Thank you for visiting nature.com. You are using a browser version with limited support for CSS. To obtain the best experience, we recommend you use a more up to date browser (or turn off compatibility mode in Internet Explorer). In the meantime, to ensure continued support, we are displaying the site without styles and JavaScript.
- View all journals
- My Account Login
- Explore content
- About the journal
- Publish with us
- Sign up for alerts
- Review Article
- Open access
- Published: 01 October 2018
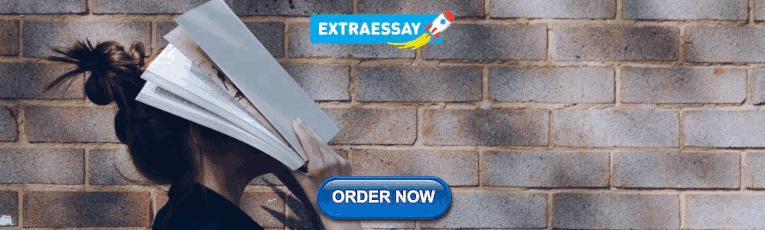
Understanding the effects of education through the lens of biology
- H. Moriah Sokolowski 1 &
- Daniel Ansari 1
npj Science of Learning volume 3 , Article number: 17 ( 2018 ) Cite this article
21k Accesses
18 Citations
150 Altmetric
Metrics details
- Human behaviour
Early educational interventions aim to close gaps in achievement levels between children. However, early interventions do not eliminate individual differences in populations and the effects of early interventions often fade-out over time, despite changes of the mean of the population immediately following the intervention. Here, we discuss biological factors that help to better understand why early educational interventions do not eliminate achievement gaps. Children experience and respond to educational interventions differently. These stable individual differences are a consequence of biological mechanisms that support the interplay between genetic predispositions and the embedding of experience into our biology. Accordingly, we argue that it is not plausible to conceptualize the goals of educational interventions as both a shifting of the mean and a narrowing of the distribution of a particular measure of educational attainment assumed to be of utmost importance (such as a standardized test score). Instead of aiming to equalize the performance of students, the key goal of educational interventions should be to maximize potential at the individual level and consider a kaleidoscope of educational outcomes across which individuals vary. Additionally, in place of employing short-term interventions in the hope of achieving long-term gains, educational interventions need to be sustained throughout development and their long-term, rather than short-term, efficacy be evaluated. In summary, this paper highlights how biological research is valuable for driving a re-evaluation of how educational success across development can be conceptualized and thus what policy implications may be drawn.
Similar content being viewed by others
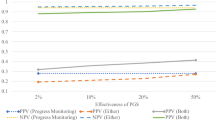
The practical utility of genetic screening in school settings
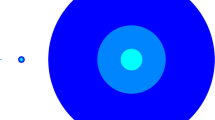
Dreams and realities of school tracking and vocational education
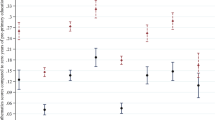
Measuring and forecasting progress in education: what about early childhood?
Introduction.
The education of children throughout their development is a key cornerstone for the creation of a successful society. 1 , 2 , 3 In order to inform educational policymakers on how to maximize the success of educational strategies, various components of education systems have been extensively studied from a variety of perspectives, such as cognitive psychology, neuroscience, and genetics. 4 , 5 , 6 , 7 , 8 , 9 Yet the causes that can help to explain why some children thrive while others fall behind in school remain unclear. A key factor in explaining why some children perform better than others in school is that children develop in heterogeneous environments and experience strikingly different education systems. 10 , 11 , 12 Within the developing world, over 200 million children below the age of five experience poverty, with limited or no healthcare, poor nutrition, and inadequate education. 13 These conditions of economic scarcity have been linked to negative outcomes across development, including decreased success in school. 14 Consequently, researchers continue to advocate for policy changes to improve equity within and across education systems. 15
The term equity has been confused with the notion of equality within the context of education. Researchers, educators, and policymakers have discussed this distinction in depth. 16 , 17 , 18 Briefly, equality in education refers to the provision of equal resources and learning opportunities to all students. Although at a glance this seems fair, it has often been highlighted that some students need more resources than others to accomplish the same achievements. Therefore, equality of resources may not be fair, given that students enter with inequalities in capabilities and opportunities. Consequently, the concept of equity is more relevant. Equity in education is the notion of redistributing resources with the goal of eliminating systematic inequality of outcome measures, for example, giving low-income students access to exceptional teachers and additional funding to provide high-quality education to this population and ideally narrow achievement gaps. Indeed, a primary goal of education is to increase equity and close the achievement gap.
It has repeatedly been advocated that educational interventions should begin in early childhood in order to improve academic achievement in the long term. 13 , 19 , 20 Economic reports suggest that applying early interventions to disadvantaged families will provide the greatest long-term rate of economic return to society. 21 In the 1960s, research teams implemented multiple early intervention programs. 20 These interventions ranged from small-scale, subject-specific interventions 22 , 23 , 24 , 25 to intensive, large-scale public programs. 26 , 27 , 28 , 29 In general, meta-analyses of effect sizes of these interventions report substantive improvements on educational outcome measures. 30 , 31
Notwithstanding, pertinent concerns about the effectiveness of early education interventions remain because group differences persist, even after interventions (e.g., between children from families with low and high socioeconomic status (SES)). 20 , 32 , 33 More specifically, although early interventions have been linked to improvements in educational achievement in children from families with low SES, 26 , 27 , 28 these improvements were not large enough to eliminate group differences (i.e., close the gap between students from families with high and low SES). 32 , 33 Additionally, individual differences within both high and low SES groups persist even following early educational interventions. 34 , 35 , 36 , 37 Taken together, there is evidence to suggest that early educational interventions are not sufficient to compensate for unfavorable learning conditions experienced by many children. Although it is possible that further refining of interventions will improve their effectiveness, differences in a child’s predispositions coupled with their early pre-natal and post-natal experiences, likely also affect a child’s responsivity to specific educational interventions. Consequently, it may be more beneficial to modify the expectations and aims of early educational interventions. So, what goals and expectations for the effects of early educational interventions might be more realistic? In what follows, we discuss what is known about the effects of educational interventions both on individuals as well as the populations of individuals undergoing educational interventions as a whole. We then discuss how the study of genetics may inform our understanding of the effects of education. We close by discussing the implications of such data for educational policy.
The alignment and misalignment between the goals and the effects of education
Educational outcome measures, such as standardized tests of reading and mathematics, capture variability in performance that falls along a normal distribution in the population. This normal distribution describes how children within a population vary along an outcome of interest (Fig. 1a ). This means that an individual child’s ability has a relative position compared to the other individuals in the population. The relative positions of an educational outcome measure for children in a population is referred to as the “rank order”. 36 , 38 There are two main goals of early educational intervention programs: one goal is to help all children improve their scores (i.e., shift the mean of the distribution) (Fig. 1b ). The other goal is to reduce the achievement gap between children on low and high ends of the distribution (i.e., narrow the distribution) (Fig. 1c ). Though a laudable goal, early educational interventions may not actually narrow the distribution of educational attainment because they do not eliminate individual differences within populations, 34 , 35 , 36 , 37 as pointed out by Scarr and McCartney 36 :
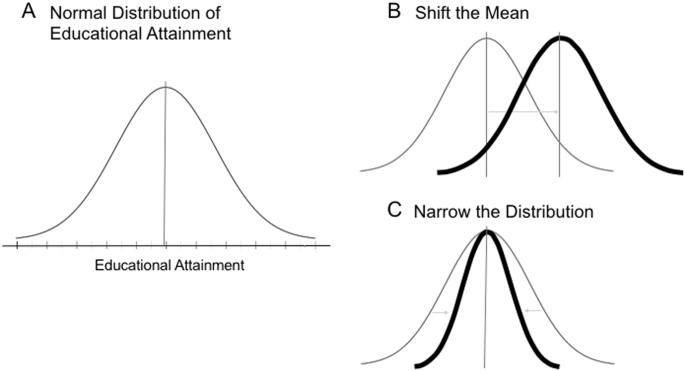
Aims of educational interventions are to shift the mean and narrow the gap of educational attainment outcomes. a Normal distribution of educational attainment. b Shift the mean. c Narrow the distribution to reduce the achievement gap between children on low and high ends of the distribution
“One must distinguish environmental events that on the average enhance or delay development for all children from those that account for variation among children. There can be ‘main effect’ that account for variation among groups that are naturally or experimentally treated in different ways. Within the groups of children there still remain enormous individual differences, some of which arise in response to the treatment. 36 ” Therefore, the existence of a rank order of abilities remains even after intervention (including when everybody has benefitted from the intervention).
Consider the follow-up study of children from low-income families who participated in the Carolina Abecedarian Project. This study revealed, even years later, that children who received a preschool educational intervention had higher academic achievement than children in an untreated control group. 39 Indeed, the means for age-referenced standard scores from the Woodcock–Johnson psychoeducational battery reported in this study revealed that standard scores for reading, math, written language, and knowledge were greater in the experimental group than in the control group by 5–7 points. However, there was little difference in variance of scores in the intervention group compared to the control group. This shows that the gap between the highest and lowest scoring children was the same regardless of whether the group of children received the intervention. 39 Although some children benefit greatly from interventions, other children do not show improvements. In other words, there are large inter-individual differences in response to interventions.
Importantly, this persistence of a rank order in the population does not mean that each individual child maintains their specific rank. Rather it means that individual differences persist. Indeed, children may respond differently to the interventions for a number of reasons. Figure 1 highlights one way that an intervention might narrow a distribution, namely, it is narrowed from both ends. However, it is certainly conceivable that interventions may change one end of the distribution. For example, in a basic reading intervention, a child who is already a proficient reader may benefit less than a child who has not yet learned how to read. This could depend on the match between the intervention and the individual’s ability. However, even children who begin an intervention with a similar skill level may respond very differently to the intervention. In empirical research on both reading and mathematics interventions, approximately 5% of the children were labeled as “treatment resisters”, because they did not respond to interventions. 34 , 35
Critically, this means that a rank order will exist in the population before and after an intervention and there will be rank order of responsivity to an intervention. In other words, educational interventions do not eliminate individual differences in a population. As such, it is realistic to expect that a good educational intervention will shift the mean of a population but not drastically narrow the actual distribution of performance in a given educational domain. When thinking about the effects of education it is important not to confound inferences about individuals with inferences about a population of students within an educational system. The focus of this paper is on population-level effects of education and their constraints.
This distinction between the effect of an intervention on the mean compared to individual differences exists beyond the classroom or children in an education system of a particular country. International comparison studies show that there are mean differences in educational achievement between countries, 11 , 12 but this does not imply that the shape of the distribution of performance on educational outcome measures is qualitatively different between countries. Indeed, at the population level, performance will be normally distributed in both countries, but the mean performance level may vary between countries. This has important implications for defining learning difficulties within and between countries. For example, if two nations with different mean achievement in mathematics both define children as having math learning difficulties when their math scores fall in the bottom 10% of the population, then the prevalence of math learning disabilities will be the same in both countries. However, the mean level of math achievement of children with so-called math learning difficulties in these two countries will differ. Thus, it is entirely possible for children who are considered to have math learning difficulties in one country to be within the normal range of performance given the distribution of another (on average more highly performing) country. Therefore, even though large differences in mean achievement between populations are possible, variability around the means likely remain unchanged.
Biological mechanism underlying individual differences
What might explain this common finding that early educational interventions shift the mean of a distribution but do not eliminate individual differences in the population? The importance of biology and specifically genetics to understanding human behavior has been brought up for decades. Indeed, multiple authors have alluded to the importance of taking biology seriously within theories of development. 40 , 41 , 42 , 43 In line with this, here we present research from the field of genetics that has the potential to shed light on the underlying biological mechanisms that may help us understand the effects of early educational interventions.
One of the oldest points of contention in the history of psychology is the nature-nurture debate. 44 , 45 , 46 The nature side of this debate maintains that variation in behavior arises from genes only (G). The nurture side of this debate argues that variation in behavior comes from experiences only (E). However, decades of research examining both genetics and early experience have determined that treating nature and nurture as a dichotomy is nonsensical and futile. 47 , 48 , 49 , 50 , 51 Currently, the question has shifted from the dichotomy of nature vs. nurture to questions about how an understanding of biology can inform our comprehension of the effects of nurture, such as in educational interventions. This has been referred to as “the nature of nurture”. 52
In the social sciences, including psychology and education, there has been a strong bias toward the “nurture” perspective and a hesitation to consider “nature” as a possible factor that can explain individual differences in responses to educational interventions. 53 One example of this strong bias toward a “nurture” perspective is the widely popular idea that changing the way an individual views their own learning can change his or her scholastic ability. Specifically, it has been argued that some individuals have a “fixed mindset”, meaning that they believe that their abilities are static, whereas other individuals have a “growth mindset”, and consequently, believe that their abilities can improve over time. Research has highlighted that individuals with a growth mindset are more likely to seek out challenges and persevere when faced with challenges. 54 Converging psychological research has explored the personality trait called grit, defined by Duckworth as perseverance and passion for long-term goals. Research has shown that grit is a predictor of school achievement and later life success. 55 , 56 These findings have led researchers and educators to try to improve academic achievement by fostering a growth mindset or encouraging grit. However, a recent meta-analysis examining the effectiveness of mindset interventions on academic achievement revealed that mindset interventions have only a weak effect on later academic achievement. 57 , 58 One explanation for these weak effects is that the notion that individual differences can be overcome with quick fixes, such as thinking differently or approaching educational challenges with more grit, is problematic as it relies on misconceptions associated with the “nurture assumption”. 59 , 60 , 61 This nurture assumption assumes that individual differences have no biological basis, and therefore can be easily overcome with interventions. However, the idea that a program can overcome individual differences contradicts what we know about the biological underpinnings of learning (see below) as well as the data on the changes induced by educational intervention (discussed above). These issues have been further highlighted in a recent blog post that discusses that “we should not forget that learners are all different and will have different strengths and weaknesses. Having a growth mindset does not mean that any weakness can be overcome”. 62
This bias toward “nurture” and a disdain for explanations of individual differences that make references to biological factors may be explained by a misconception that the consideration of biological factors implies that behavior and individual differences therein are both determined and fixed. In what follows below, we analyze some of the key biological concepts that have been used to explain individual differences and try to dispel some of the common misconceptions associated with them.
Heritability
One common misconception associated with research in genetics is that the term “heritability” means “genetic inheritance”. Heritability refers to how much variation in a trait (also called a phenotype) within a population arises from genetic variation among individuals. Heritability tells us nothing about what proportion of an individual’s phenotype is influenced by his or her genes. Moreover, measures of heritability can change even when the amount of genetic variation within a population remains constant. For example, research has revealed that SES modifies heritability of IQ in young children. 63 Specifically, heritability of IQ increased as environmental variability decreased, and likewise heritability of IQ decreased when environmental variability increased. This study provides empirical evidence for the fact that heritability is simply the proportion of variance of a specific trait that is not explained by variance of the environment or random chance.
Estimates of heritability are most commonly derived from the study of twins. Specifically, by comparing the correlations in performance of genetically identical, monozygotic twins with that of non-identical, dizygotic twins, it is possible to indirectly estimate how much of the variability between individuals is influenced by genetic similarity. In such research, heritably is quantified as twice the difference in the correlations between monozygotic and dizygotic twins. 64 While such studies provide a statistical estimate of the contribution of genetic variability to observed behavioral variability, they do not actually involve the measurement of the influence of genes. Heritability is a population measure, not a causal process within a single individual.
Another misconception is that heritability is negatively related to plasticity or modifiability. High heritability measures do not indicate that the behavioral trait within individuals is fixed and cannot be changed by the environment or that a certain behavioral trait influenced by a particular genetic variant is determined. Importantly and perhaps counter-intuitively, a higher heritability estimate implies greater equity of an environment. 65 If the heritability of a trait is 100%, this invariably means that there is no variation in the environment (i.e., the environment is equitable). It is important to note here that this notion of environmental equity does not say anything about the quality of the environment. Indeed, if a poor education system is implemented in exactly the same way across a population it is equitable and the heritability of educational achievement would be close to 100%.
It is especially important within the context of education to be cognizant of the fact that research indicating that educational achievement has high heritability does not mean that achievement is determined. Indeed, effects of heritability are probabilistic, not deterministic. Meta-analytic evidence has revealed that genetic effects vary across contexts. 66 Specifically, Tucker-Drob and Bates’ meta-analysis revealed that in the United States, where educational achievement is strongly correlated with SES, 67 heritability varies as a function of SES. In contrast, countries with social policies that ensure more uniform access to high-quality education (such as countries in Western Europe and Australia), exhibited no interaction between heritability and SES. In other words, educational achievement is reported to have greater heritability in populations from countries with greater equity in educational policy. 65 , 68
Genetics and educational achievement
The commonly replicated finding from twin studies that educational attainment is heritable has driven researchers to examine the associations between the human genome and educational outcome measures. 9 , 69 , 70 , 71 , 72 , 73 , 74 Recently, it has become possible to measure variability in genes across the entire genome of individuals and then relate this variability in the actual genes to behavioral variability. 75 , 76 , 77 , 78 Genome-Wide Association Studies (GWAS) use a complex statistical method to identify a set of genetic variants from across the entire human genome (all of our DNA) that correlate with a behavioral outcome measure such as educational attainment (for a detailed description of GWAS, see ref. 79 ). Typically, each GWAS examines millions of genetic variants (also known as single-nucleotide polymorphisms (SNP)) simultaneously. Notably, these “millions” of SNPs are not independent as they are often linked with each other via linage disequilibrium. 80 Researchers use this data to identify genetic variants that may contribute to individual differences in the population on a behavioral trait of interest. A major advantage of GWAS is that it is an unbiased approach that relies on genetic variants across the entire genome that correlate with the outcome measure, rather than a priori choosing particular genetic variants (i.e., the candidate gene approach 81 ). A critical disadvantage of GWAS has been a lack of reproducibility. Specifically, it was found that the genetic variants associated with a trait in one study were not consistently related to that same trait in other studies. 82 , 83 Reasons for this lack of reproducibility, such as insufficient sample sizes, lack of sufficient statistical tools to control for the extensive multiple comparisons, environmental differences between the cohorts, and lack of specificity in measuring behavioral outcome measures, are beyond the scope of this paper (for review, see refs. 79 , 82 , 83 , 84 ). Recently, progress has been made to overcome these limitations by increasing the sample size used to estimate the correlation between genetic variants and complex, behavioral phenotypes. Indeed, researchers have used GWAS to link genetic variants to individual differences in educational attainment measures such as number of years of schooling completed, 9 , 70 intelligence (IQ), 69 , 71 and even more specialized cognitive measures such as reading 74 and math ability. 73 The relation between “years of education” and the three SNPS from the original GWAS measuring “years of education” 70 was replicated several years later in a study that increased the sample size threefold. 9 Critically, the estimated effects sizes of these three SNPs are small (coefficient of determination R(2) ≈0.02%; this equals approximately 1 month of schooling per allele). Although, this research is in the early stages, GWAS research has revealed that contrary to previous theories based on candidate gene effects, many genes, each explaining a tiny proportion of variance, correlate with outcome measures.
More recently still, researchers have started to use a set of genetic variants (usually SNPs) selected from the entire genome using results from GWAS as predictive measures. Specifically, data from GWAS is used compute a composite genetic score for a set of genes. This is often referred to as a genome-wide polygenic score (GPS), and relates to a specific trait. 72 A GPS is a number that is composed of a set of weighted genetic variants (i.e., weighted SNPs) across many genetic loci that best predict a specific trait, such as educational attainment. Using GWAS to delineate the polygenic predictors of an outcome measure, the GPS score can be used with a different, smaller sample of individuals, to predict more refined outcome measures. For example, this method has been used to link genetic variants from a large GWAS study to specific measures of educational attainment. 9 , 70 , 72 Belsky et al. 72 computed a GPS for each individual of the “Dunedin cohort” in New Zealand for educational attainment. This GPS was derived from the GWAS that identified molecular genetic predictors of “years of education” in more than 100,000 individuals 70 and was replicated in a larger sample of almost 300,000 individuals. 9 This GPS score for educational attainment was computed by summing all alleles that were associated with “years of education” across many genetic loci that are weighted by effect sizes that were estimated from the Okbay et al. 9 GWAS study. 85 , 86 This polygenic score predicted adult economic outcomes and behavior across the life span. 72 In another study, a GPS derived from the Rietveld et al. 70 explained up to 9% of variance in educational achievement scores at ages 7, 12, and 16. 87 This GPS score was also associated with general cognitive ability and family SES. However, there was no evidence for GPS interacting with general cognitive ability or SES to predict educational achievement. Most recently, a GWAS study with 1.1 million individuals identified 1271 independent SNPs associated with educational attainment. 88 These SNPS were used to compute a polygenic prediction score that explained 11–13% of the variance in educational attainment and 7–10% of the variance in cognitive performance in independent samples. 88 Although the novel techniques used in these studies are still in their infancy, they provide the valuable insight that there is undoubtedly a relationship between individual differences in genetics and variability of educational achievement. Approaches like GPS help us to understand that biology plays a critical role in explaining individual differences in important life-span outcomes.
In the context of the present discussion, these data provide compelling evidence that the common assumption that nurture can eliminate individual differences in a population contradicts the biological mechanism associated with learning. Indeed, although the experience of educational interventions certainly affects educational outcome measures, individual differences in ability cannot be entirely attributed to the educational environment. This is due to the fact that biological factors play a key role in explaining individual differences in academic achievement. Therefore, the relation between genes and educational achievement further highlights the need to conceptualize the distinction between the two goals of educational interventions, namely, to shift the mean and narrow the distribution.
Gene–environment interplay
While GWAS studies and resulting GPS scores are useful to better understand the contributions of biology to behavioral outcomes, it is important to reiterate that genes and the environment do not operate independently. 89 Indeed, considerations of these genetic influences on individual differences in educational outcomes should still not be equated to assuming that these traits are inherited and therefore fixed. 49 A trait being 100% heritable does not mean that that this trait is completely explained by differences in individual’s DNA sequence because genes do not operate independently from environmental influences. Instead, complex dynamic interplay between genetic predispositions and environmental exposure (gene–environment interplay) lead to different developmental outcome measures. 50 , 90 For decades, scientists have explained that while genes (i.e., an individual’s DNA sequence) and the environment (i.e., experience across the life span) may seem like independent components that uniquely affect phenotypes, this is not the case. It is not either genetics or the environment that influences behavior, it is both. Moreover, the additive effect of genes plus environments (G+E) is not sufficient to explain individual differences in phenotypes. The presumed inverse relationship between genetics and environmental factors ignores large and distinct bodies of research that highlight that genes and experiences modify the effect of each other on traits. 51 , 79 Therefore, the dynamic complex relationship between genetic and environmental factors leading to specific outcomes across development lead researchers to develop the general term “gene–environment interplay”. 50 , 79 , 91 , 92
In what follows, we discuss how several components of “gene–environment interplay” relate to educational attainment. Gene–environment interplay is a broad term that incorporates the effect of genetics, gene–environment correlations, and gene–environment interactions. These biological mechanisms that underlie gene–environment interplay across developmental time will be used to help explain why individual differences remain even after early educational interventions.
Gene–environment correlations and the fade-out of educational interventions
Scarr and McCartney proposed a theory of development that genotypes direct experience. More specifically, the authors suggest that an individual’s genetics predicts their behavior across development both directly and through experience. 36 Although this important theoretical contribution is not without criticism and counterarguments, 93 , 94 it sets a critical foundation for the way that the link between genes and environments relate to predict behavior.
Indeed, an individual’s genetics and environment correlate in different ways across development. Gene–environment correlations can be passive, evocative, or active. Passive gene–environment correlations result from parents creating an environment that is influenced by their own heritable traits. Notably, under these conditions, the effect of the child’s genotype is constrained. Evocative gene–environment correlations result when an individual’s heritable behavior evokes an environmental response. Active gene–environment correlations occur when an individual possesses a heritable inclination to select a specific environment. The relative importance of these gene–environment correlations is hypothesized to change across development. 36 Passive gene–environment correlations may influence behavior more strongly in infancy and early childhood, whereas evocative and active gene–environment correlations may become more important during later childhood and adolescence. This is because, later in life, children can select niches that best fit their genotype. In contrast, a young infant is unable to select environments that fit their genotype and therefore passive gene–environment correlations dominate early in development. Consequently, in the capacity of gene–environment correlations, the environment may play a greater role in later childhood and adolescence compared to infancy and early childhood.
These developmental changes in the nature of gene–environment interplay are important to consider in the context of what is known about the long-term efficacy of early interventions. Research on the long-term effects of early education has revealed that, although interventions shift the mean of the normal distribution of an educational attainment outcome measure in the short term, the distribution eventually shifts back toward the pre-intervention mean (Fig. 1a ). Consistent with this, it has been found that the long-term results of early interventions are disappointing because the striking short-term improvements in children’s school success fades over time, 20 , 95 , 96 ,97 , 98 suggesting that investment in early educational intervention programs does not always lead to long-term improvements in later school and life success. Until recently, the mechanisms underlying fade-out effects were an enigma. However, recent work reveals that a large proportion of fade-out effects in a math intervention are explained by pre-existing differences (such as SES and academic ability before the intervention) between children, rather than school factors such as low levels of classroom instruction. 95 , 99 These data suggest that the long-term math outcomes of children are strongly influenced by individual stable traits across development. These findings highlight the need to consider how biological predispositions correlate and interact with the dynamic educational environment. In particular, it is plausible that developmental changes in gene–environment correlations could, in part, explain the frequently observed fade-out of educational interventions. Specifically, young children who, as a population, have a comparatively limited ability to select their own environment (e.g., they have to attend school, their parents have substantial control over their behavior, etc.: passive genotype–environment correlation) are more strongly influenced by environmental inputs, even if these do not align with their genotypes. This is a potential explanation for the observed short-term gains and a shift in the overall mean of the distribution following an educational intervention. Over developmental time, however, children begin to select environments that more closely fit their genotypes and thus select environments that play to their intrinsic strengths and abilities, leading to a less pronounced effect of the environment and thus the maintenance of the effects of educational interventions. This shift from passive to active gene–environment correlations across developmental time may be important for understanding the regression of the mean back to pre-intervention levels. Together, research on gene–environment correlations is among the most compelling evidence for how examining the effects of educational interventions through the lens of biology is beneficial for understanding how children experience and respond to educational interventions.
Gene–environment correlations have been reported for phenotypes associated with educational outcomes. For example, the polygenic score for educational attainment, discussed above, correlated with later life success of the individual as well as the SES of the home into which the individual was born. 72 , 100 This research supports the notion that children with certain genotypes may be more likely to receive certain kinds of parenting, evoke certain responses, and select certain aspects of environments.
In line with the notion of gene–environment correlations, a recent study has examined whether the parents’ genetic variants that are not passed on to the child affect the child’s educational attainment. 101 Specifically, a GWAS study of educational attainment 9 was used to compute polygenic scores for parents that only included genetic variants that were not passed on (i.e., non-transmitted genetic variants) to the children. The study revealed that the polygenic score that was computed using non-transmitted genetic variants accounted for approximately 30% of the variance in the children's educational achievement that was explained by the polygenic score calculated using the transmitted variants. The researchers concluded that genetic variants in parents that are not passed onto the child affect the educational attainment of the child. They call this indirect effect on educational attainment “genetic nurture”. 101 Findings such as these illustrate that it is simply impossible to separate nurture from nature. In this case parental genes, which are not passed onto the offspring, shape the environment that the child experiences. This finding is very much in agreement with the notion put forward by Scarr and McCartney 36 that “genes direct the course of human experience”.
There is no doubt that the use of polygenic scores in conjunction with environmental influences is useful for increasing understanding of the complex link between genetics and educational attainment. However, it has also been suggested that society can benefit from using information about a polygenic score in education. A recent paper indicated that polygenic scores “could be useful in both society and science to estimate genetic potential as well as risk in relation to all domains of functioning, including cognitive abilities and disabilities, personality and health and illness” (p. 1373). 102 This could be useful specifically within the context of education for identifying biological mechanisms that might help educators to understand why an individual student is struggling in a certain educational domain. Importantly, considering polygenic scores may also help educators to think more carefully about the environment. For example, one child may have a high polygenic score but nevertheless struggle in school, whereas another child may have a low polygenic score but excel at school. These contrasting examples should motivate an analysis of the environments that these children are experiencing. Moreover, knowledge of the environments experienced by these children may increase our understanding of which environments buffer against the effects of genetics or prevent the expression of the biological constraints that a learner brings to an educational setting. Unsurprisingly, there are societal fears that stem from concern that genetic scores may be used for eugenics purposes. 103 Eugenics is a term coined in the late 1800s that refers to the idea that genetics can be used to control breeding to increase the quality of a human population by increasing the occurrence of desirable heritable traits. 104 These societal fears about using polygenic scores for improving education are based in the misunderstanding that theses score are deterministic rather than probabilistic. Indeed, a base knowledge of the interplay between genetics and experience is a necessary foundation to support the valuable discussion of the application of genetic sciences to tailoring interventions to individual learners.
Gene–environment interactions
Critically, experiences, genetics, and the way that they correlate with one another is not the whole picture. Indeed, children arrive at school with more than just a set of genes and countless experiences that correlate. Specifically, in addition to gene–environment correlations, recent work has revealed complex dynamic interactions between genetic predispositions and early environments. These interactions highlight the non-deterministic way that genes affect behavioral outcomes across development. 72 , 73 , 75 , 78 , 105 , 106 , 107 Everyone has DNA sequences that respond to countless experiences (i.e., environments) across the lifespan. A span of DNA that comprises a gene is responsive to the environment. Specifically, it produces more or less of its gene product (e.g., RNA, protein) depending on experience. Early adversity has been linked to negative biological and psychological outcomes across development. 108 , 109 A growing body of research has indicated that genetic predispositions interact with environmental exposure to affect behavioral outcomes. 47 , 50 , 51 This can be conceptualized as genetic predispositions moderating the relationship between early experience and later phenotypic outcomes. The gene–environment interaction field has come under criticism in regard to sample sizes used, varying assessments of environments and behavioral outcomes, and inconsistent statistical methods. 48 Despite these important limitations, this body of research highlights that to understand individual differences in behavior, it is critical to consider the way that children’s genetic prepositions interact with their early experiences across developmental time. Therefore, it may be fruitful to explore the effect of gene–environment interactions on different components of educational attainment. Indeed, this body of research exposes the need to consider that each child arrives to the educational environment with a lifetime of the environment moderating that individual’s genetic predisposition. Therefore, it is unsurprising that a rank order of ability will exist, and that children will respond differently to educational interventions.
What role can biological research play in interpreting and refining intervention research?
This paper examined how a comprehensive understanding of gene–environment interplay can help explain individual differences in outcomes of educational interventions. In what follows, we suggest how biological research can be used to reconceptualise the effects of educational interventions.
Research has shown that countries with the greatest educational equity have the highest scores on standardized measures of achievement. 110 , 111 Consequently, an important goal of education systems across societies and countries is to improve equity. Improving educational equity is certainly a critical first step toward improving society. However, in addition to providing equitable opportunities for all children regardless of social status, perhaps the greatest insight we can gain from the field of biology is to embrace the existence of individual differences even when a high level of equity exists. When achieving equality in education, it is not surprising that children do not achieve at the same level, because different children require different inputs. Educational equity aims to provide individualized resources to achieve the same outcomes regardless of individual barriers and starting conditions. Therefore, the inherent goal of educational equity is for all children to perform at the same level on an educational outcome measure. However, research in the field of biology suggests that even with a perfectly equitable system, we will still find individual differences and therefore should not expect equal achievement. In other words, true equity is not attainable as we cannot expect an educational policy to bring all students to the same level. In this context, it is important to acknowledge that equity does not imply that everyone will be able to achieve the same educational outcome, it only means that each individual is provided with individualized inputs and environments.
The expectation that education can narrow the distribution of educational outcomes ignores the fact that the biological mechanisms (described above), that support learning across development, generate individual differences. Indeed, previous research that has used GWAS, GPS, gene–environment correlations, and gene–environment interaction methodologies to predict educational attainment has resulted in a large body of evidence that indicates that biological predispositions are directly linked to individual differences in educational outcomes. This means that equalizing the educational environment will not eliminate individual differences in educational achievement. Therefore, we must reconceptualise how we evaluate educational attainment. This is necessary in order to implement realistic and compassionate educational expectations and policies.
In order to re-evaluate educational attainment, it is important to first define the term educational attainment. Educational attainment is often measured as a single outcome measure, such as the average of school grades or the grade point average (GPA) that is thought to be most representative of general ability. However, there is no one distribution for “educational attainment”. Indeed, there are countless distributions (each of them closely approximating the normal distribution when considering a population of interest) for the many subtypes of skills associated with educational attainment. Several examples of possible educational measurement subtypes include math ability, reading ability, working memory capacity, or musical ability. If a student is performing very well in math, it does not automatically follow that this individual is also a strong reader or shines in art class. A long-term goal for evaluating the effectiveness of educational interventions should be to evaluate how an intervention relates to many different measures of educational achievement. Thus, instead of conceptualizing educational attainment on one distribution using a single measure such as GPA, it should be conceptualized on many different distributions. Therefore, educational attainment should be defined as an overarching term that includes many distinct measures of abilities related to education. We should expect individuals to fall along different parts of the distribution for each measure of educational attainment, depending on their strengths and weaknesses. Consequently, it is important to restructure educational policy to embrace individual differences and create a more diverse set of educational opportunities. 112 Beyond the teaching of basic skills in reading and math that allow individuals to become successful members of society, it is important to offer students a variety of educational opportunities in order for them to be able to find niches within the educational system that best fit their genetic predispositions and experiences within which they can therefore thrive and succeed.
Finally, research on gene–environment interplay reveals that an individual’s genetic sequence should be conceptualized as a predisposition for a range of potential behavioral outcomes. In this context it has been suggested that the environment acts as a “dimmer switch” for genetic predispositions. 113 This is important for evaluating the success of intervention research. Specifically, educational interventions should be used to help each individual child optimize their own individual range of potential (see Fig. 2 ). Critically, an individual’s position in a rank order will differ depending on the educational outcome measure. For example, one individual may be in the 80th percentile on numeracy but the 50th percentile in literacy. As such, there is no one rank order for an individual, but rather an infinite number of rank orders that depend on the specific educational outcome measure being considered. Having said this, we do want to acknowledge that there is a substantial and reproducible relationship between measures of IQ and educational achievement across many domains of learning. Furthermore, it is well established that IQ is heritable. 114 However, there is research to show that IQ does not fully explain the heritability of educational outcomes. 115 So, while it is important to recognize that IQ does predict variability in a diverse set of educational outcome measures, there do exist individual differences within and across educational domains that cannot be explained by IQ. Put differently, two individuals with the same IQ may excel in different domains of learning during their educational careers. It is these differences that we argue are necessary to consider rather than striving to equalize the performance of learners within a narrow set of learning domains. Together this suggests that an intervention that changes the mean or the position of individual children in a rank order, but does not eliminate the existence of a rank order, should not be interpreted negatively, implying that individual ability is fixed. Instead, it is time to embrace individual differences and support strengths and weaknesses through education. Additionally, education need not endeavor for every child to achieve the upper limit of their range for each educational outcome measure. Rather, beyond ensuring that children have a solid foundation in basic skills in core educational subjects, the goal of education should be to foster children’s enjoyment and motivation toward learning. In other words, a goal of educational interventions should be to maximize potential at the individual level for a wide variety of specific cognitive and emotional educational outcome measures, rather than shift the overall mean or attempt to narrow the distribution of educational achievement more broadly.
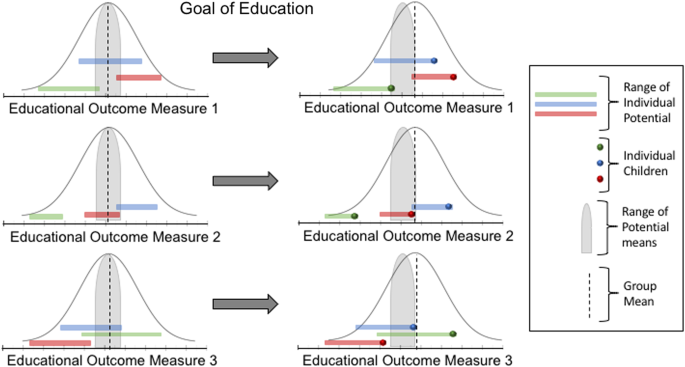
The left side of this figure depicts normal distributions of three educational outcomes measures. Individual children each have a range of scores that they may be predisposed to fall within. Ranges of three example individual children are highlighted in green, blue, and red. A hypothetical range that represents the extent to which the mean of the distribution can shift is highlighted in gray. A goal of education could be to help each child achieve the maximum score within their own individual range on these particular educational outcome measures. The right side of the figure depicts the scores of the three children and the group mean of the population if this goal of education is achieved. The educational outcome scores for the three example children are depicted with circles at the right end of each child’s range of individual potential. The dotted line on the right represents where the group mean would fall if all children achieved their maximum potential score
Conclusions
The results of educational interventions intended to improve educational outcome measures are disappointing, particularly in children from impoverished environments. Researchers and policymakers have tried to improve equity in the educational environment in an effort to reduce the achievement gap between children. However, research has consistently reported that early educational interventions do not eliminate individual differences in a population. 36 Moreover, children experience and respond to educational interventions differently. The evidence reviewed in this paper suggests that the interplay between genetic predispositions and environmental exposure across developmental time influences the way that children respond to educational interventions. For example, GWAS and GPS methodologies reveal that individual differences in genetic predispositions predict variability between children in terms of their educational achievement. 9 , 69 , 70 , 72 Moreover, these stable individual differences are a consequence of biological mechanisms that support the interplay between genetic predispositions and the embedding of experience into our biology, rather than a problem with the educational environment. Currently, some conceptualize the goals of educational interventions as an attempt to both shift the mean and narrow the distribution of particular measures of educational attainment (such as GPA) that are thought to be most representative of individual ability. We recommend reconceptualising the term “educational attainment” and the goals of educational interventions. The term “educational attainment” should be an umbrella term that encompasses a wide variety of specific measurable educational outcome measures. The primary goal of educational interventions should be to maximize each child’s potential on all educational outcome measures. Notably, maximization of potential should not simply aim to optimize a child’s achievement. Instead, the role of education should be to facilitate children’s ability to select environments that align with their genotypes. Moreover, researchers, educators, and policymakers must be cognizant of the fact that each individual child enters the education system armed with a lifetime worth of interplay between their genetic predispositions and environmental exposure. Each child’s lifetime of gene–environment interplay affects the way that the child responds to education as a whole as well as to targeted educational interventions. Moreover, gene–environment interplay is a dynamic and ongoing process across an entire lifespan, not a static event such as an educational intervention. In view of this, it is senseless to assume that one type of short-term environmental exposure (such as a short-term educational intervention) will achieve long-term gains. Instead, it is probable that implementing sustained interventions will reduce fade-out effects. Therefore, we recommend that educational interventions researchers should move toward implementing changes to the educational environment that help interventions have more long-term beneficial effects (i.e., reduce fade-out effects) at the individual child level, rather than the group level. Educational interventions should identify the range of potential for each individual child and help each child achieve their potential.
Taken together, the findings reviewed above clearly suggest that, at the population level, the most important goal of educational policy is to implement equitable systems that provide individuals within the population the opportunities to reach their individual levels of achievement across a kaleidoscope of potential educational outcome. The biological research reviewed in this paper clearly demonstrates that a system that insists that all students can reach the same educational levels of achievment both within and across different educational outcomes, severely overestimates the potential of environmental effects, and therefore lacks sufficient consideration of individual differences and human diversity.
Jamison, E. A., Jamison, D. T. & Hanushek, E. A. The effects of education quality on income growth and mortality decline. Econ. Educ. Rev. 26 , 772–789 (2007).
Article Google Scholar
Checchi, D. Inequality in incomes and access to education: a cross-country analysis (1960-95). Labour 17 , 153–201 (2003).
Hanushek, E. A. & Kimko, D. D. Schooling, labor-force quality, and the growth of nations. Am. Econ. Rev. 90 , 1184–1208 (2000).
Posner, M. I. & Rothbart, M. K. Influencing brain networks: implications for education. Trends Cogn. Sci. 9 , 99–103 (2005).
Article PubMed Google Scholar
Ansari, D. Culture and education: new frontiers in brain plasticity. Trends Cogn. Sci. 16 , 93–95 (2012).
Kroeger, La., Brown, R. D., & O’Brien, B.A. Connecting neuroscience, cognitive, and educational theories and research to practice: a review of mathematics intervention programs. Early Educ. Dev. 23 , 37–58 (2012).
Ritchie, S. J., Bates, T. C. & Deary, I. J. Is education associated with improvements in general cognitive ability, or in specific skills? Dev. Psychol. 51 , 573–582 (2015).
Article PubMed PubMed Central Google Scholar
Shakeshaft, N. G. et al. Strong genetic influence on a UK nationwide test of educational achievement at the end of compulsory education at age 16. PLoS ONE 8 , e80341 (2013).
Okbay, A. et al. Genome-wide association study identifies 74 loci associated with educational attainment. Nature 533 , 539–542 (2016).
Article PubMed PubMed Central CAS Google Scholar
Munoz, M. A. & Dossett, D. Equity and excellence: the effect of school and sociodemographic variables on student achievement. J. Sch. Leadersh. 11 , 120–132 (2001).
Google Scholar
Gorard, S. & Smith, E. An international comparison of equity in education systems. Comp. Educ. 40 , 15–28 (2004).
Alegre, M À. & Ferrer, G. School regimes and education equity: some insights based on PISA 2006. Br. Educ. Res. J. 36 , 433–461 (2010).
Engle, P. L. et al. Strategies to avoid the loss of developmental potential in more than 200 million children in the developing world. Lancet 369 , 229–242 (2007).
Ferguson, H., Bovaird, S. & Mueller, M. The impact of poverty on educational outcomes for children. Paediatr. Child Health 12 , 701–706 (2007).
Duncan, G. J., Magnuson, K. & Votruba-Drzal, E. Moving beyond correlations in assessing the consequences of poverty. Annu. Rev. Psychol . https://doi.org/10.1146/annurev-psych-010416-044224 (2017).
Espinoza, O. Solving the equity-equality conceptual dilemma: a new model for analysis of the educational process. Educ. Res. 49 , 343–363 (2007).
Omoeva, C. Mainstreaming equity in education. Issues Paper Commissioned by the International Education Funders Group. FHI 360 Education Policy and Data Center / Education Equity Research Initiative (2017).
Mann, B. Equity and equality are not equal. The Education Trust . https://edtrust.org/the-equity-line/equity-and-equality-are-not-equal/ (2014).
Karoly, L. A., Kilburn, R. M., Cannon, J. Proven benefits of early childhood interventions. Santa Monica, CA: RAND Corporation (2005).
Barnett, W. S. Effectiveness of early educational intervention. Science 333 , 975–979 (2011).
Article PubMed CAS Google Scholar
Heckman, J. J. Invest in Early Childhood Development: Reduce Deficits, Strengthen the Economy , 1–2 (The Heckman Equation Project, Chicago, 2012).
Brunyé, T. T. et al. Learning to relax: evaluating four brief interventions for overcoming the negative emotions accompanying math anxiety. Learn. Individ. Differ. 27 , 1–7 (2013).
Lovett, M. W., Lea, L., Steinbach, K. A., & Palma, M. De. Development and evaluation of a research-based intervention program for children and adolescents with reading disabilities. Perspect. Lang. Lit. 40 , 21–31 (2014).
Park, D., Ramirez, G. & Beilock, S. L. The role of expressive writing in math anxiety. J. Exp. Psychol. Appl. 20 , 103–111 (2014).
Supekar, K., Iuculano, T., Chen, L. & Menon, V. Remediation of childhood math anxiety and associated neural circuits through cognitive tutoring. J. Neurosci. 35 , 12574–12583 (2015).
Luster, T. & McAdoo, H. Family and child influences on educational attainment: a secondary analysis of the high/scope Perry Preschool data. Dev. Psychol. 32 , 26–39 (1996).
Mughal, M. K., Ginn, C. S., Perry, R. L. & Benzies, K. M. Longitudinal effects of a two-generation preschool programme on receptive language skill in low-income Canadian children to age 10 years. Early Child Dev. Care 186 , 1316–1326 (2016).
Campbell, F. A., Ramey, C. T., Pungello, E., Sparling, J. & Miller-Johnson, S. Early childhood education: young adult outcomes from the Abecedarian Project. Appl. Dev. Sci. 6 , 42–57 (2002).
Robinson, L. E., Palmer, K. K. & Bub, K. L. Effect of the children’s health activity motor program on motor skills and self-regulation in head start preschoolers: an efficacy trial. Front. Public Health 4 , 173 (2016).
Nores, M. & Barnett, W. S. Benefits of early childhood interventions across the world: (Under) investing in the very young. Econ. Educ. Rev. 29 , 271–282 (2010).
Camilli, G., Vargas, S., Ryan, S. & Barnett, W. S. Meta-analysis of the effects of early education interventions on cognitive and social development. Teach. Coll. Rec. 112 , 579–620 (2010).
Burger, K. How does early childhood care and education affect cognitive development? An international review of the effects of early interventions for children from different social backgrounds. Early Child. Res. Q. 25 , 140–165 (2010).
Dietrichson, J., Bøg, M., Filges, T. & Klint Jørgensen, A.-M. Academic interventions for elementary and middle school students with low socioeconomic status: a systematic review and meta-analysis. Rev. Educ. Res. 87 , 243–282 (2017).
Fuchs, D. & Fuchs, L. S. Introduction to response to intervention: what, why, and how valid is it? Read. Res. Q. 41 , 93–99 (2006).
Torgesen, J. K. Individual differences in response to early interventions in reading: the lingering problem of treatment resisters. Learn. Disabil. Res. Pract. 15 , 55–64 (2000).
Scarr, S. & McCartney, K. How people make their own environments: a theory of genotype –>environment effects. Child Dev. 54 , 424–435 (1983).
PubMed CAS Google Scholar
Maccoby, E. E. Parenting and its effects on children: on reading and misreading behavior genetics. Annu. Rev. Psychol. 51 , 1–27 (2000).
Tucker-Drob, E. M. & Briley, D. A. Continuity of genetic and environmental influences on cognition across the life span: a meta-analysis of longitudinal twin and adoption studies. Psychol. Bull. 140 , 949–979 (2014).
Campbell, F. A. & Ramey, C. T. Effects of early intervention on intellectual and academic achievement: a follow-up study of children from low-income families. Child Dev. 65 , 684–698 (1994).
Bjorklund, D. F. & Pellegrini, A. D. Child development and evolutionary psychology. Child Dev. 71 , 1687–1708 (2000).
Geary, D. C., Berch, D. B., Mann Koepke, K. (EDs.), Evolutionary origins and early development of number processing. In: Mathematical Cognition and Learning, vol 1. Elsevier Aademic Press, San Diego, CA (2015).
Scarr, S. Developmental theories for the 1990s: development and Individual Differences. Child Dev. 63 , 1–19 (1992).
Gottlieb, G., Wahlsten, D. & Lickliter, R. in Handbook of Child Psychology (eds Lerner, R. M. & Damon, W.) 210–258 (John Wiley & Sons, Hoboken, NJ, 2007).
Hollingworth, L. S. Gifted Children: Their Nature and Nurture (Macmillan, New York, 1926).
Pastore, N. The nature-nurture controversy. (King's Crown Press, Oxford, England, 1949).
Leahy, M. A. Nature-nurture and intelligence. Genet. Psychol. Monogr. 17 , 236–308 (1935).
Meaney, M. J. Epigenetics and the biological definition of gene x environment interactions. Child Dev. 81 , 41–79 (2010).
Charney, E. Genes, behavior, and behavior genetics. WIREs Cogn. Sci. 8 , e1405 (2016).
Moore, D. S. & Shenk, D. The heritability fallacy. WIREs Cogn. Sci. 8 , 1–8 (2016).
CAS Google Scholar
Rutter, M., Moffitt, T. E. & Caspi, A. Gene–environment interplay and psychopathology: multiple varieties but real effects. J. Child Psychol. Psychiatry 47 , 226–261 (2006).
Sokolowski, M. B. & Wahlsten, D. in Methods in Genomic Neuroscience (eds Chin, H. R. & Moldin, S. O.) 1–25 (CRC Press, Boca Raton, FL, 2001).
Miller, G. W. & Jones, D. P. The nature of nurture: refining the definition of the exposome. Toxicol. Sci. 137 , 1–2 (2014).
Pinker, S. The Blank Slate: The Modern Denial of Human Nature (Viking, New York, 2002).
Dweck, C. S. Mindset: the new psychology of success (Random House, New York, 2006).
Duckworth, A. L., Peterson, C., Matthews, M. D. & Kelly, D. R. Grit: perseverance and passion for long-term goals. J. Pers. Soc. Psychol. 92 , 1087–1101 (2007).
Duckworth, A. Grit: the power of passion and perseverance (Scribner/Simon & Schuster, New York, US, 2016).
Sisk, V. F., Burgoyne, A. P., Sun, J., Butler, J. L. & Macnamara, B. N. To what extent and under which circumstances are growth mind-sets important to academic achievement? Two meta-analyses. Psychol. Sci. 29 , 549–571 (2018).
Yeager, D. S. et al. Where and for whom can a brief, scalable mindset intervention improve adolescents’ educational trajectories? https://doi.org/10.17605/OSF.IO/MD2QA (2018).
Harris, J. R. Where is the child’s environment? A group socialization theory of development. Psychol. Rev. 102 , 458–489 (1995).
Harris, J. R. The Nurture Assumption: Why Children Turn Out the Way They Do . (Free Press, New York, 1998).
Harris, J. R. The Nurture Assumption: Why Children Turn Out the Way They Do . (Free Press, New York, 2009).
Brookman-Byrne, A. Fostering a growth mindset. Blog on Learning and Development . http://bold.expert/fostering-a-growth-mindset/ (2018).
Turkheimer, E., Haley, A., Waldron, M., D’Onofrio, B. & Gottesman, I. I. Socioeconomic status modifies heritability of IQ in young children. Psychol. Sci. 14 , 623–628 (2003).
Falconer, D. Quantitative genetics in Edinburgh: 1947–1980. Genetics 133 , 137–142 (1993).
PubMed PubMed Central CAS Google Scholar
Colodro-Conde, L., Rijsdijk, F., Tornero-Gómez, M. J., Sánchez-Romera, J. F. & Ordoñana, J. R. Equality in educational policy and the heritability of educational attainment. PLoS ONE 10 , e0143796 (2015).
Tucker-Drob, E. M. & Bates, T. C. Large cross-national differences in gene×socioeconomic status interaction on intelligence. Psychol. Sci. 27 , 138–149 (2016).
Sirin, S. R. Socioeconomic status and academic achievement: a meta-analytic review of research. Rev. Educ. Res. 75 , 417–453 (2005).
Branigan, A. R., McCallum, K. J. & Freese, J. Variation in the heritability of educational attainment: an international meta-analysis. Soc. Forces 92 , 109–140 (2013).
Sniekers, S. et al. Genome-wide association meta-analysis of 78,308 individuals identifies new loci and genes influencing human intelligence. Nat. Genet. 49 , 1107–1112 (2017).
Rietveld, C. A. et al. GWAS of 126,559 individuals identifies genetic variants associated with educational attainment. Science 340 , 1467–1471 (2013).
Marioni, R. E. et al. Molecular genetic contributions to socioeconomic status and intelligence. Intelligence 44 , 26–32 (2014).
Belsky, D. W. et al. The genetics of success: how single-nucleotide polymorphisms associated with educational attainment relate to life-course development. Psychol. Sci. 27 , 957–972 (2016).
Chen, H. et al. A genome-wide association study identifies genetic variants associated with mathematics ability. Sci. Rep. 7 , 40365 (2017).
Luciano, M. et al. A genome-wide association study for reading and language abilities in two population cohorts. Genes Brain Behav. 12 , 645–652 (2013).
Savitz, J., Solms, M. & Ramesar, R. The molecular genetics of cognition: dopamine, COMT and BDNF. Genes Brain Behav. 5 , 311–328 (2006).
Sokolowski, H. M. et al. The Drosophila foraging gene human orthologue PRKG1 predicts individual differences in the effects of early adversity on maternal sensitivity. Cogn. Dev . 42 , 62–73 (2017).
Caspi, A., Hariri, A. R., Holmes, A., Uher, R. & Moffitt, T. E. Genetic sensitivity to the environment: the case of the serotonin transporter gene and its implications for studying complex diseases and traits. Focus 8 , 398–416 (2010).
Gialluisi, A., Guadalupe, T., Francks, C. & Fisher, S. E. Neuroimaging genetic analyses of novel candidate genes associated with reading and language. Brain Lang. https://doi.org/10.1016/j.bandl.2016.07.002 (2016).
Anreiter, I., Sokolowski, H. M. & Sokolowski, M. B. Gene-environment interplay and individual differences in behavior. Mind Brain Educ. https://doi.org/10.1111/mbe.12158 (2017).
Slatkin, M. Linkage disequilibrium–understanding the evolutionary past and mapping the medical future. Nat. Rev. Genet. 9 , 477–485 (2008).
Tabor, H. K., Risch, N. J. & Myers Richard, M. Candidate-gene approaches for studying complex genetic traits: practical considerations. Nat. Rev. Genet. 3 , 1–7 (2002).
Article CAS Google Scholar
Williams, S. M. et al. Problems with genome-wide association studies. Science 316 , 1840–1842 (2007).
PubMed Google Scholar
Kraft, P., Zeggini, E. & Ioannidis, J. P. A. Replication in genome-wide association studies. Stat. Sci. 24 , 561–573 (2009).
Li, M. et al. Enrichment of statistical power for genome-wide association studies. BMC Biol. 12 , 73 (2014).
Dudbridge, F. Power and predictive accuracy of polygenic risk scores. PLoS Genet. 9 , e1003348 (2013).
Euesden, J., Lewis, C. M. & O’Reilly, P. F. PRSice: polygenic risk score software. Bioinformatics 31 , 1466–1468 (2015).
Selzam, S. et al. Predicting educational achievement from DNA. Mol. Psychiatry 22 , 267–272 (2017).
Lee, J. J. et al. Gene discovery and polygenic prediction from a genome-wide association study of educational attainment in 1.1 million individuals. Nat. Genet. 50 , 1112–1121 (2018).
Plomin, R., DeFries, J. C. & Loehlin, J. C. Genotype-environment interaction and correlation in the analysis of human behavior. Psychol. Bull. 84 , 309–322 (1977).
Boyce, W. T. et al. Social stratification, classroom climate, and the behavioral adaptation of kindergarten children. Proc. Natl Acad. Sci. USA 109 , 17168–17173 (2012).
Boyce, W. T. & Kobor, M. S. Development and the epigenome: the ‘synapse’ of gene-environment interplay. Dev. Sci. 18 , 1–23 (2015).
Caspi, A. & Moffitt, T. E. Gene–environment interactions in psychiatry: joining forces with neuroscience. Nat. Rev. Neurosci. 7 , 583–590 (2006).
Baumrind, D. The average expectable environment is not good enough: a response to scarr. Child Dev. 64 , 1299–1317 (1993).
Jackson, J. F. Human behavioral genetics, scarr’s theory, and her views on interventions: a critical review and commentary on their implications for African American children. Child Dev. 64 , 1318–1332 (1993).
Bailey, D. H. et al. Fadeout in an early mathematics intervention: constraining content or preexistingdifferences? Dev. Psychol . 52 , 1457–1469 (2016).
Protzko, J. The environment in raising early intelligence: a meta-analysis of the fadeout effect. Intelligence 53 , 202–210 (2015).
Seeman, T. et al. Education, income and ethnic differences in cumulative biological risk profiles in a national sample of US adults: NHANES III (1988–1994). Soc. Sci. Med. 66 , 72–87 (2008).
Ramey, C. T. & Ramey, S. L. Effective early intervention. Ment. Retard. 30 , 337–345 (1992).
Bailey, D. H., Watts, T. W., Littlefield, A. K. & Geary, D. C. State and trait effects on individual differences in children’s mathematical development. Psychol. Sci. 25 , 2017–2026 (2014).
Domingue, B. W., Belsky, D., Conley, D., Harris, K. M. & Boardman, J. D. Polygenic influence on educational attainment: new evidence from the national longitudinal study of adolescent to adult health. AERA Open 1 , 1–13 (2015).
Kong, A. et al. The nature of nurture: effects of parental genotypes. Science 359 , 424–428 (2018).
Krapohl, E. et al. Multi-polygenic score approach to trait prediction. Mol. Psychiatry 23 , 1368–1374 (2018).
Bliss, C. Social by nature: the promise and peril of sociogenomics. (Stanford University Press, Stanford, California, 2018)
Galton, F. Eugenics: its definition, scope, and aims. Am. J. Sociol. 10 , 1–25 (1904).
Peper, J. S., Brouwer, R. M., Boomsma, D. I., Kahn, R. S. & Hulshoff Pol, H. E. Genetic influences on human brain structure: a review of brain imaging studies in twins. Hum. Brain Mapp. 28 , 464–473 (2007).
Hill, W. D. et al. Molecular genetic contributions to social deprivation and household income in UK biobank. Curr. Biol. 26 , 3083–3089 (2016).
Canli, T., Ferri, J. & Duman, E. A. Genetics of emotion regulation. Neuroscience 164 , 43–54 (2009).
McLaughlin, K. A. et al. Causal effects of the early caregiving environment on development of stress response systems in children. Proc. Natl Acad. Sci. USA 112 , 5637–5642 (2015).
Bick, J. & Nelson, C. A. Early experience and brain development. Wiley Interdiscip. Rev. Cogn. Sci. 8 , e1387 (2017).
Sahlberg, P. Education policies for raising student learning: the Finnish approach. J. Educ. Policy 22 , 147–171 (2007).
PISA. PISA 2015 results in focus. OECD. https://doi.org/10.1787/9789264266490-en (2016).
Asbury, K. & Plomin, R. G is for Genes: The Impact of Genetics on Education and Achievement (Wiley Blackwell, Chichester, 2013).
Moore, D. S. Behavioral epigenetics. WIREs Syst. Biol. Med. 9 , 1–8 (2016).
Plomin, R. & von Stumm, S. The new genetics of intelligence. Nat. Rev. Genet. https://doi.org/10.1038/nrg.2017.104 (2018).
Rimfeld, K., Kovas, Y., Dale, P. S. & Plomin, R. Pleiotropy across academic subjects at the end of compulsory education. Sci. Rep. 5 , 11713 (2015).
Download references
Acknowledgements
This work was supported by operating grants from the Natural Sciences and Engineering Council of Canada (NSERC) (Grant no. 468721), the Canadian Institutes of Health Research (CIHR) (Grant no. 93609), the Canada Research Chairs Program, an E.W.R Steacie Memorial Fellowship from the Natural Sciences and Engineering Council of Canada (NSERC), and a Jacobs Foundation Advanced Research Fellowship to D.A. as well as an NSERC CGS-D Scholarship to H.M.S.
Author information
Authors and affiliations.
University of Western Ontario, London, ON, Canada
H. Moriah Sokolowski & Daniel Ansari
You can also search for this author in PubMed Google Scholar
Contributions
H.M.S. contributed to conceptualizing the idea, writing the first complete draft, and editing the paper. D.A. contributed to conceptualizing the idea and editing the paper.
Corresponding author
Correspondence to H. Moriah Sokolowski .
Ethics declarations
Competing interests.
The authors declare no competing interests.
Additional information
Publisher's note: Springer Nature remains neutral with regard to jurisdictional claims in published maps and institutional affiliations.
Rights and permissions
Open Access This article is licensed under a Creative Commons Attribution 4.0 International License, which permits use, sharing, adaptation, distribution and reproduction in any medium or format, as long as you give appropriate credit to the original author(s) and the source, provide a link to the Creative Commons license, and indicate if changes were made. The images or other third party material in this article are included in the article’s Creative Commons license, unless indicated otherwise in a credit line to the material. If material is not included in the article’s Creative Commons license and your intended use is not permitted by statutory regulation or exceeds the permitted use, you will need to obtain permission directly from the copyright holder. To view a copy of this license, visit http://creativecommons.org/licenses/by/4.0/ .
Reprints and permissions
About this article
Cite this article.
Sokolowski, H.M., Ansari, D. Understanding the effects of education through the lens of biology. npj Science Learn 3 , 17 (2018). https://doi.org/10.1038/s41539-018-0032-y
Download citation
Received : 21 March 2018
Revised : 02 August 2018
Accepted : 03 August 2018
Published : 01 October 2018
DOI : https://doi.org/10.1038/s41539-018-0032-y
Share this article
Anyone you share the following link with will be able to read this content:
Sorry, a shareable link is not currently available for this article.
Provided by the Springer Nature SharedIt content-sharing initiative
This article is cited by
Infrastructuring educational genomics: associations, architectures, and apparatuses.
- Ben Williamson
- Dimitra Kotouza
- Jessica Pykett
Postdigital Science and Education (2024)
On the promise of personalized learning for educational equity
- Hanna Dumont
- Douglas D. Ready
npj Science of Learning (2023)
Persistent association between family socioeconomic status and primary school performance in Britain over 95 years
- Sophie von Stumm
- Sophie Nicole Cave
- Paul Wakeling
npj Science of Learning (2022)
Genetic associations with mathematics tracking and persistence in secondary school
- K. Paige Harden
- Benjamin W. Domingue
- Kathleen Mullan Harris
npj Science of Learning (2020)
Quick links
- Explore articles by subject
- Guide to authors
- Editorial policies
Sign up for the Nature Briefing newsletter — what matters in science, free to your inbox daily.

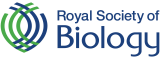
- Site Search
You are here

Journal of Biological Education
- Primary schools
- Gopher science labs
- Gopher School Registration
- SciberMonkey
- Practical Biology
- School competitions
- Scottish teacher network
- Biology in the Real World Past Presentations
- Post 16 Biology Survey 2016-17
- Post 16 Biology Survey 2017-18
- Chemistry for Biologists
- Higher education
- HUBS events
- HUBS membership
- HUBS grants
- HUBS executive committee
- HUBS news and reports
- External examiners database
- Early Career Lecturers in Biosciences
- The Bioscience Awarding Gap Network
- The Biosciences Educators' Network
- Education research
- The Biologist
- Bioscience journals
- Degree accreditation programme
The Journal of Biological Education is an international journal that publishes the latest research into the teaching, learning and assessment of biology. It is owned by the Royal Society of Biology and published by Taylor and Francis.
The journal aims to bridge the gap between research and practice, providing information, ideas and opinion, in addition to critical examinations of advances in biology research and teaching. Through the coverage of policy and curriculum developments, the latest results of research into the teaching, learning and assessment of biology are brought to the fore.
Special emphasis is placed on research relevant to educational practice, guided by educational realities in systems, schools, colleges and universities. Papers that are theoretically informed and methodologically rigorous are welcomed.
Subscription Fees
We offer a special print-only subscription rate for Royal Society of Biology members for an annual fee of £40 (complete the subscription form or contact the RSB membership team for more details). Under the RSB's Schools and Colleges Affiliation Scheme (SCAS) UK-based schools and colleges can subscribe to the journal for just £60.
Non-member subscription rates for the journal can be found on the Taylor and Francis website. Please contact the Taylor and Francis customer services team if you have any queries or would like to take out a subscription.
The archive of the journal and the current issue can be accessed at Taylor and Francis online . Details of the editorial board , instructions for authors and the journal scope are also available. Read a sample issue .
Research Guidelines
All articles that appear in the JBE must adhere to the ARRIVE guidelines for the reporting of in vivo experiments. The guidelines have been developed by the NC3Rs to improve standards of reporting of animal experiments, and ensure research featured in the JBE is based on sound and ethical experiments.
- Position paper
- Open access
- Published: 02 December 2019
Biology education research: building integrative frameworks for teaching and learning about living systems
- Ross H. Nehm ORCID: orcid.org/0000-0002-5029-740X 1
Disciplinary and Interdisciplinary Science Education Research volume 1 , Article number: 15 ( 2019 ) Cite this article
13k Accesses
17 Citations
1 Altmetric
Metrics details
This critical review examines the challenges and opportunities facing the field of Biology Education Research (BER). Ongoing disciplinary fragmentation is identified as a force working in opposition to the development of unifying conceptual frameworks for living systems and for understanding student thinking about living systems. A review of Concept Inventory (CI) research is used to illustrate how the absence of conceptual frameworks can complicate attempts to uncover student thinking about living systems and efforts to guide biology instruction. The review identifies possible starting points for the development of integrative cognitive and disciplinary frameworks for BER. First, relevant insights from developmental and cognitive psychology are reviewed and their connections are drawn to biology education. Second, prior theoretical work by biologists is highlighted as a starting point for re-integrating biology using discipline-focused frameworks. Specifically, three interdependent disciplinary themes are proposed as central to making sense of disciplinary core ideas: unity and diversity; randomness, probability, and contingency; and scale, hierarchy, and emergence. Overall, the review emphasizes that cognitive and conceptual grounding will help to foster much needed epistemic stability and guide the development of integrative empirical research agendas for BER.
Introduction
Many policy documents emphasize that student understanding of living systems requires the integration of concepts that span levels of biological organization, encompass the tree of life, and cross different fields of study (AAAS, 2011 ; NRC, 2009 ; NSF, 2019 ). Yet the institutional, disciplinary, and curricular structuring of the life sciences often works in opposition to these pursuits. More so than in physics and chemistry, “biology” encompasses an expansive array of disciplines, each of which is often housed in a different academic department (e.g., microbiology, botany, genetics). These disciplines often organize into different academic societies, communicate through different journals, embrace different methodological frameworks, and gather at separate scientific conferences. Such fragmentation is evident at many universities, which lack “biology” departments altogether, and may instead be organized by taxonomy (e.g., botany, zoology, microbiology departments), concept (e.g., genetics, ecology, evolution departments), unit or scale (e.g., cell biology, biochemistry). There is no organizational blueprint characteristic of biology departments in the United States, for example. Given that most universities have not identified a singular solution for structuring the life sciences, it is unsurprising that diverse structures also characterize biology education research. Disciplinary (and corresponding educational) fragmentation works against attempts at fostering an integrative understanding of living systems for students, which is arguably a foundational goal of biology education.
In this critical review I examine some of the conceptual challenges facing the field of Biology Education Research (BER). These challenges reflect the substantial disciplinary fragmentation of BER, but they also highlight opportunities for advancing student understanding of living systems. I focus on the conceptual foundations of the discipline because they are a unique feature of biology education and have received substantially less attention than education practices (e.g., active learning, course-based research experiences, inclusive pedagogies). I begin by documenting the disciplinary fragmentation of the biological sciences and the corresponding heterogeneity and conceptual fragmentation of BER efforts. A consequence of such compartmentalization has been the lack of attention to the development and testing of unifying conceptual frameworks for (i) living systems and (ii) student thinking about living systems (in contrast to individual concepts, such as mutation, heredity, or genetic drift). This finding aligns with prior reviews that have also noted limited empirical-theoretical coordination within BER. The lack of attention to unifying frameworks for both biology and BER has consequences for biology education. A review of Concept Inventory (CI) research is used to illustrate how the absence of robust conceptual frameworks can complicate attempts to uncover student thinking about living systems and to guide biology instruction. The reviews of BER scholarship and CIs are used to motivate discussion of possible blueprints for BER-specific frameworks. First, findings from developmental and cognitive psychology are proposed as central to the development of cognitive frameworks. Second, possible disciplinary frameworks for BER are proposed after summarizing attempts by biologists to establish unifying themes for living systems that transcend individual subdisciplines. These themes include unity and diversity; randomness, probability, and contingency; and scale, hierarchy, and emergence. The review ends by emphasizing that the most significant opportunity for strengthening and unifying BER lies in the formulation of conceptual frameworks that account for how learners make sense of living systems as they progress through ontogeny and formal education. Such frameworks are much-needed tools for organizing and executing field-specific disciplinary research agendas.
The disciplinary structures of biology and biology education research
Many journals focus on BER and have grown out of the disciplinary structures and educational needs of academic departments; this history helps to make sense of the fragmented structure currently characterizing BER. Many biological disciplines have produced associated educational journals that serve as examples: Microbiology ( Journal of Biology and Microbiology Education ), Evolution (e.g., Evolution: Education and Outreach ), and Neuroscience (e.g., Journal of Undergraduate Neuroscience Education ) (see Table 1 ). In many respects, this situation mirrors the explosion of discipline-specific journals in the life sciences.
Many of the research questions addressed within BER subdisciplines are an outgrowth of the educational contexts in which biological specialists have worked. The pressure to update curricula to reflect discipline-specific advances, for example, is a challenge inherent to all of the biological sciences (perhaps to a greater degree than in introductory physics and chemistry, where the content has remained relatively stable for the past century). Indeed, entirely new research areas (e.g., microbiomes, ancient DNA [deoxyribonucleic acid]) and methods (e.g., bioinformatics, CRISPR [clustered regularly interspaced short palindromic repeats]) emerge with increasing tempo each decade. Keeping students up-to-date with discipline-specific understanding is an ongoing challenge that has spurred educational reform, innovation, and ongoing professional development within biological subdisciplines (e.g., physiology) and their associated journals.
A second feature of the fragmented nature of biology education is the seemingly unique learning challenges that have been identified within each disciplinary context (e.g., microbiology, evolution, genetics). The challenge of addressing the student misconception that bacteria are primarily pathogenic, for example, is of particular concern within microbiology; developing approaches to tackle goal-driven reasoning about evolutionary change is central to evolution education; and helping students recognize the genetic similarity of eye cells and liver cells is foundational to genetics and genomics. Many educational efforts in biology education have arisen from attempts to tackle domain-specific learning challenges, including the development of tools for diagnosing topic-specific misunderstandings (see Student Thinking about Living Systems, below). Perhaps as a consequence of disciplinary isolation, markedly less work in BER has sought to identify common threads in the fabric of student confusion and to weave them into unified models of biological reasoning that are capable of explaining seemingly disparate educational challenges (although see Coley & Tanner, 2012 ; Opfer et al., 2012 , for cognition-based examples of such efforts).
The fragmentation of BER efforts and journals could be viewed as an historically contingent outcome of the disciplinary structure of the biological sciences and the unique challenges that characterize them. But a less myopic view might reveal cross-cutting commonalities across disciplines (see below). Indeed, recent efforts in the United States and elsewhere have attempted to reform the biology curriculum and highlight cross-cutting concepts that undergird many different subdisciplines (e.g., Vision and Change in Undergraduate Biology Education , AAAS, 2011 ). Efforts have also been made to bring different biology education communities together under new organizational arrangements (e.g., SABER: Society for the Advancement of Biology Education Research; ERIDOB: European Researchers In the Didactics Of Biology). Following these biology-specific unification efforts, the National Research Council ( 2012 ) has also attempted to define and unite the efforts of chemistry, physics, and biology education researchers under the umbrella of “Discipline-Based Educational Research” (DBER). It is clear that the disciplinary structure of biology education, like that of other educational research disciplines, is in flux. Attempts to integrate pockets of disciplinary research activity is ongoing, and it is too soon to characterize the outcomes of these efforts. But disciplinary unification is often fostered by conceptual frameworks that encompass the needs and goals of stakeholders (Miller, 1978 ). Such work will be invaluable for guiding educational integration.
In summary, the range and diversity of BER journals and research efforts (Table 1 ) continue to mirror the tangled disciplinary and academic roots from which they grew. Unifying the paradigms and perspectives being generated from multiple BER journals and scientific societies is challenging, yet a worthy goal if true conceptual unification into a “BER community” (or an even larger “DBER community”) is to be achieved. In the following sections, some cross-cutting themes from this expansive body of work are identified, reviewed, and critiqued. Much like BER itself, there are many alternative frameworks that could effectively characterize this evolving area of scholarship. But a persistent question that emerges from a review of this fractured body of work is whether there are sufficient conceptual and theoretical frameworks capable of supporting the challenge of disciplinary unification (and corresponding educational unification).
Conceptual and theoretical frameworks for biology education research
Theory building linked to causal explanation is a central goal of scientific and social-science research, although the two fields often differ in the number of theories used to explain particular phenomena. In both realms “… research emanates from the researcher’s implicit or explicit theory of the phenomenon under investigation” (Rocco & Plakhotnik, 2009 , p. 121). Therefore, clear specification of theoretical framing and grounding is essential to the research enterprise (Imenda, 2014 ). A question in need of attention is what conceptual or theoretical frameworks help to frame, ground, and unite BER as a standalone field of educational inquiry (cf. Nehm, 2014 )? Two of the more recent reviews of BER history and scholarship are notable in that they did not identify (or propose) discipline-specific educational frameworks (Dirks, 2011 ; deHaan, 2011 ). In her characterization of BER studies from 1990 to 2010, for example, Dirks ( 2011 ) identified three categories of scholarship: (1) student learning or performance, (2) student attitudes and beliefs, and (3) concept inventories and validated instruments. Within each category, Dirks examined the theoretical frameworks that were used to guide the empirical work that she reviewed. Few studies in these three categories linked empirical investigations to explicit theoretical frameworks. Instead, BER scholars framed their investigations in terms of ‘problem description.’ In cases where theoretical frameworks were hinted at, they were quite general (e.g., Bloom’s Taxonomy, Ausubel’s emphasis on prior knowledge and learning). The vast majority of studies in Dirks’s ( 2011 ) review lacked discipline-based educational framing and conceptual grounding, and no BER-specific theoretical frameworks were identified.
deHaan’s ( 2011 ) review of the history of BER also touched upon the theoretical frameworks that have been used to guide BER. Three frameworks--constructivism, conceptual change, and “others” (i.e., social interdependence and theories of intelligence)--were identified. It is notable that these frameworks did not originate within BER (they are frameworks developed in education and psychology) and they are not discipline-specific (i.e., educational frameworks unique to BER). Although not inherently problematic, one might expect (or indeed require) a discipline-focused educational enterprise to pursue and establish discipline-focused frameworks. If such frameworks are lacking, then the question arises as to what unifies and organizes the pursuits of affiliated scholars. A superficial, a-theoretical, and unsatisfying answer to this question could be that “BER focuses on biology education.” Overall, these reviews and a corresponding examination of studies from a variety of journals (Table 1 ) suggest that BER typically lacks discipline-specific conceptual or theoretical frameworks.
Although many BER studies lack explicit anchoring in conceptual or theoretical frameworks unique to living systems, some work has attempted to build such frameworks. Conceptual frameworks for the disciplinary core ideas of (i) information flow in living systems and (ii) evolutionary change illustrate how different concepts and empirical findings may be related to one another and integrated into a framework that explains, predicts, and guides research in biology education (Fig. 1 ). Shea et al. ( 2015 ), for example, elaborated on Stewart et al.’s ( 2005 ) genetics literacy model and presented a tripartite framework showing the interrelationships among content knowledge use, argumentation quality, and the role of item surface features in genetic reasoning (Fig. 1 a). This conceptual framework is biology-specific (i.e., addresses student reasoning about the disciplinary core idea of information flow at various scales) and applicable to most living systems (i.e., attends to phylogenetic diversity). The addition of argumentation to this model is valuable but not necessarily unique to this topic (argumentation is a practice central to all of science). This framework is a useful example because it (i) synthesizes prior empirical work, (ii) explains why student reasoning about information flow may fail to reach performance expectations, (iii) guides future research agendas and associated studies, (iv) applies broadly to living systems, and (v) motivates the development of particular curricular and pedagogical strategies.
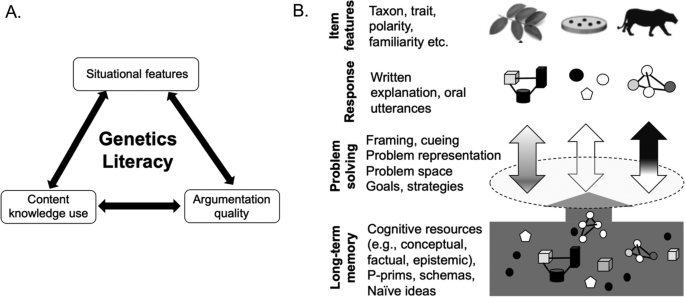
Examples of conceptual frameworks developed for biology education research. a A three-part conceptual framework for genetics literacy encompassing situational features, content knowledge use, and argumentation quality (modified from Shea et al. 2015 ). b A conceptual framework for evolutionary reasoning encompassing long-term memory, problem-solving processes, and item features (similar to the situational features of Shea et al. 2015 ). Modified from Nehm ( 2018 )
The second conceptual framework focuses on student reasoning about evolutionary change (Fig. 1 b). Nehm ( 2018 ) presents a conceptual framework that integrates aspects of Information Processing Theory, empirical findings on novice-expert evolutionary reasoning, and student challenges with evolutionary mechanisms (Fig. 1 b; see also Ha & Nehm 2014 ; Nehm & Ha, 2011 , Nehm and Ridgway 2011 ). When encountering tasks (or situations) that prompt for explanations of evolutionary change, sensitivity to item features (e.g., familiar plant species that have or lack thorns) impacts internal problem representation, which in turn affects the recruitment of individual concepts and schemas from long-term memory into working memory. The utilization of different assemblages of cognitive resources is driven by the features of the living systems. Like Shea et al.’s ( 2015 ) conceptual framework, Nehm’s ( 2018 ) conceptual framework (i) integrates existing theory (i.e., information processing theory) with prior empirical work, (ii) accounts for why student reasoning about evolutionary change may fail to reach performance expectations, (iii) guides future research agendas, and (iv) motivates the development of curricular and pedagogical strategies to address particular cognitive bottlenecks noted in the framework. Both of these frameworks attend to fundamental features of living systems (i.e., information flow, evolution) that transcend individual cases and exemplars (i.e., they consider diversity as a core feature of biological reasoning). Although both examples are simple, they organize a range of concepts central to understanding disciplinary thinking.
In summary, many factors work to maintain division among life science subfields (e.g., separate departments, conferences, journals, language; Table 1 ), and few counteracting factors promote unification (e.g., curricular cohesion, conceptual frameworks). Fragmentation of BER is an inevitable result. Interestingly, life scientists have long been concerned with a parallel challenge: the lack of attention to theoretical grounding and conceptual unification. The next section briefly reviews prior attempts to promote the development of conceptual frameworks for the life sciences. Although these frameworks do not address educational research specifically, they identify unifying concepts and principles that are essential starting points for building more robust conceptual foundations and frameworks for BER.
Conceptual frameworks for biology and biology education research
The past 60 years included several formal attempts to generate a conceptual framework for living systems and articulate a corresponding vision for the life sciences (e.g., Gerard and Stephens 1958 ; Miller, 1978 ; AAAS, 2011 ; NSF, 2019 ). The importance of theoretical foundations for biology was raised by Weiss ( 1958 , p. 93): “… the question [is] whether present-day biology is paying too little attention to its conceptual foundations, and if so, why.” In the 1950’s, the Biology Council of the U.S. National Academy of Sciences invited eminent biologists (e.g., Rollin Hotchkiss, Ernst Mayr, Sewell Wright) to explore the conceptual foundations of the life sciences given apparent disciplinary fragmentation. The report that emerged from their discussions and deliberations (NRC, 1958 ) attempted to re-envision biology through a more theoretical lens and generate a conceptual and hierarchical reconceptualization of the study of life. Conceptually, it included the broad categories of “Methods,” “Disciplines,” and “Concepts.” Methods organized life science research by the approaches used to generate understanding (e.g., immune tests, breeding, staining, factor analysis). Disciplines (structure [architecture, spatial relations, negative entropy]), and “Concepts” (history [origin]). Each of these categories—Methods, Disciplines, and Concepts--were then uniquely characterized at different biological scales (i.e., molecule, organelle, cell, organ, individual, small group, species, community/ecosystem, and total biota).
Three salient features of this early work include: (1) acknowledging the importance of conceptual grounding for the life sciences in light of disciplinary fragmentation; (2) situating academic topics and disciplines (e.g., anatomy, microbiology, ecology) within a conceptual superstructure (i.e., Structure, Equilibrium, History) and (3) highlighting the centrality of scale when considering life science Concepts, Methods, and Disciplines.
The U.S. National Research Council report Concepts of Biology ( 1958 ), while concerned with conceptual and disciplinary unification, did not lose sight of inherent connections to educational pursuits and outcomes: “Any success in improving the intellectual ordering of our subject would contribute to improved public relations, to the recruitment of more superior students, and to a better internal structure which would favor better teaching and research and in turn attract more students and support” (Weiss, 1958 , p. 95). These and many other significant efforts (e.g., Miller, 1978 ) confirm that the struggle for conceptual and educational unification of the life sciences has been ongoing, and repeated calls for unity suggest that the successes of these early efforts have been limited.
Although the history of BER illuminates the deeper roots of disciplinary challenges (deHaan 2011 ), attention to recent progress should also be noted. The efforts to develop and deploy unified conceptual and curricular frameworks for biology education that mirror expert conceptualizations are ongoing (e.g., AAAS, 2011 ; NSF, 2019 ). In the United States, for example, the past two decades have witnessed substantial progress on how to structure and reform undergraduate and K-12 biology education. Emerging from interactions among many different stakeholders and scholars (see Brownell, Freeman, Wenderoth, & Crowe, 2014 , their Table 1 ) and mirroring curricular innovations by working groups of biologists (e.g., Klymkowsky, Rentsch, Begovic, & Cooper, 2016 ) arose Vision and Change in Undergraduate Biology Education (AAAS, 2011 ) and, later, the Next Generation Science Standards (NRC, 2013 ). Both initiatives have attempted to winnow down the expansive range of biological topics that students experience and reorganize them into a more cohesive conceptual and curricular framework (much like NRC 1958 and Miller 1978 ). This framework is notable in that it continues to move the life sciences away from historically-based disciplinary structures focused on taxon (e.g., microbiology, botany, zoology) and towards more theoretical, principle-based schemes (e.g., structure and function) that transcend individual biological scales.
For example, Vision and Change reorganized biological knowledge according to five core concepts (AAAS, 2011 , pp. 12–14): (1) Evolution (The diversity of life evolved over time by processes of mutation, selection, and genetic change); (2) Structure and Function (Basic units of structure define the function of all living things); (3) Information Flow, Exchange, and Storage (The growth and behavior of organisms are activated through the expression of genetic information in context); (4) Pathways and Transformations of Energy and Matter (Biological systems grow and change by processes based upon chemical transformation pathways and are governed by the laws of thermodynamics); and (5) Systems (Living systems are interconnected and interacting). Many of these ideas are in alignment with previous conceptual work by Gerard and Stephens ( 1958 ) and Miller ( 1978 ). Vision and Change , however, provides a very limited characterization of these core concepts and does not explicitly discuss their interrelationships across biological scales (e.g., gene, organism, species, ecosystem).
The BioCore Guide (Brownell et al., 2014 ) was developed to provide more fine-grained and longer-term guidance for conceptualizing and implementing the goals of Vision and Change . Specifically, principles and statements were derived for each of the five Vision and Change core concepts in order to structure undergraduate degree learning pathways (Brownell et al., 2014 ). Efforts have also been made to stimulate change within institutions. Partnership for Undergraduate Life Science Education (PULSE Community, 2019 ), for example, has been developed to encourage adoption of these curricular innovations and self-reflection by life science departments.
Collectively, these conceptually-grounded curriculum frameworks (e.g., Vision and Change , BioCore) and associated reform efforts (PULSE) are important, new unifying forces counteracting the fragmented structure of the biological sciences. They also form necessary (but insufficient) substrates for constructing conceptual frameworks for BER. They are insufficient because, from an educational vantage point, identifying the concepts, schemas, and frameworks of a discipline is only one aspect of the challenge; these ideas must articulate in some way with how students think, reason, and learn about biological concepts and living systems. The next section reviews progress and limitations of biology educators’ attempts to understand student thinking about living systems in light of these disciplinary frameworks (e.g., NRC, 1958 ; Miller, 1978 ; AAAS, 2011 ).
Student thinking about living systems
Educational efforts to foster cognitive and practice-based competencies that align with disciplinary frameworks (such as Vision and Change ) must consider what is known about student thinking about living systems. It is therefore essential to consider how the BER community has approached this challenge, what they have learned, and what remains to be understood about living systems (e.g., NRC, 1958 ; Miller, 1978 ; AAAS, 2011 ).
The absence of robust conceptual and theoretical frameworks for the life sciences has not prevented teachers and educational researchers from different disciplinary backgrounds (e.g., microbiology, ecology) from identifying domain-specific learning challenges and misunderstandings (Driver et al. 1994 ; Pfundt & Duit, 1998 ; NRC, 2001 ). Hundreds of individual concepts (e.g., osmosis, recombination, genetic drift, trophic levels, global warming) are typically presented to students in textbooks and taught in classrooms (NRC, 1958 ). Biology teachers have correspondingly noticed, and biology researchers have empirically documented, an array of misunderstandings about these individual concepts and topics (for reviews, see Pfundt & Duit, 1998 ; Reiss and Kampourakis 2018 ). When attempting to solve biological problems, for example, many university students: convert matter into energy in biological systems; adopt use-and-disuse inheritance to explain changes in life over time; and account for differences between eye and liver cells as a result of DNA differences. Many of the same misunderstandings have been documented in young children (Driver, Squires, Rushworth, & Wood-Robinson, 1994 ; Pfundt & Duit, 1998 ).
The ubiquity and abundance of these non-normative conceptions and reasoning patterns has led biology educators in different subfields (see Table 1 ) to develop concept-specific assessment tools or instruments (so-called “Concept Inventories”) in order to document the ideas (both normative and non-normative) that students bring with them to biology classrooms (Table 2 ). For particular topics or concepts, researchers have consolidated studies of student misunderstandings by category (e.g., Driver et al., 1994 ; Pfundt & Duit, 1998 ), confirmed and refined descriptions of these misunderstandings using clinical interviews, and developed associated suites of assessment items relevant to a particular idea (i.e., concept, principle).
CIs typically contain items offering one normative scientific answer option along with a variety of commonly held misconception foils. These instruments are designed for instructors to uncover which non-normative ideas are most appealing to students and measure general levels of normative understanding. CIs have been developed for many topics in the biological subfields of cell biology, genetics, physiology, evolution, and ecology. The number of biology CIs continues to grow each year, providing valuable tools for uncovering student thinking about specific biological ideas (Table 2 ).
Biology CIs have advanced prior work on student misconceptions (Pfundt & Duit, 1998 ) by: (1) focusing attention on the core ideas of greatest importance to concept or topic learning (e.g., osmosis and diffusion), (2) attending to a broad range of common misunderstandings (previously identified in a variety of separate studies), (3) quantitatively documenting student understanding using large participant samples (in contrast to smaller-scale, qualitative studies); and (4) establishing more generalizable claims concerning students’ mastery of biology concepts (facilitated by easy administration and multiple-choice format). As noted by Dirks ( 2011 ), concept inventory development was an important advance for the BER community by helping biologists recognize the ubiquity of biology misunderstandings and learning difficulties throughout the educational hierarchy.
Given the importance of CI development to BER (Dirks, 2011 ; see above), a critical review of this work is in order. I identify six limitations in order to illustrate some of the remaining challenges to understanding student thinking about living systems. The first major limitation of BER CI development is that it continues to be largely descriptive, a-theoretical, and lacking in explicit grounding in cognitive or conceptual frameworks (BER-specific or otherwise) (e.g., NRC, 2001 ). I will illustrate the practical significance of frameworks for living systems and theoretical frameworks for measurement using the National Research Council’s ( 2001 ) “assessment triangle”. In brief, the assessment triangle encompasses the three most central and necessary features for embarking upon studies of student understanding (and CI development): cognition, observation, and interpretation (as well as interconnections thereof; see Fig. 1 ). Cognition refers to the relevant features and processes of the cognitive system that are used to frame and ground the development of assessment tasks. Observation refers to the tangible artifacts (e.g., verbal utterances, written text, diagrams) that are generated as a result of engaging with such tasks. Interpretation refers to the inferences drawn from analyses of the observations produced by the tasks.
All three corners of the assessment triangle are inextricably interrelated (Fig. 1 ). For example, interpretation relies on appropriate analyses of the observations , and the observations only have meaning when viewed in light of the cognitive models used to construct the assessment tasks. Misinterpretations and faulty inferences about student understanding may arise from implicit and unexamined (or false) assumptions at any corner of the triangle (e.g. inappropriate tasks, inappropriate analyses of observations, inappropriate theoretical grounding). The NRC assessment triangle identifies the central features involved in making inferences about student reasoning (e.g., reasoning about biological systems). Remarkably few biology CIs have attended to all of these central features.
The cognition corner of the NRC’s ( 2001 ) assessment triangle demands focused attention on what is known about how students conceptualize and process information in general and biological systems in particular. That is, theories of cognition and theories of biological reasoning should undergird and support claims about what CI tasks are seeking to capture. The majority of CIs examined lack grounding in well-established theories of cognition (e.g., information processing theory, situated cognition theory) or theories of biological thinking and reasoning (e.g., categorization of living vs. non-living; see below). As a result, the necessary features of assessment design (Fig. 2 ) are lacking; this generates an unstable base for task design, data interpretation, and claims about biological thinking (Opfer et al., 2012 ).
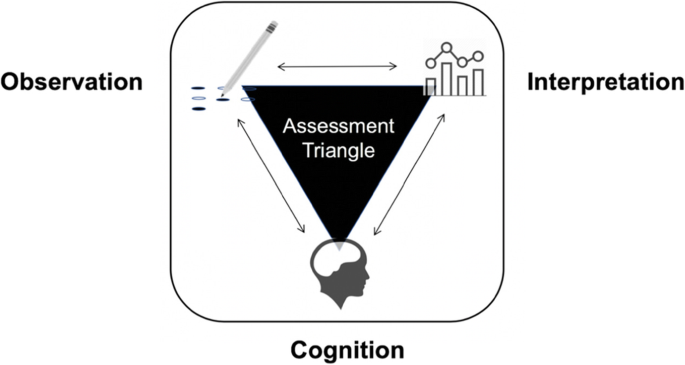
The NRC Assessment Triangle. Measurement and assessment of student understanding requires the integration of cognitive models, observations, and interpretations of observations in light of cognitive models. Models of thinking about living systems—the cognition corner—are therefore crucial to the development, application, and evaluation of assessments
A practical example may help to elucidate how the interplay among assessment triangle vertices impact claims drawn from CIs. Consider the role that the diversity of life might play in biological reasoning, for example. If the cognitive model (e.g., information processing theory) undergirding CI task design assumes that students will activate different ideas depending upon the taxon used in the assessment task (e.g., plant, non-human animal, human animal, fungus, bacteria), then multiple taxonomic contexts will be necessary in order to gather relevant observations and to draw robust inferences about how students think. If, on the other hand, the cognitive model assumes that students process information using abstractions of concepts, then attention to taxonomy in task design is unnecessary and most biological exemplars will suffice. The items that are developed and the corresponding scores that emerge from these two different cognitive perspectives are likely to be different. Cognition, observation, and interpretation (Fig. 2 ) emerge as necessary considerations in biology CI development, implementation, and score interpretations. Most CIs (Table 2 ) lack explicit alignment with the NRC’s ( 2001 ) assessment triangle, contain implicit or unexamined cognitive assumptions, and as a result may generate ambiguous or debatable claims about student thinking about living systems (and, ultimately, cloud the field’s attempt to make sense of how students think about living systems) (Tornabene, Lavington, & Nehm, 2018 ).
In addition to the lack of attention to theoretical grounding (i.e., NRC, 2001 ), a second limitation of CIs relates to their practical utility for biology education (Table 3 ). Given that hundreds of topics are typically included in textbooks and taught in biology classes (NRC, 1958 ), and dozens of CIs have now been developed (e.g., Table 2 ), the question arises as to what to do with them; what, in other words, is the broader aim of building this expansive test battery? Assessing all of the major domains for which CIs have been developed would require substantial amounts of time and effort. Devoting class time to all of the biological preconceptions and alternative conceptions uncovered by all of these instruments would require eliminating many other learning objectives or reorganizing biology instruction. The field has not developed practical strategies for aligning the numerous isolated insights generated from CIs with the practical realities of instruction, or the broader goals for BER.
One practical solution for making use of the broad array of CIs would be to develop and deploy Computer Adaptive Tests (CATs) capable of automatically diagnosing levels of conceptual understanding (as opposed to administering all assessment items from all of the CIs) and delivering personalized instructional resources aligned with documented learning difficulties. These digital tools could be provided as pre-class assignments or as supplemental resources. Another solution more closely tied with the focus of this critical review would be to identify learning challenges apparent across CIs (e.g., difficulties in reasoning about living systems) and to develop corresponding instructional materials to address these broader misunderstandings or promote cognitive coherence. This approach circles attention back to the question of how conceptual frameworks for biology and biology education could be leveraged to unify understanding of diverse misconceptions across subdisciplines (see Conceptual and Theoretical Frameworks for Biology Education Research, above).
A third limitation of biology CIs relates to the design of assessment tasks and the inferences that are drawn from their scores. When employing open-ended assessment tasks and clinical interviews, some BER research has shown that a majority of students utilize mixtures of normative and non-normative ideas together in their biological explanations (Nehm & Schonfeld, 2008 , 2010 ). Most CI instrument items nevertheless continue to employ multiple-choice (MC) formats and only permit students to choose between a normative or a non-normative answer option. This format may, in turn, introduce noise into the measurement process and weaken validity inferences. Multiple-True-False (MTF) items are one solution to this problem. Using MTF formats, students are permitted to indicate whether they consider each answer option to be correct or incorrect, thereby breaking the task design constraint evident in either-or item options. This limitation is another example of how consideration of both cognition (i.e., mixed cognitive models exist) and task design (MC vs. MTF) work together to impact the quality and meaning of inferences about biological thinking drawn from CI scores (i.e., observations).
A fourth limitation of BER CIs concerns the authenticity of the assessment tasks themselves. Most CIs assess pieces of knowledge using MC items. It is not clear if students who are able to achieve high scores (i.e., select the constellation of normative answer options across multiple items) understand the concept as a whole (Nehm & Haertig, 2012 ). For example, just because students select the normative ideas of mutation , heritability , environmental change , and differential survival from a pool of normative and non-normative item options does not necessarily mean that they would assemble these ideas in a scientifically correct manner. A student could, for example, use the aforementioned ideas to build an explanation in which environmental change in a particular habitat causes heritable mutations which in turn help these organisms differentially survive . Thus, non-normative models may be assembled from normative “pieces.” This is another example of how inferences about students’ biological understandings are tied to assessment and cognitive frameworks.
One solution to this challenge is to utilize Ordered Multiple Choice (OMC) items. These items prompt students to choose from among explanatory responses integrating many normative and non-normative combinations (as opposed to asking students to select individual ideas or conceptual fragments). These explanatory models could be designed to mirror hypothesized levels of conceptual understanding or biological expertise (e.g., learning progressions). OMC items have the potential to capture more holistic and valid characterizations of student reasoning (see Todd et al., 2017 for an example from genetics).
A fifth limitation of biology CIs centers on the “interpretation” corner of the assessment triangle (Fig. 2 ); robust validation methods aligned with contemporary psychometric frameworks are often lacking in biology CI studies (Boone, Staver, & Yale, 2014 ; Neumann, Neumann, & Nehm, 2011 ; Sbeglia & Nehm, 2018 , 2019 ). Rasch Analysis and Item Response Theory (IRT) are slowly supplanting traditional Classical Test Theory (CTT) methods for biology CI validation. In addition to psychometric limitations, validation studies of many biology instruments remain restricted to singular educational settings or demographically-restrictive samples (Mead et al. 2019 ; Campbell & Nehm, 2013 ). These methodological choices introduce uncertainty about the generalizability of CI score inferences across demographic groups, educational institutions, and international boundaries. Particular care must be made when drawing inferences from CI scores to inform instructional decisions or evaluate learning efficacy given these limitations (Table 3 ).
The sixth and final limitation of extant biology CIs returns to the topic of discipline-based conceptual frameworks. Few if any of the biology CIs and assessment instruments have been designed to target foundational disciplinary themes identified over the past 60 years (e.g., reasoning across biological scales) or the disciplinary formulations advanced in Vision and Change (AAAS, 2011 ). BER assessment tools remain aligned to concepts or topics characteristic of a particular subdiscipline, biological scale, or taxon (e.g., human animals). Despite significant progress in documenting concept understanding (and misunderstanding), biology educators have directed much less attention to assessing the foundational features of living systems that are most closely tied to disciplinary frameworks (i.e., NRC, 1958 ; Miller, 1978 ; AAAS, 2011 ). That is, analogous to many biology curricula, BER CI work has assembled a valuable but disarticulated jumble of information (in this case, lists of student learning difficulties) lacking deep structure or coherence.
In summary, a critical review of BER efforts to understand student thinking about living systems has revealed significant progress and significant limitations. Significant progress has been made in: identifying a range of important topics and concepts relevant to disciplinary core ideas; developing instruments that measure many of the learning difficulties uncovered in prior work (Table 2 ); and documenting widespread patterns of limited content mastery and numerous misunderstandings. Significant limitations have also been identified (Table 3 ). Many of the biology assessment tools lack: explicit grounding in psychometric and cognitive theory; task authenticity mirroring biological practice and reasoning; robust validation methods aligned with contemporary psychometric frameworks; robust inferences drawn from cognitively-aligned tasks; and implementation guidelines aligned with the practical realities of concept coverage in textbooks and classrooms. Collectively, much is now known about a scattered array of topics and concepts within biological subdisciplines; few if any tools are available for studying foundational and cross-disciplinary features of living systems identified by biologists over the past 60 years (e.g., identifying emergent properties across biological scales; considering stochasticity and determinism in biological causation; predicting biological outcomes using systems thinking; NRC, 1958 ; Miller, 1978 ; AAAS, 2011 ). BER requires discipline-specific frameworks that illuminate biological reasoning. Cognitive perspectives will be foundational to developing these frameworks.
What cognitive frameworks could guide BER?
A productive trend in BER involves efforts to link cognitive perspectives developed in other fields (e.g., education, psychology) with discipline-specific challenges characteristic of teaching and learning about living systems (Inagaki and Hatano, 1991 ; Kelemen and Rosset 2009 ). The fields of cognitive and developmental psychology serve as essential resources for understanding the roots of student reasoning about living systems. Developmental psychologists have generated many crucial insights into the foundations of human reasoning about living systems, including animacy, life, death, illness, growth, inheritance, and biological change (e.g., Opfer and Gelman 2010 ; Table 4 ). In particular, studies of human thinking have explored (1) whether ontogenetic development is characterized by reformulations of mental frameworks about living systems or by more continuous and less structured change, and (2) whether these early frameworks impact adult reasoning about living systems.
One of the more illuminating and well-studied examples of the linkages between cognitive and disciplinary frameworks concerns human thinking about plants (Opfer and Gelman 2010 ). Some psychologists consider the origins of biological thought to first emerge as young children ponder the question of what is alive and what is not (Goldberg & Thompson-Schill, 2009 ). For example, it is well established that young children initially conceptualize and classify plants as non-living entities. As cognitive development proceeds, plants are reclassified into an expanded category of “living” (e.g., plants + animals). An important question is whether early reasoning about biological categories and phenomena plays a significant role in later learning difficulties--including those documented in university undergraduates.
Plants provide a useful example for drawing possible connections among cognitive development, biological reasoning, and discipline-based conceptual frameworks. Plants comprise a central branch on the tree of life and are essential for human existence (i.e., sources of matter and energy). Yet, plants have posed significant challenges for life science educators (Wandersee & Schussler, 1999 ). These challenges range from students’ lack of perception of plants altogether (coined “plant blindness”) to fundamental misconceptions about how plants reproduce, transform matter and energy, and impact the chemical composition of the atmosphere (Wandersee & Schussler, 1999 ). The early reformulations of biological categories in young children--such as the reorganization of plants into the category of “living things”--appear to persist into adulthood.
A study by Goldberg and Thompson-Schill ( 2009 , p. 6) compared reasoning about plants relative to other living (e.g., animal) and non-living (e.g., rock) entities in undergraduates and biology professors. Under time pressure, it took biology professors significantly longer to recognize plants as living things (compared to animals and non-living entities). Goldberg and Thompson-Schill noted that “[t] he same items and features that cause confusions in young children also appear to cause underlying classification difficulties in university biology professors.” This case is not unique. Children’s reasoning about other biological phenomena, such as teleo-functional biases, also display continuities with adult thinking about evolutionary change (e.g., Kelemen and DiYanni, 2005 ). Work in cognitive and developmental psychology indicate that young children’s early formulations about living systems might not be “re-written”, but instead persist into adulthood, require active suppression, and impact later learning. Ongoing research in cognitive and developmental psychology has great potential for enriching our understanding of thinking in young adults, and for providing deeper insights into the causes of entrenched biology misunderstandings that often appear resistant to concerted educational efforts.
Studies at the other extreme--expert biologists--also have great potential for informing the development of unifying cognitive frameworks for BER. Comparative studies of experts and novices in different subject areas have been central to understanding domain-general and domain-specific features of problem representation and problem-solving performance for nearly a century (reviewed in Novick and Bassok, 2012 ). Novice-expert comparisons have seen comparatively little use in BER, although some notable exceptions include studies in genetics (Smith, 1983 ), evolution (Nehm & Ridgway, 2011 ), and genetically-modified organisms (Potter et al. 2017 ). These studies offer a range of insights into how novices and experts conceptualize problems, plan solutions, and utilize concepts and frameworks in problem-solving tasks. These insights could be leveraged to help elucidate expert frameworks of biological systems, as well as to identify conceptual, procedural, and epistemic barriers in novice reasoning. In a study of evolution, for example, novices performed poorly on problem-solving tasks not because of a lack of domain-specific knowledge, but because of the ways in which they used superficial task features (different organisms) to cognitively represent the problems at hand (i.e., in fundamentally different ways than the experts). Here the tension in student thinking about the unity and diversity of living systems is revealed—which is also a disciplinary idea unique to BER (Dobzhansky, 1973 ). Helping students perceive unity across the diversity of life emerges as a crucial (but often neglected) instructional goal. Comparing expert and novice problem-solving approaches could reveal unknown barriers to biology learning and illuminate potential features of a theoretical conceptualization of BER. These frameworks become central to the “cognition” corner of the assessment triangle (NRC, 2001 ) and efforts to design CIs and measure educational impact.
In addition to tracing the origination, persistence, and modification of cognitive structures about living systems through ontogeny and expertise, it is useful to ask whether the disciplinary organization of the biological sciences and associated degree programs, curricula, and textbook organizations (cf. Nehm et al., 2009 ) contribute to students’ fragmented models of living systems (e.g., Botany courses and textbooks focus on plants; Microbiology courses and textbooks focus on bacteria; Zoology courses and textbooks focus on animals). Few biologists would doubt that taxon-specific learning outcomes are essential for understanding the unique aspects of particular living systems. But an unanswered question is whether an effective balance between diversity and unity been achieved, or whether the scales have been tipped towards a focus on diversity-grounded learning (and corresponding cognitive fragmentation in biology students). It is notable that most biology textbook chapters, courses, and degree programs maintain organizational structures at odds with most conceptual reformulations of the life sciences (e.g., NRC, 1958 ; Miller, 1978 ; AAAS, 2011 ). Resolving these contradictions may help to conceptualize a more unified and principled framework for BER.
In summary, one of the most underdeveloped areas of BER concerns the formulation of conceptual and theoretical frameworks that account for how learners make sense of the similarities and differences within and across living systems as they progress through ontogeny and educational experiences. Cognitive and developmental psychology provide rich but largely untapped resources for enriching cognitively-grounded frameworks. In addition to studies of biological reasoning in young children, studies of expert thinking also offer considerable promise for uncovering barriers to expert-like conceptualizations of living systems. Collaborations with cognitive and developmental psychologists, and greater application of expert-novice comparisons, will be essential to advancing the cognitive frameworks for assessment design, curriculum development, and BER research.
What disciplinary frameworks could guide BER?
Although frameworks and models from psychology will be invaluable for crafting cognitive frameworks for BER, there are unique features of living systems that must also be explicitly considered in light of more broadly applicable cognitive models. To foster disciplinary unification and more integrative models of BER, these features should (1) span different biological subdisciplines and (2) undergird broad learning challenges about core ideas about living systems. Three areas--unity and diversity; randomness, probability, and contingency; and scale, hierarchy, and emergence—are likely to be valuable ideas for the development of discipline-grounded conceptual frameworks for BER. Each is discussed in turn below (Fig. 3 ).
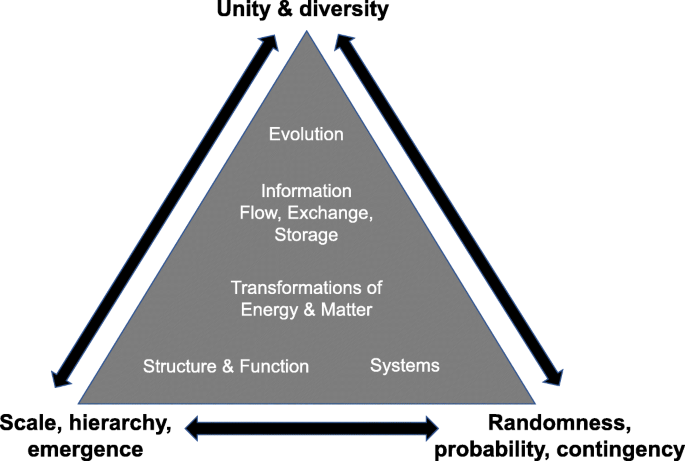
Integrating conceptual frameworks into BER: student reasoning about unity and diversity; scale, hierarchy, and emergence; and randomness, probability, and historical contingency. Note that all three ideas interact to generate understanding about living systems, including processes within them (e.g., information flow)
Unity and Diversity in biological reasoning
A foundational (yet undertheorized) disciplinary challenge inherent to BER concerns the development of conceptual models of student sensemaking about the similarities and differences within and across living systems (NRC 1958 ; Klymkowsky et al., 2016 ; Nehm, 2018 ; Nehm et al., 2012 ; Shea, Duncan, & Stephenson, 2015 ). A key argument often missed in Dobzhansky’s ( 1973 ) seminal paper expounding the importance of evolution to all of biology was “[t] he unity of life is no less remarkable than its diversity” (p. 127). Indeed, a core goal of all biological disciplines is to develop and deploy causal models that transcend particular scales, lineages, and phenomenologies. Biology educators have, for the most part, documented myriad student learning difficulties within disciplinary contexts (e.g., microbiology, heredity, evolution, ecology) that are likewise bound to particular scales, concepts, and taxonomic contexts. Much less work has explored reasoning across these areas and the extent to which conceptual unity is achieved as students progress through biology education (Garvin-Doxas & Klymkowsky, 2008 ).
A core need for BER is the development of explicit models of how student understanding of living systems changes in response to formal and informal educational experiences (e.g., exposure to household pets, gardens, books, zoos, digital media, formal schooling). Throughout ontogeny, learners experience a wide range of life forms and their associated phenomenologies (e.g., growth, function, behavior, death). As learners engage with the diversity of the living world, a foundational question for BER is whether students construct increasingly abstract models of living systems (i.e. conceptual unity) or whether their sense-making remains rooted in taxonomic contexts, experiential instances, and case examples (i.e. conceptual diversity; Fig. 4 ).
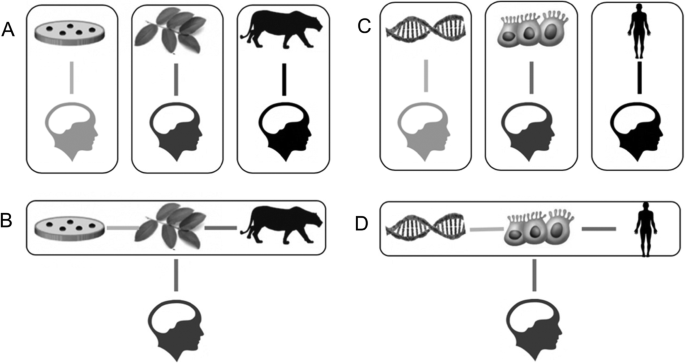
One example of unity and diversity in biological reasoning. Note that examples using a broader set of scales (e.g., ecosystem) could be utilized. a Within a biological scale (in this case, the scale of organism), reasoning about living systems lacks unification and is organized by taxonomic contexts, experiential instances, and case examples. b Within a biological scale (in this case, the scale of organism) reasoning about living systems is characterized by abstract models transcending organismal type or lineage (i.e. conceptual unity). c Among biological scales (in this case, molecule, cell, organism), reasoning about living systems lacks unification and is organized by macroscopic (organismal), microscopic (cellular), and molecular (biochemical) levels of biological organization. d Among biological scales (in this case, molecule, cell, organism), reasoning about living systems at is characterized by abstract models linking biological scales (i.e. conceptual unity)
The limited body of work exploring student reasoning about the unity and diversity of living systems has uncovered different findings. In some cases, research suggests that in older children and young adults, reasoning about living systems may remain highly fragmented and taxon-specific at particular scales (Fig. 4 a; e.g., Freidenreich et al. 2011 ; Kargbo et al., 1980 ; Nehm & Ha, 2011 ). In other cases, research has shown that student reasoning may develop into unified problem-solving heuristics within a biological scale (Fig. 4 b; e.g., Schmiemann et al., 2017 ). Much less work has explored student reasoning about biological phenomena across biological scales (Fig. 4 c, d). Work in genetics education suggests that crossing these ontological levels or scales is inherently challenging for students (Freidenreich et al. 2011 ; Kargbo et al., 1980 ; Nehm & Ha, 2011 ; Nehm, 2018 ). For example, students may develop conceptual understanding within a biological level (Fig. 4 c) but be unable to conceptually link processes as they unfold over multiple scales (e.g., molecular, cellular, organismal; Fig. 4 d). Given that unity and diversity are foundational features of living systems, the development of conceptual and theoretical frameworks guiding empirical studies about student thinking about living systems is long overdue. Such frameworks could be used to synthesize past work, connect researchers from different life science sub-disciplines, and establish a unifying research agenda for BER.
Randomness, probability, and contingency
Many students and teachers have a tacit awareness that biology is different from the physical sciences. Yet, explicit frameworks illuminating these conceptual similarities and differences are often lacking in biology education (Klymkowsky et al., 2016 ). The behavior of biological systems is complex for many reasons, although the simultaneous operation of numerous causes each of which produces weak effects is an important one (Lewontin, 2000 ). Biological systems are also impacted by multiple probabilistic interactions with and among scales (e.g., molecular, cell, organismal, ecological) (Garvin-Doxas & Klymkowsky, 2008 ). For these reasons, biological patterns and processes are characterized by “...a plurality of causal factors, combined with probabilism in the chain of events …” across scales (Mayr, 1997 , p. 68). This messy situation often stands in sharp relief to student learning experiences in physics and chemistry, where fewer causes with stronger effects and more deterministic outcomes are encountered (Lewontin, 2000 ). Given the special properties of biological systems (at least in terms of the topics explored by students), a BER research program exploring how students make sense of randomness, probability, and determinism across lineages and biological scales emerges as an essential consideration (Garvin-Doxas & Klymkowsky, 2008 ).
Student learning difficulties with randomness and probability in biology are well established (Garvin-Doxas & Klymkowsky, 2008 ). Large numbers of university undergraduates previously exposed to natural selection falsely consider it to be a “random” process (Beggrow and Nehm, 2012 ); genetic drift misconceptions--many of which are closely tied to ideas of chance--are abundant (Price et al. 2014 ); and reasoning about osmosis and diffusion, which require thinking about probability at molecular scales, remains challenging for students at advanced levels of biology education (Garvin-Doxas & Klymkowsky, 2008 ). Many fundamental but very basic biological phenomena (i.e. in terms of the number of interacting causes within and among levels of organization) pose substantial challenges. But much like the discipline-specific documentation of other learning challenges, difficulties with randomness and probability are often discussed in the context of specific biological concepts (e.g., Punnett squares, Hardy-Weinberg equilibrium) rather than as unifying features of biological systems. What is currently lacking in BER is an organizing framework that cuts across instances (e.g., diffusion, meiosis, selection, drift) and guides systematic review and synthesis of different biology learning challenges relating to randomness and probability.
Student learning difficulties may be traced to many causes, which raises the question of whether there is empirical evidence that probabilistic reasoning is responsible for the aforementioned learning difficulties. Recent work by Fiedler et al. ( 2019 ) has quantified the contribution of probabilistic reasoning to biology understanding. In a large sample of university biology students, Fiedler et al. ( 2019 ) demonstrated that statistical reasoning (in the contexts of mathematics and evolution) displayed significant and strong associations with knowledge of evolution. Although this result is perhaps unsurprising given previous work (Garvin-Doxas & Klymkowsky, 2008 ), it is notable that statistical reasoning was also found to have significant and strong associations with the acceptance of evolution. Fiedler et al. ( 2019 ) affirm the significant role of probabilistic thinking in biological reasoning, and open the door to empirical explorations of many other topics in the life sciences. Although Fiedler et al. ( 2019 ) do not propose a framework for conceptualizing randomness and probability in the life sciences, they do argue that statistical reasoning is a core feature of reasoning about living systems (as opposed to an ancillary tool for studying living systems). This perspective reformulates the role of statistics in biological competence. Clearly, the development of a conceptual framework focusing on randomness, probability, and contingency could offer great potential for uniting research efforts across biological subdisciplines (e.g., molecular biology, genetics, evolution).
Scale, hierarchy, and emergence
The hierarchical structure of life, and its corresponding biological scales (e.g., cell, tissue, organ, organism, population, species, ecosystem) are repeatedly acknowledged as important considerations about biological systems in nearly every textbook and classroom. Although most (if not all) biology education programs draw student attention to the concepts of scale and hierarchy, they rarely explore how scale and hierarchy elucidate and problematize the functioning of biological systems. For example, an understanding of the interdependence of patterns and processes across scales (e.g., upward and downward causation) as well as the emergence of novel properties at higher levels (e.g., the whole is more than the sum of its parts), is necessary for making sense of nearly all of the core ideas unifying the life sciences (e.g., information flow, matter and energy transformation, evolution). Yet, a review of the literature reveals that an explicit curriculum for helping students engage in the meaning of this hierarchical arrangement appears lacking.
Extending discussions of the unity and diversity of life (see Fig. 4 , above), reasoning about living systems may also display unity or diversity across hierarchical levels. For example, reasoning about living systems may lack unification, and knowledge structures or mental models may be organized by macroscopic (organismal), microscopic (cellular), and molecular (biochemical) levels of biological organization (Fig. 4 c). In such cases, knowledge structures and reasoning are bound to particular scales or levels , and conceptual linkages among these scales (e.g., upward and downward causation, emergent properties) may be lacking. Alternatively, reasoning about living systems may be characterized by abstract models unifying biological scales (i.e. conceptual unity) (Fig. 4 d). In such cases, knowledge structures and mental models transcend scale and utilize level-specific understanding. The main point is that hierarchical scale is an important aspect of biological reasoning that may facilitate or constrain student understanding. The principles of scale, hierarchy, and emergence are central to biological reasoning, yet BER lacks a robust conceptualization of these concepts and their role in student understanding of living systems. Theoretical and conceptual frameworks for scale, hierarchy, and emergence could help to guide systematic review and synthesis of different biology learning challenges and guide research efforts in BER.
In summary, this critical review, as well as prior reviews of BER, have found few discipline-specific conceptual or theoretical frameworks for the field (Dirks, 2011 ; deHaan, 2011 ). The fragmented disciplinary history and structure of the life sciences (see above) has been a concern noted by eminent biologists and professional organizations for at least 60 years (e.g., NRC, 1958 ). Despite progress in conceptual unification in the biological sciences, the BER community to a significant degree remains compartmentalized along historical, institutional, and disciplinary boundaries (e.g., microbiology, biochemistry, evolution). Efforts by BER researchers to understand and measure student understanding of living systems have likewise progressed along disciplinary themes, concepts, and topics.
Many core features of living systems offer opportunities for crafting discipline-specific educational frameworks for BER. Given the fragmentation of the life sciences and BER, it is presumptuous and unrealistic for any single scholar or subfield to impose such a framework. Three interconnected themes--unity and diversity; randomness, probability, and contingency; and scale, hierarchy, and emergence—have been identified in prior synthesis efforts and offered as potential starting points for a cross-disciplinary discussion of possible field-specific frameworks. Such frameworks are critical to the epistemic foundations of BER. They have immense potential for enriching a wide array of research efforts spanning different subfields, organizing the growing list of student learning difficulties, and building casual frameworks capable of grounding empirical research agendas.
Limitations
This critical review has identified significant opportunities and challenges for BER. The most pressing opportunity noted throughout this review is the development of discipline-specific conceptual and theoretical frameworks. The absence of explicit disciplinary frameworks raises questions about disciplinary identity (e.g., “What is BER?”) and encourages superficial and dissatisfying answers (e.g., “BER studies biology education”). The perspective advanced in this review is that the absence of cognitive and disciplinary frameworks generates epistemic instability (e.g., a-theoretical empiricism) and clouds our ability to rigorously understand student thinking about living systems. There are, however, alternative perspectives on the significance of discipline-specific frameworks for BER; two are discussed below.
First, if BER-affiliated scholars were to ignore or abandon the National Research Council’s ( 2013 ) conceptualization and definition of BER (and the broader topic of DBER), then biology-related educational research efforts could easily be subsumed within the field of Science Education (cf. Nehm, 2014 ). In this case, discipline-focused theoretical frameworks become less of a concern because frameworks from science education could guide epistemic aims and corresponding research agendas. Attention to the unique aspects of biological concepts (e.g., inheritance, photosynthesis, phylogenetics) would fade (but not disappear) and educational frameworks (e.g., socio-cognitive theory, constructivism) would come into sharper focus. This alternative conceptualization foregrounds educational frameworks and backgrounds disciplinary frameworks. The rationale for BER as a standalone field consequently weakens, along with arguments concerning the critical nature of discipline-focused conceptual frameworks.
A second perspective concerns the necessity of conceptual and theoretical frameworks for BER (and perhaps other scholarly efforts) altogether. Theory building linked to causal explanation is widely-recognized as a central goal of scientific and social-science research (cf. Brigandt, 2016 ; Rocco & Plakhotnik, 2009 ). Some BER scholars, however, do not appear to consider such frameworks as central epistemic features of their work (as indicated by much of the work reviewed here). Indeed, there are numerous examples of implicit or a-theoretical hypothesis testing in the BER journals listed in Table 1 . This stance minimizes the importance of conceptual or theoretical frameworks in scholarly work, and in so doing eliminates the central concern advanced in this review.
One final and significant limitation of this critical review is that it has adopted a Western, and largely American, perspective. Many of the conclusions drawn are unlikely to generalize to other nations or cultures. It is well known that the structure of biology education research differs around the world (e.g., Indonesia, China, Korea, Germany). Studies of biology learning may be situated within university education departments or biology departments (or combinations thereof). Teacher training in biology may be housed in colleges exclusively devoted to biology education, or departments focusing on general biology education (e.g., medicine, conservation).
International comparison studies (e.g., Ha, Wei, Wang, Hou, & Nehm, 2019 ; Rachmatullah, Nehm, Ha, & Roshayanti, 2018 ) are likely to offer rich insights into the relationships between biology education research agendas, institutional contexts, and the conceptual and theoretical frameworks used to make sense of student thinking about living systems. Indeed, what are the affordances and constraints of different institutional and epistemic arrangements to knowledge discovery in biology education? Collectively, how could these alternative arrangements enhance our ability to foster deeper understanding of the living world? Further reviews from a broader array of stakeholders will enhance our collective understanding of BER around the world.
This critical review examined the challenges and opportunities facing the field of Biology Education Research (BER). Ongoing fragmentation of the biological sciences was identified as a force working in opposition to the development of (i) unifying conceptual frameworks for living systems and (ii) unifying frameworks for understanding student thinking about living systems. Institutional, disciplinary, and conceptual fragmentation of the life sciences aligns with the finding that BER generally lacks unique, unifying, and discipline-focused conceptual or theoretical frameworks. Biology concept inventory research was used to illustrate the central role that conceptual frameworks (both cognitive and disciplinary) play in making sense of student thinking about living systems. Relevant insights from developmental and cognitive psychology were reviewed as potential starting points for building more robust cognitive frameworks, and prior theoretical work by biologists was leveraged to generate possible starting points for discipline-focused frameworks. Three interconnected themes--unity and diversity; randomness, probability, and contingency; and scale, hierarchy, and emergence—were identified as central to thinking about living systems and were linked to ongoing BER research efforts. The review emphasized that the development of conceptual frameworks that account for how learners make sense of similarities and differences within and across living systems as they progress through ontogeny and formal education will help to foster epistemic stability and disciplinary unification for BER.
Availability of data and materials
Not applicable as this is a review article.
Abbreviations
Biology Education Research
Computer Adaptive Test
Concept Inventory
Clustered Regularly Interspaced Short Palindromic Repeats
Classical Test Theory
Discipline-Based Education Research
Deoxyribonucleic Acid
European Researchers In the Didaktics of Biology
Item Response Theory
Multiple True False
National Research Council
Ordered Multiple Choice
Partnership for Undergraduate Life Science Education
Society for the Advancement of Biology Education Research
Abraham, J. K., Perez, K. E., & Price, R. M. (2014). The Dominance Concept Inventory: A Tool for Assessing Undergraduate Student Alternative Conceptions about Dominance in Mendelian and Population Genetics. CBE—Life Sciences Education , 13 (2), 349–358.
Article Google Scholar
American Association for the Advancement of Science (AAAS). (2011). Vision and change in undergraduate biology education. Washington, DC, 2011. http://visionandchange.org/ . Accessed 20 Feb 2018.
Au, T., Sidle, A., & Rollins, K. (1993). Developing an intuitive under-standing of conservation and contamination: Invisible particles as a plausible mechanism. Developmental Psychology , 29 , 286–299.
Bassok, M., & Novick, L. R. (2012). Problem solving . In: The Oxford Handbook of Thinking and Reasoning Edited by Keith J. Holyoak and Robert G. Morrison. Oxford University Press.
Book Google Scholar
Beggrow, E., & Nehm, R. H. (2012). Students’ mental models of evolutionary Causation: Natural Selection and Genetic Drift. Evolution Education and Outreach . https://doi.org/10.1007/s12052-012-0432-z .
Google Scholar
Boone, W. J., Staver, J. R., & Yale, M. S. (2014). Rasch analysis in the human sciences . Dordrecht: Springer.
Brigandt, I. (2016). Why the Difference Between Explanation and Argument Matters to Science Education. Science & Education , 25 . https://doi.org/10.1007/s11191-016-9826-6 .
Brownell, S. E., Freeman, S., Wenderoth, M. P., & Crowe, A. J. (2014). BioCore Guide: A Tool for Interpreting the Core Concepts of Vision and Change for Biology Majors. CBE—Life Sciences Education , 13 (2), 200–211.
Campbell, C., & Nehm, R. H. (2013). Evaluating assessment quality in genomics and bioinformatics education research. CBE-Life Sciences Education , 12 (3), 530–541. https://doi.org/10.1187/cbe.12-06-0073 .
Catley, K. M., & Novick, L. R. (2009). Digging deep: Exploring college students' knowledge of macroevolutionary time. Journal of Research in Science Teaching , 46 (3), 311–332.
Coley, J. D., & Tanner, K. D. (2012). Common Origins of Diverse Misconceptions: Cognitive Principles and the Development of Biology Thinking. CBE—Life Sciences Education , 11 (3), 209–215.
DeHaan R. L. (2011). Education Research in the Biological Sciences: A Nine-Decade Review. Paper presented at the Second Committee Meeting on the Status, Contributions, and Future Directions of Discipline-Based Education Research, Washington, DC, 2010. www7.nationalacademies.org/bose/DBER_DeHaan_October_Paper.pdf . Accessed 21 Mar 2019.
Dirks C. (2011). The Current Status and Future Direction of Biology Education Research. Paper presented at the Second Committee Meeting on the Status, Contributions, and Future Directions of Discipline-Based Education Research, Washington, DC, 2010. www7.nationalacademies.org/bose/DBER_Dirks_October_Paper.pdf . Accessed 21 Mar 2019.
Dobzhansky, T (1973). Nothing in Biology Makes Sense except in the Light of Evolution. The American Biology Teacher, Vol. 35 No. 3, Mar., 1973; (pp. 125–129). https://doi.org/10.2307/4444260 .
Driver, R., Squires, A., Rushworth, P., & Wood-Robinson, V. (1994). Making sense of secondary science: Research into Children’s ideas. New York: Routledge.
Fiedler, D., Sbeglia, G. C., Nehm, R. H., & Harms, U. (2019). How strongly does statistical reasoning influence knowledge and acceptance of evolution? Journal of Research in Science Teaching , 56 (9), 1183–1206.
Fisher, K. M., Williams, K. S., & Lineback, J. E. (2011). Osmosis and Diffusion Conceptual Assessment. CBE—Life Sciences Education , 10 (4), 418–429.
Freidenreich, H. B., Duncan, R. G., & Shea, N. (2011). Exploring middle school students’ understanding of three conceptual models in genetics. International Journal of Science Education , 33 (17), 2323–2349.
Garvin-Doxas, K., & Klymkowsky, M. W. (2008). Understanding randomness and its impact on student learning: Lessons from the biology concept inventory (BCI). CBE Life Science Education , 7 , 227–233.
Gerard, R. W., & Stevens, R. B. (1958). Concepts of Biology , National Research Council Publication 560 (). D.C.: National Academy Press. Washington.
Goldberg, R. F., & Thompson-Schill, S. L. (2009). Developmental “roots” in mature biological knowledge. Psychological Science , 20 (4), 480–487.
Ha, M., & Nehm, R. H. (2014). Darwin's difficulties and students’ struggles with trait loss: Cognitive-historical parallelisms in evolutionary explanation. Science & Education. https://doi.org/10.1007/s11191-013-9626-1 .
Ha, M., Wei, X., Wang, J., Hou, D., & Nehm, R. H. (2019). Chinese pre-service biology teachers’ evolutionary knowledge, reasoning patterns, and acceptance levels. International Journal of Science Education , 41 (5), 628–651. https://doi.org/10.1080/09500693.2019.1572936 .
Haslam, F., & Treagust, D. (1987). Diagnosing Secondary Students’ Misconceptions of Photosynthesis and Respiration in Plants Using a Two-Tier Multiple-Choice Instrument. Journal of Biological Education , 21 . https://doi.org/10.1080/00219266.1987.9654897 .
Imenda, S. (2014). Is there a conceptual difference between theoretical and conceptual frameworks? Journal of Social Science , 2 (38), 185–195.
Inagaki, K., & Hatano, G. (1991). Constrained person analogy in young children’s biological inference. Cognitive Development , 6 , 219–231.
Kalas, P., O’Neill, A., Pollock, C., & Birol, G. (2013). Development of a Meiosis Concept Inventory. CBE—Life Sciences Education , 12 (4), 655–664.
Kampourakis, K. (2013). Making sense of evolution . Oxford University Press.
Kargbo, D. B., Hobbs, E. D., & Erickson, G. L. (1980). Children’s beliefs about inherited characteristics. Journal of Biological Education , 14 (2), 137–146.
Kelemen, D., & Rosset, E. (2009). The human function compunction: Teleological explanation in adults. Cognition , 111 , 138–143.
Kelemen, D., & DiYanni, C. (2005). Intuitions About Origins: Purpose and Intelligent Design in Children's Reasoning About Nature. Journal of Cognition and Development , 6 (1), 3–31.
Klymkowsky, M. W., Rentsch, J. D., Begovic, E., & Cooper, M. M. (2016). The design and transformation of biofundamentals: A non-survey introductory evolutionary and molecular biology course. CBE—Life Sciences Education , 15 , ar70.
Lewontin, R. (2000). The triple helix . Harvard University Press.
Mayr, E. (1997). This is biology . New York: Basic Books.
McFarland, J. L., Price, R. M., Wenderoth, M. P., Martinková, P., & Cliff, W. (2017). Joel Investigating Novice and Expert Conceptions of Genetically Modified Organisms. CBE—Life Sciences Education , 16 , 3.
Mead, L. S., Kohn, C., Warwick, A., & Schwartz, K. (2019). Applying measurement standards to evolution education assessment instruments. Evolution:Education and Outreach , 12 , 5. https://doi.org/10.1186/s12052-019-0097-y .
Miller, J. G. (1978). Living Systems . McGraw Hill.
National Research Council (1958). Concepts of Biology . National Academies Press.
National Research Council (2001). Knowing what students know: the science and design of educational assessment . Washington, DC: National Academies Press.
National Research Council (2009). The New Biology . Washington, DC: National Academies Press.
National Research Council (2012). Discipline-based education research: Understanding and improving learning in undergraduate science and engineering. Washington, DC: National Academies Press.
National Research Council (2013). NGSS Lead States. Next generation science standards: for states, by states . Washington, DC: The National Academies Press.
National Science Foundation (2019). Re-Integrating Biology. https://reintegratingbiology.org/ . Accessed 5 Nov 2019.
Nehm, R. H. (2014). Discipline-based education research. Science Education. , 98 (3), 543–546.
Nehm, R. H. (2018). Evolution (chapter 14). In K. Kampourakis, & M. Reiss (Eds.), Teaching biology in schools: Global issues and trends . Taylor and Francis: Routledge.
Nehm, R. H., Beggrow, E., Opfer, J., & Ha, M. (2012). Reasoning about natural selection: Diagnosing Contextual competency using the ACORNS instrument. The American Biology Teacher. , 74 (2).
Nehm, R. H., & Ha, M. (2011). Item feature effects in evolution assessment. Journal of Research in Science Teaching , 48 (3), 237–256.
Nehm, R. H., & Haertig, H. (2012). Human vs. computer diagnosis of Students’ natural selection knowledge: Testing the efficacy of text analytic software. Journal of Science Education and Technology. , 21 (1), 56–73.
Nehm, R. H., & Mead, L. (2019). Evolution Assessment. Introduction to the Special Issue. Evolution Education & Outreach . https://doi.org/10.1186/s12052-019-0098-x .
Nehm, R. H., Poole, T. M., Lyford, M. E., Hoskins, S. G., Carruth, L., Ewers, B. E., & Colberg, P. J. (2009). Does the segregation of evolution in biology textbooks and introductory courses reinforce students’ faulty mental models of biology and evolution? Evolution Education and Outreach , 2 , 527–532.
Nehm, R. H., & Ridgway, J. (2011). What do experts and novices “see” in evolutionary problems? Evolution: Education and Outreach , 4 (4), 666–679.
Nehm, R. H., & Schonfeld, I. (2008). Measuring knowledge of natural selection: A comparison of the CINS, and open-response instrument, and oral interview. Journal of Research in Science Teaching , 1131–1160.
Nehm, R. H., & Schonfeld, I. (2010). The future of natural selection knowledge measurement. Journal of Research in Science Teaching. , 47 (3), 358–362.
Neumann, I., Neumann, K., & Nehm, R. (2011). Evaluating instrument quality in science education: Rasch-based analyses of a nature of science test. International Journal of Science Education , 33 (10), 1373–1405.
Newman, D. L., Snyder, C. W., Fisk, J. N., & Wright, L. K. (2016). Development of the Central Dogma Concept Inventory (CDCI) Assessment Tool. CBE—Life Sciences Education , 15 , 2.
Opfer, J. E., & Siegler, R. S. (2004). Revisiting preschoolers’ living things concept: A microgenetic analysis of conceptual change in basic biology. Cognitive Psychology , 49 , 301–332.
Opfer, J. E., et al. (2012). Cognitive foundations for science assessment design: Knowing what students know about evolution. Journal of Research in Science Teaching , 49 (6), 744–777.
Opfer, J. E., Gelman, S. A. (2010). Development of the Animate–Inanimate Distinction. In: Usha Goswami (Ed.). The Wiley‐Blackwell Handbook of Childhood Cognitive Development, Second edition.
Chapter Google Scholar
Partnership for Undergraduate Life Sciences Education (2019). http://www.pulse-community.org/ . Accessed 21 Mar 2019.
Pfundt, H., & Duit, R. (1998). Bibliography. Students’ Alternative Frameworks and Science Education , (2nd ed.).
Poling, D. A., & Evans, E. M. (2002). Why do birds of a feather flock together? Developmental change in the use of multiple explanations: Intention, teleology and essentialism. British Journal of Developmental Psychology , 20 , 89–112.
Potter, L. M., Bissonnette, S. A., Knight, J. D., Tanner, K. D., O’Dowd, D. K. (2017). Investigating Novice and Expert Conceptions of Genetically Modified Organisms. CBE—Life Sciences Education 16 (3):ar52.
Price, R. M., Andrews, T. C., McElhinny, T. L., Mead, L. S., Abraham, J. K., Thanukos, A., Perez, K. E., Shuster, M. (2014). The Genetic Drift Inventory: A Tool for Measuring What Advanced Undergraduates Have Mastered about Genetic Drift. CBE—Life Sciences Education , 13 (1), 65–75.
Rachmatullah, A., Nehm, R.H., Ha, M. Roshayanti, F. (2018). Evolution education in Indonesia: Pre-service biology teachers’ evolutionary knowledge levels, reasoning models, and acceptance patterns. Evolution Education around the Globe. (Eds.). Deniz, H. Borgerding, L. springer.
Raman, L., & Winer, G. A. (2002). Children’s and adults’ understanding of illness: Evidence in support of a coexistence model. Genetic, Social, and General Psychology Monographs, Washington , 128 (4), 325–355.
Reiss, M., & Kampourakis, K. (2018). Teaching Biology in Schools Global Research, Issues, and Trends . Taylor and Francis.
Rocco, T. S., & Plakhotnik, M. (2009). Literature reviews, conceptual frameworks, and theoretical frameworks: Terms, functions, and distinctions. Human Resource Development Review , 8 (1), 120–130.
Sbeglia, G., & Nehm, R. H. (2018). Measuring evolution acceptance using the GAENE: Influences of gender, race, degree-plan, and instruction. Evolution Education & Outreach. https://doi.org/10.1186/s12052-018-0091-9 .
Sbeglia, G., & Nehm, R. H. (2019). Do you see what I-SEA? A Rasch analysis of the psychometric properties of the Inventory of Student Evolution Acceptance. Science Education. https://doi.org/10.1002/sce.21494 .
Schmiemann, P., et al. (2017). Assessment of genetics understanding: Under what conditions do situational features have an impact on measures? Science Education , 26 (10), 1161–1191.
Shea, N. A., Duncan, R. G., & Stephenson, C. (2015). A tri-part model for genetics literacy: Exploring undergraduate student reasoning about authentic genetics dilemmas. Research in Science Education , 45 (4), 485–507.
Smith, M. K., Wood, W. B., & Knight, J. K. (2008). The genetics concept assessment: A new concept inventory for gauging student understanding of genetics. CBELife Sciences Education , 7 (4), 422–430.
Smith, M. U. (1983). A comparative analysis of the performance of experts and novices while solving selected classical genetics problems , Unpublished doctoral dissertation (). FL: Florida State University.
Solomon, G., Johnson, S. C., Zaitchik, D., & Carey, S. (1996). Like father like son: Young children's understanding of how and why offspring resemble their parents. Child Development , 67 , 151–171.
Stefanski, K. M., Gardner, G. E., & Seipelt-Thiemann, R. L. (2016). Development of a Lac Operon Concept Inventory (LOCI). CBE—Life Sciences Education , 15 , 2.
Stewart, J., Cartier, J. L., & Passmore, P. M. (2005). Developing understanding through model-based inquiry. In M. S. Donovan & J. D. Bransford (Eds.), How students learn (pp. 515–565). Washington D.C: National Research Council.
Todd, A., et al. (2017). Development and validation of the learning progression-based assessment of modern genetics (LPA-MG) in a high school context. Science Education , 101 (1), 32–65.
Tornabene, R. E., Lavington, E., & Nehm, R. H. (2018). Testing validity inferences for genetic drift inventory scores using Rasch modeling and item order analyses. Evolution Education & Outreach. , 11 (6). https://doi.org/10.1186/s12052-018-0082-x .
Wandersee, J. H., & Schussler, E. E. (1999). The American Biology Teacher, 61 (2) 82+84+86.
Ware, E. A., & Gelman, S. A. (2014). You get what you need: An examination of purpose-based inheritance reasoning in undergraduates, preschoolers, and biological experts. Cognitive Science , 38 (2), 197–243.
Weiss, P. (1958). Introduction , In: Gerard, R.W., Stevens, R. B. (1958). Concepts of Biology. National Research Council Publication 560 . D.C.: National Academy Press Washington.
Download references
Acknowledgements
Not applicable.
Partial funding for this work was provided by the Howard Hughes Medical Institute (HHMI) Inclusive Excellence program.
Author information
Authors and affiliations.
Department of Ecology and Evolution, Institute for STEM Education, Stony Brook University (SUNY), 650 Life Sciences Building, Stony Brook, New York, 11794-5233, USA
Ross H. Nehm
You can also search for this author in PubMed Google Scholar
Contributions
RHN wrote the manuscript. The author read and approved the final manuscript.
Authors’ information
Corresponding author.
Correspondence to Ross H. Nehm .
Ethics declarations
Ethics approval and consent to participate, consent for publication, competing interests.
The author declares that he/she has no competing interests.
Additional information
Publisher’s note.
Springer Nature remains neutral with regard to jurisdictional claims in published maps and institutional affiliations.
Rights and permissions
Open Access This article is distributed under the terms of the Creative Commons Attribution 4.0 International License ( http://creativecommons.org/licenses/by/4.0/ ), which permits unrestricted use, distribution, and reproduction in any medium, provided you give appropriate credit to the original author(s) and the source, provide a link to the Creative Commons license, and indicate if changes were made.
Reprints and permissions
About this article
Cite this article.
Nehm, R.H. Biology education research: building integrative frameworks for teaching and learning about living systems. Discip Interdscip Sci Educ Res 1 , 15 (2019). https://doi.org/10.1186/s43031-019-0017-6
Download citation
Received : 28 May 2019
Accepted : 08 November 2019
Published : 02 December 2019
DOI : https://doi.org/10.1186/s43031-019-0017-6
Share this article
Anyone you share the following link with will be able to read this content:
Sorry, a shareable link is not currently available for this article.
Provided by the Springer Nature SharedIt content-sharing initiative
- Biology education research
- Biology education
- Discipline-based education research
- Conceptual frameworks
- Life science
Change Password
Your password must have 8 characters or more and contain 3 of the following:.
- a lower case character,
- an upper case character,
- a special character
Password Changed Successfully
Your password has been changed
- Sign in / Register
Request Username
Can't sign in? Forgot your username?
Enter your email address below and we will send you your username
If the address matches an existing account you will receive an email with instructions to retrieve your username
Demystifying the Meaning of Active Learning in Postsecondary Biology Education
- Emily P. Driessen
- Jennifer K. Knight
- Michelle K. Smith
- Cissy J. Ballen
*Address correspondence to: Emily P. Driessen ( E-mail Address: [email protected] ).
Department of Biological Sciences, Auburn University, Auburn, AL 36849
Search for more papers by this author
Department of Molecular Cellular and Developmental Biology, University of Colorado, Boulder, CO 80309
Department of Ecology and Evolutionary Biology, Cornell University, Ithaca, NY 14853
Active learning is frequently used to describe teaching practices, but the term is not well-defined in the context of undergraduate biology education. To clarify this term, we explored how active learning is defined in the biology education literature ( n = 148 articles) and community by surveying a national sample of biology education researchers and instructors ( n = 105 individuals). Our objectives were to increase transparency and reproducibility of teaching practices and research findings in biology education. Findings showed the majority of the literature concerning active learning never defined the term, but the authors often provided examples of specific active-learning strategies. We categorized the available active-learning definitions and strategies obtained from the articles and survey responses to highlight central themes. Based on data from the BER literature and community, we provide a working definition of active learning and an Active-Learning Strategy Guide that defines 300+ active-learning strategies. These tools can help the community define, elaborate, and provide specificity when using the term active learning to characterize teaching practices.
INTRODUCTION
The promotion of undergraduate biology knowledge in the United States has immediate and long-term implications for increasing national science literacy, providing high-quality education to the science, technology, engineering, and mathematics (STEM) workforce, and contributing to critical scientific advances. To meet these objectives, calls to action formalized priorities and made specific recommendations aimed at improving undergraduate biology education nationwide. For example, after extensive discussions among biology faculty, students, and administrators, the American Association for the Advancement of Science (2009 ) published a formative document, Vision and Change: A Call to Action , which advocated for “student-centered classrooms” and outlined six core competencies intended to guide undergraduate biology education: 1) apply the process of science; 2) use quantitative reasoning; 3) use modeling and simulation; 4) tap into the interdisciplinary nature of science; 5) communicate and collaborate with other disciplines; and 6) understand the relationship between science and society. Another call to action came from the President’s Council of Advisors on Science and Technology (2012 ), who proposed five recommendations to change undergraduate STEM education, including the adoption of “evidence-based teaching practices.”
Although these pushes for “student-centered” and “evidence-based” practices are relatively recent, they stem from ideologies that are more than a century old. Specifically, Dewey (1916) wrote, “Learning means something which the individual does when he studies. It is an active , personally conducted affair” (p. 390). Based upon this work, Pesavento et al. (2015) identified Dewey as one of the earliest and most influential advocates of what we now know as active learning. Subsequently, others expanded on and institutionalized terms such as “student-centered” and “evidence-based” practices ( Piaget, 1932 ; Montessori, 1946 ; Vygotsky, 1987 ; Papert, 1980 ; Brown et al. , 1989 ; Turkle and Papert, 1990 ; Ackermann, 2001 , Cook et al. , 2012 ). While this body of work is critical to our understanding of active learning, the ways in which practitioners and researchers currently use the term are often vague.
Despite this ambiguity, research concerning the effectiveness of active learning in the classroom has continued. For example, a landmark meta-analysis compared student achievement and failure rates between undergraduate science, engineering, and mathematics classes that used active-learning approaches and those that used lecture ( Freeman et al. , 2014 ). Findings demonstrated that active learning decreased failure rates by 55% and increased student examination performance by approximately half a standard deviation. To define active learning for the purposes of clarity and transparency in their research, Freeman et al. (2014) developed a definition based on responses from 338 biology departmental seminar audience members: “Active learning engages students in the process of learning through activities and/or discussion in class, as opposed to passively listening to an expert. It emphasizes higher-order thinking and often involves group work” (pp. 8413–8414). This definition guided their inclusion criteria for the study, and it is one of the few examples of clearly defined parameters.
Although many articles do not define the exact parameters of active learning, the research has demonstrated the positive effects of active learning on student achievement and affect across multiple contexts. For example, researchers demonstrated that active learning yields disproportionate learning gains among the most at-risk student groups, such as first-generation college attendees and those who identify with races/ethnicities historically underrepresented in STEM fields ( Beichner et al. , 2007 ; Haak et al. , 2011 ; Eddy and Hogan, 2014 ; Ballen et al. , 2017 ; Wilton et al. , 2019 ; Bauer et al. , 2020 ). Additionally, a meta-analysis conducted by Theobald et al. (2020) demonstrated that active learning narrows achievement gaps for underrepresented students in undergraduate STEM disciplines. However, it is important to note that the definitions of active learning used in these articles vary from the antithesis of lecture ( Theobald et al. , 2020 ) to listing the specific strategies that characterize the term (e.g., in-class activities, prelecture preparation, and frequent low-risk assessment; Ballen et al, 2017 ).
Despite the varying parameters of the term, postsecondary institutions have increasingly embraced the use of the term “active learning” ( Pfund et al. , 2009 ; Aragón et al. , 2018 ). Examples include institution-wide initiatives (e.g., the Science Education Initiatives at University of Colorado and University of British Colombia, and the Active Learning Initiative at Cornell University), the Summer Institutes on Scientific Teaching ( www.summerinstitutes.org ), and the Obama Administration’s Active Learning Day ( https://obamawhitehouse.archives.gov/blog/2016/10/25/active-learning-day-america ). Additionally, more than three-fourths of colleges and universities in the United States provide some type of active-learning classrooms, defined as those that offer flexibility in design to facilitate different types of teaching ( Alexander et al. , 2019 ).
Despite these institutional supports and documented positive impacts, the term “active learning” itself is difficult to ascertain from a review of literature. For example, Eddy et al. (2015) explained that active learning is a complex process that encompasses both teaching methods and student learning. Drew and Mackie (2011) noted the meaning of active learning may be dichotomous, as it has been considered a theory of learning as well as a set of pedagogical strategies. Although attempts have been made to define active learning as a theory ( Freeman et al. , 2014 ; Connell et al. , 2016 ; Moss-Racusin et al. , 2016 ; Auerbach and Schussler, 2017 ; Jeno et al. , 2017 ) as well as a set of strategies in biology education research (BER; Tanner, 2013 ; Miller and Tanner, 2015 ), these attempts are not always 1) streamlined or easy to follow, 2) regularly used in the literature, 3) supported by literature or data, and/or 4) comprehensive. This outcome is problematic when trying to understand what exactly active learning encompasses.
Notably, the variation in the conceptualization of active learning reflects a state of scientific revolution. According to Kuhn (1970) , the development of a science has alternating phases (i.e., normal and revolutionary). Normal science, equated to puzzle-solving, comes with a reasonable chance of solution via familiar methods and can be solved by one person. On the other hand, a revolutionary phase involves a collectively negotiated revision to an existing belief or practice. While discipline-based education researchers address questions about the efficacy of recently developed teaching strategies, those strategies are commonly being binned under active learning, which is an ill-defined term. To improve our field, it is important to negotiate how the community interprets and understands this term.
Furthermore, demystifying active learning in undergraduate biology has direct applications for teaching and research. The broad interpretation of active learning may discourage instructors from trying new instructional practices and may ultimately serve as a barrier to implementation ( Kreber and Cranton, 2000 ; O’Donnell, 2008 ; Stains and Vickrey, 2017 ). It may additionally serve as a barrier to experimental replication in discipline-based education research (DBER) communities, because there are no agreed-upon standards or criteria for inclusion or exclusion. Given this, we investigated the following four questions in the context of undergraduate biology courses: 1) How does the BER literature use and define the term “active learning”? 2) How does the BER community define the term “active learning”? 3) How are active-learning strategies described in the BER literature? and 4) How are active-learning strategies described by the BER community? We addressed these research questions through a review of BER literature and a survey of the BER community. We expect that, by developing ways to efficiently communicate active learning in the context of biology education, we will encourage teaching innovations and the adoption of common research-based practices.
Analyzing the Literature
To address how the BER literature defines and uses the term “active learning,” we extracted information from peer-reviewed biology education journals. Many peer-reviewed journals publish BER, including Advances in Physiology Education , American Biology Teacher , Anatomical Science Education , BioScience , Journal of College Science Teaching , CBE−Life Sciences Education ( LSE ) and the Journal of Microbiology & Biology Education ; however, we chose to examine only two of them, acknowledging that this is an exploratory, nonexhaustive study. We chose LSE and the Journal of Microbiology & Biology Education because of their prominence, history, and readership (see websites: www.lifescied.org ; www.asmscience.org/content/journal/jmbe ). We searched for the term ”active learning” in the titles, abstracts, or text of research articles published in those two journals, and only papers that used this term were included in our analysis. To get a contemporary snapshot of how the term is used, we only included articles published over the 3 years that preceded the start of the study, from January 1, 2016, to December 31, 2018. We collected data within the same time span from CourseSource, an online journal that exclusively publishes evidence-based biology teaching materials for undergraduate classrooms and laboratories ( www.coursesource.org/about ). We included this journal, because it captures how biology instructors translate findings from the active-learning research literature into classroom practice. All CourseSource lesson articles included an Active Learning section in which authors list and/or explain their instructional approaches, so we included all published papers in the final analysis.
Once we selected articles based upon our search criteria, three of the authors (C.J.B., M.K.S., and J.K.K.) read the articles and extracted the relevant text surrounding the search term “active learning.” If active learning was defined in the article, it usually occurred after the term was first mentioned in the introduction or the methods. Articles that described specific active-learning strategies often included them in the methods section of the paper, after introducing active learning broadly as an effective form of instruction. Because this text placement could vary, we searched through each article to make sure we included any definition or strategies that the article’s authors described.
To determine how BER articles use and define the term “active learning,” we first examined to what extent, if at all, articles included a definition of active learning. Articles that met the inclusion criteria were binned into six categories, first based on whether researchers followed their definition of active learning with a citation (i.e., “literature based”) or did not include a citation (i.e., “not literature based”). We then recorded whether articles included specific active-learning strategies, either in addition to a formal definition or in place of a definition. Our final list of categories included articles that provided: 1) a definition of active learning that was literature based (i.e., included a citation) with examples of active-learning strategies; 2) a definition of active learning that was not literature based (i.e., did not include a citation) with examples of active-learning strategies; 3) a definition of active learning that was literature based with no examples of active-learning strategies; 4) a definition of active learning that was not literature based and had no examples of active-learning strategies; 5) no active literature definition with active-learning strategies; and 6) no active literature definition and no active-learning strategies ( Figure 1 ).
FIGURE 1. Ways in which articles from LSE , the Journal of Microbiology & Biology Education , and CourseSource use the term “active learning.”
Surveying the Community
In addition to combing the literature, we collected survey data from members of the Society for the Advancement of Biology Education Research (SABER; a scientific community of discipline-based education researchers and teaching practitioners who focus on improving postsecondary biology education through evidence and theory) via the Listserv. We selected SABER as a group to survey, because it is the “world’s largest organization dedicated to scientifically exploring how to teach biology most effectively” ( https://saberbio.wildapricot.org ).
Through the survey, we collected demographic information from the survey participants, including institution type, employment position (i.e., faculty, postdoc, graduate student, etc.), level of biology class (e.g., lower level, upper level, etc.), class size, country of instruction, and frequency of active-learning instruction practice ( Table 1 ). Additionally, the survey included the following two prompts: 1) “In your own words, define the term ‘active learning’ in the context of undergraduate biology classrooms”; and 2) “List the active-learning techniques that you use in biology classrooms.” All research was conducted in accordance with the Cornell University Institutional Review Board (Cornell IRB protocol no. 1810008360).
Data Categorization
After we obtained both active-learning definitions and strategies from the surveys and the literature, we analyzed the data. Specifically, we started by creating two data sets. These were created by 1) taking the active-learning definition text from the literature and from the surveys and combining it into one Excel spreadsheet, and 2) taking the active-learning strategies text from the literature and from the surveys and combining it into another Excel spreadsheet. Both data sets were then categorized.
Categorization of Active-Learning Definitions
After combining the active-learning definitions from both data sources (i.e., the literature and surveys), we analyzed the active-learning definition data. Using open coding ( Strauss, 1987 ; Strauss and Corbin, 1990 ), a method rooted in the grounded theory framework ( Glaser et al. , 1968 ), three of the authors (J.K.K., M.K.S., and C.J.B.) reviewed the responses and identified recurring themes. Using the methodology from Saldaña (2015) , the authors compared their notes and developed a final set of 10 categories: students interacting or engaging with the material, not traditional lecture, group work, scaffolding or constructivism, problem solving, individual formative assessments (e.g., through the use of personal response systems), student-centered pedagogy, application or synthesis of material, student ownership of learning, and evidence-based teaching. The authors binned each article’s use of the term into as many categories of active learning as appropriate. At first, the three coders placed 71% of the definitions in the same categories. After discussion, coders resolved all differences and shared 100% agreement. Then, we calculated how often the definitions appeared in each of the 10 categories for the surveys and the literature individually.
Categorization of Active-Learning Strategies
After merging the strategies from the literature with those obtained from the surveys, we analyzed the active-learning strategy data. Using open coding, three researchers (E.P.D. and two undergraduate students) developed a set of nine categories (Supplemental Appendix A): metacognition, discussion, group work, assessment, practicing core competencies, visuals, conceptual class design, paperwork, and games. To improve our collective ability to reliably categorize strategies, we needed definitions of each strategy listed. Because no such list of definitions existed, we defined each of the unique strategies (Supplemental Appendix B) using published literature or dictionary definitions (Supplemental Appendix C). The utility of this list, which we call the Active-Learning Strategy Guide can also be used by the education research community and disciplinary practitioners interested in learning about active-learning strategies. Using the Active-Learning Strategy Guide, we were able to categorize the strategies with an initial percentage of agreement of 75%. After discussion, the researchers resolved any differences with discussion for a final percentage of agreement of 100%.
How Does the BER Literature Use and Define the Term “Active Learning”?
Of the 148 articles that fit our search criteria, the majority did not provide a definition for the term “active learning,” but instead listed examples of specific active-learning strategies (53.42%; Figure 1 ). The second most common approach used in the articles provided less information: no definition and no list of relevant strategies (30.14%). Overall, this demonstrates the overwhelming majority of the active-learning literature (83.56%) did not define active learning.
To address how the BER literature defines active learning, we focused on articles that provided a definition of the term, with or without the inclusion of one or more references. Among the 24 articles that defined active learning ( Table 2 ), 17 articles (74%) provided literature citations and seven (26%) did not. Of the 17 articles that defined active learning using references to the literature, five of them (29%) cited Freeman et al. (2014) . There was a bit of variation in reference use, with a total of 43 different references mentioned (Supplemental Appendix D).
a Citations available in Supplemental Appendix D.
The 17 definitions obtained from the literature were categorized as previously mentioned. The most represented category defined active learning as “students interacting or engaging with the material,” followed by the category that emphasizes what active learning is not : “not traditional lecture” ( Figure 2 A).
FIGURE 2. Frequency of how the BER literature and community define active learning and describe strategies. (A) The categorized definitions of active learning from the literature ( LSE , Journal of Microbiology & Biology Education, and CourseSource ) and a survey disseminated to SABER members. Each bar shows the percentage of articles or total survey respondents who included the corresponding term in their definition of active learning or list of active-learning strategies. (B) The categorized active-learning strategies from the same BER literature and community sources. The graph is organized by increasing percentage of total survey responses in each category. The percent values represented in each figure do not add up to 100%, because each literature source and survey response could have more than one strategy or definition represented.
How Is the Term “Active Learning” Defined by the BER Community?
We received responses from 105 individuals from a range of institutions across the United States ( Table 1 ). In general, survey participants’ definitions fit into the same categories as those in the published literature surveyed ( Figure 2 A). The most common definition of active learning, from the BER community, was “interacting/engagement” with the material. The second most common categorized definition was “not lecturing/listening,” followed by “group work.”
How Are Active-Learning Strategies Described in the BER Literature?
After analyzing the qualifying articles, we found that 38% of them did not mention any specific active-learning strategy. From the papers that mentioned active-learning strategies, a total of 339 strategies were extracted, with 133 of them being unique responses. Once these strategies were categorized, the data revealed the most frequently represented strategy categories from the literature were discussion (29%), group work (22%), and metacognition (22%; Figure 2 B).
How Are Active-Learning Strategies Described by the BER Community?
We asked survey participants to respond to the prompt “List the active-learning strategies that you use in biology classrooms.” We collated a list of 681 strategies from the responses, of which 201 were unique. After categorizing these strategies, we found the most frequently represented strategy categories from the surveys were discussion (34%), group work (29%), and metacognition (45%; Figure 2 B).
Our aim was to bring clarity and transparency to the term “active learning” as it is used within the BER community. We addressed this by identifying the definitions and strategies attributed to the term by analyzing the literature and surveying a BER society. From these compiled findings, we constructed an active-learning definition (see Box 1) as well as a reference guide for 300+ defined active-learning strategies (Supplemental Appendix B).
BOX 1. Active Learning Defined
Active learning is an interactive and engaging process for students that may be implemented through the employment of strategies that involve metacognition, discussion, group work, formative assessment, practicing core competencies, live-action visuals, conceptual class design, worksheets, and/or games.
Below we propose future steps for the BER community with accompanying tools to aid in the process. First, we advocate for all BER concerning active learning to provide a cited definition. Second, we suggest authors define and describe the active-learning strategies used in the experimental research.
Define the Term
Active learning has rarely been defined in the literature. This outcome could be due to the lack of a unanimous definition for the BER community; the fact that active learning is a complex process encompassing both teaching methods and student learning ( Eddy et al. , 2015 ); the dichotomous nature of the term as both a theory and as a set of pedagogical strategies ( Drew and Mackie, 2011 ); the perception that this term is self-descriptive; and/or the notion that it is unimportant, given the majority of the research articles focused on the effects of the implementation of a specific active-learning strategy. Whatever the reason, we advocate for the inclusion of definitions in BER articles in order to clarify the author’s interpretation. This is because, based on our investigation into the contemporary literature, it is apparent that people interpret active learning in a variety of different ways (i.e., interacting/engagement, not lecturing/listening, group work, scaffolding/constructivism, individual formative assessments, application/synthesis, problem solving, student centered, and evidence based). Ultimately, providing a definition may aid in increased fidelity and reproducibility of experimental outcomes ( Stains and Vickrey, 2017 ).
When considering outcomes such as those demonstrated by Freeman et al. (2014 ; i.e., active learning decreased failure rates by 55% and increased student examination performance by approximately half a standard deviation), increased fidelity and reproducibility of experimental outcomes is important, especially because the promotion of undergraduate biology knowledge in the United States is consequential to critical scientific advances. To help in these efforts, we provide a number of resources and suggestions. First, we provided all of the definitions of active learning collected from recent BER literature in addition to the references used to support them, when applicable ( Table 2 ). We also constructed a working definition of active learning based on the summarized input from the 148 articles found in the BER literature and the 105 responses from the BER community. This definition can be used confidently by the BER community in their own research, given it is based on an average of BER literature and instructor responses.
Define the Strategies
The research papers we examined commonly listed active-learning strategies. Many of the strategies were either 1) self-descriptive, that is, the meaning could be easily deciphered from the term (e.g., applying knowledge of other subjects, circulate to check for understanding, group brainstorming); 2) defined in the literature by Tanner (2013) , Miller and Tanner (2015) , or others; or 3) easily collapsed into one of the three most common categories (i.e., metacognition, group work, or discussion). However, many strategies lacked transparency, because authors did not describe how they were implemented. We found these cases problematic, because the strategies would be difficult to replicate. To improve clarity and transparency, we share with readers our comprehensive list of unique strategies, collected from both the literature and the surveys, with definitions from the literature, when available, as well as citations of articles in which they were used in practice (Supplemental Appendix B and C).
Additionally, we have created a living-document version of Supplemental Appendix B and C that can be viewed using the following link: www.ballenlab.org/active-learning-strategies-in -biolo . Contributions or constructive feedback from the community is welcome; you can make a submission by contacting the lead author or using the following Google form: https://forms .gle/Boh6NNm1rqzHACXi8 . This feedback will be considered and used by the lead author to improve the living document going forward. Our hope is that biology education researchers and teachers use these tools to define active-learning strategies they have used or as guides to articles that previously implemented these strategies. It is important to note that the strategies used and the efficacy measured in those studies may vary based on fidelity of implementation.
Another way to increase the precision of descriptions is the use of observation protocols that can characterize classroom instruction behaviors. Some examples include the Teaching Dimensions Observation Protocol ( Hora et al. , 2013 ), the Classroom Observation Protocol for Undergraduate STEM ( Smith et al. , 2013 ), the Practical Observation Rubric to Assess Active Learning ( Eddy et al. , 2015 ), and the Measurement Instrument for Scientific Teaching ( Durham et al. , 2017 ). These protocols document the frequency of multiple instructional practices, include categories of active-learning strategies, and can be helpful both for research purposes and to provide feedback to instructors on their practices. Such information can provide valuable guidance to biology educators, especially when used in conjunction with data on student performance, attitudes, social psychological factors, and self-reflective practices.
Limitations and Future Work
One limitation of this work is that the active-learning definitions and strategies were solicited from the BER community only. While we hypothesize that these definitions and strategies may overlap with other DBER subjects (chemistry, geology, physics, etc.), we cannot generalize our results across disciplines, given results from Lund and Stains (2015) revealed differences in the factors influencing the adoption of evidence-based instructional practices among disciplinary chemistry, biology, and physics faculty. However, many of the strategies featured in the Active-Learning Strategy Guide may be useful across disciplines. Additionally, it is reasonable to expect we may have received different active-learning definitions and strategies from disciplinary biology instructors or teaching practitioners who do not have a BER background. While seeking that information is out of the scope of this research, the BER community would benefit from engaging with the larger community to see how their work is translated among practitioners.
Second, while it is important to understand how active learning is used in classroom environments—particularly those that result in improved student outcomes—we recognize this does not control for instructors’ fidelity of implementation. Fidelity of implementation is how well an intervention or activity is implemented in comparison with the original program’s intention ( O’Donnell, 2008 ; Stains and Vickrey, 2017 ), and this can strongly impact the efficacy of the pedagogy. So, as we continue to test active-learning strategies, it is critical to describe how and why certain pedagogies are enacted in the classroom.
Third, we examined only three journals that commonly publish BER. This means the findings are not representative of all BER that has been published during that time period. However, the three journals we focused on are commonly used by the BER community. For example, LSE and the Journal of Microbiology & Biology Education publish primarily research articles and have a long-standing history and a large readership; CourseSource is the only online journal that exclusively publishes evidence-based biology teaching materials for undergraduate classrooms and laboratories.
Future work will 1) identify to what extent—and how—active learning is characterized across the DBER literature; 2) characterize the definition of active learning in the context of undergraduate STEM by collecting survey data from DBER communities across STEM fields; 3) categorize the specific active-learning strategies employed across STEM disciplines through survey data; and 4) investigate to what extent, if at all, perceptions of active learning differ among DBER communities across STEM fields.
CONCLUSIONS
We support the use of active learning as a unifying term to generate awareness and collaboration among those interested in improving their teaching. The term gives DBER instructors an accessible on-ramp to engage with larger initiatives. However, because the term is rarely defined and can have many different meanings, those who use active learning should define what they mean and give examples of the strategies they are using. For example, authors could say: “We used an active-learning instructional approach focused on student engagement using group work and clicker questions with peer instruction,” followed by the appropriate citations and additional detail about the application and frequency of strategies. These additional details will allow the community to address more nuanced questions, such as: Do specific active-learning instructional strategies promote student learning in multiple environments? Which strategies increase equitable outcomes for students from diverse backgrounds? How can we maximize the effectiveness of a particular active-learning strategy in a variety of contexts? These questions can be more effectively answered when the approach and context of the learning environment is precisely defined. This clarity has the potential to make DBER communities, and their research, stronger.
ACKNOWLEDGMENTS
We are grateful to the DBER group at Auburn University for valuable feedback and to Taylor McKibben, Sara Wood, Brian Peters, and Brittany Woodruff for helping with data collection and analysis. We would also like to thank Doug Lombardi and Tim Shipley for their support and encouragement and two anonymous reviewers for critical insights that greatly improved the article.
- Ackermann, E. ( 2001 ). Piaget’s constructivism, Papert’s constructionism: What’s the difference . Future of Learning Group Publication , 5 (3), 438. Google Scholar
- Alexander, B., Ashford-Rowe, K., Barajas-Murph, N., Dobbin, G., Knott, J., McCormack, M. , … & Weber, N. ( 2019 ). In EDUCAUSE Horizon Report: 2019 Higher Education Edition (pp. 3–41). Retrieved May 10, 2020, from https://library .educause.edu/-/media/files/library/2019/4/2019horizonreport .pdf?la=en&hash=C8E8D444AF372E705FA1BF9D4FF0DD4CC6F0FDD1 Google Scholar
- American Association for the Advancement of Science . ( 2009 ). Vision and change: A call to action . Washington, DC. Retrieved January 29, 2020, from https://live-visionandchange.pantheonsite.io/wp-content/uploads/ 2011/03/VC-Brochure-V6-3.pdf Google Scholar
- Aragón, O. R., Eddy, S. L., & Graham, M. J. ( 2018 ). Faculty beliefs about intelligence are related to the adoption of active-learning practices . CBE—Life Sciences Education , 17/3 , ar47. Google Scholar
- Auerbach, A. J., & Schussler, E. ( 2017 ). A Vision and Change reform of introductory biology shifts faculty perceptions and use of active learning . CBE—Life Sciences Education , 16 (4), ar57. Link , Google Scholar
- Ballen, C. J., Wieman, C., Salehi, S., Searle, J. B., & Zamudio, K. R. ( 2017 ). Enhancing diversity in undergraduate science: Self-efficacy drives performance gains with active learning . CBE—Life Sciences Education , 16 (4), ar56. Link , Google Scholar
- Bauer, A. C., Coffield, V. M., Crater, D., Lyda, T., Segarra, V. A., Suh, K. , … & Vigueira, P. A. ( 2020 ). Fostering Equitable Outcomes in Introductory Biology Courses through Use of a Dual Domain Pedagogy . CBE—Life Sciences Education , 19 (1), ar4. Link , Google Scholar
- Becker, E. A., Easlon, E. J., Potter, S. C., Guzman-Alvarez, A., Spear, J. M., Facciotti, M. T. , … & Pagliarulo, C. ( 2017 ). The effects of practice-based training on graduate teaching assistants’ classroom practices . CBE—Life Sciences Education , 16 (4), ar58. Link , Google Scholar
- Beichner, R. J., Saul, J. M., Abbott, D. S., Morse, J. J., Deardorff, D., Allain, R. J. , … & Risely, J. S. ( 2007 ). The student-centered activities for large enrollment undergraduate programs (SCALE-UP) project . Research-Based Reform of University Physics , 1 (1), 2–39. Google Scholar
- Bentley, M., & Connaughton, V. P. ( 2017 ). A simple way for students to visualize cellular respiration: Adapting the board game Mousetrap TM to model complexity . CourseSource . https://doi.org/10.24918/cs.2017.8 Google Scholar
- Bouwma-Gearhart, J. L., Ivanovitch, J. D., Aster, E. M., & Bouwma, A. M. ( 2018 ). Exploring postsecondary biology educators’ planning for teaching to advance meaningful education improvement initiatives . CBE—Life Sciences Education , 17 (3), ar37. Link , Google Scholar
- Brown, J. S., Collins, A., & Duguid, P. ( 1989 ). Situated cognition and the culture of learning . Educational Researcher , 18 (1), 32–42. Google Scholar
- Cavanagh, A. J., Chen, X., Bathgate, M., Frederick, J., Hanauer, D. I., & Graham, M. J. ( 2018 ). Trust, growth mindset, and student commitment to active learning in a college science course . CBE—Life Sciences Education , 17 (1), ar10. Link , Google Scholar
- Connell, G. L., Donovan, D. A., & Chambers, T. G. ( 2016 ). Increasing the use of student-centered pedagogies from moderate to high improves student learning and attitudes about biology . CBE—Life Sciences Education , 15 (1), ar3. Link , Google Scholar
- Cook, B. G., Smith, G. J., & Tankersley, M. ( 2012 ). Evidence-based practices in education . In Harris, K. R.Graham, S.Urdan, T.McCormick, C. B.Sinatra, G. M.Sweller, J. (Eds.), APA handbooks in psychology®. APA educational psychology handbook, Vol. 1. Theories, constructs, and critical issues (pp. 495–527). American Psychological Association. Google Scholar Google Scholar
- Cooper, K. M., Ashley, M., & Brownell, S. E. ( 2017a ). A bridge to active learning: A summer bridge program helps students maximize their active-learning experiences and the active-learning experiences of others . CBE—Life Sciences Education , 16 (1), ar17. Link , Google Scholar
- Cooper, K. M., & Brownell, S. E. ( 2016 ). Coming out in class: Challenges and benefits of active learning in a biology classroom for LGBTQIA students . CBE—Life Sciences Education , 15 (3), ar37. Link , Google Scholar
- Cooper, K. M., Ding, L., Stephens, M. D., Chi, M. T., & Brownell, S. E. ( 2018 ). A course-embedded comparison of instructor-generated videos of either an instructor alone or an instructor and a student . CBE—Life Sciences Education , 17 (2), ar31. Link , Google Scholar
- Cooper, K. M., Haney, B., Krieg, A., & Brownell, S. E. ( 2017b ). What’s in a name? The importance of students perceiving that an instructor knows their names in a high-enrollment biology classroom . CBE—Life Sciences Education , 16 (1), ar8. Link , Google Scholar
- Dewey, J. ( 1916 ). Democracy and education . New York: McMillan. Google Scholar
- Drew, V., & Mackie, L. ( 2011 ). Extending the constructs of active learning: Implications for teachers’ pedagogy and practice . Curriculum Journal , 22 (4), 451–467. Google Scholar
- Durham, M. F., Knight, J. K., & Couch, B. A. ( 2017 ). Measurement Instrument for Scientific Teaching (MIST): A tool to measure the frequencies of research-based teaching practices in undergraduate science courses . CBE—Life Sciences Education , 16 (4), ar67. Link , Google Scholar
- Eddy, S. L., & Hogan, K. A. ( 2014 ). Getting under the hood: How and for whom does increasing course structure work? CBE—Life Sciences Education , 13 (3), 453–468. Link , Google Scholar
- Elliott, E. R., Reason, R. D., Coffman, C. R., Gangloff, E. J., Raker, J. R., Powell-Coffman, J. A., & Ogilvie, C. A. ( 2016 ). Improved student learning through a faculty learning community: How faculty collaboration transformed a large-enrollment course from lecture to student centered . CBE—Life Sciences Education , 15 (2), ar22. Link , Google Scholar
- Glaser, B. G., Strauss, A. L., & Strutzel, E. ( 1968 ). The discovery of grounded theory; strategies for qualitative research . Nursing Research , 17 (4), 364. Google Scholar
- Goff, E. E., Reindl, K. M., Johnson, C., McClean, P., Offerdahl, E. G., Schroeder, N. L., & White, A. R. ( 2017 ). Efficacy of a meiosis learning module developed for the virtual cell animation collection . CBE—Life Sciences Education , 16 (1), ar9. Link , Google Scholar
- Green, N. H., McMahon, D. G., & Brame, C. ( 2016 ). Using online active-learning techniques to convey time compensated sun compass orientation in the eastern North American monarch . Journal of Microbiology & Biology Education , 17 (3), 430. Medline , Google Scholar
- Hoefnagels, M., & Taylor, M. S. ( 2016 ). “Boost your evolution IQ”: An evolution misconceptions game . CourseSource . https://doi.org/10.24918/cs.2016.12 Medline , Google Scholar
- Hora, M. T., Oleson, A., & Ferrare, J. J. ( 2013 ). Teaching Dimensions Observation Protocol (TDOP) user’s manual . Madison: Wisconsin Center for Education Research. Google Scholar
- Jeno, L. M., Raaheim, A., Kristensen, S. M., Kristensen, K. D., Hole, T. N., Haugland, M. J., & Mæland, S. ( 2017 ). The relative effect of team-based learning on motivation and learning: A self-determination theory perspective . CBE—Life Sciences Education , 16 (4), ar59. Link , Google Scholar
- Kreber, C., & Cranton, P. A. ( 2000 ). Exploring the scholarship of teaching . Journal of Higher Education , 71 (4), 476–495. Google Scholar
- Kudish, P., Shores, R., McClung, A., Smulyan, L., Vallen, E. A., & Siwicki, K. K. ( 2016 ). Active learning outside the classroom: Implementation and outcomes of peer-led team-learning workshops in introductory biology . CBE—Life Sciences Education , 15 (3), ar31. Link , Google Scholar
- Kuhn, T. ( 1970 ). The structure of scientific revolutions . Chicago: University of Chicago Press. Google Scholar
- Lee, C. J., Toven-Lindsey, B., Shapiro, C., Soh, M., Mazrouee, S., Levis-Fitzgerald, M., & Sanders, E. R. ( 2018 ). Error-discovery learning boosts student engagement and performance, while reducing student attrition in a bioinformatics course . CBE—Life Sciences Education , 17 (3), ar40. Link , Google Scholar
- Lund, T. J., & Stains, M. ( 2015 ). The importance of context: An exploration of factors influencing the adoption of student-centered teaching among chemistry, biology, and physics faculty . International Journal of STEM Education , 2 , 13. Google Scholar
- McCourt, J. S., Andrews, T. C., Knight, J. K., Merrill, J. E., Nehm, R. H., Pelletreau, K. N. , … & Lemons, P. P. ( 2017 ). What motivates biology instructors to engage and persist in teaching professional development? CBE—Life Sciences Education , 16 (3), ar54. Link , Google Scholar
- McLean, J. L., & Suchman, E. L. ( 2016 ). Using magnets and classroom flipping to promote student engagement and learning about protein translation in a large microbiology class . Journal of Microbiology & Biology Education , 17 (2), 288. Medline , Google Scholar
- Miller, S., & Tanner, K. D. ( 2015 ). A portal into biology education: An annotated list of commonly encountered terms . CBE—Life Sciences Education , 14 (2), fe2. Link , Google Scholar
- Montessori, M. ( 1946 ). Education for a new world (Vol. 1 ). Adyar, Madras, India: Kalakshetra. Google Scholar
- Moss-Racusin, C. A., van der Toorn, J., Dovidio, J. F., Brescoll, V. L., Graham, M. J., & Handelsman, J. ( 2016 ). A “scientific diversity” intervention to reduce gender bias in a sample of life scientists . CBE—Life Sciences Education , 15 (3), ar29. Link , Google Scholar
- O’Donnell, C. L. ( 2008 ). Defining, conceptualizing, and measuring fidelity of implementation and its relationship to outcomes in K–12 curriculum intervention research . Review of Educational Research , 78 (1), 33–84. Google Scholar
- Papert, S. ( 1980 ). Mindstorms: Computers, children, and powerful ideas (pp. 607). New York: Basic Books. Google Scholar
- Pesavento, T., Klein, J., Macasaet, D., Shorter, C., & Wagstaff, S. ( 2015 ). History & context for active learning . Retrieved February 15, 2020, from https://wisc.pb.unizin.org/teachingwithtech/chapter/history-context-for -active-learning/#:∼:text=Theterm“active learning” Google Scholar
- Pfund, C., Miller, S., Brenner, K., Bruns, P., Chang, A., Ebert-May, D., Fagen, A. P. et al. ( 2009 ). Summer institute to improve university science teaching . Science , 324 (5926), 470–471. Medline , Google Scholar
- Piaget, J. ( 1932 ). The moral judgment of the child . San Diego, CA: Harcourt, Brace. Google Scholar
- President’s Council of Advisors on Science and Technology . ( 2012 ). Engage to excel: Producing one million additional college graduates with degrees in science, technology, engineering, and mathematics . Washington, DC: U.S. Government Office of Science and Technology. Google Scholar
- Saldaña, J. ( 2015 ). The coding manual for qualitative researchers . Los Angeles, CA and London: Sage. Google Scholar
- Smith, M. K., Jones, F. H. M., Gilbert, S. L., & Wieman, C. E. ( 2013 ). The Classroom Observation Protocol for Undergraduate STEM (COPUS): A new instrument to characterize university STEM classroom practices . CBE—Life Sciences Education , 12 (4), 618–627. Link , Google Scholar
- Stains, M., & Vickrey, T. ( 2017 ). Fidelity of implementation: An overlooked yet critical construct to establish effectiveness of evidence-based instructional practices . CBE—Life Sciences Education , 16 (1), rm1. Link , Google Scholar
- Stoltzfus, J. R., & Libarkin, J. ( 2016 ). Does the room matter? Active learning in traditional and enhanced lecture spaces . CBE—Life Sciences Education , 15 (4), ar68. Link , Google Scholar
- Strauss, A., & Corbin, J. ( 1990 ). Basics of qualitative research: Grounded theory procedures and techniques . Newbury Park, CA: Sage. Google Scholar
- Strauss, A. L. ( 1987 ). Qualitative analysis for social scientists . Cambridge: Cambridge University Press. Google Scholar
- Tanner, K. D. ( 2013 ). Structure matters: Twenty-one teaching strategies to promote student engagement and cultivate classroom equity . CBE—Life Sciences Education , 12 (3), 322–331. Link , Google Scholar
- Theobald, E. J., Hill, M. J., Tran, E., Agrawal, S., Arroyo, E. N., Behling, S. , … & Grummer, J. A. ( 2020 ). Active learning narrows achievement gaps for underrepresented students in undergraduate science, technology, engineering, and math . Proceedings of the National Academy of Sciences USA , 117 (12), 6476–6483. Medline , Google Scholar
- Turkle, S., & Papert, S. ( 1990 ). Epistemological pluralism: Styles and voices within the computer culture . Signs: Journal of Women in Culture and Society , 16 (1), 128–157. Google Scholar
- Vygotsky, L. S. ( 1987 ). The development of scientific concepts in childhood . In The collected works of LS Vygotsky (Vol. 1 , pp. 167–241) New York City, New York: Springer US. Google Scholar
- Wilton, M., Gonzalez-Niño, E., McPartlan, P., Terner, Z., Christoffersen, R. E., & Rothman, J. H. ( 2019 ). Improving academic performance, belonging, and retention through increasing structure of an introductory biology course . CBE—Life Sciences Education , 18 (4), ar53. Link , Google Scholar
- Carly A. Busch ,
- Parth B. Bhanderi ,
- Katelyn M. Cooper , and
- Sara E. Brownell
- Sehoya Cotner, Monitoring Editor
- Group work enhances student performance in biology: A meta-analysis 14 February 2024 | BioScience, Vol. 74, No. 3
- Promoting learning of biomechanical concepts with game-based activities 20 April 2021 | Sports Biomechanics, Vol. 23, No. 3
- Equitable Instructor Assessment Changes Amid COVID-19 Pandemic 7 March 2024 | Journal of College Science Teaching, Vol. 53, No. 2
- Baylee A. Edwards ,
- Chloe Bowen ,
- M. Elizabeth Barnes , and
- Tati Russo-Tait, Monitoring Editor
- Teaching at the intersection of science and society: An activity on healthcare disparities 5 January 2024 | Biology Methods and Protocols, Vol. 9, No. 1
- Boosting student performance with inclusive writing-to-learn assignments through graphic organizers in large enrollment undergraduate biology courses Journal of Microbiology & Biology Education, Vol. 24, No. 3
- Creating an equitable and inclusive STEM classroom: a qualitative meta-synthesis of approaches and practices in higher education 15 September 2023 | Frontiers in Education, Vol. 8
- Nicholas J. Wiesenthal ,
- Tasneem F. Mohammed ,
- Shauna Anderson ,
- Margaret Barstow ,
- Cydney Custalow ,
- Jas Gajewski ,
- Kristin Garcia ,
- Cynthia K. Gilabert ,
- Joseph Hughes ,
- Aliyah Jenkins ,
- Miajah Johnson ,
- Cait Kasper ,
- Israel Perez ,
- Brieana Robnett ,
- Kaytlin Tillett ,
- Lauren Tsefrekas ,
- Emma C. Goodwin , and
- Katelyn M. Cooper
- Stephanie Gardner, Monitoring Editor
- Community‐engaged learning to broaden the impact of applied ecology: A case study 8 January 2023 | Ecological Applications, Vol. 33, No. 5
- Tala Araghi ,
- Carly A. Busch , and
- Derek Braun, Monitoring Editor
- Inclusive-Equity Rubric and its Evaluation of Introductory Biology Course Syllabi 19 April 2023 | College Teaching, Vol. 19
- Mariel A. Pfeifer ,
- Julio J. Cordero , and
- Julie Dangremond Stanton
- Rebecca Price, Monitoring Editor
- Erika M. Nadile ,
- Madison L. Witt ,
- Cindy Vargas ,
- Missy Tran ,
- Joseph Gazing Wolf ,
- Danielle Brister , and
- Increasing retention in STEM by improving GPA through peer mentors and course enrichments in introductory biology 20 February 2023 | Journal of Biological Education, Vol. 25
- An online course on applied biochemistry and molecular biology through case‐based learning 28 September 2022 | Biochemistry and Molecular Biology Education, Vol. 51, No. 1
- A Digital Single-Session Intervention (Project Engage) to Address Fear of Negative Evaluation Among College Students: Pilot Randomized Controlled Trial 23 November 2023 | JMIR Mental Health, Vol. 10
- Perspective Chapter: Active Learning Strategies in the Veterinary Medicine Programme under the Think4Jobs Project 14 December 2022
- Conceptualizations of active learning in departments engaged in instructional change efforts 9 November 2022 | Active Learning in Higher Education, Vol. 31
- Are synchronous chats a silver lining of emergency remote instruction? Text-based chatting is disproportionately favored by women in a non-majors introductory biology course 19 October 2022 | PLOS ONE, Vol. 17, No. 10
- Claire B. Tracy ,
- Emily P. Driessen ,
- Abby E. Beatty ,
- Todd Lamb ,
- Jenna E. Pruett ,
- Jake D. Botello ,
- Cara Brittain ,
- Ísada Claudio Ford ,
- Chloe C. Josefson ,
- Randy L. Klabacka ,
- Tyler Smith ,
- Ariel Steele ,
- Min Zhong ,
- Scott Bowling ,
- Lucinda Dixon , and
- Abdi Warfa, Monitoring Editor
- Exploration of critical thinking and self‐regulated learning in online learning during the COVID‐19 pandemic 20 July 2022 | Biochemistry and Molecular Biology Education, Vol. 50, No. 5
- International survey of faculty teaching undergraduate introductory biomechanics 22 August 2022 | Sports Biomechanics, Vol. 36
- Sarah B. Wise ,
- Tim Archie , and
- Sandra Laursen
- Kimberly Tanner, Monitoring Editor
- Undergraduates' Experiences with Online and in-Person Courses Provide Opportunities for Improving Student-Centered Biology Laboratory Instruction Journal of Microbiology & Biology Education, Vol. 23, No. 1
- Perspectives on Active Learning: Challenges for Equitable Active Learning Implementation 2 March 2022 | Journal of Chemical Education, Vol. 99, No. 4
- Using an engagement lens to model active learning in the geosciences 27 April 2021 | Journal of Geoscience Education, Vol. 70, No. 2
- How Do Undergraduate Biology Instructors Engage With the Open Educational Resource Life Cycle? 1 March 2022 | Frontiers in Education, Vol. 7
- International Journal of STEM Education, Vol. 9, No. 1
- PLOS ONE, Vol. 17, No. 6
- Journal of Education, Humanities and Social Sciences, Vol. 2
- COPUS, PORTAAL, or DART? Classroom Observation Tool Comparison From the Instructor User’s Perspective 15 November 2021 | Frontiers in Education, Vol. 6
- Measuring Learning and Promoting Academic Integrity in Online Instruction Kinesiology Review, Vol. 10, No. 4
- Inclusive Instructional Practices: Course Design, Implementation, and Discourse 1 October 2021 | Frontiers in Education, Vol. 6
- Sara Odom ,
- Halle Boso ,
- Sara Brownell ,
- Sehoya Cotner ,
- Catherine Creech ,
- Abby Grace Drake ,
- Sarah Eddy ,
- Sheritta Fagbodun ,
- Sadie Hebert ,
- Avis C. James ,
- Justin R. St. Juliana ,
- Michele Shuster ,
- Seth K. Thompson ,
- Richard Whittington ,
- Bill D. Wills ,
- Alan E. Wilson ,
- Kelly R. Zamudio ,
- Min Zhong , and
- Tessa C. Andrews, Monitoring Editor
- Jeremy L. Hsu and
- Gregory R. Goldsmith
- Cynthia Brame,, Monitoring Editor
- Student perceptions of an inquiry‐based molecular biology lecture and lab following a mid‐semester transition to online teaching 10 December 2020 | Biochemistry and Molecular Biology Education, Vol. 49, No. 1

Submitted: 14 April 2020 Revised: 16 June 2020 Accepted: 30 July 2020
© 2020 E. P. Driessen et al. CBE—Life Sciences Education © 2020 The American Society for Cell Biology. This article is distributed by The American Society for Cell Biology under license from the author(s). It is available to the public under an Attribution–Noncommercial–Share Alike 3.0 Unported Creative Commons License (http://creativecommons.org/licenses/by-nc-sa/3.0).
Advertisement
The Strategies of Modeling in Biology Education
- Published: 02 December 2011
- Volume 22 , pages 119–142, ( 2013 )
Cite this article
- Julia Svoboda 1 &
- Cynthia Passmore 1
4543 Accesses
88 Citations
1 Altmetric
Explore all metrics
Modeling, like inquiry more generally, is not a single method, but rather a complex suite of strategies. Philosophers of biology, citing the diverse aims, interests, and disciplinary cultures of biologists, argue that modeling is best understood in the context of its epistemic aims and cognitive payoffs. In the science education literature, modeling has been discussed in a variety of ways, but often without explicit reference to the diversity of roles models play in scientific practice. We aim to expand and bring clarity to the myriad uses of models in science by presenting a framework from philosopher of biology Jay Odenbaugh that describes five pragmatic strategies of model use in the biological sciences. We then present illustrative examples of each of these roles from an empirical study of an undergraduate biological modeling curriculum, which highlight how students used models to help them frame their research question, explore ideas, and refine their conceptual understanding in an educational setting. Our aim is to begin to explicate the definition of modeling in science in a way that will allow educators and curriculum developers to make informed choices about how and for what purpose modeling enters science classrooms.
This is a preview of subscription content, log in via an institution to check access.
Access this article
Price includes VAT (Russian Federation)
Instant access to the full article PDF.
Rent this article via DeepDyve
Institutional subscriptions
Similar content being viewed by others
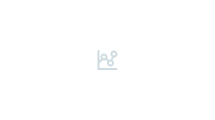
Tensions Between Learning Models and Engaging in Modeling
Using Epistemic Considerations in Teaching: Fostering Students’ Meaningful Engagement in Scientific Modeling
The black box approach: analyzing modeling strategies.
Readers interested in an example from physics might want to consider Hughes’ ( 1999 ) description of the Ising model, a model that is not faithful to reality but nevertheless has explanatory utility in physics.
See also Harre ( 1986 ) for his discussion of models that are used to explore “possibilities” and “impossibilities”.
Prediction in this context is used to refer to the practice of predicting future events with some accuracy. This is different from the reasoning strategy of imagining the implications if a given model were true, often also referred to as the predictions of a model. This type of reasoning is more closely related to exploring possibilities as discussed in Sect. 1.2.2 .
All names are pseudonyms.
Bechtel, W., & Abrahamsen, A. (2005). Explanation: A mechanist alternative. Studies in History and Philosophy of Science Part C: Studies in History and Philosophy of Biological and Biomedical Sciences, 36 (2), 421–441.
Article Google Scholar
Bechtel, W., & Abrahamsen, A. (2010). Complex biological mechanisms: Cyclic, oscillatory, and autonomous. Handbook of the Philosophy of Complex Systems, 10 , 1–26.
Google Scholar
Chinn, C., & Malhotra, B. (2002). Epistemologically authentic inquiry in schools: A theoretical framework for evaluating inquiry tasks. Science Education, 86 (2), 175–218.
Clement, J. (1989). Learning via model construction and criticism. In G. Glover, R. Ronning, & C. Reynolds (Eds.), Handbook of creativity: Assessment, theory and research (pp. 341–381). New York: Plenum Publishers.
Clement, J. C. (2000). Model based learning as a key research area for science education. International Journal of Science Education, 22 (9), 1041–1053.
Cooper, G. J. (2003). The science of the struggle for existence: On the foundations of ecology . Cambridge: Cambridge University Press.
Book Google Scholar
Darden, L. (1991). Theory change in science: Strategies from Mendelian genetics . New York: Oxford University Press.
Darden, L. (2002). Strategies for discovering mechanisms: Schema instantiation, modular subassembly, forward/backward chaining. Philosophy of Science, 69 (S3), S354–S365.
diSessa, A. (2004). Metarepresentation: Native competence and targets for instruction. Cognition and Instruction, 22 (3), 293–331.
Downes, S. (1992). The importance of models in theorizing: A deflationary semantic view. In PSA: Proceedings of the biennial meeting of the philosophy of science association (Vol. 1, pp. 142–153). Chicago: The University of Chicago Press.
Ford, M. J. (2008). Disciplinary authority and accountability in scientific practice and learning. Science Education, 92 (3), 404–423.
Ford, M. J., & Forman, E. A. (2011). Redefining disciplinary learning in classroom contexts. Educational Research, 30 (2006), 1–32.
Giere, R. N. (1988). Explaining science: A cognitive approach . Chicago: University of Chicago Press.
Giere, R. N. (2004). How models are used to represent reality. Philosophy of Science, 71 , 742–752.
Gobert, J. D. (2005). The effects of different learning tasks on model-building in plate tectonics: Diagramming versus explaining. Journal of Geoscience Education, 53 (4), 444–455.
Godfrey-Smith, P. (2006). The strategy of model-based science. Biology and Philosophy, 21 (5), 725–740.
Grandy, R., & Duschl, R. (2007). Reconsidering the character and role of inquiry in school science: Analysis of a conference. Science & Education, 16 , 141–166.
Grosslight, L., Unger, C., Jay, E., & Smith, C. L. (1991). Understanding models and their use in science: Conceptions of middle and high school students and experts. Journal of Research in Science Teaching, 28 , 799–822.
Hammer, D., Russ, R., Mikeska, J., & Scherr, R. (2008). Identifying inquiry and conceptualizing students’ abilities. In R. Duschl & R. Grandy (Eds.), Teaching scientific inquiry (pp. 138–156). Rotterdam: Sense Publishers.
Harre, R. (1986). Varieties of realism: A rationale for the natural sciences . New York: Blackwell.
Harrison, A., & Treagust, D. (2000). A typology of school science models. International Journal of Science Education, 22 (9), 1011–1026.
Hodson, D. (1996). Laboratory work as scientific method: Three decades of confusion and distortion. Journal of Curriculum Studies, 28 (2), 115–135.
Hodson, D. (1998). Science fiction: The continuing misrepresentation of science in the school curriculum. Pedagogy, Culture and Society, 6 (2), 191–216.
Hughes, R. I. G. (1999). The Ising model, computer simulation, and universal physics. In M. Morrison & M. S. Morgan (Eds.), Models as mediators: Perspectives on natural and social science (pp. 97–145). Cambridge: Cambridge University Press.
Chapter Google Scholar
Keller, E. F. (2000). Models of and models for: Theory and practice in contemporary biology. Philosophy of Science, 67 (S1), S72–S86.
Knorr-Cetina, K. (1999). Epistemic cultures: How the sciences make knowledge . Cambridge: Harvard University Press.
Knuuttila, T. (2005). Models, representation, and mediation. Philosophy of Science, 72 (5), 1260–1271.
Koponen, I. (2007). Models and modelling in physics education: A critical re-analysis of philosophical underpinnings and suggestions for revisions. Science & Education, 16 (7), 751–773.
Latour, B. (1990). Drawing things together. In M. Lynch & S. Woolgar (Eds.), Representation in scientific practice (pp. 19–68). Cambridge, MA: MIT Press.
Laubichler, M. D., & Müller, G. B. (2007). Modeling biology: Structures, behavior, evolution . Cambridge: MIT Press.
Lehrer, R., & Schauble, L. (2005). Developing modeling and argument in the elementary grades. In T. A. Rombert, T. P. Carpenter, & F. Dremock (Eds.), Understanding mathematics and science matters (Part II: Learning with understanding) . Mahway, NJ: Lawrence Erlbaum Associates.
Lehrer, R., & Schauble, L. (2006). Cultivating model-based reasoning in science education. In R. K. Sawyer (Ed.), Cambridge handbook of the learning sciences (pp. 371–388). New York: Cambridge University Press.
Lehrer, R., Schauble, L., & Lucas, D. (2008). Supporting development of the epistemology of inquiry. Cognitive Development, 23 , 512–529.
Levins, R. (1966). The strategy of model building in population biology. American Scientist, 54 (4), 421–431.
Lloyd, E. A. (1994). The structure and confirmation of evolutionary theory . Princeton: Princeton University Press.
Machamer, P., Darden, L., & Craver, C. (2000). Thinking about mechanisms. Philosophy of Science, 67 (1), 1–25.
Magnani, L., Nersessian, N. J., & Thagard, P. (Eds.). (1999). Model-based reasoning in scientific discovery . New York: Kluwer.
Matthews, M. (1994). Science teaching: The role of history and philosophy of science . New York: Routledge.
May, R. (1973). The stability and complexity of model ecosystems . Princeton: Princeton University Press.
Metz, K. (2010). Children’s understanding of scientific inquiry: Their conceptualization of uncertainty in investigations of their own design. Cognition and Instruction, 22 (2), 219–290.
Morrison, M., & Morgan, M. (1999). Models as mediating instruments. In M. Morrison & M. Morgan (Eds.), Models as mediators: Perspectives on natural and social science (pp. 10–37). Cambridge: Cambridge University Press.
Nersessian, N. J. (1992). How do scientists think? Capturing the dynamics of conceptual change in science. In R. N. Giere (Ed.), Cognitive models of science (pp. 3–44). Minneapolis: University of Minnesota Press.
Nersessian, N. J. (1999). Model-based reasoning in conceptual change. In L. Magnani, N. Nersessian, & P. Thagard (Eds.), Model-based reasoning in scientific discovery . New York: Kluwer/Plenum Publishers.
Nersessian, N. J. (2002). The cognitive basis of model-based reasoning. The cognitive basis of science (pp. 133–153). Cambridge: Cambridge University Press.
Nersessian, N. J. (2008). Model-based reasoning in scientific practice. In R. Duschl & R. Grandy (Eds.), Teaching Scientific Inquiry: Recommendations for Research and Implementation (pp. 57–79). Rotterdam, the Netherlands: Sense Publishers.
NRC. (2007). Taking science to school: Learning and teaching science in grades K-8 . Washington, DC: National Academies Press.
Odenbaugh, J. (2005). Idealized, inaccurate but successful: A pragmatic approach to evaluating models in theoretical ecology. Biology and Philosophy, 20 (2–3), 231–255.
Odenbaugh, J. (2009). Models in biology. In E. Craig (Ed.), Routledge encyclopedia of philosophy . London: Routledge.
Osbeck, L., Nersessian, N. J., Malone, K. R., & Newstetter, W. (2010). Science as psychology: Sense-making and identity in science practice . New York: Cambridge University Press.
Pluta, W. J., Chinn, C. A., & Duncan, R. G. (2011). Learners’ epistemic criteria for good scientific models. Journal of Research in Science Teaching, 48 (5), 486–511.
Rudolph, J. (2005). Epistemology for the masses: The origins of “The Scientific Method” in American schools. History of Education Quarterly, 45 (3), 341–376.
Schwarz, C., Reiser, B., Davis, E., Kenyon, L., Acher, A., Fortus, D., et al. (2009). Developing a learning progression for scientific modeling: Making scientific modeling accessible and meaningful for learners. Journal of Research in Science Teaching, 46 (6), 632–654.
Schwarz, C., & White, B. (2005). Metamodeling knowledge: Developing students’ understanding of scientific modeling. Cognition and Instruction, 23 (2), 165–205.
Smith, E., Haarer, S., & Confrey, J. (1997). Seeking diversity in mathematics education: Mathematical modeling in the practice of biologists and mathematicians. Science & Education, 6 , 441–472.
Tang, X., Coffey, J., Elby, A., & Levin, D. (2010). The scientific method and scientific inquiry: Tensions in teaching and learning. Science Education, 94 (1), 29–47.
White, B. Y. (1993). ThinkerTools: Causal models, conceptual change, and science education. Cognition and instruction, 10 (1), 1–100.
Wimsatt, W. C. (1987). False models as means to truer theories. In M. Nitecki (Ed.), Neutral models in biology (pp. 23–55). New York: Oxford University Press.
Wimsatt, W. C. (2002). Using false models to elaborate constraints on processes: Blending inheritance in organic and cultural evolution. Philosophy of Science, 69 (s3), S12–S24.
Windschitl, M., Thompson, J., & Braaten, M. (2008a). Beyond the scientific method: Model-based inquiry as a new paradigm of preference for school science investigations. Science Education , 1–27. doi: 10.1002/sce .
Windschitl, M., Thompson, J., & Braaten, M. (2008b). How novice science teachers appropriate epistemic discourses around model-based inquiry for use in classrooms. Cognition and Instruction, 26 (3), 310–378.
Wynne, C., Stewart, J., & Passmore, C. (2001). High school students’ use of meiosis when solving genetics problems. International Journal of Science Education, 23 (5), 501–515.
Download references
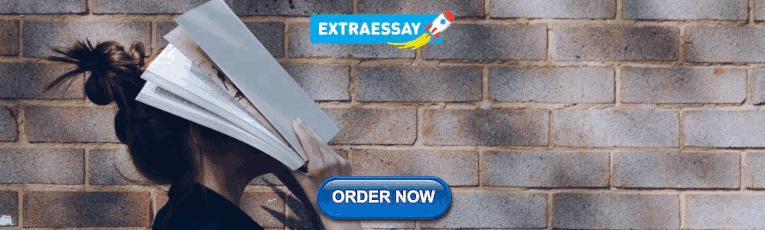
Acknowledgments
This research was supported by the National Science Foundation Interdisciplinary Training for Undergraduates in Biological and Mathematical Sciences (UBM) program under Grant No. 0531935. We would like to acknowledge CLIMB mentors and the students of the 2008–2009 CLIMB cohort for allowing us to bare witness to their learning process. We also thank Richard Lehrer and four anonymous reviewers for their helpful suggestions for improving this manuscript.
Author information
Authors and affiliations.
School of Education, University of California, Davis, Davis, CA, 95616, USA
Julia Svoboda & Cynthia Passmore
You can also search for this author in PubMed Google Scholar
Corresponding author
Correspondence to Julia Svoboda .
Rights and permissions
Reprints and permissions
About this article
Svoboda, J., Passmore, C. The Strategies of Modeling in Biology Education. Sci & Educ 22 , 119–142 (2013). https://doi.org/10.1007/s11191-011-9425-5
Download citation
Published : 02 December 2011
Issue Date : January 2013
DOI : https://doi.org/10.1007/s11191-011-9425-5
Share this article
Anyone you share the following link with will be able to read this content:
Sorry, a shareable link is not currently available for this article.
Provided by the Springer Nature SharedIt content-sharing initiative
- Science Education
- Scientific Practice
- Scientific Reasoning
- Vaccination Level
- Find a journal
- Publish with us
- Track your research

An official website of the United States government
The .gov means it’s official. Federal government websites often end in .gov or .mil. Before sharing sensitive information, make sure you’re on a federal government site.
The site is secure. The https:// ensures that you are connecting to the official website and that any information you provide is encrypted and transmitted securely.
- Publications
- Account settings
Preview improvements coming to the PMC website in October 2024. Learn More or Try it out now .
- Advanced Search
- Journal List
- CBE Life Sci Educ
- v.21(4); Winter 2022
A Vision for University Biology Education for Non-science Majors
Cara gormally.
† School of Science, Technology, Accessibility, Mathematics, and Public Health, Gallaudet University, 800 Florida Avenue NE, Washington, DC 20002
Austin Heil
‡ Marine Extension and Georgia Sea Grant, University of Georgia, Savannah, GA 31411
As college science educators, we must prepare all future college graduates to be engaged, science-literate citizens. Yet data suggest that most college biology classes as currently taught do little to make science truly useful for students’ lives and provide few opportunities for students to practice skills needed to be key decision makers in their communities. This is especially important for our non-science majors, as they represent the vast majority (82%) of college students. In this essay, we identify three critical aspects of useful college science education to prepare science literate non-science majors: prioritize local socioscientific issues; highlight communal opportunities in science that impact students’ communities; and provide students with opportunities to practice skills necessary to engage with science beyond the classroom.
INTRODUCTION: A CALL FOR ACTION
Complex scientific issues such as the COVID-19 pandemic and climate change affect our day-to-day lives both individually and collectively. We face challenging decisions related to science that have personal and societal implications, for example, whether to vaccinate and whether to support carbon offset credits. And our ability to respond is compromised by misinformation ( d’I Treen et al. , 2019 ; Puri et al. , 2020 ; Suarez-Lledo and Alvarez-Galvez, 2021 ; West and Bergstrom, 2021 ; Southwell et al. , 2022 ). For example, college graduates represent 35% of all Americans ( U.S. Census Bureau, 2019 ) and nearly one in four of these graduates believed conspiracy theories that COVID-19 was planned ( Schaeffer, 2020 ). Improving science literacy among college students is key to solving this problem of misinformation about climate change, COVID-19, and other socioscientific issues we face—and a fundamental aim of science education. As college science educators, we must better equip all students to make sound decisions in the face of misinformation.
More than eight out of 10 college students are not science majors ( National Center for Education Statistics [NCES], 2013 , 2021 ). These students are our future leaders, including our future lawyers, business owners, and politicians. However, as college educators and researchers, we tend to overlook this huge population of students who need science in lieu of preparing science, technology, engineering, and mathematics (STEM) majors to be the next generation of STEM professionals (Coley and Tanner, 2015). In reality, only five in 100 students will become our doctors, scientists, and engineers ( Sargent, 2017 ). In this essay, we present an evidence-based vision for nonmajor biology courses focused on scientific literacy for the vast majority of college-educated citizens—our non-science majors. Our positionality as instructors at 4-year institutions of higher education informs our standpoint in this essay.
DEFINING SCIENCE LITERACY
There are many definitions of science literacy in science education, and how we define it impacts how we measure it. Historically, science literacy has been defined in terms of content knowledge ( Miller, 1998 ; Allum et al. , 2008 ). Unsurprisingly, the adoption of content-focused definitions led to assessments of science literacy that focused on the general public’s ability to regurgitate scientific facts and demonstrate an understanding of scientific principles/knowledge ( Goodstein, 1992 ; Hazen, 2002 ; Johnson and Pigliucci, 2004 ; Allum et al. , 2008 ; Miller, 2010 , 2016 ). Estimates of scientific literacy varied using these content-focused assessments, but consistently pointed to high rates of science illiteracy across different populations ( Allum et al. , 2008 ; Miller 2004 , 2016 ).
More recently, science literacy has been defined as skills needed to make science knowledge useful and usable in everyday life ( Gormally et al. , 2012 ). To the best of our knowledge, there is only one freely available measure of scientific literacy that directly evaluates student skill development related to the capacity for scientifically informed action: the Test of Scientific Literacy Skills (TOSLS; Gormally et al. , 2012 ; for a review of instruments that measure scientific reasoning, please see Opitz et al. , 2017 ). There are no equivalent studies to the content-focused assessments mentioned earlier that investigate science literacy in terms of skills for the general public, as estimates of science literacy using TOSLS are often limited to pedagogical, curricular, and programmatic contexts ( Gormally et al. , 2012 ; Waldo, 2014 ; Shaffer et al. , 2019 ). However, these assessments pointed to lower scientific literacy skills for non-science majors that improved with innovative pedagogical and curricular efforts.
While a variety of definitions and subsequent assessments of science literacy exist, in this essay we adopt the definition that scientific literacy is the capacity to take scientifically informed action to make evidence-based decisions in everyday life ( American Association for the Advancement of Science [AAAS], 1990 , 1993 , 2011 ; Bybee, 1993 ; National Research Council [NRC], 1996 ; Maienschein, 1998 ; Millar et al. , 1998 ; DeBoer, 2000 ; Organisation for Economic Co-operation and Development, 2003 ). Based on this definition, we assert that our students must be able to use evidence to evaluate arguments and claims in the media and, ultimately, use scientific information to make a personally relevant decision (i.e., such as whether to get a COVID-19 vaccination or buy cereal containing genetically modified food products). Simply put, science must be useful for students ( Feinstein, 2011 ). Thus, we argue science literacy should be assessed in terms of skills ( Gormally et al. , 2012 ).
WHO ARE OUR NON-SCIENCE MAJORS?
NCES (2013) estimates that ∼85% of the 1.8 million students graduating from college annually in the United States are not science majors. Non-science majors are our future leaders, activists, teachers, lawyers, artists, counselors, voters, and parents. They must be ready to engage in discussions about important scientific issues. As individuals and as a society, they will need to evaluate climate change threats, react to genetically modified food crops, weigh health choices, and much more.
Non-science majors and science majors differ in several ways ( Table 1 ). A small study comparing non-biology ( N = 30) and biology majors ( N = 25) revealed differences existing before college, as students’ ACT scores differed significantly ( Hebert and Cotner, 2019 ). Fewer non-biology majors than biology majors reported taking advanced high school science classes ( Hebert and Cotner, 2019 ). Interestingly, the population of non-biology majors was more diverse—in terms of incoming knowledge, perceptions, backgrounds, and skills—than the biology majors’ population ( Cotner et al. , 2017 ).
Summary of characteristic differences between undergraduate science majors and non-science majors
Non-biology majors and biology majors demonstrate affective differences toward science ( Knight and Smith, 2010 ; Cotner et al. , 2017 ; Hebert and Cotner, 2019 ). Perhaps unsurprisingly, non-biology majors were less likely to describe themselves as “a science person,” were less confident in their ability to “do” science (e.g., pose questions, analyze results, and draw conclusions based on data), and had less interest in science than biology majors ( Hebert and Cotner, 2019 ). Non-biology majors also reported different perceptions of science and the usefulness of science ( Hebert and Cotner, 2019 ). While Cotner et al. (2017) reported that non-biology majors are more likely to hold misconceptions about the nature of science, Miller et al. (2010) reported that both non-science and science majors held a mix of naïve, transitional, and moderately informed views about the nature of science. Generally, however, both populations viewed science as relevant and important, similar to findings from studies by Miller (2004) and Allum et al. (2008) investigating the general public’s attitudes toward science. Earlier work by Cotner et al. (2017) reported non-biology majors were less likely to see science as personally relevant.
Multiple studies suggest that instructors should focus on improving non–science majors’ affective traits, such as interest, motivation, and attitudes toward science. For example, while non-biology majors and biology majors do not differ significantly in their abilities to “do” science, non-biology majors report a confidence gap ( Hebert and Cotner, 2019 ). Likewise, Glynn et al. (2011) reported that biology majors scored higher on every motivation component than non-biology majors. Non-science majors reported less interest in genetics, found genetics less relevant to their future careers, and were less motivated to study ( Knight and Smith, 2010 ). The authors also reported performance differences. Knight and Smith (2010) recommended addressing this disparity by focusing on changing student attitudes rather than content. Instructors may do well to connect concepts to real-world applications, so that non-science majors can connect new ideas with their mental models to form a “bigger picture.” Positive attitudes toward science must be encouraged and supported among non-science majors. Poorer attitudes toward science ( Allum et al. , 2008 ) are related to poorer outcomes in science—including decreased science literacy ( Cook and Mulvihill, 2008 ).
HOW IS UNDERGRADUATE BIOLOGY CURRENTLY TAUGHT TO OUR NON-SCIENCE MAJORS?
Science courses are often a general education requirement for non-science majors. General education, a shared core curriculum that can take many forms, provides broad exposure to multiple disciplines, including science, with opportunities for students to develop critical civic competencies ( Association of American Colleges and Universities, 2002 ). General education is the place where students come to understand that everything we teach relates to their lives ( “General Education, Finally Defined,” 2007 ) or should relate to their lives. While Vision and Change ( AAAS, 2011 ) guides learning for biology majors, at the time of writing this article, there are no national standards or recommendations for learning objectives for biology for non-science majors.
Faculty teaching non-science majors express support for teaching scientific literacy skills, especially the nature of science, research design, evaluating source validity, and evaluating the use and misuse of scientific information ( Gormally et al. , 2012 ). In fact, a large majority of faculty surveyed (≥58.7%, N = 188) reported that they teach these scientific literacy skills (with the exception of teaching understanding and interpreting basic statistics, which only 44.9% of faculty report teaching; Gormally et al. , 2012 ). However, these faculty self-report data conflict with a more recent analysis of learning objectives ( N = 872) collected from 38 faculty nationwide and from three best-selling textbooks for non-science majors ( N = 1390; Heil et al. , in press ). Few learning objectives (11.5%) from instructors or textbooks focused on science literacy skills useful for making science-informed decisions ( Heil et al. , in press ). And 80% of learning objectives for non-science majors could be classified as requiring only low levels of thinking as measured by Bloom’s taxonomy ( Heil et al. , in press ). Scientific literacy is often equated with specific content knowledge: When surveyed about skills required for scientific literacy, faculty responses categorized as specific content knowledge accounted for more responses than any one scientific literacy skill described ( Gormally et al. , 2012 ).
Science courses as currently taught do little to promote socioscientific decision making ( Feinstein, 2011 ). When it comes to class time, faculty tend to cover vast amounts of fundamental biological content but fail to see the importance of making these concepts relevant to students’ lives. For example, faculty teaching human genetics courses for non-science majors ( N = 63) rated genetics and society concepts (the application of genetics to human health, ethical implication of genetic testing, etc.) of least importance among all survey concepts ( Bowling et al. , 2007 ). Furthermore, faculty failed to take advantage of the vast amount of genetics in the popular media, reporting that they spent only 7% of class time on average on “genetics in the news” ( Bowling et al. , 2007 ). Other studies have reported similar faculty disregard of socioscientific issues for non-science major courses. For example, genetics instructors preferred a curriculum with a vast coverage of content to one that emphasized concepts that were most relevant to students’ lives ( Haffie et al. , 2000 ; Hott et al. , 2002 ). Most science courses focus on imparting practices and knowledge rather than considering the political, economic, or social dimensions of issues and how they relate to students’ lives and experiences ( Bowers, 2002 ; Fredeen, 2012 ).
Unsurprisingly, given the focus on content over skills or relevance, science instruction has not been shown to directly impact the decisions people make related to science and society ( Mulkay, 1997 ; Sadler, 2004 ; Feinstein, 2011 ; Crowell and Schunn 2014 ; Allum et al. , 2018 ) or concerns they have about socioscientific issues ( Kahan et al. , 2012 ). Students do not connect “science as a way of knowing” with the decisions they face in their daily lives ( Kuhn, 1993 ; Walker et al. , 2002 ; Rowe et al. , 2015 ) or as part of their personal knowledge ( Sadler, 2004 ). This suggests that we, as college science faculty, are not making scientific knowledge relevant to students’ lives. Our current approach to science education not only fails to foster true scientific literacy, but also alienates many students from science ( Seymour and Hewitt, 1997 ; Ede, 2000 ; Johnson, 2007 ). Ultimately, this jeopardizes America’s global competitiveness ( National Academy of Sciences, National Academy of Engineering, and Institute of Medicine, 2007 ). These findings indicate a need to revisit the goals of instruction for our non-science majors—the vast majority of college students.
WHAT CRITICAL ASPECTS SHOULD BE INCLUDED IN OUR COURSES FOR NON-SCIENCE MAJORS?
Studies from the past decade offer little evidence that the prevailing strategies in science education have an impact on how individuals use science in their daily lives. Feinstein (2011) argues: What need is there for scientific literacy, if we insist on its usefulness without demonstrating how or why it is useful for our citizens? How, then, can we make science education “useful” for all college students? Feinstein (2011) proposes that a “truly useful version of science literacy must be connected to the real uses of science in daily life (p. 168).” Focusing on “making science useful” as our overarching goal, we offer three recommendations for our fellow faculty teaching non-science major courses moving forward: 1) use local socioscientific issues as a lens for learning; 2) highlight communal opportunities that impact students’ communities; and 3) practice skills necessary for students to engage with science beyond the classroom. Each recommendation is explored in depth in the following sections.
Use Local Socioscientific Issues as a Lens for Learning
Recognizing what we know about adults’ engagement with science beyond the classroom can help us better prepare students to make use of science in their everyday lives. We live in a science-rich world situated in the activities of everyday life. Science learning encompasses an increasing amount of time in individuals’ lives beyond the classroom ( Falk et al. , 2007 ). This free-choice science learning is driven by individuals’ desire to know, intrinsic motivation rather than extrinsic motivation (e.g., grades). Beyond the classroom, adults do not necessarily engage in science-related activities simply to learn about science. Instead, people are motivated by social experiences and entertainment ( American Academy of Arts and Sciences, 2019a ), as well as current events about socioscientific issues in the media ( Falk et al. , 2007 ).
Consequently, building on what we know about how adult learners interact with science beyond the classroom suggests that contextualizing learning through socioscientific issues—especially local issues—may help students build meaningful connections to science ( Allum et al. , 2008 ; Funk et al. , 2015 ). Students enrolled in a laboratory curriculum based on socioscientific issues had increased motivation to engage in laboratory activities compared to students enrolled in a control laboratory course ( Hewitt et al. , 2019 ). Additionally, the socioscientific issues curriculum appeared to have a buffering effect on student motivation throughout the semester, as typically observed declines in motivation were not observed ( Hewitt et al. , 2019 ). Qualitative data suggest that students’ increased motivation was the result of enhanced feelings of relatedness, which could be important for student success and persistence ( Hewitt et al. , 2019 ). However, it is important to note that the socioscientific issues lab and control lab curricula also differed in that the former used an inquiry-based approach, while the latter used a traditional cookbook approach ( Hewitt et al. , 2019 ).
Moreover, teaching biology via the lens of socioscientific issues may reduce the stark differences in opinions about socioscientific issues between scientists and the general public. Scientists and the public differ in their opinions about issues such as the safety of genetically modified food; use of animals in research; human evolution; human activity as a major cause of climate change; and growth of the world population becoming a major issue ( Funk et al. , 2015 ). This difference suggests non-science major courses should use an issues-based approach as a way for our students to learn science to make informed decisions about these important socioscientific issues.
Highlight Communal Opportunities in Science That Impact Students’ Communities
Curricular interventions can also reduce differences in affective traits between non-science majors and science majors ( Knight and Smith, 2010 ; Hebert and Cotner, 2019 ). These affective traits include seeing oneself as a science person ( Gormally and Marchut, 2017 ; Hebert and Cotner, 2019 ) and seeing science as relevant to one’s life ( Cotner et al. , 2017 ). Seeing oneself as a science person requires seeing science as containing a possible future self. For many students, especially those historically underserved in science, this means seeing opportunities to achieve communal career goals, centered around working with and helping people ( Allen et al. , 2015 ; Brown et al. , 2015 ; Gormally and Marchut, 2017 ; Gormally and Inghram, 2021 ). This means careers must offer opportunities to develop interpersonal connections and to help other people or society ( Allen et al. , 2015 ; Brown et al. , 2015 ).
Science can be a vehicle for creating positive change in our communities. For example, interview studies illuminated how women of color with communal science identities redefined for themselves what it meant to be in science and whose recognition was important to them ( Carlone and Johnson, 2007 ). However, undergraduate biology education does not often contextualize curricula around the idea of science as a vehicle for communal good.
Instead, the predominant stereotypes of nerdy scientists working in isolation continue to negatively impact students’ interest in science ( Brown et al. , 2015 ; Schinske et al. , 2015 ). These stereotypes may contribute to students’ science identity. Seeing oneself as a “science person” may be impossible if one holds misperceptions about scientists ( Hazari et al. , 2013 ). Unfortunately, these stereotypes disproportionately affect students from groups underserved in science, including women, people of color, first-generation college students, and students of low socioeconomic status, who tend to value career goals focused on helping and working with people to give back to one’s community. As a result, these stereotypes preclude students’ interest in science learning, because science is not perceived as affording these communal career goals ( Diekman et al. , 2010 ; Allen et al. , 2015 ; Brown et al. , 2015 ).
There are several ways to center communal opportunities in science education. Service learning is one approach. In fact, even the mention of a service-learning project in a course description for an engineering class increased students’ beliefs that the course fulfills communal goals ( Belanger et al. , 2017 ). Likewise, emphasizing the “why” to engage in science activities helps students to identify communal opportunities in science. For example, when an opportunity to help the broader community or society was present, students reported high levels of interest in pursuing STEM ( Steinberg and Diekman, 2018 ). By highlighting communal opportunities in STEM, we, as college science educators, may disrupt common stereotypes, thus more effectively engaging students in science learning so that they are motivated to become scientifically literate.
Faculty can also directly address stereotypes about scientists and science through curricular activities. Diversifying images of computer scientists and engineers increased interest in computer science and engineering ( Cheryan et al. , 2015 ). Faculty may consider who they highlight as “scientist representatives” so that all students feel seen and valued and develop a sense of belonging—and thus are motivated to engage in science learning. For example, faculty might use resources from Scientist Spotlights ( Schinske et al. , 2016 ) or Project Biodiversify to combat stereotype threat. To address these stereotypes in computer science and engineering activities, researchers highlighted communal opportunities with direct connections to real-life scenarios. For example, in civil engineering curricular activities about building structures for water transportation and purification and bridges, educators highlighted how each project would improve community members’ lives ( Colvin et al. , 2013 ). Educators also prompted students to reflect on communal considerations of each project, for example, environmental impacts of the structure and safety, while recognizing the conflicting interests that might come into play in designing such a project ( Colvin et al. , 2013 ).
Practice Skills Necessary to Engage with Science beyond the Classroom
All college students must practice scientific literacy skills to engage with science beyond the classroom. This means faculty must consider how students engage with science beyond the classroom. The Internet is a primary source of scientific information ( Falk et al. , 2007 ; National Science Foundation [NSF], 2014 ) for 40 million Americans (20% of all Internet users in the United States), and 87% of users report having searched online about science at least once ( Horrigan, 2006 ). Both youth and adults gather much of their scientific information from online resources like WebMD, social media, and Wikipedia ( Anderson et al. , 2010 ). Social media is increasingly important as a source of scientific information, with 33% of Americans saying this is an important way they get science news ( Funk et al. , 2017 ). Adults who are relatively more frequent users of print and Internet information sources are more likely to be scientifically literate ( Miller, 2010 ). This relationship suggests that learning how to identify high-quality sources of information is an important skill for developing scientific literacy.
While Internet and social media access increase individuals’ access to scientific information, these platforms can also foster the spread of misinformation ( Burki, 2019 ; Smith and Seitz, 2019 ). An analysis of conversations on social media platforms indicated that members discussed more about the politics of vaccine use than the science, resulting in a spread of misinformation (Orr and Basam-Tsabari, 2018). The spread of scientific misinformation can be attributed to two conflicting reasons: Individuals uncritically accept most scientific information, and they reject information that fits outside their worldview (Sharon and Basam-Tsabari, 2020).
Moreover, the research is quite clear that individuals do not use scientific knowledge to make everyday decisions around science-related issues; instead, adults tend to identify and judge appropriate scientific expertise ( Hilton et al. , 2007 ; Ajzen et al. , 2011 ; Kahan et al. , 2012 ; Feinstein, 2014 ; Crowell and Schunn, 2016 ; Shauli and Baram-Tsabari, 2019 ). Consequently, creating opportunities for students to practice identifying and judging appropriate scientific expertise is critical, as this skill is useful for adults ( Feinstein, 2014 ).
While Internet use is ubiquitous, college students struggle to evaluate the relevance and reliability of information found via Web searches ( MaKinster et al. , 2002 ; Brand-Gruwel et al. , 2009 ). In fact, very few Internet users check the source and date of the information they find. Wineberg and McGrew (2017) reported that PhD historians and college students were unable to evaluate credibility of websites and even preferred sources that promoted misinformation. To support our students’ development of information literacy skills, faculty should incorporate opportunities to evaluate students’ use of Internet searches to find scientific information ( Britt and Aglinskas, 2002 ; Walraven et al. , 2009 ). Evaluating credibility becomes more challenging with little knowledge of the topic ( Braten et al. , 2011 ). Faculty can challenge students to move beyond reliance on surface markers, for example, dates of posting and the presence of details and percentages as evidence of accuracy ( Brem et al. , 2001 ). These are critical skills for our students to be capable of critiquing the quality of sources of scientific information, which is a key step in analyzing scientific arguments and evidence.
Looking to student challenges related to scientific literacy skills is also fruitful for curricular development ( Gormally et al. , 2012 ). The ability to recognize and analyze methods of inquiry that lead to scientific knowledge is at the heart of many scientific literacy skills ( Gormally et al. , 2012 ). Students must be able to critique scientific experiments, data, and results to make decisions about the ill-structured problems in their everyday lives. Essentially, students must be capable of analyzing the strength of evidenced-based arguments. However, students have trouble developing claims backed by evidence and reasoning ( Speth et al. , 2010 ), as well as linking the claims to specific evidence ( Cho and Jonassen, 2002 ). Asking students to evaluate media reports of scientific information and use scientific evidence to make decisions are ways to practice evaluating scientific arguments ( Brickman et al. , 2012 ; Rowe et al. , 2015 ). Practicing evaluating arguments helps students to identify sensationalism and oversimplification of study findings often found in media reports ( Tankard and Ryan, 1974 ; Pellechia, 1997 ; Kua et al. , 2004 ), as well as to recognize that media reports often omit study limitations ( Woloshin and Schwartz, 2006 ). Additionally, college students were found to demonstrate stronger, more sophisticated rationales around decisions regarding socioscientific issues when given opportunities to practice skills ( Dauer and Forbes, 2016 ).
It is critical to note that scientifically informed action is driven by more than just scientific literacy alone ( Crowell and Schunn, 2014 ). Individuals’ feelings of personal responsibility to the issue also matter, as well as the specific context, whether one transfers behaviors across contexts, and whether the proposed action or behavior is deemed to be practical ( Crowell and Schunn, 2014 ). And attitudes about scientific advances and innovations are more likely to vary with individuals’ social backgrounds, identities, mental models, and information sources than with knowledge ( Allum et al. , 2008 ).
PREPARING SCIENCE LITERATE NON-SCIENCE MAJORS WITH USEFUL SCIENCE EDUCATION
We argue that future non-science major courses should integrate local socioscientific issues, highlight communal opportunities in science that impact students’ communities, and provide students with opportunities to practice skills necessary to engage with science beyond the classroom to promote science literacy. Recent research reports that courses like this are still the exception, not the norm ( Heil et al. , 2021 ; A. Heil, C. Gormally, M. Brickman, unpublished data). Perhaps most importantly, effective teaching of non-science majors is dependent on the interest and commitment of individual faculty. As a result, progress depends on demanding that effective teaching of non-science majors is institutionalized and recognized as a critical professional standard in science education. Our work points to a need for policy to guide effective teaching for science literacy, such as an expansion of Vision and Change through the operationalization of learning objectives for non–science majors’ courses (M. Brickman and C. Gormally, unpublished data) and resources such as BioSkills ( Clemmons et al. , 2020 ). The development of Vision and Change– informed learning objectives for non–science majors’ courses may be useful for all faculty, and especially faculty working within institutional structures that strictly enforce content coverage due to articulation of courses and accreditation purposes (M. Brickman and C. Gormally, unpublished data). Progress is otherwise doomed if it is dependent on individual faculty heroes.
Currently, we are failing to prepare our students effectively to meet the AAAS (2011) charge to educate our citizens “for civic engagement and responsibility.” STEM majors represent only a fraction of college students. As faculty, we must consider the specific needs of college students who are not STEM majors. We face growing numbers of socioscientific issues: pandemics, vaccine resistance, climate change denial, novel technologies, and advances in genomic medicine. As science educators, we have an urgent obligation to address this pressing problem, so that our non-science majors will become avid consumers of science—using science information to make everyday decisions and, importantly, contributing to larger societal conversations. DeBoer (2000 , p. 598) notes:
Ultimately what we want is a public that finds science interesting and important, who can apply science to their own lives, and who can take part in the conversations regarding science that take place in society. Not everyone will develop the same knowledge and skill but feeling that one can continue to learn and participate are key elements to life in a democratic society.
How we teach undergraduate biology for non-science majors matters. As currently taught, most courses for non-science majors prioritize content coverage rather than making these concepts relevant to students’ lives ( Haffie et al. , 2000 ; Hott et al. , 2002 ; Bowling et al. , 2007 ; Fredeen, 2012 ). Instead, faculty must holistically rethink course structure to prioritize students’ development of science literacy. One current challenge faculty face is the lack of clearly articulated direction for what non-science majors should learn, in terms of concepts and skills. To address this barrier, operationalizing policy documents such Vision and Change into articulated learning objectives describing key concepts and skills students should learn is an important next step currently underway (M. Brickman and C. Gormally, unpublished data). A validated set of learning objectives offers faculty a clear way forward. Evidence-based articulated learning objectives may help reduce the burden for change from individual faculty members, instead supporting systemic intra- and cross-institutional change. At the intra-institutional level, articulated learning objectives describing concepts and skills critical for science literacy would support systemic change so that course sections for non-science majors are aligned.
At the cross-institutional level, adoption of common learning objectives could better support the transition from 2-year to 4-year institutions. Further, support for faculty professional development and curricular development is important for addressing barriers that might affect course reform. Professional development must offer faculty more support than onetime workshop attendance ( Dancy and Henderson, 2010 ; Singer et al. , 2012 ). Organizations such as Science Education for New Civic Engagements and Responsibilities and the Network of STEM Education Centers, as well as research coordination networks, may offer opportunities for deep engagement in faculty learning communities.
Faculty must re-envision courses so that all students have opportunities to grapple with local socioscientific issues that are relevant and meaningful to their lives. And faculty must highlight how biology affords altruistic, communal opportunities in order to engage students who continue to be marginalized in STEM. College students must be equipped with the skills necessary to be scientifically literate, to be the leaders we need, to make decisions in their personal lives, and to contribute to the ever-growing number of decisions we face as a society. We know that exposure to a college science course is a strong predictor of scientific literacy ( Miller, 2016 ). Imagine what we could accomplish collectively if we could raise the bar for numbers of students achieving actionable scientific literacy.
Acknowledgments
We thank Peggy Brickman and Scott Freeman for their thoughtful early reads of our work.
- Ajzen, I., Joyce, N., Sheikh, S., Cote, N. G. (2011). Knowledge and the prediction of behavior: The role of information accuracy in the theory of planned behavior . Basic and Applied Social Psychology , 33 ( 2 ), 101–117. [ Google Scholar ]
- Allen, J. M., Muragishi, G. A., Smith, J. L., Thoman, D. B., Brown, E. R. (2015). To grab and to hold: Cultivating communal goals to overcome cultural and structural barriers in first-generation college students’ science interest . Translational Issues in Psychological Science , 1 ( 4 ), 331. [ PMC free article ] [ PubMed ] [ Google Scholar ]
- Allum, N., Besley, J., Gomez, L., Brunton-Smith, I. (2018). Disparities in science literacy . Science , 360 ( 6391 ), 861–862. [ PubMed ] [ Google Scholar ]
- Allum, N., Sturgis, P., Tabourazi, D., Brunton-Smith, I. (2008). Science knowledge and attitudes across cultures: A meta-analysis . Public Understanding of Science , 17 ( 1 ), 35–54. [ Google Scholar ]
- American Academy of Arts and Sciences. (2019). Encountering science in America . Cambridge, MA. [ Google Scholar ]
- American Association for the Advancement of Science (AAAS). (1990). Science for all Americans . New York: Oxford University Press. [ Google Scholar ]
- AAAS. (1993). Benchmarks for science literacy . New York: Oxford University Press. [ Google Scholar ]
- AAAS. (2011). Vision and change: A call to action . Washington, DC. Retrieved December 15, 2021, from https://visionandchange.org/about -vc-a-call-to-action-2011 [ Google Scholar ]
- Anderson, A. A., Brossard, D., Scheufele, D. A. (2010). The changing information environment for nanotechnology: Online audiences and content . Journal of Nanoparticle Research , 12 ( 4 ), 1083–1094. [ PMC free article ] [ PubMed ] [ Google Scholar ]
- Association of American Colleges and Universities. (2002). Greater expectations: A new vision for learning as a nation goes to college . Washington, DC. [ Google Scholar ]
- Belanger, A. L., Diekman, A. B., Steinberg, M. (2017). Leveraging communal experiences in the curriculum: Increasing interest in pursuing engineering by changing stereotypic expectations . Journal of Applied Social Psychology , 47 ( 6 ), 305–319. [ Google Scholar ]
- Bowers, C. A. (2002). Toward an eco-justice pedagogy . Environmental Education Research , 8 ( 1 ), 21–34. [ Google Scholar ]
- Bowling, B. V., Huether, C. A., Wagner, J. A. (2007). Characterization of human genetics courses for nonbiology majors in US colleges and universities . CBE—Life Sciences Education , 6 ( 3 ), 224–232. [ PMC free article ] [ PubMed ] [ Google Scholar ]
- Brand-Gruwel, S., Wopereis, I., Walraven, A. (2009). A descriptive model of information problem solving while using Internet . Computers & Education , 53 ( 4 ), 1207–1217. [ Google Scholar ]
- Bråten, I., Strømsø, H. I., Salmerón, L. (2011). Trust and mistrust when students read multiple information sources about climate change . Learning and Instruction , 21 ( 2 ), 180–192. [ Google Scholar ]
- Brem, S. K., Russell, J., Weems, L. (2001). Science on the Web: Student evaluations of scientific arguments . Discourse Processes , 32 ( 2–3 ), 191–213. [ Google Scholar ]
- Brickman, P., Gormally, C., Francom, G., Jardeleza, S. E., Schutte, V. G., Jordan, C., Kanizay, L. (2012). Media-savvy scientific literacy: Developing critical evaluation skills by investigating scientific claims . American Biology Teacher , 74 ( 6 ), 374–379. [ Google Scholar ]
- Britt, M. A., Aglinskas, C. (2002). Improving students’ ability to identify and use source information . Cognition and Instruction , 20 ( 4 ), 485–522. [ Google Scholar ]
- Brown, E. R., Thoman, D. B., Smith, J. L., Diekman, A. B. (2015). Closing the communal gap: The importance of communal affordances in science career motivation . Journal of Applied Social Psychology , 45 ( 12 ), 662–673. [ PMC free article ] [ PubMed ] [ Google Scholar ]
- Burki, T. (2019). Vaccine misinformation and social media . Lancet Digital Health , 1 ( 6 ), 258–259. [ Google Scholar ]
- Bybee, R. W. (1993). Reforming science education. Social perspectives & personal reflections . New York, NY: Teachers College, Columbia University. [ Google Scholar ]
- Carlone, H. B., Johnson, A. (2007). Understanding the science experiences of successful women of color: Science identity as an analytic lens . Journal of Research in Science Teaching , 44 ( 8 ), 1187–1218. [ Google Scholar ]
- Cheryan, S., Master, A., Meltzoff, A. N. (2015). Cultural stereotypes as gatekeepers: Increasing girls’ interest in computer science and engineering by diversifying stereotypes . Frontiers in Psychology , 6 , 49. [ PMC free article ] [ PubMed ] [ Google Scholar ]
- Cho, K.-L., Jonassen, D. H. (2002). The effects of argumentation scaffolds on argumentation and problem solving . Educational Technology Research and Development , 50 ( 3 ), 5. [ Google Scholar ]
- Clemmons, A. W., Timbrook, J., Herron, J. C., Crowe, A. J. (2020). BioSkills guide: Development and national validation of a tool for interpreting the vision and change core competencies . CBE—Life Sciences Education , 19 ( 4 ), ar53. [ PMC free article ] [ PubMed ] [ Google Scholar ]
- Colvin, W., Lyden, S., León de la Barra, B. A. (2013). Attracting girls to civil engineering through hands-on activities that reveal the communal goals and values of the profession . Leadership and Management in Engineering , 13 ( 1 ), 35–41. [ Google Scholar ]
- Cook, M., Mulvihill, T. M. (2008). Examining US college students attitudes towards science: Learning from non-science majors . Educational Research and Reviews , 3 ( 1 ), 038–047. [ Google Scholar ]
- Cotner, S., Thompson, S., Wright, R. (2017). Do biology majors really differ from non–STEM majors? CBE—Life Sciences Education , 16 ( 3 ), ar48. [ PMC free article ] [ PubMed ] [ Google Scholar ]
- Crowell, A., Schunn, C. (2014). Scientifically literate action: Key barriers and facilitators across context and content . Public Understanding of Science , 23 ( 6 ), 718–733. [ PubMed ] [ Google Scholar ]
- Crowell, A., Schunn, C. (2016). Unpacking the relationship between science education and applied scientific literacy . Research in Science Education , 46 ( 1 ), 129–140. [ Google Scholar ]
- Dancy, M., Henderson, C. (2010). Pedagogical practices and instructional change of physics faculty . American Journal of Physics , 78 , 1056–1063. [ Google Scholar ]
- Dauer, J. M., Forbes, C. (2016). Making decisions about complex socioscientific issues: A multidisciplinary science course . Science Education and Civic Engagement , 8 , 5–12. [ Google Scholar ]
- DeBoer, G. E. (2000). Scientific literacy: Another look at its historical and contemporary meanings and its relationship to science education reform . Journal of Research in Science Teaching , 37 ( 6 ), 582–601. [ Google Scholar ]
- d’I Treen, K. M., Williams, H. T. P., O’Neill, S.J. (2019). Online misinformation about climate change . WIRES Climate Change , 11 , e665. doi: 10.1002/wcc.665 [ Google Scholar ]
- Diekman, A. B., Brown, E. R., Johnston, A. M., Clark, E. K. (2010). Seeking congruity between goals and roles: A new look at why women opt out of science, technology, engineering, and mathematics careers . Psychological Science , 21 ( 8 ), 1051–1057. [ PubMed ] [ Google Scholar ]
- Ede, A. (2000). Has science education become an enemy of scientific rationality? Skeptical Inquirer , 24 ( 4 ), 48–48. [ Google Scholar ]
- Falk, J. H., Storksdieck, M., Dierking, L. D. (2007). Investigating public science interest and understanding: Evidence for the importance of free-choice learning . Public Understanding of Science , 16 ( 4 ), 455–469. [ Google Scholar ]
- Feinstein, N. (2011). Salvaging science literacy . Science Education , 95 ( 1 ), 168–185. 10.1002/sce.20414 [ CrossRef ] [ Google Scholar ]
- Feinstein, N. W. (2014). Making sense of autism: Progressive engagement with science among parents of young, recently diagnosed autistic children . Public Understanding of Science , 23 ( 5 ), 592–609. [ PubMed ] [ Google Scholar ]
- Fredeen, D. A. (2012). Weaving a tapestry of change: Implementing SENCER on campus . In Sheardy, R., Burns, W. D. (Eds.), Science education and civic engagement: The next level (pp. 31–54). Washington, DC: ACS Publications. [ Google Scholar ]
- Funk, C., Gottfried, J., Mitchell, A. (2017). Science news and information today . Washington, DC: Pew Research Center. Retrieved on December 14, 2021, from www.journalism.org/2017/09/20/science-news-and-information-today [ Google Scholar ]
- Funk, C., Rainie, L., Page, D. (2015). Public and scientists’ views on science and society . Washington, DC: Pew Research Center. Retrieved December 15, 2021, from www.pewresearch.org/science/2015/01/29/public-and-scientists-views-onscience [ Google Scholar ]
- General education, finally defined. (2007, March–April 2007). Harvard Magazine . Retrieved December 10, 2021, from https://harvardmagazine.com/2007/03/general-education-finall.html#:∼:text=General%20education%20is%20the%20place,public%20face%20of%20liberal%20education.%E2%80%9D [ Google Scholar ]
- Glynn, S. M., Brickman, P., Armstrong, N., Taasoobshirazi, G. (2011). Science motivation questionnaire II: Validation with science majors and nonscience majors . Journal of Research in Science Teaching , 48 ( 10 ), 1159–1176. [ Google Scholar ]
- Goodstein, D. (1992). The science literacy gap: A Karplus lecture . Journal of Science Education and Technology , 1 ( 3 ), 149–155. [ Google Scholar ]
- Gormally, C., Brickman, P., Lutz, M. (2012). Developing a test of scientific literacy skills (TOSLS): Measuring undergraduates’ evaluation of scientific information and arguments . CBE—Life Sciences Education , 11 ( 4 ), 364–377. [ PMC free article ] [ PubMed ] [ Google Scholar ]
- Gormally, C., Inghram, R. (2021). Goggles and white lab coats: Students’ perspectives on scientists and the continued need to challenge stereotypes . Journal of Microbiology & Biology Education , 22 ( 1 ), ev22i1–2273. [ PMC free article ] [ PubMed ] [ Google Scholar ]
- Gormally, C. L., Marchut, A. (2017). “Science is not my thing”: Exploring deaf non-science majors’ science identities . Journal of Science Education for Students with Disabilities , 20 ( 1 ), 1–15. [ Google Scholar ]
- Haffie, T. L., Reitmeier, Y. M., Walden, D. B. (2000). Characterization of university-level introductory genetics courses in Canada . Genome , 43 ( 1 ), 152–159. [ PubMed ] [ Google Scholar ]
- Hazari, Z., Sadler, P. M., Sonnert, G. (2013). The science identity of college students: Exploring the intersection of gender, race, and ethnicity . Journal of College Science Teaching , 42 ( 5 ), 82–91. [ Google Scholar ]
- Hazen, R. M. (2002). Why should you be scientifically literate? Herndon, VA: American Institute of Biological Sciences. [ Google Scholar ]
- Hebert, S., Cotner, S. (2019). A comparison of nonmajors’ & majors’ incoming science process skills . American Biology Teacher , 81 ( 8 ), 554–560. [ Google Scholar ]
- Heil, A. D., Brickman, M., Gormally, C. (2021, July). What do faculty want non-majors to know? Characterizing the content, skills, and stated learning objectives from non-major syllabi . Paper presented at: Society of Advancement in Biology Education Research Conference (conference virtual due to COVID precautions). [ Google Scholar ]
- Heil, A. D., Gormally, C., Brickman, P. (in press). Low-level learning: Leaving behind non-science majors . Journal of College Science Teaching . [ Google Scholar ]
- Hewitt, K. M., Bouwma-Gearhart, J., Kitada, H., Mason, R., Kayes, L. J. (2019). Introductory biology in social context: The effects of an issues-based laboratory course on biology student motivation . CBE—Life Sciences Education , 18 ( 3 ), ar30. [ PMC free article ] [ PubMed ] [ Google Scholar ]
- Hilton, S., Hunt, K., Petticrew, M. (2007). Gaps in parental understandings and experiences of vaccine-preventable diseases: A qualitative study . Child Care, Health and Development , 33 ( 2 ), 170–179. [ PubMed ] [ Google Scholar ]
- Horrigan, J. B. (2006). The Internet as a resource for news and information about science . Washington, DC: Pew Research Center. Retrieved December 15, 2021, from www.pewresearch.org/internet/2006/11/20/the-internet-as-a-resource-for-news-and-information-about-science [ Google Scholar ]
- Hott, A. M., Huether, C. A., McInerney, J. D., Christianson, C., Fowler, R., Bender, … & Karp, R. (2002). Genetics content in introductory biology courses for non-science majors: Theory and practice . BioScience , 52 ( 11 ), 1024–1035. [ Google Scholar ]
- Johnson, A. C. (2007). Unintended consequences: How science professors discourage women of color . Science Education , 91 ( 5 ), 805–821. [ Google Scholar ]
- Johnson, M., Pigliucci, M. (2004). Is knowledge of science associated with higher skepticism of pseudoscientific claims? American Biology Teacher , 66 ( 8 ), 536–548. [ Google Scholar ]
- Kahan, D. M., Peters, E., Wittlin, M., Slovic, P., Ouellette, L. L., Braman, D., Mandel, G. (2012). The polarizing impact of science literacy and numeracy on perceived climate change risks . Nature Climate Change , 2 ( 10 ), 732–735. [ Google Scholar ]
- Knight, J. K., Smith, M. K. (2010). Different but equal? How nonmajors and majors approach and learn genetics . CBE—Life Sciences Education , 9 ( 1 ), 34–44. [ PMC free article ] [ PubMed ] [ Google Scholar ]
- Kua, E., Reder, M., Grossel, M. J. (2004). Science in the news: A study of reporting genomics . Public Understanding of Science , 13 ( 3 ), 309–322. [ Google Scholar ]
- Kuhn, D. (1993). Science as argument: Implications for teaching and learning scientific thinking . Science Education , 77 ( 3 ), 319–337. [ Google Scholar ]
- Maienschein, J. (1998). Scientific literacy . Science , 281 ( 5379 ), 917. [ Google Scholar ]
- MaKinster, J. G., Beghetto, R. A., Plucker, J. A. (2002). Why can’t I find Newton’s third law? Case studies of students’ use of the Web as a science resource . Journal of Science Education and Technology , 11 ( 2 ), 155–172. [ Google Scholar ]
- Millar, R., Osborne, J., Nott, M. (1998). Science education for the future . School Science Review , 80 ( 291 ), 19–24. [ Google Scholar ]
- Miller, J. D. (1998). The measurement of civic scientific literacy . Public Understanding of Science , 7 ( 3 ), 203. [ Google Scholar ]
- Miller, J. D. (2004). Public understanding of, and attitudes toward, scientific research: What we know and what we need to know . Public Understanding of Science , 13 ( 3 ), 273–294. [ Google Scholar ]
- Miller, J. D. (2010). The conceptualization and measurement of civic scientific literacy for the twenty-first century. Science and the educated American: A core component of liberal education (pp. 241–255). Cambridge, MA: American Academy of Arts & Sciences. [ Google Scholar ]
- Miller, J. D. (2016). Civic scientific literacy in the United States in 2016 . Ann Arbor, MI: International Center for the Advancement of Scientific Literacy. [ Google Scholar ]
- Miller, M. C. D., Montplaisir, L. M., Offerdahl, E. G., Cheng, F.-C., Ketterling, G. L. (2010). Comparison of views of the nature of science between natural science and nonscience majors . CBE—Life Sciences Education , 9 ( 1 ), 45–54. [ PMC free article ] [ PubMed ] [ Google Scholar ]
- Mulkay, M. (1997). Book review of: Misunderstanding science? The public reconstruction of science and technology . Science, Technology, and Human Values , 22 ( 2 ), 254–264. [ Google Scholar ]
- National Academy of Sciences, National Academy of Engineering, and Institute of Medicine. (2007). Rising above the gathering storm: Energizing and employing America for a brighter economic future . Washington, DC: National Academies Press. 10.17226/11463 [ CrossRef ] [ Google Scholar ]
- National Center for Education Statistics (NCES). (2013). Digest of education statistics, 2012 (NCES 2014-015) . Washington, DC. [ Google Scholar ]
- NCES. (2021). Digest of education statistics, 2019 (NCES 2021-009; chap. 3). Washington, DC. [ Google Scholar ]
- National Research Council. (1996). National Science Education Standards , Washington, DC: National Academies Press. [ Google Scholar ]
- National Science Foundation. (2014). Science and engineering indicators . Arlington, VA. [ Google Scholar ]
- Opitz, A., Heene, M., Fischer, F. (2017). Measuring scientific reasoning–a review of test instruments . Educational Research and Evaluation , 23 ( 3–4 ), 78–101. [ Google Scholar ]
- Organization for Economic Co-operation and Development. (2003). The PISA 2003 assessment framework—mathematics, reading, science and problem solving knowledge and skills . Paris, France: OECD
- Orr, D., Baram-Tsabari, A. (2018). Science and politics in the polio vaccination debate on Facebook: A mixed-methods approach to public engagement in a science-based dialogue . Journal of Microbiology & Biology Education , 19 ( 1 ). 19.11. 80. [ PMC free article ] [ PubMed ] [ Google Scholar ]
- Pellechia, M. G. (1997). Trends in science coverage: A content analysis of three US newspapers . Public Understanding of Science , 6 ( 1 ), 49. [ Google Scholar ]
- Puri, N., Coomes, E. A., Haghbayan, H., Gunaratne, K. (2020). Social media and vaccine hesitancy: New updates for the era of COVID-19 and globalized infectious diseases . Human Vaccines & Immunotherapeutics , 16 ( 11 ), 2586–2593. doi: 10.1080/21645515.2020.1780846 [ PMC free article ] [ PubMed ] [ Google Scholar ]
- Rowe, M. P., Gillespie, B. M., Harris, K. R., Koether, S. D., Shannon, L.-J. Y., Rose, L. A. (2015). Redesigning a general education science course to promote critical thinking . CBE—Life Sciences Education , 14 ( 3 ), ar30. [ PMC free article ] [ PubMed ] [ Google Scholar ]
- Sadler, T. D. (2004). Informal reasoning regarding socioscientific issues: A critical review of research . Journal of Research in Science Teaching , 41 ( 5 ), 513–536. [ Google Scholar ]
- Sargent, J. F., Jr. (2017). The US science and engineering workforce: Recent, current, and projected employment, wages, and unemployment. Congressional Research Service report prepared for members and committees of Congress . Washington, DC: Congressional Research Service. Retrieved September 3, 2022, from http://www.fas.org/sgp/crs/misc/R43061.pdf [ Google Scholar ]
- Schaeffer, K. (2020, July 24) A look at the Americans who believe there is some truth to the conspiracy theory that COVID-19 was planned . Washington, DC: Pew Research Center. Retrieved September 3, 2022, from https://www.pewresearch.org/fact-tank/2020/07/24/a-look-at-the-americans-who-believe-there-is-some-truth-to-the-conspiracy-theory-that-covid-19-was-planned/ [ Google Scholar ]
- Schinske, J., Cardenas, M., Kaliangara, J. (2015). Uncovering scientist stereotypes and their relationships with student race and student success in a diverse, community college setting . CBE—Life Sciences Education , 14 ( 3 ), ar35. [ PMC free article ] [ PubMed ] [ Google Scholar ]
- Schinske, J. N., Perkins, H., Snyder, A., Wyer, M. (2016). Scientist spotlight homework assignments shift students’ stereotypes of scientists and enhance science identity in a diverse introductory science class . CBE—Life Sciences Education , 15 ( 3 ), 1–18. [ PMC free article ] [ PubMed ] [ Google Scholar ]
- Seymour, E., Hewitt, N. M. (1997). Talking about leaving . Boulder, CO: Westview. [ Google Scholar ]
- Shaffer, J. F., Ferguson, J., Denaro, K. (2019). Use of the Test of Scientific Literacy Skills reveals that fundamental literacy is an important contributor to scientific literacy . CBE—Life Sciences Education , 18 ( 3 ), ar31. [ PMC free article ] [ PubMed ] [ Google Scholar ]
- Sharon, A. J., Baram-Tsabari, A. (2020). Can science literacy help individuals identify misinformation in everyday life? Science Education , 104 ( 5 ), 873–894. [ Google Scholar ]
- Shauli, S., Baram-Tsabari, A. (2019). The usefulness of science knowledge for parents of hearing-impaired children . Public Understanding of Science , 28 ( 1 ), 19–37. [ PubMed ] [ Google Scholar ]
- Singer, S. R., Nielsen, N. R., Schweingruber, H. A. (2012). Discipline-based education research: Understanding and improving learning in undergraduate science and engineering . Washington, DC: National Academies Press. [ Google Scholar ]
- Smith, C. N., Seitz, H. H. (2019). Correcting misinformation about neuroscience via social media . Science Communication , 41 ( 6 ), 790–819. [ Google Scholar ]
- Southwell, B. G., Babwah Brennen, J. S., Paquin, R., Boudewyns, V., Zeng, J. (2022). Defining and measuring scientific misinformation . Annals of the American Academy of Political and Social Science , 700 ( 1 ), 98–111. doi: 10.1177/00027162221084709 [ Google Scholar ]
- Speth, E. B., Momsen, J. L., Moyerbrailean, G. A., Ebert-May, D., Long, T. M., Wyse, S., Linton, D. (2010). 1, 2, 3, 4: Infusing quantitative literacy into introductory biology . CBE—Life Sciences Education , 9 ( 3 ), 323–332. [ PMC free article ] [ PubMed ] [ Google Scholar ]
- Steinberg, M., Diekman, A. B. (2018). Considering “why” to engage in STEM activities elevates communal content of STEM affordances . Journal of Experimental Social Psychology , 75 , 107–114. [ Google Scholar ]
- Suarez-Lledo, V., Alvarez-Galvez, J. (2021). Prevalence of health misinformation on social media: systematic review . Journal of Medical Internet Research , 23 ( 1 ), e17187. doi: 10.2196/17187 [ PMC free article ] [ PubMed ] [ Google Scholar ]
- Tankard, J. W., Jr., Ryan, M. (1974). News source perceptions of accuracy of science coverage . Journalism Quarterly , 51 ( 2 ), 219–225. [ Google Scholar ]
- U.S. Census Bureau. (2019). U.S. Census Bureau releases new educational attainment data . Retrieved December 15, 2021, from www.census.gov/newsroom/press-releases/2020/educational-attainment.html
- Waldo, J. T. (2014). Application of the test of scientific literacy skills in the assessment of a general education natural science program . Journal of General Education , 63 ( 1 ), 1–14. [ Google Scholar ]
- Walker, W. R., Hoekstra, S. J., Vogl, R. J. (2002). Science education is no guarantee of skepticism . Skeptic (Altadena, CA) , 9 ( 3 ), 24–29. [ Google Scholar ]
- Walraven, A., Brand-Gruwel, S., Boshuizen, H. P. (2009). How students evaluate information and sources when searching the World Wide Web for information . Computers & Education , 52 ( 1 ), 234–246. [ Google Scholar ]
- Weasel, L. H., Finkel, L. (2016). Deliberative pedagogy in a nonmajors biology course: Active learning that promotes student engagement with science policy and research . Journal of College Science Teaching , 45 ( 4 ), 38–45. [ Google Scholar ]
- West, J. D., Bergstrom, C. T. (2021). Misinformation in and about science . Proceedings of the National Academy of Science USA , 118 ( 15 ), e1912444117. 10.1073/pnas.1912444117 [ PMC free article ] [ PubMed ] [ CrossRef ] [ Google Scholar ]
- Wineburg, S., McGrew, S. (2017). Lateral reading: Reading less and learning more when evaluating digital information (Stanford History Education Group Working Paper No. 2017-A1) . Retrieved December 15, 2021, from https://ssrn.com/abstract=3048994
- Woloshin, S., Schwartz, L. M. (2006). Giving legs to restless legs: A case study of how the media helps make people sick . PLoS Medicine , 3 ( 4 ), e170. [ PMC free article ] [ PubMed ] [ Google Scholar ]
- Open access
- Published: 29 April 2024
Curriculum-based sexual and reproductive health education: revealing its relevance for risky sexual behaviors among secondary school students in Mwanza, Tanzania
- Ng’wamba Sitta Ngissa 1 ,
- Erica Sanga 1 ,
- Mussa Kelvin Nsanya 1 ,
- Belinda Kweka 1 ,
- Evangelista Malindisa 1 , 2 &
- Rosemarie Mwaipopo 3
Reproductive Health volume 21 , Article number: 58 ( 2024 ) Cite this article
Metrics details
Secondary school students are vulnerable to risky sexual behaviors (RSBs) which may lead to adverse health consequences, such as teenage pregnancies and sexually transmitted diseases (STDs), including HIV/AIDS. In Tanzania, the burden of teenage pregnancy was reported to be 27% in 2016. The integration of sexual and reproductive health (SRH) education into the school curriculum is one of the proven crucial interventions. However, there is limited information on the extent to which curriculum-based SRH education is relevant for fostering good practices for coping with RSBs. This study sought to describe students’ and teachers’ perceptions of the relevance of curriculum-based SRH education.
A qualitative study was conducted from May to June 2020 (involving 5 secondary schools in Ilemela district, Mwanza, Tanzania). In-depth interviews (30) were conducted among secondary school students and 10 interviews for teachers. The data were collected in Swahili and then transcribed and translated into English after which thematic content analysis was performed.
The majority (56%) of secondary school students were revealed to have a limited understanding of curriculum-based SRH education, which was limited to a few aspects of health that involved married people and pregnant women. Teachers of different subjects had different perceptions about the relevance of curriculum-based SRH education. Civics teachers had the perception that it was relevant and enough, while Biology teachers thought that it was not enough. Students reported utilizing the information taught in class to manage and navigate RSBs. Moreover, they expressed a need for additional delivery strategies to be used for a comprehensive understanding of sexual and reproductive health.
Despite the identified gaps in providing a comprehensive knowledge that builds on the appropriate attitudes and skills, the existing curriculum-based SRH education in secondary schools was utilized to help students in addressing and managing RSBs. However, there is a need for more comprehensive information and an improved delivery approach for SRH to equip students with the necessary skills when faced with RSBs.
Plain English summary
Sexual and reproductive health (SRH) education integrated into school curricula has proven effective in addressing risky sexual behaviors (RSBs) among in-school adolescents. Adolescents attending secondary schools, are susceptible to RSBs such as early sexual activity, multiple partners, unprotected intercourse, and unsafe abortions, leading to adverse health outcomes such as teenage pregnancies and HIV/AIDS. Despite the integration of SRH education into secondary school syllabi, these behaviors are still evident among students. There is a lack of data on the impact and relevance of curriculum-based SRH education on RSBs. To explore the relevance and effectiveness of SRH education in addressing RSB patterns, in-depth interviews were conducted among secondary school students and civics and biology teachers focusing on their perceptions and practical outcomes of SRH education in reducing RSBs. After conducting a thematic content analysis three main themes were discovered: (1) Student’s understanding of curriculum-based SRH education in coping with RSBs (2), Perceptions of curriculum-based SRH education in coping with RSBs and (3) Utilizing curriculum-based SRH education in coping with RSBs. Majority of secondary school students (56%) had a limited understanding of SRH narrowed to aspects concerning married individuals and pregnancy. Teachers provided varying opinions regarding the efficacy of curriculum-based SRH education, with Civics instructors believing there is relevance while Biology teachers considered it limited. Students acknowledged using the available knowledge to address RSBs but expressed a desire for supplementary strategies to achieve a more thorough understanding of SRH. Improving the SRH education provided in schools will help reduce the consequences of RSBs.
Peer Review reports
Introduction
Sexual and reproductive health (SRH) education integrated into the school curriculum is an effective intervention for in-school adolescents before risky sexual behaviors (RSBs) are firmly established [ 1 , 2 , 3 ]. Adolescents aged 10–19 years are estimated to constitute 16% and 23% of the global population and the Tanzania population, respectively, with 90% of them living in low-middle-income countries (LMICs) [ 4 , 5 , 6 , 7 ]. Adolescents are vulnerable to RSBs, including having sex at an early age, having multiple sexual partners, having sex while under the influence of alcohol or drugs, having unprotected sex, and having unsafe abortions [ 8 , 9 ]. Such behaviors may lead to adverse health consequences, such as teenage pregnancies and sexually transmitted diseases (STDs), including HIV/AIDS. For instance, in 2010 and 2016, an increase in teenage childbearing from 23% in 2010 to 27% in 2016 was reported in Tanzania [ 10 ].
Curriculum-based SRH education encompasses the contents taught in the classroom following the guidelines set forth by the secondary school syllabus prepared by the Tanzania Institute of Education (TIE). It covers various topics such as anatomy, physiology, reproductive processes, contraception, sexually transmitted infections (STIs) and healthy relationships. The curriculum-based approach ensures that SRH education is systematically integrated into academic programs, typically in secondary schools or higher education institutions. The importance of the provision of SRH education in the school setting has affected youths from a global, national and individual level in reducing the risks associated with the practice of RSBs. Schools may often be the only place where adolescents can obtain accurate information on reproductive health [ 11 ].
In 2005, TIE issued a secondary school syllabus in which sexuality content was integrated and offered along with other subjects, such as Home Economics, Biology, General Studies, and Civics, in secondary schools [ 12 ]. The syllabus content mainly focused on human reproductive anatomy, conception, sexually infectious diseases, risk behaviors, and communication skills [ 13 ]. For instance, Biology mainly focuses on general health and well-being when it comes to SRH while Civics covers the women’s role in society, economy, laws and policies relating to reproduction and reproductive health services [ 13 , 14 , 15 ]. Evidence shows that integrating comprehensive SRH education into secondary school curricula could play a vital role in enhancing adolescents’ understanding of sexuality and addressing their reproductive health concerns [ 16 ].
Despite the efforts invested in by TIE, secondary school students still practice RSBs [ 17 ]. For instance, in recent years, Mwanza city has consecutively ranked first in Tanzania, with the relatively highest proportion of secondary school dropouts due to pregnancy being 10.8% and 9% in 2018 and 2019, respectively [ 18 ]. Information regarding the impact of curriculum-based SRH education in secondary schools is not well documented, and data regarding perceptions of curriculum-based SRH education provided to secondary school attending adolescents are scarce [ 1 ]. In addition, local data on the extent to which curriculum-based SRH education has translated to reducing RSBs are scarce.
The aim of this study was therefore to examine the relevance of curriculum-based SRH education for RSB patterns among secondary school students in Mwanza, Tanzania. Specifically, we aimed to assess secondary school students’ and teachers’ perceptions of curriculum-based SRH education and how curriculum-based SRH education has helped students cope with RSBs.
Research design and study area
A qualitative cross-sectional study was conducted from May to June 2020 in the Ilemela District of the Mwanza region in northwestern Tanzania. Ilemela district is located between latitude 2.4485 0 South and 32.9663 0 East in Mwanza region in north-western Tanzania. The district is administratively divided into 19 wards with a human population of 480,779 and a population density of 1,347 per km 2 with an annual growth rate of 2.6%, and there are 50 secondary schools. According to Basic Statistics Tanzania (2019), the number of secondary school students in Ilemela District secondary schools was estimated to be 31,124. A qualitative approach was appropriate for capturing students’ and teachers’ perceptions of curriculum-based SRH education and examining how such education has helped students cope with RSBs. To clarify the relevance of the existing patterns of RSBs, this study involved selected secondary schools in Ilemela District.
Selection of schools
We purposively selected 5 secondary schools, which included public/privately owned, day/boarding, and single-sex/coeducation schools. This approach aimed to understand the perceptions of curriculum-based SRH education and coping strategies of RSBs among students with diverse backgrounds.
Selection of classes
The target population for this study was Form III and IV secondary school students who provided information on how curriculum-based SRH helps them make proper decisions when dealing with RSBs. Secondary school students at these class levels already have more than 50% of their required study years (4 years) and hence have great coverage in subjects.
Selection of students
In each school, 6 (3 males and 3 females or 6 students in single-sex schools) secondary school students were invited for interviews. Interviewed secondary school students were obtained by randomly selecting males and females from Form III and Form IV classes. A total of 30 students from 5 secondary schools in the Ilemela District (Mwanza) were invited to participate in this study.
Selection of teachers
We purposively selected and interviewed a total of 10 civics and biology teachers (2 from each school). We chose these two subjects because they had content for curriculum-based SRH education. Hence, teachers provided information on what the curriculum comprised about the coverage of SRH education curriculum content and how it was supposed to be delivered.
Data collection procedures
We separately conducted a total of 40 in-depth interviews among students (30 interviews) and 10 among teachers using interview guides, checklists, and tape recorders. The interview guides were prepared in the English language and subsequently translated to the Swahili language, which is the native language for most of the participants. The average time taken for interviews with students and teachers was 30 min. To ensure that the translated interview guide had the same meaning in both languages, it was translated back into English and still had the same meanings. Two research assistants proficient in conducting interviews, interviewed the study participants in Swahili language which is a native language for most of the participants. To ensure validity, the interview guides were pre-tested before the data collection procedures. Interviewers reviewed what the participants said to ensure clarification of anything unclear or ambiguous.
Data analysis procedures
The analysis and interpretation of findings were based on three broad themes. Under each theme, several subthemes emerged, as shown in Table 1 below.
Ethical consideration
Ethical clearance was issued by The University of Dar es Salaam with reference number AB3/12(B). Additionally, the Ilemela District municipality provided a permit to conduct this study in the selected secondary schools. Provided that curriculum-based SRH education is a sensitive issue in the Tanzanian context, throughout this study, the investigators were careful in verbal and nonverbal language to avoid any physical or emotional harm. Thus, participants were well informed in advance of the research problem under investigation and were told that they were free to participate or withdraw from the study at any time. Legal guardians/parents of participants below the age of 18 were given written informed consent. Assent was obtained from participants below the age of 18 before interviews. Prior to conducting the interviews, participants were briefed on the benefits of this research for the education sector and youth well-being. Participants were also reassured that participating in the interviews posed no risks. Moreover, participants received additional information regarding questions related to SRH and RSBs after the interviews were completed. Verbal and written consent from all study participants was sought and participants consented to the publishing of their responses anonymously. Only participants who consented to participate in the study were included. Confidentiality was also observed, as were cultural values, traditions, and taboos.
Students’ understanding of curriculum-based SRH education in coping with risky sexual behaviors
Understanding of curriculum-based srh education content.
Many of the respondents (female and male students) gave vague ideas on what they knew about SRH. In the interviews, SRH was described from various perspectives based on the type of school the respondent attended. One of the respondents stated:
“Sexual and Reproductive Health is a state where both men and women have awareness and well-being on their health in regards to their sexual and reproductive health. For example, people may be able to treat and protect themselves from diseases affecting the reproductive system.” (Male, Form IV student, private boarding coeducation school)
However, many of the female students were shy about providing their understanding of SRH which could be attributed to culture and norms, as some respondents admitted that they had no idea what SRH was. As one of them said.
“..I am truly not sure what SRH is…” (Female, Form III student, private day coeducation school)
Another subtheme on the meaning and awareness of SRH that was mentioned was the issue of pregnancy and family planning, which are associated with the issues that are involved in SRH education. This was a common response in single-sex schools, as one of the male respondents indicated that SRH is a matter that involves women.
“It is the health during and after pregnancy that helps women to have a safe delivery.” (Male, Form IV student, public boarding single-sex school)
Understanding of risky sexual behaviors contents
The majority of respondents, especially those from single-sex boarding schools, reported that RSBs are behaviors that, when practiced, may negatively impact the SRH of an individual. For students who had information on what RSBs are in particular, they would identify them in general what they are, while others could give examples of what they regard as RSBs. For instance, the respondent would say,
“…behaviors such as having sex while at school, not using a condom and having sexual relationships with many girls for boys and many boys for girls are RSBs to a student” (Female, Form IV students, private boarding single-sex school).
“…they are dangerous behaviors that lead to health disorders, particularly those of the reproductive system.” (Male, Form III student, public boarding single-sex school).
However, a few participants agreed that they knew what RSBs are but gave meaning that indicated unawareness of the related meaning of RSBs. A respondent in one of the schools said:
“These are behaviors that are done without one’s awareness.” (Male, Form IV student, Public day coeducation school)
Teachers’ perceptions of the relevance of curriculum-based SRH education for RSBs
Civics and Biology teachers also gave their opinions on whether curriculum-based SRH is relevant to students’ SRH knowledge and whether it enables them to cope with RSBs. Most of the Civics teachers explained that content coverage is sufficient to equip students with better decision-making about RSBs. The respondent (civics teacher) insisted that:
“…based on their levels, the information is quite enough for this level, and as they keep advancing to other levels more will be revealed depending on the type of setting, they will be in, but in this context, the information is enough and helpful.” (Female, Civics Teacher, private-boarding single-sex school)
The majority of biology teachers expressed that the information was too general to have any effect on RSB practices. One of the respondents (a biology teacher) replied,
“It is a sensitive topic, but the education is provided to accomplish the syllabus requirements. With such general information, it is not possible to affect a large number of students.” (Female, Biology Teacher, private-boarding single-sex school)
Teachers’ perceptions of the suitability of subjects with SRH contents for addressing RSBs
In interview sessions with civics and biology teachers, various opinions were given about whether the issues covered were suitable for providing students with knowledge of their SRH and changing their patterns of RSB practices. The relevance of these issues seems to vary among teachers in public and private schools.
“… all that is covered in Civics makes a great contribution to students since the provided guidance on teaching such issues makes it easier for teachers to deliver the information with relevant pieces of evidence from the media and everyday events.” (Male, Civics Teacher, Public boarding single-sex school)
On the other hand, most teachers in private schools believe that more needs to be done for the messages to be easy to understand; additionally, they suggest the following:
“Speaking of their relevance, I think it is quite complicated, as the contents seem to be overwhelming to students. Biology is quite broad, so I cannot guarantee that the issues covered are relevant enough to make an impact on their life in general. The general concepts including the human reproductive system, genetics, and the menstrual cycle… I would not say that are relevant as the content is not detailed enough for them to utilize the entire information related to RSBs.” (Male, Civics Teacher, private boarding single-sex school)
Utilizing curriculum-based SRH education in coping with RSBs
Students’ application of curriculum-based srh education.
Practical application of curriculum-based SRH education was revealed to contribute to some aspects of pregnancy and STDs only. Students expressed their fears for the outcomes associated with practicing RSBs. The respondents specified that.
“From what I understood in class, the effects of having bad behaviours (referring to RSBs ) may lead to pregnancy and diseases that may take a long time to cure. …. especially the issue of pregnancy at this age is what scares me the most.” (Female, Form IV student, private day coeducation school)
Another respondent added the following:
… we understand the outcomes of RSBs, and most of them may completely change one’s life. I am more interested when we are told of examples that make me think about my circumstances and I end up not doing it though sometimes it is hard to escape some mob influences. (Male, Form IV student, public boarding single-sex school)
Influence of teaching methods in establishing relevance to RSB outcomes
Teachers use various means to help deliver messages about SRH and show its relevance to RSB outcomes. One of the respondents (Biology Teacher) stated:
“…being friendly to students helps facilitate open discussions on issues that are myths received from other sources such as their fellow peers. As I teach, students are so cooperative in regard to the reproduction topic; thus, the issues I address are well understood. (Female, Biology Teacher, private boarding coeducation school)
On the other hand, the use of practical illustrations was mentioned by respondents as effective in delivering SRH education. The respondent (Civics Teacher) said,
“…sometimes diagrams in textbooks are not enough to explain how the RSBs can have negative consequences on one’s life. I prefer using references from life stories and drawing lessons from movies such as that of “Yellow Card” to help them have a clear picture of the effects of RSBs.” (Male, Civics Teacher, Private day coeducation school)
When asked about how education on SRH is given to students, students are informed about how their teacher delivers such information. Some responded that their teachers use pictures/diagrams once in a while film was used and that discussions involved questions and answers. One of the respondents specified,
“In Civics, our teacher uses live stories from the community emphasizing how we should apply life skills. (Female, Form IV student, Public day coeducation school)
In another school where some respondents were also interviewed, they explored how they were taught about the effects of SRH and RSBs. The respondent stated,
The teacher just talks by providing explanations following what is written in textbooks. (Male, Form III student, Public day coeducation school)
Secondary school students believed that the information provided by trained facilitators from nongovernmental organizations (NGOs), such as FEMA, on SRH matters is different from that provided in class. In classroom lessons, curriculum-based SRH education is delivered following what the syllabi instructs, while the information given by trained facilitators from NGOs from various programmes visiting the school consists of extensive information. Among the respondents providing this information, one mentioned the following:
“…in class, some teachers are open enough, though when experts visit, they are more open to giving further information, and most students get comfortable as they are friendly and have no exam requirements from such programs.” (Female, Form III student, private boarding coeducation school)
This study examined the relevance of curriculum-based SRH education for coping with RSBs among secondary school students in Mwanza, Tanzania. The findings reveal gaps that reduce the relevance of curriculum-based SRH education. Culture and how SRH knowledge is delivered plays a significant role in shaping the approach to RSBs. The curriculum’s relevance primarily influences student and teacher perceptions of utilizing SRH education to address RSBs.
We have revealed that knowledge of curriculum-based SRH education is limited to a few aspects, such as family planning, pregnancy issues, and topics related to women or married individuals. The explanations given were observed among most male students, especially those in single-sex schools. Students in single-sex schools were revealed to have a limited understanding which is limited to matters that mostly involve women e.g., pregnancy, childbearing and family planning. This limited understanding of SRH leaves young people exposed and vulnerable to reproductive health consequences, thus emphasizing the need for comprehensive education that can influence behavioural changes [ 19 ]. The limited understanding can be attributed to unfriendliness among teachers and students since teachers can be perceived as critical to students’ issues. This would lead to resorting to wrong sources of information on sensitive issues that involve their SRH. More effort is to be made in combating RSBs among students by encouraging better communication between teachers and students. Additionally, the delivery of information about SRH varies depending on how a particular school can afford the infrastructure such as illustration samples for SRH topics e.g.; contraceptive materials and diagrams. The use of various means used in teaching SRH has varying impacts on students’ cognitive abilities. On the other hand, female students revealed to be uncomfortable and shying away from discussing SRH issues. This accounts for the existence of cultural and religious practices that make such discussions uncomfortable. For instance, the cultural practices that provide informal SRH education, such as “Jando” and “Unyago”, are informal SRH sources of information in some regions of Tanzania [ 20 ]. Curriculum-based SRH education needs to enrich students’ knowledge by adhering to standards influencing comprehensive and rights-based programs that convey clear messages that are appropriate for age, sexual experience, gender, and culture. An important starting point is their knowledge, misconceptions, hopes, and fears [ 21 ]. The provided explanations are limited to only meanings and a few aspects and the inability to speak about SRH matters implies that the achievement of needed skills to cope with RSBs is impossible because guidance on developing practical skills is absent. The curriculum-based SRH education has a gap in matters concerning emotions and self-regulation that are not taught and applied, without addressing these aspects, individuals may struggle to resist situations that could potentially lead to engaging in RSBs. Essential information is provided at later stages i.e. Form III and IV, making it challenging to effectively address RSB practices that may already be occurring. This leaves students with the role of fulfilling the obligation of studying the content for examination and pass mark purposes. Moreover, curriculum-based SRH education has been revealed to provide a glimpse of basic knowledge rather than intensive education that will impact students’ decision-making skills, attitudes, and knowledge. Given that this information becomes more intensive in later levels i.e. Form III and Form IV, the timing becomes quite too late as by this time puberty has already kicked into a majority of secondary school students.
The difference in perceptions of the relevance of curriculum-based SRH education among civics and biology teachers reveals a gap in the content taught. Biology teachers believed that the content being taught was restrictive and did not allow for practical application, which could hinder students from understanding the relevance of the subject matter. Civics teachers’ perceptions of what is being taught suggest that the moral aspects, mostly covered in civics, are seen as complementary to the SRH information taught in other subjects. The gap becomes evident in the syllabi, where although they include information on SRH, students receive minimal knowledge, primarily focused on attaining passing grades rather than understanding the content. Analysis of secondary school curricula content concerning SRH Education, particularly addressing RSBs, has uncovered limited and generalized information. This approach leaves out crucial skills necessary for youths to effectively address RSBs. Teachers’ perceptions about the relevance of information presented in the curriculum give rise to concerns that, given their daily interactions with students, it is important to acknowledge that there might be significant instructions on coping with RSBs and SRH information that are overlooked. A qualitative study done in Nigeria also found that the teaching of biology and integrated science in the curriculum may not be sufficient for the students since the courses may have limitations of impacting the desired life skills and may have other challenges such as the availability of adequate class time and appropriateness of the class setting for effective results [ 22 ]. Depending on the teachers’ perceptions, students are likely to have unequal information about SRH education, which may affect their ability to cope with RSBs. Moreover, determining whether content coverage is effective can be strongly affected by the teacher’s comfort, values, and competence in delivering such information given that it is considered sensitive in this context. Thus, teachers require training in SRH education delivery to ensure that consistent information is provided to every student.
Private schools have taken initiative to employ alternative tactics to emphasize the application of SRH education, aligning content with existing RSBs patterns [ 23 ]. Teachers, as reliable sources of information in schools, are expected to deliver SRH education by employing various techniques and demonstrating skills that are required for effective addressing of RSBs. Teachers’ interactions with students are among the essential components that create comfortable environments for both teachers and students. UNESCO emphasizes that “teachers responsible for the delivery of comprehensive sexuality education also require training on the specific skills needed to address sexuality accurately and clearly, as well as the use of active, participatory learning methods” [ 24 ]. However, culture also influences the ability of teachers to open up talking about SRH to students. This is in line with a review of country experience in Sub-Saharan Africa which found that teachers face pressure to focus on examinable subjects, often at the expense of life skills education. Evaluations of life skills education programs revealed teachers are often inadequately trained to deliver the lessons and may feel uncomfortable discussing sexuality issues. Thus, they may not teach them or schools may encounter resistance from religious groups when addressing sexuality education that deviates from a conservative, abstinence-only approach [ 25 ]. Teachers, therefore, need to facilitate students’ ability to combine and integrate what they learn in school with other knowledge disciplines if they are to develop effective decision-making skills [ 26 ]. The Education Sector Development Plan highlights its Logical Framework Outcomes, which include the Quality of Basic and Secondary Education. The expected result is that teacher training colleges have the necessary facilities and resources, employ innovative ways to help students understand science subjects and draw more secondary teachers to better prepare them [ 27 ]. With such a plan, the quality of SRH education can be assured, although employing other forms of teaching materials depends on the school’s ability to have the infrastructure that supports the use of such materials. This reflects that when there are no or limited resources, students become deprived of SRH knowledge to address RSBs.
Secondary school students show greater excitement towards events that promote health, such as bonanzas. The presence of external facilitators in schools facilitates the delivery of accurate and precise information. When participating in these activities, students are more likely to openly share their thoughts and feelings, as they feel confident that the school will not take disciplinary action against them, unlike when confiding in teachers. This reveals that subject teachers are limited to talk about SRH in classroom interactions since it is part of the curriculum requirement. This in turn perpetuates the practice of training students to pass examinations rather than getting them interested and engaged in the subject. The encouragement of such events supported by NGOs is useful because they also fit within the established guidelines on delivering SRH information. The provision of curriculum-based SRH in schools has revealed that students depend on such information to make decisions concerning the practice of RSBs.
Study limitations
This study contributes to the emphasis placed on the significance of curriculum-based SRH education in reducing the health consequences faced by adolescents due to RSBs. Through the revelation of gaps in the contents, how the education itself is delivered and the relevance of SRH education in addressing RSBs; the study contributes to areas that call for improvement in the education sector which is the reliable source of information. Moreover, to the best of our knowledge, this study is among the few studies that provide the status of curriculum-based SRH education relevance to secondary school students in coping with RSBs. Although the study was successful, it was not without challenges. Several limitations were apparent in the process of conducting this study. When carrying out interviews and, especially given the sensitive nature of the topic in some societies, some participants, specifically secondary school students, were initially reluctant to participate, despite assurances of confidentiality and thorough explanations of the study’s purpose. Additionally, the research environment presented limitations, such as students feeling uncomfortable discussing SRH issues due to their status as students, even if they were engaging in related practices. This hesitancy to connect their experiences with what is taught could have affected the study’s outcomes.
The disparities in the relevance of curriculum-based SRH education among students and teachers reveal a gap in utilizing and presenting comprehensive knowledge that will equip students with the right attitudes and skills needed in the current context, which demands awareness of healthy sexuality and the ability to properly approach RSBs. The available information from curriculum-based SRH education has forced secondary school students to improve upon the amount of information given by putting into practice what they are taught. Therefore, curriculum-based SRH education should include more comprehensive information to better equip secondary school students with appropriate skills to cope with RSBs.
Availability of data and materials
No datasets were generated or analysed during the current study.
Cowan FM. Adolescent reproductive health interventions. Sex Transm Infect. 2002;78(5):315–8. Available from: https://sti.bmj.com/content/78/5/315 . Cited 2023 Jul 31.
Article CAS PubMed PubMed Central Google Scholar
Thepthien BO, Celyn. Risky sexual behavior and associated factors among sexually-experienced adolescents in Bangkok, Thailand: findings from a school web-based survey. Reprod Health. 2022;19(1):127. https://doi.org/10.1186/s12978-022-01429-3 . Cited 2024 Mar 18.
Article PubMed PubMed Central Google Scholar
Rangi W, Mwageni E. The role of sexual reproductive health education in adolescents: sexual behaviours in secondary schools in Morogoro municipality, Tanzania. Huria. 2012;12(1):228–42. https://www.ajol.info/index.php/huria/article/view/110782 . Cited 2024 Mar 18.
Google Scholar
UNICEF DATA. Adolescents Statistics. https://data.unicef.org/topic/adolescents/overview/ . Cited 2023 Jul 31.
Ministry of Health. Community development, gender, elderly and children national adolescent health and development strategy 2018 - 2022. 2018.
For Tanzania’s most vulnerable adolescents | UNICEF United. Republic of Tanzania. https://www.unicef.org/tanzania/stories/tanzanias-most-vulnerable-adolescents . Cited 2023 Jul 24.
DESA U. World urbanization prospects: the 2014 revision. New York: Population Division of the Department of Economic and Social Affairs of the United Nations Secretariat; 2017.
Johnson OE, Bassey BA. Sexual practices among senior students in private secondary schools in Uyo, Southern Nigeria. Afr J Reprod Health. 2019;23(4):46–53. Available from: https://www.jstor.org/stable/26891542 . Cited 2023 Oct 18.
PubMed Google Scholar
Tesfaye Y, Negash A, Gebrehiwot TT, Tessema W, Anand S, Ahmed G, Alemu D. Is there association between risky sexual behaviors and depression symptoms among youth? A case of Jimma University students, Ethiopia. Psychiatry J. 2019;2019:3757656.
Moshi FV, Tilisho O. The magnitude of teenage pregnancy and its associated factors among teenagers in Dodoma Tanzania: a community-based analytical cross-sectional study. Reprod Health. 2023;20(1):28. https://doi.org/10.1186/s12978-022-01554-z . Cited 2023 Oct 18.
Kirby DB, Laris BA, Rolleri LA. Sex and HIV education programs: their impact on sexual behaviors of young people throughout the world. J Adolesc Health. 2007;40(3):206–17.
Article PubMed Google Scholar
Birungi H, Undie CC, MacKenzie I, Katahoire A, Obare F, Machawira P. Education sector response to early and unintended pregnancy: a review of country experiences in sub-Saharan Africa. Population Council; 2015. Available from: https://knowledgecommons.popcouncil.org/departments_sbsr-rh/262 . Cited 2023 Aug 7.
Ministry of Education and Vocational Training. Syllabus for Biology O Level Form I-IV-23-1-2018Final.pdf. Available from: https://www.tie.go.tz/uploads/files/Syllabus%20for%20Biology%20O%20Level%20Form%20I-IV-23-1-2018Final.pdf . Cited 2023 Aug 7.
Ministry of Education and Vocational Training. Civics syllabus for secondary schools: form I - IV. 2nd ed. Dar es Salaam: Tanzania Inst. of Education; 2010. p. 82.
(URT) United Republic of Tanzania. Adolescent sexual and reproductive health: report on an assessment and review of training materials. - PDF Free Download. 2001. Available from: https://docplayer.net/76360132-United-republic-of-tanzania-adolescent-sexual-and-reproductive-health-report-on-an-assessment-and-review-of-training-materials.html . Cited 2024 Feb 12.
Abdul R, Gerritsen AAM, Mwangome M, Geubbels E. Prevalence of self-reported symptoms of sexually transmitted infections, knowledge and sexual behaviour among youth in semi-rural Tanzania in the period of adolescent friendly health services strategy implementation. BMC Infect Dis. 2018;18(1):229. https://doi.org/10.1186/s12879-018-3138-1 . Cited 2023 Oct 18.
Millanzi WC, Osaki KM, Kibusi SM. Attitude and prevalence of early sexual debut and associated risk sexual behavior among adolescents in Tanzania; evidence from baseline data in a randomized controlled trial. BMC Public Health. 2023;23(1):1758. https://doi.org/10.1186/s12889-023-16623-6 . Cited 2024 Jan 19.
BEST 2020 Regional. Data_Final.pdf. Available from: https://www.tamisemi.go.tz/storage/app/media/uploaded-files/BEST%202020%20Regional%20Data_Final.pdf . Cited 2023 Jul 25.
Khan M, Maqbool M, Hurrah M, Adesina M, Fekadu G. Awareness about reproductive health in adolescents and youth: a review. J Appl Pharm Sci Res. 2019;2:1–5.
CAS Google Scholar
Mbeba RM, Mkuye MS, Magembe GE, Yotham WL, Mellah A, obeidy Mellah A, Mkuwa SB. Barriers to sexual reproductive health services and rights among young people in Mtwara district, Tanzania: a qualitative study. Pan Afr Med J. 2012;13(Suppl 1):13. Available from: https://www.ncbi.nlm.nih.gov/pmc/articles/PMC3589247/ . Cited 2024 Feb 1.
PubMed PubMed Central Google Scholar
WHO. WHO recommendations on adolescent sexual and reproductive health and rights. 2018. Available from: https://www.who.int/publications-detail-redirect/9789241514606 . Cited 2023 Aug 28.
Aransiola JO, Asa S, Obinjuwa P, Olarewaju O, Ojo OO, Fatusi AO. Teachers’ perspectives on sexual and reproductive health interventions for in-school adolescents in Nigeria. Afr J Reprod Health. 2013;17(4):84–92. Available from: https://www.jstor.org/stable/24362415 . Cited 2024 Feb 12.
Mkumbo KA. Content analysis of the status and place of sexuality education in the national school policy and curriculum in Tanzania. Educ Res Rev. 2009;4(12):616–25.
United Nations Population Fund. International technical guidance on sexuality education. Available from: https://www.unfpa.org/publications/international-technical-guidance-sexuality-education . Cited 2023 Dec 29.
Birungi H, Undie CC, MacKenzie I, Katahoire A, Obare F, Machawira P. Education sector response to early and unintended pregnancy: a review of country experiences in sub-Saharan Africa. Reprod Health. 2015. Available from: https://knowledgecommons.popcouncil.org/departments_sbsr-rh/262 .
Juma ZR. Exploring the development of biological literacy in Tanzanian junior secondary school students. 2015. Available from: http://researcharchive.vuw.ac.nz/handle/10063/4246 . Cited 2023 Aug 28.
Education sector development plan 2016/17– 2020/21. Tanzania Mainland | Documents | Global Partnership for Education. Available from: https://www.globalpartnership.org/content/education-sector-development-plan-201617-202021-tanzania-mainland . Cited 2023 Dec 29.
Download references
Acknowledgements
We are thankful to the study participants for their time spent on the study. Special thanks to Dr. George PrayGod for his support throughout the preparation of this manuscript.
Not applicable.
Author information
Authors and affiliations.
Department of Clinical Research Program, National Institute for Medical Research, Mwanza, Tanzania
Ng’wamba Sitta Ngissa, Erica Sanga, Mussa Kelvin Nsanya, Belinda Kweka & Evangelista Malindisa
Department of Physiology, Catholic University of Health and Allied Sciences, Mwanza, Tanzania
Evangelista Malindisa
Department of Anthropology, University of Dar es Salaam, Dar es Salaam, Tanzania
Rosemarie Mwaipopo
You can also search for this author in PubMed Google Scholar
Contributions
NSN and RM conceptualized the study. NSN data collection and analyzed the data; NSN, ES prepared the initial draft of the manuscript. ES, MKN, BK, EM, RM reviewed and substantially edited the manuscript.
Corresponding author
Correspondence to Ng’wamba Sitta Ngissa .
Ethics declarations
Ethics approval and consent to participate.
Ethical clearance was issued by The University of Dar es Salaam with reference number AB3/12(B). Additionally, the Ilemela District municipality provided a permit to conduct this study in the selected secondary schools. Legal guardians/parents of participants below the age of 18 were given written informed consent. Assent was obtained from participants below the age of 18 before interviews. Verbal and written consent from all study participants was sought and participants consented to the publishing of their responses anonymously.
Consent for publication
Competing interests.
The authors declare no competing interests.
Additional information
Publisher’s note.
Springer Nature remains neutral with regard to jurisdictional claims in published maps and institutional affiliations.
Rights and permissions
Open Access This article is licensed under a Creative Commons Attribution 4.0 International License, which permits use, sharing, adaptation, distribution and reproduction in any medium or format, as long as you give appropriate credit to the original author(s) and the source, provide a link to the Creative Commons licence, and indicate if changes were made. The images or other third party material in this article are included in the article's Creative Commons licence, unless indicated otherwise in a credit line to the material. If material is not included in the article's Creative Commons licence and your intended use is not permitted by statutory regulation or exceeds the permitted use, you will need to obtain permission directly from the copyright holder. To view a copy of this licence, visit http://creativecommons.org/licenses/by/4.0/ . The Creative Commons Public Domain Dedication waiver ( http://creativecommons.org/publicdomain/zero/1.0/ ) applies to the data made available in this article, unless otherwise stated in a credit line to the data.
Reprints and permissions
About this article
Cite this article.
Ngissa, N.S., Sanga, E., Nsanya, M.K. et al. Curriculum-based sexual and reproductive health education: revealing its relevance for risky sexual behaviors among secondary school students in Mwanza, Tanzania. Reprod Health 21 , 58 (2024). https://doi.org/10.1186/s12978-024-01798-x
Download citation
Received : 15 February 2024
Accepted : 20 April 2024
Published : 29 April 2024
DOI : https://doi.org/10.1186/s12978-024-01798-x
Share this article
Anyone you share the following link with will be able to read this content:
Sorry, a shareable link is not currently available for this article.
Provided by the Springer Nature SharedIt content-sharing initiative
- Curriculum-based sexual and reproductive health
- Risky sexual behaviors
- Secondary school students
Reproductive Health
ISSN: 1742-4755
- General enquiries: [email protected]
- Open access
- Published: 19 April 2024
Causes and outcomes of at-risk underperforming pharmacy students: implications for policy and practice
- Alice Campbell 1 ,
- Tina Hinton 1 , 2 ,
- Narelle C. da Costa 1 ,
- Sian E. O’Brian 1 ,
- Danielle R. Liang 1 &
- Nial J. Wheate 1
BMC Medical Education volume 24 , Article number: 421 ( 2024 ) Cite this article
255 Accesses
Metrics details
This study aimed to understand the key determinants for poor academic performance of students completing a Bachelor of Pharmacy (BPharm), Bachelor of Pharmacy and Management (BPharmMgmt), or Master of Pharmacy (MPharm) degree.
Data were collected on pharmacy students who had not met academic progression requirements between 2008 and 2018 at The University of Sydney, Australia. This included: age at the start of pharmacy degree; gender; whether they transferred from another university; whether they were a domestic or international student; Australian Tertiary Admissions Rank upon entry, previous studies in biology, chemistry, or mathematics; show cause triggers (units of study failed); number of show causes; students’ written show cause responses; weighted average mark at last show cause or graduation; whether they graduated and were a registered pharmacist; and, the number of years they spent studying the degree. Descriptive studies were used to analyse student characteristics using SPSS software, and student self-reported reasons for poor performance were analysed reflexively using thematic analysis procedures using NVivo.
This study included 164 pharmacy students enrolled in a BPharm (79.3%, n = 130), BPharmMgmt (1.2%, n = 2), or MPharm (19.5%, n = 32). Of the students, 54% ( n = 88) were men, 81% ( n = 133) were domestic students, 15% ( n = 24) transferred from another degree program, and 38% ( n = 62) graduated from the course. Show cause students were less likely to graduate if they transferred from another degree program ( P = 0.0002) or failed more than three units of study (UoS; P < 0.0001). The most commonly failed UoS were related to organic or pharmaceutical chemistry, and the top student self-reported reasons for poor performance was stress/anxiety, physical health, and depression.
Pharmacy schools should aim to address student foundational knowledge in chemistry, identify at-risk students early using pre-subject testing, and provide better services to address student mental health.
Peer Review reports
Introduction
A student’s academic performance in higher education is typically defined by their achievement of learning outcomes and demonstration of their ability to apply the concepts taught. Measurement of these attributes can include assessments, quizzes, role plays, field work, practical placements, workshops, tutorials, laboratories, and examinations. In most higher education programs, a minimum standard of academic achievement is required in order to progress through the course, to ensure the student has gained adequate knowledge and skills, and that they have achieved the specified learning outcomes. In this regard, poor academic performance can be defined by instances where a student fails to meet the expected minimum academic standard. Usually this comprises a minimum overall score in a subject and/or passing a specific barrier assessment, which is ultimately linked to their retention or attrition.
Understanding the key determinants of student success, failure, retention, and attrition has become increasingly important for higher education institutions, and has been the subject of extensive research over the past few decades. Early studies on student attrition focused primarily on student characteristics [ 1 ], before attention shifted to interactions between the student and their institutions. Prominent researchers, including Spady [ 2 , 3 ], Tinto [ 4 , 5 ], and Bean [ 6 ] proposed models to explain the interplay between academic and social integration leading to underperformance, and eventually, attrition. More recently, interest has increased in examining student engagement [ 7 , 8 , 9 ], where the student and institutions have a joint responsibility for academic success. To be successful, a student needs to participate, and higher education institutions need to provide an appropriate learning environment, opportunities, and support [ 10 ].
Studies on the key determinants of student underperformance reveal an array of contributing factors. Recent systematic reviews on underperformance and dropout rates show that key determinants fall into categories relating to the institution, personal life, demographics, and social integration [ 11 , 12 ]. Within higher education institutions, studies have found that an academic’s professional knowledge and pedagogical skills, along with the institution’s learning resources, course structure, and environment, are key factors that influence academic performance and non-completion [ 13 , 14 , 15 , 16 , 17 ]. Teaching methods that higher institutions adopt have also been evaluated, with student-centered approaches that encourage active learning resulting in better performance when compared with a traditional teacher-centered approach [ 15 , 16 ].
In terms of individual factors, studies have found a lack of effort, distraction, poor time management, and no longer being interested in the course as having a negative impact on academic performance [ 14 , 15 , 18 , 19 ]. Active learning (e.g. self-quizzes, completing problem sets, and explaining concepts) has been found to yield better academic outcomes when compared with passive learning (e.g. reading lecture slides or class notes, watching lecture videos, and reading textbooks) [ 20 , 21 ]. In the same study, how early a student studied in relation to their exam did not affect their outcome, whereas students who were more distracted during the time they allocated for study, performed worst [ 20 , 22 ]. Education-related stress, poor mental health, exam anxiety, and sleep quality are also factors found to cause poor performance [ 23 , 24 , 25 , 26 , 27 ]. Other studies have shown that part-time students and those who have previously failed subjects are at risk of further poor performance and attrition [ 17 , 28 , 29 ]. Social factors including cyberbullying [ 30 ], homesickness for international students [ 31 ], and excessive socialising [ 16 ] also have a negative effect on academic performance.
Working status was found to negatively impact academic performance [ 27 ], where poor academic outcomes were correlated with a longer time spent at work [ 16 , 28 , 32 ]. Many studies have associated the lower socioeconomic status of students and their family, or financial strain with poor academic performance [ 27 , 28 , 29 ]; whereas, other studies have shown that students in families where one parent has attended higher education tend to achieve higher grades [ 31 ]. Some studies have found men and minority students are more at risk of poor performance [ 31 , 33 ]. Part-time students are much more likely cite work and family responsibilities as reasons for stopping their studies [ 17 ]. Research on students whose first language is not that of the higher education institution is mixed, with some confirming it to be a key attributor to underperformance [ 34 , 35 , 36 ], along with students with a migrant background or who are first-generation university attendees (commonly referred to as first-in-family) [ 31 , 37 , 38 ]. In contrast, other studies have found that academic performance of international students was similar, or better, than domestic students [ 39 , 40 ].
A government panel in Australia reported that the leading drivers for non-completion in higher education are both institution-related (learning environment, an academic’s ability to teach, student to staff ratios, student engagement, and support services) and student-related (health, finance, and personal responsibilities) [ 41 ]. A survey conducted by the Australian Bureau of Statistics (ABS) identified the top three reasons for attrition for students studying a bachelors degree to be: loss of interest, employment/financial reasons, and personal reasons (health, family, or other personal reasons). For postgraduate courses, reasons for attrition were highest in the order of personal reasons, employment/financial, followed by loss of interest [ 42 ].
Where a student has underperformed, they may be offered remediation assessments; to re-enroll and attempt the entire subject again, which may result in a delay in degree completion; or in some cases, be excluded from reenrolling into the same course for a period of time [ 43 , 44 ].
Consequences of poor performance vary across higher education institutions and may depend on the reasoning provided, extent of underperformance, and number of failed subjects. Key stake holders impacted by poor performance and attrition from higher education can include the students and their families, the higher education institution they are enrolled in, their community workforce, and government. Non-completion directly impacts the funding and reputation of an institution [ 17 , 45 , 46 ]. In Australia, where the cost of higher education for domestic students is subsidised by the federal government, non-completion incurs a direct cost to both the student and the tax-payer. The cost to the student includes lost time, psychological health, student debt, and forgone income [ 9 ]. From the perspective of workforce planning, a delay or non-completion of study reduces the number of employees entering into the workforce, and can lead to workforce shortages and place a burden on those currently in the field.
There are many studies that have examined the key determinants for student success or underperformance and attrition in health; however, most have focused on nursing or medical education [ 13 , 15 , 47 , 48 , 49 , 50 ]. Consequently there are limited studies that have examined the rate and reasons for attrition within pharmacy degrees. Being a degree known to be difficult in technical content, and which requires students to achieve a high level of competence, it is important to investigate reasons for attrition and potential opportunities for improvement in student teaching and engagement.
In this study we analysed 10 years of demographic data and responses to why academic progression requirements had not been met in a cohort of students enrolled in a Bachelor of Pharmacy (BPharm), Bachelor of Pharmacy and Management (BPharmMgmt), or Master of Pharmacy (MPharm) degree at The University of Sydney. Our aim was to understand the key determinants for poor performance within this group of students and identify opportunities for policy and practice to reduce underperformance in the future.
Approval for this study was granted by the Human Research Ethics Committee of The University of Sydney (2022/815).
Data collection
The inclusion criteria for this study were students enrolled in a BPharm, BPharmMgmt, or MPharm degree between the period of 2008 and 2018 (inclusive), who were required to provide a minimum of one show cause at any stage of their study. Data collected on each student included: age at the start of pharmacy degree; gender; whether they transferred from another university; whether they were a domestic or international student; Australian Tertiary Admissions Rank (ATAR) upon entry, which is a percentile score that ranks Australian students finishing secondary school in relation to their academic achievement [ 51 ]; previous studies in biology, chemistry, or mathematics; show cause triggers (units of study failed); number of show causes; students’ written show cause responses; weighted average mark (WAM) at last show cause or graduation (WAM is an average grade score indicating a student’s overall academic performance over the course of their degree and is similar to a grade point average) [ 52 ]; whether they graduated; and, the number of years they spent studying the degree. Whether those students who had graduated were currently registered as a pharmacist in Australia was retrieved using the Australian Health Practitioner Regulation Agency online registry list [accessed in 2023].
Data analysis
Researchers Da Costa, O’Brien, and Liang collected, screened, and de-identified the data, and researchers Campbell, Hinton, and Wheate analysed the data. Descriptive statistics, including mean ± SD, median, and frequencies (count and percentage) were calculated using Microsoft Excel. Mann-Whitney U tests were undertaken in GraphPad Prism 9.0 (GraphPad Software, Boston, MA, USA) to ascertain any differences between ATAR scores. Chi Square analyses were undertaken in GraphPad Prism 9.0 to compare categorical data including differences between men and women, domestic and international students, transferring and non-transferring students, and graduating and non-graduating students.
Written show cause responses were transcribed by Campbell and uploaded into NVivo (1.5.1) software (QSR International, Massachussets USA). The show cause responses were analysed reflexively using inductive thematic analysis procedures [ 53 ].This involved manually reviewing each show cause response to identify emerging themes relating to the reasons stated by the student for their poor performance. From the themes identified, a total of 43 codes were generated based on the ideas, trends, and content. Coding was conducted in a theory-driven manner, seeking to code information referencing the specific themes arising from the show cause response [ 53 ]. Themes were guided by the frequency of mention, and reported in the results if there was more than a single mention. The frequency of the subthemes was analysed to demonstrate the prevalence of stated factors that the student believed led to their poor performance.
Show cause process
Pharmacy students who do not meet the progression requirements of their degree enter one of three stages of academic intervention (Fig. 1 ). Triggers for a student not meeting the requirements for progression include: awarded a fail grade in over 50% of total units of study (subjects; UoS) taken in a semester or teaching period; an average grade (WAM) less than 50 across all UoS in a semester or teaching period; failing one, or more, barrier or compulsory UoS which includes CHEM1611, CHEM1612, PHAR2822, and any 3000 or 4000 level UoS for BPharm/BPharmMgmt; and any single UoS for MPharm; any practical component (e.g. field work or clinical work), failing the same UoS twice, having unsatisfactory attendance, or exceeding the maximum time limit allowed for the degree to be completed.
Students who fail to meet progression requirements for the first time are placed on Stage 1 of the at-risk register at which point they receive a letter from the Faculty of Medicine and Health, and are advised to complete a ‘Stay on Track’ survey and information session. At the discretion of the Associate Dean of Education, some students at Stage 1 may be required to consult an academic adviser. If a student is enrolled in a degree with a duration of less than two years full-time (e.g. MPharm), they are advised that should they fail to meet progression requirements in the following semester, they would be asked to ‘show good cause’ in order to be allowed to re-enrol in the same program; that is, they would be excluded from the degree for two years unless they could give reasons for why they should be allowed to remain studying. They are also recommended to speak to an academic advisor.
Stage 2 is triggered for a student in a 4 or 5 year undergraduate degree program (e.g. BPharm and BPharmMgmt) if they fail to meet progression requirements after being placed on Stage 1 in the previous semester, at which point the faculty sends a letter, advising the student to complete the ‘Staying on Track’ survey if they had not yet done so, and to consult an academic adviser. Stage 3 is triggered if a student fails to meet progression requirements a third time, or fails the same compulsory or barrier UoS, or any practical component twice. Students on Stage 3 are required to ‘show good cause’ and provide reasonable evidence to be allowed to re-enrol into the degree program.
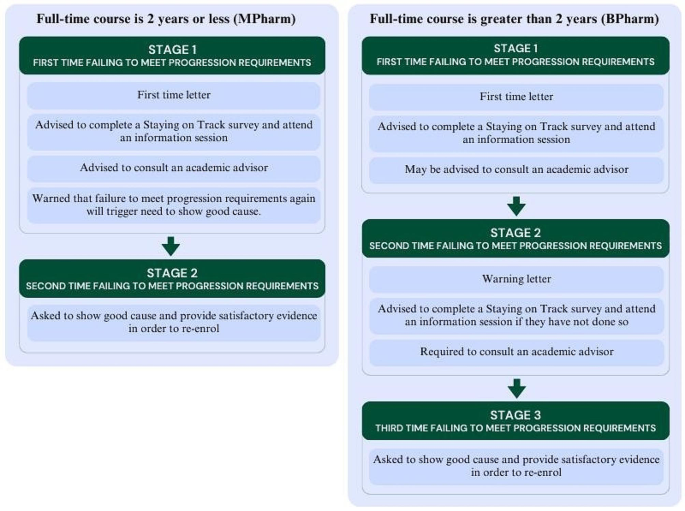
The three at-risk stages of academic intervention for students who fail to meet course progression requirements. Show cause is required at Stage 2 (MPharm) or Stage 3 (BPharm/BPharmMgmt) in order to re-enrol
Demographics
In total, 164 pharmacy students received at least one show cause notification between the period of 2008 to 2018 (inclusive) and were enrolled in a BPharm (79.3%, n = 130), BPharmMgmt (1.2%, n = 2), or MPharm (19.5%, n = 32) degree (Table 1 ). Of the students, 54% ( n = 88) were men, and 81% ( n = 133) were domestic students.
Students who transferred from another degree program made up 15% ( n = 24) of the sample, and were a median two years older than those who did not transfer (median age 21, range 19–43 years). All students who transferred from another degree, were enrolled in the BPharm. Ninety-two percent of transfer students ( n = 22) were domestic and 71% ( n = 17) were women.
The age of students at the start of their degree was positively skewed, with a median age of 19 years for BPharm and BPharmMgmt (range 17–43 years). For MPharm, the median age at commencement was 24 (range 20–24) years. The median age of domestic students at the start of their BPharm or BPharmMgmt degree was 19 (range 17–43) years compared with international students at 22 (range 18–33) years. For MPharm, the median age for domestic students at commencement was 24 (range 20–54) years while for international students it was 24.5 (range 22–38) years.
Performance on entry and exit of the degree
The ATAR scores of the students in either the BPharm or BPharmMgmt were not normally distributed ( n = 78, mean ATAR 88.8 ± 4.8) (Supplementary Figure S1 ). The average ATAR required for entry into BPharm and BPharm/Mgmt at the University of Sydney is around 90. Of the 24 students who transferred from another degree program, the ATAR score was available for four students, with an average of 78.8 ± 9.8, including two outliers who had ATAR scores of 67.80 and 74.15. The average ATAR on entry to the degree of the students who graduated was 89.4 ± 3.4, which was similar to those who did not graduate, 88.5 ± 5.4. A Mann-Whitney U test showed this difference was not statistically significant (W = 702.5, p = 0.937).
The proportion of students who graduated after receiving at least one show cause was 37.8% ( n = 62), of which 77.4% ( n = 48) were registered as pharmacists at the time of data collection (Fig. 2 ). One student did not graduate their BPharm; however, they did return and complete the MPharm degree and was registered as a pharmacist at the time of data collection. The median time taken to graduation was 7 (range 1–9) years for students enrolled in the BPharm and 3 (range 2.5-8) years for those enrolled in the MPharm. During the study period, 188 students were enrolled in the BPharmMgmt degree but only two (1.1%) were required to show cause due to poor performance. Neither of those two students graduated.
A WAM score was available for all but three of the 164 students. The overall average WAM either at last show cause, if the student had not graduated, or at degree completion was 52.1 ± 12.0. For students who graduated (38.5%, n = 62), the average WAM was 62.2 ± 5.1, while for those who did not graduate (61.5%, n = 99), the average WAM was 45.7 ± 10.5.
When the proportion of students who graduated was compared across the ATAR bands (Table S1 ), it was evident that show cause students who entered their degree with an ATAR between 85 and 89.99 were more likely to graduate (44%) when compared with those who entered their degree with lower (27%) and higher (25–35%) ATAR scores.
Units failed
Across the cohort, show cause students received between 1 and 8 show cause notifications (Fig. 1 ). When the proportion of students who graduated was compared across the number of show causes received for those who received 1–5 show causes, the rate of graduation ranged from 36 to 50%, while none of the students who received six or more show causes graduated.
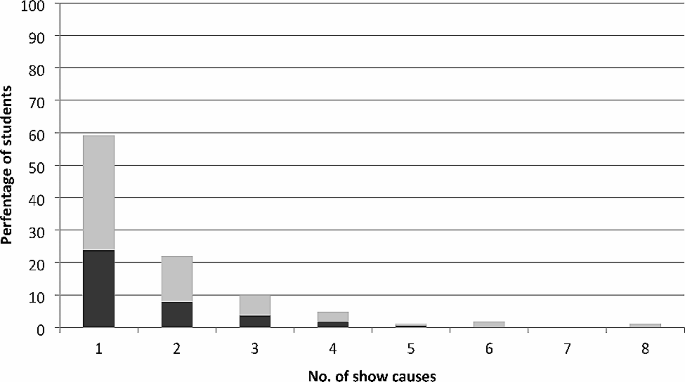
Percentage of students who graduated (black) and did not graduate (grey) by number of show causes received
Number of failed UoS
The median number of UoS failed across the three degree programs was 8 (BPharm, range 2–33), 9 (BPharmMgmt, range 5–13), and 5 (MPharm, range 2–12), respectively. In total, 8.5% ( n = 14) students were required to show cause because they failed 2 or 3 UoS, 19.5% ( n = 32) students failed 4 or 5 UoS and 72% ( n = 118) students failed more than 6 UoS. Of the 14 students who failed 2 or 3 UoS, 86% were studying the MPharm degree and the remaining were BPharm students. Students who failed 4 or 5 UoS, were studying a BPharm (66%), BPharmMgmt (3%), or MPharm (31%) degree. The majority of students who failed more than 6 units were studying BPharm (91%), followed by MPharm (8%), and BPharmMgmt (1%). Students who failed 2 or 3 UoS were significantly more likely to graduate when compared with those who failed 4 or 5 UoS, or more than 6 UoS \( (X_2^2=21.86, \text{P}<0.0001)\) (Supplementary Figure S2 ).
Type of failed UoS
The most failed UoS that triggered a show cause across students in the BPharm and BPharmMgmt degrees were a mix of pharmaceutical sciences, chemistry and biology, across the first and second years of the degree programs (Table 2 ). The top five UoS failed were Basic Pharmaceutical Sciences (8.8%, 116/1314 fails; unit code: PHAR1812), Chemistry 1B (Pharmacy) (6.9%, 91/1314 fails; unit code: CHEM1612), Drug Discovery and Design 1 (6.7%, 88/1465 fails; unit code: PHAR2811), Molecular Biology and Genetics (6.5%, 86/1314 fails; unit Code: MBLG1001), and Chemistry 1A (6.2%, 81/1314 fails; unit code: CHEM1611).
For students studying the MPharm, the majority of UoS failed were for pharmaceutical sciences in first year and one specific pharmacy practice unit (PHAR5717) in the second year. The top three UoS failed for MPharm were Pharmaceutical Chemistry 1A (12.6% 19/151 fails; unit code: PHAR5513), Pharmaceutical Science (7.9%, 12/151 fails; unit code: PHAR5515), and Pharmaceutical Chemistry 1B (7.9%, 12/151 fails; unit code: PHAR5516) (Table 3 ).
Gender, transfer and international students
There was no significant difference between the number of men and women who graduated after receiving at least one show cause \( (X_1^2=0.056, \text{P}=0.813)\) . There was also no significant difference in the number of UoS failed \( (X_2^2=2.249, \text{P}\hspace{0.17em}=\hspace{0.17em}0.325)\) or number of show causes received \( (X_6^2=2.829, \text{P}=0.830)\) between men and women.
Students who transferred from another degree program were significantly less likely to graduate \( (X_1^2=13.53, \text{P}\hspace{0.17em}=\hspace{0.17em}0.0002)\) . The likelihood of graduating was not statistically significant different between domestic and international students who received a show cause \( (X_1^2=0.88, \text{P}<0.348)\) (Supplementary Figure S3 ).
Student responses to show causes
There were 293 show causes in total, of which only 141 show cause response letters were available. Reasons given by students for their poor performance could be classified under four major themes: personal life matters, institutional aspects, social integration, and interest in the course (Fig. 3 ). Personal life matters could be further sub-divided into health, study familiarity, responsibilities, and other personal life matters.
The majority of show cause responses attributed poor performance to personal life reasons (87%, 396 responses), followed by institution-related (8.8%, 40 responses), lack of interest in the degree (2.2%, 10 responses), and social integration (2%, 9 responses). The five most mentioned personal life reasons that led to poor performance were stress and anxiety ( n = 63, 45%), physical health ( n = 51, 36%), and depression ( n = 39 28%). This was followed by family health, mentioned 37 times (26%), and reasons relating to employment or financial health, mentioned 33 times (23%). Reasons that related to the institution totalled 40, interest of the course totalled 10, and social reasons totalled 9. Personal life health-related reasons accounted for 41% of show cause responses. These included a combination of physical, mental, and unspecified health issues.
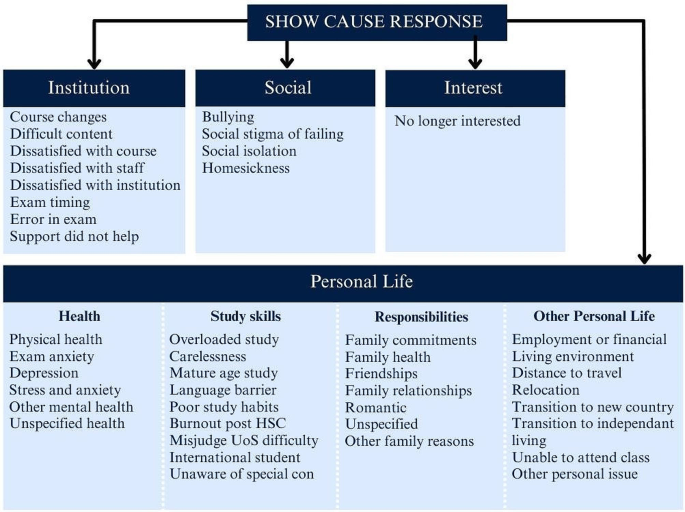
All show cause responses provided by students could be categorised into four major themes. Personal life was subcategorised into health, study skills, responsibilities, and other personal life
Some students identified a lack of study-related skills and study familiarity as a source of underperformance. Reasons included: carelessness in exams, poor study habits, language barrier, being an international student or mature age student, misjudging the course difficulty, overloading, burning out after high school, and being unaware of opportunities to apply for special consideration. Another set of reasons provided for underperformance included: needing to meet responsibilities and commitments for family, friendships, and romantic relationships. A variety of other personal life reasons were provided, which included: employment, finance, transition to independent living or a new country, living environment, distance to travel to the university, needing to relocate, and being physically unable to attend classes.
Student show cause responses that attributed poor performance to inefficiencies within the institution included UoS changes, error or poor timing of exams, dissatisfaction with the course and staff, and unhelpful support. Some students found the UoS content too difficult. Social reasons that lead to poor performance included: bullying, stigma from peers once failing, and homesickness (for those studying abroad). Another reason provided was no longer being interested or committed to the course.
This study investigated the key determinants of underperformance by pharmacy students at an Australian higher education institution. Our findings indicate that across the students enrolled in BPharm, BPharmMgmt, and MPharm degrees, those who had failed more UoS overall, were less likely to graduate. The types of UoS failed were weighted towards chemistry-based subjects, and the most frequent student-reported reasons for poor performance were related to personal health.
Our study also found that students who transferred from another higher education institution were less likely to graduate compared with students who had not transferred. Some studies in the US have found that students who transfer to bachelors programs from similar institutions or community colleges, which are US institutions that only offer two year undergraduate associate degrees that lead to a specific skilled job or can be used to transfer into a bachelor course [ 54 ], experience ‘transfer shock’ where grade point average (GPA) declines at the post transfer institution, which can eventually result in attrition [ 55 , 56 ]. In contrast, other studies have found no significant effects from transfers, and an overall lack of consensus on this as a universal experience [ 57 , 58 ]. A study that examined transferring engineering students found that students who transferred from similar degrees were more likely to graduate when compared with students who transferred from less comprehensive degrees [ 56 ]. A literature review that examined transferring student performance found factors that negatively influenced persistence and course completion included: a lack of social integration, limited transferrable credits, lower GPAs, lack of funding, distance from institution, academic rigour, and personal work/life balance [ 57 ].
Our analysis also found that students failing more than three UoS were more likely to not graduate when compared with those who failed fewer UoS. This finding parallels many studies that show students with poor academic outcomes are more likely to not complete their degree [ 59 , 60 ]. A recent study on student attrition, found that students who failed one subject were more likely to fail more subjects, and also had a four-fold higher likelihood of not graduating [ 27 ]. The Grattan Institute presents similar statistics, where students who consistently fail to meet academic progression requirements eventually decide to leave or are excluded from re-enrolling by the university [ 61 ].
The high occurrence of underperformance in relation to chemistry is consistent with other studies [ 62 , 63 ]. Pancyk et al. found that chemistry marks were correlated with attrition while biology marks predicted likelihood of delayed graduation for Master of Science (in Pharmacy) students. Another study found that the prior attainment of a Bachelor of Science degree to be a predictor of performance in a Doctor of Pharmacy program [ 64 ]. In countries, such as the US, where a specialised pre-admissions pharmacy test (Pharmacy College Admissions Test; PCAT) is used for entrance into a pharmacy program, the PCAT score correlated with student academic performance in the pharmacy course [ 65 ]. There are five areas examined by the PCAT, including: writing, biological processes, chemical processes, critical reading, and quantitative reasoning [ 66 ]. There is also evidence that better outcomes attained in pre-pharmacy biology and mathematics GPA [ 67 , 68 ], or having completed a four-year bachelor course, contributes to student performance in American pharmacy colleges [ 64 , 69 , 70 ]. Another study found prior academic achievement in secondary school, or pre-university study, can predict performance in an UK MPharm course; however, not the likelihood of graduation [ 71 ]. Other studies have found that pre-tests, for certain UoS, like biochemistry and pharmaceutical calculations conducted before starting a subject are correlated with overall subject performance, which makes these tests a good predictor for at-risk students [ 67 , 68 ].
The most common reasons reported by students for their underperformance in the present study were stress and anxiety, personal health, and depression. This is consistent with current literature [ 17 , 23 , 24 , 25 , 26 , 27 ], and the 2022 Australian Student Experience Survey [ 72 ], which reported that health or stress, followed by work/life balance were the leading causes for students attrition. A specific study in pharmacy students found that exam anxiety had a negative impact on student performance in pharmacy practical exams [ 26 ]. Psychological distress among students completing a higher education degree in Norway showed negative impacts on their self-perceived academic ability, and course progression [ 73 ]. Another study investigating students’ self-reported explanations for their poor academic performance found mental health as a contributing factor, and vice versa, where poor performance intensified mental distress [ 27 ]. Although the Australian Bureau of Statistics also reported personal health reasons as a major contributor for non-completion in bachelor programs between 2018 and 2019, the leading reason was that students were no longer interested in their chosen degree. In the same report, non-completion of masters degrees was driven by family, health, or other personal reasons [ 42 ]. Student mental health is a significant driver of attrition and is common across both private and public higher institutions in Australia [ 41 ]. The mental health burden on students is recognised at The University of Sydney and so significant mental health support is offered. All students are able to access free counselling and psychological support sessions, there is a 24/7 mental health support telephone line, and additional self-help resources (like mindfulness and relaxation) are provided through the university’s website. Mental health first health training is also included in the curricula for all pharmacy degree programs at the university.
Successful completion of a pharmacy degree requires not only academic ability, but a certain level of pre-knowledge, in particular, biology and chemistry, to decrease failure rates in these subjects, avoid delays in degree completion, and possible attrition. Institutions should aim to address these barriers by introducing pre-requisite subjects or mandate compulsory bridging courses if a prior level of knowledge attainment in these subject areas is not provided. Alternatively, pre-tests for certain UoS can be conducted prior to the course commencement to identify at-risk students, and additional academic support services can be offered.
With student poor mental health found as the most common self-reported reason for poor performance in this study, often exacerbated by academic performance pressures, institutions should implement policies for early detection and support for students going through challenging times. Such policies could include more frequent reminders for students to self-assess their mental health, and information on where to seek support services. This could take form in programs being introduced prior to lectures, access to support portals made more prominent on online learning platforms, or self-check surveys to be taken at a frequency deemed appropriate.
Limitations
The present study had a number of limitation. Not all student’s ATAR scores (or equivalent) were available. The method of collecting whether a student was registered as a pharmacist was based on them not having changed their last name which may be the case for some students who changed their name after graduation (e.g. upon marriage). Students who may be registered as a pharmacist in countries other than Australia could not be determined. Not all student show cause reasons were available because of the change from physical to electronic filing over the period studied. The limited number of students who received five or more show causes also meant the study was not powered to establish a cut-off whereby after receiving a certain number of show causes, the chance of graduating is highly unlikely.
Conclusions
This study investigated the key determinants for poor academic performance in a cohort of pharmacy students enrolled in a BPharm, BPharmMgmt, and MPharm degree. The key factors that influenced whether a show cause student completed their studies included whether they transferred from another institution, and failed more than three UoS. The UoS with the highest fail rates were chemistry based, and the most frequent student self-reported reason for poor performance was personal stress and anxiety. The results indicate that pharmacy schools should aim to address student foundation knowledge in chemistry, identify at-risk students early using pre-subject testing, and provide better access and knowledge of available services to address student mental burden. Future studies should investigate whether students who have completed chemistry and biology pre-requisites perform better in their pharmacy degree.
Data availability
The data that support the findings of this study are available on request from the corresponding author, N.J.W.
Aljohani O. A comprehensive review of the major studies and theoretical models of student retention in higher education. High Educ Stud. 2016;6:1–18.
Article Google Scholar
Spady WG. Dropouts from higher education: an interdisciplinary review and synthesis. Interchange 1984. 1970;1(1):64–85.
Spady WG. Dropouts from higher education: toward an empirical model. Interchange. 1971;2(3):38–62.
Tinto V. Dropout from higher education: a theoretical synthesis of recent research. Rev Educ Res. 1975;45(1):89–125.
Tinto V. Leaving college: rethinking the causes and cures of student attrition. 2nd ed. Chicago: University of Chicago Press; 1993.
Google Scholar
Bean JP. Dropouts and turnover: the synthesis and test of a causal model of student attrition. Res High Educ. 1980;12(2):155–87.
Tight M. Student retention and engagement in higher education. J Furth High Educ. 2020;44(5):689–704.
Carini RM, Kuh GD, Klein SP. Student engagement and student learning: testing the linkages. Res High Educ. 2006;47(1):1–32.
Thomas L, Kift S, Shah M. Student retention and success in higher education. Cham: Springer International Publishing; 2021. pp. 1–16.
Book Google Scholar
Coates H. The value of student engagement for higher education quality assurance. Qual High Educ. 2005;11(1):25–36.
Al-Tameemi RAN, Johnson C, Gitay R, Abdel-Salam A-SG, Hazaa KA, BenSaid A, et al. Determinants of poor academic performance among undergraduate students—A systematic literature review. Int J Educ Res. 2023;4:100232.
Lorenzo-Quiles O, Galdón-López S, Lendínez-Turón A. Factors contributing to university dropout: a review. Front Educ Res. 2023;8.
Wilkinson TJ, McKenzie JM, Ali AN, Rudland J, Carter FA, Bell CJ. Identifying medical students at risk of underperformance from significant stressors. BMC Med Educ. 2016;16:43.
Le HTTN, La HTT, Le TP, Nguyen TTT, Nguyen NT, Tran TP. Factors affecting academic performance of first-year university students: a case of a Vietnamese university. Int J Educ Prac. 2020;8(2):221–32.
Sharma P, Singh P, Kalhan S, Garg S. Analysis of factors affecting academic performance of MBBS students in pathology. Ann Int Med Dent Res. 2017;2.
Mascolo M, Castillo J. The origins of underperformance in higher education in America: proximal systems of influence. Pedgog Hum Sci. 2015;5(1):1–40.
Norton AC, I. and, Mackey W. Dropping out: the benefits and costs of trying university. The Grattan Institute, 2018. p. 1–65.
van Rooij E, Jansen EPWA, Van de Grift W. First-year university students’ academic success: the importance of academic adjustment. Eu J Psychol Educ. 2017;33:1–19.
Arshad M, Zaidi SM, Mahmood D. Self-esteem and academic performance among university students. J Educ Pract. 2015;6:2015.
Walck-Shannon EM, Rowell SF, Frey RF. To what extent do study habits relate to performance? CBE - Life Sci Educ. 2021;20(1):ar6.
Roick J, Ringeisen T. Students’ math performance in higher education: examining the role of self-regulated learning and self-efficacy. Learn Individ Differ. 2018;65:148–58.
Nonis SA, Hudson GI. Performance of college students: impact of study time and study habits. J Educ Bus. 2010;85(4):229–38.
Jevons C, Lindsay S. The middle years slump: addressing student-reported barriers to academic progress. High Educ Res Dev. 2018;37(6):1156–70.
Pascoe MC, Hetrick SE, Parker AG. The impact of stress on students in secondary school and higher education. Int J Adolesc Youth. 2020;25(1):104–12.
May RW, Bauer KN, Seibert GS, Jaurequi ME, Fincham FD. School burnout is related to sleep quality and perseverative cognition regulation at bedtime in young adults. Learn Individ Differ. 2020;78:101821.
Hadi MA, Ali M, Haseeb A, Mohamed MMA, Elrggal ME, Cheema E. Impact of test anxiety on pharmacy students’ performance in Objective Structured Clinical examination: a cross-sectional survey. Int J Pharm Pract. 2018;26(2):191–4.
Ajjawi R, Dracup M, Zacharias N, Bennett S, Boud D. Persisting students’ explanations of and emotional responses to academic failure. High Educ Res Dev. 2020;39(2):185–99.
Rodríguez-Hernández CF, Cascallar E, Kyndt E. Socio-economic status and academic performance in higher education: a systematic review. Educ Res Rev. 2019;29:100305.
Tomul E, Polat G. The effects of socioeconomic characteristics of ctudents on their academic achievement in higher education. Am J Educ Res. 2013;1:449–55.
Peled Y. Cyberbullying and its influence on academic, social, and emotional development of undergraduate students. Heliyon. 2019;5(3):e01393.
Sun J, Hagedorn L, Zhang Y. Homesickness at college: its impact on academic performance and retention. J Coll Stud Dev. 2016;57:943–57.
Triventi M. Does working during higher education affect students’ academic progression? Econ Educ Rev. 2014;41:1–13.
Voyer D, Voyer SD. Gender differences in scholastic achievement: a meta-analysis. Psychol Bull. 2014;140(4):1174–204.
Dafouz E, Camacho-Miñano MM. Exploring the impact of English-medium instruction on university student academic achievement: the case of accounting. Engl Specif Purp. 2016;44:57–67.
Civan A, Coskun A. The effect of the medium of instruction language on the academic success of university students. Educ Sci: Theory Prac. 2016;16:1981–2004.
Sawir E. Language difficulties of international students in Australia: the effects of prior learning experience. Int Educ J. 2005;6:567–80.
Mishra S. Social networks, social capital, social support and academic success in higher education: a systematic review with a special focus on ‘underrepresented’ students. Educ Res Rev. 2020;29:100307.
López MJ, Santelices MV, Carmen Maura T. Academic performance and adjustment of first-generation students to higher education: a systematic review. Cogent Educ. 2023;10(1).
Zheng RX, Everett B, Glew P, Salamonson Y. Unravelling the differences in attrition and academic performance of international and domestic nursing students with English as an additional language. Nurse Educ Today. 2014;34(12):1455–9.
Rienties B, Beausaert S, Grohnert T, Niemantsverdriet S, Kommers P. Understanding academic performance of international students: the role of ethnicity, academic and social integration. High Educ. 2012;63(6):685–700.
Final Report -. Improving retention, completion and success in higher education. Higher Education Standards Panel; Australian Government Department of Education; 2017.
Qualifications. and Work, 2018-19. Australian Bureau of Statistics; 2020.
Academic Progression. The University of Sydney [updated 31 March 2023; cited 2023 September ]. Available from: https://www.sydney.edu.au/students/academic-progression.html .
Failed. withheld and invalid units: Monash University; [updated 2023; cited 2023 September]. Available from: https://www.monash.edu/students/admin/enrolments/change/failed-withheld-invalid-units .
OCED. Education at a Glance 2023. Organisation for Economic Co-operation and Development Publishing 2023 [Available from: https://www.oecd-ilibrary.org/content/publication/e13bef63-en .
Yorke M, Longden B. Retention and student success in higher education. McGraw-Hill Education (UK); 2004.
Faisal R, Shinwari L, Hussain S. Academic performance of male in comparison with female undergraduate medical students in pharmacology examinations. J Pak Med Assoc. 2017;67:204–8.
van Moppes NM, Willems S, Nasori M, Bont J, Akkermans R, van Dijk N et al. Ethnic minority GP trainees at risk for underperformance assessments: a quantitative cohort study. Br J Gen Pract Open. 2023;7(1).
Liu XL, Wang T, Bressington D, Nic Giolla Easpaig B, Wikander L, Tan JB. Factors influencing retention among regional, rural and remote undergraduate nursing students in Australia: a systematic review of current research evidence. Int J Environ Res Public Health. 2023;20(5).
Caponnetto V, Dante A, Masotta V, La Cerra C, Petrucci C, Alfes CM, et al. Examining nursing student academic outcomes: a forty-year systematic review and meta-analysis. Nurse Educ Today. 2021;100:104823.
Australian Tertiary Admission Rank. Universities Admission Centre; [cited 2023 November ]. Available from: https://www.uac.edu.au/future-applicants/atar .
Weighted Average Mark (WAM). The University of Sydney; 2023 [cited 2023 November]. Available from: https://www.sydney.edu.au/students/weighted-average-mark.html .
Braun V, Clarke V. Using thematic analysis in psychology. Qual Res Psychol. 2006;3(2):77–101.
Community College. Education USA; [cited 2023 November ]. Available from: https://educationusa.state.gov/ .
Ivins T, Copenhaver K, Koclanes A. Adult transitional theory and transfer shock in higher education: practices from the literature. Ref Serv Rev. 2017;45(2):244–57.
Smith NL, Grohs JR, Van Aken EM. Comparison of transfer shock and graduation rates across engineering transfer student populations. J Eng Educ. 2022;111(1):65–81.
Aulck L, West J. Attrition and performance of community college transfers. PLoS ONE. 2017;12:e0174683.
Diaz PE. Effects of transfer on academic performance of community college students at the four-year institution community. Coll J Res Prac. 1992;16(3):279–91.
Li I, Carroll D. Factors influencing university student satisfaction, dropout and academic performance: an Australian higher education equity perspective. Perth: National Centre for Student Equity in Higher Education, Curtin University;; 2017. p. 56.
Sosu EM, Pheunpha P. Trajectory of university dropout: investigating the cumulative effect of academic vulnerability and proximity to family support. Front Educ. 2019;4.
Cherastidtham I, Norton A, Mackey W. University attrition: what helps and what hinders university completion? Grattan Institute; 2018.
Panczyk M, Rebandel H, Belowska J, Zarzeka A, Gotlib J. Risk of attrition from master of science in pharmacy degree program: 15-year predictive evaluation. Ind J Pharm Educ Res. 2016;50(1):70–9.
Houglum JE, Aparasu RR, Delfinis TM. Predictors of academic success and failure in a pharmacy professional program. Am J Pharm Educ. 2005;69(1–5):283–9.
McCall KL, Allen DD, Fike DS. Predictors of academic success in a doctor of pharmacy program. Am J Pharm Educ. 2006;70(5):106.
Meagher DG, Pan T, Perez CD. Predicting performance in the first-year of pharmacy school. Am J Pharm Educ. 2011;75(5):81.
Pharmacy College Admission Test American Association of Colleges of Pharmacy. [updated 2023; cited 2023 October]. Available from: https://www.aacp.org/resource/pharmacy-college-admission-test .
Vinall R, Khansari P, McDowell J, Ried LD, Kreys E. Impact of completion of a pre-pharmacy biochemistry course and competency levels in pre-pharmacy courses on pharmacy student performance. Pharm 2019;7(3).
Aronson BD, Eddy E, Long B, Welch OK, Grundey J, Hinson JL. Identifying low pharmaceutical calculation performers using an algebra-based pretest. Am J Pharm Educ. 2022;86(1):8473.
Chisholm MA, Cobb HH, DiPiro JT, Lauthenschlager GJ. Development and validation of a model that predicts the academic ranking of first-year pharmacy students. Am J Pharm Educ. 1999;63(4):388–93.
Chisholm MA, Cobb HH, Kotzan JA. Significant factors for predicting academic success of first-year pharmacy students. Am J Pharm Educ. 1995;59(4):364–70.
Bush J. Entry characteristics and academic performance of students in a master of pharmacy degree program in the United Kingdom. Am J Pharm Educ. 2012;76(7).
2022 Student Experience Survery. National Report. 2023.
Grøtan K, Sund ER, Bjerkeset O. Mental health, academic self-efficacy and study progress among college students - the SHoT Study, Norway. Front Psychol. 2019;10:45.
Download references
No funding was received for this research.
Author information
Authors and affiliations.
Sydney Pharmacy School, Faculty of Medicine and Health, The University of Sydney, 2006, Sydney, NSW, Australia
Alice Campbell, Tina Hinton, Narelle C. da Costa, Sian E. O’Brian, Danielle R. Liang & Nial J. Wheate
Charles Perkin Centre, Faculty of Medicine and Health, The University of Sydney NSW, 2006, Sydney, NSW, Australia
Tina Hinton
You can also search for this author in PubMed Google Scholar
Contributions
ND, SO, and DL collated and de-identified the data. AC, TH, and NW analysed the data and wrote the manuscript.
Corresponding author
Correspondence to Nial J. Wheate .
Ethics declarations
Competing interests.
The authors declare no competing interests.
Ethics approval and consent to participate
The University of Sydney Human Research Ethics Committee (HREC) reviewed and approved this study (Approval number 2022/815). All methods were carried out in accordance with relevant guidelines and regulations. As this study utilised de-identified data collected retrospectively, The University of Sydney Human Research Ethics Committee (HREC) gave ethics approval for an informed consent waiver so consent did not need to be obtained from the students whose data was used in the analysis.
Consent for publication
Not applicable.
Additional information
Publisher’s note.
Springer Nature remains neutral with regard to jurisdictional claims in published maps and institutional affiliations.
Electronic supplementary material
Below is the link to the electronic supplementary material.
Supplementary Material 1
Rights and permissions.
Open Access This article is licensed under a Creative Commons Attribution 4.0 International License, which permits use, sharing, adaptation, distribution and reproduction in any medium or format, as long as you give appropriate credit to the original author(s) and the source, provide a link to the Creative Commons licence, and indicate if changes were made. The images or other third party material in this article are included in the article’s Creative Commons licence, unless indicated otherwise in a credit line to the material. If material is not included in the article’s Creative Commons licence and your intended use is not permitted by statutory regulation or exceeds the permitted use, you will need to obtain permission directly from the copyright holder. To view a copy of this licence, visit http://creativecommons.org/licenses/by/4.0/ . The Creative Commons Public Domain Dedication waiver ( http://creativecommons.org/publicdomain/zero/1.0/ ) applies to the data made available in this article, unless otherwise stated in a credit line to the data.
Reprints and permissions
About this article
Cite this article.
Campbell, A., Hinton, T., da Costa, N.C. et al. Causes and outcomes of at-risk underperforming pharmacy students: implications for policy and practice. BMC Med Educ 24 , 421 (2024). https://doi.org/10.1186/s12909-024-05327-z
Download citation
Received : 16 January 2024
Accepted : 18 March 2024
Published : 19 April 2024
DOI : https://doi.org/10.1186/s12909-024-05327-z
Share this article
Anyone you share the following link with will be able to read this content:
Sorry, a shareable link is not currently available for this article.
Provided by the Springer Nature SharedIt content-sharing initiative
- Higher education
- Underperformance
BMC Medical Education
ISSN: 1472-6920
- Submission enquiries: [email protected]
- General enquiries: [email protected]
- Share full article
Advertisement
Supported by
Biden Administration Releases Revised Title IX Rules
The new regulations extended legal protections to L.G.B.T.Q. students and rolled back several policies set under the Trump administration.

By Zach Montague and Erica L. Green
Reporting from Washington
The Biden administration issued new rules on Friday cementing protections for L.G.B.T.Q. students under federal law and reversing a number of Trump-era policies that dictated how schools should respond to cases of alleged sexual misconduct in K-12 schools and college campuses.
The new rules, which take effect on Aug. 1, effectively broadened the scope of Title IX, the 1972 law prohibiting sex discrimination in educational programs that receive federal funding. They extend the law’s reach to prohibit discrimination and harassment based on sexual orientation and gender identity, and widen the range of sexual harassment complaints that schools will be responsible for investigating.
“These regulations make it crystal clear that everyone can access schools that are safe, welcoming and that respect their rights,” Miguel A. Cardona, the education secretary, said in a call with reporters.
The rules deliver on a key campaign promise for Mr. Biden, who declared he would put a “quick end” to the Trump-era Title IX rules and faced mounting pressure from Democrats and civil rights leaders to do so.
The release of the updated rules, after two delays, came as Mr. Biden is in the thick of his re-election bid and is trying to galvanize key electoral constituencies.
Through the new regulations, the administration moved to include students in its interpretation of Bostock v. Clayton County, the landmark 2020 Supreme Court case in which the court ruled that the Civil Rights Act of 1964 protects gay and transgender workers from workplace discrimination. The Trump administration held that transgender students were not protected under federal laws, including after the Bostock ruling .
In a statement, Betsy DeVos, who served as Mr. Trump’s education secretary, criticized what she called a “radical rewrite” of the law, asserting that it was an “endeavor born entirely of progressive politics, not sound policy.”
Ms. DeVos said the inclusion of transgender students in the law gutted decades of protections and opportunities for women. She added that the Biden administration also “seeks to U-turn to the bad old days where sexual misconduct was sent to campus kangaroo courts, not resolved in a way that actually sought justice.”
While the regulations released on Friday contained considerably stronger protections for L.G.B.T.Q. students, the administration steered clear of the lightning-rod issue of whether transgender students should be able to play on school sports teams corresponding to their gender identity.
The administration stressed that while, writ large, exclusion based on gender identity violated Title IX, the new regulations did not extend to single-sex living facilities or sports teams. The Education Department is pursuing a second rule dealing with sex-related eligibility for male and female sports teams. The rule-making process has drawn more than 150,000 comments.
Under the revisions announced on Friday, instances where transgender students are subjected to a “hostile environment” through bullying or harassment, or face unequal treatment and exclusion in programs or facilities based on their gender identity, could trigger an investigation by the department’s Office for Civil Rights.
Instances where students are repeatedly referred to by a name or pronoun other than one they have chosen could also be considered harassment on a case-by-case basis.
“This is a bold and important statement that transgender and nonbinary students belong, in their schools and in their communities,” said Olivia Hunt, the policy director for the National Center for Transgender Equality.
The regulations appeared certain to draw to legal challenges from conservative groups.
May Mailman, the director of the Independent Women’s Law Center, said in a statement that the group planned to sue the administration. She said it was clear that the statute barring discrimination on the basis of “sex” means “binary and biological.”
“The unlawful omnibus regulation reimagines Title IX to permit the invasion of women’s spaces and the reduction of women’s rights in the name of elevating protections for ‘gender identity,’ which is contrary to the text and purpose of Title IX,” she said.
The existing rules, which took effect under Mr. Trump in 2020, were the first time that sexual assault provisions were codified under Title IX. They bolstered due process rights of accused students, relieved schools of some legal liabilities and laid out rigid parameters for how schools should conduct impartial investigations.
They were a sharp departure from the Obama administration’s interpretation of the law, which came in the form of unenforceable guidance documents directing schools to ramp up investigations into sexual assault complaints under the threat of losing federal funding. Scores of students who had been accused of sexual assault went on to win court cases against their colleges for violating their due process rights under the guidelines.
The Biden administration’s rules struck a balance between the Obama and Trump administration’s goals. Taken together, the regulation largely provides more flexibility for how schools conduct investigations, which advocates and schools have long lobbied for.
Catherine E. Lhamon, the head of the department’s Office for Civil Rights who also held the job under President Barack Obama, called the new rules the “most comprehensive coverage under Title IX since the regulations were first promulgated in 1975.”
They replaced a narrower definition of sex-based harassment adopted under the Trump administration with one that would include a wider range of conduct. And they reversed a requirement that schools investigate only incidents alleged to have occurred on their campuses or in their programs.
Still, some key provisions in the Trump-era rules were preserved, including one allowing informal resolutions and another prohibiting penalties against students until after an investigation.
Among the most anticipated changes was the undoing of a provision that required in-person, or so-called live hearings, in which students accused of sexual misconduct, or their lawyers, could confront and question accusers in a courtroom-like setting.
The new rules allow in-person hearings, but do not mandate them. They also require a process through which a decision maker could assess a party or witness’s credibility, including posing questions from the opposing party.
“The new regulations put an end to unfair and traumatic grievance procedures that favor harassers,” Kel O’Hara, a senior attorney at Equal Rights Advocates. “No longer will student survivors be subjected to processes that prioritize the interests of their perpetrators over their own well being and safety.”
The new rules also allow room for schools to use a “preponderance of evidence” standard, a lower burden of proof than the DeVos-era rules encouraged, through which administrators need only to determine whether it was more likely than not that sexual misconduct had occurred.
The renewed push for that standard drew criticism from legal groups who said the rule stripped away hard-won protections against flawed findings.
“When you are dealing with accusations of really one of the most heinous crimes that a person can commit — sexual assault — it’s not enough to say, ‘50 percent and a feather,’ before you brand someone guilty of this repulsive crime,” said Will Creeley, the legal director of the Foundation for Individual Rights and Expression.
The changes concluded a three-year process in which the department received 240,000 public comments. The rules also strengthen protections for pregnant students, requiring accommodations such as a bigger desk or ensuring access to elevators and prohibiting exclusion from activities based on additional needs.
Title IX was designed to end discrimination based on sex in educational programs or activities at all institutions receiving federal financial assistance, beginning with sports programs and other spaces previously dominated by male students.
The effects of the original law have been pronounced. Far beyond the impact on school programs like sports teams, many educators credit Title IX with setting the stage for academic parity today. Female college students routinely outnumber male students on campus and have become more likely than men of the same age to graduate with a four-year degree.
But since its inception, Title IX has also become a powerful vehicle through which past administrations have sought to steer schools to respond to the dynamic and diverse nature of schools and universities.
While civil rights groups were disappointed that some ambiguity remains for the L.G.B.T.Q. students and their families, the new rules were widely praised for taking a stand at a time when education debates are reminiscent to the backlash after the Supreme Court ordered schools to integrate.
More than 20 states have passed laws that broadly prohibit anyone assigned male at birth from playing on girls’ and women’s sports teams or participating in scholastic athletic programs, while 10 states have laws barring transgender people from using bathrooms based on their gender identity.
“Some adults are showing up and saying, ‘I’m going to make school harder for children,” said Liz King, senior program director of the education equity program at the Leadership Conference on Civil and Human Rights. “It’s an incredibly important rule, at an incredibly important moment.”
Schools will have to cram over the summer to implement the rules, which will require a retraining staff and overhauling procedures they implemented only four years ago.
Ted Mitchell, the president of the American Council on Education, which represents more than 1,700 colleges and universities, said in a statement that while the group welcomed the changes in the new rule, the timeline “disregards the difficulties inherent in making these changes on our nation’s campuses in such a short period of time.”
“After years of constant churn in Title IX guidance and regulations,” Mr. Mitchell said, “we hope for the sake of students and institutions that there will be more stability and consistency in the requirements going forward.”
Zach Montague is based in Washington. He covers breaking news and developments around the district. More about Zach Montague
Erica L. Green is a White House correspondent, covering President Biden and his administration. More about Erica L. Green
Biden Title IX rules set to protect trans students, survivors of abuse
The administration’s regulations offer protections for transgender students, but do not address athletics.

The Biden administration on Friday finalized sweeping new rules barring schools from discriminating against transgender students and ordering significant changes for how schools adjudicate claims of sexual harassment and assault on campus.
The provisions regarding gender identity are the most politically fraught, feeding an election-year culture clash with conservative states and school boards that have limited transgender rights in schools, banned discussion of gender identity in classrooms and removed books with LGBTQ+ themes. Mindful of the politics, the administration is delaying action on the contentious issue of whether transgender girls and women should be allowed to compete in women’s and girls’ sports.
The long-awaited regulation represents the administration’s interpretation of Title IX, a 1972 law that bars sex discrimination in schools that receive federal funding. Title IX is best known for ushering in equal treatment for women in sports, but it also governs how schools handle complaints of sexual harassment and assault, a huge issue on many college campuses.
Now the Biden administration is deploying the regulation to formalize its long-standing view that sex discrimination includes discrimination based on gender identity as well as sexual orientation, a direct challenge to conservative policies across the country.
Ten states, for instance, require transgender students to use bathrooms and locker rooms that align with their biological sex identified at birth, according to tracking by the Movement Advancement Project. Some school districts will not use the pronouns corresponding with a trans student’s gender identity. Both situations might constitute violations of Title IX under the new regulation. In addition, if a school failed to properly address bullying based on gender identity or sexual orientation, that could be a violation of federal law.
“No one should face bullying or discrimination just because of who they are or who they love. Sadly, this happens all too often,” Education Secretary Miguel Cardona told reporters in a conference call.
The Education Department’s Office for Civil Rights investigates allegations of sex discrimination, among other things, and schools that fail to come into compliance risk losing federal funding. A senior administration official said that the office could investigate cases where schools were potentially discriminating, even if they were following their own state’s law.
The final regulation also includes provisions barring discrimination based on pregnancy, including childbirth, abortion and lactation. For instance, schools must accommodate students’ need to attend medical appointments, as well as provide students and workers who are nursing a clean, private space to pump milk.
The combination of these two issues — sexual assault and transgender rights — drew enormous public interest, with some 240,000 public comments submitted in response to the proposed version published in 2022. The new rules take effect Aug. 1, in time for the start of next school year.
The final regulation makes a number of significant changes to a Trump-era system for handling sexual assault complaints, discarding some of the rules that bolstered due process rights of the accused. Supporters said those rules were critical to ensuring that students had the opportunity to defend themselves; critics said they discouraged sexual assault survivors from reporting incidents and turned colleges into quasi-courtrooms.
Education Department officials said they had retained the elements that made sense but created a better overall framework that balanced the rights of all involved.
Under the Trump administration’s regulation , finalized in 2020, colleges were required to stage live hearings to adjudicate complaints, where the accused could cross-examine witnesses — including the students who were alleging assault.
Under President Biden’s new rules, colleges will have more flexibility. The investigator or person adjudicating the case may question witnesses in separate meetings or employ a live hearing.
The new rules also will allow schools to use a lower bar for adjudicating guilt. In weighing evidence, they are now directed to use a “preponderance of the evidence” standard, though they may opt for a higher standard of “clear and convincing evidence” if they use that standard in other similar proceedings. Under the Trump version, universities had a choice, but they were required to use the higher standard if they did so in other settings.
The proposal also expands the definition of what constitutes sexual harassment, discarding a narrower definition used under President Donald Trump . Under the new definition, conduct must be so “severe or pervasive” that it limits or denies a person’s ability to participate in their education. The prior version required it to be both severe and pervasive.
The changes were derided by conservative critics who said they would revive problems that the Trump rules had solved.
“So today’s regulations mean one thing: America’s college students are less likely to receive justice if they find themselves in a Title IX proceeding,” said Will Creeley, legal director for the Foundation for Individual Rights and Expression, a free speech advocacy group.
The group was concerned that the opportunity for cross examination would no longer be guaranteed and that schools will again be allowed to have one person investigate and adjudicate cases.
Some of the Trump provisions were retained. Schools, for instance, will continue to have the option to use informal resolution of discrimination complaints, unless the allegation involves an employee of a K-12 school.
The Biden administration’s approach was welcomed by advocates for sexual assault survivors.
The rules “will make schools safer and more accessible for young people, many of whom experienced irreparable harm while they fought for protection and support,” said Emma Grasso Levine, senior manager of Title IX policy and programs at Know Your IX, a project of the advocacy group Advocates for Youth.
“Now, it’s up to school administrators to act quickly to implement and enforce” the new rules, she added.
Sexual assault has been a serious issue on college campuses for years, and in releasing the regulation, the Biden administration said the rates were still “unacceptably high.”
But the most controversial element of the regulation involves the rights of transgender students, and it came under immediate fire.
Administration officials point to a 2020 Supreme Court ruling that sex discrimination in employment includes gender identity and sexual orientation to bolster their interpretation of the law. But conservatives contend that Title IX does not include these elements and argue that accommodations for transgender students can create situations that put other students at risk. They object, for instance, to having a transgender woman — someone they refer to as a “biological man” — using women’s bathrooms or locker rooms.
Rep. Virginia Foxx (R-N.C.), chairwoman of the House Education Committee, called the regulation an escalation of Democrats’ “contemptuous culture war that aims to radically redefine sex and gender.” The department, she said, has put decades of advancement for women and girls “squarely on the chopping block.”
Betsy DeVos, who was education secretary during Trump’s administration, criticized the gender identity protections and the new rules on handling assault and harassment allegations.
“The Biden Administration’s radical rewrite of Title IX guts the half century of protections and opportunities for women and callously replaces them with radical gender theory,” she said in a statement.
Still, the administration sidestepped the contentious issue of athletics, at least for now. A separate regulation governing how and when schools may exclude transgender students from women and girls teams remains under review, and administration officials offered no timetable for when it would be finalized. People familiar with their thinking said it was being delayed to avoid injecting the matter into the presidential campaign, where Biden faces a close race against Trump.
A senior administration official, who spoke on the condition of anonymity in a briefing with reporters Thursday, declined to comment on the politics of this decision but noted that the athletics rule was proposed after the main Title IX regulation.
Polling shows that clear majorities of Americans, including a sizable slice of Democrats, oppose allowing transgender athletes to compete on girls’ and women’s teams. Twenty-five states have statewide bans on their participation.
The proposed sports regulation disallows these statewide, blanket bans, but it allows school districts to restrict participation more narrowly defined — for instance on competitive high school or college teams. The main Title IX regulation does not address the issue, and an administration official said the status quo would remain in place for now. Still, some have argued that the new general ban on discrimination could apply to sports even though the administration does not intend it to.
Sen. Patty Murray (D-Wash.) welcomed the protections for transgender students but urged the department to issue the sports regulation as well.
“Trans youth deserve to play sports with their friends just like anyone else,” she said in a statement.
The rules governing how campuses deal with harassment complaints have changed repeatedly in recent years.
The Obama administration issued detailed guidance in 2014 for schools in handling complaints, but DeVos later tossed that out. Her department went through its own laborious rulemaking process to put a new system in place.
As a candidate for president in 2020, Biden promised to put a “quick end” to that version if elected, saying it gave colleges “a green light to ignore sexual violence and strip survivors of their rights.” Friday’s action makes good on his promise.
Ted Mitchell, president of the American Council on Education, which represents colleges and universities, praised many of the changes but bemoaned the fact that schools will have to retrain their staffs on the new rules in short order.
“After years of constant churn in Title IX guidance and regulations,” he said, “we hope for the sake of students and institutions that there will be more stability and consistency in the requirements going forward.”
Danielle Douglas-Gabriel contributed to this report.

- Bihar Board
SRM University
Jac 12th result 2024.
- TS Board Result 2024
- Punjab Board Result 2024
- JAC Board Result 2024
- WB Board Result 2024
- UK Board Result 2024
- Karnataka Board Result 2024
- Shiv Khera Special
- Education News
- Web Stories
- Current Affairs
- नए भारत का नया उत्तर प्रदेश
- School & Boards
- College Admission
- Govt Jobs Alert & Prep
- GK & Aptitude
- State Boards
HBSE 11th Biology Syllabus 2025: Download Haryana Board Biology Syllabus 2024-2025 PDF
Bseh class 11th biology syllabus 2025: here, the official haryana board class 11 biology syllabus 2025 has been presented for the students of academic session 2024-2025. also, find the free pdf download link of the hbse class 11th biology syllabus 2025 here..
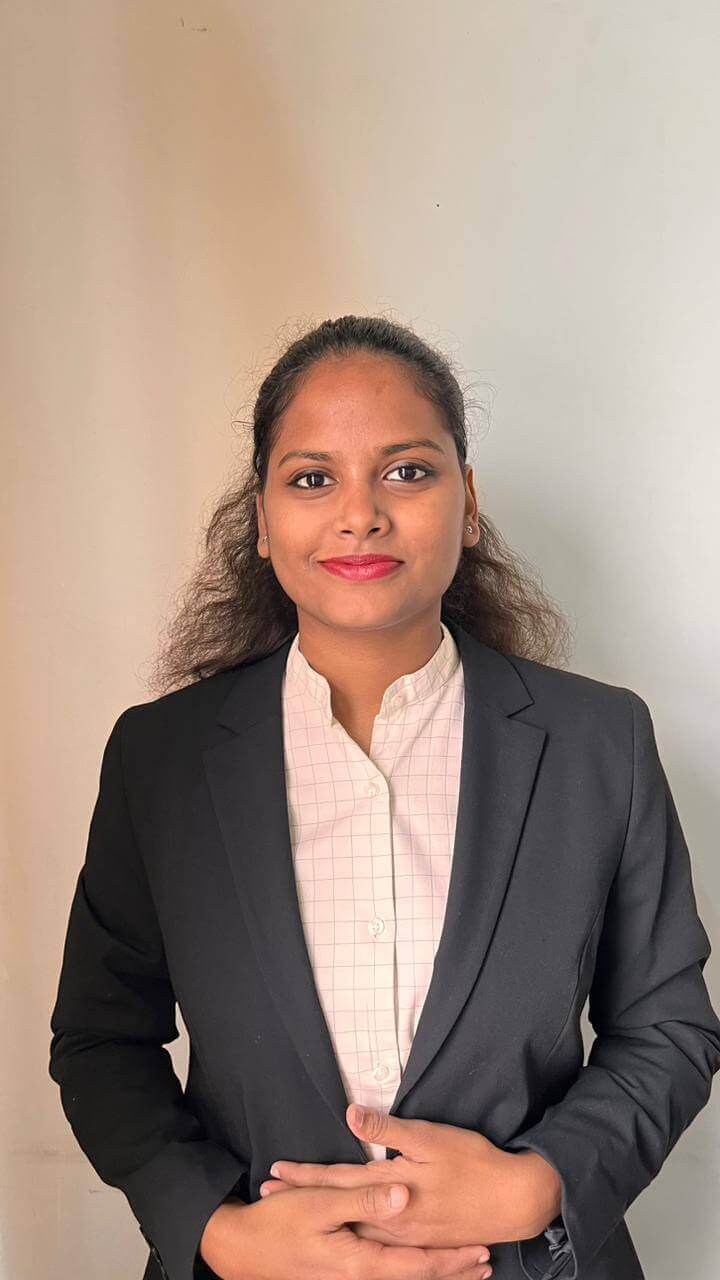
HBSE Class 11th Biology Syllabus 2025: Board of School Education Haryana (BSEH), also popularly called HBSE Board is the official education board of Haryana, that provides students with all the study materials that might be required to ace the examinations. We understand that searching the syllabus takes up a lot of time and effort for students, and hence we bring to you subject-wise syllabus articles for all subjects of Class 11. In this article, you can find Haryana Board Class 11 Biology Syllabus 2024-25 along with a free PDF download link of the same. Also, find the course structure, marking scheme, and more to know in detail about the overall course formation.
Haryana Board HBSE Class 11 Biology Syllabus 2024-25
Haryana board class 11 biology course structure 2024-2025.
More information related to the practical syllabus, exam pattern, question paper design, etc will be provided to you. Stay tuned for complete access to study materials for classes 9 to 12.
Also Check:
Get here latest School , CBSE and Govt Jobs notification in English and Hindi for Sarkari Naukari and Sarkari Result . Download the Jagran Josh Sarkari Naukri App . Check Board Result 2024 for Class 10 and Class 12 like CBSE Board Result , UP Board Result , Bihar Board Result , MP Board Result , Rajasthan Board Result and Other States Boards.
- PSEB 12ਵੀਂ ਟਾਪਰ ਸੂਚੀ 2024
- 12th, 8th ਨਤੀਜਾ 2024 PSEB
- jac.jharkhand.gov.in Class 12 Result 2024
- Uttarakhand Board Result at Jagran Josh
- UK Board 12th Toppers 2024
- JAC 12th Toppers 2024
- HBSE 12th Result 2024
- AP SSC Results 2024 Manabadi by Jagran Josh
- AP SSC Topper List 2024
Latest Education News
[Latest] IPL Points Table 2024: आईपीएल 2024 अपडेटेड पॉइंट टेबल यहां देखें, राजस्थान ऑन टॉप
GJU Admit Card 2024 OUT at gjust.ac.in; Direct Link to Download Semester Exam Hall Ticket PDF
UPMRC Admit Card 2024 OUT at lmrcl.com: Download UP Metro Call Letter Here
India T20 World Cup 2024 Squad: टी20 ताज के लिए भारत ने बनाई ‘विराट’ टीम, किस रोल में कौन देखें यहां
[LIVE] PSEB 8th, 12th Result 2024 Declared: Punjab Board 8, 12 Results Link Tomorrow At pseb.ac.in, Check Online by using Roll Number
PSEB 12th Toppers List 2024: पंजाब बोर्ड कक्षा 12,8 ਟਾਪਰ ਨਾਮ, ਅੰਕ ਅਤੇ ਪਾਸ ਪ੍ਰਤੀਸ਼ਤਤਾ
12th, 8th Result 2024 PSEB OUT: पंजाब बोर्ड 12वीं, 8वीं ਨਤੀਜਾ ਮੈਰਿਟ ਸੂਚੀ रोल नंबर से pseb.ac.in डाउनलोड करें
PSEB Topper List 2024 OUT: Check Punjab Board Class 8 and12 Exam Toppers Name, Marks, Merit List and Percentage
Punjab School Holidays 2024: Check Summer Vacation Dates and Upcoming Holidays
Uttarakhand UK Board Result 2024 Out: घोषित हुए उत्तराखंड बोर्ड के नतीजे, यहां देखें Direct Link
ubse.uk.gov.in 2024: उत्तराखंड बोर्ड 10वीं 12वीं रिजल्ट घोषित, रोल नंबर के साथ आधिकारिक वेबसाइट uaresults.nic.in पर करें चेक
Punjab Board Result 2024: Check PSEB 10th, 12th Result Link at pseb.ac.in
UPMRC Previous Year Paper: Download UP Metro Paper PDFs with Solutions
Punjab Board 12th Result 2024: Check PSEB Inter Result Link at pseb.ac.in
KPSC Group C Recruitment 2024: Apply Online for 173 Various Posts Vacancies
Find 3 differences between the pictures of a duck with an umbrella in 15 seconds!
[DECLARED] JAC 12th Result 2024 Released: Check Jharkhand Board Class 12 Results Out at jac.jharkhand.gov.in, jacresult.com, Check Online by Roll Number, Pass Percentage and Topper's List
ICSE Class 9 Economic Applications Syllabus 2024-25: Download Revised PDF
CBSE Class 9 Syllabus 2024-2025: Download in PDF
CBSE Class 12 Syllabus 2024-25: Check New Syllabus of All Subjects, Direct Download Links Here
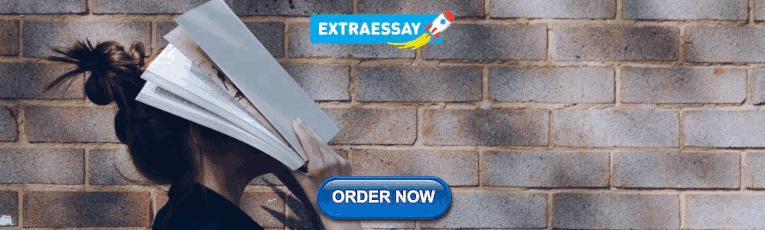
IMAGES
VIDEO
COMMENTS
Journal overview. Journal of Biological Education is firmly established as the authoritative voice in the world of biological education. The journal provides a forum where the latest advances in research into the teaching, learning and assessment of biology can contribute to policy and practice in biological education for all ages, connecting ...
Fig. 1. Aims of educational interventions are to shift the mean and narrow the gap of educational attainment outcomes. a Normal distribution of educational attainment. b Shift the mean. c Narrow ...
Biologists have long been concerned about the quality of undergraduate biology education. Indeed, some biology education journals, such as the American Biology Teacher, have been in existence since the 1930s.Early contributors to these journals addressed broad questions about science learning, such as whether collaborative or individual learning was more effective and the value of ...
Biology education research (BER) is an emerging field with increasing participation through journal publications and growing professional networks. As an example, the inaugural volume of the Journal of Microbiology and Biology Education (JMBE) in 2007 had four articles, a number that increased to almost 80 articles published in 2020 alone. ...
Taken together, the aims, ideals, and reliable processes of epistemic cognition are the ways that students gather, justify, evaluate, and construct knowledge in a particular discipline like biology. Epistemic metacognition is an individual's awareness of the knowledge, skills, and experiences related to that individual's thinking and learning.
Journal of Biological Education The Journal of Biological Education is an international journal that publishes the latest research into the teaching, learning and assessment of biology. It is owned by the Royal Society of Biology and published by Taylor and Francis. The journal aims to bridge the gap between research and practice, providing information, ideas and opinion, in addition to ...
This critical review examines the challenges and opportunities facing the field of Biology Education Research (BER). Ongoing disciplinary fragmentation is identified as a force working in opposition to the development of unifying conceptual frameworks for living systems and for understanding student thinking about living systems. A review of Concept Inventory (CI) research is used to ...
Problem-solving has been a widely accepted goal of education in biology teaching for a number of years (Nehm, 2010). Biology is the branch of science concerned with the study of the living world. It abounds in problems relating to the characteristics and behaviour of organisms and their inter-relationships, as well as the relationships between ...
Understanding how students develop biology interests and the roles interest plays in biology contexts could help instructors and researchers to increase science, technology, engineering, and mathematics students' motivation and persistence. However, it is currently unclear how interest has been defined or measured in the biology education research literature. We analyzed this body of ...
Active learning is frequently used to describe teaching practices, but the term is not well-defined in the context of undergraduate biology education. To clarify this term, we explored how active learning is defined in the biology education literature (n = 148 articles) and community by surveying a national sample of biology education researchers and instructors (n = 105 individuals). Our ...
Biology today is a popular and influential discipline that dramatically shapes our lives and affects the development and operations of societies around the world. Biology educators thus play a crucial role in ensuring the global community is made aware of the biological bases of everything we do. However, as biology teachers and educationists ...
Modeling, like inquiry more generally, is not a single method, but rather a complex suite of strategies. Philosophers of biology, citing the diverse aims, interests, and disciplinary cultures of biologists, argue that modeling is best understood in the context of its epistemic aims and cognitive payoffs. In the science education literature, modeling has been discussed in a variety of ways, but ...
Explore the latest full-text research PDFs, articles, conference papers, preprints and more on BIOLOGY EDUCATION. Find methods information, sources, references or conduct a literature review on ...
The results show that biology education in the Croatian educational system is mostly traditional, whereby students mostly practice listening to the teacher (34%) and answering the questions (18%).
He is committed to increasing understanding about the microbial sciences particularly in undergraduate and k-12 education. Editorial Board. The Journal of Microbiology & Biology Education (JMBE) offers original, previously unpublished, peer-reviewed articles that foster scholarly teaching and provide resources for all educators.
The aim of this Special Issue is to give an overview on the different branches of research that relate to these two fundamental trends relevant for biology education. In this regard the issue will be structured in four thematic sections: (1) studies addressing questions on teaching and learning biological core ideas respectively fundamental ...
In this essay, we present an evidence-based vision for nonmajor biology courses focused on scientific literacy for the vast majority of college-educated citizens—our non-science majors. Our positionality as instructors at 4-year institutions of higher education informs our standpoint in this essay. Go to:
Biochemistry and Molecular Biology Education is a journal aimed to enhance dissemination of innovative pedagogies and student learning in Biochemistry, Molecular Biology, and related sciences such as Biotechnology, Biophysics and Cell Biology, by promoting the world-wide publication of educational materials. We seek to communicate articles on a broad range of topics including innovative ...
Explore ASM Journals. The Journal of Microbiology & Biology Education® (JMBE) is rooted in the biological sciences and branches to other disciplines. JMBE topics include: Good pedagogy and design. Student interest and motivation. Recruitment and retention. Citizen science.
Vol 325, Issue 5940. pp. 541 - 542. DOI: 10.1126/science.1173876. References and Notes. eLetters (0) Advances in computing have forever changed the practice of biological research. Computational biology, or bioinformatics, is as essential for biology in this century as molecular biology was in the last. In fact, it is difficult to imagine ...
Biology is the study of life. Here, you can browse videos, articles, and exercises by topic. We keep the library up-to-date, so you may find new or improved content here over time. ... world-class education to anyone, anywhere. Khan Academy is a 501(c)(3) nonprofit organization.
Sexual and reproductive health (SRH) education integrated into the school curriculum is an effective intervention for in-school adolescents before risky sexual behaviors (RSBs) are firmly established [1,2,3].Adolescents aged 10-19 years are estimated to constitute 16% and 23% of the global population and the Tanzania population, respectively, with 90% of them living in low-middle-income ...
Ethics. Approval for this study was granted by the Human Research Ethics Committee of The University of Sydney (2022/815). Data collection. The inclusion criteria for this study were students enrolled in a BPharm, BPharmMgmt, or MPharm degree between the period of 2008 and 2018 (inclusive), who were required to provide a minimum of one show cause at any stage of their study.
The Education Department is pursuing a second rule dealing with sex-related eligibility for male and female sports teams. The rule-making process has drawn more than 150,000 comments.
The Education Department's Office for Civil Rights investigates allegations of sex discrimination, among other things, and schools that fail to come into compliance risk losing federal funding.
[8], Biology students explore the science of life in a broad sense, tapping into the inner workings of various living organisms—all of which can provide insight into health and wellness. [10] In an undergraduate biology program, you'll encounter a range of topics applicable to the best physical therapy programs, such as: Anatomy
In this article, you can find Haryana Board Class 11 Biology Syllabus 2024-25 along with a free PDF download link of the same. Also, find the course structure, marking scheme, and more to know in ...