
FREE K-12 standards-aligned STEM
curriculum for educators everywhere!
Find more at TeachEngineering.org .
- TeachEngineering
- Earthquakes Rock!
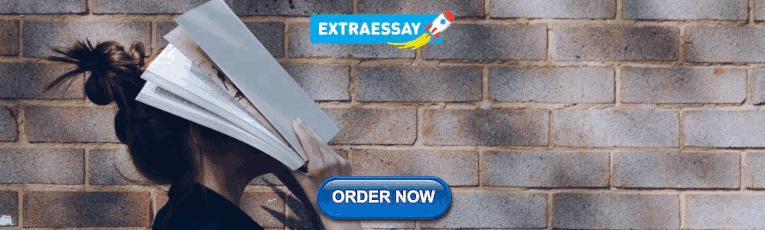
Lesson Earthquakes Rock!
Grade Level: 5 (3-5)
Time Required: 45 minutes
Lesson Dependency: None
Subject Areas: Earth and Space
NGSS Performance Expectations:
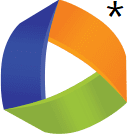
- Print lesson and its associated curriculum
Curriculum in this Unit Units serve as guides to a particular content or subject area. Nested under units are lessons (in purple) and hands-on activities (in blue). Note that not all lessons and activities will exist under a unit, and instead may exist as "standalone" curriculum.
- Engineering to Prevent Natural Disasters: Save Our City!
- Scale Model of the Earth
- Drifting Continents
- Faulty Movement
- Testing Model Structures: Jell-O Earthquake in the Classroom
- Seismology in the Classroom
- Mercalli Scale Illustrated
- Magnitude of the Richter Scale
- Ready to Erupt!
- Mini-Landslide
- Survive That Tsunami! Testing Model Villages in Big Waves
- Floodplain Modeling
- Tornado Damage!
- A Tornado in My State?
- Build It Better!
TE Newsletter
Engineering connection, learning objectives, more curriculum like this, introduction/motivation, associated activities, lesson closure, vocabulary/definitions, user comments & tips.
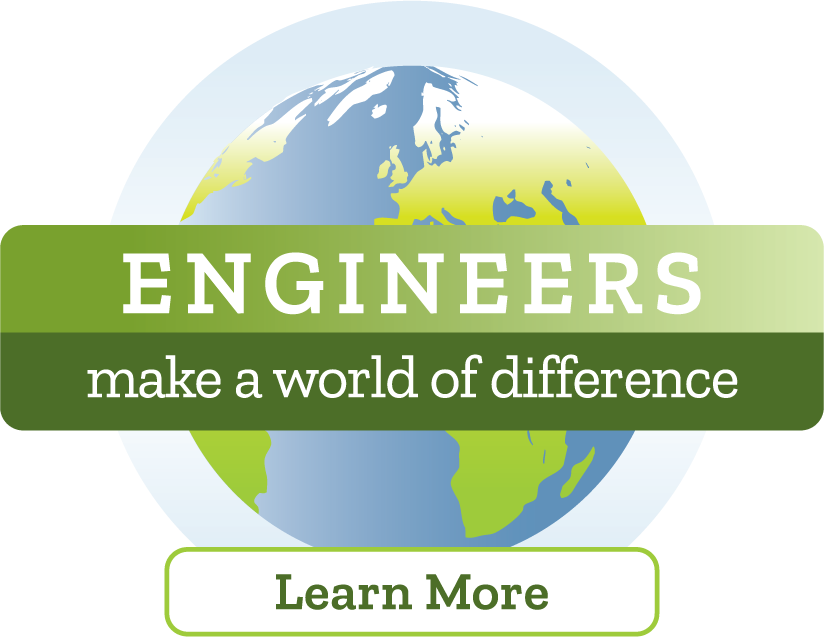
Civil, structural, mechanical and materials engineers make sure the structures we rely upon are built strong enough to keep us safe. To reduce the number of human injuries and casualties, they research and test new and improved techniques and materials that help structures withstand the tremendous earthquake forces. For example, engineers have developed shock absorbers and structure sliders—techniques that isolate the foundation of a building from the ground so the building and the earth move independently. They also create monitoring equipment to predict and measure earthquakes and warn surrounding communities.
After this lesson, students should be able to:
- Describe how humans are affected by earthquakes.
- Explain the distribution and causes of earthquakes that shape/change the Earth.
- Understand why engineers need to learn about earthquakes.
- Identify cause-effect relationships involved in earthquakes.
Educational Standards Each TeachEngineering lesson or activity is correlated to one or more K-12 science, technology, engineering or math (STEM) educational standards. All 100,000+ K-12 STEM standards covered in TeachEngineering are collected, maintained and packaged by the Achievement Standards Network (ASN) , a project of D2L (www.achievementstandards.org). In the ASN, standards are hierarchically structured: first by source; e.g. , by state; within source by type; e.g. , science or mathematics; within type by subtype, then by grade, etc .
Ngss: next generation science standards - science, international technology and engineering educators association - technology.
View aligned curriculum
Do you agree with this alignment? Thanks for your feedback!
State Standards
Colorado - science.
Although earthquakes are a natural phenomenon, they have caused billions of dollars worth of damage to buildings and other structures in the world (see Figures 1 and 2). For example, on March 27, 1964, in Prince William Sound, Alaska, an earthquake resulted in $538 million in damage (according to NOAA national data centers). The most damaging earthquake in the U.S. was the 1906 San Francisco earthquake, which measured 8.3 on the Richter scale. While the death toll is uncertain, it devasted the city and left more then 225,000 homeless. More recently, one of the deadliest natural disasers in recorded history was an undersea Indian Ocean earthquake that occurred on December 26, 2004. With an epicenter off the west coast of Sumatra, Indonesia, the earthquake triggered a series of tsunamis along the Indian Ocean coast, killing more than 225,000 people in 11 countries, and destroying coastal communities with waves up to 30 meters (100 feet). This megathrust earthquake measured 9.0 on the Richter Scale and was the fourth largest earthquake in the world since 1900 and is the largest since the 1964 Prince William Sound earthquake. Other notable earthquakes have occurred all over the world. California had the Loma Prieta earthquake in 1989 with a reading of 7.1. Alaska's earthquake in Prince William Sound in 1964 measured an 8.5 and another in 2002 in Fairbanks also measured 8.5. Other well-known and devastating earthquakes were in Tokyo in 1923 (8.2), Russia in 1952 (9.0), Alaska in 1957 (9.1), Chile in 1960 (9.5), China in 1976 (8.0) and Mexico City in 1985 (8.1).
Most earthquakes happen around the boundaries of tectonic plates ; however, the engineering feats of humans have also been known to create earthquakes. In Denver, Colorado, in 1963, pumping wastewater into deep underground holding areas caused a series of earthquakes that stopped when the pumping ended. Both the Hoover Dam in the U.S. and the Aswan High Dam in Egypt caused earthquakes because of the added pressure due to the weight of the water behind the dams.
Earthquakes cause waves of movement to occur in the ground. Waves that travel along the Earth's surface are called surface waves and waves that travel underground are called body waves . The first and fastest of the body waves to travel from the center of an earthquake are called P waves or primary waves. Secondary waves or S waves are slower than P waves and move back and forth.
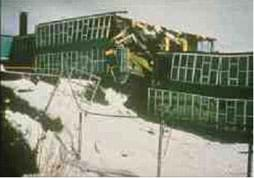
( Student activity: Have students line up to demonstrate the two types of body waves. For the P waves, or primary waves, have the first person bump shoulders with the person next in line, who bumps shoulders with the next person and so on. This demonstrates a compression wave. Go outside or into a gymnasium space to demonstrate S waves with a [gentle] game of snap the whip. Have students line up and hold hands. The first person in line runs first in one direction and then back to make the line look and move like a long snake.)
Civil engineers focus on how to make buildings and other structures more robust to withstand earthquakes — and other natural events — and reduce the loss of life and property damage. Can you imagine the consequences if the Hoover Dam in Boulder City, Nevada, cracked and crumbled apart as a result of an earthquake? The repercussions of such a disaster would be enormous! Engineers work hard to protect humans from the impacts of an earthquake and take this and similar challenges very seriously. So much so that they have designed high-tech monitoring equipment to accurately predict and measure earthquakes. In fact, engineers are continually refining and enhancing such equipment to make it better and even more accurate. Additionally, they test and research better materials and methods to make structures withstand an earthquake's tremendous force. For example, engineers have solved some damage to buildings by developing shock absorbers. These isolate the foundation of a building from the ground so the building and the earth move independently of each other.
Lesson Background and Concepts for Teachers
Where do earthquakes usually occur? Do the effects of an earthquake travel? How do scientists measure the force of earthquakes? How do scientists measure earthquake damage?
Earthquakes occur along the boundaries of the Earth's many tectonic plates , near faults . Most earthquakes occur along the Ring of Fire , which encircles the Pacific Ocean (see Figure 3). It is the boundary of the Pacific Plate that underlies the Pacific Ocean. The eastern boundary of this ring is called the San Andreas Fault , which is 800 miles long and lies along the coast of California, Washington and Alaska. The western boundary of this plate lies near Japan. Earthquakes also occur in places where tectonic plates are moving away from each other where volcanoes are forming or erupting. The force of the volcanic magma rising to the surface causes earthquakes.
The engineering feats of humans have also been known to create earthquakes. In 1963 in Denver, pumping wastewater into deep underground holding areas caused a series of earthquakes that stopped when the pumping ended. Both the Hoover Dam (see Figure 4) in the U.S. and the Aswan High Dam in Egypt caused earthquakes because of the added pressure due to the weight of the water behind the dams.
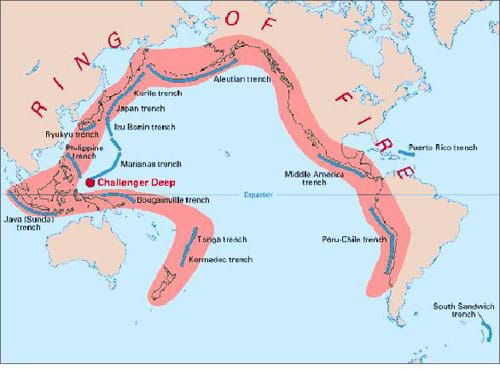
Earthquakes happen when rocks break due to high stress, usually caused by friction of tectonic plates moving by one another. The point where this rock rupture occurs is called the focus of the earthquake and is very far beneath the Earth's surface. The location on the actual surface of the Earth, directly above the focus, is called the epicenter of the earthquake. Most damage occurs at the epicenter. The release of force at the earthquake's focus creates vibrations that travel in seismic waves away from this spot. There are two main types of seismic waves: surface waves , which travel along the surface of the earth and cause little damage, and body waves , which travel underground and cause major damage. The first and fastest body waves to travel from the focus of an earthquake are called P waves or Primary waves . Like sound waves, these are compression waves. A Slinky™ spring toy demonstrates compression waves when the slinky is stretched out and one side quickly springs back or is pushed towards the other end. The rings of the slinky push against each other and travel along the outstretched spring. Secondary waves or S waves are slower than P waves and cause most of the damage in an earthquake. S waves move back and forth in a shearing motion. Secondary waves can be demonstrated by wiggling a rope in a snake-like motion along the floor. S waves cause buildings to shake and sway, and cause most of the destruction during an earthquake. Aftershocks are vibrations that occur after the main earthquake has passed. They can occur for days after the main earthquake and often cause as much or more damage than the original earthquake. Megathrust earthquakes — those that are result of two tectonic plates falling beneath each other — often cause tremendous tsunami waves that have been known to kill thousands of coastal people at one time. Unfortunately, aftershocks and tsunamis pose a real threat to clean up and rescue crews.
Seismographs measure the body and surface waves that travel from the focus of earthquakes — or simply, the amount of ground motion produced by an earthquake (Refer to the Seismology in the Classroom activity to have students conduct their own analysis of a seismograph). There are two commonly used scales to rate the strength of an earthquake, 1) the Richter Scale and 2) the Mercalli Scale. The force of an earthquake is measured using the Richter Scale , which rates the amplitude of the waves — as measured on a seismograph — on a scale from 1 (pounds of movement) to 9 (tons of movement). An earthquake rating of 1 to 2 usually cannot be detected by humans, but only by instruments. Each number on the Richter scale indicates an increase of ten times the force of the previous number. Thus, the Richter rating of 2 is ten times the force of a 1. A Richter rating of 3 is ten times a rating of 2 or one hundred times a rating of l. (See the Magnitude of the Richter Scale activity and handout for more information.) The Mercalli Scale , which measures intensity, is another method to measures earthquake damage. The 1931 Modified Mercalli scale used in the United States assigns a Roman numeral in the range I (not felt at all) to XII (buildings destroyed, nearly total damage) to each earthquake effect and measures the overall affects at certain locations. (Refer to the Mercalli Scale Illustrated Activity for an interactive opportunity for students to better understand the Mercalli Scale.)
The history of earthquakes is interesting for students. Earthquakes can occur anywhere in the world. The world's largest recorded earthquakes have all been megathrust events, occurring where one tectonic plate descends the other. The most damaging earthquake in the U.S. was the earthquake in Prince William Sound, Alaska, on March 28, 1964, with a magnitude of 9.2. This earthquake is also the second biggest earthquake in the world (the largest occurred in Chile on May 22, 1960, with a magnitude of 9.5). A recent and devastating underwater earthquake occurred on December 26, 2004, off the west coast of northern Sumatra in Indonesia. This earthquake, measuring 9.0 on the Richter Scale, is the fourth largest earthquake in the world since 1900 and is the largest since the 1964 Prince William Sound, Alaska, earthquake. The 1906 San Francisco Earthquake, measured 8.3 on the Richter Scale. More recently, California's 1992 Yucca Valley earthquake generated a reading of 7.6, and the 1994 Northridge earthquake measured 6.9. Earthquakes range from "nearly felt" to "overwhelmingly devastating" all over the world. In the U.S., California and Alaska have the most frequency of earthquakes, and North Dakota and Florida have the fewest earthquakes. Students can gain a better understanding of how engineers design structures to resist such devastation in the hands-on Testing Model Structures: Jell-O Earthquake in the Classroom activity.
Watch this activity on YouTube
- Seismology in the Classroom - Students make model seismographs and read sample seismograms.
How did your structure survive the earthquake? (Listen to student stories about how their model structures stood up to the Jell-O® earthquake. Review earthquake damage.) Are all earthquakes the same strength? Can you feel every earthquake? How do we measure earthquakes? (Review the way earthquakes are measured using the Mercalli and Richter Scales.)
Where on Earth do earthquakes happen? (Answer: Earthquakes can occur anywhere on Earth.) Where do most earthquakes occur? (Answer: Around the boundaries of tectonic plates.) What are engineers are doing to help humans survive earthquakes? (Answer: They are creating instruments for improved prediction and warning. They are designing safer structures that can better resist earthquakes). Give me some examples of what you could tell a friend or family member about earthquakes. What advice would you give to someone who lives or visits an earthquake-prone area?
Aftershocks: Shocks or tremors that occur after an earthquake. Aftershocks can cause as much or more damage than the earthquake itself.
Body waves: Waves that emanate from the focus of an earthquake that travel underground. Includes P waves and S waves.
Compression waves: Waves that travel by compressing molecules.
Epicenter: The point on the Earth's surface directly above the focus or origin of the earthquake.
Fault: A crack in the rock, caused by movement of the Earth's crust.
Focus: The point under the surface of the Earth where an Earthquake begins.
Magma: Molten or melted rock from the Earth's mantle.
Megathrust: An earthquake that occurs when one tectonic plate subducts beneath another. Megathrust earthquakes often generate large tsunamis that cause damage over a much wider area than is directly affected by ground shaking near the earthquake's rupture.
Mercalli scale: A scale from I to XII that measures the amount of damage sustained in an earthquake.
P waves: Primary body waves, which travel from the focus of an earthquake. These are compression waves.
Pacific plate: A tectonic plate underlying the Pacific Ocean.
Primary waves: The first body waves which travel from the focus of an earthquake. P waves are compression waves.
Richter scale: A scale from 1 to 9 that measures the strength of an earthquake.
Ring of Fire: The area around the Pacific Plate where many earthquakes and volcanic eruptions occur.
S waves: The slower secondary body waves that cause side-to-side movement.
San Andreas Fault: The 800 mile long fault along the edge of the Pacific Plate that runs under California, Washington and Alaska.
Secondary waves: S waves or body waves which cause side-to-side movement.
Seismogram: A zig-zag line created by vibrations read from a seismograph caused by body waves.
Seismograph: An instrument that measures how much the ground shakes or vibrates in an earthquake.
Surface waves: Waves that emanate from the epicenter of an earthquake which cause little damage.
Tectonic plate: Section of the Earth's crust.
Tsunami: A series of ocean waves generated by any rapid large-scale disturbance of the sea water. Most tsunamis are generated by earthquakes, but they may also be caused by volcanic eruptions, landslides, undersea slumps or meteor impacts.
Pre-Lesson Assessment
Formation : Have students line up to demonstrate the two types of body waves. Demonstrate P waves, or primary waves, by the first person bumping shoulders with the person next in line, who bumps shoulders with the next person and so on. This demonstrates a compression wave. Go outside or in a bigger area to demonstrate S waves with a (gentle) game of snap the whip. Have the children line up and hold hands. The first person in line runs first in one direction and then back to make the line look and move like a long snake.
Discussion Questions : solicit, summarize, and integrate student responses
- What could happen to a city when there is an earthquake?
- What could happen to our school if there was an earthquake?
- What would we do should an earthquake occur? (Review safety information for an earthquake or have students brainstorm ways to help a city in crisis. For more information see Lesson Extension section.)
Post-Introduction Assessment
Question/Answer : Ask questions and have students raise their hands to respond.
- Where do most earthquakes occur? (Answer: Around the boundaries of tectonic plates.)
- Can humans cause an earthquake? (Answer: Yes. For example, pumping wastewater in to the ground, and building large dams, such as the Hoover Dam and the Aswain High Dam have caused earthquakes due to the pressure of the water.)
- What are two types of body waves that travel underground in an earthquake? (Answer: P and S waves.)
- Which type of wave moves back and forth like a whip? (Answer: S waves.)
- Is there any way to make buildings safer from an earthquake? (Answer: One way engineers reduce building damage is to design shock absorbers for buildings so that the building foundation is isolated from the ground, which allows the building and the earth to move independently of each other.)
Lesson Summary Assessment
Earthquake Acrostic Poem : To encourage students to synthesize and evaluate their learning, have them write an acrostic poem. Have them write the word "earthquake" vertically on a piece of paper. Use each letter in the word as the first letter of a word or phrase that gives information on earthquakes. For example, E = earth, A = active movement, R = Richter scale, etc.
Engineering Poster : Using the skills they learned in the three lesson activities and the lesson on how earthquakes form, have students create a poster of a best design for a building that would withstand an earthquake. Have them title their posters with an engineering firm name that they make up. (Example: Shaky Engineering Firm). Have the students work in teams of four if possible.
Human Matching : On separate pieces of paper, write either the term or the definition of the vocabulary words listed above. Ask for volunteers from the audience to come up to the front of the room, and give each student one of the pieces of paper. Have all volunteers read what is written on their papers one at a time. Have the audience match term to definition by voting. Have students "terms" stand by their "definitions." At the end, review the concepts.
Lesson Extension Activities
Have students research famous earthquakes and reports back to the class.
Have students mark a map with currently occurring earthquake locations. The information can be researched at the following website: http://www.ngdc.noaa.gov/hazard/slideset/earthquakes/.
Prepare and present a class lesson in earthquake preparedness. Obtain information from the Federal Emergency Management Agency (FEMA), P.O. Box 70274, Washington, DC 20024. Also available for teachers: Seismic Sleuths FEMA 159, Earthquakes – A Teacher's Package for K-6, (American Geophysical Union and the Federal Emergency Management Agency P.O. Box 70274, Washington, DC 20024).
Have students search the internet for information and images from an earthquake in history that was not discussed in class. Lead a small discussion of findings during the next class and have students show the class the pictures they found.

In this activity, students learn about the Richter Scale for measuring earthquakes and make a booklet with drawings that represent each rating of the Richter Scale.

Students learn about the types of seismic waves produced by earthquakes and how they move through the Earth. Students learn how engineers build shake tables that simulate the ground motions of the Earth caused by seismic waves in order to test the seismic performance of buildings.

In this activity, students learn about the Mercalli Scale for rating earthquakes and make a booklet with drawings that represent each rating of the scale.

Students learn about the structure of the earth and how an earthquake happens. In one activity, students make a model of the earth including all of its layers. In a teacher-led demonstration, students learn about continental drift. In another activity, students create models demonstrating the di...

Clark, John, David Flint, Tony Hare, Keith Hare and Clint Twist. Encyclopedia of our Earth , New York: Shooting Star Press, 1995.
Ganeri, Anita. Science Questions and Answers: Earth Science . New York: Dillon Press, 1993.
Knapp, Brian. The Grolier Illustrated Library of the Environment. Earth , Danbury, CT: Grolier Educational Corporation, 1995.
Press, Frank and Raymond Siever. Understanding Earth , New York, NY: W.H. Freeman and Company, 1998.
Silverstein, Alvin, Virginia Silverstein and Laura Silverstein Nunn. Plate Tectonics . Brookfield, CT: Twenty-First Century Books, 1998.
Walters, Martin and Felicity Trotman. A Prentice Hall Illustrated Dictionary . Earth Sciences. New York: Prentice Hall General Reference, 1991.
World Book. Young Scientist: Planet Earth. Water . Chicago: World Book, Inc., 1991.
Modified Mercalli Scale: http://www.abag.ca.gov/bayarea/eqmaps/doc/mmi.html
Contributors
Supporting program, acknowledgements.
The contents of this digital library curriculum were developed under a grant from the Fund for the Improvement of Postsecondary Education (FIPSE), U.S. Department of Education and National Science Foundation GK-12 grant no. 0338326. However, these contents do not necessarily represent the policies of the Department of Education or National Science Foundation, and you should not assume endorsement by the federal government.
Last modified: December 4, 2019

9.2 Measuring Earthquakes
The shaking from an earthquake travels away from the rupture in the form of seismic waves . Seismic waves are measured to determine the location of the earthquake, and to estimate the amount of energy released by the earthquake (its magnitude ).
Types of Seismic Waves
Seismic waves are classified according to where they travel, and how they move particles.
Seismic waves that travel through Earth’s interior are called body waves . P-waves are body waves that move by alternately compressing and stretching materials in the direction the wave moves. For this reason, P-waves are also called compression waves. The “P” in P-wave stands for primary, because P-waves are the fastest of the seismic waves. They are the first to be detected when an earthquake happens.
A P-wave can be simulated by fixing one end of a spring to a solid surface, then giving the other end a sharp push toward the surface (Figure 9.8, top). The compression will propagate (travel) along the length of the spring. Some parts of the spring will be stretched, and others compressed. Any one point on the spring will jiggle forward and backward as the compression travels along the spring.
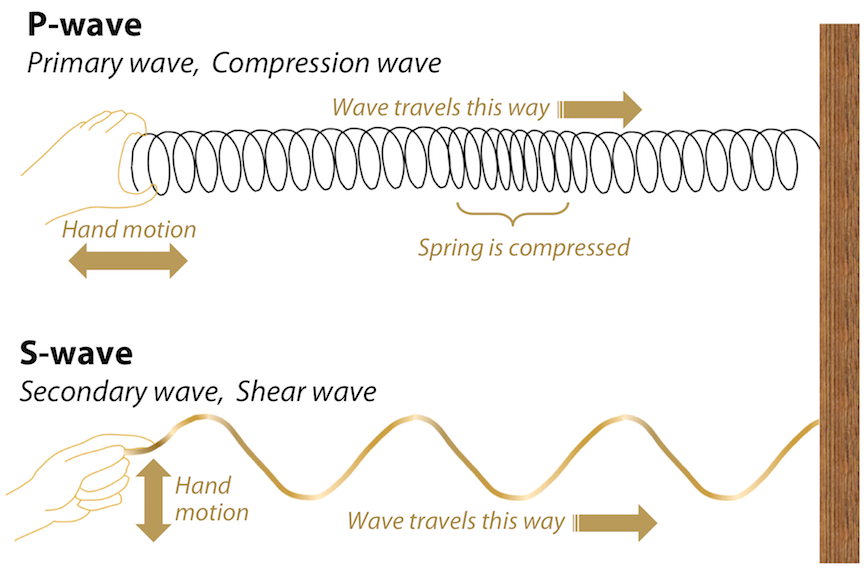
S-waves are body waves that move with a shearing motion, shaking particles from side to side. S-waves can be simulated by fixing one end of a rope to a solid surface, then giving the other end a flick (Figure 9.8, bottom). Any one point on the rope will move from side to side at a right angle to the direction in which the snaking motion is traveling. The “S” in S-wave stands for secondary, because S-waves are slower than P-waves, and are detected after the P-waves are measured. S-waves cannot travel through liquids.
P-waves and S-waves can travel rapidly through geological materials, at speeds many times the speed of sound in air.
Surface Waves
When body waves reach Earth’s surface, some of their energy is transformed into surface waves, which travel along Earth’s surface. Two types of surface waves are Rayleigh waves and Love waves (Figure 9.9). Rayleigh waves (R-waves) are characterized by vertical motion of the ground surface, like waves rolling on water. Love waves (L-waves) are characterized by side-to-side motion. Notice that the effects of both kinds of surface waves diminish with depth in Figure 9.9.
Surface waves are slower than body waves, and are detected after the body waves. Surface waves typically cause more ground motion than body waves, and therefore do more damage than body waves.
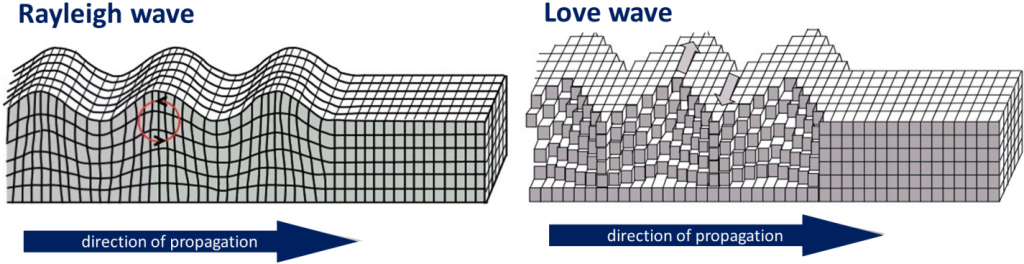
Recording Seismic Waves Using a Seismograph
A seismometer is an instrument that detects seismic waves. An instrument that combines a seismometer with a device for recording the waves is called a s eismograph . The graphical output from a seismograph is called a seismogram . Figure 9.10 (right) shows how a seismograph works. The instrument consists of a frame or housing that is firmly anchored to the ground. A mass is suspended from the housing, and can move freely on a spring. When the ground shakes, the housing shakes with it, but the mass remains fixed. A pen attached to the mass moves up and down on a rotating drum of paper, drawing a wavy line, the seismogram. The seismograph in Figure 9.10 (right) is oriented to measure vertical ground motion. The photo on the left shows a seismograph oriented to record horizontal ground motion.
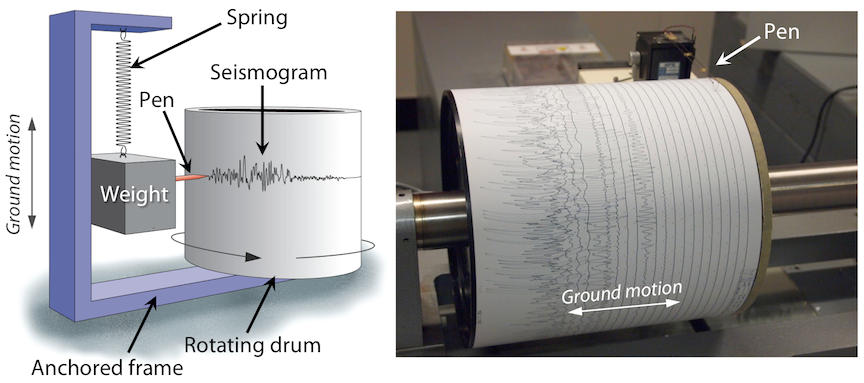
The pen and drum of a mechanical seismograph record the motion of the ground relative to the mass. However, unless an earthquake causes a large amount of ground motion directly beneath the seismograph, the height of the wave recorded on paper might be very small, making the seismogram difficult to analyze. The seismograph on the right has a device to amplify the ground motion, drawing larger waves that are easier to study.
Modern seismographs record shaking as electrical signals, and are able to transmit those signals. This means seismologists need not return to the instrument to collect recordings before the records can be examined.
Finding The Location of an Earthquake
P-waves travel faster than S-waves. As the waves travel away from the location of an earthquake, the P-wave gets farther and father ahead of the S-wave. Therefore, the farther a seismograph is from the location of an earthquake, the longer the delay between when the P-wave arrival is recorded, and the S-wave arrival is recorded. The delay between the P-wave and S-wave arrival appears as a widening gap in a diagram of P-wave and S-wave travel times (Figure 9.11, grey lines).
P-wave and S-wave arrival times can be identified on seismograms. In the three seismograms in Figure 9.11, the arrivals of the P-waves and S-waves are marked with arrows, and the interval in minutes between the P-wave and S-wave arrivals are noted. The seismograms were recorded at three different seismic stations (earthquake monitoring locations equipped with seismographs). The distance of each station from the earthquake is determined by finding the distance along the graph where the gap between the P-wave and S-wave travel-time curves matches the delay between P-wave and S-wave arrivals on the seismogram.
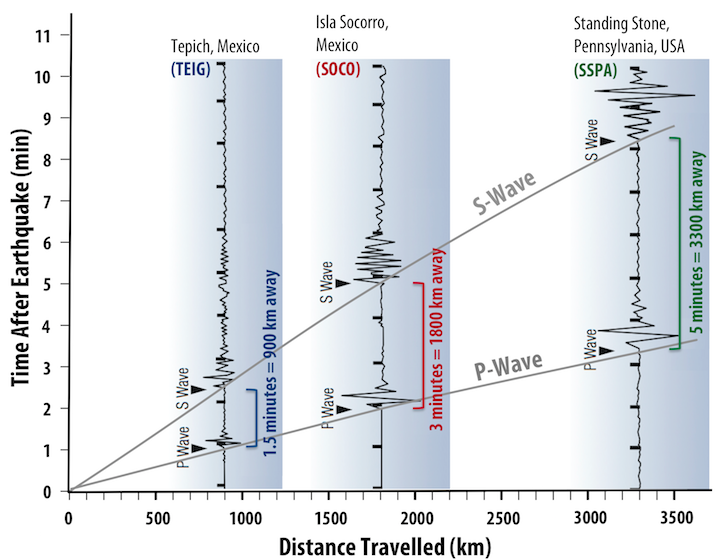
The delay between the P-wave and S-wave arrival at a seismic station can indicate how far the station is from the source of the earthquake, but not the direction from which the seismic waves travelled. The possible locations of the earthquake can be represented on a map by drawing a circle around the seismic station, with the radius of the circle being the distance determined from the P-wave and S-wave travel times (Figure 9.12). If this is done for at least three seismic stations, the circles will intersect at the origin of the earthquake.
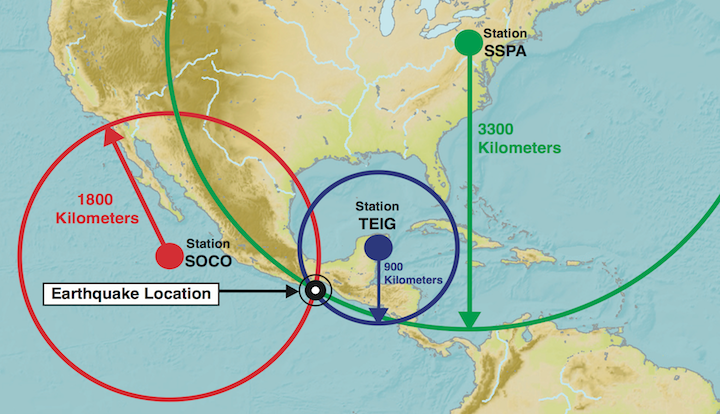
How Big Was It?
Earthquakes can be described in terms of their magnitude , which reflects the amount of energy released by the shaking. They can also be described in terms of intensity , which characterizes the impact of the shaking on people and their surroundings.
Earthquake Magnitude
Earthquake magnitudes are determined by measuring the amplitudes of seismic waves. The amplitude is the height of the wave relative to the baseline (Figure 9.13). Wave amplitude depends on the amount of energy carried by the wave. The amplitudes of seismic waves reflect the amount of energy released by earthquakes.
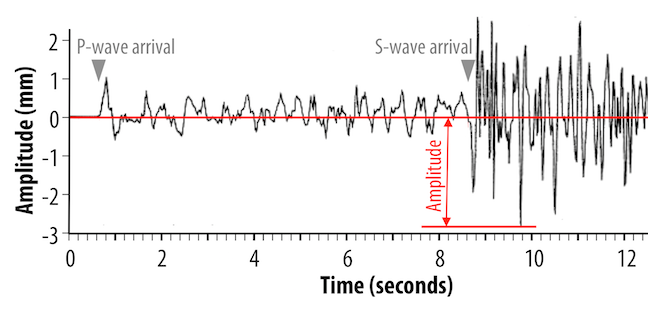
The Richter magnitude scale uses the amplitudes of S-waves, and corrects for the decrease in amplitude that happens as the waves travel away from their source. The correction depends on how seismic waves interact with the specific rock types through which they travel, and therefore on local conditions, so the Richter magnitude is also referred to as the local magnitude .
While news reports about earthquakes might still refer to the “Richter scale” when describing magnitudes, the number they report is most likely the moment magnitude . The moment magnitude is calculated from the seismic moment of an earthquake. The seismic moment takes into account the surface area of the region that ruptured, how much displacement occurred, and the stiffness of the rocks. Moment magnitude can capture the difference between short earthquakes and longer ones resulting from larger ruptures, even of both types of earthquakes generate the same amplitude of waves. The moment magnitude scale is also better for earthquakes that are far from the seismic station. Seismic wave measurements are still used to determine the moment magnitude, however different waves are used than for the local magnitude scale.
The magnitude scale is a logarithmic one rather than a linear one- an increase of one unit of magnitude corresponds to a 32 times increase in energy release (Figure 9.14). There are far more low-magnitude earthquakes than high-magnitude earthquakes. In 2017 there were 7 earthquakes of M7 (magnitude 7) or greater, but millions of tiny earthquakes.
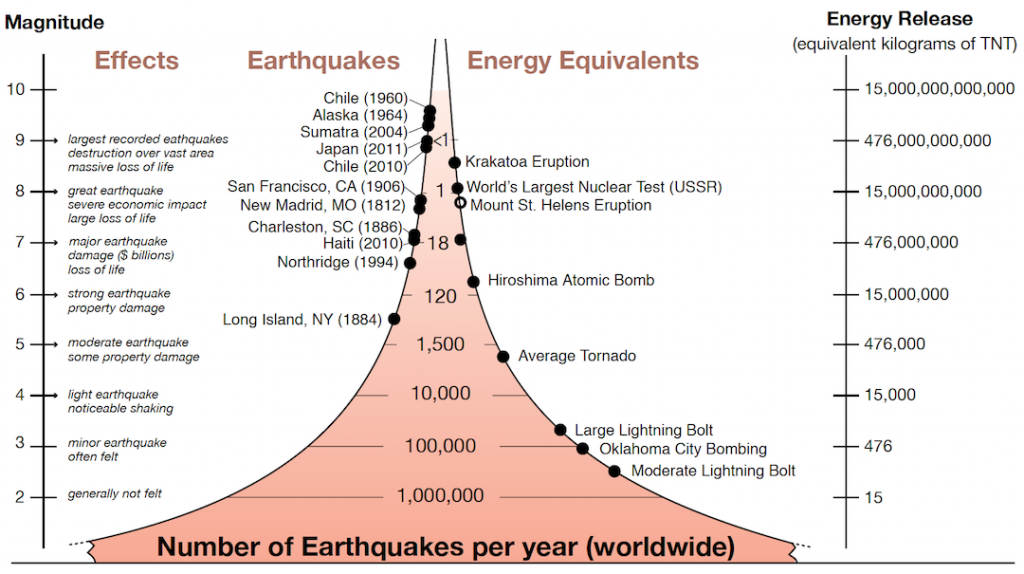
Earthquake Intensity
Intensity scales were first used in the late 19th century, and then adapted in the early 20th century by Giuseppe Mercalli and modified later by others to form what we now call the Modified Mercalli Intensity Scale (Table 9.1). To determine the intensity of an earthquake, reports are collected about what people felt and how much damage was done. The reports are then used to assign intensity ratings to regions where the earthquake was felt.
Intensity values are assigned to locations, rather than to the earthquake itself. This means that intensity can vary for a given earthquake, depending on the proximity to the epicenter and local conditions. For the 1946 M7.3 Vancouver Island earthquake, intensity was greatest in the central island region (Figure 9.15). In some communities within this region, chimneys were damaged on more than 75% of buildings. Some roads were made impassable, and a major rock slide occurred. The earthquake was felt as far north as Prince Rupert, as far south as Portland Oregon, and as far east as the Rockies, but with less intensity.
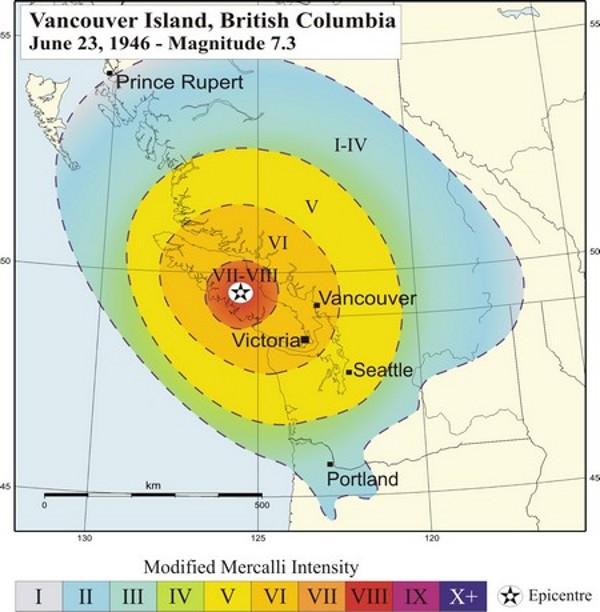
Intensity estimates are important as a way to identify regions that are especially prone to strong shaking. A key factor is the nature of the underlying geological materials. The weaker the underlying materials, the more likely it is that there will be strong shaking. Areas underlain by strong solid bedrock tend to experience far less shaking than those underlain by unconsolidated river or lake sediments.
An example of this effect is the 1985 M8 earthquake that struck the Michoacán region of western Mexico, southwest of Mexico City. There was relatively little damage near the epicenter, but 350 km away in heavily populated Mexico City there was tremendous damage and approximately 5,000 deaths. The reason is that Mexico City was built largely on the unconsolidated and water-saturated sediment of former Lake Texcoco. These sediments resonate at a frequency of about two seconds, which was similar to the frequency of the body waves that reached the city. Consequently, the shaking was amplified. Survivors of the disaster recounted that the ground in some areas moved up and down by approximately 20 cm every two seconds for over two minutes. Damage was greatest to buildings between 5 and 15 stories tall, because they also resonated at around two seconds, which amplified the shaking.
Video: Geoscience Videos – Measuring Earthquakes
Ammon, C. J. (2001). Earthquake Size Visit website
Licenses and Attributions
“Physical Geology, First University of Saskatchewan Edition” by Karla Panchuk is licensed under CC BY-NC-SA 4.0 Adaptation: Renumbering
https://openpress.usask.ca/physicalgeology/
Principles of Earth Science Copyright © 2021 by Katharine Solada and K. Sean Daniels is licensed under a Creative Commons Attribution-NonCommercial-ShareAlike 4.0 International License , except where otherwise noted.
Share This Book
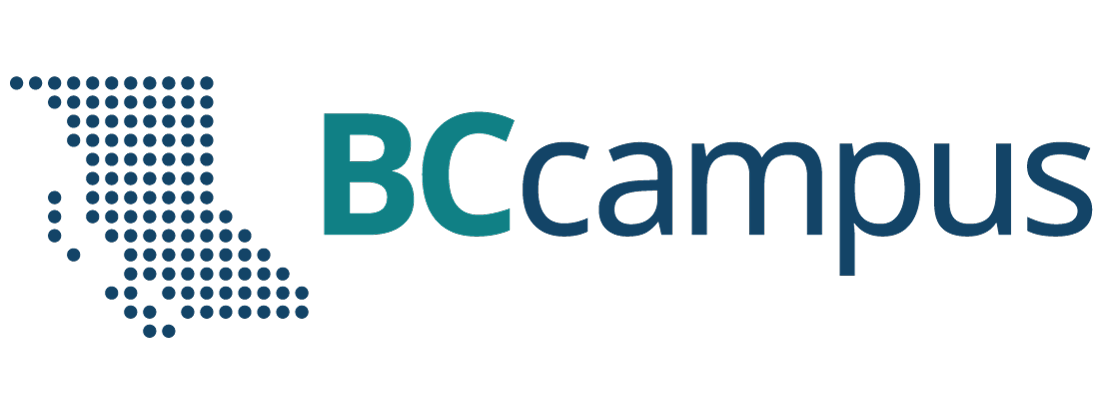
Want to create or adapt books like this? Learn more about how Pressbooks supports open publishing practices.
Chapter 11 Earthquakes
11.3 Measuring Earthquakes
There are two main ways to measure earthquakes. The first of these is an estimate of the energy released, and the value is referred to as magnitude . This is the number that is typically used by the press when a big earthquake happens. It is often referred to as “Richter magnitude,” but that is a misnomer, and it should be just “magnitude.” There are many ways to measure magnitude — including Charles Richter’s method developed in 1935 — but they are all ways to estimate the same number: the amount of energy released.
The other way of assessing the impact of an earthquake is to assess what people felt and how much damage was done. This is known as intensity. Intensity values are assigned to locations, rather than to the earthquake itself, and therefore intensity can vary widely, depending on the proximity to the earthquake and the types of materials and conditions of the subsurface.
Earthquake Magnitude
Before we look more closely at magnitude we need to review what we know about body waves, and look at surface waves. Body waves are of two types, P-waves, or primary or compression waves (like the compression of the coils of a spring), and S-waves, or secondary or shear waves (like the flick of a rope). An example of P and S seismic wave records is shown in Figure 11.13. The critical parameters for the measurement of Richter magnitude are labelled, including the time interval between the arrival of the P- and S-waves — which is used to determine the distance from the earthquake to the seismic station, and the amplitude of the S waves — which is used to estimate the magnitude of the earthquake.
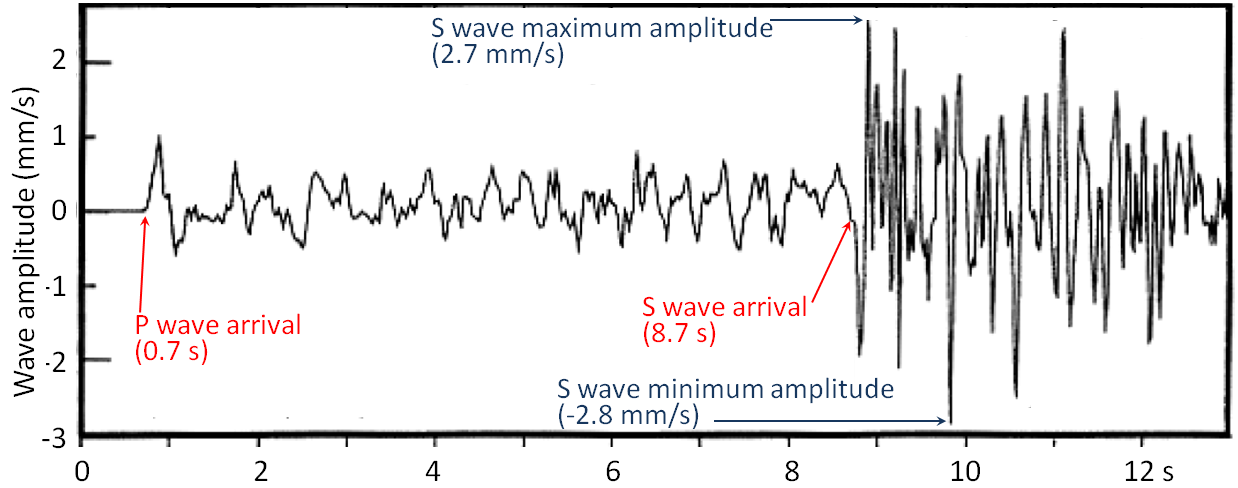
When body waves (P or S) reach Earth’s surface, some of their energy is transformed into surface waves, of which there are two main types, as illustrated in Figure 11.14. Rayleigh waves are characterized by vertical motion of the ground surface, like waves on water, while Love waves are characterized by horizontal motion. Both Rayleigh and Love waves are about 10% slower than S-waves (so they arrive later at a seismic station). Surface waves typically have greater amplitudes than body waves, and they do more damage.
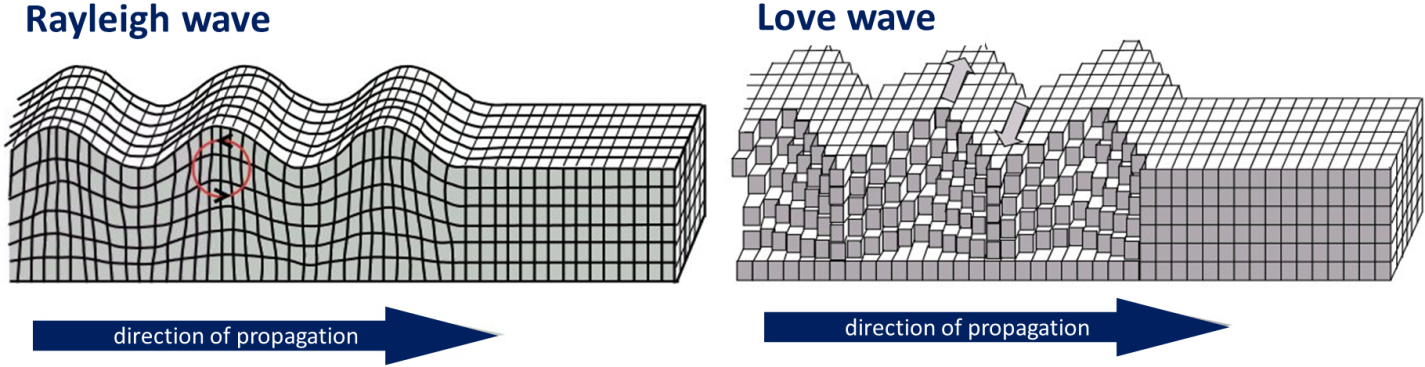
Other important terms for describing earthquakes are hypocentre (or focus ) and epicentre . The hypocentre is the actual location of an individual earthquake shock at depth in the ground, and the epicentre is the point on the land surface directly above the hypocentre (Figure 11.15).
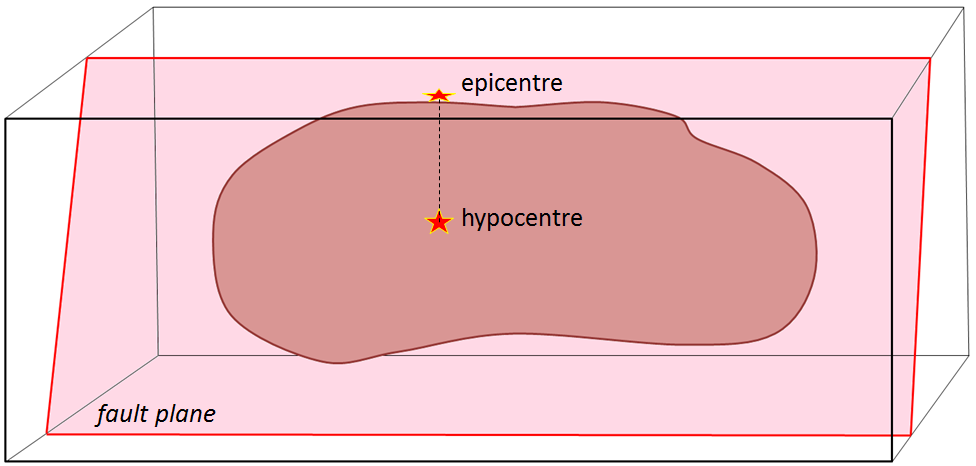
A number of methods for estimating magnitude are listed in Table 11.1. Local magnitude (ML) was widely used until late in the 20th century, but moment magnitude (MW) is now more commonly used because it gives more accurate estimates (especially with larger earthquakes) and can be applied to earthquakes at any distance from a seismometer. Surface-wave magnitudes can also be applied to measure distant large earthquakes.
Because of the increasing size of cities in earthquake-prone areas (e.g., China, Japan, California) and the increasing sophistication of infrastructure, it is becoming important to have very rapid warnings and magnitude estimates of earthquakes that have already happened. This can be achieved by using P-wave data to determine magnitude because P-waves arrive first at seismic stations, in many cases several seconds ahead of the more damaging S-waves and surface waves. Operators of electrical grids, pipelines, trains, and other infrastructure can use the information to automatically shut down systems so that damage and casualties can be limited.
Exercise 11.2 Moment Magnitude Estimates from Earthquake Parameters
A moment magnitude calculation tool is available at: http://solr.bccampus.ca:8001/bcc/items/24da5970-c4f3-4ab9-98ed-089a7caca242/1/ . You can use it to estimate the moment magnitude based on the approximate length, width, and displacement values provided in the following table:
The largest recorded earthquake had a magnitude of 9.5. Could there be a 10? You can answer that question using this tool. See what numbers are needed to make MW = 10. Are they reasonable?
The magnitude scale is logarithmic; in fact, the amount of energy released by an earthquake of M4 is 32 times higher than that released by one of M3, and this ratio applies to all intervals in the scale. If we assign an arbitrary energy level of 1 unit to a M1 earthquake the energy for quakes up to M8 will be as shown on the following chart:
In any given year, when there is a large earthquake on Earth (M8 or M9), the amount of energy released by that one event will likely exceed the energy released by all smaller earthquake events combined.
Earthquake Intensity
The intensity of earthquake shaking at any location is determined not only by the magnitude of the earthquake and its distance, but also by the type of underlying rock or unconsolidated materials. If buildings are present, the size and type of buildings (and their inherent natural vibrations) are also important.
Intensity scales were first used in the late 19th century, and then adapted in the early 20th century by Giuseppe Mercalli and modified later by others to form what we know call the modified Mercalli intensity scale (Table 11.2). Intensity estimates are important because they allow us to characterize parts of any region into areas that are especially prone to strong shaking versus those that are not. The key factor in this regard is the nature of the underlying geological materials, and the weaker those are, the more likely it is that there will be strong shaking. Areas underlain by strong solid bedrock tend to experience much less shaking than those underlain by unconsolidated river or lake sediments.
An example of this effect is the 1985 M8 earthquake that struck the Michoacán region of western Mexico, southwest of Mexico City. There was relatively little damage in the area around the epicentre, but there was tremendous damage and about 5,000 deaths in heavily populated Mexico City some 350 km from the epicentre. The key reason for this is that Mexico City was built largely on the unconsolidated and water-saturated sediment of former Lake Texcoco. These sediments resonate at a frequency of about two seconds, which was similar to the frequency of the body waves that reached the city. For the same reason that a powerful opera singer can break a wine glass by singing the right note, the amplitude of the seismic waves was amplified by the lake sediments. Survivors of the disaster recounted that the ground in some areas moved up and down by about 20 cm every two seconds for over two minutes. Damage was greatest to buildings between 5 and 15 storeys tall, because they also resonated at around two seconds, which amplified the shaking.
Exercise 11.3 Estimating Intensity from Personal Observations
The following observations were made by residents of the Nanaimo area during the M6.8 Nisqually earthquake near Olympia, Washington, in 2001. Estimate the Mercalli intensities using Table 11.2.
An intensity map for the 1946 M7.3 Vancouver Island earthquake is shown in Figure 11.16. The intensity was greatest in the central island region where, in some communities, chimneys were damaged on more than 75% of buildings, some roads were made impassable, and a major rock slide occurred. The earthquake was felt as far north as Prince Rupert, as far south as Portland Oregon, and as far east as the Rockies
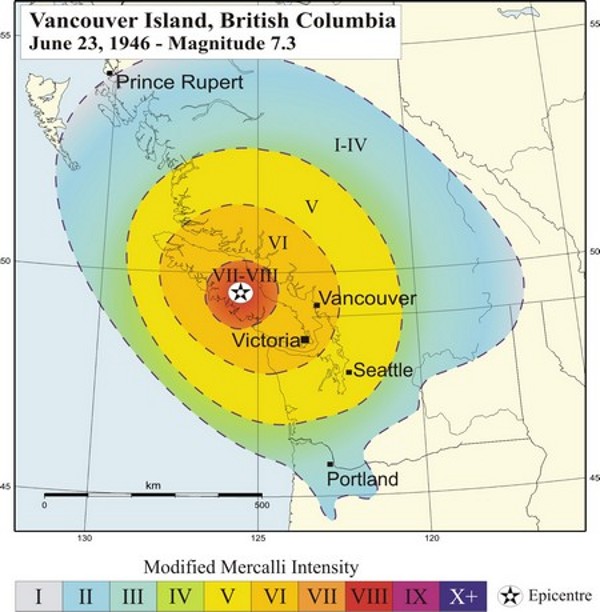
Physical Geology Copyright © 2015 by Steven Earle is licensed under a Creative Commons Attribution 4.0 International License , except where otherwise noted.
Share This Book
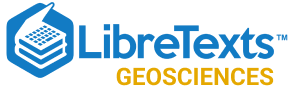
- school Campus Bookshelves
- menu_book Bookshelves
- perm_media Learning Objects
- login Login
- how_to_reg Request Instructor Account
- hub Instructor Commons
- Download Page (PDF)
- Download Full Book (PDF)
- Periodic Table
- Physics Constants
- Scientific Calculator
- Reference & Cite
- Tools expand_more
- Readability
selected template will load here
This action is not available.
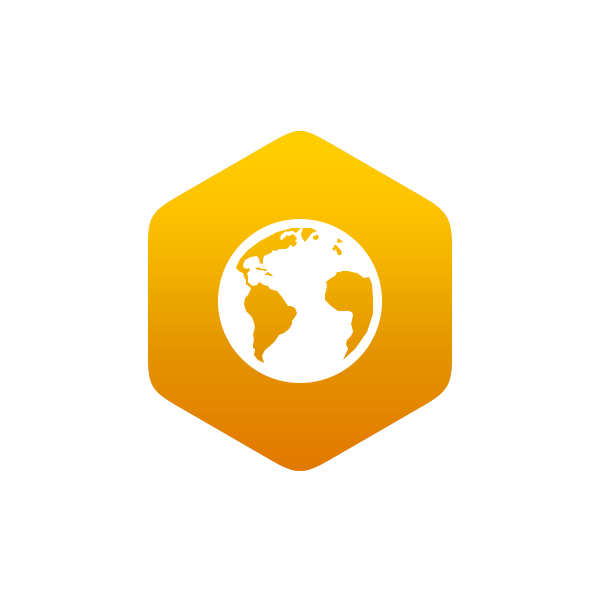
6.3: Measuring Earthquakes
- Last updated
- Save as PDF
- Page ID 25527

- Steven Earle
- Vancover Island University via BCCampus
There are two main ways to measure earthquakes. The first of these is an estimate of the energy released, and the value is referred to as magnitude. This is the number that is typically first released by the press when a big earthquake happens. It is often referred to as “Richter magnitude”, but that is a misnomer, and it should be just “magnitude”. There are many ways to measure magnitude—including Charles Richter’s method developed in 1935—but they are all ways to estimate the same number: the amount of energy released.
The other way of assessing the impact of an earthquake is to assess what people felt and how much damage was done. This is known as intensity. Intensity values are assigned to locations, rather than to the earthquake itself, and intensity can vary widely therefore, depending on the proximity to the earth quake and the type of ground underneath.
Earthquake Magnitude
Before we look more closely at magnitude we need to review what we know about body waves, and also look at surface waves. Body waves are of two types, P or primary or compression waves (like the compression of the coils of a spring), and S or secondary or shear waves (like the flick of a rope). An example of P and S seismic records is shown on Figure 6.3.1. The critical parameters for the measurement of Richter magnitude are labelled, including the time interval between the arrival of the P and S waves—which is used to determine the distance from the earthquake to the seismic station, and the amplitude of the S waves—which is used to estimate the magnitude.
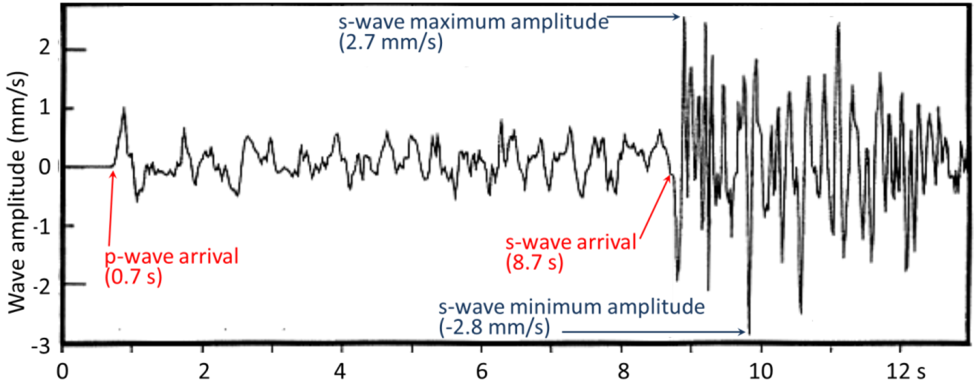
When a body wave (P or S) reaches the Earth’s surface some of its energy is transformed into surface waves, of which there are two main types, as illustrated in Figure 6.3.2. Rayleigh waves are characterized by vertical motion of the ground surface, like waves on water, while Love waves are characterized by horizontal motion. Both Rayleigh and Love waves are about 90% as fast as S waves (so they arrive later at a seismic station). Surface waves typically have greater amplitudes than body waves, and they do more damage.

Two other important terms from the perspective of describing earthquakes are hypocentre and epicenter. The hypocentre is the actual location of an individual earthquake shock at depth in the ground, and the epicentre is the point on the land surface directly above the hypocentre (Figure 6.3.4).
A number of methods for estimating magnitude are listed in Table 6.1. Local magnitude (M L ) was widely used until late in the 20th century, but moment magnitude (M W ) is now more commonly used because it gives more accurate estimates (especially with larger earthquakes) and can be applied to earthquakes at any distance from a seismometer. Surface-wave magnitudes can also be applied to large distant earthquakes.
Because of the increasing size of cities in earthquake-prone areas (e.g., China, Japan, California and Turkey) and the increasing sophistication of infrastructure, it is becoming important to have very rapid warnings and magnitude estimates of earthquakes that have already happened. This can be achieved by using P-wave data because P waves arrive first at seismic stations, in many cases several seconds ahead of the more damaging S waves and surface waves. Operators of electrical grids, pipelines, trains and other infrastructure can use the information to automatically shut systems down so that damage and casualties can be limited.
Exercise 6.2 Moment Magnitude Estimates from Earthquake Parameters
A moment magnitude calculation tool is available from the BC Campus SOL*R repository. You can use it to estimate the moment magnitude based on the approximate rupture-zone length, width and displacement values provided in the following table:
The 1989 Loma Prieta Earthquake, illustrated on Figure 6.1.4, had a magnitude of 6.9. Use that diagram to estimate the length and the width (depth) of the rupture surface, and then use the magnitude calculator to find a number for displacement that will give you the correct magnitude.
Exercise answers are provided Appendix 2 .
The magnitude scale is logarithmic, in fact the amount of energy released by an earthquake of magnitude 4 is 32 times higher than that released by one of magnitude 3, and this ratio applies to all intervals in the scale. If we assign an arbitrary energy level of 1 unit to a magnitude 1 earthquake the energy for quakes up to magnitude 8 will be as shown on the following list:
In any given year when there is a large earthquake on Earth (M 8 or 9) the amount of energy released by that one event will likely exceed the energy released by all smaller events combined.
Earthquake Intensity
The intensity of earthquake shaking at any location is determined by the magnitude of the earthquake and its distance, but also by the type of underlying rock or unconsolidated materials. If buildings are present, the size and type of building are also important.
Intensity scales were first used in the late 19th century, and then adapted in the early 20th century by Giuseppe Mercalli and modified later by others to form what we know call the Modified Mercalli Intensity scale (Figure 6.3.5). Intensity estimates are important because they allow us to characterize parts of any region into areas that are especially prone to strong shaking versus those that are not. The key factor in this regard is the nature of the underlying geological materials, and the weaker those are the more likely it is that there will be strong shaking. Areas underlain by strong solid bedrock tend to experience much less shaking that those underlain by unconsolidated river or lake sediments.
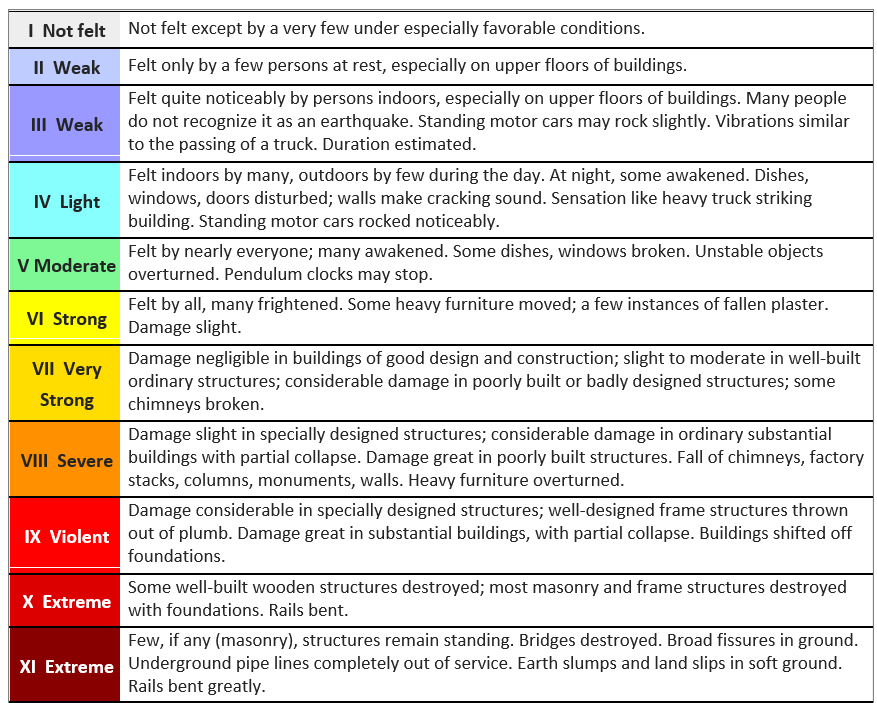
An example of this amplifying effect is provided by the 1985 M8 earthquake which struck the Pacific coast Michoacán region of western Mexico, about 350 km southwest of Mexico City. There was relatively little damage in the area around the epicentre, but there was tremendous damage and about 5000 deaths in heavily populated Mexico City. The key reason for this is that Mexico City was built largely on the unconsolidated and water-saturated sediment of former Lake Texcoco. These sediments resonate at a frequency of about 2 seconds, which was similar to the frequency of the body waves that reached the city. [1] For the same reason that a powerful opera singer can break a wine glass by singing the right note, the seismic shaking was amplified by the lake sediments. Survivors of the disaster recounted that the ground in some areas moved up and down by about 20 cm every 2 seconds for over 2 minutes. Damage was greatest to buildings between 5 and 15 stories tall, because they also resonated at around 2 seconds, and amplified the shaking.
Exercise 6.3 Estimating Intensity from Personal Observations
The following observations were made by residents of the Nanaimo BC area during the M6.8 Nisqually earthquake near to Olympia Washington in 2001. Estimate the Mercalli intensities using Figure 6.3.5.

An intensity map for the M7.3 June 1946 Vancouver Island Earthquake is shown on Figure 6.3.5. The intensity was greatest in the central island region where, in some communities, chimneys were damaged on more than 75% of buildings, some roads were made impassable and a major rock slide occurred. The earthquake was felt as far north as Prince Rupert, as far south as Portland Oregon and as far east as the Rockies.
Media Attributions
- Figure 6.3. 1 Steven Earle, CC BY 4.0, after a Open Government Licence – Canada image provided by Natural Resources Canada
- Figure 6.3.2 Modified by Steven Earle, from images via Wikipedia: https://en.Wikipedia.org/wiki/Raylei...leigh_wave.jpg , Public domain, and https://en.Wikipedia.org/wiki/Love_w...:Love_wave.jpg , CC BY 4.0
- Figure 6.3.3 Steven Earle, CC BY 4.0
- Figure 6.3.4 Steven Earle, CC BY 4.0 , based on the modified scale by the US Geological Survey ( public domain ), https://www.usgs.gov/natural-hazards...center_objects
- Figure 6.3.5 Intensity Map for 1946 M7.3 Vancouver Island Earthquake by Earthquakes Canada, Open Government Licence – Canada http://www.earthquakescanada.nrcan.g...460623-eng.php
- An earthquake creates seismic waves with a wide range of frequencies. The fast-vibrating shorter wavelength waves get absorbed by strong bedrock because strong rock has a fast natural vibration frequency. The slow-vibrating longer wavelength waves can travel a long way through the solid rocks of the crust (because they don't match its natural vibration frequency and are not absorbed), and these are the waves that reached Mexico City in 1985. Their slow frequencies matched the natural frequency of the sediments underneath the city. ↵
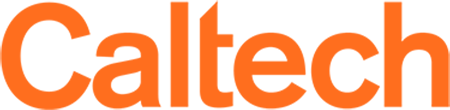
How Are Earthquakes Measured?
This article was reviewed by a member of Caltech's Faculty .
Two different viewpoints underpin the most important measurements related to earthquakes: magnitude and intensity. To scientists, an earthquake is an event inside the earth. To the rest of us, it is an extraordinary movement of the ground. Magnitude measures the former, while intensity measures the latter.
What is magnitude?
Magnitude describes the overall size of an earthquake as an event in the earth . Magnitude represents the total energy the earthquake radiates, and is calculated using information on how large an area moves, the distance that one side of the fault moves past the other, and the rigidity of the rock.
The international standard for measuring earthquake magnitude is called the moment magnitude scale and was formulated by Caltech seismologist Hiroo Kanamori with alumnus Thomas Hanks.
Caltech's Charles Richter conceptualized magnitude. He developed a method to numerically report the relative sizes of earthquakes before earthquake magnitude could be measured directly. He described the first magnitude scale, which came to be known as the Richter scale, in a paper published in 1935.
What is intensity?
Intensity describes how strong the shaking is at a given location. In the United States and many other locations, intensity values are described with Roman numerals from I (barely perceptible) to X (widespread destruction), using the Modified Mercalli Intensity scale .
This definition of intensity requires a subjective judgment by an observer. For instance, if the shaking is strong enough to awaken most people, then the intensity will be assigned a level of V. Today, scientists also can measure instrumental intensity directly, calculating and assigning intensities based on recorded ground shaking in different locations.
While an earthquake has just one magnitude, its levels of intensity decrease with distance from the epicenter . The waves get smaller as they travel away from the fault. Think of a lightbulb: It has one wattage, but the brightness that energy produces decreases with distance as the light spreads out.
Intensity also can vary depending on geology . Earthquakes may produce smaller or larger waves when they encounter different soils and where bedrock is near the surface or buried deep under sediments.
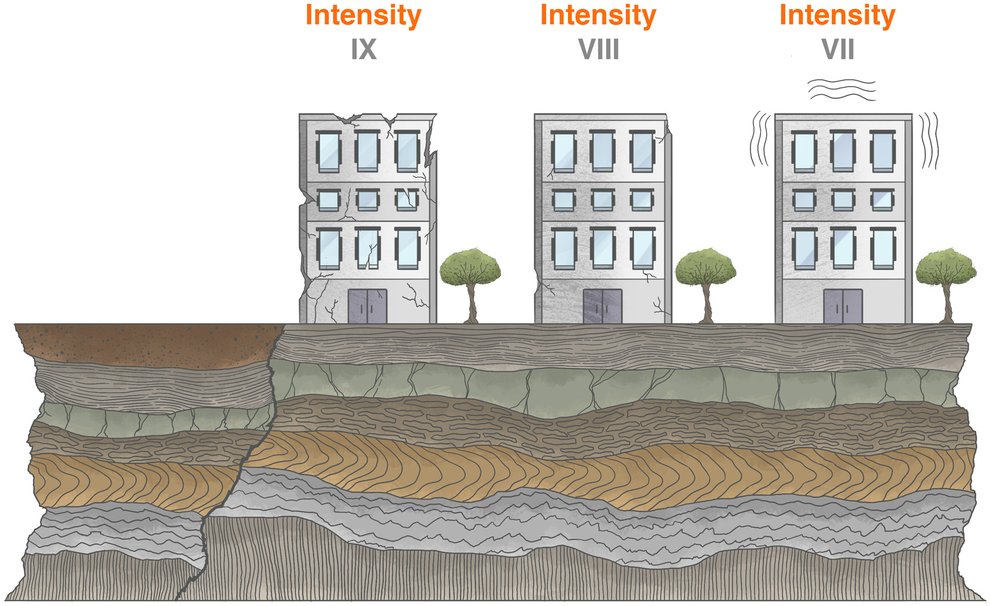
How is magnitude measured?
Magnitude is estimated using data from multiple seismograms. Estimates are made by calculating the total energy from the rupture area times the average movement on the fault. These factors can be reconstructed from seismograms or measured directly in the field by geologists, which makes the moment magnitude scale the first based on measurable physical factors.
Seismic networks detect ground motion and record it as seismograms. The most reliable data comes from sensitive seismometers installed below ground. These instruments sense and measure vibrations in the earth around them and record the amplitude of those vibrations over time as the wavy or spiky lines of seismograms . An example of one such network is the Southern California Seismic Network , operated by Caltech and the U.S. Geological Survey (USGS), which has recorded earthquakes since the 1920s.
Other seismic networks
Distributed Acoustic Sensing (DAS): These networks employ fiber-optic communications cables that are already installed underground. DAS sensors send beams of light down cables and detect differences in the travel time of reflected light when seismic waves jostle the cables . Caltech's Zhongwen Zhan and his colleagues have used this approach to track aftershocks from California's 2019 Ridgecrest earthquake sequence and create a citywide earthquake-detecting network in Pasadena.
Accelerometer arrays: In this type of network, pioneered over the past decade through Caltech's Community Seismic Network , small sensors installed in the ground and hundreds of public schools and other buildings send motion data to the cloud . The system collects continuous three-dimensional data about position and acceleration with accelerometers similar to those used in smartphones to reorient screens. The information helps researchers learn how the ground and buildings move differently during everyday disturbances, such as truck traffic, and during earthquakes. And if a building's normal movement changes after an earthquake, that data can alert engineers to potentially hidden structural damage.
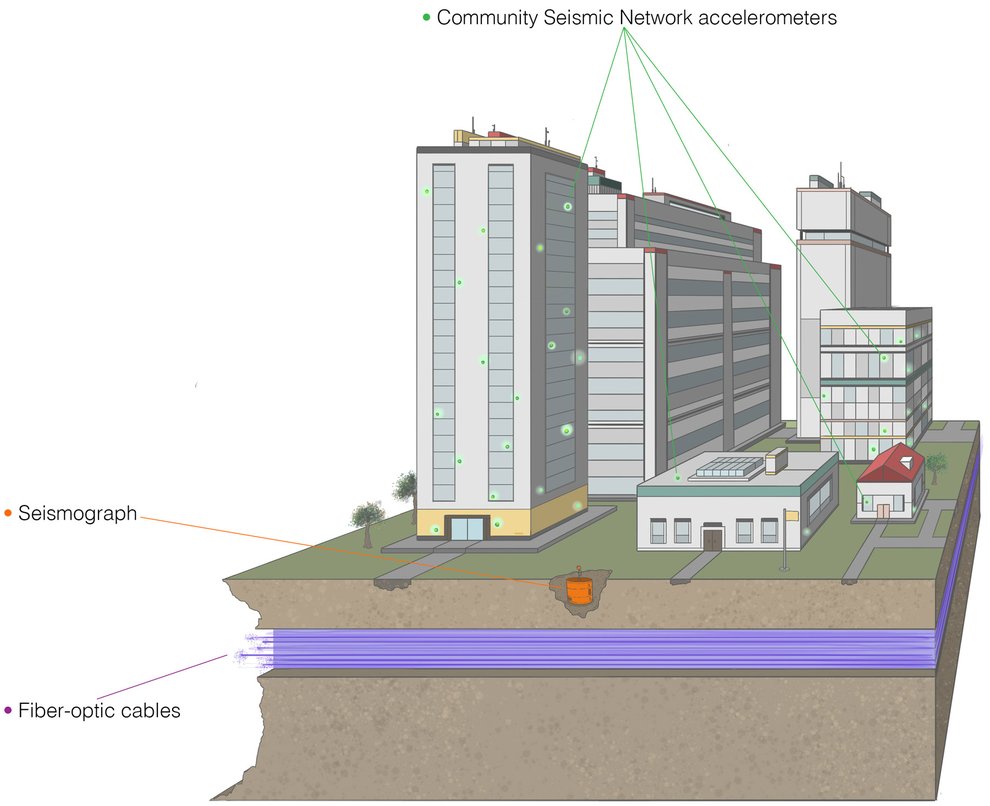
How is Intensity Measured?
To create maps of instrumental intensity, such as ShakeMaps , scientists analyze seismometer recordings of ground motion. Measures of acceleration are used to establish intensities up to VI, while the velocity is used to define the boundaries between higher intensity levels.
Qualitative measures of intensity are based on accounts from people and observations of damage in areas affected by a quake. For example, via the USGS's Did You Feel It? program, hundreds of thousands of people report their experiences of earthquakes, which the USGS filters and converts into maps called community internet intensity maps. The information from citizen science helps seismologists better understand the relationship between perceived intensity, damage, and ground motion.
Dive Deeper
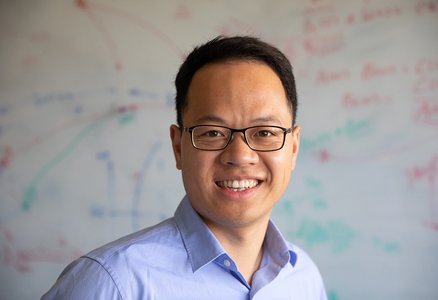
Seismologist Zhongwen Zhan: Keeping an Ear to the Ground
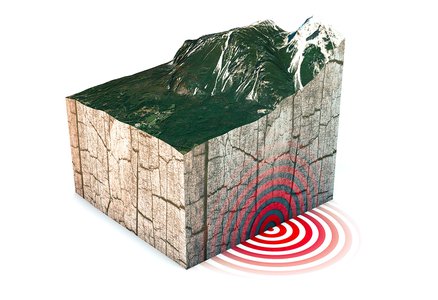
Urban Seismology in Megacities: the Los Angeles BASIN Experiment
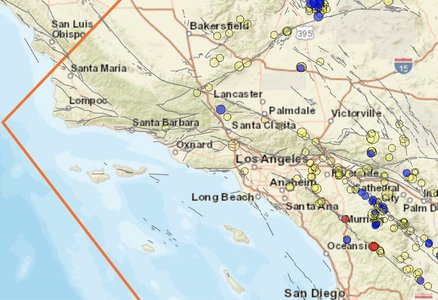
Did You Feel It? Crowdsourced maps portray people's experience

- Science Notes Posts
- Contact Science Notes
- Todd Helmenstine Biography
- Anne Helmenstine Biography
- Free Printable Periodic Tables (PDF and PNG)
- Periodic Table Wallpapers
- Interactive Periodic Table
- Periodic Table Posters
- How to Grow Crystals
- Chemistry Projects
- Fire and Flames Projects
- Holiday Science
- Chemistry Problems With Answers
- Physics Problems
- Unit Conversion Example Problems
- Chemistry Worksheets
- Biology Worksheets
- Periodic Table Worksheets
- Physical Science Worksheets
- Science Lab Worksheets
- My Amazon Books
Richter Scale and Earthquake Magnitude

The Richter scale is a logarithmic scale that measures the magnitude of an earthquake, originally developed by Charles F. Richter in 1935. It provides an objective measure of the energy an earthquake releases by quantifying the seismic waves produced. Prior to the invention of the Richter scale, the severity of earthquakes was subjective, often described based on the damage caused or eyewitness accounts, making comparisons across events and over time challenging.
Because the Richter scale is logarithmic, each whole number increase in scale is a 10x increase in the amplitude of the seismic waves.
Historical Background
Before the development of objective measures of earthquake magnitude, the assessment of earthquakes was largely descriptive. The first systematic approach to quantifying earthquakes was the Rossi-Forel scale, established in the late 19th century. This scale ranged from I (imperceptible) to X (disastrous) and was based on human perception and structural damage.
The Mercalli intensity scale, developed by Italian volcanologist Giuseppe Mercalli in the early 20th century, offered more detailed categories. It improved on the Rossi-Forel scale by incorporating modern engineering understanding into assessments of building damage. However, like its predecessor, the Mercalli scale was subjective and relied heavily on local conditions and the quality of construction in the area affected by the earthquake.
Charles F. Richter and Beno Gutenberg of the California Institute of Technology developed the Richter Scale in the 1930s to provide a more standard and objective measure. The Richter scale uses the measurements of seismic waves as recorded on seismographs. For the first time, earthquake magnitude was defined independently of the effects or damage it caused.
Calculating Richter Magnitude
Richter derived a formula to calculate the magnitude of an earthquake. It’s expressed as:
M L = log A – log A 0
- M L is the local magnitude (Richter magnitude)
- A is the maximum amplitude (in mm) of a seismic wave as recorded by the Wood-Anderson seismograph
- A 0 is the amplitude of a standard wave at 100 km from the earthquake epicenter
The value A 0 varies depending on the distance to the earthquake, the depth of the earthquake, and various other factors.
The logarithmic nature of the Richter scale means that each whole number increase in magnitude represents a tenfold increase in the measured amplitude of seismic waves and roughly 31.6 times more energy release.
Note that there are several modern adjustments to this formula, largely based on the distance to the epicenter of the quake. Also, although no earthquakes greater than 10 on the scale have been recorded, there is no upper limit to the Richter scale.
Magnitude Ranges and their Effects
The Richter scale is open-ended, but most earthquakes fall between magnitudes 2.0 and 9.0. Here is a breakdown of the categories, their descriptions, effects, and estimated annual global frequency:
- Less than 2.0 (Micro) : People don’t feel micro earthquakes, but instruments record them. There are an estimated 1.4 million of these quakes annually worldwide. Basically, they happen all the time.
- 2.0 – 2.9 (Minor) : Minor earthquakes are often felt, but rarely cause damage. There are around 1.3 million occurrences each year.
- 3.0 – 3.9 (Light) : Light earthquakes are often felt, but rarely causes significant damage. Approximately 130,000 of these quakes occur annually.
- 4.0 – 4.9 (Moderate) : A moderate earthquake causes a noticeable shaking of indoor items, accompanied by rattling noises. Significant damage is unlikely. There are about 13,000 occurrences globally each year.
- 5.0 – 5.9 (Strong) : Strong earthquakes potentially cause significant damage to buildings and other structures. There are roughly 1,300 occurrences annually.
- 6.0 – 6.9 (Major) : Major earthquakes cause a lot of damage in populated areas. There are about 100 occurrences each year.
- 7.0 and higher (Great) : These earthquakes cause serious damage. They happen around 10-20 times per year globally. There is usually only one earthquake per year with a magnitude between 8 and 10. No earthquake of 10 or higher has ever been recorded.
Some quakes with a small magnitude on the Richter scale cause more damage than large magnitude quakes. The level of the destruction depends on how deep the earthquake is and whether or not its epicenter is near a populated area. Also, some quake cause tsunamis, which add to the damage.
The Moment Magnitude Scale
While the Richter scale continues to be well-known among the general public, seismologists mainly use the moment magnitude scale (M w ) for more precise measurements, especially for extremely large earthquakes. The moment magnitude scale is also logarithmic, but it more accurately measures the total energy released by an earthquake.
The moment magnitude scale (M w ) is more complex to calculate than the Richter scale. The basic formula for calculating the moment magnitude is:
M w = 2/3 log(M 0 ) – 10.7
M 0 is the seismic moment, which is measured in dyne-cm (1 dyne-cm = 1×10 -7 joules). The seismic moment (M 0 ) is a measure of the total energy released by the earthquake. It is calculated by multiplying the shear modulus of the rocks involved (a measure of the rigidity of the material) by the area of the fault that slipped and the average amount of slip along the fault.
Let’s illustrate this with an example. In the 1906 San Francisco earthquake, the estimated slip along the fault was about 4.5 meters, the area of faulting was about 20,000 km², and the shear modulus of the Earth’s crust is about 3×10 11 dyne/cm². Thus, the seismic moment M 0 was about 2.7×10 27 dyne-cm.
Plug this into the M w formula:
Mw = 2/3 * log(2.7*10 27 ) – 10.7 ≈ 7.8
The Richter magnitude for the 1906 San Francisco earthquake was approximately 7.9. So, the magnitudes are fairly close for this particular earthquake. However, for very large earthquakes, the Richter scale underestimates the energy release, while the moment magnitude scale remains accurate. This is because the Richter scale is based on the amplitude of seismic waves, which “saturate” or fail to increase in very large earthquakes, whereas the Moment Magnitude scale considers the total energy released by the earthquake. Because the moment magnitude scale considers the area of the fault that slipped, the average amount of slip along the fault, and the rigidity of the rocks involved, it provides a more accurate and consistent measure of large earthquake magnitudes.
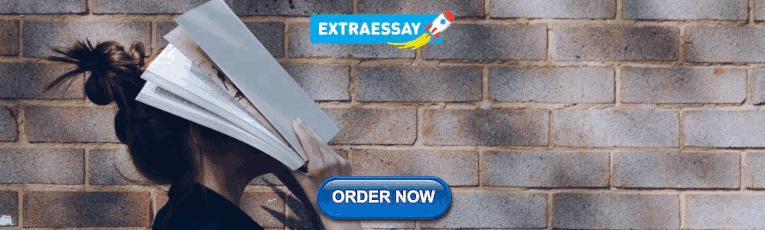
Strongest Earthquake Ever Recorded
The strongest earthquake ever recorded was the Great Chilean Earthquake that struck Chile on May 22, 1960. The earthquake reached a magnitude of 9.5 on the moment magnitude scale. This event released an immense amount of energy, causing widespread damage in Chile and triggering tsunamis that affected coastal regions as far away as Hawaii, Japan, and the Philippines.
The strongest earthquake in the United States was the March 27, 1964 earthquake in the Prince William Sound part of Alaska. Measuring 9.2 on the Richter scale, it is the second-largest quake in the world, following the 1960 earthquake. However, the June 11, 1585 earthquake in the Aleutian Islands (now Alaska) may have surpassed the 1964 quake, with an estimated magnitude of 9.25.
- Abe, Katsuyuki (1982). “Magnitude, seismic moment and apparent stress for major deep earthquakes”. Journal of Physics of the Earth . 30 (4): 321–330. doi: 10.4294/jpe1952.30.321
- Boore, D. M. (1989). “The Richter scale: its development and use for determining earthquake source parameter”. Tectonophysics . 166 (1–3): 1–14. doi: 10.1016/0040-1951(89)90200-x
- Gutenberg, B.; Richter, C. F. (1936), “Discussion: Magnitude and energy of earthquakes”. Science . 83 (2147): 183–185. doi: 10.1126/science.83.2147.183
- Gutenberg, B.; Richter, C. F. (1956). “Earthquake magnitude, intensity, energy, and acceleration”. Bulletin of the Seismological Society of America . 46 (2): 105–145.
- Hutton, L. K.; Boore, David M. (1987). “The M L scale in Southern California”. Nature . 271: 411–414. doi:10.1038/271411a0
Related Posts

- Explore Earthquakes
- Create an Earthquake
- Earthquake Locations
- Earthquakes in History
- Survivor's Story
What is an earthquake?
Where earthquakes happen, what causes earthquakes, intraplate earthquakes, faults and boundaries, predicting earthquakes, recording and locating earthquakes, measuring earthquake intensity.
An earthquake is a sudden shaking of Earth's surface caused by the movement of rocks deep underneath. Most quakes are unnoticeable by people on Earth's surface. Thousands of quakes occur every day but are too weak to be felt.
Earthquakes often happen without warning. Severe quakes can result in property damage, injury, and loss of life. They can cause fires, tsunamis, landslides and avalanches, and volcanic eruptions.
The size of an earthquake is known as its magnitude. The higher the number, the more powerful the earthquake.
Every year, on average:
Scientific instruments detect about 500,000 quakes worldwide.
A magnitude 8 quake happens somewhere on Earth.
About 50,000 people die as a result of earthquakes.
The lithosphere is the outermost layer of the Earth. It consists of the crust and uppermost mantle. The lithosphere is broken into extremely large slabs called tectonic plates. These plates move around on the molten layer beneath. For example, the North American Plate, which includes most of North America, Greenland, and part of Siberia, is approximately 75,900,000 square kilometers (29,305,000 square miles).
Looking at the map you can see the Earth’s surface divided into seven major plates and many minor plates. As you can see, earthquakes mostly (but not always) occur where these tectonic plates meet—the plate boundaries. Each boundary is made up of faults—fractures in the rock along which movement can take place. Movement of these plates is called seismic activity which is known as an earthquake.
Almost 80 percent of our planet's largest earthquakes occur along the Ring of Fire. This circles the edges of the Pacific Ocean.
Select the i for each plate to find out which direction it moves in and by how much.

- 9 cm per year
- 1 cm per year

- 3 cm per year
- 7 cm per year

- South West and North West

- 5 cm per year

The San Andreas Fault is especially visible from Earth’s surface. It is the boundary between the Pacific Plate and the North American Plate.
We already know that earthquakes occur mostly, but not always, at the boundaries of tectonic plates. Let’s look at the mechanisms behind this.

The plates do not always move smoothly past each other at faults.

Sometimes plate edges get stuck because of friction but the rest of the plate keeps moving, very slowly.

The energy and pressure that move the plates get stored up at the edges.

When the energy and pressure build up enough to overcome the friction, the plates move past each other along the fault.

The energy and pressure are released in the form of seismic waves that shake the surface as they move through it - like ripples on water.
This sudden movement along the fault can cause the ground to
- move forward and backward
- heave up and down
- shift from side to side -
Did you know?
Plates move approximately at the speeds at which human fingernails grow.
Less than 10 percent of all earthquakes occur far from plate boundaries. These are called intraplate earthquakes. These happen when pressure builds up and the earth’s crust is stretched or squeezed until it rips.

Destructive boundary - Convergent
Fault Type Observed Thrust, or reverse, faults occur where plates collide and one side of the fault is pushed up and over the other.
What Happens at Plates An oceanic plate subducts under a continental one. Earthquakes are generated within the subducting plate and where the two plates meet. Large magnitude earthquakes often occur at subduction zones, along with many small-scale ones.

Examples of Plates Involved Nazca Plate (oceanic) subducts under the South American Plate (continental).
Plate Activity Results Peru-Chile Trench
Image: A NASA Visible Earth map shows the location of a magnitude 8.0 earthquake off the coast of Peru. NASA, Map by Robert Simmon based on data from the USGS
Continental collisions - Convergent
What Happens at Plates One plate crumples over the other one, instead of being subducted. Mountain ranges are formed here.

Examples of Plates Involved Eurasian Plate and Indian Plate
Plate Activity Results The Himalayas
NASA image by Jeff Schmaltz
Fault Type Observed Normal faults are common. Plate boundaries pull apart and Earth's crust is stretched. One side of the fault slips down.
What Happens at Plates Plates move apart, and magma slowly rises toward the surface, eventually moving along fractures that appear.

Examples of Plates Involved The African Plate is splitting.
Plate Activity Results East African Rift
Astronaut photograph provided by the ISS Crew Earth Observations experiment and Image Science & Analysis Laboratory, Johnson Space Center – NASA.
Transform boundary
Fault Type Observed A strike-slip fault occurs where plates meet and slide against each other horizontally.
What Happens at Plates Plates move past each other with earthquakes generating close to the surface. Earthquakes are shallow but powerful.

Examples of Plates Involved Pacific Plate and North American Plate.
Plate Activity Results San Andreas Fault, California North Anatolian Fault, Turkey
Image: The San Andreas Fault slashes the desolate Carrizo Plain. Photograph by James P. Blair
The deadliest earthquake ever recorded occurred in 1556 and struck Shaanxi province, China. It killed an estimated 830,000 people. Afterward, buildings were made from softer materials like bamboo and wood rather than stone.
No scientists have ever predicted a major earthquake.
According to the United States Geological Survey (USGS), scientists can only calculate the probability that a significant earthquake will occur in a specific area within a certain number of years. For example, a major quake is likely in the San Francisco Bay region before 2030.
Seismologists—scientists who study earthquakes—use a range of equipment, including:
- creepmeters to check for movement along faults;
- tiltmeters to monitor changes in the slope of the land;
- satellites to detect changes in the position of plates

Rather than attempting predictions, scientists focus their efforts on helping to improve the safety of buildings and structures.
Seismologists cannot predict earthquakes but once a quake has happened, they can pinpoint its starting point, or epicenter, with accuracy.
The hypocenter (or focus), the place where the movement first occurred on the fault, can be hundreds of kilometers below the Earth's surface or just beneath it. The epicenter is the point on the surface directly above the hypocenter.
When the quake begins, seismic waves travel rapidly outward in all directions.
Primary waves (P waves) travel at the fastest speeds and spread through the crust from the hypocenter, alternately squeezing and stretching the rock as they travel through it.
Secondary waves (S waves) move slower than P waves and vibrate at right angles to the direction of wave travel. They distort the rock.
Surface waves follow at the slowest speed, causing intensive damage as they move along the Earth's surface.
Seismologists compare the arrival times of P waves and S waves at seismic stations to determine a quake's location.
The waves are measured on sensitive instruments called seismographs. Older versions are anchored to the ground and have a pen attached to them, so when the Earth moves during a quake the device moves and the pen makes zigzag tracings on paper. Modern versions are all computerized and instantly record activity at the seismic station.
The resulting pattern, or seismogram, reveals to experts:
- where the quake began
- how long it lasted
- how much energy was released
To locate the epicenter of an earthquake, we need to look at seismograms from three different recording stations. Experts compare the difference in arrival times of the P waves at the different stations. Then they compare the difference in arrival times of the S waves. This enables them to calculate the distance the earthquake is from the seismograph.
Seismologists used to assign magnitudes to earthquakes using the Richter scale.
Recently, scientists have begun to use the more precise moment magnitude scale, which measures the total energy released by a quake.
The moment-magnitude scale is logarithmic, so an increase of one unit means an earthquake is 10 times bigger, with about 30 times the energy produced.
Small earthquakes have about the same value on the Richter scale and the moment magnitude scale, so Richter is fine for those. But, larger earthquakes are better measured as moment magnitude, where Richter becomes less accurate.
All the earthquake magnitudes you will see here use the newer moment magnitude scale.
Frequency of Earthquakes per year: less than 1
Energy in Joules: 2,000,000,000,000,000,000 The amount of energy used every year in the United Kingdom.
Example: Magnitude: 9.1 Location: Sumatra-Andaman Islands Date: 12/26/2004
Frequency of Earthquakes per year: 1
Energy in Joules: 63,000,000,000,000,000 Almost half the energy a hurricane releases in one day.
Example: Magnitude: 8.8 Location: Maule, Chile Date: 02/27/2010
Frequency of Earthquakes per year: 17
Energy in Joules: 2,000,000,000,000,000 About the same energy contained in two billion candy bars.
Example: Magnitude: 7.0 Location: Haiti Date: 01/12/2010
Frequency of Earthquakes per year: 134
Energy in Joules: 63,000,000,000,000 Around the same energy as 3.3 Hiroshima-sized atomic bombs.
Example: Magnitude: 6.1 Location: Christchurch, New Zealand Date: 02/22/2010
Frequency of Earthquakes per year: 1,313
Energy in Joules: 2,000,000,000,000 Around the same energy contained in two trillion candy bars.
Frequency of Earthquakes per year: 13,000* * estimated
Energy in Joules: 63,000,000,000 Around the same energy as 6 tons of TNT.
Frequency of Earthquakes per year: 130,000* * estimated
Energy in Joules: 2,000,000,000 Twice the amount of energy in a lightning bolt.
Frequency of Earthquakes per year: 1,300,000* * estimated
Energy in Joules: 63,000,000 About the same energy contained in 63 candy bars.
Frequency of Earthquakes per year: millions
Energy in Joules: 2,000,000 About the same energy contained in two candy bars.
In March 2011, a moment magnitude 9 earthquake in Japan moved the Earth’s axis up to 25 centimeters (10 inches) and moved the main island, Honshū, 2.4 meters (7.9 feet) closer to the United States. The tsunami it triggered claimed approximately 20,000 lives.
Time to create an earthquake


- Insurance Professionals
- California Earthquake Risk Map & Faults By County
- List of Notable and Major California Earthquakes
- 2020 MMD English
- Seven Steps to Earthquake Safety
- Homeowners Coverages & Deductibles
- Condo Coverages & Deductibles
- Mobilehomes & Manufactured Homes Coverages & Deductibles
- Renters Coverages & Deductibles
- 2020 MMD Chinese
- 2020 MMD Spanish
- Nuestros Videos en Español
- Your House's Characteristics
- Inspection Professionals Search
- Dwelling Retrofit Verification Form
- Dwelling Retrofit Verification Form Instructions
- Participating Residential Insurers
- How to Make a Payment / How to Pay My Bill
- Policy Management FAQs
- How to File a Claim
- Browser Compatibility
- Other Earthquake Insurance Providers
- Stem Wall House
- Cripple Wall House
- Post and Pier House
- Living Space Over Garage
- Hillside House
- Mobilehomes & Manufactured Homes
- Standard Plan Set 1
- CEA B+B Homeowner Retrofit Guide
- Before & After Photos
- Retrofit Stories
- Steps to a CEA BB Retrofit
- Rules for Participation in the CEA Brace + Bolt Program
- How to Register
- Engineer or Design Pro Search
- Permit & Building Department Information
- FEMA Retrofit Education Course
- Program Glossary
- Ground Shaking
- Surface Rupture
- Earthquake-Induced Landslides
- Soil Liquefaction
- Preparedness Resources
- Shawna Ackerman
- Charlotte Fadipe
- Janiele Maffei
- Michael Melavic
- Zerlinia Moore
- George Sittner
- CEA Strategic Plan 2017-2019
- Governing Board
- Advisory Panel
- Stakeholder Forums
- Meeting Notifications Sign-Up
- Watch Public Meetings Live
- Meeting Materials Archive
- Policyholder Stories
- Community Stories
- CEA Advertising
- TV Commercials Archive
- CEA History
- Financial Strength
- Investment Policies
- Financial Statements
- Annual Legislative Reports
- CEA South Napa Study Report (PDF)
- South Napa Study Executive Summary (PDF)
- Research Forum Videos
- September 18, 2019 News Release - Research Forum
- Innovation in Earthquake Assessment How Technology is Changing the Industry Agenda 2018
- Research Opportunities
- Working at CEA
- Contracting with CEA
- Frequently Asked Questions
- Diversity at CEA
- Claim Professionals Resources
- 2023 Homeowners Coverage Training Registration
- CEA Residential Earthquake Damage Assessment (REDA) General Guidelines Training
- CEA Residential Earthquake Damage Assessment (REDA) Engineering Guidelines Training
- CEA Claim Manual January 2021
- CEA Deductible Calculator 2016 (ZIP)
- CEA PI Claim Liaison Reference Document
- CEA Earthquake Insurance Dwelling Retrofit Verification Form
- Inspection Professionals Registration
- Media Resources
- Press Releases
- In the News
- CEA Spokespersons
- Earthquakes, Faults and Seismology
- Public Records Requests
- Terms of Use
- Privacy & Social Media Policies
Earthquake Measurements: Magnitude vs Intensity
Earthquake magnitude vs. intensity: decoding the tremors.
You may not always feel the earth shaking, but California has earthquakes occurring all the time. Seismographic networks measure earthquakes by their magnitude, energy release and intensity.
Years ago, all magnitude scales were based on the recorded waveform lengths or the length of a seismic wave from one peak to the next. But for very large earthquakes, some magnitudes underestimated the true earthquake size. Now, scientists use earthquake measurements that describe the physical effects of an earthquake rather than measurements based only on the height of a waveform recording.
How are earthquakes recorded & detected?
When the Earth trembles, earthquakes spread energy in the form of seismic waves. A seismograph is the primary earthquake measuring instrument. The seismograph produces a digital graphic recording of the ground motion caused by the seismic waves. The digital recording is called a seismogram.
A network of worldwide seismographs detects and measures the strength and duration of the earthquake’s waves. The seismograph produces a digital graphic plotting of the ground motion of the event.
How is earthquake magnitude measured?
An earthquake has one magnitude unit. The magnitude does not depend on the location where the measurement is made. Since 1970, the Moment Magnitude Scale has been used because it supports earthquake detection all over the Earth.
Earthquake Measurements
To get a better idea of the strength of the shaking and damage, the Moment Magnitude Scale was developed to capture all the different seismic waves from an earthquake to worldwide seismic networks.
Earthquake intensity scales describe the severity of an earthquake’s effects on the Earth's surface, humans, and buildings at different locations in the area of the epicenter. There can be multiple intensity measurements. The Modified Mercalli Scale measures the amount of shaking at a particular location .
Earthquake Magnitude Scale
An important piece of information to keep in mind is that the magnitude scale is logarithmic. In other words, it is “comparing amplitudes of waves on a seismogram, not the strength, or energy, of the quakes,” according to USGS. This helps us understand that while the size (amplitude) differences between small and big quakes are big enough, it is the strength (energy) differences that are meaningful. Try out USGS’s “How Much Bigger…?” calculator to learn more about how to measure the magnitude of an earthquake.
The Richter Scale
From 1935 until 1970, the earthquake magnitude scale was the Richter scale, a mathematical formula invented by Caltech seismologist Charles Richter to compare quake sizes.
The Richter Scale was replaced because it worked largely for earthquakes in Southern California, and only those occurring within about 370 miles of seismometers. In addition, the scale was calculated for only one type of earthquake wave. It was replaced with the Moment Magnitude Scale, which records all the different seismic waves from an earthquake to seismographs across the world.
Richter's equations are still used for forecasting future earthquakes and calculating earthquake hazards.
Moment Magnitude Scale
Today, earthquake magnitude measurement is based on the Moment Magnitude Scale (MMS). MMS measures the movement of rock along the fault. It accurately measures larger earthquakes, which can last for minutes, affect a much larger area, and cause more damage.
The Moment Magnitude can measure the local Richter magnitude (ML), body wave magnitude (Mb), and surface wave magnitude (Ms).
Earthquake Magnitude Classes
Earthquakes are also classified in categories ranging from minor to great, depending on their magnitude. What’s the difference between a light and moderate quake?
These terms are magnitude classes. Classes also provide earthquake measurement. The classification starts with “minor” for magnitudes between 3.0 and 3.9, where earthquakes generally begin to be felt, and ends with “great” for magnitudes greater than 8.0, where significant damage is expected.
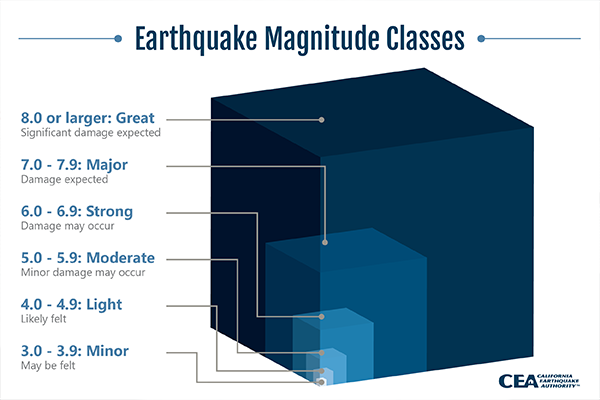
How is earthquake intensity measured?
A second way earthquakes are measured is by their intensity. Earthquake Intensity measurement is an on-the-ground description. The measurement explains the severity of earthquake shaking and its effects on people and their environment. Intensity measurements will differ depending on each location’s nearness to the epicenter. There can be multiple intensity measurements as opposed to one magnitude measurement.
The Modified Mercalli Scale
The Modified Mercalli (MM) Intensity Scale is used in the United States. Based on Giuseppe Mercalli's Mercalli intensity scale of 1902, the modified 1931 scale is composed of increasing levels of intensity that range from observable quake impacts from light shaking to catastrophic destruction. Intensity is reported by Roman numerals.
An earthquake intensity scale consists of a series of key responses that includes people awakening, movement of furniture, damage to chimneys and total destruction.
Source: USGS
How to prepare for a high magnitude quake
An earthquake is a sudden, rapid shaking of the earth caused by the shifting of rock beneath the earth’s surface. The size of an earthquake and the energy released by an earthquake will affect how much you feel it. Major earthquakes strike without warning , at any time of year, day or night.
Prepare before the next big one:
- Create an earthquake safety plan. Discuss with your family what to do, where to meet if separated, and how you will communicate when an earthquake strikes. Check work, childcare and school emergency plans.
- Practice DROP, COVER, and HOLD ON with all members of your household.
- Don’t rely on doorways for protection. During an earthquake, get under a sturdy table or desk. Hold on until shaking stops.
- Pick safe places in each room of your home.
- Create an emergency survival kit that provides you and your pets with three days of nonperishable food and water, medicines, emergency radio and first aid materials. Keep in a reachable place.
- Identify an out-of-the-area friend or relative that family members can check in by mobile texting.
- Find out if your home is in need of earthquake retrofitting and eligible for a grant.
- Identify and fix potential earthquake hazards in your home.
- Protect your home investment and bounce back from a devastating earthquake with the best choices of earthquake insurance from CEA.
Whether you are a homeowner, mobilehome owner, condo-unit owner or renter, buy peace of mind with affordable and flexible earthquake insurance now.
Understanding Geologic & Structural Risks
Every day, Californians face earthquake danger. Our state has nearly 16,000 known faults and more than 500 active faults. Most of us live within 30 miles of an active fault risk.
Visit the CEA risk map for each county to learn about faults in your area. This information will help you survive an earthquake.
Then learn about your home’s structural risks , the steps you can take to seismically strengthen your house and the benefits of retrofitting. Make your home more resistant to earthquake damage by assessing its structure, contents and need for loss of use earthquake insurance.
Avoid financial disaster with loss of use coverage if your house sufferers extensive damage—get coverage with a CEA earthquake policy .
Personal Preparedness Guidelines
Get started today on preparing for a major earthquake. Top earthquake survival tips include:
- Create an earthquake safety plan for you and loved ones, including pets.
- Identify safe places in each room of your home.
- Practice Drop, Cover, and Hold On with each member of your household.
- Make or purchase an earthquake safety kit.
Buy peace of mind with affordable and flexible earthquake insurance now.
Is your house at risk for earthquake damage?
Preparing your home BEFORE an earthquake is important to your safety. Decrease your risk of damage and injury from an earthquake by identifying possible home hazards .
A seismic retrofit by strengthening your home’s foundation makes it more resistant to shaking. CEA offers earthquake home insurance premium discounts for houses and mobilehomes that have been properly retrofitted. Find out about grants to help for retrofits under the Earthquake Brace + Bolt Program, and the CEA Brace + Bolt program.
Is earthquake insurance worth it?
While it is wise to be prepared physically when the ground shakes, it’s also important to be financially protected. Without earthquake insurance, you place yourself at risk of losing everything or sustaining damages to your personal property that you cannot afford to repair or replace.
- Pay your mortgage for a house that may need to be rebuilt?
- Cover the costs bill for temporary accommodations?
- Repair or replace your personal belongings?
CEA earthquake insurance not only helps repair damages. Loss of use coverage covers the costs of temporary shelter and additional living expenses so that families can get back on their feet quicker.
Get an Earthquake Insurance Estimate!
Contact your home insurance agent today to discuss adding a separate earthquake policy to your home insurance. You can add the coverage now, no need to wait until your home policy comes up for renewal. For the best choice of CEA earthquake insurance policies, select deductibles from 5%-25%.
We work with 20 residential insurance companies that serve the majority of California homeowners. Get an estimate now!
Sources Consulted:
- Michigan Tech University: How are earthquakes measured
- GNS Science: What is the difference between magnitude and intensity
- KQED: Measuring Earthquakes Intensity and Magnitude
- USGS: Earthquake magnitude, energy release and intensity
- USGS: How are earthquakes recorded? How are earthquakes measured?
- USGS: “How Much Bigger…?” calculator
- USGS: Modified Intensity Scale
- Physical Geology (textbook by Steven Earle): Measuring Earthquakes
- British Geological Survey: Earthquake magnitude calculations
- US Naval Academy: Earthquakes
- EGS Solutions: What is the moment magnitude scale?

An official website of the United States government
Here's how you know
Official websites use .gov A .gov website belongs to an official government organization in the United States.
Secure .gov websites use HTTPS A lock ( ) or https:// means you’ve safely connected to the .gov website. Share sensitive information only on official, secure websites.
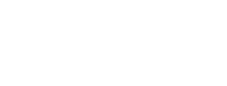
- Digg
Latest Earthquakes | Chat Share Social Media
Earthquake Magnitude, Energy Release, and Shaking Intensity
Earthquake magnitude, energy release, and shaking intensity are all related measurements of an earthquake that are often confused with one another. Their dependencies and relationships can be complicated, and even one of these concepts alone can be confusing.
Here we'll look at each of these, as well as their interconnectedness and dependencies.
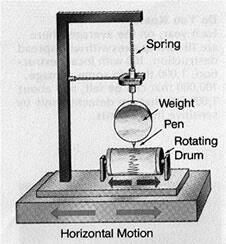
The time, location, and magnitude of an earthquake can be determined from the data recorded by seismometer. Seismometers record the vibrations from earthquakes that travel through the Earth. Each seismometer records the shaking of the ground directly beneath it. Sensitive instruments, which greatly magnify these ground motions, can detect strong earthquakes from sources anywhere in the world. Modern systems precisely amplify and record ground motion (typically at periods of between 0.1 and 100 seconds) as a function of time.
Magnitude is the size of the earthquake. An earthquake has a single magnitude. The shaking that it causes has many values that vary from place to place based on distance, type of surface material, and other factors. See the Intensity section below for more details on shaking intensity measurements.
Types of Magnitudes
Magnitude is expressed in whole numbers and decimal fractions. For example, a magnitude 5.3 is a moderate earthquake, and a 6.3 is a strong earthquake. Because of the logarithmic basis of the scale, each whole number increase in magnitude represents a tenfold increase in measured amplitude as measured on a seismogram .
When initially developed, all magnitude scales based on measurements of the recorded waveform amplitudes were thought to be equivalent. But for very large earthquakes, some magnitudes overestimate true earthquake size, and some underestimate the size. Thus, we now use measurements that describe the physical effects of an earthquake rather than measurements based only on the amplitude of a waveform recording. More on that later.
The Richter Scale (M L ) is what most people have heard about, but in practice it is not commonly used anymore, except for small earthquakes recorded locally, for which ML and short-period surface wave magnitude (Mblg) are the only magnitudes that can be measured. For all other earthquakes, the moment magnitude (Mw) scale is a more accurate measure of the earthquake size.
Although similar seismographs had existed since the 1890's, it was only in 1935 that Charles F. Richter, a seismologist at the California Institute of Technology, introduced the concept of earthquake magnitude. His original definition held only for California earthquakes occurring within 600 km of a particular type of seismograph (the Woods-Anderson torsion instrument). His basic idea was quite simple: by knowing the distance from a seismograph to an earthquake and observing the maximum signal amplitude recorded on the seismograph, an empirical quantitative ranking of the earthquake's inherent size or strength could be made. Most California earthquakes occur within the top 16 km of the crust; to a first approximation, corrections for variations in earthquake focal depth were, therefore, unnecessary.
The Richter magnitude of an earthquake is determined from the logarithm of the amplitude of waves recorded by seismographs. Adjustments are included for the variation in the distance between the various seismographs and the epicenter of the earthquakes.
Moment Magnitude (M W ) is based on physical properties of the earthquake derived from an analysis of all the waveforms recorded from the shaking. First the seismic moment is computed, and then it is converted to a magnitude designed to be roughly equal to the Richter Scale in the magnitude range where they overlap.
Moment (M O ) = rigidity x area x slip
where rigidity is the strength of the rock along the fault, area is the area of the fault that slipped, and slip is the distance the fault moved. Thus, stronger rock material, or a larger area, or more movement in an earthquake will all contribute to produce a larger magnitude.
Moment Magnitude (M W ) = 2/3 log 10 (M O ) - 9.1
See the Magnitude Types Table (below)for a summary of types, magnitude ranges, distance ranges, equations, and a brief description of each.
For More Information on Magnitudes
- Magnitude Types Table
- How much bigger is a magnitude 8.7 earthquake than a magnitude 5.8 earthquake? Try it yourself calculator
- Magnitude Educational Resources
Energy Release
Another way to measure the size of an earthquake is to compute how much energy it released. The amount of energy radiated by an earthquake is a measure of the potential for damage to man-made structures. An earthquake releases energy at many frequencies, and in order to compute an accurate value, you have to include all frequencies of shaking for the entire event.
While each whole number increase in magnitude represents a tenfold increase in the measured amplitude, it represents an 32 times more energy release .
The energy can be converted into yet another magnitude type called the Energy Magnitude (M e ) . However, since the Energy Magnitude and Moment Magnitude measure two different properties of the earthquake, their values are not the same.
The energy release can also be roughly estimated by converting the moment magnitude, M w , to energy using the equation log E = 5.24 + 1.44M w , where M w is the moment magnitude.
Whereas the magnitude of an earthquake is one value that describes the size, there are many intensity values for each earthquake that are distributed across the geographic area around the earthquake epicenter. The intensity is the measure of shaking at each location, and this varies from place to place, depending mostly on the distance from the fault rupture area . However, there are many more aspects of the earthquake and the ground it shakes that affect the intensity at each location, such as what direction the earthquake ruptured, and what type of surface geology is directly beneath you. Intensities are expressed in Roman numerals, for example, VI, X, etc.
Traditionally the intensity is a subjective measure derived from human observations and reports of felt shaking and damage. The data used to be gathered from postal questionnaires, but with the advent of the internet, it's now collected using a web-based form. However, instrumental data at each station location can be used to calculate an estimated intensity.
The intensity scale that we use in the United States is called the Modified Mercalli Intensity Scale , but other countries use other scales.
For More Information on Intensity
- The Modified Mercalli Intensity Scale
- Magnitude vs Intensity - Grades 4-12 activity: magnitude, intensity
- Intensity distribution and isoseismal maps for the Northridge, California, earthquake of January 17,1994 , USGS Open-File Report 95-92.
- Intensity Educational Resources
These examples illustrate how locations (and depth), magnitudes, intensity, and faults (and rupture) characteristics are dependent and related.
Intensity of Shaking Depends on the Local Geology
Intensity of Shaking Depends on Depth of the Earthquake
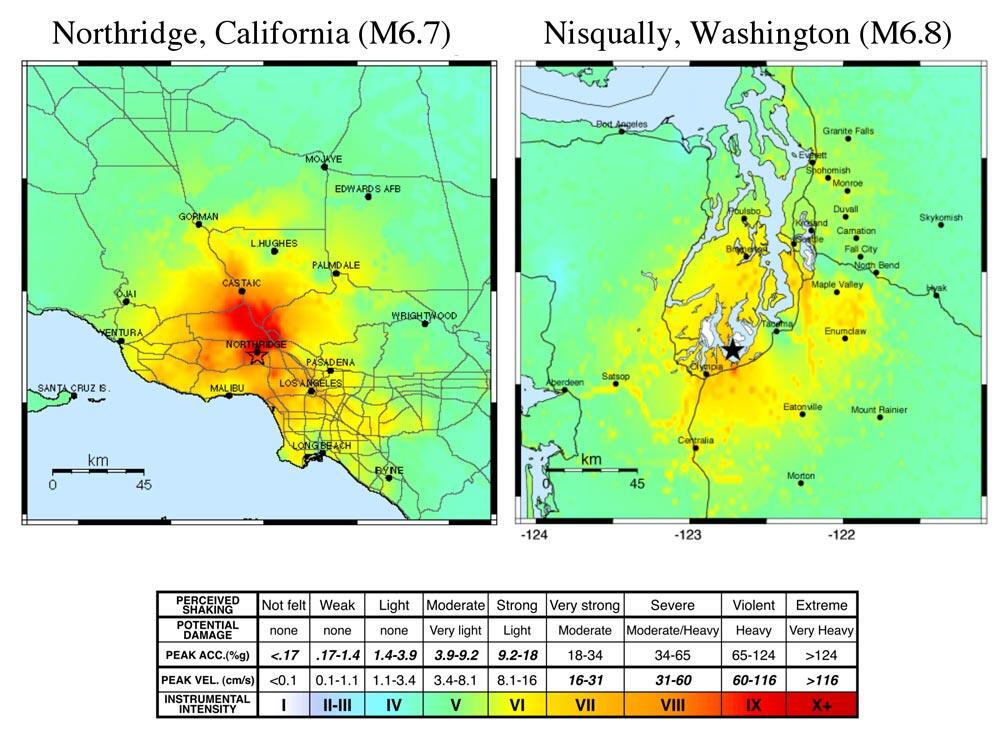
The shaking from the M6.7 Northridge, CA earthquake was more intense and covered a wider area than the slightly larger M6.8 Nisqually, WA earthquake.
The reason is shown by the two cartoon cross-sections below. There was more shaking in the Northridge earthquake because the earthquake occurred closer to the surface (3-11 miles), as opposed to the Nisqually earthquake's deeper hypocenter (30-36 miles).
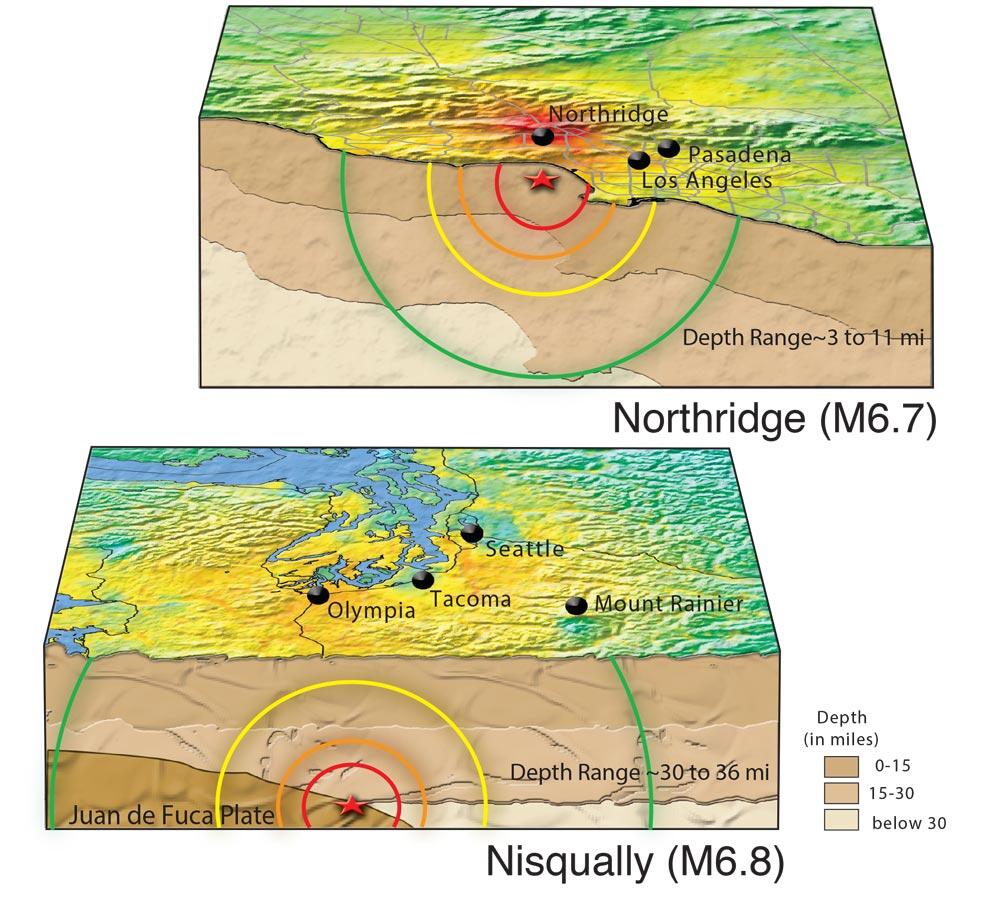
Moment Release (Energy) of Many Small Earthquakes vs. One Large Earthquake
The small- and moderate-size earthquakes that occur frequently around the world release far less energy that a single great earthquake.
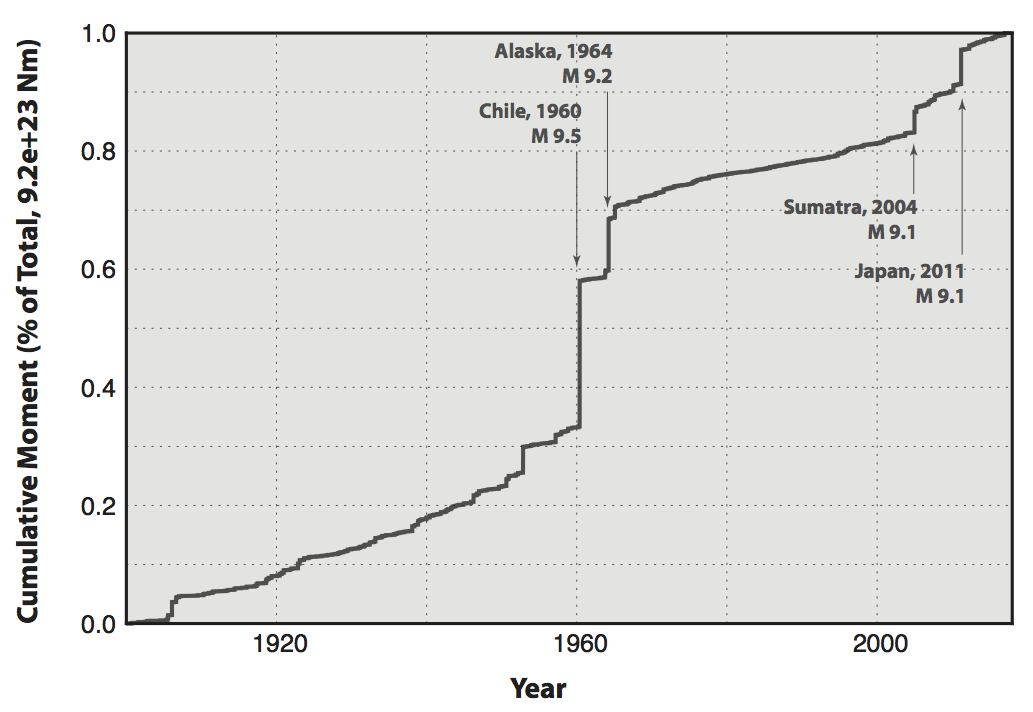
What Would it Take to Make a Magnitude N Earthquake?
If we sum all of the energy release from all of the earthquakes over the past ~110 years, the equivalent magnitude ~ Mw9.95.
If the San Andreas Fault were to rupture end-to-end (~1400km), with ~10m of average slip, it would produce an earthquake of Mw 8.47.
If the South American subduction zone were to rupture end-to-end (~6400km), with ~40m of average slip, it would produce an earthquake of Mw 9.86.
You would need ~14,000km fault length, with a seismogenic thickness averaging 40km (width of 100km), to slip and average of 30m to produce an Mw 10.
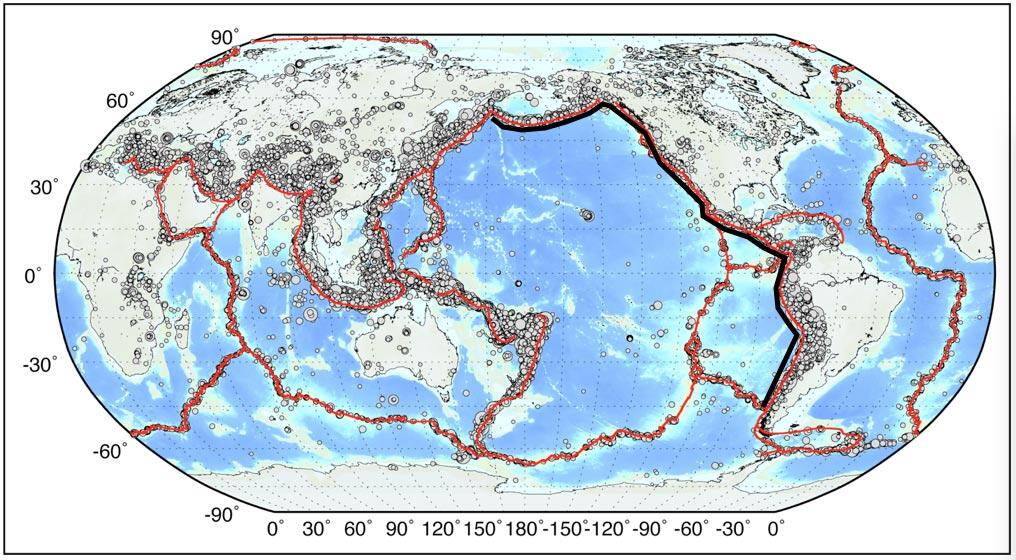
You would need ~80,000km of fault length with an average seismogenic width of 100km to produce an Mw10.5. All of the subduction zones in the World, plus some adjoining structures amount to ~40,000km, and the circumference of the Earth is ~40,000km, so an Mw 10.5 is highly unlikely.
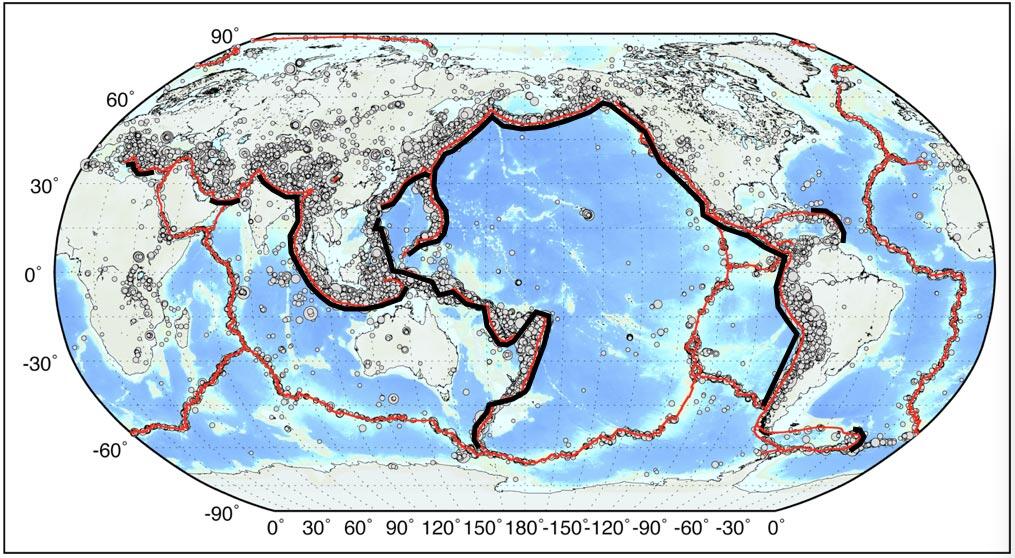
Thanks to Gavin Hayes and David Wald for providing much of the material for this page.
Watch CBS News
How are earthquakes measured? Get the details on magnitude scales and how today's event stacks up
By Kerry Breen
Updated on: April 5, 2024 / 6:43 PM EDT / CBS News
An earthquake rattled much of the Northeast on Friday morning, with shaking felt across the New York City metropolitan area and in communities from Baltimore and Philadelphia up through New England .
The quake had a magnitude of 4.8, according to the U.S. Geological Survey, and it was followed by several aftershocks, including one with a magnitude of 4.0. No major damage was reported as of midday Friday, officials said.
Here's how earthquake strength is measured and what different quake magnitudes mean.
How are earthquakes measured?
Earthquakes are recorded by a network of seismic stations that measure the movement of the ground, according to the USGS . Each station captures data about how much the earth moves and vibrates during a temblor and how much energy moves out from the hypocenter, or the site of an earthquake under the planet's surface.
Multiple scales are used to measure an earthquake. Most commonly, scientists refer to an earthquake's magnitude as a measure of its strength. Geologists once used the Richter scale, but that measure is considered outdated and is no longer used. An earthquake can also be described by its intensity, or the measure of shaking and damage caused by the quake.
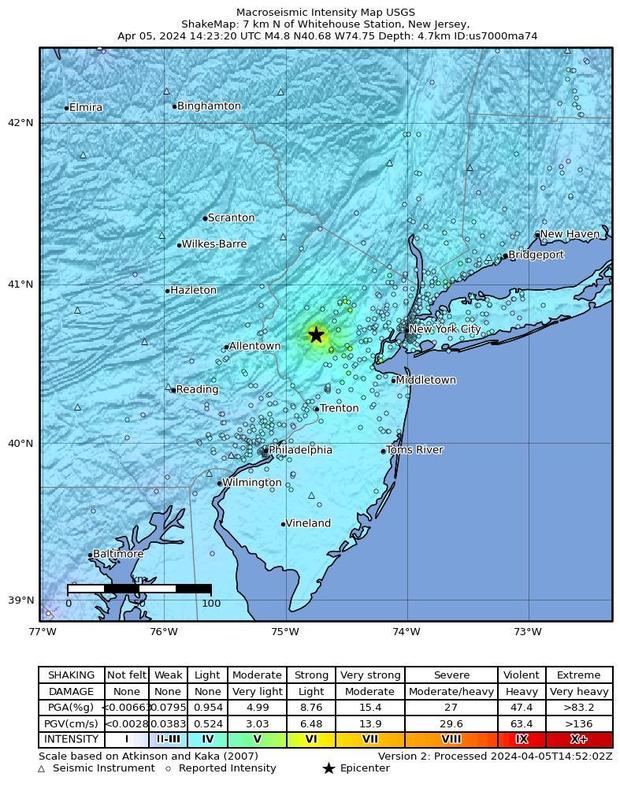
What is the magnitude scale of earthquakes?
The magnitude scale is the most common way to measure the size of an earthquake. USGS uses what's caused the moment magnitude scale to measure earthquakes.
"Moment" is a physical quantity proportional to the slip on a fault line multiplied by the area of the fault surface that slipped, USGS said. Moment can be estimated from seismograms and other geological measurements. The moment number is then converted to a scale number, which provides an estimate of the quake's size.
Going up one whole number on the scale indicates the ground motion recorded by seismic equipment increased by a factor of 10. This means a magnitude 5 earthquake would result in about 10 times as much ground movement as a magnitude 4 earthquake, according to the Department of Geological Mining Engineering and Sciences at Michigan Technological University.
The scale has no upper limit, but no fault long enough to generate a magnitude 10 earthquake is known to exist, according to USGS .
In some cases, earthquakes can be so small as to have a negative magnitude, the USGS says .
What is the Richter Scale and why is it outdated?
The Richter Scale is an older method once used for describing the size of earthquakes. The scale was developed by Charles Richter in the 1930s, but USGS says it only worked well on certain frequency and distance ranges. Newer scales were created to account for quakes outside of those ranges.
However, each scale had a limitation, so the moment magnitude scale was developed as a more thorough and uniformly applicable measure for earthquakes.
What is the strongest earthquake ever recorded?
The strongest earthquake ever recorded happened in Chile in 1960. That "megaquake" had a magnitude of 9.5 , and occurred on a thousand-mile-long fault line.
That quake lasted for over 11 minutes and triggered tsunamis around the globe. The city of Valdivia was the most affected area, so the quake is sometimes known as the Valdivia Earthquake.
Two million people were left homeless, according to the National Geographic Society . Approximately 1,655 people were killed in the initial quake, and 3,000 people were injured. Higher death tolls include those killed in tsunamis.
The strongest earthquake in U.S. history was the magnitude 9.2 Great Alaska Earthquake of 1964, which also triggered a tsunami.
So, is today's 4.8-magnitude earthquake considered big?
A quake of 2.5 magnitude or less is usually not felt, but can be recorded by a seismograph, according to the Department of Geological Mining Engineering and Sciences at Michigan Technological University.
Quakes that range from 2.5 to 5.4 are felt, but cause only minor damage in most cases. That's the range where this quake in the Northeast falls, and there have been no reports of significant damage or injuries so far. At a magnitude 4.8, it was one of the stronger quakes felt in the area over the past century, but it's not a record. A A 5.0 quake was measured in New York City in 1884, and In 2011, a 5.8 quake centered in Virginia rattled the entire East Coast .
Quakes from 5.5 to 6.0 magnitude can cause some damage.
Quakes that range from 6.1 to 6.9 magnitude can cause "a lot of damage in very populated areas," while a 7.0 to 7.9 magnitude earthquake is considered a major earthquake causing serious damage. Next is an 8.0 or greater magnitude, which is considered a massive quake that can "totally destroy communities" near its epicenter.
No tsunami activity is expected because of today's earthquake, and aftershock possibility remains low, officials said.
- Earthquakes

Kerry Breen is a reporter and news editor at CBSNews.com. A graduate of New York University's Arthur L. Carter School of Journalism, she previously worked at NBC News' TODAY Digital. She covers current events, breaking news and issues including substance use.
More from CBS News
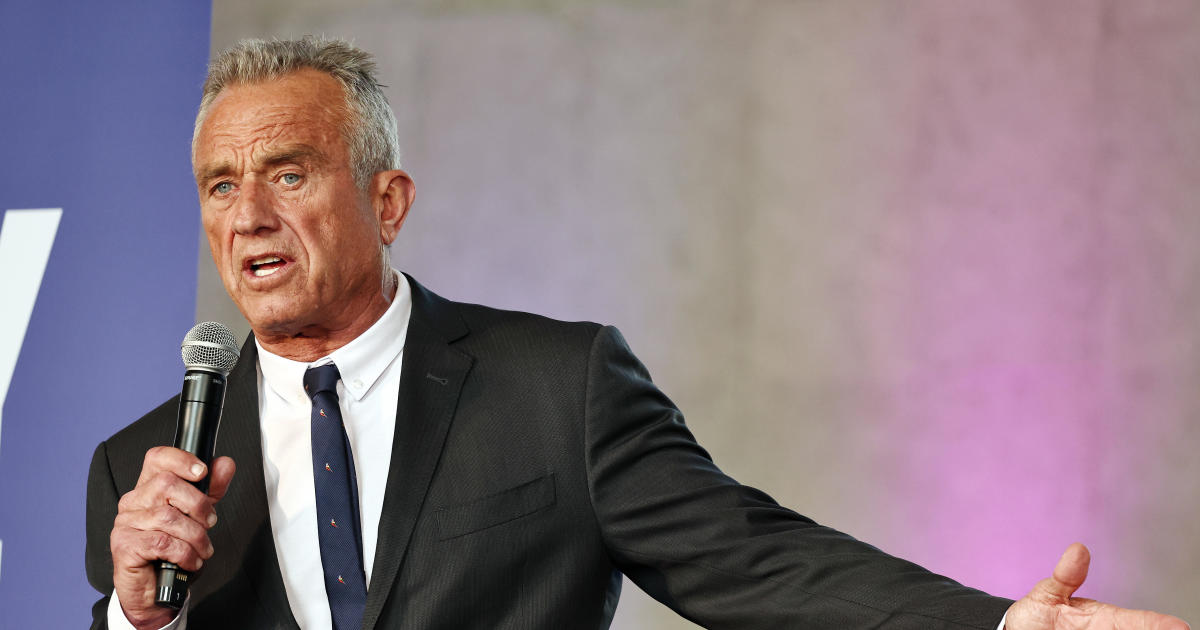
New York RFK Jr. campaign official suggests he's a spoiler who can help Trump win
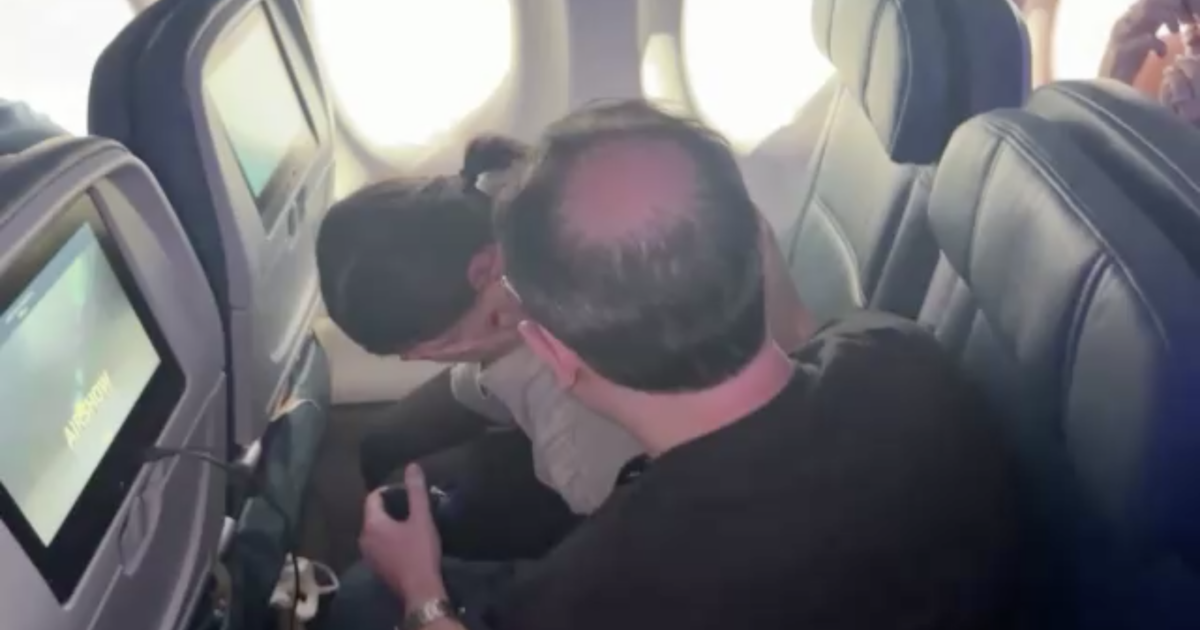
Couple gets engaged on flight to see total solar eclipse
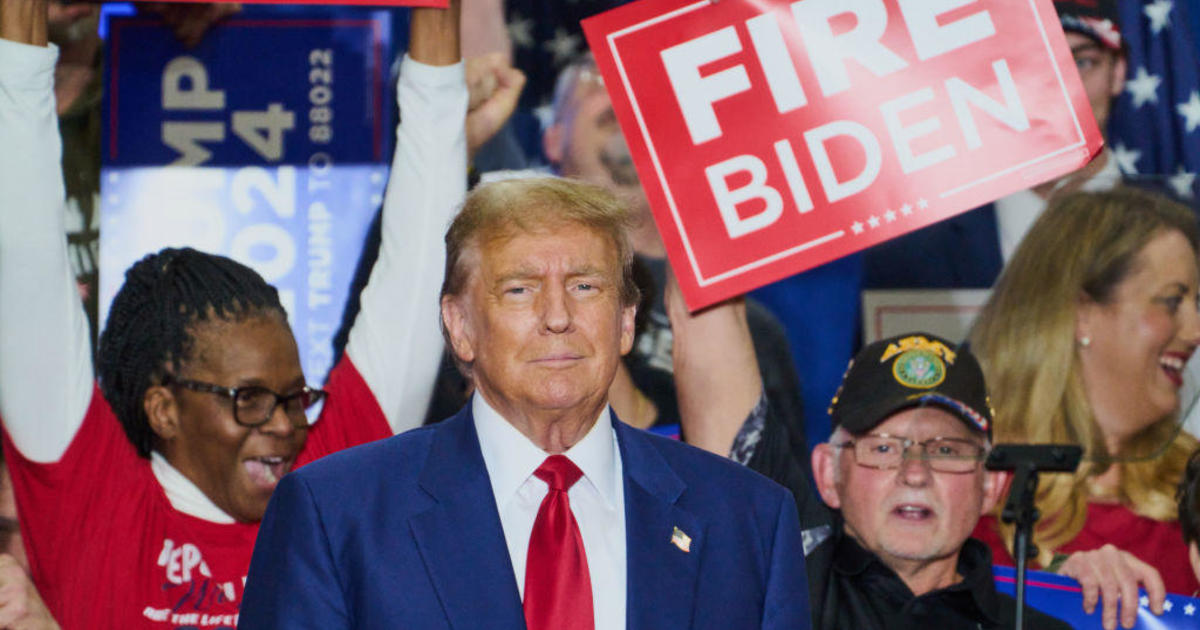
Another Trump delay effort in "hush money" trial rejected
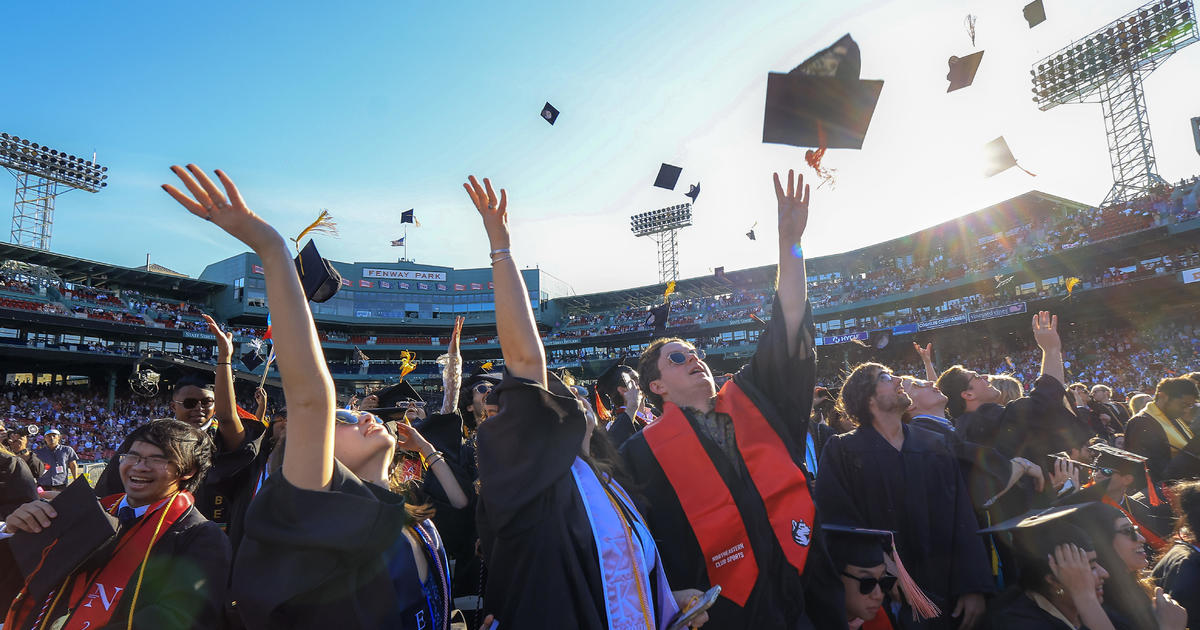
Biden to announce new student loan forgiveness proposals
Taiwan's strongest earthquake in 25 years kills 9 people, 50 missing
- Medium Text
- Earthquake kills nine, more than 900 injured
- Fifty on minibuses heading to national park missing
- Epicentre just off Taiwan's sparsely populated east coast
- Workers return to semiconductor giant TSMC facilities

FIGHTER JETS
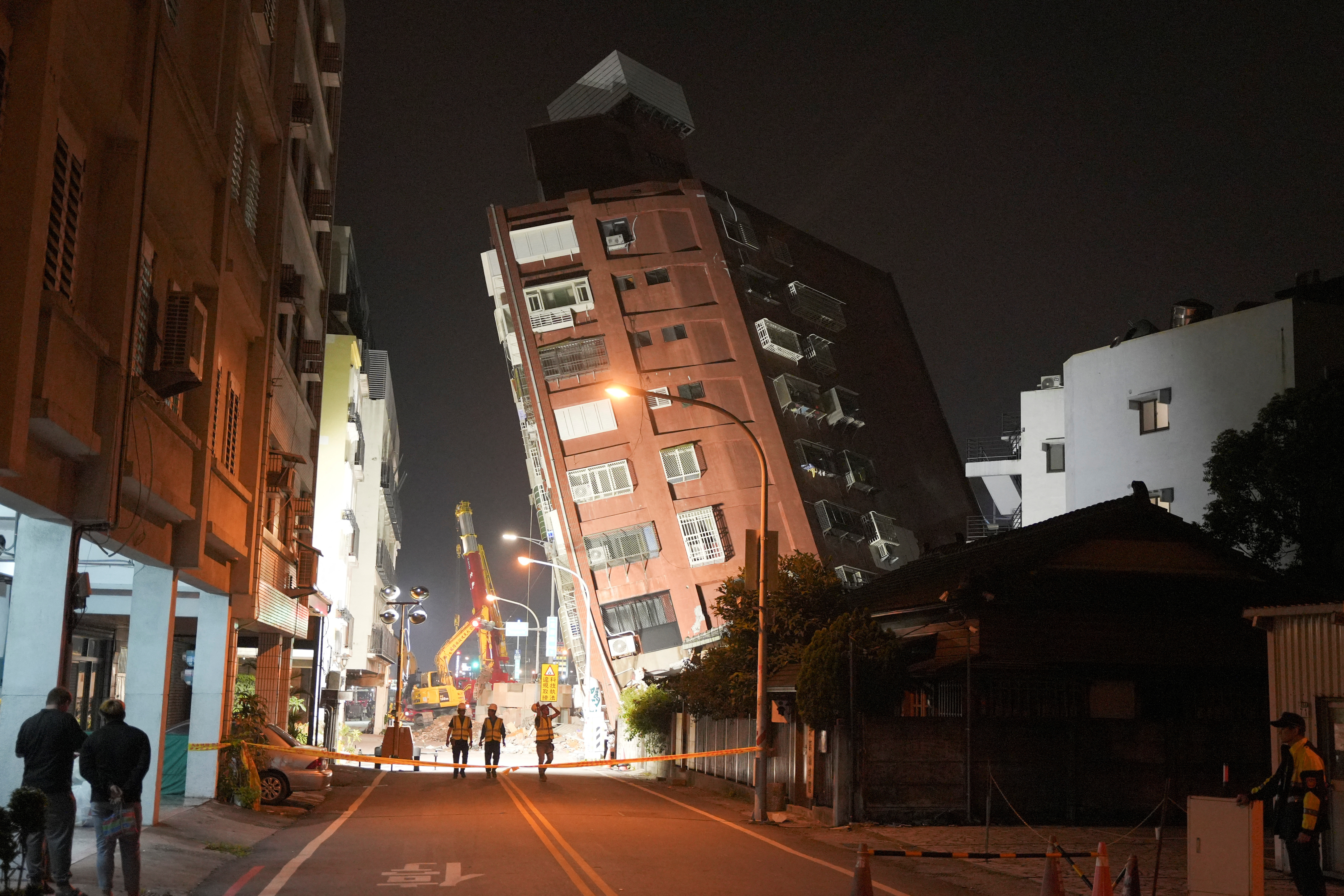
CHIP SUPPLIES

The Reuters Daily Briefing newsletter provides all the news you need to start your day. Sign up here.
Reporting by Yimou Lee and Fabian Hamacher, Shanghai and Hong Kong newsrooms; Writing by Ben Blanchard; Editing by Clarence Fernandez, Chizu Nomiyama, Alison Williams and Josie Kao
Our Standards: The Thomson Reuters Trust Principles. New Tab , opens new tab
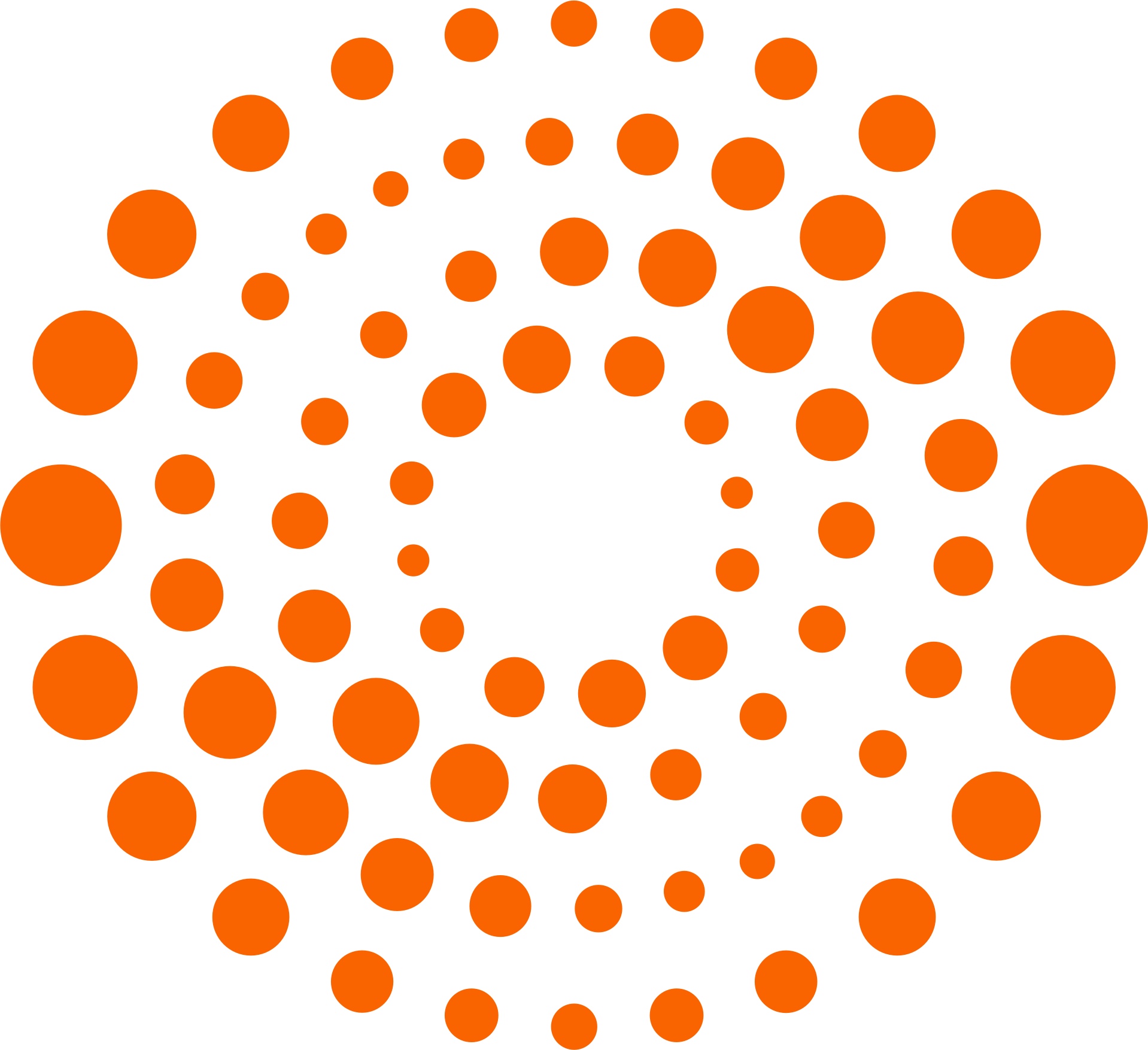
Thomson Reuters
Yimou Lee is a Senior Correspondent for Reuters covering everything from Taiwan, including sensitive Taiwan-China relations, China's military aggression and Taiwan's key role as a global semiconductor powerhouse. A three-time SOPA award winner, his reporting from Hong Kong, China, Myanmar and Taiwan over the past decade includes Myanmar's crackdown on Rohingya Muslims, Hong Kong protests and Taiwan's battle against China's multifront campaigns to absorb the island.
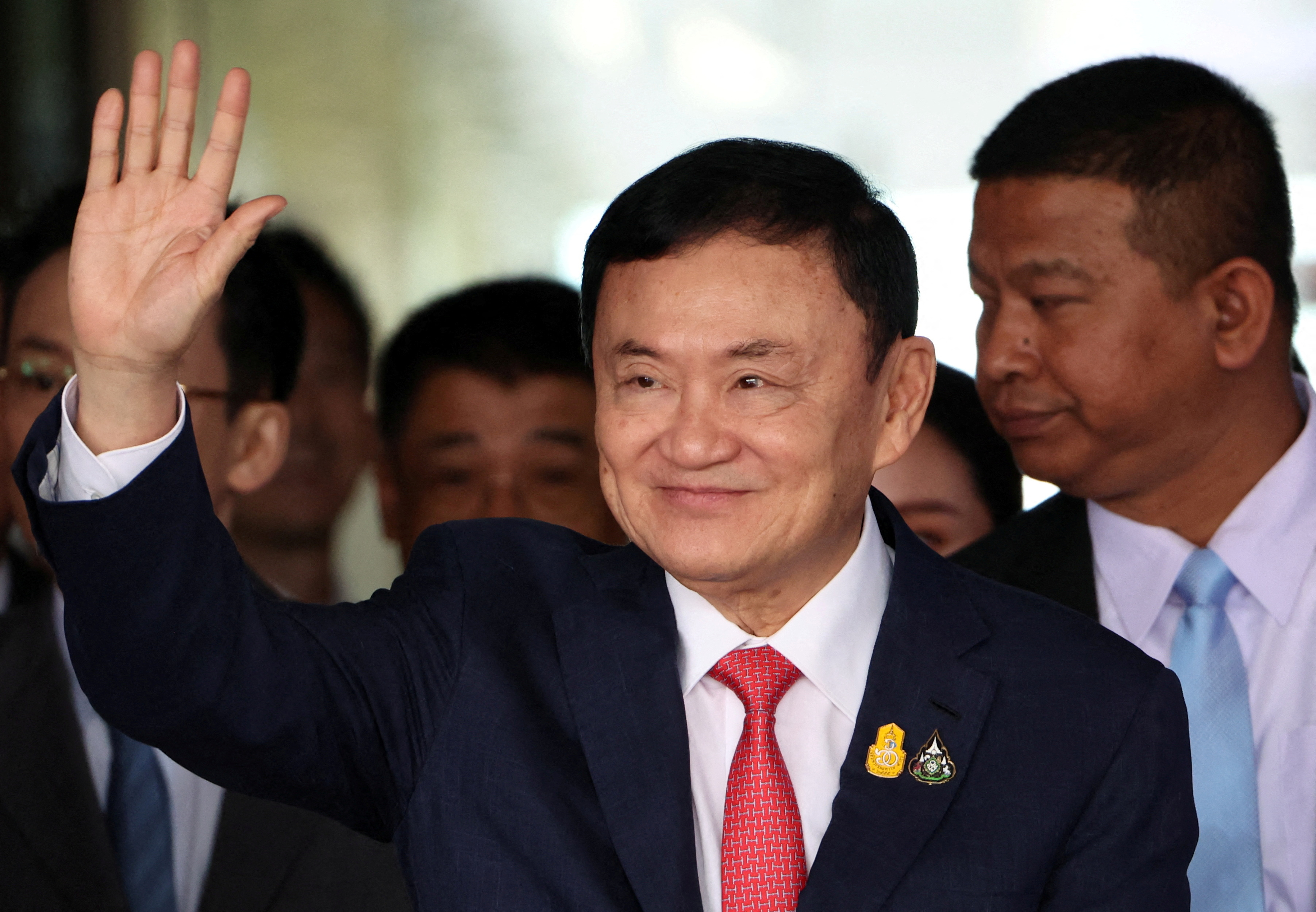
World Chevron
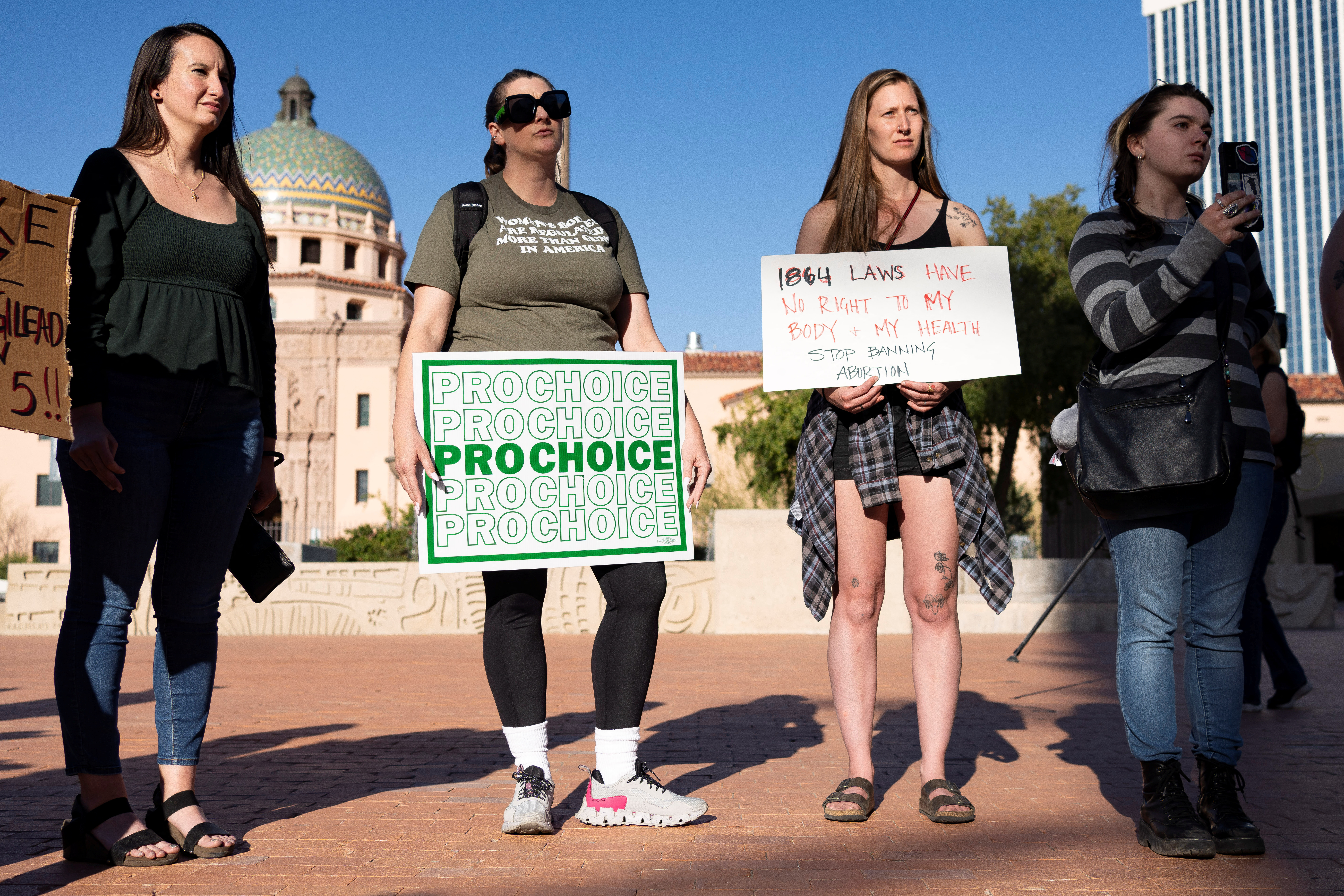
Arizona's top court revives 19th century abortion ban
Arizona's top court on Tuesday revived a ban on nearly all abortions under a law from 1864, a half century before statehood and women's suffrage, further restricting reproductive rights in a state where terminating a pregnancy was already barred at 15 weeks of gestation.
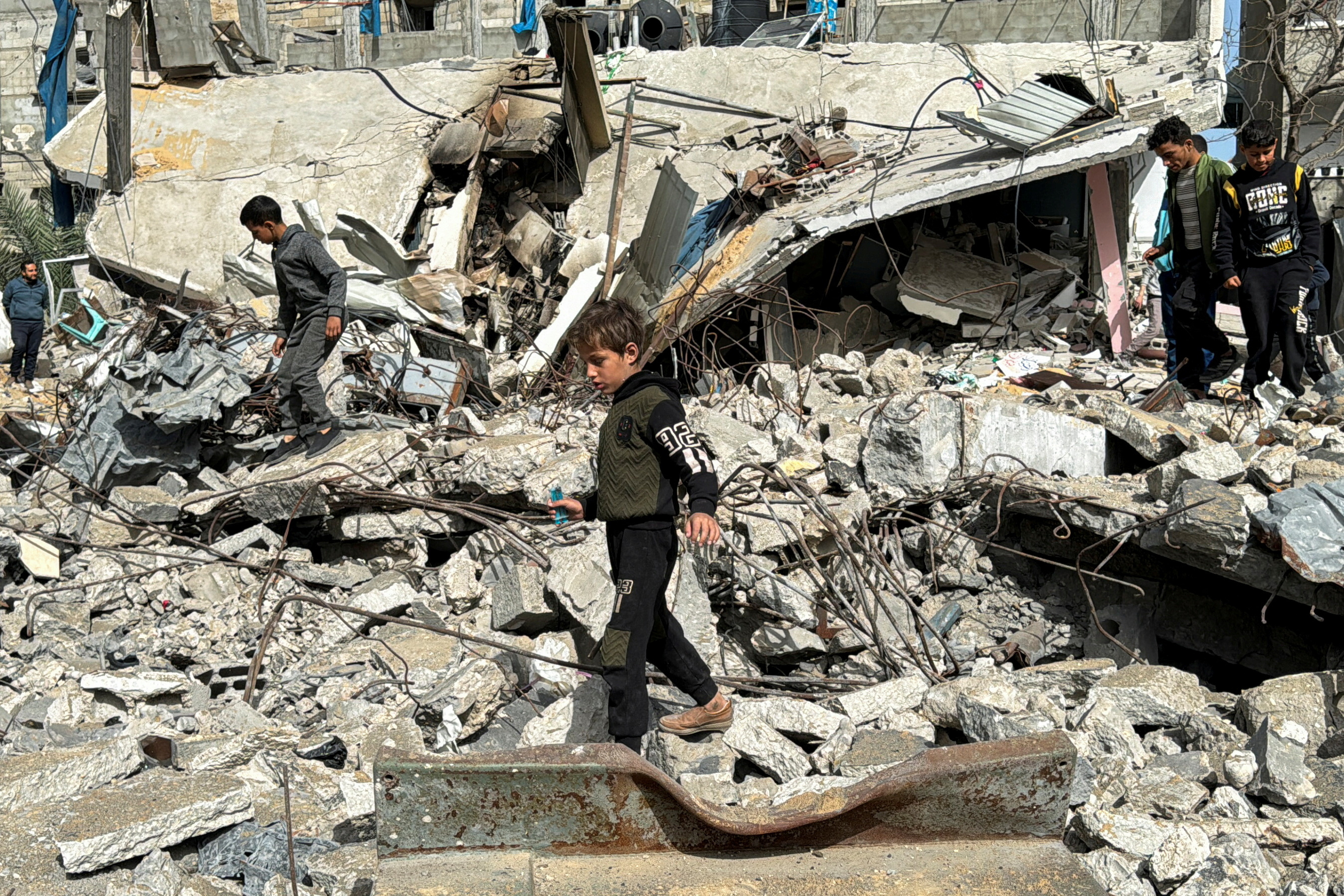
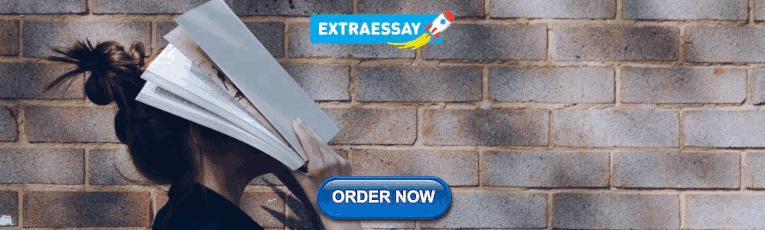
IMAGES
VIDEO
COMMENTS
Match the earthquake measuring instrument with its correct description. 1. Richter scale. 2. moment magnitude scale. 3. Mercalli intensity scale. 3 measures effects of an earthquake; measurement can vary from place to place. 1 measures strength of an earthquake; measurement is based on the size of seismic waves.
The earthquake has a magnitude _____. <2.0. Match the earthquake measuring instrument with its correct description. seismograph measures strength of an earthquake; measurement is based on the size of seismic waves. 2 measures strength of larger earthquakes; measurement is based on multiple variables. 3 measures effects of an earthquake ...
Students learn the two main methods to measure earthquakes, the Richter Scale and the Mercalli Scale. Students are challenged by the associated activities to make a model of a seismograph—a measuring device that records an earthquake on a seismogram. As well as to investigate which structural designs are most likely to survive an earthquake. And, they illustrate an informational guide to the ...
The focus , also called a hypocenter of an earthquake, is the point of initial breaking or rupturing where the displacement of rocks occurs. The focus is always at some depth below the ground surface in the crust, and not at the surface. From the focus, the displacement propagates up, down, and laterally along the fault plane.
Figure 11.3.1 11.3. 1 P-waves and S-waves from a small (M4) earthquake that took place near Vancouver Island in 1997. 11.03: Measuring Earthquakes#fig11.3.1. When body waves (P or S) reach Earth's surface, some of their energy is transformed into surface waves, of which there are two main types, as illustrated in Figure 11.3.2 11.3.
Earthquake magnitudes are determined by measuring the amplitudes of seismic waves. The amplitude is the height of the wave relative to the baseline (Figure 9.13). Wave amplitude depends on the amount of energy carried by the wave. The amplitudes of seismic waves reflect the amount of energy released by earthquakes.
In 1979, as geologists developed more accurate techniques for measuring energy release, a new scale replaced the Richter: the moment magnitude, or MW scale, which seeks to measure the energy released by the earthquake. It's also a logarithmic scale and comparable to Richter for small and medium quakes—a 5.0 on the Richter scale, for example ...
11.3 Measuring Earthquakes. There are two main ways to measure earthquakes. The first of these is an estimate of the energy released, and the value is referred to as magnitude. This is the number that is typically used by the press when a big earthquake happens. It is often referred to as "Richter magnitude," but that is a misnomer, and it ...
6.3: Measuring Earthquakes. There are two main ways to measure earthquakes. The first of these is an estimate of the energy released, and the value is referred to as magnitude. This is the number that is typically first released by the press when a big earthquake happens.
Today, scientists also can measure instrumental intensity directly, calculating and assigning intensities based on recorded ground shaking in different locations. While an earthquake has just one magnitude, its levels of intensity decrease with distance from the epicenter. The waves get smaller as they travel away from the fault.
The Richter scale (/ ˈ r ɪ k t ər /), also called the Richter magnitude scale, Richter's magnitude scale, and the Gutenberg-Richter scale, is a measure of the strength of earthquakes, developed by Charles Francis Richter in collaboration with Beno Gutenberg, and presented in Richter's landmark 1935 paper, where he called it the "magnitude scale". This was later revised and renamed the ...
6. focus. 7. liquefaction. 1smaller earthquakes that occur after a major earthquake. 4immediate return of deformed rock to its natural shape. 6specific point in the earth where the rock layers along a fault move, producing an earthquake. 5 location on the earth's surface directly over the focus of an earthquake.
The moment magnitude scale (M w) is more complex to calculate than the Richter scale. The basic formula for calculating the moment magnitude is: M w = 2/3 log (M 0) - 10.7. M 0 is the seismic moment, which is measured in dyne-cm (1 dyne-cm = 1×10 -7 joules). The seismic moment (M 0) is a measure of the total energy released by the earthquake.
Let's look at the mechanisms behind this. 1. The plates do not always move smoothly past each other at faults. 2. Sometimes plate edges get stuck because of friction but the rest of the plate keeps moving, very slowly. 3. The energy and pressure that move the plates get stored up at the edges. 4.
MMS measures the movement of rock along the fault. It accurately measures larger earthquakes, which can last for minutes, affect a much larger area, and cause more damage. The Moment Magnitude can measure the local Richter magnitude (ML), body wave magnitude (Mb), and surface wave magnitude (Ms).
Natural forces. Earthquakes are caused by the sudden release of energy within some limited region of the rocks of the Earth.The energy can be released by elastic strain, gravity, chemical reactions, or even the motion of massive bodies.Of all these the release of elastic strain is the most important cause, because this form of energy is the only kind that can be stored in sufficient quantity ...
Earthquakes are recorded by a seismographic network. Each seismic station in the network measures the movement of the ground at that site. The slip of one block of rock over another in an earthquake releases energy that makes the ground vibrate. That vibration pushes the adjoining piece of ground and causes it to vibrate, and thus the energy travels out from the earthquake hypocenter in a wave.
Magnitudes are based on a logarithmic scale (base 10). What this means is that for each whole number you go up on the magnitude scale, the amplitude of the ground motion recorded by a seismograph goes up ten times. Using this scale, a magnitude 5 earthquake would result in ten times the level of ground shaking as a magnitude 4 earthquake (and ...
A normal (dip-slip) fault is an inclined fracture where the rock mass above an inclined fault moves down (Public domain.) An earthquake is what happens when two blocks of the earth suddenly slip past one another.The surface where they slip is called the fault or fault plane.The location below the earth's surface where the earthquake starts is called the hypocenter, and the location directly ...
Intensity is a crude measure of earthquake size based on indirect, subjective descriptions, such as how strongly people reacted and the type and extent of building damage. This method was used primarily before the advent of modern seismic instruments. 2. Magnitude is a quantitative measure of earthquake size and is based on
Cross-section showing the fault area and the values that are used to compute the seismic moment. (Public domain.) Moment Magnitude (M W) is based on physical properties of the earthquake derived from an analysis of all the waveforms recorded from the shaking. First the seismic moment is computed, and then it is converted to a magnitude designed to be roughly equal to the Richter Scale in the ...
Quakes that range from 2.5 to 5.4 are felt, but cause only minor damage in most cases. That's the range where this quake in the Northeast falls, and there have been no reports of significant ...
Taiwan's biggest earthquake in at least 25 years killed nine people on Wednesday and injured more than 900, while 50 workers travelling in minibuses to a hotel in a national park were missing.