Facing dire sea level rise threat, Maldives turns to climate change solutions to survive
The islands could be 80% uninhabitable by 2050 at current global warming rates.
The Maldives are well known as a bucket list getaway. Hearing the country's name conjures up images of luxury huts overlooking an aqua blue ocean. But climate change may cross the country off the map completely.
The archipelago, which is made up of over 1,100 coral islands in the middle of the Indian Ocean, is the lowest lying nation in the world. Therefore, sea level rise caused by global climate change is an existential threat to the island nation. At the current rate of global warming, almost 80% of the Maldives could become uninhabitable by 2050, according to multiple reports from NASA and the U.S. Geological Survey.
"Our islands are slowly being inundated by the sea, one by one," Ibrahim Mohamed Solih, the president of the Maldives, told the U.N. Climate Change Conference, or COP26, earlier this week. "If we do not reverse this trend, the Maldives will cease to exist by the end of this century."
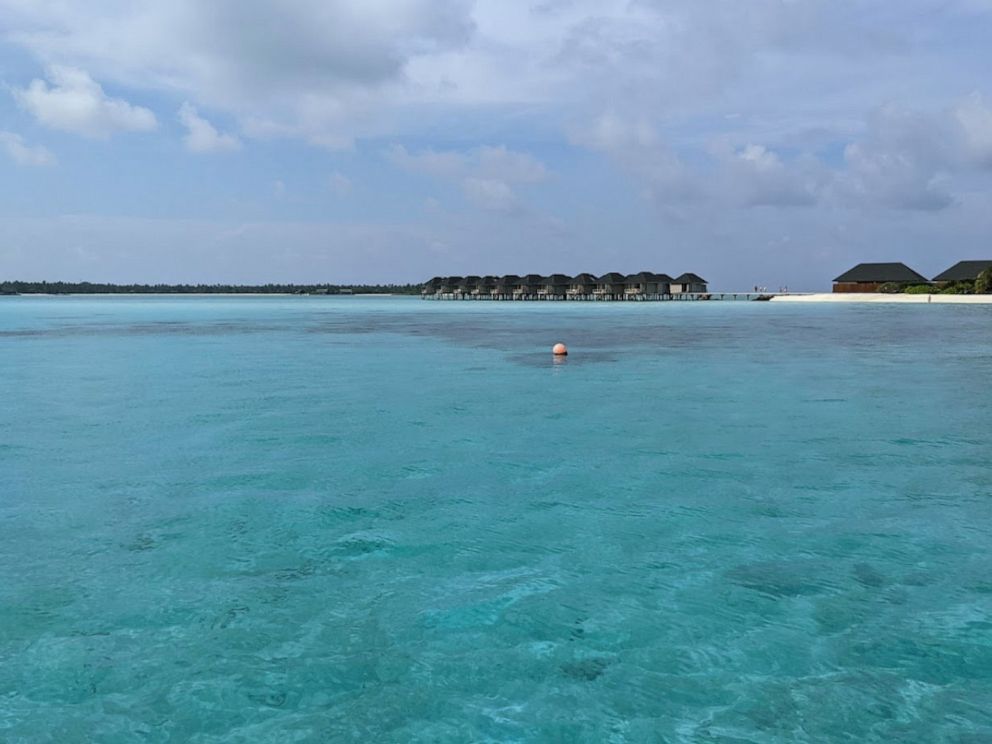
The islands that are home to local Maldivians, not the resort islands, stand to lose the most. Mohammed Nasheed, the former president of the Maldives and a leading voice for climate change equity, told ABC News that more than 90% of islands in the Maldives have severe erosion, and 97% of the country no longer has fresh groundwater.
Ibrahim Mubbasir and his family live on the island of Dhiffushi. It is suffering from severe erosion, and flooding has increased from two or three times a year to twice a month. Four years ago, the family's well became unusable because of salt water contamination, leaving them to rely on collecting rainwater. Mubbasir said they only have enough fresh water to last for three more months.
"Things that we thought would happen towards the end of the century, we are experiencing now," Aminath Shauna, the Maldives' minister of environment, climate change and technology, told ABC News' Ginger Zee.
Shauna said that more than 50% of the national budget is spent on adapting to climate change. When asked what the Maldives will look like in 2050, Shauna responded, "Are you willing to take the Maldives as climate refugees? I think that's the conversation that needs to happen."
And it's not just the Maldives. Island countries around the world have been asking developed nations for funds since 2009. Countries with the highest greenhouse gas emissions -- China, the United States and India -- are mostly responsible for the rapid sea level rise.
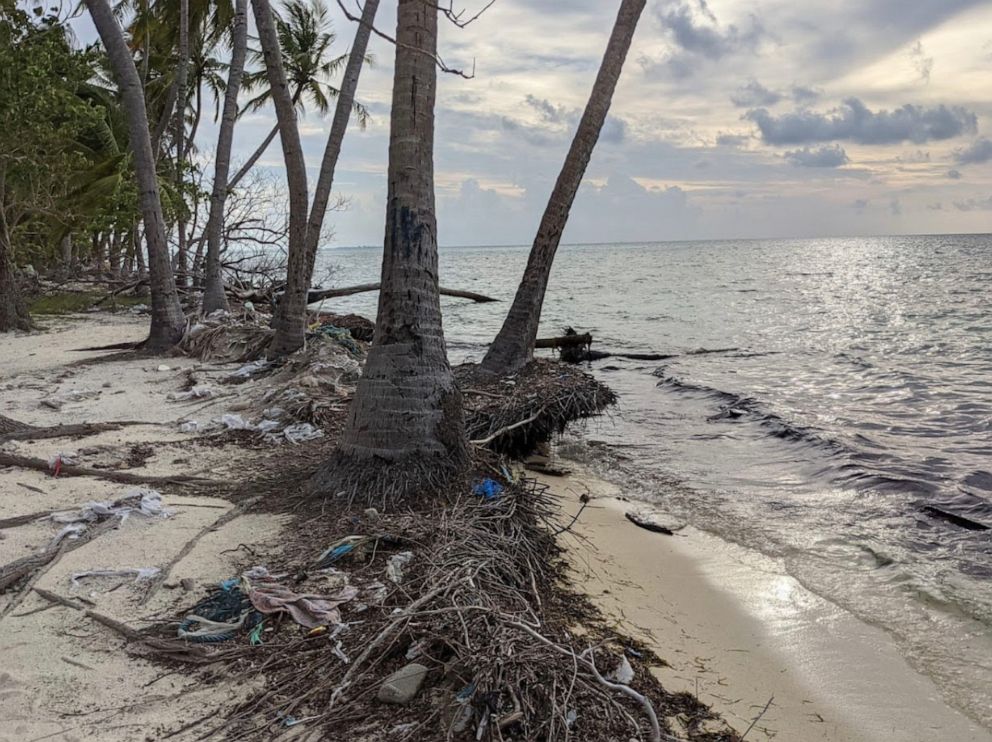
Maldives' coral reefs
At the center of the Maldives' culture are their coral reefs.
In 2016, the Maldives lost their front line of defense when a bleaching event affected about 60% of the coral reefs, according to Aya Naseem, a marine biologist and co-founder of the Maldives Coral Institute.
Without coral reefs, the islands are wide open to the rising waters. Naseem said they have one realistic choice: They need to build back and protect the reefs, "because IPCC (Intergovernmental Panel on Climate Change) is predicting that by 2050 if the temperature rises 1.5 degrees Celsius we can lose 70 to 90% of corals in the whole world."
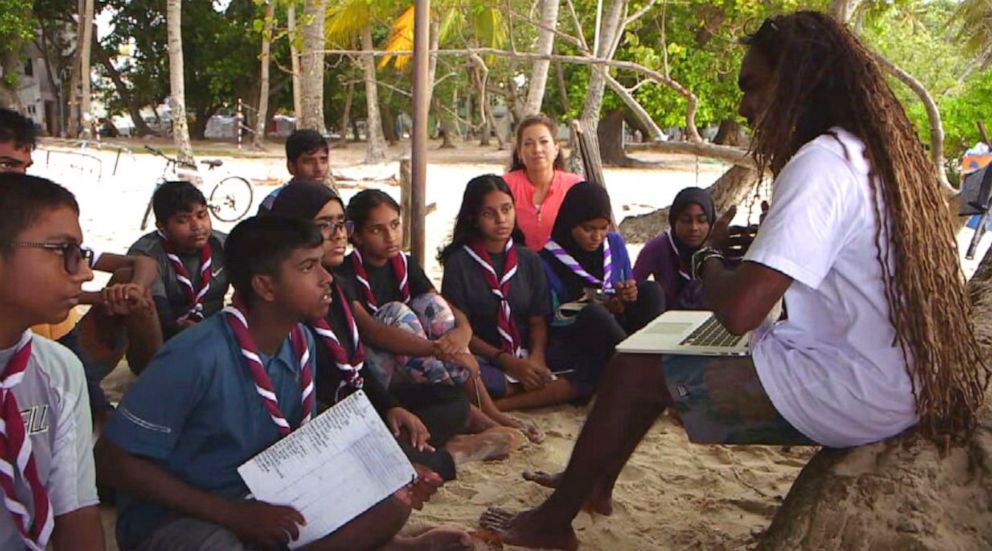
Research has previously shown that a healthy coral reef can absorb 97% of wave energy, dramatically reducing erosion, and it's affordable, Naseem said.
"It's much cheaper than building a seawall. I think it costs something like $3,000 to grow a meter of sea wall where for the coral a meter of it is about $300, including monitoring and everything," she said.
Bebe Ahmed, founder of "Save the Beach," travels island to island in the Maldives with the mission of teaching kids about restoring and protecting coral. He told ABC News that his dream is to inspire young Maldivians to want to start their own projects to restore coral reefs.
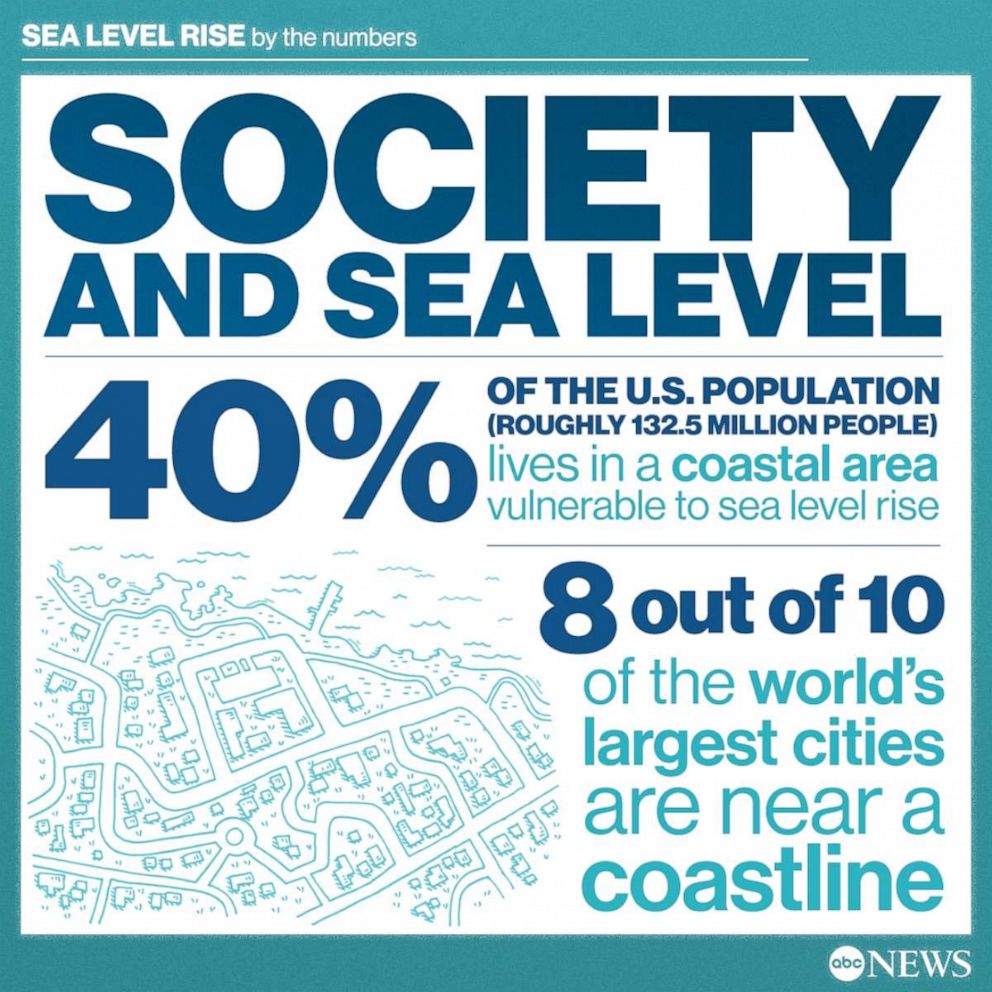
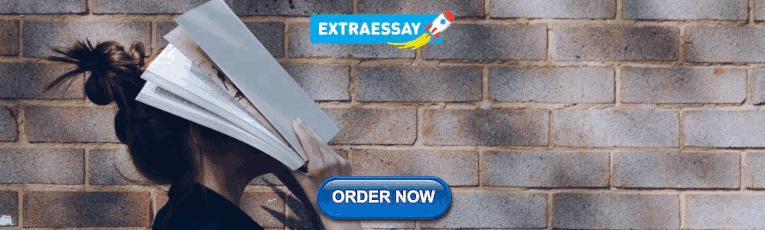
MORE: COP26 updates: 'America showed up,' Biden says of time at summit
What's next in the fight?
Technology, like 3D-printed coral structures or a floating city, may have to be part of the solution.
The Maldives is home to the Modular Artificial Reef Structure, or MARS, a coral-forming project on the resort island of Summer Island. to the coral forming project, MARS. The project has 3D-printed bases that are placed in the water with transplant corals attached to them. The hope is that the system is designed with the specific needs of the coral farm in mind, providing a permanent structure for coral to grow.
In the late '90s, the Maldives began construction on the island of Hulhumale through the process of land reclamation. Hulhumale is 6.5 feet above sea level, more than double the height of Male, the current capital of the Maldives. It is possible this island may be a future site for relocation of Maldivians suffering from sea level rise. Maldivians call their manmade island the City of Hope.
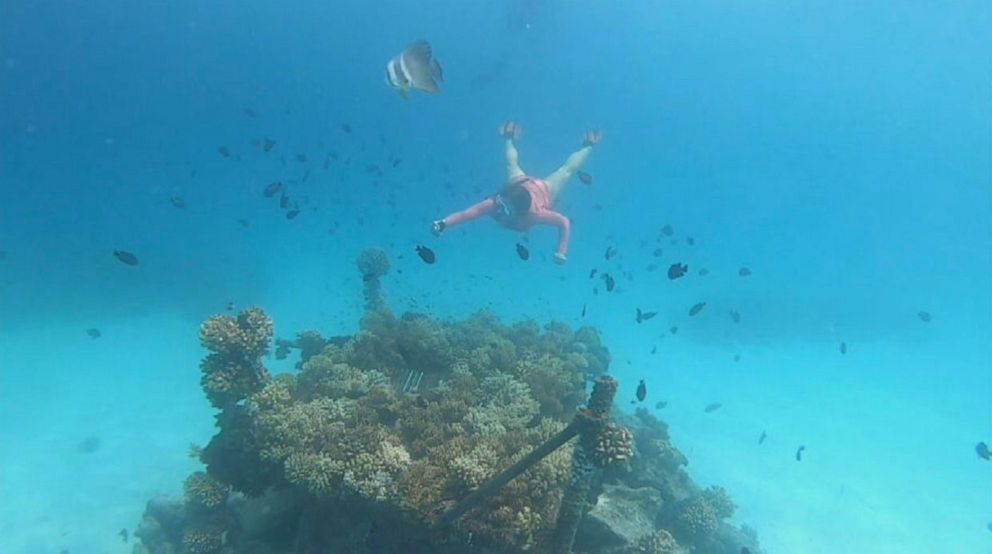
The future of the Maldives could also come in the form of a floating city. In 2022, just a few miles from the dense, capital city of Male, construction and assembly will begin on the world's first true floating city. The unique solution will not have to worry about sea level rise, because it will always be on top of the sea.
The project is being developed and led by Dutch Docklands in the Netherlands. Lead architect Koen Olthuis gave ABC News an inside look at how the floating city is designed and what it should eventually look like.
MORE: India's addicted to coal. But for how much longer?: Reporter's Notebook
The floating city has a unique pattern, modeled after the brain, both human and coral.
The entire city will shift up and down on a pile drilled into the sea floor. It will also take advantage of its environment to better provide for those living on the floating city.
"By being on the water we want to take advantage of the water -- and using the coolness of the water -- so these are water-cooled cities, for which you take cool water outside the atoll and pump water through the route and activate the air conditioning systems," Olthius said.
Since the floating city is sustainable and leaves no footprint, Olthuis called the floating cities "scarless" and said they are "renting space from nature."
Related Topics
- Climate Change
Top Stories
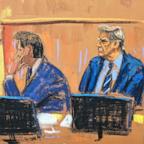
Trump hush money trial: With court off today, Trump criticizes jury selection process
- 2 hours ago
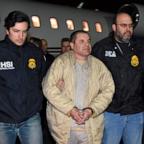
Mexican drug lord Joaquín 'El Chapo' Guzmán claims he can't get calls or visits in a US prison
- Apr 16, 5:54 PM
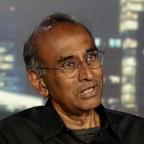
Nobel-winning molecular biologist on the consequences of aging
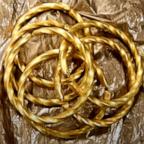
9 arrests made in $14.8 million gold heist, only fraction recovered
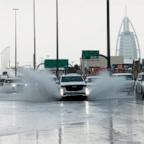
Dubai sees severe flooding after getting 2 years' worth of rain in 24 hours
- Apr 16, 7:44 PM
ABC News Live
24/7 coverage of breaking news and live events
- EO Explorer

- Global Maps
Preparing for Rising Seas in the Maldives
February 3, 1997 JPEG
February 19, 2020 JPEG
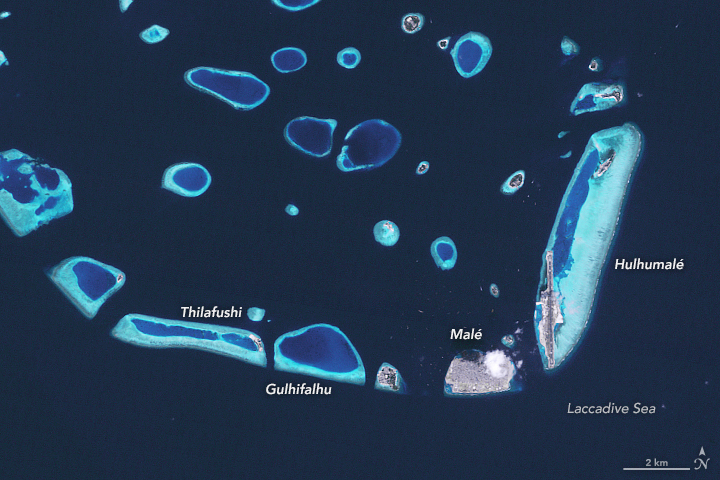
February 3, 1997
February 19, 2020
With more than 80 percent of its 1,190 coral islands standing less than 1 meter above sea level, the Maldives has the lowest terrain of any country in the world. This makes the archipelago in the Indian Ocean particularly vulnerable to sea level rise.
With global sea level rising 3 to 4 millimeters per year, and that rate expected to rise in coming decades, some analysts anticipate a grim future for the Maldives and other low-lying islands. One study concluded that low-lying islands could become uninhabitable by 2050 as wave-driven flooding becomes more common and freshwater becomes limited. The Intergovernmental Panel on Climate Changes anticipates sea level could rise by about half a meter by 2100 even if greenhouse gas emissions are sharply reduced or rise up to 1 meter if greenhouse gas emissions continue to increase strongly.
While the Maldives government has explored plans to purchase land on higher ground in other countries as an insurance policy against sea level rise, planners are also working to enhance the resilience of the country’s current islands. One example is Hulhumalé, a newly constructed artificial island northeast of the capital, Malé.
The pair of Landsat satellite images above show just how much the area has changed between 1997 and 2020. Construction of the island, designed to relieve crowding in Malé, began in 1997 in a lagoon near the airport. Since then, the island has grown to cover 4 square kilometers, making it the fourth largest island in the Maldives. Hulhumalé’s population has swollen to more than 50,000 people, with 200,000 more expected to eventually move there.
The new island, built by pumping sand from the seafloor onto a submerged coral platform, rises about 2 meters above sea level, about twice as high as Malé. The extra height could make the island a refuge for Maldivians who are eventually driven off lower-lying islands due to rising seas. It could also prove to be an option for evacuations during future typhoons and storm surges.
There is one piece of positive news: natural processes on coral reef atolls (like those in the Maldives) might make the islands more resistant to sea level rise than their low elevations might initially suggest. Multiple studies, many of which use Landsat observations, show that most coral atoll islands in the Maldives and elsewhere have remained stable or even grown larger in recent decades.
Scientists are still studying why, but some research indicates that storms and floods that wash over islands can move offshore sediment onto the island surface, building the island up in the process. Other research shows that healthy coral reefs can grow upward even when seas are rising by producing abundant sediment.
“The key thing to understand is that these islands aren’t static. They don’t sit passively as if they were in a bathtub and slowly drowning,” said Murray Ford, a geologist at the University of Auckland. “They are constantly being reshaped by oceanographic and sedimentary processes.”
These natural processes may offer only limited protection to highly developed islands, partly because the construction of sea walls can disrupt the movement of sediment and human activity often degrades the health of coral reefs. “Once an island is on an engineered pathway, it can’t easily get off it. Islands that are being built on reclaimed land must factor in sea level rise and build higher off the ground,” said Murray. “For islands that are unpopulated, or sparsely populated, care should be taken to not interfere with the natural ability of islands to adjust to changes in sea level.”
NASA Earth Observatory images by Lauren Dauphin, using Landsat data from the U.S. Geological Survey . Story by Adam Voiland .
View this area in EO Explorer
A new artificial island near Malé could be a destination for people trying to escape rising waters on lower-lying islands.
Image of the Day for April 9, 2021
Image of the Day Land Water Human Presence
View more Images of the Day:
References & Resources
- Brown et al. (2019) Land raising as a solution to sea-level rise: An analysis of coastal flooding on an artificial island in the Maldives . Journal of Flood Risk Management , 13 (S1), e12567.
- BBC (2020) A new island of hope raising from the Indian Ocean . Accessed April 8, 2021.
- Duvat, V. et al. (2018) A global assessment of atoll island planform changes over the past decades . Climate Change , 10, e557.
- Duvat, V. & Magnan, A. (2019) Rapid human-driven undermining of atoll island capacity to adjust to ocean climate-related pressures . Science Reports , 9, 15129.
- Ecowatch (2020) Coral Reefs Are Still Growing Atolls Despite Sea Level Rise . Accessed April 8, 2021.
- Ford, M. et al. (2020) Active Sediment Generation on Coral Reef Flats Contributes to Recent Reef Island Expansion . Geophysical Research Letters , 47 (23) e2020GL088752.
- Holdaway, A. et al . (2021) Global-scale changes in the area of atoll islands during the 21st century . Anthropocene , 100282.
- Intergovernmental Panel on Climate Change (2019) Special Report on the Ocean and Cryosphere in a Changing Climate . Accessed April 8, 2021.
- Masselink, G. et al . (2020) Coral reef islands can accrete vertically in response to sea level rise . Science Advances , 6 (24).
- New Scientist (2017) On front line of climate change as Maldives fights rising sea . Accessed April 8, 2021.
- Moosa, A. et al . (2020) Design response to rising sea levels in the Maldives: A study into aquatic architecture . Frontiers of Architectural Research , 10 (1), 222-223.
- NASA Sea Level Change . Accessed April 8, 2021.
- NASA EarthData (2021) Sea Level Change Data Resources . Accessed April 8, 2021.
- Storlazzi, C. et al . (2018) Most atolls will be uninhabitable by the mid-21st century because of sea-level rise exacerbating wave-driven flooding . Science Advances , 4 (4), 9741.
You might also be interested in ( view all )
Water, water everywhere.
The Maldives rise just a few meters above the surrounding ocean.
Image of the Day Land Water
Amsterdam Island
This distant, lonely volcanic rock brings new meaning to the phrase “the middle of nowhere.”
Image of the Day Land Remote Sensing
Malé Atoll, Maldive Islands, Indian Ocean
This detailed astronaut photograph features surface currents illuminated by mirror-like reflections of sunlight off the waters around the Malée Atoll in the Maldive Islands.
Image of the Day Water
Great Fox is Disappearing
Rising waters are swallowing up another island in Chesapeake Bay.
Image of the Day Land Life Human Presence
You are using an outdated browser. Upgrade your browser today or install Google Chrome Frame to better experience this site.
- IMF at a Glance
- Surveillance
- Capacity Development
- IMF Factsheets List
- IMF Members
- IMF Timeline
- Senior Officials
- Job Opportunities
- Archives of the IMF
- Climate Change
- Fiscal Policies
- Income Inequality
Flagship Publications
Other publications.
- World Economic Outlook
- Global Financial Stability Report
- Fiscal Monitor
- External Sector Report
- Staff Discussion Notes
- Working Papers
- IMF Research Perspectives
- Economic Review
- Global Housing Watch
- Commodity Prices
- Commodities Data Portal
- IMF Researchers
- Annual Research Conference
- Other IMF Events
IMF reports and publications by country
Regional offices.
- IMF Resident Representative Offices
- IMF Regional Reports
- IMF and Europe
- IMF Members' Quotas and Voting Power, and Board of Governors
- IMF Regional Office for Asia and the Pacific
- IMF Capacity Development Office in Thailand (CDOT)
- IMF Regional Office in Central America, Panama, and the Dominican Republic
- Eastern Caribbean Currency Union (ECCU)
- IMF Europe Office in Paris and Brussels
- IMF Office in the Pacific Islands
- How We Work
- IMF Training
- Digital Training Catalog
- Online Learning
- Our Partners
- Country Stories
- Technical Assistance Reports
- High-Level Summary Technical Assistance Reports
- Strategy and Policies
For Journalists
- Country Focus
- Chart of the Week
- Communiqués
- Mission Concluding Statements
- Press Releases
- Statements at Donor Meetings
- Transcripts
- Views & Commentaries
- Article IV Consultations
- Financial Sector Assessment Program (FSAP)
- Seminars, Conferences, & Other Events
- E-mail Notification
Press Center
The IMF Press Center is a password-protected site for working journalists.
- Login or Register
- Information of interest
- About the IMF
- Conferences
- Press briefings
- Special Features
- Middle East and Central Asia
- Economic Outlook
- Annual and spring meetings
- Most Recent
- Most Popular
- IMF Finances
- Additional Data Sources
- World Economic Outlook Databases
- Climate Change Indicators Dashboard
- IMF eLibrary-Data
- International Financial Statistics
- G20 Data Gaps Initiative
- Public Sector Debt Statistics Online Centralized Database
- Currency Composition of Official Foreign Exchange Reserves
- Financial Access Survey
- Government Finance Statistics
- Publications Advanced Search
- IMF eLibrary
- IMF Bookstore
- Publications Newsletter
- Essential Reading Guides
- Regional Economic Reports
- Country Reports
- Departmental Papers
- Policy Papers
- Selected Issues Papers
- All Staff Notes Series
- Analytical Notes
- Fintech Notes
- How-To Notes
- Staff Climate Notes
IMF Working Papers
Enhancing resilience to climate change in the maldives.
Author/Editor:
Giovanni Melina ; Marika Santoro
Publication Date:
April 23, 2021
Electronic Access:
Free Download . Use the free Adobe Acrobat Reader to view this PDF file
Disclaimer: IMF Working Papers describe research in progress by the author(s) and are published to elicit comments and to encourage debate. The views expressed in IMF Working Papers are those of the author(s) and do not necessarily represent the views of the IMF, its Executive Board, or IMF management.
The increased likelihood of adverse climate-change-related shocks calls for building resilient infrastructure in the Maldives. Fulfilling these infrastructure needs requires a comprehensive analysis of investment plans, including with respect to their degree of climate resilience, their impact on future economic prospects, and their funding costs and sources. This paper analyzes these challenges, through calibrating a general equilibrium model. The main finding is that there is a significant dividend associated with building resilient infrastructure. Under worsened climate conditions, the cumulative output gain from investing in more resilient technologies increases up to a factor of two. However, given the Maldives’ limited fiscal space, particularly after COVID-19, the international community should also step up cooperation efforts. We also show that it is financially convenient for donors to help build resilience prior to the occurrence of a natural disasters rather than helping finance the reconstruction ex-post.
Working Paper No. 2021/096
Environment Expenditure Infrastructure National accounts Natural disasters Private investment Public investment spending
9781513582443/1018-5941
WPIEA2021096
Please address any questions about this title to [email protected]
- Open supplemental data
- Reference Manager
- Simple TEXT file
People also looked at
Original research article, coastal flooding in the maldives induced by mean sea-level rise and wind-waves: from global to local coastal modelling.
- 1 Instituto Mediterráneo de Estudios Avanzados (UIB-CSIC), Esporles, Spain
- 2 Departament de Física, Universitat de les Illes Balears, Palma, Spain
- 3 French Geological Survey (BRGM), Orléans, France
- 4 Global Climate Forum, Berlin, Germany
- 5 Ministry of Environment, Malé, Maldives
The Maldives, with one of the lowest average land elevations above present-day mean sea level, is among the world regions that will be the most impacted by mean sea-level rise and marine extreme events induced by climate change. Yet, the lack of regional and local information on marine drivers is a major drawback that coastal decision-makers face to anticipate the impacts of climate change along the Maldivian coastlines. In this study we focus on wind-waves, the main driver of extremes causing coastal flooding in the region. We dynamically downscale large-scale fields from global wave models, providing a valuable source of climate information along the coastlines with spatial resolution down to 500 m. This dataset serves to characterise the wave climate around the Maldives, with applications in regional development and land reclamation, and is also an essential input for local flood hazard modelling. We illustrate this with a case study of HA Hoarafushi, an atoll island where local topo-bathymetry is available. This island is exposed to the highest incoming waves in the archipelago and recently saw development of an airport island on its reef via land reclamation. Regional waves are propagated toward the shoreline using a phase-resolving model and coastal inundation is simulated under different mean sea-level rise conditions of up to 1 m above present-day mean sea level. The results are represented as risk maps with different hazard levels gathering inundation depth and speed, providing a clear evidence of the impacts of the sea level rise combined with extreme wave events.
1. Introduction
Increased coastal flooding damages are among the potentially most hazardous and costliest aspects of global warming ( Hinkel et al., 2014 ), impacting populations, ecosystems and assets. Coastal flood exposure is currently increasing at rates higher than inland due to population growth, urbanisation and the coastward migration of people ( Merkens et al., 2018 ), and also due to coastal extreme water levels being raised by mean sea-level rise ( Marcos and Woodworth, 2017 ). The Special Report on the Ocean and Cryosphere in a Changing Climate (SROCC) of the Intergovernmental Panel on Climate Change (IPCC) projects that if greenhouse gas (GHG) emissions continue to rise unmitigated (i.e., RCP8.5) global-mean sea levels are likely to rise by 0.6–1.1 m by 2100, and 2.3–5.4 m by 2300 ( Oppenheimer et al., 2019 ). Projected mean sea-level rise during the twenty-first century and beyond ( Kopp et al., 2014 ) will inevitably increase the intensity of flood events and will thus exacerbate the exposure and vulnerability of coastal areas in the decades to come, with highest impacts expected in low-lying regions. Hinkel et al. (2014) estimated that, without adaptation, by 2100 almost 5% of the global population will be potentially flooded annually, with losses of up to 10% of the global GDP, under a 1.20 m mean sea-level rise. This will require the implementation of extensive and ubiquitous coastal adaptation solutions to avoid such large impacts ( Hinkel et al., 2019 ). But also if emissions are reduced to meet the goal of the Paris Agreement to limit global warming “well bellow 2°C” (i.e., RCP2.6), global mean sea-level is likely to rise by 0.3–0.6 m in 2100 and 0.6–1.1 m by 2300, which will still be a tremendous challenge, in particular for very low lying regions such as atoll states.
The threats of flooding events are particularly worrisome in low-lying coastal zones, including large deltas and sinking coastal mega-cities; but the regions with the largest expected relative impacts are small island states ( Nurse et al., 2014 ). The Maldivian archipelago is an iconic case of vulnerability to mean sea-level rise. Located in the equatorial region of the Tropical Indian Ocean, the Maldives consist of 1192 islands, dispersed across 860 km from 8° north to 1° south in latitude, of which 188 are inhabited ( NBS, 2017 ; Wadey et al., 2017 ) (see Figure 1 ). The resident population in 2014 was 437,000 people and is estimated to reach 557,000 in 2020, with 40% of the population living in the capital, Malé, and its surrounding islands Villimalé and Hulhumalé ( NBS, 2019 ). Average land elevations range from 0.5 m to 2.3 m above present-day mean sea level ( Woodworth, 2005 ), with 80% falling below 1 m. Since the 1950s several land reclamation projects have been carried out to address land scarcity, for example in the southern lagoon of Malé in 1954 ( Maniku, 1990 ). With the rapid economic development of the Maldives, land reclamation projects have also increased. The Maldivian government estimates that over 1300 hectares of reef or lagoon area have been reclaimed up until 2016 ( MEE, 2017 ). This new land is required to be elevated between 1.5 and 1.75 m above mean sea-level. However, this static approach to island elevation ignores the differing wave exposure across the archipelago.
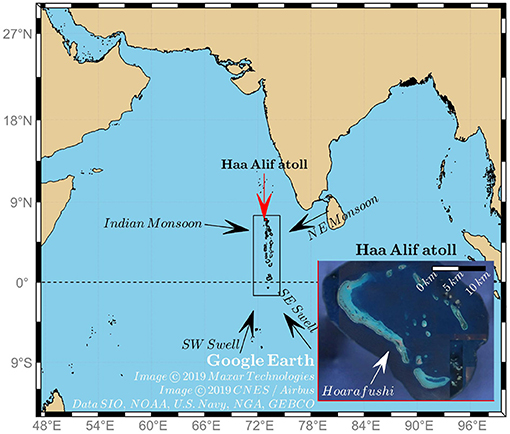
Figure 1 . Map of the Indian Ocean with the Maldivian archipelago inside the black box. The four black arrows indicate the main wave direction identified. The red arrow indicates the location of the Haa Alif atoll in which is located Hoarafushi island (satellite image in the bottom-right corner extracted from Google Earth. Image © 2019 Maxar Technologies; Image © 2019 CNES/Airbus; Data SIO, NOAA, U.S. Navy, NGA, GEBCO).
A lot of land reclamation is taking place in the Maldives and a new long-term regional development strategy is currently being prepared that prioritises islands for development ( Gussmann and Hinkel, 2021 ). While it is known that wave exposure differs across islands, this has so far not been taken into account in land reclamation and regional development. The development of adaptation plans in the framework of coastline management aimed to address flood hazards requires accurate information and a deep understanding of the driving processes. Coastal flood events are caused by extreme coastal water levels that in turn result from the combination of relative mean sea-level, tides, storm surges, wind-waves, precipitation and/or river run-off ( Woodworth et al., 2019 ). The design of adaptation strategies therefore involves the knowledge of every individual driver and their future projections at the local scale, as well as their possible interactions ( Nicholls et al., 2014 ). In the case of the Maldives, the tidal range is relatively small (<1 m of maximum high waters) and the storm surge contribution is negligible, as corresponds to an equatorial region ( Wadey et al., 2017 ). Earlier studies have pointed at wind-waves as the primary mechanism causing flooding events in the Maldives, similarly to other Indian and Pacific islands ( Hoeke et al., 2013 ). One of the first works was presented by Harangozo (2013) , who investigated an event that occurred in April 1987 that flooded Mal?é, including reclaimed land below 1 m above mean sea-level and during which the hard structures designed to protect this land were destroyed. Based on altimetric wave measurements and in-situ sea-level observations, this event was attributed to prolonged swell waves originated in the Southern Indian Ocean and reaching the island during high tides. Similarly, in 2007, the Fares island, located in the southernmost atoll of the Maldives was flooded due to a series of remotely-generated swell events reaching the island ( Wadey et al., 2017 ; Beetham and Kench, 2018 ) which also affected other areas of the eastern Indian Ocean (e.g., Lecacheux et al., 2012 in La Réunion Island). This event was particularly hazardous as it flooded almost the entire island and affected more than 1500 people as well as the limited water resources of the island. An extensive study was carried out in response to this event and a protective offshore breakwater was built to avoid future damages. For a comprehensive list of flooding events in the Maldives, the reader is referred to Wadey et al. (2017) , where the available information of several flooding events has been collected from a number of sources.
Despite the recurrent flooding episodes associated with swells, overall, in the Maldivian archipelago a complete and accurate assessment on the wind-wave climate, including extreme waves, is hindered by the lack of observations and regionalisation of model runs. Numerical wind-wave simulations are available with a global coverage, including both re-analyses (i.e., Saha et al., 2010 ) and projections (i.e., Hemer and Trenham, 2016 ; Morim et al., 2019 ), although with a coarse resolution that prevents their use for many practical purposes, such as accurate local assessments. This work intends to fill this gap by providing the necessary information on waves to perform coastal studies along the Maldivian shorelines. The objectives of the present study are three-fold: first, we fully characterise the wave climate around the Maldives on the basis of global, coarse resolution numerical wave dynamical simulations for present-day, and we further evaluate the projected changes under climate change scenarios (section 3). Secondly, we downscale the extreme wave climate through propagation of the main extreme waves from the dominant directions toward the coastlines with a much higher resolution (section 4). And finally, we illustrate how this information can be translated into a flood hazard assessment in a selected location that is exposed to the largest incoming swell waves in the archipelago. To do so, we propagate wave conditions from the nearshore to the coastline under different mean sea-level rise scenarios and quantify the flooding extent with and without land reclamation (section 5). Data, methods and numerical models are described in section 2, while all the results are discussed together in section 6.
2. Data and Methods
This section describes the global wave data that is used to characterise and downscale wave information to the nearshore in the Maldives, together with the numerical models and their implementation. Local wave modelling is used as the basis of flood hazard assessment for a case study. To do so, waves are combined with a set of mean sea-level changes using a scenario-independent approach. That is, waves are propagated toward the shoreline under prescribed mean sea-level increments of 0.25, 0.5, 0.75, and 1 m with respect to present-day averaged value. Note that these values are not necessarily interpreted as climate-induced mean sea-level rise; they can also be associated to tidal oscillations or to a combination of tides and mean sea-level rise.
2.1. Global Wind-Wave Datasets
We have used the CAWCR Global wind-wave data set that is freely distributed through the CSIRO data server ( Hemer et al., 2015 ). This set, generated with the WaveWatch III wave model (version 3.14, Tolman, 2009 ) in a common 1° × 1° resolution global grid, consists of a hindcast, historical runs (late twentieth century), and projections for the twenty-first century. The hindcast has been forced with surface wind fields from the NCEP CFSR ( Saha et al., 2010 ) and covers the period from 1979 to 2009 with a temporal resolution of 1 h (this simulation is referred to as CFSR hereinafter). The historical runs and projections were generated using the output fields of 8 different CMIP5 models (ACCESS1.0, BCC-CSM1.1, CNRM-CM5, GFDL-ESM2M, HadGEM2-ES, INMCM4, MIROC5, and MRI-CGCM3), covering three different time periods with a temporal resolution of 6 h: historical runs for 1980-2005; and projected waves for mid-(2026–2045) and late-(2081–2100) twenty-first century. The projections for mid- and late-twenty-first century were run under two different emission scenarios, RCP4.5 and RCP8.5, although we will use only the latter. A detailed description of the wave climate dataset can be found in Hemer et al. (2013) .
Global wave models are used to characterise the present-day and future projected changes of wave climate around the Maldivian archipelago, with emphasis on the extreme wave climate. Return levels of Hs for a set of prescribed return periods are calculated by fitting the top 1% waves to a Generalised Pareto Distribution. Given the coarse spatial resolution of the model configuration, we do not expect the small islands as the Maldives to be accurately represented by these global simulations. Given that the wave fields are modified by the presence of the islands (see for example Supplementary Video 1 from Amores and Marcos, 2019 ), the global fields must be downscaled in order to be usable for practical purposes. This process is described in the following.
2.2. Regional Wave Modelling
Global waves have been dynamically downscaled in the Maldives using the WaveWatchIII wave model (version 4.18, Tolman, 2014 ). The model was implemented on an unstructured mesh with 33160 nodes and 64456 elements over a domain ranging from 71.5 to 75.5° E in longitude and from −1.5° N to 8.5° N in latitude (black rectangle surrounding the Maldives in Figure 1 ). The spatial resolution of the unstructured mesh varied from 50 km along the boundaries of the domain down to 500 m in the channels between the atolls. Only the external coasts of the atoll islands were considered due to the lack of bathymetric information inside the atolls. The regional bathymetry used to build the model grid was the GEBCO bathymetry 2014 in a global 30 arc-second interval grid ( https://www.gebco.net/ ). The wave spectrum was defined by a directional resolution of 10° and 24 frequency bands ranging non-linearly from 0.0373 to 1.1 Hz. Dynamical downscaling was preferred instead of statistical approaches because there is no local information on waves that can be used to calibrate the model.
2.3. Local Wave Modelling
Nearshore downscaled waves have been propagated toward the coastline for a case study site. The selected location corresponds to Haa Alif atoll (HA) at Hoarafushi island, located at the north of the archipelago ( Figure 1 ). Hoarafushi has a maximum length of 2,500 m and a maximum width of 500 m ( Figure 1 ). This site has been chosen for two main reasons: firstly, at the start of this study a land reclamation project to build a new airport next to the island was foreseen. The development of the regional airport on the newly reclaimed island on the reef of HA Hoarafushi is part of the government's regional development and decentralisation plans, which puts extra focus on the northernmost atoll Ihavandhippolhu. We therefore aimed at evaluating the exposure of this new reclaimed land to incoming waves and how its presence can alter the wave propagation over the reef and the exposure of the current island. The process of land reclamation was started on April 16th, 2019 ( https://edition.mv/news/10159 ) and finished almost 5 months later, on September 5th, 2019 ( https://edition.mv/news/12266 ; see Supplementary Figure 1 , to see the construction process on June 15th, 2019). Secondly, information on the local bathymetry and land elevation is available and allows to simulate the wave propagation. A bathymetry around the island was generated by combining measurements on the reef flat performed by the Maldives Transport and Contracting Company, who was in charge of the design of the land reclamation project. We completed these data with reef slope measurements taken during a field trip on February 2018 (using a single beam echosounder). Our measurements included a total of 10 profiles across-slope separated around 200–500 m between them as well as several along-slope transects. The minimum depth measured in the across-slope profiles was around 3 m, that was the closest the boat could get to the reef crest, and the maximum depth recorded, that was fixed by the maximum range of the echosounder, was around 50 m. Unfortunately, there is no detailed information on the topography of the island. Instead, a constant land height of 1.5 m above present-day mean sea level has been used, according to visual inspections and in accordance with existing regulations. The coastline of the island has been represented with a constant slope, given that there are not hard structures in the oceanward side. Two topo-bathymetries have been implemented, with and without the presence of the airport. Finally, it is worth mentioning that HA Hoarafushi island is exposed to the highest incoming waves around the archipelago, as will be shown below.
The local wave propagation has used the SWASH model ( Zijlema et al., 2011 , code available at http://swash.sourceforge.net/ ) in a 2D regular grid of 6,220 m in the W-E direction and 7,320 m in the S-N direction with 10 m of spatial resolution (see the domain in Figure 8 ). This model is suitable for our purposes as it is capable of simulating wave setup and runup and predicting infragravity waves in the nearshore ( Rijnsdorp et al., 2012 ), a relevant process that contributes to the amount of flooding by raising temporary the sea level near the coast. The West and South boundaries were considered active introducing the wave forcing in the domain with Jonswap spectra with a wave dispersion of 20° and a peak enhancement parameter γ of 3.3. A different combination of wave dispersion and γ was tested (5° and 10°, respectively), resulting in essentially the same results in terms of flooding. A 150 m sponge layer was placed in the eastern boundary and 1,000 m sponge layer in the northern boundary, to avoid unrealistic wave forcing from the interior of the atoll given by spurious wave reflection from the those boundaries. The lack of in-situ measurements of wave propagation and transformation along the domain made it impossible to calibrate the Manning's friction coefficient. The values for coral reefs found in the literature vary from 0.01 to 0.2. For example, Zijlema (2012) used 0.01, Prager (1991) used 0.05, Kraines et al. (1998) used 0.1, and Cialone and Smith (2007) used spatially-varying Manning's coefficient values of 0.02, 0.19, and 0.2 depending on the region of their domain. The Manning's coefficient value was finally fixed to 0.019 following Suzuki et al. (2018) , who investigated the most suitable value for SWASH model applied to overtopping computation along a beach profile with defined defenses. In our case, there is not a complete beach profile, but the overtopping, which is the process of interest here, is occurring at the shoreline of a sandy beach.
With this configuration, the total simulated time for each combination of parameters was 70 min, with an initial integration time of 0.05 s and having outputs every 5 s. This is computationally intensive but still feasible for the range of experiments and for the two topographies (with and without the airport).
3. Characterisation of Wave Climate Around the Maldives
3.1. present-day wave climate.
The outputs of the CFSR wave hindcast at 24 grid points around the Maldives are used to describe the large-scale present-day wave climate in the archipelago ( Figure 2 ). Wave roses in Figure 2 identify, for each grid point, the direction of the dominant wave regimes with their corresponding significant wave heights ( H s ) and peak periods ( T p ). One prominent feature is that the largest significant wave heights are usually accompanied by peak periods longer than 10–12 s (and reaching up to 24 s), which suggests that these are remotely generated waves, i.e, swell waves. This is in agreement with the location of the Maldives in the Equatorial region, where winds are weak, and in a region exposed to swell waves from the Southern Ocean ( Wadey et al., 2017 ; Amores and Marcos, 2019 ) and is further examined below.
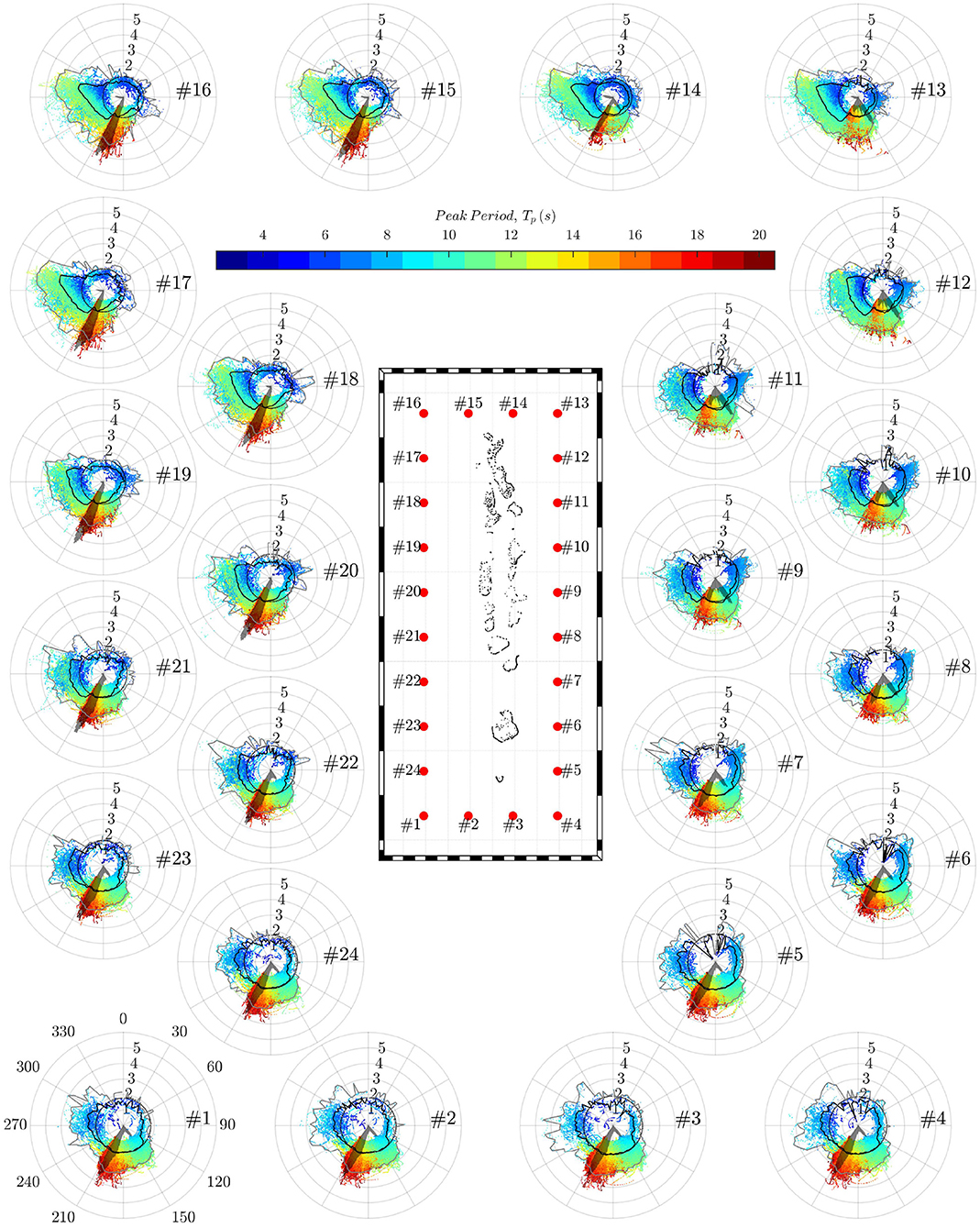
Figure 2 . Distribution of the ocean wave climate around the Maldives from the CFSR Hindcast. Each wind-rose-plot corresponds to one point of the central map. The radial distance of each single point in each wind-rose indicates the wave height (m) while the azimutal value indicates the direction that the waves are coming from in nautical convention. The colour of each point shows the peak period. The continuous black (grey) line indicates the quantile 50 (99) for each direction while the dashed black like shows the quantile 50 averaging all the directions. Shadowed areas in the wave roses indicate the most frequent incoming wave directions in a 1° bins (% referred to the radial axis).
Waves from the south-west (~205°) are the most common with H s reaching values larger than 4 m (note that the angles follow the maritime convention, as indicated by the labels in the wave rose of point #1). This finding is in line with Amores and Marcos (2019) that demonstrated that between 80 and 90% of the swell events impacting along the Maldivian coastlines are from SW and originated in a region located between south of Africa and east of South America. The second most frequent direction is the south-east (~145°). These waves reach maximum values of H s around 3 m, thus smaller than ~205° waves, and with peak periods between 10 and 12 s. In addition to these two dominant swell wave directions, two other cases much less frequent but with non-negligible H s are detected. In the north of the archipelago the largest waves with H s of up to 5 m are from the west direction (~275°, see wave-rose #17 in Figure 2 ). And finally, waves from 60° are also found in the points of the northeastern side of the archipelago (see, for example, wave-rose #10 in Figure 2 ) with peak periods smaller than 10 s and H s smaller than around 2.5 m.
The characteristics of the incoming large-scale waves are further analysed in greater detail for three grid points capturing the entire range of directions: point #17 (northwest), point #3 (south), and point #10 (northeast). Figure 3 examines the annual and seasonal distribution of incoming waves for every direction and their classification in terms of wind-seas and swells, according to the spectral partitioning provided by the global wave models. These histograms, representing the number of events per year, have been constructed with wave events separated at least 3 days to avoid over-representation of the dominant directions and with a minimum peak prominence H s of 0.2 m to remove noise from smaller waves. The three points are representative of the four incoming wave directions identified above and all register a similar number of waves during the hindcasted period (between 45 and 50 per year, as listed in the title of the panels in Figure 3 ). Their distribution in directions is, however, different, and depends on their position. The most frequent wave direction, around 205°, is evident in points #17 and #3 and is equally likely throughout the entire year (see panels d, e, g, h for comparison among seasons). A composite of the wave and wind fields corresponding to these events is mapped in Supplementary Figure 2 , demonstrating that these waves indeed correspond to remotely generated southwestern swells, in line with the findings in Amores and Marcos (2019) . Waves from the west direction, around 275°, are the second most frequent in point #17 with a marked seasonal character, being only detected between May and October (panel d) and classified as a mixed sea+swell. These waves are generated by the Indian monsoon and only affect the northernmost area of the archipelago. The corresponding composites are shown in Supplementary Figure 3 . The presence of waves generated by the Indian monsoon likely has an impact on the wave type distribution of the southwestern swell at point #17, since its percentage of sea+swell is larger between May and October; also, the wind fields of the composites corresponding to both types of waves are identical (see last rows in Supplementary Figures 2, 3 ).
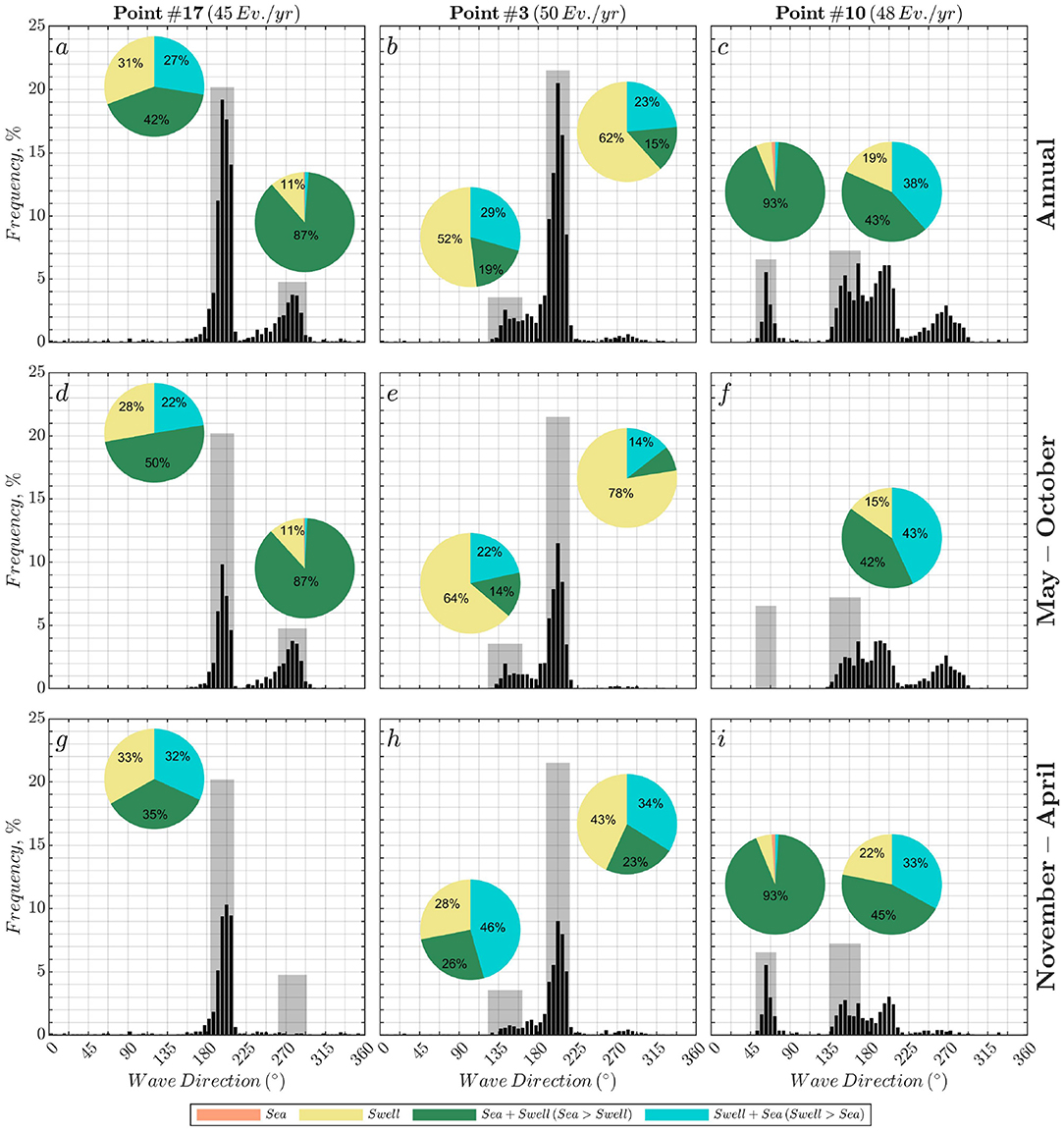
Figure 3 . Histograms of wave direction (in nautical convention) registered at the three points selected as being representative (point #17 in the first column ( a,d,g ), #3 in the second ( b,e,h ), and #10 in the third) ( c,f,i ). The first row shows the annual histograms ( a,b,c ), May-October histograms are shown in the second row ( d,e,f ) and November-April histograms are in the last row ( g,h,i ). Each pie chart indicates the spectral separation by type of wave produced by WaveWatch III model [pure sea ( H s of swell = 0), pure swell ( H s of sea = 0), sea + swell dominated by sea ( H s of sea > H s of swell) and swell + sea dominated by swell ( H s of swell > H s of sea)] corresponding to each wave component identified (grey shadows).
The second peak in point #3, seven times less frequent that the southwestern swell and also observed in point #10, corresponds to the direction around 145°, with waves detected throughout the entire year. According to the wave and wind fields composites ( Supplementary Figure 4 ) these are waves generated in the Southern Ocean, in a region off the southeastern coast of Australia ( Amores and Marcos, 2019 ). Finally, the fourth incoming direction, around 55°, is clearly detected in point #10, with a strong seasonal character. These waves correspond to the northeast monsoon ( Wadey et al., 2017 ) and are only relevant between November and April, contrasting with the Indian monsoon (panel f and i).
Return levels of H s for every direction and for the three grid points are shown in Figure 4 and listed in Supplementary Table 1 . Noteworthy are the flat tails for the southeastern swells evident in points #10 and #3. Independence among wave events is ensured with the 3-day declustering. A Generalised Pareto Distribution (GPD) has been fitted to the top 1% of the largest H s ; in the case that this subset is too small as to reliably fit the distribution, the 30 largest values (1 event per year on average) were used. The largest return levels correspond to waves generated by the Indian monsoon in point #17 ( Figure 4D ). This direction has a H s of 4.75 m for a 10 year return period that is larger than all the return levels for the 500 year return periods for all cases (with the exception of the southeastern swell affecting point #3 that has 4.99 m as H s associated with 500 year return period). On the other side, the lowest return levels correspond to the northeast monsoon affecting point #10 with a H s equal to 2.74 m for a return period of 500 years, around 1 m lower from the closest return level (3.61 m for 500 year return period for the swell coming from southeast in point #3).
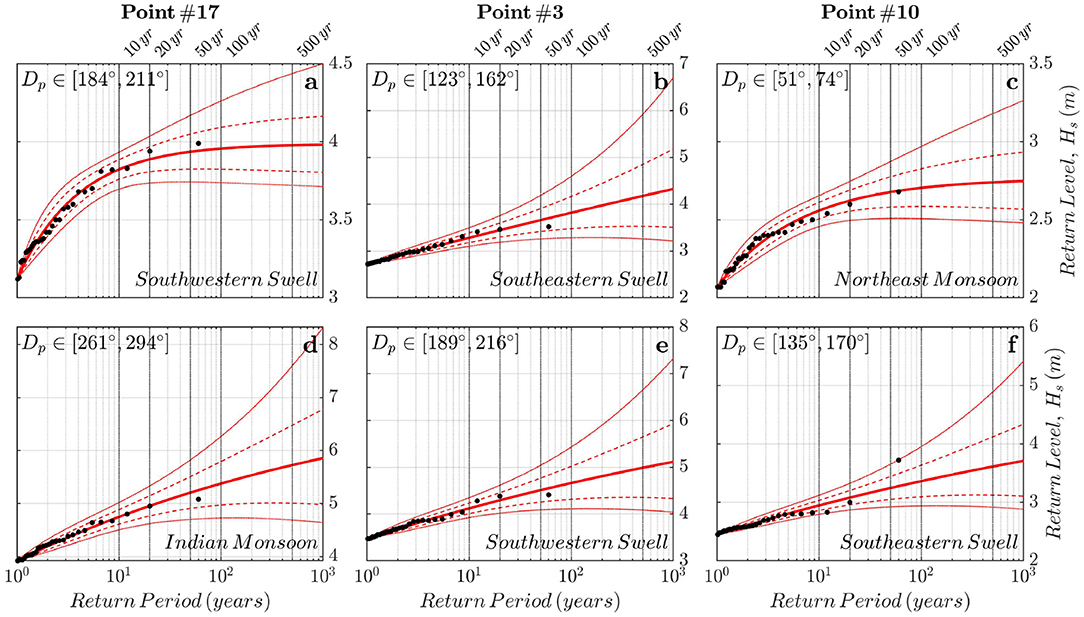
Figure 4 . Return levels associated with each return period (thick continuous lines) for the three representative points (point #17 in the first column (a,d) , #3 in the second (b,e) , and #10 in the third) (c,f) for the wave directions identified at each point (for example, panel a corresponds to the first grey shadow in Figure 3a ). The uncertainty bands correspond to ±σ (dashed lines) and the 5−95 % intervals (dotted lines) and have been computed using the delta method. The return period indicated in the top of the panels (10, 20, 50, 100, and 500 years) are the ones selected to perform the regional downscaling with WaveWatch III. Note that the y -axis are different for each panel and do not allow a direct comparison.
3.2. Wave Projections During the Twenty-First Century
The same three grid points analysed above are used as proxies to evaluate the projected changes in waves around the Maldives, using the output of historical simulations and projections during the twenty-first century. Figure 5 represents changes in the frequency of arrival of waves for each direction of propagation by the end of the twenty-first century under RCP8.5 with respect to present-day values for each point. The median of the 8 climate model projections is shown in red (blue) when the projected changes indicate an increase (decrease) in the number of wave events and the grey area represents the model spread. Global models project an increase (~3%) of the southwestern swells, consistent with the findings in Amores and Marcos (2019) , who showed a greater activity in swell generation in the region of formation of these waves later in this century. For the waves generated by the Indian monsoon, models show a smaller decrease in the number of waves. Other directions do not show robust projected changes, as the model spread is larger than the median change. The same applies to projected variations in median and extreme H s in all directions of propagation ( Supplementary Figure 8 ).
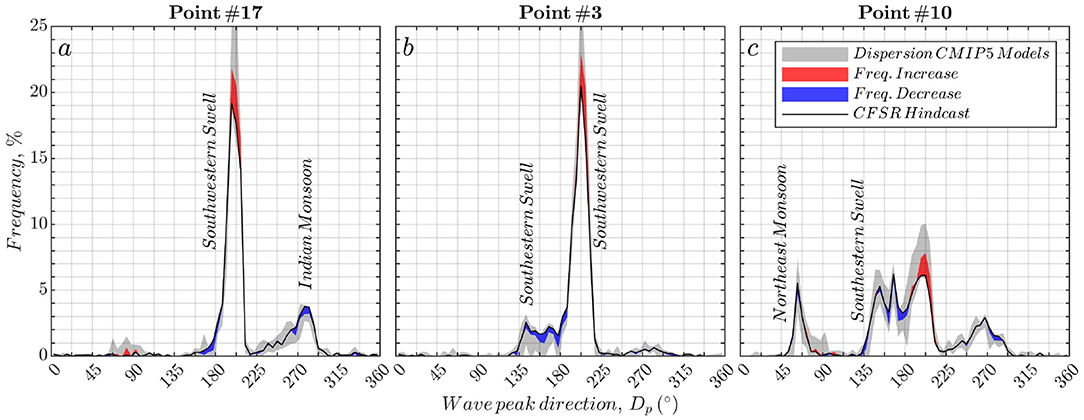
Figure 5 . Projected changes in wave direction by the CMIP5 models described in section 2.1 for each one of the representative points selected ( a point #17, b point #3, and c point #10). Black line represents the CFSR hindcast histogram (same as the first row in Figure 3 ); grey shadow indicates the spread of the CMIP5 models (RCP8.5 - Historical); red (blue) shadows show where the models agree to project a frequency increase (decrease) of a given direction.
Overall, projected changes in H s are smaller than the multi-model spread even under RCP8.5 climate scenario. Variations are expected to be even smaller under RCP4.5. In consequence, present-day significant wave height is considered to be largely representative of future wave climate for the purposes of this work and only CFSR wave fields will be downscaled and propagated toward the shorelines. It is worth noting that changes in the frequency of each wave direction ( Figure 5 ) can be relevant to the transport of sediments and could modify current erosion patterns.
4. Regional Wave Downscaling
Global wave information needs to be downscaled to become representative and usable in the nearshore; however, downscaling the full hourly 30-year CFSR hindcast is computationally too intensive. On the other hand, in terms of coastal impacts assessments and, in particular when coastal flooding is concerned, it is extreme values that are the most relevant metric. Therefore, our approach consists of dynamically downscaling the return levels for H s calculated for 6 different return periods (namely, 10, 20, 50, 100, 500, and 1000 years) and the four main wave directions that were previously identified. To do so, we have used the Wave-WatchIII model configuration described in section 2.2. The H s return level associated with a given wave direction is defined at a reference grid point and propagated along the corresponding boundary. In order to insert consistently the H s at the rest of the grid points in the same boundary, the linear relationships between simultaneous events (±24 h ), arriving from the same direction and reaching the reference point and the other boundary points were computed. This procedure is illustrated in Supplementary Figures 9–12 , where also the reference grid points at each boundary are marked. The linear relationships between the reference grid point and the others are used to scale H s at each active boundary point. The boundary points where no simultaneous events with the reference point were found or, alternatively, for which there is no correlation (we set the limit value of R 2 of the linear adjustment to 0.2), were assigned a linear slope of 0.01 in order to avoid introducing spurious waves. The peak period ( T p ) of the incoming waves associated with each return level for H s , have been determined using a linear relationship between all the ( H s , T p ) events extracted at each reference point for each of the four directions of the incoming waves ( Supplementary Figure 13 ).
The resulting downscaled wave fields consist of a set of four return level curves at every coastal grid point with a spatial resolution of ~500 m. This resolution permits to model wave propagation at the scale of the archipelago. Although it is not accurate enough to perform local assessments inside an atoll, it provides, instead, the necessary boundary condition for the forcing. The full data set is provided at the Zenodo repository under this doi: 10.5281/zenodo.3886273 . Figure 6 shows the results for the 100-year return level of the four directions over the entire domain, sorted by decreasing H s . Note that the spatial patterns of different return levels will be the same for each wave direction and only the magnitude changes. Due to the limited resolution of the GEBCO topobathymetry (~1 km ), an accurate representation of the islands and the inner part of the atolls is not feasible. Thus, the atolls have been considered as whole entities. This assumption implies that the side of the islands that faces toward the atoll's interior is not solved by our regional downscaling. Nevertheless, it is not relevant at this scale because this side of the islands is not directly impacted by waves. We consider that, given the limited depth on the atoll rims (roughly 1 m), this assumption is reasonable, especially because a more accurate assessment would require tide-current local modelling to capture lagoon/ocean interactions.
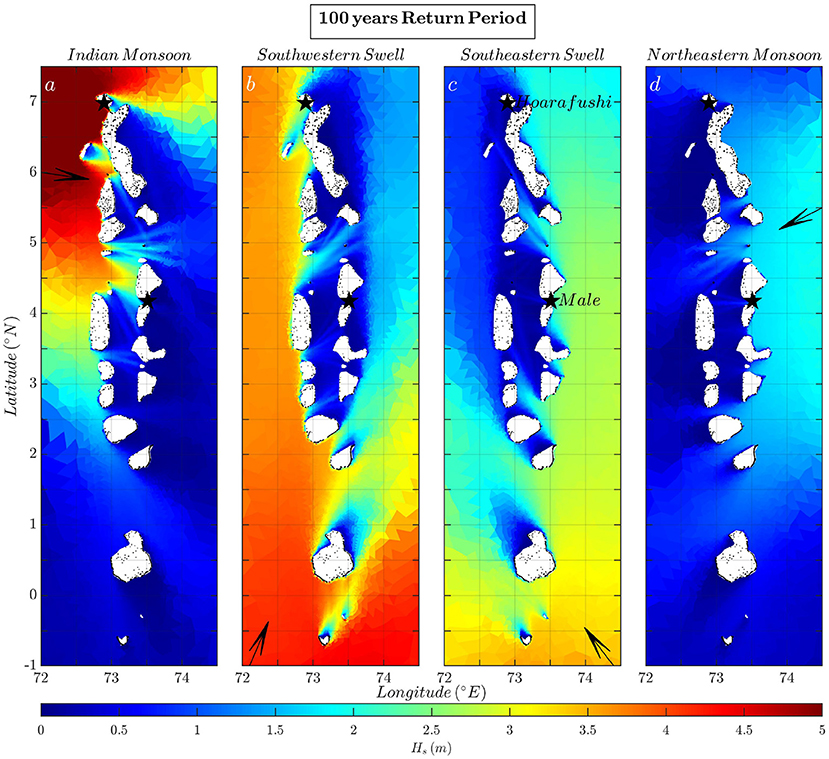
Figure 6 . Regional downscaling of the 100 year return period wave event performed with WaveWatch III model (see section 2.2 for the details) for each one of the wave components identified [ (a) Indian Monsoon, (b) Southwestern Swell, (c) Southeastern swell, and (d) Northeastern Mosoon]. Black arrow in each panel indicated the wave direction. Black stars indicate the position of Malé, the main city of the Maldives, and Hoarafushi, the island where the local modelling was done.
Figure 6 shows that the largest waves in the Maldives ( H s >5 m ) are generated by the Indian monsoon (panel a) in the northwestern part of the archipelago, with values of H s exceeding 2 m in the area northwards from 3°N (note that the same colour scale is used for the four maps). One remarkable feature is that these waves, although attenuated, reach the western side of the Kaafu atoll, the most populated atoll in the Maldives and where the capital city Malé is located. Because of the absence of shadow effects, the western coast of the Kaafu atoll, is the inner region of the Maldives exposed to waves with larger H s , reaching values between 2.5 and 3.0 m. The southwestern swell (panel b), the second direction with largest H s after the Indian monsoon, is the component that spreads larger H s to a broader scale. More precisely, it generates ocean waves with H s >3 m (even than 3.5 m) to all the western sides of the atolls comprising the Maldives. The third ocean wave direction in terms of H s is the southeastern swell (panel c), that affects all the eastern side of the Maldives with H s ranging from 2 to 3.5 m. Finally, the northeastern monsoon (panel d) is the ocean wave component with smaller H s (<2 m ). Its effects are concentrated in the central region of the eastern side of the archipelago, from 2 to 6.5°N. It does not strongly affect the northernmost part of the Maldives because this region is located under the shadow of the Indian continent to the monsoon winds ( Supplementary Figure 6 ).
Combining the results of the four wave directions shown in Figure 6 , we can identify the wave component with greater H s at each grid point along the coastlines ( Figure 7a ), the value of this greater H s ( Figure 7b ), as well as how many different directions each coastal point is exposed to Figure 7c ; H s ≥1.5 m . In relative numbers, 33% of the coastlines are exposed to the large waves from the Indian monsoon, in 25% of them the highest waves arrive from the southwestern swell, in 28% from southeastern swell, and only 14% from the northeastern monsoon. As in the case of the Kaafu atoll mentioned above, a similar effect is found in the eastern part of the Faafu and Dhaalu atolls, also located in the interior of the Maldives. Here the dominant wave component reaching the eastern coast of these atolls is the southeastern swell that penetrates in the middle of the Maldives between Thaa Atoll and Meemu Atoll.
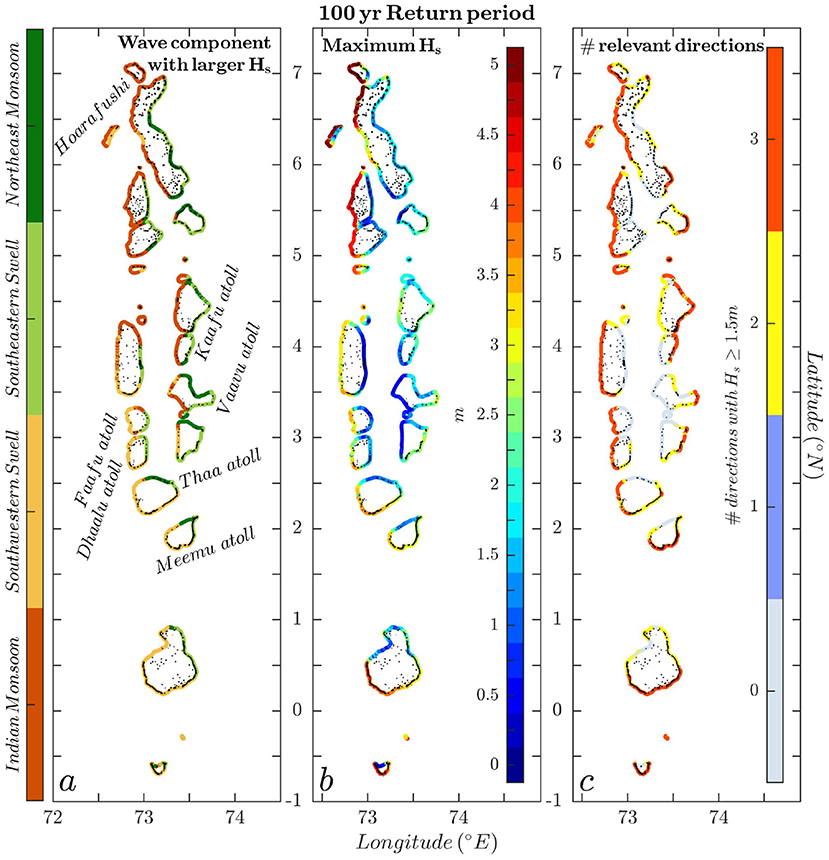
Figure 7 . Products derived by combining the regional downscaling for the 100 year return period of the 4 wave components in Figure 6 along the Maldivian coastlines. (a) shows the wave component causing the largest H s at each coastal point; (b) the maximum H s at the coast; and (c) , the number of wave directions with H s larger than 1.5 m that hit each coastal location for the 100 year return period.
In terms of maximum H s ( Figure 7b ), around 15% of the coastal points, most of them located in the interior of the archipelago, are affected by waves with 100-year return periods smaller than 1 m. The most common values are between 1 and 2 m, affecting 35% of the coastal locations. In 22% of the coasts, the 100-year return levels of H s vary between 2 and 3 m and in 17% H s between 3 and 4 m. The largest values, over 4 m, affect around 11% of the coastal points which are found, as expected, in regions where the Indian Monsoon dominates ( Figure 7b ).
Another metric for the exposure of the coasts to incoming waves is the number of swell directions reaching every coastal point. This is illustrated in Figure 7c , where we have quantified how many wave directions, from the 4 represented in Figure 6 , reach each coastal point with H s ≥1.5 m for the 100 year return period. The choice of the H s threshold and the return period selected is arbitrary and used only for illustration purposes; it is not determinant for the resulting map. We conclude that, in 32% of the coastal points, the 100-year return level of H s is always smaller than 1.5 m (grey points in Figure 7c ), with these areas located mainly in the interior of the archipelago. In 29% of the coastal points waves arrive from a single direction (blue points) and in 38% from two directions (yellow points), with the latter case mainly affecting the eastern and western side of the Maldives. In only 1% of the coastal grid points waves arrive from 3 directions (red points), but these are concentrated in the easternmost side of the Vaavu atoll.
5. Local Wave Modelling and Flood Hazard in Hoarafushi Island
The outputs of the regional wave downscaling developed in the previous section are used here in a local flood hazard assessment, illustrating its direct applicability. To do so, downscaled nearshore wave information in a coastal grid point next to Hoarafushi island is propagated toward the shoreline and used to assess coastal flooding under different mean sea-level rise scenarios. The are two reasons that make this location particularly interesting for local wave modelling: first, it is affected by the two largest wave components in the archipelago, i.e., the Indian Monsoon and the southwestern swell; and second, a new island was reclaimed to host a regional airport, which raises questions of present and future climate hazards (see section 2.3). The projected airport, that will have a length of around 1.5 km and a width of 300 m in its wider section, will be located in the reef of the island that faces toward the outer side of the atoll. This means that the shoreline of the airport will be substantially closer to the reef edge than the original island (150–200 m instead of 600 m), reducing the amount of wave energy that can be absorbed by the reef. This local-case study does not pretend to give any recommendation to stakeholders on the airport island height for this specific site. To do so, detailed local information, such as a high-resolution topo-bathymetry or ocean waves in-situ data to validate the model outputs would be required. This example illustrates the applicability of the regional wave downscaling developed here to a local study if precise local information was available.
Wave propagation with SWASH was carried out in the domains in Figure 8 . In total, 60 different runs were completed by combining 3 different return periods of H s (10, 50 and 100 years), two wave directions (Indian Monsoon and the southwestern swell), and 5 different mean sea levels (0, +0.25, +0.50, +0.75, and +1 m ) for the island configuration with and without airport. We have followed a scenario-independent approach for mean sea-level rise, with 0 m corresponding to present-day mean sea level. Mean sea-level changes with respect to the current situation may be interpreted in terms of projected mean sea-level rise (e.g., +0.50 m is the median projected mean sea-level rise in 2068 under RCP8.5 and 2088 under RCP2.6, according to Kopp et al., 2014 ) or as a combination of mean sea-level rise and high tides (e.g., +0.50 m is the mean rise in 2041 under RCP8.5 plus +0.25 m of tidal amplitude). The mean sea-level changes tested may also include, besides projected mean sea-level rise and tides, other physical processes that can cause mean sea-level variations from seasonal to decadal time scales. We recall here that tides in the Maldives reach a maximum range of around 1 m (0.7 m median range, Wadey et al., 2017 ). Note that precise geodetic references relating altitudes and tidal levels are lacking in the Maldives, so these values should be considered as an order of magnitude only. Four examples of selected simulations can be found in Supplementary Videos 1, 2 .
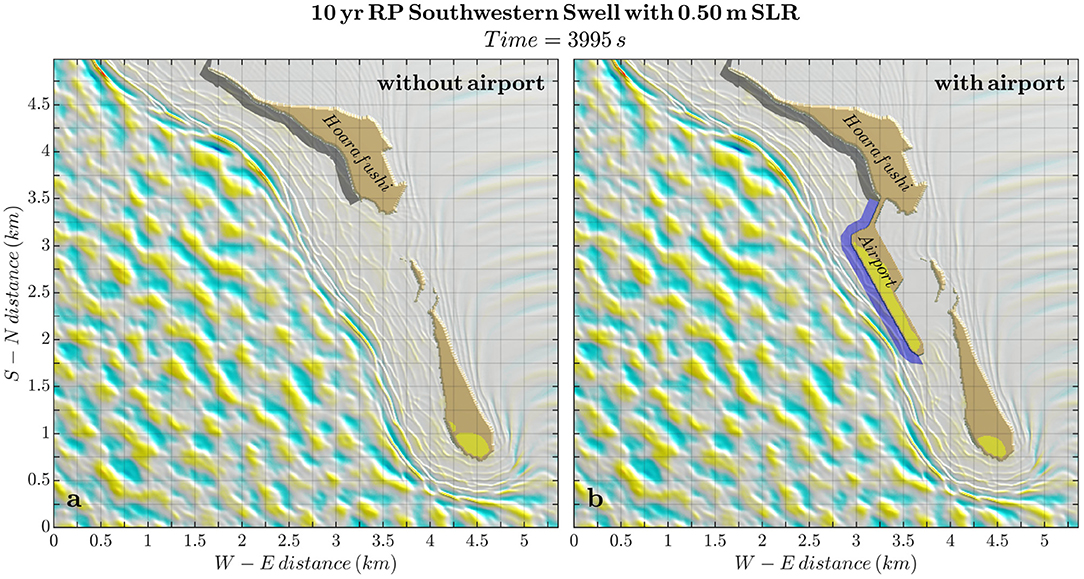
Figure 8 . Example of local downscaling simulations with SWASH model (see section 2.3 for details) for the 10 year return period southwestern swell event with 0.5 m of sea level rise (this increase in sea level could mean permanent sea level or high tide with present sea level) for the case without the airport (a) and with the new airport (b) . Grey (purple) stripe indicates the coastal region where the box plots from Figure 9 ( Supplementary Figure 15 ) are computed.
It has not been possible to validate the model outputs for the present-day situation due to the lack of observations. We are providing, nevertheless, a qualitative validation by comparing the velocity field obtained with the configuration that includes the airport to a satellite photography in which the airport is under construction ( Supplementary Figure 1 ). There is a consistency between higher current velocities in the model and the imprint of sediment transport from the new-built airport that are likely driven away by the currents.
The outputs of the first set of 30 model runs, that correspond to the spatial configuration without the airport ( Figure 8a ), are used to evaluate the exposure of the island in terms of the amount of flooding under different forcing conditions. The outputs along a 100-m wide coastal strip covering the western coast of the island (plotted as grey area in Figure 8a ) have been gathered together. To do so, the strip is divided in 25-m long sections resulting in 25 × 100 m boxes. Simulated water level time series were extracted for each box and used to compute median and maxima water levels for each model run in each of them. Figure 9 represents the boxplots along the entire coastal strip of these median (left panel) and maxima (right panel) values under all mean sea levels and return levels considered. The horizontal black thick line in both panels marks the height of the island and the two incoming directions are separated by vertical shadowed areas for comparison. Median values of total water level, that correspond to the superposition of the mean sea level and wave setup, do not reach the threshold of land elevation, indicating that there is no overflow at any point along the coastline under all the forcing conditions considered. The results also point at the southwestern swells as the potentially most hazardous waves, as these systematically induce higher water levels than the Indian monsoon waves (shadowed areas against blanked areas). The reason lies in the longer T p associated with the southwestern waves (~20 s ) in front of the monsoon waves ( ~12 s ). As expected, the larger wave setup for a given return period is obtained for the lowest mean sea level of 0 m : wave setup reaches almost 0.4 m under present-day mean sea level conditions and reduces to 0.3 m with an increase of 1 m . This is because in shallower waters the effects of wave shoaling and breaking leading to wave setup are larger. It is worth noting here that while an increased water level leads to a decreased setup, deeper water allows for larger H s on the reef flat and an increased run-up potential which could be relevant in terms of impact to infrastructures and erosion. On the other hand, maximum values along the coastal strip have been used to measure whether there has been overtopping generated by the incoming waves. Overtopping occurs whenever these values exceed the island elevation, with their magnitude indicating the severity of the flooding. The boxplots for the maximum values (right panel in Figure 9 ) point to the occurrence of overtopping under several forcing configurations. For example, 100-year return level waves from southwestern swell and +0.5 m mean sea level increase. Note that this may correspond to a 1 in 100-year events reaching the coast during the spring tides and under present-day mean sea level conditions. It also occurs for moderate extreme waves with a return period of 10-years in combination with +1 m of mean sea level (this case is also provided in the Supplementary Video 1 ) and for all the return periods for the southwestern swell with +0.75 m of mean sea level rise.
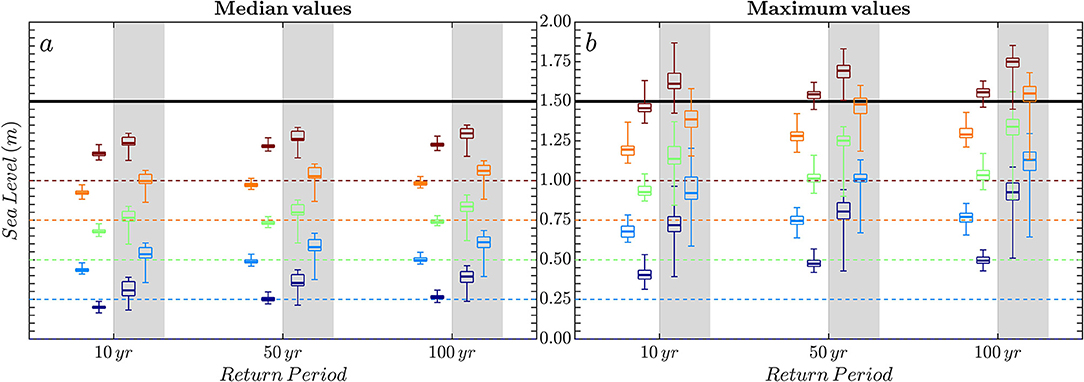
Figure 9 . Box plots along Hoarafushi coast without airport computed with time series of simulated water levels: (a) median values; (b) Maximum values. Box plot colours indicate the sea level of the simulation (dark blue 0 m; light blue 0.25 m; green 0.5 m; orange 0.75 m; dark red 1 m) and are referenced from the dashed line with the same colour. Box plots on grey (white) background are for the simulations for the southwestern swell (Indian Monsoon). The thicker line of the box plots shows the median values; the lower (upper) limit of the boxes indicates the 25th (75th) quantile; and the lower and upper whiskers indicate the minimum and maximum values. The horizontal thick black line indicates the island height.
With the construction of the airport connected to Hoarafushi ( Figure 8b ), the median and maximum water level values computed along the coast (grey area in Figure 8b ) slightly increased for all combinations of mean sea level, extreme waves and wave directions (see the equivalent figure to Figure 9 in Supplementary Figure 14 ). On average, the median values of water level along the coast increase around 0.05 m solely due to the presence of the airport, that partially blocks the channel between Hoarafushi and the island located southwards, leading to higher wave setup. The new reclaimed land is also exposed to incoming waves, and this exposure has been measured in a similar manner as for Hoarafushi, i.e., along a coastal strip on its western coast (blue area in Figure 8b ). We remark that the airport has been built 150-200 m away from the reef edge, reducing to a large extent the protection of the wave damping induced by the reef flat. Consequently, both median and maxima water level values are significantly higher than in Hoarafushi island ( Supplementary Figure 15 ). For example, with +1 m of increase in mean sea level, even moderate extreme waves would cause overtopping (e.g., 10-year return levels or less under high tide), and under current conditions a 50-year return level southwestern swell would partially flood the airport.
The flood hazard of the new reclaimed land is summarised in Figure 10 , using the set of 30 simulation runs with the airport. The flood hazard has been defined following the French standards, that define four different flooding hazard levels (low, moderate, high, and very high) that arise as combinations of inundation level and the water speed over land (see Supplementary Figure 16 ). The artificial island built for the airport is completely flooded with a high level of hazard for most part of the island for both wave directions and all return periods with an increase in mean sea level of ≥0.75 m (with the only exception of the Indian monsoon 10-year return period). It is foreseen that the reclaimed land suffers from partial flooding under a southwestern swell extreme of 50-year return period with current conditions of mean sea level. It is worth mentioning that, given the lack of topographic data for the new airport island, flooding hazard is possibly biased high. We simulated the island as being completely flat and without any coastal defenses. This is unlikely to be the case for a critical infrastructure. However, the actual defense height remains unknown, which is why we assume compliance to land reclamation regulations i.e., 1.5 m land elevation. Coastal defenses would only delay the impact of coastal flooding, but would not avoid it.
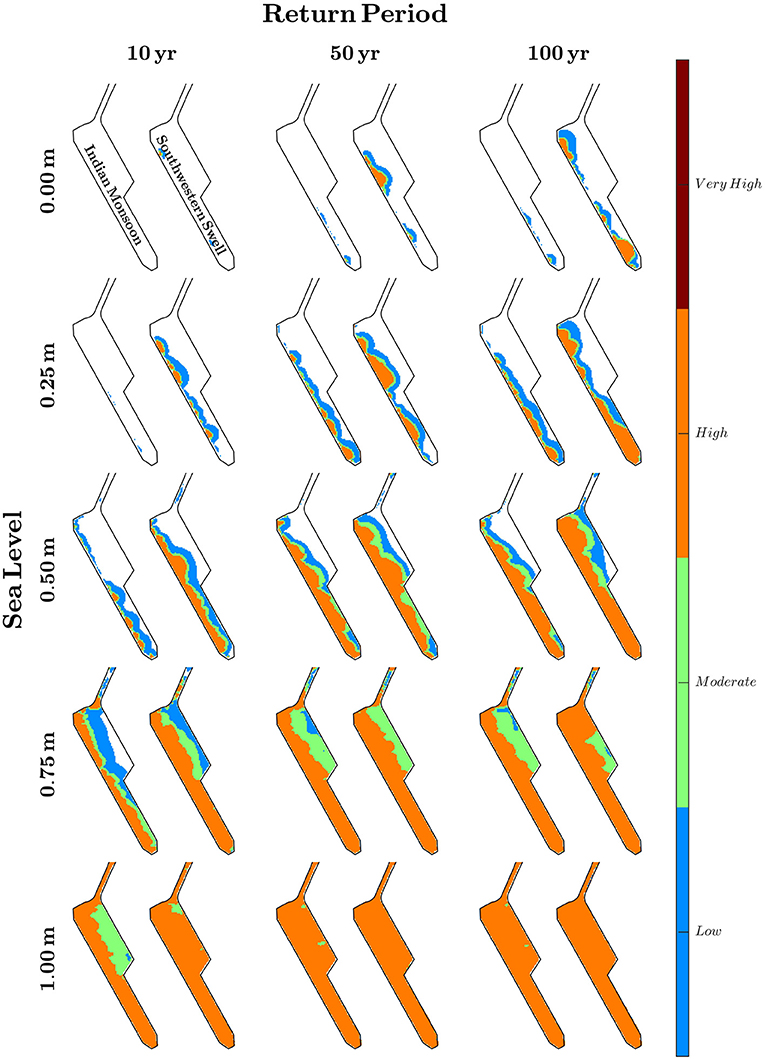
Figure 10 . Level of hazard on the new airport for all the SWASH simulations. The colourscale indicates the level of hazard defined by a combination of water height on the airport and water velocity (see Supplementary Figure 16 ). Different sea levels are represented at each row while the columns indicate return periods of H s (10, 50, and 100 years) as defined in the text for the regional wave climate. For each combination of sea level and return period, the result for the Indian Monsoon and Southwestern swell are shown at left and right, respectively.
6. Summary and Discussion
6.1. global to local coastal modelling.
Mean sea-level rise, despite having a global origin, has severe local coastal impacts, as it raises the baseline level on top of which extreme events reach the coastlines. Yet, projections of changes in mean sea-level as well as assessments of marine extremes are often provided on a large-scale basis (e.g., Vousdoukas et al., 2017 ), while understanding the causes of coastal flooding and anticipating the impacts require quantitative information at the local scale. This can be feasible to implement in regions where monitoring networks, forecasting and operational systems and development programs for sustainable coastlines are well established and mature (for example, the Flood and Coastal Erosion Risk Management Programme in the UK, or the Delta Programme in the Netherlands). In many cases, however, even local assessments rely on coarse resolution, large-scale global climate information.
In this work we have focused on the Maldivian archipelago, a region where recurrent flooding episodes occur driven by remotely generated waves. These events are, furthermore, projected to become more frequent as mean sea level rises due to the low elevation of the islands. Despite their exposure to waves, to our knowledge, the only source of wave climate information in the region so far are the outputs of global wave reanalysis with a spatial resolution of the order of a degree. Our work illustrates how these global wave fields from coarse resolution climate models can be translated into usable information for regional and local studies and how it can be combined with regionalised projections of mean sea-level rise and local topo-bathymetries.
The first step consisted of a detailed analysis and characterisation of the global wave climate around the Maldives using the closest grid points from the CFSR wave reanalysis (section 2.1). This is a prior mandatory step before the design of the regionalisation. We identified four dominant incoming wave directions from remotely generated waves: the two most common, that originate in the Southern Ocean ( Amores and Marcos, 2019 ), and swells generated by the Indian and Northwestern monsoons. In a second step, for each direction, extreme waves have been characterised in terms of H s and T p and a set of five return levels have been dynamically-downscaled using the spectral model WWIII (section 2.2). We have focused on extreme waves only because these are the most relevant for risk analyses; furthermore, the alternative of dynamically-downscaling a 35-year long reanalysis is unfeasible due to computational constraints (this worsens if historical runs and projections are considered). The regionalisation has resulted in a major product of the present work: a valuable data set of extreme waves along the Maldivian coasts with spatial resolutions down to 500 m in the points nearest to the coast. The data set is published at doi: 10.5281/zenodo.3886273 . The output of our regionalisation provides quantitative information on extreme waves, in the form of return level curves, at the regional scale in the Maldives and for the first time. This dataset is useful for coastal engineering studies, for feeding local coastal models of flooding hazards and for planning land reclamation and other regional developments. It also serves to compute the inundation potential at every location and for every incoming swell direction, that depends on wave energy, H s 2 · T p , in line with the “response approach” discussed in Sanuy et al. (2020) . Overall, it is expected to become a compelling source of scientific information that can be embedded in coastal climate services ( Le Cozannet et al., 2017 ; Kopp et al., 2019 ). The users should, nevertheless, ensure that the inherent uncertainties in the method and data are considered. This means that regional waves are representative of ocean swells in the vicinity of the atolls and that, for practical purposes, a detailed topobathymetry is needed is these regional outputs are to be used as boundary forcings. Also, the four main swell directions arriving to the archipelago are considered separately, since the generation mechanisms are independent; thus, every coastal location may be exposed to a different number of incoming wave directions, and all of them should be explored in a local case study, as illustrated in section 5 above.
There is a number of limitations in our regionalised wave fields. The bathymetry used in the regional wave model (GEBCO, see section 2.2) has a spatial resolution of ~ 1 km, which is not enough to resolve the features inside the atolls. We have therefore included every atoll as a single entity in the model domain, neglecting the wave propagation in the inner region and the exchanges between the lagoon of the atoll and the ocean. We consider, nevertheless, that this assumption is reasonable because our results provide evidence that shadow effects of the atolls to incoming waves are realistically simulated from all directions. That implies that we account for the waves that reach the external coast of the atolls everywhere in the Maldives. This limitation can be overcome in areas where mesoscale (~ 100 m resolution) bathymetric data sets exist, in which case the interactions with the inner lagoon can also be accounted for. Another caveat of the regional product is that only selected return periods of H s are provided, instead of an entire high-frequency time series at every coastal grid point. While the quantification of return levels is central to risk assessments, no information on averaged wave fields (useful for erosion studies, for example) is provided. Finally, it is worth pointing out that the regional product has not been validated against observations due to the lack of data.
6.2. Application for Coastal Flood Hazard
We have conducted a local flood hazard modelling experiment that demonstrates the applicability of the regionalised wave fields. Our case study is in the North of the archipelago, exposed to the largest incoming waves, and includes a land reclamation project. We have used the regionalised wave information to feed the wave propagation model SWASH around Hoarafushi island, where local bathymetry has been measured. We have estimated the flooding hazard under present-day conditions and also under projected future scenarios. Our analysis of the global wave climate revealed that projected changes in the large-scale wave characteristics during the twenty-first century are small in comparison to the multi-model spread even under the RCP8.5 scenario. Therefore, we rely on the downscaled regional wave reanalysis and assume that future changes in marine hazards will be driven only by mean sea-level rise. The local model does take into account the modification of the wave propagation due to higher mean sea levels, though. The set of the model experiments included the island configuration with and without the airport in order to determine how the presence of the new reclaimed land alters the flood hazard, the wave propagation and the associated currents.
Our results identified the southwestern swells as the potentially most hazardous waves in Hoarafushi, with 100-year return levels of H s up to 4 m and associated T p of ~20 s. This is, in addition, the most common wave direction that reaches this part of the archipelago, although not the one with largest H s (that are associated with the Indian monsoon). Our findings indicate that a moderate incoming southwestern swell corresponding to a return period of only 10 years will cause overtopping in Hoarafushi island if it reaches the shoreline under a mean sea level 0.75 m higher than its present-day value ( Figure 9 ). The presence of the reclaimed land slightly increases these impacts ( Supplementary Figure 14 ). The flood hazard is much stronger in the reclaimed land, that will experience overtopping episodes with sea levels only 0.25 m above present-day mean value ( Supplementary Figure 15 ). The reason is its location close to the reef that reduces the wave damping over the reef flat. We recall here that we have adopted a scenario-independent approach for mean sea level increases; this may be justified given that the range of mean sea level changes that we are considering (below 1 m) will be reached even under strong mitigation, as the maximum value lies within the committed global mean sea-level rise of past GHGs emissions ( Nauels et al., 2019 ). Thus, it is not about whether these higher mean sea levels will be reached, but when it will occur. Impact studies based on scenario-independent approaches in combination with ongoing monitoring of regional mean sea-level rise can facilitate the design of adaptive solutions to climate-induced hazards.
In addition, this approach also allows to evaluate the wave-induced flood hazard under particular tidal conditions. In the example above, mean sea level 0.75 m higher than present-day values can be interpreted as a combination of climate-induced mean sea-level rise and tidal oscillations. For instance, 0.75 m can be reached with 0.5 m of climate-induced mean sea level that, according to Kopp et al. (2014) , corresponds to the median projected value in 2068 under the RCP8.5 scenario, plus 0.25 m of tidal amplitude. In consequence, according to our estimates, the recently developed (in 2019) regional airport will be flooded under present-day mean sea-level conditions and 0.25 m of tidal amplitude if a moderate extreme swell event (10-year return period) reaches the area, that is, within the present decade. Note that we are not computing the likelihood of co-occurrence of extreme swells and high tides. The reasons for that are, firstly, that these two processes are uncorrelated (astronomical tides and remotely-generated swell events have independent driving mechanisms) which means that their joint probability could be computed as the product of their marginal probability distributions ( Pugh and Woodworth, 2014 ). However, this would require a complete set of time series of the two processes at every grid point. Although there are methods to generate a set of full synthetic time series from their statistical characterisation (e.g., Solari and Losada, 2011 ), this is a different type of product that is beyond the scope of the present work. Secondly, our approach is more flexible since it does not constrain the interpretation of the increments in mean sea level (either climate-induced sea level rise or tides or both), hence, allowing final users to tailor our approach to their needs, based on their respective risk-taking propensity.
The modification of the island configuration with the presence of the reclaimed land significantly modifies the patterns of the currents ( Supplementary Figure 1 ). Such changes are determinant for coastal erosion, as they control the sediment transport along the coastlines. Coastal erosion is considered a central problem in the Maldives, especially in densely populated islands ( Zahir et al., 2016 ; Duvat and Magnan, 2019 ). Erosion can be prevented or enhanced by many factors, including land reclamation, dredging and building coastal defenses. Here we demonstrate that our regional wave fields are a valuable tool also for anticipating possible erosion and changing spatial patterns in particular case studies.
The major limitation of our local coastal modelling exercise is the lack of a detailed topography of Hoarafushi and its nearby reclaimed airport. While we have measured bathymetric profiles during a field trip, the information on the topography is limited to the averaged elevation of the island. Likewise, the elevation of the reclaimed land (which was not yet built when the field trip took place) has been defined according to the national regulations. In consequence, we have not included coastal defenses and we have instead considered that both the island and the new reclaimed land are flat. This implies that our estimates of overtopping and flooding could be biased high; however, the presence of coastal defenses would not completely avoid the flood hazard, they would simple delay the impacts of mean sea-level rise.
Another point worthy of discussion is the assumption of static bathymetry and null reef response to changing climatic conditions. It is clear that reefs can change over time. For example, they can accrete following sea level rise ( Woodroffe and Murray-Wallace, 2012 ), they can degrade due to human activities (the construction of the airport is a good example) or they can die as a consequence of warmer temperatures ( Bruno and Selig, 2007 ) (indeed, warm reefs are projected to significantly decline even with global warming only 1.5°C above pre-industrial levels ( Bindoff et al., 2019 ) and to be virtually extinct with 2°C of warming ( Hoegh-Guldberg et al., 2018 ). In any of these cases, changes in the reef would imply changes in the wave propagation and level of protection of the island ( Sheppard et al., 2005 ). We disregard these potential changes in our local flood hazard modelling experiment because we analyse an artificially reclaimed island. Here, human activities generally have severe negative effects on the reef ( Duvat, 2020 ) and the island is protected with hard measures. This is also to urban atoll islands, as Hoarafushi, that are continuously adapting to increased hazard potential by building coastal infrastructures or artificially raising the land ( Duvat and Magnan, 2019 ; Esteban et al., 2019 ; Hinkel et al., 2019 ; Brown et al., 2020 ). This reduces the ability of the island to naturally increase its elevation by sediment deposition during overtopping events ( Kench and Beetham, 2019 ). Hence, we argue that in this case human interventions are probably more important for wave propagation than changes in the reef ( Duvat and Magnan, 2019 ). Contrastingly, in a natural island, assuming a static bathymetry and null reef response, would bias the results of model overtopping ( Beetham et al., 2017 ; Beetham and Kench, 2018 ). Nevertheless, the regional downscaling that we provide serves as a boundary condition for subsequent studies of wave-induced flooding under future conditions, which then have to account for these uncertainties of future reef responses.
7. Concluding Remarks
Our study provides the framework to fill the gap between global information of marine climate drivers, including mean sea level and extremes, and local coastal flood hazard modelling. In particular, we demonstrate the feasibility of using large-scale data sets (regionalised sea-level projections and global wind-waves simulations) to inform regional planning and local decision-making. Our work focused in the Maldives, but our technique can be applied to any coastal region, being most relevant where regional and local climate information is not available. Together with the outputs, we have discussed a number of uncertainties in regional as well as local coastal modelling that are inherent to the methodology. Some of the limitations, though, stem, to a large extent, from the lack of coastal observations (i.e., local topo-bathymetries). Our study thus advocates for improved monitoring systems and data collection to reduce uncertainties and better inform final users.
We have generated a valuable regional wave data set that fulfils the purposes of characterisation of the wave climate in a sparsely observed area. This dataset, in combination with detailed local information (e.g., high-resolution topo-bathymetries), serves as a milestone for informing adaptation policy and Maldivian decision-makers facing the challenge of adapting to rising sea-levels.
Data Availability Statement
The datasets presented in this study can be found in online repositories. The names of the repository/repositories and accession number(s) can be found below: https://zenodo.org/record/3886273#.YCFTAOhKhPY .
Author Contributions
AA, MM, GL, and JH conceived the work. AA, MM, RP, and SL designed the numerical experiments. AA, MM, and JR analysed the return periods. AS and ZK retrieved the topobathimetric data. All authors contributed to the outline and writing of the manuscript.
This study was supported by the FEDER/Ministerio de Ciencia, Innovación y Universidades Agencia Estatal de Investigación through the MOCCA project (grant no. RTI2018-093941-B-C31) and by the INSeaPTION Project that is part of ERA4CS, an ERA-NET initiated by JPI Climate, and funded by Ministerio de Economía, Industria y Competitividad Agencia Estatal de Investigación (ES) (grant no. PCIN-2017-038), BMBF (DE), NOW (NL), and ANR (FR) with co-funding by the European Union (Grant 690462). This research has been also supported by the ANR project Storisk supported by the French Research Agency. AA was funded by the Conselleria d'Educació, Universitat i Recerca del Govern Balear through the Direcció General de Política Universitària i Recerca and by the Fondo Social Europeo for the period 2014–2020 (grant no. PD/011/2019). MM was supported by the Ministerio de Ciencia e Innovación and la Agencia Estatal de Investigación through grant no. IED2019-000985-I.
Conflict of Interest
The authors declare that the research was conducted in the absence of any commercial or financial relationships that could be construed as a potential conflict of interest.
Acknowledgments
We are grateful to Dr. Aurélie Maspataud for her support with bathymetric data and Dr. Fernando Méndez for advice on numerical wave modelling. We thank the Ministry of Environment, the Environmental Protection Agency, the Maldives Transport and Contracting Company and Water Solutions for their support during the field trip and data collection. AA is grateful to M. A. Blázquez for his support and comments during the preparation of the manuscript.
Supplementary Material
The Supplementary Material for this article can be found online at: https://www.frontiersin.org/articles/10.3389/fmars.2021.665672/full#supplementary-material
Amores, A., and Marcos, M. (2019). Ocean swells along the global coastlines and their climate projections for the 21st century. J. Clim. 33, 185–199. doi: 10.1175/JCLI-D-19-0216.1
CrossRef Full Text | Google Scholar
Beetham, E., and Kench, P. S. (2018). Predicting wave overtopping thresholds on coral reef-island shorelines with future sea-level rise. Nat. Commun. 9:3997. doi: 10.1038/s41467-018-06550-1
PubMed Abstract | CrossRef Full Text | Google Scholar
Beetham, E., Kench, P. S., and Popinet, S. (2017). Future reef growth can mitigate physical impacts of sea-level rise on atoll islands. Earths Fut. 5, 1002–1014. doi: 10.1002/2017EF000589
Bindoff, N. L., Cheung, W. W., Kairo, J. G., Arístegui, J., Guinder, V. A., Hallberg, R., et al. (2019). “Changing ocean, marine ecosystems, and dependent communities,” in IPCC Special Report on the Ocean and Cryosphere in a Changing Climate , eds H. -O. Pörtner, D. C. Roberts, V. Masson-Delmotte, P. Zhai, M. Tignor, E. Poloczanska, K. Mintenbeck, A. Alegría, M. Nicolai, A. Okem, J. Petzold, B. Rama, N. M. Weyer, 477–587, in press.
Google Scholar
Brown, S., Wadey, M. P., Nicholls, R. J., Shareef, A., Khaleel, Z., Hinkel, J., et al. (2020). Land raising as a solution to sea-level rise: an analysis of coastal flooding on an artificial island in the maldives. J. Flood Risk Manag. 13:e12567. doi: 10.1111/jfr3.12567
Bruno, J. F., and Selig, E. R. (2007). Regional decline of coral cover in the indo-pacific: timing, extent, and subregional comparisons. PLOS ONE 2:e711. doi: 10.1371/journal.pone.0000711
Cialone, M. A., and Smith, J. M. (2007). “Wave transformation modeling with bottom friction applied to southeast oahu reefs,” in 10th International Workshop on Wave Hindcasting and Forecasting & Coastal Hazard Assessment , (Citeseer), 1–12.
Duvat, V. K. (2020). Human-driven atoll island expansion in the maldives. Anthropocene 32:100265. doi: 10.1016/j.ancene.2020.100265
Duvat, V. K. E., and Magnan, A. K. (2019). Rapid human-driven undermining of atoll island capacity to adjust to ocean climate-related pressures. Sci. Rep. 9:15129. doi: 10.1038/s41598-019-54659-0
Esteban, M., Jamero, M. L., Nurse, L., Yamamoto, L., Takagi, H., Thao, N. D., et al. (2019). Adaptation to sea level rise on low coral islands: lessons from recent events. Ocean Coas. Manag. 168, 35–40. doi: 10.1016/j.ocecoaman.2018.10.031
Gussmann, G., and Hinkel, J. (2021). A framework for assessing the potential effectiveness of adaptation policies: coastal risks and sea-level rise in the maldives. Environ. Sci. Policy 115, 35–42. doi: 10.1016/j.envsci.2020.09.028
Harangozo, S. A. (2013). Flooding in the Maldives and Its Implications for The Global Sea Level Rise Debate (First Published: 01 January 1992) , (American Geophysical Union (AGU)), 95–99.
Hemer, M., Trenham, C., Durrant, T., and Greenslade, D. (2015). Cawcr global wind-wave 21st century climate projections (v2). CSIRO Service Collect . doi: 10.4225/08/55C991CC3F0E8
Hemer, M. A., Katzfey, J., and Trenham, C. E. (2013). Global dynamical projections of surface ocean wave climate for a future high greenhouse gas emission scenario. Ocean Model. 70, 221–245. doi: 10.1016/j.ocemod.2012.09.008
Hemer, M. A., and Trenham, C. E. (2016). Evaluation of a cmip5 derived dynamical global wind wave climate model ensemble. Ocean Model. 103, 190–203. doi: 10.1016/j.ocemod.2015.10.009
Hinkel, J., Church, J. A., Gregory, J. M., Lambert, E., Le Cozannet, G., Lowe, J., et al. (2019). Meeting user needs for sea level rise information: a decision analysis perspective. Earths Fut. 7, 320–337. doi: 10.1029/2018EF001071
Hinkel, J., Lincke, D., Vafeidis, A. T., Perrette, M., Nicholls, R. J., Tol, R. S. J., et al. (2014). Coastal flood damage and adaptation costs under 21st century sea-level rise. Proc. Natl. Acad. Sci. U.S.A. 111, 3292–3297. doi: 10.1073/pnas.1222469111
Hoegh-Guldberg, O., Jacob, D., Bindi, M., Brown, S., Camilloni, I., Diedhiou, A., et al. (2018). Impacts of 1.5°c Global Warming on Natural and Human Systems . Global warming of 1.5°C. An IPCC Special Report.
Hoeke, R. K., McInnes, K. L., Kruger, J. C., McNaught, R. J., Hunter, J. R., and Smithers, S. G. (2013). Widespread inundation of pacific islands triggered by distant-source wind-waves. Glob. Planet. Change 108, 128–138. doi: 10.1016/j.gloplacha.2013.06.006
Kench, P., and Beetham, E. P. (2019). Evidence of vertical building of reef islands through overwash and implications for island futures. Coastal Sediments 916–929. doi: 10.1142/9789811204487_0080
Kopp, R. E., Gilmore, E. A., Little, C. M., Lorenzo-Trueba, J., Ramenzoni, V. C., and Sweet, W. V. (2019). Usable science for managing the risks of sea-level rise. Earths Fut. 7, 1235–1269. doi: 10.1029/2018EF001145
Kopp, R. E., Horton, R. M., Little, C. M., Mitrovica, J. X., Oppenheimer, M., Rasmussen, D. J., et al. (2014). Probabilistic 21st and 22nd century sea-level projections at a global network of tide-gauge sites. Earths Fut. 2, 383–406. doi: 10.1002/2014EF000239
Kraines, S., Yanagi, T., Isobe, M., and Komiyama, H. (1998). Wind-wave driven circulation on the coral reef at bora bay, miyako island. Coral Reefs 17, 133–143. doi: 10.1007/s003380050107
Le Cozannet, G., Nicholls, R., Hinkel, J., Sweet, W., McInnes, K., Van de Wal, R., et al. (2017). Sea level change and coastal climate services: The way forward. J. Mar. Sci. Eng. 5, 49. doi: 10.3390/jmse5040049
Lecacheux, S., Pedreros, R., Le Cozannet, G., Thiébot, J., De La Torre, Y., and Bulteau, T. (2012). A method to characterize the different extreme waves for islands exposed to various wave regimes: a case study devoted to reunion island. Natural Hazards Earth Syst. Sci. 12, 2425–2437. doi: 10.5194/nhess-12-2425-2012
Maniku, H. A. (1990). Changes in the Topography of the Maldives . Forum of Writers on Environment (Maldives).
Marcos, M., and Woodworth, P. L. (2017). Spatiotemporal changes in extreme sea levels along the coasts of the north atlantic and the gulf of mexico. J. Geophys. Res. 122, 7031–7048. doi: 10.1002/2017JC013065
MEE. (2017). State of the Environment 2016. Ministry of Environment and Energy . Available online at: https://www.environment.gov.mv/v2/wp-content/files/publications/20170202-pub-soe-2016.pdf
Merkens, J.-L., Lincke, D., Hinkel, J., Brown, S., and Vafeidis, A. T. (2018). Regionalisation of population growth projections in coastal exposure analysis. Clim. Change 151, 413–426. doi: 10.1007/s10584-018-2334-8
Morim, J. L. M., Wang, H. X., Cartwright, N., Trenham, C., Semedo, A., Young, I., et al. (2019). Robustness and uncertainties in global multivariate wind-wave climate projections. Nat. Clim. Change 9, 711–718. doi: 10.1038/s41558-019-0542-5
Nauels, A., Gütschow, J., Mengel, M., Meinshausen, M., Clark, P. U., and Schleussner, C.-F. (2019). Attributing long-term sea-level rise to paris agreement emission pledges. Proc. Natl. Acad. Sci. U.S.A. 116, 23487–23492. doi: 10.1073/pnas.1907461116
NBS (2017). “Statistical yearbook of maldives 2016,” in National Bureau of Statistics Ministry of Finance and Treasury, Republic of Maldives (Malé).
NBS (2019). “Maldives population projections 2014-2054, assumption and results analysis,” in National Bureau of Statistics Ministry of Finance and Treasury, Republic of Maldives (Malé)
Nicholls, R. J., Hanson, S. E., Lowe, J. A., Warrick, R. A., Lu, X., and Long, A. J. (2014). Sea-level scenarios for evaluating coastal impacts. WIRES Clim. Change 5, 129–150. doi: 10.1002/wcc.253
Nurse, L., McLean, R., Agard, J., Bibruglio, L., Duvat, V., Pelesikoti, N., et al. (2014). “Small islands (chapter 29),” in Climate Change 2014 : Impacts, Adaptation, and Vulnerability. Part B : Regional Aspects. Contribution of Working Group II to the Fifth Assessment Report of the Intergovernmental Panel on Climate Change , eds V. R. Barros, C. B. Field, D. J. Dokken, M. D. Mastrandrea, K. J. Mach, T. E. Bilir, M. Chatterjee, K. L. Ebi, Y. O. Estrada, R. C. Genova, B. Girma, E. S. Kissel, A. N. Levy, S. MacCracken, P. R. Mastrandrea, and L. L.White (Cambridge, UK; New York, NY: Cambridge University Press), 1613–1654. Available online at: https://www.ipcc.ch/site/assets/uploads/2018/02/WGIIAR5-Chap29_FINAL.pdf
Oppenheimer, M., Glavovic, B., Hinkel, J., van de Wal, R., Magnan, A., Abd-Elgawad, A., et al. (2019). “Sea level rise and implications for low-lying islands, coasts and communities,” in IPCC Special Report on the Ocean and Cryosphere in a Changing Climate , eds H.-O. Pörtner, D. C. Roberts, V. Masson-Delmotte, P. Zhai, M. Tignor, E. Poloczanska, K. Mintenbeck, A. Alegría, M. Nicolai, A. Okem, J. Petzold, B. Rama, N. M. Weyer. Available online at: https://www.ipcc.ch/srocc/chapter/chapter-4-sea-level-rise-and-implications-for-low-lying-islands-coasts-and-communities/
Prager, E. J. (1991). Numerical simulation of circulation in a caribbean-type backreef lagoon. Coral Reefs 10, 177–182. doi: 10.1007/BF00336771
Pugh, D., and Woodworth, P. (2014). Sea-Level Science: Understanding Tides, Surges, Tsunamis and Mean Sea-Level Changes . Cambridge: Cambridge University Press. doi: 10.1017/CBO9781139235778. Available online at: https://www.cambridge.org/core/books/sealevel-science/C5E551D95DA4E8AF116FED9F0DEB289B
Rijnsdorp, D. P., Smit, P. B., and Zijlema, M. (2012). Non-hydrostatic modelling of infragravity waves using swash. Coast. Eng. Proc. 1:currents.27. doi: 10.9753/icce.v33.currents.27
Saha, S., Moorthi, S., Pan, H.-L., Wu, X., Wang, J., Nadiga, S., et al. (2010). The ncep climate forecast system reanalysis. Bull. Am. Meteorol. Soc. 91, 1015–1058. doi: 10.1175/2010BAMS3001.1
Sanuy, M., Jiménez, J. A., Ortego, M. I., and Toimil, A. (2020). Differences in assigning probabilities to coastal inundation hazard estimators: Event versus response approaches. J. Flood Risk Manag. 13:e12557. doi: 10.1111/jfr3.12557
Sheppard, C., Dixon, D. J., Gourlay, M., Sheppard, A., and Payet, R. (2005). Coral mortality increases wave energy reaching shores protected by reef flats: examples from the seychelles. Estuarine Coast. Shelf Sci. 64, 223–234. doi: 10.1016/j.ecss.2005.02.016
Solari, S., and Losada, M. A. (2011). Non-stationary wave height climate modeling and simulation. J. Geophys. Res. 116:C09032. doi: 10.1029/2011JC007101
Suzuki, T., Altomare, C., De Roo, S., Vanneste, D., and Mostaert, F. (2018). Manning's Roughness Coefficient in Swash: Application to Overtopping Calculation . FHR Reports. Available online at: https://publicaties.vlaanderen.be/view-file/32905 .
Tolman, H. (2009). User manual and system documentation of wavewatch iii version 3.14. NOAA/NWS/NCEP/MMAB Technical Note 276 , (Appendices), 194.
Tolman, H. (2014). User manual and system documentation of wavewatch iii version 4.18. NOAA/NWS/NCEP/MMAB Technical Note 316 .
Vousdoukas, M. I., Mentaschi, L., Voukouvalas, E., Verlaan, M., and Feyen, L. (2017). Extreme sea levels on the rise along europe's coasts. Earths Fut. 5, 304–323. doi: 10.1002/2016EF000505
Wadey, M., Brown, S., Nicholls, R. J., and Haigh, I. (2017). Coastal flooding in the maldives: an assessment of historic events and their implications. Natural Hazards 89, 131–159. doi: 10.1007/s11069-017-2957-5
Woodroffe, C. D., and Murray-Wallace, C. V. (2012). Sea-level rise and coastal change: the past as a guide to the future. Quatern. Sci. Rev. 54, 4–11. doi: 10.1016/j.quascirev.2012.05.009
Woodworth, P. L. (2005). Have there been large recent sea level changes in the maldive islands? Glob. Planetary Change 49, 1–18. doi: 10.1016/j.gloplacha.2005.04.001
Woodworth, P. L., Melet, A., Marcos, M., Ray, R. D., Wöppelmann, G., Sasaki, Y. N., et al. (2019). Forcing factors affecting sea level changes at the coast. Surveys Geophys. 40, 1351–1397. doi: 10.1007/s10712-019-09531-1
Zahir, H., Asis, M., Rasheed, A., Musthafa, Z. M., Latheef, A. T., and Mohamed, I. (2016). “Second national communication of maldives to the united nations framework convention on climate change,” in Ministry of Environment and Energy. Republic of Maldives: Malé .
Zijlema, M. (2012). “Modelling wave transformation across a fringing reef using swash,” in ICCE 2012: Proceedings of the 33rd International Conference on Coastal Engineering, Santander, Spain, 1-6 July 2012 . Coastal Engineering Research Council. doi: 10.9753/icce.v33.currents.26. Available online at: https://journals.tdl.org/icce/index.php/icce/article/view/6479
Zijlema, M., Stelling, G., and Smit, P. (2011). Swash: an operational public domain code for simulating wave fields and rapidly varied flows in coastal waters. Coast. Eng. 58, 992–1012. doi: 10.1016/j.coastaleng.2011.05.015
Keywords: coastal flooding, wind-waves, sea-level rise, global-to-local modelling, climate services
Citation: Amores A, Marcos M, Pedreros R, Le Cozannet G, Lecacheux S, Rohmer J, Hinkel J, Gussmann G, van der Pol T, Shareef A and Khaleel Z (2021) Coastal Flooding in the Maldives Induced by Mean Sea-Level Rise and Wind-Waves: From Global to Local Coastal Modelling. Front. Mar. Sci. 8:665672. doi: 10.3389/fmars.2021.665672
Received: 08 February 2021; Accepted: 19 May 2021; Published: 21 June 2021.
Reviewed by:
Copyright © 2021 Amores, Marcos, Pedreros, Le Cozannet, Lecacheux, Rohmer, Hinkel, Gussmann, van der Pol, Shareef and Khaleel. This is an open-access article distributed under the terms of the Creative Commons Attribution License (CC BY) . The use, distribution or reproduction in other forums is permitted, provided the original author(s) and the copyright owner(s) are credited and that the original publication in this journal is cited, in accordance with accepted academic practice. No use, distribution or reproduction is permitted which does not comply with these terms.
*Correspondence: Angel Amores, angel.amores@uib.es
This article is part of the Research Topic
Climate Services for Adaptation to Sea-Level Rise
Climate Change in the Maldives
A STRING OF PEARLS
A low lying archipelago with more territorial sea than land, the Maldives is exposed to the risks of intensifying weather events. Sea level rise represents an existential threat to the country. With future sea levels projected to increase in the range of 10 to 100 centimeters by the year 2100, the entire country could be submerged.
Dr. Mohammed Waheed Hassan
MALDIVES CLIMATE CHANGE TRUST FUND
Male, April 6, 2010 - The Government of Maldives, the European Union (EU), and the World Bank Group signed a tripartite Memorandum of Understanding establishing a new Trust Fund designed to build resilience to climate change in the Maldive.
The EU has contributed $8.8 million (approximately EUR 6.5 million) to the multi-donor Maldives Climate Change Trust Fund, which the World Bank will administer over a period of three and a half years. The majority of the Trust Fund resources will be utilized by the Government of Maldives to carry out their priority projects relating to climate change adaptation and mitigation.
TRUST FUND OBJECTIVES
The development objectives of the Trust Fund Program are to:
- Strengthen knowledge and leadership in the Government of Maldives to deal with climate change issues both domestically and internationally;
- Build adaptive capacity and climate resilience in key sectors through tangible pilot interventions;
- Promote energy efficiency and increase energy access through renewable energy generation and distribution through low carbon options and public-private partnerships; and
- Improve policy and institutional capacities in the public and private sectors to deal with adaptation and mitigation interventions that bring both developmental and climate change benefits.
CLIMATE CHANGE IN MALDIVES
The Republic of Maldives comprises 1,190 islands in 20 atolls spread over 900 km in the Indian Ocean. Of these, just 199 islands are inhabited with a population of slightly over 300,000 people. The highest point of land is 2 meters or about 6 feet above sea level.
Drawn by its pristine beaches, underwater coral reefs, and spectacular marine wildlife, this small island-nation attracts some 500,000 tourists annually. Its atolls are ringed by the seventh largest coral reefs in the world and among the richest in species diversity. The reefs host over 1,900 species of fish, 187 coral species, and 350 crustaceans. In recent years, nature-based tourism has served as the engine of growth for the economy and accounts for about 70% of GDP.
“There are big challenges that come with the advantages of the islands’ tourist assets, however,” said Richard Damania, World Bank Lead Environmental Economist . “The country’s coral reefs, which protect it from storm surges and serve as the main attraction for the tourism-driven economy, are in danger of being damaged or destroyed by poorly handled waste disposal methods.”
Rising sea temperatures also threaten the coral reefs and cause bleaching and death, with the most severe damage in areas that are stressed by pollutants, or damaged by physical disturbance. Vulnerability to climate change hazards has been magnified by damage to coral reefs which has in turn impaired their protective function, thus a negative cycle of impact.
THE THREAT OF CLIMATE CHANGE
Geography has made the Maldives especially vulnerable to the consequences of climate change. Being land scarce and low lying, the country is exposed to the risks of intensifying weather events such as damage caused by inundation, extreme winds, and flooding from storms.
With the melting of polar ice caps, the Maldives is also exposed to the risks of sea-level rise. Future sea level is projected to rise within the range of 10 to 100 centimeters by the year 2100, which means the entire country could be submerged in the worst-case scenario.
“With the Maldives’ high dependence on a few key environmental assets and as pressure on these assets rises, prudent economic management calls for strengthened environmental stewardship,” said Damania . “We are working with the Government to build capacity and fill critical knowledge gaps on environmental issues. For example, an environmental management degree program at the Maldives College of Higher Education will become a reality in the near future."
WHAT CAN MALDIVES DO?
President Mohamed Nasheed has been in power for just over a year and has been proactive in raising awareness of the potential disaster facing the Maldives and the world.
In an attempt to bring the world’s attention to the problems faced by this tiny island nation, Nasheed held a cabinet meeting underwater. All cabinet officials had to be certified for scuba diving to attend and on October 17, 2009, Nasheed and his team descended around 20 feet and communicated with white boards and hand signals. After deliberating underwater for about 30 minutes, the cabinet signed a communiqué calling on all nations to cut their global emissions. Stunt or symbol, Nasheed’s iconic meeting attracted world attention.
Nasheed subsequently called for the formation of the Climate Vulnerable Forum and hosted its first meeting in the Maldives in November 2009. It was attended by 11 poor nations including Bangladesh, Bhutan, and Nepal from South Asia, all countries severely threatened by climate change.
WORLD BANK’S ROLE
Climate change and environment degradation has emerged as an important priority in the Bank’s deepening engagement in promoting responsible stewardship of global public goods.
The coral reefs of Maldives stand as the first line of defense against storms and sea-level rise. Poor solid waste management remains the most visible threat to the reefs. An estimated 248,000 tons of solid waste was generated in the Maldives in 2007 and this figure is predicted to rise by 30% to 324,000 tons over the next five years. In addition, around 510 tons of medical waste is produced in the Maldives each year.
Current arrangements for solid waste management on inhabited islands are inadequate. Most waste is dumped onto the island foreshore and burned at low combustion temperatures. This uncontrolled disposal is a threat to the coastal, marine and coral reef ecosystems and a blemish on the pristine marine landscape expected by tourists.
A World Bank-financed project aims to reverse this alarming trend. A key objective is to enhance stewardship of the assets that protect communities. Through the Maldives Environment Management Project, a $13.5 million IDA credit, the Bank is working with the Government of the Maldives to effectively manage environmental risks to fragile coral reefs and other marine habitats.
The project has three main components that are targeted to the most immediate environmental needs:
- Solid waste management;
- Capacity building for environmental management; and
- Technical assistance for monitoring and management of key natural assets.
"We are helping establish solid waste management facilities on targeted islands,” said Damania. “With its fragile marine environment, there is a need for specifically tailored solutions to develop a sustainable solid waste management system with stringent conditions for site selection, engineering, technology choice, and management."
- Maldives Environment Management Project
- Climate Change
Newsletters
You have clicked on a link to a page that is not part of the beta version of the new worldbank.org. Before you leave, we’d love to get your feedback on your experience while you were here. Will you take two minutes to complete a brief survey that will help us to improve our website?
Feedback Survey
Thank you for agreeing to provide feedback on the new version of worldbank.org; your response will help us to improve our website.
Thank you for participating in this survey! Your feedback is very helpful to us as we work to improve the site functionality on worldbank.org.
Thank you for visiting nature.com. You are using a browser version with limited support for CSS. To obtain the best experience, we recommend you use a more up to date browser (or turn off compatibility mode in Internet Explorer). In the meantime, to ensure continued support, we are displaying the site without styles and JavaScript.
- View all journals
- My Account Login
- Explore content
- About the journal
- Publish with us
- Sign up for alerts
- Open access
- Published: 07 October 2021
Considering socio-political framings when analyzing coastal climate change effects can prevent maldevelopment on small islands
- C. Gabriel David ORCID: orcid.org/0000-0002-6733-0288 1 nAff7 ,
- Arne Hennig 2 ,
- Beate M. W. Ratter ORCID: orcid.org/0000-0001-6647-4100 2 , 3 ,
- Volker Roeber 4 , 5 ,
- Zahid 6 &
- Torsten Schlurmann ORCID: orcid.org/0000-0002-4691-7629 1
Nature Communications volume 12 , Article number: 5882 ( 2021 ) Cite this article
7431 Accesses
10 Citations
74 Altmetric
Metrics details
- Climate sciences
- Environmental sciences
- Environmental social sciences
Adapting to climate change and sea level rise is challenging on small islands. False adaptation can lead to adverse impacts on natural and societal dynamics. Therefore, an interdisciplinary perspective on the interaction of natural dynamics, societal demands, and political decisions is crucial. In this sense, this study scrutinizes coastal processes and socio-political dimensions of erosion on the reef island Fuvahmulah, the Maldives. The national government and Fuvahmulah’s population have an opposed perception and attribution of the drivers and processes behind Fuvahmulah’s most pressing coastal issue – coastal erosion. To review these perceptions, natural dynamics are recreated with process-based methods and discussed regarding present and projected marine pressures. Population surveys and interviews with actors in coastal development complement the physical insights into erosion on Fuvahmulah and describe the socio-political dimension of climate change adaptation on small islands. This interdisciplinary approach demonstrates how small-islands’ adaptive capacities are typically impaired and disclose the potential of local knowledge to overcome maldevelopment.
Similar content being viewed by others
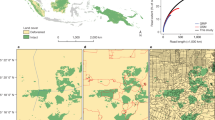
Ghost roads and the destruction of Asia-Pacific tropical forests
Jayden E. Engert, Mason J. Campbell, … William F. Laurance
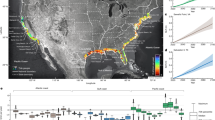
Disappearing cities on US coasts
Leonard O. Ohenhen, Manoochehr Shirzaei, … Robert J. Nicholls
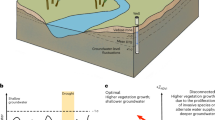
Establishing ecological thresholds and targets for groundwater management
Melissa M. Rohde, John C. Stella, … Christine M. Albano
Introduction
There is high confidence and evidence that climate-change induced sea-level rise increases the risk of small islands to suffer from ocean-borne impacts associated with high tides and swells 1 , 2 , 3 . Small low-lying islands and atolls are especially susceptible to the impacts of sea-level rise, as there is often no higher ground available for the local population to retreat to. In the upcoming decades, stronger and more frequent seaborne hazards will follow global heating, making small islands – in extreme situations – potentially uninhabitable 2 , 4 . Seaborne hazards on low-lying islands are typically rising sea levels, severe storm surges and swell waves. Likewise, these environmental pressures are constituent parts of the natural dynamics: Coastal environments react to hydrodynamic pressures with a morphodynamic response. Thus, wave-induced currents on the reef platform are an important driver of sediment transport 5 , 6 and the interplay of waves, sea level, reef platform, and sediment production are major elements of reef island genesis and evolution 5 , 7 , 8 , 9 : For example, when looking at the Maldives, monsoonal wind-wave events have an impact on locally characteristic island morphology, but, in general, distant-source swell waves are dominant in the regional wave climate 5 , 8 , 10 . Swell waves are characterized by a long wave period and play a key role in the island development on reef platforms 11 , 12 . Together with constant sediment provision by the coral reef 13 , the wave-induced sediment transport allows reef islands to mitigate erosion 14 and accrete vertically in response to sea level rise 11 , 12 . Coral reefs are important coastal protection assets 15 , because the reef dissipates most of the incoming wave energy 16 . There is evidence that reefs have grown and can continuously provide protection under rising sea levels 11 , 17 if they remain healthy. As a consequence, healthy coral reefs are a very important – if not the most important – factor of the island’s resilience to withstand sea level rise and marine extreme events. However, global warming and ocean acidification threatens the health of coral reefs 18 and with that also the important ecosystem services coral reefs provide.
For small islands, coastal protection is an important ecosystem service. Owing to their geography, small islands’ populations are more exposed to marine hazards and more vulnerable than mainland communities. In addition, marine resources deliver important ecosystem services for island communities, because they provide food and income for the island’s inhabitants 19 . However, protection from increasing risk of seaborne hazards as well as connection and exploitation of marine resources require concerted development of coastal areas, followed by construction and maintenance of infrastructure. To date, coastal structures and anthropogenic interventions have often resulted in the proliferation of engineering-type hard-coastal protection systems, defending coastal areas up to a certain level of safety from coastal hazards. These interventions are man-made disruptions of the coastal system, often undermining vital ecosystem services and interfering with the morphodynamic interchange on beaches 20 , 21 . Nevertheless, such structures are implemented widely 22 , even though they interrupt the littoral currents or deteriorate coastal environments to large extents. Anthropogenic interventions in natural dynamics put further pressures on the environment and are driven by political decision-making processes and social interests 23 .
In their first assessment report, the Intergovernmental Panel on Climate Change (IPCC) names three general response strategies to adapt to increased coastal risk and sea level rise 24 : protection, accommodation and retreat. In the meantime, further alternatives have emerged and in 2019, the Special Report Ocean and Cryosphere in a Changing Climate (SROCC) of the IPCC complements the response strategies by supporting a broader range of options 3 : protection, accommodation, advance, retreat, ecosystem-based adaptation, or no response. However, especially small island countries are often confronted with a number of issues that further complicate finding sustainable solutions to infrastructure demands 25 , 26 : on the one hand, decisions are regularly made from afar by the central government without addressing or integrating local knowledge. On the other hand, island states often lack expertize as well as human and financial resources to allow thorough planning and proper implementation 27 . Thus, development decisions are embedded in political structures on multiple levels of governance, determining how decisions are made and what actors are involved in the process 25 , 28 , 29 .
Against this background, the present study illustrates the problem of interlinked natural and societal dynamics by presenting the case study and example of erosion on the reef island Fuvahmulah, the Maldives. The study site is subject to typical aspects of increased disaster risk on small islands in the face of climate change: Fuvahmulah is located in the center of the Indian Ocean, especially exposed to seaborne hazards, such as sea level rise or wave events. In the Indian Ocean, sea level rise is accelerating and the region is prone to experience extreme climate and weather events more frequently 30 , 31 . The Maldives government addresses these challenges mainly with hard-engineered protection 20 , 22 , 32 . The resulting fortification of the coast is often an anthropogenic disturbance in the dynamic natural reef system, undermining vital ecosystem services and leading to increased vulnerability to suffer from adverse climate change effects 20 , 22 , 32 . In that context, this study investigates maladaptive developments and explicates their consequences to facilitate developing adequate and sustainable adaptation strategies – especially in the face of increasing risks due to climate change. This is achieved by an interdisciplinary approach, using methods and results from former studies on natural drivers and responses on small islands 5 and socio-political framing of climate change adaptation in the Maldives 33 . This study synthesized these results with the Drivers, Pressures, State, Impact and Response model of intervention (DPSIR) and focuses on root causes behind erosion on Fuvahmulah. Following the DPSIR’s chain of causal links, the framework facilitates identifying the oceanic-climatic and socio-political pressures leading to state changes of sensitive environments. Establishing connections between the DPSIR’s constituents allows for upscaling the lessons learned from the case of Fuvahmulah to other examples of top-down implemented coastal infrastructure in sensitive reef environments, because the island’s current adaptation pathway is an epitome of maldevelopment on small islands: the attempt to improve the socio-economic situation requires to equip the island with coastal infrastructure, which most often interferes with the natural dynamics. However, instead of addressing these adverse effects adequately, the socio-political preference and constant top-down prescription of generic hard-engineered measures is prone to lead to recurrent maladaptive actions, cascading adverse effects along the coast. Together, the socio-political framing and the top-down approach to implement infrastructure projects are anthropogenic pressures, which gradually diminish the island’s natural capacity to adjust to ocean climate-related pressures. Numerous examples exist elsewhere in the Maldives 20 , 22 , 34 and in other Small Island Developing States (SIDS) 25 , 27 with similar symptoms of maldevelopment. With an interdisciplinary perspective of coastal scientists and human geographers, this study (1) introduces the concept of maldevelopment, describing the socio-political framing leading to repeated maladaptive actions, and (2) demonstrates how local knowledge of the drivers and processes in sensitive small island environments supports low-regret development to mitigate adverse impacts on natural and societal dynamics.
Maldevelopment
The IPCC defines action or inaction as maladaptation if it “may lead to increased risk of adverse climate-related outcomes, increased vulnerability to climate change, or diminished welfare, now or in the future” (WGII AR5 35 , Glossary, page 1769). In coastal environments, maladaptation aims at reducing adverse effects to the coastal community now, but has negative implications on future response options under different climate change scenarios 36 . For example, the wide-spread anthropogenic coastal fortification in the Maldives has lead to irreversible changes to the natural coastal system, so that the only remaining future response to rising sea levels and extreme events is further armoring of the reef islands’ coasts 22 . These “anthropogenic tipping points” 22 are mainly governed by negative socio-political dynamics, which have grown historically in the Maldivian context within the last decades 26 , 37 . Despite the success of recent decentralization efforts 38 , national development policies aim at improving the socio-economic situation by fostering regional development centers (see the National Population Consolidation Program). These regional development centers are equipped with public and infrastructure facilities, and thus are considered safer and are higher populated 26 , 37 . However, these development efforts by the national government also encourage top-down implemented infrastructure projects 33 . In a top-down approach to implement infrastructure projects, decisions on these projects are generally made on a national-level, prescribing the implementation of these projects to the local level. Under this current policy pathway, anthropogenic disturbances in the natural dynamics will remain to govern coastal changes on the Maldives considerably in the future – besides climate change related pressures 26 . While recent studies found deficient coastal adaptation policies and policy compliance in the Maldives 39 , 40 , the results of this study illustrate the impact of such deficits in practice and reveal the framing of recurrent and ongoing maladaptation, which is structurally embedded within the socio-political system. In contrast to maladaptation, this “maldevelopment” is not an inadequate adaptation action leading to climate change related risks, but the socio-political driver behind repetitive maladaptation. Besides the maladaptive impact on the natural system, maldevelopment emphasizes the socio-political aspects of recurrent maladaptive actions: on the one hand, the societal factors, being the local population’s perception of and their attachment to place, their interest in economic development, and environmental protection. On the other hand, the political aspect of responsible authorities, aiming to balance the need for socio-economic development with environmental protection and issues of sustainability by means of decisions and policies. In this sense, maldevelopment is characterized by decisions or policies which are in constant, repeatedly or deliberate favor of maladaptive actions and trade-offs towards future climate change related risk – as epitomized by current coastal adaptation efforts on the reef island Fuvahmulah.
Fuvahmulah is an island in the south of the Maldives, located approximately 30 km south of the equator (latitude: − 0.30°, longitude: 73.43°, see Fig. 1 ). Unlike most other inhabited islands of the Maldives, Fuvahmulah is not part of a ring-shaped atoll and lacks the distinct protection features of oceanward islands 8 . Fuvahmulah consists of only one main island and its fringing reef. The main island has a size of about 4.4 km by 1.0 km and a coastline of ~11 km length. The island is surrounded by ridges, which are located landward behind the beaches and predominantly vegetated by a fringing palm forest. The island’s ridges reach a height of up to about 4 m above mean sea level. Together with the reef, this natural barrier mostly protects the island from storm surges, swell waves and helps mitigating overwash. Behind these natural coastal protection systems, Fuvahmulah’s inland is at mean sea level and hosts two freshwater lakes.
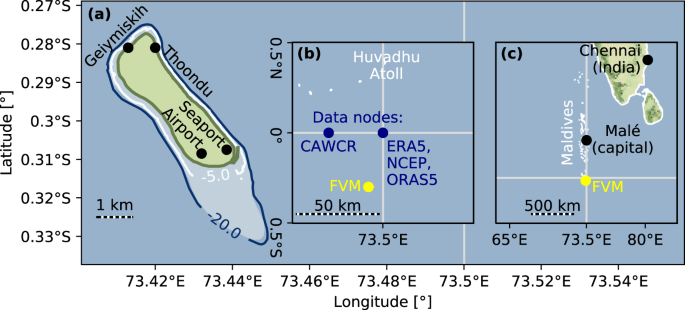
a Shows the island, the seaport, and airport location, as well as the beaches Geiymiskih and Thoondu. Contour lines show the fringing reef depth. b Location of Fuvahmulah and data nodes of the hindcast and reanalysis model, from which the wave climate was derived. c Location of Fuvahmulah within the Maldives and the Indian Ocean. Maps were generated with the Python module cartopy, using open-access © OpenStreetMap contributors data (open-access Geofabrik download server data and Stamen map tiles), as well as data from the field measurements (Fuvahmulah’s coastline and reef bathymetry).
Among islands of the Maldives, the landmark of Fuvahmulah is its sandy beach Thoondu, a coastal spit situated at the north of the island. As wave direction changes with the seasons, Thoondu responds to these natural dynamics 5 : it seasonally adjusts its landform shape, alters its sediment volume and regularly meanders from the northern part of the headland in wet season towards the north-east of the island as well as to the northwestern Geiymiskih beach in the dry season. The constant change of Thoondu is well known by locals and contributes to its uniqueness. As consequence, in 2020, the United Nations Educational, Scientific and Cultural Organization (UNESCO) designated Fuvahmulah as a Biosphere reserve.
The closest neighboring island is Hulhumeedhoo (part of Addu Atoll) located about 40 km southwest of Fuvahmulah. The 8510 inhabitants 41 of Fuvahmulah are exceptionally isolated – even when considering all 26 atolls in the Maldives, stretching over about 870 km length (north to south) and about 130 km width (west to east). The isolated location and with the reef and vegetated coastal ridges serving as the only natural coastal protection, Fuvahmulah is particularly susceptible to environmental forcing. Fuvahmulah’s location close to the equator makes it less likely to experience cyclones 10 , 42 but the island is considered to be highly exposed to monsoon winds 43 and associated wind-waves, as well as to distant-source swells 5 , 8 , 10 , 26 .
The isolated position makes Fuvahmulah dependent on transportation infrastructure, providing a safe connectivity, an access to the outside world. The transport infrastructure on the island endows the local economy and supply – especially through the shipping of goods. However, until 2002, Fuvahmulah was only accessible by small fishing boats (“dhonis”), which had to navigate through the breaking waves over the reef plateau. The “dhonis” acted as feeders, transporting people, goods, and cargo from Fuvahmulah to larger ships that were waiting offshore. In this procedure, numerous boats capsized and people died. For decades, the people of Fuvahmulah have been requesting the construction of a safe port. The new seaport was constructed on the southeast of the island opening in 2002. The seaport was followed by the construction of an airport for domestic flights in the southwest of the island that opened in 2011.
Measured coastline erosion on the east side
Ever since the port has been built, people on the island observe and report severe erosion on the east-side of Fuvahmulah 44 . The Digital Elevation Models (DEMs), derived from aerial imagery, prove this observation and quantify the observed erosion rates and its spatial extent along the east coast. Between 2017 and 2019, the cross-sections along the east side show distinct differences in morphologic changes (Fig. 2 ): while the northern area close to Thoondu (Fig. 2 a) shows a slight increase in beach volume, the other transects depict ongoing erosion (Fig. 2 b–d). The increase in sediment volume in the north at Thoondu beach is due to the typical dynamic behavior of the northern beaches in response to varying seasonal wave conditions: during dry season, Thoondu beach moves to the northeastern coast 5 . The cross-section at the end of Thoondu beach (Fig. 2 a) shows the calcareous bulk material forming the beach face. When moving further south, the coast of the central east side has a steeper profile than the northern beaches and consists of gravel to cobblestone material (Fig. 2 b) or larger boulders. The two southernmost transects near the seaport have steep edges, where the mainland meets the reef flat (Fig. 2 c, d). These transects are located about 700 m (Fig. 2 c) and 190 m (Fig. 2 d) in the north-west of the seaport’s entrance. At these locations, the subsurface insular bedrock becomes visible. The erosion process is already noticeable from field observations and has also been widely recognized and reported by locals.
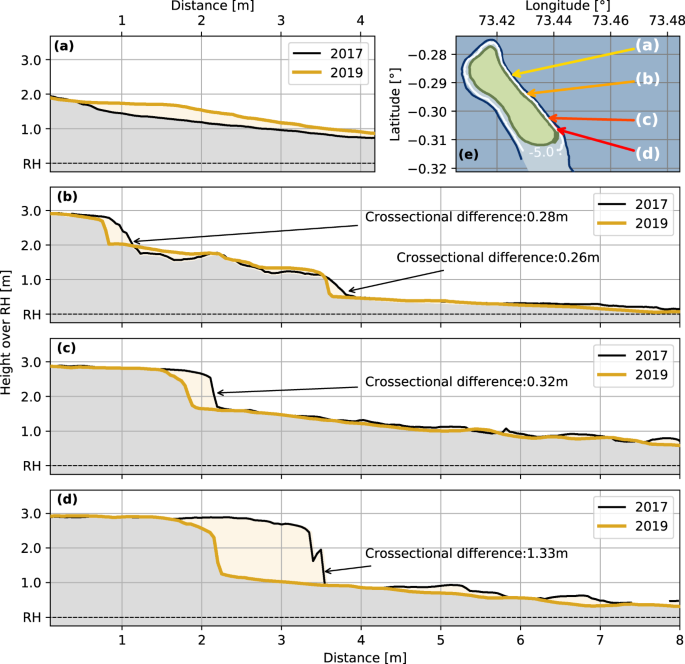
Cross-sections of four locations, showing typical coastal profiles along the east side of Fuvahmulah. The profiles originate from the Digital Elevation Models (DEMs) of the dry season of 2017 and 2019. The DEMs were produced by Agisoft Photoscan with an Structure from Motion-MultiView Stereo (SfM-MVS) approach. a marks the beginning of the beach Thoondu, showing a slight increase of the sandy beach face. b shows a transect from the central area of the east coast, suffering slight erosion of about 0.26 m to 0.28 m at the toe of the beach. Erosion like this can occasionally be measured in other areas of the central east side but it is not constantly detectable along this section of the coast. c and d show profiles of the southeastern coast adjacent to the seaport. The steep edge is the transition of the main island into the reef flat. Here, the coast suffers from constant, structural erosion of about ~0.3 m with maximum values of ~1.33 m between 2017 and 2019 (see Fig. 3 ). The map in e shows the locations of the profiles on Fuvahmulah’s east coast. The map is based on data from field measurements and adapted publicly available © OpenStreetMap contributors data, accessed through the open-access Geofabrik download server .
Along the southern stretches of the east coast, there are further signs of erosion, such as uprooted trees. For example, adjacent to the most southern cross-section was a palm tree that later fell onto the reef (see Fig. 3 ). Large parts of Fuvahmulah’s coastal ridge have a coastal forest (“heylhi”), reinforcing the sandy beach profile with its roots. On the east side, waves and currents carve out the sediment under the roots and dislocate broken bedrock material. These observations show that erosion on the east side of Fuvahmulah starts above the reef flat at the bottom of the beach profile. These observations also help to put erosion into context: while the northern transect shows slight sediment accretion, the central part shows shoreline retreat with maximum values of 0.26 m to 0.28 m between 2017 and 2019 (Fig. 2 d). However, shoreline retreat was only occasionally measurable and not present over the entire central coastline – in contrast to the southeastern coast, adjacent to the port. This area experiences substantial ongoing erosion along its entire coastline in the order of 0.3 m between 2017 and 2019 with maximum values ~1.33 m (see Fig. 3 ). The field data quantifies erosion on the southeast of Fuvahmulah in the order of decimeters over two years. Erosion rates on the east coast decline towards the north. In the north-east of Fuvahmulah, the highly dynamic morphology of Thoondu stabilizes the coast.
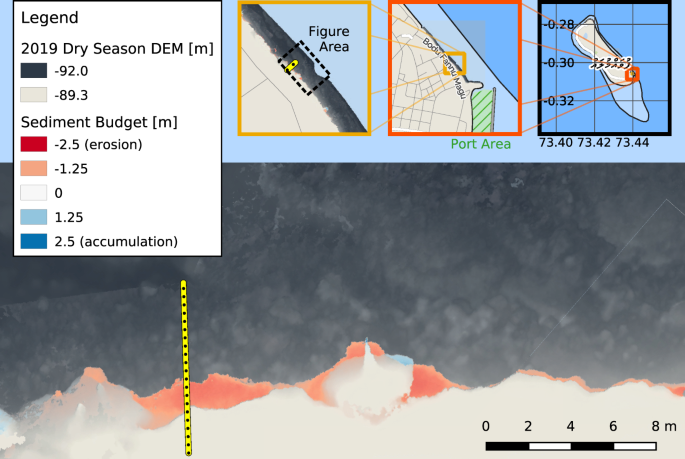
The Digital Elevation Model (DEM) of the dry season 2019 on the southeastern coast of Fuvahmulah, adjacent to the seaport. The figure shows erosion (red) and accumulation (blue) when compared to the DEM of the dry season 2017. The yellow dotted line is the most southern transect of this study with cross-sectional differences of ~1.33 m (see Fig. 2 d). Maps contain data from field measurements, mini maps also use adapted publicly available © OpenStreetMap contributors data, accessed through the open-access Geofabrik download server .
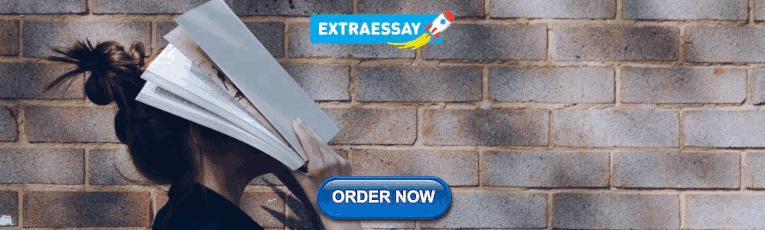
Wave climate of the southern Maldives
Both, erosion adjacent to the harbor and sediment dynamics on Thoondu beach, are effects of sediment transport dynamics on the reef. Here, the main driver behind sediment transport are wave-induced currents 5 , 7 . Therefore, the local wave climate and possible changes of the wave climate are of particular importance when assessing shoreline changes. As measured wave data for the Maldives is scarce, global wave hindcasts or reanalysis models are the only sources containing long-term wave data of directional sea states for the islands of the Maldives. Information on wave data is provided by several meteorological services, such as the National Centers for Environmental Protection (NCEP) of the American American National Oceanic and Atmospheric Administration (NOAA), the European Centre for Medium-Range Weather Forecasts (ECMWF) or the Collaboration for Australian Weather and Climate Research (CAWCR) (see Fig. 4 a). Annual time series from the considered services agree very well among each other, with mean annual correlation coefficients of R ≥ 0.89 (Fig. 4 c). To validate the hindcast and reanalysis data, this study uses harmonized and inter-calibrated Satellite Radar Altimetry (SRA) measurements from the Altimeter Data System (ADS) of the Helmholtz Centre Potsdam (GFZ) 45 and compares these to the three data sets considered for this study (Fig. 4 a). The SRA measurements are available between 1993-04-25 and 2018-06-15. Wave heights from ECMWF’s fifth generation atmospheric reanalysis of the global climate (ERA5) data set are closest to the measured SRA wave height in the region of Fuvahmulah. Even though the locations of the CAWCR output nodes differ from the other data sets, analyzing the spatial variation of the CAWCR time series shows a very high correlation of adjacent data nodes ( R ≥ 0.977 ± 0.007, see Fig. 4 d). The spatial similarity following from this high correlation of wave parameters allows the data sets to be compared among each other – despite the spatial distance. Likewise, this shows the wave climate on Fuvahmulah is typical for atolls in the south of the Maldives – as well as the associated exposure and impacts. All further analyses of this study make use of the CAWCR data, because the output node is closest to Fuvahmulah and CAWCR provides significant wave heights under Representative Concentration Pathway (RCP) 4.5, and RCP 8 for the twenty-first century 46 . These future projections allow this study to scrutinize future states of wave climate on the island as well as their impacts and triggered effects.
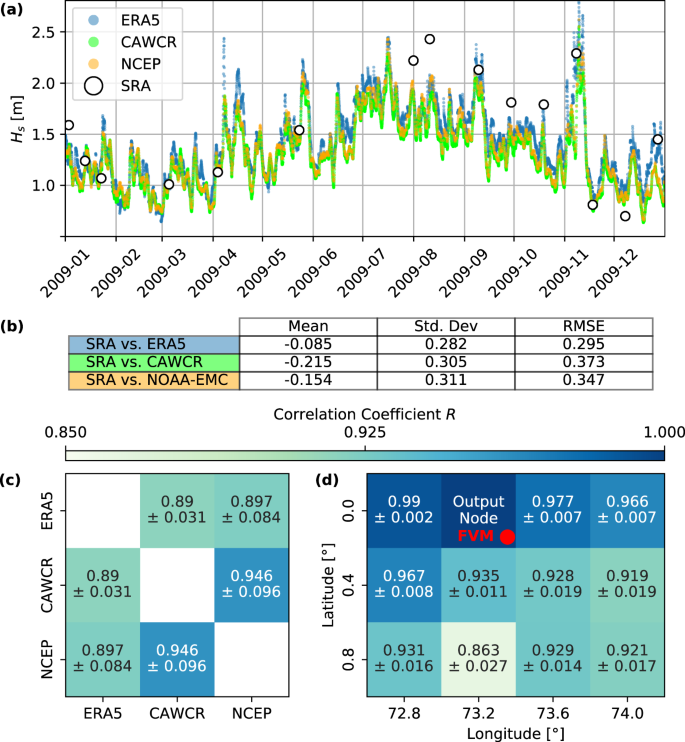
a Typical time series of the significant wave height H s for each data set over one year (here 2009). The data sets are provided through the climate data repositories of the European Centre for Medium-Range Weather Forecasts (ECMWF), the Collaboration for Australian Weather and Climate Research (CAWCR) and the National Center for Environmental Protection (NCEP) of the United States National Oceanic and Atmospheric Administration (NOAA). White dots are Satellite Radar Altimetry (SRA) measurements of H s , recorded in the vicinity of the data output node. b shows the deviation between data sets and the SRA measurements c is a cross-correlation matrix for H s from each considered data repository between 1980 and 2019-05 (sample size n = 345,504 for ECMWF’s and CAWCR’s hourly data; sample size n = 115,168 for NCEP’s 3-h data). d is a spatial cross-correlation matrix for data nodes of the CAWCR data set adjacent to the output node considered in this study. The data nodes are in the center of each cell. Values in the matrix are mean μ R and standard deviation ± σ R of the correlation coefficient R . The red dot marks the location of Fuvahmulah within the matrix.
The wave climate in the area around Fuvahmulah reflects the dry and wet season both by changed wave heights and wave direction. Median wave heights range between 0.98 m in March, as well as 1.71 m in July with a maximum wave height H s ,max of 3.32 m on June 22nd, 1987 (for more statistical data of Fig. 5 a, f, for example on boxplot mean and IQR values, see Supplementary Information File). This study defines the period between November to February as dry season and the period between April and September as wet season (Fig. 5 a). April and October are considered transition months.
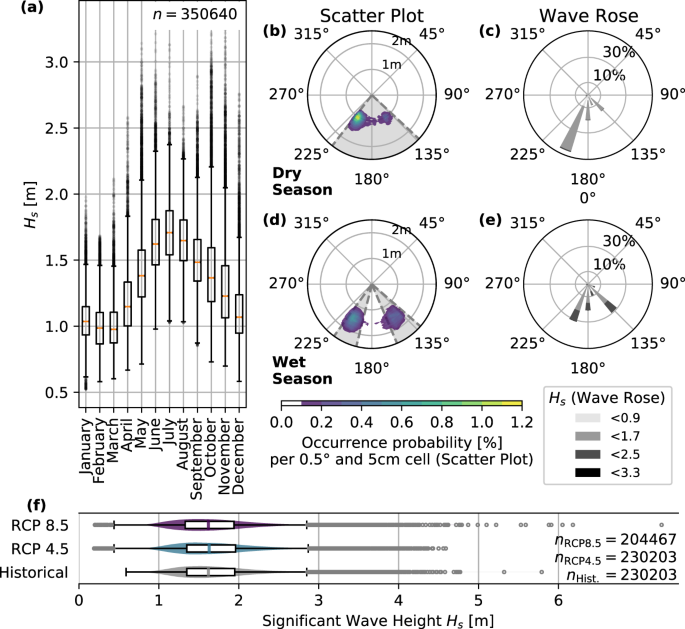
a Annual significant wave height distribution for hindcast data between 1980 to 2019 (sample size n = 350,640). b shows the discrete marginal distribution between peak wave direction θ p and significant wave height H s for the dry season, while d contains results for the wet season. Here, the colorbar shows the occurrence probability of each 0.5 ∘ wave direction and 0.05 m wave height cell. The gray areas visualize the significant peak direction range θ p , r for each subset, containing the directions which, when combined, have the highest 33% occurrence probability. c and e show wave roses for the dry and wet season. f are significant wave heights for 2010–2019 (here labeled as “Historical”; sample size n = 204,467; undefined “NaN”, or “Not a Number”, values remain unconsidered) and Representative Concentration Pathway (RCP) 4.5 and 8.5 (sample size n = 230,203; undefined “NaN” values remain unconsidered). Boxplots in a and f are done with the corresponding boxplot-function in Python’s module matplotlib. Here, the orange line of the boxplot is the median, the box limits are the upper and lower quartiles while whiskers mark the range of the non-outlier data and extend the box limits by 1.5 ⋅ IQR.
The waves are smaller in the dry season than in the wet season and are dominantly approaching the island from SSW (202.5°, see Fig. 5 b–e). There are also other southern and south western portions between November to February, but in terms of occurrence they play a minor role. Significant wave heights H s are mostly smaller than 1.7 m in the dry season (see Fig. 5 c). In the wet season, waves approach the island dominantly from southeast and south-southwest (135° and 202.5°). In the rainy season, significant wave heights increase when compared to the dry season and range between H s = 1 m − 2 m. Considering the future wave climate in the region, by using the projections of the Coupled Model Intercomparison Project Phase 5 (CMIP5), the data does not significantly differ under the respective RCPs, when compairing wave parameters between the reference time frame 1986–2005 and the last two decades of the twenty-first century (Fig. 5 f; reference time frame according to the fifth assessment report of the IPCC 47 ). These results are in line with findings of another study using CAWCR wave projection data on a global scale 48 .
Considering the results of the statistical wave climate analysis, this study concentrates on scrutinizing waves which propagate from SE and SSW into the computational domain of the numerical models in the following. These wave directions represent the two dominant shares of the wave rose for Fuvahmulah in both seasons. Considering today’s and future significant wave heights H s , the numerical models use the 99 th percentile from the reanalysis data set ( H s ,model = 2.3 m ≈ H s ,99% = 2.27 m) as boundary conditions. All conditions are therefore modeled for storm conditions.
Natural morphodynamics on the reef and anthropogenic interventions
Results from the wave climate analysis serve as boundary conditions for the regional wave models 5 . The numerical wave models also utilize the DEM data and set the observed and measured morphodynamics into broader context by considering the wave climate’s hydrodynamic forcing. With that, the wave models test the assumption of the harbor infrastructure being the root cause of erosion on the southeast coast, adjacent to seaport. While Delft3D (D3D) calculates the general sediment movement on the Fuvahmulah reef platform for different wave directions, the depth-integrated (2DH) Boussinesq-type wave model gives more detailed information on the role of the port as anthropogenic interference in the natural sediment transport system of the reef (a preceding study gives further insights into the more general dynamic pattern of seasonal and annual sediment transport on the fringing reef of Fuvahmulah 5 ).
D3D confirms that the interplay of sediment supply 13 and wave-induced currents provides sediment for the island (Fig. 6 ). It further indicates where to expect sediment deposits under the given hydrodynamic forcing. As waves approach from θ p = 202°, sediment moves towards the island and settles on the west side (Fig. 6 a). A smaller sediment depot also forms on the east and southeast of the island under the given premises. Waves from θ p = 135° will take sediment from the offshore reef and transport it towards the island (Fig. 6 b). The computations for waves from θ p = 135° disclose that the sediment stream splits at the southeastern tip of the island, approximately at today’s harbor location. The wave-induced currents distribute the sediment along the east coast of the island as well as on the southwest side. A small portion of sediment also moves around the northern tip of the island onto the lee side.
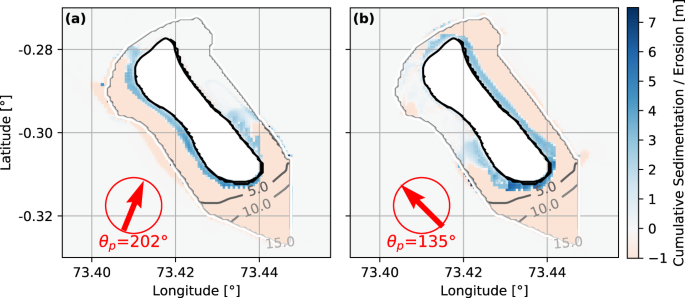
Sediment relocation calculated with Delft3D (D3D) for constant waves of one month with significant wave height H s = 2.3 m, peak period T p = 17 s approaching from a θ p = 202 ∘ and b θ p = 135 ∘ . The offshore depth is truncated to 100 m. The idealized reef has a depth of 5 m to 17.5 m. Gray contour lines mark the water depth on the reef between 5 m to 15 m in 5 m increments. The erodible sediment layer is 1 m throughout the domain. Sediment accumulation is blue, while erosion is red. The maximum erodible depth is equal to the erodible sediment layer thickness. Map data is idealized, based on measurements.
In general, the D3D computations reveal that wave-induced currents take sediment from the southern reef and transport it towards the south and east coast (without seaport structures being present). D3D also indicates the particular importance of the island’s southeast part for sediment transport along the entire east coast: waves from θ p = 202° pick up sediment at the southern reef, transport it towards the east and the southeast coast of Fuvahmulah where it finally accumulates. Then, over seasonal cycles, waves from θ p = 135° can take away the accumulated sediment and distribute it along the east side.
The 2DH model highlights the difference in wave driven current patterns leading to sediment transport around the port area. The models also facilitate scrutinizing the changes in sediment transport with and without the harbor infrastructure being present. These computations confirm the processes outlined with the D3D computations and further highlight two factors, contributing to the erosion along the east coast of the island:
The first factor is the available sediment. In both cases – with and without the port structures (breakwater and headland) – waves from θ p = 202.5° create a current in front of the port (Fig. 7 a, b), transporting sediment over the reef. At the same time, in the area of today’s harbor entrance, the current decelerates and thus allows for the sediment to accumulate in this area. However, with the breakwater present, the structure interrupts the sediment transport and sediment cannot enter this area. In addition, the breakwater reaches up to the reef’s edge. As a consequence, it deflects the current and thus redirects the sediment off the reef into deeper waters.
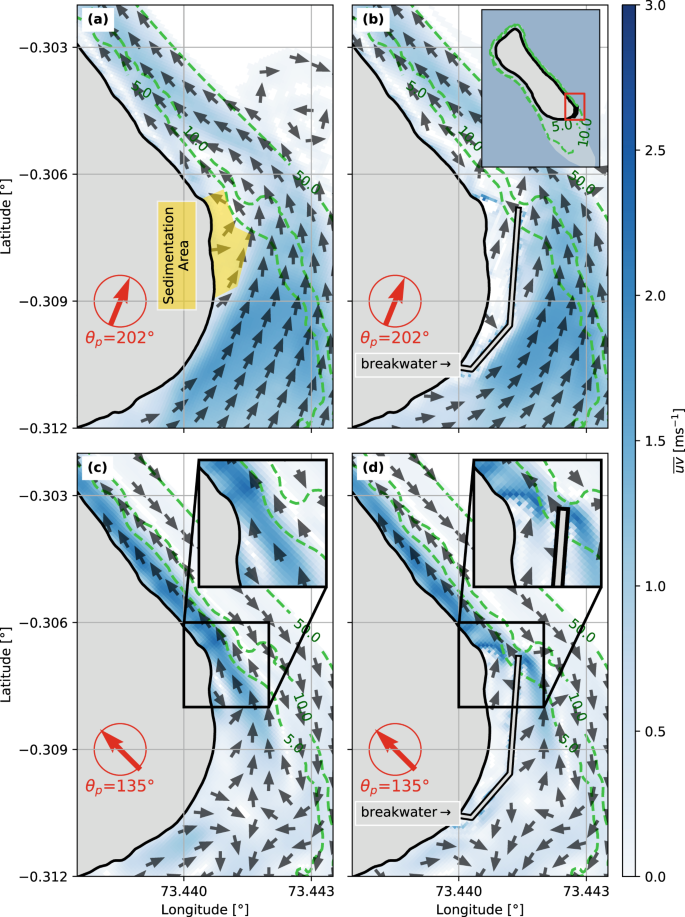
Wave-induced currents \(\overline{uv}\) from the depth-integrated (2DH) model for the seaport area in the southeast of Fuvahmulah, where the colorbar shows the magnitude of the current between 0.0 ms −1 (white) to 3.0 ms −1 (blue), while arrows depict the current direction. a shows velocity fields from waves with a peak direction of θ p = 202 ∘ for Fuvahmulah without the harbor, while b includes seaport infrastructure. Similarly, c shows wave-induced currents before the port construction, while d presents today’s situation with the harbor present for waves approaching from θ p = 135 ∘ . Green dashed contours show the water depths of the reef platform for 5 m, 10 m, and 50 m. Maps are based on measurements and adapted publicly available © OpenStreetMap contributors data, accessed through the open-access Geofabrik download server .
The second factor is the transport capacity of the east coast’s current: without breakwater, waves from θ p = 135° induce a northward current to the area of today’s port entrance (see Fig. 7 ). This is also the area, where sediment was able to settle from the θ p = 202.5° component. Instead, with the harbor present, the breakwater obstructs the emerging current in this area and deflects the velocity momentum off-shore. Yet, a wave-induced longshore current is still present along the east coast independently of the port: when waves from θ p = 135° approach the reef and break, they induce radiation stresses and subsequently create this longshore current. With missing sediment from the reef, the currents will likely take sediment from the coast, leading to erosion.
Governmental and societal framing of coastal development
On Fuvahmulah 93.8% of residents are citizens of the Maldives and as on most other inhabited islands, tourism plays a minor role 41 . Fuvahmulah’s island dwellers are mainly employed in education and commercial services 41 . Thus, together with the insights of the field campaigns, Fuvahmulah can be considered a local’s island. In the household survey, the local community perceives erosion as the most pressing issue (closed question, 27% of 345 mentions, see Fig. 8 and Supplementary Information File). At the same time, both the interviews with government officials as well as the recently issued Environmental Impact Assessments (EIAs) on coastal protection on Fuvahmulah reveal that administrations on the national and local level acknowledge erosion as a high priority issue on the island. However, while there is consensus on the need for action among all interviewed actor groups, attribution of the erosion’s root cause varied between the national government representatives and the affected community. On the one hand, the Maldives’ dominant adaptation challenge is sea level rise and the associated impacts. In fact, the Maldives national government actively promotes this narrative of being highly vulnerable to climate change induced sea level rise 49 – even in the case of Fuvahmulah. On the other hand, Fuvahmulah’s population is skeptical about the national government’s narrative. The household survey contained an open question (without pre-formulated responses) asking the participants to name visible changes of the natural environment on the island. Here, about 36% of all responses mentioned erosion and of these, 20% also attributed the erosion processes on Fuvahmulah specifically to the harbor construction (see Fig. 8 and Supplementary Information File). These diametrically opposed perceptions on root causes behind coastal adaptation originate from the historical context of national politics and local society.
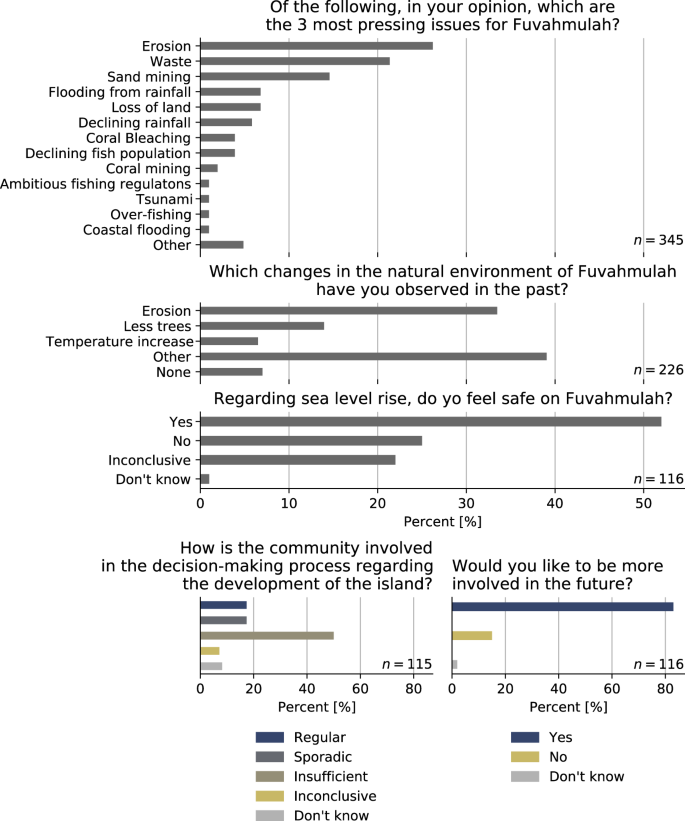
Selected questions and answer frequency from the population survey. See Supplementary Information File for the question type (open or closed question) as well as associated codes and coding rules.
First experiences with coastal infrastructure in the Maldives dates back to the 1970s, when the first modern coastal protection constructions were built on the islands 34 . However, coastal protection management was not professionalized before 1987 and 1988 when strong coastal floods caused massive damage to the Maldives’ capital Male’ and on several other islands in the middle of the country (interview with representatives of the Ministry of Environment and Energy (MEE) in 2017). At the same time, establishing coastal protection in the Maldives was accompanied with a rapid increase in population, especially in the Maldives capital Male’. In accordance with the Maldives Decentralization Act in 2010, the national government shifted its focus onto regional development centers, equipped with the necessary infrastructure 26 and limiting the migration to the capital 50 . However, the interviewees still highlight today’s accumulation of resources – economic and professional expertize – in Male’. Decisions are made at the highest level and have an impact down to the local scale. When regarding the implementation of coastal development projects in the Maldives, the planning, implementation and decision-making process are centrally executed and ministerially anchored in the national government without significant involvement of local capacities on the islands. According to actors involved in coastal governance, in general, such top-down processes in decision and implementation are applied for coastal infrastructure projects, for example seaports as well as coastal protection structures. Regional and local government institutions, such as the city council on Fuvahmulah, lack influencing power in the decision-making process. According to the interviewees, the role of local government institutions is limited to informing national-level actors about coastal problems on their island. The lack of power is also expressed by missing financial resources for coastal projects at a council-level (interview with a representative of a state environmental agency in 2017). In addition, infrastructure projects usually require external financial and knowledge resources, provided by international organizations. However, international organizations are legally bound to use the national government as an entry point and cannot initiate projects below the central national-level. Altogether, this stands in contrast to the republic’s decentralization efforts and the corresponding strengthening of local communities.
Moreover, since 2012, the EIA Regulation of the Maldives requires to assess the (adverse) impact of infrastructure projects on the environment. However, quality and compliance to EIA policies – also for example in the tourism sector 39 – are traditionally weak 40 and have extensively promulgated technical fortification of many inhabited islands in the Maldives 20 , 22 , 26 .
In contrast to the national politic perspective on coastal infrastructure, the traditions and knowledge of Fuvahmulah’s inhabitants on their environment as well as experience with coastal hazards led to a different perception on coastal adaptation. More than half of the local respondents feel safe on their island with regard to sea level rise (52% of the respondents, see Fig. 8 ). The interviews have shown this sense of safety evolves from the awareness that the lower-lying center of Fuvahmulah is protected from seaborne extreme events by the island’s fringing coastal ridges. Locals even believe Fuvahmulah to be comparatively safer than other islands in the Maldives. Some also mention the protective functions of the reef: one respondent answers an open question, asking which elements of the island’s natural environment are perceived as important: “ most important is the reef. What I’m saying is that we are protected by the reef. It’s not like the [other islands in the Maldives] that are protected by more islands in the atoll” (Survey 1 participant: 080). Others have argued about the protective function of the “heylhi”, which is the natural green belt of vegetation around the entire coastline of Fuvahmulah, stabilizing the shore and protecting local inhabitants from seaborne hazards (see Supplementary Information File). In addition, building houses was traditionally only allowed in the center of the islands behind the green belt, consisting of local trees and shrubs. However, in 1993, a 86 m long seawall was built on the central east-side as the first protection measure on the island, followed by noticeable erosion 44 . Today, the seawall is detached from the coast, substantiating this study’s earlier findings and a general propensity of adverse impacts of human-made interventions in the coastal environment. While there hasn’t been a documented response to the seawall’s impact at the time, today’s erosion adjacent to Fuvahmulah’s seaport is more severe and has a higher impact on the society. For example interviewees of the second survey discussed the effects of erosion as well as the impact on their personal lives, for example: “[…] I am very worried about the soil erosion. I think around 20 feet has gone. So, it is a huge risk for our people in our community. So, I am very much worried about it.” (Survey 2 participant: 039). Fuvahmulah has gained more attention by the national government in the last two decades, manifested through numerous development projects in that time, thus erosion – being a high priority issue on the island – is a matter dealt with on the highest governmental level nowadays.
Coastal adaptation in practice
In 2014, government actors of the MEE agreed on taking action against erosion adjacent to Fuvahmulah’s seaport. They signed a grant arrangement for an internationally sponsored infrastructure development fund and initiated the project “Coastal Protection at Gn. Fuvahmulah, Maldives”. This project includes “the development, implementation and maintenance of sustainable coastal protection to prevent erosion and flooding on […] Fuvahmulah. The main objective of this project is to decrease erosion and flooding through a possible […] combination of hard and soft coastal engineering interventions […] to protect the island of Fuvahmulah against flooding due to ongoing coastal erosion and rising sea levels” 50 . An international consultant supported the MEE together with a local sub-contractor in the site investigation, the feasibility study, the technical design 50 and the draft of the legally mandatory Environmental and Social Impact Assessment (ESIA) 51 .
The technical design report finds human interventions and changes in natural processes disturb the natural balance of the sediment supply along the coastline 50 . According to the report, these human interventions are more specifically the harbor construction, sand-mining and earlier reef blasting for beach access, while natural processes disturbing the natural balance are “changes in the natural climate” 50 , but also “may” 50 be a possible deterioration of the coral reef. The report also mentions a lacking local sediment source for the coast and predicts the fringing ridge of Fuvahmulah to breach in the next decades, causing “tremendous flood damage to the island” 50 . Because of this, the report concludes, coastal protection is required and the design should “immediately stop the ongoing erosion” 50 . A preceding feasibility study was cited in the report, identifying two possible solutions against the ongoing erosion and rendering them reasonable while considering their environmental and social impacts – an offshore breakwater and an onshore revetment. The technical report concludes the revetment to be the most viable option, after presenting preliminary designs and a cost-benefit analysis of both alternatives. The final revetment is about to cover approximately the southern 2.6 km of the 4.0 km long east side and is designed with a lifetime of 50 years, withstanding hazardous seaborne events with a return period of 100 years.
The ESIA 51 builds on the technical design report and further underlines the project’s significance in the context of future climate change risks, as well as for the national and local economy. The report also finds the project would enhance the “sense of security and safety” of Fuvahmulah’s island dwellers 51 . The ESIA initially gives five approaches to coastal adaptation, but finds ecological methods would contain a high uncertainty and that they would be ineffective. Also, the report further dismisses soft measures (also known as sediment-based measures) as unsuitable because a proper (local) sediment source is supposedly missing. The impact assessment report finally presents the same coastal protection alternatives that have been suggested in the technical design report 50 . However, in addition to the technical design report, the ESIA also contains stakeholder consultations on the project. Resulting from these consultations, the chapter “project risk mitigation” in the ESIA finds “acceptability of the Project by all stakeholders is an important factor for smooth implementation of any project” 51 . The first local stakeholders consultation took place in July 2016 on Fuvahmulah and addressed the off-shore and on-shore protection alternatives. The discussions revealed locals prefered the off-shore alternative, but the ESIA objects as “the risk of affecting [Thoondu] beach was not well understood [by the local stakeholders] at that time”. Therefore, the ESIA mentions another consultation with the local government and community in October 2016 “to explain to the stakeholders the preferred alternative that emerged following the feasibility study and proposed in the EIA report” - with the revetment being the “preferred” and to be implemented alternative. In summary, both reports dismiss feasible soft solutions due to false assumptions (missing sediment source, however the reef naturally provides marine aggregates 5 , 9 ) and undermine the participatory process by promoting the less popular alternative in the end.
In principle, public participation is a key component of EIAs 39 and when taken into the decision-making process, public participation also leads to more sustainable implementation of development projects. However, the population survey of this study from March 2017 reveals an unutilized potential to improve the participatory process based on two questions: First, when asking how far politicians work together with the community (open question), 49% of the survey’s respondents found insufficient cooperation of politicians with the community in development projects – while 19% answer to be sporadically involved and 17% report to be regularly involved (see Fig. 8 and Supplementary Information File). Second, a large majority of 83% endorses more involvement in the decision-making process regarding the development of the island, while only 15% of the interviewees do not wish for more participation in this matter. These results disclose an ongoing dissatisfaction of Fuvahmulah’s people and the lacking consideration of their interests, opinions, and knowledge – despite or because of insufficient earlier attempts to include their perspective in the ESIA.
Small island policy aims for an improved economic situation and at the same time for better provision of access. To improve this situation for the island dwellers of Fuvahmulah, a sea- and an airport were constructed at the time. As of today – almost 20 years later – climate change and sea level rise causes marine pressures and these pressures dominate the discourse on small islands. As a result, small island state governments prepare their nations for the associated adverse impacts. Coastal erosion is a pressing issue of inhabited reef islands like Fuvahmulah and adaptation measures need to address these issues under deeply uncertain future projections. Following the early example of the capital Male’, the centralized government is in favor of generic adaptation measures and implements these measures in a top-down approach 26 . In addition, the government of the Maldives – as other SIDS 27 – most often relies on external, technical knowledge and funding to realize climate change adaptation and vital development projects. As a consequence, the resulting planning efforts and constructive responses are mostly based on external design guidelines and building codes. In general, experiences from the past are mainstreamed into international design guidelines and building codes 52 , 53 , and then guidelines and bulding codes give best-practice examples under certain environmental conditions. In case of coastal structures these environmental conditions are predominantly those of mainland coasts. Endorsing hard coastal protection is common in SIDS 27 and based on both, their top-down governance structure as well as their dependency on international funding – two aspects, that are also a legacy from the colonial history of SIDS. In the Maldives, consequent consideration of hard structures and acceptance of their associated adverse impacts on the environment is historically grown, and ill-prepared policies and policy compliance is projected to continually exist 26 , 40 . In both cases, transcribing inappropriate mainland design criteria to sensitive reef environments have caused an insufficient level of coastal protection on small islands in the past. In most cases, there is a straight forward transfer from external knowledge and guidelines to local circumstances, overlooking the special features and services of the fringing coral reef. Yet, corals are important for the livelihood and protection of the accommodated reef islands. In terms of protection, the main ecosystem service of healthy coral reefs is their ability to attenuate waves 16 and to supply the reef island with coral sediments 5 , 54 . Generic hard-engineered coastal protection have led to disturbances of natural dynamics and the associated ecosystem services in the Maldives 20 , 22 , 26 , 34 and in other SIDS 25 , 55 . In a worst case scenario, generic hard-engineered approaches to coastal protection and adaptation even fail to alleviate but aggravate adverse effects on the natural environment. Also future plans for coastal protection on the Maldives 22 fail to implement a local perspective on dealing with changes. Instead, the propensity is to further fortify the island’s perimeter with traditional hard-engineered measures 26 – as in the field case example of Fuvahmulah.
The example of Fuvahmulah epitomizes planning inadequate coastal structures in a reef environment underlies special environmental features and requirements beyond conventional, generic infrastructure design rules and solutions (see the results section “Natural morphodynamics on the reef and anthropogenic interventions” and Fig. 7 ). Traditionally, human intervention beyond the natural green belt of Fuvamulah used to be highly limited, leaving the protection feature and natural sediment distribution on the reef intact. However, the first hard-engineered protection measures have already led to complications 44 . Sand-mining is discussed as a factor and cause for coastal erosion on Fuvahmulah. Sand-mining can potentially put anthropogenic pressure on small islands 25 and on Fuvahmulah, sand-mining for private purposes was frequently witnessed within the field campaigns around the island. A further reason for increased erosion could be climate variability. However, this study does not include an in-depth assessment on recent climate variability and possible effects on the east-coast of Fuvahmulah, albeit there are tendencies of changing climate impacts (for example sea surface temperature oscillations in the Indian Ocean), which could have affected the formation of Thoondu beach in 2019 5 . However, the most visible and distinct adverse changes on Fuvahmulah’s coasts is local erosion, located adjacent to the seaport in the southeast. Based on the field campaigns and the recorded high-resolution data, this study finds coastal erosion on Fuvahmulah to be a negative consequence from the seaport. The seaport is an anthropogenic intervention into the coral reef system adversely impacting the natural sediment transport and the associated wave-induced redistribution pattern around the island – much more than sand-mining or climate variability.
Together with today’s impact of the seaport, future modifications to the natural reef system will most likely have even more significant adverse effects for greater parts of the coast – especially in spatio-temporal dimensions of the project “Coastal Protection at Gn. Fuvahmulah, Maldives”. This study has shown that in the case of Fuvahmulah, there are distinctive hot-spots, which are important for the sediment supply of the island’s coast. The seaport is located at one of these hotspots, disturbing the natural sediment transport around the island (Fig. 7 ). The seaport construction was arguably a trade-off for economic development and societal well-being at the expense of natural conservation. Nevertheless it has triggered maldevelopment on Fuvahmulah, when assessing how the negative consequences on the environment are being dealt with: instead of governing this conflict 56 , future plans are going to respond to erosion associated with the seaport’s construction with further generic main-streamed adaptation measures 50 , 51 rather than acknowledging and compensating for the root causes of erosion (also being recognized by Fuvahmulah’s island dwellers). Concluding from other studies’ insights 22 , 26 , 32 and findings from this study, these measures fail to address processes of the reef’s sediment transport and thus drivers behind erosion adequately. Incorporating the topographic and reef measurements as well as the wave climate in numerical wave models helps to scrutinize the root cause behind the measured erosion on Fuvahmulah’s east coast: the seaport intervenes with the natural sediment transport and acts as barrier, deflecting suspended sediment off the reef (Fig. 7 ). Bypassing this barrier would be a suitable remedy resurrecting the natural sediment transport along the east side 57 . A bypass would nourish the beaches and act as “low-regret” adaptation to the anthropogenic disturbance, because it limits the impact on future response options under different climate change scenarios (sediment bypassing is described in typical coastal engineering manuals, such as the Shore Protection Manual 52 or the Coastal Engineering Manual 53 ). However, instead of providing a more robust and flexible approach towards coastal adaptation, the current adaptation plan is in favor of fortifying the entire coastline – a wide-spread approach in the Maldives and common pitfall, which is known to undermine the islands’ natural capacity to adjust to ocean climate-related pressures and a crucial step towards reaching an “anthropogenic tipping point” 22 . Hard-engineered responses can undermine the natural dynamics feeding the reef island with coral sediment. This study illustrates this on Fuvahmulah, but the findings are scalable to further examples in the Maldives 26 and on other SIDS 27 .
The current decisions behind the present adaptation plan on Fuvahmulah lead to a repeated violation of the reef environment’s natural prerequisites and omission of societal demands. Following the DPSIR chain of causal links, this continuously affects the current state adversely and leads to the associated maladaptive impacts on the environment and society. Scrutinizing the case study of Fuvahmulah with an interdisciplinary approach documents the significance of societal demands and political decisions leading to maladaptation. It shows that maladaptation is implemented in a socio-political context, aiming to improve the economic or societal situation by consistently trading-off ecosystem services. However, instead of compensating and mitigating these trade-offs seriously, the socio-political responses lead to cascading maladaptive activities. In the Fuvahmulah example, the constellation of funding source, client and contractor already impedes an unbiased and sincere assessment which in turn inhibits an adequate reaction to the current erosion issue: the project “Coastal Protection at Gn. Fuvahmulah, Maldives” is initiated by the MEE and funded by an infrastructure development facility of an external government. At the same time, the MEE is also consulted by experts of the funding facility’s home country. Together with the local sub-contractor Maldives Energy and Environmental Company (MEECO), these experts carry out an ESIA, which has to be acknowledged by the MEE – being contracting and supervisory authority at the same time. The subsequent ESIA and technical report dismissed a potential soft solution, such as beach nourishment, claiming no sustainable sediment source was available on the island. However, since the construction of the port, the area west of the seaport and south of the airport host large quantities of deposited sand 5 , which wasn’t present prior to the harbor construction (visible on aerial and satellite images in former reports 43 , 44 and the EIA 51 ). The external contractor disregarded the reef as sediment source and sediment transport patterns on reef islands – even though this information was made available through research in the past decades 9 and is confirmed to be valid for this island as well 5 . This research of the past decades has emphasized the reef’s natural protection capacity and its ability to feed the island in the face of sea level rise. Neglecting these vital ecosystem services and transferring generic solutions for mainland coasts beyond their scope of application is another rigorous example of building in nature – in contrast to a sustainable building with nature 58 . However, the technical report and EIA on the coastal protection project discusses only hard-engineered alternatives in the stakeholder consultation, while the measure less preferred by locals was finally chosen. By framing stakeholder consultation as project risk mitigation instead of equitable part of decision-making, the ESIA epitomizes the deficits of prescribing development projects in a top-down manner and lacks to provide a solution acceptable for the society and suitable for the environment. Somewhat arbitrary compliance with national EIA guidelines is commonplace in the Maldives, leading to questionable assessments regularly 39 , 40 and ultimately failing to address the root causes of adverse impacts on reef environments. An “unduly over-emphasis” 36 on hard protection is a symptom of maladaptation and in the Maldives this symptom originates from the socio-political history of the country and is still prevalent today. Yet such recurring and systematically provoked maladaptive actions go beyond the initial scope of maladaptation. Against this background, this study defines the concept of maldevelopment: maldevelopment is a socio-political phenomenon amplifying maladaptation which is characterized by decisions or policies in constant or deliberate favor of inadequate actions and trade-offs towards future climate change related risk. Structural maldevelopment to date impairs the potential of dealing with additional future changes, such as sea level rise, extreme wave events, and storm surges. Studying maldevelopment in its comprehensiveness leads to the conclusion that sustainable development requires an integrated analysis of political interests and societal demands within the natural boundaries, in order to adequately address future climate change stressors. This study finds structural maldevelopment being the most important human driver of undesired coastal changes on small islands. Risks evolving from maldevelopment add onto the current and future risks of the other significant natural driver, being climate change and the associated impacts. This is very likely not only true for Fuvahmulah, as many small islands in the Maldives and in the world show symptoms of maldevelopment 22 , 25 , 27 , 28 .
The perception analysis and population surveys on Fuvahmulah reveal that island dwellers are well aware of the port’s impacts and are doubting the national government’s narrative, framing coastal erosion as climate change and sea level rise impact. Local knowledge helps to identify and overcome local challenges, reflected by both the traditional primacy of island development within the borders of the “heylhi” and veritable attribution of the current coastal erosion. Analyzing the wave-induced sediment processes in numerical wave models corroborates the local people’s assumption that the harbor infrastructure interrupts coastal dynamics and acts as a sediment barrier for the east coast. Local knowledge conveys a distinct local capacity towards economic development in combination with future environmental protection on the island. Implementing and evolving these capacities into a tailored adaptation pathway plan facilitates to consider smaller and less intrusive responses beyond conventional hard-engineering approaches, discloses their potentials and limitations, and leaves further scope of action for the future. Such a more conceptual approach of adaptation pathway planning requires (1) a greater focus on the underlying process, (2) a systematic collaboration between local, national and external experts and stakeholders on the island, (3) ongoing monitoring on the natural and societal impact of the implemented measures, and based on this (4) frequent adjustments on the pathway roadmap by responsible authorities when an adaptation tipping point is reached and the current status (a policy action or actions) will fail. In this regard, dynamic adaptive policy models represent suitable, modern methods, allowing more conceptual approaches to be embedded into continuous and sustainable coastal planning 59 .
Amalgamating coastal and societal aspects leads to a comprehensive understanding of the ongoing natural and socio-political processes behind coastal issues. These interdisciplinary insights underline that adaptation strategies and measures need to be adjusted to local circumstances. This helps to (1) avoid an interruption of the coastal system, (2) undermine vital ecosystem services and (3) sustain natural coastal protection.
Digital elevation model (DEM)
Based on three field campaigns, Unmanned Aerial Vehicles (UAVs) capture the topography of Fuvahmulah and facilitate analyzing shoreline changes. A DJI Phantom 4 multicopter recorded aerial photos within two field campaigns in March and September 2017. A DJI Phantom 4 Pro was used for the coastal survey in March 2019. The aerial surveys covered the entire coast of Fuvahmulah, except the beaches adjacent to the airport, which are a no-flight-zone. After recording the aerial images of Fuvahmulah’s coast, Agisoft Photoscan Pro (version 1.4.5 build 7354) processes the photos into DEMs by means of Structure from Motion-MultiView Stereo (SfM-MVS) 60 . DEMs are three dimensional models of the area, containing height and color information, here in about 3.5 cm px −1 resolution. The resulting coastal DEMs were recorded at different points in time, facilitate analyzing temporal changes of sediment volumes on the beach, shoreline location and vegetation. The general workflow in Photoscan is to align the photos, georeference the model with Ground Control Points (GCPs), and create a dense point cloud as well as a mesh of the area 61 . Photoscan derives the DEM from the dense point cloud, while the mesh serves as base for the orthophoto (for further information on input parameters of each processing step in Photoscan, see Supplementary Materials). To position the models in the World Geodetic System (WGS84), the March 2019 DEM uses a unique set of GCPs around the island. The 2017 model uses the drone’s Global Navigation Satellite System (GNSS) antennas for initial positioning. Afterwards virtual Ground Control Points (vGCPs) of specific landmarks available in both models georeference the 2017 DEMs with the 2019 DEMs. Apart from the erosion, the position of dedicated landmarks between all three field campaigns were reproduced with the expected accuracy 61 , 62 for SfM-MVS procedures in coastal areas.
In general, the inspected erosion starts at the bottom of the beach profile. Waves and currents carve out the sediment under the roots of the coastal forest or dislocate coral rocks. Owing to the specific erosion process on Fuvahmulah, early erosion remains mostly undetected in the DEMs, mostly until the upper part slips or collapses. However, adjacent to the seaport, the erosion is advanced enough to be well visible in the DEMs.
Hydrodynamic boundary conditions
To evaluate the drivers behind sediment transport, this study uses numerical near-shore wave models. These models require regional wave climate information as hydrodynamic boundary conditions. To derive the wave climate for Fuvahmulah, this study considers data from three global hindcast and reanalysis models from the Collaboration for Australian Weather and Climate Research (CAWCR), National Centers for Environmental Protection (NCEP) of the American National Oceanic and Atmospheric Administration (NOAA), and European Centre for Medium-Range Weather Forecasts (ECMWF) (see Table 1 ). Data from wave spectra are an appropriate resource to be used as boundary condition in models of finer spatio-temporal resolution 63 , 64 . To validate the modeled wave climate data, this study compares each data set with wave heights derived from individual harmonized and inter-calibrated SRA measurements of the GFZ Altimeter Data System 45 (see Fig. 4 a). The SRA data is taken from latitude 0° and longitude 73° in a radius of 1.5 km between 1993-04-25 and 2018-06-15.
The CAWCR provides hindcast wave data 65 generated with the WAVEWATCH III ocean wave model 66 . The closest output node to Fuvahmulah and the SRA data from GFZ is at 0.0° latitude, 73.2° longitude. The model uses a grid with 0.4° zonal and meridional wide increments. CAWCR also provides estimates for wave heights under different RCP in the twenty-first century. WAVEWATCH III is a numerical model calculating wind wave generation and wave propagation based on the random phase action density balance equation for wave spectra 66 . The waves are forced by surface wind fields from atmospheric models 67 , made available by the NCEP as Climate Forecast System Reanalysis (CFSR) and Climate Forecast System Reanalysis v.2 (CFSv2). The Environmental Modeling Center (EMC) of NCEP runs an own hindcast with WAVEWATCH III and their bias corrected wind forcing. EMC maintains WAVEWATCH III and places the code at disposal. WAVEWATCH III is a fork of the ocean wave model (WAM 2.0.CO;2." href="/articles/s41467-021-26082-5#ref-CR68" id="ref-link-section-d157734856e2879">68 ). The ECMWF uses the WAM model for their ERA5 with wind forcing from their Integrated Forecasting System (IFS) 69 . The data is available through the Copernicus Climate Change Service (C3S) 70 . The closest data node to Fuvahmulah for both NCEP and ECMWF’s ERA5 are at 0.0° latitude, 73.5° longitude (see Fig. 1 ). The SRA data from GFZ were taken from the node at 0.0° latitude, 73.0° longitude (Table 1 juxtaposes the underlying models, key input parameters and output variables of each data set for this study).
To compare the data sets, this study correlates ocean wave time series from all three repositories with a cross-correlation function from the nodes closest to Fuvahmulah. The general solution for a cross-correlation function writes as:
with τ = m Δ t as the lag time for m sampling time increments and N the number of values in the correlated time series A and B , which are reduced by their respective means μ A and μ B . The normalized cross-correlation follows from considering the standard deviations σ A and σ B of each time series as
The normalized cross-correlation values range from 1 for equal signals over 0 for uncorrelated signals to –1 as a reverse or negatively correlated signal. The corresponding lag time τ of the highest absolute value, here the correlation coefficient R of the cross-correlation, gives information about the time-shift between both signals.
The wave data in each repository contains time series of over 40 years. For the analysis, the time series is split up in annual sections from 1980 until 2019-05 and used separately in the cross-correlation function (Fig. 4 b), resulting in 39 annual cross-correlation coefficients. When correlating the hourly data of CAWCR and ERA5 (sample size n = 345,504) with the 3-hourly NCEP time series ( n = 115,168), the hourly time series were reduced to the associated 3-h values of the NCEP time series. To estimate the spatial variability of the data, a correlation of different spatial output nodes of the CAWCR data set (Fig. 4 c) uses the entire CAWCR time series from 1980 to 2019 ( n = 350,640).
This study further evaluates the impact of climate change on the wave climate according to the baseline chosen in the IPCC’s fifth assessment report 47 by comparing the mean significant wave heights between the time periods 1986–2005 (historical; n = 204,467 undefined “NaN”, or “Not a Number”, values remain unconsidered) and 2081–2100 (end of the century) under RCP 4.5 and RCP 8.5 projections ( n = 230,203 for both; undefined “NaN” values remain unconsidered). These projections are part of the CAWCR repositories 71 . The data is computed with the same tools as the hindcast data. Yet, the global grid is coarser with spatial increments of 1.0° ( \({60}^{\prime}\) ) and a temporal resolution of 6 hours. The RCP 4.5 and RCP 8.5 computations for wind waves use surface wind projections of 8 atmospheric models 71 .
As the CAWCR data set has the output node closest to Fuvahmulah, boundary conditions for the numerical models used in this study were derived from the CAWCR data node north-west of the island at 0.0° latitude, 73.2° longitude (see Fig. 1 ). The boundary conditions come from the entire, available hindcast data of significant wave heights H s and associated peak directions θ p between 1979 to 2020-02. The analysis of peak directions θ p for each season introduces the range of peak directions θ p , r . This range comes from a discrete probability distribution of the entire time-series and contains the directions, which combined have the highest 33% occurrence probability (see Fig. 5 ).
In general, the performance of global ocean wave models is good in the Indian Ocean when compared to buoy data 64 , 72 . The comparison to SRA measurements shows that wave data from global models occasionally underestimates wave heights, due to their relatively coarse spatio-temporal resolution in order of kilometers and hours. This improves with higher spatio-temporal resolutions 73 . Other studies have shown that underpredicted wave heights are especially prominent in extreme events 74 . In addition, non-linear effects can mitigate increased flood risk associated with sea-level rise 75 . Since 1992 and in contrast to sea-level rise, wave heights have only increased by about 0 to ~1 cmyr −1 for the Maldives according to a most recent study 76 (see also Fig. 5 f). In front of this background, wave heights under the respective RCP scenarios are conservative estimates of hazard levels, as the ocean wave models have a resolution of 1.0° and 6 hour time steps versus 0.4° to 0.5° spatial and hourly or 3 hour temporal resolution for the hindcast models. However, even when (1) combining today’s wave heights with a projected sea level rise of about 0.4 m to 0.8 m and (2) comparing it against the topographic height of Fuvahmulah with ~3.5 m above sea level, both factors will lead to more frequent extreme events. In this context, on Fuvahmulah, historically rare extreme events will become common by 2100 under all RCPs 2 , 3 .
Numerical wave models
With the wave climate as boundary conditions, numerical wave models can reconstruct waves and wave-induced currents and thus give insights into sediment transport processes on the reef under different scenarios (for example with or without seaport infrastructure). This study uses two numerical models for different purposes. First, D3D helps to study the general sediment transport processes on the fringing reef platform under the characteristic wave climate of the region. The phase-averaging wave module of D3D uses wave spectra in its governing equations and combines them with sediment transport formulations 77 . To study the influence of the port infrastructure on the adjacent coastal areas, this study uses the depth-integrated (2DH) Boussinesq-type model BOSZ 63 . The phase-resolving wave model can directly capture wave transformation and secondary processes such as wave setup and recirculation over the reef. It is therefore suitable to study the current-induced processes in the study area in detail. While the computation of direct sediment transportation is challenging, the general wave-induced current velocities or shear stresses from wave-induced currents serve as the driving forces behind the most prominent bedload and suspended sediment transportation formulations 77 . Thus, acceleration, deceleration and presence of current fields are direct indicators for sediment accretion and sediment pick-up.
D3D has been used successfully for calculating sediment transport and demonstrated good performance in both experimental and hindcast studies 78 . The model is in general capable to outline the morphodynamic processes on the Fuvahmulah reef. To remain numerically stable and efficient in terms of computational resources, the settings in this study contain the following simplifications: the model uses a relatively coarse rectilinear grid off-shore, with a refined resolution of Δ x = 67 m, Δ y = 15 m around Fuvahmulah. To study the morphodynamic processes around the island, D3D uses both the WAVE and the FLOW module. The reef depth and island height are based on topographic and bathymetric surveys within the field campaigns, however the implementation in D3D is idealized: the off-shore section has a constant water depth of h = 100 m. The fringing reef has a constant depth of h = 4 m, which increases on the southern reef platform to h = 17.5 m. In the model, the entire island Fuvahmulah lies at 3 m above mean sea level (MSL). The off-shore reef south of the island has a similar shape in the model compared to the real reef. However, to provide enough cells to transport sediment, the virtual fringing reef in the model is wider than the physical reef around Fuvahmulah. To evaluate sediment transport pathways around the island, the reef was covered with a 1 m layer of erodible sediment at the start of each computation. The underlying assumption is that the reef platform is the main sediment supplier for the island 5 , 13 . While the simplifications allow for a larger set of computations, the implementation is still sufficiently detailed to capture the very general interaction between bathymetry, waves and sediment on the reef.
The following simulations in D3D use a PM-spectrum with H s = 2.3 m, T p = 17 s and a peak direction of θ p = 202.5° and θ p = 135° respectively. Despite the complexity of sediment transport on coral reefs 79 , for a first estimation, D3D runs only with the default (suspended and bed-load) sediment transport formulations 77 . All simulations use the same sediment properties: the sediment layer thickness is d sed = 1 m, sediment density is ρ sed = 2.0 gcm −3 (based on in-situ measurements) and sediment grain size (diameter) is D = 200 μm. The morphological scale factor is f MOR = 2.
The reef bathymetry in the 2DH model comes from records of a single-beam, dual-frequency echo-sounder (Dr. Fahrentholz LituBox 15/200) taken during the first field campaign in 2017. The echo-sounder was able to show water depths up to ~ 80−100 m. However, as water depths increase rapidly beyond the reef plateau, records from the echo-sounder exceeding the maximum depth of the Fuvahmulah reef plateau at the southern tip of ~ 20−25 m were discarded. The minimum depth of the echo-sounder was 4 m due to the draft of the boat. The 2017 Photoscan model provides the nearshore (reef) bathymetry for water depths between mean low tide to mean sea level and the island’s coastal topography (including the coastal ridges). The Generic Mapping Tool 80 interpolates the combined elevation and bathymetry data on a 7.5 m by 7.5 m grid, which was also used as a computation grid in the BOSZ model. Offshore waters are truncated to h = 60 m. Similar to the computations in D3D, the hydrodynamic boundary conditions are characterized by a TMA-wave spectrum with a significant wave height of H s = 2.3 m, a peak period of T p = 17 s and peak directions of θ p = 202.5° and θ p = 135°, respectively.
Since this study focuses on the wave driven longshore currents, ocean circulation around Fuvahmulah and the tidal current velocity was disregarded for sediment transport processes (for further information, see Supplementary Materials). This can be considered standard practice when regarding longshore sediment transport and confirms that longshore sediment transport mainly depends on incident waves, while tidal and ocean currents are of minor importance 81 .
Both numerical approaches reveal the impact of the regional wave climate on sediment transport and thus on erosion. However, some limitations apply to the results of the numerical models: the wave input is based on storm wave heights of two peak directions, while moderate seas also have an influence on sediment transport and beach restoration 82 . Furthermore, D3D is able to reconstruct experimental and hindcast data of sandy beaches 78 , but application on reef island morphodynamics is scarce 83 . For Fuvahmulah, D3D does not implement port structures in its bathymetry, to mimic the natural sediment pathways. The coarse resolution and the idealized bathymetry give a general concept of sediment movement on the reef rather than quantitative volumes of transported sediment. Still, the coarse model is able to reconstruct typical, process-based morphodynamic behavior for an environment like the Fuvahmulah reef 5 . The 2DH wave model comprises a more detailed study with and without the port structure. However, the results must be interpreted with caution: the 2DH approach calculates current velocities but does not yield transported sediment volumes. Erosion and sedimentation depend on more than only current velocities 5 . For example, research on organism scale shows that sediment transport on coral reef bottoms is complex 79 . However, current velocities are the main driver behind sediment transport phenomena and velocity gradients hint at hotspots of erosion and sedimentation 77 . With this in mind, the computed wave-induced currents are able to illustrate the role of the southeastern reef area under the given wave climate for the sediment transport around Fuvahmulah. They also facilitate a process-based understanding of the seaport’s impact on the adjacent coastline, even without increased sea level or varied wave heights.
Societal aspects
The analysis of the societal dynamics is based on a mixed-methods approach to convey the social and governance aspects influencing coastal protection and climate change related topics. The approach encompasses (1) a literature review and content analysis of climate change adaptation and coastal protection literature in the Maldives, (2) two surveys with the local population, which are complemented by (3) semi-structured interviews with relevant actors involved in coastal management on the local as well as on the national-level. All participants gave their voluntary consent to participate and have been informed about the objective of the research. The collected data of the second survey and the interviews were analyzed via a structuring content analysis in the software program MAXQDA 84 . This content analysis categorizes the interview’s responses and those of open questions in the surveys into codes and subcodes, developed in a concept-driven and data-driven approach 85 . The concept-driven or deductive approach follows a categorization structure established before the field campaign. However, the possibility to respond to open-ended questions also requires post-processing of the responses based on their content – resulting in a data-driven approach.
The first survey and interviews took place from March to April 2017 and were synchronized with the first field campaign, measuring topographic and hydrodynamic data. Intermediate results from both work packages and the associated discussions led to interdisciplinary insights, considered in the second survey (January to February 2019).
Literature review
The literature review was conducted by content analysis. The analysis focused on publications relevant to climate change adaptation and coastal protection in the Maldives, including peer-reviewed papers, legislation and regulations, as well as gray literature. Legislation and regulations were primarily identified with the support of actors in the Maldives coastal governance system through the semi-structured interviews, ensuring their relevance and completeness. The literature review also encompasses previous EIAs on coastal projects in the Maldives, as these are required to list the legislations and regulations that are applicable to the projects. Most legislation and regulations are available in an English version on the website of the respective ministry, however, documents in the local language Dhivehi have not been considered. The analyzed gray literature covered EIAs, available through the Maldives Environmental Protection Agency (EPA), as well as reports from the Government of the Maldives, Non-governmental Organizations (NGOs) and intergovernmental organizations, such as the United Nations Development Programme (UNDP) Maldives. Special attention was paid to reports, EIAs, and ESIAs concerning Fuvahmulah’s seaport or explicit climate change adaptation measures on the island (see Table 2 ). The documents were analyzed via a qualitative content analysis 85 , which focused on the following thematic codes or categories in the context of the coastal governance system in the Maldives: (1) distribution of responsibilities among actors in the context of coastal protection, (2) history of coastal protection measures, (3) framing of coastal protection in the climate change discussion and (4) framing of coastal protection in the climate change discussion framing of coastal protection in the climate change discussion. The analysis disclosed knowledge gaps in the literature and demonstrated the need for an exploratory and qualitative research approach. Therefore, the study also contains population surveys and semi-structured interviews.
Two surveys with semi-standardized questionnaires were conducted in Fuvahmulah. The first survey focused on four topics: (1) the local’s understanding of the environment and the coast, (2) their perception of environmental issues with a focus on climate change impacts and comprehension of responsibilities for action, (3) the locals’ perspectives on community life, and (4) their perspectives and attitudes towards coastal protection. The second survey focused on: (1) the locals’ perceptions on community life, (2) their potential for engagement in environmental and coastal protection activities, and (3) how politics shape the way coastal issues are addressed.
The surveys involved going from door-to-door in all eight wards of the island and were supported by local research assistants. The sample size of each survey is equivalent to about every eighth household on the island, while household selection was done by previous randomized sampling, yielding an unbiased representation of local households. Interviewees were not working in local coastal management. The first survey had 116 participants between the age of 14 and 86, while the second survey was answered by 98 inhabitants of Fuvahmulah between 14 and 84 years old. The lower threshold of 14 years results from the fact that climate change is a long-term development and coastal protection measures will have an impact for several generations. As in social science studies on future societal development, we have accordingly extended the age limit to 14 years. Furthermore, this decision is based on the experiences made on the island and made in consultation with the local research assistants – people over 14 years of age start to participate in environmental activities without the guidance of their parents and are therefore considered to be sensitive to the environmental situation of Fuvahmulah. With this in mind, and to avoid bias 86 , adolescents are considered capable of giving consent to answer the questionnaire themselves. As participants were not divided into experimental groups, the responses of all participants in the survey remain anonymous. However, to further respect their privacy, this minimal-risk study does not include direct verbatim statements from participants aged 14–18.
The questionnaire of each survey contained closed and open questions (see supplementary materials). For closed questions, the questionnaire provides predefined answers, which were analyzed with methods of descriptive analytics. In contrast to closed questions, open questions require the participant to respond freely to the question. The answers were categorized and coded 85 after the survey to quantify the results and identify important response patterns. In both surveys, the individual interview time varied strongly, ranging from 20 to 90 min. This can be explained by the qualitative approach of the surveys, utilizing open questions and the varying length and depth of answers which depended, for example, on the interviewee’s interest in the topics.
In each field trip, the interviews of Fuvahmulah’s population were complemented by semi-structured interviews with actors on the national and local level being involved in the management of coastal issues in the Maldives. The selection of interview partners is based on their profession and their expertize on the subject (purposive sampling). Using this non-random technique was necessary due to the limited number of actors in coastal governance, while obtaining the required information to compare the varying opinions and interests among those actors regarding coastal protection.
On the national-level, interview partners were representatives of the Ministry of Environment and Energy (renamed to Ministry of Environment in 2018), members of non-governmental and intergovernmental organizations that are active in the climate change adaptation and coastal management fields, as well as local researchers. Interviews with non-government actors gave in-depth and critical perspectives on the way environmental and coastal issues are addressed by government entities. This information is especially important as it helps to identify informal institutions, such as norms and attitudes towards specific responses to coastal problems that become effective and influence the way coastal problems are addressed.
On the local level, interview partners are current and former members of Fuvahmulah’s council and representatives of non-governmental organizations.
In summary, the results of this study are based on 32 interviews with 18 interviews with actors on the national-level (10 government, 8 non-governmental or intergovernmental organizations and researchers) and 14 on the local level (8 government, 6 non-governmental organizations). The interviews of the first field trip addressed (1) societal dealings with environmental problems, (2) coastal management with focus on decision-making processes, (3) community involvement and public awareness, and (4) politics and climate change. The second interview guide focused on (1) the community in the Maldives, (2) community engagement, and (3) coastal governance. The interviews lasted between 30 and 120 minutes. Owing to the political situation in the Maldives and the small number of experts in this field, not all interviewees agreed to be recorded, but memos and notes capture the interview’s statements. Recorded interviews were transcribed before analysis. In addition, the interviews were fully anonymized – except for the ministry officials – in order to protect the identity of the informants.
Reporting summary
Further information on research design is available in the Nature Research Reporting Summary linked to this article.
Data availability
The field data 87 from the aerial surveys and bathymetry for numerical modeling is publicly available through https://doi.org/10.5281/zenodo.4304049 . Wave climate data is publicly available from the respective services and homepages of CAWCR (CSIRO Data Acess Portal: https://data.csiro.au/collections/collection/CIcsiro:39819 ), ECMWF (Copernicus Climate Data Store: https://cds.climate.copernicus.eu ) and NOAA (WAVEWATCH III®Hindcast and Reanalysis Archives: https://polar.ncep.noaa.gov/waves/hindcasts/ ). For further information of the climate data, see also Table 1 . Source data supporting the social sciences’ finding are provided within the Supplementary Information File of this paper. Other raw data (for example interview transcripts) on the social sciences part that support the findings of this study are protected and not publicly available as they contain personal information that could compromise research participant privacy. Other data from the social science part can be made available from the social science team (B.M.W.R. and A.H.) upon reasonable request.
Code availability
The aerial images were processed with the Structure-from-Motion MultiView Stereo (SfM-MVS) algorithm of Agisoft Photscan Pro (version 1.4.5., build 7354). Except for the bathymetry of the numerical model, geo-data was processed with Quantum GIS 3.10 A Coruña and 3.16 Hannover on Ubuntu Linux 18.04 and 20.04 and/or Python modules rasterio v.1.1.1. to v.1.1.3. together with the module Fiona v.1.8.13. The Boussinesq Ocean and Surf Zone (BOSZ, version 01-2019) model is available through Volker Roeber upon reasonable request. The Generic Mapping Tool (GMT, version 5.4.5) was used to process the geo-data to be used as bathymetry in BOSZ. Other simulations on the morphodynamics around the reef island was carried out with Delft3D. Delft3D is open-source and available through Deltares ( oss.deltares.nl/web/delft3d ). In the Delft 3D Suite, we used the modules Deltares FLOWD3D 6.02.13.9162M, SWAN III 40.72ABCDE. Other scripts for data post processing and visualization, are written with the open-source software Python 3.7 in Jupyter Notebooks , using the modules Numpy 1.17.4, Matplotlib (pyplot) 3.1.2, Xarray 0.12.1 with NetCDF 1.5.3, Pandas 0.25.3, cartopy 0.18.0 and cdsapi 0.1.3 and later versions. Cartopy uses OpenStreetMap data (available under the Open Database License, see openstreetmap.org ) as well as Stamen open-source maps. Data of the second household survey and interviews were analyzed with the software MAXQDA.
Nurse, L. A. et al. in Climate Change 2014: Impacts, Adaptation, and Vulnerability. Part B: Regional Aspects . Contribution of Working Group II to the Fifth Assessment Report of the Intergovernmental Panel of Climate Change , (eds. Barros, V. R. et al.) 1613–1654 (Cambridge University Press, 2014).
Magnan, A. et al. Integrative cross-chapter box on low-lying islands and coasts. (eds. Pörtner, H.-O. et al.) Special Report on Ocean and Cryosphere in a Changing Climate (In press, 2019).
Oppenheimer, M. et al. Sea level rise and implications for low lying islands, coasts and communities. (eds. Pörtner, H.-O. et al.) IPCC Special Report on the Ocean and Cryosphere in a Changing Climate (In press, 2019).
Storlazzi, C. D., Elias, E. P. & Berkowitz, P. Many atolls may be uninhabitable within decades due to climate change. Sci Report 5 , https://doi.org/10.1038/srep14546 (2015).
David, C. G. & Schlurmann, T. Hydrodynamic drivers and morphological responses on small coral islands-the Thoondu spit on Fuvahmulah, the Maldives. Front. Marine Sci. 7 , 885 (2020).
Google Scholar
East, H. K., Perry, C. T., Beetham, E. P., Kench, P. S. & Liang, Y. Modelling reef hydrodynamics and sediment mobility under sea level rise in atoll reef island systems. Glob. Planet. Change 192 , 103196 (2020).
Article Google Scholar
Kench, P. S. & Brander, R. W. Response of reef island shorelines to seasonal climate oscillations: South Maalhosmadulu atoll, Maldives. J. Geophys. Res.: Earth Surface 111 , (2006). agupubs.onlinelibrary.wiley.com/doi/pdf/10.1029/2005JF000323 https://doi.org/10.1029/2005JF000323 .
Kench, P. S., Brander, R. W., Parnell, K. E. & McLean, R. F. Wave energy gradients across a Maldivian atoll: Implications for island geomorphology. Geomorphology 81 , 1–17 (2006).
Kench, P. S. & Mann, T. Reef island evolution and dynamics: insights from the indian and pacific oceans and perspectives for the spermonde Archipelago. Front. Marine Sci. 4 , 145 (2017).
Wadey, M., Brown, S., Nicholls, R. J. & Haigh, I. Coastal flooding in the Maldives: an assessment of historic events and their implications. Nat. Hazard. 89 , 131–159 (2017).
East, H. K., Perry, C. T., Kench, P. S., Liang, Y. & Gulliver, P. Coral reef island initiation and development under higher than present sea levels. Geophys. Res. Lett. 45 , 11265–11274 (2018).
Article ADS Google Scholar
Masselink, G., Beetham, E. & Kench, P. Coral reef islands can accrete vertically in response to sea level rise. Sci. Adv . 6 , eaay3656 (2020).
Ryan, E. J., Hanmer, K. & Kench, P. S. Massive corals maintain a positive carbonate budget of a Maldivian upper reef platform despite major bleaching event. Sci. Rep. 9 , (2019).
Tuck, M. E., Ford, M. R., Kench, P. S. & Masselink, G. Sediment supply dampens the erosive effects of sea-level rise on reef islands. Sci. Rep . 11 , 6515 (2021).
Beck, M. W. et al. The global flood protection savings provided by coral reefs. Nat. Commun . 9 , https://doi.org/10.1038/s41467-018-04568-z (2018).
Ferrario, F. et al. The effectiveness of coral reefs for coastal hazard risk reduction and adaptation. Nat. Commun . 5 , https://doi.org/10.1038/ncomms4794 (2014).
Beetham, E., Kench, P. S. & Popinet, S. Future reef growth can mitigate physical impacts of sea-level rise on atoll islands. Earth’s Future 5 , 1002–1014 (2017).
Hughes, T. P. et al. Coral reefs in the Anthropocene. Nature 546 , 82–90 (2017).
Article ADS CAS PubMed Google Scholar
Moustahfid, H., Marsac, F. & Gangopadhyay, A. Impacts of Climate Change on Fisheries and Aquaculture–synthesis of Current Knowledge, Adaptation and Mitigation Options, Vol. 627 , Technical Paperclimate Change Impacts, Vulnerabilities and Adaptations: Western Indian Ocean Marine Fisheries (Fisheries and Aquaculture Department of the Food and Agriculture Organization of the United Nations, Rome, Italy, 2018). ISSN 2070-7010.
Kench, P. S. Compromising Reef Island Shoreline Dynamics: Legacies of the Engineering Paradigm in the Maldives. (eds. Cooper, J. A. G. & Pilkey, O. H.) Pitfalls of Shoreline Stabilization: Selected Case Studies 165–186, (Springer Netherlands, Dordrecht, 2012) https://doi.org/10.1007/978-94-007-4123-2_11 .
Luijendijk, A. et al. The state of the world’s beaches. Sci. Rep . 8 , https://doi.org/10.1038/s41598-018-24630-6 (2018).
Duvat, V. K. E. & Magnan, A. K. Rapid human-driven undermining of atoll island capacity to adjust to ocean climate-related pressures. Sci. Rep . 9 , https://doi.org/10.1038/s41598-019-51468-3 (2019).
Patrício, J., Elliott, M., Mazik, K., Papadopoulou, K.-N. & Smith, C. J. DPSIR–two decades of trying to develop a unifying framework for marine environmental management? Front. Marine Sci. 3 , 177 (2016).
IPCC. The IPCC Response Strategies. (eds. Bernthal, F. et al.) First Assessment Report (FAR), 269 (Island Press, Cambridge, United Kingdom and New York, NY, USA, 1990).
Ratter, B. M., Petzold, J. & Sinane, K. Considering the locals: coastal construction and destruction in times of climate change on Anjouan, Comoros. Nat. Resource. Forum 40 , 112–126 (2016).
Magnan, A. K. & Duvat, V. K. E. Towards adaptation pathways for atoll islands. Insights from the Maldives. Reg. Environ. Change 20 , https://doi.org/10.1007/s10113-020-01691-w (2020).
Petzold, J. & Magnan, A. K. Climate change: thinking small islands beyond Small Island Developing States (SIDS). Climatic Change 152 , 145–165 (2019).
Petzold, J., Ratter, B. M. W. & Holdschlag, A. Competing knowledge systems and adaptability to sea-level rise in The Bahamas. Area 50 , 91–100 (2018).
Hamin, E. et al. Pathways to coastal resiliency: The adaptive gradients framework. Sustainability 10 , 2629 (2018).
Cai, W. et al. Increased frequency of extreme indian ocean dipole events due to greenhouse warming. Nature 510 , 254–258 (2014).
Dangendorf, S. et al. Persistent acceleration in global sea-level rise since the 1960s. Nat. Climate Change 9 , 705–710 (2019).
Nunn, P. D., Klöck, C. & Duvat, V. Seawalls as maladaptations along island coasts. Ocean Coastal Management 205 , 105554 (2021).
Ratter, B., Hennig, A. & Zahid. Challenges for shared responsibility–Political and social framing of coastal protection transformation in the Maldives. DIE ERDE-J. Geogr. Soc. Berlin 150 , 169–183 (2019).
MEE & UNDP. Survey of Climate Change Adaptation Measures in Maldives-Integration of Climate Change Risks into Resilient Island Planning in the Maldives Project. Final Report, Ministry of Housing and Environment, the Maldives (MEE), United Nations Development Programme (UNDP). Author: Ahmed Shaig (2011).
IPCC. Climate Change 2014: Impacts, Adaptation, and Vulnerability (Cambridge University Press, Cambridge, United Kingdom and New York, NY, USA, 2015).
Noble, I. et al. Adaptation needs and options. (eds. Field, C. et al.) Climate Change 2014: Impacts, Adaptation, and Vulnerability. Part A: Global and Sectoral Aspects. Contribution of Working Group II to the Fifth Assessment Report of the Intergovernmental Panel on Climate Change , vol. AR 5, 833–868. The Intergovernmental Panel on Climate Change (Cambridge University Press, Cambridge, United Kingdom and New York, NY, USA, 2015).
Duvat, V. K. Human-driven atoll island expansion in the Maldives. Anthropocene 32 , 100265 (2020).
Gussmann, G. & Hinkel, J. What drives relocation policies in the Maldives? Clim. Change 163 , 931–951 (2020).
Zubair, S., Bowen, D. & Elwin, J. Not quite paradise: Inadequacies of environmental impact assessment in the Maldives. Tour. Manage. 32 , 225–234 (2011).
Gussmann, G. & Hinkel, J. A framework for assessing the potential effectiveness of adaptation policies: Coastal risks and sea-level rise in the Maldives. Environ. Sci. Policy 115 , 35–42 (2021).
NBS. Maldives Population and Housing Census. Summary, National Bureau of Statistics (NBS), Ministry of Finance and Treasury, Male’ 20379, Republic of Maldives (2014).
Trigo, R. M. & Gimeno, L. Weather Pattern Changes in the Tropics and Mid-Latitudes as an Indicator of Global Changes. In Letcher, T. M. (ed.) Climate Change, chap. 8, 165–180, (Elsevier, Amsterdam, 2009) https://doi.org/10.1016/B978-0-444-53301-2.00008-7 .
MEE. Environment & Social Assessment & Management Framework Fuvahmulah. Climate Resilience and Environment Sustainability Project. Final Report, (2014). Project carried out under the Climate Change Trust Fond, the World Bank; last accessed Dec. 27 through documents.worldbank.org .
Ministry of Environment, E. & Water. Foahmulaku Beach Erosion Survey & Coastal Protection Report. Field Survey Report, (2006).
Schöne, T., Esselborn, S., Rudenko, S. & Raimondo, J.-C. Radar altimetry derived sea level anomalies–the benefit of new orbits and harmonization. (eds. Flechtner, F. M. et al.) System Earth via Geodetic-Geophysical Space Techniques , 317–324, (Springer Berlin Heidelberg, Berlin, Heidelberg, 2010) https://doi.org/10.1007/978-3-642-10228-8_25 .
Hemer, M. A., Katzfey, J. & Trenham, C. E. Global dynamical projections of surface ocean wave climate for a future high greenhouse gas emission scenario. Ocean Modell. 70 , 221 – 245 (2013).
IPCC. Summary for policymakers. (eds. Stocker, T. et al.) Climate Change 2013: The Physical Science Basis. Contribution of Working Group I to the Fifth Assessment Report of the Intergovernmental Panel on Climate Change , 1–30, (Cambridge University Press, Cambridge, United Kingdom and New York, NY, USA, 2013) https://doi.org/10.1017/CBO9781107415324.004 .
Hemer, M. A., Fan, Y., Mori, N., Semedo, A. & Wang, X. L. Projected changes in wave climate from a multi-model ensemble. Nat. Clim. Change 3 , 471–476 (2013).
Malatesta, S. & Schmidt, M. Environmental policy and climate change vulnerability in the Maldives: from the ‘lexicon of risk’ to social response to change. Island Stud. J. 12 , 53–70 (2017).
DHV, R. H. Coastal protection at Gn. Fuvahmulah. Deliverable–2e. Technical Design, Amersfoort, the Netherlands (2016).
Energy, M. & (MEECO), E. C. Environmental Impact Assessment Report For Coastal Protection at Gn. Fuvahmulah, Maldives . Technical Report, Male’, Republic of Maldives (2016). In Association with Royal HaskoningDHV.
CERC. Shore Protection Manual, vol. 2 (Coastal Engineering Research Center (CERC) Dept. of the U.S. Army, Waterways Experiment Station, Corps of Engineers, Coastal Engineering Research Center, 1984).
USACE. Coastal Engineering Manual (EM 1110-2-1100), vol. 1–6 (U.S. Army Corps of Engineers (USACE), Washington D.C., United States of America, 2002).
Perry, C. T. et al. Caribbean-wide decline in carbonate production threatens coral reef growth. Nat. Commun . 4 , https://doi.org/10.1038/ncomms2409 (2013).
Duvat, V. K. et al. Trajectories of exposure and vulnerability of small islands to climate change. WIREs Climate Change 8 , e478 (2017).
Bisaro, A. & Hinkel, J. Governance of social dilemmas in climate change adaptation. Nat. Clim. Change 6 , 354–359 (2016).
David, C. G., Schlurmann, T. & Roeber, V. Coastal Infrastructure on Reef Islands–the Port of Fuvahmulah, the Maldives as Example of Maladaptation to Sea-Level Rise? (eds. Goseberg, N. & Schlurmann, T.) Coastal Structures 2019 , 874–885, (Bundesanstalt für Wasserbau, Hannover, Germany, 2019) https://doi.org/10.18451/978-3-939230-64-9_087 .
van Slobbe, E. et al. Building with Nature: in search of resilient storm surge protection strategies. Nat. Hazard. 66 , 1461–1480 (2013).
Haasnoot, M., Kwakkel, J. H., Walker, W. E. & ter Maat, J. Dynamic adaptive policy pathways: a method for crafting robust decisions for a deeply uncertain world. Glob. Environ. Change 23 , 485–498 (2013).
Westoby, M., Brasington, J., Glasser, N., Hambrey, M. & Reynolds, J. ‘Structure-from-Motion’ photogrammetry: a low-cost, effective tool for geoscience applications. Geomorphology 179 , 300–314 (2012).
David, C. G. et al. Structure-from-motion on shallow reefs and beaches: potential and limitations of consumer-grade drones to reconstruct topography and bathymetry. Coral Reefs 40 , 835–851 (2021).
Casella, E., Drechsel, J., Winter, C., Benninghoff, M. & Rovere, A. Accuracy of sand beach topography surveying by drones and photogrammetry. Geo-Marine Lett. 40 , 255–268 (2020).
Roeber, V. & Bricker, J. D. Destructive tsunami-like wave generated by surf beat over a coral reef during typhoon haiyan. Nat. Commun . 6 , https://doi.org/10.1038/ncomms8854 (2015).
Bruno, M. F., Molfetta, M. G., Totaro, V. & Mossa, M. Performance assessment of ERA5 wave data in a swell dominated region. J. Marine Sci. Eng. 8 , 214 (2020).
Durrant, T., Hemer, M., Smith, G., Trenham, C. & Greenslade, D. CAWCR Wave Hindcast - Aggregated Collection. v1 . Last accessed: 2020-03-29, permalink: hdl.handle.net/102.100.100/137152?index=1 (2019).
Tolman, H. L. User Manual and System Documentation of WAVEWATCH III version 3.14 . Technical Note, (NOAA-NCEP, 1999).
Saha, S. et al. The NCEP Climate Forecast System Reanalysis. Bulletin of the American Meteorological Society 91 , 1015–1058 (2010).
WAMDIG. The WAM Model–A Third Generation Ocean Wave Prediction Model. J. Phys. Oceanogr . 18 , 1775–1810, (1988) https://doi.org/10.1175/1520-0485 (1988)018 < 1775:TWMTGO > 2.0.CO;2.
ECMWF. ECMWF Wave Model. In IFS Documentation - CY41R2 , chap. VII, 1–83 (European Centre for Medium-Range Weather Forecasts (ECMWF), Shinfield Park, Reading, RG2 9AX, England, 2016).
Copernicus Climate Change Service. ERA5: Fifth Generation of ECMWF Atmospheric Reanalyses of the Global Climate. Copernicus Climate Change Service Climate Data Store (CDS). Last date of access: 2019-12-06 https://doi.org/10.24381/cds.adbb2d47 (2017).
Hemer, M., Trenham, C., Durrant, T. & Greenslade, D. CAWCR Global wind-wave 21st century climate projections. v1 . Last accessed: 2020-03-29, https://doi.org/10.4225/08/55C991CC3F0E8 (2015).
Muhammed Naseef, T. & Sanil Kumar, V. Climatology and trends of the Indian Ocean surface waves based on 39-year long ERA5 reanalysis data. Int. J. Climatol. 40 , 979–1006 (2020).
Wiese, A. et al. Synergy of wind wave model simulations and satellite observations during extreme events. Ocean Sci. 14 , 1503–1521 (2018).
Hildebrandt, A., Schmidt, B. & Marx, S. Wind-wave misalignment and a combination method for direction-dependent extreme incidents. Ocean Eng. 180 , 10–22 (2019).
Arns, A. et al. Non-linear interaction modulates global extreme sea levels, coastal flood exposure, and impacts. Nat. Commun. 11 , https://doi.org/10.1038/s41467-020-15752-5 (2020).
Timmermans, B. W., Gommenginger, C. P., Dodet, G. & Bidlot, J.-R. Global wave height trends and variability from new multimission satellite altimeter products, reanalyses, and wave buoys. Geophys. Res. Lett. 47 , e2019GL086880 (2020).
van Rijn, L. C. Principles of Sedimentation and Erosion Engineering in Rivers, Estuaries and Coastal Seas . (Aqua Publications, Amsterdam, The Netherlands, 2005).
Lowe, R. J., Falter, J. L., Monismith, S. G. & Atkinson, M. J. A numerical study of circulation in a coastal reef-lagoon system. J Geophys. Res.: Oceans 114 . https://doi.org/10.1029/2008JC005081 agupubs.onlinelibrary.wiley.com/doi/pdf/10.1029/2008JC005081 (2009).
Pomeroy, A. W. M. et al. Sediment transport in the presence of large reef bottom roughness. J. Geophys. Res.: Oceans 122 , 1347–1368 (2017).
Wessel, P. & Luis, J. F. The GMT/MATLAB Toolbox. Geochem. Geophys. Geosyst. 18 , 811–823 (2017).
Burcharth, H. F., Hawkins, S. J., Zanuttigh, B. & Lamberti, A. in Environmental Design Guidelines for Low Crested Coastal Structures (eds. Burcharth, H. F., Hawkins, S. J., Zanuttigh, B. & Lamberti, A.) 203–333, (Elsevier Science Ltd, 2007).
Barnard, P. L. et al. Extreme oceanographic forcing and coastal response due to the 2015–2016 El Niño. Nat. Commun . 8 , https://doi.org/10.1038/ncomms14365 (2017).
Shope, J. B. & Storlazzi, C. D. Assessing morphologic controls on atoll island alongshore sediment transport gradients due to future sea-level rise. Front. Marine Sci. 6 , 245 (2019).
Kuckartz, U & Rädiker, S. Analyzing Qualitative Data with MAXQDA (Springer, 2019).
Kuckartz, U. Qualitative Text Analysis: A Systematic Approach. In Kaiser, G. & Presmeg, N. (eds.) Compendium for Early Career Researchers in Mathematics Education, 181-197, (Springer International Publishing, Cham, 2019) https://doi.org/10.1007/978-3-030-15636-7_8 .
Liu, C., Cox, R. B., Washburn, I. J., Croff, J. M. & Crethar, H. C. The effects of requiring parental consent for research on adolescents’ risk behaviors: A meta-analysis. Journal of Adolescent Health 61 , 45–52 (2017).
David, C. G., Ballesteros, P. & Schlurmann, T. Coastal Digital Elevation Models and Transects of the Reef Island Fuvahmulah, the Maldives [Dataset]. Zenodo (2020) https://doi.org/10.5281/zenodo.4304049 .
Download references
Acknowledgements
This study took place in the project “Dealing with change in SIDS: societal action and political reaction in sea level change adaptation in Small Island Developing States (DICES)”, grant no. SCHL 503/17-1 (C. Gabriel David, Torsten Schlurmann) and RA 585/19-1 (Arne Hennig, Beate M. W. Ratter). The project is framed within the priority programm (SPP-1889) – regional sea level change and society of the German Research Foundation (Deutsche Forschungsgemeinschaft, DFG). Volker Roeber acknowledges financial support from the Isite program Energy Environment Solutions (E2S), the Communauté d’Agglomération Pays Basque (CAPB) and the Communauté Région Nouvelle Aquitaine (CRNA) for the chair position HPC-Waves; as well as the support from the University of Hawai’i at Mānoa for the Affiliate Graduate Faculty position. Zahid was employed as Deputy Director General Climatology at the Maldives Meteorological Service (MMS) at the time of the project, but retired from his position. The authors would like to thank Ali Ahmed, Pablo Ballesteros, Tatiana Ivanova, René Klein, Nina Kohl, Manò Schütt, Ibrahim Shiyan (Panda), Jailam Zahir as well as Marion and Uwe Zander for their help in the field campaigns. In the Maldives, the authors were supported by the Maldives Meteorological Service (MMS), Fuvahmulah Island Council and Fuvahmulah DIVE School. Pablo Ballesteros and René Klein are staff of Ludwig-Franzius-Institute, Leibniz University of Hanover (LuFI-LUH) and supported the field campaign with their technical support. Nina Kohl and Manò Schütt were student assistants at LuFI, helping to record data on Fuvahmulah. Ali Ahmed and Jailam Zahir are locals from Fuvahmulah and assisted the in the coastal surveys (Ali) and in the interviews and household survey (Jailam). The authors also thank Tilo Schöne of GFZ Potsdam, providing the SRA data as well as Jean Bidlot (ECMWF), Mark Hemer (Australian Commonwealth Scientific and Industrial Research Organisation, CSIRO) and Todd Spindler (NCEP-EMC) for their help in accessing climate reanalysis data. Also, Elisa Casella and Alessio Rovere gave valuable insights and feedback to UAV-based photogrammetry, while Tobias Kersten helped with geodetic questions before the third field campaign and with post-processing the GNSS data. Furthermore, the authors acknowledge Jannek Gundlach for his feedback on and Astrid Kartes and Jonas Briese for their assistance with Delft3D. Both, Astrid and Jonas, were students at LuFI during the project.
Open Access funding enabled and organized by Projekt DEAL. This study took place within the German Research Foundation’s (DFG) priority programm “regional sea level” (SPP-1889).
Author information
C. Gabriel David
Present address: Leichtweiß-Institute for Hydraulic Engineering and Water Resources, Division Hydromechanics, Coastal and Ocean Engineering, Technische Universität Braunschweig, Beethovenstr. 51a, 38106, Braunschweig, Germany
Authors and Affiliations
Ludwig-Franzius-Institute for Hydraulics, Estuarine and Coastal Engineering, Leibniz Universität Hannover, Nienburger Straße 4, 30167, Hanover, Germany
C. Gabriel David & Torsten Schlurmann
Institute for Geography, Department for Earth System Sciences, Universität Hamburg, Bundesstraße 55, 20146, Hamburg, Germany
Arne Hennig & Beate M. W. Ratter
Helmholtz-Zentrum hereon GmbH, Department of Human Dimensions in Coastal Areas, Max-Planck-Straße 1, 21502, Geesthacht, Germany
Beate M. W. Ratter
E2S UPPA, Chair HPC-Waves, SIAME, Université de Pau et des Pays de l’Adour, Allée du parc Montaury, Anglet, France
Volker Roeber
University of Hawai’i at Mānoa, Department of Oceanography, 1000 Pope Road, Honolulu, HI, 96822, USA
Formerly Maldives Meteorological Service, Hulhule, 22000, Maldives
You can also search for this author in PubMed Google Scholar
Contributions
C.G.D. conducted the natural science/engineering part of field campaigns, research and analysis, while A.H. carried out the corresponding social science part. V.R. developed the BOSZ model used in this study and supervised the numerical modeling. T.S. and B.R. (co-)designed the research project, were responsible for funding resources and reporting and provided guidance throughout the entire research. C.G.D. wrote the manuscript with input from A.H., while B.R., V.R., T.S. and Zahid edited and contributed to the final manuscript. Zahid helped in designing the research project, coordinating the field surveys and provided a local perspective to the evaluation and analysis.
Corresponding author
Correspondence to C. Gabriel David .
Ethics declarations
Competing interests.
The authors declare no competing interests.
Ethics statement
All participants gave their informed consent and we complied with all relevant ethical regulations. Ethical affairs have been handled and declared during the funding phase of the project. Ethics committee and institutional review board approval was determined not to be required for this project.
Additional information
Peer review information Nature Communications thanks Paula Camus and the other, anonymous, reviewer(s) for their contribution to the peer review of this work. Peer reviewer reports are available.
Publisher’s note Springer Nature remains neutral with regard to jurisdictional claims in published maps and institutional affiliations.
Supplementary information
Supplementary information, peer review file, reporting summary, rights and permissions.
Open Access This article is licensed under a Creative Commons Attribution 4.0 International License, which permits use, sharing, adaptation, distribution and reproduction in any medium or format, as long as you give appropriate credit to the original author(s) and the source, provide a link to the Creative Commons license, and indicate if changes were made. The images or other third party material in this article are included in the article’s Creative Commons license, unless indicated otherwise in a credit line to the material. If material is not included in the article’s Creative Commons license and your intended use is not permitted by statutory regulation or exceeds the permitted use, you will need to obtain permission directly from the copyright holder. To view a copy of this license, visit http://creativecommons.org/licenses/by/4.0/ .
Reprints and permissions
About this article
Cite this article.
David, C.G., Hennig, A., Ratter, B.M.W. et al. Considering socio-political framings when analyzing coastal climate change effects can prevent maldevelopment on small islands. Nat Commun 12 , 5882 (2021). https://doi.org/10.1038/s41467-021-26082-5
Download citation
Received : 12 June 2020
Accepted : 01 September 2021
Published : 07 October 2021
DOI : https://doi.org/10.1038/s41467-021-26082-5
Share this article
Anyone you share the following link with will be able to read this content:
Sorry, a shareable link is not currently available for this article.
Provided by the Springer Nature SharedIt content-sharing initiative
This article is cited by
Maladaptive diffusion the spread of hard protection to adapt to coastal erosion and flooding along island coasts in the pacific and indian ocean.
- Carola Klöck
- Virginie K. E. Duvat
- Patrick D. Nunn
Regional Environmental Change (2022)
By submitting a comment you agree to abide by our Terms and Community Guidelines . If you find something abusive or that does not comply with our terms or guidelines please flag it as inappropriate.
Quick links
- Explore articles by subject
- Guide to authors
- Editorial policies
Sign up for the Nature Briefing: Anthropocene newsletter — what matters in anthropocene research, free to your inbox weekly.

- Important Information - މުހިންމު މައުލޫމާތު

- High contrast
- The Situation of Children
- UNICEF Representative
- Work for Us
- Press centre
Search UNICEF
Climate change and disaster risk reduction, because every child deserves a safe place to grow up.
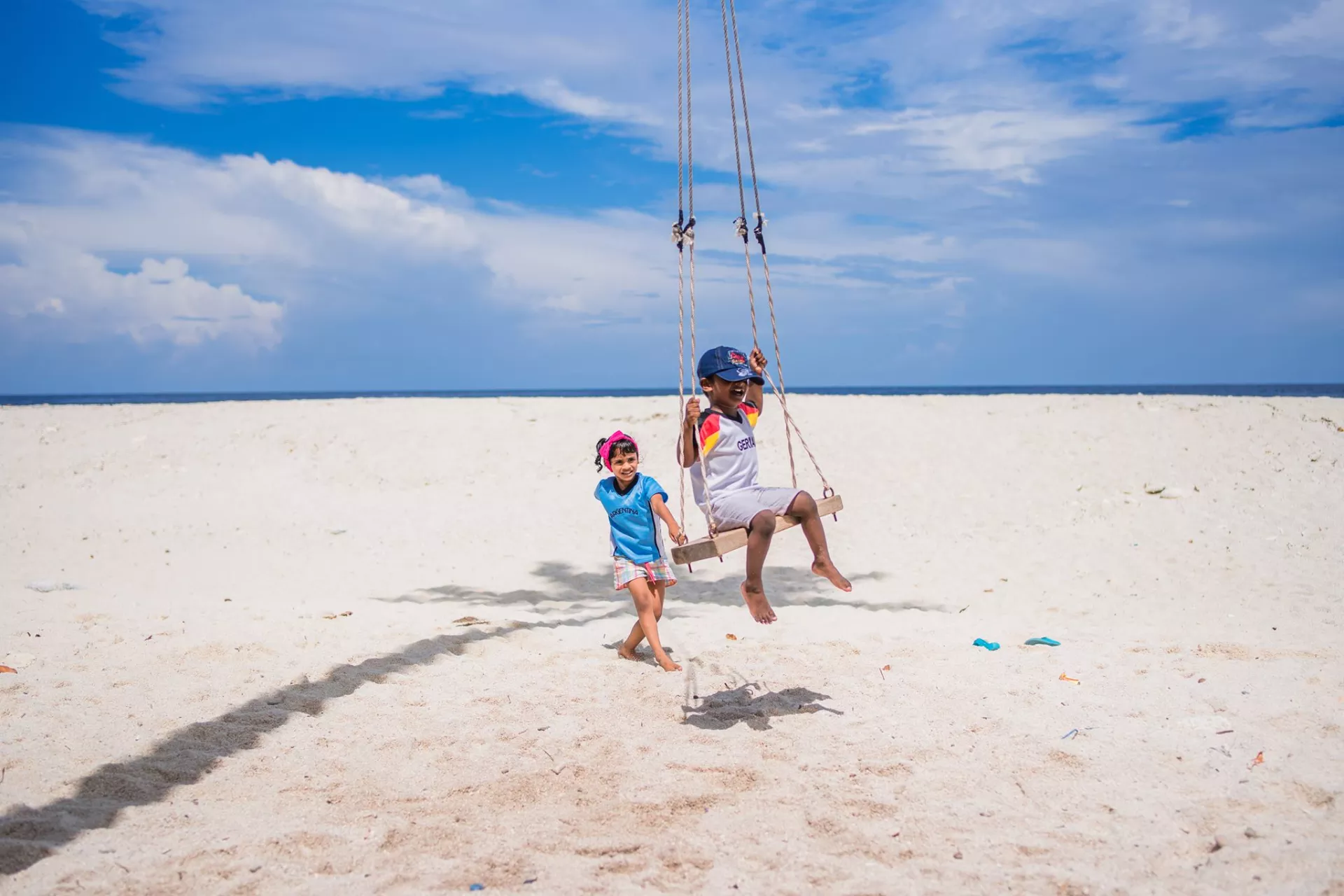
Context and challenges
The Indian Ocean is the heartbeat of the Maldives. The country is made up of 99 percent water and 1 percent land, a geographic composition that makes any change in the ocean reverberate through the islands. Because of this, the country is extremely vulnerable to natural hazards, including tsunamis, floods, intense winds and rising sea levels. Recognizing that the Maldives is one of the lowest-lying countries on earth—and as such, one of the most vulnerable to climate change—for the past three decades, the environment has been at the forefront of Maldivian political discussion. Since first convening leaders from other island states in 1989, environmental degradation and climate change have remained a priority for every administration.
On average, the Maldives rises just 1.5 meters above sea level, making any change in climate potentially deadly to its inhabitants.
In 2004, a massive tsunami swallowed 14 islands, killing over 100 people and displacing many more. The disaster touched one-third of the country’s population and destroyed one-fourth of the islands’ essential infrastructure. Before and after the tsunami, seasonal flooding and tropical storms have damaged homes, schools and communities. Flooding in the south and strong winds and rain associated with cyclonic activity in the north often stop children from attending school. Such weather events also affect health services and at times, damage homes, stores and schools. Natural hazards—and a general increase in flooding, heat and storms—also increase the incidence of water-borne diseases. When water is contaminated and mosquitoes are given a chance to spawn, children risk contracting illnesses like Dengue Fever and diarrhea.
Such circumstances are made worse by the Maldives’ limited capacity to prepare, monitor and respond to natural and man-made emergencies on the islands. Without the proper systems in place, children and families are left to deal with disasters on their own, often with little warning and a lack of resources.
Climate change is also affecting children’s daily lives. Increasing temperatures associated with global warming have affected children's schooling and health. A limited waste management system has left islands across the country littered with trash, a huge portion of which are single-use plastics. These materials end up in the ocean, inside marine life, and ultimately, on Maldivians' dinner plates. According to the Maldives' Environment Ministry, every year, 104 million non-biodegradable plastic bags are imported into the Maldives—and in the capital city alone, waste generation increased by 155 percent over the past decade.
“One day, I was almost suffocated by a plastic bag when diving with my brother.” – a 10-year-old boy in the Maldives
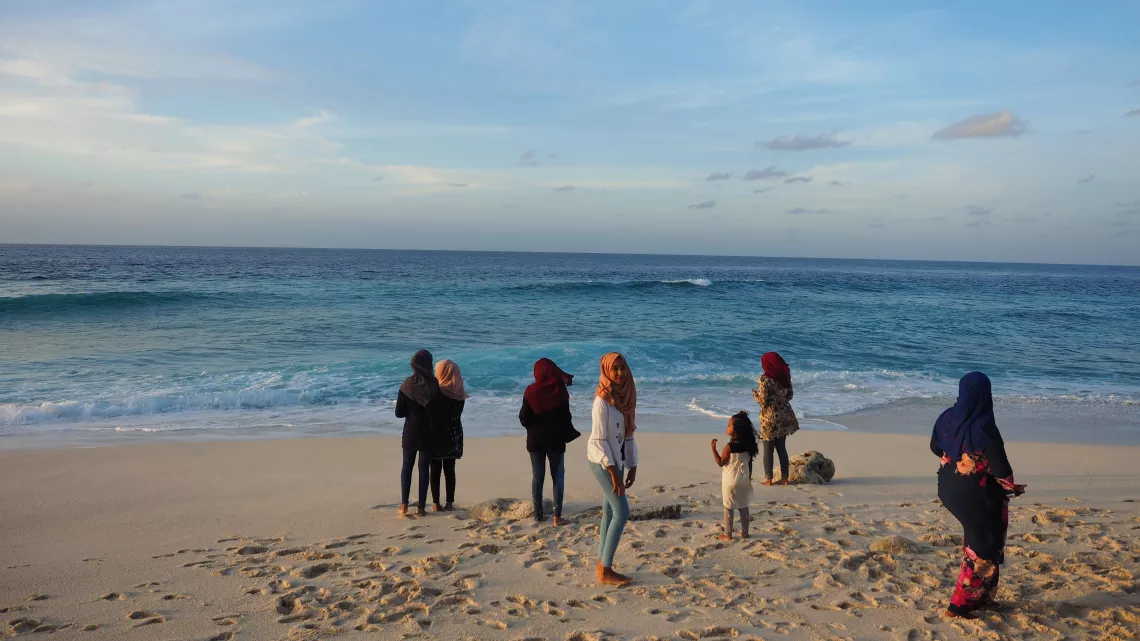
Community Emergency Response Teams (CERTs) UNICEF works with the National Disaster Management Authority (NDMA) and partners to establish Community Emergency Response Teams (CERTs) to plan for and respond to emergencies in each island. Each CERT is made up of volunteers, the majority of whom are young people interested in giving back to their communities. These volunteers are trained on first aid, basic firefighting, flood management, and water, sanitation and hygiene in emergencies. CERTs are also taught about the risks children and families face in an emergency. UNICEF supports the government to provide CERTs with the essential equipment to mitigate a crisis, such as dewatering pumps and accessories.
Prior to the development of the CERTs, if a disaster broke out on an island, communities would have to wait for a national team of responders from the Maldives National Defense Force to arrive at the scene. Now, CERTs are ready to jump into action whenever a crisis occurs. After the success of this UNICEF-supported programme in 2017, the government has committed to establishing CERTs across the country.
Promoting environmentally friendly behaviors UNICEF advocates for the promotion of sustainable, climate friendly practices in the Maldives. In 2018, for example, UNICEF provided BPA-free, reusable water bottles to first graders across the country to reduce single-use plastic water bottle use and promote drinking more water over sugary drinks. And in 2019, we distributed reusable cloth shopping bags to communities around the capital city, advocating for a reduction of single-use plastic while shopping. In addition, we are working with the private sector to install water filtration systems in schools across the country, providing a safe, environmentally conscious water source for students.
By targeting children, we draw on their ability to be change agents for families, peers and communities. These interventions are part of a national campaign to promote environmental sustainability and reduce plastic waste across the country.
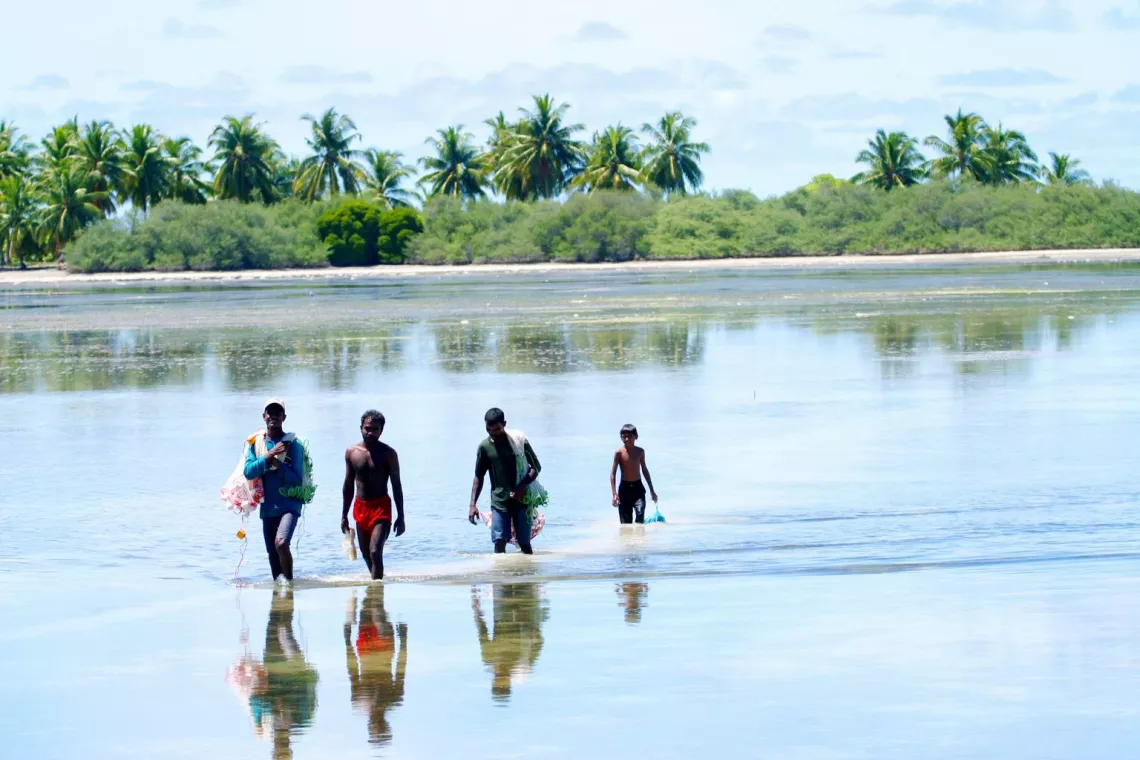
Integrating child and gender-sensitive disaster risk reduction into island planning UNICEF works with NDMA and island councils to embed disaster risk reduction planning and response into local island development strategies. We support the government to conduct participatory island disaster management planning workshops to convene people from different sectors of the island. Together, attendees brainstorm, design and launch context-specific disaster preparedness and response plans. Each of these plans consider the resources and population of an island.
By guiding this process, UNICEF ensures children are kept at the forefront of disaster risk response planning. We also ensure adolescents and young people – especially girls and women – are fully engaged in planning and response preparation, as they are often the most affected when an emergency or disaster occurs.
In addition, UNICEF works at the national level to integrate climate change adaptation and disaster risk reduction into country-wide environmental sector policies.
Studying the effects of climate change on children In 2017, together with the Ministry of Environment, we spoke to hundreds of children, parents and key community members (including teachers, health care staff and counselors) of six islands to gain a better understanding of how children view climate change, and how weather-related events are impacting their lives.
We found that children and adolescents are aware of what is happening to their environment, and that as a whole, they feel strongly about protecting their communities. They reported that high temperatures have made it difficult for them to study in school, and increased heat and sun have sparked skin disease and dehydration. They also noted a lack of potable water at home and at school. In addition, children talked about the abundance of trash and poor waste management in their communities, and how garbage flow increases disease rates. These findings help influence policies and programming at the community, atoll and national levels.
Referenced resources
Climate Change and its Effect on Children | UNICEF, 2018
Climate Change and its Effect on Children
Read about how climate change is affecting children in the Maldives.
Discover UNICEF's history in the Maldives
Research and reports
Learn more about children's lives in the Maldives
Child protection
Learn more about how UNICEF protects children in the Maldives.
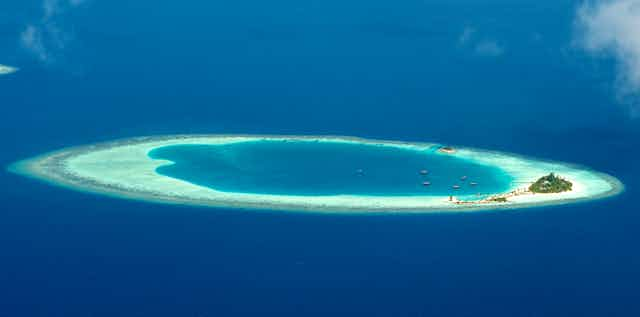
Maldives: climate change could actually help coral islands rise again – but they’re still at risk
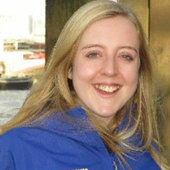
Lecturer in Physical Geography, Northumbria University, Newcastle
Disclosure statement
Holly East receives funding from NERC.
Northumbria University, Newcastle provides funding as a member of The Conversation UK.
View all partners
At the southern tip of the Maldives, on the tiny island of Villingili, a patch of ground rises to tower a whole 2.4m above the sea . It’s the world’s lowest high point.
With most islands just a metre or so above the sea level, it is often suggested that the world’s lowest country may drown beneath rising sea levels by the end of the century. For tourists, this ranks the Maldives atop bucket lists of destinations to visit before they disappear . For the 400,000 people who live on the islands, things are rather more serious: rising sea levels could render them climate change refugees.
However, such scenarios of inundation and drowning assume that the land surface remains static and unchanged. But, what if the land could build vertically as sea level rises?
This is what colleagues and I have been examining in our research, now published in Geophysical Research Letters . We studied five reef islands in the southern Maldives and found that they were actually built when sea levels were higher than they are today.
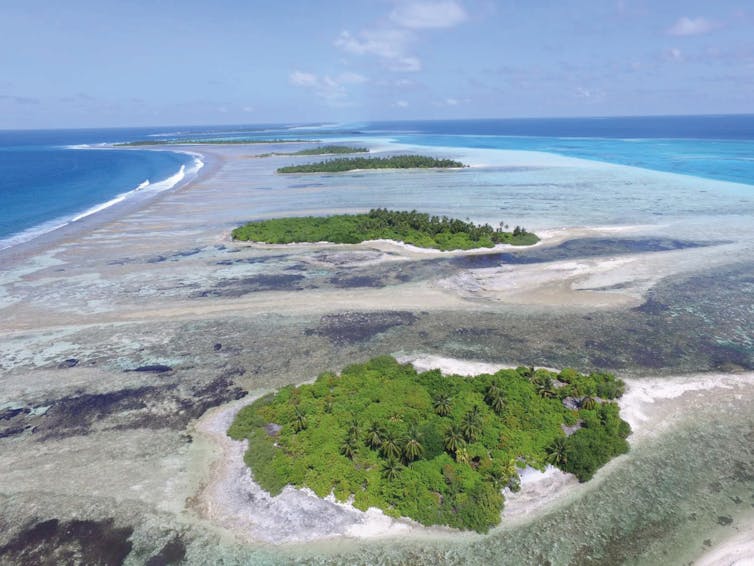
The Maldives is a nation of around 1,200 coral reef islands . Reef islands are unique landforms in that they are formed entirely of sediments produced by organisms such as corals, molluscs and gastropods that live on coral reefs in the surrounding waters.
However, this reliance on the coral reef for island-building sediments, combined with elevations rarely more than a few metres above the sea, means that reef islands are often considered among the most vulnerable environments to climate change, particularly to sea level rise . This is of particular concern for nations such as the Maldives that are built entirely on reef islands, and have nowhere else to go.
Read more: Venice flooding is getting worse – and the city's grand plan won't save it
Back to the future
To improve predictions of how reef islands may respond to future environmental change, it is important to understand how they responded to environmental change in the past. To this end, we reconstructed the island-building histories of five islands in the southern Maldives.
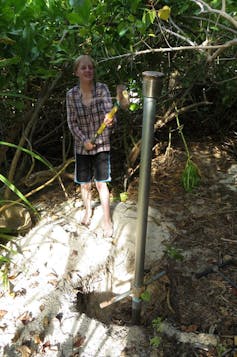
We first collected 28 reef island “cores”. This essentially involved sledgehammering an aluminium pipe into the reef island until it reached the island “foundations” – a point lower than the live coral in the surrounding ocean. The cores enabled us to access the layers of sediment that have built up throughout the island’s history. We then analysed these sediments under the microscope to find out what exactly the island is made of. In addition, we radiocarbon dated the sediments to determine the when the various layers were created.
Results showed that the key phase of reef island building occurred between 4,200 and 1,600 years ago, when sea levels reached around 0.5m higher than they are today.
In addition, this was likely under the influence of large wave events caused by distant storms. These waves would have had the power to break pieces of coral off the reef. Over time, these pieces of coral, as well as sand from the the reef, built up to form the islands.
Climate change will mean rising sea levels and even stronger large wave events. It may therefore recreate conditions that are conducive to reef island building, which may enable these islands to keep growing vertically.
This would make the islands more resilient and may even be necessary simply to keep pace with rising sea levels. Our work complements other studies which are showing that islands are in fact dynamic landforms that are able to move and adjust in response to environmental change.
So is this good news for the Maldives?
All this should make reef islands in the Maldives more physically resilient. However, large waves can also make islands less habitable for humans, for instance by damaging buildings and farmland, or by dumping salt into supplies of fresh water. Reef island nations will have to develop infrastructure that can withstand, or be adaptable to, such powerful waves. Such infrastructure must still allow natural processes to take place however, so that reef islands can maintain active connections to their surrounding coral reefs.
While our study suggests that rising sea levels could benefit reef islands in some regards, they still remain at risk. For instance, we also found the islands in our study were made predominantly (about 75%) of coral. This means a healthy reef will be vital if the islands are to keep growing in future, and the Maldives are to remain above the waves.
However, coral reefs are also threatened by climate change , not just by rising sea levels, but also by warmer and more acidic oceans. Under climate change, we may therefore end up in an odd situation where we have the perfect conditions to build coral reef islands, but an absence of any building materials.
- Climate change
- Coral reefs
- Sea level rise
- Global perspectives

Senior Enrolment Advisor

Associate Professor, Occupational Therapy

GRAINS RESEARCH AND DEVELOPMENT CORPORATION CHAIRPERSON

Faculty of Law - Academic Appointment Opportunities

Audience Development Coordinator (fixed-term maternity cover)
- Get Involved
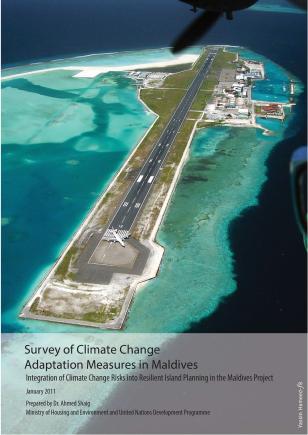
- English pdf (5.3 MB)
Survey of Climate Change Adaptation Measures in Maldives
June 17, 2015.
The ‘Integration of Climate Change Risks into Resilient Island Planning in the Maldives’ Project seeks to elaborate, demonstrate and promote community based and other climate change adaptation measures used in the Maldives.
Report of the Survey of Climate Change Adaptation Measures in Maldives is an assessment undertaken as part of this project to provide baseline information on adaptation activities in Maldives and to identify adaptation options currently being used that may be suitable for replication in the project.
The output of this survey is a compendium of adaptation measures that can be implemented by communities, highlighting the features of each measure, their strengths, weaknesses, and providing illustrated examples across Maldives. This survey was conducted in 40 islands spread across Maldives and included 25 residential islands and 12 resort islands and 3 infrastructure islands from October to December 2010.
Regions and Countries
Related publications, publications, climate induced migration in maldives: preliminary analys....
It is known that climate change is both a threat and risk multiplier. The scientific community has argued that the increase in severity and frequency of climate...
UNDP Maldives Gender Equality Strategy 2023-2026
The Gender Equality Strategy (GES) 2023 – 2026 of UNDP Maldives is futuristic and looking to advance gender equality and the empowerment of women in a digital...
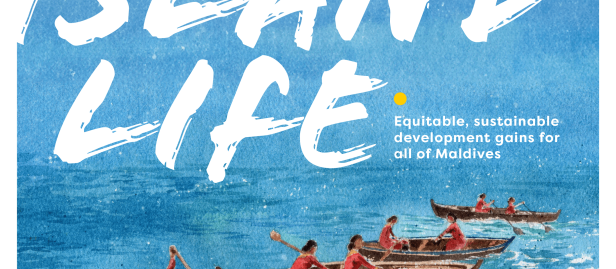
Island Life 2023
This is the 7th edition of the UNDP Maldives’ Island Life Magazine. This edition highlights our impact together in 2023 & stories that are shaping progress acro...
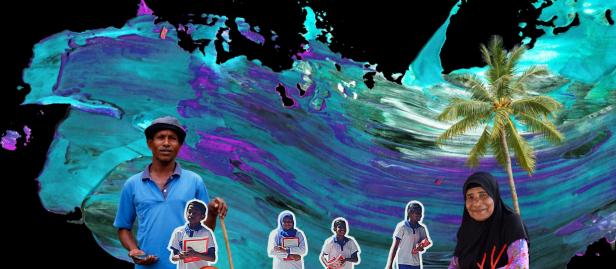
Loss and Damage and Climate Litigation
How can the Maldives and other Small Island Developing States (SIDS) position for greater climate action?
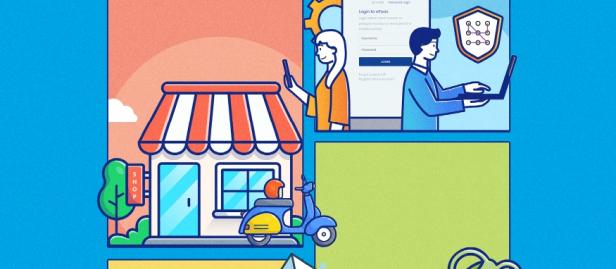
SME Handbook | Volume 1 | Introduction to SMEs
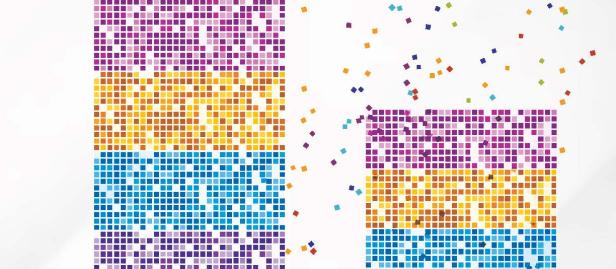
2023 GLOBAL MULTIDIMENSIONAL POVERTY INDEX (MPI)
Unstacking global poverty: Data for high impact action
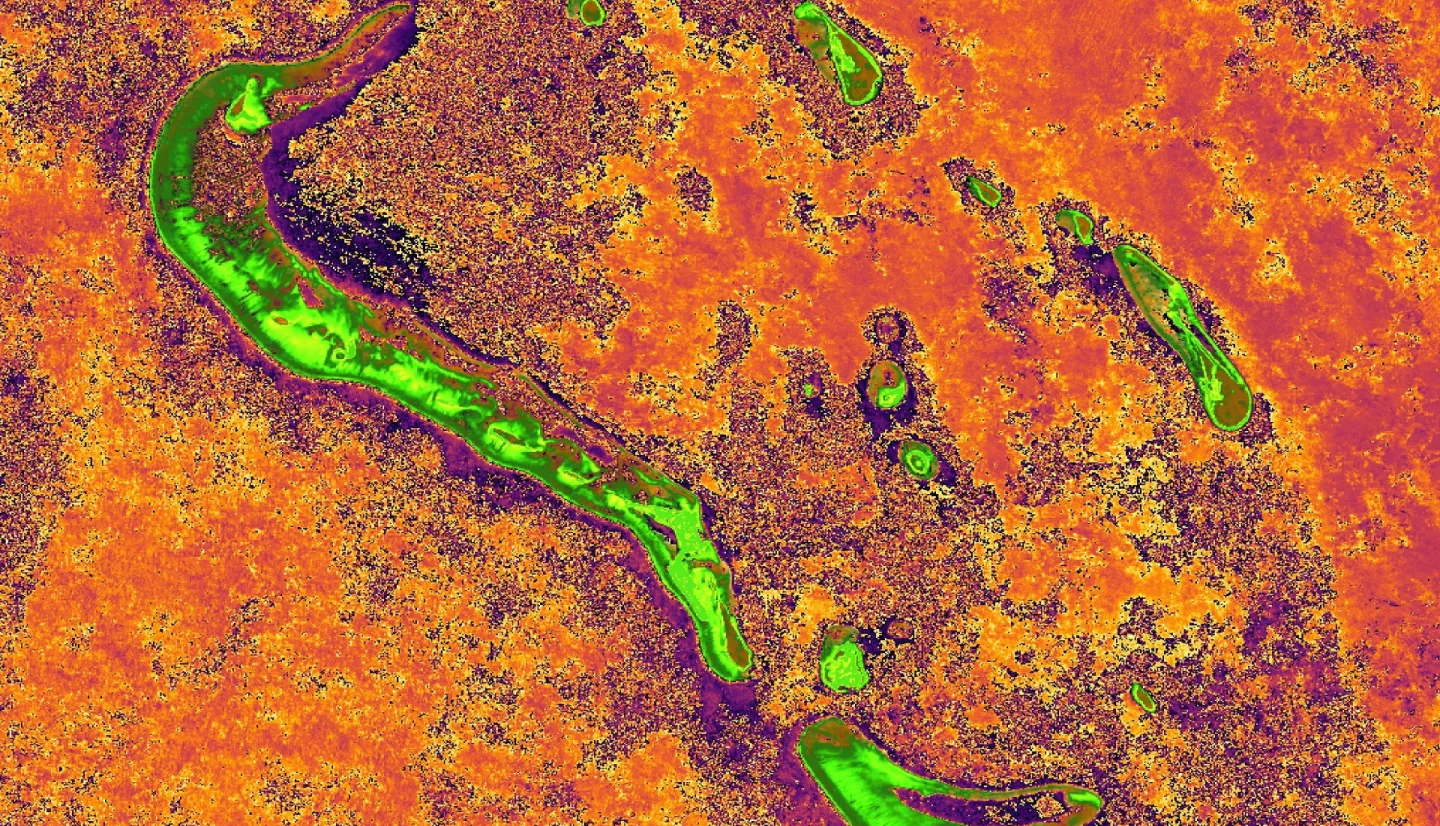
Monitoring Shoreline Change and Island Loss in Response to Climate Change
Maldives climate (fall 2022).
Team: Aidan Harvey (Project Lead), Daniel Lopez, Mitch Porter, Derek Chin
Summary: Global sea level rise as a result of climate change continues to pose a critical threat to coastal ecosystems and populations. The archipelagic country of the Maldives is of critical concern due to being one of the lowest-lying areas in the world. The development of reclaimed land in the Maldives by sand dredging has been a frequent response to both increasing sea levels and population increase. Such disturbance can lead to increased sedimentation off the coast and negatively impact coastal environments. Remote sensing tools such as satellite imagery have proved to be effective tools in observing coastal changes in response to climate change and development. The NASA DEVELOP team created a methodology to analyze water quality and shoreline erosion in the Maldives utilizing satellite imagery. Our methods relied on open-source software such as QGIS and Google Earth Engine (GEE) and Satellite Imagery from PlanetScope, Landsat 8 Operational Land Instrument (OLI) 2, Sentinel-2 Multi-Spectral Instrument (MSI), and Aqua & Terra Moderate Resolution Imaging Radiospectrometer (MODIS) to analyze the changes in shorelines and assess water quality of select atolls within the Maldives. We found that there is less shoreline change in developed parts of the island and more shoreline change in natural parts of the island. Additionally, water quality varies throughout the year, and our data did not indicate seasonal trends. Our methodology will be replicated to continue to monitor island erosion and water quality in the Maldives and will be applicable to other island and coastal systems.
Deliverables :
- Presentation
Related Impact
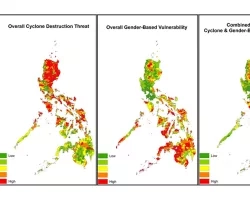
Connect with the Applied Sciences Program
With help from NASA’s Earth-observing satellites, our community is making a difference on our home planet. Find out how by staying up-to-date on their latest projects and discoveries.
Stay Connected
Study reveals: Climate change to cost global economy trillions, urgent action needed
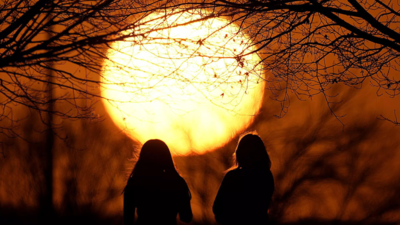
TOP TRENDING
Trending stories.
- Best Kitchen Chimneys Under Rs 20000 in India: Top Picks For Effortless Cooking with Clean Air
- ICAI CA Admit card 2024 out
- Best Single Door Refrigerators: Compare The Top Choices & Buy The Right One
- Punjab Class 10 results
- Bengaluru woman rider gets Rs 1.36 lakh fine: Honda Activa scooter seized
- 'Dry promotion': New job trend causing concern among employees
- Cloud seeding, global warming: What caused widespread flooding and brought Dubai to a grinding halt
- EXCLUSIVE: Pakistan incredibly safe for cricket tours; Rishabh Pant, Shubman Gill key for India in T20 World Cup, says New Zealand's Henry Nicholls
- Odisha man, who lost mom last month, secures second place in UPSC exam
- Wah Jamia! 31 aspirants from Delhi's Jamia Millia academy clear UPSC
- Campaigning ends in 102 seats going to LS polls in phase 1 on Friday: Key seats, candidates
- PM Modi's Ram Navami surprise for Phase 1 NDA candidates
- How Pak society has been pushed to the edge of barbarism
- Maldives prez embroiled in graft row, oppn seeks impeachment
- IPL Live: DC three down but race towards finish line
- Hamas says Iran attack on Israel 'legitimate and deserved'
- Will Modi play peacemaker to dial down tensions in W Asia?
- Google employees held post protests over Israel project; read firm's statement
- Why NPCI did not invite Google, PhonePe and Paytm for its meeting on UPI
- Ghulam Nabi Azad will not contest from J-K's Anantnag
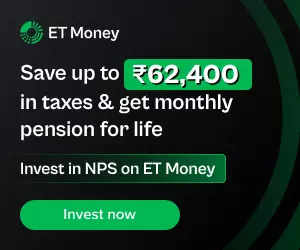
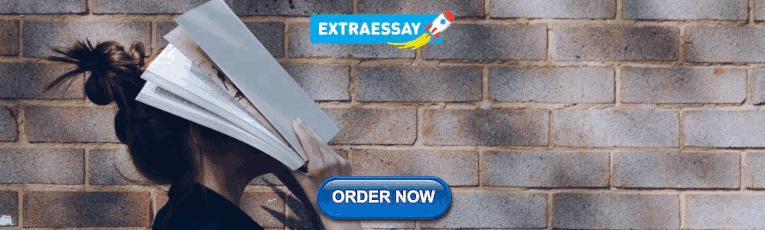
COMMENTS
Facing dire sea level rise threat, Maldives turns to climate change solutions to survive. The islands could be 80% uninhabitable by 2050 at current global warming rates. By Daniel Manzo, Ginger ...
harm the competitive position of Maldives' exports, such as fish. The case study finds that while not all the negative impacts of domestic policies were mitigated, several policy-specific flanking measures were put in place to address the impacts of climate change mitigation policies and projects.
A. INTRODUCTION. This country case study has been conducted as an input into the Second Performance Review (SPR) of the Green Climate Fund (GCF), as launched by the Board of the GCF through decision B.BM- 2021/11. The SPR is being conducted by the GCF's Independent Evaluation Unit (IEU).
With global sea level rising 3 to 4 millimeters per year, and that rate expected to rise in coming decades, some analysts anticipate a grim future for the Maldives and other low-lying islands. One study concluded that low-lying islands could become uninhabitable by 2050 as wave-driven flooding becomes more common and freshwater becomes limited.
The Maldives has a net-zero target by 2030, one of the most ambitious targets for an island nation. To help meet this target, the ASPIRE project has supported two rounds of competitive bidding of solar Photovoltaic Independent Power Producers (PV IPPs) with a total generation capacity of 6.5 megawatts (MW) in the Greater Malé region.
Referring to the IPCC AR6 report, he said, "This report is devastating news for the most climate-vulnerable countries like the Maldives. It confirms we are on the edge of extinction. Climate ...
The Maldives will be particularly vulnerable to the consequences of climate change, given that the larger quantum of precipitations will worsen the potential for damages from coastal flooding during storms, in a context of rising sea levels and enhanced salinization (IPCC 2018).
Climate Change Priorities of ADB and the WBG 24 CONTENTS. CLIMATE RISK COUNTRY PROFILE MALDIVES 1 Climate change is a major risk to good development outcomes, and the World Bank Group is committed to playing an important role in helping countries integrate climate action into their core development agendas. The World Bank Group (WBG) and the ...
The increased likelihood of adverse climate-change-related shocks calls for building resilient infrastructure in the Maldives. Fulfilling these infrastructure needs requires a comprehensive analysis of investment plans, including with respect to their degree of climate resilience, their impact on future economic prospects, and their funding costs and sources. This paper analyzes these ...
Maldives therefore can be seen as a case-study in how a changing climate not only threatens progress made to date in public health but also creates new challenges to sustainable ... Increasing climate change resilience of Maldives through adaptation in the tourism sector. Tourism Adaptation Project (TAP). Male': Ministry of Tourism; 2015
a case study. The Maldives at a glance The Maldives is a low-lying atoll nation, composed of over 1,190 islands, clustered into 26 ring-like atolls ... Robert & Duží, Barbora & Nemec, Daniel & Procházka, David. (2017). Slow Onset Climate Change Impacts in Maldives and Population Movement from Islanders' Perspective. 10 National Bureau of ...
The Maldives, with one of the lowest average land elevations above present-day mean sea level, is among the world regions that will be the most impacted by mean sea-level rise and marine extreme events induced by climate change. Yet, the lack of regional and local information on marine drivers is a major drawback that coastal decision-makers face to anticipate the impacts of climate change ...
With the Maldives facing territorial reduction, and. eventual insular inundation, and Kenya facing increased social conflict, especially. regarding water, the two case studies form an expansive model for the range of. effects that climate change has had, and is projected to have. However, both face.
As UN climate conference COP26 approaches, vulnerable nations are rightly highlighting how they are threatened by climate change. One such nation is the Maldives, for whose inhabitants sea level ...
The clear. " ". preference was to continue living in their houses with their communities in Maldives, irrespective of potential future climate change impacts. Where climate change and its impacts were noted with respect to moving, a common tone was to wait and see, with decisions being made later.
Our results support the paradigm that modern rates and magnitudes of sea-level rise due to climate change are unprecedented during the Common Era 3, 4. Radiative forcing (for example, related to ...
Climate Change in the Maldives. April 6, 2010. A STRING OF PEARLS. A low lying archipelago with more territorial sea than land, the Maldives is exposed to the risks of intensifying weather events. Sea level rise represents an existential threat to the country. With future sea levels projected to increase in the range of 10 to 100 centimeters by ...
In fact, the Maldives national government actively promotes this narrative of being highly vulnerable to climate change induced sea level rise 49 - even in the case of Fuvahmulah. On the other ...
On average, the Maldives rises just 1.5 meters above sea level, making any change in climate potentially deadly to its inhabitants. In 2004, a massive tsunami swallowed 14 islands, killing over 100 people and displacing many more. The disaster touched one-third of the country's population and destroyed one-fourth of the islands' essential ...
For instance, we also found the islands in our study were made predominantly (about 75%) of coral. This means a healthy reef will be vital if the islands are to keep growing in future, and the ...
June 17, 2015. The 'Integration of Climate Change Risks into Resilient Island Planning in the Maldives' Project seeks to elaborate, demonstrate and promote community based and other climate change adaptation measures used in the Maldives. Report of the Survey of Climate Change Adaptation Measures in Maldives is an assessment undertaken as ...
Maldives Climate (Fall 2022) Team: Aidan Harvey (Project Lead), Daniel Lopez, Mitch Porter, Derek Chin Summary: Global sea level rise as a result of climate change continues to pose a critical threat to coastal ecosystems and populations.The archipelagic country of the Maldives is of critical concern due to being one of the lowest-lying areas in the world.
Scientists have warned that climate change could alter upwelling zones where ocean currents border continents, in part by strengthening winds that help bring up colder water. In the case of the Agulhas Current, researchers in 2016 reported that since the 1990s its flow had grown broader and was more likely to create potent eddies.
The Maldives is a Group of Islands in the Indian Ocean, 199 of which are inhabited by about 300,000 people. The average island is 1.5m above sea level, but 80% of land is below 1m above sea level. Scientists think that they will be completely submerged within 50 to 100 years. Economic Impacts of Rising Sea Levels. Loss of tourism (the largest ...
A study published in "Nature" has sounded the alarm on the dire economic consequences of climate change, revealing that the global economy is poised to suffer staggering losses, with damages ...