- 0 Shopping Cart
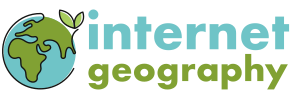
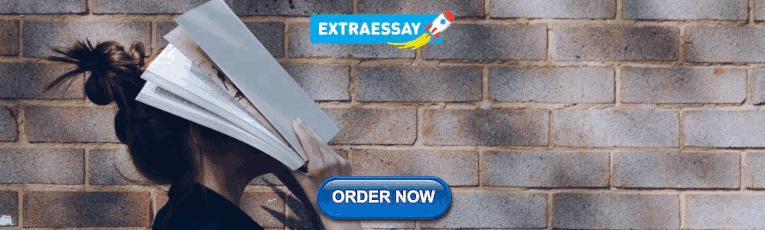
Nepal Earthquake 2015
A case study of an earthquake in a low income country (LIC).
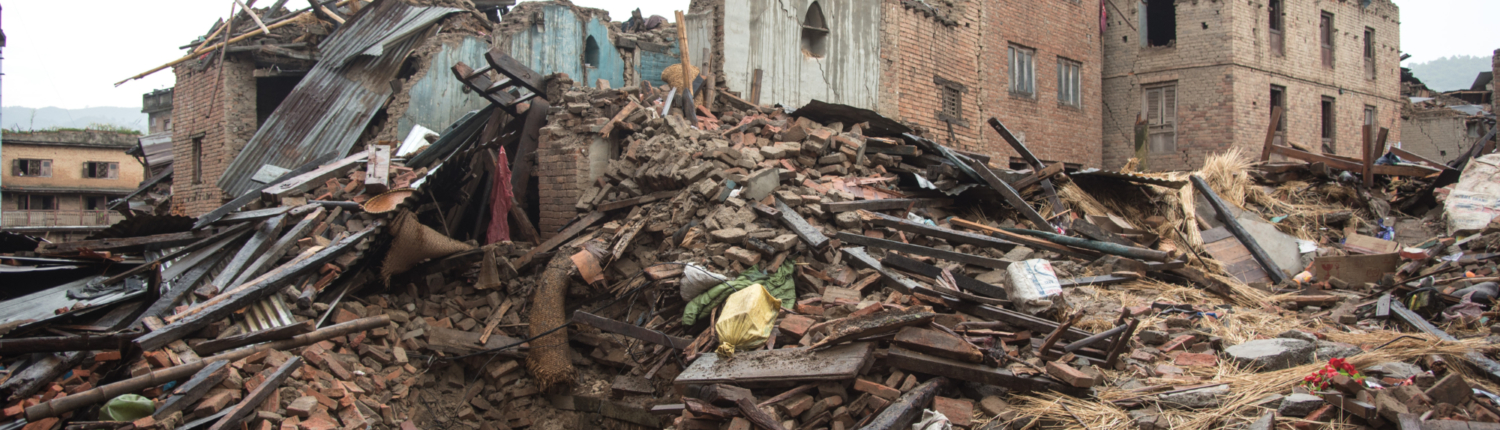
Nepal, one of the poorest countries in the world, is a low-income country. Nepal is located between China and India in Asia along the Himalayan Mountains.
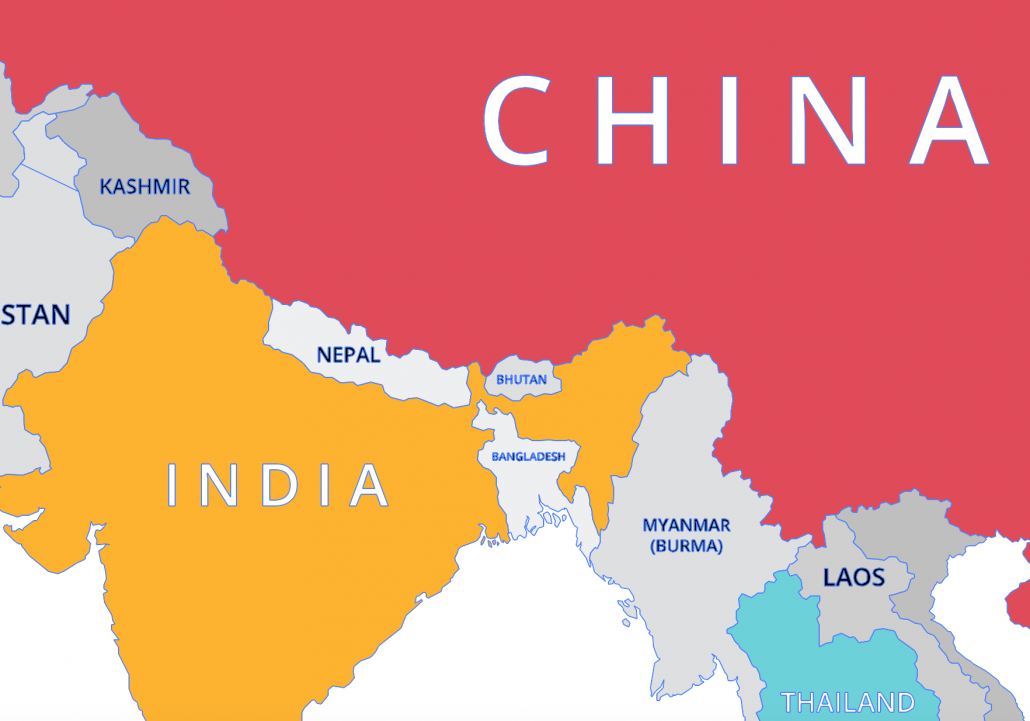
A map to show the location of Nepal in Asia
What caused the Nepal Earthquake?
The earthquake occurred on a collision plate boundary between the Indian and Eurasian plates.
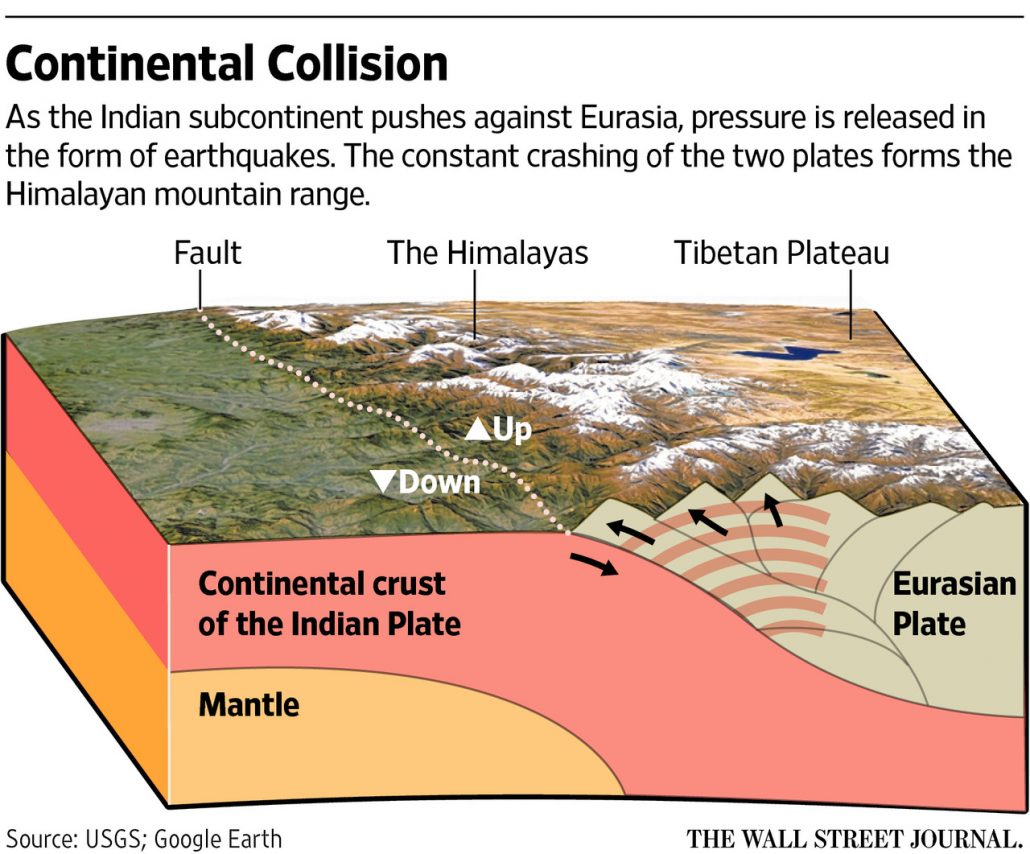
What were the impacts of the Nepal earthquake?
Infrastructure.
- Centuries-old buildings were destroyed at UNESCO World Heritage Sites in the Kathmandu Valley, including some at the Changu Narayan Temple and the Dharahara Tower.
- Thousands of houses were destroyed across many districts of the country.
Social and economic
- Eight thousand six hundred thirty-two dead and 19,009 injured.
- It was the worst earthquake in Nepal in more than 80 years.
- People chose to sleep outside in cold temperatures due to the risk of aftershocks causing damaged buildings to collapse.
- Hundreds of thousands of people were made homeless, with entire villages flattened.
- Harvests were reduced or lost that season.
- Economic losses were estimated to be between nine per cent to 50 per cent of GDP by The United States Geological Survey (USGS).
- Tourism is a significant source of revenue in Nepal, and the earthquake led to a sharp drop in the number of visitors.
- An avalanche killed at least 17 people at the Mount Everest Base Camp.
- Many landslides occurred along steep valleys. For example, 250 people were killed when the village of Ghodatabela was covered in material.
What were the primary effects of the 2015 earthquake in Nepal?
The primary effects of the 2015 earthquake in Nepal include:
- Nine thousand people died, and 19,000 people were injured – over 8 million people were affected.
- Three million people were made homeless.
- Electricity and water supplies, along with communications, were affected.
- 1.4 million people needed support with access to water, food and shelter in the days and weeks after the earthquake
- Seven thousand schools were destroyed.
- Hospitals were overwhelmed.
- As aid arrived, the international airport became congested.
- 50% of shops were destroyed, affecting supplies of food and people’s livelihoods.
- The cost of the earthquake was estimated to be US$5 billion.
What were the secondary effects of the 2015 earthquake in Nepal?
The secondary effects of the 2015 earthquake in Nepal include:
- Avalanches and landslides were triggered by the quake, blocking rocks and hampering the relief effort.
- At least nineteen people lost their lives on Mount Everest due to avalanches.
- Two hundred fifty people were missing in the Langtang region due to an avalanche.
- The Kali Gandaki River was blocked by a landslide leading many people to be evacuated due to the increased risk of flooding.
- Tourism employment and income declined.
- Rice seed ruined, causing food shortage and income loss.
What were the immediate responses to the Nepal earthquake?
- India and China provided over $1 billion of international aid .
- Over 100 search and rescue responders, medics and disaster and rescue experts were provided by The UK, along with three Chinook helicopters for use by the Nepali government.
- The GIS tool “Crisis mapping” was used to coordinate the response.
- Aid workers from charities such as the Red Cross came to help.
- Temporary housing was provided, including a ‘Tent city’ in Kathmandu.
- Search and rescue teams, and water and medical support arrived quickly from China, the UK and India.
- Half a million tents were provided to shelter the homeless.
- Helicopters rescued people caught in avalanches on Mount Everest and delivered aid to villages cut off by landslides.
- Field hospitals were set up to take pressure off hospitals.
- Three hundred thousand people migrated from Kathmandu to seek shelter and support from friends and family.
- Facebook launched a safety feature for users to indicate they were safe.
What were the long-term responses to the Nepal earthquake?
- A $3 million grant was provided by The Asian Development Bank (ADB) for immediate relief efforts and up to $200 million for the first phase of rehabilitation.
- Many countries donated aid. £73 million was donated by the UK (£23 million by the government and £50 million by the public). In addition to this, the UK provided 30 tonnes of humanitarian aid and eight tonnes of equipment.
- Landslides were cleared, and roads were repaired.
- Lakes that formed behind rivers damned by landslides were drained to avoid flooding.
- Stricter building codes were introduced.
- Thousands of homeless people were rehoused, and damaged homes were repaired.
- Over 7000 schools were rebuilt.
- Repairs were made to Everest base camp and trekking routes – by August 2015, new routes were established, and the government reopened the mountain to tourists.
- A blockade at the Indian border was cleared in late 2015, allowing better movement of fuels, medicines and construction materials.
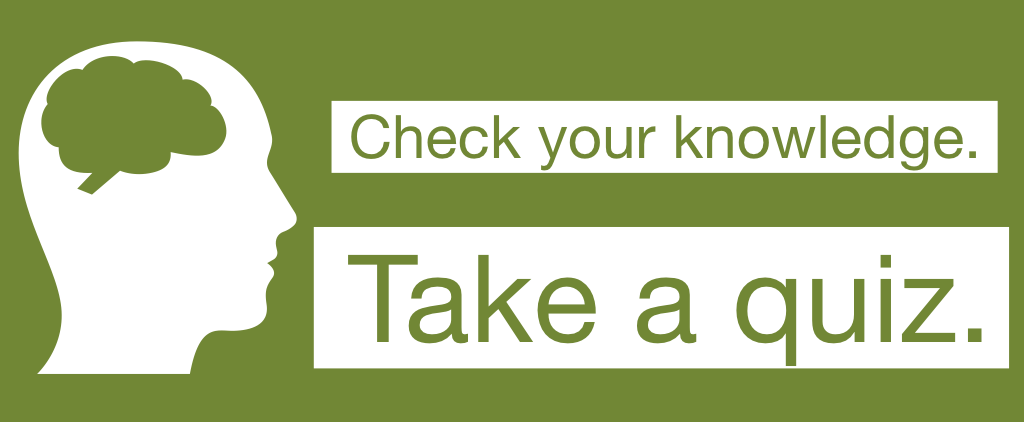
Premium Resources
Please support internet geography.
If you've found the resources on this page useful please consider making a secure donation via PayPal to support the development of the site. The site is self-funded and your support is really appreciated.
Related Topics
Use the images below to explore related GeoTopics.
Previous Topic Page
Topic home, amatrice earthquake case study, share this:.
- Click to share on Twitter (Opens in new window)
- Click to share on Facebook (Opens in new window)
- Click to share on Pinterest (Opens in new window)
- Click to email a link to a friend (Opens in new window)
- Click to share on WhatsApp (Opens in new window)
- Click to print (Opens in new window)
If you've found the resources on this site useful please consider making a secure donation via PayPal to support the development of the site. The site is self-funded and your support is really appreciated.
Search Internet Geography
Top posts and pages.

Latest Blog Entries

Pin It on Pinterest
- Click to share
- Print Friendly
- Open supplemental data
- Reference Manager
- Simple TEXT file
People also looked at
Original research article, the 2015 gorkha nepal earthquake: insights from earthquake damage survey.
- 1 Department of Civil Engineering, University of Bristol, Bristol, UK
- 2 Institute of Industrial Science, University of Tokyo, Tokyo, Japan
- 3 Department of Civil and Environmental Engineering, University of Alberta, Edmonton, AB, Canada
- 4 School of Civil Engineering and Geosciences, Newcastle University, Newcastle upon Tyne, UK
The 2015 Gorkha Nepal earthquake caused tremendous damage and loss. To gain valuable lessons from this tragic event, an earthquake damage investigation team was dispatched to Nepal from 1 May 2015 to 7 May 2015. A unique aspect of the earthquake damage investigation is that first-hand earthquake damage data were obtained 6–11 days after the mainshock. To gain deeper understanding of the observed earthquake damage in Nepal, the paper reviews the seismotectonic setting and regional seismicity in Nepal and analyzes available aftershock data and ground motion data. The earthquake damage observations indicate that the majority of the damaged buildings were stone/brick masonry structures with no seismic detailing, whereas the most of RC buildings were undamaged. This indicates that adequate structural design is the key to reduce the earthquake risk in Nepal. To share the gathered damage data widely, the collected damage data (geo-tagged photos and observation comments) are organized using Google Earth and the kmz file is made publicly available.
Introduction
An intense ground shaking struck Central Nepal on 25 April 2015 (local time 11:56 a.m.). The moment magnitude of the earthquake was M w 7.8 with its hypocenter located in the Gorkha region (about 80 km north–west of Kathmandu). The earthquake occurred at the subduction interface along the Himalayan arc between the Indian plate and the Eurasian plate ( Avouac, 2003 ; Ader et al., 2012 ). The earthquake rupture propagated from west to east and from deep to shallow parts of the shallowly dipping fault plane [ United States Geological Survey (USGS), (2015) ], and consequently, strong shaking was experienced in Kathmandu and the surrounding municipalities. This was the largest event since 1934, M w 8.1 Bihar–Nepal earthquake ( Ambraseys and Douglas, 2004 ; Bilham, 2004 ). The 2015 mainshock destroyed a large number of buildings and infrastructure in urban and rural areas, and triggered numerous landslides and rock/boulder falls in the mountain areas, blocking roads, and hampering rescue and recovery activities. Moreover, aftershock occurrence has been active since the mainshock; several major aftershocks (e.g., M w 6.7 and M w 7.3 earthquakes in the Kodari region, north–east of Kathmandu) caused additional damage to rural towns and villages in the northern part of Central Nepal. As of 26 May 2015, the earthquake damage statistics for Nepal from the 25 April 2015 mainshock stand at the total number of 8,510 deaths and 199 missing 1 . In addition, the major aftershock that occurred on 12 May 2015 caused 163 deaths/missing. Center for Disaster Management and Risk Reduction Technology (CEDIM), (2015) reports that the total economic loss is in the order of 10 billion U.S. dollars, which is about a half of Nepal’s gross domestic product. The 2015 earthquakes will have grave long-term socioeconomic impact on people and communities in Nepal [ United Nations Office for the Coordination of Humanitarian Affairs (UN-OCHA), (2015) ].
Earthquake field observations provide raw damage data of existing built environments and are useful for developing empirical correlation between ground motion intensity and damage severity for earthquake impact assessment of future events. To gain valuable lessons from this tragic event, an earthquake damage investigation team was jointly organized by the Japan Society of Civil Engineers and the Japan Geotechnical Society, and was dispatched to Nepal from 1 May 2015 to 7 May 2015. The survey trip was planned in such away that relatively large geographical areas that were affected by the earthquakes were covered to grasp spatial features of the damage in the earthquake-hit regions. A unique aspect of this damage investigation is that the data were collected at the early stage of disaster response and recovery (6–11 days after the mainshock), and thus first-hand earthquake damage observations were obtained before major repair work. The collected damage data, in the form of geo-tagged photos and some measurements (e.g., size of a landslide), are useful for other earthquake damage reconnaissance teams who visit Nepal several weeks after the mainshock, and serve as a starting point of longitudinal research of a recovery process from the earthquakes. To achieve this goal, damage photos that were taken during the survey trip are organized using Google Earth and are made publicly available; the kmz file is provided as supplementary resource of this paper. Viewers can download the photos directly and can use them for research and educational purposes; all photos are geo-tagged and are accompanied by brief comments.
This paper summarizes key findings of ground shaking damage in Nepal, and is organized as follows. To link building damage observations with available seismological data, seismotectonic setting of Nepal is reviewed, and earthquake rupture process and aftershock data, which are available from the U.S. Geological Survey (USGS), are analyzed to gain scientific insights into ground motions that were experienced during the mainshock and major aftershocks. It is important to note that strong motion observation networks in Nepal are not well developed and data are not publicly accessible. This means that the estimation of observed ground motions at building damage sites is highly uncertain. Currently, recorded time-history data of strong motion are only available at the KATNP station, which is located in the city center of Kathmandu. In this study, strong motion data at KATNP are analyzed and the results, in the form of elastic response spectra, are discussed by comparing with relevant ground motion prediction models [e.g., Kanno et al. (2006) and Boore and Atkinson (2008) ] and with well-recorded strong motion data from the 2008 M w 7.9 Wenchuan China earthquake ( Lu et al., 2010 ), seismological features of which are broadly similar to the 2015 Nepal earthquake. Furthermore, issues related to ground motion estimation for prompt earthquake impact assessment [e.g., Jaiswal and Wald (2010) and Center for Disaster Management and Risk Reduction Technology (CEDIM), (2015) ] are discussed by examining how the way source-to-site distance measures, as in ground motion prediction equations, are evaluated affects the scenario shake map of a large subduction event within a fault rupture zone (note: size of the fault rupture zone can be in the order of a few hundred kilometers for M w 8.0+ earthquakes). Such investigations provide new insights for improvements in producing more reliable scenario shake maps and prompt earthquake impact assessments ( Goda and Atkinson, 2014 ). Subsequently, building typology in Nepal is reviewed briefly, followed by earthquake damage observations in Kathmandu, Melamchi, Trishuli, and Baluwa. Finally, key lessons from the 2015 Nepal earthquake are summarized.
Regional Seismicity and Ground Motion
This section aims at providing with relevant seismological information for interpreting earthquake damage survey observations in Nepal (which are discussed in the following section). First, seismotectonic and seismological aspects of the on-going mainshock–aftershock sequence are reviewed by analyzing available earthquake catalog data and source rupture models of the mainshock. Strong ground motion recordings at KATNP are analyzed to estimate the observed ground motion intensity in Kathmandu. Subsequently, scenario shake maps are generated by considering different source-to-site distance measures to highlight the influence of finite-fault source representation for a large earthquake in applications to prompt earthquake impact assessment.
Seismotectonic Setting and Seismic Hazard in Nepal
Nepal is located along the active Main Himalayan Thrust arc, where the subducting Indian plate and the overriding Eurasian plate interact. This region accommodates approximately a half of the tectonic convergence between these two plates, i.e., about 20 mm/year ( Avouac, 2003 ; Ader et al., 2012 ). The locked part of the subduction interface has a low-dip angle (about 10°) and is located at depths of 4–18 km ( Bilham, 2004 ), and has potential to generate M w 8+ earthquakes ( Gupta, 2006 ).
Historically, Nepal hosted several large earthquakes ( Ambraseys and Douglas, 2004 ; Bilham, 2004 ). A map of Nepal and locations of major historical seismic events are shown in Figure 1 . Western Nepal experienced a M w 8.2 event in 1505. This event occurred west of the rupture zone of the 2015 earthquake and accumulated strain in this seismic gap region has not been released since then; thus, there is high potential for future large earthquakes in the western region. In Eastern Nepal, two known major earthquakes occurred in 1833 and 1934. In particular, the 1934 M w 8.1 Bihar–Nepal earthquake was destructive and caused many fatalities (+10,000 deaths). The 2015 Gorkha–Kodari earthquakes have ruptured a fault section that overlaps with the fault rupture plane of the 1934 earthquake (see Figure 1 ). It is noted that the rupture planes of the 1934 and 2015 earthquakes are directly beneath Kathmandu, although the locations of their hypocenters are east and west of Kathmandu, respectively.
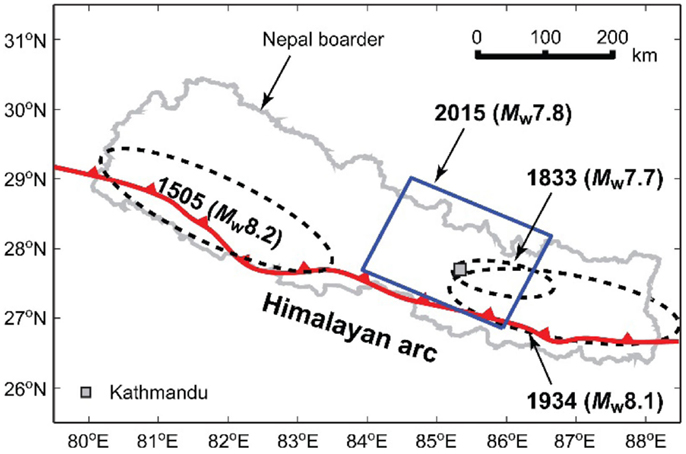
Figure 1. Major historical earthquakes in Nepal along the Himalayan arc . The locations of three historical earthquakes (1505, 1833, and 1934) are approximate. For the M w 7.8 mainshock, the fault plane model by the USGS is shown.
Recently, several probabilistic seismic hazard studies have been conducted for Nepal by employing updated seismic source zone models based on improved earthquake catalogs and modern ground motion models [e.g., Nath and Thingbaijam (2012) and Ram and Wang (2013) ]. The estimated peak ground acceleration (PGA) with 10% probability of exceedance in 50 years (i.e., return period of 475 years) in Western Nepal ranges between 0.5and 0.6 g, whereas that in Eastern Nepal ranges between 0.3 and 0.6 g. These hazard estimates are obtained for rock sites, therefore, when typical soil sites are considered (e.g., Kathmandu Valley), they need to be increased. An important observation is that the ground motion shaking in Kathmandu during the 2015 mainshock (which is discussed in detail in the following) was less than the PGA estimates with 10% probability of exceedance in 50 years, which may be considered as a basis for seismic design in Nepal.
Fault Rupture Model of the 25 April 2015 Mainshock
Several earthquake rupture models for the 2015 mainshock have been developed [e.g., United States Geological Survey (USGS) (2015) ; Yagi (2015) ]. A common feature of the estimated slip distributions is that large slips occurred north and north–east of Kathmandu, and the rupture propagated from the hypocenter (north–west of Kathmandu) toward east as well as south (deeper to shallower depth). The slip distribution of the USGS model is illustrated in Figure 2 A. The fault length and width of the rupture plane are 220 and 165 km, respectively, and its strike and dip are 295° and 10°, respectively. Figure 3 overlays the route of the survey trip over the USGS source model to put visited locations (i.e., Melamchi, Trishuli, and Baluwa) into perspective with respect to the earthquake slip distribution. The USGS source model has its maximum slip of 3.11 m (north of Kathmandu). It is also interesting to observe that the estimated slip near the hypocenter is 1.29 m, which is about 40% of the maximum slip, and its distance from the maximum slip sub-fault (i.e., asperity) is about 70 km. By analyzing numerous earthquake rupture models statistically, Mai et al. (2005) found that the rupture often nucleates in the regions of low-to-moderate slip (sub-faults with slip <2/3 of the maximum slip) and close to the maximum slip sub-fault. The rupture nucleation of the 2015 mainshock (i.e., slip and location at the hypocenter) is in good agreement with these empirical rules suggested by Mai et al. (2005) .
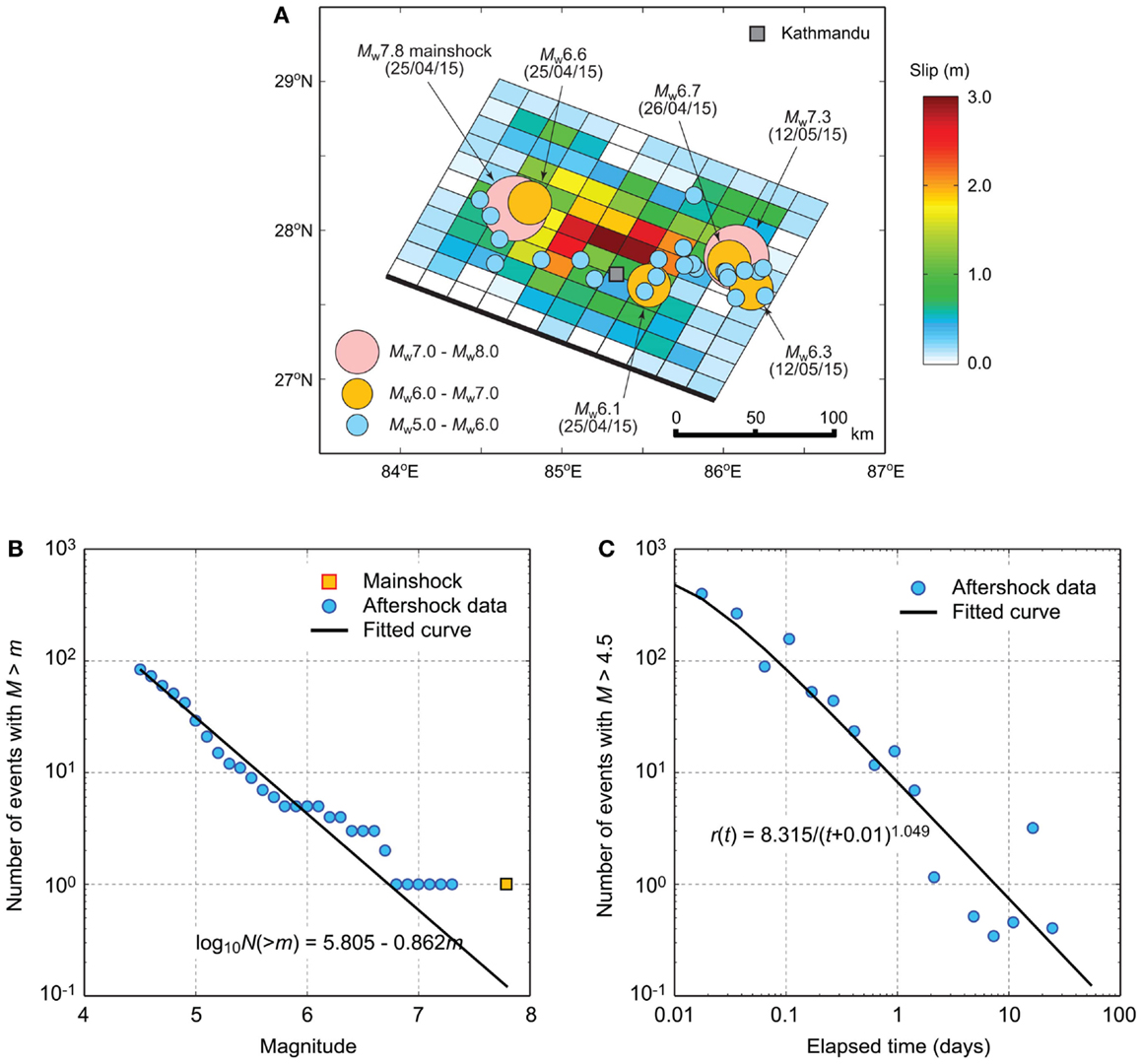
Figure 2. (A) Aftershock distribution of the 2015 earthquake sequence; an earthquake source model by the USGS is shown. (B) Gutenberg–Richter relationship of the 2015 earthquake sequence. (C) Modified Omori law of the 2015 earthquake sequence.
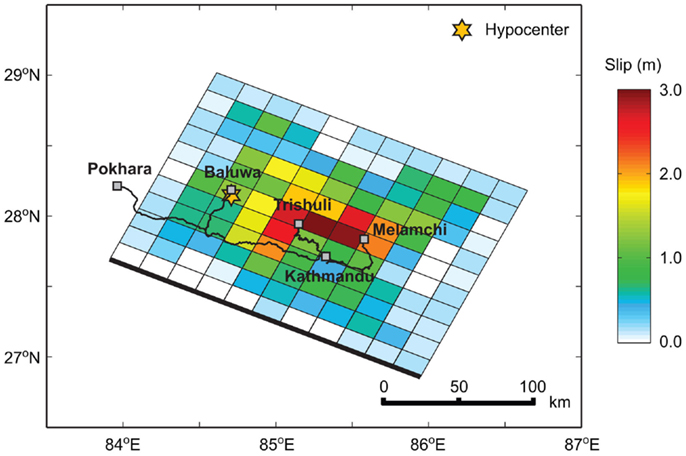
Figure 3. Earthquake damage survey locations .
Aftershocks
In post-earthquake situations, one of the major concerns for evacuees and emergency response teams is the occurrence of major aftershocks, triggering secondary hazards. Generally, a larger earthquake is followed by more aftershocks, and returning to a background level of seismic activities takes longer. Figure 2 A shows the spatial distribution of aftershocks that occurred before 25 May 2015 (30 days since the mainshock). The aftershock data are obtained from the USGS NEIC catalog 2 . Immediately after the mainshock, a moderate ( M w 6.6) aftershock occurred near the hypocenter. On the other hand, the majority of aftershocks occurred in the Kodari region (north–east of Kathmandu); a notable event was the 12 May 2015 M w 7.3 aftershock, which caused additional damage and casualties. Comparison of the aftershock distribution with respect to the slip distribution of the mainshock indicates that the major aftershocks do not occur very near to the mainshock asperity (with large slip) but they occur in the surrounding areas of the mainshock asperity. This is because the spatial and temporal characteristics of aftershocks are the manifestation of internal crustal dynamics involving the redistribution of stress and displacement fields ( Stern, 2002 ; Heuret et al., 2011 ).
To gain further insights into the aftershock occurrence process of the 2015 mainshock–aftershock sequence, statistical analysis of aftershock data is carried out by applying the Gutenberg–Richter law and the modified Omori law ( Shcherbakov et al., 2005 ); the completeness magnitude is set to 4.5 for the analyses. The Gutenberg–Richter law describes the frequency–magnitude characteristics of an aftershock sequence, whereas the modified Omori law models a temporal decay of an aftershock occurrence rate. The fitting of the 2015 Nepal aftershock data to the Gutenberg–Richter relationship is satisfactory (Figure 2 B); the estimated slope parameter (i.e., b -value) is −0.862. This slope is slightly gentler (i.e., more productive for larger aftershocks) than the typical b -value for global subduction earthquakes but within the expected range ( Shcherbakov et al., 2013 ). Figure 2 C shows that the modified Omori’s law fits well with the aftershock data. The obtained parameters are typical for global subduction earthquakes ( Shcherbakov et al., 2013 ). For example, the temporal decay parameter (i.e., p value, power parameter in the equation shown in Figure 2 C) is 1.049, which is close to the global average of about 1.2 (by taking into account inherent variability of this parameter). The above results support the applicability of well-established empirical laws for characterizing the 2015 Nepal aftershock data. This is a useful confirmation from seismic risk management viewpoints because initial estimates of aftershock-related hazard can be obtained from the empirical aftershock models immediately after the mainshock (before real-time data are collected and analyzed).
Ground Motion in Kathmandu
The accelerograms recorded at KATNP are publicly available 3 . In light of poor strong motion network in Nepal, the recorded ground motion data at KATNP are invaluable and serve as a benchmark in estimating ground motion intensity at unobserved locations in Kathmandu. Figure 4 shows the location of the KATNP station; the map also shows the locations of the earthquake damage survey sites in Kathmandu. The KATNP station is located near the historical district in the city center (e.g., Durbar Square), where severe damage and collapse of old historical buildings occurred.
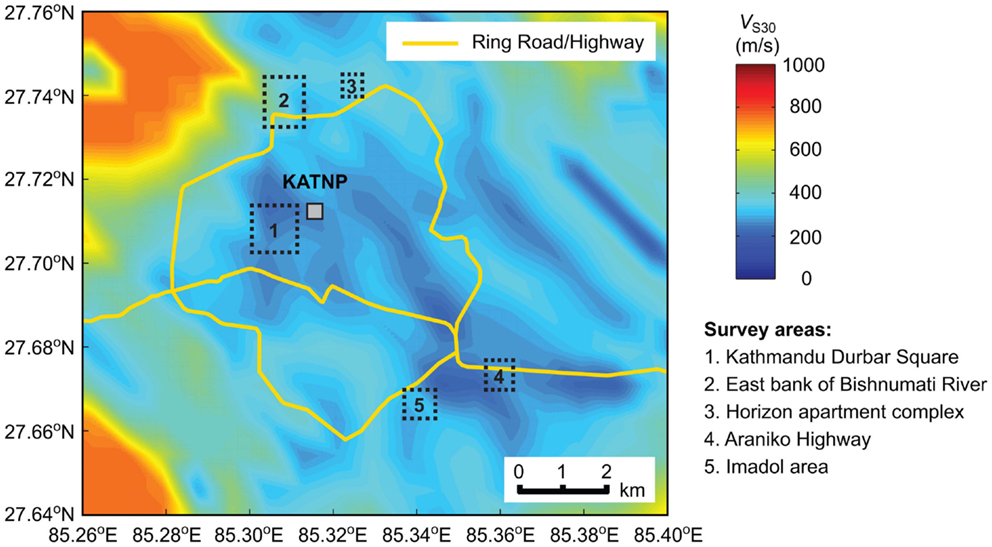
Figure 4. Location of the strong motion recording station KATNP and earthquake damage survey locations in Kathmandu .
Prior to ground motion data analysis and estimation, it is important to review typical site conditions in Kathmandu, as they affect ground motion intensity significantly. Kathmandu is located in the Kathmandu Basin, where thick lacustrine and fluvio-lacustrine sediments are deposited ( Sakai et al., 2002 ). The thickness of sediments (i.e., depth to bedrock) is in the range of 550–650 m. The setting of the Kathmandu Valley is similar to Mexico City ( Paudyal et al., 2012 ), noting that during the 1985 Michoacán earthquake, long-period ground motions were significantly amplified in Mexico City due to soft lakebed deposits and caused catastrophic damage to mid-to-high-rise buildings. A seismic microzonation study in Kathmandu, conducted by Paudyal et al. (2012) , indicates that the dominant periods of the ground at sites inside the Ring Road (see Figure 4 ) are between 1.0 and 2.0 s (i.e., high potential for resonating with long-period ground motions), and that the dominant period is correlated with the thickness of Pliocene and Quaternary deposits. The KATNP station is located within the long-dominant-period zone.
Another useful source of information in assessing site amplification potential of near-surface soil deposits in Kathmandu is the USGS global V S30 server ( Wald and Allen, 2007 ) 4 . V S30 is the average shear-wave velocity in the uppermost 30 m and is often employed as a proxy site parameter in ground motion models [e.g., Kanno et al. (2006) and Boore and Atkinson (2008) ]. Wald and Allen (2007) correlated V S30 data with topographic slope to derive the first-order estimate of the site amplification for two tectonic regimes, active and stable continental regions. The database is implemented to develop USGS ShakeMaps ( Wald et al., 2005 ) 5 , which are used for rapid earthquake impact assessment ( Jaiswal and Wald, 2010 ) 6 . Figure 4 shows the V S30 contour map in Kathmandu. The map indicates that the central part of Kathmandu has soft surface deposits (typically NEHRP site class D, V S30 between 180 and 360 m/s). The V S30 value at the KATNP station is 250 m/s. It is noteworthy that V S30 is applicable to near-surface site amplification only; amplification of long-period seismic waves due to a large-scale geological structure (e.g., basin) should be taken into account separately.
Figures 5 A,B show recorded accelerograms (three components) at KATNP for the M w 7.8 mainshock and for the M w 7.3 aftershock, respectively (note: among other recorded aftershock ground motions at KATNP, the M w 7.3 aftershock records show the most significant effects). An inspection of the time-history data indicates that the PGA of the recorded ground motions is about 150–170 and 70–80 cm/s 2 for the M w 7.8 mainshock and the M w 7.3 aftershock, respectively. These are significantly smaller than the PGA estimates with 10% probability of exceedance in 50 years from the recent regional seismic hazard studies ( Nath and Thingbaijam, 2012 ; Ram and Wang, 2013 ). It is also observed that long-period components are present in the M w 7.8 mainshock records (Figure 5 A). To further investigate the extent of ground shaking at KATNP, 5%-damped response spectra of the recorded accelerograms for the M w 7.8 mainshock and the M w 7.3 aftershock are calculated and compared in Figure 5 C. The results suggest that the amplitudes of response spectra for the mainshock are greater than those for the major aftershock (also applicable to other aftershocks). For the M w 7.8 mainshock, two large peaks of response spectra are present at vibration periods around 0.2–0.6 s (N–S component only) and around 4.0–6.0 s (both N–S and E–W components). The former is attributed to direct shaking due to near-source ruptures, whereas the latter is caused by the combination of rich long-period content of seismic waves at source (because of large moment magnitude) and site amplification due to the basin effects. Given the existing building stock in Kathmandu/Nepal (the majority of buildings are low-to-mid rise and thus are likely to have vibration periods <1.0 s; Chaulagain et al., 2015 ), the main causes of severe structural damage and collapse of buildings in Kathmandu are due to the large peak in the short vibration period range. It is important to point out that buildings in Kathmandu were largely unaffected by the long-period ground motions in the Kathmandu Valley because of non-resonance. This was fortunate in the context of the current disaster. However, earthquake engineers should pay careful attention to long-period ground motions ( Takewaki et al., 2011 ), when tall buildings are constructed in the central part of the Kathmandu Valley.
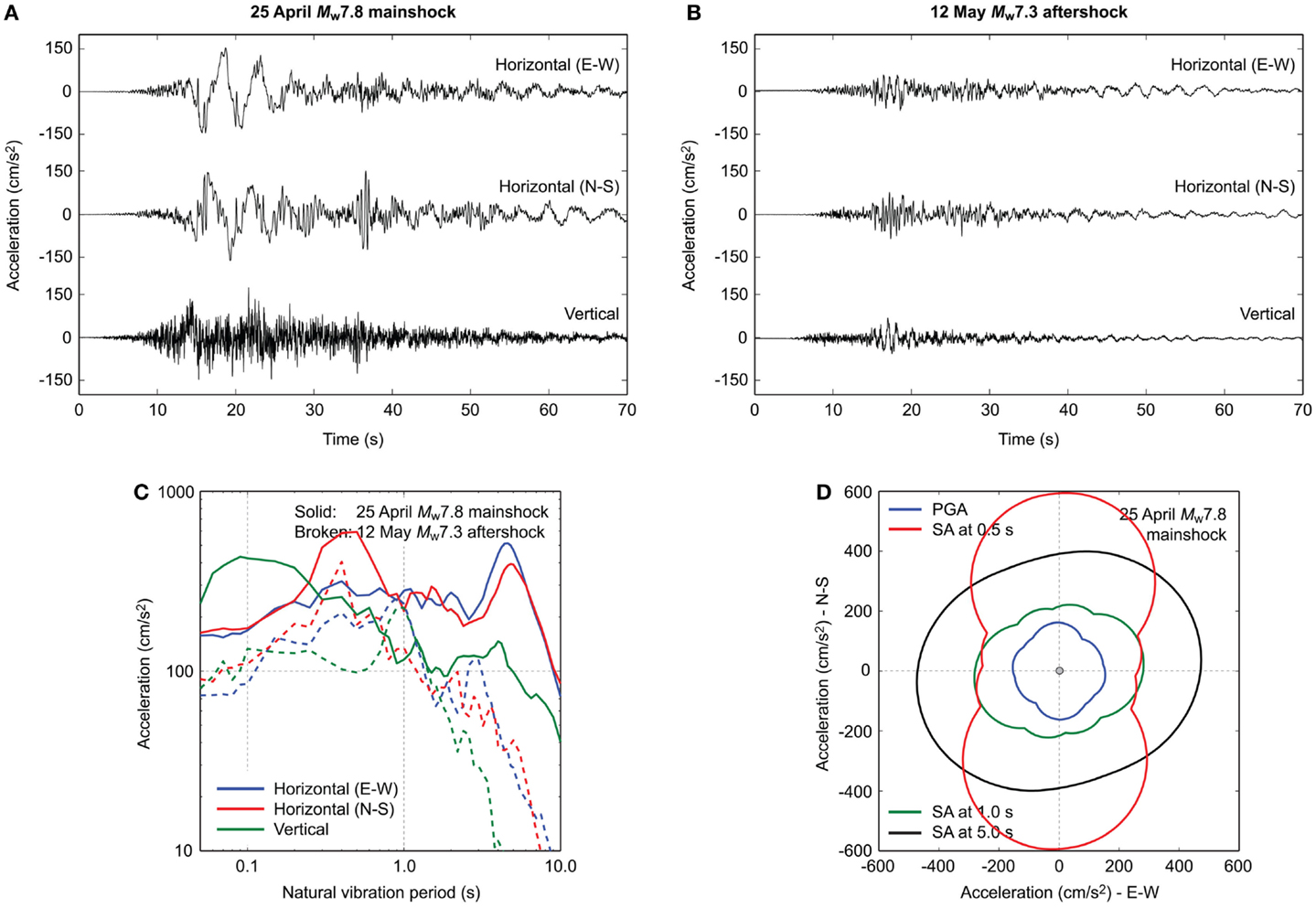
Figure 5. (A) Recorded accelerograms at KATNP for the M w 7.8 mainshock. (B) Recorded accelerograms at KATNP for the M w 7.3 aftershock. (C) 5%-damped spectral accelerations (SA) of the recorded accelerograms at KATNP for the M w 7.8 mainshock and the M w 7.3 aftershock. (D) Polar plots of the PGA and spectral accelerations at 0.5, 1.0, and 5.0 s of the rotated accelerograms at KATNP for the M w 7.8 mainshock.
To further examine the orientation of ground motion parameters at KATNP for the M w 7.8 mainshock, PGA and 5%-damped spectral accelerations are computed by rotating accelerograms recorded at KATNP from 0° to 360° ( Hong and Goda, 2007 ). The polar plots of PGA and spectral accelerations at 0.5, 1.0, and 5.0 s are shown in Figure 5 D. The results are useful for understanding the orientation dependency of the peak seismic demand in the near-fault region ( Huang et al., 2008 ). The results indicate that the spectral acceleration at 0.5 s (i.e., large response spectral peak in the short-vibration period range) is highly polarized; the ratio of the maximum-to-minimum response is about 2.5, while the degree of such polarization of the response spectra is much less pronounced at other vibration periods. Although it is beyond the scope of this study, a further insight can be gained by investigating the effects of the orientation of ground motion with regard to the structural axis of damaged versus non-damaged buildings near the KATNP station.
Comparison of Observed Ground Motion in Kathmandu with Ground Motion from the 2008 Wenchuan Earthquake and Predicted Ground Motion
Due to the limited availability of recorded ground motions in Central Nepal, ground motion estimation may need to rely on: (1) ground motion data from other seismic regions having broad similarity with the target region [e.g., Sharma et al. (2009) ], (2) empirical ground motion prediction models [e.g., Nath and Thingbaijam (2011) ], or (3) ground motion simulations [e.g., Harbindu et al. (2014) ]. In this study, the first two options are explored to gain insights into actual ground motions for the M w 7.8 mainshock.
For Option 1, ground motion data from the 2008 M w 7.9 Wenchuan earthquake ( Lu et al., 2010 ) are analyzed. This earthquake is chosen because seismotectonic settings in Nepal and Tibet (i.e., southern and eastern sides of the Tibetan Plateau) are broadly similar and their earthquake magnitudes are comparable. The Wenchuan earthquake occurred along the Longmenshan fault Sichuan, China. The amplitude–distance plots of PGA and spectral accelerations at 0.5and 5.0 s are shown in Figure 6 ; only records at soft soil sites ( V S30 <400 m/s) are considered. The rupture distance ( R rup , shortest distance from a site of interest to the fault rupture plane) for the Wenchuan data is calculated using the fault plane model by Ji (2008) .
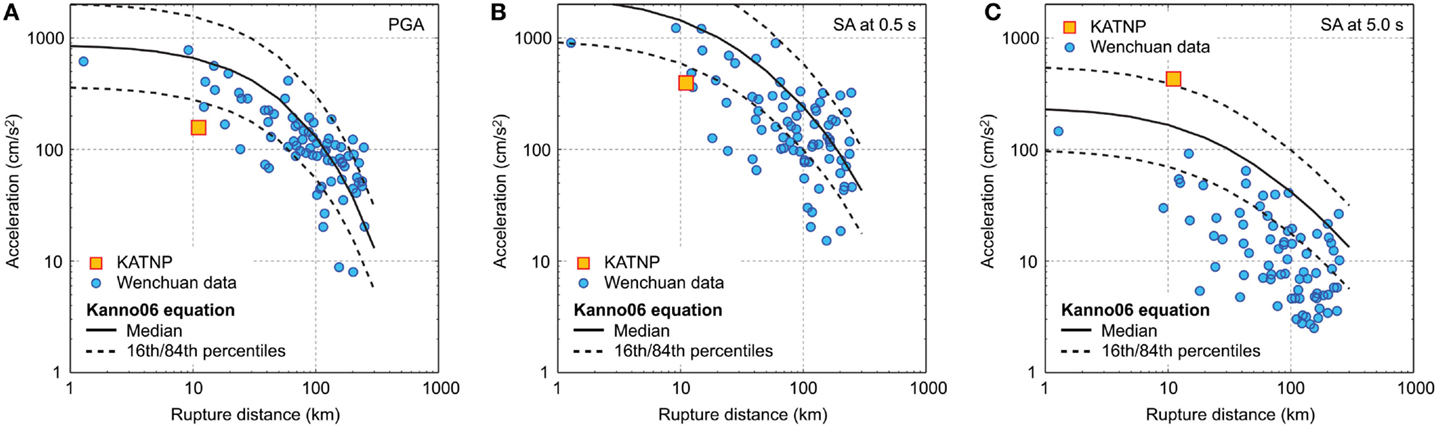
Figure 6. Comparison of the observed PGA (A) and spectral accelerations [0.5 s in (B) and 5.0 s in (C)] at KATNP with the M w 7.9 Wenchuan ground motion data and with the ground motion model by Kanno et al. (2006) .
For Option 2, a ground motion model by Kanno et al. (2006) (hereafter Kanno06) is adopted. This prediction equation was developed by using ground motion records from Japanese earthquakes and from worldwide shallow crustal earthquakes (i.e., Next Generation Attenuation database). The Kanno06 equation is selected among other applicable models [e.g., Boore and Atkinson (2008) , Sharma et al. (2009) , and Harbindu et al. (2014) ] for three reasons. The first reason is that the performance test of various ground motion models conducted by Nath and Thingbaijam (2011) indicates that the Kanno06 equation is superior to other candidate models in predicting PGA at rock sites in Northern India and Nepal. Second, the applicable moment magnitude range of the Kanno06 equation covers the moment magnitude of the 2015 Nepal earthquake; for instance, regional equations by Sharma et al. (2009) and Harbindu et al. (2014) are not applicable to M w 8-class earthquakes. Third, the Kanno06 equation adopts R rup as a representative distance measure, while the equation by Boore and Atkinson (2008) (hereafter BA08) adopts the Joyner–Boore distance ( R jb , shortest distance from a site of interest to the projected fault rupture plane on Earth’s surface). The use of R jb can be problematic because ground motion intensity for the locations above the fault rupture plane is evaluated using a uniform value of R jb = 0 km (which results in significant bias of predicted ground motion intensity). This issue is revisited in the next subsection.
Figure 6 compares observed ground motions at KATNP (i.e., Figure 5 ) with the ground motion data from the M w 7.9 Wenchuan earthquake as well as the Kanno06 model. The rupture distance for KATNP (=11.1 km) is calculated using the USGS finite-fault plane model (i.e., Figure 2 A). For the Kanno06 model, 16th and 84th percentile curves are also shown to indicate a typical range of predicted ground motion variability. Figure 6 A indicates that the observed PGA at KATNP is significantly smaller than the Wenchuan data in the similar distance range and the predicted PGA based on the Kanno06 equation (below the 16th percentile curve). The below-average trend of the observed ground motion intensity, in comparison with the Wenchuan data and the Kanno06 model, persists for spectral accelerations at vibration periods <2.0 s (Figure 6 B). These comparisons indicate that the level of short-period ground motion near KATNP during the 2015 mainshock was smaller than expected ground motion levels based on empirical data/models for similar scenarios. On the other hand, Figure 6 C shows an opposite trend: the long-period spectral acceleration at KATNP is significantly greater than the counterparts based on the Wenchuan data and the Kanno06 model. The large spectral acceleration in the long vibration period range is attributed to the basin effects. It is also interesting to note that the recent ground motion prediction model, such as Boore et al. (2014) , can take into account the basin effects using a depth-to-bedrock parameter [note: in Figure 6 , the equation by Boore et al. (2014) is not considered because it is based on R jb ]. Using the empirical model by Boore et al. (2014) , the expected site amplification due to the basin effects is a factor of two for vibration periods longer than 2.0 s; the observed long-period spectral acceleration can be better explained. Therefore, it is important to adopt advanced ground motion models that can account for major systematic components (e.g., faulting mechanism and basin amplification) in predicting ground motion intensity for future earthquakes.
Scenario Shake Map
Rapid earthquake impact reports [e.g., Center for Disaster Management and Risk Reduction Technology (CEDIM), (2015) ] are useful because emergency officers and international aiding agencies can appreciate the expected level of destruction due to an earthquake at the very early stage of a disaster. In producing rapid earthquake impact assessment, scenario shake maps are the essential input. In these applications, shake maps are generated by using a suitable ground motion model together with observed instrumental data and seismic intensity information (e.g., DYFI; Atkinson and Wald, 2007 ) 7 . In seismic regions with limited monitoring capability of strong motion, shake maps are more dependent on the accuracy of an adopted ground motion model as well as on initial estimates of the seismic event (e.g., moment magnitude). This is because there will not be many real-time observations to constrain the shake map predictions.
Modern ground motion models adopt extended-source-based distance measures, such as R rup and R jb (i.e., calculation of these distance measures requires a fault plane model). A simpler representation of an earthquake source is a point source model; in this case, hypocentral and epicentral distances, R hypo and R epi , are often used. When a slip distribution is available, another useful distance measure is the shortest distance to the asperity R asp ( Goda and Atkinson, 2014 ). For large subduction events having large fault plane dimensions, the calculated distance measures can vary significantly, depending on how a fault plane model is defined and which distance measure is adopted. For instance, for the M w 7.8 mainshock, distance measures at KATNP are evaluated as: R rup = 11.1 km, R jb = 0.1 km (numerical lower bound), R hypo = 85.3 km, R epi = 76.8 km, and R asp = 29.4 km. The influence of distance measures is particularly significant for large magnitude events.
The above-mentioned problem has an important implication on shake map generation for a large earthquake. To demonstrate this for the M w 7.8 mainshock, four scenario PGA shake maps are developed by considering different distance measures and ground motion models. The results are shown in Figure 7 . Figures 7 –C are based on the Kanno06 model together with R rup , R hypo , and R asp , respectively, whereas Figure 7 D is based on the BA08 model with R jb . For all shake maps, V S30 information at individual sites is taken into account. Strictly, R hypo and R asp should not be used in the Kanno06 model (as the distance measures and the model development process are incompatible); this is for illustration only. Figure 7 A shows the predicted PGAs at sites above the fault plane are large (0.5–0.7 g) and predicted PGA values gradually decrease toward north (i.e., the fault plane becomes deeper). Figures 7 B,C show different patterns from Figure 7 A because the distance measures are essentially defined for point source but with different source locations (i.e., hypocenter versus asperity). The predicted PGA values in Figures 7 B,C are less than those in Figure 7 A and are in more agreement with observed ground motion intensity in Kathmandu. Figure 7 D shows the most significant difference from the observed ground motion intensity in Kathmandu because for all sites above the fault plane, the distance measure is set to R jb = 0.1 km. Indeed, the USGS ShakeMap is similar to Figure 7 D in terms of amplitude and spatial pattern of the shake map. Importantly, bias in estimated ground motions propagates into rapid earthquake impact assessment. The key issue here is that the current ground motion model together with a finite-fault plane can result in biased predictions of overall earthquake impact (which may affect subsequent decisions for emergency response actions). From practical viewpoints, this issue needs to be resolved in the near future.
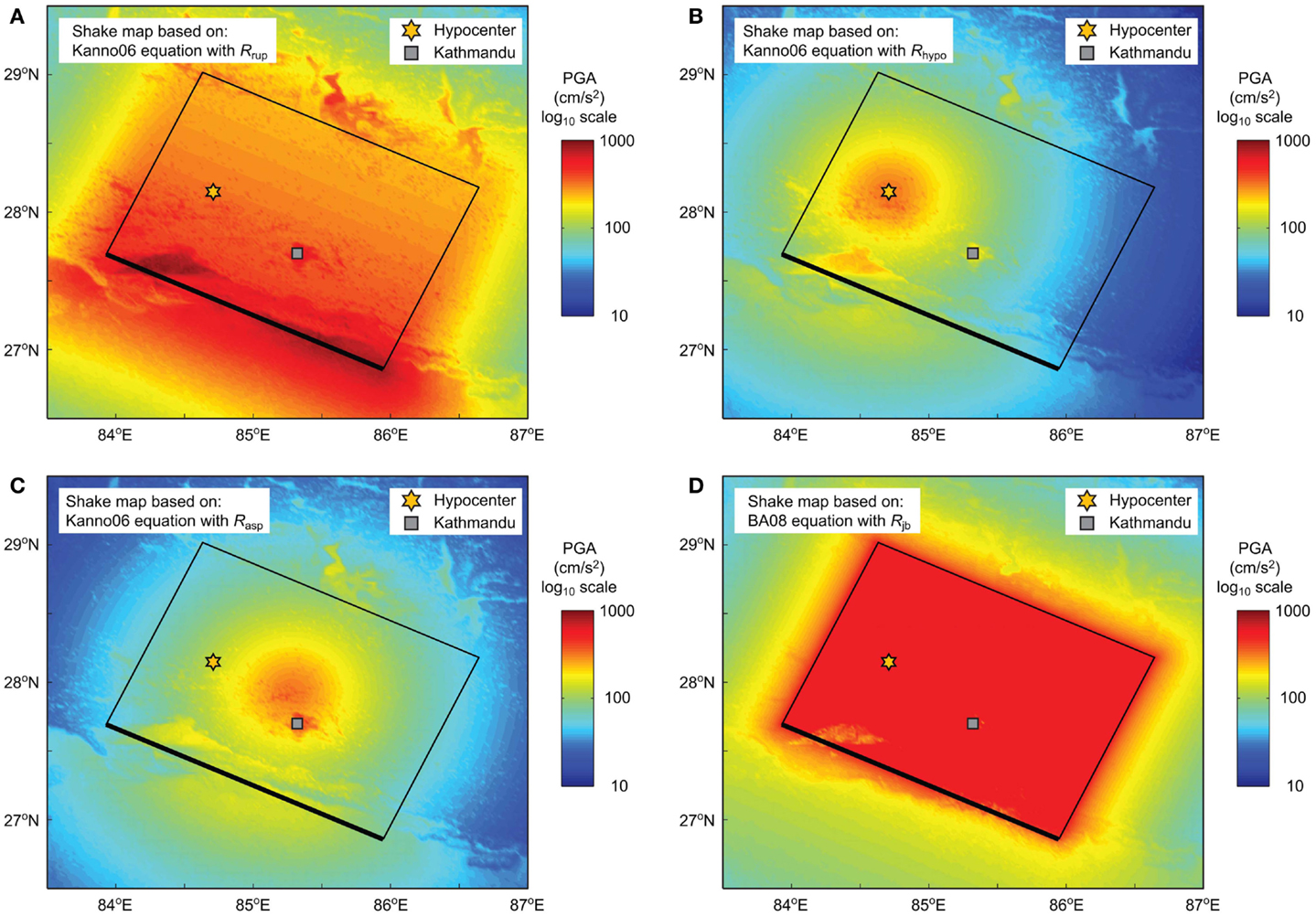
Figure 7. Comparison of scenario shake maps for the M w 7.8 mainshock using: (A) the Kanno06 equation with rupture distance ( R rup ), (B) the Kanno06 equation with hypocentral distance ( R hypo ), (C) the Kanno06 equation with distance closest to the asperity ( R asp ), and (D) the BA08 equation with Joyner–Boore distance ( R jb ) .
Earthquake Damage Survey
This section presents main observations and findings from the earthquake damage survey in Nepal. The building typology in Nepal is briefly reviewed, and then, field observations in Kathmandu, Melamchi, Trishuli, and Baluwa are discussed. The regional map of the visited locations is shown in Figure 3 , and the main survey locations in Kathmandu are indicated in Figure 4 . The cases discussed in the following are selected to highlight main observations from the survey trip. Numerous photos are available through the Google Earth file as supplementary material to this paper.
Building Typology in Nepal
Buildings in Nepal are vulnerable to seismic actions. The majority of houses and buildings are not seismically designed and constructed, lacking ductile behavior. Due to poor seismic performance, many buildings were damaged/collapsed and these structural failures caused many fatalities during the 2015 earthquake sequences. This subsection briefly summarizes general characteristics of building typology in Nepal. More complete information (e.g., statistics of building characteristics) is available in Chaulagain et al. (2015) . According to the 2011 National Population and Housing Census, the total number of individual households in Nepal is 5,423,297, while the population is 26,494,504. The census data indicate that mud-bonded brick/stone masonry buildings are the most common in all geographical regions of Nepal (44.2%), followed by wooden buildings (24.9%). In urban areas (e.g., Kathmandu Valley), buildings with cement-bounded brick/stone (17.6%) and cement concrete (9.9%) are popular.
In Nepal, many masonry buildings are constructed with walls made of sun-dried/fired bricks or stone with mud mortar, and the building frame is made of wood. These types of buildings generally have flexible floors and roof, and are prevalent in rural areas. The masonry materials are of low strength and thus are seismically vulnerable. Recently, with the advancement of the cement in Nepal, brick/stone buildings are constructed with cement mortar. The wooden buildings are popular near the forest areas in Nepal. In these buildings, wooden pillars are made out of tree trunks and walls are constructed with wooden planks or bamboo net cement/mud mortar plaster. The reinforced concrete (RC) building is a modern form of construction in Nepal, which began in late 1970s. The RC moment resisting frame assembly is comprised of cast-in-place concrete beams and columns with cast-in-place concrete slabs for floor and roof. Most of the conventional RC constructions are non-engineered (i.e., not structurally designed) and thus lack sufficient seismic resistance. Engineered RC buildings, which are relatively new, often adopt the Indian standard code with seismic provisions.
Survey Results in Kathmandu
Many historical buildings in the Kathmandu Durbar Square (in front of the Old Royal Palace of the former Kathmandu Kingdom and is a UNESCO World Heritage site) were devastated (area 1 in Figure 4 ). Figure 8 A shows the collapse of the Basantapur Tower. The complete destruction in the Durbar Square was in sharp contrast with undamaged buildings surrounding the Durbar Square (Figure 8 B; several wall cracks can be found on these buildings; however, the majority of the masonry buildings are structurally stable). This indicates that the ground shaking experienced in this area (note: this is relatively close to the KATNP station; see Figure 4 ) was sufficient to cause the collapses of the old historical buildings but was not to cause severe damage to the surrounding buildings. This observation was confirmed by walking through the Indra Chowk area (market squares near the Old Palace), where many old masonry buildings (three to six stories) were densely constructed. Nevertheless, there were several buildings that collapsed completely and some search and rescue activities were undertaken (Figures 8 C,D).
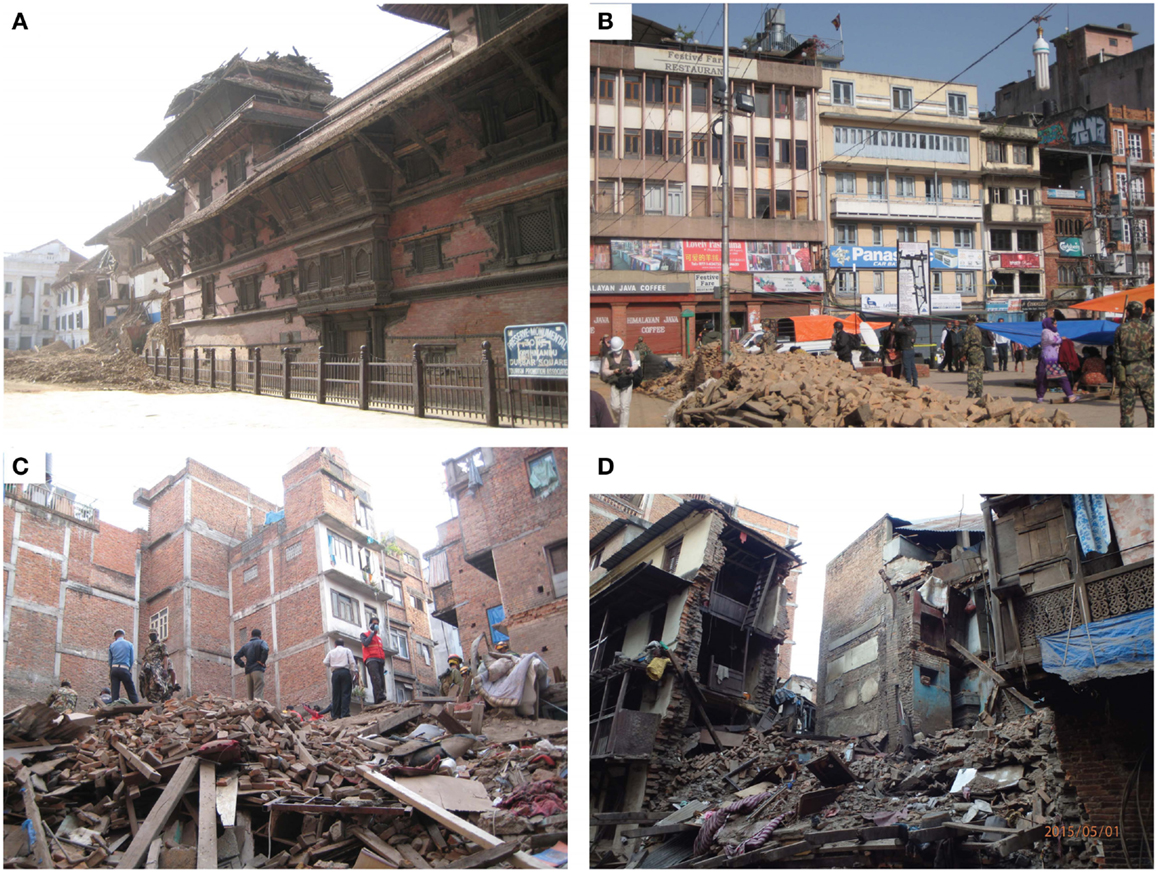
Figure 8. Damage in Kathmandu (area 1 in Figure 4 ) . (A) Collapse of the Basantapur Tower in the Kathmandu Durbar Square. (B) Undamaged buildings opposite of the Basantapur Tower in the Kathmandu Durbar Square. (C) Collapse of four 5- or 6-story old masonry buildings. (D) Collapse of a 4-story masonry building.
There were numerous building collapses in the north–west section of the Ring Road along the Bishnumati River (area 2 in Figure 4 ). According to the local geomorphological map, sites within about 300 m from the river are alluvial (Holocene) soil deposits, whereas sites farther east are Pleistocene soil deposits. Therefore, site amplification effects due to different soil conditions may be expected in this area. A walk-through survey was carried out to investigate the spatial distribution of collapsed and severely damaged buildings in this area. Out of 28 collapsed or severely damaged buildings, 19 buildings were in the alluvial deposit area (Figure 9 A), whereas 9 buildings were in the Pleistocene deposit area but nearer to the boundary (Figure 9 B). This qualitatively confirms the effects of local site conditions on the building damage and collapse.
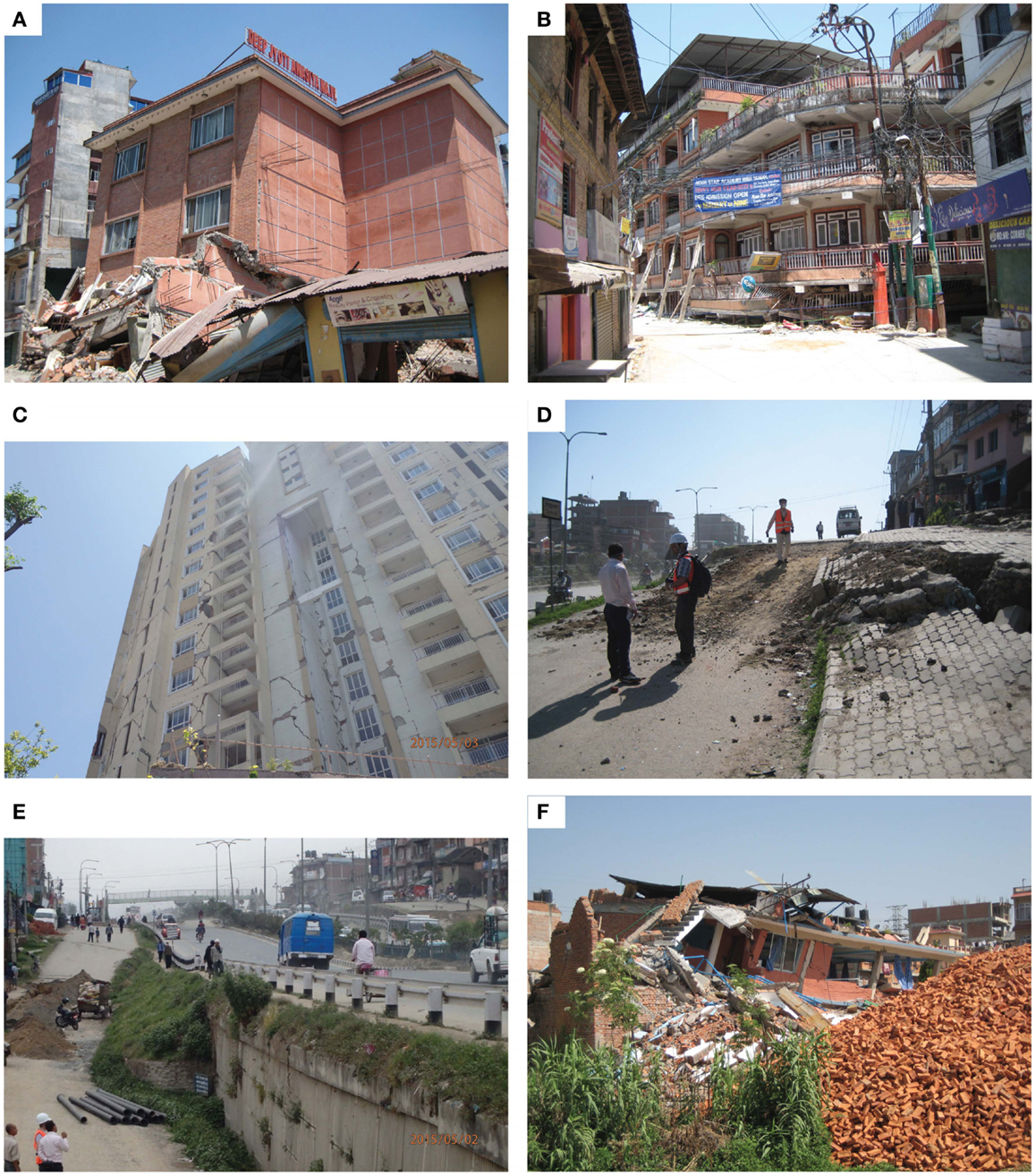
Figure 9. Damage in Kathmandu (areas 2–5 in Figure 4 ) . (A) Collapsed building along the Bishnumati River (alluvial soil deposit area; area 2 in Figure 4 ). (B) Collapsed building (soft story collapse) near the Bishnumati River (boundary between alluvial and Pleistocene soil deposit areas; area 2 in Figure 4 ). (C) Horizon apartment buildings (area 3 in Figure 4 ). (D) Settlement of the Araniko Highway (area 4 in Figure 4 ). (E) Damage to the Araniko Highway (area 4 in Figure 4 ). (F) Collapsed church in the Imadol area (area 5 in Figure 4 ).
In area 3, there was a 16-story high-rise apartment complex (Park View Horizon). The walls of this building suffered from many major cracks along its height (Figure 9 C). Currently, the apartments are unfit for living and residents have evacuated. The causes of the major damage of the Horizon apartments (and similar high-rise buildings in Kathmandu) may be attributed to the long-period ground motions (Figure 5 ). In addition, local topological features may have contributed to extensive damage there (the complex is on a hill).
Along the Araniko Highway between Kathmandu and Bhaktapur (area 4 in Figure 4 ), a section of the highway (about 200 m in length) built upon embankments was damaged due to the ground settlement. The amount of settlements was about 0.5–2.0 m, depending on locations (Figure 9 D). The central section of the highway was constructed using reinforced soil retaining wall and gravity-type retaining wall (2–3 m high and 100 m wide). The retaining walls were structurally intact and suffered from minor cracks and outward deformation only, whereas the natural slopes at both ends of the highway embankments experienced noticeable settlements (Figure 9 E). Several buildings along the highway were tilted due to the settlements. A pedestrian footbridge crossing the highway suffered from the differential settlement of foundation, resulting in a gap of 45 cm between the bridge girder and the stair steps.
In area 5 (Figure 4 ), minor liquefaction, which was evidenced by sand boils and did not cause any structural damage, was observed in a small open land near a canal. In the surveyed area, a church was collapsed due to the ground shaking (Figure 9 F). According to local residents, the church building was standing after the M w 7.8 mainshock but was collapsed due to the M w 6.7 aftershock on the following day. The extent of structural damage before the M w 6.7 aftershock is unknown. There were several houses that settled and tilted in this area. However, the degree of destruction in this area was minor.
Overall, earthquake damage in Kathmandu was not widespread but more localized. This may suggest that overall strong shaking experienced in Kathmandu was not extremely large. The areas that suffered from major destruction tend to have some local characteristics, such as soft soil conditions and structural deficiencies.
Survey Results in Melamchi
The survey was conducted along the road to Melamchi (about 30 km north–east of Kathmandu; Figure 3 ). Melamchi and the surrounding areas were close to the locations of major aftershocks (i.e., 26 April M w 6.7 aftershock and 12 May M w 7.3 aftershock; Figures 2 A and 3 ), and suffered from devastation due to these earthquakes. On the way to Melamchi, there were many small villages that suffered from earthquake damage. During interviews with local residents, they expressed serious concerns about incessant aftershocks and urgent need of repairs of the damaged houses before the arrival of rainy season. Proceeding north toward Melamchi, the occurrence of earthquake damage becomes more frequent.
Melamchi is a small town along the Indrawati River, and residents in the town have been involved with a major Melamchi Water Supply project 8 , which diverts the river and channels its water to Kathmandu through tunnels. There were several factories along the road, which make water main pipes. Overall, the earthquake damage in Melamchi was severe, mostly affecting vulnerable masonry buildings, whereas the damage to RC buildings (4- to 5-story) was limited. For instance, the main street of Melamchi was not completely destroyed (Figure 10 A); most buildings looked undamaged based on their appearances, although several buildings were collapsed. On the other hand, buildings along a side street were devastated by the earthquakes (Figures 10 B,C). The majority of the damaged buildings were made of brick and stone. Along the road, several sections of the slope suffered from shallow landsides (Figure 10 D), their debris blocked the road at one time but was removed. There was a steel truss bridge with RC deck for vehicle crossing; the bridge was not damaged (inspected from backside). It has been reported that further damage occurred in Melamchi due to the 12 May M w 7.3 aftershock. A further damage survey in Melamchi is required to investigate the effects of the aftershock with respect to the incurred damage prior to the aftershock (although it is beyond the scope of this study).
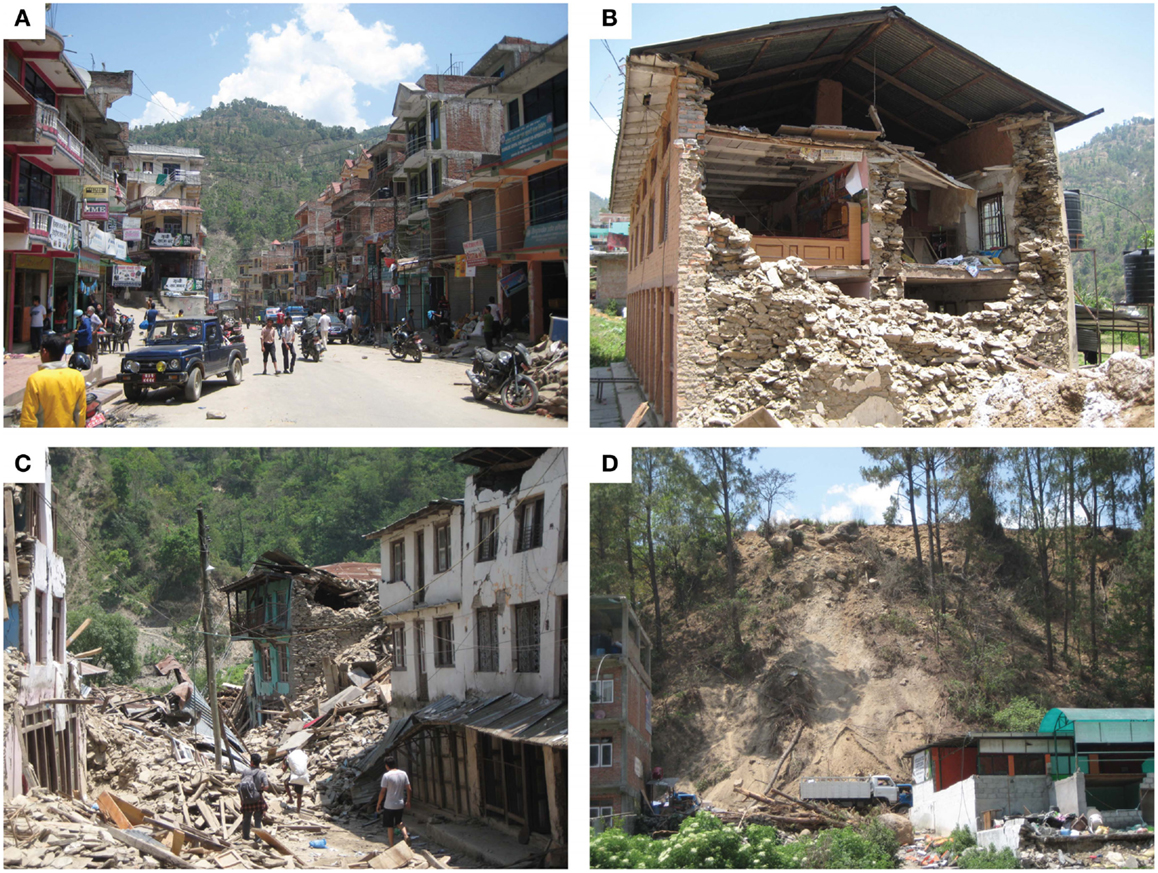
Figure 10. Damage in Melamchi (see Figure 3 ) . (A) Main street in Melamchi. (B) Damaged stone masonry house. (C) Devastated street in Melamchi. (D) Shallow landslide along the main road.
Survey Results in Trishuli
The survey was conducted along the road to Trishuli (about 30 km north–west of Kathmandu; Figure 3 ). One of the purposes of the trip was to investigate the earthquake damage near the Trishuli hydroelectric station. Trishuli was closer to the hypocenter of the M w 7.8 mainshock, and thus severer damage, in comparison with Kathmandu, was expected. Along the way to Trishuli, earthquake damage in Ranipauwa (about 15 km north–west of Kathmandu) appeared relatively minor. Proceeding further north–west, earthquake damage to houses and landslides along the mountain slopes were observed more frequently. The rock fall, as secondary hazard, can be dangerous; a bus was hit by fallen boulder and several people were killed (Figure 11 A). In Battar (about 25 km north–west of Kathmandu), a large number of brick/stone masonry buildings were collapsed (Figure 11 B). The building materials of these damaged buildings were of poor quality; for example, two different types of the fragile bricks were used in one of the damaged houses (Figure 11 C). According to local residents, many buildings were collapsed due to the 25 April M w 6.6 aftershock, which occurred 30 min after the mainshock.
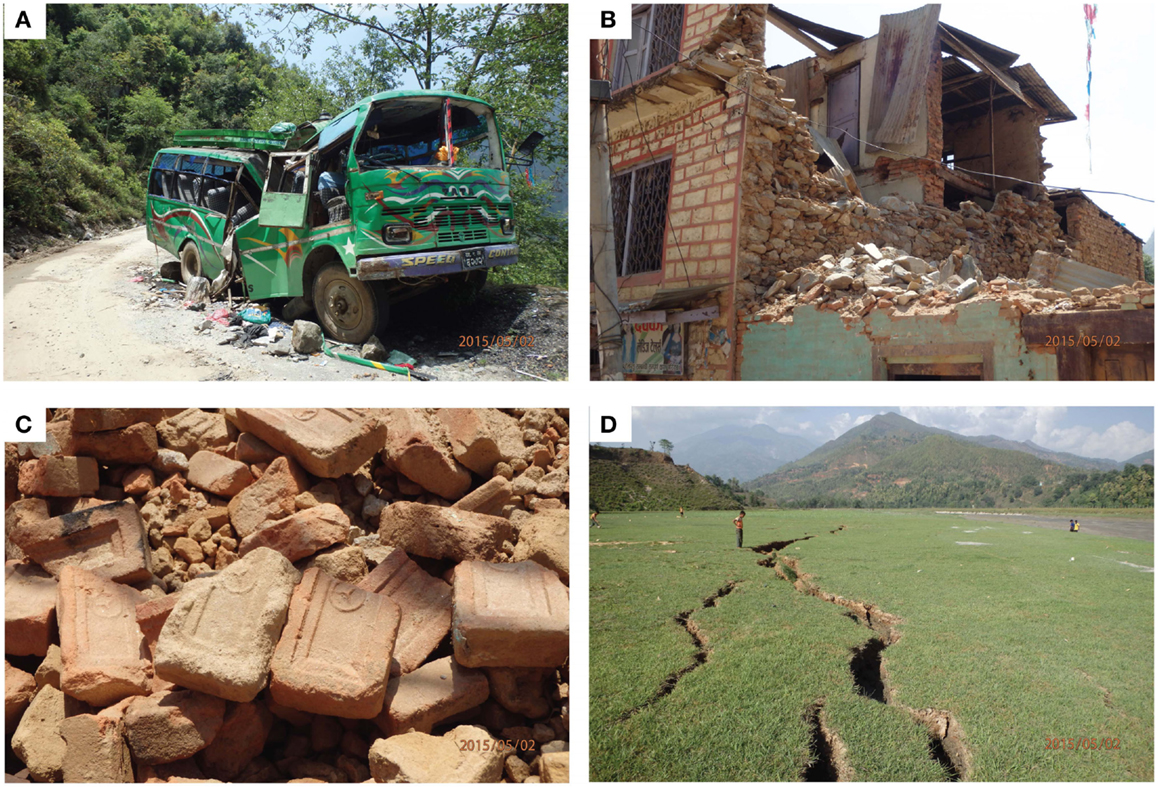
Figure 11. Damage in Trishuli (see Figure 3 ) . (A) Destroyed bus due to boulder fall. (B) Damaged brick masonry house in Battar. (C) Different types of bricks used in the damaged masonry house in Battar. (D) Ground fissures in the Trishuli dam reservoir.
In Trishuli, there was an earth fill dam for hydroelectric power generation. The main body of the dam was the excavated and compacted soil. The height of the dam was 12 m (upstream side) and 20 m (downstream side), and the crest width was about 4 m. Due to the earthquake, there were cracks at upstream side of the dam and fissures on the crest. Moreover, liquefaction (as evidenced by silt boils) and lateral spreading (Figure 11 D) occurred inside of the dam reservoir due to the earthquake. The operation of the power generation had been suspended since the following day of the mainshock; at the time of the visit, no power was available in nearby villages. Overall, the earthquake damage to the Trishuli dam will not cause severe problems immediately. However, the extent of cracking along the dam axis may suggest a deterioration of the dam body, which may be accelerated into the dam failure by future earthquakes or penetration of rain water into the dam body through cracks. It is important to mention that in worst-case scenarios (note: this earthquake is not the extreme case in terms of ground shaking intensity), catastrophic dam failures could have been caused. As there are several major hydroelectric projects along the Trishuli River as well as in other major rivers in Nepal, ensuring dam safety against large earthquakes is important.
Survey Results in Baluwa
The survey team visited Baluwa (about 70 km north–west of Kathmandu; Figure 3 ) along the Daraudi River, which is close to the epicenter of the M w 7.8 mainshock. One of the aims for this visit was to investigate the earthquake damage very near to the epicenter. Along the Kathmandu–Pokhara highway (e.g., Abu Khaireni, a town located at an intersection between the main highway and the Daraudi link road; about 30 km from the epicenter), no major earthquake damage was observed. At distances of about 18 km from the epicenter, earthquake damage to houses was observed; proceeding further north toward Baluwa, the extent of earthquake damage to houses became severer. The first stone house that was collapsed due to the earthquakes was about 4.5 km from the epicenter. Similarly, many shallow landslides and rock falls were observed along the road to Baluwa (Figure 12 A); the first middle-size landslide was observed at distances of about 15 km from the epicenter. At one location, the debris from a landslide blocked the road completely (Figure 12 B; note: detour was possible). The spatial distribution of the collapsed houses and landslides was limited to the locations near the epicenter (within 10–15 km radius), and was in contrast with Melamchi and Trishuli (i.e., farther from the epicenter). This can be understood by referring to the slip distribution of the mainshock (Figures 2 A and 3 ).
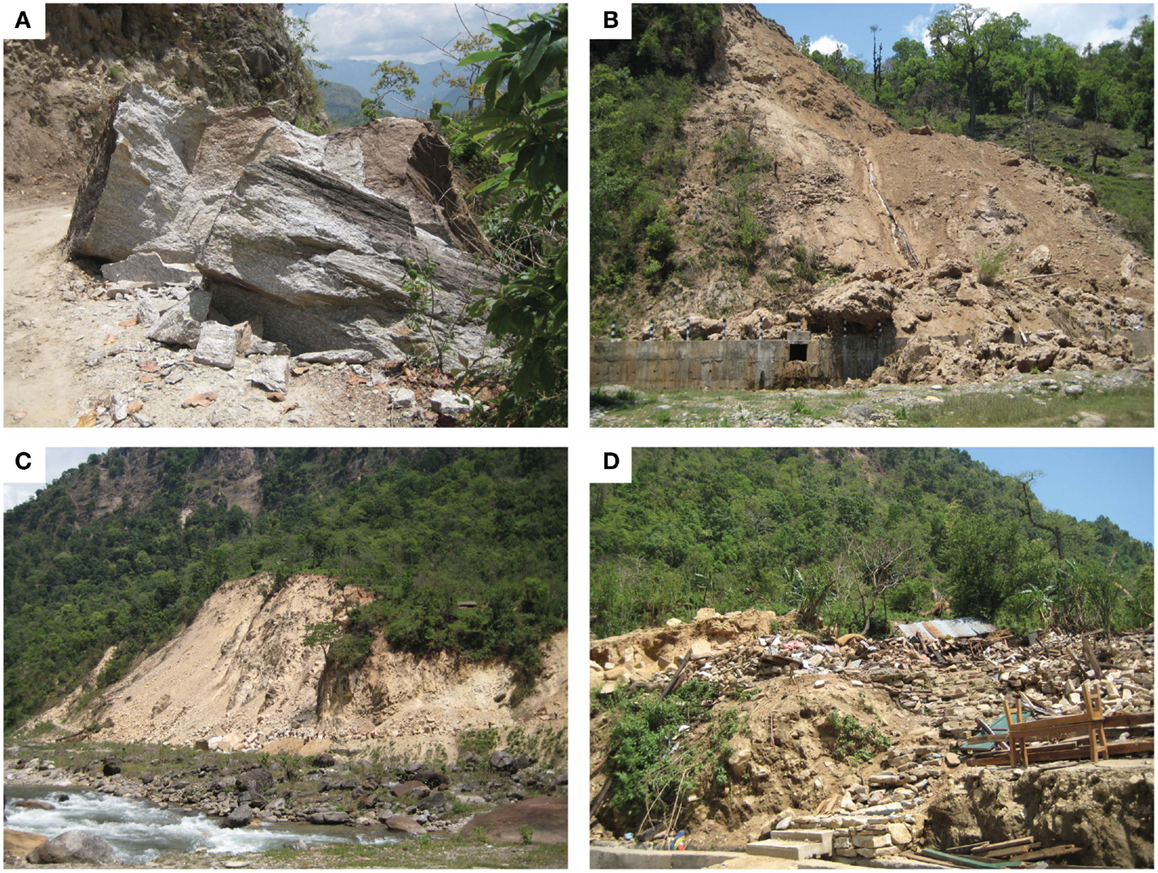
Figure 12. Damage in Baluwa (see Figure 3 ) . (A) Fallen boulder. (B) Shallow landslide; debris blocked the road. (C) Large landslide (100 m high and 300 m wide); debris blocked the road and disconnected villages further north of Baluwa. (D) Devastated houses in Baluwa.
A large slope failure was observed at the northern boundary of Baluwa (Figure 12 C); the length and height of the slope failure were 300 and 100 m, respectively. The fallen boulders and debris blocked the road completely, disconnecting villages at the upstream of the Daraudi River (e.g., Barpak, 5 km north of Baluwa); people can reach these places on foot only. This hampered rescue and recovery activities by governments and international aid teams significantly, highlighting the importance of functional critical infrastructure during the natural disaster emergency. The houses in Baluwa were devastated by the earthquakes and many residents lived in tents (Figure 12 D). Local residents mentioned that the number of fatalities in Baluwa was small because many of the residents were in the field for agricultural work at the time of the earthquake. Major concerns about the arrival of rainy season were expressed by the local residents.
The M w 7.8 subduction earthquake occurred along the Main Himalayan Thrust arc and triggered numerous major aftershocks. The earthquake damage was catastrophic, causing the fatalities of more than 8,500 and billions of dollars in economic loss. This paper presented important earthquake field observations in Nepal in the aftermath of the M w 7.8 mainshock. A unique aspect of the earthquake damage investigation is that the data were collected 6–11 days after the mainshock, and thus first-hand earthquake damage observations were obtained. To share the gathered damage data widely, geo-tagged photos with observation comments were organized using Google Earth and the kmz file was made publicly available. In the future, the updated version of the Google Earth file, containing more damage photos and measurements from follow-up investigations, will be available from http://www.gdm.iis.u-tokyo.ac.jp/index_e.html . Viewers can download the photos directly and can use them for research and educational purposes. To gain deeper understanding of the observed earthquake damage in Nepal, the seismotectonic setting and regional seismicity in Nepal were reviewed and available aftershock data and ground motion data were analyzed. In addition to ground motion data analysis, scenario shake maps were generated by trialing different combinations of applicable ground motion models and source-to-site distance measures to highlight the potential biases caused in estimated ground motion maps and prompt earthquake impact assessments for a large subduction earthquake.
The main results from the earthquake damage surveys in Nepal are as follows:
1. In Kathmandu, earthquake damage to old historical buildings was severe, whereas damage to the surrounding buildings was limited. The damaged buildings were stone/brick masonry structures with wooden frames. The RC frame buildings performed well for this earthquake. This may indicate that ground motion intensity experienced in Kathmandu was not so intense, in comparison with those predicted from probabilistic seismic hazard studies for Nepal. Therefore, a caution is necessary related to future earthquakes in Nepal because the 2015 earthquake is not necessarily the worst-case scenario.
2. The Kathmandu Basin is deposited by thick soft sediments. This has led to the generation of long-period ground motions in the Kathmandu Valley. Although the majority of the existing buildings in Kathmandu were not directly affected by the long-period ground motions, such seismic waves can pose serious risks to high-rise buildings. Adequate earthquake engineering design considerations are essential for reducing potential seismic risk to these structures.
3. The building damage in Kathmandu was localized to specific areas. It appeared that the building collapse sites were affected by local soil characteristics and/or structural deficiencies. In this regard, microzonation studies provide valuable insights into earthquake damage occurrence.
4. Some buildings that were severely damaged by the mainshock were collapsed due to major aftershocks. The capability for aftershock forecasting, building evacuation procedure, building inspection and tagging, and building repairs and retrofitting (low-cost solutions) need to be improved to mitigate the earthquake damage potential.
5. In the mountain areas, numerous villages were devastated by the earthquake sequence and major landslides were triggered. On occasion, landslides blocked roads, disconnecting remote villages. The redundancy of the local transportation network in Nepal needs to be improved for enhancing the resilience of rural communities.
Conflict of Interest Statement
The authors declare that the research was conducted in the absence of any commercial or financial relationships that could be construed as a potential conflict of interest.
Acknowledgments
The authors thank Pradeep Pokhrel for his great assistance during the field survey. The financial support by the JSPS KAKENHI (15H02631) is greatly acknowledged. The work was also funded by the EPSRC grant (EP/I01778X/1) for the Earthquake Engineering Field Investigation Team (EEFIT).
Supplementary Material
The Supplementary Material for this article can be found online at https://www.frontiersin.org/article/10.3389/fbuil.2015.00008
- ^ http://earthquake-report.com/
- ^ http://earthquake.usgs.gov/earthquakes/search/
- ^ http://www.strongmotioncenter.org/
- ^ http://earthquake.usgs.gov/hazards/apps/vs30/
- ^ http://earthquake.usgs.gov/earthquakes/shakemap/
- ^ http://earthquake.usgs.gov/earthquakes/pager/
- ^ http://earthquake.usgs.gov/earthquakes/dyfi/
- ^ http://www.melamchiwater.org/home/
Ader, T., Avouac, J. P., Liu-Zeng, J., Lyon-Caen, H., Bollinger, L., Galetzka, J., et al. (2012). Convergence rate across the Nepal Himalaya and interseismic coupling on the Main Himalayan fault: implications for seismic hazard. J. Geophys. Res. 117, B04403. doi: 10.1029/2011JB009071
CrossRef Full Text | Google Scholar
Ambraseys, N. N., and Douglas, J. (2004). Magnitude calibration of north Indian earthquakes. Geophys. J. Int. 159, 165–206. doi:10.1111/j.1365-246X.2004.02323.x
Atkinson, G. M., and Wald, D. J. (2007). Did you feel it? intensity data: a surprisingly good measure of earthquake ground motion. Seismol. Res. Lett. 78, 362–368. doi:10.1785/gssrl.78.3.362
Avouac, J. P. (2003). Mountain building, erosion and the seismic cycle in the Nepal Himalaya. Adv. Geophys. 46, 1–80. doi:10.1016/S0065-2687(03)46001-9
Bilham, R. (2004). Earthquakes in India and the Himalaya: tectonics, geodesy and history. Ann. Geophys. 47, 839–858. doi:10.4401/ag-3338
Boore, D. M., and Atkinson, G. M. (2008). Ground motion prediction equations for the average horizontal component of PGA, PGV, and 5% damped PSA at spectral periods between 0.01 s and 10.0 s. Earthquake Spectra 24, 99–138. doi:10.1193/1.2830434
Boore, D. M., Stewart, J. P., Seyhan, E., and Atkinson, G. M. (2014). NGA-West2 equations for predicting PGA, PGV, and 5% damped PSA for shallow crustal earthquakes. Earthquake Spectra 30, 1057–1085. doi:10.1193/070113EQS184M
Center for Disaster Management and Risk Reduction Technology (CEDIM). (2015). Nepal Earthquakes – Report #3 . Available at: https://www.cedim.de/english/index.php
Google Scholar
Chaulagain, H., Rodrigues, H., Spacone, E., and Varum, H. (2015). Seismic response of current RC buildings in Kathmandu Valley. Struct. Eng. Mech. 53, 791–818. doi:10.12989/sem.2015.53.4.791
Goda, K., and Atkinson, G. M. (2014). Variation of source-to-site distance for megathrust subduction earthquakes: effects on ground motion prediction equations. Earthquake Spectra 30, 845–866. doi:10.1193/080512EQS254M
Gupta, I. D. (2006). Delineation of probable seismic sources in India and neighbourhood by a comparative analysis of seismotectonic characteristics of the region. Soil Dynam. Earthquake Eng. 26, 766–790. doi:10.1016/j.soildyn.2005.12.007
Harbindu, A., Gupta, S., and Sharma, M. L. (2014). Earthquake ground motion predictive equations for Garhwal Himalaya, India. Soil Dynam. Earthquake Eng. 66, 135–148. doi:10.1016/j.soildyn.2014.06.018
Heuret, A., Lallemand, S., Funiciello, F., Piromallo, C., and Faccenna, C. (2011). Physical characteristics of subduction interface type seismogenic zones revisited. Geochem. Geophys. Geosyst. 12, Q01004. doi:10.1029/2010GC003230
Hong, H. P., and Goda, K. (2007). Orientation-dependent ground-motion measure for seismic-hazard assessment. Bull. Seismol. Soc. Am. 97, 1525–1538. doi:10.1785/0120060194
Huang, Y. N., Whittaker, A. S., and Luco, N. (2008). Maximum spectral demands in the near-fault region. Earthquake Spectra 24, 319–341. doi:10.1193/1.2830435
Jaiswal, K. S., and Wald, D. J. (2010). An empirical model for global earthquake fatality estimation. Earthquake Spectra 26, 1017–1037. doi:10.1193/1.3480331
Ji, C. (2008). Preliminary Result of the May 12, 2008 M w 7.97 Sichuan Earthquake . Available at: http://www.geol.ucsb.edu/faculty/ji/big_earthquakes/2008/05/12/ShiChuan.html
Kanno, T., Narita, A., Morikawa, N., Fujiwara, H., and Fukushima, Y. (2006). A new attenuation relation for strong ground motion in Japan based on recorded data. Bull. Seismol. Soc. Am. 96, 879–897. doi:10.1785/0120050138
Lu, M., Li, X. J., An, X. W., and Zhao, J. X. (2010). A comparison of recorded response spectra from the 2008 Wenchuan, China, earthquake with modern ground-motion prediction models. Bull. Seismol. Soc. Am. 100, 2357–2380. doi:10.1785/0120090303
Mai, P. M., Spudich, P., and Boatwright, J. (2005). Hypocenter locations in finite-source rupture models. Bull. Seismol. Soc. Am. 95, 965–980. doi:10.1785/0120040111
Nath, S. K., and Thingbaijam, K. K. S. (2011). Peak ground motion predictions in India: an appraisal for rock sites. J. Seismol. 15, 295–315. doi:10.1007/s10950-010-9224-5
Nath, S. K., and Thingbaijam, K. K. S. (2012). Probabilistic seismic hazard assessment of India. Seismol. Res. Lett. 83, 135–149. doi:10.1785/gssrl.83.1.135
Paudyal, Y. R., Bhandary, N. P., and Yatabe, R. (2012). Seismic microzonation of densely populated area of Kathmandu Valley of Nepal using microtremor observations. J. Earthquake Eng. 16, 1208–1229. doi:10.1080/13632469.2012.693242
Ram, T. D., and Wang, G. (2013). Probabilistic seismic hazard analysis in Nepal. Earthquake Eng. Eng. Vib. 12, 577–586. doi:10.1007/s11803-013-0191-z
Sakai, H., Fujii, R., and Kuwahara, Y. (2002). Changes in the depositional system of the Paleo-Kathmandu Lake caused by uplift of the Nepal Lesser Himalayas. J. Asian Earth Sci. 20, 267–276. doi:10.1016/S1367-9120(01)00046-3
Sharma, M. L., Douglas, J., Bungum, H., and Kotadia, J. (2009). Ground-motion prediction equations based on data from Himalayan and Zagros regions. J. Earthquake Eng. 13, 1191–1210. doi:10.1080/13632460902859151
Shcherbakov, R., Goda, K., Ivanian, A., and Atkinson, G. M. (2013). Aftershock statistics of major subduction earthquakes. Bull. Seismol. Soc. Am. 103, 3222–3234. doi:10.1785/0120120337
Shcherbakov, R., Turcotte, D. L., and Rundle, J. B. (2005). Aftershock statistics. Pure Appl. Geophys. 162, 1051–1076. doi:10.1007/s00024-004-2661-8
Stern, R. J. (2002). Subduction zones. Rev. Geophys. 40, 1012. doi:10.1029/2001RG000108
Takewaki, I., Murakami, S., Fujita, K., Yoshitomi, S., and Tsuji, M. (2011). The 2011 off the Pacific coast of Tohoku earthquake and response of high-rise buildings under long-period ground motions. Soil Dynam. Earthquake Eng. 31, 1511–1528. doi:10.1016/j.soildyn.2011.06.001
United Nations Office for the Coordination of Humanitarian Affairs (UN-OCHA). (2015). Available at: http://www.unocha.org/nepal
United States Geological Survey (USGS). (2015). Available at: http://earthquake.usgs.gov/earthquakes/eventpage/us20002926#scientific_finitefault
Wald, D. J., and Allen, T. I. (2007). Topographic slope as a proxy for seismic site conditions and amplification. Bull. Seismol. Soc. Am. 97, 1379–1395. doi:10.1785/0120060267
Wald, D. J., Worden, B. C., Quitoriano, V., and Pankow, K. L. (2005). ShakeMap Manual: Technical Manual, User’s Guide, and Software Guide . Golden, CO:U.S. Geological Survey, 132.
Yagi, T. (2015). Available at: http://www.geol.tsukuba.ac.jp/~yagi-y/EQ/20150425/index.html
Keywords: 2015 Nepal earthquake, earthquake damage survey, building damage, ground motion, aftershocks
Citation: Goda K, Kiyota T, Pokhrel RM, Chiaro G, Katagiri T, Sharma K and Wilkinson S (2015) The 2015 Gorkha Nepal earthquake: insights from earthquake damage survey. Front. Built Environ. 1:8. doi: 10.3389/fbuil.2015.00008
Received: 28 May 2015; Accepted: 08 June 2015; Published: 22 June 2015
Reviewed by:
Copyright: © 2015 Goda, Kiyota, Pokhrel, Chiaro, Katagiri, Sharma and Wilkinson. This is an open-access article distributed under the terms of the Creative Commons Attribution License (CC BY) . The use, distribution or reproduction in other forums is permitted, provided the original author(s) or licensor are credited and that the original publication in this journal is cited, in accordance with accepted academic practice. No use, distribution or reproduction is permitted which does not comply with these terms.
*Correspondence: Katsuichiro Goda, Department of Civil Engineering, University of Bristol, Queen’s Building, University Walk, Bristol BS8 1TR, UK, katsu.goda@bristol.ac.uk
This article is part of the Research Topic
Mega Quakes: Cascading Earthquake Hazards and Compounding Risks
Thank you for visiting nature.com. You are using a browser version with limited support for CSS. To obtain the best experience, we recommend you use a more up to date browser (or turn off compatibility mode in Internet Explorer). In the meantime, to ensure continued support, we are displaying the site without styles and JavaScript.
- View all journals
- My Account Login
- Explore content
- About the journal
- Publish with us
- Sign up for alerts
- Data Descriptor
- Open access
- Published: 20 August 2022
Strong ground motion data of the 2015 Gorkha Nepal earthquake sequence in the Kathmandu Valley
- Michiko Shigefuji ORCID: orcid.org/0000-0003-2264-6825 1 ,
- Nobuo Takai ORCID: orcid.org/0000-0001-6761-913X 2 ,
- Subeg Bijukchhen 3 ,
- Masayoshi Ichiyanagi 4 ,
- Sudhir Rajaure 5 ,
- Megh Raj Dhital 6 ,
- Lalu Prasad Paudel 7 &
- Tsutomu Sasatani 2
Scientific Data volume 9 , Article number: 513 ( 2022 ) Cite this article
3341 Accesses
4 Citations
Metrics details
- Natural hazards
Strong-motion records of earthquakes are used not only to evaluate the source rupture process, seismic wave propagation and strong ground motion characteristics, but also to provide valuable data for earthquake disaster mitigation. The Kathmandu Valley, Nepal, which is characterised by having soft sediments that have been deposited in an earthquake-prone zone, has experienced numerous earthquakes. We have operated four strong-motion stations in the Kathmandu Valley since 2011. These stations recorded the 2015 magnitude 7.8 Gorkha Nepal earthquake that occurred in the Himalayan continental collision zone. For several months after the mainshock, we deployed four additional temporary stations. Here, we describe the seismic data for 18 earthquakes over magnitude 5.0 collected by this array, including the 2015 magnitude 7.3 Dolakha earthquake of maximum aftershock and three large aftershocks of magnitude 6-class. These data are essential for validating the sedimentary structure of the basin and for evaluating the hazard and risk of future earthquakes in the Kathmandu Valley.
Similar content being viewed by others
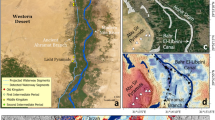
The Egyptian pyramid chain was built along the now abandoned Ahramat Nile Branch
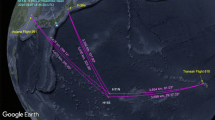
Underwater acoustic analysis reveals unique pressure signals associated with aircraft crashes in the sea: revisiting MH370
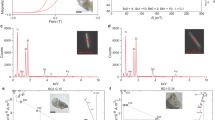
Near-collapse of the geomagnetic field may have contributed to atmospheric oxygenation and animal radiation in the Ediacaran Period
Background & summary.
The Nepal Himalaya, which formed as a result of the Indian Plate colliding with the Eurasian Plate, underthrusts at a low angle from the Main Frontal Thrust. The Himalayan continental collision zone is one of the most earthquake-prone regions and has experienced several devastating earthquakes in the last millennium (e.g., 1255, 1344, 1408, 1505, 1833, 1934, 2015) 1 , 2 , 3 . The 1934 Nepal-Bihar earthquake, which had a magnitude ( M ) of approximately 8.1, occurred in the Eastern Himalaya region and damaged 19% of buildings in the Kathmandu Valley, more than 100 km away from the epicentre 4 , 5 . It is considered that great earthquakes could potentially occur in the Central Seismic Gap of the Main Frontal Thrust in the near future 6 , 7 .
The capital city of Nepal, Kathmandu, is located in the Kathmandu Valley. The oval intermontane valley is approximately 30 km long in the east-west direction and approximately 25 km wide in the north-south direction. The valley floor is overlain by soft, thick fluvial, fluvio-lacustrine, and fluvio-deltaic sediments of Plio-Pleistocene origin 8 , which are more than 650 m thick in the central part of the valley 9 , 10 . Consequently, the valley is highly susceptible to the risks associated with site amplification 11 . In addition, the explosive population growth that has occurred in recent years has markedly increased the risk of earthquake damage in the valley 12 .
To study site amplification in the Kathmandu Valley, Hokkaido University collaborated with Tribhuvan University to install seismic stations for recording strong-motion data in four different parts of the valley, including an exposed rocky site in 2011. On 25 April 2015, the moment magnitude ( M w ) 7.8 Gorkha earthquake struck Nepal (Fig. 1 ). The hypocentral depth was shallow 13 , and the focal area was estimated to be 180 km long and 110 km wide on the low dip-angle fault plane 14 . The rupture propagated to the east, and a large slip area formed near the northern part of the valley 14 , 15 , 16 . This event caused approximately 1,700 deaths, and 13% of the buildings, including World Heritage sites, were damaged inside the valley 17 .
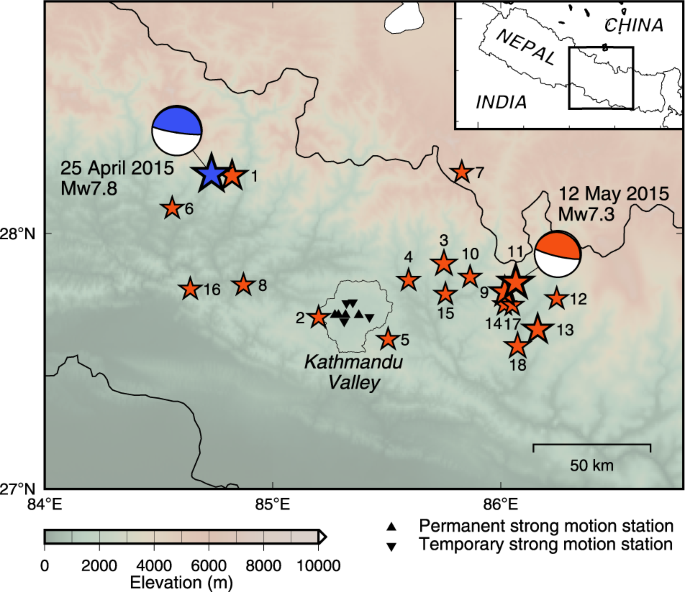
Location map of the epicentres of the 2015 Gorkha earthquake and aftershocks greater than M 5.0. The blue and red stars indicate the epicentres for the mainshock and the aftershocks from the USGS-NEIC. The focal mechanisms for the mainshock and the largest aftershock are also shown. Triangles and inverted triangles indicate permanent and temporary stations, respectively. The numbers correspond to Table 2 .
Our array in the valley successfully captured strong ground motion records 18 . During this earthquake, other two seismic observation stations located on sediments in the valley also recorded seismic data: the Kantipath station operated by the United States Geological Survey (USGS) 19 , and the station operated by the Department of Mines and Geology, Ministry of Industry 20 . These data are valuable for evaluating strong ground motion characteristics and clarifying the relationship between strong ground motions and damage. For example, the relationship between the building damage ratios based on the visual damage assessments and fragility curves was discussed 21 .
The mainshock was followed by a series of aftershocks. The ensuing aftershock activities were concentrated in the eastern part of the rupture area 22 . Ten days after the mainshock, four additional stations were installed temporarily along a north-south profile of the valley to investigate the distribution of site amplification in the valley using strong-motion records from the aftershocks. Our array captured the aftershocks 23 , including the largest aftershock ( M w 7.3) on 12 May 2015 occurred in the Dolakha region 24 (Fig. 1 ). The strong ground motion distribution for the mainshock was insufficient to estimate the site amplification and wave propagation characteristics for the entire valley. Data from additional temporary stations and strong-motion records of small and mid-sized earthquakes are useful to estimate the site amplification characteristics and validate the velocity structure models. Ichiyanagi et al . 25 investigated aftershock activity, and Bijukchhen et al . 26 constructed the initial velocity structure model for the Kathmandu Valley based on previous geological and geophysical exploration results and the strong-motion records. Mori et al . 27 proposed correction terms for the amplification by the sedimentary layers in the ground motion prediction equation.
The data were disseminated through the figshare data repository at https://doi.org/10.6084/m9.figshare.19809052 28 . These will prove to be important fundamental data for earthquake disaster mitigation activities in the Kathmandu Valley. For example, these data can be used to produce seismic hazard maps, building damage prediction maps, etc., as well as seismic design of building structures. In this study, we present observation-based strong ground motion data. We introduce our strong-motion array in the Kathmandu Valley and describe the dataset obtained after the 2015 Gorkha earthquake sequence. We also explain the quality of these data.
Strong-motion station array
On 20 September 2011, Hokkaido University and Tribhuvan University installed continuous recording accelerometers along an approximately 10-km east-west axis in four different parts of the valley: stations KTP (Kirtipur municipality office, Kirtipur), TVU (Central Department of Geology, Tribhuvan University, Kirtipur), PTN (Pulchowk Campus, Institute of Engineering, Tribhuvan University, Lalitpur), and THM (University Grants Commission Office, Sanothimi, Bhaktapur). Ten days after the 25 April 2015 Gorkha earthquake, three stations were added along an approximately 8-km north-south axis in the valley: stations RNB (Ranibu, Lalitpur), PPR (Panipokhari, Kathmandu), KPN (Kapan, Kathmandu), and one station was added at Bhaktapur (BKT), a city that suffered heavy damage during the earthquake. A total of eight stations distributed within a radius of approximately 7 km were operated from 5 May 2015 to August 2015. Figure 2 and Table 1 show the locations of the strong-motion arrays. The geological conditions 29 differed at each of the stations; for example, the KTP site was located on exposed rocky hillocks that breached through the sediments, while the TVU, PTN, THM, BKT, RNB, PPR sites were all located on lake sediments, and the KPN site was located on fluvial sediments of the Bagmati River.
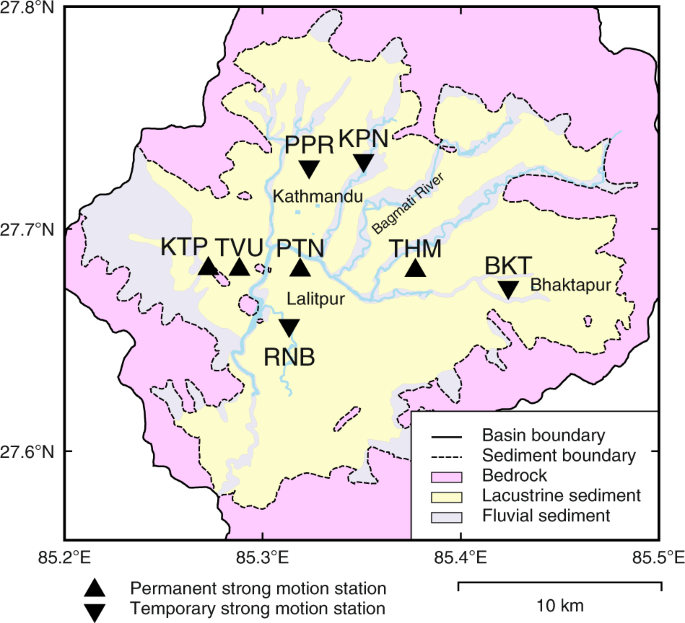
Location of strong-motion stations plotted on the simplified geological map in the Kathmandu Valley (based on Shrestha et al . 29 ). Triangles indicate permanent stations and inverted triangles are temporary stations.
Observation system
The observation equipment was selected to obtain stable records, even under conditions of unstable power supply in Kathmandu. The installed instruments consisted of a highly damped moving coil-type three-component accelerometer (JEP-6A3-2, Mitutoyo Corp., Japan) which does not require a power supply, and a 24 Analog-to-Digital bit low-power data logger (DATAMARK LS-8800, Hakusan Corp., Japan) with an external GPS antenna for time calibration. The sampling rate was 100 Hz and data were recorded continuously at all stations. The accelerometer had a flat response (−3 dB) for ground acceleration from 0.1 Hz to an aliasing frequency with a sensitivity of 0.22 V/m s −2 . The data loggers at the permanent stations were powered by a DC supply and fitted with a 12 V rechargeable car battery along with a voltage stabiliser. The data loggers at the temporary stations were powered by a 12 V car battery. Due to safety considerations and the high population density in the study area, these instruments were installed on the foundation-level floor of reinforced concrete buildings that were one to four stories high, and outside buildings for a few temporary stations. The GPS antennas were either positioned outside of the buildings or at windows so that they could receive satellite signals. The permanent accelerometers were affixed to the floor with bolts, while temporary accelerometers were affixed using two-part epoxy adhesives. Accelerometers were levelled and oriented along the building or to the magnetic north. Recorded data were stored on 16 GB SD cards and data were collected on-site. Maintenance of the permanent stations was performed at six-month intervals. Permanent stations were maintained two weeks before the 2015 Gorkha earthquake.
Strong ground motion
The strong-motion stations recorded the 2015 Gorkha Nepal earthquake sequence data. Figure 1 and Table 2 show 18 mid-to-large sized earthquakes (5.0 < M < 7.3) with the strong-motion data of signal-to-noise ratios > 2 located within the epicentral area. Source parameters were from the USGS National Earthquake Information Center (NEIC: https://www.usgs.gov/programs/earthquake-hazards/earthquakes ). As examples, Fig. 3 shows the acceleration waveforms of the M w 6.7 earthquake (Table 2 , No. 9) and the M w 7.3 maximum aftershock (Table 2 , No. 11). The mainshock records have already been published as supplementary data by Takai et al . 18 .
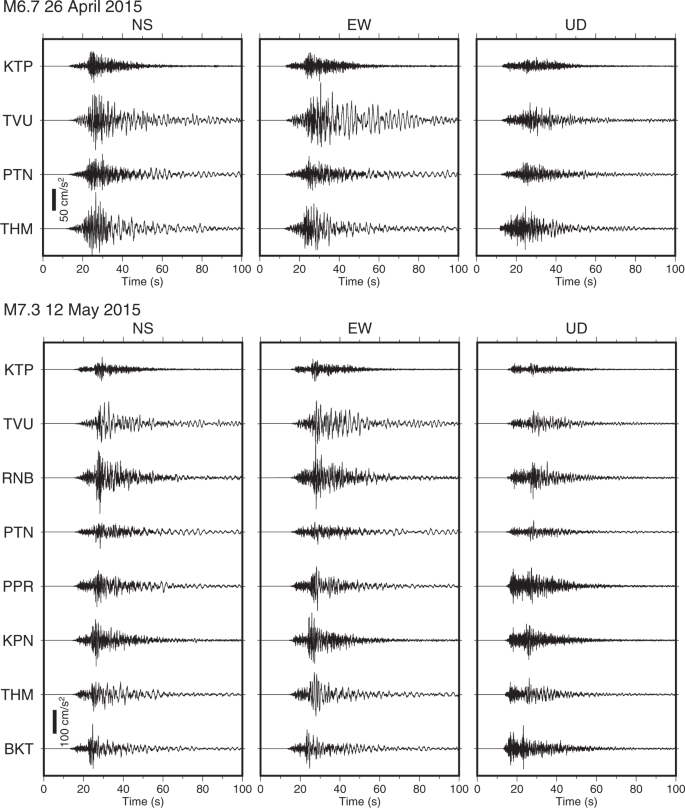
Recorded acceleration waveforms during two earthquakes. Acceleration waveforms for the M w 6.7 26 April 2015 earthquake (top) and the 2015 M w 7.3 Dolakha earthquake (bottom).
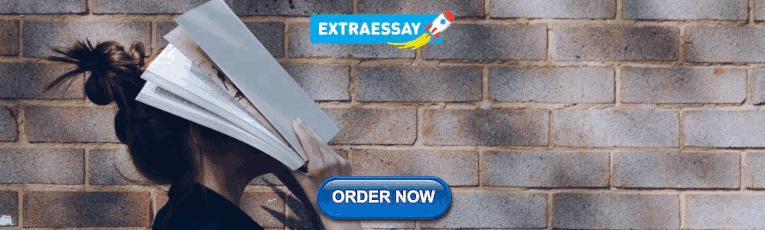
Data Records
The data files as shown in Table 2 were deposited in the figshare data repository at https://doi.org/10.6084/m9.figshare.19809052 28 . Minimum processing included converting the data from raw WIN system 30 format to acceleration waveform data and correcting the orientation. The data files consist of four columns for time and geographic north-south (NS), east-west (EW), up-down (UD) data in ASCII text format. A recording length is set to 180 s before and after the P- and S-waves. Table 3 shows the data format characteristics. The site location and recording start time (UTC) are described in the file header. The file name consists of a code, earthquake date, and file extension (.txt).
Since the JEP-6A3 accelerometer is an over-damped moving coil mechanical seismograph, it is possible to derive accurate long-period ground motions at <0.1 Hz by correcting for the sensor response. The pendulum motion is proportional to the ground velocity, h is a damping constant of 26, and the natural frequency f 0 is 3 Hz. The frequency response function Λ ( ω ) has an amplitude of 1 and can be estimated as follows:
where ω is the angular frequency and ω 0 is the resonance angular frequency of the pendulum. Therefore, the accelerometer had a flat response for ground acceleration over 0.1 Hz.
Technical Validation
After the 25 April 2015 Gorkha earthquake, we checked the permanent strong-motion stations. The instruments were undamaged and the buildings in which the equipment were installed were visually assessed as being either undamaged or only slightly damaged 21 . In addition, continuous recordings without any missing data were obtained throughout the event. However, since Kathmandu is a populous city with numerous buildings and heavy traffic, these sources of artificial ambient noise may have contaminated the observed seismic records.
Ambient noise
In populated metropolitan areas, noise levels attributed to microtremors generated by human activities such as traffic can be very high. Therefore, we estimated the vibration level from the power spectral density (PSD) of acceleration at each site. As an example, the horizontal vibration levels for one week, from 15 to 21 May 2015, are shown in Fig. 4 . Observed continuous records were subdivided into intervals of 40.96 s and the PSD of the horizontal component, its root mean square value, and the hourly mean value were calculated. The vibration levels are compared against a reference acceleration vibration level of 10 −6 m/s 2 with no weighting of the vibration sensation. The vibration levels exhibited daily cycles in the high-frequency range; specifically, the difference in the noise level between day and night differed by approximately 20 dB. The microtremors attributed to human activity affect this frequency range, and the vibration levels measured at the sedimentary stations in the central urban areas were larger than those at KTP station on the hilly rock. Conversely, although the amplitudes of the microseisms were small, predominant low-frequency peaks were observed at sedimentary sites.
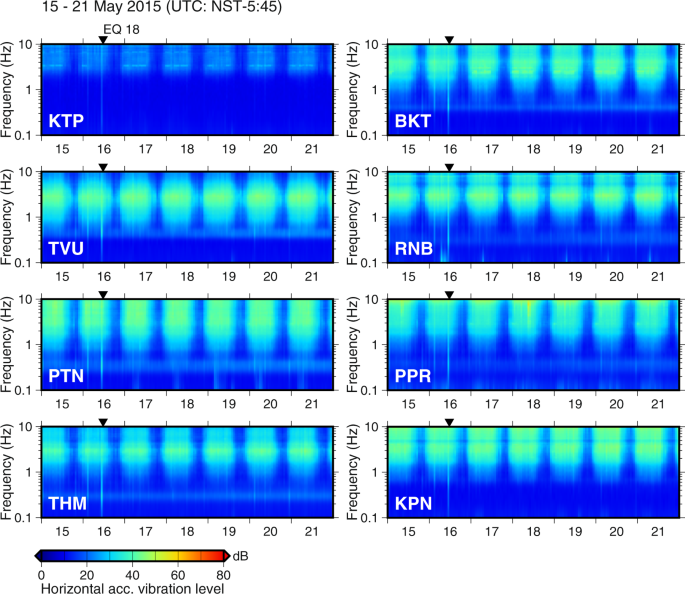
Horizontal acceleration vibration level for microtremors at each station for one week from 15 May 2015 to 21. The time is UTC (NST - 5:45). Inverted triangles indicate the origin time of the earthquake.
Figure 5 shows the average PSD for microtremors not containing seismic data over one day at each station. Compared to the results obtained from the high-noise model 31 , which uses the average value of the high background noise power obtained from broadband seismometers, the PSDs recorded by our array were slightly higher than those estimated by this model. Although the noise levels were relatively high, the PSDs for the recorded earthquake data far exceed those estimated using the model. However, the observed data may contain records from multiple earthquakes because recordings were performed immediately after large earthquakes.
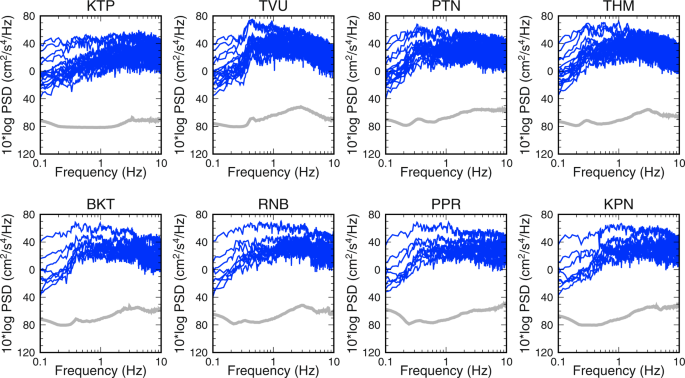
PSDs at each station. Solid grey lines show the average PSDs for microtremors over one day. Solid blue lines show the PSDs for the published earthquake data (5.0 < M < 7.3).
Sensor response
The 0.05–0.2 Hz bandpass-filtered velocity waveforms for the UD component from the M w 7.3 Dolakha earthquake are shown in Fig. 6 . We applied a correction of the sensor-response to observed records and derived accurate ground motions even at frequencies of ≤0.1 Hz. The waveforms were coherent in this low-frequency range. It corroborated the findings of Takai et al . 18 who compared the displacement waveforms of the 2015 Gorkha earthquake to the high-rate GNSS waveforms and obtained similar waveforms with static deformation.
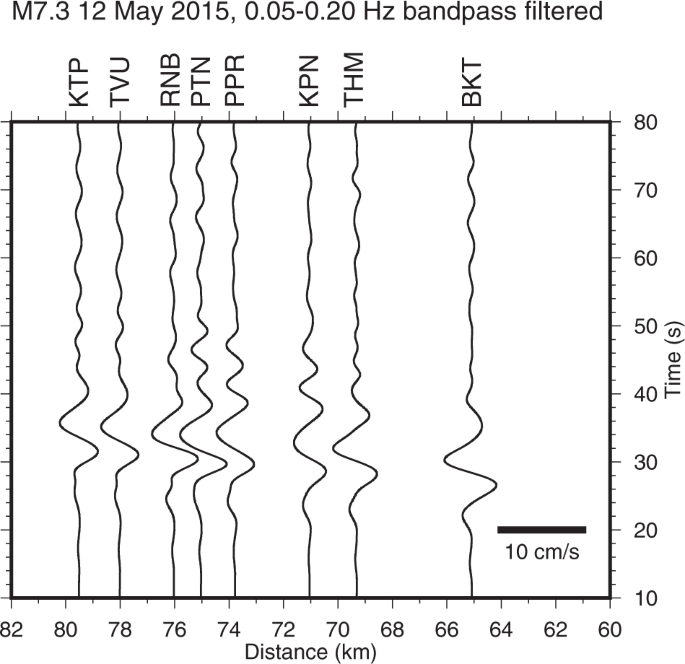
Velocity waveforms for the UD component of the M w 7.3 Dolakha earthquake bandpass filtered at 0.05–0.2 Hz.
Code availability
No specific custom code was used to generate the dataset in the manuscript. The data, which are provided in ASCII text format, can be freely downloaded from the figshare data repository and analysed using any software. We used Generic Mapping Tools 32 to compile several figures.
Mugnier, J. L. et al . Structural interpretation of the great earthquakes of the last millennium in the central Himalaya. Earth Sci. Rev. 127 , 30–47, https://doi.org/10.1016/j.earscirev.2013.09.003 (2013).
Article ADS Google Scholar
Bollinger, L. et al . Estimating the return times of great Himalayan earthquakes in eastern Nepal: Evidence from the Patu and Bardibas strands of the Main Frontal Thrust. J. Geophys. Res. 119 , 7123–7163, https://doi.org/10.1002/2014JB010970 (2014).
Sapkota, S. N. et al . Primary surface ruptures of the great Himalayan earthquakes in 1934 and 1255. Nat. Geosci. 6 , 71–76, https://doi.org/10.1038/ngeo1669 (2013).
Article ADS CAS Google Scholar
Rana, B. Nepal Ko Maha Bhukampa (Great Earthquake of Nepal) (Jorganesh Press, Kathmandu, 1935).
Pandey, M. R. & Molnar, P. The distribution of Intensity of the Bihar Nepal earthquake of 15 January 1934 and bounds on the extent of the rupture. J. Nepal Geol. Soc. 5 , 22–44, https://doi.org/10.3126/jngs.v5i1.32559 (1988).
Article Google Scholar
Bilham, R., Gaur, V. K. & Molnar, P. Himalayan seismic hazard. Science 293 , 1442–1444, https://doi.org/10.1126/science.1062584 (2001).
Article CAS PubMed Google Scholar
Stevens, V. L. & Avouac, J. P. Millenary Mw >9.0 earthquakes required by geodetic strain in the Himalaya. Geophys. Res. Lett. 43 , 1118–1123, https://doi.org/10.1002/2015GL067336 (2016).
Dhital, M. R. Geology of the Nepal Himalaya (Springer International Publishing, 2015).
Moribayashi, S. & Maruo, Y. Basement topography of the Kathmandu valley, Nepal—an application of gravitational method to the survey of a tectonic basin in the Himalayas. Jour. Japan. Soc. Eng. Geol. 21 , 80–87, https://doi.org/10.5110/jjseg.21.80 (1980).
Sakai, H. Stratigraphic division and sedimentary facies of the Kathmandu Basin sediments. J. Nepal Geol. Soc. 25 , 19–32, https://doi.org/10.3126/jngs.v25i0.32043 (2001).
Mugnier, J. L., Huyghe, P., Gajurel, A. P., Upreti, B. N. & Jouanne, F. Seismites in the Kathmandu basin and seismic hazard in central Himalaya. Tectonophysics 509 , 33–49, https://doi.org/10.1016/j.tecto.2011.05.012 (2011).
Mesta, C., Cremen, G. & Galasso, C. Urban growth modelling and social vulnerability assessment for a hazardous Kathmandu Valley. Sci. Rep. 12 , 6152, https://doi.org/10.1038/s41598-022-09347-x (2022).
Article ADS CAS PubMed PubMed Central Google Scholar
USGS. M 7.8 - 67 km NNE of Bharatpur, Nepal https://earthquake.usgs.gov/earthquakes/eventpage/us20002926/ (2015).
Kobayashi, H. et al . Joint inversion of teleseismic, geodetic, and near-field waveform datasets for rupture process of the 2015 Gorkha, Nepal, earthquake. Earth Planets Space 68 , 66, https://doi.org/10.1186/s40623-016-0441-1 (2016).
Galetzka, J. et al . Slip pulse and resonance of the Kathmandu basin during the 2015 Gorkha earthquake, Nepal. Science 349 , 1091–1095, https://doi.org/10.1126/science.aac6383 (2015).
Article ADS CAS PubMed Google Scholar
Avouac, J. P., Meng, L., Wei, S., Wang, T. & Ampuero, J. P. Lower edge of locked Main Himalayan Thrust unzipped by 2015 Gorkha earthquake. Nat. Geosci. 8 , 708–711, https://doi.org/10.1038/ngeo2518 (2015).
Government of Nepal, The Gorkha Earthquake - 2015 Experience and Learning (Ministry of home affairs, 2016).
Takai, N. et al . Strong ground motion in the Kathmandu Valley during the 2015 Gorkha, Nepal, earthquake. Earth Planets Space 68 , 10, https://doi.org/10.1186/s40623-016-0383-7 (2016).
USGS. NetQuakes Data: Station KATNP_NQ_01, 25 April 2015 https://earthquake.usgs.gov/monitoring/netquakes/viewdata/KATNP_NQ_01/20150425061138 (2015).
Bhattarai, M. et al . Overview of the large 25 April 2015 Gorkha, Nepal, earthquake from accelerometric perspectives. Seismol. Res. Lett. 86 , 1540–1548, https://doi.org/10.1785/0220150140 (2015).
Bijukchhen, S. et al . Strong-motion characteristics and visual damage assessment around seismic stations in Kathmandu after the 2015 Gorkha, Nepal, earthquake. Earthq. Spectra 33 , 219–242, https://doi.org/10.1193/042916eqs074m (2017).
Adhikari, L. B. et al . The aftershock sequence of the 2015 April 25 Gorkha–Nepal earthquake. Geophys. J. Int. 203 , 2119–2124, https://doi.org/10.1093/gji/ggv412 (2015).
Rajaure, S. et al . Characterizing the Kathmandu Valley sediment response through strong motion recordings of the 2015 Gorkha earthquake sequence. Tectonophysics 714–715 , 146–157, https://doi.org/10.1016/j.tecto.2016.09.030 (2017).
USGS. M 7.3 - Nepal https://earthquake.usgs.gov/earthquakes/eventpage/us20002ejl/executive (2015).
Ichiyanagi, M. et al . Aftershock activity of the 2015 Gorkha, Nepal, earthquake determined using the Kathmandu strong motion seismographic array. Earth Planets Space 68 , 25, https://doi.org/10.1186/s40623-016-0402-8 (2016).
Bijukchhen, S. M. et al . Estimation of 1-D velocity models beneath strong-motion observation sites in the Kathmandu Valley using strong-motion records from moderate-sized earthquakes. Earth, Planets and Space 69 , 97, https://doi.org/10.1186/s40623-017-0685-4 (2017).
Mori, T. et al . Ground motion prediction equation for the Kathmandu Valley, Nepal based on strong motion records during the 2015 Gorkha Nepal earthquake sequence. Soil Dyn. Earthq. Eng. 135 , 106208, https://doi.org/10.1016/j.soildyn.2020.106208 (2020).
Shigefuji, M. Strong ground motion datasets of the Mw7.8 2015 Gorkha earthquake aftershocks obtained at the seismograph array in the Kathmandu Valley, Nepal, figshare , https://doi.org/10.6084/m9.figshare.19809052 (2022).
Shrestha, O. et al . Engineering and environmental geological map of the Kathmandu Valley, Scale 1:50,000 . (Department of Mines and Geology, 1998).
Urabe, T. A common format for multi-channel earthquake waveform data. Prog. Abs. seismo. Soc. Japan 2 , 384 (1994).
Google Scholar
Peterson, J. R. Observations and modeling of seismic background noise. U.S. Geol. Surv. Open-File Rept . 93–322 (1993).
Wessel, P. et al . The Generic Mapping Tools Version 6. Geochemistry, Geophy. Geosystems 20 , 5556–5564, https://doi.org/10.1029/2019GC008515 (2019).
Download references
Acknowledgements
We thank Dr. Yadab Prasad Dhakal at National Research Institute for Earth Science and Disaster Resilience, Dr. Subesh Ghimire at Tribhuvan University, and former MSc students at Hokkaido University, Mr. Kosuke Sawada, Mr. Hideki Okajima, and Mr. Yuji Miyahara, who installed the strong-motion stations in 2011. We are also grateful to Dr. Sadanori Higashi, Dr. Yoshiaki Shiba, Dr. Hiroaki Sato, and Dr. Masayuki Kuriyama at Central Research Institute of Electric Power Industry for helping with the temporary observations conducted after the 2015 Gorkha earthquake. This study was supported in part by JSPS KAKENHI Grant Numbers JP23404005, JP16K16370, JP20KK0105, JP22K04655 and the J-RAPID program of the Japan Science and Technology Agency.
Author information
Authors and affiliations.
Faculty of Human-Environment Studies, Kyushu University, 744 Motooka, Nishi-ku, Fukuoka, 819-0395, Japan
- Michiko Shigefuji
Faculty of Engineering, Hokkaido University, Kita 13, Nishi 5, Kita-ku, Sapporo, Hokkaido, 060-0808, Japan
Nobuo Takai & Tsutomu Sasatani
Postgraduate Department of Earthquake Engineering, Khwopa Engineering College, Libali, Bhaktapur-8, Bagmati, Nepal
Subeg Bijukchhen
Institute of Seismology and Volcanology, Faculty of Science, Hokkaido University, Kita 10, Nishi 5, Kita-ku, Sapporo, Hokkaido, 060-0810, Japan
Masayoshi Ichiyanagi
Department of Mines and Geology, Lainchaur, Kathmandu, Nepal
Sudhir Rajaure
Central Department of Geology, Tribhuvan University, Kirtipur, Kathmandu, 44618, Nepal
Megh Raj Dhital
Tribhuvan University Service Commission, Tribhuvan University, Kirtipur, Kathmandu, 8212, Nepal
Lalu Prasad Paudel
You can also search for this author in PubMed Google Scholar
Contributions
M.S. and N.T. wrote the manuscript. All authors were involved in the study design, installation, and maintenance of the strong-motion stations.
Corresponding author
Correspondence to Michiko Shigefuji .
Ethics declarations
Competing interests.
The authors declare no competing interests.
Additional information
Publisher’s note Springer Nature remains neutral with regard to jurisdictional claims in published maps and institutional affiliations.
Rights and permissions
Open Access This article is licensed under a Creative Commons Attribution 4.0 International License, which permits use, sharing, adaptation, distribution and reproduction in any medium or format, as long as you give appropriate credit to the original author(s) and the source, provide a link to the Creative Commons license, and indicate if changes were made. The images or other third party material in this article are included in the article’s Creative Commons license, unless indicated otherwise in a credit line to the material. If material is not included in the article’s Creative Commons license and your intended use is not permitted by statutory regulation or exceeds the permitted use, you will need to obtain permission directly from the copyright holder. To view a copy of this license, visit http://creativecommons.org/licenses/by/4.0/ .
Reprints and permissions
About this article
Cite this article.
Shigefuji, M., Takai, N., Bijukchhen, S. et al. Strong ground motion data of the 2015 Gorkha Nepal earthquake sequence in the Kathmandu Valley. Sci Data 9 , 513 (2022). https://doi.org/10.1038/s41597-022-01634-6
Download citation
Received : 29 May 2022
Accepted : 11 August 2022
Published : 20 August 2022
DOI : https://doi.org/10.1038/s41597-022-01634-6
Share this article
Anyone you share the following link with will be able to read this content:
Sorry, a shareable link is not currently available for this article.
Provided by the Springer Nature SharedIt content-sharing initiative
This article is cited by
Examination of shallow and deep s-wave velocity structures from microtremor array measurements and receiver function analysis at strong-motion stations in kathmandu basin, nepal.
- Nobuo Takai
- Mukunda Bhattarai
Earth, Planets and Space (2024)
Quick links
- Explore articles by subject
- Guide to authors
- Editorial policies
Sign up for the Nature Briefing newsletter — what matters in science, free to your inbox daily.


- Chronicle Conversations
- Article archives
- Issue archives
- Join our mailing list

The Humanitarian Response to the 2015 Nepal Earthquake
About the author, puk ovesen and stine heiselberg.
May 2016, No. 1 Vol. LIII, Humanitarian Action: A Shared Responsibility
O n the morning of 25 April 2015, Nepal shook with a 7.8 magnitude earthquake. When the dust settled, thousands of people had died and buildings had come crumbling down. It was a Saturday, a day off in Nepal, which means that the offices and schools that had collapsed were closed. If it had been any other day, the death toll could have been much higher. A little more than two weeks later, on 12 May, a 7.3 magnitude aftershock struck, resulting in more casualties and destruction.
More than 8,800 lives were lost, thousands of people were injured, and over 800,000 buildings and monuments were left destroyed or damaged. Experts pegged direct damages and losses at a staggering $7 billion.
In those first few days following the earthquake, the first responders were members of local communities, along with Nepalis from across the country. Neighbours pulled neighbours out of the rubble. People shared their scarce resources with those in need. The youth of Nepal mobilized to collect relief items and travelled long distances across difficult terrain to help their fellow citizens recover. Local non-governmental organizations (NGOs) used their extensive networks to provide critical information to the military and humanitarian actors. They deployed volunteers on foot, carrying supplies on their backs to the most remote areas, places helicopters and vehicles could not reach.
With help from the United Nations, the Government of Nepal, thousands of volunteers and over 450 humanitarian agencies responded to deliver critical life-saving aid to affected communities. The United Nations, donors, international NGOs and the International Federation of Red Cross and Red Crescent Societies immediately mobilized resources and reprogrammed activities. On 29 April, the Humanitarian Country Team launched the Nepal Earthquake Flash Appeal to provide protection and relief to 2.8 million people. Under the Flash Appeal, from April to September 2015, humanitarian assistance reached 3.7 million people.
Now, as we mark one year since the 25 April 2015 earthquake, we can reflect on what went well and what could have been done better. Recent formal evaluations and reviews of the response by the humanitarian community have been positive, finding that collective humanitarian efforts were quick, well-coordinated and effective. The effectiveness of the response was facilitated by a collaborative approach among donors, as well as by the United Nations and national stakeholders that existed before the earthquake.
The fact that previous preparedness efforts capitalized on existing capacity, and that this capacity was not bypassed once the disaster occurred, likely contributed to an easier transition back to pre-existing structures when the massive international aid machinery left again. Also underlying these successes, however, is the finding that preparedness planning needs to be stepped up even further, with no time to waste. The collection of information and its strategic use needs to better guide the response, and engagement between humanitarian actors and the national Government needs to improve. Stronger linkages with in-country actors are required, and understanding and supporting community capacity are paramount. 1 The pockets of residual need require continued monitoring, as well as systematic integration into recovery and development activities to ensure that no one is left behind.
These challenges are not unique to Nepal. Natural disasters around the world affect, on average, more than 200 million people and displace more than 20 million people on a yearly basis. 2 The impact of, and preparedness and response to, natural hazards will be a central topic when the humanitarian community and world leaders gather at the World Humanitarian Summit in Istanbul in May 2016.
When disaster strikes, the humanitarian community can rely heavily on approaches that are already in place in many countries. For example, the Human Rights-Based Approach ensures that there is a concerted effort to reach and serve those who are in the most vulnerable situations; applying this approach in a crisis is critical to promoting an effective response. Only by working together with those already in-country—local communities, local government, development actors, the national Government, the private sector, civil society, the armed forces—can the humanitarian community be truly effective in addressing the needs and concerns of affected populations.
Consideration of how existing models of humanitarian response reinforce or undermine the self-recovery of communities needs special attention when reflecting on the way forward for the humanitarian community at the World Humanitarian Summit. If the core commitment to changing people’s lives, as set out in the Secretary-General’s report for the Summit, One Humanity: Shared Responsibility , is to be achieved, focusing on needs alone may not be enough.
Prior to the 2015 disaster, the United Nations Country team and the Government of Nepal laid a strong foundation for the response in the form of the Nepal Risk Reduction Consortium and the United Nations Development Assistance Framework. This foundation enabled drivers of the humanitarian response to draw on existing analysis and data from development efforts, instead of starting from scratch with humanitarian assessments to inform the response. Nepal, like many other nations, is a complex country with unique needs. Those needs were outlined in the Framework, which has a strong focus on human rights, gender equality and the social inclusion of vulnerable groups. It is underpinned by extensive disaggregated analysis and assessments. The Flash Appeal, which was based on this vital data, included an ambitious strategic objective to “protect the rights of those most affected, and promote inclusive access to humanitarian assistance, with particular attention to the most disadvantaged groups”.
From the onset of the crisis, the humanitarian community understood the importance of local engagement and accountability to the affected population, as well as gender and social inclusion issues. Feedback and outreach mechanisms were swiftly established to promote a humanitarian effort that was responsive to the needs identified by communities. This approach facilitated input from thousands of people on a regular basis, reaching 10 million people with communications products, as well as enhanced attention to sex- and age-disaggregated data and reporting.
Globally, the humanitarian system remains focused more on communicating to communities, rather than with them. Significant efforts by humanitarian actors to engage with the affected population have been undertaken, however, because of recent reforms in the humanitarian system. For example, the Inter-Agency Common Feedback Project is an innovative initiative undertaken by the Resident and Humanitarian Coordinator’s Office in Nepal to prioritize and promote community engagement.
The Feedback Project collected community feedback through periodical perception surveys, rumours and concerns tracking, as well as existing agency feedback mechanisms. That information was formulated into consolidated reports. The reports were subsequently shared with humanitarian actors, as well as civil society and district authorities, resulting in adaptive programming and planning to ensure that response efforts were aligned with community-raised concerns and needs. Using local radio and community meetings, enquiring people could gain relevant and accurate information—something highly valued by affected communities.
Nepal is one of two pilot countries in the Asia-Pacific region rolling out the new Inter-Agency Standing Committee Emergency Response Preparedness initiative. This presents a unique opportunity to ensure that systems and tools are in place to strengthen the human rights perspective and engagement with communities in future responses. As part of this ongoing work, UN-Women, together with the Humanitarian Country Team, is testing a gender package, which will contribute best practices as the Emergency Response Preparedness Guidelines are introduced globally.
The principles and commitments are in place. The challenge is now to ensure that the voices of affected people are heard and their rights are protected. This will require context-specific innovations and investment in initiatives such as the Common Feedback Project. The onus is on humanitarian actors to identify practical solutions to enhancing engagement and to promote systematically the participation and leadership of women, men and subgroups of affected communities. One step towards this paradigm shift in humanitarian action is to stop considering the people we serve as vulnerable. We must empower the individuals who, despite hardship, marginalization and/or discrimination, have no other choice but to find the strength and means to recover.
1 STAIT Learning Reviews, Global Learning from the Nepal Preparedness for Response Learning Review, Senior Transformative Agenda Implementation Team (STAIT). January 2016; Nepal Earthquake: Humanitarian Country Team After Action Report, Workshop Summary Report 2015.
2 Centre for Research on the Epidemiology of Disasters (CRED) and others, “The human cost of natural disasters 2015: a global perspective”, Research report (Brussels, Belgium, School of Public Health, Université catholique de Louvain, 2015). Available from http://reliefweb.int/sites/reliefweb.int/files/resources/PAND_report.pdf . Internal Displacement Monitoring Centre (IDMC), Global Estimates 2015: People displaced by disasters, Global disaster displacement data, July 2015. Available from http://www.internal-displacement.org/global-figures/#natural (accessed 28 April 2016).
The UN Chronicle is not an official record. It is privileged to host senior United Nations officials as well as distinguished contributors from outside the United Nations system whose views are not necessarily those of the United Nations. Similarly, the boundaries and names shown, and the designations used, in maps or articles do not necessarily imply endorsement or acceptance by the United Nations.
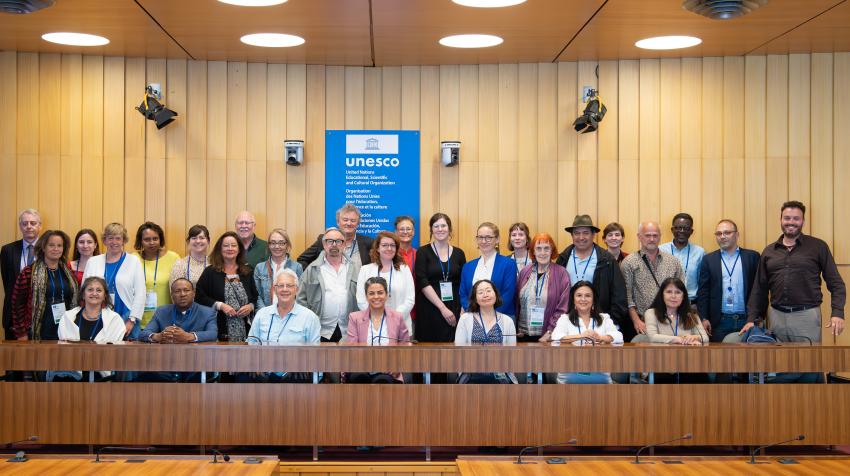
Cultural Diversity in the Digital Age: A Pillar for Sustainable Development
Two important issues affecting the protection and promotion of cultural diversity deserve our attention: the question of discoverability of local and national content, and the impact of generative artificial intelligence (AI).

“Bee Engaged with Youth” to Safeguard Bees and Other Pollinators
As we celebrate World Bee Day on 20 May, let us remember how crucial it is to prioritize efforts to protect bees and other pollinators. FAO is committed to supporting youth, who have a key role to play in fostering the transformative changes and future initiatives and activities needed to save our bees and other pollinators.
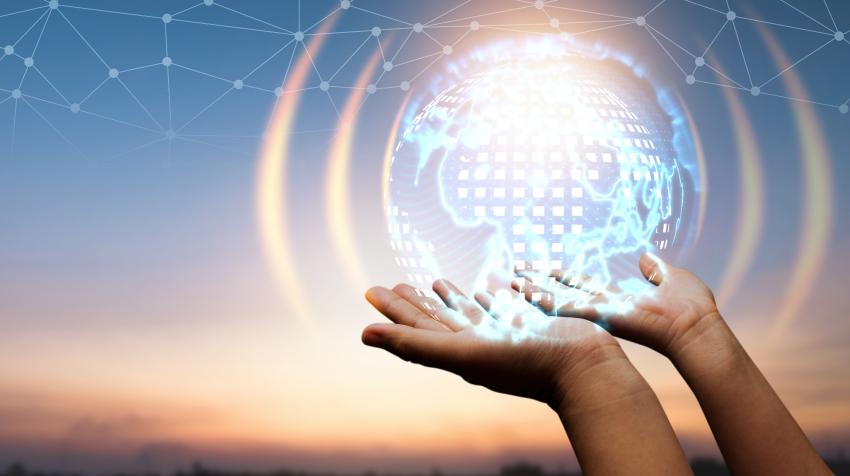
Digital Innovation—Key to Unlocking Sustainable Development
Digital tools have the potential to accelerate human progress, but those who are not online are most at risk of being left behind.
Documents and publications
- Yearbook of the United Nations
- Basic Facts About the United Nations
- Journal of the United Nations
- Meetings Coverage and Press Releases
- United Nations Official Document System (ODS)
- Africa Renewal
Libraries and Archives
- Dag Hammarskjöld Library
- UN Audiovisual Library
- UN Archives and Records Management
- Audiovisual Library of International Law
- UN iLibrary
News and media
- UN News Centre
- UN Chronicle on Twitter
- UN Chronicle on Facebook
The UN at Work
- 17 Goals to Transform Our World
- Official observances
- United Nations Academic Impact (UNAI)
- Protecting Human Rights
- Maintaining International Peace and Security
- The Office of the Secretary-General’s Envoy on Youth
- United Nations Careers
Content Search
Nepal earthquake 2015: post disaster needs assessment vol. a : key findings.
- Govt. Nepal
Attachments

Executive Summary
Background Nepal is the 11th most earthquake-prone country in the world. Ever since the first recorded earthquake of 1255 AD that killed one-third of the population of the Kathmandu Valley and its King, Abhaya Malla, Nepal has experienced a major earthquake every few generations. The last great earthquake (of magnitude 8.4) in 1934 AD resulted in more than 10,000 deaths in the Kathmandu Valley. Most of the infrastructure and major heritage sites had to be rebuilt. There have since been earthquakes causing severe human and physical loss in 1980, 1988 and 2011.
On Saturday, 25 April 2015 at 11:56 local time, a 7.6 magnitude earthquake as recorded by Nepal’s National Seismological Centre (NSC), struck Barpak in the historic district of Gorkha, about 76 km northwest of Kathmandu. Nepal had not faced a natural shock of comparable magnitude for over 80 years.
The catastrophic earthquake was followed by more than 300 aftershocks greater than magnitude 4.0 (as of 7 June 2015). Four aftershocks were greater than magnitude 6.0, including one measuring 6.8 which struck 17 days after the first big one with the epicentre near Mount Everest. To date, there are over 8,790 casualties and 22,300 injuries. It is estimated that the lives of eight million people, almost one-third of the population of Nepal, have been impacted by these earthquakes. Thirty-one of the country’s 75 districts have been affected, out of which 14 were declared ‘crisis-hit’ (see Figure 1) for the purpose of prioritizing rescue and relief operations; another 17 neighbouring districts are partially affected.
The destruction was widespread covering residential and government buildings, heritage sites, schools and health posts, rural roads, bridges, water supply systems, agricultural land, trekking routes, hydropower plants and sports facilities. The geodetic network centres including horizontal and vertical control points have been damaged in a manner that will affect reconstruction planning.
Rural areas in the central and western regions were particularly devastated and further isolated due to road damage and obstructions. In the worst hit areas, entire settlements, including popular tourist destinations like Langtang, were swept away by landslides and avalanches triggered by the earthquakes.
Due to the weakened, ruptured, and destabilized slopes and surfaces, the vulnerable areas have now become even more susceptible to flooding and landslides that can occur during the monsoon. Hundreds of historical and cultural monuments at least a century old were either destroyed or extensively damaged. Over half a million houses were destroyed. The damage exposed the weaknesses of houses that did not have any seismic-resistant features or were not in accordance with the building codes. The disaster also highlighted aspects of inequities in Nepali society spanning geography, income and gender. Poorer rural areas have been more adversely affected than towns and cities due to their inferior quality of houses. More women and girls died than men and boys, partly because of gendered roles that disproportionately assign indoor chores to women.
The time and day the first earthquake was experienced saved thousands of lives. Being a Saturday, the weekly holiday, schools across Nepal were closed on 25 April. The death toll of young people could have been much higher considering that nearly 7,000 schools were completely or significantly damaged. Similarly, if the earthquake had struck at night, and not in the middle of the day, there would certainly have been greater casualties.
Related Content
Nepal earthquake 2015: post disaster needs assessment vol. b : sector reports.
World + 15 more
Addressing loss and damage: Practical insights for tackling multidimensional risks in LDCs and SIDS (July 2022)
World + 13 more
Loss and damage case studies from the frontline: a resource to support practice and policy
Nepal + 2 more
Gender and age inequality of disaster risk
Nepal Earthquake Case Studies

About the Project
On April 25, 2015, Nepal and its people experienced a 7.8 magnitude earthquake. On May 12, another major earthquake of 7.2 magnitude hit the country. In practice, his means that millions of Nepalis have lived and died under the weight of falling buildings, landslides, floods, hunger, and homelessness brought about by massive seismic shifts across the Himalayan belt. Most will refer to this as an earthquake, singular. But this is no singular disaster. The country has experienced more than 300 seismic events since April 25, 2015, and nearly 9000 people died as a direct result of the two most major earthquakes.
For most of Nepal’s approximately 30 million people, living uncertainty is old hat. Consider the legacies of civil war (1996-2006) followed by a decade of political instability and current struggles to write a viable constitution. But the spring of 2015 has cracked open new forms of vulnerability for most Nepalis. These quakes have caused enormous destruction to the nation’s rich cultural heritage, in the Kathmandu Valley and beyond. The countryside has experienced vast devastation. More than half a million homes have been destroyed or are precariously habitable. This equates to about 2.5 million internally displaced. More than 3,500 schools have been destroyed and nearly as many health posts. There has been widespread damage to highways and road networks; glacial lakes are in danger of bursting; landslides are a constant threat, and have continued to wipe out settlements; many hydroelectric dams have been damaged; water borne illness and other public health challenges loom as monsoon has arrived. Even so, Nepalis are showing incredible resilience, creativity, and deep commitments to helping each other through this suffering.
This project – in the context of ANTH 55: Anthropology of Global Health – explores the human impacts of these disasters by asking students to engage in collective research and writing of case studies focused on specific areas of inquiry related to the earthquake.
The assumption of this project is not that students will become “experts” either on Nepal or on the health effects of earthquakes, but that they will amass sufficient knowledge about their area of inquiry so that they can contribute to an effort to expand knowledge and understanding of this event to others, and expand in the process their own conceptualization of what “global health” is, where and how it occurs, and how it links to many other aspects of human life.
Academia.edu no longer supports Internet Explorer.
To browse Academia.edu and the wider internet faster and more securely, please take a few seconds to upgrade your browser .
Enter the email address you signed up with and we'll email you a reset link.
- We're Hiring!
- Help Center
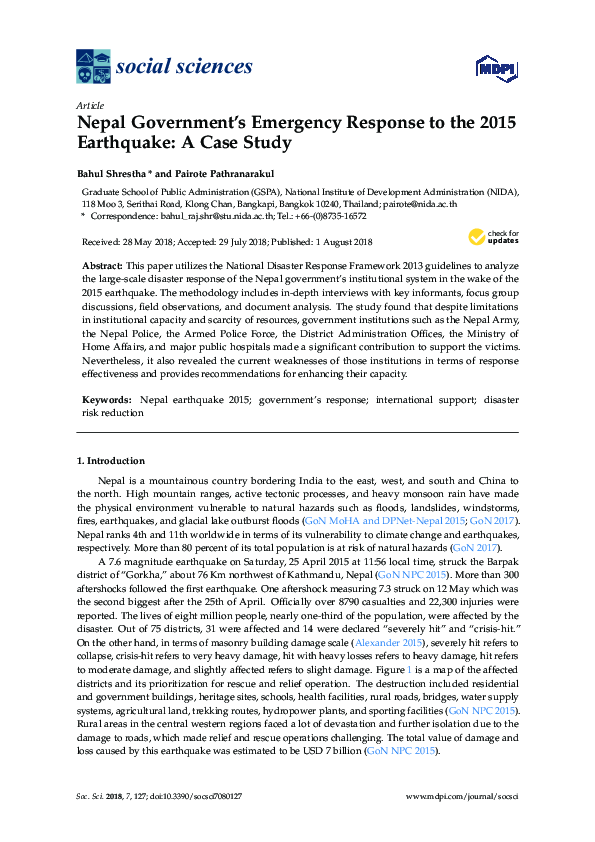
Nepal Government's Emergency Response to the 2015 Earthquake: A Case Study

2018, Social Sciences
Related Papers
David Sanderson
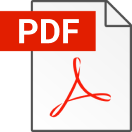
International Journal of Disaster Risk Reduction
Alistair D . B . Cook
Natural Hazards
The Humanitarian Assistance and Disaster Response (HADR) research team at the NTS Centre, S. Rajaratnam School of International Studies (RSIS) studied the international response to the 2015 Nepal earthquake to understand the dimensions and scope of the international response and to distill field observations from this particular experience. The research revealed that most international responders and parties in Nepal, who were beneficiaries of the response, considered immediate search and rescue, and relief operations a success. There was however a number of lessons which emerged from the experience for both the affected country as well as international responding parties. Through a primary focus on the immediate relief phase following the disaster, this report identifies four themes: (i) Strategic Planning; (ii) Aid Delivery; (iii) Aid Provision; and (iv) Aid Distribution. The following recommendations from the research on Nepal is to ensure greater effectiveness and efficiency for future HADR responses. While this research assessed a relatively small but critical window, and some of the lessons were context specific, it is hoped the recommendations which have emerged will help make future international humanitarian assistance and disaster response more effective.
Bulletin of the Department of Geology
Shrijan Malla
Effect of local level disaster response is always questionable in Nepal. It is because the capacity of local responding bodies' i.e. local administration, elected representatives and security forces is deficit. Overlooking own role and responsibility by public service offices such as health, water supply, road network, rural development, communication, education has overburdened the responsibility of CDO during disaster response and eroded the effectiveness of cluster approach. Similarly, over-reliance on security forces from relief and rescue to rehabilitation and reconstruction have also garnered lethargy amongst civil administration and public service offices wearing away their capacity. For that reason, it is utmost important that the prevailing tendency should be altered and derailed local level response mechanism should be brought into the right track.
Morten Wendelbo , Sebastiano Mori , Federica La China , Leonardo Taccetti , Robert Zielonka , European Institute for Asian Studies
The response to the earthquakes in Nepal was among the strongest in history, seen from an international perspective, and given the severity of the calamities. Dozens of countries came to the aid of Nepal, some of them, such as India and Pakistan, within a few short hours. Nonetheless, an unnecessary amount of people were killed, injured or otherwise had their lives permanently altered. This report details many of the challenges experienced by international and Nepalese actors before, during and after the disaster. Here, the main findings are briefly summarized.
Anastasia Aladysheva
On 25 April 2015 an earthquake measuring 7.8 on the Richter scale struck in Nepal, with its epicentre in the Lamjung district, causing destruction in 14 of the 75 districts in the country. Two weeks later, on 12 May, a second earthquake hit the country, this time 7.2 on the Richter scale, exacerbating the humanitarian situation. According to offi cial statistics, nearly 9000 people were killed, more than 21 000 injured and 188 900 temporarily displaced as a result of the earthquakes. In total, 605 254 houses were destroyed and 288 255 houses damaged, leaving many thousands homeless. According to the United Nations Offi ce for the Coordination of Humanitarian Aff airs (UNOCHA), more than 450 aid organizations are providing humanitarian assistance in the aff ected districts.1 The UNOCHA Country Team estimated that $422 million was needed to meet protection and humanitarian needs after the disaster.2 As of January 2016, two-thirds of the appeal target had been contributed, but 69 per c...
Risk Management
Meen Chhetri
Vina Ismawati
Andy Featherstone
Humanitarian response is all too often characterised by large international responses; in contrast, the approach of the Government of Nepal that required all international NGOs to work through national and district-based partners for all but the initial phase of the earthquake response offered a real-time opportunity for the humanitarian community to put principles of partnership into practice. This study draws on discussions with civil society, NGOs, UN agencies and government staff in Kathmandu and the districts of Gorkha and Sindhupalchok to harvest lessons from the experience to inform and strengthen future preparedness and response in Nepal, and to strengthen global advocacy on the need for further investment in, and support for, local and national leadership of humanitarian response.
RELATED PAPERS
Austin Foley Holmes
Littera Aperta. International Journal of Literary and Cultural Studies
Ángel Jacinto Traver Vera
Brazilian Journal of Health Review
Natane Carvalho
Vincent Terrasson
Pharmaceuticals
Nicola Hamilton
The Journal of Contemporary Dental Practice
Suma Karthigeyan
Gheorghe Savin
Peritoneal Dialysis International: Journal of the International Society for Peritoneal Dialysis
Krit Pongpirul
HAL (Le Centre pour la Communication Scientifique Directe)
Sophie Mouquin
Regiani Magalhães Yamazaki
Euromicro Conference on Real-Time Systems
Rodolfo Pellizzoni
arXiv: Commutative Algebra
Jugal Verma
Forensic-medical examination
Volodymyr Mishalov
Zbornik radova Vizantološkog instituta LX/II
Vujadin M Ivanišević , Ivan Bugarski
The Korean Journal of Orthodontics
burcu balos tuncer
Gaia Gómez Pino
Revista Cubana de Pediatría
Ester Miyuki Nakamura-Palacios
Cristian Cardozo
International Journal of Tropical Disease & Health
fuyong jiao
European Journal of Integrative Medicine
Geraldine Leydon
RELATED TOPICS
- We're Hiring!
- Help Center
- Find new research papers in:
- Health Sciences
- Earth Sciences
- Cognitive Science
- Mathematics
- Computer Science
- Academia ©2024
Earthquake Case Study: Nepal 2015
The nepal earthquake in 2015.
A shallow focus earthquake of magnitude 7.8 affected Nepal in April 2015.

- Foreign aid was needed to support recovery.
- The effects were more severe because many people lived in poor quality housing.
- The earthquake was caused by the collision of the Indo-Australian and Eurasian plates.

Primary effects
- 9,000 people were killed.
- There was US$5 billion of damage.

Secondary effects
- Flooding and damage to roads from landslides.
- An avalanche on Mount Everest which killed 19 people.

Responses to the earthquake
- Overseas aid from NGOs (non-governmental organisations) such as Oxfam.
- 300,000 people left the capital city (Kathmandu).
- An international conference to appeal for funding.
1 The Challenge of Natural Hazards
1.1 Natural Hazards
1.1.1 Types of Natural Hazards
1.1.2 Hazard Risk
1.1.3 Consequences of Natural Hazards
1.1.4 End of Topic Test - Natural Hazards
1.1.5 Exam-Style Questions - Natural Hazards
1.2 Tectonic Hazards
1.2.1 Tectonic Plates
1.2.2 Tectonic Plates & Convection Currents
1.2.3 Plate Margins
1.2.4 Volcanoes
1.2.5 Effects of Volcanoes
1.2.6 Responses to Volcanic Eruptions
1.2.7 Earthquakes
1.2.8 Earthquakes 2
1.2.9 Responses to Earthquakes
1.2.10 Case Studies: The L'Aquila & Kashmir Earthquakes
1.2.11 Earthquake Case Study: Chile 2010
1.2.12 Earthquake Case Study: Nepal 2015
1.2.13 Living with Tectonic Hazards 1
1.2.14 Living with Tectonic Hazards 2
1.2.15 End of Topic Test - Tectonic Hazards
1.2.16 Exam-Style Questions - Tectonic Hazards
1.2.17 Tectonic Hazards - Statistical Skills
1.3 Weather Hazards
1.3.1 Global Atmospheric Circulation
1.3.2 Surface Winds
1.3.3 UK Weather Hazards
1.3.4 Tropical Storms
1.3.5 Features of Tropical Storms
1.3.6 Impact of Tropical Storms 1
1.3.7 Impact of Tropical Storms 2
1.3.8 Tropical Storms Case Study: Katrina
1.3.9 Tropical Storms Case Study: Haiyan
1.3.10 UK Weather Hazards Case Study: Somerset 2014
1.3.11 End of Topic Test - Weather Hazards
1.3.12 Exam-Style Questions - Weather Hazards
1.3.13 Weather Hazards - Statistical Skills
1.4 Climate Change
1.4.1 Evidence for Climate Change
1.4.2 Causes of Climate Change
1.4.3 Effects of Climate Change
1.4.4 Managing Climate Change
1.4.5 End of Topic Test - Climate Change
1.4.6 Exam-Style Questions - Climate Change
1.4.7 Climate Change - Statistical Skills
2 The Living World
2.1 Ecosystems
2.1.1 Ecosystems
2.1.2 Ecosystem Cascades & Global Ecosystems
2.1.3 Ecosystem Case Study: Freshwater Ponds
2.2 Tropical Rainforests
2.2.1 Tropical Rainforests - Intro & Interdependence
2.2.2 Adaptations
2.2.3 Biodiversity of Tropical Rainforests
2.2.4 Deforestation
2.2.5 Case Study: Deforestation in the Amazon Rainforest
2.2.6 Sustainable Management of Rainforests
2.2.7 Case Study: Malaysian Rainforest
2.2.8 End of Topic Test - Tropical Rainforests
2.2.9 Exam-Style Questions - Tropical Rainforests
2.2.10 Deforestation - Statistical Skills
2.3 Hot Deserts
2.3.1 Overview of Hot Deserts
2.3.2 Biodiversity & Adaptation to Hot Deserts
2.3.3 Case Study: Sahara Desert
2.3.4 Desertification
2.3.5 Case Study: Thar Desert
2.3.6 End of Topic Test - Hot Deserts
2.3.7 Exam-Style Questions - Hot Deserts
2.4 Tundra & Polar Environments
2.4.1 Overview of Cold Environments
2.4.2 Adaptations in Cold Environments
2.4.3 Biodiversity in Cold Environments
2.4.4 Case Study: Alaska
2.4.5 Sustainable Management
2.4.6 Case Study: Svalbard
2.4.7 End of Topic Test - Tundra & Polar Environments
2.4.8 Exam-Style Questions - Cold Environments
3 Physical Landscapes in the UK
3.1 The UK Physical Landscape
3.1.1 The UK Physical Landscape
3.2 Coastal Landscapes in the UK
3.2.1 Types of Wave
3.2.2 Weathering & Mass Movement
3.2.3 Processes of Erosion & Wave-Cut Platforms
3.2.4 Headlands, Bays, Caves, Arches & Stacks
3.2.5 Transportation
3.2.6 Deposition
3.2.7 Spits, Bars & Sand Dunes
3.2.8 Case Study: Landforms on the Dorset Coast
3.2.9 Types of Coastal Management 1
3.2.10 Types of Coastal Management 2
3.2.11 Coastal Management Case Study - Holderness
3.2.12 Coastal Management Case Study: Swanage
3.2.13 Coastal Management Case Study - Lyme Regis
3.2.14 End of Topic Test - Coastal Landscapes in the UK
3.2.15 Exam-Style Questions - Coasts
3.3 River Landscapes in the UK
3.3.1 The River Valley
3.3.2 River Valley Case Study - River Tees
3.3.3 Erosion
3.3.4 Transportation & Deposition
3.3.5 Waterfalls, Gorges & Interlocking Spurs
3.3.6 Meanders & Oxbow Lakes
3.3.7 Floodplains & Levees
3.3.8 Estuaries
3.3.9 Case Study: The River Clyde
3.3.10 River Management
3.3.11 Hard & Soft Flood Defences
3.3.12 River Management Case Study - Boscastle
3.3.13 River Management Case Study - Banbury
3.3.14 End of Topic Test - River Landscapes in the UK
3.3.15 Exam-Style Questions - Rivers
3.4 Glacial Landscapes in the UK
3.4.1 Erosion
3.4.2 Landforms Caused by Erosion
3.4.3 Landforms Caused by Transportation & Deposition
3.4.4 Snowdonia
3.4.5 Land Use in Glaciated Areas
3.4.6 Tourism in Glacial Landscapes
3.4.7 Case Study - Lake District
3.4.8 End of Topic Test - Glacial Landscapes in the UK
3.4.9 Exam-Style Questions - Glacial Landscapes
4 Urban Issues & Challenges
4.1 Urban Issues & Challenges
4.1.1 Urbanisation
4.1.2 Urbanisation Case Study: Lagos
4.1.3 Urbanisation Case Study: Rio de Janeiro
4.1.4 UK Cities
4.1.5 Case Study: Urban Regen Projects - Manchester
4.1.6 Case Study: Urban Change in Liverpool
4.1.7 Case Study: Urban Change in Bristol
4.1.8 Sustainable Urban Life
4.1.9 End of Topic Test - Urban Issues & Challenges
4.1.10 Exam-Style Questions - Urban Issues & Challenges
4.1.11 Urban Issues -Statistical Skills
5 The Changing Economic World
5.1 The Changing Economic World
5.1.1 Measuring Development
5.1.2 Classifying Countries Based on Wealth
5.1.3 The Demographic Transition Model
5.1.4 Physical & Historical Causes of Uneven Development
5.1.5 Economic Causes of Uneven Development
5.1.6 How Can We Reduce the Global Development Gap?
5.1.7 Case Study: Tourism in Kenya
5.1.8 Case Study: Tourism in Jamaica
5.1.9 Case Study: Economic Development in India
5.1.10 Case Study: Aid & Development in India
5.1.11 Case Study: Economic Development in Nigeria
5.1.12 Case Study: Aid & Development in Nigeria
5.1.13 Economic Development in the UK
5.1.14 Economic Development UK: Industry & Rural
5.1.15 Economic Development UK: Transport & North-South
5.1.16 Economic Development UK: Regional & Global
5.1.17 End of Topic Test - The Changing Economic World
5.1.18 Exam-Style Questions - The Changing Economic World
5.1.19 Changing Economic World - Statistical Skills
6 The Challenge of Resource Management
6.1 Resource Management
6.1.1 Global Distribution of Resources
6.1.2 Food in the UK
6.1.3 Water in the UK 1
6.1.4 Water in the UK 2
6.1.5 Energy in the UK
6.1.6 Resource Management - Statistical Skills
6.2.1 Areas of Food Surplus & Food Deficit
6.2.2 Food Supply & Food Insecurity
6.2.3 Increasing Food Supply
6.2.4 Case Study: Thanet Earth
6.2.5 Creating a Sustainable Food Supply
6.2.6 Case Study: Agroforestry in Mali
6.2.7 End of Topic Test - Food
6.2.8 Exam-Style Questions - Food
6.2.9 Food - Statistical Skills
6.3.1 The Global Demand for Water
6.3.2 What Affects the Availability of Water?
6.3.3 Increasing Water Supplies
6.3.4 Case Study: Water Transfer in China
6.3.5 Sustainable Water Supply
6.3.6 Case Study: Kenya's Sand Dams
6.3.7 Case Study: Lesotho Highland Water Project
6.3.8 Case Study: Wakel River Basin Project
6.3.9 Exam-Style Questions - Water
6.3.10 Water - Statistical Skills
6.4.1 Global Demand for Energy
6.4.2 Factors Affecting Energy Supply
6.4.3 Increasing Energy Supply: Renewables
6.4.4 Increasing Energy Supply: Non-Renewables
6.4.5 Carbon Footprints & Energy Conservation
6.4.6 Case Study: Rice Husks in Bihar
6.4.7 Exam-Style Questions - Energy
6.4.8 Energy - Statistical Skills
Jump to other topics
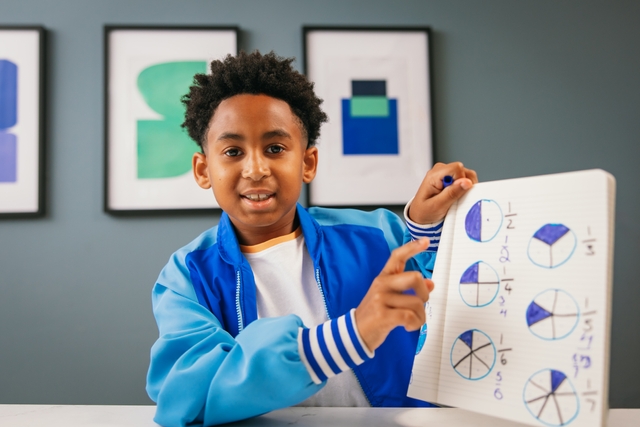
Unlock your full potential with GoStudent tutoring
Affordable 1:1 tutoring from the comfort of your home
Tutors are matched to your specific learning needs
30+ school subjects covered
Earthquake Case Study: Chile 2010
Living with Tectonic Hazards 1
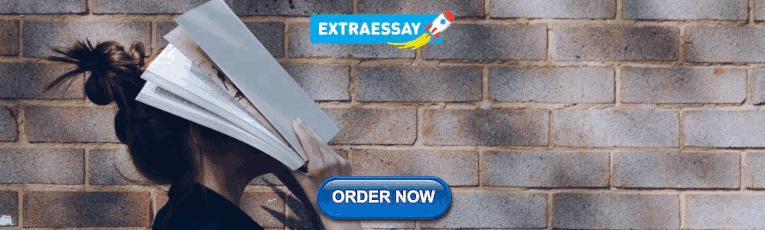
IMAGES
VIDEO
COMMENTS
Nepal Earthquake 2015 - A case study exploring the primary and secondary effects and immediate and long term responses to the Nepal earthquake. X; Facebook; ... At 11.26 am on Saturday, 25th of April 2015, a magnitude 7.9 earthquake struck Nepal. The focus was only eight kilometres deep, and the epicentre was just 60 kilometres northwest of ...
Nepal earthquake of 2015, severe earthquake that struck near Kathmandu in central Nepal on April 25, 2015. ... whether from years of experience gained by working on that content or via study for an advanced degree. ... the most recent large earthquake (that is, magnitude 6.0 or above) took place in 1988. That magnitude-6.9 event resulted in the ...
Case study: Nepal 2015 (LMIC) Tsunamis; Case study: Japan tsunami 2011 (HIC) ... Almost one in three were a magnitude of five or higher. ... It was the worst earthquake in Nepal in more than 80 years.
Abstract and Figures. The Gorkha (Nepal) earthquake of magnitude 7.8, occurred at 11:56 NST on 25 April 2015 with an epicentre 77 km northwest of Kathmandu, the capital city of Nepal, that is home ...
The April 2015 Nepal earthquake (also known as the Gorkha earthquake) killed 8,962 people in Nepal and injured 21,952 more. It occurred at 11:56 Nepal Standard Time on Saturday 25 April 2015, with a magnitude of 7.8M w or 8.1M s and a maximum Mercalli Intensity of X (Extreme).Its epicenter was east of Gorkha District at Barpak, Gorkha, roughly 85 km (53 mi) northwest of central Kathmandu, and ...
Introduction. An intense ground shaking struck Central Nepal on 25 April 2015 (local time 11:56 a.m.). The moment magnitude of the earthquake was M w 7.8 with its hypocenter located in the Gorkha region (about 80 km north-west of Kathmandu). The earthquake occurred at the subduction interface along the Himalayan arc between the Indian plate and the Eurasian plate (Avouac, 2003; Ader et al ...
On Saturday, April 25 at 11:56 am local time, an M7.8 earthquake began 82 km (51 mi) NW of Nepal's capital of Kathmandu. The event was followed by many aftershocks, the largest being an M7.3 on May 12, 17 days after the mainshock. The toll included about 9000 fatalities, 23,000 injuries, more than 500,000 destroyed houses, and about 270,000 ...
On 25 April 2015, a M w 7.8 earthquake struck near Gorka, Nepal. The earth-quake and its aftershocks caused over 8,790 deaths and 22,300 injuries; a half a million homes were destroyed; and hundreds of historical and cultural monuments were destroyed or extensively damaged ().Triggered landslides blocked access to road networks, and other lifelines were significantly impacted.
Here, we describe the seismic data for 18 earthquakes over magnitude 5.0 collected by this array, including the 2015 magnitude 7.3 Dolakha earthquake of maximum aftershock and three large ...
O n the morning of 25 April 2015, Nepal shook with a 7.8 magnitude earthquake. When the dust settled, thousands of people had died and buildings had come crumbling down. It was a Saturday, a day ...
There have since been earthquakes causing severe human and physical loss in 1980, 1988 and 2011. On Saturday, 25 April 2015 at 11:56 local time, a 7.6 magnitude earthquake as recorded by Nepal's ...
Abstract. On April 25, 2015, a 7.8 magnitude earthquake struck Nepal, destroying villages and devastating the land. Water-its uses and effects-is a recurrent theme in post-earthquake Nepal. Deforestation has prevented water absorption and land anchoring by tree roots, thus contributing to landslides and flooding.
A 7.6 magnitude earthquake on Saturday, 25 April 2015 at 11:56 local time, struck the Barpak district of "Gorkha," about 76 Km northwest of Kathmandu, Nepal (GoN NPC2015). More than 300
On April 25, 2015, Nepal and its people experienced a 7.8 magnitude earthquake. On May 12, another major earthquake of 7.2 magnitude hit the country. In practice, his means that millions of Nepalis have lived and died under the weight of falling buildings, landslides, floods, hunger, and homelessness brought about by massive seismic shifts ...
on 25 April 2015, a 7.8 magnitude earthquake struck nepal, with its epicentre 81km north-west of the capital kathmandu. this was followed on 12 May by a 7.3 magnitude earthquake that struck the district of Dolakha, leading to further loss of life and building damage, and increasing the humanitarian needs.
Email: [email protected]. ABSTRACT: The Gorkha (Nepal) earthquake of magnitude 7.8, occurred at 11:56 NST on 25 April 2015 with an epicentre 77 km northwest of Kathmandu, the capital city of ...
A second major earthquake occurred on 12 May 2015 at 12:35 IST with a magnitude of 7.3 on Richter scale. The epicentre was near the China border between Kathmandu and Mt. Everest, at Kodari around 113 kms ... A Devastating Disaster: A Case Study of Nepal Earthquake and Its Impact on Human Beings
The Asian Development Bank (ADB) worked with Nepal following the 7.8 magnitude earthquake on 25 April 2015 and the devastating aftershock on 12 May 2015 that cost 9,000 lives and destroyed thousands of structures in Kathmandu and nearby towns. The Earthquake Emergency Assistance Project (EAAP) initially provided $3 million in quick-disbursing ...
The Humanitarian Assistance and Disaster Response (HADR) research team at the NTS Centre, S. Rajaratnam School of International Studies (RSIS) studied the international response to the 2015 Nepal earthquake to understand the dimensions and scope of the international response and to distill field observations from this particular experience.
The earthquake in Nepal 2015 registered a moment magnitude of 7.8. Moment magnitude analyzes all the wavelengths recorded from the shaking for a complete picture of the scale of the earthquake ...
A shallow focus earthquake of magnitude 7.8 affected Nepal in April 2015. GCSE. ... Earthquake Case Study: Nepal 2015. Test yourself. The Nepal Earthquake in 2015. A shallow focus earthquake of magnitude 7.8 affected Nepal in April 2015. Background. Nepal is a low-income country. The lack of resources had an impact on both the effects and the ...
shelterprojects.org
On the 25th April 2015, a 7.9 magnitude earthquake hit Nepal, caused by the subduction of the Indo-Australian plate beneath the Eurasian plate (destructive plate margin). The earthquake's. epicentre was around 80km away from Nepal's capital, Kathmandu. The earthquake originated only 15km below the surface, which meant it was felt very ...
The 15 January 1934 Bihar-Nepal earthquake of a magnitude M w 8.0 rattled the ... The epicenter of the 2015 Nepal earthquake was approximately 450 km from the Uttarakhand region. ... Kamal, Mukat Lal Sharma, Ravi Sankar Jakka, and Pratibha. 2024. "Uttarakhand State Earthquake Early Warning System: A Case Study of the Himalayan Environment ...
This paper presents a multi-parameter ionospheric disturbance analysis of the total electron content (TEC), density (Ne), temperature (Te), and critical frequency foF2 variations preceding two significant earthquake events (2015 Mw 7.5 and 2023 Mw 6.3) that occurred in Afghanistan. The analysis from various ground stations and low-Earth-orbit satellite measurements involved employing the ...