnology in the field of dentistry are elaborated upon in Figure 3 .

Nanotechnology applications in field of dentistry. Nanotechnology can be largely used in dentistry to repair and treat dental issues.
2.10.2. Nanotechnology and Cosmetics Industry
The cosmetics industry, as part of the greater healthcare industry, is continuously evolving. Nanotechnology-based renovations are progressively incorporated into cosmetics industries as well. Products are designed with novel formulations, therapeutic benefits, and aesthetic output [ 123 ]. The nanocosmetics industry employs the usage of lipid nanocarrier systems, polymeric or metallic nanoparticles, nanocapsules, nanosponges, nanoemulsions, nanogels, liposomes, aquasomes, niosomes, dendrimers, and fullerenes, etc., among other such nanoparticles [ 101 ]. These nanomaterials bring about specific characteristics such as drug delivery, enhanced absorption, improved esthetic value, and enhanced shelf life. The benefits of nanotechnology are greatly captured in the improvement of skin, hair, nail, lip, and dental care products, and those associated with hygienic concerns. Changes to the skin barrier have been largely curtailed owing to the function of the nano scale of materials. The nanosize of active ingredients allows them to easily permeate skin barriers and generate the required dermal effect [ 124 ].
More profoundly, nanomaterials’ application is encouraged in the production of sun-protective cosmetics products such as sunblock lotions and creams. The main ingredient used is the rational combination of cinnamates (derived from carnauba wax) and titanium dioxide nanosuspensions which provide sun-protective effects in cosmetics products [ 125 ]. Similarly, nanoparticle suspensions are being applied in nanostructured lipid carriers (NLCs) for dermal and pharmaceutical applications [ 126 ]. They exhibit the properties of controlled drug-carrying and realizing properties, along with direct drug targeting, occlusion, and increased penetration and absorption to the skin surface. Moreover, these carrier nanoemulsions exhibit excellent tolerability to intense environmental and body conditions [ 127 ]. Moreover, these lipid nanocarriers have been researched and declared safe for potential cosmetic and pharmaceutical applications. However, more research is still required to assess the risk/benefit ratio of their excessive application [ 128 ].
2.11. Nanotechnology Industries and Environment
The environment, society, and technology are becoming excessively linked under a common slogan of sustainable development. Nanotechnology plays a key role in the 21st century to modify the technical and experimental outlook of various industries. Environmental applications cannot stand still against revolutionary applications of nanotechnology. Since the environment has much to do with the physical and chemical world around a living being, the nano scale of products greatly changes and affects environmental sustainability [ 129 ]. The subsequent introduction of nanomaterials in chemistry, physics, biotechnology, computer science, and space, food, and chemical industries, in general, directly impacts environmental sciences.
With regard to environmental applications, the remarkable research and applications of nanotechnology are increasing in the processing of raw materials, product manufacturing, contaminate treatment, soil and wastewater treatment, energy storage, and hazardous waste management [ 130 ]. In developed nations, it is now widely suggested that nanotechnology could play an effective role in tackling environmental issues. In fact, the application of nanotechnology could be implemented for water and cell cleaning technologies, drinking safety measures, and the detoxification of contaminants and pollutants from the environment such as heavy metals, organochlorine pesticides, and solvents, etc., which may involve reprocessing although nanofiltration. Moreover, the efficiency and durability of materials can be increased with mechanical stress and weathering phenomena. Similarly, the use of nanocage-based emulsions is being used for optical imaging techniques [ 131 ].
In short, the literature provides immense relevance to how nanotechnology is proving itself through groundbreaking innovative technologies in environmental sciences. The focus, for now, is kept on remediation technologies with prime attention on water treatment, since water scarcity is being faced worldwide and is becoming critical with time. There is a need for the scientific community to actively conduct research on comprehending the properties of nanomaterials for their high surface area, related chemical properties, high mobility, and unique mechanical and magnetic properties which could be used for to achieve a sustainable environment [ 132 ].
2.12. Nanotechnology—Oil and Gas Industry
The oil and gas industry makes up a big part of the fossil industry, which is slowly depleting with the rising consumption. Although nanotechnology has been successfully applied to the fields of construction, medicine, and computer science, its application in the oil and gas industry is still limited, especially in exploration and production technologies [ 133 ]. The major issue in this industry is to improve oil recovery and the further exploitation of alternative energy sources. This is because the cost of oil production and further purification is immense compared to crude oil prices. Nanotechnologists believe that they could overcome the technological barriers to developing such nanomaterials that would help in curtailing these problems.
Governments are putting millions of dollars into the exploration, drilling, production, refining, wastewater treatment, and transport of crude oil and gas. Nanotechnology can provide assistance in the precise measurement of reservoir conditions. Similarly, nanofluids have been proven to exhibit better performance in oil production industries. Nanocatalyses enhance the separation processing of oil, water, and gases, thus bringing an efficient impurity removal process to the oil and gas industry. Nanofabrication and nanomembrane technologies are excessively being utilized for the separation and purification of fossil materials [ 134 ]. Finally, functional and modified nanomaterials can produce smart, cost-effective, and durable equipment for the processing and manufacturing of oil and gas. In short, there is immense ground for the improvement of the fossil fuel industry if nanotechnology could be correctly directed in this industry [ 135 ].
2.13. Nanotechnology and Renewable Energy (Solar) Industry
Renewable energy sources are the solutions to many environmental problems in today’s world. This makes the renewable energy industry a major part of the environmental industry. Subsequently, nanotechnology needs to be considered in the energy affairs of the world. Nanotechnologies are increasingly applied in solar, hydrogen, biomass, geothermal, and tidal wave energy production. Although, scientists are convinced that much more needs to be discovered before enhancing the benefits of coupled nanotechnology and renewable energy [ 136 ].
Nanotechnology has procured its application way down the road of renewable energy sources. Solar collectors have been specifically given much importance since their usage is encouraged throughout the world, and with events of intense solar radiation, the production and dependence of solar energy will be helpful for fulfilling future energy needs. Research data are available regarding the theoretical, numerical, and experimental approaches adopted for upgrading solar collectors with the employment of nanotechnologies [ 137 ].
These applications include the nanoengineering of flat solar plates, direct absorption plates, parabolic troughs, and wavy plates and heat pipes. In most of these instruments and solar collection devices, the use of nanofluids is becoming common and plays a crucial role in increasing the working efficiency of these devices. A gap, however, exists concerning the usage of nanomaterials in the useful manufacturing design of solar panels and their associated possible efficiencies which could be brought to the solar panel industry. Moreover, work needs to be done regarding the cost-effectiveness and efficiency analyses of traditional and nanotechnology-based solar devices so that appropriate measures could be adopted for the future generation of nanosolar collectors [ 138 ].
2.14. Nanotechnology and Wood Industry
The wood industry is one of the main economic drivers in various countries where forest growth is immense and heavy industrial setups rely on manufacturing and selling wood-based products [ 139 ]. However, the rising environmental concerns against deforestation are a major cause for researchers to think about a method for the sustainable usage of wood products. Hence, nanotechnology has set its foot in the wood industry in various applications such as the production of biodegradable materials in the paper and pulp industry, timber and furniture industry, wood preservatives, wood composites, and applications in lignocellulosic-based materials [ 140 ]. Resultantly, new products are introduced into the market with enhanced performance (stronger yet lighter products), increased economic potential, and reduced environmental impact.
One method of nano-based application in the wood industry is the derivation of nanomaterials directly from the forest, which is now called nanocellulose material, known broadly for its sustainable characteristics [ 141 ]. This factor has pushed the wood industry to convert cellulosic material to nanocellulose with increased strength, low weight, and increased electromagnetic response along with a larger surface area [ 142 ]. These characteristics are then further used as reinforcing agents in different subcategories of wood-based industries, including substrate, stabilizer, electronics, batteries, sensor technologies, food, medicine, and cosmetics industries [ 143 ]. Moreover, functional characteristics such as the durability, UV absorption, fire resistance, and decreased water absorption of wood-based biodegradable products are also being improved with the application of nanomaterials such as nanozinc oxide or nanotitanium oxide [ 144 ]. Similarly, wood biodegradable properties are reduced through the application of nanoencapsulated preservatives to improve the impregnation of wood with the increasing penetration of applied chemicals and a reduced leaching effect.
Cellulosic nanomaterials exhibit nanofibrillar structures which can be made multifunctional for application in construction, furniture, food, pharmaceuticals, and other wood-based industries [ 145 ]. Research is emerging in which promising results are predicted in different industries in which nanofibers, nanofillers, nanoemulsions, nanocomposites, and nano-scaled chemical materials are used to increase the potential advantages of manufactured wood products [ 146 ]. The outstanding properties of nanocellulusice materials have largely curtailed the environmental concerns in the wood industry in the form of their potential renewable characteristics, self-assembling properties, and well-defined architecture. However, there are a few challenges related to such industries, such as cost/benefit analyses, a lack of compatibility and acceptability from the public owing to a lack of proper commercialization, and a persistent knowledge gap in some places [ 145 ]. Therefore, more effort is required to increase the applications and acceptability of nano-based wood products in the market worldwide.
2.15. Nanotechnology and Chemical Industries
Nanotechnology can be easily applied to various chemical compositions such as polymeric substances; this application can bring about structural and functional changes in those chemical materials and can address various industrial applications including medicine, physics, electronics, chemical, and material industries, among others [ 76 , 132 , 138 ]. One such industrial application is in electricity production, in which different nanomaterials driven from silver, golden, and organic sources could be utilized to make the overall production process cheaper and effective [ 147 ]. Another effective application is in the coatings and textile industry, which has already been discussed briefly. In these industries, enzymatic catalysis in combination with nanotechnology accelerates reaction times, saving money and bringing about high-quality final products. Similarly, the water cleaning industry can utilize the benefits of nanomaterials in the form of silver and magnetic nanoparticles to create strong forces of attraction that easily separate heavy material from untreated water [ 148 ]. Similarly, there is a wide range of chemicals that can be potentially upgraded, although the nano scale for application in biomedical industries is discussed under the heading of nanotechnology and medicine.
Another major application of nanotechnology in the chemical industry includes the surfactant industry, which is used for cleaning paper, inks, agrochemicals, drugs, pharmaceuticals, and some food products [ 149 ]. The traditional surfactant application was of great environmental and health concern, but with the newer and improved manufacturing and nanoscaling of surfactants, environmentally friendly applications have been made possible. These newer types may include biosurfactants obtained via the process of fermentation and bio-based surfactants produced through organic manufacturing. More research is required to establish the risks and side effects of these nanochemical agents [ 3 ].
3. Closing Remarks
Nanotechnology, within a short period, has taken over all disciplinary fields of science, whether it is physics, biology, or chemistry. Now, it is predicted to enormously impact manufacturing technology owing to the evidential and proven benefits of micro scaling. Every field of industry, such as computing, information technology, engineering, medicine, agriculture, and food, among others, is now originating an entire new field in association with nanotechnology. These industries are widely known as nanocomputer, nanoengineering, nanoinformatics, nanobiotechnology, nanomedicine, nanoagriculture, and nanofood industries. The most brilliant discoveries are being made in nanomedicine, while the most cost-effective and vibrant technologies are being introduced in materials and mechanical sciences.
The very purpose of nanotechnology, in layman’s terms, is to ease out the manufacturing process and improve the quality of end products and processes. In this regard, it is easy and predictable that it is not difficult for nanotechnology to slowly take out most of the manufacturing process for industrial improvement. With every coming year, more high-tech and more effective-looking nanotechnologies are being introduced. This is smoothing out the basis of a whole new era of nanomindustries. However, the constructive need is to expand the research basis of nanoapplications to entail the rigorous possible pros of this technology and simultaneously figure out a method to deal with the cons of the said technology.
The miniaturization of computer devices has continued for many years and is now being processed at the nanometer scale. However, a gap remains to explore further options for the nanoscaling of computers and complex electronic devices, including computer processors. Moreover, there is an immense need to enable the controlled production and usage of such nanotechnologies in the real world, because if not, they could threaten the world of technology. Scientists should keep on working on producing nanoelectronic devices with more power and energy efficiency. This is important in order to extract the maximum benefits from the hands of nanotechnology and computer sciences [ 5 ].
Under the influence of nanotechnology, food bioprocessing is showing improvement, as proven by several scientific types of research and industrial applications in food chain and agricultural fields. Moreover, the aspect of sustainability is being introduced to convert the environment, food chains, processing industries, and production methods to save some resources for future generations. The usage of precision farming technologies based upon nanoengineering, modern nano-scale fertilizers, and pesticides are of great importance in this regard. Moreover, a combined nanotechnological aspect is also being successfully applied to the food industry, affecting every dimension of packing, sensing, storage, manufacturing, and antimicrobial applications. It is pertinent to say that although the applications of nanotechnology in the food, agriculture, winemaking, poultry, and associated packaging industries are immense, the need is to accurately conduct the risk assessment and potential toxicity of nanomaterials to avoid any damage to the commercial food chains and animal husbandry practices [ 63 ].
The exposure of the nano-based building industry is immense for civil and mechanical engineers; now, we need to use these technologies to actually bring about changes in those countries in which the population is immense, construction material is depleting, and environmental sustainability problems are hovering upon the state. By carefully assessing the sustainability potential of these nanomaterials, their environmental, hazardous, and health risks could be controlled, and they could likely be removed from the construction and automobile industry all over the world with sincere scientific and technical rigor [ 150 ]. It is expected that soon, the construction and automobile industry will commercialize the nanotechnological perspectives alongside sustainability features in their branding strategies. These nano-scale materials could allow the lifecycle management of automotive and construction industries with the provision of sustainable, safe, comfortable, cost-effective, and more eco-friendly automobiles [ 32 ]. The need is to explore the unacknowledged and untapped potential of nanotechnology applications in these industry industries.
Similarly, nanotechnology-based applications in consumer products such as textile and esthetics industries are immense and impressive. Professional development involves the application of nanotechnology-based UV-protective coatings in clothes which are of utmost need with climatic changes [ 73 ]. The application of nanotechnology overcomes the limitations of conventional production methods and makes the process more suitable and green-technology-based. These properties have allowed the textile companies to effectively apply nanotechnology for the manufacture of better products [ 90 ]. With greater consumer acceptability and market demand, millions are spent in the cosmetic industry to enable the further usage of nanotechnology. Researchers are hopeful that nanotechnology would be used to further upgrade the cosmetics industry in the near future [ 123 ].
Furthermore, the breakthrough applications of nanomedicine are not hidden from the scientific community. If nanomedicine is accepted worldwide in the coming years, then the hope is that the domain of diagnosis and treatment will become more customized, personalized, and genetically targeted for individual patients. Treatment options will ultimately become excessive in number and more successful in accomplishment. However, these assumptions will stay a dream if the research remains limited to scientific understanding.
The real outcome will be the application of this research into the experimental domain and clinical practices to make them more productive and beneficial for the medical industry. For this cause, a combined effort of technical ability, professional skills, research, experimentation, and the cooperation of clinicians, physicians, researchers, and technology is imperative. However, despite all functional beneficial characteristics, work needs to be done and more exploration is required to learn more about nanotechnology and its potential in different industries, especially nanomedicine, and to take into account and curtail the risks and harms attached to the said domain of science.
Additionally, climatic conditions, as mentioned before, along with fossil fuel depletion, have pushed scientists to realize a low-energy-consuming and more productive technological renovation in the form of nanoengineered materials [ 48 ]. Now, they are employing nanomaterials to save energy and harvest the maximum remaining natural resources. There is immense ground for the improvement of the fossil fuel industry if nanotechnology could be correctly directed in this industry [ 135 ]. The beneficial applications within the solar industry, gas and oil industry, and conversion fields require comparative cost-effectiveness and efficiency analyses of traditional and nano-based technologies so that appropriate measures could be adopted for the future generation of nano-based products in said industries [ 138 ].
As every new technology is used in industries, linked social, ethical, environmental, and human safety issues arise to halt the pace of progress. These issues need to be addressed and analyzed along with improving nanotechnology so that this technology easily incorporates into different industries without creating social, moral, and ethical concerns. Wide-scale collaboration is needed among technologists, engineers, biologists, and industrials for a prospective future associated with the wide-scale application of nanotechnology in diversified fields.
4. Conclusions
Highly cost-effective and vibrant nanotechnologies are being introduced in materials and mechanical sciences. A comprehensive overview of such technologies has been covered in this study. This review will help researchers and professionals from different fields to delve deeper into the applications of nanotechnology in their particular areas of interest. Indeed, the applications of nanotechnology are immense, yet the risks attached to unlimited applications remain unclear and unpronounced. Thus, more work needs to be linked and carefully ascertained so that further solutions can be determined in the realm of nanotoxicology. Moreover, it is recommended that researchers, technicians, and industrialists should cooperate at the field and educational level to explore options and usefully exploit nanotechnology in field experiments. Additionally, more developments should be made and carefully assessed at the nano scale for a future world, so that we are aware of this massive technology. The magnificent applications of nanotechnology in the industrial world makes one think that soon, the offerings of nanotechnology will be incorporated into every possible industry. However, there is a need to take precautionary measures to be aware of and educate ourselves about the environmental and pollution concerns alongside health-related harms to living things that may arise due to the deviant use of nanotechnology. This is important because the aspect of sustainability is being increasingly considered throughout the world. So, by coupling the aspect of sustainability with nanotechnology, a prosperous future of nanotechnology can be guaranteed.
Funding Statement
K.M.’s work is supported by United Arab Emirates University-UPAR-Grant#G3458, SURE plus Grant#3908 and SDG research programme grant#4065.
Author Contributions
Conceptualization, Y.W. methodology, S.M. validation, S.M., K.M. and Y.W. formal analysis, S.M., K.M. and Y.W. investigation, S.M., K.M. and Y.W. resources, K.M. and Y.W. data curation, S.M., K.M. and Y.W. writing—original draft preparation, S.M. writing—review and editing, S.M., K.M. and Y.W. supervision, Y.W. project administration, K.M. and Y.W. funding acquisition, Y.W. and K.M. All authors have read and agreed to the published version of the manuscript.
Institutional Review Board Statement
Informed consent statement, data availability statement, conflicts of interest.
The authors declare no conflict of interest.
Disclaimer/Publisher’s Note: The statements, opinions and data contained in all publications are solely those of the individual author(s) and contributor(s) and not of MDPI and/or the editor(s). MDPI and/or the editor(s) disclaim responsibility for any injury to people or property resulting from any ideas, methods, instructions or products referred to in the content.
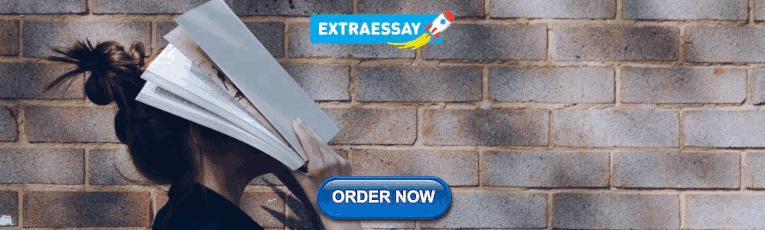
IMAGES
VIDEO
COMMENTS
Nanoparticles are particles that exist on a nanometre scale (i.e., below 100 nm in at least one dimension). They can possess physical properties such as uniformity, conductance or special optical ...
Nanotechnology-enabled Agriculture and its Environmental Impact. Xiaoping Xin. Zhenli He. Wiqar Ahmad. 534 views. An interdisciplinary journal across nanoscience and nanotechnology, at the interface of chemistry, physics, materials science and engineering. It focuses on new nanofabrication methods and their ap...
Journal of Nanoparticle Research is a peer-reviewed journal that delves into concepts, properties, phenomena, and processes of structures at the nanoscale. Covered topics include synthesis, assembly, transport, reactivity, and stability of nanoscale structures. Features applications, structures, and devices with novel functions via precursor ...
Selected Topics in Nanoscience and Nanotechnology contains a collection of papers in the subfields of scanning probe microscopy, nanofabrication, functional nanoparticles and nanomaterials, molecular engineering and bionanotechnology. Written by experts in their respective fields, it is intended for a general scientific readership who may be non-specialists in these subjects, but who want a ...
Recent research has focused on mesoporous nanomaterials for delivering therapeutic agents to tumor cells with little drug leakage into healthy cells. ... Summary of the recent topic on nanoparticles and nanostructured materials and their applications in bioimaging, biosensing, drug delivery, tissue engineering, antimicrobial, and agro-food ...
The COVID-19 mRNA vaccines put nanomedicine in the spotlight as a potential way to treat diseases like cancer and HIV. While the field isn't there yet, better design could help fulfill its promise.
Nanoparticles. Manoj Rawat, Nitin Jain, in Multifunctional Nanocarriers, 2022. 2.1.5 Particle shape. Nanoparticle attributes such as particle surface, charge, and size have an immense impact on a drug delivery system. Several authors [16-19] have conversed on the significance of nanoparticulate habit in drug delivery.Nanoparticle shapes have shown to have effects on nanoparticle drug ...
Current research into the role of engineered nanoparticles in drug delivery systems (DDSs) for medical purposes has developed numerous fascinating nanocarriers. ... Irregularities and toxicity and safety valuations will be the topic of development in the future. Nanotechnology will be in high demand. Nowadays, drug-targeted delivery through ...
2.2. Discovery of C, Ag, Zn, Cu, and Au nanoparticles. Carbon NPs were found in 1991, and Iijima and Ichihashi announced the single-wall carbon nanotube synthesis with a diameter of 1 nanometer in 1993 (Chen et al., 2021).Carbon nanotubes (CNTs), also known as Bucky tubes, are a kind of nanomaterial made up of a two-dimensional hexagonal lattice of carbon atoms.
Jul 2022. Musa Bashir. +4. Explore the latest full-text research PDFs, articles, conference papers, preprints and more on NANOPARTICLE RESEARCH. Find methods information, sources, references or ...
Nanotechnology for improving cell immunotherapy, CAR-T and others4. Using nanotechnology to deliver immunomodulatory molecules5. Using nanoparticles for diagnostics, tracking immune cells or quantification of immune signals6. Nanoparticle interaction with innate, adaptive or humoral immune mediators in the context of cancer7.
The remarkable success of two lipid nanoparticle-mRNA vaccines against coronavirus disease (COVID-19) has placed the therapeutic and prophylactic potential of messenger RNA (mRNA) in the spotlight. It has also drawn attention to the indispensable role of lipid nanoparticles in enabling the effects of this nucleic a Recent Review Articles Nanoscale 2024 Emerging Investigators
Importance and meaning. Nanoparticles are small particles ranging from 1 to 100 nm (nm) in size [].They are used in a wide range of applications and can be grouped into four types: 1) inorganic-based nanoparticles, 2) carbon-based nanoparticles, 3) organic/polymer nanoparticles, and 4) composite-based nanoparticles [].Inorganic-based nanoparticles are made up of different metal and metal oxides.
Areas to be covered in this Research Topic may include, but are not limited to: • Micro- and nanoparticles: synthesis, functionalization, characterization. • Tissue engineering and regenerative medicine. • Colloidal organic and inorganic formulations. • Cell-based therapy. • Drug and gene delivery. • Particles-based cell/transplant ...
The nanoparticles slowly release drugs that induce immunogenic cell death, or ICD, in tumors. ICD generates tumor antigens and other molecules to bring immune cells to a tumor's microenvironment.
The theoretically infinite compositional space of high-entropy alloys (HEAs) and their novel properties and applications have attracted significant attention from a broader research community. The successful synthesis of high-quality single-phase HEA nanoparticles represents a crucial step in fully unlocking the po Celebrating the scientific accomplishments of RSC Fellows
The nanoparticles slowly release drugs that induce immunogenic cell death, or ICD, in tumors. ICD generates tumor antigens and other molecules to bring immune cells to a tumor's microenvironment. The researchers have attached ATP to the nanoparticles, which also recruits immune cells to the tumor to initiate anti-tumor immune responses.
As the National Institute for Occupational Safety and Health (NIOSH) Nanotechnology Research Center (NTRC) marks its 20th anniversary, we celebrate the groundbreaking work of the Field Studies Team. Organized in 2006, the team began by evaluating potential workplace exposures to engineered nanomaterials.
Measurement Methods. Exposure Assessment. Fire and Explosion Safety. Recommendations & Guidance. Global Collaborations. Applications. Last Reviewed: May 19, 2023. Source: National Institute for Occupational Safety and Health. NIOSH has identified 10 critical topic areas to guide in addressing knowledge gaps, developing strategies, and providing ...
The anticancer potential of CuO NPs was the topic of Benguigui et al.'s research . Copper oxide(II) nanoparticles have been shown to be effective in the treatment of human pancreatic cancer in vitro and in vivo (mice model). ... The scientific community is also more interested in using well-known gold or silver nanoparticles in their research ...
Physics-based predictive tool will speed up battery and superconductor research. ScienceDaily . Retrieved April 1, 2024 from www.sciencedaily.com / releases / 2024 / 04 / 240401142457.htm
Interim Director of the Beckman Institute for Advanced Science and Technology Catherine Murphy was recently appointed to a National Academies of Sciences, Engineering, and Medicine ad hoc committee to conduct a quadrennial review of the National Nanotechnology Initiative. Nanotechnology is the branch of science and engineering devoted to designing, producing and using structures, devices and ...
Nanotechnology refers to engineered structures, devices, and systems. Nanomaterials have a length scale between 1 and 100 nanometers. At this size, materials begin to exhibit unique properties that affect physical, chemical, and biological behavior. Researching, developing, and utilizing these properties is at the heart of new technology.
Keywords: multidrug resistance, anticancer, natural products, nanoparticles, herbal medicines, synergistic effects . Important Note: All contributions to this Research Topic must be within the scope of the section and journal to which they are submitted, as defined in their mission statements.. Frontiers reserves the right to guide an out-of-scope manuscript to a more suitable section or ...
The interactive, conversational, analytical, and generative features of GenAI offer support for creativity, problem-solving, and processing and digestion of large bodies of information. Therefore ...
Abstract. Nanotechnology, contrary to its name, has massively revolutionized industries around the world. This paper predominantly deals with data regarding the applications of nanotechnology in the modernization of several industries. A comprehensive research strategy is adopted to incorporate the latest data driven from major science platforms.