Advertisement
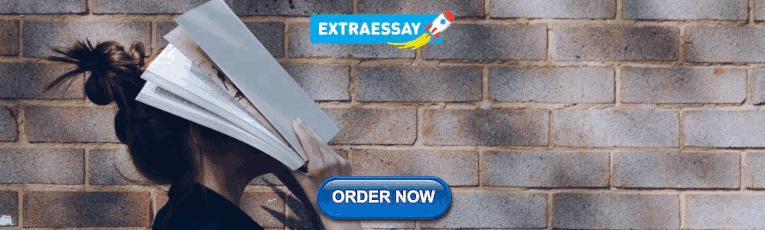
Benchmarking the Effects of Water Demand Management During Water Crisis in a Regional City
- Published: 28 July 2023
- Volume 72 , pages 1277–1292, ( 2023 )
Cite this article
- Rahul Ray Biswas 1 ,
- Tripti Ray Biswas 2 &
- Anisur Rahman 1
218 Accesses
2 Citations
Explore all metrics
Regional cities are having their unique water security challenges due to regional urban water contexts, regional socio-economic structure, and climate conditions. Regional urban community’s perceptions of water usage are expected to be different from the communities in large metropolitan cities. The city of Townsville is the largest regional city in the northern tropical region of the state of Queensland in Australia, and it is known to have its unique dry tropic climate condition. The city faced a water crisis due to a prolonged drought in 2013–2018. As part of this research, at first, a literature review was conducted to understand what water demand management (WDM) tools worked well during urban water crisis in different parts of the world. This paper then investigates how residential water usage changed with the changes in drought measures in the city of Townsville in the last decade. A minimum per capita residential water requirement is established for the study region to benchmark the effects of tools implemented in the region. The paper investigates the WDM policies implemented in the city of Townsville including when the policies were applied and the impacts and efficacy of these policies before water crisis, during water crisis and after water crisis. The most effective WDM tools identified are water restrictions, public awareness raising and education programmes. The impacts of water restriction policies and the perceptions of local water professionals on their success elements are also studied. The results are compared and the reasons behind the findings are investigated.
This is a preview of subscription content, log in via an institution to check access.
Access this article
Price includes VAT (Russian Federation)
Instant access to the full article PDF.
Rent this article via DeepDyve
Institutional subscriptions
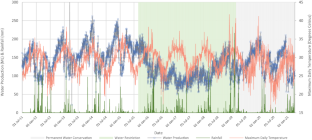
Similar content being viewed by others
Water, agriculture and food: challenges and issues, the measurement of water scarcity: defining a meaningful indicator, the wulca consensus characterization model for water scarcity footprints: assessing impacts of water consumption based on available water remaining (aware), data availability.
All data generated or analysed during this study are included in this article. Water consumption data, water production and climate data used in this research can be accessed from the public websites of Townsville City Council (TCC) and Bureau of Meteorology (BoM), Australia.
Alamanos A, Sfyris S, Fafoutis C, Mylopoulos N (2020) Urban water demand assessment for sustainable water resources management, under climate change and socioeconomic changes. Water Sci Technol Water Supply 20(2):679–687. https://doi.org/10.2166/ws.2019.199
Article CAS Google Scholar
Albannay S, Kazama S, Oguma K, Hashimoto T, Takizawa S (2021) Water demand management based on water consumption data analysis in the Emirate of Abu Dhabi. Water (Basel) 13(20):2827. https://doi.org/10.3390/w13202827
Article Google Scholar
Arbués F, Villanúa I (2006) Potential for Pricing Policies in Water Resource Management: Estimation of Urban Residential Water Demand in Zaragoza, Spain. Urban Stud 43(13):2421–2442. http://www.jstor.org/stable/43201420
Australian Bureau of Statistics (ABS) (2021) Population Projections Australia. https://www.abs.gov.au/statistics/people/population/population-projections-australia
Beal CD, Stewart RA (2011) South-East Queensland Residential End Use Study: Final Report. Urban Water Security Research Alliance Technical Report No. 47
Beer A, Clower T (2009) Specialisation and Growth: Evidence from Australia’s Regional Cities. Urban Stud 46(2):369–389
Bischoff-Mattson Z, Maree G, Vogel C, Lynch A, Olivier D, Terblanche D (2020) Shape of a water crisis: practitioner perspectives on urban water scarcity and “Day Zero” in South Africa. Water Policy 22(2):193–210. https://doi.org/10.2166/wp.2020.233
Biswas R, Sharma R, Gyasi-Agyei Y (2022) Urban water crises: making sense of climate change adaptation barriers and success parameters. Clim Serv 27:100302. https://doi.org/10.1016/j.cliser.2022.100302
Biswas R, Sharma R, Gyasi-Agyei Y, Rahman A (2023) Urban water security: water supply and demand management strategies in the face of climate change. Urban Water J 20(6):723–737. https://doi.org/10.1080/1573062X.2023.2209549
Bolorinos J, Ajami NK, Rajagopal R (2020) Consumption change detection for urban planning: monitoring and segmenting water customers during drought. Water Resour Res 56(3):e2019WR025812. https://doi.org/10.1029/2019WR025812
Braga B, Kelman J (2016) Facing the challenge of extreme climate: the case of Metropolitan São Paulo. Water Policy 18(S2):52–69. https://doi.org/10.2166/wp.2016.113
Browne OR, Gazze L, Greenstone M (2021) Do conservation policies work? evidence from residential water use. Environ Energy Policy Econ 2(1):190–225. https://doi.org/10.1086/711310
Bureau of Meteorology (BoM) (2022a) Climate data online. http://www.bom.gov.au/climate/data/
Bureau of Meteorology (BoM) (2022b) Climate change – trends and extremes. http://www.bom.gov.au/climate/change/
Caball R, Malekpour S (2019) Decision making under crisis: lessons from the Millennium Drought in Australia. Int J Disaster Risk Reduct 34:387–396
Cairns Regional Council (CRC) (2023) Water Strategy and Policy. https://www.cairns.qld.gov.au/water-waste-roads/water/strategy
Campbell HE, Johnson RM, Larson EH (2004) Prices, devices, people, or rules: the relative effectiveness of policy instruments in water conservation. Rev Policy Res 21(5):637–662. https://doi.org/10.1111/j.1541-1338.2004.00099.x
Castledine A, Moeltner K, Price M, Stoddard SW (2014) Free to choose: promoting conservation by relaxing outdoor watering restrictions. J Econ Behav Organ 107. https://doi.org/10.1016/j.jebo.2014.02.004
Connellan G (2013) Water use efficiency for irrigated turf and landscape. CSIRO PUBLISHING. https://www.perlego.com/book/1468519/water-use-efficiency-for-irrigated-turf-and-landscape-pdf
Cooper B, Burton M, Crase L (2019) Willingness to pay to avoid water restrictions in Australia under a changing climate. Environ Resour Econ 72(3):823–847. https://doi.org/10.1007/s10640-018-0228-x
Corbella HM, Sauri D (2009) What lies behind domestic water use? A review essay on the drivers of domestic water consumption. Bol Asoc Geógr Esp 50(50):297–314
Google Scholar
Domene E, Saurí D (2006) Urbanisation and water consumption: Influencing factors in the metropolitan region of Barcelona. Urban Stud 43(9):1605–1623
Dronyk-Trosper T, Stitzel B (2020) Analyzing the effect of mandatory water restrictions on water usage. B E J Econ Anal Policy 20(2):1–13. https://doi.org/10.1515/bejeap-2019-0137
DSITI (2015) Model for Effluent Disposal using Land Irrigation. Department of Science, Information Technology and Innovation (DSITI), Queensland Government
Fielding KS, Russell S, Spinks A, Mankad A (2012) Determinants of household water conservation: the role of demographic, infrastructure, behavior, and psychosocial variables. Water Resour Res 48:W10510. https://doi.org/10.1029/2012WR012398
Furlong C, Gan K, De Silva S (2016) Governance of integrated urban water management in Melbourne, Australia. Uti Policy 43:48–58. https://doi.org/10.1016/j.jup.2016.04.008
Hanak E, Lund J (2012) Adapting California’s water management to climate change. Clim Change 111(1):17–44
Inman D, Jeffrey P (2006) A review of residential water conservation tool performance and influences on implementation effectiveness. Urban Water J 3(3):127–143. https://doi.org/10.1080/15730620600961288
IPOS (2009) Code of practice for irrigated public open space. SA Water, Government of South Australia
Koop S, Van Leeuwen K (2017) The challenges of water, waste and climate change in cities. Environ Dev Sustain 19. https://doi.org/10.1007/s10668-016-9760-4
Low KG, Grant SB, Hamilton AJ, Gan K, Saphores JD, Arora M, Feldman DL (2015) Fighting drought with innovation: Melbourne’s response to the Millennium Drought in Southeast Australia. WIREs Water 2(4):315–328. https://doi.org/10.1002/wat2.2015.2.issue-410.1002/wat2.1087
Mackay Regional Council (MRC). (2016). Mackay Water Strategy. Available at https://www.mackay.qld.gov.au/__data/assets/pdf_file/0013/230116/CPPMackay_Water_Strategy_002.pdf
Maggioni E (2015) Water demand management in times of drought: what matters for water conservation. Water Resour Res 51(1):125–139. https://doi.org/10.1002/2014WR016301
March H, Saurí D (2010) The suburbanization of water scarcity in the Barcelona Metropolitan Region: sociodemographic and urban changes influencing domestic water consumption. Prof Geogr 62(1):32–45
Matikinca P, Ziervogel G, Enqvist JP (2020) Drought response impacts on household water use practices in Cape Town, South Africa. Water Policy 22(3):483–500. https://doi.org/10.2166/wp.2020.169
Meyer et al. (1985) Irrigation of turfgrass below replacement of evapotranspiration as a means of water conservation: determining crop coefficient of turfgrasses. In: Lemaire F (ed) Proc. 5th Intl. Turfgrass Research Conf., Avignon, France, July 1985. INRA Publications, Versailles, France, p 357–364
Millington N, Scheba S (2021) Day zero and the infrastructures of climate change: water governance, inequality, and infrastructural politics in Cape Town’s water crisis. Int J Urban Reg Res 45(1):116–132
Muller M (2020) Some systems perspectives on demand management during cape town’s 2015-2018 water crisis Int J Water Resour Dev 36(6):1054–1072. https://doi.org/10.1080/07900627.2019.1667754
Olmstead S, Stavins R (2009) Comparing price and non-price approaches to urban water conservation. Water Resour Res. 45. https://doi.org/10.1029/2008WR007227
Parker JM, Wilby RL (2013) Quantifying household water demand: a review of theory and practice in the UK. Water Resour Manag 27:981–1011. https://doi.org/10.1007/s11269-012-0190-2
Phipps M, Brace-Govan J (2011) From right to responsibility: sustainable change in water consumption. J Public Policy Mark 30(2):203–219
Pincetl S, Porse E, Mika KB, Litvak E, Manago KF, Hogue TS, Gillespie T, Pataki DE, Gold M (2019) Adapting urban water systems to manage scarcity in the 21st century: the case of Los Angeles. Environ Manag 63(3):293–308. https://doi.org/10.1007/s00267-018-1118-2
Queensland Government (QG) (2022) Queensland future climate dashboard. https://longpaddock.qld.gov.au/qld-future-climate/dashboard/
Rathnayaka K, Malano HM, Maheepala S, George B, Nawarathna B, Arora M, Roberts P (2015) Seasonal demand dynamics of residential water end-uses. Water 7:202–216
Sousa COM, Teixeira LV, Fouto NMMD (2022) Midterm impacts of a water drought experience: evaluation of consumption changes in São Paulo, Brazil. Water Policy 24(1):179–191. https://doi.org/10.2166/wp.2021.120
Sousa Jr W, Baldwin C, Camkin J, Fidelman P, Silva O, Neto S, Smith TF (2016) Water: drought, crisis and governance in Australia and Brazil. Water (Basel) 8(11):493
Standards Australia (SA) (2012) AS/NZS1547: 2012 On-site domestic wastewater management. https://www.standards.org.au/standards-catalogue/sa-snz/waterandwasteservices/ws-013/as-slash-nzs--1547-2012
Stauffer C (2016) Drought ends Brazil’s Sao Paolo but future still uncertain. Reuters. https://www.reuters.com/article/us-brazil-water/drought-ends-in-brazils-sao-paulo-but-future-still-uncertain-idUSKCN0VRlYJ
Stavenhagen M, Buurman J, Tortajada C (2018) Saving water in cities: assessing policies for residential water demand management in four cities in Europe. Cities 79. https://doi.org/10.1016/j.cities.2018.03.008
Taing L, Chang CC, Pan S, Armitage NP (2019) Towards a water secure future: reflections on cape town’s day zero crisis. Urban Water J 16(7):530–536. https://doi.org/10.1080/1573062X.2019.1669190
Tortajada C, González-Gómez F, Biswas A, Buurman J (2019) Water demand management strategies for water-scarce cities: the case of Spain. Sustain Cities Soc 45:649–656. https://doi.org/10.1016/j.scs.2018.11.044
Townsville City Council (TCC) (2019) Water package launches jobs, smart water use across. https://www.townsville.qld.gov.au/about-council/news-and-publications/media-releases/2019/july/water-package-launches-jobs,-smart-water-use-across-city
Townsville City Council (TCC) (2022) Role and strategy. https://www.townsville.qld.gov.au/water-waste-and-environment/water-supply-and-dams/role-and-strategy
Visser W (2018) A perfect storm: the ramifications of Cape Town’s drought crisis. TD – J Transdiscipl Res S Afr 14(1):El–El0
Wichman CJ, Taylor LO, von Haefen R (2014) Conservation policies: who responds to price and who responds to prescription? Comparative Political Economy: Regulation eJournal
Willis RM, Stewart RA, Panuwatwanich K, Williams PR, Hollingsworth AL (2011) Quantifying the influence of environmental and water conservation attitudes on household end use water consumption. J Environ Manag 92(8):1996–2009. https://doi.org/10.1016/j.jenvman.2011.03.023
Xu K, Bin L, Xu X(2019) Assessment of water resources sustainability in mainland China in terms of water intensity and efficiency. Environ Manag 63:309–321. https://doi.org/10.1007/s00267-018-1098-2
Yin RK (2009) Case Study Research: Design and Methods, 4 ed. SAGE, Los Angeles
Zapana-Churata L, March H, Sauri D (2022) Water demand management strategies in fast-growing cities. The case of Arequipa, Perú. Int J Water Resour Dev 38(3):363–387. https://doi.org/10.1080/07900627.2021.1903401
Download references
Acknowledgements
The authors would like to thank the local City Council for supporting this research work by providing valuable information and feedback. It needs to be noted that the local water distribution authority does not take any responsibility on the reliability/accuracy of the information used for this research. The authors are grateful to the water professionals who participated in this research that yielded valuable information.
This research work was supported by the Australian Government Research Training Program Scholarship provided by the Department of Education, Skills and Employment, Government of Australia.
Author information
Authors and affiliations.
School of Engineering and Built Environment, Griffith University, Gold Coast, QLD, Australia
Rahul Ray Biswas & Anisur Rahman
Engineering Research, Brisbane, QLD, Australia
Tripti Ray Biswas
You can also search for this author in PubMed Google Scholar
Contributions
RRB: assist in data collection, writing—review and editing and supervision. TRB: conceptualisation, methodology, data access and formal analysis, investigation, writing—original draft and writing—review and editing. AR: writing—review and editing and supervision.
Corresponding author
Correspondence to Rahul Ray Biswas .
Ethics declarations
Conflict of interest.
The authors declare no competing interests.
Additional information
Publisher’s note Springer Nature remains neutral with regard to jurisdictional claims in published maps and institutional affiliations.
Rights and permissions
Springer Nature or its licensor (e.g. a society or other partner) holds exclusive rights to this article under a publishing agreement with the author(s) or other rightsholder(s); author self-archiving of the accepted manuscript version of this article is solely governed by the terms of such publishing agreement and applicable law.
Reprints and permissions
About this article
Ray Biswas, R., Ray Biswas, T. & Rahman, A. Benchmarking the Effects of Water Demand Management During Water Crisis in a Regional City. Environmental Management 72 , 1277–1292 (2023). https://doi.org/10.1007/s00267-023-01862-5
Download citation
Received : 20 February 2023
Accepted : 17 July 2023
Published : 28 July 2023
Issue Date : December 2023
DOI : https://doi.org/10.1007/s00267-023-01862-5
Share this article
Anyone you share the following link with will be able to read this content:
Sorry, a shareable link is not currently available for this article.
Provided by the Springer Nature SharedIt content-sharing initiative
- Water crisis
- Water demand management
- Water restriction
- Regional cities
- Urban water
- Urban residential water
- Find a journal
- Publish with us
- Track your research
Click through the PLOS taxonomy to find articles in your field.
For more information about PLOS Subject Areas, click here .
Loading metrics
Open Access
Peer-reviewed
Research Article
Water demand management: Visualising a public good
Roles Conceptualization, Data curation, Formal analysis, Funding acquisition, Investigation, Methodology, Project administration, Resources, Validation, Visualization, Writing – original draft
* E-mail: [email protected]
Affiliation Hitotsubashi University, Kunitachi, Tokyo, Japan

Roles Conceptualization, Methodology, Validation, Writing – review & editing
Affiliation Yasuda Women’s University, Hiroshima Asaminami-ku, Hiroshima, Japan
Roles Conceptualization, Methodology, Supervision, Writing – review & editing
Affiliation University of Tokyo, Meguro, Tokyo, Japan
- Yurina Otaki,
- Hidehito Honda,
- Kazuhiro Ueda
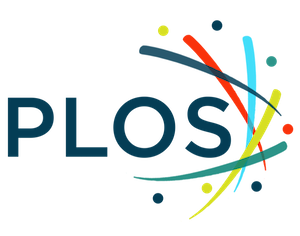
- Published: June 16, 2020
- https://doi.org/10.1371/journal.pone.0234621
- Reader Comments
Recent studies on water demand management show that providing visual information on water usage along with social comparisons with neighbouring households resulted in more efficient water usage. However, social comparisons can be discomforting for participants, especially in the case of downward or negative evaluations. To avoid this, some studies promote the use of social identity, a social norm approach that avoids comparisons. Past studies using social comparison used infographics, whereas other study types have used only textual (non-graphic) information. Therefore, in this study, we created a visualisation of water usage to highlight the importance of water as a shared resource, that is, as a public good, and feedback over six months according to the participants’ water usage. A difference-in-difference analysis indicated that the feedback was marginally significant in decreasing water consumption immediately and continuously, especially for the middle and low use households, during the summer months, which is a period of perceived water shortage. From the questionnaire survey, we found that households felt that they determined their water usage based on their preference and were satisfied with the outcome.
Citation: Otaki Y, Honda H, Ueda K (2020) Water demand management: Visualising a public good. PLoS ONE 15(6): e0234621. https://doi.org/10.1371/journal.pone.0234621
Editor: Valerio Capraro, Middlesex University, UNITED KINGDOM
Received: November 21, 2019; Accepted: May 31, 2020; Published: June 16, 2020
Copyright: © 2020 Otaki et al. This is an open access article distributed under the terms of the Creative Commons Attribution License , which permits unrestricted use, distribution, and reproduction in any medium, provided the original author and source are credited.
Data Availability: All relevant data are within the paper and its Supporting Information files.
Funding: This research was funded by JSPS: Japan Society for the Promotion of Science, Grant-in-Aid for Scientific Research (b), grant number JP18H04324 to YO, LIXIL Jyukankyo Foundation grant number 17-42 to YO, and SECOM Science and Technology Foundation grant number 2018 to KU. The funders had no role in study design, data collection and analysis, decision to publish, or preparation of the manuscript.
Competing interests: The authors have declared that no competing interests exist.
1. Introduction
The demand-side management of both energy and water has recently attracted much attention [ 1 – 3 ]. In particular, smart meters have facilitated the availability of detailed water usage data for users [ 4 – 7 ]. Many studies have examined the potential for encouraging energy- and water-saving behaviours by sharing the household’s data, as well as peer usage data. This strategy of providing usage feedback via social comparison is more effective in changing water usage behaviours than educational or awareness campaigns [ 8 ].
While providing social comparison feedback, some studies have relied on numerical information such as individual and average household usage levels, [ 3 , 9 , 10 ] as well as their rankings among the participants of field experiments conducted in a nearby region [ 9 , 11 ]. In terms of results, some of these studies reported a decrease in water usage [ 10 , 11 ], whereas others reported status quo usage [ 3 , 9 ]. Moreover, in contexts other than water and energy, some researchers reported that the provision of peer information only by text messaging leads to socially undesirable behaviour in the case of low performers (i.e. a study regarding savings reported that peer information via text led low-saving individuals to further decrease their savings [ 12 ], and another study regarding health reported that peer information sent via text led low-performers to use health equipment less [ 13 ]. Thus, to create more effective usage feedback interventions, attempts have been made to design visual feedback indicating water usage patterns of individual, average household, and efficient neighbouring household in the form of bar charts [ 2 , 11 , 14 – 16 ], emoticons [ 2 , 6 , 9 , 14 – 18 ], and circles whose size reflects the quantity consumed [ 19 ], as well as competitive framing [ 20 ]. Most of these studies reported a successful decrease in water usage by ‘high consumers’ and successful control of the boomerang effect (i.e. providing information in an attempt to reduce water usage increases water usage) for ‘low consumers’.
However, such social comparison feedback could be uncomfortable for participants, especially in the case of negative evaluations [ 21 ]. To avoid such a situation, some studies used a social norm intervention without comparisons: social identity. One’s social identity reflects an individual’s membership in a social group together with the value and emotional significance she/he attaches to it [ 22 ]. The more a person identifies with a group, the more likely her/his behaviour aligns with the group's norms [ 23 ], resulting in cooperation in the provision of public goods shared by the group [ 24 ]. A study in Cobb County, Georgia, used textual information encouraging the protection and conservation of ‘our’ environment and ‘our’ water resources and emphasize water as ‘our’ common good. This intervention was effective only for short-term water savings, as the effects failed to persist [ 25 ]. Another study targeting affluent households in Los Angeles also used textual information within a social identity approach to promote the need for water conservation and pro-environmental behaviour using the term ‘our’ city; however, ‘your’ was used in the simultaneous presentation of personal identity. The results of this study were similar to those using social comparison, as they indicated a reduction in water usage by high consumers in both the short and long term [ 18 ]. Overall, although previous studies using social comparisons used infographics, most studies that provided feedback information other than social comparisons used only textual information to emphasise the importance of environmental protection and water conservation for the common good. To fill this gap, this study proposes a visualisation of water usage information to highlight the importance of water as a resource shared by residents (that is, a public good) and observes the subsequent changes in consumption patterns.
2. Materials and methods
2.1. overview.
This study examines the efficacy of conceptualising water as a public good to encourage efficient water usage. First, a random sample of 170 households in Tokyo was drawn from a roster of survey registrants of a research company. The participants were randomly assigned into either a treatment group (hereafter, feedback group) that received feedback or into a control group that did not. Water consumption was monitored through water-meter readings and reported by the participants once every two weeks over twenty-four weeks from May 2018 to October 2018, resulting in twelve observations per participant. The first two times were used to determine the baseline consumption and the next ten were used to provide feedback information. Participants in the feedback group received e-mails informing them about their water consumption to stimulate greater public good awareness within a few days of each observation. Then, participants were given a questionnaire on how they had changed their water usage and how they felt about receiving feedback.
R version 3.6.2 was used to conduct all the statistical analyses and for drawing some figures, Microsoft excel 2016 was used for drawing some figures, and Illustrator 16.0 was used for creating the feedback visuals.
This study received approval from the Hitotsubashi University Research Ethics Committee, and participants’ consent was obtained electronically through the web-site.
2.2. Water resources in Tokyo
In Tokyo, water for residential use is stored in eleven dams. In normal years, the amount of available water is usually sufficient, but once every few years there is an acute shortage during the summer months (July to September) [ 26 ]. There was no shortage in 2018 when the survey was conducted, but the years 2001 (from 10 August to 27 August), 2012 (from 11 September to 3 October), 2013 (from 24 July to 18 September), and 2016 (from 16 June to 2 September) witnessed limited water resources.
Fig 1 shows the amount of water resources stored in Tokyo’s main dams [ 27 ]. The amount of stored water generally decreases between July and September and increases in October. The media often report water shortages in the dams. Therefore, providing information on the amount of water in the dams was assumed to be the most appropriate way to generate awareness of water as a public good among residents in Tokyo. As shown in Fig 2 , we visualised Tokyo as a vessel indicating water consumption of an individual household and then showed the amount of water in Tokyo’s main dams if all households continued to use the same amount of water as the individual household over two weeks. Before the start of the intervention, we explained to the participants that the shape of the vessel was in the form of Tokyo so that everyone could recognize it.
- PPT PowerPoint slide
- PNG larger image
- TIFF original image
https://doi.org/10.1371/journal.pone.0234621.g001
https://doi.org/10.1371/journal.pone.0234621.g002
2.3. Intervention
As shown in Fig 2 , participants in the feedback group received water vessel visuals via e-mail every two weeks from early June to mid-October, for a total of 10 times. The water level in the visual differed depending on individual consumption over the period. The lower the amount of water used, the higher the level of water in the visual vessel. We prepared eight patterns of water vessel visuals with different water levels ( S1 Fig ), and participants received the visuals depending on their usage during the prior two weeks. Daily per capita water consumption of each household was arranged in order of usage, divided into eight equal parts, and applied to each visual. As per capita water consumption is one of the important indicators in the context of water demand management [ 28 , 29 ], we used it for feedback and analysis.
2.4. Water consumption data and evaluation of changes
Per capita water consumption data were measured daily (l/capita/day) to smooth out differences across households due to household size heterogeneity. Water consumption becomes zero when the residents are away from home and becomes large when there are visitors to the home. To exclude conditions that are different from daily life from the analysis, observed values that were either extremely small or large were excluded using z values calculated from water consumption data collected during the survey period for each household. More specifically, we excluded observations with z-values greater than 2.5 or less than -2.5, that is, 1.8% of the data.
Further, each household’s month-to-month water usage was mapped to analyse how the water usage change in the previous month related to that in the following month. As shown in Fig 3 , the comparison with the previous month was categorized into three stages: increase (more than 5%), flat (more than -5% and less than 5%), and decrease (less than -5%).
↑ increase (>5%); → flat (>-5%, <5%); ↓ decrease (<-5%).
https://doi.org/10.1371/journal.pone.0234621.g003
2.5. Participants
This study was conducted among residents of Tokyo. Of the initial 170 households, 134 (75 in the control group and 59 in the feedback group) continued to participate (i.e. read the meter and received feedback) until the end of the study. The average number of members in a household was 2.65 ( SD = 1.17), with 17%, 34%, 21%, 22%, and 5% of the households having one, two, three, four, and five or more members, respectively. S2 Table provides the distribution of the number of family members. There was no statistically significant difference among groups ( t (132) = -0.25, p = 0.80). Fig 4 shows the distribution of annual household incomes, a chi-square test for its distribution indicated no evidence of a statistically significant difference between the control and feedback groups ( χ 2 (3) = 1.14, p = 0.77).
https://doi.org/10.1371/journal.pone.0234621.g004
We classified the households in terms of the baseline water consumption. It is generally understood that as the number of household members increases, water consumption per capita decreases. To eliminate the influence of household size, we converted each household’s baseline water consumption per capita into a value that is assumed for a one-person household. In this way, each household was distributed into a ‘high’, ‘middle’, or ‘low’ level of water usage [ 30 ]. Low-use households are defined as those in the bottom third of water use, and high-use households are defined as those in the top third [ 20 ].
2.6. Questionnaire
At the end of the survey, a questionnaire was conducted to confirm the following three points. The first was whether the water usage was changed consciously or unconsciously. The second was whether individuals who were guided by socially desirable behaviour (water-saving behaviour in this study) felt that their free will was infringed. The third was whether these individuals felt any dissatisfaction with the feedback information. All participants were asked the following three questions corresponding to those three points, answered using a 10-point scale: (1) By how much has your water usage changed? (2) Did you determine your water usage based on your preferences? (3) Are you satisfied with your water usage? The full questionnaire is described in S2 File .
3.1. Water consumption trends
Fig 5 shows, for each group, the change over time in the mean of LRP n (n = 5–10). The mean value of the feedback group was consistently lower than that of the control group from June to September, but the differences disappeared in October. We fitted the polynomial approximation and calculated the AIC value for each group. The AIC value was lowest in the case of n = 2 for the control group and n = 4 for the feedback group. This finding means that the water consumption change of the control group was approximated by a quadratic curve and that of the feedback group was approximated by a fourth-dimensional curve. Because approximation polynomials differ depending on the group, we conducted a monthly DID analysis.
https://doi.org/10.1371/journal.pone.0234621.g005
A difference-in-differences analysis indicated that the feedback was marginally significant to decrease water consumption from May to September ( Table 1 ). The result of the analysis with excluding outlier judged by z-value (described in 2.4) is shown in S4 Table . Basically, the outlier did not change the result of the statistical analysis. Although the trend was the same, in September, the result slightly differed. This was because there was temporary increase in water consumption more than twice the normal value in the feedback households in September. Therefore, it could be presumed that the difference in September was not due to feedback but a factor peculiar to the relevant household.
https://doi.org/10.1371/journal.pone.0234621.t001
Fig 6 presents the feedback effect for the first month (LRP 6 ) and the end of the summer months (LRP 9 ) according to the three usage levels. In the first month, the feedback was marginally significant in decreasing middle-use households’ consumption ( W = 335, p = 0.099). At the end of the summer months, the feedback tended to decrease the low water user’s consumption.
https://doi.org/10.1371/journal.pone.0234621.g006
Fig 7 categorizes the month-to-month water consumption change. As explained in Fig 3 , we described matrices of the consumption change rate from two months prior to the previous month and from the previous month to the current month for each group (control group at the top and feedback group in the middle), as well as their difference (control-feedback at the bottom). In the bottom matrices, the negative value indicates that the ratio of the feedback group is relatively low compared to that of the control group, and the positive value indicates that the ratio of the feedback group is relatively large to that of the control group, and vice versa. For example, among households of the control group who increased their consumption from May to June, 40% increased, 35% did not change, and 25% decreased their consumption from June to July (leftmost column of the leftmost matrices in Fig 7 ). Moreover, in the control group, a relatively large ratio of households increased its consumption both from May to June and June to July; in the feedback group, a relatively large ratio of households increased their consumption from May to June, and subsequently, decreased it from June to July. We coloured the cells with values less and more than 10%, respectively, in blue and pink. The leftmost matrices show that households whose usage increased in the first month also increased their usage in the following month without feedback, and the same can be said for households with flat usage. From the second to the leftmost matrices, of the households whose usage decreased from June to July, households whose usage increased in the following month were mainly in the control group, and households whose usage further decreased were mainly in the feedback group. Regardless of the previous month, the second to the rightmost matrices indicated that feedback caused a downward trend in the following month. Overall, providing feedback through visuals conceptualising water as a shared public good resulted in the immediate and continuous reduction in water consumption. However, the rightmost matrices showed that in the last month, feedback caused an upward trend regardless of the previous month’s trend.
https://doi.org/10.1371/journal.pone.0234621.g007
3.2. Questionnaire
Fig 8 shows the distribution of responses to the first question (i.e. ‘According to you, by how much has your water usage changed?’) using a violin plot. Because the distribution of responses to the question was not normally distributed, we conducted the Wilcoxon rank-sum test. It indicated that households in the feedback group felt that their water usage pattern had changed ( W = 1580.00, p = 0.01).
https://doi.org/10.1371/journal.pone.0234621.g008
If such conscious change in water consumption and behaviour results in residents’ inconvenience or dissatisfaction, the use of these infographics may be problematic. However, concerning the responses to the second question (i.e. ‘Did you determine your water usage based on your own preferences?’), Fig 9 shows that there was no significant difference between the two groups ( W = 1944.50; and p = 0.42). This result shows that people feel that they can freely determine their water use based on their preference. Concerning the responses to the third question (i.e.‘Were you satisfied with your water usage?’), Fig 10 shows that there was no significant difference between the two groups ( W = 2304.5; and p = 0.38). The results show that people did not feel dissatisfied by seeing their water use in this context.
https://doi.org/10.1371/journal.pone.0234621.g009
https://doi.org/10.1371/journal.pone.0234621.g010
4. Discussion and conclusion
We presented visual feedback in the form of infographics representing water usage over six months to generate awareness that water resources are a public good. This resulted in water usage reduction for four months. The effect of the feedback was immediate and continuous during a certain period, however, it disappeared afterward. One possible reason was due to the lack of the long-term continuity of the effect, as shown by previous studies [ 31 , 32 ]. Another possibility was that water usage was reduced only during the summer, generally the period of perceived water shortage. Although there was no water shortage during the survey year, water shortages during the summer are a general concern for the Tokyo metropolitan area [ 33 ]. Some homemakers said that they increased their water-saving awareness because of the annual summer water shortages [ 34 ]. This means that the participants may have spent the summer months of the survey year anticipating a water shortage. However, this study alone could not confidently identify the reasons, and this is one of the study’s limitations. Further research could investigate whether this occurred because there were no longer water shortage concerns or because the effect had diminished over time.
Previous studies providing water usage feedback through social comparisons reported that only high water users reduced their water consumption and low water users showed a ‘boomerang effect’ [ 6 , 17 , 35 , 36 ]. In contrast, as shown in Fig 6 , there was no ‘boomerang effect’ evident in this study, and middle and low water users reduced consumption after seeing visual feedback.
Responses to the first question indicated that participants in the feedback group consciously changed their water usage behaviour. This is different from the results of previous studies, in which participants changed their behaviour instinctively [ 9 ]. Results from the responses to the second and third questions indicated no significant differences between the two groups regarding the freedom of choice and satisfaction about their water usage. Thus, the feedback from the infographic showed that people felt they determined their water usage based on their preference; in other words, it did not threaten participants’ free will, and they were satisfied with the amount of water usage of their household.
Supporting information
S1 fig. eight patterns of water vessel visuals with different water levels..
https://doi.org/10.1371/journal.pone.0234621.s001
S1 Table. Means and 95% confidence intervals of LRP n .
https://doi.org/10.1371/journal.pone.0234621.s002
S2 Table. Distribution of the numbers of family members.
https://doi.org/10.1371/journal.pone.0234621.s003
S3 Table. Distribution of annual household income.
https://doi.org/10.1371/journal.pone.0234621.s004
S4 Table. Result of difference-in-difference analysis with excluding outlier.
https://doi.org/10.1371/journal.pone.0234621.s005
https://doi.org/10.1371/journal.pone.0234621.s006
S2 File. Full questionnaire.
https://doi.org/10.1371/journal.pone.0234621.s007
Acknowledgments
We gratefully acknowledge the provision of illustration by Ms. Yuki Amasaka, Ochanomizu University.
- View Article
- Google Scholar
- PubMed/NCBI
- 11. Erickson T, Podlasek M, Sahu S, Dai J.D, Chao T, Naphade M. The Dubuque water portal: evaluation of the uptake, use and impact of residential water consumption feedback. Proceedings of the SIGCHI Conference on Human Factors in Computing Systems, ACM. 2012
- 22. Tajfel H. Human groups and social categories. Cambridge: Cambridge University Press; 1981
- 23. Abrams D, Hogg M. Social identity theory: Constructive and critical advances. New York, NY, US: Springer-Verlag Publishing; 1990
- 27. Ministry of Land, Infrastructure, Transport and Tourism, Kanto Regional Development Bureau. Available from: http://www.ktr.mlit.go.jp/river/shihon/river_shihon00000111.html Cited 1 Oct 2019
- 34. LIXIL research report 2013. Attitude on water saving: A survey targeting homemakers living in Tokyo and Osaka. https://newsrelease.lixil.co.jp/news/2013/120_newsletter_0730_01.html (in Japanese) (accessed on Feb 17, 2020).
Participative water demand management as an adaptive response within complex socio-institutional systems: a case study of Cape Town, South Africa
Doctoral Thesis
Permanent link to this Item
Supervisors, journal title, link to journal, journal issn, volume title, description.
Viljoen, N. 2019. Participative water demand management as an adaptive response within complex socio-institutional systems: a case study of Cape Town, South Africa.
Collections
DSpace software copyright © 2002-2024 LYRASIS
- Open access
- Published: 20 April 2020
Modelling scenarios for sustainable water supply and demand in Addis Ababa city, Ethiopia
- Zinabu Assefa Alemu 1 , 2 &
- Michael O. Dioha 2
Environmental Systems Research volume 9 , Article number: 7 ( 2020 ) Cite this article
8156 Accesses
17 Citations
2 Altmetric
Metrics details
The city of Addis Ababa is under rapid development and there are enormous construction activities along with rapid urbanization, and industrialization. These anthropogenic actions combined with population growth rate are affecting the water demand of the city. The overall purpose of this study is to model water supply and demand of the city and to identify potential water management strategies that supports the sustainable development goal number six (SDG6)—clean water and sanitation.
We employed the Water Evaluation and Planning system (WEAP) modelling framework to analyze different scenarios for water demand and supply. The scenarios include population growth, living standard, as well as other supply and demand strategies.
For the modelling period, the reference scenario shows unmet water demand increases by around 48%, from 208 to 307 million cubic meter in 2015 and 2030 respectively. High population growth rate and high living standard scenarios have a great negative impact on the water supply system.
Conclusions
Satisfying the future water demand of Addis Ababa will depend on the measures which are taken today. The integrated water management practices such as reuse of water and the selected future scenarios are proposed to decrease and manage the unmet water demand of the city. Hence, future predicted scenarios which is the combination of the external factors (i.e. population growth rate and living standard) and water management strategies were considered. From the analyzed scenarios, optimistic future strategies will support the management of the existing water supply and demand system of the city. Similarly, in the integrated management strategies scenario, it was assumed that measures were taken at both the demand and supply side to improve the efficiency of water in the entire chain. Thus, if the water sector professionals and other concerned bodies consider the selected scenarios, it will go a long way to solve the water shortage problem in the city, and this will also help to promote sustainable water management.
Water supply is an important segment of development. However, in Addis Ababa, Ethiopia, the availability, adequacy and the quality of water are in danger, and the water demand of the city is unmet. Addis Ababa city population is increasing alarmingly due to internal migration and high population growth rate of the city. Furthermore, the city is under rapid development and there are enormous construction activities along with rapid urbanization, and industrialization (i.e. small-scale and large-scale industries). Sufficient and safe drinking water is a precondition for the realization of all human rights. Safe drinking water is a basic need for human development, health and well-being (OHCHR 2002 ). The growing problem of water shortage, the non-functionality of water supply sources like some of the ground water sources along with high water use, urbanization, geographical setup of the city and the high number of population are most problematic to water supply sectors, and this will be challenging to the service of giving adequate and safe drinking water supplies to the communities.
Many countries face major problems in maintaining reliable water supplies, and this is expected to continue in upcoming years (Kassa 2017 ). Addis Ababa city has very limited resources of surface and ground water which plays an important role in the support of domestic needs in mass condominium houses (Admasie 2016 ). Inadequate effort is devoted to addressing this issue in an integrated approach which combines the supply and demand side management in a sustainable approach. Integrated water supply planning is a significant issue for sustainable development to maximize economy, secure production and reduce environmental impacts. Nevertheless, the water managers and planners have given high priorities to locating, developing and managing new water resources as a result, emphasis has been given to the supply side of water development but, demand side management and improvement of patterns of water use has received less attention (Dalle 2016 ).
Climate change has become of the most important issue in the domain of sustainable development and its impacts (e.g. rising of sea levels, melting of polar ice caps, wild bush fire, intense drought etc.) can be felt in different parts of the world (Dioha and Kumar, 2019 ). Developing countries are likely to be affected mostly by climate change, and Ethiopia is one of the most vulnerable countries (Cherie and Fentaw 2015 ). Climate change, urbanization, and rapid population growth along with migration from rural to urban areas poses a major challenge for city planners; extending basic drinking water and sanitation services to each urban and slum areas (WHO and UNICEF 2006 ). The transition of people from rural areas to urban cities represents a major and permanent demographic shift. This movement to cities creates many problems, particularly when housing and infrastructure are unable to keep pace with population growth, and this makes difficulty in giving adequate and safe drinking water (Thompson et al. 2007 ). Population growth is increasing alarmingly in urban areas particularly the capital city of Addis Ababa and therefore, the population growth of the city has an excessive impact on existing water supply infrastructures. The city has always suffered from serious water shortage problems and one of the major factors affecting the public health and socioeconomic development of urban communities (WWDSE 2014 ). Therefore, residents in Addis Ababa are suffering from acute shortage of water and are often hardest hit by the disruption, and thus get water supply just once a week and sometimes once in 2 weeks (Misikir 2015 ).
As stated by the consultancy service for NRWR in 1995 and 1996, the revenue from water decreased from 65.67 to 60.02% whereas the non-revenue water (or water loss) increases from 34.43 to 39.98% in 1995 and 1996 respectively (Consultancy Service for NRWR 1997 ). One of the major bottlenecks for the water utilities is large number of water losses in the distribution network system as well as demand side, and quantification of this water loss (Ephrem 2016 ). Hence, over the period of 1990 to 2004, the number of people without access to drinking water increased by 23% and the number of people without sanitation increased by over 30% (WHO & UNICEF 2006 ).
So, the study on sustainable use of drinking water resources is geared towards achieving the United Nations sustainable development goal number 6 (Clean Water and Sanitation), and therefore there is a need for integrated water planning management in the future because, many people are exposed to lack of water in terms of quality and quantity. Water supply and sanitation situation in Ethiopia is very poor since most of the population does not have access to safe and adequate water supply and sanitation facilities (Getachew 2003 ). Hence, limited availability of water as a resource, the population pressure, the limited financial resource and other socio-economic factors, the traditional approach of endless investment is found not to be a sustainable solution to water supply problems (Dalle 2016 ). As a result, lack of clean or potable water supply and sanitation services in the country has been a serious problem and statistics show that more than 60% of health-related deaths in Ethiopia is caused by water borne diseases (Ethiopian-WFFC 2007 ). Unsafe drinking water along with poor sanitation and hygiene, are the main contributors to an estimated four billion cases of diarrheal disease annually (WHO 2005 ). Every year, at least 1.6 million children under the age of 5 years die from unsafe water, coupled with lack of basic sanitation (WHO and UNICEF 2006 ).
Many countries are entering a period of severe water shortage and the construction of new dams need very big investment as compared to the current economic situation of the city and it may be difficult to implement these projects in short period of time, rather wisely use of the water resource is becoming more important. Thus, selecting different water management system with the help of software tools is very necessary (Amsal and Mebrate 2016 ). Water Evaluation and Planning system (WEAP) software is common in scenario analysis of water supply and demand. This model helps to understand the impact of external factors on the current water supply and demand system. Hence, the WEAP model is widely documented in various literatures, as a well-organized modeling tool in effective analysis of urban water management and this model also preferred over other models because of its usefulness in analyzing the designed strategies and scenarios based on ‘What-If’ conditions (WEAP 2014 ). WEAP is a microcomputer/software tool for integrated water resources planning and it provides a comprehensive, flexible and user-friendly framework for policy analysis (SEI 2016 ). Thus, by accessing different measures in order to conserve water usage and to increase the water use efficiently, WEAP modelling study is essential to integrate both supply and demand side water management strategies.
There are numerous examples of WEAP model application such as Rheinheimer et al., who employed the WEAP model to develop a water resource management for the upper west slope of Sierra Nevada and to predict the potential effects of regional climate warming on hydropower at the watershed scale (Rheinheimer et al. 2014 ). Similarly, Al-Omari et al. used WEAP to establish water management support system for Amman Zarqa Basin by likening various management plans and creating a theoretical basis for watershed water management. The study found that in 2005, both agricultural and domestic water demand was not met (Al-Omari et al. 2009 ). Haddad et al. applied WEAP to investigate water management and water trading scenarios for the reduction of future water demand in Tulkarem basin (Haddad et al. 2007 ). The authors opined that WEAP can serve as a good decision support system to promote local water resource system. In a different context, Baharati et al. used WEAP to evaluate emerging water scarcity problems by transferring water from well-endowed to more deficient areas. The result revealed that the proposed water storage and transfer will reduce water deficit within the project command area (Bharati et al. 2009 ). Hamlat et al. used WEAP to evaluate and analyze the existing balance and expected future water resources management scenarios in watersheds of western Algeria. They found that both domestic demand and agricultural demand was not met for the base year of 2006 but argued that water demand management will be needed for managing the available resources (Hamlat et al. 2013 ).
Li et al. applied WEAP to estimate the sustainability of limited water resources management strategies in the Binhai New Area (BHNA) and to study the advantages of WEAP model for analyzing and simulating different water systems. The results indicated that the pressure on the BHNA water resources will increase in the future (Li et al. 2015 ). Kou et al. used WEAP to develop a water resource simulation by using subsystems from each district and detailed descriptions of industrial sectors and analyzing various water saving scenarios to generate water saving potentials plus water shortage problems. The study found that prevention of future water shortages requires the implementation of water-saving measures as well as the use of new water supplies (Kou et al. 2018 ). Abdelmalek et al. also employed WEAP to study the hydrology of Nabhana watershed in central Tunisia. The modeling results showed that the annual inflow to Nabhana dam for the driest year was around 16 MCM in 2011, and the maximum inflow was about 81 MCM for the hydrological year 2012 (Abdelmalek et al. 2017 ). Yates et al. used WEAP to analyze a climate-driven water resource model of California’s Sacramento River Basin and reported that the model is useful for many California water planning processes. The results displayed that the model can reproduce both local and regional water balances to track water balance changes in terms of both magnitude and seasonality in heavily managed watersheds (Yates et al. 2009 ). In a different study, Vicuña et al. applied WEAP to investigate the outcome of temperature and precipitation on surface hydrology, performance of water infrastructure, and irrigation coverage in the Limarí basin (Vicuña et al. 2012 ). Höllermann et al. used WEAP to analyze Benin’s future water situation under different scenarios of socio-economic development and climate change (Höllermann et al. 2010 ). For additional information on WEAP model usage, see selected applications for WEAP model on the website (SEI 2014 ).
Despite the numerous applications of WEAP model in different parts of the world, studies analyzing water demand and supply strategies in Ethiopia are still limited. Therefore, the overall purpose of this study is to explore scenarios for water supply and demand of Addis Ababa city that supports the realization of SDG6. Thus, this study will serve as a basis for other future studies on water management that will be conducted in the city and other towns of Ethiopia as well as other developing countries. The result of the model can be used to inform urban water management especially water sector professionals, and other concerned bodies. The water modelling tools are cost effective and are also used to predict the future water scenarios and its implications. So, study on sustainable use of drinking water resources is needed to help in conserving resources and minimizing the ground water extraction. Therefore, there is a need for integrated water planning management study to support the use of water resource in a sustainable manner.
The remainder of this paper is structured as follows. “ Features of Addis Ababa city water sector ” section discusses the specific feature of Addis Ababa city water sector. “ Methods ” section explains the research methods used in the study which incorporates the WEAP model structure, input data and scenarios development. “ Results and discussion ” section presents the results and discussions as well as further research areas, whereas the conclusions from the study are stated in “ Conclusions ” section.
Features of Addis Ababa city water sector
In this section, we review the current water scenario of Addis Ababa in order to understand the basis and methodologies for our water demand projection. Water need of the city of Addis Ababa has progressively been increasing from time to time. One of the main objectives of the AAWSA has been to provide/supply adequate and safe drinking water for the capital city by increasing deep wells water supply and combining it with the surface water sources. However, almost all of the surface water sources are located on special zone of Oromia regional administrative state. The demand for water originates from four main sources, specifically, agriculture, production of energy, industrial uses and human consumption. The research report of David and Upali concluded that the increase in demand for water by the year 2025 can be met by small dams and conjunctive use of aquifers, and medium and large dams will almost certainly be needed (David and Upali 2014 ).
The main water supply requirement of Addis Ababa city is giving its service commonly for domestic purpose, non-domestic purpose, and public fountain. Domestic purpose customers are mostly those that are registered as private users, whereas non-domestic customers are those registered as large-scale and small-scale industries, organization, and other hotels, and finally public fountain customers are people who use the service as a group most commonly known as users of bono/one pipe served for many people. The capital city of the country (Addis Ababa), has always suffered from serious water shortage problems and thus to overcome this problem, the city water supply and sewerage authority has been making effort to pursue this difficulty. Addis Ababa Water and Sewerage Authority (AAWSA) through its Water Supply and Sanitation Development and Rehabilitation Project Office different contract agreements are conducted. As per the contract agreement between AAWSA and WWDSE, many wells were drilled and the location of bore holes completed and under progress are in Legadadi, Legatafo, and Ayat ground water prospective sites (WWDSE 2011 ).
The need for proper water supply system for the city has been recognized by the Addis Ababa city administration & Addis Ababa water and sewerage authority, as one of the major factors affecting the public health and socio-economic development of urban communities. Nevertheless, the water demand of the city is still unsolved and needs different water evaluation and planning system. This will help to use the supplied water wisely and efficiently apart from increasing the number of drilled ground water sources in order to preserve the ground water aquafers and to minimize the expensive drilling costs.
Assumptions
In this paper, the WEAP model is applied to modelling of water supply and water demand for sustainable water resource management for the capital city of Addis Ababa (Ethiopia) for the design period of 2015 to 2030. Water modelling and scenario analysis are the best methods for water evaluation and planning system analysis. Efficient and sustainable use of water resources is useful in addressing the problem of access to clean water supply especially for the low-income peoples and school children in the city as well as to ensure the population in the city gets an adequate and reliable supply of water for a long period of time.
Addis Ababa city is geographically located at longitude of 38° 44′ E and latitude of 9° 1′ N. The AAWSA in Ethiopia is a public institution in the city, which is responsible for the supply of potable water. Currently, Addis Ababa gets its water supply from both surface water and ground water sources. Hence, the city water supply sources will be categorized into four clusters based on the type of water resources and geographical locations. The first cluster is Gefersa Dam-I/II which has a conventional treatment plant that is located 18 km west of Addis Ababa and initially constructed in 1943. Later, in 1996, Gefersa Dam-III was constructed to serve as silt trap and additional water storage for the main dam (Gefersa Dam-I/II) and it is located around 800 m up stream of Gefersa Dam-I/II. The second cluster is Legedadi surface water subsystem which comprised both Legedadi and Dire Dams which has a conventional treatment plant and located 25 km east of Addis Ababa. The third cluster is Akaki ground water sources which is within and near Addis Ababa and the final cluster is the spring water sources located at the foot of Entoto Mountain. Figure 1 shows the map of the study area.
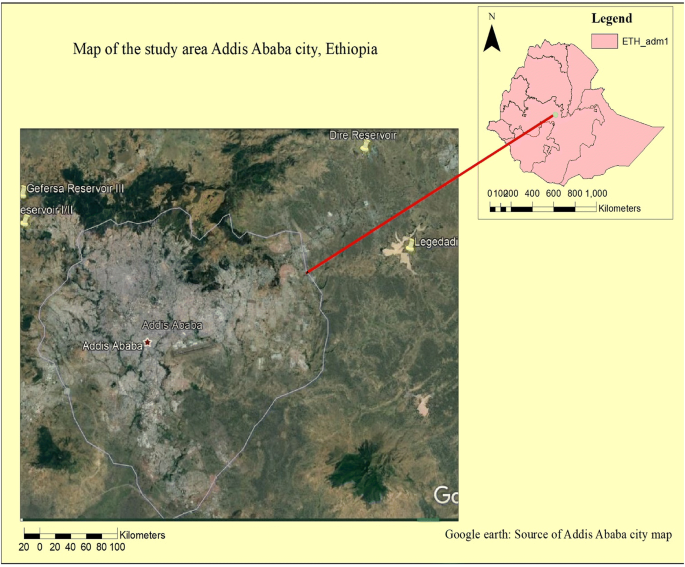
Map of the study area Addis Ababa city, Ethiopia
The WEAP model structure
WEAP is a scenario-based water evaluation and planning system modelling tool developed by Stockholm Environmental Institute ( 2019 ). The WEAP model simulates water scenarios with several segments and it is user-friendly and cost-effective. The WEAP approach works on the basic principle of a water balance. Water Evaluation and Planning system and the data structure and level of detail may be easily customized to meet the requirements of a particular analysis, and to reflect the limits imposed by restricted data. The Current Accounts, which can be viewed as a calibration step in the development of an application, provide a snapshot of actual water demand, resources and supplies for the system. Key assumptions may be built into the Current Accounts to represent factors that affect demand, pollution, supply and hydrology. Scenarios build on the Current Accounts can allow one to explore the impact of alternative assumptions or policies on future water availability and use. Finally, the scenarios are evaluated with regard to water sufficiency, costs and benefits, compatibility with environmental targets, and sensitivity to uncertainty in key variables (WEAP 2016 ).
WEAP is structured as a set of five different “views” onto the software area which is called Schematic, Data, Results, Scenario Explorer, and Notes. The Schematic View is the starting point for all activities in WEAP. In the Data View, the screen is divided into four panes. On the top left, a hierarchical tree is used to create and organize data structures under six major categories: Key Assumptions, Demand Sites, Hydrology, Supply and Resources, Environment, and Other Assumptions. The Results View displays a wide variety of charts and tables covering each aspect of the system such as demand, supply, costs, and environmental loadings. The Scenario Explorer View is used to group together “Favorite” charts (created earlier in the “Results” view) into “Overviews” for simultaneous display. Finally, the Notes View is a simple word processing tool with which we can enter documentation and references for each branch of the tree (SEI 2011 ).
A demand site’s (DS) demand for water is calculated as the sum of the demands for all the demand site’s bottom-level branches (Br). A bottom-level branch is one that has no branches below it.
The total activity level for a bottom-level branch is the product of the activity levels in all branches from the bottom branch back up to the demand site branch (where Br is the bottom-level branch, Br’ is the parent of Br, Br’’ is the grandparent of Br, etc.).
All the above Calculation Algorithms were collected and referenced from WEAP software (WEAP 2017 ).
Input parameters and data collection
The study was conducted through institutional integration/supports since this modelling of water supply and water demand needs different institutional data as input for the software. The design period for this study was simulated between the year 2015 and 2030. For that, past climatic data such as precipitation, relative humidity, sunshine duration, evaporation, maximum temperature, and minimum temperature were obtained from the NMA. Observed domestic and non-domestic water demand data plus other supportive documents were collected from AAWSA. In addition to this, the population census data for Addis Ababa city are attained from the CSA, and also, the United Nations Medium Variant Population projection is considered for the population growth of Ethiopia and this was used for calculating and estimating the population growth of Addis Ababa. The overall research data for this study were collected based on primary and secondary data sources to address the goals of the study.
Data analysis tools
The WEAP software was used to study the modelling of water supply and water demand by using different institutional data sources for predicting the future water scenarios and its implications. The Population projections and their integration graph was analyzed with Microsoft Excel. SPI Generator software and Microsoft excel was used for the analysis of Standard Precipitation Index (SPI). SPI was used long time historic climatic data (i.e. precipitation) for the characterization of hydrological water year method. In this study spi12 was used and spi12 refers to spi with a time scale of 12 month.
Population trend and non-revenue water
The population of Addis Ababa in 2015 was around 3.65 million and the population projection has been estimated to grow nearly to 4.58 million persons by 2025 and 5.06 million persons by 2030. However, as indicated in Table 1 , the population growth of Addis Ababa city increases from year to year (CSA 1984 , 1994 , 2007 ). The population projection graph was used during the formulation of the scenario in the WEAP software as well as the total population for the year 2015 were used as a current account. The water sector of the city integration with the support of Consultancy Service for NRWR reported that the non-revenue water in Addis Ababa is between the range of 34.43% and 39.98% (Consultancy Service for NRWR 1997 ).
Scenarios development
In this study, our scenarios refer to a series of assumptions or storylines depicting how the future of Addis Ababa water system might unfold. The future water demand of the city is affected by different factors such as population growth, family income, urbanization, living standard etc. The values of these factors are diverse according to the scenario configuration. Different assumptions are needed to test the effects of these factors. Hence, scenario analysis is used in this study to explore the water supply and demand of Addis Ababa city to support the water sustainability goal. In doing the same, four clusters of scenarios are developed, namely: Reference, External Driven Factor, Water Management, and Future Predicted scenarios.
Reference (Ref) scenario
This is the basic task in establishing scenarios for future water demand and supply and it is sometimes referred to as the business-as-usual scenario. In this case, all key assumption and other factors are assumed to remain the same as in the base year while socioeconomic and demographic factors are assumed to grow at the official projected rate. This scenario also serves as the benchmark for comparing the outcome of other scenarios.
External driven factor scenarios
Saraswat et al. argued that external factors will increase the pressure on the current water supply system and exacerbate the water scarcity situation (Saraswat et al. 2017 ). Therefore, to predict the effect of external factors on water demand, different assumptions are considered as indicated below:
High population growth (HPG) From the population projection result, we assume that the total population growth rate will be increasing by 4.5% annually.
Medium population growth (MPG) For this medium population growth rate, around 2.5% annual growth rate is used and this is expected and selected based on the consideration of high and low population growth rate.
Low population growth (LPG) Here, we assume the lowest population growth rate by comparing from other expected population growth rate during this study and thus the annual population growth rate is taken as 1.5%.
High living standard (HLS) As the standard of living increases, it is expected that the household demand for water will also increase. Here, we assume that the rate of water use is the only factor to be considered for high living standard. For this study, 150 LPD is taken.
High population growth + high living standard (HPG + HLS) Like the above external driven factors, the high population growth rate is assumed as 4.5% and the high living standard is also assumed as 150 LPD.
Water management scenarios
The purpose of integration of different kind of management strategies is to discover the ideal solution for achieving water security in the region (Saraswat et al. 2017 ). Thus, different strategies are identified in achieving the water demand of the city and can be summarized as follows:
Demand side management - sustainable water resource and reuse (DSM - SWSR) The water use practice is different for all the consumers and here we assume and expect that all the consumers (i.e. domestic and nondomestic) are applying good water management strategies such as recycle, reuse, and use less water in day-to-day activities.
Supply side management and non - revenue water reduction (SSM - NRW) The water supply sector faces many challenges such as the geographic set up of the city, the old pipe lines, power interruption, linkages etc. Here, we assume that the water sector applies different technologies and changes the old pipe lines by the new one in order to reduce the NRW reduction by different percentages.
Integrated management strategies (IMS) Here, we assume that the government starts to minimize the nonrevenue water and incorporates the practice of demand side management strategies by creating awareness programs. However, the result of integrated management strategies begins from the combination of the two management strategies, i.e., supply side management and NRW reduction by water sector professionals or by the supplier and the other is the demand side management strategies which is sustainable water resource and reuse by the water consumers.
Future predicted scenarios
Here, we attempt to build futures which combines the external factors and water management strategies to see how the true picture of water demand in Addis Ababa might unfold in the future. According to the analysis of different scenarios and combination of other management options, in this study, three possible cases were considered and they are explained below:
Pessimistic future (PF) In this scenario, the different factors were assumed to be changing through time based on ‘what if’ condition or question. For this scenario, different factors are considered and can be summarized as follows:
50% of NRW and we assume that there is no any measure taken to reduce the NRW.
2% sustainable water sources including reuse rate.
4.5% population growth rate estimation.
Moderate future (MS) This scenario is design based on moderate future predictions to consider different strategies based on ‘what if’ condition. We assume sustainable water use and reuse as well as supply side measure like NRW practices in such a way that the percentage change is medium; it is above the reference scenario and below the assumptions of the optimistic future. For this scenario, different factors are considered and can be summarized as follows:
29% of non-revenue water and here we undertaken that if ten percent of the nonrevenue water is reduced from the total estimated nonrevenue water.
7% Sustainable water sources including reuse rate.
2% population growth rate here we are considering the nearest medium population growth rate.
Optimistic future (OF) The scenario is formulated by considering different strategies such as supply and demand side measures and population growth control. For this scenario, different factors are considered and can be summarized as follows:
10% of NRW and 30% of the nonrevenue water reduction takes place by the supply management strategies.
15% sustainable water sources including reuse rate and the demand side management strategies are applied.
1.5% population growth rate estimation.
The overall methodological framework used in this study is shown in Fig. 2 .
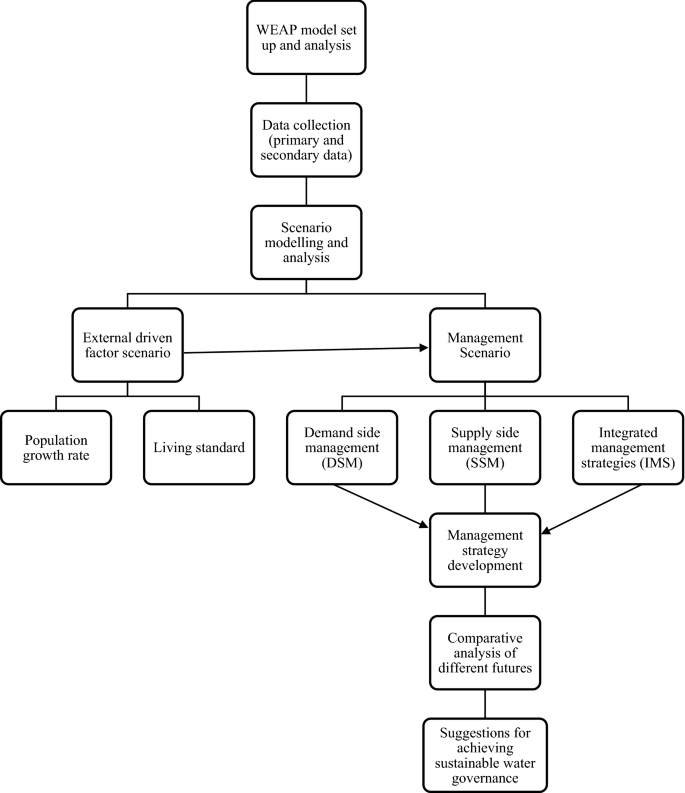
General study framework
Results and discussion
Reference scenario.
Current account and reference scenarios were assigned first before other scenarios were considered to achieve the scenario simulation and data analysis. The current accounts represent the basic definition of the water system as it currently exists, and the reference exemplifies business-as-usual scenario. The Ref scenario projection depicts a situation in which there is no improvement in water supply and demand infrastructures with respect to the base year. Figure 3 shows the simulation results of the Ref scenario. Driven by rapid population growth and urbanization, the unmet water demand of Addis Ababa is expected to grow from 208 MCM in 2015 to about 307 MCM in 2030. This result corresponds to about 48% increase in unmet water demand in the city within 15 years. The alarming increase of unmet water demand in the city therefore calls on the government to develop practical strategies to avert water crisis in the future. It is not easy to compare our results with other findings owing to the limited studies in this area for Addis Ababa. However, our analysis of the model results shows that there are significant differences in unmet water demand between current study and the work of Arsiso et al., who have attempted to use WEAP to investigate water demand and supply prospects for the City of Addis Ababa. The study developed scenarios of population growth trends and climate change (i.e. RCP 8.5 scenario and RCP 4.5 scenario) up to 2039 by applying the WEAP model. The variation in our results is because of difference in number of years and assumptions used in the two studies (Arsiso et al. 2017 ). Other studies showed that the result of some scenario studies were mostly different from the current account. According to Shahraki et al., unmet demand compared to the current account increased from 193 to 200 million cubic meters, respectively (Shahraki et al. 2016 ).
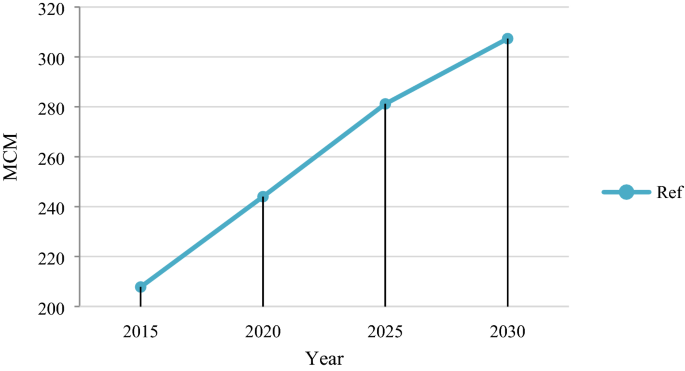
Reference scenario of unmet water demand in Addis Ababa city
External driven factor scenarios results
Figure 4 reports the projections of unmet water demand for the external driven factor scenarios. The result indicates that the projected population of Addis Ababa city using low population growth rate (1.5%) and high population growth rate (4.5%) will be about 4.57 and 7.07 million people respectively by 2030. The total unmet water demand by 2030 for the HPGR + HLS scenarios would grow to 583 MCM; this represents about 90% increase in comparison to the Ref case. However, considering only living standard; as incomes grow and the household water requirements increase, the unmet water demand of Addis Ababa in HLS scenario is expected to grow to around 410 MCM by 2030; indicating about 34% increase when compared with the Ref scenario. In the HPG scenario, a high population growth rate is assumed and the unmet water demand is seen to grow to 440 MCM by 2030. This value corresponds to 43% increase in comparison to the Ref scenario. Another study indicated that increasing number of population affects the water demand of the city. As Zhang et al. study revealed that the higher population growth rate is especially due to migration and results in an increased pressure for land and water resources (Zhang et al. 2008 ). Finally, analysis of the LPG scenario where a low population growth rate was assumed showed that unmet water demand will reach 274 MCM by 2030. In comparison with the Ref case, this value suggests a decrease of 11%. Considering the entire external driven factor scenarios, it can be observed that an increase in population growth rate coupled with an increase in living standards will create water crisis for the city in the future. Thus, proactive steps need to be taken to avert this situation.
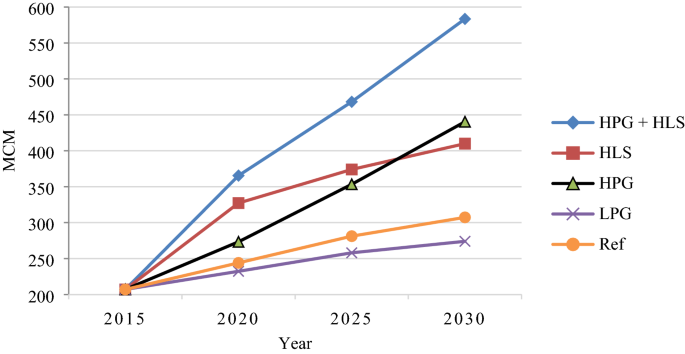
Unmet demand for reference and external driven factor scenarios
Water management scenario results
Figure 5 depicts the results of the water management scenarios. Analysis of DSM-SWSR scenario indicates that with the effective implementation of different management strategies at the demand side, unmet water demand will grow to 262 MCM by 2030. This value is about 15% decrease when compared with the Ref scenario. In the SSM-NRW scenario, it was assumed that measures were taken at the supply side to improve the efficiency of water supply system in the city. Consequently, analysis of this scenario shows that unmet water demand will reach 227 MCM by 2030, which represents about 26% reduction in unmet water demand with respect to the Ref case. Finally, in the IMS scenario, it was assumed that measures were taken at both the demand and supply side to improve the efficiency of water in the entire chain. Consequently, analysis of this integrated approach suggests that unmet water demand will reach 215 MCM by 2030 or 30% reduction in comparison to the Ref scenario. From the foregoing results, it is clear that an integrated approach is needed to reduce the unmet water demand of the city. Thus, the unmet water demand of the city will be minimized most in the IMS scenario and this will support the achievement of SDG6. Other studies showed that integrated management strategies saves more water than individual management approaches. Hence, Kou et al. study stated that industry restructuring (structural water-saving scenario) and advanced water-saving technology (technical water-saving scenario) can result in water saving potentials of 6.97% and 9.82% by 2050, respectively, while adopting both strategies (double water-saving scenario) can save 16.44% (Kou et al. 2018 ).
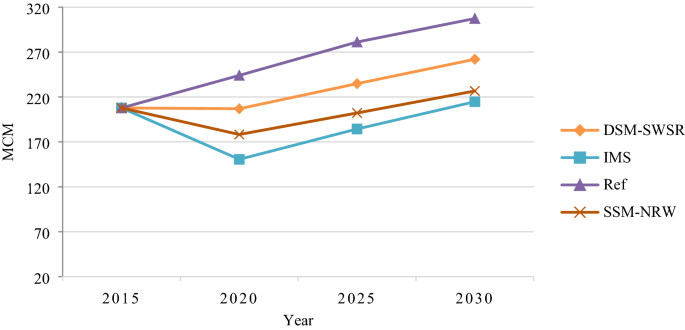
Unmet demand for management and reference
Future predicted scenario results
According to the analysis of different scenario and combination of other management options during this study, three possible cases for future water scenarios were considered (i.e. Optimistic, Moderate, and Pessimistic futures). Figure 6 presents the results of the future predicted scenarios. Based on the listed evaluation, future predicted scenario analysis and their comparative analysis with the reference scenario indicates that unmet water demand in the PF scenario will reach 728 MCM by 2030—over 130% increase compared to the Ref scenario. But, in the MF scenario, unmet water demand is expected to reach 283 MCM by 2030; about 8% reduction in comparison to the Ref scenario. On the other hand, the unmet water demand for the OF scenario grows to 164 MCM or 47% reduction in unmet water demand relative to the Ref case. This significant unmet demand reduction in OF scenario is because of the supply and demand side management improvement in in terms of percentage reduction of NRW by supply side and water reuse and recycle practice by the demand side management which in turn, reduce the amount of water wastage and increasing awareness in use of the water efficiently. Thus, from the analyzed scenarios, optimistic future strategies will support the management of the existing water supply and demand system of the city and it will also contribute to the realization of SDG6.
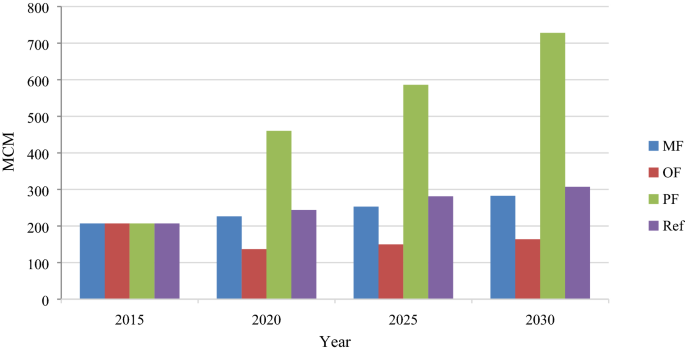
Unmet demand for future predicted scenarios
This study is not without limitations. The socioeconomic and demographic assumptions used in this study are based on historical and official projections. However, these projections are uncertain and may influence the results in long term perspectives. It is worthwhile to mention that factors like private ground water sources and water prices may affect water demand. Additionally, sedimentation and loss to ground water inside the reservoir may affect the amount of water supply. However, we did not consider these latent issues in this paper. While drawing on these limitations, it is believed that this study will go a long way to inform decision makers about the challenges and opportunities facing the country towards realizing SDG6. For future research, socioeconomic study is needed to evaluate the scenario when the price of water is changing over time as well as the investigation and acceptance of price and non-price policy. Further study could also consider private ground water sources and other non-counting water sources.
Consequently, the current study has a set of implications for the ordinary Ethiopians and the government. As can be seen in the external driven factors, increase in population and living standard will reduce water security in the country. Thus, there is a need for better synergy between the two desirable’s goals of water security and improved standard of living. At the demand side, households need to start incorporating best practices for water management. These practices include metering/measurement, replacing restroom fixtures with improved features such as sensors and low flow rate, rain water recovery among others. While these practices will improve water management, acquiring them will incur huge financial burden on the households given the current socioeconomic condition of the country. Thus, government need to provide targeted subsidies for these water smart appliances. At the supply side, government need to refurbish the infrastructures of the utility companies. This can be done by repairing the broken water pipelines, quick detection of leakages, distribution system audit and general maintenance of the water supply machines. Additionally, there will be need for massive awareness/campaign programs on different strategies to improve water security in the country. This can also be achieved via mainstream and social media. Other strategies in which the government can consider is to implement policies that will curtail population growth in the country. However, this will be very difficult to be implemented in a country like Ethiopia, where birth rate is also influenced by cultural and religious factors.
The study has used the WEAP model to explore different future strategies for improving water security in Addis Ababa, Ethiopia. The study considered different cluster of scenarios which encompasses external driven factors, water management options, and future predicted scenarios (i.e., a combination of the external driven factors and water management strategies). An increase in population growth and living standard will reduce the water security in the city and vice versa. However, the water management scenarios indicate that strategies such as recycling, reuse, better piping etc. can boost water security in the country. In fact, the study further suggests that low population growth rate combined with better demand and supply side management techniques will further boost the city’s water security as confirmed in the OF scenario of the Predicted Future scenarios cluster. Thus, to improve water security and achieve the SDG6 in Ethiopia will require the adoption of the strategies embedded in the OF scenario. In terms of policy options, government need to renovate and expand the infrastructures of the water utilities as well as provide targeted subsidies for households who cannot afford efficient potable water supply.
Availability of data and materials
Not applicable.
Abbreviations
Addis Ababa Water and Sewerage Authority
Central Statistical Agency
Demand Side Management Sustainable Water Sources and Reuse
Ethiopian Public Health Institute
High living standard
High population growth
Inter census projection
Integrated Management Strategy
Litters per day
Low population growth
Million cubic meter
Moderate future
National Meteorological Agencies
Nonrevenue water
Nonrevenue water reduction
Optimistic future
Office of the High Commissioner for Human Rights
Open Society Foundation
Pessimistic future
Sustainable Development Goal number six
Stockholm Environment Institute
Standard Precipitation Index
Supply Side Management Nonrevenue Water
United Nations International Children’s Emergency Fund
Water Evaluation and Planning
World Fit for Children
World Health Organization
Water Works Design and Supervision Enterprise
Abdelmalek MB, Nouiri I, Saidi F (2017) Application of the Water evaluation and Planning Model (WEAP) for Water Resources Management in the Nabhana Watershed, Center Tunisia. Proceedings of 1st Euro-Mediterranean Conference for Environmental Integration (EMCEI)
Admasie AM (2016) Assessment of the water demand management of condominium houses considering grey water reuse- the case of mickey Leland and Jemo Gara Addis Ababa. AAIT SCEE, Ethiopia
Google Scholar
Al-Omari A, Al-Quraan S, Al-Salihi A, Abdulla F (2009) A water management support system for Amman Zarqa basin in Jordan. Water Resour Manage 23:3165–3189. https://doi.org/10.1007/s11269-009-9428-z
Article Google Scholar
Amsal ED, Mebrate T (2016) An Integrated Approach to Water Supply Planning: Case of Addis Ababa (Nifas Silk Lafto sub city). Addis Ababa University Institute of Technology, Ethiopia
Arsiso BK, Tsidu GM, Stoffberg GH, Tadesse T (2017) Climate change and population growth impacts on surface water supply and demand of Addis Ababa, Ethiopia. Climate Risk Manag 18:21–33
Bharati L, Smakhtin VU, Anand BK (2009) Modeling water supply and demand scenarios: the Godavari-Krishna inter-basin transfer, India. Water Policy 11(Supplement 1):140–153. https://doi.org/10.2166/wp.2009.109
Cherie GG, Fentaw A (2015) Climate change impact assessment of dire dam water supply. AAUCED HES, Ethiopia
Consultancy Service for NRWR (1997) Water Audit and Benchmarking Report for Addis Ababa Water Supply System. Consultancy Service for Non-Revenue-Water reduction, Hydraulic modeling and GIS Development, Addis Ababa
CSA (1984) The 1984 population and housing census of Ethiopia, analytical report at national level, Ethiopia
CSA (1994) The 1994 Population and housing Census of Ethiopia results for Addis Ababa city administration, Volume I- Statistical report, Ethiopia
CSA (2007) The 2007 Population and Housing Census of Ethiopia: Statistical Report for Addis Ababa City Administration, third Population and Housing Census, Ethiopia
Dalle AE (2016) An Integrated Approach to Water Supply Planning: Case of Addis Ababa (Nifas Silk Lafto sub city). Addis Ababa University Institute of Technology, Ethiopia, Addis Ababa
David S, Upali A (2014) World water demand and supply, 1990 to 2025: scenarios and issues. Colorado State University, May 2014
Dioha MO, Kumar A (2019) Exploring greenhouse gas mitigation strategies for agriculture in Africa: the case of Nigeria. Ambio. https://doi.org/10.1007/s13280-019-01293-9
Ephrem M (2016) Development of a model for estimating non-revenue water in Addis Ababa (the case of bole sub city). AAiT, Ethiopia
Ethiopian-WFFC (2007) Report on Progress in Implementing the World Fit for Children Plan of Action in Ethiopia. WFFC, Addis Ababa
Getachew B (2003) Community health, water supply and sanitation. In McCornick PG, Kamara AB, Girma T, eds. Integrated water and land management research and capacity building priorities for Ethiopia. Proceedings of a MoWR/EARO/IWMI/ILRI international workshop held at ILRI, Addis Ababa, Ethiopia, 2-4 December 2002. IWMI and ILRI
Haddad A, Jayousi A, Hantash SA (2007) Applicability of WEAP as Water Management Decision Support System Tool on Localized Area of Watershed Scales: Tulkarem District in Palestine as Case Study. In proceeding of the Eleventh International Water Technology Conference (IWTC11), Sharm El-Sheikh, Egypt
Hamlat A, Errih M, Guidoum A (2013) Simulation of water resources management scenarios in western Algeria watersheds using WEAP model. Arab J Geosci 6:2225–2236. https://doi.org/10.1007/s12517-012-0539-0
Höllermann B, Giertz S, Diekkrüger B (2010) Benin 2025—balancing future water availability and demand using the WEAP ‘water evaluation and planning’ system. DOI, Water Resour Manage. https://doi.org/10.1007/s11269-010-9622-z
Book Google Scholar
Kassa M (2017) Evaluation of water supply and demand: the case of Shambu town, Western Oromia, Ethiopia. Int J Water Resour Environ Eng 9(5):96–101
Kou L, Li X, Lin J, Kang J (2018) Simulation of Urban water resources in Xiamen based on a WEAP model. Water MDPI 10:732. https://doi.org/10.3390/w10060732
Li X, Zhao Y, Shi C, Sha J, Wang Z, Wang Y (2015) Application of Water Evaluation and Planning (WEAP) model for Water resources management strategy estimation in coastal Binhai New Area, China. Ocean Coast Manag 106:97–109
Misikir T (2015) Effective groundwater resource management for Addis Ababa city. Addis Ababa University Institute of Technology, Addis Ababa
OHCHR (2002) Human rights, poverty reduction and sustainable development: health, food and water. World Summit on Sustainable Development, Johannesburg
Rheinheimer ED, Joshua H, Viers JH, Sieber J, Kiparsky M, Mehta VK, Ligare ST (2014) Simulating high-elevation hydropower with regional Climate warming in the west slope, Sierra Nevada. J Water Resour Plann Manage 140:714–723
Saraswat C, Mishra BK, Kumar P (2017) Integrated urban water management scenario modeling for sustainable water governance in Kathmandu Valley, Nepal
SEI (2011) WEAP water evaluation and planning system, users guide for WEAP21 and software help page. Stockholm Environment Institute, Boston
SEI (2014) WEAP water evaluation and planning system, users guide for WEAP21 and software help page. Stockholm Environment Institute, Boston
SEI (2016) Water Evaluation and Planning System (WEAP) Tutorial Modules. A collection of stand-alone modules to aid in learning the WEAP software. USA
Shahraki AS, Shahraki J, Hashemi Monfared SA (2016) An application of WEAP model in water resources management considering the environmental scenarios and economic assessment. Hirmand Catchment, Case Study
Stockholm Environmental Institute (2019) Water evaluation and planning system selected applications. https://www.weap21.org/index.asp?action=205&all=1 . Accessed 23 Aug 2019
Thompson T et al (2007) Chemical safety of drinking-water: assessing priorities for risk management. WHO publication, Geneva
Vicuña S, McPhee J, Garreaud RD (2012) Agriculture vulnerability to climate change in a snowmelt-driven basin in semiarid Chile. J Water Resour Plann Manage 138:431–441. https://doi.org/10.1061/(ASCE)WR.1943-5452.0000202
WEAP (2014) Water evaluation and planning. History and credits. Stockholm Environment Institute. http://www.weap21.org/index.asp?action=219 . Accessed 15 Mar 2019
WEAP (2016) WEAP water evaluation and planning system, Tutorial and users guide for WEAP21. A collection of stand-alone modules to aid in learning the WEAP software. Stockholm Environment Institute, Boston
WEAP (2017) WEAP water evaluation and planning system, a collection of stand-alone modules to aid in learning the WEAP software. Stockholm Environment Institute, Boston
WHO (2005) The World health report: 2005: make every mother and child count. WHO, Geneva
WHO & UNICEF (2006) Meeting the MDG drinking water and sanitation target: the urban and rural challenge of the decade. WHO, Geneva
WWDSE (2011) Water Works Design and Supervision Enterprise Addis Ababa Groundwater Development, Design and Supervision project, January 2011
WWDSE (2014) Addis Ababa deep wells water supply projects, phase III B completion report. WWDSE, Addis Ababa
Yates D, Purkey D, Sieber J, Huber-Lee A, Galbraith H, West J et al (2009) Climate driven water resources model of the Sacramento Basin, California. J. Water Resour Plann Manage 135:303–313. https://doi.org/10.1061/(ASCE)0733-9496(2009)135:5(303)
Zhang X, Zhang H, Chen B, Chen G, Zhao X (2008) Water resources planning based on complex system dynamics: a case study of Tianjin city. Commun Nonlinear Sci Numer Simul 13:2328–2336
Download references
Acknowledgements
We gratefully acknowledge the contributions of Dr. Prateek Sharma of TERI School of Advanced Studies, India. We want to express our greatest appreciation to Addis Ababa Water and Sewerage Authority (AAWSA) as well as National Meteorological Agencies (NMA) for providing necessary data and information for this study. We are grateful to Stockholm Environment Institute (SEI) for the license of the Water Evaluation and Planning (WEAP) system software. This research was also supported in part by Open Society Foundation (OSF). Finally, the opinions expressed herein are the authors’ own and do not necessarily express the views of OSF, AAWSA, and NMA.
Author information
Authors and affiliations.
Ethiopian Public Health Institute, P.O.Box: 1242, Addis Ababa, Ethiopia
Zinabu Assefa Alemu
Department of Energy & Environment, TERI School of Advanced Studies, 10 Institutional Area, Vasant Kunj, New Delhi, 110 070, India
Zinabu Assefa Alemu & Michael O. Dioha
You can also search for this author in PubMed Google Scholar
Contributions
ZA performed the data entry in WEAP, statistical analysis of results, data interpretation and writing the manuscript. MO performed the study design and helped to draft and edit the manuscript. Both authors read and approved the final manuscript.
Corresponding author
Correspondence to Zinabu Assefa Alemu .
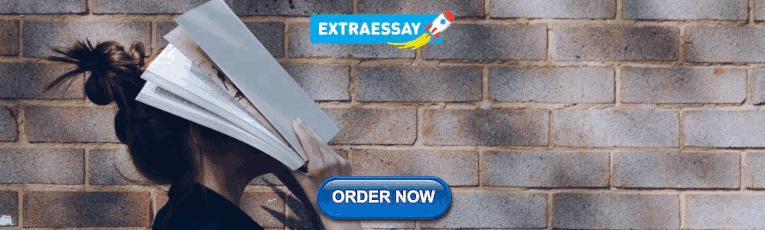
Ethics declarations
Ethics approval and consent to participant, consent to publication, competing interests.
The authors have no competing interest to declare.
Additional information
Publisher's note.
Springer Nature remains neutral with regard to jurisdictional claims in published maps and institutional affiliations.
Rights and permissions
Open Access This article is licensed under a Creative Commons Attribution 4.0 International License, which permits use, sharing, adaptation, distribution and reproduction in any medium or format, as long as you give appropriate credit to the original author(s) and the source, provide a link to the Creative Commons licence, and indicate if changes were made. The images or other third party material in this article are included in the article's Creative Commons licence, unless indicated otherwise in a credit line to the material. If material is not included in the article's Creative Commons licence and your intended use is not permitted by statutory regulation or exceeds the permitted use, you will need to obtain permission directly from the copyright holder. To view a copy of this licence, visit http://creativecommons.org/licenses/by/4.0/ .
Reprints and permissions
About this article
Cite this article.
Alemu, Z.A., Dioha, M.O. Modelling scenarios for sustainable water supply and demand in Addis Ababa city, Ethiopia. Environ Syst Res 9 , 7 (2020). https://doi.org/10.1186/s40068-020-00168-3
Download citation
Received : 08 September 2019
Accepted : 15 April 2020
Published : 20 April 2020
DOI : https://doi.org/10.1186/s40068-020-00168-3
Share this article
Anyone you share the following link with will be able to read this content:
Sorry, a shareable link is not currently available for this article.
Provided by the Springer Nature SharedIt content-sharing initiative
- Water supply
- Water demand
- Addis Ababa

- < Previous
Home > Graduate Theses, Dissertations, and Problem Reports > 9245
Graduate Theses, Dissertations, and Problem Reports
Water demand management for casablanca, morocco..
Mohamed Lahlou
Date of Graduation
Document type.
Dissertation/Thesis
Water shortages could significantly curtail economic growth and development in Casablanca, Morocco. Conservation programs in Morocco have been implemented only occasionally, usually during a water shortage crisis arising either from technical reasons or from drought. Public awareness activities suffer from a lack of effectiveness and do not fulfill the desired objectives. The purposes of this study are to examine water consumption characteristics in Casablanca and develop approaches for water management to help assure that shortages will not undermine the prospects for sustainable economic development. Research procedures used were the development and estimation of water demand models for the residential/commercial, industrial, and institutional sectors, forecasts of water demand to 2010, and simulation of the effects of a complex of water conservation methods on the forecasted demands. The analysis of water demand is made through development and estimation of regression models, while the forecasts and simulations are carried out using the Institute of Water Resources Municipal and Industrial (IWR-MAIN) system developed for the U.S. Corps of Engineers. The results indicate that residential and commercial water demand has a price elasticity which is relatively inelastic ({dollar}-{dollar}0.448). Industrial water demand, although of unexpected sign, is responsive to price (1.267) and temperature ({dollar}-{dollar}4.038). Precipitation and GDP coefficients were not statistically significant. Institutional water demand is slightly responsive to price ({dollar}-{dollar}0.648). Temperature, precipitation and GDP were not statistically significant. The appropriateness and desirability of any given conservation method must be accepted by the community and the water utility. Thus, to have positive impacts on water management, water conservation measures must be planned as part of the whole water resources management strategy. The conservation approaches used in the simulations included public education, plumbing code revisions to require use of water conservation devises, leak detection and repair, pricing policy, metering, and pressure reduction. The results indicate that considerable saving in water use can be attained through a comprehensive water demand management program.
Recommended Citation
Lahlou, Mohamed, "Water demand management for Casablanca, Morocco." (1998). Graduate Theses, Dissertations, and Problem Reports . 9245. https://researchrepository.wvu.edu/etd/9245
Since October 13, 2021
- Collections
- Disciplines
- WVU Libraries
- WVU Research Office
- WVU Research Commons
- Open Access @ WVU
- Digital Publishing Institute
Advanced Search
- Notify me via email or RSS
Author Corner
- Submit Research
Home | About | FAQ | My Account | Accessibility Statement
Privacy Copyright
Academia.edu no longer supports Internet Explorer.
To browse Academia.edu and the wider internet faster and more securely, please take a few seconds to upgrade your browser .
Enter the email address you signed up with and we'll email you a reset link.
- We're Hiring!
- Help Center
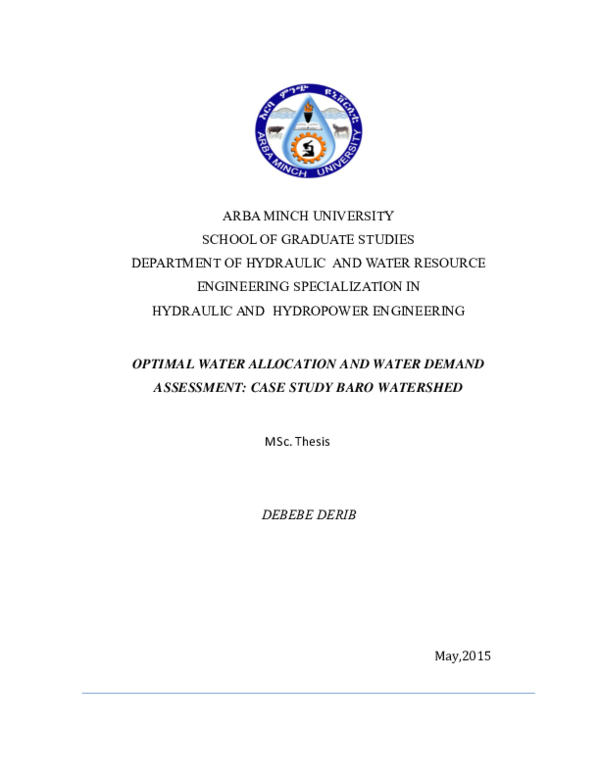
Optimal Water Allocation and Water Demand Assessment: Case Study Baro Watershed

Watershed is considered to be the ideal unit for management of the natural resources. Extraction of watershed parameters using (GIS) and use of mathematical models is the current trend for hydrologic evaluation of watersheds. The aim of this thesis is to oversee the effect future water resource development projects on the Baro watershed using SWAT models to assess water availability and WEAP mo dels in assess the water demand of the study area. The SWAT model was calibrated and validated for measured stream flow at Baro near Gambela gauging stations. The calibration and validation result showed that model performance evaluation statistics (coefficient of determination (R 2 ) and Nash-Sutcliffe model efficiency (ENS)) were in the acceptable range (R 2 =0.76 ,E NS =0.72 for calibration and R 2 =0.7,E NS=0.63 for validation). This indicates that the observed values show good agreement with simulated flow value. In this study the watershed yields average annual surface runoff of 425.07 ...
- We're Hiring!
- Help Center
- Find new research papers in:
- Health Sciences
- Earth Sciences
- Cognitive Science
- Mathematics
- Computer Science
- Academia ©2024
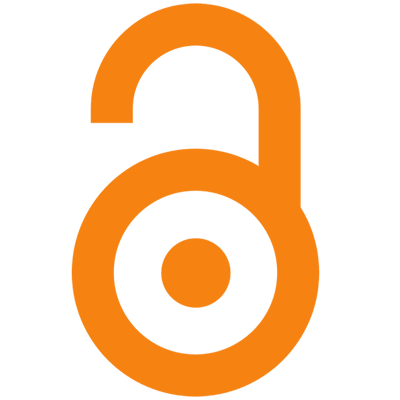
- Next Article
INTRODUCTION
Materials and methods, results and discussion, conclusions, acknowledgements, data availability statement, conflict of interest, assessment of future urban water demand and supply under socioeconomic scenarios: a case of assosa town.
- Article contents
- Figures & tables
- Supplementary Data
- Open the PDF for in another window
- Guest Access
- Cite Icon Cite
- Permissions
- Search Site
Yadeta Saketa; Assessment of future urban water demand and supply under socioeconomic scenarios: a case of Assosa town. Water Supply 1 October 2022; 22 (10): 7405–7415. doi: https://doi.org/10.2166/ws.2022.329
Download citation file:
- Ris (Zotero)
- Reference Manager
Water scarcity is becoming a progressively more serious global issue. Assosa town in Ethiopia faces serious water scarcity problems due to rapid population growth and urban expansion. This study aims to model the water demand of Assosa town using a forecasting model. Four scenarios were developed: population growth, living standards, water loss reduction, and a combination of these. The water demand and unmet demands for each scenario were evaluated. Results show that the demand for water and supply will vary significantly if the present state continues. In the base year (2018), the overall water demand is 2.07 gigalitres (GL) and the unmet demand is estimated as 0.096 GL. The water demand grows to 3.71 GL under the reference scenario in 2035. The combination of population growth and improved living standard scenarios is observed to impact greatly on water demand. The total water demand of this scenario was estimated to be 7.14 GL latterly in the projection period and the unmet demand would grow to 5.2 GL. The results confirmed that improved water management approaches are needed in the town to ensure the sustainability of water resources in the long term and outline proper water utilization policies.
Assessing the current and future urban water demand and supply gaps provides significant information.
The study analyzes the water demand and proposes options to improve the performance of the water supply system.
The study helps to inform the concerned authorities in conserving water resources for sustainable use.
Graphical Abstract

Water is an essential natural resource that plays a central role in human welfare and the environment, and is the primary resource for daily human activity, industrial operation, and agricultural production ( Pandey et al. 2019 ). The demand for water resources is rising all over the world ( Xu et al. 2017 ). Equally, water scarcity is among the limiting factors in local socioeconomic development. Water scarcity is mainly caused by climate change, rapid urbanization, population growth, increasing demand for economic development, pollution, and over-exploitation of water resources ( Divakar et al. 2011 ; MacDonald et al. 2011 ; Roozbahani et al. 2015 ). These contributory factors greatly impact the hydrological cycle and cause the decline of available water resources ( Wang et al. 2015 ; Xu et al. 2017 ).
Several countries in the world face a significant problem in sustaining reliable water supplies. This phenomenon is anticipated to continue in the future ( Kassa 2017 ). In Ethiopia, the distribution and availability of water are spatially and temporary erratic and water scarcity is the most important problem ( Seleshi 2006 ; Kassa 2017 ). In urban centers, for instance Addis Ababa, the gap between water supply and water demand is huge. The non-functionality of water supply sources, increasing problems of water shortage, high population growth and related factors are the main factors for supply–demand imbalance and are most challenging to water supply sectors ( Alemu & Dioha 2020 ).
Similarly, Assosa town is one of the water-scarce areas in Ethiopia where water management in a sustainable way is not considered in addressing the issue of increasing water demand. The inhabitants in Assosa town are in a severe water shortfall, getting water just once per week and sometimes once in two weeks. The limited freshwater resources and progressive growth in water demand are likely to increase in the future. This identifies the importance of using water demand prediction tools as a possible approach for optimizing the management of water and planning for future water status under various scenarios.
Precisely assessing the water demand plus water supply in areas with water scarcity provides significant information to support water resources management. However, water utilities directors are facing a challenge owing to the improbability of getting information about the capability of the existing water system under possible rapid increase in urban water demand. These issues inspire me to take part in the urban water planning that would provide managers with technical and more precise approaches regarding future water demand to reduce uncertainty. This involves analyzing the current water system, estimating the future unmet demand, and identifying the supply–demand gap to administer an efficient and regulated water supply system.
Researchers in different parts of the world have used different models for water resource assessments. Of these, the Water Assessment and Planning Tool (WEAP) has been widely used in different areas of the globe in recent decades ( Asghar et al. 2019 ). The WEAP model is commonly utilized due to its effectiveness in analyzing the designed strategies along with scenarios based on ‘what if’ conditions ( SEI 2012 ). Kou et al. (2018) used the WEAP to develop a water resource simulation. The authors evaluated different water-saving scenarios to produce water-saving potentials and established that preclusion of future water shortages necessitates the realization of water-saving actions and the use of new water supply sources. Albalwai (2015) investigated ways to take action over increasing water demand through a water demand management approach in Saudi Arabia.
Brown et al. (2019) also estimated the water shortages using the WEAP model in watersheds of the USA. The authors discussed the impacts of population increase and climate change, and analyzed the monthly water demand and water supply. The result of their study indicated measures such as enhancements in reservoir storage, reducing water demand, and withdrawal efficiency improvements as possible adaptations to overcome future demand shortages. Metobwa et al. (2018) used the WEAP system to assess the demand for water and the supply of the Mara River, Kenya. The study focused on scenario-based future water demand evaluation and addressed the growing water demand affecting the Mara River, and different approaches were considered to alleviate overuse practices. Alemu & Dioha (2020) employed the WEAP modeling structure to examine water demand and supply using different scenarios in the capital city of Ethiopia.
Although the applications of the WEAP system have been frequent in different parts of the world, studies that analyze water demand strategies in Ethiopia's particular urban water system are still limited. Therefore, this study evaluates the existing condition of water demand and investigates scenarios for water demand in Assosa town. The study considers different socioeconomic factors like population growth, water use rates and leak reduction in the distribution system. The result of the study can be used to inform the urban water management sector and other concerned authorities.

Location map of the study area.
Status of the present water supply sector in Assosa town
The present water supply source of Assosa town is groundwater from boreholes constructed in different years. The first borehole source was developed in the year 1984. At present, there are 11 existing boreholes; of these only eight are giving service for the town whereas the other three wells are not functioning because of low yield and drainage. The total yield of water from these well is 63.3 litres per second ( ATWSSE 2019 ). The water from these wells is pumped to service reservoirs and then distributed to consumers through private connections and public fountains.
The water demand of Assosa town has progressively increasing and it is the main task of ATWSSE to supply safe and adequate drinking water for the population. In the town, the main water supply requirement originates from domestic consumption. The domestic customers are those that are registered as private users and those that obtain water from a public fountain. Since Assosa town has experienced serious water deficit problems, ATWSSE has been attempting to conquer this problem. The need for a sufficient water supply system for the town has been acknowledged by the ATWSSE as one of the most important factors affecting public health and socioeconomic development in the town. However, the water requisite of the town is still uncertain. This makes desirable a variety of water evaluation and planning systems, which is helpful in using the available water wisely and efficiently besides increasing the number of water sources.
The WEAP model, originally developed by the Stockholm Environmental Institute, is a scenario-based water assessment and planning system tool which is very practical for water resource assessment ( Gao et al. 2017 ). Its system plus the data structure and level of detail may be easily adapted to meet the requirements of a particular study ( Alemu & Dioha 2020 ). The model applies a scenario approach to assess water resources availability and socioeconomic activities with water and their allocation for present and future periods. The WEAP model contains different approaches for modeling water balance processes ( Sieber & Purkey 2015 ). The Soil Moisture Model is considered the most efficient method for simulating the processes of the hydrological cycle ( Ougougdal et al. 2020 ). The model requires various input variables like precipitation, temperature, vegetation cover, relative humidity, and latitude; using these variables, the water balance components such as infiltration, runoff and evapotranspiration are estimated ( Olsson et al. 2017 ).
The input data in this study are grouped as water supply, water demand, and socioeconomic data. The water supply data includes climatic data such as temperature and rainfall, which are used to characterize the area. The other water supply data includes the local reservoir and groundwater data. The water demand data contains domestic water use and water loss data in the distribution system. The home water utilization rate data and other related information were collected from ATWSSE. Furthermore, the socioeconomic data, which includes the population census data for Assosa town and population growth rate, was used to project the population of the town. The standard water use rate was obtained from the Ethiopia GTP (Growth and Transformation Plan) 2 document and WHO (2006) . The population data and water use are used to develop alternative scenarios that examine how the total as well as the disaggregated water uses change in due course.
Study procedures

Study framework.
Water demand calculation algorithm
Scenario development.
Scenarios are self-consistent storylines showing how the future system might evolve under a specific set of policy improvement or technology conditions and in specific socioeconomic states ( Sieber & Purkey 2011 ). The scenario analysis enables the answering of ‘what if’ questions. The future water demand of the town might be affected by various socioeconomic factors such as population growth, the living standards of the people, water losses, and others. To understand the effects of these, different assumptions are proposed. In this study, four scenarios were set up to explore the water demand and supply of Assosa town. The water demand, water delivered, supply requirement, and the unmet demands for each scenario were evaluated using the WEAP model.
Basic assumptions
The basic assumptions in the WEAP model incorporate population number, per capita daily consumption (litre/day), annual water consumption (m 3 /capita/year), growth rate (%), and losses in the water distribution network (%). The year 2018 is taken as the base year to set up the model. The total population of the town in the base year is 93,870. The per capita daily water use rate is considered as 50 litres per day based on the Growth and Transformation Plan 2 of Ethiopia. The population growth rate is taken to be 3.4%. The current water losses in the distribution network are assumed to be 25% ( Kassa 2017 ).
Reference scenario
In the reference scenario, all assumptions remain the same. All the socioeconomic factors, population factors, and water use rates are assumed to grow at the growth rate of 3.4% with existing policies. It is used as a point of reference for comparison with other scenarios. Other scenarios are described below.
Scenario-1: high population growth (HPG)
Assosa town is characterized as having fast population growth due to natural factors and local migration from rural areas to the town. This scenario considers the consequences of an increase in population on water supply and demand. This scenario assumes an annual population growth rate of 4.5% for the years 2019 to 2035.
Scenario-2: improved living standards (ILS)
It is predictable that the water demand of the household will increase consistent with improved living standards of the people. This implies the per capita water demand will be increased and considered for the indication of improved living standards. Hence 100 litres per day per capita is taken in this scenario.
Scenario-3: high population growth plus improved living standards (HPG + ILS)
This scenario considers the combined effects of a high population growth rate and the improved living standards of the people. Thus, an annual growth rate of 4.5% and per capita water demand of 100 litres per day is used.
Scenario-4: reducing water loss (RWL)
The water supply system faces various challenges like the old pipelines of the distribution system and leakage. The current water loss in the Assosa town water distribution network is taken as 25%, caused by leaks in the distribution system. Hence, it is assumed that the water supply sector applies different water-saving technologies and replaces the old pipelines with new ones to reduce the water losses in the water distribution network. By applying different water-loss-lessening strategies this scenario considered loss reduction to be 10% in the distribution networks.
Water demand of the town
Municipal water resource evaluation is essential for increasing water efficiency, particularly in severe water scarcity areas. In this paper, the evaluation of the impact of the major socioeconomic issue on water demand is predicted through the formation of scenarios in the WEAP model which are developed in the form of key assumptions reflecting the different scenarios to be scrutinized. In the current account year, the entire water requirement is 2.07 GL and unmet demand is observed to be equal to 0.096 GL. The unmet demand, which represents the gap between water supply and water required, is the lowest accounting for 4.6% of the total demands. From the model result, the domestic water demand is 95.4% met in 2018. The water demand between months appears to be different. These monthly changes in water demand are related to the monthly variation in water consumption. The demands are larger during the months of March (0.32 GL), April (0.31 GL), and February (0.29 GL) and lower during August and September (0.17 GL). The results show that Assosa faces severe water shortfall during its dry season: February, March, and April. The monthly water requirement changes are estimated by assigning the yearly demands into months based on the exact number of days in each month. Hence, the monthly unmet water is anticipated only on the supply side. Nevertheless, the water shortage is related to both water demand and supply. The water system of the town is a significant element for optimal water resources utilization and it is imperative to collect the long-term basic data on a monthly and daily basis for WEAP modeling in the future.
Scenario evaluation
Base-case scenario.
To examine the future conditions of the municipal water system in Assosa, the WEAP model was firstly run with the reference scenario. This scenario projection illustrates a situation in which there is no water supply and demand infrastructure development. It takes into account a population growth rate of 3.4%. Using this growth rate, the population is projected to be 168,466 by 2035. Since the demand for water use is reflected in population growth, it will continue to grow at the same rate as the population. The WEAP model result for water demand indicates that the water requirement is likely to grow from 2.07 GL in 2018 to about 3.71 GL in 2035. This shows the increase in water demand will be about 44.2% in 17 years. The increase in water demand is primarily caused by an increase in future population, while the water supply remains constant. The water delivered is maintained constantly until 2035. The unmet water demand starts in 2018 at 0.096 GL and rises to 1.74 GL in 2035. This result shows an increase of unmet water demand in the town by 94.48% within 17 years. The disquieting rise of unmet demand is a call to the relevant authority to develop realistic strategies to prevent water shortage crisis in the future.
This scenario corresponds to the change in population growth rate from 3.4% to 4.5%. The result of population projection using this growth rate shows a rise in the population to 198,382 capita in 2035. This indicates that rapid population growth will expose the town to a water shortage problem in 2035. The WEAP model result of water demand analysis shows that the water demand will increase from 2.07 GL in 2018 to 4.36 GL in 2035. The sum of water demand is increased by 0.65 GL from the reference scenario. The increase in water demand affectes the supply requirements and conforms to population growth having a very important long-term impact on municipal water demand. The water demand shortfall is seen to grow to 2.4 GL by 2035, which is 0.66 GL higher than the reference scenario. This shows a need for the implementation of effective water resources management strategies and adaptation of new technologies to address the water deficit problems.

Water demand under the reference and three scenarios (MCM = 1GL).

Unmet water demand of the reference and three scenarios.
The combination of these two scenarios has significant impact on the future water demand of the town. Water demand greatly increased for this scenario. The result indicates water demand was estimated to increase from 2.07 GL in 2018 to about 7.14 GL in 2035 ( Figure 3 ). This implies that an increase in population growth together with an increase in living standards will create serious water scarcity problems for the town in the future, requiring a practical step to be taken to prevent this circumstance. The water demand shortfall for the high population growth plus improved living standard scenarios will grow to 5.2 GL ( Figure 4 ). This represents about 98.2% increase from the reference scenario.

Unmet demands under reducing water loss scenario.
The findings of this study showed that water deficiency was observed in the dry season, especially in March. All scenarios except water loss reduction showed an increase of unmet demand over all the simulation years. The shortage of water is aggravated by the high population growth and improved living standards. The population in Assosa town is likely to rise in the future and put more pressure on the limited water resources of the town. The trend of higher per capita water utilization coupled with a high population growth scenario in the town could mean that it faces more water deficit in the future. However, sustainable water management strategies in the town may conquer the practical problems that could affect the available water resources. This may be done by rainwater harvesting, the application or inclusion of a sustainable urban drainage system, developing reservoirs to store more water to use during the dry season, and creation of awareness among the public on the efficient and sustainable utilization of water. Further, additional water supply exploitation should be done to improve the water scarcity and expansion of supplementary water supply lines or reservoirs should be considered.
The WEAP model in this study could have advantages in water resources optimization studies, as it calculates water demand at all water use sites. In advance, WEAP can evaluate the realization effects of all significant policies and measures. The socioeconomic assumptions used are derived from official projections, which are uncertain and possibly will influence the results in long-term perceptions. This study will go far to notify decision-makers and relevant authorities about the challenges facing the town in realizing the supply of adequate water to its communities. The private groundwater and other non-counting water sources are not considered in this study and hence need further study. As a result, this study has a set of inferences for the municipality level or regional government and could be used as a preliminary study for future research. The results confirm the need to establish the way of integrating best practices for water resources management such as rainwater recovery and the need to renew the ageing infrastructures of the water supply utility for water loss reduction. This involves repairing the broken water distribution pipelines, water distribution system inspection, immediate detection of leakage sites, and overall maintenance works of the water supply utilities. The other basic strategy to be considered to make a safe water supply is to implement policies that will limit population growth.
The WEAP system was used to model water demand and create scenarios to assess future water availability in Assosa town. The result shows that water scarcity is a challenge in the future in the town. High population growth and improved living standards will be the main drivers of domestic water scarcity and exacerbate the difference between water demand and unmet demand. The findings of this study can be summarized as: (1) the model gives a reasonable evaluation of future water demand of the town. The results indicate that more than 3.71 GL of water will be required in 2035 to satisfy water needs with the current water supply and socioeconomic trends. (2) The model results show that the water demand would reach about 4.36 GL in 2035 at a population growth rate of 4.5%. (3) Leakage reduction in the water distribution system is a significant aspect of reducing the water supply requirement. It is observed that reducing the water loss in the distribution networks from 25% to 10% reduces the unmet demand from 1.74 to 1.22 GL in 2035 under the reference scenario. (4) In the improved living standards scenario the total water demand in 2035 is estimated as 5.17 GL and the unmet demand is 4.1 GL. (5) The water requirement under the combined effects of the high population growth and improved living standards scenario would be 7.14 GL. The unmet demand is found to be 5.2 GL in 2035. Based on the analysis, all scenarios reveal water deficiency in the future. Thus, enhanced water management plans are needed in the town such as pertaining to a water management plan to conserve water at the household level; arrangement for maintenance of the water distribution system network to reduce water losses and leakage; and supplementary development of water storage reservoirs to increase the quantity of water delivered plus storage of water during the rainy season.
This research received no external funding.
The author would like to thank those agencies who provided me with the necessary data for this study. I am grateful to SEI for the WEAP software license granted to me.
All relevant data are included in the paper or its Supplementary Information.
The author declares there is no conflict.
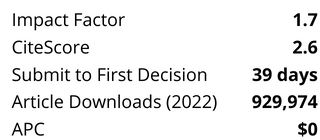
Affiliations
- ISSN 1606-9749 EISSN 1607-0798
- Open Access
- Collections
- Subscriptions
- Subscribe to Open
- Editorial Services
- Rights and Permissions
- Sign Up for Our Mailing List
- IWA Publishing
- Republic – Export Building, Units 1.04 & 1.05
- 1 Clove Crescent
- London, E14 2BA, UK
- Telephone: +44 208 054 8208
- Fax: +44 207 654 5555
- IWAPublishing.com
- IWA-network.org
- IWA-connect.org
- Cookie Policy
- Terms & Conditions
- Get Adobe Acrobat Reader
- ©Copyright 2021 IWA Publishing
This Feature Is Available To Subscribers Only
Sign In or Create an Account
- Bibliography
- More Referencing guides Blog Automated transliteration Relevant bibliographies by topics
- Automated transliteration
- Relevant bibliographies by topics
- Referencing guides
Dissertations / Theses on the topic 'Urban water demand'
Create a spot-on reference in apa, mla, chicago, harvard, and other styles.
Consult the top 43 dissertations / theses for your research on the topic 'Urban water demand.'
Next to every source in the list of references, there is an 'Add to bibliography' button. Press on it, and we will generate automatically the bibliographic reference to the chosen work in the citation style you need: APA, MLA, Harvard, Chicago, Vancouver, etc.
You can also download the full text of the academic publication as pdf and read online its abstract whenever available in the metadata.
Browse dissertations / theses on a wide variety of disciplines and organise your bibliography correctly.
Gato, Shirley, and s3024038@rmit edu au. "Forecasting Urban Residential Water Demand." RMIT University. Civil, Environmental and Chemical Engineering, 2006. http://adt.lib.rmit.edu.au/adt/public/adt-VIT20070202.113452.
Creese, Edward E. "Urban water systems, demand management and sustainable development." Thesis, National Library of Canada = Bibliothèque nationale du Canada, 2000. http://www.collectionscanada.ca/obj/s4/f2/dsk1/tape4/PQDD_0024/NQ51186.pdf.
Moreno, Cherry. "Urban water demand model: the case study of Emilia Romagna (Italy)." Master's thesis, Alma Mater Studiorum - Università di Bologna, 2013. http://amslaurea.unibo.it/5938/.
Ralston, D. Peter (David Peter). "A water demand analysis for the Massachusetts Institute of Technology." Thesis, Massachusetts Institute of Technology, 1992. http://hdl.handle.net/1721.1/69296.
Niu, Huien. "Approaches to long-term forecasting of urban water demand in China." Thesis, University of Newcastle Upon Tyne, 1993. http://hdl.handle.net/10443/400.
Feltman, Lauren E. "Effects of COVID-19 Lockdown on Urban Water Demand in Cincinnati." University of Cincinnati / OhioLINK, 2021. http://rave.ohiolink.edu/etdc/view?acc_num=ucin162635694480124.
Klein, Daniel Richard. "Supporting the implementation of effective urban water conservation and demand management strategies." Thesis, University of British Columbia, 2017. http://hdl.handle.net/2429/62881.
House-Peters, Lily. "Examining the Effects of Climate Change and Urban Development on Water Demand: A Multi-Scale Analysis of Future Water Demand in Hillsboro, Oregon." PDXScholar, 2010. https://pdxscholar.library.pdx.edu/open_access_etds/2251.
Makki, Anas Ahmad. "Development of a Domestic Urban Water End-Use Demand Statistical Forecasting Model for South-East Queensland, Australia." Thesis, Griffith University, 2015. http://hdl.handle.net/10072/366422.
Mthethwa, Nkosinathi I. "Evaluating water conservation and water demand management in an industrialised city: a case study of the City of uMhlathuze in Richards Bay." Master's thesis, University of Cape Town, 2018. http://hdl.handle.net/11427/27976.
Cole, Graham. "Smart Meter Enabled Disaggregation of Residential Peak Water Demand: Implications for Urban Water Planning and Tariff Reform." Thesis, Griffith University, 2012. http://hdl.handle.net/10072/367964.
Nambinga, Linekela Elias. "Review and gap analysis of Water Sensitive Urban Design (WSUD) in Windhoek, Namibia." Master's thesis, Faculty of Engineering and the Built Environment, 2019. http://hdl.handle.net/11427/30914.
Devi, Bhakti L. "A framework for development and evaluation of policies and programs for urban irrigation demand management." View thesis, 2009. http://handle.uws.edu.au:8081/1959.7/44535.
Maurer, Nathalie. "Modelling urban development trends and outdoor residential water demand in the Okanagan Basin, British Columbia." Thesis, University of British Columbia, 2010. http://hdl.handle.net/2429/17533.
Damkroger, Coral S. (Coral Sue). "The potential for demand side management in water and wastewater infrastructure planning : a case study from Argentina." Thesis, Massachusetts Institute of Technology, 1996. http://hdl.handle.net/1721.1/65459.
Casadei, Valentina. "Metabolism modelling of the Urban Water System of Oslo using the package UWOT." Master's thesis, Alma Mater Studiorum - Università di Bologna, 2016. http://amslaurea.unibo.it/11897/.
Ringgenberg, Sandra Lee 1961. "The proper specification of price in the demand for water in Tucson, Arizona: A case study." Thesis, The University of Arizona, 1992. http://hdl.handle.net/10150/278126.
Breyer, Elizabeth Yancey. "Household Water Demand and Land Use Context: A Multilevel Approach." PDXScholar, 2014. https://pdxscholar.library.pdx.edu/open_access_etds/1670.
Willis, Rachelle M. "Domestic Water End Use Study: An Investigation of the Water Savings Attributed to Demand Management Strategies and Dual Reticulated Recycled Water Systems." Thesis, Griffith University, 2011. http://hdl.handle.net/10072/367759.
Putnam, Merril Augusta. "Insight into the Use, Perception, and Value Surrounding Domestic Water in Peru: Envisioning Demand Management in an Intermittent, Small-City, Service Context." Scholar Commons, 2013. http://scholarcommons.usf.edu/etd/4750.
Sjömander, Magnusson Therése. "Urban Water Security – Local Conditions and Regional Context : A case study of attitudes and water use behaviour in Windhoek, Namibia." Doctoral thesis, Linköpings universitet, Tema vatten i natur och samhälle, 2005. http://urn.kb.se/resolve?urn=urn:nbn:se:liu:diva-4893.
Van, Rooijen Daniel J. "Implications of urban development for water demand, wastewater generation and reuse in water-stressed cities. Case studies from South Asia and sub-Saharan Africa." Thesis, Loughborough University, 2011. https://dspace.lboro.ac.uk/2134/8986.
Becker, Jamie Erin. "The influence of urbanization on arthropod water demand and lipid and protein consumption in mesic environments." Bowling Green State University / OhioLINK, 2017. http://rave.ohiolink.edu/etdc/view?acc_num=bgsu1510869685967938.
Qi, Cheng. "Systems Analysis for Urban Water Infrastructure Expansion with Global Change Impact under Uncertainties." Doctoral diss., University of Central Florida, 2012. http://digital.library.ucf.edu/cdm/ref/collection/ETD/id/5441.
Mutailifu, Kalibeinuer. "Water and Wastewater Management in the Mega City Istanbul : A General Analysis from a Supply-Demand-Reuse Perspective." Thesis, Linköpings universitet, Institutionen för tema, 2019. http://urn.kb.se/resolve?urn=urn:nbn:se:liu:diva-162848.
Yen, Jeffrey Lee. "A system model for assessing water consumption across transportation modes in urban mobility networks." Thesis, Georgia Institute of Technology, 2011. http://hdl.handle.net/1853/39527.
Hölscher, Marie-Therese [Verfasser], Gerd [Akademischer Betreuer] Wessolek, Thomas [Akademischer Betreuer] Nehls, Gerd [Gutachter] Wessolek, Christoph [Gutachter] Thomas, and Eva Nora [Gutachter] Paton. "Quantification of cooling effects and water demand of urban facade greenings / Marie-Therese Hölscher ; Gutachter: Gerd Wessolek, Christoph Thomas, Eva Nora Paton ; Gerd Wessolek, Thomas Nehls." Berlin : Technische Universität Berlin, 2018. http://d-nb.info/1169210457/34.
Sanchez, Diego Cesar. "Estudo para caraterização da demanda urbana de água no setor residencial da cidade de São Paulo." Universidade de São Paulo, 2007. http://www.teses.usp.br/teses/disponiveis/3/3146/tde-15022008-095605/.
Martinez, Jonathan Justen de La Vega. "Otimização estocástica na programação de bombas em redes de abastecimento urbano." Universidade Federal de São Carlos, 2014. https://repositorio.ufscar.br/handle/ufscar/3828.
Lemos, Diogo Siqueira. "Urban metabolism of Aveiro: LCA of the city demands and water cycle." Master's thesis, Universidade de Aveiro, 2011. http://hdl.handle.net/10773/7419.
Figueiredo, S?lvio Luiz de. "Estimativa da demanda residencial urbana de ?gua: o caso da cidade de Te?filo Otoni." UFVJM, 2017. http://acervo.ufvjm.edu.br/jspui/handle/1/1667.
Landa, Silvia Anastasia. "Optimizing Sustainable Integrated Use of Groundwater, Surface Water and Reclaimed Water for the Competing Demands of Agricultural Net Return and Urban Population." DigitalCommons@USU, 2016. https://digitalcommons.usu.edu/etd/5026.
Carvalho, Jano Orlando Fontes de. "Otimização do uso de água em uma unidade de TDI no pólo petroquímico de Camaçari - Bahia." Universidade Catolica de Salvador, 2009. http://ri.ucsal.br:8080/jspui/handle/prefix/430.
Liao, Wei-Chin, and 廖偉欽. "Urban Water Demand Forecasting Using Machine Learning." Thesis, 2019. http://ndltd.ncl.edu.tw/handle/358tkg.
Altai, Zulgerel. "Urban water demand management in Ulaanbaatar, Mongolia." Thesis, 2013. https://researchonline.jcu.edu.au/29889/1/29889_Altai_2013_thesis.pdf.
Barua, Suchana. "Non-residential Urban Water Demand Modelling – a Disaggregation Approach." Thesis, 2018. https://vuir.vu.edu.au/36759/.
Diaz, Cobos Carlos Augusto. "Urban water demand management : a focus on energy use." 2005. http://link.library.utoronto.ca/eir/EIRdetail.cfm?Resources__ID=370118&T=F.
(8274048), Renee L. Obringer. "Climate change effects on urban water resources: An interdisciplinary approach to modeling urban water supply and demand." Thesis, 2020.
CHEN, XIN-TING, and 沈信廷. "The demand of urban water uses:a case of study of water users on industrial water uses and residential water uses in Kaohsiung city." Thesis, 1990. http://ndltd.ncl.edu.tw/handle/57524149535849758166.
GAO, HUI-LING, and 高惠玲. "A study on the benifit of water resource conservation by forest application of household water demand in urban area." Thesis, 1992. http://ndltd.ncl.edu.tw/handle/11454796289027557337.
Ramulongo, Luvhimba. "An evaluation of urban household water demand and consumption in Vhembe District: a case study of Makhado Local Municipality, Limpopo, South Africa." Diss., 2016. http://hdl.handle.net/11602/822.
Fortier, Julia Maureen. "Examining the Social Acceptability of Cisterns in Rainwater Harvesting for Residenital Use in the Region of Waterloo, Ontario." Thesis, 2010. http://hdl.handle.net/10012/5242.
Viljoen, Nina Susara. "The feasibility of rainwater and stormwater harvesting within a winter rainfall climate context: a commercial building focus." Diss., 2014. http://hdl.handle.net/10500/14391.
Thank you for visiting nature.com. You are using a browser version with limited support for CSS. To obtain the best experience, we recommend you use a more up to date browser (or turn off compatibility mode in Internet Explorer). In the meantime, to ensure continued support, we are displaying the site without styles and JavaScript.
- View all journals
- My Account Login
- Explore content
- About the journal
- Publish with us
- Sign up for alerts
- Open access
- Published: 26 April 2024
Energy trade tempers Nile water conflict
- Mikiyas Etichia ORCID: orcid.org/0000-0001-7372-3769 1 ,
- Mohammed Basheer ORCID: orcid.org/0000-0001-9468-2249 1 , 2 , 3 ,
- Ruben Bravo ORCID: orcid.org/0000-0002-9402-5588 1 ,
- Jose Gutierrez 1 ,
- Atsede Endegnanew ORCID: orcid.org/0000-0002-7924-0773 4 ,
- Jose M. Gonzalez ORCID: orcid.org/0000-0002-4545-0692 1 ,
- Anthony Hurford ORCID: orcid.org/0000-0001-9503-2117 5 ,
- James Tomlinson 1 ,
- Eduardo Martinez 1 ,
- Mathaios Panteli ORCID: orcid.org/0000-0003-4274-529X 1 , 6 &
- Julien J. Harou ORCID: orcid.org/0000-0003-1827-6155 1 , 7
Nature Water volume 2 , pages 337–349 ( 2024 ) Cite this article
65 Altmetric
Metrics details
- Energy grids and networks
The demand for energy, water and food in Africa continues to increase, resulting in growing pressure on contentious multisector resource systems like the River Nile. The ongoing dispute over Nile resources could become a zero-sum game if addressed from a water-centric viewpoint. Understanding how energy system management impacts water infrastructure introduces new opportunities to solve water conflicts. Although benefit-sharing of water resources in the Nile Basin has been promoted to counteract water volume disputes, it has not yielded actionable solutions to the toughest negotiations over the past two decades. Here we develop a detailed and integrated energy–river basin system simulator of 13 East African countries, including the Nile Basin, and show how new electricity trade agreements between Ethiopia, Sudan and Egypt could help resolve the ongoing water dispute over the Grand Ethiopian Renaissance Dam. The results show that increasing energy trade can reduce Egyptian water deficits, reduce regional greenhouse gas emissions, increase hydropower generation in all three countries, reduce energy curtailment in Sudan and increase Ethiopia’s financial returns from electricity. This study underscores how spatial quantification of river–energy system interdependencies can help decision-makers find actionable multisector benefit-sharing solutions.
Similar content being viewed by others
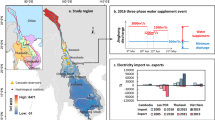
Dual water-electricity cooperation improves economic benefits and water equality in the Lancang-Mekong River Basin
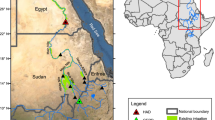
Collaborative management of the Grand Ethiopian Renaissance Dam increases economic benefits and resilience
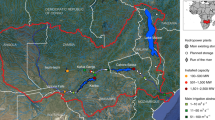
Unintended consequences of climate change mitigation for African river basins
Transboundary rivers provide essential socio-economic and environmental services, with 310 transboundary river basins globally geographically shared among 150 nations 1 . Around half the world’s population resides within transboundary river basins 1 . Managing transboundary river basins is becoming increasingly challenging due to population growth, growing demand for resources and the impacts of climate change 2 . Benefit-sharing has become the recommended strategy for fostering cooperation and creating and maintaining sustainable water infrastructure in transboundary river basins 3 , 4 , 5 . Sharing the benefits of water resources (for example, electricity, crops, fisheries) rather than allocating water volumes can increase the space for mutually beneficial agreements 6 , 7 and help solve disputes. This concept has yet to lead to acceptable large-scale solutions in the Nile region.
Benefit-sharing requires identification of non-water concerns that can be resolved through joint investment or collaborative infrastructure management 6 , 7 . Previous benefit-sharing attempts have been implemented on a project-by-project basis and do not involve multisector system-scale schemes 6 , 7 , 8 . This is partly due to the complexity of identifying such solutions at system scale and the need for multisector analytical tools that can estimate benefits and costs ex ante at the planning stage, the absence of which could lead to later discontent if the promised benefits are not delivered 7 . One challenge facing benefit-sharing aspirations in complex river basins is that it requires an agreed quantified representation of the benefits and costs of cooperation, and the rules for managing these benefits between stakeholders 7 , 9 . The design of benefit-sharing schemes requires backing from technical approaches that can accurately capture the multisector dynamics of river basins and energy systems and communicate credible solutions at high spatial and temporal resolution. To this end, the assessment approach and the solutions it investigates should be produced through a transparent process capable of securing political commitment.
The Nile is one of the longest rivers in the world and has a basin area that expands to over one-tenth of Africa and 11 countries (Fig. 1 ). Some of the Nile Basin countries suffer some of the highest levels of water stress and energy poverty in the world 10 . Several of these countries rely on hydropower as a primary source of electricity—for example, hydropower generation contributes more than 80% of the electricity mix in the Democratic Republic of Congo (DRC), Burundi, Ethiopia and Uganda. As of 2020, only 19% of the Nile Basin’s hydropower potential had been utilized 10 but large-scale hydropower development in the basin is gathering momentum. To promote system-level thinking and change existing sectorally siloed practices, regional institutions—such as the Eastern African Power Pool (EAPP) and the Nile Basin Initiative (NBI)—have been formed in recent decades as platforms for regional cooperation and coordination 11 , 12 .
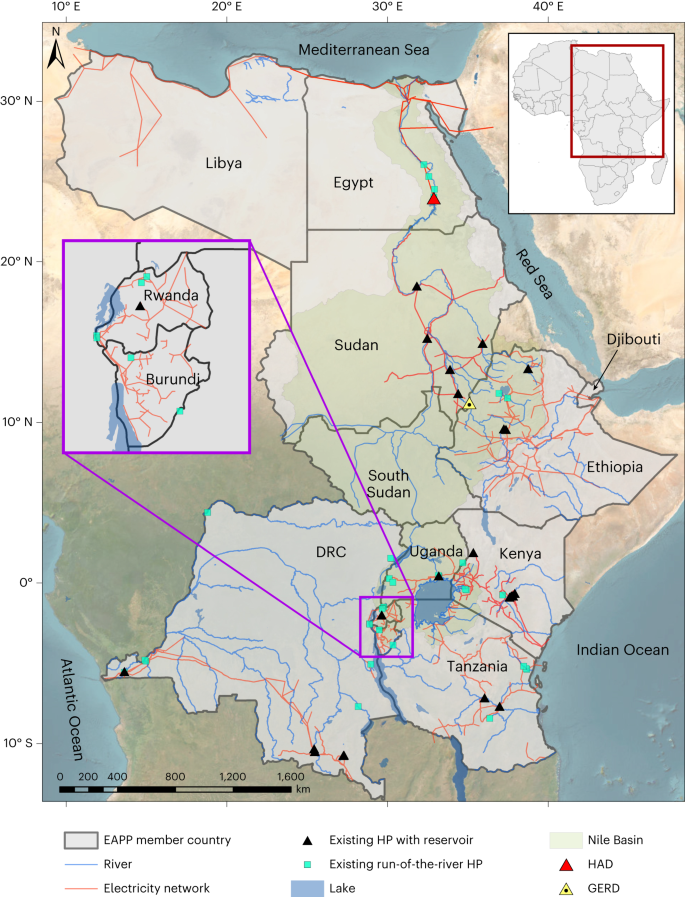
Our integrated spatial river basin–energy system model simulates all infrastructure shown in the figure (in addition to natural lakes and water consumption), except that outside of the eastern part of the DRC. HP, hydropower. The electricity network layer is based on a database from the World Bank Group; the existing hydropower and run-of-the-river hydropower layers are based on a database from Hydroshare; the river and lake layers are based on the Koordinates database; the countries’ boundary layer is based on a database from OpenDataSoft. Credit: background satellite image, ESRI.
Although benefit-sharing between riparian countries has shown promising outcomes in some transboundary river basins, including the Senegal 7 , Columbia 6 and Orange-Senqu 8 basins, its implementation in the Nile Basin has been either on a small scale or unsuccessful. An example of a successful small-scale benefit-sharing project on the Nile is the Rusumo hydroelectric plant, currently under construction on the border between Rwanda and Tanzania 13 . Rwanda, Burundi and Tanzania jointly invested in the project to generate 80 MW that will be shared among the three countries. The Joint Multi-purpose Project, initiated in 2005 between Ethiopia, Sudan and Egypt 14 , is an example of an intended benefit-sharing project that did not reach its goals. This project encountered difficulties due to water-related political disagreements between the countries that influenced the evaluation of investment options 15 . National-level water security goals have been the focus of negotiations over Nile water development for several decades, but this approach is no longer viable due to the increasing demand for, and pressure on, Nile water resources.
The construction of the Grand Ethiopian Renaissance Dam (GERD) on the Nile in Ethiopia (Fig. 1 ) offers a new opportunity for large-scale benefit-sharing in the Nile Basin. The GERD is under construction and is expected to double Ethiopia’s electricity generation. Following completion, it will have an installed power capacity of 5,150 MW and a maximum reservoir storage capacity of 74 billion m 3 (bcm) 16 , equivalent to 1.5 times the natural average annual river flow at the dam location. Filling and operating the GERD will affect both inter- and intra-annual variabilities of the Blue Nile flow, creating opportunities and challenges for Ethiopia, Sudan and Egypt. The trilateral negotiations over the initial filling and long-term operation of the GERD have so far been water-centric. For example, from November 2019 to February 2020 the United States Administration and the World Bank convened and observed several rounds of negotiations between the three countries 17 , eventually producing a draft proposal that was rejected by Ethiopia (hereafter referred to as the Washington draft proposal; further details are provided in Supplementary Information and Methods ). This proposal focused on defining water releases from the dam under different conditions to minimize disruptions to water users in Sudan and Egypt during the initial filling and long-term operation phases. From an Ethiopian viewpoint, the draft proposal would limit hydropower generation from the GERD and constrain future upstream development 18 . Subsequent negotiations led by the African Union have not yielded a publicly available draft agreement, but the scope of negotiations has remained similar to those of 2020 (ref. 19 ). Previous studies 20 , 21 , 22 did not consider the influence of energy demand on the operational dynamics of the GERD. These studies implicitly assumed sufficient electricity demands that would consume the generated power from GERD and did not dynamically adapt the dam’s operating rules based on the energy supply–demand balance. Although the GERD was initially portrayed as a benefit-sharing project in the launching speech of the late Ethiopian Prime Minister Meles Zenawi 23 , the negotiations have remained within a country-centred, water-centric approach.
In this Article we argue that large-scale benefit-sharing cooperation in the Nile Basin requires quantification of complex spatiotemporal water–energy–food–environment interdependencies. We develop an analytical approach that represents the river basin and energy systems of the EAPP and their interdependencies and variations through space and time at a high resolution. We use the case of the GERD to show that detailed multisector analyses can demonstrate the value of benefit-sharing to decision-makers by providing quantified evidence. We show that moving from country-centred, water-focused solutions to a cross-border, multisector, benefit-sharing scheme, namely electricity trade from Ethiopia to Sudan and Egypt, provides six benefits: (1) reduction in Egypt’s and Sudan’s irrigation water deficits; (2) increase in Ethiopia’s hydropower generation; (3) increase in power generation from existing hydropower stations in Sudan and Egypt; (4) reduction in Sudan’s energy demand curtailment; (5) reduction in regional CO 2 emissions; and (6) increase in Ethiopia’s financial return from power trade. Our results highlight that, with increased ties between Ethiopia, Sudan and Egypt on electricity trade, the dispute between the three countries over water releases from the GERD becomes less relevant, highlighting the potential role played by evidence-based, multisector, benefit-sharing solutions.
A multisector benefit-sharing approach for the GERD
Figure 1 shows the geographical extent of the river basin and energy system simulation framework developed in this study for the EAPP region. The framework represents major water–energy–food–environment system elements, including 51 dams (41 existing, 4 under construction and 6 planned), 89 run-of-river hydropower schemes (47 existing, 7 under construction and 35 planned), 1 large lake, 474 existing transmission lines, 12 solar (5 existing, 2 under construction and 5 planned) and 45 wind farms (11 existing and 34 planned), 197 thermal power stations (72 existing and 125 planned), 19 existing irrigation schemes and minimum environmental flows in the EAPP region. Supplementary Fig. 1 provides a schematic of the simulated integrated river basin and energy system. It enables high-resolution spatiotemporal simulation of water–energy–food–environment performance through a new iterative process that reconciles the various temporal resolutions of water and energy simulations at each simulated time step to ensure that hydropower generation in the river system model equals hydropower consumption in the energy system model. The simulation framework allows the consideration of, for example, scenarios such as energy trade, new infrastructure and dam operating rules and filling and operation of the GERD.
The potential impacts of the initial filling and long-term operation of the GERD on energy, irrigation and municipal and industrial water users in Sudan and Egypt have created political tensions. The purpose of the GERD is electricity generation, which requires the release of water through hydropower turbines. Therefore, electricity trade between Eastern Nile countries could create a demand for GERD’s electricity, ensuring sufficient water releases to Sudan and Egypt whilst providing financial benefits to Ethiopia. Such trade could take the form of a power purchase agreement (PPA), which is a long-term electricity supply agreement between electricity-producing and -consuming countries 24 . PPAs define the amount of electricity to be supplied, electricity price and penalties for non-compliance. PPAs face challenges, however, including negotiation complexity, regulatory uncertainties, financial risks, market price volatility, grid infrastructure requirements, environmental considerations, contractual disputes and operational performance risks 25 . Nevertheless, PPAs are the predominant form of electricity trade in the EAPP, as evidenced by EAPP power balance statements 26 , 27 , 28 which show that all EAPP power exchanges use PPAs. For instance, there is an existing 100 MW PPA between Ethiopia and Sudan whereby Ethiopia supplies 100 MW of firm power at US$ 0.05 kWh −1 , and there is a 100 MW PPA between Ethiopia and Djibouti at US$ 0.05 kWh −1 . To demonstrate how benefit-sharing in the form of energy trade between Ethiopia, Sudan and Egypt can help provide technical solutions to the GERD dispute, we create and examine five power trade scenarios and assess their river basin and power system implications compared with the Washington draft proposal. Although we are aware of Ethiopia’s rejection of the Washington proposal, it was selected as a baseline in this study because it is the most recent publicly accessible technical proposal on which Ethiopia, Sudan and Egypt have all negotiated.
Table 1 summarizes the scenarios and their assumptions. The baseline scenario includes existing power trade combined with the Washington draft proposal for operation of the GERD (that is, the water release constraints set within the proposal). The remainder of the scenarios are based on 100 MW (refs. 29 , 30 , 31 ) incremental increases in firm power trade between Ethiopia, Sudan and Egypt; in these scenarios, GERD water releases are governed by power supply–demand balance (that is, there are no water release constraints apart from a minimum environmental flow). Comparison of these scenarios enables the exploration of how benefit-sharing through different levels of power trade impacts river system performance. All scenarios assume that initial filling of the GERD reservoir is carried out in stages following a filling plan discussed as part of the Washington draft proposal 32 (Supplementary Table 1 ). The filling plan was modified to accommodate the progress in GERD filling achieved in 2020 and 2021, and to consider construction delays 33 . At the time of finalizing this article for publication, the initial filling phase of the GERD reservoir was nearly completed 34 and is expected to transition to long-term operations in the 2024/2025 hydrological year.
We assumed that changes to the current PPA between Ethiopia and Sudan and a new PPA between Ethiopia and Egypt will begin once the GERD reaches the steady-state operation phase (storage reaches 49.3 bcm). Trade during the steady-state phase ensures a more reliable electricity supply. To account for high interannual variability in river flows, we examined the five power trade scenarios across 30 twenty-year-long river flow sequences (‘hydrological traces’). These hydrological traces were created using the indexed sequential method 35 based on the 1972–2002 historical flow record of EAPP river systems. In the multisector simulation we use the status quo consumptive water demands in the EAPP region to quantify how benefit-sharing could impact system performance. These assumed water demands do not reflect an endorsement by the authors of status quo water allocations. Further details on how the five scenarios are implemented in the integrated river basin–energy system model of the EAPP are provided in Methods .
Impact of power trade on river system performance
Figure 2a,b shows the exceedance probability of annual irrigation water supply deficits in Sudan and Egypt for the five power trade scenarios. It illustrates how increasing power trade from Ethiopia to Sudan and Egypt reduces annual irrigation water supply deficits in Egypt and Sudan by a maximum of 5.1 and 1.3 bcm, respectively. This occurs because Ethiopia increases downstream water releases to meet electricity demand with higher power trade. Figure 2a demonstrates that, compared with the Washington draft proposal, increasing power trade reduces peak annual irrigation water supply deficit in Sudan by around 1.3 bcm. Nonetheless, the probability of an annual irrigation deficit occurring in Sudan, even under the Washington draft proposal, is low compared with Sudan’s total aggregate yearly water supply of 15.16 bcm. Supplementary Table 2 summarizes Sudan’s annual water supply deficits under different exceedance probabilities. The upstream geographical location of Ethiopia results in a consistent annual water abstraction across the five power trade scenarios under investigation.
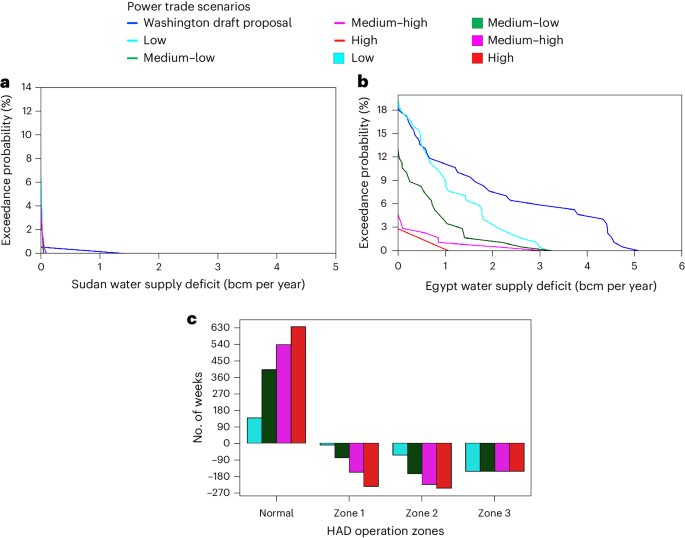
a , b , Exceedance probability of annual irrigation water supply deficits in Sudan ( a ) and Egypt ( b ). c , Change in the number of weeks in which the HAD reservoir falls within each of three zones of water management in Egypt compared with the Washington draft proposal scenario. Egypt uses the three zones shown to ration water releases from the HAD under drought conditions. In the normal zone, water is released from the HAD to fully satisfy downstream demands. In zones 1, 2 and 3, HAD water released to meet downstream demands is reduced by 5, 10 and 15%, respectively. The normal zone occurs with reservoir storage ≥60 bcm, zone 1 at 55–60 bcm, zone 2 at 50–55 bcm and zone 3 at <50 bcm (ref. 22 ).
Source data
Compared with the Washington draft proposal, the highest power trade scenario leads to the largest reduction in Egypt’s peak annual irrigation water shortage and reduces the frequency of shortages (Fig. 2b ). Figure 2b shows that Egypt’s maximum yearly irrigation water deficit would decline by 4.0, 1.87, 1.7 and 1.9 bcm in the high-, medium–high-, medium–low- and low-power trade scenarios, respectively, compared with the Washington draft proposal. Furthermore, the frequency of annual water supply deficits declines with increasing power trade. Compared with the Washington proposal, the probability of occurrence of an annual water supply deficit in Egypt would drop by 1.7, 10.3, 16.5 and 15.2% in these four power trade scenarios, respectively.
The High Aswan dam (HAD) is a large, multiyear-storage reservoir located in Egypt near the Sudan–Egypt border (Fig. 1 ). Downstream water demands in Egypt are fully satisfied when the HAD reservoir is in the normal zone, and water releases from it are reduced in Zones 1, 2 and 3 by 5, 10 and 15%, respectively. Figure 2c indicates the number of weeks in the 20-year simulation period in which the HAD reservoir fell within either normal operation or one of the three drought-management zones (Zones 1–3; Fig. 2 ). This figure shows an increase in the number of weeks in the normal zone alongside a decline in Zones 1 and 2 with increasing power trade compared with the Washington draft proposal, a trend mirrored in Zone 3 with a corresponding decline. The number of weeks in Zone 1 declines by 4, 32, 64 and 96%, and in Zone 2 by 26, 67, 90 and 98%, in the low-, medium–low-, medium–high- and high-power trade scenarios, respectively. Operation of the HAD in the normal zone more often reduces irrigation water shortages in Egypt and confirms the benefit to that country from increasing power trade.
Figure 3a,b shows reservoir storage of the GERD and HAD for the driest river flow sequence (that is, a sequence similar to historical conditions from 1977), and Fig. 3c,d shows the corresponding change in annual irrigation water deficits in Sudan and Egypt relative to the Washington draft proposal. No difference is observed between the power trade scenarios in regard to yearly water supply deficits in Sudan and Egypt during the initial filling of the GERD (2020–2024). The initial filling of the GERD reduced HAD reservoir storage in all scenarios but did not result in irrigation water deficits in Egypt during those years. This is because the HAD reservoir is currently almost full 36 and thus can supplement river flows to avoid irrigation supply deficits during the GERD’s initial filling. Results show that, during the steady-state operation phase, the GERD operates at lower levels with increasing power trade, resulting in higher storage at the HAD. Decreased levels of energy export require the GERD to operate at lower power targets due to reduced electricity exports, resulting in reduced downstream water releases.
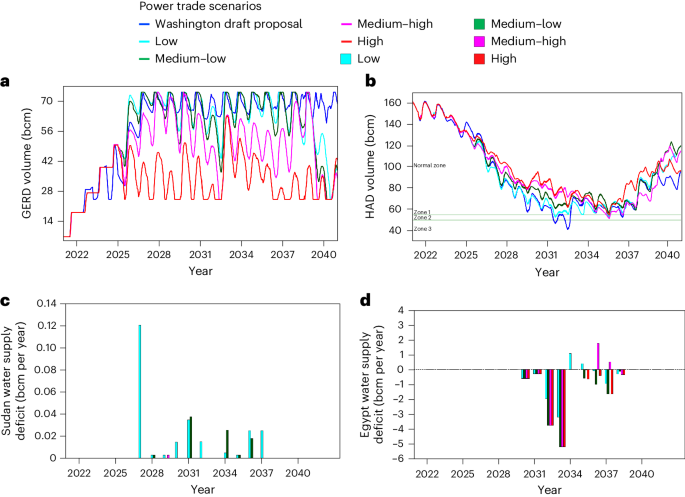
a , GERD reservoir storage. b , HAD reservoir storage. c , d , Annual change in irrigation water supply deficits for Sudan ( c ) and Egypt ( d ) compared with the Washington draft proposal trade scenario. Negative numbers denote desirable decreases in water deficits. For clarity, different maximum y axis values are used. The dry hydrologic sequence is under conditions similar to the sequence that occurred starting in 1977.
As pointed out in the Washington draft proposal 32 , besides GERD’s water releases under a normal hydrological year, additional water releases are determined by drought-mitigation measures ( Methods ). As a consequence of these mitigation measures, Fig. 3b shows that the Washington draft proposal results in higher HAD water levels in the years 2025 and 2026 during the initial filling of the GERD compared with the other four power trade scenarios. However, during normal hydrological years, GERD’s release is reduced because of the lower level of power trade in the Washington draft proposal scenario. Hence, HAD Reservoir (HADR) volume drops to a low level between the years 2029 and 2033 compared with the four power trade scenarios, which results in higher annual water supply curtailment in Egypt compared with that in scenarios with increased power trade (Fig. 3d ). Increasing power trade from the Washington proposal scenario to the high-trade scenario resulted in a yearly reduction in Egypt’s irrigation water supply deficits of up to 4.0 bcm (7.3% of annual Egyptian water demand). GERD frequently operates at lower levels in the high- and medium–high-power trade scenario, resulting in HADR volumes above its drought-management zones. Figure 3b demonstrates a notable increase in high- and medium–high-power trade scenarios in HAD volume compared with both low and medium–low. This marked difference can be attributed to the higher pressure exerted on the GERD to meet domestic and export demands.
Power trade boosts hydropower and cuts CO 2 emissions
Figure 4 shows the impact of increasing power trade on (1) the ability of each country to meet its electricity demand (expressed as demand curtailment), (2) hydropower generation and (3) CO 2 emissions associated with electricity generation. Figure 4a shows that increasing power trade reduces annual electricity demand curtailments in Sudan, with an intangible difference in Ethiopia’s curtailments compared with the Washington draft proposal. Results show that Egypt would have no electricity demand curtailments in any modelled scenario, including the Washington draft proposal. Because it was assumed that Ethiopia exports power to Sudan and Egypt only after satisfying its domestic electricity demand, that country’s electricity demand curtailment is similar in all power trade scenarios. Furthermore, the agreed power trade with Sudan and Egypt is satisfied in all scenarios.
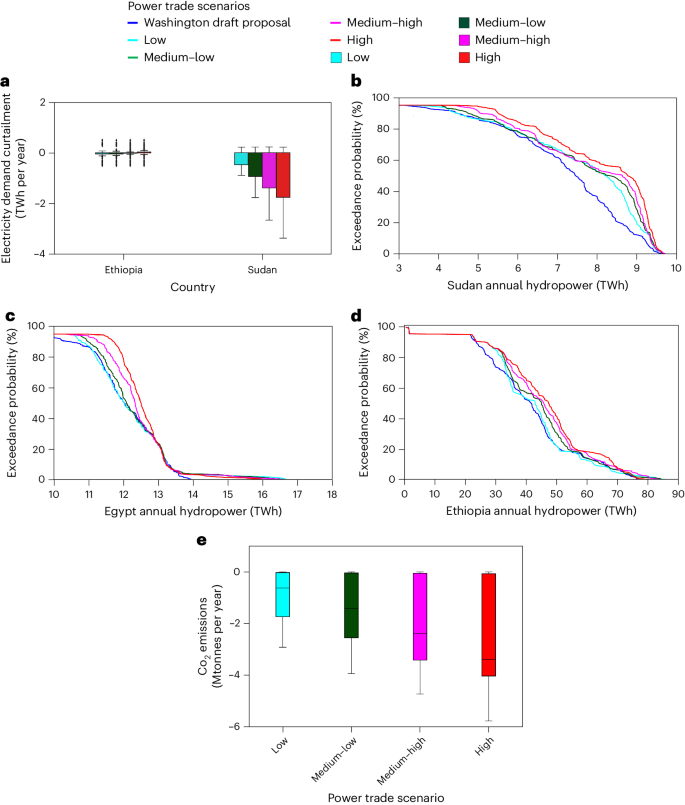
a , Annual changes in electricity demand curtailments in Ethiopia and Sudan compared with the Washington draft proposal trade scenario. b – d , Exceedance probability of hydropower generation in Sudan ( b ), Egypt ( c ) and Ethiopia ( d ). e , Annual changes in carbon emissions from electricity generation in Ethiopia, Sudan and Egypt compared with the Washington draft proposal. Electricity curtailment in Egypt is zero in all scenarios and is therefore not presented in the figure. a , e , Box plots derived from a sample size of 600.
Figure 4b–d shows a higher likelihood of hydropower generation increases in Ethiopia, Sudan and Egypt with the power trade scenarios compared with the Washington draft proposal. With increasing power trade, accumulated total hydropower generation in Sudan showed an increase of 80% of the examined river flow sequences compared with the Washington draft proposal, due to a regulated flow from the GERD (Fig. 4b ). Figure 4c shows an increase in annual hydropower generation in Egypt between the Washington draft proposal and the other examined trade scenarios in 32, 69, 73 and 82% of the examined river flow sequences in the low-, medium–low-, medium–high- and high-power trade scenario, respectively. The increase in Egypt’s hydropower occurs mainly because of an increase in the release from the GERD with increased export of electric power. Ethiopia’s annual hydropower generation increases 65, 90, 96 and 97% of river flow sequences in the four trade scenarios, respectively, compared with the Washington draft proposal. Ethiopia’s aggregated annual hydropower generation of all existing and planned capacity shows a maximum increase of 9 and 10 TWh, respectively, in the low- and medium–low-power scenarios and of 12 TWh in the medium–high- and high-power trade scenarios. Annual hydropower generation from the GERD exhibits a maximum increase of 6.0, 6.8, 9.0 and 10.1 TWh in the four trade scenarios, respectively, compared with the Washington draft proposal. The predominant increase in the GERD’s annual hydropower generation with increasing power trade compared with the Washington draft proposal results from the proposal following existing power trade levels. Because there is insufficient domestic and export demand to absorb hydropower generated from the GERD, the Washington draft proposal has to use other GERD outlets to transfer drought-mitigation releases downstream without generating hydropower.
Following increasing power trade with Sudan and Egypt, exports to Kenya and Tanzania declined, resulting in a slight increase in electricity demand curtailment and the use of thermal power generation in the latter two countries. This is because we assume that Ethiopia prioritizes power trade with Sudan and Egypt, following the sixth principle of the declaration of principles on the GERD signed between Ethiopia, Sudan and Egypt in 2015. Supplementary Fig. 2 shows the exceedance probability of Ethiopia’s power and annual energy export to Kenya and Tanzania and electricity demand curtailment in Tanzania. In all examined trade scenarios, Ethiopia supplied the agreed power trade with Kenya and Tanzania 95 and 90% of the time, respectively (Supplementary Fig. 2a,c ). Results show that, due to the decrease in export to Sudan and Egypt, Ethiopia’s export to Kenya and Tanzania is higher in the low- and medium–low-power trade scenarios compared with the Washington draft proposal. In the medium–high- and high-trade scenarios, Tanzania’s annual electricity demand curtailment increased by a maximum of 0.2 TWh compared with the Washington draft proposal (Supplementary Fig. 2e ).
Increasing power trade from Ethiopia to Sudan and Egypt reduces regional electricity generation from thermal power stations because the operational cost of hydropower is lower than that from thermal power plants. As a result, electricity generation from hydropower displaces electricity generation from thermal power plants. The change in total annual electricity generation from thermal power plants in the three countries drops by a median of 2.08, 3.48, 3.0 and 4.4 TWh in the four scenarios, respectively, compared with the Washington draft proposal (Table 2 shows the change for each country). In addition, due to an increase in both power trade and hydropower generation from the GERD and existing dams in Sudan and Egypt, annual CO 2 emissions decrease (Fig. 4c ). Supplementary Table 3 summarizes the reduction in mean annual CO 2 emissions associated with electricity generation in the three countries, showing a total decline of 1.15, 1.98, 3.20 and 4.54 million tonnes (Mtonnes) in the four scenarios, respectively, compared with the Washington draft proposal. To put these changes into perspective, the maximum annual decline in carbon emissions associated with electricity generation due to energy trade in the three countries is about 25% of Sudan and Ethiopia’s total emissions in 2020 (ref. 37 ).
Figure 5 shows Ethiopia’s annual financial income from cross-border power trade from 2026 to 2041 in the Washington draft proposal, in and the four trade scenarios, for the 30 simulated river flow sequences. Ethiopia’s annual financial income from 2022 to 2025 is similar in all trade scenarios because changes to power trade levels were assumed to start with the GERD’s steady-state operation. Supplementary Fig. 3 shows Ethiopia’s annual financial income discounted at 3%. Results show an increase in Ethiopia’s annual financial return over the period 2026–2041, depending on river flow sequence, ranging from US$71 million to US$128 million and from US$ 296 million to 498 million for the low- and high-trade scenarios, respectively, compared with the Washington draft proposal. Because Ethiopia was assumed to prioritize meeting domestic electricity demand over electricity exports, its financial return from exports is lower in some hydrological sequences for a given agreement. However, there is an overall rise in annual financial return with increasing power trade. Sudan and Egypt can achieve net annual financial savings from power trade with Ethiopia resulting from increased hydropower generation and electricity import that displace expensive thermal generators. In the low- and high-power trade scenarios these savings amount to approximately US$36 million and US$369 million per year, respectively, when discounted at 3% (Supplementary Table 4 ). Further details about the assumptions used to estimate Egypt’s and Sudan’s financial savings are provided in Methods . Following Article 6 of the Declaration of Principles on the GERD signed between Ethiopia, Sudan and Egypt that Ethiopia prioritizes satisfying electricity export to Sudan and Egypt, export demand to those two countries is always satisfied and the financial saving remains the same for the two countries in all hydrological sequences for a given agreement.
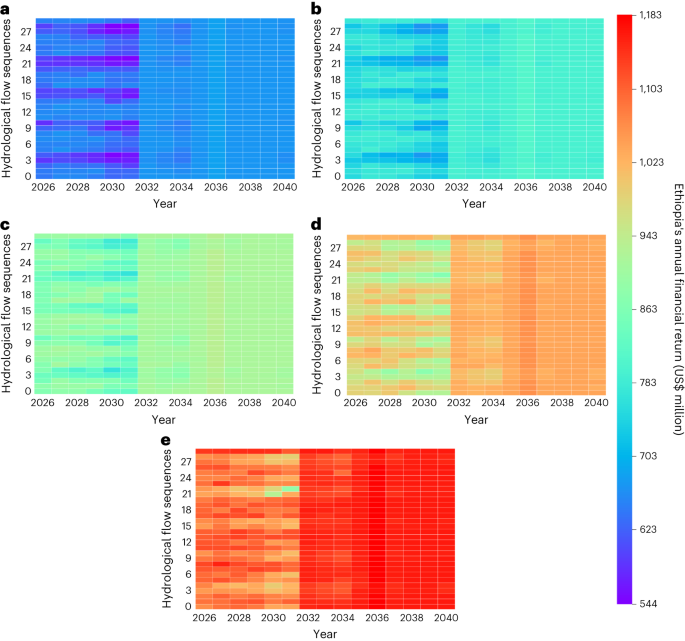
a – e , Ethiopia’s income from power trade in the period 2026–2041 under 30 river flow sequences with the Washington draft proposal ( a ) and under the low- ( b ), medium- ( c ), medium–low- ( d ) and medium–high- ( e ) power trade scenarios.
Sharing benefits in transboundary river basins offers the potential for solving water disputes and fostering cooperation. However, the absence of analytical means to quantify and investigate multisector benefit-sharing possibilities across the water, energy and food resource systems both spatially and temporally has hampered the evaluation and application of benefit-sharing in practice. Large hydropower dams on transboundary rivers often have regional implications for the water, energy and food sectors, and these implications vary both spatially and temporally 38 . Accurate spatial multisector simulation can help stakeholders better evaluate benefit-sharing proposals and explore multisectoral interdependencies within and across countries rather than focusing on volumetric water sharing.
In this study we argue that large-scale benefit-sharing negotiation and implementation in East Africa should be augmented by detailed multisector simulation of the water, energy, food and environment systems. We demonstrate how benefit-sharing among Ethiopia, Sudan and Egypt in the form of increased energy trade can benefit all three countries. Results show that GERD operating policies, including increased power trade from Ethiopia to Sudan and Egypt, can reduce agricultural water deficits in Egypt and increase hydropower generation in Ethiopia and Sudan, reduce energy demand curtailment in Sudan, increase Ethiopia’s financial return from power trade and reduce regional CO 2 emissions compared with the Washington draft proposal. Despite Egypt having nearly zero electricity deficit, increasing power trade with Ethiopia displaces expensive thermal generation yielding financial savings and contributing to a reduction in CO 2 emissions.
Pressure on the Nile water resource system is expected to grow as the population of the riparian countries reaches more than 1 billion by 2050 (ref. 39 ). Irrigation water demand is expected to increase by 15% (ref. 40 ) by 2050 and electricity demand to double by 2040 (ref. 27 ). Investments in the power sector, the evolution of future electricity demand, future PPAs and transboundary water agreements among the Eastern Nile Basin countries will influence the ability of the Nile river to sustainably provide much-needed socio-economic and ecosystem services. In addition to the benefits that increasing power trade holds for riparian countries, this study shows how power trade among the Eastern Nile countries could impact the long-term operation of the GERD.
Initiatives for benefit-sharing and electricity trade in the Nile Basin have previously been explored and studied 41 , 42 . For example, the Nile Basin Regional Power Trade Project under the NBI aimed at, among other goals, conducting basin-wide analysis of power trade opportunities to inform river basin management 41 . While such studies can foster cooperation among the Nile riparian countries, they failed to gather political momentum. The multisector impacts of power trade on Nile water resources have not been evaluated through a detailed approach that simultaneously considers river basins and energy systems. As such, the integrated water–energy method presented in this paper can help quantify the impacts of power trade on both the water and energy systems and demonstrate to decision-makers that technical win–win solutions for both regional water and energy systems are possible if the two systems are planned and operated in an integrated, interdisciplinary and collaborative manner.
This study did not explore the potential effects of increasing power trade among Eastern Nile countries under climate uncertainties. Climate change is projected to impact the mean annual naturalized flow of the Nile, but there is uncertainty in projections 29 , 30 , 31 , 43 , 44 , 45 , 46 . For instance, based on 29 CMIP6 climate simulations with some projections adjusted for the Eastern African Paradox 47 , 48 , Basheer et al. 43 estimated changes in the naturalized flow of the Nile ranging from −13 to +90% by 2050 compared with the historical flow mean. Another study on the Nile found that the long-term mean and standard deviation of runoff (based on 18 CMIP5 simulations) are expected to increase by 15 and 50%, respectively 31 . Under wetter climatic conditions an increase in hydropower generation would be expected. This, in turn, would result in a reduction in the utilization of thermal generation and a decline in electricity supply shortages. On the contrary, if the flow of the Nile decreases due to climate change, this would lead to a decrease in hydropower generation and place additional stress on water resources in the region. Exploring the potential implications of increasing power trade between the Eastern Nile countries in the context of climate uncertainties is relevant because climate change is likely to impact energy generation and water resource management in the region. Future research should look into these implications.
In this study we explore one form of benefit-sharing in the Nile Basin (that is, energy trade), but benefit-sharing can exist in many other forms. For example, Sudan is endowed with agricultural land resources that could be used to produce food to be shared among all Nile Basin countries. Lake Victoria in Kenya, Uganda and Tanzania could be a fishery source for all Nile countries, and Egypt’s industrial know-how could contribute to the manufacturing and export of products to the rest of the world through vital shipping routes such as the Suez Canal. These promising multisector solutions and others remain to be investigated and could further aid the seemingly intractable task of sharing the Nile River.
This study introduces and demonstrates a multisectoral benefit-sharing framework. The analytical approach accurately captures the complex interdependencies and spatiotemporal variations within the EAPP river and energy systems, offering a high-resolution representation. The study aims to address future energy and water resource scarcity challenges using multisector river basin–energy system analysis. Effective bidirectional communication between energy dispatch and dam operations allows the multisector river basin and energy system simulation models to be accurate and realistic. In the following sections we introduce the components of the river basin and energy systems, discuss the iterative integration process and present the integrated river basin and energy models developed for the EAPP.
River basin component
The river basin simulation component was developed using Python Water Resources (Pywr), an open-source water resource systems simulation tool 49 . Pywr uses a linear programming approach alongside system-operating rules to drive water allocations and quantify water flows through different nodes and storage at different reservoir nodes over time. Different node types can be represented using Pywr. Input (for example, catchments), output (for example, water abstraction and reservoir evaporation), storage (for example, reservoirs) and their links are among standard nodes for water resource system models in Pywr.
Power system component
The power simulator is a security-constrained, direct-current, optimal power flow model that considers intertemporal constraints and generation costs. The power simulator minimizes the total cost of generation throughout the power system at each time step (for example, hourly) subject to the physical constraints of the system (for example, thermal limits, available hydropower and so on). Different power system elements can be represented, such as various forms of generators, buses and transmission lines. The equality and inequality constraints considered in the power system model are the power balance for every bus and the capacity of transmission lines. The simulator can represent different generation technologies, such as conventional generation, renewables and runoff hydropower and hydropower with reservoir storage (for example, taking water flow inputs from a water model), and curtail demand when required. The model is used to simulate energy flows for representative days at hourly resolution to capture the intermittency of renewables.
Integrated river basin–energy simulator
The integrated water–energy simulator allocates water and energy resources through the spatial network at each time step. The spatially and temporally disaggregated water and energy system networks enable capture of cross-sector feedback. The water and power system components are linked using a soft-linking, two-way integration approach 50 using an open-source, multi-agent simulation framework called the Python Network Simulation framework (Pynsim) 51 . Pynsim manages iteration among the river basin and energy system components, model run sequencing and time stepping.
The river basin and energy components are connected at locations of hydropower production, linking a river basin model hydropower node with the corresponding bus in the power system model. This connection uses a new iterative process at each simulated time step to ensure that (1) hydropower generation in the river basin model and hydropower consumption in the power system model are equal and (2) the magnitude of hydropower generation is adjusted based on the level of energy demand curtailment. Supplementary Fig. 4 shows the iterative river basin–energy systems integration method. The iterative approach is applied using two iterations as follows.
In the first iteration, if generated hydropower from a power plant in the water model exceeds the need for hydropower at the corresponding bus in the energy model, excess hydropower value is converted to an equivalent volume of water. Based on the new water release value, a second iteration is performed whereby hydropower is simulated again in the river basin model and the hydropower value is used by the corresponding bus in the energy model.
In the second iteration, if energy demand curtailment occurs in the energy model, we apply a conditional rule whereby the water model determines how much additional hydropower to generate. The water model chooses a discrete water release value to modify the water allotted for hydropower following the level of energy demand curtailment. The volume of demand curtailment that triggers additional hydropower release is assumed to be 15% of peak power demand based on reservoir operation rules in EAPP member countries.
Multisector river basin–energy simulator of the EAPP
The spatial extent of the integrated river basin and energy system simulator covers EAPP member countries (with the exception that only the eastern part of DRC is covered). The river basin and energy simulator use a weekly time step. In this study we use an electricity trade price of US$0.05 kWh −1 for existing trade between Ethiopia and Sudan, following the agreement between the two countries. For other existing trade and planned electricity trade a price of US$0.07 kWh −1 was used, which was obtained from a power trade proposal between Ethiopia and Kenya 52 .
To implement PPA rules, the EAPP energy system model was divided into three interconnected parts (without spatial aggregation): the Ethiopian power system, the southern portion of EAPP (Kenya, Uganda, DRC, Tanzania, Rwanda and Burundi) and the northern portion of EAPP (Sudan, Egypt and Libya). Supplementary Fig. 5 shows cross-border power trade between the three parts of the EAPP energy system and hydropower transfer between the water and energy system components.
EAPP power system model
The EAPP energy system model has 300 buses. The type and number of nodes in the EAPP energy model are summarized in Supplementary Table 5 . Supplementary Fig. 1 shows a schematic of the EAPP energy model. Hourly load values for different electricity demand locations were based on 2014 load profile data obtained from EAPP; these load profiles were assumed to remain the same throughout the simulation period. The percentage of annual power demand growth for the next 20 years was obtained primarily from the EAPP and was used to project power demands (Supplementary Table 6 ).
The operating costs of power plants decrease in the following order of technology: variable renewables (such as wind and solar), run-of-river hydropower, hydropower with reservoir, geothermal, nuclear power plants and conventional thermal generators. However, conventional power plants also have different operational costs depending on the technology, fuel types and country of operation. Supplementary Table 7 summarizes the unit cost of operation and maintenance for various EAPP generation technologies and fuel types. The locations of existing and proposed variable energy sources were used to extract hourly renewable generation profiles.
The energy model uses a weekly time step with one representative day divided into 24 h. The decision to use a representative day for the weekly time step takes into account the negligible variation in hourly energy demand profiles between weekdays and weekends in the study area. The hourly profiles for wind and solar for large-scale, ground-mounted, crystalline silicon photovoltaic systems and a wind turbine of height 100 m were extracted from existing databases 53 , 54 . Hourly wind 53 and solar 54 profiles for the year 2020 were used for the entire simulation period. Available hourly wind and solar generations were calculated by multiplying profile values with the maximum generation capacity of respective solar and wind generators. The EAPP energy model was calibrated using transmission line loss against the member country’s total energy demand supplied. Total energy demand supplied data for 2020 were obtained from the EAPP database. Supplementary Table 8 shows the calibration result for each member country.
All power trade between countries within the southern and northern parts of the EAPP is assumed to be bidirectional. Planned cross-border interconnections and upgrading of existing transmission lines until 2032 are triggered during the simulation following the EAPP master plans. For the years 2032–2041 we assumed that transmission line capacity between countries will remain the same, due to a lack of data. The net financial saving from power import for Sudan and Egypt from Ethiopia was calculated considering the fuel cost of displaced thermal generators 55 and the unit price of power import.
EAPP river basin model
Supplementary Fig. 1 shows a schematic of the EAPP river basin model. The model was developed in collaboration with EAPP and NBI regional organizations. The model includes a detailed representation of storage dams, hydropower and consumptive water uses such as irrigation and domestic, and environmental flows. The historical model uses naturalized inflow data for the period 1972–2002 and simulates using a weekly time step. The river basin model has a detailed representation of existing and planned hydropower infrastructure in the EAPP region until 2041, including multiyear reservoirs such as the HAD and GERD. Hydrological inflow data, reservoir characteristics, water abstraction for irrigation, domestic and industrial water supply, hydropower characteristics and the commissioning dates of infrastructures were obtained from the NBI, EAPP Master Plan and EAPP Power Balance Study 27 , 56 .
The river basin model is divided into two components: (1) the Eastern Nile model, encompassing the eastern portion of the Nile river (Ethiopia, Sudan and Egypt) and (2) the EAPP water system outside the Eastern Nile, representing the remaining portion of the river basin that lies outside the Eastern Nile river basin but within the EAPP. Supplementary Table 11 shows the annual average water withdrawal for Ethiopia, Sudan and Egypt from the Nile river. A calibrated river basin model for the Eastern Nile was obtained from a previous study 20 and was combined with the remainder of the EAPP river basin model. The model outside the Eastern Nile, which is referred to as ‘EAPP water system outside the Eastern Nile’, was calibrated against observed river data at five key locations using historical records of river flows and reservoir water levels for 1972–1992: observed river flow data obtained from the Global Runoff Data Centre database 57 for Stieglers and Bigupu stations in Tanzania; reservoir water level data for Masinga and Kamburu and river flow data for Metara station were acquired from a study conducted on Kenya’s Tana river basin 58 . The timeframe 1972–1992 was selected due to the availability of reliable observed data. The model performance in regard to simulation of the EAPP water system outside the Eastern Nile is presented in Supplementary Fig. 6 and Supplementary Table 9 . Calibration and validation performance varies, ranging from poor to excellent. Hydropower dams outside the Eastern Nile region are simulated assuming an operation based on a constant target power that has been calibrated to closely approximate the annual hydropower generation of these projects.
Supplementary Table 9 presents the details of installed capacities for existing and planned hydropower plants, along with the corresponding annual hydropower generation. The table includes values derived from the project’s master plan and the model output. The analysis excluded hydropower plants located upstream from the hydrological inflow points and those with an installed capacity of <10 MW. Natural lakes are not included in the river basin model outside the Eastern Nile. We relied on inflow data for all hydropower plants in the equatorial lakes region to simulate hydropower generation. This approach was used to simulate hydropower plants downstream of Lake Victoria, including Owen Falls, Narubale, Kira and Bujagali, Karuma Falls and Murchison upstream of Lake Albert, and Ruzizi I, Ruzizi II, Ruzizi III and Kabu downstream of Lake Albert. Hydropower plants (such as Mpanda and Jiji) are on smaller tributaries and do not affect each other.
We generated thirty 20-year river flow sequences using the indexed sequential method (ISM) to address the interannual variability observed in river flows. These sequences were based on historical flow data for the period 1972–2002. The ISM involves the generation of multiple synthetic sequences based on past data using each year in the data as a possible starting point 35 . By shifting over each water year by one index and repeating this process n times, where n corresponds to the length of the historical record, the ISM produces a set of n synthetic sequences, making it effective in capturing the interannual variation in river flow. This method allows the model to incorporate uncertainties associated with future hydrology by considering a broad range of potential sequences that may occur in the future.
GERD filling and long-term operation
Supplementary Table 1 shows the 5-year plan for the initial filling of the GERD assuming normal or above-average hydrological conditions. For the four trade scenarios described above, the stage filling of GERD follows the plan shown in Supplementary Table 1 regardless of hydrological condition.
The operating rules of the GERD under the Washington draft proposal scenario follow the guidelines and rules annexed in a letter from Egypt to the United Nations Security Council dated 11 June 2020 (ref. 32 ). In the remaining scenarios the GERD adheres to the 5-year, stage-based filling plan outlined in the Washington draft proposal without abiding by its drought-mitigation strategies. The Washington draft proposal scenario assumes existing levels of power trade from Ethiopia to Sudan and Egypt. In all scenarios we assumed that, after achieving the water retention target of the first and second years (7.0 bcm), two 375 MW turbines would become operational. The remainder of the turbines would become operational after achieving the third year’s water retention target (18.4 bcm) 22 . Once the filling targets of the second and third years are achieved, GERD’s storage is always maintained above these targets to keep the turbines operational. Water retention is limited to July and August, with a minimum environmental release of 43 million m 3 per day.
In all power trade scenarios, the long-term operation of the GERD begins when reservoir storage reaches 49.3 bcm. It was assumed that, when reservoir storage is ≥49.3 and <72 bcm, water is released through the turbines to target 1,600 MW to increase 90% power generation reliability 59 . This power target is increased or decreased depending on the level of energy demand curtailment in Ethiopia and power trade based on feedback from the energy system model, as explained above. To reduce water spill from GERD, it was assumed that the dam targets generation at maximum capacity if storage exceeds 72 bcm. Power targets are adjusted over individual simulation time steps considering electricity demand, PPA and power network constraints to ensure electricity supply–demand balance. If power demand implies a need for lower power generation at any time step, the hydropower target is reduced, as explained above. In all scenarios, a minimum daily environmental flow of 43 Mm 3 is maintained when physically possible 32 . All scenarios assume that Ethiopia prioritizes satisfying domestic electricity demand over exports.
Karadobi is a hydropower dam upstream of GERD that is planned to start filling in 2032, according to a plan created by various countries plan and collected by EAPP. We assumed that Karadobi would start initial filling in 2032 and finish by 2036. We adopted filling stages for Karadobi similar to those for the GERD, with yearly filling targets scaled based on the ratio of the total storage capacities of the two dams. Similar to the GERD, the first two turbines of Karadobi will become operational after achieving the filling target of the second year. On achieving steady-state operation of the reservoir, the Karadobi hydropower project is assumed to adopt a target of 800 MW to maximize annual hydropower generation.
Data availability
The data that support the study’s conclusions are available via Zenodo at https://doi.org/10.5281/zenodo.7594112 (ref. 60 ). Due to proprietary restrictions, the model and data for the East African Power Pool and Eastern Africa River systems are not publicly accessible. Access to the model and data for the East African Power Pool and Eastern Africa River systems can be granted by the corresponding author upon presentation of the necessary permissions from authorities that own the data, which are the Nile Basin Initiative and the Eastern African Power Pool.
Code availability
The Pywr, Pyenr and Pynsim python libraries used to develop the integrated water and power system model are open-source and freely available via GitHub at https://github.com/pywr/pywr (ref. 61 ), https://github.com/pywr/pyenr (ref. 62 ) and https://github.com/UMWRG/pynsim (ref. 63 ).
McCracken, M. & Wolf, A. T. Updating the register of international river basins of the world. Int. J. Water Resour. Dev. 35 , 732–782 (2019).
Article Google Scholar
Zeitoun, M., Goulden, M. & Tickner, D. Current and future challenges facing transboundary river basin management. Wiley Interdiscip. Rev. Clim. Chang. 4 , 331–349 (2013).
Yu, W. et al. The benefit-sharing principle: implementing sovereignty bargains on water. Water Policy 4 , 90–100 (2009).
Google Scholar
Sadoff, C. W. & Grey, D. Beyond the river: the benefits of cooperation on international rivers. Water Policy 4 , 389–403 (2002).
Phillips, D., Daoudy, M., McCaffrey, S., Öjendal, J. & Turton, A. Trans-boundary water cooperation as a tool for conflict prevention and for broader benefit-sharing. Swedish Ministry for Foreign Affairs www.semanticscholar.org/paper/Trans-boundary-Water-Co-operation-as-a-Tool-for-and-Phillips-Daoudy/0e91451d8c789b6c7411131833aab890da7b4a50 (2006).
Tarlock, A. D. & Wouters, P. Are shared benefits of international waters an equitable apportionment. Colo. J. Int. Environ. Law Policy 18 , 523–536 (2007).
Alam, U., Dione, O. & Jeffrey, P. The benefit-sharing principle: Implementing sovereignty bargains on water. Polit. Geogr. 28 , 90–100 (2009).
Kranz, N., Interwies, E. & Vorwerk, A. Transboundary regimes in the Orange Senqu Basin (Institute for International and European Environmental Policy, 2005).
Hensengerth, O., Dombrowsky, I. & Scheumann, W. Benefit-sharing in dam projects on shared rivers. Deutsches Institut für Entwicklungspolitik 1–22 (2012).
Nile Basin Initiative. State of the River Nile Basin (Nile Basin Initiative, 2020).
Eastern African Power Pool (EAPP). Final Master Plan Report (Eastern Africa Power Pool, 2011).
M. J. K. Baitwa & M. E. Agiro. Building on Shared Benefits: Transforming Lives in the Nile Basin (Nile Basin Initiative, 2016).
Dombrowsky, I. et al. International and local benefit sharing in hydropower projects on shared rivers: the Ruzizi III and Rusumo Falls cases. Water Policy 16 , 1087–1103 (2014).
Blackmore, D. & Whittington, D. Opportunities for cooperative water resources development on the eastern Nile: risks and rewards. Nile Basin Initiative https://nilebasin.org/node/12085 (2008).
Cascão, A. E. & Nicol, A. GERD: new norms of cooperation in the Nile Basin? Water Int. 41 , 550–573 (2016).
International Panel of experts (IPoE) on Grand Ethiopian Renaissance Dam Project (Government of Ethiopia, 2013).
The foreign ministers of Egypt, Ethiopia and Sudan. Joint statement of Egypt, Ethiopia, Sudan, the United States and the World Bank (USDT, accessed 12 November 2021); https://home.treasury.gov/news/press-releases/sm827 .
Federal Democratic Republic of Ethiopia. Letter to the UN Security Council - Ethiopia (UNSC, 2020); https://digitallibrary.un.org/record/3862715/files/S_2020_409-EN.pdf .
Permanent Representative of Sudan to the United Nations. Letter from the permanent mission of the Sudan to the United Nations addressed to the President of the Security Council (UNSC, 2021); https://digitallibrary.un.org/record/3923983/files/S_2021_350-EN.pdf .
Basheer, M. et al. Collaborative management of the Grand Ethiopian Renaissance Dam increases economic benefits and resilience. Nat. Commun. 12 , 5622 (2021).
Article CAS PubMed PubMed Central Google Scholar
Wheeler, K. G., Jeuland, M., Hall, J. W., Zagona, E. & Whittington, D. Understanding and managing new risks on the Nile with the Grand Ethiopian Renaissance Dam. Nat. Commun. 11 , 5222 (2020).
Wheeler, K. G. et al. Cooperative filling approaches for the Grand Ethiopian Renaissance Dam. Water Int. 41 , 611–634 (2016).
Tawfik, R. The Grand Ethiopian Renaissance Dam: a benefit-sharing project in the Eastern Nile? Water Int. 41 , 574–592 (2016).
Badissy, M. et al. Understanding Power Purchase Agreements: Second Edition (United States Department of Commerce, 2009); https://cldp.doc.gov/sites/default/files/PPA .
Elwakil, E. & Hegab, M. Risk management for power purchase agreements. In Proc. 6th IEEE Conference on Technologies for Sustainability 1-6 (IEEE, 2018).
Eastern African Power Pool. Eastern African Power Pool Power Balance Statement , 2nd edn. (2020); https://www.ea-energianalyse.dk/wp-content/uploads/2021/06/Power-balance-statement-2020-ver-3.pdf .
Eastern African Power Pool. Eastern African Power Pool Power Balance Statement (2021).
Puig, D. et al. An action agenda for Africa’s electricity sector. Science 373 , 616–619 (2021).
Elshamy, M. E., Seierstad, I. A. & Sorteberg, A. Impacts of climate change on Blue Nile flows using bias-corrected GCM scenarios. Hydrol. Earth Syst. Sci. https://doi.org/10.5194/hess-13-551-2009 (2009).
Hirpa, F. A. et al. Streamflow response to climate change in the Greater Horn of Africa. Clim. Change 156 , 341–363 (2019).
Siam, M. S. & Eltahir, E. A. B. Climate change enhances interannual variability of the Nile river flow. Nat. Clim. Chang. 7 , 350–354 (2017).
Permanent Mission of Egypt to the United Nations. Letter from the Permanent Representative of Egypt to the United Nations (UNSC, 2020); https://digitallibrary.un.org/record/3893948/files/S_2020_566-EN.pdf .
Ethiopia completes third filling of Blue Nile mega-dam reservoir. Al Jazeera https://www.aljazeera.com/news/2022/8/12/ethiopia-says-completes-third-filling-of-mega-dam-reservoir (2022).
Filling of Grand Renaissance Dam on the Nile complete, Ethiopia says. Al Jazeera (10 September 2023).
Ouarda, T., Labadie, J. & Fontane, D. Indexed sequential hydrologic modeling for hydropower capacity estimation. J. Am. Water Resour. Assoc. 33 , 1337–1349 (1997).
Copernicus Global Land Service. Water level. Earth Observation programme of the European Commission (2020).
Crippa, M. et al. Fossil CO 2 emissions of all world countries. Publications Office of the European Union https://doi.org/10.2760/687800 (2020).
Teasley, R. L. & McKinney, D. C. Calculating the benefits of transboundary river basin cooperation: Syr Darya Basin. J. Water Resour. Plan. Manag. 137 , 481–490 (2011).
United Nation Department of Economic and Social Affairs. World Population Prospects , Vol. 2 (United Nations, 2019).
Grandi, M. The Nile river basin regime by 2050: opportunities for basin-wide integrated water management (Congrès – Société Herpétologique de France, 2017).
Council of Ministers of Water Affairs of the Nile Basin States. Nile Basin Regional Power Trade (Nile Basin Initiative, 2001); https://iwlearn.net/resolveuid/bd07def54f5bbe6cd0cd17419b5cf694 .
Economic Consulting Associates Limited. The Potential of Regional Power Sector Integration: Transmission & Trading Case Study. Nile Basin Initiative https://hdl.handle.net/10986/17520 (2009).
Basheer, M. et al. Cooperative adaptive management of the Nile River with climate and socio-economic uncertainties. Nat. Clim. Chang. 13 , 48–57 (2023).
Beyene, T., Lettenmaier, D. P. & Kabat, P. Hydrologic impacts of climate change on the Nile River Basin: implications of the 2007 IPCC scenarios. Clim. Change 100 , 433–461 (2010).
Tariku, T. B., Gan, T. Y., Li, J. & Qin, X. Impact of climate change on hydrology and hydrologic extremes of Upper Blue Nile River Basin. J. Water Resour. Plan. Manag. 147 , 2 (2020).
Yates, D. N. & Strzepek, K. M. Modeling the Nile Basin under climatic change. J. Hydrol. Eng. 3 , 98–108 (1998).
Wainwright, C. M. et al. ‘Eastern African Paradox’ rainfall decline due to shorter not less intense Long Rains. Clim. Atmos. Sci . 2 , 34 (2019).
Rowell, D. P., Booth, B. B. B., Nicholson, S. E. & Good, P. Reconciling past and future rainfall trends over East Africa. J. Clim. 28 , 9768–9788 (2015).
Tomlinson, J. E., Arnott, J. H. & Harou, J. J. A water resource simulator in Python. Environ. Model. Softw. 126 , 104635 (2020).
Fattahi, A., Sijm, J. & Faaij, A. A systemic approach to analyze integrated energy system modeling tools: a review of national models. Renew. Sustain. Energy Rev. 133 , 110195 (2020).
Knox, S., Meier, P., Yoon, J. & Harou, J. J. A python framework for multi-agent simulation of networked resource systems. Environ. Model. Softw. 103 , 16–28 (2018).
African Development Fund. Ethiopia-Kenya Electricity Highway Regional (African Development Fund, 2012); https://www.afdb.org/fileadmin/uploads/afdb/Documents/Project-and-Operations/Ethiopia-Kenya_-_Ethiopia-Kenya_Electricity_Highway_-Project_Appraisal_Report_.pdf .
Staffell, I. & Pfenninger, S. Using bias-corrected reanalysis to simulate current and future wind power output. Energy 114 , 1224–1239 (2016).
Pfenninger, S. & Staffell, I. Long-term patterns of European PV output using 30 years of validated hourly reanalysis and satellite data. Energy 114 , 1251–1265 (2016).
The World Bank. From subsidy to sustainability: diagnostic review of Sudan’s electricity sector. The World Bank https://doi.org/10.1596/33702 (2019).
Eastern African Power Pool(EAPP). Eapp Regional Power System Master Plan (Eastern African Power Pool, 2014).
Fekete, B. M., Vörösmarty, C. J. & Grabs, W. High-resolution fields of global runoff combining observed river discharge and simulated water balances. Global Biogeochem. Cycles 16 , 15-1–15-10 (2002).
Hurford, A. P. & Harou, J. J. Balancing ecosystem services with energy and food security – assessing trade-offs from reservoir operation and irrigation investments in Kenya’s Tana Basin. Hydrol. Earth Syst. Sci. 18 , 3259–3277 (2014).
Wheeler, K. G. et al. Exploring cooperative transboundary river management strategies for the Eastern Nile Basin. Water Resour. Res. 54 , 9224–9254 (2018).
Article PubMed PubMed Central Google Scholar
Etichia, M. et al. Dataset: Energy trade tempers Nile water conflict. Zenodo https://doi.org/10.5281/zenodo.7594112 (2023).
Tomlinson, J. E., Arnott, J. H. & Harou, J. J. Pywr. GitHub https://github.com/pywr/pywr (2019).
Tomlinson, J. E., Arnott, J. H. & Harou, J. J. Pyenr. GitHub https://github.com/pywr/pyenr (2021).
Knox, S., Meier, P., Yoon, J. & Harou, J. J. Pynsim. GitHub https://github.com/UMWRG/pynsim (2018).
Download references
Acknowledgements
M.E.ʼs doctoral degree was funded by the University of Manchester. We acknowledge the assistance of the NBI and EAPP in developing the Eastern Africa River and Power system models. This work was supported by the UK Research and Innovation Economic and Social Research Council (no. ES/P011373/1) as part of the Global Challenges Research Fund through the FutureDAMS research project. For the purposes of open access, the authors have applied a creative commons attribution (CC BY) licence to any author-accepted manuscript version arising.
Author information
Authors and affiliations.
School of Engineering, The University of Manchester, Manchester, UK
Mikiyas Etichia, Mohammed Basheer, Ruben Bravo, Jose Gutierrez, Jose M. Gonzalez, James Tomlinson, Eduardo Martinez, Mathaios Panteli & Julien J. Harou
Department of Civil and Mineral Engineering, University of Toronto, Toronto, Ontario, Canada
Mohammed Basheer
Albrecht Daniel Thaer-Institute, Humboldt-Universität zu Berlin, Berlin, Germany
Veritas Consulting, Addis Ababa, Ethiopia
Atsede Endegnanew
Environment Agency, Bristol, UK
Anthony Hurford
Department of Electrical and Computer Engineering, University of Cyprus, Nicosia, Cyprus
Mathaios Panteli
Department of Civil, Environmental and Geomatic Engineering, University College London, London, UK
Julien J. Harou
You can also search for this author in PubMed Google Scholar
Contributions
M.E. and M.B. wrote the original paper. M.E. and M.B. led the visualization of results. M.B., J.J.H., E.M., M.P., R.B., J.G., A.E., J.M.G., A.H. and J.T. reviewed and edited the paper. M.E. and M.B. developed the Eastern Africa River system model. M.E., R.B., J.G. and A.E. developed the Eastern Africa Power system model. M.E. integrated the river system and power system models. J.J.H., M.E., M.B., M.P. and E.M. conceptualized the study. M.E., M.B., E.M., M.P., A.E. and J.J.H. developed the power trade scenarios. J.J.H., M.P., M.B. and E.M. supervised the work. J.J.H. acquired funding for this work. All authors contributed to validation and interpretation of the results.
Corresponding author
Correspondence to Julien J. Harou .
Ethics declarations
Competing interests.
The authors declare no competing interests.
Peer review
Peer review information.
Nature Water thanks Vignesh Sridharan and the other, anonymous, reviewer(s) for their contribution to the peer review of this work.
Additional information
Publisher’s note Springer Nature remains neutral with regard to jurisdictional claims in published maps and institutional affiliations.
Supplementary information
Supplementary figs. 1–7, tables 1–11 and references., supplementary data.
Supplementary Fig. 2. Source data.
Supplementary Fig. 3. Source data.
Supplementary Fig. 6. Source data.
Supplementary Fig. 7. Source data.
Source Data Fig. 2
Statistical Source data.
Source Data Fig. 3
Source data fig. 4, source data fig. 5, rights and permissions.
Open Access This article is licensed under a Creative Commons Attribution 4.0 International License, which permits use, sharing, adaptation, distribution and reproduction in any medium or format, as long as you give appropriate credit to the original author(s) and the source, provide a link to the Creative Commons licence, and indicate if changes were made. The images or other third party material in this article are included in the article’s Creative Commons licence, unless indicated otherwise in a credit line to the material. If material is not included in the article’s Creative Commons licence and your intended use is not permitted by statutory regulation or exceeds the permitted use, you will need to obtain permission directly from the copyright holder. To view a copy of this licence, visit http://creativecommons.org/licenses/by/4.0/ .
Reprints and permissions
About this article
Cite this article.
Etichia, M., Basheer, M., Bravo, R. et al. Energy trade tempers Nile water conflict. Nat Water 2 , 337–349 (2024). https://doi.org/10.1038/s44221-024-00222-9
Download citation
Received : 13 January 2023
Accepted : 06 March 2024
Published : 26 April 2024
Issue Date : April 2024
DOI : https://doi.org/10.1038/s44221-024-00222-9
Share this article
Anyone you share the following link with will be able to read this content:
Sorry, a shareable link is not currently available for this article.
Provided by the Springer Nature SharedIt content-sharing initiative
Quick links
- Explore articles by subject
- Guide to authors
- Editorial policies
Sign up for the Nature Briefing newsletter — what matters in science, free to your inbox daily.

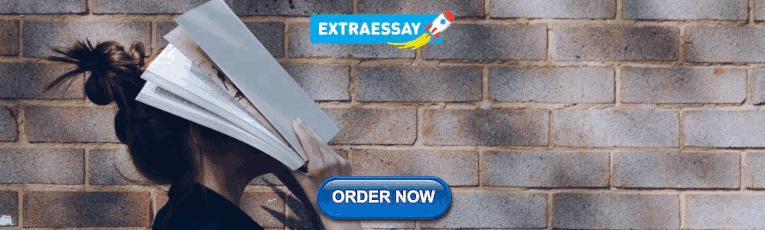
IMAGES
VIDEO
COMMENTS
This paper investigates the impact of water demand management measures (WSAs and GWR) on hydraulic profiles; this is achieved through the development and application of a simulation tool that uses water consumption data from two case studies in the UK. The key attributes of the database, the deduced consumption trends and the development ...
Water demand management (WDM) plays an important role in urban water security. The primary purpose of urban WDM is to reduce residential water consumption (Zapana-Churata et al. 2022).During a climate-induced water supply crisis, emergency water supply source augmentation is not always easy to implement especially for the cities that primarily depend on rainfall-dependent water sources.
1. Introduction. The demand-side management of both energy and water has recently attracted much attention [1-3].In particular, smart meters have facilitated the availability of detailed water usage data for users [4-7].Many studies have examined the potential for encouraging energy- and water-saving behaviours by sharing the household's data, as well as peer usage data.
several water management practices, institutional settings, and regional policies on municipal and agricultural sectors. Generally, the agent-based adoption of water management strategies can alleviate the harm of water scarcity while providing positive feedbacks to reducing municipal costs and increasing agricultural profit from production.
Makerere University, Uganda. Abstract. This paper analyses the impact of demand on water supply service delivery and. demonstrates that the higher the demand, the lower the pressure at which water ...
A comprehensive evaluation framework that can assess a wide range of water supply and demand management policy options in terms of economic, social, environmental, risk-based, and functional performance is crucial to ascertain their level of sustainability. However, such a detailed, generic, and holistic policy evaluation framework is not found in the literature. This paper reviews studies to ...
In this context, to achieve the water security in developing countries, there is an urgent need for transition from conventional water management approaches toward urban water demand management ...
Although water demand management in itself is an adaptive strategy to manage constraints on water resources, there is still a gap in finding better and more effective implementation methods to improve its acceptance by society and its success rates at reducing water demand. This thesis contributed towards new theoretical knowledge about ...
In this context, at the verge of a paradigm shift in water management [Gober et al., 2010] and at a point when the knowledge base is changing rapidly [Milly et al., 2008], a literature review of the progress of demand-side water management methods is an important contribution. A transition in water demand modeling, forecasting, and management ...
A thesis submitted in partial fulfilment of the requirements for the degree of Doctor of Philosophy in the Centre for Sustainable Infrastructure (CSI) ... and the impact of water demand management on energy use, i.e. water energy nexus, constitutes a future research direction. Keywords: DMEUW, end uses of water, integrated water demand model ...
Sustainable water resource management relates to harvesting, managing, and preserving natural resources in an adequate manner in order to sustain life and the ecosystem. 1,2 About 71% of the surface of the Earth is water, out of which 96.5% is oceans and only 3.5% is freshwater. 3 Groundwater, one of the valuable fresh water resources, provides half of all drinking water worldwide 4 and is ...
For example, the growing, transportation, processing, and trading of food products require large amounts of water and energy. A complete analysis is provided by the Comprehensive Assessment of Water Management in Agriculture [International Water Management Institute (IWMI), 2007]. This work demonstrates that in a business-as-usual scenario ...
The metropolitan city of Istanbul is becoming overcrowded and the demand for clean water is steeply rising in the city. The use of analytical approaches has become more and more critical for forecasting the water supply and demand balance in the long run. In this research, Istanbul's water supply and demand data is collected for the period during 2006 and 2014. Then, using an autoregressive ...
Abstract: This paper presents the challenges of water supply demand and enhancing water sustainability in rural areas. Data collected from field observation, regional water offices and officials, questionnaires and community responses were conducted. Data from the selected districts of the study area was discussed and analyzed.
The city of Addis Ababa is under rapid development and there are enormous construction activities along with rapid urbanization, and industrialization. These anthropogenic actions combined with population growth rate are affecting the water demand of the city. The overall purpose of this study is to model water supply and demand of the city and to identify potential water management strategies ...
Lahlou, Mohamed, "Water demand management for Casablanca, Morocco." (1998). Graduate Theses, Dissertations, and Problem Reports. 9245. https://researchrepository.wvu.edu/etd/9245. Water shortages could significantly curtail economic growth and development in Casablanca, Morocco. Conservation programs in Morocco have been implemented only ...
The hydrology of the water shade was simulated using soil and water assessment tool (SWAT) whereas the water demand and likely future scenario related to the water availability and demands were done using water evaluation and planning (WEAP) model. 1.3 Objectives of the study The main objectives of this thesis work was assessing the surface ...
The total water demand of this scenario was estimated to be 7.14 GL latterly in the projection period and the unmet demand would grow to 5.2 GL. The results confirmed that improved water management approaches are needed in the town to ensure the sustainability of water resources in the long term and outline proper water utilization policies.
In the base year (2018), the overall water demand is 2.07 gigalitres (GL) and the unmet demand is estimated as 0.096 GL. The water demand grows to 3.71 GL under the reference scenario in 2035.
Water Demand Management, Pre-water Demand Management, Total Water Scarcity, Four Representations of Potable water Scarcity, Feminisation of the ... thorough reading of my first draft of the thesis and the valuable input that I received. Thirdly I want to thank my wife for the support, and encouragement.
The goal of urban water management thesis to investigate components of urban water system and careful, economic use handling of the water in urban. The first goal of this ... Parallel with growing urban population drinking water demand especially in mega cities in the developing countries, is growing quickly and takes increasing part of total
Urban domestic water demand management has proven to be a very effective management method in the context of many difficulties in the urban water supply. UDW demand management has been applied, ... systematic research on UDW demand management. The thesis " Economic Analysis of Domestic Water Demand Management: Research on Ha Noi Area" aims to ...
This study investigated key elements of water conservation and demand management as well as interventions that were pertinent to achieving the desired outcome of efficient use of water. Respondents were required to answer questions focusing on several water conservation and water demand management related approaches and solutions.
The demand for energy, water and food in Africa continues to increase, resulting in growing pressure on contentious multisector resource systems like the River Nile. The ongoing dispute over Nile ...