- Search Menu
- Advance articles
- Editor's Choice
- Special Collections
- Author Guidelines
- Submission Site
- Open Access
- Reasons to submit
- About BioScience
- Journals Career Network
- Editorial Board
- Advertising and Corporate Services
- Self-Archiving Policy
- Potentially Offensive Content
- Terms and Conditions
- Journals on Oxford Academic
- Books on Oxford Academic

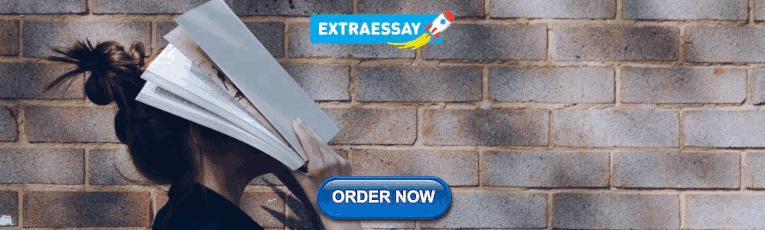
AIBS Announces Winner of the IDEAL Leadership Award
The American Institute of Biological Sciences is pleased to announce Dr. Nyeema C. Harris as the 2023 winner of its Inspiring Inclusivity, Diversity, Equity, Acceptance, and Learning (IDEAL) Leadership Award. The IDEAL Award recognizes commendable leadership in advancing inclusion, diversity, equity, acceptance, accessibility, and learning in the biological sciences community. The award was presented by past awardee Dr. Steward T. A. Pickett on 30 November 2023 at AIBS’s Council of Member Societies and Organizations meeting, entitled “Expanding the Idea.”
Latest Articles

Editor-in-Chief Charles Fenster
Senior Editor James Verdier
Impact Factor 10.1
5 year Imact Factor 10.8
Latest posts on X
Why publish with BioScience ?
Are you looking for a home for your research? Publish with BioScience and enjoy a variety of benefits, including:
- Reach a diverse and global audience
- High-impact research
- Distinguished and supportive editors
- Rigorous peer-review
I'm ready to learn more!

High-Impact Research Collection
Explore the most read, most cited, and most discussed articles published in BioScience in recent years and discover what has caught the interest of your peers.
Browse the collection

Science for Society
The AIBS collaborates with individuals and organizations to advance biology through a shared commitment to scientifically informed decision-making. In this collection of viewpoints and podcasts, we share the ongoing outreach of the past presidents of AIBS, a group that has long served society and the scientific research and education community.

BioScience Podcast Series
AIBS is pleased to announce BioScience Talks, a monthly podcast that features in-depth discussions of recently published BioScience articles and other science stories.
Listen to the latest episode

Email alerts
Register to receive table of contents email alerts as soon as new issues of BioScience are published online.

Recommend to your library
Fill out our simple online form to recommend BioScience to your library.
Recommend now

Read and Publish deals
Authors interested in publishing in BioScience may be able to publish their paper Open Access using funds available through their institution’s agreement with OUP.
Find out if your institution is participating

About the society
We are a non-profit 501(c)3 public charity organization that advances the biological sciences for the benefit of all science and society. We work with like-minded organizations, funding agencies, and political entities to promote the use of science to inform decision-making.
Related Titles
- Recommend to your Library
Affiliations
- Online ISSN 1525-3244
- Copyright © 2024 American Institute of Biological Sciences
- About Oxford Academic
- Publish journals with us
- University press partners
- What we publish
- New features
- Open access
- Institutional account management
- Rights and permissions
- Get help with access
- Accessibility
- Advertising
- Media enquiries
- Oxford University Press
- Oxford Languages
- University of Oxford
Oxford University Press is a department of the University of Oxford. It furthers the University's objective of excellence in research, scholarship, and education by publishing worldwide
- Copyright © 2024 Oxford University Press
- Cookie settings
- Cookie policy
- Privacy policy
- Legal notice
This Feature Is Available To Subscribers Only
Sign In or Create an Account
This PDF is available to Subscribers Only
For full access to this pdf, sign in to an existing account, or purchase an annual subscription.
When you choose to publish with PLOS, your research makes an impact. Make your work accessible to all, without restrictions, and accelerate scientific discovery with options like preprints and published peer review that make your work more Open.
- PLOS Biology
- PLOS Climate
- PLOS Complex Systems
- PLOS Computational Biology
- PLOS Digital Health
- PLOS Genetics
- PLOS Global Public Health
- PLOS Medicine
- PLOS Mental Health
- PLOS Neglected Tropical Diseases
- PLOS Pathogens
- PLOS Sustainability and Transformation
- PLOS Collections
- About This Blog
- Official PLOS Blog
- EveryONE Blog
- Speaking of Medicine
- PLOS Biologue
- Absolutely Maybe
- DNA Science
- PLOS ECR Community
- All Models Are Wrong
- About PLOS Blogs
Explore the latest research making an impact in your field

Our biological science research journals cover a broad range of topics, from animal biology and plant science to genetic engineering, immunology, and microbiology. Read some of the latest and most talked about research from across our portfolio.
Most mentioned
Read the most mentioned* articles from each of our journals in 2023 so far.
The manifold costs of being a non-native English speaker in science , PLOS Biology
A novel nematode species from the Siberian permafrost shares adaptive mechanisms for cryptobiotic survival with C. elegans dauer larva , PLOS Genetics
Time-travelling pathogens and their risk to ecological communities , PLOS Computational Biology
The viral origin of myalgic encephalomyelitis/chronic fatigue syndrome , PLOS Pathogens
Latest biological science research
Explore the latest articles from across our biological science journals, or browse all articles.
From PLOS Biology , Eggs of the mosquito Aedes aegypti survive desiccation by rewiring their polyamine and lipid metabolism
From PLOS Computational Biology , Rapid automated 3-D pose estimation of larval zebrafish using a physical model-trained neural network
From PLOS Pathogens , Divergence of TORC1-mediated stress response leads to novel acquired stress resistance in a pathogenic yeast
From PLOS Genetics , Wolbachia infection at least partially rescues the fertility and ovary defects of several new Drosophila melanogaster bag of marbles protein-coding mutants
Read the latest multidisciplinary research from across our journals:
*based on data from Altmetric, accessed October 2023.
Leave a Reply Cancel reply
Your email address will not be published. Required fields are marked *
Save my name and email for the next time I comment.
In this ‘behind the paper’ post, Stephanie Williams discusses how the new equipment, techniques and methods developed in her lab helped them…
In this ‘behind the paper’ post, Joe Ziminski discusses his collaborative experience while studying how our brain changes when we learn. The…
In this ‘behind the paper’ post, Olga Ponomarova discusses how testing many diverse hypothesis brought them to find the relationship between apparently…
Our researchers seek to create knowledge and advance the understanding of the life sciences and medicine and to apply this knowledge to enhance discovery and clinical outcomes.
Our faculty organize into 10 basic science and 14 clinical departments along with multiple Institutes and Centers. Co-located on a compact campus alongside world-renowned P hysical Sciences , a new Pritzker School of Molecular Engineering , the Polsky Center for Entrepreneurship and Innovation , twenty minutes from our colleagues at the Argonne National Labs and Fermi Labs , together with our affiliate Marine Biology Laboratory , the Biological Science Division provides a unique opportunity for training and research at the cutting edge of science, engineering, and medicine.
Quick Links
- Basic Science Departments
- Clinical Departments
- Office of the Dean for Basic Science
- COVID-19 Research Funding Opportunities
Research by the Numbers
Research Active Faculty
Average NIH Funding per PI in the Division
Total faculty in the Biological Sciences Division
Through our highly interdisciplinary institutes , we connect investigators from the Biological Sciences Division with faculty and students across the University and its affiliates and enable cutting edge research to thrive.
Our institutes enable groundbreaking discoveries through innovative technology and collaboration across the University which fosters innovation in the biological sciences that pushes the boundaries of traditional disciplines.
Explore our Institutes
- Duchossois Family Institute
- Neuroscience Institute
- Institute for Biophysical Dynamics
- Institute for Translational Medicine
Centers & Programs
Our Centers and programs bring together faculty, students, researchers, and staff to advance our mission through multidisciplinary research and innovative educational programs.
From centers housed within our departments to broad institutional initiatives, our Centers encompass all mission areas and bring together innovations in research, patient care, and education.
Explore our Centers
- Center for Translational Data Science
- Center for Global Health
- Center for Health and the Social Sciences
- University of Chicago Comprehensive Cancer Center
- MacLean Center for Medical Ethics
- Microbiome Center
- Program in Health Sciences Informatics
Resources for Researchers
Core facilities, office of research safety, research support, center for research informatics.
- Search by keyword
- Search by citation
Page 1 of 12
MicroRNA-148b secreted by bovine oviductal extracellular vesicles enhance embryo quality through BPM/TGF-beta pathway
Extracellular vesicles (EVs) and their cargoes, including MicroRNAs (miRNAs) play a crucial role in cell-to-cell communication. We previously demonstrated the upregulation of bta-mir-148b in EVs from oviductal...
- View Full Text
YME1L-mediated mitophagy protects renal tubular cells against cellular senescence under diabetic conditions
The senescence of renal tubular epithelial cells (RTECs) is crucial in the progression of diabetic kidney disease (DKD). Accumulating evidence suggests a close association between insufficient mitophagy and RT...
Effects of latroeggtoxin-VI on dopamine and α-synuclein in PC12 cells and the implications for Parkinson’s disease
Parkinson’s disease (PD) is characterized by death of dopaminergic neurons leading to dopamine deficiency, excessive α-synuclein facilitating Lewy body formation, etc. Latroeggtoxin-VI (LETX-VI), a proteinaceo...
Glial-restricted progenitor cells: a cure for diseased brain?
The central nervous system (CNS) is home to neuronal and glial cells. Traditionally, glia was disregarded as just the structural support across the brain and spinal cord, in striking contrast to neurons, alway...
Carbapenem-resistant hypervirulent ST23 Klebsiella pneumoniae with a highly transmissible dual-carbapenemase plasmid in Chile
The convergence of hypervirulence and carbapenem resistance in the bacterial pathogen Klebsiella pneumoniae represents a critical global health concern. Hypervirulent K. pneumoniae (hvKp) strains, frequently from...
Endometrial mesenchymal stromal/stem cells improve regeneration of injured endometrium in mice
The monthly regeneration of human endometrial tissue is maintained by the presence of human endometrial mesenchymal stromal/stem cells (eMSC), a cell population co-expressing the perivascular markers CD140b an...
Embryo development is impaired by sperm mitochondrial-derived ROS
Basal energetic metabolism in sperm, particularly oxidative phosphorylation, is known to condition not only their oocyte fertilising ability, but also the subsequent embryo development. While the molecular pat...
Fibroblasts inhibit osteogenesis by regulating nuclear-cytoplasmic shuttling of YAP in mesenchymal stem cells and secreting DKK1
Fibrous scars frequently form at the sites of bone nonunion when attempts to repair bone fractures have failed. However, the detailed mechanism by which fibroblasts, which are the main components of fibrous sc...
MSC-derived exosomes protect auditory hair cells from neomycin-induced damage via autophagy regulation
Sensorineural hearing loss (SNHL) poses a major threat to both physical and mental health; however, there is still a lack of effective drugs to treat the disease. Recently, novel biological therapies, such as ...
Alpha-synuclein dynamics bridge Type-I Interferon response and SARS-CoV-2 replication in peripheral cells
Increasing evidence suggests a double-faceted role of alpha-synuclein (α-syn) following infection by a variety of viruses, including SARS-CoV-2. Although α-syn accumulation is known to contribute to cell toxic...
Lactadherin immunoblockade in small extracellular vesicles inhibits sEV-mediated increase of pro-metastatic capacities
Tumor-derived small extracellular vesicles (sEVs) can promote tumorigenic and metastatic capacities in less aggressive recipient cells mainly through the biomolecules in their cargo. However, despite recent ad...
Integration of ATAC-seq and RNA-seq identifies MX1-mediated AP-1 transcriptional regulation as a therapeutic target for Down syndrome
Growing evidence has suggested that Type I Interferon (I-IFN) plays a potential role in the pathogenesis of Down Syndrome (DS). This work investigates the underlying function of MX1, an effector gene of I-IFN,...
The novel roles of YULINK in the migration, proliferation and glycolysis of pulmonary arterial smooth muscle cells: implications for pulmonary arterial hypertension
Abnormal remodeling of the pulmonary vasculature, characterized by the proliferation and migration of pulmonary arterial smooth muscle cells (PASMCs) along with dysregulated glycolysis, is a pathognomonic feat...
Electroacupuncture promotes neurogenesis in the dentate gyrus and improves pattern separation in an early Alzheimer's disease mouse model
Impaired pattern separation occurs in the early stage of Alzheimer’s disease (AD), and hippocampal dentate gyrus (DG) neurogenesis participates in pattern separation. Here, we investigated whether spatial memo...
Role of SYVN1 in the control of airway remodeling in asthma protection by promoting SIRT2 ubiquitination and degradation
Asthma is a heterogenous disease that characterized by airway remodeling. SYVN1 (Synoviolin 1) acts as an E3 ligase to mediate the suppression of endoplasmic reticulum (ER) stress through ubiquitination and de...
Advances towards the use of gastrointestinal tumor patient-derived organoids as a therapeutic decision-making tool
In December 2022 the US Food and Drug Administration (FDA) removed the requirement that drugs in development must undergo animal testing before clinical evaluation, a declaration that now demands the establish...
Melatonin alleviates pyroptosis by regulating the SIRT3/FOXO3α/ROS axis and interacting with apoptosis in Atherosclerosis progression
Atherosclerosis (AS), a significant contributor to cardiovascular disease (CVD), is steadily rising with the aging of the global population. Pyroptosis and apoptosis, both caspase-mediated cell death mechanism...
Prenatal ethanol exposure and changes in fetal neuroendocrine metabolic programming
Prenatal ethanol exposure (PEE) (mainly through maternal alcohol consumption) has become widespread. However, studies suggest that it can cause intrauterine growth retardation (IUGR) and multi-organ developmen...
Autologous non-invasively derived stem cells mitochondria transfer shows therapeutic advantages in human embryo quality rescue
The decline in the quantity and quality of mitochondria are closely associated with infertility, particularly in advanced maternal age. Transferring autologous mitochondria into the oocytes of infertile female...
Development of synthetic modulator enabling long-term propagation and neurogenesis of human embryonic stem cell-derived neural progenitor cells
Neural progenitor cells (NPCs) are essential for in vitro drug screening and cell-based therapies for brain-related disorders, necessitating well-defined and reproducible culture systems. Current strategies em...
Heat-responsive microRNAs participate in regulating the pollen fertility stability of CMS-D2 restorer line under high-temperature stress
Anther development and pollen fertility of cytoplasmic male sterility (CMS) conditioned by Gossypium harknessii cytoplasm (CMS-D2) restorer lines are susceptible to continuous high-temperature (HT) stress in sum...
Chemogenetic inhibition of NTS astrocytes normalizes cardiac autonomic control and ameliorate hypertension during chronic intermittent hypoxia
Obstructive sleep apnea (OSA) is characterized by recurrent episodes of chronic intermittent hypoxia (CIH), which has been linked to the development of sympathoexcitation and hypertension. Furthermore, it has ...
SARS-CoV-2 spike protein S1 activates Cx43 hemichannels and disturbs intracellular Ca 2+ dynamics
Severe acute respiratory syndrome coronavirus 2 (SARS-CoV-2) causes the ongoing coronavirus disease 2019 (COVID-19). An aspect of high uncertainty is whether the SARS-CoV-2 per se or the systemic inflammation ...
The effect of zofenopril on the cardiovascular system of spontaneously hypertensive rats treated with the ACE2 inhibitor MLN-4760
Angiotensin converting enzyme 2 (ACE2) plays a crucial role in the infection cycle of SARS-CoV-2 responsible for formation of COVID-19 pandemic. In the cardiovascular system, the virus enters the cells by bind...
Two murine models of sepsis: immunopathological differences between the sexes—possible role of TGFβ1 in female resistance to endotoxemia
Endotoxic shock (ExSh) and cecal ligature and puncture (CLP) are models that induce sepsis. In this work, we investigated early immunologic and histopathologic changes induced by ExSh or CLP models in female a...
An intracellular, non-oxidative factor activates in vitro chromatin fragmentation in pig sperm
In vitro incubation of epididymal and vas deferens sperm with Mn 2+ induces Sperm Chromatin Fragmentation (SCF), a mechanism that causes double-stranded breaks in toroid-linker regions (TLRs). Whether this mechani...
Focal ischemic stroke modifies microglia-derived exosomal miRNAs: potential role of mir-212-5p in neuronal protection and functional recovery
Ischemic stroke is a severe type of stroke with high disability and mortality rates. In recent years, microglial exosome-derived miRNAs have been shown to be promising candidates for the treatment of ischemic ...
S -Nitrosylation in endothelial cells contributes to tumor cell adhesion and extravasation during breast cancer metastasis
Nitric oxide is produced by different nitric oxide synthases isoforms. NO activates two signaling pathways, one dependent on soluble guanylate cyclase and protein kinase G, and other where NO post-translationa...
Identifying pyroptosis- and inflammation-related genes in intracranial aneurysms based on bioinformatics analysis
Intracranial aneurysm (IA) is the most common cerebrovascular disease, and subarachnoid hemorrhage caused by its rupture can seriously impede nerve function. Pyroptosis is an inflammatory mode of cell death wh...
Drosophila Atlastin regulates synaptic vesicle mobilization independent of bone morphogenetic protein signaling
The endoplasmic reticulum (ER) contacts endosomes in all parts of a motor neuron, including the axon and presynaptic terminal, to move structural proteins, proteins that send signals, and lipids over long dist...
Mucin1 induced trophoblast dysfunction in gestational diabetes mellitus via Wnt/β-catenin pathway
To elucidate the role of Mucin1 (MUC1) in the trophoblast function (glucose uptake and apoptosis) of gestational diabetes mellitus (GDM) women through the Wnt/β-catenin pathway.
Human umbilical cord mesenchymal stem cells (hUC-MSCs) alleviate paclitaxel-induced spermatogenesis defects and maintain male fertility
Chemotherapeutic drugs can cause reproductive damage by affecting sperm quality and other aspects of male fertility. Stem cells are thought to alleviate the damage caused by chemotherapy drugs and to play role...
Exploring the Neandertal legacy of pancreatic ductal adenocarcinoma risk in Eurasians
The genomes of present-day non-Africans are composed of 1–3% of Neandertal-derived DNA as a consequence of admixture events between Neandertals and anatomically modern humans about 50–60 thousand years ago. Ne...
Identification and analysis of key hypoxia- and immune-related genes in hypertrophic cardiomyopathy
Hypertrophic cardiomyopathy (HCM), an autosomal dominant genetic disease, is the main cause of sudden death in adolescents and athletes globally. Hypoxia and immune factors have been revealed to be related to ...

How do prolonged anchorage-free lifetimes strengthen non-small-cell lung cancer cells to evade anoikis? – A link with altered cellular metabolomics
Malignant cells adopt anoikis resistance to survive anchorage-free stresses and initiate cancer metastasis. It is still unknown how varying periods of anchorage loss contribute to anoikis resistance, cell migr...
Single nucleotide polymorphisms associated with wine fermentation and adaptation to nitrogen limitation in wild and domesticated yeast strains
For more than 20 years, Saccharomyces cerevisiae has served as a model organism for genetic studies and molecular biology, as well as a platform for biotechnology (e.g., wine production). One of the important eco...
Investigating the dark-side of the genome: a barrier to human disease variant discovery?
The human genome contains regions that cannot be adequately assembled or aligned using next generation short-read sequencing technologies. More than 2500 genes are known contain such ‘dark’ regions. In this st...
Hyperbaric oxygen treatment increases intestinal stem cell proliferation through the mTORC1/S6K1 signaling pathway in Mus musculus
Hyperbaric oxygen treatment (HBOT) has been reported to modulate the proliferation of neural and mesenchymal stem cell populations, but the molecular mechanisms underlying these effects are not completely unde...
Polar microalgae extracts protect human HaCaT keratinocytes from damaging stimuli and ameliorate psoriatic skin inflammation in mice
Polar microalgae contain unique compounds that enable them to adapt to extreme environments. As the skin barrier is our first line of defense against external threats, polar microalgae extracts may possess res...
Correction: Utility of melatonin in mitigating ionizing radiation‑induced testis injury through synergistic interdependence of its biological properties
The original article was published in Biological Research 2022 55 :33
Beyond energy provider: multifunction of lipid droplets in embryonic development
Since the discovery, lipid droplets (LDs) have been recognized to be sites of cellular energy reserves, providing energy when necessary to sustain cellular life activities. Many studies have reported large num...
Retraction Note: Tridax procumbens flavonoids: a prospective bioactive compound increased osteoblast differentiation and trabecular bone formation
Electroacupuncture protective effects after cerebral ischemia are mediated through mir-219a inhibition.
Electroacupuncture (EA) is a complementary and alternative therapy which has shown protective effects on vascular cognitive impairment (VCI). However, the underlying mechanisms are not entirely understood.
Topsoil and subsoil bacterial community assemblies across different drainage conditions in a mountain environment
High mountainous environments are of particular interest as they play an essential role for life and human societies, while being environments which are highly vulnerable to climate change and land use intensi...
Functional defects in hiPSCs-derived cardiomyocytes from patients with a PLEKHM2-mutation associated with dilated cardiomyopathy and left ventricular non-compaction
Dilated cardiomyopathy (DCM) is a primary myocardial disease, leading to heart failure and excessive risk of sudden cardiac death with rather poorly understood pathophysiology. In 2015, Parvari's group ident...
Human VDAC pseudogenes: an emerging role for VDAC1P8 pseudogene in acute myeloid leukemia
Voltage-dependent anion selective channels (VDACs) are the most abundant mitochondrial outer membrane proteins, encoded in mammals by three genes, VDAC1 , 2 and 3 , mostly ubiquitously expressed. As 'mitochondrial ...
ABCA1 transporter promotes the motility of human melanoma cells by modulating their plasma membrane organization
Melanoma is one of the most aggressive and deadliest skin tumor. Cholesterol content in melanoma cells is elevated, and a portion of it accumulates into lipid rafts. Therefore, the plasma membrane cholesterol ...
Acupuncture regulates the apoptosis of ovarian granulosa cells in polycystic ovarian syndrome-related abnormal follicular development through LncMEG3-mediated inhibition of miR-21-3p
The main features of polycystic ovary syndrome (PCOS) are abnormal follicular development and ovulatory dysfunction, which are caused by excessive apoptosis of ovarian granulosa cells. Acupuncture has been sho...
Cholic and deoxycholic acids induce mitochondrial dysfunction, impaired biogenesis and autophagic flux in skeletal muscle cells
Skeletal muscle is sensitive to bile acids (BA) because it expresses the TGR5 receptor for BA. Cholic (CA) and deoxycholic (DCA) acids induce a sarcopenia-like phenotype through TGR5-dependent mechanisms. Besi...
iPSCs ameliorate hypoxia-induced autophagy and atrophy in C2C12 myotubes via the AMPK/ULK1 pathway
Duchenne muscular dystrophy (DMD) is an X-linked lethal genetic disorder for which there is no effective treatment. Previous studies have shown that stem cell transplantation into mdx mice can promote muscle r...
- Editorial Board
- Manuscript editing services
- Instructions for Editors
- Sign up for article alerts and news from this journal
- Follow us on Twitter
- Follow us on Facebook
- ISSN: 0717-6287 (electronic)
Biological Research
ISSN: 0717-6287
- Submission enquiries: Access here and click Contact Us
- General enquiries: [email protected]
- Start Your Application
- Complete Your Application
- UChicago Undergrads
- Enrichment Programs
- Remote Online Programs
- Residential Programs
- Visiting Students
Research in the Biological Sciences (RIBS)
Immerse yourself in biological research, the deadline for the ribs program has passed, and we are no longer considering applications for admission to this course..
Students interested in Biology may be interested in courses in Immersion , Pre-College Connect , and Summer College .
Furthering the diagnosis and treatment of cancer, discovering how to preserve blood, laying the mathematical foundations for the theory of genetic evolution—the University of Chicago is a leader in these and in many other areas of research. This summer, learn the techniques used in cutting-edge biological research and work in person at lab facilities at a premier research university.
Program Description
This four-week intensive training program is designed to expose students to a broad range of molecular, microbiological, and cell biological techniques currently used in research laboratories. Students are immersed in the research experience, giving them a taste of "life at the bench." Using a project-based approach, the course progresses from a survey of basic lab techniques to the application of current molecular techniques in cell biology, genetics, developmental biology and cancer biology. After two weeks of basic training, students spend the remainder of the course working on an independent project. Most of a typical RIBS day is spent in lab with lectures given when needed to provide background and context for the experiments. Communication skills are important in science. RIBS students will have weekly writing assignments and keep a detailed lab notebook. Students read original research articles and participate in group presentations. Students will also attend weekly lunch time seminars given by research faculty. The course ends with a research forum in which the students present the results of their projects. Successful completion of the course will give participants the experience and confidence to work in a research laboratory.
See sample syllabus here.
Program Considerations
RIBS is a residential program and requires students to live on-campus and attend classes in person to complete the program. Students who have completed a significant amount of lab work outside of class may find this course repetitive.
Application Requirements
Eligibility: Current 10th or 11th grade students. Students must have completed one year of high school biology by the start of the program. This course is the most rigorous of the pre-college offerings in Biology, requiring that students have a demonstrated passion for science, with top grades in high school biology, math, and chemistry in honors or advanced-level courses, if available at the student's school.
The deadline to apply is our Priority Deadline, January 18, 2024. All application decisions for this program will be released at the same time at the end of February.
Students must complete the Summer Session Application and all the related requirements, which can be reviewed in the How to Apply section.
Program Overview & Dates
June 15-July 13, 2024
Move-in: Sat. Jun 15 Required Orientation: Sat. Jun 15 - Sun. Jun 16. First Class: Mon. Jun 17 Move-out: Sat. Jul 13 Class times: 9:00 a.m. - 5:00 p.m.
Course Specifics
Instructors: Christopher Schonbaum, Robert Bednarczyk
Course Code: BIOS 10010 94
Units: 200 UChicago units, which is equivalent to 2 full-time courses

An official website of the United States government
The .gov means it's official. Federal government websites often end in .gov or .mil. Before sharing sensitive information, make sure you're on a federal government site.
The site is secure. The https:// ensures that you are connecting to the official website and that any information you provide is encrypted and transmitted securely.
- Publications
- Account settings
- Browse Titles
NCBI Bookshelf. A service of the National Library of Medicine, National Institutes of Health.
National Academy of Sciences (US) Committee on Research in the Life Sciences. The Life Sciences: Recent Progress and Application to Human Affairs: The World of Biological Research Requirements for the Future. Washington (DC): National Academies Press (US); 1970.
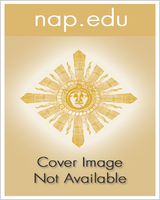
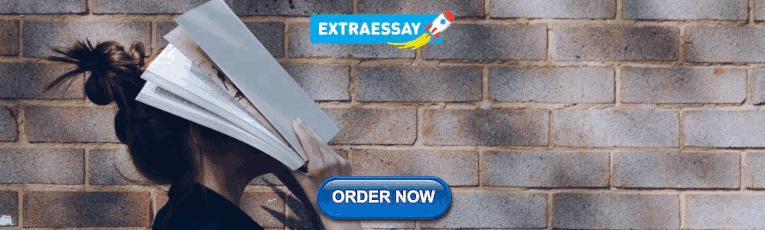
The Life Sciences: Recent Progress and Application to Human Affairs: The World of Biological Research Requirements for the Future.
- Hardcopy Version at National Academies Press
CHAPTER THREE THE WORLD OF BIOLOGICAL RESEARCH
The life sciences embrace a great array of intellectual activity, a continuum extending from the search for the origin of life and the detailed structure of the macromolecules that make life possible to understanding of the total ecology of planet Earth. The millions of micro-organisms and plant and animal species interacting in the air, the soil, freshwater ponds and streams, and the oceans afford a never-ending variety of objects of fascinating inquiry. This endeavor has enhanced man's capacity to manage and protect his environment, to feed and clothe himself, and to prolong his comfortable and fruitful years. The inquiry itself is conducted in the laboratory, in research institutes and hospitals, in experimental tracts and ponds, by walks in the woods, by surveillance from the skies, from ships at sea, and on treks through the jungle, observing both undisturbed and managed nature. Those so engaged range from amateur nature lovers to directors of large institutes. They work in and out of institutions large and small; they work with private, state, and federal resources in institutions of higher learning, nonprofit research institutes, research hospitals, federal, state, and local laboratories, and in the organized multidisciplinary teams of industry.
In 1966 the National Register of Scientific Personnel identified approximately 84,000 individuals with diverse levels of training and educational backgrounds who classified themselves as working life scientists. The identification of these people was possible through the cooperation of the two major biological research societies, the Federation of American Societies for Experimental Biology and the American Institute of Biological Sciences.
The Federation issued questionnaires to approximately 24,300 people, the great majority of whom had earned doctoral degrees. Of these, some 20,100, or 83 percent, responded to the Register questionnaires. The American Institute of Biological Sciences contributed approximately 59,800 names, but the proportion of doctorate holders among this group is lower, and hence fewer of them meet the conditions for inclusion in our survey as individual life scientists. Approximately 40,000 people, or 67 percent, responded to the Institute's questionnaire and the proportion of doctorate holders represented by those respondents is higher than that of the original 59,800 individuals surveyed by that society. The overall response to the Register from the two societies was approximately 65 percent and should comprise most working biologists. From these numbers it can be estimated that 70 to 80 percent of doctoral-degree holders responded to the National Register in 1966. However, one can only guess what fraction of American biologists, with or without doctoral degrees, this represents.
It is estimated * that, in the aggregate, $2,264 million was invested in research in the life sciences in fiscal year 1967, of which 60.3 percent came from the federal government, 7.3 percent from the resources of nonprofit institutions, and 30.0 percent from industry. In its entirety, therefore, research in the life sciences has become one of the major pursuits of American society. This chapter is devoted to a description of some of the components of the life sciences research system, based largely on information gathered from responses to our two questionnaires (Appendixes A and B).
Detailed information on the gross parameters of the total system was revealed by the first of our two questionnaires: It contains 14,362 scientists, of whom 12,383 were investigators as here defined, viz., they devoted more than 20 percent of their time to research. In 1966 they published more than 24,000 original articles, 489 books, 1,100 reviews, and 7,500 in-house reports and other contributions. The universe revealed by the second questionnaire contains 1,256 academic departments with an aggregate continuing staff of 18,608 scientists, with available research funds (direct costs only) totaling $304 million, operating in 325 acres of laboratory space in which they directed the research and training of 23,287 graduate students and 4,695 postdoctoral fellows and were assisted by 24,481 technicians, secretaries, and other personnel.
Of the 14,362 individuals who replied to the individual questionnaire, 3.4 percent were less than 30 years old and 6.3 percent were at least 60 years of age; 36.2 percent ranged from 30 to 39 years; 36.3 percent ranged from 40 to 49 years; and 17.8 percent were in the range 50 to 59 years. This distribution is fairly close to that of the scientific population at large. The average age of the group was 43.2 years, the median 41 to 42 years. Only 5.1 percent of the total population was female.
Every state of the Union was represented in the reporting of birthplaces. New York was represented by the largest number of scientists (1,989); Pennsylvania and Illinois followed with 880 and 855, respectively; and 631 were born in California; in all, 12,439 had been born in the United States, and 1,866 were foreign-born. All but 41 of the foreign-born regarded themselves as permanent residents of the United States at the time of the questionnaire. The foreign-born life scientists had come to our shores from 81 different nations. The major sources were Canada (292), Germany (236), England (162), Taiwan (142), India (97), Austria (89), Hungary (68), Poland (55), and Japan (50).
- WHERE LIFE SCIENTISTS WORK
Two thirds of the 12,383 investigators were employed by institutions of higher learning; as shown in Table 7 , 14 percent were employed by the federal government, 10 percent by industry, and the remaining 10 percent by a variety of nonprofit organizations—e.g., hospitals, clinics, museums, state and local governments—and a few are self-employed. In a general way, this pattern is relatively independent of the field in which these life scientists were trained ( Figure 33 ). With the exception of horticulturists, those trained in the agricultural sciences are more likely to work for the federal government than those trained in any other scientific area. Of the 68 percent who were trained in the basic biological sciences, biochemists are by far the largest single group, constituting 15 percent of the total population of this study, with microbiologists and physiologists 8 percent and 7 percent of the total, respectively. Although, because of their numbers, these groups are predominant on the faculties of institutions of higher education, biochemists, microbiologists, and pharmacologists are also in great demand outside these institutions. Over 40 percent of those trained in these three disciplines operate in nonacademic environments, with all three unusually well represented in the laboratories of industry.
Principal Employment of Life Scientists.
Type of employment of life scientists, by field of doctoral training. (Source: Survey of Individual Life Scientists, National Academy of Sciences Committee on Research in the Life Sciences.)
Of the 17 percent of our population who were originally trained as physicians, one third also obtained Ph.D. degrees. Seventy percent of the M.D.'s are on the faculties of universities, including virtually all the M.D.-Ph.D.'s; rather few research-performing M.D.'s are in industry, but there is unusually high representation in nonprofit institutions, particularly independent hospitals and clinics and public-health organizations. Those trained as physicians constituted 44 percent of the 3,170 reporting members of faculties of medical schools (and these schools corresponded to 39 percent of the total academic population); these were 87 percent of all reporting physicians. The remainder of the medical faculty was drawn largely from among those originally trained in the basic medical sciences; biochemists predominated in this last group (15 percent of the gross total), with major representation also from physiology, microbiology, and pharmacology.
Because of their relatively large total number, those trained in biochemistry are found throughout the system in substantial numbers. Of 1,834 trained biochemists reporting, 59 percent (1,069) were in institutions of higher learning, including 491 in medical schools, 225 on arts and sciences faculties, 126 in agricultural schools, and 37 in liberal arts colleges. Substantial numbers were also found elsewhere: 247 in the federal government, 275 in industry, and 231 in other nonacademic, nonprofit organizations. (The disciplinary designation, “biochemist,” relates only to the field of original doctoral-level training, and not to the area of science in which the scientist is currently working.)
Of the life scientists in our sample employed by institutions of higher learning, slightly less than 5 percent were at liberal arts colleges. Undoubtedly, a much larger fraction of life scientists, particularly botanists and zoologists, are on the faculties of such institutions, but relatively few engage in research on a scale sufficient to have put them within the scope of this study.
The questionnaire addressed to department chairmen yielded an aggregate faculty for all responding departments of 17,172, of whom 3,852 were on faculties of arts and sciences, 3,907 on the faculties of agricultural schools, and 8,915 on the faculties of medical schools. Although the general employment patterns in the two questionnaire files are similar, the discrepancies are of some interest. Whereas 39 percent of all individual respondents were on the faculties of medical schools, 52 percent of the total departmental faculties reported were so employed. To place this in perspective, it should be noted that, of the 1,256 departments represented in the study, 267 are in agricultural schools, 246 in faculties of arts and sciences, and 694 in medical schools. Of the medical departments, 361 were departments of the preclinical and 333 of the clinical segments of medical schools. Undoubtedly, the returns from the chairmen's questionnaire should be taken as a more valid description of the distribution of the faculties of life scientists than that provided by the individual returns.
The 1,689 individual scientists who indicated that they are employed by the federal government appear to represent a large fraction of the senior life scientists in the federal establishment. The major employers of the 1,689 reporting life scientists within the federal establishment are the Departments of Agriculture (36 percent), Health, Education, and Welfare (27 percent), Defense (15 percent), and the Veterans Administration (11 percent). The patterns of employment of scientists in the various biological disciplines reflect the character of the agency missions rather closely. Thus, 84 percent of all those trained in agricultural sciences now in the federal establishment are employed by the Department of Agriculture; 53 percent of all federally employed M.D.'s actively engaged in research work for the Department of Health, Education, and Welfare; 29 percent of the M.D.'s work in the Veterans Administration; and 18 percent of the M.D.'s work in the Department of Defense.
The disciplinary employment patterns in other areas are repeated in the federal establishment: 32 percent of all federal life scientists were trained in the basic medical sciences, varying from 12 percent in the Department of Agriculture to 55 percent in the Department of Defense. Except for the physicians employed by the Department of Health, Education, and Welfare and the Veterans Administration and the agronomists employed by the Department of Agriculture, biochemists again constitute the largest single group of scientists in all federal agencies, ranging from 7 percent in the Department of Agriculture to 16 percent in the Department of Defense, 21 percent in the Department of Health, Education, and Welfare, and 26 percent in the Veterans Administration.
An additional 135 scientists were employed in federal contract research centers, which are managed by educational or other nonprofit organizations. State governments employed 229 life scientists (1.8 percent of the grand total), largely in hospitals or state health departments and their laboratories, and approximately half as many life scientists were found in municipally controlled institutions of the same character. A significant number, 462 scientists (3.7 percent of the total), were employed by nonprofit institutes, foundations, and privately controlled museums.
There are no reliable indicators to determine whether the 1,155 individual respondents who indicated that they are employed in industry constitute either a large or a true sample of the total number of senior life scientists employed in that sector of the economy. Seventy-six percent were employed by manufacturing industries; two thirds of these were in the pharmaceutical industry. Again, those trained in the basic medical sciences predominate: 262 biochemists were the largest group, followed by 178 microbiologists and 107 pharmacologists. The low representation of other disciplines among investigators in industry is somewhat disconcerting. For example, only two embryologists, three anatomists, four cell biologists, four ecologists, eight animal pathologists, 10 biophysicists, 13 botanists, and 25 zoologists reported that they were in the employ of some industrial establishment.
Finally, in this regard, it should be remarked that of the 12,151 life scientists responding, 442 had obtained Ph.D.'s in chemistry and 114 in other fields of the physical sciences (about one half in physics), while 105 individuals were originally educated as psychologists. (No questionnaires were sent to individual practicing research psychologists or to the chairmen of either psychiatry or psychology departments.) The employment distribution of these 662 converts to the life sciences among institutions of higher learning, the federal government, industry, and other organizations was much like that of the groups described earlier.
- MOBILITY OF LIFE SCIENTISTS
Geographic mobility, so prominently a characteristic of American society, is nowhere more evident than in the scientific community. As shown in Table 8 , scientists born in each of the standard census regions can currently be found in each of the other census regions. Presumably, the direction of these migrations is dictated largely by increasing employment opportunities. This is particularly evident in the considerable migration from all other census regions to the Pacific Coast region and the South Atlantic region. Of at least equal interest, however, is the even greater tendency for relocation to regions likely to produce the least “cultural shock.” Not only is there the expected tendency of a substantial fraction of all scientists in all census regions to remain within the states or census regions within which they were born, but the most frequent move from one region to another has been to an adjoining area where life patterns are similar—e.g., from the lower South to the upper South, or within the Midwest.
Migration Patterns of Life Scientists.
For the entire population of life scientists, the average length of employment in the current position was 9.6 years, with the median 6 to 7 years. Fifty-five percent of all respondents had held at least one previous position with a different employer, quite apart from any number of postdoctoral appointments. The average length of employment in that previous position was 4.7 years, and the median was 3 to 4 years. Although 90.5 percent of all such moves had been made after less than 10 years with the previous employer, employment translocation was reported by some scientists even after as long as 40 years with the initial employer.
The pattern of these moves is of interest in itself. Although institutions of higher learning were the principal source of those who entered the employ of the federal government, private industry, and other organizations, in a general way each employing entity in the system also tended to recruit from other institutions in the same category. For example, 36 percent of all those in private industry had been employed by a different corporation, and 19 percent of those now working for an independent hospital or clinic had previously worked for some other independent hospital or clinic.
Two thirds of those who had moved to an institution of higher learning had come from another such institution. Of the remainder, 13 percent had left the federal government, 5 percent private industry, 5 percent other nonprofit organizations, and 8 percent various other state and community institutions. Perhaps the major surprise in these data is the fact that, ignoring graduate and postdoctorate education, institutions of higher learning appeared to be a net importer of scientific employees. Whereas 1,750 individuals whose previous employers had been nonacademic institutions currently were employed by the universities, only 1,260 individuals currently employed by nonacademic institutions had previously been employed by universities or colleges.
Respondents to the questionnaire were not queried about their motivation in accepting offers of new positions. It may be assumed that these were responses to offers of higher pay, of opportunity to engage in independent research or research under more desirable conditions, or to locate in geographical areas attractive to the families of the scientists concerned.
- PREVIOUS EDUCATION OF WORKING LIFE SCIENTISTS
In the foregoing summary, the initial training of working life scientists was categorized in disciplinary terms that are familiar as the titles of academic departments and that are employed in most statistical collections. However, the reader who has considered earlier chapters will have recognized that these conventional subdisciplinary titles have, in considerable measure, lost their meaning and convey false distinctions. Whereas biochemists were formerly concerned largely with elucidation of metabolic maps, they may today be concerned with macromolecular structure, the chemistry of cell-cell recognition, or the phenomena responsible for atherosclerosis. Not so long ago, microbiologists were overwhelmingly concerned with the taxonomy of microbiological forms, yet today they may be concerned with genetic mechanisms or the nature of the immune response to invasion by some specific organism. Hematologists, who only yesterday were describing changes in the morphology of blood cells in leukemia as seen with a light microscope, are now intimately involved in understanding the manner in which nucleic acids control the differentiation process among white blood cell types. Physiologists, who formerly engaged in studies of the mechanics of muscular contraction or morphological changes induced by steroid hormones, are today inquiring into mechanisms of transmembranal transport or the molecular events by which steroid hormones affect protein biosynthesis in receptor cells. Botanists, once engaged in taxonomic studies or in gross plant physiology, are today concerned with the phenomena by which plants interact with other organisms and with their environment, the cardinal aspects of ecology, while zoologists may be concerned with all those aspects of the environment that have favored rapid proliferation of new species in one set of circumstances or remarkably prolonged survival, unchanged, of other species, studies that embrace all aspects of ecology, genetics, biochemistry, and physiology. Even more dramatic have been the changes in the character of research in clinical medicine, pathology, and pharmacology. Investigators in these areas have learned to use the most recent developments in understanding such phenomena as protein structure, enzyme kinetics, transmembranal transport, neural transmission, immunochemistry, viral reproduction, lipid metabolism, and behavioral genetics as they explore disease mechanisms in man or animals, design and test new drugs, or prepare a patient for organ transplantation. And their laboratories cannot be distinguished from those of other scientists so engaged.
Because of these rapidly evolving and profound trends, it appeared desirable to reconsider individual scientists, not under classical disciplinary labels, but in relation to the nature of the research conducted during their initial formal education in graduate school and in relation to the research in which they are currently engaged. That two individuals are studying cellular structure and function is more significant than that one considers himself a zoologist and the other a botanist. The plant pathologist may have more in common with an animal pathologist than with a plant taxonomist, and similar considerations are obvious for plant and animal physiologists, or for plant, animal, and microbial geneticists, for example.
Thus, we have found it useful to recategorize life sciences research into the following dozen classifications:
Behavioral biology
Cell biology
Developmental biology
Disease mechanisms
Evolution and systematic biology
Molecular biology and biochemistry
Pharmacology
It will be evident that even these categories are somewhat arbitrary and are by no means mutually exclusive. They fail to make clear the fact that biochemistry, a research area itself, is also the common language and the tool for almost every other entry in the classification scheme. However, the questions being asked of nature by scientists within each category are sufficiently distinct to permit self-identification by our respondents, while providing a more revealing description of the life sciences endeavor than that offered by more traditional disciplinary titles.
Tables 9 and 10 summarize the current research areas of some of our respondents, comparing their current areas of involvement with the disciplines and research areas in which they had been trained as graduate students. As a consequence of an awkwardness in the design of the layout of the printed questionnaire, almost a quarter of all respondents failed to provide information concerning the research fields, as here categorized, in which they had been trained and in which they are currently engaged. However, as indicated in Appendix A , it appears fair to assume that the patterns revealed by those who did not overlook this question are representative of the total.
Comparison of Current Research Areas with Areas of Most Recent Ph.D. or D.Sc. Degree.
Comparison of Current Research Area with Disciplines in Which Life Scientists Were Trained.
As indicated by the diagonal of Table 9 , current research in any given area is conducted predominantly by individuals who were trained in that area, varying from 49 percent of those currently engaged in behavioral biology to 85 percent of those working in genetics. Equally impressive, however, is the degree of intellectual migration among research fields. Thus, 48 percent of all those trained in morphology are now engaged in some other area, as are 39 percent of those originally trained in cell biology, 33 percent of those trained in developmental biology, and 30 percent of those trained in physiology. Maximum field retention was found among those trained in pharmacology, ecology, genetics, and molecular biology and biochemistry. Perhaps the most striking fact shown by the table is that every possible crossover was reported. Noteworthy, too, are the fields that, on balance, have either attracted more investigators than they have lost, or vice versa. The “gainers” include molecular biology and biochemistry, behavioral biology, cellular biology, disease mechanisms, ecology, and pharmacology. The most significant “losers,” in absolute numbers rather than percentages, were genetics, morphology, nutrition, and physiology, with developmental biology and systematic biology remaining approximately in balance.
Many biologists currently consider that there has been a rapid growth in the opportunities for fruitful studies in behavioral and developmental biology and in ecology. But these data indicate that, although there has been some modest influx into these fields, it is not yet particularly striking, although graduate enrollments have been affected in the predicted directions. Moreover, the changes are generally immediately lateral in the sense that most of those who have changed research areas have moved into areas in which they can apply the skills and insights of their primary training. This is most certainly the case for the 184 of 287 individuals who left molecular biology and biochemistry to enter upon studies in cellular biology, disease mechanisms, pharmacology, or physiology, as it must also be true for the 317 individuals who left physiology to enter other biological categories.
Only 741 scientists were sufficiently certain of their plans to change research areas in the future to so indicate. And again, the planned changes were, in the main, relatively conservative ( Table 11 ) and into closely related areas, e.g., molecular biology to genetics, genetics to molecular biology, physiology to pharmacology, botany to ecology. Molecular biology will be the chief gainer (19 percent of all who plan to change), largely from cellular biology and physiology. However, it will lose a slightly larger number (20 percent), mainly to cell biology, developmental biology, and disease mechanisms. Disease mechanisms attracts the second largest group (15 percent), largely from among those now engaged in cellular biology, biochemistry, and physiology, while developmental biology also seems attractive to those in the same group of research areas (12 percent). The survey revealed a particularly interesting trend. Some ecologists indicated plans to enter behavioral biology, while a significant number of physiologists and students of disease were seriously considering switching to ecology.
Projected Research Areas of Some Life Scientists.
Moreover, the perhaps not unexpected conservative migratory pattern is again evident from the responses of life scientists who intended to change the biological material with which they were working. In a general way, those now seriously contemplating such a change are, in the main, thinking of switching either to the next higher or the next lower level of biological organization, e.g., from broken cell preparations to cells or tissue culture or to molecular systems; or from intact organs to either intact organisms or cellular preparations.
Table 12 relates research areas to the principal employers of the 8,139 individuals for whom such information is available. Of this subset, institutions of higher learning employed 68 percent, the federal government 14 percent, industry 9 percent, and all other nonprofit organizations, hospitals, etc., 9 percent. Noteworthy are the high levels of employment by the federal government of those studying ecology and disease mechanisms; the government shows much less interest in developmental biology, morphology, and pharmacology. Private business employs an unusually high fraction of all nutritionists and pharmacologists, but appears to have little interest in ecology, systematic biology, or morphology.
Distribution of Investigators in Various Research Areas by Principal Employer.
A small insight into the changing dynamics of the life sciences is provided by observation of the fraction of the total population within each research area under 34 years of age. This fraction is remarkably close to 21 percent for virtually all research areas, with a few interesting exceptions. Only 11 percent of those engaged in the study of disease mechanisms are within this age group, presumably reflecting the long period of residency training for physicians. In contrast, 23 percent of those in developmental biology and 28 percent of those in molecular biology and biochemistry were under the age of 34 at the time of this survey, indicating that in the recent past these two fields, as compared with the other research areas, have become increasingly attractive to young scientists. Only 18 percent of all those attracted into the life sciences from the physical sciences were within this age group, indicating that there has been no dramatic upsurge of interest in the life sciences among young chemists or physicists.
The reverse situation is in accord with the same suggestions. For the entire population, 18 percent were 50 years of age or older, but only 12 percent of those in molecular biology and biochemistry fell within that age range, in contrast with 25–28 percent in the areas of disease mechanisms, evolutionary and systematic biology, morphology, and nutrition.
Of some interest are the attributes of the group of investigators originally trained only as M.D.'s or in the other health professions. They are older, with only 15 percent under 34 years of age, but 42 percent within the age span 40–49. Logically, disease mechanisms constitute their principal single interest (27 percent of the total), but they are also represented in every other research area with the exception of systematic biology, major interests being physiology (22 percent), molecular biology and biochemistry (15 percent), cellular biology (9 percent), and pharmacology (8 percent).
The 456 women showed only a few distinct tendencies to differ from the distribution of the men. Women tended to avoid physiology, ecology, and systematic and behavioral biology, and 28 percent of all female respondents work in molecular biology and biochemistry.
- POSTDOCTORAL TRAINING
Prior to World War II, postdoctoral research training experience was a privilege granted very few young scientists. Fellowships were scarce, and only the most highly talented could aspire to such opportunity. Since available research grants were decidedly limited in size, few senior academic investigators commanded the means to support eligible new M.D.'s or Ph.D.'s desirous of embarking upon the apprentice training characteristic of the postdoctoral experience. That situation no longer obtains. Postdoctoral experience has become almost the norm rather than the exception, and we are entirely convinced that this is in the national interest.
However, the situation has given rise to concern among those less closely associated with research in these disciplines. For example, agencies that provide support for postdoctoral training are uncertain of its value. Educational institutions in which postdoctoral fellows abound are uncertain of their institutional responsibility for this enterprise. Institutions that, perhaps until 1969, have had difficulty in recruiting sufficient staff to meet teaching obligations—largely the four-year colleges and junior colleges, but also a significant number of medical schools, as well as industry and some federal laboratories—have complained that the postdoctoral system is a holdup in the pipeline that, in the steady state, keeps a substantial number of bright young investigators out of the regular job market. We appreciate these problems, but consider that the benefits of postdoctoral education far outweigh these transient difficulties. Let us consider here the postdoctoral training experience of our responding population of life scientists. In the following chapter there is a summary of the numbers and activities of postdoctoral fellows in training in 1967–1968, as well as an analysis of the contribution of postdoctoral education to the operation of the entire endeavor.
Of the 12,151 investigators in the study, 5,041 had had at least one postdoctoral appointment, including 1,402 M.D.'s who had had postdoctoral experience in which research was their major responsibility. Three fourths of those who had had postdoctoral experience are now in academic life. Indeed, 45 percent of the 8,143 scientists now employed by universities had enjoyed postdoctoral experience, compared with 21 percent of the scientists in industry and 31 percent of those in the federal establishment. Taken across all disciplines, postdoctoral experience somewhat enhances the opportunity for employment in the federal government and markedly enhances the opportunity for employment in the universities. It is our impression that in universities with major commitments to graduate education and research, measured in supporting dollars and number of graduate students, faculty appointments for individuals who have not had postdoctoral experience are probably rare indeed. According to a National Academy of Sciences study of postdoctorals, * 74 percent of all new appointees to the rank of instructor or assistant professor in 21 departments of biological sciences in 10 “leading” institutions either came from other university faculties or had just held postdoctoral appointments.
However, the trend to postdoctoral education is not universal across all biological fields. For example, of the 855 individuals with graduate training in agricultural fields, only 35 had had postdoctoral appointments. In contrast, postdoctoral training was commonplace among M.D.'s since it has become the conventional medium for obtaining research training among this group.
As shown in Table 13 , postdoctoral training was less frequent among botanists (29 percent) than among biochemists (53 percent), with the other disciplines ranging in between. Postdoctoral training was frequently taken in fields other than those in which scholars had their initial doctoral experience. Thus, of the zoologists and botanists who did take postdoctoral training, less than half did so in zoology and botany departments. Again, the biochemists appear as the other extreme. Not only did a larger fraction of biochemists than other life scientists take postdoctoral training, but a decidedly larger fraction remained within biochemistry for their postdoctoral experiences. Since an additional 540 individuals who had taken their original graduate education in fields other than biochemistry sought postdoctoral training in biochemistry, postdoctoral education is a major aspect of life in biochemistry departments. Large numbers of those trained in biochemistry in graduate school later work in other disciplinary areas, while many individuals enrich their original disciplinary education by a one- or two-year postdoctoral experience in biochemistry and then, when they become independent investigators, return to their original disciplines and research areas or enter yet other research areas.
Postdoctoral Experience of Scientists in a Limited Group of Biological Disciplines.
These data uphold one of the primary arguments in support of the trend toward postdoctoral experience as a normal component of the education of those who later will espouse careers in which research is a major activity, viz., that this constitutes a unique opportunity to broaden one's horizons, learn new techniques, and become familiar with the style of other subdisciplines, while profiting by the examples of different master scientists. The overall situation is reflected in the totals of Table 13 . Of 5,765 Ph.D.'s in this file, 2,395 undertook postdoctoral experience, of whom 1,463, or 61 percent, extended their experience in the same disciplines in which they had studied in graduate school. But the impression that postdoctoral experience is a continuation of graduate education in 61 percent of all cases is misleading, since it is weighted by the fact that more than half of all of those who did experience this continuation were biochemists. If the biochemists are excluded, only 50 percent of the remaining scientists who undertook postdoctoral training did so in their graduate disciplines. Moreover, such an experience is but rarely a mere continuation of graduate education. This is borne out by the following consideration: In a subfile of 3,234 postdoctoral fellows, only 14 percent had taken postdoctoral education in the same university in which they had obtained their doctoral degrees, and only 6 percent in the same departments that had awarded their doctoral degrees. This migratory pattern is particularly evident among the M.D. population. However, about one third of all Ph.D.'s in agriculture and forestry who undertook their postdoctoral training—a rather small group—did so in their original universities and, indeed, in the departments that had awarded their degrees. The rather small proportion of students who remained in the same department for postdoctoral study was almost twice as great in public universities as in private universities.
In sum, it is clear that the norm for postdoctoral experience, by a wide measure, consists of apprenticeship to a different set of investigators in an environment different from that in which graduate education has been completed. Further, in the experience of our panelists, the current internal heterogeneity of the classical disciplines assured that even the postdoctoral trainee who remains within his original discipline is likely to engage in a problem remote from his graduate research experience. The biochemist who studied intermediary metabolism may later become preoccupied with the mechanism of enzyme action; the physiologist who traced neural pathways as a graduate student may focus upon ion transport across the nerve membrane during his postdoctoral years. The botanist who was concerned with nutritional requirements for plant growth may later become involved in the ecology of a cornfield, while the entomologist concerned with patterns of insect distribution may switch to a study of insect sex attractants. Intellectual inbreeding is rare in the life sciences community, and the postdoctoral experience is among the chief means of assuring the hybrid vigor of the entire enterprise.
A few notes comparing the bioscience subculture with the subcultures of the physical and social sciences may be warranted. The data in support of the following statements are derived largely from the recent National Research Council study of postdoctoral education, The Invisible University . *
In the nation's leading academic institutions, postdoctoral experience has become the expected prelude to faculty appointment. In recent times, 70 to 80 percent of all initial faculty appointments at such institutions in physics, in chemistry, in biology departments of faculties of arts and sciences, and in the preclinical departments of medical schools have been made to individuals with postdoctoral experience either at the same or at some other institution. In contrast, initial faculty appointments in the social sciences, the humanities, and engineering relatively rarely require postdoctoral experience. The play of the academic marketplace is such that the frequency of postdoctoral experience among initial appointees to the faculty decreases with the general academic status of the institution. Postdoctoral experience is less frequent among the faculties of “developing” universities, is rare for scientists who are appointed to the faculties of liberal arts colleges, and is even less common among those who enter industry.
The converse is equally evident; 30 to 40 percent of all relatively young faculty at all universities who have not had postdoctoral experience feel this lack in their current professional lives. In all branches of natural science, promotion up the academic ladder occurs somewhat less rapidly for those who have not had postdoctoral experience, although this may reflect similar appraisal of human potential by the committees who select postdoctoral-fellowship recipients and those who recommend academic promotions, rather than the intellectual rewards of postdoctoral study. These trends are undoubtedly enhanced by the advice given to aspiring scientists by their mentors in graduate school, who strongly urge students in the natural sciences to undertake postdoctoral experience if they aspire to academic careers but rarely do so when this is not the case. In general, such mentors recommend a postdoctoral experience of about two years, with a specific senior scientist in a field somewhat different from that in which the student's dissertation research was conducted, thereby broadening his understanding of his discipline. When queried, postdoctoral students advance the same general purpose as their reason for undertaking postdoctoral study, but place more emphasis than do their graduate mentors upon the acquisition of additional research techniques.
Attempts by statistical means to assess the influence on subsequent scientific productivity of postdoctoral training are not revealing. Differences among those who took postdoctoral training immediately after graduate school, those who deferred such training for several years, and those who had no such training are trivial when measured by counting numbers of scientific publications, reviews, books written, and similar measures. What cannot be assessed by this means is the quality of the work or its significance to the field. One indicator has been reported in The Invisible University * : the fact that papers published by those who have had postdoctoral experience are cited about twice as frequently in the Citation Index † as are papers by those who have not had such experience. Statistically, frequency of citation of a paper is some measure of its significance or fundamentally. It is our contention that, in all scientific fields, scientific boldness—willingness to venture beyond the frontier or to undertake large and challenging problems—is established relatively early. Certainly, if this is not encouraged in graduate school or in the immediate postdoctoral years, it is rarely evident in subsequent careers. But statistical assessment of this all-important quality is not readily feasible; hence, the enhanced opportunity to develop such habits of mind is another argument that we would advance in support of a year or two of postdoctoral study, preferably not in the same institution or with the same mentor that provided the graduate experience.
Data purporting to compare the consequences of graduate or postdoctoral study in the 10 or 20 leading academic institutions with those in other institutions are probably not completely valid. The selection process that operates at the level of admission to graduate school and then to postdoctoral study in the most productive academic laboratories already serves as a screen almost sufficient to assure the ultimate outcome. It is not readily possible to distinguish between the consequences of differences in the quality of the educational experiences in such institutions and the consequences of the quality of the initial human input. Certainly it must be undeniable that those most highly qualified will benefit most from a stimulating environment in which science is being conducted at its outermost frontiers.
- EDUCATIONAL LIMITATIONS
An attempt was made to estimate the extent to which working life scientists sense deficits in the educational preparation for their careers. Respondents to the questionnaire were asked to state whether their current research programs are significantly limited by their own educational preparation in chemistry, mathematics, physics, electronics, statistics, other areas of the life sciences, or the use of computers. In all, 4,396 scientists, 30.6 percent of the entire responding population, indicated that full development of their current research effort is indeed very seriously hindered by insufficient personal training in one or more of these disciplines. Lack of knowledge of chemistry was most frequently felt to be limiting (1,766 individuals), followed by computer science (1,569), mathematics (1,427), statistics (1,136), other biological sciences (1,085), and electronics (983), with only 498 life scientists acutely aware of insufficient personal training in physics.
Scientists in academic institutions were not distinguished from those working in nonacademic institutions with respect to this pattern of perceived inadequacies, although 38 percent of academic personnel were aware of some such limitation, and only 30 percent of nonacademic scientists were. In both groups, those in the middle of the age range (35–50 years) were about 30 percent more likely to be aware of such deficits than were younger or older investigators. Again, however, age was essentially without influence on the pattern of perceived disciplinary insufficiency; the rank order of disciplines cited above for the entire population was characteristic of the youngest, oldest, and midrange investigators alike.
- WITH WHAT MATERIALS DO LIFE SCIENTISTS WORK?
The panorama of the biological universe offers such remarkable and diverse organisms, ecological situations, environmental responses, and unanswered questions at levels varying from the molecular to the cosmic that it is not surprising that research biologists employ an almost equally disparate and diverse variety of approaches to the questions they put to nature. In Table 14 is displayed a representation of primary research materials and the extent to which these are utilized by those who work in various biological research areas.
The Research Materials of Life Scientists.
It may come as a surprise to some that mathematical models are utilized by representatives of almost every research area, most frequently by those engaged in the study of physiology, molecular biology and biochemistry, genetics, or biophysics and, increasingly, in studies of ecology. Molecular models are to be found in virtually every biochemical laboratory, and the refined, precise models now available have become an extremely important tool for those seeking to relate molecular structure to biological function. Indeed, 46 individuals stated that such models constitute their primary materials.
It was somewhat surprising to find 6 percent of the entire surveyed population engaged primarily in the development of analytical procedures of various types. Study of molecular systems, utilizing highly purified materials of natural origin, engaged 10 percent of the total population, including one third of the biochemists. A somewhat greater proportion of life scientists were studying the behavior of subcellular organelles, isolated or in situ . Such materials are utilized by scientists, except the ecologists, in all research areas and, as one might expect, are a principal preoccupation of cell biologists and biochemists. A small proportion (3 percent) of our population, most notably the cell biologists, were learning to use disassociated preparations of living cells, from either plant or animal sources, as primary tools in their studies. Tissue culture was twice as popular and was utilized by at least some scientists, including behavioral biologists, in every research area, while intact tissues and organs claimed the attention of 12 percent of the total population, involving all research categories except ecology—most notably morphologists, pharmacologists, physiologists, and developmental biologists.
Intact individual organisms were the test objects of one third of all life scientists in the study, notably the behavioral biologists and those studying disease mechanisms, ecology, systematic biology, genetics, nutrition, pharmacology, and physiology. Decidedly smaller numbers of scientists addressed themselves to entire populations of organisms or to ecosystems.
Of interest is the fact that the pattern of use of materials by those with original training in the health professions cannot be distinguished from that of the remainder of the population; their primary research materials simply reflect the pattern of all others in the research areas in which they now engage. Accordingly, their major research materials are whole organisms (32 percent), tissue and organ systems (23 percent), subcellular fractions (13 percent), cell cultures (8 percent), and molecular systems (9 percent).
Within each research area a few individuals are engaged in comparative studies either within a single phylum or plant division or across several phyla or plant divisions. Although students of evolution and systematic biology were the most numerous such group, these were only 44 of the 123 individuals so engaged.
- WITH WHAT SPECIES DO LIFE SCIENTISTS WORK?
The diversity of living nature never fails to astonish. The workings of evolution have resulted in millions of distinct species of living forms, unicellular, plant, and animal, all located in the thin web of life, which is a film on the surface of our planet. These are the objects of study for life scientists. But which species should one study? The answer depends upon the question that has been raised. Some species are of interest because they are the basis of our agricultural economy. Some make the world more beautiful and exciting; some cause disease of man, plant, or animal. Sometimes even the most obscure species provide excellent models for study of complex biological phenomena. And surely a proper object for study by man is man himself! Thus there are valid reasons for the study of a great variety of species.
Some species are of interest because they are intermediate links in a food chain, because they survive under what appear to be improbable conditions, or because they represent evolutionary extremes. Still others are of interest because they offer unique opportunities to study phenomena of general importance but difficult to analyze or observe in more common species. For example, the nerve net of the crab is of interest as a prototype of the more complex nervous system of the mammal; the response of certain insects to sugars can serve as a model for some aspects of the physiological bases of behavior; the “alarm reaction” of the clam is highly instructive with respect to certain reflex activities; the photosynthetic properties of the chromatophores of purple bacteria and of certain algae are more readily studied than is photosynthesis in a higher plant; regulation of the genome of a bacterium serves as a model for the process of differentiation in a higher organism; and the giant axon of the squid is the favorite test object of numerous neurophysiologists. Nutritionists long since seized on the omnivorous white rat as a model for human nutritional requirements, but primates may be more instructive with respect to human behavior or reaction to disease. The pig offers a surface area and mass somewhat comparable to that of man, and thus should serve as a model for human response to radiation. Comparison of the properties of hemoglobins from a wide variety of species elucidates those properties of the hemoglobin molecule that are imperative to its physiological function, and frog muscle has taught us much of what we understand of muscle physiology and its molecular aspects. The list is well-nigh endless.
And so it is that life scientists continue to study or exploit the properties of a great diversity of organisms. In a highly compressed form, this is displayed in Table 15 . Each of the respondents to the questionnaire was given a choice of 58 genera, phyla, or larger divisions of the plant, animal, and microbial kingdoms and was asked to indicate no more than two that most closely described the objects of his study. Hence, the number of specific responses exceeded the number of respondents. But hundreds of investigators indicated that necessarily and properly they should indicate more than two such entries.
Biological Materials Studied by Life Scientists.
Perhaps the aggregated totals are of greatest interest: 21 percent of all scientists dealt with one or another micro-organism, 15 percent with plant forms, and 54 percent with animal forms. None of the categories of living forms was totally ignored by the current activities of life scientists but, clearly, some are more attractive than others. Viruses and bacteria are the concern of scientists in each research area, particularly those who study disease mechanisms, cell biology, and molecular biology and biochemistry. Lower plants engage the attention of all but the nutritionists and pharmacologists, while higher plants attract the attention of all but the pharmacologists. Invertebrates are of great interest to the ecologists and the systematists as well as to the behavioral biologists, who see in them models for the behavior of more advanced forms. Surprisingly little attention is being given to the species of fish that dominate our commercial harvests, whereas other fish, amphibia, reptiles, and birds are receiving greater attention. Of the mammalia, man and the common laboratory rodents are the most frequent study objects. The great utility of the latter is indicated by the fact that, whereas ecologists and systematic biologists pay them scant heed and only 6 percent of all geneticists make use of their particular attributes, these species are utilized by 12 percent of the behavioral biologists and 37 percent of the pharmacologists. Domestic mammals, i.e., cats and dogs, are particularly useful to the physiologists, pharmacologists, nutritionists, and morphologists and are used to some degree by almost all other groups.
Although 5 percent of all behavioral biologists and 4 percent of the morphologists report that they work with small primates, primates are little used by workers in other scientific areas. However, there is reason to think that this reflects not the utility of these species, but the great costs involved in their acquisition and maintenance, which have inhibited, if not prohibited, their utilization for a variety of studies in which they could be extraordinarily useful.
In contrast, millions of species currently go unstudied, and many others are under scrutiny by only one or two investigators. When, from time to time, such an investigator directs attention to some unique or remarkable attribute of a seemingly esoteric species, it can rapidly claim the attention of many other scientists, an incident that has recurred many times in the past. Thus, the bacterium Escherichia coli has become the most thoroughly studied of all cells, while both neurophysiologists and molecular biologists have recently seized upon the tiny marine organism Aplysia because of its easily studied giant nerve cells. In any case, the diversity of species under study demands an equal diversity of laboratory accommodations for their culture or maintenance. This may engender substantial expenditures and contribute much to the cost of scientific investigation, particularly in extreme instances. Elaborate facilities are required for the conduct of research employing cells in culture. Inadequate accommodations, overcrowding, or infestation can render a colony of dogs or rodents useless to the investigator and give rise to misleading data. Humane considerations demand that larger domestic mammals—cats, dogs, and primates—be housed in decent quarters, be wellnourished, and be subjected to the minimum of trauma commensurate with the purposes of study. This in turn creates further serious financial requirements, which should be borne by some institutional mechanism and not met by taking funds from personal research grants made to individual investigators. Certain plants and animals require carefully controlled environments; a continuing supply of virus may require a colony of host animals, a large-scale fermentor, or a large tissue-culture facility. Most importantly, all these demand substantial expenditures merely to assure a supply of the biological entity to be studied before the research proper can be undertaken.
- WHAT FACILITIES AND TOOLS DO LIFE SCIENTISTS USE?
The classic image of the biologist is an aging gentleman, wrapped in a dirty laboratory apron, in a musty laboratory surrounded by museum jars, an ancient, battered microscope, staining jars for microscope slides, and perhaps an unwashed dissecting table. If that image ever corresponded to reality, it no longer does. As the questions we ask of nature become more sophisticated and the information we seek becomes more remote from that which we can acquire with our naked senses, the requirements for the conduct of research in the life sciences become more complex. Today, in order to achieve his ends, the investigator may have to travel thousands of miles from his home base, armed with telemetering equipment, tape recorders, or remote sensors. He may require a floating laboratory, a deep-submersible vessel, a reconnaissance plane, or even a satellite equipped with infrared sensors. He may utilize the gadgetry of modern biochemistry— ultracentrifuges, equipment for optically following the course of kinetic processes on the scale of milliseconds or of molecular-relaxation times (10 −9 sec), for the quantitation of visible or ultraviolet light or radioactivity. His laboratory may be what amounts to a small electronics plant equipped with the complex electronic apparatus needed for the study of neurophysiology, and his experiment may be guided by an on-line computer. Increasingly, the tools of any biological subdiscipline tend to become the tools in many other areas of biology. As we have noted repeatedly, this is particularly true of the tools of the biochemist, which have become the tools of all biologists.
Specialized Biological Research Facilities
Table 16 summarizes the replies from respondents whose completed questionnaires usefully indicated their utilization of specialized research facilities. The spectrum of such activity is broad indeed. For example, we were surprised at the high rate of utilization of controlled field areas, which seemingly are employed by participants in each of the research areas. Computer centers are available to and utilized by a strikingly high fraction of all life scientists, and general animal care facilities appear to be utilized by almost half the scientists covered by our survey. Indeed, it is difficult to correlate specific types of facilities with specific research areas. Notable exceptions include the 87 percent of all systematists and 44 percent of ecologists who utilized taxonomic research collections, the 51 percent of cell biologists who employed cell- or tissue-culture facilities, and the 76 percent of all pharmacologists who made use of general animal care facilities. The existence of the specialized facilities listed here was known to the Survey Committee, but the extent of use was not anticipated.
Utilization of Specialized Biological Research Facilities.
Rarely can the cost of acquisition and maintenance of such facilities be justified by the research program of a single investigator; hence, no small or medium-sized institution can hope to have a complete selection of these opportunities for conduct of research. This has the effect of either limiting the capabilities of the staff of such institutions or so affecting their recruitment patterns that, at each institution, there are clusters of investigators whose research requires easy access to the same major research facility. For smaller institutions, this fact, in turn, may well prevent the assembly of a staff broadly representative of biology.
Major Instruments
Table 17 displays the utilization of major instruments by life scientists during 1966–1967. Like Table 16 , this table is limited to those respondents whose replies to the questionnaire were found adequate to the purpose. And, as in Table 16 , what is impressive is the extent of use of the wide variety of instruments listed and the relative amount of use without regard to specific research areas, again with a few notable exceptions. This table well illustrates how the tools developed for biochemical studies have become the tools of biology in general; this is evident in the use pattern of centrifuges, gas chromatographs, amino acid analyzers, scintillation counters, infrared and ultraviolet spectrophotometers, as well as electrophoresis apparatus. These common tools of the biochemical laboratory are now the common tools of the biological laboratory. Specialized uses of instruments will, however, be found in the table. For example, large-scale fermentors are used largely by biochemists; multichannel recorders are required by physiologists and pharmacologists; small special computers by physiologists. Biochemists are pioneering in the use of ultrasonic probes, and electron paramagnetic resonance and nuclear magnetic resonance spectrometers, as well as instruments for measuring circular dichroism. The physiologists are the major users of infrared carbon dioxide analyzers, and the clinicians interested in disease mechanisms utilize complex electronic systems for monitoring human physiology, while systematists use telemetry and sensitive tape recorders.
Utilization of Instruments by Life Scientists.
The utilization of the electron microscope is particularly revealing. This instrument, slowly introduced into biological laboratories in the years following World War II, is now used by investigators in every research area. In absolute numbers, those interested in molecular biology and biochemistry, cellular biology, disease mechanisms, and physiology are the principal users. But 48 percent of all those studying morphology and 44 percent of those studying cellular biology made use of this instrument. The great expense of acquisition and maintenance of these instruments prevents the figures for utilization from approximating 100 percent of those in both of the latter research areas.
One should not leave the subject of instruments without a tribute to the instrument-manufacturing industry. This highly competitive industry has frequently been a jump ahead of most life scientists. In general, instrument manufacturers have recognized needs and potential uses before the scientific community has. Yet, as each instrument has become available—e.g., ultraviolet spectrophotometers, electrophoresis apparatus, scintillation counters, electron microscopes, and multichannel recorders—not long thereafter the scientists involved have wondered how they had ever made progress before these commercial instruments became available. As the markets grow, the instruments become more refined, more reliable, and more versatile, thereby enormously enhancing the reliability, sophistication, and ease of performance of biological research. The availability of such instruments has been made possible by the very scale of federal support of the life sciences. By creating a sufficient market, the manufacturer has, in turn, been able to achieve economies of large-scale production, keeping the unit cost and sales price down. (It is ironic that, although the electron microscope was developed by an American firm, and this country is the major market for this instrument, no American manufacturer now supplies it.)
Nor should we fail to acknowledge our debt to our brethren in physics, chemistry, and engineering. From them came the electron microscope, spectrophotometers, the electron paramagnetic and nuclear magnetic resonance spectrometers, ultrasonic gear, the great variety of oscilloscopes, x-ray crystallographic analysis systems, the laser, telemetry, and a host of other devices. To their designers and developers, the biological community extends its gratitude.
- THE RESEARCH GROUP
Research in the life sciences is “small science”; only rarely is it organized around some very large and expensive piece of apparatus or facility. Whereas much research in other areas of science revolves about large accelerators, research vessels, telescopes, balloon-launching facilities, rocket facilities, or large magnets, for example, there are few parallels in the life sciences. Occasional exceptions include relatively elaborate hyperbaric facilities, primate colonies, colonies of germ-free animals, phytotrons or biotrons, biosatellites, museums, or marine-biology stations. But these are the exceptions rather than the rule, and even in these instances, the facilities in question are actually utilized by numbers of small research groups, each pursuing its own questions in its own way, while taking advantage of the availability of the facilities. In very few instances have the various groups that, collectively, used such a facility comprised a coordinated whole with common goals and objectives. The functional unit of research in the life sciences, therefore, usually consists of a principal investigator and the postdoctoral fellows, graduate students, and technicians who work with him. According to data collected by the Study of Postdoctoral Education of the National Academy of Sciences, * the mean such research group, in addition to the faculty member, is 6.1 members in academic biology departments, 7.6 in biochemistry departments, 5.3 in physiology departments, and 4.0 in clinical specialties. These may be compared with 5.8 members in physics and 8.3 in chemistry. When, however, research groups without postdoctoral are considered, these units are distinctly smaller, receding to 4.6, 3.9, and 4.0 in biology, biochemistry, and physiology, respectively, and 3.2 and 5.2 in physics and chemistry.
This scale of operation was borne out by reports from the individual investigators surveyed in the study. For all principal investigators, the mean was 6.5 persons per research group, in addition to the principal investigator himself, ranging from 4.4 for investigators engaged in studies of systematic biology to 8.0 for those studying disease mechanisms. Perhaps surprisingly, the sizes of groups were much the same in academic and nonacademic laboratories. Approximately equal numbers of co-investigators and professional staff are found in both classes of laboratories. The graduate students, who vary in academic laboratories from 1.5 to 4.0 students per group (the extremes being represented by morphology and behavioral biology, respectively), with an overall average for all biological disciplines of 2.2 students per group, are replaced in nonacademic laboratories by technicians and other supporting staff.
Thus, in general, the typical academic laboratory contains a principal investigator, a co-investigator, and one other scientist with a doctoral degree who may be a visiting scientist, postdoctoral fellow, or continuing research associate, two technicians, and two or three graduate students. Federal laboratories may have one or two postdoctorals in place of the graduate students, while industrial laboratories utilize additional technicians. The routine tasks of the laboratory are generally performed by the technicians, while the graduate students and postdoctoral fellows serve as junior co-investigators and colleagues for the principal investigator. In our view, such a research group does indeed constitute something close to optimal for the conduct of “small science,” particularly in the life sciences. Graduate students and postdoctorals are spared some of the drudgery of routine analyses after they have learned to perform such analyses and understand their limitations, and the total group combines a mixture of experience, expertise, ideas from other disciplines, and youthful enthusiasm. We can only conclude that, however haphazard the various mechanisms by which such an enterprise is funded, the average working unit is sufficiently large to attain an intellectual critical mass and to sustain the pace of exciting investigation while training the novice investigator for his future career.
Although this report gives emphasis to the research and education endeavor of the universities, it remains possible for dedicated scholars to pursue meaningful research in the biology departments of the independent four-year colleges. Biology is still mainly “small science,” and research in many subdisciplines can be conducted with relatively modest support. When access to major equipment is required, this is frequently arranged with the faculty of a nearby university or undertaken during the summer at some properly equipped institution. These efforts constitute a significant part of the total life sciences research endeavor.
There are, however, important exceptions to this “small science” pattern. Decidedly larger aggregates of scientists, focused on a single goal, have been brought together to design a biological experiment for a space probe or to study the ecology of a major biome. The integrated approach to environmental research, stimulated by the International Biological Program, promises to open new levels of understanding of the functioning, resilience, and critical sensitivities of man-dominated ecosystems. In this program, teams of ecologists, social scientists, and physical scientists— as many as 150 individuals—cooperate in the analysis of entire ecosystems, such as the Western grasslands, the Eastern deciduous forests, or the Southwestern desert. Their data are compiled, coordinated, and utilized to construct mathematical models of these large systems, one day to be integrated with models of the atmospheres of the same regions. These systems involve so many components and multiple interactions that realistic abstractions or simplifications must be designed for simulation on large digital computers. The model is a combination of mathematical expressions and statistical probability distributions representing the processes and interactions of the system, as from soil to plant or plant to animal, and the impact of temperature on energy flow. A properly designed model can be used to suggest the potentially most fruitful field experiments from among the multitude that might be conducted, to identify gaps in existing knowledge through deficiencies in model performance, and to suggest optimal courses of action in managing real-world ecosystems. In the medical schools, large groups with representatives from several clinical or preclinical departments coalesce to collaborate on some aspect of cardiovascular, neurological, or neoplastic disease. These groups can number from 20 to 200 scientists and may well serve as forerunners of an era of “big biology.”
- WHAT DO LIFE SCIENTISTS DO?
The average life scientist employed in an institution of higher learning devotes about half his time to research, 10 to 20 percent to administration, a fourth to a third of his time to instruction, and the balance to assorted other responsibilities. The actual distribution, of course, varies with the type of institution and the specific disciplinary field and according to whether he has clinical responsibility. This pattern is clearly in contrast with that of life scientists employed by nonacademic institutions, for whom research is, to an even greater extent, their dominant responsibility, demanding about 70 percent of their effort, while the remainder of their time is largely devoted to administrative responsibilities. Surprisingly, nonacademic scientists report that they engage in instruction that varies in percentages of their time from 0 to 10 percent—about 3 percent for the entire group but 8.5 percent for physicians. The physicians also give a sixth of their time to clinical care and hence can devote only about half their time to research. Some pertinent data in this regard are summarized in Table 18 .
Percentage Distribution of Work Time of Some Life Scientists.
The same set of respondents, 6,125 scientists in academic institutions and 3,054 scientists in nonacademic institutions, were also queried with respect to whether the research in which they were engaged was basic, clinical, or applied. It was made clear that these designations were not necessarily mutually exclusive and, indeed, that an individual could check more than one of these categories if he felt that this was appropriate, particularly if he was engaged in more than one research project. Some of the resultant data are shown in Table 19 . It is not surprising that scientists outside the academic world engage in applied and clinical research. But it may be surprising that 22 percent of all life scientists in institutions of higher learning indicated that their research is applied in some degree. By their own judgment, 76 percent of academically employed physicians indicate that they are engaged in basic research, and only 12 percent state that the research that they are doing is “applied” in some fashion. Quite logically, entomologists and the faculty of agriculture schools consider that a large fraction of their research is directed toward application. Conversely, while it was to be anticipated that 48 percent of all life scientists employed outside the academic world engage in applied research, the fact that 79 percent of all such scientists consider that they are engaged in some fundamental research was somewhat surprising. It indicates that the prejudices of many young scientists against careers outside the academic setting, for lack of opportunity to engage in basic research, may well be ill founded.
Types of Research Conducted by Some Life Scientists.
In any case, the reader will recognize that there is no meaningful close definition of the terms “basic” and “applied” in these regards and that these indications by our respondents reflect their motivation in addressing specific problems and not the character of the work. By this measure, one investigator studying sodium transport in human erythrocytes may classify it as “basic” research; another may consider the same study “clinical,” only because human cells are employed for the purpose; and a third may view it as applied, since he hopes to develop a new drug. Taking into consideration these broad caveats, the data of Table 19 provide a useful description of the world of biological research.
- FINANCIAL SUPPORT OF RESEARCH IN THE LIFE SCIENCES
Research in the life sciences is a substantial national enterprise in which the United States invested $2,264 million in fiscal year 1967*; of this, 30 percent was provided by industry, 4.1 percent by foundations and other private granting agencies, 1.2 percent by academic institutions from their own resources, 0.3 percent by local and state governments, and 60.3 percent by the federal government, principal patron of the endeavor. Table 20 summarizes federal expenditures for life science research in fiscal year 1968. Research supported by industry was largely conducted in-house. In all, biomedical research conducted within federal laboratories required the expenditure of approximately $435 million. In part because of the proprietary nature of industrial biomedical research, and largely because the “principal investigator” in industrial and federal laboratories functions with a large supporting organization for whose expenditures he is not responsible, it was patently impossible to obtain, by questionnaire, meaningful data concerning research expenditures from individual scientists in these two sectors. Our data, therefore, are restricted to information provided by individual life scientists employed by academic institutions and by academic department chairmen. Only the former are considered in this chapter; the latter are discussed in the succeeding chapter. The collected data, summarized in Tables 21 , 22 , and 23 , indicate that in fiscal year 1967 the 4,046 responding academic life scientists, each of whom was principal investigator of one or more research grants or contracts, had available to them, collectively, $162,883,000 in support of the direct costs of research. The growth of this system is indicated by the fact that, in the previous year, the same investigators had available $134,726,000 and, in the prior year, $115,319,000. It is most unfortunate that we have no data for the same group in fiscal years 1969 or 1970, and, hence, no realistic data base with which to examine the consequences of the alterations in federal funding of science that have occurred since our questionnaires were distributed.
Federal Obligations for Research in Life Sciences, by Agency and Discipline—Fiscal Year 1968 (In Thousands of Dollars).
Financial Support of Academic Research in the Life Sciences (In Millions of Dollars).
Numbers of Research Grants and Contracts Awarded to 4,046 Academic Life Scientists.
Average Size of Research Grant (Direct Costs) in Thousands of Dollars.
It will be seen that, using our categorizations of the life sciences, molecular biology and biochemistry commanded one fourth of all reported support, a substantial fraction of which went to individuals with appointments in clinical departments. Following, in rank order, were physiology (17 percent) and disease mechanisms (14 percent). Only 1 percent of the total support went to scientists who stated that they were studying morphological problems and 2 percent, each, to those engaged in behavioral biology and in the study of systematic biology and evolution, with other research areas distributed in between.
The magnitude of support reported for the research area of disease mechanisms is disturbing in that, proportionally, it is very significantly under-represented. While the relative support per research area for all other areas may be considered a reasonably fair indication of the fraction of total national support that they command, this is surely not the case for disease mechanisms, presumably due to the disproportionately low response to our questionnaire by clinical investigators. Thus, it is highly doubtful that the support of research directly concerned with disease mechanisms by the National Institutes of Health is only 15 percent of its extramural research program, since half of its total extramural research support is granted to clinical investigators.
Caution is necessary in interpreting these data, however, because of the failure of the questionnaire to be sufficiently precise in guiding the respondents. Although “disease,” broadly taken, is the concern of clinicians and pathologists, there are no aspects of the study of disease, other than access to human patients, that are unique to their endeavors. In addressing himself to cardiac disease, the clinician may actually function as a physiologist who studies vector cardiography or analyzes the composition of blood obtained by catheterization of one of the cardiac chambers; or he may be concerned with the etiology and pathogenesis of atherosclerosis and so utilize the techniques and understanding of the biochemist or nutritionist. Concerned with a hereditary disorder, he may consider himself a human geneticist; if studying changes in the architectonics of the brain, he may view himself as a morphologist or even a student of evolution. If engaged in elucidation of the causative agent of an infectious disease, he may function, variously, as a cell biologist or a biochemist, while, if he is testing a drug in the hope of finding a successful therapeutic procedure, he is, at least for the time being, a pharmacologist. Accordingly, it is entirely possible that students of disease, its etiology, pathogenesis, incidence, or therapy, may well have indicated that their current research area lies in some category other than “disease mechanisms,” thus unintentionally distorting the interpretation that might be applied to these data.
The pattern of support from the National Science Foundation contrasts with that from the National Institutes of Health. Both supported molecular biology and biochemistry more heavily than any other category, but, whereas the National Institutes of Health also contributed in a large way to the study of physiology and disease mechanisms, the National Science. Foundation was clearly the principal supporter of systematic biology. The Atomic Energy Commission and the Department of the Interior, while contributing only 4 percent and 1 percent, respectively, to the total support of these life sciences, were particularly concerned with ecology. The principal thrust of support by the National Aeronautics and Space Administration, which contributed only 1 percent of the reported federal total, was in physiology, while only the Department of Agriculture and diverse industrial contributors allocated as much as one seventh of their research funds to studies involving nutrition.
Of interest is the fact that, whereas the voluntary societies were organized to combat the dread diseases, only 22 percent of their funds went to scientists who classified their own research as bearing directly on disease mechanisms, whereas one third of their support went to investigators in molecular biology and biochemistry, and one seventh each to studies of physiology and cellular biology. Clearly, the administrators of these societies were sufficiently understanding of the problems involved in treating and preventing these diseases to recognize the need for relevant basic research.
Table 21 indicates clearly that indeed the federal government is the principal patron of these areas of scientific endeavor. Three fourths of all funds in direct support of research derived from the federal government, while one sixth of such funds was provided out of the academic institutions' own resources. The low figures quoted for support by state and municipal agencies refer to direct granting activity, but the state budgets for the public universities contributed in major degree to the 16 percent of all directly research-supporting funds that are stated to have come from the institutions' own resources.
Particularly disappointing is the low order of contribution to research support provided by industry, private foundations, voluntary societies, and individual contributors shown in Table 21 . This is the consequence not so much of a low frequency of granting activity as it is of the relatively small awards actually made by these sources, as shown in Tables 22 and 23 . Thus, the average grant from industry was only $4,000, that from the voluntary societies, $10,000, and that from private foundations, $13,000. These figures are in contrast to grants from the National Science Foundation ($14,000), the National Institutes of Health ($30,000), and the federal average of $25,000.
Of some interest is the pattern of support by discipline. Typical grants in nutrition, ecology, and systematic biology are of the order of $15,000 per year, whereas grants to investigators in most of the other research areas were about twice as large.
Utilization of Research Grants
Typically, a research grant is utilized to provide consumable supplies, major and minor equipment, salaries of technicians and clerical staff, travel and publication costs, stipends for graduate students, postdoctoral fellows, and visiting investigators, as well as a variable fraction of the salary of the principal investigator not to exceed that fraction of his annual effort invested in the research project in question. Uniquely, research grants to clinical investigators may require expenditures in support of the basic costs of maintaining patients in hospitals; other grants may provide for unusual purposes such as ship time, international travel either to meetings or for work in the field, and, increasingly frequently, computer time. The relative distribution of expenditures among these various areas from research grants in support of research in the life sciences was not ascertained by the present study. However, data describing the general patterns of funding by the National Science Foundation are summarized in Table 24 .
Utilization of Funds from an Average Two-Year Research Grant in the Life Sciences—National Science Foundation—1968.
Research Support as a Function of the Investigator's Age
In a general way, increasing research support comes to the academic investigator as he gains seniority in the system. As shown in Figure 34 , this is clearly true for investigators supported by the National Institutes of Health and most other sources. The figures shown for “all sources” represent the simple arithmetic means for all grants from all sources. Because of the relatively large number of small grants from the National Science Foundation, industry, foundations, and voluntary societies, the mean grant size for all sources is decidedly less than that shown for the National Institutes of Health. Nevertheless, the trend is quite apparent: individual research support attains a maximum at 50 to 60 years of age and declines thereafter. This phenomenon is scarcely visible for the National Science Foundation, largely because this beleaguered agency strives to stretch its available resources as far as it can to support all qualified applicant investigators whose proposals fall within its purview, thus markedly reducing the amount of money available per applicant investigator.
Research support of life scientists as a function of their age. (Source: Survey of Individual Life Scientists, National Academy of Sciences Committee on Research in the Life Sciences.)
- RESEARCH INSTITUTES
The preceding survey of the major parameters of the world of biological research fails to convey the myriad arrangements for both research and education in biology. It ignores the dozens of small research institutes in which excellent investigators quietly pursue their research, occasionally with profound impact on the conceptual development of biology. The Cold Spring Harbor Laboratory for Quantitative Biology has had a brilliant record of achievement, and its summer courses have trained virtually all those who have led the modern development of virus and bacterial genetics, a major segment of molecular biology. Developmental biology and some aspects of neurophysiology have received great stimulus from the research and education programs of marine-biology stations such as that at Woods Hole, Massachusetts. Much of the current understanding of neurochemistry and the physiology of the brain has been obtained at small research institutes under private or state auspices, while ecology has grown at a multitude of field stations remote from their parent institutions.
- NATURAL HISTORY MUSEUMS
Natural history museums, with their combinations of scientists, research collections, and field stations are unique non-degree-granting academic institutions for research and graduate training. Quite apart from its role in public education through exhibits, a natural history museum contributes to the acquisition of scientific knowledge in two principal ways.
Its staff of scientists may engage in original research in systematic biology, evolutionary biology, ecology, geophysics, astrophysics, oceanography, and many other fields of science, depending upon their academic training and scientific interests. While many museum scientists depend on specialized collections in conducting their investigations, an increasing number engage in field and laboratory experimental studies of living organisms, or of ecological problems in natural settings. Their collections provide the basis for taxonomic-classification services necessary to many other scientists and also provide a base line for ecological studies.
The combination of resident scientists, research collections, and field research facilities provides intellectually attractive settings for visiting scientists. The number of graduate students who receive part or all of their graduate training in natural history museums is impressive and increasing.
Natural history museums, as both forums and research settings for systematists, ecologists, and environmental scientists, are becoming increasingly important as a national scientific resource, despite a long history of public neglect.
- BIOLOGICAL DISCIPLINES
For brevity and conciseness, we found it useful to structure all the life sciences into a dozen research areas. But this should not conceal the rich and diverse infrastructure of the life sciences. As we have seen, classical disciplinary labels have lost their meaning, but one could readily describe a hundred or more subdisciplines based on the work of groups of likeminded scientists who have blended the approaches of several older disciplines in attacks on some specific subsets of biological problems. A few examples are cited in the following paragraph.
Photobiologists, well versed in optics and the physics of light, are variously concerned with the mechanism of vision, the events in photosynthesis, the emission of light by bacterial and animal forms (the biological purpose of light emission by all but fireflies being not at all evident), and the photoinactivation of enzymes and viruses. Neuroscientists bring the skills of electrophysiology, cellular biology, molecular biology, and communications theory to bear on studies of information processing in the nervous system. Oncologists, focusing on the essential nature of the transformation of normal cells into malignant ones, are similarly a group apart, borrowing from every major discipline that may be of help, while vascular physiologists necessarily borrow from hydrodynamics and studies of urban traffic flow as they study the operation of a capillary bed or a major blood vessel. Physical anthropology is a subdiscipline that contributes to the total endeavor while it provides a bridge from the biological to the social sciences. It is the study of the bodily manifestations of human variation—in particular, the description of human body size, shape, and function in the light of man's history—and the role of heredity, environment, and culture in bringing about man's present diversity. The biological anthropologist aims to understand human physical variation and to apply his knowledge for human betterment through medicine and engineering.
As concern with the environment grows, an increasing number of physicians and biologists of many backgrounds have generated the area of research and practice called “environmental health,” the concern of one of the panels of this survey. More sophisticated understanding of this field should permit society to enjoy the fruits of an advancing technology, a superior living environment, and freedom to develop a society with fewer restraints and tensions. Past effort is minuscule compared with the magnitude of the problem. Since the problems increase with increasing population density and developing technology, efforts at controlling the environment, and thus the health of the population, must keep pace. Indeed, in a very real sense, students of environmental health serve technology by providing the knowledge permitting its benefits to be enjoyed without adventitious adverse effects on the health of man and, more broadly, on the environment of man. Thus, support of an adequate level of competence in environmental health is indispensable to a society that elects to make optimal use of the fruits of technology. Accordingly, the environmental-health resources of the nation must first be expanded to catch up with the problems now with us and thereafter be developed, along with technological development, to provide an adequate preventive program. Current support of research in environmental health probably lies between $30 million and $50 million per year; support for training for both research and practice is between $9 million and $18 million per year and is known to support (in 1969) 974 candidates for the master's degree, 981 candidates for the Ph.D., and 148 postdoctoral fellows.
A broad federal policy is needed, with a long-range plan of attack upon the whole problem of environmental deterioration and with better identification of the separate missions and responsibilities of the several federal departments and agencies. Only with such a policy will it be possible to develop in an orderly way the required training programs to supply the personnel needed for both research and practice, both within and outside the government, necessary to build a strong foundation for effective control programs against environmental-health hazards, a foundation that must rest on the entire current understanding of the life sciences.
Thus, the world of research in the life sciences is marvelously diverse. Tens of thousands of scientists in a thousand institutions contribute to its progress. They migrate between institutions, between classes of institutions, and between subfields of biology. They are quick to seize upon any new instruments or techniques, without regard to whether these are initially devised for use in the physical sciences or for some other research area in the life sciences. Biochemistry has become the language of biology, providing the bridge to the physical sciences, but it has yet to be applied to the farthest reaches of organismal biology. The federal government is the principal sponsor of the entire endeavor and, for the indefinite future, only the federal government can sponsor an effort of this magnitude. Its success will affect all aspects of our lives, and its conduct has become one of the central purposes of our civilization.
Basic Data Relating to the National Institutes of Health 1969, Associate Director for Program Planning and Evaluation and the Division of Research Grants, National Institutes of Health. U.S. Government Printing Office, Washington, D.C., 1969, p. 4.
The Invisible University: Postdoctoral Education in the United States, Report of a Study Conducted under the Auspices of the National Research Council, National Academy of Sciences, Washington, D.C., 1969.
Science Citation Index; An International Interdisciplinary Index to the Literature of Science. (Published by Institute for Scientific Information, Philadelphia.)
- Cite this Page National Academy of Sciences (US) Committee on Research in the Life Sciences. The Life Sciences: Recent Progress and Application to Human Affairs: The World of Biological Research Requirements for the Future. Washington (DC): National Academies Press (US); 1970. CHAPTER THREE, THE WORLD OF BIOLOGICAL RESEARCH.
In this Page
Recent activity.
- THE WORLD OF BIOLOGICAL RESEARCH - The Life Sciences THE WORLD OF BIOLOGICAL RESEARCH - The Life Sciences
Your browsing activity is empty.
Activity recording is turned off.
Turn recording back on
Connect with NLM
National Library of Medicine 8600 Rockville Pike Bethesda, MD 20894
Web Policies FOIA HHS Vulnerability Disclosure
Help Accessibility Careers
Research Areas
Research activity in the Biology Department spans the full range of biological organization, from molecules to ecosystems. Main research fields are indicated here, as links to groups of faculty doing research in those areas.

Biochemistry

Cell Biology

Computational Biology
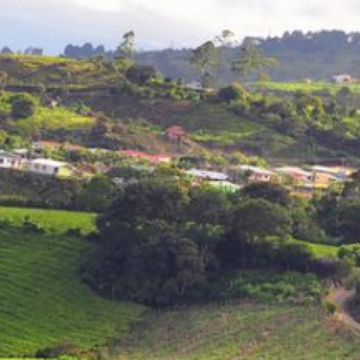
Conservation Biology

Developmental Biology

Marine Biology
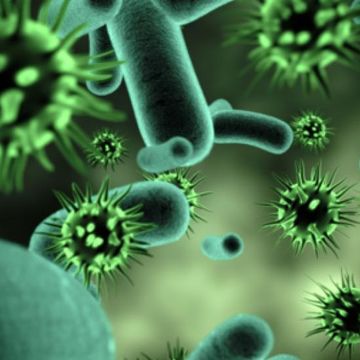
Microbiology

Molecular Biology
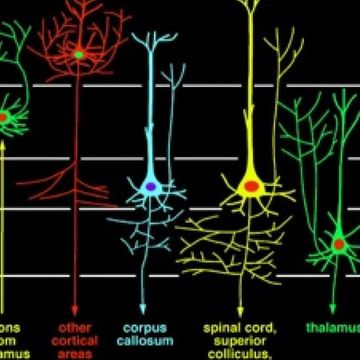
Neurobiology
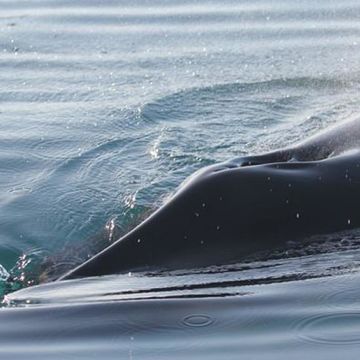
Plant Biology
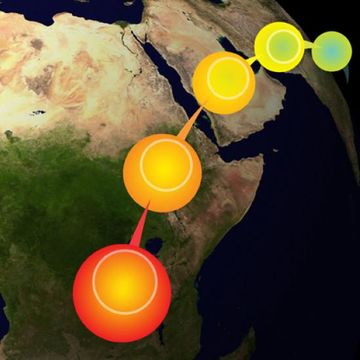
Population Biology

- Prospective Students
Biochemistry
Cell biology, computational biology, developmental biology, microbiology, molecular biology, plant biology, structural biology.
- Science Education Research
- Undergrad Research
- MCDB Program
- E & E Program
- Hot Metal Bridge Program
- How to Apply
- Financial Support
- Teaching and Teaching Minor
- Fellowships and Awards
- Career Development
- Life in Pittsburgh
- Our Current Students
- Our Past Graduates
- Docs & Forms
- Graduate Student Organization
- Intro Research Labs
- Research & Internships
- Teaching Assistant Program
- Awards and Fellowships
- Careers & Postgraduate Education
- Dept Honors
- Pedagogical Innovation
- All Faculty
- Graduate Students
- Student Employment Info
- Suggest a News item
- Upcoming Events
- Past Events
- Kohr Lecture
- Tousimis-Lauffer Distinguished Lecture Series
- In-Classroom Support
- Mentoring: A Path to Diversity
- Support & Research Opportunities
- Microscopy Facility
- Cell Culture & Virus Labs
- Greenhouses
- Molecular Imaging
- Shared Research Support Services
- Message From Our Chair
- Fiscal Office
- Main Office
The University of Pittsburgh is a leading research university, and the discovery of new scientific knowledge is a major focus of the Department of Biological Sciences. Research within the Department is aimed at understanding a broad range of biological processes, from the molecular and biochemical to the organismal and population levels. The Department fosters a highly stimulating and interactive environment for the more than 25 individual research programs, and provides a foundation for exploring novel biological phenomena that require interdisciplinary approaches.
Are you an undergraduate interested in a research experience? If so, start here .
For a recent example of the high profile, recent research being performed by our Faculty, please see Professor Boyle's collaborative work on a new placental model to measure pathogen infection
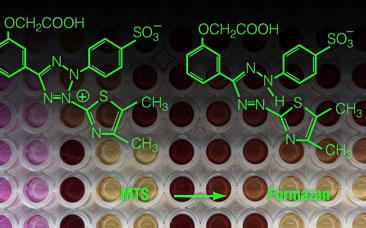
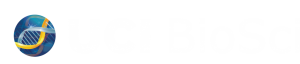
- UC Irvine Home Page

Making Biology Come Alive
Reflecting the University of California’s three-part fundamental mission of Teaching, Research and Public Service, the UCI School of Biological Sciences research programs are world-class. Faculty, graduate students and many undergraduates participate in cutting-edge research on a wide variety of subjects, including:
- Health-related issues such as cancer and infectious diseases
- Neurodegeneration, dementia, Alzheimer’s disease, learning and memory
- Stem cell therapies
- Environmental and evolutionary studies
- Science teacher education
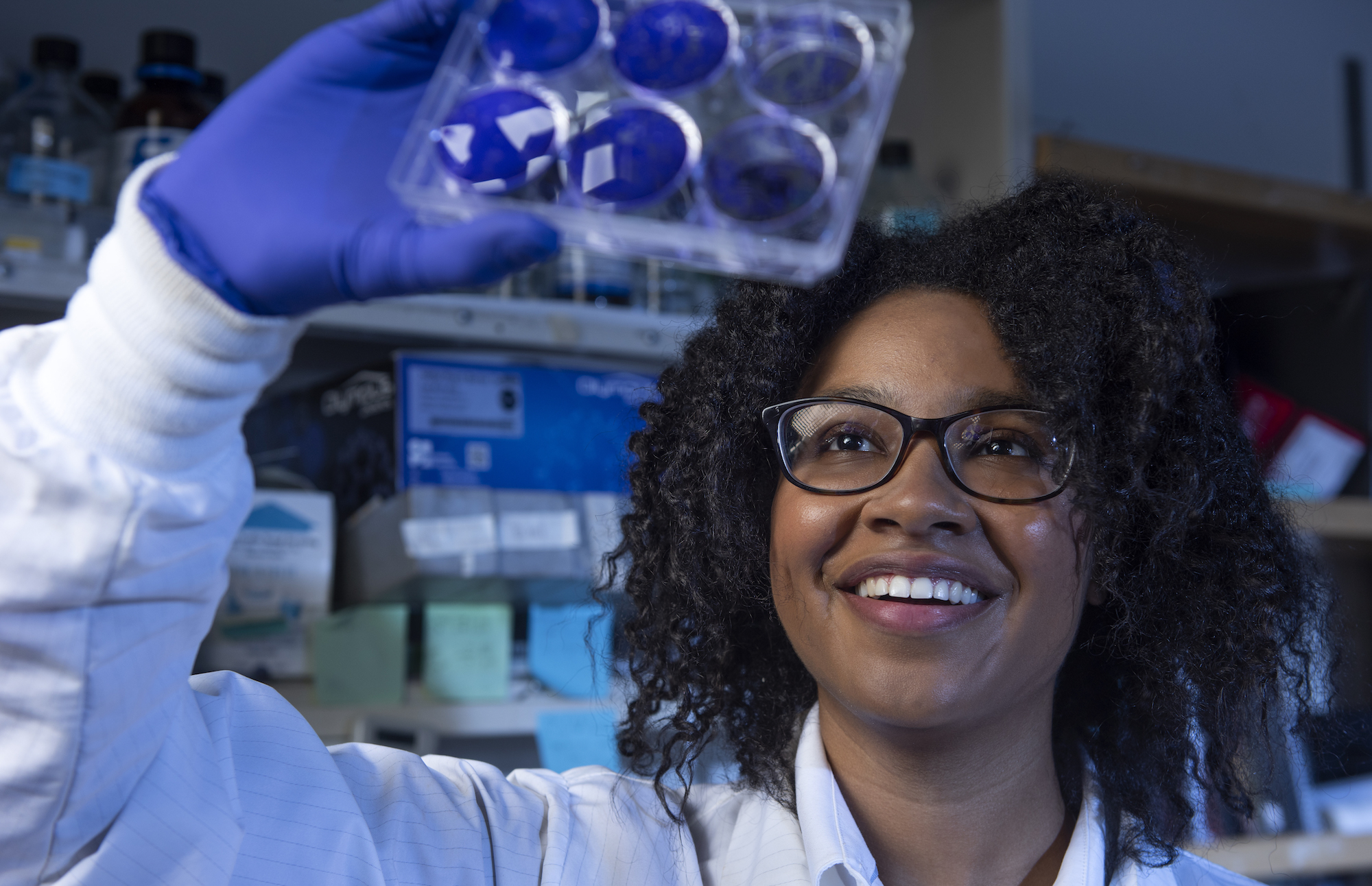
Funding Fuels Our Research
As biologists, faculty in the School of Biological Sciences investigate the underlying principles of nature and use their discoveries to bring about a better tomorrow. In order to produce groundbreaking discoveries, funding is necessary. The Research: Administration and Development unit assists with developing and submitting grant proposals and managing grant awards according to sponsor requirements.
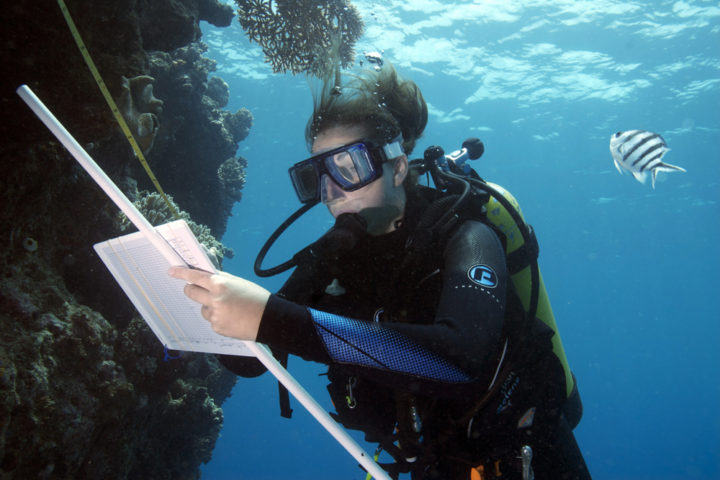
For more information on current programs, please visit the four departments here:
- Developmental and Cell Biology
- Ecology & Evolutionary Biology
- Molecular Biology and Biochemistry
- Neurobiology & Behavior
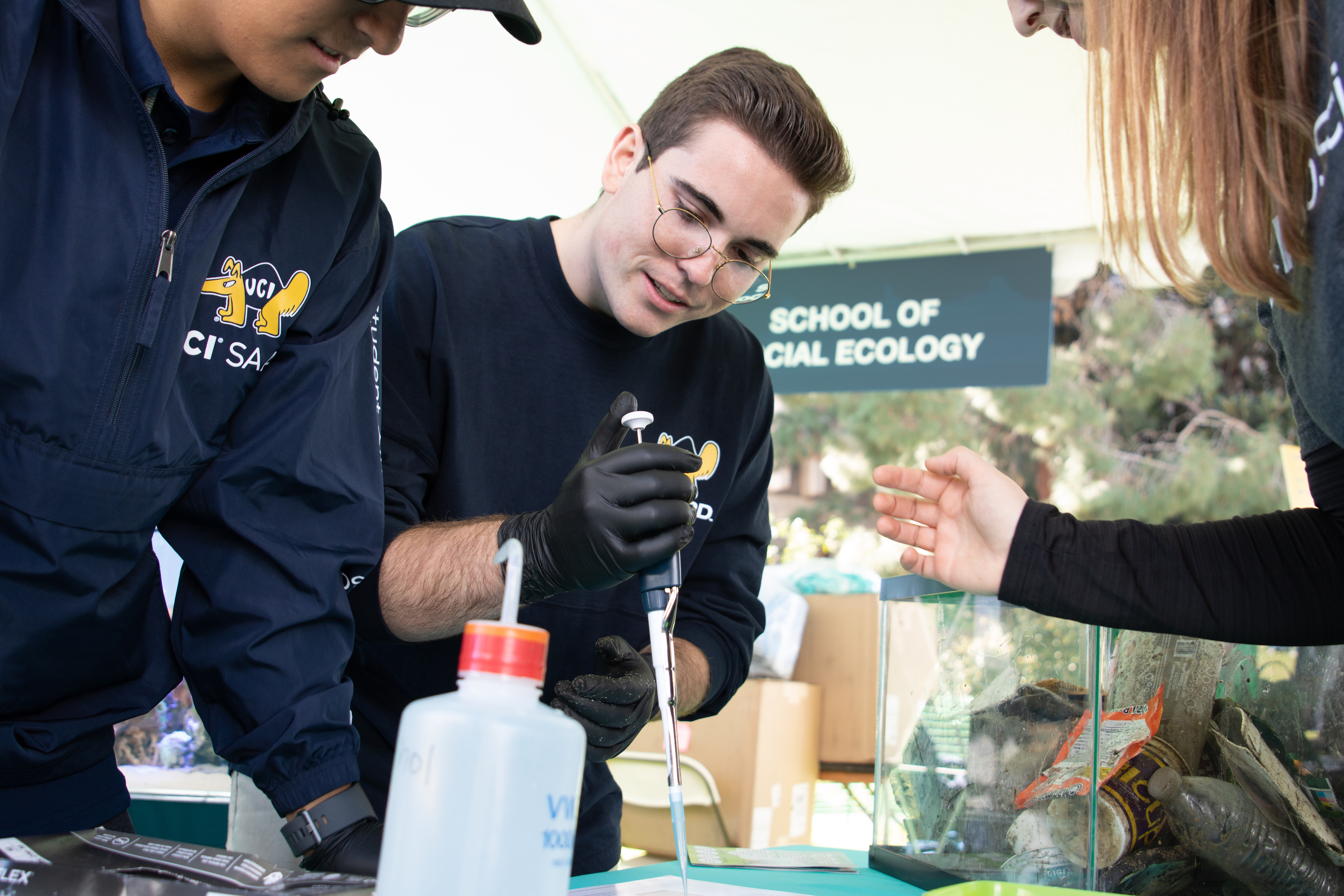
For information on student research opportunities, click on one of the following links:
- Undergraduate research >
- Graduate research
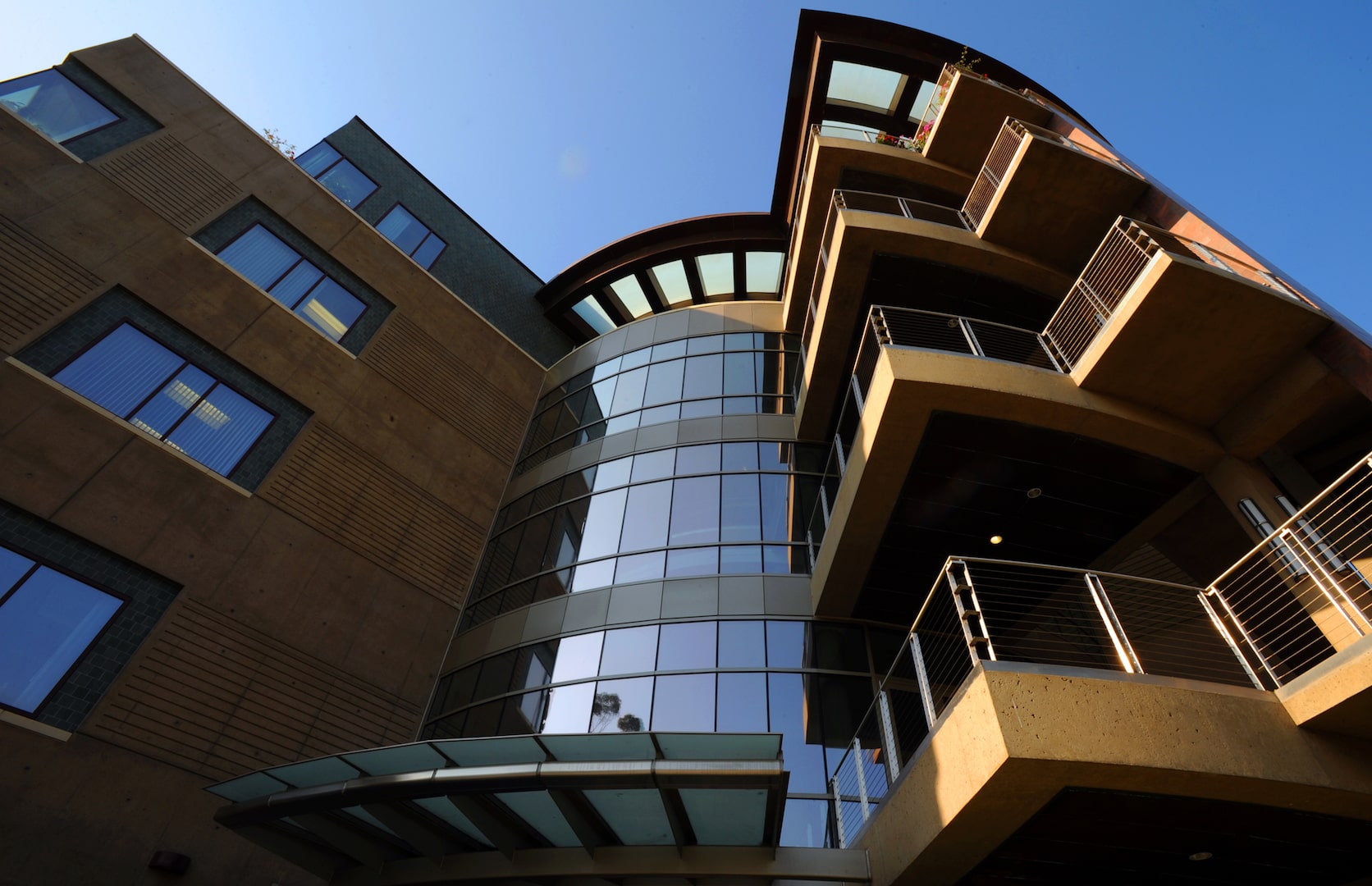
The School also offers many resources to support research including:
- Research Facilities
- Services and Resources
- Natural Reserves and Environmental Facilities
- Room Reservations
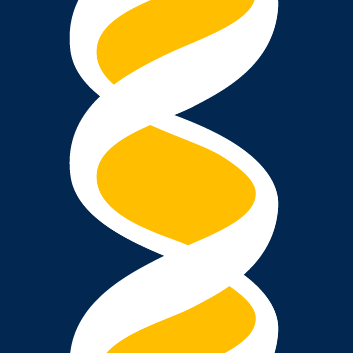
College of Biological Sciences
Student-led research reveals “off-switch” for autophagy.
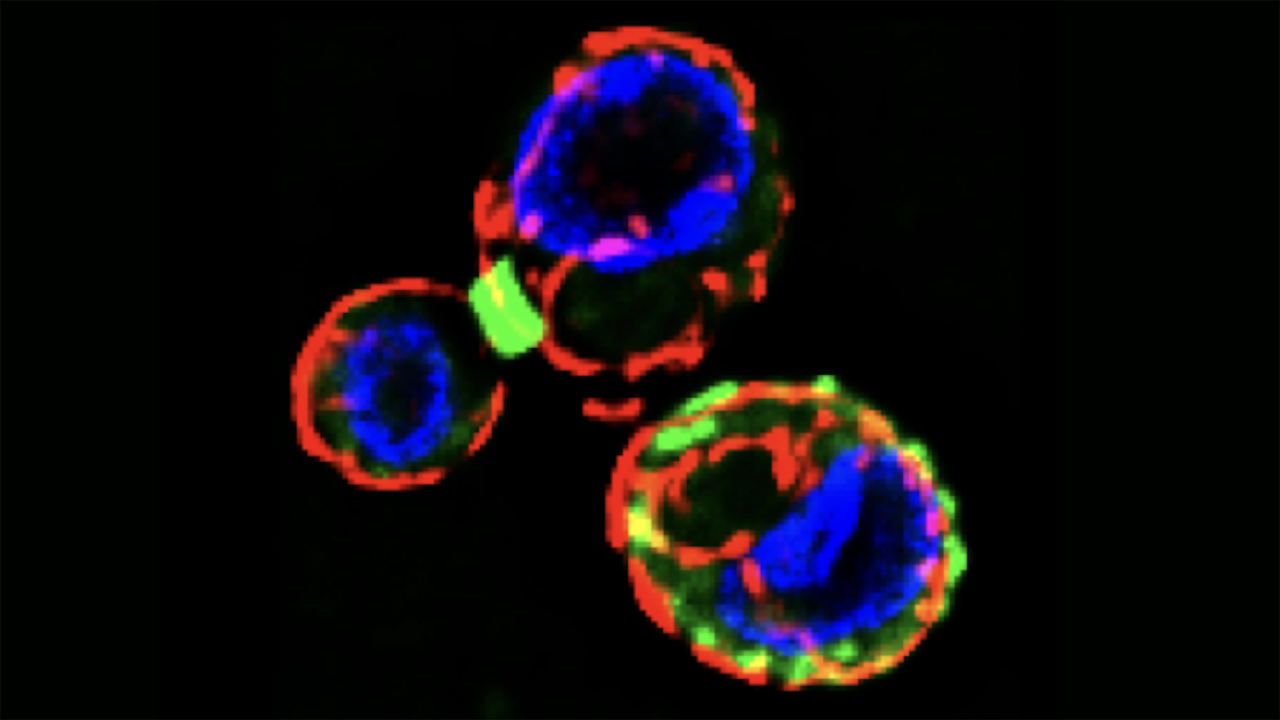
New discovery came from chance occurrence in undergraduate teaching lab
- by Liana Wait
- March 21, 2024
A chance observation in an undergraduate laboratory class has shed light on a key cleaning and recycling process carried out by all eukaryotic cells. Autophagy breaks down organelles, proteins and other molecules so their components can be reused and plays a protective role in preventing disease. However, when autophagy doesn’t work correctly, it’s associated with cancer and neurodegenerative diseases including Alzheimer’s. Previous research has uncovered how cells activate autophagy, but little is known about how it is switched off.
The new research, published Dec. 14 in Molecular Biology of the Cell, shows that cells switch off autophagy by rearranging structural proteins called septins. The researchers showed that yeast that lacked functional septins could activate autophagy but were unable to stop it.
“Our study has opened a new way to think about how autophagy can be turned off, which is something biologists have very little insight on,” said senior author Kenneth Kaplan, a professor of molecular and cellular biology. “This has important implications for understanding autophagy’s protective role in various disease progressions, and how it becomes dysregulated in other diseases.”
The study is also an example of the value of including undergraduates in research, for both students and the advancement of our collective understanding of basic science. All phases of the study were undergraduate-driven, and all three of Kaplan’s co-authors, Luis Perucho-Jaimes, Jonathan Do, and Alexandria Van Elgort, worked on the study as undergraduate research assistants.
“This paper really illustrates the serendipity of combining experiential undergraduate education with basic research,” said Kaplan. “It was an undergrad-driven project and I was really proud of them for pushing all of this forward.”
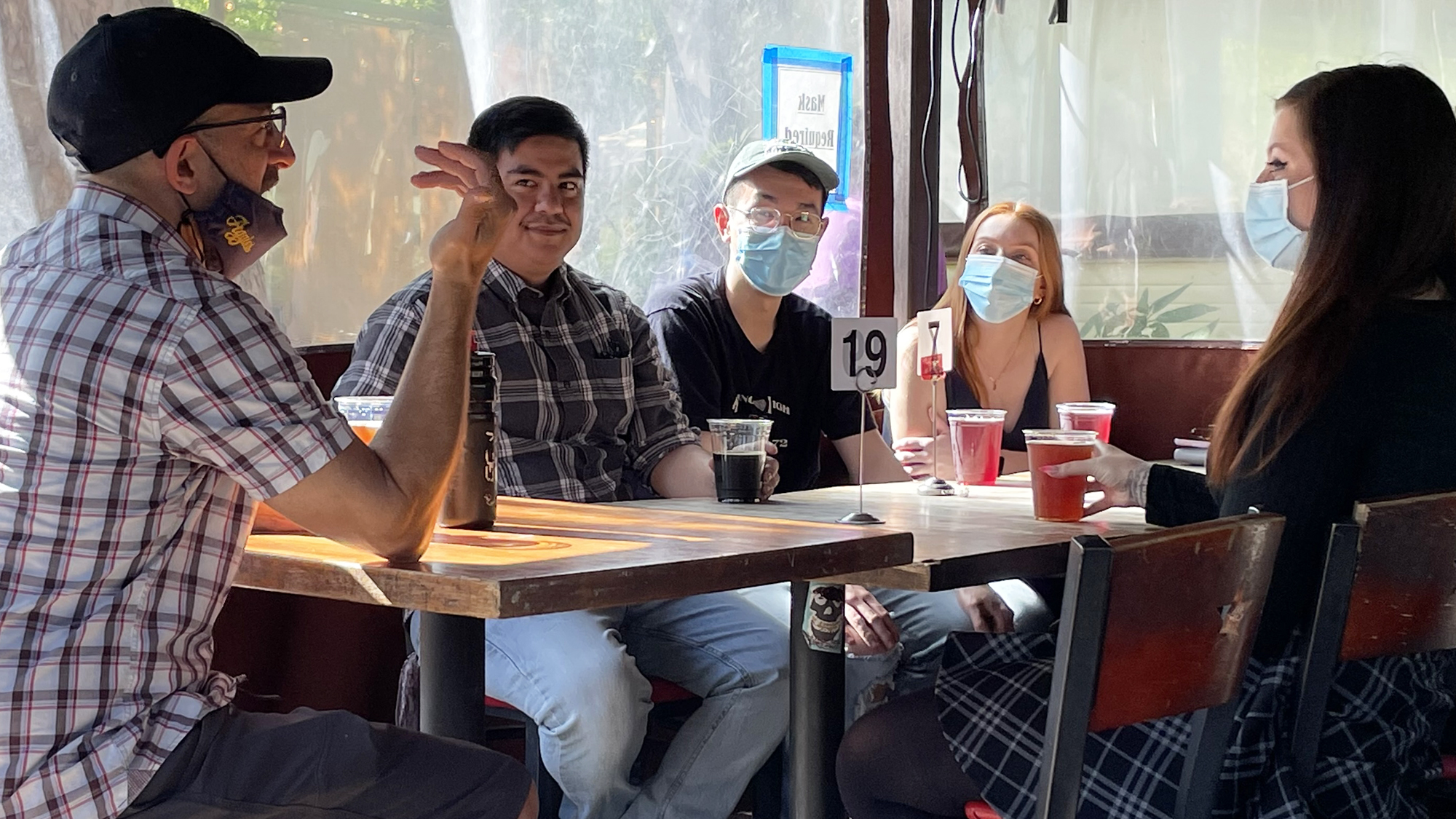
A chance observation
The study arose from a student’s observation in Kaplan’s 2016 cell biology lab class. Due to delays caused by what Kaplan describes as “class chaos,” yeast cells that the students were imaging entered a nutrient deprived state by the time they made it to the microscope.
Under the microscope, one student, Jonathan Mendez, noticed that the cells’ cytoskeletons had undergone a surprising rearrangement. Specifically, the septins—filamentous proteins usually involved in cell division—had rearranged themselves into an unusual and previously undocumented configuration.
When yeast cells divide, septins usually form a ring at the “neck” that divides the mother and daughter cells. However, in the starved yeast cells, septins formed localized patches that were scattered around the cell membrane. Initially, Kaplan and his students were unsure what this observation could mean. Mendez pushed the group to investigate further, so Kaplan took the question back to the lab.
“Jonathan definitely spurred this on—I think without his inquisitiveness we probably would have just said ‘who knows what happened’ and moved on,” said Kaplan.
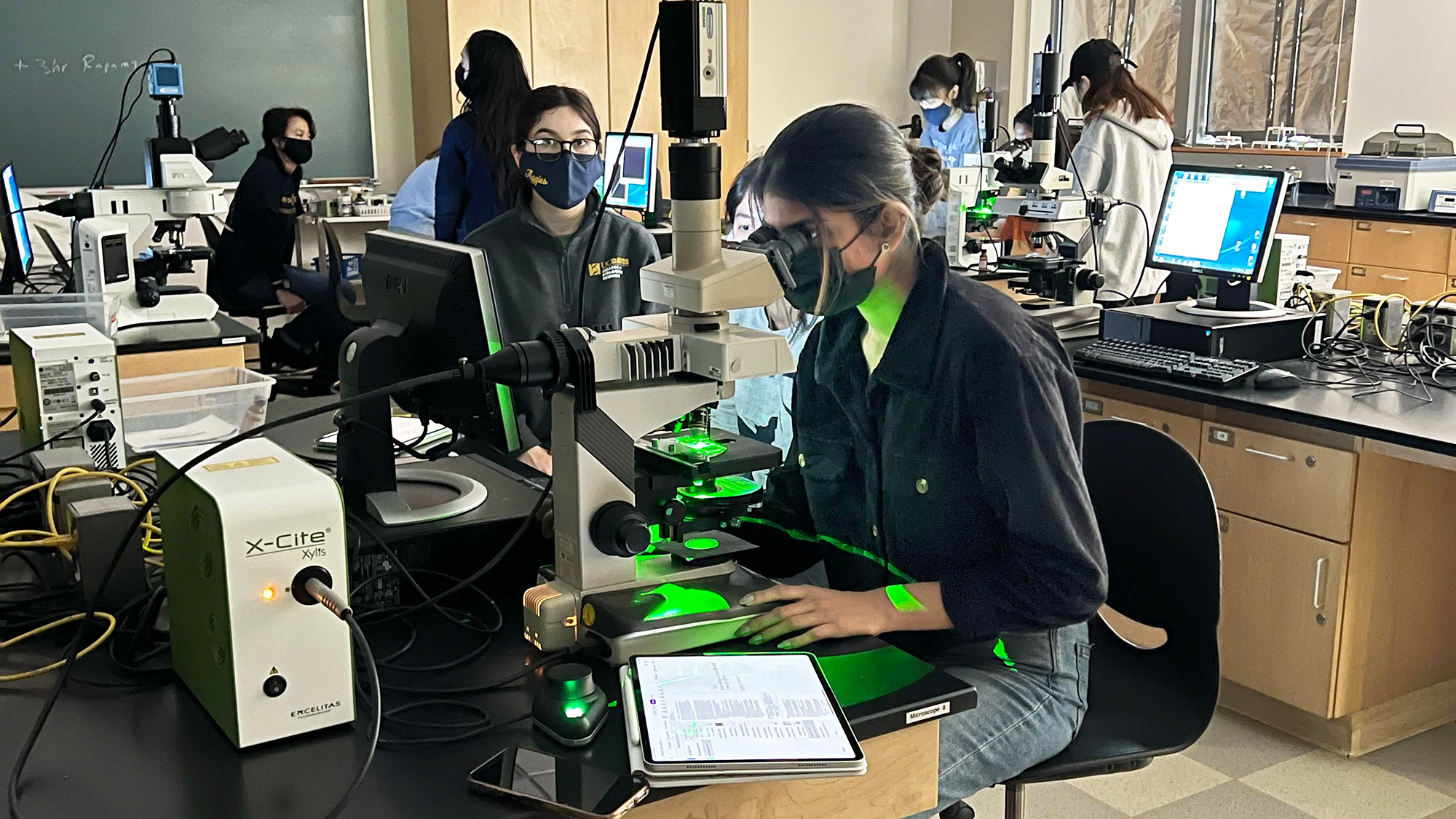
Digging deeper
Knowing that the cells must have entered starvation mode, Kaplan speculated that this rearrangement might have something to do with autophagy.
“Autophagy is one of the key responses to starvation—the cell essentially degrades some of its own structures in order to survive” Kaplan said.
However, it wasn’t clear whether the septins were involved in switching autophagy on or off. To test these two possibilities, the team used high-resolution fluorescence microscopy to make time-lapse videos of yeast cells before and after they were subjected to starvation conditions to examine the timing of the septin rearrangement. These experiments revealed that septin reorganization occurred after autophagy was activated, indicating that the septin patches are not involved in switching autophagy on.
When the researchers measured autophagy rates and duration in “septin mutant” strains of yeast that are unable to produce septin, they found that the septin-less yeast performed higher rates of autophagy than wild-type yeast, and also continued to perform autophagy long after wild type cells had switched the process off.
“Instead of turning autophagy off after 24 hours like most wild type cells, in septin mutants we saw that autophagy persists for quite a bit longer,” said Kaplan. “It seems pretty clear that without septins, autophagy fails to shut itself off.”
This finding offers one of the first clues as to how cells downregulate autophagy. For Kaplan, it’s opened new potential avenues of study, including research into neurodegenerative diseases.
“This work has led my lab to start a longer-term effort to apply our findings to the cell biology of neurodegenerative disease and the connection between septins and dysfunctional neurons,” he said.
Luis Perucho-Jaimes is now working at UC Davis; Jonathan Do is pursuing studies at the University of Arizona; and Alexandria Van Elgort is pursuing studies at Stanford Medicine.
Media Resources
- Septins modulate the autophagy response after nutrient starvation | Molecular Biology of the Cell (molbiolcell.org)
- Liana Wait is a freelance science writer based in Philadelphia. She has a Ph.D. in ecology and evolutionary biology and specializes in writing about the life sciences.
Primary Category
Secondary categories.
University of Missouri
College of Arts and Science
Biological Sciences
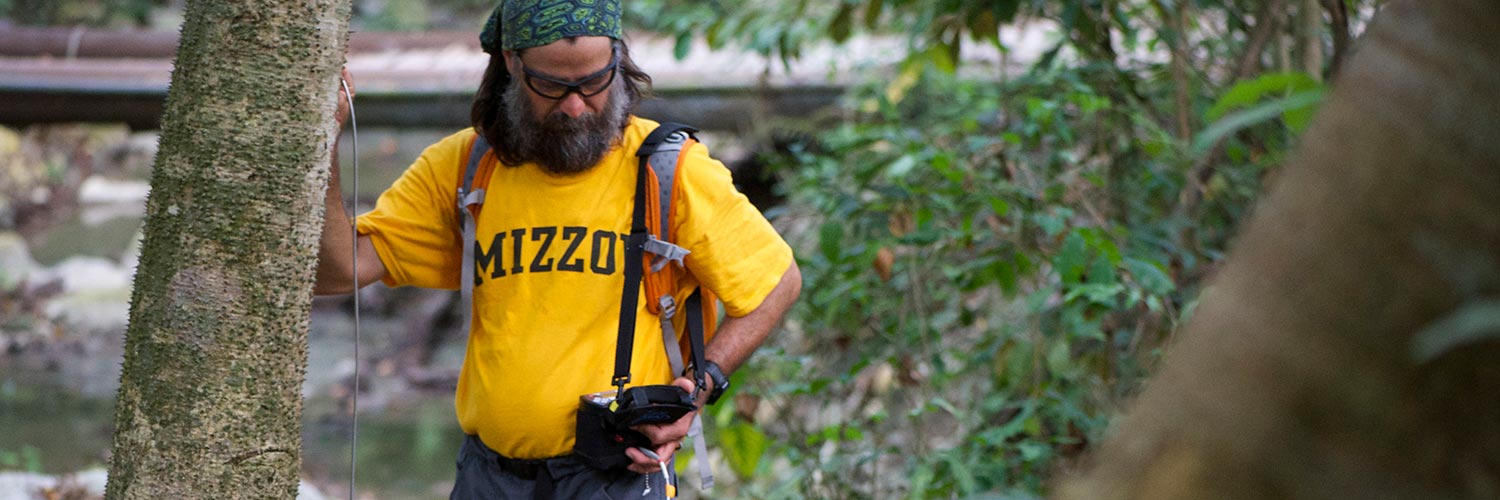
The Division of Biological Sciences at the University of Missouri is distinctive in the breadth and depth of its research. Faculty bring their special expertise in overlapping disciplines to bear on a broad range of biological questions at all levels of organization, from molecules to ecosystems, using model systems drawn from a wide range of organisms. The diversity of thought and approaches, combined with an exceptionally collegial and interdisciplinary culture, fuels new discoveries and scientific breakthroughs with impacts on human health, agriculture, and the environment. We invite you to explore our research strengths and the profiles of our faculty to get a flavor of the exciting research currently underway in the biological sciences at MU.
Research Strengths
6 Ways to Research as an Undergrad in the Biological Sciences
- by Greg Watry
- November 04, 2019
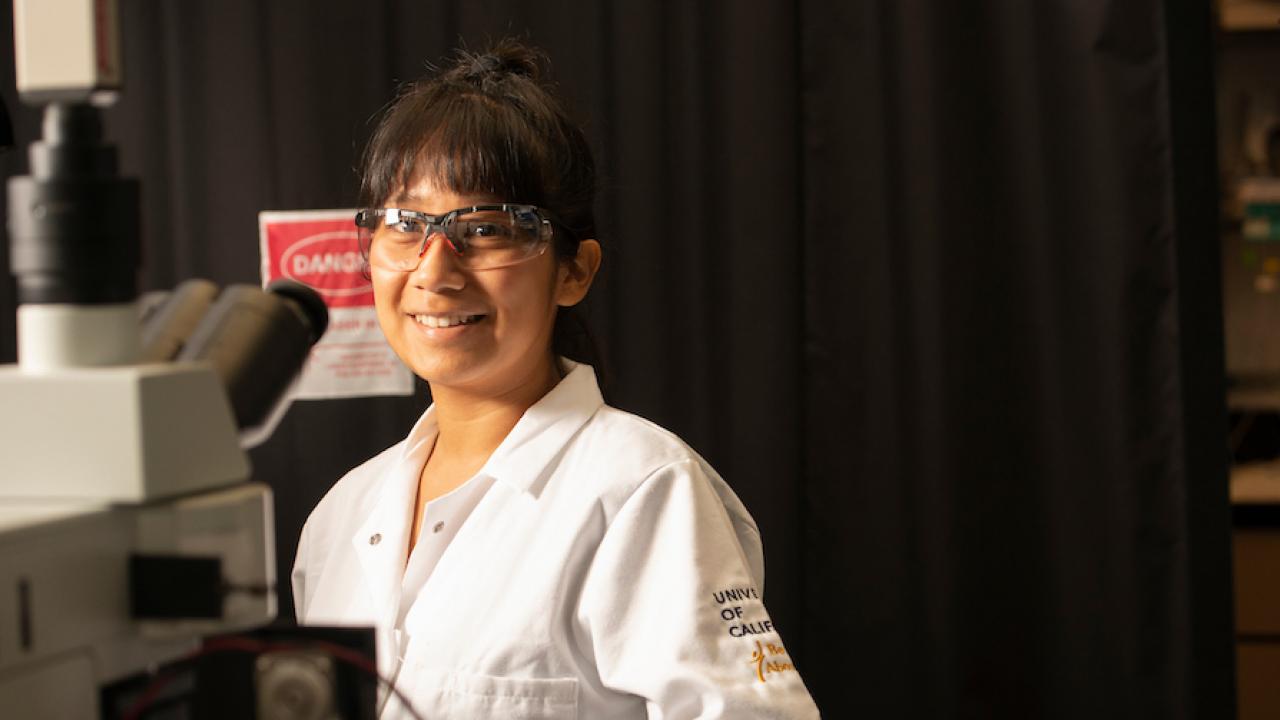
Undergraduates have many opportunities to research
Are you interested in using science to solve critical problems? Undergraduates in the College of Biological Sciences have numerous opportunities to apply knowledge from the classroom to the real world.
Our undergraduate researchers ask important questions: How do physical forces lead to cancerous cells? And what could this mean for diagnosis and treatment? How can you trace human family origins through genomics?
Working with renowned life sciences faculty, they have a close and personal view of the science leading to breakthroughs. Discoveries start with a foundation in curiosity. Here are four undergraduates who followed their interests toward meaningful questions.
1. Tracing family origins
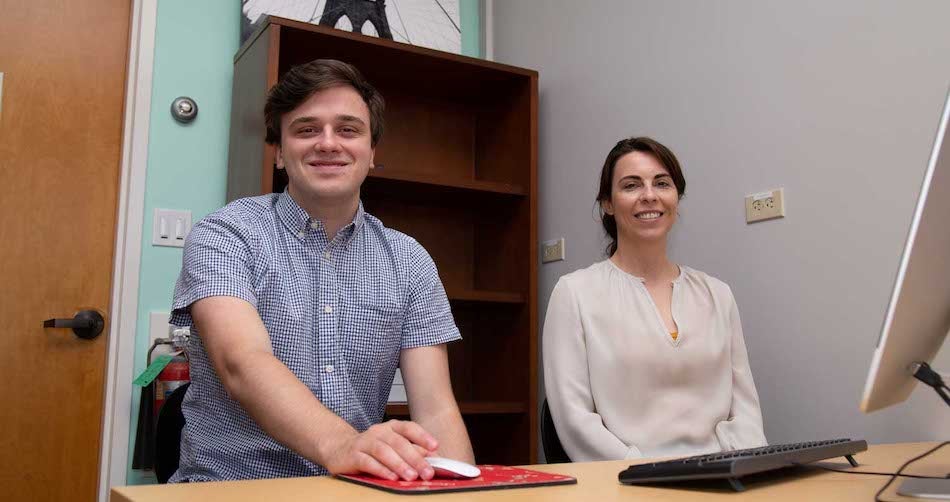
Cole Williams found a passion for genetics at an early age. Then, he became a genetics and genomics major and College of Biological Sciences Undergrad of the Year. In the lab of associate professor Brenna Henn, Department of Anthropology, Williams worked to understand familial relationships in African hunter-gatherer and pastoralist groups. But he ran into a problem. The publicly available computer algorithms Williams used couldn’t make sense of his study population’s genomic data. So Williams found a different solution. He wrote a new algorithm .
2. Experimenting to find your interests
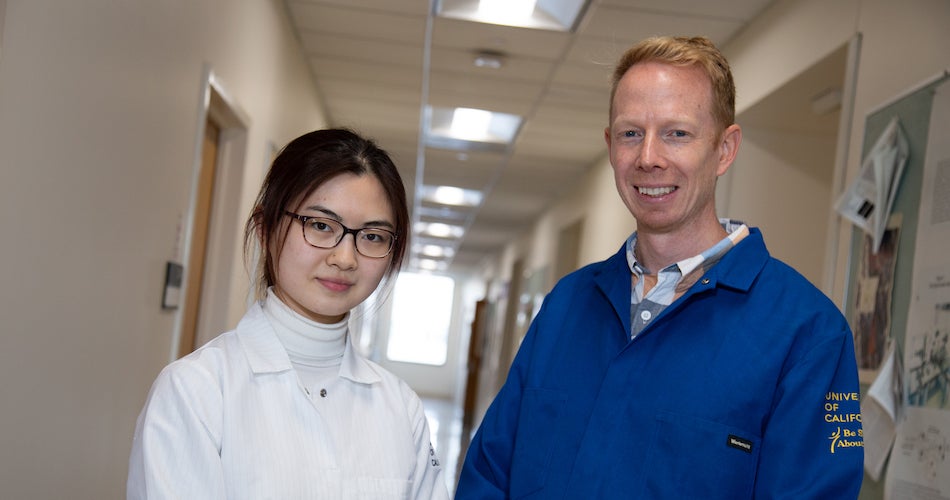
Part of the college experience is figuring out what you don’t want to do. Passion, like discovery, is found through trial and error. Biological Sciences and psychology senior Wenzhe Li rotated through two labs at UC Davis before finding a muse in cytoplasmic dynein, a motor protein used for intracellular transport. Aided by her interest in psychology, Li aims to link cellular and molecular biology processes to mind and behavior .
3. Researching in a new field
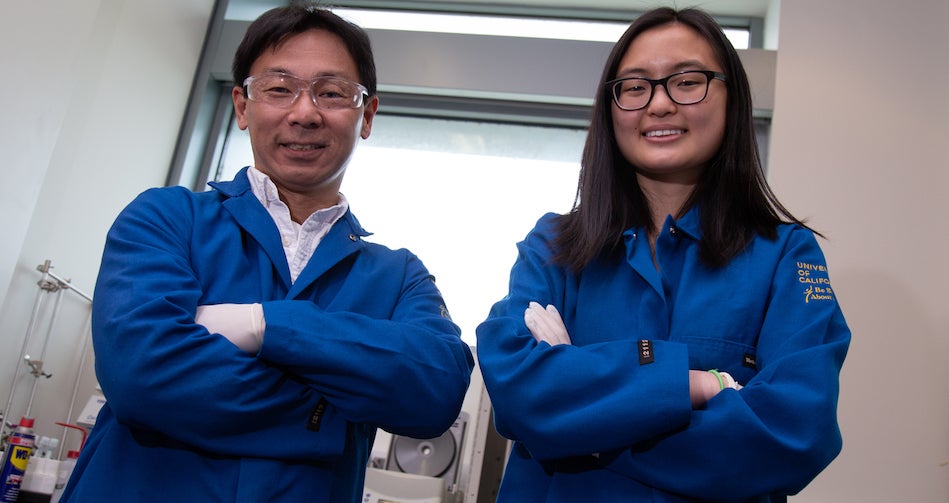
The human body is composed of trillions of cells, which are influenced by a myriad of chemical and environmental forces. But there’s another force at work here, one that’s tugged at undergraduate researcher Joleen Cheah’s curiosity. Mechanobiology is a developing field that studies how physical forces affect cells. Cheah — a senior in biological sciences — is on the ground floor , working in the lab of professor Soichiro Yamada, Department of Biomedical Engineering.
4. Switching from history to biochemistry
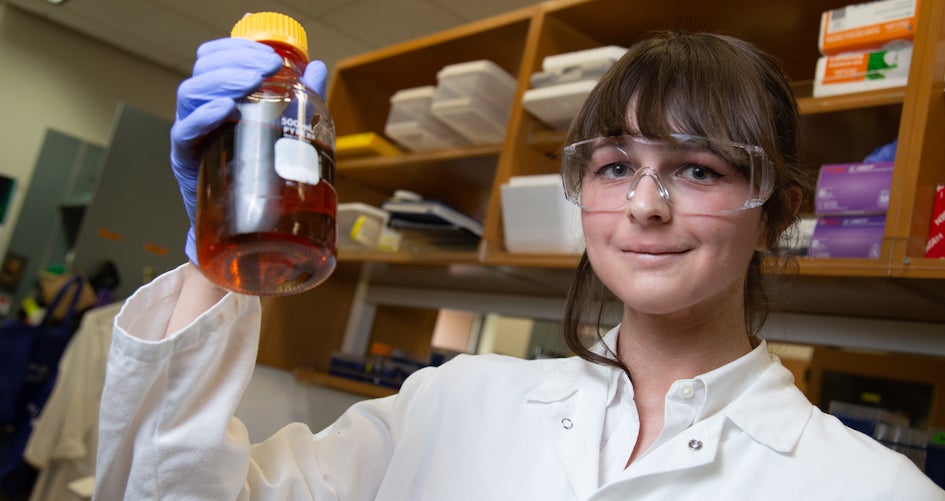
When Lynne Hagelthorn was in high school, she planned to study history as a college student. She loved speech and debate and was fascinated by how humans use language to understand and construct reality. But during her junior year, she took a biotech class and through it secured an internship in the lab of distinguished professor Richard Michelmore, director of the UC Davis Genome Center. Everything changed after that. A member of assistant professor Philipp Zerbe’s lab, Hagelthorn toured the chemical world of plants and became a biochemistry and molecular biology major , learning how plant chemical compound can be used to benefit human health.
5. Clamming at the Bodega Marine Laboratory
Every summer, the UC Davis Bodega Marine Laboratory offers summer classes to undergraduate students interested in coastal and marine sciences. Marine and coastal sciences student Tessa Filipczyk spent summer 2019 taking classes and conducting her own research on the effects of clamming. In this video, she discusses what makes the Bodega Marine Laboratory a special place and how undergraduates can help solve environmental problems with their science.
6. Tide pooling in Horseshoe Cove
After hearing about the Bodega Marine Laboratory’s summer sessions from his friends, Michael Brito knew he had to check them out. Brito, a senior in marine and coastal sciences , spent the summer tide pooling in nearby Horseshoe Cove and exploring the importance of marine protected areas. He learned from top scientists in the field at a first-class research facility.
This year, Brito is acting as BML’s recruiter. Students interested in learning about the summer sessions can contact him at [email protected]. He’s also holding office hours this quarter on Thursdays from 11 a.m. to 12 p.m. in Storer 1347.
Interested in learning more about undergraduate research opportunities at the College of Biological Sciences? Check out our undergraduate research blog .
Greg Watry is a science writer and communications specialist at the College of Biological Sciences .
Subscribe to the Majors Blog
Primary Category
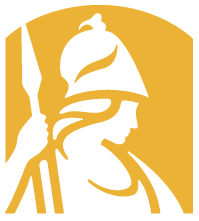
Biological Science Research
Research in biological sciences .
The faculty and students in the Department of Biological Sciences at University at Albany are actively engaged in research in highly diverse research topics and projects addressing a spectrum of issues of interest and concern to the scientific and general community.
Faculty in the Department of Biological Science work with graduate students in the Molecular, Cellular, Developmental, and Neural biology MS and Ph.D. programs and in the forensics MS program on diverse research projects spanning the mechanisms of cell signaling inside the nucleus and cytoplasm to connections between cells and with the extracellular environment in eukaryotic cells and involvement of prokaryotic organisms with disease.
Supervised undergraduate research provides students with the opportunity to gain practical experience working in actual research labs with respected faculty and the opportunity to gain experience in presenting their research and competing for awards.
Research in our lab focuses on microbial genomics and evolution as they apply to infectious diseases and public health. We ask the question: Why aren’t members of a microbial species the same? They may be clonal, but microbial populations are often composed of multiple co-circulating lineages distinguished by large phenotypic and genetic differences. Understanding the origins of genomic variation between strains is fundamental to questions critical to society and public health, such as "Are emerging diseases new species, or variants of existing ones?", “What makes a resistant strain successful?”, and "How will a pathogen respond to selective pressures?". To explore these questions, we use whole genome sequencing of closely related strains to understand the evolutionary, ecological and epidemiological dynamics of bacterial pathogens, which will inform effective, more targeted public health interventions. Current work focuses on different species of bacterial pathogens (e.g., Staphylococcus, Streptococcus, Salmonella) and antibiotic- producers (e.g., Streptomyces). Students will be trained in population genomics, phylogenetics, molecular evolution, and genomic epidemiology.
The Begley lab works on research projects that span the areas of environmental health, cancer and aging. We work to identify and characterize mechanisms of cellular stress response, with a special emphasis on reactive oxygen species detoxification and DNA repair. Specific areas of research are epitranscriptomic writers and targets, tRNA modification, and the translational regulation of protein networks. We use computational approaches, bacterial, yeast, human cell culture and mouse models in our research. We train students to utilize genetic, molecular biology and biochemical techniques to dissect the inner workings of cells and tissues, with the goal of identifying important responses to stress which are linked to human disease.
The primary goals of the Berglund lab are to understand the molecular mechanisms of pre-mRNA splicing and toxic RNA disorders including myotonic dystrophy and C9orf72 amyotrophic lateral sclerosis. We use biochemical, genomic and cellular approaches to study the fundamental rules that govern RNA processing and how these events are disrupted in disease. Knowledge gained from basic biological studies is applied to developing small molecule therapeutic strategies for these devastating neuromuscular diseases.
Our research areas are focused on molecular and cellular physiology. With combinations of conventional electrophysiology, biochemistry, molecular biology, and engineering techniques, we study mechanisms of how ion channels contribute to cellular function and are associated with cardiovascular, neuronal, and skeletal muscle diseases. Current research projects include: 1) Engineer a cell line that shows pacemaker activity and mimics cardiac and neuronal pacemaker cells; 2) Molecular mechanisms of origin of pacemaker activity (or heart beat); and 3) Roles of a group of ion channels in pacemaker activity (or heart beat) and biological clock (or circadian rhythm).
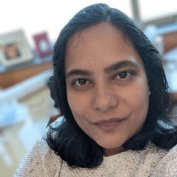
Research in the Dash Lab is focused on craniofacial development, which begins early during embryogenesis with the formation of neural crest cells. Neural crest cells are a transient population of cells that are derived in the dorsal region of the neural tube, undergo an epithelial to mesenchymal transition, migrate to various parts of the body where they interact with their surrounding tissue to undergo terminal differentiation to make up most of the craniofacial bone and cartilage as well as neurons and glia of the peripheral nervous system and melanocytes. Any defects in neural crest formation, migration or differentiation leads to developmental defects known as neurocristopathies, which often involve craniofacial anomalies. When certain subunits of the Mediator complex, which is required for transcription of all mRNA by RNA Polymerase II, are mutated, it leads to defects in neural crest cell and craniofacial development in mice and zebrafish. Our research aims to understand how and why the absence of such ubiquitously expressed genes/proteins results in tissue-specific craniofacial defects.
The focus of my laboratory is to explore the function of non-coding RNAs (microRNAs and novel small/long non-coding RNAs), epigenetics and epitranscriptomics in skeletal muscle stem cell biology, cardiac muscle biology, muscle regeneration, and muscle degenerative disease including Duchenne Muscular Dystrophy (DMD) and DMD-associated cardiomyopathy. DMD is a devastating X-linked childhood muscle degenerative disease. There is no effective treatment available that can either cure or stop the progression of DMD. The development of a successful therapy for DMD has been significantly hindered due to the lack of a complete understanding of the key myogenic processes and DMD pathophysiology at the molecular level. Our goal is to understand the fundamental molecular mechanism of muscle development and to devise new therapeutic and diagnostic platforms for DMD and related muscle degenerative diseases.
In my lab we study neurodevelopment. The focus of my research is to discover new molecular mechanisms underlying cell differentiation migration of neurons and to discover molecular principles defining neuronal identity, synaptic selectivity and neuronal circuit formation in mammals. For this we use a multidisciplinary approach that includes the generation and use of complex genetically-modified mouse models, state of the art 2D and 3D imaging, molecular biology next generation sequencing, bioinformatics, behavioral testing, and whole exome sequencing data of human patients. We have multiple national and international collaborations. Research projects in my lab received extensive federal funding.
The Fuchs lab studies the mechanism and regulation of protein biosynthesis in mammalian cells. Proteins are made by large cellular RNA-protein complexes known as ribosomes, which translate the mRNA sequence into a protein sequence. Until recently it was thought that all ribosomes within one organism were identical. However, mass spectrometry analysis revealed that ribosomes differ in composition and posttranslational modifications. Although many ribosome modifications have been identified, the impact these modifications have on protein biosynthesis is not clear. Using a combination of approaches, we are investigating different mechanisms of translation initiation and the role ribosome composition plays during translation.
The Larsen lab focuses on understanding biological mechanisms driving development, organ repair, and regeneration. Cells residing in the stroma provide signals that drive development and maintain homeostasis in the adult but alteration of signals leads to disease and loss of gland function. We are interested in defining stromal signals that drive development and that can be manipulated to facilitate gland regeneration and restoration of function. We use salivary gland organoids and in vivo models to examine cell-cell and molecular interactions driving development and disease.
The Lee lab studies cell-to-cell communications in health and disease. We develop and use quantitative methods to understand how cell-cell signaling pathways regulate tissue integrity during development, homeostasis and disease. Our current research focus centers on Notch signaling and its transcriptional regulation in stem cells. Notch signaling is a broadly conserved pathway critical for metazoan development, with Notch dysfunction leading to various human diseases, including cancer and cardiovascular disease. However, remarkably little is known about how Notch defects affect signaling at the molecular level to cause such diseases. Understanding precise molecular mechanisms of disease-causing Notch defects have potential to lead to discovery of novel therapies. We use
a multidisciplinary approach, combining biochemistry, cell biology, genetics, statistics and computational biology to understand how Notch signaling drives its transcriptional response in space and time, quantitatively and with single- molecule precision. We also aim to analyze the Notch response in disease-causing Notch states and investigate the effect of environmental, physiological, and pathological conditions on the Notch response. Our multidisciplinary, single- molecule approach put our lab in a unique vantage point to bring novel insights into quantitative understanding of cell signaling in an in vivo context.
Single molecule study of RNA folding and protein-RNA interaction. Goal: Our goal is to understand the fundamental principles that governing the folding of RNA and to protein-RNA interactions. Overview: Folding of an RNA molecule can be viewed as a biased diffusion over its folding energy landscape. Such landscape is populated with different energetic states, similar to “hills” and “valleys” on a geographic map. We survey the folding energy landscape by following folding trajectories of individual molecule. Relative levels of energetic states are reflected by folding rates as high energy barrier slows folding. Also, a molecule is more likely to take an “easy” route to fold than to follow a “difficult” one. We are particularly interested in three areas. (1) Force induced misfolding; (2) Formation of RNA kissing complexes, a type of tertiary structures formed between loops of two hairpins; (3) Ligand and protein binding to RNA structures. Approach: We use a device called optical tweezers to apply pico-Newton (10-12 Newton) force to single RNA molecules. As an RNA molecule folds and unfolds, changes in the extension of the molecule, measured with nanometer precision, reflect structural transitions in real time. Parallelly, we use biochemical and spectroscopic methods to study RNA folding at ensemble. We also employ statistical mechanical modeling to interpret experimental results both in-house and through collaboration.
The Pager lab is interested in how RNA viruses subvert different cellular RNA metabolism pathways. In our lab we study hepatitis C virus (HCV), Zika virus and Dengue virus. These are single-stranded positive-sense RNA viruses that are associated with devastating human diseases such as hepatocellular carcinoma, intense joint and muscle pain and developmental defects in new-born babies. To date there are no licensed antivirals or a vaccine. In the Pager lab applies virology, RNA biology, molecular biology, cell biology and biophysical approaches together with mass spectrometry and next generation sequencing technologies to understand the fundamental molecular biology of these viruses. In particular we are investigating the interaction and molecular mechanisms by which these RNA viruses subvert the host splicing machinery, RNA storage and decay pathways and the epitranscriptome. Additionally, we are investigating RNA structures and RNA-RNA interactions within the viral genomes to understand how these viruses regulate distinct steps in their infectious cycles. By understanding virus-host interactions our research may lead to the identification of new antiviral targets and therapies.
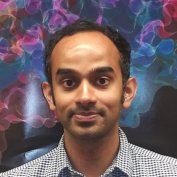
The Reddy Lab is interested in how RNA dysfunction can play a role in neuromuscular diseases and are leveraging this knowledge to develop new therapeutic strategies. We are specifically investigating RNA dysregulation in myotonic dystrophy and in human ITPase enzyme deficiency using a combination of biochemical, cellular and genomic approaches.
We are interested in understanding how DNA information is decoded by a class of proteins called transcription factors. DNA is the instruction manual for building an organism and maintaining its homeostasis. We are most interested in how cells decode this information during life or death situations, such as when cells encounter rapidly changing environmental signals. Projects in the lab will focus on using genomics, molecular biology, and high-throughput approaches to study how transcription factors interpret genetic and epigenetic information to control cell survival after exposure to DNA damage.
In our lab, we are interested in understanding the functional properties of central synapses, the specialized structures that convert the electrical activity of a neuron into a chemical signal for its target cells. We want to understand how individual molecules are distributed within the synapse and how their spatial arrangement influences the properties of neurotransmitter release. We want to know how neurotransmitters diffuse outside of the synapse and generate long-distance signals to different cells. Our ultimate goal is to gain insights into the functional consequences of changes in synaptic function caused by changes in the circadian timekeeping system and with the onset of different neuropsychiatric and neurodenegerative disorders. To perform our studies, we use a combination of experimental and theoretical approaches, including electrophysiology, optogenetics, two-photon imaging and reaction- diffusion computer simulations. We are eager to learn and develop novel experimental approaches and research tools!
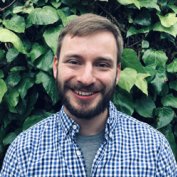
Work in our lab is centered on understanding how the brain processes visual information. We are especially interested in this question in the context of natural vision. The kinds of visual inputs encountered in the real world can be enormously complex. This poses a real challenge to nervous systems, which are tasked with extracting and representing useful information about the scene in order to guide behavior. We leverage the compact and genetically accessible fruit fly brain as a model to understand how neural circuits overcome this challenge. We use a combination of experimental and computational approaches, including in vivo two photon imaging, animal behavior, analysis of the connectome, and advanced image analysis approaches, to concretely link specific neurons, circuits and synapses to neural computations and, ultimately, to visually guided behavior. A fly’s visual system is in many respects quite different from our own, but there are powerful constraints that are shared across very different species, from flies to humans. For example, the same physical laws that underlie the statistics of scenes encountered by flies also shape the scenes humans encounter. These statistical regularities can constrain neural processing strategies. This allows us to use the fly visual system to learn general principles of natural vision that apply across species.
My laboratory is interested in the relationship between structure and function in the human microbiome; especially how the physical structure of human associated-microbial communities influence health and disease. We use the human oral microbiome as a model system for studying microbial community structure and assembly because it is well-studied and accessible. We combine molecular and DNA sequencing approaches to understand what organisms, genes and potential functions are present in a community and we use advanced imaging technologies to map the spatial distribution of organisms within their environments. With our collaborators in the Biology department as well as outside the UAlbany community we study the systems level spatial structure of oral microbial biofilms in animal models and humans. We combine this discovery based research with hypothesis testing using in vitro culture systems. A second major focus of the lab is to further develop imaging technologies to expand the number of identifiable objects in a microbial sample as well as the development of computational tools for analysis of community structure.
Visit the PhD in Biology program page for more information
Visit the MS in Biology program page for more information
Centers and Institutes
Graduate research benefits from the department’s access to state-of-the-art research facilities, including Biological Imaging facilities as well as resources available at the RNA Institute and the Core facilities (including the Tissue Culture Core, Molecular Core, Chemistry Core, Mass Spectrometry Core, Animal Labs, and Nuclear Magnetic Resonance Imaging facility).
The designation of Center, Institute, Facility, Laboratory, and Initiative denote organizational units or processes that foster collaborations.
The collaboration can involve different academic departments, different schools and colleges and even on- and off-campus investigators. Although often focusing on a particular area of research, they can also focus on teaching. The Department of Biological Sciences is involved in several such collaborations.
- Center for Achievement, Retention and Student Success
- Center for Excellence in Cancer Genomics
- Center for Neuroscience Research
- Life Sciences Research Initiative
- The RNA Institute
Skip to Content
- Undergraduate Students
- Graduate Students
- University of Denver
- College of Natural Sciences and Mathematics
Explore this Section
The Department of Biological Sciences offers extensive opportunities for graduate and undergraduate students to join research projects led by our faculty in two distinct areas: cell and molecular biology and ecology, biodiversity and evolutionary biology.
We are committed to ensuring our students feel fully supported when it comes to their research goals. We're able to supplement and grow our research community with help from our partnerships with institutions such as the National Institutes of Health, National Oceanic and Atmospheric Administration, National Science Foundation, Department of Defense and Department of Justice.
Graduate Participation in Cutting-Edge Research
Our graduate students have the opportunity to affect real change in the world, with the potential to develop new tactics for protecting our environment or find new treatments for chronic diseases.
Graduate research assistant positions are funded by a faculty mentor's research grant. Students may apply for a GRA by contacting individual faculty in the department.
Learn more about the exciting faculty research projects currently underway in our department.
- Cell & Molecular Biology Faculty Research
- Ecology, Biodiversity & Evolutionary Biology Faculty Research
- Graduate Research & Funding
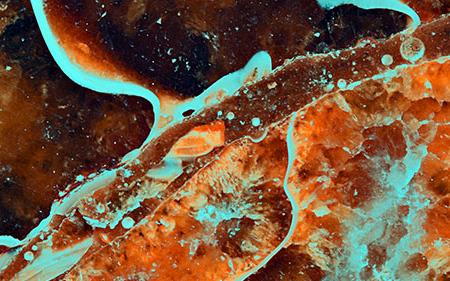
Preparing Junior Investigators
DU's Undergraduate Research Center, run by faculty mentors from the departments of biological sciences, physics and astronomy and psychology, guides undergraduates through the intricacies of high-level research and publication, approvals, travel, grants and funding, and proprietary property.
There are very few labs around the country that do forensic research and development. Within that rare group, there’s only a small subset of labs that are associated with universities, that engage students in this type of work. Dr. Phil Danielson, Professor of Molecular Biology and Forensic Genetics
Undergraduates Gaining Ground
Summer research experiences.
Through grants from DU's Undergraduate Research Center and Colorado-Wyoming Louis Stokes Alliance for Minority Participation (CO-WY AMP), a limited number of summer research experiences are awarded to undergraduate students each year.
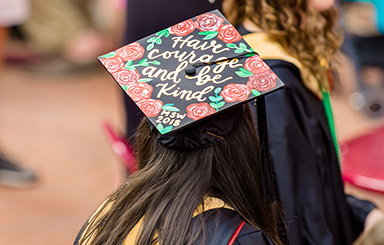
Start Your Application
Undergraduate applicants.
The Common App is a universal application that can be sent to many schools, including the University of Denver.
First-Year Students Transfer Students
Graduate Applicants
Go to the graduate admission application to submit your information. For information on admission requirements, visit the graduate academic programs page and locate your program of interest.
Graduate Application
Explore Programs
Graduate Academic Programs
The Biological Sciences major is offered in both the College of Agriculture and Life Sciences and the College of Arts & Sciences . The major is administered by The Office of Undergraduate Biology.

Biological Sciences

Research & Internships
Get started in research.
- Looking for a research opportunity at Cornell? Schedule an Exploring Research at Cornell Advising Appointment

1. Reflect: What am I looking for?
2. find opportunities, 3. contact potential research mentors, 4. meet with potential research mentors.
- What skills and knowledge am I hoping to gain from a research experience?
- What field(s) would I like to study?
- Most labs require a minimum 6-hour commitment divided in 2-3 hour blocks. Do I have the time and energy to commit to research right now?
Most research opportunities are not advertised. Students find positions by contacting researchers they would like to work with – usually by email.
- Ask your professors, TAs, or faculty advisor for advice on finding research opportunities.
- Join CURB (Cornell Undergraduate Research Board).
- Paid research opportunities may be found on the Student Employment website or by speaking with individual research mentors.
- Make a list of researchers (including faculty, research associates) with whom you would like to work by searching ForagerOne .
- build out your profile on ForagerOne , the platform for finding research at Cornell
- read the researcher’s website or the abstract of one or more papers.
- Start with a professional greeting (Dear Dr. or Professor X).
- Introduce yourself with your name, class year and major.
- Explain why you are interested in their research – be specific! Generic emails do not get much attention.
- Share why you are a good fit for the lab by providing more information about yourself. Describe your experiences (non-research experiences are great!).
- Emails should be concise; limit yourself to one to three paragraphs.
- End with a question. Ask to meet to discuss their research or getting involved in their field.
- Would you like feedback on a draft message to faculty? Meet with an OUB student advisor.
Congrats on getting meetings with researchers! Now it’s time to prepare.
- Make sure you can articulate why you’re interested in their work specifically and research in general. Practice saying it out loud .
- Prepare a list of questions. These can be about research or the lab structure (e.g., what do undergrads do each day?). Only ask questions you’re sincerely curious about. Check out these example questions for mentors from NIH.
- You do not need to understand technical details of their research or have read several of their papers.
- Be prepared to talk about time commitment. Bring your schedule.
- Aim not only to find a lab, but the right lab for you. Fit matters! Meet with several mentors if you can; finding the right mentor matters as much or more than the specific research project you will work on.
Where do bio sci majors do research?
- College of Veterinary Medicine
- Department of Animal Science
- Department of Ecology and Evolution
- Department of Molecular Biology and Genetics
- Department of Natural Resources & the Environment
- Department of Neurobiology and Behavior
- Department of Psychology
- Division of Nutritional Sciences
- Lab of Ornithology
- School of Biomedical Engineering
- School of Integrative Plant Science
- Cornell Center for Comparative and Population Genomics
Resources for undergraduate researchers
Funding your research.
Research funding can open the door to new opportunities: a summer research position, conference travel and the freedom to ask new, exciting questions. Search our database of grants and fellowships from Cornell and beyond that support undergraduate research by funding equipment and supplies, conference travel and salary or stipends. This list is not exclusive, and other opportunities may be available depending on your research area. Be sure to work with your research mentor when applying for grants.
Library guides
There are numerous opportunities at Cornell to develop your skills as a scientist outside the lab. This guide from the Cornell Libraries is designed for undergraduate researchers in biological sciences and offers advice on literature searching, citation management, data management and more.
Cornell Statistical Consulting Unit
Discover how to analyze and manage datasets in workshops from the Cornell Statistical Consulting Unit.
Research Data Management Service Group
Best practices suggested by the Research Data Management Service Group.
Science communication courses
Learn to discuss your research and other complex issues with scientists and non-scientists in science communication courses.
Cornell Undergraduate Research Board
Join the Cornell Undergraduate Research Board (CURB), take advantage of their workshops and present your work at CURB forums. Attend departmental seminars to learn about current research from around the world (check individual department websites for dates and times).
The New Science of Optimism and Longevity
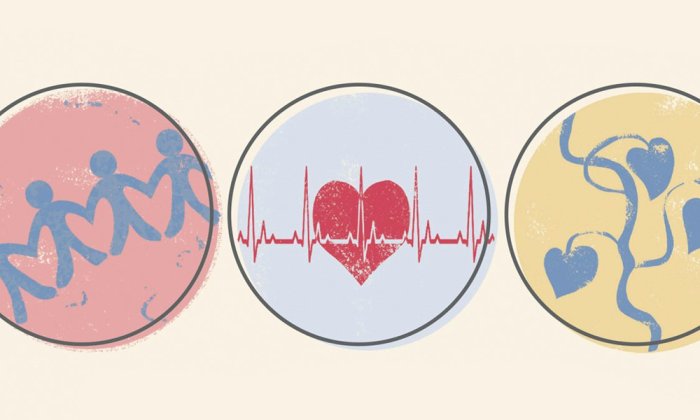
Being optimistic or pessimistic is not just a psychological trait or interesting topic of conversation; it’s biologically relevant. Indeed, there is mounting evidence that optimism may serve as a powerful tool for preventing disease and promoting healthy aging.
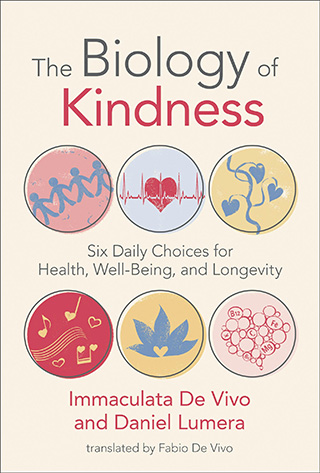
People with an optimistic mindset are associated with various positive health indicators, particularly cardiovascular , but also pulmonary , metabolic , and immunologic . They have a lower incidence of age-related illnesses and reduced mortality levels. Optimism and pessimism are not arbitrary and elusive labels. On the contrary, they are mindsets that can be scientifically measured, placing an individual’s attitude on a spectrum ranging from optimistic to pessimistic. Framing the baseline of each subject in this way, researchers are able to verify the correlation between optimism level and relative health conditions.
In 2019, a review published in JAMA Network Open by Alan Rozanski, a cardiologist at Mount Sinai Morningside hospital in New York City, compared the results of 15 different studies for a total of 229,391 participants. Rozanski’s meta-analysis showed that individuals with higher levels of optimism experience a 35 percent lower risk of cardiovascular events compared to those with lower optimism, as well as a lower mortality rate. Rozanski pointed out that the most optimistic people tend to take better care of themselves, especially by eating healthily, exercising, and not smoking. These behaviors have been found to a much lesser extent in the most pessimistic people, who tend to care less for their own well-being. But the damage produced by pessimism is also biological: The continuous wear and tear caused by elevated stress hormones like cortisol and noradrenaline leads to heightened levels of body inflammation and promotes the onset of disease. Moreover pathological pessimism can lead to depression, considered by the American Heart Association as a risk factor for cardiovascular disease.
Optimists tend to live on average 11 to 15 percent longer than pessimists and have an excellent chance of achieving “exceptional longevity.”
The same correlation has been identified in relation to minor illnesses like the common cold. A 2006 study outlined the personality profiles of 193 healthy volunteers who were inoculated with a common respiratory virus. Subjects who expressed a positive attitude were less likely to develop symptoms of the infection than their counterparts with less positive attitudes. Optimism, then, is one of the most interesting nonbiological factors involved in the mechanisms of longevity because it correlates an individual’s psychological attributes with their physical health. In this sense, it offers us a further strategy to protect our health.
Optimists tend to live longer , as revealed by research led by Lewina Lee at Harvard University analyzing 69,744 women from the NHS and 1,429 men from the aging study of the U.S. Department of Veterans Affairs. The results tell us that optimists tend to live on average 11 to 15 percent longer than pessimists and have an excellent chance of achieving “exceptional longevity” — that is, by definition, an age of over 85 years. These results are not confounded by other factors such as socioeconomic status, general health, social integration, and lifestyle because, according to Lee, optimists are better at reframing an unfavorable situation and responding to it more effectively. They have a more confident attitude toward life and are committed to overcoming obstacles rather than thinking that they can do nothing to change the things that are wrong.
A survey conducted in France in 1998 hypothesized a correlation between the death rate and group events that inspire optimism. On July 12th of that year, at the Saint-Denis stadium, the French national soccer team won the World Cup against Brazil. Data on deaths from cardiovascular events recorded that day show a singular decline compared to the average recorded between July 7th and July 17th , but this effect was limited to the male population, while for women it remained about the same. Although it’s not possible to establish a causal link, this curious coincidence suggests that the massive injection of optimism after the team’s victory may have played a role in the story.
In the Optimist’s Mind
Starting from the premise that a fundamental part of life is the pursuit of goals, it has been seen that encountering obstacles to achieving these goals can lead to different results depending on the individual’s level of optimism. If the person has a confident and positive attitude, they will try to overcome the obstacle; if they are doubtful that their efforts will succeed, they will tend to let it go, perhaps experiencing frustration from their remaining attachment to this goal, or may become completely disengaged and fail to achieve their goal. Optimism and pessimism posit this mechanism on a larger scale as a mental attitude toward not only a single goal but also the future in general.
Researchers have studied the relationship between these two attitudes and the results obtained during the course of real-life situations. It has been seen that optimists are more likely to complete university studies, not because they are smarter than others, but because they have more motivation and perseverance. And they are able to better manage the simultaneous pursuit of multiple goals — making friends, playing sports, and doing well at school — by optimizing their efforts: showing a greater commitment to priority goals and less commitment to secondary ones.
The optimist seems to invest their self-regulation resources carefully, increasing effort when circumstances are favorable and decreasing effort when they are less favorable, but also by doing more when there is a disadvantage to overcome. In a famous 1990 study by positive psychologist Martin Seligman involving college swimming teams , coaches asked athletes to compete at their best. At the end of the competitions, they were given false results about their performance, increasing their speed by about two seconds, which was low enough to be credible, but still enough for the athletes to be disappointed. After a couple of hours of rest, during which they probably mulled over their bad results in the last race, the swimmers were called to a second race, and the results between the optimists and pessimists were significantly different. The pessimists were on average 1.6 percent slower than during their first performance, while the optimists swam 0.5 percent faster. The interpretation of the experiment was that optimists tend to use failure as a goad to do better, whereas pessimists tend to be discouraged more easily and give up more readily.
Optimism, like a muscle, can be strengthened through positivity and gratitude, says cardiologist Alan Rozanski.
Results of DNA studies also seem to confirm the idea that optimism is an effective tool for slowing down cellular aging, of which telomere shortening is a biomarker . (Telomeres are the protective caps at the end of our chromosomes.) This research is still in progress, but the early results are informative. In 2012, Elizabeth Blackburn, who three years earlier shared a Nobel Prize for her work in discovering the enzyme that replenishes the telomere, and Elissa Epel at the University of California at San Francisco, in collaboration with other institutions, identified a correlation between pessimism and accelerated telomere shortening in a group of postmenopausal women. A pessimistic attitude, they found, may indeed be associated with shorter telomeres. Studies are moving toward larger sample sizes, but it already seems apparent that optimism and pessimism play a significant role in our health as well as in the rate of cellular senescence. More recently, in 2021, Harvard University scientists, in collaboration with Boston University and the Ospedale Maggiore in Milan, Italy, observed the telomeres of 490 elderly men in the Normative Health Study on U.S. veterans. Subjects with strongly pessimistic attitudes were associated with shorter telomeres — a further encouraging finding in the study of those mechanisms that make optimism and pessimism biologically relevant.
Optimism is thought to be genetically determined for only 25 percent of the population. For the rest, it’s the result of our social relationships or deliberate efforts to learn more positive thinking. In an interview with Jane Brody for the New York Times, Rozanski explained that “our way of thinking is habitual, unaware, so the first step is to learn to control ourselves when negative thoughts assail us and commit ourselves to change the way we look at things. We must recognize that our way of thinking is not necessarily the only way of looking at a situation. This thought alone can lower the toxic effect of negativity.” For Rozanski, optimism, like a muscle, can be trained to become stronger through positivity and gratitude, in order to replace an irrational negative thought with a positive and more reasonable one.
While the exact mechanisms remain under investigation, a growing body of research suggests that optimism plays a significant role in promoting both physical and mental well-being. Cultivating a positive outlook, then, can be a powerful tool for fostering resilience, managing stress, and potentially even enhancing longevity. By adopting practices that nurture optimism, we can empower ourselves to navigate life’s challenges with greater strength and live healthier, happier lives.
Immaculata De Vivo is Professor of Medicine at Harvard Medical School, Professor of Epidemiology at the Harvard School of Public Health, and Co-Director of the Science Program at the Harvard Radcliffe Institute . Her research focuses on how genetic variants interact with the environment to influence susceptibility to hormonal cancers, especially endometrial cancer. She is co-author, with Daniel Lumera, of “ The Biology of Kindness ,” from which this article is adapted.

Pushing back the limits of optical imaging by processing trillions of frames per second
Canadian research team is advancing in imaging speed with a new ultrafast camera system.
Pushing for a higher speed isn't just for athletes. Researchers, too, can achieve such feats with their discoveries. This is the case for Jinyang Liang, Professor at the Institut national de la recherche scientifique (INRS), and his team, whose research results have recently been published in Nature Communications .
The group based at INRS' Énergie Matériaux Télécommunications Research Centre has developed a new ultrafast camera system that can capture up to 156.3 trillion frames per second with astonishing precision. For the first time, 2D optical imaging of ultrafast demagnetization in a single shot is possible. This new device called SCARF (for swept-coded aperture real-time femtophotography) can capture transient absorption in a semiconductor and ultrafast demagnetization of a metal alloy. This new method will help push forward the frontiers of knowledge in a wide range of fields, including modern physics, biology, chemistry, materials science, and engineering.
Improving on past advances
Professor Liang is known around the world as a pioneer of ultrafast imaging. Already, in 2018, he was the principal developer of a major breakthrough in the field, which laid the groundwork for the development of SCARF.
Until now, ultrafast camera systems have mainly used an approach involving sequentially capturing frames one by one. They would acquire data through brief, repeated measurements, then put everything together to create a movie that reconstructed the observed movement.
"However, this approach can only be applied to inert samples or to phenomena that happen the exact same way each time. Fragile samples, not to mention non-repeatable phenomena or phenomena with ultrafast speeds, cannot be observed with this method."
Professor Jinyang Liang, expert in ultra-fast and biophotonic imaging said, "For example, phenomena such as femtosecond laser ablation, shock-wave interaction with living cells, and optical chaos cannot be studied this way."
The first tool developed by Professor Liang helped fill this gap. The T-CUP (Trillion-frame-per-second compressed ultrafast photography) system was based on passive femtosecond imaging capable of acquiring ten trillion (10 13 ) frames per second. This was a major first step towards ultrafast, single-shot real-time imaging.
Yet challenges still remained.
"Many systems based on compressed ultrafast photography have to cope with degraded data quality and have to trade the sequence depth of the field of view. These limitations are attributable to the operating principle, which requires simultaneously shearing the scene and the coded aperture."
Miguel Marquez, postdoctoral fellow and co-first author of the study said, "SCARF overcomes these challenges. Its imaging modality enables ultrafast sweeping of a static coded aperture while not shearing the ultrafast phenomenon. This provides full-sequence encoding rates of up to 156.3 THz to individual pixels on a camera with a charge-coupled device ( CCD ). These results can be obtained in a single shot at tunable frame rates and spatial scales in both reflection and transmission modes."
A range of applications
SCARF makes it possible to observe unique phenomena that are ultrafast, non-repeatable, or difficult to reproduce, such as shock wave mechanics in living cells or matter. These advances could potentially be used to develop better pharmaceutics and medical treatments.
What's more, SCARF promises very appealing economic spin-offs. Two companies, Axis Photonique and Few-Cycle, are already working with Professor Liang's team to produce a marketable version of their patent-pending discovery. This represents a great opportunity for Quebec to strengthen its already enviable position as a leader in photonics.
The work was carried out in the Advanced Laser Light Source (ALLS) Laboratory in collaboration with Professor François Légaré, Director of the Énergie Matériaux Télécommunications Research Centre, and international colleagues Michel Hehn, Stéphane Mangin and Grégory Malinowski of the Institut Jean Lamour at the Université de Lorraine (France) and Zhengyan Li of Huazhong University of Science and Technology (China).
This research was funded by the Natural Sciences and Engineering Research Council of Canada, the Canada Research Chairs Program, the Canada Foundation for Innovation, the Ministère de l'Économie et de l'Innovation du Québec, the Canadian Cancer Society, the Government of Canada's New Frontiers in Research Fund, as well as the Fonds de recherche du Québec-Nature et Technologies and the Fonds de recherche du Québec -Santé.
- Medical Technology
- Spintronics Research
- Photography
- Information Technology
- Communications
- Materials science
- Quantum mechanics
- Nanotechnology
- Security engineering
- Periodic table
Story Source:
Materials provided by Institut national de la recherche scientifique - INRS . Original written by Julie Robert. Note: Content may be edited for style and length.
Journal Reference :
- Jingdan Liu, Miguel Marquez, Yingming Lai, Heide Ibrahim, Katherine Légaré, Philippe Lassonde, Xianglei Liu, Michel Hehn, Stéphane Mangin, Grégory Malinowski, Zhengyan Li, François Légaré, Jinyang Liang. Swept coded aperture real-time femtophotography . Nature Communications , 2024; 15 (1) DOI: 10.1038/s41467-024-45820-z
Cite This Page :
Explore More
- Amazing Archive of Ancient Human Brains
- Night-Time Light and Stroke Risk
- Toward Secure Quantum Communication Globally
- Artificial Nanofluidic Synapses: Memory
- 49 New Galaxies Discovered in Under Three Hours
- Rays Surprisingly Diverse 150 Million Years Ago
- Paint Coatings That Help You Feel Cool
- A Self-Cleaning Wall Paint
- Early Human Migration Out of Africa
- Unintended Consequences of Fire Suppression
Trending Topics
Strange & offbeat.
Groundbreaking Research Establishes Biological Basis of Chronic Fatigue Syndrome: Findings from ScienceAlert
T he leading research funder in the world, the US National Institutes of Health (NIH), initiated a pivotal study into chronic fatigue syndrome (CFS) in 2016, which is alternatively known as myalgic encephalomyelitis, or ME/CFS. This was well in advance of the recognition of long COVID as a health issue.
After eight years, the findings of this comprehensive study are finally available. Focused on a small cohort of 17 individuals who contracted ME/CFS following an infection, the research delineated obvious biological discrepancies when compared to a control group of 21 healthy individuals.
NIH’s National Institute of Neurological Disorders and Stroke (NINDS) clinical director and lead researcher on the study, neurologist Avindra Nath, explained in an interview with JAMA that the results conclusively prove that ME/CFS is a biological condition, influencing multiple organ systems.
For a long time, ME/CFS was mistakenly treated by several healthcare providers as a psychological ailment. However, current research now confirms the condition is accompanied by a multitude of biological alterations.
According to Nath, “It’s a systemic disease,” and patients with ME/CFS need their experiences to be earnestly acknowledged.
During a thorough week-long assessment, subjects were subjected to various tests including brain scans, sleep studies, physical and cognitive performance evaluations, as well as blood, skin, and muscle biopsies. They were monitored under regulated dietary conditions and within metabolic chambers to measure calorie and nutrient intake in a controlled environment.
Consistent with prior research, ME/CFS patients exhibited elevated resting heart rates and signs of protracted, hyperactive immune responses resulting in T cell exhaustion. Additionally, they presented with less diverse gut microbiomes relative to the control subjects.
Despite reporting heightened cognitive challenges, the ME/CFS group displayed normal cognitive test results and no evidence of muscle fatigue.
However, the immune and microbiome variances exhibited clear effects on the central nervous system. ME/CFS patients had lowered catechol concentrations in the cerebrospinal fluid and reduced activity in the temporal-parietal junction (TPJ) of the brain during motor tasks.
The TPJ’s role in controlling motor cortex activity suggests that its malfunction may interfere with the brain’s effort exertion and fatigue perception. As summed up by Brian Walitt, the study lead author and an ME/CFS research medical scientist at NINDS, this could identify a physiological nexus for fatigue in these patients.
While the research is an important step, advocacy groups for ME/CFS have criticized some aspects such as fatigue assessments not fully encompassing the condition, particularly overlooking post-exertional malaise.
Additionally, concerns have been raised about the initial 217 patients’ reduction to merely 17 confirmed post-infection ME/CFS cases by a panel of clinicians for the study.
The goal behind selecting a smaller patient group was to facilitate the most meticulous examination feasible, thus maximizing the likelihood of uncovering significant discrepancies for further inquiry with a broader demographic. This challenge mirrors that found in understanding and treating long COVID and Alzheimer’s disease.
While the pandemic limited their target of 40 ME/CFS patients, and certain candidates were excluded based on wellness criteria and concerns about patient overwork, this study has successfully set a foundation for future exploration in this area.
These investigations have been thoroughly documented in the Nature Communications journal.
FAQ Section
What is chronic fatigue syndrome (cfs).
Chronic Fatigue Syndrome (CFS), also known as myalgic encephalomyelitis (ME), is a complex, chronic illness characterized by extreme fatigue, pain, and other symptoms that are not relieved by rest and are often exacerbated by physical or mental activity.
What did the 2016 NIH study reveal about CFS?
The 2016 study conducted by the NIH showed that CFS is unambiguously a biological condition, impacting various organ systems and not merely a psychosomatic disorder as was previously believed by some.
What kind of tests were performed in the CFS study?
Subjects underwent brain scans, sleep studies, muscle strength and cognitive performance tests, skin and muscle biopsies, blood tests, and analyses of gut microbiome and spinal fluid, among other assessments.
Why was there controversy over the study’s patient selection?
Concerns were raised about the stringent criteria that reduced an initial pool of 217 potential subjects to just 17 participants, which some argue may not reflect the broader CFS patient population.
Will there be more research on CFS?
Yes, further research is expected to build on the foundation set by this study to better understand Chronic Fatigue Syndrome and to develop effective treatments.
This landmark study represents a significant stride in chronic fatigue syndrome research, confirming its biological roots and challenging outdated misconceptions about the condition. By identifying biological differences in patients with CFS, the study paves the way for more in-depth research and potentially more targeted treatments. However, further study is necessary to explore the full scope of CFS and to translate these findings into clinical practice. Patients and advocates can now hold onto the hope that CFS is gaining the scientific attention it requires for better diagnosis, understanding, and care.
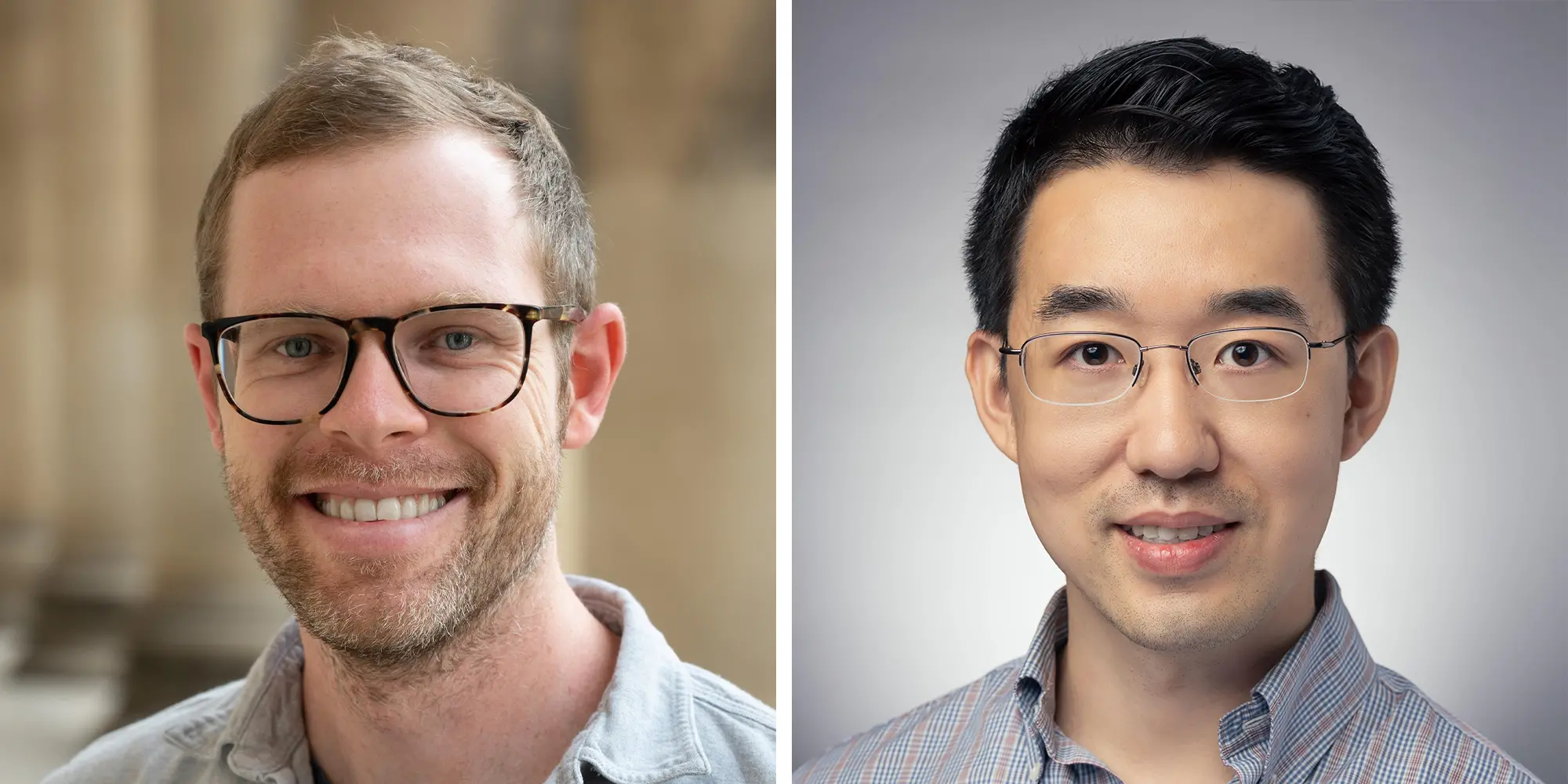
Bridges and Zhang Receive Curci Foundation Research Grants
- Share on Facebook (opens in new window)
- Share on X (opens in new window)
- Share on LinkedIn (opens in new window)
- Print this page
- Share by email
Two researchers from Carnegie Mellon University, Drew Bridges (opens in new window) , an assistant professor of biological sciences in the Mellon College of Science, and Martin Zhang (opens in new window) , an assistant professor of computational biology in the School of Computer Science, have received The Shurl and Kay Curci Foundation (opens in new window) Research Grants.
Founded in 2006, The Shurl and Kay Curci Foundation supports researchers whose work emphasizes fostering a sustainable future for humankind, focusing on projects with far-reaching and lasting implications in life sciences. The grant provides funding for two years.
Bridges said he plans to use his grant to develop new fluorescent markers to study biofilms, groups of bacteria that clump together for protection. Current markers are not effective because most require oxygen to mature, and oxygen is low in the centers of biofilms. With new markers, Bridges and other researchers will be able to see the details of bacteria’s interactions.
“In these multicellular communities, it’s thought that cells are able to specialize almost like a tissue, where you have certain cells doing a task and different cells doing another task,” Bridges said. “We’re going for a new strategy to make the cell fluorescent. The Curci Foundation grant will support students, research staff and supplies.”
The Bridges lab investigates bacteria in a variety of conditions to see how they interact.
The award will support Zhang’s research on using large-scale genetic data to decipher and link genetic variants to diseases. Zhang said he plans to use genome-wide and single-cell data to better understand cell-type-specific disease mechanisms. Support from the Curci Foundation will allow him to hire students and postdocs in his lab.
“Heritable human diseases manifest in a highly cell-type-specific manner. Studying these mechanisms is critical for our understanding of disease etiology and will inform treatment development,” said Zhang, who joined CMU’s Ray and Stephanie Lane Computational Biology Department (opens in new window) in 2023. “This funding will provide critical financial support and allow us to hire the personnel to carry out the proposed projects, greatly accelerating our research agenda.”
Zhang's lab also develops machine learning and statistical algorithms motivated by genetic applications, such as causal inference, deep learning and more.
Learn more about the Curci Foundation on its website (opens in new window) .
— Related Content —
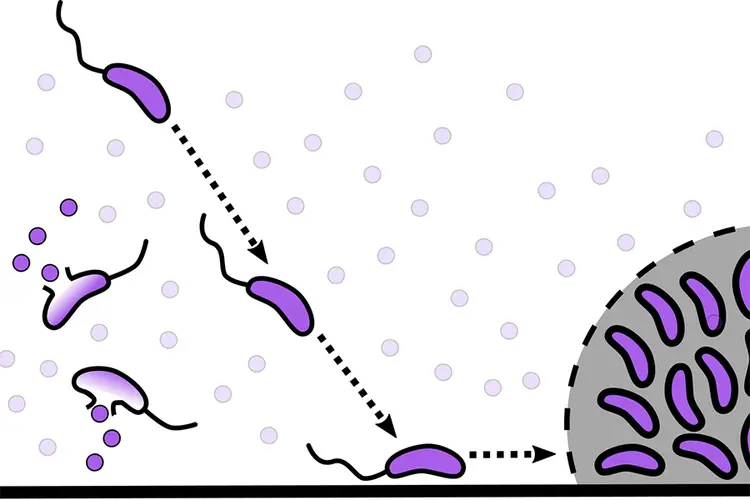
Biologists Determine Bacteria Sense Damage to Relatives
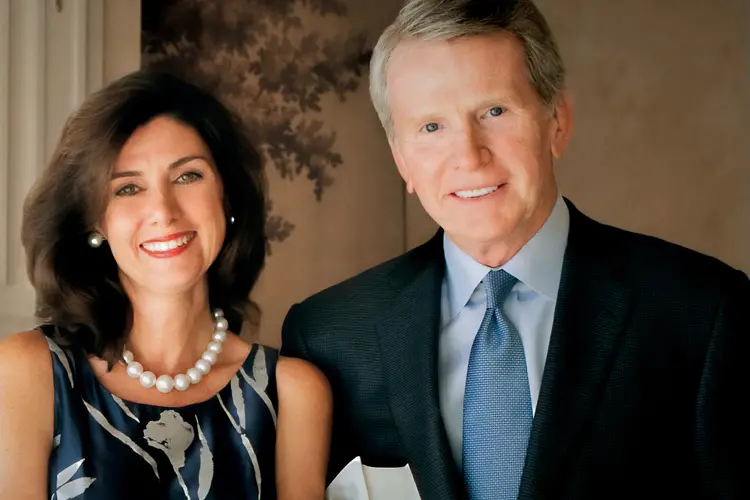
Carnegie Mellon Trustee Ray Lane and Stephanie Lane Invest $25 Million in CMU's Computational Biology Department
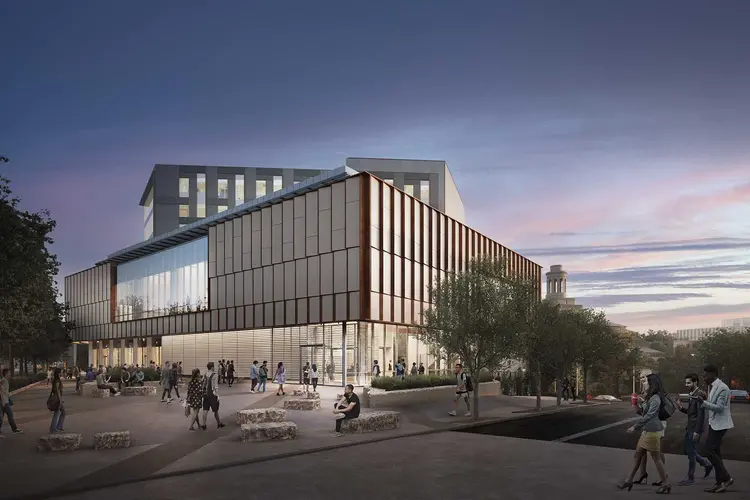
Carnegie Mellon Unveils Design for New Richard King Mellon Hall of Sciences
- The Piper: Campus & Community News (opens in new window)
- Official Events Calendar (opens in new window)
Thank you for visiting nature.com. You are using a browser version with limited support for CSS. To obtain the best experience, we recommend you use a more up to date browser (or turn off compatibility mode in Internet Explorer). In the meantime, to ensure continued support, we are displaying the site without styles and JavaScript.
- View all journals
- Explore content
- About the journal
- Publish with us
- Sign up for alerts
- 30 November 2022
In praise of research in fundamental biology
You have full access to this article via your institution.
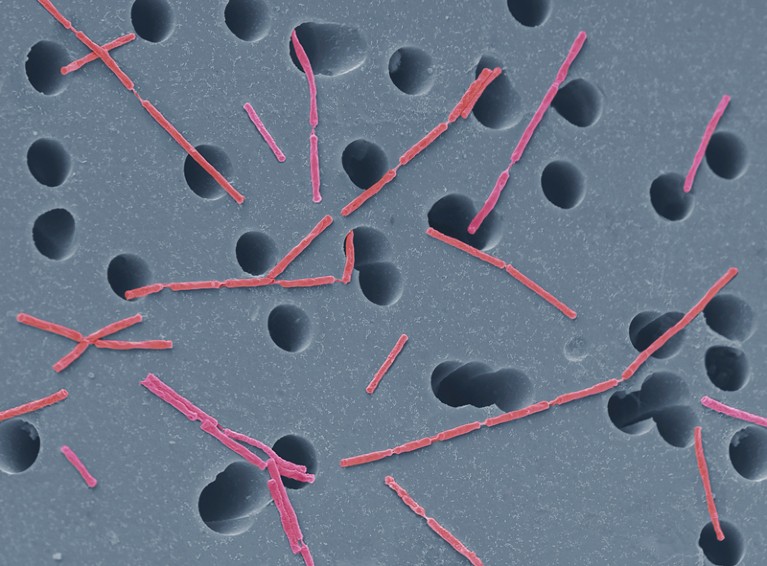
Microbes that live in extreme environments, known as extremophiles, have a range of uses in the laboratory. Credit: Steve Gschmeissner/SPL
How did eukaryotic life — the domain that includes humans — evolve? A decade or so ago, this was the question occupying Thijs Ettema and his colleagues. They found the answer in the genomes of previously undiscovered microorganisms collected from deep in the Atlantic Ocean 1 . Lurking in those sequences were hallmarks of cells from two different types of organism — archaea and eukaryotes. The former describes a group of single-celled organisms that lack a nucleus; the latter, organisms whose cells contain a membrane-bounded nucleus and other organelles. Ettema, now at Wageningen University & Research in the Netherlands, and his colleagues were surprised to find microbes that combined characteristics of both cell types.
Microbes that forge a living in the hot waters surrounding undersea vents also have uses in the laboratory. They have yielded a host of hardy enzymes. These are stable at high temperatures and resistant to degradation, and have become staples in molecular biology labs and biotechnology companies worldwide. Each newly identified microbe found thriving in extreme environments offers a fresh opportunity to discover more enzymes — but this was not Ettema’s goal.
When researchers report fundamental discoveries, their papers frequently include a sentence or more about the potential applications of their work. This is often a condition of certain types of grant. But even when it isn’t, there’s an expectation among funders (and journals, too) that fundamental science will lead to practical applications, be they in the clinic, in business or in society more broadly.
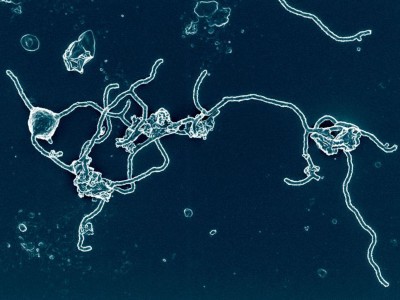
The life of archaea
It’s true that basic discoveries about cell division in yeast 2 , for example, could eventually contribute to new cancer treatments, or that a rudimentary microbial immune system 3 might provide the foundation for a genome-editing tool. (Both of these have come to pass.) But if there is a line between fundamental science and applications, it’s unlikely to be straight. Instead, it will probably resemble a drawing of a maze, with plenty of circles, U-turns and dead ends. Ettema says he never felt tempted to predict an application for his microbes — which were eventually named Asgard archaea — because it would only have been a distraction. “I think they are just fascinating organisms that can tell us something about a very important question we always ask ourselves,” he says. “Where do we come from?”
It’s not that there’s any real question that fundamental science has a wider impact. One 2018 study 4 found that a US$10-million boost in funding from the US National Institutes of Health (NIH) resulted in more private-sector patents that cite NIH work, says study co-author Pierre Azoulay, who researches innovation at the Massachusetts Institute of Technology in Cambridge.
Some fundamental research represents a landmark in its field. Take four papers published in September 5 – 8 describing the isolation of fossil fishes from the Silurian period, more than 400 million years ago. Often, only fragments of such fossils are uncovered, but these were more intact and provided researchers with a more complete picture of the early evolution of jawed vertebrates.
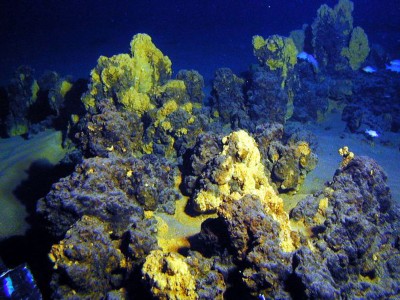
Scientists glimpse oddball microbe that could help explain rise of complex life
Fundamental discoveries can also form unexpected turning points. In 2020, a team made a detailed study of more than 20 transcription factors — proteins that can bind to DNA and switch genes on. One might expect any distortion in the usual DNA-binding site of a particular transcription factor to negatively affect how well the protein binds to the DNA. However, the researchers found that such distortions sometimes improve DNA–protein binding 9 , and, in the process, provide insight into the energetics of interactions between proteins and DNA. It cannot be stressed enough that fundamental science takes time and patience. In 2020, five years after Ettema and his colleagues reported their discovery of Asgard archaea through sequencing the DNA in seawater, a separate group of researchers who had collected undersea samples near Japan revealed that they had become the first to grow such microbes in culture 10 .
But it had taken a full 10 years — starting in 2006 — for that team to grow the Asgard archaea to sufficient quantities to study them, meaning that the researchers started their cultures before the microbes had even been identified. In fact, the scientists had been looking for ways to isolate previously uncultivated deep-sea microbes, to explore the unknown. They painstakingly nurtured their cultures for years — many deep-sea microbes are accustomed to harsh conditions and scarce nutrients, and grow at a painfully slow rate — waiting to see what they would find.
Nature has been publishing curiosity-driven research for more than 150 years, and readers will not need convincing of the work’s value. But we implore colleagues in the wider science ecosystem — the policymakers and those in science-funding agencies who expect to see direct benefits of research investments — to resist the temptation to push for quick returns. We appreciate that pressure to do so will only grow as countries confront economic recession and cost-of-living crises. But, wherever possible, this pressure needs to be resisted. Basic research must be allowed to thrive.
Nature 612 , 7 (2022)
doi: https://doi.org/10.1038/d41586-022-04172-8
Spang, A. et al. Nature 521 , 173–179 (2015).
Article PubMed Google Scholar
Basu, S., Greenwood, J., Jones, A. W. & Nurse, P. Nature 607 , 381–386 (2022).
Wiedenheft, B. et al. Nature 477 , 486–489 (2011).
Azoulay, P., Graff Zivin, J. S., Li, D. & Sampat, B. N. Rev. Econ. Stud. 86 , 117–152 (2019).
Zhu, Y.-a. et al. Nature 609 , 954–958 (2022).
Gai, Z. et al. Nature 609 , 959–963 (2022).
Andreev, P. S. et al. Nature 609 , 969–974 (2022).
Andreev, P. S. et al. Nature 609 , 964–968 (2022).
Afek, A. et al. Nature 587 , 291–296 (2020).
Imachi, H. et al. Nature 577 , 519–525 (2020).
Download references
Reprints and permissions
Related Articles
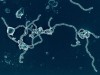
- Microbiology
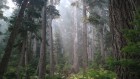
The ‘Mother Tree’ idea is everywhere — but how much of it is real?
News Feature 26 MAR 24
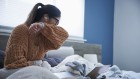
Google AI could soon use a person’s cough to diagnose disease
News 21 MAR 24
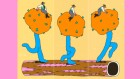
These ‘movies’ of proteins in action are revealing the hidden biology of cells
News Feature 20 MAR 24
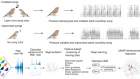
The hidden fitness of the male zebra finch courtship song
Article 20 MAR 24
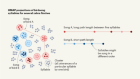
Birds convey complex signals in simple songs
News & Views 20 MAR 24
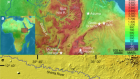
Adaptive foraging behaviours in the Horn of Africa during Toba supereruption
Principal Investigator in Modeling of Plant Stress Responses
Join our multidisciplinary and stimulative research environment as Associate Professor in Modeling of Plant Stress Responses
Umeå (Kommun), Västerbotten (SE)
Umeå Plant Science Centre and Integrated Science Lab
Postdoctoral Associate- Cellular Neuroscience
Houston, Texas (US)
Baylor College of Medicine (BCM)
Postdoctoral Associate- Cancer Biology
Recruitment of talent positions at shengjing hospital of china medical university.
Call for top experts and scholars in the field of science and technology.
Shenyang, Liaoning, China
Shengjing Hospital of China Medical University
Assistant Professor in Plant Biology
The Plant Science Program in the Biological and Environmental Science and Engineering (BESE) Division at King Abdullah University of Science and Te...
Saudi Arabia (SA)
King Abdullah University of Science and Technology
Sign up for the Nature Briefing newsletter — what matters in science, free to your inbox daily.
Quick links
- Explore articles by subject
- Guide to authors
- Editorial policies
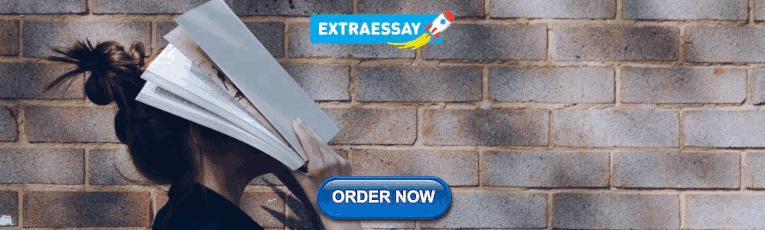
IMAGES
VIDEO
COMMENTS
Biological sciences encompasses all the divisions of natural sciences examining various aspects of vital processes. The concept includes anatomy, physiology, cell biology, biochemistry and ...
An official journal of the American Institute of Biological Sciences. Publishes overviews of current research in biology, essays, and discussion on education, public policy, history, and the conceptual underpinnings of the biological sciences.
Latest biological science research. Explore the latest articles from across our biological science journals, or browse all articles.. From PLOS Biology, Eggs of the mosquito Aedes aegypti survive desiccation by rewiring their polyamine and lipid metabolism. From PLOS Computational Biology, Rapid automated 3-D pose estimation of larval zebrafish using a physical model-trained neural network
Research. Our researchers seek to create knowledge and advance the understanding of the life sciences and medicine and to apply this knowledge to enhance discovery and clinical outcomes. Our faculty organize into 10 basic science and 14 clinical departments along with multiple Institutes and Centers. Co-located on a compact campus alongside ...
The Chilean Biology Society promotes theoretical and experimental studies and research leading to advancement in and dissemination of the biological sciences for the benefit of the community. To accomplish this, the Society organizes periodic scientific meetings in which scientists communicate, comment and discuss research carried out in ...
Areas of Research. For over 50 years, we have played a central role in the growth of molecular life sciences and the revolution in molecular and cellular biology, genetics, genomics, and computational biology. Biochemistry, Biophysics, and Structural Biology. Cancer Biology.
Citation: Biological Research 2023 56:30 Content type: Research article Published on: 8 June 2023. View Full Text View PDF iPSCs ameliorate hypoxia-induced autophagy and atrophy in C2C12 myotubes via the AMPK/ULK1 pathway. Duchenne muscular dystrophy (DMD) is an X-linked lethal genetic disorder for which there is no effective treatment. ...
This summer, learn the techniques used in cutting-edge biological research and work in person at lab facilities at a premier research university. I liked being able to apply knowledge -- just the act of doing lab work and carrying out experiments based on things we just learned made everything make much more sense, and vice versa.
The life sciences embrace a great array of intellectual activity, a continuum extending from the search for the origin of life and the detailed structure of the macromolecules that make life possible to understanding of the total ecology of planet Earth. The millions of micro-organisms and plant and animal species interacting in the air, the soil, freshwater ponds and streams, and the oceans ...
Postdoctoral research associate at Texas A&M University Borja Barbero explains the importance of studying plant telomeres in space. The findings of his research with Dr. Dorothy Shippen offer insight into the effects of spaceflight on plant chromosomes and how these findings could impact human health.
Research Areas. Research activity in the Biology Department spans the full range of biological organization, from molecules to ecosystems. Main research fields are indicated here, as links to groups of faculty doing research in those areas.
Key Features. Discusses various research topics and methods in the biological sciences in a single platform. Comprises the latest updates in advanced research techniques, protocols, and methods in biological sciences. Incorporates the fundamentals, advanced instruments, and applications of life science experiments.
The 50th anniversary of a key paper on how bird flight evolved. For a century, scientists pondered whether bird flight evolved by animals gliding down from trees or by creatures running and ...
Overview. The University of Pittsburgh is a leading research university, and the discovery of new scientific knowledge is a major focus of the Department of Biological Sciences. Research within the Department is aimed at understanding a broad range of biological processes, from the molecular and biochemical to the organismal and population levels.
Making Biology Come Alive. Reflecting the University of California's three-part fundamental mission of Teaching, Research and Public Service, the UCI School of Biological Sciences research programs are world-class. Faculty, graduate students and many undergraduates participate in cutting-edge research on a wide variety of subjects, including:
The new research, published Dec. 14 in Molecular Biology of the Cell, shows that cells switch off autophagy by rearranging structural proteins called septins. The researchers showed that yeast that lacked functional septins could activate autophagy but were unable to stop it. "Our study has opened a new way to think about how autophagy can be ...
The Division of Biological Sciences at the University of Missouri is distinctive in the breadth and depth of its research. Faculty bring their special expertise in overlapping disciplines to bear on a broad range of biological questions at all levels of organization, from molecules to ecosystems, using model systems drawn from a wide range of organisms.
Here are four undergraduates who followed their interests toward meaningful questions. 1. Tracing family origins. From left: Cole Williams and associate professor Breanna Henn. (David Slipher/UC Davis) Cole Williams found a passion for genetics at an early age. Then, he became a genetics and genomics major and College of Biological Sciences ...
The faculty and students in the Department of Biological Sciences at University at Albany are actively engaged in research in highly diverse research topics and projects addressing a spectrum of issues of interest and concern to the scientific and general community. Faculty in the Department of Biological Science work with graduate students in ...
Research. The Department of Biological Sciences offers extensive opportunities for graduate and undergraduate students to join research projects led by our faculty in two distinct areas: cell and molecular biology and ecology, biodiversity and evolutionary biology. We are committed to ensuring our students feel fully supported when it comes to ...
Research funding can open the door to new opportunities: a summer research position, conference travel and the freedom to ask new, exciting questions. ... This guide from the Cornell Libraries is designed for undergraduate researchers in biological sciences and offers advice on literature searching, citation management, data management and more.
Optimism, then, is one of the most interesting nonbiological factors involved in the mechanisms of longevity because it correlates an individual's psychological attributes with their physical health. In this sense, it offers us a further strategy to protect our health. Optimists tend to live longer, as revealed by research led by Lewina Lee ...
This new method will help push forward the frontiers of knowledge in a wide range of fields, including modern physics, biology, chemistry, materials science, and engineering. Improving on past ...
The leading research funder in the world, the US National Institutes of Health (NIH), initiated a pivotal study into chronic fatigue syndrome (CFS) in 2016, which is alternatively known as myalgic ...
Email. 412-268-1151. Two researchers from Carnegie Mellon University, Drew Bridges, an assistant professor of biological sciences in the Mellon College of Science, and Martin Zhang, an assistant professor of computational biology in the School of Computer Science, have received The Shurl and Kay Curci Foundation Research Grants.
Science funders must remember the value of addressing the intrinsic biological questions that help to explain the natural world.