20.3 Electromagnetic Induction
Section learning objectives.
By the end of this section, you will be able to do the following:
- Explain how a changing magnetic field produces a current in a wire
- Calculate induced electromotive force and current
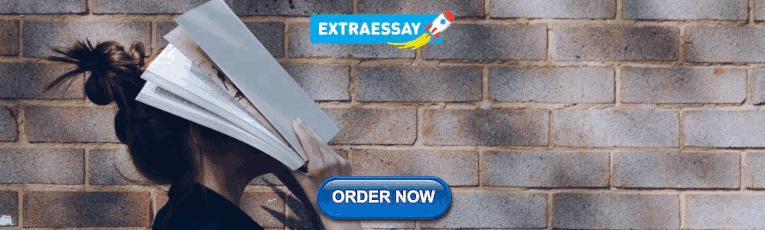
Teacher Support
The learning objectives in this section will help your students master the following standards:
- (G) investigate and describe the relationship between electric and magnetic fields in applications such as generators, motors, and transformers.
In addition, the OSX High School Physics Laboratory Manual addresses content in this section in the lab titled: Magnetism, as well as the following standards:
Section Key Terms
Changing magnetic fields.
In the preceding section, we learned that a current creates a magnetic field. If nature is symmetrical, then perhaps a magnetic field can create a current. In 1831, some 12 years after the discovery that an electric current generates a magnetic field, English scientist Michael Faraday (1791–1862) and American scientist Joseph Henry (1797–1878) independently demonstrated that magnetic fields can produce currents. The basic process of generating currents with magnetic fields is called induction ; this process is also called magnetic induction to distinguish it from charging by induction, which uses the electrostatic Coulomb force.
When Faraday discovered what is now called Faraday’s law of induction, Queen Victoria asked him what possible use was electricity. “Madam,” he replied, “What good is a baby?” Today, currents induced by magnetic fields are essential to our technological society. The electric generator—found in everything from automobiles to bicycles to nuclear power plants—uses magnetism to generate electric current. Other devices that use magnetism to induce currents include pickup coils in electric guitars, transformers of every size, certain microphones, airport security gates, and damping mechanisms on sensitive chemical balances.
One experiment Faraday did to demonstrate magnetic induction was to move a bar magnet through a wire coil and measure the resulting electric current through the wire. A schematic of this experiment is shown in Figure 20.33 . He found that current is induced only when the magnet moves with respect to the coil. When the magnet is motionless with respect to the coil, no current is induced in the coil, as in Figure 20.33 . In addition, moving the magnet in the opposite direction (compare Figure 20.33 with Figure 20.33 ) or reversing the poles of the magnet (compare Figure 20.33 with Figure 20.33 ) results in a current in the opposite direction.
Virtual Physics
Faraday’s law.
Try this simulation to see how moving a magnet creates a current in a circuit. A light bulb lights up to show when current is flowing, and a voltmeter shows the voltage drop across the light bulb. Try moving the magnet through a four-turn coil and through a two-turn coil. For the same magnet speed, which coil produces a higher voltage?
- The sign of voltage will change because the direction of current flow will change by moving south pole of the magnet to the left.
- The sign of voltage will remain same because the direction of current flow will not change by moving south pole of the magnet to the left.
- The sign of voltage will change because the magnitude of current flow will change by moving south pole of the magnet to the left.
- The sign of voltage will remain same because the magnitude of current flow will not change by moving south pole of the magnet to the left.
Induced Electromotive Force
If a current is induced in the coil, Faraday reasoned that there must be what he called an electromotive force pushing the charges through the coil. This interpretation turned out to be incorrect; instead, the external source doing the work of moving the magnet adds energy to the charges in the coil. The energy added per unit charge has units of volts, so the electromotive force is actually a potential. Unfortunately, the name electromotive force stuck and with it the potential for confusing it with a real force. For this reason, we avoid the term electromotive force and just use the abbreviation emf , which has the mathematical symbol ε . ε . The emf may be defined as the rate at which energy is drawn from a source per unit current flowing through a circuit. Thus, emf is the energy per unit charge added by a source, which contrasts with voltage, which is the energy per unit charge released as the charges flow through a circuit.
To understand why an emf is generated in a coil due to a moving magnet, consider Figure 20.34 , which shows a bar magnet moving downward with respect to a wire loop. Initially, seven magnetic field lines are going through the loop (see left-hand image). Because the magnet is moving away from the coil, only five magnetic field lines are going through the loop after a short time Δ t Δ t (see right-hand image). Thus, when a change occurs in the number of magnetic field lines going through the area defined by the wire loop, an emf is induced in the wire loop. Experiments such as this show that the induced emf is proportional to the rate of change of the magnetic field. Mathematically, we express this as
where Δ B Δ B is the change in the magnitude in the magnetic field during time Δ t Δ t and A is the area of the loop.
Note that magnetic field lines that lie in the plane of the wire loop do not actually pass through the loop, as shown by the left-most loop in Figure 20.35 . In this figure, the arrow coming out of the loop is a vector whose magnitude is the area of the loop and whose direction is perpendicular to the plane of the loop. In Figure 20.35 , as the loop is rotated from θ = 90° θ = 90° to θ = 0° , θ = 0° , the contribution of the magnetic field lines to the emf increases. Thus, what is important in generating an emf in the wire loop is the component of the magnetic field that is perpendicular to the plane of the loop, which is B cos θ . B cos θ .
This is analogous to a sail in the wind. Think of the conducting loop as the sail and the magnetic field as the wind. To maximize the force of the wind on the sail, the sail is oriented so that its surface vector points in the same direction as the winds, as in the right-most loop in Figure 20.35 . When the sail is aligned so that its surface vector is perpendicular to the wind, as in the left-most loop in Figure 20.35 , then the wind exerts no force on the sail.
Thus, taking into account the angle of the magnetic field with respect to the area, the proportionality E ∝ Δ B / Δ t E ∝ Δ B / Δ t becomes
Another way to reduce the number of magnetic field lines that go through the conducting loop in Figure 20.35 is not to move the magnet but to make the loop smaller. Experiments show that changing the area of a conducting loop in a stable magnetic field induces an emf in the loop. Thus, the emf produced in a conducting loop is proportional to the rate of change of the product of the perpendicular magnetic field and the loop area
where B cos θ B cos θ is the perpendicular magnetic field and A is the area of the loop. The product B A cos θ B A cos θ is very important. It is proportional to the number of magnetic field lines that pass perpendicularly through a surface of area A . Going back to our sail analogy, it would be proportional to the force of the wind on the sail. It is called the magnetic flux and is represented by Φ Φ .
The unit of magnetic flux is the weber (Wb), which is magnetic field per unit area, or T/m 2 . The weber is also a volt second (Vs).
The induced emf is in fact proportional to the rate of change of the magnetic flux through a conducting loop.
Finally, for a coil made from N loops, the emf is N times stronger than for a single loop. Thus, the emf induced by a changing magnetic field in a coil of N loops is
The last question to answer before we can change the proportionality into an equation is “In what direction does the current flow?” The Russian scientist Heinrich Lenz (1804–1865) explained that the current flows in the direction that creates a magnetic field that tries to keep the flux constant in the loop. For example, consider again Figure 20.34 . The motion of the bar magnet causes the number of upward-pointing magnetic field lines that go through the loop to decrease. Therefore, an emf is generated in the loop that drives a current in the direction that creates more upward-pointing magnetic field lines. By using the right-hand rule, we see that this current must flow in the direction shown in the figure. To express the fact that the induced emf acts to counter the change in the magnetic flux through a wire loop, a minus sign is introduced into the proportionality ε ∝ Δ Φ / Δ t . ε ∝ Δ Φ / Δ t . , which gives Faraday’s law of induction.
Lenz’s law is very important. To better understand it, consider Figure 20.36 , which shows a magnet moving with respect to a wire coil and the direction of the resulting current in the coil. In the top row, the north pole of the magnet approaches the coil, so the magnetic field lines from the magnet point toward the coil. Thus, the magnetic field B → mag = B mag ( x ^ ) B → mag = B mag ( x ^ ) pointing to the right increases in the coil. According to Lenz’s law, the emf produced in the coil will drive a current in the direction that creates a magnetic field B → coil = B coil ( − x ^ ) B → coil = B coil ( − x ^ ) inside the coil pointing to the left. This will counter the increase in magnetic flux pointing to the right. To see which way the current must flow, point your right thumb in the desired direction of the magnetic field B → coil, B → coil, and the current will flow in the direction indicated by curling your right fingers. This is shown by the image of the right hand in the top row of Figure 20.36 . Thus, the current must flow in the direction shown in Figure 4(a) .
In Figure 4(b) , the direction in which the magnet moves is reversed. In the coil, the right-pointing magnetic field B → mag B → mag due to the moving magnet decreases. Lenz’s law says that, to counter this decrease, the emf will drive a current that creates an additional right-pointing magnetic field B → coil B → coil in the coil. Again, point your right thumb in the desired direction of the magnetic field, and the current will flow in the direction indicate by curling your right fingers ( Figure 4(b) ).
Finally, in Figure 4(c) , the magnet is reversed so that the south pole is nearest the coil. Now the magnetic field B → mag B → mag points toward the magnet instead of toward the coil. As the magnet approaches the coil, it causes the left-pointing magnetic field in the coil to increase. Lenz’s law tells us that the emf induced in the coil will drive a current in the direction that creates a magnetic field pointing to the right. This will counter the increasing magnetic flux pointing to the left due to the magnet. Using the right-hand rule again, as indicated in the figure, shows that the current must flow in the direction shown in Figure 4(c) .
Faraday’s Electromagnetic Lab
This simulation proposes several activities. For now, click on the tab Pickup Coil, which presents a bar magnet that you can move through a coil. As you do so, you can see the electrons move in the coil and a light bulb will light up or a voltmeter will indicate the voltage across a resistor. Note that the voltmeter allows you to see the sign of the voltage as you move the magnet about. You can also leave the bar magnet at rest and move the coil, although it is more difficult to observe the results.
- Yes, the current in the simulation flows as shown because the direction of current is opposite to the direction of flow of electrons.
- No, current in the simulation flows in the opposite direction because the direction of current is same to the direction of flow of electrons.
Watch Physics
Induced current in a wire.
This video explains how a current can be induced in a straight wire by moving it through a magnetic field. The lecturer uses the cross product , which a type of vector multiplication. Don’t worry if you are not familiar with this, it basically combines the right-hand rule for determining the force on the charges in the wire with the equation F = q v B sin θ . F = q v B sin θ .
Grasp Check
What emf is produced across a straight wire 0.50 m long moving at a velocity of (1.5 m/s) x ^ x ^ through a uniform magnetic field (0.30 T) ẑ ? The wire lies in the ŷ -direction. Also, which end of the wire is at the higher potential—let the lower end of the wire be at y = 0 and the upper end at y = 0.5 m)?
- 0.15 V and the lower end of the wire will be at higher potential
- 0.15 V and the upper end of the wire will be at higher potential
- 0.075 V and the lower end of the wire will be at higher potential
- 0.075 V and the upper end of the wire will be at higher potential
Worked Example
Emf induced in conducing coil by moving magnet.
Imagine a magnetic field goes through a coil in the direction indicated in Figure 20.37 . The coil diameter is 2.0 cm. If the magnetic field goes from 0.020 to 0.010 T in 34 s, what is the direction and magnitude of the induced current? Assume the coil has a resistance of 0.1 Ω. Ω.
Use the equation ε = − N Δ Φ / Δ t ε = − N Δ Φ / Δ t to find the induced emf in the coil, where Δ t = 34 s Δ t = 34 s . Counting the number of loops in the solenoid, we find it has 16 loops, so N = 16 . N = 16 . Use the equation Φ = B A cos θ Φ = B A cos θ to calculate the magnetic flux
where d is the diameter of the solenoid and we have used cos 0° = 1 . cos 0° = 1 . Because the area of the solenoid does not vary, the change in the magnetic of the flux through the solenoid is
Once we find the emf, we can use Ohm’s law, ε = I R , ε = I R , to find the current.
Finally, Lenz’s law tells us that the current should produce a magnetic field that acts to oppose the decrease in the applied magnetic field. Thus, the current should produce a magnetic field to the right.
Combining equations ε = − N Δ Φ / Δ t ε = − N Δ Φ / Δ t and Φ = B A cos θ Φ = B A cos θ gives
Solving Ohm’s law for the current and using this result gives
Lenz’s law tells us that the current must produce a magnetic field to the right. Thus, we point our right thumb to the right and curl our right fingers around the solenoid. The current must flow in the direction in which our fingers are pointing, so it enters at the left end of the solenoid and exits at the right end.
Let’s see if the minus sign makes sense in Faraday’s law of induction. Define the direction of the magnetic field to be the positive direction. This means the change in the magnetic field is negative, as we found above. The minus sign in Faraday’s law of induction negates the negative change in the magnetic field, leaving us with a positive current. Therefore, the current must flow in the direction of the magnetic field, which is what we found.
Now try defining the positive direction to be the direction opposite that of the magnetic field, that is positive is to the left in Figure 20.37 . In this case, you will find a negative current. But since the positive direction is to the left, a negative current must flow to the right, which again agrees with what we found by using Lenz’s law.
Magnetic Induction due to Changing Circuit Size
The circuit shown in Figure 20.38 consists of a U-shaped wire with a resistor and with the ends connected by a sliding conducting rod. The magnetic field filling the area enclosed by the circuit is constant at 0.01 T. If the rod is pulled to the right at speed v = 0.50 m/s, v = 0.50 m/s, what current is induced in the circuit and in what direction does the current flow?
We again use Faraday’s law of induction, E = − N Δ Φ Δ t , E = − N Δ Φ Δ t , although this time the magnetic field is constant and the area enclosed by the circuit changes. The circuit contains a single loop, so N = 1 . N = 1 . The rate of change of the area is Δ A Δ t = v ℓ . Δ A Δ t = v ℓ . Thus the rate of change of the magnetic flux is
where we have used the fact that the angle θ θ between the area vector and the magnetic field is 0°. Once we know the emf, we can find the current by using Ohm’s law. To find the direction of the current, we apply Lenz’s law.
Faraday’s law of induction gives
Solving Ohm’s law for the current and using the previous result for emf gives
As the rod slides to the right, the magnetic flux passing through the circuit increases. Lenz’s law tells us that the current induced will create a magnetic field that will counter this increase. Thus, the magnetic field created by the induced current must be into the page. Curling your right-hand fingers around the loop in the clockwise direction makes your right thumb point into the page, which is the desired direction of the magnetic field. Thus, the current must flow in the clockwise direction around the circuit.
Is energy conserved in this circuit? An external agent must pull on the rod with sufficient force to just balance the force on a current-carrying wire in a magnetic field—recall that F = I ℓ B sin θ . F = I ℓ B sin θ . The rate at which this force does work on the rod should be balanced by the rate at which the circuit dissipates power. Using F = I ℓ B sin θ , F = I ℓ B sin θ , the force required to pull the wire at a constant speed v is
where we used the fact that the angle θ θ between the current and the magnetic field is 90° . 90° . Inserting our expression above for the current into this equation gives
The power contributed by the agent pulling the rod is F pull v , or F pull v , or
The power dissipated by the circuit is
We thus see that P pull + P dissipated = 0 , P pull + P dissipated = 0 , which means that power is conserved in the system consisting of the circuit and the agent that pulls the rod. Thus, energy is conserved in this system.
Practice Problems
The magnetic flux through a single wire loop changes from 3.5 Wb to 1.5 Wb in 2.0 s. What emf is induced in the loop?
What is the emf for a 10-turn coil through which the flux changes at 10 Wb/s?
Check Your Understanding
- An electric current is induced if a bar magnet is placed near the wire loop.
- An electric current is induced if a wire loop is wound around the bar magnet.
- An electric current is induced if a bar magnet is moved through the wire loop.
- An electric current is induced if a bar magnet is placed in contact with the wire loop.
- Induced current can be created by changing the size of the wire loop only.
- Induced current can be created by changing the orientation of the wire loop only.
- Induced current can be created by changing the strength of the magnetic field only.
- Induced current can be created by changing the strength of the magnetic field, changing the size of the wire loop, or changing the orientation of the wire loop.
As an Amazon Associate we earn from qualifying purchases.
This book may not be used in the training of large language models or otherwise be ingested into large language models or generative AI offerings without OpenStax's permission.
Want to cite, share, or modify this book? This book uses the Creative Commons Attribution License and you must attribute Texas Education Agency (TEA). The original material is available at: https://www.texasgateway.org/book/tea-physics . Changes were made to the original material, including updates to art, structure, and other content updates.
Access for free at https://openstax.org/books/physics/pages/1-introduction
- Authors: Paul Peter Urone, Roger Hinrichs
- Publisher/website: OpenStax
- Book title: Physics
- Publication date: Mar 26, 2020
- Location: Houston, Texas
- Book URL: https://openstax.org/books/physics/pages/1-introduction
- Section URL: https://openstax.org/books/physics/pages/20-3-electromagnetic-induction
© Jan 19, 2024 Texas Education Agency (TEA). The OpenStax name, OpenStax logo, OpenStax book covers, OpenStax CNX name, and OpenStax CNX logo are not subject to the Creative Commons license and may not be reproduced without the prior and express written consent of Rice University.
Revision notes for IB Physics
Topic 11: electromagnetic induction (hl).
See the guide for this topic.
11.1 – Electromagnetic induction
Electromotive force (emf).
- When a conducting wire moves through a magnetic field, a potential difference is created along the wire. This phenomenon is called electromagnetic induction.

- When the movement of the wire is perpendicular to the magnetic field, the emf (ε) induced is given by ε = Bvl where B is the magnetic field, v is the velocity of the wire, and l is the length of the wire.
Magnetic flux and magnetic flux linkage
- The magnetic flux through a surface measures the component of the magnetic field passing through the surface and is proportional to the number of magnetic field lines which intersect the surface.
- The magnetic flux (φ) through a surface is given by

where B is the magnetic field passing through the surface, A is the area of the surface, and θ is the angle between the magnetic field and the normal to the surface.

- The magnetic flux linkage of a coil measures the component of the magnetic field passing through the coil.
- For a coil with N turns, the total flux linkage is given by

where BA = φ = magnetic flux
Faraday’s law of induction
- Faraday’s law states that the magnitude of the induced emf is proportional to the rate of change of the flux linkage by
- Faraday’s law allows us to determine the induced emf by the change of magnetic flux over time.
- Lenz’s law states that the induced emf acts in the direction such that the current induced opposes the change which caused it.

11.2 – Power generation and transmission
Alternating current (ac) generators.
- The operation of a basic alternating current (ac) generator is demonstrated in the following diagram.
- A coil of wire is driven to rotate by an external force.
- As the coil rotates, the magnetic flux linkage passing through the coil changes.
- By Faraday’s law, this induces an emf and causes current to flow within the coil.
- If the rotation is at constant speed, the induced emf is sinusoidal (recurring oscillation).
- Increasing the speed of rotation increases both the frequency and the magnitude of the induced emf.

Average power and root mean square (rms) values of current and voltage
- The average power produced (or dissipated) from an alternating current cannot be computed directly using the peak values of voltage or current.
- The root mean square (rms) value of an alternating current or voltage is the value that could be substituted into the electrical power formula P=IV to calculate the average power dissipated where I and V are rms values.

where V0 is the peak value of the alternating voltage.

where I0 is the peak value of the alternating current.

- The quoted values for alternating voltage and current refer to their rms values rather than their peak values. For an example, an ac socket in Europe is rated at 220V rms.
Transformers
- A transformer is a device which can be used to transfer electrical energy from one ac circuit to another at a different voltage.
- Transformers which increase the output voltage are called step up transformers while those which decrease the output voltage are called step down transformers.
- A transformer is essentially two coil coils of wire coupled together as shown below.
- Alternating input voltage causes constant change in the magnetic field around the primary coil.
- This causes a constant change in the magnetic flux linkage in the secondary coil.
- By Faraday’s law, an emf is induced in the secondary coil.
- The ratio between the induced input voltage (rms) and the output voltage (rms) is equal to the ratio between the number of turns in the corresponding coil.

where Vp is the voltage in the primary coil, Vs is the voltage in the secondary coil, np is the number of turns on the primary coil, and ns is the number of turns on the secondary coil.
- An ideal transformer operates at 100% efficiency. In other words, its power input is equal to power output.

Diode bridges
A diode bridge is an arrangement of four (or more) diodes in a bridge circuit configuration where a diode is a two-terminal electronic component that conducts current to flow in one direction.

Half-wave and full-wave rectification
Diodes are also known as rectifiers. They can be used to change alternating current (ac) to direct current (dc) through a process called rectification.
Half-wave rectification
The final dc waveform on the screen is the positive half only of the original ac waveform. In half-wave rectification, the negative part of the current is prevented from passing.
Full-wave rectification
The four diodes connected as shown in the diagram above form a full-wave rectifier. Through this arrangement, the positive half of each cycle is allowed to pass through while the negative half of each cycle is reversed. This diode configuration is commonly known as a diode bridge.
11.3 – Capacitance
Capacitance.
Capacitance (C) is the ability to store change given in the unit farad (F) and can be expressed as
where C is capacitance, Q is charge, and V is voltage.
A capacitor is made up of two metallic plates with a dielectric material in between the plates.
When a voltage is applied over the two plates, an electric field is created with positive charge collecting in one plate and negative charge on the other.
This is what physicists mean when they say “a capacitor works by storing energy electrostatically in an electric field”.
The capacitance of a capacitor can be correlated to the area of the plates (A) and the distance of separation between the plates (d) by
The potential energy stored in a capacitor is given by

where E is the potential energy, Q is charge, V is voltage, and C is capacitance.
Dielectric materials
- Dielectric materials are insulators, such as glass, plastics, distilled water, dry air, or even a vacuum.
- Dielectric materials keep the conducting plates in contact, allowing for smaller plate separation (d), and higher capacitance (C).
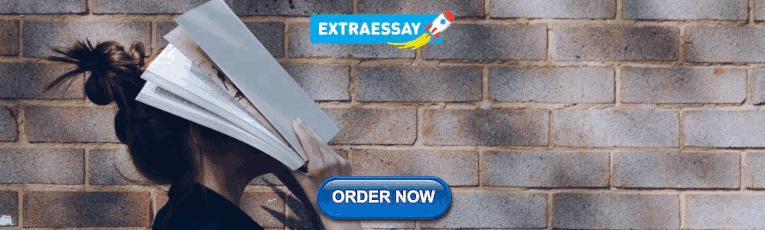
Capacitors in series and parallel

Resistor-capacitor (RC) series circuits
An RC circuit is one where you have a capacitor and resistor in the same circuit.
The charging and discharging of an RC circuit works like this:
- Assuming that C is fully discharged and that the switch is open. When the switch is closed in position 1, the battery is connected across the capacitor. Current flows and the potential difference across the capacitor begins to rise but as more and more charge build up on the capacitor plates, the current and the rate of rise of potential difference both fall until no further current flows and the potential difference across the capacitor equals that of the supply voltage (V0). The capacitor is now fully charged.
- When the switch is then closed at position 2, a large current flows and the potential difference across the capacitor drops. As charge flows from one plate to the other through the resistor, the charge is neutralized. The current and the rate of decreasing potential difference both fall. When the charge on the plates reach zero, the initial condition, the current and potential difference are also zero. The capacitor is now fully discharged.
Time constant
The RC time constant specifies the time required to charge the capacitor, through a resistor, by approximately 63.2% or to discharge the capacitor, through a resistor, by approximately 36.8% and is given by

where τ is the RC time constant in seconds, R is the circuit resistance in ohms, and C is the circuit capacitance in farads.
Share this:
Browse Course Material
Course info, instructors.
- Prof. Alan Guth
- Prof. Min Chen
Departments
As taught in.
- Electromagnetism
Learning Resource Types
Electromagnetism ii, assignments.
Problem Set 1 (PDF)
Problem Set 2 (PDF)
Problem Set 3 (PDF)
Problem Set 4 (PDF)
Problem Set 5 (PDF)
Problem Set 6 (PDF)
Problem Set 7 (PDF)
Problem Set 8 (PDF)
Problem Set 9 (PDF)
Problem Set 10 (PDF)

You are leaving MIT OpenCourseWare
- Electromagnetic Induction and its Applications
Electromagnetic Induction or Induction is a process in which a conductor is put in a particular position and magnetic field keeps varying or magnetic field is stationary and a conductor is moving. This produces a Voltage or EMF (Electromotive Force) across the electrical conductor. Michael Faraday discovered Law of Induction in 1830. Let us now study the Electromagnetic Induction in detail.
Suggested videos
Electromagnetic induction.
Suppose while shopping you go cashless and your parents use cards. The shopkeeper always scans or swipes the card. Shopkeeper does not take a photo of the card or tap it. Why does he swipe/scan it? And how does this swiping deduct money from the card? This happens because of the ‘ Electromagnetic Induction ’.
Can moving objects produce electric currents ? How to determine a relationship between electricity and magnetism? Can you imagine the scenario if there were no computers, no telephones, no electric lights. The experiments of Faraday has led to the generation of generators and transformers.

The induction of an electromotive force by the motion of a conductor across a magnetic field or by a change in magnetic flux in a magnetic field is called ‘Electromagnetic Induction’.
This either happens when a conductor is set in a moving magnetic field (when utilizing AC power source) or when a conductor is always moving in a stationary magnetic field.

This law of electromagnetic induction was found by Michael Faraday. He organized a leading wire according to the setup given underneath, connected to a gadget to gauge the voltage over the circuit. So when a bar magnet passes through the snaking, the voltage is measured in the circuit. The importance of this is a way of producing electrical energy in a circuit by using magnetic fields and not just batteries anymore. The machines like generators, transformers also the motors work on the principle of electromagnetic induction.
Browse more Topics under Magnetic Effects Of Electric Current
- Magnetic Field and Magnetic Force
- Domestic Electric Currents
Faraday’s law of Electromagnetic Induction

- First law: Whenever a conductor is placed in a varying magnetic field, EMF induces and this emf is called an induced emf and if the conductor is a closed circuit than the induced current flows through it.
- Second law: The magnitude of the induced EMF is equal to the rate of change of flux linkages.
Based on his experiments we now have Faraday’s law of electromagnetic induction according to which the amount of voltage induced in a coil is proportional to the number of turns and the changing magnetic field of the coil.
So now, the induced voltage is as follows:
e = N × d Φ d t
e is the induced voltage N is the number of turns in the coil Φ is the magnetic flux t is the time
Video on Electromagnetic Induction
Lenz’s law of Electromagnetic Induction
Lenz law of electromagnetic induction states that, when an emf induces according to Faraday’s law, the polarity (direction) of that induced emf is such that it opposes the cause of its production.
According to Lenz’s law
E = -N (dΦ/ dt) (volts)
Eddy currents
By Lenz law of electromagnetic induction, the current swirls in such a way as to create a magnetic field opposing the change. Because of the tendency of eddy currents to oppose, eddy currents cause a loss of energy. Eddy currents transform more useful forms of energy, such as kinetic energy, into heat, which isn’t generally useful. In many applications, the loss of useful energy is not particularly desirable, but there are some practical applications. Like:
- In the brakes of some trains. During braking, the brakes expose the metal wheels to a magnetic field which generates eddy currents in the wheels. The magnetic interaction between the applied field and the eddy currents slows the wheels down. The faster the wheels spin, the stronger is the effect, meaning that as the train slows the braking force is reduces, producing a smooth stopping motion.
- There are few galvanometers having a fixed core which are of nonmagnetic metallic material. When the coil oscillates, the eddy currents that generate in the core oppose the motion and bring the coil to rest.
- Induction furnace can be used to prepare alloys, by melting the metals. The eddy currents generated in the metals produce high temperature enough to melt it.

Applications of Electromagnetic Induction
Electromagnetic induction in ac generator, electrical transformers.
- Magnetic Flow Meter
You can download Magnetic Effects of Electric Current Cheat Sheet by clicking on the download button below

One of the important application of electromagnetic induction is the generation of alternating current.

The AC generator with an output capacity of 100 MV is a more evolved machine. As the coil rotates in a magnetic field B, the effective area of the loop is A cosθ, where θ is the angle between A and B. This is a method of producing a flux change is the principle of operation of a simple ac generator. The axis of rotation coil is perpendicular to the direction of the magnetic field. The rotation of the coil causes the magnetic flux through it to change, so an emf keeps inducing in the coil.
Another important application of electromagnetic induction is an electrical transformer. A transformer is a device that changes ac electric power at one voltage level to another level through the action of a magnetic field. A step-down transformer is the one in which the voltage is higher in the primary than the secondary voltage. Whereas the one in which the secondary voltage has more turns is a step-up transformer . Power companies use a step transformer to boost the voltage to 100 kV, that reduces the current and minimizes the loss of power in transmission lines. On the other end, household circuits use step-down transformers to decrease the voltage to the 120 or 240 V in them.
Solved Examples For You
Q. A straight wire length 0.20 m moves at a steady speed of 3.0 ms -1 at right angles to magnetic field of flux density 0.10 T. e.m.f induced across ends of wire is
Answer: 0.06 V
Customize your course in 30 seconds
Which class are you in.

Magnetic Effects of Electric Current
- Synchrotron
- Domestic Electric Circuits
- Magnetic field and Magnetic force
Leave a Reply Cancel reply
Your email address will not be published. Required fields are marked *
Download the App
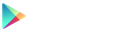
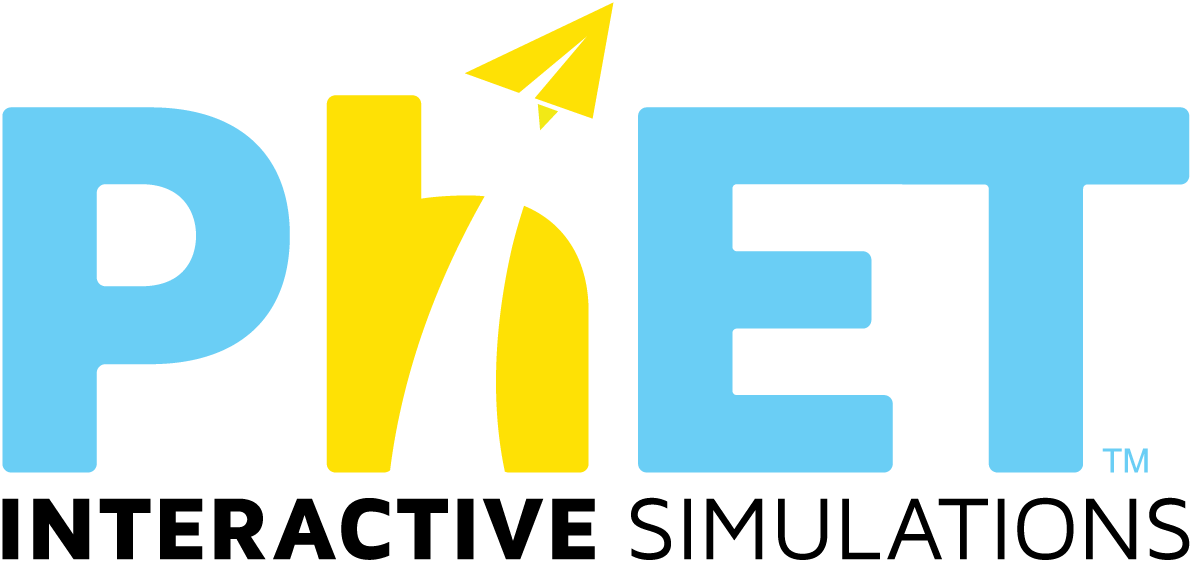
- Sign in / Register
- Administration
- Edit profile
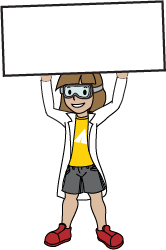
The PhET website does not support your browser. We recommend using the latest version of Chrome, Firefox, Safari, or Edge.
Electromagnetic Induction
Electromagnetic Induction is the production of voltage or electromotive force due to a change in the magnetic field. Electromagnetic induction was discovered by Michael Faraday in the 1830s. Many electrical components and types of equipment work based on the principle of electromagnetic induction. It has found many applications in technology, including electrical components such as inductors and transformers, and devices such as electric motors and generators.
Electromagnetic Induction method is based on the measurement of the change in mutual impedance between a pair of coils on or above the earth’s surface. Most Electromagnetic instruments are comprised of two or more sets of coils. These coils are electrically connected and are separated by a fixed distance. The transmitter coil is used to generate an electromagnetic field at a specific frequency. This is known as the primary field. The primary field causes electrical currents to flow in conductive materials in the subsurface. The flow of currents in the subsurface, called eddy currents, generate a secondary magnetic field, which is sensed by the receiver coil. The magnitude of the secondary field sensed by the receiver depends upon the type and distribution of conductive material in the subsurface. Both the induced secondary field, along with the primary field, is detected at the receiver coil. The number of turns in the coils/wire is directly proportional to induced voltage. The induced voltage in an electromagnetic induction is described by the following equation as:
e = N × dΦdt
e = voltage induced (measured in volts)
t = time (measured in seconds)
N = number of turns found in the coil
Φ = magnetic flux (measured in Webers)
Many types of electrical equipment such as motors, generators and transformers function based on the principle of the electromagnetic induction.
Applications of Electromagnetic Induction
Electromagnetic Induction is the production of an electromotive force (i.e., voltage) across an electrical conductor due to its dynamic interaction with a magnetic field. Induction is used in power generation and power transmission, and it’s worth taking a look at how that’s done. There are other effects with some interesting applications to consider, too, such as eddy currents.
An Electric Generator – An electric motor is a device for transforming electrical energy into mechanical energy; an electric generator does the reverse, using mechanical energy to generate electricity. At the heart of both motors and generators is a wire coil in a magnetic field. In fact, the same device can be used as a motor or a generator.
When the device is used as a motor, a current is passed through the coil. The interaction of the magnetic field with the current causes the coil to spin. To use the device as a generator, the coil can be spun, inducing a current in the coil.
Eddy Currents – An eddy current is a swirling current set up in a conductor in response to a changing magnetic field. By Lenzıs law, the current swirls in such a way as to create a magnetic field opposing the change; to do this in a conductor, electrons swirl in a plane perpendicular to the magnetic field. More accurately, eddy currents transform more useful forms of energy, such as kinetic energy, into heat, which is generally much less useful. In many applications the loss of useful energy is not particularly desirable, but there are some practical applications.
Transformers – Electricity is often generated a long way from where it is used, and is transmitted long distances through power lines. Although the resistance of a short length of power line is relatively low, over a long distance the resistance can become substantial. A power line of resistance R causes a power loss of I2R; this is wasted as heat. By reducing the current, therefore, the I2R losses can be minimized. A transformer is made up of two coils, each with a different number of loops, linked by an iron core so the magnetic flux from one passes through the other. When the flux generated by one coil changes, as it does continually if the coil is connected to an AC power source, the flux passing through the other will change, inducing a voltage in the second coil. With AC power, the voltage induced in the second coil will also be AC.
Faraday’s Law and Relativity
Faraday’s law describes two different phenomena: the motional EMF generated by a magnetic force on a moving wire, and the transformer EMF generated by an electric force due to a changing magnetic field due to the differential form of the Maxwell–Faraday equation. James Clerk Maxwell drew attention to the separate physical phenomena in 1861. This is believed to be a unique example in physics of where such a fundamental law is invoked to explain two such different phenomena.
Einstein noticed that the two situations both corresponded to a relative movement between a conductor and a magnet, and the outcome was unaffected by which one was moving. This was one of the principal paths that led him to develop special relativity.
Uncovering a Novel Process for Producing Spinning Thermal Radiation
Report on the national bank limited, emerging market economy (eme), lord of the flies, oosterboschite: properties and occurrences, lecture on inheritance, latest post, cathodic protection – a technique for controlling corrosion, electromagnetism – a discipline of physics, astronomers measure the heaviest black hole pair ever discovered, even passive smokers are extensively colonized by microbes, webb discovers proof that a neutron star powers the young supernova remnant, flyback transformer (fbt).
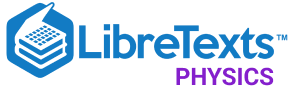
- school Campus Bookshelves
- menu_book Bookshelves
- perm_media Learning Objects
- login Login
- how_to_reg Request Instructor Account
- hub Instructor Commons
- Download Page (PDF)
- Download Full Book (PDF)
- Periodic Table
- Physics Constants
- Scientific Calculator
- Reference & Cite
- Tools expand_more
- Readability
selected template will load here
This action is not available.
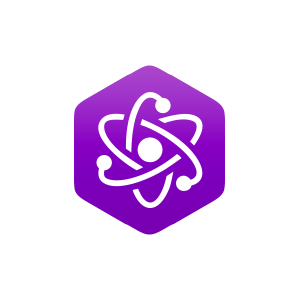
9.8: Applications of Electromagnetic Induction
- Last updated
- Save as PDF
- Page ID 32236
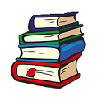
Learning Objectives
By the end of this section, you will be able to:
- Explain how computer hard drives and graphic tablets operate using magnetic induction
- Explain how hybrid/electric vehicles and transcranial magnetic stimulation use magnetic induction to their advantage
Modern society has numerous applications of Faraday’s law of induction, as we will explore in this chapter and others. At this juncture, let us mention several that involve recording information using magnetic fields.
Some computer hard drives apply the principle of magnetic induction. Recorded data are made on a coated, spinning disk. Historically, reading these data was made to work on the principle of induction. However, most input information today is carried in digital rather than analog form—a series of 0s or 1s are written upon the spinning hard drive. Therefore, most hard drive readout devices do not work on the principle of induction, but use a technique known as giant magnetoresistance . Giant magnetoresistance is the effect of a large change of electrical resistance induced by an applied magnetic field to thin films of alternating ferromagnetic and nonmagnetic layers. This is one of the first large successes of nanotechnology.
Graphics tablets, or tablet computers where a specially designed pen is used to draw digital images, also applies induction principles. The tablets discussed here are labeled as passive tablets, since there are other designs that use either a battery-operated pen or optical signals to write with. The passive tablets are different than the touch tablets and phones many of us use regularly, but may still be found when signing your signature at a cash register. Underneath the screen, shown in Figure \(\PageIndex{1}\), are tiny wires running across the length and width of the screen. The pen has a tiny magnetic field coming from the tip. As the tip brushes across the screen, a changing magnetic field is felt in the wires which translates into an induced emf that is converted into the line you just drew.
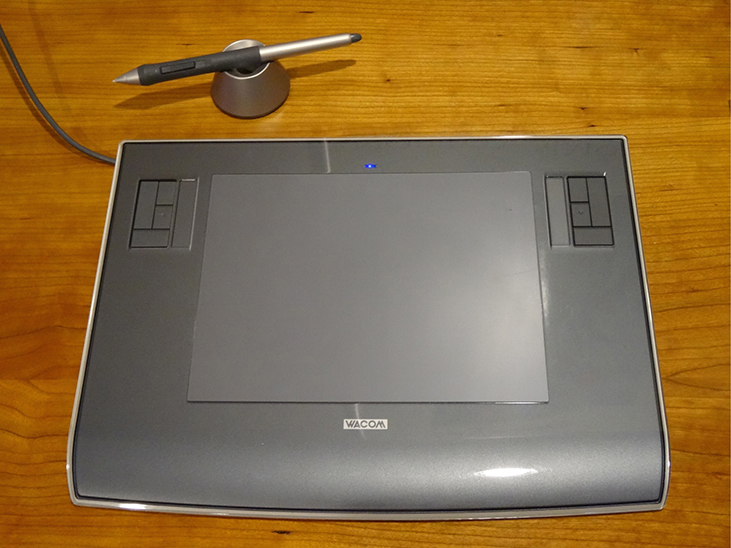
Another application of induction is the magnetic stripe on the back of your personal credit card as used at the grocery store or the ATM machine. This works on the same principle as the audio or video tape, in which a playback head reads personal information from your card.
Check out this video to see how flashlights can use magnetic induction.
A magnet moves by your mechanical work through a wire. The induced current charges a capacitor that stores the charge that will light the lightbulb even while you are not doing this mechanical work.
Electric and hybrid vehicles also take advantage of electromagnetic induction. One limiting factor that inhibits widespread acceptance of 100% electric vehicles is that the lifetime of the battery is not as long as the time you get to drive on a full tank of gas. To increase the amount of charge in the battery during driving, the motor can act as a generator whenever the car is braking, taking advantage of the back emf produced. This extra emf can be newly acquired stored energy in the car’s battery, prolonging the life of the battery.
Another contemporary area of research in which electromagnetic induction is being successfully implemented is transcranial magnetic stimulation (TMS) . A host of disorders, including depression and hallucinations, can be traced to irregular localized electrical activity in the brain. In transcranial magnetic stimulation, a rapidly varying and very localized magnetic field is placed close to certain sites identified in the brain. The usage of TMS as a diagnostic technique is well established.
Check out this Youtube video to see how rock-and-roll instruments like electric guitars use electromagnetic induction to get those strong beats.
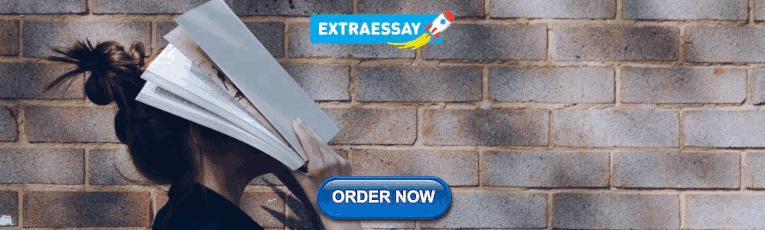
IMAGES
VIDEO
COMMENTS
Use the equation Φ = B A cos θ to calculate the magnetic flux. Φ = B A cos θ = B π ( d 2 ) 2 , 20.30. where d is the diameter of the solenoid and we have used cos 0° = 1 . Because the area of the solenoid does not vary, the change in the magnetic of the flux through the solenoid is. Δ Φ = Δ B π ( d 2 ) 2 . 20.31.
4. Motional Electromotive Force. A charged particle in rod experiences a magnetic force F = q v × B that causes free charges in rod to move, creating excess charges at opposite ends. The excess charges generate an electric field (from a to b) and electric force (F = q E) opposite to magnetic force.
13.1. 1.1 T/s. 13.2. To the observer shown, the current flows clockwise as the magnet approaches, decreases to zero when the magnet is centered in the plane of the coil, and then flows counterclockwise as the magnet leaves the coil. 13.4. ε = Bl2ω/2 ε = B l 2 ω / 2, with O at a higher potential than S. 13.5. 1.5 V.
The pen has a tiny magnetic field coming from the tip. As the tip brushes across the screen, a changing magnetic field is felt in the wires which translates into an induced emf that is converted into the line you just drew. Figure 13.8.1 13.8. 1: A tablet with a specially designed pen to write with is another application of magnetic induction.
13.7Applications of Electromagnetic Induction We have been considering electric fields created by fixed charge distributions and magnetic fields produced by constant currents, but electromagnetic phenomena are not restricted to these stationary situations. Most of the interesting applications of electromagnetism are, in fact, time-dependent. To
23049. We examine Faraday's law using a coil through which we pass a time-varying current (producing a time-varying magnetic field), and a second coaxial coil in which we measure the induced emf.
When a conducting wire moves through a magnetic field, a potential difference is created along the wire. This phenomenon is called electromagnetic induction. When the movement of the wire is perpendicular to the magnetic field, the emf (ε) induced is given by ε = Bvl where B is the magnetic field, v is the velocity of the wire, and l is the ...
More specifically, the relationship is between a changing magnetic flux and the resulting induced emf in a single closed loop of wire and is described by Faraday's law of electromagnetic induction: Induced emf = the derivative of magnetic flux with respect to time. (magnitudes) Substitute in the equation for magnetic flux:
Assignments. 100% (2) 4. Phys 2022 lab 1 - lab reports for physics lab. General Physics II ... Lab 7 Electromagnetic Induction; Lab 5 Circuits in Physics; Preview text ... The purpose of this lab was to explore the phenomenon of electromagnetic induction, the generation of an emf through a changing magnetic flux. Using Faraday's formula, the ...
Describing Electromagnetic induction and factors that influence its strength. Application of electromagnetic induction in electric generators and electric transformers. Click Create Assignment to assign this modality to your LMS.
Physics; As Taught In Fall 2012 Level Undergraduate. Topics Science. Physics. Electromagnetism; Learning Resource Types assignment Problem Sets. notes Lecture Notes. grading Exams with ... assignment Problem Sets. notes Lecture Notes. grading Exams with Solutions. Download Course.
A continually rotating magnetic field would continuously induce current. The induced current would show alternating direction. Study with Quizlet and memorize flashcards containing terms like Throughout the reflection, make sure you have a copy of the Student Guide and your data tables. Use the drop-down menus to complete the statements.
Now let us introduce a bar magnet as shown in Figure 8.2.1 8.2. 1. The magnet is centered along the axis of coil, to the right of the coil, and with its north pole facing toward the coil. The magnet is responsible for the magnetic flux density Bimp B i m p. We refer to Bimp B i m p as an impressed magnetic field because this field exists ...
Electromagnetic Induction or Induction is a process in which a conductor is put in a particular position and magnetic field keeps varying or magnetic field is stationary and a conductor is moving. This produces a Voltage or EMF (Electromotive Force) across the electrical conductor. Michael Faraday discovered Law of Induction in 1830.
Play with a bar magnet and coils to learn about Faraday's law. Move a bar magnet near one or two coils to make a light bulb glow. View the magnetic field lines. A meter shows the direction and magnitude of the current. View the magnetic field lines or use a meter to show the direction and magnitude of the current. You can also play with electromagnets, generators and transformers!
Electromagnetic induction is the process of generating electric current with a magnetic field. It occurs whenever a magnetic field and an electric conductor, such as a coil of wire, move relative to one another. As long as the conductor is part of a closed circuit, current will flow through it whenever it crosses lines of force in the magnetic ...
battery. axle. electromagnet. permanent magnet. Look carefully at the diagrams and choose the one that represents a generator. B. Explain how a generator creates electricity. An armature spins inside the magnetic field created by a permanent magnet. The magnetic field induces a current in the armature, generating electricity.
Students also viewed. Electromagnetic Induction Quiz; Magnetic Field and Force Assignment; Magnetic Field and Force Quiz; Magnets and Magnetism Quiz; Unit Test Review - Here are some notes that you can use.
Figure 8.7.1 8.7. 1: A tablet with a specially designed pen to write with is another application of magnetic induction. Another application of induction is the magnetic stripe on the back of your personal credit card as used at the grocery store or the ATM machine.
The magnetic field through the coil must change. In order that electromagnetic induction occurs in a circuit there must be a _________. change in magnetic field intensity. When a magnet is quickly pushed into a coil of wire connected to a circuit _________. work is needed to overcome a resistance to the push.
The number of turns in the coils/wire is directly proportional to induced voltage. The induced voltage in an electromagnetic induction is described by the following equation as: e = N × dΦdt. Where, e = voltage induced (measured in volts) t = time (measured in seconds) N = number of turns found in the coil. Φ = magnetic flux (measured in Webers)
Figure 9.8.1 9.8. 1: A tablet with a specially designed pen to write with is another application of magnetic induction. Another application of induction is the magnetic stripe on the back of your personal credit card as used at the grocery store or the ATM machine. This works on the same principle as the audio or video tape, in which a playback ...
Experiment 15: Electromagnetic Induction. By: Riya Shah. Partners: Andrew Lisa, Shelby Ebbighausen. Instructor Name: Matthew Melfi. PHYS 1812- AG- Physics Laboratory II. March 1, 2022. Objective: To understand the concept of electromagnetic induction and how it can be seen and applied in electric transformers and generators. Data and ...