
An official website of the United States government
The .gov means it’s official. Federal government websites often end in .gov or .mil. Before sharing sensitive information, make sure you’re on a federal government site.
The site is secure. The https:// ensures that you are connecting to the official website and that any information you provide is encrypted and transmitted securely.
- Publications
- Account settings
Preview improvements coming to the PMC website in October 2024. Learn More or Try it out now .
- Advanced Search
- Journal List
- BMJ Glob Health
- v.7(6); 2022

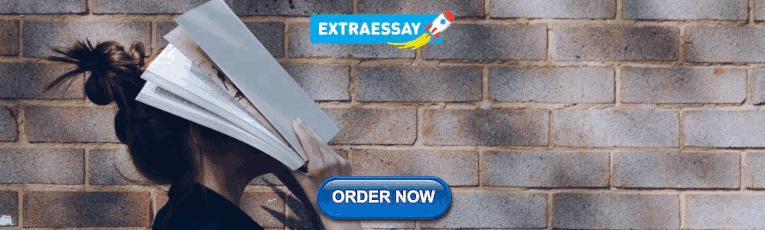
What India can learn from globally successful malaria elimination programmes
Sachin sharma.
1 ICMR-National Institute of Malaria Research, New Delhi, Delhi, India
Reena Verma
Bhawna yadav.
2 Division of Epidemiology and Communicable Diseases, Indian Council of Medical Research, Delhi, Delhi, India
Amit Sharma
3 International Centre for Genetic Engineering and Biotechnology, New Delhi, Delhi, India
Associated Data
All data relevant to the study are included in the article or uploaded as supplementary information.
India is targeting malaria elimination by 2030. Understanding and adopting the strategies employed by countries that have successfully eliminated malaria can serve as a crucial thrust in this direction for a geographically diverse country like India. This analysis is based on extensive literature search on malaria elimination policies, strategies and programmes adopted by nine countries (China, El Salvador, Algeria, Argentina, Uzbekistan, Paraguay, Sri Lanka, Maldives and Armenia) which have attained malaria-free status over the past decade. The key points which India can learn from their journey are mandatory time-bound response in the form of case reporting and management, rapid vector control response, continuous epidemiological and entomological surveillance, elevated community participation, more training and capacity building, private sector involvement, use of quality diagnostics, cross-border collaborations, inclusion of prevention of re-establishment programmes into the elimination plans, higher investment in research, and uninterrupted funds for successful implementation of malaria elimination programmes. These learnings would help India and other South Asian countries steer their programmes by devising tailor-made strategies for their own regions.
SUMMARY BOX
- By 2021, 40 countries have been certified by the WHO as malaria-free. There are 85 malaria-endemic countries, with an estimated 241 million cases in 2020.
- India continues to show a sustained decline in overall malaria but faces several challenges in its malaria elimination journey.
- An overview of malaria elimination strategies which were central to the success achieved by nine countries in the past decade is presented here. Some of these can be emulated by India and other South Asian countries to overcome the challenges in their elimination drive.
- Barriers to smooth adoption of these strategies in the Indian programme have also been described.
Introduction
The 2021 World Malaria Report (WMR) estimated 241 million cases with approximately 0.63 million malaria deaths from 85 malaria-endemic countries ( figure 1 ). Most of the deaths were reported in children under 5 years of age (~77%) and majority were in African nations (~96%). By 2021, 40 countries have been certified by the World Health Organization (WHO) as malaria-free, 1 two (Malaysia and the Islamic Republic of Iran) have achieved zero indigenous case status, and Azerbaijan and Tajikistan have applied for certification. 2 The world’s 11 highest malaria burden countries (India being one of them), accounting for 70% of global cases, have adopted the WHO’s High Burden to High Impact initiative to formulate a country-led response for malaria elimination. 3

World map depicting country-wise contribution of global malaria cases in 2020 and WHO-certified malaria-free countries so far. 1 2
India is slated for malaria elimination by 2030. 4 It contributed 83% of the estimated malaria cases and 82% of malaria deaths in South-East Asia Region (SEAR) in 2020, according to the 2021 WMR. Plasmodium falciparum and P. vivax are the major prevalent parasites in India. The country contributed 51% of the global P. vivax cases in 2016, when the country launched the National Framework for Malaria Elimination, outlining the goals, strategies, guidelines and time-bound targets to eliminate malaria in India by 2030. 4 Through concerted efforts, India has managed to sustain the decline in overall burden, with 186 532 cases and 93 deaths reported in 2020 by India’s national programme ( figure 2 ). Malaria endemicity in India is heterogeneous, with the highest endemicity regions being tribal and forested areas of the country. Analysis of epidemiological data from 2000 to 2019 revealed that forested districts contributed ~32% of malaria cases and 42% of mortality due to malaria, while harbouring ~6.6% of the country’s population. 5 The different topographies, climatic conditions and ecosystems support breeding and survival of Anopheles species. The major challenges towards malaria elimination in India are large population size, population movement across regions of different endemicities (the risk of parasite carriers moving from high endemic to low endemic areas), undetected asymptomatic and subpatent malaria cases, multiple vectors, threat of drug and insecticide resistance, shortage of skilled human resources, lack of reporting from the private sector, and unplanned expansion of urban and semi-urban areas. These factors add to the complexity of malaria transmission and make malaria elimination challenging in India.

Reported number of malaria cases and deaths in India and species-wise break-up of cases in India in the last 10 years (2011–2020). Data source: Directorate of the National Centre for Vector Borne Diseases Control, Government of India, and WHO World Malaria Report 2021. 2
Several countries share the above challenges associated with malaria control and prevention but have successfully eliminated malaria. Here, we have selected nine countries as they have successfully eliminated malaria in the last decade (2011–2021). We chose these countries for the reason that in these 10 years the most impactful interventions, namely insecticide impregnated bed nets, rapid diagnostics and artemisinin-based drug therapy, were deployed and they made a major dent in the burden of malaria in endemic countries. 6 Moreover, molecular surveillance of parasites and systematic periodic vector surveillance studies were established as the monitoring mechanisms during this decade itself. Additionally, some of these countries share a similar ecological and epidemiological scenario, such as the dominance of P. vivax cases in Sri Lanka and the southern part of India.
Therefore, we feel that studying these countries’ practices and policies would be insightful for Indian malaria programme managers and malariologists. For example, the diverse mosquito species that caused malaria in Maldives and Uzbekistan and their elimination strategies for vector control could be additional lessons for India. Environmental engineering methods as adopted by Argentina could be helpful in tackling forest malaria in India. El Salvador had successful strategies for active surveillance of migrant populations searching for employment. In India, the migrant population is a threat for reintroducing malaria in states which have reached almost zero indigenous cases. The strategy of Algeria in combating P. falciparum and the involvement of non-governmental organisations (NGOs) and private sectors in Sri Lanka and Paraguay could be inspiring strategies for India to imbibe. It is essential to reach population groups even in the hard-to-reach and conflict-ridden areas to achieve elimination. In this regard, India could learn from Sri Lanka’s elimination drive even during a civil war. If we consider China, its population size is similar to India. Also, its battle, like India, has been a long one but successful, and therefore these countries’ strategies in eliminating malaria are a great learning lesson for us.
We also realise that it is difficult to emulate all the strategies and practices of the victorious countries due to diversity in the epidemiological and entomological picture and the different population scale in India. However, we believe that India can imbibe many of the best practices followed by these countries by closely studying the factors and influences behind the successful elimination of malaria, and if possible replicate them at the appropriate level in India. In this paper, we have analysed the strategies/policies used by these countries in their fight against malaria and have listed them in the following sections, which can serve as torchbearers for India.
Key strategies adopted by some selected countries
In 2021, China, in the WHO Western Pacific Region (WHO WPR), was declared malaria-free after reporting the last indigenous malaria case in 2016 7 —this was a culmination of efforts of over ~70 years. P. vivax was the major parasite species of concern in China. At the time of transition of their programme from control to elimination in 2011, P. vivax malaria cases (2118 cases) were ~1.5 times of P. falciparum (1269 cases). 8 The policies and strategies which became the cornerstone of malaria elimination programme in China were the following: (1) In 1967, China launched a national effort called ‘the 523 project’, which resulted in the discovery of the artemisinin group of highly effective antimalarial drugs, which are the most potent antimalarial drugs to date. (2) Within the control strategy of malaria, mass drug administration (MDA) was used on a large scale (1973–1983). (3) During the decline phase (1981–2000), the country implemented the strategy of environmental management as well as protective measures for exposed population {early distribution of insecticide impregnated bednets and indoor residual spray (IRS) for vector control}, and then a foci-based response (2000-2009) to reduce the high burden of malaria in different provinces by stratification based on transmission risk and incidence. Under this phase they also introduced the National Malaria Elimination Action Plan that combined surveillance and response with real-time reporting. 9–11 (4) During the malaria elimination phase (2011–2020), the country adopted local, tailor-made, pragmatic approaches with deployment of the ‘1-3-7’ surveillance strategy, which meant prompt reporting of confirmed cases within a day to a web-based national case reporting system, further investigation within 3 days and genome sequencing to distinguish imported and indigenous cases, treatment within 3 days, and foci response and adopted reactive case detection (RACD) within 7 days to prevent further transmission. 12 Imported malaria was tackled by the ‘1-3-7’ strategy and by the collaborative approaches of health professionals at the border, with polymerase chain reaction (PCR) as an additional diagnostic tool used by the reference laboratories at the counties. For prevention of re-establishment (POR), they restructured the 1-3-7 approach to 3-3-7, where the diagnosis of case is completed within 3 days, reconfirmation and epidemiological investigation are done within another 3 days, and foci investigation and response completion is done within 7 days after diagnosis. Additionally, China in 2017 initiated a subnational malaria elimination drive for individual provinces which was in tandem with the WHO 2017 Malaria Elimination Programme. They invested in building systems such as the National Institute of Parasitic Diseases and the Chinese Center for Disease Control and Prevention (China CDC), supported by capacity building and web-based reporting system at the grass-roots level. They also collaborated with the Global Fund to Fight AIDS, Tuberculosis and Malaria (GFATM), which contributed to global malaria elimination efforts through collaborative projects which created an opportunity for mutual learning. 12
El Salvador
In 2021, El Salvador became the first country in the Central America of the WHO Region of the Americas (WHO AMR) to be certified as malaria-free. The last indigenous malaria case of P. falciparum in El Salvador was reported in 1995, while the last P. vivax cases were reported in 2016. 13 The key programmatic activities which possibly paved the way for elimination were the following: (1) geographical stratification using altitude and slide positivity rate data; (2) decentralisation for diagnosis facilities and data reporting; (3) weekly reporting systems and analysis; (4) computerised malaria information system; (5) decision on MDA and IRS at the local and regional level; (6) mandatory reporting by the private sector and (7) outbreak response on detection of two or more cases. 14
In 2019, Algeria, in the WHO African Region (AFR), was declared malaria-free by the WHO, with the last case of indigenous malaria reported in 2015. P. falciparum was the dominant parasite species. Geographical information system (GIS) mapping to identify imported cases of malaria, epidemiological surveys around each positive case and entomological surveillance to document the movement of mosquito vectors helped in curtailing imported malaria. 15
Argentina, in the WHO AMR, was certified by the WHO as a malaria-free country in 2019. In this South American country, the last indigenous case was reported in 2010. The most prevalent parasites in the country were P. falciparum and P. vivax . The key elements were IRS including at border areas, collaboration between border countries, prompt IRS by brigades on diagnosis of a malaria case and surveillance within the 500 meter radius of the identified case. Management of estuaries as breeding sites via infrastructural development and reintroduction of vertical vegetation, removal of green algae, and IRS with dichloro-diphenyl-trichloroethane (DDT) were especially helpful. 16
Uzbekistan, in the WHO European Region (WHO EUR), was declared malaria-free in 2018. The last locally acquired malaria case in Uzbekistan was reported in 2010. Both P. falciparum and P. vivax infections were prevalent in the country. Private sector involvement, supervised treatment for P. vivax cases, MDA, larvivorous fish Gambusia for vector control, and annual surveys to identify and liquidate water bodies acting as mosquito breeding grounds helped the country achieve malaria-free status in 2018. 17
Paraguay, in the WHO AMR, was declared malaria-free by the WHO in 2018, with the last indigenous case reported in 2011. 18 P. falciparum was the major reported parasite. Reporting of cases within 24 hours of detection and timely treatment, investigation of outbreaks within 24 hours of a case, GIS and establishment of a behavioural change communications plan for at-risk populations were some of the key steps.
Sri Lanka, which belongs to the WHO SEAR, was declared malaria-free in 2016, with the last case of indigenous P. vivax infection reported in 2012. 19 20 P. vivax and P. falciparum were the most prevalent parasites. Some of the crucial steps taken were the following: (1) the ‘1, 2, 3 approach’ that is confirmation within 24 hours (1 day) of malaria case by either a public or a private facility, investigation within 48 hours (2 days) and RACD within 72 hours (3 days); (2) close and periodic follow-up upto one year to tackle the resurgence of P. vivax cases in malaria camps and prevention of relapse due to lack of treatment compliance by directly observed primaquine (PQ) treatment was adopted 20 21 ; (3) public–private partnerships; (4) mobile clinics and (5) stringent vigilance on imported malaria, which is key to POR of malaria in Sri Lanka. 22 23 Despite facing a civil war, Sri Lanka achieved the elimination of malaria. The realisation that malaria is deadly but can be prevented and cured, the motivation and cooperation of conflicting groups to work with the government to protect the populations, and the involvement of neutral organisations played crucial roles. 24
Maldives became the first country within the WHO SEAR to eliminate malaria in 2015. The last case of indigenous P. vivax was reported in 1984, after which the reported cases were only from imported malaria. There are a total of 1200 islands in Maldives with large forested areas, out of which 198 are habitable. Important interventions which helped the country were the following: (1) hospital boat, called Golden Ray , which was equipped with medicines moved between the islands to treat patients (2) epidemiological and entomological surveys along with efforts to wipe out malaria vectors and (3) vigilance of imported malaria cases and vectors. 25
Armenia, in the WHO EUR, attained malaria-free status in 2011. The country reported its last indigenous case in 2009. P. vivax was the dominant parasite species and P. falciparum was via imported malaria. Mandatory notification, hospitalisation (in no later than 1–3 days), treatment of asymptomatic cases by supervised treatment, prophylaxis among military personnel and follow-up of patients for a period of 3 years for monitoring relapses were important steps. 26 27
Current challenges to India’s malaria elimination programme
India has managed to sustain the decline in overall malaria burden, but some of the significant current challenges which make malaria intractable in India are the following:
- Incomplete understanding of the actual burden of malaria as the private sector is not involved in data reporting, although it caters to the healthcare needs of a large section of the population in India.
- Inaccessible and remote areas of India are malaria-endemic (with persistent malaria), and providing health services to these communities becomes extremely difficult especially during monsoon and post-monsoon (transmission seasons) when these areas are cut off from the usual mode of communication and transportation.
- Uncertain contribution of asymptomatic and low-density malaria infections to continued transmission of malaria.
- Inadequate coverage and use of vector control products due to huge target population and time lag in replacements through a single channel of national control programme.
- Cross-border malaria and internal migration.
- Substantial burden of P. vivax malaria and weak mitigation policies and tools, such as missed diagnosis by the current methods, poor compliance to PQ’s 2-week course and lack of monitoring of relapses.
- Emergence of drug and insecticide resistance in India’s neighbouring countries and border areas poses a threat of introduction of resistant parasites and vectors in the country.
- Lack of skilled human resource in the national programme has been a long-standing challenge and more so with the integration of malaria programme with the general health services. Grass-root-level workers and healthcare staff are shared between several healthcare schemes and programmes and malaria may not be given the prioritisation it needs, more so when the target to report the last indigenous case is 2027 and elimination certification by 2030.
Lessons India can learn in its programme to accelerate malaria elimination
The national strategies for malaria elimination in the nine countries were mainly based on WHO guidelines and included intensified surveillance, vector control programmes, early diagnosis, rigorous case investigations, free and prompt treatment of patients based on malaria cases, and follow-ups. The key strategies of the nine countries are summarised in table 1 .
Countries certified as malaria-free by the WHO from 2011 to 2021 and their key elimination strategies
POR, prevention of re-establishment.
India, in its national programme for malaria elimination, has all the major and essential elements well documented, and perhaps most of the steps are in the right direction to make malaria elimination possible. Additionally, certain strategies/policies/activities of the successful countries can be emulated in the following facets of the Indian elimination programme:
Strengthening of surveillance
- Time-bound response by countries, such as the 1-3-7 strategy of China and the 1, 2, 3 strategy of Sri Lanka: a strong surveillance system was the most important pillar that played a crucial role in malaria elimination for most countries. The pivotal strategy, which could be adopted in our context, could be similar to those used in China and Sri Lanka. This will need to be supported by a strong web-based system that connects the public and private sectors at the level of healthcare to a central portal system. In India, at present, there is no time-bound strategy for mitigation of malaria cases on detection of a case. Hence, adopting some time frame would be useful in early management and thus in curtailing transmission. 28
- Modernisation of surveillance system, that is, digital near real-time surveillance and smart surveillance systems: in China, a smart web-based health information system called the Chinese information system for disease control and prevention used time-bound alert Short Message Service (SMS) for follow-up and control measures. El Salvador also used computerised management information system to overcome delays in manual reporting system. 14 29 Such strong real-time surveillance and data-based decision making at the local level were also applied in Uzbekistan and Maldives. Countries like China and El Salvador overcame barriers by integrating digital technology and mobile SMS systems in their malaria elimination programme. Although surveillance has been strengthened in the malaria elimination drive in India, it still needs to be more inclusive and comprehensive. Therefore, this is the right time for India to adopt smart digital tools for surveillance, 30 and discussions at the national malaria elimination programme have already begun in this direction. Following the same, the ICMR-National Institute of Malaria Research has developed a Malaria Dashboard that is ready for data reporting, collation, visualisation and research. Case-based and foci-based examinations are much required, particularly in low transmission areas to achieve elimination in India. 31 Hence, implementation of a robust surveillance system (digital methods such as electronic dashboards) is very important. 30 32 It is therefore an opportunity for India to revamp its surveillance strategies from the archaic paper-based and aggregated systems to near real-time, digital and technology-backed integrated systems. 33 Such changes may fasten the process of malaria elimination.
- Annual Parasite Index (API)-based stratification: India has stratified the states and districts (unit of implementation) based on their API. The subnational plan for elimination, although adopted by India, is yet to be fully deployed to certify states as malaria-free as and when they achieve this status.
- Focus on high transmission areas: India, in its national strategic plan, has focused on high-burden endemic regions. Regular process monitoring, innovative research and prompt translation in policy along with increased community mobilisation will play an important role in reducing malaria cases.
- Involvement of the private sector in reporting of malaria case data: Sri, Lanka, El Salvador and Paraguay have led by example and allowed active involvement of the private sector in the mainstream of malaria surveillance and management. In India, participation of the private sector is crucial because it provides 60%–70% of healthcare. The inclusion of this sector in India is in the nascent stages as there is no concrete roadmap towards this aim. We have suggested ways to involve the private sector in our previous work. 34 Additionally, India could gain insights from the national programmes of Sri Lanka, El Salvador and Paraguay which have actively involved private providers. India has made malaria a notifiable disease in 31 states, but it is only an initial step towards tackling under-reporting and underestimation of malaria burden in the country. Rapid diagnostics as field diagnostics have been the cornerstone of early identification and thus timely management of cases. Over-the-counter availability of rapid diagnostic tests (RDTs) in the commercial sector can empower people to self-diagnosis and reporting to the healthcare system. 34
Use of molecular tools of diagnosis and national reference laboratories
Prompt and accurate diagnosis of malaria cases, including the hidden burden of asymptomatic and subpatent infections, is important as it will help in treatment and thus cessation of transmission. Adoption of molecular methods for diagnosis in the national programme would be a way forward. The WHO recommends microscopy as the gold standard and RDT as field diagnostics. Use of molecular tools like PCR and loop-mediated isothermal amplification for RACD, as adopted by China, was useful in the identification and resolution of all malaria cases. India suffers from considerable burden of low-density infections which escape detection by routine diagnostics. 31 The development of field-friendly, point-of-care/collection molecular tests could help bring out the burden and management of subpatent malaria. 35 District-level healthcare facilities have been empowered in terms of infrastructure and expertise owing to the COVID-19 pandemic and these can be co-opted for diagnosis of submicroscopic malaria. 36 37 In India, the barrier to adopting molecular tools in its routine programme would be the prohibitive cost of infrastructure and training of the laboratory workforce. However, countries like China and Sri Lanka have shown the way that it is possible to use these more sensitive tools as routine diagnostics. The establishment of the National Reference Laboratory for quality assurance was adopted by China, El Salvador, Uzbekistan, Paraguay and Armenia. Having such central hub that connects all states and district-level laboratories can step up India’s centralized diagnostic structure.
- India, in its national guidelines, has a special emphasis on P. vivax elimination. The foreseeable barriers for India are poor compliance of PQ treatment, inadequate follow-up of patients with P. vivax malaria, cross-border and migration issues. Compliance to antimalarials, especially for P. vivax malaria, which needs 14-day treatment with PQ is a daunting challenge in India. Almost half of India’s malaria burden is P. vivax malaria. It is widely acknowledged that it will be difficult to achieve elimination of P. vivax as compared with P. falciparum . Adoption of single-day treatment with tafenoquine may be considered by India after due regulatory consideration. 38 The issue of compliance can be overcome if the P. vivax antimalarial therapy is administered as directly observed treatment (DOT). We could follow the Sri Lankan example which to tackle the P. vivax resurgence in army camps adopted PQ directly observed treatment for infected army personnel. 20 21 El Salvador and Uzbekistan supervised the PQ treatment. Similarly, Armenia had also introduced DOTs for both P. falciparum and P. vivax control in their national programme. Good compliance to 14-day radical treatment with primaquine along with estimation of glucose-6-phosphate dehydrogenase (G6PD) deficiency in the population, addressing low-density infections and tackling asymptomatics will play pivotal role in P. vivax elimination.
- Follow-up of P. vivax cases to capture relapse cases: P. vivax malaria is characterised by latent hypnozoites which can get activated in variable durations. Therefore, it is important to follow these cases so as to capture relapse cases and treat them on time. In the national guidelines of Sri Lanka, El Salvador, Armenia, Algeria and Maldives, at least 6 months to 1 year of follow-up was practised.
- Cross-border malaria: countries which have achieved malaria elimination have robust mitigation strategies against imported malaria. Cross-country cooperation is at the centre of the elimination programme. Strict surveillance of cross-border transmission as embraced by China, Algeria, El Salvador, Sri Lanka, Armenia, Paraguay and Uzbekistan with deployment of mobile teams and examination of travellers from malaria-endemic countries through RACD could be possible steps which need induction in our control guidelines. India has porous borders with many neighbouring malaria-endemic countries and also poses a threat to the neighbouring countries which are at the cusp of elimination such as Bhutan. 39 Therefore, India should institute strong parasite and vector surveillance programmes to curtail exchange. Cross-border malaria issues have been handled well by China, Algeria, El Salvador, Sri Lanka, Armenia, Paraguay and Uzbekistan by intense surveillance of migrants and travellers.
- Internal migration: El Salvador had carried out active surveillance and chloroquine+primaquine (CQ+PQ) single-dose prophylaxis for migrant populations in employments such as cotton production, coffee fields or factories. 13 In India, the interstate movement of people is high for employment, tourism and other purposes. Special attention is needed in areas where malaria elimination status has progressed from control to pre-elimination phase. For example, the state of Punjab has shown a drastic decline in the number of malaria cases. 40 However, there is always a risk of re-establishment owing to the constant influx of migrant labourers for construction/agriculture activities in the state. This mobile population is very often from malaria-endemic states such as Bihar, Chhattisgarh and Jharkhand and thus could act as reservoirs for the parasite resulting in re-establishment of infection. Thus, India could also consider devising plans for screening, treating and reporting of malaria cases among such migrant populations.
- Many malaria elimination demonstration projects have successfully shown that adopting certain strategies can dent the malaria endemicity situation. One of the programmes, the Comprehensive Case Management Plan (CCMP), has been adopted by the government of Odisha as the Durgama Anchalare Malaria Nirakarana (DAMaN) programme for mitigation of malaria in inaccessible areas and has contributed to a remarkable decline in malaria. Biannual screening of malaria in mass surveys and subsequent treatment has been adopted as a programme strategy in this state’s malaria plan. 41
- In order to implement public–private partnerships, Indian policy makers should devise a well-defined strategy to work locally and focally at panchayat/district levels with emphasis on common platform for reporting, regular communication and assessment of progress.
Vector control and management of resistance
- Robust vector surveillance using smart tools: China, Algeria and Sri Lanka adopted a robust vector surveillance with the use of GIS and spatiotemporal analysis. Similar to these platforms, a web-based database of vector surveillance should be adopted in India.
- Insecticide resistance management (IRM): Resistance to routinely used insecticides (DDT and some synthetic pyrethroids) has been reported in the malaria-endemic areas of India. Frequency of insecticide resistance should be monitored at sentinel sites periodically. IRM with rotational or mosaic pattern with insecticides of different mechanisms of action has been deployed by Sri Lanka. Focal IRS in areas such as plantations, factories and along countries’ border with high endemicity regions have been adopted by China, Sri Lanka and El Salvador. Environmental engineering methods were adopted by Argentina. All these strategies and tools could be useful to India with its diverse geographical regions. Laboratory studies conducted in India are indicative that newer tools like attractive toxic sugar baits (ATSBs) could be a promising vector control. 42
- Integrated vector management (IVM): IRM and IVM, although discussed in India, are not holistically implemented. In Maldives, elimination of malaria vectors was one of the key contributing factors to maintaining a malaria-free status since 1984. El Salvador and Armenia implemented water management projects to reduce mosquito breeding sites and also planted neem trees to prevent mosquitoes in the surrounding areas. India should consider IVM as an umbrella vector management programme.
Role of partners
Currently, the different partners and stakeholders in India are working in silos towards the common goal of malaria elimination. There is a lack of cohesion at the central level and thus at the peripheral levels. Experiences of other countries can be leveraged on using the strengths of partner organisations and taking them in the fold of the national programme. Sri Lanka’s successful elimination was achieved despite facing a civil war. Indian policy makers could involve NGOs, private partners and voluntary collaborative network, as adopted by Sri Lanka, El Salvador, Armenia, Paraguay and Algeria, to enhance successful deployment of all components of malaria elimination. Involvement of intersectoral ministries and uninterrupted funding were adopted in Sri Lanka to eliminate the disease. National and international partners are crucial to achieving malaria elimination, especially for overcoming the last-mile challenges. 39
Prevention of re-establishment strategies
POR of malaria transmission in a malaria-free country is a daunting task. After elimination, active case detection (ACD) with mobile malaria clinics is still maintained in Sri Lanka. Algeria has taken stern steps in quickly identifying any imported malaria, followed by appropriate POR actions. Obligatory notification and reporting of malaria and timely epidemiological investigation of each imported case and focus are followed by Paraguay, Uzbekistan, El Salvador and Maldives. These activities would need to be undertaken by the states which have eliminated malaria to prevent its re-establishment from other states. India would need robust alert systems and prompt surveillance and diagnostics to mitigate the threat of imported malaria, as done by other countries.
Domestic funding and sustained political commitment
In addition to global and international funding {GFATM, World Bank, President’s Malaria Initiative (PMI)/United States Agency for International Development (USAID)}, China, Sri Lanka, Algeria, Armenia, Uzbekistan and El Salvador allotted heavy domestic funding for malaria elimination. 2 In India, the national programme is mainly funded by the Government of India, but the Global Fund is the major financial source for procurement of long-lasting insecticidal nets (LLINs) in India. From 2016 to 2018, ~80% (~40 million) of the LLINs distributed in the country were procured by the Global Fund financial resources. Political and financial commitment from the government is vital to maintain the momentum of the malaria elimination programme in India. 43 India needs to pledge substantial funds for sustenance of the national programme for malaria elimination and beyond. Provision of funds in the scenario of withdrawal of the Global Fund also needs to be created.
Promoting research
Research and Development (R&D) need constant thrust as the limitations of the currently available tools can hinder the achievement of malaria elimination, and threats like drug and vector resistance need investment in research to discover newer tools. Malaria-free countries have invested in research to identify the most optimum strategies, tools and operations. China invested in R&D and artemisinin derivatives have been the cornerstone of malaria treatment. In 1967, the CDC Division of Parasitic Diseases was set up in San Salvador. 44 Efforts should be put in to develop and validate non-histidine-rich protein (non-HRP) 2/3-based RDTs, feasibility of a single dose of tafenoquine for P. vivax malaria 38 , field-friendly molecular tools, and robust research to assess the impact of climate change on malaria transmission. 45 Research into the possible zoonotic transmission of malaria parasites in India should also be considered. Innovative approaches to mosquito control like ATSB, 42 insecticide impregnated clothing, hammocks etc could be explored to address the challenges associated with forest malaria, 5 outdoor biting and insecticide resistance. Concerted efforts have been initiated in this direction by a nodal research body of the Government of India. 46 Consolidation and implementation of the research findings into policy and practice would provide the necessary thrust to malaria elimination in India. However, poor investment and lack of priority to continue research once elimination is near or achieved can derail research programmes on promising tools. Advocacy for continued and breakthrough research needs to be made at the highest level.
India’s track record in elimination of other infectious diseases
Despite enormous population and diverse geographical conditions, India has successfully eliminated polio (2014), 47 smallpox (1977) 48 and guinea worm disease (2000) 49 50 and is possibly on track to eliminate tuberculosis by 2025.
India was certified polio-free in 2014. Considering the scale at which the polio vaccination drive was conducted, it can be considered as one of the biggest success stories. However, the path was not easy for India. The challenges ranged from arranging logistics for a large population and reaching even the hard-to-reach areas, building trust, changing perceptions and convincing the communities to accept vaccines amidst anti-vaccine movements. 51 This was achieved by enhanced communication within the community, by involving local representatives and religious leaders, and by providing basic health packages to address immediate concerns regarding other health issues. Screening of migrant and mobile populations and international travellers played a crucial role in identifying potential sources of continued transmission. In addition to government officials, several partners worked collectively in the polio elimination drive. Defining the role and accountability of each of the public and private stakeholder was also done to avoid redundancy in the tasks performed. Further, the accuracy of data was monitored to get reliable information on disease prevalence. The need for training healthcare workers was identified and addressed by capacity building and repeated trainings. 52
As India is hurtling towards malaria elimination, it is the most appropriate time to review and assess the strategies and practices by countries that have been successful in achieving malaria elimination. The same can be tailored according to India and neighbouring South Asian countries as they share several commonalities in the context of environmental conditions, vectors, parasites, community behaviour and health infrastructures. These shared features could be the basis of cross-learnings and can help India and others steer their malaria elimination programme.
Acknowledgments
We acknowledge Bhabani Shankar Muduli and Mansi Arora for their contribution and help in generating the world map depicting country-wise contribution to global malaria cases (figure 1).
Handling editor: Seye Abimbola
Contributors: MR and AS conceived the idea and framed the manuscript. SS wrote the initial draft. RV, BY and AK did review of literature, analysis and graphics. All authors read and approved the manuscript.
Funding: The authors have not declared a specific grant for this research from any funding agency in the public, commercial or not-for-profit sectors.
Map disclaimer: The inclusion of any map (including the depiction of any boundaries therein), or of any geographic or locational reference, does not imply the expression of any opinion whatsoever on the part of BMJ concerning the legal status of any country, territory, jurisdiction or area or of its authorities. Any such expression remains solely that of the relevant source and is not endorsed by BMJ. Maps are provided without any warranty of any kind, either express or implied.
Competing interests: None declared.
Provenance and peer review: Not commissioned; externally peer reviewed.
Data availability statement
Ethics statements, patient consent for publication.
Not required.
Thank you for visiting nature.com. You are using a browser version with limited support for CSS. To obtain the best experience, we recommend you use a more up to date browser (or turn off compatibility mode in Internet Explorer). In the meantime, to ensure continued support, we are displaying the site without styles and JavaScript.
- View all journals
- My Account Login
- Explore content
- About the journal
- Publish with us
- Sign up for alerts
- Open access
- Published: 28 December 2023
The effectiveness of malaria camps as part of the malaria control program in Odisha, India
- Danielle C. Ompad 1 ,
- Timir K. Padhan 2 na1 ,
- Anne Kessler 3 na1 ,
- Yesim Tozan 1 ,
- Abbey M. Jones 1 ,
- Anna Maria van Eijk 3 , 4 ,
- Steven A. Sullivan 3 ,
- Mohammed A. Haque 2 ,
- Madan Mohan Pradhan 5 na2 ,
- Sanjib Mohanty 2 na2 ,
- Jane M. Carlton 1 , 3 , 6 na2 &
- Praveen K. Sahu 2 na2
Scientific Reports volume 13 , Article number: 22998 ( 2023 ) Cite this article
1125 Accesses
6 Altmetric
Metrics details
- Epidemiology
Durgama Anchalare Malaria Nirakaran (DAMaN) is a multi-component malaria intervention for hard-to-reach villages in Odisha, India. The main component, malaria camps (MCs), consists of mass screening, treatment, education, and intensified vector control. We evaluated MC effectiveness using a quasi-experimental cluster-assigned stepped-wedge study with a pretest–posttest control group in 15 villages: six immediate (Arm A), six delayed (Arm B), and three previous interventions (Arm C). The primary outcome was PCR + Plasmodium infection prevalence. The time (i.e., baseline vs. follow-up 3) x study arm interaction term shows that there were statistically significant lower odds of PCR + Plasmodium infection in Arm A (AOR = 0.36, 95% CI = 0.17, 0.74) but not Arm C as compared to Arm B at the third follow-up. The cost per person ranged between US$3–8, the cost per tested US$4–9, and the cost per treated US$82–1,614, per camp round. These results suggest that the DAMaN intervention is a promising and financially feasible approach for malaria control.
Similar content being viewed by others
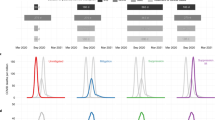
The potential public health consequences of COVID-19 on malaria in Africa
Ellie Sherrard-Smith, Alexandra B. Hogan, … Thomas S. Churcher
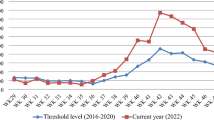
Malaria surveillance, outbreak investigation, response and its determinant factors in Waghemra Zone, Northeast Ethiopia: unmatched case–control study
Habtu Debash, Marye Nigatie, … Alemu Gedefie
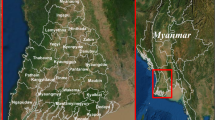
Factors associated with the decline of malaria in Myanmar’s Ayeyarwady Region between 2013 and 2017
Sarah Gallalee, Abigail V. Ward, … Myat Min Tun
Introduction
India has made noteworthy progress towards malaria elimination, with cases decreasing from 20 million in 2000 to approximately 4.1 million cases in 2020 1 . Despite this decline over the past two decades, it remains an important public health problem. India was one of eleven countries accounting for 70% of the global burden of malaria in 2020 1 , and it continues to account for 79% of all malaria cases and 83% of all malaria deaths in the South-East Asia region 2 . Within India, the state of Odisha has the highest burden of malaria, accounting for 22.4% of all cases in the country in 2020, of which 91.4% were Plasmodium falciparum infections 3 . The malaria burden in Odisha has been persistently high in the remote, forested areas of the state. In response to this, the Government of Odisha implemented the Durgama Anchalare Malaria Nirakaran (DAMaN; ‘malaria control in inaccessible areas’) program in 2017. DAMaN was designed to supplement existing and routine malaria control programs that serve approximately 5000 inaccessible villages and hamlets in the rural parts of the state 4 .
A key activity of the DAMaN program is the implementation of ‘malaria camps’ (MCs). In the MC model, teams of health workers visit villages and hamlets to provide the intervention which includes seven key activities in each cycle: (1) one round of mass screen and treat (MSAT) before the monsoon season conducted with point-of-care rapid diagnostic tests (RDTs), (2) one or two rounds of fever screen and treat (FSAT) during or after the monsoon season, (3) IRS (indoor residual spraying), (4) other vector control methods, (5) LLIN (long-lasting insecticidal net) distribution (not on an annual basis), (6) educational programming, and (7) maternal and child health visits and screenings. The intervention has been described in detail 4 , 5 , 6 , 7 . The control condition, as a part of the National Vector Control Strategy, is standard of care (SOC) whereby Accredited Social Health Activists (ASHAs) conduct door-to-door fever surveillance weekly. Fever cases are tested with RDTs and treated; severe cases are referred to hospitals 8 . IRS is conducted twice in a year during the transmission season in selected high risk areas having an annual parasite index (API) > 5, and LLINs are distributed in high risk areas having API > 2 9 . Thus key distinguishing features of MCs versus SOC include MSAT (everyone is offered malaria screening regardless of fever status in the MCs), educational programming, and maternal and child health visits and screenings.
Odisha state has seen a > 80% decline in malaria cases since 2017, attributed to the large-scale distribution of LLINs and the DAMaN program 10 . The aim of our project was to evaluate the effectiveness of the DAMaN MCs through a quasi-experimental cluster-assigned (i.e., non-randomized) stepped-wedge study with a pretest–posttest control group design.
We enrolled 2463 participants into three study arms: six immediate interventions (Arm A), six delayed interventions (Arm B), and three previous interventions (Arm C), and sampled them at baseline, with three follow-ups from August 2019 to December 2020. The primary outcome was PCR + Plasmodium infection prevalence. A time (i.e., visit) \(\times\) study arm interaction revealed statistically significant lower odds of PCR + malaria in Arm A versus B at the third follow-up. Our results suggest that the DAMaN program’s malaria camps were associated with lower malaria incidence relative to standard-of-care.
Study population and study design
Fifteen villages were selected in the northern districts of Keonjhar and Jharsuguda in Odisha state, India, in consultation with the Odisha Malaria Control Program (Fig. 1 ). The villages were distributed between three study arms: six villages in Arm A ‘new-MC’ (communities receiving MCs for the first time in year one), six villages in Arm B ‘delayed-MC’ (communities undergoing routine malaria control in year one and receiving MCs for the first time in year two), and three villages in Arm C ‘old-MC’ villages, where MCs had already been implemented prior to the study period. A flow chart of the study is shown in Fig. 2 .
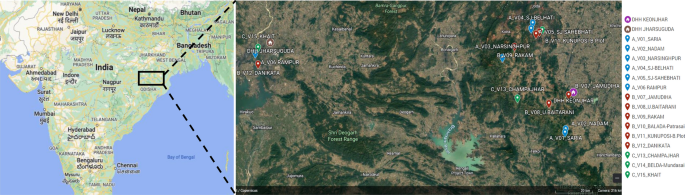
Location of 15 hard-to-reach villages in Odisha, India. The two DHH (district headquarter hospitals) are indicated, and each village is named with its study arm letter (A, B, C) and village number (V1–15) provided. Maps created with Google©2023, INEGI.
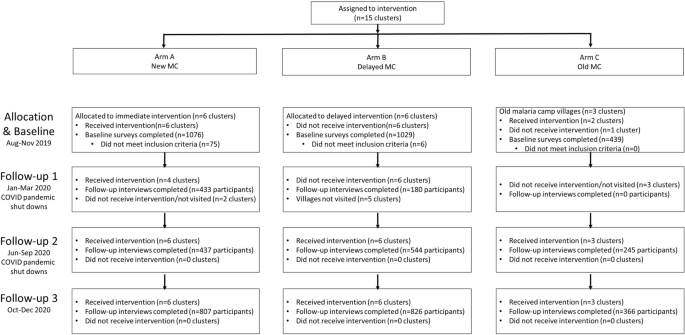
Cluster-assigned quasi-experimental study of malaria camps as part of the DAMaN malaria elimination program in Odisha, India. Flow chart providing a summary of the 15 clusters (villages) distributed between three arms and sampled at baseline and three follow-ups. A timeline of the activities is also given, and whether COVID-19 shutdowns are known to have occurred.
We enrolled 2463 participants in the study and Table 1 presents their baseline demographic characteristics. A total of 14.1% of the participants were aged 5 years or younger, 29.4% were aged 6 to 17, 23.6% were 18–34, and 32.8% were aged 35 or older, with females comprising 56.1% of the participants. The participants generally had low educational attainment: almost half (49.3%) had no schooling/less than primary school whereas 5.7% had higher secondary school or more. With respect to occupation, 28.8% were housewives, 34.9% were students, 5.6% were farmers/agricultural laborers, 18.6% were employed in another trade, 9.6% were children that were not in school, and 2.6% did not have an occupation.
Across the study arms there were statistically significant differences in age, educational attainment, and occupation, but not sex. As evidenced by the age category distribution and the mean age for each arm, Arm A was older (mean age [M age ] = 27.2, standard deviation [SD] = 19.0) and Arm C was younger (M age = 22.2, SD = 18.8) than Arm B (M age = 25.7, SD = 19.6, p ANOVA < 0.001). A higher proportion of participants in Arm A had higher secondary education or higher while a higher proportion in Arm B had middle, secondary, or matric and in Arm C a high proportion had primary education. There were a higher proportion of farmers/agricultural laborers and people with other employment/trades in Arm A, a higher proportion of housewives in Arm B, and higher proportions of students and children not in school in Arm C. Given the differences across arms, adjusted odds ratios (aORs) control for demographics.
Intent-to-treat analysis for primary and secondary malaria outcomes
The MC intervention was revised from our initial protocol paper 7 with two major and consistent differences. First, all MCs conducted MSAT rather than FSAT in all rounds of the intervention. Second, LLINs were not distributed in our study villages because their 3-yearly replenishment distribution scheduled for 2020 was delayed due to the Covid-19 pandemic.
Table 1 presents the malaria outcomes at baseline and the third follow-up (FU3) visit; associations are considered statistically significant if p < 0.013, based on a Bonferroni correction. At baseline, 9.8% of the sample had PCR + Plasmodium infection, with statistically significant differences across study arms. Arm A had the highest prevalence of Plasmodium infection (14.2%), followed by Arm B (8.1%) and Arm C (3.9%, p < 0.001) (Fig. 3 , panel A). Prevalence of RDT + Plasmodium infection was 1.9% and there were no significant differences across arms; Arm A again had the highest prevalence of Plasmodium infection (2.7%), followed by Arm B (1.8%) and Arm C (0.5%, p = 0.014). When malaria was defined by any positive PCR or RDT test, prevalence overall was 9.7% at baseline. With respect to species, 236 (98.3%) were P. falciparum cases and 9 (3.8%) were P. vivax ; 5 (2.1%) were co-infected with P. falciparum and P. vivax [data not shown]. Approximately 48.8% of Plasmodium infections were asymptomatic (defined as RDT + or PCR + and no fever) at baseline with Arm C having the highest proportion (94.1%) of asymptomatic cases as compared to Arms A and B (Fig. 3 , panel C: 40.4 and 53.7%, respectively; p < 0.001). A substantial majority (80.3%) of Plasmodium cases were subpatent (i.e., RDT- and PCR +), with no significant differences across arms (Fig. 3 , panel D).
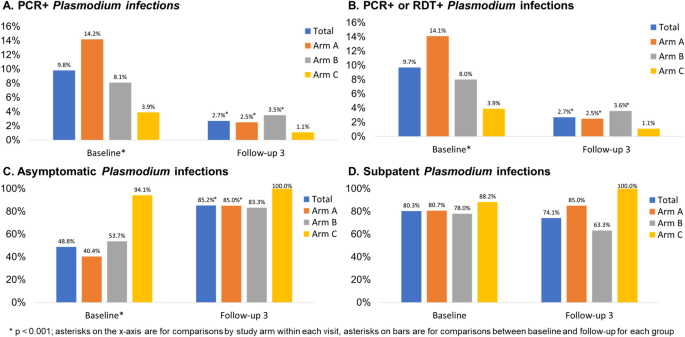
Plasmodium infection outcomes at baseline and follow-up 3 by study arm, Odisha, India. Four panels of data showing: (A) PCR + and (B) PCR + or RDT + Plasmodium infection prevalence at baseline (n = 2463) and follow-up 3 (n = 1999); (C) asymptomatic Plasmodium infection prevalence at baseline (among n = 240 total infections) and follow-up 3 (among n = 54 total infections); (D) subpatent Plasmodium infection prevalence at baseline (among n = 240 total infections) and follow-up 3 (among n = 54 total infections).
At FU3, 2.7% of the sample had PCR + Plasmodium infection, with no significant differences across arms (Fig. 3 , panel A). Prevalence of RDT + Plasmodium infection was 0.7% overall at follow-up, with 0.4% prevalence in Arm A, 1.3% prevalence in Arm B, and no observed cases in Arm C Table 1 , p = 0.017). When malaria was defined by any positive PCR or RDT test, prevalence overall was 2.7% at FU3 (Fig. 3 , panel B). When we compared malaria outcomes from baseline to follow-up for the entire sample as well as each arm, we found that there was a statistically significant reduction in both RDT + and PCR + Plasmodium infection in the sample overall and for Arm A. We observed a statistically significant reduction in PCR + but not RDT + Plasmodium infection in Arm B and no significant differences in Arm C. With respect to species, 53 (98.1%) were P. falciparum cases and 1 (1.9%) was P. vivax ; none were co-infected with P. falciparum and P. vivax at FU3. Approximately 85.2% of Plasmodium infections were asymptomatic at FU3 with no significant differences across arms but statistically significant increases in the proportion of cases that were asymptomatic from baseline to FU3 for the entire sample (Fig. 3 , panel C, p < 0.001), Arm A (p = < 0.001), and Arm B (p = 0.004) but not Arm C. A substantial majority (74.1%) of Plasmodium cases were subpatent at FU3, with no significant change from baseline to FU3 (Fig. 3 , panel D).
The overall follow-up rate for FU3 was 81.2% with a range of 80.6 to 83.4% across arms, with no significant differences across arms (Table 1 ). The sociodemographic and malaria outcome correlates of participants lost-to-follow-up (LTFU) is shown in Supplemental Table 1. There were no significant differences in LTFU by study arm or sex. Younger people were more likely to be LTFU as were those with lower educational attainment, students, and children not in school. Those with malaria, regardless of detection method, were statistically significantly more likely to be LTFU but there were no significant differences by asymptomatic or subpatent cases.
Table 2 presents the crude and adjusted multilevel mixed effects logistic regression models for the primary and secondary malaria outcomes. The adjusted models controlled for baseline Plasmodium infection, age, and gender given baseline differences in Plasmodium infection prevalence and demographics across study arms. Education and occupation were not included in the model, although they were statistically significant in the bivariate analyses, due to multicollinearity. The time (i.e., baseline vs. FU3) x study arm interaction term shows that there were statistically significant lower odds of PCR + Plasmodium infection in Arm A (AOR = 0.36, 95% CI = 0.17, 0.74) but not Arm C as compared to Arm B at follow-up. The adjusted model for PCR + or RDT + Plasmodium infection showed similar results. For RDT + Plasmodium infection, odds ratios could not be calculated due to small numbers of RDT + Plasmodium infections at FU3. Village-specific Plasmodium infection detected by PCR at baseline and FU3 are provided in Supplemental Table 2.
Intent-to-treat analysis for secondary outcomes
The anthropometric and clinical outcomes at baseline and the third follow-up visit are shown in Table 3 ; associations are considered statistically significant if p < 0.013. At baseline, 2.4% were severely underweight, with no differences across study arms. At follow-up, the prevalence of severe underweight decreased to 1.5%; there were still no differences across arms in underweight at follow-up. Few (2.2%) were severely anemic, with significantly more anemia in Arm A (5.1%) as compared to Arms B and C (0.1 and 0.7%, respectively; p < 0.001). At follow-up there were no significant differences in anemia across arms, but there were statistically significant decreases from baseline to follow-up for the total sample and Arm A.
At baseline, prevalence of fever was 24.2%. Arm B had the highest prevalence (38.0%) as compared to Arm A (14.6%) and Arm C (13.9%, p < 0.001). There were no significant differences in fever across arms at follow-up, although there were statistically significant reductions from baseline to follow-up overall and for each arm (p < 0.001). Prevalence of malnutrition measured by mid-upper arm circumference (MUAC) was 19.3% at baseline, with higher prevalence in Arm C (26.4%) as compared to Arms A (18.2%) and B (17.2%, p < 0.001). At follow-up there were statistically significant differences in malnutrition across arms but no significant differences from baseline to follow-up. Crude and adjusted multilevel mixed effects logistic regression models were not estimated for these secondary outcomes due to small numbers (i.e., severe underweight, severe anemia, fever) and/or few significant differences in the bivariate analyses comparing baseline to follow-up (i.e., severe anemia and MUAC malnutrition).
Malaria exposure and serology in a subset of study participants
We assayed 285 baseline samples (238 PCR + and 47 PCR-) for anti- Plasmodium antibodies to 13 P. falciparum -specific antigens and four P. vivax -specific antigens using the Luminex MAGPIX platform and xPONENT software to estimate mean fluorescence intensity (MFI). Data were missing for six markers (n = 174 missing for PvMSP10; n = 20 missing for PfEBA140 R3-5, PfETRAMP5_ag1, PfMSP2CH150, PfRh2_2030, and PvMSP8). There were no significant differences by gender for P. falciparum and P. vivax antibodies, but the proportion positive increased as age increased (data not shown).
Table 4 summarizes the antibody results by study arm and infection status. There were no significant differences between Arms A and B in terms of P. falciparum antibodies at baseline; a higher proportion of Arm B participants had P. vivax antibodies at baseline compared to Arm A participants. There were statistically significant differences in antibodies across all three arms, with a lower proportion of Arm C participants having Plasmodium antibodies. With respect to baseline infection status, a higher proportion of symptomatic participants had P. falciparum antibodies and P. vivax antibodies as compared to uninfected and asymptomatic participants.
Village-level costs of MCs
We conducted an analysis of the implementation costs of MCs in the study villages from the service provider perspective. The results of the cost analysis are presented in Table 5 . In the study villages, the cost per person participated ranged between US$3–8, the cost per tested between US$4–9, and the cost per treated between US$82–1614 per camp round.
In this quasi-experimental study conducted in rural villages in Odisha, intent-to-treat analyses suggest that the DAMaN program’s malaria camps were associated with lower malaria incidence relative to standard-of-care. This is suggested by the reductions in Plasmodium infection prevalence at baseline versus FU3. We note that all villages received MCs at some point, with Arm A receiving MCs four times, Arm B receiving it twice, and Arm C receiving it prior to the study’s inception and three times during the study. Intervention activities were impacted by the COVID-19 pandemic-related shutdowns and, as a result, 9 villages (2 of 6 in Arm A, 6 of 6 in Arm B, and 2 of 3 in Arm C) did not receive any malaria camp activities during the first follow-up. There were no serious adverse events.
Our findings are in contrast to a recent meta-analysis that noted a marginal and non-significant pooled effect size for MSAT interventions on Plasmodium infection prevalence and incidence 11 . One major limitation of previous evaluations of MSAT is low sensitivity of the diagnostic methods (i.e., RDTs and light microscopy) 11 . The Odisha DAMaN MCs use RDTs for MSAT while this evaluation used both an RDT and PCR, overcoming some previous evaluations’ limitations.
There were no significant differences in the subset of Arm A and B participants tested for Plasmodium antibodies, suggesting that Arms A and B had similar malaria exposure prior to the intervention. There were statistically significant differences in Plasmodium antibodies across all 3 arms, with a lower proportion of Arm C participants having antibodies; this suggests lower transmission of Plasmodium in these villages and/or impact of the previous exposure to the MC intervention.
At baseline, more symptomatic (i.e., febrile) participants had P. falciparum and P. vivax antibodies as compared to uninfected and asymptomatic participants. These results suggest possible cross-reactivity of P. falciparum and P. vivax antibodies and antigens in the assay as well as potential previous exposure to P. vivax . The lower proportions of participants with P. vivax antibodies relative to P. falciparum antibodies is consistent with RDT and PCR data demonstrating lower prevalence of P. vivax in this study sample, as well as data from the National Center for Vector Borne Diseases Control showing that 91.4% of cases in 2021 were P. falciparum infections 3 .
The Malaria Control Program implemented MSAT across all malaria camp rounds rather than FSAT in the subsequent rounds. This likely contributed to the impact of the intervention, as 49 of the 69 RDT + cases (71.0%) detected in the study were afebrile [data not shown]. In other words, they treated more Plasmodium infections than they otherwise would have if they had only tested and treated febrile cases each time.
The cost per person for the MCs ranged between US$3–8, the cost per tested person between US$4–9, and the cost per treated person between US$82–1,614 per MC round. According to our systematic literature review on the effectiveness and cost-effectiveness of intermittent mass MSAT interventions for malaria 11 , these results are comparable to the costs reported in the published literature, ranging between US$3.5–14.3 per person tested per round, and corresponds roughly to 20–35% of the per-capita domestic general health expenditure in India (US$19.6 in 2018) 12 .
We note several important study limitations. First, as discussed in our study protocol 7 , the villages were not randomized to study arms, which may result in potential selection bias. Second, COVID-19 pandemic shutdowns resulted in disruption in delivery of the MCs at FU1 which meant that several villages may not have received all or any of the activities in that MC round. Third, while the follow-up was over 80%, people with Plasmodium infection at baseline were less likely to be followed-up but there were no significant differences in follow-up among asymptomatic or subpatent cases.
Collectively, our quasi-experimental cluster-assigned stepped-wedge study results suggest that the DAMaN malaria camp intervention is associated with reductions in malaria in rural villages and thus is a promising, financially feasible approach for malaria control in rural settings. However, given the quasi-experimental design and COVID-related study challenges, we cannot infer causality. This is timely considering that Odisha State government extended the DAMaN initiative in October 2022 for five more years in 21 districts in a bid to achieve malaria elimination in Odisha by 2030 13 .
Ethics statement
Ethical approval for the trial was obtained from the Odisha State Research and Ethics Committee (Odisha, India, dated 24 June 2019), institutional ethics committee at Community Welfare Society Hospital (Rourkela, Odisha, India) and the institutional review board at New York University (New York, NY, USA). All research was performed in accordance with relevant guidelines/regulations and the Declaration of Helsinki.
Study design and enrollment procedures
The quasi-experimental cluster-assigned stepped-wedge study design, power analysis, and recruitment/enrollment procedures are described in a protocol paper 7 and the study was registered at ClinicalTrials.gov (NCT03963869; first posted 28 May 2019). Briefly, 12 intervention villages were selected in two districts of Odisha state, Keonjhar and Jharsuguda, and distributed in equal numbers between two study arms: Arm A ‘new-MC’ (communities receiving MCs for the first time in year one) and Arm B ‘delayed-MC’ (communities undergoing routine malaria control in year one and receiving MCs for the first time in year two); control Arm C contained three ‘old-MC’ villages, where MCs had already been implemented prior to the study period. A cohort was planned with target enrollment of 2,700 individuals across all 15 villages (i.e., 1100 in Arms A and B; 500 in Arm C).
Study participants in each village were recruited and enrolled at baseline before the initial administration of the intervention by the Government of Odisha DAMaN team and subsequently surveyed prior to each round of their screen and treat program. RDT diagnosis and treatment of the study participants followed the Government of Odisha Department of Health and Family Welfare Standard Treatment Guidelines ( https://health.odisha.gov.in/sites/default/files/2020-02/STG-2018.pdf ). Study participants in the three villages not receiving the intervention were recruited and enrolled in parallel temporally. Residents of the study villages aged 1–69 were eligible for the study. Written informed consent was obtained in the local language from all adult study participants aged 18–69 years with apparent full comprehension of the study procedures. Assent was obtained in addition to parental or legal guardian informed consent for participants aged 7–17 years. Parental informed consent was obtained for children aged 1–6 years. During each survey round, all subjects completed a health questionnaire that captured demographic, malaria knowledge and prevention, and Plasmodium infection and treatment history data and provided a blood sample for Plasmodium parasite detection.
Intervention adaptations due to COVID-19 pandemic
Arm A (new MC) and C (old MC) villages were to receive two cycles of MCs, each with two rounds of MSAT. The first MC cycle was from August to November 2019 (Round 1) and January to March 2020 (Round 2). We note that the Round 2 MSAT was interrupted by COVID-19 pandemic shutdowns. The second MC cycle was from June to September 2020 (Round 1) and October to December 2020 (Round 2). We note that Round 1 MSAT was also interrupted by COVID-19 pandemic shutdowns. Arm B (delayed MC) villages were to receive SOC in the first cycle (August 2019–March 2020) and one MC cycle with two rounds of MSAT from June to September 2020 (Round 1) and October to December 2020 (Round 2). Again, Round 1 was interrupted by the pandemic; see Supplemental Fig. 1 for details.
Primary outcomes
The primary outcome measures were any Plasmodium as detected by PCR and Plasmodium species (i.e., P. falciparum and/or Plasmodium vivax ) as detected by PCR. Secondary malaria outcome measures included Plasmodium infection as detected by RDT and Plasmodium -specific serology. Plasmodium infection by RDT is defined as RDT negative (RDT−) or RDT positive (RDT+). We further classified infections as ‘asymptomatic’, defined as PCR+ and/or RDT+ for any Plasmodium species with absence of documented fever or self-reported fever in the last 48 h, and/or ‘subpatent’, defined as RDT− and PCR+. Plasmodium -specific serology was analyzed as a continuous antibody titer variable as well as a categorical (seropositive vs. seronegative) variable.
Blood sample collection and processing
Blood samples for blood smears, blood spots (Whatman FTA cards), a small volume microvette, and micro volumes required for point of care hemoglobin and RDTs (FalciVax), were collected from consenting study participants by sterile lancet finger prick as previously described 7 . Vacutainers (5 ml; BD Vacutainer glass blood collection tubes with acid citrate dextrose) of venous blood were requested from study participants found to be positive by RDT. Microvette and vacutainer samples were refrigerated until separated into plasma and infected red blood cell (iRBC) components by centrifugation. Plasma was stored at −80 °C until assayed for Plasmodium immunoglobulin G (IgG), and total DNA was extracted from the iRBC pellet using QIamp DNA mini kit (QIAGEN) with a final elution volume of 50 μl.
Molecular detection of Plasmodium parasites by species-specific PCR
DNA samples were tested for Plasmodium species by a PCR assay targeting multi-copy loci Pfr364 and Pvr47 14 as previously described 7 . Five μl of DNA was used in a total reaction volume of 30 μl, and amplification products were visually confirmed by ethidium bromide-stained gel electrophoresis using a Gel Doc EZ documentation system (BioRad Laboratories, Inc.).
Detection of anti-Plasmodium IgG by Luminex MAGPIX cytometric bead array
Plasma isolated from a subset of microvette samples of finger prick blood was assayed for 13 anti- P. falciparum and four anti- P. vivax antibodies as previously described. 7 The panel included antigens whose corresponding antibodies have been suggested as indicators of protection from clinical disease 15 , 16 , long-term or cumulative exposure 17 , 18 , 19 , 20 , and recent infection 17 , 18 , 19 , 20 . Briefly, n = 300 plasma samples were diluted 1/200 and assayed in duplicate according to standard procedures 21 , 22 . xPONENT software was used for data acquisition (Luminex Corp., Austin, TX). Specifically, the net Mean Fluorescence Intensity (MFI; net MFI Ag = raw MFI Ag − background MFI Ag where background MFI Ag is the mean MFI of a given antigen in the blank wells) was calculated for each antigen in each sample. The presence of antibody to each antigen (e.g., seropositivity) was defined as the mean net MFI naive pool plus three standard deviations. Composite variables for any P. falciparum , any P. vivax , and any P. falciparum or P. vivax antibodies were created.
Secondary anthropometric and clinical outcomes
We report on several anthropometric measurements, including severe underweight, severe anemia, fever, and malnutrition as measured by mid-upper arm circumference (MUAC). For adults aged 18 and older, severe underweight was defined as a BMI < 16. For children, severe underweight was defined as three standard deviations below the median based on the WHO growth charts used for children < 5 years and the revised Indian Academy of Pediatrics (IAP) growth charts for Indian children and adolescents aged 5–17 23 . Severe anemia was defined as hemoglobin < 5 g/dl for persons aged < 12 and 7 g/dl among those aged 12 and older 24 . Fever was defined as a temperature of 99.5°F or higher. MUAC malnutrition was defined as < 23 cm for adults and adolescents aged 15 and older 25 , 26 , < 16 cm for 10–14 year olds 27 , < 12.9 cm for 5–9 year olds 27 , and < 11.5 cm for children 6 to 60 months 28 .
Costing study design, data collection, and analyses
Using an activity-based costing method, we conducted an analysis of the implementation costs of malaria camps (MCs) in the study villages from the service provider perspective 29 . Interviews were conducted with the malaria camp supervisors who led the MCs in the study villages, and programmatic documentation from the Odisha State malaria control program were reviewed to identify the activities related to the planning and implementation of DAMaN at the village-level. These included: (1) Training activities (training of public health supervisors and workers as well as village volunteers, including the village specific ASHAs; (2) Micro-plan development for MCs; (3) Planning and sensitization activities (identification of village volunteers, selection of camp venue and time); (4) Community sensitization activities; (5) Community mobilization activities; (6) Health activities (malaria testing and treatment, child health, maternal health); (7) Monitoring and supervision activities; and (8) Follow-up activities. Resource use was linked to specific activities associated with an intervention. During interviews, the MC supervisors identified the time dedicated by MC staff to different activities for each camp round. Time costs of MC staff were calculated based on average annual gross wage rates, apportioned according to time devoted to DAMaN activities per camp round, and summed across all MC staff. Unit costs of rapid diagnostic kits and antimalarial drugs were extracted from the DAMaN Operational Guidelines 30 . The costs of these commodities were calculated by multiplying the quantities used during a camp round by their unit costs based on participation in each camp round in each village. Personnel and implementation costs per round were summed and divided by the population that participated in the MC, the population tested for malaria, and the population treated for malaria, in each study village to calculate cost per person participated, cost per person tested, and cost per person treated per camp round. All costs were collected in local currency and converted to and presented in 2020 US dollars (US$), using the average exchange rate for 2020 (1 US$ = 74.27 Indian Rupees) 31 .
Statistical analyses
The primary outcome was Plasmodium presence and species as detected by PCR at the third follow-up visit. Secondary outcomes included Plasmodium presence and species as detected by RDT and anthropometric and clinical outcomes including underweight, anemia, fever, and malnutrition as measured by mid-upper arm circumference (MUAC) at the third follow-up visit. Missing data was minimal among the analytic sample and ranged from 0 to 1.5% depending on the variable.
Bivariate analyses compared outcomes and key covariates across study arms with Pearson’s χ 2 or Fishers exact tests (if cell sizes were < 5) at baseline and outcomes at follow-up 3. We use a Bonferroni adjusted α of 0.013 to account for multiple comparisons in the bivariate analyses comparing baseline to follow-up outcomes. Follow-up rates were calculated as the proportion of baseline participants that were followed up at the third time point.
Multilevel mixed-effects logistic regression was used to estimate the intervention effects, which enabled us to account for the repeated measure and nesting of individuals within the 15 villages. Mixed models are recommended when there are at least 10 clusters and fewer than 40 clusters 32 , 33 . The equation is:
where \({y}_{ij}^{*}\) is the expected logit of plasmodium infection, \(i\) is the individual, \(j\) is the cluster (i.e., village), \(\beta\) s are the fixed effects, \({u}_{j}\) is the random intercept for the villages, and \({\epsilon }_{ij}\) is the error term. Study arm, age, sex, education, and occupation are time-invariant covariates. Study arm is intent-to-treat, so even if a participant moved to another village, they remain assigned to the village where they were initially enrolled. Visit number in the only time-varying covariate. An interaction term (study arm x visit [baseline vs. FU3]) was used to estimate time effects for each arm and village was included as a random effect. We first estimated the crude associations for each outcome by study arm. We then adjusted these estimates for age, sex, education, and occupation. Odds ratios with 95% confidence intervals are reported. All analyses were conducted with Stata 17.0 (StataCorp, College Station, TX, USA) and were intent-to-treat. The study was registered at ClinicalTrials.gov (#NCT03963869).
Data availability
Epidemiology study data are available in the Clinical Epidemiology Database 34 ( https://clinepidb.org ) under the ‘India ICEMR DAMaN Quasi-experimental Stepped-wedge’ study title.
World malaria report 2021. Geneva: World Health Organization; 2021. Licence: CC BY-NC-SA 3.0 IGO. ( Geneva, 2021).
World malaria report 2022. Geneva: World Health Organization; 2022. Licence: CC BY-NC-SA 3.0 IGO. (2022).
National Vector Borne Disease Control Program, D. G. o. H. S., Ministry of Health and Family Welfare, Government of India. (2020).
Rajvanshi, H. et al. Learnings from two independent malaria elimination demonstration projects in India. Trans. R. Soc. Trop. Med. Hyg. (2021)
Pradhan, M. M. et al. Comprehensive case management of malaria: Operational research informing policy. J. Vect. Borne Dis. 56 , 56–59 (2019).
Article CAS Google Scholar
Bal, M. et al. Assessment of effectiveness of DAMaN: A malaria intervention program initiated by Government of Odisha, India. PLoS ONE 15 , e0238323 (2020).
Article CAS PubMed PubMed Central Google Scholar
Ompad, D. C. et al. The effectiveness of malaria camps as part of the Durgama Anchalare Malaria Nirakaran (DAMaN) program in Odisha, India: Study protocol for a cluster-assigned quasi-experimental study. Glob. Health Action 14 , 1886458 (2021).
Article PubMed PubMed Central Google Scholar
National Vector Borne Disease Control Programme. (2007).
Department of Health & Family Welfare (India). National Vector Borne Disease Control Programme (NVBDCP). https://health.odisha.gov.in/NVBDCP.asp . (Accessed 16 Dec 2021).
Pradhan, M. M. & Meherda, P. K. Malaria elimination drive in Odisha: Hope for halting the transmission. J. Vect. Borne Dis. 56 , 53–55 (2019).
Article Google Scholar
Kim, S., Luande, V. N., Rocklov, J., Carlton, J. M. & Tozan, Y. A Systematic Review of the Evidence on the Effectiveness and Cost-Effectiveness of Mass Screen-and-Treat Interventions for Malaria Control. Am. J. Trop. Med. Hyg. 105 , 1722–1731 (2021).
World Health Organization. Global Health Expenditure database , https://apps.who.int/nha/database (2021).
Service, E. N. in The New Indian Express (2022).
Demas, A. et al. Applied genomics: data mining reveals species-specific malaria diagnostic targets more sensitive than 18S rRNA. J. Clin. Microbiol. 49 , 2411–2418 (2011).
Osier, F. H. et al. Naturally acquired antibodies to polymorphic and conserved epitopes of Plasmodium falciparum merozoite surface protein 3. Parasite Immunol. 29 , 387–394 (2007).
Osier, F. H. et al. Opsonic phagocytosis of Plasmodium falciparum merozoites: Mechanism in human immunity and a correlate of protection against malaria. BMC Med. 12 , 108 (2014).
Elliott, S. R. et al. Research priorities for the development and implementation of serological tools for malaria surveillance. F1000Prime Rep. 6 , 100 (2014).
Achan, J. et al. Serologic markers of previous malaria exposure and functional antibodies inhibiting parasite growth are associated with parasite kinetics following a plasmodium falciparum controlled human infection. Clin Infect Dis 70 , 2544–2552 (2020).
Article CAS PubMed Google Scholar
Wu, L. et al. Sero-epidemiological evaluation of malaria transmission in The Gambia before and after mass drug administration. BMC Med. 18 , 331 (2020).
Wu, L. et al. Antibody responses to a suite of novel serological markers for malaria surveillance demonstrate strong correlation with clinical and parasitological infection across seasons and transmission settings in The Gambia. BMC Med. 18 , 304 (2020).
Wu, L. et al. Optimisation and standardisation of a multiplex immunoassay of diverse Plasmodium falciparum antigens to assess changes in malaria transmission using sero-epidemiology. Wellcome Open Res. 4 , 26 (2019).
Article PubMed Google Scholar
Helb, D. A. et al. Novel serologic biomarkers provide accurate estimates of recent Plasmodium falciparum exposure for individuals and communities. Proc. Natl. Acad. Sci. USA 112 , E4438-4447 (2015).
Indian Academy of Pediatrics Growth Charts, C. et al. Revised IAP growth charts for height, weight and body mass index for 5- to 18-year-old Indian children. Indian Pediatr 52 , 47–55 (2015)
Malaria, S. Trop Med Int Health 19 , 7–131 (2014).
Tang, A. M. et al. Determining a global mid-upper arm circumference cut-off to assess underweight in adults (men and non-pregnant women). Public Health Nutr 23 , 3104–3113 (2020).
Van Tonder, E. et al. Mid-upper arm circumference (MUAC) as a feasible tool in detecting adult malnutrition. South Afr. J. Clin. Nutrit. 32 , 93–98 (2019).
WHO. Guidelines for an Integrated Approach to the Nutritional Care of HIV-Infected Children (6 Months-14 Years) . (2009).
Roberfroid, D. et al. Utilization of mid-upper arm circumference versus weight-for-height in nutritional rehabilitation programmes: A systematic review of evidence. WHO commissioned white paper (2013). https://www.who.int/nutrition/publications/guidelines/updates_management_SAM_infantandchildren_review1.pdf .
Drummond, M. F., Sculpher, M. J., Torrance, G. W., O’Brien, B. J. & Stoddart, G. L. Methods for the Economic Evaluation of Health Care Programmes . (Oxford University Press, 2005).
Secretary, P. (ed Health &Family Welfare Department) (National Vector Borne Disease Control Programme, 2016–2020).
Fund, I. M. in Exchange Rates (2020).
Snijders, T. A. B. & Bosker, R. J. Multilevel analysis : an introduction to basic and advanced multilevel modeling . 2nd edn, (Sage, 2012).
McNeish, D. & Stapleton, L. M. Modeling clustered data with very few clusters. Multivar. Behav. Res. 51 , 495–518 (2016).
Ruhamyankaka, E. et al. ClinEpiDB: An open-access clinical epidemiology database resource encouraging online exploration of complex studies. Gates Open Res. 3 , 1661 (2019).
Download references
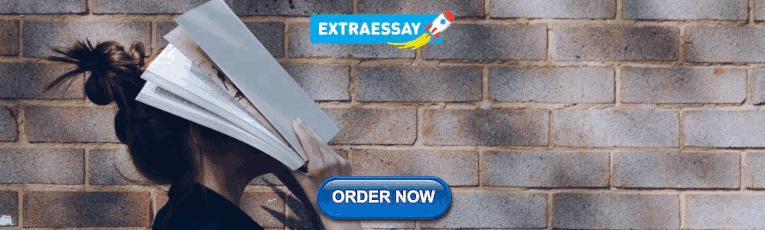
Acknowledgements
We thank study participants and the DAMaN teams in the study villages of Keonjhar and Jharsuguda districts for their cooperation, and Dr Deben Das, Mr. Baladev Nanda, and Mr. Birat R Padhan of the Odisha State Malaria Control Programme for their support. We thank field team members Asit Samal, Anna Bage, Pratibha Ekka, Satyanarayana Panigrahi, Sahil Ekka, John P Lakra, Sobharani Toppo, and Madhusmita Parichha and lab technicians Swagatika Dash and Satyaranjan Chhatria at Community Welfare Society Hospital for their efforts, and Dr. Kevin Tetteh, Ms. Elin Dumont, and Ms. Catriona Patterson of the London School of Hygiene and Tropical Medicine (England, UK) for providing serology assay support. We also thank Dr. James Beeson (Burnet Institute, Australia) and Dr. Simon Draper (University of Oxford, UK) for providing serology assay antigens. This study was supported by the National Institute of Allergy and Infectious Diseases (NIAID) of the National Institutes of Health (NIH) under Award Number U19AI089676 as part of the International Centers of Excellence for Malaria Research. The content does not necessarily represent the official views of the US NIH or NIAID.
Author information
These authors contributed equally: Timir K. Padhan and Anne Kessler.
These authors jointly supervised this work: Madan Mohan Pradhan, Sanjib Mohanty, Jane M. Carlton and Praveen K. Sahu.
Authors and Affiliations
School of Global Public Health, New York University, New York, NY, 10003, USA
Danielle C. Ompad, Yesim Tozan, Abbey M. Jones & Jane M. Carlton
Department of Molecular Biology and Infectious Diseases, Community Welfare Society Hospital, Rourkela, Odisha, 769042, India
Timir K. Padhan, Mohammed A. Haque, Sanjib Mohanty & Praveen K. Sahu
Center for Genomics and Systems Biology, Department of Biology, New York University, New York, NY, 10003, USA
Anne Kessler, Anna Maria van Eijk, Steven A. Sullivan & Jane M. Carlton
Liverpool School of Tropical Medicine, Liverpool, United Kingdom
Anna Maria van Eijk
Department of Health and Family Welfare, State Vector Borne Disease Control Programme, Bhubaneswar, Odisha, 751001, India
Madan Mohan Pradhan
Johns Hopkins Malaria Research Institute, Johns Hopkins Bloomberg School of Global Public Health, Baltimore, MD, 21205, USA
Jane M. Carlton
You can also search for this author in PubMed Google Scholar
Contributions
D.O., A.K., A.V.E., Y.T., S.A.S., P.K.S., S.M., M.M.P., and J.M.C. conceptualized and developed the study design and methodology with input from all authors. P.K.S. led the field and laboratory work in Odisha, India with support from T.K.P., M.A.H., S.M., and M.M.P. A.K. undertook the serology assay. D.O. and S.A.S. managed the data. D.O., A.J., and Y.T. completed the data analyses. J.M.C. acquired the funding. D.O., A.K., Y.T., J.M.C., and P.K.S. wrote the manuscript with input from all authors.
Corresponding author
Correspondence to Danielle C. Ompad .
Ethics declarations
Competing interests.
Dr. Madan M. Pradhan is employed by the Odisha State Vector Borne Disease Control Programme, which is the organization that is implementing the intervention. He has a potentially self-serving stake in the research results via potential promotion or career advancement based on outcomes. The other authors have no competing interests to declare.
Additional information
Publisher's note.
Springer Nature remains neutral with regard to jurisdictional claims in published maps and institutional affiliations.
Supplementary Information
Supplementary information., rights and permissions.
Open Access This article is licensed under a Creative Commons Attribution 4.0 International License, which permits use, sharing, adaptation, distribution and reproduction in any medium or format, as long as you give appropriate credit to the original author(s) and the source, provide a link to the Creative Commons licence, and indicate if changes were made. The images or other third party material in this article are included in the article's Creative Commons licence, unless indicated otherwise in a credit line to the material. If material is not included in the article's Creative Commons licence and your intended use is not permitted by statutory regulation or exceeds the permitted use, you will need to obtain permission directly from the copyright holder. To view a copy of this licence, visit http://creativecommons.org/licenses/by/4.0/ .
Reprints and permissions
About this article
Cite this article.
Ompad, D.C., Padhan, T.K., Kessler, A. et al. The effectiveness of malaria camps as part of the malaria control program in Odisha, India. Sci Rep 13 , 22998 (2023). https://doi.org/10.1038/s41598-023-46220-x
Download citation
Received : 10 January 2023
Accepted : 30 October 2023
Published : 28 December 2023
DOI : https://doi.org/10.1038/s41598-023-46220-x
Share this article
Anyone you share the following link with will be able to read this content:
Sorry, a shareable link is not currently available for this article.
Provided by the Springer Nature SharedIt content-sharing initiative
By submitting a comment you agree to abide by our Terms and Community Guidelines . If you find something abusive or that does not comply with our terms or guidelines please flag it as inappropriate.
Quick links
- Explore articles by subject
- Guide to authors
- Editorial policies
Sign up for the Nature Briefing newsletter — what matters in science, free to your inbox daily.

- Open access
- Published: 16 April 2020
Surveillance based estimation of burden of malaria in India, 2015–2016
- Ashwani Kumar 1 ,
- Himanshu K. Chaturvedi 2 ,
- Ajeet Kumar Mohanty 1 ,
- Surya Kant Sharma 3 ,
- Mantoshkumar S. Malhotra 3 &
- Arvind Pandey 2
Malaria Journal volume 19 , Article number: 156 ( 2020 ) Cite this article
6584 Accesses
11 Citations
1 Altmetric
Metrics details
India has launched the malaria elimination initiative in February 2016. Studies suggest that estimates of malaria are useful to rationalize interventions and track their impact. Hence, a national study was launched to estimate burden of malaria in India in 2015.
For sampling, all 624 districts of India were grouped in three Annual Parasite Incidence (cases per thousand population) categories, < two (low); two-five (moderate) and > five (high) API. Using probability proportional to size (PPS) method, two districts from each stratum were selected covering randomly 200,000 persons per district. Active surveillance was strengthened with 40 trained workers per study district. Data on malaria cases and deaths was collated from all health care providers i.e. pathological laboratories, private practitioners and hospitals in private and public health sectors and was used for analysis and burden estimation.
Out of 1215,114 population under surveillance, 198,612 (16.3%) tests were performed and 19,386 (9.7%) malaria cases were detected. The malaria cases estimated in India were 3875,078 (95% confidence interval 3792,018–3958,137) with API of 3.05 (2.99–3.12) including 2789,483 (2740,577–2838,389) Plasmodium falciparum with Annual Falciparum Incidence of 2.2 (2.16–2.24). Out of 8025 deaths investigated, 102 (1.27%) were attributed to malaria. The estimated deaths in India were 29,341 (23,354–35,327) including 19,067 (13,665–24,470) confirmed and 10,274 (7694–12,853) suspected deaths in 2015–2016.
Conclusions
Estimated malaria incidence was about four folds greater than one million reported by the national programme, but three folds lesser than thirteen million estimated by the World Health Organization (WHO). However, the estimated deaths were 93 folds more than average 313 deaths reported by the national malaria programme in 2015–2016. The 29,341 deaths were comparable with 24,000 deaths in 2015 and 22,786 deaths in 2016 estimated by the WHO for India. These malaria estimates can serve as a benchmark for tracking the success of malaria elimination campaign in India.
The World Health Organization (WHO) has reported 22% decline in malaria from the estimated 271 (177–382) million cases in the year 2000 to 212 (144–294) million in 2015 [ 1 ]. The reduction in estimated malaria attributable mortality is even more impressive from 856,728 (594,760–1204,220) deaths down to 426,791 (218,780–630,698). With these trends, the WHO has advocated elimination of malaria in at least 35 countries by the year 2030 [ 2 ]. Following the WHO path, India has launched the malaria elimination initiative in 2016.
The first set of global disease burden modelling studies was carried out a couple of decades ago for estimation of communicable and non-communicable diseases, injuries and deaths [ 3 , 4 ]. Many studies have also been conducted using country data and subjecting it to different methodologies, assumptions and epidemiological models to generate estimates of malaria burden [ 1 , 5 , 6 , 7 , 8 , 9 , 10 , 11 ]. However, wide gaps between the estimates and the reported incidence have been the subject of intense debate calling to question not only deficiencies in surveillance and reporting systems, but also methodologies adopted to arrive at such estimates.
Outside of Africa, India is the main contributor to malaria related morbidity and mortality in the South-East Asia. Hence, several attempts have been made to estimate malaria burden in India from time to time using secondary data [ 1 , 8 , 12 ]. Mortality estimates for the year 2002 were provided by Dhingra et al. based on cause of death by verbal autopsy (COD VA) data of the Million Death Study from 2001 to 2003 [ 13 , 14 ]. It was estimated that below the age of 70 years, there were 205,000 deaths attributable to malaria/annum; < 5 years of age-55,000, 5–14 years of age-30,000 and 15–69 years of age-120,000 deaths. As the death estimates were about 300 times greater than the deaths reported by the Indian national programme, this publication triggered intense debate on the methodology adopted. It was surmised that besides issues related to the time gap between the death and the verbal autopsy, the overlapping of symptoms of other diseases with malaria could have influenced responses of the respondents [ 15 , 16 ]. Further, based on Vital Registration System and Medical Certification of Cause of Death, Kumar et al. estimated about 146,000 and 141,000 deaths due to malaria in India respectively in 1997 and 1998 [ 17 ]. A committee constituted by the Government of India arrived at an estimate of 9.751 million cases and 40,297 deaths due to malaria (30,014–48,660) in the year 2010 [ 18 ].
More recent global malaria mortality trends published suggest 46,970 (14,757–94,945) deaths for individuals of all ages in India in 2010 [ 5 ]. These included 4826 deaths (781–14,437) in children less than 5 years of age and 42,145 (11,340–88,615) deaths for individual of 5 years and older. Curiously, malaria ranked 7th among 291 causes of death and injuries in both 1990 and 2010 [ 9 ].
Malaria burden estimates at national and sub-national levels are vital not only as benchmark for priority setting and resource allocation but also to gauge programmatic achievements during disease elimination process. As malaria burden estimates based on a nationally representative sample of primary morbidity and mortality data are lacking, the present study is first such attempt globally which was carried out in three different malaria-endemic zones representing India in 2015–2016.
Sampling frame and sample size
A national sampling frame was prepared based on data provided by National Vector Borne Diseases Control Programme (NVBDCP) for stratification and selection of the clusters as a basic requirement of a sampling design. A list of all 624 districts of India with annual parasite incidence (API) of last 3 years (2011–2013), which was obtained from NVBDCP, served as the sampling frame. Based on the maximum API of last 3 years, all the districts were divided into three strata (S1, S2 and S3) of endemicity, i.e., high (S1: API ≥ 5), moderate (S2:2 ≥ API < 5) and low (S3: API < 2).
Selection of study districts
The sample size was worked out to provide the reliable estimate of API for each region and death rate due to malaria at the national level. It was based on the median API of the malaria endemic strata (7/1000 in S1, 3/1000 in S2 and 0.5/1000 in S3) with 10% margin of error in S1 and S2 and 20% margin of error in S3, 95% confidence interval, 10% non-response and design effect-2. As the median API of low endemic region (districts with API < 2) was low, the computed required sample size worked out to 400,000 persons. The same sample size was uniformly applied to the other two regions to maximize the possibility of capturing both malaria and death cases. The total sample size was thus 1200,000 from all the three regions. In this manner, two representative districts each from low, moderate and high burden districts and overall 6 study districts were selected in the country (Fig. 1 ). Further, three Primary Health Centres (PHCs) were selected randomly from the list of all PHCs of each selected district so that study population size within each selected PHC was approximately 70,000. In case the selected PHC was smaller (i.e. population was < 60,000), some population of the adjacent PHC or a sub-Centre was included in the study area to obtain the desired sample size. Similarly, the larger PHC (i.e. with a population > 80 000) was divided to select a contiguous segment of required population size. Overall study population of surveillance area was about 0.2 million/district. For concurrent death enumeration, an adjacent PHC of similar size and epidemiological features matching the surveillance PHC area was also selected.
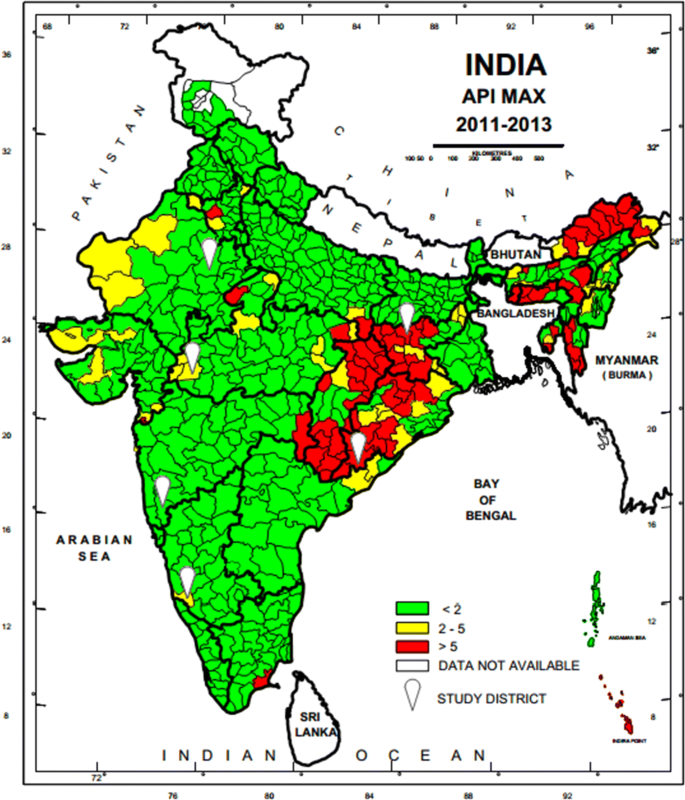
Map of India showing geographical location of six study districts (white balloons) for capturing malaria morbidity and mortality. Firstly district level stratification of India was done on the basis of three Annual Parasite Incidence (API) classes, < 2, 2–5 and > 5 taking into consideration APImax of malaria from 2011–2013 and then two study districts from each of the three strata were randomly selected as per PPS sampling method to conduct malaria burden estimation study
Study personnel
Six Technical Assistants (one in each district), 18 Field Workers (three in each district) and 240 (40 in each district) Voluntary Surveillance Monitors (VSMs), a statistical Assistant, an Epidemiologist, Consultant Biostatistician and 6 Data Entry Operators along with Co-Is and PIs were engaged for managing the project activities. The VSMs were chosen from the study or neighbouring villages/wards where they were assigned surveillance work. Field staff was trained in performing Rapid Diagnostic Test (RDT), preparing blood smears, filling up of study forms and record keeping. The VSMs worked in close collaboration with village Accredited Social Health Activists (ASHAs) and Multi-Purpose Workers (MPWs) and were instrumental in finding fever cases and testing their blood for malaria followed by treatment of malaria cases.
Surveillance
Active surveillance.
The community level active surveillance was carried out in each selected villages of PHC areas in rural and municipal areas or ward in urban area. House listing and complete enumeration of the study population was carried out in each study area (PHCs/Municipal wards) before initiation of surveillance by the 12 to 15 VSMs with help of ASHAs. One VSM was assigned a population of about 5000 or 1000 houses (one or more villages of PHCs or section of municipal areas) fortnightly to carry out the active surveillance of fever cases for the period of 12 months. During the surveillance, all the fever cases were recorded by the VSMs and their blood test for malaria was performed by the surveillance team. They were also referred to the PHCs or Sub-centres for confirmation of malaria and treatment. All the fever cases identified during the active surveillance were recorded with the result of blood test in the active surveillance format (A) and compiled at the end of every month. Field Workers (FW) were supervising VSMs, solving day to day problems, cross-checking all the cases reported by the VSMs every month, and maintaining the supplies of study related material and carrying out of the verbal autopsy of each death case using prescribed formats (D). Technical Assistants (TA) were responsible for overall field activities, logistics, and coordination with state health officials, solving of local problems, supervision, data collation and reporting to the project co-investigators.
Passive surveillance
The information related to passive cases detection was collated to ascertain morbidity and mortality due to malaria in the study PHC areas in rural areas and municipal wards in the urban areas (Figs. 2 and 3 ). All government and private health facilities in the study area and the vicinity were listed and empanelled to capture malaria cases coming from the study surveillance area and accessing these facilities. All records of fever and malaria cases were cross checked in both active and passive lists to avoid duplication. Blood tests were performed by bivalent RDTs (for both Plasmodium vivax and Plasmodium falciparum ) and by making thin and thick blood smear of fever cases encountered in the study population. Treatment of confirmed malaria cases was done by study personnel following the current national anti-malarial drug policy [ 19 ]. Data of all fever cases and their blood tests results collected through active and passive surveillance and also the VA of death cases were finally checked with name and address for confirmation of cases belonging to the surveillance study population by the project team (VSMs, FW, TA) during the district level monthly meeting and finally confirmed by the project co-investigator before sending the data to the central team for analysis.
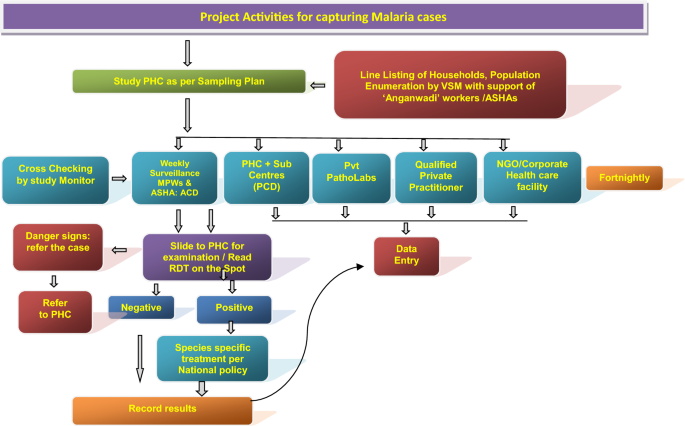
Flow diagram of activities carried out in the surveillance areas of the study districts to capture malaria cases by instituting surveillance and from enlisted health facilities, diagnostic laboratories, private practitioners and institutions
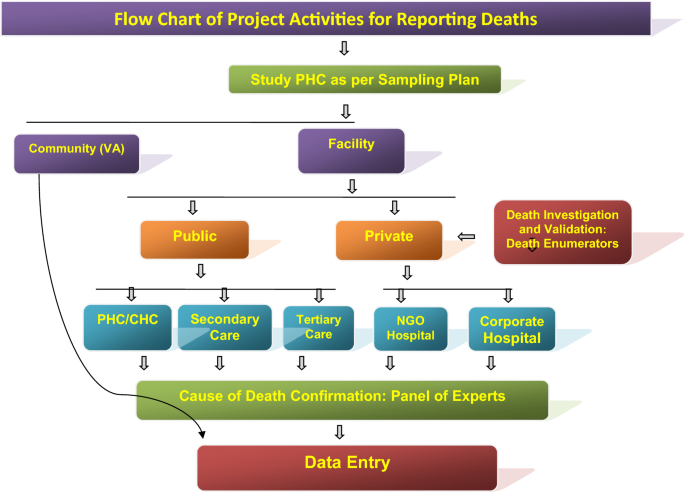
Flow diagram of the surveillance activities carried out in the study areas of the study districts to capture death cases by eliciting information from ‘Panchayats’ (Local self-Government bodies in the study villages), Municipal Councils/corporations, burial/cremation ground, public, community leaders, schools, shops, ASHAs, hospitals, etc. Verbal Autopsy of death cases was done after a fortnight of death occurrence by visiting residence of the deceased and information was captured on standard VA instrument in local language. Each VA report was examined by two medical experts independently for labeling the cause of death viz., probably due to malaria, confirmed due to malaria, cause other than malaria and unclassified death (cause cannot be discerned)
Deaths and cause of death assignment
Information on deaths captured through different sources (including hospital, death registry, cremation/burial records) was recorded. A pre-designed and tested verbal autopsy (VA) tool was filled up for all the death cases by visiting household of the deceased on day 15 post death. Two independent physicians after auditing all VA forms assigned the cause of death. In case of disagreement between the two physicians, a third physician was consulted and final cause of death based on agreement between any two physicians was assigned. If available, the medical records related to the death cases were also taken into consideration for cause of death assignment.
Data analysis
Data collected during the surveillance period of 1 year was analysed to obtain the crude and weighted estimates of annual incidence rates, death rates. The weights were calculated according to the study design adjusting the differences in sample coverage in each study area of district and overall estimates were obtained using the population weight of three endemicity strata (S1–S3).
Malaria morbidity
In Koraput district with high malaria endemicity, 15,563 cases with test positivity rate (TPR) of 19.69% and in Chatra district 916 cases (TPR 3.06%) were detected from study population. In the moderate endemic districts, malaria cases were 1947 with TPR of 10.01% in Jhabua, but in Dakshin Kannada 791 cases (TPR: 2.45%) were captured. In the low endemic areas, 36 and 133 malaria cases were captured in Jaipur and Kolhapur districts respectively with < 1 TPR (Fig. 4 , Table 1 ). Koraput and Jhabua districts showed predominance of P. falciparum (55–88%), while remaining 4 districts viz., Chatra, Dakshin Kannada, Jaipur and Kolhapur, showed predominance of P. vivax (44–95%). Mixed infections were reported in all the districts except Jaipur and Kolhapur which had extremely low incidence (Fig. 5 ). The observed Annual Parasite Incidence (API) which denotes malaria cases per 1000 population in Koraput being 74.5, was two-four folds higher than reported API in the previous 3 years, but observed API was within the range of reported API in Chatra, Dakshin Kannada and Jaipur districts. However, it was higher (9.3) in Jhabua compared to 3.6–8.0 reported in the previous years. In Kolhapur, though API of 0.7 was low in general, yet it was 17–35 folds higher when compared with reported API (0.02–0.04) in the years 2012–2014 (Table 1 ).
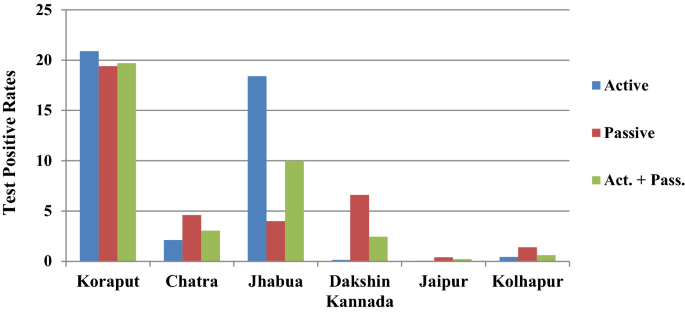
Test Positive Rates for malaria showed high variaility in active and passive collections in six study districts
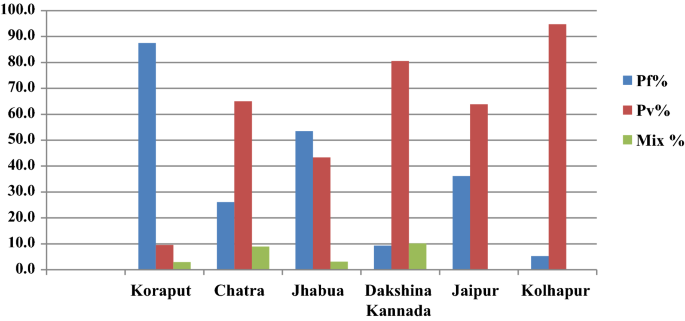
The proportion of P. falciparum and P. vivax varied in the 6 study districts
Malaria attributable deaths
From the six study districts, 8025 deaths were investigated (Table 2 ). Ten physicians assigned cause of death based on verbal autopsy (VA) narratives and available medical records. In high-malaria endemic Koraput district, out of 946 verbal autopsies performed, 60 and 35 deaths were labelled as attributed to malaria by the medical experts as confirmed and suspected deaths, respectively. In Chatra district only two deaths, one confirmed and one suspected were caused by malaria. In moderately malaria-endemic region, one suspected death due to P. falciparum malaria in Jhabua district and three malaria deaths (all due to P. vivax ) in Dakshin Kannada district were captured from the hospital records. In low malaria-endemic region, only one confirmed death due to complicated P. falciparum was reported in Kolhapur district (Table 2 ). Of these 102 total malaria deaths, 65 (63.7%) were among males and the rest 37 (36.3%) were among females in a male: female ratio of 1.75:1. The number of deaths was greater among males as compared to females in all the age groups except in children 1–14 years of age (Fig. 6 ). The number of deaths (62) was greatest in the broad age group of 15–70 years. In this age group, deaths were twice greater in males than in females. However, 18 deaths occurred among persons over 70 years of age involving both sexes almost equally.
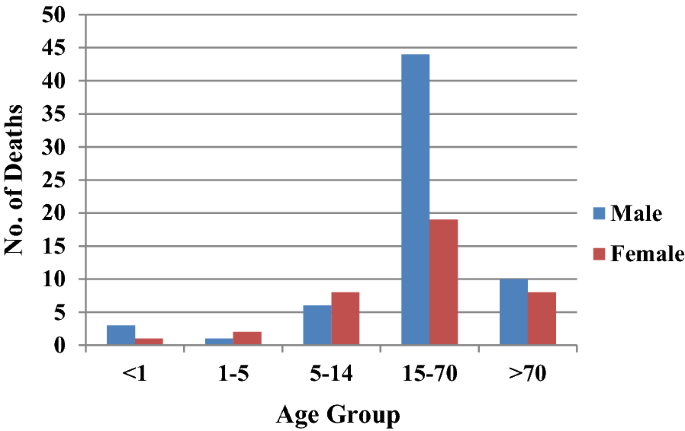
Age and sex distribution of deaths in the study districts
Plasmodium falciparum prevalence and mortality rates
When computed P. falciparum prevalence rate ( Pf PR) was highest (17.8%) in Koraput district followed by Jhabua (5.66%). In the remaining 4 districts, Pf PR was low from 0.03 to 1.0% (Table 3 ). Plasmodium falciparum specific mortality rates ( Pf MR) showed wide variation. In high malaria-endemic district, Pf MR was 0.67% and 0.62% respectively in Koraput and Chatra, while in Jhabua district Pf MR was low at 0.09% and in Dakshin Kannada district it was nil as all 3 deaths were due to P. vivax . Incidentally, in Kolhapur district with only 7 P. falciparum cases, one P. falciparum -attributable death was confirmed and hence and Pf MR rate stood abnormally high at 14.2%. The crude death rate was 47.5/100,000 persons in case of Koraput but < 1 in the remaining districts and the overall rate was 5.01/100,000 persons (Table 3 ).
Estimation of malaria morbidity and mortality burden
Based on the sample data of 6 districts, the number of malaria cases and deaths attributable to malaria were estimated for India by the weighted estimates of various rates such as annual fever rates and annual incidence of malaria and death rates due to malaria (Additional files 1 , 2 , 3 , 4 : Table S1–S4). As per Expert Group of Population Projection of India report, the projected population of India as on March 1, 2016 (mid of study period 2015–16) was worked out as 1.268 billion which was used to arrive at population-based malaria morbidity and mortality estimates. The population share of the three strata to the total population of India was 5.2% in case of high, 8.4% in moderate and 86.4% in case of low malaria endemic districts in strata S1, S2 and S3 respectively. This categorization was as per the initial sampling frame prepared for this study design.
The estimated Annual Parasite Incidence (API) was 41.66 for high, 6.53 for moderate and 0.39 for low malaria endemic areas (Table 4 ). Overall, the weighted estimate of API for the country worked out to 3.05 (2.99–3.12) per thousand population. Based on weighted estimates of API and standard error, the estimated number of malaria cases in the country ranged from 3792,018 to 3958,137 during the study period of one year with point estimate of 3875,078 malaria cases in India (Table 4 ).
The estimated AFI (Annual Falciparum Incidence) based on P . f malaria cases (both P. f & Mix P. f + P. v ) for the three regions was 36.57 for high, 3.0 for medium and 0.05 for low endemic area (Table 4 ). Overall, the weighted estimate of AFI for the country was 2.20 (2.16–2.24) per thousand populations. Based on weighted estimates of AFI and standard error of estimate, the estimated number of Pf malaria cases in the country was worked out between 2740,577 and 2838,389 with point estimate of 2789,483 P. falciparum cases (including mix infections).
Estimation of deaths due to malaria
All deaths attributed to malaria were categorized as confirmed and suspected deaths. In high malaria-endemic areas, the death rate due to confirmed malaria was estimated at 25.44/100,000 population and death rate due to suspected malaria as 15.0/100,000 population with overall death rate due to malaria as 40.44/100,000 population (Table 5 ). In high malaria prevalence area which embodies a population of 0.066 billion, the estimated deaths due to confirmed malaria were 16,789 and estimated deaths due to suspected malaria were 9901 hence 26,690 total deaths. In moderate malaria prevalence area, the death rate due to confirmed malaria was 0.5368/100,000 of population and death rate due to suspected malaria was 0.34/100,000. Hence in moderate prevalence area with 0.107 billion population, the estimated deaths were 945 of which 572 were due to confirmed malaria and 373 were due to suspected malaria. In low malaria burden area, the death rate due to confirmed malaria was 0.1556/100,000 and death rate due to suspected malaria was nil. In low malaria prevalence areas of India which had 1.096 billion populations, the estimated deaths due to confirmed malaria were 1706 and suspected malaria deaths were nil. Hence, the overall point-estimates of deaths due to confirmed malaria were 19,067 (13,665–24,470) and the point estimate of deaths due to suspected malaria was 10,274 (7694–12,853) with total deaths of 29,341 (23,354–35,327) due to malaria in a population of 1.269 billion in India (Table 5 ).
The foregoing effort on estimation of morbidity and mortality attributable to malaria has been made by conducting surveillance based prospective study for the first time in India. The findings of the study confirm that malaria scenario is highly diverse in the country. The observed malaria incidence in high endemic Koraput district situated in Odisha state of India was two-four folds greater than expected. On the other hand, Jhabua which represented districts of the country with moderate incidence showed much higher incidence of malaria than reported in the earlier years (Table 1 ). In the remaining districts, however, the observed incidence was quite as expected with over all indication that the sample districts could capture wide spectrum of variability of malaria normally observed in India. The same was also true for P. falciparum and P. vivax variability found between the study districts (Fig. 5 ). The overall contribution of P. falciparum and P. vivax was 72% and 28%, respectively in the present study as opposed to two-third and one-third ratio reported by the national programme, showing a differential of about 5% in both parasite species in 2015 [ 20 ].
The total number of estimated cases of malaria were about four folds more than about 1 million reported to the National Malaria Control Programme of India in the years 2015–2016 [ 20 ]. However, they were about one-third of 13 million (9.9–18 million) estimated by WHO for India for the year 2015 and 2016 [ 1 , 23 ]. Interestingly, 71% of the incident cases were contributed by only 4.7% of the total 1.268 billion population spread across eastern and north-eastern states of India. These states also contributed to 86.5% of the total estimated P. falciparum cases (Table 4 ).
During the present study, 93% of the reported deaths were in Koraput district of Odisha, a state which is highly malaria endemic with predominance of P. falciparum . It may be mentioned that Odisha state with only about 4% (43.7 million) population contributed 42% to the total reported malaria cases and 55.1% to the total P. falciparum cases in the country. Odisha also contributed to 31.8% of the total reported deaths due to malaria in India in 2015 [ 20 ]. The age-gender composition of deaths as seen in Fig. 6 confirms previously reported trends [ 5 , 12 ].
Malaria deaths in children in south and south-east Asia have been steadily decreasing since 1980 and accounted for a small proportion of the global deaths in this age group in 2010 [ 5 ]. Many studies have suggested that adult mortality due to malaria in India far exceeds in proportion than earlier known [ 5 , 12 , 13 ]. Even in Africa, adult absolute mortality is greater than child mortality than it was previously believed [ 23 ]. This has implications on the distribution of resources among affected populations both for surveillance as well as for vector control. Accordingly, the intervention focus needs to be widened covering both children as well as adults. This has epidemiological significance too. The re-enforced immunity after repeated infections in lower ages, which is expected to reduce adult malaria mortality, is short lived or not strong enough to prevent complications and deaths in adults in India [ 23 ].
India contributed 6% to the global estimated malaria cases and 49% to P. vivax cases in the year 2015 [ 1 ]. The country also contributed 6% to total deaths estimated and 51% to P. vivax mortality figures [ 1 ]. The time trends of malaria mortality estimated for India and endemic countries for 1980–2010 have also been recently published [ 5 ]. The WHO has reported a decline in the estimated number of malaria cases in India by 38% from 21 million in 2010 to 13 million in 2015 and by 55% (9.59 million) in 2017 and malaria deaths by 27%, i.e., from 33 000 in 2010 to 24 000 in 2015 and by 50% (16,733) in 2017 [ 1 , 24 ]. It is pertinent to mention that in the last decade, various programmatic changes have been introduced in India viz., improvement in the health infrastructure under National Health Mission and health care delivery over time through 600,000 village level health workers known as Accredited Social Health Activists (ASHAs). These workers have been providing better on-the-spot diagnosis (with RDT) of malaria at the doorsteps of the local people and enhanced ACT (artemisinin-based combination therapy) access for the treatment of P. falciparum malaria besides decentralized procurement and improving supply chain of LLINs to the communities in malaria high risk areas, etc. All these factors must have impacted trends of malaria morbidity and mortality in the country as observed in this study.
The estimated 29,341 (23,354–35,327) deaths from the primary data were comparable with WHO estimates of 24,000 (1500–47,000) for the year 2015; 22,786 (1580–45,300) for the year 2016 and 16,733 (1200–31,900) for the year 2017 [ 1 , 24 ]. However, these estimates were significantly lesser than 46,970 (14,757–94,945) estimated for India by Murray et al. and 205,000 by Dhingra et al. [ 5 , 13 ] while the deaths estimated in the present study were 76 folds greater than 384 deaths reported in India in 2015 [ 20 ].
The well cited limitations of the VA, notwithstanding, the observed crude P. falciparum mortality rate of 0.63% was on expected lines [ 21 , 22 ]. Najera and Hempel have reported that outside of Africa, malaria mortality has been estimated to be 1% of the estimated P. falciparum malaria incidence [ 23 ]. In the present study, malaria mortality rate was similarly 1.05% which was estimated taking 29,341 deaths as numerator and 2789,483 estimated P. falciparum cases as denominator in this study. Further, this agreement in P. falciparum mortality rates of the current study with that of earlier studies suggests that the methodology adopted in the current study for the burden estimation was quite appropriate. The recall period during VA was kept the shortest possible as 15 days during the study to elicit accurate information from the respondents. Another caveat of the study is that study population was enumerated just before initiation of surveillance and all the households and individuals were listed for follow up, but there is no information recorded about their movement or lost to follow up during the surveillance period.
Most importantly, this study has provided estimates of malaria cases and deaths in India at a time when they are most needed, i.e., at the inception of the malaria elimination campaign in the country. For such a vast and diverse country as India, the investigators recommend estimation of malaria burden at suitable intervals during the entire phase of malaria elimination till the target year 2027 and possibly beyond. This will offer distinct advantages as (1) the progress towards malaria elimination could be tracked when the current annual incidence is compared with the baseline numbers of malaria cases and deaths in different strata; (2) in the pre- and post-elimination phases, if any setbacks are observed in the targets, they could be timely addressed and (3) the regional priorities for resource allocation could be appropriately set to address residual transmission when the country is approaching malaria elimination targets to accelerate transmission control efforts and prevention of resumption of active transmission of malaria in areas of the country where malaria is eliminated.
The incidence of malaria in India were estimated at about 4 million in the year 2015–16. The estimates were four-fold improved over the number of malaria cases reported by the National Malaria Control Programme. Though they were about the three-fold lower than those which were estimated by the WHO for India for the same year, but the present estimates were based on an active surveillance sample survey design. The estimates depicted that over 70% of the total incident cases of malaria and 87% of the falciparum malaria cases were from about 5% of India’s population spread over eastern and north-eastern states of India.
The survey, during the year, estimated 29,341 (23,354–35,327) deaths due to malaria. They were the improved estimates over the earlier estimates and were comparable to the estimates provided by the WHO for the same period. The present estimates can serve as the benchmark for tracking the success of malaria elimination campaign in India.
Availability of data and materials
All the data and documents supporting the results of this study have been archived in ICMR-National Institute of Malaria Research, Dwarka, New Delhi. The raw and analysed data are available with ICMR-National Institute of Medical Statistics, Ansari Nagar, New Delhi.
Abbreviations
Annual parasite incidence
Annual falciparum incidence
Probability proportion to size
World Health Organization
Cause of death
Verbal autopsy
National Vector Borne Diseases Control Programme
Voluntary Surveillance Monitor
Rapid diagnostic test
Acredited Social Health Activist
Multipurpose health worker
Test positive rate
Plasmodium falciparum prevalence rate
Plasmodium falciparum mortality rate
WHO. World Malaria Report 2016: Geneva: World Health Organization; 2016. Accessed 18 July 2018.
WHO. Eliminating malaria. Geneva: World Health Organization; 2016. http://apps.who.int/iris/bitstream/10665/205565/1/WHO_HTM_GMP_2016.3_eng.pdf . Accessed 18 July 2018.
Murray CJ, Lopez AD. Evidence-based health policy-lessons from the Global Burden of Disease Study. Science. 1996;274:740–3.
Article CAS Google Scholar
Murray CJ, Lopez AD. The Global Burden of Disease 1990–2020: alternative projections of mortality and disability by cause for eight regions. Lancet. 1997;349:1498–504.
Murray CJ, Rossenfeld LC, Lim SS, Andrew KG, Foreman KJ, Haring D, et al. Global malaria mortality between 1980 and 2010: a systematic analysis. Lancet. 2012;379:413–31.
Article Google Scholar
Cibulskis RE, Aregawi M, Williams R, Otten M, Dye C. Worldwide incidence of malaria in 2009: estimates, time trends, and a critique of methods. PLoS Med. 2011;8:e1001142.
Hay SI, Okiro EA, Gething PW, Patil AP, Guerra CA, Snow RW. Estimating the Global Burden of Plasmodium falciparum malaria in 2007. PLoS Med. 2010;7:e1000290.
Korenromp EL. Malaria incidence estimates at country level for the year 2004—proposed estimates and draft report. Geneva: World Health Organization, Roll Back Malaria, 2005. http://mosquito.who.int/docs/incidence_estimations2.pdf . Accessed 15 June, 2018.
Murray CJ, Vos T, Lozano R, Naghavi M, Flaxman AD, Michaud C, et al. Disability-adjusted life years (DALYs) for 291 diseases and injuries in 21 regions, 1990–2010: a systematic analysis for the Global Burden of Disease Study 2010. Lancet. 2012;380:2197–223.
Mendis K, Sina BJ, Marchesini P, Carter R. The neglected burden of Plasmodium vivax malaria. Am J Trop Med Hyg. 2001;64:97–106.
Snow RW, Guerra CA, Noor AM, Myint HY, Hay SI. Malaria risk: estimation of the malaria burden. Nature. 2005;434:214–7.
Kumar A, Valecha N, Jain T, Dash AP. Burden of malaria in India: retrospective and prospective view. Am J Trop Med Hyg. 2007;77(Suppl 6):69–78.
Dhingra N, Jha P, Sharma VP, Cohen AA, Jotkar RM, Rodriguez PS, et al. Adult and child malaria mortality in India: a nationally representative mortality survey. Lancet. 2010;376:1768–74.
Registrar General India. Centre for Global Health Research. Causes of Death in India, 2001–2003: sample registration system. New Delhi: Government of India, (2009). Accessed 10 June 2018.
Shah NK, Dhariwal AC, Sonal GS, Gunasekara A, Dye C, Cibulskis R. Malaria—attributed death rates in India. Lancet. 2011;337:991.
Valecha N, Staedke S, Filler S, Mpimbaza A, Greenwood B, Chandarmohan D. Malaria –attributed death rates in India. Lancet. 2011;337:992–3.
Kumar A, Dua VK, Rathod P. Malaria–attributed death rates in India. Lancet. 2011;337:991–2.
Anonymous. Report of Expert Committee for estimating malaria mortality in the country. Ministry of Health and Family Welfare, Govt. of India; 2011, p. 1–53.
National Vector Borne Diseases Programme http://www.mrcindia.org/Diagnosis%20of%20Malaria%20pdf/Guidelines%202014.pdf . Accessed 15 June 2018.
National Vector Borne Diseases Programme. http://nvbdcp.gov.in/Doc/malaria-situation-March17.pdf . Accessed 15 June 2018.
Lozano R, Lopez AD, Atkinson C, Naghavi M, Flaxman AD, Murray CJ, Population Health Metrics Research Consortium (PHMRC). Performance of physician-certified verbal autopsies: multisite validation study using clinical diagnostic gold standards. Popul Health Metr. 2011;9:32.
Snow R, Armstrong J, Forster D, Winstanley MT, Marsh VM, Newton CR, et al. Childhood deaths in Africa: uses and limitations of Verbal Autopsies. Lancet. 1991;340:351–5.
Najera JA, Hempel J. The burden of malaria. Geneva: World Health Organization 1996; CTD/MAL/96.10. http://citeseerx.ist.psu.edu/viewdoc/download?doi=10.1.1.597.5367&rep=rep1&type=pdf . Accessed 10 June 2019.
WHO. World Malaria Report 2018. Geneva: World Health Organization; 2018. Accessed 29 September 2019.
Download references
Acknowledgements
Authors are grateful to Secretary to the Govt. of India at the Department of Health Research & Director General, Indian Council of Medical Research; and the Directorate of National Vector Borne Diseases Control Programme for facilities and evincing keen interest in the study. They thank Director General of Health Services, Govt. of India for funding the study. They also thank Dr. Neena Valecha, the then Director ICMR-NIMR New Delhi, Dr. Manju Rahi, Scientist F, ECD, ICMR, New Delhi, Technical Review Group and the Chairman Dr. Shiv Lal and Dr. P.L. Joshi for technical, scientific and administrative advice. The logistic support from Dr. A. C. Dhariwal, Dr. G. S. Sonal and Dr. Sher Singh Kashyotia from National Vector Borne Diseases Control Programme was laudable. The advice of Dr. S. Sridhar is also sincerely acknowledged. Assistance received from the state and district Health officials, Drs. Mahesh Khalipe, Mahendra Jagtap, Arun Kumar, O. P. Thakan, P. Aswal, Narottam Sharma, Hari Shankar, S. Barve, D. Sisodia, P. K. Behra and S. N. Singh and NIMR colleagues Drs. S.K. Ghosh and Hemanth Kumar is thankfully acknowledged. The government and private health sectors, Municipal Corporations, Village bodies, burial/cremation staff, school teachers, Accredited Social Health Activists (ASHAs), community leaders provided information on cases and deaths which is gratefully acknowledged. The panel of doctors who assigned cause of death are also thanked. Finally, investigators thank NVBDCP, NIMS and NIMR staff for support and successful conclusion of the study. The manuscript is approved by the publication committee of NIMR and bears approval No. 019/2018.
The study was funded by the Ministry of Health and Family Welfare, Government of India.
Author information
Authors and affiliations.
Indian Council of Medical Research, National Institute of Malaria Research, Field Unit, Campal, Panaji, 403 001, Goa, India
Ashwani Kumar & Ajeet Kumar Mohanty
Indian Council of Medical Research, National Institute of Medical Statistics, Ansari Nagar, Medical Enclave, New Delhi, 110 029, India
Himanshu K. Chaturvedi & Arvind Pandey
Indian Council of Medical Research, National Institute of Malaria Research, Sector 8, Dwarka, 110 077, New Delhi, India
Surya Kant Sharma & Mantoshkumar S. Malhotra
You can also search for this author in PubMed Google Scholar
Contributions
AP planned and administered the study and arranged for its critical appraisal by Technical Review Group constituted by Ministry of Health and Family Welfare, Govt. of India. AK, AKM, SKS, MSM, HKC, prepared study formats, trained field teams, launched and supervised field study. MSM coordinated logistical support and provided administrative backing to all study teams, ensured timely supplies in all the six study districts and coordinated COD VA analysis and the cause of death assignment by medical experts. HKC and AP prepared sampling plan and performed analysis of data. HKC and AK interpreted data and prepared the manuscript and coordinated with all the authors. The opinion expressed and inferences drawn are exclusively of authors and not the organizations they belong to. All authors read and approved the final manuscript.
Corresponding author
Correspondence to Ashwani Kumar .
Ethics declarations
Ethics approval and consent to participate.
The study was approved by the Institutional Ethics Committee of ICMR-National Institute of Malaria Research, New Delhi. However, during the study, no human subject was recruited for any drug trial. The blood test for malaria diagnosis by either microscopy or rapid diagnostic test was done as per standard procedures during routine fortnightly surveillance followed by treatment as per the national drug policy guidelines.
Consent for publication
Not applicable
Competing interests
The authors declare we have no competing interests.
Additional information
Publisher's note.
Springer Nature remains neutral with regard to jurisdictional claims in published maps and institutional affiliations.
Ashwani Kumar and Himanshu K. Chaturvedi are equal first authors
Supplementary information
Additional file 1: table.
S1. Estimated Fever Rate in high, moderate and low malaria strata.
Additional file 2: Table
S2 . Estimated Fever Rate for all the study districts.
Additional file 3: Table
S3. Estimated Test Positive Rate Crude).
Additional file 4: Table
S4. Estimated Malaria Mortality Rate (weighted).
Rights and permissions
Open Access This article is licensed under a Creative Commons Attribution 4.0 International License, which permits use, sharing, adaptation, distribution and reproduction in any medium or format, as long as you give appropriate credit to the original author(s) and the source, provide a link to the Creative Commons licence, and indicate if changes were made. The images or other third party material in this article are included in the article's Creative Commons licence, unless indicated otherwise in a credit line to the material. If material is not included in the article's Creative Commons licence and your intended use is not permitted by statutory regulation or exceeds the permitted use, you will need to obtain permission directly from the copyright holder. To view a copy of this licence, visit http://creativecommons.org/licenses/by/4.0/ . The Creative Commons Public Domain Dedication waiver ( http://creativecommons.org/publicdomain/zero/1.0/ ) applies to the data made available in this article, unless otherwise stated in a credit line to the data.
Reprints and permissions
About this article
Cite this article.
Kumar, A., Chaturvedi, H.K., Mohanty, A.K. et al. Surveillance based estimation of burden of malaria in India, 2015–2016. Malar J 19 , 156 (2020). https://doi.org/10.1186/s12936-020-03223-7
Download citation
Received : 14 June 2019
Accepted : 06 April 2020
Published : 16 April 2020
DOI : https://doi.org/10.1186/s12936-020-03223-7
Share this article
Anyone you share the following link with will be able to read this content:
Sorry, a shareable link is not currently available for this article.
Provided by the Springer Nature SharedIt content-sharing initiative
- Malaria burden
- Estimation of cases
- Test positivity rate
Malaria Journal
ISSN: 1475-2875
- Submission enquiries: [email protected]
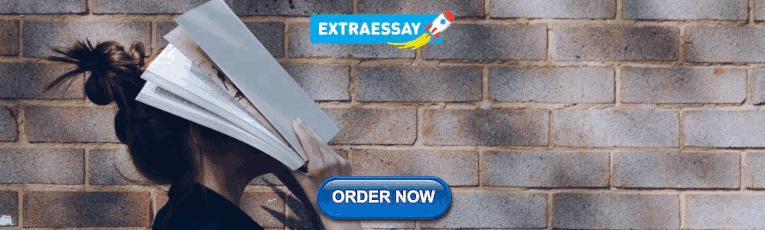
IMAGES
COMMENTS
The National Vector Borne Disease Control Program of India reported ~1.6 million cases and ~1100 malaria deaths in 2009. Some experts argue that this is a serious underestimation and that the actual number of malaria cases per year is likely between 9 and 50 times greater, with an approximate 13-fold underestimation of malaria-related mortality.
This study analysed historical malaria cases in India from 1990 to 2022 to assess the annual trends and the impact of key anti-malarial interventions on malaria incidence. Factors associated with malaria incidence were identified using univariate and multivariate linear regression analyses.
Summary of World Malaria Report 2021. In 2020, 29 of the 85 countries that were malaria-endemic accounted for 96% of malaria cases. India contributed 1.7% of malaria cases and 1.2% deaths globally. Between 2019 and 2020, all high burden to high impact (HBHI) countries except India reported increases in cases and deaths (and in India, the rate ...
The WHO’s World Malaria Report 2020 highlighted India’s gains in the path to elimination. India recorded impressive 60% reduction in reported cases compared with 2017, and a 46% reduction in cases compared with 2018, which built momentum to reach the goal of zero indigenous malaria cases by 2027. India’s National Strategic Plan for ...
The country contributed 51% of the global P. vivax cases in 2016, when the country launched the National Framework for Malaria Elimination, outlining the goals, strategies, guidelines and time-bound targets to eliminate malaria in India by 2030. 4 Through concerted efforts, India has managed to sustain the decline in overall burden, with 186 ...
Background Even though malaria cases have drastically come down in the last decade, malaria remains a serious public health concern in many parts of India. National Framework for Malaria Elimination in India (2016–2030) has been launched with the goal to eliminate malaria by 2030. Understanding the socio-economic and household determinants of malaria at the national level will greatly aid ...
In our clinic studies (passive case detection), malaria prevalence as detected by PCR was 21.1% (222 infections) ... Malaria in India: the Center for the Study of Complex Malaria in India.
India was one of eleven countries accounting for 70% of the global burden of malaria in 2020 1, and it continues to account for 79% of all malaria cases and 83% of all malaria deaths in the South ...
Globally, four people die due to malaria every 3 minutes. Malaria is caused by the protozoan parasite Plasmodium, with five species (P falciparum, P vivax, P malariae, P ovale, and P knowlesi) causing infections in humans. In 2020, the South-East Asia region was the second largest contributor of malaria cases worldwide after the African region, with around 5 million cases and 9000 deaths.
Background India has launched the malaria elimination initiative in February 2016. Studies suggest that estimates of malaria are useful to rationalize interventions and track their impact. Hence, a national study was launched to estimate burden of malaria in India in 2015. Methods For sampling, all 624 districts of India were grouped in three Annual Parasite Incidence (cases per thousand ...